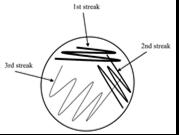
26 minute read
The impacts of eye drop storage conditions on the microbial growth of eye surfaces
The impacts of eye drop storage conditions on the microbial growth of eye surfaces
Rosie Kerruish – Willoughby Girls High School
Abstract
Increasing eye-related concerns in patients has led to a heightened need for treatments in the form of over-the-counter eye lubricants. The misconduct in the storage of these medications is known to increase the microbial contamination of the bottles and solutions, however, the impacts on the eye were yet unknown. This study aimed to investigate how differing storage conditions of eye drop bottles affected the microbial growth on eye surfaces. This was done by manually counting the number of microbial colonies on nutrient agar cultures of normal, open, half-open and expired eye drop bottles and treated cows’ eye surfaces. It was found that moving away from the standardised storage conditions of eye drop bottles produced greater microbial growth on cow’s eyes. Expired eye drops cultured the highest number of colonies, both in the bottle and on the treated eye surface. The averages of all growths were plotted to determine the R2 value, which was found to be 0.938, indicating a very strong, positive correlation between the variables. Thus, the correct storage of eye drops has high importance to prevent or limit microbial growth on patient eye surfaces.
Literature Review
Tetrahydrozoline
Tetrahydrozoline (C13H16N2) is a decongestant drug due to its induction of vasoconstriction, the temporary narrowing of blood vessels, in the nose and conjunctiva. This occurs from an imitation of the sympathetic nervous system on adrenergic transmission, which involves adrenergic nerve tissue using adrenaline, noradrenaline or dopamine neurotransmitters across neuron synapses (Farzam, et al., 2022). Without decongestant drugs, alpha-adrenergic receptors (α1-receptors) found at the postsynaptic target cells naturally bind to these neurotransmitters (Perez, 2020). However, the introduction of α1-receptors agonists, including tetrahydrozoline (THZ), can mimic neurotransmitters to activate receptors (Hosten and Snyder, 2020), stimulating the same contraction of the inner layer of blood vessels, vascular endothelial cells, causing vasoconstriction. Due to this, THZ is typically found in eye drops treating burning and irritation in eyes in the form of Tetrahydrozoline HCl 0.05%, from such vasoconstriction, narrowing the prominent blood vessels. The disorder of red eyes is most commonly associated with bacterial infections such as conjunctivitis and can result in discharge, prominent red blood vessels, pain, itching and blurry vision. Dry eye is an abnormality in lacrimal gland activity that reduces secretion of lacrimal fluid, the supply of lubricating fluids, to the conjunctival fornix and cornea. Sympathetic nerves in lacrimal glands stimulate the ocular surface for tear production (Conrady, et al., 2016), meaning that THZ can again act as α1- receptors agonists, artificially replicating the acetylcholine and norepinephrine neurotransmitters. These send chemical and electrical signals to duct cells to regulate and produce lacrimal gland protein secretion, relieving dry eye symptoms (Bhattacharya, et al., 2018). It was found that the most frequent ocular disorder primary care physicians encounter is red eye and dry eye (Leibowitz, 2000, Wirbelauer, 2006), underlining their significance in medicinal research, particularly in synthesising and understanding treatments, including THZ.
Microbial Contamination in Eye Drop Bottles
The frequency of microbial contamination within eye drop bottles has been assessed from hospital and pharmaceutical company samples, with rates ranging from 5.3% (Teuchner, et al., 2015) to 45.9% (Feghhi, et al., 2008). The 2015 Teuchner study compared different types and brands of eye drops, outside the defined independent variable, which restricts the validity of the experiment due to a lack of controlled variables. Additionally, the study focused on the percentage of contaminated eye drops and did not go into further detail surrounding the specific storage conditions affecting bacterial growth, despite its significant contribution to the results. This inconsistency means that additional research must be conducted to understand the factors that affect microbial growth in eye drop bottles. The shelf life of unopened and opened Autologous Serum Eye Drops (ASEDs) has been found to be between 1 to 6 months and 3 hours to 1 week, respectively, (Lee, et al., 2014). As well as this, a 2001 study found the contamination rate of eye drop bottles to be 10.2% in unopened bottles and 34.8% in opened bottles, (Taşli and Coşar, 2001). These differing rates highlight the importance of closing eye drop bottles to both preserve eye solutions and potentially decrease microbial contamination, however, both the 2014 and 2001 studies focused on other active ingredient-based eye solutions in place of THZ. Staphylococcus aureus (S. aureus), a natural flora found in the nasal cavity and skin surface, has the highest contamination rate (Tsegaw, et al., 2017, Nisar, et al., 2018) in eye drop bottles than other bacterial colonies, due to accessible transfer from skin to bottles. Kruszewska (2006) exhibited THZ’s ability to inhibit S. aureus growth, in concentrations of 0.05 mg/ml, however, this is significantly below the 5-100 mg/ml concentrations of other non-antibiotic drugs. This is due to THZ’s ineffective prevention of translation as part of bacterial polypeptide synthesis, as it struggles to bind to a ribosomal aminoacyl active site in place of the bacterial amino-acyl tRNA. Kruszewska’s study did not include findings on the likelihood of contamination of THZ, rather it focuses on its general antimicrobial properties, meaning there is limited knowledge surrounding the conditions in which THZ products have increased microbial growth and how this may affect treated eyes. No similar studies have been conducted in relation to any microbial growth virulence in THZ solutions, which leaves an opening for further research, specifically surrounding the introduction of undesirable microbes to such THZ products. The studies suggested THZ’s lesser antibiotic properties, indicating the increased potential for contamination of tetrahydrozoline products.
Microbial Growth on Eyes
Dry eyes has been shown to increase the prevalence of microbes on eye surfaces due to the lack of antibacterial lacrimal fluid coverage, including S. aureus and other closely related bacterial species. This occurs as a result of a significant decrease in immunoglobulin A (IgA), an antibody produced by plasma b cells in mucous membranes, and an increase in harmful lipase activity and toxins from ocular bacteria, causing cellular damage (Li, et al., 2019). Dry eyes inducing an increased microbial ocular growth, combined with THZ’s limited antimicrobial activity producing contaminated eye drop bottles, significantly increases the chances of disease, such as keratitis, blepharitis and endophthalmitis. Many studies have injected bacteria samples directly into the conjunctival sac or cornea (O’Callaghan, 2018) to determine the effects, however, there are minimal methodologies surrounding surface level contamination, due to time constraints. In relation to other external sources infecting the ocular surface with microbes, contaminated contact lenses’ impact on ocular health has been thoroughly researched. Contact lenses act as vectors for microorganisms to adhere to the cornea and transfer to the internal elements of the eye (Shin, et al., 2016). However, there are few studies within the past two decades that relate to eye drops as vectors for microbes, and minimal information is available regarding the species and number of any such microorganisms.
The incorrect storage conditions of eye drop solutions have been shown to increase the contamination rate of many bottles in specific settings, however, studies lack depth on further applications of this information. There are many connections yet to be discovered between contaminated THZ eye drop bottles and growth on treated eye surfaces, leaving a gap in scientific research regarding this correlation.
Research Question
How do tetrahydrozoline eye drops held in non-standardized conditions affect the microbial growth on eyes?
Hypothesis
As microbial growth increases inside eye drop bottles with a greater risk of pathogen contamination, microbial growth on treated eye surfaces will increase.
Methodology
Preparation of Eye Drop Bottles:
Four 15mL Visine(R) Red Eye Comfort eye drop bottles were collected for the Tetrahydrozoline HCl 0.05% active ingredient. One eye drop bottle was stored according to all safety instructions on the product box and was labelled “Bt 1” (indicating Bottle 1) with a permanent marker. A second bottle was stored according to all safety instructions on the product box, however, the lid was placed onto the nozzle, not screwed on and labelled “Bt 2”. A third bottle was stored according to all safety instructions on the product box, however, the lid was left off, placed next to the bottle and labelled “Bt 3”. A fourth bottle was stored according to all safety instructions on the product box, however, had expired for five months and labelled “Bt 4”. The four eye drop bottles were stored together for five weeks in a cool, dry cupboard before the study began.
Preparation of Nutrient Agar plates:
800mL of water was measured at eye level using a 1L measuring cylinder which was poured into a 2L beaker on a hotplate set to maximum heat output. Four solid beef stock cubes were added and stirred using a plastic stirring rod for 3 minutes or until dissolved to make the beef broth. Two sterilised spatulas weighed 50g of salt and 50g of sugar to a watch glass on an electronic balance, to then be added to the broth. This solution was heated until the boiling point where 15g of solid agar, measured by the electronic balance, was added and stirred until dissolved. This solution was transferred to a 750mL autoclave bottle, where any excess not evaporated during boiling was poured down a suitable drain. The solution was sterilised overnight in an Autoclave and was collected the next morning using heat-resistant gloves. Antibacterial dish soap was combined with water to clean and rinse 20 plastic Petri dishes. The agar solution was then poured from the autoclave bottle into each Petri dish until three-quarters of the plate was full. The lids were immediately placed onto the agar plate to prevent air contamination and were left for 10 minutes to solidify, before being placed upside down in a refrigerator. One agar plate was placed in an incubator at 30oC for 72 hours to determine if any contamination occurred during preparation.
Data Collection:
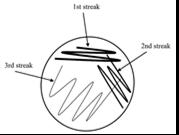
Figure 1. Method of culture swab
Four cotton swabs were dipped in sterile water to dampen. These each swabbed one of the four eye drop bottles; in the lid, around the rim of the nozzle for thirty seconds and a single drop from the eye solution. These were cultured onto separate agar plates using three short zig-zag patterns, decreasing the pressure of the swab each time. Refer to Figure 1. They were then sealed with two layers of masking tape and labelled “1” (indicating bottle 1), “2”, “3” and “4”, respectively, with a permanent marker. These were left in an incubator upside down at 30oC for seventy-two hours. After these plates were stored, five cow eyes were placed into a container, where three drops of each bottle were added to a cow eye, leaving one eye with no additional eye drop solution. Each cow eye was labelled 1 through to 5 using a permanent marker on the side of the container, which was sealed and placed into a refrigerator. The next day the cow eyes were removed from the refrigerator, swabbed again using damp cotton swabs and cultured onto agar plates using the same method (Figure 1), being labelled “Eye 1” through to “Eye 5”. These were left in an incubator at 30oC for seventy-two hours. Three more drops of each bottle were added to the eyes before being stored again in the refrigerator. This was repeated for one more day. After the final swab, the cow eyes were placed in a ziplock bag and safely disposed of in a bin.
Data Analysis:
After seventy-two hours, each culture was taken out of the incubator and photographed. The size, shape, colour and number of each type of growth were counted and arranged into qualitative data in a table. The total number of observable colonies was counted for each agar culture, meaning that only the distinct colonies were recorded. A column graph was produced to graphically represent both the averages of the number of colonies in the eye drop bottles as well as the number of colonies on the cow’s eyes. Using excel, a single factor ANOVA test was conducted to find if there was a statistical significance between microbial growth in both the eye drop bottles and the cow’s eyes as well as the variance in each data set. If a statistically significant result was found, individual two-sample unpaired T-tests were run between groups, using ‘assuming equal variances’ or ‘assuming unequal variances’ where applicable. A scatter plot was also generated between the number of colonies in the bottles versus the eyes to produce Pearson’s Correlation Coefficient to determine the strength of the relationship between the variables.
Experimental Repetition:
The whole study was repeated four times to check for reliability, using different eye drop bottles and cow’s eyes in each repetition.
Results:
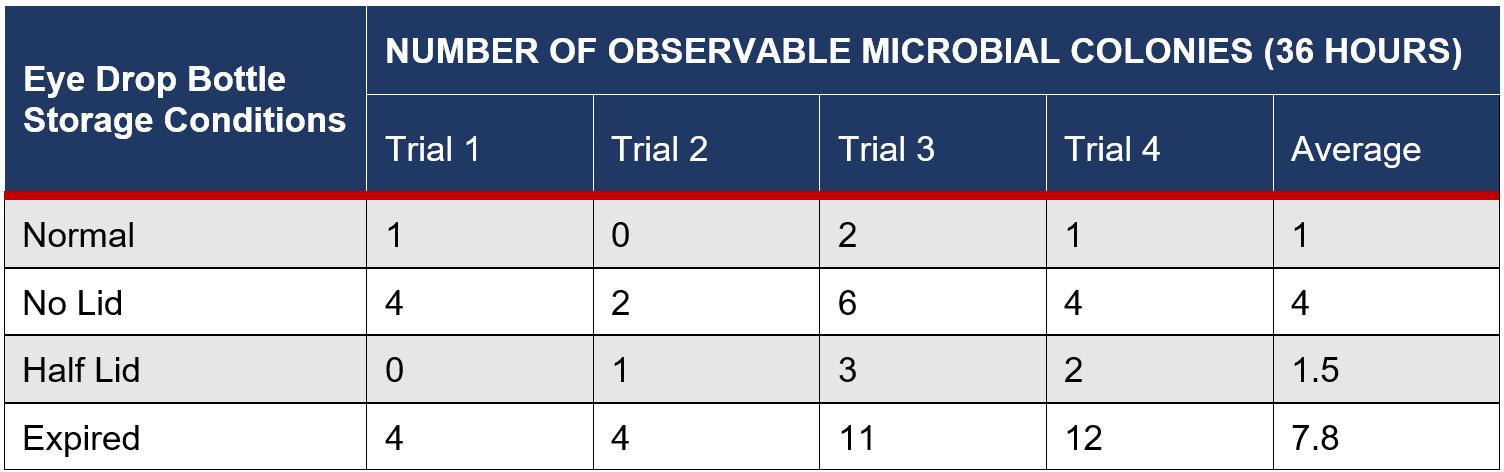
Table 1: Number of Colonies in Eye Drop Bottles after 72 Hours
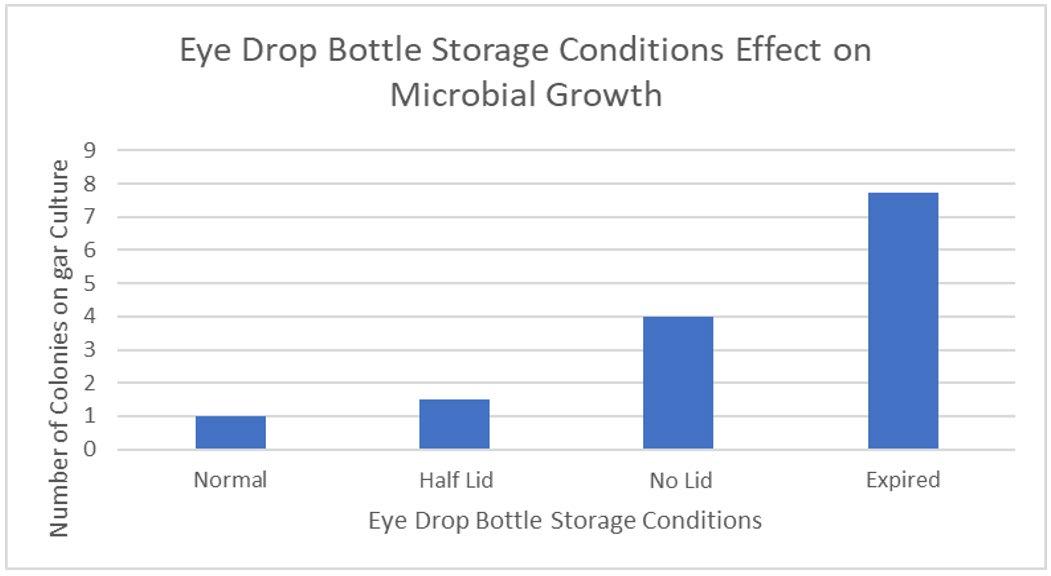
Figure 2: Average Number of Colonies of Microbes For Different Storage Conditions of Eye Drop Bottles
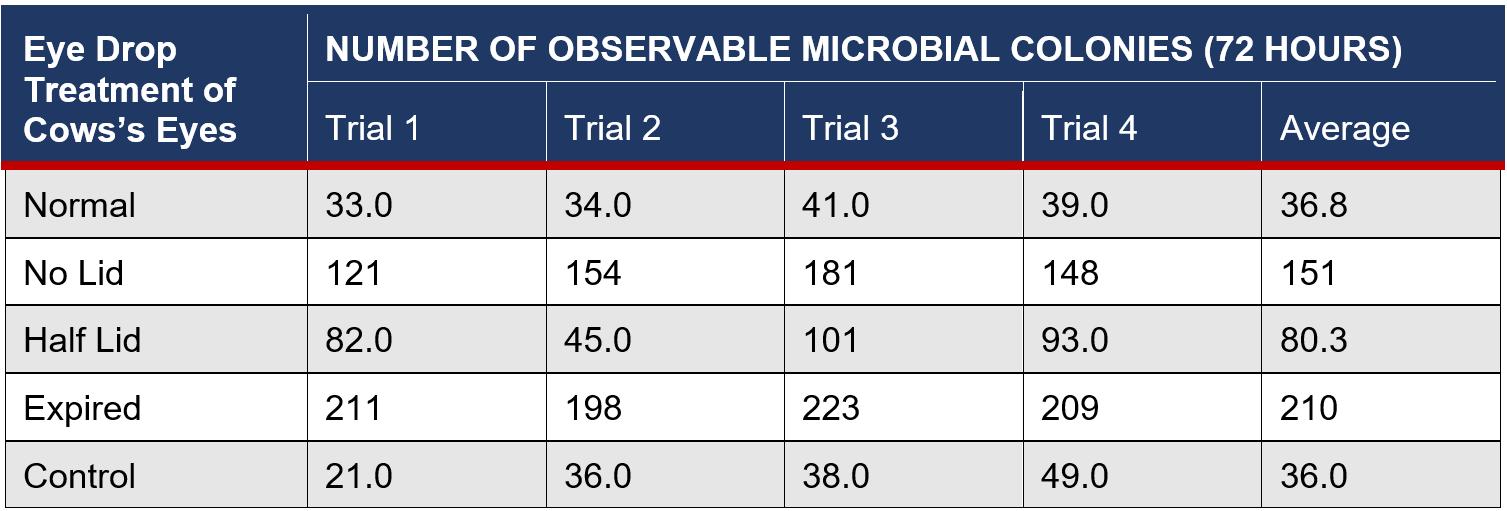
Table 2: Number of Colonies on Cows’ Eyes with Treatment From Eye Drop Bottles after 72 Hours
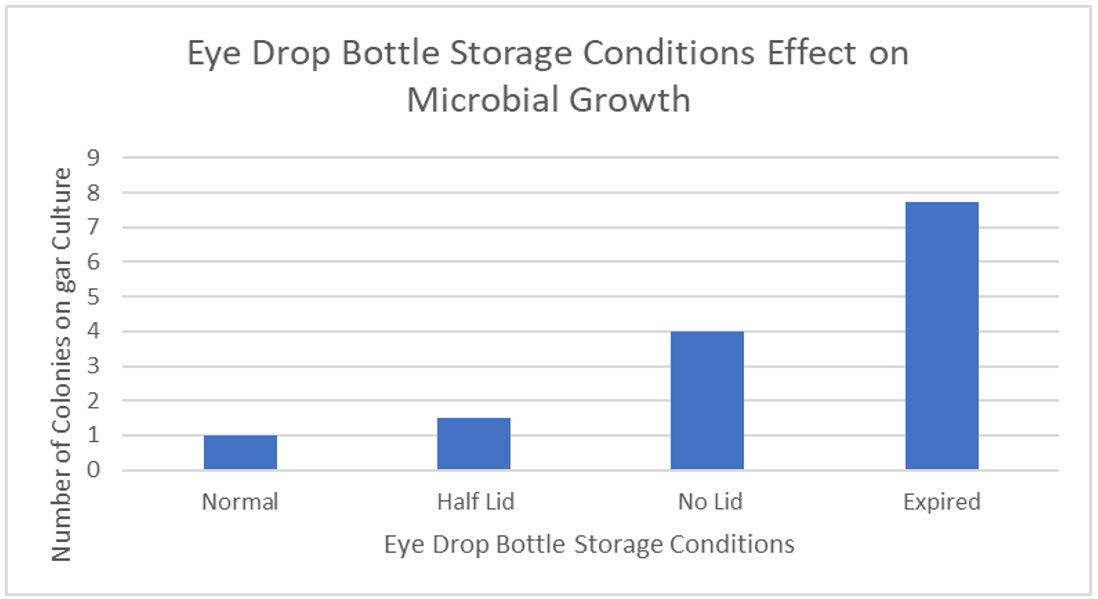
Figure 3: Average Number of Colonies of Microbes For Cows’ Eyes Treated with Differently Stored Eye Drops
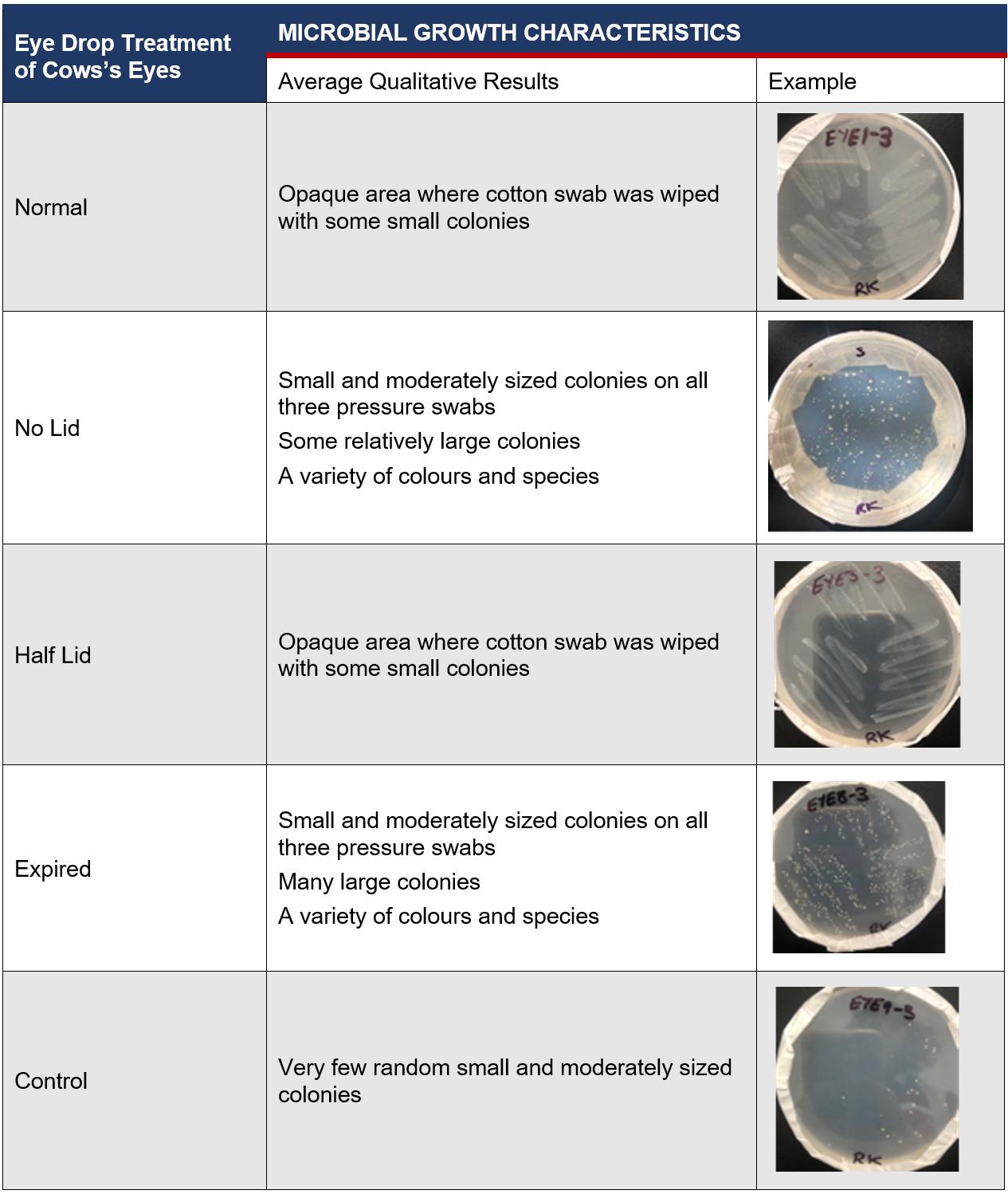
Table 3: Average Qualitative Results of Physical Characteristics of Microbes on Cow’s Eyes after 72 Hours
Statistical Analysis
Eye Drop Bottles Test:
Alpha Significance Level = 0.05
H0: There is no statistical significance in the means of the microbial growth of the eye drop bottles across different storage conditions
H1: There is a statistical significance in the means of the microbial growth of the eye drop bottles across different storage conditions
An ANOVA Single factor test was used to determine if there was a significant difference between the means of the number of microbial colonies in eye drop bottles. This is a suitable test as there are more than two samples.
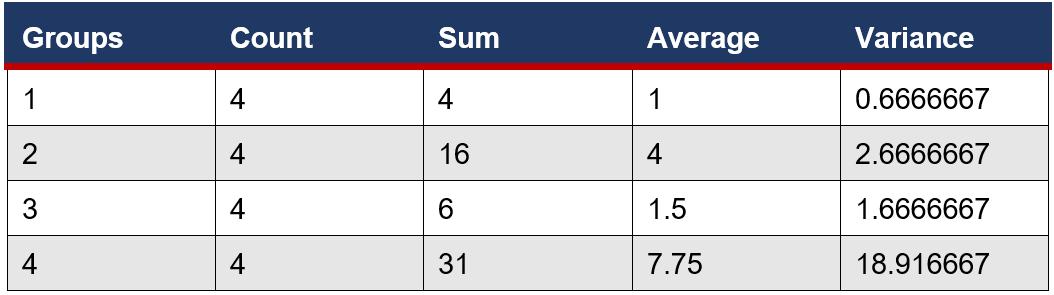
SUMMARY
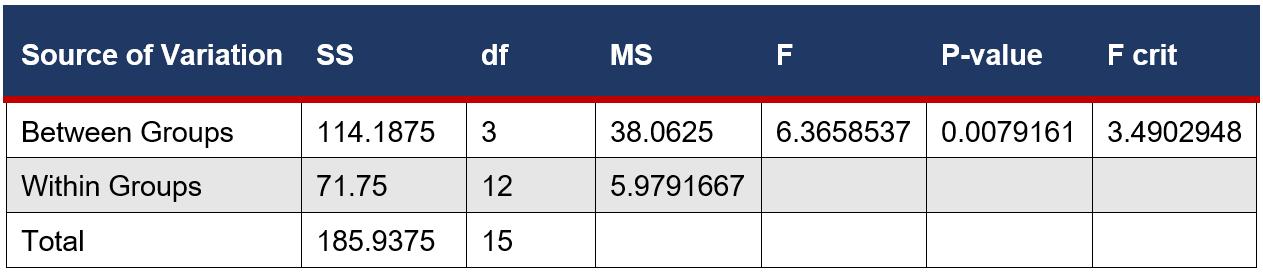
ANOVA
This was followed by 2 sample, unpaired T-tests between each variable assuming equal variance from the ANOVA Single Factor test. This is a suitable test as it compared the means between individual groups and such groups were not related.
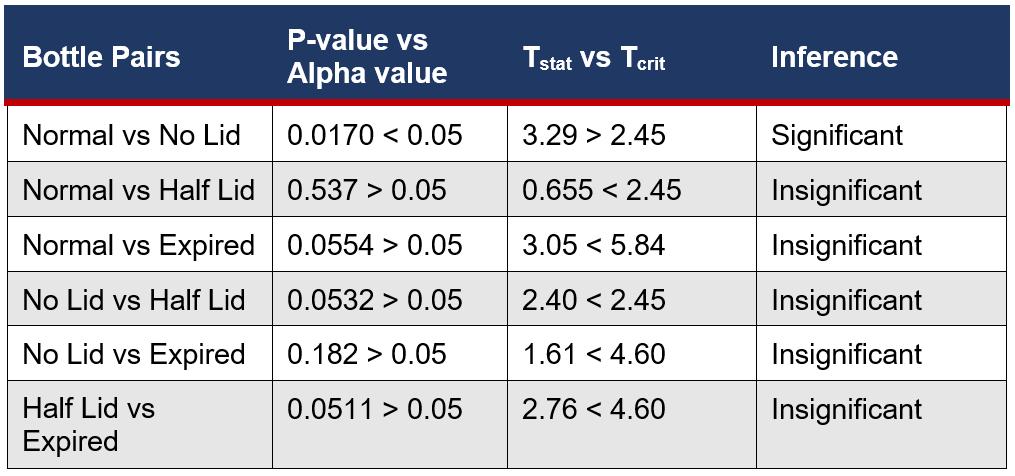
Table 4: Summary of the Statistical Difference Between Eye Drop Bottle Storage Conditions and Microbial Growth
Cows’ Eye Test:
Alpha Significance Level = 0.05
H0: There is no statistical significance in the means of the microbial growth on the cow eyes treated with of eye drops in different storage conditions
H1: There is a statistical significance in the means of the microbial growth on the cow eyes treated with of eye drops in different storage conditions
An ANOVA Single factor test was used to determine if there was a significant difference between the means of the number of microbial colonies on eye surfaces. This is a suitable test as there are more than 2 samples.
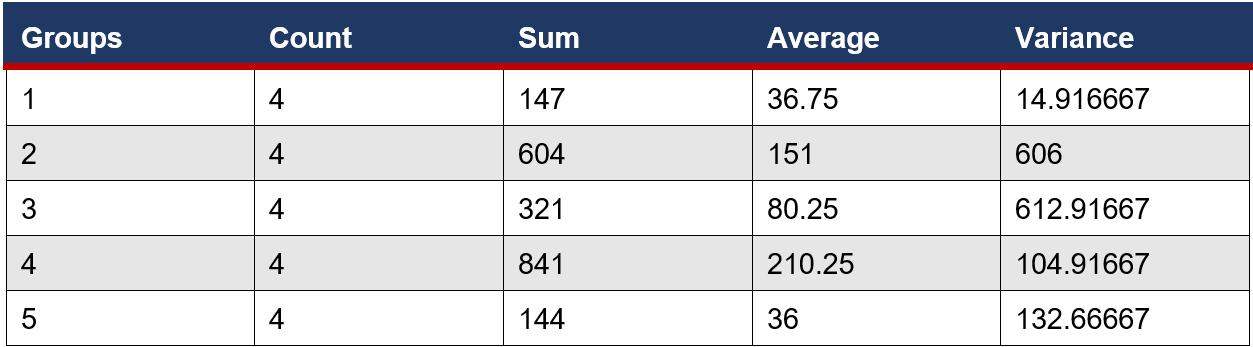
Figure 5: ANOVA single factor test performed on the microbial growth of on cows’ eyes – SUMMARY

Figure 5: ANOVA single factor test performed on the microbial growth of on cows’ eyes - ANOVA
This was followed by 2 sample, unpaired T-tests between each variable assuming equal or unequal variances, depending on the given variance from the ANOVA Single Factor test. This is a suitable test as it compared the means between individual groups and such groups were not related.
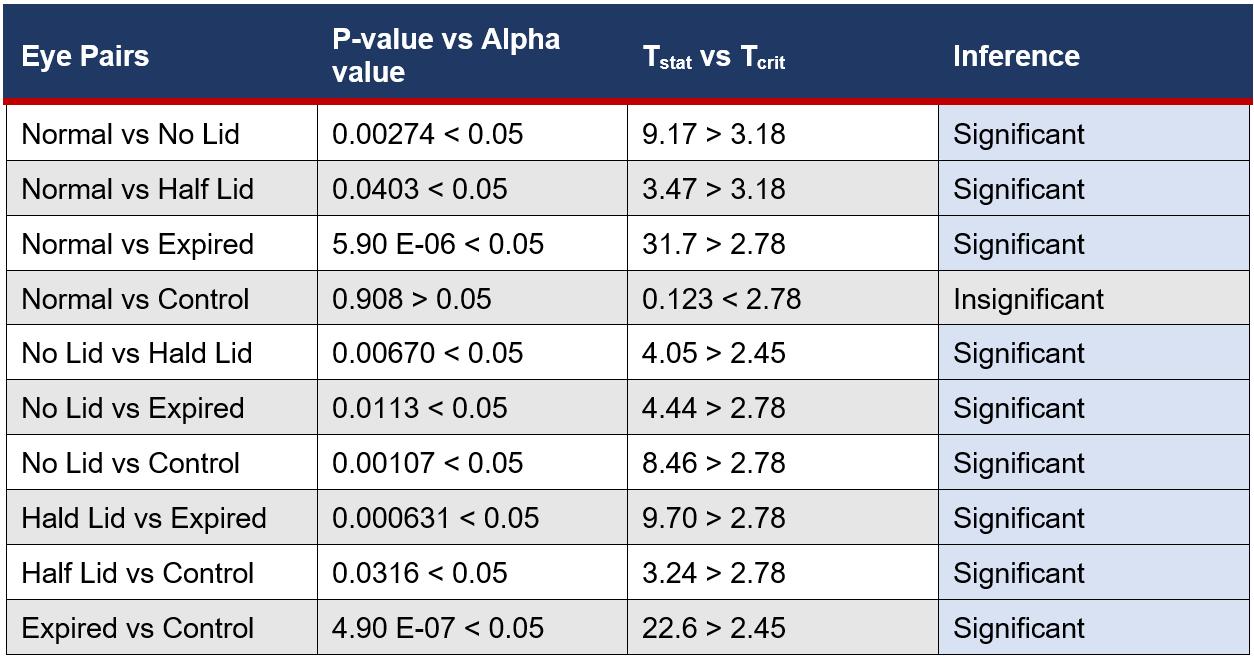
Table 5: Statistical Difference Between Eye Drop Bottle Groups for Microbial Growth on Eyes
Correlation Between Variables:
A Pearson Correlation was used to analyse the relationship between the growth of microbes inside the eye drop bottle and the subsequent growth on cows’ eyes. The averages were plotted against each other to determine the R2 value.
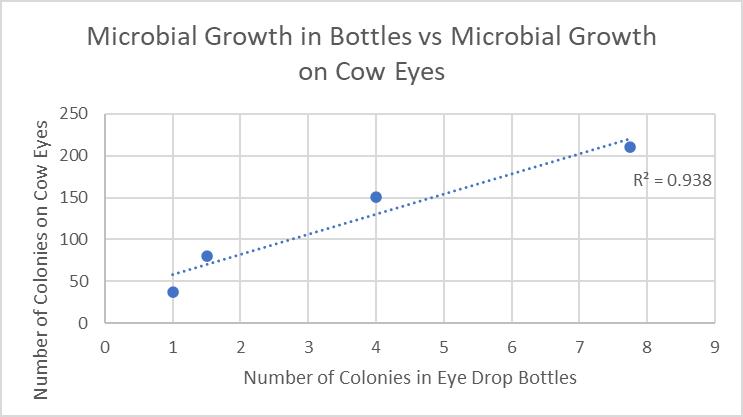
Figure 6: Growth in Eye Drop Bottles Plotted Against Growth on Cows’ Eyes
Discussion
This study aimed to show the relationship between microbial growth inside eye drop bottles stored in varying conditions and growth on eye surfaces. Stagnant liquids are ideal conditions for processes of binary fission and spore reproduction, meaning that open eye drop solutions, especially that of THZ with low antimicrobial properties, will produce more growth than bottles stored with the lid both thoroughly and partially closed. Furthermore, exposure to oxygen can accelerate the degeneration of chemical ingredients, causing the weakening of antimicrobial properties, exhausting essential processes, including vasoconstriction, and decreasing expiration dates. The open eye drop bottle versus the normal bottle had the only statistically significant result (Table Figure 6F4), meaning that the microbial growth is directly impacted by the storage conditions, not due to random chance. Eye drops that have already expired have undergone physical and chemical degradation, including oxidation and decarboxylation (Inam and Batool, 2017). This chemical change alters the properties of THZ, where long periods of time allow multiple stages of oxidation to occur and an increased risk of microbial contamination. This correlated with experimental results, the expired eye drops having the highest microbial growth with an average of 7.8 (Table 1), due to this fundamental deterioration and greater susceptibility to growth. However, as a result of the high variance between trials, 18.9 (Figure 4), there was no statistical significance with other variables, meaning that it has no meaningful impact on microbial growth.
The placement of eye drops onto the surface of cows’ eyes allows microbes inside eye drop solutions to transfer and absorb into the eye, increasing contamination of the cornea. This suggests that increasing the microbial growth inside eye drop bottles will increase the growth in eyes, supported by the found R2 value of 0.938 (Figure 6), indicating that 93.8% of the variance within the growth is explained by the different storage conditions of eye drop bottles. This is seen in the expired eye drops producing the highest average of 210 colonies on the eye (Table 2), from the transfer of its high growth within the bottle. This value was compared against other bottles’ influence on microbial growth in eyes and a statistically significant outcome was found between each group (Table 5), indicating that there is a correlation between growth in eyes and contamination inside eye drop bottles. Furthermore, the expired eye drops had the greatest variety of colour, size and type of microbial colonies, indicating its high virulence rate. While the control experiment, the eye without solution, did produce microbial growth, this was expected due to the high contamination rate of raw meat in the agricultural industry (Bantawa, et al., 2018). This growth, 36 colonies (Table 2), is notably less than the growth from the no lid, half lid and expired treated eyes, producing a statistically significant difference for each one. However, the control had no statistical significance to the eye treated with eye drops stored under normal conditions, attributable to the lesser growths inside both bottles. The normal bottle had an average of 1 colony (Table 1), indicating low contamination from limited exposure to pathogens in the air, meaning it transfers few microorganisms across to the eye during treatment. Similarly, tetrahydrozoline’s limited antibiotic properties would not prevent nor restrict growth, indicating its similarity to the control experiment.
Accuracy:
The results from this experiment were compared to expected values from secondary resources and the identified trend was found to be very accurate. The linear regression figure between the two variables, R2 = 0.938 (Figure 6), was exceptionally high indicating a very strong, positive correlation, which reflects expected results that increased exposure to pathogens within bottles increases growth on treated eyes. The equipment was accurate to determine microbial growth, using an electronic balance and a measuring cylinder with small error margins of 0.01g and 5mL, respectively. Parallax error did not affect water volume in the measuring cylinder, as measurements were taken at a flat angle. However, total counts of microorganisms were lower than expected, and this is most likely due to a combination of limited sensitivity of manual counting and the use of cows’ eyes. Despite this decreased value of microbe colonies, this study is concluded to be accurate due to the correct correlation identification and the use of precise equipment and techniques.
Reliability:
Four trials were conducted for each of the eye drop bottles and cow eye samples to check for reliability in the results. Very consistent data was obtained from eye drop bottle storage conditions trials, with the expired eye drops having the highest variance of 18.9. Moderately consistent data was obtained from the cow eyes trials, with the open eye drops having the highest variance at 613 (Figure 5) and a range of 60 (Table 2). However, this is expected due to microbial growth having a known high variability, with multiple factors affecting development of different organisms. An average was taken between the four trials to overcome this high variance, producing an accurate linear relationship. The sample size of this study was moderate, using four storage conditions and five treatments of cow eyes. Due to the consistent results in each data range, it can be concluded that this study was reliable.
Validity:
The controlled variables include, but are not limited to, the type of agar plate cultured, the time spent in the incubator, the set temperature of the incubator, location of sourced cows' eyes, and the culturing method. A control experiment was used to see if any contamination had occurred, allowing the plate to incubate normally, and no colonies were identified. A control experiment was utilised for the cow eye trials, with one eye having no eye drops added, to determine that the drops were increasing the microbial growth. The method of this study was valid as the independent variable of the storage conditions of eye drop bottles was changed and the dependent variable of the microbial growth on treated cow eyes was measured appropriately using manual counting of observable microbe colonies. There were some colonies that were too small to be accurately measured, meaning that they were discounted, however, this method was held constant for each plate, meaning that it is valid in its consistency. Due to the accurate results and valid methods, it can be concluded that this study was valid.
Limitations/Errors and Improvements:
Deceased cow eyes are utilised due to ethical and health concerns of human eye experimentation, however, there are a variety of differences between them. The number of microbial colonies found, a maximum of 210 (Table 2), was significantly lower than the predicted 0.06 bacteria per conjunctival cell (Shivaji, 2022), for each of the 50 million cells on the eye surface. This may be attributed to a lack of sensitive equipment, however, this large range indicates another variable influencing the results, which is likely attributable to the use of cow eyes instead of human eyes. Cow eyes can be more resistant to S.aureus due to the Panton-Valentine Leukocidin (PVL) toxin, a secreted polypeptide that increases the virulence potential in hosts, present in approximately 5% of S.aureus colonies. PVL toxins bind to anaphylatoxin chemoreceptors, which are protein byproduct receptors that mediate acute inflammatory processes, to disrupt such processes, such as neutrophil differentiation into B, T and dendritic cells. Cow neutrophils are resistant to PVL toxins, whereas human cells are not (Astley, et al., 2019), indicating a potential for negatively skewed results, with the total capacity of bacteria not being grown on the cow’s eyes where it would on human eyes.
• The use of animal eyes more closely related to humans, such as monkeys or chimpanzees, should be used to prevent systematic errors in the prevention of microbial growth. If ethical parameters are managed, deceased human eyes could be used to produce the most accurate data.
The measurements of microbial growth were taken by counting the colonies on agar plates, meaning that small or indistinguishable colonies were not recorded.
• Automated microbe counters are machinery that counts microscopic colonies with much greater precision than the naked eye. Smartphone apps are being developed that allow microscopic and macroscopic counting of colonies on agar plates, therefore, future research may use cheap and accurate measurement methods (Austerjost, et al., 2017)
The agar method was changed for trials 3 and 4 due to limited time and inaccessibility to an autoclave, meaning that the solution was not sterilised and there was an increased chance of contamination. This can be seen in the microbial growth of expired eye drop bottles increasing from 4 to 12 (Table 1) and the no lid bottle cow eye increasing from 121 to 181 (Table 2).
• The agar solution was boiled, however, it should have been stored in an airtight container until the autoclave was available
Future Implications:
The results from this study provide various opportunities for further investigation into the effects of other eye drop active ingredients, such as brimonidine tartrate, on microbial growth on eyes. Distinctive microorganisms, such as E. coli or fungal species, could be further identified on each cow eye to determine the impact of tetrahydrozoline eye drops on specific microbes. Another study may involve determining the change in dryness or moisture content levels on cows' eyes with the treatment of eye drop bottle storage conditions to increase understanding of the treatment of dry eyes with tetrahydrozoline.
Conclusion
This study was conducted to investigate how tetrahydrozoline eye drops held in non-standardized conditions affect microbial growth on eye surfaces. It was hypothesised that ‘As microbial growth increases inside eye drop bottles with a greater risk of pathogen contamination, microbial growth on treated eye surfaces will increase’. It was found that moving away from the standardised storage conditions of eye drop bottles produced greater microbial growth on cow’s eyes. The expired eye drops cultured the highest number of colonies, both in the bottle and on the treated eye surface, followed by the open and half-open bottles. This is most likely a result of a change in the chemical composition of solutions and increased exposure to pathogens in the air causing greater microbial contamination. Statistical tests of single factor ANOVA and 2-sample, unpaired T-tests were performed to confirm that there was a statistically significant difference in the means of the number of microbial colonies. The main issue that arose during this study regarded the use of cows’ eyes in place of human eyes, where differing structures and physiological processes caused a negatively skewed growth of microorganisms. The inquiry question was answered by finding the R2 value when plotting the growth inside bottles versus the growth on eye surfaces, which was found to be 0.938. This indicates there is a very strong, positive correlation between the two variables and that the storage conditions of bottles do directly affect microbial growth on eyes. It can be concluded that the hypothesis was supported by many qualitative and quantitative results.
Reference List
Astley, R, Miller, FC, Mursalin, MH, Coburn, PS & Callegan, MC 2019, ‘An Eye on Staphylococcus aureus Toxins: Roles in Ocular Damage and Inflammation’, Toxins, vol. 11, no. 6.
Austerjost, J, Marquardt, D, Raddatz, L, Geier, D, Becker, T, Scheper, T, Lindner, P & Beutel, S 2017, ‘A smart device application for the automated determination of E. Coli colonies on agar plates’, Engineering in Life Sciences, vol. 17, no. 8, pp. 959–966.
Bantawa, K, Rai, K, Subba Limbu, D & Khanal, H 2018, ‘Food-borne bacterial pathogens in marketed raw meat of Dharan, eastern Nepal’, BMC Research Notes, vol. 11, no. 1.
Bhattacharya, S, García-Posadas, L, Hodges, RR, Makarenkova, HP, Masli, S & Dartt, DA 2018, ‘Alteration in nerves and neurotransmitter stimulation of lacrimal gland secretion in the TSP-1−/− mouse model of aqueous deficiency dry eye’, Mucosal Immunology, vol. 11, no. 4, pp. 1138–1148.
Conrady, CD, Joos, ZP & Patel, BCK 2016, ‘Review: The Lacrimal Gland and Its Role in Dry Eye’, Journal of Ophthalmology, vol. 2016, pp. 1–11.
Farzam, K & Lakhkar, A 2019, Adrenergic Drugs, in A Kidron (ed.), Nih.gov, StatPearls Publishing.
Inam, R & Batool, H 2017, ‘Bacterial Appraisal in Expired and Unexpired Pharmaceutical Products’, RADS Journal of Biological Research & Applied Sciences, vol. 8, no. 2, pp. 25–30.
Feghhi, M, Mahmoudabadi, AZ & Mehdinejad, M 2008, ‘Evaluation of fungal and bacterial contaminations of patient-used ocular drops’, Medical Mycology, vol. 46, no. 1, pp. 17–21.
Hosten, LO & Snyder, C 2020, ‘Over-theCounter Ocular Decongestants in the United States – Mechanisms of Action and Clinical Utility for Management of Ocular Redness’, Clinical Optometry, vol. Volume 12, pp. 95–105.
Kruszewska, H, Zaręba, T, Kociszewska, A & Tyski, S 2006, ‘Activity of selected non-antibiotic medicinal preparations against standard microorganisms including bacterial probiotic strains’, Acta Poloniae Pharmaceutica - Drug Research, vol. 78, no. 2, pp. 179–186.
Lee, HR, Hong, YJ, Chung, S, Hwang, SM, Kim, TS, Song, EY, Park, KU, Song, J & Han, KS 2014, ‘Proposal of standardized guidelines for the production and quality control of autologous serum eye drops in Korea: based on a nationwide survey’, Transfusion, vol. 54, no. 7, pp. 1864–1870.
Leibowitz, HM 2000, ‘The Red Eye’, New England Journal of Medicine, vol. 343, no. 5, pp. 345–351.
Li, ZH, Gong, Y, Chen, S, Li, S, Zhang, Y, Zhong, H, Wang, Z, Chen, Y, Deng, Q, Jiang, Y, Li, L, Fu, M & Yi, G 2019, ‘Comparative portrayal of ocular surface microbe with and without dry eye’, Journal of Microbiology, vol. 57, no. 11, pp. 1025–1032.
Nisar, S, Rahim, N & Maqbool, T 2017, ‘Bacterial Contamination of Multi-Dose Ophthalmic Drops’, Hamdard Medicus, vol. Vol. 61, no. 3 & 4.
O’Callaghan, R 2018, ‘The Pathogenesis of Staphylococcus aureus Eye Infections’, Pathogens, vol. 7, no. 1, p. 9
Perez, DM 2020, ‘α1-Adrenergic Receptors in Neurotransmission, Synaptic Plasticity, and Cognition’, Frontiers in Pharmacology, vol. 11.
Shin, H, Price, K, Albert, L, Dodick, J, Park, L & Dominguez-Bello, MG 2016, ‘Changes in the Eye Microbiota Associated with Contact Lens Wearing’, mBio, vol. 7, no. 2.
Shivaji, S 2022, Human Ocular Microbiome: Bacteria, Fungi and Viruses in the Human Eye, Google Books, Springer Nature.
Taşli, H & Coşar, G 2001, ‘Microbial contamination of eye drops’, Central European Journal of Public Health, vol. 9, no. 3, pp. 162–164.
Teuchner, B, Wagner, J, Bechrakis, NE, Orth-Höller, D & Nagl, M 2015, ‘Microbial Contamination of Glaucoma Eyedrops Used by Patients Compared With Ocular Medications Used in the Hospital’, Medicine, vol. 94, no. 8, p. e583.
Tsegaw, A, Tsegaw, A, Abula, T & Assefa, Y 2017, ‘Bacterial Contamination of Multi-dose Eye Drops at Ophthalmology Department, University of Gondar, Northwest Ethiopia’, Middle East African Journal of Ophthalmology, vol. 24, no. 2, pp. 81–86.
Wirbelauer, C 2006, ‘Management of the Red Eye for the Primary Care Physician’, The American Journal of Medicine, vol. 119, no. 4, pp. 302–306.