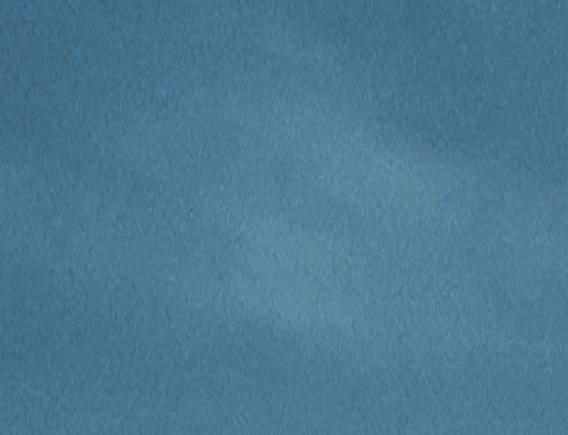
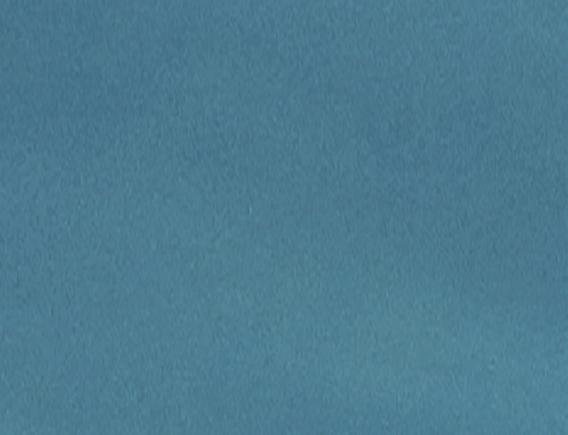
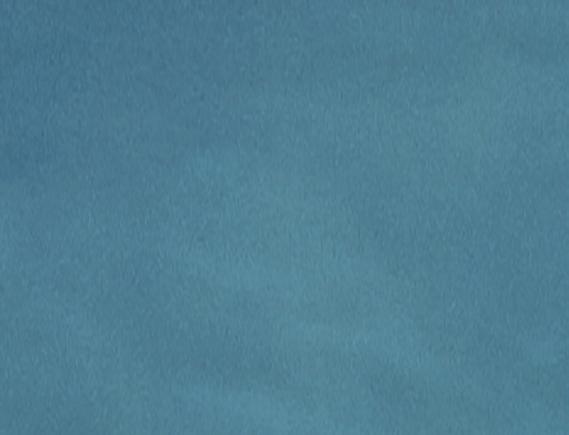
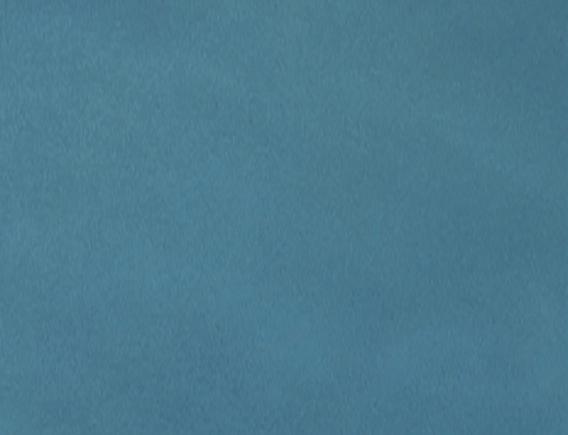
Producing enough green hydrogen is the key to decarbonizing businesses that can’t be directly electrified. Our power-to-X processes convert renewable electricity and water into green hydrogen and its derivate net-zero fuels. Together with our subsidiary Quest One we are mass-producing PEM electrolyzers and scaling up production of green hydrogen –moving big things to zero in the energy, heavy industry, and transport sectors.
with green hydrogen from PEM electrolyzers
Introducing the Damen CSOV 9020 E, a fully electric Service Operations Vessel for the offshore wind industry of tomorrow. With its revolutionary offshore charging capabilities, the CSOV E takes a significant step towards zero emissions maintenance of offshore wind farms. Connecting to a turbine offshore, the vessel is able to safely and efficiently charge without manual intervention – making the generation of green energy even more sustainable and providing a solution fit for the future.
03. Guest comment
04. Negative electricity prices: Blessing or curse?
Jesse Hettema and Simon De Clercq, Aurora Energy Research, explain how negative electricity prices are on the rise across Europe, and how they can hinder progress in Europe’s energy transition by discouraging investment in renewables.
10. Powering progress: The UK's subsea system opportunity
Neil Gordon, CEO, Global Underwater Hub, highlights the issue of cable failures and explores how the UK could lead in improving performance and reliability.
14. Unlocking insurability: Data's value to offshore wind insurance
Danny Constantinis, Executive Chairman and CEO of EM&I, Malta, reviews how lessons from oil and gas can aid the development of risk criteria for offshore wind structures and emphasises the value of risk data for offshore wind insurance.
20. Lightning in a bottle
Dr Neal Fine, Arctura Wind, USA, discusses how coatings can protect wind turbine blades against lightning damage.
26. Driving green innovations with SAR technology
Dr Motoyuki Arai, CEO, Synspective, details how synthetic aperture radar technology can help tackle global sustainability challenges.
enquiries [enquiries@energyglobal.com]
32. What does the future hold for Europe's offshore wind industry?
Cristina Fernández, Germany Managing Director and Continental Europe Sales Director, K2 Management, examines the progress of offshore wind in Europe and the likelihood of meeting future targets in a Q&A with Jessica Casey, Editor of Energy Global
36. Time to stop organised crime getting high on Europe's supply
Kelly Barnes, Senior Intelligence Analyst, DeterTech, maps out the emerging patterns followed by organised criminals targeting solar farms across the UK and Europe and provides data-based prevention techniques.
42. Cybersecurity in renewables: The key to energy security
Uri Sadot, Cybersecurity Program Director, SolarEdge Technologies, showcases why an increased focus on cybersecurity amongst vendors, commercial businesses, grid operators, and governments is critical to safeguarding future energy security in a Q&A with Jessica Casey, Editor of Energy Global
46. Illuminating future innovation in solar power generation
Dongyoung Kim, Technical Assistant, Reddie & Grose, outlines the trend towards technological developments in the solar photovoltaics sector seen through increased patent filings and details the current legal and developmental barriers to progress.
50. Packed for Evolution!
Shwu Tyng Goh, Global Product Manager of Packings, Sulzer, considers how increasing the efficiency of distillation processes through innovative structured packing can help to dramatically reduce the energy consumption of distillation processes.
54. Global news
Producing enough green hydrogen is the key to decarbonising businesses that cannot be directly electrified. MAN’s power-to-X processes convert renewable electricity and water into green hydrogen and its derivate net-zero fuels. Together with its subsidiary, Quest One, the company is mass-producing PEM electrolysers and scaling up production of green hydrogen – moving big things to zero in the energy, heavy industry, and transport sectors. Learn more at www.man-es.com
MANAGING EDITOR
James Little james.little@palladianpublications.com
SENIOR EDITOR
Elizabeth Corner elizabeth.corner@palladianpublications.com
EDITOR
Jessica Casey jessica.casey@palladianpublications.com
EDITORIAL ASSISTANT
Abby Butler abby.butler@palladianpublications.com
SALES DIRECTOR
Rod Hardy rod.hardy@palladianpublications.com
SALES MANAGER
Will Powell will.powell@palladianpublications.com
PRODUCTION DESIGNER
Amy Babington amy.babington@palladianpublications.com
HEAD OF EVENTS
Louise Cameron louise.cameron@palladianpublications.com
EVENT COORDINATOR
Chloe Lelliott chloe.lelliott@palladianpublications.com
DIGITAL EVENTS COORDINATOR
Merili Jurivete merili.jurivete@palladianpublications.com
JUNIOR VIDEO ASSISTANT
Amélie Meury-Cashman amelie.meury-cashman@palladianpublications.com
DIGITAL ADMINISTRATOR
Nicole Harman-Smith nicole.harman-smith@palladianpublications.com
DIGITAL CONTENT COORDINATOR
Kristian Ilasko kristian.ilasko@palladianpublications.com
ADMINISTRATION MANAGER
Laura White laura.white@palladianpublications.com
Editorial/Advertisement Offices: Palladian Publications Ltd
15 South Street, Farnham, Surrey, GU9 7QU, UK +44 (0) 1252 718 999 www.energyglobal.com
The renewable energy transition race has well and truly begun and, as part of the UK government, UK Export Finance (UKEF) is eager to play our part. New players in the renewables space are appearing at an ever-increasing rate; however, while many are thriving in the domestic market, their potential to have an international impact remains unrealised. This is where UKEF comes in: by supporting UK-based companies with loans, guarantees, or insurance, enabling access to international trade opportunities, we ensure no business is left behind. For UKEF, it is vital no viable UK export fails because of a lack of finance or insurance, doing so sustainably and at no net-cost to the taxpayer.
A great example of a UK business thriving is Hampshire-based Hive Energy. Hive wanted to expand its operations overseas, particularly into markets crucial to the global energy transition. Initially serving exclusively to the UK market, Hive became an established figure in the green energy sector. Consequently, Hive now strives to make its mark on an international scale, building on its existing domestic growth.
Hive specialises in developing greenfield sites into renewable energy facilities, with this process spanning from the initial site identification process to eventual grid-connection and power generation. These spaces are then sold upon completion and the proceeds are reinvested into new projects.
With support from UKEF’s General Export Facility (GEF) scheme, Hive Energy secured a £19 million export finance package from Santander.
Given Hive Energy’s business model of continually selling and rebuying, factors such as guarantees can be a challenge.
Local authorities and power grid operations frequently require a financial guarantee from developers, evidencing that the company can pay the connection fees initially. This is where UKEF came in, helping Hive secure the financing they needed when they needed it most.
Through UKEF’s General Export Facility, UKEF provided a partial guarantee to Hive’s bank,
helping them secure the trade finance they needed. By bridging the gap between lenders and businesses such as Hive, UKEF ensures that UK businesses continue to grow and overcome the hurdles of funding that they often face.
With UKEF’s support in place, Hive not only overcame the hurdles from other lenders, but is now participating in the renewable energy transition race without any financing barriers – finally free to continue on its growth trajectory.
Hive’s business growth has not just seen expansion into new markets, but also the expansion of products. For example, they are now offering green hydrogen and green ammonia. With support from the UK government, their operations have been extended into Italy, Spain, Türkiye, Serbia, Chile, and South Africa.
Markets such as Türkiye are crucial for reaching the global goal of net zero, precisely because of Türkiye’s location. Situated at the crossroads of Europe and Asia, Türkiye can take advantage of its location and has the potential to become a major influence. This gives Hive an opportunity to make a real impact by exporting to promising markets with abundant renewable energy resources and a growing economy.
Another key location for Hive is Serbia. Given that this is less traversed, exporting to Serbia will allow Hive to diversify their energy mix, reducing reliance on fossil fuels and enhancing overall energy security.
Since Hive’s launch in 2010, the business has developed 2.8 GW of solar capacity around the world. Hive Energy is just one example of a business UKEF has helped support on the road to the renewable energy transition.
The potential for UK businesses to have a true impact on the goal to net zero by 2050 is growing. The UK is one of the leading countries in clean energy and is ready to collaborate and support other countries in reaching this joint goal. UKEF is keen to support UK renewables businesses to unlock their export potential and we will do so through improved cashflow management and access to capital.
Jesse Hettema and Simon De Clercq, Aurora Energy Research, explain how negative electricity prices are on the rise across Europe, and how they can hinder progress in Europe’s energy transition by discouraging investment in renewables.
fter years of high inflation, negative electricity prices might seem like a positive. However, the reality is more complex. Without adjustments to pricing structures and subsidy schemes, negative prices could slow down Europe’s energy transition.
In the past two years, the number of negative price hours on the European electricity market has increased significantly. Power consumers are now paid to use electricity for hundreds of hours annually in multiple countries. This is a phenomenon that was almost unheard of five years ago. Aurora’s analysis for 2024 shows that negative price hours are coming up across all of Europe. The Netherlands stands out through having one of the highest numbers of negative prices on the continent, with over 450 recorded negative price hours in 2024, and some of the most extreme negative price levels. This makes the Netherlands an appropriate case study to understand what is going on with negative prices in Europe and what might lie ahead for other markets.
Essentially, negative prices on the day-ahead market occur when electricity supply exceeds demand at a given moment. During these periods of surplus, electricity producers must pay to offload their excess power onto the market. So why not just stop producing?
One of the main drivers for negative prices is the inflexibility of solar energy systems, which continue to produce during periods with negative price hours. In the Dutch day-ahead market,
negative price hours mainly occur during spring and summer, between 9 am and 3 pm, when solar panels generate electricity on a large scale. Solar capacity in the Netherlands has quintupled in five years, from 5 GW in 2018, to 24 GW in 2023. On sunny days, the power production of solar energy often exceeds demand, which averages around 13 GW. Neighbouring countries such as Germany and Belgium face similar challenges with solar overproduction, which limits the Netherlands’ ability to export the excess power. In 2024, this led to prices as low as -€200/MWh during midday hours. Dutch households lack incentives to stop producing during times with negative prices due to the net metering policy. Over 10 GW of solar capacity in the Netherlands
is residential capacity installed behind the meter. Under the current net metering scheme, households can offset their annual generation against their annual consumption and they do not pay taxes or grid fees on this production. Moreover, if they generate more than they consume, they receive compensation for the surplus. This means that they will maximise their production, even when prices go negative. Large scale installations have more incentives to curtail generation during negative price hours, but many installations either lack remote control capabilities or are bound by contractual obligations that prevent flexibility. Most large scale installations in the Netherlands are under the SDE++ subsidy programme, which provides a ‘feed in’ premium on top of the day-ahead market revenues. Under this framework, renewable energy producers do not receive subsidies during periods of negative electricity prices – a rule designed to discourage production during oversupply. However, many smaller parks lack the technology or expertise to be remotely controlled and to scale down production. As a result, these installations continue producing even when they do not receive subsidies. This contrasts some other markets, such as Spain, where large solar parks automatically shut down as soon as prices drop below zero. While prices in Spain only go a few euros negative at most, in the Netherlands they can drop to below -€100/MWh.
It is not just solar panels that contribute to negative prices. Between 2018 and 2023, wind power in the Netherlands increased from 4 GW to 12 GW, of which almost 5 GW is installed offshore. While wind generation alone is usually insufficient to push prices into negative territory, its relatively consistent output throughout the day, combined with high solar production during sunny periods, can result in an electricity surplus.
Thermal generation, including coal and gas generation, also adds to negative price hours because they operate based on must-run conditions and face ramping constraints. Currently, system flexibility in the Netherlands is mainly provided by gas and coal fired power plants. These plants traditionally operate at minimum output levels even during periods with low demand, to remain available for rapid deployment when solar and wind generation drop. These must-run conditions suppress power prices and contribute to moments of oversupply. When prices go negative, the high costs associated with shutting down and restarting thermal plants are an additional incentive to remain operational, even if this means paying to generate power. In the Netherlands, most gas and coal plants have been installed over the last 15 years and are more flexible compared to older plants. The plants have relatively short start up times and can ramp up and down quickly, but their operation still contributes to negative pricing. In countries with much older thermal fleets, like Poland, the impact of fossil generation on negative prices will be even larger.
Negative prices pose a significant concern for project developers and investors. Large solar and wind farms benefit from the SDE++ subsidy, which provides financial stability by protecting them from low prices and guaranteeing predictable revenues. This makes it easier to secure bank loans and reduces the financing costs of projects. However, during periods of negative prices these projects receive no subsidy. In 2024, over 20% of solar generation occurred during times with negative prices and therefore was not covered by the SDE. This has led to a loss of revenue for projects which, in turn, increases financial risks.
Consequently, banks are becoming more cautious about lending to renewable projects. Lenders are reviewing their portfolios to confirm the performance of the existing assets and are deciding whether the Dutch solar market remains attractive. For new projects, they raise requirements to account for the lost revenue during negative price hours. This includes setting higher expectations for debt service coverage ratios and requiring stronger operational and management capabilities within project teams. This makes it more difficult to develop new projects, since developers need to raise more equity, which requires higher returns than debt.
As the share of renewable energy in the Netherlands increases, the impact of negative price hours could further increase. This will make it more difficult to mobilise the investments that are needed to achieve the country’s climate goals. In 2024, solar power contributed less than 20% of the Dutch electricity mix. Achieving a net-zero power system will require a substantial increase in solar capacity in the coming years. Without updates to market design and support mechanisms, the frequency of negative prices is expected to rise, potentially becoming a key barrier to the Netherlands’ energy transition.
This being said, negative prices are not inherently problematic. They provide the incentive needed for producers and consumers to become more flexible with energy use. Electricity producers are encouraged to scale back production during negative price periods, which also reduces carbon dioxide emissions from fossil fuel plants. By optimising their production during negative prices, solar parks can increase their earnings. For consumers, negative prices offer benefits when they concentrate their consumption during these times, leveraging storage or demand-response systems.
Exposing more households, particularly those with solar panels, to negative prices would be beneficial. It would encourage them to charge their electric vehicles (EVs) or invest in home batteries rather than exporting surplus energy to an already overloaded grid. Dynamic energy contracts, in which prices differ throughout the day, can be an enabler. In these contracts, prices are directly coupled to the prices on the day-ahead market on which power is traded. To make sure that households react to these prices, energy suppliers can remotely manage the household solar panels,
thereby unburdening customers. This allows them to optimise across their portfolios and share the resulting revenues. This would be far more efficient than the fixed monthly ‘feed in’ fees that energy providers currently impose.
For wind and solar projects, an adjusted subsidy scheme could help. One option is a financial contract for difference (CfD), in which the government pays the producer a fixed hourly lump sum, independent of output or prices. Unlike conventional CfDs tied to the production of a specific asset, a financial CfD bases payments on an independent benchmark. This contract offers a fixed remuneration linked to the installation’s capacity, supplemented with variable payments based on a reference, such as average regional production. Since revenues are not dependent on real-time production, compensation remains stable, even when producers curtail output during periods of negative market prices. Operators have an incentive to outperform the benchmark, which allows them to further optimise revenues. The CfD based on potential production that Belgium is implementing for its new offshore wind park could be an example.
A regulatory framework that supports the transition from thermal plants to batteries and demand side response, such as smart EV charging, is essential to reducing the number of negative price hours. In a volatile market, batteries can make money by charging during times with surplus solar generation and selling this power back to the grid when prices are high. Negative prices in the Netherlands create significant price spreads offering substantial revenue opportunities. However, high Dutch grid fees remain a major barrier for the battery investment case. While several grid fee proposals are being implemented to lower costs for installations that provide flexibility, Dutch grid fees remain among the highest in Europe, even under the proposed changes. To unlock the gigawatts of battery capacity currently in development, a more ambitious grid fee reform will be required. On demand response, strengthening mandates for the connectivity of large energy consumers, such as EVs, can significantly enhance market flexibility. Besides their impact on power markets, EVs place a significant strain on the grid. Therefore, enforcing higher standards for smart charging will be essential to enable widespread EV adoption without overburdening power markets and grids. Such measures will help achieve a better balance between supply and demand, particularly during periods of high solar generation.
In conclusion, negative price hours play an important role in balancing the energy system and preventing oversupply. By leveraging these price fluctuations effectively, both producers and consumers can benefit. This fosters more flexible production and consumption, which is essential in a system predominantly powered by solar and wind. To achieve this, pricing structures need to be improved for household solar energy, revamped subsidy schemes for solar and wind projects, and better regulation on grid usage. This way, negative prices can support the energy transition without unnecessary delays.
2-3 April 2025
ExCeL, London
Explore solar and energy storage solutions, along with complementary products and services from over 200 global exhibitors.
Hear from over 250 speakers on top case studies, market trends, policies, training, certification and more.
Connect with over 10,000 solar and energy storage professionals at exciting networking events.
Visit the dedicated zones to connect with local UK installers, project advisors, insurers, banks, and legal experts for their services.
Neil Gordon, CEO, Global Underwater Hub, highlights the issue of cable failures and explores how the UK could lead in improving performance and reliability.
Globally, over US$800 billion of investment in offshore wind farms is anticipated by 2030 and, for the world to hit net-zero emissions by 2050, the generating capacity from offshore wind must increase by a staggering 1120 GW. In the UK, the government has set ambitious targets of 50 GW of installed capacity by 2030.
Subsea cables are essential components of infrastructure for both fixed and floating offshore wind farms and this scale of expansion in offshore wind can only be achieved by installing and maintaining thousands of miles of reliable underwater cables.
The performance and reliability of subsea cables are therefore paramount not only to developers and the industry, but to the whole country which will increasingly rely on the power that passes through them.
With an extensive installed base of offshore wind capacity and a strong project pipeline, combined with a stable policy framework, there is a significant opportunity for the UK’s underwater supply chain to lead in subsea power cable systems. Indeed, the subsea cable sector for offshore wind has a potential, estimated value of over £100 billion across manufacture and installation over the next 10 years.
In 2024, the ‘Offshore Wind Industrial Growth Plan’ identified future electrical systems and cables as two of five areas in which the UK should be excelling, as well as offshore installation, operation, and maintenance. To achieve this, the report highlighted the need to triple manufacturing capacity and double research and development.
These areas were chosen because of the size and growth of the domestic and international markets, existing UK capabilities, and its track record of developing solutions to complex technical challenges. Examples include the UK’s deployment of the world’s largest fixed offshore wind farms, coupled with the expertise honed in the oil and gas industry over the last five decades. However, there are major challenges varying from supply chain constraints to cost escalation and cable reliability.
Global Underwater Hub (GUH), the trade and development body which represents the UK’s £9.2 billion underwater industry, has identified cable performance and reliability as a major issue which needs to be solved, especially as floating offshore wind, which requires even more complex dynamic cables, becomes an increasing percentage of the UK’s overall installed base.
Continuous power supply is crucial, and any cable failures can lead to significant disruption to power availability and energy security. Reliable subsea cable systems ensure that the electricity generated offshore is consistently and continuously delivered to the grid.
Failures in subsea power cables also result in prolonged downtime and high repair costs. The economic viability of offshore wind projects is therefore inextricably linked to the reliability of subsea power cables. However, high failure rates and associated repair costs can deter investment.
Unfortunately, failure of these cables is common – to the point where the cost of insuring them is becoming prohibitive. Insurance claims have increased significantly in recent years, with available data pointing to 80% of failures related to the cable system and repairs ranging from £10 million – £40 million, not to mention the costly downtime of at least 40 – 60 days.
The challenge of insuring cables was laid bare by insurance experts at a recent GUH event, one expert claimed that 85% of the total value of offshore wind claims relate to subsea cables. Most insurers are losing money underwriting cables
with the average settlement claim being £9 million. Another broker warned that the high number of cable claims is affecting capacity and coverage, noting that the cost of repairs typically runs into the millions with warranties rarely covering the high cost of business interruption.
According to one developer, the cost of insuring a 1.2 GW offshore wind farm over its lifetime is in the region of £350 million and insurance brokers estimate that the costs of floating offshore wind will be 30% higher than fixed bottom wind farms. If these critical components become uninsurable, offshore wind projects around the world will be derailed, rendering global 2050 net-zero targets unachievable.
With the shift from fixed to floating offshore wind, where the dynamic nature of floating cables is even more challenging, GUH is working to improve the performance and reliability of subsea power cables with a view to developing the UK’s expertise in this field.
GUH is bringing together other trade and standards bodies to explore the reasons for cable failure and to address the lack of standards and guidelines across the lifecycle of offshore wind subsea power cables. Unlike fixed offshore wind, floating offshore wind has few standards and no established supply chains. This means that research into these issues starts with a clean slate and provides the UK with an enormous opportunity to create a new generation of products, services, and standards to meet a domestic market of £270 billion and export market of £1 trillion.
The critical issue of cable reliability, and therefore insurability, must be addressed as a matter of urgency and GUH is taking a holistic approach to finding solutions which can be implemented as floating offshore wind increases in scale and technical capability with higher voltages and dynamic elements. The organisation believes the UK can and should become a global leader in subsea cable technology, particularly with regards to dynamic cables for floating offshore wind.
At the annual GUH-organised subsea cable insurance workshop in October 2024, it became apparent that there are inherent issues affecting the performance and reliability of subsea cables that are within the industry’s control. Failures could stem from any stage in the cable lifecycle – from design to manufacture, handling and installation, through to operation and maintenance.
Identifying potential weak points along the lifespan of the cable is imperative to ensuring these key components of offshore infrastructure operate robustly and efficiently to bring clean, green renewable energy ashore.
Representatives from government, cable insurers, developers and operators, cable manufacturers, risk management consultancies, and engineering, installation and specialist maintenance companies attended the workshop. Common themes were identified across each stage of the lifespan, as well as concerns which may originate in one stage, but have an impact on another.
These common themes and concerns demonstrated the need – and the desire – for a set of industry led and recognised standards, encompassing the life of the cable that
would be adopted by developers, suppliers, contractors, warranty surveyors, and others whilst also being accepted by insurance bodies.
Adoption of a systems-based design methodology is essential as the industry moves into floating offshore wind. The development of clear standards that tackle the system as a whole must be integrated across the supply chain and all phases of the cable lifecycle.
Data sharing is key. There is an urgent need for better information sharing and a move away from a siloed approach that is often secrecy-driven and NDA heavy. This data sharing is one of the main challenges that must be overcome. Introducing shared learning, data logging, and increased transparency will create a more open environment for best practice to be developed. By bringing the industry together, GUH anticipates that this will improve access to the data that is critical to driving long-term improvements and ensuring a focused approach.
It goes without saying that quality control is paramount and that there is a need to focus resources on ‘critical to quality’ parameters that will drive control and improvement. This quest for quality and reliability needs to be driven at the same pace as the drive for efficiency and speed, and considered from both a capital and operational cost perspective.
Risk allocation needs to be addressed as there are challenges around the disproportionate allocation of risk between stakeholders with the need to incentivise failure prediction to reduce insurance claims. With more effective
Wind Turbine Automation
More than 150,000 wind turbines automated
Condition Monitoring
Comprehensive protection for your operating assets
Retrofit
Extend turbine lifecycles
Grid Protection and Control
Safe, fast and stable power management
Wind Power SCADA
Access protection and operation control
industry collaboration across the entire cable system supply chain, these common themes can be tackled.
This collaboration must extend to the innovation and manufacturing ecosystem including, but not limited to, universities, catapult centres (high value manufacturing, offshore wind and energy systems), and Renewable UK – the trade association that advocates for the renewable industry in the UK. It must also include governments and governmental organisations so that the supply chain’s experience and expertise can influence, shape, and help deliver clean power and industrial strategies.
Forums, such as GUH’s recently established subsea cables forum, whose members are drawn from companies involved in every stage of the cable lifecycle, will help establish an industry wide approach to best practice and improve the quality, reliability, and therefore insurability, of cables which is paramount to achieving our offshore wind ambitions, particularly in the nascent floating offshore wind arena.
GUH will engage with the industry to ensure that the whole supply chain has a voice and a co-ordinated approach to influence standards and policy, driving reliability, cost-effectiveness, and quality into cables systems supplied in the UK. This will ensure that the UK becomes well-positioned as an authoritative centre for reliable subsea cable systems and solutions that enhance the economic viability of offshore wind projects and help accelerate the development of floating offshore wind.
Danny Constantinis, Executive Chairman and CEO of EM&I, Malta, reviews how lessons from oil and gas can aid the development of risk criteria for offshore wind structures and emphasises the value of risk data for offshore wind insurance.
The global floating offshore wind market size was valued at US$0.29 billion in 2021 and is projected to grow to US$15.64 billion by 2029.1 Significant and rapid growth towards commercialisation requires investment and leadership, not least in harnessing emerging technology, but also in assuring financiers and banks that their investments are safe. In summary, insurance provides assurance to financiers and makes projects ‘bankable’.
In turn, insurers need reliable and comprehensive information on the risks they are covering, ranging from design through to construction, assembly, installation, operation, and decommissioning. At the moment, insurers do not have sufficient life cycle data to give them certainty of these risks needed to provide cost-effective and
comprehensive insurance while current regulation and classification societies do not fully cover their needs. This is mainly because although in the floating offshore wind sector, human and environmental risks are low, the industry has little experience of the design, construction, and operating risks of large scale floating offshore wind arrays. Ultimately, as indicated by the World Forum of Offshore Wind: “Insurability rhymes with bankability, rhymes with credibility and, above all, rhymes with feasibility.” 2
Are there alternative means of getting the necessary evaluation of risk?
In the absence of floating offshore wind experience, EM&I is adapting methodology from the innovation phase of the floating oil and gas world to develop guidance on risk that
helps both insurers (and projects needing insurance) to move forward.
Insurance risks in operations are difficult to evaluate. For example, replacing a failed mooring chain may not seem to be a costly item in itself, yet the costs of getting the floating offshore wind unit back online may well be substantially increased and operations much delayed by the absence of suitable towing vessels or yard availability.
EM&I has over four decades of asset integrity management services provision in the floating energy production sector, hitherto in oil and gas. From this, risk assessment processes specific to the floating offshore wind sector have been developed. Working with DNV, EM&I will present and test these guidelines to NIORD, an insurance organisation for the floating offshore wind sector, in 2025.
Floating wind assets are usually unmanned, they typically have negligible hydrocarbon inventory and a series construction of multiple identical or near identical units. Additionally, they often have low target operating costs, frequently quoted as approximately 2% of CAPEX annually.
However, the floating oil and gas sector is moving quickly towards reduced manning and the robotic remote inspection and maintenance of their assets – a development of great interest to the floating offshore wind sector. Remotely operated vehicles (ROVs) capable of cleaning, inspecting, and maintaining underwater assets are already being deployed by EM&I, piloted from onshore bases hundreds of kilometres from the worksite. Similar remotely operated robots are also being trialled for energy majors in Brazil to carry out similar tasks.
Working with a national oil company, EM&I continues to develop diver-less inspection techniques utilising ROVs in extreme ocean conditions, minimising persons on board (POB), mitigating human safety risk, and enhancing data quality. EM&I utilises small, manoeuvrable ‘inspection class’ ROVs to prevent risk of damage to both the asset under inspection or to a larger, more expensive model of ROV. Developing the capability further and incorporating rapidly progressing communication technology, POB is further reduced with the ROVs being flown from onshore control hubs.
Lessons from the floating offshore oil and gas sector can be implemented in the inspection, thickness measurement, and the cleaning of mooring chains within the floating offshore wind sector. These lessons are progressing to non-destructive testing and to the inspection of several alternative materials being developed for floating wind mooring systems and dynamic power cables.
Integrity management of the mooring systems of floating offshore wind arrays is the focus of a new joint industry project (JIP) within the Floating Energy Research Forum. This JIP will focus on the methods, procedures, and technologies for integrity management, conducting trials and providing leadership. Whilst such tools are useful, this usefulness stems from the fact that parallel developments in other industries can provide shortcuts leading to better understanding of risks in floating offshore wind.
The oil and gas industry, and other established industries, use principles set out following the Piper Alpha incident. They are essentially based on a safety case which sets out the risks created by a project as well as the proposed mitigation measures from stakeholders. However, the floating offshore wind sector needs to set out its own risk guidelines based on reliability rather than safety – mainly because the human safety and environmental risks are much lower. The floating offshore wind risk is largely commercial and is primarily related to how the failure of equipment causes financial loss.
Regulatory focus is less intense in these circumstances and looks through a more financial lens, resulting in a fundamental change in how risk is assessed, evaluated, and regulated. The guidelines currently being tested by EM&I set out how best to assess and mitigate the risk of
floating offshore wind assets throughout their lifecycle, from design, construction, assembly, installation, and then through to the design service life, life extension, and decommissioning. EM&I’s guidelines are generic and are not focused on any specific floating offshore wind project design although common methods of construction, materials, and other elements have been considered.
There are currently more than 50 different floating offshore wind asset designs identified in the public domain, 30 of which are semi-submersible with varying structural arrangements. Most of the designs are based on steel fabrication and only 10 are based on concrete fabrication or an option between steel and concrete. Most current designs are based on spread mooring systems. The floating offshore wind market also draws on experience from the fixed wind installation industry.
The International Association of Engineering Insurers, a network of experts in engineering insurance from around the world, has noted: “Experience from the fixed offshore wind industry has shown that certification against recognised industry standards is a generally accepted way to establish stakeholder confidence and ensure bankability as well as insurability. Certification according to an accepted assessment scheme demonstrates compliance with relevant industry standards and shows that technical risks have been understood and managed across all phases of a project.” 3
Currently, there are no common standards for assessing the risk during the construction, assembly, installation, or in-service phases and there is also potential for series losses of floating wind assets in an array rather than a single oil and gas asset loss.
It is unknown under which regulatory regime any of the floating offshore wind asset designs will come. It is likely that there will be some national or coastal state regulatory requirements, but these are also currently unknown. There may also be a survey, inspection, and monitoring requirement led by a certification body or
classification society, likely to be particularly related to design and construction.
Several classification societies, such as DNV, ABS, Bureau Veritas, and Lloyd’s Register, have standards and guides for offshore wind assets, but most do not issue rules for operations, in-service surveys, and inspections. These standards and guides are generally based on the pre-existing classification rules for offshore oil and gas units.
Since there are few, if any, rules relating to in-service survey and inspection requirements, it is currently unclear what such requirements would entail. However, for offshore oil and gas units, in-service surveys and inspections may be either prescriptive, to the rules, or a risk-based approach. Given the multiple identical, or near identical, assets within floating wind arrays, regular inspection and monitoring of all assets is unlikely. It is more likely that sample inspections and monitoring will be carried out on critical areas or on selected assets within the array, which would suggest a risk-based approach.
The risk-based inspection (RBI) process focuses on the optimisation of structural assessment inspections or monitoring programmes of floating assets. The RBI process recognises that the purpose of inspection and monitoring is to prevent incidents that impair the safety and reliability of assets. Unlike the oil and gas sector, reliability is becoming a highly critical aspect of inspection. As a risk-based approach, the process provides a means of evaluating the risk of failure from specific areas or degradation mechanisms. This develops an inspection or monitoring approach that will effectively reduce the associated risk of failure in critical components. Resources that focus on the areas of greater concern or criticality will provide a methodology for determining the optimum combination of methods and frequencies for maintenance by prioritising structural and mooring components. Meanwhile, planning of inspection and monitoring activities must aim to minimise operational disruption.
RBI usually combines qualitative and semi-quantitative risk assessment and management techniques for all inspection and monitoring activities, helping to develop plans which direct inspections and monitoring towards areas of highest risk. Processes should include reviews and feedback to the RBI system to provide continuous improvement and to allow for the reassessment of risk and the refocusing of inspection activities where required. The RBI process aims to prevent failures that lead to safety, environmental, or business concerns by planning the inspection and monitoring activities based on information obtained from a risk analysis. Risk analyses for components need to identify potential causes of failure alongside its likelihood and consequences.
Periodic in-service inspections consist of three levels of inspection: general visual inspection (GVI), close visual inspection (CVI), and non-destructive testing
or monitoring. These in-service inspections and monitoring cover both the above and below-water floating foundation structure, tower structure, the station keeping mooring system, turbine assembly, and cables.
For some designs of floating foundations, in-service GVI and CVI are limited to external only as there is no access to the internal structures. For example, above-water internal visual inspections of floating foundation structures are limited to floating foundations which have access to the internal structure. In many designs, only part of the floating foundation structure is accessible above water for inspection whilst the remainder of the structure is submerged when in-service. Additionally, certain designs, including above-water pinned connections and their bearings, as well as grouting on pinned floating foundations, may not be accessible for inspection. Lastly, access to above-water structures for inspection may be limited during bad weather or sea state conditions. These limitations add an extra dimension of uncertainty for insurers, particularly given the lack of reliability data from the outset. Below-water external visual inspections of the floating foundation structures can be carried out by ROVs.
Whilst floating offshore wind mooring systems might appear relatively simple compared to mooring chains in spread-moored floating oil and gas production units, there are challenges which remain. For example, little redundancy, interdependence in designs, the large areas of seabed needed, scouring, the influence of currents, and varied
environmental influences through an array are all potential rapid degradation factors.
The World Forum of Offshore Wind has said: “For the acceleration of new technology deployment, investment is a prerequisite. It is therefore necessary to gain the trust of investors and financiers to enable projects to move from a demonstrator to a commercial scale. Insurance is the security on which both investors and lenders rely when making their investment decisions and which makes investment possible.”2
Lessons must be learned from oil and gas experiences. There is no doubt that insurers need more data and not just from more prototypes, but before this data materialises, greater assurance can be given by following agreed guidelines which clarify the challenges and identify the methods for risk mitigation whilst also giving transparency for credibility and feasibility.
1. ‘Floating Wind Power Market Size, Share & COVID-19 Impact Analysis, by water depth, turbine capacity, and regional forecast’, Fortune Business Insights (17 February 2025), www.fortunebusinessinsights.com/industry-reports/floatingwind-power-market-101488
2. SKOWRONNEK, R., ‘Insurability of Floating Offshore Wind’, World Forum Offshore Wind, (October 2021), https://wfo-global.org/wp-content/uploads/2023/01/WFO_ Insurance-WhitePaper-October2021-FINAL.pdf
3. D’ANDREA, F. et al., ‘Floating Offshore Wind: Risk Management & Insurance’, IMIA Working Group, (October 2023), www.imia.com/wp-content/uploads/2023/10/ Floating-Offshore-Wind_-Risk-management-insurance-for-publication.pdf
(ALP 20), a solution designed to enhance the performance of lightning protection systems and significantly reduce the frequency of blade damage caused by strikes.
In the moments preceding a typical lightning strike, a highly conductive channel of ionised gas, called a leader,
propagates downward from a charged storm cloud in discrete steps. As the stepped leader approaches a wind turbine blade, its strong electric field induces the formation of upward leaders that emanate from grounded parts of the blade. An upward leader then connects mid-air to the downward stepped leader from the cloud, as shown in Figure 1. With a continuous conductive pathway now formed between the cloud and ground, a large amount of potentially damaging electrical charge is transferred in a short period of time.
Modern turbines are equipped with lightning protection systems (LPS) that use surface-mounted receptors connected to down conductors to channel lightning safely to the ground. However, these systems are not infallible. In many cases, the upward lightning leaders emanate directly from internal components such as the down conductor rather than the surface-mounted receptors. Often these internal leaders will reach through the blade shell and outpace the leaders from the receptor to connect to the downward leader from the cloud. These leaders can puncture the blade skin, leading to costly damage which includes structural weakness, delaminations, and, in some cases, catastrophic failure. Repairs are often complicated by the remote locations of wind farms and the height of turbines, making lightning damage one of the most expensive maintenance challenges for operators.
ALP 20, engineered by Arctura and manufactured by Mankiewicz, is an advancement in the field of lightning protection. Whilst other aftermarket products directly conduct lightning through internal pathways, ALP 20 promotes the formation of ionised channels above the blade’s surface, guiding the strike safely to the receptor without damaging the coating or the blade.
The technology relies on a proprietary formulation of conductive particles embedded within a polyurethane-based topcoat. These particles disrupt the electric field on the blade’s surface, encouraging early ionisation and enhancing leader activity around the receptors (Figure 2). This results in upward leaders at the receptor forming earlier and reaching farther when the coating is applied. Extensive high voltage testing has shown that ALP 20 enables leaders to grow over four times faster. Stronger leaders at the receptor can outcompete the unwanted leaders forming inside the blade in reaching the downward leader from the cloud. The result is a more reliable pathway for lightning strikes to follow to the receptor, minimising the likelihood of damaging punctures elsewhere on the blade.
This innovative approach not only enhances the existing LPS, but also introduces several unique benefits:
All-Energy is the ultimate platform for renewable energy professionals, bringing together the entire clean energy community to share ideas, discover innovative solutions, and shape the future of sustainability.
Gain invaluable insights into renewable energy from industry-leading conference speakers.
Discover the latest innovations and solutions driving progress in the renewable energy sector.
Connect with industry leaders and suppliers across the entire clean energy supply chain.
> Non-sacrificial performance: The coating does not conduct current directly, ensuring that it remains intact even after multiple strikes. Repeat testing with full-threat lightning strike current shows no damage to the coating.
> Ease of application: Suitable for both retrofit and new turbine blades, the coating integrates into existing blade maintenance workflows through a simple two-coat, roll-on application.
> Longevity: The coating is designed to last the lifetime of the blade without the need for maintenance or replacement.
The development of the ALP 20 coating involved four years of empirical research funded by the U.S. Department of Energy. Testing was conducted at Lightning Technologies Inc. under the auspices of Andy Plumer, an experienced lightning expert, where the coating’s effectiveness was assessed under both high voltage and high current conditions.
Validation was performed using initial leader attachment tests in accordance with IEC 61400-24, the international standard for lightning protection in the wind industry. Field-used blades with a standard LPS were obtained for testing. The blades were coated with and without ALP 20; blades that were not coated experienced multiple punctures in several testing scenarios where the blades coated with ALP 20 did not, as shown in Figure 3. Based on hundreds of tests, Arctura predicts a 73% decrease
in the number of damaging strikes when blades are coated with ALP 20.
To evaluate the coating’s durability, high current tests simulated full scale lightning strikes with currents up to 200 kA. Under these extreme conditions, the ALP 20 coating showed no signs of degradation. This non-sacrificial property ensures long term reliability, making the coating a cost-effective solution for wind farm operators.
The ALP 20 coating has been piloted at multiple wind farms in the US since 2022. The coating’s material performance has been monitored via annual drone inspections, which show no signs of degradation over the pilot period; the coating is expected to last the lifetime of the blade.
One of the features of the ALP 20 coating is its straightforward application process, which can be completed in just three steps:
1. Preparation: Begin by addressing any existing blade damage, followed by sanding with 120 grit sandpaper and cleaning.
2. Application: Apply the two-coat system using a simple roll-on technique, with a 45-minute flash-off time between coats.
3. Cure: Allow the coating to cure for a minimum of four hours, depending on environmental conditions.
The process is efficient and accessible, easily managed by a rope crew or bucket truck. This versatility makes it suitable for both retrofit applications on operational turbines and new or repowered blades.
The ALP 20 coating comes as a two-component system packaged in a single canister. Mixing of the base and hardener together in the container, with optional thinning for precise application, ensures a hassle-free on-site process.
Given its performance in both laboratory and field testing, application, and durability, ALP 20 represents an impactful solution in the wind energy sector. By enhancing lightning protection systems, preventing costly damage, and extending blade longevity, this coating can address some of the most pressing challenges in the industry. ALP 20 can be applied as a retrofit on existing turbines or on new construction blades.
As turbines grow taller and lightning events become more frequent, coatings such as ALP 20 are positioned to become an industry standard, safeguarding the future of renewable energy.
Delivering a clean energy future
Save the date
Dr
Motoyuki Arai, CEO,
Synspective, details how synthetic aperture radar technology can help tackle global sustainability challenges.
s global temperatures rise and natural resources deplete, governments, business, and communities are forced to search for ways to mitigate climate risks. The ability of synthetic aperture radar (SAR) to cut through adverse weather or light conditions and provide detailed insights into the landscape has consequently become more important than ever.
SAR technology provides the raw data that enables informed decision-making and supports the sustainable development practices needed to tackle crucial global issues. By helping address key challenges such as
offshore wind energy development and dam site monitoring, SAR has become an invaluable tool in the transition to renewable energy and ecological preservation.
The expansion of offshore wind energy has been a driving force in the transition to greener energy. However, the development and maintenance of offshore wind farms comes with significant challenges, including environmental concerns and the harsh conditions of marine environments.
SAR addresses these challenges effectively, playing a crucial role in identifying optimal locations for wind turbine installations, ultimately ensuring that project completion is sustainable and efficient. SAR data acquired through the monitoring of ocean currents, wave heights, and wind patterns, equips developers with important insight into marine conditions that allow for the most precise site selection. By highlighting sites with favourable conditions, SAR minimises energy loss and ensures the long-term viability of wind farms.
Besides site allocation, offshore wind projects must also balance the optimisation of energy generation with the implementation of strategies that help preserve the integrity of marine ecosystems. SAR technology’s ability to detect extremely subtle changes in seabed conditions enables project developers to mitigate potential environmental impacts, such as sediment displacement or disturbances to marine life. By tracking changes in marine habitats before, during, and after construction, SAR ensures wind farms operate harmoniously with their surrounding ecosystems. This long-term environmental monitoring also provides support to adaptive strategies, minimising the cumulative effects of wind farm operations on marine biodiversity.
Dams play a critical role in water resource management and hydropower generation. However, if unsuitably monitored, dams can also pose significant risks to surrounding landscapes and communities. SAR technology has revolutionised methods of assessing and managing these risks, providing actionable insights into land displacement and environmental impacts.
SAR’s capacity for large scale, consistent monitoring makes it a cost-effective tool in long-term environmental studies, empowering an integrated approach to infrastructure planning.
green initiatives has the potential to become more impactful. Through the delivery of accurate, frequent, and scalable data, SAR enables informed decision-making that supports global environmental goals, from combating deforestation to addressing biodiversity loss and climate change.
The technology’s ability to capture high-frequency data and produce precise, high-resolution images makes it a critical tool in tackling urgent environmental challenges. Offering a detailed and
Spain’s Dedicated Solar and Storage Trade Exhibition
25th - 26th June 2025 | Feria Valencia, Spain
100+ exhibitors
Hear industry leaders share their expertise on residential solar systems, utility-scale, commercial & industrial, and storage.
Coming from across the globe to present new ways of thinking and showcase innovative solutions to boost the energy transition.
150+ speakers networking
Plenty of networking opportunities available through our dedicated networking app and meet-ups organised by our strategic partners.
https://www.terrapinn.com/solarespana/energyglobal
up-to-date picture of environmental conditions, SAR supports effective planning and intervention strategies. It assists governments, organisations, and researchers in ecosystem monitoring, land use tracking, and extreme weather damage assessment. Real-time insights are indispensable for the implementation of timely, impactful solutions that boost resilience against climate change and environmental degradation.
Moreover, SAR’s ability for providing consistent, global coverage ensures that regions traditionally underrepresented or underserved by other monitoring technologies are not left behind. This inclusive access to vital environmental datasets supports a more equitable approach to sustainability, enabling all corners of the world to track their environmental status and align with green initiatives. With its global reach, SAR ensures that decision-makers from developing and developed regions alike can access the data needed to safeguard natural resources and plan for sustainable futures.
SAR technology is tied to several of the United Nations Sustainable Development Goals (SDGs) particularly those focused on climate action, affordable and clean energy, and sustainable infrastructure. Its contribution to the safe efficient development of offshore wind farms and its support for responsible dam site management align with SDG targets related to renewable energy and sustainable infrastructure. By offering a reliable means of monitoring these industries, SAR helps ensure that large scale infrastructure projects are developed in a way that minimises environmental distress and maximises long-term sustainability.
SAR’s potential for supporting climate risk mitigation reinforces its importance as a tool for advancing towards a greener, more resilient global future. Its application across a variety of sectors, including agriculture, urban planning, and disaster management, further highlight this potential. In agriculture, for example, SAR aids in crop monitoring, water management, and land use optimisation, which all play crucial roles in building sustainable food systems. In urban planning, SAR helps cities monitor air quality, track emissions, and assess
the impacts of urbanisation, enabling more sustainable growth. When it comes to disaster management, SAR can detect and map the extent of damage caused by natural disasters like floods, hurricanes, and earthquakes, aiding in efficient recovery and resilience-building efforts.
Through its diverse applications, SAR technology stands as an important tool in the global push towards sustainability, helping address some of the most pressing challenges of our time. Its ability to collect, analyse, and efficiently share information across various sectors is shaping the future of environmental stewardship, driving systemic changes that align with both local and global sustainability objectives.
SAR technology is redefining the way sustainability and energy development are being approached, offering solutions to the pressing environmental challenges humanity currently faces. From optimising offshore wind projects to enhancing the safety and sustainability of dam sites, SAR has already proven its usefulness as a tool for industries striving to balance development with environmental responsibility. As it continues to evolve, its integration with other rising technologies such as artificial intelligence (AI) holds the potential to unlocking even greater possibilities, expanding SAR’s role beyond traditional applications into new and diverse fields.
The integration of image sensing technologies such as SAR with AI-powered algorithms and machine learning is dramatically improving efficiency and precision across various industries. In Earth Observation, AI-driven techniques such as automated analysis, pattern recognition, and predictive modelling are transforming the way actionable insights are being extracted, making large scale environmental monitoring more effective than before.
Beyond these applications, AI introduces a new dimension to disaster prediction and mitigation. Leveraging extensive datasets on global disaster occurrences, machine learning models can refine predictive intelligence, helping authorities anticipate damages and take proactive measures to minimise impact. In the near future, AI-driven SAR analysis is expected to play a crucial role in infrastructure resilience, providing critical data for designing disaster-resistant structures while improving response strategies in the face of natural catastrophes. SAR technology can provide real-time, or near real-time, imaging and map imagery during emergencies, helping with recovery and relief. SAR can also provide critical information for conservation efforts, such as data on deforestation and rising sea levels.
With continued investment in research and development, SAR is poised to expand across industries, solidifying its place as a transformative force in environmental sustainability, disaster management, and beyond. As adoption grows, these advancements will not only meet evolving industry demands but also contribute to a future where technology empowers progress while preserving the planet for the future.
Cristina
Fernández (CF), Germany Managing Director and Continental Europe Sales Director, K2 Management , examines the progress of offshore wind in Europe and the likelihood of meeting future targets in a Q&A with Jessica Casey (JC), Editor of Energy Global.
JC: How has Europe’s offshore wind industry developed over the last few years?
CF: Europe has become a global offshore wind leader through rapid capacity growth and embracing the latest wind innovations. In the last year alone, Europe installed 2.3 GW of offshore wind capacity, awarded developers over 37 GW in new projects, and France announced its first major commercial floating projects that are due to be installed over the next few years. This demonstrates Europe’s commitment to accelerating the renewable energy transition and decarbonising industries – the EU and regional governments have long recognised the importance of taking a unified approach to achieving net zero by 2050. Europe has done this by taking a number of critical steps:
> Introducing bold policy frameworks and initiatives in recent years – including the EU Wind Power Package, Net-Zero Industry Act, European Wind Charter, and REPowerEU – that have helped to streamline the development of offshore wind projects by ensuring legal stability, creating a resilient supply chain, and enhancing grid networks.
> Pursuing country-specific investment strategies and committing substantial capital that have de-risked and ensured the financial viability of offshore wind projects.
> Facilitating collaboration between offshore wind leaders like Germany, the Netherlands, and the UK, and emerging leaders, such as Poland, the Baltic States, Norway, and southern European countries. For example, the Netherlands and Estonia recently partnered together to create agreements and share industry best practices that will enable their offshore wind industries to flourish.
What does the future hold for Europe’s offshore wind industry?
All of this has solidified Europe’s position at the forefront of the global renewable energy transition, but adherence to these regulations – and sharing knowledge and skills between countries – is critical to ensuring Europe continues to expand its offshore wind industry.
JC: What does 2025 have in store for Europe’s offshore wind industry?
CF: There is significant positive momentum in Europe’s offshore wind industry, with strong political awareness and commitment. The focus is no longer on whether offshore wind should be deployed, but rather on determining how much capacity needs to be installed annually to meet net-zero targets.
According to the European Commission, the region needs to install up to 450 GW of offshore wind by 2050 – making it a crucial part of the energy mix alongside onshore wind. To achieve this, Europe will need to increase its current capacity from 16 GW and install 12 GW/y on average to hit the new targets. Numerous large offshore wind tenders have been announced or are expected to be announced in 2025, including from emerging markets such as Malta, Portugal, and Estonia, which will go a long way in enabling Europe to realise its offshore wind ambitions.
However, announcing major tenders does not guarantee success or the further development of offshore wind energy. Recent cases, such as the Danish tender that failed to attract any bidders, highlight that political will alone is insufficient to create a thriving offshore wind industry. A constructive dialogue between policymakers and the industry is essential and, without legal frameworks that align with industry requirements and enable viable business models, no-show tenders are likely to persist.
JC: Which European countries are due to announce big offshore wind tenders in 2025? Will the predicted surge in offshore wind auctions lead to a supply chain crunch, labour shortages and other challenges?
CF: Most European countries involved in offshore wind are set to initiate or award major tenders in 2025. Notable examples include Belgium, Germany, Norway, Poland, the UK, the Netherlands, and France. These tenders vary in scope and size, with some focusing solely on seabed leasing that allows developers and investors to begin exploring potential projects, while others include provisions for grid connection and potential remuneration. As a result, the timelines will differ from project to project, with those announced in 2025 unlikely to be completed until the early-to-mid 2030s. Nonetheless, critical challenges that require a co-ordinated, multinational strategy – such as supply chain constraints, labour shortages, financial stability, and grid capacity, among others –still persist.
JC: Is Europe’s port infrastructure sufficient to support the development of large scale offshore wind projects?
CF: Limited port infrastructure is also another critical area of concern that will require significant investment from all parties, such as local governments, port authorities,
and project developers, to ensure it can support large scale offshore wind projects.
If Europe’s offshore wind industry is to realise its full potential, the industry and governments must commit significant investments to projects and infrastructure to facilitate alignment across regions.
JC: How is the shifting political landscape in countries like France, the UK, and Germany impacting offshore wind developers and investor appetite? Is the election of Trump having an impact?
CF: It is still too early to determine whether political changes in France, Germany, and the UK will have any material impact on the future of Europe’s offshore wind industry. While short-term setbacks are possible, offshore wind is a long-term industry and we expect continued growth – especially since decarbonisation is an important and shared objective across Europe, regardless of political shifts.
In the US, Trump has stated that no wind farms will be built during his second term. Currently, the US offshore wind industry is approaching this with caution and some projects have been temporarily paused while others have been terminated. Several developers are also withdrawing from the US or holding back to assess the impact of Trump’s policies. If these political dynamics hinder offshore wind progress in the US, some developers may choose to retreat from the market and refocus their efforts on more established European markets.
However, Trump’s administration has stated that offshore wind projects that have already been approved and “make sense” will continue to be developed. Offshore wind projects are also often approved at a state, not federal, level – meaning that the US offshore wind industry is likely to expand going forward, given that many states like New York and California are in favour of offshore wind.
CF: Generally, developing offshore wind projects is becoming increasingly challenging due to difficulties in securing financing, the risks associated with larger projects, and the use of larger wind turbines further offshore. These factors directly affect the profitability not only of project developers, but also the entire supply chain.
The impact on floating wind projects is particularly evident. Developers and the supply chain tend to favour fixed-bottom projects which are more mature and less risky compared to floating wind that requires significant development at scale. On top of this, the wide variety of floating wind designs has driven up projects cost, ports are unable to support the large structures, and there is very low investor appetite for floating wind projects. It is therefore anticipated that large scale floating offshore wind development will not unfold as initially planned. The first major commercial floating wind projects are now expected to be installed by the early to mid-2030s. However, the industry can speed up the adoption of floating wind in a number of ways. For instance: > Floating wind designs are currently complex, numerous, and expensive. Designs will need to be standardised to bring costs down. Zeroing in on a set of uniform models and designs will also be key to scaling the sector.
> Many investors and developers still see floating wind projects as risky and low-return, meaning new financing structures and government investment programmes will need to be created to de-risk projects and stimulate investment appetite.
> Local ports and supply chains are not yet mature enough to support all the developments in floating wind projects and significant investment in both will be needed to get projects off the ground and ensure consistent long-term growth.
> The permitting process for floating wind projects is frequently haphazard and inefficient. It must be streamlined to make the process more effective and attractive for developers.
How far are development ambitions in Asia Pacific, the US, and other global markets being realised?
CF: Asia Pacific, the US, and other markers are still in the early stages of their offshore wind journeys. Due to the fact that Europe is seen as a leader and pioneer in offshore wind, there are a lot of lessons Europe has learned that these markets should take on board to avoid the same pitfalls and mistakes. This includes:
> Creating clear legal frameworks and streamlining the permitting process. This is not only critical to giving developers certainty around the construction of offshore wind projects, but also to making the industry more attractive to investors.
> Ensuring close collaboration between developers, governments, and local interest groups – including fishermen, environmental groups, and local communities – around the development of offshore wind projects. In turn, this will help
make sure any concerns are addressed and ports and local supply chains are updated to support the development of offshore wind farms.
> De-risking offshore wind projects through introducing public-private partnerships and government-backed investment packages.
By taking these steps, APAC, US, and other markets around the world will be able to create flourishing offshore wind industries that help to accelerate the global renewable energy transition.
CF: According to WindEurope, Europe installed 13 GW of offshore wind capacity in 2024. However, to achieve the EU’s 2030 wind targets, the region needs to install 30 GW of new wind farms –both offshore and onshore – each year. This clearly indicates that progress is off track, and achieving the 2030 targets appears unlikely until certain challenges are addressed such as inadequate legal frameworks, limited grid connection capacity, supply chain bottlenecks, port infrastructure constraints, and a lack of financial security. To do this, all EU countries will need to create working groups to oversee the implementation of the EU Wind Power Package, Net-Zero Industry Act, European Wind Charter, and REPowerEU acts. This will help to create a level field for all developers, give legal certainty to offshore wind projects, and ensure the necessary infrastructure, including ports, supply chains, and grids, is in place to support projects.
Kelly Barnes, Senior Intelligence Analyst, DeterTech, maps out the emerging patterns followed by organised criminals targeting solar farms across the UK and Europe and provides data-based prevention techniques.
Solar is the fastest growing energy source in the EU. In 2024, Germany alone added 14.1 GW of additional solar capacity to its portfolio, setting a new record for the most installed by an EU country in a single year. For 2025, the EU is expected to add 62 GW, rising to 93 GW in 2026, and needs to be consistently adding 70 GW/y if it is to hit 2030 targets.
This has proved positive news for the developers and operators of solar farms and battery storage facilities as both benefit from the predictable demand for services. However, it must be acknowledged that another group has taken a keen interest in this element of the clean energy revolution. Crime data conclusively shows that organised criminal gangs (OCGs) have identified solar farms – both under construction and in operation – as an easy target.
This is mostly driven by cable theft. Shockingly, in 2024, enough cable was stolen in the UK to stretch the length of the UK and back. OCG activity is so industrialised that international disposal routes have emerged across Europe to facilitate the sale of stolen material. The result is unexpected delays, unplanned downtime, and costly remediation work to restore output. Site security is therefore an area that should be reviewed and enhanced to mitigate risk, protect revenue, and successfully meet projected electricity generation forecasts.
DeterTech operates a crime intelligence portal for the police and critical infrastructure companies which helps to track the movements and modus operandi of OCGs. In the UK, where a data sharing agreement that covers all 43 police forces is in place, a comprehensive picture of theft from solar sites is possible. It shows that, while panels can be a target, it is the large quantity of cable that can be found on solar farms that is driving OCG activity. From this, lessons can and should be applied throughout Europe where the picture is much the same.
The most striking finding from DeterTech’s data is the significant ambition and scale of the cable thefts being carried out. From January – August 2024, there were at least 70 reported offences against UK solar farms and, in over one-fifth of these, more than 20 km of cable was stolen. Just as concerning is the high likelihood of impacted sites being retargeted again within a matter of months, often as soon as the cable has been replaced. In total,
approximately 750 km of copper cable was recorded as stolen from UK solar farms over a period of eight months. Contextually, this amounts to the distance of around 18 marathons. The general pattern for acquisitive crimes such as these shows that OCGs tend to exploit the darkness of winter months, striking most commonly at night.
The implication of these figures when applied to the EU, where external estimates suggest there are around 5000 major solar thefts per year, is significant. The theft of such huge volumes of cable points to the involvement of both local and cross-border OCGs and reveals the presence of a massive criminal operation that is both well established and persistent.
Cable theft is the most significant issue DeterTech has seen in its data – it is responsible for two-thirds of all reported offences against solar farms this year. Of course, other concerns also persist. Though solar panel theft accounts for just under one in five recorded offences, around 10% relates to damage to perimeter fencing, main gates, or locks, and 5% involves the theft of other assets from solar energy sites. Given these diverse and persistent threats, combined with the high rates of repeat victimisation in the sector, it is imperative to regularly review site security and crime prevention measures across all facilities. In particular, it is advisable to establish standard operation procedures that trigger an immediate and visible security upgrade for impacted sites.
OCGs will typically reconnoitre sites in advance of an attack meaning any suspicious activity should be treated seriously and acted upon. These groups will also commonly revisit recently attacked sites to determine whether replacement cable and equipment can be easily retargeted. This is where a rapid response solution becomes vital. In both cases, it is essential to ensure that any site receiving unwanted attention receives an obvious security upgrade. This acts as a clear deterrent that has been shown to prevent up to 80% of further intrusion. Flexibility is key, requiring levels of security capable of stepping up or down quickly based on an evolving risk assessment. Additionally, deployment time, and the promised response time for an intrusion being detected and escalated, is crucial.
So, what do the current methods and activities of OCGs show about the steps that can be taken? While every site will have its own unique vulnerabilities, there is a level of predictability to the types of location favoured by OCGs. Thus, no site can ever be considered 100% secure. Sites can however be made much less desirable, increasing the likelihood OCGs move on to other targets.
Risk levels are constantly in flux and do not remain static across the lifetime of a site, meaning options should be capable of being relocated, scaled up, or scaled down as necessary. For example, in the initial construction phase, the equipment under the greatest threat is likely to be plant and machinery, fuel bowsers, and material stores, including cable drums. In light
of this, careful consideration should be given to where this equipment is stored, especially out of hours.
As the fit-out stage is reached, a whole new category of risk materialises. During these periods, more permanent security systems are unlikely to be fully implemented yet, adding to the vulnerability of the site. In these circumstances, consideration must be given to the storage of solar panels and cable drums, both of which prove particular favourites for OCGs. The same drums and pallets that make life easier for installers can also assist criminals in quickly stealing large quantities of assets. It is essential that responsible steps are taken to protect these tools – by keeping their presence and locations subtle, for instance.
Finally, in the operation phase, the typically rural nature and low on-site staffing of solar farms must be considered. At these sites, there is little natural surveillance as offenders are unlikely to be seen or
reported by passers-by. OCGs must be deterred from initially entering a site and, if criminals do enter, alert-based CCTV must be fully functional. This means footage with no gaps and a rapid escalation process when a threat is detected. Regular checks of perimeter fencing for possible signs of malicious activity is also a pertinent measure to schedule into improving site security.
In the fit-out phase, data also shows that offenders are most likely to focus on cable above ground, with the current trend being towards string cable theft. Looking back at past incidents, evidence of offenders planning routes through nearby fields, allowing them to bring vehicles to an identified access point, is significant. As such, signs of these behaviours should be urgently escalated and considered an early warning to sites that they have attracted the undesired attention of OCGs.
Deploy prominent signage
Clearly communicate that advanced security measures such as CCTV and forensic marking are in place. The first and most valuable impact of any site security technology is its known deterrent value.
Regularly check the perimeter for any signs of unusual activity. Report anything suspicious and quickly repair fencing that has been damaged or cut.
A determining factor in the likelihood and size of resulting thefts is how closely criminals can park a vehicle to targeted materials.
Regularly review whether CCTV is in good working order and whether the evolution of activity on site has created any new blind spots. Address any damage or interference to CCTV cameras immediately as this could be a precursor to an attack.
Notify the police of all incidents and thefts regardless of the value of the loss. Otherwise, it is impossible for government and law enforcement to fully understand the scale of the problem when allocating the appropriate resources for preventing it.
Avoid leaving cable, materials, and plant equipment in view of the site perimeter to avoid attracting unwanted attention.
Once targeted, sites are highly likely to be revisited by the same OCGs who will now be familiar with the existing layout and security measures. Ensure there is a visible
security upgrade at these premises to help break this vicious cycle.
Employ visually verified intruder detection
Incident response times are critical in deterring and limiting thefts. Ensure the capabilities are in place to identify and respond to intrusions in real time. Unmonitored CCTV can only help establish liability after the fact.
Use forensic marking
SmartWater, a traceable liquid and forensic asset marking system, serves as a powerful deterrent with its 100% conviction rate in contested court cases. It is an easy and cost-effective measure that ensures the best chances of recovery for any stolen items.
Leverage local eyes and ears
Make emergency procedures and contacts clear to staff and the community in the event that suspicious activity is witnessed in the vicinity of a solar farm. Instruct potential witnesses not to directly challenge offenders, but to note details such as clothing or license plate details that could be of interest to security and the police.
Greentech is a leading player in Europe’s renewable energy sector. As a fully integrated photovoltaic (PV) specialist
with around 200 employees, the company is committed to delivering a reliable and growing supply of clean energy, crucial for meeting the ambitious renewable energy targets across the continent. The company’s services include the project development and design, construction, operation, and management of PV power plants in Europe.
For Greentech, site security on an undeveloped construction site became an urgent issue following a mistake made by a logistics company which resulted in a large quantity of solar panels being delivered 10 days earlier than scheduled. Thus, the site needed to be secured sooner than expected. DeterTech was able to get a real-time visually verified system for CCTV and intruder detection in place within 24 hours.
Greentech, 18 months later, has a framework agreement in place that has seen this site security solution successfully deployed on a further nine construction sites. The standard model used to protect Greentech’s compounds involves immediately deploying between 4 – 6 PID 360 intrusion detection devices, sometimes supported by two DT Sentinel CCTV towers. These can be delivered, installed, and in place within 72 hours as part of a visible security upgrade. Additionally, these devices sufficiently provide an immediate deterrent and security upgrade, with their rapid deployment allowing time for a comprehensive site security survey to be conducted where further units can be deployed if additional risks are identified.
Uri Sadot (US), Cybersecurity Program Director, SolarEdge Technologies, showcases why an increased focus on cybersecurity amongst vendors, commercial businesses, grid operators, and governments is critical to safeguarding future energy security in a Q&A with Jessica Casey (JC), Editor of Energy Global
hile renewable energy is a key component to achieving the world’s net zero goals, the reality is that there is still work to do to ensure it can be relied upon as a secure energy source.
JC: What is the current state of the solar industry, and what are the key factors driving its growth and development?
US: The rise of solar energy in recent years has seen it become an increasingly important
component of the global energy landscape, providing homeowners and businesses with a clean energy source to lower energy bills, while enabling grid operators to tap into a vast distributed energy source to support the grid. By 2022, over 1300 TWh of solar energy had been installed worldwide, accounting for just over 5% of global electricity consumption.1 Since then, drivers such as cost reductions and innovation have accelerated solar deployment, making it the fastest growing power generation technology.2
Energy demands on the grid have reach unprecedented levels and are expected to rise further due to digitisation, artificial intelligence (AI), and the mass rollout of electric vehicles and heat pumps. Governments are also intensifying sustainability efforts to meet their net-zero targets. Meanwhile, the invasion of Ukraine highlighted the threat to energy security when dependence on one’s energy supply lies outside its borders.
These factors have propelled solar and other renewable energy sources to the forefront of future energy security strategies. Yet, with the shift of oil and gas dependency to renewables, questions arise. For instance, how secure are renewable energy supplies? How cybersecure are systems connected to homes, businesses, and grid infrastructure and who else has access?
JC: Does solar cybersecurity need to be prioritised now or can the industry wait until solar deployment is more widespread?
US: The development of cybersecurity threats in solar closely mirrors what was seen with the rise of the internet three decades ago. If, in 1995, time had been taken to design the basic protocols to make the internet cybersecure from the bottom up, the industry would have saved hundreds of billions of dollars in reactive fixes. With the benefit of hindsight, the solar industry should prioritise cybersecurity in its product designs now, before mass mainstream deployment occurs – waiting could result in catastrophic cyber events or extortionate costs to implement retrospective security measures.
JC: How serious is the threat to energy security posed by cyberattacks and is it expected to increase as hackers become more sophisticated? Can you provide any real-world examples of such incidents?
US: The sophistication of cyberattacks has increased hugely in recent years, with energy networks and grid infrastructure a potentially crippling target. One example is the September 2024 cyberattack on Lithuanian energy infrastructure which exploited vulnerabilities in solar monitoring software, enabling a hacker to gain unauthorised access to data from 22 facilities, including critical sites such as hospitals and military academies.3
In the past six months alone, solar inverters from nine different global manufactures have reportedly been targeted by hackers. In most of these cases, the breach was caused by an ‘ethical hacker’ – an individual seeking to expose security
vulnerabilities rather than acting with harmful or criminal intent. However, with two maliciously motivated incidents also reported, including the event in Lithuania, concern is rising.
Regardless of the hackers’ intentions, the fact that they were able to breach these systems is a significant concern. These incidents have raised alarms among organisations such as the FBI, the White House, the Lithuanian Government, SolarPower Europe, and the European Solar Manufacturing Council, all of whom have expressed concerns about the lack of adequate cybersecurity regulations for solar and other distributed energy resources.
Why are solar inverter manufacturers targeted?
US: The solar inverter is a critical component of the solar system, converting power produced by solar panels into usable electricity. It is also the part that connects to a home or business’ energy network and the grid. Without adequate cybersecurity, inverters can be vulnerable to hacking, potentially allowing remote control of an energy supply. Homeowners, business owners, and grid operators must consider who has access to these inverters and ensure the products they use are produced by manufacturers who prioritise cybersecurity.
Recent events like the 2021 Texas freeze and the 2022 California heatwave have shown the devastating effects of grid failures, with widespread power outages disrupting millions of lives.
Grid restoration can take days or more, and in the event of a cyberattack, even longer. Operators must first identify the cause and location of the attack and then eliminate intruders before restoring the grid. Only then can a black start process be initiated to gradually restore the grid and carefully bring assets back online to balance supply and demand. When the consequences of a cyberattack on the grid are laid out in these terms, the solar industry’s 5% share of global energy production becomes more significant, highlighting the critical need to prioritise cybersecurity from the top down.
US: Defending against today’s sophisticated cyberattacks requires increased awareness among homeowners, businesses, grid operators, and governments that the cybersecurity of solar products varies significantly by manufacturer. Understanding the risk to energy security, a shift in mindset is needed across the energy value chain to a ‘prevention is better than a cure’ approach – this approach is no different to the robust cybersecurity measures built into phones or cars as standard.
Manufacturers currently set their own security levels without regulation, leading to inconsistent standards. This is comparable to car manufacturers individually deciding their own safety standards. The technology required to enhance cybersecurity during product development exists and vendors should prioritise investment in these technologies over cost-cutting and higher margins. These standards should be as non-negotiable as fire or electrical safety.
Government regulation is crucial to enforce strict cybersecurity standards in the industry. This includes mandating basic cybersecurity standards for all connected devices, including distributed energy resources and encouraging solar manufacturers to implement both physical and software security measures, monitoring capabilities, and mitigation plans for cyberattacks.
JC: In your opinion, are governments worldwide doing enough to address the threat that cyberattacks pose to energy security?
US: Recent developments show promising progress. For instance, the UK’s introduction of the PSTI cybersecurity standard in April 2024 set a global precedent, requiring compliance from all manufacturers of connected consumer devices – including solar inverters – on password strength, support period, and technical documentation. Similarly, the EU’s Cyber Resilience Act establishes cybersecurity obligations for all products with digital elements, including solar inverters, with full implementation expected by December 2027. The act addresses thousands of Internet of Things products, including solar inverters. While this is a good starting point, solar cybersecurity requires its own legislative category and priority focus, especially in regions where solar is key to reducing reliance on foreign oil and gas.
JC: Are there any final thoughts you would like to share?
US: Whether it is solar, wind, or other renewable sources, it is clear that abundant clean energy is critical to improving lives and the health of the planet. However, as consumption of renewable energy grows, it is crucial to address the security of underlying technology to protect against potential threats before it is too late. Even if the likelihood of an event is low, robust cybersecurity measures are necessary to mitigate the consequences if an attack were to occur. While governments are beginning to recognise this, tackling cybersecurity in renewables requires international collaboration, especially across Europe where cross-country electricity trading is widespread. Top-down legislation must be met halfway with bottom-up pressure, requiring both the homeowners and businesses investing in solar to demand high cybersecurity standards as a prerequisite.
It goes back to the timeless wisdom: investing in prevention is better than investing in the cure.
1. ‘Solar PV’, International Energy Agency, www.iea.org/energy-system/renewables/ solar-pv
2. DREVES, H., ‘At a glance: How renewable energy is transforming the global electricity supply’, National Renewable Energy Laboratory, www.nrel.gov/news/program/2023/ how-renewable-energy-is-transforming-the-global-electricity-supply.html
3. ‘Solar monitoring solutions in hacktivists’ crosshairs’, Cyble, https://cyble.com/blog/ solar-monitoring-solutions-in-hacktivists-crosshairs/
& Grose, outlines the trend towards technological developments in the solar photovoltaics sector seen through increased patent filings and details the current legal and developmental barriers to progress.
he solar energy industry has seen rapid development over the past three decades, with significant progress in the past 10 years. In 2024, global solar capacity reached a record 2 TW, with 260 GW of new photovoltaic (PV) power generation capacity installed in 2023 alone. This exponential growth highlights the increasing importance of solar energy in global electricity generation, especially as PV technology now accounts for a substantial share of renewable energy production worldwide. In particular, the solar industry witnessed dramatic advances in the efficiency of solar cells and modules in the first two decades of the 21st Century, making large scale PV power generation technically and economically feasible. This, in turn, brought about further cost reductions through economies of scale and, as a result, solar energy is now cheaper than many fossil fuels, with costs ranging between US$0.90 – US$1.50/W.
These can often serve as a useful proxy for the level and focus of innovation within a specific sector. In the PV sector, they have traditionally reflected the industry’s focus on advancements in efficiency. For instance, between 2002 and 2019, there was a dramatic 678% increase in the number of Patent Cooperation Treaty (PCT) applications related to solar power and, by 2019, over half of all renewable energy-related PCT applications were linked to solar technologies, compared to just over one-quarter in 2002.
However, as silicon-based panels approach their theoretical maximum efficiency limit, the margin for improvement is becoming increasingly narrow. This theoretical maximum efficiency cap arises due to a fundamental physical constraint, known as the Shockley-Queisser limit, which caps the maximum solar conversion efficiency of silicon solar
Figure 1 . Patent filings directed to structural details of photovoltaic (PV) modules other than those related to light conversion (IPC: H02S30/00+). Search covers: “Major authorities” (CA, CN, DE, EP, FR, GB, JP, KR, RU, US, WO). Note: The plateau in growth in 2021 is most likely attributable to global slow-down during the COVID-19 pandemic.
Figure 2 . Patent filings directed to (i) Automated monitoring for PV module-cleaning (IPC: H02S 40/10 – Cleaning arrangements; and H02S 50/00 - Monitoring or testing of PV systems); (ii) Predictive PV maintenance technologies (IPC: G01R31/00 – Arrangements for testing electric properties, arrangements for locating electric faults, and arrangements for electrical testing; AND H02S50/00+ – Monitoring or testing of PV systems).
Figure 3 Grant rate* by jurisdiction for patent applications directed to (i) Automated monitoring for PV module cleaning and/or (ii) Predictive PV maintenance technologies (IPC: H02S 40/10 –cleaning arrangements; H02S 50/00 – Monitoring or testing of PV systems; G01R31/00 – arrangements for testing electric properties, arrangements for locating electrical faults, and arrangements for electrical testing; and H02S50/00+ – monitoring or testing of PV systems). Search covers applications having priority date from 2019 to the date of search (21.08.2024). *Grant rate as of the date of search.
cells at 29% under standard testing conditions (AM 1.5 solar spectrum). Current state-of-the-art monocrystalline silicon cells have already achieved efficiencies of approximately 26%, leaving very little room for further gains. These intrinsic efficiency boundaries of conventional silicon-based solar cells have not only made additional improvements increasingly challenging, but have also prompted a shift in innovation priorities within the industry. Currently, there is growing interest in exploring novel materials, such as space PV materials and nanostructures, to overcome these constraints, while also expanding innovation into non-power generating technologies that enhance the overall performance, reliability, and cost-effectiveness of solar energy systems.
Recent patent filing trends reflect a growing emphasis on such non-power generating technologies. For example, as shown in Figure 1, the number of patent filings directed to structural details of PV modules, other than those related to light conversion, have nearly doubled in the past five years.
One of the key advancements in such non-power generation aspects of the solar power sector can be found in the digitalisation of automated monitoring and maintenance systems. These are important technologies as they enhance the efficiency and prolong the working lifespan of existing equipment. This extends the anticipated return on investment and encourages faster adoption, which can in turn fund further research and development activity, enabling innovation.
Furthermore, predicting the maintenance needs of large scale solar farms using automated systems, such as SCADA-based systems, drones, and IoT-enabled devices, not only enhances the performance of the solar farms, but also significantly reduces operational costs by preventing issues escalating into costly problems.
In particular, the use of automated monitoring for PV module cleaning has gained considerable attention in recent years. This is evidenced by a steady rise in patent filings in this area, as shown in Figure 2, reflecting growing commercial interest in the efficient, long-term maintenance and cleaning of solar panels. It not only promises a longer working life for panels, but also more optimal performance for every day, month, and year that they remain in operation.
Predictive maintenance using advanced algorithms and artificial intelligence (AI) to forecast equipment failures can also minimise downtime and reduce maintenance costs. As illustrated in Figure 2, patent filings relating to predictive maintenance technologies in the solar sector have also surged dramatically in the current decade, reflecting the industry’s shift toward integrating AI and Internet of Things (IoT) into large scale solar power generation.
However, despite this surge in innovation, securing patents for digitalisation-related technologies in the solar sector, which can serve as useful tools for protecting commercial interests, presents unique challenges. In UK and European patent law
in particular, applicants must overcome the hurdle that computer programs and algorithms ‘as such’ are excluded from patent protection. Thus, applicants seeking patents for AI, software, and algorithm-based inventions need to ensure their claimed invention is carefully scoped in the application to avoid falling into these exclusions. Furthermore, to strengthen their chances of meeting the patentability requirements in the UK and Europe, they must clearly articulate the ‘technical problem’ solved by their invention, as this will be closely examined during the prosecution of the patent application.
This requirement means that patent applications directed to such inventions need to clearly convey a technical effect beyond the software or algorithm itself. This may explain the considerably lower grant rate for patent applications directed to automated monitoring for PV module cleaning and predictive PV maintenance technologies (the same group of applications discussed in Figure 2) compared to other IP5 jurisdictions, as depicted in Figure 3.
Therefore, building a successful patent portfolio in the field of solar digitalisation requires not only innovative technologies, but also careful legal considerations regarding how these innovations are presented in patent applications. For example, an AI-powered system designed to predict solar panel degradation by analysing environmental data, operational history, and performance metrics could help optimise maintenance schedules and reduce downtime, offering measurable technical benefits. To obtain patent protection for such an innovation, it is important to clearly articulate its technical contributions and the specific improvements over existing methods. This can, for example,
be accomplished by describing how the AI model achieves greater prediction accuracy by incorporating real-time and historical data, surpassing conventional manual or rule-based approaches. It is often advantageous to include detailed information about the algorithms and training data, particularly when these elements are critical to achieving the technical effect of the invention. Providing comprehensive descriptions of the system’s architecture, data processing workflows, training methodologies, and integration into solar farm infrastructure can further support the case for patentability by highlighting its practical and technical contributions.
Whilst the era of rapid efficiency improvements in silicon-based PV modules and cells may be nearing its limit, the solar industry remains far from reaching a plateau in innovation. The focus and likely direction of that innovation are simply shifting as the community seeks new routes to greater cost-effectiveness, performance, and efficiency.
Of specific note, innovations utilising AI, IoT, and automation are expected to continue growing, as these technologies become increasingly vital for the efficient and reliable operation of large-scale solar plants. Digital innovations in automated monitoring, predictive maintenance, and grid management are good examples of new frontiers in solar energy, and it is becoming clear that the future of solar power will be shaped not only by advances in power generation but also by the digital technologies that support and enhance the solar power industry.
+44 (0)1252 718999 reprints@energyglobal.com
Shwu Tyng
Goh, Global
Product
Manager
of Packings, Sulzer, considers how increasing the efficiency of distillation processes through innovative structured packing can help to dramatically reduce the energy consumption of distillation processes.
s the world pushes towards a more sustainable future, it is tempting to assume that energy producers alone are responsible for switching to renewable sources while industrial users continue with their usual practices. However, achieving ambitious targets for reduced emissions and environmental impacts requires collaboration on both sides. For meaningful progress, energy producers and industrial users must share the responsibility and actively work together towards these goals.
The industry must explore innovative approaches not only to source energy responsibly, but also to reduce overall consumption. This involves implementing smarter strategies for existing operations and ensuring that current processes are optimised for maximum efficiency.
Many sectors can be optimised to achieve significant reductions in emissions and energy costs, particularly in the chemical processing industry which relies on energy-intensive processes such as the distillation of hydrocarbon and chemical components. According to the U.S. Department of Energy, distillation consumes a staggering 40% of the processing energy used in refining and continuous chemical processes worldwide, working out to around 4.8 quadrillion Btu/y.1
Contextually, this is approximately four times the annual energy consumption of Switzerland. 2 Therefore, designing an efficient distillation process is essential to optimising energy usage – including the selection of the optimal correct column internals – and mass transfer components to achieve maximum efficiency.
Structured packing plays a vital role in the process intensification of distillation columns. Structured packing was invented with the preliminary idea of having a regular structure and providing low pressure drop per theoretical stage. Since the introduction of the first metal sheet structured packing Mellapak TM by Sulzer in the 1970s, this type of mass transfer component has increasingly gained prominence in the distillation industry, providing higher efficiency at lower column pressure drop as compared to other available options such as random packing and tray.
The second-generation structured packing MellapakPlus TM increases the useful capacity of a column by up to 50%, with a critical change in the packing
geometry which minimises the local pressure drop at the interface between two packing elements.
As Sulzer’s customer base expanded and requirements grew more specialised, the company began addressing these precise process needs through the development of a range of structured packings, including Mellacarbon TM for corrosive environments and MellapakCC TM for carbon capture applications.
Recently, Sulzer launched the third-generation structured packing, the MellapakEvo TM structured packing (Figure 1). This structured packing offers a high effective interfacial area to promote mass transfer between the vapour and liquid phase to separate components in a distillation column. Wettability on the packing surface has a significant effect on efficiency. Superior surface texture ensures that the liquid film on the wetted surface is created, maintained, and continually renewed to maximise the utilisation of the packing surface. At the same time, the pressure drop is kept to a minimum due to the packing’s low resistance to the gas flow, thereby extending the useful capacity of the packing.
Tested and proven in Sulzer’s in-house research and development facility in Oberwinterthur, Switzerland, and an independent testing facility located in the US, MellapakEvo offers up to 40% higher separation at the same useful capacity and pressure drop as MellapakPlus 252.Y. Compared to MellapakPlus 452.Y, the new packing offers similar separation efficiency with approximately 20% higher capacity (Figure 2).
The MellapakEvo structured packing offers appreciable mass transfer and hydraulic performance, delivering significant benefits to distillation columns.
The dimensions of a separation column, including its diameter and height, are typically determined by the number of required separation stages (efficiency) and the energy input (reboiler duty). By increasing the number of theoretical stages within a column, the reflux ratio can be lowered, enabling the same product quality to be achieved with reduced energy demand and at the same column pressure drop. An optimised column design balances the capital costs of a taller column, resulting from increased packed bed height, with the operating costs of energy for the reboiler. MellapakEvo enhances column design by increasing separation stages without necessitating taller columns, helping to avoid the higher capital expenses
associated with them. In an era of rising energy prices, these improvements translate into substantial economic benefits for operators.
Distillation columns in the chemical and petrochemical industries are typically built to last for decades, often with a lifespan of 20 – 30 years or more. While replacing an entire column can be prohibitively expensive, upgrading internal components during maintenance cycles is far more practical and cost effective. By thoroughly analysing the existing configuration of packed columns, advanced packing like MellapakEvo and internals such as MellaTech TM , can be applied to achieve higher separation efficiency at the same throughput or higher throughput at the same separation efficiency, without increasing the total column pressure drop. This approach retains the current column setup and minimises the CAPEX required for a complete process overhaul.
For vacuum distillation columns, minimising column pressure drop is an effective strategy to lower energy consumption. MellapakEvo’s design achieves the required degree of separation while reducing pressure drop, translating to lower reboiler duty. This capability is critical in energy-efficient process designs, such as heat pump-assisted vacuum distillation, heat-integrated distillation systems, and mechanical vapour recompression units. By reducing pressure drop,
packing such as MellapakEvo also minimises energy losses and improves the temperature gradient across the column, enhancing the performance of heat pumps and compressors.
All industries are actively seeking ways to minimise their environmental footprint, with many recognising that the path to sustainability begins by optimising process efficiency. For operations involving distillation, selecting the right structured packing technology is critical to achieve these goals.
MellapakEvo represents an advancement in this area, establishing a benchmark for performance and efficiency. Ongoing investment in research and development enables industries to adopt more sustainable solutions without sacrificing operational performance. This commitment unlocks promising opportunities in all chemical processing industries.
1. ‘Distillation Column Modeling Tools: Advanced computational and experimental techniques will optimize distillation column operation’, Office of Industrial Technologies, (September 2001), www1.eere.energy.gov/manufacturing/resources/ chemicals/pdfs/distillation.pdf
2. ‘Switzerland energy: Energy consumption in Switzerland’, Worldometer www.worldometers.info/energy/switzerland-energy
Ocean Installer has been appointed by Cerulean Winds to join the alliance of companies developing the Aspen floating offshore wind project in the North Sea.
Ocean Installer’s addition to the alliance is key to driving down the cost of installation, a critical component within floating offshore wind development. The company’s existing fleet of vessels and a skilled workforce, developed over decades working in subsea oil and gas construction, can be transferred to the floating offshore wind sector without significant adaptation.
Furthermore, Ocean Installer will work in conjunction with fellow alliance member, Haventus, owners of the Port of Ardersier, on the dry storage of the structures, batched installation, and quick connect/disconnect systems to optimise processes and create a blueprint for how future floating offshore wind projects are installed.
Ocean Installer will provide engineering expertise and installation services, post-FID for the mooring system installation, inter-array cables system, and marshalling of the floating units during the fabrication phase, tow-out, and hook up.
Once built, Aspen, Beech, and Cedar will comprise over 300 turbines. The 1 GW Aspen site will be developed first, providing new offshore wind capacity helping to meet the UK government’s target of 50 GW by 2023.
European Energy and TotalEnergies have launched three vessels to conduct seabed surveys in the area surrounding the planned Jammerland Bugt offshore wind farm, located between Kerteminde on Funen and Kalundborg on Zealand, Denmark.
With a capacity of 240 MW, Jammerland Bugt offshore wind farm will be capable of supplying more than 240 000 European households once it becomes operational, expected in 2029.
The three vessels will carry out seabed measurements in the areas designated for the offshore wind projects. These measurements will provide a detailed insight into the seabed’s shape, geological composition, and overall condition – crucial data for designing wind turbine foundations, planning cable routes, and other installations. Geophysical data is essential to ensure a stable and secure placement of the wind turbines.
The three vessels will dock in Kerteminde and Nyborg on Funen, using these ports as their base until the surveys are completed in August 2025. The results will then be analysed to support the further planning of the offshore wind farms.
The same vessels will be used for geophysical surveys for the Lillebælt Syd wind farm, located between Sønderborg and Assens, also in Denmark. These surveys are set to begin in April 2025.
In addition to vessel-based surveys, drone flights from the coast will also be conducted to gather further data.
Leading renewable energy partners, FuturEnergy Ireland and SSE Renewables, have been granted planning permission by An Bord Pleanála for the development of Gort Uí Rathaile wind farm close to the Cork/Kerry border.
The onshore wind development, which has received consent for 13 turbines and is located approximately 4 km from Ballyvourney in County Cork, would support both national and global climate action. The clean, home-produced electricity generated would also boost Ireland’s energy security and
reduce dependence on expensive fossil fuels.
Delivery of the wind farm will be subject to the project securing a route to market as well as a final investment decision by co-developers FuturEnergy Ireland and SSE Renewables.
During construction, up to 60 jobs will be created while six long-term roles will be required to run the wind farm during operations. Demand for a wide range of products and services will also create indirect employment and boost the local community.
The University of Sheffield study assessed how agrivoltaics technology – integrating solar panels into farmland in a way that maintains agricultural activities – could help the UK reach its solar energy targets (PV) and meet its commitment to reach net zero by 2050.
Current government plans to significantly increase ground-mounted solar parks, which are already in use across the UK, have proven contentious and unpopular with farmers and the public alike. Concerns about the loss of high-quality agricultural land, potential impacts on food production and cost of living, and the visual impact on landscapes are among factors fuelling the criticism.
The Great Britain-focused study demonstrates that the deployment of agrivoltaics, which would see solar photovoltaic panels installed in ways to allow for farming activities underneath or between panels, could enable the simultaneous production of crops, livestock, and renewable energy. Agrivoltaics has such high coverage potential that it could meet UK government PV targets on its own, while avoiding land use conflicts.
Energi Coast Regional Supply Chain Showcase
02 April 2025
Newcastle, UK
www.nof.co.uk/events/energi-coast-regional-supply-chain-showcase
Solar & Storage Live London 2025
02 – 03 April 2025
London, UK
www.terrapinn.com/exhibition/solar-storage-live-london
Pulse 2025
03 – 04 April 2025
Madrid, Spain
https://ratedpower.com/pulse
WindEurope 2025
8 – 10 April 2025
Copenhagen, Denmark windeurope.org/annual2025
The US installed a record-breaking 50 GW of new solar capacity in 2024, the largest single year of new capacity added to the grid by any energy technology in over two decades.
According to the U.S. Solar Market Insight 2024 Year in Review report released by the Solar Energy Industries Association and Wood Mackenzie, solar and storage account for 84% of all new electric generating capacity added to the grid in 2024.
In addition to historic deployment, surging US solar manufacturing emerged as a landmark economic story in 2024. Domestic solar module production tripled in 2024, and at full capacity, US factories can now produce enough to meet nearly all demand for solar panels in the US. Solar cell manufacturing also resumed in 2024, strengthening America’s energy supply chain and cementing its place as a solar powerhouse.
Texas led all states for new solar capacity additions in 2024, replicating a record-setting 2023 with 11.6 GW of new installations. In total, 21 states set new annual installation records, with 13 states adding over 1 GW of new solar capacity in 2024.
Large Scale Solar USA 29 – 30 April 2025
Texas, USA
https://lssusa.solarenergyevents.com
The smarter E Europe 2025 07 – 09 May 2025
Munich, Germany www.thesmartere.de/home
All-Energy Exhibition and Conference 2025
14 – 15 May 2025
Glasgow, Scotland www.all-energy.co.uk
Solar & Storage Live España 2025
25 – 26 June 2025
Valencia, Spain
www.terrapinn.com/exhibition/solar-storage-live-espana
Root-Power reaches new milestone on construction at BESS site
Root-Power, which launched Summer 2024 with the backing of the YLEM Group, has received its battery energy storage systems (BESS) at its flagship 11 MW/22 MWH site at Coryton Energy Park in Corringham, Essex.
Supplied by Canadian Solar Inc. e-STORAGE SolBank technology, the BESS is expected to go live later this year and has been designed with a two-hour storage duration, enough to power around 22 000 homes.
Root-Power announced it had begun construction on the site in September 2024 and also confirmed it had partnered with Eclipse Power Networks as the independent distribution network operator and RJ Power as an independent connection provider on the project.
Spearmint Energy has established an initiative to wrap its commercially operating battery energy storage systems (BESS) projects in artwork.
The initiative serves to visually bring awareness to the importance of BESS/BESS technology in securing safe and dependable power globally.
In connection with the establishment of its BESS artwork initiative, Spearmint has unveiled its inaugural art installation at Revolution, the company’s commercially operating 150 MW/300 MWh BESS project in West Texas. Designed by street and mural artist, Sophie Mess, Revolution’s artwork depicts a bold and colourful floral pattern – a nod to biological carbon sequestration and the importance of nature preservation – and features the slogan: “Join the Revolution.” The slogan represents a call to action for all to participate in the mission to deliver affordable energy solutions through grid-stabilising battery storage.
Spearmint collaborated with art consultant, Maxence Doytier, Twenty6North, to select Sophie Mess as the artist for its inaugural art installation. The company partnered with signage expert, Mathew Crane, Coastal Vibes Graphics & Signs, to install 22 mural panels across the west face of Revolution’s battery modules and one panel facing south. Spearmint co-ordinated closely with Revolution’s battery system manufacturer to ensure the art installation would not impact the safe operation of the battery.
Renewable Power Capital (RPC) has signed key construction and supply contracts for their 50 MW battery energy storage system (BESS) facility in Finland.
This is RPC’s first BESS and is planned to be operating in summer 2026. Located in Uusikaupunki, Finland, the project will bring 50 MW/100 MWh of storage to the system. The timelines were confirmed alongside the announcement of key project partners that will see the delivery of the project to the Finnish energy system.
Batteries for the site will be supplied by Sungrow, who will install a 2-hour solution with their newest model PowerTitan 2.0 liquid-cooled technology, as part of a 15-year contract with RPC.
Balance of plant services will be provided by Suvic Oy, encompassing civil works, substation construction, and BESS equipment mechanical and electrical installation.
The project is one of the largest of its kind in Finland, and will provide grid services such as frequency response and participate in energy trading on wholesale power markets.
> Siemens Gamesa and Hull College collaborate for renewable energy apprenticeships
> Eco Wave Power commences infrastructure enhancements for Portugal wave energy project
> Google Cloud, SLB, and Project Innerspace, collaborate to accelerate global geothermal energy adoption
Follow our website and social media pages for more updates, industry news, and technical articles. www.energyglobal.com
The first of three new sets of high-voltage electricity cables connecting the Dinorwig hydroelectric power station in Gwynedd to National Grid’s transmission network has been energised.
Engineers from National Grid and contractors, Morgan Sindall Infrastructure and Siemens Energy, achieved this as part of the Dinorwig to Pentir project to replace cables originally installed in the 1970s that are now coming to the end of their operational life.
The first new circuit, comprising three 12.2 km cables each made from 14 sections joined together and weighing an estimated 1500 t, has been installed under sections of the A4086 and A4244, in nearby fields and inside Dinorwig.
Each new circuit takes a different route from the original cables, ensuring the existing connection can remain in service and continue transmitting vital clean power from Dinorwig while the upgrades take place.
ANDRITZ has received an order from BC Hydro to supply four 60 MVA generators for the Bridge River 1 hydropower plant in British Columbia, Canada.
The value of the order will not be disclosed.
After more than 70 years of operation, the upgrade project will enhance the reliability of the plant and increase the installed generator capacity by 15%, contributing to British Columbia’s renewable energy goals.
The Bridge River 1 plant is located in the core St’át’imc territory, adjacent to the Tsal’alh community on Seton Lake.
The upgrade project is expected to create jobs, stimulate economic growth, and ensure a reliable supply of clean energy for British Columbia residents.
ANDRITZ looks forward to working with the local First Nations communities in the execution of the project, continuing its tradition of strong relationships with Indigenous peoples in renewable energy projects.