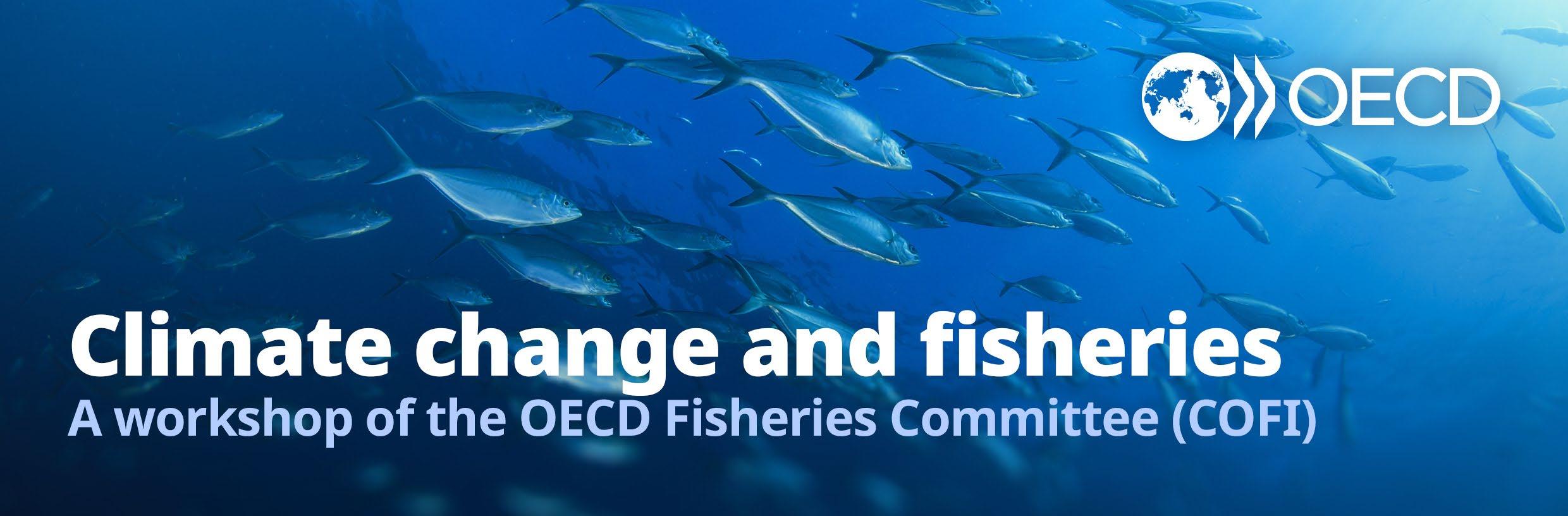
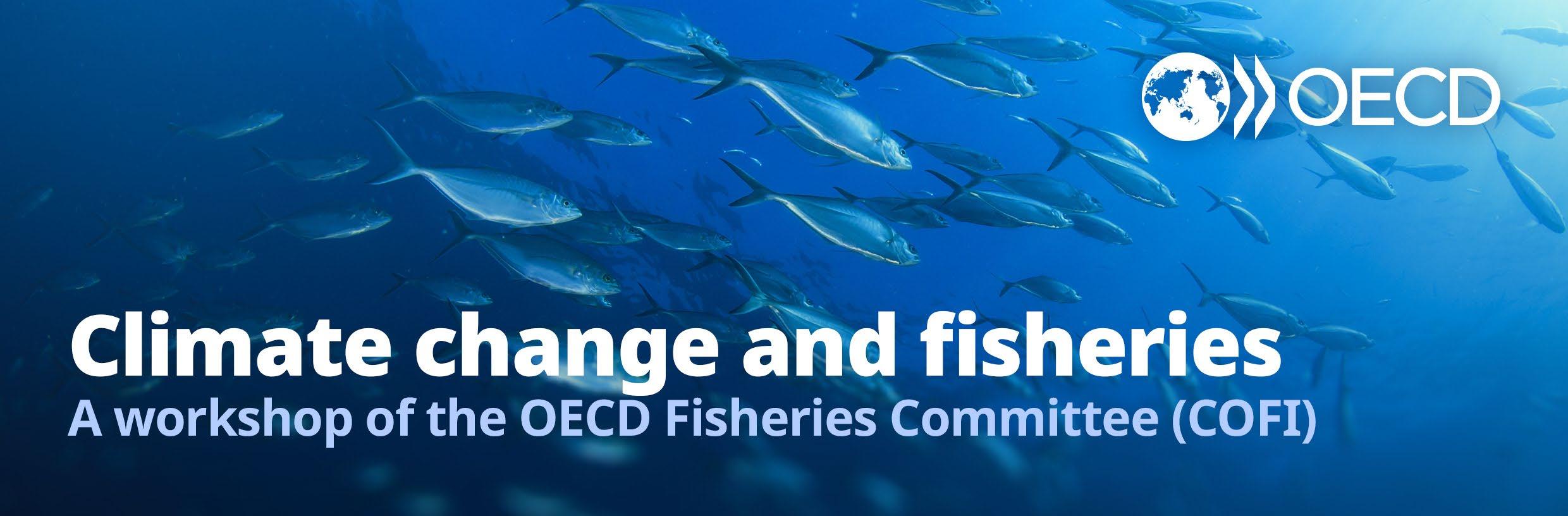
Mitigating the impact of fisheries on climate change
Issues note
This note explores the issue of climate change mitigation in capture fisheries. At a global scale, fisheries’ greenhouse gas (GHG) emissions are estimated to account for around 4% of all emissions from food production and 0.5% of total emissions. Fuel use during fishing is estimated to account for between 60% and 90% of the sector’s emissions up to the point of landing.
Reducing GHG emissions from fisheries can help governments reach economy-wide emissions reduction targets and potentially reduce fishers’ reliance on fossil fuels. Policy options include:
• Implementing management measures to restore the health and productivity of fish stocks, and encourage more efficient fishing, thereby improving the volume and the value of catch per unit of fishing effort and reducing capture fisheries’ emissions and emissions intensity.
• Promoting technology adoption and innovation through regulation or government support to increase the use of existing fuel efficiency technologies and encourage the development of zero or low-emissions vessels and gear.
• Reforming government support policies to avoid encouraging the consumption of fossil fuels in fisheries or encouraging unsustainable fishing.
• Economy-wide policies, such as carbon pricing, could also help to reduce emissions in fisheries.
A fundamental question facing policy makers is the type of policy intervention and the sequence in which they should be applied to reduce GHG emissions from a fishery in the most effective way, while also reducing any distributional impacts. Further, any intervention that reduces the costs of fishing – by increasing the energy efficiency of fishing – poses a high risk of encouraging unsustainable fishing in the absence of effective management. Thus, good fisheries management is needed to ensure policies introduced to reduce emissions do not result in unsustainable fishing as fishers increase effort in response to reduced costs.
This note provided background material for the “Climate Change and Fisheries” workshop, that was held back-to-back with the 132nd meeting of the OECD Fisheries Committee, on 21 November 2023. 1 It gives a brief overview of the role of capture fisheries in GHG emissions, the sources of emissions in capture fisheries, and the range of policy responses available to help fisheries reduce these emissions.
1 The OECD Secretariat warmly thanks all presenters and workshop participants, as well as OECD colleagues from the Agriculture and Resources Policies Division (TAD/ARP) and the Climate, Biodiversity and Water Division (ENV/CBW), for their input and feedback in the preparation of this note. The programme of the workshop is provided at the end of this note for reference. This note does not necessarily represent the view of the OECD or that of its members
Introduction
1. At a global scale, fisheries’ greenhouse gas (GHG) emissions are estimated to account for around 4% of emissions from all food production and 0.5% of total emissions (Parker et al., 2018[1]; Kristofersson, Gunnlaugsson and Valtysson, 2021[2]). However, emissions vary widely both within and between fisheries, notably depending on the vessel and gear used, as well as the abundance and catchability of the target species – i.e. how far, deep and ‘findable’ fish are (Parker and Tyedmers, 2014[3])
2. This note explores the issue of climate change mitigation in capture fisheries, focusing on how to reduce GHG emissions from fishing activities (and leaving aside the issue of mitigation in the post-harvest steps of fish product value chains, such as transport, processing and storage, or the issue of mitigation in aquaculture production). 2 It gives a brief overview of the sources of GHG emissions in capture fisheries, and discusses a range of policy responses available to help fisheries reduce these emissions.
GHG emissions in fisheries
3. Compared to other foods, the production of fish is generally less emissions intensive, i.e. fewer GHGs are emitted to produce a certain quantity (or value) of food (or protein) (Figure 1). Although there has been a global increase in fisheries’ emissions intensity in recent decades, average emissions from fish production (both capture fisheries – the focus of this note – and aquaculture) are lower than for most other animal protein sources (IPCC, 2023[4]) 3
4. Fuel use during fishing is the main source of emissions from fisheries. It is estimated to account for 60-90% of emissions up to the point of landing (Parker et al., 2018[1]; Tyedmers, 2004[5]; FAO, 2015[6]) Further, several studies estimate that up to the point of retail sale, fishing activities altogether account for 75% to 95% of overall GHG emissions, with transport, processing and storage accounting for the rest (Ziegler et al., 2016[7]) Fuel costs are also significant for many fishers, typically accounting for 5% to 45% of operating costs (STECF, 2022[8]; Parker et al., 2015[9]; Greer et al., 2019[10]). This section compares the emissions intensity of capture fish to other foods, gives an overview of recent trends in global fisheries emissions and reviews the differences in GHG emissions from different fisheries.
2 This issue was discussed previously at the OECD, with the 2013 report on Green Growth and Energy Use in Fisheries and Aquaculture outlining emissions from different fisheries and potential mitigation methods (OECD, 2013[27])
3 These calculations however depend on what is compared and how. Food life-cycle carbon emissions assessments (LCAs) are highly influenced by methodological choices, so standardisations across products compared are needed. For example, Gephart et al. (2021[11]) provide a harmonised comparison of fish and chicken, which confirms that many fed aquaculture groups outperform industrial chicken, the most efficient major terrestrial animal-source food They find that capture fisheries vary widely in their GHG emissions (with some species having a higher GHG emissions intensity than chicken, and some lower; see Figure 2).
Figure
1. The GHG emissions intensity of protein production
Source: (IPCC, 2023[4])
Fish are a relatively low-emissions food source
5. In general, fish from wild-catch or aquaculture have a lower emissions intensity of production than other animal products, both by live weight and by grams of protein (Figure 2). The main exceptions are certain demersal flat-fish and crustaceans, which can have relatively high emissions because of the fuelintensive fishing methods used, namely bottom trawling and boats using pots and traps.
6. From a nutritional perspective, while plant-based proteins are less emissions intensive (in GHG/kg of protein) than almost all animal products, the lowest emissions-intensive fish, such as small pelagics, are comparable to the emissions intensity of plant-based protein. Aside from protein, fish is also an important source of essential vitamins, minerals and fatty acids. Some recent studies – such as Hallström (2019[12]) – compare fisheries emissions based on an overall nutrition rating. 4 This decreases the relative emissions intensity of some highly nutritious species, such as small pelagic fish and oysters, while increasing it for some finfish species with very high salt content. Finally, if emissions are considered on the basis of landed value rather than production weight (e.g. GHG/dollar), crustaceans are less emissions-intensive than landbased animal products in most cases (Parker and Tyedmers, 2014[3]; Parker et al., 2015[9])
4 The overall nutrition rating can compare positive elements such as vitamins, minerals, proteins and fatty acids against negative elements such as excessive salt and unhealthy fats. Nutrition scores can vary depending on the weightings given to different factors.
Figure 2. Emissions intensity of fisheries products kg CO2-equivalent per 100g of protein
Note: Grey bar represents emissions from chicken.
Source: (Gephart et al., 2021[11])
Emissions have increased
7. Overall, the emissions intensity of capture fisheries has increased in recent decades. Parker (2018[1]) estimated that global fisheries emissions increased by 1.2% annually between 1990 and 2011, while catches remained steady (Figure 3).
Figure 3. Global fisheries catches and emissions intensity by fleet segment
8. The increase in emissions is explained by changes in the nature of fishing activities, notably:
• higher catches in fuel-intensive crustacean fisheries
• increased fuel use per kilogramme (kg) of landed catch in large pelagic fisheries, primarily tuna 5
• increased fuel use per kg of landed catch in bottom trawl fisheries (Parker et al., 2018[1]) 6
• growing motorisation of the global fleet (Greer et al., 2019[10]) 1
9. Despite the overall increase in emissions from fisheries, since 1990 some individual fisheries have experienced a substantial decline in emissions intensity and total emissions, largely due to increased fuel efficiency from improved technology or healthier stocks. Consequently, fishers have been able to catch the same amount of fish with less fishing effort, and therefore with lower GHG emissions (Parker and Tyedmers, 2014[3]). For examples of this, see the section further below on reduc ing fisheries emissions.
Emissions vary both between and within fisheries
10. Fuel use during fishing is the main source of emissions in most fisheries. A number of factors influence fuel-use intensity, measured in litres of fuel used per kilogramme of catch. The most important of these are vessel and gear type, as well as the characteristics of target species, with some fish more “catchable” than others As a result, emissions intensity varies widely across fisheries: noting these differences can help fisheries managers to prioritise policy and management effort to achieve GHG emissions reduction objectives.
11. Bottom trawl fisheries and pot/trap fisheries have some of the highest emissions intensities (measured by GHG emissions per kg of captured fish) (Figure 4) The picture is different if emissions intensity is measured relative to value, not weight. However, even by this measure, higher value trawlcaught flat-fish and pot and trawl-caught crustaceans still have the highest emissions intensity, although the difference is smaller (Parker and Tyedmers, 2014[3]). Bottom trawl fishing is emissions-intensive due to the resistance of dragging nets through the water, while pot and trap fishing is highly emissions intensive
5 Fuel use has increased on average in global tuna fisheries; however, it is unclear what has driven this overall change. It has likely been affected by changes to fishing methods, distance travelled by fleets, types of vessels and abundance of stocks, but these effects will be different across different fisheries (Parker, Vázquez-Rowe and Tyedmers, 2015[73])
6 An increase in fuel use in trawl fisheries could be due to decreased abundance; however, other factors such as fishing restrictions to protect stocks can also increase fuel use in the short term (Ziegler and Hornborg, 2014[14])
due to the long distances travelled between crustacean pots and traps compared to the relatively low weight of catches (Bastardie et al., 2022[13]). These gear types also account for a large share of fishing emissions globally (Parker and Tyedmers, 2014[3]). Together, pot and trawl fisheries for crustaceans are estimated to account for around 6% of global catches but 22% of emissions (Parker et al., 2018[1])
12. Purse seine, gillnet and pelagic trawl fisheries, on the other hand, are significantly less emissions intensive. As a comparison, purse seine and pelagic trawl fisheries targeting small pelagics account for around 20% of global catches but only 2% of emissions.
Figure 4. Fuel use per tonne of catch, by species and gear type
Note: Figure shows range of fuel intensity between different fisheries.
Source: (Parker and Tyedmers, 2014[3])
Some of the variation can be explained by other characteristics of fisheries
13. There are important local differences in the emissions intensity of different gear types, even within and between fisheries using the same gear. These differences are driven by a variety of factors, including species characteristics, fishing practices or management measures that influence fishing practices (Table 1) (Ziegler and Hornborg, 2014[14]; Waldo and Paulrud, 2016[15]; Driscoll and Tyedmers, 2010[16]; CEFAS, 2022[17]; Parker et al., 2015[9]; Bastardie et al., 2022[13]) As a result, it is possible for the emissions intensity of production for one fisher to be double that of another despite using the same gear and targeting
the same species (Table 2). 7 Where differences are explained by factors that can be influenced by management measures, such as abundance, vessel type or fishing style, fisheries managers have an opportunity to bring down emissions.
Table 1. Factors influencing variations in emissions between similar fisheries
Factors
Examples
Species characteristics Abundance and catchability can influence the efficiency of fishing operations and their emissions intensity. Fishing practices Vessel choice, fishing gear and distance to fishing grounds all impact emissions intensity. Management measures Management measures can decrease efficiency by mandating vessel size or fishing days, or it can increase it by improving allocation of fish such as through total allowable catches (TACs), reducing inefficient competition for fish between fishers.
Source: (Ziegler and Hornborg, 2014[14]; Waldo and Paulrud, 2016[15]; Driscoll and Tyedmers, 2010[16]; CEFAS, 2022[17]; Parker et al., 2015[9]; Bastardie et al., 2022[13])
Table 2. Variations in fuel intensity within fisheries
Litres/tonne of catch
Source: (Parker and Tyedmers, 2014[3])
Carbon release from bottom trawling
14. In addition to emissions from fuel use, bottom trawling fisheries also release CO2 from sediments on the sea floor (Sala et al., 2021[18]). While some authors have estimated these emissions to be of a similar scale to all other emissions from fishing, further data and research are needed before drawing conclusions. Indeed, the magnitude of these releases, and the fraction released into the atmosphere, remain subject to debate because of uncertainties around the theoretical assumptions underpinning the estimates (Hiddink et al., 2023[19]; Atwood et al., 2023[20]). Epstein et al. (2022[21]) compare various studies on emissions released from sediments during trawling, with some showing emissions increases, others showing decreases, and still others showing no change, depending on the location and study methods
7 It is important to note that some of these differences may be attributable to different data collection methods or quality.
Reducing fisheries’ emissions
Managing fish stocks sustainably
15. There are two main ways in which fisheries managers can reduce the intensity of fisheries’ GHG emissions, and often fuel costs. In both cases, capping the fishing pressure at sustainable levels is essential. Fisheries managers can:
1. Implement stock management measures and avoid government support that may inadvertently encourage unsustainable fishing to restore or maintain the health and productivity of fish stocks and encourage more efficient fishing. This will improve the volume and value of catch per unit of fishing effort (CPUE)
2. Use regulation and government support to promote the adoption of existing fishing technologies and practices that reduce emissions and encourage the development of zero or low-emissions vessels
16. As emissions reduction technologies and practices also have the potential to reduce fishing costs by increasing energy efficiency, their promotion should be backed by strong management and well targeted and to ensure additional profits do not result in over-capacity and unsustainable fishing (OECD, 2022[22]) Any increase in fishing effort driven by climate mitigation policies, beyond sustainable limits, would both jeopardise the health of fish stocks and undermine the impacts of the policy itself, as emissions reductions from greater efficiency could be offset by increased emissions from greater effort.
17. Carbon pricing, which may not be fisheries specific, can also play a role in reducing GHG emissions by increasing the costs of using emissions-intensive fishing techniques and practices, and encouraging the adoption of efficient technologies and practices
Using data to design effective energy transition strategies for specific fisheries
18. The specific context of individual fisheries dictates the extent to which a set of energy-saving techniques and practices can reduce emissions and their associated costs, and, ultimately, determines whether it is economically efficient to implement them, and whether and how public policy can accelerate the transition. In extreme cases, for which policies may not be able to reduce emissions cost-effectively, managers may also want to consider whether continuing with current fishing activity is in line with broader economy-wide climate change objectives.
19. The ability of different measures to reduce emissions is likely to depend on the health and productivity of the harvested stocks, the type of vessels used and fishing activity taking place, the availability of low-emissions technologies, as well as the management and support policies in place For example, older vessels with less modern equipment are likely to have greater scope to increase efficiency through technical improvements. However, these vessels may have a shorter useful life over which to benefit from investment in fuel savings. The opportunity for quick improvements to reduce emissions may be greater in some fisheries, while in others further innovation will be required. In many cases there are also trade-offs that may limit the adoption of effective measures. For example, the uptake of speed limitation strategies may be limited by increased labour costs due to longer fishing days offsetting any fuel savings (Ziegler and Hornborg, 2023[23]). Understanding these trade-offs will be important for designing effective incentive policies.
20. To prioritise policy interventions and measure their effectiveness, it is necessary to accurately measure fuel use in fisheries. Currently, global measures of fuel use are lacking (Parker and Tyedmers, 2014[3]). However, many jurisdictions are making progress on collecting fuel data. A notable example is the European Union, which publishes up-to-date fuel use data by country and type of fishing gear as part of their Blue Economy Observatory (European Commission, 2023[24])
21. A fundamental question facing policy makers is the type of policy intervention and the sequence in which they should be applied to most effectively reduce GHG emissions from fisheries while limiting any distributional impacts. The remainder of this section provides discusses a range of measures that can be considered as part of fisheries energy transition strategies
Restoring stocks to optimal levels and encouraging efficient fishing
22. Restoring overfished stocks to biomass levels that allow for catches to be maximised sustainably, and maintaining all harvested stocks at these levels while encouraging efficient fishing, can be an effective way of reducing emissions, particularly in overfished stocks (Hornborg and Smith, 2020[25]). Management measures to increase biomass can reduce emissions by increasing the catch per unit of fishing effort. By increasing the density and/or size of the stocks, search times are reduced and fishers can reduce the effort, and fuel, used to catch the same amount of fish (Bastardie et al., 2022[26])
23. A review of the literature conducted by the OECD showed that, in many cases, optimising management could be the most effective emissions reduction policy to date (OECD, 2013[27]). For example, Duy et al. (2014[28]) estimated that optimising fisheries management to achieve maximum sustainable yields (MSY) would reduce fuel consumption by 29% and increase economic returns by 100%. Applying emissions taxes and trading systems to the optimised fishery would result in relatively modest additional reductions to fuel use of between 0.2% and 11.3%. 8 In general, management measures have a higher potential for fuel savings than technical and behavioural interventions (Figure 5).
Figure 5. Fuel savings from different efficiency measures (%)
Note: ITQ stands for individual transferrable quotas. References to 2 different stock status improvement strategies refers to two studies of improved stock status in Swedish and Icelandic fisheries. References to 3 different ITQ schemes relate to the introduction of widespread ITQs in Iceland, and the introduction of ITQs in Canadian and United States demersal and shellfish fisheries. Source: (OECD, 2013[27]; Arnason, 2010[29]; Brandt, 1999[30]; Driscoll and Tyedmers, 2010[16]; IMARES, 2009[31]; Repetto, 2001[32]; Sigler and Lunsford, 2001[33]; Wilson, 1999[34])
8 When the model excluded Icelandic fisheries, which were already managed with a relatively high level of efficiency, the reduction in fuel use in the remaining countries was almost 50%.
24. There is currently significant scope to rebuild stocks and implement management plans to increase fishing efficiency and reduce emissions (OECD, 2022[22]) Among the stocks assessed in the 32 countries covered in the 2022 edition of the OECD Review of Fisheries (OECD, 2022[22]), only 30% were known to be meeting productivity targets for optimal harvest efficiency (Figure 6, bottom panel) About one-third of stocks were not even meeting sustainability targets (top panel).
Figure 6. Sustainability and productivity of assessed stocks reported by COFI Members and Partners for the OECD Review of Fisheries 2022
Note: The figure was based on recent Source:
25. reductions, usually 4 to 26 2012
26. fishery
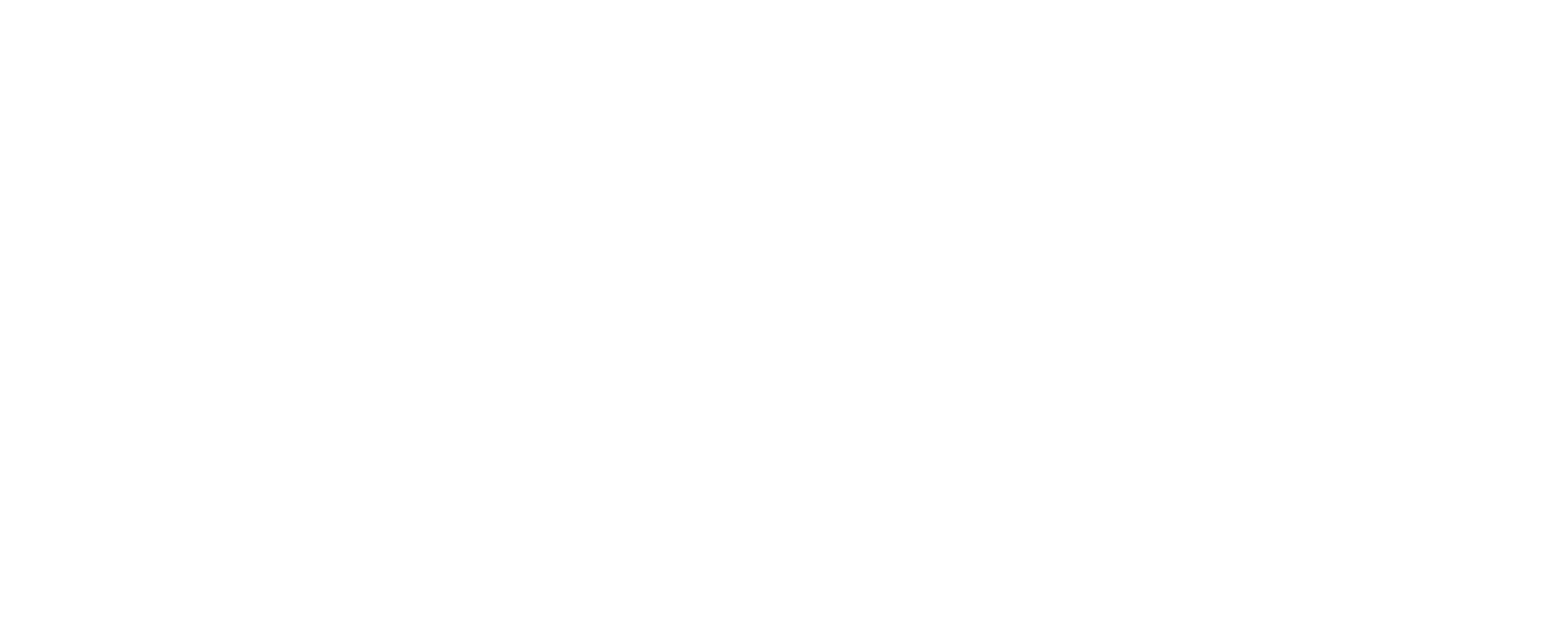
On re and reduce overexploitation. This area of the fishery a corresponding 75% drop in fuel intensity. management practices saw no change in CPUE or fuel use intensity over this time (Bloor et al., 2021[39]). Another example is the implementation of a transferable quota system in Icelandic fisheries in 1991, which allowed depleted fish stocks to rebuild. Stocks of cod – the most important species in Icelandic fisheries, representing around 45% of total value in 2019 – have consistently increased following the introduction of quota management. A 2021 study showed that fuel use in Icelandic fisheries decreased by 40% between 1997 and 2008, mostly due to higher CPUE from rebuilt fish stocks (Kristofersson, Gunnlaugsson and Valtysson, 2021[2]) Box 1 also describes a third example – the introduction of total allowable catch quotas in a New Zealand rock lobster fishery.
27. The target for effective stock rebuilding can vary depending on the fishery. While MSY is widely accepted as the minimum biological target for sustainable stocks, a biomass higher than that required to support MSY can also result in higher CPUE, reduced costs and reduced emissions intensity. The extent to which increased biomass will lead to reduced emissions depends on both the biological characteristics of the stock and the economic and technical aspects of individual fishing operations, such as vessel capacity and overall operating costs.
Box 1. Stock rebuilding reduces emissions in a New Zealand rock lobster fishery
Rebuilding depleted stocks can be an efficient way to improve catch per unit of effort (CPUE), increase profitability and reduce emissions. In the early 1990s, rock lobster catches in New Zealand had fallen to historic lows after overfishing depleted stocks (OECD, 2012[40]). A quota management system was introduced to control overfishing, and management plans were introduced to rebuild stocks to levels that could support maximum sustainable yields (MSY). While the main goal of stock rebuilding was to increase catches, it also led to an increased CPUE and an estimated two-thirds reduction in fuel use intensity.
New Zealand rock lobster are of catchable size between 8-13 years after breeding occurs (Fisheries New Zealand, 2023[41]). Fourteen years after a TAC and stock rebuilding plan were introduced in two specific management areas in 1990, the CPUE began to climb, and more than tripled in the three years between 2005 and 2008. Since then, fishable biomass has increased to almost 1.5 times the biomass required to support MSY, and CPUE has gradually increased over time, stabilising at around six times the CPUE when the TAC was introduced (Fisheries New Zealand, 2022[42]; Fisheries New Zealand, 2021[43]; Holland, 2010[44])
Further, a 2017 study of fuel use in lobster fisheries in New Zealand and Australia demonstrates the significant relationship between increased CPUE and decreased fuel use intensity (Figure 7) (Parker et al., 2017[45])
Based on the average estimated relationship between CPUE and fuel use in New Zealand lobster fisheries, the implementation of the TAC and stock rebuilding plan described above is estimated to have reduced fuel-use intensity by over two-thirds in these management zones. This demonstrates the effectiveness of both reaching and surpassing MSY management goals for significantly decreasing emissions while improving catches and profitability.
Source: (Parker et al., 2017[45]).
Targeting support to address the socio-economic impacts of stock rebuilding
28. In some fisheries, measures reducing the overall fishing effort to rebuild stocks and increase CPUE may lead to fishers leaving the fishery, or catches decreasing, at least in the short term, while other fishers may expand their operations, and change their business structures. For example, in the Nordic fisheries, the transition to optimal fisheries management was accompanied by a 45% decrease in the number of fishing vessels (Duy et al., 2014[28]). The political economy dimension of biologically optimal management may thus need to be addressed upfront. Fisheries managers could consider implementing specific policies to ensure these changes do not have adverse impacts on particular groups of fishers or their communities. Social safety-nets, training support to develop alternative activities and adjustment programmes, such as carefully-designed licence buyouts, have proven successful in addressing the distributional impacts of changes in fisheries conditions in several COFI Member and Partner fisheries. Such policies may be helpful to address the impacts of climate change mitigation policies (Teh, Hotte and Sumaila, 2017[46]; Squires, 2010[47]; Melnychuk et al., 2021[37]; Squires, Joseph and Groves, 2006[48]; FAO, 2018[36]; Graff Zivin and Mullins, 2015[49]).
29. It is also important to note that management measures may need to be adapted to take into account new behaviours stemming from increased fuel prices or fuel efficiency. For example, fishers in multi-species fisheries have been observed to change their fishing grounds to fish closer to port and target higher value species in response to higher fuel prices (Abernethy et al., 2010[50]) On the other hand, increased fuel efficiency can lead to increased effort in fisheries where catch in not constrained. In the absence of effective measures to constrain catches when required, increased profitability can have a potentially cascading effect on other stocks as fishing effort shifts to focus on new stocks leading to progressive depletion of multiple stocks (Beckensteiner, Boschetti and Thébaud, 2023[51])
30. Finally, while improved fisheries management can be an effective method for reducing emissions in many fisheries, there may be some exceptions, as not all stocks will see a strong relationship between CPUE and fuel-use intensity (Bastardie et al., 2022[26]; Bastardie et al., 2022[13]; FAO, 2018[36]). For example, this is the case for species where dense aggregations allow for high rates of catchability even as populations decline. Notable examples include Atlantic cod in Canada (Rose et al., 2000[52]) and orange roughy in Australia and New Zealand (AFMA, 2022[53]). For both these stocks, density and CPUE remain steady, even as stocks grow or decline; reducing emissions would therefore require technological innovation or even a change in fishing practices. 9
Improving the fuel efficiency of fishing
31. Notwithstanding actions to maintain fish stocks’ productivity, the fuel efficiency and the GHGintensity of fishing can be improved by both technical measures that affect vessels and gear, and behavioural measures through which fishers change the way they use their vessels and gear. Such fuel efficiency measures can generate significant savings, which can be realised immediately. Increases in fuel prices have already been seen to result in behavioural changes. However, fuel-efficiency measures may not be sufficient to meet emissions reductions targets in all fisheries, and more drastic changes in practices, such as shifting away from the most emissions-intensive fishing techniques, may be required to meet goals for the sector.
9 Even where CPUE does not increase under a stock rebuilding plan, economic returns can increase as quota are consolidated to improve economies of scale, fishers are required to change their practices to compete, less economically efficient fishers exit the industry or prices increase in response to reduced supply. This can have mixed outcomes for fuel use (Parker and Tyedmers, 2014[3]) (Parker et al., 2015[9])
Making use of existing fuel efficiency strategies
32. In terms of technical measures, energy efficiency improvement techniques can result in fuel savings for existing vessels of between 5% and 20%, depending on the current state of the vessel and the type of fishing it engages in (see Figure 5) (IRENA, 2021[54]; ISMAR, 2012[55]; BIM, 2009[56]; AMARREE, 2021[57]; Scottish Government, 2022[58]; OECD, 2023[59]). For example, one of the many technical measures to reduce fuel use, and one of the more effective and relatively low-cost technological measures, is the use of a ducted propeller, also known as a nozzle. A ducted propeller is essentially a ring which fits around the propeller, and has been shown to reduce overall fuel use by up to 15% in some applications (Notti, 2012[60]; OECD, 2013[27]). Replacing existing auxiliary engines with more efficient models or replacing trawl nets with the most efficient available are other lower cost ways to increase efficiency and can each deliver fuel savings of up to 15% (OECD, 2013[27]; IMARES, 2009[31])
33. Another example is the use of a hybrid diesel-electric motor, which can reportedly generate fuel savings of between 10% and 25% (Gabrielii and Jafarzadeh, 2020[61]). Diesel engines operate most efficiently at a certain design speed, which is ideal for vessels operating at a constant cruising speed. However, during fishing, engines are often required to operate at different speeds and with different loads, while also providing power for auxiliary loads such as ice and cooling which can lead to inefficient operation. A hybrid diesel-electric motor uses diesel generators to power batteries that then run electric motors, which can provide varying levels of power without significant efficiency losses. This allows the diesel motors to run at their most efficient speeds to generate power, while the electric motors can efficiently adjust their output to power the vessel and auxiliary loads. In addition to efficiency savings from a hybrid system, where vessel charging facilities are available in port, estimated fuel savings are up to 70%. However, case studies on this are rare. Savings will be lower for vessels that have high energy use during fishing or spend longer periods at sea (Ziegler and Hornborg, 2023[23]; Gabrielii and Jafarzadeh, 2020[61]) 10
34. Among the behavioural fuel-efficiency measures, one of the more straightforward is to optimise vessel speed to reduce fuel use. One way of achieving this is to install a fuel meter that displays real-time fuel use, allowing vessel operators to optimise fuel when travelling to and from fishing grounds. A 2021 programme in France funded the installation of fuel meters on 181 vessels and encouraged fishers to optimise speed when going to and from fishing grounds, achieving reported average fuel savings of 5% (AMARREE, 2021[57])
Developing low-emissions vessels
35. While policy changes can reduce emissions from fishing, to achieve a low-emissions, and ideally a zero-emissions, fishing industry, major transformations to vessels would be needed. Diesel is currently the most widely available and used fuel for fishing vessels and alternative fuels with lower emissions, such as green methanol, hydrogen, ammonia, e-fuels and biodiesel, are all potential candidates to replace fossil fuels. However, prices for all these fuels are significantly higher than for diesel or liquified natural gas (LNG), and current supplies are limited (Figure 8) (IRENA, 2021[54])
36. There are also greater technical challenges to their adoption by fishing vessels than for other types of ocean-going vessels. Most of these fuels require more space for on-board storage than diesel and are best suited for operation at constant speeds. However, the fishing fleet is characterised by relatively small vessels which operate at a range of different speeds during fishing and steaming. This reduces both the space for alternative fuel systems on board fishing vessels and the potential energy savings from these fuels during operation (Ziegler and Hornborg, 2023[23]; Gabrielii and Jafarzadeh, 2020[61]). These
10 Aquaculture vessels that travel at low speeds for short distances with relatively small loads have been fully electrified in some cases (Ziegler and Hornborg, 2023[23]; Gabrielii and Jafarzadeh, 2020[61])
challenges are illustrated by the adoption of LNG to replace traditional fuel oils. This transition has driven emissions reductions in global shipping in recent years. In 2022, 44% of larger, non-fishing vessels under construction globally, such as cargo ships, were LNG or dual fuel diesel/LNG driven, compared to 14% in 2017 (OECD, 2023[59]). Emissions reductions from LNG compared to diesel fuel are estimated to be around 20%. However, most fishing vessels are too small to accommodate sufficient LNG storage or have a speed profile which does not suit LNG engines (Gabrielii and Jafarzadeh, 2020[61])
37. With changes in diesel prices, taxes, subsidies or increased investment in alternative fuel production, these fuels may become price competitive in the coming decades (Solakivi, Paimander and Ojala, 2022[62]; EMSA, 2022[63]; EMSA, 2022[64]; IEA, 2019[65]). Given the current gap in prices between diesel and alternative low-emissions fuels, policies designed to develop low- emissions fishing vessels and encourage innovation in alternative fuels are likely to be necessary for governments wanting to move to zero emissions It is also important to observe alternative fuel developments in the wider shipping industry, which will drive changes to fuel provision and port infrastructure. As with other sectors, governments will have to weigh the possible emissions reductions against the cost of investment in developing lowemissions fishing vessels and adapt their objectives in light of more economically efficient emissions reductions in other sectors.
Figure 8. Prices for conventional and low-emissions shipping fuels
Note: The price of diesel, used in almost all fishing vessels, is typically similar to or lower than the price of LNG, the lowest priced fuel on this graph.
Source: (IEA, 2019[65])
38. Despite the potential of better management and behavioural measures, reaching the emissions reduction targets set for the sector may be either too difficult or too costly in some of the highest emitting fisheries. In these cases, fisheries managers may want to consider shifting fishing activity away from certain emissions-intensive techniques
Reforming fisheries support to accelerate the energy transition and help make it fair
39. Well-targeted support policy packages can be key in leveraging the energy transition and making it socially beneficial and acceptable. Support provided as part of fisheries management programmes will be required to limit the socio-economic impacts of stock rebuilding programmes and ensure the management systems are effectively funded. Further, moving away from supporting fossil fuel use and towards supporting fuel-saving technologies and practices could provide a price signal for fishers and induce behavioural change, thereby reducing emissions with limited impact on fishing profitability.
40. In addition, aside from reducing emissions, reducing support to fossil fuel consumption could leave some fishers better off in the long term, as fuel support has a low transfer efficiency, with less than 50% of the value of government payments being captured by the fisher themselves (Martini, 2022[66]). Support to fuel also tends to be disproportionately captured by large-scale fishing operations (Martini and Innes, 2018[67]; Martini, 2022[66])
41. However, it is important to note that in the absence of effective management, many forms of direct support provided to fishers and fishing companies risk encouraging unsustainable fishing (Figure 9). By increasing fishing efficiency and reducing fishing costs, support granted to fuel-saving measures, or GHG emissions-reduction technology adoption, could also inadvertently create incentives for unsustainable fishing if unconstrained by management (Palomares and Pauly, 2019[68]; OECD, 2022[22]) Policy makers should carefully consider the risks of encouraging overfishing posed by GHG emissions-reducing support and how to mitigate these risks through careful targeting and effective management of recipient fisheries (OECD, 2022[22]) Further, they may want to favour re-purposing existing support towards GHG emissions reduction rather than adding additional support measures.
42. More generally, overfishing and illegal, unreported and unregulated fishing lead to a reduction in the biomass of stocks and greater fishing effort, which would see fisheries emissions and emissions intensities increase. Therefore, reforming support policies to move away from support that can pose a risk of unsustainable fishing is a smart strategy for countries looking to reduce emissions from their fisheries. Importantly, support to improve stock health and productivity, which leads to improved catch efficiency and lower emissions intensity, has the advantage of not presenting any risk to resource sustainability. This type of support should be considered as low-hanging fruit when it comes to climate mitigation and should be prioritised in climate mitigation strategies for fisheries.
Figure 9. Risk of encouraging unsustainable fishing depending on the type of support policy and the context in which it is disbursed
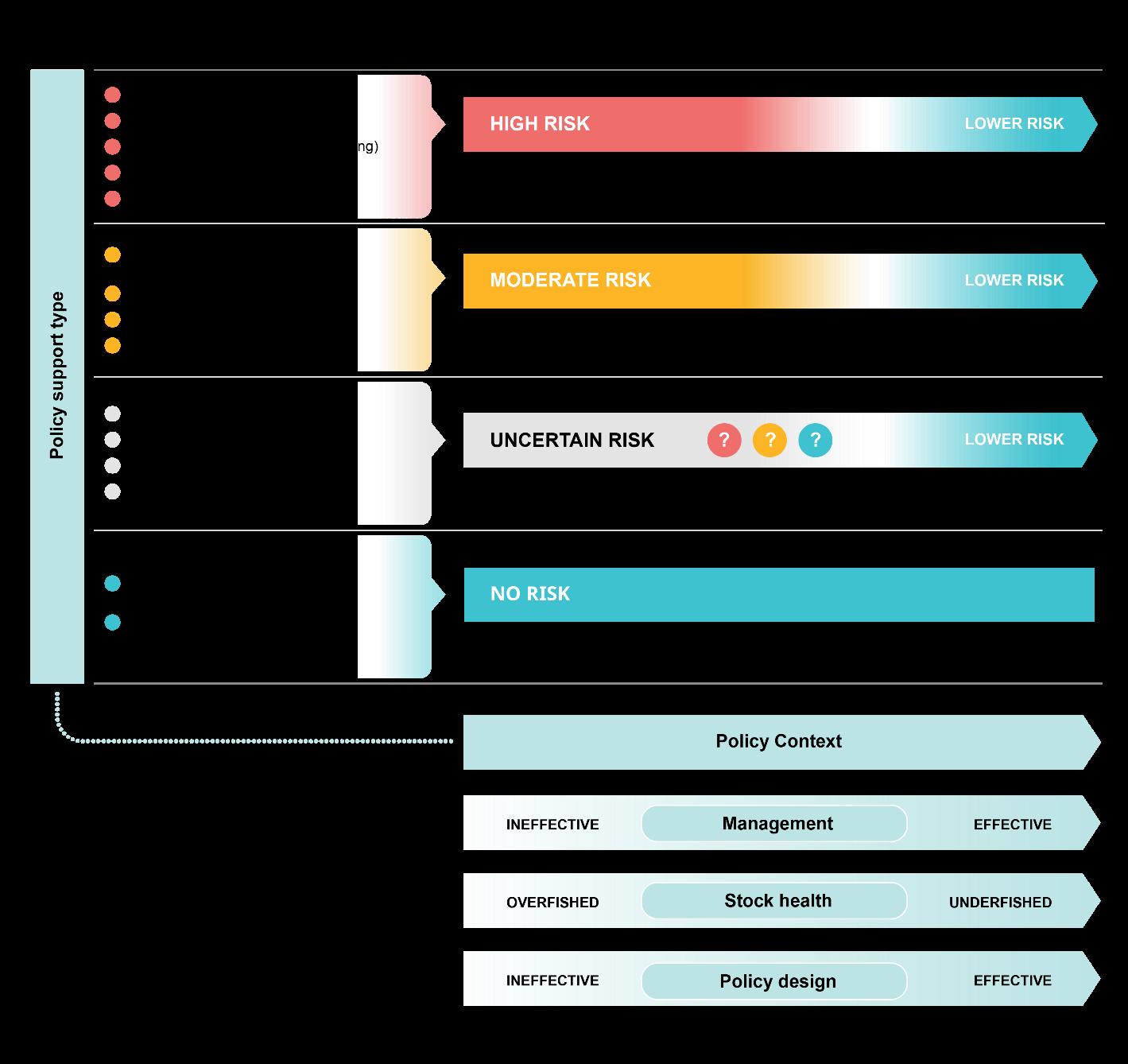
Source: (OECD, 2022[22]).
Putting a price on carbon
43. Carbon pricing can be an effective way of reducing emissions (OECD, 2021[69]) as taxes and payment policies for GHG emissions can significantly change investment decisions. There are several mechanisms for putting prices on carbon, most notably taxes and cap and trade systems. 11 The use of carbon prices is increasing and is now relatively common. In 2021, over 40% of emissions from
11 As described on OECD Emissions trading systems webpage, “there are two main types of emissions trading systems: “Capand-trade systems” and “baseline-and-credit systems”. In a cap-and-trade system, an upper limit on emissions is fixed, and emission permits are either auctioned out or distributed for free according to specific criteria. Under a baseline-and-credit system, there is no fixed limit on emissions, but polluters that reduce their emissions more than they otherwise are obliged to can earn ‘credits’ that they sell to others who need them in order to comply with regulations they are subject to. A cap-and-trade system could ” Emissions trading systems can apply to one or multiple industries.
71 countries (accounting for 80% of global emissions) were covered by carbon prices of some kind, up from 32% in 2018 (OECD, 2022[70]).
44. The pricing of carbon in the fisheries sector, either as part of a specific mechanism (e.g. tax or cap and trade) or as part of a wider system, has the potential to promote more energy efficient practices and technologies, thereby reducing emissions in an economically efficient way. How much such a system can actually reduce GHG emissions in fisheries would depend on the scope for emissions reduction, the responsiveness of fuel use to changes in price, the capacity for fishers to support any additional financial burden, and the administrative costs of implementing and enforcing the system (OECD, 2016[71]; Parry, Black and Zhunussova, 2022[72]; Ziegler and Hornborg, 2023[23])
45. However, to date, negative carbon prices resulting from fossil fuel subsidies are most common in the agriculture and fisheries sector (OECD, 2022[70]), although disaggregated information is lacking on how much fuel for use in fisheries is subsidised through non-specific policies (OECD, 2022[22])
References
Abernethy, K. et al. (2010), “Fuelling the decline in UK fishing communities?”, ICES Journal of Marine Science, Vol. 67/5, pp. 1076-1085, https://doi.org/10.1093/icesjms/fsp289
AFMA (2022), Orange Roughy (Hoplostethus atlanticus) Stock Rebuilding Strategy, Australian Fisheries Management Authority, https://www.afma.gov.au/sites/default/files/2023-02/orangeroughy-rebuilding-strategy-2022.pdf
AMARREE (2021), Programme AMARREE, https://amarree.fr/
Arnason, R. (2010), Saving Fuel in Fisheries the Painless Way
Atwood, T. et al. (2023), “Reply to: Quantifying the carbon benefits of ending bottom trawling”, Nature, Vol. 617/7960, pp. E3-E5, https://doi.org/10.1038/s41586-023-06015-6
Bastardie, F. et al. (2022), “Ten lessons on the resilience of the EU common fisheries policy towards climate change and fuel efficiency - A call for adaptive, flexible and well-informed fisheries management”, Frontiers in Marine Science , Vol. 9, https://doi.org/10.3389/fmars.2022.947150.
Bastardie, F. et al. (2022), “Reducing the fuel use intensity of fisheries: through efficient fishing techniques and recovered fish stocks”, Frontiers in Marine Science, Vol. 9, https://doi.org/10.3389/fmars.2022.817335
[13]
Beckensteiner, J., F. Boschetti and O. Thébaud (2023), “Adaptive fisheries responses may lead to climate maladaptation in the absence of access regulations”, npj Ocean Sustainability, Vol. 2/1, https://doi.org/10.1038/s44183-023-00010-0 [51]
BIM (2009), Improving fuel efficiency on fishing vessels, Bord Iascaigh Mhara, Dublin, https://bim.ie/wpcontent/uploads/2021/02/Improving,Fuel,Efficiency,on,Fishing,Vessels,(User,Friendly,Guide). pdf
Bloor, I. et al. (2021), “Boom not bust: Cooperative management as a mechanism for improving the commercial efficiency and environmental outcomes of regional scallop fisheries”, Marine Policy, Vol. 132, p. 104649, https://doi.org/10.1016/j.marpol.2021.104649
Brandt, S. (1999), “Productivity and Industrial Structure Under Market Incentives and Traditional Regulation”, University of California, Berkeley, Department of Agricultural and Resource Economics, CUDARE Working Papers, https://ageconsearch.umn.edu/record/198683.
[56]
[39]
[30]
CEFAS (2022), Carbon emissions in UK fisheries: recent trends, current levels, and pathways to Net Zero, https://www.cefas.co.uk/media/x2wh5q45/final-report-zero-carbon-fisheriesfinal.pdf?e=v3pgcZ
Costello, C. et al. (2012), “The Economic Value of Rebuilding Fisheries”, OECD Food, Agriculture and Fisheries Papers, No. 55, OECD Publishing, Paris, https://doi.org/10.1787/5k9bfqnmptd2-en
Driscoll, J. and P. Tyedmers (2010), “Fuel use and greenhouse gas emission implications of fisheries management: the case of the new england atlantic herring fishery”, Marine Policy, Vol. 34/3, pp. 353-359, https://doi.org/10.1016/j.marpol.2009.08.005.
[17]
[35]
Duy, N. et al. (2014), Reducing Climate Impact from Fisheries: A Study of Fisheries Management and Fuel Tax Concessions in the Nordic Countr ies, TemaNord, Nordic Council of Ministers, Copenhagen K, https://doi.org/10.6027/TN2014-533 [28]
EMSA (2022), Potential of ammonia as fuel in shipping, European Maritime Safety Agency, http://https:www.emsa.europa.eu/newsroom/latest-news/download/7322/4833/23.html
EMSA (2022), “Update on potential of biofuels in shipping”, European Maritime Safety Agency, https://www.google.com/url?sa=i&rct=j&q=&esrc=s&source=web&cd=&cad=rja&uact=8&ved= 0CAIQw7AJahcKEwjQsOGX1cqBAxUAAAAAHQAwww.emsa.europa.eu/publications/reports/ download/7321/4834/23.html
Epstein, G. et al. (2022), “The impact of mobile demersal fishing on carbon storage in seabed sediments”, Global Change Biology, Vol. 28/9, pp. 2875-2894, https://doi.org/10.1111/gcb.16105
European Commission (2023), Fishing fleet fuel analysis, https://blue-economyobservatory.ec.europa.eu/fishing-fleet-fuel-analysis_en
FAO (2018), Rebuilding of marine fisheries. Part 1: Global review, https://www.fao.org/3/ca0161en/ca0161en.pdf
FAO (2015), Fuel and energy use in the fisheries sector. Approaches, inventories and strategic implications., https://www.fao.org/3/i5092e/i5092e.pdf
Fisheries New Zealand (2023), New Zealand Rock Lobster, https://www.mpi.govt.nz/dmsdocument/45625-New-Zealand-rock-lobster-biology
Fisheries New Zealand (2022), Rock lobster catch and effort data: 1979-80 to 2021-22, https://www.mpi.govt.nz/dmsdocument/52609-FAR-202242-Rock-lobster-catch-and-effortdata-197980-to-202122
Fisheries New Zealand (2021), Review of rock lobster sustainability measures for 2022/23, https://www.mpi.govt.nz/dmsdocument/49075-Review-of-sustainability-measures-for-rocklobster-CRA-17-and-8-for-2022
Gabrielii, C. and S. Jafarzadeh (2020), Alternative fuels and propulsion systems for fishing vessels, SINTEF Energy Research, https://www.sintef.no/contentassets/a582d1444c244557be4a64b765dbe9a4/coolfish-reportpropulsion_and_fuels-signed.pdf
Gephart, J. et al. (2021), “Environmental performance of blue foods”, Nature, Vol. 597/7876, pp. 360-365, https://doi.org/10.1038/s41586-021-03889-2 [11]
Graff Zivin, J. and J. Mullins (2015), “Vessel buybacks in fisheries: The role of auction and financing structures”, Marine Policy, https://doi.org/10.1016/j.marpol.2014.10.004.
Greer, K. et al. (2019), “Global trends in carbon dioxide (CO2) emissions from fuel combustion in marine fisheries from 1950 to 2016”, Marine Policy, Vol. 107, p. 103382, https://doi.org/10.1016/j.marpol.2018.12.001
Hallström, E. et al. (2019), “Combined climate and nutritional performance of seafoods”, Journal of Cleaner Production, Vol. 230, pp. 402-411, https://doi.org/10.1016/j.jclepro.2019.04.229
[49]
[10]
[12]
Hiddink, J. et al. (2023), “Quantifying the carbon benefits of ending bottom trawling”, Nature, Vol. 617/7960, pp. E1-E2, https://doi.org/10.1038/s41586-023-06014-7 [19]
Holland, D. (2010), “Management Strategy Evaluation and Management Procedures: Tools for Rebuilding and Sustaining Fisheries”, OECD Food, Agriculture and Fisheries Papers, No. 25, OECD Publishing, Paris, https://doi.org/10.1787/5kmd77jhvkjf-en
Hornborg, S. and A. Smith (2020), “Fisheries for the future: greenhouse gas emission consequences of different fishery reference points”, ICES Journal of Marine Science, https://doi.org/10.1093/icesjms/fsaa077
IEA (2019), The Future of Hydrogen, Seizing Today’s Opportunities, International Energy Agency, https://iea.blob.core.windows.net/assets/9e3a3493-b9a6-4b7d-b4997ca48e357561/The_Future_of_Hydrogen.pdf.
IMARES (2009), Energy Saving in Fisheries, (ESIF) FISH/2006/17 LOT3-Final Report, https://edepot.wur.nl/3509
IPCC (2023), “Central and South America”, in Climate Change 2022 – Impacts, Adaptation and Vulnerability, Cambridge University Press, https://doi.org/10.1017/9781009325844.014.
[4]
IRENA (2021), A Pathway to Decarbonsie the Shipping Sector by 2050, International Renewable Energy Agency, https://www.irena.org//media/Files/IRENA/Agency/Publication/2021/Oct/IRENA_Decarbonising_Shipping_2021.pdf [54]
ISMAR (2012), Information collection in energy efficiency for fisheries (ICEEF): Effects of engine replacement on the fuel consumption reduction in fisheries, https://stecf.jrc.ec.europa.eu/c/document_library/get_file?uuid=28b58802-3f2e-4c4d-9a95d60696c3a894&groupId=12762
Kristofersson, D., S. Gunnlaugsson and H. Valtysson (2021), “Factors affecting greenhouse gas emissions in fisheries: evidence from Iceland’s demersal fisheries”, ICES Journal of Marine Science, https://doi.org/10.1093/icesjms/fsab109
[55]
[2]
Martini, R. (2022), “International effects of fisheries support policies”, OECD Food, Agriculture and Fisheries Papers, No. 188, OECD Publishing, Paris, https://doi.org/10.1787/984b0ce6en [66]
Martini, R. and J. Innes (2018), “Relative effects of fisheries support policies”, OECD Food, Agriculture and Fisheries Papers, No. 115, OECD Publishing, Paris, https://doi.org/10.1787/bd9b0dc3-en [67]
Melnychuk, M. et al. (2021), “Identifying management actions that promote sustainable fisheries”, Nature Sustainability, Vol. 4/5, pp. 440-449, https://doi.org/10.1038/s41893-02000668-1
[37]
Notti, E. (2012), “On the opportunity of improving propulsion system efficiency Italian fishing vessels.”, Second International Symposium on Fishing Vessel Energy Efficiency E-Fishing, https://www.researchgate.net/publication/267784276_On_the_opportunity_of_improving_pro pulsion_system_efficiency_for_italian_fishing_vessels
OECD (2023), Analysis of the maritime equipment industry and its challenges, https://one.oecd.org/document/C/WP6(2022)15/FINAL/en/pdf
OECD (2022), OECD Review of Fisheries 2022, OECD Publishing, Paris, https://doi.org/10.1787/9c3ad238-en
OECD (2022), Pricing Greenhouse Gas Emissions: Turning Climate Targets into Climate Action, OECD Series on Carbon Pricing and Energy Taxation, OECD Publishing, Paris, https://doi.org/10.1787/e9778969-en
OECD (2021), Effective Carbon Rates 2021: Pricing Carbon Emissions through Taxes and Emissions Trading, OECD Series on Carbon Pricing and Energy Taxation, OECD Publishing, Paris, https://doi.org/10.1787/0e8e24f5-en
OECD (2016), Effective Carbon Rates: Pricing CO2 through Taxes and Emissions Trading Systems, OECD Series on Carbon Pricing and Energy Taxation, OECD Publishing, Paris, https://doi.org/10.1787/9789264260115-en.
OECD (2013), Green growth and energy use in fisheries and aquaculture, https://one.oecd.org/document/TAD/FI(2012)2/FINAL/En/pdf
OECD (2012), Rebuilding Fisheries: The Way Forward, OECD Publishing, Paris, https://doi.org/10.1787/9789264176935-en
Palomares, M. and D. Pauly (2019), “On the creeping increase of vessels’ fishing power”, Ecology and Society, Vol. 24/3, https://doi.org/10.5751/es-11136-240331
Parker, R. et al. (2018), “Fuel use and greenhouse gas emissions of world fisheries”, Nature Climate Change, Vol. 8/4, pp. 333-337, https://doi.org/10.1038/s41558-018-0117-x
Parker, R. et al. (2017), “Drivers of fuel use in rock lobster fisheries”, ICES Journal of Marine Science, https://doi.org/10.1093/icesjms/fsx024
Parker, R. et al. (2015), “Environmental and economic dimensions of fuel use in Australian fisheries”, Journal of Cleaner Production, Vol. 87, pp. 78-86, https://doi.org/10.1016/j.jclepro.2014.09.081
Parker, R. and P. Tyedmers (2014), “Fuel consumption of global fishing fleets: current understanding and knowledge gaps”, Fish and Fisheries, Vol. 16/4, pp. 684-696, https://doi.org/10.1111/faf.12087
Parker, R., I. Vázquez-Rowe and P. Tyedmers (2015), “Fuel performance and carbon footprint of the global purse seine tuna fleet”, Journal of Cleaner Production, Vol. 103, pp. 517-524, https://doi.org/10.1016/j.jclepro.2014.05.017
Parry, I., S. Black and K. Zhunussova (2022), Carbon Taxes or Emissions Trading Systems? Instrument Choice and Design, International Monetary Fund, https://www.imf.org//media/Files/Publications/Staff-Climate-Notes/2022/English/CLNEA2022006.ashx
Repetto, R. (2001), “A natural experiment in fisheries management”, Marine Policy, Vol. 25/4, pp. 251-264, https://doi.org/10.1016/s0308-597x(01)00018-5.
Rose, G. et al. (2000), “Distribution shifts and overfishing the northern cod (Gadus morhua): a view from the ocean”, Canadian Journal of Fisheries and Aquatic Sciences, Vol. 57/3, pp. 644-663, https://doi.org/10.1139/f00-004
[32]
[52]
Sala, E. et al. (2021), “Protecting the global ocean for biodiversity, food and climate”, Nature, Vol. 592/7854, pp. 397-402, https://doi.org/10.1038/s41586-021-03371-z [18]
Scottish Government (2022), Review of fishing practices which have been adapted in commercial fisheries around the world in order to help mitigate the sector’s contribution to climate change, https://www.gov.scot/publications/review-fishing-practices-adaptedcommercial-fisheries-around-world-order-help-mitigate-sectors-contribution-climatechange/documents/
Sigler, M. and C. Lunsford (2001), “Effects of individual quotas on catching efficiency and spawning potential in the Alaska sablefish fishery”, Canadian Journal of Fisheries and Aquatic Sciences, Vol. 58/7, pp. 1300-1312, https://doi.org/10.1139/f01-074
Solakivi, T., A. Paimander and L. Ojala (2022), “Cost competitiveness of alternative maritime fuels in the new regulatory framework”, Transportation Research Part D: Transport and Environment, Vol. 113, p. 103500, https://doi.org/10.1016/j.trd.2022.103500
Squires, D. (2010), “Fisheries buybacks: a review and guidelines”, Fish and Fisheries, Vol. 11/4, pp. 366-387, https://doi.org/10.1111/j.1467-2979.2010.00365.x
Squires, D., J. Joseph and T. Groves (2006), Buybacks in fisheries, FAO, https://www.fao.org/3/a1338e/a1338e14.pdf
STECF (2022), The 2022 annual economic report on the EU fishing fleet (STECF 2206)Economic Report on the EU Fishing Fleet (STECF 22-06), Scientific, Technical and Economic Committee for Fisheries (STECF), European Commission, https://stecf.jrc.ec.europa.eu/reports/economic//asset_publisher/d7Ie/document/id/41304484?inheritRedirect=false&redirect=https%3A%2F% 2Fstecf.jrc.ec.europa.eu%2Freports%2Feconomic%3Fp_p_id%3D101_INSTANCE_d7Ie%26 p_p_lifecycle%3D0%26p_p_state%3Dnormal%26p_
Sumaila, U. et al. (2012), “Benefits of Rebuilding Global Marine Fisheries Outweigh Costs”, PLoS ONE, https://doi.org/10.1371/journal.pone.0040542
Teh, L., N. Hotte and U. Sumaila (2017), “Having it all: can fisheries buybacks achieve capacity, economic, ecological, and social objectives?”, Maritime Studies, Vol. 16/1, https://doi.org/10.1186/s40152-016-0055-z
Tyedmers, P. (2004), “Fisheries and Energy Use”, in Encyclopedia of Energy, Elsevier, https://doi.org/10.1016/b0-12-176480-x/00204-7.
[8]
[38]
Waldo, S. and A. Paulrud (2016), “Reducing Greenhouse Gas Emissions in Fisheries: The Case of Multiple Regulatory Instruments in Sweden”, Environmental and Resource Economics, Vol. 68/2, pp. 275-295, https://doi.org/10.1007/s10640-016-0018-2 [15]
Wilson, J. (1999), “Fuel and financial savings for operators of small fishing vessels”, FAO Fisheries technical papet 383, https://www.fao.org/3/x0487e/x0487e.pdf
Ziegler, F. and S. Hornborg (2023), Decarbonising the fishing sector: Energy efficiency measures and alternative energy solutions for fishing vessels, https://www.europarl.europa.eu/RegData/etudes/STUD/2023/740225/EPRS_STU(2023)7402 25_EN.pdf
Ziegler, F. and S. Hornborg (2014), “Stock size matters more than vessel size: The fuel efficiency of Swedish demersal trawl fisheries 2002–2010”, Marine Policy, Vol. 44, pp. 72-81, https://doi.org/10.1016/j.marpol.2013.06.015
Ziegler, F. et al. (2016), “Expanding the concept of sustainable seafood using Life Cycle Assessment”, Fish and Fisheries, Vol. 17/4, pp. 1073-1093, https://doi.org/10.1111/faf.12159.
[23]
[14]
[7]
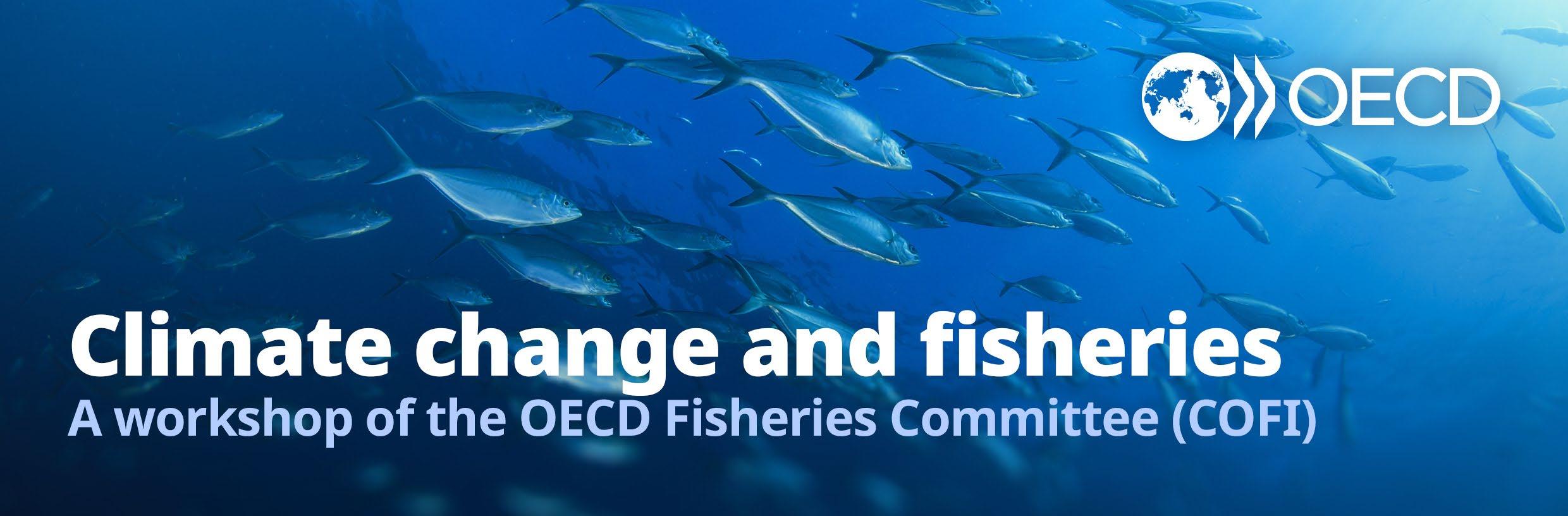
Draft Agenda
Date: 21 November 2023 – 10:00-18:00
Room CC2, OECD La Muette Conference Centre
Session 1: What’s the issue?
10:00-10:45
Chair: Björn Åsgård (Deputy Director in the Division for Fisheries, Game Management and Reindeer Husbandry, Swedish Ministry of Rural Affairs and Infrastructure and Chair of the OECD COFI)
• Welcome and introductory remarks
Björn Åsgård
• Introductory remarks
Ulrik Vestergaard Knudsen (OECD, Deputy Secretary General)
• Why is it important that climate change be discussed in the context of COFI?
Angel Calvo (European Commission)
• Discussion
Session 2: What do we know about climate change and fisheries and what else do we need to know?
10:45 – 12:15
Chair: Olivier Thébaud (Economist, AMURE - Centre for Law and Economics of the Sea and IFREMER and President of the International Institute of Fisheries Economics and Trade, Oregon State University)
• Climate change impacts on Mediterranean fisheries: A sensitivity and vulnerability analysis for main commercial species
Nathalie Hilmi (Section Head of Environmental Economics, Centre Scientific de Monaco)
• How do fisheries contribute to climate change?
Sara Hornborg (Researcher Sustainable Seafood, Research Institute of Sweden)
• The policy-makers perspective: Adaptation of Australian fisheries management to climate change
Harry Beeson (Senior advisor - Permanent delegation of Australia to the OECD)
• Discussion
Session 3: Mitigating the impact of fisheries on climate change
14:00-15:30
Chair: Justine Garrett (Co-ordinator of Inclusive Forum on Carbon Mitigation Approaches, OECD, General Secretariat)
• The potential of different fisheries’ emissions reduction strategies
François Bastardie (Senior Researcher, Technical University of Denmark)
• Energy transition in the making: the example of shipbuilding
Laurent Daniel (Head of Shipbuilding Unit, OECD Directorate for Science, Technology and Innovation) and Emilie Berger (Policy Analyst, OECD Science, Technology and Innovation)
• Climate mitigation: what have we learned across sectors and how could the COFI potentially contribute to horizontal work on climate change?
Cian Montague (Policy Analyst, OECD Environment Directorate)
• The policy-makers perspective: Overview of current policies and evidence-based pathways to reach net zero in the fishing sector
Gemma Cripps (Department for Environment Food and Rural Affairs, United Kingdom)
• Discussion
Session 4: How to adapt to the effects of climate change on fisheries?
16:00 - 17:30
Chair: Nathalie Hilmi
• The potential of climate-smart fisheries management and good governance in climate adaptation Olivier Thébaud
• The policy-makers perspective: Experiences in climate change fisheries adaptation policies
Jo Heon-Ju (Korean Maritime Institute, Director for Fisheries Management and Resource Research Division)
Eleanor Bors (Foreign Affairs Specialist, National Marine Fisheries Service of the United States)
Paul Tacuri (Fisheries Resource Analyst, Instituto del Mar del Perú)
Megan Linwood (Principal Advisor, Fisheries and Aquaculture Science and Management Branch, Fisheries New Zealand) and Jean Davis (Senior Scientist, Fisheries New Zealand)
Carl-Christian Schmidt (Vice-Chair of the Nordic Marine Think Tank and former Head of the OECD Fisheries Division)
• Discussion
Session 5: Conclusion on what the COFI could do on climate change
17:30 – 18:00
Chair: Guillaume Gruère (Head of Resources Policy Division, OECD, Trade & Agriculture Directorate)
• The COFI Chair’s perspective on lessons learned Björn Åsgård
• Final comments from the floor