I J S PT
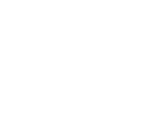
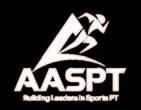
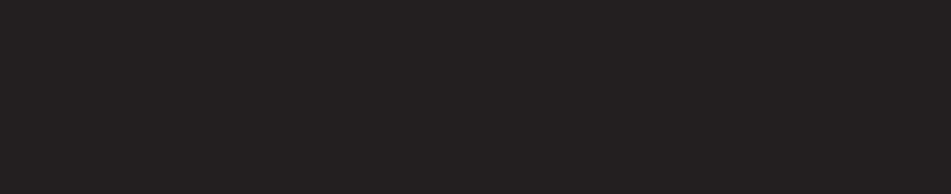
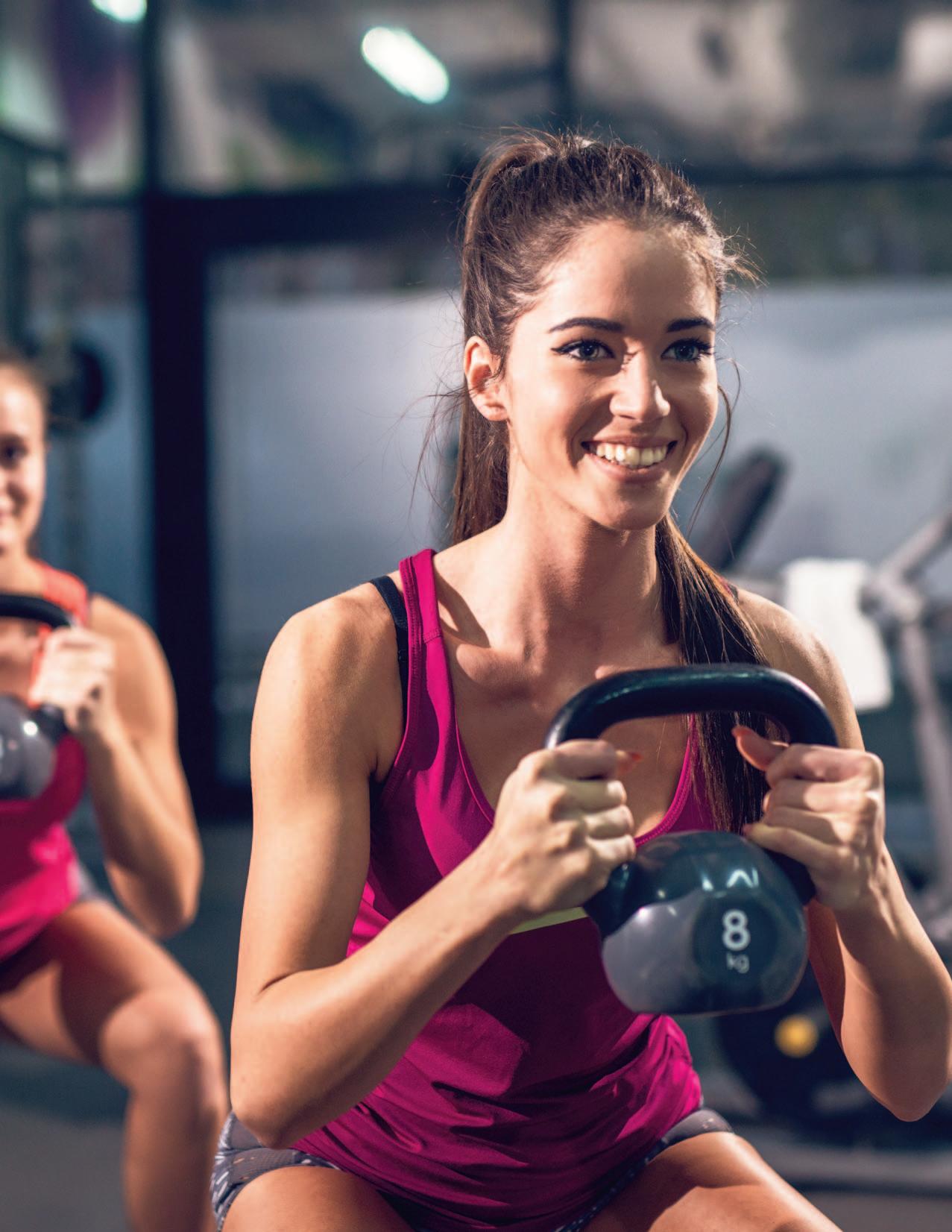
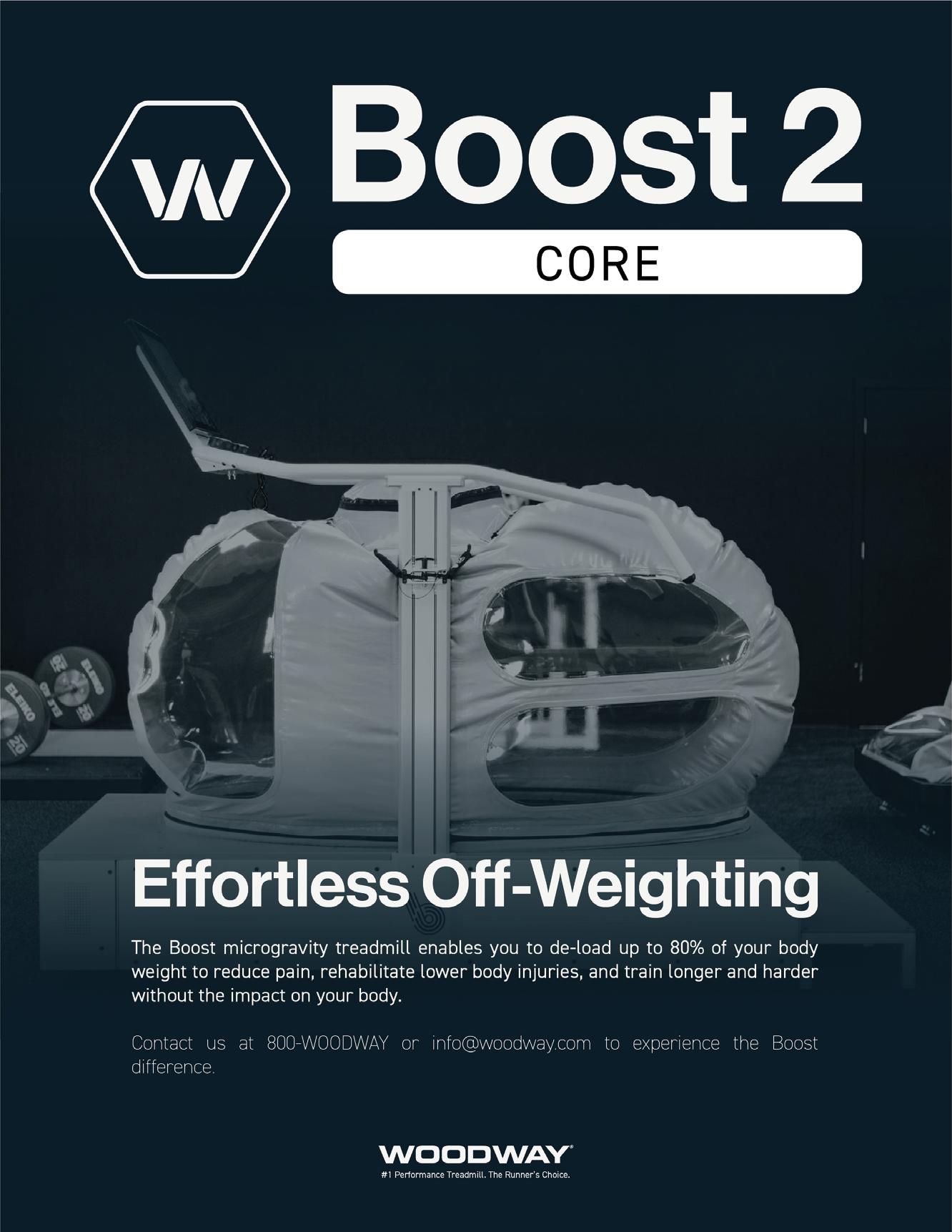
LightForce® Therapy Lasers empower you to treat soft tissue with confidence. Harnessing photobiomodulation (PBM)—a powerful form of light therapy—our lasers stimulate cellular metabolism to help treat muscle and joint pain from acute and chronic conditions.
Equipped with smart features like dosing recommendations, real-time visual and haptic feedback, and convenient portability, our range of therapy lasers combines a fusion of power with intelligence to enhance the patient and user experience. With the ability to reach deep tissues, LightForce lasers can cut the time needed by clinicians to treat patients—making light work of pain.
More than 250 professional and collegiate sports teams around the world trust LightForce Therapy Lasers to provide rehabilitation and pain management.
Scan the QR code to request a demo, or visit https://learn.chattanoogarehab.com/ijspt-journal
We are pleased to provide an overview of the remarkable achievements and sustained growth of the International Journal of Sports Physical Therapy (IJSPT) over the past two decades. Since its inception, the IJSPT has evolved into a globally recognized resource for clinicians and researchers in sports physical therapy, continually fulfilling its mission of advancing knowledge and practice within the field. The IJSPT continues to serve as a cornerstone in advancing the fields of sports physical therapy, sports medicine, and rehabilitation sciences.
• Global Reach and Recognition: The journal's inclusion in major indexing platforms underscores our credibility and reach. Indexed in PubMed, the Library of Congress, SCOPUS, and SCRIMAGO, IJSPT serves as an official publication for the International Federation of Sports Physical Therapy (representing 40 countries), ICCUS, and AASPT.
• Expanding Circulation: Our reach continues to expand, with an official circulation now encompassing approximately 30,000 physical therapists. This audience is steadily growing to include professionals from other disciplines, such as sports medicine physicians (AOSSM), athletic trainers, and chiropractors.
• Digital Engagement: In 2023-2024, IJSPT recorded over 1.9 million unique user IP visits and 2.2 million article downloads via the National Library of Medicine, with an additional 250,000 downloads from our website, Scholastica portal, Crossref, and Portico, our archiving portal. These metrics demonstrate our significant influence and affirm our role as a vital resource in the global healthcare community.
• Academic Metrics: With a 2023 Impact Factor of 1.6, a 5-year Impact Factor of 2.0, and an H-index of 18, IJSPT articles have been cited in over 340 sports medicine and rehabilitation journals worldwide, underscoring our influence in advancing the field. These milestones are evidenced by the increasing number of manuscript submissions we receive each year. We anticipate these metrics will continue to improve moving forward.
IJSPT adheres to the highest publication ethics, following the best practices and guidelines of the Committee on Publication Ethics (COPE), the International Committee of Medical Journal Editors (ICMJE), and the World Association of Medical Editors (WAME). These partnerships ensure that our editorial processes are transparent, ethical, and aligned with international standards.
In 2022, the IJSPT received the prestigious "Gold Seal" from the Directory of Open Access Journals (DOAJ), a distinction awarded to only 10% of indexed journals. This recognition highlights our dedication to author rights, long-term archiving, and accessibility. Further solidifying our standing, IJSPT was accepted into the Open Access Scholarly Publishers Association (OASPA), signifying compliance with the highest standards of transparency and best practices in scholarly publishing.
The IJSPT is considered a Gold Open Access journal, meaning that we publish all our content open access under a Creative Commons license and do not work
under a subscription or hybrid (subscription journal that offers an option for the author to pay for their manuscript to be open access) model.
Open access publishing provides several benefits, including increased visibility of research, wider readership due to free access for anyone with an internet connection, higher citation rates, greater potential for collaboration, and compliance with many funding agency mandates, ultimately promoting wider dissenation of knowledge and societal impact by making research readily available to a global audience.
The cost of journal publishing continues to increase. To maintain and continue this level of service to our readership and those who receive our disseminated knowledge, changes will need to be made in 2025. However, our goal is to make publishing in an open access format as affordable as possible for our membership.
Beginning January 1, 2025, the IJSPT will be charging an APC. Any new submissions made after this date will be asked to pay the APC if accepted for publication. The portal submission charge will remain in place. Manuscripts currently under review will not be subject to the APC. This APC covers the cost of publication and ensures that your article will be freely available online in perpetuity under a Creative Commons license.
The maximum APC for the IJSPT will be $1250 USD at this time.
*The IJSPT is committed to advancing equity in open access publishing internationally. We will offer full and partial waivers for the APC for authors from low-
income countries using World Bank Income Levels. Authors can reach out to the editorial office to managing editor Ashley Campbell for inquires prior to submission.
**The IJSPT will also offer “other” discount categories for those who serve as reviewers, as well as for affiliated society members. Additional details will be available on the IJSPT website but please reach out to Ashley Campbell if you have immediate questions.
Our manuscript submissions continue to increase annually, reflecting the trust and confidence of authors worldwide. This growth serves as a testament to the journal’s impact on global clinical practice and research in sports physical therapy.
We are proud of the strides the IJSPT has made in promoting knowledge dissemination and fostering a global community of professionals dedicated to sports physical therapy. We remain committed to upholding the highest standards of publication ethics, accessibility, and quality as we continue to expand our influence.
We look forward to continuing our mission of providing valuable resources to professionals across the globe.
Thank you for your continued support of IJSPT’s mission and contributions to the field.
Sincerely,
The IJSPT Editorial Committee
Barb Hoogenboom, Editor in Chief
Michael L. Voight, Publisher and Editor Emeritus
Ashley Campbell, Managing Editor
Mary Wilkinson, Executive and Marketing Director
IJSPT Board of Directors Advisory Board
Turner A. Blackburn
Robert Mangine
Robert Manske
Phil Page
Russ Paine
Kevin Wilk
Turner A Blackburn, APTA Life Member, AT-Ret, AOSSM-Ret President
Mary Wilkinson Executive Director
Michael Voight Executive Editor and Publisher
Joe Black, PT, DPT, SCS, ATC
Eric Fernandez
Jay Greenstein, DC
Skip Hunter, PT, ATC-Ret
Russ Paine, PT, DPT
Tim Tyler, PT, ATC
Sports Legacy Advisory Board
Turner A. Blackburn, PT, ATC
George Davies, PT, DPT, MEd, SCS, ATC, LAT, CSCS, PES, FAPTA
Terry Malone, PT, PhD
Bob Mangine, PT
Barb Sanders, PT, PhD
Tim Tyler, PT, ATC
Kevin Wilk, PT, DPT, FAPTA
Executive Editor/Publisher
Michael L. Voight, PT, DHSc, OCS, SCS, ATC, CSCS
Executive Director/Operations and Marketing
Mary Wilkinson
Editor in Chief
Barbara Hoogenboom, PT, EdD, SCS, ATC
Managing Editor
Ashley Campbell, PT, DPT, SCS
Manuscript Coordinator
Casey Lewis, PTA, ATC
Publisher
Contact Information
International Journal of Sports Physical Therapy 6011 Hillsboro Pike Nashville, TN 37215, US, http://www.ijspt.org
IJSPT is a monthly publication, with release dates on the first of each month.
ISSN 2159-2896
Founding Sponsors
Enovis Hyperice Woodway
Platinum Sponsors ATI Elvation
Gold Sponsors
Hawkgrips Kayezen
Structure + Function Education Winback Partners
Northeast Seminars Academy of Human Movement
American Academy of Sports Physical Therapy
IJSPT is an official journal of the International Federation of Sports Physical Therapy (IFSPT). Countries with access to IJSPT as a member benefit. Reach us at www.ifspt.org.
IJSPT is an official journal of the ICCUS Society for Sports Rehabilitation. www.iccus.org
Stand out in your community with a diversified patient experience. Designed to improve outcomes, attract new patients, and increase revenue through insurance, cash-based services, and retail sales.
Gain access to a robust library of research, clinical education, and marketing tools including:
• On-demand clinical education courses
• Written treatment protocols
• Over 50 research studies specific to Hyperice technology
• Marketing tips and best practices including social media content, videos, and more
• Live trainings
Executive Editor/Publisher
Michael L. Voight, PT, DHSc, OCS, SCS, ATC, CSCS
Belmont University
Nashville, Tennessee – USA
Editor in Chief
Barbara Hoogenboom, PT, EdD, SCS, ATC
Grand Valley State University Grand Rapids, Michigan - USA
Managing Editor
Ashley Campbell, PT, DPT, SCS
Nashville Sports Medicine and Orthopaedic Center Nashville, Tennessee – USA
Manuscript Coordinator
Casey Lewis, PTA, ATC
Nashville Sports Medicine and Orthopaedic Center
Nashville, Tennessee – USA
Executive Director/Marketing
Mary Wilkinson
Indianapolis, Indiana – USA
Editors
Robert Manske PT, DPT, Med, SCS, ATC, CSCS
University of Wichita Wichita, KS, USA
Terry Grindstaff, PT, PhD, ATC, SCS, CSCS
Creighton University Omaha, NE, USA
Phil Page PT, PhD, ATC, CSCS
Franciscan University DPT Program Baton Rouge, LA, USA
Kevin Wilk PT, DPT, FAPTA
Clinical Viewpoint Editor Champion Sports Medicine Birmingham, AL, USA
International Editors
Luciana De Michelis Mendonça, PT, PhD UFVJM
Diamantina, Brazil
Colin Paterson PT, MSc PGCert(Ed), MCSP, RISPT, SFHEA
University of Brighton Brighton, England, UK
Chris Napier, PT, PhD
Clinical Assistant Professor
University of British Coumbia, Vancouver, BC, Canada
Nicola Phillips, OBE, PT, PhD, FCSP
Professor School of Healthcare Sciences Cardiff University, Cardiff, Wales, UK
Associate Editors
Eva Ageberg, PT, PhD
Professor, Lund University Lund, Sweden
Lindsay Becker, PT, DPT, SCS, USAW Buckeye Performance Golf Dublin, Ohio, USA
Keelan Enseki, PT, MS, OCS, SCS, ATC University of Pittsburgh Pittsburgh, PA, USA
John Heick, PT, PhD, DPT, OCS, NCS, SCS
Northern Arizona University
Flagstaff, AZ, USA
Julie Sandell Jacobsen, MHSc, PhD
VIA University
Aarhus, Denmark
RobRoy L. Martin, PhD, PT, CSCS
Duquesne University Pittsburgh, PA, USA
Andrea Mosler, PhD, FACP, FASMF
La Trobe Sport and Exercise Medicine Research Centre, School of Allied Health, Human Services and Sport, La Trobe University
Melbourne, Victoria, Australia
Brandon Schmitt, DPT, ATC
PRO Sports Physical Therapy Scarsdale, NY, USA
Barry Shafer, PT, DPT
Elite Motion Physical Therapy Arcadia, CA, USA
Laurie Stickler, PT, DHSc, OCS
Grand Valley State University
Grand Rapids, MI, USA
Editorial Board
James Andrews, MD
Andrews Institute & Sports Medicine Center
Gulf Breeze, AL, USA
Amelia (Amy) Arundale, PT, PhD, DPT, SCS
Red Bull/Ichan School of Medicine
Salzburg, Austria/New York, NY, USA
Gary Austin, PT PhD
Belmont University Nashville, TN, USA
Roald Bahr, MD
Oslo Sports Trauma Research Center Oslo, Norway
Lane Bailey, PT, PhD
Memorial Hermann IRONMAN Sports Medicine Institute
Houston, Texas, USA
Gül Baltaci, PT,Ph.D. Professor, CKTI, FACSM
Private Guven Hospital Ankara, Turkey
Asheesh Bedi, MD
University of Michigan
Ann Arbor, MI, USA
David Behm, PhD Memorial University of Newfoundland St. John's, Newfoundland, Canada
Barton N. Bishop, PT, DPT, SCS, CSCS Kaizo Clinical Research Institute Rockville, Maryland, USA
Mario Bizzini, PhD, PT Schulthess Clinic Human Performance Lab Zürich, Switzerland
Joe Black, PT, DPT, SCS, ATC Total Rehabilitation Maryville, Tennesse, USA
Turner A. "Tab" Blackburn, APTA Life Member, ATC-Ret, AOSSM-Ret NASMI Lanett, AL, USA
Lori Bolgla, PT, PhD, MAcc, ATC Augusta University Augusta, Georgia, USA
Matthew Briggs The Ohio State University Columbus, OH, USA
Tony Brosky, PT, DHSc, SCS Bellarmine University Louisville, KY, USA
Brian Busconi, MD UMass Memorial Hospital Boston, MA, USA
Robert J. Butler, PT, PhD St. Louis Cardinals St. Louis, MO, USA
Duane Button, PhD Memorial University St. Johns, Newfoundland, Canada
J. W. Thomas Byrd, MD Nashville Sports Medicine and Orthopaedic Center Nashville, TN, USA
Lyle Cain, MD Andrews Institute & Sports Medicine Center Birmingham, AL, USA
Gary Calabrese, PT, DPT Cleveland Clinic Cleveland, Ohio, USA
Meredith Chaput, PT, DPT, SCS Ohio University Athens, OH, USA
Rita Chorba, PT, DPT, MAT, SCS, ATC, CSCS United States Army Special Operations Command Fort Campbell, KY, USA
John Christoferreti, MD Texas Health Dallas, TX, USA
Richard Clark, PT, PhD Tennessee State University Nashville, TN, USA
Juan Colado, PT, PhD University of Valencia Valencia, Spain
Brian Cole, MD Midwest Orthopaedics at Rush Chicago, IL, USA
Ann Cools, PT, PhD
Ghent University Ghent, Belgium
Andrew Contreras, DPT, SCS Washington, DC, USA
George Davies, PT, DPT, MEd, SCS, ATC, LAT, CSCS, PES, FAPTA
Georgia Southern University Savannah, Georgia, USA
Pete Draovich, PT
Jacksonville Jaguars Footbal Jacksonvile, FL, USA
Jeffrey Dugas, MD Andrews Institute & Sports Medicine Center Birmingham, AL, USA
Jiri Dvorak, MD Schulthess Clinic Zurich, Switzerland
Todd Ellenbecker Rehab Plus Phoenix, AZ, USA
Carolyn Emery, PT, PhD University of Calgary Calgary, Alberta, Canada
Ernest Esteve Caupena, PT, PhD University of Girona Girona, Spain
Sue Falsone, PT, MS, SCS, ATC, CSCS, COMT Structure and Function Education and A.T. Still University Phoenix, Arizona, USA
J. Craig Garrison, PhD, PT, ATC, SCS Texas Health Sports Medicine Fort Worth, Texas, USA
Maggie Gebhardt, PT, DPT, OCS, FAAOMPT Fit Core Physical Therapy/Myopain Seminars Atlanta, GA and Bethesda, MD, USA
Lance Gill, ATC
LG Performance-TPI Oceanside, CA, USA
Phil Glasgow, PhD, MTh, MRes, MCSP Sports Institute of Northern Ireland Belfast, Northern Ireland, UK
Robert S. Gray, MS, AT Cleveland Clinic Sports Health Cleveland, Ohio, USA
Jay Greenstein, DC Kaizo Health Baltimore, MD, USA
Martin Hagglund, PT PhD
Linkoping University Linkoping, Sweden
Allen Hardin, PT, SCS, ATC, CSCS
University of Texas Austin, TX, USA
Richard Hawkins, MD
Professor of surgery, University of South Carolina
Adjunct Professor, Clemson University
Principal, Steadman Hawkins, Greenville and Denver (CU)
John D.Heick, PT, PhD, DPT, OCS, NCS, SCS
Northern Arizona University Flagstaff, AZ, USA
Tim Hewett, PhD
Hewett Consulting Minneapolis, Minnesota, USA
Per Hølmich, MD
Copenhagen University Hospital Copenhagen, Denmark
Kara Mae Hughes, PT, DPT, CSCS
Wolfe PT Nashville, TN, USA
Lasse Ishøi, PT, MSc
Sports Orthopedic Research Center
Copenhagen University Hospital Hvidovre, Denmark
Jon Karlsson, MD Sahlgrenska University Goteborg, Sweden
Brian Kelly, MD Hospital for Special Surgery New York, NY, USA
Benjamin R. Kivlan, PhD, PT, OCS, SCS Duquesne University Pittsburgh, PA, USA
Dave Kohlrieser, PT, DPT, SCS, OCS, CSCS
Ortho One Columbus, OH, USA
Andre Labbe PT, MOPT
Tulane Institute of Sports Medicine New Orleans, LA USA
Henning Langberg, PT, PhD University of Copenhagen Copenhagen, Denmark
Robert LaPrade, MD Twin Cities Orthopedics Edina, MN, USA
Lace Luedke, PT, DPT University of Wisconsin Oshkosh Oshkosh, WI, USA
Phillip Malloy, PT, PhD
Arcadia University/Rush University Medical Center Glenside, PA and Chicago, IL, USA
Terry Malone, PT, EdD, ATC, FAPTA University of Kentucky Lexington, KY, USA
Robert Mangine, PT University of Cincinnati Cincinnati, OH, USA
Eric McCarty, MD University of Colorado Boulder, CO, USA
Ryan P. McGovern, PhD, LAT, ATC Texas Health Sports Medicine Specialists Dallas/Fort Worth, Texas, USA
Mal McHugh, PhD
NISMAT
New York, NY, USA
Joseph Miller, PT, DSc, OCS, SCS, CSCS
Pikes Peak Community College Colorado Springs, CO, USA
Havard Moksnes, PT PhD
Oslo Sports Trauma Research Center Oslo, Norway
Andrew Murray, MD, PhD
European PGA Tour Edinburgh, Scotland, UK
Andrew Naylor, PT, DPT, SCS
Bellin Health
Green Bay, WI, USA
Stephen Nicholas, MD NISMAT New York New York, NY, USA
John O'Donnel, MD
Royal Melbourne Hospital Melbourne, Australia
Russ Paine, PT McGovern Medical School Houston, TX, USA
Snehal Patel, PT, MSPT, SCD
HSS Sports Rehabilitation Institute New York, NY, USA
Marc Philippon, MD
Steadman-Hawkins Clinic Vail, CO, USA
Kevin Plancher, MD, MPH, FAAOS
Plancher Orthopedics and Sports Medicine
New York, NY USA
Marisa Pontillo, PT, PhD, DPT, SCS
University of Pennsylvania Health System Philadelphia, PA, USA
Matthew Provencher, MD
Steadman Hawkins Clinic Vail, CO, USA
Charles E. Rainey, PT, DSc, DPT, MS, OCS, SCS, CSCS, FAAOMPT
United States Public Health Service Springfield, MO, USA
Alexandre Rambaud, PT PhD Saint-Etienne, France
Carlo Ramponi, PT Physiotherapist, Kinè Rehabilitation and Orthopaedic Center Treviso, Italy
Michael Reiman, PT, PhD Duke University Durham, NC, USA
Mark F. Reinking, PT, PhD, SCS, ATC Regis University Denver, CO, USA
Mark Ryan, ATC Steadman-Hawkins Clinic Vail, CO, USA
David Sachse, PT, DPT, OCS, SCS USAF San Antonio, TX, USA
Marc Safran, MD Stanford University Palo Alto, CA, USA
Alanna Salituro, PT, DPT, SCS, CSCS New York Mets Port Saint Lucie, FL, USA
Mina Samukawa, PT, PhD, AT (JSPO) Hokkaido University Sapporo, Japan
Barbara Sanders, PT, PhD, FAPTA, Board Certified Sports Physical Therapy Emeritus Professor and Chair, Department of Physical Therapy Texas State University Round Rock, TX, USA
Felix “Buddy” Savoie, MD, FAAOS Tulane Institute of Sport Medicine New Orleans, LA, USA
Teresa Schuemann, PT, DPT, ATC, CSCS, Board Certified Specialist in Sports Physical Therapy Evidence in Motion Fort Collins, CO, USA
Timothy Sell, PhD, PT, FACSM Atrium Health Musculoskeletal Institute Charlotte, NC, USA
Andreas Serner, PT PhD
Aspetar Orthopedic and Sports Medicine Hospital Doha, Qatar
Ellen Shanley, PT, PhD ATI Spartanburg, SC, USA
Karin Silbernagel, PT, PhD University of Delaware Newark, DE, USA
Holly Silvers, PT, PhD Velocity Physical Therapy Los Angeles, CA, USA
Lynn Snyder-Mackler, PT, ScD, FAPTA STAR University of Delaware Newark, DE, USA
Alston Stubbs, MD Wake Forest University Winston-Salem, NC, USA
Amir Takla, B.Phys, Mast.Physio (Manip), A/Prof
Australian Sports Physiotherapy The University of Melbourne Melbourne, Australia
Charles Thigpen, PhD, PT, ATC ATI
Spartanburg, SC, USA
Steven Tippett, PT, PhD, ATC, SCS Bradley University Peoria, IL, USA
Tim Tyler, PT, ATC NISMAT New York, NY, USA
Timothy Uhl, PT, PhD, ATC University of Kentucky Lexington, KY, USA
Bakare Ummukulthoum, PT University of the Witswatersrand Johannesburg, Gauteng, South Africa
Yuling Leo Wang, PT, PhD Sun Yat-sen University Guangzhou, China
Mark D. Weber, PT, PhD, SCS, ATC Texas Women’s University Dallas, TX, USA
Richard B. Westrick, PT, DPT, DSc, OCS, SCS US Army Research Institute Boston, MA, USA
Chris Wolfe, PT, DPT Belmont University Nashville, TN, USA
Tobias Wörner, PT, MSc Lund University Stockholm, Sweden
PAGE TITLE
IJSPT 2025 NEWS
Highlights, Accomplishments and the Future for the International Journal of Sports Physical Therapy.
SCOPING REVIEW
1 Examination of the Clinical Utility of Eating Disorder and Disordered Eating Screening Tools in Young Athletes: A Scoping Review.
Capulong Z, Teeter M, Hoogenboom BJ.
ORIGINAL RESEARCH
15 Effectiveness of a Foam Roller Warm-Up in Professional Basketball Players: A Randomized Controlled Trial.
Casado D, Nacher I, Pardo J, et al.
30 Effects of Kettlebell Swing Style and Mass on Female Hip Joint Kinetics.
Murphy KM, Riemann BL
40 The Influence of Cognitive Dual Tasking on the Outcomes of the Triple Hop Test following Anterior Cruciate Ligament Reconstruction.
Ricupito R.
47 Augmented Feedback Response Prediction by Peak Vertical Ground Reaction Force in Adolescent Female Athletes.
Jamie H, Rutherford D, Heinert B, et al.
55 The Relationship Between Self-Efficacy, Aerobic Fitness, and Traditional Risk Factors for Musculoskeletal Injuries in Military Training: A Prospective Cohort Study.
Kreisel BR, Scott KM, Florkiewicz EM, et al.
70 Range of Motion and Muscle Stiffness Differences in Junior Tennis Players with and without a History of Shoulder Pain.
Colomar J, Peña J, Vicens J, et al.
78 Accuracy of Two Methods in Estimating Target Muscle Force During Shoulder Submaximal Isometric Contractions.
Griech S, Karagiannopoulos C.
86 High Prevalence of Low Back Pain in College Rifle Athletes.
Urbach B, Mansfield C, Harr M, et al.
CASE SERIES
96 Low-Load Blood-Flow Restriction Training for Medial Tibial Stress-Syndrome in Athletes: A Case Series.
Brekke AF, Bjørklund J, Holse RC, et al.
CLINICAL COMMENTARY
105 Early Mobility and Rehabilitation Protocol after Internal Brace Ankle Stabilization.
Vesely B, Challa S, Moyer B, et al.
111 Evaluation and Treatment of Baseball Pitchers: There’s More to Assess than the Arm.
Gauthier M, Unverzagt C, Davies GJ.
125 Posterior Medial Meniscus Root Tears: Clinical Implications, Surgical Management, and Postoperative Rehabilitation Considerations.
Monson JK, LaPrade RF.
MSK ULTRASOUND BITES: TIPS AND TRICKS
135 Diagnostic Musculoskeletal Ultrasound for the Evaluation of the Lateral Elbow: Implications for Rehabilitation Providers.
Manske RC, Wolfe C, Page P, Voight M.
Most Advanced Electrotherapy Device: Powerful, intuitive and user-friendly
Treat up to three body zones at once on all types of tissues
Effective in less than 10 minutes Enter A New Era of Therapy
TECAR
HIGH FREQUENCY
Metabolic Action at Cell Level
Hi-TENS
LOW FREQUENCY IN PULSED HIGH FREQUENCY
Ultimate Pain Management
Hi-EMS
MEDIUM FREQUENCY
Deep Muscle Contraction
Scoping Review
Hoogenboom BJ,
Z,
M. Examination of the Clinical Utility of Eating Disorder and Disordered Eating Screening Tools in Young Athletes: A Scoping Review. IJSPT. 2025;20(1):1-14. doi:10.26603/001c.126965
Barbara J. Hoogenboom1a , Zachary Capulong2 , Megan Teeter3
1 Physical Therapy and Athletic Training, Grand Valley State University, 2 Physical Therapy, Corewell Health, 3 i'Move Physical Therapy
Keywords: athletes, disordered eating, eating disorders, screening tools
https://doi.org/10.26603/001c.126965
International Journal of Sports Physical Therapy
Vol. 20, Issue 1, 2025
Background
Many screening tools are used to identify eating disorders (ED) and disordered eating (DE) in individuals. The purpose of this scoping review was to identify the most commonly used ED/DE screening tools for young male and female athletes.
Study Design
Scoping Review
Methods
Following the Johanna Briggs Institute scoping review guidelines, PubMed, CINAHL Complete, PsycInfo, SPORTdiscus, and Web of Science Core Collection databases were searched using keywords related to eating disorder, disordered eating, athletes, and screening. Included articles were randomized controlled trials, cohort studies, or cross-sectional studies published in English between 2011-2023; included primarily non-aesthetic athletes aged 14-24 years; and utilized an ED/DE screening tool for diagnostic purposes. Articles were excluded if analysis of ED/DE was a secondary purpose or < 20 athletes participated. Tools utilized and demographic and outcomes data were extracted and qualitatively analyzed.
Results
Thirty articles were included. The Eating Attitudes Test-26 (EAT-26), the Sick, Control, One, Fat, Food (SCOFF) questionnaire, and the Eating Disorder Inventory (EDI) were most used among all included articles. Three articles examined only males and used a variety of tools. Five articles utilized a tool specifically designed for athletes: the Eating Disorder Screen for Athletes (EDSA), Brief Eating Disorder in Athletes Questionnaire (BEDA-Q) or the ATHLETE questionnaire.
Discussion
While the EAT-26 is most used for diagnosing ED/DE risk within young athletes, clinical utility of screening tools for male athletes is varied. Combinations of tools utilized for examining ED/DE risk in athletes are not agreed upon. Continued research is needed to assess the clinical utility of screening tools that identify ED/DE risk specifically in athletes. Tool adjustment or development for male athletes may be necessary
Corresponding Author: Barbara J. Hoogenboom, ORCID-0000-0003-0754-6404
Grand Valley State University
Cook-DeVos Center for Health Sciences, Rm 246
301 Michigan St. NE
Grand Rapids, MI 49503
Phone: 616-331-2695
Email: hoogenbb@gvsu.edu
Eating disorders (EDs) and disordered eating (DE) are clinically relevant issues in both male and female athletes1‑4 The majority of the current research focuses on female athletes, while emerging evidence includes male athletes.1,5
The prevalence rates of EDs and DE are higher in athletes than in non-athletes, but vary across age and sports, as well as by the screening tool used to identify eating pathology.1, 3 Beals and Hill found that 25% of female collegiate athletes from seven different sports met the criteria for DE or ED.6 Karrer et al. assert that despite having been “largely ignored by research, the number of elite male athletes with EDs is growing, surpassing that of male non-athletes.”7(p1)
The Diagnostic and Statistical Manual of Mental Disorders, Fifth Edition (DSM-5) defines EDs as “ a persistent disturbance of eating or eating-related behavior that results in the altered consumption of food and that significantly impairs physical health or psychological functioning.”8(p329) Wells et al. describe DE in athletes as being “ on a spectrum between optimized nutrition and ED.”9(p1) Athletes may suffer from an undiagnosed ED, engaging in DE behaviors that are not recognized until further manifestation. Kennedy et al. defined DE as, “intentional chronic abnormal, unhealthy eating/drinking behaviors that can lead to clinically relevant problems and do not necessarily meet DSM-5 criteria for eating disorders.”10(p2) Both EDs and DE behaviors in the athletic setting can occur due to the negative effects of low energy intake in highly active individuals. DE can lead to longer recovery times after workouts, more frequent musculoskeletal injuries, and extended time away from sport.9 DE behaviors may be triggered by teammates and/or coaches through body-focused comments or observed DE behaviors among teammates.2,3,9 Thus, it is important to look beyond the definition of EDs to be ready and able to screen for unsafe eating habits in the athletic population, with the intention of preventing worsening effects and facilitating referrals for nutritional guidance.
Low energy availability (LEA) occurs when an athlete does not consume an adequate number of calories to match their energy needs. Relative energy deficiency in sport (RED-S) is used to describe the multiple system effects of LEA in athletes. The International Olympic Committee originally defined RED-S as, “impaired physiological functioning caused by relative energy deficiency, and includes but is not limited to impairments of metabolic rate, menstrual function, bone health, immunity, protein synthesis, and cardiovascular health.”11(p316) It is essential that all members of the healthcare team, including physicians, physical therapists, nurses, athletic trainers, sports psychologists, and other relevant health professionals have the ability to recognize signs of and screen for ED/DE risk, for the overall health and safety of the athlete. Members of the healthcare team should work collaboratively to ensure that early identification of ED/DE occurs12 and that athletes are
referred to the appropriate providers, each operating within their area of expertise and scope of practice.
The most commonly used measures in previous research appear to be the Eating Attitudes Test (EAT), the Eating Disorder Inventory (EDI), the Bulimia Test Revised (BULITR), the Questionnaire for Eating Disorder Diagnosis (QEDD), and the Eating Disorder Examination Questionnaire (EDE-Q).13 Pope et al.13 assert that these measures have been used more frequently for assessing EDs in athletes than several measures that have been specifically developed for athletes, such as the Weight Pressure Sport Scale for Male Athletes, the Athlete Questionnaire (ATHLETE), and the Athletic Milieu Direct Questionnaire.
According to the American Psychology Association (APA) Dictionary of Psychology, clinical utility is defined as “the extent to which diagnostic testing is useful in facilitating beneficial health outcomes from interventions that are initiated based on test results.”14(p194) Another definition from the APA’s Criteria for Evaluating Treatment Guidelines states that clinical utility is, “the applicability, feasibility, and usefulness of the intervention in the local or specific setting where it is to be offered.”15(p1053) Tyson and Connell define clinical utility as the “cost, time taken to complete, need for specialist training or equipment, and the portability.”16(p826) Thus, clinical utility is herein operationally defined as the ability of a diagnostic screening tool to recognize disorders relevant to the health outcomes of the patient from a holistic standpoint. Clinical utility includes, but is not limited to, the feasibility, usefulness, applicability, and time/cost-effectiveness of relevant screening tools.
As the clinical utility of ED/DE screening tools within the non-aesthetic athletic population has not been wellstudied, a scoping review was chosen to cast a wide net to capture the breadth of current available literature. The purpose of this scoping review was to identify the most commonly used ED/DE screening tools for young male and female athletes.
This scoping review was conducted following the Johanna Briggs Institute Scoping review protocol and the associated PRISMA-ScR framework. This scoping review was not registered.
For articles to be included they had to be randomized controlled, cohort, or cross-sectional study articles published between January of 2010 and April of 2023, written in English, that utilized a DE/ED screening tool for diagnostic purposes. The articles also had to include over half of the athletes participating in non-aesthetic National Collegiate Athletic Association (NCAA) sponsored sports, between the ages of 14 to 24 (herein defined as “young athletes”). Less than 50% of subjects could have participated in aesthetic sports. The focus on athletes from non-aesthetic sports was chosen because athletes within these sports have been
studied less regarding their risk of ED/DE than those in aesthetic sports. Non-aesthetic sports were operationally defined for this scoping review as sports without a subjective scoring system, or sports in which athletes play together or contribute to an overall team score.
Excluded from this scoping review case reports, case series, and narrative reviews/clinical commentary Other exclusion criteria were articles including athletes from only non-NCAA-recognized sports, only aesthetic sports, and articles where establishing an ED/DE diagnosis was not the primary purpose. Finally, research that included less than 20 athletes was excluded due to the potential for decreased internal validity.
In collaboration with a university research librarian, five electronic databases (PubMed, CINAHL Complete, PsycInfo, SPORTDiscus, and Web of Science Core Collection) were initially searched in April 2021, with an updated search completed in April of 2023 using keywords related to eating disorders, disordered eating, athletes, and screening. A detailed summary of the search strategy used for each of the databases can be found in Appendix A. Due to the purpose of this scoping review, a gray literature search was not performed.
After the searches were completed, duplicate articles were manually removed by two reviewers. Once the duplicates were removed, titles and abstracts were screened independently by two reviewers to ensure that the inclusion and exclusion criteria were met. Titles and abstracts were divided into three groups and each reviewer screened two groups; thus, two reviewers independently screened each abstract, and if the reviewers were unsure of the disposition of an article, a third reviewer was consulted. If disagreements were not resolved, a fourth reviewer (BJH, senior author) performed an additional review to reach consensus. All articles included after title and abstract screening went on to full-text assessment, using the same procedures for determining inclusion as during title and abstract screening. All 30 of the final articles were included unanimously.
Data were extracted by a single author, confirmed by a second author, and entered on a spreadsheet including publication year, number and type of participants, sports included, ages, setting/location, screening tools used, study objectives, phenomenon of interest, and objective outcomes/results. The compiled results were qualitatively analyzed to develop the synthesized tables and narrative.
The initial broad search of the literature produced 4,341 articles. After removing duplicates, 2,419 articles remained. After title and abstract screening, 96 articles remained for full-text screening. Thirty articles were included following the full-text screening. Figure 1 outlines the study selection process.
Athlete demographics and study characteristics of the 30 articles are summarized in Table 1 and Appendix C. A total
of 12,009 athletes were included in the studies contained in this scoping review, 5,202 of which were male, 6,806 female, and one that identified neither male nor female. The articles included athletes from 74 different non-aesthetic and aesthetic (e.g cheerleading, dance, gymnastics, and synchronized swimming) and non-aesthetic (e.g rugby, soccer, track and field, volleyball) sports and 15 different countries.
A total of 13 different ED/DE screening tools were utilized as well as 17 other screening tools that examined a variety of psychosocial factors such as body image, perfectionism, and self-esteem (Appendix B). Table 2 provides the screening tools utilized and in which studies they were used.
The most commonly used tool was a version of the EAT (n=15), the majority of studies used the EAT-2617‑19,25,26, 29,34,35,37‑39,42‑44,46 and only studies by Hernandez and Karaağaç et al. used the EAT-40.25,26 Several authors combined the EAT with another ED screening tool such as the SCOFF,34 and the ATHLETE questionnaire,38,44 and one study utilized both the ORTO-15 and the YBC-EDS.37
Five articles utilized the SCOFF questionnaire.27,30,32,34, 36 Two authors utilized the SCOFF as an accessory tool to the EDI.27,32 Pustivšek et al. were the only authors to use the SCOFF questionnaire as a stand-alone tool.36
The EDI was used in two forms (EDI-3 and EDI-2) in five studies.23,27,32,33,41 Muia et al. combined the EDI with the TFEQ while Gapin and Kearns combined the EDI with the QEDD.23,33 The EDE-Q was utilized in four studies.22,24,30, 40 The ATHLETE and ORTO-15 were utilized in three studies each and one study utilized ORTO-1126 instead of the ORTO-1521,37,38,43‑45
The Q-EDD was also utilized in two studies.20,23 Chatterton and Petrie utilized the Q-EDD due to its validity and because it has been used to assess ED in male collegiate athletes.20 Each of the following tools was used only once: the EDSA, the ESP, the TFEQ, the BULIT-R, the YBC-EDS, and the BEDA-Q.20,24,28,31,33,37
In three studies that included only males the Q-EDD,20 BULIT-R,20 EDE-Q,22 and EAT-2644 and ATHLETE44 were utilized. In the 19 studies where both male and female athletes were examined, the trends followed those seen in the overall results, the EAT-26/EAT-40 was used most commonly,17,19,25,26,29,39,43 followed by the SCOFF.27,30,36 None of the studies including athletes and non-athletes used an athlete-specific questionnaire within the screening process.
Overall, 10 articles used a single tool,19,21,22,28,29,35,36, 40,41,45 12 articles used two tools,17,20,23,24,30,32‑34,38,42,43, 46 and eight articles used three or more tools.18,25‑27,31, 37,39,44 (Appendix B) When a single tool was used, it was most commonly the EAT-26 (n=3) or the EDE-Q (2), while all other stand-alone tools were used in a single study (5). When more than a single tool was chosen, multiple combinations of tools were used, no patterns emerged; some were grouped to test similar constructs of ED/DE, whereas in other cases the selected tools tested very different con-
Figure 1. Preferred Reporting Items for Systematic Reviews and Meta-Analyses (PRISMA) diagram demonstrating study selection process for inclusion into the scoping review.
structs (e.g. ED/DE and exercise, motivation, or psychological factors).
A variety of studies are available in the literature regarding screening young athletes for ED/DE risk, and the results of this scoping review highlight significant findings in the research regarding clinical utilization of specific tools for young athletes in general as well as males and females.
The most commonly utilized tool in this systematic review was the EAT-26 (with two instances of the EAT-40),17‑19,25,26,29,34,35,37‑39,42‑44,46 possibly because it has the longest history of utilization in both clinical and non-clinical settings and is easily administered. The EAT was reportedly selected for its prevalence in practice,18,34, 46 and because the test has commonly been used with athletes.17,38,44 Wollenberg et al., Segura-García et al., Lanfranchi et al., and Soubliere and Gitimu incorporated the EAT-26 due to its reliability, validity, and internal consistency 29,37,38,46 However, this tool developed to screen for eating disorder risk in the general population was not developed specifically for athletes, possibly affecting its usefulness in athletic populations, which could be a drawback to its use.
The second most utilized tool was the SCOFF,27,30,32, 34,36 consisting of only five “yes or no” questions, which makes this test appealing due to the short amount of time required for completion. Petisco-Rodriguez et al. assert that while the SCOFF is often utilized due to the ease of application, whether the SCOFF is sensitive enough to detect ED/ DE in the athletic population (as compared to the general population) remains unexplored.34 Kutz et al. claim that the SCOFF questionnaire is the most widely used screening measure for EDs within the general population; however, they concluded that while this test is effective for screening for AN and BN, it may not be clinically useful for detecting the vast array of EDs experienced by athletes and described in the DSM-5.47 While utilization of a short, uncomplicated tool such as the SCOFF is feasible and might decrease the common problem of under-reporting, it may also miss key DE/ED’s in athletes.
Multiple articles included utilized tools that focus on identification of specific types of EDs and therefore may have limited the clinical applicability for identifying overall ED/DE patterns in athletes. For example, the BULIT-R focuses on the diagnosis of BN, and not EDs or DE in general as compared to the EAT-26 and other tests.48 The authors of this review believe the BULIT-R may lack usefulness for screening for the variety of EDs and DE seen within the
Table 1. Article Demographics (n=30)
Author/ Year Total number of participants
Abbot et al. (2021)17
n=406
Athletes/ Non-Athl
Athletes (n=227)
Controls (n=179)
Aleksić Veljković et al. (2020)18
Boudreault et al. (2022)19
Chatterton and Petrie (2013)20
n=120
n=424 (total) n=314 (who completed EAT-26)
n=732
Aesthetic
Athletes (n=54) NonAesth Athletes (n=66)
Athletes (n=424)
Gender
Males (n=198)
Females (n=208)
Females (n=120)
Mean age (yrs +SD)
Males
Athletes 21+/-5
Controls 25+/-6
Females
Athletes 23+/-4
Controls 26+/-6
Aesthetic Athletes 19+3
Non-Aesth Athletes 20+3
Females (n=197)
Males (n=116)
Other (n=1)
Athletes (n=732)
Sports included*
Soccer
Artistic & Rhythmic Gymnastics, Dance , Synchronized Swimming, Volleyball, Soccer, Basketball
Athletes 21.83 +/2.6
Males (n=732) Athletes 19.91
Soccer, Volleyball, Ultimate, Rugby, Cheerleading, Cross-country running, Swimming, Track and field, Badminton, Basketball, Ice Hockey
Technical (Bowling, Golf, Fencing, Skiing) ; Endurance (Swimming, Track and Cross-Country) ; Aesthetic (Diving) ;Weight class (Crew/Rowing, Wrestling) ; Ball game (Baseball, Basketball, Football, Hockey, Lacrosse, Soccer, Tennis, Volleyball)
Clifford and Blyth (2019)21
n=215
Athletes (n=116) Non-Athl (n=99)
Males (n=74)
Females (n=141)
Athletes 21+1 Non-Athl 21+2
Track, Basketball, Cheerleading, Cricket, Cycling, Dance, Diving, Football, Golf, Gymnastics, Hockey, Judo, Korfball, Lacrosse, Netball, Olympic Weightlifting, Orienteering, Rowing, Rugby, Sepak Takraw, Swimming, Taekwondo, Table Tennis, Trampolining, Triathlon, Ultimate Frisbee, Volleyball, Water Polo
Gorrell et al. (2019)22
n=611
Competitive Athletes (n=429) Non-Comp Athletes (n=183)
Gapin and Kearns (2013)23
Hazzard et al. (2020)24
n=133
Part 1
n=434
Part 2 n=862
Athletes (n=133)
Part 1
Division I Athletes (n=434)
Part 2
Majority reported competing at DI (n=588) or club (n=257) levels
Males (n=611) Total
Swimming, Rugby, Rowing/Crew, Lacrosse, Football, Ultimate Frisbee, Soccer, Cycling, Wrestling, Ice Hockey, Triathlon, Basketball, Running/Cross Country/Track/ Field, Volleyball, Fencing, Gymnastics, Cheerleading, Baseball, Water Polo
Males (n=57)
Females (n=76) Total
Part 1
Males (n=229)
Females (n=205)
Part 2
Males (n=312)
Females (n=550)
Rowing
Cross Country, Gymnastics, Rowing, Swim and Dive, Track and Field, Baseball, Basketball, Football, Golf, Soccer, Volleyball
Author/ Year Total number of participants
Hernández et al. (2021)25
Karaağaç, et al. (2022)26
Kraus et al. (2018)27
Athletes/ Non-Athl
n=167 Athletes n=167
n=168
n=113
Krebs et al. (2019)28
Lanfranchi et al. (2014)29
n=638
n=770
Athletes (n=66) Non-Athl (n=102)
Competitive Athletes
Lightweight (n=45)
Heavyweight: (n=32)
Non-Comp Athletes (n=37)
Athletes (n=638)
Athletes (n=335) NonAthl (n=435)
Gender
Females (n=70)
Males (n=97)
Females (n=63)
Males (n=105)
Males (n=71)
Females (n=42)
Total Population 24+ 2.12
Lichtenstein et al. (2022)30
Magee et al. (2023)31
n=28
n=94
Males (n=366)
Females (n=272)
Males (n=341)
Females (n=429)
Males 19.99+.07
Molnár et al. (2016)32 n=130
Muia et al. (2016)33 n=110
PetiscoRodriguez et al. (2020)34
Prather et al. (2016)35
Athletes (n=28)
Athletes (n=94)
Athletes (n=65) NonAthl (n=65)
Athletes (n=61) Non-Athl (n=49)
Females (n=25)
Males (n=3)
Females (n=42)
Males (n=52)
Females (n=130)
Females (n=110)
n=120
Sports included*
Cross-Country and Track running
Football, Volleyball, Basketball, Sailing
Rowing
Track (800m or greater distances) , Cross Country
Dancing, Hiphop, Figure Skating, Gymnastics; Boxing, Kickboxing, French Savate, Wrestling, Aïkido, Judo, Karate, Kung Fu Taekwondo, Fitness, Rowing. Swimming, Cycling, Jogging, Mountain-Biking, Rock Climbing, Twirling, Olympic Walk. Alpine Skiing, Badminton, Tennis, Water Polo, American Football, Rugby, Basketball, Soccer, Handball, Volleyball, Shooting, Archery, Pétanque, Fishing, Golf, Rollerblading, Horse Riding, Pelota, Powerlifting, Sailing, Scuba Diving, Skateboard, Table Tennis, Tai-Chi-Chuan
Athletes 23
Athletes 18.09+2.44
Athletes
23.27±5.13
Non-Athl 22.11±2.04
Athletes 16.5** Non-Athl Does not report
Athletes (n=80) Non-Athl (n=40)
n=225
Athletes (n=225)
Females (n=120)
Gymnasts 16.60+2.62
Footballers 17.98+3.42
Non-Athl 17.13+2.16
Females (n=225)
Athletes 16.4+4
Track And Field, Rowing, Cycling, Triathlon, Swimming, Orienteering, Martial Arts, Ice Skating
Soccer, Football, Track/CrossCountry, Wrestling, Baseball, Volleyball, Gymnastics, Basketball, Weight/Power Lifting, Dance, Softball, Tennis, Crossfit
Soccer (Football)
Mid- to Long Distance Running
Gymnastics, Football
Soccer
Author/ Year Total number of participants
Pustivšek et al. (2016)36
n=583
SeguraGarcía et al. (2012)37
Soubliere and Gitimu (2012)38
Stephens et al. (2021)39
TheinNissenbaum et al. (2011)40
TorresMcGehee et al. (2020)41
Uriegas et al. (2023)42
n=794
n = 56
n=101
n=311
Athletes/ Non-Athl Gender
Athletes (n=337)
Controls (n=246)
Athletes (n=577)
Controls (n=217)
Athletes (n=56)
Athletes (n=101)
Athletes (n=311)
Females (n=273)
Males (n=310)
Females (n=268)
Males (n=526)
Females (n=56)
Females (n=74)
Males (n=27)
Females (n=311)
Athletes 15.87
Controls 16.09
Male
Athletes 23.2 +/- 5.5
Female
Athletes 21.3 +/- 7.0)
Reported age range 18-22 y.o.
Athletes 20.34+1.38
Uriegas et al. (2021)43
n=121
n=1885 .
Athletes (n=121)
Athletes (n=1885)
Females (n=121) Athletes 19.8+2.0
Females (n=1312)
Males (n=573)
Athletes 19.8±1.4
Sports included*
Track And Field; Gymnastics/ Rhythmic Gymnastics; Judo; Mountain Running;, Cycling; Swimming; Xc Skiing;, Triathlon, Rowing, Alpine Skiing, Ski Jumping; Basketball; Football; Volleyball; Dodgeball; Handball; Tennis
Taekwondo, Boxing, Judo, Bodybuilding; Volleyball, Basketball, And Soccer; Etc.
Soccer, Softball, Track and Field, Swimming
Baseball, Basketball, Cross Country, Track And Field, Football, Golf, Rowing, Soccer, Softball, Tennis, Volleyball
Tennis, Volleyball, Swimming, Softball, Golf, Basketball, Track And Field, Cross Country, Soccer, Cheerleading, Pom-Pom Squad, Dance Team, Diving, And Gymnastics
Equestrian, Soccer, Beach Volleyball, Softball, Volleyball, Ballet
Endurance (e.g., Cross Country, Track: middle and long distance, Swimming) , Aesthetic [e.g., Cheerleading, Diving, Dance, Equestrian) , Power (e.g., Football, Track Sprints, Track and Field; NonLean Events [Discus, Shot-Put, Hammer]) , Ball/Team (e.g. Baseball, Softball, Basketball, Soccer, Volleyball, Beach Volleyball) And Technical Sports (e.g. Golf, Tennis, Track and Field Lean Events [High Jump, Javelin]) .
n=1090
Athletes (n=1090)
Females (n=756)
Males (n=334)
Cross Country, Track: middle and long distance, Swimming, Cheerleading, Diving, Dance, Equestrian, Football, Track Sprints, Throws, Baseball, Softball, Basketball, Soccer, Volleyball, Beach Volleyball, Lacrosse, Golf, Tennis, Track Jumps
Wadas and Debeliso (2014)44
n=68
Wells et al. (2015)45 n=83
Wollenberg et al. (2015)46
Athletes (n=68)
Male (n=68)
Non-aesth= non-aesthetic athlete; Non-Athl= non-athletes; Non-comp= non-competitive * >50% of athletes in the study participated in NCAA-recognized sports (and therefore met the inclusion criteria) **mean age averaged from reported median age of athletes and nonathletes
Cross Country
Cheer, Swimming, Volleyball, Cross Country, Basketball, Softball, Soccer, Golf
Soccer, Cross-Country, Track and Field, Basketball, Cheer/Pom/ Dance, Equestrian, Tennis, Golf, Softball
Eating Disorder/Disordered Eating Screening Tool
Eating Attitudes Test (EAT-26 or EAT-40)
Eating Disorder Inventory (EDI-2 or 3)
Sick, Control, One, Fat, Food Questionnaire (SCOFF)
Eating Disorder Examination Questionnaire (EDE-Q)
ORTO-15/ORTO-11
ATHLETE
Questionnaire for Eating Disorder Diagnosis (QEDD)
Eating Disorder Screen in Primary Care (ESP)
Three Factor Eating Questionnaire (TFEQ)
Eating Disorder Screen for Athletes (EDSA)
Bulimia Test Revised (BULIT-R)
Yale-Brown-Cornell-Eating Disorder Scale (YBC-EDS)
Brief Eating Disorder in Athletes Questionnaire (BEDA-Q)
athletic population. Another test, the TFEQ focuses on the cognitive “restraint” around food as compared to diagnosing EDs or DE in general.49 Therefore, while it may be useful in identifying DE related to the restriction of caloric intake, it is likely less useful for detecting a wide scope of EDs. Finally, the ORTO-15 assesses risk for a specific condition, orthorexia nervosa, instead of EDs or DE in general. Due to the limited research and applicability associated with the BULIT-R, TFEQ, and ORTO-15 tools, the authors of this review do not currently recognize them as having good clinical utility
The findings of this review were inconsistent regarding which screening tools were able to identify differences in ED/DE risk between male and female athletes, and to the authors’ knowledge no tools exist for identifying ED/DE
Studies that utilized the tool
Abbott et al.17
Aleksić Veljković et al.18
Boudreault et al.19
Hernández et al.25
Karaağaç et al.26
Lanfranchi et al.29
Petisco-Rodriguez et al.34
Prather et al.35
Segura-García et al.37
Soubliere and Gitimu38
Stephens et al.39
Uriegas et al.42
Uriegas et al.43
Wadas and DeBeliso44
Wollenberg et al.46
Gapin and Kearns23
Kraus et al.27
Molnár et al.32
Muia et al.33
Torres-McGehe et al.41
Kraus et al.27
Liechtenstein et al.30
Molnár et al.32
Petisco-Rodriguez et al.34
Pustivšek et al.36
Gorrell et al.22
Hazzard et al.24
Lichtenstein et al.30
Thein-Nissenbaum et al.40
Clifford and Blyth21
Karaağaç et al.26
Segura-García et al.37
Uriegas et al.43
Soubliere and Gitimu38
Wadas and DeBeliso44
Wells et al.45
Chatterton and Petrie20
Gapin and Kearns23
Krebs et al.28
Muia et al.33
Hazzard et al.24
Chatterton and Petrie20
Segura-García et al.37
Magee et al.31
specifically in males. When using tools not developed for males, it may be important to take in consideration that male and female athletes may display different presentations of/driving factors toward ED which could impact how athletes answer questions. This seems to be consistent with suggestions from Lanfranchi et al. that certain statements like think they are too thin or if they are striving to become thinner on the EAT-26 are more likely to be negatively answered by male athletes. Lanfranchi et al. state that this could be due to males being significantly less likely to be concerned about the idea of being thin and more thoughtful about their body composition.29 Perhaps future research should focus on adaptation of existing tools or development of new tools to better assess ED/DE in male athletes.
Although self-report measures such as the EAT-26, SCOFF, and EDI are well-studied and have been used to identify ED risk in a variety of competitive athletes, none of these tools were developed specifically for athletes. Pope et al. have reported that the use of questionnaires developed for the general population among athletes is more common than the use of athlete-specific questionnaires,13 which is supported by the results of this scoping review
Regarding athlete-specific tools, the ATHLETE, EDSA, and BEDA-Q questionnaires may be useful in formulating a targeted approach to screening athletes for ED/DE risk and may expose different results in athlete/non-athlete comparisons of risk. The ATHLETE questionnaire provides athlete-specific measures that assess psychological predictors of DE.45 The ATHLETE may be a strong option to consider as multiple studies included in this scoping review used the ATHLETE for its utility in examining the etiology of DE in athletes.44 The ATHLETE contains specific measures for psychological predictors of ED45 and questions aimed at gaining information regarding other factors leading to DE, which may positively affect its usefulness in athletes.38
Although the EDSA was not widely utilized, Hazzard et al. examined the validity of the EDSA, to assess the risk of EDs within male and female athletes, specifically 24 The EDSA has demonstrated excellent criterion validity when compared to the EDE-Q, and was accurate in predicting ED risk status for both male and female collegiate athletes across several levels of competition and sports types.24 With a reported sensitivity of 96% and specificity of 80% for male athletes, and a sensitivity of 96% and specificity of 64% for female athletes,24 the authors of this review suggest that the EDSA should be considered when assessing athletes based on its applicability Finally, Magee et al. utilized BEDA-Q, to assess the ED risk in athletes.31 The BEDA-Q has been reported to have a sensitivity of 82.9% and specificity of 84.6%31 and reports of acceptable accuracy for identifying athletes with and without an ED, supporting the consideration of its use in athletes versus other tools.31
Because referral of athletes to other members of the healthcare team is made possible by screening, healthcare professionals should be well versed in the most current, commonly utilized ED/DE screening tools (the EAT-26/40, SCOFF, EDI, and EDE-Q) for clinical use and/or interpretation, including their limitations. Of the most utilized tools, the SCOFF is the shortest and most time-efficient and would be easily integrated into a clinical examination of an athlete. Lesser known and studied tools including the ATHLETE, EDSA, and BEDA-Q should be considered for more in-depth screening of athletes or be utilized in concert with another tool. The clinical utility of these newer tools needs to be further documented and studied. Regardless of the screening tool utilized, it is incumbent upon the PT as a primary health provider to refer to the appropriate mental health or medical provider when issues are identified by screening.
The use of clinical interviewing to assess ED/DE risk is considered the gold standard in diagnosis of EDs.50 Trained medical providers can verify information reported in a questionnaire and gather additional information that the ED/DE screening tools may have missed.13,20,22,23,29,34 Furthermore, according to Wells et al., the recommendation for assessing for DE is to utilize the ED/DE screening tools, and then to follow up with a clinical interview 9 Only one study by Lichtenstien et al, utilized a clinical interview following a screening tool to assess the external validity of the tool and to look at the subjective aspect of symptoms.30 The other 29 studies did not utilize clinical interviews to verify the quantitative responses of the screening tools and gain additional qualitative information that may have been missed with only the use of a screening tool. Of all the tools used in studies in this review, only the SCOFF has been described as having sensitivity (84.6%) and specificity (89.6%) compared to a clinical interview.34 Regarding athlete versus non-athlete comparisons, Martisen and Sundgot-Borgen found that when self-report questionnaires (including EDI-2) were used alone to detect EDs in adolescent male and female athletes and a non-athletic control group, the prevalence was higher in the controls.50 However, in their two-part study, when “at-risk” and matched controls participated in a clinical interview including assessing for DSM-IV criteria of EDs, athletes had a higher prevalence of EDs than among the controls. More athletes reported pathogenic weight control methods in clinical interviews than within screening methods.50 Therefore, the authors of this review believe that clinical interviews should be frequently considered as part of a comprehensive ED/DE assessment of athletes, following the use of each/any of screening tools, to gain additional information and verify the responses.
There are several limitations of this scoping review The authors recognize that relevant data about ED/DE prevalence in different sports and athletes versus non-athletes, may have been excluded from the scoping review due to article inclusion and exclusion criteria. Excluding sports not recognized by the NCAA, athletes participating in aesthetic sports, and articles with a primary focus other than diagnosis of ED/DE, may have limited the available data for this review
As is inherent to any systematic or scoping review, there is a potential for bias in the selection, evaluation, and interpretation of the relevant articles. To diminish the effect of personal bias in the selection of articles, 100% consensus for inclusion/exclusion was achieved by the authors during review of articles.
Most of the studies included in this scoping review were cohort studies, often with convenience samples from specific populations/teams, which varied significantly in their methodology This may have contributed to the lack of racial/ethnic diversity within studies.38,44 Several of the studies had small sample sizes, despite the decision to exclude studies with less than 20 participants.18,23,25,29,44 Small and homogenous (athletes of only a specific sport, or from a specific country or region) samples make the re-
sults less generalizable to the global athletic community The authors acknowledge that the variability of strength of evidence and potential for risk of bias for the articles included may make it difficult to justify the strength of the conclusions made in this scoping review
Finally, the possibility of athletes under-reporting DE/ ED symptoms is a limitation that was stated by many of the included articles.19,20,22‑24,27‑30,34,36,39‑43,45,46 The self-report nature of screening tools is believed to impact the accuracy of risk assessment as many athletes may be apprehensive in making their ED/DE symptoms known.24 Even if the responses are kept anonymous, the possibility of athletes being untruthful with their about responses about DE/ ED in surveys remains.40
The EAT-26/40, EDI, and SCOFF questionnaires are the most broadly clinically utilized and researched ED/DE screening tools. Of these, the SCOFF questionnaire is the shortest test to administer The main limitation of each of these three commonly used tools is that they were not specifically developed for use in the athletic population. Relatively new, athlete-specific questionnaires such as the ATHLETE, EDSA, and BEDA-Q questionnaires may be useful for identifying ED/DE risk in young athletes and may be used to supplement efficient, well-known, and studied tools
such as the EAT-26, EDI, or SCOFF questionnaires. Healthcare professionals should consider the use of various tools for assessment of young male and female athletes to assess risk of ED/DE.
The authors have no conflicts of interest related to this manuscript.
No financial support was received in the preparation of this manuscript.
The authors would like to acknowledge the assistance of research librarians Betsy Williams and Emily Metcalf for development of the search strategy and assistance with the project. Sarah Neumar PT, DPT participated in several aspects of this scoping review.
Submitted: May 07, 2024 CST, Accepted: November 24, 2024 CST
© The Author(s)
This is an open-access article distributed under the terms of the Creative Commons Attribution 4.0 International License (CCBY-NC-4.0). View this license’s legal deed at https://creativecommons.org/licenses/by-nc/4.0 and legal code at https://creativecommons.org/licenses/by-nc/4.0/legalcode for more information.
1. Sundgot-Borgen J, Torstveit MK. Prevalence of eating disorders in elite athletes is higher than in the general population. Clin J Sport Med. 2004;14(1):25-32. doi:10.1097/ 00042752-200401000-00005
2. DiPasquale LD, Petrie TA. Prevalence of disordered eating: a comparison of male and female collegiate athletes and nonathletes. J Clin Sport Psychol 2013;7(3):186-197. doi:10.1123/jcsp.7.3.186
3. Bratland-Sanda S, Sundgot-Borgen J. Eating disorders in athletes: overview of prevalence, risk factors and recommendations for prevention and treatment. Eur J Sport Sci 2013;13(5):499-508. doi:10.1080/17461391.2012.740504
4. Giel KE, Hermann-Werner A, Mayer J, et al. Eating disorder pathology in elite adolescent athletes. Int J Eat Disord 2016;49(6):553-562. doi:10.1002/ eat.22511
5. Petrie TA, Greenleaf C, Reel J, Carter J. Prevalence of eating disorders and disordered eating behaviors among male collegiate athletes. Psychol Men Masc. 2008;9(4):267-277 doi:10.1037/a0013178
6. Beals KA, Hill AK. The prevalence of disordered eating, menstrual dysfunction, and low bone mineral density among US collegiate athletes. Int J Sport Nutr Exerc Metab 2006;16(1):1-23. doi:10.1123/ ijsnem.16.1.1
7. Karrer Y, Halioua R, Mötteli S, et al. Disordered eating and eating disorders in male elite athletes: a scoping review BMJ Open Sport Exerc Med 2020;6(1). doi:10.1136/bmjsem-2020-000801
8. Diagnostic and Statistical Manual of Mental Disorders: DSM-5 Fifth edition. American Psychiatric Publishing; 2013. Accessed April 13, 2022. https:// search.ebscohost.com/ login.aspx?direct=true&AuthType=ip,sso&db=cat091 95a&AN=gvsu.111b0a8e.8091.4a14.8de5.cb3f106be8 d&site=eds-live&scope=site
9. Wells KR, Jeacocke NA, Appaneal R, et al. The Australian Institute of Sport (AIS) and National Eating Disorders Collaboration (NEDC) position statement on disordered eating in high performance sport. Br J Sports Med. 2020;54:1247-1258. doi:10.1136/bjsports-2019-101813
10. Kennedy SF, Kovan J, Werner E, Mancine R, Gusfa D, Kleiman H. Initial validation of a screening tool for disordered eating in adolescent athletes. J Eat Disord 2021;9(1):21. doi:10.1186/s40337-020-00364-7
11. Mountjoy M, Sundgot-Borgen J, Burke L, et al. International Olympic Committee (IOC) consensus statement on relative energy deficiency in sport (RED-S): 2018 update. Int J Sport Nutr Exerc Metab 2018;28(4):316-331. doi:10.1123/ijsnem.2018-0136
12. Reinking MF, Alexander LE. Prevalence of disordered-eating behaviors in undergraduate female collegiate athletes and nonathletes. J Athl Train 2005;40(1):47-51.
13. Pope Z, Gao Y, Bolter N, Pritchard M. Validity and reliability of eating disorder assessments used with athletes: a review. J Sport Health Sci. 2015;4(3):211-221. doi:10.1016/j.jshs.2014.05.001
14. VandenBos GR. APA Dictionary of Psychology Second edition. American Psychological Association; 2015. Accessed April 13, 2022. https:// search.ebscohost.com/ login.aspx?direct=true&AuthType=ip,sso&db=cat091 95a&AN=gvsu.f462fcd2.8a3a.4bde.b4e7.a793260def8 &site=eds-live&scope=site
15. American Psychological Association. Criteria for evaluating treatment guidelines. Am Psychol 2002;57(12):1052-1059. doi:10.1037/ 0003-066x.57.12.1052
16. Tyson SF, Connell LA. How to measure balance in clinical practice. a systematic review of the psychometrics and clinical utility of measures of balance activity for neurological conditions. Clin Rehabil 2009;23:824-840. doi:10.1177/ 0269215509335018
17. Abbott W, Brett A, Brownlee TE, et al. The prevalence of disordered eating in elite male and female soccer players. Eat Weight Disord. 2021;26(2):491-498. doi:10.1007/s40519-020-00872-0
18. Aleksić Veljković A, Đurović D, Biro F, Stojanović K, Ilić P Eating attitudes and body image concerns among female athletes from aesthetic sports. Ann Kinesiol Published online 2020:3-16. doi:10.35469/ ak.2020.242
19. Boudreault V, Labossière S, Gauthier V, et al. Symptoms of mental illness among university student-athletes during the second wave of the COVID-19 pandemic lockdown in Canada. Front Sports Act Living 2022;4:1017376. doi:10.3389/ fspor.2022.1017376
20. Chatterton JM, Petrie TA. Prevalence of disordered eating and pathogenic weight control behaviors among male collegiate athletes. Eat Disord 2013;21(4):328-341. doi:10.1080/ 10640266.2013.797822
21. Clifford T, Blyth C. A pilot study comparing the prevalence of orthorexia nervosa in regular students and those in University sports teams. Eating and Weight Disord 2019;24:473-480. doi:10.1007/ s40519-018-0584-0
22. Gorrell S, Nagata JM, Hill KB, et al. Eating behavior and reasons for exercise among competitive collegiate male athletes. Eat Weight Disord 2019;26(1):75-83. doi:10.1007/s40519-019-00819-0
23. Gapin JI, Kearns B. Assessing prevalence of eating disorders and eating disorder symptoms among lightweight and open weight collegiate rowers. J Clin Sport Psychol 2013;7:198-214. doi:10.1123/ jcsp.7.3.198
24. Hazzard VM, Schaefer LM, Mankowski A, et al. Development and validation of the eating disorders screen for athletes (EDSA): a brief screening tool for male and female athletes. Psychol Sport Exerc. 2020:50. doi:10.1016/j.psychsport.2020.101745
25. Hernández Monserrat M, Arjona Garrido Á, Checa Olmos JC, Salguero García D. Relationship between negative running addiction and eating disorder patterns in runners. Nutrients 2021;13(12):4344. doi:10.3390/nu13124344
26. Karaağaç Y, İrem Çetinkaya F, Bellikci Koyu E. Comparison of exercise dependence, eating attitude disorder and risk of orthorexia nervosa among regular students and student athletes. Turk J Sports Med 2022;57(4):171-176. doi:10.47447/tjsm.0675
27. Kraus U, Holtmann SC, Legenbauer T. Eating disturbances in competitive lightweight and heavyweight rowers. J Clin Sport Psychol 2018;12(4):630-646. doi:10.1123/jcsp.2016-0042
28. Krebs PA, Dennison CR, Kellar L, Lucas J. Gender differences in eating disorder risk among NCAA division I cross country and track student-athletes. J Sports Med 2019;2019:1-5. doi:10.1155/2019/ 5035871
29. Lanfranchi MC, Maïano C, Morin AJS, Therme P Prevalence and sport-related predictors of disturbed eating attitudes and behaviors: moderating effects of sex and age. Scand J Med Sci Sports. 2014;24(4):622-633. doi:10.1111/sms.12044
30. Lichtenstein MB, Johansen KK, Runge E, Hansen MB, Holmberg TT, Tarp K. Behind the athletic body: A clinical interview study of identification of eating disorder symptoms and diagnoses in elite athletes. BMJ Open Sport Exerc Med 2022;8(2). doi:10.1136/ bmjsem-2021-001265
31. Magee MK, Jones MT, Fields JB, et al. Body composition, energy availability, risk of eating disorder, and sport nutrition knowledge in young athletes. Nutrients. 2023;15(6):1502. doi:10.3390/ nu15061502
32. Molnár AH, Vidiczki-Dóczi A, Petrovszki Z, Győri F. Prevalence of eating disorders and menstrual irregularities among female football players. Arena J Phys Act 2016;(5):16-27
33. Muia EN, Wright HH, Onywera VO, Kuria EN. Adolescent elite kenyan runners are at risk for energy deficiency, menstrual dysfunction and disordered eating. J Sports Sci 2016;34(7):598-606. doi:10.1080/ 02640414.2015.1065340
34. Petisco-Rodríguez C, Sánchez-Sánchez LC, Fernández-García R, Sánchez-Sánchez J, GarcíaMontes JM. Disordered eating attitudes, anxiety, selfesteem and perfectionism in young athletes and nonathletes. Int J Environ Res Public Health 2020;17(18):6754. doi:10.3390/ijerph17186754
35. Prather H, Hunt D, McKeon K, et al. Are elite female soccer athletes at risk for disordered eating attitudes, menstrual dysfunction, and stress fractures? Am J Phys Med Rehabil 2016;8:208-213. doi:10.1016/j.pmrj.2015.07.003
36. Pustivšek S, Hadžić V, Dervišević E, Carruthers J. Risk for eating disorders and body composition among adolescent female and male athletes and nonathlete controls. Int J Adolesc Med Health. 2020;32(4):1-8. doi:10.1515/ijamh-2017-0190
37 Segura-García C, Papaianni MC, Caglioti F, et al. Orthorexia nervosa: a frequent eating disordered behavior in athletes. Eat Weight Disord 2012;17(4):e226-33. doi:10.3275/8272
38. Soubliere D, Gitimu PN. Female athletes and eating disorders. Sport J 2012;1.
39. Stephens LE, Bowers EP, Schmalz DL, Duffy LN, Lenhoff J. A mixed method approach to evaluating eating-related psychopathologies in collegiate student-athletes. J Am Coll Health Published online 2021:1-14. doi:10.1080/07448481.2021.1947304
40. Thein-Nissenbaum JM, Rauh MJ, Carr KE, et al. Associations between disordered eating, menstrual dysfunction, and musculoskeletal injury among high school athletes. J Orthop Sports Phys Ther. 2011;41(2):60-69. doi:10.2519/jospt.2011.3312
41. Torres-McGehee TM, Emerson DM, Pritchett K, Moore EM, Smith AB, Uriegas NA. Energy availability with or without eating disorder risk in collegiate female athletes and performing artists. J Athl Train 2020;56(9):993-1002. doi:10.4085/jat0502-20
42. Uriegas NA, Moore K, Torres-McGehee TM. Prevalence and association between exercise dependence and eating disorder risk in collegiate student-athletes. J Athl Train. Published online February 24, 2023. doi:10.4085/1062-6050-0553.22
43. Uriegas NA, Winkelmann ZK, Pritchett K, TorresMcGehee TM. Examining eating attitudes and behaviors in collegiate athletes, the association between orthorexia nervosa and eating disorders. Front Nutr. 2021;8. doi:10.3389/fnut.2021.763838
44. Wadas G, DeBeliso M. Disordered eating, eating attitudes, and reasons for exercise among male high school cross country runners. Sport J. 2014;17.
45. Wells EK, Chin AD, Tacke JA, Bunn JA. Risk of disordered eating among division I female college athletes. Int J Exerc Sci. 2015;8(3):256-264. doi:10.70252/RAQK3996
46. Wollenberg G, Shriver LH, Gates GE. Comparison of disordered eating symptoms and emotion regulation difficulties between female college athletes and non-athletes. Eat Behav. 2015;18:1-6. doi:10.1016/j.eatbeh.2015.03.008
47 Kutz AM, Marsh AG, Gunderson CG, Maguen S, Masheb RM. Eating disorder screening: a systematic review and meta-analysis of diagnostic test characteristics of the SCOFF. J Gen Intern Med 2020;35(3):885-893. doi:10.1007/s11606-019-05478-6
48. Anglé S, Engblom J, Eriksson T, et al. Three factor eating questionnaire-R18 as a measure of cognitive restraint, uncontrolled eating and emotional eating in a sample of young Finnish females. Int J Behav Nutr Phys Act 2009;6(1):41. doi:10.1186/1479-5868-6-41
49. Black DR, Larkin LJS, Coster DC, Leverenz LJ, Abood DA. Physiologic screening test for eating disorders/disordered eating among female collegiate athletes. J Athl Train 2003;38(4):286-297
50. Martinsen M, Sundgot-Borgen J. Higher prevalence of eating disorders among adolescent elite athletes than controls. Med Sci Sports Exerc 2013;45(6):1188-1197. doi:10.1249/ mss.0b013e318281a939
Download: https://ijspt.scholasticahq.com/article/126965-examination-of-the-clinical-utility-of-eating-disorder-anddisordered-eating-screening-tools-in-young-athletes-a-scoping-review/attachment/ 256753.docx?auth_token=-2wgJZRmD4iHAAw8WsWC
Download: https://ijspt.scholasticahq.com/article/126965-examination-of-the-clinical-utility-of-eating-disorder-anddisordered-eating-screening-tools-in-young-athletes-a-scoping-review/attachment/ 256752.docx?auth_token=-2wgJZRmD4iHAAw8WsWC
Download: https://ijspt.scholasticahq.com/article/126965-examination-of-the-clinical-utility-of-eating-disorder-anddisordered-eating-screening-tools-in-young-athletes-a-scoping-review/attachment/ 256751.docx?auth_token=-2wgJZRmD4iHAAw8WsWC
Daniel Casado
1 ,
Ivan Nacher
2a ,
Juan Pardo
3
, Javier Reina
2
1 Health Sciences PhD Program, Universidad Católica San Antonio de Murcia, 2 Physiotherapy Department, Universidad Católica San Antonio de Murcia, 3 Department of Mathematics, Physics and Technological Sciences, Universidad Cardenal Herrera CEU
Keywords: Athletic performance, balance, physical conditioning, range of motion, team sports https://doi.org/10.26603/001c.127266
International Journal of Sports Physical Therapy
Vol. 20, Issue 1, 2025
Background
Fax: 0034968482358 a Casado D, Nacher I, Pardo J, Reina J.
The foam roller is considered a versatile tool. Along with an active warm-up, it appears to positively affect range of motion, stability, muscle stiffness, and perceived exertion with no reductions in performance.
Hypothesis/Purpose
The main purpose of the study was to observe the effects of the utilization of a foam roller during the warm-up on ankle mobility and lower limb stability, and secondarily, to assess if any induced effects were sustained over time.
Study design
Randomized controlled trial.
Methods
Twenty-two healthy male subjects were randomly assigned to two groups: the control group, which only carried out a general warm-up over a period of four months, and the foam roller group, which followed a specific warm-up routine using a foam roller for a period of three months plus one month of follow-up in which no foam rolling was performed. Two outcome measurements were taken pre- and post- intervention to assess ankle mobility: the Dorsi-Flexion Lunge test and the Y-Balance test (YBT) for the lower quarter. Outcomes were measured at three time points: before the protocol was initiated, at the end of the protocol (at 12 weeks), and after a one-month follow-up period (at 16 weeks).
Results
A significant increase was observed in the dorsiflexion of the right (p < 0.001) and left (p < 0.001) ankles in the experimental group. Significant increases were also noticed in the anterior (p < 0.003), posteromedial (p < 0.050), and posterolateral (p < 0.050) reach distances of the right leg and in the anterior (p < 0.002), posteromedial (p < 0.010), and posterolateral (p < 0.030) reach distances of the left leg during the YBT in the experimental group. The control group also showed significant differences in the right (p < 0.007) and left (p < 0.010) anterior reach distances on the YBT. At the one-month follow-up period, the improvements that had been obtained in both groups were lost, except for the dorsiflexion of the right ankle (p < 0.050) and right (p < 0.010) and left (p < 0.030) anterior reach distance on the YBT in the experimental group.
Corresponding author: Ivan Nacher
Physiotherapy Department, Catholic University San Antonio of Murcia (UCAM) Guadalupe, 30107, Murcia, Spain inacher@ucam.edu
Tel.: 0034 652687182
The foam roller can be used as a part of a pre-training warm-up routine to enhance the dorsiflexion range of motion and performance on the YBT
3. Registered as a clinical trial at ClinicalTrials.gov with registration number: NCT05971316.
A “warm-up” is defined as a period of exercise that precedes the physical activity or sports practice, and the intention is to gradually adapt the body both physically and mentally.1 This process improves neuromuscular performance, reduces the risk of injuries during the sport activity,2 and is essential to achieve optimal performance. Regarding the physiological mechanisms that are responsible for the effects of warm-up,3 both active4,5 and passive6 warm-up have been shown to result in an increase in the range of movement (ROM)7 and a reduction in muscle stiffness.8,9 These beneficial effects can be attributed to an increase in the rate of metabolic chemical reactions and muscle temperature, alterations in viscoelastic and thixotropic properties, and an enhanced sensitivity of the nerve receptors in the muscle.10 Furthermore, the increase in the elasticity of muscle fibers that follows the warm-up period enhances the muscle’s capacity to absorb energy within the muscletendon unit, thereby supporting an increase in tension and preventing injuries to muscle and tendon fibers.5 The most recent research on the efficacy of various warm-up methods, both active and passive, indicates that a general warmup and a sport-specific warm-up are recommended in team sports such as soccer, basketball, handball and rugby, amongst others.11 However, since the sport-specific warmup is primarily based on trial and error, identifying the most effective warm-up methods for each sport can prove challenging.12
The foam roller (FR) has been identified as a potentially beneficial tool for use as part of a warm-up routine to exercise or pre-competition,13 both in amateur and professional athletes. The primary objective of incorporating the FR into a warm-up routine is to enhance athletic performance.14‑16 The FR technique involves the athlete using a roller, typically made of high- to medium-density foam, to apply a direct pressure to a specific muscle group.17 The use of the FR has been demonstrated to have a wide range of beneficial effects, including an increase in flexibility,9 improved muscle recovery,18,19 reduced soft tissue adhesion, decrease in delayed-onset muscle soreness,20 modulation of the nervous system function,21 enhanced vascular response,22,23 increased ROM,7 and improved muscle performance before and after exercise.24,25 In contrast, meta-analytic evidence on lower limb performance in parameters related to strength, speed, and jump height continues to show controversial data on a significant improvement when a FR routine is applied.26
At the present time, there is no standardized FR application protocol. However, recent studies have provided some notable guidelines. For instance, the use of FR in combina-
tion with an active warm-up before a training session has been shown to be more effective in improving ROM and flexibility 27 When combined with other techniques, such as dynamic stretching or cycling, significant improvements have been observed.5,9,13 The minimum application time to induce physiological changes appears to be 90 seconds, as reported in several series.28 Additionally, the athlete’s experience with this tool29 and the possibility of rolling at higher speed30 should be taken into account, as they may contribute to a more pronounced decrease in tissue stiffness.
The sport of basketball is defined by a series of continuous movements, which include sprints, changes of direction, and high-impact jumps. These actions underscore the significance of both aerobic and anaerobic physical capacities, as well as lower limb strength, agility, and ROM. Limitations of ROM have been demonstrated to be a main predictor of lower limb injuries,31 and consequently, are a crucial element in the reduction of the risk of injury and the optimization of the athletic performance.32 Within the context of basketball, lateral ankle sprains represent a particularly prevalent musculoskeletal injury.33 The primary underlying cause may be attributed to a limitation of ankle dorsiflexion ROM, which affects the biomechanics of the foot34 and of running and landing, alters balance, and increases the risk of injury to more proximal joints such as the knee or the hip.35,36 Foam rolling has demonstrated a significant impact on ROM, not only in the dorsiflexion of the ankle,28,37‑40 but also in the knee7,18,25 and hip.41,42 It has been shown that ankle dorsiflexion is intimately connected to lower limb balance. A reduction in ankle dorsiflexion has been linked to a decline in lower limb balance, whereas an increase in ankle dorsiflexion has been associated with an enhanced lower limb balance.33 The FR can be used as an unstable surface and presents a challenge to maintain the stability and balance of to the body. Use of body weight and postures during rolling may be associated with secondary effects on core stability.43
This is the first study to examine the impact of FR as a component of the warm-up routine in professional basketball players over a three-month period of time. The main purpose of the study was to observe the effects of the utilization of a foam roller during the warm-up on ankle mobility and lower limb stability, and secondarily, to assess if any induced effects were sustained over time.
MATERIALS AND METHODS
A randomized controlled study with two parallel groups was carried out. The sample for this study of healthy male professional basketball players was recruited from the Spanish national division and had to be between 19 and 29 years of age. In order to be included in the study, participants were required to meet several criteria. These included engaging in a minimum of three training sessions per week, participating in weekend competitions, having prior experience in the use of a FR, not having sustained significant musculoskeletal injuries or undergone surgery in the lower limbs within the year preceding the study, and not having a diagnosed orthopedic or neurological pathology in the lower limbs. Injuries that impeded compliance with the prescribed minimum training sessions per week or the established warm-up routine lead to exclusion. The study was conducted in accordance with the Declaration of Helsinki and approved by the Ethics Committee of the Catholic University San Antonio of Murcia (UCAM) (reference no.: CE052314, ClinicalTrials.gov registration number: NCT05971316). Informed consent was obtained from all the subjects involved in the study.
The 24 participants were randomly assigned to two study groups using the GraphPad Software (https://www.graphpad.com/quickcalcs/randomize1/). The control group (CG) consisted of 12 participants who underwent a general basketball warm-up. The experimental group, the foam roller group (FRG), consisted of 12 participants who performed a foam roller warm-up (FRWU), which added to the general warm-up performed by the CG.
There were three data collection timepoints for both groups. Initial pre-FRWU measurement (T0, week 0) was conducted. Subsequently, FRG participants were instructed on the FRWU they were to perform over the subsequent three-month period (weeks 1 to 12). In contrast, CG subjects only engaged in a general basketball warm-up, as prescribed by the strength and conditioning coach. This warmup compromised mobility exercises, ballistic stretches, changes of direction and ball exercises. A second postFRWU measurement (T1) was conducted at week 12. Following a four-week follow-up period during which neither the FRWU nor the general warm-up was performed for either group, a third post-FRWU measurement (T2, week 16) was conducted (Figure 1).
Prior to the completion of the primary tests, anthropometric data including body mass and height, were obtained. Body mass was determined using a TANITA BC-601 scale (Tanita®, Illinois, USA) with an accuracy of 0.1 kg. Height was assessed with a TANITA HR001 stadiometer (Tanita®, Illinois, USA), with a graduation of 1 mm, a measurement range from 0 to 210 cm, and an accuracy of 0.1 cm. Subsequently, the body mass index (BMI) of each of the participants was calculated (kg/m2).
In order to assess ankle dorsiflexion, the dorsiflexion lunge test (DLT)44 was utilized. Data were recorded using the MyRom app from MyJumpLab for iOS. This method has been fully validated, exhibiting a standard error of estimate of 0.48°, an intraclass correlation coefficient of 0.976, and a coefficient of variation of 5.1 ± 2.3%, which is comparable to the results obtained using a digital inclinometer (coefficient of variation = 4.9 ± 2.5%).45
The subject, barefoot and facing a wall, positioned the first toe to be measured on a line marked on the floor at a 15 cm distance from the wall. A strap was utilized to secure the mobile phone at the tibial midline, located 15 cm distal to the tibial tuberosity44 (Figure 2). Participants were instructed to attempt to contact the wall using their knee, thereby performing a maximal dorsiflexion without lifting the heel off the ground, on three attempts. In the event that the subject contacted the wall during the initial attempt, he was instructed to withdraw his foot from the starting line and to repeat the three attempts. The degrees of both ankles were automatically obtained using the inclinometer of the mobile phone. The same procedure was then repeated for both ankles, and the mean value from the three attempts subsequently utilized for data analysis.
The YBT was selected to assess lower limb stability. This is a valid adaptation of the star excursion balance test (SEBT) that evaluates coordination, balance, flexibility, and lower limb strength, specifically through the anterior reach (AR), posteromedial reach (PMR), and posterolateral reach (PLR) distances. The protocol established by Plisky et al.46 was utilized employing the Y-Balance Test Kit™ (Functional Movement Systems, Chatham, VA, USA) The YBT intraclass correlation coefficient for intrarater reliability ranges from 0.85 to 0.91, whereas the interrater reliability ranged from 0.99 to 1.00; additionally, composite reach score reliability was 0.91.47 Subject was positioned in a unipedal, barefoot position, with the leg to be tested situated at the center of the inverted Y test equipment. With the hands firmly placed on the hips, athletes were instructed to slide the block as far as possible with the foot, not lifting the heel, and return to the initial upright position. Once three successful reaches had been achieved, athletes went on to the next foot. The order of performance was as follows: right AR, left AR, right PMR, left PMR, right PLR, and left PLR (Figure 3). For the purpose of data analysis, relative distance was employed. This was defined as the percentage obtained by dividing the absolute reach distance achieved in each of the directions by the limb length to be evaluated and multiplying the result by 100.
FRWU was conducted exclusively by subjects of the FRG for a period of three months, prior to their training sessions and in combination with the general warm-up routine prescribed by the strength and conditioning coach. In contrast,
Note:
participants or the CG only performed a general warm-up routine that was identical to the one that was carried out by the experimental group, and which included mobility exer-
cises, ballistic stretching, changes of direction and ball exercises.
FRWU was designed with a special focus on the lower limb musculature and was developed based on adaptations from previous studies.39,48,49 Participants were instructed to perform three sets of 60 seconds of work with a 30 second rest period between sets, three times per week (during training sessions), using a smooth, high-density foam roller (33 centimeters long and with a diameter or 13 centimeters), which is commonly available on the market. Exercises were bilateral for calf muscles, hamstrings, quadriceps, and gluteal muscles, and unilateral for the iliotibial band. Participants only applied their body weight with a moderate pressure ranging a self-report of between 5 and 7 on a numerical subjective scale (where 0 represents the absence of discomfort and 10 represents the maximum level of discomfort), and they avoided muscle spasms or cramps.50
For each segment of the lower limb, participants rolled the foam roller in a continuous motion from the top to the bottom of the muscle or muscle group, returning to the starting position at the conclusion of the movement.
For hamstrings, calves, and gluteal muscles, participants adapted a seated position with the roller positioned beneath the targeted muscle group, with the legs elevated for the hamstrings and calves and supported for the glutes. Hands were placed on the ground with fingers pointing toward the body, thereby supporting a portion of the participants’ body weight. In the case of the quadriceps, subjects assumed a prone position, with forearms on the ground in a plank position, and the foam roller was positioned under their thighs. Finally, for the iliotibial band, participants assumed a lateral position, with one leg crossed in front of the other and the bottom leg slightly elevated off the ground (Figure 4).
All data were stored in a database that had been specifically designed for this study A review was conducted to identify any inaccurate data entries or extreme outliers, i.e., data that were considered not to be representative of the study variables and none were found. All descriptive and inferential statistical analyses were performed using various libraries within the RStudio advanced statistical processing program.51 An independent sample t-test was used to assess for significant differences between the two groups. Paired t-tests were used to assess the mean difference before and after the intervention and to assess if significant differences had occurred. Data normality was examined through the Shapiro-Wilk test. In cases where these assumptions were not met, a non-parametric equivalent test was employed, such as the Wilcoxon signed-rank test. Significance level was set at p < 0.05 for all of the tests.
Moreover, to describe the magnitude of the difference or the relationship between the means of two paired groups, the effect sizes were calculated using the Cohen’s d which provides an indication of the practical significance, or the strength of observed the relationship, which is independent of the sample size. Finally, to calculate the study power, a post-hoc analysis was performed using the results obtained through the G*Power program.52 A significance level of α < 0.05 was established, employing a one-tailed test, as improvement was obtained, and a large effect size was assumed for the variables of interest in the study.
Twenty-four subjects were recruited and enrolled in the study Subsequently, two subjects were excluded from the study due to an injury and their inability to comply with the routine, resulting in a final study sample of 22 participants. (Table 1). There were no statistically significant differences between the groups.
The comparison of the ankle dorsiflexion means between both groups at the three measurement points revealed no statistically significant differences. Both groups exhibited non-significantly different values at T0 (pre-FRWU) (for the FRG: p = 0.355; and for the CG: p = 0.227). Whereas at T1 (post-FRWU), the FRG (p = 0.092) displayed higher mean values than the CG (p = 0.379), again without significant differences. Results were not statistically significant, with the FRG (p = 0.583) mean being lower than that of the CG (p = 0.086). (Table 2).
Table 1. Participant characteristics. Values are reported as mean and standard deviation (SD).
foam roller group; CG: control group. aWilcoxon rank sum test. bTwo sample t-test.
Table 2. Ankle dorsiflexion means for the foam roller group and the control group over time. All are reported in degrees.
Figure 5. Comparison of medians in ankle dorsiflexion between T0 and T1 in the FRG. Med.: median; density: proportion of individuals.
A significant increase was observed between T0 and T1 between groups for dorsiflexion of both the right (p = 0.001) and left (p = 0.001) ankles, with a large effect size (d ˃ 0.8) observed exclusively in the FRG. However, a significant increase was observed only in the FRG for the ROM of the right ankle (p = 0.041) in the T0-T2 (pre-FRWU to followup) comparison, with a medium effect size (d = 0.6). No statistically significant differences were observed in the CG. (Table 3).
Figure 5 presents a comparison of medians exclusively for the FRG between T0 and T1. Left and right ankle dorsiflexion is shown. A shift to the right is observed in both the density curve and the median of both ankles, in the left ankle grades (medT0 = 46.6° versus medT1 = 51.45°; p = 0.149) and in the right ankle grades (medT0 = 47.1° versus medT1 = 53.5°; p=0.336).
A comparison of the means of the YBT outcomes between the two groups at the three measurement points revealed a significant increase in percentage distance only at T1 for the FRG for right PMR (p = 0.003) and left PLR (p = 0.005) and also for the CG for right PMR (p = 0.014) and left PLR (p = 0.024) (Table 4).
A significant increase was observed in all of the YBT distances within the FRG between T0 and T1, exhibiting a large effect size for right (p = 0.003, d = 1.1) and left (p = 0.002, d = 1.3) AR, for right (p = 0.050, d = 1.3) and left (p = 0.010, d = 0.8) PMR, and for right (p = 0.050, d = 0.8) and left (p = 0.030, d = 0.9) PLR. In contrast, CG showed a significant increase only in right (p = 0.007, d = 1.3) and left (p = 0.010, d = 1.2) AR between T0 and T1. With regard to the improvements observed between T0 and T2, a significant increase was identified only in the FRG for right (p =
Effectiveness of a Foam Roller Warm-Up in Professional Basketball Players: A Randomized Controlled Trial
Table 3. Comparison and intragroup differences in ankle dorsiflexion across different time points, with associated effect sizes. All differences are reported in degrees.
0.010, d = 1.3) and left (p = 0.030, d = 1.2) AR, with a large effect sizes (Table 5).
Figure 6 presents a comparison of medians exclusively for FRG between T0 and T1, for all of the distances of the YBT A shift to the right is observed in the density curve and the median of both lower limbs in left AR (medT0 = 63.73% versus medT1 = 78.43%; p = 0.002), right AR (medT0 = 63.73% versus medT1 = 78.1%; p = 0.003), left PMR (medT0 = 73.64% versus medT1 = 80%; p = 0.015), right PMR (medT0 = 69.09% versus medT1 = 80.87%; p = 0.052), left PLR (medT0 = 73.53% versus medT1 = 84.55%; p = 0.035) and right PLR (medT0 = 73.91% versus medT1 = 83.33%; p = 0.050).
Finally, post-hoc statistical power of 0.84 was achieved, which is the minimum common convention for statistical power required for a study, although the sample was small.
Based on the literature review that was conducted, and to the best of the authors’ knowledge, this is the first study to evaluate the potential benefits of FR as a component of an active warm-up routine prior to the sport practice over a 12-week period of time, specifically in terms of enhancing ankle mobility and lower limb balance. Furthermore, the study sought to ascertain whether these outcomes were maintained over time, with a four-week follow-up period. The obtained results indicated a significant improvement in the right and left ankle dorsiflexion for the FRG following the FRWU. With regard to the lower limb balance, significant improvements were observed in all of the variables of the YBT for the FRG following the FRWU. However, these improvements were not sustained during the follow-up period for any of the tests. Significant improvements were only observed in the CG in the right and left AR in the YBT The observed increase in the ankle dorsiflexion degrees is supported by a substantial body of evidence from previous studies, which demonstrates that foam rolling results in an immediate enhancement of the ROM, not only in the ankle, but also in other joints such as the hip41,42 and the knee.18,25,53 Results of the current study indicate that the foam roller group exhibited an increase in the ankle dorsiflexion of 6.5° on the left side and 7.1° on the right after 12 weeks of foam roller warm-up. Prior research has demonstrated that FR can facilitate a 3.4° increase in plantar flexion following its application to the calf, for three sets of 60 seconds on, 30 seconds off, for 4 weeks, every day of the week37 and a 9° enhancement in acute ankle ROM was seen with a 4.5° increase observed on the dominant leg and a 4.4° increase on the non-dominant leg , following 1 minute of foam rolling to the quadriceps and calf 38 Additionally, a 7% increase in the ankle ROM after 3 sets of 30 seconds of application and 30 seconds of rest,39 and an enhancement in the ankle dorsiflexion were both noted without modifying the maximal muscle torque, regardless of the performance of one set, 3 sets or 10 sets of 30 seconds.28 On the other hand, the implementation of FR over a six-week intervention period has been demonstrated to result in an enhanced ankle ROM and targeted movement
patterns during the athletic activities, thereby reducing the risk of injury and enhancing athletic performance.54 A notable 11% increase in the ankle ROM was observed after five weeks of intervention.50 Furthermore, incorporating FR into the warm-up for eight weeks resulted in an enhanced ankle ROM without compromising the lower limb stability 55 The obtained results and a recent review indicate that an FR intervention longer than four weeks and with a minimum of three sessions per week induces a significant effect on gains in the ankle ROM.56 Therefore, based on the aforementioned studies and the data obtained in this study, the use of the FR on the lower limb as a part of an active warmup over a long period of time (three months) can be used to improve the ankle dorsiflexion ROM of both ankles. Thus, this is the only study that corroborates the improvements seen and provides evidence that prolonged use of FR is associated with enhanced ankle range of motion.
Currently, there are no published references on the influence of FR on lower limb stability Hence, this article, makes a novel contribution to the existing literature on the topic. The importance of the improvements in lower limb stability, which appear to be closely related to the improvements in the ankle dorsiflexion ROM, cannot be overstated. Previous authors have demonstrated that a reduction in the ankle dorsiflexion results in a decline in lower limb balance.33 Additionally, the performance of FR interventions can provide core muscular activation levels that are comparable to or even higher than those achieved with plank exercises.55 This is because the postures that are applied during the use of FR, such as those adopted for the quadriceps, are similar to the ones that are applied in the plank position. This could exert an enhancing and activating effect on core stability, thus indirectly contributing to improving the lower limb balance as measured by the YBT.57 The findings of the current study indicate that improvements were observed in all of the lower limb balance parameters, which occurred with enhanced ankle dorsiflexion range ROM in both ankles.
In addition to being a valid method for measuring lower limb balance, the YBT is also considered to be a reliable test for assessing the risk of lower limb injury 46,58 In a study performed by Engquist et al.,59 the YBT was administered to university athletes and non-athlete university students, and results demonstrated that the athlete group exhibited a superior performance in the anterior reach (75% better), posteromedial reach (85-90% better), and posterolateral reach (90-95% better) compared to the non-athlete group. Following the application of the FRWU in this study, the mean distances achieved by the FRG nearly reached the benchmarks established by Engquist et al.59 for all of the reach distances in both legs. Athletes in the current study demonstrated improved reaches which may relate to enhanced sensory integration, enabling optimal responses to diverse motor tasks and offering a potential for reduction of lower extremity injuries.
Following the four-week follow-up period (T2), during which FR was not employed, the enhancements achieved by the FRG exhibited a notable decline, approaching the values observed at T0. This is not surprising as the main
Effectiveness of a Foam Roller Warm-Up in Professional Basketball Players: A Randomized Controlled Trial
Table 5. Comparison and intragroup differences in lower limb balance across different time points. All values are reported as percentages (normalized to limb length).
Cohen’s values (d) are indicated as follows: L for large, M for medium, S for small, and N for negligible. MD: mean difference; CI: confidence intervals; FRG: foam roller group; CG: control group; AR: anterior reach; PMR: posteromedial reach; PLR: posterolateral reach. *Statistically significant differences at p < 0.05.
Table 4. Reach outcomes between the foam roller group and the control group over time. All values are reported as percentages (normalized to limb length).
benefits of foam rolling are acute adaptations of soft tissue elasticity, pain threshold, and stretch tolerance.60 This is a result of the rich sensory innervation of soft tissue, which plays an important role in specific tissue adaptations, including increased skin, muscle, and fascia temperature,24 improvements in vascular function,23 and reduced muscle stiffness.61 Additionally, FR contributes to systemic adaptations, which are related to the inhibition of the mechanoreceptors. These adaptations are thought to result in increased muscle relaxation after approximately fifteen minutes of use.62 It can be reasonably assumed that the altered perception of pain, which is a result of the sensory stimulation and the acute local systemic response, is the reason for the observed increase in ROM rather than muscle stiffness.63 Therefore, this would suggest that a FR is suitable tool for use during the warm-up prior to explosive actions due to its acute potential. However, it should be noted that the effects of this technique can affect endurance actions, as improvements are maintained for thirty minutes40 and then begin to decline drastically after sixty minutes,64 with values similar to the initial values being observed at three hours.65 This would underpin the assertion that, after using FR for a period of three consecutive months, discontinuing its application, even for a brief time interval (one month), results in a regression to the initial values observed at the onset of the first three months.
The findings of this study indicate that the regular practice of FR for a minimum of three sessions per week over an extended period of time, with no interruptions, results in an enhancement in ankle dorsiflexion ROM and lower limb balance, which can positively impact the biomechanics of running and landing after jumping, as well as altering the balance of lower extremities.35 ROM and balance factors are considered to be the main causes of ankle injuries in basketball.66 As previously hypothesized, alterations in such factors can result in a reduction in the probability of lower extremity injuries by up to 85% and an enhancement in athletic performance.67
It must be acknowledged that this study is not without limitations. Firstly, the FR is a relatively novel tool, that presents certain challenges in establishing a standardized protocol for its use. Secondly, the sample size was small, primarily due to the inclusion of professional basketball players who were required to possess proficiency in the use
of the FR, which presented a challenge in terms of recruitment. However, adequate post-hoc power was achieved. Thirdly, a follow-up period of an equal duration to that of the intervention would allow for the clarification of whether the observed improvements are maintained over time or diminish rapidly Due to the small sample size, this work can only be considered to be an exploratory study with the aim of generating new hypotheses that should lead to additional trials. Accordingly, further research with a larger sample size and beyond male basketball players is required to validate these findings.
The results of the present study demonstrate the effectiveness of the foam roller as a component of a warm-up routine for male basketball athletes, with a particular emphasis on ankle dorsiflexion and lower limb balance. Enhancement in the degrees of dorsiflexion of both ankles and in all of the distances of the YBT was seen post intervention in the experimental group, after a three-month period of continuous use. The FR could be a valuable tool to incorporate into the pre-training warm-up routine for basketball athletes to improve ankle dorsiflexion and lower limb stability
The authors declare no conflicts of interest. This research received no external funding.
We appreciate the voluntary participation of all of the players and their invaluable kindness.
Submitted: May 20, 2024 CST, Accepted: November 18, 2024 CST
© The Author(s)
Figure 6. Comparison of medians in the reaches of the balance test between T0 and T1 in the FRG.
Med.: median; density: proportion of individuals.
This is an open-access article distributed under the terms of the Creative Commons Attribution 4.0 International License (CCBY-NC-4.0). View this license’s legal deed at https://creativecommons.org/licenses/by-nc/4.0 and legal code at https://creativecommons.org/licenses/by-nc/4.0/legalcode for more information.
1. Hedrick A. Physiological responses to warm-up. Natl Strength Cond Assoc J 1992;14(5):25. https:// doi.org/10.1519/ 0744-0049(1992)014%3C0025:PRTWU%3E2.3.CO;2
2. Hedrick A. Learning from each other: warming up. Strength Cond J. 2006;28. https://doi.org/10.1519/ 1533-4295(2006)28[43:LFEOWU]2.0.CO;2
3. McGowan CJ, Pyne DB, Thompson KG, Rattray B. Warm-up strategies for sport and exercise: mechanisms and applications. Sports Med 2015;45(11):1523-1546. doi:10.1007/ s40279-015-0376-x
4. Bishop D. Warm up II: performance changes following active warm up and how to structure the warm up. Sports Med. 2003;33(7):483-498. doi:10.2165/00007256-200333070-00002
5. Morales-Artacho AJ, Lacourpaille L, Guilhem G. Effects of warm-up on hamstring muscles stiffness: cycling vs foam rolling. Scand J Med Sci Sports. 2017;27(12):1959-1969. doi:10.1111/sms.12832
6. Opplert J, Babault N. Acute Effects of dynamic stretching on mechanical properties result from both muscle-tendon stretching and muscle warm-up. J Sports Sci Med 2019;18(2):351-358.
7. MacDonald GZ, Penney MDH, Mullaley ME, et al. An acute bout of self-myofascial release increases range of motion without a subsequent decrease in muscle activation or force. J Strength Cond Res. 2013;27(3):812-821. doi:10.1519/ JSC.0b013e31825c2bc1
8. Fradkin AJ, Zazryn TR, Smoliga JM. Effects of warming-up on physical performance: a systematic review with meta-analysis. J Strength Cond Res 2010;24(1):140-148. doi:10.1519/ JSC.0b013e3181c643a0
9. Su H, Chang NJ, Wu WL, Guo LY, Chu IH. Acute effects of foam rolling, static stretching, and dynamic stretching during warm-ups on muscular flexibility and strength in young adults. J Sport Rehabil 2017;26(6):469-477. doi:10.1123/jsr.2016-0102
10. Axelson HW, Hagbarth KE. Human motor control consequences of thixotropic changes in muscular short-range stiffness. J Physiol. 2001;535(Pt 1):279-288. doi:10.1111/j.1469-7793.2001.00279.x
11. Van Den Tillaar R, Lerberg E, von Heimburg E. Comparison of three types of warm-up upon sprint ability in experienced soccer players. J Sport Health Sci 2019;8(6):574-578. doi:10.1016/j.jshs.2016.05.006
12. Bishop D Warm up I: potential mechanisms and the effects of passive warm up on exercise performance. Sports Med 2003;33(6):439-454. doi:10.2165/00007256-200333060-00005
13. D’Andrea J, Wicke J, Kleber F. Foam rolling as a warm-up technique for anaerobic power activities. Clinmedjournals.org 2017;3(5). doi:10.23937/ 2469-5718/1510077
14. Peacock CA, Krein DD, Silver TA, Sanders GJ, Von CarloWitz KPA. An acute bout of self-myofascial release in the form of foam rolling improves performance testing. Int J Exerc Sci 2014;7(3):202-211. doi:10.70252/DTPM9041
15. Ferreira RM, Martins PN, Goncalves RS. Effects of self-myofascial release instruments on performance and recovery: an umbrella review Int J Exerc Sci 2022;15(3):861-883.
16. Healey KC, Hatfield DL, Blanpied P, Dorfman LR, Riebe D The effects of myofascial release with foam rolling on performance. J Strength Cond Res 2014;28(1):61-68. doi:10.1519/ JSC.0b013e3182956569
17 Freiwald J, Baumgart C, Kühnemann M, Hoppe M. Foam-rolling in sport and therapy – Potential benefits and risks. Sports Orthop Traumatol Published online July 1, 2016. doi:10.1016/ j.orthtr.2016.07.002
18. Macdonald GZ, Button DC, Drinkwater EJ, Behm DG. Foam rolling as a recovery tool after an intense bout of physical activity. Med Sci Sports Exerc. 2014;46(1):131-142. doi:10.1249/ MSS.0b013e3182a123db
19. Rey E, Padrón-Cabo A, Costa PB, Barcala-Furelos R. Effects of foam rolling as a recovery tool in professional soccer players. J Strength Cond Res 2019;33(8):2194-2201. doi:10.1519/ JSC.0000000000002277
20. Romero-Franco N, Romero-Franco J, JiménezReyes P. Jogging and practical-duration foam-rolling exercises and range of motion, proprioception, and vertical jump in athletes. J Athl Train 2019;54(11):1171-1178. doi:10.4085/ 1062-6050-474-18
21. Kim S, Bleakney R, Boynton E, et al. Investigation of the static and dynamic musculotendinous architecture of supraspinatus. Clin Anat 2010;23(1):48-55. doi:10.1002/ca.20896
22. Hotfiel T, Swoboda B, Krinner S, et al. Acute effects of lateral thigh foam rolling on arterial tissue perfusion determined by spectral doppler and power doppler ultrasound. J Strength Cond Res. 2017;31(4):893-900. doi:10.1519/ JSC.0000000000001641
23. Okamoto T, Masuhara M, Ikuta K. Acute effects of self-myofascial release using a foam roller on arterial function. J Strength Cond Res 2014;28(1):69-73. doi:10.1519/JSC.0b013e31829480f5
24. Beardsley C, Škarabot J. Effects of self-myofascial release: A systematic review J Bodyw Mov Ther 2015;19(4):747-758. doi:10.1016/j.jbmt.2015.08.007
25. Cheatham SW, Kolber MJ, Cain M, Lee M. The effects of self-myofascial release using a foam roll or roller massager on joint range of motion, muscle recovery, and performance: a systematic review Int J Sports Phys Ther 2015;10(6):827-838.
26. Konrad A, Nakamura M, Behm DG. The effects of foam rolling training on performance parameters: a systematic review and meta-analysis including controlled and randomized controlled trials. Int J Environ Res Public Health 2022;19(18):11638. doi:10.3390/ijerph191811638
27. Hendricks S, Hill H, Hollander S den, Lombard W, Parker R. Effects of foam rolling on performance and recovery: A systematic review of the literature to guide practitioners on the use of foam rolling. J Bodyw Mov Ther 2020;24(2):151-174. doi:10.1016/ j.jbmt.2019.10.019
28. Nakamura M, Onuma R, Kiyono R, et al. The acute and prolonged effects of different durations of foam rolling on range of motion, muscle stiffness, and muscle strength. J Sports Sci Med. 2021;20(1):62-68. doi:10.52082/jssm.2021.62
29. Mayer I, Hoppe MW, Freiwald J, et al. Different effects of foam rolling on passive tissue stiffness in experienced and nonexperienced athletes. J Sport Rehabil 2020;29(7):926-933. doi:10.1123/ jsr.2019-0172
30. Wilke J, Niemeyer P, Niederer D, Schleip R, Banzer W Influence of foam rolling velocity on knee range of motion and tissue stiffness: a randomized, controlled crossover trial. J Sport Rehabil 2019;28(7):711-715. doi:10.1123/jsr.2018-0041
31. Gonzalo-Skok O, Serna J, Rhea MR, Marín PJ. Relationships between functional movement tests and performance tests in young elite male basketball players. Int J Sports Phys Ther. 2015;10(5):628-638.
32. Bonnel F, Toullec E, Mabit C, Tourné Y, Sofcot. Chronic ankle instability: biomechanics and pathomechanics of ligaments injury and associated lesions. Orthop Traumatol Surg Res OTSR. 2010;96(4):424-432. doi:10.1016/j.otsr.2010.04.003
33. Padua E, D’Amico AG, Alashram A, et al. Effectiveness of warm-up routine on the ankle injuries prevention in young female basketball players: a randomized controlled trial. Med Kaunas Lith. 2019;55(10):690. doi:10.3390/medicina55100690
34. Bohannon RW, Tiberio D, Zito M. Selected measures of ankle dorsiflexion range of motion: differences and intercorrelations. Foot Ankle. 1989;10(2):99-103. doi:10.1177/107110078901000209
35. Lazarou L, Kofotolis N, Pafis G, Kellis E. Effects of two proprioceptive training programs on ankle range of motion, pain, functional and balance performance in individuals with ankle sprain. J Back Musculoskelet Rehabil. 2018;31(3):437-446. doi:10.3233/ BMR-170836
36. Bolívar YA, Munuera PV, Padillo JP Relationship between tightness of the posterior muscles of the lower limb and plantar fasciitis. Foot Ankle Int 2013;34(1):42-48. doi:10.1177/1071100712459173
37. Aune AAG, Bishop C, Turner AN, et al. Acute and chronic effects of foam rolling vs eccentric exercise on rom and force output of the plantar flexors. J Sports Sci. 2019;37(2):138-145. doi:10.1080/ 02640414.2018.1486000
38. Phillips J, Diggin D, King DL, Sforzo GA. Effect of varying self-myofascial release duration on subsequent athletic performance. J Strength Cond Res 2021;35(3):746-753. doi:10.1519/ JSC.0000000000002751
39. Smith JC, Pridgeon B, Hall MC. Acute effect of foam rolling and dynamic stretching on flexibility and jump height. J Strength Cond Res. 2018;32(8):2209. doi:10.1519/JSC.0000000000002321
40. Kasahara K, Konrad A, Yoshida R, et al. Comparison of the prolonged effects of foam rolling and vibration foam rolling interventions on passive properties of knee extensors. J Sports Sci Med 2022;21(4):580-585. doi:10.52082/jssm.2022.580
41. Romero Moraleda B, López Rosillo A, González J, Morencos Martínez E. Efectos del foam roller sobre el rango de movimiento, el dolor y el rendimiento neuromuscular: revisión sistemática. Retos Nuevas Tend En Educ Física Deporte Recreación 2020;(38):879-885. doi:10.47197/retos.v38i38.75532
42. Behara B, Jacobson BH. Acute effects of deep tissue foam rolling and dynamic stretching on muscular strength, power, and flexibility in division I linemen. J Strength Cond Res. 2017;31(4):888. doi:10.1519/JSC.0000000000001051
43. Lukas C. Faszienbehandlung mit der Blackroll [Treatment of fascia with the blackroll] BoD - Books on Demand; 2012. https://books.google.es/ books?hl=es&lr=&id=_rxgbIWaR0C&oi=fnd&pg=PA19&ots=KEcQh1SQkq&s ig=sv2_NAJnI-JmTJHjv2lzmC0Sk4&redir_esc=y#v=onepage&q&f=false
44. Bennell KL, Talbot RC, Wajswelner H, Techovanich W, Kelly DH, Hall AJ. Intra-rater and inter-rater reliability of a weight-bearing lunge measure of ankle dorsiflexion. Aust J Physiother. 1998;44(3):175-180. doi:10.1016/ s0004-9514(14)60377-9
45. Balsalobre-Fernández C, Romero-Franco N, Jiménez-Reyes P. Concurrent validity and reliability of an iPhone app for the measurement of ankle dorsiflexion and inter-limb asymmetries. J Sports Sci. 2019;37(3):249-253. doi:10.1080/ 02640414.2018.1494908
46. Plisky PJ, Rauh MJ, Kaminski TW, Underwood FB. Star excursion balance test as a predictor of lower extremity injury in high school basketball players. J Orthop Sports Phys Ther. 2006;36(12):911-919. doi:10.2519/jospt.2006.2244
47 Plisky PJ, Gorman PP, Butler RJ, Kiesel KB, Underwood FB, Elkins B. The reliability of an instrumented device for measuring components of the star excursion balance test. North Am J Sports Phys Ther. 2009;4(2):92-99.
48. Baumgart C, Freiwald J, Kühnemann M, Hotfiel T, Hüttel M, Hoppe MW Foam solling of the calf and anterior thigh: biomechanical loads and acute effects on vertical jump height and muscle stiffness. Sports. 2019;7(1):27 doi:10.3390/sports7010027
49. Madoni SN, Costa PB, Coburn JW, Galpin AJ. Effects of foam rolling on range of motion, peak torque, muscle activation, and the hamstrings-toquadriceps strength ratios. J Strength Cond Res 2018;32(7):1821-1830. doi:10.1519/ JSC.0000000000002468
50. Kiyono R, Onuma R, Yasaka K, Sato S, Yahata K, Nakamura M. Effects of 5-week foam rolling intervention on range of motion and muscle stiffness. J Strength Cond Res. 2022;36(7):1890-1895. doi:10.1519/JSC.0000000000003757
51. RStudio Team. RStudio: integrated development for R. 2020. http://www.rstudio.com/
52. Faul F, Erdfelder E, Lang AG, Buchner A. G*Power 3: a flexible statistical power analysis program for the social, behavioral, and biomedical sciences. Beh Res Methods. 2007;39:175-191. doi:10.3758/bf03193146
53. Mohr AR, Long BC, Goad CL. Effect of foam rolling and static stretching on passive hip-flexion range of motion. J Sport Rehabil. 2014;23(4):296-299. doi:10.1123/jsr.2013-0025
54. Guillot A, Kerautret Y, Queyrel F, Schobb W, Di Rienzo F. Foam rolling and joint distraction with elastic band training performed for 5-7 weeks respectively improve lower limb flexibility J Sports Sci Med. 2019;18(1):160-171.
55. Junker D, Stöggl T. The training effects of foam rolling on core strength endurance, balance, muscle performance and range of motion: a randomized controlled trial. J Sports Sci Med 2019;18(2):229-238.
56. Konrad A, Nakamura M, Tilp M, Donti O, Behm DG. Foam rolling training effects on range of motion: a systematic review and meta-analysis. Sports Med. 2022;52(10):2523-2535. doi:10.1007/ s40279-022-01699-8
57. Zahiri A, Alizadeh S, Daneshjoo A, Pike N, Konrad A, Behm DG. Core muscle activation with foam rolling and static planks. Front Physiol 2022;13:852094. doi:10.3389/fphys.2022.852094
58. Butler RJ, Lehr ME, Fink ML, Kiesel KB, Plisky PJ. Dynamic balance performance and noncontact lower extremity injury in college football players: an initial study Sports Health 2013;5(5):417-422. doi:10.1177/ 1941738113498703
59. Engquist KD, Smith CA, Chimera NJ, Warren M. Performance comparison of student-athletes and general college students on the Functional Movement Screen and the Y-balance test. J Strength Cond Res. 2015;29(8):2296-2303. doi:10.1519/ JSC.0000000000000906
60. Behm DG, Wilke J. Do self-myofascial release devices release myofascia? rolling mechanisms: a narrative review Sports Med 2019;49(8):1173-1181. doi:10.1007/s40279-019-01149-y
61. Heiß R, Mayer I, Hüttel M, et al. Evaluation of tissue stiffness in athletes with different experience in foam rolling assessed by acoustic radiation force impulse elastography. Sem Musculoskel Radiol. 2019;23. doi:10.1055/s-0039-1687708
62. Ketelhut S, Möhle M, Hottenrott K. Acute Effects of self-myofascial release using a foam roller on arterial stiffness in healthy young adults. Artery Res. 2020;26. doi:10.2991/artres.k.200615.001
63. Wilke J, Müller AL, Giesche F, Power G, Ahmedi H, Behm DG. Acute effects of foam rolling on range of motion in healthy adults: a systematic review with multilevel meta-analysis. Sports Med 2020;50(2):387-402. doi:10.1007/s40279-019-01205-7
64. Kasahara K, Konrad A, Yoshida R, et al. Comparison of the effects of different foam rolling durations on knee extensors function. Biol Sport. 2024;41(2):139-145. doi:10.5114/ biolsport.2024.131820
65. Giovanelli N, Vaccari F, Floreani M, et al. Shortterm effects of rolling massage on energy cost of running and power of the lower limbs. Int J Sports Physiol Perform. 2018;13(10):1337-1343. doi:10.1123/ ijspp.2018-0142
66. Lebleu J, Mahaudens P, Pitance L, et al. Effects of ankle dorsiflexion limitation on lower limb kinematic patterns during a forward step-down test: A reliability and comparative study J Back Musculoskelet Rehabil. 2018;31(6):1085-1096. doi:10.3233/BMR-171063
67 Desai P, Jungmalm J, Börjesson M, Karlsson J, Grau S. Effectiveness of an 18-week general strength and foam-rolling intervention on running-related injuries in recreational runners. Scand J Med Sci Sports. Published online January 11, 2023. doi:10.1111/sms.14313
Kasey M Murphy, BS1 , Bryan L Riemann, PhD, ATC1a
1 Health Sciences and Kinesiology, Georgia Southern University
Keywords: strength and conditioning, power, resistance training, kettlebell training https://doi.org/10.26603/001c.127464
International Journal of Sports Physical Therapy
Vol. 20, Issue 1, 2025
Background
Kettlebell (KB) swing exercises, whether performed using shoulder height (SHS) or overhead (OHS) swing variations in therapeutic or strength and conditioning settings, are posterior chain dominant exercises that require hip extension contributions when performed correctly.
Purpose/ Hypothesis
The primary purpose of this study was to evaluate the effect of swing style (SHS, OHS) and KB mass on hip extension kinematics and kinetics in young adult females. A secondary purpose was to determine the effects of swing style and KB mass on the forces applied to the total body center of mass and KB. It was hypothesized that velocity, power, and work would be greater for the OHS compared to the SHS, as well as for the heavier compared to the lighter KB’s.
Study Design
Crossover study design.
Methods
Fifteen physically active females performed 15 swings under four conditions, SHS/12kg, SHS/16kg, OHS/12kg, and OHS/16kg, while three-dimensional dominant limb foot, shank, thigh, pelvis, and KB kinematic and ground reaction force (GRF) data were collected.
Results
KB distance (95%CIDiff: 17.5–25.1 %BH), time (95%CIDiff: 0.067–0.023 s), and peak velocity (95%CIDiff: 0.56–0.74 m‧s-1) were significantly greater during the OHS (p<0.05). During OHS, significantly greater hip joint peak power (95%CIDiff: 1.6–4.1 W kg-1), work (95%CIDiff: 0.104–0.527), peak velocity (95%CIDiff: 7.6–40.5 ° ‧s-1) occurred compared to SHS, although the time and hip angular position of peak velocity and peak power were not statistically different between styles.
Conclusions
These results may influence choices of KB progressions, suggesting that one may first consider changes in KB mass prior to changing from the SHS style to the OHS style.
Corresponding Author: Bryan L Riemann, PhD, ATC Biodynamics and Human Performance Center Georgia Southern University- Armstrong Campus 11935 Abercorn Street Savannah, GA 31419 briemann@georgiasouthern.edu a
3
Kettlebells (KB’s) are commonly used in strength and conditioning programs to train various aspects of fitness and performance and offer an additional technique for sports rehabilitation practitioners to consider during terminal phases of rehabilitation with patients seeking to return to high activity levels. The kettlebell (KB) is composed of a ball and handle,1 with a center of mass that extends past the hand. The off-center mass distribution challenges grip, stability, and muscle engagement, and enables a different kind of training compared to traditional dumbbells or barbells.2 Kettlebells are available in various masses and sizes, allowing for a wide variety of movements such as carries, presses, swings, snatches, cleans, and jerks. Studies have demonstrated that KB training facilitates development of lower body strength and power, endurance, and cardiorespiratory fitness,3‑5 as well as a contribution to a variety of performance training metrics such as the vertical jump,5,6 and one-repetition maximum strength in the deadlift, squat, and power clean.3,5,6 Additionally, due to the ability of KB swings to activate the medial hamstrings,7,8 they have been used in rehabilitation settings to manage pain and musculoskeletal disorders of the lower back9,10 and in anterior cruciate ligament injury rehabilitation programs.11,12 In practical application, the integration of KB exercises can enhance rehabilitation and exercise protocols by promoting functional hip extension movement patterns that are crucial for athletes returning to sport.
Popular variations of KB exercises include the shoulder height swing (SHS) and overhead swing (OHS). Both swings are ballistic in nature and involve a rapid cyclical sequence of eccentric and concentric actions of the hip extensor musculature.3,7,10 Strong and powerful hip extensor muscles are essential to the maintenance of an upright posture and many functional movements (e.g., vertical jump). The sequence of muscle activation begins with active flexion of the hip during the eccentric phase of the swing. This is followed by the concentric swing phase that requires activation of the trunk extensors, abdominal co-contraction for spinal stability, while the gluteal muscles primarily produce the forces needed to elevate the KB to the desired height.13, 14
Shoulder height kettlebell swings impose large compressive and shear forces on the body 1 There is an inertial component of the KB that causes higher posterior shear forces in relation to compressive forces.1 This requires sufficient dynamic spinal stability and is one of the factors that differentiate KB swings from traditional resistance exercises like the back squat or deadlift. Objective evidence concerning the mechanical demands of an exercise,1 as well as how mechanical demands change with various progressions (e.g., external mass changes),1,15 is a necessary prerequisite for practitioners to make informed decisions about exercise prescription and progression.3,5,6,13 Several investigations have used EMG,7,14,16,17 ground reaction force
(GRF) characteristics,1,13,18,19 and joint kinetics1,14,15,18,19 to quantify the mechanical demands of the different KB swing styles and masses, but few have conducted a comprehensive analysis of hip biomechanics that includes consideration of kinematics (e.g., angular distance and velocity) and kinetics (e.g., impulse, work and power). From a specificity of training perspective, understanding the timing and angular positions of peak kinematic and kinetic events is also important. For example, if the timing, angular positions, and event magnitudes are similar between the SHS and OHS swings, then the SHS may be preferred over OHS to lower injury risk while eliciting a similar training stimulus. Each of these characteristics reflect different perspectives of the demands and stimulus imposed on the hip by KB swing exercise and their documentation may better assist practitioners with exercise prescription. In addition to changing the KB load1,15 KB swing style is an additional mechanism for exercise progression.19 In their comparison of OHS, SHS, and Indian club swings, Bullock et al19 reported the SHS and OHS to be mechanically similar despite moderate differences in peak hip flexion, impulse and cycle time. It is important to note that their study used a mixed sex sample in which the males used a 20kg KB and the females used a 12kg KB for the OHS and SHS. However, to date there has not been a direct comparison of KB load effects between the OHS and SHS styles. As males and females appear to demonstrate different responses to kettlebell masses during SHS,1 studies examining KB load effects need to consider sex. The primary purpose of this study was to evaluate the effect of swing style (SHS, OHS) and KB mass on hip extension kinematics and kinetics in young adult females. A secondary purpose was to determine the effects of swing style and KB mass on the forces applied to the total body center of mass and KB as reflected by GRF and overall KB swing characteristics. It was hypothesized that velocity, power, and work would be greater for the OHS compared to the SHS, as well as for the heavier compared to the lighter KB’s. It has also been hypothesized that GRF characteristics (peak, average, and impulse) would be greater with the higher mass. The results of this research can directly inform practitioners on the most effective KB movements and progressions to implement during rehabilitation and performance enhancement programs to maximize patient outcomes while ensuring safety and effectiveness.
Fifteen physically active females (29.8 ± 5.3 years, 64.9 ± 10.5 kg, 1.65 ± 0.07 m) with a minimum of six months KB swing training experience volunteered to participate in this study 13,15,19 Prior to data collection, participants were given an overview of the study procedures, signed informed consent documents, and completed demographic
and health history questionnaires related to musculoskeletal injuries and surgeries. All participants were free from any lower extremity, glenohumeral, or spinal pain or injuries that restricted their participation in physical activities within the prior six months. Participants were asked to abstain from vigorous physical activity 24 hours prior to the data collection session. The university’s Institutional Review Board approved the study protocol, and all participants were informed of the risks and benefits of testing prior to data collection. A previous investigation examining the effects of KB loads (8kg, 12kg, 16kg) on similar hip joint kinetic variables as the current investigation reported linear effect sizes (Hedges g) between 0.91 and 1.11 (median=1.01),15 which correspond to a Cohen’s f of 0.505. As the primary purpose of the current investigation sought to compare KB swing style differences, a smaller effect size was anticipated than the previously reported KB load effect sizes. Power analysis using G*Power (α=.05, β=.2, f=.45) yielded a minimal sample size of 12; thus 15 participants were targeted to provide an allowance for any data collection or reduction issues (e.g., missing markers, etc.).
This cross-over study was conducted in resistance-trained females experienced with KB swing exercise. In a randomly assigned order, KB swings were performed under four conditions: SHS 12 kg, SHS 16 kg, OHS 12 kg, and OHS 16 kg. These two KB loads were chosed because they have been described as appropriate loads for females.2 Participants performed one trial under each condition and each trial consisted of fifteen continuous swings. Trials were separated by a 2-minute rest period. Pilot work indicated that 15 swings was a sufficient number of swings to obtain a block of five clean consecutive swings for analysis (i.e. no missing markers) while avoiding the risk of inducing fatigue with too many swings under each condition. Participants were cued to initiate each swing by extending their hips as hard as they could, swinging the KB as fast as they could, and actively pulling the KB back down during the eccentric portion of the swing. Prior to completing the KB swings, each data collection session began with a standardized warm-up consisting of five minutes on an arm ergometer (60-80 rpm), air squats, and 10 repetitions of 4.5 kg KB swings. Next, reflective marker clusters, each with three to five markers in unique asymmetrical and nonlinear patterns, were attached to the participant’s left and right feet, shanks, thighs, as well as their sacrum and thorax.
Three-dimensional kinematic data of the reflective marker clusters were captured (100Hz) by a 12 infrared camera system (Vicon, Oxford, UK). Additional marker clusters were secured to the 12kg and 16kg KB. The camera data was streamed into The Motion Monitor acquisition software (IST, Chicago, IL, USA) where it was synchronized with GRF data collected (1000Hz) from two forceplates (AMTI, Watertown, MA, USA), one under each foot. Participant calibration involved digitizing the proximal and distal end of each foot, shank, thigh (distal end), pelvis, and trunk segment (centroid of contralateral points) using calibrated stylus. The hip joint center was established by com-
puting the apex of femoral rotation during a series of circumduction movements.20 The KB center of mass was also computed relative to the attached cluster by taking the centroid of two antipodal digitized points. Participant mass and height were recorded for the anthropometric computations for locating each segment’s center of mass.
Hip flexion/extension joint angles and net joint moments (NJM) using inverse dynamics were computed within The Motion Monitor. Prior to exporting as text files for further data reduction using MATLAB-based scripts (The Mathworks, Inc., Natick, MA, USA), the KB and hip kinematic and NJM data were low pass filtered at 10Hz while the GRF data were low pass filtered at 35Hz. All data were visually examined to verify integrity (i.e., no missing markers, etc.) and five consecutive swings within each set, typically between the 5th and 11th swings, were selected for analysis. The criteria used for swing selection included no missing marker data and the swings completed continuously (no pauses between swings) with similar KB displacements. The start of the concentric phase and end of the eccentric phase for each selected swing was defined by the posterior KB displacement. The concentric-eccentric transition was defined by the maxima of the combined vertical and anterior-posterior KB displacement vector:
where x2 AP is the anterior-posterior displacement and x2 V is the vertical displacement
The hip NJM were normalized to body mass and the polarity was reversed to make extension moments positive. Hip joint angular velocity was computed using the central finite difference method with the angular displacement time series data. Hip net joint powers were computed as the product of the normalized NJM and angular velocity (radians‧sec-1) and net joint work was computed by integrating the net joint power-time data. Hip NJM impulse (integral of NJM and time), peak power and work were computed for the concentric phase for each of the five selected swings.
In addition, three KB based variables were also computed for the concentric phase of each selected swing: KB travel distance (normalized to body height), concentric swing time, and peak KB vector velocity KB travel distance and peak velocity were based upon the combined vertical and anterior-posterior KB compositive vector 15 GRF were summed across the two plates followed by the computation of the combined vertical and anterior-posterior GRF vector. Three GRF variables were computed from the combined vector for the concentric phase of each selected swing: average GRF, peak GRF, and GRF impulse (integral of GRF vector and time).
Statistical analyses were conducted with IBM SPSS Statistics (Version 27.0. Armonk, NY: IBM Corp). All outcome measures were averaged across the five trials and were examined for normality using QQ plots and Shapiro-Wilk tests. Two factor (style by mass) repeated measures analyses were conducted on all outcome measures. Partial eta squared effect sizes were used to indicate interaction and
Table 1. Descriptive statistics (means ± standard deviation) for the kettlebell swing characteristics variables.
*Overhead swing significantly greater than shoulder height swing; †12 kg significantly greater than 16 kg
main effect size magnitudes and were interpreted as 0.01, 0.06, and 0.14 for small, medium and large, respectively Significant interactions were followed by Bonferroni adjusted simple main effect post analyses.21 Additionally, standardized effect sizes (d) were computed using Hedges’ g method, adjusted for small samples.22 The standardized effect sizes were interpreted as 0.2, 0.6, 1.2, 2.0, and 4.0 for small, moderate, large, very large, and extremely large, respectively 23 Significance for all inferential statistics was set a priori to α<0.05.
Kettlebell travel distance (Table 1) was 23.4% significantly greater for the OHS compared to the SHS (p<0.001, d=2.7, 95%CIDiff: 17.5–25.1 %BH). Kettlebell travel distance was not significantly influenced by KB mass as evidenced by the interaction (p =0.636, η2 p=0.016) and mass main effect (p=0.970, d=0.01). The concentric swing time was 15.5% greater for the OHS compared to the SHS (p=0.001, d=0.94, 95%CIDiff: 0.067–0.203s). Kettlebell mass had no significant effect on concentric swing time based on the interaction (p =0.741, η2 p=0.008) and mass main effect (p=0.517, d=0.10). While the style by mass interaction for peak KB velocity was not statistically significant (p=0.057, η2 p=0.235), there was a significant style difference (p<0.001, d=2.1, 95%CIDiff: 0.56–0.74 m‧s-1) with 16.6% greater peak velocity for the OHS and a significant mass difference (p=0.003, d=0.36, 95%CIDiff: 0.05–0.18 m s-1) with 2.7% greater peak velocity for the 12 kg KB compared to the 16 kg KB.
For a randomly chosen participant, hip angular velocity, net joint moments, and net joint power ensemble average graphs against time (percent swing cycle) and angular position are presented in Figure 1. Based on the interaction (p=0.634, η2 p=0.017) and style (p=0.140, d=0.08) and mass (p=0.920, d=0.01) effects, there were no significant differences in the hip angular position at start of the swing (Table 2). At the end of the OHS, the hip was significantly more flexed (95%CIDiff: -9.9—4.8°) compared to the SHS (p<0.001, d=1.2). The hip angular distance was 9.8% greater (95%CIDiff: 5.5–10.6°) for the OHS compared to the SHS (p<0.001, d=0.44) and the angular distance was 4.1% greater (95%CIDiff: 0.8°–6.1°) for the 16kg mass compared to the 12kg mass (p=0.014, d=0.19). The OHS peak hip extension
velocity was 9.7% greater (95%CIDiff: 7.6–40.5 ° s-1) relative to the SHS (p=0.007, d=0.46). Peak hip extension velocity was not significantly influenced by KB mass based upon the interaction (p=0.460, η2 p=0.040) and mass main effect (p=0.281, d=0.09). The time to reach peak hip extension velocity was not statistically different between the two swing styles (p=0.232, d=0.13) nor was the time to reach peak hip extension velocity significantly influenced by KB mass based upon the interaction (p=0.572, η2 p=0.068) or mass main effect (p=0.358, d=0.12). The angular position of peak hip extension velocity was not statistically different between the two swing styles (p=0.849, d=0.02) nor was the angular position of peak hip extension velocity significantly influenced by KB mass based upon the interaction (p=0.688, η2 p=0.012) and mass main effect (p=0.427, d=0.10).
The OHS peak hip extension power (Table 3) was 42.1% greater (95%CIDiff: 1.6–4.1 W kg-1) compared to the SHS (p<0.001, d=0.73) and peak hip extension power was 34.1% greater (95%CIDiff: 0.93–5.8 W kg-1) during the 16kg mass compared to the 12kg mass (p=0.010, d=0.62). The interaction for time to peak hip extension power was not significant (p=0.469, η2 p=0.038). Additionally, neither swing style (p=0.311, d=0.04) nor KB mass (p=0.107, d=0.06) had significant effects on the time to peak hip extension power. There was a significant style by mass interaction (p=0.037, η2 p=0.274) for the angular position of peak power. There were no significant differences in angular position of peak power between the swing styles for either the 12 kg (p=0.062, d=0.25) or 16kg (p=0.655, d=0.03) mass. There was no significant difference between the two masses for the OHS (p=0.197, d=0.16). In contrast, during the SHS, the hip was significantly more flexed (95%CIDiff: -10.2 to -3.3°) at peak power for the 12 kg compared to the 16 kg (p=0.001, d=0.47).
Hip joint work was 21.9% greater (95%CIDiff: 0.104–0.527 J kg-1) for the OHS compared to the SHS (p=0.006, d=0.38). Hip joint work was not significantly influenced by KB mass based upon the interaction (p=0.134, η2 p=0.153) and mass main effect (p=0.117, d=0.37). The interaction for hip impulse was not significant (p=0.527, η2 p=0.029). Additionally, neither swing style (p=0.608, d=0.10) nor KB mass (p=0.259, d=0.31) had significant effects on hip impulse.
Figure 1. Ensemble averages of hip angular velocity (A), net joint moment (B), and power (C) against normalized time and hip angular velocity (D), net joint moment (E) and power (F) against hip angular position of five trials for a randomly selected participant completing the concentric portion of overhead swings (black lines) and shoulder height swings (grey lines) with 12 kg (solid) and 16 kg (dashed) kettlebells.
Error bars represent one standard deviation across the five trials.
Table 2. Descriptive statistics (means ± standard deviation) for the hip joint kinematic and kinetic variables.
*Overhead swing significantly greater than shoulder height swing; †12 kg significantly greater than 16 kg; ‡ 12kg significantly greater than 16kg for shoulder height swing
Table 3. Descriptive statistics (means ± standard deviation) for the hip joint kinetic variables.
PP: peak power
*Overhead swing significantly greater than shoulder height swing; †12 kg significantly greater than 16 kg; ‡ 12kg significantly greater than 16kg for shoulder height swing
Table 4. Descriptive statistics (means ± standard deviation) for the ground reaction force variables.
GRF: ground reaction force, BW: body weight
*12 kg significantly greater than 16 kg; †overhead swing significantly greater than shoulder height swing for 12 kg; ‡16 kg significantly greater than 12 kg for shoulder height swing
GROUND REACTION FORCES
There was a significant style by mass interaction (p=0.009, η2 p=0.398) for peak GRF (Table 4). With the 12 kg mass, the peak VGRF was 7.0% greater (95%CIDiff: 0.08–0.20 BW) for the OHS compared to the SHS (p<0.001 d=0.49), whereas with the 16kg there was no significant difference between swing styles (p=0.109, d=0.18). While there was no significant difference in peak GRF between masses for the OHS (p=0.384, d=0.09), the peak GRF was 6.3% greater (95%CIDiff: 0.07–0.17 BW) for the 16 kg mass compared to the 12 kg (p<0.001, d=0.44) during the SHS.
The average GRF was 4.4% greater (95%CIDiff: 0.08–0.35 BW) for the 16 kg mass compared to the 12 kg mass (p<0.001, d=0.88). There were no significant differences between the styles based upon the interaction (p=0.131, η2 p=0.155) and style main effect (p=0.178, d=0.19).
There was a significant style by mass interaction (p=0.032, η2 p=0.289) for GRF impulse. With the 12kg mass, the peak GRF was 14.0% greater (95%CIDiff: 1.4–4.8 BW‧s) for the OHS compared to the SHS (p=0.002, d=0.73), whereas with the 16kg there was no significant difference between swing styles (p=0.445, d=0.20). While there was no significant difference in GRF impulse between masses for the OHS (p=0.645, d=0.10), for the SHS the GRF impulse was 15.2% greater (95%CIDiff: 0.53–4.8 BW s) for the 16 kg mass compared to the 12 kg (p=0.018, d=0.63).
With the aim of assisting practitioners to make informed KB swing exercise prescription decisions in their training protocols, this study primarily sought to quantify the magnitude of the differences between the SHS and OHS with re-
spect to KB swing characteristics, hip joint kinematics and kinetics, and GRF characteristics in resistance trained females. KB distance during the OHS was ~25% greater, coupled with ~16% greater time and peak velocity At the hip joint, the most profound swing style effect was on peak power (~42%) followed by work (~22%) and peak velocity (~10%). Remarkably, the time and angular position of attaining peak velocity and power were similar between the swing styles. This result suggests that the hip movement patterns, and muscle activations are similar between the two styles but the OHS requires greater effort. The GRF peak and impulse were also greater for the OHS compared to the SHS, but the relative changes were substantially less than the hip joint changes. Compared to the swing style differences, there were fewer and smaller magnitude changes associated with a 4 kg KB mass increase. Thus, based on the biomechanical demands identified in the current study, these results may influence choices of KB progressions, suggesting that one may first consider changes in KB mass prior to changing from the SHS style to the OHS style.
Based on the results, both styles are mechanically similar in regard to the hip joint. The starting position for both swing styles occurred at similar degrees of hip flexion. While peak velocity and peak power were higher for the OHS, their location and timing were similar between the two swing styles. These results suggest that the mechanical demands and active musculature are similar between styles. The findings of the current study more closely align with those of McGill and Marshall.14 Although McGill and Marshall compared single arm swings and single arm snatches, the start and end hip joint positions they reported are like the current SHS and OHS results. Additionally, McGill and Marshall measured EMG activity of the gluteal muscles, which play a critical role in hip extension, and demon-
strated the KB swings promoted gluteal muscle activation. Collectively, these results indicate that both KB swings are appropriate exercise selections for the development of posterior chain power.
It comes as no surprise that the OHS was characterized by a greater overall travel distance and swing time than the SHS based on the KB reaching an overhead versus shoulder height position. The higher peak KB velocity is explained upon the relative change and standardized effect size between the swing styles being greater for the KB travel distance compared to the concurrent increase in swing time. Based on the KB swing trajectory, it is also important to note that KB travel distance and peak velocity were based upon the vertical and anterior-posterior composite displacement vector 15 This operational definition is different than previous research that just considered vertical displacement19 or composite displacement for just the propulsion phase of the swing.13 It is worthwhile to note that the current OHS KB distance and peak velocity are similar to a previous OHS report15 but slightly less than an investigation considering SHS.3 The discrepancy with the latter report is likely explained by participant differences (i.e., males versus females), KB masses, and consideration of just the propulsion phase of the swing.
Remarkably, in the current study, both swing styles began with near identical hip positions that were consistent across both KB masses. The statistical results revealed the hip to be more flexed at the end of the OHS compared to the SHS. As illustrated by the example ensemble average graph (Figure 1), towards the end of the OHS, the hip attained ~10° of hip extension, but then returned to a more flexed position by the end of the swing. This pattern of hip motion at the end of the swing was consistent for most participants. The end of the swing was defined by the KB displacement. Thus, the movement back to a slightly flexed position at the end of the OHS can be attributed to a postural stability component that allows the KB to attain an overhead position when the KB displacement vector reached a maximal value. While controlled hip extension can be a beneficial training stimulus, practitioners should be aware that repeated bouts of excessive hip extension coupled with poor form or excessive loading may pose an injury risk to the hip and lower back.
A previous investigation reporting hip kinematics reported females began two-handed7 SHS with 85.6±9.0° of hip flexion and ended in 10.9±8.1° of hip hyperextension. In contrast, the current SHS results revealed the participants beginning the swings with ~6° less flexion and ending close to neutral hip extension. The difference between these results may be explained by the previous study precisely controlling the start/end position of the KB as well as the swing cadence. Consistent with the current results, an additional previous study using a mixed sex sample with different KB masses reported similar peak hip flexion angles between the OHS and SHS styles at the beginning of the swing19; however, the starting hip flexion angle was substantially less flexion (~61°) than either the current or previous study Like the current study, participants were not restricted in start position or cadence, but the different methods used to
define the start of the swing may explain the starting hip position difference.
Impulse provides insight regarding net torque production over time while work provides insight on torque production through the range of motion. Joint power, the product of torque and angular velocity, reflects the rate at which mechanical energy is being produced. Collectively, the three metrics provide a comprehensive understanding of the training stimulus to the hip extensors. The current results show OHS was associated with greater peak hip extension velocity and power which is likely attributable to greater effort required to swing the KB overhead. A previous report by Bullock et al19 observed no difference between styles for peak hip extension velocity, moment, or power It should be noted however, that they utilized a mixed sex population, in which the males used a 20 kg KB and females used 12 kg. On the other hand, Lake and Lauder3 saw significant increases in velocity as KB mass increased. Their KBs were much heavier (16, 24, and 32 kg) and their population was entirely male.
In contrast to the hip velocity and power, the current results revealed hip impulse to be the same for both swing styles. It is important to recall that the start and end of the interval of interest was defined by the position and velocity of the KB. It is evident upon review of the ensemble average graphs that the role of the hip was predominant in the first ~50% of the swings, with less involvement during the later part of the swing. With the computation of hip impulse being based on the product of NJM and overall concentric phase time, the presumable higher NJM required for the OHS may have been diluted because of the longer concentric phase time.
As mentioned previously, the greater hip angular distance and more flexed terminal hip position during the OHS was attributed to the act of decelerating the KB and regaining stability during the terminal swing phase. As evidenced by the ensemble average graphs, starting hip angular position, and angle of peak power, the hip displacement during the concentric phase was similar between the two styles. As a result, the greater work produced during the OHS can be attributed to a greater average net joint moment compared to the SHS. Thus, the OHS may offer the hip extensor musculature a greater sustained training stimulus through the range of motion utilized in performing KB swings.
As it pertains to mass, significant differences were observed in swing characteristics, other than a small (2.7%) increase in peak velocity for the 12 kg KB during the OHS. Hip angular distance was 4.1% greater for the 16 kg KB than the 12 kg KB. Hip extension velocity, time to reach peak hip extension velocity, and the position of peak hip extension velocity were similar across both masses. Watts et al15 saw significant linear increases in peak power across 8 kg, 12 kg, and 16 kg masses, which is similar to the increased power and velocity observed in the 16 kg in the current study. This demonstrates that both masses are appropriate exercise selections for the development of posterior chain power, but intensity increases as mass increases.
Analysis of GRF provides a mode to compare the overall mechanical demands of the two swing styles under the two
masses. Like previous research,3,15 the composite vector of the vertical and anterior-posterior GRF was used. While mass prompted a % increase in mean GRF, there were no mean GRF differences between the swing styles. This result is consistent with Ross et al18 who reported no significant vertical or anterior-posterior mean GRF forces associated with changes in KB swing height. The peak and impulse GRF results revealed greater values for the OHS compared to the SHS only when swings were completed with the 12 kg KB. This finding suggests the mechanical demand to swing the 12 kg KB to shoulder height is less than the requirements to perform a 12 kg OHS and both swing styles with the 16kg KB. Previous research has also reported similar peak vertical GRF values between the OHS and SHS.19 The lack of GRF differences between the swing styles is contradictory to previous research documenting a 20% change in swing height was a sufficient stimulus to prompt GRF impulse differences.18 The discrepancy in impulse results can be attributed to differences in the KB masses used; Ross et al18 has male participants perform the swings with a 56 kg KB whereas the current investigation used 12 kg and 16 kg KB with female participants.
Additionally, it is worthwhile to note there were more significant results related to swing style compared to KB mass. Of those results that were significant for both factors, the swing style effect size magnitudes were generally larger than the mass effect size magnitudes. This outcome can be attributed to using 12 kg and 16 kg KB with females who regularly performed KB swing training. Thus, biomechanical research utilizing KB with greater mass, particularly when examining experienced individuals, is recommended. This study has a few limitations. First, this study only considered females. Given the differential effects of KB load between males and females,1 this limits the ability to speculate if similar results would be attained with males. Furthermore, despite requiring that all participants had at least six months of KB swing training experience, exact training protocols or participants’ varying degrees of technique were not controlled for There is a possibility that hip kinetic patterns change with advancements in KB swing training. Additionally, KB mass was limited to 12 kg and 16 kg and relative strength was not accounted for The 12 kg KB mass for females has been described as an appropriate starting weight for strength-trained females by Steve Cotter in his book Kettlebell Training2 and 16 kg is a standard prescribed mass for females in high-volume CrossFit workouts and competition. Because the participants were recruited from CrossFit gyms, it is likely that they were accustomed to these KB masses, and they may not have been great enough to stimulate maximal power production. Future studies should consider incorporating greater masses to evaluate their participants under more challenging KB mass conditions.
An additional limitation to the current investigation was only considering the concentric (propulsion) phase of the
swing, defined by the start and end position of the KB. The rationale for limiting the focus on the concentric portion of the KB swing was the similarity of the training stimulus relative to many functional athletic movements such as vertical jump and Olympic style weightlifting. The ensemble graphs illustrate that only about 50% of the swing for both styles involve active propulsion effort by the hip. As mentioned previously, perhaps more subtle swing style outcome measure differences would have been evident if only the active propulsion portion of the swing had been considered. For example, Lake and Lauder13 defined the propulsion phase based on the peak resultant KB velocity This study opted to consider the full concentric phase because of the timing and location uncertainty of differences in KB velocity, as well as the underlying hip joint kinetics. Based upon the current results showing similarity in the time and location of hip joint kinetics, future research comparing swing styles has some rationale for limiting consideration to the active propulsion portion of the swing. It is worth noting that altering swing height by 20% of shoulder height had more influence on the eccentric (braking) phase GRF characteristics than the concentric (propulsion) phase.18 Thus, it is recommended future research consider swing style differences during the eccentric phase of the KB swing.
At the hip, the OHS promoted greater peak power, work, and peak velocity compared to the SHS. Interestingly, despite these kinetic differences, the time and angular position of attaining peak velocity and power were not statistically different between the two swing styles. The GRF peak and impulse were also greater for the OHS compared to the SHS, but the relative changes were substantially less than the hip joint changes. Kettlebell mass had fewer and smaller magnitude changes than swing style. In conclusion, the results of the current study may have application regarding choices of KB progressions. Specifically, practitioners may want to first consider changes in KB mass prior to changing from the SHS style to the OHS style.
The authors report no conflicts of interest.
The authors thank Drs. Kellen Krajewski and Kelsey Piersol for their help with data collection.
Submitted: June 27, 2024 CST, Accepted: October 20, 2024 CST
© The Author(s)
This is an open-access article distributed under the terms of the Creative Commons Attribution 4.0 International License (CCBY-NC-4.0). View this license’s legal deed at https://creativecommons.org/licenses/by-nc/4.0 and legal code at https://creativecommons.org/licenses/by-nc/4.0/legalcode for more information.
1. Levine NA, Hasan MB, Avalos MA, Lee S, Rigby BR, Kwon YH. Effects of kettlebell mass on lower-body joint kinetics during a kettlebell swing exercise. Sports Biomech 2022;21(9):1032-1045. doi:10.1080/ 14763141.2020.1726442
2. Cotter S. Kettlebell Training. Human Kinetics; 2021. doi:10.5040/9781718225343
3. Lake JP, Lauder MA. Kettlebell swing training improves maximal and explosive strength. J Strength Cond Res 2012;26(8):2228-2233. doi:10.1519/ JSC.0b013e31825c2c9b
4. Farrar RE, Mayhew JL, Koch AJ. Oxygen cost of kettlebell swings. J Strength Cond Res. 2010;24(4):1034-1036. doi:10.1519/ JSC.0b013e3181d15516
5. Otto WH 3rd, Coburn JW, Brown LE, Spiering BA. Effects of weightlifting vs. kettlebell training on vertical jump, strength, and body composition. J Strength Cond Res. 2012;26(5):1199-1202. doi:10.1519/JSC.0b013e31824f233e
6. Maulit MR, Archer DC, Leyva WD, et al. Effects of kettlebell swing vs. explosive deadlift training on strength and power Int J Kinesiol Sports Sci 2017;5(1):1-7 doi:10.7575//aiac.ijkss.v.5n.1p.1
7. Van Gelder LH, Hoogenboom BJ, Alonzo B, Briggs D, Hatzel B. EMG analysis and sagittal plane kinematics of the two-handed and single-handed kettlebell swing: a descriptive study. Int J Sports Phys Ther 2015;10(6):811-826.
8. Del Monte MJ, Opar DA, Timmins RG, Ross JA, Keogh JWL, Lorenzen C. Hamstring myoelectrical activity during three different kettlebell swing exercises. J Strength Cond Res 2020;34(7):1953-1958. doi:10.1519/JSC.0000000000002254
9. Edinborough L, Fisher JP, Steele J. A comparison of the effect of kettlebell swings and isolated lumbar extension training on acute torque production of the lumbar extensors. J Strength Cond Res 2016;30(5):1189-1195. doi:10.1519/ JSC.0000000000001215
10. Jay K, Frisch D, Hansen K, et al. Kettlebell training for musculoskeletal and cardiovascular health: a randomized controlled trial. Scand J Work Environ Health 2011;37(3):196-203. doi:10.5271/ sjweh.3136
11. Zebis MK, Andersen CH, Bencke J, et al. Neuromuscular coordination deficit persists 12 months after ACL reconstruction but can be modulated by 6 weeks of kettlebell training: a case study in women’s elite soccer Case Rep Orthop 2017;2017:4269575. doi:10.1155/2017/4269575
12. Zebis MK, Skotte J, Andersen CH, et al. Kettlebell swing targets semitendinosus and supine leg curl targets biceps femoris: an EMG study with rehabilitation implications. Br J Sports Med 2013;47(18):1192-1198. doi:10.1136/ bjsports-2011-090281
13. Lake JP, Lauder MA. Mechanical demands of kettlebell swing exercise. J Strength Cond Res 2012;26(12):3209-3216. doi:10.1519/ JSC.0b013e3182474280
14. McGill SM, Marshall LW Kettlebell swing, snatch, and bottoms-up carry: back and hip muscle activation, motion, and low back loads. J Strength Cond Res 2012;26(1):16-27 doi:10.1519/ JSC.0b013e31823a4063
15. Watts CQ, Boessneck K, Riemann BL. Effects of kettlebell load on joint kinetics and global characteristics during overhead swings in women. Sports 2022;10(12). doi:10.3390/sports10120203
16. Sørensen B, Aagaard P, Malchow-Møller L, Kreutzfeldt Zebis M, Bencke J. Medio-lateral hamstring muscle activity in unilateral vs. bilateral strength exercises in female team handball players - a cross-sectional study. Int J Sports Phys Ther. 2021;16(3):704-714. doi:10.26603/001c.24150
17 Lyons BC, Mayo JJ, Tucker WS, Wax B, Hendrix RC. Electromyographical comparison of muscle activation patterns across three commonly performed kettlebell exercises. J Strength Cond Res 2017;31(9):2363-2370. doi:10.1519/ JSC.0000000000001771
18. Ross JA, Keogh JW, Lorenzen C, Lake J. Effects of 56-kilogram kettlebell swing endpoint on total body mechanics. J Strength Cond Res. 2022:10.1519. doi:10.1519/JSC.0000000000004568
19. Bullock GS, Schmitt AC, Shutt JM, Cook G, Butler RJ. Kinematic and kinetic variables differ between kettlebell swing styles. Int J Sports Phys Ther 2017;12(3):324-332. Effects
20. Leardini A, Cappozzo A, Catani F, et al. Validation of a functional method for the estimation of hip joint centre location. J Biomech 1999;32(1):99-103. doi:10.1016/S0021-9290(98)00148-1
21. Richardson JTE. Eta squared and partial eta squared as measures of effect size in educational research. Educ Res Rev 2011;6(2):135-147 doi:10.1016/j.edurev.2010.12.001
22. Hedges LV, Olkin I. Chapter 5 - Estimation of a single effect size: parametric and nonparametric methods. In: Hedges LV, Olkin I, eds. Statistical Methods for Meta-Analysis. Academic Press; 1985:75-106. doi:10.1016/ B978-0-08-057065-5.50010-5
23. Hopkins WG, Marshall SW, Batterham AM, Hanin J. Progressive statistics for studies in sports medicine and exercise science. Med Sci Sports Exerc 2009;41(1):3-13. doi:10.1249/MSS.0b013e31818cb278
Ricupito R,
A,
M,
P. The Influence of Cognitive Dual Tasking on the Outcomes of the Triple Hop Test Following Anterior Cruciate Ligament Reconstruction. IJSPT. 2025;20(1):40-46. doi:10.26603/001c.127511
Roberto Ricupitoa , Alberto Grassi1 , Matteo Zanuso2 , Paolo Torneri3
1 II Clinic Rizzoli Orthopaedic Institute, 2 University of Verona, 3 F3 Physiotherapy Clinic
Keywords: ACL, Assessments, Neurocognitive, RTP, Hop https://doi.org/10.26603/001c.127511
International Journal of Sports Physical Therapy
Vol. 20, Issue 1, 2025
Introduction
Anterior cruciate ligament (ACL) reconstruction involves prolonged rehabilitation, with Return to Sport (RTS) as a key goal for athletes. Integrating Dual Task (DT) strategies, which combine cognitive and physical tasks, is critical, as multitasking mirrors real-world and sports-specific demands. Assessing how distractions affect performance is essential to optimize RTS outcomes for both the reconstructed and healthy limbs.
Purpose
To analyze the influence of DT on the performance of the Triple Hop Test for distance (THD) in individuals’ status post ACL reconstruction.
Study type
Cross Sectional
Materials and Methods
Seventeen patients post-ACL were recruited and performed THD under two conditions: single task (standard condition) and dual task (with an added neurocognitive task).
Assessments were conducted on both the healthy and the previously injured limb over six meters, measured via a standard measuring tape. Paired t-tests and Mann-Whitney-Wilcoxon or Kruskal-Wallis tests were applied to investigate differences. Categorical variables were compared using chi-squared tests.
Results
There was a significant difference in average distance between single task performances in the healthy and previously operated limbs, with a difference of 20.71 cm (p=0.016). A significant difference was also observed in DT performance, with a distance variation of 10.41 cm (p=0.038). Comparing performances, both the healthy and the ACL-reconstructed limbs showed performance deterioration under DT conditions, with a greater percentage decline in the healthy limb.
Conclusions
Dual Task conditions appear to hinder performance in the THD in both the healthy and post-ACL reconstructed limbs.
a
Correspondig author:
Roberto Ricupito
Via Felice Frossi Gondi 35, 00162, Rome, Italy
Robertoricupito90@gmail.com, +393290946509
The post-operative rehabilitation after anterior cruciate ligament (ACL) injuries is an important topic in sports medicine and ACL reconstruction is commonly undertaken to restore knee stability and return the individual to function. Neuroplasticity, or the brain’s ability to reorganize itself, allows the central nervous system to adapt to both external (environmental) and internal factors, such as injuries. In some cases, patients may struggle with adopting effective motor-learning strategies, or traditional rehabilitation may not provide sufficient stimulation to promote motor skill recovery This could help explain why, after an ACL injury, patients do not always regain full motor function, as current rehabilitation programs may not fully engage the neuroplastic potential needed for optimal recovery.1 The efficacy of this recovery process is primarily evaluated through functional tests like the Triple Hop for distance (THD), which is one means to assess the dynamic movement capabilities of individuals. Despite the routine nature of this test, daily life and sports often require multitasking, and the impact of dual-tasking on THD performance remains an underexplored area.2,3
The safe return to sports activities following ACL reconstruction is a critical chapter in the multidisciplinary management of patients undergoing this procedure. Scientific interest in this area has increased over the years, highlighting that despite growing knowledge, the re-injury rate remains high. An area of interest that could have implications for a safer return to sports is the investigation of neurocognitive performance.
The prevalence of individuals experiencing a second injury to the same knee within the first five years after ACL reconstruction exceeds 15%, while it is around 8% for the contralateral knee.4,5 In a recent study, Della Villa et al.6 reported an impressive 42% rate of isolated ACL injuries with non-contact mechanisms experiencing re-injury within five years. According to research by Paterno et al.,7 this risk is up to 15 times higher, with a fourfold increased risk for female individuals of sustaining a homolateral ACL injury and six times for the contralateral knee.
Limited evidence suggests that deficits in neurocognitive functions are associated with an increased risk of re-injury 2,8‑10 While the mechanisms through which neurocognitive demands influence injury risk are not fully understood, it is known that performing a motor action under the influence of a dual cognitive task exposes the athlete to a more realistic context that requires cognitive processing and decision-making.11‑13 In sports activities, where cognitive demands are high, motor deficits may become more apparent, thereby exposing the patients to a higher risk of injury The introduction of neurocognitive distraction elements into testing protocols is advocated to provide a more realistic and comprehensive assessment of an athlete’s readiness to return to sport, potentially mitigating
the high risk of re-injury associated with ACL rehabilitation.14,15
Emerging studies suggest that current functional tests may not adequately simulate the complex environments encountered during sports, which often leads to injuries. These environments typically involve cognitive distractions that can impact performance, highlighting a gap in standard testing protocols.14,15
Furthermore, neuroplastic changes following ACL injuries can lead to maladaptations such as arthrogenic muscle inhibition, influenced by altered sensory feedback and disrupted homeostasis.16,17 Authors have assessed the effect of cognitive challenge during functional tasks18,19 but this is the first to assess the effect of a dual task during a triple leg hop for distance on ACLR patients. This manuscript explores incorporating dual-task scenarios into the rehabilitation assessment as this could better reflect true functional capacity by mimicking the cognitive and motor tasks athletes face in competitive environments.
This study aims to explore the influence of DT on the execution of the THD, comparing the healthy limb to the surgically repaired limb in patients post-ACL reconstruction.14
The hypothesis was that the injured knee will show a greater decline in performance during dual-task conditions compared to the healthy limb.
Between March 2023 and February 2024, patients who had undergone anterior cruciate ligament (ACL) reconstruction surgery were recruited from one physiotherapy clinic for a normal testing procedure after completing the rehabilitation programs. All patients undergoing ACL reconstruction between 2022 and 2023 were screened for inclusion in the study.
Inclusion criteria were: patients who had undergone an ACL reconstruction at least six months but not greater than 12 months prior (with full passive range of motion and absence of swelling) and participated in neurocognitive training during rehabilitation, being 18 years of age or older, of activity level 1 or 2, and having a stable contralateral knee with no history of injury or surgery in the prior two years, The exclusion criteria included the presence of concurrent musculoskeletal conditions (e.g., back, hip, or ankle pain) impairing the ability to perform jumps on both lower limbs, advanced degenerative changes (grade III or higher per the Kellgren-Lawrence classification based on pre-operative or intra-operative radiography), and an inability to speak, read, write, or understand Italian.
Each patient underwent a protocol to evaluate their performance on THD under two different conditions: a single task (standard condition) and a dual task (incorporating a neuro-cognitive task), on both the healthy limb and the post-operative limb. Testing protocols were uniformly ap-
plied across all testing sessions and were administered by the same researcher to ensure consistency
The testing session was conducted in a private musculoskeletal clinic during a single test session following the usual clinical practices. All patients had already completed rehabilitation programs before the testing session, and the testing procedure did not modify any component of the existing rehabilitation pathways. The data were collected as part of routine clinical practice and did not introduce experimental research elements.
All procedures were performed following written informed patient consent and in accordance with the ethical standards of the institutional and national research committee and the 1964 Declaration of Helsinki. Since the approval was obtained as part of established clinical practice, no specific code is required. The approval date corresponds to the session held on May 24, 2022. All participants signed an informed consent form before participating in the study
The THD was performed on a concrete floor with a 6-meter-long and 15 cm-wide tape (Figure 1).
Prior to the test, participants underwent a five minute warm-up on a stationary bicycle, followed by 10 bipedal squats, 10 right/left lunges, a one minute break, 10 bipedal vertical jumps, 10 bipedal horizontal jumps, a two minute break, and five vertical and horizontal jumps on each side. Participants then familiarized themselves with the THD by performing it twice per leg without any additional cognitive stimuli.
For each leg, three trials were performed for each test condition (single and dual task). Trials were always conducted starting with the healthy leg followed by the injured leg. To qualify as successful, the landing of the THD had to be maintained for two seconds. If the attempt was unsuccessful, verbal feedback was provided, and the test was repeated without additional instructions, typically requiring one or two extra trials. The mean distance from the three trials was used for analysis.
In the dual task condition, cognitive interference was introduced through the use of two smartphones (iPhone X) placed on tripods at eye level to the right and left of the
participant and an iPad in front showing a sequence of 8 numbers every 0.3 seconds through the switch-ON app. The phones displayed a color signal changing between blue, yellow, and green every 0.3 seconds. Participants began the test when the signal turned green, regardless of phone position. During the THD, participants were instructed to prioritize the cognitive task of observing the number sequence and recalling it in reverse order after completion of the physical test. The accuracy of the neuro-cognitive task was not assessed (Figure 2).
In research contexts, the effect of the dual task can be quantified as the percentage change in performance parameters between the dual task and single task, known as the Dual Task Cost (DTC).3 The dual task cost, as proposed by Grooms and colleagues,3refers to the decline in performance observed when an individual is required to simultaneously execute both a cognitive task and a physical task, such as during neurocognitive testing for ACL rehabilitation. In the context of ACL patients, this cost is typically evaluated by comparing performance on functional motor tasks (e.g., jumping, cutting, or balancing) with and without the addition of a concurrent cognitive task, such as responding to visual or auditory stimuli, or engaging in working memory challenges. The dual task cost is calculated by comparing performance on a task when it is performed in isolation (single task) to performance when it is paired with a concurrent cognitive task (dual task). The formula is typically According to the formula, a greater distance covered in the jump indicates better performance; thus, a DTC value below 100% indicates a performance deterioration in the dual-
Table 1. Demographic and clinical characteristics of the included patients.
Injured Leg
task condition, while a value above 100% reflects improved performance under dual-task conditions.
The sample size for this observational descriptive study was determined based on a hypothesized 15% reduction in jump height post-intervention from a literature-based mean (μ) of 547 cm to 464 cm, with a population standard deviation (σ) of 97 cm. The calculated effect size (d) was 0.8556701, indicating an expected 15% decrease. Thus, a sample size of 17 subjects was determined a priori to ensure 95% power (1−β), reflecting the likelihood of detecting a true effect.
All statistical analyses were performed using Stata version 18. Paired Student’s t-tests were used to compare the means of continuous variables with normal distributions. For continuous variables not meeting normality assumptions, nonparametric tests (Mann-Whitney-Wilcoxon or Kruskal-Wallis) were applied. Frequency distributions of categorical variables were compared using 2x2 contingency tables and chi-square tests.
Seventeen patients were included (mean age of 23.2 ± 5.1 years), 12 males and 5 females Detailed demographic and clinical characteristics of the patients are shown in Table 1
During the THD under ST conditions, a statistically significant difference in the average distance was observed between the healthy and operated limbs, with a mean difference of 20.7 +/- 3.64 cm (p= 0.016). A statistically significant difference was also noted under DT conditions, with a difference of 10.41 cm ± 22.6, (p= 0.038) between the healthy and operated limbs.
When comparing the differences in performance between the healthy and operated limbs DTC results were utilized and the results are presented in Table 2.
A higher percentage drop in performance for the healthy limb is demonstrated compared to the operated limb.
The most important finding of the present study is that patients who have undergone anterior cruciate ligament (ACL) reconstruction showed disparities between the healthy limb and the operated limb in the THD. Performance measurements were significantly better in the healthy limb versus the post operative limb.
An unexpected outcome was that the sample demonstrated a better dual task cost value on the post-operative limb compared to the healthy one. All subjects included in the study integrated traditional rehabilitation with motor learning that incorporated elements of distraction from the earliest stages. This allows us to hypothesize that the administered interventions might have addressed deficits that would have been expected. One possible explanation for the better result in DTC condition, is that the majority of the ‘neurocognitive’ training was performed on the postoperative leg. Patients generally engaged in strength and conditioning exercises for both limbs; however, the injured leg was more frequently targeted during dual-task conditions and cognitive challenges. These included tasks such as maintaining balance while tracking the movement of a laser pointer with their gaze or performing exercises like jumps and running drills while memorizing sequences of numbers displayed on a screen. Notably, there was a more pronounced performance decline in the healthy limb compared to the operated limb, further underscoring the complexity of the relationship between ACL reconstruction and motor skills. A second reason could be that neuroplastic alterations occur bilaterally, potentially affecting the healthy limb as well or strength deficit that could persist after completing rehabilitation program.20 Finally, due to deconditioning, the healthy limb may not always represent a realistic target for recovery It is important to note that In this research design, only the distance of the hop was measured, which do not incorporate metrics pertaining to quality, such as knee valgus motion or trunk displacement. Cognitive proficiency/scores, such as the accurate recall of numerical sequences were also not measured.
1=Average performance, 2=Best performance
Despite the modest magnitude of these disparities, the findings are crucial from both clinical and scientific perspectives. Clinically, they underscore the need to develop specific rehabilitative approaches that can precisely measure the effects of neurocognitive interventions based on the pathophysiology of ACL reconstruction that involves neuroplastic changes in the brain. In research, the examination of performance assessments assists in deepening the understanding of the effects of specific rehabilitation protocols, enhancing knowledge in a critical area of medical research. A previous study had investigated the effects of dual tasking in the context of the triple hop test, focusing on healthy subjects and finding no significant performance variations. In the current study, substantial differences were observed not only in healthy participants but also in those with ACLR, suggesting that both lower limbs could be negatively affected in terms of overall performance.
A strength of the study is its novelty in evaluating the effects of dual tasking on the outcomes of the triple hop test between six and 12 months after ACL reconstruction. This indicates that after ACL surgery, limited cognitive resources may not only impact the affected limb but also the uninjured limb. This consideration is crucial, especially given that a significant percentage of re-injuries occur in the contralateral limb.
Due to limited resources and the small number of patients recruited, it was not feasible to test subjects who had not undergone neuro-cognitive training as part of their rehabilitation. A limitation relates to the neuro-cognitive skills prescribed during the rehabilitation period prior to the tests. Rehabilitation tasks and progressions for these subjects were not reported.
Different variables could provide deeper insights into the observed differences between the performance of the healthy and operated limbs, which, while statistically significant, remained below a 15% threshold. As mentioned, this study considered performance only qualitatively (distance), while the influences of dual tasking on biomechanical quality (kinematics) were not accounted for due to resource limitations. Cognitive distraction could impact the quality of performance during activities and the subsequent risk of injury, highlighting the need for further exploration of the relationship between these variables. For instance, while performing a jump, the patient might recall numbers
displayed on a screen. If the uninjured leg achieves 4/4 correct responses but the injured leg achieves only 2/4, this may indicate a decline in cognitive performance during functional tasks.
Finally, strength data were not included in the testing session so it cannot be assumed that deficits in performance are a consequence of a decline in strength. Including a cognitive task evaluation within the functional assessment could provide valuable insights. The relatively small sample size and low proportion of female participants limit the generalizability of these findings to the broader population undergoing ACL reconstruction.
Although RTS testing impacts the risk of reinjury, it cannot fully predict who may sustain a second ACL injury.21, 22 Future research should evaluate dual-task effects in this population, focusing on both performance quantity (e.g., distance) and quality (e.g., kinematics and cognitive function). Investigating the impact of neurocognitive training or graft type on dual-task cost could provide further insights.
The results of this study demonstrate significant disparities in performance on the THD between the healthy and operated limbs of patients following anterior cruciate ligament (ACL) reconstruction, under both single and dual task conditions. Notably, the healthy limb exhibited a greater decline in performance, suggesting that both limbs are vulnerable to the effects of cognitive load post-surgery These insights suggest the potential value of incorporating neurocognitive elements in future research to better address the complex demands of real-world activities and explore strategies to reduce the risk of re-injury.
The results underscore the importance of considering both limbs in the rehabilitation process, reflecting the interconnectedness of bodily systems and the widespread impact of cognitive factors on motor performance. Further research is encouraged to explore the broader implications of dual task training in ACL rehabilitation and its potential to improve long-term outcomes for patients returning to sport.
Submitted: July 22, 2024 CST, Accepted: November 18, 2024 CST
© The Author(s)
This is an open-access article distributed under the terms of the Creative Commons Attribution 4.0 International License (CCBY-NC-4.0). View this license’s legal deed at https://creativecommons.org/licenses/by-nc/4.0 and legal code at https://creativecommons.org/licenses/by-nc/4.0/legalcode for more information.
1. Kakavas G, Malliaropoulos N, Pruna R, Traster D, Bikos G, Maffulli N. Neuroplasticity and anterior cruciate ligament injury. Indian J Orthop. 2020;54:275-280.
2. Ness BM, Zimney K, Kernozek T, Schweinle WE, Schweinle A. Incorporating a dual-task assessment protocol with functional hop testing. Int J Sports Phys Ther 2020;15(3):407-420. doi:10.26603/ ijspt20200407
3. Grooms DR, Chaput M, Simon JE, Criss CR, Myer GD, Diekfuss JA. Combining neurocognitive and functional tests to improve return-to-sport decisions following ACL reconstruction. J Orthop Sports Phys Ther 2023;53(8):415-419. doi:10.2519/ jospt.2023.11489
4. Wright RW, Magnussen RA, Dunn WR, Spindler KP Ipsilateral graft and contralateral ACL rupture at five years or more following ACL reconstruction: a systematic review J Bone Joint Surg Am 2011;93(12):1159-1165. doi:10.2106/JBJS.J.00898
5. Schiffner E, Latz D, Grassmann JP, et al. Anterior cruciate ligament ruptures: return to play and longterm outcome in elite handball players. Knee Surg Sports Traumatol Arthrosc. 2018;26(1):192-198.
6. Della Villa F, Hägglund M, Della Villa S, Ekstrand J, Waldén M. High rate of second ACL injury following ACL reconstruction in male professional footballers: An updated longitudinal analysis from 118 players in the UEFA Elite Club Injury Study Br J Sports Med 2021;55(23):1350-1356. doi:10.1136/ bjsports-2020-103555
7 Paterno MV, Rauh MJ, Schmitt LC, Ford KR, Hewett TE. Incidence of contralateral and ipsilateral anterior cruciate ligament (ACL) injury after primary ACL reconstruction and return to sport. Clin J Sport Med 2012;22(2):116-121. doi:10.1097/ JSM.0b013e318246ef9e
8. Herman DC, Barth JT Drop-jump landing varies with baseline neurocognition: Implications for anterior cruciate ligament injury risk and prevention. Am J Sports Med 2016;44(9):2347-2353. doi:10.1177/ 0363546516657338
9. Arden CL, Webster KE, Taylor NF, Feller JA. Return to sport following anterior cruciate ligament reconstruction surgery: a systematic review and meta-analysis of the state of play. Br J Sports Med. 2011;45(7):596-606. doi:10.1136/bjsm.2010.076364
10. Bertozzi F, Fischer PD, Hutchison KA, Zago M, Sforza C, Monfort SM. Associations between cognitive function and ACL injury-related biomechanics: a systematic review Sports Health 2023;15(6):855-866. doi:10.1177/19417381221146557
11. Mache MA, Hoffman MA, Hannigan K, Golden GM, Pavol MJ. Effects of decision making on landing mechanics as a function of task and sex. Clin Biomech. 2013;28(1):104-109. doi:10.1016/ j.clinbiomech.2012.10.001
12. Kipp K, Brown TN, McLean SG, Palmieri-Smith RM. Decision making and experience level influence frontal plane knee joint biomechanics during a cutting maneuver J Appl Biomech 2013;29(6):756-762. doi:10.1123/jab.29.6.756
13. Seymore KD, Cameron SE, Kaplan JT, Ramsay JW, Brown TN. Dual-task and anticipation impact lower limb biomechanics during a single-leg cut with body borne load. J Biomech 2017;65:131-137 doi:10.1016/ j.jbiomech.2017.10.021
14. Chaaban CR, Turner JA, Padua DA. Think outside the box: incorporating secondary cognitive tasks into return to sport testing after ACL reconstruction. Front Sports Act Living. 2023;4:1089882. doi:10.3389/ fspor.2022.1089882
15. Davies WT, Myer GD, Read PJ. Is it time we better understood the tests we are using for return to sport decision making following ACL reconstruction? A critical review of the hop tests. Sports Med 2017;47(5):763-775.
16. Abrams GD, Harris JD, Gupta AK, et al. Functional performance testing after anterior cruciate ligament reconstruction: a systematic review. Orthop J Sports Med. 2014;2(1):2325967113518305. doi:10.1177/ 2325967113518305
17 Lepley LK, Gribble PL, Thomas AC, Tevald MA, Sohn DH, Pietrosimone BG. Longitudinal evaluation of quadriceps strength and corticospinal excitability following anterior cruciate ligament reconstruction. Phys Ther. 2019;99(1):35-46.
18. Mazaheri M, Negahban H, Soltani M, et al. Effects of narrow-base walking and dual tasking on gait spatiotemporal characteristics in anterior cruciate ligament-injured adults compared to healthy adults. Knee Surg Sports Traumatol Arthrosc. 2017;25(8):2528-2535. doi:10.1007/ s00167-016-4014-4
19. Akhbari B, Salavati M, Ahadi J, et al. Reliability of dynamic balance simultaneously with cognitive performance in patientss with ACL deficiency and after ACL reconstructions and in healthy controls. Knee Surg Sports Traumatol Arthrosc 2015;23(11):3178-3185. doi:10.1007/ s00167-014-3116-0
20. Sherman DA, Rush J, Glaviano NR, Norte GE. Knee joint pathology and efferent pathway dysfunction: Mapping muscle inhibition from motor cortex to muscle force. Musculoskel Sci Pract 2024;103204. doi:10.1016/j.msksp.2024.103204
21. Millikan N, Grooms DR, Hoffman B, Simon JE. The development and reliability of 4 clinical neurocognitive single-leg hop tests: Implications for return to activity decision-making. J Sport Rehabil. 2019;28(5):536-544. doi:10.1123/jsr.2018-0037
22. Losciale JM, Zdeb RM, Ledbetter L, Reiman MP, Sell TC. The association between passing return-tosport criteria and second anterior cruciate ligament injury risk: A systematic review with meta-analysis. J Orthop Sports Phys Ther. 2019;49(2):43-54. doi:10.2519/jospt.2019.8190
Hannah
Jaime1,2 , Drew Rutherford2 , Becky Heinert3 , C. Nate Vannatta4,5 , Sherwin Toribio6 , Thomas W Kernozek2a
1 Sports Medicine, Gundersen Health System, 2 Health Professions, University of Wisconsin–La Crosse, 3 Health, Exercise and Rehabilitation Science, Winona State University, 4 Sports Medicing, Gundersen Health System, 5 Health Professions, University of Wisconsin-La Crosse, 6 Mathematics and Statistics, University of Wisconsin-La Crosse
Keywords: kinetics, motor learning, landing, knee
https://doi.org/10.26603/001c.127139
International Journal of Sports Physical Therapy
Vol. 20, Issue 1, 2025
Anterior cruciate ligament (ACL) tears often occur due to non-contact mechanisms in landing within females. Impact loading and aberrant landings may be addressed with augmented feedback training. The purpose of this study was to identify which female athletes most readily respond to a single session of augmented feedback to attenuate vGRF, by considering baseline peak vGFR and change in vGRF during training.
Design
Repeated Measures
Methods
One hundred, forty-seven female athletes landed from 50 cm onto two force platforms with and without augmented feedback of vertical ground reaction force (vGRF), vGRF symmetry, and lower extremity position. Each performed six baseline trials and two sets of six training trials with cues. Following training, athletes completed six post-feedback trials (with no feedback) and six dual-task (transfer) trials where they randomly caught a basketball during landing. Peak vGRF was measured. Mean responses were reported for the sets of six trials. Participants were grouped based on their responses to training. Linear regression was used to indicate how well initial performance and response predicted the final response.
Results
Four groups were identified, with 107 participants showing high baseline ground reaction forces and response to training. Only 23 participants (16.4%) did not respond to training. Baseline vGRF predicted post-feedback vGRF and transfer task vGRF (R2=0.508 and R2=0.400) across all participants. When change in vGRF was assessed following two blocks of augmented feedback training, prediction of responders improved with post-feedback vGRF and transfer vGRF (R2=0.911 and R2=0.761).
Conclusions
The combination of both baseline ground reaction force and response to initial training is more accurate than baseline measures alone in identifying those who respond to training. Assessing initial response to training may be necessary to more accurately identify individuals most likely to benefit from augmented feedback training and who may require further evaluation and training.
Corresponding Author: Thomas W. Kernozek, PhD, FASCM University of Wisconsin-La Crosse, Department of Health Professions 1300 Badger Street, La Crosse, WI 54601 e-mail: kernozek.thom@uwlax.edu a
Anterior cruciate ligament (ACL) injuries are common, with approximately 100,000 incidences occur annually 1‑3 Rehabilitation is lengthy,4 prognosis for developing osteoarthritis is high after ACL reconstruction5 which poses a significant annual healthcare burden.6 Over half of all sports-related ACL tears occur with a non-contact mechanism, with female incidence being two to eight times greater than males.7,8 Insufficient motor control and poor landing mechanics, such as increased dynamic knee valgus and shallow knee flexion angles, are hypothesized to increase ACL injury risk.9 Additionally, stiff landings,10 often characterized by increased vertical ground reaction force (vGRF)11 and axial compressive forces,12 have been associated with a greater risk of ACL injury. Many of these factors in non-contact injuries are movement-related which are more likely modifiable with training. Identifying and integrating strategies to address these factors may reduce ACL injury risk.13 Although the cause of injury is multifactorial and complex, in a recent prospective study vGRF was found to have the strongest association with sustaining an ACL injury.11 Furthermore, vGRF is easily measurable via force plates and may be a salient measure that is readily modifiable by athletes as previous work has demonstrated improved vGRF and knee alignment following augmented feedback.14
Since jumping and landing pose ACL injury risks to athletes in sport, motor learning approaches and strategies can be used to modify technique where four processes need to occur in rapid succession. Each athlete must strategically decide on the environment-specific goals of the task, integrate their motor perception, sequence any observed spatial targets, and effectively interpret the sequence of special targets into the desired muscle activation.15,16 The use of both knowledge of performance and knowledge of results provided via augmented feedback may better serve to facilitate the scaffolding of a cognitive bridge to more efficiently modify a given motor pattern.17
Insufficient neuromuscular coordination and execution during drop landing tasks place athletes at an increased risk of ACL injury 9,11,12,18,19 Incorporating two-dimensional (2-D) video footage and the use of augmented feedback has been an effective modality in manipulating the aberrant movement patterns reflected by neuromuscular deficits, displayed during landing.18,20‑22 Furthermore, it’s postulated that the inclusion of randomized, reactive movement patterns throughout training may further improve motor planning and skill acquisition integration into game-like scenarios.23 These understandings drive the foundational salience of a drop landing movement pattern as well as the importance of simultaneously completing a task that demands the athlete’s attention. The use of augmented feedback to train landing mechanics in adolescent female athletes suggests that individuals who experience initial vGRF > 3.77 body weight (BW) demonstrated the most change af-
ter training.24 Therefore, there seems to be a floor effect to the responsiveness of this type of training. Such a phenomenon may partially explain the difficulty of implementing large-scale and generalized preventative programs. It has been estimated that approximately 122 female athletes would need to be trained in a preventative program for one ACL injury to be avoided.13 Although such preventative training programs appear to be effective in reducing injury, if individual athletes with specific risk factors can be readily identified, this may increase the efficacy of such efforts. Since vGRFs have been identified as a risk factor for ACL injury risk, and it has been shown that some athletes respond more favorably to augmented feedback to reduce vGRFs, establishing a means to identify those individuals that may respond best to augmented feedback may improve the efficient delivery of preventative training. Therefore, the purpose of this study was to identify which female athletes most readily respond to a single session of augmented feedback to attenuate vGRF, by considering baseline peak vGFR and change in vGRF during training.
This study was approved by the University Institutional Review Board. Before participation, all participants or a parent/guardian provided their written informed consent following university guidelines. One hundred forty-seven females at regional high schools between the ages of 13-18 years participated in a single session. Age, height, body mass index, and Tegner level25 were recorded. All were actively participating female athletes in competitive organized sports. Exclusion criteria for the study included (1) any current lower extremity injury, (2) knee pain at rest or during running or jumping, (3) pregnancy, (4) any cardiovascular abnormalities or medical condition that limited training as indicated by a physical activity readiness questionnaire (PAR-Q).26 All participants utilized their own athletic footwear and wore comfortable athletic clothing.
Participants were asked to complete a drop landing from a 50-centimeter (cm) box onto two portable force plates after a self-selected warm-up and three trials for familiarization. Before testing, athletes were given verbal instructions for the landing task including: 1) jump forward with both feet and land with both feet on one of the force platforms, (2) jump bilaterally rather than stepping off the box, (3) land as to not to step from the force platforms, (4) return to standing after the landing. During data collection, trials were repeated if those requirements were not met. Data were collected generally in a gymnasium or common space on site with a ceiling height that could accommodate the experimental setup.
Athletes completed 30 landing trials divided into five blocks of six trials with a one-minute rest period between each trial block. During the initial block (Pre-test), participants were blinded to feedback to determine their baseline landing performance. Before beginning the training blocks, the athletes were provided with a brief orientation of the type of visual feedback they would receive on the visual feedback display following each trial. This information included peak vGRF displayed in body weight (BW), symmetry of lower extremity vGRF demonstrated through a bar graph, and frontal plane video that was replayed by the investigator in a slower motion to depict a qualitative impression of the performer’s body position during landing. No quantitative data was gathered from the video and presented during training.
Specific feedback was provided using the visual display by instructing participants to lower the overall height of the graph, try to make the graphs representing each leg even, and how knee position may have changed between trials (Figure 1). The baseline test was followed by the first training block where participants received post-trial feedback based on these data coupled with cues for improving their landing performance. Athletes were provided with both externally focused feedback first (e.g., “try to reduce the vGRF value”, “try to land quieter or more softly”) and internally focused feedback (e.g., “try to keep your knees over your toes,” “try to land with your knees out”). This feedback was individualized based on what was observed from the peak vGRF, vGRF symmetry, and impression from the frontal plane video of the landing. The feedback was based on the order of processing and displaying these data (peak vGRF, vGRF symmetry first, and then video performance). Data appeared within 20 seconds of the landing. The video was viewed at the same time in a separate window.
Athletes were given time to review the data with an investigator and relevant cues addressing their performance were provided. The next trial was then immediately performed such that the entire testing and feedback provided took approximately 20 minutes for all trial blocks. The general training and feedback provided were systematic where once vGRF showed substantial improvement, the next variable (symmetry or body positioning) was addressed. During the second training block, the feedback from the investigator was gradually withdrawn so that the athletes were asked to self-evaluate their peak vGRF, loading symmetry, and body position projected to the display
Following the completion of both training blocks, a posttest (six trial block) was completed without any verbal or visual feedback. Finally, a transfer task was examined where a dual task landing was performed without feedback. During the transfer task, participants were required to complete the landing task with a distracting element. This distraction was accomplished by having to respond to a ball being passed in their direction to catch it, or the pass would be faked. A completed or faked pass was completed in a randomized order Six trials were performed. Outcome data from the transfer condition performance trials were averaged.
Figure 1. Computer display depicting peak vertical ground reaction force in body weight, bar graph of the peak vertical ground reaction force in body weight on each leg, and a mirrored frontal plane video of each participant that is replayed to show body position and limb motion.
Kinetic data were collected in situ from two custom, highimpact force plates. Each force platform was positioned adjacent to one another and 25 cm in front of the 50 cm box. Each force platform had 4 calibrated load cells (ntep-1klb shear beam load cell) capable of measuring vertical force. Vertical ground reaction force data were sampled at 2000 Hz from each plate and data were normalized to each participant’s body weight. The system was validated in a pilot investigation of 20 participants where the custom force platforms were placed directly on top of two commercially available force platforms (Model 4080, Bertec Corporation, Columbus, OH) and sampled at 2000 Hz on both systems. The peak vGRF was well within 5% peak vGRF obtained with commercially available force platforms.14 This level of accuracy is similar to other portable force plate systems that have been validated against traditional force platforms.27 During each performance trial, a frontal plane video displaying each participant’s lower extremity was recorded from a high-speed camera at 100 Hz (DFK 23UV024, The Imaging Source, LLC, Charlotte, NC, USA). The camera was positioned 65 cm atop a tripod and located 130 cm from the force plates. Custom scripts were implemented within Visual Basic (Microsoft, Redmon, WA, USA) to calculate vGRF parameters and portray the video.
Participants were classified by initial baseline vGRF and response to augmented feedback training following training block 2. Previous work has suggested that impact force of greater than 3.77 BWs may indicate individuals most likely to benefit from augmented feedback training.24 Additionally, when investigating response to training over four weeks, a change of 0.25 BWs was the smallest change observed during this training.28 Thus, participants were grouped into four types of data: (1) those showing >3.77
Table 1. Participant numbers and demographics.
BWs initially and demonstrating an improvement >0.25 BW in training (High Baseline-Responders (HB-R)), (2) those showing >3.77 BWs initially and demonstrating an improvement <0.25 BWs in training (High Baseline-Non-responders (HB-NR)), (3) those showing <3.77 BWs initially and demonstrating an improvement >0.25 BWs in training (Low Baseline-Responders (LB-R)), and (4) those showing <3.77 BWs initially and demonstrating an improvement of <0.25 BWs in training (Low Baseline-Non-responders (LBNR)).
Linear regression was then used to quantify how well initial baseline vGRF measures and change in vGRF measures could be used to predict response to augmented feedback training. Pre-test vGRF, change following training block 1, and change following training block 2 were included in a hierarchical regression model to predict mean post-test vGRF and transfer vGRF using SPSS (Version 28, IBM, Aramonk, NY).
A total of 147 athletes participated and were classified into these four groups (Table 1). The first group consisted of 107 participants with high baseline vGRFs and responded to augmented feedback training (HB-R). The second group consisted of 11 participants with high baseline vGRFs but did not respond to augmented feedback training (HB-NR). The third group was comprised of 17 participants with low baseline vGRFs and responded to augmented feedback training (LB-R). The final group contained 12 participants with low baseline vGRFs but did not respond to augmented feedback training (LB-NR).
Baseline vGRFs, changes in vGRFs with augmented feedback training, post-test vGRFs, and transfer vGRFs are reported in Table 2 and displayed in Figure 2 Twenty-three athletes did not appear to respond to augmented feedback training and initial measures of vGRF were not sufficient to identify these athletes. There was a nearly equal number of low baseline non-responders (LB-NR) and high baseline non-responders (HB-NR) (12 vs. 11, respectively).
In the HB-R group, individuals showed a reduction of 26.7% in vGRF after training block 1 of augmented feedback. This was followed by a further improvement of 7.01% after training block 2. These participants were able to reduce their vGRF during the post-test and transfer tasks compared to baseline scores by 27.17% and 16.82%, respectively Those with high baseline vGRFs but did not respond to augmented feedback training (HB-NR) showed less than 4% improvement in vGRF at any point during training and
even increased 8.78% compared to baseline vGRFs during the transfer task.
In those with low baseline vGRFs, 17 participants athletes demonstrated further reductions in vGRF with augmented feedback training (LB-R). They exhibited a 11.95% reduction in vGRF after training block 1 of augmented feedback, with vGRF exhibiting another 5.96% reduction after training block 2. This subset of participants reduced their vGRFs by 17.96% and 7.19% during the posttest and transfer tasks, respectively Those not responding to augmented feedback training but demonstrating an initial low baseline vGRF, demonstrated less than 5% change across all blocks (LB-R).
Linear regression revealed that baseline vGRF explained over 50% of the variance in post-test vGRF (R = 0.713; R2 = 0.508). Adding the change score from augmented feedback training block 1 improved the model (R = 0.883; R2 = 0.780) and including the change score from training block 2 improved the model further (R = 0.955; R2 = 0.911). When assessing the transfer task, linear regression revealed that baseline vGRF explained 40% of the variance in vGRF (R = 0.633, R2 = 0.400). Utilizing the change score from augmented feedback training block 1 improved the model (R = 0.797, R2 = 0.635) with the change score from training block 2 resulted in further improvements in the model (R = 0.872, R2 = 0.761).
The purpose of this study was to assess how baseline impact force and single session response to augmented feedback training can be used to identify those most likely to benefit from this training. Baseline ground reaction force appears to be a relatively strong predictor of those that will respond to augmented feedback training explaining 50% of the variance in reductions in vGRF as a stand-alone measure. However, there appears to be a subset of athletes who, despite showing high baseline vGRFs, do not respond to augmented feedback training. Assessing their response to at least one round of augmented feedback training appears necessary to identify these individuals. Thus, attempts to identify those most likely to benefit from augmented feedback training will likely need to incorporate both screenings of baseline vGRFs and at least one trial of augmented feedback training.
An athlete’s responsiveness to augmented feedback training appears to be different based on an athlete’s baseline vGRF in landing. Heinert et al.24 observed when dividing participants into quartiles according to their baseline peak vGRF, those in the highest quartile, with an initial
Table 2. Means (standard deviation) of six trials of vertical ground reaction force (vGRF) in Body Weight following each testing or training block.
Testing Block
block 1
block 2
Figure 2. Mean peak vertical ground reaction force for each grouping of athletes of testing blocks. Legend: All = All athletes, High baseline -responders (HB-R), High baseline - non-responders (HB-NR). Low baseline –responders (LB-R), and Low baseline – non-responders (LB-NR)
vGRF ≥ 4.30 times BW displayed a 32% reduction in their peak vGRF while those in the lowest quartile (vGRF<3.77 BW) demonstrated only a 15% decrease in vGRF. Those who showed such small improvements after training were largely unchanged during the transfer task suggesting that there may be limited efficacy for some individuals as they had minimal room for improvement. This quartile data was used to inform our threshold for those athletes who appeared to have greater room for improvement in reducing vGRF. Similarly, athletes were classified in this manner who exhibited a reduction in vGRF > 0.25 BW from augmented feedback training. This was based on Heinert et al.28 who reported the retention in the reduction of vGRF > 0.25 BW after the completion of 4 weeks of augmented feedback training. Considering these distinct differences in responses of athletes, terminology that refers to these differ-
ences can be introduced. A “responder” referred to an athlete who responded favorably to augmented feedback and a “non-responder” referred to an athlete who displayed minimal to no change following the training intervention.29 When utilizing both metrics (vGRF and change in vGRF from a single session of augmented feedback training), an athlete’s likelihood of vGRF improvement during the postfeedback block and transfer task block was greater
Most athletes were classified as high baseline-responders (HB-R) (n=107) and appeared to respond favorably to a single session of augmented feedback training to reduce vGRF. Kiminski et al.30 similarly used augmented feedback with Division III female collegiate athletes that explored deceleration with landing during a sport-specific movement (blocking for volleyball or rebounding for basketball) both before and following training. These individuals reported a 0.63 g or 11.5% reduction in the mean peak deceleration following training, reflecting the additional transfer to a more game-like activity related to vGRF.
Myer et al.18 (2013) reported female athletes under the age of 18 demonstrated a larger reduction in risk (72%) compared to those over 18 years old who had a risk reduction of 16%. Their risk was determined by evaluating biomechanical abnormalities in landing mechanics such as increased knee valgus, diminished trunk stability, and increased lateral trunk motion. Their data supports the notion that there may be a particular age range where skill acquisition and aptitude for skill refinement may be optimal and that this may occur before the integration of neuromuscular deficits and maturation. The augmented feedback training provided for these individuals may be effective based on their baseline landing performance as well as their concurrent affinity for improvement during training. The athletes classified in our study demonstrated a near 50% reduction in vGRF on the post-test and readily responded to augmented feedback within training block 1. There seems to be a large portion of athletes who may benefit and respond to augmented feedback training.
Additionally, 17 athletes were classified as “low baseline vGRF” and were deemed less likely to benefit from aug-
mented feedback training, but still showed changes greater than 0.25 BW (low baseline-responders (LB-R)). This group showed a lower magnitude of change overall with augmented feedback training and showed posttest and transfer task vGRF values nearly identical to the low baseline-nonresponder (LB-NR) group. Although on average this group showed a reduction in vGRF of nearly 18% it is uncertain how this may translate to reducing injury risk given their low baseline vGRF. Thus, the utility of this type of augmented feedback training in this group remains an area to further investigate.
Low baseline-non-responders (LB-NR) athletes (n=12) had a low vGRF during baseline testing, so there was likely a floor effect regarding their ability to improve beyond these relatively low-impact values. These athletes appear to be non-responders to augmented feedback training; however, they also may be at relatively low risk due to the reported inverse relationship between vGRF and ACL injury risk.11, 19,31 These athletes may not require much, if any, drop landing augmented feedback training to reduce their vGRF.
The high baseline-non-responders (HB-NR) athletes (n=11) did not demonstrate marked improvement with augmented feedback trials despite having high baseline vGRFs. These athletes may require additional attention. It is possible they may need more augmented feedback training, another type of training or feedback mechanism, or may have other impairments (i.e., lack of strength or proprioception) that preclude their ability to respond to a single session of training. A meta-analysis by Sugimoto et. al.32 reported a larger volume, characterized by both session duration and frequency, of preventative training (>30 min/week) increased the effectiveness of ACL injury risk reduction. As such, these individuals may benefit from additional practice or assessment. However, it may be worth noting, that the studies analyzed in this meta-analysis provided intervention throughout an entire pre-season and/or season. As such, while we’re addressing peak vGRF, the further assessment of neuromuscular control may require supplementary evaluation of other constructs, such as gross multi-directional hip strength,33 quadriceps limb symmetry index,34 and rate of force development.34 More robustly evaluating female athletes may require multi-modal interventions to ameliorate injury risk.
While the ideal integration for more autonomous motor learning would require a robust multi-session program, the time and financial cost of this approach makes this less feasible for universal integration in high school sports. The proposed single-session evaluation and augmented feedback training may provide a targeted means to stratify
those who better respond to such training based on their specific presentation. Equipping adolescent athletes, their coaches, strengthening and conditioning staff, and athletic trainers with data that identifies “responders” and “ nonresponders”, may allow better understanding of each athlete’s landing performance. Assessing baseline vGRF and response to initial augmented feedback training appears to be a feasible avenue to identify those most responsive to training and those who may benefit from additional evaluation or intervention within a single session.
This study was performed over a single session with the inclusion of a transfer task block to assess for carryover of learning into a more game-like scenario; however, without a follow-up retention measure at a secondary point in time, the long-term efficacy of the augmented feedback training is speculative. Heinert et al.28 performed a study with a similar training protocol that examined the efficacy of a four-week training program on retention in NCAA Division III volleyball players, who were able to demonstrate weekto-week carryover, with an overall 28.8% reduction in vGRF. In this sample of female athletes, it is unknown how many, if any, of the athletes incurred an ACL injury throughout the year However, this was not the point of this study developed to assess ACL injury risk mitigation, and injury data were not collected. Finally, the methodology did not include a control group, so the performance improvements may be due to the augmented feedback training, further investigation may be needed using a randomized controlled trial design.
Assessing baseline vGRF alone does not entirely capture who may or may not respond to augmented feedback training. The combination of baseline vGRF and change in vGRF during augmented feedback training may be used to identify those most apt to respond to a single session of training, and those who may require further evaluation and/or focused training beyond augmented feedback. These findings may be important to sports health professionals regarding the implementation and use of augmented feedback training for female athletes related to ACL injury risk mitigation during landing.
Submitted: April 16, 2024 CST, Accepted: October 20, 2024 CST
© The Author(s)
This is an open-access article distributed under the terms of the Creative Commons Attribution 4.0 International License (CCBY-NC-4.0). View this license’s legal deed at https://creativecommons.org/licenses/by-nc/4.0 and legal code at https://creativecommons.org/licenses/by-nc/4.0/legalcode for more information.
1. Flynn RK, Pedersen CL, Birmingham TB, Kirkley A, Jackowski D, Fowler PJ. The familial predisposition toward tearing the anterior cruciate ligament: a case control study Am J Sports Med 2005;33(1):23-28. doi:10.1177/0363546504265678
2. Griffin LY, Albohm MJ, Arendt EA, et al. Understanding and preventing noncontact anterior cruciate ligament injuries: a review of the Hunt Valley II meeting, January 2005. Am J Sports Med. 2006;34(9):1512-1532. doi:10.1177/ 0363546506286866
3. Renström PA. Eight clinical conundrums relating to anterior cruciate ligament (ACL) injury in sport: recent evidence and a personal reflection. Br J Sports Med. 2013;47(6):367-372. doi:10.1136/ bjsports-2012-091623
4. Grindem H, Snyder-Mackler L, Moksnes H, Engebretsen L, Risberg MA. Simple decision rules can reduce reinjury risk by 84% after ACL reconstruction: the Delaware-Oslo ACL cohort study Br J Sports Med 2016;50(13):804-808. doi:10.1136/ bjsports-2016-096031
5. Lohmander LS, Ostenberg A, Englund M, Roos H. High prevalence of knee osteoarthritis, pain, and functional limitations in female soccer players twelve years after anterior cruciate ligament injury Arthritis Rheum. 2004;50(10):3145-3152. doi:10.1002/ art.20589
6. Herzog MM, Marshall SW, Lund JL, Pate V, Spang JT. Cost of outpatient arthroscopic anterior cruciate ligament reconstruction among commercially insured patients in the United States, 2005-2013. Orthop J Sports Med. 2017;5(1):2325967116684776. doi:10.1177/2325967116684776
7 Arendt EA, Agel J, Dick R. Anterior cruciate ligament injury patterns among collegiate men and women. J Athl Train 1999;34(2):86-92.
8. Agel J, Arendt EA, Bershadsky B. Anterior cruciate ligament injury in national collegiate athletic association basketball and soccer: a 13-year review. Am J Sports Med 2005;33(4):524-530. doi:10.1177/ 0363546504269937
9. Larwa J, Stoy C, Chafetz RS, Boniello M, Franklin C. Stiff landings, core stability, and dynamic knee valgus: A systematic review on documented anterior cruciate ligament ruptures in male and female athletes. Int J Environ Res Public Health 2021;18(7):3826. doi:10.3390/ijerph18073826
10. Laughlin WA, Weinhandl JT, Kernozek TW, Cobb SC, Keenan KG, O’Connor KM. The effects of singleleg landing technique on ACL loading. J Biomech. 2011;44(10):1845-1851. doi:10.1016/ j.jbiomech.2011.04.010
11. Leppänen M, Pasanen K, Kujala UM, et al. Stiff landings are associated with increased ACL injury risk in young female basketball and floorball players. Am J Sports Med. 2017;45(2):386-393. doi:10.1177/ 0363546516665810
12. Boden BP, Sheehan FT Mechanism of noncontact ACL injury: OREF Clinical Research Award 2021. J Orthop Res 2022;40(3):531-540. doi:10.1002/ jor.25257
13. Pfile KR, Curioz B. Coach-led prevention programs are effective in reducing anterior cruciate ligament injury risk in female athletes: A numberneeded-to-treat analysis. Scand J Med Sci Sports. 2017;27(12):1950-1958. doi:10.1111/sms.12828
14. Kernozek TW, Rutherford D, Heinert B, et al. Posttrial feedback alters landing performance in adolescent female athletes using a portable feedback system. Int J Sports Phys Ther 2021;16(1):87-95. doi:10.26603/001c.18808
15. Willingham DB. A neuropsychological theory of motor skill learning. Psychol Rev 1998;105(3):558-584. doi:10.1037/ 0033-295x.105.3.558
16. Masaki H, Sommer W Cognitive neuroscience of motor learning and motor control. J Phys Fit Sports Med. 2012;1(3):369-380. doi:10.7600/jpfsm.1.369
17 Moinuddin A, Goel A, Sethi Y The role of augmented feedback on motor learning: A systematic review. Cureus. 2021;13(11):e19695. doi:10.7759/ cureus.19695
18. Myer GD, Sugimoto D, Thomas S, Hewett TE. The influence of age on the effectiveness of neuromuscular training to reduce anterior cruciate ligament injury in female athletes. Am J Sports Med 2013;41(1):203-215. doi:10.1177/0363546512460637
19. Hewett TE, Myer GD, Ford KR, et al. Biomechanical measures of neuromuscular control and valgus loading of the knee predict anterior cruciate ligament injury risk in female athletes: a prospective study Am J Sports Med 2005;33(4):492-501. doi:10.1177/0363546504269591
20. Onate JA, Guskiewicz KM, Sullivan RJ. Augmented feedback reduces jump landing forces. J Orthop Sports Phys Ther 2001;31(9):511-517 doi:10.2519/jospt.2001.31.9.511
21. Welling W, Benjaminse A, Gokeler A, Otten B. Enhanced retention of drop vertical jump landing technique: A randomized controlled trial. Hum Mov Sci. 2016;45:84-95. doi:10.1016/j.humov.2015.11.008
22. Oñate JA, Guskiewicz KM, Marshall SW, Giuliani C, Yu B, Garrett WE. Instruction of jump-landing technique using videotape feedback: altering lower extremity motion patterns. Am J Sports Med 2005;33(6):831-842. doi:10.1177/0363546504271499
23. Yadollahi A, Zarei M, Gheiitasi M. The effect of eight weeks reactive neuromuscular training on lower extremity kinematics during landing in female basketball players. Stud Sport Med. 2022;14(33):59-80. doi:10.22089/ smj.2022.12556.1594
24. Heinert B, Rutherford D, Kernozek TW. Effectiveness of augmented feedback on drop landing using baseline vertical ground reaction in female athletes. Int J Athl Ther Train. 2022;28(1):40-45. doi:10.1123/ijatt.2021-0132
25. Tegner Y, Lysholm J. Rating systems in the evaluation of knee ligament injuries. Clin Orthop. 1985;(198):43-49. doi:10.1097/ 00003086-198509000-00007
26. Shephard RJ. PAR-Q, Canadian Home Fitness Test and exercise screening alternatives. Sports Med Auckl NZ 1988;5(3):185-195. doi:10.2165/ 00007256-198805030-00005
27. Mylonas V, Chalitsios C, Nikodelis T. Validation of a portable wireless force platform system to measure ground reaction forces during various tasks. Int J Sports Phys Ther 2023;18(6):1283-1289. doi:10.26603/001c.89261
28. Heinert B, Rutherford D, Cleereman J, Lee M, Kernozek TW. Changes in landing mechanics using augmented feedback: 4-Week training and retention study. Phys Ther Sport. 2021;52:97-102. doi:10.1016/ j.ptsp.2021.08.007
29. Taylor JB, Nguyen AD, Shultz SJ, Ford KR. Hip biomechanics differ in responders and nonresponders to an ACL injury prevention program. Knee Surg Sports Traumatol Arthrosc 2020;28(4):1236-1245. doi:10.1007/ s00167-018-5158-1
30. Kiminski R, Williams C, Heinert B, et al. Transfer of post-trial feedback on impacts during drop landings in female athletes. Sports Biomech. Published online August 30, 2022:1-15. doi:10.1080/ 14763141.2022.2114931
31. Bates NA, Ford KR, Myer GD, Hewett TE. Impact differences in ground reaction force and center of mass between the first and second landing phases of a drop vertical jump and their implications for injury risk assessment. J Biomech 2013;46(7):1237-1241. doi:10.1016/j.jbiomech.2013.02.024
32. Sugimoto D, Myer GD, Foss KDB, Hewett TE. Dosage effects of neuromuscular training intervention to reduce anterior cruciate ligament injuries in female athletes: meta- and sub-group analyses. Sports Med 2014;44(4):551-562. doi:10.1007/s40279-013-0135-9
33. Collings TJ, Diamond LE, Barrett RS, et al. Strength and biomechanical risk factors for noncontact ACL injury in elite female footballers: A prospective study. Published online August 1, 2022. doi:10.1249/MSS.0000000000002908
34. Zore MR, Kregar Velikonja N, Hussein M. Pre- and post-operative limb symmetry indexes and estimated preinjury capacity index of muscle strength as predictive factors for the risk of ACL reinjury: A retrospective cohort study of athletes after ACLR. Appl Sci 2021;11(8):3498. doi:10.3390/app11083498
Kreisel BR, Scott KM, Florkiewicz EM, et al. The Relationship Between Self-Efficacy, Aerobic Fitness, and Traditional Risk Factors for Musculoskeletal Injuries in Military Training: A Prospective Cohort Study. IJSPT. 2025;20(1):55-69. doi:10.26603/001c.127137
Brian R Kreisel1,2a , Kelly M Scott1 , Erin M Florkiewicz1,3 , Michael S Crowell1,4 , Jamie B Morris1 , Paige A McHenry1 , Timothy M Benedict1
1 Baylor University-Keller Army Community Hospital Division 1 Sports Physical Therapy Fellowship, 2 Department of Rehabilitative Services, Martin Army Community Hospital, 3 PhD in Health Sciences, Rocky Mountain University of Health Professions, 4 Doctoral Physical Therapy Program, University of Scranton
Keywords: self-efficacy, military training injuries, musculoskeletal injury risk factor, aerobic fitness, prior injury https://doi.org/10.26603/001c.127137
International Journal of Sports Physical Therapy
Vol. 20, Issue 1, 2025
Background
The United States military strives to prepare soldiers physically and mentally for war while preventing injury and attrition. Previous research has focused on physical injury risk factors but has not prospectively examined psychological risk factors.
Purpose
This study’s purpose was to investigate whether self-efficacy is a risk factor for musculoskeletal injury in an initial military training environment and compare it to other known risk factors.
Study Design
Prospective, Longitudinal Cohort Study
Materials and Methods
Shortly after starting cadet basic training, new cadets rated self-efficacy by an 11-point questionnaire. Other risk factor data including injury history, sex, height, weight, body mass index, age, aerobic fitness, upper body muscular endurance, core muscular endurance and previous military experience were collected by self-report questionnaire and military fitness testing. The primary dependent variable was musculoskeletal injury that originated during the seven-week course. Independent variables were compared between participants who were and were not injured using Chi-squared test, t-tests, Cox regression analysis and time to injury was evaluated using Kaplan-Meyer survival analyses.
Seven hundred eighty-one (65.1%) new cadets were eligible and consented to participate. Injured cadets had significantly lower self-efficacy scores (p=0.003 and p=<0.001), shorter height (p=<0.001), lower weight (p=0.036), lower push-up and plank performance (p=<0.001), slower two-mile run performance (p=<0.001), and females sustained a proportionally higher number of injuries than males (p=<0.001). Cadets with low self-efficacy, shorter height, lower hand release push-up performance, lower plank performance and slower two-mile run performance were at greater risk for
a
Corresponding Author:
Brian R. Kreisel
6600 Van Aalst BLVD
Fort Moore, GA 31905
Email Address: brian.kreisel@gmail.com Telephone Number: 762-408-1641
musculoskeletal injury Cadets with less self-efficacy were also less likely to continue uninjured throughout cadet basic training according to a Kaplan-Meier survival analysis (log rank test<0.002). Multivariable Cox regression revealed that only aerobic fitness predicted musculoskeletal injury (HR=1.005 [1.003-1.006], p=<0.001).
Participants with less self-efficacy sustained injuries earlier and more often than those with greater self-efficacy However, aerobic fitness alone predicted future injury after controlling for all risk factors. Resolved prior injury was not a risk factor for future injury
Level of Evidence 2b
Individual cohort study
The steady decline in national physical activity levels and increases in overweight and obese adolescents1,2 has decreased the number of individuals eligible for military service3 and widens the physical readiness gap between the general population and military physical demands.4 This disparity complicates training physically capable recruits without injuring those who are less fit and highlights the need of minimizing preventable attrition such as those from musculoskeletal injury (MSK-I). Despite efforts to improve soldier readiness, more than half of all soldiers experience an injury annually.5 As many as 58,400 soldiers were not able to deploy in April 2020, which is the equivalent of 13 brigade combat teams.6 MSK-Is are the leading cause of non-deployability, lost duty days (LDD), medical encounters, military discharge, and disability 7,8 In 2018 the U.S. Army spent $434 million in direct medical costs to treat MSK-Is.7
The leading causes of MSK-Is are the same activities that leaders deliberately conduct to develop physical resilience9 including running, load carriage, other physical fitness training activities (besides running) and work-related tasks.8 Physical training results in the highest number of total injuries, while load carriage causes greater injuries per exposure.10 Other common causes of MSK-Is include overexertion, falls/slips, and occupational accidents.11
Researchers have identified multiple physical and behavioral MSK-I risk factors including prior injury, low aerobic endurance,12‑16 high or low body mass index (BMI),17 and low muscular strength and endurance.18,19 Multiple previous studies have demonstrated that prior injury strongly predicts future injury14,16; however, it is unclear whether these previous injures had fully resolved or not. Female biological sex is a long-accepted risk factor for MSK-I,20‑22 but recent research suggests this may be due to differences in average physical fitness rather than biological sex-exclusive differences.12,23‑26 Military leaders have used these and similar study outcomes to develop injury prevention programs including physical fitness training regimens, MSK-I risk screening, and leader education with mixed results.10,27‑29
These epidemiological studies summarize the dilemma: service members with prior injury and lower fitness levels tend to get injured while training to improve their physical fitness. It is possible that other modifiable risk factors exist
that, if trained, may reduce injuries without such high injury risk.
Albert Bandura (1977) introduced self-efficacy as one’s confidence to perform a specific task. In behavioral psychology, this concept helps patients and clinicians establish a therapeutic path by accomplishing individualized, increasingly difficult tasks.30 Multiple subsequent publications have proposed positive relationships between selfefficacy and self-regulation,31 performance,31‑33 goal-setting,34 and motor learning.35
Researchers have also found that psychological factors predict military performance outcomes.32,36,37 Gruber et al. found that for every one point increase on the new general self-efficacy scale, participants were 77.7% less likely to voluntarily withdraw assuming the other factors remained constant. Self-efficacy did not predict medical withdrawal; however, this study did not report injury statistics.36 In an initial entry Army training environment, Moran et al. found that psychological burnout was predictive of MSK-I, but did not measure self-efficacy 38 To date, no studies have reported the prospective relationship between self-efficacy, other known risk factors, and MSK-I in an initial entry military training environment.
Cadet basic training at the United States Military Academy (USMA) is a seven-week initial entry training course with physical demands similar to basic combat training. Many traditional risk factors for MSK-I have been investigated previously in service academy cadets, but the relationship between self-efficacy and injury has not. This study’s purpose was to investigate whether self-efficacy is a risk factor for MSK-I in an initial military training environment and compare it to other known risk factors. The researchers hypothesized that self-efficacy (overall and taskspecific) would be lower among new cadets who sustain an MSK-I and negatively associated with MSK-I over time throughout cadet basic training.
This was a prospective cohort study investigating the relationships between self-efficacy, other known intrinsic and extrinsic risk factors and MSK-I in an initial entry military setting.
All 1,200 incoming USMA new cadets were eligible to participate in this study The Portsmouth Naval Medical Center Institutional Review Board (IRB) and USMA administrators reviewed and approved the study procedures prior to initiation. Participation was voluntary.
All participants were USMA new cadets completing cadet basic training from June to August 2022. Cadet basic training introduces new cadets to military skills while physically and psychologically preparing them to be service academy cadets and future officers. Some of the most strenuous and injury-causing events include running and strength training during morning physical fitness training (every week), the Army Combat Fitness Test (ACFT) (Weeks 5-6), land navigation (Weeks 3-4), and the final 12-mile ruck march (Week 7). Cadets also practice tactical skills including individual movement techniques, basic marksmanship, water survival, communication skills, and emergency medical treatment. The researchers recruited participants and collected all demographic, injury history, and self-efficacy data on Day 8 of 44 following a verbal study explanation and written description.
All English-speaking new cadets qualified to begin cadet basic training were eligible for inclusion. Cadets were excluded only if they declined to participate, had not fully recovered from a previous injury, were already injured at the time of the consent/survey, or attritted from cadet basic training for reasons other than MSK-I. All participants under 18 years old were considered emancipated minors and did not require parental consent. All participants who consented for this study also consented participation to a randomized controlled trial by Scott et al. related to back pain beliefs and maximum deadlift. All randomization and interventions for this study were conducted separately and included same-day back pain resilience education for all participants to prevent subsequent pain avoidance behaviors during Week 5 or 6 of cadet basic training.39
Reporting prior injuries is a sensitive topic due to fear of administrative disqualification, so the researchers assured the new cadets’ confidentiality whenever asked to self-report prior or current injury All participants who reported a history of injury within the past year classified themselves as either completely recovered (1), mostly recovered (2), or not recovered (3) and completed the Single Assessment Numeric Evaluation (SANE). The SANE required the participants to rate the previously injured body part on a scale of 0% to 100% and has demonstrated reliability and concurrent validity with other injury-specific outcome measures.40‑43 Patient Acceptable Symptom State (PASS) thresholds for SANE scores range from 65.5% to 82.5%, but there is no evidence-based threshold when an injured individual has fully recovered.44‑46 In the present study, participants were excluded if they described themselves as
“not recovered” regardless of SANE score or if they described themselves as “mostly recovered” or “completely recovered” with a SANE score of less than 80%. This was to ensure the unresolved prior injuries (or a currently injured state) did not affect participants’ self-efficacy to complete CBT and specific events without injury.
The primary outcome of interest was either new injury or recurrence of a previously resolved musculoskeletal or neurological injury Systemic conditions, skin reactions, and infections were excluded as well as blisters and ingrown toenails which are commonly treated by on-site U.S. Army medics without duty limitations. The researchers operationally defined injuries as a neuromusculoskeletal condition which required care at a medical treatment facility, with or without duty limitations.20,21,47 This definition eliminated recall bias and the difficulty of obtaining selfreported injury status, which might not have been serious enough to seek medical treatment. Time was recorded as the number of days from study consent to the initial medical encounter
The primary independent variable of interest was the participants’ self-efficacy to complete cadet basic training without injury and self-efficacy to complete the three most injury-causing and physically demanding events without injury (land navigation physical demands, the 12-mile ruck march, and the ACFT). Although scales for general and pain self-efficacy exist, these scales lack task-specific concordance with the cadet basic training events.48 The researchers developed task-specific self-efficacy questions (Appendix A) following guidance from Bandura and other previous studies.32,49 The consent form and survey were piloted among a group of first-year cadets to ensure clarity, accuracy and cultural appropriateness. The participants rated their self-efficacy prospectively on Day 8 via the questionnaire with an 11-point numerical rating scale from 0 (no confidence) to 10 (fully confident). The combined event-specific self-efficacy item combined the three scores from land navigation athletic demands, the 12-mile ruck march, and the ACFT for a maximum score of 30 (items 2-4, Appendix A). Prior to collecting data, the researchers selected approximately 70% as the cut-off for low self-efficacy based on previous research.50,51 We also planned on visually inspecting the data in quartiles and tertiles in comparison with our cut-off score.32,52 Participants completed this questionnaire immediately after consenting to participate. The baseline questions survey demonstrated good internal consistency according to Cronbach’s alpha (0.817).
Other independent variables included age, biological sex, height, weight, previous military experience, performance motivation, athletic intentions, history of injury within the past year, current level of relative function (only for those with a history of previous injury), baseline aerobic fitness (2-mile run time), baseline upper body muscular endurance (hand release push-ups in 2 minutes), and anterior
core muscular endurance (maximum plank duration). Age and sex were both self-reported and verified with the participants’ medical record. Injury history, previous military experience, academy overall performance motivation, athletic intentions (intending to participate in division 1, club or intramural athletics), height, and weight were also collected by self-report. Self-reported height and weight has previously demonstrated validity in the military population.53 The service academy cadet basic training cadre and instructors conducted the 2-mile run, hand release push-up and plank performance assessments according to military testing standards54 on days 3 to 5.
The researchers received demographic and performance information from USMA administrators. Participants self-reported additional demographic information, previous experiences, previous injury, and self-efficacy using a paper-based survey The researchers collected new injury data individually using the Armed Forces Health Longitudinal Technology Application (AHLTA) and the Cadet Injury and Illness Tracking System (CIITS). CIITS is a program unique to this service academy for all participation-limiting injuries. To protect from bias, all other variable data (including self-efficacy data) were hidden during injury surveillance. Additionally, 10% of all questionnaire responses and injury designation data were checked by another researcher for accuracy
The injury study period lasted 36 days during cadet basic training and the injury surveillance additionally lasted for the entire following semester (174 total days from start to finish). This additional surveillance time was to capture injuries that began during cadet basic training but either received delayed medical care, did not resolve with time alone or worsened during the fall semester The additional surveillance time did not include any injuries that occurred after cadet basic training ended. The researchers also captured days until the approximate onset of symptoms and days between the onset of symptoms until the medical encounter
After excluding ineligible participants (Figure 1), the researchers conducted descriptive analyses to compare the independent variables between injured and non-injured participants. Missing values were excluded with pairwise deletion. The researchers recorded and analyzed all data using Statistical Package for the Social Sciences (SPSS), version 28 (IBM Corps, Armonk, NY, 2021).
The independent samples t-test was used to compare continuous data, chi-square test was used to compare categorical data, and effect sizes were calculated with Cohen’s d and phi.
The researchers used a Kaplan-Meier survival analysis to determine whether the risk of injury throughout cadet basic training was different between those with low or high self-efficacy New cadets who reported self-efficacy of 7/10 or less to complete all of cadet basic training or 24/30 or
less to complete the three combined specific events without injury were identified as having low self-efficacy and compared to new cadets with 8/10 or 25/30 or higher self-efficacy, respectively. The new cadet population was a fixedsized, closed cohort that began and ended training on the same days. There were some differences in daily training schedules, but the cohort completed training events within the same week. Kaplan-Meier analysis assumptions were met by excluding all new cadets with current or unresolved injuries, beginning the surveillance period simultaneously, defining the censoring event as the first medical encounter date, and by terminating survival at the day of the first medical encounter or right-censoring at 174 days. Subsequent injuries sustained by the same participant were not included in the analysis. The researchers used the log-rank test (α < 0.05) to determine whether the time to event was statistically significant between new cadets with high versus low self-efficacy.
Individual variables predictive of future injury were identified using univariable Cox proportional hazard ratios. The researchers purposefully selected variables similar to methods in previous literature based on previously established risk factors.32,55 Variables that were statistically significant (α < 0.05) were compared using correlation coefficients (Pearson’s and Spearman rho for continuous and categorical data, respectively) to identify potential collinearity. Variables that did not statistically predict MSKI or demonstrated moderate to high (> 0.5) correlation were considered for omission from the multivariable analysis. Finally, a multivariable Cox proportional hazard ratio was used to construct a model that predicted MSK-I between all variables of interest and the dependent variable of time to injury
Of 1200 new cadets, 903 (75.3%) consented to participate in the study. The baseline demographic information revealed that 22 participants had “not recovered” from pre-existing injuries and 43 reported an injury within the last year with SANE of less than 80%. Injury surveillance revealed that 49 new cadets received medical treatment for injuries prior to consent and self-efficacy data collection. Eight included participants left cadet basic training for either non-musculoskeletal medical reasons or by voluntary withdrawal. In total, 122 participants were excluded, leaving 781 new cadets for analysis (Figure 1).
During the 36 days following the survey, 136 (17.4%) new cadets sustained at least one MSK-I that required medical attention with or without work limitations. The most injured regions were the knee (38, 27.9%), ankle (27, 19.9%), lower leg (22, 16.1%), foot (13, 9.6%), and hip/pelvis (9, 6.6%). The most common injury natures were ligament sprains (33, 24.3%), bone stress injuries (23, 16.9%), joint synovitis (20, 14.7%), and tendinopathy (20, 14.7%). The most common injury causes were aerobic physical training/ running (41, 30.1%), ruck marching (24, 17.6%), land navigation (19, 14.0%), gradual onset (14, 10.3%), and orga-
Figure 1. Graphic depicting the total number of cadets attending cadet basic training in 2022 (n = 1200), consented participants (n = 903), excluded participants due to injury at the time of the initial survey or attrition (n = 122) and the total number of participants included (n = 781).
nized athletics (8, 5.9%). Appendix B contains the complete injury and associated activity analysis.
The average class self-efficacy score was 8.2/10 to complete cadet basic training without injury and 26.0/30 to complete the combined task-specific events without injury. The lowest tertile responded at or below 7/10 to complete all of cadet basic training without injury and at or below 24/ 30 to complete the three combined specific events without injury The researchers used these cutoff scores to differentiate between low and high self-efficacy.
Statistical results comparing injured and uninjured participants are summarized in Table 1. Two-mile run performance was the only statistically significant variable with a large (Cohen’s d>0.8) effect size (t(764)=-9.40, p=<0.001). Independent variables with a positive and statistically significant but small effect size (Cohen’s d<0.5) included selfefficacy to complete cadet basic training without injury (t(773)=2.94, p=0.002), combined event-specific self-efficacy (t(770)=4.08, p=<0.001), sex (X2(1, N=781)=41.28, p=<0.001), hand release push-up performance (t(773)=4.00, p<0.001), plank performance (t(772)=3.88, p=<0.001), height (t(765)=5.00, p=<0.001), and weight (t(762)=2.01, p=0.036). Age, BMI, injury history (only including those above an 80% SANE), athletic intentions, personal performance goals, and prior military experience were not statistically different between those who experienced an injury and those who did not. Although attending the service academy preparatory school was found to be significantly different between injured and uninjured new cadets, it was not carried forward in the analysis due to internal differ-
ences (higher athletics participation) between cadets with and without this extra year of training.21
The Kaplan-Meier survival curves in Figures 2 and 3 display MSK-I occurrences over time by participants with low (blue line) and high self-efficacy (green line) to complete cadet basic training uninjured (Figure 2) and the combined specific events uninjured (Figure 3). Under both conditions, new cadets with self-efficacy scores of 7/10 or less (Figure 2) or task-specific combined self-efficacy of 24/30 or less (Figure 3) demonstrated a significantly reduced probability (p=0.004 and p=<.001, respectively) to continue training without injury over time according to the log-rank test.
All variables that demonstrated statistically significant difference between new cadets with and without MSK-I resulted in statistically significant univariable hazard ratios (HR). History of a resolved prior injury again was not a hazard for injury during cadet basic training. Correlation analysis (Table 2) demonstrated a moderate (>0.5) correlation between height and weight (r=0.68), sex and height (r=0.60), and sex and hand release push-up (r=0.58). Using a selective stepwise method, height and weight were excluded from the subsequent multivariable analysis due to correlation with each other, sex, and hand release push-up. There was also a moderate to strong correlation between self-efficacy to complete cadet basic training and combined specific events (r=0.63). The multivariable Cox proportional hazard ratio analysis was conducted using two different models to account for collinearity between the self-efficacy variable, each with a self-efficacy variable included.
Tables 3 and 4 depict results from the univariable and multivariable Cox proportional hazard analyses. The univariable analysis demonstrated that height, weight, hand release push-up performance, plank performance, and both self-efficacy measures were all individually protective against MSK-I risk over time. Compared to males, females had a greater injury risk over time. Lastly, new cadets with slower 2-mile run times were also at a greater MSK-I risk over time (Table 3). Of the other variables statistically significant in the univariable models, only slower 2-mile run time predicted injury risk over time (HR=1.004 [1.003-1.006], p=<0.001) in the multivariable model (Table 4).
Although injury history did not demonstrate a statistical significance, the researchers decided to include it in the multivariable analysis due to strong historical prediction for future injury When included in the multivariable analysis, it was again not predictive of future MSK-I (HR=1.19 [0.83-1.70], p=0.335).
The primary purpose of this study was to test the strength of association and predictive value between self-efficacy and future MSK-I. The results support the hypothesis that new cadets who sustained an MSK-I had significantly less self-efficacy than those who remained uninjured and had a shorter survival time during cadet basic training. However, when controlling for other known risk factors, only aerobic fitness (2-mile run time) predicted MSK-I.
Table 1. Demographics and Personal Characteristics of
These results are the first to prospectively demonstrate that self-efficacy to complete strenuous military training without injury was lower among new cadets who sustained an MSK-I. Those with lower self-efficacy also had reduced
time to injury compared to new cadets with high self-efficacy However, self-efficacy is not predictive of future MSKIs when controlling for other important covariates. This is not surprising considering that the six sources of self-
Table 2. Correlations Between Independent Risk Factors with Significant Univariable Cox Proportional Hazard Ratios
Self-efficacy (SE)
**Correlation is significant at the 0.01 level (2-tailed)
*Correlation is significant at the 0.05 level (2-tailed)
Bold = Moderate to high correlation (>0.5)
efficacy56 might interact with the other risk factors. For instance, individuals with lower aerobic fitness might report lower self-efficacy due to the perceived level of necessary exertion to perform specific tasks. Future research is needed to determine how previous injury affects self-efficacy, the interactions between self-efficacy sources and performance, and how self-efficacy affects injury, performance, and other important risk factors.
These findings support prior research that low aerobic physical fitness, as identified in a one to two mile running assessment, is the strongest predictive risk factor for MSKI during initial entry training.13,14,24,57,58 In this cohort, a one second increase in 2-mile run time increased the MSK-I hazard ratio by 0.5%. This can be interpreted as for every one minute increase in 2-mile run time, there was a 30% increased risk of sustaining an MSK-I. This study’s injury surveillance echoes the classic training conundrum: aerobic physical training (running) and ruck marching accounted for 47.1% of all training injuries. This implies that military leadership and instructors should continue to use low aerobic fitness performance to identify at-risk populations, conduct appropriately programed training such as ability group-based running,59,60 and consider improving the other independent factors that were associated, although not predictive of MSK-I.
Previous injury is one of the most widely accepted risk factors for future injury, but was not significantly different between the injured and non-injured population in this cohort. This may have been due to either the previous injury timeframe (one year) or distinguishing between resolved and unresolved previous injuries. The researchers surveyed previous injuries within the past year in order to capture relatively common athletic injuries such as anterior cruci-
ate ligament tears, which can take a year to fully resolve. Previous studies surveyed previous injuries within the prior three to six months.18,47 The longer timeframe might have resulted in including more positive responses for mild to moderate previous injuries with more time to fully resolve.
This study uniquely distinguished between resolved and unresolved previous injuries by requiring participants to rate their current level of function according to the SANE. The researchers chose this method to control for unresolved previous injuries at the time of the survey but expected previous injury again to strongly predict future injury This finding presents a potential need to differentiate between resolved and unresolved previous injuries at the beginning of an injury surveillance study and might emphasize the importance of “finishing” rehabilitation to prevent future injury The plethora of previous research on prior injury as an MSK-I risk factor necessitates future research with the same and related populations before elucidating the relationship between prior injury, injury resolution, and future injury.
Other independent variables that were significantly different between the injured and non-injured groups (associated with MSK-I) but did not collectively predict MSK-I included height, weight, biological sex, plank, and hand release push-up performance. Biological sex and height are both intrinsic factors which have previously demonstrated association with MSK-I during military training. This study adds to a substantial body of evidence that when controlling for other injury risk factors, biological sex alone does not predict future injury 12,14,24,26,57 However, females may exhibit higher injury risk due to physiological traits that predispose all soldiers to injury, such as slower 2-mile run
Figure 3. Kaplan-Meier Survival Curve to MSK-I By Self-Efficacy to Complete Combined Specific Events Without MSK-I.
Table 3. Univariable Cox Proportional Regression Analysis and MSK-I
Confidence
times, shorter height, lower weight, and lower hand-release push-up performance.18,22
Hand release push-up and plank performance were significantly different between the injured and non-injured groups and were predictive of MSK-I individually, but not when controlling for the other risk factors. De la Mott et al. previously found strong and moderate evidence of relation-
ships between upper body and core muscular endurance respectively and both have demonstrated value as part of a prospective injury screen.18,60 However, when controlling for other risk factors, Moran et al. also did not find pushup endurance to be predictive of injury 38 Because of this association, but not predictive value, military instructors should continue to train upper body muscular endurance, but it is unclear whether the hand release push-up nor the plank should be included in injury prevention screening. This is the first known study to identify hand release pushup performance as a potential MSK-I risk factor based on univariable analysis.
It is important to emphasize how self-efficacy concepts should and should not be incorporated into training. First, this study did not find that low self-efficacy was able to predict future injury when controlling for other known risk factors and therefore, should not be used in isolation to identify at-risk populations. However, based on mean differences and univariable relationships, self-efficacy may be a modifiable extrinsic risk factor. Self-efficacy is a psychological concept, but training could include strategies beyond talking and thinking about confidence. Training strategies could include leveraging the six sources of selfefficacy: enhancing mastery experiences (prior success), vicarious experiences (seeing others succeed), physiological state (including nutrient timing and adequate sleep), affective state, verbal persuasion, and mental imagery.56 More specific intervention strategies are beyond the scope of this analysis and future studies should explore intervention effectiveness.
One strength of this study was the researchers’ thorough injury surveillance through multiple electronic medical record reviews, including all musculoskeletal patient en-
counters, radiology reports, and physical screenings. Working in the same facility as the other medical providers allowed the authors to clarify injury diagnoses when medical documentation was unclear. This resulted in very few classifications as “other” or “unknown” injury causes. Injury trends were otherwise similar to prior initial entry training environment research.20,21
The researchers chose to develop a task-specific self-efficacy assessment to ensure concordance48 with cadet basic training events and it demonstrated strong internal consistency. The low self-efficacy cutoff scores based on the lowest tertiles were consistent with findings on the Pain SelfEfficacy Questionnaire (40/60 [67%])48 and the Return to Work Self-Efficacy (RTW-SE) Questionnaire (4.6/6 [77%]).49 Although the items were developed following guidance by Bandura, a limitation of our study is that it was not validated with another accepted self-efficacy scale. Future studies should consider validating custom self-efficacy questionnaires with either the Pain50 or General36 Self-Efficacy Scales.
Another limitation to this study included consent and survey timing. Due to the demanding cadet basic training schedule, study consent and survey took place 1 week after cadet basic training commencement, which was the soonest available opportunity. Although this occurred prior to highdemand physical training events except for a portion of the ACFT (only push ups, plank and 2-mile run), 26% of all known injuries during cadet basic training occurred prior to the beginning of data collection and therefore were not a part of the analyzed data set. It is reasonable to assume that these cadets, who were the first to sustain injuries, may have been a valuable addition. Future studies should prioritize surveying participants as early as possible for overall self-efficacy.
Self-selection bias both when consenting to the study and in seeking medical care was another considerable limitation. The reasons for only 903 (75.3%) of the 1200 new cadets agreeing to participate in this study despite no additional time requirements could have been related to reporting prior injuries, consenting to medical records review, or having to consent to two separate studies simultaneously Also, new cadets had the ability to choose whether or to seek medical care except in emergency situations.
Lastly, this study was limited to the relationship between self-efficacy and MSK-I, but as a psychological trait, may have a broader reach. Individuals with lower self-efficacy might exhibit decreased resilience to illness or psychological stress, leading to more medical or mental health encounters. Future studies might consider examining the relationship between self-efficacy and all medical encounters.
There was a significant, although small relationship between new cadets who reported low self-efficacy and future MSK-I during initial entry training. Self-efficacy could potentially be a modifiable risk factor, but further research is necessary to validate these findings and identify beneficial interventions. At this time, self-efficacy assessments should not be used alone to identify those with higher MSKI risk. Additionally, this study strongly supports the body of literature that aerobic performance can be used to identify at-risk populations and proposes using the SANE to differentiate between resolved and unresolved injuries for injury surveillance research.
Submitted: May 22, 2024 CST, Accepted: October 20, 2024 CST
© The Author(s)
This is an open-access article distributed under the terms of the Creative Commons Attribution 4.0 International License (CCBY-NC-4.0). View this license’s legal deed at https://creativecommons.org/licenses/by-nc/4.0 and legal code at https://creativecommons.org/licenses/by-nc/4.0/legalcode for more information.
1. Center for Disease Control and Prevention. Trends in the prevalence of obesity and dietary behaviors, National Youth Behavior Survey 1991-2019. Division of Adolescent and School Health, National Center for HIV/AIDS, Viral Hepatitis, STD, and TB Prevention. 2020. Accessed February 4, 2022. https:// www.cdc.gov/healthyyouth/data/yrbs/pdf/trends/ 2019_obesity_trend_yrbs.pdf
2. Knapik JJ, Sharp MA, Steelman RA. Secular trends in the physical fitness of United States Army recruits on entry to service, 1975–2013. J Strength Cond Res 2017;31(7):2030-2052. doi:10.1519/ JSC.0000000000001928
3. Maxey H, Bishop-Josef S, Goodman B. Unhealthy and unprepared. Council for a Strong America. 2018. Accessed April 24, 2023. https:// strongnation.s3.amazonaws.com/documents/484/ 389765e0-2500-49a2-9a67-5c4a090a215b.pdf?153961 6379&inline;%20filename=%22Unhealthy%20and%2 0Unprepared%20report.pdf%22
4. Alemany JA, Pierce JR, Bornstein DB, Grier TL, Jones BH, Glover SH. Comprehensive physical activity assessment during U.S. Army basic combat training. J Strength Cond Res. 2022;36(12):3505-3512. doi:10.1519/JSC.0000000000004114
5. U.S. Army Public Health Center 2021 Health of the Force. 2022. Accessed April 24, 2022. https:// phc.amedd.army.mil/topics/campaigns/hof
6. U.S. Army Center for Initial Military Training. The U.S. Army Holistic Health and Fitness Operating Concept U.S. Army Training and Doctrine Command; 2020. Accessed September 8, 2024. https:// www.army.mil/e2/downloads/rv7/acft/ h2f_operating_concept.pdf
7 Molloy JM, Pendergrass TL, Lee IE, Chervak MC, Hauret KG, Rhon DI. Musculoskeletal injuries and United States Army readiness part I: Overview of injuries and their strategic impact. Mil Med 2020;185(9-10):e1461-e1471. doi:10.1093/milmed/ usaa027
8. Roy LTC, Faller TN, Richardson MD, Taylor KM. Characterization of limited duty neuromusculoskeletal injuries and return to duty times in the U.S. Army during 2017-2018. Mil Med 2021;00:9.
9. Jones BH, Hauschild VD, Canham-Chervak M. Musculoskeletal training injury prevention in the U.S. Army: Evolution of the science and the public health approach. J Sci Med Sport 2018;21(11):1139-1146. doi:10.1016/j.jsams.2018.02.011
10. Lovalekar M, Hauret K, Roy T, et al. Musculoskeletal injuries in military personnel—Descriptive epidemiology, risk factor identification, and prevention. J Sci Med Sport. 2021;24(10):963-969. doi:10.1016/ j.jsams.2021.03.016
11. U.S. Army Public Health Center. Health of the Force 2020. 2021. Accessed January 20, 2022. https:// phc.amedd.army.mil/Periodical%20Library/2020-hofreport.pdf
12. Rappole C, Grier T, Anderson MK, Hauschild V, Jones BH. Associations of age, aerobic fitness, and body mass index with injury in an operational Army brigade. J Sci Med Sport 2017;20:S45-S50. doi:10.1016/j.jsams.2017.08.003
13. Blacker SD, Wilkinson DM, Bilzon JLJ, Rayson MP. Risk factors for training injuries among British Army recruits. Mil Med 2008;173(3):278-286. doi:10.7205/ MILMED.173.3.278
14. Dos Santos Bunn P, de Oliveira Meireles F, de Souza Sodré R, Rodrigues AI, da Silva EB. Risk factors for musculoskeletal injuries in military personnel: A systematic review with meta-analysis. Int Arch Occup Environ Health 2021;94(6):1173-1189. doi:10.1007/ s00420-021-01700-3
15. Lisman PJ, de la Motte SJ, Gribbin TC, Jaffin DP, Murphy K, Deuster PA. A systematic review of the association between physical fitness and musculoskeletal injury risk: Part 1—Cardiorespiratory endurance. J Strength Cond Res 2017;31(6):1744-1757. doi:10.1519/ JSC.0000000000001855
16. Molloy JM. Factors influencing running-related musculoskeletal injury risk among U.S. Military recruits. Mil Med. 2016;181(6):512-523. doi:10.7205/ MILMED-D-15-00143
17 Hruby A, Bulathsinhala L, McKinnon CJ, et al. BMI and lower extremity injury in U.S. Army Soldiers, 2001–2011. Am J Prev Med 2016;50(6):e163-e171. doi:10.1016/j.amepre.2015.10.015
18. de la Motte SJ, Gribbin TC, Lisman P, Murphy K, Deuster PA. Systematic review of the association between physical fitness and musculoskeletal injury risk: Part 2-Muscular endurance and muscular strength. J Strength Cond Res 2017;31(11):3218-3234. doi:10.1519/JSC.0000000000002174
19. Jones BH, Hauret KG, Dye SK, et al. Impact of physical fitness and body composition on injury risk among active young adults: A study of Army trainees. J Sci Med Sport. 2017;20:S17-S22. doi:10.1016/ j.jsams.2017.09.015
20. Epstein Y, Fleischmann C, Yanovich R, Heled Y Physiological and medical aspects that put women soldiers at increased risk for overuse injuries. J Strength Cond Res 2015;29 Suppl 11:S107-110. doi:10.1519/JSC.0000000000001079
21. Hearn DW, Kerr ZY, Wikstrom EA, et al. Lower extremity musculoskeletal injury in US Military Academy cadet basic training: A survival analysis evaluating sex, history of injury, and body mass index. Orthop J Sports Med 2021;9(10):232596712110398. doi:10.1177/ 23259671211039841
22. Kucera KL, Marshall SW, Wolf SH, Padua DA, Cameron KL, Beutler AI. Association of injury history and incident injury in cadet basic military training. Med Sci Sports Exerc 2016;48(6):1053-1061. doi:10.1249/MSS.0000000000000872
23. Anderson AB, Braswell MJ, Pisano AJ, et al. Factors associated with progression to surgical intervention for lumbar disc herniation in the military health system. Spine. 2021;46(6):E392-E397. doi:10.1097/BRS.0000000000003815
24. Bell N. High injury rates among female Army trainees: A function of gender? Am J Prev Med. 2000;18(1):141-146. doi:10.1016/ S0749-3797(99)00173-7
25. Bunn PDS, Rodrigues AI, Bezerra da Silva E. The association between the functional movement screen outcome and the incidence of musculoskeletal injuries: A systematic review with meta-analysis. Phys Ther Sport Off J Assoc Chart Physiother Sports Med 2019;35:146-158. doi:10.1016/ j.ptsp.2018.11.011
26. Schram B, Canetti E, Orr R, Pope R. Injury rates in female and male military personnel: A systematic review and meta-analysis. BMC Womens Health. 2022;22(1):310. doi:10.1186/s12905-022-01899-4
27 Canham-Chervak M, Cowan DN, Pollack KM, Jackson RR, Jones BH. Identification of fall prevention strategies for the military: A review of the literature. Mil Med 2015;180(12):1225-1232. doi:10.7205/MILMED-D-14-00673
28. Molloy JM, Pendergrass TL, Lee IE, Hauret KG, Chervak MC, Rhon DI. Musculoskeletal injuries and United States Army readiness. Part II: Management challenges and risk mitigation initiatives. Mil Med. 2020;185(9-10):e1472-e1480. doi:10.1093/milmed/ usaa028
29. Terry AC, Thelen MD, Crowell M, Goss DL. The musculoskeletal readiness screening tool- Athlete concern for injury and prior injury associated with future injury. Int J Sports Phys Ther. 2018;13(4):595-604. doi:10.26603/ijspt20180595
30. Bandura A. Self-efficacy: Toward a unifying theory of behavioral change. Psychol Rev 1977;84(2):191-215. doi:10.1037/0033-295X.84.2.191
31. Bandura A. Self-efficacy mechanism in human agency Am Psychol 1982;37(2):122-147 doi:10.1037/ 0003-066X.37.2.122
32. Benedict TM, Grier TL, Roy TC, Toussaint MN, Jones BH. Demographic, psychosocial, and physical fitness predictors of successful graduation from U.S. Army Ranger School. Mil Psychol 2023;35(2):180-191. doi:10.1080/ 08995605.2022.2094174
33. Feltz DL, Chow GM, Hepler TJ. Path analysis of self-efficacy and diving performance revisited. J Sport Exerc Psychol. 2008;30(3):401-411. doi:10.1123/ jsep.30.3.401
34. Brinkman C, Baez SE, Genoese F, Hoch JM. Use of goal setting to enhance self-efficacy after sportsrelated injury: A critically appraised topic. J Sport Rehabil 2020;29(4):498-502. doi:10.1123/ jsr.2019-0032
35. Beattie S, Fakehy M, Woodman T. Examining the moderating effects of time on task and task complexity on the within person self-efficacy and performance relationship. Psychol Sport Exerc 2014;15(6):605-610. doi:10.1016/ j.psychsport.2014.06.007
36. Gruber KA, Kilcullen RN, Iso-Ahola SE. Effects of psychosocial resources on elite soldiers’ completion of a demanding military selection program. Mil Psychol 2009;21(4):427-444. doi:10.1080/ 08995600903206354
37 Ledford AK, Dixon D, Luning CR, et al. Psychological and physiological predictors of resilience in Navy SEAL training. Behav Med 2020;46(3-4):290-301. doi:10.1080/ 08964289.2020.1712648
38. Moran DS, Evans R, Arbel Y, et al. Physical and psychological stressors linked with stress fractures in recruit training: Stress fractures in combat recruits. Scand J Med Sci Sports. 2013;23(4):443-450. doi:10.1111/j.1600-0838.2011.01420.x
39. Scott KM, Kreisel BR, Florkiewicz EM, et al. The effect of cautionary versus resiliency spine education on maximum deadlift performance and back beliefs: A randomized control trial. J Strength Cond Res 2024;38(7):e341-e348. doi:10.1519/ JSC.0000000000004783
40. Dumont GD, Glenn RL, Battle NC, Thier ZT Correlation of the Single-Assessment Numeric Evaluation (SANE) score with hip-specific patientreported outcome measures. Arthrosc Sports Med Rehabil. 2021;3(2):e435-e440. doi:10.1016/ j.asmr.2020.10.008
41. Thigpen CA, Shanley E, Momaya AM, et al. Validity and responsiveness of the single alphanumeric evaluation for shoulder patients. Am J Sports Med 2018;46(14):3480-3485. doi:10.1177/ 0363546518807924
42. Williams GN, Gangel TJ, Arciero RA, Uhorchak JM, Taylor DC. Comparison of the single assessment numeric evaluation method and two shoulder rating scales. Am J Sports Med. 1999;27(2):214-221. doi:10.1177/03635465990270021701
43. Williams GN, Taylor DC, Gangel TJ, Uhorchak JM, Arciero RA. Comparison of the single assessment numeric evaluation method and the Lysholm score. Clin Orthop 2000;373:184-192. doi:10.1097/ 00003086-200004000-00022
44. Gowd AK, Charles MD, Liu JN, et al. Single Assessment Numeric Evaluation (SANE) is a reliable metric to measure clinically significant improvements following shoulder arthroplasty J Shoulder Elbow Surg 2019;28(11):2238-2246. doi:10.1016/j.jse.2019.04.041
45. Puzzitiello RN, Gowd AK, Liu JN, Agarwalla A, Verma NN, Forsythe B. Establishing minimal clinically important difference, substantial clinical benefit, and patient acceptable symptomatic state after biceps tenodesis. J Shoulder Elbow Surg 2019;28(4):639-647. doi:10.1016/j.jse.2018.09.025
46. O’Halloran B, Cook CE, Oakley E. Criterion validation and interpretability of the single assessment numerical evaluation (SANE) of selfreported recovery in patients with neck pain. Musculoskelet Sci Pract 2021;56:102467 doi:10.1016/ j.msksp.2021.102467
47 Helton GL, Cameron KL, Zifchock RA, et al. Association between running shoe characteristics and lower extremity injuries in United States Military Academy cadets. Am J Sports Med. 2019;47(12):2853-2862. doi:10.1177/ 0363546519870534
48. Moritz SE, Feltz DL, Fahrbach KR, Mack DE. The relation of self-efficacy measures to sport performance: A meta-analytic review Res Q Exerc Sport. 2000;71(3):280-294. doi:10.1080/ 02701367.2000.10608908
49. Bandura A. Guide for Construction Self-Efficacy Scales. In: Urdan T, Pajares F, eds. Self-Efficacy Beliefs of Adolescents. Vol 5. Information Age Publishing; 2006:307-337
50. Ferrari S, Vanti C, Costa F, Fornari M. Can physical therapy centred on cognitive and behavioural principles improve pain self-efficacy in symptomatic lumbar isthmic spondylolisthesis? A case series. J Bodyw Mov Ther. 2016;20(3):554-564. doi:10.1016/j.jbmt.2016.04.019
51. Gjengedal RGH, Lagerveld SE, Reme SE, Osnes K, Sandin K, Hjemdal O. The Return-To-Work SelfEfficacy questionnaire (RTW-SE): A validation study of predictive abilities and cut-off values for patients on sick leave due to anxiety or depression. J Occup Rehabil 2021;31(3):664-673. doi:10.1007/ s10926-021-09957-8
52. Isaac V, Wu CY, McLachlan CS, Lee MB. Associations between health-related self-efficacy and suicidality BMC Psychiatry 2018;18(1):126. doi:10.1186/s12888-018-1705-z
53. Martin RC, Grier T, Canham-Chervak M, et al. Validity of self-reported physical fitness and body mass index in a military population. J Strength Cond Res 2016;30(1):26-32. doi:10.1519/ JSC.0000000000001026
54. Department of the Army. Holistic Health and Fitness Testing.; 2020. Accessed March 29, 2023. https://armypubs.army.mil/epubs/DR_pubs/DR_a/ ARN35869-ATP_7-22.01-002-WEB-5.pdf
55. Bursac Z, Gauss CH, Williams DK, Hosmer DW Purposeful selection of variables in logistic regression. Source Code Biol Med. 2008;3(1):17. doi:10.1186/1751-0473-3-17
56. Shipherd AM, Renner KB, Samson A, Duncan CK. An examination of the sources of self-efficacy in runners throughout training: A mixed methods study J Sport Behav 2021;44(1):144-164. Accessed March 29, 2023. https://www.journalofsportbehavior.org/ index.php/JSB/article/view/65
57 Anderson MK, Grier T, Dada EO, Canham-Chervak M, Jones BH. The role of gender and physical performance on injuries: An Army study Am J Prev Med. 2017;52(5):131-138. doi:10.1016/ j.amepre.2016.11.012
58. Jones BH, Bovee MW, Harris JMcA, Cowan DN. Intrinsic risk factors for exercise-related injuries among male and female Army trainees. Am J Sports Med 1993;21(5):705-710. doi:10.1177/ 036354659302100512
60. Sefton JM, Lohse KR, McAdam JS. Prediction of injuries and injury types in Army basic training, infantry, armor, and cavalry trainees using a common fitness screen. J Athl Train. 2016;51(11):849-857. doi:10.4085/1062-6050-51.9.09 The Relationship Between
59. Knapik JJ, Scott SJ, Sharp MA, et al. The basis for prescribed ability group run speeds and distances in U.S. Army basic combat training. Mil Med 2006;171(7):669-677. doi:10.7205/MILMED.171.7.669
Download: https://ijspt.scholasticahq.com/article/127137-the-relationship-between-self-efficacy-aerobic-fitness-andtraditional-risk-factors-for-musculoskeletal-injuries-in-military-training-a-prospective/attachment/ 257106.docx?auth_token=qHy98LkAtY80fiYYm_8h
Download: https://ijspt.scholasticahq.com/article/127137-the-relationship-between-self-efficacy-aerobic-fitness-andtraditional-risk-factors-for-musculoskeletal-injuries-in-military-training-a-prospective/attachment/ 257107.docx?auth_token=qHy98LkAtY80fiYYm_8h
Joshua Colomar1,2a , Javier Peña1,2 , Jordi
Vicens-Bordas
1,2 , Ernest
Baiget
3
1 University of Vic - Central University of Catalonia, Sport, Exercise and Human Movement Research Group (SEaHM), Vic, Spain, 2 Sport and Physical Activity Studies Centre (CEEAF), University of Vic – Central University of Catalonia, Vic, Spain, 3 National Institute of Sport and Physical Education (INEFC), University of Barcelona, Barcelona, Spain
Keywords: injury, prevention, contractile properties, performance
https://doi.org/10.26603/001c.127263
International Journal of Sports Physical Therapy
Vol. 20, Issue 1, 2025
Background
Due to its significant unilateral predominance, tennis can provoke functional and morphological asymmetries that develop over time and may result in undesired morphological alterations.
Hypothesis/Purpose
The goals of this study were a) to assess glenohumeral range of motion and muscular stiffness in young tennis players with and without a history of shoulder pain and b) to examine interlimb asymmetries in these variables in both groups. It was hypothesized that players with a history of shoulder pain would show a reduced glenohumeral internal rotation (IR) and total arc of motion (TAM) and increased stiffness in internal rotator muscles compared to those without shoulder pain.
Study Design
Cross-sectional observational study
Methods
Twenty-five participants participated in the study (11 with a history of shoulder pain and 14 without pain). Participants performed stiffness measurements on muscles involved in the main tennis stroke motions alongside range of motion examinations on the dominant (D) and non-dominant (ND) extremities including IR, external shoulder rotation (ER), and TAM. A two-way mixed-design ANOVA analyzed group and limb effects, with effect sizes classified as small, medium, or large. Significant effects were further examined using Bonferroni post hoc tests.
Results
There were significant differences between the shoulder pain and no shoulder pain group in the D IR (-3.1º, 6.43%, p = 0.048; effect size [ES] = 0.58) and D TAM (-6.1º, 3.01%, p = 0.024; ES = 0.66). Moreover, significant differences were found between the D and ND extremities in IR in both groups (-9.2º, 14.94%, p < 0.001; ES = -1.72) and TAM in the shoulder pain group (-5.6º, 2,77%, p = 0.038; ES = 0.61). Stiffness measurements showed no significant differences between groups or extremities.
Corresponding author:
Name: Joshua Colomar
Institution: University of Vic - Central University of Catalonia
Address: C/ Sagrada Família, 7, 08500, Vic
Phone: +34 679260619
Email: joshua.colomar@uvic.cat
Significantly lower values of D IR and TAM and higher IR asymmetries in the shoulder pain group suggest that a deficit in these parameters could be associated with shoulder pain history in junior competitors.
2
Tennis has a significant unilateral predominance, as most game-play actions are performed with the dominant extremity 1 Because of this, functional and morphological asymmetries develop due to continued training and match play 2‑4 Young competitors generally show specific values in shoulder strength, humeral retroversion (HRV), and range of motion (ROM) as a chronic alteration derived from competitive development.1,5‑7 Specifically, regarding ROM in uninjured athletes, the dominant arm typically shows a decreased shoulder internal rotation (IR), increased shoulder external rotation (ER), and a reduced total arc of motion (TAM) compared to the other extremity 8 These adaptations are typically produced because of soft tissue pathophysiological alterations such as shoulder posterior capsule tightness9 or protective adaptations like humeral retroversion that can start developing at young ages.7 When analyzing professional athletes with a history of shoulder pain compared to uninjured participants, Marcondes et al.10 and Moreno-Pérez et al.11 found significant differences in ROM values that indicated greater and more evident alterations (i.e., decreased IR) in the shoulder pain group (SHP). These findings, alongside previous works in throwing/overhead athletes, suggest that a deficit in the IR of the dominant (D) shoulder compared to the non-dominant (ND) extremity (i.e., glenohumeral internal rotation deficit or GIRD) could be a relevant injury risk factor 12 Specifically, differences higher than 20º could be associated with injury, and players may be at a higher risk of developing specific overuse problems that can impair progress, adequate training availability, and competition time.11
Muscle stiffness has been established as a contractile property that can aid in the stretch-shortening cycle (SSC) and be beneficial for high-speed sporting actions such as tennis strokes and on-court movements like sprinting and changing direction.13,14 Nevertheless, high muscle stiffness values may also be counterproductive, as increased stiffness can raise peak shock forces and compromise technical and kinematic needs.15 Specific stiffness values are yet to be studied and confirmed to establish detailed beneficial and detrimental standards for professional athletes.16 However, similar to alterations in ROM, stiffness can influence performance and injury incidence and should be considered.17 Specifically, Pruyn et al.17 found that a high bilateral difference in leg muscle stiffness was related to a greater incidence of lower body soft tissue injury in Australian football players. Although comparing lower body and upper extremity results may have limitations, literature is scarce when studying muscle stiffness around the glenohumeral joint. Certain stiffness levels may influence the
glenohumeral joint’s overall performance and, to some extent, affect shoulder ROM or interlimb asymmetries.
Some investigations have analyzed young tennis competitors’ injury risk factors18 and shoulder ROM profiles of injured and uninjured professional players.10,11 Nevertheless, no studies have focused on this issue in adolescent athletes or have included muscle stiffness testing. This perspective seems relevant since, as serious competitors, young players deal with high playing volumes that involve many actions that may place the player’s body structures under stress.19‑21
The goals of this study were a) to assess ROM and stiffness characteristics in young tennis players with and without a history of shoulder pain and b) to examine interlimb asymmetries in both groups. The authors’ hypothesized that ROM values would be significantly altered in players with a history of shoulder pain, including reduced glenohumeral internal rotation and total arc of motion compared to players without shoulder pain. Regarding stiffness values, it was hypothesized that players with shoulder pain would present significantly greater stiffness of the internal rotator muscles (pectoralis major) compared to the nonpain history group.
Twenty-five male junior (under 18 years old) tennis players from a high-performance tennis academy were recruited to participate in this study Inclusion criteria required at least one year of participation in a structured strength and conditioning program and a minimum background of five years of tennis training and competition. Also, the inclusion criteria of the SHP group consisted of reporting shoulder pain or discomfort that prevented them from training or playing during the two months before the start of the study. The no-pain participants (NSHP) did not present any shoulder pain, and they had not undergone surgery or taken medication to relieve discomfort in the six months before the data collection. The diagnosis of shoulder pain and recommendations to drop out of training and competition were established by the accredited medical staff of the tennis academy All participants were informed about the particularities of the study and signed informed consent. In the case of being underage, their legal guardians signed the arrangement. The study was conducted following the ethical principles for biomedical research with human beings, established in the Declaration of Helsinki of the World Medical Association (WMA) It was approved by a local Ethics Committee (19/2019/CEICEGC).
Table 1. Participant characteristics and group differences.
The testing was divided into two sessions, which were performed on the same day and separated by 10 minutes. Participants performed the ROM testing followed by the muscle stiffness assessment. Participants did not exercise for at least 18 hours before the protocol. They were asked to maintain their routine and to avoid moderate to intense exercise or consuming excitatory substances (i.e., caffeine) during the hours prior to the testing sessions. All measurements were performed in the morning, approximately from 8:00 am to 9:00 am. Data collection was conducted during the competition period.
RANGE OF MOTION (ROM)
Glenohumeral rotation was measured following previous research11,22 using a manual inclinometer (Farway, Shenzhen Dobiy Electronic Co., Ltd, Shenzhen, China). Each participant lay supine on a bench, with both shoulders abducted to 90º and the dominant arm elbow flexed 90°. A coauthor stabilized the participant’s proximal shoulder region to prevent undesired scapular movements and to record passive IR and ER. Values in degrees were recorded and used to calculate TAM (summation of IR and ER values). The following formula was used to calculate between limb differences: ER – IR/ER * 100.23 Two measurements per extremity and participant were performed with no rest and in a randomized order. The best value was used for data analyses.
Stiffness, the resistance of the muscle to an external force that changes its initial shape,24 was measured while in anatomical position in four muscle groups in both extremities using a handheld myometer (Myoton-Pro, Myoton AS, Tallinn, Estonia), following previous protocols.13 Before the assessment, body marks were established for the measurement points using the SENIAM electrode placement guidelines.25 The muscle groups chosen were the biceps brachii, infraspinatus, deltoids, and pectoralis major, which are all involved in the kinetic chain of tennis strokes.26,27 The tip of the Myoton Pro was sampled at 15 ms with a force of 0.58 N. The accelerometers operated at 3200 Hz, offering an average value of five consecutive measurements. Interlimb absolute and relative stiffness differences were calcu-
lated using ROM measurements. The reliability of the measurements showed excellent test-retest values in previous investigations (ICC = 0.956 – 0.998; CV = 0.3 - 0.9; SEM = 3.2 – 6.5).13
Descriptive data were reported as mean ± standard deviation (SD). The distributions’ normality and the variances’ homogeneity were assessed with the Shapiro-Wilk test. Independent t-tests were used to check players’ characteristics/demographic differences. Differences between groups (between-subject factor: NSHP and SHP) and limbs (withinsubject factor: D and NDs) and interactions were tested with a two-way mixed-design ANOVA model, including the variables of interest (IR, ER, TAM, and muscle stiffness). The partial eta-square (η2) effect sizes were calculated to evaluate the main and interaction effects of the ANOVA. The η2 values of 0.01–0.05, 0.06–0.13, and >0.14 indicate small, medium, and large effect sizes, respectively 28 When a significant difference was found for either main effect (limb or group), a Bonferroni post hoc analysis was performed, presenting the mean difference with 95% CI and Cohen’s d effect size (ES). The magnitude of the effects was interpreted as follows: < 0.2 trivial; 0.2–0.5 small; 0.5–0.8 medium; >0.8 large.28 All statistical analyses were performed with JASP (JASP 0.16.1, University of Amsterdam, The Netherlands).
Participants had a weekly training volume of 20 hours per week, including three hours of specific tennis sessions and one hour of physical fitness workouts daily from Monday to Friday. Two subjects were left-handed, while all other participants played using their right arms. Both groups were homogeneous and presented no significant differences in biometric measurements (Table 1).
Table 2 presents between group and limb differences, D shoulder ROM comparative descriptive data in the SHP and NSHP, and inter-limb asymmetry differences in both groups.
Regarding ROM, medium significant group-by-limb interactions were observed in the IR (F = 6.554, p = 0.014, η2 = 0.125) whereas no interactions were found in ER (F = 0.129, p = 0.721, η2 = 0.003) and TAM (F = 3.283, p = 0.077, η2 = 0.067). Medium significant main effect of group was
Table 2. Glenohumeral range of motion and stiffness differences between no shoulder and shoulder pain groups.
Biceps brachii stiffness
Pectoralis major stiffness
a Significantly different from non-dominant shoulder (p < 0.05)
b Significantly different from the no-pain group (p < 0.05)
found in IR (F = 4.146, p = 0.048, η2 = 0.083) and TAM (F = 5.437, p = 0.024, η2 = 0.106) and a large main effect of limbs was found in TAM (F = 4.587, p = 0.038, η2 = 0.091) and IR (F = 36.333, p = <0.001, η2 = 0.441). The post hoc tests showed significant differences between D and ND extremities in the IR ROM (-9.2º, 14.94%, p < 0.001; ES = -1.72) and TAM (-5.6º, 2,77%, p = 0.038; ES = 0.61), and between SHP and NSHP in the IR ROM (-3.1º, 6,43%, p = 0.048; ES = 0.58) and TAM (-6.1º, 3.01%, p = 0.024; ES = 0.66).
Regarding stiffness, no significant group-by-limb interactions or main effects of group and limb were found for any of the variables analyzed.
This study aimed to assess glenohumeral ROM and muscle stiffness differences in young tennis players with and without a history of shoulder pain, alongside examining the ex-
istence of interlimb asymmetries in both groups. The study hypothesis was partially confirmed, as results indicated significantly lower D IR and D TAM values in the SHP compared to the NSHP participants. Additionally, when comparing the D to the ND extremity, reduced values in the IR were found in both groups, while the SHP group also showed significant asymmetries regarding TAM. These results demonstrate that glenohumeral ROM alterations exist (i.e., reduced IR and TAM) irrespective of injury history. However, players with SHP have a greater IR deficit in the D arm and a higher level of asymmetry between limbs than NSHP participants.
Tennis practice and exposure to match-play is considered a highly asymmetrical activity, which will may induce glenohumeral strength and ROM alterations. Players generally show an increased ER and a reduced IR and TAM of the D extremity as an adaptation to upper limb load induced by training and competition.1,6 Normative values of junior players’ ROM show how these alterations are already present in U14 participants, and in the case of male athletes, these seem to increase progressively with age and level.8 These increases appear as a consequence of an inevitable intensification in training and match-load, triggered by a higher number of overhead motions performed at higher competitive levels and older ages.29 The observed differences in this study reaffirm that limb asymmetry is highly prevalent in sports performance but, although existent, previous research indicates it is not necessarily linked to injury risk.30 For instance, in other prior studies, tested groups showed significant GIRD irrespective of their injury history 11,18 In fact, and worth noting, the NSHP group here presented significant shoulder IR interlimb variances (5.29º; 8.05%) that may be expected in these participants but were below clinically meaningful thresholds (5 –15%).30
Nevertheless, the SHP group showed differences of around 13.09º (21.96%), which are not close to typical injury risk thresholds regarding absolute values11 but may account for percentual asymmetry levels that exceed normal morphological alterations and are of sufficient magnitude to be considered relevant.12,30 In short, specific ROM differences can be expected in junior tennis players, and they may be regarded as ‘normal’ sport-specific alterations unrelated to a higher injury incidence.18 However, values that exceed normative shoulder ROM profiles8 may have to be carefully addressed because they play a role in subsequent injuries as external risk factors.
Following the idea of inherent side-to-side differences in sports performance, some authors suggest using dominant/ non-dominant glenohumeral ROM comparison between groups rather than analyzing interlimb asymmetry as an indicator of injury risk.11 The current results show similar values of ER between groups but a significantly higher deficit in the D IR and, consequently, in the D TAM of the SHP over the NSHP group. Factors such as humeral retrotorsion or capsular tightness can increase IR deficit, and while some are typical protective adaptations (i.e., humeral retrotorsion), some may evolve into undesired pathologies over time.7,9 The mentioned deficits have been linked to
years of practice, greater age, and level, which can increase the load (competitions and shots) and trigger specific morphological chronic adaptations2‑4 that may increase injury risk. Previous research relating glenohumeral shoulder ROM values and injury incidence has mainly concluded these ideas based on the analysis of professional players with extensive experience and accumulated training and competitive load (> 15 years of playing or < 5 years of professional experience).11 These previous results add value to the current findings, in which competitive junior players with a history of SHP showed higher levels of IR deficit, a reduced TAM, and a greater IR interlimb asymmetry compared to players with NSHP. These results indicate that alterations in the glenohumeral ROM of the dominant limb appear early in athletes’ careers, and U18 players with training loads of around 20h/week can present a significantly increased interlimb asymmetry in consequence. Early detection, prevention programs, and strategies to counteract the specificity of training are highly recommended to reduce the effects of age and years of tennis practice.
Regarding stiffness, none of the muscle groups tested showed significantly different values from one side to another As hypothesized, limitations in shoulder glenohumeral ROM may also lead to an affected stiffness in the surrounding muscles. This effect was not seen since both groups’ observed D IR asymmetries were not linked or accompanied by relevant differences in stiffness values when comparing extremities (Table 2). Although some previous investigations have found relatively high stiffness interlimb differences related to soft tissue injury incidence,17 these were found in the lower limbs and when using active muscle versus passive testing. Results here align with previous investigations in junior tennis players in which passive stiffness measurements failed to find significant inter-limb differences, most likely due to the active nature of tennis training and match-play 31 Also, comparisons between stiffness levels of the SHP and NSHP groups did not show relevant differences, suggesting passive measurements of this variable do not seem to discriminate between competitors with and without a history of shoulder pain. Active measurements are recommended for future investigations.
This study had some limitations. First, ‘pain’ can be a vague term that includes many possible discomforts and pathologies. Although the participants in this study were diagnosed by a health professional, different pathologies can affect shoulder ROM and stiffness adaptations. Second, not all participants were tested on the same day Instead, measurements were gathered the following week to diagnose, which could have affected the results.
The results of this study indicate that ROM limb differences (i.e., decreased D IR and D TAM) are common in most junior tennis competitors, especially as age and level increase. However, players with a history of SHP show higher differences between limbs than their non-injured peers, suggesting the magnitude of these asymmetries should be
Range of Motion and Muscle Stiffness Differences in Junior Tennis Players with and without a History of Sh…
carefully assessed if they exceed what can be considered normal thresholds.8 Establishing specific injury prevention programs is highly recommended for junior players to reduce the effects of high loads of tennis exposure..
The authors declare no conflicts of interest.
Submitted: June 14, 2024 CST, Accepted: November 22, 2024 CST
This is an open-access article distributed under the terms of the Creative Commons Attribution 4.0 International License (CCBY-NC-4.0). View this license’s legal deed at https://creativecommons.org/licenses/by-nc/4.0 and legal code at https://creativecommons.org/licenses/by-nc/4.0/legalcode for more information.
1. Cools AM, Palmans T, Johansson FR. Age-related, sport-specific adaptions of the shoulder girdle in elite adolescent tennis players. J Athl Train. 2014;49(5):647-653. doi:10.4085/1062-6050-49.3.02
2. Sanchis-Moysi J, Idoate F, Dorado C, Alayón S, Calbet JAL. Large asymmetric hypertrophy of rectus abdominis muscle in professional tennis players. Lucia A, ed. PLoS ONE 2010;5(12):e15858. doi:10.1371/journal.pone.0015858
3. Sanchis-Moysi J, Idoate F, Izquierdo M, Calbet JAL, Dorado C. Iliopsoas and gluteal muscles are asymmetric in tennis players but not in soccer players. Konigsberg L, ed. PLoS ONE 2011;6(7):e22858. doi:10.1371/journal.pone.0022858
4. Sanchis-Moysi J, Idoate F, Olmedillas H, et al. The upper extremity of the professional tennis player: muscle volumes, fiber-type distribution and muscle strength: The tennis player upper extremity. Scand J Med Sci Sports 2009;20(3):524-534. doi:10.1111/ j.1600-0838.2009.00969.x
5. Cools AM, Johansson FR, Cambier DC, Velde AV, Palmans T, Witvrouw EE. Descriptive profile of scapulothoracic position, strength and flexibility variables in adolescent elite tennis players. Br J Sports Med 2010;44(9):678-684. doi:10.1136/ bjsm.2009.070128
6. Gillet B, Begon M, Sevrez V, Berger-Vachon C, Rogowski I. Adaptive Alterations in shoulder range of motion and strength in young tennis players. J Athl Train. 2017;52(2):137-144. doi:10.4085/ 1062-6050.52.1.10
7 Hannah DC, Scibek JS, Carcia CR, Phelps AL. Bilateral asymmetries of humeral retroversion in junior and collegiate tennis players. J Athl Train. 2021;56(12):1321-1326. doi:10.4085/ 1062-6050-0686.20
8. Johansson F, Asker M, Malmberg A, FernandezFernandez J, Warnqvist A, Cools A. Eccentric and isometric shoulder rotation strength and range of motion: Normative values for adolescent competitive tennis players. Front Sports Act Living 2022;4:798255. doi:10.3389/fspor.2022.798255
9. Dines JS, Bedi A, Williams PN, et al. Tennis injuries: Epidemiology, pathophysiology, and treatment. J Am Acad Orthop Surg. 2015;23(3):181-189. doi:10.5435/JAAOS-D-13-00148
10. Marcondes FB, Jesus JF de, Bryk FF, Vasconcelos RA de, Fukuda TY Posterior shoulder tightness and rotator cuff strength assessments in painful shoulders of amateur tennis players. Braz J Phys Ther 2013;17(2):185-193. doi:10.1590/ S1413-35552012005000079
11. Moreno-Pérez V, Moreside J, Barbado D, VeraGarcia FJ. Comparison of shoulder rotation range of motion in professional tennis players with and without history of shoulder pain. Man Ther 2015;20(2):313-318. doi:10.1016/j.math.2014.10.008
12. Kibler WB, Sciascia A, Thomas SJ. Glenohumeral internal rotation deficit: Pathogenesis and response to acute throwing. Sports Med Arthrosc Rev 2012;20(1). doi:10.1097/JSA.0b013e318244853e
13. Colomar J, Baiget E, Corbi F. Influence of strength, power, and muscular stiffness on stroke velocity in junior tennis players. Front Physiol. 2020;11:196. doi:10.3389/fphys.2020.00196
14. Sheehan WB, Watsford ML, Pickering Rodriguez EC. Examination of the neuromechanical factors contributing to golf swing performance. J Sports Sci 2019;37(4):458-466. doi:10.1080/ 02640414.2018.1505185
15. Brazier J, Maloney S, Bishop C, Read PJ, Turner AN. Lower extremity stiffness: Considerations for testing, performance enhancement, and injury risk. J Strength Cond Res. 2019;33(4):1156-1166. doi:10.1519/JSC.0000000000002283
16. Pickering Rodriguez EC, Watsford ML, Bower RG, Murphy AJ. The relationship between lower body stiffness and injury incidence in female netballers. Sports Biomech. 2017;16(3):361-373. doi:10.1080/ 14763141.2017.1319970
17 Pruyn EC, Watsford ML, Murphy AJ, et al. Relationship between leg stiffness and lower body injuries in professional Australian football. J Sports Sci 2012;30(1):71-78. doi:10.1080/ 02640414.2011.624540
18. Hjelm N, Werner S, Renstrom P. Injury risk factors in junior tennis players: a prospective 2-year study: Injury risk factors in junior tennis players. Scand J Med Sci Sports. 2012;22(1):40-48. doi:10.1111/ j.1600-0838.2010.01129.x
19. DiFiori JP, Benjamin HJ, Brenner JS, et al. Overuse injuries and burnout in youth sports: a position statement from the American Medical Society for Sports Medicine. Br J Sports Med. 2014;48(4):287-288. doi:10.1136/bjsports-2013-093299
20. Johansson F, Cools A, Gabbett T, FernandezFernandez J, Skillgate E. Association between spikes in external training load and shoulder injuries in competitive adolescent tennis players: The SMASH cohort study. Sports Health Multidiscip Approach. 2022;14(1):103-110. doi:10.1177/19417381211051643
21. Johansson F, Tranaeus U, Asker M, Skillgate E, Johansson F. Athletic identity and shoulder overuse injury in competitive adolescent tennis players: The SMASH cohort study Front Sports Act Living 2022;4:940934. doi:10.3389/fspor.2022.940934
22. Fernandez-Fernandez J, Nakamura FY, MorenoPerez V, et al. Age and sex-related upper body performance differences in competitive young tennis players. Boullosa D, ed. PLOS ONE. 2019;14(9):e0221761. doi:10.1371/ journal.pone.0221761
23. Bishop C, Read P, Lake J, Chavda S, Turner A. Interlimb asymmetries: Understanding how to calculate differences from bilateral and unilateral tests. Strength Cond J. 2018;40(4):1-6. doi:10.1519/ SSC.0000000000000371
24. Ko CY, Choi HJ, Ryu J, Kim G. Between-day reliability of MyotonPRO for the non-invasive measurement of muscle material properties in the lower extremities of patients with a chronic spinal cord injury. J Biomech. 2018;73:60-65. doi:10.1016/ j.jbiomech.2018.03.026
25. Hermens HJ, Freriks B, Disselhorst-Klug C, Rau G. Development of recommendations for SEMG sensors and sensor placement procedures. J Electromyogr Kinesiol. 2000;10(5):361-374. doi:10.1016/ S1050-6411(00)00027-4
26. Kibler WB, Chandler TJ, Shapiro R, Conuel M. Muscle activation in coupled scapulohumeral motions in the high performance tennis serve. Br J Sports Med 2007;41(11):745-749. doi:10.1136/ bjsm.2007.037333
27. Rogowski I, Rouffet D, Lambalot F, Brosseau O, Hautier C. Trunk and upper limb muscle activation during flat and topspin forehand drives in young tennis players. J Appl Biomech. 2011;27(1):15-21. doi:10.1123/jab.27.1.15
28. Cohen J. Statistical Power Analysis for the Behavioral Sciences. 2nd ed. L. Erlbaum Associates; 1988.
29. Myers NL, Aguilar KV, Mexicano G, Farnsworth JL, Knudson D, Kibler WB. The acute:chronic workload ratio is associated with injury in junior tennis players. Med Sci Sports Exerc 2020;52(5):1196-1200. doi:10.1249/MSS.0000000000002215
30. Afonso J, Peña J, Sá M, Virgile A, García-deAlcaraz A, Bishop C. Why sports should embrace bilateral asymmetry: A narrative review. Symmetry. 2022;14(10):1993. doi:10.3390/sym14101993
31. Colomar J, Corbi F, Baiget E. Inter-limb muscle property differences in junior tennis players. J Hum Kinet 2022;82(in press). doi:10.2478/ hukin-2022-0026
Sean F. Griech1a , Christos Karagiannopoulos1
1 Doctor of Physical Therapy Program, DeSales University
Keywords: isometric, shoulder, hand-held dynamometry, submaximal https://doi.org/10.26603/001c.127141
International Journal of Sports Physical Therapy Vol. 20, Issue 1, 2025
Background
Submaximal isometric exercises are used for pain control and neuromuscular facilitation. Typically, an ipsilateral maximal voluntary isometric contraction (MVIC) is used as a reference; however, when this is not clinically feasible, an alternative must be considered. Two options are (1) the no reference (NR) method (submaximal contraction at a self-perceived effort level without reference) and (2) the reciprocal reference (RR) method (MVIC on the contralateral side serves as a reference for a submaximal effort-level on the ipsilateral side). No research evidence exists as to which alternative method is more accurate at the shoulder
Purpose
To determine the accuracy of the NR and RR methods in estimating target muscle force during shoulder ER and IR submaximal isometric contractions among healthy adults.
Study Design
Observational cross-sectional
Methods
Isometric shoulder force was measured via a hand-held dynamometer on 48 healthy participants (36 females and 12 males) mean age of 27.4 ±1.6 years. Both methods (NR and RR), direction of force (IR and ER), and starting test-side (right or left) were randomized. RR testing involved a contralateral MVIC (reference) prior to a 50% submaximal contraction. NR testing entailed a 50% submaximal contraction with no prior reference MVIC.
Results
Actual submaximal efforts were compared to MVIC-based estimated submaximal efforts. Significant moderate - good correlations existed for both the RR (r = 0.691) and NR (r = 0.620) methods, regardless of test-side or shoulder motion. Significant moderate - good correlations were found between both methods for both ER [RR (r = 0.717) and NR (r = 0.614)] and IR [RR (r = 0.669) and NR (r = 0.628)].
Conclusion
Both methods had moderate - good accuracy levels and were not influenced by the test side or direction of force. Either method (RR or NR) can be equally useful for shoulder isometric exercise prescription when an ipsilateral reference cannot be determined.
Corresponding Author:
Sean F. Griech, PT, DPT, Ph.D.
E-mail: sean.griech@desales.edu | a
Address: DeSales University College of Health Professions, Department of Physical Therapy 2755 Station Ave. Center Valley, PA 18034.
Telephone: 610-282-1100 ext. 2784
Isometric exercises are commonly prescribed for patients/ clients with various musculoskeletal pathologies, including tendinopathies, fractures, and post-operative care.1‑7 Often, submaximal [i.e., 25-50% of maximal voluntary isometric contraction (MVIC)] isometric contractions are prescribed in the early stages of rehabilitation, including for the shoulder, in an effort to effectively manage pain and improve functional recovery with minimal muscle overload.8‑10 In such cases, a degree of uncertainty may exist on whether an individual is accurately exerting the appropriate amount of submaximal muscle force prescribed by a clinician. The apparent clinical concern is whether a patient safely exerts an isometric muscle effort level appropriate for a given level of physiological tissue healing.
A direct way to improve accuracy on exercises that utilize submaximal isometric muscle efforts could be having the patient first perform an MVIC in the same direction and the same side in order to establish an ipsilateral muscle force reference criterion. This ipsilateral muscle-force criterion (IMFC) develops a conscious sense of how an MVIC muscle effort feels first and may help a patient’s acuity in more accurately estimating a prescribed submaximal isometric muscle-force level. Previous research has established the accuracy of the IMFC approach described above for both shoulder external rotation (ER) and internal rotation (IR) (ICC for ER=0.909 and IR=0.849).11
Despite these results, producing an ipsilateral MVIC for reference may not always be possible, such as in the acute phases of post-operative management, fracture, or highly irritable tendinopathy. In these cases, another instructional method for producing a targeted submaximal isometric muscle force must be used. Two possible alternative techniques include a no reference (NR) method and a reciprocal reference (RR) method. The NR method is done through simple verbal commands directing the individual to perform a given percentage of one’s MVIC level (i.e., “only use 50% of your total effort level”). This submaximal contraction is done without the patient first performing an MVIC as a reference to base their effort level. Instead, the person is only asked to imagine and then perform what a hypothetical submaximal muscle contraction at the given target would be. The RR method, on the other hand, is done by asking the patient to perform an MVIC on the contralateral (uninvolved) side to serve as the baseline reference criterion. In this case, developing a sense of how an MVIC muscle effort feels first on the opposite side helps a patient’s ability to apply the targeted force on the opposite (reciprocal) side by downgrading the intended submaximal muscle contraction force during exercise.12 Both of these alternative methods may potentially be clinically useful approaches among patients when an ipsilateral muscle MVIC is not advisable or practical.
Currently, there are no studies, in the shoulder or elsewhere, that examine the accuracy of these alternative
methods of isometric force production when an ipsilateral reference criterion is not available. Exploring this research gap is clinically meaningful, especially for the initial rehabilitation stages of various shoulder pathologies requiring submaximal isometric exercises’ application and progression. These exercises have been shown to reduce pain threshold, increase pain tolerance, and safely improve neuromuscular control.8,9,13,14 Additionally, training-specific adaptations to targeted submaximal isometric exercises may include improvements in muscular endurance, muscle hypertrophy, and muscle strength.15 The level of these physiological muscle adaptations may be influenced by the patient’s accuracy with which submaximal muscle force production can be estimated and applied during exercises.13 The purpose of this study was to determine the accuracy of the NR and RR methods in estimating target muscle force during shoulder ER and IR submaximal isometric contractions among healthy adults. The first aim of the study was to determine and compare the overall accuracy of the NR and RR in producing a targeted submaximal shoulder isometric force (50% of MVIC) as compared to the IMFC regardless of the test side or shoulder motion (ER and IR). The second aim was to determine the accuracy between the NR and RR methods as compared to the IMFC in producing a targeted submaximal isometric force (50% of MVIC) specifically for shoulder ER and IR, regardless of the test side.
This was a cross-sectional observational study, which was approved by the DeSales University Institutional Review Board (ED60-110821). A convenience sample of healthy participants were recruited from the University community via word of mouth and electronic communication. All participants signed informed consent and were screened for eligibility through a questionnaire that was completed by the participant with a researcher present. Inclusion criteria included healthy adults over the age of 18 years. Exclusion criteria included those with known current shoulder or neck pain and associated weakness of the shoulder within the past six months, a history of shoulder or neck surgery within the past two years, any history of neurologic or rheumatologic conditions that may be affecting the upper extremities, and inability to communicate in English.
Force production was measured using the ActivForce (AF; Activbody, San Diego, CA) digital dynamometer. Although the isokinetic dynamometer is considered the gold-standard measurement of isometric force production,16,17 hand-held dynamometry (HHD) has been shown to be strongly correlated (ρ = .65–.82) with force production out-
Figures 1A (upper figure) and 1B (lower figure). Modified permuted block randomization flow for determining the direction of force and starting test side.
puts compared to isokinetic dynamometry, including across different clinician experience levels.18,19 The AF was chosen due to its lighter weight compared to other HHDs, making it much easier to fixate the dynamometer at specific heights on the wall. Additionally, the lower profile design did not impede the normal alignment for shoulder ER and IR isometric contraction. Specifically, the AF HHD has been previously shown to be reliable for different shoulder movements (ER, IR, forward elevation) and across different experience levels (intra-rater ICC = 0.97, inter-rater ICC =0.91).19
All subjects’ active range of motion was screened for functional IR (ability to place the hand behind their back) and ER (ability to place the hand behind their head/neck) prior to participating. Participants were asked to perform a series of maximal (MVIC) and submaximal (50% of MVIC) isometric contraction efforts in pre-determined randomly selected directions (IR or ER). This predetermined randomization enabled NR and RR methods to be assessed in each direction on both sides (Figure 1).
Values were determined by the peak force over a fivesecond timeframe. A single tester placed the AF HHD be-
tween the subject’s distal forearm and the wall, with the location of the dynamometer set by the participant having the shoulder at 0º of abduction and the elbow flexed to 90°. The dynamometer location was fixed to the wall throughout testing for each subject (Figure 2).
Standardized verbal instructions were given to the subject to perform a maximum isometric contraction for a fivesecond hold into either ER or IR. The direction of force and the starting test side were randomized using a permuted block randomization. This randomized process was determined by a simple coin flip, and then required participants to follow either procedure A or B (Figure 1). Each procedure consisted of two series (based on which side was tested first) of six isometric contractions (100% MVICs and submaximal 50% MVICs) with a one-minute break between each contraction. The 100% MVIC recorded initially was used as the IMFC to establish the accuracy of both the RR and NR at a submaximal effort by dividing this value by two. After each series, a five-minute break was given, and the patient was asked to report their fatigue based on the Modified Borg Rate of Perceived Exertion (RPE) 0-10 scale.20 It has been previously established that a 1-minute rest in between consecutive five-second MVICs is ample time for recovery with no signs of fatigue.8,20 Completing the testing sequences within either procedure A or B enabled partici-
Figure 2. Set up to measure isometric force of shoulder external rotation (A) and internal rotation (B) using dynamometer fixed to the wall
pants to perform both methods (RR and NR) in a different order on both sides. Both the tester and participant were blinded to the results of their force production. This was achieved because the HHD utilized does not have a digital display It requires a remote connection with a wireless device. In this study, it was remotely connected to a device managed by an independent researcher who recorded participant force production for all isometric contractions. An additional researcher timed each trial and rest period throughout the data collection process.
Descriptive and inferential statistical analysis was performed using SPSS version 27 (IBM Corp., Armonk, NY). Data were assessed for normality by using the K-S statistic and examining Q-Q plots. The assumptions of Pearson correlation were met; and, therefore, were used to analyze both study aims. Pearson correlations were intended to assess the accuracy of the participants’ ability to perform a self-estimated 50% submaximal effort for both the NR and RR methods compared to their actual submaximal effort, which was used as a reference criterion. The actual submaximal effort was calculated as 50% of the MVIC (actual MVIC divided by 2) produced by each participant during procedures A or B. Pearson correlations were interpreted as: > 0.75 strong, 0.50 - 0.75 moderate - good, and < 0.50 fairpoor.21 Statistical significance for this study was based on an alpha value of p = 0.05.
Thirty-sic females and 12 males with a mean age of 27.4 ±1.6 years participated (Table 1). The majority of the 48 participants were right-hand dominant females. For study-aim 1, significant correlations existed between the actual and estimated submaximal efforts for both the RR [r (190) = .69, p <0.001] and NR [r (190) = .62, p<0.001] methods, regardless of test-side or shoulder motion. The
Table 1. Demographic Information for Participants
Variable
y, mean (SD)
(10.9) Gender
Male, n (%)
(25) Female, n (%)
(75) Hand Dominance Right, n (%)
(6.25) RPE
Note. RPE – rate of perceived exertion on a 0-10 scale; Middle – fatigue between testing series; post–fatigue immediately after both testing series
mean difference between the actual and estimated efforts was -1.36% and 2.25% for the RR and NR methods, respectively (Table 2). A 2-tailed power analysis (utilizing GPower) of aim 1 results indicated that a 0.99 power was achieved based on a medium effect size at a .05 alpha level and a sample size of 192 data comparison points (two methods were compared using both sides of 48 participants for both shoulder ER and IR).
For study-aim 2, significant moderate - good correlations were found between the actual and estimated submaximal efforts for the RR method [ER r (94) = .72, p <0.001 and IR r (94) = .67, p <0.001] and the NR method [ER r (94) = .61, p <0.001 and IR r (94) = .63, p <0.001], regardless of test-side. A 2-tailed power analysis (utilizing G-Power) of aim 2 results indicated that a 0.85 power was achieved based on a medium effect size at a .05 alpha level and a sample size of 96 data comparison points (two methods were compared for shoulder ER and IR using both sides of 48 participants). The mean differences between the actual
Table 2. No Reference Versus Reciprocal Reference Methods
between actual and calculated (%)
Note. *Correlation is significant <0.001 (2-tailed)
a Sample size includes 48 subjects tested into ER and IR for both left and right sides.
Table 3. ER versus IR for both No Reference versus Reciprocal Reference Methods compared to the Ipsilateral Muscle-Force Criterion Method
and
Note. *Correlation is significant <0.001 (2-tailed)
a Positive % infers an overestimation of muscle effort; a negative % infers an underestimation of
b Percent of change for ER, regardless of the method, was 4.63%
c Percent of change for IR, regardless of the method, was -3.74%
d Sample size includes 48 subjects tested on both left and right sides
and estimated efforts for the NR method IR and ER ranged between 3.76% and -6.48%, and for the RR method, IR and ER ranged between 5.50% and - 0.99% (Table 3). Negative mean difference values in muscle effort inferred an underestimation while positive mean difference values in muscle effort represented an overestimation of the actual muscle force compared to the self-estimated submaximal muscle force levels. RPE scores on the modified Borg scale (0-10 scale) were assessed at the midway point (mean 1.35, SD 0.2) and again at the end of testing (mean 1.42, SD 0.21) of both procedures A and B.
DISCUSSION
This study aimed to determine how accurately a healthy adult could recreate a targeted submaximal (50% of MVIC) isometric force of the shoulder regardless of the test side (left or right) or shoulder motion (IR and ER) when IMFC is not possible. It was determined that both the NR and RR methods may have statistically meaningful accuracies at moderate - good (r > .60) levels among healthy adults for shoulder ER and IR motions bilaterally To the best of the authors’ knowledge, the accuracy of these two methods has yet to be determined for the shoulder
Instead, what has already been reported in the literature is the reliability in estimating submaximal isometric shoulder IR and ER force efforts using an already performed ipsilateral MVIC effort as a reference criterion. A single study by Maenhout et al.11 determined a high agreement (ICCs range .84 -.90) for shoulder IR and ER among healthy adults
and patients with shoulder tendinopathy Although these values indicate a higher accuracy level than this study’s results, this discrepancy is expected when comparing these methods. Using an ipsilateral MVIC effort as a reference may result in a stronger perception of muscle force and memorization than methods in which a contralateral side MVIC effort (RR method) or having no previous muscle effort reference (NR method) is utilized. Thus, the RR and NR methods may be expected to offer slightly lower accuracy levels, as shown in the current study However, the advantage of the RR and NR methods is their clinical utility in shoulder patient populations where an ipsilateral MVIC is unsafe or undesirable, especially during the early postoperative stages when only submaximal isometric exercises may be beneficial. In such cases, the RR or NR methods may be clinically meaningful viable options in estimating submaximal shoulder IR and ER isometric efforts with small but expected error levels (-6.48 – 5.50%). In the current study, the error levels for the RR or NR methods were determined by calculating the mean difference between the actual and targeted submaximal (50% of MVIC) performed efforts. These error levels are comparable to those (-5.76 – 6.04%) reported in the Maenhout et al.11 study. The results indicate that errors in IR consistently had a negative value, indicating an underestimation of the target muscle force. Conversely, ER consistently had errors with a positive value, indicating an overestimation tendency.
Other strengths of the study are worth noting, including its randomized testing protocol, well-standardized testing conditions, and fatigue-level monitoring during testing. The two testing procedures, A and B, helped to randomize
the initial testing side and shoulder motion. This randomization helped to mitigate the effect of dominant versus non-dominant extremity as all participants were tested bilaterally. This randomization and the utilization of a wellstandardized blinded testing protocol across all participants may also have resulted in the reported low estimated error of measurements, leading to greater trustworthiness of the current study’s results. The estimated high study power (≥ 0.85) also adds credibility to the findings. Finally, monitoring participants’ fatigue levels during and immediately after testing via the Modified Borg RPE scale,20 a previously validated outcome measure, confirmed the stability of the study’s result against any influence from muscle fatigue during testing. The average levels of perceived exertion were minimal during (1.2/10) and after (1.4/10) testing across all participants for both methods. This indicated that the implemented 1- and 5-minute resting periods between testing trials effectively controlled fatigue, as only a 4.4% level of exertion increase was noted by the end of each testing series. No adverse effects were reported by the participants during or after data collection.
The results of this study should be interpreted with some caution due to the following limitations. Participants’ age and activity levels were not accounted for as independent variables, and therefore, their possible effect on isometric force production and determining the accuracy of the NR and RR methods in estimating target muscle force during shoulder ER and IR was not determined. Activity level prior to testing was not controlled in this study, and the RPE scale was utilized only at the end of the testing series. Thus, the effect of possible increased baseline physical fatigue from performing daily activities prior to testing was not accounted for in this study. Unfortunately, this study’s sample consisted predominantly of young adults, and the results of this study cannot be extrapolated in middle age or older adult populations. Future studies should include a broader age spectrum to allow for age-appropriate comparisons. The lack of gender stratification was another weakness worth noting in this study as the majority of participants were females. Our study’s gender disproportion (75% female) did not allow for a reliable investigation of gender-based differences in the accuracy of the NR and RR methods. A more balanced gender representation between
males and females might be a useful aim of future similar studies. A trend of males over-estimating isometric target forces as compared to females has been reported in a previous study evaluating lower extremity musculature.13 Finally, this study focused on only estimating submaximal isometric force levels of 50% among healthy participants. Thus, the results of this study may not be fully generalized at different other levels or individuals with shoulder injuries. Future studies should examine these methods’ accuracy at different submaximal isometric force levels (e.g., 25% or 75% of MVIC) as well as if accuracy is affected by underlying shoulder pain or pathology
This study sought to determine the accuracy of alternative methods for targeted submaximal (50% of MVIC) isometric contractions of the shoulder when an ipsilateral reference could not be obtained. This study’s results indicate that, overall, the RR or NR are adequate methods in estimating submaximal target muscle forces with moderate - good accuracy levels. Both may be viable alternatives for clinicians working with individuals and athletes with shoulder pathology whose ipsilateral upper extremities should not be engaged in maximum isometric efforts during testing or exercises.
The authors report no conflicts of interest.
The authors would like to thank our DeSales DPT students: Salvatore Pagano, Frank McHugh, Kevin Danilowski, Marissa Corso, Abdalrazzag Albokhari, and Grace Merritt for their vital contributions and commitment during the entire data collection process, as well as our volunteer participants.
Submitted: June 07, 2024 CST, Accepted: October 20, 2024 CST
© The Author(s)
This is an open-access article distributed under the terms of the Creative Commons Attribution 4.0 International License (CCBY-NC-4.0). View this license’s legal deed at https://creativecommons.org/licenses/by-nc/4.0 and legal code at https://creativecommons.org/licenses/by-nc/4.0/legalcode for more information.
1. Nejati P, Ghahremaninia A, Naderi F, Gharibzadeh S, Mazaherinezhad A. Treatment of subacromial impingement syndrome: platelet-rich plasma or exercise therapy? A randomized controlled trial. Orthop J Sports Med 2017;5(5):2325967117702366. doi:10.1177/2325967117702366
2. Marlow K, Morris R, Fogarty J, Gretsch Z, Riemann B, Davies G. Torque steadiness measurements of the shoulder in normal subjects and subjects with shoulder pain. Presented at: American Physical Therapy Association-Combined Sections Meeting; 2020; Denver, CO. Accessed April 20, 2023. https:// digitalcommons.georgiasouthern.edu/healthkinesiology-facpres/290/
3. Foxen-Craft E, Dahlquist L. Brief submaximal isometric exercise improves cold pressor pain tolerance. J Behav Med 2017;40(5):760-771. doi:10.1007/s10865-017-9842-2
4. van Ark M, Cook JL, Docking SI, et al. Do isometric and isotonic exercise programs reduce pain in athletes with patellar tendinopathy in-season? A randomised clinical trial. J Sci Med Sport 2016;19(9):702-706. doi:10.1016/j.jsams.2015.11.006
5. Rio E, Kidgell D, Purdam C, et al. Isometric exercise induces analgesia and reduces inhibition in patellar tendinopathy Br J Sports Med 2015;49(19):1277-1283. doi:10.1136/ bjsports-2014-094386
6. Khosrojerdi H, Tajabadi A, Amadani M, Akrami R, Tadayonfar M. The effect of isometric exercise on pain severity and muscle strength of patients with lower limb fractures: A randomized clinical trial study. Med Surg Nurs J. 2018;7(1). doi:10.5812/ msnj.68104
7 Sgroi TA, Cilenti M. Rotator cuff repair: postoperative rehabilitation concepts. Curr Rev Musculoskelet Med 2018;11(1):86-91. doi:10.1007/ s12178-018-9462-7
8. MK HB, Dicapo J, Rasiarmos R, Hunter SK. Dose response of isometric contractions on pain perception in healthy adults. Med Sci Sports Exerc 2008;40(11):1880-1889. doi:10.1249/ MSS.0b013e31817eeecc
9. Lemley KJ, Drewek B, Hunter SK, MK HB. Pain relief after isometric exercise is not task-dependent in older men and women. Med Sci Sports Exerc. 2014;46(1):185-191. doi:10.1249/ MSS.0b013e3182a05de8
10. Lewis J, McCreesh K, Roy JS, Ginn K. Rotator cuff tendinopathy: navigating the diagnosis-management conundrum. J Orthop Sports Phys Ther. 2015;45(11):923-937 doi:10.2519/jospt.2015.5941
11. Maenhout AG, Palmans T, De Muynck M, De Wilde LF, Cools AM. The impact of rotator cuff tendinopathy on proprioception, measuring force sensation. J Shoulder Elbow Surg 2012;21(8):1080-1086. doi:10.1016/j.jse.2011.07.006
12. Ting LH, Raasch CC, Brown DA, Kautz SA, Zajac FE. Sensorimotor state of the contralateral leg affects ipsilateral muscle coordination of pedaling. J Neurophysiol 1998;80(3):1341-1351. doi:10.1152/ jn.1998.80.3.1341
13. Keller JL, Housh TJ, Smith CM, Hill EC, Schmidt RJ, Johnson GO Sex-related differences in the accuracy of estimating target force using percentages of maximal voluntary isometric contractions vs. ratings of perceived exertion during isometric muscle actions. J Strength Condit Res 2018;32(11):3294-3300. doi:10.1519/JSC.0000000000002210
14. Clifford C, Challoumas D, Paul L, Syme G, Millar NL. Effectiveness of isometric exercise in the management of tendinopathy: a systematic review and meta-analysis of randomised trials. BMJ Open Sport Exerc Med 2020;6(1):e000760. doi:10.1136/ bmjsem-2020-000760
15. Lum D, Barbosa TM. Brief Review: Effects of Isometric Strength Training on Strength and Dynamic Performance. Int J Sports Med. 2019;40(6):363-375. doi:10.1055/a-0863-4539
16. Sole G, Hamrén J, Milosavljevic S, Nicholson H, Sullivan SJ. Test-Retest Reliability of Isokinetic Knee Extension and Flexion. Arch Phys Med Rehabil. 2007;88(5):626-631. doi:10.1016/j.apmr.2007.02.006
17 Toonstra J, Mattacola CG. Test-retest reliability and validity of isometric knee-flexion and-extension measurement using 3 methods of assessing muscle strength. J Sport Rehabil. 2013;22(1). doi:10.1123/ jsr.2013.TR7
18. Croteau F, Robbins SM, Pearsall D Hand-held shoulder strength measures correlate with isokinetic dynamometry in elite water polo players. J Sport Rehabil 2021;30(8):1233-1236. doi:10.1123/ jsr.2020-0277
19. Karagiannopoulos C, Griech S, Leggin B. Reliability and validity of the ActivForce digital dynamometer in assessing shoulder muscle force across different user experience levels. Int J Sports Phys Ther 17(4):669-676. doi:10.26603/001c.35577
20. Williams N. The Borg rating of perceived exertion (RPE) scale. Occup Med. 2017;67(5):404-405. doi:10.1093/occmed/kqx063
21. Portney LG. Foundations of Clinical Research: Applications to Evidence-Based Practice. 4th ed. F. A. Davis; 2020.
Bailey Urbach1 a , Cody Mansfield2,3 , Mica Harr1 , Matthew S. Briggs4,5,6 , James Onate2,5 , Laura Boucher2,5
1 Neuroscience, The Ohio State University, 2 School of Health & Rehabilitation Sciences, The Ohio State University, 3 Sports Medicine, The Ohio State University Wexner Medical Center, 4 Rehabilitation Services, The Ohio State University Wexner Medical Center, 5 Sports Medicine Research Institute, The Ohio State University Wexner Medical Center, 6 Department of Orthopedics, The Ohio State University Wexner Medical Center
Keywords: low back pain, collegiate athletics, rifle, rehabilitation, Olympic https://doi.org/10.26603/001c.127385
International Journal of Sports Physical Therapy
Vol. 20, Issue 1, 2025
Background
The sport of rifle places unique physical demands on its athletes relative to other collegiate sports, including maintaining lumbar-straining positions for extended time. Anecdotal reports of low back pain (LBP) are common among collegiate rifle athletes, but the prevalence of LBP in this population has not yet been established in the literature.
The purpose of this study was to survey collegiate rifle athletes to quantify the prevalence and impact of low back pain in this population and identify possible contributing factors including the COVID-19 pandemic. It was hypothesized that over 50% of athletes would report an episode of LBP due to participation in collegiate rifle events.
Study Design
Cross-Sectional Study
Methods
Athletes at least 18 years of age who were members of a collegiate rifle program during the 2019-2020 season were surveyed via an online questionnaire. The questionnaire included four sections: (a) demographics, (b) presence of LBP, (c) the impact of pain episodes on daily activities, and (d) shooting stance and training characteristics.
Results
Responses from 114 athletes were collected. A total of 101 (89%) respondents indicated having experienced at least one episode of LBP. Of those, 24% also missed at least one day of training or competition during the 2019-2020 season because of LBP During the COVID-19 pandemic, 60% were unable to receive the same level of medical care while 69% experienced equal or greater pain levels. An association between sex and LBP was statistically significant (p<0.001).
Conclusion
There is a high prevalence of LBP in collegiate rifle athletes. It is necessary for Athletic Training staff and other medical professionals to be aware of this for prevention and treatment, as it has significant impacts including missed playing time. More research on predisposing factors such as sex and training duration or positional characteristics would benefit management of this injury.
Corresponding Author:
Bailey Urbach, MD1
Address: c/o Graduate Medical Education, 2500 MetroHealth Drive, Cleveland OH 44109
Phone: (603) – 566 – 1751
Email: bxu36@case.edu
Low back pain (LBP) is the most common form of musculoskeletal pain and is a major cause of activity limitations, disability, health provider visits, and health care costs.1 Athletes have been observed to experience higher rates of LBP than the general population.2‑5 While the one-year prevalence of LBP for the general population has been reported as high as 56%, for elite athletes in any sport at the university and Olympic levels the one-year prevalence has been reported to be as high as 81%.1‑5 Anecdotally, many elite rifle athletes including those competing at the collegiate level have been cited to suffer from bouts of acute and persistent LBP.6‑9
LBP can impact these athletes’ participation, performance amongst teammates, scholarship acquisition, and potential Olympic berths.7,9 However, there is a paucity of evidence in the scientific literature regarding injury surveillance and injury prevention for this unique athletic population. A systematic review found only three studies focused exclusively on injury surveillance in summer Olympic-style shooting events like those contested at the collegiate level, and only two studies focused on adolescent or universityaged athletes that include shooting athletes.3,4,6,8,10,11
Though previous studies have examined the rate of injury and prevalence of LBP for shooting sports athletes, they have important limitations. The investigation by Volski et al. is the only study that distinguishes between shooting disciplines (rifle, pistol, shotgun) in its methodology, rather than simply listing “shooting” as the sport, however, this study does not distinguish between events within disciplines.6 None of the former studies pertain specifically to NCAA athletes or events, though multi-sport studies by Muller et al. and Noormohammadpour et al. focus on adolescent and university athletes outside of the United States.3,4 This lack of specificity allows for the reported prevalence of LBP in rifle athletes, particularly within the collegiate population, to be influenced by athletes in the other shooting disciplines whose injury rate may differ due to the athletic and equipment requirements unique to their events.8
Low back pain in athletes has been shown to correlate with a lack of strength of the trunk muscles (i.e., internal and external obliques, transversus and rectus abdominis).2, 12 Other muscles that have been implicated as dysfunctional in LBP include the posterior spinal muscles, including the erector spinae and multifidi.13,14 Olympic-style rifle shooting requires strength in the trunk muscles for uses that are atypical compared to other sports.9 Rifle athletes utilize their trunk in a static manner, requiring these muscles to provide stabilization and static control for optimal performance, rather than coordination with arm and leg movement as seen in other sports.7,15‑17 This positioning requires lumbar rotation and hyperextension which places stress on the spine and pelvis, illustrated in Figure 1.6 Further, athletes may hold these positions for over an hour at
Figure 1. Depiction of a traditional Olympic-style rifle shooting stance.
A traditional right-handed shooting stance with a .22 caliber smallbore rifle that would be used in collegiate competition. The same position is used for the .177 caliber air rifle event.15 16
a time.15,18 These unique physical demands of rifle athletes may predispose them to a higher risk of LBP and potential recurrent episodes of LBP Improper form or trunk strength deficits, particularly in the aforementioned muscle groups, may exacerbate this risk.6
Given that LBP may both have a significant impact on athletic opportunities as well as result in a chronic injury with potentially lifelong physical and financial consequences, obtaining information regarding prevalence and relevant factors can guide medical professionals, coaching staff, and sports governing bodies in the surveillance, prevention, and treatment of LBP in these athletes. The purpose of this study was to survey NCAA and collegiate club rifle athletes nationally to quantify the prevalence and impact of LBP in this population and identify possible contributing factors of LBP, including the COVID-19 pandemic which occurred during the time of data collection. The COVID-19 pandemic was caused by the novel SaRS-CoV-2 virus and resulted in vast but varying term shutdowns, and modifications of colleges, athletics programming, and nonemergent medical services.19
This is the first known study to investigate injury patterns of LBP specifically in collegiate rifle athletes, particularly the prevalence of this injury. Ultimately this work will help guide future research investigating the prevalence of LBP and its effects on rifle athlete performance. It is hypothesized that over 50% of NCAA and collegiate club ri-
fle athletes will report an episode of LBP due to participation in collegiate rifle events, thereby warranting further investigation and surveillance of injury in this understudied athletic population. This study also seeks to investigate the effects of the novel COVID-19 pandemic on LBP in this population, including factors such as access to medical care or training facilities which may have influenced such impact. It is hypothesized that greater than half of respondents would report changes to their baseline LBP and reduced access to medical care previously received for LBP during the pandemic.
This was a cross sectional, observational study, using an online survey via Qualtrics Survey Software (QualtricsXM, Provo, UT) available from July 8, 2020 to March 27, 2021. The project and study protocol were reviewed and approved by the Institutional Review Board. Consent was obtained via affirmative response to a required element on the first page of the questionnaire. All survey data were stored in a secure cloud-based folder accessible only to study personnel. No identifying information was connected to the results of the survey
The survey was designed to best reflect the most pertinent consequences of LBP for a student athlete, including impact on both training and other team related obligations as well as day to day activities, and factors relevant specifically to the sport of rifle. Two authors developed the initial survey, which was further refined utilizing feedback from the remainder of the study team. The drafted survey was developed from: 1) relevant questions selected from several validated LBP questionnaires (Japanese Orthopaedic Association Back Pain Evaluation Questionnaire, Örebro Musculoskeletal Pain Questionnaire, Oswestry Low Back Pain Disability Questionnaire)20‑22 and 2) concepts deemed important by the authors to evaluate in the study population, which included questions related to shooting position characteristics and how they may influence the prevalence or severity of LBP Additionally, as the survey was being administered during the early COVID-19 pandemic at a time which significant activity restrictions were in place in many locations, several questions were developed which focused on the impact of the pandemic on LBP in the study population and were included throughout the questionnaire. Following development, study personnel accessed the survey to verify functionality and usability on both mobile and desktop devices.
The survey was comprised of four sections: Personal and Athletic Background, Pain Background, Pain Influence on Activity, and Athletic Information. The first section contained demographic information regarding age, overall time spent participating in the shooting sports, and other activities
that may be pertinent to the presence of back pain including COVID-19 pandemic related changes to physical activity and enactment of stay-at-home orders in the athlete’s primary location of residence during the pandemic. The first of these pandemic-specific questions was adapted from a study by University College London entitled “Health Behaviours During the COVID-19 Pandemic,” as changes to an athlete’s physical activity routine were considered during survey design as playing a potential role in pandemicrelated changes to pain.23 The Pain Background section assessed the presence and parameters of a participant’s back pain, while the Pain Influence on Activity section assessed the extent of influence a participant’s back pain had on daily activities. The Athletic Information section built a general description of the participant’s standing position during rifle competition. As there is no known validated survey regarding rifle shooting positions, the authors designed the questions in the two sport-related sections of the survey specifically for this study
For this survey, LBP was defined as pain “in the area between the inferior margin of the 12th rib and inferior gluteal folds.”1 In the Pain Background section, respondents were asked whether they had ever experienced LBP in conjunction with rifle training or competition, along with an accompanying image illustrating the defined “low back” region.24 This section also contained two questions pertaining to the COVID-19 pandemic and its effects on both severity of pain and access to medical care. The latter of these two questions was based on data from a Kaiser Family Foundation poll indicating as many as 48% of respondents or members of their households have experienced delayed healthcare due to the pandemic, as this would be likely to extend to collegiate athletes who are no longer able to access their campus resources.25
Those individuals responding affirmatively to having experienced LBP in conjunction with rifle training or competition were next given access to the Pain Influence on Activity section. Those who responded negatively to having a history of LBP were not able to access this section and were directed to the final Athletic Information segment. This section also contained a question regarding changes in the athlete’s planned training routine during the COVID-19 pandemic, which added context to perceived changes to pain increases or decreases. Presence of LBP history was the only required element of the study questionnaire following consent to participate.
A web link to complete the survey was disseminated via email by program coaches to potential participants. Inclusion criteria of participants were: 1) any current member of an NCAA or collegiate club level rifle program, 2) at least 18 years of age, and 3) former members of such teams who participated in the 2019-2020 season but had since completed their athletic eligibility or graduated from the university Subjects were not excluded on any other basis provided they met the eligibility criteria.
To recruit participants, the Head Coach of each team, identified using the “Team Homepages” at ncaarifle.org, the “Collegiate Shooting Sports Directory” at compete.nra.org, and the individual web pages for each rifle program, was emailed to request participation of their program.26,27 Following approval, a link to access the study questionnaire was then sent to the coach who distributed this to their team members via a forwarded email. A total of 23 programs received this link. The online survey was active and available to participants for a period of approximately 8.5 months, a time frame which encapsulated one complete NCAA athletic season.
Descriptive and frequency analyses were performed on the survey responses. The presence of LBP, rifle shooting career length, and training volume was expressed as a percent rounded to the nearest tenth. The extent to which LBP impacted everyday activities and characteristics of the rifle shooting position were expressed through either percent or mean, median and standard deviation, dependent on whether the survey question yielded quantitative or qualitative responses. All surveys with at least one question of the study questionnaire, excluding the consent page, containing a response were included. Non-responders at the item level were not included in analyses of individual question responses, so as to avoid potential bias from imputation on quantitative survey items.
A tertiary analysis to examine the association between sex (male, female) and the presence of low back pain (yes, no) was conducted via a chi-square test of independence. Due to small expected frequencies in 50% of the cells, with a minimum expected count of 0.11, the Fisher-FreemanHalton Exact Test was employed to ensure valid results. This test is suitable for contingency tables where the assumption of the standard Pearson Chi-Square test is violated due to low cell counts. The significance level was set at 0.05 for all statistical tests.
The questionnaire was sent to 23 NCAA and collegiate club level rifle programs (15 NCAA; seven collegiate club), a participation rate of 50% of contacted programs (46 total; 27 NCAA, 19 collegiate club). A total of 117 individual responses were collected from athletes affiliated with these programs. Three responses were discarded: two due to ineligibility and the third being a duplicate response, resulting in a total of 114 surveys included in analysis. This pathway of response collection is illustrated in Figure 2
Over 60% (70/113) of respondents to the gender question identified as female, with one participant electing not to respond to this question. The gender breakdown of respondents can be seen in Table 1 The median age of respondents was 20 years old, with more than 50% (63/114) indicating being in either their first or second year of col-
2. Flow diagram of recruitment and participation.
lege. At the collegiate level, the median number of years participated in rifle shooting was two, out of a maximum possible of five. For overall career length in rifle shooting, inclusive of participation in non-NCAA type rifle disciplines such as BB gun and sporter programs, the median was six years. These characteristics in addition to training details are also depicted in Table 1
At least one episode of LBP in conjunction with training or competition was indicated by 89% (101/113) of athletes (Figure 3). One participant did not answer this question. Of the remaining 12 athletes who reported not having experienced LBP, only one identified as female, with the other 11 identifying as male. Thus, 74% (32/43) of male respondents reported having experienced LBP in comparison to 99% (69/ 70) of female respondents. The results of the Fisher-Freeman-Halton Exact Test demonstrated this to be a statistically significant difference in the presence of LBP between the sexes (χ²(2) = 16.37, p < 0.001). Of athletes who had experienced LBP, 73% (73/100) of those indicated the location of their pain was bilateral. The median percentage of the previous collegiate rifle season for which respondents estimated that they experienced episodes of LBP was 40.
As many as 24% (33/98) of those experiencing LBP reported having missed at least one day of training or competition during the previous collegiate rifle season due to their LBP Of those who experienced LBP, the mean duration of the 2019-2020 playing season which these athletes estimated themselves to have experienced pain was 45% (n=100). This pain also impacted activities critical to team participation such as travel, with 49% (48/98) reporting increased LBP with travel. Additionally, 61% (60/98) endorsed occasional sleep disturbances due to pain and 27% (26/98)
Table 1. Demographics and Training Characteristics
reported having to modify their work, training, or competition routine because of LBP
With respect to shooting position characteristics, 88% (100/114) reported using a right-handed stance. Over 75% (79/105) of those who answered further questions about their shooting position reported a support hand position using an open palm, with several variations in hand and forearm rotation among these responses. Additionally, 59% (62/105) place the support elbow directly on top of the hip, and 63% (66/105) report thrusting their leading hip fully toward the target (Figure 4, Figure 5). An overview of participant shooting position characteristics in more detail is shown in Table 2.
Since the onset of the COVID-19 pandemic in March 2020, 69% (69/99) of respondents who had experienced LBP reported continuing to experience the same or higher levels of pain as previously reported in this period. One participant who had experienced LBP elected not to answer this question. Of those who reported receiving medical care for their LBP, 60% (27/45) indicated being able to receive only some or none of the care they had received prior to the pandemic.
Figure 4. Typical support hand positions for Olympicstyle rifle competition.
(A) Fist-style hand position in which the rifle rests on the knuckles. (B) Open palm hand position with fingers pointing in the direction of the target. This was selected as the most common hand position among both athletes with and without history of LBP (C) Open palm hand position with fingers pointing behind the athlete, parallel to the firing line.
Figure 5. Typical support elbow placements for Olympic-style rifle competition.
(A) Elbow position ahead of the hip. (B) Elbow position directly on top of the hip. This was selected as the most common elbow position among both athletes with and without history of LBP (C) Elbow position behind the hip.
The purpose of this study was to assess the prevalence of low back pain in the collegiate rifle athlete population and investigate factors which may contribute to its presence or absence. This is the first study to identify the prevalence of LBP in this group. Most respondents (89%) indicated a history of LBP in conjunction with training or competition in rifle. Though the present study reflects the experiences of only a portion of this population, these athletes represent a large number of its members based on roster sizes of the programs identified during the recruitment
process.26,27 These rosters indicate fewer than 400 athletes compete in rifle through the NCAA annually; the total number of non-NCAA collegiate club athletes is less standardized year-to-year and therefore unclear, but between 60 and 80 additional athletes from as many as 19 identified club programs compete in the Intercollegiate Rifle Club National Championships annually, the highest level nonNCAA collegiate rifle competition.
The results of this study suggest a high prevalence of LBP in collegiate rifle athletes. These results align with a 2011 study on injuries in Turkish Shooting Championship participants which asserted that one of the most common areas of injury during shooting competition was the lower back.10 Only one study, published in 1986, specifically investigated LBP in rifle and pistol athletes, and found a significant correlation between performance on a functional screen and LBP 6 When viewed in the context of the current study, this may provide a framework for future research regarding the role muscular dysfunction or weakness of the trunk plays in this injury.
There are no known studies regarding LBP in the collegiate rifle athlete population, though there are several multi-sport studies regarding athlete injury rates and incidence of LBP that include shooting sports. One of these multi-sport studies, focusing on elite German athletes, indicated a 12-month prevalence of LBP in as many as 87% of respondents from the shooting sports and lifetime prevalence of 95%, which is consistent with the 89% prevalence rate determined by the present study.2 This is in contrast, however, with the results of a systematic review by Harr et al. showing a low prevalence of overall injury in shooting athletes at the Summer Olympic Games, less than 10%. It is likely that injuries reported at competition events are more acute and not reflective of chronic conditions such as LBP, a limitation which is also noted by Harr et al.8
Nearly one quarter (24%) of athletes with LBP in this study have had to miss playing time in the form of training or competition for this injury Overall, athletes with LBP spent an average of 45% of the previous playing season experiencing this pain. These results suggest that this injury places a high burden on the collegiate rifle athlete population, as many athletes are missing time or otherwise struggling with LBP throughout a large portion of their competitive season both on and off the playing field. Details regarding the traditional NCAA Rifle playing season timeline and that of the 2020-2021 season impacted by COVID-19 pandemic restrictions are highlighted in Figure 6 and Figure 7.
This study also sought to examine factors that may be implicated in LBP, including characteristics of the shooting position. The most common shooting position characteristics among respondents indicated the use of a right-handed shooting stance with significant hip thrust towards the target, elbow placement ahead of the hip towards the anterior ribcage, and a variant of the open palm support hand position. Despite the fact that these positions can be highly variable athlete to athlete, the listed characteristics are representative of those found in instructional materials for both beginner and high level athletes.16,29,30 These shooting positions place increased unilateral strain on the lumbar spine and require adequate trunk strength and proper technique and standing positioning (Figure 1).7 Though there are athletes able to use these positional variations without subsequent LBP, this study shows that many athletes using these positions do experience LBP Therefore, it may be worth examining the common positions for alternative options that maintain accuracy while reducing physical strain. It may also be of use for athletic training and strength training professionals who work with these athletes to design workout and rehabilitation programs that account for these common positions and the unilateral
Figure 6. Timeline of a typical NCAA Rifle Playing Season prior to 2020-2021.
The season is separated into a fall segment and a spring segment, with a maximum number of 13 regular season competitions followed by the NCAA Championships Qualifying Match and NCAA Championship Tournament.28 Many collegiate rifle athletes additionally participate in the U.S. National Junior Olympics and U.S. National Championships following the NCAA-sanctioned season, resulting in year-round competition.
Figure 7 Timeline of the 2020-2021
The NCAA Rifle playing season operated under an approximately normal timeline, though notably the fall half of the season ended about two weeks earlier than during other years.26 Additionally, the number of teams competing in NCAA competition on any given weekend was much fewer than in the 2019-20 season.
strain they place on the lower back and joints. More specifically, it may be prudent for these programs to focus on core strength and stability, as dysfunction or weakness of the trunk muscles has been implicated in LBP. It may also be of
benefit for coaching staff to focus on making athlete-specific positional adjustments based on reported pain to mitigate the risk of further injury
Worth noting is that although the pool of respondents with no history of LBP is small, as only 12 out of 113 who indicated a sex, 92% (11/12) of these individuals identified as male, a relationship which was found to be statistically significant via Fisher-Freeman-Halton Exact Test (χ²(2) = 16.37, p < 0.001). The higher prevalence of LBP in females seen in this study is compatible with the results of a systematic review by Harr et al., in which the incidence of all injuries in summer Olympic-style shooting events, like those used in NCAA competition, was higher in women than men.8 The Olympic-style standing shooting position relies heavily on pelvic positioning and stability It is possible that these positions induce more strain in women due to the wider pelvic structure seen in female anatomy, and differences in muscle, fat, and bone density between the sexes, as suggested by Fett et al.2 Although rifle is a coed sport for championship purposes at the collegiate level, no less than seven out of the nearly 30 current NCAA Rifle programs sponsor female-only teams while others maintain female-dominant co-ed rosters.26 This pattern suggests a need for further investigation into the significance of this relationship, given the large proportion of female participants in the sport.
The study also investigated the impact of the Covid-19 pandemic on LBP in the collegiate rifle athlete population. During the first year of the pandemic, spanning from March 2020 until closure of the survey in March 2021, most participants with history of LBP reported the same or increased levels of pain. While not all respondents with history of LBP were receiving medical care for this injury at the time of the pandemic, more than half of such athletes were unable to receive the same level of medical care compared to prior to March 2020. These results highlight that the Covid-19 pandemic may have been detrimental to the rehabilitation of this chronic musculoskeletal injury
There were several limitations to this study that should be considered. First, the cross-sectional nature of the study design limits the potential interpretation of factors relating to the development of LBP Athletes are likely to change their techniques and training volume over time, something that cannot be fully captured by a cross-sectional survey Future cohort studies may assess the incidence or recurrence of LBP in rifle athletes over the course of a collegiate season or career while also tracking such changes.
Second, recall bias is an important consideration in any cross-sectional study which uses a survey tool for measurement, as it relies on the memory of the participant to obtain accurate data.31 However, it is unlikely that participants who responded affirmatively to having experienced LBP incorrectly recalled this information. Rather, it is more
likely that those who stated they did not experience LBP failed to recall an episode of LBP that did not result in substantial cost or activity limitation and thus may have led to under-reporting. Additionally, selection bias may have played a role in the distribution of respondents with and without history of low back pain.32 Athletes who have experienced low back pain may be more likely to participate in a study exploring this phenomenon than those who do not have such history. Despite this potential for selection bias, these respondents still represent a substantial portion of rifle athletes competing at the NCAA and collegiate club level, making awareness of this data an important step. Additionally, there are limitations to the tertiary statistical analysis regarding the relationship sex and LBP The overall sample size of respondents with no LBP is quite small, only 12 athletes. There was also an unequal distribution of respondents of each sex, with nearly double the number of female respondents compared to male. This makes generalization of these results challenging despite a statistically significant relationship being identified. However, this relationship is worth acknowledgement as nearly a quarter of NCAA Rifle programs feature exclusively female rosters.
Lastly, this survey was administered during the early months of the COVID-19 pandemic.19 This may have negatively impacted the response rate as many programs were still under various pandemic-related restrictions, and some programs did not compete at all in the season during which responses were collected.26
The results of this survey indicate a high prevalence of LBP in the collegiate rifle athlete population, particularly in female athletes. LBP resulted in impacts to both athletes’ daily lives and their sport participation, including loss of training and competition time. The COVID-19 pandemic additionally impaired these athletes’ ability to maintain medical care for LBP Future studies investigating novel stance modifications and further characterizing the epidemiology and time course of this injury in collegiate rifle athletes should be pursued. Further investigation of the relationship between sex and prevalence of LBP in this population may also be of value, given the large proportion of female participants in the sport.
The authors report no conflicts of interest.
Submitted: April 10, 2024 CST, Accepted: October 20, 2024 CST
© The Author(s)
This is an open-access article distributed under the terms of the Creative Commons Attribution 4.0 International License (CCBY-NC-4.0). View this license’s legal deed at https://creativecommons.org/licenses/by-nc/4.0 and legal code at https://creativecommons.org/licenses/by-nc/4.0/legalcode for more information.
1. Hoy D, Brooks P, Blyth F, Buchbinder R. The Epidemiology of low back pain. Best Pract Res Clin Rheumatol. 2010;24(6):769-781. doi:10.1016/ j.berh.2010.10.002
2. Fett D, Trompeter K, Platen P Back pain in elite sports: A cross-sectional study on 1114 athletes. PLoS One 2017;12(6). doi:10.1371/ journal.pone.0180130
3. Noormohammadpour P, Rostami M, Mansournia MA, Farahbakhsh F, Pourgharib Shahi MH, Kordi R. Low back pain status of female university students in relation to different sport activities. Eur Spine Jl. 2016;25(4):1196-1203. doi:10.1007/ s00586-015-4034-7
4. Müller J, Müller S, Stoll J, Fröhlich K, Otto C, Mayer F. Back pain prevalence in adolescent athletes. Scand J Med Sci Sports 2017;27(4):448-454. doi:10.1111/sms.12664
5. Schulz SS, Lenz K, Büttner-Janz K. Severe back pain in elite athletes: a cross-sectional study on 929 top athletes of Germany. Eur Spine J. 2016;25(4):1204-1210. doi:10.1007/ s00586-015-4210-9
6. Volski RV, Bourguignon GJ, Rodgriguez HM. Lower Spine Screening in the Shooting Sports. Phys Sports Med 1986;14(1):101-106. doi:10.1080/ 00913847.1986.11708968
7. Arnot C. Postural Considerations for Rifle and Pistol Shooters. USA Shooting News Published online 2010:10-11.
8. Harr MR, Mansfield CJ, Urbach B, Briggs M, Onate J, Boucher LC. Prevalence and incidence of injury during Olympic-style shooting events: A systematic review. Int J Sports Phys Ther. 2021;16(5):1235-1249. doi:10.26603/001c.28231
9. Krilling W Shooting For Gold Midland Georgia; 1986.
10. Kabak B, Karanfilci M, Ersoz T, Kabak M. Analysis of sports injuries related to shooting. J Sports Med Phys Fitness. 2016;56(6):737-743.
11. Zeman V, Pitr K. Dysfunction of the motor system in sports shooters. Sports Med Train Rehabil 2001;10(1):1-11. doi:10.1080/15438620109512092
12. Critchley DJ, Coutts FJ. Abdominal muscle function in chronic low back pain patients. Physiotherapy. 2002;88(6):322-332.
13. Fortin M, Macedo LG. Multifidus and paraspinal muscle group cross-sectional areas of patients with low back pain and control patients: A systematic review with a focus on blinding. Phys Ther 2013;93(7):873-888. doi:10.2522/ptj.20120457
14. Freeman MD, Woodham MA, Woodham AW. The role of the lumbar multifidus in chronic low back pain: A review PM&R 2010;2(2):142-146. doi:10.1016/j.pmrj.2009.11.006
15. Engle N. 2021 USA Shooting Rules for 50m Rifle and 10m Air Rifle. Adapted for Use in NCAA Rifle Competitions. 2021. Accessed January 5, 2024. https:/ /ncaaorg.s3.amazonaws.com/championships/sports/ rifle/rules/2021-22PRXRI_USAShootingRules.pdf
16. Bühlmann G, Reinkemeier H, Eckhardt M. Ways of the Rifle. Verlag/MEC; 2009.
17 Kibler WB, Press J, Sciascia A. Current Opinion: The Role of Core Stability in Athletic Function. 2006;36. doi:10.2165/00007256-200636030-00001
18. ISSF. Official Statutes Rules and Regulations Edition 2013. 2013. http://www.issf-sports.org/ documents/rules/2013/ISSFRuleBook2013-3rdPrintENG.pdf
19. Nicola M, O’Neill N, Sohrabi C, Khan M, Agha M, Agha R. Evidence based management guideline for the COVID-19 pandemic - Review article. Int J Surg. 2020;77:206-216. doi:10.1016/j.ijsu.2020.04.001
20. Fukui M, Chiba K, Kawakami M, et al. Japanese Orthopaedic Association back pain evaluation questionnaire. Part 3. Validity study and establishment of the measurement scale: Subcommittee on low back pain and cervical myelopathy evaluation of the clinical outcome committee of the Japanese Orthopaedic Association, Japan. J Orthop Sci 2008;13(3):173-179. doi:10.1007/ s00776-008-1213-y
21. Linton SJ, Boersma K. Early identification of patients at risk of developing a persistent back problem: The predictive validity of the Örebro musculoskeletal pain questionnaire. Clin J Pain. 2003;19(2):80-86. doi:10.1097/ 00002508-200303000-00002
22. Fairbank JCT, Pynsent PB. The Oswestry disability index. Spine. 2000;25(22):2940-2953. doi:10.1097/ 00007632-200011150-00017
23. Shahab L, Herbec A. UCL HEBECO Study Baseline (Core). 2020. https://osf.io/bja7g/
24. Dionne CE, Dunn KM, Croft PR, et al. A consensus approach toward the standardization of back pain definitions for use in prevalence studies. Spine. 2008;33(1):95-103. doi:10.1097/ BRS.0b013e31815e7f94
25. Hamel L, Kearney A, Kirzinger A, Lopes L, Munana C, Brodie M. KFF Health Tracking Poll - May 2020.; 2020.
26. NCAA Rifle Dashboard. NCAA Rifle. http:// ncaarifle.org/
27 Competitive Shooting Programs. NRA Explore. https://competitions.nra.org/competitions/nranational-matches/collegiate-championships/ collegiate-shooting-sports-directory/
28. National Collegiate Athletic Association. NCAA 2021-22 Division I Manual. NCAA; 2021.
29. Ihalainen S, Kuitunen S, Mononen K, Linnamo V Determinants of elite-level air rifle shooting performance. Scand J Med Sci Sports. 2016;26(3):266-274. doi:10.1111/sms.12440
30. Meili L. Rifle: Steps to Success Human Kinetics, Inc.; 2008.
31. Jager KJ, Tripepi G, Chesnaye NC, Dekker FW, Zoccali C, Stel VS. Where to look for the most frequent biases? Nephrology. 2020;25(6):435-441. doi:10.1111/NEP.13706
32. Kamper SJ. Bias: Linking evidence with practice. J Orthop Sports Phys Ther 2018;48(8):667-668. doi:10.2519/jospt.2018.0703
Brekke AF, Bjørklund J, Holse RC, Larsen C, Hjortshoej MH. Low-Load Blood-flow Restriction Training for Medial Tibial Stress-Syndrome in Athletes: A Case Series. IJSPT 2025;20(1):96-104. doi:10.26603/001c.126963
Anders F. Brekke1,2,3a , Johanne Bjørklund4 , Rosa C. Holse4 , Christian Larsen4 , Mikkel H. Hjortshoej4
1 Department of Physiotherapy, Centre for Health and Rehabilitation, University College Absalon, Denmark, 2 Department of Orthopaedics and Traumatology, Odense University Hospital, Denmark, 3 Department of Clinical Research, University of Southern Denmark, Denmark, 4 Department of Physiotherapy, Centre for Health and Rehabilitation, University College Absalon
Keywords: Medial tibial stress syndrome, shin splint, blood-flow restriction training, running, Athlete https://doi.org/10.26603/001c.126963
International Journal of Sports Physical Therapy
Vol. 20, Issue 1, 2025
Background
Medial tibial stress syndrome (MTSS) is a common overuse injury characterized by activity-induced pain along the distal medial tibial border. Current best practice includes rest and progressive resistance training. However, some patients with MTSS may be unable to tolerate the loading during exercise. Blood-flow restriction training using low loads (LL-BFR) may induce similar physiological and structural adaptations as heavy resistance training but without peak loads. This could potentially allow the athlete to continue sports activities during rehabilitation.
Purpose
The purpose of this case series was to describe an exercise program utilizing LL-BFR training for athletes with running-related MTSS.
Study design
Case series
Methods
Six recreational athletes (one handball player, one soccer player, and four runners) with MTSS were recruited. Inclusion criteria included pain along the distal two-thirds medial tibial border occurring during or after activity. Exclusion criteria were symptoms of compartment syndrome, tibial stress fracture, or contraindications for BFR training. Participants underwent a progressive six-week home-based LL-BFR training intervention with three sessions per week and were allowed to continue sports activities if pain was ≤ NRS 5. Outcome measures included change in standardized running performance (distance and pain level), pain pressure threshold (algometry), and self-reported physical function.
Results
Five athletes experienced improvements in running performance (pain and/or distance) and self-reported function. One athlete sustained an injury unrelated to the LL-BFR training, and therefore the running post-test could not be completed. Adherence to exercise was high, and post-test interviews revealed positive feedback on the training method, with no side effects reported.
Corresponding Author
Anders Falk Brekke
Address: University College Absalon, Parkvej 190, 4700 Næstved, Denmark
Phone: +45 7248 2626
Email: afr@pha.dk
This case series demonstrated that following a therapeutic exercise program utilizing LL-BFR training improvements in pain and function were seen in athletes with MTSS. BFR may allow clinicians to prescribe lower-load exercises, facilitating continued sports participation. Future research should compare the effectiveness of exercise programs for MTSS with and without LL-BFR training.
Level of Evidence
Level V
Medial tibial stress syndrome (MTSS) is characterized as activity-induced pain located at the distal two-thirds of the posteromedial tibial border, with recognizable pain reproduced with palpation with an extent larger than 5 cm in length.1 MTSS is one of the most frequent overuse injuries of the lower extremities related to sports that involve running or jumping.2,3 MTSS is the most frequent running-related musculoskeletal injury, accounting for an incidence of 13.6-20% and a prevalence of 9.5%.3 The severity of MTSS can range from brief suspensions from activity to careerending.4
The etiology of MTSS is unclear; however, different mechanisms have been proposed. MTSS has been characterized as micro-damage of the tibia due to repeated bending (absorption of energy) during weight-bearing activities, leading to osteopenia, with decreased bone mineral density at the injury site.5,6 It has also been suggested that MTSS is related to muscular traction-induced periostitis.7
Current best practice for treatment of MTSS includes rest and discontinuing activities that provoke symptoms.8 An additional recommended treatment for MTSS is a progressive training intervention divided into three phases.9 The three phases include rest and modified activity levels, isometric and concentric resistance training, and eccentric and plyometric exercises. There is, however, no high-quality evidence for the effect of any intervention in treating MTSS.5,10
Low-load blood-flow restriction (LL-BFR) training utilizes low loads of 20-40% of 1-repetition maximum (1-RM), and is accomplished by the use of a strap or an inflated cuff that restricts the arterial inflow and occludes the venous outflow to an extremity during exercise.11 Despite the low load, LL-BFR training has been demonstrated to induce similar structural outcomes, such as muscle hypertrophy and improved muscle strength compared to traditional heavy-load resistance training (HL-RT) (70-80% of 1RM).12 LL-BFR has been used in clinical rehabilitation of various musculoskeletal conditions (i.e. anterior cruciate ligament injuries, knee osteoarthritis, patellofemoral pain) and has demonstrated superior physical function and structural outcomes compared to intensity-matched resistance training13 and comparable outcomes to those of HL-RT 14 A potential mechanism is that LL-BFR training seems to induce increased levels of hormone (i.e. growth hormone) and immune responses of similar magnitude compared to conventional HL-RT.15 Increased growth hormone has been reported to positively influence Type I collagen synthesis16
which is a key component in bone cell activity via the effect on osteoblasts. Furthermore, LL-BFR training has also been demonstrated to induce similar increases in bone metabolism compared to traditional strength training.17 Therefore, athletes rehabilitating with a LL-BFR training program may increase bone mass and connective tissue healing without exposing the body to the same amount of physical stress as would occur during HL-RT 18 The purpose of this case series was to describe an exercise program utilizing LL-BFR training for athletes with running-related MTSS.
CASE DESCRIPTION: PATIENT HISTORY, PHYSICAL EXAMINATION, AND OUTCOME SCORES
PATIENT HISTORY
One handball player, one soccer player, and four runners, all recreational athletes with symptoms corresponding to MTSS, were recruited through posters, social media, friends, and fellow athletes in Region Zealand Denmark. Included athletes were aged 15-50 years with a history of pain at the distal two-thirds medial border of the tibia during or after activity. Their pain had to have been reproduced with palpation in a continuous area larger than 5 cm in length. Athletes with history and symptoms corresponding to compartment syndrome, tibial stress fracture, or contraindication for LL-BFR training were excluded.
Athlete 1 (runner) was a 22-year-old male with symptoms corresponding to right-sided MTSS for two months. The pain was present when running but not at rest. He had a history of anterior lateral stress syndrome one year ago in the same leg. Due to MTSS, he reduced his running level to three times a week for a total of 2-3 hours. He had not tried structured treatment for MTSS, provided by a healthcare professional.
Athlete 2 (runner) was a 21-year-old female with bilateral symptoms corresponding to MTSS for more than five years. The pain was present primarily when running but not at rest. She had stopped running and walked for 45 min twice a day instead. She also felt pain in the lower extremities during resistance training. She used to play badminton years ago but had to stop this, too. She was advised by a physician to do muscle stretching exercises, use analgesics, and reduce activity, but with no effect.
Athlete 3 (soccer) was a 22-year-old male with bilateral symptoms corresponding to MTSS for the prior year. The pain was present during activities related to soccer but not
at rest. He played soccer and ran 5 km twice a week, respectively. Despite experiencing pain during exercise, he did not discontinue or use analgesics. He had not tried structured treatment for MTSS, provided by a healthcare professional.
Athlete 4 (runner) was a 24-year-old female with bilateral symptoms corresponding to MTSS for more than five years. The pain was present during activities related to running but not at rest. She was running two times per week for approximately 30 min despite experiencing pain during running; she did not discontinue or use analgesics. She had not tried structured treatment for MTSS, provided by a healthcare professional.
Athlete 5 (handball) was a 16-year-old female with bilateral symptoms corresponding to MTSS for the prior four years. The pain was present primarily during activities related to handball but not at rest. She played handball twice a week and participated in gymnastics once a week. She had not tried structured treatment for MTSS, provided by a healthcare professional.
Athlete 6 (runner) was a 24-year-old female with bilateral symptoms corresponding to MTSS for more than five years. The pain was more intense in the left leg. The pain was present primarily during activities related to running but not at rest. Due to MTSS, she reduced her running level. She was running one time per week for 30 min and participating in CrossFit training three times per week, respectively. She was using analgesics in connection with running. She had not tried structured treatment for MTSS, provided by a healthcare professional.
All participants underwent a thorough examination to ensure they were eligible for participation. The potential for compartment syndrome was assessed by history using the algorithm suggested by Winters,19 which included the following symptoms: cramping, burning pain over the posterior compartment, and numbness, pins, and needles in the foot during exercise Indications of tibial stress fracture were: known palpation pain less than 5 cm in length, a positive percussion test of the medial and lateral malleolus with referred pain to the tibia or fibula bone, respectively, or a local pain to the tibia or fibula by a jump test.20‑22 Participants would also be excluded if there was a history of diseases related to blood circulation, e.g., swelling or varicose veins.11
Written informed consent was obtained from all participants. The study conformed to the standards set by the Declaration of Helsinki as well as by the procedures stated by The Danish National Center for Ethics. The study was approved by the local research ethics committee, University College Absalon, Region Zealand Denmark. In addition, all participants gave written informed consent that the data concerning the case would be submitted for publication.
A standardized and individualized running test was developed with inspiration from a protocol described by Schütte et al. 2018.23 The running test took place at a 400 m athletic
track. The test was standardized by having the participants stop running after a maximum of eight laps, equivalent to 3200 m, or if they experienced a perceived level of pain of NRS=7 (numeric rating scale; from 0: no pain to 10: maximum pain). A change in NRS pain score by 2 points is considered the minimal clinically important difference (MCID) in chronic pain conditions.24 The test was individualized and reproducible by the participants, who were instructed to maintain a consistent self-chosen speed throughout the test, measured with a GPS watch. The running distance covered and pain level before and after running were recorded.
The participants’ local pressure pain threshold (NRS 0: no pain to 10: maximum pain) was measured using a handheld digital algometer (Figure 1: CommanderTM Algometer, JTECH Medical, Midvale, Utah, USA) following a standardized protocol with inspiration from Aweid et al. 2013.25
The pressure surface was 1 cm2 , and the pressure threshold was a maximum of 30 Newton (N) or when stopped before that level by the participant. By testing healthy individuals before the study, it was determined that 30 N was the threshold for experiencing discomfort by standardized algometry at the area of MTSS. Aweid et al. reported intrarater reliability from moderate to excellent (ICC 0.53–0.90) and concluded that pain pressure threshold algometry could be incorporated into MTSS clinical assessment to assess pain and monitor progress objectively. The participants were supine on the examination table with support under both feet; thus, the calf muscles did not touch the surface. Algometry was assessed bilaterally at the most tender point located by palpation, in a horizontal and posterior-lateral direction, for evaluating the periosteum and muscle tissue, respectively The distance from the most tender point to the apex of the medial malleolus was recorded to ensure the exact location for the post-test.
Change in self-reported physical function was assessed by the Lower Extremity Functional Scale (LEFS) questionnaire26 and the Patient Specific Functional Scale (PSFS).27 LEFS has a total score ranging from 0: extreme difficulty or inability to perform activity to 80: no difficulty LEFS is valid and reliable for assessing change in persons with chronic conditions.26 The MCID for LEFS is 9 points.26 The PSFS was used accordingly to an individual running-related ac-
Figure 2. The LL-BFR training calf raise exercise for Levels 1 and 2 from A) standing with flat feet on the floor to B) heel-lift. The Level 3 exercise was carried out on one leg. The occlusion band is visible proximally on the right thigh.
tivity The scoring scale (NRS) ranges from 0: unable to perform the activity to 10: able to perform the activity as before the problem arose PSFS is valid, reliable, and responsive in individuals with a limited number of acute, subacute, and chronic conditions.27 The MCID for PSFS is 2-3 points across various conditions.27
Pre-tests were collected on the day of the beginning of the intervention, and post-tests took place one week after the last training session to avoid possible influence from the previous training session.
The participants completed a six-week home-based progressive LL-BFR training intervention with three training sessions per week (a total of 18 sessions). A training session consisted of calf raises, given the association between reduced plantar flexor strength and endurance and MTSS (Figure 2).28 This included four sets of LL-BFR training with a 30-15-15-15 repetition scheme (75 total repetitions) and 30 seconds of rest between sets.11
The participants were instructed to keep the standard elastic occlusion band (width 5 cm) tightened throughout all four sets and placed most proximally on the symptomatic leg.29 The occlusion band was tightened corresponding to NRS=7 (0: no sensation of tightness to 10: maximal sensation of tightness).30 Before starting the home-based training, all participants were instructed to tighten the occlusion band under the practical guidance of a physiotherapist, who ensured proper application until the participants felt comfortable and confident in performing the procedure
independently This training ensured the occlusion of venous backflow (visually dilated superficial veins) without causing arterial occlusion (palpable posterior tibial artery pulsation). Regarding participants with bilateral MTSS symptoms, the participant performed the training protocol on each leg separately.
The calf raise exercise was carried out as continuous cyclic movements from standing with flat feet on the floor to the heel(s) being lifted as much as possible for 1-2 seconds and then lowered for 1-2 seconds until the entire foot was placed on the floor again. The exercise was performed wearing training shoes. The participants were allowed to use their hands to support their balance and keep an upright position, with their feet pointing straight forward, hipwidth distance apart.
The LL-BFR training progression was developed using table values on load (% of 1RM) and the corresponding number of repetitions.31 Accordingly, 30 repetitions of oneleg calf raises (Level 3) corresponded to 45% of 1RM and thus deemed acceptable for the 30-15-15-15 repetitions LLBFR training protocol. The LL-BFR training intervention followed a standardized progression every second week. Level 1 (weeks one and two): both heels were lifted simultaneously with even weight bearing. Level 2 (week three and four): both heels were lifted simultaneously with more weight on the occluded leg. Level 3 (week five and six): the exercise was performed on one leg. Two persons from the author group, one with MTSS, conducted the three levels of LL-BFR training in parallel with the participants for first-hand expression of the progression of the intervention. Data from these persons were not included in the present study
After three weeks of training, individual mid-term interviews were held with the participants regarding potential side effects, adherence, practical matters (such as correct tightness of the occlusion band), experience of progression from Level 1 to 2, and the opportunity to ask questions. The participants were encouraged to contact the project team by phone or e-mail if they had questions about the training. If deemed necessary, a physical meeting would be arranged. Continuation of training (soccer, handball, and running) was allowed as long as the pain level did not exceed NRS 5. The participants filled in a training diary with a record of all training sessions, the progression of exercises, and any potential side effects of the training.
An overview of the individual results is presented in Table 1.
Athlete 1 (runner) had a post-test pain reduction of 2 NRS points in the running test and completed the total distance without pain. Overall, there was no change in pain by algometry in the periosteum or muscle. The self-reported improvement in LEFS was 7 points, and PSFS 6 points.
Athlete 2 (runner) had no post-test pain change in the running test and completed with NRS 7 However, the running distance was increased by 600 m (86%). Overall, the post-test pain by algometry was unchanged (NRS 8) for
both periosteum and muscle. The self-reported improvement in LEFS was 13 points, and PSFS 4 points.
Athlete 3 (soccer) had no post-test pain change in the running test and completed with NRS 7. However, the running test was terminated prematurely due to pain caused by a calf muscle strain from playing soccer a few days before the post-test. Overall, the post-test pain by algometry increased with 3 NRS points to NRS 5-7 for both periosteum and muscle. The self-reported change in LEFS was a reduction of 1 point and an improvement in PSFS by 4 points.
Athlete 4 (runner) had a post-test pain reduction of 7 NRS points in the running test and completed the total distance without pain. Additionally, the running distance was increased by 250 m (26%). Overall, the post-test pain by algometry decreased with 1-4 NRS points to approximately NRS 5 for both periosteum and muscle. The self-reported improvement in LEFS was 4 points and PSFS 7 points, respectively. The LEFS post-test score was 77 points, and thus close to the highest possible score of 80 points.
Athlete 5 (handball) had a post-test pain reduction of 1 NRS point in the running test and completed the total distance with NRS 4. Overall, the post-test pain by algometry was unchanged (NRS 6-7) for both periosteum and muscle. The self-reported improvement in LEFS was 21 points, and PSFS 5 points.
Athlete 6 (runner) had a post-test pain reduction of 2 NRS points in the running test and completed the total distance without pain. Overall, the post-test pain by algometry decreased with 1-5 NRS points to NRS 2-6 for both periosteum and muscle. The self-reported improvement in LEFS was 7 points, and PSFS 5 points. The LEFS post-test score was 80 points, and thus, the highest possible score.
The participants expressed that it required practice to find the correct tightness of the occlusion band, but after a few attempts, it worked well. Athlete 1 felt the first level of progression was too easy Athlete 2 had experienced cramps in the feet during the first week of Level 1 LL-BFR training. Consequently, an expert in LL-BFR training was consulted (MHH). The athlete was advised to do calf raises without the occlusion band to test if the cramping was related to the restriction in blood flow. The cramping of the feet continued without the use of the occlusion band. After a short period of treatment of the plantar muscles of the foot in week two of the intervention, the symptoms stopped, and the LLBFR training continued.
Individual interviews were conducted one week after the last training session, on the same day as the post-tests. The athletes were asked about their experiences with the LL-BFR training intervention. All expressed that they were generally positive about the training method.
Athletes 1 and 4 found the training challenging but did not experience severe muscle fatigue or subsequent muscle soreness. Athlete 1: “It has been hard, but I haven’t felt it afterward. I also haven’t had muscle soreness during the
process ” Athlete 4: " I only had a bit of muscle soreness the first few times after training."
Athletes 4 and 6 highlighted the sensation of training with restricted blood flow. Athlete 4: “You just have to get used to it. The way the lactic acid builds up. It is a little surprising if you are not used to it.” Athlete 6: “The lactic acid buildup with occlusion feels much stronger than regular strength training, but it disappears as soon as the band is loosened. ” Athlete 5: “It was nice that it wasn’t 30 all the way through. It was a bit of a relief that you only had to do 15 repetitions afterward. ”
The participants agreed that Level 1 (week one and two) was easy to complete, and the following weeks, especially the last two (Level 3), were challenging. Athlete 1: “The first two weeks were easy when standing on both legs. I wasn’t tired in my calves afterward. Weeks 3-4 and 5-6 were hard. During the last two weeks of training, I was on the verge of needing a little help with the other foot for the last two sets’ last repetitions.” Only Athlete 2 had difficulties completing the number of repetitions. “The only thing I have considered is whether there were too many sets. It has always been those last two sets where I have thought, now I can’t do it anymore. ”
There was a consensus that it was easy to do LL-BFR training at home and not time-consuming, making it easier to complete the training. Athlete 6: “You can train at home, and you don’t need a lot of weights or machines to do the training. ” One participant expressed that training with the occlusion band could sometimes be uncomfortable on the thigh as it fits very tightly. Athlete 3: “ … you have to be willing to do it because it can be uncomfortable that it tightens ”
All participants, except for athlete 2, completed all 18 training sessions as prescribed. Athlete 2 did not train in week two of the intervention and, thus, completed 15 training sessions. No side effects were reported to the LL-BFR training. Athlete 3 did not report difficulties in conducting the training despite the strained calf muscle.
This report presents six cases utilizing LL-BFR training to treat running-related MTSS. The athletes had chronic MTSS symptoms for 4-5 years, except for athlete 1, who had symptoms for two months.
Five athletes improved their running performance after completing the six-week LL-BFR training intervention, except for athlete 3, who sustained an acute calf muscle strain during the intervention period while playing soccer. Thus, the results from athlete 3 are not included in the following summary of change in the pre- to post-test outcomes scores. Three athletes achieved an MCID decrease in pain of 2 NRS from pre- to post-test and completed the total running distance with no pain (no. 1, 4, and 6). Athlete 2 did not change pain level but increased the running distance, and athlete 5 reduced pain slightly.
There was no difference in pain pressure algometry between the periosteum and muscle. Thus, this case series found no tendency regarding whether MTSS pain originated from one or the other tissue. Furthermore, the relation between performance in the running test and pain
Table 1. Pre-test and post-test outcome scores for each athlete with MTSS
Athlete 1
Running test (NRS-pain 0-10)
Not applicable PROMS: Patient-reported outcome measures
PSFS:
pressure algometry was inconsistent. Regarding athletes 1, 2, and 5, there was a relation between low (NRS 0), high (NRS 7), and moderate pain (NRS 4) after the running test and low (NRS: periosteum 1 and muscle 0), high (NRS: periosteum 8 and muscle 8), and moderate pain (NRS: periosteum 6-7 and muscle 6-7) by the pressure algometry test, respectively This relation is supported by evidence suggesting a relationship between increased muscle pain by
Athlete 2
Athlete 3
pressure algometry and decreased physical performance.32 Conversely, athletes 4 and 6 experienced no pain (NRS 0) after the running test but had moderate pain (NRS: periosteum 4-6 and muscle 2-6) by the pressure algometry test. However, However, both athletes experienced reduced posttest pressure pain (NRS: periosteum -2 to -4 and muscle -1 to -5), which potentially influenced the running test positively (NRS reduction of -7 and -2, respectively). This in-
consistent finding may indicate that running is a function that involves many muscles and can be performed with individual techniques, which involve muscles differently Overall, the evidence is sparse on the effect of LL-BFR training in patients with chronic musculoskeletal conditions related to the connective tissue, such as tendinopathies and MTSS.33
All five athletes improved their self-reported level of function by 4-21 points, and two athletes reported a MCID of ≥ 9 points, with final LEFS scores between 70 and 80 points (80 points= No difficulty to perform activity). There was a ceiling effect when using the LEFS for athletes 4 and 6. The pre-test score was 73 points; thus, achieving a MCID of 9 points was impossible. All five athletes improved their self-reported running-related activities by 4-7 NRS points (exceeding the MCID of 2-3 points), with final PSFS scores between 5 and 9 points (10 points= Able to perform the activity as before the problem arose). This result is consistent with all participants’ improvement in the standardized running test.
In the interviews, the six athletes said they were generally positive about the training method. They found the progression easy at the beginning and strenuous at the end. The training was easy to perform once they gained experience in tightening the occlusion band optimally. There were no side effects or adverse events from the training. All participants continued their sport throughout the intervention period.
This case series details a novel therapeutic home-based progressive exercise program utilizing LL-BFR training for athletes with running-related MTSS along with a standardized and individualized running test, which has some methodological strengths and weaknesses.
LL-BFR training was evaluated as a novel treatment method for athletes with running-related MTSS in a clinical practice setting. The study presents the process beginning with the systematic differential diagnostic examination, which minimizes the potential risk associated with LL-BFR training. Then, continuing with an intervention tailored to each participant’s body weight, facilitating a pragmatic approach to load progression without the need for additional strength training equipment. The intervention consisted of a progressive single, technically simple exercise, which enhanced participant adherence. The LL-BFR training was sufficiently manageable, considering the athletes’ MTSS, allowing them to continue their sports activities. The standardized running test was individualized based on each athlete’s natural running speed and monitored with a GPS watch, enabling reproducibility of the running speed during the post-test. This approach allowed for the assessment of changes in pain and running distance.
There are some limitations to the study. Firstly, this is a case series with a low number of participants which limits the generalizability to a broader population.34 The findings may be specific to the particular cases and not applicable to other people with the same condition. Thus, there is a higher risk of bias and random chance. Due to the non-randomized, non-controlled, and non-blinded design, the results cannot infer a causal effect, and the results
of this case series should be interpreted with caution. Additionally, there was no follow-up after the intervention post-test, so it remains uncertain if the changes in MTSS are permanent. Therefore, a future high-quality randomized controlled trial, with a long-term follow-up period on the level of function and pain, is needed in testing the effectiveness of an exercise program utilizing LL-BFR training for athletes with running-related MTSS. Further, it is unclear what impact limiting the participants to practice their sport only up to a pain level of a maximum of NRS 5, thereby reducing their activity during the intervention period, has had on pain reduction and improved function. Since this study did not monitor and record the participants’ sporting activities the potential variability between cases could have influenced the results of this case series. There is no consensus on the duration (weeks) and frequency (sessions per week) of LL-BFR training, but a training volume of three training sessions per week for six weeks is frequently reported.15,33 Therefore, it is unclear if the applied intervention for this study is optimal, and how extra practices or exercise (dose-response) could affect the findings. As a result of the pragmatic choice of an occlusion band instead of an inflatable thigh cuff with an attached sphygmomanometer, it is not known to what extent blood flow was restricted.35 However, there are wide variances in occlusion pressures throughout studies, with recommended pressures typically ranging between 40–80% of total arterial occlusion pressure.33 Furthermore, with the approach presented in the present study with very tight occlusion bands (NRS 7), visually dilated superficial veins, and the statements from the participants regarding training intensity, it must be assumed that all athletes have performed LL-BFR training and not only performed calf raises. Nonetheless, the use of non-standardized occlusion pressures is a less reliable method compared to an inflatable thigh cuff with an attached sphygmomanometer. The intervention was carried out as unsupervised home-based training. Although participants completed an exercise diary and reported good adherence, there is potentially greater variability compared to supervised exercise.
This case series presents a therapeutic exercise program utilizing LL-BFR training, implemented in conjunction with sports activities, that reduced pain and increased function in some of the athletes with running-related MTSS. MTSS is a condition with pain potentially limiting the ability to participate in a rehabilitation program. LL-BFR training may allow clinicians to prescribe exercises at a lower load, decreasing the chance of pain during exercise. Future research should investigate the effectiveness of an intervention program with and without the use of LL-BFR training.
Submitted: July 11, 2024 CST, Accepted: October 20, 2024 CST
© The Author(s)
This is an open-access article distributed under the terms of the Creative Commons Attribution 4.0 International License (CCBY-NC-4.0). View this license’s legal deed at https://creativecommons.org/licenses/by-nc/4.0 and legal code at https://creativecommons.org/licenses/by-nc/4.0/legalcode for more information.
1. McClure CJ, Oh R. Medial Tibial Stress Syndrome. In: StatPearls StatPearls Publishing; 2024.
2. Brown AA. Medial tibial stress syndrome: Muscles located at the site of pain. Scientifica 2016;2016:7097489. doi:10.1155/2016/7097489
3. Lopes AD, Hespanhol Júnior LC, Yeung SS, Costa LO. What are the main running-related musculoskeletal injuries? A Systematic Review Sports Med. 2012;42(10):891-905. doi:10.1007/bf03262301
4. Beck BR. Tibial stress injuries: an aetiological review for the purposes of guiding management. Sports Med 1998;26:265-279. doi:10.2165/ 00007256-199826040-00005
5. Winters M, Eskes M, Weir A, Moen MH, Backx FJ, Bakker EW Treatment of medial tibial stress syndrome: a systematic review. Sports Med. 2013;43(12):1315-1333. doi:10.1007/ s40279-013-0087-0
6. Magnusson HI, Westlin NE, Nyqvist F, Gärdsell P, Seeman E, Karlsson MK. Abnormally decreased regional bone density in athletes with medial tibial stress syndrome. Am J Sports Med. 2001;29(6):712-715. doi:10.1177/ 03635465010290060701
7. Gaeta M, Minutoli F, Scribano E, et al. CT and MR imaging findings in athletes with early tibial stress injuries: comparison with bone scintigraphy findings and emphasis on cortical abnormalities. Radiology. 2005;235(2):553-561. doi:10.1148/radiol.2352040406
8. Mihalko SL, Cox P, Ip E, et al. Severity of overuse injury impacts self-efficacy and quality of life in runners: A 2-year prospective cohort study J Sport Rehabil 2021;30(7):1073-1079. doi:10.1123/ jsr.2020-0326
9. Khan K, Cook J, Cools A, et al. Brukner and Khan’s Clinical Sports Medicine: Injuries McGraw-Hill Education (Australia) Pty Limited; 2017.
10. Newman P, Witchalls J, Waddington G, Adams R. Risk factors associated with medial tibial stress syndrome in runners: a systematic review and metaanalysis. Open Access J Sports Med. 2013;4:229-241. doi:10.2147/oajsm.S39331
11. Patterson SD, Hughes L, Warmington S, et al. Blood flow restriction exercise: Considerations of methodology, application, and safety Front Physiol 2019;10:533. doi:10.3389/fphys.2019.00533
12. Grønfeldt BM, Lindberg Nielsen J, Mieritz RM, Lund H, Aagaard P Effect of blood-flow restricted vs heavy-load strength training on muscle strength: Systematic review and meta-analysis. Scand J Med Sci Sports 2020;30(5):837-848. doi:10.1111/sms.13632
13. Hughes L, Paton B, Rosenblatt B, Gissane C, Patterson SD Blood flow restriction training in clinical musculoskeletal rehabilitation: a systematic review and meta-analysis. Br J Sports Med. 2017;51(13):1003-1011. doi:10.1136/ bjsports-2016-097071
14. Jørgensen SL, Kierkegaard-Brøchner S, Bohn MB, Høgsholt M, Aagaard P, Mechlenburg I. Effects of blood-flow restricted exercise versus conventional resistance training in musculoskeletal disorders-a systematic review and meta-analysis. BMC Sports Sci Med Rehabil 2023;15(1):141. doi:10.1186/ s13102-023-00750-z
15. Hjortshoej MH, Aagaard P, Storgaard CD, et al. Hormonal, immune, and oxidative stress responses to blood flow-restricted exercise. Acta Physiol. 2023;239(2):e14030. doi:10.1111/apha.14030
16. Doessing S, Heinemeier KM, Holm L, et al. Growth hormone stimulates the collagen synthesis in human tendon and skeletal muscle without affecting myofibrillar protein synthesis. J Physiol 2010;588(Pt 2):341-351. doi:10.1113/jphysiol.2009.179325
17. Wang X, Wang Y, Yang X, et al. Effects of blood flow restriction training on bone metabolism: a systematic review and meta-analysis. Front Physiol. 2023;14:1212927 doi:10.3389/fphys.2023.1212927
18. Early KS, Rockhill M, Bryan A, Tyo B, Buuck D, McGinty J. Effecte of blood flow restriction training on muscular performamce, pain and vascular function. Int J Sports Phys Ther 2020;15(6):892-900. doi:10.26603/ijspt20200892
19. Winters M. The diagnosis and management of medial tibial stress syndrome: An evidence update. Der Unfallchirurg. 2020;123(Suppl 1):15-19. doi:10.1007/s00113-019-0667-z
20. Milgrom C, Zloczower E, Fleischmann C, et al. Medial tibial stress fracture diagnosis and treatment guidelines. J Sci Med Sport. 2021;24(6):526-530. doi:10.1016/j.jsams.2020.11.015
21. Kahanov L, Eberman LE, Games KE, Wasik M. Diagnosis, treatment, and rehabilitation of stress fractures in the lower extremity in runners. Open Access J Sports Med. 2015;6:87-95. doi:10.2147/ oajsm.S39512
22. Bradley LN, Blakey SC. Single-leg hop test in the evaluation of stress injuries. Athl Train Sports Health Care. 2014;6(5):201-202. doi:10.3928/ 19425864-20140916-11
23. Schütte KH, Seerden S, Venter R, Vanwanseele B. Influence of outdoor running fatigue and medial tibial stress syndrome on accelerometer-based loading and stability Gait Posture 2018;59:222-228. doi:10.1016/j.gaitpost.2017.10.021
24. Farrar JT, Young JP Jr, LaMoreaux L, Werth JL, Poole MR. Clinical importance of changes in chronic pain intensity measured on an 11-point numerical pain rating scale. Pain 2001;94(2):149-158. doi:10.1016/s0304-3959(01)00349-9
25. Aweid O, Gallie R, Morrissey D, et al. Medial tibial pain pressure threshold algometry in runners. Knee Surg Sports Traumatol Arthrosc 2014;22(7):1549-1555. doi:10.1007/ s00167-013-2558-0
26. Binkley JM, Stratford PW, Lott SA, Riddle DL. The Lower Extremity Functional Scale (LEFS): Scale development, measurement properties, and clinical application. Phys Ther 1999;79(4):371-383.
27. Horn KK, Jennings S, Richardson G, Vliet DV, Hefford C, Abbott JH. The patient-specific functional scale: psychometrics, clinimetrics, and application as a clinical outcome measure. J Orthop Sports Phys Ther 2012;42(1):30-42. doi:10.2519/jospt.2012.3727
28. Madeley LT, Munteanu SE, Bonanno DR. Endurance of the ankle joint plantar flexor muscles in athletes with medial tibial stress syndrome: a casecontrol study J Sci Med Sport 2007;10(6):356-362. doi:10.1016/j.jsams.2006.12.115
29. Wortman RJ, Brown SM, Savage-Elliott I, Finley ZJ, Mulcahey MK. Blood flow restriction training for athletes: A systematic review Am J Sports Med 2021;49(7):1938-1944. doi:10.1177/ 0363546520964454
30. Lorenz DS, Bailey L, Wilk KE, et al. Blood flow restriction training. J Athl Train 2021;56(9):937-944. doi:10.4085/418-20
31. Nuzzo JL, Pinto MD, Nosaka K, Steele J. Maximal number of repetitions at percentages of the one repetition maximum: A meta-regression and moderator analysis of sex, age, training status, and exercise. Sports Med 2024;54(2):303-321. doi:10.1007/s40279-023-01937-7
32. Henriksen M, Klokker L, Bartholdy C, GravenNielsen T, Bliddal H. The associations between pain sensitivity and knee muscle strength in healthy volunteers: a cross-sectional study Pain Res Treat 2013;2013(1):787054. doi:10.1155/2013/787054
33. Burton I. Blood flow restriction training for tendinopathy rehabilitation: A potential alternative to traditional heavy-load resistance training. Rheumatol. 2022;3(1):23-50. doi:10.3390/ rheumato3010003
34. Nissen T, Wynn R. The clinical case report: a review of its merits and limitations. BMC Res Notes. 2014;7:264. doi:10.1186/1756-0500-7-264
35. Bell ZW, Dankel SJ, Spitz RW, Chatakondi RN, Abe T, Loenneke JP. The perceived tightness scale does not provide reliable estimates of blood flow restriction pressure. J Sport Rehabil 2019;29(4):516-518. doi:10.1123/jsr.2018-0439
Bryanna Vesely1a , Shanthan Challa2 , Benjamin Moyer2 , Blake Gereb3 , Troy Watson4
1 Orthopedics, Desert Orthopedic Center, 2 Orthopedic Surgery, Valley Hospital Medical Center, 3 Physical Therapy, Atrium Health Wake Forest Baptist, 4 Orthopedic Surgery, Desert Orthopedic Center
Keywords: Internal Brace, Brostrom, rehabilitation, postoperative protocol, lateral ankle instability, ankle sprain https://doi.org/10.26603/001c.127849
International Journal of Sports Physical Therapy
Vol. 20, Issue 1, 2025
Chronic lateral ankle instability is a common diagnosis in foot and ankle clinics. Internal Brace (IB) augmentation is a surgical procedure that utilizes fibertape augmentation of the lateral ankle ligaments. Studies have shown the superiority of fibertape augmentation over traditional lateral ankle stabilization procedures such as the Brostrom or Brostrom-Gould. The IB procedure has been described elsewhere and the fixation involves placing bone anchors with attached suture tape at each end of the Brostrom repair augmenting the fixation. Various studies exist that support the mechanical advantage of the Brostrom with IB over non augmented repair This technique allows for earlier weight bearing and range of motion which translates into earlier return to activity and sport. While there are guidelines for rehabilitation after Brostrom procedures, there are currently no guidelines regarding rehabilitation after the IB. The purpose of this clinical commentary is to describe an early mobility and rehabilitation protocol after IB augmentation for the ankle. The post operative protocol provides treatment goals, weight bearing status recommendations, and rehabilitation intervention suggestions after IB augmentation.
V
Ankle sprains are one of the most common orthopedic injuries that occur in the general population and among athletes.1,2 Injuries to the lateral ankle complex are the most common type of ankle sprains.1 Acute lateral ankle sprain injuries are initially treated with conservative treatment including modalities to address pain, swelling, and inflammation. These modalities include ice, rest, and anti-inflammatory medication. In severe cases, immobilization may be necessary Physical therapy is recommended, focusing on muscle strengthening, balance training, and proprioception. These therapy treatments are essential parts of conservative treatment.3 Despite conservative management, patients may subsequently develop chronic lateral ankle instability (CLAI). In a study by Doherty et al. 40% of the 82 patients included who sustained a first-time acute lateral ankle sprain developed CLAI.4
Freeman et al. described two types of CLAI. Functional instability describes a patient who reports the foot feels unstable or “gives out.”5,6 Mechanical instability occurs when there is pathological laxity of the ankle with increased varus tilt of the talus under inversion stress.5,6 In acute ankle sprains, the anterior talo-fibular ligament (ATFL) is the most commonly injured ligament followed by the calcaneofibular ligament (CFL) which occurs in more severe sprains.1,7 Therefore, surgical treatment for patients who fail conservative treatment targets stabilizing the ATFL and occasionally the CFL as well.8 The Brostrom repair was first introduced in 1966 to reapproximate the ATFL.9 Later Gould created a modification which included the extensor retinaculum which was used to augment the Brostrom repair 10
The Internal Brace (IB) was first created as a “fibertape” (suture tape) augmentation for ligament reinforcement to act like an “internal seat belt.” While it was first used in
Corresponding author:
Bryanna Vesely DPM MPH
2772 Alameda Dr, Virginia Beach, VA, 23456
bdvesely@gmail.com
the upper extremity, it was first used for CLAI in 2012. In CLAI there has been clinical endorsement of fibertape augmentation to maintain stability and allow for earlier range of motion and mobilization.11 This variation of the traditional Brostrom procedure has shown promise with improved stability with quicker return to function.11 Biomechanical studies have shown superior strength of the repair at post-op time zero when comparing the Brostrom augmented with fibertape and the Brostrom-Gould repair.12 Clinical studies also support outcomes after using fibertape augmentation over the Brostrom-Gould repair.11,13 Because of the emerging superiority in the literature of fibertape augmentation regarding graft strength and return to function, the authors’ recommend early mobility and more aggressive rehabilitation compared to patients who have undergone a Brostrom or Brostrom-Gould repair
While surgical technique and the biomechanics of the IB has been studied, no specific rehabilitation protocol exists when IB is used during surgical intervention.14,15 Previous authors have discussed rehabilitation protocols specifically for patients treated with a modified Brostrom; however, the authors recommend earlier mobilization and return to activity with the IB augmentation compared to the protocols created for Brostrom-Gould repairs.14,15 Therefore, the purpose of this clinical commentary is to describe an early mobility and rehabilitation protocol after IB augmentation for the ankle.
The ankle IB surgical technique has been thoroughly described in the literature.14,15 Briefly, an ankle arthroscopy to treat intra-articular pathology is performed prior to performing the lateral ankle stabilization with IB augmentation. After the ankle arthroscopy is performed, the lateral ankle incision is performed in a linear fashion across the distal fibula 1 cm from the proximal for the fibula tip in line with the ATFL. Sharp dissection is performed through skin and subcutaneous tissue in layered fashion retracting the anterolateral fat pad. The peroneal tendons are visualized and peroneal pathology is addressed appropriately.
The ATFL is identified and removed from the distal fibula in a cuff of tissue with the inferior extensor retinaculum. In standard fashion, a 4.5mm Swivelock biocomposite suture Anchor (Arthrex, Naples, FL, USA) is placed into the talar body in a trajectory to avoid violation of the tibiotalar or subtalar joint and the attached sutures are passed through the ATFL and extensor retinaculum cuff (Figure 1). Two 3.5mm BioSuture Tak suture anchors (Arthrex) are implanted into the anterolateral aspect of the distal fibula (Figure 2) and the FiberTape is passed from the articular side to the extra articular side being secured with hand ties. We recommend placing the foot in neutral dorsiflexion and slight eversion for the tying of the sutures for the Brostrom procedure.
The fibertape from the first anchor is passed through the eyelet of the second anchor in the IB augmentation prior to implanting it on the anterolateral aspect of the fibula, with careful attention to avoid penetration of the ankle joint or
fracture of the fibula. The anchor is inserted with the ankle in resting plantarflexion position to avoid significant decrease in motion secondary to an over tensioned augmentation (Figure 3). Vicryl is used to repair the sleeve of tissue to the neighboring periosteum (Figure 4). Non-absorbable suture is recommended for the skin followed by a posterior splint application.14
Figure 4. Final Internal Brace augmentation
Post–operative management of patients who undergo IB augmentation of Brostrom lateral ankle instability is outlined in Table 1 These guidelines are modified for each patient based on post–operative exam and radiographic findings. The current program, spanning 12 weeks, utilizes early mobilization with no casting to improve return to sport time and reduce complications from stiffness. Wound healing should be evaluated at every exam. There should be open communication between the surgeon and physical therapist if there are any concerns of wound dehiscence, infection, or other post– operative complications. If any signs of wound dehiscence exist, it may be necessary to decellerate rehabilitation and weight bearing status until the wound is adequately healed. After sutures are removed, the wound should be covered and well-padded in the brace or boot to prevent dehiscence and infection.
Table 1 presents treatment goals, weight bearing status, and physical therapy rehabilitation interventions after IB augmentation. Initially, patients are immobilized in a nonweight bearing splint immediately after surgery for 3-5 days and utilize the appropriate assistive device. The patient is then allowed to progress into full weight bearing in a CAM boot after day 3-5, assuming the wound is healing without complications. As early as three weeks postoperatively, the patient may transition into an ASO brace and a supportive tennis shoe dependent on the patient’s progress in physical therapy and wound evaluation. Inversion exercises are restricted until week six to not stress the lateral ankle repair Patients may be able to return to sport as early as 8-10
weeks after surgery, although this protocol outlines interventions through 12 weeks post-op.
Controversy regarding immobilization and early mobilization after lateral ankle stabilization has been discussed in the literature. Petrera et al. analyzed patients who underwent a modified Brostrom with two double-loaded suture anchors and early mobilization. They concluded that this construct allowed for immediate weight-bearing with a low failure rate and improved patient functional outcomes. Vopat et al. compared studies examining early and delayed mobilization after primary lateral ankle ligament stabilization.16 They found early mobilization had improved functional outcomes; however, patients demonstrated increased objective laxity and higher complication rates.15 Of note, both of these authors excluded patients who underwent IB fixation and therefore comparisons and conclusions regarding outcomes after augmention with the IB cannot be made.
Schuh et al. performed a biomechanical study comparing the Brostrom technique to suture anchor repair and to IB augmentation.12 They found the Brostrom alone and the suture anchor group failed at a 24.1 degree rotary displacement angle, while the IB group failed at 46.9 degrees (p=0.02). They also found that the IB group had a larger torque at failure of 11.2 Nm compared to 8.0 Nm for suture anchor group and 5.7 Nm for the Brostrom alone group. They concluded that the IB group had significantly superior performance in regards to angle at failure as well as failure torque.12 These findings suggest that the IB augmentation can support early weight-bearing and return to activity compared to what has previously been described in the literature for a modified Brostrom.
Improved strength and functional outcomes have been described with IB augmentation over traditional Brostrom or Brostrom-Gould repair 11‑14 Therefore, the authors’ believe that early mobilization and progressive therapy is indicated given the strength of the IB augmentation. Specifically, Jain et al. compared 148 patients who underwent either a Modified Brostom-Gould repair versus IB augmentation. They found IB augmentation had better outcomes of early rehabilitation, earlier return to pre-injury activity, and better functional outcomes and VAS scores.11 Flaherty et al. performed a similar study comparing the Modified Brostrom-Gould versus IB augmentation. They found those who underwent surgery with IB augmentation had better overall function and fewer complications with lower recurrence of pain and quicker return to walking than those who underwent the Modified Brostrom.13 Finally, Kulwin et al. performed a randomized control trial of 119 patients who either received a modified Brostrom procedure alone or the modified Brostrom with suture tape augmentation. They used an accelerated rehabilitation protocol similar to what is described in the current paper They found an average return to preinjury level of activity to be achieved at 17.5 weeks after modified Brostrom repair versus 13.3 weeks after suture tape augmentation. Only one patient in the suture tape group was unable to complete the accelerated re-
Table 1. Proposed post–operative rehabilitation protocol after Internal Brace augmentation
Phase 1: Days 0-5
Rehab Goals
Weight Bearing Interventions
Phase 2: Days 5-21
Rehab Goals
Weight Bearing Interventions
• Protect repair
• Edema and pain control
• Incision healing
• Non-weight bearing in a posterior splint, with appropriate assistive device
• Rest, elevate, ice, compression
• Avoid all ankle PROM/AROM
• Edema and pain control
• Protect lateral ankle wound
• Progress weight bearing in protective boot in order to transition to ankle stabilizing orthosis (ASO) brace after 21 days post-op
• Begin range of motion with active dorsiflexion, plantarflexion, eversion, should avoid inversion motion
• Advance to full weight bearing in controlled ankle motion (CAM) boot
• No inversion exercises
• Begin AROM/PROM in DF, PF and Eversion.
• Blood flow restriction therapy may begin if candidate is appropriate
• Stationary bike/NuStep with boot on
• Upper body exercises
• Cardiovascular exercise for increased nonspecific blood flow to surgical site
• Bilateral closed chain LE movements to improve functional strength.
• Intrinsic foot strengthening (towel scrunches, toe yoga, windshield wipers etc)
• Taping for management of bruising/swelling
Phase 3: Weeks 3-4
Rehab Goals
Weight Bearing Interventions
• Normalize gait pattern and reduce antalgic gait
• Increase ankle ROM and strength to achieve full ankle dorsiflexion, plantar flexion, eversion
• Transition into ASO brace and supportive tennis shoe if wound is healed
• No inversion exercises
• Begin resisted DF, PF, Eversion (Theraband)
• Calf raises and calf exercises
• Leg press, knee extension, hip abduction and adduction machines/strengthening
• Begin bilateral lower extremity proprioceptive exercises(airex squats, tilt board for DF/PF only, etc)
• Stationary bike, NuStep, or elliptical trainer
• Continue upper body exercise program
• Aquatic therapy once incision is closed
• Alter G training may begin
habilitation protocol while four in the modified Brostrom group failed to complete the protocol.15
IB is still a novel surgical approach without established rehabilitation protocols. A well-constructed rehabilitation protocol that leverages the improved biomechanical characteristics of IB technique is necessary In this commentary the authors present a rehabilitation protocol that describes an appropriate approach for early mobilization with the
goal of early return to activity that has proved to be beneficial in returning patients to function in an expedited fashion.
Submitted: May 30, 2024 CST, Accepted: November 18, 2024 CST
© The Author(s)
Phase 4: Weeks 4-6
Rehab Goals
Weight Bearing Interventions
Phase 5: Weeks 6-8
Rehab Goals
Weight Bearing Interventions
• Increase strength and restore normal functional LE movement
• Improve proprioception
• Slowly wean out of ASO brace to patient’s tolerance but continue to wear during athletics or strenuous activity
• Increase speed and incline on elliptical
• May begin treadmill to tolerance
• Single leg calf raises, squats, deadlifts
• Farmer’s carry, suitcase carry
• Single leg proprioception
• Single leg stance on airex, staggered sit to stand
• Closed chain LE movements
• Eccentric loading of bilateral lower extremities
Phase 6: Weeks 8-12
Rehab Goals
Weight Bearing Interventions
• Progress strength and activity
• Return to prior level of function (PLOF)
• Ambulate in regular shoes
• Begin inversion AROM/PROM exercises
• Introduce plyometric exercises
• Begin interval jogging
• Change of direction, jumping exercises
• Lateral lunges, single leg presses, squat to chair
• Progress activity to sport
• Return to PLOF
• Ambulate in regular shoes
• Begin sport specific training programs
• Sports Ladder
• Interval running
• Agility training
• Continue to progress plyometric movements
This is an open-access article distributed under the terms of the Creative Commons Attribution 4.0 International License (CCBY-NC-4.0). View this license’s legal deed at https://creativecommons.org/licenses/by-nc/4.0 and legal code at https://creativecommons.org/licenses/by-nc/4.0/legalcode for more information.
1. Hertel J, Corbett RO An updated model of chronic ankle instability J Athl Train 2019;54(6):572-588. doi:10.4085/1062-6050-344-18
2. Miklovic TM, Donovan L, Protzuk OA, Kang MS, Feger MA. Acute lateral ankle sprain to chronic ankle instability: a pathway of dysfunction. Phys Sportsmed. 2018;46(1):116-122. doi:10.1080/ 00913847.2018.1409604
3. Dhillon MS, Patel S, Baburaj V. Ankle sprain and chronic lateral ankle instability: Optimizing conservative treatment. Foot Ankle Clin 2023;28(2):297-307. doi:10.1016/j.fcl.2022.12.006
4. Doherty C, Bleakley C, Hertel J, Caulfield B, Ryan J, Delahunt E. Recovery from a first-time lateral ankle sprain and the predictors of chronic ankle instability: A prospective cohort analysis. Am J Sports Med 2016;44(4):995-1003. doi:10.1177/0363546516628870
5. Freeman MA. Treatment of ruptures of the lateral ligament of the ankle. J Bone Joint Surg Br. 1965;47(4):661-668. doi:10.1302/0301-620X.47B4.661
6. Freeman MA, Dean MR, Hanham IW The etiology and prevention of functional instability of the foot. J Bone Joint Surg Br 1965;47(4):678-685. doi:10.1302/ 0301-620X.47B4.678
7. Swenson DM, Collins CL, Fields SK, Comstock RD. Epidemiology of U.S. high school sports-related ligamentous ankle injuries, 2005/06-2010/11. Clin J Sport Med. 2013;23(3):190-196. doi:10.1097/ JSM.0b013e31827d21fe
8. Drakos M, Hansen O, Kukadia S. Ankle instability Foot Ankle Clin. 2022;27(2):371-384. doi:10.1016/ j.fcl.2021.11.025
9. Broström L. Sprained ankles. VI. Surgical treatment of “chronic” ligament ruptures. Acta Chir Scand. 1966;132(5):551-565.
10. Gould N, Seligson D, Gassman J. Early and late repair of lateral ligament of the ankle. Foot Ankle 1980;1(2):84-89. doi:10.1177/107110078000100206
11. Jain NP, Ayyaswamy B, Griffiths A, Alderton E, Kostusiak M, Limaye RV Is Internal brace augmentation a gold standard treatment compared to isolated Modified Brostrom Gould repair for chronic lateral ligament ankle instability? Effect on functional outcome and return to preinjury activity: A retrospective analysis. Foot 2022;50:101865. doi:10.1016/j.foot.2021.101865
12. Schuh R, Benca E, Willegger M, et al. Comparison of Brostrum technique, suture anchor repair, and tape augmentation for reconstruction of the anterior talofibular ligament. Knee Surg Sports Traumatol Arthrosc 2016;24:1101-1107 doi:10.1007/ s00167-015-3631-7
13. Flaherty DJ, McGuigan J, Cullen SE, Pillai A. Brace for impact: A retrospective analysis of the Modified Broström-Gould procedure with and without internal brace augmentation. Cureus. 2023;15(9):e44563. doi:10.7759/cureus.44563
14. Kulwin R, Suhling M, Rigby R, Vora AM, Watson TS. Preliminary results of a multicenter prospective randomized trial: Traditional modified Brostrom versus InternalBraceTM augmentation. Foot Ankle Orthop. 2020;5(4):2473011420S0005. doi:10.1177/ 2473011420S00051
15. Kulwin R, Watson TS, Rigby R, Coetzee JC, Vora A. traditional modified Broström vs suture tape ligament augmentation. Foot Ankle Int 2021;42(5):554-561. doi:10.1177/1071100720976071
16. Vopat ML, Tarakemeh A, Morris B, et al. Early versus delayed mobilization postoperative protocols for lateral ankle ligament repair: A systematic review and meta-analysis. Orthop J Sports Med. 2020;8(6):2325967120925256. doi:10.1177/ 2325967120925256
Matthew L Gauthier1a , Casey A Unverzagt2 , George J Davies3
1 Department of Physical Therapy, University of Illinois at Chicago, 2 Department of Physical Therapy, Baylor University, 3 Department of Physical Therapy, The University of Texas Rio Grande Valley
Keywords: overhead athlete, baseball, biomechanics, kinetic chain https://doi.org/10.26603/001c.127461
International Journal of Sports Physical Therapy
Vol. 20, Issue 1, 2025
Injuries are commonplace in the overhead athlete, with many occurring to the shoulder and elbow The increasing prevalence of injuries to the shoulder and elbow has been discussed at length in recent years, with increased research focus being placed on arm care for rehabilitation and prevention strategies. Even though the pitching motion is well-established as a whole-body kinetic chain movement, most attention in rehabilitation and prevention efforts is often placed on the upper extremity The purpose of this clinical commentary is to highlight the impact the lower extremity and trunk have on the upper extremity during the throwing motion, better equipping clinicians to treat this patient population. Additionally, this clinical commentary highlights the current literature and recommendations regarding examination of the entire kinetic chain and concludes with a review of current evidence and recommendations regarding treatment of impairments and functional limitations identified in the proximal kinetic chain. In leaving no stone unturned, clinicians can address all components of the kinetic chain in throwers, maximizing performance and mitigating risk of injury.
Level of Evidence: 5
Throughout every level of overhead athletics, upper extremity injuries are a common occurrence. Given its high injury rates, few overhead sports have been researched as much as baseball. At the collegiate and professional levels, shoulder pathology accounts for up to 35% of all baseballrelated injuries,1‑3 with pitchers making up as much as 60% of injured players.4 Elbow pathology in this population accounts for 7-16% of all injuries,3 with pitchers making up 40% of injured players.5 At the high school level, injury surveillance studies estimate that shoulder pathology accounts for 13-21% of all baseball-related injuries, with pitchers making up 40% of injured players.6,7 Elbow pathology in this population is estimated to account for 11-12% of all injuries, with pitchers making up 60% of injured players.6,7
With the significant prevalence of shoulder and elbow injuries, much attention has been given to the upper extremity in rehabilitation and prevention strategies.8‑11 However, despite the fact that the overhead throwing mo-
tion has been well-established as a full-body kinetic chain motion, less attention seems to be paid to the trunk and lower quarter in the clinical setting. The intention of this current concept review is to highlight the importance of the trunk and lower extremity in mitigating load through the upper extremity in the overhead athlete. With this knowledge, clinicians can better evaluate and treat the entire throwing athlete, not just the injured segment.
The impact of the kinetic chain in the overhead throwing motion was detailed by Atwater et al. in 1979, discussing how the larger, slower moving proximal segments of the lower extremity and trunk progressively developed kinetic energy that was later released by the smaller, rapidly-moving distal segments of the arm.12 Subsequent research would later organize the throwing motion into six phases: wind-up, stride, arm cocking, arm acceleration, arm decel-
Corresponding Author:
Matthew L Gauthier
Department of Physical Therapy, College of Applied Health Sciences
University of Illinois at Chicago, Chicago, IL 60608, USA
P: 312.355.1465 E: mgauthie@uic.edu
Figure 1. Joint motion and muscle action during foot contact, acceleration, and deceleration phases of the throwing motion.
eration, and follow-through.13 In 1995, Glenn Fleisig highlighted two “critical instants” of the throwing motion in which the upper extremity undergoes the greatest stress: just before maximum external rotation and just after ball release (Figure 1).14 Years later, Aguinaldo and Escamilla further elaborated on the impact these proximal segments had on the upper extremity in their study on segmental energy flow through the kinetic chain.15 In this study, they found that trunk rotation power played a significant role in the development of elbow valgus torque and ball speed. By synthesizing these descriptions of the kinetics and kinematics of the overhead throwing motion, we can better understand the impact that the trunk and lower extremity has on the performance and injury risk of the upper extremity In the past decade, several studies have offered insight into how impairments of the trunk and lower quarter in each phase of throwing can impact the upper extremity
The wind-up phase of the throwing motion, occurring from a position of rest to the position of maximum lead leg lift, is the phase in which the athlete prepares the body to generate force. Since the athlete is in a position of single leg stance, lumbopelvic control is paramount in this phase. As described by Chaudari et al., throwing athletes demonstrating decreased lumbopelvic control in single leg stance were significantly more likely to miss over 30 days of a baseball season due to injury 16 Similarly, Laudner et al. found that decreased lumbopelvic control on an athlete’s drive leg was correlated with increased torque at the anterior shoulder and medial elbow 17 These data underscore the impor-
tance of lumbopelvic stability on the drive limb during the windup phase of throwing.
The stride phase of the throwing motion, occurring from the position of maximum lead leg lift to the position of front foot contact, is the phase in which the athlete uses their drive leg to propel their body toward home plate. During this phase, as the drive limb is pushing laterally against the pitching rubber to initiate the propulsion toward home plate, strength of the drive leg hip abductors is of considerable importance. Laudner et al. compared hip performance in position players versus pitchers in professional baseball and found that pitchers exhibited significantly decreased drive leg hip abductor strength compared to position players, requiring increased demand on the trunk and upper extremity to generate force.18 This strength deficit takes on greater importance when considering a study by Hamano et al. that found that high school pitchers experiencing an upper extremity injury were significantly more likely to present with decreased hip abduction strength of the drive limb compared to their uninjured peers.19 Drive leg hip abduction strength has been shown to impact performance as well, as peak ground reaction force (GRF) of the drive leg is strongly associated with ball speed.20 This suggests that a stronger push off the pitching rubber via hip abduction increases pitch velocity. These studies highlight the importance of hip abduction strength to optimize lower extremity contribution to the throwing motion.
The arm cocking phase of the throwing motion, occurring from the position of front foot contact to the position of maximum external rotation of the shoulder, is the phase in which the kinetic energy developed through lower extremity and trunk motion is transferred to potential energy of the shoulder In other words, the energy developed from the legs, which has been augmented and transferred by the trunk, is now ready to be released from the arm through elastic energy. During this phase, the lead leg experiences considerable eccentric loading as it accepts the force of the body as it accelerates toward home plate. As a result, knee flexion at front foot contact has been identified as an important link in the kinetic chain. Chalmers et al. indicate that knee flexion angle at front foot contact was significantly correlated with shoulder and elbow strain.4 A strong eccentric quadriceps contraction of the lead leg is required to provide a braking impulse and allow propagation of energy up the kinetic chain from the legs to the trunk.21,22 Inadequate braking impulse alters the timing of trunk rotation, potentially leading to increase strain at the anterior shoulder and medial elbow.15 These studies underscore the importance of lead leg strength and eccentric control to promote the transfer of energy up the kinetic chain and to maintain optimal timing of trunk rotation.
The arm acceleration phase of the throwing motion, occurring from the position of maximum external rotation of the shoulder to the position of ball release, is the phase in which kinetic energy generated by the body is transferred to the ball. When beginning in the position of maximum shoulder external rotation, the first of Fleisig’s two aforementioned “critical instants” occurs, as there is significant internal rotation torque at the shoulder, valgus torque at the elbow, and anterior force at the humeral head on the glenoid.14 Trunk motion is the key for generating power in the throwing motion, highlighting the importance of trunk rotation mobility, strength, control, and timing. Aguinaldo and Escamilla found that roughly 86% of the total energy transferred through the elbow during the throwing motion was due to trunk motion.23 Other researchers have also found that high school pitchers do not generate as much trunk rotation as professional pitchers, resulting in increased strain to the shoulder and elbow during acceleration.15,24 Trunk motion in the frontal plane during the throwing motion has been found to significantly impact strain to the upper extremity as well, and Oyama et al. found that increased contralateral trunk tilt during the acceleration phase was associated with increased velocity as well as increased joint loading to the upper extremity 25 Subsequently Solomito et al. found that increased contralateral trunk tilt resulted in only a 1.5% increase in ball velocity, but a 3.2 and 4.8% increase in anterior shoulder and medial elbow torque, respectively 26 These validate the importance of trunk motion related to developing elbow torque and ball velocity
The arm deceleration phase of the throwing motion, occurring from the position of ball release to the position of maximum shoulder internal rotation, is the phase in which the arm must decelerate after a full-body acceleration. The follow-through phase is a continuation of this deceleration, but at lower speeds. When beginning in the position of ball release, the deceleration phase is where the second of Fleisig’s two “critical instants” occurs, as the posterior shoulder is placed under large stresses to decelerate the arm and resist distractive force.14 Just as accelerating the upper extremity in preparation for ball release was a result of the kinetic chain, deceleration of the upper extremity after ball release will require the kinetic chain as well. During this phase, the athlete is decelerating their body over the lead leg in single leg stance. As such, adequate hip internal rotation range of motion is needed to decelerate the trunk and pelvis over a stationary femur Several studies have investigated hip range of motion norms among pitchers, with 30-35° of hip internal rotation being commonly described amongst healthy individuals.27,28 Decreased hip internal rotation has been correlated with hip, groin, back, and abdominal injuries in professional pitchers27,28 which suggests that an inability to decelerate over the lead leg may put more stress on the trunk to decelerate the upper extremity following ball release.
With this detailed understanding of how deficits along the kinetic chain can impact the upper extremity, clinicians can better evaluate the throwing athlete and create a plan of care that will mitigate injury risk and optimize performance.
Considering the importance of the aforementioned trunk and lower extremity contributions to the throwing motion in an overhead athlete, it is imperative that a systematic and comprehensive physical examination be conducted. This manuscript will highlight tests that can be performed in a “traditional” clinical setting without access to advanced testing equipment (motion capture, force plates, etc.). Various tests of range of motion, muscle performance testing, proprioceptive/kinesthetic testing, upper extremity functional performance tests and qualitative functional movement performance assessment will be listed.
When examining the entire kinetic chain, the concept of “Test, Don’t Guess” applies. The primary reason being that the kinetic chain is a complicated link system and if there is a deficit at one link in the kinetic chain it can impair performance of the movement pattern. The only way to determine if there are potential deficits is to test using appropriate methods and techniques.
Although the shoulder complex and elbow receive a lot of attention due to the significant injuries that occur with pitching, as indicated throughout this manuscript, various components of the kinetic chain can be a major cause or a contributing factor to the high-profile injuries.
A classic example of the impact of the kinetic chain is the case of Dizzy Dean, a Hall of Fame pitcher from the 1930s.29 In 1937, Dean was struck in the foot by a line drive, fracturing his great toe. This injury and the subsequent impairments resulted in altered throwing mechanics leading to an eventual career-altering arm injury. This example highlights the need to evaluate the pitcher and the kinetic chain “from the toes to the nose” as the Dizzy Dean injury illustrates what can stem from an impairment very distant from the shoulder Specifically, Dean’s injury demonstrates the significance of evaluating the lower extremity range of motion of specific joints. Because of the function of the hip extensors contributing to the power of the acceleration and the quadriceps eccentrically contracting during the deceleration phase, a thorough exam includes evaluation of the muscle performance of the entire kinetic chain.
The frequency of oblique strains occurring in pitchers is another example of the need to evaluate the entire kinetic chain.30‑32 In this case, the trunk/core needs to be evaluated for range of motion, flexibility, and muscle strength. Tables 1-4 provide specific details of various assessments that can be used throughout the kinetic chain and the potential effects on performance. Figure 2 features the mobility and strength considerations at various points along the kinetic chain.
While distinct impairments are commonly seen in pitchers, it is vital that clinicians pursue treatment only after a thorough history and physical examination. Interventions must be targeted to each athlete; otherwise, cognitive biases such as availability bias, anchoring bias, confirmation bias, authority bias, and illusory correlations will lead to a shotgun approach to interventions. For example, if lumbopelvic or lower extremity impairments are discovered, they must receive as much attention, if not more, than anything in the upper quarter. A regional approach to treatment is important for these athletes.
Trunk motion (thoracic, lumbar, and pelvic) is key to generating power. Without adequate motion in the various components of the kinetic chain, joints above and below the hypomobility are likely to become hypermobile as a means of compensation. If impairments are found with thoracic extension and rotation, clinicians are encouraged to consider the use of thoracic mobilization/manipulation, self-mobilization, assisted ROM in quadruped, and dynamic ROM (Figure 3). Particular attention should be paid to the soft tissue as well, including, but not limited to, the length of the hip flexors and hip rotators. Soft tissue deficits can often be addressed by dry needling, soft tissue mobilization, instrument-assisted soft tissue mobilization, and/or with active or passive stretching.
If the athlete has adequate trunk mobility but appears to be lacking stability, the authors recommend assessing
and treating the trunk in all planes, with an emphasis on the transverse plane. While the trunk may have sufficient ROM and control in the frontal and sagittal planes it is not uncommon to lack rotational control. In these cases, clinicians are encouraged to focus on foundational movement patterns such as segmental rolling (Figure 4) pelvic diagonals, and/or proper pelvic floor recruitment before moving to more advanced interventions. Segmental rolling can also be progressed by shortening the lever arm used by the patient (Figure 5). Long-moment isometrics, such as resisted bird dogs, Pallof press, sustained battle ropes, or corrective side planks are examples. Cardinal plane movements, such as a prone extension progression, landmine press, and/or overhead squats can then be considered. In the final phase of interventions aimed at improving trunk-related impairments, power production should be emphasized using explosive medicine ball training, Turkish getups, and explosive diagonal squats and lifts. Regardless of the naming convention used to describe the exercise, trunk interventions should follow a logical progression, starting with mobility followed by motor control, isometrics, cardinal plane activities, transverse plane activities, and finally, interventions aimed at improving power
Hip rotation is imperative for both acceleration (external rotation) and deceleration (internal rotation) during the throwing motion. If the deficit articular in nature, long axis hip distraction, lateral distractions, and lateral distractions with ER and IR can be effective. Prone pelvic mobilizations with the femur positioned in internal rotation (mobilizing pelvis on femur) as shown in Figure 6 (to restore hip internal rotation). Self-mobilizations, such as an inferior-posterior glide with step back, lateral femoral glides, and kneeling rotation with active internal rotation58 serve as a home exercise program to reinforce the ROM gained via manual therapy in the clinic. Finally, active exercises using the new ROM are imperative and can include shin box hip thrust (sitting exercise emphasizing internal rotation of one leg and external rotation of the other), hip controlled articular rotation (CARS), plie squats, etc.
If the athlete is found to demonstrate decreased lumbopelvic control in single leg stance, it is recommended that the athlete be progressed through a drop squat progression similar to that reported by Andreyo et al.59 If a pitcher demonstrates reduced strength of their drive limb hip abductors, a targeted strengthening and neuromuscular program should be implemented. While classic exercises such as clamshells, side-lying hip abduction, “monster walks” and unilateral standing hip abduction effectively target the weakened musculature, it is unlikely there will be sufficient carryover from these interventions into the throwing motion. The authors recommend initiating a strength and neuromuscular progression using a side-lying technique in which the athlete learns the movements associated with anterior elevation of the pelvis (Figure 7) followed by posterior depression of the pelvis (Figure 8). Once the athlete has adequate proprioception and motor control of the hip, the athlete should be progressed to more weightbearing and pitching-specific interventions, such as resisted abduction using a SportCord® (JDP Products, Peo-
Table 1. Range of Motion Assessments. All ROM assessments can be made with a goniometer, inclinometer, and/ or tape measure. Expectation is for >90% LSI for all assessments.
Joint/Region ROM
1st MTP Flexion/Extension
Ankle Plantarflexion/Dorsiflexion
STJ Inversion/Eversion
Knee Flexion/Extension
Hip Flexion/Extension
Hip Adduction/Abduction
Hip Internal/External Rotation
Trunk Flexion/Extension
Trunk Lateral Flexion
Trunk Rotation
Scapular Protraction/Retraction
Scapular Upward/Downward Rotation
Scapular Elevation/Depression
Glenohumeral Flexion/Extension
Glenohumeral Adduction/Abduction
Glenohumeral Internal/External Rotation
Elbow Flexion/Extension
Elbow Pronation/Supination
Wrist Flexion/Extension
Wrist Radial/Ulnar Deviation
Hand/Fingers Flexion/Extension
Hand/Fingers Rotation
Impact on Performance if Deficient
Limits push-off
Limits push-off
Stability of LE; decreased ability push-off of pitching rubber
Flexion: Limits windup
Extension: Limits eccentric braking
Flexion: Limits windup
Extension: Limits power in push-off and velocity of ball
Stability of lower extremity
Limits rotation power and ability to decelerate trunk
Extension: Limits “lay-back” position
Flexion: Limits power and ball speed
Stability of trunk
Limits rotational power and ball speed
Limits normal scapular motion33
Limits normal scapular motion
Limits normal scapular motion
Flexion: Limits achievement of “lay-back” position
Abduction: Effects acceleration and delivery
Adduction: Effects follow-through
External Rotation: Limits achievement of “lay-back” position
Internal Rotation: May affect ball speed and ability to follow-through34,35
Flexion: Increased elbow torque at ball release
Effects “quality” of pitches, i.e., spin rate
Effects “quality” of pitches, i.e., spin rate
Effects “quality” of pitches, i.e., spin rate
Effects “quality” of pitches, i.e., spin rate
Effects “quality” of pitches, i.e., spin rate
ria, AZ), resisted abduction with rotation using a SportCord® , and breakaway abduction with resistance using a SportCord® Explosive side hops, side-lying hops on a Shuttle, and explosive resisted single leg squats all serve appropriate advanced interventions.
Arguably the most common knee-related impairment seen in pitchers is that of a loss of lead leg eccentric quadriceps control. It is this eccentric braking mechanism of the lead leg that contributes to the transition of linear power created by the drive leg to angular power This transition occurs as the lead leg serves as an “anchor” over which the body rotates, creating a “catapult” effect of the trunk and arm.60‑62 Furthermore, if the pitcher lacks eccentric control of the lead leg, this will limit the braking mechanism needed to quickly and efficiently position himself in a ready position after he throws the ball. Additionally, the lack of force attenuation will place additional stress on the articular cartilage and meniscus, possibly increasing a pitcher’s risk of injury Interventions to address this should start with loaded bilateral squats, focused on the deceleration
phase, progressing to single leg squats. The athlete should be quickly progressed to more demanding and sport-specific interventions including plyometric training emphasizing the deceleration/eccentric phase as well as lateral movements that mimic the throwing motion.
A lack of dorsiflexion ROM, as well as a loss of great toe extension, are deficits commonly seen in pitchers. While these deficits may appear insignificant at first, a prospective cohort study of 228 baseball players found that a loss of trail leg dorsiflexion strongly correlated with shoulder and elbow injuries in youth baseball players.63 Additionally, a loss of dorsiflexion ROM has been shown to have a strong influence on dynamic balance.64 Manual therapy aimed at improving closed chain dorsiflexion should include ankle long axis distraction, posterior talar glides, lateral tibial glides, and mobilizations with movement using a strap/belt (Figure 9). Self-mobilizations can serve as a great means to reinforce manual therapy delivered in the clinic. Kneeling knee-to-wall mobilizations, mobilization with movement with talar fixation, and rearfoot eversion self-mobi-
Table 2. Muscle Strength Assessments. All strength assessments can be made with manual muscle testing or hand-held dynamometry. Expectation is for >90% LSI for all assessments.
Joint/Region Muscle Assessment
Ankle Plantarflexion/Dorsiflexion
Knee Flexion/Extension *
Hip Flexion/Extension
Hip Adduction/Abduction
Hip Internal/External Rotation
Trunk Flexion/Extension
Trunk Lateral Flexion
Trunk Rotation
Scapular Protraction/Retraction
Scapular Upward/Downward Rotation
Scapular Elevation/Depression
Glenohumeral Flexion/Extension *
Glenohumeral Adduction/Abduction *
Impact on Performance if Deficient
May limit push-off during acceleration phase
Extension: Impairs eccentric braking mechanism
Extension: Impairs leg drive
May limit motion and dynamic stability for optimum performance
May limit motion and dynamic stability for optimum performance
Flexion: Impairs trunk power generation
May increase stresses to shoulder/elbow
Rotation: Impairs trunk power generation and ball speed
Protraction: Impairs eccentric braking mechanism of scapular movements36
Upward Rotation: May limit ability to get into the cocking position
May limit motion and dynamic stability for optimum performance
May limit motion for optimum performance36,37
May limit motion and dynamic stability for optimum performance
Glenohumeral Internal/External Rotation * Internal Rotation: Affects shoulder power generation38,39
Elbow Flexion/Extension
Elbow Pronation/Supination
Wrist Flexion/Extension
May limit motion for optimum performance
Affects “quality” of pitches, i.e., spin rate
Affects “quality” of pitches, i.e., spin rate
Wrist Radial/Ulnar Deviation Affects “quality” of pitches, i.e., spin rate
Hand/Fingers Flexion/Extension ǂ Affects “quality” of pitches, i.e., spin rate
Hand/Fingers Rotation ǂ
* indicates isokinetic testing as option
ǂ indicates hand grip dynamometer as option
Table 3. Joint Proprioception
Joint/ Region
Affects “quality” of pitches, i.e., spin rate
Impact on Performance if Deficient
All joints May impair the player’s ability to get into the proper position for throwing, or decrease reproducibility of pitches40 42
Table 4. Upper Extremity Physical Performance Tests. Expectation is for >90% LSI for all bilateral assessments. Normative data and cut-scores are available for several tests.
Physical Performance Test
Single Arm Shot Put Test
Prone Medicine Ball Drop Test in Glenohumeral Abduction
Prone Medicine Ball Drop Test in Glenohumeral 90/90
Kneeling Medicine Ball Throwing on wall in Glenohumeral 90/90
Closed Kinetic Chain Upper Extremity Stability Test
Purpose Impact on Performance if Deficient
Assess single arm power
Assess power and endurance of posterior shoulder complex
Assess power and endurance of posterior shoulder complex
Assess power and endurance of shoulder complex
Assess power of upper extremity and trunk
lizations are all paramount to restoring and maintaining closed chain dorsiflexion. Soft tissue deficits can often be addressed via dry needling of the gastrocnemius and/or
May impair patient’s ability to maximize their power output43 51
May impair patient’s ability to decelerate arm following ball release52 53
May impair patient’s ability to decelerate arm following ball release52 54
May indicate an endurance deficit with in functional position of throwing52 53
May indicate deficits in power of upper extremity and trunk. May predict increased injury risk52 54 57
soleus, soft tissue mobilization, instrument-assisted soft tissue mobilization, and with active or passive stretching. Finally, active exercise should be utilized in order to de-
Figure 2. (a) Mobility considerations at each joint along the kinetic chain. (b) Muscle strength/control at each region along the kinetic chain.
KEY: Add/Abd = Adduction/Abduction, E/D = Elevation/Depression, F/E = Flexion/Extension, I/E = Inversion/Eversion, IR/ER – Internal/External Rotation, Lat F = Lateral Flexion, P/R = Protraction/Retraction, P/S = Pronation/Supination, PF/DF = Plantarflexion/Dorsiflexion, RD/UD = Radial/Ulnar Deviation, UR/DR = Upward/Downward Rotation,
velop new motor patterns. Active exercises emphasizing closed chain dorsiflexion include lunges, deep squats, heel raises off a step, and single leg Romanian deadlifts with a wedge under the forefoot. A loss of great toe extension can be addressed in a similar fashion via manual therapy followed up by a home self-mobilization program, soft tissue treatment, stretching, and finally, active engagement of the gained ROM.
While evaluation and treatment of mobility and strength deficits are critical to both the prevention and treatment of injuries in pitchers, clinicians should appreciate the neuroplastic changes that occur in overhead athletes both before and after an injury. Clinicians should consider incorporating reactive neurocognitive (RNC) testing and treatment into an athlete’s testing and treatment. These tests should be dynamic, sport specific, non-invasive, and require reactive agility.65 They can assess reaction time, attention, memory, perception, as well as a player’s ability to multitask.65 Recently, a testing battery was proposed by Wilk et al. which includes: 1) reactive closed kinetic chain upper extremity test (CKCUEST+); 2) reactive triangle CKCUEST+; 3) reactive right vs. left CKCUEST+; and 4) reactive OKC 90/90 wall throws. Neurocognitive tests utilizing reactive agility pods such as Blaze Pods (Blaze Pods, Tel Aviv, Israel) can quantify athlete speed, reaction time, left to right asymmetries, etc. Should deficits be discovered through the testing, interventions must be tailored accordingly Often, the plan of care can simply be modified, as opposed to overhauled, to meet the athlete’s specific needs. Emphasis can be placed on dual-tasking, dyad training, reacting to an un-
predictable stimulus, or completing the activity with different visual stimulus.
While the authors strongly believe that the trunk and lower extremity are grossly under-evaluated and treated in baseball players, it would not be wise to not bring to light the fact that the biggest predictor of injuries in pitchers is not necessarily biomechanical but is likely overuse. As such, readers are encouraged to digest this information with the healthy understanding that preventing and treating injuries goes far beyond range of motion, strength, and pitching technique. Every component of the kinetic chain as well as biopsychosocial considerations, and training loads must be addressed: neglecting any one of these factors, may lead to decreased performance at best and injury at worst.
The authors report no conflicts of interest.
The authors would like to express their sincere thanks to Sam Bond (Figure 1) and Emily Schaedle (Figure 2) for their contributions of original artwork within this commentary
Submitted: July 05, 2024 CST, Accepted: October 20, 2024 CST
© The Author(s)
6. Anterolateral graded mobilizations provided to pelvis as the contralateral leg is held in internal rotation, producing a relative hip internal rotation mobilization.
Figure 7 Facilitating anterior elevation of the pelvis using manual and verbal cueing
8. Facilitating posterior depression of the pelvis using manual and verbal cueing
9. Manual interventions aimed at restoring ankle dorsiflexion range of motion. a) long axis distraction; b) posterior talar glides; c) mobilization with movement; d) lateral tibial glide mobilization with movement.
This is an open-access article distributed under the terms of the Creative Commons Attribution 4.0 International License (CCBY-NC-4.0). View this license’s legal deed at https://creativecommons.org/licenses/by-nc/4.0 and legal code at https://creativecommons.org/licenses/by-nc/4.0/legalcode for more information.
1. Laudner K, Sipes R. The incidence of shoulder injury among collegiate overhead athletes. J Intercolleg Sport. 2009;2(2):260-268. doi:10.1123/ jis.2.2.260
2. Fares MY, Fares J, Baydoun H, Fares Y Prevalence and patterns of shoulder injuries in Major League Baseball. Phys Sportsmed 2020;48(1):63-67 doi:10.1080/00913847.2019.1629705
3. Posner M, Cameron KL, Wolf JM, Belmont PJ, Owens BD Epidemiology of major league baseball injuries. Am J Sports Med 2011;39(8):1675-1691. doi:10.1177/0363546511411700
4. Chalmers PN, Wimmer MA, Verma NN, et al. The relationship between pitching mechanics and injury: a review of current concepts. Sports Health. 2017;9(3):216-221. doi:10.1177/1941738116686545
5. Ciccotti MG, Pollack KM, Ciccotti MC, et al. Elbow injuries in professional baseball: epidemiological findings from the Major League Baseball injury surveillance system. Am J Sports Med 2017;45(10):2319-2328. doi:10.1177/ 0363546517706964
6. Wasserman EB, Sauers EL, Register-Mihalik JK, et al. The first decade of web-based sports injury surveillance: descriptive epidemiology of injuries in us high school boys’ baseball (2005–2006 through 2013–2014) and National Collegiate Athletic Association men’s baseball (2004–2005 through 2013–2014). J Athl Train 2019;54(2):198-211. doi:10.4085/1062-6050-239-17
7. Saper MG, Pierpoint LA, Liu W, Comstock RD, Polousky JD, Andrews JR. Epidemiology of shoulder and elbow injuries among United States high school baseball players: school years 2005-2006 through 2014-2015. Am J Sports Med 2018;46(1):37-43. doi:10.1177/0363546517734172
8. McElheny K, Sgroi T, Carr JB. Efficacy of arm care programs for injury prevention. Curr Rev Musculoskelet Med. 2021;14(2):160-167. doi:10.1007/ s12178-021-09694-8
9. Matsel KA, Butler RJ, Malone TR, Hoch MC, Westgate PM, Uhl TL. Current concepts in arm care exercise programs and injury risk reduction in adolescent baseball players: a clinical review Sports Health. 2021;13(3):245-250. doi:10.1177/ 1941738120976384
10. Mullaney M, Nicholas S, Tyler T, Fukunaga T, McHugh M. Evidence based arm care: the Throwers 10 revisited. Int J Sports Phys Ther. 2021;16(6):1379-1386. doi:10.26603/001c.29900
11. Matsel KA, Weiss EE, Butler RJ, et al. A nationwide survey of high school baseball coaches’ perceptions indicates their arm care programs play a role in injury prevention. Int J Sports Phys Ther 2021;16(3):816-826. doi:10.26603/001c.24247
12. Atwater AE. Biomechanics of overarm throwing movements and of throwing injuries. Exerc Sport Sci Rev. 1979;7(1):43. doi:10.1249/ 00003677-197900070-00004
13. Werner SL, Fleisig GS, Dillman CJ, Andrews JR. Biomechanics of the elbow during baseball pitching. J Orthop Sports Phys Ther 1993;17(6):274-278. doi:10.2519/jospt.1993.17.6.274
14. Fleisig GS, Andrews JR, Dillman CJ, Escamilla RF. Kinetics of baseball pitching with Implications about injury mechanisms. Am J Sports Med 1995;23(2):233-239. doi:10.1177/ 036354659502300218
15. Aguinaldo A, Escamilla R. Segmental power analysis of sequential body motion and elbow valgus loading during baseball pitching: comparison between professional and high school baseball players. Orthopaedic Journal of Sports Medicine. 2019;7(2):2325967119827924. doi:10.1177/ 2325967119827924
16. Chaudhari AMW, McKenzie CS, Pan X, Oñate JA. Lumbopelvic control and days missed because of injury in professional baseball pitchers. Am J Sports Med. 2014;42(11):2734-2740. doi:10.1177/ 0363546514545861
17 Laudner KG, Wong R, Meister K. The influence of lumbopelvic control on shoulder and elbow kinetics in elite baseball pitchers. J Shoulder Elbow Surg. 2019;28(2):330-334. doi:10.1016/j.jse.2018.07.015
18. Laudner KG, Moore SD, Sipes RC, Meister K. Functional hip characteristics of baseball pitchers and position players. Am J Sports Med 2010;38(2):383-387 doi:10.1177/0363546509347365
19. Hamano N, Shitara H, Tajika T, et al. Relationship between upper limb injuries and hip range of motion and strength in high school baseball pitchers. J Orthop Surg. 2021;29(1):23094990211003347. doi:10.1177/23094990211003347
20. McNally MP, Borstad JD, Oñate JA, Chaudhari AMW. Stride leg ground reaction forces predict throwing velocity in adult recreational baseball pitchers. J Strength Cond Res. 2015;29(10):2708. doi:10.1519/JSC.0000000000000937
21. Dun S, Fleisig GS, Loftice J, Kingsley D, Andrews JR. The relationship between age and baseball pitching kinematics in professional baseball pitchers. J Biomech 2007;40(2):265-270. doi:10.1016/ j.jbiomech.2006.01.008
22. Milewski MD, Õunpuu S, Solomito M, Westwell M, Nissen CW Adolescent baseball pitching technique: lower extremity biomechanical analysis. J Appl Biomech. 2012;28(5):491-501. doi:10.1123/ jab.28.5.491
23. Aguinaldo AL, Escamilla RF. Induced power analysis of sequential body motion and elbow valgus load during baseball pitching. Sports Biomech 2022;21(7):824-836. doi:10.1080/ 14763141.2019.1696881
24. Luera MJ, Dowling B, Magrini MA, Muddle TWD, Colquhoun RJ, Jenkins NDM. Role of rotational kinematics in minimizing elbow varus torques for professional versus high school pitchers. Orthop J Sports Med 2018;6(3):2325967118760780. doi:10.1177/2325967118760780
25. Oyama S, Yu B, Blackburn JT, Padua DA, Li L, Myers JB. Effect of excessive contralateral trunk tilt on pitching biomechanics and performance in high school baseball pitchers. Am J Sports Med 2013;41(10):2430-2438. doi:10.1177/ 0363546513496547
26. Solomito MJ, Garibay EJ, Woods JR, Õunpuu S, Nissen CW Lateral trunk lean in pitchers affects both ball velocity and upper extremity joint moments. Am J Sports Med 2015;43(5):1235-1240. doi:10.1177/ 0363546515574060
27. Li X, Ma R, Zhou H, et al. Evaluation of hip internal and external rotation range of motion as an injury risk factor for hip, abdominal and groin injuries in professional baseball players. Orthop Rev. 2015;7(4):6142. doi:10.4081/or.2015.6142
28. Camp CL, Spiker AM, Zajac JM, et al. Decreased hip internal rotation increases the risk of back and abdominal muscle injuries in professional baseball players: Analysis of 258 player-seasons. J Am Acad Orthop Surg. 2018;26(9):e198. doi:10.5435/JAAOSD-17-00223
29. National Baseball Hall of Fame. Dizzy Dean | Baseball Hall of Fame. National Baseball Hall of Fame. Accessed June 20, 2024. https:// baseballhall.org/hall-of-famers/dean-dizzy
30. Camp CL, Conte S, Cohen SB, et al. Epidemiology and impact of abdominal oblique injuries in major and minor league baseball. Orthop J Sports Med 2017;5(3):2325967117694025. doi:10.1177/ 2325967117694025
31. Camp CL, Dines JS, van der List JP, et al. Summative report on time out of play for Major and Minor League Baseball: an analysis of 49,955 injuries from 2011 through 2016. Am J Sports Med 2018;46(7):1727-1732. doi:10.1177/ 0363546518765158
32. Chalmers PN, Mcelheny K, D’Angelo J, Ma K, Rowe D, Erickson BJ. Is workload associated with oblique injuries in professional baseball players? Orthop J Sports Med 2024;12(6):23259671241250066. doi:10.1177/23259671241250066
33. Ellenbecker TS, Ben Kibler W, Bailie DS, Caplinger R, Davies GJ, Riemann BL. Reliability of scapular classification in examination of professional baseball players. Clin Orthop Relat Res. 2012;470(6):1540-1544. doi:10.1007/ s11999-011-2216-0
34. Ellenbecker TS, Roetert EP, Bailie DS, Davies GJ, Brown SW Glenohumeral joint total rotation range of motion in elite tennis players and baseball pitchers. Med Sci Sports Exerc. 2002;34(12):2052-2056. doi:10.1097/00005768-200212000-00028
35. Manske R, Wilk KE, Davies G, Ellenbecker T, Reinold M. Glenohumeral motion deficits: friend or foe? Int J Sports Phys Ther 2013;8(5):537-553.
36. Turner N, Ferguson K, Mobley BW, Riemann B, Davies G. Establishing normative data on scapulothoracic musculature using handheld dynamometry J Sport Rehabil 2009;18(4):502-520. doi:10.1123/jsr.18.4.502
37 Ellenbecker TS, Davies GJ. The application of isokinetics in testing and rehabilitation of the shoulder complex. J Athl Train. 2000;35(3):338-350.
38. Riemann BL, Davies GJ, Ludwig L, Gardenhour H. Hand-held dynamometer testing of the internal and external rotator musculature based on selected positions to establish normative data and unilateral ratios. J Shoulder Elbow Surg 2010;19(8):1175-1183. doi:10.1016/j.jse.2010.05.021
39. Ellenbecker TS, Davies GJ, Rowinski MJ. Concentric versus eccentric isokinetic strengthening of the rotator cuff. Objective data versus functional test. Am J Sports Med 1988;16(1):64-69. doi:10.1177/ 036354658801600112
40. Davies GJ, Dickoff-Hoffman S. Neuromuscular testing and rehabilitation of the shoulder complex. J Orthop Sports Phys Ther 1993;18(2):449-458. doi:10.2519/jospt.1993.18.2.449
41. Hatterman D, Kernozek T, Palmer-McLean K, Davies G. Proprioception and its application to shoulder dysfunction. Crit Rev Phys Rehabil Med 2003;15:47-64. doi:10.1615/ CritRevPhysRehabilMed.v15.i1.40
42. Fox JA, Luther L, Epner E, LeClere L. Shoulder proprioception: A review. J Clin Med. 2024;13(7):2077. doi:10.3390/jcm13072077
43. Negrete RJ, Hanney WJ, Kolber MJ, et al. Reliability, minimal detectable change, and normative values for tests of upper extremity function and power J Strength Cond Res 2010;24(12):3318-3325. doi:10.1519/ JSC.0b013e3181e7259c
44. Negrete RJ, Hanney WJ, Kolber MJ, Davies GJ, Riemann B. Can upper extremity functional tests predict the softball throw for distance: a predictive validity investigation. Int J Sports Phys Ther 2011;6(2):104-111.
45. Riemann BL, Davis SE, Huet K, Davies GJ. Intersession reliability of upper extremity isokinetic push-pull testing. Int J Sports Phys Ther. 2016;11(1):85-93.
46. Riemann BL, Johnson W, Murphy T, Davies GJ. A bilateral comparison of the underlying mechanics contributing to the seated single-arm shot-put functional performance test. J Athl Train 2018;53(10):976-982. doi:10.4085/1062-6050-388-17
47. Riemann BL, Davies GJ. Association between the seated single-arm shot-put test with isokinetic pushing force. J Sport Rehabil. 2020;29(5):689-692. doi:10.1123/jsr.2019-0140
48. Watson MD, Davies GJ, Riemann BL. Relationship between seated single-arm shot put and isokinetic shoulder flexion and elbow extension strength. J Sport Rehabil 2020;30(3):521-524. doi:10.1123/ jsr.2020-0069
49. Davies G, Rowe J, Collins B, Riemann B. Relationships between upper extremity functional test and patient reported outcome in patients completing shoulder rehabilitation. Med Sci Sports Exerc 2021;53:380-380. doi:10.1249/ 01.mss.0000763680.39347.0f
50. Riemann BL, Davies GJ. Limb dominance effects on seated single-arm shot-put limb symmetry indices following shoulder rehabilitation. J Sport Rehabil 2023;32(2):215-219. doi:10.1123/jsr.2022-0225
51. Barber P, Pontillo M, Bellm E, Davies G. Objective and subjective measures to guide upper extremity return to sport testing: A modified Delphi survey Phys Ther Sport. 2023;62:17-24. doi:10.1016/ j.ptsp.2023.05.009
52. Wilk KE, Bagwell MS, Davies GJ, Arrigo CA. Return to sport participation criteria following shoulder injury: a clinical commentary. Int J Sports Phys Ther 2020;15(4):624-642. doi:10.26603/ ijspt20200624
53. Riemann BL, Wilk KE, Davies GJ. Reliability of upper extremity functional performance tests for overhead sports activities. Int J Sports Phys Ther 2023;V18(3):687-697. doi:10.26603/001c.74368
54. Schwank A, Blazey P, Asker M, et al. 2022 bern consensus statement on shoulder injury prevention, rehabilitation, and return to sport for athletes at all participation levels. J Orthop Sports Phys Ther 2022;52(1):11-28. doi:10.2519/jospt.2022.10952
55. Collins BK, Watson MD, Davies GJ, Riemann BL. Relationship between closed kinetic chain upper extremity stability test and isokinetic shoulder flexion and elbow extension work. Athl Train Sports Health Care 2021;13(6):e383-e387 doi:10.3928/ 19425864-20210401-03
56. Goldbeck T, Davies G. Test-retest reliability of the Closed Kinetic Chain Upper Extremity Stability Test: a clinical field test. J Sport Rehabil 2000;9:35-45. doi:10.1123/jsr.9.1.35
57 Welch ES, Watson MD, Davies GJ, Riemann BL. Biomechanical analysis of the closed kinetic chain upper extremity stability test in healthy young adults. Phys Ther Sport 2020;45:120-125. doi:10.1016/j.ptsp.2020.06.010
58. Reiman MP, Matheson JW. Restricted hip mobility: clinical suggestions for self-mobilization and muscle re-education. Int J Sports Phys Ther 2013;8(5):729-740.
59. Andreyo E, Unverzagt C, Tompkins J. A rehabilitation progression for a baseball athlete following anterior cruciate ligament reconstruction: a case report. JOSPT Cases 2022;2(2):64-84. doi:10.2519/josptcases.2022.10804
60. Liu JM, Knowlton C, Gauthier M, et al. Roles of each leg in impulse generation in professional baseball pitchers: preliminary findings uncover the contribution of the back leg towards whole-body rotation. Sports Biomech Published online August 16, 2022. doi:10.1080/14763141.2022.2108490
61. MacWilliams BA, Choi T, Perezous MK, Chao EYS, McFarland EG. Characteristic ground-reaction forces in baseball pitching. Am J Sports Med 1998;26(1):66-71. doi:10.1177/ 03635465980260012801
62. Howenstein J, Kipp K, Sabick M. Peak horizontal ground reaction forces and impulse correlate with segmental energy flow in youth baseball pitchers. J Biomech 2020;108:109909. doi:10.1016/ j.jbiomech.2020.109909
63. Shitara H, Tajika T, Kuboi T, et al. Ankle dorsiflexion deficit in the back leg is a risk factor for shoulder and elbow injuries in young baseball players. Sci Rep. 2021;11(1):5500. doi:10.1038/ s41598-021-85079-8
64. Hoch MC, Staton GS, McKeon PO Dorsiflexion range of motion significantly influences dynamic balance. J Sci Med Sport 2011;14(1):90-92. doi:10.1016/j.jsams.2010.08.001
65. Wilk KE, Thomas ZM, Mangine RE, Fuller P, Davies GJ. Neurocognitive and reactive return to play testing protocol in overhead athletes following upper extremity injury. Int J Sports Phys Ther. 2023;18(6):1364-1375. doi:10.26603/001c.89926
Clinical Commentary/Current Concept Review
Jill K. Monson1a , Robert F. LaPrade2
1 Physical Therapy, Twin Cities Orthopedics, 2 Orthopedic surgery, Twin Cities Orthopedics
Keywords: knee, meniscus, meniscus root tear, meniscus repair, post-operative rehabilitation https://doi.org/10.26603/001c.126967
International Journal of Sports Physical Therapy
Vol. 20, Issue 1, 2025
Tears of the posterior medial meniscus root (PMMR) are common in older patients and reportedly contribute to rapid joint degeneration over time. Recognition of these tear types and the appropriate diagnosis through clinical exam and diagnostic imaging have improved significantly in recent years, as have surgical techniques to address them. Standardized post-operative rehabilitation protocols specific to PMMR repair have not been established or well understood in the scientific literature. Thus, clinicians have little guidance for managing post-operative care. Upon diagnosis of a root tear, appropriate surgical treatment, with appreciation for native anatomy and function of the meniscus root, is a critical first step for recovery. Post-operative rehabilitation protocols should account for biomechanical considerations unique to root tears, because they can destabilize the entire meniscus and compromise its native function. Rehabilitation specialists can apply known science regarding the influence of joint motion, muscle strengthening activities, gait, squatting, and progressive loading activities on the healing meniscus to guide post-operative recovery This knowledge, complemented with existing foundational principles for sound post-operative rehabilitation, can optimize recovery for patients following PMMR repair The purpose of this clinical commentary is to explore clinical questions related to 1) PMMR injury epidemiology, 2) diagnosis and surgical management of PMMR tears, and 3) critical considerations for safe and effective post-operative rehabilitation.
Level of Evidence: 5
The menisci are critical structures that contribute to the function and health of the knee joint. Each meniscus is anchored to the tibia through an anterior and posterior root attachment within each compartment. Radial tears span across and sever the circumferential fibers of the meniscus and disrupt the meniscus’s native hoop stress. Hoop stress is the mechanism through which the meniscus manages axial joint load by creating an elastic containment at the joint that allows the meniscus to “give but not give way,”
thus dispersing load. A radial tear located within one centimeter of the meniscus’s tibial attachment is referred to as a root tear1 and compromises the overall stability of the meniscus. Disruption of the circumferential fibers (and hoop stress) allows the meniscus adjacent to the tear to extrude outside of the joint space.2,3 Meniscal extrusion outside of the compartment reduces the contact area between the tibia and femur, normally buffered and broadened by the meniscus, leading to increased pressure on the articular cartilage. Notable extrusion creates an environment comparable to a total meniscectomy A reduction of contact area by 40 to 75% may increase joint contact stresses by as
Corresponding Author: Jill Monson, PT, OCS
Lead Physical Therapist – Complex Knee Injury Clinic
Twin Cities Orthopedics – Eagan
2700 Viking Circle
Eagan, MN, USA 55121
Email: jillmonson@tcomn.com
Telephone: 612.964.5443
Fax: 952.456.7638
much as 200 to 300%, transferring significant burden to the articular cartilage.4‑6 To this end, accelerated osteoarthritis (OA) progression has been observed in association with meniscus root tears and meniscal extrusion among patients with no prior evidence of radiographic OA four years prior 7 More dramatic joint changes have also been observed over shorter time periods.8 For this reason, there is a justified sense of urgency in the orthopedic community to improve the management of this pathology.
The purpose of this clinical commentary is to explore clinical questions related to 1) posterior medial meniscus root epidemiology, 2) diagnosis and surgical management of posterior medial meniscus root tears, and 3) critical considerations for safe and effective post-operative rehabilitation.
Meniscus root tears account for up to 10 to 21% of all meniscus tears.1,9 Posterior medial meniscus root (PMMR) tears comprise approximately 52% of all root tears and occur more commonly in females, typically in the 4th or 5th decade of life.9,10 These tears often occur with deep knee flexion or deep squatting with knee rotation.11 In general, tears of the PMMR are considered degenerative in nature with associated chondral defects observed 5.8 times more often with medial compared to lateral meniscus root tears.9 The posterior root of the medial meniscus is adjacent to the tibial attachment of the PCL and has been reported to be acutely injured with PCL tunnel drilling.12
Meniscus root tears are classified by tear location and tear pattern details. Type II tears, radial tears within 9 millimeters of the root attachment, are the most common.1 Tears at the posterior root attachments are more common at both the medial and lateral meniscus, perhaps related to lower loads to failure compared to the anterior root attachments.13,14
The term “non-operative management” is not consistently synonymous with “physical therapy” in orthopedic literature. Rather, non-operative management typically refers to oral pain medication, corticosteroid injections, use of an assistive device, use of an unloader knee brace, bed rest, or other palliative and passive interventions and these treatments have not shown favorable outcomes for PMMR tears.15 Well-designed physical therapy protocols, however, have demonstrated equivocal or superior outcomes to meniscectomy among older and younger patients with other meniscal tear types, but it is not known if the same can be true for meniscus root tears.16 Surgical repair is typically advised per concerns about rapid OA progression associated with PMMR tears in relatively young patients with previously healthy knees,7 but there is more to be learned
from prospective longitudinal studies comparing surgery to physical therapy.
Up until the past decade, PMMR tears were often debrided rather than repaired. Emerging literature, however, has sounded the alarm for the critical need for repair rather than resection, because patients undergoing meniscectomy exhibit more rapid progression of radiographic arthritic joint changes and significantly earlier pursuit of a total knee arthroplasty (TKA) when compared to a root repair.15, 17‑20 The anatomic two-tunnel transtibial repair technique has emerged as the gold standard for PMMR tears due to biomechanical evidence showing restoration of joint compartment stress to near normal levels.21,22
Most patients who present with meniscal root tear pathology have medial knee pain which is exacerbated by deep squatting. Many can recall a specific episode, such as kneeling in the garden or climbing stairs and hearing a pop in their knee, while others may have no specific history and report a gradual onset of symptoms. In general, the longer patients have symptoms, the more often they experience joint effusions with activities, which usually indicates that the medial compartment has developed varying degrees of chondromalacia due to the meniscal root tear
On physical examination, some will have medial joint line pain, where others may only have deep knee pain with full knee flexion or when squatting. In thin patients, when performing a valgus stress test at 30 degrees of knee flexion, meniscal extrusion may be felt along the margins of the medial collateral ligament. In addition, the meniscus may be extruded somewhat anteriorly, and this can be painful with deep palpation in patients.
Plain radiographs are essential to determine the status of the articular cartilage of the effected medial compartment when there is suspicion for a PMMR tear. Specifically, anterior posterior (AP), lateral, and Rosenberg views should be obtained in all patients to assess their degree of cartilage wear In addition, a long leg alignment radiograph should be obtained to determine if the knee is in varus alignment. Patients in varus alignment have been noted to have poorer surgical outcomes, and this may be because they have extra stress on the healing root tear.23,24 MRI scans are essential to diagnose a PMMR tear Most commonly, there is loss of signal within 1 cm of the posterior root attachment. This discontinuity can be seen on the axial and coronal views. On the sagittal views, there may be a loss of signal at the normal root attachment posteriorly, which has been called a “ghost sign.” This occurs because the meniscus root has been detached and it extrudes medially so there is a loss of signal where there would normally be a dark triangle shape of the meniscus at its posterior root attachment.
First, it is important to ensure that the patient is an appropriate candidate for a meniscus root repair This can include ensuring that they have no more than grade 1 or 2 Kellgren-Lawrence changes on plain radiographs and do not have any limitations or pathologies which could limit their ability to be compliant in their postoperative rehabilitation program. Root repair may be contraindicated for patients who are unable to use crutches or a walker to follow the initial weight bearing (WB) restrictions.
The most common surgical technique is a transtibial tunnel root repair In this type of technique, the bone where the meniscus root would normally attach is decorticated to provide a bony surface to maximize healing potential of the root reattachment. In addition, it is important to release the meniscus peripherally from the capsule when it is extruded, or the meniscus function will not be restored.25 This may require a release of the meniscofemoral and meniscotibial capsule all the way up to the level of the deep medial collateral ligament. Once the extruded meniscus has been sufficiently released, then tibial tunnel drilling is performed. The authors’ preferred technique is a two-tunnel technique which provides a broad-based healing surface of the meniscus. Self-capture meniscal suturing devices are used to place sutures or suture tapes into the meniscus substance and then shuttle them down these tunnels. When they are pulled out the anteromedial tibia, one can judge the ability of the meniscus to be pulled back to its attachment site and to reduce the meniscal extrusion. Once satisfactory reduction is achieved, the surgical sutures or suture tapes used to repair the meniscus substance are fixed to the tibia. The most common technique is to utilize a cortical button and tie the sutures over the button to the tibia. Postoperatively, radiographs are obtained to assess the position of the cortical button because an early fall after surgery could tear the sutures through the meniscus repair and result in button loosening. In cases where concomitant ligament reconstructions are performed, such as cruciate ligament reconstructions, it is important to assess tunnel trajectories for both the ligament reconstruction and the meniscus root repair to ensure that there is no convergence of the tunnels during the procedure.26
Post-operative physical therapy can commence immediately after PMMR repair and is divided into four distinct phases (Table 1): Phase I – Recovery, Phase II – Transition to WB, Phase III – Rebuild, and Phase IV – Restore. Each phase has unique rehabilitation priorities, precautions (Table 2), and goals that align with timelines related to tissue healing and adaptation to physiological demands of the limb and joint. This review will focus on precautions following PMMR repair and the rationale behind them.
Post-operative precautions aim to minimize joint loading or movements that contribute to excessive distraction or displacement of the repair. Gradual activity progressions, with incrementally increasing meniscal demand, should align with biological healing timeframes and maturation of the repair Rehabilitation progressions are not only guided by time and task mastery, but also by observations of joint irritability, utilizing algorithms such as the Pain Monitoring Model27 or Soreness Rules.28 Rehabilitation following PMMR repair differs from the more commonly seen repairs of traumatic vertical longitudinal tears in younger active or athletic patients. A more conservative progression is followed due to the fact that a PMMR tear is typically a radial tear type that severely disrupts meniscal hoop stress in an older patient with potentially more fragile or degenerative meniscus tissue. With these concerns in mind, there are multiple rehabilitation considerations that may be unique or amplified in the context of a PMMR repair
Patients remain non-weight bearing (NWB) during the Recovery phase to minimize hoop stress through the meniscus and tension on the repair This precaution is based on the known function of the meniscal root as an anchor against axial loading and findings from a laboratory study showing transtibial repair site loosening and displacement following cyclical joint loading to simulate WB.29 Weight bearing can be initiated after six weeks, but a gradual progression (two to four weeks) toward full weight bearing (FWB) is recommended to minimize joint reactivity and maximize normal gait mechanics. Once FWB is achieved, graded increases in daily step counts can be monitored through use of wearable technologies (smart phone/watch, pedometer) to avoid sudden spikes in joint loading which may contribute to increased irritability
Joint ROM exercises can begin immediately after surgery; however, flexion angles are initially limited and the motion is performed passively to avoid potentially harmful tension through the repair. (Figure 1) The medial meniscus natively exhibits slight posterior translation with knee flexion.30 In the setting of a root tear, the posterior meniscus has been observed to exhibit increased posterior extrusion with progression into deeper knee flexion angles (90 degrees).31 Flexion ROM is limited to 90 degrees for the first two weeks after surgery and then patients can gradually progress, but should not push aggressively into end range flexion (Table 1). Passive flexion ROM can be advanced with different patient positioning and belt placement during the first four to six weeks after surgery (Figure 1) Low rates of repair failure have been observed, upon second look arthroscopy, among patients following this ROM protocol.32 Full joint extension should be achieved immediately after surgery and preserved throughout recovery
Table 1. Rehabilitation phases with associated goals per post-operative week
Extension
ROM
Flexion
ROM 90° 120°
Walking NWB
Quadriceps muscle recovery
Work toward: 1) Full, active knee extension, 2) SLR x 20+ reps with no lag
Hamstring muscle recovery NO
Immediate full extension (hyperextension), symmetrical to opposite limb
Gradually work toward full, symmetrical end-range flexion
Wean from crutches
Measured progressions with walking distances, speed, and elevation per joint irritability (utilize wearable technology)
Work toward: 1) 70% LSI, 2) >50% peak torque/BW
AROM flexion, hiphinging drills, Fixed angle ISO
Squatting No squatting ≤70 degrees
Return to run criteria
Work toward: 1) 90% LSI, 2) 70-90% peak torque/BW (per lifestyle goals)
Gradual progression with resisted hamstring curling
Work toward: ≤8 cm SSD (YBT squat)
Work toward: 1) ≤4 cm SSD (YBT squat), 2) Relative squat depth ≥60% of LL
1. Prior history of regular running, 2. Near-normal knee ROM (KE ROM ≤5 deg SSD, KF ROM ≤15 SSD), 3. Minimal joint irritability (effusion consistently ≤trace, pain consistently ≤2/10), 4. Quad LSI ≥70%, 5. Quad peak torque relative to body weight ≥60%, 6. YBT squat SSD ≤8 cm , 7. Tolerance for ≥1-mile brisk walking without increased joint irritability, 8. Tolerance for foundational strength training/loading program without increased joint irritability *No sooner than 6 months post-op to allow for sufficient recovery of strength and function after hamstring strength and squat depth precautions lifted at 4 months
Abbreviations: (AROM) active range of motion, (BW) body weight, (ISO) isometric, (KE) knee extension, (KF) knee flexion, (LL) leg length, (LSI) limb symmetry index, (NWB) non weight bearing, (ROM) range of motion, (SLR) straight leg raise, (SSD) side to side difference, (YBT) Y-balance test
Table 2. Post-surgical precautions following PMMR repair
Weight bearing NWB 6 weeks
Minimize hoop stresses inducing extrusion at repair site Bracing
immobilizer
unloader brace *For patients with varus limb alignment
and protect limb during WB restriction
weeks Manage elevated medial compartment loads associated with varus limb alignment
Range of motion 90-degree flexion limit 2 weeks Reduce stress at posterior meniscus associated with deeper flexion angles OKC hamstring muscle activation Passive knee flexion
First minimize, then gradually progress trans-capsular pulling of semimembranosus as repaired meniscus matures Delay heavy, resisted, isolated hamstring curling 16 weeks Squatting Limit depth to 70 degrees (upon initiating squatting at 8 to 10 weeks post-op) 16 weeks Minimize increased posterior meniscus stress associated with increased squat depth Rotation/ cross-legged sitting Avoid 16 weeks Minimize rotational knee joint stress in flexed knee
Abbreviations: (OKC) open kinetic chain, (NWB) non weight bearing, (WB) weight bearing
Early muscle strengthening activities should focus on restoring a strong volitional quadriceps contraction. Quadriceps strength has been identified as a critical factor
for maximizing long term meniscal and articular cartilage health at the knee joint.33,34 Addressing joint pain and swelling is critical to minimize arthrogenic muscular inhibition (AMI).35 Traditional symptom management strategies such as limb/joint cryo-compressive therapy, elevation
Figure 1. Passive knee flexion range of motion (PROM) progressions: A. Seated PROM <90 degrees with belt under thigh, B. Seated PROM ≥90 degrees with belt at foot, C. Long sitting PROM into end range flexion with belt at foot and compression garments can be supplemented with specific techniques to minimize AMI (Examples: focal joint cooling, and transcutaneous electrical nerve stimulation [TENS]).35,36 These strategies can be coupled with modalities to enhance quadriceps muscle contraction specificity, intensity and/or awareness such as neuromuscular electrical stimulation (NMES), surface electromyography (EMG) and vibration.35,36 The goal is to minimize muscular atrophy and maximize recovery of strength with exercises that are low-load and safe during the early recovery period. Blood flow restriction (BFR) therapy (with patients who do not exhibit any contraindications) can be added to minimize muscle strength loss and atrophy during this most restrictive phase. It may be advisable to perform open chain strengthening activities in a manner that minimizes tibial shear immediately following root repair This would include isometric contractions at fixed angles or isotonic strengthening through a small arc of motion. As healing progresses into the remodeling and maturation phases after the initial three to four weeks, unloaded shear at the joint, with a broader arc of motion, may be tolerated.37,38
Hamstring strengthening, or even repetitive hamstring pulling with flexion ROM exercises, may warrant caution initially after PMMR repair. There are no biomechanical studies expressly examining the impact of open chain hamstring strengthening on meniscal repair, but caution stems from the known anatomy of the semimembranosus with its relatively broad attachment at the posteromedial joint capsule (Figure 2) and its function to mobilize the meniscus posteriorly with knee flexion.39 Theoretically, this could be disruptive, or at the very least irritating, to a healing posteromedial meniscus repair, because of this shared capsular attachment zone (Figure 3). When the meniscus is surgically released from the posteromedial capsule to address chronic extrusion, healing must occur at the meniscocapsular interval (Figure 3) and could be compromised by repeti-
tive traction forces at the posteromedial capsule caused by hamstring pulling with knee flexion or strengthening activities. Further research is warranted to understand the effect of hamstring forces on PMMR repair
The role of the meniscus in managing joint compressive forces increases with greater knee flexion angles in WB as the articular cartilage contact surface area reduces between the femur and tibia.40‑42 Increases in posterior horn meniscal pressure, joint compressive forces and joint shear have been observed with increasing squat depth.43,44 These biomechanical factors justify early squat depth restrictions following meniscus repair, particularly for radial and/or root tears at the posterior meniscus. Shallow squatting (<70 degrees) can commence once WB through an extended knee with gait is well tolerated, typically no sooner than 9 to 10 weeks post-surgery Leg press or 2-legged squatting is initially well tolerated but does not control for off-loading of the surgical limb. To prevent off-loading, patients can initially employ strategies such as isometric holds in a triple flexion lunge position at the surgical limb (Figure 4A) or staggered stance squatting with the non-surgical limb up on a box or step. As recovery progresses and coordination permits, bilateral squatting on a BOSU provides excellent immediate input to help correct surgical limb off-loading. (Figure 4B) Progressions with squat depth or load should occur independently to allow for clear observations of joint reactivity before progressing both variables (depth plus load). Deep squatting is generally not advised following PMMR repair, especially in patients with no prior exposure to deep squatting or those with more degenerative tissue quality observed at the time of surgery. This precaution is based on prior research reporting increased risk of degenerative meniscus pathology in individuals with higher
3. Meniscocapsular interval at posteromedial knee
(occupational) demand for deep knee flexion (squatting, kneeling, stairs).45,46 Younger, more active patients, with healthier tissue quality and a non-degenerative tear mechanism, can progress squat depth and external loads gradually with ongoing observation for symptom response; however, this is not the primary demographic seeking care for PMMR tears.
Guidelines for returning to running or higher impact/sport activity are not well defined for PMMR repairs in part because the patient demographic is older, and the condition is often considered degenerative. In contrast, patients who undergo posterior lateral meniscus root repair are often expected to return to sporting activities, because lateral root tears are typically an acute injury that often occurs
Figure 4. Lunge and squat modifications: A. Triple flexion isometric hold for focused surgical limb loading, B. BOSU for bilateral squat WB symmetry
in the context of anterior cruciate ligament rupture in a younger, sporting population.9 Counseling on post-operative activities should account for the patient’s pre-operative activity exposure (activity type, intensity, frequency), underlying joint health, time-based healing guidelines, objective recovery criteria, and knee joint reactivity in response to activity progressions. Objective criteria that should be achieved prior to considering return to run are described in Table 1 Such criteria stem from the ACL reconstruction literature, including patients with concomitant meniscus repair The goal is to maximize the likelihood of normal, symmetrical running kinematics and kinetics to reduce the potential for elevated or abnormal knee joint loading with running.47‑49 Given the paucity of literature on this topic specific to this population, return to run and sport counseling should be approached on a case-by-case basis with shared decision-making between the patient, physical therapist, and surgeon.
Research on long term outcomes following root repair is increasing, but existing studies are often underpowered, retrospective in nature, and generally lower quality and should be interpreted with caution. Most studies compare root repair to arthroscopic debridement of the meniscus/root. Post-operative patient reported outcome measures show improvement from the pre-operative state following PMMR repair,50 with results superior to those reported for menis-
cectomy 51 A higher rate of conversion to TKA is reported following meniscectomy compared to root repair.52 Progression of OA is observed and meniscal extrusion persists following root repair, but the degree of OA progression is reduced compared to that observed following meniscectomy.50,52,53 Varus limb alignment and a higher degree of baseline articular cartilage disease are associated with worse post-operative outcomes, whereas age and body mass index are not consistently identified as poor prognostic indicators.51,54
Meniscus root tears are an increasingly recognized diagnosis that has been reported to be associated with a deterioration of knee joint health.7,52,53,55 Surgical techniques to repair PMMR tears yield superior outcomes compared to debridement51‑53,56 and may slow OA progression and delay the need for future TKA.7,52,53,55 Post-operative rehabilitation protocols are not well defined in the literature and the degree of physical recovery to be expected or advised following PMMR repair is not yet well understood. Post-operative rehabilitation should focus on resolving fundamental deficits in joint motion, restoring muscle strength to symmetry and a relative level appropriate to the patient’s lifestyle, and coaching patients through a gradual return to activity, perhaps lower impact, per joint tolerance. Future research will help define best practices for post-operative rehabilitation and expectations for long term patient outcomes.
STATEMENT OF FINANCIAL DISCLOSURE AND CONFLICT OF INTEREST
Jill Monson, PT, OCS has no conflicts of interest. Dr Robert F. LaPrade, MD, PhD discloses the following relationships: Consultant: Ossur, Smith & Nephew, Responsive Arthroscopy, Royalties: Ossur, Smith & Nephew, Elsevier, Arthrex, Research Grants: Ossur, Smith & Nephew, AANA, AOSSM, Committees: ISAKOS, AOSSM, AANA, Editorial Board: AJSM, JEO, KSSTA, JKS, JISPT, OTSM, Education: Foundation Medical
Submitted: August 15, 2024 CST, Accepted: October 25, 2024 CST
© The Author(s)
This is an open-access article distributed under the terms of the Creative Commons Attribution 4.0 International License (CCBY-NC-4.0). View this license’s legal deed at https://creativecommons.org/licenses/by-nc/4.0 and legal code at https://creativecommons.org/licenses/by-nc/4.0/legalcode for more information.
1. LaPrade CM, James EW, Cram TR, Feagin JA, Engebretsen L, LaPrade RF. Meniscal root tears: a classification system based on tear morphology. Am J Sports Med 2015;43(2):363-369. doi:10.1177/ 0363546514559684
2. Moatshe G, Chahla J, Slette E, Engebretsen L, Laprade RF. Posterior meniscal root injuries. Acta Orthop 2016;87(5):452-458. doi:10.1080/ 17453674.2016.1202945
3. Tachibana Y, Mae T, Fujie H, et al. Effect of radial meniscal tear on in situ forces of meniscus and tibiofemoral relationship. Knee Surg Sports Traumatol Arthrosc 2017;25:355-361. doi:10.1007/ s00167-016-4395-4
4. Allaire R, Muriuki M, Gilbertson L, Harner CD. Biomechanical consequences of a tear of the posterior root of the medial meniscus. Similar to total meniscectomy. J Bone Joint Surg Am. 2008;90(9):1922-1931. doi:10.2106/JBJS.G.00748
5. Schillhammer CK, Werner FW, Scuderi MG, Cannizzaro JP. Repair of lateral meniscus posterior horn detachment lesions: a biomechanical evaluation. Am J Sports Med 2012;40(11):2604-2609. doi:10.1177/0363546512458574
6. Marzo JM, Gurske-DePerio J. Effects of medial meniscus posterior horn avulsion and repair on tibiofemoral contact area and peak contact pressure with clinical implications. Am J Sports Med. 2009;37(1):124-129. doi:10.1177/0363546508323254
7 Foreman SC, Liu Y, Nevitt MC, et al. Meniscal root tears and extrusion are significantly associated with the development of accelerated knee osteoarthritis: data from the osteoarthritis initiative. Cartilage. Published online 2020:1947603520934525. doi:10.1177/1947603520934525
8. Floyd ER, Rodriguez AN, Falaas KL, et al. The natural history of medial meniscal root tears: A biomechanical and clinical case perspective. Front Bioeng Biotechnol. 2021;9:744065. doi:10.3389/ fbioe.2021.744065
9. Kennedy MI, Strauss M, LaPrade RF. Injury of the meniscus root. Clin Sports Med 2020;39(1):57-68. doi:10.1016/j.csm.2019.08.009
10. LaPrade RF, Floyd ER, Carlson GB, Moatshe G, Chahla J, Monson JK. Meniscal root tears: Solving the silent epidemic. J Arthros Surg Sports Med. 2021;2:47-57 doi:10.25259/jassm_55_2020
11. LaPrade CM, Ellman MB, Rasmussen MT, et al. Anatomy of the anterior root attachments of the medial and lateral menisci: a quantitative analysis. Am J Sports Med 2014;42(10):2386-2392. doi:10.1177/0363546514544678
12. Kennedy NI, Michalski MP, Engebretsen L, LaPrade RF. Iatrogenic meniscus posterior root injury following reconstruction of the posterior cruciate ligament: a report of three cases. JBJS Case Connector. 2014;4(1):e20. doi:10.2106/JBJS.CC.M.00175
13. Ellman MB, LaPrade CM, Smith SD, et al. Structural properties of the meniscal roots. Am J Sports Med 2014;42(8):1881-1887 doi:10.1177/ 0363546514531730
14. Hauch KN, Villegas DF, Donahue TLH. Geometry, time-dependent and failure properties of human meniscal attachments. J Biomech 2010;43(3):463-468. doi:10.1016/ j.jbiomech.2009.09.043
15. Krych AJ, Reardon PJ, Johnson NR, et al. Nonoperative management of medial meniscus posterior horn root tears is associated with worsening arthritis and poor clinical outcome at 5-year follow-up. Knee Surg Sports Traumatol Arthrosc. 2017;25(2):383-389. doi:10.1007/s00167-016-4359-8
16. Fernández-Matías R, García-Pérez F, GavínGonzález C, Martínez-Martín J, Valencia-García H, Flórez-García MT. Effectiveness of exercise versus arthroscopic partial meniscectomy plus exercise in the management of degenerative meniscal tears at 5-year follow-up: a systematic review and metaanalysis. Arch Orthop Trauma Surg 2023;143(5):2609-2620. doi:10.1007/ s00402-022-04579-y
17 Chung KS, Ha JK, Yeom CH, et al. Comparison of clinical and radiologic results between partial meniscectomy and refixation of medial meniscus posterior root tears: a minimum 5-year follow-up. Arthroscopy: J Arthrosc Rel Surg. 2015;31(10):1941-1950. doi:10.1016/ j.arthro.2015.03.035
18. Bernard CD, Kennedy NI, Tagliero AJ, et al. Medial meniscus posterior root tear treatment: A matched cohort comparison of nonoperative management, partial meniscectomy, and repair. Am J Sports Med 2020;48(1):128-132. doi:10.1177/ 0363546519888212
19. Chung KS, Ha JK, Ra HJ, Yu WJ, Kim JG. Root repair versus partial meniscectomy for medial meniscus posterior root tears: comparison of longterm survivorship and clinical outcomes at minimum 10-year follow-up. Am J Sports Med 2020;48(8):1937-1944. doi:10.1177/ 0363546520920561
20. Kim SB, Ha JK, Lee SW, et al. Medial meniscus root tear refixation: comparison of clinical, radiologic, and arthroscopic findings with medial meniscectomy J Arthrosc Rel Surg 2011;27(3):346-354. doi:10.1016/j.arthro.2010.08.005
21. Padalecki JR, Jansson KS, Smith SD, et al. Biomechanical consequences of a complete radial tear adjacent to the medial meniscus posterior root attachment site: in situ pull-out repair restores derangement of joint mechanics. Am J Sports Med 2014;42(3):699-707 doi:10.1177/0363546513499314
22. Bhatia S, LaPrade CM, Ellman MB, LaPrade RF. Meniscal root tears: significance, diagnosis, and treatment. Am J Sports Med 2014;42(12):3016-3030. doi:10.1177/0363546514524162
23. Chung KS, Ha JK, Ra HJ, Kim JG. Preoperative varus alignment and postoperative meniscus extrusion are the main long-term predictive factors of clinical failure of meniscal root repair. Knee Surg Sports Traumatol Arthrosc Published online 2021:1-9. doi:10.1007/s00167-020-06405-7
24. Ridley TJ, Ruzbarsky JJ, Dornan GJ, et al. Minimum 2-year clinical outcomes of medial meniscus root tears in relation to coronal alignment. Am J Sports Med. 2022;50(5):1254-1260. doi:10.1177/ 03635465221080167
25. LaPrade CM, Foad A, Smith SD, et al. Biomechanical consequences of a nonanatomic posterior medial meniscal root repair Am J Sports Med 2015;43(4):912-920. doi:10.1177/ 0363546514566191
26. Gursoy S, Perry AK, Brady A, et al. Optimal tibial tunnel placement for medial and lateral meniscus root repair on the anteromedial tibia in the setting of anterior and posterior cruciate ligament reconstruction of the knee. Am J Sports Med 2022;50(5):1237-1244. doi:10.1177/ 03635465221074312
27 Silbernagel KG, Thomeé R, Eriksson BI, Karlsson J. Continued sports activity, using a pain-monitoring model, during rehabilitation in patients with Achilles tendinopathy: a randomized controlled study Am J Sports Med. 2007;35(6):897-906. doi:10.1177/ 0363546506298279
28. Adams D, Logerstedt D, Hunter-Giordano A, Axe MJ, Snyder-Mackler L. Current concepts for anterior cruciate ligament reconstruction: a criterion-based rehabilitation progression. J Orthop Sports Phys Ther. 2012;42(7):601-614. doi:10.2519/jospt.2012.3871
29. Steineman BD, LaPrade RF, Donahue TLH. Loosening of transtibial pullout meniscal root repairs due to simulated rehabilitation is unrecoverable: A biomechanical study J Arthrosc Rel Surg 2019;35(4):1232-1239. doi:10.1016/ j.arthro.2018.11.041
30. Thompson WO, Thaete FL, Fu FH, Dye SF. Tibial meniscal dynamics using three-dimensional reconstruction of magnetic resonance images. Am J Sports Med 1991;19(3):210-216. doi:10.1177/ 036354659101900302
31. Masuda S, Furumatsu T, Okazaki Y, et al. Medial meniscus posterior root tear induces pathological posterior extrusion of the meniscus in the kneeflexed position: An open magnetic resonance imaging analysis. Orthop Traumatol Surg Res 2018;104(4):485-489. doi:10.1016/j.otsr.2018.02.012
32. DePhillipo NN, Dekker TJ, Aman ZS, Bernholt D, Grantham WJ, LaPrade RF. Incidence and healing rates of meniscal tears in patients undergoing repair during the first stage of 2-stage revision anterior cruciate ligament reconstruction. Am J Sports Med 2019;47(14):3389-3395. doi:10.1177/ 0363546519878421
33. Kawada K, Furumatsu T, Fukuba M, et al. Increased quadriceps muscle strength after medial meniscus posterior root repair is associated with decreased medial meniscus extrusion progression. BMC Musculoskelet Disord. 2023;24(1):727. doi:10.1186/s12891-023-06858-0
34. Øiestad BE, Juhl CB, Culvenor AG, Berg B, Thorlund JB. Knee extensor muscle weakness is a risk factor for the development of knee osteoarthritis: an updated systematic review and meta-analysis including 46 819 men and women. Br J Sports Med. 2022;56(6):349-355. doi:10.1136/ bjsports-2021-104861
35. Norte G, Rush J, Sherman D Arthrogenic muscle inhibition: Best evidence, mechanisms, and theory for treating the unseen in clinical rehabilitation. J Sport Rehabil. 2021;31(6):717-735. doi:10.1123/ jsr.2021-0139
36. Harkey MS, Gribble PA, Pietrosimone BG. Disinhibitory interventions and voluntary quadriceps activation: a systematic review. J Athl Train. 2014;49(3):411-421. doi:10.4085/1062-6050-49.1.04
37 Broughton G II, Janis JE, Attinger CE. The basic science of wound healing. Plast Reconstr Surg. 2006;117(7S):12S-34S. doi:10.1097/ 01.prs.0000225430.42531.c2
38. Reinke J, Sorg H. Wound repair and regeneration. Eur Surg Res 2012;49(1):35-43. doi:10.1159/ 000339613
39. Beltran J, Matityahu A, Hwang K, et al. The distal semimembranosus complex: normal MR anatomy, variants, biomechanics and pathology Skeletal Radiol. 2003;32:435-445. doi:10.1007/ s00256-003-0641-1
40. Walker PS, Erkiuan MJ. The role of the menisci in force transmission across the knee. Clin Orthop Rel Res 1975;109:184-192. doi:10.1097/ 00003086-197506000-00027
41. Seedhom B, Dowson D, Wright V. Proceedings: Functions of the menisci. A preliminary study Ann Rheum Dis 1974;33(1):111. doi:10.1136/ard.33.1.111
42. Yao J, Lancianese SL, Hovinga KR, Lee J, Lerner AL. Magnetic resonance image analysis of meniscal translation and tibio-menisco-femoral contact in deep knee flexion. J Orthop Res. 2008;26(5):673-684. doi:10.1002/jor.20553
43. Becker R, Wirz D, Wolf C, Gopfert B, Nebelung W, Friederich N. Measurement of meniscofemoral contact pressure after repair of bucket-handle tears with biodegradable implants. Arch Orthop Trauma Surg. 2005;125(4):254-260. doi:10.1007/ s00402-004-0739-5
44. Escamilla RF. Knee biomechanics of the dynamic squat exercise. Med Sci Sports Exerc 2001;33(1):127-141. doi:10.1097/ 00005768-200101000-00020
45. Snoeker BA, Bakker EW, Kegel CA, Lucas C. Risk factors for meniscal tears: a systematic review including meta-analysis. J Orthop Sports Phys Ther 2013;43(6):352-367 doi:10.2519/jospt.2013.4295
46. Bahns C, Bolm-Audorff U, Seidler A, Romero Starke K, Ochsmann E. Occupational risk factors for meniscal lesions: a systematic review and metaanalysis. BMC Musculoskelet Disord. 2021;22(1):1042. doi:10.1186/s12891-021-04900-7
47 Knurr KA, Cobian DG, Kliethermes SA, StifflerJoachim MR, Heiderscheit BC. The influence of quadriceps strength and rate of torque development on the recovery of knee biomechanics during running after anterior cruciate ligament reconstruction. Am J Sports Med 2023;51(12):3171-3178. doi:10.1177/ 03635465231194617
48. Knurr KA, Kliethermes SA, Stiffler-Joachim MR, Cobian DG, Baer GS, Heiderscheit BC. Running biomechanics before injury and 1 year after anterior cruciate ligament reconstruction in division i collegiate athletes. Am J Sports Med 2021;49(10):2607-2614. doi:10.1177/ 03635465211026665
49. Pairot-de-Fontenay B, Willy RW, Elias AR, Mizner RL, Dubé MO, Roy JS. Running biomechanics in individuals with anterior cruciate ligament reconstruction: a systematic review Sports Med 2019;49:1411-1424. doi:10.1007/s40279-019-01120-x
50. Chang PS, Radtke L, Ward P, Brophy RH. Midterm outcomes of posterior medial meniscus root tear repair: A systematic review Am J Sports Med 2022;50(2):545-553. doi:10.1177/0363546521998297
51. Eseonu KC, Neale J, Lyons A, Kluzek S. Are outcomes of acute meniscus root tear repair better than debridement or nonoperative management? A systematic review. Am J Sports Med. 2022;50(11):3130-3139. doi:10.1177/ 03635465211031250
52. Krivicich LM, Kunze KN, Parvaresh KC, et al. Comparison of long-term radiographic outcomes and rate and time for conversion to total knee arthroplasty between repair and meniscectomy for medial meniscus posterior root tears: A systematic review and meta-analysis. Am J Sports Med. 2022;50(7):2023-2031. doi:10.1177/ 03635465211017514
53. Perry AK, Lavoie-Gagne O, Knapik DM, et al. Examining the efficacy of medial meniscus posterior root repair: A meta-analysis and systematic review of biomechanical and clinical outcomes. Am J Sports Med 2023;51(7):1914-1926. doi:10.1177/ 03635465221077271
54. Jiang EX, Abouljoud MM, Everhart JS, et al. Clinical factors associated with successful meniscal root repairs: A systematic review Knee 2019;26(2):285-291. doi:10.1016/j.knee.2019.01.005
55. Ahmed I, Dhaif F, Khatri C, et al. The meniscal tear outcome (METRO) review: A systematic review summarising the clinical course and outcomes of patients with a meniscal tear. Knee. 2022;38:117-131. doi:10.1016/j.knee.2022.07.002
56. Ahmed AF, Rinaldi J, Noorzad AS, Zikria BA. Return to Sports Following Meniscal Allograft Transplantation Is Possible but Remains Questionable: A Systematic Review Arthroscopy 2022;38(4):1351-1361. doi:10.1016/ j.arthro.2021.11.003
Robert C. Manske, PT, DPT, MEd, SCS, ATC, CSCS, FAPTA
Chris
Wolfe, PT, DPT, OCS, Cert MDT
Phil
Page, PT, PhD, ATC, CSCS, FACSM
Michael
Voight, PT, DHSC, SCS, OCS, ATC, CSCS, FAPTA
Abstract
Musculoskeletal ultrasound (MSKUS) has emerged as a valuable diagnostic tool in the evaluation and management of lateral elbow pathologies. This imaging modality provides high-resolution, dynamic visualization of superficial soft tissue structures, making it particularly advantageous for assessing conditions such as lateral epicondylitis (tennis elbow), ligamentous injuries, radial tunnel syndrome, and other common disorders. This article reviews the utility of MSKUS for rehabilitation providers, focusing on its role in accurately identifying pathoanatomical changes, guiding treatment strategies, and monitoring therapeutic outcomes. Specific emphasis is placed on the integration of diagnostic ultrasound in clinical practice, imaging techniques, and its advantages compared to other modalities. By enhancing diagnostic accuracy and facilitating targeted interventions, MSKUS serves as a critical adjunct in the comprehensive care of lateral elbow conditions.
Key Words: Lateral elbow, lateral epicondylitis, radial collateral ligament, diagnostic MSK ultrasound
Introduction
Lateral elbow pain is a frequent complaint in clinical practice, with diverse etiologies that range from overuse injuries to degenerative and inflammatory conditions.1-3 Accurate diagnosis is pivotal to tailoring effective rehabilitation interventions. Musculoskeletal ultrasound (MSKUS) has gained prominence as a diagnostic modality due to its ability to provide realtime, high-resolution imaging of soft tissues, including tendons, ligaments, and nerves, without the radiation exposure inherent in other imaging techniques. This article explores the application of MSKUS in evaluating lateral elbow disorders, focusing on its diagnostic capabilities, procedural benefits, and integration into rehabilitation settings.
Understanding anatomy is essential for accurate ultrasound evaluation. The lateral elbow comprises several key structures, including
•. The lateral epicondyle of the humerus, serving as the origin for the common extensor tendon (CET).
•. The radial collateral ligament complex providing lateral stability.
•. The radial head and its articulation with the humerus and ulna.
•. Adjacent neurovascular structures, including the radial nerve.
Benefits of MSK Ultrasound
MSKUS offers several benefits in the assessment, diagnosis, and management of lateral elbow pain.
•. Real-Time Imaging: Compared to other imaging modalities, MSKUS enables dynamic assessment and immediate correlation with clinical findings. Standardized protocols for static4 and dynamic5 elbow assessment are available.
•. High Resolution: MSKUS is excellent for superficial structures like tendons and ligaments, making it an ideal imaging modality for lateral epicondylitis.6
•. Cost-Effective and Accessible: Compared to magnetic resonance imaging (MRI), MSKUS is more widely available and affordable.Researchers reported moderate agreement of MSKUS with MRI in detecting tears of the CET.7
•. Guidance for Interventions: MSKUS facilitates precision in procedures such as corticosteroid injections or platelet-rich plasma (PRP) therapy.8
Common Pathologies of the Lateral Elbow
MSKUS provides clear visualization of the anatomical details required for precise diagnosis, which is critical for forming appropriate rehabilitation strategies. Several pathologies can cause lateral elbow pain.
•. Lateral Epicondylitis: Characterized by degeneration of the extensor carpi radialis brevis (ECRB) tendon, lateral epicondylitis is the most prevalent cause of lateral elbow pain.9-11 MSKUS can reveal tendon thickening, hypoechoic regions, and neovascularization, providing critical insights into the extent of tendon pathology. Dynamic scanning5 can aid in identifying pain generators.
•. Tendon Tears: MSKUS is adept at identifying partial tendon disruptions and other degenerative changes. Sensitivity, specificity, and accuracy of MSKUS in detecting CET tears were reported as 64.5%, 85.2%, and 72.2%, respectively.7
• Radial Collateral Ligament, or Lateral Ulnar Collateral Ligament Injury (Posterolateral Rotatory Instability):12-14 MSKSUS can identify ligamentous sprains or tears through visualization of discontinuity, thickening, or hypoechoic changes in the ligament. Varus stress testing during ultrasound can confirm functional instability.
•. Radial Tunnel Syndrome:15–17 MSKUS aids in the evaluation of compression or irritation of the posterior interosseous nerve, often caused by fibrosis or muscular hypertrophy.
•. Olecranon Bursitis and Synovitis:18–20 Fluid accumulation and synovial thickening in bursae can be detected with MSKUS, allowing differentiation between inflammatory and infective processes.
•. Elbow Fractures: Fluid accumulation demonstrating a lipohemarthrosis is children with acute elbow fractures may be seen.21,22 However, poor ultrasound penetration through bone makes it difficult to fully characterize the type of fracture.
Ultrasound Techniques for the Lateral Elbow MSKUS imaging of the lateral elbow involves proper positioning, probe selection, and protocol selection.
•. Patient Positioning: The patient is seated with the elbow flexed to 90 degrees, the forearm pronated or supinated depending on the target structure, and the hand resting on a flat surface. This position optimizes exposure of the lateral elbow structures.
•. Probe Selection: A high-frequency linear transducer (10-15 MHz) is recommended for optimal resolution.
•. Scanning Protocol:
Extensor Tendon Evaluation: Place the transducer longitudinally over the lateral epicondyle to assess the common extensor tendon for hypoechoic areas, calcifications, or tears. Longitudinal and transverse views of the ECRB and extensor digitorum tendons are essential.
Radial Collateral Ligament: Scan the lateral joint line in a longitudinal plane, looking for ligament thickening, disruptions, or instability during valgus stress testing.
Nerves: Trace the posterior interosseous nerve for evidence of entrapment.
Dynamic Assessment: Real-time imaging during active or resisted movements aids in diagnosing subtle abnormalities, such as snapping syndromes or dynamic nerve impingement.
MSKUS supports rehabilitation of lateral elbow pain through several methods.
•. Guiding Interventions: MSKUS enhances the precision of dry needling and injection therapies, including corticosteroids or platelet-rich plasma by confirming target localization.
•. Monitoring Progress: Periodic imaging allows for assessment of tendon healing, ligament repair, or resolution of inflammation, supporting adjustments to rehabilitation protocols.
•. Real-time Feedback: Dynamic ultrasound enables visualization of biomechanical alterations during therapeutic exercises, facilitating biomechanical retraining.
•. Operator Dependence: MSKUS is operatordependent, necessitating adequate training and experience to achieve optimal diagnostic accuracy.
•. Limited Visualization of Deep Structures: MSKUS is less effective for evaluating deep osseous or joint structures.
•. Artifacts: Improper technique can lead to imaging artifacts, potentially misguiding diagnosis.
Conclusion
MSKUS is an invaluable tool for rehabilitation providers managing lateral elbow conditions. Its ability to deliver high-resolution, real-time imaging facilitates accurate diagnosis, guides therapeutic
interventions, and monitors patient progress. As expertise in MSKUS continues to grow, its integration into routine clinical practice promises to enhance the quality and outcomes of care for patients with lateral elbow pathologies. Future research should focus on standardizing ultrasound protocols and further exploring its efficacy in diverse rehabilitation settings.
1. Nirschl RP, Ashman ES. Elbow tendinopathy: tennis elbow. Clin Sports Med. 2003;22(4):813-836. doi:10.1016/S0278-5919(03)00051-6
2. Gilmor R, Remily EA, Ingari JV. Management of lateral epicondylosis. J Hand Surg. 2024;49(11):1124-1128. doi:10.1016/j.jhsa.2024.07.003
3. Sidhu GAS, Amanullah N, Kaur H, et al. Persistent lateral elbow pain from overlooked posterolateral impingement of the elbow: a literature review and guidance for treatment. Clin Shoulder Elb. 2024;27(4):487-495. doi:10.5397/cise.2023.01081
4. Özçakar L, Kara M, Chang KV, et al. EUROMUSCULUS/USPRM Basic scanning protocols for elbow. Eur J Phys Rehabil Med. 2015;51(4):485-489.
5. Ricci V, Güvener O, Chang KV, et al. EUROMUSCULUS/USPRM Dynamic ultrasound protocols for elbow. Am J Phys Med Rehabil. 2022;101(6):e83-e92. doi:10.1097/PHM.0000000000001915
6. Ricci V, Cocco G, Mezian K, et al. Anatomy and sonographic examination for lateral epicondylitis: EURO-MUSCULUS/USPRM approach. Am J Phys Med Rehabil. 2023;102(4):300-307. doi:10.1097/PHM.0000000000002090
7. Bachta A, Rowicki K, Kisiel B, et al. Ultrasonography versus magnetic resonance imaging in detecting and grading common extensor tendon tear in chronic lateral epicondylitis. PloS One. 2017;12(7):e0181828. doi:10.1371/journal.pone.0181828
8. Ricci V, Mezian K, Cocco G, et al. Ultrasonography for injecting (around) the lateral epicondyle: EUROMUSCULUS/USPRM Perspective. Diagn Basel Switz. 2023;13(4):717. doi:10.3390/diagnostics13040717
9. Ikonen J, Lähdeoja T, Ardern CL, et al. Persistent tennis elbow symptoms have little prognostic value: a systematic review and meta-analysis. Clin Orthop 2022;480(4):647-660. doi:10.1097/CORR.0000000000002058
10. Kraushaar BS, Nirschl RP. Tendinosis of the elbow (tennis elbow). Clinical features and findings of histological, immunohistochemical, and electron microscopy studies. J Bone Joint Surg Am. 1999;81(2):259-278.
11. Descatha A, Albo F, Leclerc A, et al. Lateral epicondylitis and physical exposure at work? A review of prospective studies and meta-analysis. Arthritis Care Res. 2016;68(11):1681-1687. doi:10.1002/acr.22874
12. Koso R, Logli A, Mirvish A, et al. Arthroscopic evaluation for posterolateral rotatory instability of the elbow. Shoulder Elb. Published online November 14, 2024:17585732241293326. doi:10.1177/17585732241293326
13. Kniesel B, Huth J, Bauer G, et al. Systematic diagnosis and therapy of lateral elbow pain with emphasis on elbow instability. Arch Orthop Trauma Surg. 2014;134(12):1641-1647. doi:10.1007/s00402-014-2087-4
14. O’Driscoll SW. Classification and evaluation of recurrent instability of the elbow: Clin Orthop. 2000;370:34-43. doi:10.1097/00003086-200001000-00005
15. McCourt A, Pazik M, Roach RP, et al. Radial tunnel syndrome in a collegiate baseball pitcher: a case report. Sports Health. Published online June 13, 2024:19417381241258479. doi:10.1177/19417381241258479
16. Bolster M a. J, Bakker XR. Radial tunnel syndrome: emphasis on the superficial branch of the radial nerve. J Hand Surg Eur Vol. 2009;34(3):343-347. doi:10.1177/1753193408099832
17. Moradi A, Ebrahimzadeh MH, Jupiter JB. Radial tunnel syndrome, diagnostic and treatment dilemma. Arch Bone Jt Surg. 2015;3(3):156-162.
18. Nassar AY, Hanna B, Abou Chahine Y, et al. Chronic bilateral olecranon bursitis: a case report. Cureus. 2024;16(7):e65881. doi:10.7759/cureus.65881
19. Nchinda NN, Wolf JM. Clinical management of olecranon bursitis: a review. J Hand Surg. 2021;46(6):501-506. doi:10.1016/j.jhsa.2021.02.006
20. Del Buono A, Franceschi F, Palumbo A, et al. Diagnosis and management of olecranon bursitis. Surg J R Coll Surg Edinb Irel. 2012;10(5):297-300. doi:10.1016/j.surge.2012.02.002
21. Thomas JM, Chang EY, et al. Expert panel on musculoskeletal imaging, ACR Appropriateness Criteria® chronic elbow pain. J Am Coll Radiol JACR. 2022;19(11S):S256-S265. doi:10.1016/j.jacr.2022.09.022
22. Zuazo I, Bonnefoy O, Tauzin C, et al. Acute elbow trauma in children: role of ultrasonography. Pediatr Radiol. 2008;38(9):982-988. doi:10.1007/s00247-008-0935-5
Figure 1A: Patient Position. The patient is supine with the elbow in mild flexion to 35-45 degrees. The forearm is pronated. Place a bolster under the elbow to help keep the arm level for greater comfort and ease of the exam. The Green Box outlines the oblique longitudinal/LAX position of transducer.
Figure 1B: Long Axis Transducer Placement. The transducer is in long axis orientation (LAX). Long axis orientation (LAX) at the lateral epicondyle region with slight angulation with beam direction lateral to medial not anterior to posterior.
Figure 1c: Short Axis (SAX) Transducer Placement:
The transducer is in short axis orientation (SAX) and placed in a transverse plane on top of the lateral epicondyle. Starting over the radial head and then sweeping the transducer proximally will provide a good indication of the tendon as it inserts on the lateral epicondyle.
Figures 2A and 2B: The superficial common extensor tendon should be distinguishable from the deeper radial collateral ligament. Depending upon on the individual being scanned, differentiating the lateral collateral ligament from the common extensor tendon can be difficult. Normal thickness of the common extensor tendon ranges from 4.41 mm-5.34 mm.
Figures 3A and 3B: The hyperechoic tendon fibers can be seen in a cylindrical fascicle bordered by the hypoechoic visualization of the extensor musculature. \
PATHOLOGY: LATERAL EPICONDYLITIS OF EXTENSOR MASS WITH CALCIFICATIONS.
Figure 4A: Long Axis View (LAX) of Extensor Mass with an indication of tendinopathy and calcifications (Blue Arrow). The calcifications are visualized as hyperechoic and the common extensor tendon is measured as thickened due to the chronic tendinopathy.
Figure 4b: Short Axis View (SAX) of Extensor Mass with calcifications seen as hyperechoic (Blue arrow).
Figures 5A and 5B: Long Axis View (LAX) of thickened common extensor tendon with diffuse heterogeneity and areas of focal hypoechogenicity. The most common findings in a patient with lateral epicondylitis are focal areas of hypoechogenicity.