I J S PT
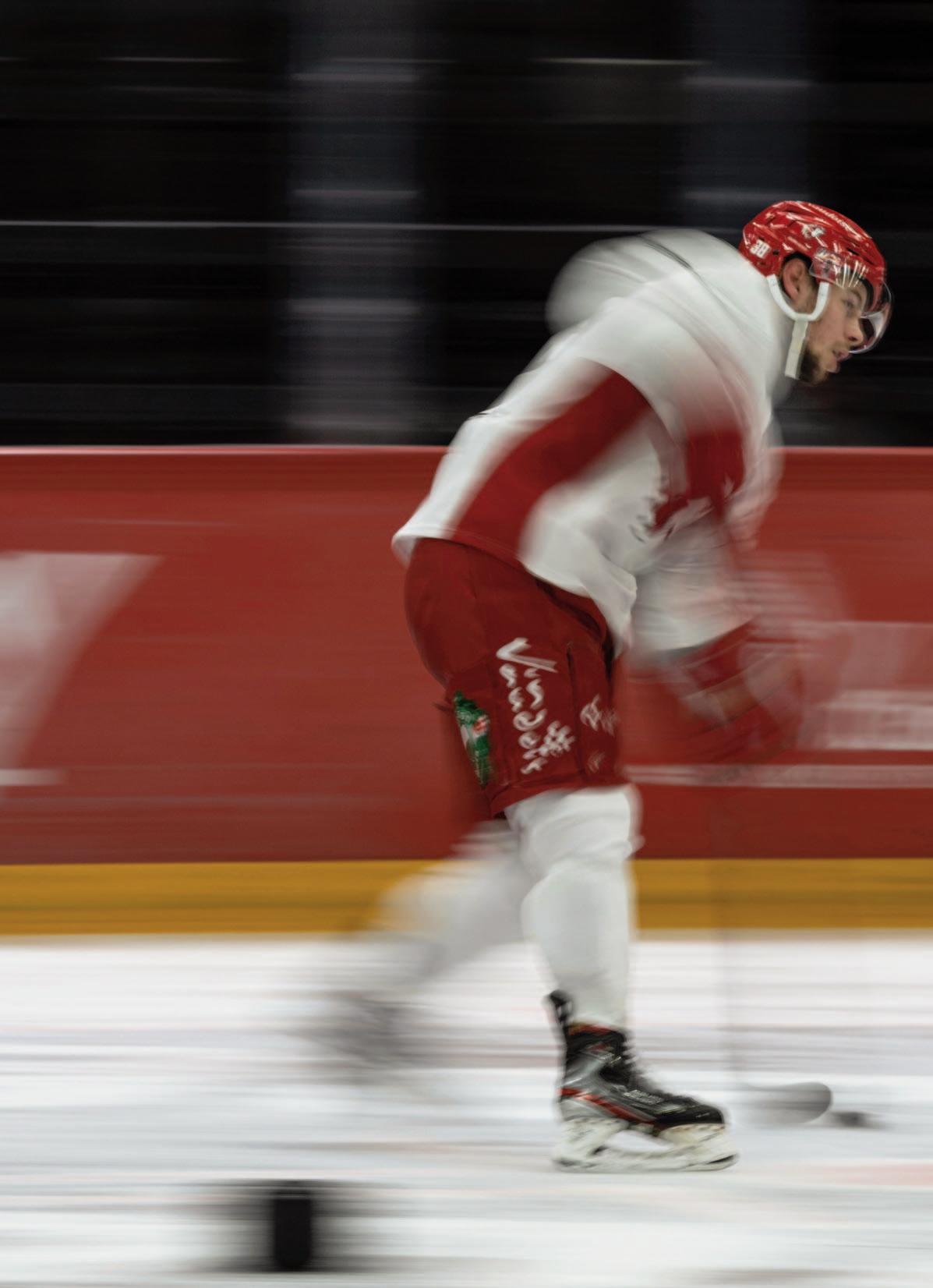
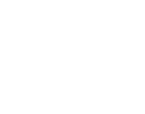
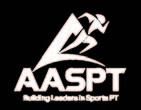
IN COLLABORATION WITH

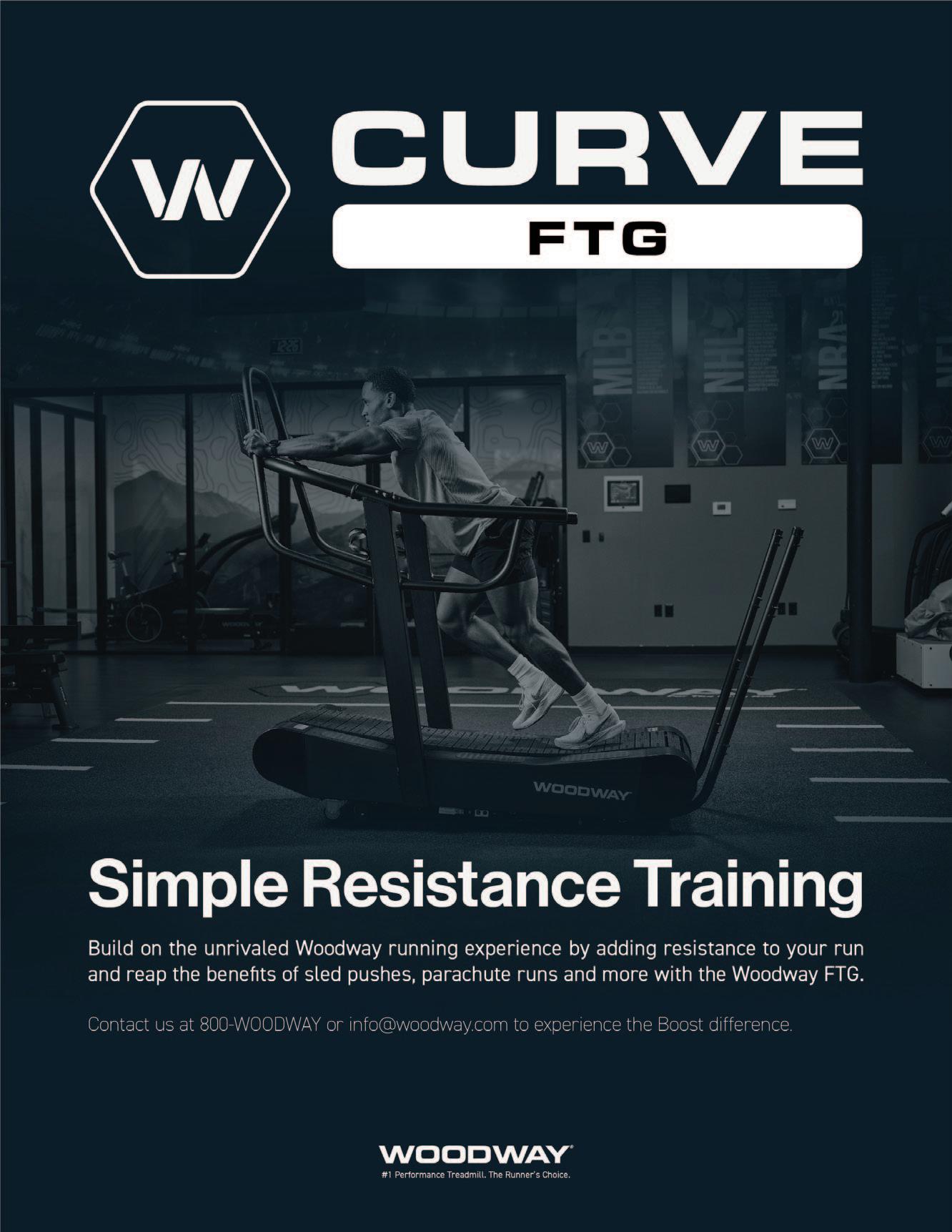
LightForce® Therapy Lasers empower you to treat soft tissue with confidence. Harnessing photobiomodulation (PBM)—a powerful form of light therapy—our lasers stimulate cellular metabolism to help treat muscle and joint pain from acute and chronic conditions.
Equipped with smart features like dosing recommendations, real-time visual and haptic feedback, and convenient portability, our range of therapy lasers combines a fusion of power with intelligence to enhance the patient and user experience. With the ability to reach deep tissues, LightForce lasers can cut the time needed by clinicians to treat patients—making light work of pain.
TRUSTED GLOBALLY
More than 250 professional and collegiate sports teams around the world trust LightForce Therapy Lasers to provide rehabilitation and pain management.
Scan the QR code to request a demo, or visit https://learn.chattanoogarehab.com/ijspt-journal-2024.
Turner A Blackburn, APTA Life Member, AT-Ret, AOSSM-Ret President
Mary Wilkinson Executive Director
Michael Voight Executive Editor and Publisher
Joe Black, PT, DPT, SCS, ATC
Eric Fernandez
Jay Greenstein, DC
Skip Hunter, PT, ATC-Ret
Russ Paine, PT, DPT
Tim Tyler, PT, ATC
Turner A. Blackburn, PT, ATC
George Davies, PT, DPT, MEd, SCS, ATC, LAT, CSCS, PES, FAPTA
Terry Malone, PT, PhD
Bob Mangine, PT
Barb Sanders, PT, PhD
Tim Tyler, PT, ATC
Kevin Wilk, PT, DPT, FAPTA
Executive Editor/Publisher
Michael L. Voight, PT, DHSc, OCS, SCS, ATC, CSCS
Executive Director/Operations and Marketing
Mary Wilkinson
Editor in Chief
Barbara Hoogenboom, PT, EdD, SCS, ATC
Managing Editor
Ashley Campbell, PT, DPT, SCS
Manuscript Coordinator
Casey Lewis, PTA, ATC
Publisher
Contact Information
International Journal of Sports Physical Therapy 6011 Hillsboro Pike Nashville, TN 37215, US, http://www.ijspt.org
IJSPT is a monthly publication, with release dates on the first of each month.
ISSN 2159-2896
Underwriting Sponsor Genie Health
Founding Sponsors Enovis Exertools Hyperice Trazer Woodway
Platinum Sponsors ATI Elvation
Gold Sponsors Hawkgrips Kayezen Structure + Function Education Winback Partners
Northeast Seminars Academy of Human Movement American Academy of Sports Physical Therapy
IJSPT is an official journal of the International Federation of Sports Physical Therapy (IFSPT). Countries with access to IJSPT as a member benefit. Reach us at www.ifspt.org.
IJSPT is an official journal of the ICCUS Society for Sports Rehabilitation. www.iccus.org
Stand out in your community with a diversified patient experience. Designed to improve outcomes, attract new patients, and increase revenue through insurance, cash-based services, and retail sales.
Gain access to a robust library of research, clinical education, and marketing tools including:
• On-demand clinical education courses
• Written treatment protocols
• Over 50 research studies specific to Hyperice technology
• Marketing tips and best practices including social media content, videos, and more
• Live trainings
Executive Editor/Publisher
Michael L. Voight, PT, DHSc, OCS, SCS, ATC, CSCS
Belmont University
Nashville, Tennessee – USA
Editor in Chief
Barbara Hoogenboom, PT, EdD, SCS, ATC
Grand Valley State University Grand Rapids, Michigan - USA
Managing Editor
Ashley Campbell, PT, DPT, SCS
Nashville Sports Medicine and Orthopaedic Center Nashville, Tennessee – USA
Manuscript Coordinator
Casey Lewis, PTA, ATC
Nashville Sports Medicine and Orthopaedic Center
Nashville, Tennessee – USA
Executive Director/Marketing
Mary Wilkinson
Indianapolis, Indiana – USA
Editors
Robert Manske PT, DPT, Med, SCS, ATC, CSCS
University of Wichita Wichita, KS, USA
Terry Grindstaff, PT, PhD, ATC, SCS, CSCS
Creighton University Omaha, NE, USA
Phil Page PT, PhD, ATC, CSCS
Franciscan University DPT Program Baton Rouge, LA, USA
Kevin Wilk PT, DPT, FAPTA
Clinical Viewpoint Editor Champion Sports Medicine Birmingham, AL, USA
International Editors
Luciana De Michelis Mendonça, PT, PhD UFVJM
Diamantina, Brazil
Colin Paterson PT, MSc PGCert(Ed), MCSP, RISPT, SFHEA
University of Brighton Brighton, England, UK
Chris Napier, PT, PhD
Clinical Assistant Professor
University of British Coumbia, Vancouver, BC, Canada
Nicola Phillips, OBE, PT, PhD, FCSP
Professor School of Healthcare Sciences Cardiff University, Cardiff, Wales, UK
Associate Editors
Eva Ageberg, PT, PhD
Professor, Lund University Lund, Sweden
Lindsay Becker, PT, DPT, SCS, USAW Buckeye Performance Golf Dublin, Ohio, USA
Keelan Enseki, PT, MS, OCS, SCS, ATC University of Pittsburgh Pittsburgh, PA, USA
John Heick, PT, PhD, DPT, OCS, NCS, SCS
Northern Arizona University
Flagstaff, AZ, USA
Julie Sandell Jacobsen, MHSc, PhD
VIA University
Aarhus, Denmark
RobRoy L. Martin, PhD, PT, CSCS
Duquesne University Pittsburgh, PA, USA
Andrea Mosler, PhD, FACP, FASMF
La Trobe Sport and Exercise Medicine Research Centre, School of Allied Health, Human Services and Sport, La Trobe University
Melbourne, Victoria, Australia
Brandon Schmitt, DPT, ATC
PRO Sports Physical Therapy Scarsdale, NY, USA
Barry Shafer, PT, DPT
Elite Motion Physical Therapy Arcadia, CA, USA
Laurie Stickler, PT, DHSc, OCS
Grand Valley State University
Grand Rapids, MI, USA
Editorial Board
James Andrews, MD
Andrews Institute & Sports Medicine Center
Gulf Breeze, AL, USA
Amelia (Amy) Arundale, PT, PhD, DPT, SCS
Red Bull/Ichan School of Medicine
Salzburg, Austria/New York, NY, USA
Gary Austin, PT PhD
Belmont University Nashville, TN, USA
Roald Bahr, MD
Oslo Sports Trauma Research Center Oslo, Norway
Lane Bailey, PT, PhD
Memorial Hermann IRONMAN Sports Medicine Institute
Houston, Texas, USA
Gül Baltaci, PT,Ph.D. Professor, CKTI, FACSM
Private Guven Hospital Ankara, Turkey
Asheesh Bedi, MD
University of Michigan
Ann Arbor, MI, USA
David Behm, PhD Memorial University of Newfoundland St. John's, Newfoundland, Canada
Barton N. Bishop, PT, DPT, SCS, CSCS Kaizo Clinical Research Institute Rockville, Maryland, USA
Mario Bizzini, PhD, PT Schulthess Clinic Human Performance Lab Zürich, Switzerland
Joe Black, PT, DPT, SCS, ATC Total Rehabilitation Maryville, Tennesse, USA
Turner A. "Tab" Blackburn, APTA Life Member, ATC-Ret, AOSSM-Ret NASMI Lanett, AL, USA
Lori Bolgla, PT, PhD, MAcc, ATC Augusta University Augusta, Georgia, USA
Matthew Briggs The Ohio State University Columbus, OH, USA
Tony Brosky, PT, DHSc, SCS Bellarmine University Louisville, KY, USA
Brian Busconi, MD UMass Memorial Hospital Boston, MA, USA
Robert J. Butler, PT, PhD St. Louis Cardinals St. Louis, MO, USA
Duane Button, PhD Memorial University St. Johns, Newfoundland, Canada
J. W. Thomas Byrd, MD Nashville Sports Medicine and Orthopaedic Center Nashville, TN, USA
Lyle Cain, MD Andrews Institute & Sports Medicine Center Birmingham, AL, USA
Gary Calabrese, PT, DPT Cleveland Clinic Cleveland, Ohio, USA
Meredith Chaput, PT, DPT, SCS Ohio University Athens, OH, USA
Rita Chorba, PT, DPT, MAT, SCS, ATC, CSCS United States Army Special Operations Command Fort Campbell, KY, USA
John Christoferreti, MD Texas Health Dallas, TX, USA
Richard Clark, PT, PhD Tennessee State University Nashville, TN, USA
Juan Colado, PT, PhD University of Valencia Valencia, Spain
Brian Cole, MD Midwest Orthopaedics at Rush Chicago, IL, USA
Ann Cools, PT, PhD
Ghent University Ghent, Belgium
Andrew Contreras, DPT, SCS Washington, DC, USA
George Davies, PT, DPT, MEd, SCS, ATC, LAT, CSCS, PES, FAPTA
Georgia Southern University Savannah, Georgia, USA
Pete Draovich, PT
Jacksonville Jaguars Footbal Jacksonvile, FL, USA
Jeffrey Dugas, MD Andrews Institute & Sports Medicine Center Birmingham, AL, USA
Jiri Dvorak, MD Schulthess Clinic Zurich, Switzerland
Todd Ellenbecker Rehab Plus Phoenix, AZ, USA
Carolyn Emery, PT, PhD University of Calgary Calgary, Alberta, Canada
Ernest Esteve Caupena, PT, PhD University of Girona Girona, Spain
Sue Falsone, PT, MS, SCS, ATC, CSCS, COMT Structure and Function Education and A.T. Still University Phoenix, Arizona, USA
J. Craig Garrison, PhD, PT, ATC, SCS Texas Health Sports Medicine Fort Worth, Texas, USA
Maggie Gebhardt, PT, DPT, OCS, FAAOMPT Fit Core Physical Therapy/Myopain Seminars Atlanta, GA and Bethesda, MD, USA
Lance Gill, ATC
LG Performance-TPI Oceanside, CA, USA
Phil Glasgow, PhD, MTh, MRes, MCSP Sports Institute of Northern Ireland Belfast, Northern Ireland, UK
Robert S. Gray, MS, AT Cleveland Clinic Sports Health Cleveland, Ohio, USA
Jay Greenstein, DC Kaizo Health Baltimore, MD, USA
Martin Hagglund, PT PhD
Linkoping University Linkoping, Sweden
Allen Hardin, PT, SCS, ATC, CSCS
University of Texas Austin, TX, USA
Richard Hawkins, MD
Professor of surgery, University of South Carolina
Adjunct Professor, Clemson University
Principal, Steadman Hawkins, Greenville and Denver (CU)
John D.Heick, PT, PhD, DPT, OCS, NCS, SCS
Northern Arizona University Flagstaff, AZ, USA
Tim Hewett, PhD
Hewett Consulting Minneapolis, Minnesota, USA
Per Hølmich, MD
Copenhagen University Hospital Copenhagen, Denmark
Kara Mae Hughes, PT, DPT, CSCS
Wolfe PT Nashville, TN, USA
Lasse Ishøi, PT, MSc
Sports Orthopedic Research Center
Copenhagen University Hospital Hvidovre, Denmark
Jon Karlsson, MD Sahlgrenska University Goteborg, Sweden
Brian Kelly, MD Hospital for Special Surgery New York, NY, USA
Benjamin R. Kivlan, PhD, PT, OCS, SCS Duquesne University Pittsburgh, PA, USA
Dave Kohlrieser, PT, DPT, SCS, OCS, CSCS
Ortho One Columbus, OH, USA
Andre Labbe PT, MOPT
Tulane Institute of Sports Medicine New Orleans, LA USA
Henning Langberg, PT, PhD University of Copenhagen Copenhagen, Denmark
Robert LaPrade, MD Twin Cities Orthopedics Edina, MN, USA
Lace Luedke, PT, DPT University of Wisconsin Oshkosh Oshkosh, WI, USA
Phillip Malloy, PT, PhD
Arcadia University/Rush University Medical Center Glenside, PA and Chicago, IL, USA
Terry Malone, PT, EdD, ATC, FAPTA University of Kentucky Lexington, KY, USA
Robert Mangine, PT University of Cincinnati Cincinnati, OH, USA
Eric McCarty, MD University of Colorado Boulder, CO, USA
Ryan P. McGovern, PhD, LAT, ATC Texas Health Sports Medicine Specialists Dallas/Fort Worth, Texas, USA
Mal McHugh, PhD
NISMAT
New York, NY, USA
Joseph Miller, PT, DSc, OCS, SCS, CSCS
Pikes Peak Community College Colorado Springs, CO, USA
Havard Moksnes, PT PhD
Oslo Sports Trauma Research Center Oslo, Norway
Andrew Murray, MD, PhD
European PGA Tour Edinburgh, Scotland, UK
Andrew Naylor, PT, DPT, SCS
Bellin Health
Green Bay, WI, USA
Stephen Nicholas, MD NISMAT New York New York, NY, USA
John O'Donnel, MD
Royal Melbourne Hospital Melbourne, Australia
Russ Paine, PT McGovern Medical School Houston, TX, USA
Snehal Patel, PT, MSPT, SCD
HSS Sports Rehabilitation Institute New York, NY, USA
Marc Philippon, MD
Steadman-Hawkins Clinic Vail, CO, USA
Kevin Plancher, MD, MPH, FAAOS
Plancher Orthopedics and Sports Medicine
New York, NY USA
Marisa Pontillo, PT, PhD, DPT, SCS
University of Pennsylvania Health System Philadelphia, PA, USA
Matthew Provencher, MD
Steadman Hawkins Clinic Vail, CO, USA
Charles E. Rainey, PT, DSc, DPT, MS, OCS, SCS, CSCS, FAAOMPT
United States Public Health Service Springfield, MO, USA
Alexandre Rambaud, PT PhD Saint-Etienne, France
Carlo Ramponi, PT Physiotherapist, Kinè Rehabilitation and Orthopaedic Center Treviso, Italy
Michael Reiman, PT, PhD Duke University Durham, NC, USA
Mark F. Reinking, PT, PhD, SCS, ATC Regis University Denver, CO, USA
Mark Ryan, ATC Steadman-Hawkins Clinic Vail, CO, USA
David Sachse, PT, DPT, OCS, SCS USAF San Antonio, TX, USA
Marc Safran, MD Stanford University Palo Alto, CA, USA
Alanna Salituro, PT, DPT, SCS, CSCS New York Mets Port Saint Lucie, FL, USA
Mina Samukawa, PT, PhD, AT (JSPO) Hokkaido University Sapporo, Japan
Barbara Sanders, PT, PhD, FAPTA, Board Certified Sports Physical Therapy Emeritus Professor and Chair, Department of Physical Therapy Texas State University Round Rock, TX, USA
Felix “Buddy” Savoie, MD, FAAOS Tulane Institute of Sport Medicine New Orleans, LA, USA
Teresa Schuemann, PT, DPT, ATC, CSCS, Board Certified Specialist in Sports Physical Therapy Evidence in Motion Fort Collins, CO, USA
Timothy Sell, PhD, PT, FACSM Atrium Health Musculoskeletal Institute Charlotte, NC, USA
Andreas Serner, PT PhD
Aspetar Orthopedic and Sports Medicine Hospital Doha, Qatar
Ellen Shanley, PT, PhD ATI Spartanburg, SC, USA
Karin Silbernagel, PT, PhD University of Delaware Newark, DE, USA
Holly Silvers, PT, PhD Velocity Physical Therapy Los Angeles, CA, USA
Lynn Snyder-Mackler, PT, ScD, FAPTA STAR University of Delaware Newark, DE, USA
Alston Stubbs, MD Wake Forest University Winston-Salem, NC, USA
Amir Takla, B.Phys, Mast.Physio (Manip), A/Prof
Australian Sports Physiotherapy The University of Melbourne Melbourne, Australia
Charles Thigpen, PhD, PT, ATC ATI
Spartanburg, SC, USA
Steven Tippett, PT, PhD, ATC, SCS Bradley University Peoria, IL, USA
Tim Tyler, PT, ATC NISMAT New York, NY, USA
Timothy Uhl, PT, PhD, ATC University of Kentucky Lexington, KY, USA
Bakare Ummukulthoum, PT University of the Witswatersrand Johannesburg, Gauteng, South Africa
Yuling Leo Wang, PT, PhD Sun Yat-sen University Guangzhou, China
Mark D. Weber, PT, PhD, SCS, ATC Texas Women’s University Dallas, TX, USA
Richard B. Westrick, PT, DPT, DSc, OCS, SCS US Army Research Institute Boston, MA, USA
Chris Wolfe, PT, DPT Belmont University Nashville, TN, USA
Tobias Wörner, PT, MSc Lund University Stockholm, Sweden
VOLUME 19, NUMBER 11
PAGE TITLE
THE BRAIN AND SPORTS: Neurocognitive Enriched Rehabilitation
In Collaboration with Sportfisio Switzerland.
EDITORIAL
1289 The Power of International Cooperation.
Voight M, Hoogenboom B, Campbell A, Bizzini M.
1290 Monitoring Cortical and Neuromuscular Activity: Six-month Insights into Knee Joint Position Sense Following ACL Reconstruction.
Busch A, Gianotti LRR, Mayer F, et al.
1304 Increased Visual Attentional Demands Alter Lower Extremity Sidestep Cutting Kinematics in Male Basketball Players.
Rikken KTH, Panneman T, Vercauteren F, et al. (listed as Benjaminse)
1314 Proprioceptive Reweighting and Postural Control are Impaired Among Elite Athletes Following Anterior Cruciate Ligament Reconstruction.
Attalin B, Sagnard T, Laboute E, et al.
1324 Advanced Neuromuscular Training Differentially Changes Performance on Visuomotor Reaction Tests and Single-leg Hop Tests in Patients with ACL Reconstruction.
Chmielewski T, Obermeier M, Meierbachtol A, et al. (Listed as Tompkins)
1333 The Impact of Visual Perturbation Neuromuscular Training on Landing Mechanics and Neural Activity: A Pilot Study.
Wohl TR, Criss CR, Haggerty AL, Rush JL, Simon JE, Grooms DR.
1346 Task-Driven Neurophysiological qEEG Baseline Performance Capabilities in Healthy, Uninjured Division-I College Athletes.
Mangine RE, Palmer TG, Tersak JA, et al.
1362 Neurocognitive and Ecological Motor Learning Considerations for the 11+ ACL Injury Prevention Program: A Commentary.
Grooms DR, Bizzini M, Silvers-Granelli H, et al.
INVITED CLINICAL COMMENTARY
1373 Neurocognitive and Neuromuscular Rehabilitation Techniques after ACL Injury, Part 1: Optimizing Recovery in the Acute Post-Operative Phase- A Clinical Commentary.
Wilk KE, Ivey M, Thomas ZM, Lupowitz L.
ORIGINAL RESEARCH
1386 Agreement Between 2D Visual and 3D Motion Capture-Based Assessment of Foot Strike Pattern. Goto H, Kamikubo T, Yamamoto R, et al.
1397 Intra-rater and Inter-rater Reliability of the KangaTech (KT360) Fixed Frame Dynamometry System During Maximal Isometric Strength Measurements of the Knee Flexors.
Woolhead E, Partner R, Parsley M, et al. (Lead author is listed as Jones)
1407 Position- and Sex-Related Differences in Sagittal and Frontal Plane Concentric Isokinetic Hip Muscle Peak Torques and Agonist-Antagonist Ratios.
Hoglund LT, Schiffino MC, Freels JE, et al.
VOLUME 19, NUMBER 11 (Continued)
1417 Hip Stability Isometric Test (HipSIT): Concurrent Validity and Reference Values for CrossFit® Participants.
Santos TRT, Rodrigues ALR, Faria HMP, et. al. (listed as lead author Ocarino)
1426 Hip and Groin Problems in Female Team-Sport Athletes: A Cross-Sectional Study. Stadelmann JD, Reichman F, Franceschini-Brunner R.
1439 Dominant Arm Internal Rotation Strength is Related to Arm Pain in Youth Baseball Players. Paskewitz J, Breidenbach F, Malloy P, et al.
1447 Relationship Between Lumbar Locked Rotation, Trunk Rotation During Pitching, and Pitch Velocity in High School Baseball Players. Okamura S, Gakuin B.
1455 The Quality of the Functional Movements and the Back Squat in Amateur and Professional Bodybuilders.
Iljinait� V, Šiupšinskas L, Berškien� K.
1465 Epidemiology and Influencing Factors in Davis Cup Retirements Over the Past TwentyYears. Casals M, Cortés J, Llenderrozos D, et al.
CLINICAL COMMENTARY
1477 Physical Therapy Utilization Prior to Biceps Tenodesis or Tenotomy for Biceps Tendinopathy. McDevitt AW, Cleland JA, Hiefield P, et al.
MSK ULTRASOUND BITES: Tips and Tricks
The Utilization of Diagnostic Musculoskeletal Ultrasound in the Evaluation for Ischiofemoral Impingement: A Perspective for Rehabilitation Providers. Manske RC, Wolfe C, Page P, Voight M, Bardowski B.
Mario Bizzini, PhD, PT (SSPA)
Ashley Campbell, PT, DPT, SCS, (IJSPT)
Barb Hoogenboom, PT, EdD, SCS, ATC (IJSPT)
Michael Voight, PT, DHSC, SCS, OCS, ATC, CSCS, FAPTA (IJSPT)
The International Journal of Sports Physical Therapy (IJSPT) is proud to present you with this special issue, in collaboration with the Swiss Sports Physical Therapy Association (SSPA), and in conjunction with the 2024 annual conference November 8, in Bern, Switzerland.
The topic Neurocognitive Enriched Rehabilitation has seen recent advances in neuroscience and motor learning. In the last decade, research groups in the US and in Europe have provided ground-breaking knowledge to better understand the role of the brain in preventing and recovering from ACL injuries. We're pleased and thankful that some of these researchers, such as Anne Benjaminse and Dustin Grooms, who are also among the speakers at the SSPA conference, have actively contributed to this special issue!
The SSPA and the IJSPT have been partners for more than 20 years. We firmly believe that open access is one of the keys to effective dissemination and promotion of clinically relevant evidence, as well as sharing trends and practices in sports physical therapy worldwide. We also believe that only with true open international cooperation we can together advance the rehabilitation and care of our athletes worldwide.
IJSPT readers will be able to relive the 2024 SSPA conference on the SSPA YouTube channel (sportfisioswiss - YouTube), a free educational resource for the sports PT community worldwide. We also remind our readers that they can find our popular Journal Club series on the IJSPT Youtube channel (https://www.youtube.com/@IJSPT/videos).
We hope you'll enjoy reading this IJSPT special issue, and "may the international power be with you!"
Student Programs and Internships
• Clinical Rotation Programs
-In 24 states
-Designed for PT, PTA, OT, OTA or ATC students
Request placement through your clinical coordinator
Development Programs
• Sports and Orthopedic Residencies
• Upper Extremity Athlete Fellowship
• Research Fellowship
• 18-Month New Clinician Support
• Leadership Development Pathways
BE THE CLINICIAN YOU’RE INSPIRED TO BE! LEARN MORE! Sponsorship opportunities available.
Most Advanced Electrotherapy Device: Powerful, intuitive and user-friendly
Treat up to three body zones at once on all types of tissues
Effective in less than 10 minutes Enter A New Era of Therapy
TECAR
HIGH FREQUENCY
Metabolic Action at Cell Level
Hi-TENS
LOW FREQUENCY IN PULSED HIGH FREQUENCY
Ultimate Pain Management
Hi-EMS
MEDIUM FREQUENCY
Deep Muscle Contraction
Mario Bizzini, PhD, PT, Ashley M Campbell, PT, DPT, SCS, Barb Hoogenboom, PT, EdD, SCS, ATC, Michael Voight, PT, DHSC, SCS, OCS, ATC, CSCS, FAPTA
https://doi.org/10.26603/001c.125160
International Journal of Sports Physical Therapy Vol. 19, Issue 11, 2024
The International Journal of Sports Physical Therapy (IJSPT) is proud to present you with this special issue, in collaboration with the Swiss Sports Physical Therapy Association (SSPA), and in conjunction with the 2024 annual conference November 8, in Bern, Switzerland.
The topic Neurocognitive Enriched Rehabilitation has seen recent advances in neuroscience and motor learning. In the last decade, research groups in the US and in Europe have provided ground-breaking knowledge to better understand the role of the brain in preventing and recovering from ACL injuries. We’re pleased and thankful that some of these researchers, such as Anne Benjaminse and Dustin Grooms, who are also among the speakers at the SSPA conference, have actively contributed to this special issue!
The SSPA and the IJSPT have been partners for more than 20 years. We firmly believe that open access is one of the keys to effective dissemination and promotion of clinically relevant evidence, as well as sharing trends and practices in sports physical therapy worldwide. We also believe that only with true open international cooperation we can together advance the rehabilitation and care of our athletes worldwide.
IJSPT readers will be able to relive the 2024 SSPA conference on the SSPA YouTube channel (sportfisioswissYouTube), a free educational resource for the sports PT community worldwide. We also remind our readers that they can find our popular Journal Club series on the IJSPT Youtube channel (https://www youtube.com/@IJSPT/ videos).
We hope you’ll enjoy reading this IJSPT special issue, and “ may the international power be with you!”
Mario Bizzini, PhD, PT (SSPA)
Ashley Campbell, PT, DPT, SCS (IJSPT)
Barb Hoogenboom, PT, EdD, SCS, ATC (IJSPT)
Michael Voight, PT, DHSC, SCS, OCS, ATC, CSCS, FAPTA (IJSPT)
© The Author(s)
This is an open-access article distributed under the terms of the Creative Commons Attribution 4.0 International License (CCBY-NC-4.0). View this license’s legal deed at https://creativecommons.org/licenses/by-nc/4.0 and legal code at https://creativecommons.org/licenses/by-nc/4.0/legalcode for more information.
Busch A, Gianotti LRR, Mayer F, Baur H. Monitoring Cortical and Neuromuscular Activity: Six-month Insights into Knee Joint Position Sense Following ACL Reconstruction. IJSPT. 2024;19(11):1290-1303. doi:10.26603/001c.124840
Aglaja Busch1,2a , Lorena R. R. Gianotti3 , Frank Mayer2 , Heiner Baur1
1 School of Health Professions, Bern University of Applied Sciences, 2 Sports Medicine & Sports Orthopeadics, University of Potsdam, 3 Department of Social Neuroscience and Social Psychology, University of Bern
Keywords: Anterior cruciate ligament, electroencephalography, surface electromyography, brain, proprioception https://doi.org/10.26603/001c.124840
International Journal of Sports Physical Therapy
Vol. 19, Issue 11, 2024
Background
Changes in cortical activation patterns after rupture of the anterior cruciate ligament (ACL) have been described. However, evidence of these consequences in the early stages following the incident and through longitudinal monitoring is scarce. Further insights could prove valuable in informing evidence-based rehabilitation practices.
To analyze the angular accuracy, neuromuscular, and cortical activity during a knee joint position sense (JPS) test over the initial six months following ACL reconstruction. Study design: Cohort Study
Methods
Twenty participants with ACL reconstruction performed a JPS test with both limbs. The measurement time points were approximately 1.5, 3-4 and 6 months after surgery, while 20 healthy controls were examined on a single occasion. The active JPS test was performed seated with a target angle of 50° for two blocks of continuous angular reproduction (three minutes per block). The reproduced angles were recorded simultaneously by an electrogoniometer. Neuromuscular activity of the quadriceps muscles during extension to the target angle was measured with surface electromyography Spectral power for theta, alpha-2, beta-1 and beta-2 frequency bands were determined from electroencephalographic recordings. Linear mixed models were performed with group (ACL or controls), the measurement time point, and respective limb as fixed effect and each grouping per subject combination as random effect with random intercept.
Results
Significantly higher beta-2 power over the frontal region of interest was observed at the first measurement time point in the non-involved limb of the ACL group in comparison to the control group (p = 0.03). Despite individual variation, no other statistically significant differences were identified for JPS error, neuromuscular, or other cortical activity
Aglaja Busch
E-mail: agbusch@uni-potsdam.de a
Outpatient Clinic, University of Potsdam Am Neuen Palais 10, Building 12 14469 Potsdam, Germany
Variation in cortical activity between the ACL and control group were present, which is consistent with published results in later stages of rehabilitation. Both indicate the importance of a neuromuscular and neurocognitive focus in the rehabilitation.
An anterior cruciate ligament (ACL) rupture represents a significant and long-lasting sports injury. Surgical reconstruction is frequently selected as a means of restoring joint stability.1 Nonetheless, neither reconstruction nor rehabilitation can fully restore knee function.2 It has been stated that the ACL contains mechanoreceptors that are responsible for detecting position, movement, and force within the joint.3 These contribute to the proprioceptive information that is provided for the central nervous system (CNS) in order to maintain function.4,5 An evaluation of these proprioceptive senses is the joint position sense (JPS) test, which assesses the ability to reproduce actively or passively a previously presented angle by a body segment.6 Systematic reviews and meta-analyses have demonstrated tendencies of greater knee JPS errors after ACL rupture in the injured limb compared to the non-injured side as well as to healthy controls,7‑10 however, the clinical significance of these errors is still a matter of debate.11 In addition to changes in proprioception, sensory dysfunction can result in deficits in balance, coordination, and joint stability.12,13 Neuromuscular activity, which encompasses both voluntary and involuntary muscle activation for the purpose of performing functional tasks and maintaining or restoring joint stability, can be quantified through the use of surface electromyography (EMG).4 In patients with anterior cruciate ligament (ACL) injuries, there is evidence that neuromuscular activity is impaired.14 Thus, proprioceptive changes might translate to errors in the coordination of movement, potentially leading to an elevated secondary injury risk.11, 15
The loss of mechanoreceptors resulting from an ACL rupture is likely to impact the adaptability of the CNS in regulating joint function.15,16 Consequently, studies have examined brain activation following ACL rupture during a knee JPS test. In their functional magnetic resonance imaging (fMRI) study, Strong et al.17 reported no significant differences in JPS error or brain response in the ACL group, with an average follow-up of 23 months post-surgery, compared to a healthy control group. However, greater JPS errors were significantly associated with higher brain activity in some brain regions (ipsilateral anterior cingulate, supramarginal gyrus and insula) in both groups.17 Baumeister et al.18 examined brain activity via electroencephalography (EEG) during a JPS test in ACL patients with an average follow-up of 12.5 months after reconstruction. The authors observed a higher JPS error in the ACL injured limb in comparison to the healthy controls, accompanied by a significantly higher theta-power recorded by the electrodes over the frontal cortical region of interest and a significantly
higher alpha-2 power in the electrodes over the parietal cortical region of interest.18 Additionally, they identified significant differences in brain activity between the contralateral limb and healthy controls. Moreover, a review article discusses that bilateral alterations in other tasks may be attributable to the functional reorganization of motor networks, which is not confined to somatosensory and mechanical deficiencies subsequent to unilateral injury.15
The aforementioned studies have only investigated participants with an ACL rupture and reconstruction crosssectionally after completion of the standard rehabilitation programme. This leaves uncertainty regarding early adaptations and potential time points for specified interventions during the rehabilitation phase.16,19 Therefore, the purpose of this investigation was to analyze the angular accuracy, neuromuscular, and cortical activity during a knee joint position sense (JPS) test over the initial six months following ACL reconstruction.
The hypothesis was that no significant proprioceptive deficiencies measured by the angular error would be detectable between the groups, measurement time points, and limbs10 Although the performance outcome may remain unchanged, alterations in the underlying neuromuscular and cortical activity were assumed. It was hypothesized that there would be differences in the neuromuscular activity during the extension to the target angle.20 Furthermore, higher theta frequency was expected in the frontal region of interest and lower alpha-2 power in the parietal region of interest. Conversely, no differences in the beta frequency were anticipated in the ACL group compared to the healthy control group, regardless of the region of interest.18
In this prospective observational study, 20 participants with reconstructed ACL were recruited in collaboration with their corresponding orthopaedic surgeon between March 2022 and September 2023. Additionally, 20 healthy controls participated voluntarily The participants were matched according to sex, age, height, weight and leg dominance. The subsequent analyses were conducted on a single leg of the healthy control group. This was identified as the leg corresponding to the involved limb of the ACL participant, representing the matched involved leg. General inclusion criteria were: age between 18 and 50 years, physically active (at least 2x 45 min per week21) currently or before the ACL rupture, and no former knee pathology. The ACL patient group was required to have a clinically
confirmed ACL rupture and have undergone reconstructive surgery with a quadriceps tendon graft within eight weeks of the incidence. No restriction regarding concomitant injuries was set. General exclusion criteria were: cardiac, neurological or peripheral vascular diseases, acute infection, alcohol abuse, current pain medication, other injuries of the lower extremity or trunk, back pain, thrombosis, pregnancy, dementia, or other musculoskeletal disorders limiting successful execution of the test protocol. An a priori sample size calculation was not feasible, due to missing data in a previous publication. Nonetheless, based on findings of previous studies examining EEG after ACL rupture, it was estimated that a sample size of 20 participants per group would be required.18,22 The study complied with the criteria of the declaration of Helsinki and was ethically approved by the local legal authority Kantonale Ethikkommision für die Forschung (KEK Bern, CH, No. 2020-02200). All participants gave their informed consent before participation. The study was pre-registered at the German Clinical Trials Register (DRKS-ID: 00023002).
The ACL group was invited to participate in a series of measurements at the Bern Movement Lab (Bern University of Applied Sciences, Bern, Switzerland) at three defined time points: 1.5 months, 3-4 months and 6 months following the reconstructive surgery The healthy control group was invited to participate in a single measurement session. Following clarification of the inclusion criteria, anthropometric data, leg dominance, the side of the ACL rupture and reconstruction and any concomitant injuries of the ACL group were recorded. Additionally, the Tegner activity score (TAS)23 (before the injury in the ACL group) and the current Knee Osteoarthritis Outcome Score (KOOS)24 were obtained at the first measurement. The ACL group reported if they received preoperative rehabilitation and provided details of their current main rehabilitation program content and the amount of rehabilitation they received at each measurement time point.
Next, the participants were prepared for the JPS test in the knee with recordings of the cortical activity using electroencephalography (EEG; see EEG recording and processing section), neuromuscular activity using electromyography (EMG; see EMG recording and processing section) as well as the angular error using an electrogoniometer (see electrogoniometer recording and processing). The procedure of the active-active JPS test as well as the recording and processing of the neuromuscular activity and angular deviation has been described in detail elsewhere.25 In short, following preparation, the participants’ actual general well-being and pain levels were assessed using a visual analogue scale with 100 mm in length.26 Then, the participants completed five minutes of level walking on a treadmill (Quasar® med, h/p/cosomos sports & medical GmbH, Nussdorf-Traunstein, Germany) at a speed of 3 km*h-1 as a standardized warm-up with additional recording of the neuromuscular activity during the final minute for later submaximal normalization of the EMG signals.27 Subsequently, the resting EEG activity during the period of seated
rest with the eyes open was recorded for three minutes and later used for the normalization of the EEG signals.28 Following this, the active-active JPS test was performed. Participants were seated with a hip angle of 100° flexion and a gap of approx. 5 cm between the posterior aspect of the knee and the chair surface, in an open kinetic chain (Figure 1).
During familiarization with the task, the participants actively flexed their knee into the starting position of 90° knee flexion (0° = full extension) and extended it to the target angle of 50° knee flexion (movement range of 40°) as indicated by the instruction of the examiner and visual feedback of the knee angle on a screen.25 While the vision of the participants towards their leg was obstructed by a hanging curtain throughout the five familiarization trials, they were allowed to use the feedback displayed on a screen. During the actual testing, the visual feedback was no longer available. The testing phase comprised two blocks of 3-minutelong active continuous angular reproduction per leg at a self-selected pace. Between the blocks, a 3-minute rest was taken and the starting leg was randomised for each measurement time point. The participants were instructed to reproduce the target angle as accurately as possible, hold it for three seconds and then return to the starting position (90° flexion).25 During the execution of the JPS test, the angular changes and neuromuscular and cortical electrical activity were recorded.
The electrogoniometer (Potentiometer RP20, Megatron Elektronik GmbH & Co.KG, Munich, Germany) was attached with the center of rotation placed at the knee joint
space, in the midline between the lateral femoral and tibial epicondyle of the participant’s leg. The goniometer arms were affixed with Velcro strips in a superior/inferior orientation, aligned proximally with the greater trochanter and distally with the lateral malleolus. The electrogoniometer data were captured at a rate of 4000 Hz and then underwent analogue-to-digital conversion (NI PCI 6255 device from National Instruments®, Austin, USA; 1.25 MS/s, 16 bits). Subsequently, the signals were recorded ustilizing LabVIEW®-based software Imago Record (Pfitec®, Endingen, Germany). The electrogoniometer data were processed using Imago Process Master (Pfitec®, Endingen, Germany). The angle reproduced for each trial was determined as the midpoint of the 3-second holding phase, in accordance with the specified procedure. These angles were then exported to a Microsoft® Excel spreadsheet (Windows 10, Microsoft Corporation, Redmond, WA, USA). The angular error, defined as the difference between the targeted and reproduced angles, was calculated for each trial. Moreover, the constant angular error (CE) and absolute angular error (AE) were computed.29 The CE quantifies the directional bias of the error, with positive or negative arithmetical differences considered in the calculation. Negative arithmetic differences indicate an underestimation of the reproduced angle in comparison to the targeted angle, which is characterised by lesser knee extension. Conversely, positive values signify an overestimation, indicating greater knee extension. Additionally, the variable error (VE) was calculated in order to assess the consistency of the constant error.30
For the surface EMG measurements, the electrodes were positioned meticulously on the vastus medialis (VM), vastus lateralis (VL), and rectus femoris (RF) of both limbs, adhering to the guidelines provided by SENIAM.31 Prior to electrode placement, the skin was prepared by shaving, sandpaper abrasion, and alcohol cleaning in order to optimize muscle signal detection. Bipolar electrodes (Type P-00-S, Blue Sensor®, Ambu, Ballerup, Denmark, interelectrode distance: 20 mm) were utilized to ensure that interelectrode impedance remained below 2 kΩ (Impedance meter: D175, Digitimer®, Hertfordshire, UK). The data acquisition methodology was consistent with that described previously The neuromuscular activity during the JPS test was analyzed in the extension movement phases based on angular recordings obtained from the electrogoniometer. The extension phase spanned from the commencement of the extension until reaching the anticipated target angle. EMG data underwent processing using the Imago Process Master (Pfitec®, Endingen, Germany). The raw signals were subjected to full-wave rectification and band-pass filtering within the range of 10–500 Hz (Butterworth, 2nd order). The root mean squares (RMS) of the amplitudes were computed for each muscle and movement phase and exported to a Microsoft® Excel spreadsheet (Windows 10, Microsoft Corporation, Redmond, WA, USA). The submaximal normalization of neuromuscular activity involved utilising the mean gait cycle activity of each muscle during level walking.27
Cortical electrical activity was recorded continuously throughout each block of JPS testing using a dry electroencephalography-system (DSI-24, Wearable Sensing, San Diego, CA, USA) and the corresponding software (DSISTREAMER–V.1.08.44), operating at a sampling rate of 300 Hz. The dry-EEG headset comprised 19 electrodes arranged in accordance with the international 10:20 system across the scalp and with two electrodes positioned at the ear lobules. The electrodes were mounted and secured following the instructions provided in the device manual to ensure optimal scalp contact.32 The impedances of each electrode were carefully monitored to maintain levels below 5kΩ. The recorded cortical activity signals were processed in MATLAB (Version R2020a, Mathworks Inc., Natick, MA, USA) utilising the EEGLAB toolbox (eeglab2022.1),33 following a standardized pipeline. Initially, the two blocks of JPS test per leg were combined. To mitigate line noise, the CleanLine software plugin34 was employed. Subsequently, the cortical activity signals were band-pass filtered within the range of 1 to 30 Hz. An “Artifact Subspace Reconstruction bad burst correction”35 was implemented to rectify any erroneous data periods. Following manual identification and rejection of undesirable episodes or channels, an adaptive mixture independent component analysis (AMICA)36 was conducted to discern and eliminate non-brain signals originating from muscle activity, eye movements, or electrocardiogram interference. The EEG data were then rereferenced to a common average, with the reference channel Pz reinstated in the dataset. Power spectra for the following frequency bands – theta (4-7.5 Hz), alpha-1 (8-10 Hz), alpha-2 (10.5-12.5 Hz), beta-1 (13-18 Hz), and beta-2 (18.5-25 Hz) – were computed using Fast-Fourier-Transformation and extracted for each trial and participant. Regions of interest (ROI) were formed for the frontal (F3, Fz, F4), central (C3, Cz, C4), and parietal (P3, Pz, P4) brain areas, as per current literature.18,28 The spectral power values per ROI were normalised to the resting cortical activity baseline for further statistical analysis.
Statistical data analysis was carried out using R (R Core Team, Version 4.3.2, 2023).37 Participants’ characteristics were tested for significant group differences using a MannWhitney U test and a one-way repeated measures ANOVA to identify any significant differences in the general wellbeing and pain levels before and after each measurement time point performed. Post-hoc analysis was utilized for multiple comparisons. The electrogoniometer, neuromuscular, and cortical activity data were examined to ensure their plausibility Individual values exceeding two standard deviations from the overall mean were identified and traced back to the original dataset. A comprehensive examination of the data processing procedures pertaining to these outliers was conducted, and adjustments were made if deviations from standard procedures were identified. Otherwise, they were excluded from the dataset in accordance with the
relevant criteria.27 The results section presents descriptive statistics as mean, standard deviation (SD) and 95% confidence intervals (95% CI). Inferential statistics were conducted on the independent variable “Group”, which consisted of the three factors: group (ACL patients or healthy controls), measurement time point, and whether the task was executed with the involved or non-involved leg. A linear mixed model was fitted to each dependent variable (namely the absolute error of the electrogoniometric data, normalized neuromuscular activity during extension to the target angle and normalised cortical activity for the defined frequency bands per ROI frontal theta, parietal alpha-2 and beta-1 and beta-2 within the frontal, central, and parietal ROI) with Group as the fixed effect and using different random effect structures, that is random intercept and slope per Group-subject combination, random intercept per Group-subject combination and random intercept for both Group and subject. Model comparison using the Akaike information criterion (AIC) indicated that the optimal model was the one with “Group:subject” as a random intercept, thereby allowing each Group-subject combination to have a random intercept. From the fitted model, contrasts of interest were computed, that is, the effects relative to the reference category (matched-involved leg of the healthy control group), with standard errors and 95% CI’s including p-value adjustment for multiple comparisons using the Tukey’s Honest Significance Difference method. A residual analysis was performed to ascertain the validity of the model assumptions. The R lme4 package38 was used for model fitting and emmeans package39 was used for computing contrasts.
A total of 40 participants were enrolled in this study (20 participants after ACL rupture and reconstruction and 20 healthy matched controls). The initial measurement time point (M1) was 1.5 ± 0.2 months post-surgery, the second (M2) was 3.5 ± 0.3 months post-surgery and the third measurement time point (M3) was 6.1 ± 0.3 months postsurgery. There were no dropouts. Table 1 provides an overview of the participants’ characteristics. Half of the ACL participants had a rupture in their dominant leg. Only one participant reported no associated injuries. Four participants received preoperative rehabilitation, and all participants in the ACL group underwent standard rehabilitation procedures following surgery over the course of the six month measurement period. Details on associated injuries and the rehabilitation content and frequency can be found in the supplement. One ACL participant was unable to perform the JPS test at the first measurement time point due to discomfort in the targeted range of motion in the involved limb. Data of one non-involved limb of an ACL participant at the first measurement time point and the second block of the non-involved limb at the third measurement time point were excluded from the analysis due to technical issues. The pre- and post-measurement self-reported well-
being scores were comparable between the two groups (p = 0.39; Table 1). Significant differences were observed in pain levels between the measurement time points (p = 0.004). A post-hoc analysis revealed that the ACL group exhibited significantly elevated pain levels following the first measurement time point when compared to both pre- and postmeasurements in the control group (p = 0.017 and p = 0.006, respectively). The remaining mean pain levels were higher among the ACL group than the control group, though they did not reach a statistically significant difference (0.22 < p < 1).
The mean number of repetitions performed by the participants was 48 repetitions (overall range of repetitions: 8-99; Con-matched-involved limb: 25-84; ACL-M1-involved limb range: 39-99; ACL-M1-non-involved limb range: 40-77; ACL-M2-involved limb range: 35-75; ACL-M2-non-involved limb range: 22-75; ACL-M3-involved limb range: 27-90; ACL-M3-non-involved limb range: 8-88). The highest mean absolute errors were observed in the control group with (9.1 ± 5.9°), while the lowest was noted in the non-involved leg of the ACL group at M3 (6.3 ± 4.2°). Conversely, the mean constant error was found to be lowest in the control group (4.9 ± 9.7°) and highest in the involved limb of the ACL group at M3 (7.5 ± 6.1°). The mean variable error did not exceed 1°. A comprehensive presentation of the descriptive statistics for the constant, variable and absolute error are presented in Table 2
The linear mixed model revealed no statistically significant difference in the absolute error in the fixed effects estimates (0.06 < p < 0.77, AIC = 33943.0; R2 = 0.56) (Table 3). The residual analysis provided no evidence against model assumptions.
Descriptive statistics of the normalised neuromuscular activity during the extension to the target angle are displayed in Table 4 Residual analysis revealed no evidence to contradict the model assumptions. Vastus medialis presented no statistical difference in the fixed effect estimates (0.80 < p < 0.98; AIC = 52333.5; R2 = 0.84). No statistically significant difference in the fixed effect estimates was found for the vastus lateralis (0.64 < p < 1; AIC = 48910.8; R2 = 0.85). The rectus femoris demonstrated no statistically significant difference in the fixed effect estimates (0.31 < p < 0.99, AIC = 49927.3; R2 = 0.78) (Table 4).
Descriptive statistics of the normalized cortical activity over the entire JPS test per frontal, central and parietal region of interest, as well as frequency bands, are displayed in Table 5 Residual analysis demonstrated no evidence against model assumptions. No significant differences were observed in the fixed effects estimates for frontal theta (0.16 < p < 0.99; AIC = 3613.4; R2 = 0.34) and frontal beta-1 (0.06 < p < 0.47; AIC = 3550.5; R2 = 0.35) (Table 5). A signif-
Table 1. Participant characteristics displayed in mean ± standard deviation.
VAS well-being (mm)
VAS pain (mm)
anterior cruciate ligament group; n number; KOOS Knee injury and Osteoarthritis Outcome Score; max maximum; VAS visual analogue scale
Table 2. Constant, variable and absolute error of the JPS test per measurement time point and leg.
M mean; SD standard deviation; Con control group; ACL anterior cruciate ligament group; M1-M3 measurement time point 1-3
Table 3. Linear mixed model output for the absolute error with Group as fixed effect and Group:subject as random effect with random intercept.
Absolute Error Model output
= standard error; CI = confidence interval; Con = control group; ACL = anterior cruciate ligament group; M1-M3 = measurement time point 1-3
Table 4. Normalized neuromuscular activity during extension to the target angle. Descpritive statistic and linear mixed model output with Group as fixed effect and Group:subject as random effect with random intercept.
Vastus lateralis
Rectus femoris
icantly different fixed effect estimate was found for frontal beta-2 when comparing the ACL-M1-non-involved leg to the control group (p = 0.03). The remaining comparison of frontal beta-2 did not yield a statistically significant difference (0.32 < p < 0.75, AIC = 3468.2; R2 = 0.27) (Table 5).
No statistically significant differences were detected in the fixed effects estimates for central beta-1 (0.06 < p < 0.47; AIC = 3550.5; R2 = 0.35) and central beta-2 (0.81 < p < 1, AIC = 3595.9; R2 = 0.46) (Table 5).
Furthermore, no statistically significant differences were evident in the fixed effects estimates for parietal alpha-2 (0.15 < p < 0.99; AIC = 3623.3; R2 = 0.49), parietal beta-1 (0.50 < p < 0.95, AIC = 3391.1; R2 = 0.50) and parietal beta-2 (0.62 < p < 0.99, AIC = 3365.3; R2 = 0.54) (Table 5).
This is the first study to examine the angular accuracy, neuromuscular, and cortical activity during a JPS test longitudinally over the first six months following ACL reconstruction. No significant differences were observed between the measurement time points or limb in comparisons to the
healthy control group, with the exception of the beta-2 frequency band in the frontal region of interest for the noninvolved leg of the ACL group, which demonstrated a difference at the first measurement time point in comparison to the control group.
The mean constant error was observed to be the smallest in the control group, although this was accompanied by a high standard deviation and no statistically significant differences when compared to the ACL group. It could be suggested that the mean precision presented by the constant error is marginally better in the control group when compared to the ACL group. Nevertheless, the mean absolute errors were found to be higher in the healthy control group than in the ACL group for each measurement time point and limb, with no significant difference observed. The non-significant difference confirms the authors’ hypotheses. Furthermore, a systematic review focusing on the active JPS test among ACL reconstructed participants in the early phase following surgery demonstrated nonhomoge-
Table 5. Normalized cortical activity during joint position sense test. Descpritive statistic and linear mixed model output with Group as fixed effect and Group:subject as random effect with random intercept.
Parietal Alpha-2
Frontal Beta-1
Frontal Beta-2
Central Beta-1
Central
Parietal Beta-1
Parietal Beta-2
neous results.10 Contrary, in the literature, JPS errors have most often been reported to be higher among the ACL patient groups compared to the contralateral side or a healthy control group,7‑9 however, no clear consensus exists.40
The psychometric properties of the present study setup were investigated within a healthy control group, and a minimal detectable change of 6.8° was identified.25 Therefore, presented differences found within the current study are likely to be the result of systematic error and demonstrate no clear influence of the measurement time point or limb in the ACL group. Moreover, it has been proposed that differences should exceed 5° to be considered clinically meaningful.8,9,11 The absolute errors presented exceed the minimal clinically important difference, yet no significant differences were observed between the ACL group and the healthy control group.
No significant differences were found between the healthy control group and the ACL group at each time point and for each limb. In the literature, only a few studies investigating the neuromuscular activity in the early postoperative period following ACL reconstruction exist. A general reduction in voluntary quadriceps activation has been reported in the literature,14,20,41 as well as alterations in the neural pathways responsible for this activation.42 Studies have discussed whether the quadriceps deficits can be attributed to arthrogenic muscle inhibition.14,43 In the present study, it can be hypothesized that the open-chain and non-weight-bearing JPS task may not have elicited differences in the neuromuscular activity due to the absence of sensory feedback and task functionality
A higher mean frontal theta power was observed in both limbs of the ACL group in the first two measurement time points, although this did not reach statistical significance when compared to a healthy control group. Baumeister et al.18 reported significantly higher theta power over the frontal cortical areas in both limbs of participants on average one year after ACL reconstruction compared to healthy controls.18 Elevated frontal theta power might represent a higher need for attention during the JPS test execution.44, 45
No significantly different alpha-2 power was detected in recordings from the electrodes over the parietal region at both limbs of the ACL group in comparison to the healthy control group. The mean alpha-2 power over the parietal region demonstrated a slight increase over the measurement time points in the ACL group. Higher alpha-2 power over parietal cortical brain regions indicates less task-specific demands.46 It can be hypothesized that the slight increase in alpha-2 power may be influenced by a learning effect with the repeated measures, reflecting a reduction in the need for cortical activation during the task.46 However, this hypothesis was not supported by the findings of the present study The study by Baumeister et al.18 presented a significantly lower alpha-2 power from the parietal electrodes during JPS testing with the involved limb of the ACL group in comparison to healthy controls.18 Reduced activity within the alpha-2 frequency band in sensorimotor areas has been found to be associated with an increase in movement-related information and sensory processes.47
The literature suggests that frontal theta and parietal alpha-2 activities play an important role in the executive functions of working memory 46,48 The findings of the present study did not reveal any alterations in these executive functions within the ACL group while maintaining performance in the JPS test. It is worth considering, whether the study protocol, which included an open-chain JPS test, creates a sufficiently challenging environment for typically physically active, young participants. Moreover, the primary focus of attention during the execution of a JPS test is internally. It is possible that the ACL group has undergone a process of training whereby they have focused predominantly on their knees and limbs over the past six months of rehabilitation. which could result in a different basis in comparison to a healthy control group. It would be of interest to examine whether similar results would be found if the JPS test was conducted with external visual cues or under dual-task conditions. Herewith, it may be beneficial to investigate attention control in addition to working memory.49 Furthermore, recent clinical commentaries have recommended adapting ACL rehabilitation to allow for motor re-learning and support neuroplasticity, during which an external focus during the execution of movements is emphasized.50,51
Beta oscillations are thought to be involved in top-down processing and sensorimotor integration and are linking different brain regions, including the pre-motor and somatosensory cortex, the supplementary motor areas and the cerebellum.44,52 The literature indicates that fluctuations in beta oscillations can be divided into movementbased reductions, with a decrease in beta power slightly prior to and during movement, and post-movement beta rebound, which is characterized by an elevation of beta power following movement.52 It should be noted that the referenced study did not distinguish between beta-1 and beta-2. Apart from frontal beta-2 in one comparison, no other significant results were found in the present study 51 A detailed analysis regarding the movement phase would provide valuable insights into the differences in movement planning, execution and the integration of sensory information. Moreover, examining cortical activity in repetitions with higher angular error could prove beneficial. One fMRI study reported a significantly positive correlation between angular error and brain activity 17 Further investigation could clarify how proprioceptive error detection and integration contribute to the execution of precise movements and the association with cortical activities. This could be used to evaluate the high re-injury rates among patients with ACL reconstruction.
It is possible that the statistically significant differences, which were not observed during the initial six month period following ACL reconstruction, may become apparent at a later stage.14 This is supported by a systematic review, reporting neurocognitive changes and alterations to the central nervous system that persist after athletes return to sport.53 It is further recommended that a neurocognitive test battery be implemented in the return to sport testing process to enhance the detection of neurocognitive reliance in patients returning to sport following ACL reconstruc-
tion.54 Nevertheless, it remains ambiguous whether differences in cortical activities and functional connectivity precede ACL injury as a cause or emerge as a consequence thereof.55,56
The characteristics of the participants were not significantly different between the groups, with the exception of the (preinjury) Tegner score and the current KOOS total score. Both groups exhibited a mean Tegner score of greater than 4, indicating that they were a physically active cohort in total. Nevertheless, the preinjury Tegner score of the ACL group was slightly higher than that of the healthy control group. The influence of physical activity on proprioceptive capacity is a topic that has been discussed in the literature.57,58 It is possible that a less active ACL patient group would yield more distinct results.17 Moreover, the presence of joint effusion and potential pain, particularly at the first measurement time point for the ACL group, may have exerted additional influence on the outcomes. This is, however, unavoidable in measurements taken during the early phase following injury and reconstruction. Nonetheless, it is possible that the observed effects within the ACL group may have been influenced by the rehabilitation process, which was not controlled. A sub-analysis of patients with a higher frequency of rehabilitative appointments in comparison to a lower frequency might provide further insights into the potential effect on the neuromuscular or cortical activity Nevertheless, the number of patients included in the present study was insufficient for the performance of meaningful sub-analyses. The influence of limb dominance on JPS quality has been the subject of previous research.25, 57,58 A recent fMRI study has proposed the existence of a right hemisphere lateralization of proprioceptive processing test in healthy persons during a JPS.59 Although the present study included only one participant per group defined as left-footed and ACL patients with equally paired ACL injuries in their non-dominant limb, future research should evaluate the potential influence of limb dominance. The self-selected pace during testing resulted in different repetitions per execution of the JPS test. The use of a metronome would have ensured greater standardisation of the procedure, although it is possible that this would have resulted in alterations to individual movement preferences. Nonetheless, the range of repetitions was comparable between the two groups and measurement time points, particularly in order to minimize the impact of potential pain or discomfort during the first measurement time points in the involved limb of the ACL group. Moreover, the rather long duration of angular reproduction may have resulted in a decline in motivation or focus. Yet, the variable error was minimal and the numerous repetitions ensured an accurate assessment of angular reproduction performance.60 As previously stated, the clinical relevance of the JPS test is a topic of ongoing debate.6,11 The limitation of the test to a single joint does not accurately reflect the planning and execution of movement in functional tasks, which often involve multiple joints and movement directions.60 Accordingly, a test simulating weight-bearing movement or ob-
stacle clearance test has been proposed.61,62 However, the feasibility of these tests within a study population in the early stages after surgery, with the measurement of brain activity using EEG, remains unknown and would be subject to further investigation. Moreover, the JPS test enables the explicit measurement of the participant’s ability to perceive the position of the knee joint. A comprehensive understanding of the changes observed during the execution of this test may provide valuable insights into the various affected parts of the sensorimotor system. This could offer a differentiated view of the observed alterations during functional tasks and assist in determining the original causes of the changes.
It should be noted that this study is not without limitations. The rehabilitation process of the ACL group was not subject to control, but was documented. While the primary rehabilitation content was largely consistent across the participants, there were also notable differences in terms of frequencies. Furthermore, the degree of independent training varied considerably between participants, depending on their motivation. This may have had an impact on the results and a more structured and balanced rehabilitation scheme may facilitate more effective clinical implications. Nevertheless, the recruited patients represent patients in their regular therapy setting, which lends to a high external validity to the study and corresponds well with procedures in everyday practice. The target angle consisted of solely 50° knee flexion. Despite this angle representing the range of typical daily activities and having been used in previous studies,63,64 a variation of movement directions and angles would provide more comprehensive insight.25
No statistically significant differences in acuity, neuromuscular, or cortical activity were observed during the first six months after ACL reconstruction, except for the beta-2 frequency band in the frontal region of interest for the noninvolved leg in comparison to the control group at the first measurement time point. Differences might become evident in later phases following ACL reconstruction and neurocognitive testing should be incorporated into the return to sport evaluation process. In an active study population, the JPS test may not align with the requirements of functionality or difficulty, particularly in terms of eliciting observable changes in neuromuscular activity. An extension with visual cues or under dual-task conditions may yield a distinct evaluation.
The authors report no conflicts of interest.
We express our gratitude to all participants for their time and dedication to this study
We would like to thank Philipp Henle for his support in informing and recruiting the ACL volunteers.
Submitted: July 18, 2024 CDT, Accepted: September 23, 2024 CDT
© The Author(s)
This is an open-access article distributed under the terms of the Creative Commons Attribution 4.0 International License (CCBY-NC-4.0). View this license’s legal deed at https://creativecommons.org/licenses/by-nc/4.0 and legal code at https://creativecommons.org/licenses/by-nc/4.0/legalcode for more information.
1. Thomas AC, Villwock M, Wojtys EM, PalmieriSmith RM. Lower extremity muscle strength after anterior cruciate ligament injury and reconstruction. J Athl Train 2013;48:610-620. doi:10.4085/ 1062-6050-48.3.23
2. Wiggins AJ, Grandhi RK, Schneider DK, Stanfield D, Webster KE, Myer GD Risk of secondary injury in younger athletes after anterior cruciate ligament reconstruction: A systematic review and metaanalysis. Am J Sports Med 2016;44(7):1861-1876. doi:10.1177/0363546515621554
3. Johansson H, Sjölander P, Sojka P. A sensory role for the cruciate ligaments. Clin Orthop Rel Res 1991;268:161-178.
4. Riemann BL, Lephart SM. The sensorimotor system, part I: the physiologic basis of functional joint stability J Athl Train 2002;37(1):71-79. http:// www.ncbi.nlm.nih.gov/pubmed/16558670
5. Riemann BL, Lephart SM. The Sensorimotor System, part II: The role of proprioception in motor control and functional joint stability. J Athl Train. 2002;37(1):80-84. http://www.ncbi.nlm.nih.gov/ pubmed/16558671
6. Hillier S, Immink M, Thewlis D Assessing proprioception: A systematic review of possibilities. Neurorehabil Neural Repair 2015;29(10):933-949. doi:10.1177/1545968315573055
7. Strong A, Arumugam A, Tengman E, Röijezon U, Häger CK. Properties of knee joint position sense tests for anterior cruciate ligament injury: A systematic review and meta-analysis. Orthop J Sports Med 2021;9(6). doi:10.1177/23259671211007878
8. Kim HJ, Lee JH, Lee DH. Proprioception in patients with anterior cruciate ligament tears: A metaanalysis comparing injured and uninjured limbs. Am J Sports Med 2017;45(12):2916-2922. doi:10.1177/ 0363546516682231
9. Relph N, Herrington L, Tyson S. The effects of ACL injury on knee proprioception: a meta-analysis. Physiotherapy. 2014;100:187-195. doi:10.1016/ j.physio.2013.11.002
10. Busch A, Blasimann A, Mayer F, Baur H. Alterations in sensorimotor function after ACL reconstruction during active joint position sense testing. A systematic review PLoS One 2021;16(6 June):1-14. doi:10.1371/journal.pone.0253503
11. Gokeler A, Benjaminse A, Hewett TE, Lephart SM, Engebretsen L, Ageberg E, et al. Proprioceptive deficits after ACL injury: Are they clinically relevant? Br J Sports Med 2012;46(3):180-192. doi:10.1136/ bjsm.2010.082578
12. Cooper R, Taylor N, Feller JA. A systematic review of the effects of proprioceptive and balance exercise on people with an injured or reconstructed ACL. Res Sports Med. 2005;13(2):163-178. doi:10.1080/ 15438620590956197
13. Fridén T, Roberts D, Ageberg E, Waldén M, Zätterström R. Review of knee proprioception and the relation to extremity function after an anterior cruciate ligament rupture. J orhop Sports Phys Ther 2001;31(10):567-576. doi:10.2519/ jospt.2001.31.10.567
14. Tayfur B, Charuphongsa C, Morrissey D, Miller SC. Neuromuscular Function of the Knee Joint Following Knee Injuries: Does It Ever Get Back to Normal? A Systematic Review with Meta-Analyses. Sports Medicine. 2021;51(2):321-338. doi:10.1007/ s40279-020-01386-6
15. Needle AR, Lepley AS, Grooms DR. Central nervous system adaptation after ligamentous injury: a summary of theories, evidence, and clinical interpretation. Sports Med 2017;47(7):1271-1288. doi:10.1007/s40279-016-0666-y
16. Neto T, Sayer T, Theisen D, Mierau A. Functional brain plasticity associated with ACL injury: A scoping review of current evidence. Neural Plast. 2019;2019:2019. doi:10.1155/2019/3480512
17 Strong A, Grip H, Boraxbekk CJ, Selling J, Häger CK. Brain response to a knee proprioception task among persons with anterior cruciate ligament reconstruction and controls. Front Hum Neurosci 2022:16. doi:10.3389/fnhum.2022.841874
18. Baumeister J, Reinecke K, Weiss M. Changed cortical activity after anterior cruciate ligament reconstruction in a joint position paradigm: An EEG study. Scand J Med Sci Sports. 2008;18(4):473-484. doi:10.1111/j.1600-0838.2007.00702.x
19. Criss CR, Melton MS, Ulloa SA, Simon JE, Clark BC, France CR, et al. Rupture, reconstruction, and rehabilitation: A multi-disciplinary review of mechanisms for central nervous system adaptations following anterior cruciate ligament injury. Knee. 2021;30:78-89. doi:10.1016/j.knee.2021.03.009
20. Blasimann A, Busch A, Henle P, Bruhn S, Vissers D, Baur H. Neuromuscular control during stair descent and artificial tibial translation after acute ACL rupture. Orthop J Sports Med. 2022;10(10). doi:10.1177/23259671221123299
21. Booth FW, Lees SJ. Physically active subjects should be the control group. Med Sci Sports Exerc 2006;38(3):405-406. doi:10.1249/ 01.mss.0000205117.11882.65
22. Baumeister J, Reinecke K, Schubert M, Weiß M. Altered electrocortical brain activity after ACL reconstruction during force control. J Orthop Res 2011;29(9):1383-1389. doi:10.1002/jor.21380
23. Tegner Y, Lysholm J. Rating systems in the evaluation of knee ligament injuries. Clin Orthop Relat Res Published online 1985:43-49. doi:10.1097/ 00003086-198509000-00007
24. Roos EM, Lohmander LS. The Knee injury and Osteoarthritis Outcome Score (KOOS): from joint injury to osteoarthritis. Health Qual Life Outcomes. 2003;1(1):64. doi:10.1186/1477-7525-1-64
25. Busch A, Bangerter C, Mayer F, Baur H. Reliability of the active knee joint position sense test and influence of limb dominance and sex. Sci Rep 2023;13(1). doi:10.1038/s41598-022-26932-2
26. Scott J, Huskisson EC. Graphic representation of pain. Pain. 1976;2(2):175-184. doi:10.1016/ 0304-3959(76)90113-5
27 Busch A, Blasimann A, Henle P, Baur H. Neuromuscular activity during stair descent in ACL reconstructed patients: A pilot study Knee 2019;26(2):310-316. doi:10.1016/j.knee.2018.12.011
28. Gebel A, Busch A, Stelzel C, Hortobágyi T, Granacher U. Effects of physical and mental fatigue on postural sway and cortical activity in healthy young adults. Front Hum Neurosci. 2022;16. doi:10.3389/fnhum.2022.871930
29. Arvin M, Hoozemans MJM, Burger BJ, Verschueren SMP, van Dieen JH, Pijnappels M. Reproducibility of a knee and hip proprioception test in healthy older adults. Aging Clin Exp Res 2015;27(2):171-177. doi:10.1007/s40520-014-0255-6
30. Schmidt R, Lee T Motor Control and Learning 3rd ed. Human Kinetics; 1999.
31. Hermens H, Freriks B, Disselhorst-Klug C, Rau G. Development of recommendations for SEMG sensor and sensor placement procedures. J Electromyogr Kinesiol. 2000;10:361-374. doi:10.1016/ S1050-6411(00)00027-4
32. Wearable Sensing. DSI-24 EEG Headset User Manual.; 2017.
33. Delorme A, Makeig S. EEGLAB: An open source toolbox for analysis of single-trial EEG dynamics including independent component analysis. J Neurosci Methods 2004;134(1):9-21. doi:10.1016/ j.jneumeth.2003.10.009
34. Mullen T. CleanLine EEGLAB Plugin. Neuroimiging Informatics Toolsand Resources Clearinghouse (NITRC).
35. Kothe C, Miyakoshi M, Delorme A. clean_rawdata. Published online January 19, 2019.
36. Palmer J. AMICA - Adaptive Mixture ICA.
37 R Core Team. R: a language and environment for statistical computing. 2023. http://www.r-project.org
38. Bates D, Maechler M, B B. Fitting linear mixedeffects models using lme4. J Stat Softw 2015;67(1):1-48. doi:10.18637/jss.v067.i01
39. Lenth R. Estimated Marginal Means, Aka LeastSquares Mean.; 2023.
40. Nakamae A, Adachi N, Ishikawa M, Nakasa T, Ochi M. No evidence of impaired proprioceptive function in subjects with anterior cruciate ligament reconstruction: a systematic review J ISAKOS 2017;2(4):191-199. doi:10.1136/jisakos-2016-000087
41. Busch A, Henle P, Boesch L, Blasimann Schwarz A, Baur H, Blasimann A, et al. Neuromuscular control in patients with acute ACL injury during stair ascent – a pilot study Orthop Traumatol 2018;35(2):158-165. doi:10.1016/j.orthtr.2019.04.002
42. Lepley AS, Gribble PA, Thomas AC, Tevald MA, Sohn DH, Pietrosimone BG. Quadriceps neural alterations in anterior cruciate ligament reconstructed patients: A 6-month longitudinal investigation. Scand J Med Sci Sports 2015;25(6):828-839. doi:10.1111/sms.12435
43. Hopkins JT, Ingersoll CD. Arthrogenic muscle inhibition: A limiting factor in joint rehabilitation. J Sport Rehabil 2000;9(2):135-159. doi:10.1123/ jsr.9.2.135
44. Cheron G, Petit G, Cheron J, Leroy A, Cebolla A, Cevallos C, et al. Brain oscillations in sport: Toward EEG biomarkers of performance. Front Psychol. 2016;7(FEB). doi:10.3389/fpsyg.2016.00246
45. Cavanagh JF, Frank MJ. Frontal theta as a mechanism for cognitive control. Trends Cogn Sci 2014;18(8):414-421. doi:10.1016/j.tics.2014.04.012
46. Sauseng P, Klimesch W, Schabus M, Doppelmayr M. Fronto-parietal EEG coherence in theta and upper alpha reflect central executive functions of working memory. Int J Psychophysiology. 2005;57:97-103. doi:10.1016/j.ijpsycho.2005.03.018
47 Babiloni C, Del Percio C, Arendt-Nielsen L, Soricelli A, Romani GL, Rossini PM, et al. Cortical EEG alpha rhythms reflect task-specific somatosensory and motor interactions in humans. Clin Neurophys. 2014;125(10):1936-1945. doi:10.1016/j.clinph.2014.04.021
48. Sauseng P, Griesmayr B, Freunberger R, Klimesch W Control mechanisms in working memory: A possible function of EEG theta oscillations. Neurosci Biobehav Rev 2010;34(7):1015-1022. doi:10.1016/ j.neubiorev.2009.12.006
49. Pak R, McLaughlin AC, Engle R. The relevance of attention control, not working memory, in human factors. Human Factors: The Journal of the Human Factors and Ergonomics Society. 2024;66(5):1321-1332. doi:10.1177/ 00187208231159727
50. Faltus J, Criss CR, Grooms DR. Shifting Focus: A Clinician’s Guide to Understanding Neuroplasticity for Anterior Cruciate Ligament Rehabilitation. Curr Sports Med Rep. 2020;19(2):76-83. doi:10.1249/ JSR.0000000000000688
51. Gokeler A, Neuhaus D, Benjaminse A, Grooms DR, Baumeister J. Principles of motor learning to support neuroplasticity after ACL injury: Implications for optimizing performance and reducing risk of second ACL injury. Sports Med. 2019;49(6):853-865. doi:10.1007/s40279-019-01058-0
52. Barone J, Rossiter HE. Understanding the role of sensorimotor beta oscillations. Front Syst Neurosci. 2021:15. doi:10.3389/fnsys.2021.655886
53. Piskin D, Benjaminse A, Dimitrakis P, Gokeler A. Neurocognitive and neurophysiological functions related to ACL injury: A framework for neurocognitive approaches in rehabilitation and return-to-sports Tests. Sports Health. 2022;14(4):549-555. doi:10.1177/19417381211029265
54. Grooms DR, Chaput M, Simon JE, Criss CR, Myer GD, Diekfuss JA. Combining neurocognitive and functional tests to improve return-to-sport decisions following ACL reconstruction. J Orthop Sports Phys Ther. 2023;53(8):415-419. doi:10.2519/ jospt.2023.11489
55. Diekfuss JA, Grooms DR, Yuan W, Dudley J, Barber KD, Thomas S, et al. Does brain functional connectivity contribute to musculoskeletal injury? A preliminary prosperctive analysis of a neural biomarker of ACL injury risk. J Sci Med Sport 2019;22(2):169-174. doi:10.1016/j.jsams.2018.07.004
56. Diekfuss JA, Grooms DR, Nissen KS, Schneider DK, Foss KDB, Thomas S, et al. Alterations in knee sensorimotor brain functional connectivity contributes to ACL injury in male high-school football players: a prospective neuroimaging analysis. Braz J Phys Ther 2020;24(5):415-423. doi:10.1016/ j.bjpt.2019.07.004
57. Azevedo J, Rodrigues S, Seixas A. The influence of sports practice, dominance and gender on the knee joint position sense. Knee. 2021;28:117-123. doi:10.1016/j.knee.2020.11.013
58. Cug M, Wikstrom EA, Golshaei B, Kirazci S. The effects of sex, limb dominance, and soccer participation on knee proprioception and dynamic postural control. J Sport Rehabil 2016;25(1):31-39. doi:10.1123/jsr.2014-0250
59. Strong A, Grip H, Arumugam A, Boraxbekk CJ, Selling J, Häger CK. Right hemisphere brain lateralization for knee proprioception among rightlimb dominant individuals. Front Hum Neurosci. 2023:17 doi:10.3389/fnhum.2023.969101
60. Han J, Waddington G, Adams R, Anson J, Liu Y Assessing proprioception: A critical review of methods. J Sport Health Sci 2016;5(1):80-90. doi:10.1016/j.jshs.2014.10.004
61. Grinberg A, Strong A, Häger CK. Does a knee joint position sense test make functional sense? Comparison to an obstacle clearance test following anterior cruciate ligament injury. Phys Ther Sport. 2022;55:256-263. doi:10.1016/j.ptsp.2022.05.004
62. Mir SM, Hadian MR, Talebian S, Nasseri N. Functional assessment of knee joint position sense following anterior cruciate ligament reconstruction. Br J Sports Med 2008;42(4):300-303. doi:10.1136/ bjsm.2007.044875
63. Strong A, Srinivasan D, Häger CK. Development of supine and standing knee joint position sense tests. Phys Ther in Sport. 2021;49:112-121. doi:10.1016/j.ptsp.2021.02.010
64. Tian F, Zhao Y, Li J, Wang W, Wu D, Li Q, et al. Test–retest reliability of a new device versus a longarm goniometer to evaluate knee proprioception. J Sport Rehabil 2021;31(3):368-373. doi:10.1123/ jsr.2021-0146
Rikken KTH, Panneman T, Vercauteren F, Gokeler A, Benjaminse A. Increased Visual Attentional Demands Alter Lower Extremity Sidestep Cutting Kinematics in Male Basketball Players. IJSPT. 2024;19(11):1304-1313. doi:10.26603/001c.124804
Koen
T.H. Rikken1 , Tom Panneman1 , Fabian Vercauteren1 , Alli Gokeler2 , Anne Benjaminse3 a
1 a. Department of Human Movement Sciences, University Medical Center Groningen, University of Groningen, 2 Exercise and Neuroscience unit, Department Exercise & Health, Faculty of Science, Paderborn University, 3 Department of Human Movement Sciences, University Medical Center Groningen, University of Groningen
Keywords: Anterior cruciate ligament, basketball, cognitive load, sidestep cutting, visual attention https://doi.org/10.26603/001c.124804
International Journal of Sports Physical Therapy
Vol. 19, Issue 11, 2024
Background
In basketball, changing direction is one of the primary mechanisms of anterior cruciate ligament (ACL) injury, often occurring within complex game situations with high cognitive demands. It is unknown how visual attention affects sidestep cutting kinematics during the entire energy absorption phase of the cut in an ecologically valid environment.
Purpose
The purpose of this research was to study the effect of added cognitive load, in the form of increased visual attentional demands, on sidestep cutting kinematics during the energy absorption phase of the cut in an ecologically valid environment.
Study Design
Crossover Study
Methods
Fifteen male basketball players (aged 22.1 ± 2.3) performed ten sidestep cutting movements without (BASE) and with (VIS) a visual attention dual task. 3D kinematics of the hip, knee and ankle were recorded utilizing Xsens IMU motion capture. Temporal kinematics were analyzed using Statistical Parametric Mapping. Discrete time point kinematics were additionally analyzed at initial contact (IC) and at peak knee flexion utilizing paired t-tests. Effect sizes were calculated.
Results
Hip flexion was significantly reduced in the VIS condition compared to the BASE condition (p<0.01), including at IC (VIS 35.0° ± 7.2°, BASE 40.7° ± 4.9°, p=0.02, d=0.92) and peak (VIS 37.8° ± 9.7°, BASE 45.5° ± 6.9°, p=0.001, d=0.90). Knee flexion was significantly reduced in the VIS condition, in comparison to the BASE condition (p<0.01), at peak (VIS 59.9° ± 7.5°, BASE 64.1° ± 7.4°, p=0.001, d=0.55).
Conclusion
The addition of visual attention during sidestep cutting altered lower limb kinematics, which may increase ACL injury risk. It is suggested that ACL injury risk screening and
Corresponding author:
Dr A. Benjaminse
W: http://www.rug.nl/staff/a.benjaminse/cv +31(0)50-3616015 a
Department of Human Movement Sciences, University Medical Center Groningen, University of Groningen. Antonius Deusinglaan 1 (Room 3215-325), 9713 AV Groningen, The Netherlands
E: a.benjaminse@umcg.nl
prevention should include sidestep cutting with visual attentional demands, in order to mimic the cognitive demands of the sports environment.
Injuries of the anterior cruciate ligament (ACL) have increased in incidence over the last decennia, with an increase of 14% in basketball specifically 1,2 Sustaining an ACL injury is associated with numerous adverse health effects3‑7 and impaired athletic performance.8‑10 These facts indicate the need for better primary prevention of ACL injuries and justify the current study.
Of all ACL injuries in basketball 58-67% are non-contact in nature,11,12 of which sidestep cutting is one of the primary injury mechanisms.13 One of the primary playing situations in basketball in which ACL injuries happen is the first step after picking up the ball when attacking.14 During the energy absorption phase (i.e. initial contact (IC) to peak knee flexion) of sidestep cutting several factors may contribute to high knee joint loading, such as low knee flexion angles,15 high initial knee abduction angle,13,16 low hip flexion angle,17 high hip abduction angle,15 and low initial hip internal rotation.18 These unfavorable kinematics are especially prevalent during unplanned sidestep cutting maneuvers.19,20
The aforementioned sidestep cuts are made during games in a dynamically changing environment in which athletes are exposed to numerous stimuli.14,21 Visual attention, defined as the goal-directed allocation of cognitive resources to external stimuli,22 aids the uptake of relevant information in this dynamically changing environment.23 While visual attention is a lower order cognitive function, it facilitates higher order cognitive functions such as working memory, inhibitory control and cognitive flexibility 24 Increased cognitive task demands, which challenge the aforementioned cognitive functions, are consistently linked to an increase in at-risk biomechanics for lower extremity injury 25
A deficit or delay in attentional processing can contribute to a decreased ability to coordinate movement, which could result in the aforementioned unfavorable knee joint loading.26 This has been demonstrated in studies where primarily female basketball players performed sidestep cuts with a passing or dribbling task which divided their attention, which elicited reduced knee flexion,27 increased knee abduction angles,27 increased knee abduction moments,28,29 and increased hip abduction angles compared to when they did not perform the task.29
These findings show the influence of cognitive function, in the form of decision making and divided visual attention, on lower limb biomechanics and the need to include these factors in ACL injury screening and prevention practices.30, 31 The effect of decision-making and visual attention has been studied more directly in various sports, eliciting unfavorable biomechanics.32‑34 However, the effects of increased visual attentional demands during the entire en-
Table 1. Subject characteristics (mean ± standard deviation).
(kg)
Leg dominance left/right 14/1
Hours of basketball played per week 6.4 ± 2.9
Playing level
National (N=5)
Regional (N=2)
Local (N=8)
ergy absorption phase of the sidestep cut in male basketball players remain unknown. Previous research has been limited to studying biomechanics at initial contact and peak values in a lab setting, as opposed to in the natural sporting environment.35 Furthermore, previous research has shown that discrete time point analysis failed to identify statistically significant biomechanical differences which were uncovered with full waveform analysis.36 Gaining insight into the kinematics of the entire energy absorption phase of the sidestep cut could thus provide valuable information on how the movement execution is affected by including a temporal element in the analysis, as opposed to an analysis limited to two discrete timepoints.
37
This study will investigate kinematic changes during a sport-specific task and will be conducted on an indoor basketball court, increasing its ecological validity.35 The purpose of this research was to study the effect of added cognitive load, in the form of increased visual attentional demands, on sidestep cutting kinematics during the energy absorption phase of the cut in an ecologically valid environment in male basketball players. The hypothesis was that increased visual attentional demands alter sidestep cutting kinematics unfavorably in the context of ACL injury risk.
Fifteen male subjects were recruited from different basketball clubs from the north of the Netherlands (Table 1). Inclusion criteria were male, player in an official basketball team, 18-30 years, >3 hours of team training and game time per week. Exclusion criteria were recent lower-limb injury (<6 months), ACL injury in history, alcohol or caffeine 24h before measurements and color blindness. All subjects had normal or corrected-to-normal vision. Leg dominance was defined as the leg with which a participant pushes off during a lay-up.38,39
The Central Ethical Review Board of the University of Groningen approved this study under ID number #11257 All subjects provided informed consent prior to participating. First, anthropometric measures were taken in order to scale the Xsens Motion Tracking System (XSens Techonolgies, Enschede, Netherlands) proprietary biomechanical model and calibrate the motion capture in Xsens MVN Analyze (v.2021.2.0).40 Joint angles were defined using the Euler sequence ZXY. Xsens MVN has fair to excellent agreement on sagittal plane movements in relation to gold-standard optoelectronic motion capture and allows for sport specific on-field movements to be captured.40,41 Subjects were required to wear their own basketball shoes.
The kinematics of each basketball player were recorded on an indoor basketball court. Before starting the actual trials, subjects were granted familiarization trials until they indicated that they were ready to start. The sidestep cutting kinematics were recorded during a 90° near full speed sidestep cut. The subjects were asked to perform the route at near full speed (80-90% of maximum effort) since this mimics the demands of a basketball game more realistically and because kinematics of near full speed cutting elicit biomechanical risk factors for ACL injury more clearly than cutting at 60% speed.42 Furthermore, 90° sidestep cutting results in the highest knee internal rotation and knee abduction moments and angles compared to 45° or 180° cuts; indicating this might be the cutting angle with the greatest inherent risk.43
Each trial started with a participant initiated 8.35m forward sprint followed by a sidestep cut with the non-dominant leg in the direction of the dominant leg. This was followed by a 3.5m forward sprint resulting in a 90° sidestep cut with the dominant leg, which was recorded for analysis. Each trial ended with a 3.5m forward sprint immediately after this last sidestep cut to finish the route. (Figure 1)
First, each subject performed ten trials of baseline sidestep cutting, without cognitive load (BASE). The Fitlights (FITLIGHT Corp, Canada, 2022, v2.17) placed upon the boxes on this predefined route would light up before and during the whole duration of the trial.
Second, each subject performed around fifteen trials of sidestep cutting in the cognitively loaded condition (VIS). The difference with the BASE and the VIS condition is that the subject did not know the route before the trial. The subject had to pay attention to, and then respond to, the Fitlights which dictated the direction. In the VIS condition the subject was tasked to perform distractor trials and actual capturing trials, intertwined with each other The ratio between actual capturing and distractor trials was 2:1 respectively In the distractor trials any of the directions was possible. In the actual capturing routes of the VIS condition the directions were exactly the same as the BASE condition, with the only difference being the added cognitive load through the increased visual attentional demands. Ultimately ten valid trials were performed in which the subject
Figure 1. Left, in red: schematic route of sidestep cut for participants with left sided leg dominance. Right: experimental set-up on the basketball court with FitLights.
thus had to visually scan the environment on approach to (at Fitlight #2) and during (at Fitlight #3) the sidestep cut which was recorded.
An initial a priori sample size estimate was performed using Gpower 3.1,44 with a paired t-test where α was set at 0.05, β at 0.20. With an effect size of 0.7 this analysis indicated that 15 subjects were sufficient to achieve adequate power. While sample size calculation using this method is valid for traditional 0D outcome data, the estimated sample size is in line with recommendations for 1D power analysis in biomechanical variables using a paired t-test with medium to large effect sizes.45,46 The region of interest in this study is 0% to 50% of the sidestep cut, since the ACL is most at risk during this energy absorption phase.15,17 The energy absorption phase in this study is defined as from the instance of IC to peak knee flexion angle, in line with previous research.18,47,48 While the analysis focuses on the energy absorption phase, the kinematics of the entire sidestep cut are shown for clarity.
Trials were cropped from initial contact (IC) to toe off (TO). IC was defined as the instant where the vertical acceleration of the foot Inertial Measurement Unit (IMU) reached its minimum value.49 TO was defined as the instant where peak knee extension was achieved.50 All trials were time normalized at IC on a scale of 1-100% of the stance phase to allow for statistical analysis.
A customized Python script (Python 3.9, Python Software Foundation, Delaware, USA) was used in order to perform Statistical Parametric Mapping (SPM) (Spm1D 0.435, http://www.spm1d.org). A two tailed paired t-test was performed to compare hip, knee and ankle kinematics between the BASE and VIS conditions.
The kinematic outcome measures were flexion/extension angles of the hip and knee, dorsi- and plantarflexion of the ankle, abduction/adduction angles of hip and knee, and internal/external rotation angles of the hip, during the entire stance phase of the sidestep cut.
Alpha was set at 0.05 for all analyses. Effect sizes for statistically significant differences between conditions at IC
and at peak values were indicated with Cohen’s d, small (d=0.2), medium (d=0,5), large (d=0,8).51 As the timing of peak values can differ inter-individually this may not be visible in the SPM figures, which average every participant on a set window of 0-100%. The differences between conditions at peak values (i.e. discrete time point) are thus additionally analyzed and reported separately, to allow comparison with other research.
KINEMATICS BASE VS VIS
Hip flexion was significantly reduced over the entire sidestep cut during the VIS condition in comparison to the BASE condition (p<0.01). At IC the hip flexion angle in the VIS condition (35.0° ± 7.2°) was significantly reduced compared to the BASE condition (40.7° ± 4.9°) (t(14)=3.717, p=0.02, d=0.92). The peak hip flexion angle in the VIS condition (37.8° ± 9.7°) was also significantly reduced compared to the BASE condition (45.5° ± 6.9°) (t(14)=4.344, p=0.001, d=0.90). Hip external rotation was significantly increased during the entire sidestep cut during the VIS condition in comparison to the BASE condition (p<0.01). Peak hip external rotation was significantly increased in the VIS condition (13.2° ± 8.8°) compared to the BASE condition (8.2° ± 9.3°) (t(14)=2.798, p=0.014, d=0.56). Results for the hip joint can be found in Figure 2 and Table 2
In the VIS condition, knee flexion was significantly reduced from 10.7% of the sidestep cut and onwards compared to the BASE condition (p<0.01). Peak knee flexion was reduced in the VIS condition (59.9° ± 7.5°) compared to the BASE condition (64.1° ± 7.4°) (t(14)=4.028, p=0.001, d=0.55). Knee abduction was significantly reduced from 17.9% of the cut and onwards in the VIS condition, in comparison to the BASE condition (p<0.01). Results for the knee joint can be found in Figure 2 and Table 2
At the ankle no significant differences were observed between the BASE and VIS conditions. Results for the ankle joint can be seen in Figure 2 and Table 2
Time spent during the sidestep cut between IC and TO in the VIS condition (251.12ms ± 58.23ms) did not differ significantly from the BASE condition (259.20ms ± 40.95ms) (t(149)=1.596, p=0.113).
The addition of visual attentional demands to sidestep cutting resulted in reduced hip flexion and increased hip external rotation angles, and reduced knee flexion and knee abduction angles in the energy absorption phase of the cut. The difference in sidestep cutting kinematics between conditions primarily occurred in the sagittal plane for the hip and knee joint.
The current findings are in line with previous research, which indicates that divided visual attention can lead to reduced hip and knee flexion angles.27 At baseline, participants in the current study performed the sidestep cut with 40.7° of hip flexion at IC whereas the participants in the
Figure 2. Means (solid line) and standard deviations (cloud) joint kinematics during the stance phase (IC=0%, TO=100%) of the sidestep cut (1,2,3,4,5,6). Significance graph of Statistical Parametric Mapping where grey indicates a statistically significant difference between conditions (1a, 2a, 3a, 4a, 5a, 6a).
study of Almonroeder et al.27 performed the sidestep cut with 53.3° of hip flexion at IC. The magnitude of change differs however In the study of Almonroeder et al.27 hip flexion at IC only decreased by 2.5° under the influence of divided visual attention, compared to 5.7° in the current study This indicates that the participants in the current study started with lower hip flexion angle, from which they reduced the hip flexion angle even further in the VIS condition. This finding, in combination with a reduced knee flexion angle in the VIS condition in the current study, could increase ACL injury risk since it could limit the ability to actively absorb energy during the sidestep cut through range of motion and ultimately transfer more load to the ACL.16,18,52‑54 A possible explanation for the differences between the studies is that in the current study subjects were required to perform the visual attention task during the sidestep cut, whereas in the study of Almonroeder et al.27 the subjects had to perform the divided visual attention task directly after the sidestep cut. This could allow the participant to still focus on executing the sidestep cut,27 something which was not the case in the current study Additionally it should be noted that the study by Almonroeder et al.,27 was performed with female basketball players, whereas the current study was performed with male basketball players.
Almonroeder et al.27 reported no effect of visual attention on knee abduction angle at IC (6.4° at baseline vs 7.0° with divided visual attention). Other researchers have shown an increase in knee abduction angle from 7.4° to 8.5°
Table 2. Joint angles during the sidestep cut
and an increase in hip internal rotation angle from 4.6° to 6.4°, but this was measured during sidestep cuts of 45°.28 Comparing kinematics of 45° cuts with 90° cuts, as per the current study, is futile since baseline kinematics differ based on the angle of the cut.43,55 In addition to the effect of the cutting angle, it has also been shown that the type of visual stimulus matters in altering kinematics during sidestep cutting.56,57 The differences in findings between the studies, in all three planes, highlight the specificity of task constraints.
Since the subjects were asked to perform the route at near full speed (80-90% of maximum effort), the altered kinematics during the VIS condition, can be linked to the performance-injury risk trade-off hypothesis during sidestep cutting.58 This hypothesis states that an increase in performance often results in suboptimal kinematics when related to ACL injury risk.58 It is possible that in the current study these dual-task costs came into effect. In order to maintain performance (i.e. perform the route at 80%-90% of their speed) with the increased dual-task constraints in the VIS condition, the sidestep cutting kinematics changed as a function of the increased visual attentional demands.
Altered higher-risk kinematics are undesirable when related to ACL injury risk, but it has been shown that specifically training sidestep cutting for six weeks can improve both sidestep cutting performance and kinematics in male football players.53 In semi-elite basketball players it has also been shown that including cognitive-motor dual-task training using LED-displays during dribbling tasks can improve dribbling performance in five weeks of training, to a greater extent than motor training without the cognitive dual task.59 Furthermore, the cognitive-motor dual-task training also improved cognitive performance by allowing a faster connection between sensory encoding and response execution.59 These findings indicate that an improvement in cognitive or sidestep cutting performance when trained properly does not have to occur at the expense of sport performance. These promising results may be important for the secondary prevention of ACL injuries, since due to neu-
roplasticity an ACL reconstructed athlete may shift towards visual-motor control of movement instead of sensory-motor control of movement.60 This is shown during sidestep cutting when ACL reconstructed basketball players exhibited different hip and knee coordination when a simulated visual attention dual task was added, compared to healthy matched basketball players who did not show altered coordination.61 These combined findings highlight the importance of including integrated sidestep cutting execution and visual attention in training, in order to improve primary and secondary prevention of ACL injuries.
A strength of the current study is the investigation of the combined effects of unplanned change of direction and a visual attention task on sidestep cutting kinematics in basketball players in a gym environment. Prior research indicates that sidestep cutting kinematics in a laboratory setting differ from actual on field kinematics.62 Since the current study took place on an indoor basketball court the athlete-environment relationship was preserved resulting in increased ecological validity 35
Another strength of the current study was the statistical analysis. The area most of interest in ACL injury risk research is the energy absorption phase during the sidestep cut, which roughly corresponds with the first 50% of the cut.15,17,18,47,48 Focusing only on kinematic values at IC and peak generally discards a plethora of information in between these timepoints.36,63 Since this study utilized Statistical Parametric Mapping we were able to statistically analyze the entire stance phase of the sidestep cut instead of being limited to fixed time points only. Analyzing the entire absorption phase allows for a more thorough interpretation of the kinematics, since temporal differences are included.37
A limitation of the current study is that some caution is warranted when interpreting the kinematic results. IMU based 3D motion capture systems allow for sport specific on field measurement, but are less accurate than gold standard infrared optoelectronic 3D measurement systems.38, 39,64 IMU based systems are quite accurate at capturing
movement in the sagittal plane, however, the accuracy of IMU based systems is acceptable in movements in the frontal plane, and questionable in the transverse plane.38, 64 During change of direction, IMU based knee abduction/ adduction angles could be up to 11° off and hip rotation angles could be more than 8° off compared to optoelectronic measurement systems.38,39 During the current study these movements had ranges of motion of 5.0 degrees and 7.5 degrees respectively. Since the ranges of motion of these movements are relatively small and measurement errors can be relatively large, caution is warranted when interpreting the outcomes of the current study with regards to these movements. IMU-based motion capture was chosen to accommodate the on-court movements needed for an ecologically valid task, but may have only maintained accuracy in the study of sagittal plane movements.
Another limitation of the current study lies within the practical design of the measurements. In the VIS condition subjects did not know the route which they had to follow, the FitLights dictated this such that they had to scan their environment. Some routes would be eligible for capturing the sidestep cutting kinematics and other distractor routes would not be eligible. To guarantee that the subject would not know the route beforehand, these distractor routes were added. The ratio between actual recording routes and distractor routes was 2:1 respectively We limited the current study to a ratio of 2:1 so that fatigue would not be a factor within the design. However, there was still a possibility that subjects would be able to guess the route beforehand, although the authors saw no signs of this.
Additionally, while the current study did analyze the duration of the sidestep cut in the BASE and VIS conditions, timing gates were not utilized to analyze the approach speed of the participants.
Caution is warranted with generalizing the results of the current study to other populations as the participants were all male and did not differ greatly in skill level. Finally, it could be that the sample size was too limited (small) to uncover more nuanced effects on sidestep cutting execution. The sample size in the current study was similar to comparable research27 and was calculated on the basis of the power analysis with medium to large effects, as found in the hip and knee. While adequate for medium to large effects, the current sample size could be too small to uncover more nuanced kinematic changes.46 Finally, caution is warranted with generalizing the results of the current study to other populations as the participants were all male and did not differ greatly in skill level.
Consistent with the findings of this study, as a practical implication, it can be advised that sidestep cutting execution with cognitive load should be incorporated in injury prevention practices. There are several ways to incorporate visual attention in sport specific prevention training. For
example, cognitive load can be added by performing unanticipated cutting on the sports field by tasking the athlete with mirroring or chasing a leading teammate. A teammate can also approach the athlete and when together, the teammate decides to cut to the left or right, and the athlete must cut the opposite direction. Third, the teammate approaches the athlete dribbling with a ball and when together, the teammate decides to cut to the left or right with the ball, and the athlete cuts and tries to intercept the ball. And lastly, the teammate passes a ball (direction and speed selfchosen) and the athlete has to change direction to chase for the ball. In these examples, temporal variability (timing of stimuli), spatial variability (location of stimuli) and complexity of stimuli can be decreased or increased, thus less or more mirroring the demands of the actual sports environment.65,66
Future research could study the effect of specifically training sidestep cutting with increased visual attentional demands on performance and kinematics, in order to generate methods to improve ACL injury prevention. Furthermore, future research could study the effect of variability (e.g. timing, location, type, complexity) of the visual stimuli on sidestep cutting performance and kinematics. In line with this, future research could investigate whether questionnaires more suited to monitoring the attentional demand could provide more information. Finally, future research could study the possible mediating impact of skill level, alongside the possible effects of age and gender, on the effect of increased visual attentional demands and study the effect of dribbling during sidestep cutting as this is a common ACL injury mechanism in basketball.14
The results of the current study demonstrate that the addition of a visual attention dual-task alters sidestep cutting kinematics in male basketball players. Increased visual attentional demands primarily result in less hip and knee flexion during the absorption phase and the increased cognitive demand could lead to an increased ACL injury risk. Professionals in sports and healthcare are encouraged to consider implementing visual attention in their injury prevention paradigms in order to improve ecological validity and facilitate transfer to the actual sports environment.
The authors declare that they have no conflicts of interest.
Submitted: July 31, 2024 CDT, Accepted: October 08, 2024 CDT
© The Author(s)
This is an open-access article distributed under the terms of the Creative Commons Attribution 4.0 International License (CCBY-NC-4.0). View this license’s legal deed at https://creativecommons.org/licenses/by-nc/4.0 and legal code at https://creativecommons.org/licenses/by-nc/4.0/legalcode for more information.
1. Agel J, Rockwood T, Klossner D Collegiate ACL Injury rates across 15 sports: National Collegiate Athletic Association Injury Surveillance System Data update (2004-2005 Through 2012-2013). Clin J Sport Med 2016;26(6):518-523. doi:10.1097/ JSM.0000000000000290
2. Paudel YR, Sommerfeldt M, Voaklander D Increasing incidence of anterior cruciate ligament reconstruction: a 17-year population-based study. Knee Surg Sports Traumatol Arthrosc 2023;31(1):248-255. doi:10.1007/s00167-022-07093-1
3. Ardern CL, Webster KE, Taylor NF, Feller JA. Return to sport following anterior cruciate ligament reconstruction surgery: A systematic review and meta-analysis of the state of play. Br J Sports Med. 2011;45(7):596-606. doi:10.1136/bjsm.2010.076364
4. Kuenze C, Collins K, Pfeiffer KA, Lisee C. Assessing physical activity after ACL injury: moving beyond return to sport. Sports Health 2022;14(2):197-204. doi:10.1177/19417381211025307
5. Webster KE, Hewett TE. Anterior cruciate ligament injury and knee osteoarthritis: An umbrella systematic review and meta-analysis. Clin J Sport Med. 2022;32(2):145-152. doi:10.1097/ JSM.0000000000000894
6. Wiggins AJ, Grandhi RK, Schneider DK, Stanfield D, Webster KE, Myer GD. Risk of secondary injury in younger athletes after anterior cruciate ligament reconstruction: A systematic review and metaanalysis. Am J Sports Med. 2016;44(7):1861-1876. doi:10.1177/0363546515621554
7 MacAlpine EM, Talwar D, Storey EP, Doroshow SM, Lawrence JTR. Weight gain after ACL reconstruction in pediatric and adolescent patients. Sports Health. 2020;12(1):29-35. doi:10.1177/1941738119870192
8. Saltzman BM, Cvetanovich GL, Nwachukwu BU, Mall NA, Bush-Joseph CA, Bach BR Jr. Economic Analyses in anterior cruciate ligament reconstruction: A qualitative and systematic review. Am J Sports Med. 2016;44(5):1329-1335. doi:10.1177/ 0363546515581470
9. DeFroda SF, Patel DD, Milner J, Yang DS, Owens BD. Performance after anterior cruciate ligament reconstruction in National Basketball Association Players. Orthop J Sports Med. 2021;9(2):2325967120981649. doi:10.1177/ 2325967120981649
10. Kester BS, Behery OA, Minhas SV, Hsu WK. Athletic performance and career longevity following anterior cruciate ligament reconstruction in the National Basketball Association. Knee Surg Sports Traumatol Arthrosc 2017;25(10):3031-3037 doi:10.1007/s00167-016-4060-y
11. Chia L, De Oliveira Silva D, Whalan M, et al. Noncontact anterior cruciate ligament injury epidemiology in team-ball sports: A systematic review with meta-analysis by sex, age, sport, participation level, and exposure type. Sports Med 2022;52(10):2447-2467. doi:10.1007/ s40279-022-01697-w
12. Takahashi S, Nagano Y, Ito W, Kido Y, Okuwaki T A retrospective study of mechanisms of anterior cruciate ligament injuries in high school basketball, handball, judo, soccer, and volleyball. Medicine 2019;98(26). doi:10.1097/MD.0000000000016030
13. Koga H, Nakamae A, Shima Y, et al. Mechanisms for noncontact anterior cruciate ligament injuries: knee joint kinematics in 10 injury situations from female team handball and basketball. Am J Sports Med 2010;38(11):2218-2225. doi:10.1177/ 0363546510373570
14. Gill VS, Tummala SV, Boddu SP, Brinkman JC, McQuivey KS, Chhabra A. Biomechanics and situational patterns associated with anterior cruciate ligament injuries in the National Basketball Association (NBA). Br J Sports Med Published online 2023. doi:10.1136/bjsports-2023-107075
15. Donelon TA, Dos’Santos T, Pitchers G, Brown M, Jones PA. Biomechanical determinants of knee joint loads associated with increased anterior cruciate ligament loading during cutting: A systematic review and technical framework. Sports Med Open 2020;6(1):53. doi:10.1186/s40798-020-00276-5
16. Jones PA, Herrington LC, Graham-Smith P Technique determinants of knee joint loads during cutting in female soccer players. Hum Move Sci. 2015;42:203-211. doi:10.1016/j.humov.2015.05.004
17 Koga H, Nakamae A, Shima Y, Bahr R, Krosshaug T. Hip and ankle kinematics in noncontact anterior cruciate ligament injury situations: Video analysis using model-based image matching. Am J Sports Med 2018;46(2):333-340. doi:10.1177/0363546517732750
18. Havens KL, Sigward SM. Cutting mechanics: relation to performance and anterior cruciate ligament injury risk. Med Sci Sports Exerc 2015;47(4):818-824. doi:10.1249/ MSS.0000000000000470
19. Florian G, Felix S, David AG, Jan W Effect of unplanned athletic movement on knee mechanics: a systematic review with multilevel meta-analysis. Br J Sports Med 2021;55(23):1366. doi:10.1136/ bjsports-2021-103933
20. Almonroeder TG, Garcia E, Kurt M. The effects of anticipation on the mechanics of the knee during single-leg cutting tasks: A systematic review Int J Sports Phys Ther. 2015;10(7):918-928.
21. Dingenen B, Gokeler A. Optimization of the return-to-sport paradigm after anterior cruciate ligament reconstruction: A critical step back to move rorward. Sports Med 2017;47(8):1487-1500. doi:10.1007/s40279-017-0674-6
22. Pashler H, Johnston JC, Ruthruff E. Attention and performance. Annu Rev Psychol 2001;52(1):629-651. doi:10.1146/annurev.psych.52.1.629
23. Vaughan RS, Laborde S. Attention, workingmemory control, working-memory capacity, and sport performance: The moderating role of athletic expertise. Eur J Sport Sci. 2021;21(2):240-249. doi:10.1080/17461391.2020.1739143
24. Diamond A. Executive functions. Annu Rev Psychol. 2013;64:135-168. doi:10.1146/annurevpsych-113011-143750
25. Reyes MA, Probasco MO, Worby TN, Loertscher DE, Soderbeck LK, Huddleston WE. Lower kinetic chain, meet the thinking brain: A scoping review of cognitive function and lower extremity injury risk. Int J Sports Phys Ther. 2022;17(5):787-815.
26. Swanik CB. Brains and sprains: The brain’s role in noncontact anterior cruciate ligament injuries. J Athl Train 2015;50(10):1100-1102. doi:10.4085/ 1062-6050-50.10.08
27 Almonroeder TG, Kernozek T, Cobb S, Slavens B, Wang J, Huddleston W Divided attention during cutting influences lower extremity mechanics in female athletes. Sports Biomech 2019;18(3):264-276. doi:10.1080/14763141.2017.1391327
28. Chan MS, Huang CF, Chang JH, Kernozek TW. Kinematics and kinetics of knee and hip position of female basketball players during side-step cutting with and without dribbling. J Med Biol Eng. 2009;29(4):178-183.
29. Fadie R, Carlstedt K, Willson JD, Kernozek TW Effect of attending to a ball during a side-cut maneuver on lower extremity biomechanics in male and female athletes. Sports Biomech. 2010;9(3):165-177 doi:10.1080/ 14763141.2010.502241
30. Hughes G, Dai B. The influence of decision making and divided attention on lower limb biomechanics associated with anterior cruciate ligament injury: a narrative review. Sports Biomech. 2023;22(1):30-45. doi:10.1080/ 14763141.2021.1898671
31. Gokeler A, Benjaminse A, Della Villa F, Tosarelli F, Verhagen E, Baumeister J. Anterior cruciate ligament injury mechanisms through a neurocognition lens: implications for injury screening. BMJ Open Sport Exerc Med 2021;7(2):e001091. doi:10.1136/ bmjsem-2021-001091
32. Bedo BLS, Cesar GM, Moraes R, et al. Influence of side uncertainty on knee kinematics of female handball athletes during sidestep cutting maneuvers. J Appl Biomech 2021;37(3):188-195. doi:10.1123/ jab.2020-0141
33. Whyte EF, Richter C, O’connor S, Moran KA. The effect of high intensity exercise and anticipation on trunk and lower limb biomechanics during a crossover cutting manoeuvre. J Sports Sci. 2018;36(8):889-900. doi:10.1080/ 02640414.2017.1346270
34. Weinhandl JT, Earl-Boehm JE, Ebersole KT, Huddleston WE, Armstrong BS, O’Connor KM. Anticipatory effects on anterior cruciate ligament loading during sidestep cutting. Clin Biomech. 2013;28(6):655-663. doi:10.1016/ j.clinbiomech.2013.06.001
35. Bolt R, Heuvelmans P, Benjaminse A, Robinson MA, Gokeler A. An ecological dynamics approach to ACL injury risk research: a current opinion. Sports Biomech. Published online 2021:1-14. doi:10.1080/ 14763141.2021.1960419
36. Marshall B, Franklyn-Miller A, Moran K, et al. Biomechanical symmetry in elite rugby union players during dynamic tasks: an investigation using discrete and continuous data analysis techniques. BMC Sports Sci Med Rehabil. 2015;7:1-13. doi:10.1186/ s13102-015-0006-9
37 Thomas C, Dos’Santos T, Warmenhoven J, Jones PA. Between-limb differences during 180 degrees turns in female soccer players: Application of statistical parametric mapping. J Strength Cond Res 2022;36(11):3136-3142. doi:10.1519/ JSC.0000000000004022
38. Zech A, Steib S, Hentschke C, Eckhardt H, Pfeifer K. Effects of localized and general fatigue on static and dynamic postural control in male team handball athletes. J Strength Cond Res. 2012;26(4):1162-1168. doi:10.1519/JSC.0b013e31822dfbbb
39. Nijmeijer EM, Elferink-Gemser MT, Otten E, Benjaminse A. Optimal and suboptimal video instructions change movement execution in young talented basketball players. Int J Sports Sci Coaching 2022:17479541221118882. doi:10.1177/ 17479541221118882
40. Nijmeijer EM, Heuvelmans P, Bolt R, Gokeler A, Otten E, Benjaminse A. Concurrent validation of the Xsens IMU system of lower-body kinematics in jumplanding and change-of-direction tasks. J Biomech 2023;154:111637. doi:10.1016/ j.jbiomech.2023.111637
41. Di Paolo S, Lopomo NF, Della Villa F, et al.
Rehabilitation and return to sport assessment after anterior cruciate ligament injury: Quantifying joint kinematics during complex high-speed tasks through wearable sensors. Sensors. 2021;21(7). doi:10.3390/ s21072331
42. Dai B, Garrett WE, Gross MT, Padua DA, Queen RM, Yu B. The effect of performance demands on lower extremity biomechanics during landing and cutting tasks. J Sport Health Sci 2019;8(3):228-234. doi:10.1016/j.jshs.2016.11.004
43. Dos’Santos T, Thomas C, Jones PA. The effect of angle on change of direction biomechanics: Comparison and inter-task relationships. J Sports Sci 2021;39(22):2618-2631. doi:10.1080/ 02640414.2021.1948258
44. Faul F, Erdfelder E, Lang AG, Buchner A. G*Power 3: a flexible statistical power analysis program for the social, behavioral, and biomedical sciences. Behav Res Methods 2007;39(2):175-191. doi:10.3758/ bf03193146
45. Robinson MA, Vanrenterghem J, Pataky TC. Sample size estimation for biomechanical waveforms: Current practice, recommendations and a comparison to discrete power analysis. J Biomech 2021;122:110451. doi:10.1016/ j.jbiomech.2021.110451
46. Luciano F, Ruggiero L, Pavei G. Sample size estimation in locomotion kinematics and electromyography for statistical parametric mapping. J Biomech 2021;122:110481. doi:10.1016/ j.jbiomech.2021.110481
47. Jones PA, Herrington L, Graham-Smith P. Braking characteristics during cutting and pivoting in female soccer players. J Electromyogr Kinesiol 2016;30:46-54. doi:10.1016/j.jelekin.2016.05.006
48. McBurnie AJ, Dos’Santos T, Jones PA. Biomechanical associates of performance and knee joint loads during a 70-90 degrees cutting maneuver in subelite soccer players. J Strength Cond Res. 2021;35(11):3190-3198. doi:10.1519/ JSC.0000000000003252
49. Mo S, Chow DHK. Accuracy of three methods in gait event detection during overground running. Gait Posture 2018;59:93-98. doi:10.1016/ j.gaitpost.2017.10.009
50. Fellin RE, Rose WC, Royer TD, Davis IS. Comparison of methods for kinematic identification of footstrike and toe-off during overground and treadmill running. J Sci Med Sport. 2010;13(6):646-650. doi:10.1016/j.jsams.2010.03.006
51. Cohen J. Statistical Power Analysis for the Behavioral Sciences. Academic press; 2013. doi:10.4324/9780203771587
52. Beaulieu ML, Ashton-Miller JA, Wojtys EM. Loading mechanisms of the anterior cruciate ligament. Sports Biomech 2023;22(1):1-29. doi:10.1080/14763141.2021.1916578
53. Dos’ Santos T, McBurnie A, Comfort P, Jones PA. The effects of six-weeks change of direction speed and technique modification training on cutting performance and movement quality in male youth soccer players. Sports 2019;7(9):205.
54. Dos’ Santos T, Thomas C, McBurnie A, Donelon T, Herrington L, Jones PA. The cutting movement assessment score (CMAS) qualitative screening tool: Application to mitigate anterior cruciate ligament injury risk during cutting. Biomech. 2021;1(1):83-101. doi:10.3390/biomechanics1010007
55. Dos’Santos T, Thomas C, Comfort P, Jones PA. The effect of angle and velocity on change of direction biomechanics: An angle-velocity trade-off Sports Med 2018;48(10):2235-2253. doi:10.1007/ s40279-018-0968-3
56. Schroeder LE, Peel SA, Leverenz BH, Weinhandl JT Type of unanticipated stimulus affects lower extremity kinematics and kinetics during sidestepping. J Sports Sci 2021;39(6):618-628. doi:10.1080/02640414.2020.1837481
57 Lee MJ, Lloyd DG, Lay BS, Bourke PD, Alderson JA. Effects of different visual stimuli on postures and knee moments during sidestepping. Med Sci Sports Exerc. 2013;45(9):1740-1748. doi:10.1249/ MSS.0b013e318290c28a
58. Dos’Santos T, Thomas C, McBurnie A, Comfort P, Jones PA. Biomechanical determinants of performance and injury risk during cutting: A performance-injury conflict? Sports Med. 2021;51(9):1983-1998. doi:10.1007/ s40279-021-01448-3
59. Lucia S, Bianco V, Di Russo F. Specific effect of a cognitive-motor dual-task training on sport performance and brain processing associated with decision-making in semi-elite basketball players. Psych Sport Exerc 2023;64:102302. doi:10.1016/ j.psychsport.2022.102302
60. Grooms DR, Page SJ, Nichols-Larsen DS, Chaudhari AM, White SE, Onate JA. Neuroplasticity associated with anterior cruciate ligament reconstruction. J Orthop Sports Phys Ther. 2017;47(3):180-189. doi:10.2519/jospt.2017.7003
61. Heidarnia E, Letafatkar A, Khaleghi-Tazji M, Grooms DR. Comparing the effect of a simulated defender and dual-task on lower limb coordination and variability during a side-cut in basketball players with and without anterior cruciate ligament injury. J Biomech 2022;133:110965. doi:10.1016/ j.jbiomech.2022.110965
62. Di Paolo S, Nijmeijer E, Bragonzoni L, Dingshoff E, Gokeler A, Benjaminse A. Comparing lab and field agility kinematics in young talented female football players: Implications for ACL injury prevention. Eur J Sport Sci 2023;23(5):859-868. doi:10.1080/ 17461391.2022.2064771
63. Pataky TC, Robinson MA, Vanrenterghem J. Region-of-interest analyses of one-dimensional biomechanical trajectories: bridging 0D and 1D theory, augmenting statistical power. Peer J. 2016;4:e2652. doi:10.7717/peerj.2652
64. Al-Amri M, Nicholas K, Button K, Sparkes V, Sheeran L, Davies JL. Inertial measurement units for clinical movement analysis: Reliability and concurrent validity Sensors 2018;18(3). doi:10.3390/ s18030719
65. Spiteri T, McIntyre F, Specos C, Myszka S. Cognitive training for agility: The integration between perception and action. Strength Condit J 2018;40(1):39-46. doi:10.1519/ SSC.0000000000000310
66. Buchel D, Gokeler A, Heuvelmans P, Baumeister J. Increased cognitive demands affect agility performance in female athletes - Implications for testing and training of agility in team ball sports. Percept Mot Skills. 2022;129(4):1074-1088. doi:10.1177/00315125221108698
Attalin B, Sagnard T, Laboute E, Forestier N, Rémy-Néris O,
B. Proprioceptive Reweighting and Postural Control are Impaired Among Elite Athletes Following Anterior Cruciate Ligament Reconstruction. IJSPT. 2024;19(11):1314-1323.
doi:10.26603/001c.124802
Benoit Attalin1a , Telma Sagnard2 , Eric Laboute1,3 , Nicolas Forestier2 , Olivier Rémy-Néris4 , Brice Picot2,5
1 C.E.R.S, Ramsay-santé, Capbreton, France, 2 Interuniversity Laboratory of Human Movement Biology, Savoie Mont-Blanc University, Chambéry, France, 3 Société Française de Traumatologie du Sport (SFTS), France, 4 Université Brest, CHU Brest, INSERM, UMR 1101, F29200 Brest, France, 5 French Society of Sports Physical Therapist (SFMKS Lab), Pierrefitte-sur-Seine, France
Keywords: ACL rehabilitation, central nervous system, postural control, proprioception, proprioceptive flexibility, vibration, ACL injury-induced neuroplasticity.
https://doi.org/10.26603/001c.124802
International Journal of Sports Physical Therapy
Vol. 19, Issue 11, 2024
Background
After anterior cruciate ligament reconstruction (ACLR), the risk of recurrence can reach 20%, partially due to poor postural control and impaired sensory processing. Lack of flexibility in proprioceptive postural strategy has recently been shown to be a potential risk factor for ACL injury.
Hypothesis/Purpose
This study aimed to compare proprioceptive reweighting and postural control between ACLR and controls elite athletes. It has been hypothesized that athletes with ACLR exhibit impaired proprioceptive reweighting and poor postural control.
Study design
Cross-sectional study
Methods
Fifty-two ACLR and 23 control elite athletes (50 males and 25 females, mean age 24.7 years) were included. Proprioceptive reweighting was determined using the evolution of proprioceptive weighting (eRPW), calculated from the center of pressure (CoP) displacements generated by tendon vibration during bilateral standing tasks on firm and foam surfaces. An eRPW <95% classified individuals as flexible (i.e., able to reweight proprioceptive signals from the ankle to the lumbar region), whereas an eRPW >105% classified individuals as rigid (i.e., maintaining an ankle dominant strategy). CoP velocity (vCoP) and CoP ellipse area (EA) were used to characterize postural control. Independent sample t-test and a Chi-squared test were used to compare eRPW, vCoP, EA, and the proportion of flexible and rigid athletes between groups.
Results
The eRPW was higher in the ACLR group (100.9±58.8 vs. 68.6±26.6%; p=0.031; Rank biserial correlation=0.314; medium), with a greater proportion of rigid athletes than in the control group (38.5 vs. 4.4%; p=0.010), reflecting lower proprioceptive reweighting. The ACLR group had greater EA on foam surface (8.0±4.6 vs. 6.3±4.4cm²; p=0.019), revealing poorer postural control.
Corresponding author:
Benoît Attalin
Centre Européen de Rééducation du Sportif (CERS)
Groupe Ramsay Santé, Capbreton France
Email: benoit.attalin@gmail.com
Elite athletes with ACLR showed impaired proprioceptive reweighting and poor postural control on an unstable surface. This reflects an inability to adapt proprioceptive weighting when balance conditions are changing and suboptimal postural strategies.
Anterior cruciate ligament (ACL) injuries are common in sports and the most common treatment for athletes is surgery and rehabilitation.1,2 In this specific population, the rate of reinjury can reach up to 20% and only 65% of athletes returned to their preinjury level.3,4 One possible explanation for these poor outcomes is the persistence of some defiencies that are not targeted during the rehabilitation such as proprioception, proprioceptive adjustment, or neuromotor deficits.
It has been shown that postural control impairments related to a central origin persist in the short and long term after ACL reconstruction (ACLR) and are considered as potential risk factors for reinjury 5‑9 Recent evidence has identified neuroplastic adaptation of the central nervous system (CNS) following ACL injuries in particular in afferent pathways.10‑12 Ligament injuries significantly disrupt proprioceptive signals and induce nociceptor hyperactivity due to associated inflammatory processes. These factors alter sensory information and its processing by the CNS, leading to cortical reorganization in injured athletes.13‑15
A recent studies has revealed that six weeks post-surgical reconstruction, patients show increased cortical connectivity during an unipedal balance tasks without vision compared to healthy subjects.5 This heightened functional connectivity in somatosensory and visual areas may indicate a compensatory mechanism to control postural stability of the injured leg. Long-term (up to 20 years post-injury) injured individuals exhibit reduced adaptability in postural control and greater effort to maintain balance compared to healthy individuals.16 This decreases motor control flexibility in unexpected situations.17,18 These postural control deficits are primarily attributed to alterations in sensory reweighting.
A fundamental contributor to optimal postural control is sensory reweighting.19 It reflects the ability of the CNS to identify and select the most appropriate signal according to balance conditions. For instance, in eyes-closed postural conditions, the CNS mainly relies on proprioception to maintain optimal postural control while in eyes-open balance situations, a reweighting towards vision is observed. The systematic review by Wikstrom et al. confirms an overreliance on visual information during postural tasks in ACL injured athletes.20 This increased dependence on visual signals is considered as a central mechanism of sensory reorganization, compensating for degraded proprioceptive signals due to ligamentous damage to maintain effective postural control and joint stability 21,22
In addition to this intermodal reweighting (proprioception vs vision), an intramodal modality has also been iden-
tified (i.e. proprioceptive reweighting). Proprioceptive reweighting is defined as the ability of the CNS to integrate sensory signals from different anatomical locations and select the most appropriate cue according to balance conditions. For example, when standing on a firm surface individuals mainly rely on ankle signals. Conversely, on a foam surface a shift toward a lumbar proprioceptive strategy is observed.19,23,24 However, it appears that in certain pathological conditions such as chronic low back pain or diabetic neuropathy, this ability to reweight proprioceptive signals when postural conditions are changing is impaired.25‑28 In addition, recent investigations revealed that a large heterogeneity exists among healthy athletes regarding this proprioceptive strategies.29,30 Most athletes are indeed able to switch from ankle to lumbar proprioceptive signals when moving from a firm to a foam surface (i.e. flexible athletes). However, some individuals seem to adopt an ankle-steered strategy, even on unstable surface (i.e. rigid athletes). It could be considered as a suboptimal strategy since it has been shown that rigid athletes exhibited at-risk determinants for ACL injuries during side-cutting maneuvers.29
Therefore, the primary aim of this study was to compare proprioceptive reweighting between ACLR and control elite athletes. The authors hypothesized that following ACLR, athletes would exhibit a lower ability to switch from ankle to lumbar signals than healthy athletes. The secondary aim of this study was to compare postural control between control and ACLR elite athletes. It was hypothesized that ACLR athletes would exhibit poor balance control.
This cross-sectional study was conducted in the European Sports Rehabilitation Center of Capbreton, France, from June to September 2023. Based on the primary aim of this study, a priori calculation of the number of subjects required to obtain a statistical power of 0.90 and type 1 error of 0.05 with an effect size of 0.8, showed that at least 68 subjects were needed.29
The inclusion criteria for the ACLR group were as follows. Patients were high-level athletes (top national and international division) who had undergone ACL reconstruction (for first or reinjury) and had to be able to stand on two legs with their eyes closed without pain or instability For these reasons, the minimum postoperative inclusion period was set at 30 days. Individuals in the control group were high-level athletes free from any recent (six months) lower limb injuries but involved in a rehabilitation process primarily for shoulder, elbow, or wrist injury/surgery The ex-
clusion criteria for both groups were known neurological or vestibular impairments and spine or lower limb injuries in the prior six months, and pain during the procedure or significant destabilization leading the examiner to prevent the athlete from falling. This study was performed in accordance with the principles of the Declaration of Helsinki. All subjects provided written informed consent, and this study received Institutional Ethics Approval (IRBA02114-49).
The procedure was the same as previously detailed by Picot et al.29,30 Participants stood barefoot in a bipedal stance with their arms relaxed by their sides and their head in neutral. Feet position was standardized and vision was prevented using opaque goggles. Two conditions were evaluated: “firm” (standing on the force plates) and “foam” (Physiopad®; 50x41x5cm; 52 kg/m3) surfaces. Four muscle vibrators (VB115, Techno Concept, France) were placed bilaterally on the triceps surae (TS) and the lumbar paravertebral muscles (LPM) by the same experienced experimenter.25 Vibration frequency was set at 80 Hz with an amplitude of 0.5 mm to stimulate the muscle spindles. Computer software automatically and randomly triggered the site of vibration. This also ensured that neither the participant nor the experimenter could anticipate the next vibration site. Each trial lasted for 60s. Recordings began at 20s prior to vibration, then vibration was applied for 20s and the recording continued for another 20s during the restabilization period. Force and moment data were collected using portable, uniaxial, dual force plates (Force Decks, FDLite, V.2, VALD, Brisbane, Australia, 200 Hz) to determine center of pressure (CoP) displacement. Raw data were extracted by Force Decks software. Signals were filtered using a Butterwoth low-pass, fourth order filter with a cut-off frequency of 10 Hz. Anterior/Posterior CoP displacement (dCoP) was calculated using custom software developed in Matlab (The MathWorks, Inc., Version: 9.13.0 (R2022b).
PROPRIOCEPTIVE STRATEGY
Relative Proprioceptive Weighting (RPW) is the ratio between the effects of vibration of the TS and the LPM (absolute TS / (absolute TS + absolute LPM)) on CoP displacement. It provides a reliable indication of individual proprioceptive strategies: an RPW of 1 indicates 100% reliance on ankle afferent input while an RPW of 0 indicates a 100% reliance on lumbar afferent input.
RPW has been shown to be the most reliable indicator of the response to muscle vibration and to establish proprioceptive strategy.25,27,31 The evolution of the relative proprioceptive weighting (eRPW) between the firm and foam surfaces (expressed as a percentage of the RPW on the firm support) was then calculated.29,30
Participants were then dichotomized according to their proprioceptive profile using the eRPW by a second blind assessor. A change < 95% corresponded to a reallocation of signals from ankle to lumbar when standing on foam surface and indicated a flexible proprioceptive profile. Conversely, a change ≥ 105% indicated a rigid profile with an
inability to reweight proprioceptive reliance reflecting ankle-dominated strategy.26,29,30 Participants with an eRPW value between 95% and 105% were not characterized to avoid incorrect characterization due to variability in the index.25,29
Postural stability was determined by analyzing the CoP ellipse and CoP velocity area during the 20s of the pre-vibration period on the two different surfaces (firm and foam).32 The data were averaged for trials performed on the same surface and under the same conditions. Higher values indicated poorer postural stability 33
Normality and equality of variances were assessed using the Shapiro-Wilk and Levene’s tests. For the primary aim of the study, eRPW was compared between the groups using a nonparametric independent t-test. The proportions of flexible and rigid subjects in each group were tested using the chi-square (Chi²) test. RPW values were compared using a two-way (Group x Surface) repeated measure ANOVA and post-hoc analysis (Scheffé’s correction) were performed if needed. As postural control data (vCoP and EA) were not normally distributed, they were compared using independent (between-group) and paired (between-surface) nonparametric t-tests. The level of significance was set at 0.05. Effect sizes (Rank biserial correlation, Cramer V or partial η2) were calculated for all comparisons and compared using the Hopkins scale. The statistical analysis was performed using JASP (Amsterdam 0.12.2.0) and G*Power (Version 3.1, University of Dusseldorf, Germany).
Fifty-two ACLR (17 females and 35 males, 23.2±4.9 years) and 23 control elite athletes (8 females and 15 males, 28.2±5.3 years) were included (Table 1). Among ACLR athletes, five underwent reinjury surgery (9.6%). The mean post-operative delay was 162.1 days (SD: 65.1) (Table 1).
The ACLR group exhibited a higher eRPW value than the control group (100.9 ± 58.8% vs. 68.6 ± 26.6%; p=0.031; Rank biserial correlation=0.314; medium) (Figure 1). In addition, the ACLR group had a significantly lower proportion of flexible athletes and a higher proportion of rigid athletes than the control group (p=0.01, Cramer’s V=0.351, large) (Table 2).
There was a significant interaction (Group x Surface) for RPW values (p=0.05; partial η2=0.014; small). Post-hoc analysis revealed differences only in the control group where RPW values were significantly lower on the foam compared to the firm surface (0.51 ± 0.26% vs. 0.71 ± 0.20%; p=0.002, Cohen’s d=0.845; big) (Figure 2).
Table 1. Mean (±SD) baseline characteristics.
Figure 1. Individual change in eRPW from the firm to the foam condition: RPW increased for the rigid strategy (≥105%) and decreased for the flexible strategy (≤95%).
RPW: Relative Proprioceptive Weighting; ACLR: Anterior Cruciate Ligament Reconstruction; *: p<0.05
Table 2. Distribution of athletes (%) according to their proprioceptive postural strategy and groups.
Group
Control (N=23) ACLR (N=52)
Flexible (N, % of group) 19 (82.6%) 27 (51.9%)
Rigid (N, % of group) 1 (4.4%) 20 (38.5%)
Not characterized (N, % of group) 3 (13.0%) 5 (9.6%)
p=0.01
POSTURAL CONTROL
On the foam surface, the ACLR group showed increased mean CoP ellipse area compared to the control group (7.95 ± 4.57 cm² vs. 6.25 ± 4.40 cm²; p=0.019; Rank biserial correlation=0.343; medium) indicating poorer postural control. No other differences were observed between the two groups (Table 3). CoP velocity was significantly higher on the foam than on the firm surface for both ACLR (p<0.001) and con-
Figure 2. Mean ± SD and individual RPW values for the firm and foam support surfaces of the ACLR and control groups.
RPW: Relative Proprioceptive Weighting; ACLR: Anterior Cruciate Ligament Reconstruction; *: p=0.001
trol groups (p<0.001), as well as the CoP ellipse area for both ACLR (p<0.001) and control groups (p<0.001) confirming the higher difficulty to maintain postural control on foam surface (Table 3).
The primary aim of this study was to compare proprioceptive reweighting between elite athletes following ACL reconstruction and a control group. Results revealed higher eRPW values in the ACLR group indicating an inability to reweight proprioceptive signals according to balance conditions.29,30 More specifically, it appears that only the control group was able to significantly shift from an ankle strategy on the firm surface to a lumbar strategy when standing on foam surface (i.e. decrease RPW value, Figure 2). On the contrary, the ACLR group maintained an ankle-dominated strategy regardless of the surface stability The mean eRPW of ACLR elite athletes was 100.9%, reflecting a strategy close to a rigid profile for the entire group. On the contrary, mean eRPW value of the control group (69%) was similar to values from a previous study obtained from a group of flexible athletes (72%).29 The higher eRPW values found in ACLR athletes can be explained by lower RPW on firm surfaces, which do not decrease on foam surface (Figure 2). It
Table 3. Mean ± SD postural parameters in the control and ACLR groups
P-values refer to the independent t-test between the
reveals an inability to shift from ankle reliance when the reliability of the signals from this joint is altered by the instability of the support.23‑25,34 Conversely, more than 80% of controls were able to operate a proprioceptive reweighting to lumbar signal on foam surface. This reveals a suboptimal proprioceptive postural strategy in ACLR group.30, 35 When examining RPW values on firm and foam surfaces, both groups exhibit higher values than those previously reported among healthy populations.26,27,31,36,37 This might be explained by the characteristics of our population, since high level athletes tend to increase reliance on ankle proprioceptive information.33,38
When comparing the proprioceptive strategies between the two groups, a significantly higher proportion of rigid athletes was observed in the ACLR group (38.5% vs. 4.4%). Additionally, the control group exhibited a remarkably high proportion of flexible athletes (83%). Only one study among young healthy athletes reported the proportion of flexible/rigid individuals with 43% of rigid and 57% of flexible 30
Contrary to Picot et al. who used an eRPW cut-off value of 100% to distinguish between rigid and flexible individuals, in the present study, the dichotomization was made using an eRPW > 105% for rigid and eRPW < 95% for flexible athletes in order to avoid incorrect characterization of participants due to the variability of this index.25,29,30 In addition, the population studied by Picot et al. was composed of young subelite healthy handball players.30 Both type of sport and level of practice may play a role in proprioceptive reweighting strategy.24,33,38,39 It might be possible that most healthy elite athletes exhibit flexible strategy compared to non-expert individuals. Further studies with larger sample sizes are needed to better understand the specific proportion of flexible/rigid and the role of sports expertise in proprioceptive postural strategies according to the type of population.
The secondary aim of this study was to compare postural performance between ACLR athletes and a control group. Data confirm previous results revealing bilateral deficits in both static and dynamic postural control, in single-leg and double-leg stances following ACLR. Single-leg assessments showed impairment for both the affected and unaffected limb. These bi and unilateral deficits are found in the short and long term.7,40‑43 It is worth mentioning that only significant differences between the two groups were observed on foam surfaces. The moderate effect size as well as the absence of difference on firm surface between the
two groups could be explained by the fact that individuals were elite athletes and bipedal tasks might not be challenging enough.44 It seems assessment of postural control deficits in challenging tasks, such as unstable surfaces, single-leg tasks may improve the characterization of postural control deficits.44,45
Overall, the results indicate possible functional modifications of the CNS in elite athletes following ACLR, especially in areas involved in postural control. Over-reliance on visual signals after ACL injury have been identified as a probable consequence of altered proprioceptive signal.20 Indeed, an hyperactivation of lingual gyrus has been shown after ACLR which could be considered as an adaptation mechanism of the CNS to maintain postural control by reweighting altered proprioceptive signals to visual signals.46‑48 Although the CNS attempts to compensate for this proprioceptive impairment, central alterations persist, which may explain the ongoing postural control deficit after an ACL injury Indeed, Grooms et al. found greater activation of the contralateral cerebellum and the pre-motor area, and the ipsilateral secondary somatosensory cortex in individuals who underwent an ACLR, all of which are highly implicated in postural control.47 The recent scoping review from Vitharana et al. confirmed impaired central processing within the somatosensory and visual systems and underlined the impact of these neuroplastic changes on balance control.22 These results suggest that cortical and subcortical deficiencies after ACLR may be involved in the persistence of a postural control deficit after ACLR.6,46,47 Further imaging studies are needed to better understand which brain regions are involved in the proprioceptive reweighting process and whether functional connectivity between brain regions responsible for posture and sensory integration is impaired among rigid individuals.
It has been shown that several regions of the CNS involved in proprioceptive reweighting such as the somatosensory, pre-motor and motor cortex exhibit altered activity after an ACL rupture.46,47,49 This could explain the lack of proprioceptive reweighting found in ACLR subjects in this study. In addition, impaired functional connections between the left primary sensory cortex and the right posterior lobe of the cerebellum were observed in athletes who go on to suffer an ACL injury. These connections are considered essential for motor control, thus functional impairments of the CNS especially between brain regions implicated in sensory integration during postural control might
increase the risk of ACL injury 50,51 It has been suggested that these central adaptations could be responsible for reinjury after ACLR, since it limits the ability of athletes to adapt to complex and unplanned game situations.52
Recently, Picot et al. showed that lack of proprioceptive reweighting in postural strategy is associated with at-risk biomechanics and neuromuscular control for ACL injuries during sidecutting maneuvers.29,53,54 Poorer postural control was also observed among rigid athletes during bipedal stance tasks which is also a major risk factor for primary and secondary ACL injuries.8,9,30,40
These results suggest that following ACLR elite athletes exhibiting suboptimal (i.e. rigid) proprioceptive strategies could be more likely to suffer from a reinjury Further studies are needed to determine whether a lack of proprioceptive reweighting is a risk factor of ACL (re)injury
This is the first study to evaluate proprioceptive reweighting abilities in athletes undergoing ACLR. It is also the first to use vibration as a method to probe the CNS in this population without imaging or electroencephalography, which is more functional and closely approximates postural control. This could be considered as a CNS assessment tool that can be easily used in clinical practice to personalize rehabilitation and be part of return-to-sport criterion.
This study had several limitations. First, due to the design of this study, it is not possible to determine whether the lack of proprioceptive reweighting observed in ACLR athletes was present before the injury. Future studies should examine preinjury data to confirm the link between proprioceptive reweighting and ACL rupture.
Furthermore, the exact effects of rehabilitation on proprioceptive reweighting remains to be established. The mean post-operative delay for the ACLR group was five months. It remains unclear whether proprioceptive reweighting impairments persist in the long term. Thus, further research is needed to determine the progression or potential recovery of these proprioceptive deficits over time. Future longitudinal studies should evaluate the evolution of proprioceptive reweighting during rehabilitation, and whether exercising can transform rigid to flexible patients. Since athletes who suffer an ACL lesion are 30 to 40 times more prone to recurrences than those who did not, lack of proprioceptive reweighting and impaired postural control should be evaluated and targeted during the rehabilitation.3,55
On another note, as bipedal testing on a unilateral injury may have limitations and could fail to reveal important asymmetries in weight-bearing strategies for each individ-
ual limb, it would be valuable to conduct a bilateral singleleg assessment to identify potential differences in proprioceptive reweighting capabilities between the injured and healthy limbs.55
Additionally, the authors did not conduct a subgroup analysis based on the type of graft (hamstring or quadriceps), associated injuries (antero-lateral ligament suture or lateral tenodesis, meniscal resection or suture), or first or reinjury surgery because of the low number of athletes. Future studies should include larger cohorts to evaluate the effect of these differences on proprioceptive reweighting.56
Moreover, the small sample size likely contributed to the high variability observed. This variability is especially in the ACL group and is possibly due to variable postoperative times. Such variability in proprioceptive reweighting is common with this methodology
The average age of the ACLR group was lower than that of the control group (23.2 vs 28.2 years). Even if it has been shown that age could influence proprioceptive reweighting capabilities in elderly, a difference of five years seems insufficient to induce variations in proprioceptive reweighting capabilities.24,39 Furthermore, given that the proprioceptive system is fully matured at this age it seems unlikely that this could have influenced the results.57
Elite athletes who underwent ACL reconstruction exhibit a lack of proprioceptive reweighting, and a higher proportion of rigid individuals compared to a control group. More specifically, they show a lack of ability to reweight proprioceptive signals when moving from a firm to a foam surface. In addition, poorer postural control was observed on foam surface among ACLR athletes. These results highlighted central alterations associated with suboptimal strategy that could increase the risk of reinjury. Further studies are needed to assess whether these alterations exist prior to injury and if these can be considered potential risk factors for reinjury
The authors report no conflicts of interest.
Submitted: September 01, 2024 CDT, Accepted: October 12, 2024 CDT
© The Author(s)
This is an open-access article distributed under the terms of the Creative Commons Attribution 4.0 International License (CCBY-NC-4.0). View this license’s legal deed at https://creativecommons.org/licenses/by-nc/4.0 and legal code at https://creativecommons.org/licenses/by-nc/4.0/legalcode for more information.
1. Sanders TL, Maradit Kremers H, Bryan AJ, et al. Incidence of anterior cruciate ligament tears and reconstruction: A 21-year population-based study. Am J Sports Med 2016;44(6):1502-1507 doi:10.1177/ 0363546516629944
2. Kotsifaki R, Korakakis V, King E, et al. Aspetar clinical practice guideline on rehabilitation after anterior cruciate ligament reconstruction. Br J Sports Med. 2023;57(9):500-514. doi:10.1136/ bjsports-2022-106158
3. Wiggins AJ, Grandhi RK, Schneider DK, Stanfield D, Webster KE, Myer GD. Risk of secondary injury in younger athletes after anterior cruciate ligament reconstruction: A systematic review and metaanalysis. Am J Sports Med. 2016;44(7):1861-1876. doi:10.1177/0363546515621554
4. Ardern CL, Taylor NF, Feller JA, Webster KE. Fiftyfive per cent return to competitive sport following anterior cruciate ligament reconstruction surgery: an updated systematic review and meta-analysis including aspects of physical functioning and contextual factors. Br J Sports Med 2014;48(21):1543-1552. doi:10.1136/ bjsports-2013-093398
5. Lehmann T, Büchel D, Mouton C, Gokeler A, Seil R, Baumeister J. Functional cortical connectivity related to postural control in patients six weeks after anterior cruciate ligament reconstruction. Front Hum Neurosci 2021;15:655116. doi:10.3389/ fnhum.2021.655116
6. Lehmann T, Paschen L, Baumeister J. Single-Leg Assessment of postural stability after anterior cruciate ligament injury: A systematic review and meta-analysis. Sports Med - Open 2017;3(1):32. doi:10.1186/s40798-017-0100-5
7. Dauty M, Collon S, Dubois C. Change in posture control after recent knee anterior cruciate ligament reconstruction? Clin Physiol Funct Imaging 2010;30(3):187-191. doi:10.1111/ j.1475-097X.2010.00926.x
8. Oshima T, Nakase J, Kitaoka K, et al. Poor static balance is a risk factor for non-contact anterior cruciate ligament injury Arch Orthop Trauma Surg 2018;138(12):1713-1718. doi:10.1007/ s00402-018-2984-z
9. Collings TJ, Bourne MN, Barrett RS, Du Moulin W, Hickey JT, Diamond LE. Risk factors for lower limb injury in female team field and court sports: A systematic review, meta-analysis, and best evidence synthesis. Sports Med 2021;51(4):759-776. doi:10.1007/s40279-020-01410-9
10. Gokeler A, Benjaminse A, Hewett TE, et al. Feedback techniques to target functional deficits following anterior cruciate ligament reconstruction: implications for motor control and reduction of second injury risk. Sports Med 2013;43(11):1065-1074. doi:10.1007/ s40279-013-0095-0
11. Needle AR, Lepley AS, Grooms DR. Central nervous system adaptation after ligamentous injury: A summary of theories, evidence, and clinical interpretation. Sports Med 2017;47(7):1271-1288. doi:10.1007/s40279-016-0666-y
12. Roy JS, Bouyer LJ, Langevin P, Mercier C. Beyond the joint: The role of central nervous system reorganizations in chronic musculoskeletal disorders. J Orthop Sports Phys Ther 2017;47(11):817-821. doi:10.2519/jospt.2017.0608
13. Chaput M, Onate JA, Simon JE, et al. Visual cognition associated with knee proprioception, time to stability, and sensory integration neural activity after ACL reconstruction. Journal Orthopaedic Research 2022;40(1):95-104. doi:10.1002/jor.25014
14. Neto T, Sayer T, Theisen D, Mierau A. Functional brain plasticity associated with ACL injury: A scoping review of current evidence. Neural Plast 2019;2019:3480512. doi:10.1155/2019/3480512
15. Laboute E, Verhaeghe E, Ucay O, Minden A. Evaluation kinaesthetic proprioceptive deficit after knee anterior cruciate ligament (ACL) reconstruction in athletes. J Exp Orthop. 2019;6(1):6. doi:10.1186/ s40634-019-0174-8
16. Stensdotter AK, Tengman E, Häger C. Altered postural control strategies in quiet standing more than 20 years after rupture of the anterior cruciate ligament. Gait Posture 2016;46:98-103. doi:10.1016/ j.gaitpost.2016.02.020
17. Latash M. There is no motor redundancy in human movements. There is motor abundance. Latash M, ed. Motor Control. 2000;4(3):259-261. doi:10.1123/mcj.4.3.259
18. Latash ML. The bliss (not the problem) of motor abundance (not redundancy). Exp Brain Res. 2012;217(1):1-5. doi:10.1007/s00221-012-3000-4
19. Peterka RJ. Sensory integration for human balance control. Handb Clin Neurol. 2018;159:27-42. doi:10.1016/B978-0-444-63916-5.00002-1
20. Wikstrom EA, Song K, Pietrosimone BG, Blackburn JT, Padua DA. Visual utilization during postural control in anterior cruciate ligamentdeficient and -reconstructed patients: Systematic reviews and meta-analyses. Arch Phys Med Rehabil. 2017;98(10):2052-2065. doi:10.1016/ j.apmr.2017.04.010
21. Grooms D, Appelbaum G, Onate J. Neuroplasticity following anterior cruciate ligament injury: a framework for visual-motor training approaches in rehabilitation. J Orthop Sports Phys Ther. 2015;45(5):381-393. doi:10.2519/jospt.2015.5549
22. Vitharana TN, King E, Moran K. Sensorimotor dysfunction following anterior cruciate ligament reconstruction- an afferent perspective: A scoping review Int J Sports Phys Ther 2024;19(1). doi:10.26603/001c.90862
23. Ivanenko YP, Solopova IA, Levik YS. The direction of postural instability affects postural reactions to ankle muscle vibration in humans. Neuroscience Letters 2000;292(2):103-106. doi:10.1016/ S0304-3940(00)01438-5
24. Brumagne S, Cordo P, Verschueren S. Proprioceptive weighting changes in persons with low back pain and elderly persons during upright standing. Neurosci Lett. 2004;366(1):63-66. doi:10.1016/j.neulet.2004.05.013
25. Kiers H, Brumagne S, Van Dieën J, Van Der Wees P, Vanhees L. Ankle proprioception is not targeted by exercises on an unstable surface. Eur J Appl Physiol 2012;112(4):1577-1585. doi:10.1007/ s00421-011-2124-8
26. Claeys K, Brumagne S, Dankaerts W, Kiers H, Janssens L. Decreased variability in postural control strategies in young people with non-specific low back pain is associated with altered proprioceptive reweighting. Eur J Appl Physiol 2011;111(1):115-123. doi:10.1007/s00421-010-1637-x
27. Brumagne S, Janssens L, Knapen S, Claeys K, Suuden-Johanson E. Persons with recurrent low back pain exhibit a rigid postural control strategy. Eur Spine J 2008;17(9):1177-1184. doi:10.1007/ s00586-008-0709-7
28. Bonnet CT, Lepeut M. Proximal postural control mechanisms may be exaggeratedly adopted by individuals with peripheral deficiencies: a review J Mot Behav. 2011;43(4):319-328. doi:10.1080/ 00222895.2011.589415
29. Picot B, Lempereur M, Morel B, Forestier N, Rémy-Néris O Lack of proprioceptive strategy modulation leads to at-risk biomechanics for anterior cruciate ligament in healthy athletes. Med Sci Sports Exerc. 2024;56(5):942-952. doi:10.1249/ MSS.0000000000003378
30. Picot B, Rémy-Neris O, Forestier N. Proprioceptive postural control strategies differ among non-injured athletes. Neurosci Lett. 2022;769:136366. doi:10.1016/j.neulet.2021.136366
31. Johanson E, Brumagne S, Janssens L, Pijnenburg M, Claeys K, Pääsuke M. The effect of acute back muscle fatigue on postural control strategy in people with and without recurrent low back pain. Eur Spine J 2011;20(12):2152-2159. doi:10.1007/ s00586-011-1825-3
32. Pinsault N, Vuillerme N. Test-retest reliability of centre of foot pressure measures to assess postural control during unperturbed stance. Med Eng Phys 2009;31(2):276-286. doi:10.1016/ j.medengphy.2008.08.003
33. Paillard T, Noé F. Techniques and methods for testing the postural function in healthy and pathological subjects. Biomed Res Int. 2015;2015:891390. doi:10.1155/2015/891390
34. Lubetzky AV, McCoy SW, Price R, Kartin D Response to tendon vibration questions the underlying rationale of proprioceptive training. J Athl Train 2017;52(2):97-107 doi:10.4085/ 1062-6050-52.1.06
35. Brumagne S, Janssens L, Claeys K, Pijnenburg M. Altered variability in proprioceptive postural strategy in people with recurrent low back pain. In: Spinal Control Elsevier; 2013:135-144. doi:10.1016/ B978-0-7020-4356-7.00012-4
36. Forestier N, Terrier R, Teasdale N. Ankle muscular proprioceptive signals’ relevance for balance control on various support surfaces: an exploratory study Am J Phys Med Rehabil. 2015;94(1):20-27. doi:10.1097/ PHM.0000000000000137
37 Claeys K, Dankaerts W, Janssens L, Pijnenburg M, Goossens N, Brumagne S. Young individuals with a more ankle-steered proprioceptive control strategy may develop mild non-specific low back pain. J Electromyogr Kinesiol. 2015;25(2):329-338. doi:10.1016/j.jelekin.2014.10.013
38. Han J, Anson J, Waddington G, Adams R, Liu Y The role of ankle proprioception for balance control in relation to sports performance and injury BioMed Research International. 2015;2015:1-8. doi:10.1155/ 2015/842804
39. Ito T, Sakai Y, Yamazaki K, Nishio R, Ito Y, Morita Y Postural strategy in elderly, middle-aged, and young people during local vibratory stimulation for proprioceptive inputs. Geriatrics 2018;3(4):93. doi:10.3390/geriatrics3040093
40. Paterno MV, Schmitt LC, Ford KR, et al. Biomechanical measures during landing and postural stability predict second anterior cruciate ligament injury after anterior cruciate ligament reconstruction and return to sport. Am J Sports Med 2010;38(10):1968-1978. doi:10.1177/ 0363546510376053
41. Hirjaková Z, Šingliarová H, Bzdúšková D, et al. Postural stability and responses to vibrations in patients after anterior cruciate ligament surgical reconstruction. Physiol Res 2016;65(Suppl 3):S409-S416. doi:10.33549/physiolres.933437
42. Zouita Ben Moussa A, Zouita S, Dziri C, Ben Salah FZ. Single-leg assessment of postural stability and knee functional outcome two years after anterior cruciate ligament reconstruction. Ann Phys Rehabil Med 2009;52(6):475-484. doi:10.1016/ j.rehab.2009.02.006
43. Culvenor AG, Alexander BC, Clark RA, et al. Dynamic single-leg postural control is impaired bilaterally following anterior cruciate ligament reconstruction: Implications for reinjury risk. J Orthop Sports Phys Ther 2016;46(5):357-364. doi:10.2519/jospt.2016.6305
44. Lion A, Gette P, Meyer C, Seil R, Theisen D. Effect of cognitive challenge on the postural control of patients with ACL reconstruction under visual and surface perturbations. Gait Posture. 2018;60:251-257. doi:10.1016/j.gaitpost.2017.12.013
45. Howells BE, Ardern CL, Webster KE. Is postural control restored following anterior cruciate ligament reconstruction? A systematic review Knee Surg Sports Traumatol Arthrosc 2011;19(7):1168-1177 doi:10.1007/s00167-011-1444-x
46. Grooms DR, Page SJ, Onate JA. Brain activation for knee movement measured days before second anterior cruciate ligament injury: Neuroimaging in musculoskeletal medicine. J Athl Train 2015;50(10):1005-1010. doi:10.4085/ 1062-6050-50.10.02
47 Grooms DR, Page SJ, Nichols-Larsen DS, Chaudhari AMW, White SE, Onate JA. Neuroplasticity associated with anterior cruciate ligament reconstruction. J Orthop Sports Phys Ther. 2017;47(3):180-189. doi:10.2519/jospt.2017.7003
48. Macaluso E, Driver J. Spatial attention and crossmodal interactions between vision and touch. Neuropsychologia. 2001;39(12):1304-1316. doi:10.1016/s0028-3932(01)00119-1
49. Kapreli E, Athanasopoulos S, Gliatis J, et al. Anterior cruciate ligament deficiency causes brain plasticity: a functional MRI study Am J Sports Med 2009;37(12):2419-2426. doi:10.1177/ 0363546509343201
50. Diekfuss JA, Grooms DR, Yuan W, et al. Does brain functional connectivity contribute to musculoskeletal injury? A preliminary prospective analysis of a neural biomarker of ACL injury risk. J Sci Med Sport 2019;22(2):169-174. doi:10.1016/ j.jsams.2018.07.004
51. Manto M, Bower JM, Conforto AB, et al. Consensus paper: roles of the cerebellum in motor control--the diversity of ideas on cerebellar involvement in movement. Cerebellum 2012;11(2):457-487 doi:10.1007/s12311-011-0331-9
52. Grooms DR, Onate JA. Neuroscience application to noncontact anterior cruciate ligament injury prevention. Sports Health 2016;8(2):149-152. doi:10.1177/1941738115619164
53. Donelon TA, Dos’Santos T, Pitchers G, Brown M, Jones PA. Biomechanical determinants of knee joint loads associated with increased anterior cruciate ligament loading during cutting: A systematic review and technical framework. Sports Med - Open 2020;6(1):53. doi:10.1186/s40798-020-00276-5
54. Zebis MK, Aagaard P, Andersen LL, et al. Firsttime anterior cruciate ligament injury in adolescent female elite athletes: a prospective cohort study to identify modifiable risk factors. Knee Surg Sports Traumatol Arthrosc 2022;30(4):1341-1351. doi:10.1007/s00167-021-06595-8
55. Spiliopoulou SI, Amiridis IG, Hatzitaki V, Patikas D, Kellis E. Tendon vibration during submaximal isometric strength and postural tasks. Eur J Appl Physiol 2012;112(11):3807-3817 doi:10.1007/ s00421-012-2319-7
56. Laboute E, James-Belin E, Puig PL, Trouve P, Verhaeghe E. Graft failure is more frequent after hamstring than patellar tendon autograft. Knee Surg Sports Traumatol Arthrosc. 2018;26(12):3537-3546. doi:10.1007/s00167-018-4982-7
57 Sá CDSCD, Boffino CC, Ramos RT, Tanaka C. Development of postural control and maturation of sensory systems in children of different ages a crosssectional study. Braz J Phys Ther. 2018;22(1):70-76. doi:10.1016/j.bjpt.2017.10.006
Chmielewski T, Obermeier M, Meierbachtol A, et al. Advanced Neuromuscular Training Differentially Changes Performance on Visuomotor Reaction Tests and Single-leg Hop Tests in Patients with ACL Reconstruction tional Test Results in Patients with ACL Reconstruction. IJSPT. 2024;19(11):1324-1332. doi:10.26603/001c.124807
Terese Chmielewski1 , Michael Obermeier2 , Adam Meierbachtol1 , Asher Jenkins3 , Michael Stuart4 , Robby Sikka5 , Marc Tompkins6a
1 Tria Orthopedic Center, 2 Orthopedic Surgery, University of Minnesota, 3 University of Minnesota, 4 Orthopedics and Sports Medicine, Mayo Clinic, 5 COVID Sports and Society Workgroup/SMART, Mayo Clinic, 6 TRIA Orthopaedic Center
Keywords: ACL, Rehabilitation, Sports, functional test, Reaction time https://doi.org/10.26603/001c.124807
International Journal of Sports Physical Therapy
Vol. 19, Issue 11, 2024
Background
Advanced neuromuscular training prepares patients with anterior cruciate ligament reconstruction (ACLR) for sport participation. Return-to-sport testing often includes single-leg hop tests, yet combining motor and cognitive tasks (i.e., dual-task) might reveal neurocognitive reliance.
Purpose/Hypothesis
This study examined changes in performance on visuomotor reactions tests and single-leg hop tests following advanced neuromuscular training in patients with ACLR. The hypothesis was that performance would improve less on reaction tests than on single-leg hop tests.
Study Design
Quasi experimental, Pretest-Posttest
Methods
Twenty-five patients with ACLR (11 males) completed 10 sessions of advanced neuromuscular training and pre-and post-training testing. Reaction tests outcomes were from a platform and visual display. The double-leg reaction test involved touching target dots with either leg for 20 seconds; correct touches and errors were recorded. The single-leg reaction test involved hopping on the test leg to 10 target dots; hop time and errors were recorded. Single-leg hop tests included forward, triple, crossover triple, and timed hop; limb symmetry index was recorded. Effect sizes were calculated for corrected touches on the double-leg reaction test, surgical side hop time on the single-leg reaction test, and surgical side hop distance or time on single-leg hop tests.
Corresponding Author: Marc A. Tompkins, MD
marc.tompkins@tria.com a
Department of Orthopedic Surgery
University of Minnesota
2450 Riverside Avenue South Suite R200
Minneapolis, MN 55455
Ph: 612-273-1177
Fax: 612-273-7959
Correct touches on the double-leg reaction test significantly increased from pre- to post-training (20.4 +/- 4.3 vs. 23.9 +/- 2.8, p<0.001). Hop time on the single-leg reaction test significantly decreased from pre- to post-training (Surgical leg 13.2 vs. 12.3 seconds, non-surgical leg 13.0 vs. 12.1 seconds, p=0.003). Mean errors did not significantly change on either reaction test (p> 0.05). Cohens d effect sizes in descending order was single-leg hop tests (d=0.9 to 1.3), double-leg reaction test (d=0.9), and single-leg reaction test (d=0.5).
Motor performance improved after advanced neuromuscular training, but the effect size was less on visuomotor reaction tests than single-leg hop tests. The results suggest persistence of neurocognitive reliance after ACLR and a need for more dual-task challenges in training.
of Evidence 3
Anterior cruciate ligament (ACL) injuries are prevalent in the United States, with 1 in 3000 persons sustaining an ACL rupture, and the incidence appears to be increasing.1 The current standard of care for athletes and active individuals with an ACL injury is arthroscopic ACL reconstruction (ACLR), and roughly 100,000 ACLR surgeries are performed annually 2 Patients with ACLR participate in months of rehabilitation to address impairments in knee range of motion, lower extremity strength, and lower extremity neuromuscular control before attempting to return to activity and/or sport participation.3 Despite extensive rehabilitation after ACLR, the risk for a secondary ACL injury is high.4‑6
A patient’s readiness to return to sport participation after ACLR is often determined from clinical tests, including single-leg hop tests.7 Research suggests that patients might demonstrate good performance on motor tasks like singleleg hop tests but require greater attention and neural effort to perform the motor task.8 This neural compensation, called neurocognitive reliance,9 could put patients at risk for secondary injury during sport participation because visual, auditory, and tactile information in the sporting environment must also be processed. Neurocognitive reliance can be exposed by pairing a motor task with a cognitive task (i.e., dual task training), such as reaction tests.9 Few studies have administered reaction tests to patients with ACLR, and these have focused on reaction time during simulated driving10 and tapping lights while standing.11
Advanced neuromuscular training is recommended to prepare patients for the physical demands of sport participation.12‑14 Typical interventions including plyometrics, dynamic stability exercises, and agility drills to provide physical challenges that exceed traditional rehabilitation and address residual neuromuscular deficits that could increase risk for re-injury.12‑14 Post-training improvements include better hop test performance, agility, and self-reported knee function.14‑16 Dual-task challenges, such as ball catches, may be implemented during training to challenge motor control and improve dynamic knee joint sta-
bility while simulating sport conditions, but these are not the primary focus. It is unknown if advanced neuromuscular training improves performance on reaction tests, including physical and cognitive measures.
This study examined changes in performance on visuomotor reactions tests and single-leg hop tests following advanced neuromuscular training in patients with ACLR. The hypothesis was that performance would improve less on reaction tests than on single-leg hop tests. Study findings can provide insight into dual-task performance after ACLR and the impact of advanced neuromuscular training.
This study used a quasi-experimental pretest-posttest design. Study participants completed testing immediately before and after participating in an advanced neuromuscular training program.
Patients with ACLR who enrolled in an advanced neuromuscular training program between January 2014 and June 2015 were eligible for this study Inclusion criteria were: (1) age 14–30 years at the time of surgery; (2) primary ACLR; (3) participated in organized sports that require cutting, jumping, or pivoting before injury; (4) intention to return to pre-injury activity level at the time of study participation. In addition, patients had to meet eligibility criteria for participating in advanced neuromuscular training: (1) at least five months postoperative; (2) symmetrical knee extension and knee flexion within five degrees of the contralateral knee; (3) able to hop vertically on the surgical leg without knee instability or pain; (4) trace effusion or less; and (5) surgeon approval. Exclusion criteria included previous lower extremity surgery or concomitant surgical procedures that alter postoperative rehabilitation (i.e., concomitant meniscal or ligamentous repair or cartilage stimulating procedure). Study criteria were meant to identify patients
with good potential to return to sport after ACLR. The study protocol was approved by the Institutional Review Board at the authors’ facility
Demographic variables collected from the electronic medical records included age, gender, body mass index (BMI), graft source, surgical side, concomitant surgeries, pre-injury sport, and time from surgery to pre-training and posttraining testing.
The advanced neuromuscular training program has been described previously 16 Briefly, the training program is cash-based (USD 300 at the time of the study) and offered to patients after they finish standard rehabilitation and received surgeon clearance to initiate a return to sport participation. Patients in the study were enrolled in the training program and were not compensated for the cost of the training. Training sessions were held in group format at a frequency of two times per week over five weeks and duration of two hours per session for a total of 20 hours of training. Groups consisted of up to six patients supervised by a physical therapist and athletic trainer who provided feedback on movement patterns.16,17 Each session began with a dynamic warm-up that lasted approximately 20 minutes, then patients performed the neuromuscular exercises prescribed for that session for approximately one hour, and the session ended with cool down exercises of stretching or foam rolling. Neuromuscular exercises were categorized as dynamic stability, plyometric, agility, and strengthening, and exercises were progressed in physical demand over the course of training (Table 1). If necessary, exercises were modified for patient safety Some exercises incorporated a partner ball toss (e.g. ball catch while kneeling on or jumping on/off the BOSU ball) or were performed with reaction to a verbal command (e.g. cone drills) or ball-bounce (e.g., partner race to reaction ball), but this was intended to improve dynamic knee stability,18 not to resolve neural compensation. The volume of dual-task challenges in the advanced training program was low and did not exceed more than two exercises per session.
Reaction tests were administered on a commercially available system consisting of a platform with five yellow dots on the surface connected to an electronic visual display (The QuickBoard, QuickBoard LLC, Memphis, TN) (Figure 1). The arrangement of the dots is two on the right side of the platform, two on the left side of the platform, and one in the center as on the number five side of a dice. Force sensors are located underneath the dots, and the arrangement of the dots is replicated on the visual display For the double-leg reaction test, patients stood with their feet positioned on either side of the center dot. Target dots were randomly presented on the visual display. The patient used the right leg to touch targets on the right side, the left leg
Table 1. Exercises in the Advanced Neuromuscular Training Program.
• Hop & hold
• Superman and swimmers on BOSU® ball
• Jump on/off BOSU® ball
• Hop & hold on Airex pad
• Kneeling on stability ball
• Hamstring curl on stability ball
• Tuck jumps (with or without hurdle)
• Box jump
• Scissor jump
• Lateral line hop (single- & double-leg)
• Lunge jump
• Various drills that incorporate running and shuffling with or without cones
• Dead lift
• Barbell squat
• Lunge
• Side plank
• Abdominal crunch
• Sidelying Copenhagen
• Rolling T (core strength progressions)
• Bridge (single- & double-leg)
1. Patient Position for Double-Leg (A) and Single-Leg (B) Reaction Tests.
to touch targets on the left side, and either of the legs to touch the center target. After the patient touched a target correctly, the next target was shown on the visual display. The test was performed for 20 seconds, and the number of correct touches and incorrect touches (i.e., errors) were recorded. The single-leg reaction test was first completed with the non-surgical leg. Patients stood on the nonsurgical leg positioned on the ipsilateral side of the center dot (e.g., right side of the center dot when the right leg was tested). Target dots were randomly shown on the visual display, and the patient hopped to the corresponding dot on the platform. When the target dot was touched, the next target was shown on the visual display. The test was complete when the patient had correctly hopped to 10 targets. Hop time was recorded in seconds, and the number of errors (i.e., incorrect touches) was recorded. The test was then repeated with the surgical leg.
The single leg hop tests were forward hop for distance, triple hop for distance, crossover triple hop for distance, and a six-meter timed hop.19 The non-surgical leg was tested first. For each hop test, patients received one or more practice trials and then performed three maximal effort test trials. The best performance was recorded in centimeters. A limb symmetry index was calculated as [(surgical limb performance/non-surgical limb performance) x 100] for distance measures and [(non-surgical limb performance/surgical limb performance) x 100] for the timed hop test. Testing was then repeated on the surgical leg.
No power analysis was conducted for this study. Data were analyzed with SPSS version 28 (IBM Statistics,). Descriptive statistics were computed for all variables, including means and standard deviations for continuous variables and frequency distributions for categorical variables. The ShapiroWilk test assessed normality of the measures.20 The double-leg reaction test measures were found to have non-normal distribution due to outliers with a high number of errors; however, the data points were confirmed and the variables are continuous, so parametric statistics were used for all analyses. Alpha was set at 0.05 to determine statistical significance.
Pre- to post-training changes in the number of correct touches and errors on the double-leg reaction test were examined with paired t-tests. Separate repeated measures general linear models (limb x time) examined changes in hop time and the number of errors on the single-leg reaction test. Although not the primary purpose of this study, pre-to-post training differences in the limb symmetry indexes on each single leg hop test were examined with paired t-tests. This analysis was intended to confirm expected improvements in physical performance following advanced neuromuscular training.15 Effect size (Cohen’s d) was computed for the pre- to post-training difference in number of correct touches on the double-leg reaction test, surgical side hop time on the single-leg reaction test, and surgical side hop distance or hop time on the single-leg hop tests. An effect size of 0.2 is considered a small effect size, 0.5 is considered moderate, and > 0.8 is considered large.21
A total of 43 patients were enrolled in the study and completed pre-training testing, but four patients were excluded from analysis for missing data at pre-training and an additional 14 patients were excluded for missing data at posttraining (Figure 2). The missing data resulted from a combination of technological issues and a change in the testing protocol. Thus, only the data from the 25 patients who completed the entire study were included in the analysis.
Demographic information is found in Table 2 No significant differences were found in gender or age between patients analyzed and those excluded from the analysis
2. Participant flow diagram.
(p>0.05). The patients in the analysis included 54% females and 73% had ACLR with bone-patellar tendon-bone autograft.
PRE- TO POST-TRAINING CHANGE IN PERFORMANCE ON REACTION TESTS
Scores on the reaction tests are found in Table 3. For the double-leg reaction test, the number of correct touches significantly increased from pre- to post-training (p<0.001), but there was no significant change in the number of errors (p=0.637). The highest number of errors at pre-training and post-training was 12. For the single-leg reaction test, the main effect of time was significant for hop time (p=0.003), indicating that hop time improved in both the surgical and non-surgical legs. No significant main effect was found for number of errors on the single-leg reaction test (p>0.05). At pre-training, the highest number of errors on the surgical side was 9 and on the non-surgical side was 12. At posttraining, the highest number of errors on the surgical side was n=6 and on the non-surgical side was n=9.
EFFECT SIZES FOR CHANGE IN PERFORMANCE ON REACTION TESTS AND SINGLE-LEG HOP TESTS
Scores on the single-leg hop tests are shown in Table 4 Limb symmetry indexes on each single-leg hop test significantly improved from pre- to post-training (p<0.05). Effect sizes for the pre-to post-training change in test performance varied according to the difficulty of the test; specifically, effect sizes decreased in magnitude from single-leg hop tests to double-leg reaction test to single-leg reaction test (Table 5). All effect sizes were moderate to large.
Table 2. Demographic Information
Patellar Tendon
Graft source, n
Surgical side, n
Concomitant surgeries, n
Values for continuous variables are mean (SD).
Mass Index
Table 3. Pre- and Post-Training Performance on the Double-Leg and Single-Leg Reaction Tests.
*p<0.05
**Main effect for time, p<0.05
EXPLORATORY POST-HOC ANALYSIS
In the dataset, 13 patients had post-training limb symmetry indexes ≥90% for all single-leg hop tests and 12 patients failed to achieve the criteria for at least one single-leg hop test (one hop test: n=3; two hop tests: n=2; three hop tests: n=3; four hop tests: n=4). Independent samples ttests compared post-training results on the double-leg reaction test and single-leg reaction test (surgical side only) between groups. No significant group differences were found in correct touches (p=0.737) or errors (p=0.771) on the double leg reaction test or in surgical side hop time (p=0.073) or errors (p=0.237) on the single-leg reaction test (Table 6).
This study examined changes in performance on reaction tests (dual-task) and single-leg hop tests (single-task) following advanced neuromuscular training in patients with ACLR. The study hypothesis was supported. Performance
improved from pre-training to post-training on both reaction tests and single-leg hop tests, but the effect sizes were generally lower for reaction tests than for single-leg hop tests. Additionally, the mean number of errors on the reaction tests did not significantly change. The findings indicate that advanced neuromuscular training improves motor task performance in patients with ACLR but does not prepare them as well for performing motor tasks that include a cognitive challenge (i.e, visuomotor reaction test).
Results of this study support the concept of neural compensation, specifically neurocognitive reliance, after ACLR.8 Neural cognitive reliance is demonstrated in the current study by improvement in motor performance on the reaction tests without a significant improvement in the number of errors. The highest number of errors in any patient on the single-leg reaction test was lower following training, and this indicates that individual results might differ from overall group results. Future work is needed to understand if neurocognitive reliance occurs in all, or only some, patients with ACLR and how advanced neuromuscu-
Table 4. Pre- and Post-Training Performance on Single Leg Hop Tests. Reported as mean (SD)
Forward Hop
Triple Hop
Crossover Triple Hop
Timed Hop
Table 5. Effect Sizes for the Pre- to Post-training Change in Performance on Reaction Tests and Single-Leg Hop Tests.
Table 6. Post-Training Performance on Reaction Tests in Patients Categorized by Criteria for Single-Leg Hop Tests (≥90% Limb Symmetry Index on All Hop Tests). Reported as mean (SD).
lar training should be augmented to reduce neurocognitive reliance.
The dual-task test used in this study was a lower extremity visuomotor reaction test. Study results add to a small body of literature on reaction tests in patients with ACLR and could be used as comparative data in future studies. The exploratory analysis found no significant difference in post-training reaction test performance based on clinical criteria for return-to-sport (i.e., ≥90% limb symmetry index
on single-leg hop tests). The finding adds to knowledge that single-task motor performance and dual-task motor performance are not mirrored.22,23 It is yet unknown which dual-task testing methods provides meaningful insight into readiness for sport participation after ACLR. Visuomotor tests have been recommended, and a variety of reaction tests demonstrate good reliability8,24; however, interpretation of reaction test performance requires a benchmark. Alternatively, a motor task (e.g., single-leg hop tests) can
be administered with and without a cognitive challenge so that a “dual-task cost” metric can be computed.9 For this method, the cognitive challenge requires thoughtful selection, and equipment may be needed to acquire an objective measure. As visuomotor tests are developed, it will be important to understand the association with return-to-sport outcomes to determine their contribution within a comprehensive testing battery
It has previously been posited that neuromuscular training with a focus on biomechanical factors (i.e., movement control) might be inadequate to address neural compensation after ACLR.8 This is supported in the current study by the smaller effect size change in reaction test performance compared to single-leg hop test performance. The advanced neuromuscular training program incorporated some dualtasks conditions, such as catching a ball while balancing on an unstable surface, to challenge postural stability and dynamic joint stability, but a greater volume of dual-task challenges appears to be needed. It is yet unclear what training methods might be most effective. Positive changes in dual-task performance been reported in uninjured athletes after visuomotor25 and cognitive training on a computer,26 suggesting different methodologies for providing neurocognitive tasks in rehabilitation may be effective in minimizing neurocognitive reliance.
The strengths of this study are the application of novel reaction tests in patients with ACLR before and after participation in an advanced neuromuscular training program. The results of this study provide insight into dual-task visuomotor performance after ACLR as well as the impact of advanced neuromuscular training. The single-leg hop tests in the testing protocol are commonly used in return-tosport testing7 and provided a comparator to the reaction tests. The primary limitation of this study is the small sample size, which is partly due to the relatively large attrition from the initially included population. The attrition could
contribute to bias in the study if patient characteristics were different between those who did or did not complete the study Study bias may also be present because the advanced training program was cash-based, which means patients needed to be motivated and have financial means to participate. Another limitation is potential confounding from the dual-task challenges in the advanced neuromuscular training program that may have contributed to improved performance on the reaction tests. More of these dual-task challenges, or different challenges, may be needed for improving errors on the reaction tests. An additional limitation is the lack of a control group that would aid the understanding of performance changes that could be attributed to recovery over time. A final limitation is the lack of isokinetic quadriceps strength data. At the time of this study, the clinic did not own an isokinetic dynamometer Knowledge of quadriceps strength would aid the interpretation of neural compensation as patients with lower quadriceps strength (i.e., better motor reserve) are expected to demonstrate greater neural compensation.9
Motor performance in patients with ACLR improved after advanced neuromuscular training but the effects on visuomotor reaction tests were less than those effects seen on single-leg hop tests. The results suggest the persistence of neurocognitive reliance after ACL and for more dual-task challenges in advanced neuromuscular training to minimize neurocognitive reliance that might increase the risk for re-injury when returning to sport.
The authors declare that they have no conflict of interest.
Submitted: February 15, 2022 CDT, Accepted: October 03, 2024 CDT
©
The Author(s)
This is an open-access article distributed under the terms of the Creative Commons Attribution 4.0 International License (CCBY-NC-4.0). View this license’s legal deed at https://creativecommons.org/licenses/by-nc/4.0 and legal code at https://creativecommons.org/licenses/by-nc/4.0/legalcode for more information.
1. Beck NA, Lawrence JTR, Nordin JD, DeFor TA, Tompkins M. ACL tears in school-aged children and adolescents over 20 Years. Pediatrics. 2017;139(3). doi:10.1542/peds.2016-1877
2. Cimino F, Volk BS, Setter D Anterior cruciate ligament injury: diagnosis, management, and prevention. Am Fam Physician 2010;82(8):917-922.
3. Della Villa F, Andriolo L, Ricci M, et al. Compliance in post-operative rehabilitation is a key factor for return to sport after revision anterior cruciate ligament reconstruction. Knee Surg Sports Traumatol Arthrosc. 2020;28(2):463-469. doi:10.1007/ s00167-019-05649-2
4. Di Stasi S, Myer GD, Hewett TE. Neuromuscular training to target deficits associated with second anterior cruciate ligament injury J Orthop Sports Phys Ther 2013;43(11):777-792. doi:10.2519/ jospt.2013.4693
5. Grindem H, Snyder-Mackler L, Moksnes H, Engebretsen L, Risberg MA. Simple decision rules can reduce reinjury risk by 84% after ACL reconstruction: the Delaware-Oslo ACL cohort study Br J Sports Med 2016;50(13):804-808. doi:10.1136/ bjsports-2016-096031
6. Wiggins AJ, Grandhi RK, Schneider DK, et al. Risk of Secondary injury in younger athletes after anterior cruciate ligament reconstruction: A systematic review and meta-analysis. Am J Sports Med. 2016;44(7):1861-1876. doi:10.1177/ 0363546515621554
7. Turk R, Shah S, Chilton M, et al. Return to sport after anterior cruciate ligament reconstruction requires evaluation of >2 functional tests, psychological readiness, quadriceps/hamstring strength, and time after surgery of 8 months. Arthroscopy. 2023;39(3):790-801. doi:10.1016/ j.arthro.2022.08.038
8. Grooms D, Appelbaum G, Onate J. Neuroplasticity following anterior cruciate ligament injury: a framework for visual-motor training approaches in rehabilitation. J Orthop Sports Phys Ther 2015;45(5):381-393. doi:10.2519/jospt.2015.5549
9. Grooms DR, Chaput M, Simon JE, et al. Combining neurocognitive and functional tests to improve return-to-sport decisions following ACL reconstruction. J Orthop Sports Phys Ther. 2023;53(8):415-419. doi:10.2519/jospt.2023.11489
10. Salem HS, Park DH, Friedman JL, et al. Return to driving after anterior cruciate ligament reconstruction: A systematic review. Orthop J Sports Med 2021;9(1):2325967120968556. doi:10.1177/ 2325967120968556
11. Reiche E, Collins K, Genoese F, et al. Lower extremity reaction time in individuals with contact versus noncontact anterior cruciate ligament injuries after reconstruction. J Athl Train. 2024;59(1):66-72. doi:10.4085/1062-6050-0428.22
12. Buckthorpe M. Optimising the late-stage rehabilitation and return-to-sport training and testing process after ACL reconstruction. Sports Med 2019;49(7):1043-1058. doi:10.1007/ s40279-019-01102-z
13. de Mille P, Osmak J. Performance: Bridging the gap after ACL surgery Curr Rev Musculoskelet Med 2017;10(3):297-306. doi:10.1007/s12178-017-9419-2
14. Nyland J, Greene J, Carter S, et al. Return to sports bridge program improves outcomes, decreases ipsilateral knee re-injury and contralateral knee injury rates post-ACL reconstruction. Knee Surg Sports Traumatol Arthrosc 2020;28(11):3676-3685. doi:10.1007/s00167-020-06162-7
15. Meierbachtol A, Obermeier M, Yungtum W, et al. Advanced training enhances readiness to return to sport after anterior cruciate ligament reconstruction. J Orthop Res. 2022;40(1):191-199. doi:10.1002/ jor.25072
16. Meierbachtol A, Yungtum W, Paur E, Bottoms J, Chmielewski TL. Psychological and functional readiness for sport following advanced group training in patients with anterior cruciate ligament reconstruction. J Orthop Sports Phys Ther. 2018;48(11):864-872. doi:10.2519/jospt.2018.8041
17 Myer GD, Paterno MV, Ford KR, Hewett TE. Neuromuscular training techniques to target deficits before return to sport after anterior cruciate ligament reconstruction. J Strength Cond Res. 2008;22(3):987-1014. doi:10.1519/ JSC.0b013e31816a86cd
18. Williams GN, Chmielewski T, Rudolph K, Buchanan TS, Snyder-Mackler L. Dynamic knee stability: current theory and implications for clinicians and scientists. J Orthop Sports Phys Ther. 2001;31(10):546-566. doi:10.2519/ jospt.2001.31.10.546
19. Noyes FR, Barber SD, Mangine RE. Abnormal lower limb symmetry determined by function hop tests after anterior cruciate ligament rupture. Am J Sports Med. 1991;19(5):513-518. doi:10.1177/ 036354659101900518
20. Mishra P, Pandey CM, Singh U, et al. Descriptive statistics and normality tests for statistical data. Ann Card Anaesth. 2019;22(1):67-72. doi:10.4103/ aca.ACA_157_18
21. Sullivan GM, Feinn R. Using effect size-or why the P value is not enough. J Grad Med Educ. 2012;4(3):279-282. doi:10.4300/JGME-D-12-00156.1
22. Kiani Haft Lang M, Mofateh R, Orakifar N, Goharpey S. Differences in neurocognitive functions between healthy controls and anterior cruciate ligament-reconstructed male athletes who passed or railed return to sport criteria: A preliminary study. J Sport Rehabil 2023;32(6):645-654. doi:10.1123/ jsr.2022-0288
23. Wilke J, Vogel O, Ungricht S. Traditional neuropsychological testing does not predict motorcognitive test performance. Int J Environ Res Public Health. 2020;17(20). doi:10.3390/ijerph17207393
24. Wilke J, Vogel O, Ungricht S. Can we measure perceptual-cognitive function during athletic movement? A framework for and reliability of a sports-related testing battery. Phys Ther Sport. 2020;43:120-126. doi:10.1016/j.ptsp.2020.02.016
25. Lucia S, Bianco V, Di Russo F. Specific effect of a cognitive-motor dual-task training on sport performance and brain processing associated with decision-making in semi-elite basketball players. Psychol Sport Exerc. 2023;64:102302. doi:10.1016/ j.psychsport.2022.102302
26. Wilke J, Vogel O Computerized cognitive training with minimal motor component improves lower limb choice-reaction time. J Sports Sci Med 2020;19(3):529-534.
Wohl TR,
CR,
AL,
DR. The Impact of Visual Perturbation Neuromuscular Training on Landing Mechanics and Neural Activity: A Pilot Study. IJSPT. 2024;19(11):1333-1347. doi:10.26603/001c.123958
Timothy R Wohl1,2 , Cody R Criss3,4,5 , Adam L Haggerty5,6 , Justin L Rush5,7 , Janet E Simon5,6 , Dustin R Grooms5,6,7a
1 Department of Physical Therapy, The Ohio State University, 2 Honors Tutorial College, Ohio University, 3 Translational Biomedical Sciences Program, Ohio University, 4 Heritage College of Osteopathic Medicine, Ohio University, 5 Ohio Musculoskeletal & Neurological Institute, Ohio University, 6 Department of Athletic Training, Ohio University, 7 Department of Physical Therapy, Ohio University
Keywords: anterior cruciate ligament, neuroimaging, fMRI, injury prevention https://doi.org/10.26603/001c.123958
International Journal of Sports Physical Therapy Vol. 19, Issue 11, 2024
Background
Athletes at risk for anterior cruciate ligament (ACL) injury have concurrent deficits in visuocognitive function and sensorimotor brain functional connectivity
Purpose
This study aimed to determine whether visual perturbation neuromuscular training (VPNT, using stroboscopic glasses and external visual focus feedback) increases physical and cognitive training demand, improves landing mechanics, and reduces neural activity for knee motor control.
Design
Controlled laboratory study
Methods: Eight right leg dominant healthy female athletes (20.4±1.1yrs; 1.6±0.1m; 64.4±7.0kg) participated in four VPNT sessions. Before and after VPNT, real-time landing mechanics were assessed with the Landing Error Scoring System (LESS) and neural activity was assessed with functional magnetic resonance imaging during a unilateral right knee flexion/extension task. Physical and cognitive demand after each VPNT session was assessed with Borg’s Rating of Perceived Exertion (RPE) for both physical and cognitive perceived exertion and the NASA Task Load Index. Descriptives and effect sizes were calculated.
Results
Following VPNT, LESS scores decreased by 1.5 ± 1.69 errors with a large effect size (0.78), indicating improved mechanics, and reductions in BOLD signal were observed in two clusters: 1) left supramarginal gyrus, inferior parietal lobule, secondary somatosensory cortex (p=.012, z=4.5); 2) right superior frontal gyrus, supplementary motor cortex (p<.01, z=5.3). There was a moderate magnitude increase of cognitive RPE between the first and last VPNT sessions.
Conclusion
VPNT provides a clinically feasible means to perturbate visual processing during training that improves athletes’ real-time landing mechanics and promotes neural efficiency for lower extremity movement, providing the exploratory groundwork for future randomized controlled trials.
Corresponding Author: Dustin Grooms
Grover Center W283, 1 Ohio University, Athens OH 45701-2979 groomsd@ohio.edu
Level 3
The anterior cruciate ligament (ACL) is a static knee stabilizer that is commonly injured in sports.1,2 The incidence of ACL injury has continued to rise over the years, with young female athletes being at an increased risk for noncontact ACL injuries compared to males.3‑6 Injury prevention programs (IPP) are a common tool used to reduce the incidence of ACL tears, but these programs have limited efficacy, as they require ~100 participants to prevent a single ACL injury and may not target all aspects of injury risk.7,8 Typical IPPs target biomechanical risk factors associated with ACL injuries, such as knee valgus and stiff-legged landing patterns.9,10 However, the efficacy of IPPs may be limited by treatment approaches targeting biomechanical outputs without accounting for critical nervous system processes that contribute to injury risk.11‑13
Dynamic knee valgus, or collapse of the knee toward midline during landing, is a specific and sensitive biomechanical risk factor for ACL injury,9 which has been associated with neurocognitive deficits14 (e.g., visual attention, reaction time) and increased sensorimotor and visuospatial neural activity12,13 for knee movement during functional magnetic resonance imaging (fMRI). Taken together, these studies suggest athletes at increased risk of ACL injury employ sensorimotor control strategies highly influenced by the visuocognitive system. Further support comes from three prospective studies: one that identified baseline visuospatial deficits in uninjured athletes who later sustained an ACL injury15; and two studies that used resting state MRI to identify decreased sensorimotor brain functional connectivity in uninjured athletes who later sustained an ACL injury 11,16 Taken together, these neurophysiological and neurocognitive data support the influence of sensory and visuospatial cognition on injuryrisk. Therefore, incorporating visuocognitive training into IPPs may improve their efficacy by preparing athletes for the visually and cognitively demanding dynamic sport environment.
A simple and relatively cost-effective way that IPPs could add visuocognitive training is by incorporating stroboscopic glasses (SG).17 SG allow for portable visual perturbation by alternating between clear and opaque lens states at controllable frequencies. This high degree of control enables clinicians/athletic trainers/coaches to incrementally perturb the amount of visual information available to their athletes during neuromuscular training. SG has previously been used in a research setting to assess training effects on behavioral performance, neurocognitive function, and sport-specific abilities,18‑28 but there is a paucity of research quantifying neural activity changes following visuocognitive training with SG. Therefore, we developed visual perturbation neuromuscular training (VPNT, injury prevention exercises29,30 overlayed with visual perturbation via SG and external focus feedback to direct attention to the environment) to fill this gap. The purpose
of this study was to determine changes in landing mechanics, neural activity (quantified using blood-oxygen-leveldependent [BOLD] signal) for knee motor control, and physical and cognitive training demands in young female athletes following VPNT
This study was approved by Ohio University’s Institutional Review Board, and all experiments were performed in accordance with the approved protocol. Pre-/post-intervention procedures and VPNT were carried out by one researcher (TW). Female recreational athletes (at least 3 hours of moderate-to-vigorous exercise per week, including one hour of running, cutting, pivoting, or decelerating every week) aged 18-30 were included. Eight female participants (8 F; age = 20.4 ± 1.1 years, height = 1.6 ± 0.1 m, mass = 64.4 ± 7.0 kg) from Ohio University (Athens, OH) were enrolled to participate in this study, and informed written consent was obtained prior to participation. All participants were right leg dominant and met the exercise requirement criteria, as determined by the Marx Activity Rating Scale,31 which assesses one’s general level of activity on four metrics indicating involvement in activities requiring running and rapid change of direction (run=2.6±0.7, cut=1.9±1.6, decelerate=1.9±1.6, pivot=2.1±1.5). No formal power analysis was completed as these data are exploratory and meant to be hypothesis-generating.
Participants were excluded who were contraindicated for fMRI (e.g., pregnancy, implanted metal devices, claustrophobia, and any other criteria as determined by the MRI operator), had visual impairments, had a history of seizures or epilepsy, or had a history of surgery on the back, hip, leg, knee, etc. Other screening criteria included: primary sport, leg dominance, and exclusion for previous leg injury or medical history of anxiety disorder, ADHD, depression, diabetic neuropathy, concussion or traumatic brain injury, cerebral palsy, balance disorder, vertigo, Parkinson’s disease, multiple sclerosis, substance abuse or dependence, heart disease/defect.
PRE-/POST-INTERVENTION LANDING ERROR SCORING SYSTEM (LESS) ASSESSMENT
The LESS is a clinical tool used to assess lower extremity injury risk by identifying injury risk landing mechanics during a jump-landing task.32,33 To ensure participants were enrolled that would benefit from the training, potential subjects were screened and only enrolled participants with high injury risk landing mechanics (LESS score of 6+). This cutoff was selected as prior work validated the 6+ error threshold with 3D motion capture to discriminate between high and low risk biomechanics.34 A total of 20 athletes were screened and eight met the enrollment criteria.
A designated experimenter (TW) was trained to evaluate jump-landing mechanics in real-time using the LESS. For training, TW rated the landing biomechanics of athletes using the LESS while watching 50+ videos from frontal and sagittal views. TW’s ratings were compared with an expert’s ratings (with 10+ years of clinical experience) until TW’s ratings were reliable and valid (over 90% agreement).35‑37 The LESS methods have previously demonstrated good to excellent intra- (ICC: .82-.99) and interrater (ICC: .83-.92) reliability as well as good intersession reliability (ICC: .81).36,38
Participants performed the jump-landing task for the LESS four times: twice with a face-forward frontal view and twice with a right (dominant) sagittal view Participants performed the jump-landing task on a 30 cm tall box, landed at a distance equal to half their height, and were given unlimited practice trials until they could perform the task correctly and explicitly follow test instructions.32 Participants received the following instructions and no additional feedback: 1) stand at the edge of the box in a neutral position, 2) jump forward so both your legs leave the box simultaneously, 3) land just past the target line, and 4) jump for maximal height immediately after landing Trials were considered successful if the participant jumped from the box using both feet, cleared the minimum distance and performed the task in a fluid motion. All unsuccessful trials (e.g., the participant jumped vertically from the box) were excluded and repeated until four successful jumps were completed.32 After the final VPNT training session, participants completed the same jump-landing task to determine intervention efficacy of reducing high injury risk landing biomechanics. Total LESS score was used for statistical analyses pre- and post-intervention.
All participants completed a pre- and post-intervention fMRI neuroimaging session (~45 minutes including set-up, instruction, and scan time). During imaging, all participants wore standardized shorts and socks without shoes to reduce the possibility of altered skin tactile feedback. Participants also wore a splint to lock their right (dominant leg) ankle at neutral (~0 degrees) to minimize ankle movement throughout the scan. Headphones and hearing protection were provided for safety and communication. While lying supine in the scanner, participants were strapped down to the table with four straps: one across the thighs at the mid-point between the greater trochanter and knee joint line, one across the hips at the anterior superior iliac spines, and two in an X pattern across the chest from each shoulder to the pelvis. Participants were also fitted with customized padding to reduce head motion. This padding was high-density MRI-safe foam that was inserted around the sides and top of the head to remove space between the skull and head coil. This was customized based on skull size. The straps and customized padding were employed to reduce head motion during imaging. A priori head motion threshold for exclusion was set at >0.35 mm of relative motion to ensure high quality data.
The methods used for the fMRI knee motor control task are based on previous literature that mapped whole brain activity and lateralization of the brain activity during isolated lower extremity movements.39,40 Similar knee specific motor tasks have demonstrated good to excellent reliability across days to weeks.41‑43 Standardized auditory cues informed the participants when to move and rest, and the frequency of the movement (1.2 Hz). The participants’ dominant (right) leg rested upon a foam roller and alternated from ~40 degrees flexion to full extension, while the nondominant (left) leg rested at full extension (0 degrees). This motion was completed continuously for 30 seconds with 30 seconds of rest (right leg relaxed at ~40 degrees flexion) for four total cycles. The participants were given an opportunity to ask questions and practice the task with feedback from the experimenter
Prior to data collection at the MRI, participants completed a mock MRI session where they familiarized themselves with the MRI environment, restraints to reduce head motion, and the lower extremity motor task. The participants were permitted to ask questions and practice the tasks with feedback from the experimenter. The practice session included three practice blocks (30 seconds each) of the motor task with examiner cueing to ensure the participant understood the task, followed by a complete run of the task with the same feedback and timing as during the actual MRI data collection session. This standardized training and mock scanning session played a vital role in reducing head motion during the task, performing the task correctly, and ensuring participants were not claustrophobic.
fMRI scans were collected at Holzer Health (Athens, OH) using a 16-channel head coil. Prior to the functional data collection, a 3-diminsional high-resolution T1-weighted image (repetition time: 2000 ms, echo time: 4.58 ms, field of view: 256×256 mm; matrix: 256×256; slice thickness 1 mm, 176 slices, 8° flip-angle) was collected for image registration (~8 minutes). fMRI collection parameters included 10 whole-brain gradient-echo-echo planar scans per block (4 movement blocks, 5 rest blocks) acquired with a 3 second TR with anterior-posterior phase encoding and a 3.75×3.75 in plane resolution, 5 mm slice thickness for 38 axial slices with a 35 ms TE, 90° flip angle, field of view 240 mm and 64×64 matrix. The functional knee extension/flexion run lasted 4 minutes and 30 seconds. fMRI measured regional brain activity during rest and motor control conditions, which were contrasted to isolate the regional brain activity to the knee flexion/extension task.
All participants completed four separate one hour-long training sessions within two to three weeks. These sessions combined agility, balance, and plyometric exercises with visual perturbation training. VPNT exercises were developed based on a previous clinical commentary and methodology paper,44 detailing how chosen exercises were selected to offset the risk of initial ACL rupture and further modified to incorporate a movement goal that required the participants to interact with an external visual object or target.30,
45,46 Each training session spanned one hour with rest interspersed throughout the session to avoid fatigue.
I. AGILITY EXERCISES
• Exercise 1: The T-test involved running 6 m to tap a cone, cutting to the right or left for 3 m to tap another cone, cutting the opposite direction for 6 m to tap a third cone, returning to the center by cutting 3 m to tap the first cone, then running 6 m back to the start position – thereby running in a “T” shape. The experimenter verbally instructed the participant which direction to cut after the participant left the start position, but prior to reaching the first cone reducing ability to plan direction and anticipatory time.
• Exercise 2: Agility ladder drills required the participant to quickly match specified foot placement patterns within the agility ladder The experimenter used five visual aids to instruct each participant on how to perform the foot placement pattern to minimize direct explicit feedback (cones, lines etc.) and increase the salience of the SG visual perturbation.
II. BALANCE EXERCISES
• Exercise 1: Single-leg Romanian deadlifts required the participant to pick up and gently set down a cup at one of three locations marked on the ground (-30, 0, +30 degrees from center) at a distance equal to his or her max volitional reaching distance. The experimenter verbally instructed the participant of which location to place the cup at random. The participant alternated legs for each trial.
• Exercise 2: Single-leg stance required the participant to stand on a foam surface while holding a lightweight bar horizontally for 30 seconds. The experimenter instructed the participant to maintain the bar’s horizontal orientation and to quickly reset her single-leg stance if the participant placed a second limb on the ground. The participant alternated legs for each trial.
III PLYOMETRIC EXERCISES
• Exercise 1: The vertical jump task required participants to reach 80% of their max vertical jump height as measured by a Vertec, a vertical jump tester that serves as the external target during the task. Additionally, the vertical jump task incorporated an unanticipated unilateral landing, where the experimenter called out the desired landing leg as soon as the participant began the flight phase of the jump.
• Exercise 2: The jump squat task incorporated a cognitive challenge: participants had to perform a jump squat and land facing a target in one of four locations (0, 90, 180, 270 degrees from the participant in the center); the specified location was quickly called out at random by the experimenter
Table 1. Error scoring system used to assess behavioral performance.
Exercise Error Count T-test
1. Miss a cone
2. Cut to the wrong direction
Agility Ladder Drills
Single-leg Deadlifts
Single-leg Stance (on foam)
Vertical Jumps Squat Jumps
1. Hit the ladder
2. Incorrect foot placement
1. Opposite foot touches ground
2. Either hand touches ground
3. Object placed in wrong location
1. Opposite foot touches ground
2. Either hand touches ground
1. Miss the target
2. Land on wrong foot
1. Land facing wrong direction
All participants initially performed all exercises without SG to familiarize themselves with the task at every training session. SG were then worn throughout all other trials for each exercise. For the first training session, SG remained at level 1 throughout the whole training session (highest frequency of fluctuation between clear and opaque states and the lowest level of visual perturbation). For the second, third, and fourth training sessions, SG remained at levels 2, 3 and 4, respectively, for the duration of each training session. SG’s opaque state duration progressively lengthened with each subsequent training session (25 ms for level 1, 43 ms for level 2, 67 ms for level 3, and 100 ms for level 4) while the clear state duration remained constant across SG levels (100 ms), thereby creating greater interruptions in the availability of visual information for the participants. This approach standardizes SG difficulty exposure for each participant.
An error scoring system (Table 1) assessed behavioral performance during training sessions. Scores are reported as counts, which reflect the number of errors incurred during each exercise. All errors are counted equally (one count per error).
The Rating of Perceived Exertion (RPE) scale47 and NASA Task Load Index (NASA TLX)48‑51 were presented to the athletes after each training session to assess perceived levels of difficulty performing exercises with SG. RPE assesses perceived exertion level on a scale from 0 (Nothing at All) to 10 (Very, Very Hard). Both physical and cognitive RPE were assessed. Physical exertion refers to the physical work
of the athlete to perform the exercise. Cognitive exertion refers to the mental work required of the athlete to complete the exercise goal. NASA TLX assesses mental workload on six separate scales (mental demand, physical demand, temporal demand, performance, effort, frustration), each with 21-point scale gradations.
The fMRI technique used in this study quantified the BOLD signal via the hemodynamic response (blood flow) by contrasting the lower extremity motor control condition with interspersed rest conditions.52 The BOLD response quantified via fMRI collection and analysis has been validated against actual neural recordings, demonstrating a very high correlation between blood flow and neural activity 53,54 The reliability of fMRI quantification of the BOLD signal is generally high and specific to knee movement and has high inter-session reliability.43,55
The fMRI image analyses and statistical analyses were performed using the Oxford Centre for Functional MRI of the Brain Software Library.56 Image analysis began with standard pre-statistic processing applied to individual data in the standardized FSL recommended order,57 which included nonbrain removal, slice timing correction, standard motion correction and realignment parameters (3 rotations and 3 translations) as covariates to limit confounding effects of head movement and spatial smoothing at 6 mm before statistical analysis.58 High-pass temporal filtering at 100 Hz and time-series statistical analyses were carried out using a linear model with local autocorrelation correction. Functional images were coregistered with the respective high-resolution T1 image and the standard Montreal Neurological Institute template 152 using linear image registration. This registration process allowed data from each participant to be spatially aligned on a standardized brain template for comparison.
The subject-level analysis of knee sensorimotor control relative to rest was completed using a cluster corrected zscore greater than 3.1 and significance threshold of p<.05 (corrected). The cluster correction for multiple comparisons uses a variant of the Gaussian random field theory to decrease type I error in statistical parametric mapping of imaging data by evaluating the activation not only at each voxel, but also at the surrounding voxel cluster (as it is unlikely that the voxel tested and surrounding voxels are active above the threshold due to chance).59‑61 The paired contrast between the pre- and post-intervention brain activity was cluster corrected with z scores greater than 3.1 and a significance level of p<.05 (corrected). This pair-wise analysis compares each individual’s pre-/post-intervention brain activity and averages those differences at the group level.
Descriptive statistics including means, standard deviations, 95% confidence intervals (CIs), and Hedge’s g effect sizes were calculated for the LESS, error scores during each training session, RPE, and NASA TLX. With this being a proof-of-concept study with a small sample size, inferential statistics were not calculated. To evaluate the impact of VPNT on these variables, Hedge’s g effect sizes were used
because of the small sample size and interpreted as 0.0-0.3 as small, 0.4-0.5 as medium, and 0.6-0.8 as large.62
Descriptive statistics including 95% CIs and effect sizes for the LESS, error scores, RPE, and NASA TLX are provided in Table 2 All re-assessments were completed within 23 ± 4.3 days from initial assessments. LESS scores decreased by 1.5 ± 1.69 errors with a large effect size after VPNT Regarding individual participant’s changes in LESS scores, 5 participants’ scores decreased, 3 did not change, and 0 increased after VPNT (Figure 1). Agility errors from day 1 to day 4 of VPNT had a medium effect size with decreased errors of -2.6±5.2. Lastly, RPE-C had a large effect size between day 1 and day 4 of VPNT with an increase of 1.5±2.2 points.
Regional brain activity are reported that were identified in FSLeyes based on peak-voxel with the Harvard-Oxford Cortical & Subcortical Structural Atlas,63 Juelich Histological Atlas,64,65 and the Cerebellar Atlas in MNI152 space after normalization with FNIRT66 and with FSL tool atlasquery.57 The atlasquery function from FSL utilizes the averaged probability across all voxels in the cluster to identify probabilistic anatomy across the cluster ensuring reporting of peak voxel location and overall cluster spatial representation.
The results are presented as a z-score (activation level relative to the contrast of pre- vs post-intervention) and percent signal change for each group from baseline to sensorimotor control in Table 3 By comparing pre- and postintervention regional brain activity, reduced BOLD signals were demonstrated in two clusters following VPNT (Figure 2, Table 3): 1) left supramarginal gyrus, inferior parietal lobule, secondary somatosensory cortex (158 voxels; 4.51 zstat; p=0.0122 cluster corrected; MNI -50,-26,34); 2) right superior frontal gyrus, supplementary motor cortex (314 voxels; 5.33 z-stat; p=0.000159 cluster corrected; MNI 16,-4,72). The clusters are the group average of the paired contrasts of each participant at pre/post timepoints (n=7). One participant was excluded from the fMRI analysis due to excessive head motion (>0.35 mm relative head motion and task correlated). Average relative head motion across the remaining seven participants was 0.11±0.05 mm and absolute head motion was 0.35±.19 mm.
Each participant’s landing mechanics were evaluated with the LESS at pre-/post-intervention timepoints to assess VPNT effects on biomechanical ACL injury risk. There was a decrease in LESS score after VPNT, indicating that VPNT can improve landing mechanics and potentially reduce injury risk. The mean difference in LESS score after VPNT (1.5±1.69) is similar to other reports of improved LESS scores after injury prevention programs, despite the relatively shorter intervention of only two weeks.67‑71 For example, an aquatic injury prevention program that only in-
Table 2. Descriptive statistics of the LESS (yellow), error scoring system (blue), RPE (green), and NASA-TLX (orange). Values for each training session (days 1, 2, 3, 4) were averaged across all participants (n=8) and reported as mean ± standard deviation (95% CI). The difference between the first and last session (Day 4 – Day 1) is reported as mean ± standard deviation (effect size). *For the LESS, “Day 1” and “Day 4” correspond to testing days before and after Days 1 and 4 of training, respectively Abbreviations: SLD (single-leg deadlift); SLS (single-leg stance); RPE (Rating of Perceived Exertion); RPE–C (RPE, Cognitive); RPE–P (RPE, Physical); NASA (NASA Task Load Index); NASA–M (NASA, Mental); NASA–Ph (NASA, Physical); NASA–T (NASA, Temporal); NASA–PE (NASA, Performance); NASA–E (NASA, Effort); NASA–F (NASA, Frustration).
(0.08) SLS 8.0 ± 5.9 (3.9, 12.1) 5.0 ± 4.2 (2.1, 7.9) 4.0 ± 4.6 (0.8, 7.2) 5.4 ± 6.37 (1.0, 9.8) -2.6±9.9 (-0.37)
Jump Squat 4.6 ± 3.5 (2.2, 7.0) 4.4 ± 2.4 (2.7, 6.1) 4.9 ± 1.5 (3.9, 5.9) 4.1 ± 2.5 (2.4, 5.8) -0.5±2.7 (-0.15)
Vertical Jump 5.1 ± 3.0 (3.0, 7.2) 3.9 ± 2.4 (2.2, 5.6) 4.5 ± 3.7 (1.9, 7.1) 3.9 ± 3.2 (1.7, 6.1)
(-0.34) RPE–C 3.0 ± 1.1 (2.2, 3.8) 2.9 ± 1.1 (2.1, 3.7) 3.8 ± 2.6 (2.0, 5.6) 4.5 ± 2.5 (2.8, 6.2) 1.5±2.2 (0.69) RPE–P 3.6 ± 1.5 (2.6, 4.6) 3.9 ± 1.8 (2.7, 5.2) 3.6 ± 1.7 (2.4, 4.8) 4.1 ± 2.0 (2.7, 5.5) 0.5±2.5 (0.25) NASA–M 9.0 ± 4.3 (6.0, 12.0) 8.5 ± 4.3 (5.5, 11.5) 10.0 ± 6.1 (5.8, 14.2) 10.8 ± 6.3 (6.4, 15.2) 1.8±5.0 (0.30)
NASA–Ph 9.6 ± 4.1 (6.8, 12.4) 9.9 ± 4.2 (6.7, 12.8) 9.3 ± 3.9 (6.6, 12.0)
cluded female participants and lasted for six weeks improved LESS scores by 1.68±1.68 (p=0.004).67 Another study that included mixed-gender youth soccer teams implemented a prevention training program led by athletic trainers for eight weeks and yielded similar results (1.29±0.34, p=0.01).68 The ability of VPNT to achieve similar behavioral outcomes as traditional IPPs in a shorter timeframe could improve athlete/coach compliance for teams with limited resources and time.
It is important to note that while most participants (n=5) in the present study experienced improved landing mechanics after the intervention, some participants (n=3) did not change scores and no participants experienced worsened (higher) LESS scores. Therefore, while VPNT may preferentially improve some athletes’ landing mechanics more than others, no athlete experienced worsened per-
formance after training. In comparison to previous studies67,71 that used LESS to measure an injury prevention program’s efficacy and reported an increase in LESS score post-intervention for some select participants, the current study may lack such findings due to the individualized nature of the training sessions, the addition of SG and external focus feedback, or small sample size. Further, Gholami et al.26 showed that training with visual information disruption improved landing mechanics in a larger study with athletes who already completed post-op ACL reconstruction rehabilitation.
Motor performance errors were tracked during each training session that consisted of objective, clinicalfriendly measures of motor performance (e.g., the number of times an athlete placed their opposite foot down during a single-leg balance task). As SG difficulty increased with
Figure 1. Left: Individual participant LESS scores at pre- and post-VPNT timepoints; two subjects with identical scores (pre/post LESS of 6) overlap and share the same circle/line. Right: Within-subject differences, with the mean of difference represented by the solid horizontal line.
Figure 2. Cross-sections of composite brains that reflect the two clusters with statistically significant decreased neural activity following VPNT. Cluster 1: left supramarginal gyrus, inferior parietal lobule, secondary somatosensory cortex. Cluster 2: right superior frontal gyrus, supplementary motor cortex.
each training session, the error scores did not substantially change between Day 1 and Day 4 of training for all exercises except for the agility ladder drills, which had a medium effect size of decreased errors (Table 2). Taken together, the training effects on error scores indicate that progressing SG difficulty between levels 1 through 4 does not impair motor performance as assessed by our clinician error scoring system (Table 1). Therefore, decreasing the amount of vi-
sual information available to athletes with SG did not hinder participant’s ability to complete their desired movement goals/objectives during training.
Another aim of this study was to quantify the brain activation changes after VPNT during a knee flexion/extension task. Cluster 1 of decreased activation included the left supramarginal gyrus, inferior parietal lobule and secondary somatosensory cortex, indicating increased neural efficiency (i.e., reduced neural activity demands) in visuospatial/visuomotor processing,72‑76 somatosensory integration, and attention.77‑79 Cluster 2 of decreased activation included the right superior frontal gyrus and supplementary motor cortex, indicating increased neural efficiency in motor planning.80,81 Overall, neural efficiency in both clusters may reflect training effects from VPNT, which aimed to improve neuromuscular control and the neural activity associated with baseline high injury risk landing mechanics (e.g., increased visuospatial and sensorimotor neural activity).82 Decreased activity in both clusters aligns with motor skill learning83‑85 and improved expertise86,87 specific to lower extremity motor control. Recently, Grooms et al.,88 similarly examined brain activation changes during a knee flexion/extension task in healthy female athletes who underwent neuromuscular training with implicit augmented biofeedback and identified an association between sensory neural activity changes and improved landing mechanics.
The current results are also similar to prior work by Seidel et al.87 that measured brain activity with functional near-infrared spectroscopy during balance training, finding decreased neural activity pre to post-training in the primary motor cortex and inferior parietal lobe to maintain postural stability. The similar regions of decreased activity secondary to improvements in lower extremity motor control (the current study) and postural control (Seidel et al.87), despite the differences in intervention (multimodal balance training87 vs. VNPT) and testing (fNIRS vs. fMRI), may support the key role of proprioceptive processing and motor planning neural efficiency to improve motor performance.
In addition to decreased localized neural activity demands, neural efficiency can be a function of enhanced connectivity between supporting regions. Previous work using resting-state fMRI identified increases in fronto-parietal connectivity following dynamic balance training, where participants had to maintain the horizontal positioning of an unstable stabilometer platform for repeated 30 second intervals.85 After two ~45 minute training sessions spread over two weeks, intrinsic functional connectivity increased between the supplementary motor cortex and parietal cortex. These results following balance training align with the results of the current study, as VPNT (four, 1-hour training sessions spread over two weeks) facilitates functional activity changes in fronto-parietal regions (clusters 1 and 2 of the current study) that could support enhanced connectivity.
Table 3. Regions of brain activity are reported that were identified in FSLeyes with the Harvard-Oxford Cortical & Subcortical Structural atlas, Julich histological atlas and the Cerebellar Atlas in MNI152 space after normalization with FNIRT and with FSL tool atlasquery. *Indicates identified on peak voxel.
There were no substantial changes in physical or cognitive training demands across the intervention as assessed with the RPE-P and NASA TLX. There was an increase in RPEC, indicating that VPNT preferentially increases cognitive demand over physical demand. Overall physical and cognitive training demands were largely maintained by increasing the visual perturbation dosage each session. One might expect a decrease in training difficulty and demand over time if the SG were not worn, as the athletes experience practice and learning effects for the exercises. Furthermore, of the six metrics measured with the NASA TLX, “frustration” tended to score the lowest. This suggests SG is not aversive to athletes during exercise, which is important for clinicians who want to ensure that their patients have adequate “buy in” and do not become frustrated with the technology.
This study utilized SG to perturbate vision during agility, balance, and plyometric exercises in female recreational athletes. By comparing pre-/post-intervention brain activity during a knee flexion/extension task, the results show that VPNT has the potential to modulate somatosensory, visual, and motor neural activity. Promoting increased neural efficiency in visuomotor and visuospatial regions of the brain, VPNT may have the ability to improve neuromuscular control and movement efficiency Additionally, subjective reports of frustration with SG were low, and our athletes’ motor performance (error count) was not substantially worsened by progressing SG difficulty Therefore, SG is an attractive, novel modality that warrants further research in future studies that apply visual perturbation training to populations with a maladaptive, increased reliance on vision for motor control (e.g., patients with ACLR).89,90
While the reliability of fMRI quantification of the BOLD signal is generally high and specific to knee movement has high inter-session reliability,43,55 this study is limited by the lack of a control group and small sample size (n=8). Thus, the analyses included 95% CIs and effect sizes instead of inferential statistics to illustrate this proof-of-concept pilot study
The inclusion of a control group, who underwent the same intervention without SG, would allow the authors to delineate whether the results were driven by visual perturbation, neuromuscular training, or their combination. However, published reliability data indicates that no change in the neural activity metrics should be expected across this timeframe if engaged in regularly daily activity, increasing probability that the intervention induced the changes.41 The inclusion of only high injury risk female athletes may prevent the generalization of these findings to non-female athletes and athletes with low injury risk landing biomechanics. And it is reasonable that VPNT may not
be as effective in athletes who already display good landing mechanics. Finally, due to practice trials and performance of four or more recorded trials for the LESS, there was potentially a learning effect of the LESS which could have influenced the scores. However, the practice trials are necessary to ensure the LESS is being performed correctly to complete the assessment, and these methods have demonstrated high reliability 32 Controlling the total number of trials completed (practice and recorded) may be beneficial for a larger clinical trial.
Future research may explore VPNT training effects with a longer timeframe (such as six or eight weeks), VPNT training effects in other populations (such as males, the aging, or patients with ACL injuries), or whether the behavioral and neuroimaging effects of VPNT are resilient against time (e.g., 3-month follow-up). Patients with ACL injuries may preferentially benefit from VPNT as they exhibit increased LESS scores relative to uninjured controls91 and an increased reliance on visual information processing to guide motor control.92,93 Future research is needed to examine task-based connectivity following VPNT, as the current study provides support for the use of primary and secondary motor regions and parietal cortex as key regions of interest. Also, the incorporation of 3D motion capture would enable future researchers to increase their sensitivity to movement metrics altered by VPNT Future research is needed to compare an intervention group to a control group to provide further validation for this proof-of-concept study Finally, future clinical trials with larger sample sizes should also consider potential factors that could limit reproducibility and reliability of the collection including the learning curve of examiners training individuals, the length of training sessions, and the cost of MRI.
This proof-of-concept pilot study evaluates the potential of VPNT to alter landing mechanics, neural activity, and physical/cognitive training demands in uninjured female recreational athletes. The findings suggest that visual perturbation training improves landing mechanics and visuocognitive abilities simultaneously by promoting neural efficiency in brain regions responsible for sensorimotor integration, visuomotor/visuospatial processing, and motor planning.
The authors declare no competing interests.
This study was supported in part by the US Department of Defense Congressionally Directed Medical Research Program Peer Reviewed Orthopaedic Research Program Re-
search Award (81XWH-18-1-0707) and NIH/National Institute of Arthritis and Musculoskeletal and Skin Diseases NIH/NIAMS; Awards NIAMS; R01AR076153 and R01AR077248. Opinions, interpretations, conclusions, and recommendations are those of the author and are not necessarily endorsed by the Department of Defense and NIH/ National Institute of Arthritis and Musculoskeletal and Skin Diseases.
We thank the Holzer Health system and Holly Henry MRI technologist and Dr. Phillip B. Long radiologist for MRI support.
The datasets generated during and/or analyzed during the current study are available from the corresponding author on reasonable request.
Submitted: February 12, 2024 CDT, Accepted: August 28, 2024 CDT
© The Author(s)
This is an open-access article distributed under the terms of the Creative Commons Attribution 4.0 International License (CCBY-NC-4.0). View this license’s legal deed at https://creativecommons.org/licenses/by-nc/4.0 and legal code at https://creativecommons.org/licenses/by-nc/4.0/legalcode for more information.
1. Sanders TL, Maradit Kremers H, Bryan AJ, et al. Incidence of anterior cruciate ligament tears and reconstruction: A 21-year population-based study. Am J Sports Med 2016;44(6):1502-1507 doi:10.1177/ 0363546516629944
2. Montalvo AM, Schneider DK, Webster KE, et al. Anterior cruciate ligament injury risk in sport: A systematic review and meta-analysis of injury incidence by sex and sport classification. J Athl Train. 2019;54(5):472-482. doi:10.4085/1062-6050-407-16
3. Beynnon BD, Vacek PM, Newell MK, et al. The effects of level of competition, sport, and sex on the incidence of first-time noncontact anterior cruciate ligament injury Am J Sports Med 2014;42(8):1806-1812. doi:10.1177/ 0363546514540862
4. Montalvo AM, Schneider DK, Yut L, et al. “What’s my risk of sustaining an ACL injury while playing sports?” A systematic review with meta-analysis. Br J Sports Med 2019;53(16):1003-1012. doi:10.1136/ bjsports-2016-096274
5. Mall NA, Chalmers PN, Moric M, et al. Incidence and trends of anterior cruciate ligament reconstruction in the United States. Am J Sports Med. 2014;42(10):2363-2370. doi:10.1177/ 0363546514542796
6. Bram JT, Magee LC, Mehta NN, Patel NM, Ganley TJ. Anterior cruciate ligament injury incidence in adolescent athletes: A systematic review and metaanalysis. Am J Sports Med. Published online October 22, 2020:0363546520959619. doi:10.1177/ 0363546520959619
7. Sugimoto D, Myer GD, Bush HM, Klugman MF, Medina McKeon JM, Hewett TE. Compliance with neuromuscular training and anterior cruciate ligament injury risk reduction in female athletes: a meta-analysis. J Athl Train 2012;47(6):714-723. doi:10.4085/1062-6050-47.6.10
8. Grindstaff TL, Hammill RR, Tuzson AE, Hertel J. Neuromuscular control training programs and noncontact anterior cruciate ligament injury rates in female athletes: a numbers-needed-to-treat analysis. J Athl Train 2006;41(4):450-456.
9. Hewett TE, Myer GD, Ford KR, et al. Biomechanical measures of neuromuscular control and valgus loading of the knee predict anterior cruciate ligament injury risk in female athletes: a prospective study Am J Sports Med. 2005;33(4):492-501. doi:10.1177/ 0363546504269591
10. Leppänen M, Pasanen K, Kujala UM, et al. Stiff landings are associated with increased ACL injury risk in young female basketball and floorball players. Am J Sports Med 2017;45(2):386-393. doi:10.1177/ 0363546516665810
11. Diekfuss JA, Grooms DR, Yuan W, et al. Does brain functional connectivity contribute to musculoskeletal injury? A preliminary prospective analysis of a neural biomarker of ACL injury risk. J Sci Med Sport 2019;22(2):169-174. doi:10.1016/ j.jsams.2018.07.004
12. Grooms DR, Diekfuss JA, Slutsky-Ganesh AB, et al. Preliminary report on the train the brain project, Part I: Sensorimotor neural correlates of anterior cruciate ligament injury risk biomechanics. J Athl Train 2022;57(9-10):902-910. doi:10.4085/ 1062-6050-0547.21
13. Grooms DR, Diekfuss JA, Criss CR, et al. Preliminary brain-behavioral neural correlates of anterior cruciate ligament injury risk landing biomechanics using a novel bilateral leg press neuroimaging paradigm. Di Giminiani R, ed. PLOS ONE 2022;17(8):e0272578. doi:10.1371/ journal.pone.0272578
14. Herman DC, Barth JT Drop-jump landing varies with baseline neurocognition: Implications for anterior cruciate ligament injury risk and prevention. Am J Sports Med 2016;44(9):2347-2353. doi:10.1177/ 0363546516657338
15. Swanik CB, Covassin T, Stearne DJ, Schatz P. The relationship between neurocognitive function and noncontact anterior cruciate ligament injuries. Am J Sports Med. 2007;35(6):943-948. doi:10.1177/ 0363546507299532
16. Diekfuss JA, Grooms DR, Nissen KS, et al. Alterations in knee sensorimotor brain functional connectivity contributes to ACL injury in male highschool football players: a prospective neuroimaging analysis. Braz J Phys Ther. Published online July 17, 2019. doi:10.1016/j.bjpt.2019.07.004
17 Grooms D, Appelbaum G, Onate J. Neuroplasticity following anterior cruciate ligament injury: a framework for visual-motor training approaches in rehabilitation. J Orthop Sports Phys Ther 2015;45(5):381-393. doi:10.2519/jospt.2015.5549
18. Appelbaum LG, Schroeder JE, Cain MS, Mitroff SR. Improved visual cognition through stroboscopic training. Front Psychol. 2011;2:276. doi:10.3389/ fpsyg.2011.00276
19. Appelbaum LG, Cain MS, Schroeder JE, Darling EF, Mitroff SR. Stroboscopic visual training improves information encoding in short-term memory Atten Percept Psychophys. 2012;74(8):1681-1691. doi:10.3758/s13414-012-0344-6
20. Smith TQ, Mitroff SR. Stroboscopic training enhances anticipatory timing. Int J Exerc Sci 2012;5(4):344-353. doi:10.70252/OTSW1297
21. Mitroff S, Friesen P, Bennett D, Yoo H, Reichow WA. Enhancing ice hockey skills through stroboscopic visual training: A pilot study. Athl Train Sports Health Care 2013;5:261-264. doi:10.3928/ 19425864-20131030-02
22. Wilkins L, Gray R. Effects of stroboscopic visual training on visual attention, motion perception, and catching performance. Percept Mot Skills 2015;121(1):57-79. doi:10.2466/22.25.PMS.121c11x0
23. Hülsdünker T, Rentz C, Ruhnow D, Käsbauer H, Strüder HK, Mierau A. The effect of 4-week stroboscopic training on visual function and sportspecific visuomotor performance in top-level badminton players. Int J Sports Physiol Perform 2019;14(3):343-350. doi:10.1123/ijspp.2018-0302
24. Wilkins L, Appelbaum L. An early review of stroboscopic visual training: insights, challenges and accomplishments to guide future studies. Int Rev Sport Exerc Psychol Published online March 1, 2019:1-16. doi:10.1080/1750984X.2019.1582081
25. Bennett SJ, Hayes SJ, Uji M. Stroboscopic vision when interacting with multiple moving objects: Perturbation is not the same as elimination. Front Psychol. 2018;9:1290. doi:10.3389/fpsyg.2018.01290
26. Gholami F, Letafatkar A, Moghadas Tabrizi Y, et al. Comparing the effects of differential and visuomotor training on functional performance, biomechanical, and psychological factors in athletes after ACL reconstruction: A randomized controlled trial. J Clin Med. 2023;12(8):2845. doi:10.3390/ jcm12082845
27 Lee H, Han S, Hopkins JT Balance training with stroboscopic glasses alters neuromechanics in chronic ankle instability patients during single leg drop. J Athl Train Published online July 1, 2023. doi:10.4085/1062-6050-0605.22
28. Lee H, Han S, Hopkins JT. Altered visual reliance induced by stroboscopic glasses during postural control. Int J Environ Res Public Health. 2022;19(4):2076. doi:10.3390/ijerph19042076
29. Rössler R, Donath L, Verhagen E, Junge A, Schweizer T, Faude O. Exercise-based injury prevention in child and adolescent sport: a systematic review and meta-analysis. Sports Med Auckl NZ 2014;44(12):1733-1748. doi:10.1007/ s40279-014-0234-2
30. Myer GD, Ford KR, Hewett TE. Methodological approaches and rationale for training to prevent anterior cruciate ligament injuries in female athletes. Scand J Med Sci Sports. 2004;14(5):275-285. doi:10.1111/j.1600-0838.2004.00410.x
31. Marx RG, Stump TJ, Jones EC, Wickiewicz TL, Warren RF. Development and evaluation of an activity rating scale for disorders of the knee. Am J Sports Med 2001;29(2):213-218. doi:10.1177/ 03635465010290021601
32. Padua DA, DiStefano LJ, Beutler AI, de la Motte SJ, DiStefano MJ, Marshall SW The Landing Error Scoring System as a screening tool for an anterior cruciate ligament injury-prevention program in eliteyouth soccer athletes. J Athl Train 2015;50(6):589-595. doi:10.4085/1062-6050-50.1.10
33. Kim SY, Spritzer CE, Utturkar GM, Toth AP, Garrett WE, DeFrate LE. Knee kinematics during noncontact anterior cruciate ligament injury as determined from bone bruise location. Am J Sports Med 2015;43(10):2515-2521. doi:10.1177/ 0363546515594446
34. Padua DA, Marshall SW, Boling MC, Thigpen CA, Garrett WE, Beutler AI. The Landing Error Scoring System (LESS) Is a valid and reliable clinical assessment tool of jump-landing biomechanics: The JUMP-ACL study Am J Sports Med 2009;37(10):1996-2002. doi:10.1177/ 0363546509343200
35. Onate J, Cortes N, Welch C, Van Lunen BL. Expert versus novice interrater reliability and criterion validity of the landing error scoring system. J Sport Rehabil 2010;19(1):41-56. doi:10.1123/jsr.19.1.41
36. Hanzlíková I, Hébert-Losier K. Is the Landing Error Scoring System reliable and valid? A systematic review Sports Health 2020;12(2):181-188. doi:10.1177/1941738119886593
37. Markbreiter JG, Sagon BK, Valovich McLeod TC, Welch CE. Reliability of clinician scoring of the landing error scoring system to assess jump-landing movement patterns. J Sport Rehabil. 2015;24(2):214-218. doi:10.1123/jsr.2013-0135
38. Padua DA, Boling MC, Distefano LJ, Onate JA, Beutler AI, Marshall SW. Reliability of the landing error scoring system-real time, a clinical assessment tool of jump-landing biomechanics. J Sport Rehabil 2011;20(2):145-156. doi:10.1123/jsr.20.2.145
39. Kapreli E, Athanasopoulos S, Papathanasiou M, et al. Lower limb sensorimotor network: issues of somatotopy and overlap. Cortex J Devoted Study Nerv Syst Behav. 2007;43(2):219-232. doi:10.1016/ S0010-9452(08)70477-5
40. Kapreli E, Athanasopoulos S, Papathanasiou M, et al. Lateralization of brain activity during lower limb joints movement. An fMRI study. NeuroImage. 2006;32(4):1709-1721. doi:10.1016/ j.neuroimage.2006.05.043
41. Grooms DR, Diekfuss JA, Ellis JD, et al. A novel approach to evaluate brain activation for lower extremity motor control. J Neuroimaging 2019;29(5):580-588. doi:10.1111/jon.12645
42. Jaeger L, Marchal-Crespo L, Wolf P, Riener R, Kollias S, Michels L. Test-retest reliability of fMRI experiments during robot-assisted active and passive stepping. J Neuro Engineering Rehabil 2015;12(1):102. doi:10.1186/s12984-015-0097-2
43. Newton JM, Dong Y, Hidler J, et al. Reliable assessment of lower limb motor representations with fMRI: use of a novel MR compatible device for realtime monitoring of ankle, knee and hip torques. NeuroImage 2008;43(1):136-146. doi:10.1016/ j.neuroimage.2008.07.001
44. Wohl TR, Criss CR, Grooms DR. Visual perturbation to enhance return to sport rehabilitation after anterior cruciate ligament injury: A clinical commentary. Int J Sports Phys Ther. 2021;16(2):552. doi:10.26603/001c.21251
45. Myer GD, Paterno MV, Ford KR, Quatman CE, Hewett TE. Rehabilitation after anterior cruciate ligament reconstruction: criteria-based progression through the return-to-sport phase. J Orthop Sports Phys Ther. 2006;36(6):385-402. doi:10.2519/ jospt.2006.2222
46. Grooms DR, Chaudhari A, Page SJ, Nichols-Larsen DS, Onate JA. Visual-motor control of drop landing after anterior cruciate ligament reconstruction. J Athl Train 2018;53(5):486-496. doi:10.4085/ 1062-6050-178-16
47 Borg G. Perceived exertion as an indicator of somatic stress. Scand J Rehabil Med 1970;2(2):92-98. doi:10.2340/1650197719702239298
48. Hart SG, Staveland LE. Development of NASATLX (Task Load Index): Results of empirical and theoretical research. In: Human Mental Workload. Advances in psychology, 52. North-Holland; 1988:139-183. doi:10.1016/S0166-4115(08)62386-9
49. Sherman DA, Lehmann T, Baumeister J, Grooms DR, Norte GE. Somatosensory perturbations influence cortical activity associated with single-limb balance performance. Exp Brain Res. 2022;240(2):407-420. doi:10.1007/ s00221-021-06260-z
50. McWethy M, Norte G, Bazett-Jones D, Murray A, Rush J. Cognitive-motor dual-task performance of the Landing Error Scoring System. J Athl Train Published online March 6, 2024. doi:10.4085/ 1062-6050-0558.23
51. Smith EM, Sherman DA, Duncan S, et al. Testretest reliability and visual perturbation performance costs during 2 reactive agility tasks. J Sport Rehabil. 2024;33(6):444-451. doi:10.1123/jsr.2023-0433
52. Friston KJ, Frith CD, Turner R, Frackowiak RS. Characterizing evoked hemodynamics with fMRI. NeuroImage 1995;2(2):157-165. doi:10.1006/ nimg.1995.1018
53. Logothetis NK, Pauls J, Augath M, Trinath T, Oeltermann A. Neurophysiological investigation of the basis of the fMRI signal. Nature 2001;412(6843):150-157. doi:10.1038/35084005
54. Goense JBM, Logothetis NK. Neurophysiology of the BOLD fMRI signal in awake monkeys. Curr Biol CB. 2008;18(9):631-640. doi:10.1016/ j.cub.2008.03.054
55. McGregor KM, Carpenter H, Kleim E, et al. Motor map reliability and aging: a TMS/fMRI study. Exp Brain Res 2012;219(1):97-106. doi:10.1007/ s00221-012-3070-3
56. Smith SM, Jenkinson M, Woolrich MW, et al. Advances in functional and structural MR image analysis and implementation as FSL. NeuroImage 2004;23 Suppl 1:S208-219. doi:10.1016/ j.neuroimage.2004.07.051
57 Jenkinson M, Beckmann CF, Behrens TEJ, Woolrich MW, Smith SM. FSL. NeuroImage 2012;62(2):782-790. doi:10.1016/ j.neuroimage.2011.09.015
58. Jenkinson M, Bannister P, Brady M, Smith S. Improved optimization for the robust and accurate linear registration and motion correction of brain images. NeuroImage 2002;17(2):825-841. doi:10.1006/nimg.2002.1132
59. Poldrack RA, Mumford JA, Nichols TE. Handbook of Functional MRI Data Analysis Cambridge Core doi:10.1017/CBO9780511895029
60. Woolrich MW, Ripley BD, Brady M, Smith SM. Temporal autocorrelation in univariate linear modeling of FMRI data. NeuroImage 2001;14(6):1370-1386. doi:10.1006/nimg.2001.0931
61. Woolrich MW, Behrens TEJ, Beckmann CF, Jenkinson M, Smith SM. Multilevel linear modelling for FMRI group analysis using Bayesian inference. NeuroImage. 2004;21(4):1732-1747. doi:10.1016/ j.neuroimage.2003.12.023
62. Cohen J. Statistical Power Analysis for the Behavioral Sciences. Academic Press; 2013. doi:10.4324/9780203771587
63. Desikan RS, Ségonne F, Fischl B, et al. An automated labeling system for subdividing the human cerebral cortex on MRI scans into gyral based regions of interest. NeuroImage 2006;31(3):968-980. doi:10.1016/j.neuroimage.2006.01.021
64. Eickhoff SB, Heim S, Zilles K, Amunts K. Testing anatomically specified hypotheses in functional imaging using cytoarchitectonic maps. NeuroImage. 2006;32(2):570-582. doi:10.1016/ j.neuroimage.2006.04.204
65. Eickhoff SB, Paus T, Caspers S, et al. Assignment of functional activations to probabilistic cytoarchitectonic areas revisited. NeuroImage 2007;36(3):511-521. doi:10.1016/ j.neuroimage.2007.03.060
66. Diedrichsen J, Balsters JH, Flavell J, Cussans E, Ramnani N. A probabilistic MR atlas of the human cerebellum. NeuroImage 2009;46(1):39-46. doi:10.1016/j.neuroimage.2009.01.045
67 Scarneo SE. The Effects of an Injury Prevention Program in an Aquatic Environment on Landing Technique University of Conneticut; 2014.
68. Pryor JL, Root HJ, Vandermark LW, et al. Coachled preventive training program in youth soccer players improves movement technique. J Sci Med Sport 2017;20(9):861-866. doi:10.1016/ j.jsams.2017.01.235
69. Pfile KR, Gribble PA, Buskirk GE, Meserth SM, Pietrosimone BG. Sustained improvements in dynamic balance and landing mechanics after a 6-week neuromuscular training program in college women’s basketball players. J Sport Rehabil 2016;25(3):233-240. doi:10.1123/jsr.2014-0323
70. Owens BD, Cameron KL, Duffey ML, et al. Military movement training program improves jump-landing mechanics associated with anterior cruciate ligament injury risk. J Surg Orthop Adv 2013;22(1):66-70. doi:10.3113/jsoa.2013.0066
71. DiStefano LJ, Padua DA, DiStefano MJ, Marshall SW. Influence of age, sex, technique, and exercise program on movement patterns after an anterior cruciate ligament injury prevention program in youth soccer players. Am J Sports Med 2009;37(3):495-505. doi:10.1177/0363546508327542
72. de Azevedo Neto RM, Amaro Júnior E. Bilateral dorsal fronto-parietal areas are associated with integration of visual motion information and timed motor action. Behav Brain Res. 2018;337:91-98. doi:10.1016/j.bbr.2017.09.046
73. Reader AT, Royce BP, Marsh JE, Chivers KJ, Holmes NP Repetitive transcranial magnetic stimulation reveals a role for the left inferior parietal lobule in matching observed kinematics during imitation. Eur J Neurosci. 2018;47(8):918-928. doi:10.1111/ejn.13886
74. Crottaz-Herbette S, Fornari E, Clarke S. Prismatic adaptation changes visuospatial representation in the inferior parietal lobule. J Neurosci Off J Soc Neurosci. 2014;34(35):11803-11811. doi:10.1523/ JNEUROSCI.3184-13.2014
75. Andersen RA. Inferior parietal lobule function in spatial perception and visuomotor integration. In: Comprehensive Physiology American Cancer Society; 2011:483-518. doi:10.1002/cphy.cp010512
76. Assmus A, Marshall JC, Ritzl A, Noth J, Zilles K, Fink GR. Left inferior parietal cortex integrates time and space during collision judgments. NeuroImage. 2003;20(Suppl 1):S82-88. doi:10.1016/ j.neuroimage.2003.09.025
77. Chen TL, Babiloni C, Ferretti A, et al. Human secondary somatosensory cortex is involved in the processing of somatosensory rare stimuli: an fMRI study. NeuroImage. 2008;40(4):1765-1771. doi:10.1016/j.neuroimage.2008.01.020
78. Hoechstetter K, Rupp A, Meinck HM, et al. Magnetic source imaging of tactile input shows taskindependent attention effects in SII. Neuroreport 2000;11(11):2461-2465. doi:10.1097/ 00001756-200008030-00024
79. Forss N, Jousmäki V Sensorimotor integration in human primary and secondary somatosensory cortices. Brain Res. 1998;781(1-2):259-267. doi:10.1016/s0006-8993(97)01240-7
80. Borggraefe I, Catarino CB, Rémi J, et al. Lateralization of cortical negative motor areas. Clin Neurophysiol Off J Int Fed Clin Neurophysiol 2016;127(10):3314-3321. doi:10.1016/ j.clinph.2016.08.001
81. Hoshi E, Tanji J. Differential roles of neuronal activity in the supplementary and presupplementary motor areas: from information retrieval to motor planning and execution. J Neurophysiol. 2004;92(6):3482-3499. doi:10.1152/jn.00547.2004
82. Criss CR, Grooms DR, Diekfuss JA, et al. Simulated landing neural correlates of anterior cruciate ligament injury risk niomechanics. Anterior Cruciate Ligament Research Retreat VIII Published online March 2019. doi:10.4085/1062-6050-54.081
83. Lohse KR, Wadden K, Boyd LA, Hodges NJ. Motor skill acquisition across short and long time scales: a meta-analysis of neuroimaging data. Neuropsychologia. 2014;59:130-141. doi:10.1016/ j.neuropsychologia.2014.05.001
84. Hardwick RM, Rottschy C, Miall RC, Eickhoff SB. A quantitative meta-analysis and review of motor learning in the human brain. NeuroImage 2013;67:283-297 doi:10.1016/ j.neuroimage.2012.11.020
85. Taubert M, Lohmann G, Margulies DS, Villringer A, Ragert P Long-term effects of motor training on resting-state networks and underlying brain structure. NeuroImage 2011;57(4):1492-1498. doi:10.1016/j.neuroimage.2011.05.078
86. Yang J. The influence of motor expertise on the brain activity of motor task performance: A metaanalysis of functional magnetic resonance imaging studies. Cogn Affect Behav Neurosci. 2015;15(2):381-394. doi:10.3758/s13415-014-0329-0
87 Seidel O, Carius D, Kenville R, Ragert P Motor learning in a complex balance task and associated neuroplasticity: a comparison between endurance athletes and nonathletes. J Neurophysiol 2017;118(3):1849-1860. doi:10.1152/jn.00419.2017
88. Grooms DR, Diekfuss JA, Slutsky-Ganesh AB, et al. Preliminary report on the train the brain project, Part II: Neuroplasticity of augmented neuromuscular training and improved injury-risk biomechanics. J Athl Train 2022;57(9-10):911-920. doi:10.4085/ 1062-6050-0548.21
89. Criss CR, Lepley AS, Onate JA, et al. Brain activity associated with quadriceps strength deficits after anterior cruciate ligament reconstruction. Sci Rep 2023;13(1):8043. doi:10.1038/s41598-023-34260-2
90. Chaput M, Onate JA, Simon JE, et al. Visual cognition associated with knee proprioception, time to stability, and sensory integration neural activity after ACL reconstruction. J Orthop Res. 2022;40(1):95-104. doi:10.1002/jor.25014
91. Bell DR, Smith MD, Pennuto AP, Stiffler MR, Olson ME. Jump-landing mechanics after anterior cruciate ligament reconstruction: a landing error scoring system study J Athl Train 2014;49(4):435-441. doi:10.4085/1062-6050-49.3.21
92. Grooms DR, Page SJ, Nichols-Larsen DS, Chaudhari AMW, White SE, Onate JA. Neuroplasticity associated with anterior cruciate ligament reconstruction. J Orthop Sports Phys Ther 2016;47(3):180-189. doi:10.2519/jospt.2017.7003
93. Kapreli E, Athanasopoulos S, Gliatis J, et al. Anterior cruciate ligament deficiency causes brain plasticity: a functional MRI study Am J Sports Med 2009;37(12):2419-2426. doi:10.1177/ 0363546509343201
Mangine RE, Palmer TG, Tersak JA, et al. Task-Driven Neurophysiological qEEG Baseline Performance Capabilities in Healthy, Uninjured Division-I College Athletes. IJSPT 2024;19(11):1348-1361. doi:10.26603/001c.124935
Robert E. Mangine, PT, ATC, Med1,2 , Thomas G. Palmer, PHD, ATC, CSCS*D, TSAC-F3 , James A. Tersak1,2 , Michael Mark, PsyD, BNC4 , Joseph F Clark, Ph.D, Marsha Eifert-Mangine, PT, ATC, EdD, CERT1,3a , Audrey Hill-Lindsay, PhD4 , Brian M Grawe, MD5,6
1 NovaCare Rehabilitation, A Select Medical Company, Mechanicsburg, PA, USA, 2 University of Cincinnati Athletics, Cincinnati, OH, USA, 3 Mount St. Joseph University, Cincinnati, OH, USA, 4 CLR Neurosthenics, Inc., Manhattan, CA, USA, 5 University of Cincinnati, College of Medicine, Cincinnati, OH, USA, 6 Team Physician, Cincinnati Bearcats & Cincinnati Bengals, Cincinnati, OH USA
Keywords: Neurophysiological assessment, Neuroplasticity, Quantitative electroencephalography/qEEG, Brain Mapping, Mirror Neuron Network, Neural networks
https://doi.org/10.26603/001c.124935
International Journal of Sports Physical Therapy
Vol. 19, Issue 11, 2024
Athletic performance can be measured with a variety of clinical and functional assessment techniques. There is a need to better understand the relationship between the brain’s electrical activity and the body’s physiological performance capabilities in real-time while performing physical tasks related to sport. Orthopedic functional assessments used to monitor the neuroplastic properties of the central nervous system lack objectivity and/or pertinent functionality specific to sport. The ability to assess brain wave activity with physiological metrics during functional exercises associated with sport has proven to be difficult and impractical in real-time sport settings. Quantitative electroencephalography or qEEG brain mapping is a unique, real-time comprehensive assessment of brain electrical activity performed in combination with physiometrics which offers insight to neurophysiological brain-to-body function. Brain neuroplasticity has been associated with differences in musculoskeletal performance among athletes, however comparative real-time normal data to benchmark performance capabilities is limited.
Purpose/Design
This prospective, descriptive case series evaluated performance of task-driven activities using an innovative neurophysiological assessment technique of qEEG monitored neurophysiological responses to establish a comparative benchmark of performance capabilities in healthy, uninjured Division-I athletes.
Methods
Twenty-eight healthy uninjured females (n=11) and males (n=17) NCAA Division-I athletes participated in real-time neurophysiological assessment using a Bluetooth, wireless 21-channel dry EEG headset while performing functional activities.
Email: marshamangine@gmail.com a
Corresponding Author: Marsha Eifert-Mangine PT, ATC, EdD, CERT
Associate Professor Emeritus
Physical Therapy Program
School of Health Sciences
Mount St. Joseph University
5701 Delhi Road | Cincinnati, OH 45233-1672 United States of America
Cell: 859 802-5714
Results
Uninjured athletes experienced standard and regulated fluctuations of brain wave activity in key performance indicators of attention, workload capacity and sensorimotor rhythm (SMR) asymmetries.
Conclusion
qEEG neurophysiological real-time assessment concurrent with functional activities in uninjured, Division-I athletes may provide a performance capability benchmark. Real-time neurophysiological data can be used to monitor athletes’ preparedness to participate in sport, rehabilitation progressions, assist in development of injury prevention programs, and return to play decisions. While this paper focuses on healthy, uninjured participants, results underscore the need to discen pre-injury benchmarks.
4
Injury to the musculoskeletal system perpetuates concurrent and responsive neuroplastic alteration to the central nervous system that impacts quality of function.1,2 Recent objective real-time quantitative electroencephalogram (qEEG) neurophysiological assessment techniques have been identified to monitor neural adaptive structural and functional changes of the brain that impact functional movement patterns in pre- and post-injury status.1,3 Measuring neural activity of the brain during functional tasks offers clinicians objective data to evaluate and monitor regulatory functional properties of the brain-to-body connection offering insight to assist in identification of disturbances in musculoskeletal function leading to less than optimal biomechanical utility.4 Disturbances in the neural excitability and neuroplastic properties of the brain impacts neurophysiological function of the Central Nervous System (CNS) leading to altered motor responses during functional activities.1‑4 Targeting the neuroplastic properties of the brain and CNS have become a primary goal for sports medicine professionals and athletes during both training and injury rehabilitation progressions. The ability to objectively track and monitor neurological structural and functional changes in the brain’s state that affect musculoskeletal function may allow for the optimal management of training and rehabilitation protocols. qEEG has been suggested as a metric for monitoring brain states and brain function as they relate to functional motor performance.1
Similar to functional Magnetic Resonance Imaging (fMRI) of the brain, qEEG reflects changes in the state of the brain related to workload of the different brain regions.5 However, fMRI techniques are not practically applicable for the assessment of dynamic and functional movements.1,3 In addition, the static fMRI images provide limited time windows of brain activity which limits the conclusive alterations associated with musculoskeletal function.5 qEEG offers consistent objective assessments of brain state and the ability to adapt to the changing environment.6
Assessing qEEG brain activity while performing functional movement in healthy uninjured athletes will provide
normal objective performance indicators of neurophysiological function. Such baselines can serve as real-time performance properties that assume the brain state is adequately in sequence with the peripheral neurological properties.5,6 Such performance benchmarks can be used as comparative norms to help establish standards for athlete readiness to participate in sport. Therefore, this prospective investigation evaluated neurophysiological responses to performance of task-driven activities using an innovative neurophysiological assessment technique of qEEG monitored neurophysiological responses to establish a comparative benchmark of performance capabilities in healthy, uninjured Division-I athletes. Such baseline data may be used to measure neurophysiological changes as related to degradation and/or improvement of brain state over time.
Twenty-eight uninjured NCAA Division-I athletes qualified and consented to participate in this IRB approved prospective case series designed study. Athletes were excluded from participation if they presented with a current injury, a current history of an attention deficit, anxiety, or history of injury that resulted in disqualification from play in the prior six months. The twenty-eight athletes (10 females and 18 males) participated in a variety of competitive sports including both contact and non-contact.
Quantitative electroencephalography (qEEG) is a modern clinical digital assessment used to measure electrical patterns at the surface of scalp which reflect a continuous measure of cortical activity and are referred to as “brainwaves” and assess the central nervous system processing efficiency, power spectra, amplitude, and connectivity. qEEG was used to investigate real-time brain electrical patterns and neurophysiological function as it relates efficiency, power spectra, amplitude, and brain connectivity during functional movement tasks associated with sport. qEEG data were collected during a single testing session of baseline measures where each participant performed a uniform series of cognitive, motor imagery, reaction time and
Figure 1. Depiction of the planned Neurophysiological assessment tasks.
physical functional motor tasks (Figure 1). Baseline data were established by monitoring qEEG brain wave activity during periods where participants sat with eyes closed and eyes open. Once the qEEG baseline was established, participants performed a variety of tasks, including a cognitive test, overt imagery and a corresponding covert activity, functional movement exercises (balance, single limb, and agility tasks) and a reaction time test. Motor imagery was performed before and after five functional movement tasks as previously published.1,7 Functional movement tasks emphasized balance, gait, mobility and lower extremity symmetry.8‑10
1. EO/EC Visual Arrest Baseline – Participants sat in a resting state for one minute with their Eyes Closed (EC) and one minute with their Eyes Open (EO), followed by two minutes with eyes open EO and two minutes with EC. CLR AdvantageTM then compared raw EEG signals collected during EC vs EO periods to provide a neurologic baseline from which to process data collected during subsequent assessment tasks. This baseline provided analytics derived from EEG channels, including frequency band power and ratios, band and ratio topo-plots, and the performance of brain Regions of Interest (ROIs).
2. Stroop Cognitive Test – Participants viewed a 30-second series of congruent and incongruently colored words (“Blue”, “Green”, “Red”, “Yellow”) while using a PC mouse to click on particular words correctly rendered in matching colors. CLR AdvantageTM recorded the number of correct and incorrect responses to assess participants’ speed and ability to discern incongruencies. The Stroop Cognitive Test is a common method to measure psychological performance in athletes, and is used to measure speed of
processing, executive function and selective attention.
3. Positive Imagery Exercise – Participants imagined a pleasing scene or memory for 30 seconds. Positive Imagery is important to either recreate a good past performance or create a current positive new experience which can optimize performance, enhance focus and concentration, and improve self- regulation of heart rate, breath rate, and galvanic skin response.
4. Anticipation Response Exercise – Participants waited 30 seconds for a stressful audiovisual interruption after being told to expect such by investigators. The exercise is used to measure stress, arousal levels, Galvanic Skin Response, diaphragmatic breathing, and how quickly a participant can recover from a perceived stressor.
5. Covert Imagery Task A – Participants focused on a screen-centered white star then responded to a 30-second display of randomly alternating colors (green, red) by imagining tossing a ball into a basket with their right (green) or left (red) hand.
6. Overt Motor Task B – Participants focused on a screen-centered white star then responded to a 30-second display of randomly alternating colors (green, red) by actually tossing a ball into a basket with their right (red) or left (green) hand. Together, Covert Imagery and corresponding Overt Motor Tasks are used to stimulate and measure Mirror Neuron Network activation. Overt is the act of physically directing eyes to a stimulus and covert is mental shift of attention without physical movement. This indicates whether the participant learned from the covert action to better replicate movement during the overt action.
7 Unloaded Squat – Participants repetitiously (3X) squatted into a 90-degree knee flexion position while maintaining an upright torso and holding an unloaded rod overhead with arms fully extended and feet shoulder width apart in a sagittal plane. Part of the Functional Movement Screen, the Unloaded Squat is used to measure a subject’s bilateral, symmetrical functional mobility of hips, knees and ankles.
8. Single Leg Step-Down Right – Participants repetitiously (3X) stepped down from a 10" pedestal with their right leg.
9. Single Leg Step-Down Left – Participants repetitiously (3X) stepped down from a 10" pedestal with their left leg. Part of the Functional Movement Screen, the Single Leg Step Down exercises are used to measure a subject’s dynamic knee function, hip and trunk strength and biomechanical and kinematic deficiencies.
10. Single Leg Balance Right – Participants repetitiously (3X) maintained balance for 30 seconds, while raising their right leg to a perpendicular angle with a rest period of 15 seconds between each repetition.
11. Single Leg Balance Left – Participants repetitiously (3X) maintained balance for 30 seconds while raising their left leg to a perpendicular angle with a rest period of 15 seconds between each repetition. Single Leg Balance tasks were initiated with the nonweightbearing leg flexed to approximately 30 degrees from a hands-on-hips position. Part of the Functional Movement Screen, the Single Leg Balance tasks are used to measure a subject’s balance, joint stability and proprioception.
12. Sidestep Right – Participants repetitiously (3X) led with their right leg to step over a set of three 6" low hurdles before stepping back to the starting position.
13. Sidestep Left – Participants repetitiously (3X) led with their left leg to step over a set of three 6" low hurdles before stepping back to the starting position. Part of the Functional Movement Screen, the Sidestep tasks are used to measure a subject’s gait mechanics, compensation and asymmetry
14. Single Leg Squat Right – Participants repetitiously (3X) maintained balance for 30 seconds while squatting from a hands-on-hip position to a 90-degree knee flexion angle on their right leg with the left leg extended.
15. Single Leg Squat Left – Participants repetitiously (3X) maintained balance for 30 seconds while squatting from a hands-on-hip position to a 90-degree knee flexion angle on their left leg with the right leg extended. Part of the Functional Movement Screen, the Single Leg Squat tasks are used to measure a subject’s balance and control, strength of lower body, postural malalignments and kinematic and biomechanical deficiencies.
16. Reaction Time – Participants focused on a screencentered white star then responded to a 30-second display of randomly alternating colors (green, red) by
clicking a PC keyboard with their right (red) or left (red) index finger. CLR AdvantageTM recorded the speed and accuracy of responses. This Reaction Time Test is a common method to measure responsiveness in athletes
Cognitive tasks, including a Stroop Test, Positive Imagery Exercise, and Anticipation Challenge were designed to stimulate and measure cognitive functions. The Covert Imagery Task and companion Overt Motor Task were designed to stimulate and measure Mirror Neuron Network (MNN) activation. MNN is a critical component of the brain’s social cognitive function in action recognition, imitation, learning, and understanding the intentions and observations behind others’ actions. These neurons are excited during incidences when an individual performs or observes a motor skill which stimulates exclusive motor functions. Nine physical tasks including: an unloaded squat; single leg step down (right and left); single leg balance (right and left); sidestep right, sidestep left; and single leg squat (right and left), as well as a scored Reaction Test, were designed to stimulate Mirror Neuron Network activity while measuring regional connectivity and hemispherical asymmetry These tasks also enabled measurement of attention levels and workload capabilities.11,12
Investigators utilized a CGX9 (Cognionics Company, San Diego CA) Quick-20r v2, 21-channel dry EEG head set to collect continuous electrical brain activity while simultaneously completing the the functional movements. Chi, et al.7 found this system to be a reliable and valid method to measure evoked response potentials as repeatable signals were seen when a standardized test protocol approach is used as compared to traditional wet, wired EEG systems. The dependent variables of cognitive function, attention, workload capability, and Sensorimotor Rhythm (SMR) asymmetries were monitored.1,12 Each measure accounted for acute real-time neurophysiological compensation and accommodations to physical and cognitive tasks.13,14 Additional physiological measures were conducted as secondary dependent variables to examine participants’ physical performance during each Neurophysiological Assessment Task. These measures included: heart rate to examine stress, anxiety and ability to relax15; heart rate variability to examine the ability regulate emotion, attention and breathing16; respiration rate to examine stress, concentration and ability to minimize distraction17; trapezius muscle tension to examine asymmetry and injury predisposition18; galvanic skin response to examine fatigue, emotional arousal and anticipation19; and peripheral temperature to examine the participant’s ability to regulate stress response.20 Physiometric data was collected with the CGX AIMTM (CGX, a Cognionics Company, San Diego CA) physiological device. The CLR AdvantageTM (CLR Neurosthenics® Manhattan Beach, CA) Neurophysiological Assessment Platform and the CGXAIM were utilized to simultaneously collect, process and analyze neurophysiological data from the brain and physiological monitoring through electrical data from
Figure 2. Data collection and output process steps
1. Selection of tasks from a remote assessment screen during data collection
2. Performance of physical task (in this case, the single leg squat) with visual cues and instructions for participants displayed on a separate screen
3. Output of analytic reports of EEG data representing asymmetry of Mirror Neuron Network Regions of Interests (ROIs)
the body. The CGX devices were then used to stream continuous biometric data via wireless connection to CLR Advantage. This configuration allowed participants to perform various physical tasks without restriction. After confirming the quality and consistency of CGX signal data, CLR Advantage was used to guide participants through the series of preprogrammed neurophysiologic assessment tasks. As investigators selected each task from a remote assessment screen (Figure 2, Step 1), CLR Advantage would display corresponding visual cues and instructions for participants to follow on a separate screen (Figure2, Step 2). Upon completion of each assessment, CLR Advantage would utilize Intheon NeuroscaleTM to generate analytic reports for each participant (Figure2, Step 3). CLR Advantage was also used to collect preassessment profile and medical history data from each participant.1
The EEG and physiologic data screen were designed to capture the most relevant and incisive athletic performance metrics. With 21 channels of continuously streaming EEG, investigators were able to collect data to determine participants’ neural network connectivity, activation, asymmetry and frequency bands levels during each neurophysiological assessment task. The data collected supported sufficient Power Spectral Density (PSD) levels to measure performance across multiple networks and regions of interest including: a) Default Mode Network (medial prefrontal cortex, posterior cingulate cortex, Hippocampus, precuneus, inferior parietal lobe, parietal regions and temporal lobe); b) Salience Network (anterior insula and dorsal anterior cingulate cortex); c) Mirror Neuron Network (inferior frontal cortex and in the inferior parietal cortex, d) Attention (dorsal frontoparietal); e) Sensorimotor Cortex (primary somatosensory cortical area and the primary motor
cortical area); and, f) Occipital Lobe (visual processing center ). PSD levels also provided sufficient data to calculate performance within EEG frequency bands, Including: g) Delta (0.5 to 4Hz); h) Theta (4 to 7Hz); i) Alpha (8 to 12Hz); j) SMR(12 to 15Hz); and k) Beta1-3 (12 to 30Hz
Power spectral analysis (PSA) is a common and wellestablished method for analyzing EEG signals.19 PSA uses a power spectrum to quantify the amplitude of each frequency component in the EEG waveform. PSA estimates the power of a signal at different frequencies.
Spectral analysis comparison between power and frequency bands was measured at the change between Eyes Open (EO) and Eyes Closed (EC). The Welsh20 method was used for spectral density estimation and used for estimating the power spectral density analysis and then used 1/frequency (F) normalization to convert to decibels. The raw EEG compared EO versus EC during resting states and analytics based on measurements per channel, across all channels, right and left hand as well as different brain regions, frequency bands, frequency band ratios and Regions of Interest (ROI). Figure 3 represents a sample Power Spectral Density (PSD) assessment of a male participant.
Upon completion of each participant assessment session, collected data was further processed to calculate individual performance metrics, data aggregation, exponential smoothing (by task) and generation of sub-cohort (uninjured, male/female) analytics. The CLR Advantage Neurophysiological Assessment Platform was utilized to analyze participants’ Individual Performance Reports (IPRs) then compare those results to that of the study sub-cohort (uninjured male and female athletes). IPRs may be used to identify neurophysiological deficiencies and provide clinically valuable information to the rehabilitation specialists,
This example was captured from a male participant during the Visual Arrest portion of the qEEG Baseline Neurophysiologic Assessment Task. Each trace (separate color line) represents one of the 19 EEG channels of the regions of interest measured as an electrical frequency of brain activity Each trace shows Mean +/- 95% confidence intervals.
Figure 4. Examples of athletes performing physical and cognitive tasks during data collection.
coach, or athlete themselves about how the reacts and accommodates based on the demands of their sport and/or position. Four reports generated include:
1. Pre vs Post Motor Training Task Report provides results from the cognitive metrics as a comparison from motor imagery baseline periods before (pre) and after (post) the motor training tasks. The report includes: SMR Asymmetry (the average S MR (13-15 Hz) Asymmetry for the Mirror Neuron Network Regions of Interest (ROIs) averaged together and computed from
Figure 5. Graphic depiction of data collection methods
The figure on the left depicts EEG electrode sensor placement. The center and right figures depict physiologic data collection points including:
• ECG Paired Electrocardiagraph (ECG) electrodes (Output:Heart Rate BPM, Heart Rate Variability ms/BPM, Respiration BRPM)
• Peripheral Temperature (Temp) sensor (C0 , F0) positioned on the inner bicep as shown to allow free movement by participants’ hands
• Paired Galvanic Skin Response (GSR) electrodes (ms), positioned on the forearm as shown to allow free movement of participants’ hands
• Paired Electromyography (EMG) electrodes positioned as shown to measure Trapezius muscle activity with ground (Grnd) (Output: Left, Right Trapezoid mV, Trapezoidal Imbalance ms).
the motor imagery periods before and after the motor training tasks); Analysis by Channels (analyses and statistics for all channels with power spectral analysis plots for pre motor imagery vs post motor task); Analysis by Sources (analyses and statistics using Regions of Interests (ROIs) as determined by source localization); Cortex Activity (plots showing the difference in pre vs post motor imagery task frequency band powers as T-scores computed for all ROIs, by each hand, and mapped onto a 3D cortex [Figure 6]).
2. Pre vs Post Mirror Neuron Network Connectivity Report provides Connectivity Analyses (MNN Network), including connectogram plots21 (visual representations of neural connections in the brain) showing Pre vs Post (motor imagery) differences in effective connectivity (a multivariate Granger Causaliy22 measure) between selected cortical regions of interest following standardized Low Resolution Electromagnetic Tomography23 sLORETA source localization.
3. Motor Training Task Session Report provides Cognitive Metrics (showing the average Attention and Workload metrics computed across the entire session); SMR Asymmetry Over Time (showing the average SMR [13-15 Hz] Asymmetry for the Mirror Neuron Network ROIs averaged together over time); and Power Bands (with line plots of the frequency band powers (dB) for all channels across the entire session).
4. Individual Session Visual Arrest Report provides: Analysis by Channels (with Power Spectra Channels,
BandPower Channels, BandPower Bands / Ratio Topoplots); and Analysis by Sources (with Power Spectra Sources, BandPower Sources, BandPowerRatio Cortex Plots).
The mean age of participants was 19.37 ± 1 years (females 19.8 years; males 19.1 years); height = 176.75cm ± 8.05 cm (females 167cm; males 186cm); weight = 79.38 ± 14.36 kg females 67kg; males 84kg). (Figure 7)
Analysis of the qEEG data of the male and female athletes in this case series demonstrated asymmetries during motor strategies during the step down left, single leg squat and the unloaded squat. Females performed better in the single leg squat and unloaded squat while males performed better on the step-down landing left task. These findings were also supported by the SMR Cortex plots. These cortex plots illustrate characteristics for both male and female, regions of interest, frequency bands of the EEG and network activation during assessment of motor tasks that emphasize balance, gait, mobility and lower extremity symmetry. (Figure 8)
The Attention metric indicates the ability to maintain goaldirected behavior in the face of distractions. The metric composites were measured during the performance of the functional movement tasks, covert and overt imagery, and
Figure 6. Sample cortex plots in the Pre vs Post Motor Training Task Report generated for a male participant.
Visualizations illustrate the difference in Pre and Post Motor Imagery Task frequency band powers as T-scores (masked for significant values only) computed for all ROIs, by each hand, and mapped onto a 3D cortex. Positive (yellow to red) values represent hyperactivity, where negative values (green to violet) represent hypoactivity
The leftmost column shows the entire cortex, while the middle and right images independently represent the two hemispheres of the cortex.
Figure 7. Subject demographic and sport participation data, including a summary of contact versus non-contact athletic participation.
cognitive tasks. The attention metric is calculated utilizing frequency band ratios of frontal theta and beta/alpha. Attention increased consistently for both females and males until the single leg balance task as represented in Figure 9
Brain workload is related to the brain region(s) of interest engaged through electrical connections during the performance of tasks being performed. The workload metric in-
Figure 8. Represents SMR Asymmetry data (depicted above in decibels (db)
This figure illustrates the average SMR Asymmetry for the Mirror Neuron Network Regions of Interests (ROI’s). Data compare the motor imagery periods before and after the motor imagery tasks.
Figure 9. The ability to maintain goal-directed behavior in the face of distractions indicating attention during performance tasks.
This composite of brain activity results (measured in db) of all male participants versus all female participants was measured during the performance of the functional movement tasks, covert and overt imagery tasks, and cognitive tasks.
dicates how the brain responds to the activities being engaged. Results from previous studies have shown that there is a significant difference between men and women in terms of brain workload capability.24 Figure 10 indicates the brain
workload metric by task for both females and males. Females’ cognitive workload capability was higher than males beginning at the initial baseline task. Monitoring brain workload in tandem with other key components, such as,
Figure
This depicts the cognitive ability to interact with complex environments in a goal-directed manner The metric composite results of all male participants versus all female participants measured during the performance of functional movement tasks, covert and overt imagery and cognitive tasks.
attention and focus provide insight as to the effect certain tasks may tax the brain state.
Resting state utilized a spectral analysis comparison between power and frequency bands measuring the delta between EO and EC. The EEG cortex plots illustrate characteristics of various networks for both males and females, and activity in both left and right hemispheres during select functional movement tasks. The EEG cortex plots demonstrate longitudinal EEG for the initial brain map baseline, cognitive tasks, motor tasks and mental imagery Females exhibited more symmetrical pre- and post- motor task. (Figure 11)
The primary objective of this case series was to utilize neurophysiologic assessment data, including brain hemisphere asymmetry, attention levels, and brain workload analytics to quantify performance outcomes in healthy, uninjured athletes during functional movements.1,12 The results demonstrate variances in functional tasks between uninjured Division-I athletes (males and female) in key performance indicators of cognitive function, attention, brain workload capability and SMR asymmetry were observed. Musculoskeletal biomechanical asymmetries or disfunction have been previously reported to be associated with variations in muscle and brain symmetry between left and right hemispheres.25 The reported data affords a visual representation of neurophysiological performance observed dur-
ing with qEEG monitoring during performance of task driven assessments. This provides researchers and clinicians alike with a possible mechanism to explore neural behaviors, brain symmetries, and brain state regulation associated with normal movements.
Current applications in rehabilitation have increasingly embraced the concept of neural-oriented rehabilitation methods to facilitate neuroplastic adaptation. The brain has multiple cell types that divide and grow, thus developing new connections throughout a lifespan.26 Plasticity is a hallmark of the adaptability of the brain to remodel, adapt, and repair the central nervous system as a result of purposeful interventions using environmental modifications and brain exercises to stimulate neurofeedback improvements.27,28 In a similar fashion, neurological assessments provide insight into the functioning properties of the neural brain-to-body connection.
Sports medicine professionals are familiar with the concept that skeletal muscle cells do not divide with conditioning, but brain cells can divide and precipitate plasticity 26 It is incumbent upon the rehabilitation specialist to be cognizant of the role of the brain’s adaptability and changes that are seen in the pre- and post-injury periods. Dysregulation and rebuilding of neural networks during functional development and during the rehabilitation process are the hallmarks of neuroplasticity. Mangine et al.1 used high fidelity real-time qEEG and physiometric monitoring software to demonstrate simultaneous linear improvements in neurophysiological and musculoskeletal performance in a case report of an athlete after anterior cruciate ligament reconstruction and rehabilitation during a return to play progression. Although in a single subject, these findings sug-
Figure 11. Depicts Pre/Post-motor task SMR asymmetry between female and male athletes measured in decibels. Females exhibited less left to right asymmetry than males in pre/post Motor Task SMR Asymmetry Analysis than males pre/post Motor Task Asymmetry
gest changes in the brain’s neuroplastic properties impact musculoskeletal function.1 Thus, clinicians should seek to objectively evaluate brain state during functional training and/or rehabilitation progressions.
Division I athletes possess elite levels of human performance capabilities in strength, agility, balance, reaction time and focus.24 Until recently, measuring these capabilities was largely limited to sport statistics, kinematic observation (time trials, jumping distance, etc.)24 and various strength assessments (bench press, leg press, etc.).29 Over time, the proliferation of sports related injuries has warranted investigations into the role the of the brain-to-body connection30 in athletic performance, including both psychological factors31,32 and neurological function.33
There is a need for methods to support assessment of facets of neuroplasticity as part of functional rehabilitation and the development of athletic skills. The current case series provides information gained from neurophysiologic assessment that demonstrates a foundation utilizing analytics from task-driven exercises to evaluate and benchmark athletic performance capabilities and may assist optimize rehabilitation outcomes within the sports medicine field.
Embracing rehabilitation interventions designed to optimize brain and body performance seems ideal for monitoring athlete preparedness in both clinical rehabilitation and sports performance. Recent findings1 have reported dysregulation in qEEG brain mapping occurs following anterior-cruciate injury and/or reconstruction. Mangine et al.1 demonstrated a functional correction in brain state regulation to be related to improved neurophysiological outcomes, such as, reaction time and task completion during a rehabilitative return to play process following an anterior cruciate ligament repair in a single subject. A dysregulated
brain state appears to disrupt neuroprocessing necessary to maintain biomechanical and functional stability associated with sport performance and injury prevention mechanics.7, 33 Future studies could utilize neurophysiological baseline data and progressive assessment information to aid in decision making concerning management and rehabilitation of the injured athlete** **
This case series is a first requisite step in building a body of evidence connecting physical activities and brain functional responses among healthy athletes. Using a combination of qEEG, physiometrics, psychometric, and kinematic applications to monitor change in neurophysiological performance post musculoskeletal injury seems warranted but requires more specialized targeted programs for behaviors associated with brain process for motor control, skill development, and biomechanical sport functions. Future studies should investigate the use of neurophysiological assessments to help determine brain regulatory status and functional readiness to return to athletic participation. Additionally, advanced understanding of brain activity to coordinate neuromuscular function during sports participation may assist sports medicine professionals in examining strategies to mitigate injuries.
The neurophysiologic assessments were performed on healthy non-fatigued, uninjured Division 1 athletes using musculoskeletal movements associated with sport and rehabilitation. Fatigue factors have been shown to have a relationship with functional performance34 and were not accounted for in this case series. qEEG data were not collected during actual sport participation, so maximal strength and
maximal speed likely not reached by each participant. The task driven activities were limited to controlled movements requiring the brain and body functioning together supporting clean analytics by limiting extraneous EEG “noise” during data collection. Notwithstanding, outcome measures from the current study are unique in combining qEEG, physiometric, and physical movements** **
The data collected in this case series supports the potential use of the combination of qEEG and physiometric data as a novel neurophysiological real-time measurement to serve as a clinical assessment for establishing comparative baseline normative data for athlete performance. In addition to the unique utility of qEEG and neurophysiologic as an as-
sessment for baseline data, qEEG assessment could provide meaningful data to support clinical decision making and clinical intervention choices. Performing qEEG assessments in tandem with functional movements may allow clinicians to gain insight into the athlete’s potential readiness for participation and safe return to play, related to brain health and neurophysiological function. The authors hope that this work will be to empower sports medical professionals to consider quantitative information concerning the brain’s role in motor function as it relates to motor performance and rehabilitation in athletic or functionally active populations.
Submitted: July 23, 2024 CDT, Accepted: September 25, 2024 CDT
©
The Author(s)
This is an open-access article distributed under the terms of the Creative Commons Attribution 4.0 International License (CCBY-NC-4.0). View this license’s legal deed at https://creativecommons.org/licenses/by-nc/4.0 and legal code at https://creativecommons.org/licenses/by-nc/4.0/legalcode for more information.
1. Mangine RE, Tersak J, Palmer T, et al. The longitudinal neurophysiological adaptation of a Division I female Lacrosse player following anterior cruciate rupture and repair: a case report. Int J Sports Phys Ther 2023;18(2):467-476. doi:10.26603/ 001c.73179
2. Schutte MJ, Dabezies EJ, Zimny ML, Happel LT Neural anatomy of the human anterior cruciate ligament. J Bone Joint Surg Am. 1987;69(2):243-247. doi:10.2106/00004623-198769020-00011
3. Grooms DR, Diekfuss JA, Criss CR, et al. Preliminary brain-behavioral neural correlates of anterior cruciate ligament injury risk landing biomechanics using a novel bilateral leg press neuroimaging paradigm. PloS one. 2022;17(8):e0272578. doi:10.1371/ journal.pone.0272578
4. Bruns A, Eckhorn R. Task-related coupling from high- to low-frequency signals among visual cortical areas in human subdural recordings. Int J Psychophysiol. 2004;51(2):97-116. doi:10.1016/ j.ijpsycho.2003.07.001
5. Eklund A, Nichols TE, Knutsson H. Cluster failure: Why fMRI inferences for spatial extent have inflated false-positive rates. Proc Natl Acad Sci USA 2016;113(28):7900-7905. doi:10.1073/ pnas.1602413113
6. Simon JE, Millikan N, Yom J, Grooms DR. Neurocognitive challenged hops reduced functional performance relative to traditional hop testing. Phys Ther Sport 2020;41:97-102. doi:10.1016/ j.ptsp.2019.12.002
7. Chi YM, Wang Y, Wang YT, Jung TP, Kerth T, Cao Y. A practical mobile dry EEG system for human computer interfaces. In: Schmorrow DD, Fidopiastis CM, eds. Foundations of Augmented Cognition. Lecture notes in Comput Sci. Springer; 2013. doi:10.1007/ 978-3-642-39454-6_69
8. Mantashloo Z, Letafatkar A, Moradi M. Vertical ground reaction force and knee muscle activation asymmetries in patients with ACL reconstruction compared to healthy individuals. Knee Surg Sports Traumatol Arthrosc 2020;28(6):2009-2014. doi:10.1007/s00167-019-05743-5
9. Myer GD, Paterno MV, Ford KR, Quatman CE, Hewett TE. Rehabilitation after anterior cruciate ligament reconstruction: criteria-based progression through the return-to-sport phase. J Orthop Sports Phys Ther 2006;36(6):385-402. doi:10.2519/ jospt.2006.2222
10. Bakal DR, Morgan JJ, Lyons SM, Chan SK, Kraus EA, Shea KG. Analysis of limb kinetic asymmetry during a drop vertical jump in adolescents post anterior cruciate ligament reconstruction. Clin Biomech 2022;100:105794. doi:10.1016/ j.clinbiomech.2022.105794
11. Clark JF, Ellis JK, Burns TM, Childress JM, Divine JG. Analysis of central and peripheral vision reaction times in patients with postconcussion visual dysfunction. Clin J Sport Med 2017;27(5):457-461. doi:10.1097/JSM.0000000000000381
12. Kamzanova AT, Kustubayeva AM, Matthews G. Use of EEG workload indices for diagnostic monitoring of vigilance decrement. Hum Factors 2014;56(6):1136-1149. doi:10.1177/ 0018720814526617
13. Swanik CB. Brains and sprains: the brain’s role in noncontact anterior cruciate ligament injuries. J Athl Train 2015;50(10):1100-1102. doi:10.4085/ 1062-6050-50.10.08
14. Della Villa F, Buckthorpe M, Grassi A, et al. Systematic video analysis of ACL injuries in professional male football (soccer): injury mechanisms, situational patterns and biomechanics study on 134 consecutive cases. Br J Sports Med 2020;54(23):1423-1432. doi:10.1136/ bjsports-2019-101247
15. Caruana-Montaldo B, Gleeson K, Zwillich CW. The control of breathing in clinical practice. Chest 2000;117(1):205-225. doi:10.1378/chest.117.1.205
16. Thayer JF, Ahs F, Fredrikson M, Sollers JJ 3rd, Wager TD A meta-analysis of heart rate variability and neuroimaging studies: implications for heart rate variability as a marker of stress and health. Neurosci Biobehav Rev 2012;36(2):747-756. doi:10.1016/ j.neubiorev.2011.11.009
17. Kaplan J, Colgan DD, Klee D, Hanes D, Oken BS. Patterns of respiration rate reactivity in response to a cognitive stressor associate with self-reported mental health outcomes. Psychol Rep. 2023:332941231171887 doi:10.1177/ 00332941231171887
18. Peper E, Gibney KH. A teaching strategy for successful hand warming. Somatics. 2003;XIV(1):26-30. Accessed May 11, 2024. https:// biomedical.com/media/support/ teaching_strategy_for_successful_hand_warming.pdf
19. Demuru M, La Cava SM, Pani SM, Fraschini M. A Comparison between power spectral density and network metrics: An EEG Study. Biomed Signal Process Contr 2020;57:101760. doi:10.1016/ j.bspc.2019.101760
20. Shumway RH, Stoffer DS. Time Series Analysis and Its Applications 4th ed. Springer Cham. Berlin, Heidelberg, Dordrecht, and New York City; 2017 doi:10.1007/978-3-319-52452-8
21. Bassett DS, Sporns O Network neuroscience. Nat Neurosci 2017;20(3):353-364. doi:10.1038/nn.4502
22. Bayley PJ, Tully K. A multivariate Granger causality approach to the analysis of brain networks. J Neurosci Meth 2018;300:27-35. doi:10.1016/ j.jneumeth.2018.01.013
23. Pascual-Marqui RD, Lehmann D. Error estimates for EEG source localization. Electroencephalogr Clin Neurophysiol. 1999;109(5):493-497. doi:10.1016/ S0013-4694(99)00134-0
24. Rentz LE, Brandmeir CL, Rawls BG, Galster SM. Reactive task performance under varying loads in Division I collegiate soccer athletes. Front Sports Act Living 2021;3:707910. doi:10.3389/fspor.2021.707910
25. Olajos AA, Takeda M, Dobay B, Radak Z, Koltai E. Freestyle gymnastic exercise can be used to assess complex coordination in a variety of sports. J Exerc Sci Fit 2020;18(2):47-56. doi:10.1016/ j.jesf.2019.11.00
26. Power JD, Schlaggar BL. Neural plasticity across the lifespan. Wiley Interdiscip Rev Dev Biol 2017;6(1). doi:10.1002/wdev.216
27 Tassani S, Font-Llagunes JM, Gonzalez-Ballester MA, Noailly J. Muscular tension significant affects stability in standing posture. Gait Posture 2019;68:220-226. doi:10.1016/j.gaitpost.2018.11.034
28. Kumar J, Patel T, Sugandh F, et al. Innovative approaches and therapies to enhance neuroplasticity and promote recovery in patients with neurological disorders: a narrative review. Cureus. 2023;15(7):e41914. doi:10.7759/cureus.41914
29. McGuine TA, Post EG, Herzel SJ, Brooks MA, Trigsted S, Bell DR. A prospective study on the effect of sport specialization on lower extremity injury rates in high school athletes. Am J Sports Med 2017;45(12):2706-2712. doi:10.1177/ 0363546517710213
30. Spreng RN, Stevens WD, Chamberlain JP, Gilmore AW, Schacter DL. Default network activity, coupled with the frontoparietal control network, supports goal-directed cognition. Neuroimage 2010;53(1):303317. doi:10.1016/ j.neuroimage.2010.06.016
31. Junge A. The influence of psychological factors on sports injuries. Review of the literature. Am J Sports Med 2000;28(5 Suppl):S10-S15. doi:10.1177/ 28.suppl_5.s-10
32. Webster KE, Feller JA. Psychological readiness to return to sport after anterior cruciate ligament reconstruction in the adolescent athlete. J Athl Train 2022;57(9-10):955-960. doi:10.4085/ 1062-6050-0543.21
33. Grooms DR, Page S, Onate JA. Brain activation for knee movement measured days before second anterior cruciate ligament injury: Neuroimaging in musculoskeletal medicine. J Athl Train 2015;50(10):1005-1010. doi:10.4085/ 1062-6050-50-10-02
34. Verschueren J, Tassignon B, De Pauw K, et al. Does acute fatigue negatively affect intrinsic risk factors of the lower extremity injury risk profile? A systematic and critical review Sports Med 2020;50(4):767-784. doi:10.1007/s40279-019-01235-1
Kevin E. Wilk, PT, DPT, FAPTA1,2a , Morgan Ivey, PT, DPT, SCS3 , Zachary M. Thomas, PT, DPT, OCS, SCS, CSCS4,5 , Lewis Lupowitz, PT, DPT, SCS, CSCS6 1 Champion Sports Medicine, Select Medical, 2 Director of Rehabilitative Research, American Sports Medicine Institute, 3 Sports Physical Therapist, Sports Medicine Fellow, Champion Sports Medicine, 4 Sports Physical Therapist, University of Georgia, 5 Piedmont Orthopedics & Sports Medicine, 6 Sports Physical Therapist, Northwell Health
Keywords: Anterior cruciate ligament reconstruction, neurocognitive training, neuroplasticity, proprioception, motor control https://doi.org/10.26603/001c.124945
Anterior cruciate ligament (ACL) injury rates are on the rise, despite improved surgical techniques and prevention programs. While traditional rehabilitation emphasizes the restoration of motion, strength, and physical performance, emerging research highlights the importance of addressing neurocognitive deficits that can persist after injury. These deficits, including altered proprioception, impaired motor control and muscle recruitment, as well as heightened reliance on visual feedback, can significantly increase the risk of re-injury and impede return to sport. The purpose of this clinical commentary is to outline a proposed comprehensive approach to rehabilitation that challenges the neurocognitive system to optimize rehabilitation outcomes and reduce reinjury risk. Thus, this clinical commentary discusses the rationale for integrating neurocognitive training into all phases of ACLR rehabilitation, from initial injury to eight weeks post-surgery. It details the neurophysiological changes caused by ACL injury and presents evidence supporting the use of exercises that challenge visual attention, decision-making, and motor planning. A comprehensive rehabilitation framework incorporating both physical and neurocognitive components is proposed, aiming to improve long-term outcomes and reduce re-injury risk.
Level of Evidence: 5
Each year, an estimated 300,000 ACL injuries occur annually in the United States, resulting in about 225,000 to 250,000 surgeries.1 Approximately 70% of ACL injuries occur from a non-contact mechanism, such as running and pivoting, cutting, or landing from a jump.2‑4 Unfortunately, between 10 and 25% of individuals will sustain a second ACL injury.5‑7
Female athletes in sports like soccer, basketball, and volleyball, as well as gymnasts, and male football players, face a heightened risk of injury. These injuries are often triggered by unanticipated or decelerating movements, and the injury that is sustained disrupts the body’s normal sensory input and motor control, leading to neuroplastic changes in the brain.8 Research has shown that these changes can
manifest as decreased reaction times, processing speeds, and proprioception, further contributing to the risk of injury.9‑13
Neuroplasticity, the brain’s ability to form new neural connections, plays a crucial role in recovery from ACL injury. This process can involve modification of cognitive strategies, recruitment of new and different neural networks (neural patterning), or adjusting the strength of existing connections in brain areas in charge of carrying out particular tasks.14 Neuroplasticity can be both beneficial and deleterious. While positive effects can enhance motor control, negative effects can lead to inefficient and compensatory movement patterns.14 This central nervous system
Corresponding Author: Kevin Wilk
200 Montgomery Hwy, Suite 150 Birmingham, AL, 35216 kwilkpt@hotmail.com
re-wiring combined with biomechanical alterations in loading strategies could explain the reason some ACL patients are unable to recruit their quadriceps muscle leading to a quadriceps avoidance gait. As Wexler et al. describe, this maladaptation or negative neuroplastic change involves walking with a slightly flexed knee, marked by overactive hamstring musculature and inhibited quadriceps.15 If not corrected, this could lead to a delay in the return of quadriceps muscle strength, and poorer knee function.
Neuroplastic changes that occur following ACL injury are likely due to altered afferent input which affects the efferent output. The alteration of afferent input is often a result of a loss of somatosensory signal from the ruptured ligament and increased nociceptor activity associated with pain, swelling, and inflammation.11 The altered efferent output leads to a disruption in gamma-motor neuron feedback loops,16 delayed long latency reflexes,17 and altered spinal excitability.18 Therefore, increased cortical excitability is required to generate a muscle contraction after ACLR.19,20 This mechanism results in the shift from what used to be a feedforward or semi-automatic movement, to movements that now require increased volitional control. Grooms et al. demonstrated this paradigm utilizing fMRI with individuals following ACLR, finding that ACLR patients demonstrated increased cortical drive, visual dependency, and decreased neuromuscular control compared to controls during a simple supine knee extension exercise triggered by a visual prompt.10
Often, the regions of the brain most affected following ACL injury are those involved in visual and cognitive function.21 This results in an overreliance on visual processing/ feedback and motor planning to maintain neuromuscular control and dynamic stabilization of the knee. Baumeister et al, utilizing electroencephalography, demonstrated that individuals following ACLR had greater brain activation in attention and sensory areas, however this did not correlate to improvements in proprioceptive performance.22 They concluded that the increased activation may be attributed to decreased efficiency and/or increased excitability required to complete the same task. Each of these studies highlight the complexity of neuroplastic changes that occur after ACL injury, therefore it is important to address these factors in rehabilitation.
NEUROCOGNITIVE REHABILITATION: A COMPREHENSIVE APPROACH
Given the prevalence of non-contact, unanticipated injuries and their impact on the brain, the authors suggest implementing early neurocognitive training into ACL rehabilitation immediately following injury and/or surgery This approach aims to improve neuromuscular control and dynamic stabilization, with the ultimate goal of reducing reinjury risk.
Neurocognitive rehabilitation can be thought of as any task that occupies the patient/athlete’s attention through visual, mental, auditory, verbal and/or kinesthetic stimuli, while simultaneously performing a movement, task, exercise, or skill.12 As the patient demonstrates more automatic
control with decreased response time and proper mechanics, the complexity of the task is increased. The neurocognitive variables could include the following: speed, dual tasking, verbal, visual tracking, perturbations, directions, obstacles, and surfaces.
Traditional ACLR rehabilitation often focuses on physical aspects such as limb symmetry, range of motion, and strength, with less emphasis on neuromuscular and neurocognitive adaptations. Additionally, there is a reliance on an internal focus of control (i.e. “ squeeze your quad”, “don’t let your knee go in” or “bend your knee”) as opposed to an external control (i.e. responding to a visual stimulus, hitting a target or catching a ball). While this may be necessary in the early stages for focusing attention to complete activation of specific muscles, it doesn’t fully prepare athletes for the dynamic and reactive environment of their sport. Internal focus can overload cognitive resources, leading to decreased neuromuscular control during complex tasks. Shifting towards an external focus, where athletes respond to environmental stimuli, promotes more automatic movements and better prepares them for the demands of their sport.
Sports are filled with neurocognitive challenges that expand far beyond just the physical demands placed on an individual. Chaput et al. explain that visual-cognitive processes in sport require components of both divided and selective visual attention.21 Divided attention is described as the ability to simultaneously provide attention to or switch between multiple stimuli (i.e. dual tasking). Divided attention can further be described by the limited or multiple resource theory 23,24 When both stimuli rely on the same sense, such as auditory, they become competitive in nature (limited resource theory) versus when two stimuli affect different senses, such as vision and auditory, it is easier to multi-task (multiple resource theory). Selective attention is being able to ignore irrelevant stimuli to focus on a singular task which can occur by volitional control (topdown) or reactionary due to an un-anticipatory event (bottom-up). It has been mentioned that neurocognitive challenges for athletes do not have to be sport specific if they challenge the same underlying neurocognitive demands of sport (i.e. working memory, rapid decision making, visual processing, or postural perturbations).12,21
With increased task complexity, neuromuscular control deteriorates in those following ACLR as compared to healthy controls, possibly due to an overload of the motor planning resources.11 Sherman et al. found that individuals had greater cortical inhibition and committed more decision accuracy errors to maintain reaction time following ACLR, compared to healthy controls during a Go/No Go task stimulated by a visual target.25 Recently Chaput et al.21 have described implementing the Visual-Cognitive Control Chaos Continuum (VC-CCC) within ACLR rehabilitation which is an expansion from the original Control Chaos Continuum described by Taberner et al.26 In the VC-CCC, the
authors proposed a five-phase progression beginning at high control and stability and ending at a high chaotic environment mimicking the visual cognitive demands of sport. As task complexity increases, if performance is errorless then the challenge may not be difficult enough to elicit learning.27
In the authors’ experience, a comprehensive approach that includes neurocognitive training is necessary to address neuroplasticity changes affecting proprioception and motor control deficits post-ACLR. The senior author has found that incorporating a combination of neuromuscular and neurocognitive training has led to significantly improved outcomes for ACL patients. Therefore, the purpose of this clinical commentary is to outline a proposed comprehensive approach to rehabilitation that challenges the neurocognitive system to optimize rehabilitation outcomes and reduce reinjury risk.
It is imperative to include exercises that address these deficits both immediately after injury and throughout the continuum of care to prevent the deleterious effects of neuroplasticity. Performing a motor task while altering visual input through external factors (e.g., blindfolds/goggles, targets, balls, defenders, visual signals) has been shown to negatively impact neuromuscular control in ACLR patients compared to performing motor tasks alone.20 In addition, vibration can positively impact efferent motor output by enhancing muscle activation and proprioception.28,29 Moreover, many studies indicate no difference at one and two-year follow up with the addition of neuromuscular training alone,28,29 indicating the need for a cognitive component to be included during the exercise. Finally, deficits in both unilateral and contralateral limb proprioception and neuromuscular control30 exist after injury; thus it is important to include education and training for the entire neurocognitive system. The authors’ detailed program including variables and progressions is outlined in Table 1
The pre-operative phase of ACL reconstruction is crucial for preparing the patient physically and mentally for surgery and subsequent rehabilitation and is ideally incorporated immediately following injury The primary goals during this phase include reducing inflammation and pain to achieve a “quiet knee” prior to surgery, normalizing ROM (especially extension and flexion), quadriceps muscle activation, preventing significant quadriceps atrophy, and normalizing gait. A critical component of this phase is the incorporation of neurocognitive exercises to decrease early motor compensations and quadriceps muscle inhibition. The specific exercises are recommended as soon as possible.
Motor and cognitive dual-tasking exercises are implemented, where patients perform functional and cognitive tasks simultaneously This approach promotes agonist/an-
tagonist muscle coactivation while stimulating brain centers responsible for motor planning and proprioception.20 Patients who initiate gait training early have been shown to have decreased compensations and increased functional outcomes compared to those who initiate gait training later in rehab.31 Gait training drills such as stepping over cones is initiated to develop symmetry between the lower extremities. Symmetrical hip, knee, and ankle flexion over the cone, quadriceps and hip control during weight acceptance, gastroc/soleus symmetry during push-off, and equal stance time between limbs are all vital components in this exercise. This drill is progressed to tax the neurocognitive system by performing at various speeds, in multiple directions (forward, backward, lateral), (Figure 1a, 1b) adding serial counting/memory recall drills, ball tossing/catching, or using strobe goggles.
Additional exercises include squats on a tilt board while throwing/catching a ball or balancing on an unstable surface while catching a randomly colored stick and reacting to the correct color. These exercises target balance, reaction time, and visual processing. Furthermore, active joint repositioning drills can be performed with eyes closed to enhance proprioception. These early intervention drills are designed to minimize and prevent negative neuroplastic adaptations. Consistent implementation of these exercises prior to surgery is crucial to optimize beneficial neuroplastic changes and prevent maladaptive patterns.
The immediate post-operative phase echoes the goals of the pre-operative phase and focuses on achieving a “quiet knee” quickly to facilitate early quadriceps activation, protect the surgical graft, and aid in regaining extension ROM. From day one, quadriceps activation exercises are implemented using neuromuscular electrical stimulation (NMES) and, for those with difficulty in efferent firing, electromyographic (EMG) visual biofeedback devices. These techniques attempt to override quadriceps inhibition by increasing action potentials in motor units (NMES)32 or enhancing real time visual feedback (EMG), allowing patients to adjust their muscle activation patterns during exercise. A limitation of using NMES alone is that, while many patients experience improved quadriceps contraction during individual sessions, it does not directly improve knee extensor strength. Additionally, there is often minimal carryover (voluntary control) to the next session, indicating limited beneficial neuroplasticity Grooms et al. found that increased demands on the central nervous system during complex, high-stakes activities can override normal efferent inhibition mechanisms.11 To accomplish this, the authors advocate for the use of exercises such as quad sets (QS), straight leg raises (SLR), and knee extensions from 90-40 degrees with NMES to assist patients in quadriceps activation. These can be also performed with the patient visualizing EMG data for biofeedback to improve contraction or combined with a reactive cognitivemotor task, such as performing a QS while throwing/catch-
Exercise/Drill Variables/ progressions to enhance neuroplasticity
Quad sets
Straight leg raises (3 way)
Knee extensions (90-40 deg)
Mini squats Standing TKEs
NMES EMG biofeedback
Reactive cognitive motor task (BlazePods)
Surface (i.e. foam, rocker board)
Resistance
Dual task (i.e ball toss or HecoStix)
Reactive cognitive motor task (BlazePods, Neurocognitive Sensory station)
Perturbations
Cognitive task (counting, memory, etc.)
Altered vision Contralateral limb/ UE task
Gait/stepping drills:
Weight shifts
Cone stepping
Lateral lunges
Lateral stepping (slides)
Quick Board stepping drills
CKC neuromuscular exercises:
Anterior/ lateral step
downs
Multidirectional lunges
Speed Direction (ant/ post/lateral)
Dual task (i.e ball toss or HecoStix)
Cognitive task (counting, memory, etc.)
Reactive cognitivemotor task
Resistance Surface
Dual task (i.e ball toss or HecoStix)
Perturbation/ resistance from CLX band
Cognitive task (counting, memory, etc.)
Reactive cognitive motor task (BlazePods)
Resistance Surface
Single leg balance:
SL RDL with UE task
SL balance with band perturbations
Dual task (i.e. ball toss)
Direction (star drill on floor)
Visual targets
Perturbation (KB exchange/ resistance band)
UE task (i.e cable row, med ball chop, reach)
Dynamic shuffling Speed Direction
Neurocognitive Demand
Visual Cognitive Efferent firing
Cognitive Dual task
External focus of control Reaction time
Examples:
Quad set with NMES
Straight leg raises while using UE to react to indicated BlazePod color
Knee extensions using visual EMG biofeedback Virtual Reality Glasses
Mini squats on rocker board with external perturbations while throwing/catching a ball
Standing TKE while using UE to react to indicated quickboard target
Visual Cognitive Decision making
Memory
Dual task
External focus of control Reaction time
Visual Cognitive Decision making
Memory
Dual task
External focus of control
Reaction time
Visual Cognitive Decision making
Memory
Dual task
External focus of control Reaction time
Weight shifts on force plates with visual feedback
Multi-directional cone stepping counting backward by 7’s and tossing HecoStix
Lateral lunges with sport cord perturbation and ball toss, landing on foam surface
Lateral slides with resistance band around knees, reacting to verbal cues (direction, speed) and catching ball
Rapid stepping in place with visual target, reacting to correct location on the board or surface (Quickboard)
Anterior step down from box with CLX band pulling into femoral IR and dynamic LE valgus (figure 8)
Lateral lunges with sport cord perturbation and ball toss toward indicated direction. Athlete must react to indicated direction using light up pods (i.e. antero-lateral or posterolateral)
Visual Cognitive Decision making
Memory
Dual task
External focus of control Reaction time
Cognitive
SL RDL standing on foam while catching a ball and performing RDL to the indicated cone (5 cones set up in front of the patient)
Single-leg balance with band resistance and valgus perturbations: The patient stands on a rocker board while resisting external forces, dribbling a basketball, and catching Hecostix with contralateral UE (figure 9)
Exercise/Drill Variables/ progressions to enhance neuroplasticity
Visual targets (with/without distraction targets)
Auditory cues (i.e direction/speed)
Height/location of target
Sport specific task (i.e catching basketball or lacrosse ball with stick)
Neurocognitive Demand
Decision making
Memory
Dual task
External focus of control
Reaction time
Figure 1a. Forward/Backward Cone Stepping: Patient steps over cones, focusing on symmetry and 90-degree hip/knee/ankle flexion.
ing a ball or tapping light-up pods (BlazePods, Blazepod, San Diego, CA) with the upper extremity (Figure 2/Supplemental File 1). Patients can also perform knee extensions with NMES from 90 to 40 degrees with light resistance while performing hand taps with Blazepods (Figure 3/Supplemental File 2). Finally, standing terminal knee extensions with a resistance band behind the knee can be implemented using BlazePods to reinforce neural patterning (Figure 4/Supplemental File 3).
The acute phase of rehab builds upon the immediate postoperative phase, focusing on managing residual inflamma-
Examples:
Figure 1b. Lateral Cone Stepping: Patient steps laterally over cones, emphasizing controlled movement and core engagement.
tion, progressing range of motion, gait training, optimizing quadriceps firing. During this time, proprioceptive/balance training is introduced to increase neuromuscular control and prepare for more advanced stages of rehabilitation. An external focus of control is utilized to reinforce motor learning, as this has been shown to increase intracortical inhibition, which plays a role in increasing quadriceps firing.9
Double leg exercises such as mini squats and weight shifts are performed first advancing to single leg exercises such as balance and stepping drills as a progression. The mini squat is performed from 0 to 45 degrees of flexion for maximal quadriceps and hamstring co-activation. A neurocognitive challenge can easily be added by introducing
See Supplemental File 1 for video.
See Supplemental File 2 for video.
an unstable surface such as foam or a tilt board under the patient’s feet. The clinician can perturbate the surface so
See Supplemental File 3 for
that the patient must react and right the rocker board (Figure 5). As proprioception improves, the patient can catch objects or perform cognitive tasks such as counting, math problems, or responding to visual stimuli during the task. If the patient continues to demonstrate quad avoidance gait, standing terminal knee extension (TKE) exercises with Blazepods can be performed.
Reinforcing proper gait patterns in this phase is vital as soon as possible as the patient will be transitioning off crutches and utilizing the drop-lock brace only for ambulation. Gait compensations that commonly occur after ACLR include reduced knee flexion, increased hip flexion/circumduction, reduced knee extension moment, altered quadriceps-hamstring co-activation during stance, along with reduced ipsilateral stance time.33‑35 Stepping drills over cones can be utilized again here to challenge the neurocognitive system and reinforce proper mechanics.
Once the patient demonstrates appropriate gait mechanics and control with double leg exercises, lunging and stepping movements can be implemented to work towards single leg activity Lunges are performed as the patient steps to the side and lands on a flexed knee to approximately 30-45 degrees for maximal stability Each of these tasks are initiated straight in the frontal plane on a solid surface. In this phase, a ball toss or slight external perturbation may be added to incorporate additional core and lower extremity musculature with feedforward mechanisms.
Single leg exercises begin with eyes open, solid surface, and static positions, progressing with visual disturbances, varied surfaces, and dynamic UE tasks. Examples include
provides perturbations.
single leg balance on the floor with the knee slightly flexed to 30-45 degrees. This can be performed on various unstable surfaces with external perturbations, and/or a reactive sensorimotor task on a television screen in front of them (ex: using Senaptec Sensory station [Senaptec, Beaverton, OR], reacting to animals or solving math problems on the screen – Figure 6). Each of these exercises are vital to include early in the rehabilitation process in order to reinforce fundamental movement patterns and prepare the patient for more complex movements in the later stages.
The intermediate phase of ACLR rehabilitation focuses on restoring full knee range of motion (ROM) to 0°-135°-145°, advancing lower extremity strength/endurance, balance, and neuromuscular control. Most patients will unlock and discharge their knee immobilizer in this phase, so it is imperative to prepare the neuromuscular system for dynamic environments in their daily life while focusing on limb confidence and closed kinetic chain (CKC) function.
CKC neuromuscular control exercises in the sagittal plane are initiated with an anterior step down on level ground. The patient is instructed to place their hands on their hips and tap the heel of the uninvolved leg on the ground in front of them while controlling dynamic lower extremity (LE) valgus and pelvic pronation. Initially, a mirror is used as an external focus of control. This can be progressed to an elevated surface using weights, and eventually with spiral band resistance to enhance the control of rotational patterns (Figure 7). The front step-down exercise can also be enhanced with the utilization of blazepods for
resistance band applying adduction and internal rotation force.
reactive stability. These progressions will be described in greater detail in Part 2 of this commentary
Figure 8a. Quickboard (Memphis, TN) Lateral Step: Patient performs lateral step onto Quickboard, focusing on knee stability and target reaction.
See Supplemental File 4 for video.
Frontal plane movements are progressed with lateral lunges and lateral stepping drills. Lateral stepping is introduced once the patient demonstrates appropriate sagittal plane control. This is performed with a resistance band placed around the knees while the patient steps laterally at the assigned speed (slow, medium, or fast) and direction. A ball is then thrown for additional neurocognitive engagement as the patient must react to both the verbal direction and direction of the ball. Faster speeds and reactive directions are reserved for the later stages.
Lunges are progressed to diagonal lunges in both the anterior and posterior direction and upon unstable surfaces. Rotation may be added when the patient is further along in the rehab process. Multi-directional lunges are progressed with resistance using a sport cord or manual external perturbation. A visual stimulus on the ground can also be added using target lights or the QuickBoard Device (Figure 8a). The patient can lunge onto the indicated surface, reacting to the correct position on the board (Figure 8b).
Stepping exercises can be advanced in this stage to emphasize speed, coordination, and endurance. Patients perform in-place stepping drills while reacting to visual targets on the Quick Board (Figure 9a/Supplemental File 6). This can also be performed with a reaction target (Figure 9b/Supplemental File 7). The Quick Board may facilitate various single and dual-leg coordination exercises while assessing accuracy, reaction time, and limb symmetry.
Finally, single leg tasks can also be combined into a functional task such as a single leg Romanian Deadlift (SL RDL). This can be performed on various surfaces, unweighted or weighted with kettlebells (KB). Tasks such as
Lunge with Catch: Patient performs lateral lunge towards Blazepod (San Diego, CA) while catching a ball.
See Supplemental File 5 for video.
9a. Quickboard (Memphis, TN) Foot Fire: Patient performs rapid stepping in place while tapping targets on Quickboard.
See Supplemental File 6 for video.
SL RDL with KB exchanges may be incorporated, where the patient performs an RDL holding the bottom position while
Figure 9b. Quickboard (Memphis, TN) Foot Fire with Reactive Taps: Patient performs rapid stepping in place while reacting to visual targets on the screen.
See Supplemental File 7 for video.
exchanging a weighted KB from hand to hand to challenge the limits of stability. Another option is the SL RDL with star drill, where the patient catches a ball and touches a series of 5 cones placed on the floor while in the bottom of the RDL position, allowing for incorporation of UE, visual system, and SL stability SL RDL with CLX tubing wrapped around the thigh is used for proprioceptive input to control dynamic LE valgus, adduction and internal rotation. These single-leg balance exercises with extremity movement are designed to engage multiple muscle groups while enhancing dynamic stabilization.
As exercise complexity increases, patients often exhibit deteriorating movement patterns, which can heighten their risk of injury For those demonstrating significant pelvic drop and hip internal rotation on the stance leg during functional tasks, a targeted exercise using a resistance band can be implemented. The band is strategically placed around the athlete’s limb to replicate adduction and internal rotation forces commonly experienced during dynamic movements. The athlete then performs sport-specific tasks, such as dribbling a basketball or throwing/kicking a soccer ball, while balancing on an unstable surface (e.g., rocker board). Simultaneously, the practitioner applies external perturbations by pulling on the resistance band and tapping the board (Figure 10). Alternatively, the practitioner can pull the band medially to recreate knee valgus forces. To increase the neurocognitive challenge, BlazePods can be introduced on the floor for the athlete to tap with their contralateral extremity during the task.
To introduce dynamic lateral movements focused on rapid visual processing and change of direction movements,
lateral shuffling is introduced with BlazePods. Four Blazepods are placed in a straight line in front of the patient on top of cones for enhanced visibility As the BlazePod lights up, the athlete performs a lateral shuffle towards the illuminated pod while staying low and maintaining balance. Simultaneously, the clinician tosses a ball towards the athlete. The athlete must catch the ball mid-shuffle, continue moving towards the BlazePod, and tap the lit pod with the ball before shuffling back to the starting position. This drill can be progressed by placing the pods on the floor so that the athlete must maintain a lower stance and with quicker speeds (Figure 11/Supplemental File 8). The exercise is designed to enhance reaction time, hand-eye coordination, lateral speed, and agility while keeping the athlete engaged in multiple tasks simultaneously Each progressive variation simulates the visual-cognitive demands of sport, training the athlete to maintain control and react effectively to external stimuli while executing complex movements.
In conclusion, incorporating neurocognitive training into ACL rehabilitation is essential to reduce re-injury rates and promote optimal recovery. Traditional rehabilitation methods that focus on physical aspects such as strength and range of motion, are missing a key element. Addressing neuromuscular control and cognitive function is equally crucial. Neurocognitive exercises that focus on dual-tasking and reactive/feed forward activities enhance proprioception, dynamic stability, and motor planning, and better
Figure 11. Reactive Lateral Shuffle Drill: Patient performs lateral shuffles towards illuminated BlazePods (San Diego, CA) while catching and tapping the pod with a ball.
See Supplemental File 8 for video.
mimic the real world and sporting environment, which is when most ACL injuries and re-injuries occur These exercises not only improve the immediate functional outcomes,
but also foster beneficial neuroplasticity, ensuring better long-term joint stability and movement efficiency. Clinically, it is vital to understand that neuro-cognitive training is a dynamic principle and should not be isolated to the beginning stages of rehabilitation. Instead, integrating neurocognitive training from the pre-operative phase through full recovery with sport specific drills is vital and provides a comprehensive approach, addressing the multifaceted demands of returning to both sport and competition, but it does not end here. It is essential to use neurocognitive training as an injury prevention mechanism and should be incorporated in both injured and non-injured athletes at any level of competition. Ultimately, the authors’ goal with this strategy is to bridge the gap between rehabilitation and performance, while enhancing the long-term resilience of the knee joint. Overall, the authors believe by including neuromuscular and neurocognitive exercises early and throughout the rehabilitation process, it reduces the likelihood of re-injury and supports patients in achieving a higher quality of life post-ACLR.
No funding was provided for this manuscript. Kevin Wilk serves on the medical advisory board for BlazePods and receives educational grant funds from Quickboard. No other relevant author disclosures.
Submitted: August 12, 2024 CDT, Accepted: October 01, 2024 CDT
©
The Author(s)
This is an open-access article distributed under the terms of the Creative Commons Attribution 4.0 International License (CCBY-NC-4.0). View this license’s legal deed at https://creativecommons.org/licenses/by-nc/4.0 and legal code at https://creativecommons.org/licenses/by-nc/4.0/legalcode for more information.
1. Wilk KE. Anterior cruciate ligament injury prevention & rehabilitation: Let’s get it right. J Orthop Sports Phys Ther. 2015;45(10):729-730. doi:10.2519/jospt.2015.0109
2. Griffin LY, Albohm MJ, Arendt EA, Dick RW, Garrett WE, Garrick JG, et al. Noncontact anterior cruciate ligament injuries: Risk factors and prevention strategies. J Am Acad Orthop Surg 2000;8(3):141-150. doi:10.5435/00124635-200005000-00001
3. Boden BP, Sheehan FT, Torg JS, Hewett TE. Noncontact anterior cruciate ligament injuries: mechanisms and risk factors. J Am Acad Orthop Surg. 2010;18(9):520-527 doi:10.5435/ 00124635-201009000-00003
4. Boden BP, Dean GS, Feagin JA Jr, Garrett WE Jr. Mechanisms of anterior cruciate ligament injury Orthopedics 2000;23(6):573-578. doi:10.3928/ 0147-7447-20000601-15
5. Paterno MV, Rauh MJ, Schmitt LC, Ford KR, Hewett TE. Incidence of contralateral and ipsilateral anterior cruciate ligament (ACL) injury after primary ACL reconstruction and return to sport. Clin J Sport Med 2012;22(2):116-121. doi:10.1097/ JSM.0b013e318246ef9e
6. Wiggins AJ, Grandhi RK, Schneider DK, Stanfield D, Webster KE, Myer GD Risk of secondary injury in younger athletes after anterior cruciate ligament reconstruction: A systematic review and metaanalysis. Am J Sports Med 2016;44(7):1861-1876. doi:10.1177/0363546515621554
7. Barber-Westin S, Noyes FR. One in 5 athletes sustain reinjury upon return to high-risk sports after ACL reconstruction: A systematic review in 1239 athletes younger than 20 years. Sports Health. 2020;12(6):587-597 doi:10.1177/1941738120912846
8. Swanik CB, Covassin T, Stearne DJ, Schatz P The relationship between neurocognitive function and noncontact anterior cruciate ligament injuries. Am J Sports Med. 2007;35(6):943-948. doi:10.1177/ 0363546507299532
9. Gokeler A, Neuhaus D, Benjaminse A, Grooms DR, Baumeister J. Principles of motor learning to support neuroplasticity after ACL injury: Implications for optimizing performance and reducing risk of second ACL injury. Sports Med. 2019;49(6):853-865. doi:10.1007/s40279-019-01058-0
10. Grooms DR, Page SJ, Nichols-Larsen DS, Chaudhari AMW, White SE, Onate JA. Neuroplasticity associated with anterior cruciate ligament reconstruction. J Orthop Sports Phys Ther 2017;47(3):180-189. doi:10.2519/jospt.2017.7003
11. Grooms DR, Page SJ, Onate JA. Brain activation for knee movement measured days before second anterior cruciate ligament injury: Neuroimaging in musculoskeletal medicine. J Athl Train. 2015;50(10):1005-1010. doi:10.4085/ 1062-6050-50.10.02
12. Walker JM, Brunst CL, Chaput M, Wohl TR, Grooms DR. Integrating neurocognitive challenges into injury prevention training: A clinical commentary. Phys Ther Sport. 2021;51:8-16. doi:10.1016/j.ptsp.2021.05.005
13. Huston L, Wojtys EM. Neuromuscular performance characteristics in elite female athletes. Am J Sports Med 1996;24(4):427-436. doi:10.1177/ 036354659602400405
14. Sharma N, Classen J, Cohen LG. Neural plasticity and its contribution to functional recovery Handb Clin Neurol 2013;110:3-12. doi:10.1016/ B978-0-444-52901-5.00001-0
15. Wexler G, Hurwitz DE, Bush-Joseph CA, Andriacchi TP, Bach BR Jr Functional gait adaptations in patients with anterior cruciate ligament deficiency over time. Clin Orthop Relat Res. 1998;(348):166-175. doi:10.1097/ 00003086-199803000-00026
16. Konishi Y, Aihara Y, Sakai M, Ogawa G, Fukubayashi T Gamma loop dysfunction in the quadriceps femoris of patients who underwent anterior cruciate ligament reconstruction remains bilaterally Scand J Med Sci Sports 2007;17:393-399. doi:10.1111/j.1600-0838.2006.00573.x
17. Madhavan S, Shields RK. Neuromuscular responses in individuals with anterior cruciate ligament repair. Clin Neurophysiol. 2011;122:997-1004. doi:10.1016/j.clinph.2010.09.002
18. Héroux ME, Tremblay F. Corticomotor excitability associated with unilateral knee dysfunction secondary to anterior cruciate ligament injury. Knee Surg Sports Traumatol Arthrosc 2006;14:823-833. doi:10.1007/s00167-006-0063-4
19. Kuenze CM, Hertel J, Weltman A, Diduch D, Saliba SA, Hart JM. Persistent neuromuscular and corticomotor quadriceps asymmetry after anterior cruciate ligament reconstruction. J Athl Train. 2015;50:303-312. doi:10.4085/1062-6050-49.5.06
20. Grooms DR, Onate JA. Neuroplasticity following anterior cruciate ligament injury: a framework for visual-motor training approaches in rehabilitation. J Orthop Sports Phys Ther 2016;46(5):384-393.
21. Chaput M, Simon J, Taberner M, Grooms D From control to chaos: Visual-cognitive progression during recovery from ACL reconstruction. J Orthop Sports Phys Ther 2024;54:1-26. doi:10.2519/ jospt.2024.12443
22. Baumeister J, Reinecke K, Schubert M, Weiss M. Altered electrocortical brain activity after ACL reconstruction during force control. J Orthop Res. 2011;29(9):1383-1389. doi:10.1002/jor.21380
23. Wickens CD Multiple resources and mental workload. Hum Factors. 2008;50(3):449-455. doi:10.1518/001872008X288394
24. Pashler H. Dual-task interference in simple tasks: Data and theory. Psych Bull. 1994;116(2):220-244. doi:10.1037/0033-2909.116.2.220
25. Sherman DA, Baumeister J, Stock MS, Murray AM, Bazett-Jones DM, Norte GE. Inhibition of motor planning and response selection after anterior cruciate ligament reconstruction. Med Sci Sports Exerc. 2023;55(3):440-449. doi:10.1249/ MSS.0000000000003072
26. Taberner M, Allen T, Cohen DD Progressing rehabilitation after injury: Consider the ‘controlchaos continuum.’ Br J Sports Med. 2019;53(18):1132-1136. doi:10.1136/ bjsports-2018-100157
27. Leech KA, Roemmich RT, Gordon J, Reisman DS, Cherry-Allen KM. Updates in motor learning: Implications for physical therapist practice and education. Phys Ther. 2022;102(1). doi:10.1093/ptj/ pzab250
28. Risberg MA, Holm I, Myklebust G, Engebretsen L. Neuromuscular training versus strength training during first 6 months after anterior cruciate ligament reconstruction: a randomized clinical trial. Phys Ther. 2007;87(6):737-750. doi:10.2522/ptj.20060041
29. Moksnes H, Snyder-Mackler L, Risberg MA. Individuals with an anterior cruciate ligamentdeficient knee classified as noncopers may be candidates for nonsurgical rehabilitation. J Orthop Sports Phys Ther. 2008;38(10):586-595. doi:10.2519/ jospt.2008.2750
30. Urbach D, Nebelung W, Weiler HT, Awiszus F. Bilateral deficit of voluntary quadriceps muscle activation after unilateral ACL tear. Med Sci Sports Exerc 1999;31(12):1691-1696. doi:10.1097/ 00005768-199912000-00001
31. Gokeler A, Benjaminse A, van Eck CF, Webster KE, Schot L, Otten E. Return of normal gait as an outcome measurement in ACL reconstructed patients. A systematic review. Int J Sports Phys Ther. 2013;8(4):441-451.
32. Hauger AV, Reiman MP, Bjordal JM, Sheets C, Ledbetter L, Goode AP. Neuromuscular electrical stimulation is effective in strengthening the quadriceps muscle after anterior cruciate ligament surgery. Knee Surg Sports Traumatol Arthrosc. 2018;26(2):399-410. doi:10.1007/s00167-017-4669-5
33. Lewek MD, Rudolph KS, Axe MJ, Snyder-Mackler L. The effect of insufficient quadriceps strength on gait after anterior cruciate ligament reconstruction. Clin Biomech 2002;17(1):56-63. doi:10.1016/ S0268-0033(01)00097-3
34. Paterno MV, Schmitt LC, Ford KR, et al. Biomechanical measures during landing and postural stability predict second anterior cruciate ligament injury after anterior cruciate ligament reconstruction and return to sport. Am J Sports Med 2010;38(10):1968-1978. doi:10.1177/ 0363546510376053
35. Pietrosimone B, Blackburn JT, Padua DA, et al. Walking gait asymmetries 6 months following anterior cruciate ligament reconstruction predict 12-month patient-reported outcomes. J Orthop Res 2018;36(11):2932-2940. doi:10.1002/jor.24056
Figure 2/Supplemental File 1
Download: https://ijspt.scholasticahq.com/article/124945-neurocognitive-and-neuromuscular-rehabilitationtechniques-after-acl-injury-part-1-optimizing-recovery-in-the-acute-post-operative-phase-a-clinical/attachment/ 250700.mov?auth_token=KYwfPePOkQ1n4VpJ4-4K
Figure 3/Supplemental File 2
Download: https://ijspt.scholasticahq.com/article/124945-neurocognitive-and-neuromuscular-rehabilitationtechniques-after-acl-injury-part-1-optimizing-recovery-in-the-acute-post-operative-phase-a-clinical/attachment/ 250701.mov?auth_token=KYwfPePOkQ1n4VpJ4-4K
Figure 4/Supplemental file 3
Download: https://ijspt.scholasticahq.com/article/124945-neurocognitive-and-neuromuscular-rehabilitationtechniques-after-acl-injury-part-1-optimizing-recovery-in-the-acute-post-operative-phase-a-clinical/attachment/ 250702.mov?auth_token=KYwfPePOkQ1n4VpJ4-4K
Figure 8a/Supplemental
Download: https://ijspt.scholasticahq.com/article/124945-neurocognitive-and-neuromuscular-rehabilitationtechniques-after-acl-injury-part-1-optimizing-recovery-in-the-acute-post-operative-phase-a-clinical/attachment/ 250703.mov?auth_token=KYwfPePOkQ1n4VpJ4-4K
Figure 8b/Supplemental file 5
Download: https://ijspt.scholasticahq.com/article/124945-neurocognitive-and-neuromuscular-rehabilitationtechniques-after-acl-injury-part-1-optimizing-recovery-in-the-acute-post-operative-phase-a-clinical/attachment/ 250704.mov?auth_token=KYwfPePOkQ1n4VpJ4-4K
Figure 9a/Supplemental file 6
Download: https://ijspt.scholasticahq.com/article/124945-neurocognitive-and-neuromuscular-rehabilitationtechniques-after-acl-injury-part-1-optimizing-recovery-in-the-acute-post-operative-phase-a-clinical/attachment/ 250705.mov?auth_token=KYwfPePOkQ1n4VpJ4-4K
Figure 9b/Supplemental file 7
Download: https://ijspt.scholasticahq.com/article/124945-neurocognitive-and-neuromuscular-rehabilitationtechniques-after-acl-injury-part-1-optimizing-recovery-in-the-acute-post-operative-phase-a-clinical/attachment/ 250706.mov?auth_token=KYwfPePOkQ1n4VpJ4-4K
Figure 11/Supplemental file 8
Download: https://ijspt.scholasticahq.com/article/124945-neurocognitive-and-neuromuscular-rehabilitationtechniques-after-acl-injury-part-1-optimizing-recovery-in-the-acute-post-operative-phase-a-clinical/attachment/ 250707.mov?auth_token=KYwfPePOkQ1n4VpJ4-4K
Kevin E. Wilk, PT, DPT, FAPTA1,2a , Morgan Ivey, PT, DPT, SCS3 , Zachary M. Thomas, PT, DPT, OCS, SCS, CSCS4,5 , Lewis Lupowitz, PT, DPT, SCS, CSCS6 1 Champion Sports Medicine, Select Medical, 2 Director of Rehabilitative Research, American Sports Medicine Institute, 3 Sports Physical Therapist, Sports Medicine Fellow, Champion Sports Medicine, 4 Sports Physical Therapist, University of Georgia, 5 Piedmont Orthopedics & Sports Medicine, 6 Sports Physical Therapist, Northwell Health
Keywords: Anterior cruciate ligament reconstruction, neurocognitive training, neuroplasticity, proprioception, motor control https://doi.org/10.26603/001c.124945
Anterior cruciate ligament (ACL) injury rates are on the rise, despite improved surgical techniques and prevention programs. While traditional rehabilitation emphasizes the restoration of motion, strength, and physical performance, emerging research highlights the importance of addressing neurocognitive deficits that can persist after injury These deficits, including altered proprioception, impaired motor control and muscle recruitment, as well as heightened reliance on visual feedback, can significantly increase the risk of re-injury and impede return to sport. The purpose of this clinical commentary is to outline a proposed comprehensive approach to rehabilitation that challenges the neurocognitive system to optimize rehabilitation outcomes and reduce reinjury risk. Thus, this clinical commentary discusses the rationale for integrating neurocognitive training into all phases of ACLR rehabilitation, from initial injury to eight weeks post-surgery. It details the neurophysiological changes caused by ACL injury and presents evidence supporting the use of exercises that challenge visual attention, decision-making, and motor planning. A comprehensive rehabilitation framework incorporating both physical and neurocognitive components is proposed, aiming to improve long-term outcomes and reduce re-injury risk.
Level of Evidence: 5
Each year, an estimated 300,000 ACL injuries occur annually in the United States, resulting in about 225,000 to 250,000 surgeries.1 Approximately 70% of ACL injuries occur from a non-contact mechanism, such as running and pivoting, cutting, or landing from a jump.2‑4 Unfortunately, between 10 and 25% of individuals will sustain a second ACL injury 5‑7
Female athletes in sports like soccer, basketball, and volleyball, as well as gymnasts, and male football players, face a heightened risk of injury These injuries are often triggered by unanticipated or decelerating movements, and the injury that is sustained disrupts the body’s normal sensory input and motor control, leading to neuroplastic changes in the brain.8 Research has shown that these changes can
200 Montgomery Hwy, Suite 150 Birmingham, AL, 35216 kwilkpt@hotmail.com a Wilk KE, Ivey M, Thomas ZM, Lupowitz L. Neurocognitive and Neuromuscular Rehabilitation Techniques after ACL Injury, Part 1: Optimizing Recovery in the Acute Post-Operative Phase- A Clinical Commentary. IJSPT. Published online November 2, 2024:1373-1385. doi:10.26603/001c.124945
Corresponding Author: Kevin Wilk
manifest as decreased reaction times, processing speeds, and proprioception, further contributing to the risk of injury.9‑13
Neuroplasticity, the brain’s ability to form new neural connections, plays a crucial role in recovery from ACL injury. This process can involve modification of cognitive strategies, recruitment of new and different neural networks (neural patterning), or adjusting the strength of existing connections in brain areas in charge of carrying out particular tasks.14 Neuroplasticity can be both beneficial and deleterious. While positive effects can enhance motor control, negative effects can lead to inefficient and compensatory movement patterns.14 This central nervous system
re-wiring combined with biomechanical alterations in loading strategies could explain the reason some ACL patients are unable to recruit their quadriceps muscle leading to a quadriceps avoidance gait. As Wexler et al. describe, this maladaptation or negative neuroplastic change involves walking with a slightly flexed knee, marked by overactive hamstring musculature and inhibited quadriceps.15 If not corrected, this could lead to a delay in the return of quadriceps muscle strength, and poorer knee function.
Neuroplastic changes that occur following ACL injury are likely due to altered afferent input which affects the efferent output. The alteration of afferent input is often a result of a loss of somatosensory signal from the ruptured ligament and increased nociceptor activity associated with pain, swelling, and inflammation.11 The altered efferent output leads to a disruption in gamma-motor neuron feedback loops,16 delayed long latency reflexes,17 and altered spinal excitability.18 Therefore, increased cortical excitability is required to generate a muscle contraction after ACLR.19,20 This mechanism results in the shift from what used to be a feedforward or semi-automatic movement, to movements that now require increased volitional control. Grooms et al. demonstrated this paradigm utilizing fMRI with individuals following ACLR, finding that ACLR patients demonstrated increased cortical drive, visual dependency, and decreased neuromuscular control compared to controls during a simple supine knee extension exercise triggered by a visual prompt.10
Often, the regions of the brain most affected following ACL injury are those involved in visual and cognitive function.21 This results in an overreliance on visual processing/ feedback and motor planning to maintain neuromuscular control and dynamic stabilization of the knee. Baumeister et al, utilizing electroencephalography, demonstrated that individuals following ACLR had greater brain activation in attention and sensory areas, however this did not correlate to improvements in proprioceptive performance.22 They concluded that the increased activation may be attributed to decreased efficiency and/or increased excitability required to complete the same task. Each of these studies highlight the complexity of neuroplastic changes that occur after ACL injury, therefore it is important to address these factors in rehabilitation.
NEUROCOGNITIVE REHABILITATION: A COMPREHENSIVE APPROACH
Given the prevalence of non-contact, unanticipated injuries and their impact on the brain, the authors suggest implementing early neurocognitive training into ACL rehabilitation immediately following injury and/or surgery This approach aims to improve neuromuscular control and dynamic stabilization, with the ultimate goal of reducing reinjury risk.
Neurocognitive rehabilitation can be thought of as any task that occupies the patient/athlete’s attention through visual, mental, auditory, verbal and/or kinesthetic stimuli, while simultaneously performing a movement, task, exercise, or skill.12 As the patient demonstrates more automatic
control with decreased response time and proper mechanics, the complexity of the task is increased. The neurocognitive variables could include the following: speed, dual tasking, verbal, visual tracking, perturbations, directions, obstacles, and surfaces.
Traditional ACLR rehabilitation often focuses on physical aspects such as limb symmetry, range of motion, and strength, with less emphasis on neuromuscular and neurocognitive adaptations. Additionally, there is a reliance on an internal focus of control (i.e. “ squeeze your quad”, “don’t let your knee go in” or “bend your knee”) as opposed to an external control (i.e. responding to a visual stimulus, hitting a target or catching a ball). While this may be necessary in the early stages for focusing attention to complete activation of specific muscles, it doesn’t fully prepare athletes for the dynamic and reactive environment of their sport. Internal focus can overload cognitive resources, leading to decreased neuromuscular control during complex tasks. Shifting towards an external focus, where athletes respond to environmental stimuli, promotes more automatic movements and better prepares them for the demands of their sport.
Sports are filled with neurocognitive challenges that expand far beyond just the physical demands placed on an individual. Chaput et al. explain that visual-cognitive processes in sport require components of both divided and selective visual attention.21 Divided attention is described as the ability to simultaneously provide attention to or switch between multiple stimuli (i.e. dual tasking). Divided attention can further be described by the limited or multiple resource theory 23,24 When both stimuli rely on the same sense, such as auditory, they become competitive in nature (limited resource theory) versus when two stimuli affect different senses, such as vision and auditory, it is easier to multi-task (multiple resource theory). Selective attention is being able to ignore irrelevant stimuli to focus on a singular task which can occur by volitional control (topdown) or reactionary due to an un-anticipatory event (bottom-up). It has been mentioned that neurocognitive challenges for athletes do not have to be sport specific if they challenge the same underlying neurocognitive demands of sport (i.e. working memory, rapid decision making, visual processing, or postural perturbations).12,21
With increased task complexity, neuromuscular control deteriorates in those following ACLR as compared to healthy controls, possibly due to an overload of the motor planning resources.11 Sherman et al. found that individuals had greater cortical inhibition and committed more decision accuracy errors to maintain reaction time following ACLR, compared to healthy controls during a Go/No Go task stimulated by a visual target.25 Recently Chaput et al.21 have described implementing the Visual-Cognitive Control Chaos Continuum (VC-CCC) within ACLR rehabilitation which is an expansion from the original Control Chaos Continuum described by Taberner et al.26 In the VC-CCC, the
authors proposed a five-phase progression beginning at high control and stability and ending at a high chaotic environment mimicking the visual cognitive demands of sport. As task complexity increases, if performance is errorless then the challenge may not be difficult enough to elicit learning.27
In the authors’ experience, a comprehensive approach that includes neurocognitive training is necessary to address neuroplasticity changes affecting proprioception and motor control deficits post-ACLR. The senior author has found that incorporating a combination of neuromuscular and neurocognitive training has led to significantly improved outcomes for ACL patients. Therefore, the purpose of this clinical commentary is to outline a proposed comprehensive approach to rehabilitation that challenges the neurocognitive system to optimize rehabilitation outcomes and reduce reinjury risk.
It is imperative to include exercises that address these deficits both immediately after injury and throughout the continuum of care to prevent the deleterious effects of neuroplasticity. Performing a motor task while altering visual input through external factors (e.g., blindfolds/goggles, targets, balls, defenders, visual signals) has been shown to negatively impact neuromuscular control in ACLR patients compared to performing motor tasks alone.20 In addition, vibration can positively impact efferent motor output by enhancing muscle activation and proprioception.28,29 Moreover, many studies indicate no difference at one and two-year follow up with the addition of neuromuscular training alone,28,29 indicating the need for a cognitive component to be included during the exercise. Finally, deficits in both unilateral and contralateral limb proprioception and neuromuscular control30 exist after injury; thus it is important to include education and training for the entire neurocognitive system. The authors’ detailed program including variables and progressions is outlined in Table 1
The pre-operative phase of ACL reconstruction is crucial for preparing the patient physically and mentally for surgery and subsequent rehabilitation and is ideally incorporated immediately following injury The primary goals during this phase include reducing inflammation and pain to achieve a “quiet knee” prior to surgery, normalizing ROM (especially extension and flexion), quadriceps muscle activation, preventing significant quadriceps atrophy, and normalizing gait. A critical component of this phase is the incorporation of neurocognitive exercises to decrease early motor compensations and quadriceps muscle inhibition. The specific exercises are recommended as soon as possible.
Motor and cognitive dual-tasking exercises are implemented, where patients perform functional and cognitive tasks simultaneously This approach promotes agonist/an-
tagonist muscle coactivation while stimulating brain centers responsible for motor planning and proprioception.20 Patients who initiate gait training early have been shown to have decreased compensations and increased functional outcomes compared to those who initiate gait training later in rehab.31 Gait training drills such as stepping over cones is initiated to develop symmetry between the lower extremities. Symmetrical hip, knee, and ankle flexion over the cone, quadriceps and hip control during weight acceptance, gastroc/soleus symmetry during push-off, and equal stance time between limbs are all vital components in this exercise. This drill is progressed to tax the neurocognitive system by performing at various speeds, in multiple directions (forward, backward, lateral), (Figure 1a, 1b) adding serial counting/memory recall drills, ball tossing/catching, or using strobe goggles.
Additional exercises include squats on a tilt board while throwing/catching a ball or balancing on an unstable surface while catching a randomly colored stick and reacting to the correct color. These exercises target balance, reaction time, and visual processing. Furthermore, active joint repositioning drills can be performed with eyes closed to enhance proprioception. These early intervention drills are designed to minimize and prevent negative neuroplastic adaptations. Consistent implementation of these exercises prior to surgery is crucial to optimize beneficial neuroplastic changes and prevent maladaptive patterns.
The immediate post-operative phase echoes the goals of the pre-operative phase and focuses on achieving a “quiet knee” quickly to facilitate early quadriceps activation, protect the surgical graft, and aid in regaining extension ROM. From day one, quadriceps activation exercises are implemented using neuromuscular electrical stimulation (NMES) and, for those with difficulty in efferent firing, electromyographic (EMG) visual biofeedback devices. These techniques attempt to override quadriceps inhibition by increasing action potentials in motor units (NMES)32 or enhancing real time visual feedback (EMG), allowing patients to adjust their muscle activation patterns during exercise. A limitation of using NMES alone is that, while many patients experience improved quadriceps contraction during individual sessions, it does not directly improve knee extensor strength. Additionally, there is often minimal carryover (voluntary control) to the next session, indicating limited beneficial neuroplasticity Grooms et al. found that increased demands on the central nervous system during complex, high-stakes activities can override normal efferent inhibition mechanisms.11 To accomplish this, the authors advocate for the use of exercises such as quad sets (QS), straight leg raises (SLR), and knee extensions from 90-40 degrees with NMES to assist patients in quadriceps activation. These can be also performed with the patient visualizing EMG data for biofeedback to improve contraction or combined with a reactive cognitivemotor task, such as performing a QS while throwing/catch-
Exercise/Drill Variables/ progressions to enhance neuroplasticity
Quad sets
Straight leg raises (3 way)
Knee extensions (90-40 deg)
Mini squats Standing TKEs
NMES EMG biofeedback
Reactive cognitive motor task (BlazePods)
Surface (i.e. foam, rocker board)
Resistance
Dual task (i.e ball toss or HecoStix)
Reactive cognitive motor task (BlazePods, Neurocognitive Sensory station)
Perturbations
Cognitive task (counting, memory, etc.)
Altered vision Contralateral limb/ UE task
Gait/stepping drills:
Weight shifts
Cone stepping
Lateral lunges
Lateral stepping (slides)
Quick Board stepping drills
CKC neuromuscular exercises:
Anterior/ lateral step
downs
Multidirectional lunges
Speed Direction (ant/ post/lateral)
Dual task (i.e ball toss or HecoStix)
Cognitive task (counting, memory, etc.)
Reactive cognitivemotor task
Resistance Surface
Dual task (i.e ball toss or HecoStix)
Perturbation/ resistance from CLX band
Cognitive task (counting, memory, etc.)
Reactive cognitive motor task (BlazePods)
Resistance Surface
Single leg balance:
SL RDL with UE task
SL balance with band perturbations
Dual task (i.e. ball toss)
Direction (star drill on floor)
Visual targets
Perturbation (KB exchange/ resistance band)
UE task (i.e cable row, med ball chop, reach)
Dynamic shuffling Speed Direction
Neurocognitive Demand
Visual Cognitive Efferent firing
Cognitive Dual task
External focus of control Reaction time
Examples:
Quad set with NMES
Straight leg raises while using UE to react to indicated BlazePod color
Knee extensions using visual EMG biofeedback Virtual Reality Glasses
Mini squats on rocker board with external perturbations while throwing/catching a ball
Standing TKE while using UE to react to indicated quickboard target
Visual Cognitive Decision making
Memory
Dual task
External focus of control Reaction time
Visual Cognitive Decision making
Memory
Dual task
External focus of control
Reaction time
Visual Cognitive Decision making
Memory
Dual task
External focus of control Reaction time
Weight shifts on force plates with visual feedback
Multi-directional cone stepping counting backward by 7’s and tossing HecoStix
Lateral lunges with sport cord perturbation and ball toss, landing on foam surface
Lateral slides with resistance band around knees, reacting to verbal cues (direction, speed) and catching ball
Rapid stepping in place with visual target, reacting to correct location on the board or surface (Quickboard)
Anterior step down from box with CLX band pulling into femoral IR and dynamic LE valgus (figure 8)
Lateral lunges with sport cord perturbation and ball toss toward indicated direction. Athlete must react to indicated direction using light up pods (i.e. antero-lateral or posterolateral)
Visual Cognitive Decision making
Memory
Dual task
External focus of control Reaction time
Cognitive
SL RDL standing on foam while catching a ball and performing RDL to the indicated cone (5 cones set up in front of the patient)
Single-leg balance with band resistance and valgus perturbations: The patient stands on a rocker board while resisting external forces, dribbling a basketball, and catching Hecostix with contralateral UE (figure 9)
Exercise/Drill Variables/ progressions to enhance neuroplasticity
Visual targets (with/without distraction targets)
Auditory cues (i.e direction/speed)
Height/location of target
Sport specific task (i.e catching basketball or lacrosse ball with stick)
Neurocognitive Demand
Decision making
Memory
Dual task
External focus of control
Reaction time
Figure 1a. Forward/Backward Cone Stepping: Patient steps over cones, focusing on symmetry and 90-degree hip/knee/ankle flexion.
ing a ball or tapping light-up pods (BlazePods, Blazepod, San Diego, CA) with the upper extremity (Figure 2/Supplemental File 1). Patients can also perform knee extensions with NMES from 90 to 40 degrees with light resistance while performing hand taps with Blazepods (Figure 3/Supplemental File 2). Finally, standing terminal knee extensions with a resistance band behind the knee can be implemented using BlazePods to reinforce neural patterning (Figure 4/Supplemental File 3).
The acute phase of rehab builds upon the immediate postoperative phase, focusing on managing residual inflamma-
Examples:
Figure 1b. Lateral Cone Stepping: Patient steps laterally over cones, emphasizing controlled movement and core engagement.
tion, progressing range of motion, gait training, optimizing quadriceps firing. During this time, proprioceptive/balance training is introduced to increase neuromuscular control and prepare for more advanced stages of rehabilitation. An external focus of control is utilized to reinforce motor learning, as this has been shown to increase intracortical inhibition, which plays a role in increasing quadriceps firing.9
Double leg exercises such as mini squats and weight shifts are performed first advancing to single leg exercises such as balance and stepping drills as a progression. The mini squat is performed from 0 to 45 degrees of flexion for maximal quadriceps and hamstring co-activation. A neurocognitive challenge can easily be added by introducing
See Supplemental File 1 for video.
See Supplemental File 2 for
an unstable surface such as foam or a tilt board under the patient’s feet. The clinician can perturbate the surface so
See Supplemental File 3 for
that the patient must react and right the rocker board (Figure 5). As proprioception improves, the patient can catch objects or perform cognitive tasks such as counting, math problems, or responding to visual stimuli during the task. If the patient continues to demonstrate quad avoidance gait, standing terminal knee extension (TKE) exercises with Blazepods can be performed.
Reinforcing proper gait patterns in this phase is vital as soon as possible as the patient will be transitioning off crutches and utilizing the drop-lock brace only for ambulation. Gait compensations that commonly occur after ACLR include reduced knee flexion, increased hip flexion/circumduction, reduced knee extension moment, altered quadriceps-hamstring co-activation during stance, along with reduced ipsilateral stance time.33‑35 Stepping drills over cones can be utilized again here to challenge the neurocognitive system and reinforce proper mechanics.
Once the patient demonstrates appropriate gait mechanics and control with double leg exercises, lunging and stepping movements can be implemented to work towards single leg activity Lunges are performed as the patient steps to the side and lands on a flexed knee to approximately 30-45 degrees for maximal stability Each of these tasks are initiated straight in the frontal plane on a solid surface. In this phase, a ball toss or slight external perturbation may be added to incorporate additional core and lower extremity musculature with feedforward mechanisms.
Single leg exercises begin with eyes open, solid surface, and static positions, progressing with visual disturbances, varied surfaces, and dynamic UE tasks. Examples include
provides perturbations.
single leg balance on the floor with the knee slightly flexed to 30-45 degrees. This can be performed on various unstable surfaces with external perturbations, and/or a reactive sensorimotor task on a television screen in front of them (ex: using Senaptec Sensory station [Senaptec, Beaverton, OR], reacting to animals or solving math problems on the screen – Figure 6). Each of these exercises are vital to include early in the rehabilitation process in order to reinforce fundamental movement patterns and prepare the patient for more complex movements in the later stages.
The intermediate phase of ACLR rehabilitation focuses on restoring full knee range of motion (ROM) to 0°-135°-145°, advancing lower extremity strength/endurance, balance, and neuromuscular control. Most patients will unlock and discharge their knee immobilizer in this phase, so it is imperative to prepare the neuromuscular system for dynamic environments in their daily life while focusing on limb confidence and closed kinetic chain (CKC) function.
CKC neuromuscular control exercises in the sagittal plane are initiated with an anterior step down on level ground. The patient is instructed to place their hands on their hips and tap the heel of the uninvolved leg on the ground in front of them while controlling dynamic lower extremity (LE) valgus and pelvic pronation. Initially, a mirror is used as an external focus of control. This can be progressed to an elevated surface using weights, and eventually with spiral band resistance to enhance the control of rotational patterns (Figure 7). The front step-down exercise can also be enhanced with the utilization of blazepods for
resistance band applying adduction and internal rotation force.
reactive stability. These progressions will be described in greater detail in Part 2 of this commentary
Figure 8a. Quickboard (Memphis, TN) Lateral Step: Patient performs lateral step onto Quickboard, focusing on knee stability and target reaction.
See Supplemental File 4 for video.
Frontal plane movements are progressed with lateral lunges and lateral stepping drills. Lateral stepping is introduced once the patient demonstrates appropriate sagittal plane control. This is performed with a resistance band placed around the knees while the patient steps laterally at the assigned speed (slow, medium, or fast) and direction. A ball is then thrown for additional neurocognitive engagement as the patient must react to both the verbal direction and direction of the ball. Faster speeds and reactive directions are reserved for the later stages.
Lunges are progressed to diagonal lunges in both the anterior and posterior direction and upon unstable surfaces. Rotation may be added when the patient is further along in the rehab process. Multi-directional lunges are progressed with resistance using a sport cord or manual external perturbation. A visual stimulus on the ground can also be added using target lights or the QuickBoard Device (Figure 8a). The patient can lunge onto the indicated surface, reacting to the correct position on the board (Figure 8b).
Stepping exercises can be advanced in this stage to emphasize speed, coordination, and endurance. Patients perform in-place stepping drills while reacting to visual targets on the Quick Board (Figure 9a/Supplemental File 6). This can also be performed with a reaction target (Figure 9b/Supplemental File 7). The Quick Board may facilitate various single and dual-leg coordination exercises while assessing accuracy, reaction time, and limb symmetry.
Finally, single leg tasks can also be combined into a functional task such as a single leg Romanian Deadlift (SL RDL). This can be performed on various surfaces, unweighted or weighted with kettlebells (KB). Tasks such as
Lunge with Catch: Patient performs lateral lunge towards Blazepod (San Diego, CA) while catching a ball.
See Supplemental File 5 for video.
9a. Quickboard (Memphis, TN) Foot Fire: Patient performs rapid stepping in place while tapping targets on Quickboard.
See Supplemental File 6 for video.
SL RDL with KB exchanges may be incorporated, where the patient performs an RDL holding the bottom position while
Figure 9b. Quickboard (Memphis, TN) Foot Fire with Reactive Taps: Patient performs rapid stepping in place while reacting to visual targets on the screen.
See Supplemental File 7 for video.
exchanging a weighted KB from hand to hand to challenge the limits of stability. Another option is the SL RDL with star drill, where the patient catches a ball and touches a series of 5 cones placed on the floor while in the bottom of the RDL position, allowing for incorporation of UE, visual system, and SL stability SL RDL with CLX tubing wrapped around the thigh is used for proprioceptive input to control dynamic LE valgus, adduction and internal rotation. These single-leg balance exercises with extremity movement are designed to engage multiple muscle groups while enhancing dynamic stabilization.
As exercise complexity increases, patients often exhibit deteriorating movement patterns, which can heighten their risk of injury For those demonstrating significant pelvic drop and hip internal rotation on the stance leg during functional tasks, a targeted exercise using a resistance band can be implemented. The band is strategically placed around the athlete’s limb to replicate adduction and internal rotation forces commonly experienced during dynamic movements. The athlete then performs sport-specific tasks, such as dribbling a basketball or throwing/kicking a soccer ball, while balancing on an unstable surface (e.g., rocker board). Simultaneously, the practitioner applies external perturbations by pulling on the resistance band and tapping the board (Figure 10). Alternatively, the practitioner can pull the band medially to recreate knee valgus forces. To increase the neurocognitive challenge, BlazePods can be introduced on the floor for the athlete to tap with their contralateral extremity during the task.
To introduce dynamic lateral movements focused on rapid visual processing and change of direction movements,
lateral shuffling is introduced with BlazePods. Four Blazepods are placed in a straight line in front of the patient on top of cones for enhanced visibility As the BlazePod lights up, the athlete performs a lateral shuffle towards the illuminated pod while staying low and maintaining balance. Simultaneously, the clinician tosses a ball towards the athlete. The athlete must catch the ball mid-shuffle, continue moving towards the BlazePod, and tap the lit pod with the ball before shuffling back to the starting position. This drill can be progressed by placing the pods on the floor so that the athlete must maintain a lower stance and with quicker speeds (Figure 11/Supplemental File 8). The exercise is designed to enhance reaction time, hand-eye coordination, lateral speed, and agility while keeping the athlete engaged in multiple tasks simultaneously Each progressive variation simulates the visual-cognitive demands of sport, training the athlete to maintain control and react effectively to external stimuli while executing complex movements.
In conclusion, incorporating neurocognitive training into ACL rehabilitation is essential to reduce re-injury rates and promote optimal recovery. Traditional rehabilitation methods that focus on physical aspects such as strength and range of motion, are missing a key element. Addressing neuromuscular control and cognitive function is equally crucial. Neurocognitive exercises that focus on dual-tasking and reactive/feed forward activities enhance proprioception, dynamic stability, and motor planning, and better
Figure 11. Reactive Lateral Shuffle Drill: Patient performs lateral shuffles towards illuminated BlazePods (San Diego, CA) while catching and tapping the pod with a ball.
See Supplemental File 8 for video.
mimic the real world and sporting environment, which is when most ACL injuries and re-injuries occur These exercises not only improve the immediate functional outcomes,
but also foster beneficial neuroplasticity, ensuring better long-term joint stability and movement efficiency. Clinically, it is vital to understand that neuro-cognitive training is a dynamic principle and should not be isolated to the beginning stages of rehabilitation. Instead, integrating neurocognitive training from the pre-operative phase through full recovery with sport specific drills is vital and provides a comprehensive approach, addressing the multifaceted demands of returning to both sport and competition, but it does not end here. It is essential to use neurocognitive training as an injury prevention mechanism and should be incorporated in both injured and non-injured athletes at any level of competition. Ultimately, the authors’ goal with this strategy is to bridge the gap between rehabilitation and performance, while enhancing the long-term resilience of the knee joint. Overall, the authors believe by including neuromuscular and neurocognitive exercises early and throughout the rehabilitation process, it reduces the likelihood of re-injury and supports patients in achieving a higher quality of life post-ACLR.
No funding was provided for this manuscript. Kevin Wilk serves on the medical advisory board for BlazePods and receives educational grant funds from Quickboard. No other relevant author disclosures.
Submitted: August 12, 2024 CST, Accepted: October 01, 2024 CST
© The Author(s)
This is an open-access article distributed under the terms of the Creative Commons Attribution 4.0 International License (CCBY-NC-4.0). View this license’s legal deed at https://creativecommons.org/licenses/by-nc/4.0 and legal code at https://creativecommons.org/licenses/by-nc/4.0/legalcode for more information.
1. Wilk KE. Anterior cruciate ligament injury prevention & rehabilitation: Let’s get it right. J Orthop Sports Phys Ther. 2015;45(10):729-730. doi:10.2519/jospt.2015.0109
2. Griffin LY, Albohm MJ, Arendt EA, Dick RW, Garrett WE, Garrick JG, et al. Noncontact anterior cruciate ligament injuries: Risk factors and prevention strategies. J Am Acad Orthop Surg 2000;8(3):141-150. doi:10.5435/00124635-200005000-00001
3. Boden BP, Sheehan FT, Torg JS, Hewett TE. Noncontact anterior cruciate ligament injuries: mechanisms and risk factors. J Am Acad Orthop Surg. 2010;18(9):520-527 doi:10.5435/ 00124635-201009000-00003
4. Boden BP, Dean GS, Feagin JA Jr, Garrett WE Jr. Mechanisms of anterior cruciate ligament injury Orthopedics 2000;23(6):573-578. doi:10.3928/ 0147-7447-20000601-15
5. Paterno MV, Rauh MJ, Schmitt LC, Ford KR, Hewett TE. Incidence of contralateral and ipsilateral anterior cruciate ligament (ACL) injury after primary ACL reconstruction and return to sport. Clin J Sport Med 2012;22(2):116-121. doi:10.1097/ JSM.0b013e318246ef9e
6. Wiggins AJ, Grandhi RK, Schneider DK, Stanfield D, Webster KE, Myer GD Risk of secondary injury in younger athletes after anterior cruciate ligament reconstruction: A systematic review and metaanalysis. Am J Sports Med 2016;44(7):1861-1876. doi:10.1177/0363546515621554
7. Barber-Westin S, Noyes FR. One in 5 athletes sustain reinjury upon return to high-risk sports after ACL reconstruction: A systematic review in 1239 athletes younger than 20 years. Sports Health. 2020;12(6):587-597 doi:10.1177/1941738120912846
8. Swanik CB, Covassin T, Stearne DJ, Schatz P The relationship between neurocognitive function and noncontact anterior cruciate ligament injuries. Am J Sports Med. 2007;35(6):943-948. doi:10.1177/ 0363546507299532
9. Gokeler A, Neuhaus D, Benjaminse A, Grooms DR, Baumeister J. Principles of motor learning to support neuroplasticity after ACL injury: Implications for optimizing performance and reducing risk of second ACL injury. Sports Med. 2019;49(6):853-865. doi:10.1007/s40279-019-01058-0
10. Grooms DR, Page SJ, Nichols-Larsen DS, Chaudhari AMW, White SE, Onate JA. Neuroplasticity associated with anterior cruciate ligament reconstruction. J Orthop Sports Phys Ther 2017;47(3):180-189. doi:10.2519/jospt.2017.7003
11. Grooms DR, Page SJ, Onate JA. Brain activation for knee movement measured days before second anterior cruciate ligament injury: Neuroimaging in musculoskeletal medicine. J Athl Train. 2015;50(10):1005-1010. doi:10.4085/ 1062-6050-50.10.02
12. Walker JM, Brunst CL, Chaput M, Wohl TR, Grooms DR. Integrating neurocognitive challenges into injury prevention training: A clinical commentary. Phys Ther Sport. 2021;51:8-16. doi:10.1016/j.ptsp.2021.05.005
13. Huston L, Wojtys EM. Neuromuscular performance characteristics in elite female athletes. Am J Sports Med 1996;24(4):427-436. doi:10.1177/ 036354659602400405
14. Sharma N, Classen J, Cohen LG. Neural plasticity and its contribution to functional recovery Handb Clin Neurol 2013;110:3-12. doi:10.1016/ B978-0-444-52901-5.00001-0
15. Wexler G, Hurwitz DE, Bush-Joseph CA, Andriacchi TP, Bach BR Jr Functional gait adaptations in patients with anterior cruciate ligament deficiency over time. Clin Orthop Relat Res. 1998;(348):166-175. doi:10.1097/ 00003086-199803000-00026
16. Konishi Y, Aihara Y, Sakai M, Ogawa G, Fukubayashi T Gamma loop dysfunction in the quadriceps femoris of patients who underwent anterior cruciate ligament reconstruction remains bilaterally Scand J Med Sci Sports 2007;17:393-399. doi:10.1111/j.1600-0838.2006.00573.x
17. Madhavan S, Shields RK. Neuromuscular responses in individuals with anterior cruciate ligament repair. Clin Neurophysiol. 2011;122:997-1004. doi:10.1016/j.clinph.2010.09.002
18. Héroux ME, Tremblay F. Corticomotor excitability associated with unilateral knee dysfunction secondary to anterior cruciate ligament injury. Knee Surg Sports Traumatol Arthrosc 2006;14:823-833. doi:10.1007/s00167-006-0063-4
19. Kuenze CM, Hertel J, Weltman A, Diduch D, Saliba SA, Hart JM. Persistent neuromuscular and corticomotor quadriceps asymmetry after anterior cruciate ligament reconstruction. J Athl Train. 2015;50:303-312. doi:10.4085/1062-6050-49.5.06
20. Grooms DR, Onate JA. Neuroplasticity following anterior cruciate ligament injury: a framework for visual-motor training approaches in rehabilitation. J Orthop Sports Phys Ther 2016;46(5):384-393.
21. Chaput M, Simon J, Taberner M, Grooms D From control to chaos: Visual-cognitive progression during recovery from ACL reconstruction. J Orthop Sports Phys Ther 2024;54:1-26. doi:10.2519/ jospt.2024.12443
22. Baumeister J, Reinecke K, Schubert M, Weiss M. Altered electrocortical brain activity after ACL reconstruction during force control. J Orthop Res. 2011;29(9):1383-1389. doi:10.1002/jor.21380
23. Wickens CD Multiple resources and mental workload. Hum Factors. 2008;50(3):449-455. doi:10.1518/001872008X288394
24. Pashler H. Dual-task interference in simple tasks: Data and theory. Psych Bull. 1994;116(2):220-244. doi:10.1037/0033-2909.116.2.220
25. Sherman DA, Baumeister J, Stock MS, Murray AM, Bazett-Jones DM, Norte GE. Inhibition of motor planning and response selection after anterior cruciate ligament reconstruction. Med Sci Sports Exerc. 2023;55(3):440-449. doi:10.1249/ MSS.0000000000003072
26. Taberner M, Allen T, Cohen DD Progressing rehabilitation after injury: Consider the ‘controlchaos continuum.’ Br J Sports Med. 2019;53(18):1132-1136. doi:10.1136/ bjsports-2018-100157
27. Leech KA, Roemmich RT, Gordon J, Reisman DS, Cherry-Allen KM. Updates in motor learning: Implications for physical therapist practice and education. Phys Ther. 2022;102(1). doi:10.1093/ptj/ pzab250
28. Risberg MA, Holm I, Myklebust G, Engebretsen L. Neuromuscular training versus strength training during first 6 months after anterior cruciate ligament reconstruction: a randomized clinical trial. Phys Ther. 2007;87(6):737-750. doi:10.2522/ptj.20060041
29. Moksnes H, Snyder-Mackler L, Risberg MA. Individuals with an anterior cruciate ligamentdeficient knee classified as noncopers may be candidates for nonsurgical rehabilitation. J Orthop Sports Phys Ther. 2008;38(10):586-595. doi:10.2519/ jospt.2008.2750
30. Urbach D, Nebelung W, Weiler HT, Awiszus F. Bilateral deficit of voluntary quadriceps muscle activation after unilateral ACL tear. Med Sci Sports Exerc 1999;31(12):1691-1696. doi:10.1097/ 00005768-199912000-00001
31. Gokeler A, Benjaminse A, van Eck CF, Webster KE, Schot L, Otten E. Return of normal gait as an outcome measurement in ACL reconstructed patients. A systematic review. Int J Sports Phys Ther. 2013;8(4):441-451.
32. Hauger AV, Reiman MP, Bjordal JM, Sheets C, Ledbetter L, Goode AP. Neuromuscular electrical stimulation is effective in strengthening the quadriceps muscle after anterior cruciate ligament surgery. Knee Surg Sports Traumatol Arthrosc. 2018;26(2):399-410. doi:10.1007/s00167-017-4669-5
33. Lewek MD, Rudolph KS, Axe MJ, Snyder-Mackler L. The effect of insufficient quadriceps strength on gait after anterior cruciate ligament reconstruction. Clin Biomech 2002;17(1):56-63. doi:10.1016/ S0268-0033(01)00097-3
34. Paterno MV, Schmitt LC, Ford KR, et al. Biomechanical measures during landing and postural stability predict second anterior cruciate ligament injury after anterior cruciate ligament reconstruction and return to sport. Am J Sports Med 2010;38(10):1968-1978. doi:10.1177/ 0363546510376053
35. Pietrosimone B, Blackburn JT, Padua DA, et al. Walking gait asymmetries 6 months following anterior cruciate ligament reconstruction predict 12-month patient-reported outcomes. J Orthop Res 2018;36(11):2932-2940. doi:10.1002/jor.24056
Figure 2/Supplemental File 1
Download: https://ijspt.scholasticahq.com/article/124945-neurocognitive-and-neuromuscular-rehabilitationtechniques-after-acl-injury-part-1-optimizing-recovery-in-the-acute-post-operative-phase-a-clinical/attachment/ 250700.mov?auth_token=KYwfPePOkQ1n4VpJ4-4K
Figure 3/Supplemental File 2
Download: https://ijspt.scholasticahq.com/article/124945-neurocognitive-and-neuromuscular-rehabilitationtechniques-after-acl-injury-part-1-optimizing-recovery-in-the-acute-post-operative-phase-a-clinical/attachment/ 250701.mov?auth_token=KYwfPePOkQ1n4VpJ4-4K
Figure 4/Supplemental file 3
Download: https://ijspt.scholasticahq.com/article/124945-neurocognitive-and-neuromuscular-rehabilitationtechniques-after-acl-injury-part-1-optimizing-recovery-in-the-acute-post-operative-phase-a-clinical/attachment/ 250702.mov?auth_token=KYwfPePOkQ1n4VpJ4-4K
Figure 8a/Supplemental
Download: https://ijspt.scholasticahq.com/article/124945-neurocognitive-and-neuromuscular-rehabilitationtechniques-after-acl-injury-part-1-optimizing-recovery-in-the-acute-post-operative-phase-a-clinical/attachment/ 250703.mov?auth_token=KYwfPePOkQ1n4VpJ4-4K
Figure 8b/Supplemental file 5
Download: https://ijspt.scholasticahq.com/article/124945-neurocognitive-and-neuromuscular-rehabilitationtechniques-after-acl-injury-part-1-optimizing-recovery-in-the-acute-post-operative-phase-a-clinical/attachment/ 250704.mov?auth_token=KYwfPePOkQ1n4VpJ4-4K
Figure 9a/Supplemental file 6
Download: https://ijspt.scholasticahq.com/article/124945-neurocognitive-and-neuromuscular-rehabilitationtechniques-after-acl-injury-part-1-optimizing-recovery-in-the-acute-post-operative-phase-a-clinical/attachment/ 250705.mov?auth_token=KYwfPePOkQ1n4VpJ4-4K
Figure 9b/Supplemental file 7
Download: https://ijspt.scholasticahq.com/article/124945-neurocognitive-and-neuromuscular-rehabilitationtechniques-after-acl-injury-part-1-optimizing-recovery-in-the-acute-post-operative-phase-a-clinical/attachment/ 250706.mov?auth_token=KYwfPePOkQ1n4VpJ4-4K
Figure 11/Supplemental file 8
Download: https://ijspt.scholasticahq.com/article/124945-neurocognitive-and-neuromuscular-rehabilitationtechniques-after-acl-injury-part-1-optimizing-recovery-in-the-acute-post-operative-phase-a-clinical/attachment/ 250707.mov?auth_token=KYwfPePOkQ1n4VpJ4-4K
Goto H, Kamikubo T, Yamamoto R, Tsutsui T, Torii S. Agreement between 2D Visualand 3D Motion Capture-based Assessment of Foot Strike Pattern. IJSPT 2024;19(11):1386-1396. doi:10.26603/001c.123952
Haruhiko Goto1,2a , Toshinao Kamikubo2 , Ryota Yamamoto2 , Toshiharu Tsutsui3 , Suguru Torii3
1 Department of Sports Sciences, Japan Institute of Sports Sciences, 2 Graduate School of Sport Sciences, Waseda University, 3 Faculty of Sport Sciences, Waseda University
Keywords: foot strike pattern, forefoot strike, midfoot strike, non-rearfoot strike, rearfoot strike, strike angle, strike index https://doi.org/10.26603/001c.123952
International Journal of Sports Physical Therapy
Vol. 19, Issue 11, 2024
Background
Foot strike patterns during running are typically categorized into two types: non-rearfoot strike (NRFS) and rearfoot strike (RFS), or as three distinct types: forefoot strike (FFS), midfoot strike (MFS), and RFS, based on which part of the foot lands first. Various methods, including two-dimensional (2D) visual-based methods and three-dimensional (3D) motion capture-based methods utilizing parameters such as the strike index (SI) or strike angle (SA), have been employed to assess these patterns. However, the consistency between the results obtained from each method remains debatable.
Hypothesis/Purpose
The purpose of this study was to examine the agreement for assessing foot strike patterns into two (NRFS and RFS) or three types (FFS, MFS, and RFS) between 2D visual- and 3D motion capture-based methods. The authors hypothesized that using two description types (NRFS and RFS) would have high inter-method reliability; however, using three description types (FFS, MFS and RFS) would have lower inter-method reliability because of the difficulty in distinguishing between FFS and MFS.
Study design
Controlled Laboratory Study
Methods
Overall, 162 foot strikes from four healthy runners with various foot strike patterns were analyzed. Running kinematics and kinetics were recorded using a 3D motion capture system with a force platform. Each foot strike was filmed at 240 fps from the sagittal perspective. The visual, SI, and SA methods were used, and the kappa values for each method were calculated.
Results
An assessment of the two types of foot strike: NRFS and RFS, revealed almost perfect kappa values (κ = 0.89–0.95) among the visual, SI, and SA methods. In contrast, an assessment of the three types: FFS, MFS, and RFS, revealed relatively low kappa values (κ = 0.58–0.71). Kappa values within the NRFS category, which includes MFS and FFS, ranged from fair to slight (κ = 0.08–0.33).
Corresponding author: Haruhiko Goto
Department of Sports Sciences, Japan Institute of Sports Sciences
Tell: +3-5963-0286
Fax: +3-5963-0252
Email: go-to.3-15@akane.waseda.jp
Conclusion
Previous laboratory findings that categorized foot strike patterns into two distinct types may be applied in observational studies, clinical practice, and training situations.
Level of evidence
Level 2
Foot strike patterns during running are classified into two or three types depending on the specific part of the foot that makes initial contact with the ground. A forefoot strike (FFS) occurs when the ball of the foot or the front third part of the foot lands before the heel. Midfoot strike (MFS) involves the simultaneous landing of the whole foot, and rearfoot strike (RFS) is characterized by the heel or rear third of the foot landing first.1,2 In certain studies, FFS and MFS have been classified together as one group: non-rearfoot strike (NRFS), in which the heel does not land first.3 RFS runners have demonstrated higher initial vertical loading rates than NRFS runners; thus, RFS runners were considered to have a higher risk of running-related injury (RRI) such as stress fracture of the lower extremities or plantar fasciitis.4‑7 In contrast, NRFS runners demonstrated higher force applied around the ankle joint than RFS runners; thus, they were considered to have a higher risk of RRI occurring in the Achilles tendon or lower leg.8,9
The strike index (SI), initially proposed by Cavanagh and Lafortune1 approximately 40 years ago, has become the gold standard method for assessing foot strike patterns. They measured the location of the center of pressure (COP) at initial contact relative to the foot length as the SI, and then, classified it into three patterns as follows: 66.7–100 % from the heel as FFS, 33.4–66.6 % as MFS, and 0–33.3 % as RFS. Many previous studies have used three-dimensional (3D) motion capture systems with force platforms1, 10 or pressure plate systems11,12 in laboratories to measure the COP location and then calculated the SI for assessing foot strike patterns.
The strike angle (SA), which is the angle between the foot and the running surface at initial contact relative to static posture, is also commonly used for assessing foot strike patterns.13‑15 A negative SA value indicates that the ankle joint is more plantar flexed, whereas a positive SA value indicates that it is more dorsiflexed. Altman and Davis13 previously proposed specific cut-off values of -1.6° to differentiate between FFS and MFS and 8.0° to distinguish MFS from RFS. These values were calculated based on kinematic data collected via a 3D motion capture system. Thus, the SI and SA settled each value on a certain figure, and assessment results of those methods were highly correlated.13 Consequently, SA is primarily used in laboratorybased research, similar to the SI.
While two laboratory 3D motion capture-based methods exist for evaluating foot strike patterns, there is also a visual classification method available for assessing foot strike patterns in situ 2,3 Using a high-speed digital video camera at 240 or 180 frames per second (fps), foot strike patterns were visually classified into two or three types based on
the initial contact point of the foot with the surface. Visual classification is predominantly utilized in field-based observational studies to assess the incidence of RRI for each foot strike pattern type,16,17 or to analyze step variables in track events or road races.2,3 In these earlier studies, participants were filmed from a sagittal perspective, often from outside the running track, and foot strike patterns were assessed using two-dimensional (2D) images. Intraand inter-rater reliabilities of the visual classification results were excellent or very good on a treadmill or overground.18,19 However, the categorization of foot strike patterns into two (NRFS and RFS) or three types (FFS, MFS, and RFS) has been inconsistent, and there is no consensus regarding whether NRFS should be further subdivided into FFS and MFS.
Although the basics of the theory of classification methods are the same for the SI, SA, and visual classification, studies examining the agreement among them are limited. For example, in certain observational studies that examined the agreement among the SI, SA, and visual classification involving overground running, the camera’s frame rate was only 30 fps,20 or the pressure plate had a low sampling frequency compared with the 3D motion capture systems used in previous studies.1,13,21 These limitations can result in an unclear representation of the foot touchdown during running. Consequently, the consistency between the results of each classification method remains debatable, and it remains uncertain whether the findings from laboratorybased studies can be readily applied to observational in situ studies. Previous observational studies, which used 2D visual classification for foot strike patterns, have revealed differences in the type of RRI between foot strike patterns.16, 17 While these studies referenced the aforementioned 3D motion capture-based investigations in their discussions, it is worth noting that the methods used for foot strike pattern assessments varied across the studies. Therefore, it is essential to clarify the degree of agreement between the SI, SA, and visual methods for foot strike assessment to apply laboratory findings to visual-based observational studies or clinical practice.
The present study aimed to examine the agreement for assessing foot strike patterns into two (NRFS and RFS) or three types (FFS, MFS, and RFS) between 2D visual- and 3D motion capture-based methods. The authors hypothesized that classifying foot strike patterns into two types (NRFS and RFS) have high inter-method reliability; however, using three description types (FFS, MFS and RFS) would have lower inter-method reliability because of the difficulty in distinguishing between FFS and MFS using 2D methods.
MATERIALS AND METHODS
Four healthy recreational male runners without lower-extremity injuries in the prior six months participated in the study. Their age, height, and monthly running mileage were verbally confirmed and entered into a Microsoft Excel (Microsoft Corp., Washington, USA) file on site. The study was approved by the Ethics Committee of Waseda University (#2021-384) and was conducted in accordance with the tenets of the Declaration of Helsinki. All participants provided written informed consent before participation.
During all data collecting trials, participants used their own running shoes. All of their shoes were standard running shoes, and there were no low drop shoes or minimal footwear. Before the data collection, participants had 15–30 min for both warm up, including static and/or dynamic stretching and familiarization trial for running in their preferred speeds. Ten reflective markers (three tracking markers with red boundaries and seven anatomical markers) were attached to the bottom and middle of the heel; upper heel; lateral heel; first, third, and fifth metatarsal heads; toe; medial and lateral malleolus of the right shoes; and toes10,13 (Figure 1).
Prior to the running trial, a static standing calibration trial was performed for at least 2 sec. Subsequently, the running trial was performed on a 20 m runway using a 3D motion capture system and force platforms (Figure 2). The participants were instructed to run at their preferred running speeds and land on one of the force platforms with their right foot under the following four conditions: uncontrolled habitual foot strike; FFS, to land with the third part of the foot; MFS, to land with the mid-third part of the foot or simultaneously land the whole foot; and RFS, to land with the rear third part of the foot. The sequence of the conditions was randomized, and the participants ran 10 trials for each condition. If participants stepped force platforms twice by their right feet in one trial, the analysis included both steps.
The marker trajectories and ground reaction force were recorded using a seven-camera 3D motion capture system (T40-S, Vicon, Oxford, UK; 250 Hz) and three built-in force platforms (9287C, Kistler Instrumente AG, Winterthur, Switzerland; 1000 Hz, 0.9 m × 0.6 m), respectively The x-, y-, and z-axes of the global coordination system were defined as the medial-lateral, anterior-posterior, and vertical directions of the participants, respectively
A high-speed digital video camera (GC-LJ25BM, Sports Sensing, Fukuoka, Japan; 240 fps) was mounted on a stationary tripod at a height of 0.8 m perpendicular to the runway All running trials were filmed from the participants’ sagittal perspective. Photocells (NT7728A, NISHI Sports, Tokyo, Japan; 100 Hz) were placed at 15 m intervals along the center of the runway to capture running times. Trials were rejected if the participant obviously targeting the
force platforms, or if the participant accelerated or decelerated during a trial.
The marker trajectories were reconstructed and labeled using Vicon Nexus 2.11 (Vicon Motion System Limited, Oxford, UK). The marker trajectories and ground reaction force data were filtered using a fourth-order low-pass Butterworth filter at 8 and 50 Hz, respectively.10 The body weights (BW) of the participants were calculated using the ground reaction force in a static standing calibration trial. The point of initial contact was defined as the moment at which the ground reaction force exceeded 20 N.20 All foot strikes were classified as RFS, MFS, or FFS using the SI and SA methods.
The SI was calculated using the location of the COP at the initial contact relative to the foot length, which is the distance between the heel and toe. Subsequently, foot strike patterns were classified into three types: FFS, 66.7–100%; MFS, 33.4–66.6%; and RFS, 0–33.3%.1,12 Furthermore, FFS and MFS were combined as NRFS: SI of 33.3–100%.
The SA was calculated by subtracting the foot angle during the static standing trial from that at the initial contact during the running trial in sagittal plane. The foot was defined as the line connecting the third metatarsal and bottom of the heel, with the foot angle representing the angle between the foot and the ground. Foot strike patterns were classified into three types: FFS, <-1.6°; MFS, between -1.6° and 8.0°; and RFS, >8.0°.13 Furthermore, FFS and MFS were combined into the NRFS category, defined as SA <8.0°.
All calculations were performed using Microsoft Excel and MATLAB R2021b (MathWorks Inc., Natick, MA, USA).
All images filmed during data collection were processed using QuickTime for Windows (Apple Inc., CA, USA), and an experienced researcher performed visual classification into the following three types according to which part of the foot landed first: RFS, the rear-third part of the foot lands first; MFS, the mid-third part of the foot lands first or whole foot lands simultaneously; and FFS, the front third part of the foot lands first.2 NRFS was defined as FFS and MFS (Figure 3).3
The running time to pass the runway was measured using photocells, which were placed at 15 m intervals. This time was then converted to running speed using the following formula:
Prior to the analysis, a weighted kappa was computed for the visual foot strike assessment to evaluate inter-rater reliability This involved assessing 30 randomly selected foot
Figure 1. Ten reflective markers (three tracking markers with red boundaries and seven anatomical markers) on the right shoes of the participant: A. lateral view and B. medial view.
Figure 2. Data collection arrangement.
strikes three times. The resulting value was 0.933, indicating almost perfect reliability.
Afterward, a simple kappa value along with a 95% confidence interval (95%CI) was calculated to confirm the reliability between the SI and visual methods, as well as between the SA and visual methods, for assessing foot strike patterns. This analysis was performed for both two type
classification (NRFS and RFS), three type classification (FFS, MFS, and RFS), and within the NRFS (FFS and MFS).
Kappa values were categorized as almost perfect when > 0.81, substantial from 0.61 to 0.80, moderate from 0.41 to 0.60, fair from 0.21 to 0.40, and slight when < 0.20. All statistical analyses were performed using IBM SPSS Statistics for Windows version 28 (IBM Corp., Armonk, NY, USA).
3A. Examples of 2D visual assessments for each foot-strike pattern: Forefoot strike (FFS).
3B. Examples of 2D visual assessments for each foot-strike pattern: Midfoot strike (MFS).
The participants’ characteristics and running speeds during data collection are presented as means ± standard deviations in Table 1 Overall, 162 foot strikes from four runners were analyzed, including two trials of two steps in one trial.
3C. Examples of 2D visual assessments for each
The number and values of each foot strike assessment are presented as means ± standard deviations in Table 2.
In the foot strike assessment using SI, there were 44 instances of FFS (SI, 78.0 ± 8.4%), 38 instances of MFS (SI, 55.3 ± 9.8%), and 80 instances of RFS (SI, 15.9 ± 8.1%).
In the foot strike assessment using SA, there were 40 instances of FFS (SA, -5.2 ± 2.6°), 39 instances of MFS (SA, 1.7 ± 2.6°), and 83 instances of RFS (SA, 21.8 ± 7.7°). For the foot strike patterns obtained through 2D visual assessment, there were 43, 37, and 82 instances of FFS, MFS, and RFS, respectively. The SI and SA values for FFS, MFS, and RFS based on 2D visual assessment were as follows: FFS, 71.1 ± 8.4% and -5.2 ± 2.6°; MFS, 55.3 ± 9.8% and 1.7 ± 2.6°; RFS, 15.9 ± 8.1% and 21.8 ± 7.7° (Figures 4A and B).
For foot strike classification into two types (NRFS and RFS), kappa values and 95%CI between the visual and both SI and SA methods were almost perfect (κ = 0.90 [0.82, 0.98] and 0.95 [0.90, 1.00], respectively) (Table 3A and B). The kappa value and 95%CI between the SI and SA methods were almost perfect (κ = 0.91 [0.85, 0.98]).
In the classification of foot strike patterns into three types (FFS, MFS, and RFS), kappa values and 95%CI between the visual and SI or SA methods ranged from moderate to substantial (κ = 0.58 [0.48, 0.68] or κ = 0.71 [0.62, 0.80]) (Tables 4A and B). In particular, kappa values and 95%CI between the methods for FFS and MFS were found to be fair or slight (κ = 0.08 [-0.15, 0.31] or κ = 0.33 [0.11, 0.54]) (Tables 5A and B).
To the best of the authors’ knowledge, this study represents the first attempt to analyze the concordance between foot
Table 1. Means ± standard deviations for the participants’ characteristics and running speeds during data collection.
FFS: forefoot strike, MFS: midfoot strike, RFS: rearfoot strike, SI: strike index, SA: strike angle
strike assessments utilizing both a high-speed video camera and a 3D motion capture system. The agreement among the visual, SI, and SA classification methods was investigated for evaluating foot strike patterns. Overall, 162 foot strikes, including various foot strike patterns, were analyzed. In the running trials, participants were asked to use uncontrolled habitual foot strike, FFS, MFS, and RFS, and the numbers of NRFS and RFS were nearly the same, regardless of the assessment method.
As hypothesized, the kappa values of the agreement between the visual and both SI and SA methods for the two types of foot strike patterns, NRFS and RFS, were almost perfect (κ = 0.89 and 0.95, respectively). In contrast, the kappa values of the agreement between those methods for the three types of foot strike patterns, FFS, MFS, and RFS, were relatively low compared with those for the two types (κ = 0.58–0.71). Particularly among NRFS, between FFS and MFS, the kappa values indicating agreement between those methods were fair or slight (κ = 0.08–0.33).
In a prior study, the assessment of the two types of foot strike patterns, NRFS and RFS, demonstrated both validity and reliability when using a visual method with a standard video camera capturing footage at 30 fps, as well as the SA method during overground running.20 Another study indicated high agreement between the visual method using a high-speed video camera and the SI method with a pressure plate of 120 Hz.21 However, in those studies, the frame rate of the video camera or sampling frequency of the pressure plate system was lower than that of previous studies.2,10,22 This reduced frame rate or sampling frequency was considered insufficient to capture the timing of foot strikes accurately 20,23 In this study, a 240 fps high-speed video camera, equivalent to those employed in previous analyses of athletic events,2,3 was utilized alongside a 3D motion capture system operating at 250 Hz. This sampling frequency significantly surpassed that of earlier studies with lower sampling rates.20,21 The findings imply that foot strike as-
sessment in NRFS and RFS demonstrates a rational approach to the methods, and the laboratory-based evaluations of foot strike patterns for these two types can be extrapolated to observational studies or clinical practice. Previous observational studies have revealed that NRFS runners are three times more likely to experience RRI at their Achilles tendon than RFS runners17 and that RFS runners have a two-fold higher rate of RRI than NRFS runners.16 These studies utilized 2D visual-based methods for assessing foot strike patterns via high-speed movies, while also referencing 3D motion capture-based studies in their discussions. NRFS runners exhibited greater force applied around the Achilles tendon and triceps surae compared to RFS runners in those studies, suggesting a heightened risk of Achilles tendinopathy The current findings reinforce their conclusions by elucidating the consistency of assessment methods, indicating the potential extension of laboratory-based findings regarding the disparity between NRFS and RFS to observational in situ studies. Consequently, physiotherapists and athletic trainers might be able to evaluate the risk of RRI according to the foot strike patterns using high-speed movies. This could eliminate the need for time- and cost-consuming 3D motion capture systems.
When assessing the three types of foot strike: FFS, MFS, and RFS, relatively lower agreement was noted compared with that observed for the two types, as previously mentioned. Specifically, when comparing the 2D visual method with the SI as the gold standard, the kappa value for the three types was moderate (κ = 0.58), whereas for the two types, it was nearly perfect (κ = 0.90). A previous study, which examined the intra- and inter-rater reliability of foot strike pattern assessment across various types of foot strike patterns, demonstrated a high level of reliability, particularly for two types: NRFS and RFS (κ > 0.80); however, there was relatively low reliability for classifying foot strike patterns into three or more types (κ = 0.41–0.69).24 The pre-
Figure 4. SI and SA for each foot strike pattern using 2D visual assessment. A: Strike index for each foot-strike pattern using 2D visual assessment. B: Strike angle for each foot strike pattern using 2D visual assessment. FFS, forefoot strike; MFS, midfoot strike; RFS, rearfoot strike.
sent study also exhibited low agreement among the three types. The discrepancy in actual values between FFS and MFS, with an SI of 78.0% vs. 55.3%, translates to a 5 cm difference, assuming a foot size of 26 cm. Alternatively, for SA, the difference was only 6.9°. This subtle distinction can pose challenges in visual discernment, particularly when capturing the entire body; therefore, identifying FFS and MFS may be difficult, irrespective of the methods or devices employed. Thus, assessing the three types of foot strike patterns might not be appropriate when applying these findings to in situ studies or clinical practice.
Table 3. Contingency table of agreement for foot strike pattern for two types—NRFS and RFS. A: strike index and 2D visual assessment, B: strike angle and 2D visual assessment.
NRFS: non-rearfoot strike, RFS: rearfoot strike, SA: strike angle
Table 4. Contingency table of agreement for foot strike pattern for three types. A: strike index and 2D visual assessment, B: strike angle and 2D visual assessment.
= 0.71 [0.62, 0.80], p < 0.001
FFS: forefoot strike, MFS: midfoot strike, RFS: rearfoot strike, SI: strike index
A previous study that classified foot strike patterns into three types by the visual method indicated that MFS runners had a higher prevalence of Achilles tendon injury and FFS runners had a higher prevalence of posterior lower leg injuries.25 The force applied around the ankle joints, such as the Achilles tendon and triceps surae, has been reported to be higher for NRFS runners, including both FFS and MFS,8,9 and this might increase the risk for both Achilles tendon and lower leg injury In the present study, foot strike patterns were classified into three types by visual method with 125 fps high-speed movies.25 However, categorizing foot strike patterns into three types may have lacked reliability, and it is important to note that a previous study
might not have accurately distinguished between FFS and MFS. Consequently, caution is warranted when applying the results of studies that classify foot strike patterns into three types, particularly in observational studies and training scenarios. In the contemporary context, the widespread availability of smartphones and tablets enables the convenient capture of high-speed movies at 240 fps. Scientific discoveries are frequently utilized by physiotherapists, athletic trainers, and coaches to mitigate RRI or improve performance, extending to clinical practice and training alike. Notably, the current findings indicate that classifying foot strike patterns into two types, NRFS and RFS, is more suit-
Table 5. Contingency table of agreement for foot strike pattern for two types—FFS and MFS. A: strike index and 2D visual assessment, B: strike angle and 2D visual assessment.
able when utilizing high-speed movies in clinical practice and/or training settings.
The present study has some limitations. The participants ran at their preferred speeds, and the velocity of their feet might have influenced the assessment of foot strike patterns if the sampling frequency was inadequate. Nonetheless, it is crucial to highlight that in this study, the video camera operated at a high frame rate of 240 fps. This is akin to the video cameras typically used in analyzing athletic events, which are marked by faster running speeds than those observed in the present study 3 Thus, the running speed of the participants might have a limited impact on the results of the present study Another limitation is that the results of the present study is based on a small and homogenous sample from the participants of four male runners. The number of foot strikes allowed for statistical analysis, however, a wider range of participants’ demographics or running experience might lead to more robust results.
For foot strike assessments for NRFS and RFS, the kappa values of agreement between the visual, SI, and SA methods were nearly perfect. However, agreement values for the
three types (FFS, MFS, and RFS) was relatively low, especially between FFS and MFS. The laboratory findings that assessed foot strike patterns for NRFS and RFS can be applied to observational studies or clinical and coaching situations; however, caution is advised when applying the findings of foot strike patterns for the three types.
The authors report no conflicts of interest.
This research did not receive specific grants from public, commercial, or not-for-profit funding agencies. The authors thank the Gifu Pref Sports Science Center, a former affiliation of author H. G. for using their 3D motion capture systems.
Submitted: April 12, 2024 CDT, Accepted: September 05, 2024 CDT
© The Author(s)
This is an open-access article distributed under the terms of the Creative Commons Attribution 4.0 International License (CCBY-NC-4.0). View this license’s legal deed at https://creativecommons.org/licenses/by-nc/4.0 and legal code at https://creativecommons.org/licenses/by-nc/4.0/legalcode for more information.
1. Cavanagh PR, Lafortune MA. Ground reaction forces in distance running. J Biomech 1980;13(5):397-406. doi:10.1016/ 0021-9290(80)90033-0
2. Hasegawa H, Yamauchi T, Kraemer WJ. Foot strike patterns of runners at the 15-km point during an elite-level half marathon. J Strength Cond Res 2007;21(3):888-893. doi:10.1519/R-22096.1
3. Hanley B, Tucker CB, Bissas A, Merlino S, Gruber AH. Footstrike patterns and race performance in the 2017 IAAF World Championship men’s 10,000 m final. Sports Biomech. 2024;23(3):314-323. doi:10.1080/14763141.2020.1856916
4. Pohl MB, Hamill J, Davis IS. Biomechanical and anatomic factors associated with a history of plantar fasciitis in female runners. Clin J Sport Med 2009;19(5):372-376. doi:10.1097/ JSM.0b013e3181b8c270
5. Zadpoor AA, Nikooyan AA. The relationship between lower-extremity stress fractures and the ground reaction force: a systematic review. Clin Biomech 2011;26(1):23-28. doi:10.1016/ j.clinbiomech.2010.08.005
6. Hamill J, Gruber AH. Is changing footstrike pattern beneficial to runners? J Sport Health Sci. 2017;6(2):146-153. doi:10.1016/j.jshs.2017.02.004
7 Futrell EE, Jamison ST, Tenforde AS, Davis IS. Relationships between habitual cadence, footstrike, and vertical load rates in runners. Med Sci Sports Exerc 2018;50(9):1837-1841. doi:10.1249/ MSS.0000000000001629
8. Kulmala JP, Avela J, Pasanen K, Parkkari J. Forefoot strikers exhibit lower running-induced knee loading than rearfoot strikers. Med Sci Sports Exerc. 2013;45(12):2306-2313. doi:10.1249/ MSS.0b013e31829efcf7
9. Kuhman D, Melcher D, Paquette MR. Ankle and knee kinetics between strike patterns at common training speeds in competitive male runners. Eur J Sport Sci. 2016;16(4):433-440. doi:10.1080/ 17461391.2015.1086818
10. Mo S, Huang M, Ng L, Cheung RTH. Footstrike angle cut-off values to classify footstrike pattern in runners. Res Sports Med 2023;31(2):181-191. doi:10.1080/15438627.2021.1954516
11. Hatala KG, Dingwall HL, Wunderlich RE, Richmond BG. Variation in foot strike patterns during running among habitually barefoot populations. PLOS ONE 2013;8(1):e52548. doi:10.1371/ journal.pone.0052548
12. Breine B, Malcolm P, Frederick EC, De Clercq D. Relationship between running speed and initial foot contact patterns. Med Sci Sports Exerc 2014;46(8):1595-1603. doi:10.1249/ MSS.0000000000000267
13. Altman AR, Davis IS. A kinematic method for footstrike pattern detection in barefoot and shod runners. Gait Posture 2012;35(2):298-300. doi:10.1016/j.gaitpost.2011.09.104
14. Lieberman DE. Strike type variation among Tarahumara Indians in minimal sandals versus conventional running shoes. J Sport Health Sci 2014;3(2):86-94. doi:10.1016/j.jshs.2014.03.009
15. Forrester SE, Townend J. The effect of running velocity on footstrike angle—a curve-clustering approach. Gait Posture. 2015;41(1):26-32. doi:10.1016/j.gaitpost.2014.08.004
16. Daoud AI, Geissler GJ, Wang F, Saretsky J, Daoud YA, Lieberman DE. Foot strike and injury rates in endurance runners: a retrospective study. Med Sci Sports Exerc 2012;44(7):1325-1334. doi:10.1249/ MSS.0b013e3182465115
17. Goto H, Torii S. Foot strike patterns and runningrelated injuries among high school runners: a retrospective study J Sports Med Phys Fitness 2022;62(12):1668-1674. doi:10.23736/ S0022-4707.22.13445-6
18. de Oliveira FCL, Fredette A, Echeverría SO, Batcho CS, Roy JS. Validity and reliability of 2-dimensional video-based assessment to analyze foot strike pattern and step rate during running: A systematic review. Sports Health. 2019;11(5):409-415. doi:10.1177/1941738119844795
19. Murray L, Beaven CM, Hébert-Losier K. Reliability of overground running measures from 2D video analyses in a field environment. Sports 2018;7(1):8. doi:10.3390/sports7010008
20. Esculier JF, Silvini T, Bouyer LJ, Roy JS. Videobased assessment of foot strike pattern and step rate is valid and reliable in runners with patellofemoral pain. Phys Ther Sport. 2018;29:108-112. doi:10.1016/ j.ptsp.2016.11.003
21. Santuz A, Ekizos A, Arampatzis A. A pressure plate-based method for the automatic assessment of foot strike patterns during running. Ann Biomed Eng 2016;44(5):1646-1655. doi:10.1007/ s10439-015-1484-3
22. Pipkin A, Kotecki K, Hetzel S, Heiderscheit B. Reliability of a qualitative video analysis for running. J Orthop Sports Phys Ther. 2016;46(7):556-561. doi:10.2519/jospt.2016.6280
23. de Almeida MO, Saragiotto BT, Yamato TP, Lopes AD. Is the rearfoot pattern the most frequently foot strike pattern among recreational shod distance runners? Phys Ther Sport 2015;16(1):29-33. doi:10.1016/j.ptsp.2014.02.005
24. Damsted C, Larsen LH, Nielsen RO Reliability of video-based identification of footstrike pattern and video time frame at initial contact in recreational runners. Gait Posture. 2015;42(1):32-35. doi:10.1016/ j.gaitpost.2015.01.029
25. Hollander K, Johnson CD, Outerleys J, Davis IS. Multifactorial determinants of running injury locations in 550 injured recreational runners. Med Sci Sports Exerc 2021;53(1):102-107 doi:10.1249/ MSS.0000000000002455
Woolhead E, Partner R, Parsley M, Jones A. Intra-rater and Inter-rater Reliability of the KangaTech (KT360) Fixed Frame Dynamometry System During Maximal Isometric Strength Measurements of the Knee Flexors. IJSPT. 2024;19(11):1397-1406. doi:10.26603/001c.124121
Ellie
Woolhead1 , Richard Partner1 , Megan Parsley1 , Ashley Jones1a
1 School of Health, Leeds Beckett University
Keywords: Inter-rater reliability, intra-rater reliability, fixed frame dynamometry, maximal isometric strength https://doi.org/10.26603/001c.124121
International Journal of Sports Physical Therapy Vol. 19, Issue 11, 2024
Background
Fixed-frame dynamometry systems are used worldwide to assess isometric strength in both general and athletic populations. There is currently a paucity of published work where reliability estimates for fixed-frame dynamometry systems have been estimated. The aim of this study was to determine the inter-and intra-rater reliability of the KangaTech (KT360) fixed frame dynamometry system when measuring maximal isometric strength of the knee flexor muscles.
Study Design
Inter and intra-rater reliability single cohort study.
Methods
Twenty healthy university-level athletes (age= 21.65 ± 3 years, weight= 74.465 ± 30kg, height= 170.1 ± 7.0cm) took part in two testing sessions where two raters collected data during a 90° hip and knee flexion protocol. Participants performed each test twice, building to a maximal isometric contraction holding over a 5 second period with 30 second rest between sets. Data were checked for normality using a Shapiro-Wilk test. Intraclass correlation coefficient (ICC), standard error of measurement (SEM) and minimal detectable change (MDC) were calculated. Finally, a Bland-Altman analysis was used to determine the levels of agreement for intra-and inter-rater measurements.
High levels of agreement were demonstrated between left and right knee flexion as 95% of the differences were less than two standard deviations away from the mean. ‘Almost perfect’ intraclass correlation coefficient (ICC) values were demonstrated (Knee flexion: Inter-rater: Left, 0.99; Right, 0.99; Intra-rater: Left, 0.99; Right:0.99). Standard error of measurement (SEM) for inter-and intra-rater strength ranged from 0.26-0.69 kg, SEM% ranged from 1.34-2.71% and minimal detectable change (MDC) ranged from 1.14-2.31kg.
Conclusion
Overall, high level of inter-and intra-rater reliability were demonstrated when testing maximal isometric knee flexion. Therefore, the KT360 fixed frame dynamometry system may be considered a viable tool for measuring maximal isometric contraction of the knee flexors when repeat measures are required in clinical settings.
Corresponding Author
Email: Ashley.D.Jones@leedsbeckett.ac.uk a
Dr Ashley Jones
School of Health, Leeds Beckett University, CL602, City Campus Leeds, LS1 3HE. Phone: 0113 8123764
Isometric strength testing is used extensively in sport and healthcare settings to test a participant’s physical capabilities during clinical decision-making processes.1,2 As hamstring strain injuries are one of the most prevalent injuries in sports that require high-speed running3,4 measurements of maximal isometric knee flexion are important to assess for hamstring dysfunction. This measure can be used to highlight muscle imbalances between limbs,5 as well as monitor for risk of re-injury 6 Having highly reliable and repeatable measures to work with is important to justify return to play decisions that practitioners are required to make, as well as guide progression through rehabilitation protocols.
A plethora of different dynamometry devices are available on the market which are often selected according to ease of use, cost, and portability.7,8 Isokinetic dynamometry (IKD) is currently suggested to be the “gold standard” measurement of strength in research and clinical practice,9 however, this equipment is not portable, which may limit its use in certain field-based settings. To address this potential issue, portable fixed-framed dynamometry systems have recently come to market. Kangatech (KT360) (KangaTech Ltd, Melbourne, Victoria, Australia) is a portable fixed-frame dynamometry system that utilizes a stainlesssteel frame with detachable dynamometers and can be positioned to assess varied muscle groups across different planes of motion.10 The system has a multitude of standardized protocols to measure the isometric strength in different fixed positions. Because the KT360 system is a novel device, there is a paucity of evidence surrounding its reliability as has been established for other portable or fixed frame dynamometry measurement tools.11 A recent study evaluated the test-retest reliability of the KT360 system prior to the addition of a 360° rotational frame.10 This study found that Interclass correlation coefficient (ICC) for left knee flexion was 0.927 and 0.923 for right knee flexion. More recently, high test-retest reliability (ICC 0.895-0.974) was found in hip adductor strength with standard error of measure (SEM) and minimal detectable change (MDC) % ranging from 0.59-2.25 and 1.63-6.26, respectively 10 While this provides some initial reliability estimates for the portable fixed frame system, there are currently no studies available estimating the reliability since the 360° rotational frame was released into the market, and none that have considered the testing protocol of 90° hip and knee flexion. Furthermore, there are currently no studies that have estimated the inter-rater reliability of the equipment. As the pressure of keeping athletes in play and delivering results continues to increase within sporting environments, sports teams have a strong economic incentive to invest in injury prevention and rehabilitation.12 Having reliability estimates will help develop practitioners to make an informed decision on whether to integrate such equipment into multidisciplinary sports science and medical departments.
It was hypothesised that the KT360 system would demonstrate a high threshold of reliability when measuring maximal strength produced during the isometric knee flexion protocol based on the results of previous literature.10, 11 The aim of this study was to determine the inter-and intra-rater reliability of the KT360 fixed frame dynamometry system when measuring maximal isometric strength of the knee flexor muscles.
A cross-sectional study design was used. Ethical approval was granted by the School of Health Ethics committee at Leeds Beckett University (ethics number: 98113) and complied with the Declaration of Helsinki on Human Research international standards.
Healthy, university level athletes were recruited to take part in the study using a convenience sampling method. All participants had to be over the age of 18, have membership with University Athletic Union, train >2 times a week, and regularly take part in sporting competitions. Participants were excluded from the study if they had any current or recent lower limb injury (within the prior six months), had induced caffeine sixty minutes prior to any measurements being taken, had completed strenuous exercise forty-eight hours prior to the day of testing, or did not score a pass on the health screening questionnaire as recommended in previous research.13 Prior to the commencement of the study, participants were provided with a participant information sheet which fully explained the study process. All participants were required to complete a study consent form.
Both raters were present for all testing days. Prior to the commencement of the study, both raters had undertaken two days of familiarization and training with the KT360 equipment from an experienced user who had undergone training from the manufacturer. To prevent bias, a method of simple randomization by flipping a coin was implemented to select which rater began the testing process. A computerized random number generator (using Microsoft random number generator software) picked the order in which measurements were taken for each participant. Prior to strength measurements, demographic and anthropometric data was collected. Body mass was measured using an electronic scale (SECAAlpha770, Birmingham, UK) to the nearest 0.1kg, pre-calibrated with a 5Kg dumbbell. Height was measured using a stadiometer (SECA Alpha, Birmingham, UK) to the nearest 0.1 cm. Limb length measurements were then collected and inputted into the KT360 system. Left and right leg length was measured laying in a supine position, by measuring from the anterior -supe-
Figure 1. Knee flexion protocol set-up and 90° hip and knee flexion positioning. A: front view, B: Side view.
rior-iliac spine (ASIS) to the medial malleolus.14 Fibular length was measured from the apex of the head of the fibula to the apex of the lateral malleolus while laying supine.15 Participants were identified by a numerical value to ensure anonymity
A standardized warm-up was then completed. This consisted of a 15-minute stationary cycle (40% VO2 max), followed by 3 sets of 10 reps bodyweight squats and 2 sets of 8 reps of isometric hamstring curls (90° hip, 90° knee; 50% 1RM load), holding the contraction in mid-range for 5 seconds using the KT360 pads to meet the participants resistance.16 The participants knee flexion was then tested in both the right and left limbs. This was measured in supine with arms across the chest, aligned pelvis, and neutral knee position, with knees over toes. The leg being tested was placed at 90° of hip and knee flexion measured with a goniometer and positioned so the ankle of the cuff was aligned with the lateral malleolus, the housing was rotated so the cuff was flush with the achilles tendon, this angle was noted for the re-test. The resting foot was flat with the heel pressed against the base of the platform. Images of the testing position is shown in Figure 1. The test was performed at 90° knee flexion as previous studies including Electromyography (EMG) measurements indicate that maximum hamstrings EMG amplitude is achieved in the midrange knee flexion angle.17The 90° hip flexion angle is associated with producing optimal range for force production through the knee joint.18 In this position, the knee flexors are not fully elongated or contracted, enabling the muscles to produce peak torque.18
Each participant had a familiarization repetition where they sustained a five second contraction at 50% effort, followed by 30 second rest. The knee flexors were tested twice with the participant accelerating into a maximal isometric contraction as quickly as possible and then maintaining for a five second period with a 30 second rest between tests.11 The rater not collecting the data was blinded to the results by waiting outside the testing room. The resting period be-
tween the second test for Rater 1 and the single test for Rater 2 was five minutes as previous studies have suggested that this is sufficient time to facilitate recovery between tests.19
Motivational cues given by both raters during testing included ‘push’ to maximal effort as soon as possible straight after the ‘3, 2, 1 Go’ visual display on the KT360 tablet. This verbal encouragement has been suggested in previous research to increase motivation to encourage subjects to exert maximal strength.20 A standardized script of instructions to explain the protocol was used to ensure consistency within and between raters. A schematic of the study testing procedure can be found in Figure 2.
Data were transferred to Microsoft Excel, (Microsoft Corp, Redmond, WA, USA) and statistical analysis was conducted using SPSS version 27. The distribution of data was assessed for normality using the Shapiro-Wilk test. The intraclass correlation coefficient (ICC) was used for the analysis of relative reliability 21 The type of ICC model used for this was the two-way design as multiple raters obtained measures of each participant.22 Interpretations of the results were as follows: a <0.1= trivial; >0.1 to 0.3= small; >0.3 to 0.5= moderate: >0.5 to 0.7= large; >0.7 to 0.9= very large; and >0.9 to 1.0,= almost perfect.23 Standard error of measurement (SEM) was calculated using the following equation: standard deviation (sd)*(√1-ICC), the SEM% was: (SEM/ mean)*100.11 The minimal detectable change (MDC) was calculated using the formula: 1.96*√2*SEM.11 Finally, Bland-Altman analyses were used to determine the levels of agreement for intra-and inter-rater measurements.23 The difference of the two paired measurements was plotted against the mean of the two measurements. The limits of agreement were calculated as follows: Lower Limit, mean difference – (1.96 x standard deviation of difference); Upper Limit, mean of difference + (1.96 x standard deviation of
Figure 2. An illustration of the study procedure.
Table 1. Intra-rater and inter-rater reliability analysis for isometric strength measures of the knee flexors. Values are presented as the mean ± standard deviation.
left; R,
KF, knee flexion (90°); kg, kilogram; SD, standard deviation, ICC, interclass correlation coefficient; CI, confidence
MDC, minimal detectable change
difference). Data was interpreted as recommended by previous research19 whereby 95% of the data points should lie within ±2SD (2 standard deviations) of the mean difference.
A total of twenty (n=20) participants completed the study (age= 21.65 ± 3 years, weight= 74.465 ± 30kg, height= 170.1 ± 7.0cm).
INTRA-RATER RELIABILITY
The ICC for the data was ‘almost perfect’ between all measurements. Table 1 presents the ICC, SEM, SEM%, and MDC values. Figure 3 displays a Bland-Altman analysis. Bilateral levels of agreement were found to be +/- 2 standard deviations (SD) of the interval of agreement bilaterally for knee flexion. Levels of agreement (LOA) and bias are as follows, left knee flexion: upper 2.596, lower -1.136, bias: 0.73; right knee flexion: upper 1.881, lower: -0.471, bias: 0.705.
INTER-RATER RELIABILITY
The ICC values were all ‘almost perfect’ between Rater 1 and Rater 2. The results are shown in table 1. Figures 3-6 show the results of the Bland Altman analyses. Bilateral levels of agreement were found to be +/- 2 standard deviations (SD) of the interval of agreement bilaterally for knee flexion. Levels of agreement (LOA) and bias are as follows left knee flexion: upper 2.080, lower -0.780, bias: 0.65; right knee flexion: upper 1.410, lower: -0.325, bias: 0.542.
The aim of the study was to evaluate the intra-rater and inter-rater reliability of the KT360 dynamometry system when measuring the maximal isometric strength of the knee flexors. The ICC analysis shows principal findings that data collected on two occasions by the same rater and by two different raters had values between 0.98-0.99 on inter-
class correlational analysis. The SEM, SEM%, and MDC values ranged from 0.45-0.69 kg, 1.72-2.71%, and 1.87-2.31kg, respectively High levels of agreement for inter-rater and intra-rater reliability were demonstrated in all measurements, with 95% of the differences shown to be less than two standard deviations away from the mean.
Prior to the commencement of this study, it was hypothesised that reliability would be high, as previous studies using the KangaTech system prior to the addition of a 360° had yielded excellent test-retest reliability when measuring isometric hip and knee flexor strength.10,11 Prior researchers have suggested that the original KangaTech model had very high reliability for left and right knee flexion (ICC 0.92),11 which is in line with the present study where ICC values of 0.99 were recorded in both left and right limbs. Further research comparing the reliability of the KT360 when testing in different positions such as those used in these previous studies10,11 (prone with 30° and 45° hip and knee flexion with a foam roller under the pelvis and 90° hip and knee flexion) would also be a welcome addition to the KT360 literature.
Previously, researchers have considered handheld dynamometry (HHD) as a portable and low-cost alternative to estimate maximal isometric strength.24,25 The efficacy of using HHD at joints such as the knee where larger forces can be produced has been questioned,26 although implementing best-practice techniques such as external stabilization when using HHD has been shown demonstrate acceptable reliability.24 Researchers measured the inter-rater and intra-rater reliability of maximal isometric knee flexion at a 90° hip and knee position in both seated and prone positions with clinician stabilization, using the Hoggan MircroFET2 HHD 26 The authors reported ICC values of 0.92 (0.75-0.97) and 0.89 (0.69-0.96) for the seated protocol and 0.90 (0.76-0.96) and 0.94 (0.82-0.98) for the prone protocol.26 The MDC values were for the seated protocol were 27.3N (2.78kg) and 29.4N (2.99kg) and for the prone protocol were 24.3N (2.47kg) and 22.7N (2.31kg). In the present study, ICC values of 0.99 (0.98-0.99) were reported for both inter- and intra-rater data, showing that when comparing both studies, the KT360 produces higher relative reliability
Figure 3. Bland-Altman analysis for intra-rater measures of left knee flexion. Displaying the mean difference between test-retest and 95% confidence limits for the measures.
Figure 4. Bland-Altman analysis for intra-rater measures of right knee flexion. Displaying the mean difference between test-retest and 95% confidence limits for the measures.
for both inter-rater and intra-rater reliability. Furthermore, the present study reported MDC values of 2.13-2.31kg and 1.87-2.10kg, indicating that the fixed-frame device produces a smaller amount of measurement error compared to a HHD system. Future studies are needed to directly compare the level of agreement between KT360 and HHD in a range of muscle groups and testing positions.
Isokinetic dynamometry (IKD) is suggested to be the gold standard measurement of strength within sports science and clinical practice.9 When comparing results from the present study and previous studies that have measured isometric knee flexion strength with other dynamometry devices, the KT360 device shows higher values. ICC values for intra-rater reliability of isometric knee flexor strength
using the Cybex II IKD was 0.93 compared to 0.92 when measured with the ‘BTE technologies’, a portable fixed frame dynamometer.27 This highlights that the ICC values were 0.06 and 0.1 higher when using the KT360 compared to the IKD and 0.07 and 0.03 compared to ‘BTE technologies’ dynamometer used in the study 27 This shows that the KT360 is a reliable tool for measuring the strength of knee flexors and is comparable to IKD devices. Further research evaluating the level of agreement between the KT360 to IKD at a fixed angle on the same subjects would provide further evidence regarding validity regarding whether the different types of equipment could be used interchangeably in sporting and healthcare settings.
Figure 5. Bland-Altman analysis for inter-rater measures of left knee flexion. Displaying the mean difference between test-retest and 95% confidence limits for the measures.
Figure 6. Bland-Altman analysis for inter-rater measures of right knee flexion. Displaying the mean difference between test-retest and 95% confidence limits for the measures.
Despite this study representing reliable results of high absolute agreement, inter-rater and intra-rater reliability, limitations require highlighting. The 90° hip and knee protocol was selected from the variety of standardized protocols available on the KT360 system. Although specific joint angles were chosen in relation to previous research suggesting these testing positions serve to achieve peak torque,13,28 some hip extension movement may have occurred during testing due to the nature of the maximal contraction of the hip flexors, which may affect the results found in the study Attempts were made to mitigate this with verbal cues and familiarization. However, further research using this protocol with an additional fixation around the hips and trunk would be required to discover if
this did influence the data collected in the present study. Furthermore, the sample utilized was a convenience sample of healthy university level athletes between the ages of 19-23. Therefore, data may not be extrapolated to nonsporting or injured populations. Further investigations are required to determine if equivalent findings exist in different age groups or populations.
Almost perfect inter-and intra-rater reliability was demonstrated when testing isometric knee flexion with the KT360 as well as low SEM, SEM%, and MDC values. This suggests
that the protocol used in the study is worthy of consideration when repeat measurements of isometric knee flexion are required in non-elite sports populations.
The data used to support the findings of this study are available from the corresponding author upon request.
The authors declare that they have no conflicts of interest.
This research did not receive any specific funding. However, the research was performed as part of the authors employment and/or academic qualification at Leeds Beckett University
Submitted: January 09, 2024 CDT, Accepted: September 05, 2024 CDT
© The Author(s)
This is an open-access article distributed under the terms of the Creative Commons Attribution 4.0 International License (CCBY-NC-4.0). View this license’s legal deed at https://creativecommons.org/licenses/by-nc/4.0 and legal code at https://creativecommons.org/licenses/by-nc/4.0/legalcode for more information.
1. Croisier JL, Ganteaume S, Binet J, Genty M, Ferret JM. Strength imbalances and prevention of hamstring injury in professional soccer players: a prospective study Am J Sports Med 2008;36(8):1469-1475. doi:10.1177/0363546508316764
2. van Dyk N, Witvrouw E, Bahr R. Interseason variability in isokinetic strength and poor correlation with Nordic hamstring eccentric strength in football players. Scand J Med Sci Sports. 2018;28(8):1878-1887. doi:10.1111/sms.13201
3. Ekstrand J, Waldén M, Hägglund M. Hamstring injuries have increased by 4% annually in men’s professional football, since 2001: A 13-year longitudinal analysis of the UEFA Elite Club injury study. Br J Sports Med. 2016;50(12):731-737. doi:10.1136/bjsports-2015-095359
4. Ekstrand J, Krutsch W, Spreco A, et al. Time before return to play for the most common injuries in professional football: a 16-year follow-up of the UEFA Elite Club Injury Study Br J Sports Med 2020;54(7):421-426. doi:10.1136/ bjsports-2019-100666
5. Nara G, Samukawa M, Oba K, et al. The deficits of isometric knee flexor strength in lengthened hamstring position after hamstring strain injury Phys Ther Sport 2022;53:91-96. doi:10.1016/ j.ptsp.2021.11.011
6. De Vos RJ, Reurink G, Goudswaard GJ, Moen MH, Weir A, Tol JL. Clinical findings just after return to play predict hamstring re-injury, but baseline MRI findings do not. Br J Sports Med 2014;48(18):1377-1384. doi:10.1136/ bjsports-2014-093737
7. Gonosova Z, Stastny P, Belka J, Bizovska L, Lehnert M. Muscle strength variations of knee joint muscles in elite female handball players after pre-season conditioning. J Hum Kinet 2018;63(1):105-115. doi:10.2478/hukin-2018-0011
8. Katoh M, Hiiragi Y, Uchida M. Validity of isometric muscle strength measurements of the lower limbs using a hand-held dynamometer and belt: a comparison with an isokinetic dynamometer. J Phys Ther Sci 2011;23(4):553-557 doi:10.1589/jpts.23.553
9. Kambič T, Lainščak M, Hadžić V Reproducibility of isokinetic knee testing using the novel isokinetic SMM iMoment dynamometer. PLoS One. 2020;15(8):e0237842. doi:10.1371/ journal.pone.0237842
10. Dunne C, Callaway AJ, Thurston J, Williams JM. Validity, reliability, minimal detectable change, and methodological considerations for HHD and portable fixed frame isometric hip and groin strength testing: A comparison of unilateral and bilateral testing methods. Phys Ther Sport. 2022;57:46-52. doi:10.1016/j.ptsp.2022.07.002
11. Ransom M, Saunders S, Gallo T, et al. Reliability of a portable fixed frame dynamometry system used to test lower limb strength in elite Australian Football League players. J Sci Med Sport 2020;23(9):826-830. doi:10.1016/j.jsams.2020.03.006
12. Eckard TG, Kerr ZY, Padua DA, Djoko A, Dompier TP Epidemiology of quadriceps strains in National Collegiate Athletic Association athletes, 2009–2010 through 2014–2015. J Athl Train 2017;52(5):474-481. doi:10.4085/1062-6050-52.2.17
13. Price OJ, Tsakirides C, Gray M, StavropoulosKalinoglou A. ACSM pre-participation health screening guidelines: a UK university cohort perspective. Med Sci Sports Exerc. Published online 2018. doi:10.1249/MSS.0000000000001868
14. Vogt B, Gosheger G, Wirth T, Horn J, Rödl R. Leg length discrepancy—treatment indications and strategies. Dtsch Arztebl Int 2020;117(24):405. doi:10.3238/arztebl.2020.0405
15. Ide Y, Matsunaga S, Harris J, Connell DO, Seikaly H, Wolfaardt J. Anatomical examination of the fibula: digital imaging study for osseointegrated implant installation. J Otolaryngol Head Neck Surg. 2015;44(1):1. doi:10.1186/s40463-015-0055-9
16. Barroso R, Silva-Batista C, Tricoli V, Roschel H, Ugrinowitsch C. The effects of different intensities and durations of the general warm-up on leg press 1RM. J Strength Cond Res 2013;27(4):1009-1013. doi:10.1519/JSC.0b013e3182606cd9
17. Keerasomboon T, Mineta S, Hirose N. Influence of altered knee angle and muscular contraction type on electromyographic activity of hamstring muscles during 45 hip extension exercise. J Sports Sci Med. 2020;19(4):630.
18. Kellis E, Blazevich AJ. Hamstrings force-length relationships and their implications for angle-specific joint torques: a narrative review BMC Sports Sci Med Rehabil. 2022;14(1):166. doi:10.1186/ s13102-022-00555-6
19. Neves PP, Alves AR, Marinho DA, Neiva HP Warming-Up for Resistance Training and Muscular Performance: A Narrative Review Cont Adv Sports Sci Published online 2021. doi:10.5772/intechopen.96075
20. Midgley AW, Marchant DC, Levy AR. A call to action towards an evidence-based approach to using verbal encouragement during maximal exercise testing. Clin Physiol Funct Imaging. 2018;38(4):547-553. doi:10.1111/cpf.12454
21. Buczinski S. Reliability Associated with the Measurement of Continuous Variables in Veterinary Medicine: What the Different Possible Indicators Tell, and How to Use and Report Them. Animals 2023;13(17):2793. doi:10.3390/ani13172793
22. Ribeiro B, Pereira A, Neves P, Marinho D, Marques M, Neiva HP The effect of warm-up in resistance training and strength performance: a systematic review Motricidade 2021;17(1):87-94.
23. Bland JM, Altman DG. Statistical methods for assessing agreement between two methods of clinical measurement. Int J Nurs Stud 2010;47(8):931-936. doi:10.1016/j.ijnurstu.2009.10.001
24. Mentiplay BF, Perraton LG, Bower KJ, Adair B, Pua YH, Williams GP, et al. Assessment of lower limb muscle strength and power using hand-held and fixed dynamometry: a reliability and validity study. PloS one 2015;10(10):e0140822. doi:10.1371/ journal.pone.0140822
25. Stark T, Walker B, Phillips JK, Fejer R, Beck R. Hand-held dynamometry correlation with the gold standard isokinetic dynamometry: a systematic review. PM R. 2011;3(5):472-479. doi:10.1016/ j.pmrj.2010.10.025
26. Larson D, Lorenz D, Melton B. Can clinicianstabilization with hand-held dynamometry yield a reliable measure of knee flexion torque? Int J Sports Phys Ther 2022;17(6):1095. doi:10.26603/001c.37907
27 Toonstra J, Mattacola CG. Test-retest reliability and validity of isometric knee-flexion and-extension measurement using 3 methods of assessing muscle strength. J Sport Rehabil 2013;22(1). doi:10.1123/ jsr.2013.TR7
28. Maloney SJ. The relationship between asymmetry and athletic performance: A critical review J Strength Cond Res. 2019;33(9):2579-2593. doi:10.1519/ JSC.0000000000002608
Lisa
T. Hoglund1a , Matthew C. Schiffino2 , James
E. Freels
3 ,
Isabella G. Romano
4
1 Physical Therapy, Thomas Jefferson University, 2 Aurora, Colorado, Orthopedic Centers of Colorado , 3 Garden City, New York, Professional Physical Therapy, 4 Cherry Hill, New Jersey, Penn Therapy & Fitness
Keywords: adult, hip, isokinetics, strength https://doi.org/10.26603/001c.124117
International Journal of Sports Physical Therapy
Vol. 19, Issue 11, 2024
Background
Hip muscle weakness is associated with many musculoskeletal conditions and athletic injuries. Isokinetic testing is often performed to measure a patient’s strength or to assess treatment response. Patient sex and positioning during isokinetic strength testing may influence peak torque production and the agonist-antagonist peak torque ratios. However, different test positions are used clinically and in research to measure hip muscle strength. It is unknown how patient test position or sex impacts test results.
Hypothesis/Purpose
The purpose of this study was to determine whether concentric isokinetic peak torques of sagittal and frontal plane hip muscles differ when tested in recumbent versus standing positions and if results were impacted by patient sex.
Study Design
Cross-sectional observational
Methods
Forty healthy adults (20 male, 20 female) participated. Concentric isokinetic hip torques of the hip flexors, extensors, abductors, and adductors were measured with participants in two positions (recumbent versus standing; 60°/second angular velocity). Peak torque values were normalized by body mass and height. Data were analyzed with mixed-model ANOVAs (sex x position). Effect sizes were examined using partial eta squared.
Results
No significant interactions or main effects for sex were found. Significant main effects for position were found for hip extensor and abductor peak torques and for hip flexor-extensor ratio. Hip extensor peak torque was greater when tested supine versus standing with a large effect size (p=.02, effect size =.14). Hip abductor peak torque was greater when tested sidelying versus standing with a medium effect size (p=.03, effect size =.12). Hip flexor-extensor agonist-antagonist ratio was greater when tested standing versus supine with a large effect size (p<.001, effect size =.27).
Corresponding Author:
Lisa T. Hoglund, PT, PhD Thomas Jefferson University, 901 Walnut Street, 5th floor, Department of Physical Therapy, Philadelphia, PA 19107
E-mail: lisa.hoglund@jefferson.edu Office telephone: 1-215-503-6016
Hip extensor and abductor concentric isokinetic peak torques were greater when tested in recumbent versus standing positions. In contrast, hip flexor-extensor ratio was greater when tested in standing versus supine.
Adequate hip muscle strength is critical for proper function of the hip joint and performance of many activities of daily living and sport.1 The hip muscles stabilize the pelvis on the lower extremity (LE) and provide forces for propulsion during activities including walking, running, vertical jumping, and landing from jumps.1,2 Hip muscle weakness is associated with many conditions affecting athletes including patellofemoral pain,3,4 iliotibial band syndrome,5 anterior cruciate ligament injury,6,7 femoroacetabular impingement,8,9 groin injury in sport,10 and low back pain.11 Hip muscle weakness is also reported in patients with knee osteoarthritis,12 and hip osteoarthritis.13,14
The hip muscles that control the sagittal and frontal planes of motion are reported to be particularly important to function. The hip extensors (EXT) and hip abductors (ABD) generate large torques to propel the body forward and stabilize the pelvis on the LE.1,13 Female athletes with weakness of hip EXT and hip ABD were found to have excessive lumbopelvic motion and velocity during single-leg jump landing indicating possible risk for injury.2 Reduced preseason hip ABD strength was a risk factor for noncontact anterior cruciate ligament injury in male and female collegiate athletes.7 Male athletes with hip EXT weakness had lower peak heights during vertical jump testing.15 Reduced hip adductor (ADD) strength is a risk factor for groin injury in sport.10 Since hip muscle weakness is a common impairment for many patients, it is important for clinicians to quantify hip muscle strength to determine if weakness is present and to assess response to treatment.
Altered ratios of hip agonist and antagonist muscle strength have also been reported to be factors associated with painful musculoskeletal conditions. Agonist-antagonist strength imbalances of hip muscles were found in injured athletes and reported to be a risk factor for injury.16, 17 Reduced hip ADD strength relative to hip ABD strength is a risk factor for groin injury in sport.10 Eccentric hip ADD-ABD peak torque ratios were altered in females with patellofemoral pain such that hip ABD strength was reduced relative to hip ADD strength.16 Weakness of an agonist relative to its antagonist may result in reduced joint stability and aberrant movement, for example collapse into femoral adduction and knee valgus due to weakness of the hip ABD.18
Isokinetic dynamometry is used to measure muscle torque and is considered the “gold standard” for strength measurement.19,20 Peak isokinetic torque is often used as a measure of muscle strength to determine if weakness is present or to measure response to rehabilitation.6,21,22 Use of an instrumented dynamometer is particularly important
for hip muscle strength testing due to reported inaccuracy for hand-held dynamometry strength test results for large muscles.23 Many factors may impact isokinetic strength test results including testing mode (concentric, eccentric, isometric), angular velocity, dynamometer lever arm alignment, participant positioning, and participant sex.22,24 An angular velocity of 60°/second for hip flexor (FLEX) and EXT peak torque assessment was reported to be commonly used and recommended as the most reliable testing velocity 22,24 Studies testing hip ABD and ADD strength were reported to use 60°/second or 30°/second.24
Patient position during hip muscle isokinetic testing lacks consensus on the optimal position.22,25 Hip FLEX and EXT isokinetic testing were reported to be performed with the patient lying supine, standing, and in a forward bent semi-standing position.22,24 Hip ABD and ADD isokinetic testing has been performed in sidelying and standing positions.24 Both recumbent and standing position tests are used clinically as well as in research.22,24‑26 Alteration of body position during testing may alter results of peak hip torques due to altered muscle length and consequent altered muscle length-tension relationships.1 In addition, stabilization of adjacent body regions may be altered in different testing positions. Proponents of standing test positions report this to be preferable since the upright posture more closely approximates trunk and LE position during walking, running, and other functional activities.27 But other researchers and clinicians support a recumbent testing position since it may better stabilize non-tested body segments, enable the patient to exert greater peak torques, and reduce compensatory movement.22 To the authors’ knowledge no study to date has directly compared the recumbent and standing positions for isokinetic peak torque of the hip FLEX, EXT, and ADD muscles. Only one recent study with healthy male adults has examined concentric and eccentric peak torques of the hip ABD tested in sidelying and standing positions and found no difference between positions.28 No study to date has compared the agonist-antagonist peak torque ratios of the hip FLEX-EXT and hip ABD-ADD obtained in recumbent and standing positions. It is unknown how use of the recumbent compared to the standing position during testing will impact concentric isokinetic hip muscle peak torque as well as the agonist-antagonist ratios.24 In addition, it is unknown if changes in body position will have the same impact on peak torques and agonist-antagonist ratios for males and females. Males have been reported to have greater isokinetic peak torque for some hip muscles than females, which may yield different agonist-antagonist torque ratios.24 Thus, the purpose of this study was to determine whether concentric isokinetic peak torques of sagittal and frontal plane hip muscles
differ when tested in recumbent versus standing positions and if results were impacted by patient sex.
This cross-sectional observational study was approved by the Institutional Review Board of Thomas Jefferson University and carried out according to the Declaration of Helsinki Code of Ethics. All participants were given written copies of the consent form and the opportunity to ask questions about the study procedures prior to giving their informed consent. The study was conducted in a research laboratory at Thomas Jefferson University between January 30, 2021 and November 4, 2022. The Strengthening the Reporting of Observational Studies in Epidemiology (STROBE) guidelines were followed in reporting the study findings.29
Participants were recruited from Thomas Jefferson University and the surrounding community through flyers and word of mouth. Inclusion criteria included age 18-40 years and the ability to walk without an assistive device. Exclusion criteria included a history of hip arthroplasty, hip dislocation, or hip deformity; hip or knee joint surgery within the previous year; LE or low back pain rated 3 or greater on a numeric pain rating scale (0-10, 10 = worst pain); LE or low back pain or injury in the prior six months that limited function more than two days; fracture of the pelvis or LE bones within the previous year; any condition that might cause LE pain or muscle weakness (eg, rheumatoid arthritis); current known pregnancy due to possible difficulty stabilizing the pelvis during testing and to avoid any possible adverse effects from maximal muscle contractions; any condition that might make it unsafe for a person to exert maximal hip muscle force (eg, cardiac conditions); and body weight > 400 lbs due to weight limits of the instrumentation.
Demographic and anthropometric data were collected at the beginning of testing. Participants completed a questionnaire reporting demographics, relevant health history, hours of weekly physical activity, and LE dominance. Participant sex was determined from self-report. The LE used to kick a ball was defined as dominant.30 The Tegner Activity Scale (TAS) was used to measure physical activity level.31 The TAS is reliable and valid for use with patients with LE musculoskeletal conditions.32,33 Participant height and weight were measured using a medical scale.
Isokinetic muscle strength of participants’ dominant LE was measured using the Biodex System 4 Pro™ instrumented dynamometer (Biodex Medical Systems, Shirley, New York). Concentric-concentric peak torque of hip EXT, FLEX, ABD, ADD were assessed at 60°/second.24 The cushion setting was “hard” as recommended during peak torque testing. The dynamometer was calibrated according to
manufacturer instructions prior to each participant’s testing. Peak torques for agonists and antagonists were collected during the same test but when the hip was moving in opposite directions. Hip FLEX and EXT were tested in supine, as per the manufacturer’s instructions, and also while standing on the non-tested LE. Hip ABD and ADD were tested in sidelying with the tested LE uppermost, per manufacturer’s instructions, and also while standing on the non-tested LE. During hip FLEX-EXT testing, the dynamometer axis of rotation was aligned opposite the superior border of the patient’s greater trochanter. During hip ABD-ADD testing, the dynamometer axis of rotation was positioned opposite the center of the tested LE buttock in the same transverse plane as the superior border of the greater trochanter The dynamometer lever arm resistance pad was firmly attached to the participant’s tested LE using rigid fabric straps with hook and loop connectors. The resistance attachment was positioned with its distal edge 1" proximal to the superior patella for FLEX-EXT tests and 1" proximal to the lateral femoral epicondyle for ABD-ADD testing. Participants were stabilized with rigid fabric straps placed over the pelvis, trunk, and non-tested LE during supine testing and over the pelvis during sidelying tests. Participants were stabilized during standing tests by grasping a stable support. Testing bench position, dynamometer platform position, height, tilt, rotation, and resistance attachment length were recorded. The distance from the center of the dynamometer axis of rotation to the center of the resistance attachment pad was measured and recorded as the lever arm length. The tested limb weight was measured according to the manufacturer’s instructions. Gravity correction for mitigating the impact of limb weight on torque results was automatically applied in the data acquisition software. Testing order was randomized using a random sequence generator
Participants performed a warm-up activity of ten repetitions of tested LE swings in hip FLEX-EXT and hip ABDADD. The participant was positioned either standing adjacent to the dynamometer head or lying on the dynamometer padded bench, dependent upon the randomly determined starting test. The dynamometer head, platform, and resistance lever arm were adjusted to properly align the dynamometer axis of rotation with the participant’s tested hip axis of rotation at the superior border of the greater trochanter. The participant’s tested LE was firmly attached to the resistance lever arm with rigid straps. Participants moved their LE through the tested motions of hip FLEX-EXT or hip ABD-ADD for several submaximal reciprocal practice trial repetitions to become familiarized with the motions and isokinetic resistance. Standardized scripts were used to instruct participants “to move (their) leg back and forth as hard as (they could), pushing against the machine.” Following practice trials, participants performed five maximal reciprocal repetitions. Verbal encouragement was given during testing to encourage maximal muscle force in both directions. Participants were given 60 seconds to rest following each set of maximal repetitions. Three sets of five repetitions in each motion direction were recorded. Following completion of testing in one position,
the participant and dynamometer were moved to perform testing of the other motions and positions.
Eight participants (four male, four female) returned for a second test session within one month of the first testing session to assess intrarater test-retest reliability The recorded settings for the dynamometer, bench, and resistance lever arm from the first session were used to position the instrumentation at the second session. Muscle group testing was performed in the same order as randomly determined for the first session. Intrarater test-retest reliability for normalized peak torque values was examined using intraclass correlation coefficients (3,k) (ICC3,k). The ICC3,k results were as follows: FLEX (recumbent =.925, standing =.761), EXT (recumbent =.861, standing =.903), ABD (recumbent =.606, standing =.893), ADD (recumbent =.727, standing =.829) These were interpreted as moderate – excellent reliability with ratings of ICC ≤.5 = poor, .5<ICC≤.75 = moderate, .75<ICC≤.9 = good, and ICC>.9 = excellent reliability 34
An a priori power analysis was conducted using the software program G* Power version 3.1.9.2.35 For a medium effect size of .50, α=.05, and 80% power, 34 participants were necessary to find a significant difference. Forty participants were enrolled to ensure adequate sample size in case of participant drop-out or data loss.
Descriptive statistics were calculated for demographic, physical activity, and anthropometric data including means and standard deviations (SD). Participant mean peak torques were calculated for each muscle group in both test positions. Mean peak torques were normalized according to body mass and height (Nm / kg * m) as recommended for hip muscle strength assessment.36 Group mean (SD) normalized peak torques were calculated for the two tested positions in males and females. Mixed-model analyses of variance (ANOVA), 2-tailed (factors of sex and position) were used to examine normalized peak torque variables. Significant interactions were examined followed by simple main effects using a Bonferroni correction for multiple comparisons. Agonist-antagonist ratios were calculated from sagittal plane (hip FLEX-EXT) and frontal plane (hip ABD-ADD) muscle peak torques in recumbent and standing positions. Agonist-antagonist ratios were compared with mixedmodel ANOVAs (sex x position), 2-tailed. Examination included significant interactions followed by planned post hoc comparisons for simple main effects with Bonferroni correction, when no interactions were significant. The significance level was set a priori at p=.05 for all comparisons. Effect sizes were examined using partial eta squared (ηp 2) with ηp 2 <.01 considered a negligible effect, .01≤ ηp 2 <.06 a small effect, .06≤ ηp 2 <.14 a medium effect, and ηp 2 ≥.14 a large effect size.37 Statistical analyses were conducted using SPSS statistical software version 28 (SPSS Inc, Chicago, IL).
Study participants included 20 males and 20 females. There were no significant differences between sexes for age, TAS, and reported hours of physical activity per week (Table 1). The males were significantly heavier, taller, and had a greater body mass index than females. All male participants were right LE dominant while 18 of the females were right LE dominant and two females were left LE dominant.
No significant sex x position interactions were found for any normalized muscle group torques (p>.05) (Table 2). Planned post hoc comparisons for sex and position revealed no significant main effects for sex. Significant main effects for position were found for hip EXT and hip ABD normalized peak torques (Table 2). Hip EXT normalized peak torque was greater when tested in the recumbent position than in the standing position with a large effect size (ηp 2 =.14). Hip ABD normalized peak torque was also greater when participants were tested in the recumbent position compared to standing, with a medium effect size (ηp 2 =.12) (Table 2).
No significant sex x position interactions were found for any agonist-antagonist peak torque ratio. Planned post hoc comparisons revealed no significant main effects for sex. A significant main effect for position was found for hip FLEXEXT ratio in which the ratio is greater when participants were tested in the standing position than in the recumbent position with a large effect size (ηp 2 =.27) (Table 3). That is, the hip FLEX peak torque was greater in relation to the hip EXT peak torque when tested with the participant standing compared to when participants were recumbent.
The primary aim of this study was to compare concentric isokinetic normalized peak torques of the hip FLEX, EXT, ABD, and ADD muscle groups when patients were tested in recumbent compared to standing positions, and to determine if any relationships were modified by sex. A secondary aim was to determine if the agonist-antagonist ratios were different according to testing position and sex. The study results showed that the normalized peak torques of the hip EXT and hip ABD were greater when patients were tested in recumbent positions compared to the standing position. In addition, the hip FLEX-EXT ratio was significantly greater when patients were tested in the standing position rather than while recumbent.
Previous studies examining isokinetic peak torques of hip muscles have used recumbent, standing, and semistanding positions. It is acknowledged in the scientific literature that comparison of hip muscle peak torques ob-
Table 1. Demographic Characteristics
Activity
Values are presented as group mean ± standard deviation.
* Results in bold are significant, p <0.05.
Abbreviations: SD, standard deviation; n, number
Table 2. Normalized Concentric Isokinetic Peak Torque, Effect of Test Position and Participant Sex Normalized Muscle Torque (Nm
Hip ABD Recumbent
± 0.23 (0.93-1.13)
Recumbent
Hip ADD Standing 0.66 ± 0.27 (0.53-0.78) 0.57 ± 0.28 (0.45-0.70)
Hip FLEX Recumbent
± 0.22 (0.92-1.09)
± 0.16 (0.81-0.98)
Hip FLEX Standing 1.01 ± 0.16 (0.92-1.10) 0.99 ± 0.23 (0.90-1.08)
Hip EXT Recumbent 1.06 ± 0.33 (0.91-1.20) 1.04 ± 0.32 (0.89-1.19)
Hip EXT Standing 0.85 ± 0.32 (0.69-1.01) 0.94 ± 0.37 (0.79-1.10)
Values are presented as mean ± standard deviation and (95% confidence interval).
* Bold results are significant, p <0.05.
Abbreviations: SD, standard deviation; ANOVA, analysis of variance; Nm/kg*m, newton-meters normalized by weight in kilograms and height in meters; CI, Confidence interval; ABD, Abductors; ADD, Adductors; FLEX, Flexors; EXT, Extensors.
tained in one position should not be directly compared to results obtained in a different position.24 Despite this, only one study to date has directly compared results of hip isokinetic peak ABD torque obtained with patients standing compared to in a recumbent position.28 Isokinetic hip ABD peak torques of healthy adult males were found to be no different when tested with concentric-eccentric ABD resistance in standing and sidelying positions (median concentric peak torque = 2.1 Nm/kg standing and 2.0 Nm/kg sidelying).28 This is in contrast to the current study results in which concentric isokinetic hip ABD peak torque results were significantly greater when healthy males and females were tested in sidelying compared to standing (Table 2).
Differences in study results may be due to the different isokinetic resistance modes used. In the current study, participants were tested in the concentric-concentric mode of reciprocal resistance to hip ABD and ADD rather than resistance to only the hip ABD using concentric-eccentric resistance. It may be that alternating resistance to agonist and antagonist muscle groups was more challenging to participants while they were required to be in single-leg standing on the non-tested LE, thus yielding lower ABD peak torques in that position.
No previous studies have examined how isokinetic peak torque test results obtained with participants recumbent compared to in standing test positions for hip ADD, FLEX,
Table 3. Concentric Isokinetic Peak Torque Agonist-Antagonist Ratios, Effect of Test Position and Participant Sex
Agonist-Antagonist Ratios, Muscle Torque
Mean ± SD (95% CI)
Hip ABD/Hip ADD, Recumbent 1.47 ± 0.29 (1.13-1.81) 1.87 ± 1.02 (1.53-2.21)
Hip ABD/Hip ADD, Standing 1.65 ± 0.75 (1.28-2.01) 2.00 ± 0.85 (1.64-2.37)
Hip FLEX/ EXT, Recumbent 1.00 ± 0.25 (0.89-1.12)
Mixed-Model ANOVA p values & Effect Sizes
± 0.26 (0.81-1.04)
Hip FLEX/ EXT, Standing 1.36 ± 0.61 (1.13-1.60) 1.17 ± 0.42 (0.93-1.40)
Values are presented as mean ± standard deviation and (95% confidence interval). * Bold results are significant, p <0.05.
Abbreviations: SD, standard deviation; ANOVA, analysis of variance;
Confidence interval; ABD, Abductors; ADD, Adductors; FLEX, Flexors; EXT, Extensors.
or EXT The results of the current study indicate that concentric isokinetic hip EXT peak torques obtained with patients in supine were greater than those obtained when patients were standing. This finding supports previous recommendations to avoid comparison of hip muscle isokinetic strength tests performed with different test positions.24
The current study did not find a significant main effect for sex for any of the normalized muscle group torques. This differs from previous studies for the hip FLEX, EXT, and ABD muscle groups. Borges et al.38 found that young males had significantly greater absolute concentric peak torque of the hip FLEX and hip EXT than young females when tested in standing at 60°/second (mean [SD]: FLEX male = 161.9 [30.0] Nm; FLEX female = 94.7 [15.8] Nm; EXT male = 155.0 [40.0] Nm; EXT female = 83.6 [30.5] Nm). Sugimoto et al.39 found that young males had significantly greater body weight-normalized concentric hip ABD than young females tested in standing at 60°/second (mean [SD]: ABD male = 1.29 [0.24] Nm/kg; ABD female = 1.13 [0.20] Nm/kg). However, hip ADD body weight- normalized concentric torque was no different between sexes, which is in agreement with the results of the current study (standing position, 60°/second, mean [SD]: ADD male = 0.75 [0.32] Nm/kg ADD female = 0.72 [0.27] Nm/kg).39 Differences between the findings of the current study and earlier studies may be due to different methods for analysis of muscle torque. Previous studies either did not normalize peak torque or normalized torque using body weight only 38,39 The current study normalized peak torque by body mass and height, as recommended for hip muscles.36 Differences between study findings may also be due to differing physical activity level of participants.
A significant difference between the hip FLEX-EXT ratio for results determined from supine and standing test posi-
tions was an additional finding of this study The FLEX-EXT ratio in standing (male FLEX = 136% of EXT, female FLEX = 117% of EXT) was greater than in supine (male FLEX = 100% of EXT, female FLEX = 93% of EXT) tests since the peak torque of hip FLEX was greater relative to the hip EXT in the standing test. The difference between these ratios was likely as a result of greater hip EXT peak torque in supine compared to the standing position in our participants. The hip FLEX-EXT ratios from the current study are different from a study that examined this ratio in a sample of male and female CrossFit participants who were tested in supine.19 The study by Rodrigues, et al.19 found that hip FLEX torques were much lower compared to hip EXT torques (male FLEX = 67.24% of EXT, female FLEX = 62.39% of EXT). The dissimilar findings may be due to differences in physical activity level, overall strength, and regular exercise regimens of participants in the two studies.
One interesting finding from this study was that isokinetic testing position seemed to have a particular impact on the hip EXT and ABD muscles. Weakness of these muscle groups has been found to be associated with or a risk factor for a variety of musculoskeletal conditions including patellofemoral pain,3 anterior cruciate ligament injury,7 femoroacetabular impingement,9 and iliotibial band syndrome.5 It may be that adequate strength of the hip EXT and ABD muscles is particularly critical for athletes due to their important role in stabilization of the pelvis on the LE.1
This study has some limitations that must be acknowledged. The participants were all young, healthy adults so generalization of study findings to persons with pathologies or of different age ranges should be cautioned. Only the dominant LE was examined so there are no results for
the non-dominant LEs. But previous authors have shown that there is no significant impact of LE dominance on concentric isokinetic hip muscle strength in healthy adults.40 Participants may not have given maximal effort during all tests, but consistent, scripted directions and verbal encouragement were given to encourage maximal effort throughout testing. Activation of tested muscle groups is unknown since electromyography was not used. Therefore, participants may have used complementary muscles to increase torque, which may vary depending upon test positions. There may have been a learning effect with repetitions of test movements, but attempts were made to minimize this through use of practice trials prior to recorded trials. Examiners were not blinded to torque values during testing, although participants were blinded to torque values. This study did not include examination of the hip internal and external rotator muscle groups, which should be considered for future research.
Concentric isokinetic peak torque test results for the sagittal and frontal plane hip muscles were different when tested with participants in recumbent compared to standing positions. Normalized hip EXT and hip ABD peak torques were greater when tested in recumbent positions.
The agonist-antagonist ratio of hip FLEX-EXT was greater when tested in standing. There were no differences between test position results for hip FLEX and hip ADD There were also no differences between sexes for body-mass-andheight-normalized peak torques for any sagittal or frontal plane muscle group. This information will be useful to clinicians when comparing their patients’ concentric isokinetic test results to normative data, taking the test position into consideration. Since many athletic injuries and conditions are associated with weakness of the hip EXT and hip ABD, this information will be particularly relevant to clinicians treating athletes. Future studies should be conducted to determine if these relationships are present in patients with pathologies or sports injuries.
The authors declare no conflicts of interest.
Submitted: April 02, 2024 CDT, Accepted: September 09, 2024 CDT
© The Author(s)
This is an open-access article distributed under the terms of the Creative Commons Attribution 4.0 International License (CCBY-NC-4.0). View this license’s legal deed at https://creativecommons.org/licenses/by-nc/4.0 and legal code at https://creativecommons.org/licenses/by-nc/4.0/legalcode for more information.
1. Neumann DA. Kinesiology of the hip: A focus on muscular actions. J Orthop Sports Phys Ther 2010;40(2):82-94. doi:10.2519/jospt.2010.3025
2. Popovich JM Jr, Kulig K. Lumbopelvic landing kinematics and EMG in women with contrasting hip strength. Med Sci Sports Exerc. 2012;44(1):146-153. doi:10.1249/MSS.0b013e3182267435
3. Nunes GS, de Oliveira Silva D, Crossley KM, Serrao FV, Pizzari T, Barton CJ. People with patellofemoral pain have impaired functional performance, that is correlated to hip muscle capacity Phys Ther Sport 2019;40:85-90.
4. Van Cant J, Pineux C, Pitance L, Feipel V. Hip muscle strength and endurance in females with patellofemoral pain: A systematic review with metaanalysis. Int J Sports Phys Ther 2014;9(5):564-582.
5. Fredericson M, Cookingham CL, Chaudhari AM, Dowdell BC, Oestreicher N, Sahrmann SA. Hip abductor weakness in distance runners with iliotibial band syndrome. Clinical Journal of Sport Medicine 2000;10(3):169-175. doi:10.1097/ 00042752-200007000-00004
6. Petersen W, Taheri P, Forkel P, Zantop T Return to play following ACL reconstruction: A systematic review about strength deficits. Arch Orthop Trauma Surg 2014;134(10):1417-1428. doi:10.1007/ s00402-014-1992-x
7. Khayambashi K, Ghoddosi N, Straub RK, Powers CM. Hip muscle strength predicts noncontact anterior cruciate ligament injury in male and female athletes: A prospective study. Am J Sports Med. 2016;44(2):355-361. doi:10.1177/0363546515616237
8. Freke MD, Kemp J, Svege I, Risberg MA, Semciw A, Crossley KM. Physical impairments in symptomatic femoroacetabular impingement: A systematic review of the evidence. Br J Sports Med 2016;50(19):1180-096152. doi:10.1136/ bjsports-2016-096152
9. Bizzini M, Schaub G, Ferrari E, et al. Hip muscle strength in male and female patients with femoroacetabular impingement syndrome: Comparison to healthy controls and athletes. Phys Ther Sport. 2023;61:142-148. doi:10.1016/ j.ptsp.2023.03.010
10. Whittaker JL, Small C, Maffey L, Emery CA. Risk factors for groin injury in sport: An updated systematic review. Br J Sports Med. 2015;49(12):803-809. doi:10.1136/ bjsports-2014-094287
11. Nadler SF, Malanga GA, Feinberg JH, Prybicien M, Stitik TP, DePrince M. Relationship between hip muscle imbalance and occurrence of low back pain in collegiate athletes: A prospective study. Am J Phys Med Rehabil 2001;80(8):572-577 doi:10.1097/ 00002060-200108000-00005
12. Hislop A, Collins NJ, Tucker K, Semciw AI. Hip strength, quadriceps strength and dynamic balance are lower in people with unilateral knee osteoarthritis compared to their non-affected limb and asymptomatic controls. Braz J Phys Ther 2022;26(6):100467 doi:10.1016/j.bjpt.2022.100467
13. Loureiro A, Mills PM, Barrett RS. Muscle weakness in hip osteoarthritis: A systematic review Arthritis Care Res 2013;65(3):340-352. doi:10.1002/ acr.21806
14. Burgess LC, Taylor P, Wainwright TW, Swain ID Strength and endurance deficits in adults with moderate-to-severe hip osteoarthritis, compared to healthy, older adults. Disabil Rehabil 2022;44(19):5563-5570. doi:10.1080/ 09638288.2021.1939797
15. Tsiokanos A, Kellis E, Jamurtas A, Kellis S. The relationship between jumping performance and isokinetic strength of hip and knee extensors and ankle plantar flexors. Isokinetics Exerc Sci 2002;10(2):107-115. doi:10.3233/ies-2002-0092
16. Baldon R de M, Nakagawa TH, Muniz TB, Amorim CF, Maciel CD, Serrao FV. Eccentric hip muscle function in females with and without patellofemoral pain syndrome. J Athl Train. 2009;44(5):490-496. doi:10.4085/1062-6050-44.5.490
17 Vosburg E, Hinkey M, Meyers R, et al. The association between lower extremity strength ratios and the history of injury in collegiate athletes. Phys Ther Sport 2022;55:55-60. doi:10.1016/ j.ptsp.2022.02.004
18. Claiborne TL, Armstrong CW, Gandhi V, Pincivero DM. Relationship between hip and knee strength and knee valgus during a single leg squat. J Appl Biomech. 2006;22(1):41-50. doi:10.1123/jab.22.1.41
19. Rodrigues ALR, Resende RA, Pogetti LS, et al. Trunk, mass grasp, knee, and hip muscle performance in CrossFit participants: Reference values according to participants’ sex and limb dominance. Int J Sports Phys Ther 2023;V18(3):726-736. doi:10.26603/ 001c.75222
20. Tuominen J, Leppänen M, Jarske H, Pasanen K, Vasankari T, Parkkari J. Test-retest reliability of isokinetic ankle, knee and hip strength in physically active adults using biodex system 4 pro. Methods Protoc 2023;6(2):26. doi:10.3390/mps6020026
21. Barbic S, Brouwer B. Test position and hip strength in healthy adults and people with chronic stroke. Arch Phys Med Rehabil. 2008;89(4):784-787. doi:10.1016/j.apmr.2007.10.020
22. Zapparoli FY, Riberto M. Isokinetic evaluation of the hip flexor and extensor muscles: A systematic review J Sport Rehabil 2017;26(6):556-566. doi:10.1123/jsr.2016-0036
23. Lipovšek T, Kacin A, Puh U. Reliability and validity of hand-held dynamometry for assessing lower limb muscle strength. Isokinetics Exerc Sci 2022;30(3):231-240. doi:10.3233/IES-210168
24. Castro MPd, Ruschel C, Santos GM, Ferreira T, Pierri CAA, Roesler H. Isokinetic hip muscle strength: A systematic review of normative data. Sports Biomech 2020;19(1):26-54. doi:10.1080/ 14763141.2018.1464594
25. Chamorro C, Armijo-Olivo S, De la Fuente C, Fuentes J, Javier Chirosa L. Absolute reliability and concurrent validity of hand held dynamometry and isokinetic dynamometry in the hip, knee and ankle joint: Systematic review and meta-analysis. Open Med 2017;12:359-375. doi:10.1515/med-2017-0052
26. Contreras-Díaz G, Chirosa-Ríos LJ, Chirosa-Ríos I, Intelangelo L, Jerez-Mayorga D, Martinez-Garcia D Reliability of isokinetic hip flexor and extensor strength measurements in healthy subjects and athletes: A systematic review and meta-analysis. Int J Environ Res Public Health 2021;18(21):11326. doi:10.3390/ijerph182111326
27 Claiborne TL, Timmons MK, Pincivero DM. Testretest reliability of cardinal plane isokinetic hip torque and EMG. J Electromyogr Kinesiol. 2009;19(5):345. doi:10.1016/j.jelekin.2008.07.005
28. de Castro MP, Sanchotene CG, Costa GV, et al. Comparison between side-lying and standing positions for hip abductor strength assessment. J Bodyw Movement Ther 2022;30:181-186. doi:10.1016/ j.jbmt.2022.02.011
29. von Elm E, Altman DG, Egger M, et al. The strengthening the reporting of observational studies in epidemiology (STROBE) statement: Guidelines for reporting observational studies. Ann Intern Med. 2007;147(8):573-577 doi:10.7326/ 0003-4819-147-8-200710160-00010
30. Hoglund LT, Wong AL, Rickards C. The impact of sagittal plane hip position on isometric force of hip external rotator and internal rotator muscles in healthy young adults. Int J Sports Phys Ther. 2014;9(1):58-67
31. Briggs KK, Steadman JR, Hay CJ, Hines SL. Lysholm score and tegner activity level in individuals with normal knees. Am J Sports Med. 2009;37(5):898-901. doi:10.1177/0363546508330149
32. Chamorro-Moriana G, Perez-Cabezas V, EspunyRuiz F, Torres-Enamorado D, Ridao-Fernández C. Assessing knee functionality: Systematic review of validated outcome measures. Ann Phys Rehabil Med 2022;65(6):101608. doi:10.1016/j.rehab.2021.101608
33. Terwee CB, Bouwmeester W, van Elsland SL, de Vet HC, Dekker J. Instruments to assess physical activity in patients with osteoarthritis of the hip or knee: A systematic review of measurement properties. Osteoarthritis Cartilage 2011;19(6):620-633. doi:10.1016/j.joca.2011.01.002
34. Koo TK, Li MY A guideline of selecting and reporting intraclass correlation coefficients for reliability research. J Chiropr Med. 2016;15(2):155-163. doi:10.1016/j.jcm.2016.02.012
35. Faul F, Erdfelder E, Lang AG, Buchner A. G*Power 3: A flexible statistical power analysis program for the social, behavioral, and biomedical sciences. Behav Res Methods 2007;39(2):175-191. doi:10.3758/ bf03193146
36. Bazett-Jones DM, Cobb SC, Joshi MN, Cashin SE, Earl JE. Normalizing hip muscle strength: Establishing body-size-independent measurements. Arch Phys Med Rehabil 2011;92(1):76-82. doi:10.1016/j.apmr.2010.08.020
37. Cohen J. Statistical Power Analysis for the Behavioral Sciences 2nd ed. Lawrence Erlbaum Associates; 1988.
38. Borges VS, Domingues JM, Dias RC, Garcia PA, Dvir Z. Strength and torque consistency of the hip and knee flexors and extensors: A comparative study of elderly and young individuals. Isokinetics Exerc Sci. 2015;23:45-51. doi:10.3233/IES-140563
40. Abdelmohsen AM. Leg dominance effect on isokinetic muscle strength of hip joint. J Chiropr Med. 2019;18(1):27-32. doi:10.1016/j.jcm.2018.03.009 Position- and Sex-Related Differences
39. Sugimoto D, Mattacola CG, Mullineaux DR, Palmer TG, Hewett TE. Comparison of isokinetic hip abduction and adduction peak torques and ratio between sexes. Clin J Sport Med. 2014;24(5):422-428. doi:10.1097/JSM.0000000000000059
Thiago R T Santos1 , Ana Luiza R Rodrigues2 , Henrique M P Faria2 , Stéphane M Teixeira2 , Livia S Pogetti2 , Andressa Silva2 , Renan A Resende2 , Juliana M Ocarino2a
1 Universidade Federal de Uberlândia, 2 Universidade Federal de Minas Gerais
Keywords: strength, athletes, validity, psychometric properties, sports https://doi.org/10.26603/001c.124119
International Journal of Sports Physical Therapy
Vol. 19, Issue 11, 2024
Background
The Hip Stability Isometric Test (HipSIT) is a practical clinical assessment of posterolateral hip muscle performance. There is no information regarding the validity of the HipSIT in participants exposed to high-intensity training, such as CrossFit®.
Purpose
The purpose of this study was to investigate the Hip Stability Isometric Test (HipSIT) concurrent validity with the isokinetic assessment in CrossFit® participants. A secondary purpose was to characterize posterolateral hip muscular performance with HipSIT according to sex and lower limb dominance in athletes who participate in CrossFit®.
Study Design
Cross-sectional.
Methods
One-hundred and eleven CrossFit® participants were evaluated. The posterolateral hip muscles were evaluated using the HipSIT with a hand-held dynamometer. The hip extensors and abductors’ peak torque and maximum work were assessed with the Biodex System® 4 Pro isokinetic dynamometer at 60º/s. Concurrent validity between measurements was assessed with the Spearman correlation coefficient and Bland-Altman analyses. The comparison of results between sexes and between limbs was also performed.
Results
Spearman analyses indicated a significant positive correlation with medium effect size between HipSIT and isokinetic variables ( = 0.36 to 0.49). Bland-Altman analyses showed that most measures were within the 95% limits of agreement. The HipSIT was greater in males than females (p < 0.001) and greater in the dominant than non-dominant limb (p = 0.03).
Conclusion
The findings support using HipSIT in the clinical assessment of CrossFit® participants. Clinicians can use the data as reference values for athletes who participate in CrossFit® and should consider the difference between sexes and lower limbs.
Corresponding author.
Juliana Melo Ocarino
Departament of Physical Therapy, Universidade Federal de Minas Gerais (UFMG)
Av. Presidente Antônio Carlos, 6627 Campus Pampulha, CEP 31270-901
Belo Horizonte, Minas Gerais, Brazil
E-mail: julianaocarino@gmail.com
The Hip Stability Isometric Test (HipSIT) is a clinical examination of hip posterolateral muscle strength (i.e., hip abductors, lateral rotators, and extensors).1 This test is considered more functional and quicker to perform than the traditional uniplanar assessment of hip muscles.1 The hip posterolateral muscles are commonly considered during sports assessment since their deficits in torque favor altered hip motion and greater stress on the musculoskeletal system, such as the anterior cruciate ligament, increasing the risk of sports injuries.2,3 In support of that, a previous study showed that the HipSIT outcome was one of the variables that classified mountain bikers with and without anterior knee pain.4 Therefore, the HipSIT is a practical clinical test for sports assessments.
The HipSIT has been previously investigated regarding measurement properties in females with and without patellofemoral pain, showing appropriate reliability and validity 1 Although this test is valid for a population with low force generation capacity, there is no information regarding its measurement properties for assessing participants with greater muscular performance due to high-intensity training. For this reason, CrossFit® participants were chosen for this investigation as this sport involves high-intensity strength and power activities,5,6 typically requiring the recruitment of hip muscles to contribute to proper body alignment, propulsion, and force transmission among segments.7,8
The validity of the HipSIT among CrossFit® participants can be examined by correlating its results to the results from isokinetic assessment, commonly considered the standard reference measure of muscle performance. If valid, the HipSIT may be viewed as a preferable alternative to the gold-standard isokinetic dynamometer within the context of sports applications. The isokinetic dynamometer is a non-portable device that requires more time and training of the evaluator to assess hip muscle performance than the HipSIT assessment. These characteristics prohibit the use of the isokinetic dynamometer for quick evaluations on the field, and perhaps during preseason assessments with a large number of athletes. Also, the cost of an isokinetic dynamometer is higher than that of a hand-held dynamometer, such as that used with the HipSIT Therefore, despite the isokinetic dynamometer providing objective muscle performance data with remarkable quality, the HipSIT could be a good option for a quick, easy, and valid clinical assessment of hip muscle performance, especially in clinical and sports settings involving large-scale assessments.
In addition to validity, the HipSIT can be used to assess any differences in hip muscle performance between males and females and between dominant and non-dominant lower limbs. Thus, the purpose of this study was to investigate the Hip Stability Isometric Test (HipSIT) concurrent validity with the isokinetic assessment in CrossFit® partic-
ipants. A secondary purpose was to characterize posterolateral hip muscular performance with HipSIT according to sex and lower limb dominance in athletes who participate in CrossFit®
Participants
CrossFit® practitioners (58 males and 53 females) were recruited by posting announcements in CrossFit® gyms. The inclusion criteria were age between 18 and 40 years, at least one year of CrossFit® practice, absence of musculoskeletal injuries in the last six months, and absence of surgery in the previous year. The musculoskeletal injury was defined as time loss of the practice equal to or greater than seven days or 14 days with reduced training capability 9 The exclusion criteria were any pain during the procedures or inability to perform the tests. No participants were excluded. The sample size was estimated based on a priori power analysis with the following parameters: significance level of 0.05, statistical power of 80%, and medium effect size (ρ = 0.30). This analysis revealed that a minimum sample size of 84 participants would be necessary to ensure that the correlation between variables would differ from 0. All the participants provided written informed consent before participation. The ethics in research committee of the Universidade Federal de Minas Gerais approved this study (Protocol number: CAAE 93670418.9.0000.5149).
The participants answered a questionnaire about the characteristics of their CrossFit® training, and then their body mass and height were measured. To warm up, the participants did jump jacks for one minute. Subsequently, they performed one submaximal repetition of the HipSIT and isokinetic assessment for familiarization. The dominant lower limb was assessed first, followed by the non-dominant one. The dominant lower limb was defined as the preferred leg to kick a ball as far as possible.10
The HipSIT was performed as described by Almeida et al 1 The participant was positioned side-lying, with 45° of hip flexion, 20° of hip abduction, and 90° of knee flexion (Figure 1A). A manual dynamometer (microFET2; Hoggan Scientific, LLC, Salt Lake City, UT, USA) was positioned with a rigid strap at 5 cm superior to the lateral femoral epicondyle. The participants were instructed to lift the knee of the upper leg, keeping contact between heels, and pushing the dynamometer with maximum force for five seconds. Three measures were performed on each limb, with one minute of rest between the attempts. The mean peak torque was considered for analysis. If any compensation was observed during the test, the attempt was discarded, and the trial was repeated. The intra-rater reliability was examined in a pilot study with 10 participants assessed during two
sessions seven days apart. The results showed excellent intra-rater reliability (overall ICC = 0.921, 95% Confidence Interval = 0.790-0.978; Standard Error of Measurement - SEM = 6.86 N m).
Isokinetic assessment of hip extensors and abductors was performed with the Biodex System® 4 Pro dynamometer (Biodex Medical System Inc., Shirley, NY, EUA) in concentric mode.11,12 Previous authors have demonstrated that the isokinetic assessment presented adequate measurement properties.13,14 The hip extensors were assessed in a supine position; the trunk was stabilized with two straps crossed over it, and another strap was placed proximal to the iliac crest (Figure 1B). The range of motion was set at 110° (10° to 120° of flexion). The hip abductors were assessed in a side-lying position,15 with the hip parallel to the ground at a neutral position; the trunk was stabilized with a belt proximal to the iliac crest; the contralateral hip and knee were flexed and stabilized with straps (Figure 1C). The range of motion was set at 45° (0° to 45° of hip abduction). For both tests, the rotational axis was aligned with the greater trochanter of the femur, the attachment was positioned on the distal third of the thigh, and the participants performed five repetitions at 60º/s. The assessed limb was weighted to correct the gravity effects on the torque measured.16 The peak torque and maximum work (i.e., extracted from the repetition with the greatest amount of work) were selected for analysis.
Descriptive statistics were used to characterize HipSIT data (non-normalized and normalized by body weight) according to sex and limb dominance. Muscle strength normalized by body weight is usually recommended to compare the performance of different subjects and, thus, helpful in the clinical setting as reference data. Kolmogorov-Smirnov test revealed that the data showed a significant non-normal distribution. The comparison of the non-normalized HipSIT data between sexes was performed with the Mann-Whitney U test. The comparison between limbs was performed with the Wilcoxon test.
The consistency between the HipSIT and isokinetic variables was investigated with the Spearman correlation coef-
ficient (rs).17 The closer rs is to -1 or +1, the stronger the relationship is compared to values closer to zero. Values of rs = ±0.1 indicate a small effect size, rs = ±0.3 a medium effect size, and rs = ±0.5 a large effect size.18 Also, the BlandAltman analysis and its 95% limits of agreement (LoA) were used to graphically show the agreement between HipSIT and isokinetic peak torque measurements.17,19 This analysis reveals the spread of the difference between methods according to each participant against the mean score.17,19
The Bland-Altman analysis was performed only with the isokinetic peak torque measurement since this variable is expressed in the same unit of HipSIT output. A significance level of 0.05 was used for all analyses.
The anthropometric and training variables are presented in Table 1 Descriptive data for the HipSIT are shown in Table 2. The HipSIT performance was greater in males than in females for the dominant (p < 0.001) and non-dominant limbs (p < 0.001). Also, the HipSIT was greater in the dominant than non-dominant limb (p = 0.03).
Spearman analyses indicated a significant positive correlation with medium effect size between HipSIT and isokinetic variables (ρ = 0.36 to 0.49) (Table 3). The relationship between variables is shown in scatter plots (Supplementary material).
Bland-Altman analyses showed that most measures were within the LoA (Figure 2). The analyses showed the following trend: the difference between measures tended to be greater with greater peak torque values.
This study investigated the validity of the HipSIT with the isokinetic assessment in CrossFit® practitioners and showed a positive correlation with medium effect size between these measurements. Furthermore, this study provides data using the HipSIT that can be used as reference values for future research and clinical practice. Hip muscular performance was greater for males than females in both limbs. Also, the performance was greater in the dom-
Table 1. Characteristics of the CrossFit® practitioners (n = 111)
Variable
(5.5)
(12.1)
(0.08)
Note: All variables except the sex and category are presented as mean (standard deviation). The sex and category are presented as the observed frequency RX: workout performed as prescribed for each exercise; Intermediate: a modified version of the RX workout for more experienced athletes; Scale: workout adapted for athletes with little experience.
Table 2. Descriptive data of hip posterolateral muscle performance according to the HipSIT Non-normalized
Females (n = 53)
All participants (n = 111)
Note: DOM Dominant, NDOM Non-dominant, IQR Interquartile range, SD Standard Deviation, CI Confidence Interval.
inant than the non-dominant limb. Thus, the hip posterolateral muscle strength measured by HipSIT showed adequate properties to be used with CrossFit® practitioners. The HipSIT and isokinetic assessment were positively correlated, however may assess different properties of muscle performance. The isokinetic test analyzes the hip muscle performance according to the muscle actions of the
tested groups (i.e., hip extensors and abductors) during a constant angular speed throughout the range of motion.20 On the other hand, the HipSIT measures the isometric torque generated by three hip muscle groups simultaneously at a single point in the range of motion.1 Despite these differences between the tests, the HipSIT demands hip posterolateral muscles to generate a combined isomet-
Table 3. Correlation between HipSIT and isokinetic variables
Isokinetic variables
60º/s
Peak of torque
Hip extensors ρ = 0.45, p <0.001
Hip abductors ρ = 0.37, p <0.001
Maximum work
Hip extensors ρ = 0.44, p <0.001
Hip abductors ρ = 0.36, p <0.001
The level of significance was 0.05.
= 0.49, p <0.001
= 0.48, p <0.001
= 0.49, p <0.001
= 0.42, p <0.001
Figure 2. Bland-Altman plots between HipSIT and the following isokinetic variables: peak of torque of the dominant hip extensors (A), non-dominant hip extensors (B), dominant hip abductors (C), non-dominant hip abductors (D).
ric torque related to each hip muscle’s capacity to generate torque, explaining the moderate correlations seen. The HipSIT could be used as a valid clinical way to measure the hip extensors’ and abductors’ combined performance of CrossFit® practitioners.
The HipSIT presented a slightly greater strength of association with hip extensors (ρ = 0.44 to 0.49) than abductors (ρ = 0.36 to 0.48). These results suggest that HipSIT may better represent the performance of hip extensors than abductors. In support of this interpretation, authors have previously described the greater activity of the gluteus maximum than medius during clamshell exercise, a position like the one adopted to perform the HipSIT test.21,22
The HipSIT was correlated with both peak torque and maximum work. A previous study indicated that the maximum work best represented the torque generation capac-
ity, and the peak torque should not be used solely to represent this capacity 23 The isokinetic peak torque represents the maximum torque generated at a single point of the entire range of motion, whereas maximum work represents the capacity to generate muscle torque throughout the full range of the movement.24 Bland-Altman analysis revealed that most data were within the LoA. Furthermore, the plots showed a tendency for greater differences between measurements in the participants that generated the greatest torque mean values. Therefore, it appears that the HipSIT measure is mainly related to the hip extensors’ capacity to generate torque in CrossFit® practitioners, and the measurements tend to show greater differences in those that produced greater torque.
The measurement properties investigated in the current study complemented the previous research that examined
reliability and validity of the HipSIT The prior study validated HipSIT with isometric hand-held dynamometry in 49 recreational physically active females and found a large correlation with hip abductors (r = 0.535), extensors (r = 0.514), and lateral rotators (r = 0.536).1 The present study investigated validity with a reference standard device for assessing hip muscle performance in a larger sample, including both males and females practicing CrossFit® Since the HipSIT maintained a medium correlation to the reference measurement, the test may be considered a practical clinical assessment for participants in high-intensity conditioning programs.
The second aim of this study was to characterize the hip posterolateral muscle muscle performance according to sex and limb dominance. Males presented greater performance than females, which is not surprising. A similar finding has been shown for shoulder and knee muscles assessed with an isokinetic dynamometer in participants enrolled in highintensity training programs.25 The HipSIT also differed between lower limbs: the dominant limb presented greater performance than the non-dominant. This result also corroborates the observed difference between limbs for knee flexors’ peak torque, as shown previously 25 Clinicians assessing CrossFit® participants should consider the difference between sexes and limbs, and the current results could be used as reference data.
This study showed some limitations. The findings of this study are generalizable to healthy CrossFit® practitioners. Despite the inclusion criterion of an absence of injury in the prior six months, a limitation would be that any injury history beyond this period could influence hip performance. However, due to the cross-sectional design of the current study, the reporting of this information could be affected by memory bias. Future studies may be designed to investi-
gate HipSIT in other samples and other measurement properties (e.g., responsiveness). In addition, future prospective investigations may look for the capacity of this clinical test outcome to predict sports injuries.
In CrossFit® practitioners, the hip posterolateral performance assessed using HipSIT showed acceptable concurrent validity with the peak torque and maximum work of the hip extensors and abductors assessed isokinetically. The observed correlations and their medium association suggest that the relationship between these measures occurs mainly with hip extensors’ performance. Furthermore, posterolateral hip performance values as measured by the HipSIT were greater in males than females and in the dominant versus the non-dominant limb.
The authors were partially supported by the following agencies: Fundação de Amparo à Pesquisa do Estado de Minas Gerais (FAPEMIG), Conselho Nacional de Desenvolvimento Científico e Tecnológico (CNPq), and Coordenação de Aperfeiçoamento de Pessoal de Nível Superior (CAPES –Grant code 001).
Submitted: January 25, 2024 CDT, Accepted: September 09, 2024 CDT
© The Author(s)
This is an open-access article distributed under the terms of the Creative Commons Attribution 4.0 International License (CCBY-NC-4.0). View this license’s legal deed at https://creativecommons.org/licenses/by-nc/4.0 and legal code at https://creativecommons.org/licenses/by-nc/4.0/legalcode for more information.
1. Almeida GPL, Rodrigues HLN, Freitas BW, Lima POP Reliability and validity of the hip stability isometric test (HipSIT): a new method to assess hip posterolateral muscle strength. J Orthop Sports Phys Ther 2017;47(12):906-913. doi:10.2519/ jospt.2017.7274
2. Khayambashi K, Ghoddosi N, Straub RK, Powers CM. Hip muscle strength predicts noncontact anterior cruciate ligament injury in male and female athletes: a prospective study Am J Sports Med 2016;44(2):355-361. doi:10.1177/0363546515616237
3. Mendonça LD, Ocarino JM, Bittencourt NFN, Macedo LG, Fonseca ST Association of hip and foot factors with patellar tendinopathy (jumper’s knee) in athletes. J Orthop Sports Phys Ther. 2018;48(9):676-684. doi:10.2519/jospt.2018.7426
4. Branco GR, Resende RA, Bittencourt NFN, Mendonça LD. Interaction of hip and foot factors associated with anterior knee pain in mountain bikers. Phys Ther Sport 2022;55:139-145. doi:10.1016/j.ptsp.2022.04.001
5. Wagener S, Hoppe MW, Hotfiel T, et al. CrossFit® –development, benefits and risks. Sports Orthopaedics and Traumatology. 2020;36(3):241-249. doi:10.1016/ j.orthtr.2020.07.001
6. Glassman G. A theoretical template for CrossFit’s programming. The Crossfit Jornal. 2013;(6):1-5.
7. Baldon RM, Lobato DFM, Carvalho LP, Wun PYL, Presotti CV, Serrão FV Relationships between eccentric hip isokinetic torque and functional performance. J Sport Rehabil. 2012;21(1):26-33. doi:10.1123/jsr.21.1.26
8. Stone MH, Sands WA, Pierce KC, Carlock J, Cardinale M, Newton RU. Relationship of maximum strength to weightlifting performance. Med Sci Sports Exerc 2005;37(6):1037-1043.
9. Weisenthal BM, Beck CA, Maloney MD, DeHaven KE, Giordano BD Injury rate and patterns among CrossFit athletes. Orthop J Sports Med 2014;2(4):2325967114531177. doi:10.1177/ 2325967114531177
10. van Melick N, Meddeler BM, Hoogeboom TJ, Nijhuis-van der Sanden MWG, van Cingel REH. How to determine leg dominance: The agreement between self-reported and observed performance in healthy adults. PLoS One. 2017;12(12):e0189876. doi:10.1371/ journal.pone.0189876
11. Belhaj K, Meftah S, Mahir L, Lmidmani F, Elfatimi A. Isokinetic imbalance of adductor–abductor hip muscles in professional soccer players with chronic adductor-related groin pain. Eur J Sport Sci 2016;16(8):1226-1231. doi:10.1080/ 17461391.2016.1164248
12. Ghareeb DM, McLaine AJ, Wojcik JR, Boyd JM. Effects of two warm-up programs on balance and isokinetic strength in male high school soccer players. J Strength Cond Res 2017;31(2):372-379. doi:10.1519/JSC.0000000000001509
13. Caruso JF, Brown LE, Tufano JJ. The reproducibility of isokinetic dynamometry data. Isokinet Exerc Sci 2012;20(4):239-253. doi:10.3233/ IES-2012-0477
14. Drouin JM, Valovich-mcLeod TC, Shultz SJ, Gansneder BM, Perrin DH. Reliability and validity of the Biodex system 3 pro isokinetic dynamometer velocity, torque and position measurements. Eur J Appl Physiol 2004;91(1):22-29. doi:10.1007/ s00421-003-0933-0
15. Baldon RM, Lobato DFM, Carvalho LP, Santiago PRP, Benze BG, Serrão FV Relationship between eccentric hip torque and lower-limb kinematics: gender differences. J Appl Biomech 2011;27(3):223-232. doi:10.1123/jab.27.3.223
16. Biodex Medical Systems. Biodex Advantage Software (V.4X) Operation Manual. Biodex Medical Systems Inc.
17 Portney LG. Foundations of Clinical Research: Applications to Evidence-Based Practice. 4th ed. F. A. Davis Company; 2020.
18. Cohen J. Statistical Power Analysis for the Behavioral Sciences. 2nd ed. Lawrence Erbaum Associates; 1988.
19. Bland JM, Altman DG. Statistical methods for assessing agreement between two methods of clinical measurement. Lancet 1986;1(8476):307-310.
20. Perrin DH. Isokinetic Exercise and Assessment Human Kinetics; 1993.
21. Selkowitz DM, Beneck GJ, Powers CM. Which exercises target the gluteal muscles while minimizing activation of the tensor fascia lata? Electromyographic assessment using fine-wire electrodes. J Orthop Sports Phys Ther 2013;43(2):54-64. doi:10.2519/jospt.2013.4116
22. Boren K, Conrey C, Le Coguic J, Paprocki L, Voight M, Robinson TK. Electromyographic analysis of gluteus medius and gluteus maximus during rehabilitation exercises. Int J Sports Phys Ther. 2011;6(3):206-223.
23. Amaral GM, Marinho HVR, Ocarino JM, Silva PLP, de Souza TR, Fonseca ST Muscular performance characterization in athletes: a new perspective on isokinetic variables. Braz J Phys Ther 2014;18(6):521-529. doi:10.1590/bjpt-rbf.2014.0047
24. Kannus P Isokinetic evaluation of muscular performance: implications for muscle testing and rehabilitation. Int J Sports Med 1994;15(Suppl 1):S11-8. doi:10.1055/s-2007-1021104
25. Motta C, Lira CAB, Vargas VZ, Vancini RL, Andrade MS. Profiling the isokinetic muscle strength of athletes involved in sports characterized by constantly varied functional movements performed at high intensity: a cross-sectional study PM R 2019;11(4):354-362. doi:10.1016/j.pmrj.2018.08.380
Supplementary material
Download: https://ijspt.scholasticahq.com/article/124119-hip-stability-isometric-test-hipsit-concurrent-validity-andreference-values-for-crossfit-participants/attachment/247575.docx
Stadelmann JD, Reichmann F, Franceschini-Brunner R, Mosler A, Maffiuletti NA,
M. Hip and Groin Problems in Female Team-Sport Athletes: A Cross-Sectional Study. IJSPT. 2024;19(11):1426-1438. doi:10.26603/001c.123946
Juan Diego Stadelmann1,2a , Freddy Reichmann1,2 , Romana Franceschini-Brunner2 , Andrea Mosler3 , Nicola A. Maffiuletti2 , Mario Bizzini2
1 School of Health Professions, Institute of Physiotherapy, ZHAW Zurich University of Applied Sciences, 2 Human Performance Lab, Schulthess-Klinik, 3 Sport and Exercise Medicine Research Centre, La Trobe University
Keywords: hip problems, groin problems, female athletes, epidemiology, clinical entities
https://doi.org/10.26603/001c.123946
International Journal of Sports Physical Therapy
Vol. 19, Issue 11, 2024
Background
Hip and groin problems are common among team-sport athletes. However, few studies have been conducted in female athletes that have used the Doha Agreement classification system to categorize these problems.
Purpose
The purpose of this study was to examine the preseason point prevalence of hip and groin problems in elite female team-sport athletes. Secondary aims were to categorize the groin problems according to the Doha Agreement classification system and to explore the association between hip muscle strength and self-reported hip and groin function.
Study Design
Cross-sectional study.
Methods
Female athletes who participated in various sports underwent preseason assessment of isometric hip adduction and abduction strength, with pain levels recorded during respective contractions, and self-reported function on the Copenhagen Hip and groin Outcome Score (HAGOS). If any athlete reported current hip and groin problems during this screening assessment, a full, standardized clinical hip and groin examination was undertaken. These hip and groin problems were then categorized according to the Doha Agreement classification system. Comparisons between the participants with vs without hip and groin problems were made, and correlational statistics were used to examine relationships between strength and HAGOS scores.
Results
Ninety-one elite female athletes from six different sports were included in the study
Thirteen athletes reported current hip and groin problems, with an overall prevalence rate of 14.3%. Iliopsoas-related groin pain was the most common category diagnosed, accounting for 58.8% of cases, followed by hip-related groin pain (17.6%), adductor-related (11.8%), and inguinal- and pubic-related pain (both 5.9%). No association was found between strength and function.
Conclusion
The hip and groin point prevalence in female athletes documented during preseason appears to be similar to those reported in male athletes. Iliopsoas-related groin pain was
Corresponding Author: Juan Diego Stadelmann jd.stadelmann.pt@gmail.com / +41 78 691 37 80
the most common clinical entity reported in female athletes in this study There was no association between hip muscle strength and self-reported hip and groin function.
Hip and groin problems are a common concern among athletes participating in multi-directional team-sports involving high-intensity, repetitive, and forceful hip movements.1‑3 Movements such as kicking, skating, and rapid changes of direction can lead to trauma or overuse, causing pain around the hip and groin area and long-standing and debilitating conditions1‑5 that may affect sports participation and performance.6,7
Hip and groin problems are particularly common in professional male athletes, with high prevalence reported in soccer players ranging from 21-59%7‑11 and 12-29%9,12 for seasonal and weekly prevalence, respectively In addition, seasonal prevalence of hip and groin problems of 53% have been reported in professional ice hockey players,13 while other sports such as basketball, Australian football, and field hockey have reported prevalences ranging from 17% to 22%.8,14,15 In contrast, there is less research examining the epidemiology of hip and groin problems in female athletes. Studies in female football (soccer) and ice hockey, report a cumulative prevalence of 27-45%9,16,17 and 62%,18 respectively Additionally, to date, very few studies have applied the Doha Agreement classification system to this population17 or examined the association between hip and groin function and hip muscle strength. As women’s sports continue to grow rapidly in popularity and the number of professional athletes is increasing, it is crucial to address this gap about hip and groin problems in female athletes.
Hip and groin problems can be classified as either timeloss or non-time-loss, depending on whether the athlete is able to fully participate in training and competition despite the problem. While time-loss injuries account for only a small proportion of all groin problems in elite and subelite athletes (9-38%),9,12,13,16,19,20 it is essential to examine non-time-loss problems to better appreciate the overall burden of hip and groin pain.
Factors such as previous groin injury, higher level of play, reduced hip adduction strength, and lower levels of sport-specific training have been associated with increased risk of groin injury in sport.21‑23 Higher body mass index, age, and reduced hip range of motion (ROM) have also been reported as intrinsic risk factors for groin injury, although with conflicting evidence.21‑23
The inconsistent use of terminology and classifications in the literature and the complexity of the clinical examination can make diagnosing hip and groin problems in athletes challenging.24 To address this, the Doha Agreement meeting proposed a classification system to clarify and standardize terminology and clinical taxonomy.25 Accurate and standardized classification can enhance the comprehension of these problems and improve the quality and synthesis of research to improve management and pre-
vention strategies.24 Previous research in male athletes using the new taxonomy has shown that most groin problems are classified as adductor-related groin pain,10,11,14,20,26 as did a recent study in female soccer players.17
The purpose of this study was to examine the preseason point prevalence of hip and groin problems in elite female team-sport athletes. Secondary aims were to categorize the groin problems according to the Doha Agreement classification system and to explore the association between hip muscle strength and self-reported hip and groin function.
This project was an exploratory, observational, cross-sectional cohort study
This research project was conducted among female teamsport athletes competing in the highest league of their respective sport (basketball, floorball, handball, ice hockey, soccer, and volleyball) during the 2022/2023 preseason in Switzerland, as this appears to be the period with the greatest exposure to hip and groin problems.27,28
Ethical approved for the study was provided by the Zurich Ethics Committee (ID# 2022-00409).
The recruitment period for the different teams ran from April to June 2022, and the data were collected in the summer of 2022 within the first three weeks of each sport’s preseason: June for ice hockey, July for floorball and soccer, August for handball, and September for basketball and volleyball.
The study coordinator contacted the managers of 20 teams verbally or by e-mail to provide them with an overview of the study and an information sheet detailing its objectives. After receiving verbal or written participation confirmation, an informed consent form was sent to the manager for distribution to the athletes. The manager then introduced the project to the athletes and ensured that each participant understood the voluntary nature of their participation and their right to withdraw at any time during the study. Written informed consent was obtained from all participants prior to participation.
Included participants underwent a two-stage assessment. First, all players underwent hip adduction and abduction muscle strength assessment and completed the Copenhagen Hip and Groin Outcome Score (HAGOS) to describe
• Female athlete
• > 18 years old
• Team-sport athlete in Switzerland (basketball, floorball, handball, ice-hockey, soccer, volleyball)
• Highest National League
• German, French or English-speaking
their hip and groin function. Secondly, those players who reported hip and groin problems underwent a full standardized clinical hip and groin examination (further described in the Clinical Entities section). All assessments were performed by two physiotherapists (JDS, FR) who were trained in the assessment procedure.
PARTICIPANTS
Out of possible 20 team, six teams from six different sports agreed to participate in the study, and participants were included based on the criteria outlined in Table 1
STUDY OUTCOMES AND MEASUREMENTS
STAGE-1 MEASUREMENTS
The following assessments were conducted during the first phase of data collection.
PARTICIPANTS’ CHARACTERISTICS
All participating athletes were asked to provide personal information, including their age, height, weight, sport, playing experience (measured as the number of years competing at the highest level), and dominant leg (defined as the preferred leg for kicking, regardless of the sport).
HIP MUSCLE STRENGTH
Hip adduction and abduction muscle strength was assessed isometrically in the supine position (with 45° of hip flexion and 90° of knee flexion) by means of a measurement device (ForceFrame, Vald Performance, Albion, Australia) that has been shown to be valid and reliable for the assessment of hip muscle strength.29,30 The strength measurement protocol used in this study was based on the methodology described by Oliveras and colleagues and is detailed in Appendix A.31
Briefly, participants were first asked to perform a submaximal contraction of each muscle group for warm-up and familiarization purposes. Then, they completed three bilateral maximal voluntary contractions of the hip adductor and abductor muscles. The assessment order for the two muscle groups was randomized to avoid systematic bias. Only the highest peak force trial was retained for each muscle group and side. The primary outcomes were absolute and relative (normalized to body weight) hip abduction and adduction strength, and the ratio of hip adduction to ab-
• Issue that does not allow them to perform strength assessments:
◦ Acute problems
◦ Illness
◦ Pregnancy
• Lower limb operation in the last year
• Unable to participate in team training due to pain
• Those who spoke no German, French or English
duction strength for each side. For the analyses, the average of the left and right side was calculated for participants without pain or with bilateral pain, while only the value of the affected side was used for participants with pain.
Immediately after each contraction of the strength tests, athletes were asked to rate their groin pain using a 0-10 Visual Analog Scale (VAS), where 0 and 10 corresponded respectively to no pain and maximum pain, and to indicate which side was painful (right, left or both). A cut-off score of >2 was used to differentiate between players with and without pain.32,33
Before the strength tests, hip and groin pain was also assessed using a subjective complaint question20: “Have you recently had hip or groin pain that has limited your performance while doing your sport?”. If the answer was positive, an additional question was asked: “Which limb was symptomatic (dominant, non-dominant, both)?”. Hip and groin pain was defined as any physical symptom located in the groin region that prevented a player from fully participating in training or match or reduced performance in the previous week.
Hip and groin function was measured using the HAGOS, a self-report questionnaire that has been validated for assessing hip and groin disability and is recommended for use in young to middle-aged individuals.34 It consists of 37 items divided into six subscales assessing: symptoms, pain, physical function in daily living, physical function in sport and recreation, participation in physical activities, and hip and/or groin-related quality of life. Items are answered on a 5-point Likert scale ranging from 0 to 4, with 0 indicating no hip and groin problem and 4 indicating extreme hip and groin problems. Subscale scores range from 0 to 100, with 100 indicating no hip and groin problems and 0 indicating severe hip and groin problems. The questionnaire has been found to be reliable, internally consistent, and valid in young athletes.35
The most interesting and relevant subscale for teamsport athletes is the sport subscale because it focuses on activities such as sprinting, twisting/pivoting, and kicking.32, 36 Based on previous studies, a cut-off score of <86 was used on the sport subscale to differentiate between players with and without problems.20,32
Clinical entities Symptoms and examination findings
Adductor-related groin pain
Iliopsoas-related groin pain
Inguinal-related groin pain
Pubic-related groin pain
Additional categories
Hip-related groin pain
Other
Adductor tenderness AND pain on resisted adduction testing
Iliopsoas tenderness, more likely if pain on resisted hip flexion AND/OR pain on hip flexor stretching
Pain located in the inguinal canal region and tenderness of the inguinal canal. No palpable inguinal hernia is present. More likely if pain aggravated by abdominal resistance or valsalva/cough/sneeze
Local tenderness of the pubic symphysis and the immediately adjacent bone
Clinical suspicion that the hip joint is the source of groin pain, either through history or through clinical examination (Flexion-abduction-external rotation (FABER) and Flexion-adduction-internal rotation (FADIR) test
Any other medical issue that results in discomfort within the groin area.
Participants were defined as having hip and groin problems and assessed for a complete clinical examination of the hip and groin area if that had at least two of the following responses: VAS score >2 during hip muscle strength tests AND/OR a score of <86 on the HAGOS sports subscale AND/ OR a response of “yes” to the Subjective Complaint Question. These defined criteria were based on a previous crosssectional study conducted in athletes.32
STAGE-2 MEASUREMENTS
Following the guidelines of the Doha Agreement classification system, a clinical examination protocol was conducted to assess the hip and groin area. The assessment was performed by a physiotherapist (JDS or FR) trained specifically for the protocol.
CLINICAL ENTITIES
The clinical entity approach consists of categorizing the hip and groin problems using standardized, reproducible examination techniques and classifying the problems into four defined clinical entities: (1) adductor-related, (2) iliopsoasrelated, (3) inguinal-related, (4) pubic-related groin pain, and the two additional categories of: hip-related groin pain or other (Table 2).6,25,37 The examination is preceded by a screening to exclude causes other than hip and groin.38 The intra- and inter-observer reliability of several elements of this physical examination approach are acceptable for use in practice.25,39 Since the definition of the iliopsoasrelated clinical entity is open to interpretation, prevalence rates were calculated with “tenderness” as the only criterion, and also with “tenderness AND pain on resisted hip flexion AND/OR pain on hip flexor stretching” as the criteria.
Prior to the assessment, the two physiotherapists who conducted the examination (5 years of clinical practice) were trained by two other experienced physiotherapists (MB, RFB) to perform the clinical examination using the above-mentioned protocol. The examination was always
performed bilaterally (randomized order) and the time available for the entire testing procedure was approximately 15 minutes per subject.
According to the Doha Agreement, tenderness was defined as discomfort or pain felt on palpation of the area and identified by the athlete as her specific pain.25
The detailed examination protocol is described in Appendix B.
Continuous variables (participants’ characteristics data, hip strength) are described using means and standard deviations (SD), ordinal variables (HAGOS subscale scores) using medians and interquartile ranges, and categorical variables (prevalence) using numbers and percentages. For all variables, comparisons between the participants with vs without hip and groin problems were made using non-parametric Mann-Whitney U tests due to unequal group sizes and non-normally distributed data, with a significance level of less than 0.05 or 0.001, as indicated. Box plots were generated to illustrate between-group comparisons for strength and functional parameters. The overall point prevalence of hip and groin problems was calculated by dividing the number of participants with hip and groin problems by the total number of participants. For the calculation of the hip-related entity prevalence, only athletes with clear hip-related pain as a single entity were reported, as highly specific tests to include hip-related pain have not been described.40
The strength of association between hip adductor/abductor strength and hip function (each HAGOS subscale) was estimated using Spearman’s rank correlation coefficients. Correlations were arbitrarily interpreted as negligible (0.0-0.10), weak (0.10-0.39), moderate (0.40-0.69), strong (0.70-0.89), or very strong (0.90-1.00).41All analyses were performed using R Statistical Software (v4.2.2, R Core Team 2022).
Figure 1. Selection of participants. Excluded: Players who did not meet the inclusion criteria or who were absent on the day of measurement.
Table 3. Demographic Data
RESULTS
Complete participants’ characteristics data, hip adduction/ abduction muscle strength, and hip and groin function data were collected from 91 participants from six different team sports in Switzerland (Figure 1, Table 3, detailed strength and function data in Appendix C).
The overall point prevalence of hip and groin problems was 14.3% (n=13). The prevalence for each sport was 30.8% for volleyball, 20.0% for ice hockey, 14.3% for soccer, 7.7% for floorball, and 0% for basketball (Table 4).
Of the 13 participants with hip and groin problems, one participant withdrew from the study (absent on the day of measurement) leaving 12 participants who underwent the complete clinical examination. Among these 12 participants, there were a total of 17 clinically defined entities, with three cases of bilateral groin pain, seven cases of sin-
gle entity, and two cases of multiple entities (two entities). There were three cases of hip-related groin pain, and there were no cases defined as ‘other’ The clinical entity-specific prevalence, calculated by dividing the number of a given entity by the total number of entities, was 58.8% for iliopsoas-related, 11.8% for adductor-related, 5.9% for inguinaland pubic-related groin pain. The hip-related groin pain prevalence was 17.6% (Table 5). Detailed information on distribution of clinical entities by sports can be found in Appendix D
No group differences were found between the participants with and without problems in participants’ characteristics data, absolute and relative hip abduction and adduction strength, or hip adduction to abduction ratio (all, p>0.05, Tables 6 and 7).
However, the two groups differed in all HAGOS subscales (p<0.001) (Appendix E).
Table 4. Overall and Sport-Specific Prevelences
Table 5. Clinical Entities Prevalence
*Hip-related only if no other clinical entity present, †Doha Agreement Definition: Iliopsoas-related clinical entity defined as “iliopsoas tenderness” ‡Definition 2: Iliopsoas-related clinical entity defined as"iIiopsoas tenderness AND pain on resisted hip flexion AND/OR pain on hip flexor stretching"
Table 6. DEMOGRAPHIC DATA COMPARISON
HAG: Hip/groin; Values are mean ± SD; y = years; cm = centimeters; kg = kilogram; m2 = square meters; *Mann Whitney U Test
Internal and external rotation hip ROM were recorded for the 12 participants in the hip and groin problems group. Due to the insufficient sample size, no statistical analysis was performed.
STRENGTH-FUNCTION CORRELATION
No associations were found between hip strength and hip function (Table 8).
Of the 91 female athletes included in this study, 14.3% were found to be affected by hip and groin problems in preseason assessment. This represents a non-negligible proportion as these are athletes who train “normally” but have impairments that could affect their performance.
Studies investigating the prevalence of hip and groin problems in female athletes are limited and diverse in methodology.9,16‑18 In addition, the published literature
Table 7. Strength Data comparison
mostly refers to a time-loss definition of hip and groin problems and included athletes from only one sport, making it difficult to compare with the current findings.
Of the studies that investigated a female athlete population, Wörner et al.18 conducted a study of 69 elite female ice hockey players, reporting a seasonal prevalence of hip and groin problems of 62%. The study was conducted retrospectively over an extended period based solely on selfreported questionnaire results, and reported a cumulative prevalence, which may explain the high prevalence values compared to this study Caution should be taken when comparing the results of the current study with those of Wörner and colleagues, because they defined seasonal prevalence as having experienced at least one hip and groin problem in the previous season, so that 62% represents the cumulative proportion of hip and groin problems. Therefore, it cannot be compared with the findings of this study, in which the prevalence referred to a specific time (point prevalence). Similarly, Langhout et al.16 reported a cumulative prevalence of hip and groin problems of 27% in the preseason (eight weeks) among 434 amateur female soccer players, using an online self-reported questionnaire. This is a lower value than the one reported by Wörner et al.,18 probably given the shorter period assessed, but again it is not comparable with the values of this study Haroy et al.9 with a sixweek prospective study reported a cumulative prevalence of 45% and an average weekly prevalence of 14% among 45 elite female soccer players. The results of the present study seem to be consistent with those of Haroy and colleagues. In a recent paper, Thorarinsdottir et al.17 report a cumulative prevalence of 42% and a weekly prevalence of 4% in a two-season prospective study in female soccer players. This study reported a time-loss injury rate of 80%, which
may indicate that less severe cases of hip and groin injuries were not identified, thus possibly explaining the 4% weekly prevalence.
The accuracy of diagnosing hip and groin problems is influenced by data collection methods, observation period length, and assessment criteria. Retrospective studies using self-reported data can be less accurate due to recall bias. The length of observation impacts precision in prospective studies. Criteria for defining hip and groin issues affect prevalence calculation, possibly leading to over/underestimation. The aforementioned studies mainly used questionnaires based on past events, where subjective factors such as personal experience, pain perception and emotions could potentially affect recall. Since the aim of this study was to define the point prevalence as accurately as possible, a combination of three criteria for past and present events was used, adding a pain reproduction test. As shown in Table 4, this resulted in lower percentages, but seems to have led to a more accurate selection of subjects with hip and groin problems, since all HAGOS subscales (and not just the sport subscale) showed a significant difference between the hip and groin problem and no problems groups, thereby indicating discomfort in all aspects of daily life and a real burden.
Several research studies have been published on hip and groin problems in males, with varying prevalence’s depending on the sport and level of practice.7,9,12‑15,20,42,43 As with studies in female athletes, there is a large difference between prospective and retrospective studies. When the results of studies using either point-in-time or prospective measurements (weekly prevalences) are compared with those of this study, the prevalence is found to be similar (11-29%).9,12,14,15,20 Therefore, the prevalence of hip and
groin problems in athletes seems to be similar between men and women. However, further high-quality prospective studies in female populations are needed to confirm this trend.
As expected, the HAGOS Sports and Recreation subscale differed between the hip and groin problem and no problems groups as this was part of the criteria to differentiate these groups. Interestingly, all the other scales of the HAGOS questionnaire also differed between the hip and groin problem and no problems groups indicating that all subscales of the HAGOS questionnaire were sensitive to detect hip and groin problems in female athletes of various sports.
The results indicate found no association between hip muscle strength and hip and groin problems nor between participants’ characteristics data and hip and groin problems, in line with other recent investigations in male athletes.15,27,44,45 However, with only 17 cases, it may have been underpowered to detect an association between these variables and hip and groin pain. Furthermore, the current results may be biased by the fact that participants come from sports with different player profiles and the distribution between the two groups was not homogeneous (e.g., there were no basketball players in the hip and groin problems group). It should also be considered that strength tests were performed in a single position using an isometric contraction, for adduction and abduction movements only. The iliopsoas muscle, for example, was not specifically measured, despite seeming to be a major source of pain in female athletes. It would therefore be interesting for future studies in a female population to also include an assessment of hip flexion strength.
To the best of the authors’ knowledge, this study is one of the first to categorize the hip and groin problems in a female athlete population using the Doha Agreement classification system. The most frequent entity identified during the clinical examination was iliopsoas-related groin pain. One possible reason for the large difference between the iliopsoas and adductor entity rates (58.8 % vs 11.8%) is that the criterion for the iliopsoas entity is “iliopsoas tenderness, more likely if pain on resisted hip flexion and/or pain on hip flexor stretching.”25 The “ more likely” is an ambiguous element, indicating that a simply iliopsoas tenderness could be sufficient to define the entity, which may have led to different interpretations and methodologies by the authors and contributed to a different number of iliopsoas entities in the studies (Table 5).
Several studies have investigated the epidemiology of hip and groin pain in male athletes using the classification system proposed by the Doha Agreement.10,11,20,26,46,47 In contrast to the findings of the present study, where the most common clinical entity in a female population was iliopsoas-related groin pain, the most frequent hip and groin problem in males seem to be adductor-related groin pain.
This is further supported by Serner et al.48 who found a predominance of acute adductor injuries in male athletes using a combination of clinical examination, magnetic resonance imaging and ultrasound. Only one study reported a majority of iliopsoas entities, which were present in 89% of participants.47 This may be due to a less strict classification approach with vague boundaries between pain and tenderness, allowing more entities to be identified, and indeed a median of 3 entities per athlete was reported.47 DeLang et al.20 highlighted the importance of defining this boundary as their study showed that 50% of athletes who did not report hip and groin problems still had tenderness.
These findings also raise the question of the reliability of clinical examination in identifying clinical entities. Heijboer et al.49 showed that the inter-examiner reliability of the Doha agreement classification ranged from slight to substantial. However, given the variability of results in previous studies, a more precise and standardized approach to clinical examination could increase the reliability and accuracy of diagnosis, thereby minimizing the confusion.
In female athletes, only one other study used the Doha Agreement classification system and examined female soccer players, highlighting a majority of adductor-related cases but with an increase in hip flexor cases (iliopsoasand rectus femoris-related groin pain) compared to male athletes.17 Despite differences in methodology, both studies found a greater number of hip flexor-related problems, indicating their relevance in female athletes with hip and groin pain. More research is needed to confirm this trend.
Analysis of a potential association between hip muscle strength and function in the 91 participants in this study showed no significant correlation. To the authors’ knowledge, no other studies have examined this relationship in a female population, but the current results are consistent with a study by Beddows and colleagues15 in a male population that found no clear association between HAGOS symptom severity and hip muscle strength. Again, the heterogeneity of the sample, consisting of athletes from different sports and therefore with diverse backgrounds, may have influenced the results. An analysis of a single sport population and a larger sample would be valuable to obtain more conclusive results.
The present study has several strengths. First, it focuses on a population of female athletes that is still largely underrepresented in the scientific literature. Therefore, this study provides new insight into the point prevalence of hip and groin problems in this specific population. In addition, it is one of the first to use the clinical entity approach described in the Doha consensus statement in this same population, which also provides new information. Finally, based on previous scientific studies, this study proposes a new approach and definition for the selection of hip and groin problems, including pain reproduction test as a
criterion. This approach could be used for future studies in this field.
A major limitation of this study is the small sample size within each sport, which prevented the authors from conducting a detailed statistical analysis by sport. This was due to constraints such as limited availability of participating teams, which led us to evaluate only those athletes who were present at the time of the scheduled interventions. Another limitation relates to the limited time available for on-site data collection, which was a maximum of 15 minutes per participant. To optimize the time, compromises were made by having two examiners instead of one, as inter-examiner reliability was estimated to be acceptable,49 and by collecting participants’ characteristics data by selfreport, which may have slightly biased the accuracy of the data.
A further limitation, is that, due to time and financial constraints, no imaging was performed on participants of the hip and groin problems group to confirm the exact location of pain.
A pre-season point prevalence of 14.3% for non-time-loss hip and groin problems was documented during preseason in elite female athletes, which is similar to that previously reported in male athletes. This study is one of the first to use the clinical entity approach in a population of female
athletes highlighting that most hip and groin problems appear to be iliopsoas-related followed by adductor-related and inguinal- and pubic-related. This contrasts with findings in males where adductor-related entities predominate. While as expected, the HAGOS scores differed between the groups, hip muscle strength and participants’ characteristics data did not. Furthermore, no association was found between hip muscle strength and HAGOS scores. This study provides valuable information allowing a better understanding of hip and groin problems in female athletes. More research should be conducted in this population to confirm these findings and to further increase knowledge in this topic.
The authors report no conflicts of interest.
The authors thank the players and staff members of the different teams involved in the study for their dedication during the study.
Submitted: April 25, 2024 CDT, Accepted: September 09, 2024 CDT
© The Author(s)
This is an open-access article distributed under the terms of the Creative Commons Attribution 4.0 International License (CCBY-NC-4.0). View this license’s legal deed at https://creativecommons.org/licenses/by-nc/4.0 and legal code at https://creativecommons.org/licenses/by-nc/4.0/legalcode for more information.
1. Eckard TG, Padua DA, Dompier TP, Dalton SL, Thorborg K, Kerr ZY Epidemiology of hip flexor and hip adductor strains in National Collegiate Athletic Association athletes, 2009/2010-2014/2015. Am J Sports Med 2017;45(12):2713-2722. doi:10.1177/ 0363546517716179
2. Orchard JW Men at higher risk of groin injuries in elite team sports: a systematic review Br J Sports Med. 2015;49(12):798-802. doi:10.1136/ bjsports-2014-094272
3. Sedaghati P, Alizadeh MH, Shirzad E, Ardjmand A. Review of sport-induced groin injuries. Trauma Mon. 2013;18(3):107-112. doi:10.5812/traumamon.12666
4. Clarsen B, Bahr R, Heymans MW, et al. The prevalence and impact of overuse injuries in five Norwegian sports: Application of a new surveillance method. Scand J Med Sci Sports 2015;25(3):323-330. doi:10.1111/sms.12223
5. Waldén M, Hägglund M, Ekstrand J. The epidemiology of groin injury in senior football: a systematic review of prospective studies. Br J Sports Med 2015;49(12):792-797 doi:10.1136/ bjsports-2015-094705
6. Hölmich P Long-standing groin pain in sportspeople falls into three primary patterns, a “clinical entity” approach: a prospective study of 207 patients. Br J Sports Med. 2007;41(4):247-252. doi:10.1136/bjsm.2006.033373
7 Thorborg K, Rathleff MS, Petersen P, Branci S, Hölmich P Prevalence and severity of hip and groin pain in sub-elite male football: a cross-sectional cohort study of 695 players. Scand J Med Sci Sports 2017;27(1):107-114. doi:10.1111/sms.12623
8. Mercurio M, Corona K, Galasso O, et al. Soccer players show the highest seasonal groin pain prevalence and the longest time loss from sport among 500 athletes from major team sports. Knee Surg Sports Traumatol Arthrosc 2022;30(6):2149-2157. doi:10.1007/ s00167-022-06924-5
9. Harøy J, Clarsen B, Thorborg K, Hölmich P, Bahr R, Andersen TE. Groin problems in male soccer players are more common than previously reported. Am J Sports Med 2017;45(6):1304-1308. doi:10.1177/ 0363546516687539
10. Mosler AB, Weir A, Eirale C, et al. Epidemiology of time loss groin injuries in a men’s professional football league: a 2-year prospective study of 17 clubs and 606 players. Br J Sports Med 2018;52(5):292-297 doi:10.1136/bjsports-2016-097277
11. Werner J, Hägglund M, Waldén M, Ekstrand J. UEFA injury study: a prospective study of hip and groin injuries in professional football over seven consecutive seasons. Br J Sports Med. 2009;43(13):1036-1040. doi:10.1136/ bjsm.2009.066944
12. Esteve E, Clausen MB, Rathleff MS, et al. Prevalence and severity of groin problems in Spanish football: A prospective study beyond the time-loss approach. Scand J Med Sci Sports. 2020;30(5):914-921. doi:10.1111/sms.13615
13. Wörner T, Thorborg K, Eek F. High prevalence of hip and groin problems in professional ice hockey players, regardless of playing position. Knee Surg Sports Traumatol Arthrosc 2020;28(7):2302-2308. doi:10.1007/s00167-019-05787-7
14. Drew MK, Lovell G, Palsson TS, Chiarelli PE, Osmotherly PG. Do Australian Football players have sensitive groins? Players with current groin pain exhibit mechanical hyperalgesia of the adductor tendon. J Sci Med Sport 2016;19(10):784-788. doi:10.1016/j.jsams.2015.12.516
15. Beddows TPA, Weir A, Agricola R, et al. Hip and groin pain in male field hockey players: Prevalence, incidence and associations with patient reported outcome scores and hip muscle strength. Phys Ther Sport 2023;61:66-72. doi:10.1016/j.ptsp.2023.02.010
16. Langhout R, Weir A, Litjes W, et al. Hip and groin injury is the most common non-time-loss injury in female amateur football. Knee Surg Sports Traumatol Arthrosc. 2019;27(10):3133-3141. doi:10.1007/ s00167-018-4996-1
17 Thorarinsdottir S, Amundsen R, Larmo A, et al. Groin injuries in women’s premier league football in Norway: A two-season prospective cohort study describing clinical and imaging characteristics. Scand J Med Sci Sports. 2024;34(4):e14611. doi:10.1111/ sms.14611
18. Wörner T, Thorborg K, Eek F. Hip and groin problems in the previous season are associated with impaired function in the beginning of the new season among professional female ice hockey players - a cross sectional study. Int J Sports Phys Ther. 2020;15(5):763-769. doi:10.26603/ijspt20200763
19. Bahr R. No injuries, but plenty of pain? On the methodology for recording overuse symptoms in sports. Br J Sports Med 2009;43(13):966-972. doi:10.1136/bjsm.2009.066936
20. DeLang MD, Garrison JC, Hannon JP, et al. Midseason screening for groin pain, severity, and disability in 101 elite American youth soccer players: A cross-sectional study. Clin J Sport Med. Published online November 10, 2021. doi:10.1097/ JSM.0000000000000987
21. Kloskowska P, Morrissey D, Small C, Malliaras P, Barton C. Movement patterns and muscular function before and after onset of sports-related groin pain: A systematic review with meta-analysis. Sports Med. 2016;46(12):1847-1867 doi:10.1007/ s40279-016-0523-z
22. Ryan J, DeBurca N, Creesh KM. Risk factors for groin/hip injuries in field-based sports: a systematic review Br J Sports Med 2014;48(14):1089-1096. doi:10.1136/bjsports-2013-092263
23. Whittaker JL, Small C, Maffey L, Emery CA. Risk factors for groin injury in sport: an updated systematic review. Br J Sports Med. 2015;49(12):803-809. doi:10.1136/ bjsports-2014-094287
24. Serner A, van Eijck CH, Beumer BR, Hölmich P, Weir A, de Vos RJ. Study quality on groin injury management remains low: a systematic review on treatment of groin pain in athletes. Br J Sports Med. 2015;49(12):813. doi:10.1136/bjsports-2014-094256
25. Weir A, Brukner P, Delahunt E, et al. Doha agreement meeting on terminology and definitions in groin pain in athletes. Br J Sports Med 2015;49(12):768-774. doi:10.1136/ bjsports-2015-094869
26. Taylor R, Vuckovic Z, Mosler A, et al. Multidisciplinary assessment of 100 athletes with groin pain using the Doha agreement: High prevalence of adductor-related groin pain in conjunction with multiple causes. Clin J Sport Med 2018;28(4):364-369. doi:10.1097/ JSM.0000000000000469
27 Esteve E, Rathleff MS, Vicens-Bordas J, et al. Preseason adductor squeeze strength in 303 Spanish male soccer athletes: A cross-sectional study Orthop J Sports Med 2018;6(1):2325967117747275. doi:10.1177/2325967117747275
28. Ralston B, Arthur J, Makovicka JL, et al. Hip and groin injuries in National Collegiate Athletic Association women’s soccer players. Orthop J Sports Med 2020;8(1):2325967119892320. doi:10.1177/ 2325967119892320
29. Desmyttere G, Gaudet S, Begon M. Test-retest reliability of a hip strength assessment system in varsity soccer players. Phys Ther Sport 2019;37:138-143. doi:10.1016/j.ptsp.2019.03.013
30. O’Brien M, Bourne M, Heerey J, Timmins RG, Pizzari T A novel device to assess hip strength: Concurrent validity and normative values in male athletes. Phys Ther Sport. 2019;35:63-68. doi:10.1016/ j.ptsp.2018.11.006
31. Oliveras R, Bizzini M, Brunner R, Maffiuletti NA. Field-based evaluation of hip adductor and abductor strength in professional male ice hockey players: Reference values and influencing factors. Phys Ther Sport. 2020;43:204-209. doi:10.1016/ j.ptsp.2020.03.006
32. Thorborg K, Branci S, Nielsen MP, Langelund MT, Hölmich P. Copenhagen five-second squeeze: A valid indicator of sports-related hip and groin function. Br J Sports Med 2017;51(7):594-599. doi:10.1136/ bjsports-2016-096675
33. Wörner T, Thorborg K, Eek F. Five-second squeeze testing in 333 professional and semiprofessional male ice hockey players: How are hip and groin symptoms, strength, and sporting function related? Orthop J Sports Med 2019;7(2):232596711982585. doi:10.1177/ 2325967119825858
34. Thorborg K, Tijssen M, Habets B, et al. Patientreported outcome (PRO) questionnaires for young to middle-aged adults with hip and groin disability: a systematic review of the clinimetric evidence. Br J Sports Med 2015;49(12):812. doi:10.1136/ bjsports-2014-094224
35. Thorborg K, Holmich P, Christensen R, Petersen J, Roos EM. The Copenhagen Hip and Groin Outcome Score (HAGOS): development and validation according to the COSMIN checklist. Br J Sports Med 2011;45(6):478-491. doi:10.1136/bjsm.2010.080937
36. Delahunt E, Fitzpatrick H, Blake C. Pre-season adductor squeeze test and HAGOS function sport and recreation subscale scores predict groin injury in Gaelic football players. Phys Ther Sport. 2017;23:1-6. doi:10.1016/j.ptsp.2016.07.002
37 Hölmich P, Thorborg K, Dehlendorff C, Krogsgaard K, Gluud C. Incidence and clinical presentation of groin injuries in sub-elite male soccer Br J Sports Med 2014;48(16):1245-1250. doi:10.1136/bjsports-2013-092627
38. Thorborg K, Reiman MP, Weir A, et al. Clinical examination, diagnostic imaging, and testing of athletes with groin pain: An evidence-based approach to effective management. J Orthop Sports Phys Ther 2018;48(4):239-249. doi:10.2519/ jospt.2018.7850
39. Hölmich P, Holmich L, Bjerg A. Clinical examination of athletes with groin pain: an intraobserver and interobserver reliability study Br J Sports Med. 2004;38(4):446-451. doi:10.1136/ bjsm.2003.004754
40. Ishøi L, Nielsen MF, Krommes K, et al. Femoroacetabular impingement syndrome and labral injuries: grading the evidence on diagnosis and nonoperative treatment-a statement paper commissioned by the Danish Society of Sports Physical Therapy (DSSF). Br J Sports Med 2021;55(22):1301-1310. doi:10.1136/ bjsports-2021-104060
41. Schober P, Boer C, Schwarte LA. Correlation coefficients: Appropriate use and interpretation. Anesth Analg. 2018;126(5):1763. doi:10.1213/ ANE.0000000000002864
42. Thorborg K, Serner A, Petersen J, Madsen TM, Magnusson P, Hölmich P Hip Adduction and abduction strength profiles in elite soccer players: Implications for clinical evaluation of hip adductor muscle recovery after injury. Am J Sports Med. 2011;39(1):121-126. doi:10.1177/0363546510378081
43. Hanna CM, Fulcher ML, Elley CR, Moyes SA. Normative values of hip strength in adult male association football players assessed by handheld dynamometry J Sci Med Sport 2010;13(3):299-303. doi:10.1016/j.jsams.2009.05.001
44. van Klij P, Langhout R, van Beijsterveldt AMC, et al. Do hip and groin muscle strength and symptoms change throughout a football season in professional male football players? A prospective cohort study with repeated measures. J Sci Med Sport Published online April 2021:S1440244021000839. doi:10.1016/ j.jsams.2021.03.019
45. Wörner T, Thorborg K, Clarsen B, Eek F. Hip and groin function and strength in male ice hockey players with and without hip and groin problems in the previous season- a prospective cohort study Phys Ther Sport 2021;52:263-271. doi:10.1016/ j.ptsp.2021.10.005
46. Mosler AB, Weir A, Serner A, et al. Musculoskeletal screening tests and bony hip morphology cannot identify male professional soccer players at risk of groin injuries: A 2-year prospective cohort study Am J Sports Med 2018;46(6):1294-1305. doi:10.1177/0363546518763373
47. Nielsen MF, Ishøi L, Juhl C, Hölmich P, Thorborg K. Pain provocation tests and clinical entities in male football players with longstanding groin pain are associated with pain intensity and disability Musculoskelet Sci Pract Published online January 10, 2023:102719. doi:10.1016/j.msksp.2023.102719
48. Serner A, Tol JL, Jomaah N, et al. Diagnosis of acute groin injuries: A prospective study of 110 athletes. Am J Sports Med. 2015;43(8):1857-1864. doi:10.1177/0363546515585123
49. Heijboer WMP, Weir A, Vuckovic Z, et al. Interexaminer reliability of the Doha agreement meeting classification system of groin pain in male athletes. Scand J Med Sci Sports doi:10.1111/sms.14248
Appendix A
Download: https://ijspt.scholasticahq.com/article/123946-hip-and-groin-problems-in-female-team-sport-athletes-across-sectional-study/attachment/247012.docx
Appendix B
Download: https://ijspt.scholasticahq.com/article/123946-hip-and-groin-problems-in-female-team-sport-athletes-across-sectional-study/attachment/247013.docx
Appendix C
Download: https://ijspt.scholasticahq.com/article/123946-hip-and-groin-problems-in-female-team-sport-athletes-across-sectional-study/attachment/247011.docx
Appendix D
Download: https://ijspt.scholasticahq.com/article/123946-hip-and-groin-problems-in-female-team-sport-athletes-across-sectional-study/attachment/247010.docx
Appendix E
Download: https://ijspt.scholasticahq.com/article/123946-hip-and-groin-problems-in-female-team-sport-athletes-across-sectional-study/attachment/247009.docx
Jeffrey Paskewitz1 , Fred Breidenbach1 , Philip Malloy1 , P
Michael Eckrich
2 , Ryan Zarzycki1a
1 Physical Therapy, Arcadia University, 2 Physical Therapy, University of Delaware
Keywords: baseball, injury, youth, strength, throwing, arm pain
https://doi.org/10.26603/001c.124447
International Journal of Sports Physical Therapy
Vol. 19, Issue 11, 2024
Background and Purpose
The prevalence of arm pain in youth baseball players is high with approximately half reporting arm pain during the season, and the number of ulnar collateral ligament reconstructions in youth baseball players is increasing. Few studies have examined the relationship between shoulder strength and passive range of motion (ROM) with arm pain (either shoulder or elbow pain) during throwing, especially in athletes in early adolescence. The purpose of this study was to determine the relationship between shoulder ROM and strength and the presence of arm pain during throwing in youth baseball players. It was hypothesized that less internal rotation (IR) ROM, less total rotational ROM, and lower IR and external rotation (ER) strength would be associated with arm pain.
Study Design
Observational cohort study
Methods
Sixty-five youth male baseball players between the ages of 12-15 were enrolled. Shoulder strength (ER, IR, ER/IR strength ratio, scaption) and passive ROM (ER, IR, flexion, horizontal adduction) were collected prior to the start of the spring 2021 baseball season. Players self-reported their arm pain frequency during throwing as never, rarely, sometimes, often, or always. The relationship between reported arm pain frequency during throwing and shoulder ROM and strength measurements was examined.
Results
ER (ρ= -0.289, p=0.020) and IR strength (ρ= -0.262, p=0.035) were weakly and negatively correlated with reports of arm pain during throwing. No other clinical variables were associated with reports of arm pain (p ≥ 0.124)
Conclusion
In youth baseball players, greater IR and ER strength were associated with less arm pain frequency during throwing, while ROM was not associated with arm pain frequency Future research should explore these variables prospectively to determine if changes in ROM and strength are related to the development of arm pain in youth baseball players.
Level of Evidence
3
a
Corresponding author: Ryan Zarzycki
Department of Physical Therapy, Arcadia University, 450 S Easton Rd, Glenside, PA 19038
Phone: 215-572-8527
Email address: ZarzyckiR@arcadia.edu
Fax: 215-517-2342
The prevalence of arm pain in youth baseball players is high with approximately half reporting arm pain (either shoulder or elbow pain) during the season.1,2 In addition, the number of ulnar collateral ligament reconstructions has increased disproportionately in youth baseball players.3 Several factors related to throwing load (i.e. pitch counts, innings pitched, and pitching greater than 8 months per year) have been identified as potential risk factors for arm pain and injuries.4,5 However, fewer studies have examined the relationship between arm pain and clinical measures, especially in athletes in early adolescence (<16 y/o). Understanding these relationships could help inform rehabilitation and injury risk reduction for these athletes as these clinical measures are commonly addressed treatment targets in physical therapy
There is evidence that suggests passive range of motion (ROM) and strength may be factors associated with arm injury and/or arm pain in baseball players. However, the majority of literature examining these factors evaluate high school, collegiate, and professional baseball players.6‑11 Few studies have evaluated the association of these variables in younger baseball players, and conflicting findings are reported among these studies.12‑15
In youth baseball players, two studies have examined the associations between shoulder internal and external rotator strength and arm injury and/or pain with varying results.12,13 A large prospective cohort study by Sakata12 evaluated risk factors for medial elbow injury in 353 youth baseball players (pitchers and nonpitchers) aged 6-12 and used handheld dynamometry of both the dominant and nondominant arms to measure shoulder internal and external rotation (ER) strength and used this strength ratio (dominant arm strength/nondominant arm strength) as the variable characterizing strength in their analysis. These authors characterized medial elbow injury as medial elbow pain during throwing with either an abnormal ultrasonography finding or the presence of pain during the clinical assessment of the elbow Using these operational definitions, neither shoulder internal rotation (IR) nor shoulder ER strength were identified as risk factors for medial elbow pain. One cross sectional study by Harada13 evaluated 294 youth baseball players (pitchers and nonpitchers) aged 9-12 and used handheld dynamometry to measure shoulder internal and ER strength. Harada characterized elbow injury as having an ultrasonographic abnormality They reported that increased shoulder internal and ER strength were each associated with greater odds of elbow injury Given the variation in methodology and reported findings, it remains unclear if shoulder IR or ER strength are important factors contributing to arm injury in youth baseball players. Four studies have examined the association of shoulder ROM and arm injury and/or pain.12‑15 Sakata12 also evaluated risk factors for medial elbow injury in youth baseball players aged 6-12 and used goniometry to measure passive total shoulder IR and ER ROM. These authors characterized medial elbow injury as medial elbow pain during throwing with either an abnormal ultrasonography finding or the
presence of pain during the clinical assessment of the elbow. They reported significantly less passive total shoulder rotation ROM in those with medial elbow injury compared to those without shoulder or elbow injury. Another prospective cohort study by Shanley14 used digital inclinometry to measure glenohumeral horizontal adduction and IR ROM in a cohort of youth baseball pitchers aged 8-12. Shanley14 reported that side-to-side differences in either measure were associated with increased injury risk, as defined by an upper extremity overuse injury that occurred during any baseball team–sponsored activity (from the beginning of preseason through the last postseason game) to any muscle, joint, tendon, ligament, bone, or nerve. A cross sectional study by Harada13 evaluated 294 youth baseball players (pitchers and nonpitchers) aged 9-12 by assessing shoulder internal and external ROM using goniometry These authors characterized elbow injury as ultrasonographic abnormality. They reported that shoulder IR ROM was not significantly associated with greater odds of elbow injury, but players with shoulder ER range motion <130 degrees had significantly greater odds of elbow injury Another cross-sectional study by Tajika15 evaluated 229 baseball players (pitchers and nonpitchers) aged 9-14 and used goniometry to measure shoulder external and IR ROM. The authors characterized elbow injury as ultrasonographic abnormality of the elbow but also analyzed whether shoulder ROM was associated with symptoms in those with sonographic abnormalities. They reported that while shoulder IR and ER ROM were not significantly different between those with and without sonographic abnormality, significant decreases in IR compared to the nondominant arm of the players were reported in those with sonographic abnormality In those with sonographic abnormality, no associations were found between shoulder rotation ROM or sideto-side differences and symptoms.
Given the variation in methodology and reported findings, it remains unclear if shoulder IR or ER ROM are important factors contributing to arm injury in youth baseball players. An important limitation to consider is that two of these studies13,15 have characterized arm injury with ultrasonographic findings, but it is evident that imaging findings at the shoulder and elbow are not necessarily indicative of symptoms in baseball players.16‑18 When evaluating risk factors for arm injury in baseball players, it is critical to evaluate the associations between potential risk factors and symptoms, because symptoms (pain, instability, etc.) directly contribute to functional limitation and missed baseball participation. Furthermore, it is likely that some degree of pain precedes overuse injuries commonly seen in baseball players, so early detection of arm pain may allow for earlier intervention and may minimize disabling arm injuries.
Therefore, the purpose of this study was to determine the relationship between shoulder ROM and strength and the presence of arm pain during throwing in youth baseball players. The authors hypothesized that less shoulder IR strength, less ER strength, and decreased ER/IR strength ratio would each be associated with greater arm pain frequency during throwing. It was also hypothesized that less
shoulder IR ROM, ER ROM, total rotational (ER ROM + IR ROM), and horizontal adduction ROM would each be associated with greater arm pain frequency during throwing.
Sixty-five youth male baseball players between the ages of 12-15 were recruited for this study Subjects were recruited and enrolled in February 2021 from several local youth baseball leagues, and all provided informed consent to participate in the study prior to data collection. The study was approved by the Arcadia University institutional review board. Height and weight were recorded using a standard balance scale and wall mounted tape measure, respectively.
Shoulder strength, ROM, height, weight, and reported arm pain during throwing were collected at the time of enrollment, which was in February 2021, prior to the start of the spring 2021 baseball season.
Measurement of isometric shoulder strength was measured with a hand-held dynamometer (HHD) (Lafayette Instrument, Lafayette, IN) for shoulder ER (ER), IR, and elevation in the scapular plane (scaption). All isometric strength measures were taken in the supine position and all measurements were taken by a single assessor with 20 years of combined clinical and research experience. For shoulder ER
and IR strength measures, the shoulder was placed at 90 degrees abduction and in a neutral rotation position with the elbow flexed to 90 degrees. The pad of the HHD was placed at the dorsal surface of the wrist for ER strength measurement, and the volar surface of the wrist for IR measurements (Figure 1).
Shoulder elevation in the scapular plane was measured with the shoulder flexed to 90 degrees and the elbow in full extension with the forearm in neutral pronation and supination. To familiarize the subject with the strength testing procedures one submaximal and one maximal isometric contraction was performed to demonstrate the use of the HHD. Three maximal isometric strength trials were collected, and the ensemble average of the trials was recorded for analysis. Verbal encouragement was provided during the testing.
Measurement of shoulder passive ROM consisted of goniometric assessment of glenohumeral ER, IR, horizontal adduction, and flexion ROM with each subject in a supine position. Measurements were collected using a two-person method as described previously and using a standard goniometer with attached bubble-level.9 Glenohumeral ER and IR measurements were performed with the shoulder placed at 90 degrees of abduction and elbow flexed to 90 degrees. Horizontal adduction was measured with the arm placed in 90 degrees of shoulder flexion and neutral shoulder rotation. The participant’s shoulder was horizontally adducted and the angle between the humerus and the vertical was measured. Pure glenohumeral flexion ROM measurements were collected by first palpating the lateral bor-
Figure 2. Glenohumeral flexion ROM testing position
Figure 2 legend: Goniometric assessment of glenohumeral flexion passive ROM was performed with two examiners and with subjects positioned supine.
der of the scapula in a neutral position. The shoulder was then flexed until movement of the scapula was noted by the examiner, and pure glenohumeral flexion was measured in this position (Figure 2).
To characterize arm pain while throwing, all subjects completed a written questionnaire at one timepoint.19 The questionnaire included the following question that included a Likert based ordinal rating scale, “Does your arm hurt when you throw?” Subjects responded with one of the following options: “never”, “rarely”, “sometimes”, “often”, or “always” The questionnaire did not specify any reference time for subjects recall. Answers were recorded and then converted ordinally into a 0-4 scale for statistical analysis.
Spearman correlations were used to examine the relationship between the players self-reported arm pain during throwing and the following clinical measures: height, weight, body mass index, dominant arm flexion ROM, IR ROM, ER ROM, horizontal adduction ROM , total rotational ROM (IR ROM + ER ROM), IR strength, ER strength, ER/ IR strength ratio, and scaption strength. Statistical significance was set at p ≤ 0.05.
Demographic information for the participating subjects is provided in Table 1
Correlations between reported arm pain during throwing and shoulder strength are summarized in Table 2.
Weak negative correlations with reported arm pain frequency were observed for both ER (r = -0.289 , p = 0.020) and IR (r = -0.262 , p = 0.035) strength. No other significant correlations were found between reported arm pain and any other strength variable (p ≥ 0.118).
Correlations between arm pain frequency during throwing and anthropometrics and shoulder ROM are summarized in Table 3
No significant correlations were observed between any anthropometric or ROM variables (p ≥ 0.124).
The purpose of this study was to examine the relationships between reported arm pain frequency, shoulder ROM, and strength in youth baseball players. The hypotheses were partly supported as significant although weak negative correlations were found between ER and IR strength and reported arm pain frequency during throwing. There were no significant correlations between arm pain and any ROM measurements. The negative correlations between arm pain frequency and shoulder strength indicate that greater frequency of arm pain was associated with less shoulder ER and IR strength. These findings suggest that strength of the shoulder internal and external rotators may be relevant modifiable factors that contribute to arm pain during throwing and imply that these factors are treatment targets that should be optimized in youth baseball players.
Future work would be helpful to clarify the mechanisms that explain why increased shoulder rotator strength is associated with less frequent arm pain during throwing. The authors speculate that given the relatively high torques at the shoulder during throwing motions, sufficient dynamic stability that is provided by the shoulder rotators may be necessary to minimize stress on other passive shoulder stabilizers.20 In addition, higher shoulder rotator strength may reflect superior mechanical, neurophysiological, and vascular qualities of the musculotendinous structures that may be sources of overuse-related arm pain during throwing.21,22 Despite significant negative correlations between arm pain frequency and shoulder rotator strength, the weak strength of these correlations suggest that other factors apart from strength may also be contributing to arm pain frequency during throwing.
The findings of this investigation somewhat differ from the findings of previously reported studies which have reported associations with shoulder ROM and arm injury or pain. The current findings differ from those of Shanley14 who reported significantly increased risk of overuse injury sustained during baseball activity in those with limited IR and horizontal adduction ROM, but evaluated only pitchers who were younger than the players (pitchers and nonpitchers) which were evaluated in our study In addition, shoulder ROM was evaluated using inclinometry, rather than goniometry which was used in the current study The study by Shanley14 also evaluated arm injuries prospectively in baseball pitchers throughout the course of a season, whereas as-
Table 1. Demographics
Table 2. Correlations between arm pain and shoulder strength
Table 3. Correlations between arm pain and shoulder ROM and player anthropometrics
sessment of reported arm pain in baseball players was performed only at one time point in the current study. While the findings reported by Shanley14 that shoulder ROM is associated with higher injury risk may be more specific to pitchers, the findings in the current study that ROM is not significantly associated with arm pain frequency may be more generalizable to nonpitching baseball players. In addition, Sakata12 found that total passive shoulder rotation ROM was significantly different in those with medial elbow injury The current study’s methodology differs from that of Sakata as older baseball players were included and did not characterize elbow injury using clinical tests nor ultrasonographic findings. Instead, elbow injury was characterized according to arm pain frequency during throwing. The
authors believe that classifying arm injury according to reported arm pain frequency during throwing may give additional insight because it takes into account shoulder, elbow, and other arm pain that may contribute to and precede injury Given the high number of baseball players participating despite arm pain, it is likely that arm pain precedes participation-restricting and otherwise disabling injury
The current findings somewhat differ from the findings of previous studies which have examined associations with shoulder strength and arm injury or pain in youth baseball players. One large prospective study12 reported that neither shoulder ER nor IR weakness are risk factors for elbow injury, but they only assessed for medial elbow injuries, and evaluated baseball players that were 6-12 years old,
which is younger than the current sample of baseball players which were 12-15 years old. It may be that shoulder strength has less association with medial elbow injury but is more associated with shoulder and other arm injuries. Contrary to the current study which found that increased shoulder IR and ER strength was associated with less frequent arm pain, another cross sectional study13 reported that increases in shoulder IR and ER strength were associated with elbow injury. However, the prior study used ultrasonography to characterize elbow injury, which may or may not be associated with arm pain which was measured in the current study
There are limitations to the current study. Correlational analysis was utilized to examine the relationships between clinical measures and arm pain, so causal relationships between these variables cannot be assumed. While causation cannot be established with the current study design, the findings from this study imply that more shoulder rotation strength may be considered positive, because it was related to less frequent arm pain. More research is needed to discern the relationship between shoulder strength and arm pain in youth athletes. Future longitudinal studies may help further clarify the relationship between shoulder ROM and strength and arm pain in youth baseball players. Another limitation to the current study is that shoulder strength measurements were not normalized and thus do not account for confounding anthropometric variables (height and weight) between participants which are thought to correlate with strength.23 Despite this limitation, associations were found between non-normalized shoulder rotation strength and reported arm pain frequency, but no significant associations between reported arm pain frequency and either height or weight. The current study consisted of a relatively small sample size compared to other studies examining range of motion and strength as risk factors for arm injuries in youth baseball players. Studies with larger samples may be more helpful to confirm whether or not these factors are associated with
reported arm pain. Lastly, reliance on self-reported data at one timepoint should be considered a limitation. Given that pain is in part a subjective measure, it may be difficult to characterize pain using strictly objective measures, but more frequent assessment of pain that is consistently measured shortly following throwing may be a more comprehensive method to characterize arm pain frequency which minimizes variability in recall of throwing experiences.
In youth baseball players, shoulder ER and IR strength is weakly associated with self-reported arm pain frequency. Non-normalized shoulder ER and IR strength may be more associated with reported arm pain frequency than ER/IR strength ratio or shoulder flexion, ER, IR, or horizontal adduction ROM in youth baseball players. These findings may help guide arm care program development. Future research should prospectively evaluate the relationship between shoulder ROM and strength and the development of arm pain by exploring these measures longitudinally
The authors declare no conflicts of interest
The authors would like to acknowledge the contributions of the following individuals during data collection and analysis: David LaPlaca, PhD, SPT, Selena Belohoubek, SPT, James Lenzi, SPT, and Abbey Finkill SPT.
Submitted: March 28, 2024 CDT, Accepted: September 09, 2024 CDT
© The Author(s)
This is an open-access article distributed under the terms of the Creative Commons Attribution 4.0 International License (CCBY-NC-4.0). View this license’s legal deed at https://creativecommons.org/licenses/by-nc/4.0 and legal code at https://creativecommons.org/licenses/by-nc/4.0/legalcode for more information.
1. Makhni EC, Morrow ZS, Luchetti TJ, et al. Arm pain in youth baseball players: A survey of healthy players. Am J Sports Med. 2015;43(1):41-46. doi:10.1177/ 0363546514555506
2. Matsel KA, Butler RJ, Malone TR, et al. Current concepts in arm care exercise programs and injury risk reduction in adolescent baseball players: A clinical review Sports Health 2021;XX(X):1-6. doi:10.1177/1941738120976384
3. Mahure SA, Mollon B, Shamah SD, Kwon YW, Rokito AS. Disproportionate trends in ulnar collateral ligament reconstruction: Projections through 2025 and a literature review J Shoulder Elb Surg 2016;25(6):1005-1012. doi:10.1016/j.jse.2016.02.036
4. Erickson BJ, Chalmers PN, Axe MJ, Romeo AA. Exceeding pitch count recommendations in little league baseball increases the chance of requiring Tommy John surgery as a professional baseball pitcher Orthop J Sport Med 2017;5(3):1-6. doi:10.1177/2325967117695085
5. Norton R, Honstad C, Joshi R, et al. Risk Factors for Elbow and shoulder injuries in adolescent baseball players: A systematic review Am J Sports Med 2019;47(4):982-990. doi:10.1177/0363546518760573
6. Tyler TF, Mullaney MJ, Mirabella MR, Nicholas SJ, McHugh MP Risk factors for shoulder and elbow injuries in high school baseball pitchers: The role of preseason strength and range of motion. Am J Sports Med 2014;42(8):1993-1999. doi:10.1177/ 0363546514535070
7. Dines JS, Frank JB, Akerman M, Yocum LA. Glenohumeral internal rotation deficits in baseball players with ulnar collateral ligament insufficiency. Am J Sports Med. 2009;37(3):566-570. doi:10.1177/ 0363546508326712
8. Shanley E, Rauh MJ, Michener LA, et al. Shoulder range of motion measures as risk factors for shoulder and elbow injuries in high school softball and baseball players. Am J Sports Med. 2011;39(9):1997-2006. doi:10.1177/ 0363546511408876
9. Wilk KE, MacRina LC, Fleisig GS, et al. Deficits in glenohumeral passive range of motion increase risk of elbow injury in professional baseball pitchers: A prospective study. Am J Sports Med. 2014;42(9):2075-2081. doi:10.1177/ 0363546514538391
10. Wilk KE, Macrina LC, Fleisig GS, et al. Correlation of glenohumeral internal rotation deficit and total rotational motion to shoulder injuries in professional baseball pitchers. Am J Sports Med 2011;39(2):329-335. doi:10.1177/0363546510384223
11. Shitara H, Kobayashi T, Yamamoto A, et al. Prospective multifactorial analysis of preseason risk factors for shoulder and elbow injuries in high school baseball pitchers. Knee Surg Sports Traumatol Arthrosc 2017;25(10):3303-3310. doi:10.1007/ s00167-015-3731-4
12. Sakata J, Nakamura E, Suzukawa M, Akaike A, Shimizu K. Physical risk factors for a medial elbow injury in junior baseball players: A prospective cohort study of 353 players. Am J Sports Med. 2017;45(1):135-143. doi:10.1177/0363546516663931
13. Harada M, Takahara M, Mura N, et al. Risk factors for elbow injuries among young baseball players. J Shoulder Elbow Surg 2010;19(4):502-507 doi:10.1016/j.jse.2009.10.022
14. Shanley E, Kissenberth MJ, Thigpen CA, et al. Preseason shoulder range of motion screening as a predictor of injury among youth and adolescent baseball pitchers. J Shoulder Elbow Surg. 2015;24(7):1005-1013. doi:10.1016/j.jse.2015.03.012
15. Tajika T, Kobayashi T, Yamamoto A, et al. A clinical and ultrasonographic study of risk factors for elbow injury in young baseball players. J Orthop Surg. 2016;24(1):45-50. doi:10.1177/230949901602400112
16. Wright RW, Steger-May K, Klein SE. Radiographic findings in the shoulder and elbow of Major League Baseball pitchers. Am J Sports Med 2007;35(11):1839-1843. doi:10.1177/ 0363546507304493
17 Lesniak BP, Baraga MG, Jose J, et al. Glenohumeral findings on magnetic resonance imaging correlate with innings pitched in asymptomatic pitchers. Am J Sports Med 2013;41(9):2022-2027 doi:10.1177/ 0363546513491093
18. Gutierrez NM, Granville C, Kaplan L, Baraga M, Jose J. Elbow MRI findings do not correlate with future placement on the disabled list in asymptomatic professional baseball pitchers. Sports Health 2017;9(3):222-229. doi:10.1177/ 1941738117701769
19. Makhni EC, Morrow ZS, Luchetti TJ, et al. Arm pain in youth baseball players: a survey of healthy players. Am J Sports Med 2015;43(1):41-46. doi:10.1177/0363546514555506
20. Wilk KE, Arrigo CA, Andrews JR. Current concepts: the stabilizing structures of the glenohumeral joint. J Orthop Sports Phys Ther 1997;25(6):364-379. doi:10.2519/jospt.1997.25.6.364
21. Seitz AL, McClure PW, Finucane S, Boardman ND 3rd, Michener LA. Mechanisms of rotator cuff tendinopathy: intrinsic, extrinsic, or both? Clin Biomech 2011;26(1):1-12. doi:10.1016/ j.clinbiomech.2010.08.001
22. Rio E, Moseley L, Purdam C, et al. The pain of tendinopathy: physiological or pathophysiological? Sports Med 2014;44(1):9-23. doi:10.1007/ s40279-013-0096-z
23. Arnold AJ, Thigpen CA, Beattie PF, et al. Normalized Isometric Shoulder Strength as a Predictor of Ball Velocity in Youth Baseball Players. Int J Sports Phys Ther. 2022;17(2):259-269. doi:10.26603/001c.31045
Okamura S, Iida K. Relationship Between Lumbar Locked Rotation, Trunk Rotation During Pitching, and Pitch Velocity in High School Baseball Players. IJSPT 2024;19(11):1447-1454. doi:10.26603/001c.123954
Shun Okamura1a , Kai Iida2
1 Department of Physical Therapy, Faculty of Health Science Technology, Bunkyo Gakuin University, 2 Department of Physical Therapy, Faculty of Health Science Technology, Bunkyo Gakuin University
Keywords: baseball, trunk rotation, lumbar locked rotation, ball velocity https://doi.org/10.26603/001c.123954
International Journal of Sports Physical Therapy Vol. 19, Issue 11, 2024
Background
Trunk rotation during pitching correlates with pitching velocity and load on the joints of the upper limb. Trunk rotation is often focusing on during teaching the pitching motion. Athletes who exhibit early trunk rotation during pitching often have low thoracic spine rotational range of motion. The purpose of this study was to investigate the relationship between the range of thoracic spine rotation measured using the lumbar locked rotation test (LLR-t), hip-shoulder separation (H/S) and thoracic rotation angle at stride foot contact (SFC), and ball velocity
Study design
Descriptive laboratory study.
Methods
Fifteen healthy male high school students belonging to a baseball club were recruited for participation. The throwing side and non-throwing side LLR-t was performed using an inclinometer Hip-shoulder separation and thoracic rotation angle during SFC were measured using a pitching motion analysis application: Pitch AI. In addition, a tracking device specifically designed for pitching:Pitching2.0 was employed to measure ball velocity Correlations between LLR angle, H/S, trunk rotation angles at SFC, and ball velocity were examined using Pearson’s correlation coefficient.
Result
There was a positive correlation(r=0.52,p=0.047) between the LLRt angle and H/S. Additionally, there was a negative correlation(r=-0.62, p =0.01) between the LLRt angle on the throwing side and thoracic rotation angle. There was a positive correlation(r=0.54, p=0.04) between ball velocity and H/S and a negative correlation(r=-0.56, p=0.03) between ball velocity and thoracic rotation angle during SFC.
Conclusion
The LLR-t measures thoracic rotation angle with the pelvis-lumbar spine fixed. The results indicated that throwing side LLR was related to the thoracic angle during SFC and hip-shoulder separation.
Level of evidence
3
s-okamura@bgu.ac.jp a
Corresponding Author:
Shun Okamura, MS
Department of Physical Therapy, Faculty of Health Science Technology, 1196 Kamekubo, Fujimino-shi, Saitama 356-8533, Japan
+81-49-261-7973
+81-49-261-8923
The pitching motion in baseball involves not only the upper limbs but also coordinated energy generation from the trunk.1 Therefore, during pitching, various tension and compression loads are applied to the shoulder and elbow joints.2 In baseball, particularly for pitchers, ball velocity serves as an indicator of pitching performance, and enhancing ball velocity is crucial for coaching.
However, ball velocity is influenced not only by physical factors, such as muscle strength, but also by various other factors, including the pitching form.3 Although ball velocity is affected by various factors, it can be improved by efficiently transmitting energy from the lower limbs to the trunk and upper limbs. Therefore, when an injured player returns to competition, it is necessary not only to address symptoms such as joint pain and anxiety, but also to focus on pitching form, considering the aspects of pitching performance such as ball velocity
The throwing motion generally comprises 1) wind-up, 2) stride, 3) cocking, 4) acceleration, 5) deceleration, and 6) follow-through.4 When teaching the pitching motion, it is easy to confirm the movement of the pelvis and trunk that occurs from the stride to the cocking period. The relationship between ball velocity and trunk motion during pitching has been widely reported; Manzi et al.5 and Solomito et al.6 reported that an increase in the angle of forward trunk tilt during ball release increases ball velocity Oyama et al.7 also reported that ball velocity increases as the lateral flexion angle to the body increases during maximum external rotation of the shoulder joint. Thus, the authors believe that the trunk motion during pitching is closely related to ball velocity However, prior studies have usee a three-dimensional motion analysis device, which is difficult to use in clinical practice. A method that uses artificial intelligence (AI) for motion analysis and measures the knee joint angle during gait without using a 3-dimentional motion analyzer has been reported.8 With the development of AI, pitching motion analysis can now be performed without using infrared reflective markers (marker-less). The disadvantage of marker-less motion analysis is that the joint positions are estimated based on videos and images and the joint angles can be calculated. However, the advantage of this analysis is that it only uses videos and eliminates complex equipment operations. In addition, the measurement space is no longer restricted, making using use in clinical and instructional settings easier
Focusing on trunk rotation to the non-throwing side (observed during stride foot contact) is one of the key points when evaluating pitching form. Davis et al. previously reported that throwing efficiency (normalized shoulder joint internal rotation torque or normalized elbow valgus torque/ball velocity) was poor in athletes who had elbow drop and trunk rotation to the non-throwing side that occurred early during the throwing motion.9 Sgroi et al.10 reported that the hip-shoulder separation (H/S) is a factor that influences ball velocity. Furthermore, because the trunk rotation angular velocity is also related to ball velocity,11 the rotation angle of the thorax at stride foot con-
tact (SFC) is often a necessary factor for analysis. Therefore, elucidating the factors related to early trunk rotational movements is crucial to effectively guide pitching mechanics.
H/S and thoracic rotation angles require spinal mobility, particularly of the thoracic spine. There have been various reports on methods for measuring thoracic spine rotation range of motion in the past,12,13 including the use of the lumbar locked rotation test (LLR-t).14 Regarding the relationship between LLR-t and baseball, Okamura et al.15 reported that an increase in the angle of LLR-t to the throwing side decreases maximal elbow valgus torque. Thus, LLR-t throwing side angle may correlate with H/S and SFC thoracic rotation angle during the throwing motion, which is the approximate limb position during LLR-t. Therefore, The purpose of this study was to investigate the relationship between the range of thoracic spine rotation measured using the lumbar locked rotation test (LLR-t), hip-shoulder separation (H/S) and thoracic rotation angle at stride foot contact (SFC), and ball velocity. The hypothesis was that players with higher throwing side angles measures in the LLR-t would exhibit smaller trunk rotation angles at SFC, larger H/S, and higher ball velocity
Fifteen healthy male students (twelve right-handed and three left-handed, who belonged to a high school baseball team were included in this study The exclusion criteria included individuals who had experienced shoulder or elbow pain during pitching in the past year and those who had a sidearm or underhand pitching motion. This study was approved by the Ethics Review Committee for Health and Medical Sciences Research at the Bunkyo Gakuin University (Approval Number 2022-009). The participants were provided with an explanation of the research objectives and measurements were taken after obtaining their consent.
The thoracic spine rotation range of motion was measured using the method described by Johnson et al.,16 following their approach for the LLR-t. The measurement posture involved adopting a quadruped position with the buttocks resting on the heels and forearms in contact with the floor. The upper limb on the side being measured was placed behind the head, and trunk rotation was performed through voluntary movement (Figure 1A, B). The thoracic spine rotation angle was measured using an inclinometer (Shinwa Rules Co., Ltd., Japan). The inclinometer was positioned between the first and second thoracic vertebrae, and measurements were taken in one-degree increments. If compensatory movements due to lateral spinal flexion were observed, re-measurements were performed. Measurements were taken three times on both the left and right sides, and the average value was considered the representative measurement. The throwing and non-throwing sides were de-
Figure 1. Lumbar locked rotation test (LLR-t) measurement start position (A) and maximum rotation position (B).
fined as the dominant and nondominant sides, respectively Furthermore, Feijen et al reported the intra-rater reliability of this measurement method to be 0.91 for right rotation and 0.96 for left rotation, while the inter-rater reliability was 0.89 for right rotation and 0.86 for left rotation.17 All LLR-t angles were measured by a physical therapist with 15 years of experience (S.O ).
Pitch motion analysis was conducted using a tablet device (iPad Pro, Apple Inc, Cupertino, CA, USA) and a single camera marker-less motion capture solution (Pitch AI, 3MotionAI Inc. Oakville, ON, Canada). The measurement environment was an outdoor field with a pitching mound. The tablet device was positioned in the direction of the third base from the pitching plate on the mound. The distance from the pitching plate to the tablet device was set to align with the height of the pitcher’s belt, following the recommendation of the Pitch AI application, where a line appears on the screen.
First, the players were instructed to warm up before the measurement in the way that each player normally did. A total of 30 minutes was allotted for warming up: 15 min for stretching and 15 min for playing catch. After warming up, the players were asked to throw a pitch to a catcher sitting 18.44 m away as a measurement task. The throwing motion was started from the set position. The instructions for pitching were “Please pitch as you would normally do in a game” The trial with the highest ball velocity among the five ball pitches measured was included in the analysis. The analysis utilized Pitch AI to calculate the H/S and thoracic rotation angle at SFC (Figure 3). This pitching motion
analysis app estimates joint positions using AI by capturing videos from the front plane with a tablet device, enabling the measurement of joint angles. Of note, Dobos et al.18 assessed the measurement error of this app, by comparing it with three dimensional motion capture, and reported an r² of 0.92 and a root mean squared error of 6.04°for the trunk segment.
For right-handed pitchers, the calculation of H/S and trunk angle was defined using the third base direction as 0° and the home plate direction as 90°, with positive values indicating rotation towards the non-throwing side and negative values indicating rotation towards the throwing side. Calculation of thoracic rotation angle during H/S and SFC using the Pitch AI was performed by a physical therapist with 11 years of experience (K.I.). To assess pitching performance, ball velocity was measured using a pitching tracking device Pitching2.0 (Rapsodo LLC, Kanagawa, Japan). The pitching tracking device was positioned 4.75 m from the front edge of the home plate, as recommended by the usage instructions. The reliability of this pitch tracking device has not determined.
All statistical analyses were performed using SPSS version 25 (IBM Corporation, Chicago, IL, USA). After confirming normality using the Shapiro–Wilk test, correlations between LLR angle, H/S, trunk rotation angles at SFC, and ball velocity were examined using Pearson’s correlation coefficient. Based on the report by Alveiero et al.,19 correlations were assessed as weak (0.1< r < 0.3), moderate (0.3 < r < 0.5), or strong (r.>0.5). Correlations were deemed significant at p<0.05.
Figure 2. Thoracic rotation angle during stride foot contact
Table 1. Characteristics of the participants (n = 15)
±
(m) 1.74.1±5.8
Experience (years) 8.9±1.5
SD, standard deviation
Table 2. Mean values of each measurement
Mean ±SD
Throwing side LLR(degrees)
53.7±8.3
Non-throwing side LLR (degrees) 51.2±8.5
Hip-shoulder separation (degrees) 9.1±8.1
Thoracic rotation angle during SFC (degrees) 31.2±19.4
Ball velocity (km/h) 123.5±4.6
SD, standard deviation
Characteristics of the participant are shown in Table1 The average values of each measurement are presented in Table 2 The throwing side LLR, non-throwing side LLR, H/S, and trunk rotation (at SFC) angles were 53.7±8.3°, 51.2±8.5°, 31.2±19.4°, and 9.1±8°, respectively, and the ball velocity was 123.5±4.6 km/h.
Table 3 presents the correlation coefficients for each measurement. There was a positive correlation between the LLR angles and H/S (r=0.52, p=0.04). The LLR angle on the
throwing side and the thoracic rotation angle exhibited a negative correlation (r=-0.62, p=0.01). There was a positive correlation between the ball velocity and H/S (r=0.54, p=0.04). Ball velocity and thoracic rotation angle at SFC showed anegative correlation (r=-0.56, p=0.03). No other significant correlations were observed.
In this study, the authors utilized LLR-t and Pitch AI to elucidate the correlation between the thoracic spine rotational range and trunk rotational movement during pitching. Regarding the interplay between LLR and pitching mechanics, the results revealed that a greater throwing side angle in LLR-t was associated with an increased H/S and decreased trunk rotation angle. In terms of ball velocity, no correlation was observed with the LLR angle. However, ball velocity increased as H/S increased and thoracic rotation angle decreased during pitching.
First, regarding the angle of gyration during pitching, Nissen et al.20 reported pelvic and thoracic rotation angles to be -64±12° and -92±11°, respectively at the time of SFC. These angle differences were approximately 30°. In the current study using the Pitch AI, the H/S was approximately 31.2±19.4°, yielding a result that closely approximated those reported by Nissen. However, considering the measurement accuracy of the trunk rotation angle of Pitch AI used in this study, considering the possibility of an error from the actual angle is necessary
A positive correlation was observed between LLR on the throwing side and H/S, whereas a negative correlation was found with the thoracic rotation angle at SFC. In other words, players with higher LLR angles on the throwing side tended to exhibit higher H/S values at SFC, allowing them
Table 3. Relationship between each measurement result
to maintain a position with the trunk directed towards third base. Motion between the trunk and H/S can be characterized as “opening up” or not “opening up” when providing instruction. The necessary H/S angle is approximately 30° and considering that the average LLR angle on the throwing side in this study was 53.7±8.3°, it can be inferred that players had a sufficient thoracic rotation range relative to the pelvis. Bullock et al.21 reported a lack of correlation between the seated thoracic rotation angle and H/S. However, the authors attribute the observed relationship to differences in the measurement positions for the thoracic rotation angles in the current study. This study involved fixing the pelvis and lumbar spine while elevating the upper limb of the measured side. This differs from seated thoracic rotation, and the authors believe that the increased influence of the extensibility of the pectoralis major and minor muscles plays a significant role in contributing to the discrepancies observed in previous reports.
Aguinaldo et al.22 reported that the initiation timing of trunk rotation during pitching, which is related to the thoracic rotation angle at SFC, tends to be delayed in professional players compared to college-level players and younger age groups. Therefore, in the high school age group, the initiation timing of trunk rotation may occur earlier, potentially leading to an increase in the thoracic rotation angle towards the non-throwing side. However, the participants in this study, although high school students, had an average athletic experience of 8.9±1.5 years. Hence, we speculate that the observed relationship was present because the participants could effectively utilize their own LLR angles in thoracic rotation during pitching.
The observed relationship between H/S, thoracic rotation angle, and ball velocity may be attributed to the significant torsional difference between the pelvis and thorax driven by the stretch-shortening cycle (SSC) in the core muscle groups, particularly the external oblique on the throwing side and the internal oblique on the non-throwing side. The increase in H/S may have been related to the non-throwing side rotation movement that occurs after SFC
because the SSC works by stretching the external oblique muscles on the pitching side and the internal oblique muscles on the non-throwing side.
The low thoracic rotation angle during SFC means that the right-handed pitcher’s thoracic is facing toward third base. Lin et al.23 reported that players exhibiting early rotational movements towards the non-throwing side tended to have slower ball velocity Therefore, it was thought that a relationship would be found between ball velocity and thoracic rotation angle, which was confirmed.
There are four limitations of this study This study is fails to consider of the relationship between the pelvis and lower limb joints. Because the H/S is determined by the relative positions of the pelvis and thorax, it may be influenced by factors such as pelvic and hip joint movements of the stance leg. Dowling et al.24 have previously reported relationships between the pelvic rotation and knee joint angles on the stepping side, highlighting the need for future investigations to address these aspects. Next, the measurement accuracy of the Pitch AI and the pitch tracking device is discussed. It was 11.16° and 8.64° RMS for H/S and trunk rotation angle during SFC compared to the 3D motion analysis analyzer, respectively.18 Thus, the Pitch AI should be used understanding that the results are not the same as those that would be achieved using 3D motion capture. In addition, the accuracy of the pitch tracking device used in the ball velocity measurement was unknown. Finally, the sample size and the analysis test were only for the highest ball velocity In this study, 15 participants were selected because the individuals were either pitchers or experienced pitchers; the pitching motion other than overthrow was also an exclusion criterion. In addition, the throwing motion by pitchers is a repetitive one: the joint angles may differ from test to test. Therefore, increasing the sample size and number of trials in the future is necessary.
The results of this study indicate that as the LLR angle on the throwing side increased, the H/S increased and the thoracic rotation angle at the SFC decreased. This suggests that evaluating the LLR angle on the throwing side could be beneficial for players who exhibit early rotation towards the non-throwing side during pitching.
The authors report no conflicts of interest.
Submitted: March 15, 2024 CDT, Accepted: September 05, 2024 CDT
© The Author(s)
This is an open-access article distributed under the terms of the Creative Commons Attribution 4.0 International License (CCBY-NC-4.0). View this license’s legal deed at https://creativecommons.org/licenses/by-nc/4.0 and legal code at https://creativecommons.org/licenses/by-nc/4.0/legalcode for more information.
1. Howenstein J, Kipp K, Sabick MB. Energy flow analysis to investigate youth pitching velocity and efficiency. Med Sci Sports Exerc. 2019;51:523-531. doi:10.1249/mss.0000000000001813
2. Fleisig GS, Andrews JR, Dillman CJ, Escamilla RF. Kinetics of baseball pitching with implications about injury mechanisms. Am J Sports Med 1995;23:233-239. doi:10.1177/036354659502300218
3. Kew ME, Koo A, Manzi JE, et al. Kinematic parameters predictive of pitch velocity in youth to professional baseball pitchers: A qualitative systematic review. Orthop J Sports Med. 2023;11:23259671231196539. doi:10.1177/ 23259671231196539
4. Chalmers PN, Wimmer MA, Verma NN, et al. The relationship between pitching mechanics and injury: A review of current concepts. Sports Health 2017;9(3):216-221. doi:10.1177/1941738116686545
5. Manzi JE, Wang Z, Wright-Chisem J, Nicholson A, Dennis E, Dines JS. Elbow varus torque and ball velocity associations in high school and professional pitchers with increased sagittal-plane trunk tilt. J Shoulder Elbow Surg 2022;31:151-158. doi:10.1016/ j.jse.2021.07.028
6. Solomito MJ, Garibay EJ, Nissen CW. Sagittal plane trunk tilt is associated with upper extremity joint moments and ball velocity in collegiate baseball pitchers. Orthop J Sports Med. 2018;6:2325967118800240. doi:10.1177/ 2325967118800240
7. Oyama S, Yu B, Blackburn JT, Padua DA, Li L, Myers JB. Effect of excessive contralateral trunk tilt on pitching biomechanics and performance in high school baseball pitchers. Am J Sports Med. 2013;41:2430-2438. doi:10.1177/0363546513496547
8. Saiki Y, Kabata T, Ojima T, et al. Reliability and validity of Open Pose for measuring hip-knee-ankle angle in patients with knee osteoarthritis. Sci Rep 2023;13:3297. doi:10.1038/s41598-023-30352-1
9. Davis JT, Limpisvasti O, Fluhme D, et al. The effect of pitching biomechanics on the upper extremity in youth and adolescent baseball pitchers. Am J Sports Med. 2009;37(8):1484-1491. doi:10.1177/ 0363546509340226
10. Sgroi T, Chalmers PN, Riff AJ, et al. Predictors of throwing velocity in youth and adolescent pitchers. J Shoulder Elbow Surg 2015;24:1339-1345. doi:10.1016/ j.jse.2015.02.015
11. Cohen AD, Garibay EJ, Solomito MJ. The association among trunk rotation, ball velocity, and the elbow varus moment in collegiate-level baseball pitchers. Am J Sports Med 2019;47:2816-2820. doi:10.1177/0363546519867934
12. Hwang D, Lee JH, Moon S, Park SW, Woo J, Kim C. The reliability of the nonradiologic measures of thoracic spine rotation in healthy adults. Phys Ther Rehabil Sci. 2017;6:65-70. doi:10.14474/ ptrs.2017.6.2.65
13. Furness J, Schram B, Cox AJ, Anderson SL, Keogh J. Reliability, and concurrent validity of the iPhone (®) Compass application to measure thoracic rotation range of motion (ROM) in healthy participants. PeerJ 2018;6:e4431. doi:10.7717/peerj.4431
14. Welbeck AN, Amilo NR, Le DT, et al. Examining the link between thoracic rotation and scapular dyskinesis and shoulder pain amongst college swimmers. Phys Ther Sport 2019;40:78-84. doi:10.1016/j.ptsp.2019.08.013
15. Okamura S, Iida K, Inoue S, Kizugawa K, Chiba M. The relationship between the combined rotational range of motion of the thoracic spine and scapulothoracic joint and elbow joint valgus torque during pitching in high school baseball players. Japanese J Sports Phys Ther 2023;1:1-6. doi:10.57495/ jjspt.1.1_1
16. Johnson KD, Kim KM, Yu BK, Saliba SA, Grindstaff TL. Reliability of thoracic spine rotation range-ofmotion measurements in healthy adults. J Athl Train. 2012;47:52-60. doi:10.4085/1062-6050-47.1.52
17 Feijen S, Kuppens K, Tate A, Baert I, Struyf T, Struyf F. Intra- and interrater reliability of the “lumbar-locked thoracic rotation test” in competitive swimmers ages 10 through 18 years. Phys Ther Sport 2018;32:140-144. doi:10.1016/j.ptsp.2018.04.012
18. Dobos TJ, Bench RWG, McKinnon CD, et al. Validation of pitchAITM markerless motion capture using marker-based 3D motion capture. Sports Biomech. Published online 2022:1-21. doi:10.1080/ 14763141.2022.2137425
19. Albiero ML, Kokott W, Dziuk C, et al. Hip Flexibility and Pitching Biomechanics in Adolescent Baseball Pitchers. J Athl Train 2022;57(7):704-710. doi:10.4085/1062-6050-0103.21
20. Nissen CW, Westwell M, Ounpuu S, et al. Adolescent baseball pitching technique: a detailed three-dimensional biomechanical analysis. Med Sci Sports Exerc. 2007;39:1347-1357. doi:10.1249/ mss.0b013e318064c88e
21. Bullock GS, Schmitt AC, Chasse PM, Little BA, Diehl LH, Butler RJ. The relationship between trunk rotation, upper quarter dynamic stability, and pitch velocity J Strength Cond Res 2018;32:261-266. doi:10.1519/jsc.0000000000001772
22. Aguinaldo AL, Buttermore J, Chambers H. Effects of upper trunk rotation on shoulder joint torque among baseball pitchers of various levels. J Appl Biomech. 2007;23:42-51. doi:10.1123/jab.23.1.42
23. Lin YC, Lin HT, Lu CC, Pei-Hsi Chou P, Su FC. Is early trunk rotation really hazardous for shoulder biomechanics in baseball throwing? J Shoulder Elbow Surg. 2022;31:1282-1293. doi:10.1016/ j.jse.2021.12.042
24. Dowling B, Knapik DM, Luera MJ, Garrigues GE, Nicholson GP, Verma NN. Influence of pelvic rotation on lower extremity kinematics, elbow varus torque, and ball velocity in professional baseball pitchers. Orthop J Sports Med. 2022;10(11):23259671221130340. doi:10.1177/ 23259671221130340
VERONIKA ILJINAITĖ1a , LAIMONAS ŠIUPŠINSKAS2 , KRISTINA BERŠKIENĖ2
1 Movement Diagnostics Clinic, 2 Department of Sports Medicine, Lithuanian University of Health Sciences
Keywords: Functional Movement Screen, bodybuilding, back squat assessment, back squat performance, fundamental movements. https://doi.org/10.26603/001c.124998
International Journal of Sports Physical Therapy
Vol. 19, Issue 11, 2024
Background
It is important to assess the quality of fundamental movements, to discover deficits, evaluate mobility, balance, and stability, and identify movement dysfunction and asymmetries. However, little research has been performed on the assessment of fundamental movements with bodybuilders.
Purpose
The purpose of this research was to examine the quality of professional and amateur bodybuilders’ functional movements and the quality of the back squat performance. A secondary purpose was to discern whether greater experience in bodybuilding was associated with better scores on the back squat assessement (BSA).
Study design
Cross-Sectional Cohort
Methods
Twenty-six athletes were recruited to participate. The group of professional bodybuilders consisted of five men and six women, a total of 11 athletes. The group of amateur bodybuilders consisted of seven men and eight women, a total of 15 athletes. The Functional Movement Screen™ (FMS™) was used to assess the seven included fundamental patterns that evaluate an individual’s neuromuscular control, mobility, balance, and stability The BSA was used to assess the quality of movement, dysfunction, deficit, or compensation during the squat exercise. Statistical analyses applied non-parametric tests (Wilcoxon, Mann-Whitney U, and Friedman’s) for dependent and independent samples, with significance set at p<0.05, and the Spearman correlation coefficient and Chi-square test were used to assess relationships between quantitative and qualitative variables.
Results
Overall, athletes with a higher total FMS™ score performed better on the BSA as well. The professional athletes scored 2.58 points higher than the amateurs on total FMS™ scores (p<0.001).
Professional athletes scored better on the BSA than amateurs (p<0.001). A statistically significant, positive moderate correlation was revealed between the FMS™ total score and the squat total score (r=0.68; p=0.005).
Corresponding Author: Veronika Iljinaitė, Partizanų gatvė 33-4, Kaunas, Lithuania +37067793369, jdkveronika@gmail.com
A higher FMS™ score in bodybuilders is associated with a higher BSA score. Professional bodybuilders have higher FMS™ scores and higher BSA scores than amateurs. Greater experience in bodybuilding is associated with the compliance with several BSA criteria: trunk position, frontal knee alignment, tibial translation angle, foot position in all three back squat variations with different external loads, and descent with the training weight.
3b
The competence of fundamental movements may relate to safe participation in physical activities and reduction of the risk of injuries. Development and training of these movements should be essential elements in sports,1,2 e.g., in bodybuilding. Bodybuilders perform many exercises/movements consistently,3 and the squat is one of the most important core movements that contributes to the enhancement athletic performance,1 and is widely used by amateur and professional athletes. The back squat recruits most of the major muscles of the lower body, including the quadriceps, hamstrings, glutes, and calves, while also engaging the trunk muscles, such as the abdominals and lower back, to ensure coordinated timing and activation of all muscles involved in the movement.1,4 The dynamics of the squat exercise under different circumstances have been thoroughly investigated in the past.4 For instance, Kristiansen et al.4 explored the squat of skilled weightlifting athletes and their inter- and intra-individual variability while performing this exercise, and Fry et. al.5 examined the effect of knee position on hip and knee torques during the back squat, while Escamilla et al.6 explored biomechanical parameters while performing the back squat with varying stance widths, and Swinton et. al.7 compared the biomechanics of the box squat, traditional squat, and powerlifting squat.
To further assess and identify biomechanical movement deficiencies, Myer and colleagues proposed the Back Squat Assessment (BSA).1,8 This screening tool allows for evaluation of an athlete’s strength, mobility, and neuromuscular control.1,9‑13 Another popular screening tool used in sports is the Functional Movement Screen™ (FMS™).14,15 The FMS™ assesses seven distinct fundamental patterns, to identify functional movement deficiencies, asymmetry, and compensatory mechanisms, and to evaluate neuromuscular control, mobility, balance, and stability.16‑18 Both the FMS™ and BSA screening tools offer simple methods for the evaluation of movement.
The purpose of this research was to examine the quality of professional and amateur bodybuilders’ functional movements and the quality of the back squat performance. A secondary purpose was to discern whether greater experience in bodybuilding was associated with better scores on the BSA. It was hypothesized that professional bodybuilders would demonstrate higher back squat and FMS™ scores, and would squat closer to the BSA standard, as professionals have more experience in bodybuilding than amateurs.
The permission of the Center for Bioethics (No. BECSR(M)-197) was obtained to conduct the study. From October 8, 2020 to November 10, 2020 athletes from four Lithuanian cities participated in the study 19
Twenty-six bodybuilders who were between 20 and 35 years old were recruited to participate in the study as shown in Table 1 Athletes were divided into two groups: professionals and amateurs as indicated in.
FUNCTIONAL MOVEMENT SCREEN™ (FMS™)
The FMS™ is widely used in sports practice to assess foundational movement patterns. Seven functional movements are evaluated during the test, including the deep squat, inline lunge, hurdle step, active straight leg raise and shoulder mobility, trunk stability push-up, and rotary stability After performing these movements, the athletes also had to perform three clearing tests, which included spinal flexion, extension, and a shoulder test.14,17 Each of the seven functional movements was performed three times, and the best performance was scored.14,17,20 All of the movement patterns were scored separately from 0 to 3 points. If the athlete felt pain in any part of the body, 0 points were given. A score of 1 was given when the athlete was unable to fully complete the movement, and a score of 2 was given when the athlete was able to complete the movement but performed the task with compensation of adjacent regions. If the athlete was able to perform the movement without a visible deficit or compensation, they were given a maximum rating of 3 points.14,20 If the athlete received a different number of points while performing a movement on both sides of the body, the lower score of the two was used for the total FMS™ score. The sum of the athlete’s performance scores for all seven movement patterns resulted in a total FMS™ score. The highest possible total FMS™ score is 21.14
The BSA is used to assess the quality of movement, dysfunction, deficit, or compensation during the back squat exercise. After identifying the deficiencies during the funda-
Table 1. List of criteria for the selection, inclusion, and exclusion of athletes, and the recruitment method of the study. Recruitment method
Time since the last competition
Age
Official invitation to participate in the study by the Lithuanian Fitness and Bodybuilding Federation
Injuries
Participation in competitions
mental movement pattern of the back squat, clinicians can prescribe corrective exercises1 and reduce existing asymmetries, deficits, and compensations, which can affect the quality of training and athletes’ performances. In total, the BSA screening tool has 10 criteria. During the back squat performance, the clinician assesses three movement mechanics criteria, three upper body criteria, and four lower body criteria. When evaluating the movement mechanics, attention is paid to the recruitment, timing, and coordination; when evaluating the criteria of descent, the depth, and ascent are observed. When assessing the upper body, the head position, thoracic position, and torso position are monitored. And finally, when assessing the lower body, the hip position, frontal plane knee alignment, tibial translation angle, and foot position are inspected.1 Every professional and amateur athlete performed three back squats. The back squats were performed with different external loads: first with no weight, then with an Olympic bar weighing 20 kilograms, and finally with which the weight that the athlete was currently training. Each of the ten criteria was scored as either 0 or 1. If the criterion was fulfilled correctly, it was scored as 1 point. If the criterion did not meet the standard, the athlete was given 0 points. There were three back squat attempts. The repetition with the highest score was evaluated. A maximum score of 10 indicated the best back squat performance.19 A total back squat score was calculated by adding and averaging the scores for the weightless back squat, the back squat with the Olympic bar, and the back squat with which the weight that the athlete was currently training. Back squat performance trials were recorded from two angles. One of the two tripods (BRAUN Lightweight BLT 100S) was placed in front of the athlete and captured the frontal plane, while the second tripod was set to the right side of the athlete and captured performance from the sagittal plane. Two iPhone X devices were placed on the tripods. In order to start recording the video in the two planes with two devices at the same time, two remote controls were used. A certified physiotherapist performed the evaluation of the quality of the back squat.
Less than 3 years prior
Between 18 and 35 years old
No injuries and/or conditions that could affect the study
Participation in International Fitness and Bodybuilding Federation (IFBB) competitions
More than 3 years prior
Younger than 18 and older than 35 years old
Had injuries and/or conditions that may have affected the study
Participation in other than IFBB federation competitions
It is important for conditioning experts and athletic health care professionals to ensure precise estimations of variables to reduce uncertainty and avoid incorrect conclusions in the monitoring process.18 It is known that tester’s experience can affect scoring of screening tests. For that reason, the BSA screening tool was chosen to reduce subjectivity as much as possible, and the use of structured, detailed, verbal instructions assist in promoting inter-rater reliability Since the FMS™ scoring is standardized, clinicians across varying professions can use this tool,15,21 and to reduce error in scoring, the professional should have been instructed in and be familiar with the screening tool (>100 trials).18 Despite the known shortcomings of the tests, the BSA and FMS™ were chosen because they are easy to use, low-cost, time-efficient, and widely accessible to personal trainers, physical therapists, and other practitioners.
Head position. For the athlete to meet the first criterion, their head must maintain a neutral alignment, which is in a slight physiological extension, and the neck is in line with the torso.1 It is equally important to pay attention to the athlete’s gaze. The gaze should be directed straight or slightly above the midline (Figure 2, criterion 1).1,22 The athlete should avoid placing their head too far forward or backwards, and tilting it to either side.1,23
Thoracic position The thoracic spine should be vertical during the entire back squat movement (Figure 2, criterion 2).1,24 The glenohumeral joint should be externally rotated, in a slightly depressed position, and the forearms in line with the torso help to keep the thoracic spine straight. The athlete’s scapulas must be in a depressed and retracted position.1
Trunk position It is necessary that the lumbar spine maintains a neutral, slightly lordotic position while keeping the core stable throughout the squat (Figure 2, criterion 3).1 A mistake that should be avoided is excessive forward lean of the trunk because it increases the shear forces in the lumbar region.1,25 The correct position of the trunk is ensured by the presence of the trunk and tibia in parallel
Figure 1. Squat with different load intensities in the sagittal plane at the end of the movement. On the left side (A) - without weight, in the middle (B) - with the Olympic bar, and on the right side (C) with the training weight. alignment when viewed from the side (Figure 2, criterion 6).1
Hip position. Throughout the exercise, the position of the hips should be symmetrical (Figure 2, criterion 4),1,23 malpositioning can be seen when one is higher or lower than the other, or rotated to one side or the other The incorrect position of the hips can also be visualized by the non-horizontal and inclined position of the barbell when viewed from the front. The position of the pelvis is equally important, i.e., it must also remain in a neutral, anterior pelvic tilt position throughout the squat. Athletes often break this rule by demonstrating a posterior pelvic tilt or so-called “butt wink” at the end of the descent phase.1
Frontal plane knee alignment. Throughout the movement, the hip, knee, and ankle joints must remain in one vertical line when viewed from the front (Figure 2, criterion 5), and the knee joint with the tibia should be perpendicular to the floor A knee valgus position that occurs when the knee joint and tibia cross a vertical line inward, and a knee varus position, that occurs when the knee joint and tibia cross a vertical line outward, are considered deficits.1,10
Tibial translation angle. The tibial translation angle can be greater or lesser depending on the ratio of the length of the femur to the torso of the athlete. However, regardless of body composition, it is recommended that the angle of the tibia is parallel with the angle of the trunk when viewed from the side (Figure 2, criterion 3, 6).1
Foot position. The feet are supposed to be tightly placed on the ground throughout the entire back squat motion. The feet tilting inwards, outwards, or lifting of the heel or toes off the ground are considered a deficit (Figure 2, criterion 7).1,24
Descent This phase should begin with the hip hinge.1, 26 One of the criteria that determines that an athlete performs a descent well is maintaining the same distance be-
tween the shoulders and hips during the movement. The tempo should be at least 2:1, meaning the descent should be slower than the ascent due to gravity assistance when descending. A failure to comply with this criterion is considered when athletes descend too quickly, unevenly, haphazardly, and without smoothness.1
Depth The recommended depth is reached when the athlete descends until the upper part of the thighs is perpendicular to the floor (Figure 2, criterion 9).1,23 One of the main mistakes athletes make is incomplete squats that lack required depth.1
Ascent. Like the descent phase, the ascent should start from the hips. When performing a reverse hip hinge, the hips and shoulders should go up at an equal speed, maintaining the same distance throughout the entire phase.1
IBM SPSS 21.0 software was used to assess the research data. Due to the small sample sizes in the study, non-parametric criteria were chosen. The Wilcoxon test was used to compare two dependent samples, and the Mann-Whitney U test was used to compare two independent samples. To equate three dependent samples, Friedman’s test was selected. Quantitative data are represented as mean ( ) and standard deviation (± SD) – ( ± SD). Qualitative data are presented in absolute and relative frequencies. To estimate the relationship between two quantitative variables, the Spearman correlation coefficient was computed. The Chisquare (χ2) test analyzed qualitative variables. The differences at p<0.05 were considered statistically significant.
Figure 2. Ten back squat criteria: on the left side in a sagittal plane (A) 1 – head position and gaze direction; 2 –thoracic position; 3 – trunk position; 6 – tibial translation angle; 7 – foot position; 9 – depth; on the right side in a frontal plane (B) 4 – hip position; 5 – frontal knee alignment; 7 – foot position; 8 – ascent; 10 – descent.
The study involved 26 athletes. The group of professional bodybuilders consisted of five men and six women, a total of 11 athletes. The group of amateur bodybuilders consisted of seven men and eight women, a total of 15 athletes. The number of professional and amateur athletes in the study was not statistically different (p=0.557), nor was the number of males and females among the amateurs (p=1) and professionals (p=1). The sports experience of amateur athletes in bodybuilding was 5.33 ± 4.62 years, and the sports experience of professional athletes in bodybuilding was 11.27 ± 4.54 years. The comparison of these two groups of athletes revealed that the years of experience in this sport were statistically significantly different (U=21.5; p<0.001), with the professionals having more experience in bodybuilding than the amateurs.
In the process of analyzing all bodybuilders, regardless of their experience, it was established that the participants with a higher total FMS™ score performed better on the BSA as well. A statistically significant, positive, and moderate correlation was revealed between the FMS™ total score and the BSA total score (r=0.68; p=0.005).
While evaluating the total FMS™ score between the groups, it was found that more experienced professional athletes scored 2.58 points higher than less experienced amateurs. A statistically evident difference (U=11.5; p<0.001) was found while comparing the results of FMS™ between amateur and professional bodybuilders (Figure 3).
The professional athletes scored 8.64 ± 1.01 points on the BSA during the weightless back squat, while the amateurs scored 6.27 ± 0.96 points, which was statistically significantly different (U=6.5; p<0.001). The amateurs with the Olympic bar scored 6.07 ± 0.88 points, and the professionals scored 8.64 ± 1.03 points, which was also significantly different (U=5; p<0.001). Finally, using the training weight, the amateurs scored 5.93 ± 1.1 points while the professionals scored 8.82 ± 0.98, again, significantly different (U=4; p<0.001).
A statistically significant difference was found within the amateur’s group (χ2(2)=7.6; p=0.022) when comparing the back squat performance without weight, the squat with the Olympic bar, and the squat with the training weight. Post hoc analyses demonstrated that only a statistically significant result when comparing the performance without weight and squat performance with the training weight (Z= -2.236; p=0.031), while no statistically significant differences were revealed between the squat performance without weight and with the Olympic bar (Z= -1.732; p=0.125), or the squat performance with the Olympic bar and with the training weight (Z= -1.414; p=0.250). Comparing professionals’ weightless back squat, back squat with an Olympic bar, and using training weight, no statistically significant differences were found (χ2(2)=4; p=0.135).
A detailed analysis of the compliance with the criteria during the performance of the back squat between the amateur and professional bodybuilders revealed that the athletes equally performed the following criteria while squatting without weight: head position (p=0.218), which was
Figure 3. Comparison of the FMS™ results between amateur and professional bodybuilders.
*-p<0.001
met by 80% of the amateurs and 54.5% of the professionals; thoracic position, which was met by 80% of the amateurs and 72.7% of the professionals (p=1); hip position, which was met by 86.7% of the amateurs and all of the professionals (p=0.492); descent which was met by 66.7% of the amateurs and 90.9% of the professionals (p=0.197); depth, which was met by 80% of the amateurs and 81.8% of the professionals (p=1); ascent, which was met by 60% of the amateurs and by 81.8% of the professionals (p=0.395). During the squat with the Olympic bar, the athletes also complied with the following criteria equally: head position, which was met by 80% of the amateurs and 54.4% of the professionals (p=0.218); thoracic position, which was met by 80% of the amateurs and 72.7% of the professionals (p=1); hip position, which was met by 86.7% of the amateurs and all of the professionals (p=0.492); descent, which was met by 60% of the amateurs and 90% of the professionals (p=0.178); depth, which was met by 73.3% of the amateurs and 81.8% of the professionals (p=1); ascent, which was met by 53.3% of the amateurs and 81.8% of the professionals (p=0.217). Finally, during the squat with a training weight, the athletes also equally performed the following criteria: head position, which was met by 80% of the amateurs and 54.5% of the professionals (p=0.218); thoracic position, which was met by 80% of the amateurs and 72.7% of the professionals (p=1); hip position, which was met by 86.7% of the amateurs and all of the professionals (p=0.492); depth, which was met by 66.7% of the amateurs and 90.9% all of the professionals (p=0.197), and ascent, which was met by 53.3% of the amateurs and 81.8% of the professionals (p=0.217).
Further analysis of the results revealed that during the squat without weight the following criteria were performed
differently between the amateurs and the professionals: trunk position (p=0.005), frontal plane knee alignment (p=0.024), tibial translation angle (χ2(1)=8.11; p=0.004), and foot position (p=0.010) (Figure 4). The same results were obtained during the squat with the Olympic bar, i.e., the professional and amateur athletes performed the following criteria differently: trunk position (p=0.005), frontal plane knee alignment (p=0.024), tibial translation angle (χ2(1)=8.11; p=0.004), foot position (p=0.010) (Figure 4). Both groups of athletes during the squat with a training weight also fulfilled the following criteria contrastly: trunk position (p=0.005), frontal plane knee alignment (p=0.024), tibial translation angle (χ2(1)=8.11; p=0.004), foot position (p=0.010), and descent (p=0.010) (Figure 4).
According to Wang et al.27 and Vehrs et al.,16 the FMS™ method is used to assess the quality of principal movements, to discover deficits, evaluate mobility, balance, and stability, and to identify movement disorganization, as well as asymmetries in athletes of different sports. However, much less research has been done on this topic in the area of bodybuilding.
The first aim of this study was to assess the links between the quality of functional movements and the quality of back squat performance in bodybuilders. It is already known that higher FMS™ scores are associated with higher lower extremity strength in professional soccer players,28, 29 and that a strong association exists between flexibility and the capacity of movement that is observed.29,30 Equally important is the link between morphology, trunk strength,
Figure 4. The distribution of the squat criteria not met by the amateurs and the professionals.
Am – amateurs
Pro – professionals
*Indicates significant difference at p<0.05
and the quality of movement patterns.29,31,32 Thus, in general the findings of this study indicate that regardless of experience, the bodybuilders who got higher total FMS™ scores demonstrated higher rates of the back squat performances as well (r=0.68; p=0.005). These findings suggest that as an athlete’s FMS™ score increases, their squat score also improves. Therefore, it can be assumed that athletes with a higher FMS™ score perform the back squat with higher quality.
The second aim of this study was to measure the quality of the functional movements as scored by the FMS™ between amateur and professional bodybuilders. It was found that the less experienced amateur athletes scored 15.87 ± 1.3 points and more experienced professional athletes scored 18.45 ± 1.13 points, which was statistically significantly different (U=11.5; p<0.001). The higher FMS™ scores observed in professionals may suggest that they have better neuromuscular control, balance, postural stability, and functional mobility, although each of these specific constructs were not measured. Tafuri et al.,33 analyzed FMS™ outcomes among CrossFitters, weightlifters, and amateur bodybuilders, finding that CrossFitters scored 15.2 ± 1.7 points, weightlifters scored 14.8 ± 2 points, and amateur bodybuilders scored 14.2 ± 1.9 points, and that all three groups of athletes results were not statistically different (F=2.28; p=0.10). This suggests that the level of skill and experience play an important role, because in the current study the professionals had more experience in bodybuilding than the amateurs did (U=21.5; p<0.001), while in the study of Tafuri et al.,33 CrossFitters, weightlifters, and amateur bodybuilders had experience that was not significantly different (p=0.15). Despite the current study analyzing the total FMS™ score, Vehrs’ et al.16 have suggested
that it is valuable to pay attention to scores on individual screening tests, deficits, asymmetry, compensations or other deviations from the standard of movement patterns and their possible causes. Such an approach could help athletes not just to understand their strengths and weaknesses and also how to evolve or correct them.
The third aim of this study was to compare amateur and professional bodybuilders’ quality of the back squat performance with different intensities of load between groups and within groups. The comparison of the results between the groups revealed that more experienced professional bodybuilders meet more BSA criteria while performing the back squat than less experienced amateurs did in all three back squat variations (weightless, with an Olympic bar, and with a training weight). These results suggest that amateur bodybuilders who include the back squat exercise in their daily training routine and participate in international competitions still lack the quality while performing the back squat. To perform the squat qualitatively, i.e. meeting the back squat criteria requires time and experience. This is an ongoing process, which cannot be simplified and reached easily with 100 percent quality In line with this, a comparison of the results within the groups revealed that amateurs performed the squat differently from professionals, with amateurs showing varying quality in their back squats. In contrast, the professional athletes performed the back squat with consistent quality, regardless of the additional weight. The results suggest that the back squat, especially with the additional loads, should be supervised by the clinicians, physical therapists or personal trainers more intentionally While squatting with a heavier weight, and not meeting BSA criteria, bodybuilders may compromise quality even more, which can put athletes at a higher risk for
injuries or chronic conditions. A similar focus on the back squat can be found in the study by Kristiansen et al.,4 which explored the variability in performance among skilled weightlifters. Their results demonstrated that experienced weightlifting athletes showed evident inter- and intra-individual variability during successfully performed back squats.4 It is possible that the different results between the professional bodybuilders in the current study and the experienced weightlifters in the study by Kristiansen et al.4 exists due to the small sample size in the current study Even though the authors’ utilized mathematical statistical methods to minimize the influence of sample size to the findings, this remains a limiting factor of this study. Nevertheless, it should be pointed out that the training goals and characteristics of athletes in bodybuilding and weightlifting are also distinct. Bodybuilders are ranked on aesthetics, and their accomplishments depend on the symmetry, size, physical presentation, depth, and definition of the muscles,34‑36 while weightlifters’ achievements depend on characteristics such as explosive power, speed, and strength.37‑39 Also, it must be noted that prior to the evaluation the authors’ were unable to monitor bodybuilders’ training load, differences in training method, volume, and frequency, and these types of data can enrich further studies.
Lastly, the fourth aim of this study was to find out whether more experience in bodybuilding is associated with meeting the BSA criteria. After analyzing the athletes’ back squat performance, it was found that less experienced amateurs and more experienced professionals performed some criteria equally, and some criteria differently The following criteria were met equally and are not associated with compliance to the BSA: head position, thoracic position, hip position, depth, ascent in all three back squat variations with different external loads, and descent without weight or with the Olympic bar, as both amateur and professional athletes met these criteria equally At the same time, the criteria that were different among athletes were the following: trunk position, frontal knee alignment, tibial translation angle, foot position in all three back squat variations with different external loads, and descent with the training weight. These results indicate that greater experience in bodybuilding is related to meeting the mentioned BSA criteria, as professionals performed back squats better, overall, than amateurs did. Naturally, these criteria are interdependent, and often, when one of them is not met, deficits can also be observed in adjacent regions as well. The correct position of the trunk is ensured by the presence of the trunk and tibia in parallel when viewed from the side, but these guidelines require correct feet and knee joint positions too.1 From an observational standpoint, the knee varus position, while assessing frontal plane knee alignment criterion, is a much less frequent deficiency compared to the knee valgus position.1 Lorenzetti et al.40 found that the athletes with less squatting experience were more likely
to exhibit knee varus position when squatting without additional load, and knee varus was less common in the athletes with more squatting experience, while in the current study, the amateurs tended toward more knee valgus alignment. However, the alignment of lower structures, such as the feet, can also be influenced by the positioning of higher ones.1 Regarding the last criterion, it should be noted that monitoring uneven, too rapid, and unsmooth movement while descending often means insufficiency of back squat performance, which can be noticed more often in the athletes who have less squatting experience.1,11 The analysis of amateur bodybuilders’ descent in the current study allowed the observation of similar results. Interestingly, Miletello et al.11 found that skilled competitive collegiate powerlifters take longer while descending than powerlifting athletes who attended high school and who were beginners.4 The comparison of the performance of world-class and less experienced athletes of powerlifting revealed that more skilled athletes also take longer to descend, indicating more control.4,41
The results of the current study indicate that higher FMS™ scores in bodybuilders are associated with a better BSA scores during the performance of the back squat. Professional bodybuilders have higher FMS™ scores and higher BSA scores during back squat performances than amateurs. Amateur bodybuilders perform back squats with varying quality, depending on the amount of additional load, whereas professional bodybuilders, regardless of the weight, perform back squats with consistent quality Greater experience in bodybuilding is associated with the compliance with the following BSA criteria: trunk position, frontal knee alignment, tibial translation angle, foot position in all three back squat variations with different external loads, and descent with the training weight, as professionals met these criteria more commonly than amateurs. Further studies are needed to evaluate training load, method, volume, frequency, and other parameters that can influence the quality of the back squat exercise performance.
The authors report no conflicts of interest.
Submitted: February 22, 2024 CDT, Accepted: September 09, 2024 CDT
© The Author(s)
This is an open-access article distributed under the terms of the Creative Commons Attribution 4.0 International License (CCBY-NC-4.0). View this license’s legal deed at https://creativecommons.org/licenses/by-nc/4.0 and legal code at https://creativecommons.org/licenses/by-nc/4.0/legalcode for more information.
1. Myer GD, Kushner AM, Brent JL, et al. The back squat: A proposed assessment of functional deficits and technical factors that limit performance. Strength Cond J 2014;36(6):4-27 doi:10.1519/ SSC.0000000000000103
2. Lubans DR, Morgan PJ, Cliff DP, et al. Fundamental movement skills in children and adolescents: review of associated health benefits. Sports Med 2010;40(12):1019-1035. doi:10.2165/ 11536850-000000000-00000
3. Coratella G, Tornatore G, Caccavale F, et al. The activation of gluteal, thigh, and lower back muscles in different squat variations performed by competitive bodybuilders: implications for resistance training. Int J Environ Res Public Health. 2021;18(2):1-11. doi:10.3390/ijerph18020772
4. Kristiansen M, Rasmussen GHF, Sloth ME, et al. Inter- and intra-individual variability in the kinematics of the back squat. Hum Mov Sci 2019;67(1):1-10. doi:10.1016/j.humov.2019.102510
5. Fry AC, Smith JC, Schilling BK. Effect of knee position on hip and knee torques during the barbell squat. Strength Cond J 2023;17(4):629-633.
6. Escamilla RF, Fleisig GS, Lowry TM, et al. A threedimensional biomechanical analysis of the squat during varying stance widths. Med Sci Sports Exerc 2001;33(6):984-998. doi:10.1097/ 00005768-200106000-00019
7 Swinton PA, Ray L, Keogh JWL, et al. A biomechanical comparison of the traditional squat, powerlifting squat, and box squat. Strength Cond J. 2012;26(7):1805-1816. doi:10.1519/ JSC.0b013e3182577067
8. Hirth CJ. Clinical movement analysis to identify muscle imbalances and guide exercise. Athl Ther Today 2007;12(4):10-14. doi:10.1123/att.12.4.10
9. Clark M, Lucett S. NASM’s Essentials of Corrective Exercise Training 1st ed. Wolters Kluwer Health/ Lippincott Williams & Wilkins; 2011.
10. Escamilla RF, Fleisig GS, Zheng N, et al. Effects of technique variations on knee biomechanics during the squat and leg press. Med Sci Sports Exerc 2001;33(9):1552-1556. doi:10.1097/ 00005768-200109000-00020
11. Miletello WM, Beam JR, Cooper ZC. A biomechanical analysis of the squat between competitive collegiate, competitive high school, and novice powerlifters. J Strength Cond Res 2009;23(5):1611-1617 doi:10.1519/ JSC.0b013e3181a3c6ef
12. Myer DG, Ford RK, Palumbo PJ. Neuromuscular training improves performance and lower-extremity biomechanics in female athletes. J Strength Cond Res. 2005;19(1):51-60.
13. Myer DG, Paterno VM, Ford RK. Neuromuscular training techniques to target deficits before return to sport after anterior cruciate ligament reconstruction. J Strength Cond Res 2008;22(3):987-1014. doi:10.1519/JSC.0b013e31816a86cd
14. Silva B, Clemente FM, Martins FM. Associations between Functional Movement Screen scores and performance variables in surf athletes. J Sports Med Phys Fit 2018;58(5):583-590. doi:10.23736/ S0022-4707.17.07154-7
15. Frost MD, Beach TAC, Callaghan JP. Using the Functional Movement ScreenTM to evaluate the effectiveness of training. J Strength Cond Res 2012;26(6):1620-1630. doi:10.1519/ JSC.0b013e318234ec59
16. Vehrs PR, Uvacsek M, Johnson AW Assessment of dysfunctional movements and asymmetries in children and adolescents using the Functional Movement Screen–A narrative review Int J Environ Res Public Health. 2021;18(23):1-19. doi:10.3390/ ijerph182312501
17 Cook G, Burton L, Hoogenboom B. Preparticipation screening: the use of fundamental movements as an assessment of function – Part 2. N Am J Sports Phys Ther 2006;1(3):132-139.
18. Kraus K, Schrutz E, Taylor WR, et al. Efficacy of the Functional Movement Screen: a rewiev. J Strength Cond Res 2014;28(12):3571-3584. doi:10.1519/ JSC.0000000000000556
19. Iljinaitė V, Poškaitis V, Berškienė K. The comparison and correlation among the quality of functional movements, the perception of dynamic stereotype of movement, and the regularity of performance in bodybuilding. In: The 6th International Scientific Congress: Exercise for Health and Rehabilitation. ; 2020. https:// www.healthrehabconference.eu/wp-content/uploads/ 2020/12/Book-of-Abstracts-2020.pdf
20. Cook G, Burton L, Hoogenboom BJ, et al. Functional Movement Screening: the use of fundamental movements as an assessment of function – Part 1. Int J Sports Phys Ther. 2014;9(3):396-409.
21. Minthorn LM, Fayson SD, Stobierski LM, et al. The Functional Movement Screen’s ability to detect changes in movement patterns after a training intervention. J Sport Rehabil 2015;24(3):322-326. doi:10.1123/jsr.2013-0146
22. Donnelly DV, Berg WP, Fiske DM. The effect of the direction of gaze on the kinematics of the squat exercise. J Strength Cond Res 2006;20(1):145-150.
23. Branta CF. Sport specialization: developmental and learning issues. J Phys Educ Recreat Dance 2010;81(8):19-28. doi:10.1080/ 07303084.2010.10598521
24. Brocki KC, Bohlin G. Executive functions in children aged 6 to 13: a dimensional and developmental study. Dev Neuropsychol. 2004;26(2):571-593. doi:10.1207/s15326942dn2602_3
25. Mcgill SM, Norman RW Dynamically and statically determined low back movements during lifting. J Biomech 1985;18(12):877-885. doi:10.1016/ 0021-9290(85)90032-6
26. Myer GD, Chu DA, Brent JL, et al. Trunk and hip control neuromuscular training for the prevention of knee joint injury Clin Sports Med 2008;27(3):425-448. doi:10.1016/j.csm.2008.02.006
27. Wang D, Lin XM, Kulmala JP, et al. Can the Functional Movement Screen method identify previously injured wushu athletes? Int J Environ Res Public Health. 2021;18(2):1-9. doi:10.3390/ ijerph18020721
28. Sannicandro I, Giacomo C, Traficante P Lower limb strength asymmetry and Functional Movement Screen values in professional soccer players. MOJ Sports Med 2019;3(3):59-62. doi:10.15406/ mojsm.2019.03.00081
29. Koźlenia D, Domaradzki J. Effects of combination movement patterns quality and physical performance on injuries in young athletes. Int J Environ Res Public Health 2021;18(11):1-10. doi:10.3390/ ijerph18115536
30. Chimera NJ, Knoeller S, Cooper R, et al. Prediction of Functional Movement ScreenTM performance from lower extremity range of motion and core tests. Int J Sports Phys Ther. 2017;12(2):173-181.
31. Silva B, Rodrigues LP, Clemente FM, et al. Association between motor competence and Functional Movement Screen scores. PeerJ 2019;7(8):1-18. doi:10.7717/peerj.7270
32. Campa F, Semprini G, Messina G, et al. Anthropometry, physical and movement features, and repeated-sprint ability in soccer players. Int J Sports Med. 2018;40(2):100-109.
33. Tafuri S, Notarnicola A, Monno A, et al. CrossFit athletes exhibit high symmetry of fundamental movement patterns: a cross-sectional study. Muscles Ligaments Tendons J 2016;6(1):157-160. doi:10.32098/mltj.01.2016.19
34. Alves RC, Prestes J, Enes A, et al. Training programs designed for muscle hypertrophy in bodybuilders: a narrative review Sports 2020;8(11):1-15. doi:10.3390/sports8110149
35. Cyrino ES, Sobrinho JMS, Maestá N, et al. Perfil morfológico de culturistas Brasileiros de elite em período competitivo. Rev Bras de Medicina do Esporte. 2008;14(5):460-465.
36. Hackett DA, Johnson NA, Chow CM. Training practices and ergogenic aids used by male bodybuilders. J Strength Cond Res 2013;27(6):1609-1617 doi:10.1519/ JSC.0b013e318271272a
37. Huebner M, Meltzer D, Ma W, et al. The masters athlete in olympic weightlifting: training, lifestyle, health challenges, and gender differences. PLoS ONE. 2020;15(12):1-19. doi:10.1371/journal.pone.0243652
38. Garhammer J. Power production by olympic weightlifters. Med Sci Sports Exerc 1980;12(1):54-60. doi:10.1249/00005768-198021000-00011
39. Storey A, Smith HK. Unique aspects of competitive weightlifting: performance, training and physiology. J Sports Med. 2012;42(9):769-790. doi:10.1007/BF03262294
40. Lorenzetti S, Ostermann M, Zeidler F, et al. How to squat? Effects of various stance widths, foot placement angles and level of experience on knee, hip and trunk motion and loading. BMC Sports Sci Med Rehabilitation. 2018;10(1):1-11.
41. McLaughlin T, Dillman CJ, Lardner TJ. A kinematic model of performance in the parallel squat by champion powerlifters. Med Sci Sports Exerc. 1997;9(2):128-133.
Martí Casals1a , Jordi Cortés2 , Daniel Llenderrozos3 , Miguel Crespo4 , Timothy E. Hewett5 , Lorena Martin6 , Ernest Baiget7 1 Health Sciences, National Institute of Physical Education of Catalonia , 2 Department of Statistics and Operations Research, Universitat Politècnica de Catalunya, 3 National Institute of Physical Education of Catalonia, 4 International Tennis Federation, 5 Orthopaedics , Marshall University, 6 University of Southern California, 7 Performance, National Institute of Physical Education of Catalonia
Keywords: tennis, injuries, teams competition, tennis surface, sports health
https://doi.org/10.26603/001c.123948
International Journal of Sports Physical Therapy
Vol. 19, Issue 11, 2024
Background
The demands of professional tennis, including physical and psychological aspects, contribute to the frequency of retirements at elite levels of the sport.
Purpose
The aim of this study was to explore the frequency of injuries and the factors that influence the retirements of professional tennis players competing in the Davis Cup over the last two decades.
Study Design
Retrospective cohort study
Methods
The data set includes data from 6,060 men’s singles matches that included 1,814,141 games from Davis Cup ties played between 2000 to 2019. Factors that might influence the retirements were studied by means of generalized linear models using Poisson distribution. Incidence rates by 1000 games and incidence rate ratios of retirements are provided as association measures.
Results
The retirement incidence was 1.05 per 1000 games [95% CI: 0.90, 1.21]. The main risk factors associated with retirements were matches played on hard courts (IRR: 2.52 [95% CI: 1.32, 4.83]) and matches played in the final two matches of the tie and in a best-of-5-set format (IRR: 2.63 [95% CI: 1.69, 4.09] and IRR: 5.52 [95% CI: 3.50, 8.69], respectively). The most common injuries that led to retirements were those affecting the lower extremities, specifically involving muscular or tendinous tissues.
Conclusion
This study provides valuable insights for coaches, players, support teams, and epidemiologists regarding retirements and their associated risk factors in Davis Cup tournaments. These findings may guide future research and inform strategies aimed at managing player health and performance in professional tennis.
Level of evidence
Level 2b.
Corresponding Author:
Martí Casals
Sport and Physical Activity Studies Centre (CEEAF), University of Vic – Central University of Catalonia (Uvic-UCC). Address: C. Dr. Antoni Vilà Cañellas, s/n, 08500 Vic, Spain. Tel: +34 647053785. E-mail: marticasals@gmail.com, marti.casals1@umedicina.cat a
The Davis Cup is an annual national team competition with more than 100 years of history organized by the International Tennis Federation (ITF), in which male players represent their respective national teams in a knock-out format.1 Until 2020, the format of the competition was structured in four groups based on the teams’ results in previous years. These national teams were promoted or relegated according to the results obtained in the different phases of the competition in a home and away schedule. In each tie, five matches were played to the best of five sets in three consecutive days (Friday to Sunday); with two singles matches played on the first day, the doubles match on the second day, and the last two singles matches on the third day The playing surface for each tie was selected by the hosting team. This format was used over a long period of time, with players competing in different countries and playing surfaces at different periods of the season. A new format of the competition, which was introduced in 2019, consists of two World Groups (I and II) which include an additional play-off round, and provides opportunities for more nations to compete.2
Although the competitive load in men’s professional tennis is variable depending on the result or round reached in the tournament, due to the extensive competitive calendar of the Association of Tennis Professionals (ATP) and the ITF professional circuit, high-performance players are exposed to a high weekly volume of training and competition (17-19 hours per week).3 In addition to the professional tour tournaments, many of the players also play in the Davis Cup for their respective national teams. Unlike other sports, a tennis match does not have a predefined duration, it varies according to the number of games and sets to be played to determine the winner of the match, and in particular in Davis Cup and Grand Slam matches can be well over five hours of play 4 There is also a broad consensus that over the last two decades there has been a continuous trend in the increase in the speed of strokes and movements and consequently in the overall intensity of play in professional tennis.5‑7 Players cover about 3 m per stroke, 8-12 m per point and a total of 600-800 m per set played, with about 70% of strokes being the sum of first serves, forehand and backhand rallies.4,8,9 This activity occurs at physiological intensities ranging from 12% to 80% of maximal oxygen consumption (VO2max) and 47% to 86% of maximum heart rate (HRmax), depending on the specific demands of each point or rally.10,11
Regarding health problems associated with the sport, it has been found that playing long matches on consecutive days generates neuromuscular and perceptual fatigue in the player, as well as an increase in muscle damage markers.12 There are also factors that increase the competitive stress of the player, as matches are usually played outdoors in high temperatures or humidity conditions, which have been associated with increased medical problems and retirements in Grand Slam players.13 A number of authors have explored the incidence of injuries in professional male tennis.14‑18 Incidence rates of 8.25, 3.34 and 6.64 per 1000
match exposures (EP) have been observed in ATP tournaments, on the United States Tennis Association (USTA) professional circuit and in Davis Cup respectively 15,17,19 A retirement in tennis means that a player stops playing once the match has started, and the victory is automatically awarded to the opponent. Retirements in professional tennis and their causes have also been studied.15,17,19,20 However, these studies included data from 1968 to 2013. Given the specific competition features of the Davis Cup, only one of the studies analyzed the reasons for retirements in this competition.19 Consequently, it is relevant to determine whether the increase in the intensity of play, as described above, may have led to a change in the patterns of retirements in the Davis Cup. Therefore, the aims of this study were to describe the pattern of the epidemiology of retirements, and to determine the factors that influence match retirements over the last twenty years in Davis Cup.
The Strengthening the Reporting of Observational Studies in Epidemiology statement21 was utilized to control potential biases in the design phase (Table S1 in Supplemental material). The data set contains 6060 Davis Cup singles matches played between 2000 and 2019. Six thousand thirty-six matches were analyzed after exclusion of those matches that were walk-overs (W/O; n = 24). These matches were played by professional male tennis players from all qualifying groups and regions in which the ITF structures this competition. The matches were played throughout the year (i.e., at different times of the season), and on all four types of surfaces (i.e., clay, hard, carpet, and grass). The study focused on Davis Cup singles matches and relied solely on publicly available data. As there was no direct interaction with human subjects or animals, ethical approval was not applicable for this research.
According to the current rules, a tennis match is officially recorded as a retirement when a player cannot continue due to illness or injury after the match has started.22 Only retirements due to medical conditions (injuries and illnesses) were included, not taking into account a considerable number of retirements that occur due to non-medical reasons, such as match suspensions due to weather conditions, unsportsmanlike behavior of a player, and other reasons.
For this study, data of 20 years of Davis Cup singles matches played between 2000 and 2019 were included from the datasets collected by GitHub, which are available at https://github.com/JeffSackmann. The four competition groups were unified into a world group (WG I and WG II) and a non-world group (G III and G IV) for the analysis. Specifically, the following variables were evaluated: anthropometric data (players’ age and height), match characteris-
tics (surface, number of sets played: best of three or five), group (World Group or not), round (final rounds: finals, semi-finals, quarterfinals, or other), number of matches, total number of games played per match, average age of the players in the match, and retirements (including reasons, and anatomy and body region of the injuries).
Furthermore, new variables were added to the original data set: type of retirements (medical conditions, nonmedical conditions, unknown reasons), sources of information on retirements (location of the player: home, away, neutral) which were obtained following an exhaustive search for each retirement using the Google search engine and specialized websites such as https://www.daviscup.com/; https://www.savannahsworld.blogspot.com; https://www.eurosport.com, and websites such as https://www.newspapers.com and https://www.theguardian.com For the search strategy, keywords such as “Davis Cup”, “injury”, “retirement” (accompanied by the identifying data of the match for which information was sought) were used, and sometimes adding the full name of the retired player
For the complementary analysis of medical conditions (i.e., injuries and diseases), the classification determined in the tennis-specific extension of the International Olympic Committee consensus statement, developed specifically for tennis was utilized.23 Medical conditions were classified in terms of location in four body regions: head/neck, upper extremities, trunk, and lower extremities. The type of injury was classified as: muscle/tendon, bone, joint/ligament, skin, central/peripheral nervous system or other Illnesseswere classified according to the organ system affected as: cardiovascular, respiratory, gastrointestinal, renal, urogenital, metabolic/endocrinological, haematological, dermatological, neurological, psychiatric, ophthalmic/otorhinolaryngological, dental, rheumatological, allergic, infectious, environmental and others.24
A descriptive analysis was conducted using the data from 6036 Davis Cup matches played between 2000 and 2019. This involved the calculation of both absolute and relative frequencies for all qualitative variables. Measures of central tendency (mean and median) and dispersion statistics (standard deviation and range) were determined for the quantitative variables. Furthermore, various potential influencing factors associated with match outcomes were explored. These factors included the type of playing surface, the ‘best of sets’ format, group classifications, stages of the competition (round), and the sequential number of each match. These variables were examined at a bivariable level, with group comparisons examined using chi-squared tests or Fisher’s exact tests, depending on data distribution and sample size. The significance level was set at 0.05.
EPIDEMIOLOGICAL MEASURES
Retirement incidence rate was calculated as the number of retirements per 1000 games. To calculate the unadjusted incidences, the epi.2by2 function of the R package epiR25
was mainly used, setting method as cohort.time. The number of retirements, exposure (no. games), incidence rate and the 95% confidence interval (95%CI) are provided. The raw incidence of retirements over time was studied representing their values during the range of years included in this study. In addition, adjusted relative and absolute measures of association are given as incidence rate ratio (IRR) and risk difference (with 95%CI’s). At the multivariable level, a generalized linear model was fitted, assuming the frequency of the retirements followed a Poisson’s distribution. The relationship of the incidence of retirements with age was studied, observing different behavior in two groups (< 27 years, ≥ 27 years; (Figure S1 in Supplementary Material). Consequently, two different slopes for both groups were considered in the model.
The model expression for i-th match is the following: log (λi) = log(gi)+ Xi β+ ui where λi is the expected number of retirements following a Poisson distribution, gi is the number of game exposures of matches, which in turn is the offset of this model, and Xi is the matrix containing all the independent variables of interest. Model selection was based on the Akaike Information Criterion (AIC). The fitted models were tested for overdispersion with the dispersion test function in the R package AER.26 The final model considered the interaction between the variables related to the number of sets (three or five) and the number of matches (1st; 2nd; 3rd; 4th). In addition, a logistic model was adjusted by variables prior to the match with the aim of providing the probabilities of retirement under different scenarios.
All analyses were carried according to the checklist for statistical assessment of medical papers (CHAMP) statement.27 All analyses were performed using version 4.1.3 of the R statistical software.28 The R code of the analysis carried out in this study are available at: https://github.com/ marticasals/Davis_Cup_Retirements/tree/main
DATA AVAILABILITY STATEMENT
Data and the reproducible code used in this study are available on a publicly accessible GitHub repository (https://github.com/marticasals/Davis_Cup_Retirements/ tree/main), allowing for the transparency and replicability of the statistical analysis. This ensures that other researchers can reproduce the findings of the study and build upon it for further research.
GENERAL CHARACTERISTICS OF DAVIS CUP MATCHES ACCORDING TO RETIREMENTS
A total of 6036 Davis Cup matches were included during the period from 2000 to 2019. The players had a median age of 24 years, with ages ranging from 15 to 46 years, and a median height of 185 cm, varying from 160 to 208 cm. Table 1 presents a descriptive overview of Davis Cup matches during this 20-year period, with a categorization based on the presence or absence of retirements. Of all matches, 5845
Table 1. Descriptive of the Davis Cup matches (2000-2019) based on the occurrence or not of retirements.
*Mean (SD) values were calculated for the variables “Games” and “Mean ages” (96.8%) concluded without retirements, while 191 (3.2%) had retirements.
In terms of playing surfaces, most matches were played on clay (37.6% of matches: 97.0% without retirements, 2.96% with retirements) and hard (49.5 % of matches: 96.4% without retirements, 3.62% with retirements), with grass (2.9% of matches) and hard courts experiencing a higher frequency of retirements. The most common competition format was to best of five sets (96.0% without retirements, 4.02% with retirements). Regarding the competition stage, most of the analysed matches were seen in the preliminary rounds, although there were similar percentage of retirements in the qualifying and the final rounds (3.18% and 3.10%, respectively).
The analysis of the distribution of the retirements according to the match order in each tie showed a higher percentage of retirements occurring in the last two matches played (3.72% and 3.74%) compared to the first two matched played (2.42% and 2.87%). On the other hand, a noticeable disparity in the number of games played per match in the matches with (20.6 ± 11.3 games played) and without (30.4 ± 10.7 games played) retirements was observed. In fact, there were 34% fewer games played in matches with retirements, highlighting the potential impact of retirements on match length and intensity The average age of the players in the matches with retirements
was 24.4 years (SD: 3.36) while players in the matches without retirement 24.7 (SD: 3.04) (Table 1).
Through the period analyzed, there was an exposure time of 181414 games. The general retirement incidence was of 1.05 retirements per 1000 games (95% CI: 0.90, 1.21) during Davis Cup play Three specific years (2012, 2018 and 2019) had a remarkably lower retirement incidence (Figure 1).
Both relative and absolute measures of retirement incidence in Davis Cup matches reveal a higher risk of retirements on matches played on hard courts, to best-of-five-set matches, and in the last two Davis Cup matches of the tie (Table 2). For instance, the fourth match showed the highest incidence rate in the Davis Cup, with 1.54 retirements per 1000 games. Conversely, the first match displayed the lowest retirement incidence, with 0.73 retirements per 1000 games. This indicates an IRR of retirements that is 2.10 (95% CI: 1.35, 3.28) times higher, which implies a greater risk of experiencing a retirement in the fourth match compared to the first. The risk difference between them was 0.80 (95% CI: 0.32, 1.29) retirements per 1000 games (Table 2).
Figure 1. Evolution of the incidence rate over the year-period 2000-2019 in Davis Cup. The incidence rate is expressed as the number of retirements per 1000 games played.
The IRR for the different factors of the final model chosen in matches of Davis Cup during 2000-2019 is shown in Figure 2
Regarding play surfaces, the incidence of retirements on clay, hard, or grass court was more than double than on the carpet surface, which was statistically significantly different. The incidence of retirements is also different depending on the age of the players. If they are younger (mean age below 27), the incidence rate decreases by half over fiveyear period. Meanwhile, if the mean age of the players is at least 27 years, the incidence rate doubles over a five-year period, but without reaching a statistically significant difference.
Finally, the number of the singles matches also plays a role in the number of retirements, depending on the number of sets for the tie: If the matches are at best of three sets, retirements are less usual in the third and fourth matches. On the contrary, if the matches are at best of five sets, retirements are more likely to happen in the last matches of the tie.
A table showing the retirement probabilities per match based on the variables available prior to the start of the match was generated to assess the likelihood of retirements in tennis matches (Table 3). For instance, on hard courts, approximately one out of every 50 matches results in a retirement, whereas on carpet, retirements occur in approx-
imately one out of every 125 matches. In terms of age, the highest probability of retirement is observed at the extremes of the age spectrum, such as 18 and 36 years old, with a probability of approximately one retirement in every 18 matches. Conversely, for ages between 26 and 28, the probability of retirement decreases to one in every 56 matches. Regarding the number of matches, the highest retirement probability is found in a five-game tie during the last match, where there is one retirement in every 15 matches.
Information on the reason for retirements was obtained for 74 (38.7%) of the 191 retirements in the period analyzed, while 61.3% of the retirements (n=117) were due to unknown causes. Of these 74 retirements, 73.0% (n=54/74) were due to injury, 14.9% (n=11/74) were for non-medical reasons, and 12.2% (n=9/74) were due to illness. Of the injuries, 53.7% (n=29/54) occurred when the injured player was playing away, and 46.3% (n=25/54) when playing at home. The most frequent injuries were to the lower extremities (n=34/54; 63%), were to the muscle/tendon (n=29/ 54; 54%) and were located in the thigh (n=15/54; 28%), as shown in Table 4 In terms of retirements due to illness (n=9), the most recurrent type of illness was metabolic causes with 55% (n=5/9) of the total number of illnesses,
Table 2. Retirement incidence rates, incidence rate ratio, risk difference, and the 95% confidence intervals, expressed per 1000 games played.
Davis Cup Retirements
Surface Carpet 10
Group
followed by infectious diseases (n=2/9; 22%), gastrointestinal diseases (n=1/9; 11%), and respiratory diseases (n=1/9; 11%).
This study aimed to describe the frequency of injuries and the main match factors that influence player retirements in Davis Cup matches. The analysis of 6036 Davis Cup matches spanning two decades (2000-2019) revealed a player retirement incidence of 3.2% or 1.05 retirements per 1000 games which was reasonably constant during the analyzed period. The hard-court surfaces, the matches played in best-of-five-sets, and the last two matches played in the tie were the variables that showed the highest incidence rate. Moreover, the youngest and the oldest players had higher probability of retirement. The most common reason for the retirement was injury, especially in the lower-extremities due to muscular and tendon injuries.
Previous authors have reported that the playing surface significantly affects the external and the internal match load,11,29 and the different court characteristics and game styles of tennis players might affect their retirement rate.30 The present study highlights hard court surfaces as a key factor contributing to the high incidence of retirements, as has been previously observed.31 Playing on hard courts leads to high-intensity changes of direction, resulting in high rates of acceleration and deceleration, which consequently increases the load on muscles and tendons.32
These surfaces, coupled with the endurance challenge of the best-of-five set matches, seem to increase the physical strain on players. These factors have a clear impact on retirements, as the hitting and movement workload in bestof-five matches is significantly greater than in best-ofthree-set matches.9 Furthermore, the knockout format played througout the year seems to also play a critical role, underlining the multifaceted nature of challenges faced by athletes in this elite competition.
The injury pattern found, which predominantly affected the lower extremities (62.96%), and was often related to muscular or tendinous (53.7%) and joint or ligament (42.6%) issues, aligns with the existing literature on injury trends in professional tennis.19,33 However, it is important to note that while acute injuries are more prevalent in the lower body, chronic injuries tend to affect the upper extremity 34 Most tennis injuries occur in the lower extremity (31%–67%), followed by the upper extremity (20%–49%), and lastly, the trunk (3%–21%),14 highlighting the importance of understanding the specific regions of the body that are more vulnerable to injuries in tennis. While the current study focused on retirements in Davis Cup matches, the broader context of tennis injuries highlights the need for adopting comprehensive injury prevention measures that consider the unique demands tennis places on different body regions.
A novel finding is that the incidence of retirements occurred during the preliminary rounds (3.18%) and the final phase (3.10%) of the Davis Cup are similar. This finding might show that the pressures faced by players in different
Figure 2. Incidence rate ratio for different factors of Davis Cup matches.
competitive stages (preliminary vs. final stages) in which retirements occur are similar On one hand, preliminary rounds often serve as a gateway for players striving to secure a spot in the main draw of the Davis Cup, and the pressure to perform at their best can be immense. Furthermore, players in the preliminary rounds may have already navigated through multiple matches to reach this stage, potentially leading to physical and mental fatigue.35 On the other hand, retirements during the final phase of the Davis Cup may reflect the culmination of a long and gruelling journey over the course of a whole season. The fact that the final phase is played late in the season, when players may already be experiencing cumulative physical and mental fatigue, could be considered a contributing factor. Players who have advanced to this stage have already demonstrated their skill and endurance, but they now face the added pressure of representing their country on a prestigious international stage. The desire to perform exceptionally for their nation, coupled with the accumulated physical and psychological toll of earlier matches, could be contributing factors to retirements during this phase. Future research in this area should delve deeper into understanding the specific factors that influence retirements during these critical phases of the Davis Cup. This could include examining the psychological aspects of player performance, assessing the impact of match scheduling and recovery strategies, and exploring the role of team dynamics and national pride in influencing players’ decisions to retire.
Limitations of this study include lack of comprehensive data regarding the reasons for retirement in 110 cases. One specific aspect that warrants attention is the documentation of injuries. The authors acknowledge that this study lacks detailed information on how injuries were documented during the matches. Understanding the precise nature and severity of injuries is essential for a comprehensive analysis of retirements in professional tennis. While efforts were made to gather data on retirements, the absence of detailed injury documentation is a limitation that future research should aim to address. This data gap suggests an opportunity for more granular data collection in future research to enable a more detailed exploration of retirement causes in professional tennis. Ensuring consistency in injury surveillance in tennis would greatly improve the understanding and management of injuries in the sport.
Additionally, it is important to note that while the current findings suggest an association between certain match conditions (such as hard courts and best-of-five-set formats) and a higher incidence of retirements, the study design does not allow the determination of specific factors underlying these associations. For example, hard courts might increase the risk due to their surface properties, but other factors such as match duration, player fatigue, or tournament schedule could also contribute to the higher retirement rates observed. Future research should aim to
Table 3. Retirement probabilities per match with
explore these specific factors to better understand the underlying causes of retirements.
PRACTICAL APPLICATIONS
This study presents a comprehensive analysis of retirements in Davis Cup tennis matches over a 20-year period (2000-2019), filling a notable research gap. By examining this extensive timeframe, it unveils potential shifts in retirement patterns and sheds light on the evolving landscape of player injuries and match retirements, contributing significantly to the understanding of athlete health in professional tennis tournaments. The findings from this study can help coaches better understand the risk factors associated with high-level team competitions and lays the groundwork for more targeted and effective policies aimed at safeguarding athlete health in the competitive realm of Davis Cup tennis.
This results of this study indicate a retirement incidence of 3.2% or 1.05 retirements per 1000 games, with a consistent trend throughout the analyzed period. Higher retirement rates were observed on hard courts, during best-offive-set matches, and in the last two matches of elimination rounds, highlighting specific conditions that increase the risk of retirements. Younger and older players were found to have a higher probability of retirement, indicating that
the extremes of the age spectrum are more vulnerable. The most common cause of retirement was injury, particularly affecting the lower extremities, muscles, and tendons, underlining the need for targeted injury prevention.
The authors report no conflicts of interest.
FUNDING STATEMENT/ACKNOWLEDGMENTS
This research was funded by the Ministerio de Ciencia e Innovación (Spain) (PID2019-104830RB-I00) and the Departament de Recerca i Universitats de la Generalitat de Catalunya (Spain) [2021 SGR 01421 (GRBIO)]. The funders had no role in study design, data collection and analysis, decision to publish, or preparation of the manuscript.
Submitted: April 28, 2024 CDT, Accepted: September 05, 2024 CDT
© The Author(s)
Table 4. Characteristics of the 54 Davis Cup injuries between 2000-2019* according to anatomy and body region. Variable
Injury anatomy
This is an open-access article distributed under the terms of the Creative Commons Attribution 4.0 International License (CCBY-NC-4.0). View this license’s legal deed at https://creativecommons.org/licenses/by-nc/4.0 and legal code at https://creativecommons.org/licenses/by-nc/4.0/legalcode for more information.
1. Gayton WF, Steinroeder W, Bonnica C, Loignon AC. An investigation of home disadvantage in Davis Cup tennis. Percept Mot Skills. 2009;109(2):382-386. doi:10.2466/pms.109.2.382-386
2. Davis Cup – The World Cup of Tennis. Accessed April 25, 2024. https://www.daviscup.com/en/ home.aspx
3. Vescovi JD Acute:chronic training loads in tennis: which metrics should we monitor? Br J Sports Med. 2017;51(18):1321-1322. doi:10.1136/ bjsports-2017-097596
4. Reid M, Duffield R. The development of fatigue during match-play tennis. Br J Sports Med. 2014;48(Suppl 1):i7-i11. doi:10.1136/ bjsports-2013-093196
5. Colomar J, Corbi F, Brich Q, Baiget E. Determinant physical factors of tennis serve velocity: A brief review Int J Sports Physiol Perform 2022;17(8):1159-1169. doi:10.1123/ijspp.2022-0091
6. Fernandez-Fernandez J, Kinner V, Ferrauti A. The physiological demands of hitting and running in tennis on different surfaces. J Strength Cond Res. 2010;24(12):3255. doi:10.1519/JSC.0b013e3181e8745f
7 Gale-Watts AS, Nevill AM. From endurance to power athletes: The changing shape of successful male professional tennis players. Eur J Sport Sci 2016;16(8):948-954. doi:10.1080/ 17461391.2016.1192690
8. Reid M, Morgan S, Whiteside D. Matchplay characteristics of Grand Slam tennis: implications for training and conditioning. J Sports Sci. 2016;34(19):1791-1798. doi:10.1080/ 02640414.2016.1139161
9. Whiteside D, Reid M. External match workloads during the first week of Australian Open tennis competition. Int J Sports Physiol Perform 2017;12(6):756-763. doi:10.1123/ijspp.2016-0259
10. Baiget E, Fernández-Fernández J, Iglesias X, Rodríguez FA. Tennis play intensity distribution and relation with aerobic fitness in competitive players. PLOS ONE. 2015;10(6):e0131304. doi:10.1371/ journal.pone.0131304
11. Fernandez-Fernandez J, Sanz-Rivas D, MendezVillanueva A. A review of the activity profile and physiological demands of tennis match play Strength Cond J. 2009;31(4):15. doi:10.1519/ SSC.0b013e3181ada1cb
12. Gescheit DT, Cormack SJ, Reid M, Duffield R. Consecutive days of prolonged tennis match play: performance, physical, and perceptual responses in rrained players. Int J Sports Physiol Perform 2015;10(7):913-920. doi:10.1123/ijspp.2014-0329
13. Smith MT, Reid M, Kovalchik S, Woods TO, Duffield R. Heat stress incident prevalence and tennis matchplay performance at the Australian Open. J Sci Med Sport. 2018;21(5):467-472. doi:10.1016/ j.jsams.2017.08.019
14. Abrams GD, Renstrom PA, Safran MR. Epidemiology of musculoskeletal injury in the tennis player Br J Sports Med 2012;46(7):492-498. doi:10.1136/bjsports-2012-091164
15. Hartwell MJ, Fong SM, Colvin AC. Withdrawals and retirements in professional tennis players. Sports Health 2016;9(2):154-161. doi:10.1177/ 1941738116680335
16. McCurdie I, Smith S, Bell PH, Batt ME. Tennis injury data from The Championships, Wimbledon, from 2003 to 2012. Br J Sports Med. 2017;51(7):607-611. doi:10.1136/ bjsports-2015-095552
17 Okholm Kryger K, Dor F, Guillaume M, et al. Medical reasons behind player departures from male and female professional tennis competitions. Am J Sports Med. 2015;43(1):34-40. doi:10.1177/ 0363546514552996
18. Pluim BM, Staal JB, Windler GE, Jayanthi N. Tennis injuries: occurrence, aetiology, and prevention. Br J Sports Med. 2006;40(5):415-423. doi:10.1136/bjsm.2005.023184
19. Maquirriain J, Baglione R. Epidemiology of tennis injuries: An eight-year review of Davis Cup retirements. Eur J Sport Sci 2016;16(2):266-270. doi:10.1080/17461391.2015.1009493
20. Breznik K, Batagelj V. Retired matches among male professional tennis players. J Sports Sci Med 2012;11(2):270-278.
21. Elm E von, Altman DG, Egger M, Pocock SJ, Gøtzsche PC, Vandenbroucke JP. The strengthening the reporting of observational studies in epidemiology (STROBE) statement: guidelines for reporting observational studies. J Clin Epidemiol 2008;61(4):344-349. doi:10.1016/ j.jclinepi.2007.11.008
22. International Tennis Federation | ITF. January 2, 2024. Accessed April 25, 2024. https:// www.itftennis.com/en/
23. Verhagen E, Clarsen B, Capel-Davies J, et al. Tennis-specific extension of the International Olympic Committee consensus statement: methods for recording and reporting of epidemiological data on injury and illness in sport 2020. Br J Sports Med. 2021;55(1):9-13. doi:10.1136/bjsports-2020-102360
24. Pluim BM, Fuller CW, Batt ME, et al. Consensus statement on epidemiological studies of medical conditions in tennis, April 2009. Clin J Sport Med 2009;19(6):445. doi:10.1097/JSM.0b013e3181be35e5
25. Stevenson M, Sergeant E, Heuer C, et al. epiR: Tools for the analysis of epidemiological data. March 11, 2024. Accessed April 7, 2024. https://cran.rproject.org/web/packages/epiR/index.html
26. Kleiber C, Zeileis A. Applied Econometrics with R Springer Science & Business Media; 2008. doi:10.1007/978-0-387-77318-6
27. Mansournia MA, Collins GS, Nielsen RO, et al. A CHecklist for statistical Assessment of Medical Papers (the CHAMP statement): explanation and elaboration. Br J Sports Med 2021;55(18):1009-1017 doi:10.1136/bjsports-2020-103652
28. R: The R project for statistical computing. Accessed April 25, 2024. https://www.r-project.org/
29. Pluim BM, Jansen MGT, Williamson S, et al. Physical demands of tennis across the different court surfaces, performance levels and sexes: a systematic review with meta-analysis. Sports Med. 2023;53(4):807-836. doi:10.1007/s40279-022-01807-8
30. Alexander S, Naaz N, Fernandes S. The incidence of injuries across various tennis surfaces: a systematic review. ITF Coach Sport Sci Rev. 2022;30(88):39-44. doi:10.52383/ itfcoaching.v30i88.353
31. Pluim BM, Clarsen B, Verhagen E. Injury rates in recreational tennis players do not differ between different playing surfaces. Br J Sports Med 2018;52(9):611-615. doi:10.1136/ bjsports-2016-097050
32. Damm L, Starbuck C, Stocker N, Clarke J, Carré M, Dixon S. Shoe-surface friction in tennis: influence on plantar pressure and implications for injury Footwear Sci 2014;6(3):155-164. doi:10.1080/ 19424280.2014.891659
33. Perkins RH, Davis D Musculoskeletal injuries in tennis. Phys Med Rehabil Clin 2006;17(3):609-631. doi:10.1016/j.pmr.2006.05.005
34. Fu MC, Ellenbecker TS, Renstrom PA, Windler GS, Dines DM. Epidemiology of injuries in tennis players. Curr Rev Musculoskelet Med. 2018;11(1):1-5. doi:10.1007/s12178-018-9452-9
35. Girard O, Racinais S, Micallef JP, Millet GP Spinal modulations accompany peripheral fatigue during prolonged tennis playing. Scand J Med Sci Sports 2011;21(3):455-464. doi:10.1111/ j.1600-0838.2009.01032.x
Supplemental File 1
Download: https://ijspt.scholasticahq.com/article/123948-epidemiology-and-factors-influencing-davis-cupretirements-over-the-past-twenty-years/attachment/247025.docx
McDevitt A, Cleland J, Hiefield P, Bravman J, Snodgrass S. Physical Therapy Utilization Prior to Biceps Tenodesis or Tenotomy for Biceps Tendinopathy. IJSPT 2024;19(11):1477-1489. doi:10.26603/001c.123950
Amy
McDevitt1a , Joshua Cleland2 , Paisley
Hiefield
1 , Jonathan
Bravman
3 , Suzanne
Snodgrass
4
1 Physical Medicine and Rehabilitation, University of Colorado Denver, 2 Department of Public Health and Community Medicine, Tufts University School of Medicine, 3 CU Sports Medicine, University of Colorado Health, 4 Discipline of Physiotherapy, The University of Newcastle
Keywords: biceps tendinopathy, physical therapy, biceps tenodesis https://doi.org/10.26603/001c.123950
International Journal of Sports Physical Therapy
Vol. 19, Issue 11, 2024
Introduction
Surgery for the management of individuals with long head of the biceps tendon (LHBT) tendinopathy is common. Little is known about physical therapy (PT) utilization prior to surgery The purpose of this review was to investigate the use of PT prior to biceps tenodesis and tenotomy surgeries by assessing the number of visits and the types of interventions. A secondary objective was to report on themes of PT interventions.
Methods
A retrospective observational cohort study design was used to analyze medical records and report on patient visits, procedure codes based on active or passive interventions, and themes of interventions utilized by PT
Results
Patient records (n=308) were screened for eligibility, n=62 (20.1%) patients attended PT prior to surgery The median number of PT visits was four (IQR=3.5), and 39/62 (63%) patients had four or more visits to PT Active interventions were used in 54.5% (533/978) of the codes billed; passive interventions were used in 45.5% (445/978) of the codes. There was high utilization of therapeutic exercise [93.4% (498/533) of active procedure codes] including muscle performance/resistance, functional activity, motor control and stretching. Manual therapy [84.3% (375/445) of passive procedure codes] included soft tissue mobilization, non-thrust manipulation (glenohumeral joint and cervical spine) and thrust manipulation (thoracic spine).
Conclusions
PT was not commonly utilized prior to undergoing biceps tenodesis and tenotomy surgery Further research is needed to understand the reasons for low utilization.
3b
INTRODUCTION
Shoulder pain related to pathology of the long head of the biceps tendon (LHBT) can be debilitating. The long head of the biceps tendon (LHBT) is a known pain generator of the shoulder and can interfere with an individual’s activity and participation.1‑3 Tendinopathy of the LHBT may start as an inflammatory condition or tenosynovitis1‑3 and may progress to a degenerative tendinopathy (character-
Corresponding Author:
Amy McDevitt PT, DPT, PhD, OCS, FAAOMPT University of Colorado School of Medicine 13121 E 17th Avenue C-244 Aurora, Colorado 80045 amy.mcdevitt@cuanschutz.edu 303-902-3312 a
ized by tendon thickening, disorganization, and irregularity of the tissue including the presence of hemorrhagic adhesions and scarring).3 The overall incidence of LHBT tendinopathy remains uncertain due to its presence as a secondary shoulder condition associated with other shoulder pathology including rotator cuff disease and subacromial impingement.1,4 Overall, there remains a paucity of literature regarding diagnosis, and appropriate management of disorders related to the LHBT, including physical therapy (PT) management and surgical intervention.1,4‑6
There is little consensus regarding the optimal approach to treating chronic anterior shoulder pain due to the LHBT tendinopathy.2,3 Conservative management including PT, activity modification, NSAIDS, and steroid injections in the biceps sheath are often recommended prior to more invasive interventions.3,7‑9 However, conservative management may be suboptimal and provide only partial/temporary relief of symptoms and many individuals go on to seek more invasive surgical procedures including biceps tendon reattachment (tenodesis) or release (tenotomy).1,10
Physical therapy management of anterior shoulder pain (including LHBT tendinopathy) may involve a multimodal approach addressing impairments of the shoulder, scapular region and cervicothoracic spine. Further, interventions may include therapeutic exercise, joint and soft tissue mobilization as well as retraining of dysfunctional movement patterns.3 Information on the management of subacromial shoulder pain is robust,11‑14 however, there remains a lack of high-quality literature describing PT management of individuals with LHBT tendinopathy in isolation.15‑20 Most randomized controlled trials exploring PT management for LHBT pain involve the utilization of biophysical agents (ultrasound, electrotherapy, extracorporeal shockwave therapy and iontophoresis) and are of questionable study quality 12,15‑20 Invasive surgical intervention is one approach to managing chronic biceps tendinopathy pain, therefore, to potentially avoid such procedures it is essential for physical therapists to recognize other interventions that may be effective in treating LHBT tendinopathy 21 A retrospective chart review is a first step in determining the typical PT interventions utilized in this population to support next steps, which may include the development of randomized intervention trials. The purpose of this retrospective chart review was to investigate the use of PT prior to biceps tenodesis and tenotomy surgeries by assessing the number of visits and use of different interventions and whether they were active or passive. A secondary objective was to report on the themes of PT interventions used in treatment.
STUDY DESIGN
This was a retrospective observational cohort study of patients who underwent biceps tenodesis or tenotomy for biceps tendinopathy in a large healthcare system from March 15, 2016, through March 15, 2020, with presurgical physical therapy utilization (active and passive billing codes) extracted for each individual up to 24 months prior to surgery.
To guide study reporting, the REporting of studies Conducted using Observational Routinely-collected health Data (RECORD)22 statement was utilized, an extension of the Strengthening the Reporting of Observational Studies in Epidemiology (STROBE) statement.23
Data were extracted from electronic medical records of the UC Health University of Colorado Hospital system. These data include person-level data for all outpatient physical therapy visits. They also included information about physical therapy procedures and subsequent biceps tenodesis or tenotomy surgery
This retrospective medical chart review study was approved by the Colorado Multiple Institutional Review Board (Protocol 20-2235) and the Human Research Ethics Committee at the University of Newcastle (H-2021-0009).
The medical records of eligible patients between the ages of 18 and 85 years of age who underwent biceps tenotomy or tenodesis surgery at the UC Health University of Colorado Hospital between March 15, 2016, and March 15, 2020 were included in the cohort. The study cohort was identified using current procedural terminology (CPT) codes most used for biceps tenodesis (23430) and biceps tenotomy (23405) within a four-year period while excluding patients who underwent concomitant procedures such as rotator cuff repair (29827), distal clavicle excision (29824), and labral repair (23455 and 29807). Arthroscopic biceps tenodesis (29828) was also excluded since this surgery is typically performed in conjunction with another surgery listed above. Subacromial decompression (29826) was not excluded since it is presumed not to interfere with the outcomes of biceps tenodesis or tenotomy Patients who saw a physical therapist within two years prior to surgery for shoulder pain related to LHBT tendinopathy were included in the analysis. Data were initially filtered by an orthopedic research administrative staff by surgical CPT codes to track which patients had the surgery of interest (23430 and 23405) and dates of surgery to determine preliminary eligibility and determine patients who had the surgery of interest without other associated surgeries. March 15, 2020, served as the cut-off date as all ambulatory services and elective surgeries in the hospital system were significantly impacted by the COVID pandemic after this time period.
Two investigators (AM, PH) completed the next phase of detailed chart reviews on all patient records meeting inclusion criteria based on filtering of CPT codes and dates. Individual charts were reviewed to verify further inclusion based on rehabilitation billing codes, operative reports and rehabilitation notes.
Table 1. Description of Surgical and Rehabilitation Procedure Codes
Description of Surgical Procedure CPT Codes
Biceps tenodesis 23430
Biceps tenotomy 23405
Subacromial decompression 29826
Description of Rehabilitation Procedure CPT Codes
Physical Therapy Visit 97010-97799
Physical Therapy Evaluation-low complexity 97161
Physical Therapy Evaluation-moderate complexity 97162
Physical Therapy Evaluation-high complexity 97163
Physical Therapy Re-evaluation 97164
Active Physical Therapy Codes
Passive Physical Therapy Codes
Other
Therapeutic exercise
Therapeutic activity
Neuromuscular Re-ed Self-care home management 97110 054299 97530 054301 97112 97535 54303
Manual Therapy
Dry Needling
Ultrasound/Phonophoresis
Hot/cold Iontophoresis
Electrical stimulation
TENS/ES 997140 054329 97799 600245 570629 97035 054282 97010 97033 97014 97032 054311
Vasopneumatic Device
Mechanical Traction Physical Performance Test 97016 054309 054305
Abbreviations: CPT, Current Procedural Terminology; Re-ed, Re-education; TENS, Transcutaneous Electrical Nerve Stimulation; ES, Electrical Stimulation.
Billing code data were initially used to screen which patients had the surgery of interest (23430 and 23405). Following the screening of surgery codes, CPT codes for rehabilitation informed the research team to which patients engaged with physical therapy prior to surgery (within two years of surgery). The use of PT prior to surgery was of interest including the number of individual rehabilitation visits. To satisfy the criteria for physical therapy utilization, patients needed to have at least one PT evaluation (97161-97163) specifically for a shoulder diagnosis (on the same side as the operative side) within 24 months years prior to surgery All rehabilitation visits based on physical therapy procedure codes were also identified (Table 1). The research team did not record or analyze codes related to PT evaluation or assessment of the patient as the research question involved active and passive intervention codes.
Data obtained from general chart review included patients’ age, sex, procedural side (right or left) and baselines scores for patient reported outcome measures including the nu-
meric pain rating scale (NPRS) and The Shortened Disabilities of the Arm, Shoulder and Hand Questionnaire (QuickDASH). Surgical data collected included procedure(s) performed, date of surgery, and relevant perioperative and operative notes. Chart review of the treating physical therapists’ notes included date of PT episode relative to the surgery date, PT problem list (to determine if the patient had anterior shoulder pain vs low back pain or other complaints), shoulder physical examination findings (pain localization, palpation, range of motion, Speeds and Yergason’s tests), and all PT notes in the episode of care (to document specific interventions related to the active and passive codes). Information from PT chart notes included examination and all follow-up treatments which were recorded. Data were extracted using a data collection template created in an excel spreadsheet.
The aim of the data analysis was to describe the frequency of patients who attended PT prior to surgery, therefore, the total number of visits patients attended physical therapy was calculated. A secondary aim was to describe the PT in-
terventions received. Active and passive procedure codes were identified from chart review. Active procedure codes were procedure codes used when the patient is actively participating as a component of the PT intervention; these included therapeutic exercise, therapeutic activity, neuromuscular re-education and self-care home management. Passive procedure codes are codes used when the patient is passively receiving an intervention void of patient participation; these included manual therapy, therapeutic modalities, dry needling and other The percentage of active and passive codes were calculated. Further, thematic analysis of the interventions within each code, based on chart review of rehabilitation notes, was performed for both active and passive procedure codes. Two investigators individually categorized them to organize the qualitative, intervention data into themes. The two investigators (AM, PH) then came together to reach a consensus. Inconsistencies between the reviewers were resolved by discussion, and, if needed, a third reviewer was consulted (SS). The types of interventions performed within each theme were tabulated. Due to the heterogeneous nature of the physical therapy interventions, a quantitative analysis of PT interventions was not feasible.
Of 308 eligible patients who underwent biceps tenodesis or tenotomy surgery 79.9% (246/308) of the total cohort did not receive PT prior to surgery; 20.1% (62/308) patients attended PT for LHBT pain within two years of surgery, and met the inclusion criteria for further analysis (Figure 1). Demographics of the cohort are described in (Table 2). The 62 patients who attended physical therapy had a total of 355 visits for their reported shoulder pain. Of the 62 patients who initiated physical therapy, 11.3% (7/61) received no additional care beyond the initial evaluation. The median number of PT visits for patients was four (IQR=3.5), 22 patients had three visits or less and 64.5% (40/62) participants had four or more visits of PT (Figure 2). After tabulation of active and passive procedure codes, 54.5% (533/ 978) of the codes were active and 45.5% (445/978) of the codes were passive. There was high utilization of the active codes for therapeutic exercise and activity [96.4% (514/ 533)] and the passive procedure code of manual therapy [84.3% (375/445)]. Among the remaining 3.6% (19/445) of active codes, interventions included neuromuscular re-education [2.3% (12/533)] and self-care home management [1.3% (7/533)]. Among the remaining 15.7% (70/445) of passive codes, interventions included therapeutic modalities [10.6% (47/445)], dry needling [3.4% (15/445)] to the shoulder region, and other [1.8% (8/445)] (Figure 3).
Theme and coding synthesis of the chart notes within the active code of therapeutic exercise, revealed themes of resistance exercise/muscle performance (subthemes: tendon loading techniques and progressive resistance exercise) and muscle length/mobility (subthemes: stretching and flexibility and range of motion). Theme and coding synthesis of the chart notes within the active code of therapeutic activity included the theme of functional activity.
The active code of neuromuscular re-education revealed the theme of motor control training (subthemes: stabilization and muscle re-education). The active code of self-care home management revealed the theme of motor control training (subthemes: stabilization and muscle re-education). Interventions specific to identified themes and subthemes of chart notes based on active codes can be found in Table 3
Theme and coding synthesis of the chart notes within the passive code of manual therapy included themes of joint mobility (subthemes: non-thrust manipulation and thrust manipulation), soft tissue mobilization (subthemes: general techniques and specific techniques) to the shoulder region and LHBT and range of motion (subthemes: passive range of motion and active assisted range of motion). Among the other passive codes, theme analysis of chart notes included the use of biophysical agents which was the theme for the following billing codes (electrical stimulation, transcutaneous electrical nerve stimulation, iontophoresis, ultrasound/phonophoresis, and hot/cold therapy) typically applied to the shoulder region and/or LHBT Synthesis of chart notes within the passive code of dry needling revealed the themes of dry needling without electrical stimulation and with electrical stimulation to LHBT, glenohumeral and scapular muscles in the shoulder region. Additional themes, subthemes and interventions derived from chart notes of passive codes can be found in Table 4.
The purpose of this retrospective review was to report on the use of PT for the treatment of LHBT tendinopathy by describing the number of visits and the use of active and passive interventions as defined by procedure codes. A secondary objective was to report on the interventions utilized as described in the PT chart notes. The results indicate overall low utilization of PT prior to surgery for individuals with LHBT (62 patients over four years in a large hospital system). Treating therapists utilized active interventions slightly more than passive interventions, as defined by the procedural codes they selected. The most common interventions were therapeutic exercise (progressive resistance exercise, tendon loading techniques and stretching) and manual therapy (joint mobility, soft tissue mobilization and range of motion), suggesting that a multi modal approach is being utilized. However, there is a lack of evidence for the treatment of LHBT tendinopathy in isolation, therefore these findings provide a first step in understanding how physical therapists manage patients with LHBT tendinopathy.
The following sections aim to better explain these findings, interpret the findings in the context of PT care for shoulder pain including LHBT tendinopathy, and highlight clinical implications, limitations and future directions for research.
Figure 1. Flow Diagram of Eligiblity
Abbreviations: CPT, current procedural terminology; EMR, electronic medical records; PT, physical therapy; SLAP, superior labrum anterior and posterior; sx, surgery
Of patient records meeting eligibility criteria, only 20.1% of patients attended PT for LHBT pain within two years prior to biceps tenodesis or tenotomy surgery. Similarly, in a study of patients who had arthroscopic rotator cuff repair, only 21% of patients received some form of PT in the year prior to their surgery24 which is consistent with the current results. The combined results of the current medical records review and the study by Malik et al.24 are surprising, given contemporary evidence has shown PT is effective for the management of shoulder pain.11,12 Patients may lack knowledge of the benefits of PT for shoulder pain, patients may have barriers to attending PT or may not want to attend due to unknown reasons. According to a recent article on patient expectations, one factor that may affect patients seeking PT care may be that patients lack understanding of PT care including the role of PT 25 Further, patients may not be referred for PT by their general practi-
tioner or specialist, or patients may have had prior PT for LHBT with limited success. In addition, LHBT tendinopathy is difficult to diagnose3,7,26 which may further complicate management pathways.
The median number of PT visits for participants was four (IQR=3.5), and 40 (64.5%) patients had four or more visits to PT. In a study of Medicare beneficiaries with just under 2000 episodes of care for low back, shoulder, or knee pain, patients attended PT for 6.8 visits (SD=4.7) on average over a median of 27 days.27 The results of this medical records review demonstrated a lower number of visits per patient for LHBT which may relate to 1) access issues in a large hospital-based system, 2) other patient-specific reasons stated above, 3) patients in the sample are active and younger (mean age was 43 years +/-13.7), 4) patients may feel equipped to manage their care independently through a home program or other avenues.
Table 2. Descriptive Characteristics of Patient Sample (n=62)
Variable Data
Age
43.32 (+/- 13.73)
Sex 30 (male), 32 (female)
Days prior to surgery†
250.74 (+/- 195.87)
Biceps sheath injection prior to PT 11/62 (17.7%)
Surgical procedure information
Side of surgery 38 (right), 24 (left)
Biceps tenodesis 61/62 (98.4%)
Biceps tenotomy 1/62 (1.6%)
Patient reported outcome
NPRS maximum (0-10)a 6.02 (+/- 2.48)
NPRS minimum (0-10)a 2.10 (+/- 2.15)
NPRS current (0-10)a 3.02 (+/- 2.32)
NPRS average (0-10)a 3.76 (+/- 2.37)
QuickDASH (0-100)a 42.78 (+/- 21.67)
Values are presented as mean +/- SD unless otherwise indicated.
Days prior to surgery†, days between physical therapy visit and date of surgery
Abbreviations: NPRS, Numeric Pain Rating Scale; PT, Physical Therapy; QuickDASH, The Shortened Disabilities of the Arm, Shoulder and Hand Questionnaire
a Lower Scores are better b Higher Scores are better
PROCEDURE CODES AND INTERVENTION THEMES
The results of this review demonstrate high utilization of active interventions (54.5% of procedure codes) with therapeutic exercise and activity (96.4%) being the most utilized intervention codes. Therapeutic exercise are activities that include specific muscles at specific joints while therapeutic activities are dynamic activities used to increase func-
tional performance. A recent update of systematic reviews made a strong recommendation for “exercise therapy” as the first-line treatment to improve pain, mobility, and function in patients with subacromial pain syndrome (SAPS),11 however, it is difficult to determine if these recommendations extend to managing pain specific to LHBT tendinopathy. These conditions of the shoulder have some symptoms in common, and in some patients present concurrently
Figure 3.
Abbreviations: CPT=current procedural terminology; ESTIM=electrical stimulation; TENS=transcutaneous electrical nerve stimulation; Re-ed=re-education
Therefore exercise-based interventions recommended for patients with SAPS such as strengthening, flexibility, and range of motion may also have benefits for LHBT pathology. Intervention themes related to therapeutic exercise included resistance exercise/muscle performance, progressive resistance exercise, and stretching. Several interventions were utilized in the exercise subtheme of tendon loading techniques such as heavy slow load activities which are well supported in the literature for the treatment of tendinopathy28,29 therefore, therapists may be practicing in alignment with guidelines for managing tendinopathies. The main interventions related to the procedural code of therapeutic activity were functional activities (such as reaching, lifting, occupation, and sport-specific activities). Overall, utilization of therapeutic exercise and activity by the clinicians who treated this sample, is in alignment with current recommendations for SAPS.11,12,30
Passive interventions represented 45.5% of the procedure codes, with high utilization of manual therapy (86.2%) among the passive codes. In an update of systematic reviews specific to patients with shoulder pain, manual therapy (joint mobilization and manipulation, soft tissue techniques, neurodynamic mobilizations, and mobilizations with movement) was an intervention with a strong recommendation especially when combined with exercise.11 Manual therapy interventions utilized by PTs in this medical chart review, included soft tissue mobilization, nonthrust and thrust manipulation of the glenohumeral joint,
thoracic spine, and cervical spine which are consistent with contemporary evidence for managing SAPS.11,12,31 While it is encouraging that PT interventions were consistent with contemporary evidence for the treatment for shoulder pain, it is unknown if these evidence-based recommendations are applicable to LHBT tendinopathy. A recent Delphi study on PT interventions for treating individuals with LHBT tendinopathy included the following themes within the manual therapy recommendation: soft tissue mobilization (including deep transverse friction and trigger point release), and thrust and non-thrust manipulation to the glenohumeral joint, thoracic spine and cervical spine32 which do align with the findings of this medical records review Additional Delphi study recommendations included the use of a multimodal approach including exercise combined with manual therapy which again, is consistent with our current findings.32
Among the 20% of passive codes in the current study not attributed manual therapy, interventions included therapeutic modalities (10.5%) and dry needling (3.4%) to the shoulder region and LHBT Several studies have investigated the use of therapeutic modalities to treat pain specific to the LHBT including iontophoresis, ultrasound, low level laser, and extracorporeal shock wave therapy, with reported improvements in pain or function.15,18‑20 A case series of ten individuals with LHBT tendinopathy reported reduced pain and disability and avoided surgery after dry needling, stretching and tendon loading techniques.21 Pas-
Table 3. Themes and subthemes from active procedural codes
Procedure Code Theme
Therapeutic Exercise
Resistance Exercise/ Muscle Performance
Therapeutic Activity
Neuromuscular Reeducation
Muscle Length/Mobility
Self-Care Home Management
Functional Activity
Motor Control Training
Patient Education
Load modification Physical
Subthemes and Interventions
Tendon Loading Techniques (target tissue and/or action)
Isometric tendon loading (biceps brachii muscle)
Concentric tendon loading (biceps brachii muscle/shoulder flexion)
Concentric tendon loading (biceps brachii muscle/elbow flexion)
Eccentric tendon loading (biceps brachii muscle/shoulder flexion)
Eccentric tendon loading (biceps brachii muscle/elbow flexion)
Progressive Resistance Exercise (target tissue or action)
Elbow flexion and shoulder flexion (biceps brachii muscle)
Scapular muscles (upper/middle/lower trapezius, serratus anterior, rhomboid major/minor muscles)
Rotator cuff internal (medial) rotation
Rotator cuff external (lateral) rotation
Stretching and Flexibility (target tissue)
Pectoralis major and minor muscles
Upper trapezius muscle
Biceps brachii muscle
Posterior rotator cuff muscles
Glenohumeral medial (internal) rotators
Latissimus dorsi muscle
Range of Motion (region or joint)
Active range of motion (shoulder)
Active assisted range of motion with equipment (shoulder)
Passive range of motion (shoulder)
Active range of motion (thoracic spine)
Functional Activities
Reaching, lifting and overhead activity
Occupation specific
Sport specific
Stabilization
Lumbar, hip, and abdominal stabilization
Scapular stabilization
Muscle Re-education
Shoulder re-education (unspecified)
Scapular re-education (unspecified)
Proprioceptive neuromuscular re-education to scapula
Kinesio® Taping techniques
Posture Education
Postural control (static) activity
Postural control (with movement) activity
Activity Related Education
Activity modification
Avoid aggravating activities
Active recovery
Rest
Procedure
Subthemes and Interventions
Shoulder positioning for decreased pain (with activity and at rest)
Expectations anticipating and following surgery
sive interventions utilizing therapeutic modalities including iontophoresis, electrical stimulation and ultrasound therefore appear to be consistent with available evidence.
A limitation to this study was that we were unable to identify patients with LHBT tendinopathy who did not have biceps tenodesis or tenotomy surgery. We did not use the ICD-10 diagnosis codes M75.21 and M75.22 for bicipital tendinitis of the right and left shoulder respectively, because in the medical records system analyzed in this study, diagnoses of the shoulder are often coded more broadly using the ICD-10 code for shoulder pain M25.51. There are a number of reasons clinicians may use this code, one of which is that LHBT pathology often accompanies other primary shoulder pathologies and LHBT is difficult to definitively diagnose2 and may not be diagnosed at an initial visit. However, this makes it difficult to track patients with a specific diagnosis in electronic medical records. The only mechanism to track patients with the pathology of interest (LHBT tendinopathy) was to follow them retrospectively from their date of surgery. As a result, another related limitation is the inability to report on patients who went to PT prior to surgery and improved and therefore did not elect to have surgery Again, the use of ICD-10 codes that are more general such as “shoulder pain” creates a barrier to identifying patients with a specific diagnosis. This retrospective chart review would have been more comprehensive if LHBT tendinopathy was more explicitly diagnosed; this would have afforded us the ability to track patients who went through a course of PT regardless of whether they had surgery We are therefore unable to determine if the number of visits and PT-based interventions provided would have been different for those who did not have surgery and we are unable to determine if our studied sample is more inclusive of those who “failed” conservative management. Further, it is possible that patients excluded from our sample received physical therapy care outside of the healthcare system which is challenging to track due to a lack of documentation including billing codes.
Physical therapy is underutilized prior to biceps tendon surgeries and few guidelines exist to guide clinical care for LHBT tendinopathy. Guidelines exist for the management of SAPS and for tendinopathy, including tendinopathies of the rotator cuff, which may serve as a guide due to the paucity of recommendations specific to LHBT tendinopathy Based on this review, when PT was utilized, active interventions were utilized more often than passive interven-
tions, and the common themes from clinician records of exercise, manual therapy, and therapeutic modalities were all consistent with evidence-based recommended interventions used to treat SAPS and tendinopathy Further research in the form of randomized controlled trials is needed to determine if these intervention approaches provide optimal effective care for patients with LHBT tendinopathy
Physical therapy was not commonly utilized prior to undergoing biceps tenodesis and tenotomy surgery by patients seeking care in a large hospital-based health system. Further research is needed to understand the reasons for poor utilization and whether the PT interventions commonly utilized provide optimal care for patients with LHBT tendinopathy.
All authors do not have conflicts of interest to report.
This work was supported by The American Academy of Orthopedic Manual Physical Therapists (AAOMPT) under a grant from Cardon Rehabilitation (Ontario, Canada). Neither AAOMPT nor the funding agency had any role in the study design, analysis, interpretation, or decisions about publication.
Submitted: May 02, 2024 CDT, Accepted: September 05, 2024 CDT
© The Author(s)
Table 4. Themes and subthemes from passive procedure codes
Procedure Code Theme
Joint Mobility
Subthemes and Interventions
Non-thrust Manipulation (grade I-IV) Region
Glenohumeral joint
Thoracic spine
Cervical spine
Acromioclavicular joint
Thrust Manipulation (grade V) Region
Thoracic spine
Cervical spine
Specific Techniques (target tissue)
Deep transverse friction (biceps brachii muscle, LHBT)
Trigger point therapy (biceps brachii muscle)
Manual Therapy
Soft Tissue Mobilization
Range of Motion
Electrical Stimulation
TENS
Iontophoresis
Ultrasound/ Phonophoresis
Hot/Cold
Therapeutic Modalities
Dry Needling without Electrical Stimulation
Dry Needling
Dry Needling with Electrical Stimulation
Instrument-assisted soft tissue mobilization (biceps brachii muscle, shoulder)
General Techniques (target tissue)
Soft tissue techniques (biceps brachii muscle)
Soft tissue mobilization (periscapular muscles)
Soft tissue mobilization (scapular muscles)
Soft tissue mobilization (rotator cuff muscles)
Soft tissue mobilization (cervical region)
Passive Range of Motion (region or joint)
Passive range of motion (shoulder)
Passive range of motion (scapula)
Active Assisted Range of Motion (region or joint)
Active assisted range of motion (shoulder)
Electrical Stimulation (target tissue)
Shoulder and anterior shoulder (with heat or ice and without heat or ice)
TENS (target tissue)
Shoulder
Iontophoresis (target tissue)
Iontophoresis with dexamethasone (anterior shoulder/LHBT)
Ultrasound/Phonophoresis (target tissue)
Ultrasound (long head of biceps tendon and anterior shoulder)
Thermal Agents (target tissue)
Moist heat (shoulder or cervical region)
Cold pack (shoulder)
Ice massage (anterior shoulder)
Dry Needling without Electrical Stimulation (target tissue)
Dry needling (long head of the biceps tendon)
Dry needling (biceps brachii muscle)
Dry needling (rotator cuff muscles)
Dry needling (upper trapezius)
Dry needling (deltoid)
Dry Needling with Electrical Stimulation Location (target tissue)
Dry needling (deltoid)
Procedure Code Theme
Subthemes and Interventions
Dry needling (rotator cuff muscles)
Dry needling (upper trapezius)
Dry needling (biceps brachii muscle)
Dry needling (pectoralis major)
Dry needling (latissimus dorsi)
Abbreviations: LHBT=long head of the biceps tendon; TENS=transcutaneous electrical nerve stimulation
This is an open-access article distributed under the terms of the Creative Commons Attribution 4.0 International License (CCBY-NC-4.0). View this license’s legal deed at https://creativecommons.org/licenses/by-nc/4.0 and legal code at https://creativecommons.org/licenses/by-nc/4.0/legalcode for more information.
1. Nho SJ, Strauss EJ, Lenart BA, et al. Long head of the biceps tendinopathy: diagnosis and management. J Am Acad Orthop Surg. 2010;18(11):645-656. doi:10.5435/00124635-201011000-00002
2. Ahrens PM, Boileau P The long head of biceps and associated tendinopathy. J Bone Joint Surg Br. 2007;89(8):1001-1009. doi:10.1302/ 0301-620X.89B8.19278
3. Krupp RJ, Kevern MA, Gaines MD, Kotara S, Singleton SB. Long head of the biceps tendon pain: differential diagnosis and treatment. J Orthop Sports Phys Ther. 2009;39(2):55-70. doi:10.2519/ jospt.2009.2802
4. Murthi AM, Vosburgh CL, Neviaser TJ. The incidence of pathologic changes of the long head of the biceps tendon. J Shoulder Elbow Surg 2000;9(5):382-385. doi:10.1067/mse.2000.108386
5. Griffin JW, Cvetanovich GL, Kim J, et al. Biceps tenodesis is a viable option for management of proximal biceps Injuries in patients less than 25 years of age. Arthroscopy. 2019;35(4):1036-1041. doi:10.1016/j.arthro.2018.10.151
6. Pogorzelski J, Horan MP, Hussain ZB, Vap A, Fritz EM, Millett PJ. Subpectoral biceps tenodesis for treatment of isolated type II SLAP lesions in a young and active population. Arthroscopy 2018;34(2):371-376. doi:10.1016/j.arthro.2017.07.021
7. Lewis RB, Reyes BA, Khazzam MS. A review of recent advances in the diagnosis and treatment modalities for long head of bicep tendinopathy Clinical Medicine Insights: Trauma and Intensive Medicine 2016;7:CMTIM.S39404. doi:10.4137/ CMTIM.S39404
8. Paynter KS. Disorders of the long head of the biceps tendon. Phys Med Rehabil Clin N Am 2004;15(2):511-528. doi:10.1016/ S1047-9651(03)00131-1
9. Schickendantz M, King D Nonoperative management (including ultrasound-guided injections) of proximal biceps disorders. Clin Sports Med 2016;35(1):57-73. doi:10.1016/ j.csm.2015.08.006
10. Becker DA, Cofield RH. Tenodesis of the long head of the biceps brachii for chronic bicipital tendinitis. Long-term results. J Bone Joint Surg Am 1989;71(3):376-381. doi:10.2106/ 00004623-198971030-00011
11. Pieters L, Lewis J, Kuppens K, et al. An update of systematic reviews examining the effectiveness of conservative physical therapy interventions for subacromial shoulder pain. J Orthop Sports Phys Ther 2020;50(3):131-141. doi:10.2519/jospt.2020.8498
12. Diercks R, Bron C, Dorrestijn O, et al. Guideline for diagnosis and treatment of subacromial pain syndrome: a multidisciplinary review by the Dutch Orthopaedic Association. Acta Orthop. 2014;85(3):314-322. doi:10.3109/ 17453674.2014.920991
13. Christiansen DH, Frost P, Frich LH, Falla D, Svendsen SW The use of physiotherapy among patients with subacromial impingement syndrome: Impact of sex, socio-demographic and clinical factors. PLoS One 2016;11(3):e0151077 doi:10.1371/ journal.pone.0151077
14. Page MJ, Green S, McBain B, et al. Manual therapy and exercise for rotator cuff disease. Cochrane Database Syst Rev 2016;(6):CD012224. doi:10.1002/14651858.CD012224
15. Alizadeh A, Mardani-Kivi M, Ebrahimzadeh MH, Rouhani A, Hashemi K, Saheb-Ekhtiari K. A randomized prospective comparative study of four methods of biceps tendonitis treatment: Ultrasound, low-level laser+ ultrasound, intra-sheath, and extrasheath corticosteroid guided injection. Shiraz E Med J. 2018;19(11). doi:10.5812/semj.67138
16. Barbosa RI, Goes R, Mazzer N, Fonseca MCR. The influence of joint mobilization on tendinopathy of the biceps brachii and supraspinatus muscles. Brazilian Journal of Physical Therapy 2008;12:298-303. doi:10.1590/ S1413-35552008000400008
17 Živanović S, Zgradić I, Jevtić M, Petrović-Rackov L. Combined therapy of exudative and stenosing tenosynovitis. Medicus 2007;8(1):23-27 http:// scindeks.ceon.rs/ article.aspx?artid=1450-79940701023Z
18. Liu S, Zhai L, Shi Z, Jing R, Zhao B, Xing G. Radial extracorporeal pressure pulse therapy for the primary long bicipital tenosynovitis a prospective randomized controlled study Ultrasound Med Biol 2012;38(5):727-735. doi:10.1016/ j.ultrasmedbio.2012.01.024
19. Taskaynatan MA, Ozgul A, Ozdemir A, Tan AK, Kalyon TA. Effects of steroid iontophoresis and electrotherapy on bicipital tendonitis. J Musculoskelet Pain 2007;15(4):47-54. doi:10.1300/J094v15n04_06
20. Xiao L, Zou J, Fang F. Study of the therapeutic effects of betamethasone injection combined with musculoskeletal ultrasonography compared with radial shock wave therapy in the treatment of tenosynovitis of the long head of the biceps brachii. Am J Transl Res 2021;13(3):1734-1741. https:// www.ncbi.nlm.nih.gov/pubmed/33841696
21. McDevitt AW, Snodgrass SJ, Cleland JA, Leibold MBR, Krause LA, Mintken PE. Treatment of individuals with chronic bicipital tendinopathy using dry needling, eccentric-concentric exercise and stretching; a case series. Physiother Theory Pract 2020;36(3):397-407. doi:10.1080/ 09593985.2018.1488023
22. Benchimol EI, Smeeth L, Guttmann A, et al. The REporting of studies conducted using observational routinely-collected health data (RECORD) statement. PLoS Med 2015;12(10):e1001885. doi:10.1371/ journal.pmed.1001885
23. von Elm E, Altman DG, Egger M, et al. The strengthening the reporting of observational studies in epidemiology (STROBE) statement: guidelines for reporting observational studies. Int J Surg 2014;12(12):1495-1499. doi:10.1016/ j.ijsu.2014.07.013
24. Malik AT, Sridharan M, Bishop JY, et al. Health care utilization and costs in the year prior to arthroscopic rotator cuff repair. Orthopaedic Journal of Sports Medicine 2020;8(7):232596712093701. doi:10.1177/2325967120937016
25. Subialka JA, Smith K, Signorino JA, Young JL, Rhon DI, Rentmeester C. What do patients referred to physical therapy for a musculoskeletal condition expect? a qualitative assessment. Musculoskelet Sci Pract 2022;59:102543. doi:10.1016/ j.msksp.2022.102543
26. Ejnisman B, Monteiro GC, Andreoli CV, de Castro Pochini A. Disorder of the long head of the biceps tendon. Br J Sports Med 2010;44(5):347-354. doi:10.1136/bjsm.2009.064139
27. Fritz JM, Hunter SJ, Tracy DM, Brennan GP. Utilization and clinical outcomes of outpatient physical therapy for medicare beneficiaries with musculoskeletal conditions. Phys Ther. 2011;91(3):330-345. doi:10.2522/ptj.20090290
28. Cardoso TB, Pizzari T, Kinsella R, Hope D, Cook JL. Current trends in tendinopathy management. Best Pract Res Clin Rheumatol 2019;33(1):122-140. doi:10.1016/j.berh.2019.02.001
29. Mascaró A, Cos MÀ, Morral A, Roig A, Purdam C, Cook J. Load management in tendinopathy: clinical progression for Achilles and patellar tendinopathy Apunts Medicina de l’Esport. 2018;53(197):19-27. doi:10.1016/j.apunts.2017.11.005
30. Kelley MJ, Shaffer MA, Kuhn JE, Michener LA. clinical practice guidelines linked to the international classification of functioning, disability, and health from the Orthopaedic Section of the American Physical Therapy …. sports physical therapy. Published online 2013. doi:10.2519/jospt.2013.0302
31. Mintken PE, McDevitt AW, Cleland JA, et al. Cervicothoracic manual therapy plus exercise therapy versus exercise therapy alone in the management of individuals with shoulder pain: a multicenter randomized controlled trial. J Orthop Sports Phys Ther 2016;46(8):617-628. doi:10.2519/ jospt.2016.6319
32. McDevitt AW, Cleland JA, Addison S, Calderon L, Snodgrass S. Physical therapy interventions for the management of biceps tendinopathy: an international Delphi study. Int J Sports Phys Ther. 2022;17(4):677-694. doi:10.26603/001c.35256
Robert C. Manske, PT, DPT, MEd, SCS, ATC, CSCS, FAPTA
Chris Wolfe, PT, DPT, OCS, Cert MDT
Phil Page, PT, PhD, ATC, CSCS, FACSM
Michael Voight, PT, DHSC, SCS, OCS, ATC, CSCS, FAPTA
Beth Bardowski, MSN, APN, ACNP-BC
Abstract
Ischiofemoral impingement (IFI) is a significant but often underdiagnosed source of deep posterior hip pain and functional impairment, frequently associated with narrowing of the ischiofemoral space (IFS) between the ischial tuberosity and the lesser trochanter of the femur. Traditionally, diagnostic approaches for IFI have relied on clinical examination and magnetic resonance imaging (MRI). However, the advent of diagnostic musculoskeletal ultrasound (MSKUS) has transformed the evaluation process. MSKUS offers a non-invasive, cost-effective, and dynamic method for assessing the IFS and associated musculature in real time, allowing for high-resolution visualization of soft tissues, bones, and neurovascular structures. For rehabilitation providers, understanding the application, strengths, and limitations of MSKUS can enhance diagnostic accuracy, guide targeted therapeutic interventions, and potentially expedite recovery. This article reviews the anatomy of the IFS, the pathophysiology of IFI, and the use of MSKUS in diagnosing IFI, equipping rehabilitation professionals with the knowledge to integrate this valuable diagnostic tool into clinical practice.
Key Words: Ischiofemoral impingement, deep hip rotators, quadratus femoris, diagnostic MSK ultrasound.
Introduction
The integration of musculoskeletal ultrasound (MSKUS) into clinical practice has significantly transformed the diagnostic landscape for rehabilitation providers, offering a detailed, real-time view of musculoskeletal structures in a cost-effective and non-invasive manner. This modality is especially beneficial for conditions like ischiofemoral impingement (IFI), a condition characterized by the narrowing of the ischiofemoral space (IFS), which lies between the ischial tuberosity and the lesser trochanter of the femur. Narrowing of this space can lead to compression of adjacent soft tissue structures, most notably the quadratus femoris muscle, resulting in posterior hip pain that often radiates down the leg.
IFI may result from congenital abnormalities, acquired anatomical changes, or post-surgical complications such as hip arthroplasty. It is often underdiagnosed due to its subtle presentation and overlapping symptoms with other hip pathologies, such as hamstring tendinopathy or piriformis syndrome. When it is diagnosed correctly it is commonly based on reproduction of the known pain patterns during movement that lead to a further shrinking of the already reduced ischiofemoral space. These movement patterns include long stride-walking,1 the flexion-abduction-external rotation (FABER test),2 or a during motions of extensionadduction-external rotation known as the posterior impingement test.3 Traditionally, magnetic resonance imaging (MRI) has been the gold standard for diagnosing IFI, offering detailed visualization of the ischiofemoral space and associated pathologies. However, MSKUS has gained increasing recognition for its utility in musculoskeletal imaging, providing several advantages over MRI, including portability, dynamic real-time imaging, and the absence of ionizing radiation.
This article explores the application of MSKUS for evaluating the IFS in patients with suspected IFI, emphasizing its potential role in early diagnosis, accurate management, and tailored therapeutic interventions for rehabilitation specialists. By using MSKUS, clinicians can enhance diagnostic accuracy and improve patient outcomes by facilitating timely and targeted treatment strategies.
Hip disorders are prevalent, affecting about a third of adults aged 38 to 77, with higher rates observed in older adults, especially women. IFI specifically is more common in females and often occurs bilaterally.4,5 Although the precise prevalence of IIS is unknown, it is frequently overlooked as a potential cause of hip pain.
Anatomy of the Ischiofemoral Space
The IFS is the anatomical interval between the lateral cortex of the ischial tuberosity and the medial cortex of
the lesser trochanter of the femur. Within this space lies the quadratus femoris muscle, along with the sciatic nerve and associated vascular structures, all of which are crucial to hip function. Narrowing of the IFS, typically defined as a distance below 1.5 cm, can lead to IFI, a condition characterized by pain and restricted motion in the posterior hip. In addition to the IFS, the quadratus femoris space (QFS) the distance between the hamstring origin on the ischial tuberosity and the insertion of the quadratus femoris muscle — is another critical measurement in assessing IFI.
When the IFS becomes narrowed, compression of the quadratus femoris muscle and the sciatic nerve can occur, leading to the hallmark symptoms of IFI, including deep posterior hip pain and potential nerve-related symptoms. Normal IFS values range from 2 to 2.5 cm, and accurate measurement of this space is crucial for diagnosis. Given that the actual space is highly variable based on the gait cycle or what position the hip is in during testing, and MRI tends to overestimate the measurement,6 MSKUS is the perfect tool for assessing this space either statically or dynamically. Dynamic hip MSKUS can corroborate IFI and space with the change in position of the lower extremity, as well as to assess whether the symptoms are due to other potential causes.7 MSKUS is an invaluable tool for visualizing these structures, offering dynamic, real-time imaging that enhances diagnostic accuracy in cases of suspected IFI.
Pathophysiology of Ischiofemoral Impingement IFI is thought to result from repetitive mechanical compression of the quadratus femoris muscle as the femur moves into extension and external rotation, which decreases the IFS. Over time, this chronic impingement can lead to muscle edema, atrophy, and in severe cases, tendinosis or tearing of the quadratus femoris muscle. Patients typically present with vague posterior hip pain that is exacerbated by hip extension or external rotation, making clinical diagnosis challenging due to symptom overlapping with other posterior hip pathologies.
An important anatomical factor influencing the IFS is the femoral neck anteversion angle, which refers to the angle between the femoral neck and the shaft of the femur. Individuals with excessive femoral anteversion (a larger angle) are at an increased risk for IFI, as this greater angle shifts the lesser trochanter posteriorly, further restricting the already limited space. This anatomical variation, coupled with the repetitive mechanical stress on the quadratus femoris, exacerbates the impingement and contributes to the clinical presentation of IFI.8
Given the non-specific nature of the symptoms and the complexity of posterior hip pain, imaging techniques such as MSKUS are crucial for accurately diagnosing IFI and differentiating it from other hip pathologies, thereby preventing misdiagnosis and guiding appropriate treatment interventions.
Diagnosing IIS is challenging since its symptoms can mimic those of other conditions affecting the lower back, sacroiliac joint, or sciatic nerve. A thorough physical examination can aid in identifying potential cases. Two common physical tests include
•. Long-stride walking test:9,10 The patient is asked to walk with long strides while holding the affected buttock. Pain during this activity suggests IFI, especially if it resolves with shorter strides.
•. Ischiofemoral impingement test:11,12,13 With the patient lying on their side, the examiner applies pressure to the buttock near the ischium while passively extending the hip. Pain in neutral or adducted positions — but not in abduction—indicates the likelihood of IFI.
Imaging studies are essential for confirming IFI. MRI remains the gold standard, as it provides accurate measurements of the IFS and can detect abnormalities in the quadratus femoris muscle.14
Musculoskeletal Ultrasound (MSK US) in Evaluating the Ischiofemoral Space
While MRI has traditionally been the primary diagnostic tool for IFI syndrome, MSKUS is gaining recognition as a viable, radiation-free alternative. MSKUS offers real-time, dynamic visualization of soft tissues and bony structures in the posterior hip, making it a valuable tool for assessing the anatomy involved in IFI syndrome, including the IFS and quadratus femoris muscle.
MSKUS uses high-frequency transducers to provide detailed imaging of muscles, tendons, and spaces, allowing for a dynamic assessment of the ischiofemoral and quadratus femoris spaces during hip movement. This ability to visualize soft tissues in real time makes MSKUS particularly useful in diagnosing conditions like IFI, where the impingement is influenced by changes in hip position.
Protocol for MSK Ultrasound of the IFS
To ensure consistent and accurate evaluation of the IFS and surrounding structures, a standardized scanning protocol should be followed. Typically, the patient is positioned either in a prone or lateral decubitus position, with the hip in neutral or slight extension and external rotation to optimize visualization of the ischiofemoral region.
• Transverse View: The ultrasound transducer is placed transversely across the gluteal region, oriented to visualize both the ischial tuberosity and the lesser trochanter. This view allows for direct measurement of the IFS and identification of the quadratus femoris muscle.
• Longitudinal View: The probe is also oriented along the long axis of the quadratus femoris muscle to evaluate for signs of edema, atrophy, or tendinopathy
within the muscle, which are commonly associated with IFI.
By adhering to these protocols, MSKUS provides a detailed and dynamic evaluation of the IFS, enabling clinicians to assess anatomical variations and soft tissue changes that contribute to IFI, thereby improving diagnostic accuracy and guiding treatment decisions.
Key Findings - Visualization of Ischiofemoral Space MSKUS plays a pivotal role in identifying key diagnostic features of IFI by providing detailed imaging of the deep external rotator muscles and associated structures. Several hallmark findings can be observed with ultrasound:
• Narrowing of the IFS: A key indicator of IFI is the decreased distance between the ischial tuberosity and the lesser trochanter. Ultrasound allows for precise measurement of the IFS, particularly at the point of greatest narrowing, which is critical for diagnosing impingement. IFS measurements by MSKUS have been shown to be very similar to those obtained with MRI.15
• Quadratus Femoris Edema or Atrophy: MSKUS can identify increased echogenicity in the quadratus femoris muscle, which suggests edema or early degeneration. This muscle is most involved in IFI and serves as a crucial diagnostic marker.
•. Dynamic Testing: One of the strengths of MSKUS is its ability to assess the IFS during hip movement, such as extension and external rotation. This real-time imaging allows clinicians to directly observe impingement of the quadratus femoris during dynamic motion, further aiding in diagnosis.
In addition to these findings, MSKUS provides detailed visualization of the deep external rotator muscles, which are critical in the context of IFI:
• Quadratus Femoris: The primary muscle involved in IFI, located between the ischial tuberosity and the lesser trochanter.
• Obturator Externus: Situated beneath the quadratus femoris and often affected in cases of IFI.
• Piriformis, Superior Gemellus, and Inferior Gemellus: Alongside the quadratus femoris and obturator externus, these muscles form the deep external rotators of the hip and are located near the sciatic nerve, making them relevant in differential diagnosis.
• Hamstring Muscles: Although not external rotators, the proximity of their attachment to the ischial tuberosity can complicate the distinction between hamstring pathologies and IFI.
By enabling detailed imaging and dynamic assessment, MSKUS enhances the clinician’s ability to accurately diagnose IFI and differentiate it from other posterior hip pathologies, particularly those affecting the deep rotator and hamstring muscles.
Clinical Relevance for Rehabilitation Providers
For rehabilitation providers, incorporating MSKUS into clinical practice offers several benefits:
• Early Diagnosis and Prevention: Early identification of IFS narrowing and quadratus femoris pathology allows for prompt intervention with conservative measures such as physical therapy, activity modification, or injection therapies.
• Non-Invasive Diagnostic Tool: MSKUS is a noninvasive, patient-friendly imaging modality that can be used in the outpatient setting, providing real-time feedback without the need for advanced imaging centers.
•. Guidance for Treatment: MSKUS can guide therapeutic interventions, such as percutaneous quadratus femoris injections or physical therapy designed to modify movement patterns that exacerbate IFI.
MSKUS is a highly practical and effective tool for evaluating the IFS in patients presenting with posterior hip pain. Its ability to provide high-resolution, dynamic imaging of soft tissue structures makes it an invaluable adjunct to clinical examination, significantly enhancing diagnostic accuracy. MSKUS allows for a detailed assessment of the IFS, visualization of deep gluteal muscles, and evaluation of sciatic nerve dynamics, making it particularly useful in diagnosing IFI, a condition often overlooked due to its subtle presentation.
For rehabilitation providers, including physical therapists, physiatrists, and orthopedic specialists, the integration of MSKUS into the diagnostic process enables earlier detection and more targeted treatment of IFI, ultimately leading to improved patient outcomes. Its radiation-free nature and capacity for real-time dynamic assessment further establish MSKUS as a promising, essential tool in the management of posterior hip pathologies.
References
1. Li YP, Li GP, Liu K, et al. Interpretation of Ischiofemoral impingement via a clinical test using hip triaxial dynamic magnetic resonance imaging. Quant Imaging Med Surg. 2022;12:384-394.
2. Bagwell J, Bauer L, Gradoz M, Grindstaff T. The reliability of FABER test hip range of motion measurements. Int J Sports Med. 2016;11:1101-1105.
3. Heimann AF, Wagner M, Vavron P, et al. CT-guided infiltration of the ischiofemoral space in young patients with ischiofemoral impingement is an effective diagnostic tool. Insights Imaging. 2024;15:235. https://10.1186/s13244-024-01815-4
4. Taneja A, Taneja A, Bredella M, Torriani M. Ischiofemoral impingement. Magn Reson Imaging Clin N Am. 2013;21:65-73.
5. Bredella M, Azevedo D, Oliveira A, et al. Pelvic morphology in Ischiofemoral impingement. Skeletal Radiol. 2015;44:249-253.
6. Mayo-Juanatyey A, Ferrer LG, Ballester-Suarez A, et al. Ischiofemoral impingement syndrome: A five-case series report. Reumatol Clin. 2024;20:162-165.
7. Gabrielli AS, Tisherman RT, Curley AJ, et al. Open ischiofemoral impingement decompression. Arthrosc Tech. 2022;11(7):e1149-1155.
8. Yanagishita CMA, Falotico GG, Rosario DAV, et al. Ischiofemoral impingement - an etiology of hip pain: a case report. Rev Bras Ortop. 2015;47:780-783.
9. Martin HD, Kivlin BR, Palmer IJ, et al. Diagnostic accuracy of clinical tests for sciatic nerve entrapment in the gluteal region. Knee Surg Sports Traumatol Arthrosc. 2014;22(4):882-888.
10. Martin RL, Schroder RG, Gomez-Hoyos J, et al. Accuracy of 3 clinical tests to diagnose proximal hamstring tears with and without sciatic nerve involvement in patients with posterior hip pain. Arthroscopy. 2018;34(1):114-121.
11. Battaglia PJ, D’Angelo K, Kettner NW. Posterior, lateral, and anterior hip pain due to musculoskeletal origin: a narrative literature review of history, physical examination, and diagnostic imaging. J Chiropr Med. 2016;15(4):281-293.
12. Martin HD, Khoury A, Schroder R, et al. Ischiofemoral impingement and hamstring syndrome as causes of posterior hip pain: where do we go next. Clin Sports Med. 2016;35(3):469-486.
13. Martin RL, Martin HD, Kivlin B. Nerve entrapment in the hip region: current concepts review. Int J Sports Phys Ther. 2017;12(7):1163-1173.
14. Torriani M, Souto SCL. Thomas BJ, et al. Ischiofemoral impingement syndrome: An entity with hip pain and abnormalities of the quadratus femoris muscle. Am J Radiol. 2009;193:186-190.
15. Bo L, Heping D, Baicheng C, et al. The accuracy assessment of ultrasound for the diagnosis of ischiofemoral space - A validation study. J X-Ray Sci Tech. 2019;27(4):605-614.
Figure 1A: Patient Position. The patient lies prone with the hip positioned in neutral extension, while the clinician maintains the affected knee in flexion throughout the examination.
Figure 1B: Long Axis View (LAX) Transducer Placement with Hip in Neutral. For this LAX view, the transducer is aligned parallel to the muscle fibers. It is initially positioned perpendicular to the femoral shaft (Figure 1b) for reference. Once the femur is visualized, the transducer is translated along the inferior gluteal region in an axial plane to locate the medial cortex of the lesser trochanter and the lateral cortex of the ischial tuberosity. A ‘heel-toe’ maneuver is then used to angle the transducer medially, allowing clear visualization of the ischiofemoral space.
Figure 1C: Long Axis View (LAX) Transducer Placement with Hip in Abduction and Internal Rotation. Begin with the hip in a neutral position, then externally rotate or abduct the hip to visualize the width of the space. To reduce the width, adduct or internally rotate the hip during the exam. Optimal measurements for maximizing the space typically involve 15 degrees of hip abduction and 30 degrees of internal rotation, while minimizing the space to demonstrate ischiofemoral impingement typically requires 15 degrees of hip adduction and 30 degrees of external rotation. The narrowest interval between the two osseous landmarks is identified and measured using the ultrasound machine’s measurement software. Real-time assessment can also be performed by rotating the hip internally and externally to observe impingement of the quadratus femoris muscle or a bony impingement between the lesser trochanter and ischial tuberosity.
Figures 2A and 2B. Long Axis View. The quadratus femoris originates from the deep surface of the ischium and the inferior border of the semimembranosus, inserting on the intertrochanteric crest. Beneath the quadratus femoris lies the obturator externus, while the gluteus maximus is positioned above it. When performing the exam, identify the hyperechoic outline of the medial cortex of the lesser trochanter and the lateral cortex of the ischial tuberosity. The space between these two landmarks can be dynamically adjusted, narrowing or widening as described above.
Figures 3A and 3B. Muscular Ischiofemoral Impingement of the Quadratus Femoris. In this image, the hip is shown in real-time internal rotation, with narrowing of the space between the lateral aspect of the ischium and the medial aspect of the lesser trochanter. The quadratus femoris is visibly compressed and displaced superiorly out of the ischiofemoral space. This finding confirms a positive test for ischiofemoral impingement in a symptomatic patient.
Figure 4A: Bony Ischiofemoral Impingement of the Ischial Tuberosity and Lesser Trochanter. Ischiofemoral impingement can occur when the ischial tuberosity and the medial border of the lesser trochanter come into direct contact, reducing the space between them. Although this condition typically involves compression of the quadratus femoris, in some cases, the impingement is purely osseous, with the two bony structures colliding. This bone-on-bone impingement limits hip movement and can still be classified as ischiofemoral impingement, even in the absence of direct muscle involvement, as the narrowed space disrupts normal biomechanics and may cause pain or restricted mobility. As demonstrated above, hip internal rotation narrows the ischiofemoral space, bringing the lesser trochanter and ischial tuberosity into contact, resulting in a classification of bony impingement.