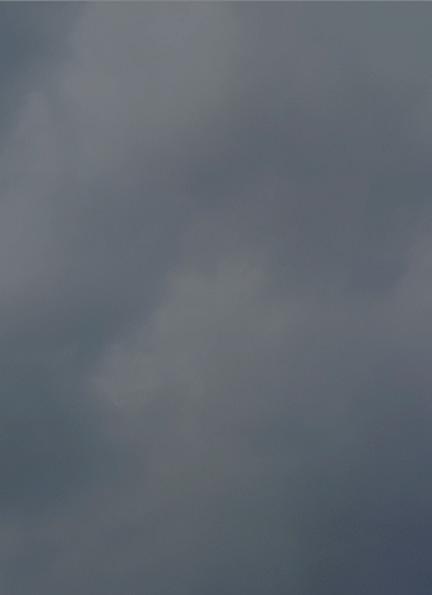
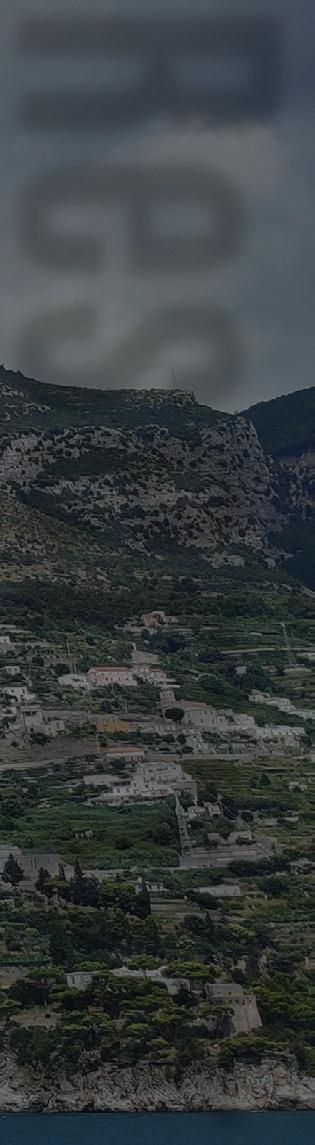
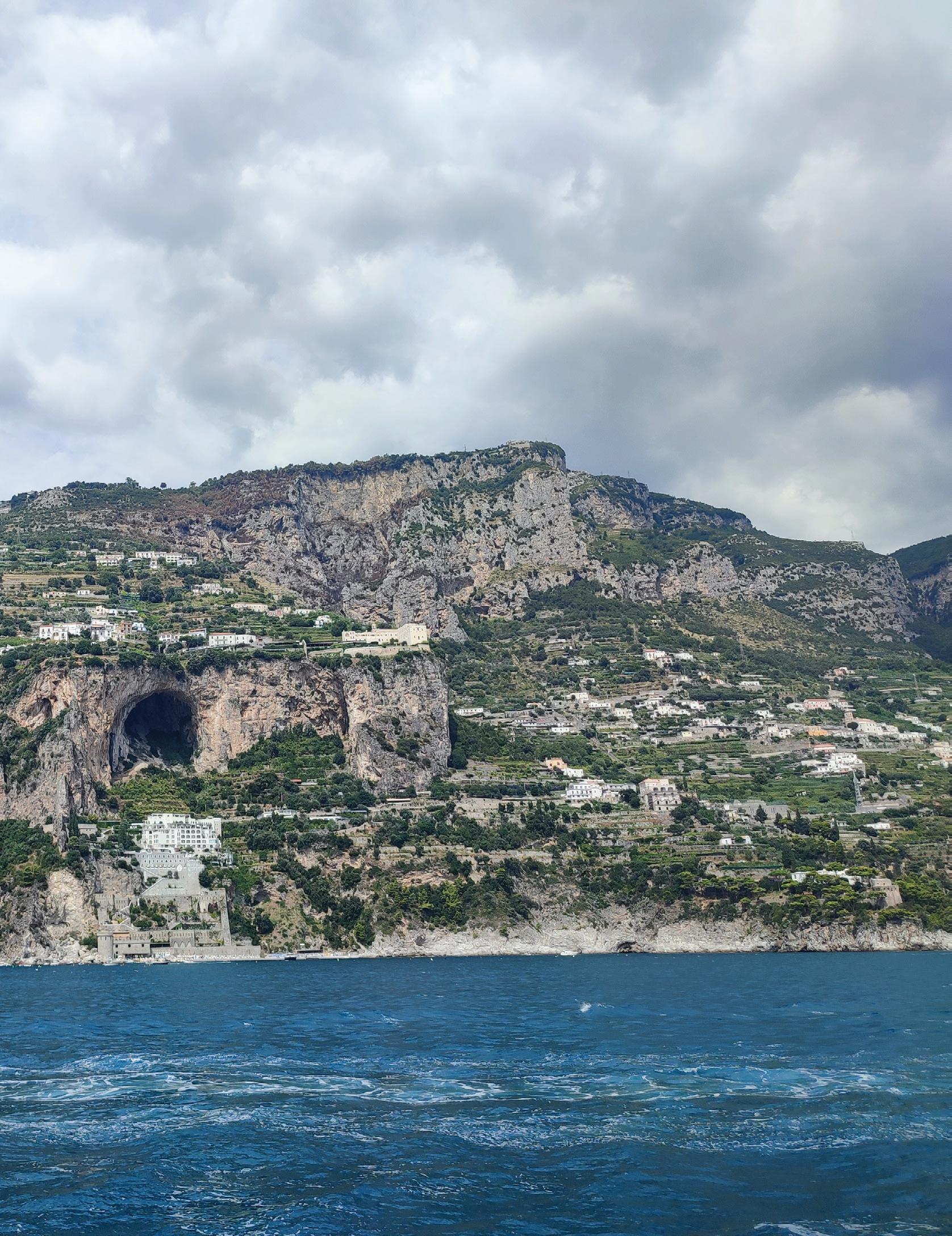
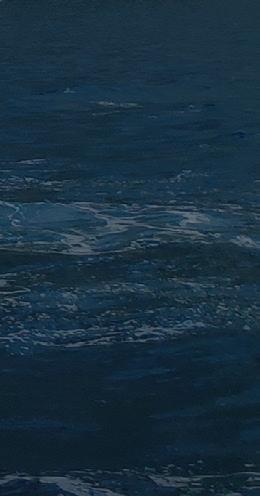
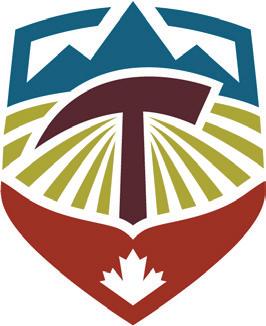
Thursday, February 6 - Friday, February 7, 2025
8:30 AM - 4:30 PM
Instructor: Byron Veilleux, Conjugate Geologic Services
Location: New CEGA Classroom
Online registration closes January 30, 2025
Thursday, February 27 - Friday, February 28, 2025
8:30 AM - 4:30 PM
Instructor: Ryan Mardani, Data Energy
Location: New CEGA Classroom
Online registration closes February 20, 2025
Effects of Warfare on Geology
From the Desk of the AER
The 2024 CEGA Golf 32nd Anniversary Tournament
Flat Pebble Conglomerates: A Cambrian Enigma 23 In Memorium – Dr. Raymond Alexander Price (1933-2024)
The 33rd Annual Sweat the Subsurface Road Race
2024 Field Trip Season in Review 28 The Blue View: Industry Trends Through Woodmac’s Lens
AMALFI COAST, Italy.
Mesozoic carbonates are well-exposed along the Amalfi Coast that lies on the southern side of the Sorrentine Peninsula to the west of Salerno, overlooking the Tyrrhenian Sea. These strata have been karstified including the development of large caves that are locally broached at the surface as shown in this view. Geotechnically, this is a hazardous region, in particular given its proximity to Vesuvius, only 25 km to the north. Where caves occur at sea level, the flooded cavities are described as grottos and are popular tourist attractions. The extreme topography combined with the precarious but widespread construction on the cliffs has led to this area being designated as a UNESCO World Heritage Site in 1997.
SARAH SCHULTZ , TECHNICAL EDITOR FOR THE RESERVOIR
to our November and December issue of the CEGA Reservoir!
CEGA has a lot of excellent technical luncheon and technical division talks scheduled for the remainder of 2024 and beginning of 2025. Please check the CEGA website to register for these talks and short courses.
The CEGA Core Conference committee is now accepting abstracts for the 2025 conference. Please check out the core conference website to submit an abstract or to get more information regarding this event.
In this issue we present the continuation of our regular articles:
• Geology in Motion: The Effects of Warfare of Geology (Part 3: Sediment)
• From the Desk of the AER: 2024 Summer Student Project Summaries
• The Blue View: Industry Trends Through Woodmac’s Lens
In this issue we present the following technical articles:
• Jon Noad: Flat Pebble Conglomerate: A Cambrian Enigma
In this issue we showcase the CEGA Summer Events:
• 2024 CEGA Golf 32nd Anniversary Tournament
• 33rd Annual Sweat the Subsurface Road Race
• 2024 Field Trip Season in Review
CEGA would like to offer its condolences to the family, friends and colleagues of Dr. Raymond Price who passed away this October. In this issue, we include a memorial tribute written by his son, Paul.
We look forward to receiving your manuscripts for the upcoming 2025 issues of the CEGA Reservoir.
The RESERVOIR is published 6 times per year by the Canadian Energy Geoscience Association. The purpose of the RESERVOIR is to publicize the Association’s many activities and to promote the geosciences. We look for both technical and non-technical material to publish.
The contents of this publication may not be reproduced either in part or in full without the consent of the publisher.
No official endorsement or sponsorship by the CEGA is implied
for any advertisement, insert, or article that appears in the RESERVOIR unless otherwise noted. All submitted materials are reviewed by the editor. We reserve the right to edit all submissions, including letters to the Editor. Submissions must include your name, address, and membership number (if applicable). The material contained in this publication is intended for informational use only.
While reasonable care has been taken, authors and the CEGA make no guarantees that any of the equations, schematics, or
devices discussed will perform as expected or that they will give the desired results. Some information contained herein may be inaccurate or may vary from standard measurements. The CEGA expressly disclaims any and all liability for the acts, omissions, or conduct of any third-party user of information contained in this publication. Under no circumstances shall the CEGA and its officers, directors, employees, and agents be liable for any injury, loss, damage, or expense arising in any manner whatsoever from the acts, omissions, or conduct of any third-party user.
PRESIDENT
Andrew Vogan
ConocoPhillips Canada Ltd. andrew.vogan@cegageos.ca
FINANCE DIRECTOR ELECT
Rachel Lea
Suncor Energy director nanceelect@cegageos.ca
DIRECTOR
Scott Leroux Taggart Oil Corp. education@cegageos.ca Linkedin
CEGA OFFICE
#415, 500 4th Ave SW
Calgary Alberta, Canada T2P 2V6
Tel: 403-264-5610 | cegageos.ca
PAST PRESIDENT
Simon Haynes Rocklocker LLC
simon.haynes@cegageos.ca
DIRECTOR
Gary Bugden Cabra Consulting Ltd membershipdirector@cegageos.ca Linkedin
DIRECTOR
Michael Livingstone GLJ Ltd
technicaldivisions@cegageos.ca LinkedIn
PRESIDENT ELECT
Shelley Leggitt
Kiwetinohk Energy Corp. shelley.leggitt@cegageos.ca
DIRECTOR
Ross Kukulski
Chevron Canada publications@cegageos.ca
DIRECTOR
Michelle Thoms Freehold Royalties Ltd. outreach@cegageos.ca Linkedin
MEMBERSHIP INQUIRIES
Tel: 403-264-5610
Email: membership@cegageos.ca
ADVERTISING INQUIRIES
Britney Tang
Tel: 403-513-1230
Email: britney.tang@cegageos.ca
FINANCE DIRECTOR
Scott Norlin Wood Mackenzie director nance@cegageos.ca Linkedin
DIRECTOR
Marcelina Labaj
Central European Petroleum Ltd.
eldtrips@cegageos.ca Linkedin
DIRECTOR
Kevin Webb
Aquitaine Energy Ltd. conferences@cegageos.ca Linkedin
CONFERENCE INQUIRIES
Kristy Casebeer
Tel: 403-513-1234
Email: kristy.casebeer@cegageos.ca
MANAGING DIRECTOR
Emma MacPherson
Tel: 403-513-1235
Email: emma.macpherson@cegageos.ca
DALLIN LAYCOCK, SEAN FLETCHER, HAN BYUL WOO, PAUL BREMNER, ERIN PEMBERTON, RICHARD MACKENZIE
Previously, we have examined the impact of warfare on geology. Part one of this series looked at examples of how bombing scarred the landscape (Laycock et al. 2024). Part two examined how deliberate flooding in warfare affected the landscape (Fletcher et al. 2024). For this final installment, we will examine the effect of warfare on the sediment itself. Examples of the effects of warfare on sediment can generally be divided into two categories: 1) Sediment creation (examples 1 and 2 below), and 2) Sediment redistribution (examples 3 and 4 below). All of these examples display how sedimentary environments are important parts of warfare, and how they can change or be manipulated in the context of the most intense varieties of human intervention.
Normandy Beach Sand: In August of 1942, the allies learned how difficult a seaborne invasion of Nazi occupied France would be, and how important the geologic setting of the landing location would be. An Anglo-Canadian force launched an amphibious attack on the German-occupied port of Dieppe with the objective of testing German defenses and gaining a foothold in Western Europe. The operation suffered from lack of intelligence on the beach terrains, which proved unsuitable for disembarking landing vehicles. The loose gravel on the beach caused many vehicles to bog down (Figure 1) preventing support for the infantry. The hard chert nodules also commonly got wedged in the tracks of the tanks and broke them apart, rendering the tanks immobile. As a result, 900 allied soldiers were killed, and nearly 3,000 more were either wounded or captured.
Armed with knowledge gained from Dieppe, allied geologists set out to identify beaches with better suitability to support their assault. In preparation for D-Day, the Allied forces employed various methods to obtain critical geologic and geomorphic data in enemy territory. One step included confirming the geomorphology of the beaches of Normandy via aerial photography (Morgan, 2020).
In January 1944, British divers launched from midget submarines to collect samples of the sediment just offshore of the beaches of Normandy (Morgan, 2020, Bressan 2018). Samples were then analyzed by geologists to confirm the composition of the sand, to assess the suitability for landing crafts, and ensure that vehicles wouldn’t get bogged down in sticky clay-rich sediment. They observed that the sand consisted mostly of quartz, with lesser amounts of feldspar, limestone, and fragmented shells. British geologists mapped out beaches that would be ideal to support
the weight of landing crafts (Rose et al. 2006).
On D-Day, June 6, 1944, the Allied forces launched a massive amphibious assault on the beaches of Normandy. The sand provided an ideal substrate for the soldiers and adapted vehicles in addition to artificial harbors to stage their invasion. The battle was intense enough to leave behind a significant amount of shrapnel. In 1988, geologists collected a sand sample from Omaha Beach, and discovered the presence of shrapnel in the sand (McBride and Picard, 2011) (Figure 2). They identified shrapnel fragments that ranged in size from 0.06-1.0 mm in diameter (very fine sand to coarse sand), and that the grains display various degrees of roundness (McBride and Picard, 2011). Small glass beads in their sample also likely have a WWII origin, and displayed higher degrees of roundness. Their diameters ranged from 0.5 to 0.6 mm. Interestingly, the authors suggested that the glass fragments were formed in battle, not by breaking of glass,
but by melting of sediment from the extreme heat of munitions explosions (McBride and Picard, 2011). Their results suggested that up to 4% of the sand in some places consisted of both metal and glass fragments (McBride and Picard, 2011). The exact distribution of shrapnel among the D-Day beaches is not certain, but the presence of shrapnel up to 44 years after the battle is significant.
Depositional Remnants of Fires: Fires are a common byproduct of warfare, both indirectly through bombing, or directly through strategic burning. In ancient Asian warfare, strategic burning appears to have been commonly used in the military and was even developed into a treatise. In The Art of War, Sun Tzu identified 5 ways to use fire in warfare: “The first is to burn soldiers in their camp; the second is to burn stores; the third is to burn baggage trains; the fourth is to burn arsenals and magazines; the fifth is to hurl dropping fire amongst the enemy” (Tzu, 2010, originally written 5th century BCE).
Ancient fires were also caused by population growth and agricultural practices. However, by examining charcoal concentration records from deltaic sediments, Li et al. (2009) showed that periods of intensified fire frequency over the past 1500 years coincided with those periods of dynastic changes that involved warfare, often overwhelming the indicators of other contributing factors. These and similar fires produced black carbon sediment that is often preserved in lacustrine, fluvio-deltaic, and marine depositional environments. Zhang et al (2023) examined sediment cores from Tianchi Lake that represented ~6000 years of deposition. By analyzing fire-derived sediment, they found a correlation between the frequency of warfare along the Silk Road and the frequency of fires (Figure 3). Both of these studies illustrate how warfare has directly impacted sediment composition and how the sediment deposited in these catchment areas can help us interpret the past.
In modern warfare, a similar process occurred in the Gulf War in 1991, where the region suffered an environmental catastrophe. The retreating Iraqi army set fire to more than 780 oil wells in Kuwait, some of which burned for 250 days before finally being controlled (Figure 4) (Udin et al., 2021). In other instances, oil was spilling directly onto the desert floor, which mixed with the desert sand, and soot from the fires
FIGURE 1: Abandoned Daimler Dingo armoured car, along with two churchill tanks, stuck in the loose gravel during the failed Dieppe Raid. Image credit: Bundesarchiv, Bild 101I-362-2211-12 / Jörgensen / CC-BY-SA 3.0, CC BY-SA 3.0 de, https://commons.wikimedia.org/w/index.php?curid=5411278
Figure 2: SEM image of sand sized particles from Omaha Beach. Red chevrons indicate pieces of shrapnel or polished glass leftover from the battle. Modified from McBride and Picard (2011).
FIGURE 3: Illustration showing the correlation between warfare and soot influx in Tianchi Lake, located in NW China. The coordinates of the lake are: 43°53’29”N, 88°07’45”E. The amount of herb pollen functions as a proxy for agricultural activity. The orange bands show the major peaks of fire activity. The amount of herb pollen and population change displays an inconsistent or complex correlation with soot influx. However, the number of wars shows a strong correlation to the soot flux. Adapted from Figure 4 of Zhang et al. 2023.
FIGURE 4: A) Image of oil fires started by the Iraqi army, taken on March 2, 1991. B) Image of a bird mummified in Tarcrete. Image Credit: Aljawad - Own work, CC BY-SA 3.0, https://commons.wikimedia.org/w/index.php?curid=6006712.
C) Google Earth image of oil contamination in the Burgan Oilfield likely caused by the environmental sabotage of the Iraqi armed forces in the Gulf War. Coordinates: 28°59’25”N, 47°56’49”E.
created a substance referred to as “tarcrete”, which at one point covered approximately 5% of Kuwait’s landmass, and is still visible today (Figure 4).
Moreover, a total of 11 million barrels of oil were spilled into the Persian Gulf, contaminating the shallow marine environment. The Massive oil fire and oil spill resulted in a significant increase in various hydrocarbons and heavy metal pollutants concentrated in the Kuwait bay and eventually settling in the Gulf marine sediment, especially within 400 km of the release sites. The sediment in the Gulf is still reported to be a highly polluted marine environment, requiring specific attention (Udin et al, 2021).
Alexander the Great and the Isle of Tyre: In 332 BCE, during his campaigns against the Persians, Alexander the Great faced the formidable island city of Tyre in what is now modern Lebanon. Tyre’s offshore location, formidable navy, and impregnable city walls posed a challenge where conventional siege tactics would be ineffective. Undeterred, Alexander embarked on an audacious project: constructing a causeway across the channel to reach the island (Figure 5).
The rise of agricultural activity on the mainland in the vicinity of Tyre, including deforestation after about 3,000 years ago, accelerated the rate of sediment supply to the coast where the original island of Tyre served as a breakwater (Nir, 1996). This protected the shelf from wave erosion, resulting in a shallow proto-tombolo that sat less than 5 m below mean sea-level. Accounts from the time indicate Alexander had his engineers destroy the mainland centre of Tyre and used its timber and ruins to build a causeway exploiting the natural land bridge (Marriner et al. 2008). In the Library of History, Diodorus describes the causeway as being a kilometre-long and 80 m wide, thus allowing Alexander’s artillery and siege towers to get in range of the walls. Eventually using a naval fleet and battering rams to breach Tyre’s defenses, Alexander’s army conquered the city after many months.
The new causeway eventually created an unintended change to the natural migration of sand along the beach. The predominant direction of longshore sediment transport is from south to north, but occasional storm swells also transport sediment from the north, creating a bi-directional drift (Marriner et al. 2008, Rosen and Kit 1981, Goldsmith and Sofer, 1983) (Figures 5 and 6). Over the last 2,300 years since the siege, this sediment
movement reinforced the tombolo with at least 10 million cubic meters of sediment, mostly composed of sand from nearby beaches forming bays on either side of the causeway (Nir, 1996) (Figure 6).
This strategic use of the shallow proto-tombolo from then, without the understanding of geological processes, is one of the earliest examples of human ingenuity altering a landscape in a profound way still seen today. Today’s modern city of Tyre occupies the territory of the original island city, as well as the isthmus itself, which has expanded by some 65,000 square meters and is a prominent site on the present day Lebanese coastline.
Sediment Redistribution as Ballast: One common aspect of warfare has been the redistribution of sediment as ballast in both marinecraft and aircraft. Sedimentologists were able to trace the origin of these ballast systems from their composition and characteristics related to their original depositional environment (Bar et al, 2019, Fournier et al, 2024). One such example was during World War II, in which Japan developed an innovative weapon known as the Fu-Go
FIGURE 5: Illustration showing the mole constructed by Alexander the Great, connecting the Isle of Tyre to what is now mainland Lebanon. This would eventually provide a barrier to sand migration, both to sediment from the south as well as the north, as indicated by the arrows in the diagram.
FIGURE 6: A) Aerial photograph of Tyre taken in 1934. Notice how the sand accumulation is greater on the south side of the tombolo (pink arrow), as the predominant direction of longshore drift is from south to north. Additional storms occasionally transport sediment from the north (green arrow). Image adapted from Poidebard and Cayeux (1939). B) Satellite image of Tyre (yellow arrow). White arrow indicates the Litani River delivering a plume of sediment to the Mediterranean Sea, highlighting what a major sediment plume appears like in aerial photographs. Image courtesy of the Earth Science and Remote Sensing Unit, NASA Johnson Space Center.
7: A) Simplified illustration of the Fu-Go Balloons. Red box near the bottom shows the part of the device shown in panel B. B) Remnants of a carriage recovered from a Fu-Go Balloon on display at the Hangar Flight Museum in Calgary, Canada.
balloon bomb. The objective of these devices was to initiate widespread forest fires and cause panic among the American public. These balloon bombs were filled with hydrogen, each approximately 33 feet in diameter, designed to carry a payload of four incendiary bombs and one high-explosive anti-personnel bomb. The engineering behind these balloons was quite sophisticated for the time, incorporating an altimeter and a ballast system of sandbags to maintain altitude during their trans-Pacific journey (Figure 7).
The balloons were launched using a simple yet effective mechanism. Hydrogen gas was used to fill the balloons, providing the necessary lift. Once airborne, the balloons relied on the strong westerly winds of the jet stream to travel across the Pacific Ocean. As hydrogen leaked out, the altimeter would trigger the release of sandbags to keep the balloon at a consistent height, allowing it to be carried by the jet stream from Japan to North America. Engineers of the device designed a time-delay fuse system that would release the bombs after a predetermined period, ideally when the balloon had reached North America.
Ultimately the balloons were ineffective, failing to start any forest fires. Tragically, 6 civilians were killed by one of the bombs in Oregon, marking the only mainland deaths in the United States during the war. Despite the limited effect of the balloons, the Military Geology Unit of the US Geologic Survey was
given samples of sand recovered from the balloons’ ballast system, hoping they could identify the origin of the balloons. Sand samples were recovered from balloon crash sites at Glendo Wyoming, and Holy Cross Alaska (Mikesh, 1973) (Figure 8). The task fell to a group led by Julia A. Ganer, which became known as “The Dungeon Gang” (USGS Communications and Publishing, 2024). Gardner specialized in mollusks. Other members of the group included Kathryn Lohman (specializing in foraminifera), Clarence S. Ross (specializing in mineralogy), and Kenneth Lohman (specializing in diatoms) (Mikesh 1973, McPhee 1996, and Tewksbury 2013).
Key observations of the group included the following: 1) Lack of coral fragments. 2) The sand contained over 100 species of both fossil and modern diatoms. Fossil diatoms were primarily Pliocene age. 3) The sand contained abundant mollusk fragments. 4) Foraminifera species identified in the samples aligned with those documented in Japanese geologic papers describing the eastern beaches of Honshu. 5) Siliciclastic grains were primarily of non-granitic origin, but with a unique suite of trace minerals. These included hypersthene, augite, hornblende, and garnet (Tewksbury 2013, and Rogers, no date).
The mineralogy and micropaleontology helped them identify certain beaches that were candidates for the sand collection sites,
Bar, A., Olivarius, M., Boldreel, L. O., Sheldon, E., Mart, Y., & Cvikel, D. (2019). Analyses of ballast stones from the Akko Tower Wreck, Israel: A clue to the sailing route of the ship. Journal of Archaeological Science: Reports, 26, 101911. Bressan, D. (2018). How Geology Influenced The Landing In Normandy On D-Day. Forbes. https://www.forbes.com/sites/ davidbressan/2018/06/06/how-geologyinfluenced-the-landing-in-normandy-on-d-day/# Fournier, F., Léonide, P., Marié, L., Quillévéré, F., Margerel, J.P., Miholjek, I., Dugonjić, P., M.B., Carre, Cavassa, L., Morsilli, M., Boetto, G. (2024). Provenance of the ballast stones from the Roman Republican ship Ilovik-Paržine 1: A hypothesis about its place of construction. Journal of Archaeological Science: Reports, v. 57 Li, Z., Saito, Y., Dang, P. X., Matsumoto, E., & Vu, Q. L. (2009). Warfare rather than agriculture as a critical influence on fires in the late Holocene, inferred from northern Vietnam. Proceedings of the National Academy of Sciences, 106(28), 11490-11495.
Marriner N., Morhange C. & Meulé S. Proc. Natl Acad. Sci. USA, doi:10.1073.pnas.0611325104 (2007).
McBride, E. F., & Picard, M. D. (2011). Shrapnel in Omaha Beach Sand. The Sedimentary Record, 9(3), 4-8.
McPhee, J.(1996), Balloons of War, in The Gravel Page: The New Yorker (magazine), v. 71, n. 46, January 29, 1996, p. 52-60.
Mikesh, Robert C., 1973, Japan’s World War II Balloon Bomb Attacks on North America: Smithsonian Institute Press, National Air and Space Museum, Wash., D.C., 85 pages. Morgan, N. (2020). Safe Landings. Geoscientist, v. 30(10). p.27.
Nir Y., et al. 1996. The city of Tyre, Lebanon and its semi-artificial tombolo. Geoarchaeology, 11. 235 - 250.
Poidebard, A., Cayeux, L. (1939). Un grand port disparu, Tyr ; Recherches aériennes et sousmarines (1934-1936). Bibliothèque Archéologique et Historique, 29
Rogers, J. no date. How geologists unraveled the mystery of Japanese vengeance balloon bombs in World War II. https://web.mst.edu/~rogersda/ forensic_geology
Rose, E. P., Clatworthy, J. C., & Nathanail, C. P. (2006). Specialist maps prepared by British military geologists for the D-Day landings and operations in Normandy, 1944. The Cartographic Journal, 43(2), 117-143.
and the lack of coral helped eliminate areas of Japan that had nearby reefs. The group narrowed on two sites on Japan’s east coast, a beach at Shiogama, and Ninety-nine League Beach at Ichinomiya. Subsequently, aerial reconnaissance planes photographed the sites in 1945, and the surveys succeeded in identifying two of the three hydrogen plants responsible for the Fu-Go Balloons. These were destroyed by American B-29 bombers in April 1945 (Rogers, no date). Post-war investigations showed 2 additional launch sites further north along the coast of Honshu. Ultimately, none of the sand samples provided to the “Dungeon Gang” came from these other sites, and as such the northern sites went unidentified by the group (Rogers, no date).
This article demonstrates the significant effect that warfare can have on sediment. In the cases of D-Day and battles along the silk road, sediment can be created and record details of the battles. In other cases sediment can be deliberately transported, such as the Fu-Go balloons and ancient marine ballast systems. Warfare can even change the sedimentary depositional systems themselves, such as the Isle of Tyre. When considered with the previous editions of this series about bombturbation and flooding, the impact that warfare has on our planet is measurable and persistent.
Rose, E. P. F., Clatworthy, J. C. (2008). Fred Shotton: a ‘hero’ of military applications of geology during World War II. Quarterly Journal of Engineering Geology and Hydrogeology, 41(2), 171-188.
Tewkesbury, D.A. 2008. Japan’s balloon bomb attacks on North America - a GIS exercise for forensic geology. Geological Society of America Abstracts with Programs 40(6): 490
Tzu, S. (2010). The art of war. Capstone Publishing. (Originally written 5th century BCE)
Uddin, S., Fowler, S. W., Saeed, T., Jupp, B., & Faizuddin, M. (2021). Petroleum hydrocarbon pollution in sediments from the Gulf and Omani waters: Status and review. Marine Pollution Bulletin, 173, 112913.
USGS Communications and Publishing, 2024. Julia Gardner: 5 Things to Know About a Pioneering Woman with the USGS. https://www.usgs.gov/ news/featured-story/julia-gardner-5-things-knowabout-a-pioneering-woman-usgs
Zhang, S., Liu, H., Li, G., Zhang, Z., Chen, X., Shi, Z., ... & Dong, G. (2023). Warfare impact overtakes climate-controlled fires in the eastern Silk Roads since 2000 BP. PNAS Nexus, 2(12), pgad408.
The Alberta Energy Regulator and Alberta Geological Survey, a branch within the regulator, offer a range of interesting and challenging geoscience summer student projects each year. The projects help to meet research objectives while also providing development opportunities to undergraduate and graduate students keen on pursuing a career in the geosciences. In some cases, these are their very first “jobs” of a geological nature and we value not only their technical contributions but also the opportunity to support university students as they work towards their individual goals. Herein each student has provided a short summary of what they worked on this summer.
Nancy Pearl Osei Boakye, Ph.D. Program University of Manitoba
Rare earth elements (REEs) refer to a set of fifteen elements from Lanthanum to Lutetium in the periodic table. They are also known as Lanthanoids. Yttrium and Scandium are often associated with REEs. Their use in electric engines, defense equipment, rechargeable batteries among others has drawn attention to this resource. Research has shown coal is a notable host of rare earth elements making it important for coal to be explored in this regard. Over the summer, I explored an XRF dataset from 40 wells, analyzing and providing preliminary interpretation of the lithologic and stratigraphic geospatial distribution of REEs and other proxy elements. Tasks included QAQC, analyses of elemental distributions, highlighting relevant correlations and anomalies and informing on notable lithologic and stratigraphic intervals. From the results, wells that indicated potential REE enrichment were sampled for further geochemical analyses.
Ashley Ewing, Hydrogeology Undergraduate Program at University of Alberta
The Oil Sands Monitoring (OSM) project aims to assess the baseline and recent hydraulic head values around the oil sands and quantify their changes resulting from anthropogenic actions. The project has covered bedrock units, primarily the Mannville Group, and currently is being expanded to the surficial units in both the Athabasca and Cold Lake Oil Sands areas.
For my time in the role with the AGS this summer, my work has focused on exploring different ways to assess the vertical hydraulic head gradients in these surficial units. Using primarily monitoring wells, I have
been able to calculate vertical head gradients between aquifer units, create pressure vs depth plots and hydraulic head vs elevation plots, of which I have displayed visually and assessed using GIS software. This work is extremely useful for delineating hydrostratigraphic units (HSUs) and assessing recharge and discharge areas for aquifers, both of which greatly improve our understanding of groundwater flow patterns within the subsurface.
Colleen Lamb, B.Sc Double Major, University of Alberta
This summer I have been updating the surficial maps of the House River and Wandering River areas of northeastern Alberta, which were produced before we had access to LiDAR imagery. The majority of my work has been desk-based interpretation of the landforms visible from our LiDAR data, but I also completed fieldwork to confirm both previous mapping interpretations and my own. For this, I sampled sediment using soil augers, and examined roadcut exposures and gravel pits when available (Figure 1). This map area contains prominent icecontact glaciofluvial features, proglacial lake shorelines and a fluting field. Notably, we found during fieldwork that an area of the fluting field previously mapped as bedrock contained glaciotectonized sediment and was not in situ.
Mark Mlella, M.Sc. Student at University of Alberta
This summer, I undertook three pivotal projects aimed at enhancing seismic monitoring and analysis in Alberta. The first project involved creating an email notification system to alert seismologists about seismic events in the region. This tool required real-time database interaction to
Hot Disk M1 Thermal Conductivity Analyzer measuring thermal properties of rock samples.
Upper right: Canadian Shield Sample from the AGS Godfrey Collection. Lower Right: Precambrian Basement Sample from the University of Alberta Core Collection.
extract and report seismic data, significantly improving response times for seismic events.
In the second project, I enhanced the existing Alberta Energy Regulator (AER) system by developing tools to extract valuable information from digitized operator reports on seismic events. This experience demonstrated the potential of using machine learning models, like large language models (LLMs), to assist geoscientists in data analysis.
The third project focused on determining the focal mechanisms of seismic events by updating and refining the existing codebase. These improvements hope to facilitate more accurate and efficient identification of earthquake source characteristics, contributing to a better understanding of seismic activities in Alberta.
Dora Poon Matsune, B.Sc. Program, University of Alberta
While sedimentary formations of the Western Canadian Sedimentary Basin hold potential for conventional geothermal development, the Precambrian Basement is key for advancing modern geothermal technologies like closed-loop systems. Advancing these technologies depends on understanding subsurface thermal properties like thermal conductivity, which dictates heat transfer, and thermal diffusivity, which governs the rate of heat spread and long-term extraction sustainability.
At the Alberta Geological Survey, we focused on the Precambrian Basement and its proxy, the Canadian Shield in northeastern Alberta, to determine potential viability for advanced geothermal systems. Using a Hot Disk M1 thermal conductivity meter and the Transient Plane Source method (Figure 2), we measured thermal conductivity, diffusivity, and specific heat capacity in various low-porosity and low-permeability rocks to further understand these principles. For comparison, we also used Voigt and Reuss mixing laws to predict thermal conductivity values
using mineralogy data from X-ray diffraction analysis. These results are expected to assist in further evaluating Alberta’s geothermal resource viability.
Andrea Pezzera, Ph.D. Candidate at University of Alberta
My summer internship was varied and instructive. During the first half I described ~500 thin sections of rocks from the Canadian Shield in Alberta using a petrographic microscope at the Mineral Core Research Facility in Edmonton. The descriptions consisted of major and minor mineral abundances, indication of the presence of radiogenic minerals and notes on textural features. The rocks were than classified using standard nomenclature and consist mostly of granitoid and amphibolitic lithologies. Pictures of characteristic minerals and assemblages were taken, and the thin sections were scanned both in plane and crossed polarized light. The second half of the internship consisted of fieldwork in the Rocky Mountains. In the Nordegg area (Figure 3) the fieldwork was a stratigraphic-diagenetic study aimed at assessing the Carbon Capture Use and Storage (CCUS) potential of time-equivalent subsurface
Devonian carbonates of the Leduc formation, and in the Crowsnest Pass area, to assess its base and precious metal potential. Thanks AGS for this amazing experience!
After last year’s successful event, the 32nd Annual CEGA Golf tournament was held on August 14th at Lynx Ridge Golf Course. The midweek afternoon outing best ball scramble benefited from blue skies and warm temperatures. Our attendance peaked at the maximum 132 golfers attending this year, and we were pleased to host an excellent and fun tournament. We hope to see everyone back in 2025.
The Low Net Trophy and tournament winner was the team of Nick Mason, Shannon Balzer, Erik Munson and Troy Miller with a net score of 48. The Low Gross Score Trophy with a score of -13 was taken by the team of Darren Hiscott, Shane Caravan, Ryan Buis & Tony Evens who won on a countback. The Spirit Award “Fossil Coprolite” Trophy went to the most honest team of Erik Ditzler, Jon Horner, Peter Nyman and Benjamin Hollands at +17.
We recognize how our great sponsors came through this year, and we appreciate each and every one of them. We would like to thank: Platinum sponsors geoLOGIC systems ltd. and Haliburton; Silver sponsors AGAT Laboratories Ltd., PetroCraft, RigSat and Weatherford; and in-kind sponsors by Little Rock Printing and Market Wines Calgary. Hospitality tents were hosted by Pro Geo Consultants on Hole 5 with a $10,000 hole-in-one contest and beat the hack for Flames game tickets (James Ablett). RPS Energy was on Hole 2, Copoint was on Hole 8, AGAT Laboratories Ltd. on Hole 10, GLJ Consulting on Hole 11, Chinook Consulting Services and Weatherford were on Hole 14, and Halliburton was on Hole 17.
Additionally, our hole sponsors were Belloy Petroleum Consulting, Beryl Mining Services, GLJ, Pro Geo Consultants and RPS Energy. The fantastic prize donation by Belloy Petroleum Consultants (Jeff Kriz) of a cooler filled with steaks was won by Bob Sephton.
During the delicious steak banquet dinner teams relived the good times and the missed shots that could have put them in contention. In the end, the members, guests, sponsor players and representatives enjoyed a great day catching up with colleagues, making new friends, and succeeded in delivering $3370 to support the CSPG Foundation activities for geoscience. We have received both positive and constructive feedback from participants and sponsors and will look to improve the tournament even more for next year!
I would like to thank the many sponsors, golfers, and the greenskeeping, pro shop and catering team at Lynx Ridge Golf course and especially the fine organizing committee of co-chair Rachel Lea, Brenda Pearson (prizes), Norm Hopkins (trophies), Darren Hiscott (sponsorship), and Nash Hayward (course prizes) and our CEGA office support from Julie Beally. A big thank you to Audrey Monlevade who volunteered to take all the team pictures and candid shots.
Attending members and sponsors overwhelmingly expressed their gratitude to be back this year, and we look forward to your attendance at next year’s tournament.
Many years ago, I was given two beautiful stone frogs carved from a conglomeratic stone. The texture of the rock was striking, composed of a flat pebble conglomerate (FPC) - unlike anything I had seen in the field. Looking further into its origin, I found that this sedimentary rock has been used extensively for carving and decoration in China where it is termed Bamboo Stone. The clasts are extremely fine grained, are tabular and are multi-coloured. They typically occur in a mud grade matrix and may reach 8 cm in length.
I had not considered the genesis of this deposit until I started working on Carbon Sequestration (see the previous edition of the Reservoir: Noad 2024). The Deadwood Formation is the primary target interval for Carbon Capture and Sequestration in the Aquistore Project in Saskatchewan, so I had selected some Deadwood cores drilled in SE Alberta to log, in preparation for presentation at the CEGA Core Conference (Noad 2022a). Imagine my surprise to see the same FPC in three of the four cores. More FPCs were exposed in Cambrian Deadwood core drilled for the Aquistore Project and this led me to investigate this enigmatic facies further. These FPCs are generally thought to be restricted to Silurian and older sedimentary rocks (Kim and Lee 1995), although they have occasionally been observed in younger rocks, so I started by exploring Cambrian palaeoenvironments to see if there was a convincing reason for this.
FIGURE 1: The frogs that sparked my interest in Cambrian Flat Pebble Conglomerates. The bigger one is around 12 cm long.
The Cambrian was refreshingly different to the world we live in today. There were no land plants, which meant that rivers did not meander. Instead, they were low sinuosity, braided rivers that flowed straight down to the sea. They carried a relatively coarse bedload and sourced some of the most extensive shoreface deposits in the Earth’s history. This is demonstrated by the presence of Cambrian sandstones (and equivalent quartzites) beneath a significant proportion of the North American continent (American Geoscience Institute 2024). Shallow epeiric seas existed across much of the planet.
Climate, and possibly sea temperatures, were significantly higher than today. This is likely to have led to stronger convective currents and much larger storms. This should in theory lead to thicker and more prevalent storm deposits, although similar temperatures in the Cretaceous did not deposit any FPCs. Greenhouse conditions were extant, with high levels of CO2 and low levels of oxygen in the atmosphere (Pruss and Gill 2024).
The Cambrian year was likely to have been about 420 days long (Hughes and Heim 2005). Tidal ranges may have been 12 to 17% higher than today (Halamski 2017, comment on Researchgate. net), while other authors do not think that they were very different to now, with the Moon only slightly closer to the Earth (Evans 2010). The absence of tidal structures in many Cambrian deposits do not suggest high tidal ranges. There was an overall transgression through much of the Cambrian (possibly due to rapid sea floor spreading) and high rates of subsidence, leading to high sedimentation rates (Hughes and Heim 2005).
Surface seawater temperatures were higher in the Archean, while the initial salinity of the oceans was 1.5–2x the modern value and remained high throughout the Archean in the absence of long-lived continental cratons required to sequester giant halite beds and brine. Lower ocean salinities probably occurred in the latest Precambrian (Knauth 2005).
Marine salinity is thought to have remained relatively stable during the Phanerozoic, with Cambrian marine salinities similar to modern day seawater. However, the chemical composition of brines has oscillated significantly between Ca-rich and SO4-rich over geological time. In the Cambrian, Ca2+ concentrations were higher and SO42- concentrations lower than in modern seawater (Petrychenkoa et al 2005). This may have driven the onset of biocalcification.
It is estimated that more than 95% of the world’s salt is locked up as halite. If this was dissolved in seawater, it would more than double the current salinity (Bielenberg 2019). Locking up water in ice caps only changes salinities by around 2‰. Hay et al (2006) suggest that salinities may have reached 50‰ in the early Cambrian, gradually decreasing to the present day. Such an increase in salinity results in greater water density which may have increased circulation and mixing. There is also the potential for extensive brackish water environments where thick argillites would be deposited (Lui et al 2024). The presence of marine fossils, such as trilobites, may provide some indication of full salinity.
Flat-pebble conglomerates are intraformational limestone conglomerates (calcirudites) composed of rounded, tabular intraclasts. These conglomerates are common in shallow-water carbonate facies throughout the geologic column but are especially abundant in early Paleozoic strata (Kim and Lee 1995).
The FPC beds range from a few centimetres to a few metres in thickness. They have sharp to erosive bases and are generally massive. They are poorly sorted with spherical to tabular intraclasts at random orientations. Most have gradational upper boundaries (Pittenger et al 1994; Kim and Lee 1995). They are typically interbedded with fine grained sediments such as marl and lime mudstone which may contain tempestite layers (Kim and Lee 1995).
Chinese workers describe bamboo stone as a nodular limestone composed of clay and calcite. These deposits originated long ago during the Ordovician period. Bamboo stones are excavated from the Yanwashan Mountains in Zhejiang Province and have been used decoratively since at least the Qing Dynasty (Vsana 2024). Bamboo Stone is apparently recommended for weight management as it signals the body to call upon stored energy - fat - and to find the inner discipline to hold the course of a new exercise regime (Life is a Gift Shop 2024).
FPCs have been reported from Alberta (Noad 2022a), Saskatchewan (Dixon 2007; Vaisblat 2022), many localities in the US, and China, in fact in many parts of the world (Kim and Lee 1995). The author has recorded them in cores from the Deadwood Formation in both Saskatchewan and Alberta, and in the field in Precambrian deposits in Waterton (both clastic and carbonate settings). Carved samples of bamboo stone,
FIGURE 2: Two examples of polished bamboo stone spheres, the first around 10 cm, and the second 5 cm in diameter.
originating in China, are for sale on several commercial websites such asEBayandEtsy.
Flat pebble conglomerates are very common in the Cambrian, formed from thin limestone beds that have been ripped up and redeposited, mostly during storms. Episodic deposition, rapid lithificationandsubsequenterosionandredepositionwillfacilitatetheir creation.InfaunalexpansionintheOrdovicianmayhaveeliminatedthe potential for rapid marine cementation of thin carbonate layers (Sepkoski 1982). A storm origin is also favoured for FPCs in the Cambrian Furong Zhaimidian Formation in Luxi, China, where gravels of tabular, chondrite grained limestone were created by storms and transported
3: Examples of Flat Pebble Conglomerate in outcrop from Waterton National Park, Alberta
4: Examples of FPC in Deadwood Formation cores from Alberta and Saskatchewan
across the cemented seafloor to form mounds (Chen 2023). The activity of storms breaking up microbial mats is proposed for Proterozoic FPC deposits in India (Sarkar et al 2018). FPCs in the Deadwood Formation in Saskatchewan are interpreted as having been deposited by very large storms, as sediment gravity flows or by tsunamis, although little sedimentological evidence is presented to support any of these models (Dixon 2007).
In the Middle Proterozoic Castner Marble of West Texas, the FPCs are interbedded with rhythmites, which are either intertidal (tidal or algal) or subtidal (glacial varves, seasonal influx into a marine basin or distal
storm or turbidite deposits) (Pittenger et al 2014). The authors attribute the FPCs to outer ramp, distal storm deposits but do not otherwise propose a model for their creation and deposition.
FPCs have been identified in both Precambrian to Ordovician shallow water cyclic and non cyclic strata (Myrow et al 2004). Suggested origins include storm dominated shoreface deposits, with failure induced by storm or seismic waves. Clasts are thought to have developed along cm scale laminae within thick, amalgamated hummocky cross stratified (HCS) beds, possibly transported in a viscous flow dominated by laminar shear. The clasts are not reworked (not rounded) and have no coatings.
FPCs are also seen at the top of upward coarsening, metre scale cycles and may represent lowstand or transgressive deposits. These clasts are well rounded with iron-rich coatings and are considered as condensed deposits. A third type of FPC represents reworking of carbonatenodulestolags.
Other authors attribute FPCs to tidal currents. Bimodal pebble imbrication recorded in Cambrian core from the Powder River Basin, Wyoming, supports a tidal current origin, although the completeness of the clasts suggests only limited transport. The pebbles were not cemented early. Burrowing is absent which may indicate high salinities. Wilson (1985) proposes abnormally high tides as a possible mechanism to initiate and deposit FPCs, although later work may refute this theory by indicating that tides were of only slightly greater magnitude than today (Evans 2010; see also the earlierdiscussiononCambrianenvironments).
Another model suggests that the FPCs were formed by tsunamis (Kazmierczak and Goldring 1978; Pratt 2002; Luczynski et al 2014). Pratt examined Upper Cambrian outcrops in Montana, postulating that post depositional cracking associated with calcite cementation opened fractures that were then exploited by tsunamis, ripping clasts free from the seabed. Luczynski and co-authors (2014) examined FPCs associated with stromatoporoids, stretching for more than 20 km oblique to the palaeo-shoreline in Silurian rocks in SW Ukraine. They interpreted the sedimentary rocks as peritidal and noted that the stromatoporoids were often overturned and fragmented. The material had been transported landward and deposited in lagoons, with FPCs composed of micritic clasts lithologically identical to the underlying beds. Similar beds have been seen by the author in the Upper Devonian Moberly Member (Waterways Formation) around Fort McMurray and reported elsewhere (Schneideretal2013).
A structural origin is inferred for the stretch pebble conglomerate beds of the Proterozoic Kingston Peak Formation exposed in Death Valley National Park (Walker et al 2002). The original conglomeratic clasts have been metamorphosed, thinned and stretched. These deposits have been dated to the time of Snowball Earth and are not considered to have a similar origin to any of the FPCs described in this article. Similar stretch pebble conglomerates can be found near Prescott, Arizona (Highlands CenterforNaturalHistory2024).
Deposition of the Cambro-Ordovician, of which the Deadwood Formation forms part, in the subsurface of the Western Canada Sedimentary Basin in Alberta took place within an intracontinental basin inboard from, and flanking the western passive margin of ancestral North America. In Alberta, the Deadwood comprises green and purple, micaceous, fissile shales interbedded with siltstone, and fine to coarse grained, micaceous and locally glauconitic sandstone (HeinandNowlan1996).
A limited number of core penetrations into the Deadwood have been drilled in Alberta, many with poor recovery and/or currently unslabbed. These cores, drilled mainly in the southeastern part of the Province, expose conglomerate, sandstone, siltstone and mudstone beds (Noad 2022a). Some of the sandstone beds include glauconite and are bioturbated. Contacts to overlying Devonian sediments and to underlying regolith and gneiss have also been recorded (although the latter beds may immediately underlie the Cambrian Basal Sandstone Unit). Depositional settings are interpreted as dominantly shallow marine to tidal, with trough, ripple and herringbone cross-bedding seen in core. A marine setting is indicated by rare brachiopod and trilobite fossils in the core (Greggs 2000).
Location
FIGURE 6: of the four logged cores: A, B, C and D. Note also the location of 16-35-2-5W4 (green circle) which tested 1.1% Helium in the Deadwood
The author logged four cores to establish a depositional framework (Noad 2022a). The following lithofacies associations were identified:
Basement RegolithGranular feldspathic arenite
Gneiss Igneous rock
Alluvial fan Pebbly sandstone, red in colour
Red, trough cross-bedded sandstone
Weathered gneiss forming soil
Debris flowsFan top
Channel deposits
Channels crossing the fan
Tidal Algal siltstoneAlgal laminaeTidal flat
Flat pebble conglomerate Flattened clasts in sandy matrix with high glauconite concentrations
Shallow Marine Stacked, trough cross-bedded and planar sandstone (St, Sp)
Shoreface parasequences (Sc)
Upper shoreface; sediments may be mottled
Sequences grade up from mudstone to wavy laminated sandstone (HCS?) to trough cross-bedded sandstone
Mudstone Distal or quiet depositional conditions
Dolomite DolomiteMassive carbonate, unconformably overlying the Deadwood Formation
Sandy tidal flat
Shallow marine setting
Shallow marine setting
Embayment or distal lower shoreface
Shallow marine
TABLE 1: Lithofacies scheme erected for the logged Deadwood cores
FIGURE 7: Logged section through well B.14-35-34-17W4: 2066 to 2077 m. Note the extensive intervals of Flat Pebble Conglomerate, shown in orange (see also Figure 4).
Three of the four cores included intervals with FPCs. The facies association (with algal laminites) indicated a possible tidal flat origin, similar to FPCs in the Middle Cambrian Gros Ventre Formation of Wyoming (Lynn 2012). It is postulated that thin, laminated mudstone beds, deposited on the flats, dried out and formed upward curling flakes that were mobilized and redeposited during high tides. This model contrasts with those of most authors (see previous section, for example Dixon 2007) and takes into consideration the relative weakness and hence ephemeral nature, of the mudstone flakes making up the FPCs.A tidal origin for FPCs is also suggested by Kim and Lee (1995).
FIGURE 8: Palaeo-reconstruction of the Cambrian Deadwood Formation with core examples.
It is interesting that FPCs have not been reported (to my knowledge) from the Basal Cambrian Sandstone (Dixon 2007; Desjardins et al 2013; Weides et al 2014; Herbers and Hauck 2022). Similarly, they have not been readily identified in a series of outcrops of the Gog Quartzite around Lake Louise and Kicking Horse Pass (Desjardins 2010). Possibly the heavy influx of clastic sediment at the time of deposition of these sand dominated formations choked deposition of the calcirudites that may source the FPC.
More “classical” conglomeratic facies, with rounded to angular extraformational clasts, are noted in the Basal Cambrian Sandstone in Saskatchewan (Dixon 2007) and in the Gog Quartzite. These include regolith deposits near the base of the Formation as well as beds higher in the stratigraphy. Conglomeratic quartzites are noted in the Deadwood Formation in the Hartville Uplift, Wyoming (Beikman 1962).
American Geoscience Institute. 2024. Interactive map of carbon dioxide storage potential in the United States. https://www.americangeosciences. org/critical-issues/maps/us-co2-storage. Accessed October 2024.
Beikman, H.M. 1962. Geology of the Powder River Basin, Wyoming and Montana, with reference to subsurface disposal of radioactive wastes. Trace Elements Investigations Report TEI-823, USGS publication.
Bielenberg, A.P. How Salty Has The Sea Been Over the Past 541 Million Years? Blog: https:// alioshabielenberg.com/how-salty-has-the-seabeen-over-the-past-541-million-years/ Chen, J. 2023. Origin of Cambrian Flat-Pebble Conglomerate Mounds in the North China Platform. Chenji xuebao, 2023, Vol.41 (6), p.1662-1672.
The more you read about the Cambrian, the more of an alien world it seems when compared to the modern world. One tiny example is the presence of common Cambrian flat pebble conglomerates, thought to be absent in today’s sedimentary deposits. The genesis of this facies remains unclear, but new evidence from the Deadwood Formation indicates a tidal rather than a storm or tsunami origin for at least some of the numerous examples described from around the world. More detailed sedimentological analysis of well exposed outcrops would go a long way to answering the question of their origin. What can certainly be said is that, like so many early Palaeozoic and older rocks, they would make a lovely tabletop.
Desjardins, P.R. 2010. Sedimentology, ichnology and sequence stratigraphy of the Lower Cambrian Gog Group, southern Rocky Mountains, Canada. Unpublished PhD. thesis, University of Saskatchewan.
Desjardins, P., Smith, M., 2013. The Basal Cambrian Sandstone in the subsurface of Alberta: Quest Carbon Capture and Storage Injection Target. CSPG Geoconvention, Oral Presentation.
Dixon, J. 2007. Stratigraphy and facies of Cambrian to Lower Ordovician strata in Saskatchewan. Bulletin of Canadian Petroleum Geology, vol. 56, no. 2, Pages 93-117.
Evans, R. 2010. Magnitude of tides. The Geological Society magazine. https://www.geolsoc.org.uk/ Education-and-Careers/Ask-a-Geologist/PlanetaryGeology/Magnitude-of-Tides2
Greggs, D.H. (2000): The stratigraphy, sedimentology, and structure of the lower Paleozoic Deadwood Formation of western Canada; M.Sc. thesis, University of Calgary, Calgary, Alberta, 349p. Halamski, A.T. 2017. Personal comment on Researchgate website in answer to question: https://www.researchgate.net/post/How-highwere-tides-during-Cambrian-times
Hay, W.W., Migdisov, A., Balukhovsky, A.N., Wold, C.N., Flogel, S. and Solding, E. 2006. Evaporites and the salinity of the ocean during the Phanerozoic: Implications for climate, ocean circulation and life. Palaeogeography, Palaeoclimatology, Palaeoecology, Volume 240, Issues 1–2, 6 October 2006, Pages 3-46.
Hein, F.J. and Nowlan, G.S. 1996. Regional sedimentology, conodont biostratigraphy and correlation of Middle Cambrian – Lower
Ordovician(?) strata of the “Finnegan” and Deadwood formations, Alberta subsurface, Western Canada Sedimentary Basin. Bull. Can. Pet. Geol. Vol. 46, no. 2, pages 166-188.
Herbers, D. and Hauck, T. 2022. Sedimentary Facies and Depositional Systems of the Basal Cambrian Sandstone at the Industrial Heartland and Cold Lake Regions. Abstract, Core Conference, Calgary, Alberta.
Highlands Center for Natural History. 2024. StretchPebble Loop Nature Trail, Prescott, Arizona. Undated field guide. https://highlandscenter.org/ trails/ accessed October 2024.
Hughes, N.C. and Heim. N.A. 2005. Palaeozoic I Cambrian. Chapter in Encyclopedia of Geology. Kazmierczak, J. and Goldring, R. 1978. Subtidal flat-pebble conglomerate from the Upper Devonian of Poland: a multiprovenant high-energy product. Geol. Mag. 115 (5), 1978, pp. 359-366.
Kim, J.C. and Lee, Y.I. 1995. Flat-pebble conglomerate: a characteristic lithology of Upper Cambrian and Lower Ordovician shallow water carbonate sequences. Ordovician Odyssey: Short Papers for the Seventh International Symposium on the Ordovician System, 1995. Copyright © 2012 Pacific Section, SEPM (Society for Sedimentary Geology).
Knauth, L.P. 2005. Temperature and salinity history of the Precambrian ocean: implications for the course of microbial evolution. Palaeogeography, Palaeoclimatology, Palaeoecology 219 (2005) 53 – 69.
Life is a Gift Shop website (accessed October 2024): https://lifeisagiftshop.com/collections/bamboostone-jasper
Liu, Z., Algeo, T., Arefifard, S., Wei, W., Brett, C.., Landing, E. and Lev, S.M. 2024. Testing the salinity of Cambrian to Silurian epicratonic seas. Journal of the Geological Society (2024) 181 (3).
Łuczyński, P., Skompski, S. and Kozłowski, W. 2014. Stromatoporoid beds and flat-pebble
conglomerates interpreted as tsunami deposits in the Upper Silurian of Podolia, Ukraine. Acta Geologica Polonica, 64 (3), 261–280. Warszawa. Lynn, H.B. 2012. Structural controls on subsurface fluid migration through thrust sheets of the Stewart Peak culmination, Northern Salt Range, Wyoming. MSc. thesis submitted to the University of Montana.
Myrow, P., Tice, L., Aechuleta, B., Clark, B., Taylor, J.F. and Ripperdan, R.L. 2004. Flat-pebble conglomerate: Its multiple origins and relationship to metre-scale depositional cycles. Sedimentology 51(5):973 – 996.
Noad, J. 2022a. The Mighty Deadwood: Alberta’s Most Versatile Reservoir. Abstract, Core Conference, Calgary, Alberta.
Noad, J. 2022. The Rise of Cambrian Quartzites: Sedimentology of Carbon Sequestration in Alberta.
Noad, J. 2024. The Sedimentology of High Perm Streaks and Reservoir Heterogeneity: Implications for CCS. Reservoir, issue 5, Sept/Oct 2024. CEGA.
Petrychenkoa, O.Y., Peryt, T.M. and Chechel, T.E.I. 2005. Early Cambrian seawater chemistry from fluid inclusions in halite from Siberian evaporites. Chemical Geology 219 (2005) 149 – 161.
Pittenger, M.A., Marsaglia, K.M. and Bickford, M.E. 1994. Depositional history of the middle Proterozoic Castner Marble and basal Mundy Breccia, Franklin Mountains, West Texas. Journal of Sedimentary Research (1994) 64 (3b): 282–297.
Pruss, Sara B.; Gill, Benjamin C. (30 May 2024). “Life on the Edge: The Cambrian Marine Realm and Oxygenation”. Annual Review of Earth and Planetary Sciences. 52 (1). doi:10.1146/annurevearth-031621-070316. hdl:10919/117422. ISSN 0084-6597.
Sarkar, S., Choudhuri, A., Mandal, S. and Bose, P.K. 2018. Flat pebbles and their edge-wise fabric in relation to 2-D microbial mat. Geological Journal. 2019;54: 2566–2587.
Sarnoski, Anthony Henry, 2015. “The Stratigraphy And Depositional History Of The Deadwood
Formation, With A Focus On Early Paleozoic Subsidence In The Williston Basin” (2015). University of North Dakota. MSc. Theses and Dissertations. 1957.
Schneider, C.L. Grobe, M. and Hein, F.J. 2013. Geology of the Upper Devonian Moberly Member (Waterways Formation, Beaverhill Lake Group) Outcrops in Fort McMurray, Alberta (NTS 74D/11). Open File Report 2012-19. Alberta Geological Survey.
Sepkoski, J.J. 1982. Flat-Pebble Conglomerates, Storm Deposits, and the Cambrian Bottom Fauna. In: Cyclic and Event Stratification, ed. by Einsele/ Seilacher, Springer 1982.
Vaisblat, N., Hayes, B., Lake, J., Chalaturnyk, R. and Scott, J. 2022. Depositional Models for the Basal Deadwood Sandstone. Abstract, Core Conference, Calgary, Alberta.
Vsana website (accessed October 2024): https:// www.vsana.org/som-2012-08
Walker, J.D., Martin, M.W., and Glazner, A.F., 2002, Late Paleozoic to Mesozoic development of the Mojave Desert and environs, California: in Glazner, A.F., Walker, J.D., and Bartley, J.M., eds., Geological Evolution of the Mojave Desert and Southwestern Basin and Range: Boulder, Colorado, Geological Society of America, Memoir 195, p. 1-18.
Weides, S., Moeck, I., Majorowicz, J. and Grobe, M. 2014. The Cambrian Basal Sandstone Unit in central Alberta – an investigation of temperature distribution, petrography and hydraulic and geomechanical properties of a deep saline aquifer. Canadian Journal of Earth Sciences 51, 783-796.
Wilson, M.D. 1985. Origin of Upper Cambrian flat pebble conglomerates in the northern Powder River basin, Wyoming (in Rocky Mountain carbonate reservoirs; a core workshop, Mark W. Longman (editor), Keith W.Shanley (editor), Robert F. Lindsay (editor) and David E. Eby (editor)). SEPM Core Workshop (1985) 7: 1-50.
On behalf of the Price family, It is with deep sadness that I wish to inform the CEGA membership of the passing of our father, Dr. Raymond Alexander Price OC PhD DSc LLD FRSC NAS PEng. Dad passed on Oct 16, 2024 in Kingston Ontario. He was 91 years old.
Dr. Price had many awards and honorary degrees including being an Officer of the Order of Canada. Amongst his most cherished awards was the life/honorary membership bestowed on him by the CEGA (formerly CSPG) - an organization that he deeply cared for and believed in. Dr Price was a past president of the GSA and one of only a handful of foreign members of the US National Academy of Sciences. Ray was a giant amongst geoscientists in Canada. His good friend and colleague at Queens, Dr Peter Roeder, called him an "extra-ordinary" Ray (an inside petrology joke in case you did not get it). He was a mentor to many students, grad students and colleagues.
His pioneering work in mapping the southern Canadian foothills and Rocky Mountains, in the 1950s to 1970s, paved the way for many oil and gas discoveries, provided new paradigms regarding structural geology and plate tectonics and led to a more comprehensive understanding of the geology of Western Canada. His many students and graduate students blazed trails amongst energy companies, mineral companies and geotechnical companies
in Canada and around the world. He continued to think about and write about geology right up to his passing.
Although his accomplishments were many to our family he was first and foremost a loving/gentle husband, a loving and wise father, a grandfather and a great-grandfather. He was also a good friend and colleague to many. He will be sorely missed. He leaves behind his wife of 68 years Mina Price, his son Paul Price PGeol/PGeo (Carole), and his daughters Trish Van Huesen and Linda Price-Bennett, numerous grandchildren and great-grandchildren.
His full obituary can be found at the following link: https://www.arbormemorial.ca/en/reid/obituaries/raymondalexander-price/130850.html
In lieu of flowers or other tokens of respect, if you feel inclined, donations can be made to a local Alzheimers Society or to the CSPG Foundation.
Rest in Peace Dad.
Thank you for all you gave us and to what you gave the worldwide geoscience community.
By Paul Raymond Price
The 33rd annual Sweat the Subsurface Road Race took place on the evening of September 26, 2024, against the stunning backdrop of a fall evening in Calgary. This year’s event was a sell-out success, with 120 participants tackling the looped course along the scenic Bow River Pathway, starting at Sien Lok Park.
Runners and walkers alike embraced the challenge of both the 5K and 10K distances, navigating course markers that creatively highlighted the Phanerozoic time scale. The race brought together a vibrant mix of geologists from the Canadian Energy Geoscience Association (CEGA), geophysicists from the Canadian Society of Exploration Geophysicists (CSEG), and landmen from the Canadian Association of Land Energy Professionals (CALEP), along with many members of the public.
The competition was spirited yet friendly, as participants vied for the title of the fastest runner in the industry. Volunteers lined the course, ensuring a safe and enjoyable experience for all, and participants were easy to spot in the brightly colored shirts provided by Heritage Royalty. Meanwhile, Katalyst Data Management representatives were on hand at the lively Cheer Station near the Peace Bridge, where energy and enthusiasm helped keep spirits high.
None of this would have been possible without the incredible support of our sponsors and the dedicated volunteers who devoted
their time to making the event a success. After crossing the finish line and hydrating at Headwater Seismic’s water table, participants headed to the Calgary Curling Club for the post-race social. Many prizes were awarded, and the crowd eagerly awaited the return of the fan-favorite sock toss, provided by Echo Seismic and Pro Geo Consultants! You can check out the race results here.
As the evening unfolded, stories were shared, old friendships rekindled, and new connections made, underscoring the strong sense of community within our industry. We also would like to take a chance to thank our Platinum Sponsors, Athabasca Oil Corporation, CNOOC International, Key Seismic Solutions Ltd., and Teine Energy. Our Gold Sponsors were Ikon Science Canada and lxl consulting ltd. Thank you to our Silver Sponsors, BJV 3D Design, Bow Tie Club, Chinook Consulting Services, DMT Geosciences Ltd., Earth Signal Processing Ltd, GeologicAI, Qeye Labs, and Synterra Technologies. We appreciate our Bronze Sponsors, Cordax Evaluation Technologies, Eagle Canada Seismic Services ULC, ROGII Inc., SIGMA Explorations Inc., and Stack Technologies Ltd.
A heartfelt thank you goes out to the organizing committee, CEGA, CSEG, CALEP, and our generous sponsors for their continued support. We’re already excited to begin planning for next year’s race and look forward to making it even bigger and better!
November 6th In Person
12:00-1:00 pm MST
International Technical Division
Seismic facies differentiation using a multivariate process of the Early Jurassic, Browse Basin, North West Shelf, Australia
Speaker: Ryan Green
Location: New CEGA Classroom, 500 4 Ave SW, +15 level, Calgary, AB
November 14th Online + In Person
12:00-1:00 pm MST
Canadian Well Logging Technical Division
Title: Extracting and using Hi-Resolution data from borehole image logs and core photos to better characterize reservoir properties
Speaker: Gerry Peters, CNRL
Location: New CEGA Classroom, 500 4 Ave SW, +15 level, Calgary, AB
November 20th Online + In Person
12:00-1:00 pm MST
BASS Technical Division
Title: Geological Characterization of Organic-Rich Mudstones with Petroleum Generative Potential from The Montney Formation, West-central Alberta
Speaker: Daniela Becerra
Location: New CEGA Classroom, 500 4 Ave SW, +15 level, Calgary, AB
December 3rd In Person
11:30 am -1:00 pm MST
Technical Luncheon
Exploring for Alberta’s Other ReservesThe Scenic Geology of Alberta
Speaker: Dr. Dale Leckie
Location: Calgary Petroleum Club, Devonian Ballroom, 319 5 Ave SW, Calgary, AB, T2P 0L5
December 4th In Person
12:00-1:00 pm MST
Heavy Oil/Oil Sands Technical Division
Organic and isotope geochemistry of oil sands
Speaker: Jaime Cesar
Location: New CEGA Classroom, 500 4 Ave SW, +15 level, Calgary, AB
December 18th In Person
Holiday Social!
4:00-6:00 pm MST
International Technical Division
Exploration in the Cuu Long Basin, VietnamFrom Fractured Granites to Clastic plays
Speaker: Dr. Nick Fry, Gran Tierra Energy
Location: Bottlescrew Bill’s Pub 140 10 Ave SW, Calgary, AB
It was another busy field trip season with an increase in the number and variety of field trips offered. From May to October, CEGA provided a range of field trip opportunities including guided geoscience trips focused on relevant industry plays, a GeoTour to the Grand Canyon, a visit to the renowned Burgess Shale Walcott Quarry, and free field trips to geological points of interest as part of the series Go Take a Hike Together.
The season began in May with the Williston Lake Field Seminar led by JP Zonneveld to study the sedimentology and ichnology of the Upper Triassic Baldonnel Formation in northeastern British Columbia. In June, there was another amazing Grand Canyon GeoTour, organized by Astrid Arts. Highlight reels of the trip can be viewed here. If you missed the 2024 GeoTour, we are currently gauging interest for 2026/2027. Please reach out to the field trip committee to reserve your spot. Also in June,
there was the popular half-day hike to Jura Creek with Pavel Kabanov to observe the Drowning Unconformity and Anoxic Sediments at the Devonian-Carboniferous Boundary.
July provided our members with two options in the Go Take a Hike Together series. Tako Koning led the Tyndall Stone walking tour to view Ordovician fossils around the inner city of Calgary. A more challenging hike to Nihahi Ridge in Kananaskis was led by Andre Chow and Mark Mallamo. Besides the spectacular views of the Front Ranges, the Nihahi Ridge trail allowed participants to cross the McConnell thrust fault and travel in time from the Cretaceous back to the Mississippian and Devonian. Good vibes were felt by all as the group crossed the thrust fault and entered the fossiliferous carbonates of the Palliser (Wabamun), Exshaw, Banff and Pekisko Formations.
In August, Simon Haynes led a vigorous hike up to the Burgess Shale fossils at Walcott Quarry, a UNESCO World Heritage site. The Burgess shale is worldfamous for its high frequency of soft tissue preservation and taxonomic diversity in the Cambrian. These exquisitely preserved 508-million-year-old fossils have influenced the scientific view of the evolution of life on Earth.
September held the chance for members to put their finger on the K/Pg Mass Extinction Boundary on Knudsen’s Farm near Huxley, Alberta. The trip leader, Tako Koning, arranged special permission with the landowners, Kent and Marion Knudsen, to access the outcrop, which is on the south side of the Red Deer River valley, south of Dry Island Buffalo Jump Provincial Park. Later in September, members, as well as friends and family, participated in the Go Take a Hike Together: Red Rock Coulee, led by Rachelle Pinnow. The trails were a little muddy due to rain the day before, but we hiked carefully and enjoyed a sunny afternoon exploring hundreds of giant spheroid concretions. There was a great turnout, and it was wonderful to see some Saskatchewan members make the trip to Red Rock. Together, we found the largest intact concretion in the coulee (so far), with a diameter measuring 4.41 meters!
The last field trip of the season, Montney Sedimentary Facies and Environments and Relations to Fractures, was led by Per Pedersen on October 1st. This field trip was offered in conjunction with the 2024 Gussow conference in Banff, Alberta, this year focusing on the cutting-edge geoscience work being undertaken to optimize fluid recovery within the Triassic Montney Formation.
The 2024 field trip season had excellent participant attendance. Special thanks to all the trip leaders, especially the volunteer leaders hosting free hikes for the CEGA community. Trip leaders will continue to hone future itineraries to accommodate participant interests. The Field Trip Committee is currently working on the lineup for the 2025 season. We are open to suggestions from our members and are always looking for more volunteers. Please click the link if you would like to submit a suggestion for our Go Take a Hike Together series.
SUBMIT HERE
The CEGA Field Trip program provides opportunities for members to gain field experience, including GeoTours to international locations, the free series Go Take a Hike Together, and guided trips to geological points of interest in Alberta and British Columbia. There was plenty of opportunity to get outside this season, connect with your geo-community, and see some great outcrops. We hope to see you on our 2025 field trips!
Explosive growth in the Clearwater
n Explosive growth in the Clearwater
2.
Tourmaline bolsters BC Montney position via Crew acquisition
The Clearwater play has evolved into one of the most dynamic growth stories in the Canadian upstream sector, surging from zero production in 2016 to become a major contributor to the country’s oil output. Production is set to exit 2024 at 130,000 bbl/d, but overall production growth in 2023 slowed to 20% annual growth, relative to 70% growth in both 2022 and 2021. This remarkable growth has been underpinned by continued innovation in drilling and completion techniques, particularly the adoption of Open Hole Multi Laterals (OHML). These advancements have allowed operators to achieve significant productivity at highly competitive costs, making Clearwater one of the most economically resilient oil plays even at lower price thresholds.
Key players like Spur Petroleum, Tamarack Valley, and Headwater Exploration have capitalized on this opportunity, driving impressive production growth through an aggressive drilling schedule and strategic expansion into new subplays, including the Peavine and Marten Hills regions. While early phases saw exponential growth, production rates are expected to moderate as mature areas of the play experience natural declines. Nonetheless, operational improvements and longer lateral designs continue to yield strong results, extending well productivity and enhancing project economics.
M&A activity has also been a pivotal force in shaping Clearwater’s landscape. Strategic acquisitions, including Tamarack Valley’s consolidation of assets in 2022, have positioned key operators to maximize synergies and further exploit the play’s potential. While Clearwater’s high-growth phase may plateau toward the late 2020s, waterflooding and further technical enhancements promise to sustain long-term production, reinforcing the play’s status as a cornerstone of Alberta’s oil industry. The current outlook for Clearwater remains robust, with operators continuing to push boundaries in cost efficiency and well performance across the play’s sub-regions.
Tourmaline Oil Corp. announced its agreement to acquire Crew Energy in an all-share deal valued at Cdn$1.3 billion in August, 2024. This acquisition will involve the issuance of 18.8 million Tourmaline shares, and includes Cdn$240 million of Crew’s debt. Crew’s Montney assets are particularly attractive to Tourmaline, with 30,000 boe/d of production and 700 tier-one drilling locations included in the deal. The strategic move will strengthen Tourmaline’s holdings in the southern Montney region, positioning the company for long-term growth. The transaction is expected to close by early October 2024, pending regulatory approval.
3.
Expand Energy emerges as North America’s largest gas producer
Crew Energy’s asset base of 218,240 net acres adjacent to Tourmaline’s South Montney acreage significantly enhances Tourmaline’s production portfolio. The acquisition follows several years of consolidation in the Montney region, reflecting Tourmaline’s strategy of opportunistic asset acquisitions. Although the deal’s Cdn$44,440 per flowing barrel price is higher than previous Tourmaline transactions, it underscores the premium nature of Crew’s assets, with a strong liquids component and significant development potential. Tourmaline’s shares saw a 4% rise on the announcement, reinforcing the market’s positive reception to the deal and its alignment with Tourmaline’s long-term LNG and condensaterich inventory strategy.
n Expand Energy emerges as North America’s largest gas producer
The combination of Chesapeake (CHK) and Southwestern (SWN) has displaced EQT as the largest gas producer in North America. With productive capacity of nearly 8 bcf/d it amounts roughly 8% of total US gas production. That’s almost 2 bcf/d more than Appalachian gas giant EQT, who previously held the title.
This unmatched scale among North American gas producers will provide new options to create value including lower per-unit costs, greater marketing options, and an investment grade credit rating. Although both producers have operations split between the Marcellus and Haynesville, there are differences in these portfolios that mean greater optionality once they are joined together.
CHK and SWN had differing reactions to low prices in 2024. SWN has operated a maintenance level development program while CHK has, since Q1, curtailed production and built up its backlog of DUC and behind pipe wells. SWN’s greater liquids weighting (15% vs CHK’s 0%) and relatively stronger hedge position are factors. During Q2, CHK saw an uplift of US$0.62/mcf and SWN realized an uplift of US$0.80/mcf from hedging. We estimate that CHK’s hedge book will net nearly US$400 million in gains during H2 2024.
Given the low liquids weighting of the combined portfolio, we anticipate that the newly formed company will take after CHK, opting to defer production in the face of continuing low prices. Apart from a brief surge during Winter, we forecast sub-$3/mcf Henry Hub pricing through Q4 2025. Under this price outlook, our analysis suggests that delaying spud timing from early 2025 until late 2026 boosts single well Haynesville NPVs by more than 45%. Having production behind pipe that can easily be brought to sales is key so we don’t anticipate that the combined 13 active rigs (down from 19 in February) will drop substantially.
There are a few questions outstanding on the path forward for Expand. What role will hedges play versus LNG feedstock and other long-term market agreements? At present, the companies have hedged 35% of combined 2025 gas volumes but clarity on feedstock contracts is lacking. CHK has nonbinding agreements to provide 3 mmtpa of LNG supply by 2031 to projects owned by Gunvor and Vitol. How much will its
Scott joined the Canadian Upstream Research team at Wood Mackenzie in June 2019. He is responsible for providing financial asset valuation and objective commercial analysis on company and play activity across Canada. His coverage ranges from North American large caps to junior private producers. He also covers CNRL and Cenovus for the corporate analysis team, providing high level company valuation and strategy analysis.
contiguous acreage allow for opex and capex improvements? CHK has proposed target of 20% of pre-deal production linked to international price contracts. It remains to be seen to what extent Expand will grow into the LNG space. We anticipate that integration efforts will focus on these strategic elements in the months following deal close.
Xin's responsibilities include financial asset valuation and emissions modelling for Canadian and Alaskan assets. She also contributes to corporate analysis for Canadian and international mid caps and large caps such as Tourmaline Oil and Chevron.
DISCLAIMER – THE VIEWS AND OPINIONS STATED BELOW ARE BASED ON WOOD MACKENZIE’S DATA, SOURCED FROM PUBLIC SOURCES ACROSS THE GLOBE AND OUR PROPRIETARY TOOLS SUCH AS LENS.
RigSat
CNOOC International
Teine Energy
Athabasca Oil Corporation
Belloy Petroleum Consultants Ltd.
GVERSE GeoGraphix
Heritage Royalty
Key Seismic Solutions Ltd.
Spectrum Geosciences
Tallman Geological Consulting
Torys LLP
Beryl Mining Services Ltd.
Canadian Stratigraphic Services Ltd.
Echo Seismic
lxl consulting ltd
DMT Geosciences Ltd.
Ampco Pipe Ltd.
BJV 3D Design
Bow Tie Club
Earth Signal Processing Ltd
Freehold Royalties
GeologicAI
Katalyst Data Management
Qeye Labs
Synterra Technologies
XRF Solutions
SIGMA Explorations Inc.
Barrel Eye Tools
Eagle Canada Seismic Services ULC
Headwaters Seismic Processing
Cordax Evaluation Technologies
Stack Technologies Ltd