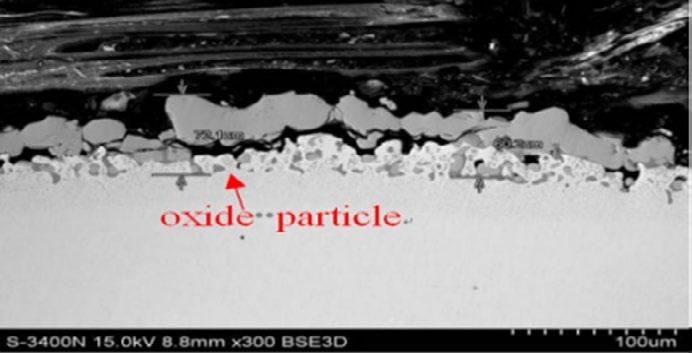
19 minute read
Effect of si-cr-mo on oxidation behavior of duplex steel and strip surface quality
C. Wang, Y. Yu, K. Liu, L. Wang, Z. Guo, T. Niu
The interactive effects of Si-Cr-Mo alloy elements on oxidation behavior at scale/metal interface in dual-phase steel are studied in present paper, through thermal simulation, scanning electron microscopy (SEM) and electron probe analysis. The influence of alloy elements on hot coil surface quality under industrial production conditions are also discussed. Results show that the oxidation resistance of Si-Cr steel is stronger than that of Si-Cr-Mo steel, because of Cr-rich protective oxide film at scale/metal interface under elevated temperature. Comparing with steel alloyed by Si, the primary scale of the Si-Cr and Si-Cr-Mo steel are easier to be removed, owing to the reduction of anchor structure at scale/metal interface. However, Si and Cr element will form dense oxide film under 1,100°C and above, which increases the difficulty of finishing descaling. Owing to the accumulation of Mo on the scale surface, the diffusion channel of Si and Cr elements is blocked, resulating in the increase of oxidation resistance and the decrease of SiCr oxide film at scale/metal interface. Therefore the surface quality of Si-Cr-Mo steel is significantly improved under industrial production. Suggestions conclued from present study can provide useful guidance for production of cold rolled dual-phase steel.
KEYWORDS: OXIDATION BEHAVIOR, ELEMENT ENRICHMENT, INTERACTIVE EFFECT, SCALE, DESCALING
Introduction
Basing on the phase transformation strengthening mechanism, the dual-phase steel composed of ferrite and martensite has been developed as advanced highstrength stamping steel for automobile. The cold rolled dual-phase steel are mainly classsfied by C-Si-Mn steel and C-Mn-Cr steel [1,2], which sometimes is micro-alloyed with Nb, Mo and B elements. In the widely used C-SiMn dual-phase steel, the mass percent of Si element is controlled between 0.2% and1.5%, which can improve the austenitic harden ability and improve the purity of the ferrite. However, when the mass percent of Si is more than 0.2% ,the red scale defect are easily generated during hot rolling process resultiing in surface color difference defect on the finished product[3,4]. According to the high surface quality standards, the Si is replaced by Cr or CrMo as an option to produce C-Mn-Cr/C-Mn-Cr-Mo dual phase steel.
The effect of Si on oxidation resistance at high temperature has been adequately studied by predecessors.The Si element exists as hydrothermal Fe2SiO4 phase at scale/ metal interface at elevated temperatures above 1,173°C. When the temperature drops to 1173°C and below, the
Shougang Research Institute of Technology China - Beijing Key Laboratory of Green Recyclable Process for Iron & Steel Production Techology, China scale will tranformed to eutectoid structure in which FeO grains will be surrounded by Fe2SiO4. The FeO particles could be tightly sticked on the slab surface by the solidified eutectoid structure, which is difficult to be removed by descaling. The residual FeO will be squashed by following hot rolling process, leading to the increase of contact area with oxygen and giving rise to the red Fe2O3 phase[5, 6]. In the range of 700~1,000°C [7], with higher Si content, oxidation mass gain and scale thickness of the steel with high Si content are both reduced because of improved oxidation resistance. At present, some papers focusing on oxidation behavior of Cr-Mo in stainless steel and heat-resistant steel at elevated temperatures. Chen WY found that Cr2O3 protective oxide film accumulated at the strip surface of 3Cr13 stainless steel, in which the Cr content is about 13.46%, significantly improveing antioxidation properties[8]. Zhao Q H concluded that the SiO2 and MnCr2O4 oxide film was formed under 1100°C and SiO2 oxide film adjacent to metal matrix can obviously decrease the oxidation mass gain rate basing on one kind of ferritic heat resistant steel[9]. However, few reports have been publised focusing oxidation behavior of the coldrolled dual-phase steel at elevated temperature, basing on C-Mn-Cr/C-Mn-Cr-Mo system. For the oxidation behvior of Mo element, it is reported that Mo element is easy to be oxidized in air when the temperature is heated up to 300°C. When the temperature increases up t0 600°C and above, Mo will be oxidized to volatile oxides with dark green color. Xie Hong-bo studied the influence of Mo on oxidation behvior of high-entropy alloy at elevated temperature and found that the oxidation resistance was seriously deteriorated because of volatile Mo oxide[10]. In the referenced papers listed above, the content of Cr/Mo in mass percent is around 10-20%, the scale of given steel grade mainly consists of compact single-layer oxidation film. However, the Si/Cr/Mo content in cold rolled dualphase steel range from 0.2~1.15%, the interreaction of SiCr-Mo on oxidation behavior should be interesting and not be neglected.
In present work, the interreaction of Si-Cr-Mo elements on oxidation characteristics at scale/metal interfacei of dual-phase steel are studied. In addition, the effect of alloyed elements on coil surface quality under industrial production condition is also discussed, which can provide useful guidance for production of dual-phase steel.
Experiment Method
Three types of composition are designed for present study. The first sample contains1.15%Si without Cr-Mo; the second sample contains 0.55% Cr and 0.2% Si without Mo; and the third sample contains 0.2%Si in combination with 0.2% Cr and 0.25%Mo (as shown in Tab. 1).
Tab.1 - Designed omposition of high strength DP steel with different alloyed element (wt. %).
NO. C Si Mn P≤ S≤ Alt [N]≤ Cr Mo
Steel 1 0.76 1.15 1.7 0.015 0.005 0.035 0.0030
Steel 2 0.12 0.20 1.6 0.015 0.005 0.035 0.0030 0.55
Steel 3 0.10 0.20 1.5 0.015 0.005 0.035 0.003 0.20 0.25
The oxidation behavior of given three steels are studied under two different temperature ranges by STA449C type thermo gravimetric analyzer. To simulate the oxidation behavior in reheating furnace, the samples were heated up to 1250°C in the atmosphere contains 10% CO 2,5% O2 and other argon gas. The samples were heated under given heating rate of 10°C/min and were kept for minutes under aimed temperature. Secondly, we simulated the oxidation during hot rolling process in the temperature range of 900-1150°C. In order to oxidation behavior during hot rolling process, the samples were heated up to 1150°C, 1100°C. 1050°C, 1000°C, 950°C and 900°C, respectively, in protective argon gas with the heating rate of 10°C / min. Then, the samples were kept in the air at the target temperature for 1 min. Finally, the samples will be put into the protective atmosphere again and be cooled to room temperature at the cooling rate of 10°C / min. The surface topography, scale/metal interface topography and energy spectrum, were investigated with the scanning electron microscopy (SEM). The elements distribution quantitative analysis at scale/metal interface was detected by EPMA-1720 electronic probe analysis .
The surface quality difference between differernt dual phase steels under industrial production condition was studied in a domestic hot rolling production line by Parsytec surface inspection equipment adopting CCD scanning digital camera with the resolution ratio of 500µm*1000µms. One of the key equipment for the surface quality control is high pressure descaling system, of which the pressure could achieve 20-22MPa. For the three designed steels, the rolling process parameters is the same in order to investigate the effects of alloyed elements on coil surface quality.
Results
Oxide film morphology under heating furnace conditon
As shown in Fig. 1, the oxide film micromorphology of thres different steels under 1250°C are given. The oxidation film of steel No.1 at scale/metal interface shows typical anchor pinning morphology of Si alloyed steel, in which the ferrous oxide (FeO) is surrounded by Fe2SiO4.
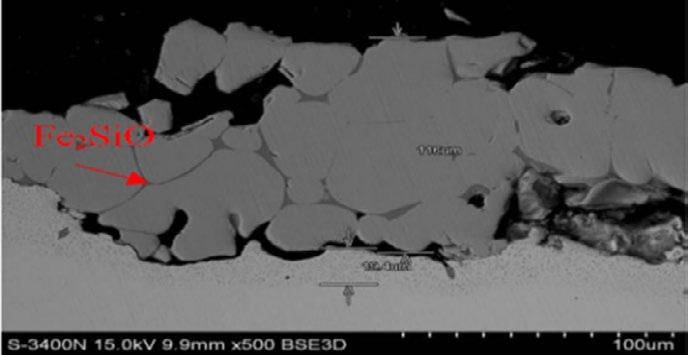
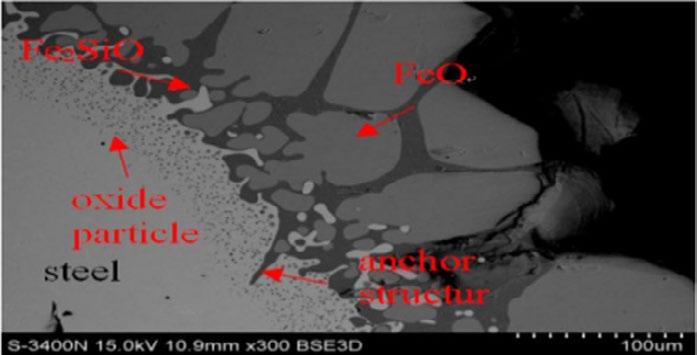
The anchor made by theFe2SiO4 is nailed into the metal matrix with a depth of 50 µ m. Because of the anchor structure,the inner layer of scale has good adhesive force. In addition, the oxide particle layer under interface is about 50 µ m. The anchor pinning morphology is originated from the melt behavior of Fe2SiO4 at the preset temperature and the molten Fe2SiO4 will infiltrate into the grain boundary of scale and the steel substrate. Steel No.2 contains 0.2%Si and 0.55% Cr element. The mesh scale morphology and anchor pinning morphology in oxide film are significantly reduced. Compared with steel 1, the depth of the liquid Fe2SiO4 infiltrated into the matrix decreased to about 20 µ m. During the samples’ preparation process, due to the weakened anchor pinning structure, the scale is easy to crack and peel off. Cr content of steel No.3 is reduced to 0.2% on the basis of steel No.2 with an addition of 0.25% Mo. The mesh scale morphology and anchor pinning morphology is not obvious, but large oxide particle with a particle-layer thickness of 50 µ m present at sacle/metal interface. The outermost layer scale of steel No.3 was also easy to peel off during sample preparation process.
(b) Steel 2
(a) Steel 1 (c) Steel 3
Fig.1 - Scale/interface morphology of given three steels under heating furnace condition.
The distribution of Cr–Si element at scale/metal interface of steel No.2 is shown in Fig. 2 in detail. Si enrichment taking place in the mesh scale structure, anchor pinning structure and small oxide particles. The content of Si element in the mesh structure is highest illustrated as red color. Si-rich in the oxide particles originated from the internal oxidation of alloying element and the Si content in oxide particles is lower than that in the mesh scale. Cr element accumulates greater in the scale than that in metal matrix. In the oxide particles, Cr element enrichment could also be found. Complementary relationship between the distribution of Cr and Si is found in the scale. In the region of the mesh structure where the Si element is rich, the Cr element is poor. Because the content of Cr element in scale is higher than Si element, the anchor pinning morphology formed by Fe2SiO4 nearly disappeared.
(b) Si distribution (a) scale morphology (c) Cr distribution
Fig.2 - The element distribution of Cr – Si in oxidation film of steel 2.
Oxidation scale morphology of steel 3 is given in Fig. 3. The mesh structure in the scale of steel No.3 is significantly weakened compared with steel 2, especially in the inner oxidation layer adjacement to matrix. The mesh scale almost disappeared. Complementary relationship between content of Cr and that of Si element in oxidation scale. In steel 3, the content of Cr and Si element are both about 0.2%. According to the free energy rule, Si is oxidized earlier than Cr, inducing Si-rich in oxide particles. Mo-rich layer distributed in the metal matrix around scale/metal interface, the depth of which is the same with internal oxidation zone. Mo is not involved in the formation of the internal oxide particles.
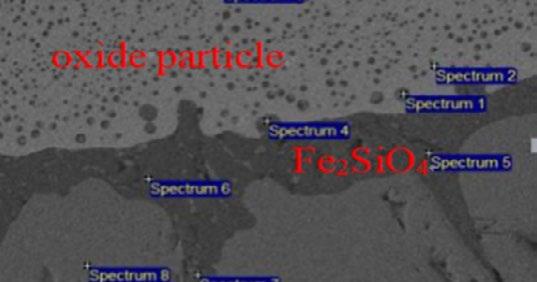
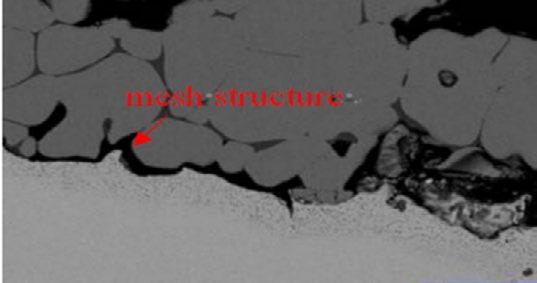
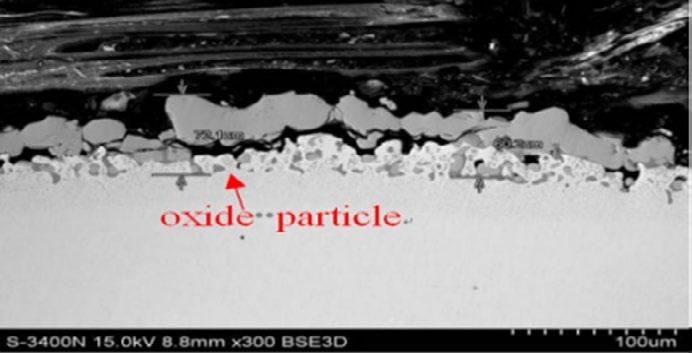
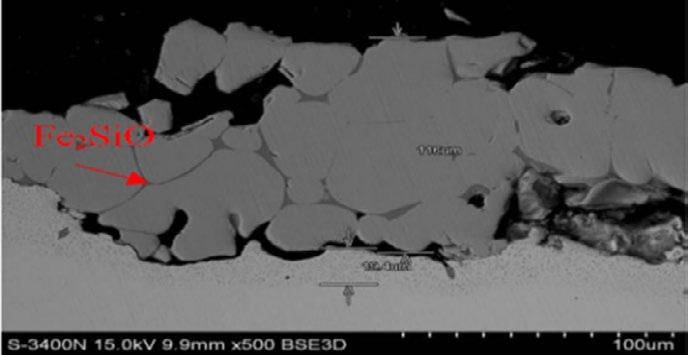
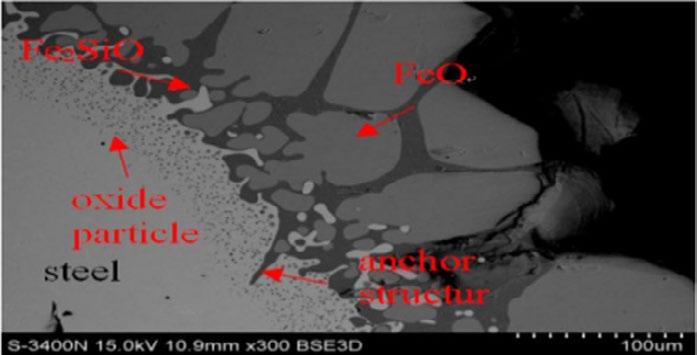
(b) Si element distribution (a) scale (c) Cr element distribution (d) Mo element distribution
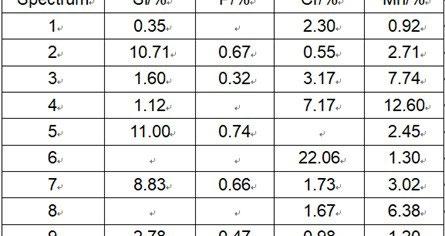
Fig.3 - The Cr-Si-Mo element distribution at the interface in oxidation film of steel 3.
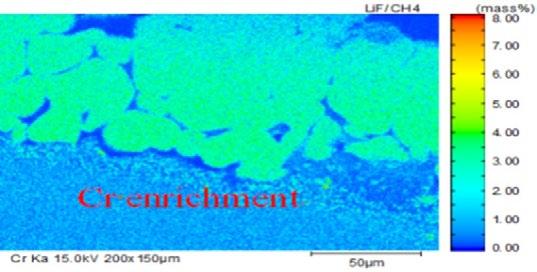
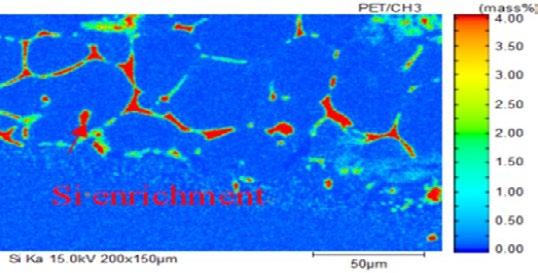
As shown in Fig. 4, energy spectrum analysis of the oxidation particles was applied at the interface of steel No.1 and steel No.2. The Si content in the band of molten Fe2SiO4 is as high as 10-13%. Meanwhile, with the internal oxidation of Mn, a series of oxides of low melting point are formed. With increase of Cr content and decrease of Si content, the width of the molten band decreases. It is inferred by the energy spectrum analysis that competition oxidation mechanism resuts in the complementary relationship between Si content and Cr content in scale.
(a) Steel No.1
(b) Steel No.2
Fig.4 - Energy spectrum analysis of scale/metal interface in steel 1 and steel 2.
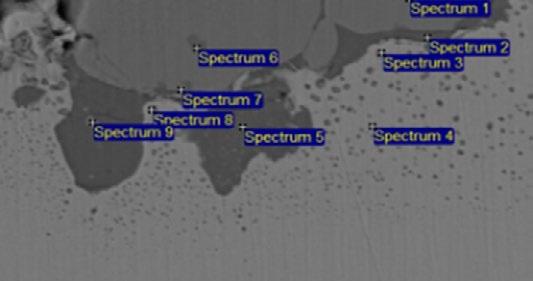
Oxide morphology under hot rolling condition
The surface morphology of oxidation scale of given three types of dual phase steel under different temperature was simulated by thermo gravimetric analyzer. The scale morphology transformation patterns of the three given steels under different temperature is consistent. Fig. 5 illustrates the oxide film morphology of steel No.2 at different temperatures. Under tested condition, the scale is thin and smooth at 900°C and becomes rough and uneven when the temperature heated up to 950°C. The scale surface looks like tumor morphology at 1050°C. When the temperature rises to 1100°C, preferential growth of oxide particles is found on the surface and the scale mainly consists of coarse particles. Under the condition of 1150°C, oxide particles present obvious granular structure with a grain size of 50 μ m.
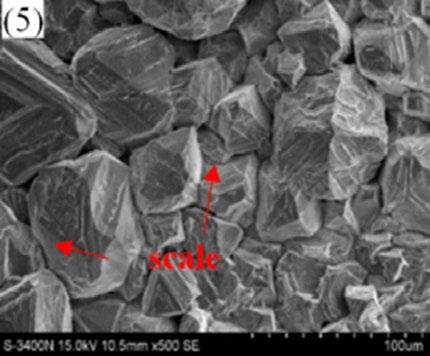
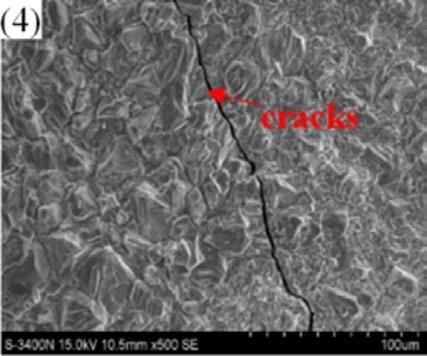
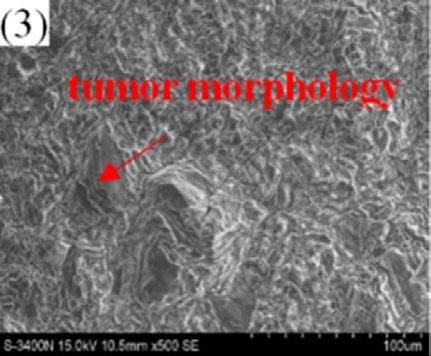
More and more cracks can be observed on the scale surface as the test achieved higher and higher aimed temperature because thermal expansion coefficient difference between scale and matrix becomes larger and larger, inducing internal stress growth at scale/ metal interface and surface scale spalling during sample preparation, as shown in Fig. 6[11] .
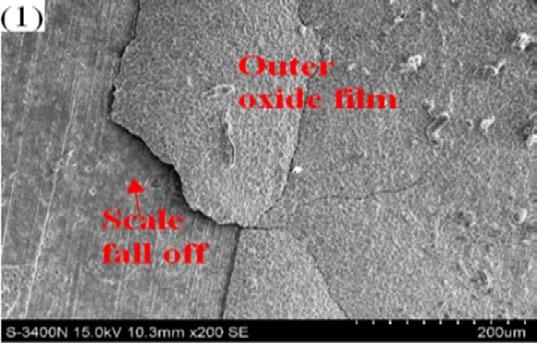
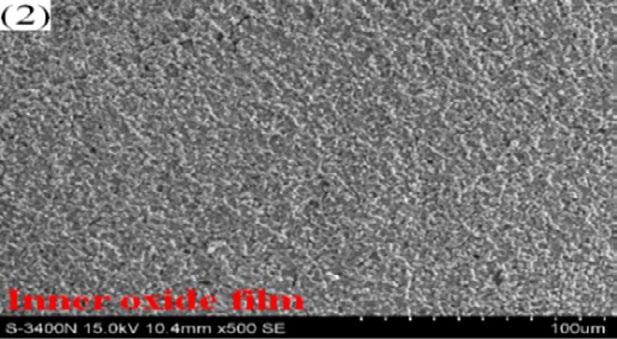
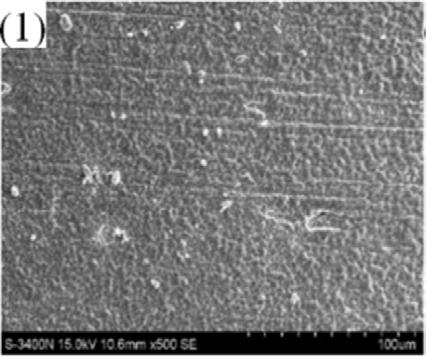
Fig.6
Element enrichment in oxidation film under hot rolling condition
SEM was applied to determine scale thickness under different test temperature. Results is given in Fig. 7. steel No.1 alloyed with Si content of 1.15 mass% possesses the best oxidation resistance and thinnest scale thickness at 1150°C among the three predesigned steels. Benifit from the dense Si - Cr composite oxide film at scale/metal interface, steel No.2 alloyed with Si- Cr has better oxidation resistance than that of steel No.3 alloyed with Si-Cr-Mo. The scale thickness of steel No.3 is the thickest. The scale thickness of steel No.3 is about 20 µ m thicker than that of steel No.1 at 1150°C.
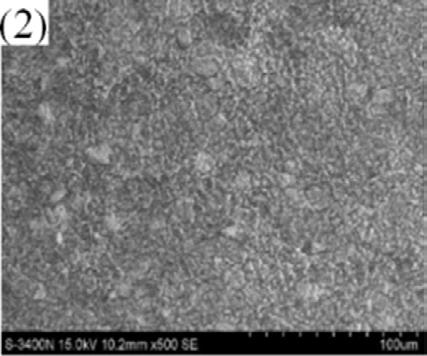
Fig.7 - Scale thickness of three given steels under different temperature.
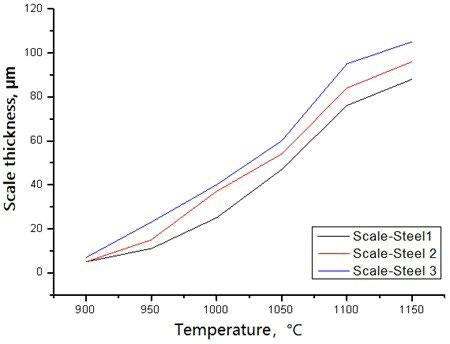
The cross section morphology and energy spectrum results upon oxidation particles of steel No.1 under 105°C is illustrated in Fig. 8. The inner oxidation particles in metal matrix beneath the scale/metal interface are made of Si-Mn compound oxide, labeled as spectrum 1 to 3. The oxidation film of Fe-Si-O thickness is about 7-8 µ m and consists of small dense particles resulting in good oxidation resistance. Compared with the mesh scale and anchor pinning morphology under 1250°C as shown in Fig.1, under the tested condition of 1050°C, Fe-Si-O composite oxide located between outermost scale and metal matrix remains solid state without infiltration into metal matrix or outermost scale, in which the enriched Si content reaches up to 9%. The enriched Si content in the mesh scale is about 12% on average under 1250°C.
(a) Cross-section morphology
(b) Energy spectrum
Fig.8 - Cross section analysis of steel 1 (high Si steel) at a simulated temperature of 1050°C.
The element distribution at scale/metal interface of the steel No.2 under 1050°C was detected by electron microprobe analysis, as shown in Fig. 9. A clear dense alloyed elements enrichment zone located at the inner side of oxidation film, in which enriched Cr content reaches up to 13% and enriched Si content is only 3%. Higher content of Cr in the dense alloy element enrichment layer than that of Si originates from the different content of Cr and Si in metal matrix inducing better oxidation kinetics for Cr than that of Si.
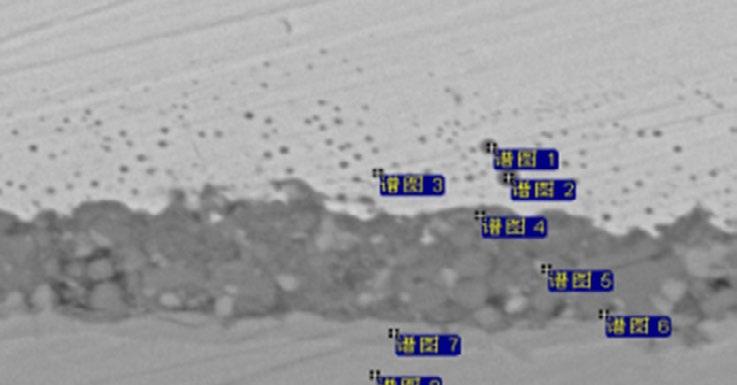
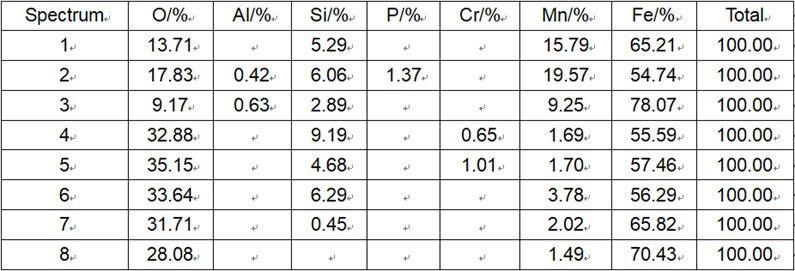
(b) Si
(a) Scale morphology (c) Cr
Fig.9 - Map scanning elements analysis of steel2 at 1050 °C.
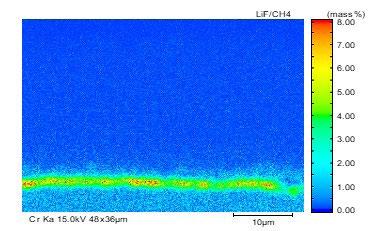
The element distribution at scale/metal interface of the steel No.3 alloyed with Si-Cr-Mo under 1050 °C is detected by electron microprobe analysis, as shown in Fig. 10. All of these three kinds of alloy element enrich at the inner side of oxidation film. As element diffusion distance order is Si > Cr > Mo, Mo-rich layer at the scale/ metal interface located nearby the matrix. Because of higher oxidation free energy of Mo than that of metal matrix, the presentation of Mo-rich layer in the inner layer of oxidation scale break the continuity of Si-Cr oxide film, resulting in the decrease of oxidation resistance and the increase of scale thickness.
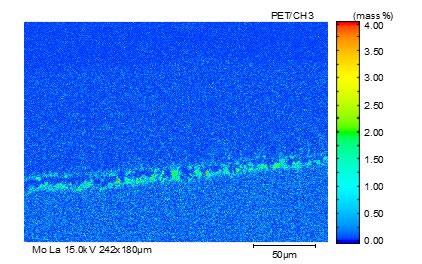
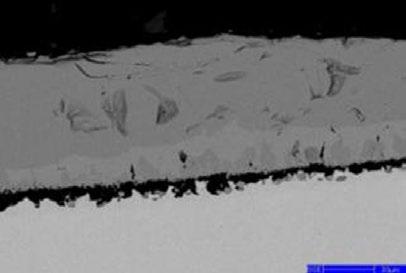
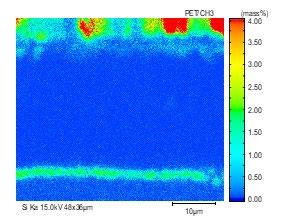
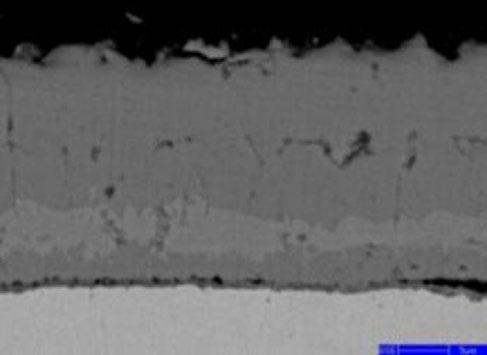
(b) Si (a) Scale morphology (c) Cr (d) Mo
Fig.10 - Map scanning elements analysis of steel3 at 1050 °C.
Surface quality of duplex steel in practice
Influenced by the formation of fayalite phase and strong adhesion force of primary scale under high Si content condition, it is difficult to remove this kind of scale by present roughing and finish descaling system. Severe red scale defects distrubution is found on the hot rolling coil surface of steel No.1 in industrial produciton practice, the area ratio of which reaches up to 70~90%, as shown in Fig.11. In the produciton of steel No.2, the pattern of red scale defects tranformed as bands like, the pitch of which matched with spacing of finishing descaling nozzles very well. The descaling difficulty for steel No.2 upon the temperaure range of 1000-1100°C originates from the dense Si - Cr composite oxide layer at scale/metal interface, as shown in Fig. 6. Basing on present finishing descaling system, as illustrated in Fig. 12, the spacings and angles of descaling nozzles should be meticulously designed to gurrantee constant impact force upon the interact area of neighbouring nozzles. Otherwise, the impact forces sharply decreases upon the overlapping area of descaling water, leading to residual banded red scale. The area ratio of banded red scale decreases to 5~10% while applying the Si-Cr alloying pattern for duplex steel. While applying the Si-Cr-Mo alloying receipt, the dense oxidation film in the inner side of scale is broken by the presence of Morich layer. Under the same production parameters, the proportion of red scale area on hot rolling coil surface is significantly decreased to less than 5% for Steel No.3 and good surface quality of duplex steel is achieved.
(a) Steel 1 (Si)
(b) Steel 2 (Si-Cr)
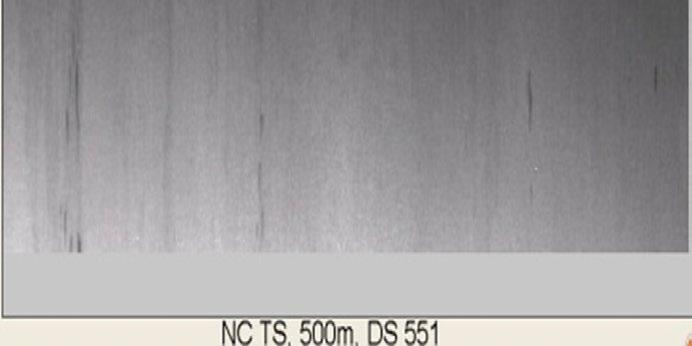
(c) Steel 3 (Si-Cr-Mo)
Fig.11 - Surface quality of hot rolling coil with different alloy systems.
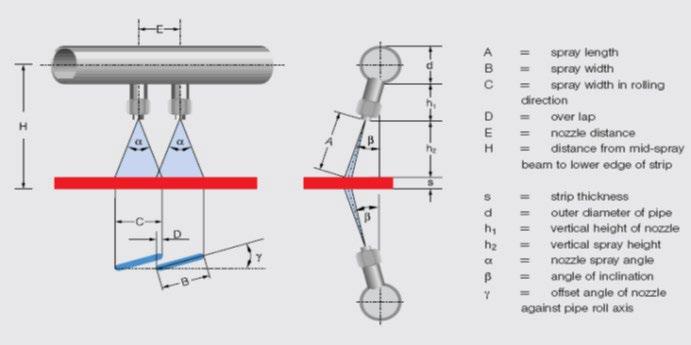
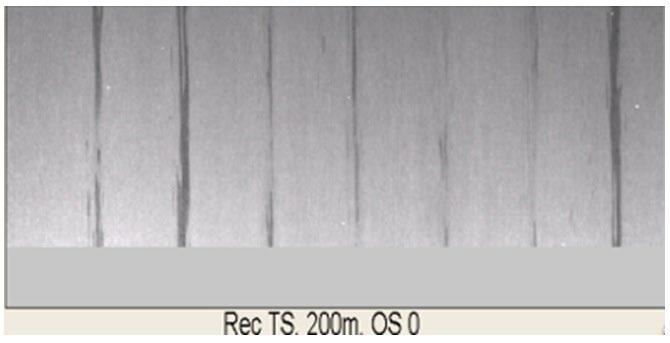
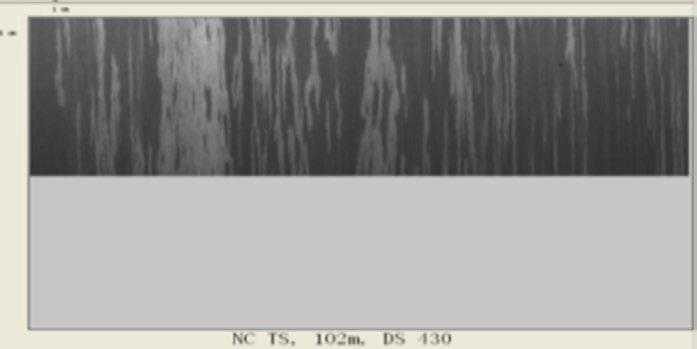
Fig.12 - Schematic diagram of the descaling nozzle.
Discussion
As typical alloyed elements in metal matrix, such as Cr, Al, Si, have strong cohesive force with oxygen. From the thermodynamic side, the diffusion coefficient of metal ions in these oxide films is limited,stable Cr, Al, Si et al. oxide could be formed during oxidation process, covering the strip surface as compact and uniform oxidation film, acting as protective film[12]. In present study, the conclusion was proved that the scale thickness decreased with the addition of Si and Cr elements. The dense oxide film can inhibit the process of steel oxidation and reduce scale thickness.
According to the thermodynamic calculation with the equation listed in Tab.2[13], the Gibbs free energy of oxidation reaction between alloyed elements or ferrus and oxygen is ranked as: Δ G (SiO 2) < ΔG (MnO) < Δ G (Cr 2O3) < Δ G (Mn3O4)< Δ G (FeO) < Δ G (Fe3O4) < Δ G (Fe2O3) < Δ G (MoO). The oxidation serial of typical alloy elements (Si, Cr, Mo, and Mn) in steel and ferrus under elevated temperature is Si, Mn, Cr, Fe, and Mo.
Tab.2 - Relationship between ΔG of oxidation reaction and temperature for different alloy elements.
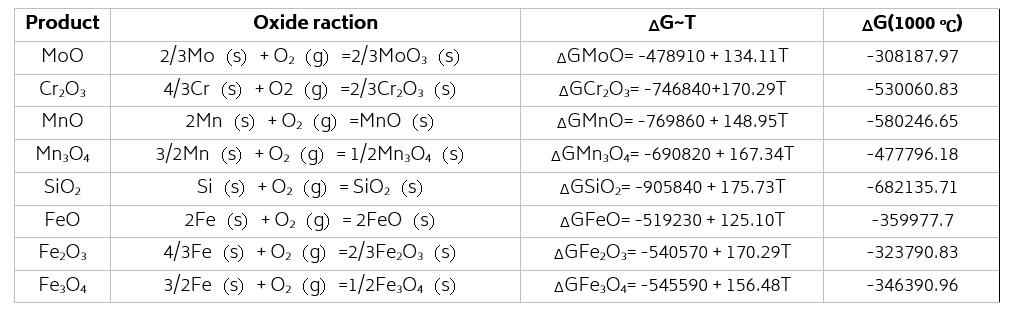
As the generation of SiO2 andCr2O3 will reduce the oxygen partial pressure at the scale/metal interface. The "oxygen absorption effect" effectively inhibits the oxygen atom diffusing into the substrate, prevents internal oxidation and promotes the formation of compound oxidation film of SiO2 and Cr2O3. According to present study, at the temperature ranged from 900 to 1150°C, Fe 2SiO4 and Fe2SiO4-FeCr2O4 spinel at scale/metal interface in steel 1 and 2 enhance the compactness of oxide film and perform excellent oxidation resistance, slowing down the growth of scale. In steel No.2, because of higher content of Cr is designed, the activity of Si is obviously decreased, Cr is prior to be oxidized first, reducing the generation of Si oxide. Thus, the anchoring morphology in scale of steel No.1 at 1250°C is eliminated by modifying the alloyed receipt by addtion of Cr and preventing the generation of eutectoid product of Fe2SiO4.
At the initial stage of oxidation, the sufficient oxide film on the surface has not been established, and the oxidation rate is governed by the interface reaction between atomsphere and substrate. Along with time extension, the oxidation film gets denser and denser acting a barrier layer to protect the substrate from being oxidized. The further growth of the oxide film depends on the diffusion of alloyed elements. In solid state, diffusion is the only mode of mass transport. Fick studied this The diffusion phenomenon in solid state was studied by Fick and described as first Fick law, listed as follows:
In which, J is the diffusion flux, D is the diffusion coefficient, dc/dx is the mass concentration gradient along the x direction and D s is the self-diffusion coefficient.It can be seen from the above formula that the self-diffusion coefficient of a certain element in the alloy is the diffusion coefficient when its mass concentration gradient goes to zero.Diffusion rate depends on the diffusivity of alloyed elments in oxidation film at elevated temperature[14, 15] The diffusion coefficient of the oxide film reflects the difficulty of the alloying element and oxygen passing through the oxide film. The smaller the diffusion coefficient is ,the more obvious barrier effect of oxide film on element diffusion is, and the better protection of the film is. The diffusion coefficients of various ions in typical oxides are given in Tab. 3. It can be found that the self-diffusion coefficient of SiO2 is the smallest, followed by Cr2O3 and α -Fe2O3. According to the thermodynamic calculation results, the oxidation particles generated from typical alloyed elements are very stable. Combined with the extremely low self-diffusion coefficient, all of these oxides can act as protective oxide film.
Tab.3 - Self-diffusion coefficients of different oxide.
Oxide Self-diffusion coefficients
FeO 9×10-8
Fe3O4 2×10-9
Fe2O3 2×10-15
Cr2O3 1×10-14
SiO2 1.3×10-18
MnO 1×10-10
Based on the relevant experimental results and the above thermodynamic and kinetic analysis, steel No.1 has the best oxidation resistance because of Si-Mn-Fe oxidation film at scale/metal interface during oxidation process. The Si-Mn-Fe oxidation film acting as best protection barrier with smallest self-diffusion coefficient. The formation mechanism of the anchor morphology can be summarized as followiig. Firstly, Si element is enriched at the scale/ metal interface. When the temperature is below 1170°C, a layer made of oxide particles is formed at the interface. When the temperature is heated up to 1170°C or above,the particles made of FeO-SiO2 transform from solid to liquid, and then the molten Fe2SiO4 phase penetrated into the matrix along the grain boundary or defects in the matrix and finally forms the typical anchor morphology. In the oxidation process of steel No.2, the major oxides in scale are Cr2O3, MnO, SiO2 and Fe x Oy. Basing on the thermodynaic of Cr oxidation reaction and higher Cr content in steel No.2, Cr is prior to be oxidized first and tranforms as protective Cr2O3, reducing the generation of Si oxide. Since the content of Si in steel No.2 is decreased and Si4+ has the smallest diffusion coefficient in the oxide film, Si element could only be oxidized at the Cr2O3 film/metal interface. The oxidation film of Si element distribution is illustratd in Fig. 12. Owing to the stronger cohesive force between Cr and O than that between Fe and O, Cr-rich oxidation film is first formed on the surface, acting as protective film at scale/metal interface, just like Si oxidation behavior. Under actual production condition, at the elevated temperature in the heating furnace, the interfacial oxidation of Cr weakens the enrichment of Si element at the interface, decreases the anchor depth of Fe2SiO4 and makes the primary scale easy to be removed by descaling, combined with the preset temperature below the melting point of FeCr2O4 phase. As the compact Si-Cr composite oxide layer at the interface has a strong adhesive ability to resist descaling impact, the spacings and angles of descaling nozzles should be meticulously designed to gurrantee constant impact force upon the interact area of neighbouring nozzles and to eliminate the banded like secondary scale.
FIG.13 - Interface oxidation mechanism of Cr-Si element in steel No.2.
Because of higher Gibbs free energy of Mo oxidation reaction, Mo is the last element in the given threee steels to be oxidized during hot rolling process. Mo-rich laye locates at the inner side of oxidation film, reduces the activity of Si and Cr and prevent the formation of dense
Si-Cr oxide film and Fe2SiO4 at scale/metal interface. Therefore, the scale adhesion of steel No.3 is reduced, and the surface quality of hot rolling coil of steel No.3 is obviously improved.
Conclusion
1) Steel No.1 alloyed with Si content of 1.15 mass% possesses the best oxidation resistance and thinnest scale thickness among the three predesigned steels. Benifit from the dense Si - Cr composite oxide film at scale/metal interface, steel No.2 alloyed with Si- Cr has better oxidation resistance than that of steel No.3 alloyed with Si-Cr-Mo. The scale thickness of steel No.3 is the thickest.
2) Influenced by the formation of fayalite phase and strong adhesion force of primary scale under high Si content condition, it is difficult to remove this kind of scale by present roughing and finish descaling system. Severe red scale defects distrubution is found on the hot rolling coil surface of steel No.1 in industrial produciton practice, the area ratio of which reaches up to 70~90%.
3) In the produciton of steel No.2, the pattern of red scale defects tranformed as bands like. The area ratio of banded red scale decreases to 5~10% while applying the Si-Cr alloying pattern for duplex steel. The descaling difficulty for steel No.2 originates from the dense Si - Cr composite oxide layer at scale/metal interface. The spacings and angles of descaling nozzles should be meticulously designed to gurrantee constant impact force upon the interact area of neighbouring nozzles
4) While applying the Si-Cr-Mo alloying receipt, the dense oxidation film in the inner side of scale is broken by the presence of Mo-rich layer. Under the same production parameters, the proportion of red scale area on hot rolling coil surface is significantly decreased to less than 5% for Steel No.3 and good surface quality of duplex steel is achieved.
References
[1] Song S, Yan C, Jiang J, Li Z. Application of QP980 in lightweight development of body in white. Modern Manufacturing Engineering. 2017.
[2]. Basabe VV, Szpunar JA. Growth Rate and Phase Composition of Oxide Scales during Hot Rolling of Low Carbon Steel. ISIJ International. 2004;44(9):1554-9.
[3]. Okada H, Fukagawa T, Ishihara H, Okamoto A, Azuma M, Matsuda Y. Prevention of Red Scale Formation during Hot Rolling of Steels. ISIJ International. 1995;35(7):886-91.
[4]. Fukagawa T, Okada H, Maehara Y. Mechanism of Red Scale Defect Formation in Si-added Hot-rolled Steel Sheets. ISIJ International 1994;34(11):906-11.
[5]. Zhang M, Shao G. Characterization and properties of oxide scales on hot-rolled strips. Materials Science and Engineering A, Structural Materials: Properties, Microstructure and Processing. 2007;A452/53(Apr):p.189-93.
[6]. Kizu T, Nagataki Y, Inazumi T, Hosoya Y. Effects of Chemical Composition and Oxidation Tempera-ture on the Adhesion of Scale in Plain Carbon Steels. ISIJ International. 2001;41(12):1494-501.
[7]. Yang Y-L, Yang C-H, Lin S-N, Chen C-H, Tsai W-T. Effects of Si and its content on the scale for-mation on hot-rolled steel strips. Materials Chemistry and Physics - MATER CHEM PHYS. 2008;112:566-71.
[8]. Chen WY, Wang SQ, Wei MX, Feng N. Study on high temperature oxidation resistance of 3Cr13 steel. Foundary. 2012;61(03):296-9.
[9]. Zhao QH, Liu JY, Liu J. Research on anti-oxidation properies of Cr13Si5 ferritic heat resistant steel under 1100°C. Foundary Technology. 2011;32(02):179-83.
[10]. Xie HB, Liu GZ, Guo JJ. Effects of Mn, V, Mo, Ti, Zr elements on microstructure and high temperature oxidation performance of AlFe-C-rCo-Cu-X high-entropy alloys. Zhongguo Youse Jinshu Xuebao/Chinese Journal of Nonferrous Metals. 2015;25:103-10.
[11]. Min K, Kim K, Kim SK, Lee D-J. Effects of oxide layers on surface defects during hot rolling processes. Metals and Materials International. 2012;18(2):341-8.
[12]. Chatterjee-Fischer R. Internal oxidation during carburizing and heat treating. Metallurgical Transactions A. 1978;9(11):1553-60
[13]. Takada J, Yamamoto S, Kikuchi S, Adachi M. Determination of diffusion coefficient of oxy-gen in γ-iron from measurements of internal oxidation in Fe-Al alloys. Metallurgical Transactions A. 1986;17(2):221-9.
[14]. Carl, E., Lowell, Charles, A., Barrett, et al. COSP: A computer model of cyclic oxidation. Oxidation of Metals. 1991.
[15]. Auping J. COSP for windows—Strategies for rapid analyses of cyclic-oxidation behavior. Oxidation of Metals. 2002.