The Spectrum of Simulation
Written by Dr. Nora Colman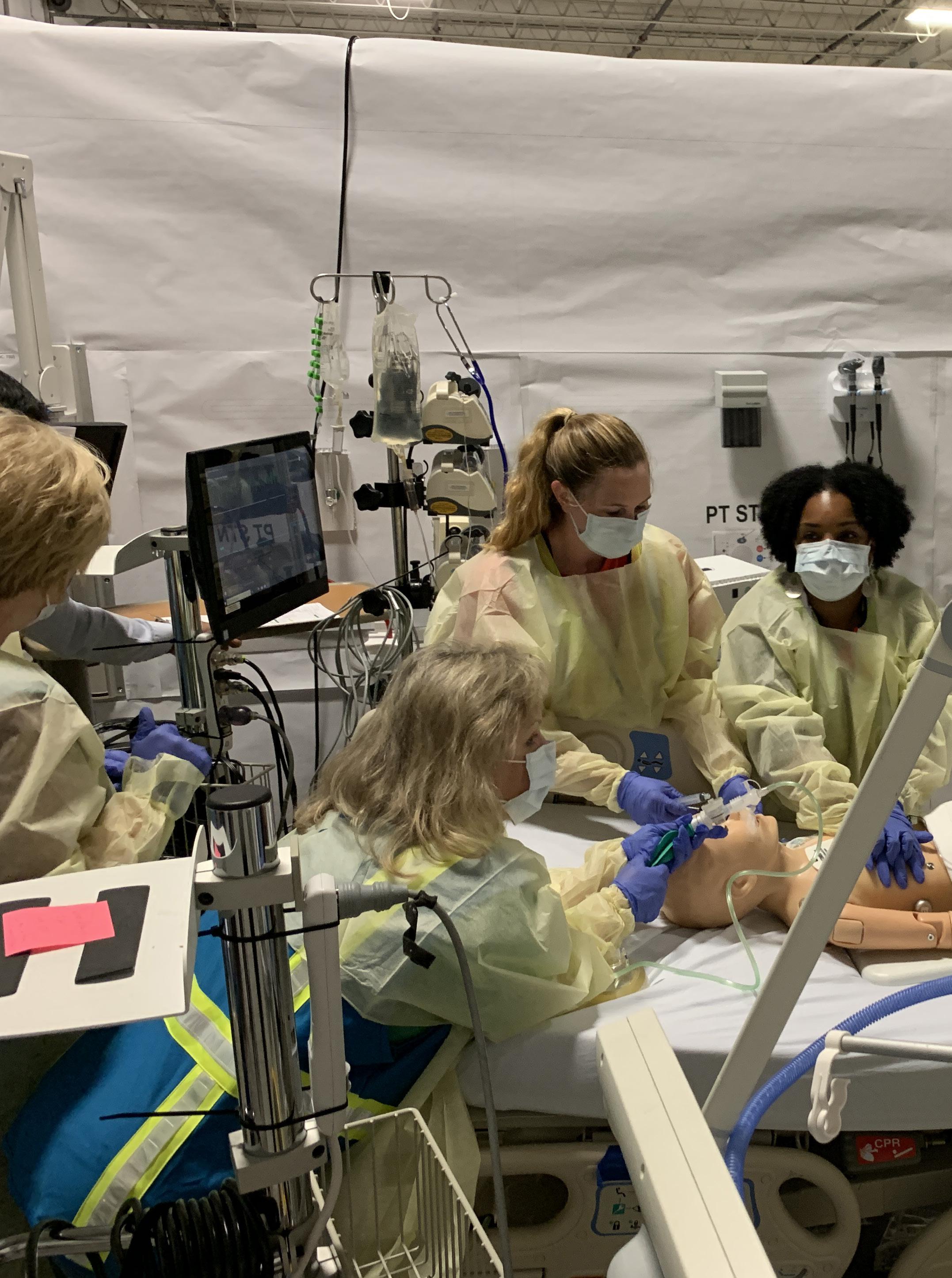
In the past several years there has been a major boom in the rates of hospital construction to replace aging facilities, support population shifts, and accommodate advancing healthcare technologies1. Despite advances in technology and design, healthcare errors continue to be one of the leading causes of patient deaths1. Hospital- acquired infections, medical errors, falls, length of stay, privacy, communication, patient satisfaction, staff injuries, work effectiveness and satisfaction – all are linked, at least in part, to the built environment1. The Joint Commission, the Institute of Medicine (IOM), and the Agency for Healthcare Research and Quality (AHRQ), urge healthcare systems to better understand the complex interactions between people and their environment that contribute to patient safety and quality2,3.
Simulation is a risk-mitigation strategy, and when aligned with evidence-based design, system engineering and human factors modeling, it augments how we reliably identify latent safety threats (LSTs) to understand system failures and eliminate, facilitate, or mitigate errors. Lean strategies to improve workflow require teams to imagine how work is conducted. This approach can be directly misleading because work as imagined does not accurately reflect local microsystem work culture or actual bedside practice. Such failures result in wasted resources, recurrent errors, development of workarounds, and drift from best practices. Simulation demonstrates work as done to identify flaws and devise opportunities for improvement2, thereby having a better chance of successful integration and error reduction.
The development of a new healthcare facility starts with design planning, but it does not end there. Simulation-based evaluation is a methodology applicable to the life cycle of a facility development project from design through occupancy. In working systems, it is applied as an iterative process improvement tool. [Figure 1]
Design Development
Simulation-based Hospital Design Testing
Simulation-based Design Prototype Testing Design Prototype Testing
Transition Planning
Pre-Occupancy Evaluation
Simulation-based Clinical Systems Testing
Post-Occupancy Evaluation
Ongoing Process Improvement
TWISTS; Translational Work Integrating Systems Testing and Simulation
Pre-Construction: Architectural Planning
Post-Construction: Systems and Operational Planning
Post-Construction: Process planning and ongoing process improvement
This review discusses how simulation is uniquely poised to bring together evidence-based design, human factors, systems engineering, and patient safety theory to proactively identify and mitigate risk. We describe how simulation was applied from design through post occupancy using a case example from pediatric, cardiac, and neonatal intensive care units (ICUs).
The business case for building better, safer, hospitals is strengthened by an advanced understanding of the relationship between the built environment and patient safety, quality, workforce safety, and satisfaction4. The ability to address safety and mitigate risk during the facility’s design stage is imperative, practical, and cost effective5. Flaws in design require costly retrofitting and design enhancements later. The ability to impact quality and patient safety has an upfront cost but results in long-term savings. A one-time incremental cost to ensure safe care delivery is paid back in two to three years, in the form of operational savings and increased revenues6. The timing of simulation on the cost-influence curve5 allows for major modifications to be made in a phase of hospital design planning where long-term impact is high and cost is low.7
Assessing patient safety and designing systems that produce positive patient outcomes rely on systems engineering and the study of human factors to understand complexities of healthcare delivery. When the interaction of system elements is suboptimal, incomplete, or conflicting, errors and inefficiencies arise2
Reason’s Swiss cheese model is the most recognized systems framework that describes the relationship between system errors and healthcare design. It illustrates how defenses, barriers, and safeguards may be penetrated by an accident8. Latent safety threats (LSTs) are holes or weakness in the work system. When multiple “holes” align there is an opportunity for failure that may impact patients or staff negatively. An active failure is an error related to the LSTs that occurs at the level of a frontline operator where the effect is immediate9. Despite exhaustive planning, inevitably weaknesses or latent conditions are introduced into a system8,10. When a LST aligns with an active failure harm occurs8,10 [Figure 2]
HAZARDS
Latent Conditions in Design
Staff fatigue, poor communication, interruptions and distractions, cross traffic of sterile and dirty supplies, lack of privacy, lack of family involvement
Unsafe practices, lack of compliance with best practices, unsafe practices, cognitive failures, procedural violations, delays in care, workflow inefficiencies
Evidence-based safe design principles defined by AHRQ and the Center for Health Design 2,11 provide the framework to probe the environment for latent conditions 9,12. Simulation grounded in evidence-based design offers the ability to detect a wide range of LSTs related to the built environment. This framework ensures that debriefings focus on specific errorprovoking design elements that if mitigated and redesigned early have the potential to impact outcomes. Pre-identified testing objectives are applied in scenario development to ensure participant interaction with specific design features in question during simulation. Evidence-based design principles also guide the discussion during the debrief.
[Figure 3]
Evidence-based Safe Design Principles
When not effectively employed
Potential Active Failures
Unsafe practices
Slips, falls
In the planning of a new healthcare facility and the evaluation of the work system, there is a practical need to “imagine” (work as imagined) how work would be conducted as part of design, administrative, and operational planning13. It is impossible, however, to precisely predict how work should be done, making work as imagined misleading. Work as done on the other hand, reflects actual care delivery and realities of the work system. Ability to experience work as done illuminates how work is shaped by the environment and is necessary to identify hazards and make improvements13
Clinicians see care delivery through the lens of process and task completion. When shown two-dimensional drawings, clinicians must imagine work in the space: what should happen, what they think will happen14. The complex ways in which humans interact with their work system makes space utilization unpredictable, as work as imagined often misrepresents work as done. On the other hand, simulation is participatory: clinicians actively perform work and experience how clinical care would be conducted in their proposed new space. This creates a shared experience that bridges gaps in perceptions among stakeholders.
Simulation testing includes high fidelity simulated scenarios of routine and high-risk care episodes, SAFEE debriefing, and Failure Mode and Effect Analysis (FMEA) scoring session15. Summarize, Anchor, Facilitate, Explore, Elicit (SAFEE) debriefing16 facilitates the identification of LSTs in work system elements and clinical processes. FMEA risk stratifies the LSTs identified during debriefing.15 Stakeholders use the categorized and stratified FMEA report to review LSTs and identify solutions and opportunities for improvement.9
Simulation-based Hospital Design Testing is intended to probe and stress the built environment. High-fidelity simulations are conducted in a mockup or fabricated space during schematic and/or detail design development. Clinical areas may include patient rooms, operative spaces, emergency departments, radiologic imaging, or interventional radiology. Frontline staff including physicians, nurses, respiratory therapists, and technicians participate in simulated scenarios that represent both routine and high-risk care episodes. High-fidelity simulation manikins are used, and the mockup is staged with real equipment, equipment mocked in cardboard, and disposable supplies to simulate patient care.
In the design of a 400+ bed new children’s hospital, Simulation-based Hospital Design Testing was conducted in two rounds of testing during schematic and detail design development to stress the environment for Latent Safety Threats (LSTs) and mitigate risk through innovative design strategies. Fifteen clinical areas including the bed tower and diagnostic and treatment areas were tested. Overall, 883 staff members participated in 146 scenarios conducted over 41 days of testing. 722 LSTs were identified and 456 of those were mitigated through design modification, with an estimated cost avoidance of $90 million.
Case Example of SbHDT: During Simulation-based Hospital Design Testing in the PICU, NICU, and CICU, teams verified adjacencies of support areas (nourishment, clean supply, equipment, medication, and utility rooms) during schematic design testing. Teams tested usability and functionality of the space when gathering supplies and equipment for patient admissions or changes in patient clinical status. By actively responding to changes in vital signs, teams evaluated sightlines and visibility from nursing alcoves and care team areas. In detail design SbHDT teams evaluated placement, accessibility, and usability of mounted fixtures, equipment, and in-room furnishings. Paths of movement that impacted cross contamination were evaluated while simulating procedures. Location and orientation of electrical outlets and medical gases were evaluated for obstructions to path of movement and environmental hazards. LSTs were highimpact;3 frequently used design elements that staff interact with frequently (electrical outlets, sinks, cabinets, storage space, countertops, doorway sizes); and high-risk design elements that impact patient or staff safety (code blue buttons, sharps disposal containers). Cardiac arrest and cannulation to ECMO were clinical situations used to test adaptability of the space. Challenges were related to clearances around the surgical tables during ECMO cannulation, access to the head of the bed, and ergonomic positioning of personnel and equipment. In evaluation of all three ICUs during schematic and detail design, 79 latent conditions were identified; changes made to mitigate these conditions avoided $20 million in costs.
Examples of design changes made following Simulation-based Hospital Design Testing
Changes to millwork
Relocation of code blue button
Repositioning of mounted fixtures
Removal of fixed documentation station
Additional outlets added to footwall
Redesign of nurse server to accommodate bedside cart
Repositioning of paper towel, soap dispenser, and glove box
722 Latent Safety Threats Identified
456 Latent Safety Threats Mitigated with Design Changes
$90 Million in Cost Avoided
Simulation-based Prototype Testing is an iterative phase of testing that occurs after finalization of architectural drawings, during hospital construction, but prior to finalization of medical equipment planning. Its purpose is to evaluate design features that are not feasibility tested in a warehouse or fabricated space, such as noise, lighting, medical equipment, and furniture. This full-scale mockup is constructed in dry wall and includes millwork, medical equipment, mounted fixtures, and functional lighting features and electrical outlets. The same methodology including simulated scenarios, SAFEE debriefing and Failure Mode and Effect Analysis is used to identify LSTs.
Case Example:
During testing of the ICU rooms, 78 new latent safety threats were identified. These LSTs were related to the location of the boom mount in the ceiling and its impact on flexibility and functionality of the patient care zone. Teams evaluated the orientation and layout of medical gas and outlet positioning on boom panels. Design challenges in the family area were related to space flexibility and usability of the space. During high-risk procedures such as intubation, teams identified inadequate lighting panels at the head of the bed and obstructed lighting panels by boom arms when they were repositioned to accommodate respiratory equipment. Recurrent themes around resuscitation ergonomics and the ability to safely perform bedside surgical procedures required further operational and process development that would need to take place as part of transition planning. 107 latent conditions from Simulation-based Hospital Design Testing (median criticality = 20) were rescored during Simulation-based Prototype Testing (mean criticality = 8). A 40% reduction in median FMEA score demonstrated that changes made to address safety concerns during hospital design testing did not result in the introduction of unintended downstream latent safety threats. Cost avoidance of $24 million was related to renovations including changes to millwork, relocation of mounted fixtures (sharps disposal containers, gloves boxes, hand foam), and reconfiguration of electrical outlets, the nurse server, and breakaway doors.
78 Latent Safety Threats Identified
40% Reduction in Safety Score with design changes
$23 Million in Cost Avoided
Simulation-based Clinical Systems Testing (SbCST) is conducted in-situ, pre- or post-occupancy. At this time point in facility development, clinical processes and policies are nearly completely developed.17 SbCST recreates system complexities to test all five components of the work system and new operational models. It ensures operational readiness and eases transitioning healthcare systems by promoting preparedness18. SbCST focuses less on the built environment since major modifications at this point are costly or even cost prohibitive. LSTs identified during SbCST are categorized as resource, process/workflow, facility issues or gaps in clinical performance. LSTs may be related to personnel, medication, and equipment that is missing or malfunctioning, policies or procedures that do not work as anticipated, limitations in the built environment not conducive to efficient and safe care, or clinical gaps in knowledge, technical skills, or institutional processes18. Opportunities for improvement may be additional education (people), adjustments in staffing (organization), or purchasing of equipment (tasks). Technologies are modified, and processes are optimized 3,19,20 to improve organizational health 2,21,22
Case Example:
During SbHDT the ICU teams identified process challenges during ECMO cannulation related to the A/B mirrored room configuration. Prior to simulation, the ICU team conducted a process mapping exercise where they devised multiple ergonomic map options for the configuration of the patient bed, personnel (ICU, surgical, ECMO team members), equipment (pumps, ventilators, surgical tables), and ECMO equipment (ECMO pump) in the A and B room configurations. These mapping options were then tested in SbCST where a high-risk ECMO cannulation was simulated. During simulation, lines of communication, visibility to team members and the physiologic monitor, ability to preserve sterile field clearances and maintain open travel paths for movement of surgical equipment, ECMO equipment, and personnel were evaluated. During SbCST, 31 latent conditions were identified. None of the maps created during the process mapping exercise entirely mitigated all latent safety threats. Therefore, the best and final mapping plan for ECMO cannulation reflected the clinical process that mitigated threats that posed the highest risk to patient safety. The finalized ergonomic map designated bed orientation, boom rotation, equipment, and personnel placement for cannulation. This approach standardized bed position for both A/B room configurations and accommodated multiple surgical approaches, mitigating 13 latent safety threats.
31 Latent Safety Threats Identified
13
Latent Safety Threats Mitigated by ergonomic mapping
Translational Work Integrating Simulation and Systems Testing (TWISST) is a process improvement tool that couples Simulationbased Clinical Systems Testing (SbCST)18 with simulation-based training in a working system. This method specifies simulation as the iterative process improvement tool. Embedding solutions in simulation-based training ensures optimal integration into clinical workflow before widespread implementation, hardwires system improvements, and drives change management.23
Case Example:
In a working ICU system, TWISST was applied to optimize resuscitation ergonomics. SbCST was conducted to identify limitations and barriers to optimal resuscitation ergonomics. Equipment positioning, sight lines to team members, visibility of the physiologic monitors and defibrillator machine, medication preparation, team communication, and CPR quality were evaluated. Three one-hour SbCST sessions were conducted, and 40 latent conditions were identified. System improvements included the purchase of an organizing tray to categorize drugs for resuscitation, a stand-alone cart for the defibrillator, and a mobile pharmacy table for medication preparation. An ergonomic map was created to standardize the location and positioning of personnel and equipment during resuscitation events. Thirty simulation-based training workshops of three hours each, involving 160 ICU staff members were conducted over a nine-month period where systems improvements were hardwired into practice.
ICU team members actively perform resuscitation to test and evaluate the proposed ergonomic map in a working system
Work as imagined does not equate to work as done. During simulation, teams identified latent safety threats that they did not anticipate. This led to revisions and a new mapping diagram following simulation testing.
The ability to achieve seamless care delivery in a highly complex healthcare system is challenging given the diversity of tasks, patient care needs, and infinite combinations of care activities. Human factors are consistently in play in health care and the complex ways in which humans interact with the work system make space utilization and process implementation unpredictable. Opportunities for clinical teams to improve safety evaluation through all stages of architectural and system development are at hand. Simulation is a safety and process improvement tool that can be applied in a continuum across the life cycle of facility planning, from design development through evaluation of working systems. Simulation is uniquely poised to integrate improvement science, safety, systems engineering, human factors, and evidence-based design to augment how we discover, investigate, and remediate threats. Simulation is arguably the only modality that fully recreates system complexities and dynamic care episodes to accurately reflect work as done.
A well-designed built environment and work system supports best practices, reduces variation in care through standardization, minimizes staff fatigue through optimal adjacency layout, provides flexibility, and can adapt to varying staff and patient care needs9. Employment of evidence-based design and lean solutions to remediate latent conditions identified in Simulation-based Hospital Design Testing, Simulation-based Prototype Testing, or Simulation-based Clinical Systems Testing optimizes the built environment, rectifies system gaps, improves system functioning, and engages multidisciplinary teams. The entire system is then better able to support productive processes so that performance, safety, health, organizational outcomes, and work satisfaction are optimized 2,11. Furthermore, resiliency is cultivated, creating a buffer so that when disturbances occur the system does not collapse 24
Simulation is a tool that effectively identifies latent safety threats from the very beginning of facility planning. From identification of inefficiencies during design development through evaluating a working system, proactive identification and early mitigation reduce the potential for harm. Simulation impacts how health facilities are evaluated and built and provides an avenue for continuous and iterative system and process improvements through the entirety of a facility’s life cycle.
1. Ulrich RS, Zimring C, Zhu X, et al. A review of the research literature on evidence-based healthcare design. HERD 2008;1:61-125.
2. Carayon P, Schoofs Hundt A, Karsh BT, et al. Work system design for patient safety: the SEIPS model. Qual Saf Health Care 2006;15 Suppl 1:i50-8.
3. Dube MM, Reid J, Kaba A, et al. PEARLS for Systems Integration: A Modified PEARLS Framework for Debriefing SystemsFocused Simulations. Simul Healthc 2019.
4. Berry LL, Parker D, Coile RC, Jr., Hamilton DK, O’Neill DD, Sadler BL. The business case for better buildings. Healthc Financ Manage 2004;58:76-8, 80, 2-4 passim.
5. Taylor E, Hignett S, Joseph A. The environment of safe care: considering building design as one facet of safety. International Symposium on Human Factors and Ergonomics in Healthc Care: Advacning the Cause 2014:123-7.
6. Henriksen K, Isaacson S, Sadler BL, Zimring CM. The role of the physical environment in crossing the quality chasm. Jt Comm J Qual Patient Saf 2007;33:68-80.
7. Wingler D, Machry H, Bayramzadeh S, Joseph A, Allison D. Comparing the Effectiveness of Four Different Design Media in Communicating Desired Performance Outcomes With Clinical End Users. HERD 2019;12:87-99.
8. Reason J. Human error: models and management. West J Med 2000;172:393-6.
9. Joseph A, Quan X, Taylor E, Jelen M. Designing for Patient Safety: Developing Methods to Integrate Patient Safety Concerns in the Design Process. Center for Healthcare Design. 2012; Appendix V. 105–116.
10. Joseph A, Rashid M. The architecture of safety: hospital design. Curr Opin Crit Care 2007;13:714-9.
11. Holden RJ, Carayon P, Gurses AP, et al. SEIPS 2.0: a human factors framework for studying and improving the work of healthcare professionals and patients. Ergonomics 2013;56:1669-86.
12. High Reliability. In Agency for Research Healthcare and Quality. 2019. https://psnet.ahrq.gov/primer/high-reliability. Accessed 16 May 2020.
13. Hollnagel E. Prologue: Why do our expectations of how work should be done never correspond exactly to how work is done? In: Braithwaite J, Wears R, Hollnagel E, editors. Resiliant Health Care III: Recon- ciling work-as-imagined and workas-done. Boca Ra- ton: CRC Press, Taylor & Francis Group; 2017.
14. Catchpole K, Neyens DM, Abernathy J, Allison D, Joseph A, Reeves ST. Framework for direct observation of performance and safety in healthcare. BMJ Qual Saf 2017;26:1015-21.
15. Colman N, Stone K, Arnold J, et al. Prevent Safety Threats in New Construction through Integration of Simulation and FMEA. Pediatr Qual Saf 2019;4:e189.
16. Colman N, Dalpiaz A, Walter S, Chambers MS, Hebbar KB. SAFEE: A Debriefing Tool to Identify Latent Conditions in Simulation-based Hospital Design Testing. Adv Simul (Lond) 2020;5:14.
17. Agency for Healthcare Research and Quality. Improve Patient Safety Through Simulation Research. Agency for Healthcare Research and Quality. 2018. https:// www.ahrq.gov/research/findings/factsheets/errors- safety/simulproj11/index.html. Accessed 16 May 2020.
18. Colman N, Doughty C, Arnold J, et al. Simulation-based clinical systems testing for healthcare spaces: from intake through implementation. Adv Simul (Lond) 2019;4:19.
19. Barlow M, Dickie R, Morse C, Bonney D, Simon R. Documentation framework for healthcare simulation quality improvement activities. Adv Simul (Lond) 2017;2:19.
20. Geis GL, Pio B, Pendergrass TL, Moyer MR, Patterson MD. Simulation to assess the safety of new healthcare teams and new facilities. Simul Healthc 2011;6:125-33.
21. Colman N, Figueroa J, McCracken C, Hebbar K. Simulation-Based Team Training Improves Team Performance among Pediatric Intensive Care Unit Staff. J Pediatr Intensive Care 2019;8:83-91.
22. Colman N, Stone K, Arnold J, Doughty C, Reid J, Younker S, et al. Prevent safety threats in new con- struction through integration of simulation and FMEA. Pediatr Qual Saf. 2019;4(4):e189.
23. Colman N, Saldana C, Craig K, et al. Simulation-based User-centered Design: An Approach to Device Development during COVID-19. Pediatr Qual Saf 2021;6:e427.
24. Paige JT, Terry Fairbanks RJ, Gaba DM. Priorities Related to Improving Healthcare Safety Through Simulation. Simul Healthc 2018;13:S41-S50.