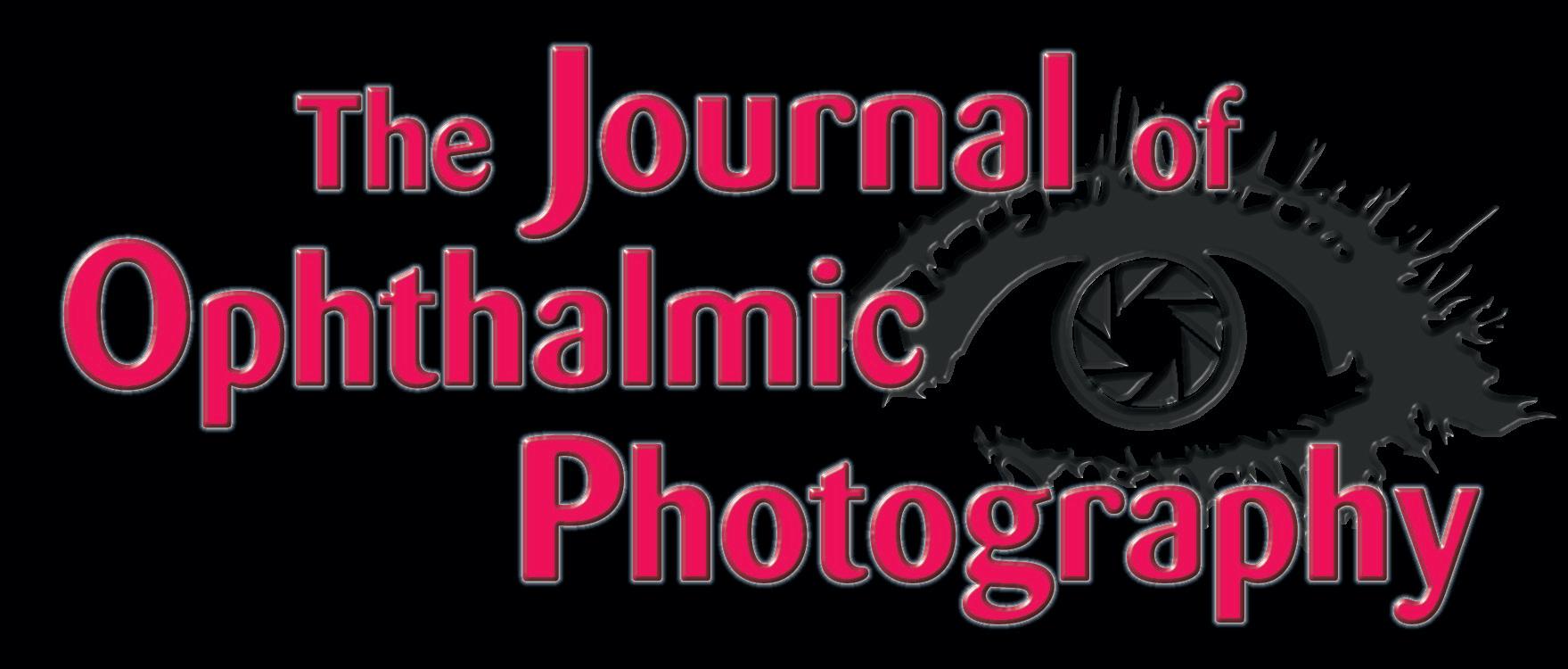
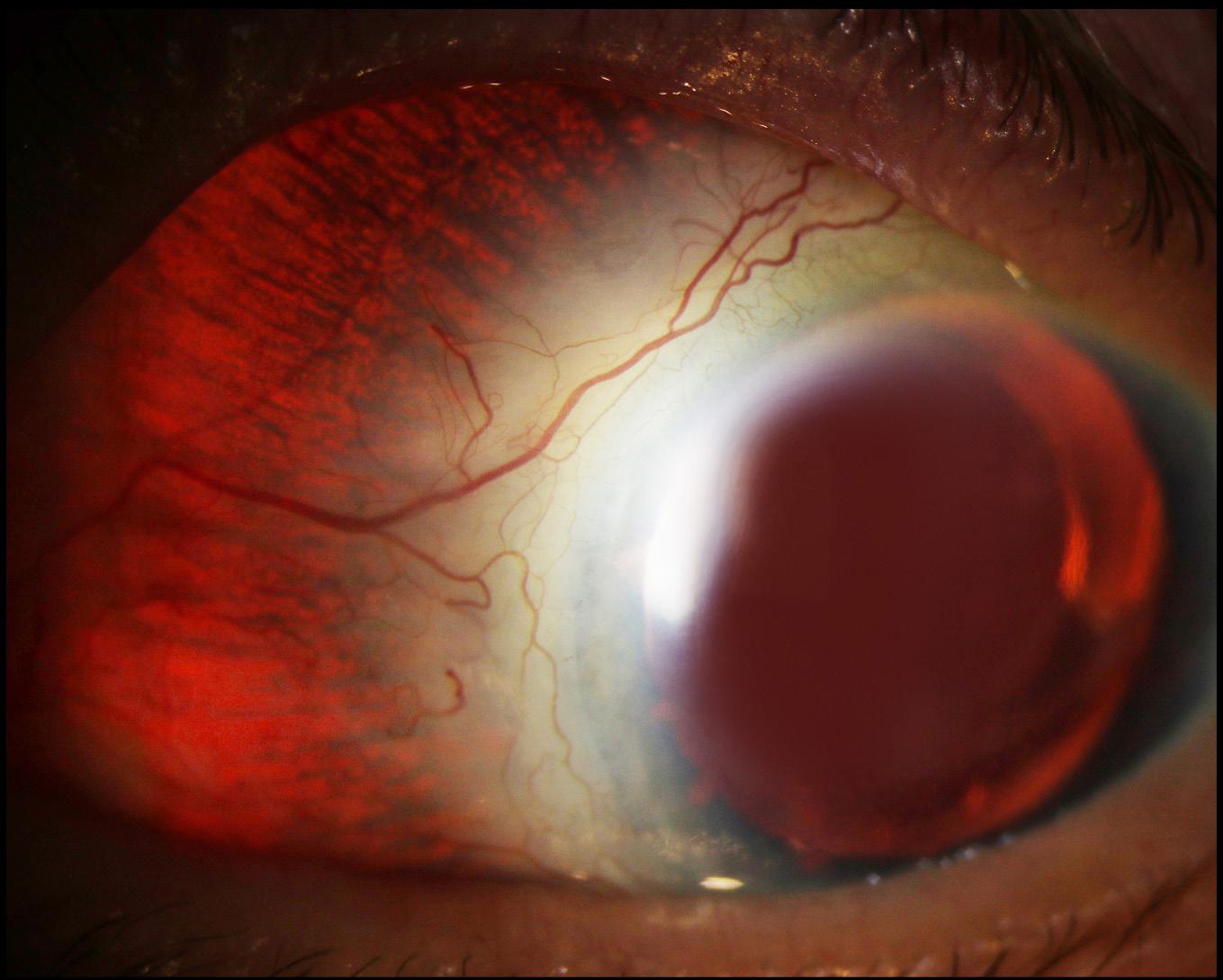
Amazing things can happen when you see the full picture. The BX 900® LED Slit Lamp from Haag-Streit provides exceptional high-resolution ophthalmic photography for publications, research institutions, and educational facilities. With an integrated electronic flash allowing you to capture images with an extended depth of field and high dynamic range, the BX 900® allows you to see, and discover, more.
BX 900® LED Slit Lamp for ophthalmic photography
Editorial: Who Let Her in Here?
Kathleen Warren . . . . . . . . . . . . . . . . . . . . . . . . . . . . . . . . . . . . . .
Original Article: Screening for Sickle Cell Retinopathy (SCR): Why we do Widefield Imaging, OCT/OCT-A for SCR Richard Bell
Original Article: The Clinical Utility of OCT Angiography
Eduardo Novais, MD; Caroline Baumal, MD . . . . . . . . . . . . . . . . . . . . . .
Original Article: How COVID-19 Affects the Eyes
Rohini Radhakrishnan, ENT . . . . . . . . . . . . . . . . . . . . . . . . . . . . .
2022 ASCRS Ophthalmic Photography Exhibit . . . . . . . . . . . . . . . . .
Original Article: A Review of Optical Coherence Tomography Angiography (OCTA)
Talisa E de Carlo; Nadia K Waheed; Jay S Duker; Andre Romano . . . .
INDEX
Haag-Streit. 50
Carl Zeiss Meditec, Inc. 85 Heidelberg Engineering . . . . . . . . . . . . . . . . . . . . . . . . . . . . . . . . . . . 86
Kathleen Warren
Duke University Eye Center Department of Ophthalmology 2351 Erwin Road Durham, NC 27710 kathleen.warren@duke.edu
Jennifer Manning 5682 Dunnigan Road Lockport, NY 14094 jenmanningJOP@gmail.com
Michael P. Kelly, FOPS
Duke University Eye Center 2351 Erwin Road, Suite 209 Durham, NC 27710 michaelpkellydukeeye@gmail.com
Elizabeth Affel, MT, MS, OCT-C, FOPS
Jefferson University Hospital Philadelphia, PA president@opsweb.org
Lisa Dennehy, BS
Mass Eye and Ear Boston, MA lisa_dennehy@meei.Harvard.edu
Alan Frohlichstein, BFA, BS, CRA, FOPS
Retinal Angiography Services Morton Grove, IL alanfroh@gmail.com
Christiaan Lopez-Miro
Duke University Eye Center Durham, NC christiaan.lopezmiro@duke.edu
Monica Motta, SRA III, Supervisor I, BS, RVT, RLAT UC Davis
School of Veterinary Medicine MRLT Vision Science Lab Davis CA Ocular Services on Demand Madison, WI mjmotta@ucdavis.edu
Barbara McCalley
1621 East Jody Circle, Republic, MO 65738 Phone: 417/725-0181 Fax: 417/724-8450 ops@opsweb.org
Akbar Shakoor, MD John A. Moran Eye Center University of Utah School of Medicine Salt Lake City, UT Akbar.Shakoor@hsc.utah.edu
Paula Morris, BS, CRA, FOPS
John A. Moran Eye Center Salt Lake City, UT paula.morris@hsc.utah.edu
Kasi Sandhanam
Singapore National Eye Centre Singapore kasi.sandhanam@snec.com.sg
Robert G. Shutt, CRA, OCT-C Connecticut Eye Consultants Danbury, CT shutteye@optimum.net
Paola Torres, COT, CPT, CRA, OCT-C
Duke University Eye Center Durham, NC paola.belleau-torres@duke.edu
Instructions to Authors can be found at http://www.opsweb.org/?page=journal
Duke University Eye Center Department of Ophthalmology
2351 Erwin Road Durham, NC 27710 kathleen.warren@duke.edu
Hello everyone, please allow me to introduce myself - I am Kathleen Warren and I am quite excited to be joining the OPS family as Editor-InChief for the JOP.
How did I get here? Whose big idea was this? I am going to write a strongly-worded email to someone about this… maybe even send in a professional headshot, just to avoid confusion as to my identity.
Ever since I can remember, I wanted to be a photog rapher. The urge to create images from what my visual senses were showing me, ran deep within my soul. As a young padawon, I took art and photography classes in school, using any type of SLR camera I could get my hands on. On Saturdays my mom would let me drop off the rolls of film for processing… and waiting. The 2-4 day wait was always such torture for me, but the big reveal was even more exciting. That is something I foundationally miss; the days before smartphones and editing software, where an image is seen, captured, and developed, hoping for a refrigerator-worthy outcome. It was pure magic.
My first professional grade camera was a Minolta X-9, and let me tell you, I ran that thing into the ground. I learned how to develop my own film in the dark rooms of junior college, spending countless hours learn ing the process, hoping to perfect my skills. It was always a labor of love for me. Actually, my friend I worked in the darkrooms with is the same person who brought me into ophthalmology (hold that thought… we will circle around to that).
I went off to study photojournalism at Brooks Institute of Photography in California, acting as the com mencement speaker for my graduating class, right next to Leonard Nemoy (yes, Spock.) I spoke on the journey of life vs. the destination. It is natural for a person to want to have their whole life set in their sites, to plan for the final destination. In my opinion, embracing the journey, its interactions, its lessons, its opportunities – this is some thing you wouldn’t want to miss out on.
I worked in the field for over 15 years as a freelance
photographer, journalist, editor, and imaging capture extroardinaire, if you will. I traveled parts of the world working on documentaries, editorial pieces, and personal work. I have collaborated with some of the most talented artists and visionaries, gained the experience, took away the knowledge, and continued to stimulate this over-thinking brain of mine. It seems like that was another lifetime ago.
OK, so back to my college friend. He worked as an ophthalmic photographer at Tufts Medical Center – the New England Eye Center – in the Theater District of Boston. He reached out about a job opening and asked if I was interested in doing something totally different. Considering it was about time to switch gears, I agreed to go in for an interview… but not before Googling what exactly an ophthalmic photographer was. I was blown away with the amount of information I retained that day; my mind was like a sponge. Considering all the cool cameras I got to utilize, I was completely fascinated by the types of information these machines could offer a doctor for diagnosis and research. A person’s quality of life, their camera lenses to the world, could possibly be salvaged with a few swift clicks and a few strong flash pops. All of a sudden, I could see myself helping people in this way. That was 9 1/2 years ago. What started at New England Eye Center, led to Massachusetts Eye and Ear, and now Duke Eye Center in Durham, NC. I have trained and worked beside some of the finest retina surgeons, trained on some of the newest coming of age equipment, witnessed breakthrough events in eye medi cine, and put in long hours with some really amazing people, to help our most deserving patients.
I have been involved with OPS since late 2013, starting with social media posts and weekly photo chal lenges. Now, I sit at the big kids table as a member of the BOD, member of the marketing committee, editorial committee, and now, present day, Editor of the JOP. I am truly honored to be a part of the OPS journey, and even more excited to see what an already prestigious journal has in store for the future.
Senior Medical Photographer/Ophthalmic Photography Research Coordinator
Newcastle Eye Centre
Royal Victoria Infirmary
Queen Victoria Road
Newcastle upon Tyne, England NE1 4LP richard.bell15@nhs.net 0191 2825422
Ihave been working in ophthalmology for over five years as a photographer and research coordinator, and it is fascinating, rewarding and interesting to learn all about the different diseases associated with the eye. One condition that has been a particular favourite is sickle cell disease and why screening is so important to prevent visual loss for the patients.
Sickle Cell Disease (SCD) is a worldwide important condition usually associated more commonly with those of African descent, but can also be found in people from Indian, Mediterranean or Middle Eastern, South and Central American descent. In the UK, it is now the most common newly inherited disease, with estimates that there are now 15,000 affected people.1
SCD is a disease of haemoglobin which carries oxygen in the red blood cells. It consists of four haem molecules containing iron. Most adult haemoglobin in patients with out a haemoglobin disorder is haemoglobin A. It is made up of two alpha (a) and two beta (b) chains. During foetal development, we make other forms of haemoglobin which are still present in adult life and these are different combi nations of the two alpha and two beta molecules. To put it simply, a haemoglobin molecule consists of two conforma tions, a deoxygenated and oxygenated conformation.
Sickle haemoglobin carries oxygen normally, but there is a mutation in the beta (b) chain, so that the haemo globin can polymerise when deoxygenated which results in red cell breakdown and aggregation of red cells in the blood vessels thereby causing a blockage, which in turn leads to ischaemia and tissue damage.1,2 SCD is associated with chronic anaemia and episodes of recurring acute pain and fatigue. Further complications include renal/lung dis ease, stroke, pregnancy loss, avascular necrosis of hips or shoulder joints, leg ulcerations and retinopathy.1
Sickle retinopathy is the most common ophthalmic condition that is associated with SCD which can lead to significant visual impairment. Like diabetic retinopathy,
sickle retinopathy is classified as non-proliferative (NPSR) and proliferative (PSR). It is an occlusive vascular condi tion which can lead to retinal ischaemia and infarction.1
Non-proliferative sickle retinopathy (NPSR) does not normally lead to visual loss unless the vaso-occlu sive process involves the macula. This can be picked up on an OCT or OCTA, which can detect macular ischaemia and loss of the capillary bed. Other features of NPSR include salmon patch like haemorrhages (pink ish-red retinal haemorrhages), which ,when resolved, can lead to coruscating spots (intra-retinal deposits of haemosiderin) or black sunbursts (patches of retinal pigment epithelial hyperplasia). Other findings include retinal vein occlusion, retinal arteriolar occlusion, dilated dark capillaries on the optic disc and android streaks. Proliferative sickle retinopathy (PSR), as the name suggests, is more severe just like proliferative diabetic retinopathy. It is illustrated by the development of peripheral retinal neovascularisation, sometimes known as ‘sea fans’, and can develop visual loss from a vitreous haemorrhage and/or retinal detachment.3
At the Newcastle upon Tyne Hospitals NHS Foundation Trust, we have developed a screening programme to make it easier for patients to attend. Previously, patients would not always attend for their eyes to be screened as we used to do visual acuity, dilation and then photograph them by just doing a widefield Optos image. Patients were put off by having dilation as sickle cell does cause acute pain and dilation can really hurt the patient if they are already suffering. We only saw about 5% of the 43 patients currently registered with ophthalmology. When a patient did attend, then once imaged, a report was then produced by Mr. James Talks (Consultant Ophthalmologist – Medical Retina) and sent to haematology.
Our screening programme was created in con junction with Dr. Brigit Greystoke (Consultant Haematologist), Melanie Hall (Haemoglobinopathy Nurse Specialist) and Mr. James Talks. Our imaging protocol was developed to include the patient’s visual acuity, OCT and OCTA of the macula using the Heidelberg Spectralis along with an ultra-widefield colour Optos image, all of which can be done without dilation, is noninvasive and can be performed quickly without delaying the patient. Before coming to photography the patient would be seen in haematology first so everything is coordinated in one visit, therefore minimising repeated vis its. We now screen over 85% of the patients.
Ultra-widefield imaging up to 200° is proven as a sensi tive tool and is far superior in comparison to a dilated fundus examination4 for detecting capillary occlusions associated with SCR. It is very quick and makes an easier examination, especially for children, and allows for docu mentation. It also allows for retinal vascular peripheral non-perfusion and macular ischemia/non-perfusion.
SD-OCT is quick and easy to acquire. It can lead to a better understanding of sickle cell maculopathy. One sim ple fast scan (20°x20° box, 9 ART, 25 sections) is obtained on the Spectralis in the central macular. It can find tempo ral macular atrophy/inner retinal thinning which is thought to be related to occlusions in the deep capillary plexus.
OCTA is a highly sensitive method to diagnose sickle cell maculopathy and identifies vascular abnormali
ties before the onset of macular thinning. Its findings mainly in the temporal area can identify non-perfusion in the superficial and deep capillary plexus, irregularity or increase of the foveal avascular zone (FAZ), increased vascular tortuosity and vascular diameter and shows a decrease in vascular density.
Figure 1 shows the RE and LE, respectively, of a wide field colour image of a 36yr old female with sickle cell anaemia, which is usually the most severe form of the disease. The visual acuity was 6/9 in RE and 6/6 in LE. In the RE, there are a couple of small coruscating spots (intra-retinal deposits of haemosiderin) resolved from retinal haemorrhages superiorly temporal and inferiorly. There is also a large patch of retinal pigment epithelial hyperplasia (black sunburst) temporally. In the superior temporal arcade there is an area of ischaemia and a vas cular loop. In the LE, there are a couple of coruscating spots nasally and inferiorly and a large area of ischaemia temporally and a white without pressure sign illustrated by the zig-zag appearance. These widefield images pro vide a good indicator of progression of the disease.
The OCT’s of the RE and LE are shown in Figure 2. Both eyes show macular thinning in the nerve fibre layer leading into the inner nuclear and plexiform layers. The RE, in particular, demonstrates irregularity in the foveal avascular zone. This is highlighted by how the layers thin out from the fovea towards the temporal arcade, which corresponds to the Optos images with the ischaemia.
Figure 3 shows the retinal thickness maps and this thin ning can be seen easily from the maps illustrated by the violet colour and the differential in the patterned ripple indentation line. The OCTA’s in Figure 4 highlight some capillary dropout shown in the deep capillary plexus within the foveal avascular zone, which could lead to some visual loss. This area should show capillaries but the blackened area indicates the dropout which ties in with the retinal thickness map.
To conclude, our protocol works extremely well. From the example shown, this patient has not suffered any visual loss indicated by her visual acuity. Although there is some macular thinning and capillary dropout, the OCT’s and OCTA can be followed up and used to track any further changes.
1. Taken from an interview with Dr Brigit Greystoke (Consultant Haematologist) on 02Nov2021
2. Sickle Cell Society. Standards for the Clinical Care of Adults with Sickle Cell Disease in the UK. 2018. https://www.sicklecellsociety. org/sicklecellstandards/ Accessed October 2021.
3. Moriarty, BJ, Acheson, RW, Condon, PI, Serjeant, GR. Patterns of visual loss in untreated sickle cell retinopathy. Eye 1988; 2(3):330-335.
4. Alabduljalil, T, Cheung, CS, VandenHoven, C, Mackeen, LD, Kirby-Allen, M, Kertes, PJ, Lam, W-C. Retinal ultra-widefield colour imaging versus dilated fundus examination to screen for sickle cell retinopathy. Br J Ophthalmol 2020; 105(8):1121-1126.
Eduardo Novais, MD, PhD
Federal University of São Paulo
R. Sena Madureira
1500 - Vila Clementino
São Paulo - SP, 04021-001, Brazil
Eduardo@novais.md
New England Eye Center
Tufts Medical Center
800 Washington St, Box 450 Boston, MA 02116 cbaumal@tuftsmedicalcenter.org 617/636-7950 617/636-4866 fax
TThe retinal and choroidal vasculature can be the site of pathology in many ocular diseases, and dye-based angiography has been the gold standard diagnostic test for assessing vascular disorders such as choroidal neovascularization, retinal vascular occlusion, diabetic retinopathy, macular telangiectasia and central serous chorioretinopathy.1,2 Though dye-based approach es such as fluorescein angiography (FA) and indocyanine green angiography (ICG) allow for direct visualization of the dynamic properties of vascular flow such as leak age, pooling or staining, they’re not without limitations: Visualization of small vascular details can be difficult, since they may become obscured due to late-phase dye leakage. Furthermore, dye-based tests are time-consum ing, require intravenous access and have the potential for systemic side effects, including rare reports of anaphylax is.3 Optical coherence tomography angiography (OCTA) is an imaging technique that may be able to overcome some of the limitations of dye-based approaches—though it has some limitations as well. In this article, we’ll discuss the clinical utility of OCTA.
As we know, OCTA is a noninvasive imaging modality that allows for detection of blood flow and three-dimen sional reconstruction of blood vessels using signal decor relation between consecutive transverse cross-sectional OCT scans. An OCTA image is computed by comparing, on a pixel-by-pixel basis, repeated B-scans acquired at the same retinal location in rapid succession. The rationale behind OCTA imaging is that in non-mobile tissue the reflected signal will be stationary, and thus the repeated B-scans will be identical. Inside vasculature, however, moving erythrocytes cause a time-dependent backscat tering of the OCT signal, which manifests as differences among the repeated B-scans.4-6
Basically, OCT angiograms of the posterior pole can be obtained by using one or a combination of two meth odologies: amplitude decorrelation and phase-variance.
Amplitude decorrelation analyzes amplitude changes in the OCT signal. Split-spectrum amplitude decorrelation partitions the spectrum into smaller spectra and per forms the repeated B-scan decorrelation separately for each sub-spectrum, which improves the signal-to-noise ratio.7 Doppler OCT is a phase-based technology from which OCTA has its origins.8,9 It can quantify axial blood flow that’s parallel to the direction of the imaging acquisi tion device.7,10,11
In addition, it is possible to view OCTA images in tandem with the corresponding structural en face and cross-sectional OCT B-scans. This allows the correlation of clinical and structural features of retinal and choroidal diseases with microvascular features seen on OCTA.
The retinal capillary network is arranged into morphologi cally distinct layers. The superficial retinal capillary plexus is located predominantly within the ganglion cell layer; and the deep retinal capillary plexus is located at the outer boundary of the inner nuclear layer, with a smaller intermediate retinal capillary plexus at the inner margin of the inner nuclear layer. The vascular layers of the retina are connected by perpendicularly positioned ves sels.12 With the ability to analyze each vascular plexus sep arately, OCTA has become an important tool for studying retinal vascular diseases such as macular telangiectasia (MacTel), retinal vein occlusion (RVO), diabetic retinopa thy (DR) and others. For example, OCTA was used to demonstrate that the superficial and deep retinal capillary plexuses may be affected differently by retinal vascular diseases such as retinal artery and vein occlusion.13-15
Another advantage of OCTA is its superior abil ity to precisely delineate the vessels surrounding the foveal avascular zone (FAZ) (See Figure 1). In contrast, perifoveal leakage of fluorescein dye may blur the FAZ margins, and FA is limited to primarily delineating the superficial vascular plexus. Increased FAZ size has been correlated to reduced visual acuity prognosis in eyes with
Figure 1: Multimodal imaging of a 65-year-old patient with mod erate non-proliferative diabetic retinopathy (a) Color photograph shows intraretinal blot-dot hemorrhages and microaneurysms (b) Yellow-dashed frame identifies the corresponding 3x3-mm area imaged on OCTA (c) OCTA segmented at the superficial retinal plexus shows an enlarged foveal avascular zone (FAZ) Microaneurysms can also be easily identified (yellow arrow) (d) OCTA segmented at the deep retinal plexus shows further enlarge ment of the FAZ (e) and (f) represent corresponding OCT B-scan segmentation of the superficial and deep plexuses, respectively, with decorrelation signal overlay
retinal vascular disease.16 A recent study demonstrated that the perifoveal intercapillary area on OCTA appears to increase in size as the level of diabetic retinopathy progresses.17 Also, neovascularization of the optic nerve, which can occur in proliferative DR and ischemic reti nopathy, can be easily detected using OCTA by viewing the inner retina/optic nerve surface at the most superfi cial level (See Figure 2).18
It has been hypothesized that OCTA may show reti nal microcirculation impairment in the macula prior to the development of retinopathy.19 One study demonstrat ed that diabetic individuals without clinical evidence of diabetic retinopathy have larger FAZ sizes as well as FAZ remodeling and subtle capillary non-perfusion compared to normal, non-diabetic control eyes.20 Microaneurysms imaged with FA may not always be apparent on OCTA. This may result from stagnant erythrocytes blocking flow, or the flow in the microaneurysms may be below the threshold of detection of OCTA. Microaneurysms on FA may also correspond to capillary loops or preretinal neo vascular tufts on OCTA.21
Figure 2: Multimodal imaging of a 60-year-old patient with supe rotemporal branch retinal vein occlusion (a) Color photograph shows neovascularization of the disc (b) Late-phase FA shows hyperfluorescence of the optic nerve due to neovascularization leakage Optical coherence tomography angiography of the vitre ous (c) and optic nerve head (d) demonstrates some well-delin eated, fine, abnormal vessels (e) and (f) represent corresponding OCT B-scan segmentation of the optic nerve head and vitreous The yellow arrow points to the presence of the decorrelation sig nal on the vitreous interface, indicative of neovascularization flow
Evaluation of choroidal neovascularization (CNV) using OCTA is one of the most important applications of this modality.4,22,23 OCTA can be used to determine the loca tion of the CNV, its morphology and its response to anti-VEGF (vascular endothelial growth factor) therapy. As a baseline, in a normal eye, the “outer retina” OCTA scan segmenting between the outer plexiform layer and Bruch’s membrane will be devoid of signal, as there’s no vascular flow in this layer. However, this may not be the case in eyes with CNV.
There are different CNV morphologies on OCTA, but the clinical relevance of this is yet to be determined. CNV can be identified as several small filamentous vessels that form anastomoses (known as “seafan” or “lacy-wheel”), or as vessels associated with a central trunk of vessels (“Medusa”) (Figure 3). Recently, researchers analyzed OCTA patterns of CNV and their potential correspon dence to quiescent and progressive CNV characteristics seen on multimodal imaging (FA, Indocyanine green angi ography (IGCA) and spectral-domain OCT). In this study,
the investigators imaged CNV using OCTA and graded it according to the presence or absence of the following features: welldefined CNV; presence of tiny capillaries; presence of anastomoses and loops; morphology of the ves sels termini as opposed to a “dead tree” aspect; and presence of a hypo-intense halo surrounding the CNV. They found a 95-percent agreement between OCTA and the traditional multi modal imaging protocol for a “treatment required” decision in eyes with at least three out of the five fea tures. There was a 91-per cent agreement for “treat ment not required” when patients presented with fewer than three features.24
Depending on the clinical presentation, OCTA’s sensitivity to visu alize abnormal vascular networks may vary. When massive hemorrhage, exu date or fibrotic tissue is present, OCTA signals can be blocked, limiting visu alization of CNV. Because CNV secondary to chronic central serous chorio retinopathy (CSCR) and myopic CNV are rarely associated with large sub retinal hemorrhages that limit penetration of the OCT signal, the abnormal vascular network may be identified with a screening OCTA in such cases.
Figure 3: Multimodal imaging of a 63-year-old patient with choroidal neovascularization secondary to central serous chorioretinopathy (a) Color photograph shows a subretinal hemorrhage at the center of the macula surrounded by retinal pigment epithelium clumps Early (b) and late-phase (c) fluorescein angiography show leakage from CNV (d) and (e) represent corresponding OCT B-scan segmentation of the outer retinal and choriocapillaris, respectively Yellow arrowheads point to the decorrelation signal below the RPE detachment suggestive of CNV (f) OCT angiogram segmented at the level of the outer retina reveals CNV . (g) OCT angiogram segmented at the level of the choriocapillaris The yellow arrow highlights the hypo-intense halo surrounding the CNV
Figure 4: Multimodal imaging of a 54-year-old patient with branch retinal vein occlusion of the inferior temporal arcade (a) Color fundus photography shows non-perfused vessels and hyperpigmented laser scars on the inferior temporal retina (b) Fluorescein angiography Yellow-dashed frame identifies the corresponding 6x6-mm area imaged on optical coherence tomography angiography . White asterisks denote an ischemic retina not captured due to the small-field coverage (c) En face OCTA segmented at the superficial retinal plexus shows a non-perfused area of the retina inferior-temporal to the fovea .
Choroidal neovascu larization can be classified according to the abnormal vascular plexus location in vari ous retinal and choroidal layers: below the retinal pigment epithelium (type 1 CNV); above the RPE (type 2 CNV); and intraretinal (type 3 CNV). On OCTA, CNV classifica tion depends on where the evidence of a vascular decor relation signal is located. It can be located immediately above the retinal pigment epithelium (RPE) (type 2 CNV), and between Bruch’s membrane and the RPE (type 1 CNV).24 Since there can be OCT signal attenuation from
the RPE-Bruch’s membrane complex,25,26 it is possible that visualization beneath the RPE may influence the type 1 CNV identification. Thus, differences in signal penetration may play an important role in this imaging modality.
SpectRal domain (Sd) VS. Swept-SouRce octS (SS octa) foR cnV
There are currently three FDA-approved OCTA sys tems. Two of them use a spectral-domain platform
operating at ~840-nm wavelength. The third uses swept-source OCTA technology with a longer ~1050nm wavelength and is in limited commercial release in the United States. It’s possible that SS-OCTA may be less affected by ocular opacity and allow a deeper pen etration into the choroid.25-28
SS-OCTA also has less variation in sensitivity with depth (sensitivity roll-off) compared to SD-OCTA. In SD-OCTA, the imaging system is most sensitive to sig nals coming from reflectors close to what is known as the zero-delay line; as a reflector is moved away from the zero-delay, the system becomes less sensitive to the back-reflected signals.29 This sensitivity roll-off is due to the limited spectral resolution of the spectrometers that are used in SD-OCTA systems. In contrast, SS-OCTA systems don’t use spectrometer-based detection. Instead, in SS systems, the instantaneous linewidth of the swept light source, along with the analog-to-digital acquisition rate, determine the sensitivity roll-off of the system. This difference enables SS-OCTA systems to have improved sensitivity roll-off compared to SD-OCTA systems,30 which improves visualization of the choroid both on cross-sectional as well as en face OCTA imaging, and may also improve visualization of CNV, especially the sub-RPE component of the membrane.31
There are some limitations to OCTA imaging in its cur rent configuration. One drawback is its restricted field of view for high-definition scans (3x3 mm) (See Figure 4). In order to generate an OCT angiogram, OCT scans need to be repeated at least twice at each position in a volumetric raster scan. However, since the number of cross-sectional OCT scans is limited by the scan ning speed of the instrument, a larger field of view will have reduced density and resolution. However, with the increase in acquisition speed in the newer OCTA devic es, this limitation has become less relevant, and widefield OCTA can be used to access vascular changes in the periphery of the retina.
OCTA is prone to several artifacts during acquisi tion or post-acquisition image processing. In order to interpret OCTA images, it is necessary to be aware of the potential image artifacts that can occur so as not to interpret these as part of the actual anatomy. OCTA works by detection of erythrocyte motion, so any extra neous movement during the image-capturing process may result in motion artifacts, which appear as white or black lines in the flow angiograms, and/or misalignment of the retinal vasculature.
Additionally, superficial vessels may erroneously appear in segmented views of deeper layers, such as the outer retina and choriocapillaris.32 These are termed “pro jection artifacts,” and may lead to incorrect diagnosis if not promptly identified (i.e., the retinal vessels’ projection may be misinterpreted as CNV).
Another issue is that commercially available OCTA
devices are currently expensive, although this may change as the technology becomes more common. Also, there is currently no code beyond the standard OCT imaging code with regards to reimbursement. Finally, in terms of utility, while OCTA provides additional clinical informa tion for the practitioner, such as noninvasive identification of CNV and retinal vascular abnormalities, its usefulness with regards to therapeutic monitoring is unclear.
FA and ICGA demonstrate the dynamic properties of dye within vascular networks, such as leakage in dis orders that produce vascular incompetence and exuda tion, and dye-based angiography is still an important tool for diagnosis and management of chorioretinal diseases, especially when assessing the periphery of the fundus. OCTA is a promising technology, however, as it allows simultaneous assessment of both structural and vascular flow. OCTA is also safer, faster, more easily repeated and more comfortable for the patient than dye-based angi ography, and it may provide more detailed information about blood flow in retinal and choroidal vasculature. The ability to rapidly obtain images of vascular plexuses and assess the integrity of retinal and choroidal perfusion should prove invaluable as a screening and diagnostic strategy for chorioretinal disorders.
1. Stanga PE, Lim JI and Hamilton P. Indocyanine green angiography in chorioretinal diseases: Indications and interpretation: An evi dence-based update. Ophthalmology 2003; 110:15-21;quiz 22-13.
2. Spaide RF, Klancnik JM, Cooney MJ. Retinal vascular layers imaged by fluorescein angiography and optical coherence tomog raphy angiography. JAMA ophthalmology 2015;133:45-50.
3. Ha SO, Kim DY, Sohn CH, Lim KS. Anaphylaxis caused by intra venous fluorescein: Clinical characteristics and review of literature. Intern Emerg Med 2014;9:325-330.
4. de Carlo TE, de Carlo TE, Bonini Filho MA, Chin AT, et al. Spectral-domain optical coherence tomography angiography of choroidal neovascularization. Ophthalmology 2015;122:1228.
5. Jonathan E, Enfield J, Leahy MJ. Correlation mapping method for generating microcirculation morphology from optical coherence tomography (OCT) intensity images. J Biophotonics 2011;4:583-587.
6. An L, Wang RK. In vivo volumetric imaging of vascular perfusion within human retina and choroids with optical micro-angiography. Optics Express 2008;16:11438-11452.
7. Jia Y, O Tan, J Tokayer, et al. Split-spectrum amplitude-decorre lation angiography with optical coherence tomography. Optics Express 2012;20:4710-4725.
8. Fingler J, D Schwartz, C Yang, SE Fraser. Mobility and transverse flow visualization using phase variance contrast with spectral domain optical coherence tomography. Optics Express 2007; 15:12636-12653.
9. Kim DY, J Fingler, JS Werner. In vivo volumetric imaging of human retinal circulation with phase-variance optical coherence tomography. Biomedical Optics Express 2011;2:1504-1513.
10. White B, Pierce MC, Nassif N, et al. In vivo dynamic human retinal blood flow imaging using ultra-high-speed SD-OCT. Optics Express 2003;11:3490-3497.
11. Leitgeb RA, Werkmeister RM, Blatter C, et al. Doppler optical coherence tomography. Prog Retin Eye Res 2014;41:26-43.
12. Snodderly DM, Weinhaus RS, Choi JC. Neural-vascular relation ships in central retina of macaque monkeys (Macaca fascicularis). J Neurosci 1992;12:1169-1193.
13. Sarraf D, Rahimy E, Fawzi AA, et al. Paracentral acute middle maculopathy: a new variant of acute macular neuroretinopa thy associated with retinal capillary ischemia. JAMA Ophthalmol 2013;131:1275-1287.
14. Freiberg FJ, Pfau M, Wons J, et al. Optical coherence tomography angiography of the foveal avascular zone in diabetic retinopathy. Graefes Arch Clin Exp Ophthalmol 2016;254:6:1051.
15. Bonini Filho MA, Adhi M, de Carlo TE, et al. Optical coher ence tomography angiography in retinal artery occlusion. Retina 2015;35:2339.
16. Parodi MB, Visintin F, Della Rupe P, et al. Foveal avascular zone in macular branch retinal vein occlusion. Int Ophthalmol 1995;19:25-28.
17. Salz DA, de Carlo TE, Adhi M, et al. Select features of diabetic retinopathy on swept-source optical coherence tomographic angi ography compared with fluorescein angiography and normal eyes. JAMA Ophthalmol 2016;134:644-650.
18. de Carlo TE, Bonini Filho MA, Baumal CR, et al. Evaluation of preretinal neovascularization in proliferative diabetic retinopathy using optical coherence tomography angiography. Ophthalmic Surg Lasers Imaging Retina 2016;47:115-119.
19. Takase N, Nozaki M, Kato A, et al. Enlargement of foveal avascu lar zone in diabetic eyes evaluated by en face optical coherence tomography angiography. Retina 2015;35:2377.
20. de Carlo TE, Chin AT, Bonini Filho MA, et al. Detection of micro vascular changes in eyes of patients with diabetes but not clinical diabetic retinopathy using optical coherence tomography angiogra phy. Retina 2015;35:2364-2370.
21. Hwang TS, Jia Y, Gao SS, et al. Optical coherence tomog raphy angiography features of diabetic retinopathy. Retina 2015;35:11:2371-6.
22. Kuehlewein L, Bansal M, Lenis TL et al. Optical coherence tomography angiography of type 1 neovascularization in agerelated macular degeneration. Am J Ophthalmol 2015;160:4:739.
23. Baumal CR, de Carlo TE, Waheed NK, et al. Sequential optical coherence tomographic angiography for diagnosis and treatment of choroidal neovascularization in multifocal choroiditis. JAMA Ophthalmol 2015;133:1087-1090.
24. Coscas GJ, Lupidi M, Coscas F, et al. Optical coherence tomogra phy angiography versus traditional multimodal imaging in assess ing the activity of exudative age-related macular degeneration: A new diagnostic challenge. Retina 2015;35:2219.
25. Saito M, Iida T, Nagayama D. Cross-sectional and en face optical coherence tomographic features of polypoidal choroidal vascu lopathy. Retina 2008;28:459-464.
26. Ueno C, Gomi F, Sawa M, et al. Correlation of indocyanine green angiography and optical coherence tomography findings after intravitreal ranibizumab for polypoidal choroidal vasculopathy. Retina 2012;32:2006-2013.
27. Povazay B, Hermann B, Unterhuber A, et al. Three-dimensional optical coherence tomography at 1050 nm versus 800 nm in reti nal pathologies: Enhanced performance and choroidal penetration in cataract patients. J Biomed Opt 2007;12:041211.
28. Unterhuber, Povazay B, Hermann B A, et al. In vivo retinal optical coherence tomography at 1040 nm—enhanced penetration into the choroid. Optics Express 2005;13:3252.
29. Imamura Y, Fujiwara T, Margolis R, Spaide RF, et al. Enhanced depth imaging optical coherence tomography of the choroid in central serous chorioretinopathy. Retina 2009;29:1469-1473.
30. Grulkowski I, Liu JJ, Potsaid B, et al. Retinal, anterior segment and full eye imaging using ultrahigh speed swept-source OCT with vertical-cavity surface emitting lasers. Biomedical Optics Express 2012;3:2733-2751.
31. Novais EA, Adhi M, Moult EM, et al. Choroidal neovasculariza tion analyzed on ultra high-speed swept-source optical coher ence tomography angiography compared to spectral-domain optical coherence tomography angiography. Am J Ophthalmol 2016;164:80-88.
32. de Carlo TE, Romano A, Waheed NK, Duker JS, et al. A review of optical coherence tomography angiography (OCTA). International Journal of Retina and Vitreous 2015;1:1:1-15.
Bayshore Health Centre 97/ 31 A, Ormes Rd
Kilpauk, Chennai, Tamil Nadu 600010, India rohini.radhakrishnan@ideas2it.com
Recent studies have found that COVID-19 can affect multiple organs, including the eyes. In some cases, the virus can enter the body through the eyes when rubbed with infected hands or if respiratory droplets from an affected person happen to land on the eye. Ocular symptoms can occur at any stage during the course of the illness, appearing within two weeks of other COVID-19 symptoms and lasting less than two weeks. Medications administered to COVID-19 patients for treatment can also lead to serious side effects associ ated with the eyes.
fiVe paRtS of the eyeS affected by coVid-19
Conjunctiva: Conjunctivitis or tearing of eyes may be the first symptom of a viral infection. This is caused by inflammation of the clear mucus membrane that envel ops the eye. The primary symptom of conjunctivitis is a pink or reddish color that occurs when the blood vessels in the conjunctiva become inflamed, making them more visible. Other symptoms of conjunctivitis include eye redness, irritation, soreness, itchiness, tearing, watery discharge, eyelid swelling, congestion, and chemosis. Though these symptoms rarely present as an initial symp tom, it is more commonly seen in patients with severe systemic symptoms of COVID-19.
Sclera: Episcleritis is a possible complication of COVID19. It is an inflammatory condition that affects the epi scleral tissue between the conjunctiva and the sclera, or white part of the eye. The link between COVID-19 and episcleritis may include vasculo-immunological factors and coagulation disorders.
Retina: Retinal microvascular changes have been noted on ocular imaging in people with COVID-19. These signs were even seen in asymptomatic patients. Various fundoscopic findings of the retina include retinal micro hemorrhages, retinal vascular tortuosity, retinal vascu lar occlusion, cotton wool spots, and hyper-reflective
plaques in the ganglion cell-inner plexiform layer. Retinal involvement of the infection may present in the form of a decrease in vision or blindness.
Optic Nerve: A wide variety of neuro-ocular symp toms have been found in people with COVID-19 infection. The hypotheses proposed for this include direct neuronal attack by viral particles, endothelial cell dysfunction leading to ischemia, and coagulopathy, or a widespread inflammatory cytokine storm induced by the virus. Optic neuritis, which is inflammation of the optic nerve, has been noted in several cases. The condition leads to a sudden loss of vision, relative afferent pupillary defect, pain with eye movements, and optic disc edema. Orbit: Mucormycosis, a severe fungal infection, pres ents with symptoms that include eye swelling or red ness, double vision, loss of vision, eye pain, and drooping eyelid. The infection mainly affects individuals with a compromised immune system along with decreased CD4+ and CD8+ lymphocytes, those with comorbidities (such as diabetes mellitus and decompensated pulmonary functions), and those on immunosuppressive therapy (corticosteroids).
Medications that have been used to treat COVID-19 have been associated with ocular toxicities. Chloroquine and hydroxychloroquine, when used long-term, can lead to retinal toxicity. Lopinavir or Ritonavir can lead to the reactivation of autoimmune conditions. Ribavirin has been seen to cause retinopathy, retinal vein occlusion, serous retinal detachment, and nonarteritic ischemic optic neuropathy. Interferon has been reported to cause reti nopathy, conjunctivitis, uveitis, optic neuropathy, corneal ulcers, and epithelial defects. Tocilizumab has been asso ciated with retinal hemorrhages and cotton wool spots.
make this competition a reality. We look forward to seeing your entries for the 2023 exhibit in San Diego, CA. For further information, see https://www.opsweb.org/page/ SEC_OPSASCRS or contact Stuart Alfred at ops@ascrs.org. Fluorescein
Angiography
Clinical Setting Photography
HM: Gross Dissection, Denice Barsness
Composite
3rd: Boat Shaped Hemorrhage, Sarah L. Skiles
HM: Retinal Detachment with Multiple Retinal Holes, John Leo
Cross Categories
3rd: Retinoschisis, Sean Grout, OCT-C
HM: Optic Disc Atrophy, Sarah L Skiles
External Photography
3rd: Before and after K-Pro Removal, Sarah L Skiles
HM: Argyrosis, Denice Barsness
Fluorescein Angiography
HM: Tubercular Serpiginous-like Choroiditis, Sara Ash
HM: Florid PDR, Denice Barsness
Fundus Autofluorescence
3rd: Stargardts, Darrin A Landry, CRA, OCT-C
Fundus Photography Wide Angle 45°+
3rd: Papilledema, Sarah L Skiles
HM: VMT, Derick Ashley
Gonio Photography
HM: Kelman IOL with Iris Neovascularisation, Surinder Kumar
Indocyanine Green Angiography
3rd: Gleaming in the Gloom - Vogt-Koyanagi-Harada Disease, Prasanna Venkatesh Ramesh
Instrumentation Photography
HM: The 3D Holographic Metaverse with Hololens 2 - Future of Ophthalmic Counseling, Prasanna Venkatesh Ramesh
Monochromatic Photography 3rd: MEWDS, Lina K . Olsauskas
Optical Coherence Tomography 3rd: Subretinal Hemorrhage Resolution, Sean Grout, OCT-C HM: Lamellar Hole, Anahli Jazhmin Vazquez-Galvan
Optical Coherence Tomography Angiography
3rd: Y in the Well - Glaucomatous Optic Atrophy, Shruthy Vaishali Ramesh
Photo/Electron Micrography
HM: Oestrus Ovis - Hook and Eye Closure, Prasanna Venkatesh Ramesh
Slit Lamp Photography 3rd: Corneal Nerves, Denice Barsness HM: Silicone Oil Droplet in Anterior Chamber, Darrin A Landry, CRA, OCT-C
The Eye as Art
3rd: Fovea, Darrin A Landry, CRA, OCT-C
HM: The Optic Nerveheads Diagnosis: Rockeyetis, Sarah L . Skiles
Ultra-Widefield Imaging
3rd: Pigmented Paravenous Retinochoroidal Atrophy, Sean Grout, OCT-C
HM: Gaucher’s Disease, Sean Grout, OCT-C
HM: PDR, Bradley Stern
Talisa E de Carlo, MD
Assistant Professor of Ophthalmology Medical Director of Imaging
Children’s Hospital Colorado
13123 E. 16th Avenue, Aurora, CO 80045
talisa.e.decarlo@gmail.com
Nadia K Waheed, MD Jay S Duker, MD Andre RomanoTufts University School of Medicine Department of Ophthalmology 145 Harrison Ave, Boston, MA 02111
Optical coherence tomography angiography (OCTA) is a non-invasive imaging technique that generates volumetric angiography images in a matter of seconds. This is a nascent technology with a potential wide appli cability for retinal vascular disease. At present, there are more devices that do OCTA with varying imaging speeds and different postprocessing. In this paper, we introduced the technology, reviewed the available English language publications regarding OCTA, and compared it with the current angiographic gold stan dards, fluorescein angiography (FA) and indocyanine green angiography (ICGA). Finally, we summarized its potential application to retinal vascular diseases. OCTA is quick and non-invasive, and provides volumetric data with the clinical capability of specifically local izing and delineating pathology along with the ability to show both structural and blood flow information in tandem. Its current limitations include a relatively small field of view, inability to show leakage, and proclivity for image artifact due to patient movement/blinking. Published studies hint at OCTA’s potential efficacy in the evaluation of common ophthalmologic diseases such age-related macular degeneration (AMD), diabetic retinopathy, artery and vein occlusions, and glaucoma. OCTA can detect changes in choroidal blood vessel flow and can elucidate the presence of choroidal neovascu larization (CNV) in a variety of conditions but especially in AMD. It provides a highly detailed view of the retinal vasculature, which allows for accurate delineation of the foveal avascular zone (FAZ) in diabetic eyes and detection of subtle microvascular abnormalities in diabetic and vascular occlusive eyes. Optic disc perfu sion in glaucomatous eyes is notable as well on OCTA. Further studies are needed to more definitively deter mine OCTA’s utility in the clinical setting and to estab lish if this technology may offer a non-invasive option of visualizing the retinal vasculature in detail.
Age-related macular degeneration, diabetic retinopathy, fluorescein angiography, glaucoma, indocyanine angiog raphy, optical coherence tomography angiography, optic disc, retina, retinal vessel occlusion
Optical coherence tomography angiography (OCTA) is a new non-invasive imaging tech nique that employs motion contrast imaging to high-resolution volumetric blood flow information generating angiographic images in a matter of seconds. OCTA compares the decorrelation signal (differences in the backscattered OCT signal intensity or amplitude) between sequential OCT b-scans taken at precisely the same cross-section in order to construct a map of blood flow. Axial bulk motion from patient movement is eliminated so sites of motion between repeated OCT b-scans represent strictly erythrocyte movement in reti nal blood vessels.1-4
OCTA requires higher imaging speeds than most currently available OCT systems can provide in order to obtain a densely sampled volume. Conventional OCT device scanning speeds would result in too much tradeoff between decreased field of view, lower image quality, and greatly increased scanning time.
compaRing octa with fa and icga Fluorescein angiography (FA) and indocyanine green angiography (ICGA) are both invasive test that require intravenous administration of dye and imaging up to 10–30 minutes.5-9 They provide two-dimensional image sets that allow for dynamic visualization of blood flow with a wide field of view. Therefore, patterns of dye leakage, pooling, and staining can be appreciated and are well-documented in the literature.10 FA remains the gold standard for the detection of choroidal neovascularization (CNV), as well as retinal neovascularization such as neo vascularization of the disc (NVD) and neovascularization
elsewhere (NVE).11-13 However, retinal pathology can be obscured by this leakage as well as hemorrhage or media opacities, and localization of the depth of the lesion and size delineation of neovascularization can be difficult due to dye leakage and poor stereopsis, and because the imaging modalities are not depth resolved. As a result, segmentation of different layers is not routinely possible with FA or ICGA. Therefore, identification of the axial location of pathology requires an understanding of pat terns of blockage and leakage.10 For example, differentia tion between type 1 CNV, which is found between the retinal pigment epithelium (RPE) and Bruch’s membrane, and type 2 CNV, which is found in the subretinal space above the RPE, requires understanding that the RPE blocks underlying fluorescence so type 1 CNV requires a larger amount of dye to accumulate before hyperfluores cence is apparent.14
FA and ICGA have other drawbacks that can limit their widespread use. Since they are invasive, relatively expensive, and time-consuming, they are not ideal tech niques to use on a regular basis in a busy clinical setting. Although considered safe, the dyes pose risks ranging from nausea to allergic reactions, including anaphylaxis in rare instances. Aside from allergic reactions of which the likelihood increases with frequency of use, indo cyanine green dye is contraindicated in pregnancy and kidney disease.15-17 For the evaluation of patients requir ing frequent follow-up exams or of those that may not tolerate injection of intravenous dye, a rapid non-inva sive technique to visualize retinal and choroidal vessels would be beneficial.
OCTA in comparison is a non-invasive technique that acquires volumetric angiographic information with out the use of dye. Each three-dimensional scan set takes approximately six seconds to obtain. The en-face images (OCT angiograms) can then be scrolled outward from the internal limiting membrane (ILM) to the choroid to visualize the individual vascular plexus and segment the inner retina, outer retina, choriocapillaris, or other area of interest. The en-face acquisition areas currently range from 2 × 2 mm to 12 × 12 mm with the scan quality greatly decreased with a widened field of view since the same number of OCT b-scans is used for all scanning areas. The 12 x 12 mm scan is only available on research prototypes. The 3 × 3 mm OCT angiograms appear to be higher resolution than the currently available FA/ ICGA images, and a study by Matsunaga et al. deduced that they were at least equivalent in showing important vascular detail.18 Use of the montage technique allows for a larger field of view much like FA/ICGA while main taining this improved resolution (Figure 1; de Carlo TE et al., unpublished data in review). Carl Zeiss, Inc (Carl Zeiss Meditec, Dublin, CA) is developing an automatic widefield montage software, which employs motion tracking to track the eyes and stitch images together.
OCTA provides flow information at a fixed point in time. Although leakage is not appreciable, exact delin
eation and size measurements can be performed for pathology such as CNV (de Carlo TE et al., unpublished data in review).19 This is especially useful for identifica tion of type 1 CNV where localization is inferential and therefore may be inaccurate with FA/ICGA. Retinal blood flow on OCTA can be obscured by hemorrhage as this decreases the ability of light to penetrate into the deeper layers of the eye.
OCTA provides both structural and functional (i.e. blood flow) information in tandem. The “corresponding” OCT b-scans can be co-registered with the simultaneous OCT angiograms so the operator is able to scroll through the OCT angiogram like a cube scan. As a result, the precise location of pathology can be viewed on the cor responding OCT b-scans. The axial resolution of the corresponding OCT b-scans are lower quality than the typical highly-sampled line scans and are similar to the resolution of individual OCT b-scans within a volumetric cube scan. Both the retinal and the choroidal microvas culature can be visualized using OCTA while FA is used for seeing the retinal vessels and ICGA is more ideal for imaging the choroid.
Using the present technology, OCTA is more prone to artifact than FA or ICGA. The larger retinal vessels cause a “ghost image” referred to as a shadow artifact, when segmenting deeper layers, especially the outer retina. This can make it more difficult to appreciate the presence of abnormal vasculature in the deeper layers.
Figure 1:
(OCTA) wide-field montage of the normal right eye of a 56-year-old Caucasian man Images were acquired using the AngioVue software of the RTVue XR Avanti (Optovue, Inc., Fremont, CA) and montaged using Adobe Photoshop (San Jose, CA) This allows for a larger field of view much like fluorescein and indocyanine green angiography while maintaining improved resolution (de Carlo TE et al., unpublished data in review)
b f
c g
a e i j
XR
) (a) Full-thickness (internal limiting membrane to Bruch’s membrane) 3 x 3 mm OCT angiogram . (b) Full-thickness 6 x 6 mm OCT Angiogram (c) Full-thickness 8 x 8 mm OCT Angiogram (d) Fluorescein angiography cropped to approximately 8 x 8 mm or 30 degrees demonstrates less capillary detail than A-C (e) 3 x 3 mm OCT angiogram of the “Superficial” inner retina (f) 3 x 3 mm OCT angiogram of the “Deep” inner retina (g) 3 x 3 mm OCT angiogram of the outer retina shows absence of vasculature The white represents noise (h) 3 x 3 mm OCT angiogram of the choriocapillaris is generally homogenous . There is black shadowing from retinal vessels (i) En-face intensity OCT image (j) Highly-sampled OCT b-scan image
Because OCTA uses the principle that movement in the back of the eye represents blood flow, it is prone to motion artifact. White lines (representing decorrela tion signal over the entire b-scan) appear in areas of bulk patient movement such as when the patient loses fixation or moves. Conversely, blinks appear as a black line across the OCT angiogram because the OCT signal is blocked from reaching the retina and the software, therefore, detects no movement. Although erythrocytes should be the only moving object in the retina, some non-vascular structures such as fine tissue may also cause a decorrelation signal, especially if the patient is moving. For example, the edges of a retinal pigment epithelial detachment (RPED) often show up on OCTA as white noise artifact in cases of increased patient movement. It
is postulated that because the RPE is a fine structure, in areas of disruption such as a RPED, it can presumably move and therefore be detected on the OCT angiogram.
On the other hand, OCTA can also miss areas of slow blood flow such as in microaneurysms or fibrotic CNV. Since OCTA relies on change between consecu tive b-scans, it will detect flow only above a minimum threshold, the slowest detectable flow, which is deter mined by the time between the two sequential OCT b-scans. Lesions that have flow below the slowest detectable flow would therefore not be visualized using this imaging technique. Increasing the time between consecutive OCT b-scans could allow for increased flow detection but would offer a trade-off due to increased movement artifact. One of the advantages of a higher
d h
a d
b e
Figure 3: OCT Angiogram Fields of View and Segmentation Layers on the SS-OCT Protype . The normal right eye of a 26-year-old Caucasian woman using a prototype swept source optical coherence tomog raphy angiography (OCTA) system (Department of Electrical Engineering and Computer Science and Research Laboratory of Electronics, Massachussetts Insitute of Technology, Cambridge, MA) (a) Fullthickness (internal limiting membrane to Bruch’s membrane) 3 x 3 mm OCT angiogram . (b) Fullthickness 6 x 6 mm OCT angiogram (c) Corresponding OCT b-scan (d) 3 x 3 mm OCT angiogram of the retinal nerve fiber layer plexus of the inner retina . (e) 3 x 3 mm OCT angiogram of the ganglion cell layer plexus of the inner retina (f) 3 x 3 mm OCT angiogram of the “deep” inner retina
speed system is that multiple volumetric sets can be obtained at each cross-section so the threshold can be altered later by selecting different time frames between the OCT b-scans to determine the optimal image quality. Therefore, if a low-flow vessel is undetectable by using the first and second OCT b-scans at a given cross section, the image may be processed using the first and third OCT b-scans to increase the time between the OCT b-scans thereby decreasing the minimum threshold.
A couple of publications have qualitatively compared OCTA with FA. Spaide et al. described the peripapillary retinal vascular layers in 12 normal eyes, finding that OCTA provided improved visualization of all the vascu lar layers including the radial peripapillary and deep cap illary networks that were not well-distinguished on FA.3 OCTA imaging of the perifoveal region was reported by Matsunaga et al., demonstrating that the ability to see the normal retinal vasculature was equivalent to that of FA.18
The most widely available prototype OCTA system is the AngioVue software of the RTVue XR Avanti spectral-domain OCT (SD-OCT) (Optovue, Inc, Fremont, CA), which uses a split-spectrum amplitude decorrela tion angiography (SSADA) algorithm. The device obtains
volumetric scans of 304 × 304 A-scans at 70,000 A-scans per second in approximately 3.0 seconds. The software offers the option of 2 × 2 mm, 3 × 3 mm, 6 × 6 mm, and 8 × 8 mm OCT angiograms (Figure 2a-c) and auto mated segmentation of these full-thickness retinal scans into the “superficial” and “deep” inner retinal vascular plexuses, outer retina, and choriocapillaris (Figure 2e-h). The OCT angiogram segmentation of the superficial inner retina contains a projec tion of the vasculature in the retinal nerve fiber layer (RNFL) and ganglion cell layer (GCL) (Figure 2e). The deep inner retina OCT angiogram segmenta tion shows a composite of the vascular plexuses at the border of the inner plexiform layer (IPL) and inner nuclear layer (INL) and the border of the INL and outer plexiform layer (OPL) (Figure 2f).
c f
The OCTA prototype with the fastest acquisition rate was developed by the Massachusetts Institute of Technology using a swept-source OCT (SS-OCT) device (Department of Electrical Engineering and Computer Science and Research Laboratory of Electronics, Massachussetts Institute of Technology, Cambridge, MA). This ultra-high-speed prototype employs a verti cal cavity surface emitting laser (VCSEL) operating at 1060 nm wavelength which allows increased light pen etration into pigmented tissues and improved choroidal blood flow visualization compared to the light source used in SD-OCT. The SS-OCTA system obtains scans of 500 × 500 A-scans at 400,000 A-scans per second in approximately 3.8 seconds. This ultra-high speed allows for imaging of wider fields of view. The prototype can be manipulated to obtain OCT angiograms up to 12 × 12 mm, however, it is most commonly used to create 3 × 3 mm and 6 × 6 mm OCT angiograms of great detail (Figure 3a-b). Full-thickness scans are manually segment ed into the superficial (plexus at the RNFL), intermediate (plexus at the GCL), and deep (plexuses at IPL/INL and INL/OPL borders) inner retinal vascular plexuses, outer retina, choriocapillaris, and choroidal layers (Figure 3d-f). Using this OCTA system, the choriocapillaris and choroi dal vessels were described in normal eyes by Choi et al.2
Figure 4: OCTA and Color Fundus Photo of Drusen in Non-Neovascular AMD The left eye of a 72-year-old Caucasian man with non-neovas cular age-related macular degeneration using the AngioVue optical coherence tomography angiography (OCTA) software of the RTVue XR Avanti (Optovue, Inc., Fremont, CA) (a) Color fundus photo zoomed in to an approximately 3 x 3 mm area centered at the macula show ing hard and soft drusen (b) 3 x 3 mm OCT angiogram of the cho riocapillaris centered at the macula as in A The green and red lines represent the x and y axis OCT b-scans respectively which cross at a soft druse demonstrating an area of decreased signal in the chorio capillaris underlying the druse (c) 3 x 3 mm en-face structural OCT of the choriocapillaris centered at the macula as in A-B This image was simultaneously obtained during the same scan as the OCT angio gram in B This structural OCT is still able to show the choriocapillaris changes at the location of the soft drusen in B, but detail is overall lim ited (d) Corresponding x axis OCT b-scan at the cross-section demon strated by the green line in B showing the soft druse The correspond ing OCT b-scans were simultaneously obtained during the same scan as the OCT angiogram in B
(
CA) (a) 6 x 6 mm en-face images of the right eye (a1) En-face structural OCT at the level of the RPE demonstrat ing GA The area of GA is circumscribed in yellow, which is overlaid over the images in a2 and a3 (a2) En-face structural OCT at the level of the choriocapillaris demonstrating alteration in a similar area as the GA (a3) OCT angiogram at the level of the choriocapillaris demonstrat ing flow impairment in a similar area as the GA Larger choroidal vessels have been push inward into the area of choriocapillaris alteration so are seen in this 10micrometer slice . Detail is greatly improved over that of the en-face structural OCT (a4) Corresponding OCT b-scan shows the loss of RPE causing increased intensity below Bruch’s membrane which is characteristic of GA (b) 3 x 3 mm en-face images of the left eye (b1) En-face structural OCT at the level of the RPE demonstrating GA The area of GA is circumscribed in yellow, which is overlaid over the images in b2 and b3 (b2) En-face structural OCT at the level of the choriocapillaris demonstrating alteration in a similar area as the GA . (b3) OCT angiogram at the level of the choriocapillaris demonstrating flow impairment in a similar area as the GA Larger choroidal vessels have been push inward into the area of choriocapillaris alteration so are seen in this 10micrometer slice Detail is greatly improved over that of the en-face structural OCT . (b4) Corresponding OCT b-scan shows the loss of RPE causing increased intensity below Bruch’s membrane which is characteristic of GA
Figure 6: OCTA and FA/ICGA of CNV in Neovascular AMD The left eye of a 67-year-old Caucasian man with choroidal neovascularization (CNV) due to neovascular age-related macular degeneration using the AngioVue optical coher ence tomography angiography (OCTA) software of the RTVue XR Avanti (Optovue, Inc., Fremont, CA) (a) 6 x 6 mm OCT angiogram segmented so both the choriocapillaris and the outer retina are shown A circular net of abnormal vessels are shown surrounded by relatively homogenous choriocapillaris The abnormal vessels exist both below and above Bruch’s membrane (in the outer retina) (b) En-face structural OCT with a red line corresponding to the highly-sampled OCT b-scan in c . (c) 12 mm highly sampled OCT b-scan through the fovea demonstrates a large retinal pigment epithelial detachment, subretinal fluid, disruption of Bruch’s membrane, and hyper-reflective material characteristic of CNV (d) Indocyanine green angiography early, intermediate, and late frames show increasing hyper-fluorescence and pooling of dye in the CNV . (e) Fluorescein angiography intermediate and late frames show increasing hyper-fluores cence and pooling of the CNV .
Dry age-related macular degeneration (AMD) is char acterized by drusen, pigmentary changes, and photore ceptor and RPE loss, called geographic atrophy (GA). Decreased foveolar choroidal blood flow is associated with AMD and increased drusen extent, and it has been hypothesized that the choroidal blood flow may pre dict disease progression.14 Choi et al. (unpublished data, presented in part at the Association for Research in Vision and Ophthalmology annual meeting, May 2014, Orlando, Florida) demonstrated OCTA findings in dry AMD. Areas of impaired choriocapillaris flow typically extended beyond the borders of the GA. Eyes with dry AMD were shown to have a generalized decrease in choriocapillaris density, which was sometimes associated with drusen. Figure 4 demonstrates discrete areas of decreased sig nal at the choriocapillaris level below many but not all drusen in three eyes. These areas of alteration did not appear to be due to shadowing (from material in the drusen), and some choroidal vessels were appreciated below these areas. However, further studies would be necessary to determine if the choriocapillaris changes associated with the drusen are true areas of flow impair
ment. Choriocapillaris flow alterations are also shown in two eyes along the border of GA in Figure 5.
OCTA of wet (Neovascular) AMD
Several publications concerning OCTA of eyes with wet AMD appear in the literature. In July 2014 Jia et al. first described the ability of a prototype SS-OCTA system to visualize and quantify CNV that had been seen on FA in five eyes.19 Then in November 2014, Moult and Choi et al. described CNV in 16 of 19 eyes with neovasculariza tion, noting that the majority of these eyes (14/16, 88%) also demonstrated choriocapillaris alteration surround ing the CNV.20 De Carlo et al. described qualitative and quantitative characteristics of CNV in 48 eyes.21 The group determined sensitivity and specificity of the proto type AngioVue software, using FA as the ground truth, to be 50% (4/8) and 91% (20/22) respectively, hypoth esizing that the low sensitivity was due to small sample size and blockage from large amounts of retinal hemor rhage in some patients. Figures 6 and 7 illustrate three examples of CNV, including one type 3 CNV (retinal angiomatous proliferation, RAP), on OCTA confirmed with FA/ICGA, using the Angiovue OCTA software of the RTVue XR Avanti (Optovue, Inc., Fremont, CA).
Figure 8 shows two OCTA examples of CNV, one of which was treatment naïve, using the SS-OCT prototype (Department of Electrical Engineering and Computer Science and Research Laboratory of Electronics, Massachussetts Insitute of Technology, Cambridge, MA).
There are few published papers as of early 2015 on OCTA of diabetic retinopathy. Choi et al. (unpublished data) demonstrated that OCTA of diabetic eyes ranging from no retinopathy to proliferative diabetic retinopathy (PDR) demonstrated choriocapillaris abnormalities and/ or retinal microvascular abnormalities such as micro aneurysms, vascular remodeling adjacent to the foveal avascular zone (FAZ), enlarged FAZ, and capillary
Figure 7: OCTA and FA of CNV in Neovascular AMD (a) The right eye of a 63-year-old Caucasian man with choroidal neovascularization (CNV) due to neovascular age-related macular degeneration (AMD) using the AngioVue optical coherence tomography angiography (OCTA) software of the RTVue XR Avanti (Optovue, Inc., Fremont, CA) (a1) 3 x 3 mm OCT angio gram segmented so both the choriocapillaris and the outer retina are shown Two nets of abnormal vessels are shown surrounded by relatively homogenous choriocapillaris The abnormal vessels exist both below and above Bruch’s membrane (in the outer reti na) (a2-3) En-face structural OCT with a red line corresponding to a 12 mm highly sam pled OCT b-scan (cropped to 3 mm) through the macula The OCT b-scan demonstrates a retinal pigment epithelial detachment (RPED), subretinal fluid, an intraretinal cyst, and hyper-reflective material characteristic of CNV (a4-6) Fluorescein angiography (FA) early, intermediate, and late frames showing increasing hyper-fluorescence and staining of the CNV . (b) The left eye of an 89-yearold Caucasian woman with CNV type three (retinal angiomatous proliferation, RAP) due to neovascular AMD using the AngioVue OCTA software of the RTVue XR Avanti (Optovue, Inc., Fremont, CA) (b1) 6 x 6 mm OCT angiogram segmented at the outer retina showing a round RAP lesion (yellow arrow) A feeder vessel from a retinal vessel was noted (not shown) (b2) Color fundus photo demonstrating hemorrhage in the region of the RAP lesion (b3) 6 mm highly sampled OCT b-scan through the macula shows subretinal and intraretinal fluid and a round ball of hyper-reflective tissue above a serous RPED (b4-6) FA early, intermediate, and late frames showing increasing hyperfluorescence and pooling in the CNV
tortuosity and dilation. OCTA and FA were compared in unpublished data by Salz et al. The group supported the utility of OCTA in evaluating FAZ and the perifove al intercapillary area, showing that they were sequential ly enlarged in each stages of diabetic retinopathy (nor mal eyes to PDR). The data showed that OCTA visual ized the majority but not all of the microaneurysms visu alized by FA likely because OCTA is limited by the prin ciple of slowest detectable flow. However, OCTA was able to appreciate some microaneurysms that were not detected by FA. OCTA also successfully detected other abnormalities that were not evident on FA such as areas of retinal non-perfusion, reduced capillary density, and increased vessel tortuosity. de Carlo et al. (unpublished
a1 b1
data in review) described a wide-field OCTA montage of an eye with newly proliferative diabetic retinopathy. The wide-field montage OCTA image also successfully allowed visualization of an enlarged FAZ, perifoveal intercapillary area, and multiple microaneurysms. It also provided a larger field of view allowing more peripheral
Figure 8: OCTA of CNV in Neovascular AMD . (a) The left eye of an 89-year-old Caucasian man with choroidal neovascularization (CNV) due to neovascular age-related macular degeneration (AMD) using the swept source optical coherence tomography angiography (OCTA) proto type (Department of Electrical Engineering and Computer Science and Research Laboratory of Electronics, Massachussetts Insitute of Technology, Cambridge, MA) (a1) 3 x 3 mm OCT angiogram of the outer retina with manual removal of the retinal vessel ghost artifact A sea-fan appearing CNV is seen (a2) Corresponding OCT b-scan showing a retinal pigment epithelial detach ment, disruption of Bruch’s membrane, and hyperreflective material characteristic of CNV (b) The left eye of a 70 year old Caucasian man with treatment-naïve choroidal neovascularization (CNV) due to neovascu lar age-related macular degeneration (AMD) using the swept source OCTA prototype (Department of Electrical Engineering and Computer Science and Research Laboratory of Electronics, Massachussetts Insitute of Technology, Cambridge, MA) (b1) 3 x 3 mm OCT angio gram of the outer retina with manual removal of the retinal vessel ghosting artifact A sea-fan appearing CNV is seen (b2) Red-free fundus photo exhibiting a lesion of the same shape and location as the CNV seen in b1
a2 b2
Figure 9: OCTA and FA of Microaneurysms in NPDR The right eye (a) and left eye (b) of a 45-year-old Caucasian man with non-proliferative diabetic retinopathy using the swept source optical coherence tomography angiography (OCTA) prototype (Department of Electrical Engineering and Computer Science and Research Laboratory of Electronics, Massachussetts Insitute of Technology, Cambridge, MA) (a1) Fluorescein angiography (FA) cropped to approximately 6 x 6 mm Aneurysms are circled in yellow (a2) Full-thickness (internal limiting membrane to Bruch’s membrane) 6 x 6 mm OCT angio gram FAZ appears enlarged Aneurysms that are seen on FA in a1 that are also seen on OCTA are circled in yellow Aneurysms on FA that are seen as areas of capil lary non-perfusion on OCTA are circled in blue Areas where aneurysms are seen on FA, but show normal vasculature on OCTA are circled in red (b1) FA cropped to approximately 3 x 3 mm Aneurysms are circled in yel low (b2) Full-thickness 3 x 3 mm OCT angiogram, which provides improved detail over 6 x 6 mm OCT angio grams, demonstrates higher sensitivity in detecting micro vascular abnormalities FAZ appears enlarged Aneurysms that are seen on FA in b1 that are also seen on OCTA are circled in yellow Aneurysms on FA that are seen as areas of capillary non-perfusion on OCTA are circled in blue
detection of microvascular changes, early NVE, and areas of capillary non-perfusion including areas too small to visualize on FA.
Figure 9 shows an enlarged FAZ on OCTA and compares OCTA and FA in the identification of micro aneurysms in two eyes with non-proliferative diabetic
Figure 10: OCTA of NPDR The right eye (a) and left eye (b) of a 58-year-old Caucasian man with non-proliferative diabetic reti nopathy and diabetic macular edema (DME) using the AngioVue optical coherence tomography angiography (OCTA) software of the RTVue XR Avanti (Optovue, Inc., Fremont, CA) (a1) Full-thickness (internal limiting membrane to Bruch’s membrane) 6 x 6 mm OCT angiogram shows microvascular abnormalities such as areas of capillary non-perfusion (yellow arrows), capillary loops, and micro aneurysms (a2) En-face structural OCT with a red line correspond ing to the highly-sampled OCT b-scan in a3 (a3) 12 mm highly sampled OCT b-scan through the fovea demonstrating DME and hard exudates (b1) Full-thickness 3 x 3 mm OCT angiogram, which provides improved detail over 6 x 6 mm OCT angiograms, shows microvascular abnormalities such as areas of capillary non-perfusion (yellow arrows), capillary loops, and microaneurysms (b2) En-face structural OCT with a red line corresponding to the highly-sampled OCT b-scan in b3 (b3) 12 mm highly sampled OCT b-scan through the fovea demonstrating DME and hard exudates
Figure 11: OCTA of Neovascularization in PDR (a) The right eye of a 74-year-old African woman with neovascularization of the disc (NVD) due to proliferative diabetic retinopathy (PDR) using the Angiovue opti cal coherence tomography angiography (OCTA) software of the RTVue XR Avanti (Optovue, Inc., Fremont, CA) (a1) Color fundus photo demonstrating fine neovas cular vessels over the optic disc (a2) Fullthickness (internal limiting membrane to Bruch’s membrane) 3 x 3 mm OCT angio gram at the optic disc Wispy NVD is difficult to appreciate (a3) 3 x 3 mm OCT angio gram at the optic disc segmented with the inner boundary in the vitreous above the NVD and the outer boundary slightly below the internal limiting membrane (ILM) The NVD is clearly appreciable (a4) En-face structural OCT showing abnormal tissue at the optic disc (A5) Highly-sampled OCT b-scan of the optic disc where abnormal tissue is observed extending above the ILM into the vitreous cavity (b) The right eye of a 46-year-old African woman with neovas cularization elsewhere (NVE) due to pro liferative diabetic retinopathy (PDR) using the Angiovue optical coherence tomogra phy angiography (OCTA) software of the RTVue XR Avanti (Optovue, Inc., Fremont, CA) . (b1) 3 x 3 mm OCT angiogram with the inner boundary in the vitreous and the outer boundary at Bruch’s membrane . Abnormal vessels are seen in an area of capillary non-perfusion . Image quality is limited by artifact from movement (horizon tal and vertical lines) (b2) Corresponding OCT b-scan showing abnormal tissue above the ILM extending into the vitreous cavity .
Figure 12: OCTA of BRAO and CRAO (a) The right eye of a 70-year-old Caucasian man with an acute branch retinal artery occlusion using the Angiovue optical coherence tomography angiography (OCTA) software of the RTVue XR Avanti (Optovue, Inc., Fremont, CA) (a1) Fullthickness (internal limiting membrane to Bruch’s membrane) 4 5 x 4 5 mm OCT angiogram of the optic disc showing decreased capillary perfusion superotemporal and nasal to the disc (yellow arrows) (a2) 4 5 x 4 .5 mm en-face OCT thickness map showing retinal thickening in red and thinning in blue (yellow arrows) that correspond to the decreased capillary perfusion in a1 (a3) Full-thickness 6 x 6 mm OCT angiogram illustrating decreased capillary perfusion superotemporal and nasal to the disc (yellow arrow) as in A1 . (a4) 6 x 6 mm en-face OCT thickness map showing retinal thickening in red (yellow arrow) that correspond to the decreased capillary perfusion in a3 (b) The right eye of an 81-year-old Caucasian man with a subacute central retinal artery occlusion using the AngioVue optical coherence tomogra phy angiography (OCTA) software of the RTVue XR Avanti (Optovue, Inc., Fremont, CA) (b1) Full-thickness 4 5 x 4 5 mm OCT angiogram of the optic disc showing diffusely decreased peripapillary capillary perfusion (b2) Full-thickness 6 x 6 mm OCT angiogram illustrating decreased capillary perfusion in the macula Only the large retinal and peripapillary vessels demonstrate blood flow (B3) Fluorescein angi ography is hypo-fluorescent in the macula and peripapillary region due to the decreased ability for the fluorescein dye to reach these areas because of low blood flow The vessels appear attenuated (b4) Red-free fundus photo demonstrates attenuation of the vessels especially in the macular and peripapillary regions
retinopathy (NPDR). Capillary non-perfusion and other retinal microvascular abnormalities are demonstrated in Figure 10. OCTA examples of NVD and NVE in PDR eyes are shown in Figure 11.
OCTA of Artery and Vein Occlusion
Retinal vascular occlusions have yet to be described in the literature using OCTA as an imaging modality. However, preliminary work at the New England Eye Center of Boston, MA shows that OCTA may be useful for evaluating these diseases. Unpublished data in review by de Carlo et al. described a case of branch retinal vein occlusion (BRVO) using a wide-field montage technique. The OCTA showed a large wedge-shaped area of capil lary non-perfusion in the inferotemporal macula with clear delineation of the boundary of ischemia, and vas cular abnormalities such as microaneurysms, telangiecta sis, and anastamoses.
Figure 12 shows OCT angiograms of an acute branch retinal artery occlusion (BRAO) and a subacute central retinal artery occlusion (CRAO). The BRAO demonstrates wedge-shaped areas of capillary non-
perfusion that correlate to areas of abnormalities on the retinal thickness map. This illustrates the potential use of OCTA in pinpointing areas of ischemia and edema. The CRAO shows diffuse capillary non-perfusion in areas supplied by the central retinal artery as seen on the same-day FA. Flow is still seen in the major retinal vessels. Around the optic disc, there is an absence of blood flow in the superficial disc vasculature supplied by the central retinal artery but the lamina cribosa blood flow remains intact. As OCTA provides a snap shot in time, it does not demonstrate delayed arteriove nous transit time as FA does.
A case of BRVO and a case of central retinal vein occlusion (CRVO) are illustrated in Figure 13. OCTA of the BRVO shows capillary non-perfusion superotempo rally along the superior arcade extending into the FAZ, and telangiectatic vessels, capillary loops, and possible microaneurysms at the border of the ischemic areas.
The OCTA of the chronic CRVO demonstrates diffuse capillary non-perfusion continuous with the FAZ and telangiectatic vessels.
Figure 13: OCTA
BRVO
(a) The left eye of a 61
with a chronic branch retinal vein occlusion using the Angiovue optical coherence tomography angiography (OCTA) software of the RTVue XR Avanti (Optovue, Inc., Fremont, CA) (a1) Fullthickness (internal limiting membrane to Bruch’s membrane) 3 x 3 mm OCT angiogram showing capillary non-perfusion superotemporal extending into the foveal avascular zone (FAZ) and telangiectatic vessels at the border of the ischemic areas (a2) Full-thickness 6 x 6 mm OCT angiogram demonstrating that the capillary non-perfusion is along the superior arcade . The edges of the ischemia are bordered by telangiectatic vessels, capillary loops, and possible microaneurysms (a3) En-face structural OCT with a retinal thickness map and a red line corresponding to the highly-sampled OCT b-scan in a4 . (a4) 12 mm highly sampled OCT b-scan through the fovea which appears rela tively unaffected (a5) Retinal thickness map demonstrating superior thickening due to edema (b) The left eye of a 72 year old Caucasian man with a chronic central retinal vein occlusion using the AngioVue OCTA software of the RTVue XR Avanti (Optovue, Inc., Fremont, CA) (b1) Full-thickness 3 x 3 mm OCT angiogram showing diffuse capillary non-perfusion continuous with the FAZ and telangiectatic vessels (b2) Full-thickness 6 x 6 mm OCT angiogram demonstrating telangiectatic vessels and diffuse capillary non-perfusion especially along the inferior arcade (b3) En-face structural OCT with a retinal thickness map and a red line corresponding to the highly-sampled OCT b-scan in b4 (b4) 12 mm highly sampled OCT b-scan through the fovea which shows macular edema and disruption of the photoreceptor layer (b5) Retinal thickness map demonstrating thickening that is greatest inferiorly
OCTA is a useful tool for evaluating optic disc perfusion in glaucomatous eyes. The normally dense peripapillary microvascular network is attenuated in both the super ficial disc vasculature and the deeper lamina cribosa. Averaging the decorrelation signal in OCT angiograms approximates the area of microvasculature and allows the user to calculate the flow index, which is decreased in eyes with glaucoma. The flow index has been shown to have both a very high sensitivity and specificity in dif ferentiating glaucomatous eyes from normal eyes.22,23
OCTA is a new technology that has great potential for use in the clinical setting. Compared with FA and ICGA, the current retinal angiographic gold standards, OCTA advantages are that it is non-invasive, acquires volu metric scans that can be segmented to specific depths, uses motion contrast instead of intravenous dye, can be obtained within seconds, provides accurate size and localization information, visualizes both the retinal and
choroidal vasculature, and shows structural and blood flow information in tandem. Disadvantages of OCTA are its limited field of view, inability to view leakage, increased potential for artifacts (blinks, movement, vessel ghosting), and inability to detect blood flow below the slowest detectable flow.
OCTA has been shown to be a useful imaging modal ity for the evaluation of common ophthalmologic diseases such AMD, diabetic retinopathy, artery and vein occlu sions, and glaucoma. In some cases OCTA has even been shown to detect pathology not seen on FA. In the future, faster scanning speeds would be crucial to obtain larger fields of view with higher resolution. More studies are needed to determine OCTA’s utility in the clinical setting and to determine if this technology may offer a non-inva sive option of visualizing the retinal vasculature in detail.
The authors gratefully acknowledge Dr. James G. Fujimoto, Eric Moult, Chen Lu, Jonathan Liu, Alex Cable, and Vijaysekhar Jayaraman for developing the prototype
SS-OCTA system and processing the images used in three of our figures. Additionally we gratefully acknowledge Dr. Caroline R. Baumal and Dr. Andre J. Witkin who each provided one patient case included in this review.
financial SuppoRt and diScloSuRe
This work was supported in part by a Research to Prevent Blindness Unrestricted grant to the New England Eye Center/Department of Ophthalmology, Tufts University School of Medicine, and the Massachusetts Lions Clubs. Jay S. Duker is a consultant for and receives research support from Carl Zeiss Meditech, Inc. and OptoVue, Inc.; André Romano is a consultant for and receives research support from OptoVue, Inc.
1. Kim DY, Fingler J, Zawadzki RJ, Park SS, Morse LS, Schwartz DM, et al. Optical Imaging of the chorioretinal vasculature in the living human eye. Proc Natl Acad Sci 2013;110:14354–9. doi: 10.1073/pnas.1307315110. [PMC free article] [PubMed] [CrossRef] [Google Scholar]
2. Choi W, Mohler KJ, Potsaid B, Lu CD, Liu JJ, Jayaraman V, et al. Choriocapillaris and Choroidal Microvasculature Imaging with Ultrahigh Speed OCT Angiography. Plos One 2013;8:e81499. doi: 10.1371/journal.pone.0081499. [PMC free article] [PubMed] [CrossRef] [Google Scholar]
3. Schwartz DM, Fingler J, Kim DY, Zawadzki RJ, Morse LS, Park SS, et al. Phase-variance optical coherence tomography: a technique for noninvasive angiography. Ophthalmology 2014;121:180–7. doi: 10.1016/j.ophtha.2013.09.002. [PMC free article] [PubMed] [CrossRef] [Google Scholar]
4. Spaide RF, Klancnik JM, Cooney MJ. Retinal Vascular Layers Imaged by Fluorescein Angiography and Optical Coherence Tomography Angiography. JAMA Ophthalmol. 2014; E1-6. doi:10.1001/jamaophthalmol.2014.3616. [PubMed]
5. Novotny HR, Alvis DL. A method of photographing fluorescence in circulating blood in the human retina. Circulation 1961;24:82–6. doi: 10.1161/01.CIR.24.1.82. [PubMed] [CrossRef] [Google Scholar]
6. Novotny HR, Alvis D. A method of photographing fluorescence in circulating blood of the human eye. Tech Doc Rep SAMTDR USAF Sch Aerosp Med 1960;60–82:1–4. [PubMed] [Google Scholar]
7. Kogure K, Choromokos E. Infrared absorption angiography. J Appl Physiol 1969;26(1):154–7. [PubMed] [Google Scholar]
8. Yannuzzi LA, Slakter JS, Sorenson JA, Guyer DR, Orlock DA. Digital indocyanine green videoangiography and cho roidal neovascularization. Retina 1992;12(3):191–223. doi: 10.1097/00006982-199212030-00003. [PubMed] [CrossRef] [Google Scholar]
9. Staurenghi G, Bottoni F, Giani A. Clinical Applications of Diagnostic Indocyanine Green Angiography. In: Ryan SJ, Sadda SR, Hinton DR, editors. Retina London: Elsevier Saunders; 2013. pp. 51–81. [Google Scholar]
10. Johnson RN, Fu AD, McDonald HR, Jumper M, Ai E, Cunningham ET, et al. Fluorescein Angiography: Basic Principles and Interpretation. In: Ryan SJ, Sadda SR, Hinton DR, et al., editors. Retina London: Elsevier Saunders; 2013. pp. 2–50. [Google Scholar]
11. Do DV, Gower EW, Cassard SD, Boyer D, Bressler NM, Bressler SB, et al. Detection of New-Onset Choroidal Neovascularization Using Optical Coherence Tomography: the AMD DOC Study. Ophthalmology 2012;119:771–8. doi: 10.1016/j.oph tha.2011.10.019. [PubMed] [CrossRef] [Google Scholar]
12. Kotsolis AI, Killian FA, Ladas ID, Yannuzzi LA. Fluorescein Angiography and Optical Coherence Tomography Concordance for Choroidal Neovascularization in Multifocal Choroiditis. Br J Ophthalmol. 2010;94:1506–8. doi: 10.1136/ bjo.2009.159913. [PubMed] [CrossRef] [Google Scholar]
13. Do DV. Detection of New-Onset Choroidal Neovascularization. Curr Opin Ophthalmol. 2013;24:224–7. doi: 10.1097/ ICU.0b013e32835fd7dd. [PubMed] [CrossRef] [Google Scholar]
14. Bressler NM, Bressler SB. Neovascular (Exudative or “Wet”) AgeRelated Macular Degeneration. In: Ryan SJ, Sadda SR, Hinton DR, editors. Retina London: Elsevier Saunders; 2013. pp. 1183–212. [Google Scholar]
15. Yuan A, Kaiser PK. Branch Vein Occlusion. In: Ryan SJ, editor. Retina 5. China: Saunders; 2013. pp. 1029–38. [Google Scholar]
16. Kwiterovich KA, Maquire MG, Murphy RP, Schachat AP, Bressler NM, Bressler SB, et al. Frequency of adverse systemic reactions after fluorescein angiography. Results of a prospective study. Ophthalmology 1991;98:1139–42. doi: 10.1016/S01616420(91)32165-1. [PubMed] [CrossRef] [Google Scholar]
17. Lopez-Saez MP, Ordoqui E, Tornero P, Baeza A, Sainza T, Zubeldia JM, et al. Fluorescein-Induced Allergic Reaction. Ann Allergy Asthma Immunol 1998;81:428–30. doi: 10.1016/S10811206(10)63140-7. [PubMed] [CrossRef] [Google Scholar]
18. Matsunaga D, Puliafito CA, Kashani AH. OCT Angiography in Healthy Human Subjects. Ophthalmic Surg Lasers Imaging Retina 2014;45(6):510–5. doi: 10.3928/23258160-2014111804. [PubMed] [CrossRef] [Google Scholar]
19. Jia Y, Bailey ST, Wilson DJ, Tan O, Klein ML, Flaxel CJ, et al. Quantitative Optical Coherence Tomography Angiography of Choroidal Neovascularization in Age-Related Macular Degeneration. Ophthalmology 2014;121:1435–44. doi: 10.1016/j.ophtha.2014.01.034. [PMC free article] [PubMed] [CrossRef] [Google Scholar]
20. Moult E, Choi W, Waheed NK, Adhi M, Lee B, Lu CD, et al. Ultrahigh-Speed Swept-Source OCT Angiography in Exudative AMD. Ophthalmic Surg Lasers Imaging Retina 2014;45(6):496–505. doi: 10.3928/23258160-20141118-03. [PMC free arti cle] [PubMed] [CrossRef] [Google Scholar]
21. de Carlo TE, Bonini Filho MA, Chin AT, Adhi M, Ferrara D, Baumal CR, et al. Spectral Domain Optical Coherence Tomography Angiography (OCTA) of Choroidal Neovascularization. Ophthalmology. In press. [PubMed]
22. Jia Y, Morrison JC, Tokayer J, Tran O, Lombardi L, Baumann B, et al. Quantitative OCT Angiography of Optic Nerve Head Blood Flow. Biomed Opt Express 2012;3(12):3127–37. doi: 10.1364/ BOE.3.003127. [PMC free article] [PubMed] [CrossRef] [Google Scholar]
23. Jia Y, Wei E, Wang X, Zhang X, Morrison JC, Parikh M, et al. Optical Coherence Tomography Angiography of Optic Disc Perfusion in Glaucoma. Ophthalmology 2014;7(121):1322–32. doi: 10.1016/j.ophtha.2014.01.021. [PMC free article] [PubMed] [CrossRef] [Google Scholar]
The Journal of Ophthalmic Photography (ISSN 0198-6155) is published biannually by the Ophthalmic Photographers’ Society, Inc. Membership in the Ophthalmic Photographers’ Society includes an annual subscription to the The Journal of Ophthalmic Photography Membership requests, subscription inquiries, past issue requests and changes of address should be sent to Barbara McCalley, Executive Director, 1621 East Jody Circle, Republic, MO 65738; Phone: 417/725-0181; Email: ops@opsweb.org.
The Ophthalmic Photographers’ Society (OPS) is a nonprofit international organization dedicated to the advancement of pho tography as applied to ophthalmology and visual science. Our membership, numbering over 1000, includes a broad spectrum of professionals in eye health care, including ophthalmologists, optometrists, photographers, nurses, ophthalmic medical personnel, and basic researchers. The Society’s educational programs, designed to supplement the professional expertise of our members, have earned the OPS recognition as being an organization dedicated to the elevation of standards in the craft. The Society’s major publication, The Journal of Ophthalmic Photography, presents tutorials and articles on photographic techniques, instrumentation, and related topics in ophthalmology. Visit us online at www.opsweb.org.
The Ophthalmic Photographers’ Society. All rights reserved. Printed in the U.S.A. None of the contents may be reproduced by mechani cal or electronic processes, stored in a retrieval system or transmitted by any means without prior written permission from the Editor.
71-year-old male with non-proliferative diabetic retinopathy and drusen.
The CLARUS ® True Color Ultra-widefield image (figure 1) shows scattered dot and blot hemorrhages, mainly located outside the posterior pole. The CLARUS covers the ETDRS 7 standard fields in one single widefield image (figure 2), making it a valuable tool for diabetic eye exams and documenting retinopathy.
The zoomed-in image of the posterior pole (figure 3) shows the excellent resolution and color of CLARUS images, allowing clear visualization of diabetic retinopathy, as well as the optic disc and macular drusen in a single image.
Each month, our clinical team will select a case submitted by you or one of your peers to be showcased. This is your opportunity to highlight a unique and challenging case, demonstrating your technical expertise and clinical insight using the latest technologies. Submit your case today:
CAP-en-US_31_030_0115I
Images and diagnoses courtesy of Jesse Jung, MD 1Zhao D, Cho J, Kim MH, Friedman DS, Guallar E. Diabetes, Fasting Glucose, and the Risk of Glaucoma. Ophthalmology. 2015 Jan;122(1):72-8. The diagnoses provided by the healthcare professionals reflect only their personal opinions and experiences and do not necessarily reflect the opinions of any institution with whom they are affiliated. The healthcare professionals credited in this case study have a contractual relationship with Carl Zeiss Meditec, Inc., and have received financial compensation. © Carl Zeiss Meditec USA, Inc., 2021. All rights reserved.Welcome to a platform where every image is remarkably accurate. Reliably repeatable. Proven to enable confident clinical decision-making. And ready for the advanced image analytics necessary for success in a changing healthcare environment. It’s a platform like no other. And now, one that’s accessible for any practice.
Experience powerful images that empower you to do more for your patients and your practice. Experience Heidelberg Image Quality.
To learn more, please call 800-931-2230 or visit HeidelbergEngineering.com.