32 minute read
The New Pollution: A Scientific Review of the Effects of Anthropogenic Chemical Contaminants on Several Avian Physiological and Molecular Systems Across Species
The New Pollution: A Scientific Review of the Effects of Anthropogenic Chemical Contaminants on Several Avian Physiological and Molecular Systems Across Species
Rachel Goodman
Introduction
Although not considered a looming epidemic by the average person, various sources of pollution—and the human mortality associated with them—are increasing. According to the most recent Lancet commission on pollution and health, pollution is the leading cause internationally of disease and premature death in humans, and—in the most severely affected countries—pollution-related diseases account for more than one in four human deaths daily (Landrigan et al., 2018). Although household air pollution is decreasing, ambient air pollution is on the rise; major sources of ambient air pollution can be found in every country, and include fuel-powered vehicles, the generation of electricity, and mechanized agricultural industries (Landrigan et al., 2018).
Chemical pollution is also a growing concern. Since 1950, more than 140,000 new chemicals and pesticides have been synthesized, 5,000 of which are present at pathological concentrations within human environments (Landrigan et al., 2018). Synthetic chemical testing and pre-market evaluation have only become mandatory procedures within the last ten years, and are only required in certain high-income countries; otherwise, most synthetic compounds are produced and sold without any safety or toxicity testing (Landrigan et al., 2018). Less than half of the 5,000 chemicals in the environment have undergone any toxicity evaluation or harm prevention testing whatsoever (Landrigan et al., 2018).
The effects of pollution on human societies are known. In 2012, 7 million people worldwide died from outdoor and indoor
air pollution-related illnesses (Jasarevic, Thomas, & Osseiran, 2014). Of the outdoor air pollution-related deaths, 40% died from ischemic heart disease; 40% died from stroke; 11% died from chronic obstructive pulmonary disease (COPD); 6% died from lung cancer; and 3% died from acute pediatric respiratory infections (Jasarevicet al., 2014). In 2015, 16% of all deaths worldwide were due to diseases
caused by pollution (Landrigan et al., 2018).
The sources of the pollution are also known. According to the United Nation’s World Water Assessment Programme of 2003, 2 million tons of sewage and agricultural and industrial
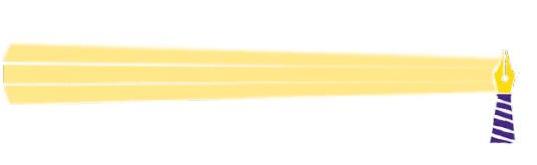
wastes are deposited into the world’s waters every day (“The natural water cycle,” 2003). In 2016, 78 million tons of pollution were emitted into the atmosphere in the United States alone (“Air quality trends,” 2016). Of this airborne particulate pollution, 85% is due to the combustion of fossil fuels (Landrigan et al., 2018).
Multiple studies have been completed on the effects of pollutants on human physiology. Rats and amphibians, along with birds, which are considered excellent indicators of ecological status, have been made common test subjects in an effort to deduce environmental health.
However, an intriguing question has been raised: What are the effects of anthropogenic pollutants—man-made pollutants—on the physiological and molecular systems within the avian body? What effects do various types of pollutants—including large-scale agricultural pesticides and herbicides, polycyclic aromatic hydrocarbons produced from incomplete fossil fuel combustion, and crude oil—have on the circulatory, nervous, endocrine, renal, and digestive physiologies within birds across species? How do these mutagen compounds transform or potentially damage bird DNA?
How pollutants affect the avian circulatory system
One way in which the avian circulatory system may be affected by anthropogenic chemical pollutants is the morphological distortion and misshaping of erythrocytes and the nuclei within erythrocytes. Erythrocytes are red blood cells that contain hemoglobin, the molecule responsible for shuttling oxygen and waste products, such as carbon dioxide, to and from tissues and organs of the body. Although human red blood cells do not have nuclei, bird erythrocytes are nucleated.
In one study on female Japanese quails (Coturnix japonica), a commercial pesticide containing abamectin (ABA), an insecticide belonging to the avermectin chemical group of pesticides widely used in the production of citrus fruits, potatoes, and maize, was fed to 3 experimental groups of specimens; the control received only drinking water, while groups 2 and 3 received 1% of the median lethal dose (15.5 mg a.i./L ABA), and 10% of the median lethal dose (155.0 mg a.i./L ABA), respectively (De Faria et al., 2018). The effects of the pesticide on the nuclei of the quails’ erythrocytes were assessed through blood smears fixed, stained, and inspected under a light microscope. A dose-dependent trend was observed during the course of the experiment; the higher the concentrations of abamectin fed to the quail through their drinking water, the higher the incidence of nuclear abnormalities within the quails’ erythrocytes (De Faria et al., 2018). These abnormalities included notched nuclei, moved
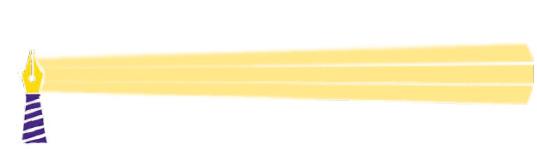
nuclei, binucleated erythrocyte cells, and asymmetric constriction of nuclei, and were detected in significantly larger numbers in the erythrocytes collected from both treatment groups as compared to the control group (De Faria et al., 2018).
In another experiment conducted on broiler chicks (Gallus gallus domesticus), who were treated with 92% technical grade cypermethrin—a type II pyrethroid insecticide used to control ectoparasite infections in domestic pets and livestock—and attenuated with antioxidant selenium and vitamin E, chicks who received cypermethrin only in physiologic saline produced erythrocytes with nuclear abnormalities at a significantly higher frequency than all other groups, including the control group (Sharaf et al., 2010). Blood from the control group demonstrated erythrocytes with normal configuration and shape; in all treatment groups, however, immature erythrocytes, spindle-shaped erythrocytes, nuclear segmentation within erythrocytes, notched nuclear erythrocytes, and spindle and pear-shaped erythrocytes were found (Sharaf et al., 2010). In addition, cytoplasm from the red blood cells was easily detached during the smear preparation of the blood from the treatment groups (Sharaf et al., 2010). In an experiment in which male Japanese quails (Coturnix japonica), were given varying levels of butachlor—a pre-emergent chloroacetanilide herbicide—mixed in corn oil and administered
daily for 30 days, the treatment groups that received 75 mg/kg b.w., 100 mg/kg b.w., 150 mg/kg b.w., and 250 mg/kg b.w. (i.e. all treatment groups except one given the lowest dose of 50 mg/kg b.w.), displayed erythrocytes with significantly increased levels of lobed and notched nuclei relative to the control
group (Hussain, Khan, Mahmood, Rehan, & Ali, 2014).
De Faria et al. (2018) hypothesized that abamectin and other agricultural pesticides may cause chemical defects within the cell which affect the erythrocyte’s ability to fully and efficiently separate its daughter cells and daughter nuclei during the end stages of cell division and cytokinesis, which could produce abnormalities such as asymmetrically constricted nuclei, notched nuclei, and indentations within both the erythrocyte cell membrane and nuclear membrane. In addition, the authors posit that the individual moved nuclei within each damaged erythrocyte could be caused by chemical damage to the cell structures responsible for maintaining the centralization of the nucleus, such as the cytoskeleton (De Faria et al., 2018).
Another mechanism by which chemical pollutants may affect the avian circulatory system is the micronucleation of erythrocytes. Micronuclei are remnants of chromosomes
found within small satellite bodies in the cell
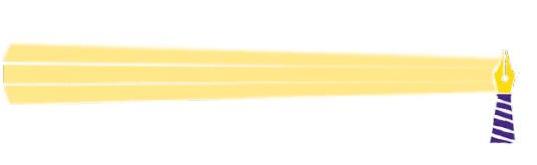
cytoplasm after exclusion from the daughter nucleus during cell separation in mitosis. When micronuclei are found within cells, it is a signal of cell division malfunction, and thus are indicators of cell damage (Sharaf et al., 2010). In an experiment conducted on five groups of broiler chicks (Gallus gallus domesticus) treated with cypermethrin and antioxidants, 2,100 cells from each chick were examined for micronuclei on three separate blood sampling days at the beginning (day 10), middle (day 20), and end (day 30), of the experiment; on days 20 and 30, the experimental group that were fed only cypermethrin displayed the most significant increase of micronucleated erythrocytes relative to all other experimental groups, demonstrating progressive accumulation of cell damage due to non-lethal pesticidal exposure (Sharaf et al., 2010). Interestingly, in three of the five treatment groups dosed with butachlor in the study on herbicide exposure in male Japanese quails, the ratio of micronucleated erythrocytes to normal erythrocytes in 1,000 red blood cells taken from each bird also increased dramatically on days 20 and 30 (Hussain, Riaz et al. 2014). The most frequent erythrocyte abnormality observed in all treatment groups was the formation of micronuclei (Hussain et al., 2014).
The mechanisms by which chemical pollutants disturb the inclusion of the chromosomal bodies within cell nuclei in red blood cells are still unknown. Cypermethrin, a highly toxic insecticide, enters the circulation system because it is highly lipophilic (easily dissolved in fats and lipids) and crosses the blood-brain barrier’s semipermeable phospholipid bilayer membrane; pyrethroid insecticides are easily absorbed into hematological systems of animals due to the biochemistry of these compounds (Sharaf et al., 2010). One theory put forth by experimenters as to how micronuclei are formed within hemocytes damaged by lipophilic pesticides is that chemical damage causes chromosomal lagging during anaphase; as the chromatids move towards the poles and separate from their sister chromatids, some may lag behind and become part of the cytoplasmic bodies excluded from the nuclei in the individual daughter cells (Sharaf et al., 2010).
Three final ways in which chemical pollutants may alter the functioning of the circulatory system in birds are by (1) lowering the concentration of hemoglobin within erythrocytes, (2) decreasing the total number of erythrocytes within the blood system, and (3) diminishing the percentage volume of red blood cells within whole blood
volume (hematocrit). In the experiment that treated male Japanese quail (Coturnix japonica), with the chloroacetanilide herbicide butachlor and examined blood samples from
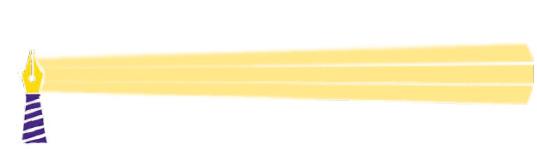
culled specimens on experimental days 10, 20, and 30, the total erythrocyte count was significantly reduced in the 3 most highly dosed groups of the 5 total treatment groups, in the most severely affected group showing significant reduction as early as day 10 of treatment (Hussain et al. 2014). However, as day 10 was the earliest sampling day, it is also possible that reduction in erythrocytes may have begun earlier in the treatment. On days 20 and 30, both the hematocrit percentages and hemoglobin concentrations decreased significantly in the experimental groups with the highest and second-highest dosages prescribed (Hussain et al. 2014). The total erythrocyte count, hemoglobin concentration, and hematocrit percentage also decreased significantly relative to control for all experimental groups treated with cypermethrin on all sampling days, despite other pathophysiological symptoms of cypermethrin exposure being attenuated by combined selenium and vitamin E antioxidant
treatment (Sharaf et al., 2010). Additionally, the chicks also showed an increase in erythrocyte sedimentation rate, which is usually indicative of infection or inflammation as blood cells
clump together, thus increasing their weight (Sharaf et al., 2010).
Interestingly, the broiler chicks experimentally treated with cypermethrin and antioxidants demonstrated an increase in the mean corpuscular volume of their red blood cells, despite a decrease in hemoglobin concentration and independent of treatment with selenium and vitamin E (Sharaf et al., 2010). Therefore, their red blood cells swelled and became larger, but the constituent proteins became less concentrated. The experimenters posit that this may have been an indication of macrocytic hypochromic anemia (Sharaf et al., 2010).
How pollutants affect avian DNA
Anthropogenic pollutants alter the physical structures of DNA strands. In herring gulls (Larus argentatus) taken from various regions of the highly polluted Baltic Sea, the frequency of DNA adducts was found at significantly increased levels in gulls from urban centers compared to gulls from rural areas (Skarphedinsdottir et al., 2010). DNA adducts are formed when chemical mutagens are metabolized to form highly reactive metabolites that covalently bond to DNA molecules within cells; these bonded metabolites may become carcinogenetic or lead to mutations in DNA sequences if not removed by DNA repair proteins (Skarphedinsdottir, Halldora et al., 2010). In the study on herring gulls, adult herring gulls were collected from three regions in Sweden and two regions in Iceland; the county of Skåne in Sweden was the most highly polluted site, while the two other
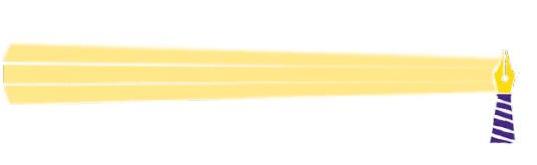
Swedish sites (Blekinge county and Södermanland county) were considered intermediate sites between the severely polluted site and control. The two Icelandic sites (Reykjanes peninsula and Djúpivogur), were considered controls, as the areas are extremely rural and have no known anthropogenic pollution sources in close proximity (Skarphedinsdottir et al., 2010). Herring gull chicks were also collected from two intermediate sites in Sweden (Värmland county and Södermanland county), and one rural control site in Iceland (Reykjanes peninsula.) Of the adult specimens, 100% of the Swedish birds had DNA adducts detected in their
tissues, and 88.2% of the Icelandic birds had DNA adducts at detectable levels within their
tissues; the highest DNA adduct levels were found in the gulls from the most polluted site, Skåne county (Skarphedinsdottir et al., 2010). In chicks, DNA adducts were detected in 71.4% of the intermediate Swedish specimens’ tissues, but none were detected in the rural Icelandic
specimens tissues (Skarphedinsdottir et al., 2010). That DNA adducts were found in Icelandic adult gulls from Reykjanes peninsula but not in chicks from Reykjanes peninsula seems to indicate that even when the chicks are born
into a rural area with a “clean genome,” being reared in the same remote spot does not necessarily preserve this result into adulthood. The authors theorize that this conundrum may be due to background exposure to ambient particu- late pollution (Skarphedinsdottir et al., 2010).
DNA methylation status—and therefore, transcription rates of lengths of DNA strands—may also be affected by pollutants. In double-crested cormorants (Phalacrocorax auritus) colonizing highly polluted Pier 27 in Hamilton Harbour in Ontario, a statistically significant 0.5-fold increase of 5-mC levels was detected in lung tissues relative to the less polluted Centre Island nearby on the same harbor (Wallace et al., 2018). 5-mC is the methylated form of the DNA base cytosine, and is formed after a DNA methyltransferase enzyme transfers a methyl group to a DNA molecule during DNA methylation. The process of methylation typically results in a decreased rate of transcription of portions of methylated DNA as the configuration of the strand becomes less accessible to transcription factors. Certain chemical compounds, such as polycyclic aromatic compounds (including polycyclic aromatic hydrocarbons), have been shown to potentially cause hypermethylation or hypomethylation of DNA, both of which can lead to carcinogenesis through the suppression or overexpression of antioncogene P53, respectively (Wallace et al., 2018). Hamilton Harbour—specifically Pier 27—has a significantly elevated level of polycyclic aromatic hydrocarbons in the surrounding atmosphere due to its location downwind of coking ovens, industrial complexes, and the
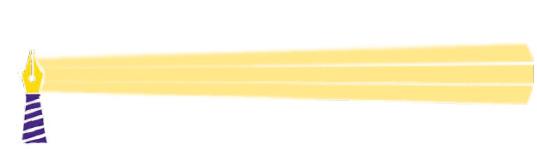
two largest steel mills in Canada (Wallace et al., 2018; King,de Solla, Small, Sverko, & Quinn, 2014). In addition, the pier is near a major highway with vehicles that produce substantial gas and diesel emissions (King et al., 2014).
Byproduct pollutants, such as polycylic aromatic hydrocarbons, have also been shown to cause mutations in DNA nucleotide
sequences. In another study on double-crested cormorants in the Hamilton Harbour
area of Ontario, scientists studied germline
microsatellite
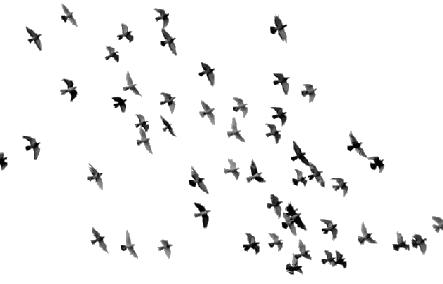
DNA mutation
rates in adults
and their chicks in several
colonies, including a control colony on the pristine Mohawk Island in Lake Erie. In total, 9.3% of all cormorant families had chicks with mutations in the short tandem
repeats amplified by polymerase chain reaction analysis; of that 9.3%, 78% were from Pier 27, the site with both the highest number of unique mutations (11 of the 14 total novel mutations discovered) and the most significant levels of the hydroxylated polycyclic aromatic hydrocarbon benzo[a]pyrene-7,8-diol within samples tissues (King et al., 2014). Pier 27 cormorants were also found to have a
mutation rate 9.7 times higher than that of the cormorants from the control site; in one case, two chicks from one family were found to each have separate mutations at two different loci, which were not found in the parent birds (King, L.E. et al., 2014). The authors of the study theorize the hydroxylation of mutagens may be responsible for microsatellite mutations. Hydroxylation, though important for the catabolism of various chemical
compounds, may result in excess hydroxyl groups when imbalanced, which could potentially lead to a radical interaction
between the hydroxylated metabolite and
an organism’s
DNA molecules, resulting in
oxidized bases and possible
DNA mutations (Albers, 2006; King et al., 2014). Therefore, the oxidative degradation of certain polycyclic aromatic compounds may account for mutated DNA sequences.
Proof of damage to DNA can also be illustrated by the increased response of DNA repair and maintenance proteins and
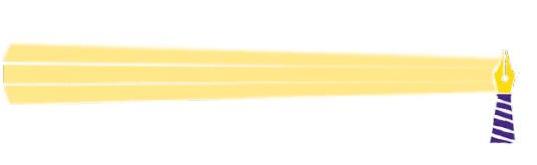
the presence of these proteins’ progenitor mRNA molecules within a bird’s molecular
physiology. P53R2 mRNA levels—messenger RNA transcribed from a gene with significant importance in the repair of damaged DNA by producing proteins that supply dNTPs to fragmented sites—were found to be increased by 1.5-fold in both liver and lung tissue in the double-crested cormorants (Phalacrocorax auritus) living and breeding on Hamilton Harbour’s heavily PAC-polluted Pier 27, as compared to the control birds from the pristine Mohawk Island on Lake Erie (Wallace et al., 2018). GADD45α transcript concentrations were also significantly increased in Pier 27 cormorants’ lung tissues—a full 2-fold change relative to the Mohawk Island control birds (Wallaceet al., 2018). GADD45α is another gene involved in DNA repair and demethylation; and, perhaps not coincidentally, both GADD45α and P53R2 are two of many downstream genes within the pathway of antioncogene P53, a gene responsible for the suppression and prevention of tumors in numerous multicellular organisms and triggered by mutagen exposure (Wallace et al., 2018). Additionally, MDM2 mRNA levels were significantly increased in the tissues of the birds exposed to greater numbers of polycyclic aromatic hydrocarbons from local gas and diesel emissions (Wallace, S.J. et al., 2018). The MDM2 gene is transcribed to produce proteins that participate in a negative feedback loop when P53 protein concentrations become too high and must be reduced to prevent apoptosis of healthy cells; thus, it is not surprising that MDM2 proteins were induced to stabilize the presumably rampant P53 pathway in the cells of the significantly exposed birds.
Lastly, anthropogenic environmental pollutants may affect avian DNA by catalyzing enzymatic reactions that produce the potential for future damage to DNA and possible DNA mutations. Cytochrome P450 proteins—hemeproteins involved in phase I reactions of avian and mammal metabolisms—create chemical changes in compounds that make them more readily dissolvable in water and thus, more able to be eliminated as a waste product from the body. Specifically, these metabolism enzymes induce biotransformation of chemical
species through the process of oxidation (Alexander et al., 2017; Kubota, Eun-Young, & Hisato, 2009; Nebert & Dalton, 2006). Although the metabolism of both natural and synthetic chemicals is essential to healthy physiological functioning, some cytochromes’ processing of foreign compounds can result in the production of reactive oxygen species—in particular, cytochromes from the CYP1A subfamily (Alexander et al., 2017; Schlezinger, Struntz, Goldstone, & Stegeman,
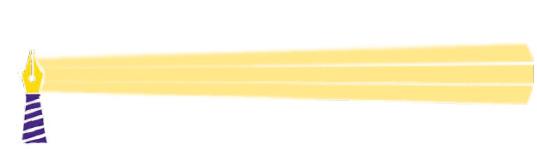
2006; Stegeman et al., 1992). When balanced with cellular antioxidants, reactive oxygen species are vital for various functions within cells. However, environmental stress can cause a maladaptive increase in the production of reactive oxygen species, resulting in oxidative damage to DNA due to the unstable—and now hypothetically unencumbered—reactive oxygen molecule’s tendency to steal electrons from DNA bases (Alexander et al., 2017; Jena, 2012). Therefore, the overexpression of CYP1A subfamily proteins could potentially lead to DNA damage.
In both European quails (Coturnix coturnix cotunix) treated with the chloros-triazine herbicide atrazine and double
crested cormorants experimentally dosed with Mississippi Canyon 252 crude oil, CYP1A mRNA and protein levels increased relative to control groups; in the double-crested cormorants, the increase was significant and found in all four treatment groups (Alexander et al., 2017; De la Casa-Resino, I. et al., 2012). Astonishingly, in the cormorant treatment group given only a single dose of 20 mL/kg b.w. crude oil, a 71-fold increase in CYP1A protein expression was detected in hepatic cells as compared to the control group given vehicle only (Alexander et al., 2017). Although the increase in concentration of CYP1A
mRNA within the quail hepatic cells of the affected experimental groups was not found to be significant, it should be noted that the doses administered in the experiment were lower than the lowest-observed-adverse
effect-concentration (675 mg/kg), and the no-observed-adverse-effect-concentration
(225 mg/kg) for atrazine in target avian organisms; in addition, the duration for which the quails were treated with the herbicide was insubstantial compared to the actual amount of time organisms are exposed to ambient herbicidal particulate matter in the wild (De la Casa-Resino, Valdehita, Soler, Navas, & Pérez-López, 2012). Atrazine concentrations post-application can exceed a staggering 960 mg/kg (De la Casa-Resino et al., 2012).
In the double-crested cormorants
treated with MC252 crude oil, all four treatment groups also demonstrated significant inductions of ethoxyresorufin-Odeethylase activity (Alexander et al., 2017). Ethoxyresorufin-O-deethylase (EROD) activity assays measure induction rates of various types of cytochromes by quantifying the amount of resorufin (a fluorescent compound) produced from the dealkylation of the substrate 7-ethoxyresorufin, which is an activity mediated by cytochrome enzymes (Whyte & Tillitt, n.d.). Thus, the amount of resorufin detected by an EROD assay is a direct indicator of how much of a specific cytochrome protein is present within the cell (Whyte & Tillitt, n.d.). In addition to
producing potentially harmful reactive oxygen species through the metabolism of foreign chemical compounds, cytochromes from the P450 superfamily also make excellent bioindicators of environmental pollution due to their tendency to increase in response to mutagenic contaminants; therefore, EROD assays also provide an approximate indication of how substantially the tested organism has been exposed to physiologically harmful pollutants (Whyte & Tillitt, n.d.). This is because certain chemical substances—such
as polycyclic aromatic hydrocarbons—bind to aryl hydrocarbon receptors within the cell, which have been found to regulate the transcription rate of various P450 cytochromes (Alexander et al., 2017; Walker, Heid, Smith, & Swanson, 2000; Whitlock, 1999; Whyte & Tillitt, n.d.). Shockingly, EROD activity levels were still significantly increased in birds such as pigeon guillemots (Cepphus columba) exposed to crude oil after the Exxon Valdez oil spill ten years later (Alexander et al., 2017; Golet et al., 2002).
How pollutants affect the avian nervous system
One way in which chemical pollutants affect the avian nervous system is by crossing the semipermeable blood-brain barrier and lowering the activation threshold of action potentials within neurons. The blood-brain barrier is a filtering mechanism located within the capillaries that carry blood to the brain and spinal cord. One function of the blood-brain barrier
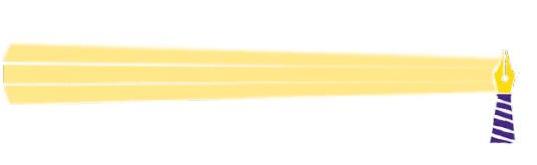
is to prevent potentially neurotoxic substances from entering the brain and altering the body’s neurological system. A protective phospholipid bilayer also surrounds the blood-brain barrier. Many pesticides, such as pyrethroid insecticides, are specifically formulated to be lipophilic to ensure their absorption into fatty tissues and through lipid-rich membranes (Sharaf et al., 2010). Abamectin, a pesticide which belongs to the avermectin chemical group of pesticides and is widely used in the production of citrus fruits, potatoes, and maize, displays antihelminthic, nematicidal, and insecticidal properties due to its neurotoxic method of action—it actively crosses the blood-brain barrier of its target and causes
paralysis (De Faria et al., 2018). Although itended only as a pesticide, abamectin can leak from holes in layers of the soil where it has collected and poison sources of drinking water for birds due to spread by rainfall; many other pesticides have been known to leak into bodies of water, such as rivers, and poison aquatic organism as well (De Faria et al., 2018). Pyrethroid insecticides such as cypermethrin also enter the nervous system through the bloodbrain barrier and cause oxidative damage to cells through the release of free radicals,
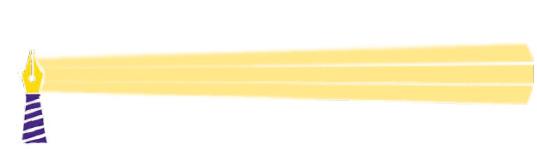
which damage plasma membranes by means of lipid peroxidation (Sharaf et al., 2010). Once inside the nervous system, pyrethroids alter sodium ion channels, effectively lowering the activation threshold of action potentials; this decrease in the threshold minimum within
sensory nerve fibers ultimately leads to overactivation of the transfer system of neuronal electrical signals and premature hyperexcitation of the nervous system (Sharaf et al., 2010). Because cypermethrin is considered less toxic to birds and mammals by environmental regulatory institutions than other pesticides such as organochlorides and carbamates, birds may be more likely to be exposed to cypermethrin and its highly neurotoxic effects (Sharaf et al., 2010). Lastly, pyrethroid insecticides have also been shown to inhibit gamma aminobutyric acid (GABA) receptors—the neurotransmitter responsible for reducing excitability within the nervous system (Sharaf et al., 2010). This byproduct consequence of its neurotoxic method of action surely reduces the avian body’s ability to remedy the effects of the insecticide’s damage to sodium ion channels within neurons.
How pollutants affect the avian endocrine system
Environmental pollutants may overstimulate or otherwise increase concentrations of sex hor
mones within the endocrine system, resulting in excessive production of endocrine-regulated biosynthetic proteins and possible sex-specific behaviors. In female European quail (Coturnix coturnix coturnix) given the herbicide atrazine, a possible endocrine-disrupting chemical that inhibits photosynthesis in plants, the expression of estrogen receptor alpha increased significantly in both treatment groups relative to control on sampling day 30 (20 days after the final dosing day), before reverting back to levels maintained on the previous sampling day (De la Casa-Resino et al., 2012). Perhaps not coincidentally, plasma estradiol concentration in both treatment groups also increased only on sampling day 30, though not significantly (De la Casa-Resino et al., 2012). Similarly, vitellogenin protein levels demonstrated no significant difference between experimental groups on any sampling days except sampling day 30, when the group with the highest prescribed dose of atrazine (which was still substantially lower than the no-observed-adverse-effect-concentration and the lowest-ob
served-adverse-effect-concentration), reached levels a full three times higher than control (De la Casa-Resino et al., 2012). Thus, it can be speculated that estrogen receptor alpha, the sex hormone estradiol, and the yolk protein vitellogenin must be linked and directly affected by endocrine-disrupting chemicals, which alter the normal functioning of the endocrine system; estradiol has been found to activate estrogen receptors and indirectly control the
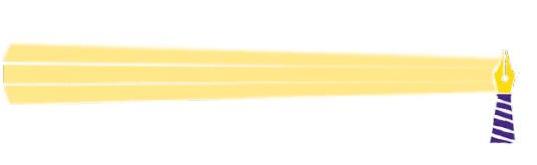
production of vitellogenin in oviparous vertebrates (De la Casa-Resino et al., 2012; Matozzo, Gagné, Marin, Ricciardi, & Blaise, 2008). As endocrine-disrupting chemicals have a proclivity to mimic the configurations and actions of sex hormones and bind to hormone receptors such as estrogen receptor alpha, it is possible that endocrine-disrupting herbicides such as atrazine may modify the molecular composition of sex-specific tissues and concentrations of endocrine-catalyzed biosynthetic proteins within cells (Markman, Müller, Pascoe, Dawson, & Buchanan, 2011). Disturbingly, even at the lowest-observed-adverse-effect-concentration, the Environmental Protection Agency still warns of observed lower viability of embryos, lower egg production, and an increase in the ratio of defective eggs to total clutch size in bobwhites and mallards (De la Casa-Resino et al., 2012; EPA, 2006). Furthermore, endocrine-disrupting chemicals seem to affect birds at a macroscopic level, manifesting in modifications of sex-specific behaviors. In a study on bioaccumulation of estrogenic chemicals, Eisenia fetida earthworms living in the biofilm of sewage sites contaminated with sewage treatment chemicals were shown to accumulate endocrine-disrupting chemicals stored within the affected soil systems of the filter beds; compared to controls, the experimental earthworms had significantly higher levels of estradiol, dibutyl phthalate, and bisphenol A (Markman et al., 2007). In a related experiment conducted a year later, the same research group dosed European starlings (Sturnis vulgaris) with the same estrogens found in the E. fetida earthworms; interestingly, this dosing of starlings resulted in increased song complexity, hypothesized to be due to hypermasculinization of the brain (Markman, 2008). As these particular earthworms are a major prey item of the starlings in the area, it stands to reason that the current population and its successor populations will display this and potentially other progressively more concerning behaviors associated with endocrine disruption as pollution levels continue to rise.
How pollutants affect the avian renal and digestive systems
Environmental contaminants may affect avian renal systems through damage to the glomerulus of the kidneys, impairing their ability to filter metabolic wastes from the blood. In male
Japanese quails (Coturnix japonica) given varying doses of pre-emergent herbicide butachlor daily for 30 days, the group prescribed the highest amount of the herbicide exhibited significantly higher serum urea concentrations on all sampling days; the group prescribed the second highest amount of the herbicide presented significantly increased serum urea concentrations on the final two sampling days; and, the group given the third highest amount of the herbicide showed significantly in
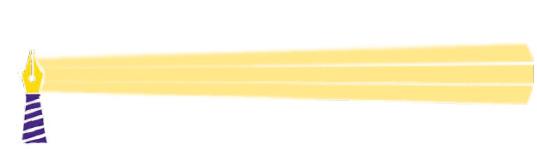
creased serum urea concentrations on the final
sampling day (Hussain et al. 2014). Urea is the main nitrogenous waste product of protein catabolism; therefore, the author theorizes, the increase in serum urea concentration is indica
tive of damage to the glomerulus, which filters metabolic wastes from the blood before the
blood is recirculated through the cardiovascular vessels and the waste products are further filtered and excreted by the kidneys (Hussain et al. 2014).
Chemical pollutants may also injure hepatocytes in the digestive system and cause liver enzymes to leak into the bloodstream. In the same experiment on male Japanese quails treated with sublethal doses of butachlor, the treatment group given the highest dose of the herbicide (250 mg/kg b.w.) exhibited significantly increased serum levels of lactate dehydrogenase, aspartate transaminase, and alanine transaminase on all sampling days, while the treatment group given the second highest concentration of butachlor (150 mg/kg b.w.) also showed significantly increased levels of all three liver enzymes relative to control on two of the three sampling days (Hussain et al. 2014). Lactate dehydrogenase catalyzes the conversion of lactate to pyruvic acid, and is released after damage to tissues; aspartate transaminase is important in the metabolism of amino acids, and is frequently measured in conjunction with alanine transaminase to differentiate between causes of hepatotoxicity or liver damage. Injured liver cells leak more of their constituent enzymes into the bloodstream, resulting in increased serum hepatic enzyme levels; thus, Hussain et al. (2014) posit that the observed increase in all serum lactate dehydrogenase, alanine transaminase, and aspartate transaminase levels may ultimately indicate damage to hepatocytes.
Conclusion
From the evidence cited, it is plain to see that anthropogenic environmental contaminants have a significant effect on avian physiological and molecular systems. In the circulatory system, various pollutant molecules alter the morphological phenotypes of erythrocytes and the nuclei within the erythrocytes, as well as increase the frequency of micronuclei within erythrocytes by mechanisms still relatively unknown. It can be presumed that physical changes in phenotypic structures of red blood cells and the organelles within red blood cells could potentially ignite or delay biochemical reactions due to the modification in the configuration of the proteins.
At the molecular level within avian DNA, chemical pollutants appear to have significant effects on the nucleotide sequences and future backbone of DNA strands by catalyzing mutations and enzymatic reactions that create
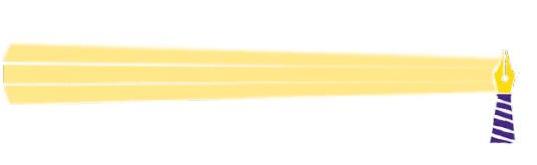
potential for oxidation of bases through the production of reactive oxygen species. In addition, pollutants seem to alter methylation statuses of DNA ready to be transcribed. The nervous system is affected by the alteration of gated sodium ion channels within the membranes of neuronal cells, increasing action potentials of electrical signals through the reduction of activation thresholds in signaling pathways.
Environmental contaminants mimic the
actions of sex hormones in the endocrine
system, and have been shown to attach to hormone receptors responsible for sexspecific biosynthetic protein production; consequently, these rapid increases of sex hormones also appear to affect behaviors in birds that are thought of as being sexspecific, such as songbirds singing for mates. Lastly, avian renal systems are affected by pollutants through damage to the glomerulus of the kidney, as well as injury to hepatocyte cells in the liver; the consequences of which produce significantly increased levels of liver enzymes in the bloodstream and a pathological increase in serum urea concentrations. These
systems have been shown to be affected across various bird species, including herring gulls (Larus argentatus) Japanese quails (Coturnix japonica), European quails (Cortunix coturnix coturnix), pigeon guillemots (Cepphus columba), European starlings (Sturnus vulgaris), mallard ducks (Anas platyrhynchos), Northern bobwhites (Colinus virginianus), double-crested cormorants (Phalacrocorax auritus), and numerous others. With the human data exhibiting rising death rates due to pollution-related diseases and rapidly increasing levels of pollutants in human and animal environments, it seems to be only a matter of time before bird species across the globe experience correlated death and disease rates as well; or, at least, before humans stop denying the impact of pollution and actively work to depollute the planet that we are defiling. n
Rachel Goodman is an undergraduate student of Biology at East Carolina University. She is passionate about animals, kindness, learning, problemsolving, and her lovely mom Jane, brother Max, and babushka Dorothy. She enjoys hiking, gardening, reading, studying, and spending time with her pet children. She loves to work hard and achieve goals, and she is on a lifelong journey to gather as much knowledge and experience as possible. She is particularly fascinated by the studies of genetics, physiology, molecular biology, electrochemistry and organic chemistry, the Russian language and its dialects, mathematics, the atom, biological evolution, and psychopathology.
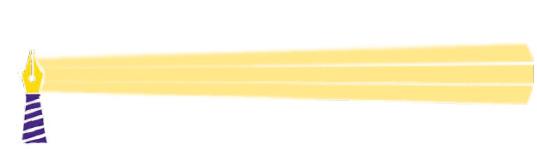
Works Cited
Albers, P. H. (2006). Birds and polycyclic aromatic hydrocarbons. Avian and Poultry
Biology Reviews, 17(4), 125–140.
Alexander, C. R., Hooper, M. J., Cacela, D., Smelker, K. D., Calvin, C. S., Dean, K.
M., . . . Godard-Codding, C. A. J. (2017). CYP1A protein expression and catalytic activity in double crested cormorants experimentally exposed to Deepwater Horizon
Mississippi Canyon 252 oil. Ecotoxicology and Environmental Safety, 146, 68–75.
De Faria, D. B. G., Montalvão, M. F., de Souza, J. M., Mendes, B. D., Malafaia, G., & de
Lima Rodrigues, A. S. (2018). Analysis of various effects of abamectin on erythrocyte morphology in Japanese quails (Cortunix japonica). Environmental Science and
Pollution Research, 25(3), 2450–2456.
De la Casa-Resino, I., Valdehita, A., Soler, F., Navas, J. M., & Pérez-López, M. (2012).
Endocrine disruption caused by oral administration of atrazine in European quail (Coturnix coturnix coturnix). Comparative Biochemistry and Physiology, 156, 159–165.
EPA. (2006). Finalization of atrazine IRED, and completion of tolerance reassessment and reregistration eligibility process. United States Environmental Protection Agency.
Science Chapter for Atrazine: Environmental Fate and Effects, Office of Prevention,
Pesticides and Toxic Substances, Washington, DC.
EPA. (2016). Air quality—National summary. United States Environmental Protection
Agency. Retrieved from https://www.epa.gov/air-trends/air-quality-national-summary
Albers, P. H. (2006). Birds and polycyclic aromatic hydrocarbons. Avian and Poultry
Biology Reviews, 17(4), 125–140.
Golet, G. H., Seiser, P. E., McGuire, A. D., Roby, D. D., Fischer, J. B., Kuletz, K. J., . . .
Newman, S. H. (2002). Long-term direct and indirect effects of the ‘Exxon Valdez’ oil spill on pigeon guillemots in Prince William Sound, Alaska. Marine Ecology Progress
Series, 241, 287–304.
Hussain, R., Khan, A., Mahmood, F., Rehan, S., & Ali, F. (2014). Clinico-hematological and tissue changes induced by butachlor in male Japanese quail (Coturnix japonica).
Pesticide Biochemistry and Physiology, 109, 58–63.
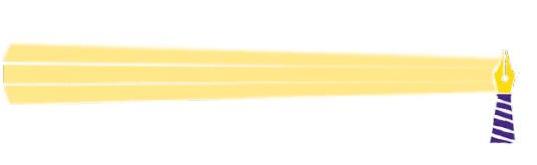
Jasarevic, T., Thomas, G., & Osseiran, N. (2014). 7 million premature deaths annually linked to air pollution. World Health Organization. Retrieved from http://www.who. int/mediacentre/news/releases/2014/air-pollution/en/
Jena, N. R. (2012). DNA damage by reactive species: mechanisms, mutation, and repair.
Journal of Biosciences, 37(3), 503–517.
King, L. E., de Solla, S. R., Small, J. M., Sverko, E., & Quinn, J. S. (2014). Microsatellite
DNA mutations in double-crested cormorants (Phalacrocorax auritus) associated with exposure to PAH-containing industrial air pollution. Environmental Science and
Technology, 48(19), 11637–11645.
Kubota, A., Eun-Young, K., & Hisato, I. (2009). Alkoxyresorufin (methoxy-, ethoxy-, pentoxy- and benzyloxyresorufin) O-dealkylase activities by in vitro-expressed cytochrome P450 1A4 and 1A5 from common cormorant (Phalacrocorax carbo).
Comparative Biochemistry and Physiology, 149(4), 544–551.
Landrigan, P. J., Fuller, R., Acosta, N. J. R., Adeyi, O., Arnold, R., Basu, N., . . . Zhong,
M. (2018). The Lancet commission on pollution and health. The Lancet, 391(10119), 462–512.
Markman, S., Guschina, I. A., Barnsley, S., Buchanan, K. L., Pascoe, D., Müller, C. T. (2007). Endocrine disrupting chemicals accumulate in earthworms exposed to sewage effluent. Chemosphere, 70, 119–125.
Markman, S., Leitner, S., Catchpole, C., Barnsley, S., Müller, C. T., Pascoe, D., &
Buchanan, K. L. (2008). Pollutants increase song complexity and the volume of the brain area HVC in a songbird. PLos ONE, 3, 1–6.
Markman, S., Müller, C. T., Pascoe, D., Dawson, A., & Buchanan, K. L. (2011).
Pollutants affect development in nestling starlings Sturnus vulgaris. Journal of Applied
Ecology, 48, 391–397.
Matozzo, V., Gagné, F., Marin, M. G., Ricciardi, F., & Blaise, C. (2008). Vitellogenin as a biomarker of exposure to estrogenic compounds in aquatic invertebrates: a review.
Environment International, 34(4), 531–545.
Nebert, D. W., & Dalton, T. P. (2006). The role of cytochrome P450 enzymes in endogenous signaling pathways and environmental carcinogenesis. Nature Reviews
Cancer, 6(12), 947–960.
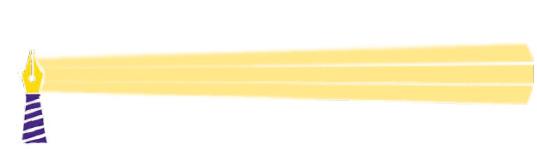
Schlezinger, J. J., Struntz, W. D. J., Goldstone, J. V., & Stegeman, J. J. (2006).
Uncoupling of cytochrome P450 1A and stimulation of reactive oxygen species production by co-planar polychlorinated biphenyl congeners. Aquatic Toxicology, 77(4), 422–432.
Sharaf, S., Khan, A., Khan, M. Z., Aslam, F., Saleemi, M. K., & Mahmood, F. (2010).
Clinico-hematological and micronuclear changes induced by cypermethrin in broiler chicks: their attenuation with vitamin E and selenium. Experimental and Toxicologic
Pathology, 62(4), 333–341.
Skarphedinsdottir, H., Hallgrimsson, G. T., Hansson, T., Hägerroth, P. A., Liewenborg,
B., Tjärnlund, U., . . . Balk L (2010). Genotoxicity in herring gulls (Larus argentatus) in Sweden and Iceland. Mutation Research/Genetic Toxicology and Environmental
Mutagenesis, 702(1), 24–31.
Stegeman, J. J., Marius, B., Di Giulio, R. T., Lars, F., Fowler, B. A., Sanders, B. M., Van
Veld, P. A. (1992). Molecular responses to environmental contamination: enzyme and protein systems as indicators of chemical exposure and effect. In Biomarkers:
Physiological and Histological Markers of Anthropogenic Stress (pp. 235–335). Taylor & Francis.
United Nations. (2003). The natural water cycle. World Water Assessment Programme:
Executive Summary. The United Nations World Water Development Report (pp. 8–9).
Walker, M. K., Heid, S. E., Smith, S. M., & Swanson, H. I. (2000). Molecular characterization and developmental expression of the Aryl hydrocarbon receptor from the chick embryo. Comparative Biochemistry and Physiology, 126(3), 305–319.
Wallace, S. J., de Solla, S. R., Thomas, P. J., Harner, T., Eng, A., & Langlois, V. S. (2018).
Airborne polycyclic aromatic compounds contribute to the induction of the tumoursuppressing P53 pathway in wild double-crested cormorants. Ecotoxicology and
Environmental Safety, 150, 176–189.
Whitlock, J. P. (1999). Induction of cytochrome P4501A1. Annual Review of
Pharmacology and Toxicology, 39, 103–125.
Whyte, J. J., & Tillitt, D. E. (n.d.) EROD activity. United States Geological Survey
Columbia Environmental Research Center. Retrieved from https://www.cerc.usgs.gov/ pubs/BEST/EROD.pdf