RADIANT COOLING I An Investigation into Cooling Comfort
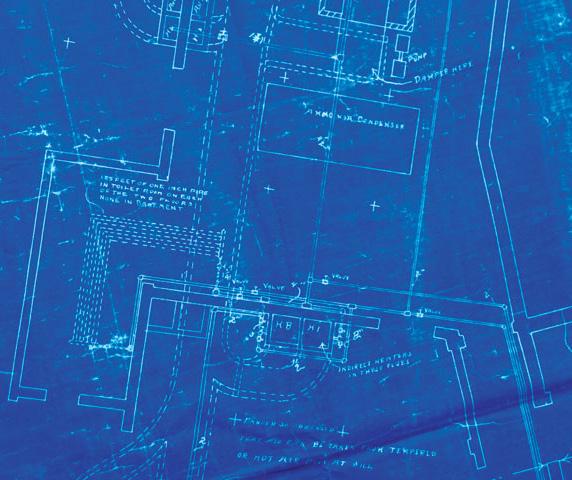
2 Image: The sandstone caves of Ennedi, Chad where early paintings and petroglyphs have been found Source: Travel-Tour-Guide.com
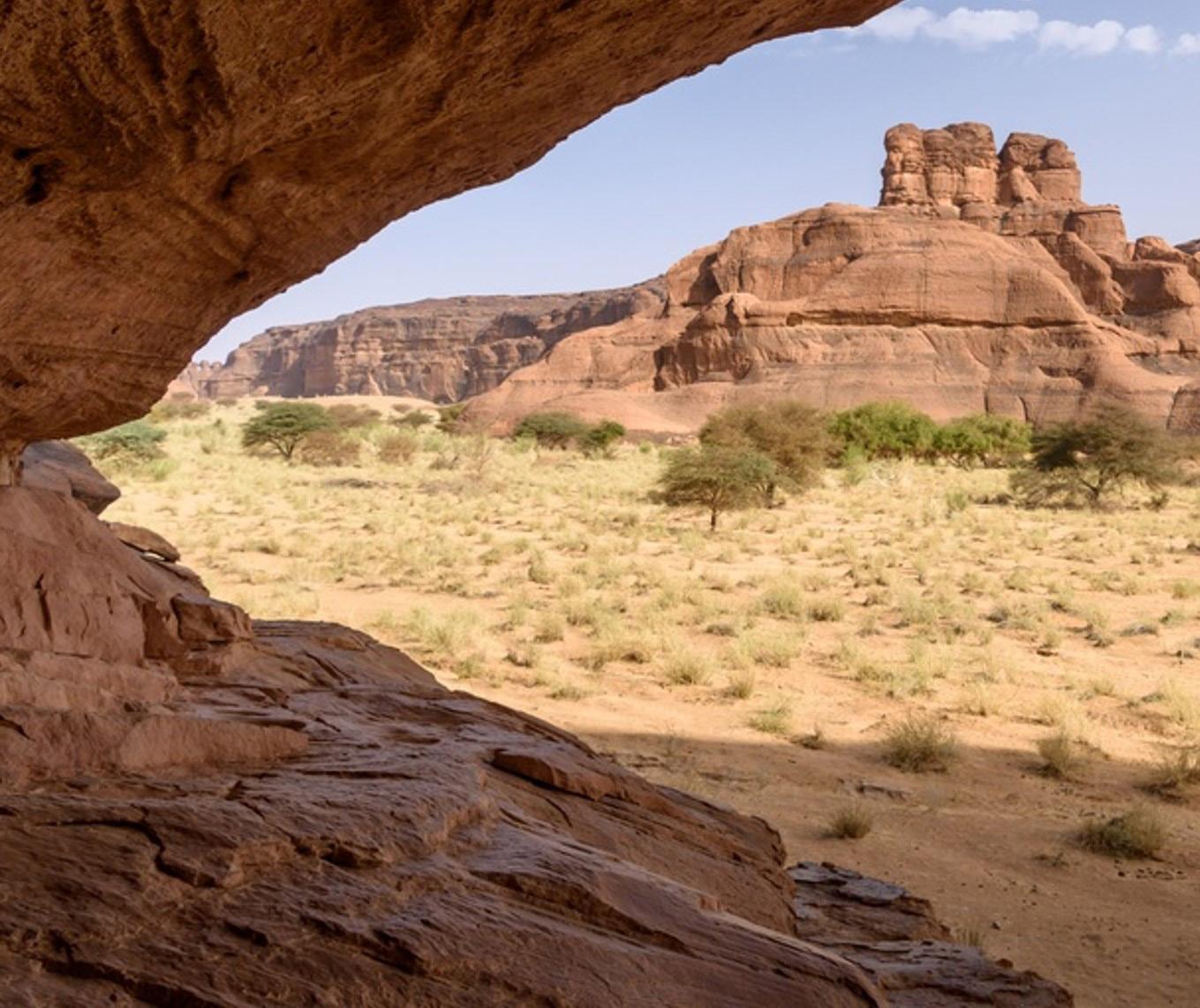
3 RADIANT COOLING I
Adaptive Thermal Comfort, Indoor Environmental Quality (IEQ), Thermal Alliesthesia, Hedonic Response, Hydronic Cooling, Radiant Cooling
Optimal thermal comfort is hard to define and even harder to achieve. But this quest for comfort is central to our human journey on earth. The first part of this two-part investigation explores the history of cooling, thermal comfort basics and hydronic cooling, with a focus on Radiant Cooling. It contextualizes the emergence of this technology through surveying the history of thermal comfort and highlighting experts’ evolving thinking around thermal comfort and how radiant systems are part of this evolution. The emergence of radiant cooling promises a lower-energy cooling method while optimizing thermal comfort and integrating with architectural systems. Finally, this investigation provides guidelines to aid architects in incorporating these types of systems into their projects.
An Investigation into Cooling Comfort Samantha Rusek 2021
ABSTRACT
KEYWORDS
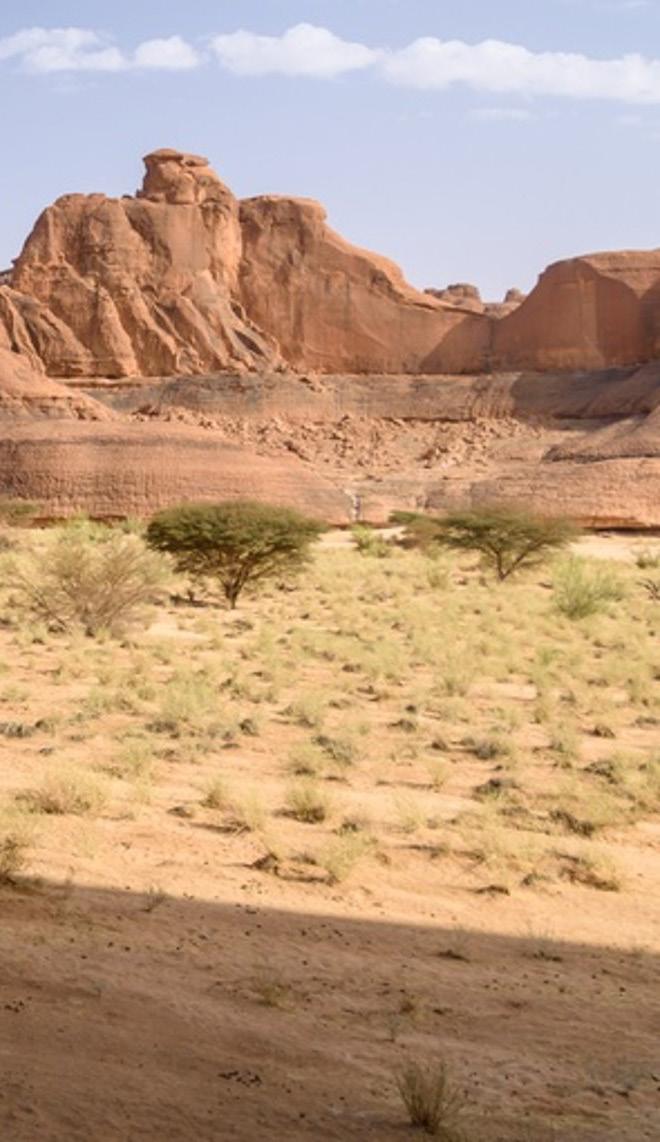
Above: Korean ondol floor heating and cooking system
4
Spanning the history of human civilization, we have developed clever methods to keep us comfortable in various climates across the world. Our ability to make and contain fire was a pivotal skill that laid the foundation for many developments throughout our history.
From the 2nd century CE, a Korean ondol system under a hanok style house used fire to simultaneously heat the stone floors of a building and cook. Similar in its underfloor positioning, a Roman hypocaust system circulated hot air below the floor of the house to heat the space above, sometimes also using pipes as pathways through the walls to provide further heat to the home. Containing the Cold
A BRIEF HISTORY OF COOLING COMFORT
Pre-Industrial Methods of Keeping Comfortable
Early methods of preservation and refrigeration allowed us to prolong our food stores. Clay pots that used evaporative cooling to keep food fresh and water chilled have been found across hot, dry climates in the ancient world, some still in use today. Called zeer in some Arabic speaking nations and búcaro in Egypt, these pot-in-pot “refrigerators” help to keep perishable food fresh, helping it to last significantly longer than without these cooling containers.
Surahi, matka and ghara in India, and botijos in Spain were used to keep water cool. Botijos were traditionally designed fully enclosed with only a spout for drinking and an opening for adding water.
A type of early Chinese ice chest, jian, was first made of ceramic and then copper. These ice boxes were built to contain a block of ice to be used as a space-cooling device where small holes would allow the cool air to flow out (Nan, 2017). The variety and specifics of these early methods and technologies of bringing heat and cool to make our homes and public spaces more comfortable, when sampled from across the world, could easily be a research project in themselves.
Persian yakhchals were a structure both for making ice in the winter and storing it through the summer. Water was channeled from the aqueduct to the structure and frozen over the winter, or ice was brought from the mountains and stored. Two-meter-thick walls made of a unique water-resistant mortar composed of sand, clay, egg whites, lime, goat hair, and ash in specific proportions were used to line the structure (Pochee & Gunstone, 2017).
Source: Korea Tourism Organization and Cultural Heritage Administration of Korea
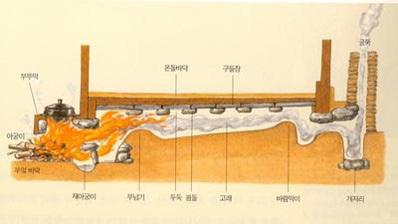
5 Images of Heating and Cooling Inventions A Yakhchal in Iran Source: Quintin Lake Photography B Yakhchal Section Illustration Source: Solaripedia, Hemming Jorgensen C Illustration of a Thermoscope (thermometer) Source: Robert Fludd, 1638 D Depiction of a Hearth, Albert Anker 1867 Source: WikiArt, Die Bauern und die Zeitung E Jian ice chest, China Source: Artron.net F Modern Botijo Source: filtroshop.pt G Ice Cellar from the Forbidden City Source: line.17qq.com H Solar powered absorption refridgeration Source: ASHRAE, Augustin Mouchot 1878 A B C E F GD H
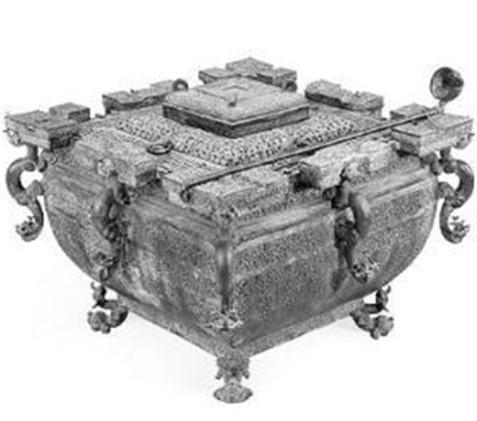
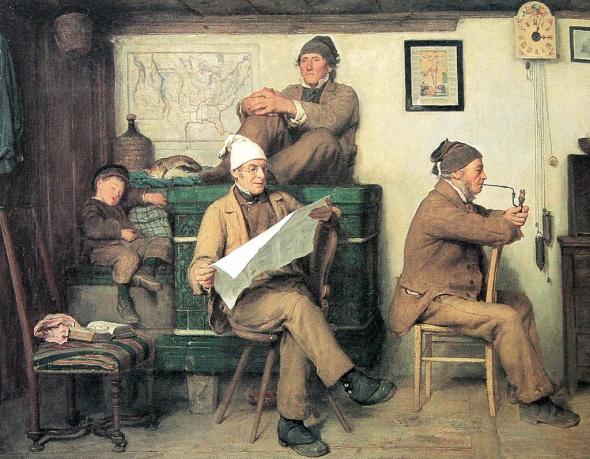
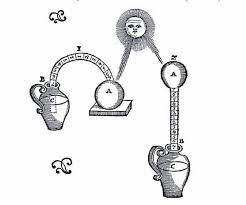
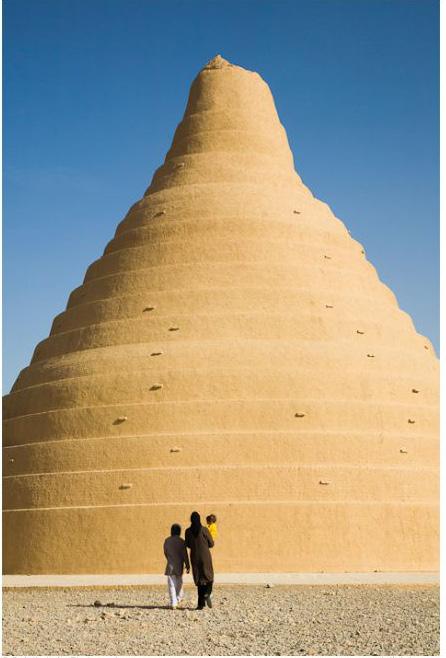
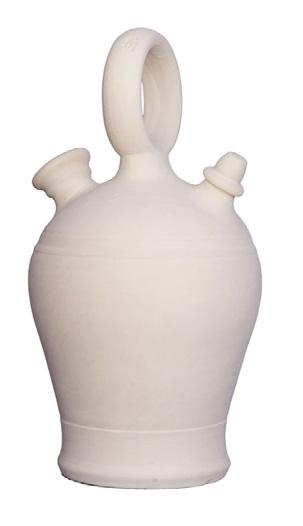
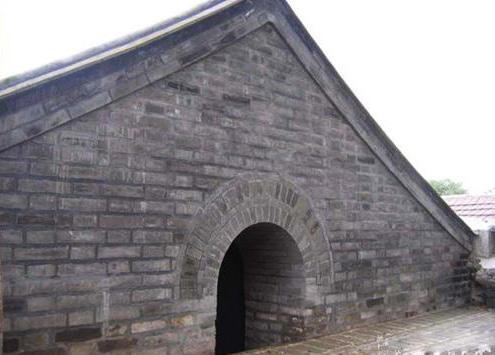
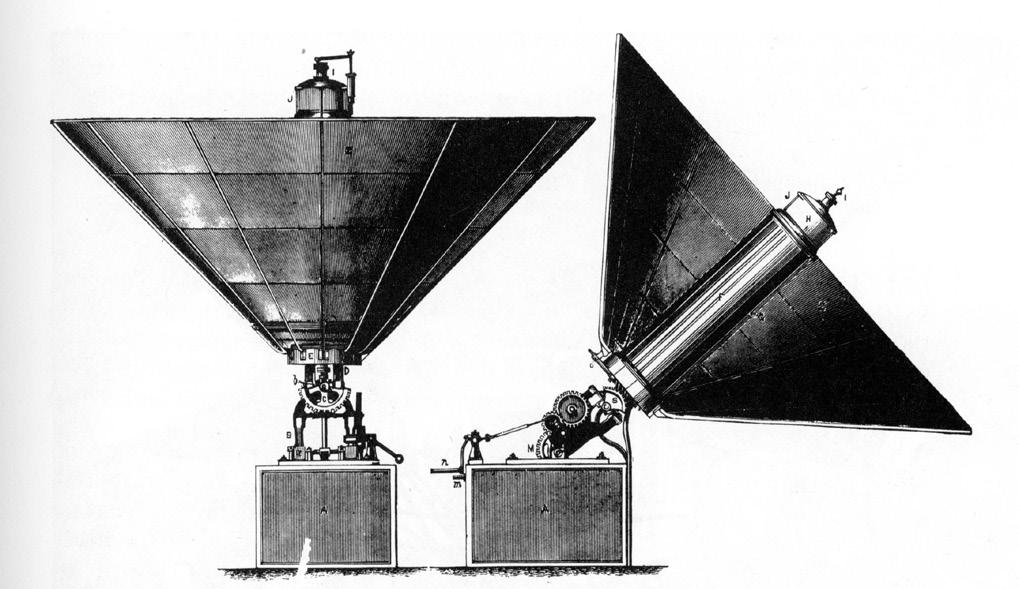
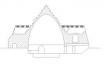
6 Above: An illustration of ice harvesting Source: Wikimedia Commons Opposite page: Top: The Rivoli Theater promoting their interior refrigerated space. Bottom: A confectionary utilizing air conditioning to help stabalize their wares Source: Willis Carrier Archive
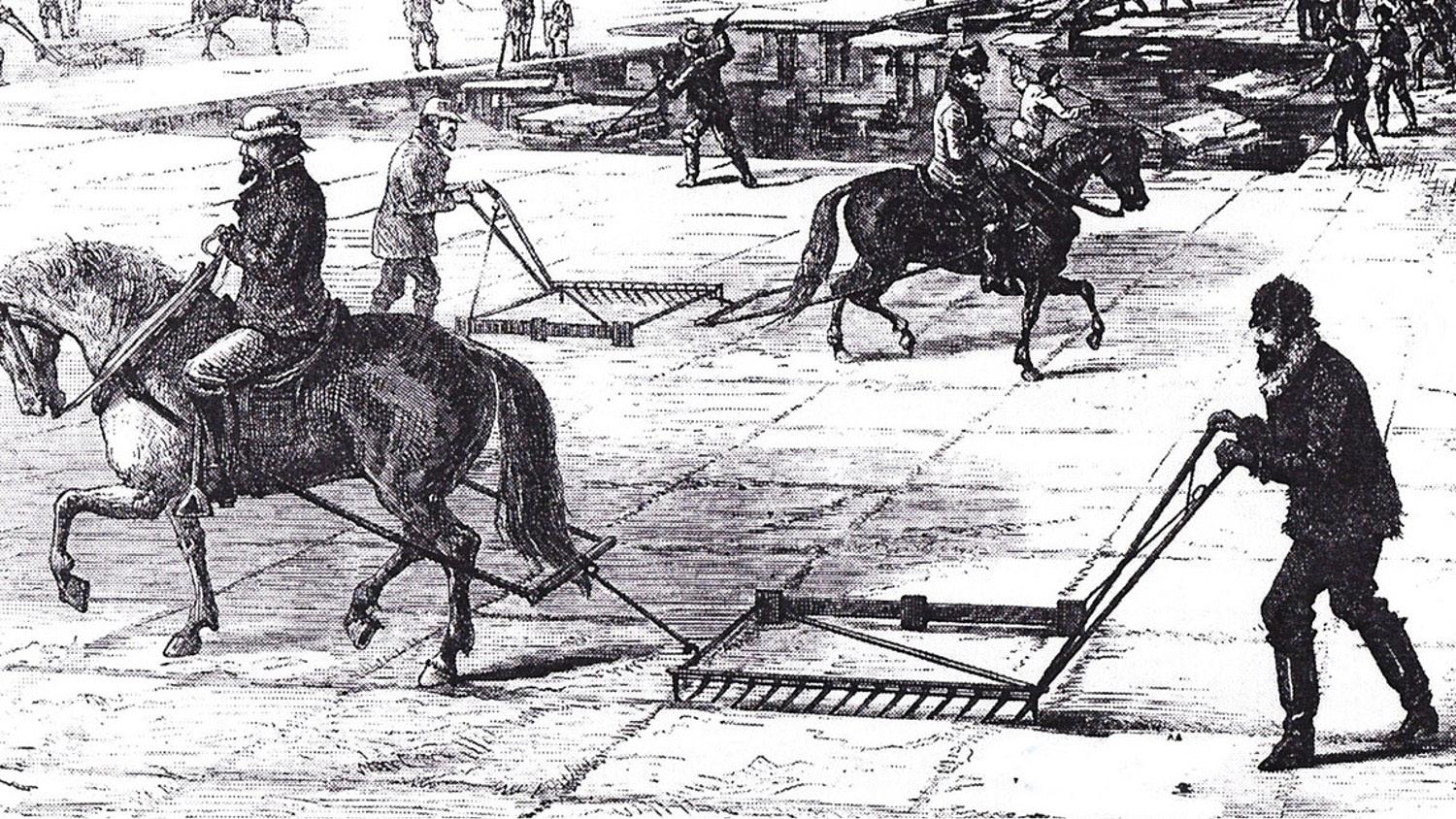
7
Seeking the Cold Ice harvesting, shipping and storage was a method used across the world for cooling beverages and as an early method of central space cooling (Mitenbuler, 2013). In the mid-1800s, ice in the United States was a luxury good and The Tudor Ice Company, helmed by Frederic Tudor, nicknamed the ‘Ice King,’ was a Boston entrepreneur trying to move this highly sought after and heat sensitive commodity to the southern regions of the world. After a few massive failures to move large shipments of ice and make money from its transport, Tudor discovered years later how to ship the ice without melting and store it in hot climates for months, without the aid of modern refrigerants (Johnson, 2015).
The invention of the air conditioning system as we know it was at first considered a luxury. Some of the earliest publicly accessible spaces with air conditioning, outside of places of work, were movie theaters. By the 1950s, these cooling systems were considered must-have household appliances for modern life in the United States, and would have a massive impact on how we thought about comfort. Architectural design decisions were no longer dictated by exterior climatic conditions.
During the Industrial Revolution in the early 1900s a young engineer, Willis Carrier, signed a set of drawings for the Sackett & Wilhelms Lithography and Printing Company in Brooklyn, New York which was the design for a system to remove enough humidity from the air to allow the ink to dry (Johnson, 2015). This was one of the first modern large-scale air conditioning systems.
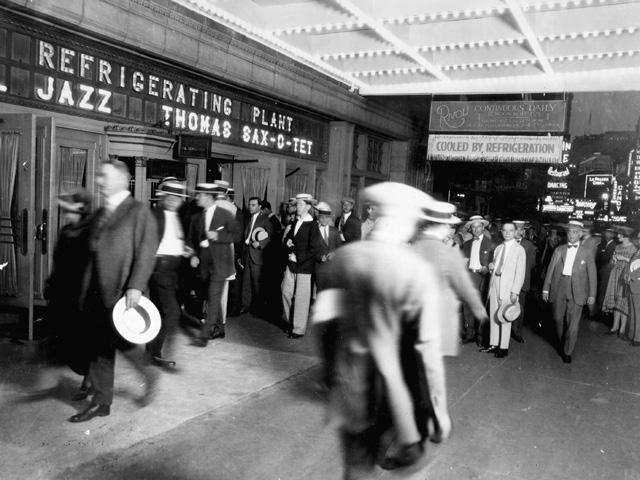
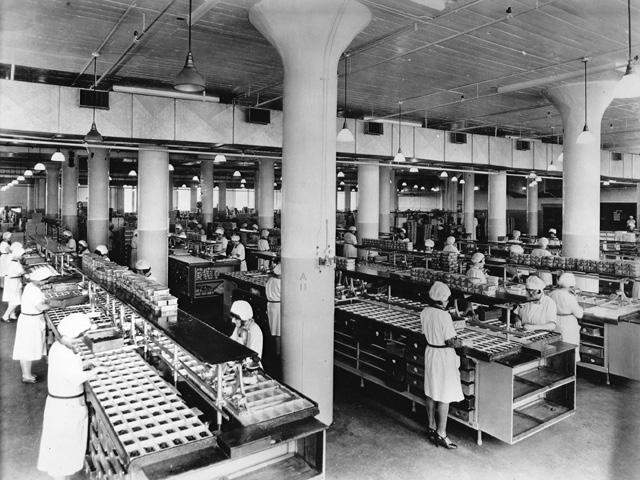
According to the ASHRAE 55 standard, there are six factors affecting one’s thermal comfort, four physical or environmental factors and two personal factors: Factors
8 THERMAL COMFORT: A REVIEW Thermal Comfort Basics
Upper:
Clo is a value that describes the degree of insulation provided by an article of clothing. A clo value of 1 is equal to the amount of clothing required by a resting human to maintain thermal comfort in a room temperature of 71 degrees F (KieranTimberlake Architects, RoastSurvey).
Environmental
Air HumidityAirRadiantVelocityTemperatureTemperature Personal Factors Clothing MetabolicInsulationRate
2019 Lower:
Thermal Comfort is defined by the ASHRAE 55 and the EN 15251 standard for Commercial and Residential. The conventional way to meet these thresholds is to create a highly predictable, controlled environment using energyintensive mechanical equipment. The six factors of thermal comfort. Source: simulationHub blog , Jan 25, Visual examples of Clo factor. Source: University of Oregon Baker Lighting Lab AND ABBREVIATIONS
DEFINITIONS
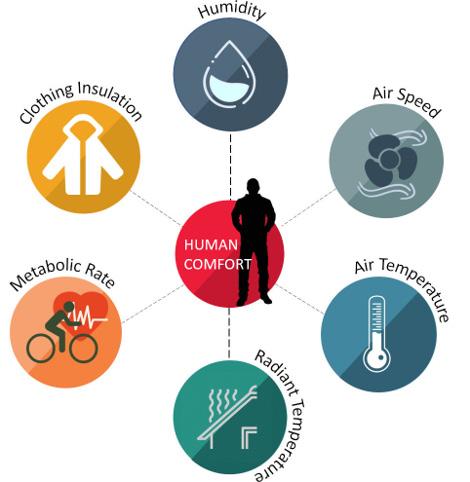
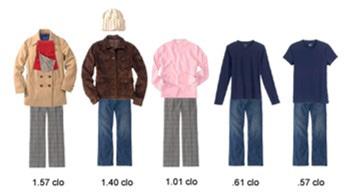
Fanger wanted to present a method of prediction for heating and air conditioning engineers to use for an indoor environement ‘s thermal comfort (Hoof, 2008). This method would account for any type of activity and clothing that an occupant could be doing or wearing and could apply to the various combinations of thermal factors in an environment for which the largest possible percentage of a given group of people would experience thermal comfort. This is still the most recognized model in thermal comfort research today.
Lower Left: photo of P. Ole Fanger at Syracuse University and his 1970 ‘Thermal Comfort: Analysis and Applications in Environmental Engineering’ Source: Syracuse COE
The ASHRAE standard is used for identifying a combination of factors that produce thermally comfortable environmental conditions that will be acceptable to a majority of the occupants.
The ASHRAE 55 standard foundational study was performed in 1967 by P. Ole Fanger at the Technical University of Denmark (Hoof, 2008). The study indicated that the sensation of thermal comfort was most significantly determined by the Predictive Mean Vote (PMV), a model predicting the average thermal sensation of a group of people on a seven-point scale that was correlated with a narrow range of recorded skin temperature and sweat evaporation rates, depending on activity level (Fanger, 1967).
9
Lower Right: Comfort-meter developed by Korsgaard and Madsen. Upper Right: Subjects wearing standard uniform, 0.6 clo, during Fanger’s 1973 study at Technical Univeristy of Denmark. Source: Fanger, 1973
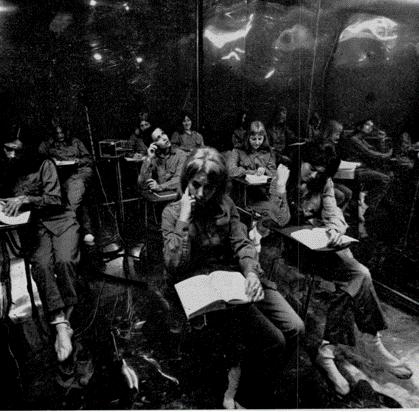
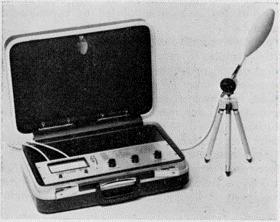
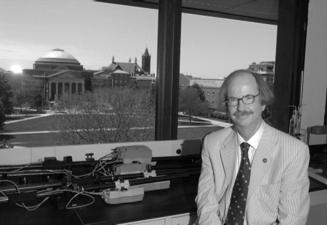
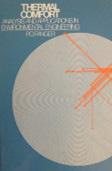
In Fig.1, any single thermal condition, even in an optimized thermal environment (PMV=0), 5% of occupants are expected to be dissatisfied with their thermal environment. This is due to variation in individuals’ personal temperament and preferences; thus, the only way to further reduce PPD is to provide individual control of the thermal environment.
IEQ - Indoor Environmental Quality describes the conditions inside a building and includes air quality, access to daylight and views, acoustic conditions as well as occupant control over lightings and thermal comfort (ASHRAE). Adaptive Thermal Comfort - the gradual diminution of an organism’s response to environmental thermal stimulation through Behavioral Adjustment, Physiological and Psychological (de Dear & Brager, 1998).
The WELL standard uses both PMV and the Predicted Percentage of Dissatisfied (PPD). PPD is a function of the PMV and came out of a Fanger study in 1970 of 1300 subjects (Fanger, 1970).
10
Thermal comfort is achieved according to ASHRAE 55 when 80% occupant satisfaction is achieved. This also means that 20% of occupants are regularly dissatisfied with their thermal environment even when, according to the standard, thermal comfort is Throughoutachieved. his work on thermal comfort standards Dr. Fanger continued to strive for better Environmental Quality (IEQ) systems, envisioning a comfort standard that might satisfy one hundred percent of occupants instead of just the eighty percent to meet the new ASHRAE standard (Bogucz, 2015). Even as he was studying to standardize thermal comfort, it seemed that he longed for a better solution. Fig. 1 Predicted Percentage of Dissatisfaction (PPD) graph and Predicted Mean Vote (PMV) index Source: simulationHub.com DEFINITIONS AND ABBREVIATIONS
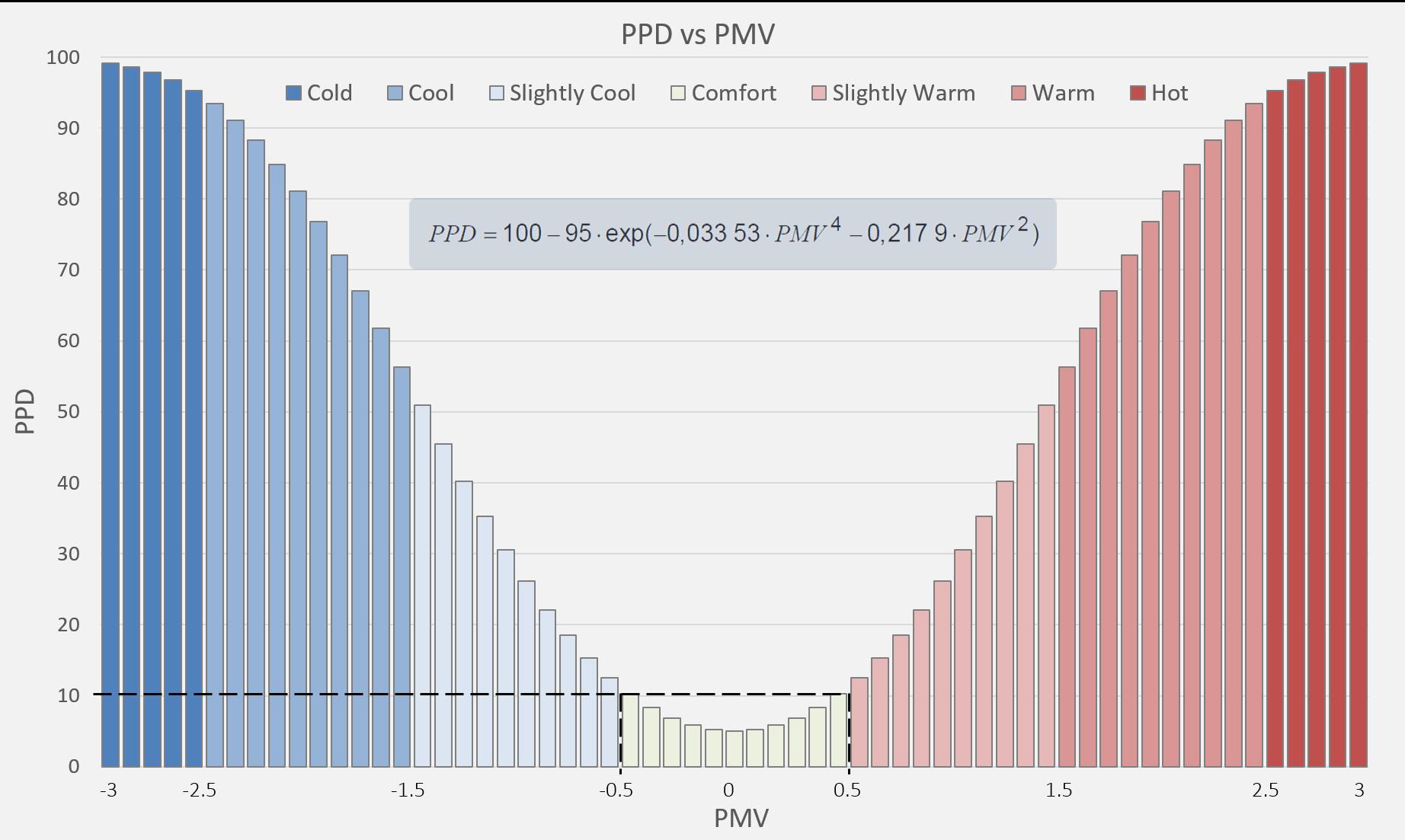
If the PMV with PPD model is considered the ‘standard’ thermal comfort model, then the alternative is the Adaptive Thermal Comfort Model. The theory suggests that a connection to the outdoors and control over one’s immediate environment allows humans to adapt to, and even prefer, a wider range of thermal conditions than is generally considered comfortable by the standard model (de Dear & Brager, 1998).
11
Thermal Delight and Adaptive Comfort Models
When talking about thermal variation in spaces, Lisa Heschong (1979) posits that a thermal environment could be likened to our eating habits, habits that do not simply fulfill our nutritional needs: “Our level of understanding makes it theoretically possible to provide for all of our nutritional needs with a few pills and injections...(but) A few tubes of an astronaut’s nutritious goop are no substitute for a gourmet meal. They lack sensuality - taste, aroma, texture, temperature, color. They are disconnected from all the customs that have developed around eating...” (p.17). If uniformly conditioned interior environments are boring and rarely satisfactory to a majority of occupants, what is the alternative? For about 10 years of our one-hundred-twenty-year foray into manipulating our environment’s mechanically, many have come to the conclusion that variation is delightful.
This idea of variation in our thermal interior environments has led some in the engineering field to challenge the longaccepted ASHRAE 55 standard by expanding the criteria for thermal comfort for specific climate zones.
Air motion across an occupants’ exposed skin can cause a personal sensation of cooling, even when the ambient air tempertaure exceeds the recomended set points of the ASHRAE 90.1 standard for a particular indoor space. This sensation can be experienced in hot humid climates where ceiling fans can provide a localized cooling effect on exposed body surfaces. These localized solutions that prioritize the occupant rather than the volume of air that the occupant is within, provides an opportunity for variation in thermal comfort within a building’s spaces. This thermal variation can enhance thermal pleasure and a person’s experience within a building while offering more diverse and potentially lower-energy solutions to how we heat and cool our buildings, an anticdote to the brute-force mono-culture thermal environements that many indoor environmental engineering has subscribed to in recent years. There are currently many adaptive thermal comfort models, and they are being developed in response to specific climate zones. For example, the Center for the Built Environment’s (CBE) Thermal Comfort Tool from the University of California, Berkeley, is applicable for occupant-controlled naturally conditioned spaces that meet all of the following criteria:
(a) There is no mechanical cooling system installed. No heating system is in operation; (b) Metabolic rates range from 1.0 to 1.3 met; and (c) Occupants are free to adapt their clothing to the indoor and/or outdoor thermal conditions within a range at least as wide as 0.5-1.0 clo.
Hedonic Response - a shift in disposition to a behavior or immediate behavioral outcome, relating to pleasant or unpleasant sensations (Davis, 2018).
Georgina Davis describes alliesthesia as being traditionally used as a medical term to describe a physiological response to a stimulus on a part of the body not necessarily at the source of the stimulus; a more widely used definition describes scenarios in which a person’s current internal state affects how a stimulus is perceived (if it provides pleasure or not, otherwise known as the “hedonic response”). When used in a design context, it refers more specifically to the “...influence that dynamic thermal environments can have on creating enhanced levels of occupant satisfaction compared to static and uniform environments, especially moving beyond ‘neutrality’ to create ‘thermal pleasure” (Davis, 2010).
Thermal Alliesthesia and the Hedonic Response
The knowledge of how our personal thermal state, both physiological and psychological, affect our perception of thermal comfort continues to evolve. One study from Concordia University in Quebec sought to map brain activity, in particular the hemodynamic correlates of hedonic experience, using functional magnetic resonance imaging (fMRI)
Thermal Alliesthesia is a conceptual framework to understand the hedonics (dealing with pleasant and unpleasant states of consciousness) of a larger spectrum of thermal environments than the more thoroughly researched concept of thermal neutrality or thermal monotony. This concept poses that the hedonic qualities of the thermal environment are determined as much by the thermal state of the occupant as by the environment itself (Parkinson, 2014).
12
Fig. 2 Adaptive Thermal Comfort Model, CBE Thermal Comfort Tool Source: Center for the Built Environment, UC Berkeley https://comfort.cbe.berkeley.edu/ DEFINITIONS AND ABBREVIATIONS
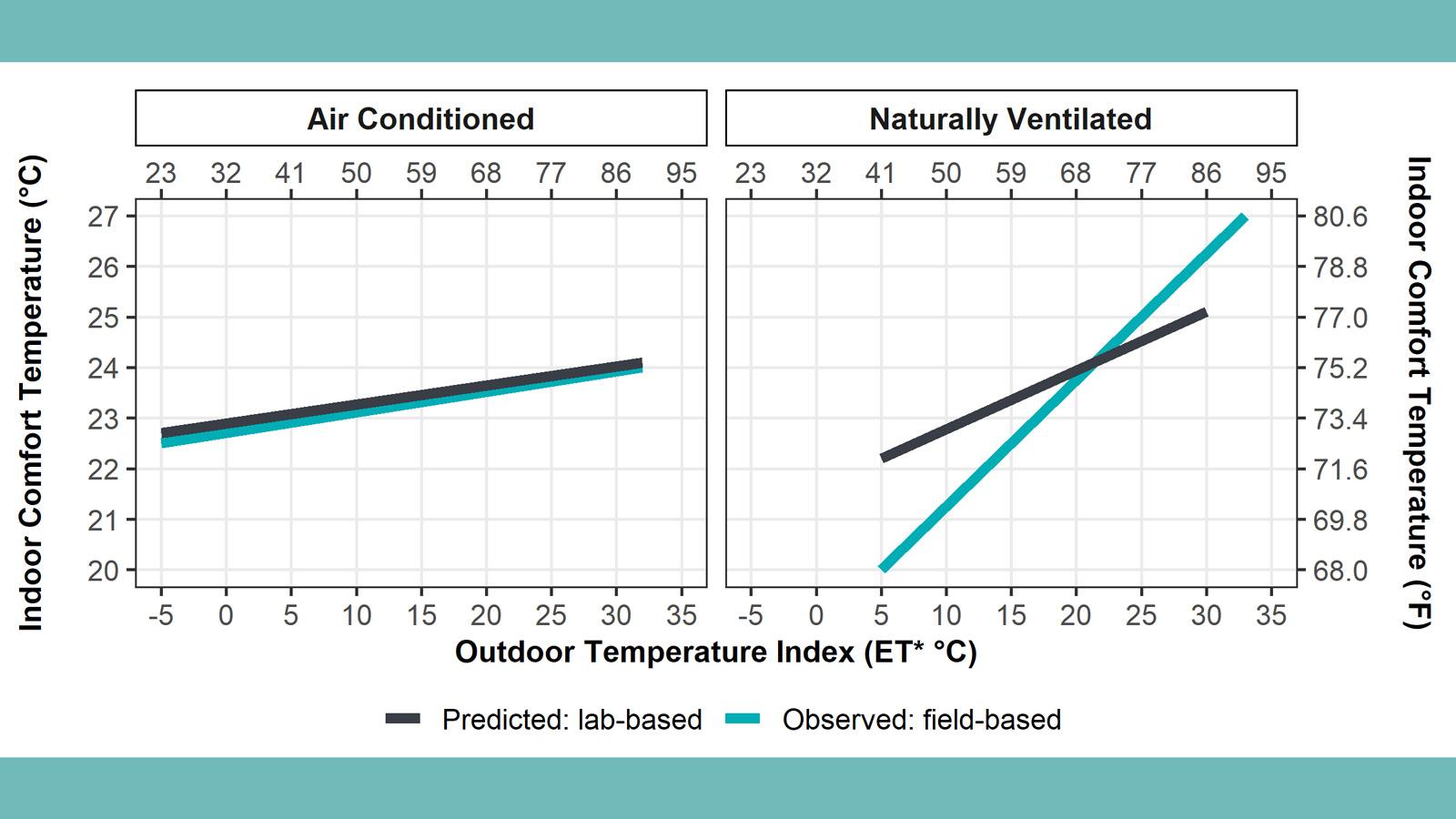
13 during thermal alliesthesia. To encourage a response, participants wore a water-perfused suit through which water at hot and cold temperatures was circulated at intervals (Dunn, 2010). It was found that all participants modulated hedonic responses to peripheral thermal stimuli through core temperature deviations. Through studies like these, we build a deeper understanding of how internal and external stimuli affect our personal comfort and how this understanding can influence how we design our indoor environments.
Upper Right: Water perfused suit and fMRI images from Hedonic Response during Thermal Alliesthesia study Source: Dunn, 2010 Lower: Outdoor circulation at Ciudad Universitaria de Caracas. Source: worldarchitecture.org
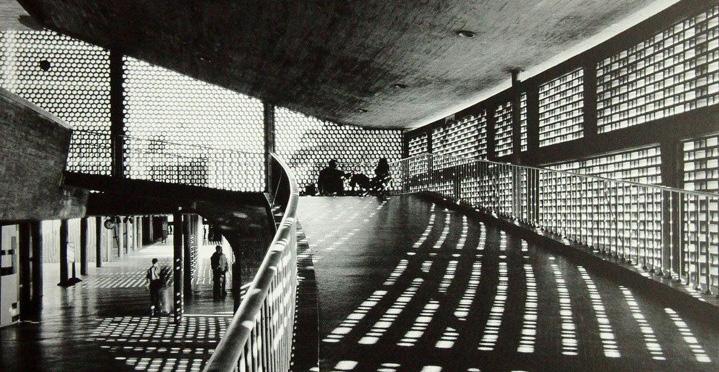
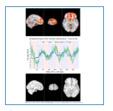
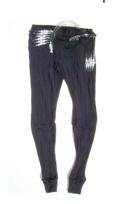
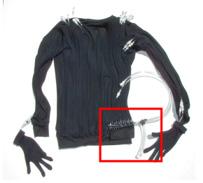
Upper: Example of an Active Chilled Beam with diagram. Source: Taco Advanced Hydronics
RADIANT COOLING Types of Hydronic Cooling Chilled beams were developed in Norway in the mid-1970s.
are specific sensory structures that enable animals to detect thermal changes. These free nerve endings reside in human skin, liver and skeletal muscles, with cold thermoreceptors 3.5 times more common than heat receptors (Moe, 2010).
14
Active Chilled Beams use forced air blown over a cooling coil to bring cool air into a space. Passive Chilled Beams use the convective cycle to drop cool air into a space. Though both of these systems use chilled water, chilled beams rely on passing air over these hydronically chilled tubes to deliver coolth to the occupants. So although these systems are using hydronic or water cooling, they are not radiant cooling systems because they don’t use radiant transfer to cool occupants.
Lower: Example of a Passive Chilled Beam with diagram. Source: Taco Advanced Hydronics DEFINITIONS AND ThermoreceptorsABBREVIATIONS
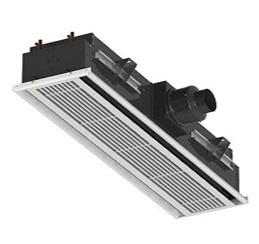
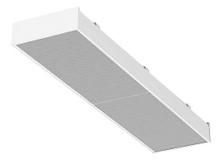
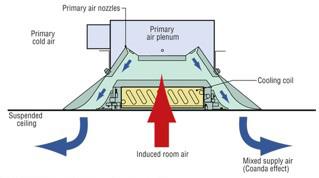
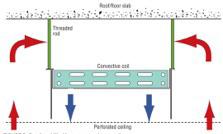
This Page: Image of office worker with thermal image displaying window surface temperature and skin surface temperature. Source: Author
Radiant heat transfer is the transfer of energy through electromagnetic waves emitted by any material based on their temperature. Radiant thermal exchange operates with warmth radiating to cool where warmth is absorbed by a cooler surface. Hydronic radiant heating and cooling systems operate at temperatures emitting within the infrared spectrum, invisible to the human eye. Though we can’t see these emissions, we perceive their effects as a sensation of warmth or cool through receptors in our skin, our thermoreceptors. For example, a hot windowpane in the summer will radiate towards a colder body.
15
For instance, this employee is sitting near a single pane window in the summer in San Antonio, Texas. Her skin’s surface at 8689 degrees adjacent to the window at 96 degrees will absorb the heat from this window glass through radiant transfer. We most often experience the sensation of radiant transfer as a pleasant experience when we are cold and enjoy heating up around a hearth, the hearth being the thermal mass that absorbs heat from the fire and radiates to warm our body. A less common experience is the enjoyment of radiant cooling, perhaps this might happen if you are on a long walk in warm weather and you come across a shaded and cool cave system where you can duck in and allow yourself to be cooled by the cool mass of stone in the cave
Conditioning the Occupant, Not the Air
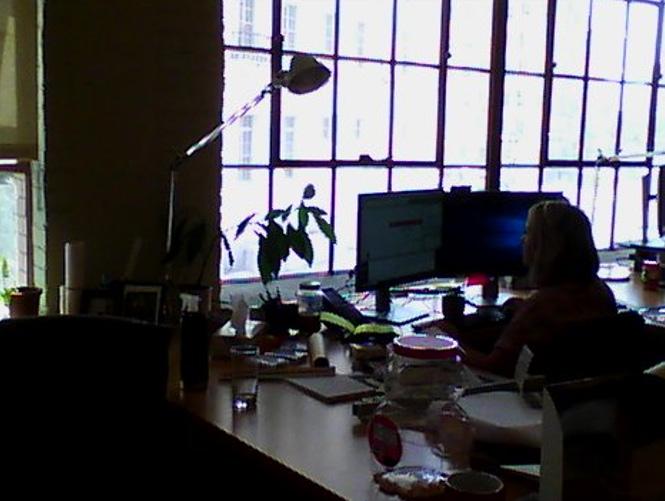
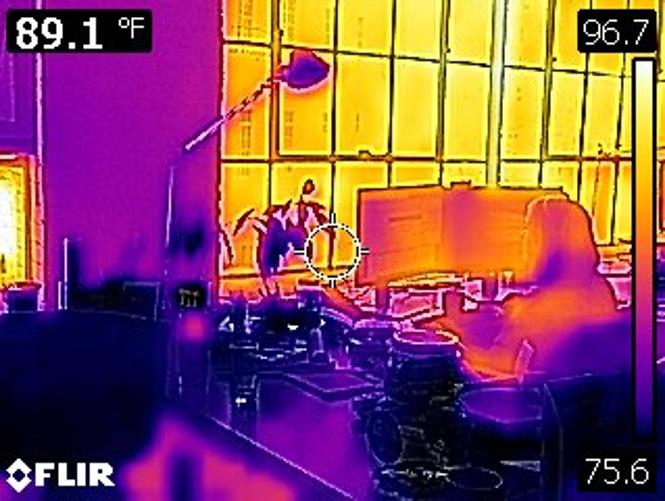
Typical skin temperatures in a comfortable environment vary at different parts of the body as illustrated in Fig. 3. Our core tends to stay around 91 degrees F at rest, our extremities are at lower temperatures which can vary. We tend to find a 4-degree difference from our skin’s surface temperature to adjacent radiant surfaces comfortable (Grondzik 2006). Anything above that 4-degree delta in surface temperatures can start to cause discomfort. A key energy-efficiency feature of radiant surface systems is the unique ability to utilize lower temperatures for heating and higher temperatures for cooling in comparison to traditional forced-air HVAC systems. We’ll discuss this in the section ‘Energy Efficient and Space Saving’ below.
Hydronic Radiant panel systems use a thin thermal mass diffuser or conductive surface depending on the application to radiate cooling or heating to the occupant. The panel is insulated from behind to direct the heated or cooled water to radiate towards
16
Left: Traditional forced air system average mean radiant temperature delta. Source: Messana Right: Thermally active surface average mean radiant temperature. Source Messana
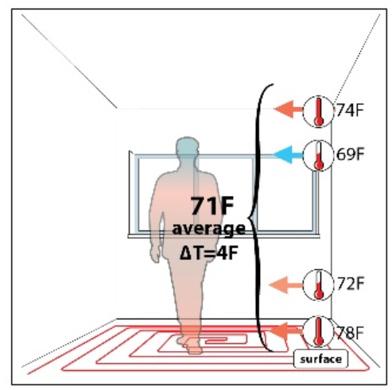
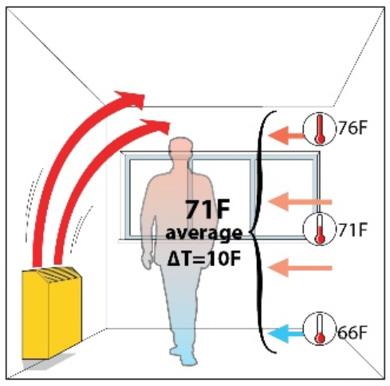
All forced-air systems attempt to maintain comfort by controlling air temperature to deliver comfort to the occupant. The modulation of the body’s own production of heat energy is central to human comfort.
Thermally active surface systems are compelling in that more than any other thermal comfort system, they more closely mimic our own physiology, our skin’s surface operating as our own radiant transfer surface. Additionally, radiant transfer is unique in that it is almost instantaneous.
Fig. 3 Human Surfaces Temperature Source: Moe, 2010
The body produces about 400 BTUs per hour from its metabolic processes (Fig. 4). Ninety BTUs are used to maintain life functioning. The additional energy must be dispersed from the system, or the body overheats. In most common situations inside a building, the body transfers about 190 BTUs through radiant transfer. About 110 BTUs are transferred by convection. The remainder is transferred via respiration (Moe, 2010).
Superior Thermal Comfort
17 the distributing surface and chilled or hot water is pumped through the pex or copper tubing. These systems are particularly nice in laboratory or hospital environments to reduce unwanted airflow. All of these hydronic systems should be considered in projects for cooling, in particular if that project already requires chilled water.
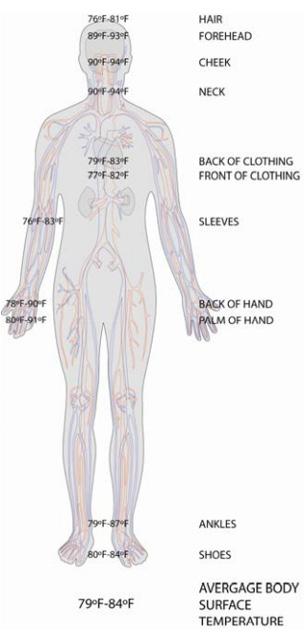
Air is also much more difficult to control in terms of distribution as well as issues with infiltration, drafts within a designed space, air buoyancy and convective flows around objects and bodies in a space. In another measurement, to provide 100 tons of refrigeration worth of cooling, you would need to supply 40,000 cfm of air in comparison to 32 cfm of water. The air-cooled system would need four to six times more energy than the water-cooled system for just fans and pumping (Moe, 2010).
The body’s largest organ, the skin, regulates surface and deep body temperature through hydronic exchange. Our skin is highly absorptive and emissive at (0.97) which consequently makes us highly responsive to shifts in radiant temperature. Given that our bodies are continuously using radiant transfer as a means to regulate our thermal comfort, we are sensitive to the temperature of other radiant surfaces in a space. Designing around people in terms of radiant cooling works well in that the system delivers comfort directly to the occupant. This is different than conditioning the Fig. 4 Human Heat Transfer Modes Source: Moe, 2010
18
Water is 832 times denser than air with four times the heat capacity, as illustrated in Fig. 5. “Energy Density is directly related to the density of a material. Water can capture and channel far more energy per unit volume than air. Thermally active surfaces are built around this basic principle“ (Moe, 2010).
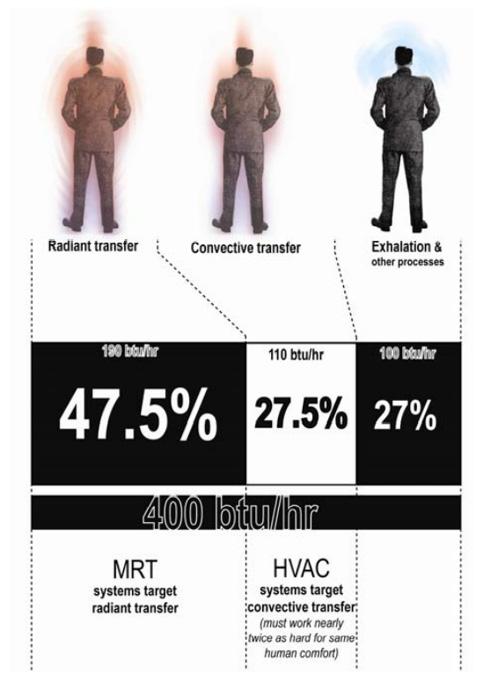
Above: Air and Water Cooling Strategies. Source: Moe, 2010 Fig. 4 Energy Density of Water vs. Air Source: Moe, 2010
Forcedvs. Air Cooling Setpoints: 30 F (refrigerant coil) Radiant Heating Setpoints: 80-85 F Forcedvs. Air Heating Setpoints: 140 F
19 air that then delivers comfort to the occupant through convective transfer. In this way, mean radiant temperature is important, being the average temperature of all the radiant surfaces in the room which in total affect our perception of comfort, in particular in indoor spaces.
Energy-Efficient and Space-Saving
Radiant Cooling Setpoints: 60-65 F
As mentioned previously, a key energy-efficiency feature of radiant surface systems is the unique ability to utilize lower temperatures for heating and higher temperatures for cooling in comparison to traditional forced air HVAC systems.
A study by the Lawrence Berkeley National Lab of a simulated building shows the potential reduction in Sensible Load Cooling with the aid of Radiant Cooling providing significant overall energy savings. These systems utilize efficient operating modes, higher room setpoints, and experience lower transmission losses.
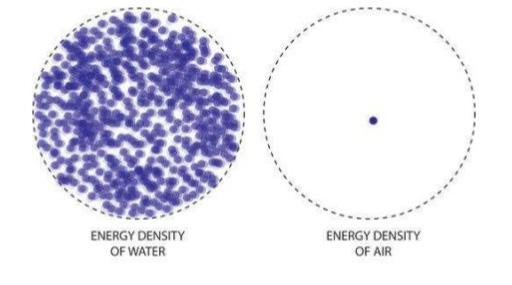
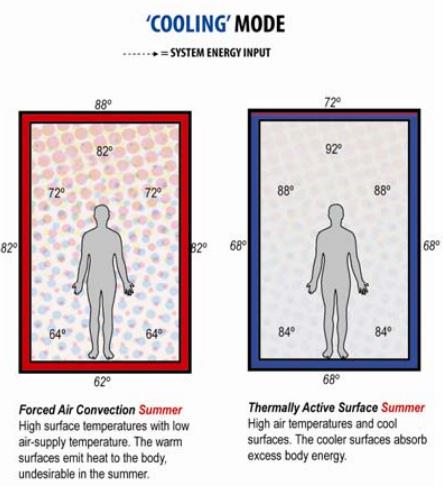
Opposite Page: Peter Zumthor’s Kunsthaus Bergenz with Radiant Cooling ceiling panels. Integrated Design Opportunities
This Page: Lawrence Berkeley National Lab, Energy Use Comparison between Conventional and Radiant Systems
The most significant reduction in these systems appears to come from fan and motor requirements to move air around the building. This equation changes a bit when we move to a more humid climate due to the need for dedicated dehumidification as noted here as a 17% savings in cold humid climates.
One other thought on resiliency of these systems per PAE’s David Mead, one of Project Drawdown’s most impactful carbon-dioxide-reducing solution, is the reduction in use and management of refrigerants, which is focused on stopping leaking refrigerants from entering the Accordingatmosphere.toa 2016 study from the Berkeley National Laboratory, the world is set to install some 700 million air conditioners by 2030, and 1.6 billion by 2050. Most of that increase will happen in developing countries with hot climates, like India, Mexico and Brazil, where populations and incomes are rising. Using a more efficient cooling medium reduces the refrigerant volume needed in each newly installed system.
20
The design advantage with a radiant cooling system in the floor slab or within a ceiling or wall is that the visible components, such as air handlers, ductwork and grilles, can be smaller, allowing greater flexibility in the architectural design. The space requirements for the mechanical systems, for instance mechanical rooms or ceiling space for ductwork, can be compacted, potentially reducing floor-to-floor heights or increasing ceiling heights. Because a radiant slab can much more effectively deal with direct solar loads, temperatures in areas with ample fenestration such as lobbies, can be more easily controlled with less mechanical noise or high air velocity from forced air systems.
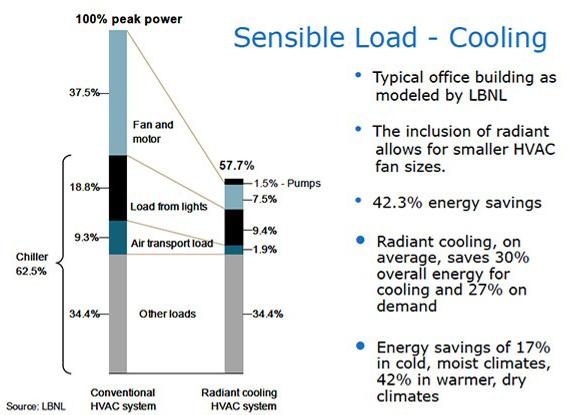
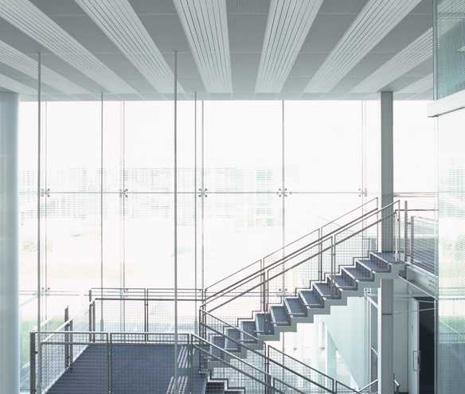
Define your Thermally Active Surfaces: Ceiling and wall locations provide about twice the cooling effect as floor systems. Coordinate your strategy to integrate with your design and consult your MEP engineer about required areas depending on the selected location.
Consider Surface Material Covering Loss: A less conductive material such as wood will reduce the cooling available on a surface when compared with a thin mass surface such as concrete or tile. Make sure to consider this loss in your overall strategy.
Coordinate your Thermally Active Surfaces: Whether using the ceiling, walls or floor for your radiant cooling system, coordinate other systems such as lighting, electrical, fire suppression, plumbing and other mechanical systems with your radiant panels/surfaces. These panels should be located in a more permanent location that will not be modified in the near future.
Radiant cooling does not stand alone and onl y works well when paired with other systems to deliver comfort to building occupants. Secondary ventilation is required to achieve high IEQ. Depending on the climate, additional mechanical dehumidification may be needed.
Understand the Role of Radiant Cooling:
Take Advantage of ‘Free Cooling’: Consider a radiant cooling system for your project especially when chilled water is already a project requirement. Use a geothermal system if project size appropriate.
Humid Climates and Radiant Cooling: Surface condensation can be avoided in humid climates when paired with a well designed indoor environment. Indoor relative humidity should be kept to 60% or less. Energy savings in a humid climate can be expected to be 17%-30% from a traditional forced air system. Include humidity sensors in your system strategy to verify interior conditions.
AN ARCHITECT’S GUIDE TO RADIANT COOLING
Third Party Verify the System Installation: Commission the system by an experienced professional to verify that it was properly installed.
Verify the System Components: Modern Radiant Cooling systems utilize PEX tubing. A good guide for installation is 5/8” pipe six inches on center. If embedded in a slab, it if recommended to build a mockup. Pressure test before pouring concrete.
Reducing Energy Demand as an Opportunity: Due to the reduction in air volume requirements, a radiant cooling system can reduce energy consumption by up to 50% when compared with a traditional forced air system. This reduction changes in humid climates but is still significant.
Consider the Reduction in Forced Air System Size: When using radiant cooling, significant reduction in air ducting can be achieved, between 60-70% of ducts when compared with a traditional forced-air system. Depending on project requirements, air system volume can be reduced by 70%.
RESOURCES Messana Radiant Cooling. (2016, May 8). Radiant Cooling for Residential and Commercial SolutionsRadiantMessanaSpacePakRadiantOffhttps://www.youtube.com/watch?v=dYjMBfORftkRadiantAvailableCoffeewell-v2-thermal-comfort/https://resources.wellcertified.com/articles/what-s-new-in-What’sWELLradiant-cooling-chilled-beams/https://tacoadvancedhydronics.wordpress.com/category/TourTacofor-residential-and-commercial-applicationshttps://www.slideshare.net/AlessandroArnulfo/radiant-cooling-Applications.AdvancedHydronics.(2013,February19).theMechanicalRoom.Standard.(2019,January22).NewinWELLv2:ThermalComfort.withCaleffi™WebinarsNOWonYouTube|CaleffiCoolingAnimation-YouTubetheShelfHydronicCoolingSystems(viega.us)|HydronicHeatingAndCoolingRadiantCoolingandHeatingceilingsolutionsHeating&Cooling|Clean,Reliable&EfficientHeating|ZehnderRittling(zehnder-rittling.com) CASE STUDY BUILDINGS Kunsthaus Bregenz Peter Zumthor Zollverein School of Management and Design SANAA Südwestmetall Regional Headquarters Dominik Dreiner Architekt Linked Hybrid Steven Holl Architects Charles Hostler Student Recreation Center VJAA Housing for Kripalu Center for Yoga and Health Peter Rose and Partners The Fred Kaiser Building, University of British Columbia architectsAlliance Terrence Donnelly Centre for Cellular and Biomolecular Research architectsAlliance / Behnisch Architekten Klarchek Information Commons, Loyola University Solomon Cordwell Buenz The Graham Resource Center, Crown Hall, Illinois Institute of Technology Tom Brock Architect, P.C.
Cooper,https://www.simscale.com/blog/2018/02/ashrae-standard-55-thermal-comfort/Churazova,https://www.bdcnetwork.com/21-tips-executing-radiant-cooling-systems-aia-courseCassidy,http://insight.gbig.org/fit-for-a-princess-next-generation-personal-controls-deliver-whole-building-energy-savings-and-precision-ieq/IEQ’.R.(2016,March21).21TipsforExecutingRadiantCoolingSystems.A.(2021,February24).ASHRAE55ThermalComfortAssessmentwithSimulation.G.(2002).
Alliesthesia: A Functional Magnetic Resonance Imaging Study. https://spectrum.library.concordia.ca/7035/1/DUNN_MA_S011.pdf
Savings and ‘Precision
Alter, L. (2020, May 31). Looking Up to Radiant Ceilings for Heating and Basile,https://www.treehugger.com/sustainable-product-design/looking-radiant-ceilings-heating-and-cooling.htmlCooling.S.(2014,September1).
BIBLIOGRAPHY
Cool: How Air-Conditioning Changed Everything. Fordham University Press. Belluk, P. (2015, August 3). Chilly at Work? Office Formula Was Devised for Men. The New York Bogucz,https://www.nytimes.com/2015/08/04/science/chilly-at-work-a-decades-old-formula-may-be-to-blame.htmlTimes.E.(2015,March9).FitforaPrincess:Next-GenerationPersonalControlsDeliverWhole-BuildingEnergy
Air-Conditioning America:Engineers and the Controlled Environment, 1900-1960. John Hopkins University Press. Cubick, R. (2016, June 10). Radiant Cooling vs. Forced Air Systems. Radiant Cooling vs Forced Air Systems - Uponor Blog Davis, G. (2018, January 29). So Sweet/ And So Cold: Sensation, Perception, and Hedonic Response. dehttps://www.terrapinbrightgreen.com/blog/2018/01/5008/Dear,R.,Brager,G.S.(1998).DevelopingandAdaptiveModel of Thermal Comfort and Preference. Center for the Built Environment, University of California, Berkeley. Djebbara, Z. (2016, June 24). Beyond Thermal Comfort Part Dunn,https://issuu.com/zakariadjebbara/docs/thermal_bath_theory_for_libraryI.B.J.(2010,November).HumanHedonicExperienceDuringThermal
Egg, J. (2018, February 18). Radiant Floors and Ceilings Make Geothermal Even Better. Radiant Floors and Ceilings Make Geothermal Even Better | 2018-02-28 | phcppros Edelstein, K. (2017, September 6). Of DOAS, Displacement, Desiccants, and Godbole,https://www.osti.gov/servlets/purl/6214501Feustel,Fanger,Fanger,https://oem.bmj.com/content/oemed/30/4/313.full.pdfFanger,https://livingbuilding.kendedafund.org/2018/01/08/pae-david-mead-on-net-zero-refrigerants-and-project-drawdown/Edelstein,https://livingbuilding.kendedafund.org/2017/09/06/doas-displacement-desiccant-radiant-flooring/Radiance.K.(2018,January8).PAE’sDavidMeadonNetZero,RefrigerantsandProjectDrawdown.P.O.(1973).AssessmentofMan’sThermalComfortinPractice.P.O.(1967).CalculationofThermalComfort-IndtroductionofabasicComfortEquation.ASHRAETransacions.P.O.(1970).ThermalComfort:AnalysisandApplicationsinEnvironmentalEngineering.McGraw-HillInc.H.E.,(1993).HydronicRadiantCoolingOverviewandPreliminaryPerformanceAssessment.S.(2018).InvestigatingTheRelationshipBetweenMeanRadiantTemperature(MRT)AndPredictedMeanVote (PMV): A case study in a university Grondzik,https://www.diva-portal.org/smash/get/diva2:1254389/FULLTEXT01.pdfbuilding.W.,Kwok,A.(2006).MechanicalandElectricalEquipmentforBuildings.John Wiley & Sons Inc. He, Y., Li, N., Wang, X., He, M., & He, D. (2017, November 17). Comfort, Energy Efficiency and Adoption of Personal Cooling Systems in Warm Environments: A Field Experimental Heiz,https://pdfs.semanticscholar.org/f103/c6d6909b81f14879f8b7b08e3314978d6710.pdfStudy.B.P.V.,Su,L.,Pan,Z.,&Wondraczek,L.(2018).Fluid-IntegratedGlass–Glass Laminate for Sustainable Hydronic Cooling and Indoor Air Conditioning. Sustainable_Hydronic_Cooling_and_Indoor_Air_Conditioninghttps://www.researchgate.net/publication/326510851_Fluid-Integrated_Glass-Glass_Laminate_for_
Kare, V. R., Mathur, A., Mathur, J., Bhandari, M. (2015, December 7). Development of Personalized Radiant Cooling System for an Office Room. http://www.ibpsa.org/proceedings/BS2015/p3071.pdf
Johnson,https://www.slideshare.net/PeteJefferson/rocky-mountain-green-thermal-comfort-radiant-energy-and-regionalismJefferson,https://onlinelibrary.wiley.com/doi/full/10.1111/j.1600-0668.2007.00516.xP.(2013,July28).ThermalComfort,RadiantEnergyandRegionalism.S.(2015,September22).
Mui, K. W., Tsang, T. W., and Wong, K.T. (2020, May). Bayesian Updates for Indoor Thermal Comfort Models. Nan,https://www.sciencedirect.com/science/article/abs/pii/S2352710219314147B.(2017,July12).CultureInsider:HowDidtheAncientPeopleEscape the Summer Heat? China Daily. https://www.chinadaily.com.cn/culture/2017-07/12/content_26240433_2.htm
How We Got to Now: Six Inventions That Made the Modern World. Riverhead Books.
Heschong, L. (1979). Thermal Delight in Architecture. MIT Press. Hoof, J.V. (2008, March 24). Forty Years of Fanger Model of Thermal Comfort: Comfort for All?
Kim, J., Schiavon, S., Brager, G. (2018). Personal Comfort Models - A New Paradigm in Thermal Comfort for Occupant-Centric Environmental Control. Building and Moe,https://www.theatlantic.com/national/archive/2013/02/the-stubborn-american-who-brought-ice-to-the-world/272828/Mitenbuler,https://www.researchgate.net/publication/305755583_Practical_evaluation_of_the_thermal_comfort_parametersMarkov,https://www.scientificamerican.com/article/how-heat-from-the-sun-can-keep-us-all-cool1/Lim,https://www.sciencedirect.com/science/article/abs/pii/S0360132318300350Environment.X.(2017,January31).HowHeatfromtheSunCanKeepUsAllCool.D.(2002,October)PracticalEvaluationoftheThermalComfortParameters.R.(2013,February5).TheStubbornAmericanWhoBroughtIcetotheWorld.K.(2010).
Thermally Active Surfaces in Architecture. Princeton Architectural Press.
Nicol, F. & Humphreys, M. (2002, July). Adaptive Thermal Comfort and Sustainable Thermal Standards for Buildings. Oxford Brookes University. https://www.pnas.org/content/early/2020/06/09/2001802117/tab-figures-data?versioned=trueFlexibleZhang,https://ntrs.nasa.gov/api/citations/19930073077/downloads/19930073077.pdfWolverton,https://www.hpacmag.com/features/radiant-cooling/Waters,https://www.architectural-review.com/essays/fragments-the-history-of-architecture-in-14-fireplacesStilwell,https://www.pmengineer.com/articles/94860-john-siegenthaler-details-for-small-scale-hydronic-coolingSiegthaler,http://www.iapmo.org/media/3489/wwnl-3-15.pdfSiegenthaler,https://www.maxfordham.com/research-innovation/the-physics-of-freezing-at-the-iranian-yakhchal/Pochee,https://www.tandfonline.com/doi/abs/10.1080/09613218.2015.989662ResearchParkinson,Zones.Parkinson,https://www.wbur.org/hereandnow/2016/06/02/world-air-conditionersNPRfor_Buildingshttps://www.researchgate.net/publication/222402882_Adaptive_Thermal_Comfort_and_Sustainable_Thermal_Standards_(2016,June2).WorldonPacetoInstall700MillionMoreAirConditionersby2030.T.,Candido,C.,&deDear,R.(2015,May).ThermalPleasureinBuiltEnvironments:AlliesthesiainDifferentThermoregulatory(PDF)Thermalpleasureinbuiltenvironments:alliesthesiaindifferentthermoregulatoryzones(researchgate.net)T.&deDear,R.(2014,December15).ThermalPleasureinBuiltEnvironments:PhysiologyofAlliethesia.Building&Information.H.,andGunstone,J.(2017).ThePhysicsofFreezingAttheIranianYakhchal.MaxFordham.J.(2015,March).SmallScaleHydronicCooling.J.(2020,July2).DetailsforSmall-ScaleHydronicCooling.D.(2015,January31).Fragments:TheHistoryofArchitecturein14Fireplaces.R.(2017,May16).RadiantCooling:EmergingTechnologyShowsPromise.B.C.,Johnson,A.,&Bounds,K.(1989).InteriorLandscapePlantsforIndoorAirPollutionAbatement.H.,Ly,K.C.S.,Liu,X.,Chen,Z.,Yan,M.,Wu,Z.,Wang,X.,Zheng,Y.,Zhou,H.,Fan,T.(2020,June15).BiologicallyInspiredPhotonicFilmsforEfficientPassiveRadiativeCooling.