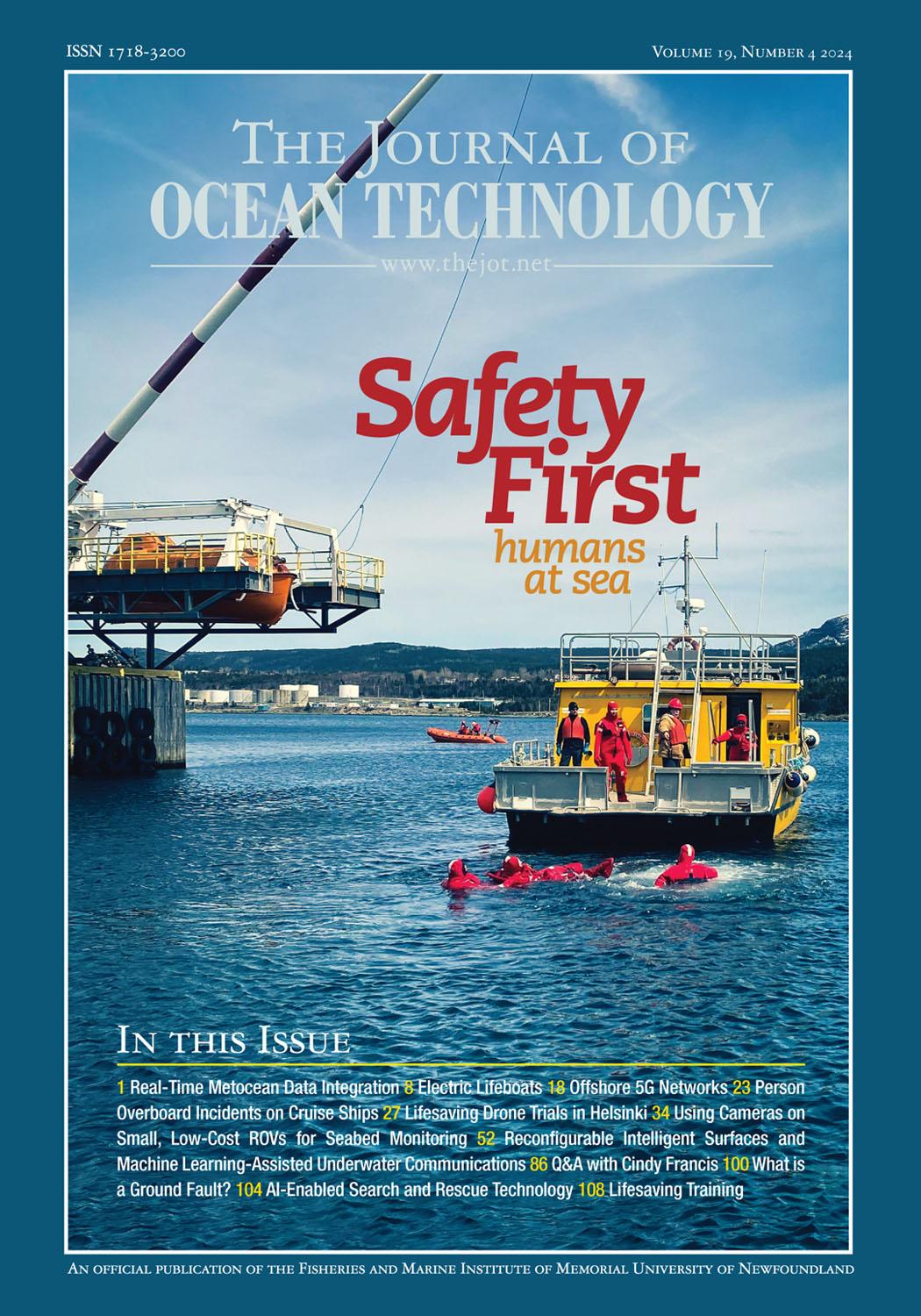
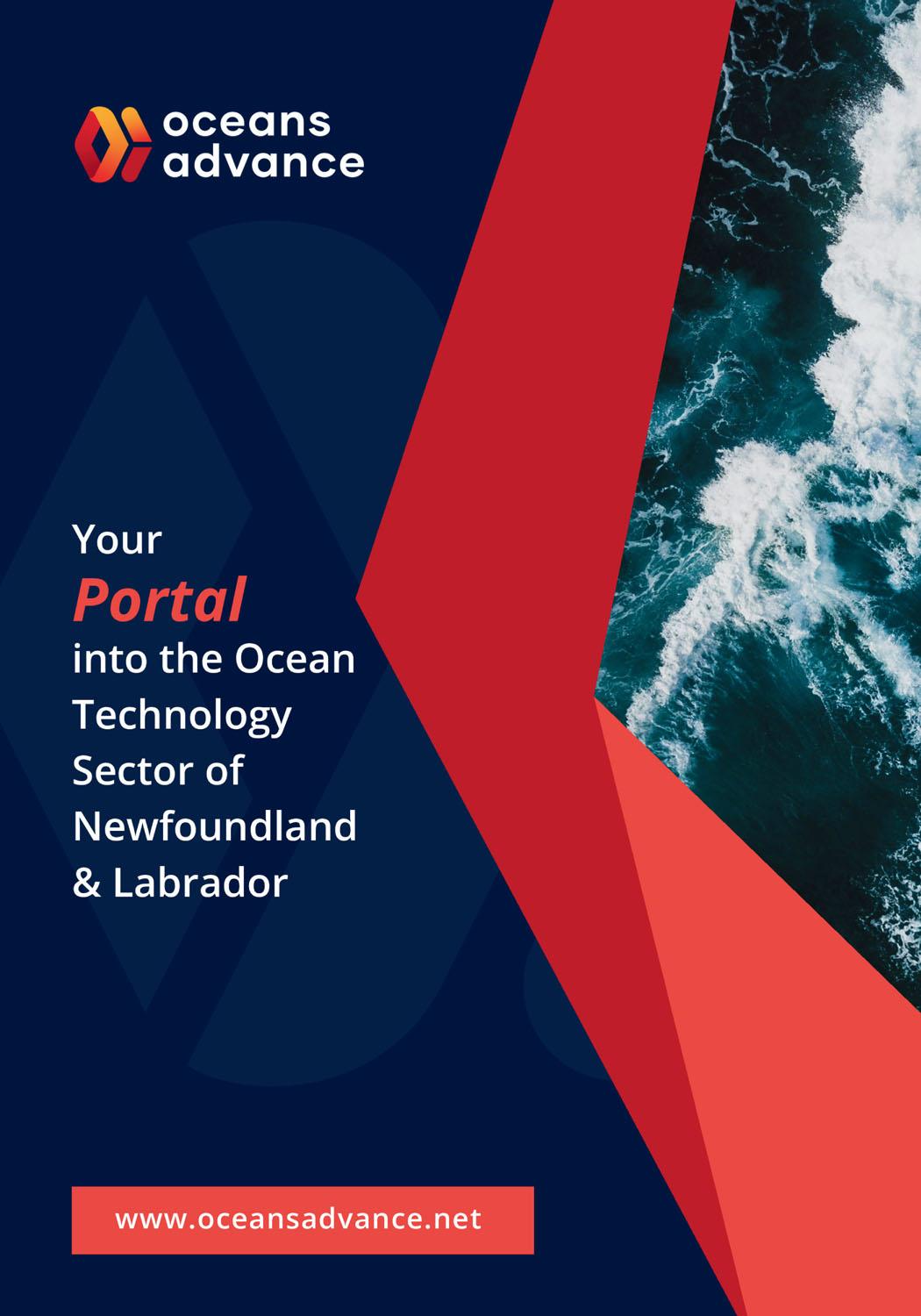
ii Editorial Board
iii On the Cover
iv Publishing Schedule and Advertiser ’s Index
v Guest Editor ’s Note from Steven Mallam
1 Sight from Shore: Enhancing Maritime Safety and Efficiency through Real-Time Metocean Data Integration
Becky Conway OceanWise Ltd.
8 Electric Lifeboats Lead the Charge to Offshore Safety
Erik Mostert, Georgios Nikoltsis, Joe Dawes
VIKING Life-Saving Equipment
18 Offshore 5G Networks for Safety at Sea
Izzy Taylor
JET Connectivity
23 Person Overboard Incidents on Cruise Ships: How Detection Technology is Transforming Passenger Safety at Sea Frederik Giepmans, Mike Collier MARSS
27 A New Era in Water Rescue Operations: Lifesaving Drone Trials in Helsinki
Zacharoula Syrivli Forum Virium Helsinki
Peer-Reviewed Papers
34 Advantages and Limitations of Using Cameras on Small, Low-Cost ROVs for Seabed Monitoring
Amanda Frederikke Irlind, Alex Jørgensen,
Jonathan Eichild Schmidt, Anders Skaarup
Johansen, Thomas B. Moeslund, Karen Ankersen
Sønnichsen, Niels Madsen
Aalborg University
50 Lodestar … Ankan Bhattacharjee, Antonios Andreadakis
52 A Review of Reconfigurable Intelligent Surfaces and their Application to Machine Learning-Assisted Underwater Communications
Mohamed A. Ouf
Arab Academy for Science, Technology and Maritime Transport
Abdelrahman Elkhateeb
Orange Business
Abdelmoneim Soliman, Mervin A. Marshall
Fisheries and Marine Institute
Md Safiqur Rahaman
King Fahd University of Petroleum and Minerals
Sara Mobarak University of Ottawa
86 Q&A with Cindy Francis
90 Trade Winds … Hefring Marine, Ocean Safety Research Unit, Ocean Business 2025, Offshore Safety and Survival Centre
100 Inside Out … What is a Ground Fault?
Daniel Gomez-Ibanez
Woods Hole Oceanographic Institution
102 Perspective … Virtual Marine
104 Reverberations … Offshore Trials for AI-Enabled Search and Rescue Technology
Sam Mayall, Zelim
108 Homeward Bound … Washington Sea Grant Provides Lifesaving Training to Commercial Fish Harvesters
Samantha Larson, Washington Sea Grant
110 Parting Notes … the puddle that was once you
Katherine Percival
PUBLISHER
Kelley Santos info@thejot.net
MANAGING EDITOR
Dawn Roche
Tel. +001 (709) 778-0763 info@thejot.net
ASSISTANT EDITOR
Bethany Randell
Tel. +001 (709) 778-0769 bethany.randell@mi.mun.ca
TECHNICAL CO-EDITORS
Dr. David Molyneux Director, Ocean Engineering Research Centre Faculty of Engineering and Applied Science Memorial University of Newfoundland
WEBSITE AND DATABASE Scott Bruce
GRAPHIC DESIGN/SOCIAL MEDIA
Danielle Percy Tel. +001 (709) 778-0561 danielle.percy@mi.mun.ca
Dr. Katleen Robert Canada Research Chair, Ocean Mapping School of Ocean Technology Fisheries and Marine Institute
FINANCIAL ADMINISTRATION Michelle Whelan
Dr. Keith Alverson University of Massachusetts USA
Dr. Randy Billard Virtual Marine Canada
Dr. Safak Nur Ertürk Bozkurtoglu Ocean Engineering Department Istanbul Technical University Turkey
Dr. Daniel F. Carlson Institute of Coastal Research Helmholtz-Zentrum Geesthacht Germany
Dr. Dimitrios Dalaklis World Maritime University Sweden
Randy Gillespie Windover Group Canada
S.M. Asif Hossain National Parliament Secretariat Bangladesh
Dr. John Jamieson Dept. Earth Sciences Memorial University Canada
Paula Keener Global Ocean Visions USA
Richard Kelly Centre for Applied Ocean Technology Marine Institute Canada
Dr. Peter King University of Tasmania Australia
Dr. Sue Molloy Glas Ocean Engineering Canada
Dr. Kate Moran Ocean Networks Canada Canada
EDITORIAL ASSISTANCE Paula Keener, Randy Gillespie
Kelly Moret Hampidjan Canada Ltd. Canada
Dr. Glenn Nolan Marine Institute Ireland
Dr. Emilio Notti Institute of Marine Sciences Italian National Research Council Italy
Nicolai von OppelnBronikowski Memorial University Canada
Dr. Malte Pedersen Aalborg University Denmark
Bethany Randell Centre for Applied Ocean Technology Marine Institute Canada
Prof. Fiona Regan School of Chemical Sciences Dublin City University Ireland
Dr. Mike Smit School of Information Management Dalhousie University Canada
Dr. Timothy Sullivan School of Biological, Earth, and Environmental Studies University College Cork Ireland
Dr. Jim Wyse Maridia Research Associates Canada
Jill Zande MATE, Marine Technology Society USA
The Journal of Ocean Technology is a scholarly periodical with an extensive international editorial board comprising experts representing a broad range of scientific and technical disciplines. Editorial decisions for all reviews and papers are managed by Dr. David Molyneux, Memorial University of Newfoundland, and Dr. Katleen Robert, Fisheries and Marine Institute.
The Journal of Ocean Technology is indexed with Scopus, EBSCO, Elsevier, and Google Scholar. Such indexing allows us to further disseminate scholarly content to a larger market; helps authenticate the myriad of research activities taking place around the globe; and provides increased exposure to our authors and guest editors. All content in the JOT is available online in open access format. www.thejot.net
The Journal of Ocean Technology, ISSN 1718-3200, is protected under Canadian Copyright Laws. Reproduction of any essay, article, paper or part thereof by any mechanical or electronic means without the express written permission of the JOT is strictly prohibited. Expressions of interest to reproduce any part of the JOT should be addressed in writing. Peer-reviewed papers appearing in the JOT and being referenced in another periodical or conference proceedings must be properly cited, including JOT volume, number and page(s). info@thejot.net
At The Launch in Holyrood, NL, Canada, students complete the “Sea-Day” component of the Basic Survival Training (BST) course, which is required of individuals working offshore. The BST provides a basic understanding of the hazards associated with working in an offshore environment, the knowledge and skills necessary to react effectively to offshore emergencies, and the ability to care for themselves and others in a survival situation.
Read more about the training offered at the Offshore Safety and Survival Centre in the “Trade Winds” section of this issue.
The JOT production team invites the submission of technical papers, essays, and short articles based on upcoming themes. Technical papers describe cutting edge research and present the results of new research in ocean technology or engineering, and are no more than 7,500 words in length. Student papers are welcome. All papers are subjected to a rigorous peer-review process. Essays present well-informed observations and conclusions, and identify key issues for the ocean community in a concise manner. They are written at a level that would be understandable by a non-specialist. As essays are less formal than technical papers, they do not include abstracts, listing of references, etc. Typical essay lengths are up to 3,000 words. Short articles are between 400 and 800 words and focus on how a technology works, evolution or advancement of a technology as well as viewpoint/commentary pieces. All content in the JOT is published in open access format, making each issue accessible to anyone, anywhere in the world. Submissions and inquiries should be forwarded to info@thejot.net.
All themes are approached from a Blue Economy perspective.
Spring 2025 Marine tourism
Summer 2025 Ocean monitoring
Fall 2025 Maritime security
Winter 2025 Technologies in Indigenous-led Projects
Each issue of the JOT provides a window into important issues and corresponding innovation taking place in a range of ocean sectors –all in an easy-to-read format with full colour, high-resolution graphics and photography. CIOOS 16,17
The Journal of Ocean Technology c/o Marine Institute P.O. Box 4920 155 Ridge Road St. John's, NL A1C 5R3 Canada +001 (709) 778-0763 info@thejot.net www.thejot.net
Safety at sea begins with its people. The maritime industry has unquestionably improved its safety record in recent decades. Even as ocean and water-related activities on, under, and near bodies of water have grown, the domain is safer and more reliable than it has ever been. However, incidents and accidents continue to occur, and reoccurring themes contribute to arise. This is communicated by Transportation Safety Board of Canada’s biennial maritime watchlist and accident investigations where human factors and organizational safety are continuously highlighted.
As we move into a new era of maritime operations, advancements in automation and autonomy, artificial intelligence, and digitalization are changing the way people work and engage in operations and how tasks are organized and implemented. Furthermore, the push for natural resource exploration and development in offshore energy and mining and increasing maritime commercial and pleasure activities further north in remote areas of the Arctic are changing where and how we work. This brings new requirements across complex and safety-critical maritime sectors and the people working within them.
However, even with the rapid developments in new technologies and their ripple effects through a system, the basics must still be addressed and evolve with established legacy systems and sectors currently in place. People are still at the centre of safety. People are still at the centre of creating and maintaining safe and reliable systems, and this is true for highly automated and even autonomous systems. It is a fallacy that removing the person removes the “human error”; rather, we reduce and/or reorganize how and where humans work within the system. Cultural change is required here, including the expansion of traditional views on safety, with the incorporation and move toward increasing human performance and organizational safety.
In recent years there has been argument for a shift away from the more static view on safety compliance and risk reduction. There has been more emphasis on learning, flexibility, and adaptability across the system rather than on unwanted events and accidents. This ensures that as much as possible goes right in a much more dynamic safety management perspective. There is great value in highlighting success and learning from normal work. Historically, a reactive approach of “learning from accidents, training for extremes” has been the typical approach for risk assessment and training. Of course there is value to this, as the adage goes: we must learn from history in order not to repeat our
Dr.
and organizational performance in the maritime domain.
mistakes. However, the move toward learning from the successes in normal work is an increasingly important area.
Working within safety-critical maritime systems, whether in commercial fishing, offshore energy, or commercial shipping, is incredibly complex, and the majority of one’s work and tasks are successfully completed and carried out as planned. These systems are extremely safe and reliable the vast majority of the time. This is something that should not be overlooked or taken for granted, which includes the human contributions to safety. Highly trained and experienced humans can make good decisions and allow for flexibility that contributes to and supports complex safety critical systems and operations. This is about utilizing the strengths of humans, mitigating their weaknesses, and designing systems that take advantage of the current strengths and weaknesses of both humans and technologies. This concept is referred to as human-automation teaming (HAT) or, more broadly, the initiative of human-centred design as a process and conceptualization of how to view the design and operation of systems. This is increasingly important as we move toward a more digitalized work ecosystem where fitting people to the technology and understanding underlying processes and decision-making in automated and autonomous functioning will be ever more critical to keep humans “in the loop” of complex systems and processes.
I have enjoyed the editorial process in this edition with the theme “safety first: humans at sea.” The collection of articles and issues presented by our authors focuses on how new technologies, and particularly how leveraging differing data streams, can help enhance people and system safety. Topics range from utilizing enhanced connectivity and data integration for increased safety monitoring and reactions, new technology development in lifeboats and lifesaving equipment, emerging uses for AI in differing search and rescue and detection technologies, augmented reality for maritime navigation support, offshore safety training facilities and programs, and advanced training simulators.
I hope you enjoy this edition and that it engages you in some pressing issues and differing approaches taken to enhance safety at sea. We must remember, humans are at the centre of safety, and we must empower and create systems and technologies that aid the people working within these complex and safety critical maritime sectors. In this we can truly develop system safety by leveraging strengths of humans and technologies together.
Enhancing Maritime Safety and Efficiency through Real-Time Metocean Data Integration
by Becky Conway
A pilot boat and its crew must undertake a sometimes-risky journey to reach vessels in the port approaches, with some journeys aborted due to unsafe environmental conditions. What if these important decisions could be made before the pilots leave the shore?
Introduction
As global maritime activities intensify, maintaining the safety and efficiency of maritime operations throughout the year has never been more imperative. With a rise in the utilization of the ocean and coastal seas, maritime operators now must contend with increasingly extreme and unpredictable environmental conditions.
With vessel sizes and the quantity of marine traffic growing, access to accurate real-time meteorological and oceanographic (metocean) data becomes vital to ensure efficient cargo operations, safe berthing of commercial ships, and adherence to project deadlines. This, in turn, helps prevent logistical backlogs and shortages across the world’s ports.
The Requirement
OceanWise, a Hampshire based smallmedium enterprise specializing in marine data, collaborated with Associated British Ports (ABP) Southampton’s Marine Pilots to enhance their “sight from shore.” The objective was to provide access to accurate, real-time metocean data to support time-critical decision-making processes during pilotage operations.
Since 2014, OceanWise has been working closely with ABP Southampton to establish a comprehensive network of environmental monitoring sensors and telemetry systems. These systems provide critical data to the port’s pilots, Vessel Traffic Services (VTS) operators, hydrographers, and the wider port team. Utilizing OceanWise’s Port-Log online data platform, this network facilitates efficient vessel planning and decision-making.
As the UK’s leading hub for deep-sea trade and a critical link in the supply chains
servicing the UK, as well as being Europe’s leading turnaround cruise port, ABP Southampton’s marine team operates 24/7 to manage the transit of some of the world’s largest vessels. To ensure the smooth movement of commodities, the team relies on accurate, real-time environmental data to assess conditions and conduct safe passage plans for some of the world’s largest vessels. Each pilotage trip involves substantial costs and carbon emissions and comes with certain risks to the personnel involved. For each vessel requiring pilotage support, a pilot boat (Figure 1) and its crew must undertake a sometimesrisky journey to reach vessels in the port approaches, with some journeys aborted due to unsafe environmental conditions.
What if these important decisions could be made before the pilots leave the shore?
The effectiveness of any technological solution relies on capturing the end-user requirements and involving stakeholders throughout the design and implementation processes. OceanWise adopted a usercentric approach by developing a dedicated webpage which is easily accessible on any smart device via OceanWise’s cloud-based data platform, Port-Log. This webpage offers marine pilots a tailored view of key real-time metocean and environmental data from the safety of the shore.
Given the time-critical nature of these decisions, which must often be made during busy operational periods, operators require clear, concise, and reliable information in a single, easily accessible location. The new custom webpage ensures data-driven decisions can be made quickly, and that operators stay fully informed about offshore conditions.
As an existing OceanWise customer, ABP Southampton already utilized an array of internal and third-party metocean data feeds from sensors situated around the Port of Southampton and the Solent. These data feeds
are integrated into Port-Log, providing the foundation for this project. The dedicated webpage consolidates key data feeds, including meteorological and wave buoy data from various locations within the Solent. ABP Southampton’s marine pilots and launch crews monitor the webpage via a live stream at the launch station or access it remotely on smart devices (Figure 2).
The availability of up-to-date environmental data enables quick decisions regarding embarking and disembarking of pilots, reducing lost time, effort, and costs associated with aborted pilotage journeys. It also improves safety for all involved and reduces carbon emissions.
According to ABP Southampton, the Pilots page (Figure 3) has been invaluable. It provides data on the critical environmental conditions that determine the safe embarkation and disembarkation of pilots in the boarding grounds. With this data, determinations can be made from the VTS Centre and the launch station on whether to suspend or continue operations in parts of the district without putting pilots or launch crew at undue risk.
To further support the decision-making process, OceanWise has integrated an operational safety matrix established by ABP Southampton into the webpage. This matrix provides colour-coded values based on defined operational thresholds relating to ABP
Southampton’s risk assessment and enhances situational awareness.
Looking ahead, the integration of real-time metocean data with predictive weather forecast data services offers further opportunities for improvement in the situational awareness of operators and aids data-driven decisionmaking. When operating under fine timescales, having a good understanding of the speed and intensity of local weather fronts and how these may impact operations is key. While weather forecasts provide a perspective on future prevailing conditions, they can often be subject to change as weather systems evolve. Accurate real-time data on meteorological conditions, such as wind speed, direction, and gusts, from additional locations, would enhance decision-making by providing insights into approaching weather systems.
Expanding data availability through broader sensor networks would offer pilots and other port users a more comprehensive understanding of the environmental and oceanographic climate, combining forecasts with real-time and historical data. This approach would empower personnel, both shore-side and waterborne, with the
information needed to make safe, datadriven decisions.
The technology required for such advancements already exists; the challenge now lies in encouraging data sharing, changing mindsets, and expanding networks to fully realize the potential.
Environmental data sharing among ports, shipping companies, and other maritime stakeholders offers several key benefits. By expanding the scope of data available to pilots, VTS operators, and port authorities, ports can build a more comprehensive understanding of the environmental conditions impacting their operations. Access to a wider range of real-time and historical data, combined with forecasts, enables a holistic view of the maritime environment, allowing for better anticipations and management of potential disruptions.
Furthermore, data sharing fosters greater collaboration and coordination across the maritime community. When multiple stakeholders have access to the same high-quality data, it helps establish common situational awareness, reduces miscommunication, and ensures more
consistent decision-making. This shared approach enhances safety by enabling parties to respond more effectively to rapidly changing conditions and to coordinate responses to adverse weather conditions or other environmental hazards.
Moreover, environmental data sharing contributes to sustainability goals by optimizing ship routing and port operations. By minimizing the number of aborted or delayed journeys and reducing idle times due to unforeseen environmental conditions, data sharing reduces fuel consumption, emissions, and overall carbon footprints. This is increasingly important in the context of global efforts to combat climate change and achieve carbon neutrality in the maritime sector.
The integration of real-time metocean data has proven invaluable in enhancing operational safety and efficiency at ABP Southampton. By developing a user-focused solution that provides access to critical environmental data, OceanWise has enabled more informed decision-making, reducing risks, costs, and emissions. As the demand for maritime operations grows, the continued development and sharing of data resources beyond a port’s
boundary will be essential in supporting sustainable and safe maritime activities worldwide. The future of maritime operations will significantly benefit from fostering an open environment for data sharing and exchange, driving innovation, and building a resilient maritime infrastructure. u
Becky Conway is the business development and oceanographic engineer at OceanWise Ltd. This role involves developing business and customer relations while supporting the marketing function with business and product development as well as supporting field activities in Scotland. She has a master’s degree in oceanography and a post graduate diploma in advanced hydrography for professionals along with over 10 years of experience working in the marine environment and specifically the ports and harbours sector where she was principal hydrographic surveyor for Associated British Ports at the Port of Southampton. In addition to her role at OceanWise, she is also the chief executive officer of The Hydrographic Society UK and Ireland (THS:UKI) where she provides strategic leadership for the promotion of the Society and the importance of the science of surveying and related disciplines.
ERIK
A quiet revolution is underway as offshore platform developers bring a new generation of all-electric free-fall lifeboats into service. Marine battery power improves reliability, performance, and safety, as well as sustainability.
Introduction
When Aker Kvaerner began work to extend the life of Equinor’s Njord A floating and drilling platform in the Norwegian North Sea to 2040, the upgrade included the replacement of the existing evacuation system with a set
of modern 70-person free-fall lifeboats and a custom designed davit system.
Part way through the project, developers further updated their thinking, in a switch away from conventional diesel engines, which also brought VIKING Life-Saving Equipment its first order for E-GES-52 (Electrical Gravity Escape System) free-fall lifeboats (Figure 1). The technology had become available after a successful threeyear project between Statoil (now Equinor)
and Norsafe (now part of VIKING LifeSaving Equipment) to establish a technology qualification and viability evaluation of electrical propulsion for lifeboats.
Fast forward and the Njord A installation features lifeboats that are documented to meet DNV Battery Installations on vessels of less than 15 m in length – including full fire propagation testing – and SOLAS Novel Design Resolution A.520(13), as documented by the Norwegian Maritime Authority.
Confirming that the project was no one-off, VIKING has since gone on to secure allelectric lifeboat projects for an operator in the western Australia offshore sector and for Aker BP’s Hugin A and Hugin B platforms –part of the Yggdrasil Norwegian Continental Shelf development 160 km west of Kollsnes, near Bergen.
All-electric power is increasingly accepted as a viable solution for boat operators, with advances in battery technology also pointing toward more powerful performance from similarly sized units as power density increases. VIKING already offers the E-Mako-655 (Figure 2) – the world’s first all-electric SOLAS rescue boat, whose 63 kWh battery pack offers four hours of continuous operations at 6 knots with a crew of three personnel.
Electric propulsion also offers operational safety benefits that lifeboat specifiers in particular will wish to consider. These include lower levels of vibration, a quieter cabin with much better air quality, increased acceleration, and higher top speeds – thereby transporting evacuees more comfortably and quickly away from the platform in an emergency when compared to diesel powered boats.
Battery power allows the asset owner to dispense with the need to transport, store, and handle diesel fuel on the platform, and avoids issues with fuel contamination altogether. A further benefit for operators working in areas affected by H2S gas clouds (sour gas environments) is that, as the battery is the entire power resource, there is no need to draw exterior air when the lifeboat is in operation. This means the boat can operate autonomously for up to two hours, compared to 10 minutes for a diesel boat.
But these factors are only part of a broader set of safety-based reasons that suggest all-electric propulsion is worthy of wider consideration.
Many market watchers will know that innovations in Norway’s well-funded and safety aware offshore industry are often adopted by others once they have proved their relevance. One basis for wider adoption is the forward-looking stance Norwegian stakeholders take with respect to safety standards and certification.
In an indicative development, the structural part of VIKING’s GES free-fall lifeboats provides an early example of the new generation of designs which evolved out of the NOROG (Norske Oil and Gas –formerly OLF) industry group in 2005. The most extensive study ever undertaken of conventional and free-fall lifeboat safety, this project eventually resulted in DNVGLST-E406 – the lifeboat performance standard that goes far beyond the Convention of the Safety of Life at Sea (SOLAS) and specifies no harmful accelerations in up to 100-year storm conditions.
The compact, low weight davit installations (Figure 3) provided by VIKING Norsafe for the Njord A and for the Hugin A and B projects also merit separate attention in the safety context. Both have been built to the NORSOK 002 standard – whose methodology includes dynamic considerations that build on crane-based safety rules, as well as being rooted in maritime regulations and VIKING’s commitment to include operator preferences for simplified operations.
It is in this context that the shift to electric lifeboat propulsion should be further studied because the selection of battery power has also been made on grounds of improved reliability, reduced accident risk, easier maintenance, and through-life cost savings.
Pressure to reduce the number of personnel working at sea or offshore is nothing new, but recent developments in connectivity,
digitalization, and remote management techniques have allowed asset managers to step up efforts to automate processes, and control others from afar. Today, many offshore platforms are not normally crewed.
At the same time, in being formulated for diesel engines, the maintenance procedures for lifeboats within SOLAS demand that engines are turned over for a five-minute run test every 14 days to ensure readiness for action.
The specificity of the requirement brings into focus the accident risks that maintenance engineers are exposed to in landing on the platform, and when working on lifeboats in their tilted launch position.
Where readiness for action is concerned, it is also understood that an engine tilted at an
angle of up to 35 degrees and cold-started every two weeks will lose performance over time. It is fair to assert that the use of allelectric propulsion would mitigate risk.
The E-GES-45 free-fall lifeboats, for 60 person, and E-GES-52 free-fall lifeboats are typically powered by three Akasol batteries, which are contained in robust, waterproof cases, with individual cells thermally insulated. The solution also includes a ventilation system, gas/ smoke detection, and a water sprinkler system.
With electric systems wholly compatible with remote maintenance, equivalence also means land-based checks of operating systems are sufficient to give assurance that the boat’s propulsion system is available on arrival on board not normally crewed platforms.
The lifeboats on board Njord A have selfdiagnostic programmable logic controller systems, with each boat connected to an individual email address that alerts maintenance teams of any issues, or trend deviations on temperature, charge, gases, or fumes (Figure 4). In a real incident, the boat informed the team that attendance was needed to deal with a malfunctioning battery charger.
As well as eliminating the risks brought by physically entering the lifeboat, the use of remote diagnostics, maintenance, and control methods can extend the requirement for service engineers to attend the lifeboat from once every 14 days into an annual visit.
Overall, the maintenance work required to support a diesel-powered lifeboat is estimated at over 300 hours in a year. With tests of the deluge systems, steering systems, and hook release systems also remote, and the boat launch simulated, analysis indicates that the same procedures for an electric lifeboat can be accomplished in 90% less time. u
Erik Mostert is the manager of both innovation and boats and davits with VIKING Life-Saving Equipment. He has worked with electrical lifeboats for nearly 10 years. Georgios Nikoltsis is the senior manager, servitization and innovation with a broad remit in development and is based in Arendal, Norway. He joined the company in 2005. Joe Dawes is the senior sales area sales manager and is based in Arendal, Norway. With huge experience across the maritime and offshore industries covering boats and davits, he joined the company in 1998. All three have worked together at VIKING Life-Saving Equipment on free-fall lifeboats for well over 10 years on projects across the offshore industry, overseeing deliveries to floating and fixed installations around the world.
by Izzy Taylor
In today’s interconnected world, access to reliable internet connectivity is becoming increasingly critical, not just for convenience but for safety and well-being, especially for those working in remote and hazardous environments. Among these, seafarers are a particularly vulnerable group – often isolated from terrestrial networks while engaged in crucial offshore industries like shipping, fishing, and energy production.
Updates to the 2006 Maritime Labour Convention in 2022 solidified the right of seafarers to social connectivity, including access to the internet, as a mandatory provision. This shift has accelerated the push for better and more affordable communication technologies at sea in order for employers to provide this necessary asset to their staff.
Traditionally, offshore connectivity has been achieved through either a localized radio link or specialized satellite systems, which require advanced equipment and can be cost prohibitive. However, the emergence of offshore 5G networks is changing the game. They offer a new level of resilience, enabling safety-critical communications and other services on an offshore site.
Here we will explore the rise of offshore 5G networks and other options for connecting people at sea.
There has been a shift in business needs for the internet over the past few years. In 2018 it would have been largely sufficient for offices to have connection for emails, online research, accessing websites, and the occasional online call. During the COVID-19 pandemic, there was a major shift to online meetings which has increased the bandwidth requirements, and we have become accustomed to video calling colleagues and customers, spending all day jumping from one meeting to the next.
From smart hubs which control the doorbell and lighting to precision machinery
controlled remotely, the majority of workplaces are continuously ramping up the use of fast connectivity. In well-connected areas on land, a broadband connection and fibre are covering these needs, but there is a significant gap in remote areas and at sea where people are working daily without the capability of sending emails or images without internet – something which the majority of us take for granted.
The importance of communication networks for safety in offshore environments cannot be overstated. Taking into account those working offshore and holiday makers using water transport, it is estimated that on average 1-2 million people are at sea at any time around the globe.
Whether on a vessel far from the nearest port or working on an offshore wind turbine, reliable access to communication can mean the difference between life and death. Immediate access to emergency services, weather updates, and vessel tracking are vital to the safety of seafarers and offshore workers. Additionally, the mental well-being of workers can be significantly impacted by prolonged isolation, making access to the internet a crucial aspect of maintaining a healthy team.
Until recently, offshore communication relied heavily on satellite links, which have limitations in terms of bandwidth, latency, and cost. Satellite connections like Inmarsat or Iridium provide global coverage but are often expensive and require specialized equipment to operate. For instance, a maritime satellite communication system can cost thousands of dollars to install, with monthly fees high for even basic data packages. The need for a more efficient, scalable, and cost-effective solution has led to the uptake of Low Earth Orbit satellite connectivity, such as Starlink and OneWeb, as well as offshore terrestrial networks, designed specifically to overcome these challenges.
Figure 1: High-speed, reliable connectivity at sea saves lives, protects the environment, and ensures stable growth of a global blue economy and digital ocean. JET Connectivity’s resilient 5G connectivity provides real-time data acquisition and transmission, allowing users to connect from their usual devices and operate offshore as on land, without the infrastructure or costs of fibre or satellite communications.
Fifth-generation (5G) networks are often associated with faster speeds and lower latency in urban environments, but they have significant potential offshore as well. 5G is a broad term that encompasses many different network setups. In their formation, 5G standards were largely set around three areas:
• Connecting machines to machines, e.g., smart cities and roads
• Providing ultra-low latency speeds, e.g., for autonomous vehicles
• High bandwidth capacity, e.g., for ultraHD video with virtual reality
Each of these has a slightly different setup depending on the need of the user and are geared toward businesses and devices rather than people.
A key advantage of 5G is its ability to support a wide range of devices and applications simultaneously. In an offshore setting, this includes everything from smartphones and laptops to advanced sensors, drones, and autonomous vessels. Crucially, 5G can support mission-critical communication with low latency, enabling real-time data transmission
between land-based operations and offshore platforms or vessels. This can be especially important for emergency situations, where every second counts.
The use of 5G can go beyond just providing internet access; it plays a pivotal role in enhancing safety. For instance, in the case of an accident or equipment failure, realtime video and data feeds can be transmitted to onshore control centres, enabling quick response and decision-making. Additionally, automated monitoring systems can continuously relay information on vessel positions, weather conditions, and machinery health, allowing potential issues to be identified and addressed before they escalate into emergencies.
JET Connectivity has developed a specialist 5G solution which is flexible in its ability to work in ultra-remote areas without reliance on fibre-optic cables, due to its advanced meshing capability. This is achieved through purpose-built 5G radio equipment that can be deployed on JET’s floating platforms, offshore rigs, or wind turbines, extending network coverage far beyond the reach of conventional networks (Figure 1).
While 5G is a revolutionary step forward in offshore communications, it is important to recognize that it is not the only technology available. Traditional communication systems, like TETRA (Terrestrial Trunked Radio), have long been a staple for safety communications in industries like shipping and oil and gas. TETRA radios are designed for missioncritical voice communication and are known for their resilience in harsh environments. They operate on dedicated frequencies, ensuring that communication channels remain open even in the event of network congestion. However, TETRA systems are often limited in terms of data transmission, making them less suitable for the high-bandwidth needs of modern work operations.
Satellite communications, as previously mentioned, remain a key player in offshore safety. Satellites provide global coverage, making them ideal for vessels that operate far from shore and beyond the reach of terrestrial networks. However, their high cost issue makes them less suited for real-time data transmission and video feeds. In combination with 5G networks, however, satellites can serve as a backup option or additional data route, ensuring continuous communication with added resilience.
One of the most promising applications of offshore 5G technology is in the renewable energy sector, particularly offshore wind farms. The UK, with its extensive coastline and favourable wind conditions, is home to one of the largest offshore wind farm industries in the world. These wind farm sites are often located dozens of miles from the nearest shore, making traditional communication systems inadequate. However, by deploying 5G networks directly on wind turbines or floating platforms, operators can maintain real-time communication with onshore control centres.
These wind farms are already embracing 5G technology and the services that it
enables, including high-speed, low-latency communication between the turbines, maintenance vessels, and onshore control rooms. In addition to improving safety by providing real-time video feeds and sensor data, the networks also support robotic blade inspections and autonomous vessel operations, reducing the need for human workers to be exposed to dangerous conditions.
By using 5G technology, the wind farm operators can monitor the performance of each turbine in real time, quickly responding to any issues that arise and fixing issues immediately as opposed to waiting for routine checks. This not only improves the efficiency of the wind farm but also reduces the risk of accidents, as potential problems can be addressed before they pose a danger to workers or equipment.
Resilient 5G or hybrid networks represent a significant leap forward in providing reliable, high-speed communication for seafarers and offshore workers. As industries like shipping and renewable energy continue to expand their operations into increasingly remote locations, the need for resilient communication systems becomes ever more critical.
A combination of connectivity for wellbeing and safety, along with the technology requirements for more data, is pushing the development of robust and reliable communications and JET is delighted to be a part of this advancement in technology by providing real-time data and connectivity between shore and site. u
Izzy Taylor is head of business development and marketing at JET Connectivity. She is responsible for managing relationships with customers and ensuring solutions and end user needs are aligned. Her background has been in innovative uses of data in the satellite and space industry before moving to telecoms and maritime in 2022 where she found a new passion for utilizing data in different sectors.
How Detection Technology is Transforming Passenger Safety at Sea –A Timeline
by Frederik Giepmans and Mike Collier
Person overboard (POB) incidents remain an unfortunate, and persistent, reality in the cruise industry. With an average of more than 20 people going overboard on cruise ships every year, they remain the leading single cause of death for passengers on board
When a passenger or member of the crew falls overboard (Figure 1), a fast response time is crucial for a successful rescue or recovery, and the presence or absence of advanced POB detection technologies can significantly influence the way these incidents play out.
This essay explores the timelines of a POB event through two contrasting scenarios: one without POB detection technology, and another with a dedicated POB system in place.
Without a dedicated detection system – unless the individual has been witnessed going overboard – most POB incidents begin as
missing person cases, with the initial confusion significantly delaying any search and rescue attempts.
The majority of passengers who go overboard do so at night. Typically, the crew will not hear about it until a friend or relative alerts them to the passenger’s absence in the morning. This normally takes between one and seven hours – though there are recorded incidents of this taking days or even longer.
When people go missing on cruise ships, only a very small percentage of these are eventually identified as POB incidents. As such, only limited POB measures will ensue until any incident has been confirmed. Typically, this is limited to the Bridge recording GPS coordinates from the time the person has been identified as missing –which, combined with wind, wave, and drift measurements/forecasts, can predict where a person might be carried to some degree.
2. Confirmation of POB Incident (2-6 hours – total 3-13 hours)
Once the crew is alerted to a missing individual, a headcount will be initiated – a time-consuming process, considering the largest cruise ships can carry well over 7,000 passengers and 2,000 crew members.
Simultaneously, the crew will comb through many hours of CCTV footage, from thousands of cameras, to identify the individual, confirm where they might have gone, and if they have fallen overboard.
3. Search and Rescue Efforts (24+ hours –total 3 hours-multiple days)
If the missing individual cannot be located from the headcount, and/or is identified as going overboard from the CCTV footage, then a search and rescue will commence, and responsibility handed to the relevant coast guard authority (Figure 2).
In most eventualities, this will see the cruise ship initiating an emergency U-turn, to join
the wider search party. The cruise ship will have shared the Bridge’s drift information with the coast guard – though, the reliability of the modelling declines exponentially over time – particularly with uncertainty of when the individual went missing. As such, even with this system, the coast guard could end up searching nearly 13,000 square kilometres of sea.
The Incident
In ships equipped with automated POB detection systems, like MARSS’ MOBtronic, the timelines differ dramatically. MOBtronic combines radar, thermal imaging, and video analytics to automatically detect and alert the crew when a person goes overboard.
1. Immediate Detection (0 minutes)
The moment a person falls overboard, their fall is recorded and analyzed by the MOBtronic unit. A near-immediate alert is then sent to the Bridge, accompanied by looped video/ infrared footage of the event, enabling the crew
to confirm a POB incident within a matter of seconds (Figure 3). MOBtronic immediately records the GPS position to provide the most accurate drift analysis.
2. Instant Response (within minutes)
Within minutes of the fall, the crew can initiate a search and rescue operation, and deploy lifesaving equipment, such as transponders, buoys, lifeboats, or even drones. Because of the immediacy of the response, it is much more likely that the individual will be spotted by the crew, with the ship’s systems then able to track their movements.
3. Coordinated Rescue (tens of minutes)
Once a POB incident has been verified, the officer in charge will immediately inform the coast guard authorities, which, in turn, will dispatch search and rescue vehicles and alert nearby vessels, which can be directed to the person’s last known position.
It is immediately evident that the difference between the two incidents can be many hours – during which time the chances of a successful search and rescue attempt are dramatically reduced.
In addition to the human tragedy, the scenario without a detection system involves major cost and effort by way of fuel, delay, diverted routes, port rearrangement charges, and the professional resources involved in the recovery.
No Longer an Option, but
Sadly, POB incidents will continue to present a serious challenge for the cruise industry – and, without detection systems in place, response times will remain slow and typically unsuccessful. However, the integration of automated detection systems is revolutionizing this process by providing immediate alerts, enabling precise tracking, and greatly enhancing the chances of a successful rescue.
As the cruise industry continues to evolve, the adoption of such technology will no longer be seen as an option, but a necessity for passenger safety at sea. u
Frederik Giepmans is the managing director at MARSS Safety & Security. He has more than 15 years of experience delivering projects in offshore oil and gas, yachting, and security sectors, from concept engineering and design to completion. He joined MARSS in 2019 and has been instrumental in its rapid development in recent years.
Mike Collier is the business development manager with MARSS and has more than 30 years of experience across defence, government, and commercial sectors. With a background in combat systems, sensors, and effectors, he supports MARSS across the full portfolio of products. He has specific responsibility for person overboard detection (MOBtronic), supporting the cruise industry to improve safety.
BY ZACHAROULA SYRIVLI
Water rescue operations can be hazardous. The unwavering dedication of rescue workers to duty often calls them to put their own lives at risk in water. Flotation devices, life vests, protective suits, boats, and ropes are what rescue teams have had at their disposal in their endeavours so far.
Adverse weather conditions, limited resources, and, above all, time can jeopardize the success of water rescue operations or even the lives of rescue workers. Water rescue is ultimately a matter of life and death that necessitates immediate response.
In Finland, the land of 188,000 lakes, the rescue departments are frequently called to duty in the wintertime when lakes are frozen. The ice can easily break when walking or skating over a lake and incidents of water emergencies increase. Nevertheless, the accidents do not diminish when ice melts, days get longer, and seasons change.
In the Finnish capital Helsinki, water rescue incidents have spurred a need to investigate the role of technology in rescue missions. Drone technology has lately emerged as a very promising technical solution in these challenging missions with growing evidence of its efficiency in related operations. Equipped with thermal cameras, the so-called lifesaving drones can locate people in distress at sea, access hard-to-reach areas, and deliver first aid supplies to those in need before the rescue teams arrive. They can provide first aid in frigid and turbulent waters without putting the lives of rescuers in jeopardy.
Lifesaving drones (Figure 1) were trialled in Helsinki on a windy morning in August 2024, in a simulated life-threatening situation. A staff member of the Helsinki City Rescue Services acting as a victim jumped into the waters of the city’s Eastern Harbour. The drone came to his rescue by dropping a floating device safely
near him that inflated upon contact with water (Figure 2). The victim could hold on to this until the rescue boat arrived. The drone was flown within visual range from approximately half a kilometre away.
Piloting water rescue by drone at the Port of Helsinki offered an excellent opportunity to bring together emergency services and authorities, enhance understanding of the promising drone technology, and the importance of cooperation among all parties. Innovative technology and its validation require efficient collaboration among the different key players and this case was not an exception. The pilot project was actualized in collaboration between the city’s innovation company, the City Rescue Department, the Port of Helsinki, and the Technical Research Centre of Finland VTT that acted as the drone operator.
The potential of drones in water rescue has not yet been utilized or tested previously by the local rescue department despite the deployment of drones in some of its operations (e.g., fire monitoring). Thus, it offered valuable lessons to all on the importance of safe operations in challenging environments, such as the one in the harbour area. It gave additional evidence to the City of Helsinki for setting necessary conditions to accommodate the drone technology in its urban environment as the pilot was coordinated by its innovation company, Forum Virium Helsinki, as part of its leading EU project, CITYAM.
The pilots in Helsinki showed that drones can support water rescue. Nevertheless, drone technology per se is not a panacea for successful rescue operations. Its potential should be first utilized in rescue systems and further optimized for making future water rescue missions faster and safer. Access to hard-to-reach areas, increased situational awareness, rapid response, and safety are all listed as the greatest advantages of drones in addition to the traditional means of rescue
1: Lifesaving drones can locate people in distress at sea and deliver floating devices, for example, to help those in need before rescue teams arrive.
The Technical Research Centre of Finland VTT operated the drone in this pilot.
missions. These distinct advantages, though, cannot be actualized without efficient communication, collaboration, and coordination among emergency services and authorities.
In the critical moments involved in water rescue operations, nothing is more essential than the effective communication among relevant parties (e.g., rescue workers, police, paramedics, coast guard). Inaccurate positioning information of the drone and GNSS interferences can put the entire rescue operation at risk. A holistic approach to water emergency response can optimize this potential alongside solid regulatory frameworks and essential digital and physical infrastructure to facilitate drone operations. Automation and the possibility of flying the drone out of sight range can furthermore make drones an integral part of the water rescue emergency system and increase its efficiency.
The potential of drones in water rescue is great, yet not boundless. Collaboration, context, and resources are its boundaries and limits. Drones can evidently complement rescue efforts; however, emergency response mechanisms cannot be built rigorously only on drones. They can be built on a holistic approach and context analysis that encompasses regulations and resources. All these can open the skies for the lifesaving drones and provide rescue departments with an indispensable tool in their water rescue missions.
This pilot was carried out in Helsinki on August 27, 2024, and was funded by the Interreg Baltic Sea Region (co-funded by the European Union) as part of the CITYAM project – Preparing Cities for Sustainable Urban Air Mobility (2023-2025). u
Zacharoula Syrivli is a project planner at Forum Virium Helsinki, the innovation company of the City of Helsinki. With an academic background in public management, social and computer sciences, her current work is focused on urban air mobility under the umbrella of the Interreg Baltic Sea Region-funded project CITYAM that aims to support and empower cities on their way to a wider-scale deployment of drones in the most responsible, acceptable, and sustainable way. The mission of Forum Virium Helsinki is to develop Helsinki into the most functional smart city in the world in cooperation with other public sector organizations, companies, research institutes, Helsinki residents, and cities within and beyond the Finnish borders.
• Special events
• Reunions
• Degree frames
• Alumni and campus news
• Group rates on insurance services
• Alumni credit card
• Retail discounts
• University library access
instagram.com/marine.institute SERVICES AND
• Resume/CV critique and evaluation
• Mock interviews
• Job search skills
– personal branding, networking, job search resources
• Resume referral services
• Job postings
• Access to career events
Stay connected and up to date on new alumni services, events, latest news and how you can become involved.
www.mi.mun.ca/alumni/reconnectwithmi alumni@mi.mun.ca
1 800 563 5799, ext. 0367
linkedin.com/edu/ FisheriesandMarineInstituteofMemorialUniversity
youtube.com/marineinstitutepr facebook.com/ MarineInstituteAlumni twitter.com/ MarineInstitute
Advantages and Limitations of Using Cameras on Small, Low-Cost ROVs for Seabed Monitoring Amanda Frederikke Irlind, Alex Jørgensen, Jonathan Eichild Schmidt, Anders Skaarup Johansen, Thomas B. Moeslund, Karen Ankersen Sønnichsen, Niels Madsen
A Review of Reconfigurable Intelligent Surfaces and their Application to Machine Learning-Assisted Underwater Communications Mohamed A. Ouf, Abdelrahman Elkhateeb, Abdelmoneim Soliman, Md Safiqur Rahaman, Sara Mobarak, Mervin A. Marshall
Researchers from Denmark investigate the potential of a non-invasive and simple underwater remotely operated vehicle to enhance seabed monitoring.
This paper is of interest to marine researchers involved in monitoring the marine environment. Readers are introduced to the advantages and limitations of using cameras on small, low-cost ROVs, which can help with decision-making on monitoring equipment. Policy-makers and marine managers can also benefit from understanding the practicality of using these ROVs for ecological monitoring in areas with limited resources. Additionally, this paper addresses the shortcomings of ROVs and camera solutions, which can be helpful for the camera industry and underwater image enhancement research.
The paper focuses on evaluating the performance of a small, low-cost ROV for monitoring marine environments. The study demonstrates how these eyeball-class ROVs can provide valuable data across various substrates, including challenging conditions like strong currents and turbid, murky waters. It emphasizes the flexibility of ROVs compared to traditional tools like towed cameras or sampling equipment. Additionally, it highlights the potential to revolutionize species identification through post-processing tools and eDNA integration, sparking excitement about the advancements in lowcost monitoring and marine research.
As the first study to use cameras in this part of the Greater North Sea, sparse taxa communities were observed in stone reefs and sandy substrate. These findings provide a deeper understanding of marine habitats, which can guide future research and contribute to better ecological management, biodiversity conservation, and the development of informed protection measures for critical marine habitats.
Amanda Frederikke Irlind (M.Sc. in biology) is a PhD fellow at Aalborg University. Her research is focused on the impact of fisheries on the marine environment. She has previously studied bycatch in small-scale fishing in Greenland fisheries.
Alex Jørgensen (M.Sc. in biology, research assistant) has worked on research projects on discard survival in fisheries and the environmental impact of fishing gear.
Jonathan Eichild Schmidt holds a three-year PhD scholarship at the Technical University of Denmark, specializing in the assurance of
perception systems for autonomous vessels. He earned his M.Sc. in robotics with a focus on computer vision for navigation. His current research focuses on using computer vision at sea.
Dr. Anders Skaarup Johansen is a postdoc at Aalborg University. He studies applications of object-centric computer vision algorithms for real-world applications. He is interested in how to retain the performance of machine learning-based vision systems in adverse conditions.
Professor Thomas B. Moeslund (PhD) is currently the head of the Visual Analysis and Perception Laboratory, head of Section for Media Technology, and head of AI for the People Center, all at Aalborg University, Denmark. His overall research interest is building intelligent systems that make sense out of data with a special focus on computer vision and AI.
Karen Ankersen Sønnichsen’s (M.Sc. in biology, research assistant) research interests are primarily focused on the ecology and population biology of marine macro and megafauna, particularly the impact of human disturbance on these communities. She has also conducted research on marine mammals.
Dr. Niels Madsen is a professor at Aalborg University (Denmark) and a member of the Danish Biodiversity Council. His main research areas are marine biology and technology. His current research focus is on marine protected areas and the environmental effects of fishing gear.
Amanda Frederikke Irlind1*, Alex Jørgensen1, Jonathan Eichild Schmidt2, Anders Skaarup Johansen2, Thomas B. Moeslund2, Karen Ankersen Sønnichsen1, Niels Madsen1
1Section for Biology and Environmental Science, Department of Chemistry and Bioscience, Aalborg University, Fredrik Bajers Vej 7H, 9220 Aalborg, Denmark
2Visual Analysis and Perception Lab, Media Technology, Aalborg University, Rendsburggade 14, 9000 Aalborg, Denmark
*Corresponding author: aifr@bio.aau.dk
ABSTRACT
Monitoring methods, such as seabed bottom-towed cameras, sediment grabs, and benthic sledges, have limitations in spatial coverage, cause seabed disturbance, are restricted to soft-bottom substrates, and offer low flexibility for marine seabed monitoring. In this study, we investigate the potential of a non-invasive and simple underwater remotely operated vehicle (ROV) to enhance marine seabed monitoring. A tethered ROV equipped with a GoPro camera was deployed in three areas of Skagerrak at depths from 15-34 m to assess accuracy in species identification and substrate classification identified from still frames. The quality of still frames varied between areas due to turbidity, motion blur, and marine snow, which reduced the number of high-quality frames by approximately 20%. Classification of substrates and taxa identification were possible in the remaining still frames. Two different substrates were detected: sand and stone reef. Stone reefs had a lower occurrence compared to sand. A total of 10 taxa were detected in the two substrate types. The highest abundance was observed in the stone reef substrate compared to the sand substrate. Identification at the species level was limited due to the quality of the still frames, which affected the detectability of morphological traits. This study demonstrates that a widely accessible ROV can be used for marine monitoring. The ROV can be used in different substrates, and still frames provide valuable information on species composition, which can enhance the replicability of monitoring programs.
Keywords: Drones, Greater North Sea; Image quality; Marine habitats; Remotely operated vehicle; Species identification; Underwater video camera
Monitoring marine environments is essential as it provides valuable information that enhances scientific understanding of marine ecosystems and supports management and sustainable development. The marine environment is currently under pressure from climate change, pollution, and exploitation of marine resources [1] and comprehensive monitoring programs are important for marine protection. Within the European Union (EU), the Marine Strategy Framework Directive (MSFD) requires all member states to achieve Good Environmental Status for the marine environment [2], [3]. Since its implementation, EU nations have adopted monitoring programs that often overlap with other Marine Protected Area programs, such as the European Nature 2000 and EU Habitat Directive, which are implemented to protect rare, endangered, and characteristic species and habitats [4]. Member states are free to choose the sampling design and assessment methodology, which can limit the robustness and replicability of the monitoring program. In MSFD, methods such as seabed towed cameras, sediment cores, grabs, and sonars are deployed from vessels, or data is collected by observations from divers [5], [6], [7]. These methods either require a high expertise level, are spatially restricted in terms of coverage, have difficulties operating in hard substrates, lack flexibility in movement, or disturb flora, fauna, and substrate when towed on the seabed [8], [9], [10], [11], [12].
As an alternative monitoring tool, remotely operated vehicles (ROVs) – such as underwater drones and drop cameras – have been successfully used in marine monitoring,
providing marine scientists with a powerful tool that enhances monitoring capabilities [13], [14], [15], [16], [17], [18]. These camera solutions are non-invasive with limited disturbances to the seabed, function on hard substrates, can be deployed in remote locations, provide long-term monitoring that ensure replicability, and provide information of an environment [14], [16]. However, these methods are not accessible to all monitoring parties due to their high cost, the need for larger vessels for deployment, and the logistical challenges. An emerging option is affordable open-source underwater ROVs that are small and lightweight, typically weighing only 2 kg. These ROVs have adjustable speeds ranging from about 3 knots to standstill, can dive down to 200 m (depth rating), and have tether lengths that allow ranges up to 400 m. The tether ensures a live video feed that can be used to inspect and avoid collision. Launching from the surface is easy with a simple tether system, and the ROVs are easy to control remotely, offering high manoeuvrability due to the fast-responsive thruster. As a standard, ROVs come equipped with 4K cameras, and most include floodlights, allowing for monitoring in dark and turbid waters. These ROVs have been used experimentally [19], in the aquaculture context [20], and for ecological purposes [21], all proving to be useful.
In this study, we tested if substrate type and species composition could be detected from frames provided by cameras mounted on a low-cost ROV system and how information hereof can be used in seabed monitoring. We focused on an area in the Greater North Sea in the southern Skagerrak with strong current and murky turbid waters. These conditions can
reduce the quality of frames and complicate extracting ecological information. Moreover, comprehensive monitoring programs to support effective management decisions are missing in this area, and we aim to identify the advantages and limitations of small, low-cost ROVs for seabed monitoring.
2.1
The study was conducted in the Greater North Sea (Skagerrak region, ICES area 3a) (Figure 1). The study area features shallow coastal waters influenced by tides. The North Atlantic current runs from south to north, mixing the water and maintaining salinity between 3335‰ and temperatures ranging from 6 to 17°C depending on the time of year. The depth in the study area gradually decreases from the coast to approximately 40 metres. This area is one of the most intensively fished areas globally, utilizing various bottom-towed fishing gears [22], and it also includes Marine Protected Areas such as Natura 2000 [4].
The sampling activities targeted three areas with varying depth profiles and distances from the coast. Area A was 12-16 nautical miles from the coast with depths between 31-34 metres, Area B was 5-7 nautical miles from the coast with depths between 15-23 metres, and Area C was 7-10 nautical miles from the coast with depths between 24-28 metres (Figure 1). The ROV was deployed at four sites within each of these areas.
The seabed examination was conducted using a tethered ROV, specifically the Chasing m2 Pro Max (Chasing, China) (Figure 2a). The ROV
has a maximum speed of 3 knots and a battery life of approximately 1.5 hours, depending on the duration of recordings, use of lights, and thruster activity. It features a standard 4K resolution camera and is equipped with two 4000-lumen floodlights. This camera was used solely for navigation, as configuring its settings to our needs was challenging.
A GoPro Hero Black 11 camera (GoPro, USA) was mounted and tilted at a 45° angle from the ROV (Figure 2a), providing an oblique view of the seabed. The GoPro was used as the main camera and provided the frames used in substrate and fauna classification. The GoPro camera records GPS data at one-second intervals, enabling geolocation of collected data by interpolating the last position recorded before submerging the ROV with the first after resurfacing, thus allowing the ROV’s path estimation. The lights were positioned to limit backscatter in the GoPro camera recordings.
The ROV was deployed at predetermined sites and positioned approximately 0.5-1.5 metres above the seabed, allowing it to drift with the survey vessel for approximately a 15-minute interval.
Only one frame per 10-second recording was retained to ensure comprehensive data without overlap in frames, thus covering most of the area. The first frame in each block was selected for annotation. Additionally, the analyst reviewed the frame directly before and after the selected frame to identify potentially hidden organisms. All frames were annotated using the open-source software LabelMe (https://github. com/wkentaro/labelme).
Figure 1: Sites for recording with underwater ROV in the Greater North Sea off the northwest coast of Denmark. The sampling sites are shaped per area: A) circle, B) square, and C) triangle. The number adjacent to the point shows the site number. The lilac line is the 12-nautical-mile line that indicates where the Danish territory ends. Made with QGIS.
In cases where the entire frame and video were blurry, had a high level of marine snow, or the camera angle was off the seabed, the whole block was labelled low quality. When the seabed was visible, taxa were noted and classified. Frames were labelled as low quality if taxa could not be detected.
Each frame was labelled according to its substrate, either stone reef or sand, as per the definition in section 2.4.
The substrates observed in the frames were classified based on the revised European Nature Information System (EUNIS) habitat
classification [23]. EUNIS is a comprehensive classification system for European terrestrial and marine substrates based on descriptors and environmental parameters. The classification system is hierarchically structured and includes an online key [24]. Using this key, two substrate types are identified:
• Sand (MC52 Atlantic circalittoral sand [25]) are substrates predominantly made up of sand and, to some degree, affected by wave actions or tidal currents. These are found between 0-50 metres. Sand substrates must have a minimum of 70% cover in this study and have mussel shells and stone with less than 30% cover.
• Stone reef (MC12 Atlantic circalittoral rock [26]) is classified as predominantly bedrock and boulders at 10-50 metres depth and subject to tidal stream. This study classified areas with more than 30% rock cover (determined by visual inspection) as stone reefs.
Every frame was annotated for all fauna taxa to the most specific taxonomic level possible. A cautious approach was taken in cases where there was uncertainty in classification due to low resolution, obscured views (e.g., behind objects or in the background), or morphological similarities that made it difficult to distinguish taxa based solely on
the frames. In such instances, annotations of taxa were kept to lower-taxonomic resolutions (e.g., genus, family) to avoid incorrect classifications.
Quantitative measures of taxa included taxa richness and abundance to determine taxa composition. Taxa richness was defined as the total number of unique taxa and abundance as the total number of individuals. Both were determined by counting the total number of different taxa and individuals observed within each site. Taxa richness and abundance were determined in two groups: one included all individuals at the highest taxonomic level, and the second included only taxa identified at the species level. The second group was included
Table 1: Overview of sampling efforts with the ROV at each site with the corresponding date (DD-MM-YY), depth (in metres), and the data output in the number of frames extracted from the camera, recording time, number of frames annotated as high- or low-quality frames, and substrate (sand, stone reef, non-identifiable). All frames are publicly available at https://www.kaggle.com/amandairlind/datasets.
to assess the extent at which taxa can be classified at the species level using frames.
2.6 Visualization
Visualizations were made in R version 4.3.1 via RStudio version 2023.06.2+561 (http:// www.rstudio.com) using the packages dplyr [27], Tidyverse [28], and ggplot2 [29], and in QGIS version 3.32.1.
3.1
Overall, the ROV was easy to handle when launching, manoeuvring, live video feed, and retrieving. The live video feed and the remote control allowed adjustments of the ROV’s direction or distance from the seabed when obstacles, mainly large stones, appeared in its path. It was possible to maintain the ROV’s stability while drifting at 1-2 knots with minimum thruster usage. Stronger currents (above 2 knots) resulted in shorter
recording times and increased motion blur (unclear focus of objects). The thrusters were used to counteract the strong current, but this increased battery consumption. In strong current sites, particularly in Area C, half of the battery was depleted after 30 minutes, whereas approximately 25% of battery power remained in sites with less current (Area A). Significant battery consumption occurred during the ROV’s deployment and retrieval to counteract drifting caused by waves and swells. Generally, we observed that battery power consumption correlated with current strength but not depth. In Area A (depth 26-36 m) (Table 1), the ROV had more battery power remaining after retrieval than in Area B (depth 15-24 m) and Area C (depth 24-27 m).
Sampling resulted in 976 frames covering 163 minutes of seabed recordings, with 763 high-quality frames and 213 low-quality frames (Table 1). Areas A and B had similar
frames (412 and 413 frames), while Area C had fewer frames (151 frames). The quality of frames differed between areas: Area A had 338 high-quality frames and 74 low-quality frames, whereas Areas B and C had almost one-third of their frames categorized as low-quality (127 low-quality frames for Area B and 69 lowquality frames for Area C) (Figure 3).
Sand was the most abundant substrate, observed in all areas and sites (representing 513 out of 976 frames and 85.5 minutes) (Table 1, Figure 3). Stone reef substrate was observed in all areas but only in five sites (Sites 1, 7, 8, 9, and 10), accounting for a total of 327 frames. The higher number of sand frames compared to stone reef frames is not due to a higher quality of frames in the sand substrate but because sand was the dominant substrate in the entire study area, while stone reefs occurred in patches. Classifying stone reef substrate was
easier than sand substrate in murky water (dark or cloudy water), as the stone reef structure and the fauna’s colour contrasted with the water. The sand substrate was less apparent in murky water and primarily classified based on ripples.
Of the 213 low-quality frames, 136 were labelled “not identifiable” (Table 1) as the substrate could not be determined. These frames were not allocated to a single site but were found in nine of the 12 sites. The three sites with no “not identifiable” frames were all in Area A (Table 1), which had an overall higher visibility. Area B had 86 frames labelled “not identifiable,” with 58 of these frames from a single site (Site 5). Similarly, Area C had one site (Site 10) accounting for half of the area’s “not identifiable” frames (26 out of 44 frames).
3.4 Detection and Identification of Taxa
Out of the 763 frames classified as high-quality with identified substrates, 73 frames contained
detectable organisms (see all frames at https:// www.kaggle.com/amandairlind/datasets).
Taxa were observed in all three areas and in nine out of the 12 sites (except for sites 3, 5, and 12). No detectable organisms were found in five sand sites (Sites 3, 5, 9, 10, and 12), while organisms were observed at all stone reef sites, though not in every frame. Area C had a lower abundance than Areas A and B, with only 13 individuals detected in Area C. One soft coral species, dead man’s fingers (Alcyonium digitatum), accounts for 12 of the 13 individuals. Area A had six taxa, while Area B had the highest richness with seven taxa.
A total of 10 unique taxa were detected. Of these, nine were found in the sandy substrate,
and six of the 10 taxa were found in the stone reef substrate (Figure 4). The abundance differed greatly, with 69 individuals detected in the sand substrate, while the stone reef substrate had an abundance four times higher (319 individuals). The abundance in the sand and stone reef substrates was dominated by A. digitatum (59 individuals in sand and 263 individuals in stone reef). Stone reef substrates also had a high abundance of Bryzoan (flustra foliacea and gymnolaemata) while one Bryzoan was observed in sand. In the sand substrate, two worm taxa were observed (polychaete sp. and Lanice conchilega).
The quality of frames, particularly the resolution, affected the detectability of sizes.
All individuals were categorized as macrofauna (retained by >1 mm mesh) and epifauna, except for the worm taxa (infauna). The frame resolution also affected the taxonomic resolution, with only five of 10 taxa classified at the species level.
In this study, we tested the monitoring potential of a low-cost ROV in various areas and substrates subject to turbidity and strong currents. The ROV performed well at depths from 15-34 m. Given that models like ours have a depth rating to 200 m, it is expected that it can provide data at greater depths, though potentially limited in battery capacity. Compared to other monitoring tools, such as seabed towed camera systems, grabs, and sledges, which are limited to soft-bottom substrates, the ROV functioned well in both sand and stone reef substrates. Considering the ROV’s versatility, it is expected to function in other substrates such as mussel beds, muddy areas, and eelgrass beds. The ROV’s easy manoeuvring and live video feed help avoid collisions, which is especially important in eelgrass beds, where the thruster could become entangled in the leaves and cause damage to both the eelgrass bed and the ROV.
Compared to seabed-towed or larger camera systems and scientific ROVs, small eyeball class ROVs are easy to use, require little training, and can be deployed from smaller vessels or even from the coast in coastal shallow areas where a vessel cannot be used. Smaller drop cameras can, in many aspects, be used similarly to the small ROV; however,
the drop cameras are less flexible to instantly adjust heading and rely on drifting to cover the same area as the ROV.
The greatest challenge encountered in this study and with underwater cameras, in general, is the occurrence of colour distortions because red wavelengths are absorbed faster than the blue and green, which reduces the visible colours [30]. Other challenges are the environmental factors such as murky water and organic matter in the water column (marine snow) and strong currents, which reduce frame quality [13], [31], [32]. These conditions are common in most marine habitats, especially in depths from 0 to 100 metres. Coincidentally, such areas require extensive monitoring due to concentrated human activities (e.g., fishing, sailing, coastal infrastructure), habitat diversity (e.g., stone reef, coral reef, mangroves), and high exposure to pollution and contamination [33].
Environmental factors cannot be controlled; hence, frame quality must be improved by adjusting the camera and floodlight in a position and with a lumen to minimize backscatter from marine snow [13], [34]. Complete avoidance of backscatter in turbid water is not possible, as seen in this study. Therefore, post-processing is essential to improve frame quality. Several post-processing tools have shown promising results in enhancing frame quality [30], [35], [36], [37]. By improving the frame quality, more accurate and detailed information can be extracted [30], [37]. In our study, 14% of the frames were of such low quality that the substrate could not be identified, and organisms could not be
detected in 22% of the frames. Implementing a post-processing tool to remove marine snow and enhance colour will increase the number of frames suitable for substrate and species-level identification, thereby strengthening the ROV’s monitoring potential.
Enhanced frame quality could potentially improve the taxonomic resolution [37]. Only 50% of the different taxa could be classified at the species level due to issues such as organisms being too small, cryptic, or partially obscured in complex structural habitats (e.g., Bryzoan in stone reefs) to be accurately identified from frames alone [14], [31], [32]. This limitation is common to all camera systems but not a problem for sampling equipment such as grabs, survey trawls, and sledges, which collect specimens, allowing for species-level classification. These sampling methods also enable the sampling of smaller and infauna species that are not visible with low-cost ROVs. However, these issues are not unique to small, low-cost ROVs, as even industry cameras are unable to penetrate the sediment and observe infauna.
Manual data processing is time-consuming and laborious. Annotation protocol [32], [38] and automated recognition or analysis models have been successfully developed for other ecosystems to determine spatial cover (e.g., of stone reefs) [39], identify taxa [40], [41], and highlight salient objects [42]. These models require a strong ground truth to be effective. No models have been specially designed for our environment, but existing models can be modified and trained on our data, thereby increasing the efficiency and replicability of
annotation and monitoring capabilities. Our data allowed us to determine taxa richness and abundance, but overcoming the challenges and limiting the laborious task will enhance the effectiveness of ROVs in marine monitoring.
The difference in macrofauna composition between the sand and stone substrate is primarily due to the substrate itself. Bryzoans and dead man’s finger, which dominate in stone reefs, require hard surfaces to attach to. This explains their lower abundance in sand substrates, where these taxa were only observed if hard substrates were present, such as smaller stones. As a result of these two taxa, the abundance was higher in stone reefs compared to the sand substrates. Despite that they cover a smaller proportion of the seabed than the sandy substrate, as seen in our study, they are ecologically important as they provide substrate and shelter for a variety of species [43], [44].
Counting dead man’s fingers and Bryzoans as individuals can be problematic because they have multiple branches, overlap with each other, or are layered. Therefore, determining these species in terms of biomass would be more informative. Biomass determination can be achieved by mounting a laser pointer on the ROV (Figure 2b), which allows for measuring the size of organisms or spatial coverage [45]. Size can be correlated with biomass and used to estimate age. This method can also be applied to other species to determine their size and biomass. Furthermore, data can be converted into biodiversity measures or coupled with traits and functionality to provide ecological information about the ecosystem.
ROVs are effective and versatile monitoring tools, but several challenges must be addressed to maximize their potential. The most important improvement needed is the frame quality to enhance the accuracy of the data. This can be achieved by adjusting the system itself or enhancing quality through post-processing. For ROVs to be widely adopted in monitoring programs, post-processing tools must be approachable to individuals with varying professional backgrounds.
Improving frame quality can enhance species identification, which is currently challenging due to morphological similarities and motion blur that limit the ability to identify at the species level. However, some species are morphologically similar and can only be distinguished by having a physical specimen or DNA samples. Combining camera observations with eDNA can provide the final information at the species level and additional information on species present in the environment that might not have been observed. eDNA samples can be taken simultaneously with the ROV’s recordings by mounting a filter that collects particles in the water or a water/sediment sampler.
Additionally, higher resolution on the camera is needed to enable the detection of smaller organisms. To demonstrate the ROV’s versatility, further studies in various substrates, depths, and marine environments are needed to ensure replicability and to streamline the sampling method.
Our study demonstrated the effectiveness of a low-cost ROV for monitoring two marine
substrates in low visibility due to turbid and murky water. Enhancing the image quality could improve organism detection and taxa classification, and methods are needed to make versatile and replicable ecological assessments. This study provides essential information that can be used in a management context regarding substrates, species composition, biodiversity, and seabed state.
The funding for this study was provided by the European Maritime and Fisheries Fund and the Ministry of Environment and Food of Denmark (Grant numbers 33113-B-23-190). We want to express our gratitude to Thorupstrand Kystfiskerlaug, particularly Thomas Højrup and Tommy. We also wish to thank the crew of Skarreklit: Jørgen Nissen, Jørgen Legård, Palle Jensen, Lars Monczuk, Villy Petersen, and Erik Troelsen. A special thanks goes to Per Vilsen for his additional and valued help in coordination. Furthermore, a thank you should go to Malte Pedersen for his assistance with the frame recordings.
[1] H. Frigstad et al., Andersen, H. Trannum, M. McGovern, L. Naustvoll, O. Kaste, A. Deininger og D. Hjermann, “Three decades of change in the Skagerrak coastal ecosystem, shaped by eutrophication and coastal darking,” Estuarine, Coastal, and Shelf Sci., vol. 283, p. 108193, 2023, doi: 10.1016/j.ecss.2022.108193.
[2] A. Palialexis et al., “Monitoring biodiversity for the EU Marine Strategy Framework Directive: Lessons learnt from evaluating the official reports,” Mar. Policy, vol. 128, p. 104473, 2021, doi: 10.1016/j.marpol.2021.104473.
[3] European Union, “Directive 2008/56/EC of the European Parliament and of the Council of 17 June 2008 establishing a framework for community
action in the field of marine environmental policy” (Marine Strategy Framework Directive), 2008.
[4] European Union, “Council Directive 92/43/EEC of 21 May 1992 on the conservation of natural habitats and of wild fauna and flora,” Official Journal of the European Union, vol. 206, no. 7, p. 50, 1992.
[5] G. Van Hoey et al., “Methodological elements for optimising the spatial monitoring design to support regional benthic ecosystem assessments,” Environ. Monit. Assess., vol. 191, no. 7, pp. 423-13, 2019, doi: 10.1007/s10661-019-7550-9.
[6] S. Birk et al., “Three hundred ways to assess Europe's surface waters: An almost complete overview of biological methods to implement the Water Framework Directive,” Ecological Indicators, vol. 18, pp. 31-41, 2012, doi: 10.1016/j. ecolind.2011.10.009.
[7] N. Zampoukas et al., “Technical guidance on monitoring for the Marine Stategy Framework Directive,” 2014.
[8] H. Rumohr, “Soft bottom macrofauna: Collection, treatment, and quality assurance of samples,” ICES Techn. In Mar. Environ. Sci., no. 43, 2009, doi: 10.25607/OBP-238.
[9] R. Rosenberg and H.C. Nilsson, ”Deterioration of soft-bottom benthos along the Swedish Skagerrak coast,” J. Sea Res., vol. 54, no. 3, pp. 231-242, 2005, doi: 10.1016/j.seares.2005.04.003.
[10] M. Obst et al., “Marine long-term biodiversity assessment suggests loss of rare species in the Skagerrak and Kattegat region,” Mar. Biodiv., vol. 48, no. 4, pp. 2165-2176, 2018, doi: 10.1007/ s12526-017-0749-5.
[11] D. Morris, D. Speirs, A. Cameron, M. Heath, “Global sensitivity analysis of an end-to-end marine ecosystem model of the North Sea: Factors affecting the biomass of fish and benthos,” Ecological Model., vol. 273, pp. 251-263, 2014, doi:10.1016/j.ecolmodel.2013.11.019.
[12] I. Kröncke et al., “Changes in North Sea macrofauna communities and species distribution between 1986 and 2000,” Estuarine, Coastal and Shelf Sci., vol. 94, no. 1, pp. 1-15, 2011, doi: 10.1016/j.ecss. 2011.04.008.
[13] E. Sheehan et al., “An experimental comparison of three towed underwater video systems using species metrics, benthic impact and performance,” Methods in Ecol. and Evol., vol. 7, no. 7, pp. 843852, 2016, doi: 10.1111/2041-210X.12540.
[14] A. Williams, F. Althaus, T. Schlacher, ” Towed camera imagery and benthic sled catches provide different views of seamount benthic diversity,” Limnol. and Oceanogr. Methods, vol. 13, no. 2, pp. 62-73, 2015, doi: 10.1002/lom3.10007.
[15] M. Lacharité and C. Brown, “Utilizing benthic
habitat maps to inform biodiversity monitoring in marine protected areas,” Aquatic Conservation, vol. 29, no. 6, pp. 938-951, 2019, doi: 10.1002/ aqc.3074.
[16] S. de Mendonça and A. Mextaxas, “Comparing the performance of a remotely operated vehicle, a drop camera, and a trawl in capturing deep-sea epifaunal abundance and diversity,” Frontiers in Mar. Sci., vol. 8, 2021, doi: 10.3389/fmars.2021.631354.
[17] R. Easton, S. Heppell, R. Hannah, “Quantification of habitat and community relationships among nearshore temperate fishes through analysis of drop camera video,” Mar. and Coastal Fisheries, vol. 7, no. 1, pp. 87-102, 2015, doi:10.1080/19425 120.2015.1007184.
[18] N. Bethoney and K. Stokesbury, “Methods for image-based surveys of benthic macroinvertebrates and their habitat exemplified by the drop camera survey for the Atlantic Sea Scallop,” J. Vis. Exper., vol. 137, 2018, doi: 10.3791/57493.
[19] A. Yusof, M. Nor, M. Aras, S. Shamsudin, “Opensource platform comparison for research and development in underwater drones,” Proceedings of the 12th National Technical Seminar on Unmanned System Technology 2020, Singapore, 2021, pp. 139-151.
[20] R. Pacunski, W. Palsson, H. Greene, H. Gunderson, “Conducting visual surveys with a small ROV in shallow water,” Marine Habitat Mapping Technology for Alaska, 2008, pp. 109-128, doi: 10.4027/mhmta.2008.08.
[21] E. Buscher, D. Mathews, C. Bryce, K. Bryce, D. Joseph, N. Ban, “Applying a low cost, mini remotely operated vehicle (ROV) to assess an ecological baseline of an indigenous seascape in Canada,” Frontiers in Mar. Sci., vol. 7, doi: 10.3389/fmars.2020.00669.
[22] O. Eigaard et al., “The footprint of bottom trawling in European waters: distribution, intensity, and seabed integrity,” ICES J. Mar. Sci., vol. 74, no. 3, pp. 847-865, 2017, doi: 10.1093/ICESJMS/ FSW194.
[23] C. Davies, D. Moss, M. Hill, “EUNIS Habitat Classification Revised 2004,” 2004, pp. 127-143.
[24] European Environment Agency. “Habitat types key navigation.” EUNIS. Accessed: April 4, 2024. [Online]. Available: https://eunis.eea.europa.eu/ habitats-key.jsp
[25] European Environment Agency. “Atlantic circalittoral sand.” EUNIS. Accessed: April 4, 2024. [Online]. Available: https://eunis.eea.europa. eu/habitats/31202p
[26] European Environment Agency. “Atlantic circalittoral rock.” EUNIS. Accessed: April 4, 2024. [Online]. Available: https://eunis.eea.europa. eu/habitats/30972.
[27] H. Wickham, R. François, L. Henry, K. Müller, “dplyr: A grammar of data manipulation,” R package version 0.4, vol. 3, p. 156, 2015.
[28] H. Wickham et al., “Welcome to the Tidyverse,” J. Open Source Softw., vol. 4, no. 43, p. 1686, 2019, doi: 10.21105/JOSS.01686.
[29] H. Wickham, ggplot2 : Elegant graphics for data analysis, 1st ed., New York, NY, USA: Springer, 2009.
[30] Y. Wang, W. Song, G. Fortino, L. Qi, W. Zhang, A. Liotta, “An experimental-based review of image enhancement and image restoration methods for underwater imaging,” Access, vol. 7, pp. 140233-140251, 2019, doi: 10.1109/ACCESS. 2019.2932130.
[31] C. Jac et al., “Assessing the impact of trawling on benthic megafauna: comparative study of video surveys vs. scientific trawling,” ICES J. Mar. Sci., vol. 78, no. 5, pp. 1636-1649, 2021, doi: 10.1093/ icesjms/fsab033.
[32] B. Taormina et al., “Optimizing image-based protocol to monitor macroepibenthic communities colonizing artificial structures,” ICES J. Mar. Sci., vol. 77, no. 2, pp. 835-845, 2020, doi: 10.1093/ icesjms/fsz249.
[33] R. Seitz, H. Wennhage, U. Bergström, R. Lipcius, T. Ysebaert, “Ecological value of coastal habitats for commercially and ecologically important species,” ICES J. Mar. Sci., vol. 71, no. 3, pp. 648665, 2014, doi: 10.1093/icesjms/fst152.
[34] R. Taylor et al., “Evolution of a benthic imaging system from a towed camera to an automated habitat characterization system,” in OCEANS 2008, Quebec City, QC, Canada, 2008, pp. 1-7 doi: 10.1109/OCEANS.2008.5152032.
[35] Z. Lyu, A. Peng, D. Wang, D. Ding, “An efficient learning-based method for underwater image enhancement,” Displays, vol. 74, p. 102174, 2022, doi: 10.1016/j.displa.2022.102174.
[36] B. Cyganek and K. Gongola, “Real-time marine snow noise removal from underwater video sequences,” J. Electron. Imag., vol. 27, no. 4, p. 043002, 2018, doi: 10.1117/1.JEI.27.4.043002.
[37] J. Ahn, S. Yasukawa, T. Sonoda, T. Ura, K. Ishii, “Enhancement of deep-sea floor images obtained by an underwater vehicle and its evaluation by crab recognition,” J. Mar. Sci. Technol., vol. 22, no. 4, pp. 758-770, 2017, doi: 10.1007/s00773-017-0442-1.
[38] F. Althaus et al., “A standardised vocabulary for identifying benthic biota and substrata from underwater imagery: The CATAMI Classification Scheme,” PloS One, vol. 10, no. 10, p. e0141039, 2015, doi: 10.1371/journal.pone.0141039.
[39] G. Raber and S. Schill, “A low-cost small unmanned surface vehicle (sUSV) for very high-resolution mapping and monitoring of shallow marine habitats,” Proceedings of Remote Sensing of the Ocean, Coastal Waters, and Large Water Regions,
vol. 11150, 2019, doi: 10.1117/12.2531361.
[40] M. Ahmed, L. Peña-Castillo, A. Vardy, P. Gagnon, “Improving detection and localization of green sea urchin by adding attention mechanisms in a convolutional network,” J. Ocean Technol., vol. 19, no. 2, pp. 82-97, 2024. [Online]. Available: www.thejot. net/article-preview/?show_article_preview=1532
[41] M. Pedersen, J. Bruslund Haurum, R. Gade, T. Moeslund, N. Madsen, “Detection of marine animals in a new underwater dataset with varying visibility,” in Proc. of the IEEE/CVF Conf. on Comput. Vis and Pattern Recognit. (CVPR) Workshops, Long Beach, California, USA, Jun. 2019, pp. 18-26.
[42] N. Kumar, H. Sardana, S. Shome, N. Mittal, “Saliency subtraction inspired automated event detection in underwater environments,” Cogn. Comput., vol. 12, no. 1, pp. 115-127, 2020, doi: 10.1007/s12559-019-09671-x.
[43] C. Stenberg, et al., “Ecological benefits from restoring a marine cavernous boulder reef in Kattegat, Denmark,” DTU Aqua Rep., vol. 49, 2015.
[44] J. Svendsen et al., “The importance of reef habitats for fish, harbor porpoise and fisheries management,” DTU Aqua Rep., vol. 82, 2020.
[45] F. Dias et al., “Area estimation of deep-sea surfaces from oblique still images,” PloS One, vol. 10, no. 7, p. e0133290, 2015, doi: 10.1371/journal.pone. 0133290.
MASTER'S STUDENT MARITIME STUDIES (SAFETY: THE HUMAN ELEMENT) FISHERIES AND MARINE INSTITUTE OF MEMORIAL UNIVERSITY OF NEWFOUNDLAND
ST. JOHN'S, NL, CANADA
Ankan Bhattacharjee works with the OpenAR project, which aims to develop an augmented reality (AR)-based application interface tailored for advanced maritime operations, such as vessel navigation, icebreaker assistance, and cargo operations. The objective is to reduce human error and enhance safety at sea. His primary focus is the evaluation of maritime navigation operators’ performance by measuring situational awareness and mental workload in an AR-aided navigational interface.
By creating an open platform, the project enables companies to develop consistent, usercentred AR interfaces that integrate across diverse systems, ensuring safe, cost-effective, and adaptable solutions for both current and future autonomous maritime operations.
The safety of life at sea is paramount. By promoting marine safety and efficiency, this research benefits maritime operators, system designers, autonomous developers, regulators, maritime companies, and academia. Further, by reducing human error, it contributes to the protection of marine ecosystems and supports sustainable navigation.
sea, where he witnessed two major accidents: a deck crew member tragically died during cargo handling and a second engineer broke his fingers during exhaust valve maintenance. These incidents profoundly motivated him to contribute to maritime safety from an academic perspective. After transitioning as a marine engineer from the maritime industry into academia and marine education and training, he became interested in different advanced technologies (AR, virtual reality) related to the maritime field. This also motivated him to join the OpenAR project.
Ankan’s interest in maritime safety research was influenced by his first-hand experience at https://www.mi.mun.ca/programsand courses/programs/masterofsciencein maritimestudiessafetythehumanelement/ ankanb@mun.ca
PHD CANDIDATE
WORLD MARITIME UNIVERSITY
MALMo, SWEDEN
Originally from Greece, Antonios Andreadakis graduated from the Greek Merchant Marine Academy. He holds a Captain Class C certificate as well as an M.Sc. in maritime safety and environmental administration from the World Maritime University in Sweden. Before continuing his studies, he gained valuable experience working on tanker ships as a Second Officer.
As a PhD candidate, Antonios focuses on identifying the key factors that contribute to unsuccessful evacuations on cruise and passenger ships. He aims to investigate these factors in depth and explore the countermeasures that can be taken to improve evacuation outcomes. Additionally, he is examining the role of training, psychological factors, ship design, human nature, and new technologies to determine how they can be applied to enhance evacuation times and efficiency. The ultimate goal is to provide actionable insights that can help shape safer maritime practices and reduce the risks to passengers and crew during emergencies at sea.
The impact of this research is significant as it directly addresses the safety of millions of people who travel by sea in large passenger carriers each year. By improving evacuation procedures on cruise and passenger ships, this work seeks to reduce life-losses during maritime emergencies, safeguarding human
lives. In addition, the research has broader implications for the shipping industry, promoting a culture of safety and preparedness, which can reduce the likelihood of catastrophic events.
For Antonios, knowing that his research has the potential to save lives and could uncover key insights to prevent future tragedies is incredibly fulfilling. By investigating the factors contributing to unsuccessful evacuations and exploring innovative solutions, he hopes to make a meaningful impact in preventing such tragedies. He is also driven by the potential to leverage new technologies to enhance evacuation processes, ensuring a safer future for all who travel by sea.
www.wmu.se aan@wmu.se
Researchers propose using Reconfigurable Intelligent Surfaces in machine learning-assisted underwater communication, supported by a comprehensive bibliometric analysis.
This study is for anyone interested in the cutting-edge developments of Reconfigurable Intelligent Surfaces (RISs) and their revolutionary applications in machine learning-assisted underwater communications.
RIS is an emerging technology that uses principles from electromagnetics, signal processing, and antenna theory to control the transmission of electromagnetic waves in wireless communication channels. When merged with machine learning, RISs could revolutionize wireless underwater communication by offering a more efficient, cost-effective, and adaptable solution.
The authors examine potential signalling technologies in a RIS-assisted underwater environment, including the Internet of Underwater Things (IoUWT), various RIS implementation approaches, and hardware architecture. They also investigate machine learning-enabled optimization techniques for RIS-aided networks. Their pioneering systematic bibliometric analysis on RISs gives insight into research trends and citation patterns in the published literature on RISs.
The findings will establish a baseline for evaluating the future path for RIS publications. They raise awareness of the critical areas of research that demand immediate exploration, especially in the application of Artificial Intelligence in RISs and the profound influence of RISs on machine learningassisted underwater communications.
Mohamed Ashraf Ouf earned his B.Sc. in computer engineering from Arab Academy for Science, Technology, and Maritime Transport in July 2023. His passion revolves around integrating cutting-edge technologies to revolutionize industrial standards. With a focus on harnessing the power of AI, he is dedicated to unlocking new possibilities across diverse sectors. Continuously seeking innovative ways to merge ML, he aims to tackle complex challenges and elevate operational efficiency to unprecedented heights.
Abdelrahman Elkhateeb earned his B.Sc. and M.Sc. from the German University in Cairo in information engineering and technology with a focus on communications. He currently works at Orange Business in Egypt, specializing in network and SD-WAN solutions. His interests include 5G and 6G technologies, Non-Orthogonal Multiple Access, Multiple Input
Multiple Output systems, and RIS, driving his contributions to nextgeneration mobile communications solutions.
Abdelmoneim Soliman achieved his B.Eng. in mechanical engineering in 2016 from Arab Academy for Science, Technology, and Maritime Transport. He delved into the intricacies of mechatronics during his coursework. Currently, he is on the verge of attaining his B.Tech. in engineering and applied science from the Marine Institute of Memorial University, Newfoundland and Labrador. His areas of focus encompass the seamless integration of cutting-edge technologies such as AI, machine learning, and Digital Twins into the dynamic operations of marine vessels.
Dr. Md Safiqur Rahaman is a well-regarded leader in library affairs. He currently serves as the dean of library affairs at King Fahd University of Petroleum and Minerals in Dhahran, Saudi Arabia. With over 17 years of experience as a librarian and a PhD in library and information science, he provides exceptional research support services, including literature search, training, reference and information services, and research guidance. His bibliometric and scientometric studies specialization places him at the forefront of library and information science research.
Dr. Sara Mobarak earned her B.Sc. (Hons.) in information engineering and technology with a major in communications from the German University in Cairo (GUC) in 2018. She completed her M.Sc. in 2019 and her PhD in 2023, both from GUC. Currently, she is pursuing postdoctoral studies at the University of Ottawa, Canada, focusing on mobile and wireless communications, resource allocation, power control, and machine learning.
Dr. Mervin A. Marshall, P.Eng., is a faculty member at the Marine Institute of Memorial University in Newfoundland and Labrador. With a strong focus on structural integrity monitoring using stochastic processes, finite element analysis, hydro-elastic modelling, and applied mechanics, he brings a wealth of expertise to his work. His current research delves into the application of AI, ML, and Digital Twin technology to optimize the operational phase of marine vessels and offshore structures.
Mohamed A. Ouf1, Abdelrahman Elkhateeb2, Abdelmoneim Soliman3, Md Safiqur Rahaman4, Sara Mobarak5, Mervin A. Marshall3*
1Arab Academy for Science, Technology and Maritime Transport, Cairo, Egypt
2Orange Business, Nile City, Egypt
3Fisheries and Marine Institute of Memorial University of Newfoundland, St. John’s, NL, Canada
4King Fahd University of Petroleum and Minerals, Dhahran, Kingdom of Saudi Arabia
5University of Ottawa, ON, Canada
*Corresponding author: mervin.marshall@mi.mun.ca
ABSTRACT
Underwater communication systems face unique challenges that require advanced research and technologies. Environmental factors such as surface scattering, harsh sea conditions, water currents, and marine life can disrupt the propagation of acoustic signals. Integrating Reconfigurable Intelligent Surfaces (RISs) into underwater communication systems is a promising solution to address these challenges. RISs enhance signal propagation by creating optimal environments through passive beamforming and phase tuning, reducing scattering and absorption. This research proposes integrating RIS technology with machine learning (ML) techniques in underwater communication systems, leveraging recent advancements in both fields. This paper is the first to combine RIS technology with ML techniques in underwater communications while offering a comprehensive bibliometric analysis of RISs.
Keywords: Reconfigurable Intelligent Surfaces (RISs); Bibliometric Analysis; Wireless Communication; Underwater Communications; Machine Learning (ML); Artificial Intelligence (AI); Research Productivity; Internet of Underwater Things (IoUWT); Challenge State Information (CSI); Non-Orthogonal Multiple Access (NOMA); Multiple Input Multiple Output (MIMO)
1.1
Reconfigurable Intelligent Surfaces (RISs) represent a groundbreaking technology that merges principles from electromagnetics, signal processing, and antenna theory to dynamically manage the transmission of electromagnetic waves in wireless communication channels [1], [2], [3], [4]. RISs consist of an array of passive reflecting elements, each capable of independent tuning to introduce phase shifts to incident signals [5], [6], [7], [8]. These meticulously designed planar structures feature reconfigurable properties enabled by integrated electronic circuits [9]. By programming these circuits, we can control the reflection of incoming incident electromagnetic waves, creating an innovative and adaptable wireless communication environment [10], [11]. The key advantages of RIS technology are:
1. Energy Efficiency: RIS elements consume minimal power, enhancing wireless network performance without significantly increasing power consumption [12].
2. Cost-Effectiveness: The technology can be implemented using low-cost materials and integrated with existing infrastructures, providing an economical solution for improving wireless communication systems [13], [14], [15].
3. Spectrum Optimization: RIS technology optimizes spectrum use by mitigating interference, enhancing the signal-to-noise ratio (SNR), and enabling more efficient utilization of available frequency resources [16].
Overall, RIS technology offers a promising approach to creating more efficient,
cost-effective, and adaptable wireless communication networks.
RISs, which are made from cost-effective materials, have lightweight and low-profile designs that make them easy to deploy in modern networks and attach to various surfaces such as building facades, walls, ceilings, and windows [17]. By optimizing the phase shifts of the RISs, the signals transmitted via the reflecting channel link can be added constructively at the intended receiving user or destructively at the interferer, resulting in a higher rate compared to not deploying RISs [9]. Nonetheless, conventional passive RISs can only reflect the incident signal without introducing any gain [18]. Also, the capacity gain achieved by passive RISs is limited because of the multiplicative fading effect, which is very significant in communication scenarios with strong line-of-sight (LOS) links between the base station (BS) and the users [14], [19]. To address these issues, active RISs have been suggested. In active RISs, the incident electromagnetic signal is amplified, and its phase shift is favourably adjusted simultaneously. Compared with amplify-andforward (AF) relays, active RISs operate in full-duplex (FD) mode and rely on low-power reflection amplifiers instead of power-hungry radio frequency chains [20].
RIS technology is recommended for underwater communications because of its ability to create favourable propagation environments using passive beamforming and wave manipulation. However, there are challenges. This technology can effectively address these challenges, such as severe scattering and absorption, high path
loss, multipath fading, non-line-of-sight (NLoS) scenarios, harsh sea conditions, and the dynamic nature of the underwater environment, by reducing signal scattering and absorption [21], [22].
Although RIS technology holds great promise for underwater communication deployment, it also faces several obstacles [23]. These obstacles range from the need for physical robustness to withstand harsh underwater conditions to dynamic reconfiguration in response to constantly changing underwater topography. Moreover, integrating RISs with existing underwater communication infrastructure requires careful consideration to ensure compatibility and realize the full benefits of this technology. Nonetheless, RISs remain an effective technology for improving signal propagation characteristics by concentrating signal power in the desired direction, making the communication environment reconfigurable [24]. One of the most effective approaches to reconfiguring RISs in underwater communications is to use different machine-learning techniques [1].
In the literature, there are several works that explore the use of RISs in communication networks. These works can be categorized into three main groups:
1. RIS-Assisted Wireless Communication Networks: In reference [9], the authors discussed the use of an unmanned aerial vehicle (UAV) with an active RIS operating in the terahertz (THz) band. They considered a scenario involving a cellular user and multiple device-to-device (D2D) links.
The authors proposed a joint power allocation and active RIS precoding matrix optimization scheme to maximize the uplink sum rate, considering coexisting D2D links. Their simulations demonstrated that their proposed active RIS-aided approach outperformed other schemes, achieving the highest sum rate. In [25], the authors proposed a dynamic RIS subarray structure to enhance the performance of a THz downlink multiple-input-multipleoutput (MIMO) communication system. They introduced a weighted minimum mean square error-RIS local search (WMMSELS) scheme with limited RIS phase shift accuracy and an adaptive block coordinate descent (BCD)-aided joint beamforming approach to improve throughput performance for the investigated RIS THz system. In [26], the authors developed an RISenhanced uplink user-centric network (UNC), where RISs are used to enhance the signal quality of uplink transmission for users with minimal additional energy consumption. To achieve optimal energy efficiency, the authors jointly performed reflect beamforming at the RISs and uplink power control at users. The numerical results showed that their proposed algorithm could achieve significant gains in energy and spectral efficiency compared to the benchmark algorithm.
2. RISs and ML-Assisted Wireless Communication Networks: In reference [27], the authors propose a deep learning (DL)-based rate-splitting multiple access (RSMA) scheme for RIS-aided THz multi-user MIMO systems. Their approach involves a hybrid data-model driven DL-
based RSMA precoding scheme that includes passive precoding at the RISs, as well as analog active precoding and RSMA digital active precoding at the BS. The authors tested their scheme and demonstrated its advantages, showing that the proposed DL-based RSMA scheme enhances the robustness against challenge state information (CSI) imperfections and achieves higher spectral efficiency with lower signalling overhead in RIS-aided THz MIMO systems. In reference [28], the authors provide an overview of RISs and explain the operations and implementations of reinforcement learning (RL) algorithms for optimizing RIS technology parameters. They highlighted the significant performance improvements in communication systems when RL algorithms are implemented for RIS technology in wireless communications.
3. RISs in Underwater Communications:
Several papers in the literature discuss the use of reconfigurable intelligent surface (RIS) technology in underwater communications [29], [30], [31], [32]. In the first paper [29], the authors used RIS-assisted optical links to minimize the effects of skip zones and enable highspeed, efficient communication. They also proposed an RIS-assisted dual-hop underwater wireless optical communication system and derived mathematical expressions for outage probability and bit error rate for various modulation techniques. The authors validated their results using Monte-Carlo simulations and asymptotic analysis. In the second paper [30], they focused on analyzing the performance
of an RIS-assisted underwater optical communication system using a decode-andforward (DF) relaying protocol. The authors derived mathematical expressions for the total outage probability and average bit error rate (ABER) for various modulation schemes. Their findings highlighted the significant impact of the number of RIS elements, detection techniques, and optical turbulence on the system’s performance. In the third paper [31], the authors developed specific hardware to create an acoustic RIS system to address challenges in underwater communications. Their proposed acoustic RIS system demonstrated, through simulations, the ability to function as an underwater infrastructure enabling beamforming capabilities for various devices, such as small robots and lowcost sensors, by efficiently reflecting acoustic waves and significantly increasing communication data rates and distances.
In the fourth paper [32], the authors designed three key components of the acoustic RISs to realize the underwater RIS concept, including new acoustic RIS hardware, ultra-wideband beamforming (UWB), and a practical operation protocol. Additionally, the authors developed a practical operation protocol to implement acoustic RIS functionalities in complex underwater environments, and they validated their acoustic RIS design through COMSOL multi-physics simulations and end-to-end Bellhop-based simulations.
Based on the preceding discussions, it is evident that prior research has not explored the potential of deploying machine learning (ML) in RIS-assisted underwater communications.
This presents an exciting opportunity to harness the combined capabilities of ML and RIS technology in underwater communications, an area that is ripe for significant research and technological advancements.
Therefore, the focus is to combine the benefits of ML techniques and RIS technology in underwater communications, which poses significant propagation challenges. The goal is to integrate RIS technology and ML techniques in underwater communications, as previous literature has yet to do so. Initially, we aimed to include a comprehensive bibliometric analysis to integrate ML techniques with RIS technology. Nevertheless, the total number of publications considering RIS and ML techniques was less than 300, which is insufficient for a reliable bibliometric analysis. As a result, this paper will incorporate a detailed systematic bibliometric analysis of RISs; as far as we know, it represents the first comprehensive systematic bibliometric analysis of RISs.
The remainder of this paper is structured as follows: Section 2 introduces possible signalling technologies in RIS-Assisted Underwater Environments. Section 3 provides an overview of the Internet of Underwater Things (IoUWT). In Section 4, the joint power allocation and phase shift optimization are introduced, along with different approaches to deploying RISs underwater and hardware architectures. Section 5 summarizes MLenabled optimization techniques for RISaided networks. In Section 6, a systematic bibliometric analysis provides insights into research directions and citation patterns in the RIS field. Finally, Section 7 includes the conclusion and suggestions for future work.
In RIS-assisted communication systems, RISs play a crucial role in shaping the electromagnetic signal path between the transmitter (Tx) and receiver (Rx). As shown in Figure 1, the RIS consists of multiple passive reflecting elements that intelligently mould the wireless environment. The Smart controller allows precise control over each RIS element to introduce specific phase shifts, thus steering the beam toward the receiver with amplified signal strength and minimized interference.
In underwater environments, electromagnetic waves struggle to provide a strong signal because of challenges such as scattering and absorption. Therefore, alternative signalling technologies have been suggested to overcome these obstacles. Two suitable signalling technologies in underwater environments are acoustic communication and magnetic induction (or magnetic resonance):
1. Acoustic Communication. Transmitting signals underwater relies on acoustic signalling due to the high absorption of electromagnetic waves by water, especially saline water. This absorption significantly reduces the range and quality of electromagnetic signals. In contrast, acoustic waves can travel much longer distances underwater with minimal attenuation, making them more practical for communication in underwater environments. Speakers are used to transmit acoustic signals, which are then received using hydrophones. These signals
have frequencies ranging from a few Hertz to a few Megahertz (ultrasound). Acoustic signal propagation underwater is affected by scattering at uneven surfaces, water currents, and fish, resulting in severe frequency selectivity and high pass loss due to multipath, leading to a low effective data rate. While conventional RIS technology has been recommended for electromagnetic waves, deploying acoustic waves has also been proposed to address the challenges alluded to earlier [33].
In underwater environments using acoustic signalling, RISs are deployed to overcome the multipath effect, even in line-of-sight
(LOS) propagation, owing to frequency selectivity fading. Recent literature has suggested acoustic RIS technology, where uneven surfaces are concealed to eliminate scattering [34], [35], [36], [37].
Underwater communications present unique challenges that differ from those found in land-based environments. Signal transmission becomes more complex because there are many paths it can take, including direct paths, surface reflections, and bottom reflections (as shown in Figure Furthermore, ambient noise from passing vessels and biological noise from marine
life add to the complexity of signal reception. These impediments (when combined) make designing reliable communication systems for underwater applications challenging.
2. Magnetic Induction (or Magnetic Resonance). Magnetic induction or magnetic resonance involves creating nonpropagating quasi-static magnetic fields using induction coils within resonance circuits rather than traditional EM antennas [38]. Magnetic induction typically operates at low frequencies, ranging from a few kHz to around 13.56 MHz, resulting in a narrow frequency band. Due to the low frequency, the signal wavelength is very large and does not experience reflections or scattering. Consequently, it is not practical to deploy RIS technology alongside magnetic induction [39]. Nonetheless, utilizing magnetic induction waveguides
can achieve a similar effect through the passive relaying of magnetic fields [40]. When passive magnetic induction coils align in a wave, they induce secondary magnetic fields without consuming power. This phenomenon is analogous to pseudoreflections. By adjusting the impedance of the resonance circuit of each magnetic conduction relay, these pseudo-reflections become both tunable and reconfigurable. Therefore, passive magnetic induction relays represent a unique case of magnetic RISs, where each device functions as a single reflective element [21].
3.1 Overview of IoUWT
The Internet of Underwater Things is a
concept that aims to create large-scale wireless networks connecting devices below the water’s surface, like the Internet of Things (IoT) above the surface [41]. The IoUWT involves various underwater devices such as submarines, autonomous underwater vehicles (AUVs), sensors, and ships [42]. The main goal is to ensure that these devices have reliable connectivity in the challenging underwater environment, which differs significantly from terrestrial networks attributable to their impact on signal propagation and path loss. This is particularly evident in the underwater environment’s conductivity, which varies based on salinity, temperature, and pressure, significantly affecting the absorption of electromagnetic fields, and causing high path loss. This limits data rates to below 8 kbit/s for distances greater than 10 metres in seawater. It is also important to note that the conductivity in freshwater is 0.01 S/m and can reach 4-5 S/m in seawater.
Underwater communications primarily use acoustic waves because they are less susceptible to absorption compared to electromagnetic and other signalling techniques [43]. Acoustic communication transmits through pressure variations in the water, allowing signals to travel tens of kilometres in open water. However, this communication method faces challenges such as interference from marine life, signal scattering, and reflections caused by the heterogeneity of the underwater environment, including varying water currents with different pressures and temperatures. These factors lead to multipath propagation, frequency-selective, and time-variant channel responses [44].
Although some alternative communication methods (utilizing very low signal frequencies through magnetic induction or optical communications) have been proposed, they have not yet been implemented due to similar path loss issues [41].
There are various deployment scenarios for IoUWT, such as monitoring maritime animals, observing seismic activities to predict natural disasters like tsunamis, and communicating with underwater vehicles [43]. Some networks also incorporate floating buoys that gather data from submerged sensors. The primary challenge in all these scenarios is dealing with the time-variant multipath channels when using acoustic waves for communication. IoUWT is an area of significant research interest and has seen substantial advancements in recent years [45].
In this section, we introduce the various methods for deploying RISs underwater and discuss the hardware architecture for RIS-assisted networks in underwater communications.
For deploying RISs in the underwater medium, particularly within the context of IoUWT, the approaches that have been considered are:
• Stationary Deployment. The simplicity and minimal design challenges of this method make it particularly beneficial
for integrating into IoUWT [46]. One of its key advantages is that the static geometrical configuration ideally leads to relatively stable communication channels. On the contrary, this stability is generally only applicable for short distances. For longer distances, especially over 100 metres, the rapidly changing channels in the underwater medium present significant challenges [47].
• Autonomous Underwater Vehicles (AUVs). AUVs are expected to support the distributed nodes of the IoUWT by moving from one node to another like traditional mobile relaying [48]. Their key role is to improve connectivity and recharge node batteries, and they are also well-suited for carrying RISs, contributing to the smart underwater environment. This type of mobility can be adjusted to enhance signal propagation using the RIS’s phase shifting capabilities, in addition to the vehicles’ mobility [21]. The trajectory of the AUVs in this context is of particular interest, and one challenge related to their mobility scenarios is channel prediction. Unlike stationary deployment, the system configuration’s geometry is not fixed but changing, making communication channels time-varying. However, the relatively slow motion of the AUVs results in a long motion-related coherence time, meaning that the motion is not critical for the RISequipped AUVs. On the other hand, the AUVs’ surfaces are likely designed to reduce friction in water, which could otherwise be a primary issue for vehicle control. Therefore, RISs should cover only relatively small areas to minimize this effect while maintaining control over the smart environment [46].
• Floating RISs. The floating deployment is a strategic option for RISs that hold great promise. In this approach, the RISs are tethered to the seabed with cables, allowing them to be positioned at an optimal depth between underwater sensors and the surface. This method limits the maximum distance between the RISs and their anchor position on the ocean floor. By using this strategy, control over the position of the RISs is maintained, and it also helps stabilize the communication channels by maintaining a certain level of geometrical configuration [21].
N.B.: These different deployment methods limit the adaptability of RISs for underwater communication challenges, aiming to maximize communication effectiveness within underwater constraints.
In underwater communications, the hardware architecture for RISs is designed for autonomous operation. RISs extend beyond simply reflecting signals to include advanced features such as wireless sensors and actuators [23].
The proposed architecture is a modified version of traditional wireless sensor node structures. Each RIS node integrates the RIS as a front-end and can also have conventional antennas for extra functions like active relaying and signal buffering [49]. Traditional antennas and RISs work in parallel and have the same connections to other hardware components. To address energy limitations underwater, a power
unit with a battery power all components, including the tunable reflective elements of the RISs. Energy harvesting modules, supporting the idea of self-sustainable RISs, capture signals not reflected by the RISs [50]. This approach, which involves simultaneous signal reflection and energy harvesting clusters, aligns with potential advances in simultaneous wireless information and power transfer (SWIPT) technology [51].
A processing unit, consisting of a processor and memory storage, manages phase shifts in the RISs. It operates as a transmitter or receiver through a dedicated transceiver, allowing communication with other RISs [52]. For improved signal processing capabilities, the processing unit can link to external processors and memory blocks from neighbouring RIS patches.
This architecture aims to reduce the computational complexity per Reconfigurable Intelligent Surface (RIS) while allowing for functional extensions. It ensures that the nodes of the RIS in underwater environments can not only reflect signals but also have advanced functionalities and efficient power management systems.
Machine learning (ML), when used with RIS technology, can significantly enhance network performance in RIS-aided networks. By using various ML-based optimization techniques, such as dynamic spectrum
allocation and interference mitigation, we can optimize predictive resource management to improve overall network capacity. In this context, we will explore several ML techniques that can be used within RIS, including supervised learning, unsupervised learning, reinforcement learning, federated learning, graph learning, transfer learning, and hierarchical learning.
• Supervised
In Supervised Learning algorithms, the model learns to map input data to the correct output labels using a labelled dataset. A technique described in [53] aims to maximize the power received in an RIS-enabled network. In a scenario involving multiple users and a multiple-input single-output (MISO) system, the downlink is examined, and a deep neural network (DNN) is utilized to learn the mapping between the users’ locations and the RIS reflecting elements. The goal is to enhance the signal quality at each predicted user position in indoor environments. The proposed DNN architecture consists of five layers, with each output layer employing a nonlinear function. In another study [54], a communication link between two nodes is supported by multiple RISs. Lowcomplexity supervised learning algorithms are used to configure the phase shifts of the RISs. A multi-layer perceptron neural network is proposed, which can be trained with positioning values or instantaneous channel coefficients. The study explores centralized and individual training of the RISs and their coordination and computational requirements. Simulation results in the paper demonstrate the
advantages of using individual neural networks at the RISs to improve the link budget performance.
• Unsupervised Learning. Unsupervised Learning involves identifying patterns or structures in data without using explicit labels. Unsupervised learning techniques work with unlabelled input data to discover connections between data points and form clusters [55]. In a paper referenced as [56], the authors introduced a low-complexity unsupervised learning scheme, named a learning-phase-neural network, to maximize spectral efficiency in RIS-aided MIMO networks. Their simulation results demonstrate that the proposed scheme significantly improves network performance in terms of spectral efficiency while reducing system complexity. Also, in reference [57], the authors proposed an unsupervised learning-based joint active and passive beamforming design for RIS-aided wireless networks. Their proposal involved a deep learning-based algorithm for joint active and passive beamforming design. They trained a two-stage neural network offline without supervision and implemented it online for real-time prediction. Their simulation results showed that their proposed scheme significantly reduces computational complexity compared with existing methods in the literature.
• Reinforcement Learning. Reinforcement Learning (RL) teaches a model to make sequences of decisions by rewarding desired actions and punishing undesired ones. It includes model-free algorithms such as Q-learning and deep Q-learning (DQN) and model-based algorithms like dynamic programming [58]. In a study
referenced as [59], an RIS-assisted cellular network, supported by an RIS reflector powered via energy harvesting technologies, was considered, and the energy efficiency optimization problem was investigated. The study proposed a deep reinforcement learning algorithm to optimize the BS transmit power allocation and RIS phase shift configuration by deploying a neural network. The simulation results indicated significant improvements in energy efficiency when the number of RIS elements increased from 9 to 25. Besides, in reference [60], UAVs were integrated with RISs to passively communicate information sampled by Internet of Things Devices (IoTDs) to the BS. The study aimed to minimize the expected sum age-of-information (AoI) by optimizing the altitude of the UAV, the phase shifts of the RIS elements, and the communication schedule. The authors developed a proximal policy optimization algorithm, a deep reinforcement learning approach, to solve the optimization problem. Their numerical results demonstrated the superiority of the authors’ suggested scheme over all other counterparts presented in the literature.
• Federated Learning. Federated Learning (FL) is an approach to training machine learning models across multiple decentralized devices or servers without directly sharing the data. In a wireless FL system described in reference [61], many edge devices synergize to train a shared model. This process is coordinated by a centralized base station (BS) using overthe-air computation. The authors used an RIS to adjust the wireless environment and
focused on minimizing the gap between the model’s performance and its optimal state. They also addressed differential privacy and transmit power constraints. To achieve these goals, they jointly optimized the transmit power of the devices, the phase shifts of the RIS, and the artificial noise, using a two-step alternating minimization framework. Their research demonstrated that the proposed scheme outperformed the benchmarks regarding accuracy and privacy. Reference [62] introduces a framework for balancing the accuracy and integrity of over-the-air FL, utilizing an RIS to optimize multiantenna devices and the BS. The system aimed to minimize the distortion of the aggregated model by optimizing the transmit beamformers of the devices, the receive beamformers of the BS, and the phase shifts of the RIS. The research considered both perfect and imperfect channel state information (CSI). Simulations confirmed that the proposed scheme achieved a robust design of the beamformers and RIS configuration, even around imperfect CSI. Furthermore, experimental results indicated that the framework achieved accuracy close to the ideal FL.
• Graph Learning. The field of Graph Learning involves using machine learning techniques on graph data. Some of these techniques include graph attention networks (GAN), graph neural networks (GNN), and graph convolution networks (GCN). In a study referenced as [63], the authors addressed the joint optimization problem involving user scheduling, RIS configuration, and base station (BS) beamforming in an RIS-assisted downlink network with limited
pilot overhead. The authors found that GNN, characterized by permutation invariance and equivalent properties, was an effective approach for scheduling users and optimizing RIS phase shifts, thus enhancing system performance in terms of throughput while ensuring fairness among users. The proposed scheme first optimizes the user schedule and then the RIS phase shifts using a second GNN. Subsequently, the BS beamformers are designed based on the overall effective channel. The results presented by the authors indicated that their approach utilizes received pilots more efficiently than conventional channel estimation-based approaches. In another study referenced as [64], a smart RIS-THzMIMO-NOMA framework was proposed to reconfigure hybrid beams effectively through the cooperation between access points (APs) and the RIS. The authors employed a decentralized partially observable Markov decision process (Dec-POMDP) to optimize the network’s energy efficiency while meeting diverse user performance requirements. The authors jointly optimized the RIS element selection and power allocation strategy and coordinated discrete phase-shift control. They proposed a multiagent deep reinforcement learning (MADRL) algorithm to solve the non-convex and strongly coupled optimization problem. Their numerical results demonstrated that their proposed algorithm outperforms traditional MADRL algorithms.
• Transfer Learning. Transfer Learning allows knowledge gained in one problem domain to be applied to a different but related domain. In the context of RISs, transfer learning can be beneficial when
there are limited labelled datasets for specific conditions. It can be used to expedite the training of machine learning algorithms in RIS-assisted wireless networks, where fast decision-making is crucial. However, low sampling efficiency may impede the deployment of machine learning in RISassisted wireless communication networks [58]. In a study [65], transfer learning was used to jointly optimize resource allocation for network slicing. The authors proposed a deep transfer reinforcement learning (DTRL) scheme for joint radio and cache resource allocation for 5G RAN slicing. Their proposed algorithms were compared with bonus deep Q-learning, model-based priority proportional fairness, and time-to-live (PPF-TTL) algorithms, and their scheme achieved lower delay and higher throughput. In another study [66], the authors addressed interference mitigation in a 5G millimeter-wave (mm Wave) communication network by using beamforming and NOMA techniques to improve the network’s aggregate rate. They considered jointly optimizing the user-cell association and the number of beams to maximize the aggregate network capacity. Three machine learning-based approaches were deployed: Q-learning, transfer Q-learning (TQL), and Best SINR association with density-based spatial clustering of applications with noise (BSDC) algorithms. The authors compared the performance of these approaches under mobility and stationary scenarios. Moreover, transfer learning can be used in machine learning-enabled RIS wireless networks to achieve prompt phase-shift responses and faster convergence [58].
• Hierarchical Learning. Hierarchical Learning is a powerful approach in reinforcement learning that enhances exploration efficiency by breaking down long-term tasks into multiple sub-tasks [67]. This method allows for more manageable and focused learning processes, leading to better performance and faster convergence. In a comprehensive survey, the authors of [68] discussed various approaches to hierarchical reinforcement learning. They identified several critical open problems that could inspire future research directions in this field. These open problems highlight the potential for further advancements and innovations in hierarchical reinforcement learning, motivating researchers to explore new methodologies and applications.
The integration of Reconfigurable Intelligent Surface (RIS) technology and machine learning (ML) techniques in underwater communications is an emerging area with limited existing literature. With fewer than 300 publications addressing RISs and ML techniques simultaneously, conducting a comprehensive bibliometric analysis on this specific intersection is challenging.
As a result, this section will present a systematic bibliometric analysis focusing solely on RISs. To our knowledge, it is the first comprehensive systematic bibliometric analysis in the field of RIS. This analysis aims to provide valuable insights into research trends, key contributors, and significant developments within the RIS domain, laying the groundwork for future studies and innovations.
We used the Scopus database to examine the publishing trends and patterns in the global literature on RISs. The Scopus database is known for indexing and abstracting scholarly publications and provides extensive bibliometric data through a straightforward extraction method, making it well-suited for comprehensive analyses [69].
To extract relevant data, we designed a comprehensive search strategy that focused on specific aspects such as titles, author keywords, and abstracts using the advanced search feature of the Scopus database with the following search query: TITLE-ABSKEY (“Reconfigurable Intelligent Surface*” OR “Reconfigurable Intelligent Surface (RIS)”).
The initial search yielded 4,632 publications. After refining the search by limiting it to English language documents and specific document types (articles, conference papers, reviews, conference reviews, and book chapters), as well as excluding irrelevant documents, the final dataset consisted of 4,537 publications. This data was downloaded in CSV and Research Information System formats for further analysis and visualization.
For data analysis, we utilized Microsoft Excel, VOSviewer [70], and Biblioshiny [71]. Additionally, to ensure the accuracy and consistency of the data and information, we standardized discrepancies in organization names, author details, source titles, and countries. The accuracy of the data was verified by replicating the process with two other members of the research group.
In the field of data analysis, various terms are used to quantify research output and impact. TP stands for total publications, TC stands for total citations, and TC/TP represents the average citation per publication. Table 1 provides valuable information about RIS publications from 2019 to 2024. A bibliometric study of RISs reveals that there were 4,537 publications and 54,012 citations from 625 diverse sources during this period. The data indicates an annual growth rate of 169.59% and an average age of 1.48 years for the publications. On average, each paper received 11.90 citations and had 72,185 references.
The field of RISs includes a variety of keywords, with 10,223 keywords and 6,243 author keywords identified. Collaboration in RIS research is evident, with 5,023 contributing authors, including 123 who have authored only one paper. On average, there are 4.43 authors per publication, and international co-authorships account for 41.92% of collaborations. The analysis also shows the types of research conducted; out of 4,537 publications, articles were the most usual form (2,609 publications), followed by conference papers (1,802), reviews (58), conference reviews (38), and book chapters (30).
Figure 3 provides a detailed overview of the
research productivity and citation trends for Reconfigurable Intelligent Surfaces (RISs) from 2019 to 2024. The analysis shows that RIS is a relatively new field of study, with the first research paper being published in 2019 (TP = 5) and receiving 4,425 citations, resulting in the highest average citation per article at 885, TC/TP. There has been an exponential increase in publication growth over the years, with a significant surge in 2020, which saw 153 publications and a certain number of citations. In 2023, there was the highest number of research papers, with 1,882 publications and 4,065 citations, followed by 1,172 publications and a certain number of citations in 2022, 613 publications and a certain number of citations in 2021,
and 712 publications and 125 citations in 2024. The year 2021 recorded the highest total citations with a certain number, followed by 2020 with a certain number, 2022 with a certain number, 2019 with 4,425, and 2023 with 4,065; the year with the oldest data had the highest average number of citations per article, while the most recent year experienced a decline in the average number of citations per article. The decrease in citations can be attributed to the additional two to three years needed to attain significance and impact. The year 2024 had the lowest number of publications and citation impact, likely because the data were acquired on May 8, 2024, before the year was complete.
The significant increase in RIS research publications and citations from 2019 to 2024 underscores the rapid growth and growing significance of the field. The collaborative aspect of the research, along with the high impact of early publications, highlights the dynamic and evolving nature of RIS
studies. As the field continues to develop, the findings from this analysis will provide a crucial foundation for future research and advancements in RIS technology.
Table 2 is an exhaustive overview of the primary sources in RISs. From 2019 to 2024, 625 sources (journals and books) have produced 4,537 publications. The top ten leading sources have contributed approximately 30% of these papers. Among them, IEEE Transactions on Vehicular Technology (JIF = 6.8) has published 291 papers with 3,596 citations, followed by IEEE Transactions on Wireless Communications (JIF = 10.4) with 285 papers and 8,329 citations. Other prominent sources include IEEE Wireless Communications Letters, IEEE Communications Letters, and IEEE Transactions on Communications. Notably, all the top ten sources are from the USA and published by IEEE. Besides, the table reveals
other journals with significant h-index values, PY Start, journal impact factors, publisher, and country of publication. This overview can help researchers identify key publication platforms in RISs and concentrate on influential outlets for sharing their research.
Table 3 presents a comprehensive review of the top ten authors who have made significant contributions to the field of RISs between 2019 and 2024.
The leading author in the top ten list is Pan C from Southeast University, China, with 109 publications and 3,444 citations. Next is Liu Y from Queen Mary University of London, the UK, with 98 publications and 3,195 citations. Followed closely is Jin S from Southeast University, China, with 95 publications and 1,825 citations. Yuen C from Nanyang Technological University, Singapore, is the fourth most productive author with 89 publications and 6,076 citations, closely followed by Alexandropoulos, G.C from National and Kapodistrian University of Athens, Greece, with 88 publications and 4,815 citations. Hanzo, L from the University of Southampton, the UK, has 64 publications
and 2,617 citations, making him the least productive on the list. Also, Di Renzo, M, of Laboratoire des Signaux et Systèmes, France, received the highest citation count with 6,968 TC for 76 publications, followed by Yuen, C from Nanyang Technological University, Singapore, with 6,076 citations. Notably, Di Renzo, M from Laboratoire des Signaux et Systèmes and Yuen, C from Nanyang Technological University also have impressive citation impacts of 91.68 and 68.27, respectively.
Most of the top ten productive authors conduct their research in China (4), the UK (2), and one each from Singapore, Greece, France, and the USA, respectively. This assessment facilitates the identification of leading authors who have contributed to the field, their affiliations, and their impact on literature. As a result, it helps scholars comprehend the prominent persons and institutions affecting the subject of discussion in RIS literature.
Table 4 provides an overview of the top ten most productive organizations in the field of RIS during 2019-2024. The leading
organization was Southeast University in China, with 406 publications, 6,706 citations, and an average of 16.52 citations per publication. Following was the University of Electronic Science and Technology of China with 215 publications and 4,979 citations, Queen Mary University of London with 199 publications and 6,596 citations, and Beijing University of Posts and Telecommunications with 182 publications and 2,675 citations.
Laboratoire des Signaux et Systèmes in France had the lowest number of publications on the list (125) but the highest number of citations at 9,402. Centrale Supélec-Paris-Saclay received the highest number of citations with 13,084 and a TC/TP ratio of 89.62.
Other organizations (e.g., Université ParisSaclay and Laboratoire des Signaux et Systèmes) had high total citation numbers, demonstrating their significant impact in the field of RIS. The analysis also indicates that the top ten organizations are predominantly from China (5), followed by France (4) and the UK (1), suggesting that organizations from China and France are leading in RIS literature. This comprehensive analysis provides valuable insights into the importance and global
distribution of institutions in RIS literature, aiding scholars in understanding research trends and the contributions of different universities.
Please note the following information: Figure 4 shows the countries with the highest number of publications in RISs from 2019 to 2024. China had the most publications with 2,301, along with 30,258 citations, followed by the United Kingdom with 703 publications and 14,206 citations, the United States with 524 publications and 8,841 citations, Canada with 315 publications and 4,210 citations, and South Korea with 300 publications and 3,140 citations. Other significant countries include India, France, Singapore, Australia, Greece, Germany, Taiwan, Italy, Sweden, Saudi Arabia, Turkey, and Finland, each with over 100 publications. On the other hand, Indonesia, the Netherlands, Vietnam, and Zimbabwe had the fewest publications, each with five. China, Cyprus, Germany, the United Kingdom, and South Africa had the highest number of citations of 30,258, 29,341, 14,625, 14,206, and 10,306, respectively. The average citation per publication (i.e., TC/TP) varies across the countries. Cyprus, Chile, South Africa, Indonesia, and the USA have the highest
averages of 1,630.06, 722.00, 515.30, 205.20, and 205.16, respectively.
This analysis provides valuable insights into the global distribution of research efforts, allowing scholars to understand the geographical contributions to the subject. It also helps identify the leading nations in RIS literature.
In Figure 5, we can see the classification of authorship patterns in RIS publications. The analysis shows 18 diverse types of authorship, resulting in 4,537 publications. These ranged
from single publications to as many as 22 for a single author. The fourth authorship pattern contributed to the highest number of research papers, totalling 986 publications and 9,844 citations, followed by the fifth with 945 publications and 13,135 citations. Three authorship patterns held the third position with 815 publications and 6,805 citations, while the sixth authorship pattern contributed 682 publications and 8,834 citations.
Meanwhile, the authorship patterns of the 16th and 21st had the lowest number of publications, with only one publication
each. Furthermore, patterns 15, 12, 21, 10, and 18 had the highest average citations per publication, with 229.00, 216.50, 71.00, 45.43, and 39.50, respectively. The analysis suggests that 99% of researchers in the field are highly interested in collaborative work rather than pursuing solo research (TP = 123).
Figure 6 portrays the results from a comprehensive evaluation of the most used author keywords in the field of RISs. VOSviewer, a bibliometric software, was used to create the illustration. The investigation focused on keywords that co-occurred at least 15 times out of 6,177 instances. In total, 88 keywords met this threshold and were included in the analysis. These top 88 keywords were selected based on their overall link strength and were categorized into 12 clusters or themes, each represented by a distinct colour.
Each bubble size reflects the frequency of keyword usage, and the connections between the bubbles show the number of research publications featuring these keywords. The analysis revealed the top five most frequently used keywords by RIS researchers to be “Reconfigurable Intelligent Surface,” “Wireless Communication,” “Non-Orthogonal Multiple Access,” “Energy Efficiency,” and “Unmanned Aerial Vehicle.” The figure illustrates 12 distinct clusters of keywords, each corresponding to a specific research area:
• Cluster 1. This cluster is the largest and consists of 24 keywords. The primary topics covered in this cluster include Wireless Communication, Non Orthogonal Multiple Access, Physical Layer Security, Internet of Things, Simultaneous Wireless Information and Power Transfer, Active Reconfigurable Intelligent Surface, Simultaneously Transmitting and
Reflecting Reconfigurable Intelligent Surface (STAR-RIS), Fading Channels, Quality of Service, Wireless Networks, Manifold Optimization, Receivers, SumRate Maximization, Hardware, Interference Cancellation, Mutual Coupling, Protocols, Backscatter, Fractional Programming, Backscatter Communication, Communication System Security, Jamming, MISO Communication, and Full-Duplex (FD).
• Cluster 2. The primary focus of this cluster includes Metasurfaces, Beyond 5G, Federated Learning, Intelligent Reflecting Surfaces, Metamaterials, Artificial Intelligence, Reliability, Smart Radio Environments, Sensing, and Visible Light Communication.
• Cluster 3. The significant topics in this cluster include Modulation, Symbols, Index Modulation, Precoding, Reflection
Coefficient, Index Modulation (IM), Orthogonal Frequency Division Multiplexing (OFDM), Cell-Free Network, and Wideband.
• Cluster 4. The main topics in this cluster include Millimeter Wave, Estimation, Compressive Sensing, Training, Location Awareness, 6G Mobile Communication, Beam.
• Cluster 5. The main topics in this cluster include Reconfigurable Intelligent Surfaces, Energy Efficiency, Cognitive Radio, Convex Optimization, Outage Probability (OP), Smart Radio Environment, Imperfect Channel State Information (CSI), MultipleInput Multiple-Output, Training, Antenna Arrays, and Terahertz Communication.
• Cluster 6. The main topics in this cluster include Successive Convex Approximation, Non-Convex Optimization, Phase Shift
Optimization, Trajectory Design, PhysicalLayer Security, Secure Communication, ISAC, and UAV Communication.
• Cluster 7. The main topics in this cluster include Intelligent Reflecting Surface (IRS), Positioning, Reflectarray, mmWave Communications, and Compressed Sensing.
• Cluster 8. Topics in this cluster are Integrated Sensing and Communication, Radar, Robust Beamforming, Relay, and Active RISs.
• Cluster 9. Includes topics like Ergodic Rate, Coverage Probability, and UAVs.
• Cluster 10. Covers Unmanned Aerial Vehicle (UAV), Deep Reinforcement Learning, and SWIPT.
• Cluster 11. Focuses on mmWave and Power Control.
• Cluster 12. Addresses Cell-free Massive MIMO.
Figure 6 provides a comprehensive summary of the main ideas and research topics in RISs, making it a significant source of information. It demonstrates that RISs are a rapidly expanding domain with numerous practical applications.
Table 5 provides an in-depth analysis of the top ten most highly cited research papers on Reconfigurable Intelligent Surfaces (RISs) published between 2019 and 2024. These papers have made significant contributions and gained substantial recognition in the academic community. The papers received varying citations, with the highest being 2,158 and the lowest being 458. Notably, three of the publications received over 1,000 citations.
Among the top ten papers, four were published in “IEEE Transactions on Wireless Communications,” two in “IEEE J Sel Areas Commun,” and one each in “IEEE Open J Commun Soc,” “IEEE Access,” “IEEE Trans Commun,” and “IEEE Commun Surv Tutor.”
The most cited paper is “Reconfigurable Intelligent Surfaces for Energy Efficiency in Wireless Communication” by Huang C (2019), published in “IEEE Transactions on Wireless Communications,” with 2,158 citations [72]. It is followed by “Wireless Communications through Reconfigurable Intelligent Surfaces” by Basar E (2019), published in “IEEE Access,” with 1,834 citations [73]. “Smart Radio Environments Empowered by Reconfigurable Intelligent Surfaces: How it Works, State of Research, and the Road Ahead” by Di Renzo M (2020) has 1,452 citations [74]. The fourth most cited paper is “Wireless Communications with Reconfigurable Intelligent Surface: Path Loss Modeling and Experimental Measurement” by Tang W (2021) with 736 citations [75], and “Multicell MIMO Communications Relying on Intelligent Reflecting Surfaces” by Pan C (2020) ranks fifth with 616 citations [76].
The least cited paper in the top ten list is “Reconfigurable Intelligent Surfaces vs. Relaying: Differences, Similarities, and Performance Comparison” by Di Renzo M (2020) with 458 citations [77].
This study aims to highlight impactful research in the field of RISs to help researchers gain a deeper understanding of important topics.
Figure 7 depicts the results from a detailed analysis of trends in RIS research. It shows the frequency and distribution of different themes in RIS research over time. The most frequently occurring themes include reconfigurable (n = 3,545), Reconfigurable Intelligent Surface (n = 2,552), interlocking signals (n = 939), beamforming (n = 859), wireless communications (n = 856), signal-to-noise ratio (n = 706), 5G
mobile communication systems (n = 306), and wireless networks (n = 265). Other significant topics common in RIS research are performance, outage probability, reflecting elements, optimization problems, propagation environment, closed-form expression, modulation, task analysis, and job analysis.
The analysis reveals the emerging research themes in RISs during 2021, which include interlocking signals, reflecting elements,
optimization problems, propagation environment, closed-form expression, and modulation. In 2022, the emerging research themes are Reconfigurable Intelligent Surface, beamforming, wireless communications, signal-to-noise ratio, 5G mobile communication systems, wireless networks, performance, and outage probability. The emerging trends in recent years (2023 and 2024) include task analysis, job analysis, and backscatter.
This thorough review highlights the dynamic nature of RIS research, with themes changing over time to address new opportunities and challenges in the field.
Factorial analysis is a method used to examine the dimensions and clusters of a conceptual structure map derived from Multiple Correspondence Analysis (MCA). It provides valuable insight into the primary patterns of data variation, the impact of specific variables, and the interconnections
among categorical variables. In Figure 8, two distinct clusters are visually represented with varying colour shades. Each cluster is linked to a set of keywords that symbolize a specific theme.
Cluster 1 includes keywords such as Reconfigurable Intelligent Surface, Interlocking Signals, Beamforming, Wireless Communications, Signal-to-Noise Ratio, MIMO Systems, Optimizations, Energy Efficiency, Antennas, Channel State Information, Millimeter Waves, Array Signal Processing, Channel Estimation, Array Processing, Iterative Methods, Deep Learning, Fading Channels, X5G Mobile Communication Systems, Spectrum Efficiency, Internet of Things, Wireless Networks, Unmanned Aerial Vehicles (UAV), Network Layers, Quality-ofService, Bit Error Rate, Optimization, Reinforcement Learning, Performance, Millimeter-wave Communications, Outage Probability, Physical Layer Security, Communications Systems, Monte Carlo
Methods, Aerial Vehicle, Metasurface, Mobile Telecommunication Systems, Signal Receivers, Interference, Multiple Inputs, Mean Square Error, Multiple Outputs, Intelligent Systems, Convex Optimization, and Reflecting Surface. This cluster highlights the multifaceted nature of RIS technology and its potential to revolutionize wireless communications through various optimization and enhancement techniques.
Cluster 2 is a comprehensive collection of topics encompassing Multiple Access, Non-Orthogonal Multiple Access, Stars, and Resource Management. This cluster signifies a central theme associated with advanced multiple-access techniques, potentially referring to the implementation of multiple access-schemes in 5G and resource management strategies within the field of RISs.
The data illustrated in Figure 9 underscores the extensive international collaboration in RIS research. It shows that Chinese authors had the most frequent collaborations with other countries among the top ten on the list. The highest number of research collaborations was observed between China and the United Kingdom, resulting in 427 publications. Next are the collaborations between China and the USA (197 publications), China and Singapore (177 publications), China and Australia (145 publications), and China and Canada (114 publications). On the other hand, the USA and Korea had the lowest level of collaboration, with only 54 publications. Noteworthy collaborations also existed between the UK and the USA, China and France, China and Korea, and China and Hong Kong. The map
depicts the global nature of RIS research and knowledge exchange across diverse cultures. This international network of collaborations highlights the mutual interest and expertise in RIS across different countries.
The visual representation of these connections provides valuable insights into how various nations contribute to and benefit from RIS research, fostering a rich exchange of knowledge and innovation.
In our research, we focused on RISs and ML-assisted underwater communications. We discussed potential signalling technologies in an RIS-assisted underwater environment, including IoUWT, various RIS implementation
approaches, and hardware architecture. Furthermore, we explored ML-enabled optimization strategies for RIS-aided networks. Our systematic bibliometric analysis provided an in-depth look at research trends and citation patterns in the RIS field. This analysis revealed 4,537 publications indexed in the Scopus database over six years between 2019 and 2024, highlighting that RISs is a relatively new research topic. It also highlighted leading figures in the field, trustworthy sources for research dissemination, publication and citation trends, prolific authors, organizations, countries, authorship patterns, key concepts and contributions, highly cited articles, and level of country collaboration.
The analysis of research publications on RISs revealed a remarkable surge in the number
of papers over the years, with an astounding annual growth rate of 169.59%. The year 2023 stood out with the highest number of research papers (1,882 publications) and citations (4,065). Additionally, the study unveiled the top three sources favoured by scholars and highlighted the three most prolific authors in the field. Notably, a strong trend toward collaborative authorship emerged, with the fourth authorship pattern contributing the highest count of research papers (986 publications) and citations (9,844), signifying an overwhelming preference for collaborative work (99%) over solo research (1%). It is worth emphasizing that our study is a pioneering effort to conduct a comprehensive bibliometric analysis of the published literature on RISs.
Therefore, our research will establish a baseline for evaluating the future path for RIS publications. The findings of this study will not only pave the way for future research into the many aspects of this subject but also provide valuable insights to guide further studies, inspiring new research directions and methodologies. This study is significant because it relies on high-quality literature indexed by the Scopus database. While our findings already enhance the understanding of research patterns in this domain, including additional bibliographic databases like Web of Science, PubMed, Dimensions, and Google Scholar could impact and supplement our research findings. Scholars, practitioners, and policy-makers interested in RISs will find the analysis presented in this paper beneficial. Our research also highlights areas of study that will require attention in the future, including the application of Artificial Intelligence in RISs
and the influence of RISs on machine learningassisted underwater communications.
The authors extend appreciation to the Marine Institute (MI) of Memorial University in Newfoundland and Labrador, Canada; King Fahd University of Petroleum and Minerals in the Kingdom of Saudi Arabia; Arab Academy of Science, Technology and Maritime Transport in Cairo, Egypt; and the University of Ottawa in Ontario, Canada; for allowing them to use their facilities. Their work was made possible by the funding support from Mitacs through a Lab2Market grant. They are also grateful to Capt. Kristopher Drodge, Capt. Fabian Lambert, and Joe Singleton for their support and encouragement. Capt. Drodge is the head, Capt. Lambert is the assistant head of the School of Maritime Studies, and Mr. Singleton is the head of the School of Ocean Technology at MI.
[1] Megahed, M. M. Elmesalawy, I. I. Ibrahim, and A. M. A. El- Haleem, “Deep learning-assisted reconfigurable intelligent surface for enhancing 6g mobile networks,” Transactions on Emerging Telecommunications Technologies, vol. 35, no. 1, p. e4895, 2024.
[2] Al-Hilo, M. Samir, M. Elhattab, C. Assi, and S. Sharafeddine, “Reconfigurable intelligent surface enabled vehicular communication: Joint user scheduling and passive beamforming,” IEEE Transactions on Vehicular Technology, vol. 71, no. 3, pp. 2333-2345, 2022.
[3] S. Farrag, E. A. Maher, A. El-Mahdy, and F. Dressler, “Outage probability analysis of uplink RIS and UAV-assisted thz mobile communications,” in 2022 14th International Congress on Ultra Modern Telecommunications and Control Systems and Workshops (ICUMT). IEEE, 2022, pp. 14-21.
[4] Khaleel and E. Basar, “Phase shift-free passive beamforming for reconfigurable intelligent surfaces,”
IEEE Transactions on Communications, vol. 70, no. 10, pp. 6966-6976, 2022.
[5] E. Björnson, H. Wymeersch, B. Matthiesen, P. Popovski, L. Sanguinetti, and E. de Carvalho, “Reconfigurable intelligent surfaces: A signal processing perspective with wireless applications,” IEEE Signal Processing Magazine, vol. 39, no. 2, pp. 135-158, 2022.
[6] Y. Liu, X. Liu, X. Mu, T. Hou, J. Xu, M. Di Renzo, and Al-Dhahir, “Reconfigurable intelligent surfaces: Principles and opportunities,” IEEE communications surveys & tutorials, vol. 23, no. 3, pp. 1546-1577, 2021.
[7] J. Chen, Y.-C. Liang, H. V. Cheng, and W. Yu, “Channel estimation for reconfigurable intelligent surface aided multi-user mmWave mimo systems,” IEEE Transactions on Wireless Communications, 2023.
[8] B. Rana, S.-S. Cho, and I.-P. Hong, “Review paper on hardware of reconfigurable intelligent surfaces,” IEEE Access, 2023.
[9] S. Farrag, E. A. Maher, A. El-Mahdy, and F. Dressler, “Sum rate maximization of uplink active RIS and UAV-assisted thz mobile communications,” in 2023 19th International Conference on the Design of Reliable Communication Networks (DRCN). IEEE, 2023, pp. 1-7.
[10] H. Guo, Y.-C. Liang, J. Chen, and E. G. Larsson, “Weighted sum-rate maximization for reconfigurable intelligent surface aided wireless networks,” IEEE transactions on wireless communications, vol. 19, no. 5, pp. 3064-3076, 2020.
[11] Z. Zhang, L. Dai, X. Chen, C. Liu, F. Yang, R. Schober, and H. V. Poor, “Active RIS vs. passive RIS: Which will prevail in 6g?” IEEE Transactions on Communications, vol. 71, no. 3, pp. 1707-1725, 2022.
[12] E. Basar and H. V. Poor, “Present and future of reconfigurable intelligent surface-empowered communications [perspectives],” IEEE Signal Processing Magazine, vol. 38, no. 6, pp. 146-152, 2021.
[13] S. Basharat, S. A. Hassan, H. Pervaiz, A. Mahmood, Z. Ding, and M. Gidlund, “Reconfigurable intelligent surfaces: Potentials, applications, and challenges for 6g wireless networks,” IEEE Wireless Communications, vol. 28, no. 6, pp. 184-191, 2021.
[14] K. Liu, Z. Zhang, L. Dai, S. Xu, and F. Yang, “Active reconfigurable intelligent surface: Fullyconnected or sub-connected?” IEEE Communications Letters, vol. 26, no. 1, pp. 167-171, 2021.
[15] X. Yuan, Y.-J. A. Zhang, Y. Shi, W. Yan, and H. Liu, “Reconfigurable-intelligent-surface empowered wireless communications: Challenges and opportunities,” IEEE Wireless Communications, vol. 28, no. 2, pp. 136–143, 2021.
[16] S. Hassouna, M. A. Jamshed, J. Rains, J. u. R. Kazim, M. U. Rehman, M. Abualhayja, L. Mohjazi,
T. J. Cui, M. A. Imran, and Q. H. Abbasi, “A survey on reconfigurable intelligent surfaces: Wireless communication perspective,” IET Communications, vol. 17, no. 5, pp. 497-537, 2023.
[17] Y. Feng, Q. Hu, K. Qu, W. Yang, Y. Zheng, and K. Chen, “Reconfigurable intelligent surfaces: Design, implementation, and practical demonstration,” Electromagnetic Science, vol. 1, no. 2, pp. 1-21, 2023.
[18] L. Dong, H.-M. Wang, and J. Bai, “Active reconfigurable intelligent surface aided secure transmission,” IEEE Transactions on Vehicular Technology, vol. 71, no. 2, pp. 2181-2186, 2021.
[19] M. Ahmed, A. Wahid, S. S. Laique, W. U. Khan, A. Ihsan, F. Xu, S. Chatzinotas, and Z. Han, “A survey on STAR-RIS: Use cases, recent advances, and future research challenges,” IEEE Internet of Things Journal, 2023.
[20] Z. Peng, X. Liu, C. Pan, L. Li, and J. Wang, “Multipair D2D communications aided by an active RIS over spatially correlated channels with phase noise,” IEEE Wireless Communications Letters, 2022.
[21] S. Kisseleff, S. Chatzinotas, and B. Ottersten, “Reconfigurable intelligent surfaces in challenging environments: Underwater, underground, industrial and disaster,” IEEE Access, vol. 9, pp. 150 214-150 233, 2021.
[22] Z. Wei, Z. Wei, J. Fang, J. Pan, L. Wang, and Y. Dong, “Impulse response modeling and dynamic analysis for SIMO UOWC systems enhanced by RIS-equipped UUV,” IEEE Transactions on Vehicular Technology, 2023.
[23] S. Aboagye, A. R. Ndjiongue, T. M. Ngatched, O.A. Dobre, and H. V. Poor, “RIS-assisted visible light communication systems: A tutorial,” IEEE Communications Surveys & Tutorials, vol. 25, no. 1, pp. 251-288, 2022.
[24] S. Li, L. Yang, D. B. da Costa, M. Di Renzo, and M.-S. Alouini, “On the performance of RIS-assisted dual-hop mixed RF-UWOC systems,” IEEE transactions on Cognitive communications and Networking, vol. 7, no. 2, pp. 340-335, 2021.
[25] Y. Liu, W. Li, and Z. Lin, “A dynamic subarray structure in reconfigurable intelligent surfaces for terahertz communication systems,” arXiv preprint arXiv:2206.14968, 2022.
[26] C. Zhang, H. Lu, and C. W. Chen, “Reconfigurable intelligent surfaces-enhanced uplink user-centric networks on energy efficiency optimization,” IEEE Transactions on Wireless Communications, 2023.
[27] M. Wu, Z. Gao, Y. Huang, Z. Xiao, D. W. K. Ng, and Z. Zhang, “Deep learning-based rate-splitting multiple access for reconfigurable intelligent surface-aided tera-hertz massive MIMO,” IEEE Journal on Selected Areas in Communications, vol. 41, no. 5, pp. 1431-1451, 2023.
[28] A. A. Puspitasari and B. M. Lee, “A survey on reinforcement learning for reconfigurable intelligent
surfaces in wireless communications,” Sensors, vol. 23, no. 5, p. 2554, 2023.
[29] P. N. Ramavath and W.-Y. Chung, “Performance evaluation of re-configurable intelligent surfaceassisted underwater and free-space wireless optical communication in the skip-zones,” ICT Express, vol. 10, no. 2, pp. 320-329, 2024.
[30] K. O. Odeyemi, P. A. Owolawi, and O. O. Olakanmi, “Performance analysis of reconfigurable intelligent surface assisted underwater optical communication system,” Progress In Electromagnetics Research M, vol. 98, pp. 101-111, 2020.
[31] Z. Sun, H. Guo, and I. F. Akyildiz, “High-data-rate long-range underwater communications via acoustic reconfigurable intelligent surfaces,” IEEE Communications Magazine, vol. 60, no. 10, pp. 96102, 2022.
[32] H. Wang, Z. Sun, H. Guo, P. Wang, and I. F. Akyildiz, “Designing acoustic reconfigurable intelligent surface for underwater communications,” IEEE Transactions on Wireless Communications, 2023.
[33] J. Zhao, B. Li, Z. N. Chen, and C.-W. Qiu, “Redirection of sound waves using acoustic metasurface,” Applied Physics Letters, vol. 103, no. 15, 2013.
[34] S.-W. Fan, S.-D. Zhao, L. Cao, Y. Zhu, A.-L. Chen, Y.-F. Wang, K. Donda, Y.-S. Wang, and B. Assouar, “Reconfigurable curved metasurface for acoustic cloaking and illusion,” Physical Review B, vol. 101, no. 2, p. 024104, 2020.
[35] X.-S. Li, Y.-F. Wang, A.-L. Chen, and Y.-S. Wang, “An arbitrarily curved acoustic metasurface for three-dimensional reflected wave-front modulation, Journal of Physics D: Applied Physics, vol. 53, no. 19, p. 195301, 2020.
[36] S.-W. Fan, S.-D. Zhao, A.-L. Chen, Y.-F. Wang, B. Assouar, and Y.-S. Wang, “Tunable broadband reflective acoustic metasurface,” Physical Review Applied, vol. 11, no. 4, p. 044038, 2019.
[37] J. He, X. Jiang, D. Ta, and W. Wang, “Experimental demonstration of underwater ultrasound cloaking based on metagrating,” Applied Physics Letters, vol. 117, no. 9, 2020.
[38] H. Griffiths, “Magnetic induction tomography,” Measurement Science and Technology, vol. 12, no. 8, p. 1126, 2001.
[39] L. Ma and M. Soleimani, “Magnetic induction tomography methods and applications: A review,” Measurement Science and Technology, vol. 28, no. 7, p. 072001, 2017.
[40] R. Syms, E. Shamonina, and L. Solymar, “Magneto-inductive waveguide devices,” Proceedings of the IEEE Microwaves, Antennas and Propagation, vol. 153, no. 2, pp. 111-121, 2006.
[41] S. A. H. Mohsan, Y. Li, M. Sadiq, J. Liang, and M. A. Khan, “Recent advances, future trends, applications and challenges of internet of
underwater things (IOUT): A comprehensive review,” Journal of Marine Science and Engineering, vol. 11, no. 1, p. 124, 2023.
[42] J. Jiang, G. Han, and C. Lin, “A survey on opportunistic routing protocols in the internet of underwater things,” Computer Networks, vol. 225, p. 109658, 2023.
[43] R. A. Khalil, N. Saeed, M. I. Babar, and T. Jan, “Toward the internet of underwater things: Recent developments and future challenges,” IEEE Consumer Electronics Magazine, vol. 10, no. 6, pp. 32-37, 2020.
[44] M. F. Ali, D. N. K. Jayakody, Y. A. Chursin, S. Affes, and S. Dmitry, “Recent advances and future directions on underwater wireless communications,” Archives of Computational Methods in Engineering, vol. 27, pp. 1379-1412, 2020.
[45] S. A. H. Mohsan, A. Mazinani, N. Q. H. Othman, and H. Amjad, “Towards the internet of underwater things: A comprehensive survey,” Earth Science Informatics, vol. 15, no. 2, pp. 735-764, 2022.
[46] R. Chen, M. Liu, Y. Hui, N. Cheng, and J. Li, “Reconfigurable intelligent surfaces for 6g IOT wireless positioning: A contemporary survey,” IEEE Internet of Things Journal, vol. 9, no. 23, pp. 23570-23582, 2022.
[47] B. Feng, J. Gao, Y. Wu, W. Zhang, X.-G. Xia, and C. Xiao, “Optimization techniques in reconfigurable intelligent surface aided networks,” IEEE Wireless Communications, vol. 28, no. 6, pp. 87-93, 2021.
[48] M. Al-Bzoor, E. Al-assem, L. Alawneh, and Y. Jararweh, “Autonomous underwater vehicles support for enhanced performance in the internet of underwater things,” Transactions on Emerging Telecommunications Technologies, vol. 32, no. 3, p. e4225, 2021.
[49] B. Smida, A. Sabharwal, G. Fodor, G. C. Alexandropoulos, H. A. Suraweera, and C.-B. Chae, “Full-duplex wireless for 6g: Progress brings new opportunities and challenges,” IEEE Journal on Selected Areas in Communications, 2023.
[50] K. Ntontin, A.-A. A. Boulogeorgos, E. Björnson, W. A. Martins, S. Kisseleff, S. Abadal, E. Alarcón, A. Papazafeiropoulos, F. I. Lazarakis, and S. Chatzinotas, “Wireless energy harvesting for autonomous reconfigurable intelligent surfaces,” IEEE Transactions on Green Communications and Networking, vol. 7, no. 1, pp. 114-129, 2022.
[51] M. Munochiveyi, A. C. Pogaku, D.-T. Do, A.-T. Le, M. Voznak, and N. D. Nguyen, “Reconfigurable intelligent surface aided multi-user communications: State-of-the-art techniques and open issues,” IEEE Access, vol. 9, pp. 118 584-118 605, 2021.
[52] Z. Chu, J. Zhong, P. Xiao, D. Mi, W. Hao, R. Tafazolli, and A. P. Feresidis, “RIS assisted wireless powered IOT networks with phase shift error and transceiver hardware impairment,” IEEE Transactions
on Communications, vol. 70, no. 7, pp. 4910-4924, 2022.
[53] C. Huang, G. C. Alexandropoulos, C. Yuen, and M. Debbah, “Indoor signal focusing with deep learning designed reconfigurable intelligent surfaces,” in 2019 IEEE 20th International Workshop on Signal Processing in Wireless Communications (SPAWC). IEEE, 2019, pp. 1-5.
[54] G. C. Alexandropoulos, S. Samarakoon, M. Bennis, and M. Debbah, “Phase configuration learning in wireless networks with multiple reconfigurable intelligent surfaces,” in 2020 IEEE Globe-Com Workshops (GC Wkshps.) IEEE, 2020, pp. 1-6.
[55] K. Faisal and W. Choi, “Machine learning approaches for reconfigurable intelligent surfaces: A survey,” IEEE Access, vol. 10, pp. 27 343-27 367, 2022.
[56] N. T. Nguyen, L. V. Nguyen, T. Huynh-The, D. H. Nguyen, A. L. Swindlehurst, and M. Juntti, “Machine learning-based reconfigurable intelligent surfaceaided MIMO systems,” in 2021 IEEE 22nd International Workshop on Signal Processing Advances in Wireless Communications (SPAWC). IEEE, 2021, pp. 101-105.
[57] H. Song, M. Zhang, J. Gao, and C. Zhong, “Unsupervised learning-based joint active and passive beamforming design for reconfigurable intelligent surfaces aided wireless networks,” IEEE Communications Letters, vol. 25, no. 3, pp. 892896, 2020.
[58] H. Zhou, M. Erol-Kantarci, Y. Liu, and H. V. Poor, “A survey on model-based, heuristic, and machine learning optimization approaches in RIS-aided wireless networks,” IEEE Communications Surveys & Tutorials, 2023.
[59] G. Lee, M. Jung, A. T. Z. Kasgari, W. Saad, and M. Bennis, “Deep reinforcement learning for energyefficient networking with reconfigurable intelligent surfaces,” in ICC 2020-2020 IEEE International Conference on Communications (ICC). IEEE, 2020, pp. 1-6.
[60] M. Samir, M. Elhattab, C. Assi, S. Sharafeddine, and A. Ghrayeb, “Optimizing age of information through aerial reconfigurable intelligent surfaces: A deep reinforcement learning approach,” IEEE Transactions on Vehicular Technology, vol. 70, no. 4, pp. 3978-3983, 2021.
[61] Y. Yang, Y. Zhou, T. Wang, and Y. Shi, “Reconfigurable intelligent surface assisted federated learning with privacy guarantee,” in 2021 IEEE International Conference on Communications Workshops (ICC Workshops). IEEE, 2021, pp. 1-6.
[62] J. Zheng, H. Tian, W. Ni, W. Ni, and P. Zhang, “Balancing accuracy and integrity for reconfigurable intelligent surface-aided over-the-air federated learning,” IEEE Transactions on Wireless Communications, vol. 21, no. 12, pp. 10964-10980, 2022.
[63] Z. Zhang, T. Jiang, and W. Yu, “Learning based user scheduling in reconfigurable intelligent surface assisted multiuser downlink,” IEEE Journal of Selected Topics in Signal Processing, vol. 16, no. 5, pp. 1026-1039, 2022.
[64] X. Xu, Q. Chen, X. Mu, Y. Liu, and H. Jiang, “Graph-embedded multi-agent learning for smart reconfigurable thz mimo-noma networks,” IEEE Journal on Selected Areas in Communications, vol. 40, no. 1, pp. 259-275, 2021.
[65] H. Zhou, M. Erol-Kantarci, and H. V. Poor, “Learning from peers: Deep transfer reinforcement learning for joint radio and cache resource allocation in 5g ran slicing,” IEEE Transactions on Cognitive Communications and Networking, vol. 8, no. 4, pp. 1925-1941, 2022.
[66] M. Elsayed, M. Erol-Kantarci, and H. Yanikomeroglu, “Transfer reinforcement learning for 5g new radio mmWave networks,” IEEE Transactions on Wireless Communications, vol. 20, no. 5, pp. 2838-2849, 2020.
[67] S. Pateria, B. Subagdja, A.-h. Tan, and C. Quek, “Hierarchical re-inforcement learning: A comprehensive survey,” ACM Computing Surveys (CSUR), vol. 54, no. 5, pp. 1-35, 2021.
[68] H. Zhou, L. Kong, M. Elsayed, M. Bavand, R. Gaigalas, S. Furr, and M. Erol-Kantarci, “Hierarchical reinforcement learning for RIS-assisted energyefficient ran,” in GLOBECOM 2022-2022 IEEE Global Communications Conference. IEEE, 2022, pp. 3326-3331.
[69] F. Lopez Muñoz, A. García-Perez, V. O. Cárdenas Concha, S. Meramo, D. Mainardi, L. RicardezSandoval, Á. D. González-Delgado, and J. LeonPulido, “A bibliometric study of chitosan applications: Insights from processes,” Revista Ion, vol. 36, no. 1, pp. 59-78, 2023.
[70] N. Van Eck and L. Waltman, “Software survey: Vosviewer, a computer program for bibliometric mapping,” Scientometrics, vol. 84, no. 2, pp. 523538, 2010.
[71] M. S. Rahaman and K. M. Ansari, “Quantitative analysis of quality research output in the field of physics: A bibliometric evaluation of literature in Bahrain and Kuwait,” International Journal of Information Studies & Libraries, vol. 6, no. 1, 2021.
[72] C. Huang, A. Zappone, G. C. Alexandropoulos, M. Debbah, and C. Yuen, “Reconfigurable intelligent surfaces for energy efficiency in wireless communication,” IEEE transactions on wireless communications, vol. 18, no. 8, pp. 4157-4170, 2019.
[73] E. Basar, M. Di Renzo, J. De Rosny, M. Debbah, M.-S. Alouini, and R. Zhang, “Wireless communications through reconfigurable intelligent surfaces,” IEEE access, vol. 7, pp. 116 753-116 773, 2019.
[74] M. Di Renzo, A. Zappone, M. Debbah, M.-S. Alouini, C. Yuen, J. De Rosny, and S. Tretyakov,
“Smart radio environments empowered by reconfigurable intelligent surfaces: How it works, state of research, and the road ahead,” IEEE Journal on Selected Areas in Communications, vol. 38, no. 11, pp. 2450-2525, 2020.
[75] W. Tang, M. Z. Chen, X. Chen, J. Y. Dai, Y. Han, M. Di Renzo, Y. Zeng, S. Jin, Q. Cheng, and T. J. Cui, “Wireless communications with reconfigurable intelligent surface: Path loss modeling and experimental measurement,” IEEE Transactions on Wireless Communications, vol. 20, no. 1, pp. 421-439, 2020.
[76] C. Pan, H. Ren, K. Wang, W. Xu, M. Elkashlan, A. Nallanathan, and L. Hanzo, “Multicell MIMO communications relying on intelligent reflecting surfaces,” IEEE Transactions on Wireless Communications, vol. 19, no. 8, pp. 5218-5233, 2020.
[77] M. Di Renzo, K. Ntontin, J. Song, F. H. Danufane, X. Qian, F. Lazarakis, J. De Rosny, D.-T. PhanHuy, O. Simeone, R. Zhang et al., “Reconfigurable intelligent surfaces vs. relaying: Differences, similarities, and performance comparison,” IEEE Open Journal of the Communications Society, vol. 1, pp. 798-807, 2020.
Cindy Francis commenced work with the Australian Maritime Safety Authority (AMSA) in 2003 as a search and rescue operator. Her current position is as a maritime senior search and rescue officer within the AMSA Response Centre. In this role, she is responsible for the supervision, mentoring, and development of a team of specialists in the coordination of search and rescue operations and oversight of operational responses on a 24/7 basis from AMSA’s Joint (aviation and maritime) Rescue Coordination Centre (JRCC).
Why did you choose this occupation?
Being a senior search and rescue mission coordinator in the AMSA is an operational role that makes use of my previous skills and allows me to help people.
Where has your career taken you?
I started out in the Australian Navy and had the opportunity to travel widely across Southeast Asia and the South West Pacific. During my time with JRCC Australia, I have had the good fortune to travel to the Maldives, Mauritius, New Zealand, Philippines, Singapore, and Sri Lanka, along with numerous locations across Australia. Most of these trips have been training opportunities from which I learned a lot.
If you had to choose another career, what would it be? I have not imagined any other career. I have loved being involved in search and rescue for 21 years now and cannot imagine that there is a better career out there. When I was young, I had dreams of being an archaeologist or an architect, but the path I ended up on went in quite a different direction.
What is your personal motto?
I struggle with this question as I do not think I have a personal motto. The closest I can come is “if you are going to do something, do it properly.” I do not believe in doing anything half-heartedly, whether in a work sense or in my personal life. I try to do the right thing and make the most of every opportunity I am given.
Where do you like to vacation?
I love to travel and go to different places. The world is such a big place and my wish list of countries to visit is long. I have not travelled enough yet to start revisiting places, but my dream would be to spend a couple of years in Italy after I retire.
What has been the highlight of your career?
It is difficult to pick one highlight from 21 years. I feel fortunate to do the job I am doing, and every time we rescue someone, I feel pride that my team has been directly involved in saving a life. I have also received a great deal of job satisfaction from training others to do search and rescue; it is a privilege to see the success of those I have helped train.
What do you like most about working in this field?
I work with a team of professional and dedicated people who are focused on doing their absolute best to help others in their time of need. Every day and every search are slightly different, and there are new challenges with each job, which keeps it interesting. I find search and rescue highly rewarding as you are directly involved in saving people and returning them to their families.
What are some of the biggest challenges your job presents?
Shift work is getting harder the longer I do it. I find night shifts pose the biggest challenges. As far as coordinating search and rescue, the biggest challenges are posed by the size of the Australian search and rescue region and the relative lack of assets to respond; those challenges are also what makes the job interesting. Australia’s search and rescue region is over 53 million square kilometres, covering 10% of the world’s surface, ranging from the tropics to Antarctica, the Tasman Sea to halfway to Africa.
What technological advancements have you witnessed?
There have been so may advances in communications and our ability to connect to ships at sea and aircraft in flight has never been easier. There has also been a proliferation of systems designed to track and provide distress alerting for not only commercial vessels and aircraft, but also recreational pursuits at sea and in remote areas. This has made locating people in distress much quicker as we either have a relatively accurate last known position, or we can narrow down the search area to a much smaller locale. The quicker we can get help to people in trouble, the better the outcomes are for everyone.
What does the future hold for this industry? For you?
The need to conduct a search for a boat or an aircraft with no last known position is decreasing and will continue to decrease as communications and connectivity keeps improving. We are more connected than we have ever been before; however, there are still circumstances where search planning and wide area search operations are still necessary. Maintaining our ability, knowledge, and skills
to respond to those low occurrence, high impact jobs will be a challenge as we increasingly rely on technology to solve the problem quickly. For me, I am close to retirement, and I am looking forward to the opportunity to do more travel. I also hope to continue to help my community in whatever way I can; I will not be stopping work completely, just moving to a different kind of work.
What new technologies would you like to see?
Global integrated satellite coverage that enables faster and more reliable communications no matter where you are in the world or what service provider you use. Also, more integration of data to provide search and rescue authorities the ability to access and interrogate information from multiple sources more efficiently. There are so many stand-alone systems and service providers
that we need a more efficient way to search for information across multiple platforms quickly and have that information integrated into our incident management systems to allow better decisionmaking. As I said earlier, the quicker we can get help to people in trouble, the better the outcomes – having the most accurate and timely information is key to that.
What advice do you have for those just starting their careers?
Technology is better supporting the job every year, but industry experience and judgement is still necessary and valuable. Search and rescue is a fulfilling job; there is no better feeling than knowing you have been directly involved in saving someone’s life and returning them to their families and community.
by Karl Birgir Björnsson AI-Powered Support
Over the years, advancements in technology have significantly improved maritime safety, yet the number of accidents and incidents at sea remains a concern. Hefring Marine believes that artificial intelligence (AI) is the next step toward revolutionizing safety for vessel operators and their crews.
Hefring’s flagship solution, the Intelligent Marine Assistance System (IMAS), is designed to tackle many of the critical safety issues that arise in maritime operations. By harnessing the power of AI, IMAS provides real-time decision-making support that not only enhances safety at sea, but also mitigates the risks associated with human error, one of the leading contributors to maritime accidents.
IMAS empowers vessel operators in making real-time decisions to help ensure safer and more efficient journeys while at sea. The system continuously collects and analyzes data from onboard sensors, environmental factors, and vessel performance metrics (Figure 1). Based on this data, IMAS provides recommendations for optimal speed that continuously improves as the AI learns. In doing so, the system helps to avoid potentially hazardous situations, such as harsh wave conditions, which could lead to accidents or injuries on board.
A key advantage of IMAS is its ability to reduce wave slamming impacts by an impressive 87% when the recommended safety speed is followed within +-5% versus when you are above recommended safety speed. By adjusting speed based on realtime wave data, IMAS minimizes these
impacts, ensuring a smoother, safer ride. This is particularly valuable for high-speed vessels, such as patrol boats, rescue vessels, and offshore supply vessels, where the risk of injury from wave slamming is high. This 87% reduction is achieved while only reducing the average speed by less than 1%. This maintains operational effectiveness while still improving overall safety.
Situational awareness is a critical component of maritime safety. In high-stress or complex environments, the ability to quickly and accurately assess the situation can be the difference between a safe voyage and a disaster.
IMAS enhances situational awareness by continuously monitoring vessel conditions, the surrounding environment, and operational data, ensuring that the crew has access to real-time insights. These insights allow operators to make informed decisions, reducing the likelihood of accidents caused by poor visibility, changing sea states, or unexpected hazards.
Moreover, human error is a leading cause of maritime accidents, often resulting from fatigue, miscommunication, or cognitive overload. By providing AI-driven recommendations, IMAS reduces the cognitive burden on vessel operators, allowing them to focus on critical tasks while the system handles complex data analysis. This synergy between human expertise and AI support leads to better decision-making and, ultimately, improved safety outcomes.
Fatigue is a pervasive issue in the maritime industry, especially on long voyages or during extended periods of high activity. Exhausted crew members are more likely to make mistakes, which can lead to accidents, equipment failure, or environmental damage. The AI-driven capabilities of IMAS help
mitigate the effects of fatigue by taking over some of the decision-making responsibilities from the crew. By offering continuous monitoring and data-driven recommendations, the system reduces the workload on operators, allowing them to focus on key tasks and ensuring they remain alert and responsive when necessary.
In addition to improving decision-making, IMAS contributes to the overall well-being of the crew by reducing the physical strain of operating vessels in rough sea conditions. As the system optimizes the vessel’s speed to minimize wave impacts, the crew experiences a smoother ride, which translates to less physical fatigue and stress. In turn, this promotes better health and performance over extended periods at sea.
Beyond safety, Hefring Marine’s IMAS offers substantial operational benefits. By optimizing speed and route based on real-time data, the system can help vessel operators reduce fuel consumption by up to 20% – a significant cost
factor in maritime operations. Reduced fuel consumption also leads to lower emissions, which IMAS also helps contribute to by a 20% reduction. This reduction in pollution helps contribute to the industry’s sustainability goals of creating a cleaner maritime industry. This makes IMAS not only a safety tool but also a solution for improving operational efficiency and reducing the environmental impact of maritime activities.
The system’s predictive capabilities also extend to maintenance. By monitoring the performance of key vessel components, IMAS can identify potential issues before they become critical, allowing operators to perform maintenance proactively. This reduces the risk of unexpected equipment failures, improving uptime and reducing the cost associated with emergency repairs. Furthermore, having the IMAS system in place on board a vessel can also help in savings on insurance premiums by 20%.
by Steven Mallam Applied Research and Education
The Ocean Safety Research Unit (OSRU) at the Fisheries and Marine Institute strives to improve safety and performance for persons engaging in work and pleasure activities on and near bodies of water through a variety of applied research and education approaches. It takes an inherently multidisciplinary approach in tackling these challenges aiming to better understand and optimize interactions between people, technology, and environments to enhance maritime operations and activities. As technologies advance, so do the tasks, organization, and required skills of the work and workers within evolving systems. This requires constant updating in approaches and understanding of these evolving demands within already complex and safety-critical systems.
Currently, the unit has five faculty members actively engaging in applied research, supervising graduate student projects, and teaching across a variety of topics. Dr. Rob Brown, Dr. Francis Obeng, Dr. Elizabeth Sanli, Dr. Rose Ricciardelli, and Dr. Steven Mallam represent a diverse set of backgrounds, expertise, and research approaches, including in the areas of engineering and naval architecture, sociology, applied psychology, human factors, and kinesiology.
Sitting within the School of Maritime Studies at the Marine Institute (MI), the OSRU has direct access to world leading technologies and training facilities and centres. These include MI’s Centre for Marine Simulation, Offshore Safety and Survival Centre, and the Safety and Emergency Response Training Centre, with further collaboration with MI’s School of Ocean Technology, School of Fisheries, and other departments and experts at Memorial University, throughout Canada and internationally. This creates a rich platform for
interactions, access to world-class equipment and facilities, and subject-matter experts across differing fields creating a living lab experience for our faculty and students alike.
Some examples of current OSRU research topics include human performance and evacuation modelling for large passenger ships and naval ships; thermal protection of humans in survival situations, including survival and evacuation equipment analysis; search and rescue modelling throughout Arctic regions; risk analysis and modelling of commercial fishing and shipping industries; maritime education and training of seafarers and offshore energy workers; simulator training procedures and efficacy; land and marinebased firefighting; autonomous and remote shipping; complex skill training and retention; safety and survival training; public safety at sea and first responders; and mental health and well-being of maritime workers.
Most importantly, the OSRU has the privilege of managing MI’s graduate programs in Maritime Studies, offering both master’s and doctorate level programs in Safety: The Human Element and Public Safety. These degrees are designed as thesis-based programs, requiring students to complete independent research projects in the field of maritime studies working within and being supervised by OSRU faculty. This creates a hands-on, applied approach to real-world, relevant matters to address current issues and gaps in knowledge and ultimately to identity solutions and create change.
The OSRU continues to grow and expand its research portfolio, aiming to address current and future issues related to improving human and system safety of the people engaging in water-related work and pleasure activities.
Dr. Steven Mallam is the academic director of the Maritime Studies graduate programs at the Fisheries and Marine Institute. www.mi.mun.ca/departments/oceansafetyresearchunitosru
The global ocean technology community will gather for Ocean Business 2025 at the National Oceanography Centre in Southampton, UK, from April 8-10. Recognized as a premier event for the marine industries, the show has already sold out all exhibitor spaces.
Ocean Business 2025 will showcase emerging technologies from new and established manufacturers and innovators. Attendees can experience live demonstrations, test-drive equipment, and connect with key industry players in an interactive environment.
Highlights include the renowned Training and Demonstration Program, featuring the latest developments in autonomous vehicles and sensor technologies, with live dockside and onboard demonstrations.
The event will also see the return of the Ocean Careers program, a platform for students to network with potential employers and gain valuable insights from professionals in the field.
Visitors will have the opportunity to attend a two-day conference again this year, addressing critical industry issues, alongside on-site meetings.
Additionally, Ocean Business 2025 will host MARELEC, the world’s only conference dedicated to marine electromagnetics. Delegates will hear from leading experts on advancements in geophysics, oceanography, naval warfare, and geotechnical engineering.
There will be plenty of networking opportunities, with both formal and informal social events, including the popular Wine Trail, creating a lively setting for industry connections.
FREE registration for Ocean Business opens in January, when the program details will be released.
For further information: www.oceanbusiness.com
by Randy Hart
Inherently our daily lives are full of risk. Depending on the day, some days are more dangerous than others. As a result, we rely on emergency response professionals to manage and mitigate our emergencies. If you are woken at night by a fire alarm and discover a fire in your home, chances are you would call a universal emergency number (such as 911) and depend on emergency professionals. However, what if calling 911 was not an option? This is the reality faced by offshore and maritime workers. These workers, in most cases, are the first responders and have emergency response responsibilities secondary to their primary roles. This makes high-quality safety and emergency response training essential. Greater fidelity in safety and emergency response training with deeper objectives can make all the difference.
Newfoundlanders and Labradorians are all too familiar with the consequences of maritime related incidents. A tragic example is the sinking of the Ocean Ranger, an offshore drilling rig, in the North Atlantic Ocean on February 15, 1982, which resulted in no survivors among its 84 crew members. Similarly, a 1985 helicopter crash near Argentia, NL, led to six fatalities, and more recently the Cougar 491 helicopter crash in 2009. These incidents each highlighted an urgency for improved training standards within the local offshore jurisdiction. For example, the Royal Commission on the Ocean Ranger Marine Disaster (1984) emphasized the significance of robust lifeboat training, recommending that:
• Lifeboat crews be trained in the use and operation of the type of lifeboat to which they are assigned.
• Such training should include actual launching and operation of the lifeboat in the sea.
• Lifeboat crews be required to launch and operate the lifeboat in the sea at least twice each year.
• If this training could not be conveniently or safely done from the drilling unit, then it should be done from a shore-based installation.
Similarly, the Transportation Safety Board of Canada (TSB) highlights the crucial role of thorough training in its safety investigation reports, such as the final safety investigation report (M21A0041) regarding the catastrophic machinery failure, subsequent fire, and sinking of the fishing vessel Atlantic Destiny that occurred 120 nautical miles south of Yarmouth, Nova Scotia, in March 2021. The TSB notes that when individuals encounter uncertain situations – especially under time pressure and with serious potential consequences –they tend to prioritize immediate responses over evaluating the situation. The board emphasizes that to address this, it is essential for crew members to undergo adequate training and practice various emergency scenarios and tasks. With sufficient practice, actions can become automatic, requiring minimal cognitive effort, which can be compromised by external stressors, such as emergencies and distractions. The report also underscores that in an emergency, there is no time to learn how to operate firefighting equipment, and the pressure of the situation can make it even harder to remember the necessary procedures and techniques needed for a quick response. These conclusions highlight the significance of robust training, particularly for those offshore workers who have been delegated emergency responsibilities that we would expect emergency professionals to manage onshore.
As a result of the recommendations from the Royal Commission, the Offshore Safety and Survival Centre (OSSC) was established in 1989 to ensure offshore workers receive
adequate training for their preparedness for offshore emergencies. It has since become recognized as the gold standard for survival and emergency response training at both provincial and international levels. The OSSC is dedicated to continually improving its training courses, facilities, and equipment to provide offshore workers with the highest quality training, not just meeting the minimum requirements for training. The instructional team, which is vital to the quality of the OSSC programming and training, primarily consists of professionals from offshore and maritime industries. Many instructional members hold senior officerlevel certifications from Transport Canada and possess extensive experience either at sea or in the offshore energy sector. Members of the team effectively bring the training standards to life, sharing their knowledge and personal experiences to provide relevance and realism for students. They utilize world-class facilities and equipment to meet and exceed the learning objectives set by regulatory bodies. It is not just a tick in the box.
A centre within the Fisheries and Marine Institute at Memorial University, the OSSC offers a wide range of safety and emergency response training for the energy, marine transportation, fishing, and land-based
industries. Prioritizing quality and safety management, the OSSC provides modern, high-fidelity emergency training within a riskmanaged environment. The OSSC’s facilities and equipment represent strategic investments that have stemmed from lessons learned during incidents such as the Ocean Ranger and Cougar 491 tragedies. Among its features is a marine evacuation facility equipped with a twin-fall davit system, a PROD (Preferred Orientation and Displacement) davit system with 50-person lifeboats (the only such facility in the world), fast rescue craft davits and vessels, and a slewing arm davit equipped with SOLAS-approved rescue boats and davit raft launching capabilities.
In accordance with the Royal Commission recommendations, the OSSC ensures that industry partners have access to world-class facilities designed for training lifeboat crews (Figure 1). This training includes the actual launching and operation of lifeboats at sea. Additionally, the OSSC offers a coxswain lifeboat and a full-motion free-fall lifeboat simulator to enhance its marine emergency evacuation programs.
The OSSC features an Environmental and Survival Training Theatre which includes
a Helicopter Underwater Escape Trainer (HUET), wind and wave generation, and realistic sound effects, creating an immersive training environment. This theatre also offers a variety of marine and offshore evacuation systems, including a fully functional lifeboat and davit arrangement. To enhance HUET training and improve the robustness of offshore helicopter safety and emergency training, the OSSC has incorporated a full-motion S92 helicopter cabin simulator (Figure 2). This simulator allows students to practice high-fidelity helicopter-controlled ditching scenarios, ensuring realistic practice for crew members dealing with different emergencies and tasks.
Setting the gold standard for offshore firefighting training in the province, the OSSC boasts the most comprehensive firefighting training facility on the East Coast of Canada. It features a CSA/NFPA-approved, three-
storey propane-fueled firefighting system, complete with marine, offshore energy, and industrial training props. Moreover, the facility is equipped for low-emission, environmentally controlled fuel oil firefighting operations and includes various firefighting props, such as large pan fires, helicopter firefighting systems, and interior firefighting setups. The OSSC also offers unique training opportunities with a three-storey high-angle rescue tower, complete with vertical rescue capabilities to enhance fire emergency response and rescue programming.
By training at the OSSC today, companies are further lowering the risk in their workplaces and mariners are investing in their safety for tomorrow.
www.mi.mun.ca/departments/ offshoresafetyandsurvivalcentreossc
by Daniel Gomez-Ibanez
When you touch your ocean technology, do you feel a tingling sensation? Does everything work fine until it hits the water? Do you see unexpected corrosion or tiny bubbles on metal objects? These conditions may be symptoms of a ground fault.
Ground faults are unintentional connections between active electric circuits and other electrically conductive materials. With conductive seawater all around, ground faults are common in ocean technology and easy to overlook. This article reviews several adverse consequences of ground faults, including damage to equipment, diminished functionality, and potential for personnel injury. Finally, strategies are introduced to prevent, detect, and repair ground faults, including robust sealing, water alarms, ground fault monitoring, and partitioning.
Equipment can be ruined by ground faults. In the presence of seawater, electric currents drive chemical changes in metal objects. Accelerated corrosion can occur, eating away solid material (Figure 1). If material is removed from a sealed housing, corrosion can create a path for rapid water ingress, leading to loss of buoyancy or implosion.
Even without obvious physical changes, electronic signals can be corrupted. Ground faults provide a path for interference to reach vulnerable circuit nodes which may lead to loss of critical functions. Ground fault hygiene supports clear and consistent measurements and images.
Ground faults can be dangerous. Common sense and safety rules require that exposed ship or vehicle structures must not be intentionally energized and shall instead be earthed. Away from land, however, ground faults can reroute electric currents so exposed parts become energized. When the system voltage is less than 50 volts, a ground fault may barely provoke a tingling sensation, but higher voltages can cause muscle spasms or burns. Ground fault monitoring helps avoid these dangerous conditions before they cause harm.
If a ground fault is allowed to persist, an explosion is possible. Water will dissociate into its elements in the presence of as little as 1.5 volts. A single AA battery has enough energy to produce about a litre of explosive hydrogen and oxygen. In a closed space, a large volume of this dangerous gas mixture can accumulate, and the resulting explosions at sea have caused serious injuries. Awareness of the underlying ground fault could have prevented some of these incidents.
The first line of defence against ground faults is robust mechanical design which seals out water from electronic enclosures using O-rings, gaskets, or moulded materials. Cable assemblies exposed to water pressure can be constructed inside oil-filled tubing or with polymer-filled interstices to block water ingress. Not only water but pinched wires and metallic debris also cause ground faults.
Water alarms can prevent some ground faults. An electrical enclosure, filled with air or oil,
can be fitted with a water probe, consisting of a pair of closely spaced electrical contacts located at the low point of the enclosure. Water entering the enclosure will pool at the bottom and bridge the gap between the contacts. This circuit is detected by a remote alarm which announces the presence of water. An operator or mariner can then go to the indicated location and repair the source of the leak before water reaches other circuits. A large system may have many leak probes, each protecting a separate volume. An autonomous system might be programmed to abort its mission when a leak is detected.
For complete coverage of active circuits, a ground fault monitor can keep watch over conductors even where a water alarm is not practical. Also known as an insulation monitor, this type of alarm works by establishing a test-voltage between active circuits and the surrounding chassis or vessel. The monitor continuously measures electric current flow between these domains. If the insulation is perfect, there is no ground fault and measured current will be zero. If the current rises beyond an established threshold such as 1 mA, it indicates that there is contact between active circuits and the environment somewhere in the system. For consistent insulation measurements, all chassis and enclosure parts should be reliably bonded to each other.
Once a ground fault is detected, it may be difficult to find its location. Typically, branch circuits are switched off one by one to exonerate sections of the electrical network. Alternatively, components may be dipped in and out of the water to find the offending part. This process is facilitated if the electrical system includes switches that completely isolate branch circuits instead of just interrupting one conductor.
It is also possible to use multiple insulation monitors. By using transformers, isolated converters, or optocouplers, a vessel or vehicle can be broken into multiple ground domains. Each section can then be monitored separately, allowing a targeted response to a detected fault.
Ground faults are common in ocean vehicle systems and may seem insignificant. With time, however, failures compound, and the effects can be catastrophic. Robust insulation, water alarms, and active monitoring help keep ground faults at bay, supporting reliable operation of electronic systems and personnel safety.
Daniel Gomez-Ibanez is a senior engineer at the Woods Hole Oceanographic Institution. He is interested in underwater vehicle system design and reliability. www.whoi.edu
Virtual Marine pioneers maritime safety with state-of-the-art training simulators, helping coxswains train safely in realistic weather conditions for real emergencies. With over 20 years of expertise, we deliver high-fidelity simulation solutions that enhance the safety of personnel and assets worldwide. Located in Newfoundland and Labrador, Canada, we integrate advanced science into unique, risk-free training systems that build seafarers’ confidence and competence. As the first to offer IMO-approved digital lifeboat and fast rescue boat training for the offshore oil and gas sector, we proudly lead the shift toward immersive, performancefocused maritime safety training.
Artificial intelligence and machine learning continue to expand the capabilities of virtual trainers. Simulator software can now measure performance and observe behaviours as trainees complete tasks in simulator scenarios. The data collected in these exercises is used to form models of performance that can be used to diagnose skill level. The simulator captures data and expert knowledge that is input into numerical models to set training targets. As new participants practice in the simulator, a digital signature is captured that measures their performance and identifies where additional training is needed. Training is then adapted to individual training needs resulting in a customized learning experience.
The outcome of the training is operational readiness. We are continuing to find better ways to build the competence and confidence needed to prepare workers for their jobs on the ocean. www.virtualmarine.ca
by Sam Mayall
With an estimated 1.9 million seafarers working offshore and over 31 million cruise passengers setting sail each year, safety at sea is of paramount importance. Tragically though, some 40% of all person overboard incidents result in fatality. For recovery to be successful, rescue must happen fast. Survival windows can be short – it takes less than 11 minutes, on average, before someone who has fallen into cold water becomes unresponsive.
Advancements in artificial intelligence (AI), machine learning, satellite communication networks, and other related technologies are underpinning the development of more effective rescue methods, reducing risk for both the casualty and the rescuers.
As an additional link in the rescue chain, uncrewed search and rescue (SAR) systems can reach people in peril faster, without endangering the lives of others. Uncrewed SAR solutions are now seeing increasing demand from rescue agencies and governments looking to support and improve existing operations and, crucially, increase the number of lives saved.
With first-hand experience of tragic loss of life at sea from my early career as a mariner, I founded Zelim in 2017 to develop ways to use advancing technology to assist in offshore emergencies and, ultimately, make uncrewed search and rescue the norm. Zelim’s approach is threefold – find, recover, and protect –effectively creating a complete SAR ecosystem.
Find Them – Eyes on the Water
Zelim’s AI-enabled person overboard detection, tracking, and alerting solution, ZOE, was developed in collaboration with the US Coast Guard. ZOE automatically detects a person overboard using AI-enabled camera tracking technology (Figure 1). A suite of cameras monitors the area around a vessel, raising alerts if someone falls into the water.
In the rare incidence of a person overboard, ZOE transmits an automatic alarm to the user, who can see a 10-second clip of when the alert was triggered while simultaneously viewing live footage. ZOE continues to track the person in the water with around 97% accuracy to a distance of over 330 metres, thereby increasing their chances of a successful rescue. The system logs the position of the vessel and the person overboard at the point of detection for mayday call geo-location. It also provides the user with a mayday script and actions checklist, for fast and accurate reporting under stressful circumstances.
ZOE recently received Approval in Principle from Lloyd’s Register for Person Overboard Detection and is currently undergoing testing on a cruise ship to secure full Type Approval. ZOE has been trained on over 5.4 million maritime rescue images across a wide range of ocean conditions and additions are constantly being added to the dataset. The technology is highly extensible, with other potential uses including automatic security threat detection and alerting for small vessels, such as rigid-hulled inflatable boats used by criminal gangs and pirate vessels which can be missed by traditional radar and AIS systems, as well as safety zone monitoring.
ZOE is currently at work on WindFloat Atlantic, Ocean Winds’ floating offshore wind farm in Portugal, on a drone for CASARA, Canada’s Civil Air Search and Rescue Association, on a cruise ship, and on
the jackup rig VALARIS Stavanger in the North Sea. Initial feedback from VALARIS is very positive, with ZOE’s 360-degree view from the rig removing potential reliance on the human eye to find and maintain sight of someone in an emergency.
In search and rescue situations, the key point of difference between a human and a computer is accuracy and consistency. According to US Coast Guard reports, the human searcher offers a probability of detection of just 20% in a single search pass; ZOE, by contrast, offers 97% probability.
Sea survival is hugely dependent on the time it takes to retrieve people from the water, but inclement weather can prohibit the launch of crewed rescue craft and lives are needlessly lost.
In September 2024, Zelim unveiled its 8.5 metre fast rescue craft (FRC) GUARDIAN at the SMM tradeshow in Hamburg,
Germany. GUARDIAN is the world’s first rescue vessel that can operate uncrewed, via remote control, or with crew on board. The aluminium-hulled craft is lightweight and designed to be rapidly deployed from a mother ship to recover casualties. It has a free-fall launch capability of 25 metres or can be mobilized using LARS davits on deck.
With no crew onboard, GUARDIAN can speed along at 30 plus knots to reach people in the water fast. It has a range of 15 nautical miles, a six-hour endurance, and can accommodate 11 survivors in uncrewed mode. The FRC also incorporates the ZOE detection and tracking solution for optimal search.
During early sea trails in Holyhead, North Wales, the vessel successfully retrieved multiple unconscious casualties (mannequins) from the water without a human required to pull the casualty on board. The next phase of testing will start to increase the sea state, proving the capability in evermore challenging conditions.
Protect Them – Rescue from the Water Ship crews have little time to carry out an effective person overboard recovery before maritime conditions prevent the casualty from assisting in their own rescue. When rescue vessels approach, many survivors simply do not have the strength or use of their limbs to pull themselves to safety due to the effects of cold water on the human body. This is obviously exacerbated when casualties are unconscious or unresponsive. GUARDIAN’s design also incorporates Zelim’s type approved SWIFT rapid rescue conveyor (Figure 2), enabling people to be rescued quickly without any human effort required even in harsh weather conditions.
The SWIFT conveyor carries the casualty upwards onto the rescue vessel out of harm’s way, without the need for the casualty to physically climb on board, reducing the time to recover them from the sea surface and the risk of injury during rescue. During extensive sea trials, SWIFT has operated effectively in 40 knot wind speeds and wave heights of 3 metres, retrieving mannequins from the water in under a minute.
Following initial sea trials, feedback from the Port of Milford Haven in the UK indicated that the SWIFT system has the potential to become a market leader in person overboard recovery. The design and operation of the system also presented advantages in reducing potential further injury to personnel being recovered, particularly when recovering in a swell.
SWIFT received full Type Approval from classification society Lloyd’s Register earlier this year, paving the way for the maritime industry’s widespread use of the sea survival safety system.
The advancement of AI and sensor technologies has been a significant enabler to take SAR into the next generation, where computer vision systems and remotely operated rescue craft work alongside human searchers, helping them to find and recover people in danger, faster and more effectively than ever before.
Sam Mayall is the founder and CEO of Zelim. He has spent his life working at sea, starting out as a dinghy instructor, then joining the Merchant Navy and working for several years as a deck officer on platform supply vessels.
Commercial fishing is an incredibly dangerous job. According to the US Centers for Disease Control and Prevention, the fatality rate for commercial fishing in 2019 was 40 times higher than the national average. Extreme working conditions are often the norm, as fish harvesters frequently contend with long hours, physical labour, and heavy equipment, often within the context of treacherously cold water and inclement weather. Emergencies such as a limb caught in machinery, a person falling overboard, or a fire in the galley are all too common.
When it comes to preventing injuries and deaths in the trade, one of the oldest technologies is also one of the most effective: passing down lifesaving knowledge from one fish harvester to another.
For more than 30 years, Washington Sea Grant (WSG) has delivered high-quality instruction in first aid and sea safety for commercial fish harvesters. This program meets fish harvesters where they are up and down Washington’s coast, including providing instruction to Tribes.
WSG is one of 34 Sea Grant programs supported by the National Oceanic and Atmospheric Administration in coastal and Great Lakes states that encourage the wise stewardship of our marine resources through research, education, outreach, communications,
and technology transfer. Housed within the College of the Environment on the University of Washington’s Seattle campus, WSG maintains regional offices throughout Western Washington. Through its outreach activities, WSG staff provide training, education, and technical assistance to a variety of stakeholders and communities on issues ranging from aquaculture to coastal hazards resilience to preventing small oil spills.
WSG’s safety at sea training is one of the organization’s longest-running and bestknown programs. Since the mid-1990s, WSG classes on Puget Sound, Washington’s Pacific coast, and the Columbia River have been credited with saving lives in several fisheries and helping participants confidently handle emergency situations.
WSG offers two types of training in this vein: a First Aid at Sea course and a Drill Instructor course. The First Aid at Sea course covers topics including CPR, hypothermia, and fractures. The Drill Instructor course covers emergency procedures and how to develop appropriate emergency drills for participants’ own vessels. Both courses include a combination of lecture and handson experience. The activities are particularly valuable: it is impossible to know how it will feel jumping into the water with a survival suit on until that person has done it (Figure 1). Being able to anticipate the experience can help to prevent panic when it matters most.
These courses also help fish harvesters meet the United States Coast Guard requirement that all commercial fishing vessels must have someone on board who has these certifications. Vessels are required to do their own safety drills every 30 days. The reality is that the more people on board who have received this type of training, the safer. However, fish harvesters often struggle to find courses that fit into their seasonal schedule and are at a price point that fits within their budget. One
of WSG’s goals is to make these courses affordable and accessible enough so that captains feel they can enroll their entire crew.
Robert Maw, himself a fish harvester and vessel owner, has recently taken the helm of the WSG sea safety program. He first learned about WSG’s work through taking the course with two of his crew members. With a background in education in addition to more than 40 years of fishing experience, when the opportunity arose to take over the program at WSG he jumped on it.
In his first year, Mr. Maw became an Alaska Marine Safety Education Association-certified instructor, so he can now directly teach the First Aid at Sea and Drill Instructor courses (previously, WSG organized the courses but contracted out the actual instruction). Having this type of expertise in-house at WSG is invaluable, as it enables the organization to be more nimble in responding to requests from the community. For example, Mr. Maw recently received a request from the Makah Tribe to
provide Drill Instructor training, which he provided near their home in Neah Bay.
Mr. Maw sees additional opportunities to strengthen WSG’s safety at sea program, for example, by further integrating the First Aid at Sea and Drill Instructor coursework. In real situations, the first aid and emergency response would go hand-in-hand: if someone fell overboard the crew would need to retrieve them and treat them for potential hypothermia, or if there were a fire on board the crew would need to put it out and potentially treat burns.
The most important goal is to expose as many people to the scenarios and lifesaving information as possible. For commercial fish harvesters, the odds are that it is not a question of if they will experience an emergency – the question is when, and whether they have the practice and knowledge to quickly and calmly respond.
Samantha Larson is a science writer at Washington Sea Grant, covering issues related to the state’s ocean and coasts.
the puddle that was once you Katherine Percival
This work explores the psychological pull of the “call of the void” –the sudden urge to leap from high places – and its parallels with the magnetism of depression. Like these impulses, the urge fades, leaving behind self-reflection and the challenge of coexisting with inner darkness. Drawing from personal experiences and inspired by hiking the East Coast Trail in Newfoundland, the watery hues reflect the emotional journey of navigating intense feelings and surfacing from the depths of our own hidden currents.
https://kpercivalfineart.weebly.com/