

A Comparative Assessment of C-ITS Technologies

Dr. Shuo Ding
Krishnamoorthy Muthuswamy
Erik van Vulpen
Prof. Aniruddha Desai

May 2024
This research is funded by iMOVE CRC and supported by the Cooperative Research Centres program, an Australian Government initiative.
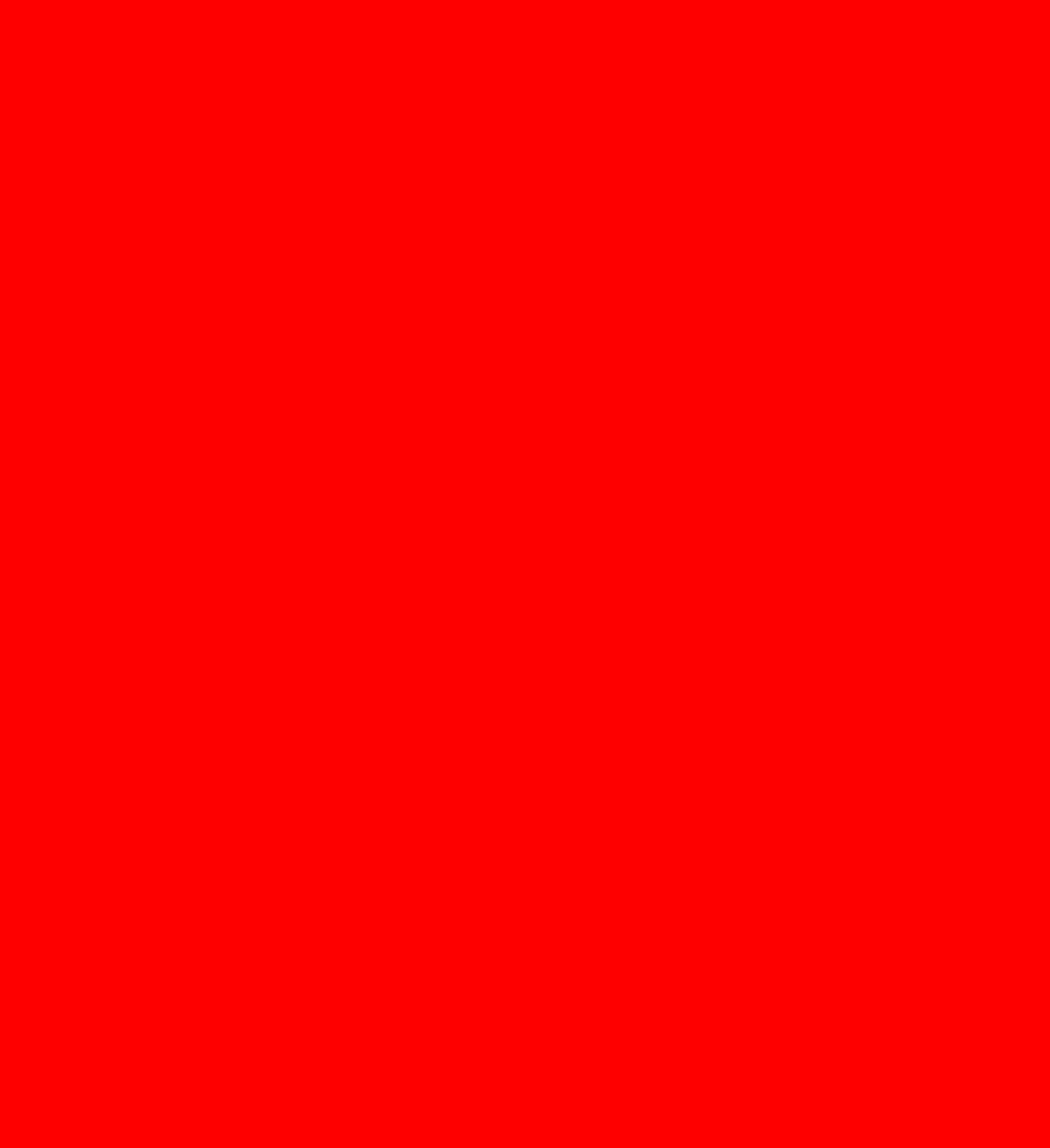





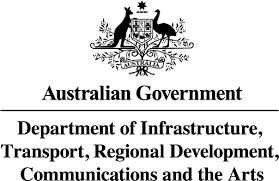

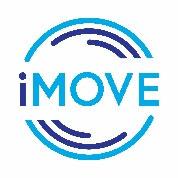

Abbreviations
3GPP 3rd Generation Partnership Project
4G Fourth Generation Wireless Broadband
5G Fifth Generation Wireless Broadband
5GAA 5G Automotive Alliance
5G-V2X 5G Vehicle-to-Everything or 5G New Radio (NR) Vehicle-to-Everything (V2X)
802.11p/bd IEEE standard for direct communication between road users and roadside infrastructure
ACMA Australian Communications and Media Authority
AV Autonomous Vehicle
BPSK Binary Phase-Shift Keying
Car2X Car-2-Anything
C2C-CC Car 2 Car Communication Consortium
CAM Cooperative Awareness Message
CAV Connected and Automated Vehicle
CBR Constant Bit Rate
CCAM Cooperative, Connected and Automated Mobility
CEN European Committee for Standardization
C ITS Cooperative Intelligent Transport Systems
CMC Connected Motorcycle Consortium
C-V2X Cellular Vehicle-to-Everything
dB Decibel
DCM Dual Connectivity Mode
DENM Decentralized Environment Notification Message
DSRC Dedicated Short-Range Communications
ETSI European Telecommunications Standards Institute
EU European Union
FCC Federal Communications Commission
FCAI Federal Chamber of Automotive Industries
GHz Gigahertz
GLOSA Green Light Optimal Speed Advisory
GM General Motors
GNSS HARQ Global Navigation Satellite System
Hybrid Automatic Repeat reQuest
IEEE Institute of Electrical and Electronics Engineers
ISO International Organization for Standardization
ITS Intelligent Transport Systems
ITS-G5 European standard for vehicular communications based on the IEEE-1609.x and IEEE-802.11p standards
LTE Long-Term Evolution or 4G Cellular Communication
LTE-V2X 3GPP standard for Vehicle-to-Everything communication
ITU International Telecommunication Union
MHz Megahertz
MIMO Multiple-Input Multiple-Output
NTC National Transport Commission
NR New Radio (5G)
OEM Original Equipment Manufacturer (broader than only vehicle manufacturers)
OBU On Board Unit
PC5 Interface for direct communication between road users and roadside infrastructure as specified in C-V2X standards
PKI Public Key Infrastructure
QPSK Quadrature Phase-Shift Keying
QAM Quadrature Amplitude Modulation
RSU Roadside Unit
SAE Society of Automotive Engineers
SCMS C ITS Security Credential Management System
SDO Standard Development Organisation
SPAT Signal Phase and Timing
TMR Queensland Department of Transport and Main Roads
USA United States of America
USDOT United States Department of Transportation
Uu Interface for communication between vehicles and mobile networks in C-V2X
V2I Vehicle-to-Infrastructure
V2N Vehicle-to-Network
V2P Vehicle-to-Pedestrian (in this report this also includes cyclists)
V2V Vehicle-to-Vehicle
V2X Vehicle-to-Everything
VRU Vulnerable Road User
Technology Terms for Non-Technologists
This section aims to explain some of the fundamental concepts of Cooperative Intelligent Transport Systems (C-ITS), with a particular focus on a comparison between the two prominent technologies, Dedicated Short-Range Communications (DSRC) and Cellular Vehicle-to-Everything (C-V2X).
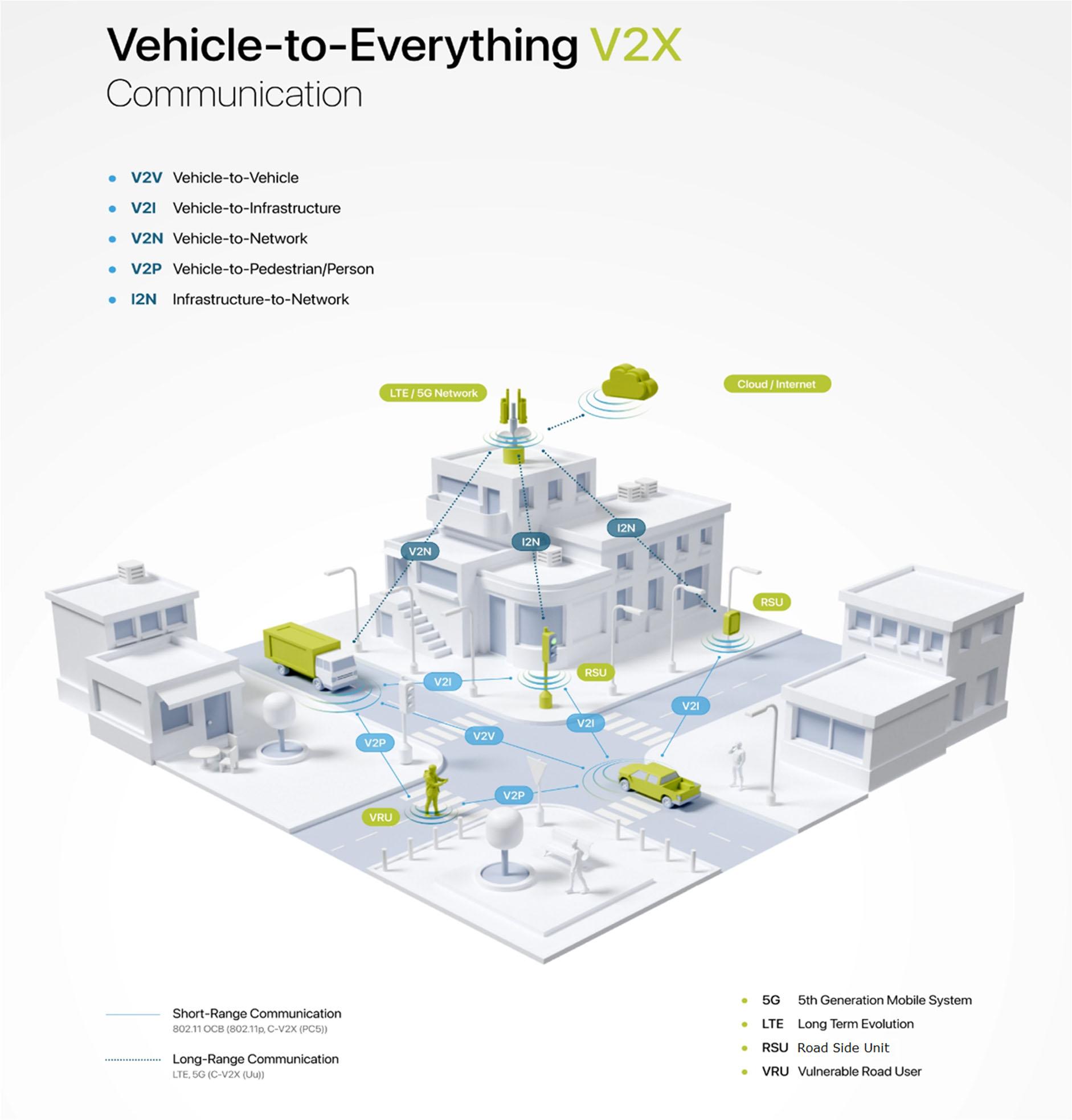
Figure 1 V2X overview (Source: La Trobe University)
Key C-ITS Technology Terms
Table 1 Key C-ITS technology terms
Tech Terms
C-ITS
ITS band
Short-range communication
Description
Cooperative Intelligent Transport Systems (C-ITS) enable vehicles, roads, and infrastructure to work together to share information and communicate with each other. The aim of this is to improve the safety, efficiency, and overall user experience of transportation.
Unlike mobile phones that use commercial frequencies for communication, most countries have a dedicated free, highfrequency spectrum range (expressed in gigahertz [GHz], in this case, 5.9 GHz) for C-ITS devices. This is known as the ITS band. The 5.9 GHz frequency band is divided into multiple channels, allowing vehicles and roadside infrastructure to exchange safety-related information and improve road safety and traffic efficiency.
Short-range communication, which normally operates at the 5.9 GHz ITS band, provides direct, rapid communication between vehicles and infrastructure. Dedicated Short-Range Communications (DSRC) systems are a typical short-range communication technology, operating within approximately a 400-meter range. This technology is used for various safetycritical applications. For example, it facilitates Vehicle-to-Vehicle (V2V) communications such as collision warnings about sudden braking or an impending crash. Similarly, in Vehicle-toInfrastructure (V2I) scenarios, it allows Roadside Units to send traffic signals to vehicles to optimise traffic flow or provide alerts about congestion conditions. These communications are vital for enhancing road safety and efficiency. Cellular Vehicle-toEverything (C-V2X), a newer short-range communication technology provided by cellular communication standards, also offers a direction mode (PC5 mode) at the 5.9 GHz ITS band. However, it's important to note that DSRC devices are not compatible with C-V2X devices even though they both operate at the 5.9 GHz band as their access technologies are different.
Long-range communication
In the context of C-ITS, long-range communication is essential for extending reach beyond immediate proximity, typically involving cellular network technology. DSRC short-range communication may integrate with cellular networks for broader data sources, enabling applications like global traffic condition status, traffic priority requests, and trip route discovery. Conversely, C-V2X inherently includes long-range communication in its standards, leveraging cellular networks (like
Tech Terms Description
4G or 5G) for Vehicle-to-Network (V2N) communications, thereby enhancing short-range communication reliability and coverage. For advanced use cases such as autonomous driving and connected vehicles, long-range communication facilitates functionalities convenient for road operators, such as highdefinition maps (HD maps) data collection and sharing.
C-ITS stack or OSI model
A C-ITS stack refers to a layered architecture or protocol stack that enables cooperative communication and data exchange between vehicles, infrastructure, and other road users. The choice of DSRC or C-V2X impacts the so-called physical layer (the raw data communication), however, the other layers of the C-ITS system can be the same and can support both technologies.
The Open Systems Interconnection (OSI) model is a concept that describes how different network protocols interact and work together to provide network services.
Application Layer: provides services directly to end-users and enables the communication between network services and applications, such as web browsers, email clients, and file transfer programs.
Presentation Layer: translates, encrypts, and compresses data.
Session Layer: manages communication sessions between computers.
Transport Layer: manages data transport, including error checking and recovery.
Network Layer: responsible for data routing and determining the best path for data transfer.
Data Link Layer: manages the physical addressing and the error-free transmission of data frames.
Physical Layer: deals with the physical characteristics of the network hardware interface to the transmission mediums (e.g. coaxial cables, fibre optics, etc.).
Day 1 use cases
Day 1 services in the context of the CAR 2 CAR Communication Consortium focus on the initial phase of implementing Cooperative V2X (Vehicle-to-Everything) communication systems. These services are centred around enhancing foresighted driving by exchanging crucial status data such as vehicle position, speed, driving direction, and incidents like vehicle defects. This phase is critical for establishing a basic set of information and warning services that support drivers by making them aware of potential risks not immediately visible. Examples of Day 1 services include Intersection Collision Warning, Emergency Vehicle Warning, and Traffic Jam
Tech Terms Description
Warning. These services are fundamental in supporting lower penetration rates of C-ITS during the early stages of market introduction and are already operational on European roads.
Day 2 use cases
Day 3 use cases
Day 2 services represent an evolution in the Cooperative V2X communication framework, aiming to improve service quality and enhance shared perception and awareness among road users. In this phase, beyond the basic status data, vehicles and infrastructure can share observations obtained through advanced sensors and environmental information. This additional layer of information helps in protecting all road users, including non-communicating participants, in various traffic scenarios. Services in this category include Extended Intersection Collision Warning, Vulnerable Road User Warning, and Cooperative Adaptive Cruise Control. Day 2 services mark a significant step towards more integrated and interactive road safety solutions.
Day 3 services are the most sophisticated in the Cooperative V2X communication system, incorporating advanced functionalities like sharing intentions and supporting negotiation and cooperation among vehicles. This phase is instrumental in paving the way toward cooperative, accident-free automated driving. It involves the exchange of not only status and sensor data but also intention data, enabling intelligent interaction and coordinated behaviour in complex traffic situations. Examples include Platooning, Cooperative Merging, and Cooperative Lane Change. Day 3 services are essential for the realisation of highly automated and autonomous driving, requiring extensive research, development, and standardisation efforts to ensure their effectiveness and safety.
Modulation technique
Network resource efficiency
In electronics, modulation is like giving a regular wave (the carrier) a special twist using another signal that has the actual message (data, voice, or video). The two are mixed to make the information ride on the fast wave. Modulation also allows for the use of different ‘channels’ using the same medium without interference.
Network resource efficiency denotes the optimal use of available resources in a communication network, emphasising enhanced latency, bandwidth utilisation, and communication quality.
Major Players
Table 2 Main organisations involved in C-ITS technology development and standardisation
Organisations
ETSI
Descriptions
The European Telecommunications Standards Institute (ETSI) is an independent, not-for-profit organisation that develops globally applicable standards for information and communication technologies (ICT) in Europe and beyond. ETSI was established in 1988 and is headquartered in Sophia Antipolis, France. As an officially recognised European Standards Organisation (ESO), ETSI plays a crucial role in standardisation efforts for various technologies, including telecommunications, broadcasting, multimedia, cybersecurity, and other ICT-related fields. ETSI’s standards cover a wide range of technologies and services, including mobile communications (e.g., GSM, UMTS, 5G), Internet of Things (IoT), 5G, next-generation networks (NGN), and many others.
IEEE The Institute of Electrical and Electronics Engineers (IEEE). IEEE originally developed the DSRC standard in the late 1990s.
3GPP
5GAA
The 3rd Generation Partnership Project (3GPP) is an umbrella term for several standards organisations that develop protocols for mobile telecommunications, including 5G-V2X, which is the next generation C-V2X.
The 5G Automotive Association (5GAA) is a global cross-industry organisation that brings together leading companies from the automotive, telecommunications, and technology sectors to develop, test, and promote communication solutions for connected and autonomous vehicles using 5G and other advanced technologies.
Key Differences between DSRC and C-V2X
Table 3 Key differences between DSRC and C-V2X
Characteristic DSRC
Safety applications
Origin
Current standards
C-V2X
Both DSRC and C-V2X use ETSI ITS standards for safety-centric applications, which means that the syntax of the warnings – that is, the key information shared (referred to as CAM and DENM1 messages) – is the same. Although DSRC and C-V2X utilise different access layer technologies for message transmission, with DSRC employing Wi-Fi technology and C-V2X using cellular communication, the content of the messages, once received and processed by ITS safety applications, remains identical. This means that regardless of the transmission method, the critical safety information conveyed in these messages is consistent across both technologies.
Developed by IEEE in the USA in the late 1990s based on Wi-Fi 802.11 standards.
USA: IEEE 802.11p / Wireless Access in Vehicular Environments (WAVE)
Europe: ETSI ITS-G5
Next-generation standards IEEE 802.11bd
Short-range communication
Long-range communication
DSRC is allocated a spectrum of either 70 MHz or 75 MHz in the 5.9 GHz ITS band, where each channel spans 10 MHz. This setup is akin to multiple lanes on a highway, with each 10 MHz channel acting as a separate lane within the broader 5.9 GHz frequency range.
In DSRC, the primary focus is on short-range, direct communication for low-latency and time-critical applications such as collision warnings. However, this is effectively complemented by long-range
Introduced by 3GPP in 2016-2017, based on cellular technology.
4G LTE-V2X (3GPP Release 14-15)
5G-V2X (3GPP Release 16-18), also known as 5G New Radio (NR) V2X
Typically, LTE-V2X PC5 mode utilises the upper 20 MHz band of the 5.9 GHz ITS band, ranging from 5.905 GHz to 5.925 GHz. As for 5GV2X, it is expected to require a broader spectrum, potentially 40 MHz or more, to accommodate its advanced capabilities, although this specification has not been definitively confirmed yet.
In C-V2X, long-range communication, such as 4G/5G, is inherently included in the C-V2X standard suites, referred to as network or Uu mode. This facilitates vehicle-to-network (V2N) communications, enhancing the
1 CAM (Cooperative Awareness Messages) and DENM (Decentralised Environmental Notification Messages) are two fundamental message types defined within C-ITS, which enable vehicles and infrastructure to communicate with each other and detect road hazard.
Characteristic DSRC C-V2X
communication for non-critical information, like speed zone updates. This integration, known as DSRC-Cellular Hybrid, allows both short-range and long-range communications to operate in coordination, each within their respective coverage areas, ensuring a hybrid communication network for various vehicular use cases.
reliability and coverage of shortrange communication. The latest 5GV2X specification incorporates Quality of Service in the network service, specifically optimising C-ITS message communication for longrange scenarios. For autonomous and connected vehicles, road operators often require the benefits of long-range communication, such as high bandwidth and low latency, to facilitate advanced applications like High-Definition (HD) Maps Data Collection and Sharing.
Modulation technique
Network resource efficiency
Orthogonal frequency division multiplexing (OFDM) is a method used in many wireless communications systems, including DSRC, to send many signals simultaneously over the same communication channel. It offers robust communication in a dense and dynamic environment. OFDM can be compared to a multi-lane freeway where each small chunk of data is like a car, and every car gets its lane (or frequency) to drive in. So, it is comparable to dispatching numerous small cars, each in their own lanes, all at the same time.
OFDM is specifically designed for high-reliability and lowlatency data delivery.
Traditionally, this has not been the focus of DSRC as it assumes a dedicated channel.
Single-carrier frequency division multiplexing (SC-FDM) is used in digital communications to transmit large amounts of data. SC-FDM can be compared to a single, large truck carrying a big load (all packages) on a single lane (frequency) at once, instead of sending many small cars at once (as in OFDM).
SC-FDM is designed to excel in network environments with less complex technical device requirements and less stringent power requirements.
As C-V2X has been designed with network resources in mind, it includes a comprehensive set of standards that features both Uu mode and PC5 mode. This aims to improve integration, compatibility, and network resource efficiency. For example, vehicles can seamlessly switch between Uu mode and PC5 mode.
Characteristic DSRC C-V2X
Message delivery verification
Error protection coding
DSRC does not verify if a sent message is received. It uses broadcast mode for highfrequency transmission in a dedicated channel, making the need for verification redundant.
Hybrid Automatic Repeat reQuest (HARQ) is a technique used in communication systems, particularly in wireless networks, to improve the reliability of data transmission. By using a combination of the original and previous data, as well as adding extra error correction information, the receiver has a better chance of correctly decoding the packet even if some parts were lost or corrupted during transmission. HARQ facilitates the retransmission of only those messages containing errors.
Data transmission
Convolutional coding is an error correction method of adding redundancy (extra information) to data before it is transmitted over a communication channel. This redundancy helps in detecting and correcting errors that might occur during transmission. It involves using a special mathematical operation called convolution to generate the redundant bits.
Convolutional coding is used in various communication systems, including satellite communication, wireless networks, and digital broadcasting, to improve the reliability of data transmission by detecting and correcting errors.
Carrier-Sense Multiple Access with Collision Avoidance (CSMA-CA) is a protocol used in wireless networks to manage how multiple devices share the same communication channel to avoid sending data simultaneously, which could lead to data collisions and loss. CSMA-CA helps wireless devices take turns using the
Turbo coding is an advanced error correction technique that combines multiple convolutional codes to achieve even higher levels of error correction performance. It was developed in the 1990s and has proven to be highly effective in improving communication system reliability. Turbo coding is used in various modern communication standards, such as 3G, 4G LTE, and some digital broadcasting systems. Turbo codes play a crucial role in ensuring data integrity and reliability, especially in noisy or error-prone communication channels.
Semi-persistent scheduling is a way to manage wireless communication by dividing devices into priority categories and reserving resources at fixed, regular intervals. This can help improve overall system performance and meet the diverse communication needs of different devices.
Characteristic DSRC C-V2X
communication channel to reduce the chance of data collisions and improve overall network efficiency.
Time synchronisation
Predictive quality of service (QoS)
DSRC employs a loose asynchronous timing, utilising the Global Navigation Satellite System (GNSS) for time synchronisation:
Loose Timing: DSRC communication may not rely on strict, synchronised timing between devices. Unlike some real-time communication systems where data must be transmitted and received at precise moments, DSRC may allow for some variation in the timing of messages.
Asynchronous Communication: DSRC devices can send and receive data independently without the need for strict coordination. Asynchronous communication means that devices don't have to wait for each other to finish transmitting before sending their own data.
While DSRC may not inherently provide predictive QoS features, it can still achieve reliable communication through techniques such as message repetition, error correction, and adaptive transmission power control.
Tight synchronous requirements, also utilising GNSS. When GNSS is unavailable, a device utilises other sources for synchronisation, including base stations, other vehicles, and roadside units. In the context of C-V2X, ‘tight synchronous requirements’ means that the communication between vehicles and infrastructure, or between vehicles themselves, is highly synchronised. In other words, there are strict timing standards that devices must adhere to when transmitting and receiving data.
Utilising the enhanced feature of predictive QoS, C-V2X can adaptively boost performance. For instance, during cooperative lane merges, if latency crosses the 20 milliseconds threshold, the QoS monitor will intervene and reallocate network resources to ensure lower latency and more secure lane merges.
Executive Summary
Introduction
Cooperative Intelligent Transport Systems (C-ITS) hold significant promise to enhance road safety, sustainability, and productivity. C-ITS uses wireless technology to enable real-time vehicle-to-vehicle (V2V) and vehicle-to-infrastructure (V2I) communication. This, in turn, enables far greater coordination between road users and allows for the creation of safer and more efficient traffic flows, reducing the impact on the environment.
C-ITS requires short-range communication focused on critical safety warnings between vehicles, as well as long-range communication aimed at enhancing productivity and service levels. With its ‘view-around-the-corner’ capability, C-ITS enables the detection of hazardous situations earlier than the human eye can see, thereby also overcoming the line-of-sight limitations of onboard vehicle sensors such as cameras and LiDAR.
Dedicated Short-Range Communications (DSRC) is one of the key C-ITS technologies that facilitate short-range direct communication between vehicles (V2V) and between vehicles and infrastructure (V2I). DSRC has been developed specifically for short-range communications. DSRC solutions, therefore, often integrate with cellular communication to form DSRC-Cellular Hybrid systems. Developed in the late 1990s, DSRC has undergone extensive development, testing, and trials, thus making it a mature C-ITS standard. However, DSRC-based solutions have not yet achieved global deployment.
In 2016-2017, Cellular Vehicle-to-Everything (C-V2X) technology emerged as a competitor to DSRC, which also provides direct communication. In contrast to DSRC, C-V2X focuses on both direct short-range communication (PC5 mode) as well as network modes (Uu mode) via cellular networks.
The Principles for a National Approach to C-ITS in Australia [1] advocate a nationally consistent approach and were endorsed by Infrastructure and Transport Ministers in December 2023.2 Adopting a nationally consistent C-ITS standard ensures seamless integration and compliance across all states and territories. The question now is: which of the two technologies should the Australian Government support going forward?
This report evaluates the two competing technologies, DSRC and C-V2X, and aims to provide the Australian Government with guidance for the consistent, nationwide deployment of C-ITS. It is based on 40 in-depth interviews with senior industry and government representatives globally, mostly in the USA and Europe. Further to this, a literature review was conducted to gather a robust body of evidence, supporting, and contrasting the stakeholder views obtained during the consultation process.
Findings
Both technologies have active standardisation bodies that are releasing regular updates. The key standardisation bodies for DSRC are the European Telecommunications Standards Institute (ETSI) in Europe and the Institute of Electrical and Electronics Engineers (IEEE) in
2 The Principles for a National Approach to C-ITS in Australia were endorsed by all Australian governments, including the Commonwealth, States, and Territories, as part of the Infrastructure and Transport Ministers' Meetings (ITMM) in December 2023.
the USA, while for C-V2X, the global standardisation organisation is the 3rd Generation Partnership Project (3GPP). Both technologies, serving as access-layer communication technologies, are not interoperable but can share ITS safety applications (such as those standardised by ISO, CEN, ETSI, ITU, and SAE). The stable edition of DSRC is 802.11p, while C-V2X’s equivalent is LTE-V2X (3GPP Release 14/15). Both technologies have already been adopted for commercial original equipment manufacturer (OEM) manufacturing and C-ITS deployment in the market for basic safety applications, namely ‘Day 1’ use cases, which include collision avoidance, road work warnings, accident warnings, intersection warnings, emergency vehicle warnings, and more. The latest edition of DSRC is IEEE 802.11bd, released in early 2023, for enhancing transmission speed and reliability. The latest edition for C-V2X is 5G-V2X (3GPP Release 16/17), released in 2022. The latest upgrades of DSRC and C-V2X are intended to provide advanced performance for more complex use cases such as automated driving, namely ‘Day 2’ and ‘Day 3’ use cases.
Global division
At the moment, the global landscape is far from settled on this issue: China has made its choice for C-V2X, the USA has all but abandoned DSRC in favour of C-V2X, but Europe is investing in DSRC. Car manufacturers are also split on the choice of a standard. Several leading car manufacturers have aligned themselves with the 5G Automotive Association (5GAA), endorsing C-V2X for inclusion in their new vehicles. Volkswagen, however, has launched cars with built-in C-ITS devices based on DSRC.
DSRC vs. C-V2X: Common arguments
DSRC proponents assert that it is a proven technology, specifically designed for road scenarios that require high reliability and very low latency communication to deliver ultra-fast C-ITS warnings. The technological strengths are rooted in its design principles: to create a dedicated channel for very low latency ultra-fast connectivity that is free from interference. DSRC proponents further point out that upgrades from 802.11p to 802.11bd maintain backward compatibility, while the next generation of C-V2X (5G-V2X) will not be compatible with its current version (LTE-V2X).
C-V2X proponents highlight that C-V2X has been designed to integrate direct short-range communications and cellular communications. It aims to deliver ultra-fast warnings, passing on packets of information seamlessly from one network mode (direct communications) to the other (cellular communications). It is also designed to interconnect a variety of end users and devices, not only car devices, but also smartphones, wearables, and other devices. As a result, compared to DSRC, C-V2X is designed to offer advantages when using the cellular network. For instance, the latest 5G-V2X specifications include the ability to predict and review network conditions (such as congestion) before initiation, optimising C-ITS message transmission. Additionally, 5G-V2X is specifically designed to cater to the future needs of automated vehicles. These vehicles require not only ultra-low latency communications for critical safety warnings but also high data volumes for complex road scenarios, such as highdefinition mapping. C-V2X proponents emphasise that the integration of short-range and cellular connectivity in C-V2X positions it well to meet these advanced requirements.
Large body of academic evidence for DSRC, C-V2X performance increasingly substantiated We examined numerous academic literature and industry reports to gather evidence to support the technical performance claims of both technologies and concluded that both
options meet the globally accepted standard requirements. DSRC has proven itself over many years in numerous real-world trials. C-V2X trials are of a more recent nature; however, they are starting to build critical mass. DSRC and C-V2X can meet the required standard of latency of 20 milliseconds for critical warnings. Tests of warnings delivered via cellular networks show promising results with latencies below 100 milliseconds. However, attaining the 20-millisecond latency needed for a critical V2V warning remains a challenge for cellular long-range communication.
The C-ITS governance model, value chain and business case are unclear
Probably the most important factor driving the adoption of either technology is the value and governance model for C-ITS. Almost all our interviewees concur that the slow adoption rates to date can be attributed to a lack of clarity in the value and governance model of C-ITS. The questions ‘Who will invest in the required infrastructure?’, ‘What is the role of the government and regulators?’ and ‘What is in it for the telecommunication companies (telcos) and the car manufacturers?’ have not been sufficiently addressed. With the advancement of connected cars using long-range communications, a compelling revenue opportunity has emerged for both car manufacturers and telecommunications companies (telcos). Specifically, providing non-critical safety warnings and other data services through these networks offers a lucrative prospect. Both telcos and car manufacturers claim that short-range communications between vehicles are hard to monetise. ‘You can’t charge for safety’ is a frequently heard, but debatable claim. Another common argument is that DSRC’s cost of deployment is high due to the need for roadside infrastructure whereas commercial telcos could bear a larger share of the investment for infrastructure requirements for their business-as-usual commercial model. C-V2X would therefore require much lower levels of investment from the government side. This assumption should be treated with a degree of caution. It is very likely that in both cases, additional roadside units will be required, for instance at critical intersections in built-up environments.
DSRC vs. C-V2X affects a portion of the C-ITS system
The choice at hand affects only a portion of the C-ITS system: the so-called physical layer, which is responsible for the way messages are transmitted. However, the rest of the C-ITS system, including safety applications and security, adheres to globally accepted ITS standards and other common ICT standards. Therefore, at this stage, it is feasible to plan and construct higher-layer infrastructure, such as the C-ITS central station, data platform, and security Public Key Infrastructure (PKI), without concern over the specific choice of access layer technology between DSRC and C-V2X. Another commonality between the two technologies lies in the deep private-public partnership that is required to address matters such as data sharing, regulation, and security.
Our Point of View
Both technologies are viable options. Focusing on the technologies, irrespective of intricate global interdependencies, the choice between DSRC and C-V2X is about deciding between one of the following:
An ‘open’ strategy: This approach emphasises the interconnectivity and integration of various devices and communication modes. It implies that the government will promote a more flexible and adaptable C-ITS ecosystem that accommodates a wide range of technologies and devices. This vision is closely aligned with C-V2X.
A dedicated C-ITS system strategy, supported by a cellular network: In this approach, the government could decide to manage C-ITS as a dedicated system, similar to, for instance, the traffic light system. This system would prioritise road users with DSRC devices. In this case, DSRC and DSRC-Cellular Hybrid provide solid solutions.
DSRC has intentionally been developed as a dedicated system, aimed at optimising its operational efficiency and reliability. However, in our view, the emphasis C-V2X places on interconnectivity and seamless integration aligns more closely with the evolving dynamics of roadways and road users. Drawing upon the discussion in this report, we envision a transformative future for C-ITS, aimed at significantly enhancing both safety and the overall driving experience for road users. In this future landscape, 5G connectivity is anticipated to be a cornerstone, empowering connected vehicles with remote intervention capabilities and consistent software updates, thereby aligning with the evolving regulations for Connected and Autonomous Vehicles (CAVs). The vision extends to the integration of in-vehicle shortrange communication devices, multi-technology connectivity, and seamless integration with the Internet of Things (IoT). A special emphasis is placed on the protection of Vulnerable Road Users (VRUs) through specialised C-ITS devices, mobile phones, and wearables. Central to this vision is the development of a robust, adaptable, and diverse multi-technology ecosystem that accommodates the unique connectivity requirements of a broad spectrum of end users. Currently, standards organisations (such as ETSI), consortiums (like ITS America, C2C-CC, and CMC), and industry leaders (e.g., Bosch, Vodafone, Verizon, Nissan, and Cisco) are all showing heightened interest in standardising and technically implementing VRU protections.
Recommendations
Our recommendations are to:
1. defer a conclusive decision on the preferred short-range C-ITS technology until Europe and the USA finalise their respective decisions (China has already decided to adopt C-V2X), which is anticipated to occur in the next 1 to 2 years (this is crucial, as car manufacturers are unlikely to adopt separate technology for a small Australian market versus the EU and USA)
2. closely monitor the progress of the next generation of C-V2X short-range communication technology, expected to reach maturity by 2029, to assess if the backward compatibility issue becomes redundant or is solved. 5G-V2X may maintain backward compatibility at higher layers, and technological advancements, particularly in chip design, could alleviate compatibility concerns by enabling adjacent channel coexistence between 5G-V2X and LTE-V2X
3. adopt a technology-neutral stance in the meantime, in line with the 2020 European Union ITS spectrum plan. It is recommended that consideration be given to allocating the upper 20 MHz of the ITS 5.9 GHz spectrum to LTE-V2X for greater flexibility and future resilience in developing C-ITS in Australia.
4. determine an optimal operating framework, followed by the resulting funding and revenue model and the governance structure and stakeholders’ accountability to establish a resilient cornerstone for the development of investment business cases
5. establish the necessary platforms for public-private collaboration, lead the development of a nationwide C-ITS ecosystem, and foster collaborative business models with telcos and other stakeholders to accelerate large-scale C-ITS deployment
6. start the development of the C-ITS ecosystem and create high-value safety use cases that can be delivered using cellular technology in compliance with the global C-ITS standards (e.g., ETSI and ISO C-ITS standards) which can be shared by both technologies.
Report Summary
Background
Cooperative Intelligent Transportation Systems (C-ITS) are systems that allow for shortrange communication and the real-time exchange of information between vehicles (V2V), roadside infrastructure (V2I), and other users of the road such as pedestrians and cyclists (V2P). The primary aim of adopting and expanding C-ITS is to enhance road safety, boost transport productivity, promote sustainability, and decrease emissions. This includes aiding the growth and development of emerging technologies like Connected and Automated Vehicles (CAVs).
C-ITS primarily utilise short-range communication methods to provide low-latency responses directly between vehicles and roadside infrastructure, critical for situations such as collision warnings. For less urgent warnings, like traffic congestion alerts, and to tap into diverse traffic data such as work zone alerts, a nationwide C-ITS system can combine long-range communication, like 5G, with these short-range communications.
Currently, the market for C-ITS standards is witnessing competition between two technologies: Dedicated Short-Range Communications (DSRC), also known as ITS-G5 in Europe, and Cellular Vehicle-to-Everything (C-V2X), which appears to be the chosen technology in the USA and China. Besides offering a short-range communication capacity similar to DSRC, the C-V2X standard efficiently combines both short-range communication (PC5 sidelink) and long-range communication in its standard suites. Despite the widespread interest and trialling of DSRC over the past two decades, DSRC has not been deployed on a wide scale. Alternatively, the C-V2X standard, which has experienced fast growth in the last five years, has been attracting increasing attention from industries.
Emerging technologies like autonomous driving and 5G are promoting countries to reevaluate their strategies for C-ITS to align with worldwide trends. The goal of this project is to provide the Australian Government with a reference report that aids in making wellinformed decisions about a uniform national approach to C-ITS in Australia [1].
Methodology
The two competing technologies have their own standards. A technology standard is to CITS what grammar and vocabulary are to a language. Our research objective was primarily to review the standard suites of the two competing technologies. We also want to provide an overview of the considerations that the government should take into account with regard to a consistent nationwide approach to C-ITS.
We conducted interviews with 40 high-ranking representatives from various global sectors, including C-ITS equipment manufacturers, chip makers, automobile manufacturers, telecommunication companies, government bodies, policymakers, and standardisation institutes. These interviews served to incorporate their critical viewpoints into our report. During our discussions, we primarily focused on the question, 'What should the Australian Government take into consideration?' and consequently sought evidence to support these considerations. The majority of these interviews went beyond the allotted one-hour timeslot, necessitating follow-up meetings in some instances. Additionally, we examined international standards, scholarly articles, technical white papers, and prospective roadmaps issued by
influential alliances like the 5G Automotive Association (5GAA) and the CAR 2 CAR Communication Consortium (C2C-CC). This summary provides a high-level outline, with further supporting evidence detailed in the following chapters of this report. For those interested in a more in-depth exploration of the technical aspects, we have included references and appendices.
DSRC vs. C-V2X: A Quick Glance
DSRC, a technology that employs the IEEE 802.11p protocol, allows precise, real-time transmission of safety messages between vehicles and Roadside Units (RSUs) within about 400 meters. Despite over two decades of development and testing, DSRC has not achieved widespread global deployment, and its standards vary between regions such as Europe and the USA. The IEEE 802.11p with Wireless Access in Vehicular Environments (WAVE) protocols [2-4], designed to facilitate reliable communication for swift vehicles, is the most used standard. There is also the more recent iteration, IEEE 802.11bd, which was released in Feb 2023 [5]. This updated standard is anticipated to provide better efficiency, lower latency, and improved reliability for vehicular communications. It aims to enhance the performance of DSRC, particularly for highly automated and connected vehicles and is expected to be backward compatible with 802.11p.
C-V2X, which encompasses 4G Long-Term Evolution (LTE) V2X and the 5G-V2X technologies (also referred to as 5G New Radio V2X), is emerging as a solution for integrating Vehicle-to-Vehicle (V2V), Vehicle-to-Infrastructure (V2I), and Vehicle-toEverything (V2X) communication. The standards required for C-V2X are gradually introduced through 3GPP Releases 14-17, with Release 18 also in development [6, 7]. Despite being a relatively new technology with limited global deployment, the future roadmap of C-V2X has attracted substantial attention and interest.
By leveraging the cellular network infrastructure, C-V2X provides a solution for communication between vehicles, infrastructure, and other devices (for instance, phones of Vulnerable Road Users). C-V2X incorporates both direct communications through PC5 mode at the free 5.9 GHz ITS band and network communications using Uu mode via cellular base stations, which is anticipated to extend the communication range and facilitate highcapacity data transmission [7]. Under a unified 3GPP standard, both Uu mode and PC5 mode in C-V2X enhance integration and compatibility. The Uu mode of C-V2X facilitates efficient use of network resources by prioritising traffic and managing congestion effectively. Vehicles equipped with C-V2X can seamlessly switch between Uu mode and PC5 mode, dynamically adjusting based on cellular coverage availability and the types of messages to be transmitted.
Due to fundamental differences in their underlying technologies and communication protocols, DSRC and C-V2X are not compatible with each other and lack interoperability. This means that they cannot seamlessly work together or exchange information.
DSRC vs. C-V2X: Common Arguments
Our findings revealed three distinct groups: DSRC proponents, C-V2X advocates, and those who remain neutral, awaiting a firm decision. This last group primarily consists of C-ITS device manufacturers. Both DSRC and C-V2X supporters employ a regular series of arguments, which we have condensed in Table 4.
C-ITS Standards
C-ITS Readiness
C-ITS Performance
DSRC Proponents
DSRC standards have been developed and tested for 20 years and are well-suited for the task.
C-V2X Advocates
C-V2X standards have been rapidly developed to meet new requirements and can leverage existing ITS standards.
C-V2X is unproven. DSRC is outdated.
DSRC is custom-designed for reliability. C-V2X’s strict synchronicity requirements make it vulnerable.
C-ITS Roadmaps
Global Rollout
Optimising ITS Band
Autonomous Vehicle
Cost, Value Model and Governance
Security and Privacy
DSRC has a shorter range. Design is rigid, not flexible.
C-V2X is not backward-compatible. Backward compatibility may be redundant in a few years and can be achieved at the Application Layer.3
DSRC has proven to be effective in basic safety applications in Europe.
DSRC is more reliable as a decentralised system.
The next generation of DSRC will offer higher speeds and lower latency, expanding its suitability for a broader range of applications.
DSRC has the least dependency on telco networks. Private companies may not be the best fit for serving the public good as they often prioritise commercial profits.
DSRC is limited to V2V/V2I and does not take the future of C-ITS into account.
DSRC makes inefficient use of the ITS band. In the USA, DSRC didn’t make full use of the bandwidth allocated to it.
C-V2X is designed to leverage network capabilities for automated driving and remote operation.
Telcos' involvement can be advantageous for both the government and end-users in terms of quick deployment of the C-ITS ecosystem.
Security and privacy are essential. Security and privacy are essential.
3 The Open Systems Interconnection model (OSI model) is a conceptual model from the International Organisation for Standardisation (ISO). In the OSI reference model, the communications between a computing system are split into seven different abstraction layers: Physical, Data Link, Network, Transport, Session, Presentation, and Application.
Table 4 Interviewees' common arguments
Technology Intrinsic Factors
The question is: To what extent can these arguments be validated, and which arguments should the government take into consideration? Below we review the key aspects, beginning with the considerations that are intrinsic to the technology, followed by derived aspects that we recommend governments to consider.
C-ITS Standards
Short-range communication technology is a crucial driver of low latency, direct V2V and V2I communications, primarily using the class-licensed ITS band (predominantly in the 5.9 GHz band) within the broader C-ITS landscape. Over the past two decades, the DSRC standard, centred around short-range communications, but complemented by cellular networks (DSRC Hybrid), has undergone rigorous development and testing. The emerging C-V2X technology is increasingly gaining attention due to its unique feature of offering both a direct short-range communication mode (PC5) and a network mode (Uu) that leverages cellular network capacity, standardised by 3GPP.
The creation of C-ITS standards represents an international, collaborative effort between multiple Standards Development Organisations (SDOs) such as ISO, CEN, ETSI, ITU, and SAE, focusing on the core C-ITS standards. Experts from member organisations primarily volunteer to write and update these C-ITS standards. While both DSRC and C-V2X utilise these C-ITS standards, they have each tailored these standards to fit their unique requirements. ETSI mainly develops and maintains DSRC standards, whereas 3GPP is the principal developer of C-V2X standards. Each standard's working groups are committed to customising and integrating mature ITS standards into their respective domains. DSRC and C-V2X function as access layer technologies, meaning their radio channel communication mechanisms are distinct and not interoperable. However, they can share safety applications like safety warning algorithms and message formats.
DSRC's stable edition is 802.11p, and C-V2X's equivalent is LTE-V2X (3GPP Release 14/15). Both have already been adopted for commercial OEM manufacturing and C-ITS deployment in the market. DSRC's latest edition (IEEE 802.11bd) was published in early 2023 and aims to enhance transmission speed and reliability.
For C-V2X, the latest edition (5G-V2X, 3GPP Release 16/17) was released in 2022. While 3GPP Release 14/15-based 4G LTE-V2X focuses on basic safety message exchanges, Release 16/17-based 5G-V2X offers advanced performance for more complex use cases like automated driving. Work on 5G Advanced Release 18 commenced in Q2-2022.
C-ITS Technology Readiness
DSRC has a proven record of reliable performance, having been successfully trialled over the last two decades. Its hardware and standards represent a mature and readily available solution. On the other hand, C-V2X has risen as a strong competitor in the last 6 years. It has undergone extensive testing for basic safety applications, known as ‘Day 1’ use cases, in countries such as Germany, the USA, Italy, and China. Especially in China, commercial deployment of LTE-V2X devices supporting direct communication is underway. Advocates of C-V2X argue that current cellular networks can deliver many use cases today, while DSRC coverage is yet to be fully implemented.
However, it's important to note that presently, C-ITS equipment only supports DSRC 802.11p and LTE-V2X. At the time of this report, C-ITS devices compatible with NextGeneration DSRC 802.11bd standard and 5G-V2X are not commercially available.
Experts we've consulted concur that both DSRC 802.11p and LTE-V2X PC5 short-range communications sufficiently meet the requirements of Day 1 basic safety applications; the predominant opinion is that there is no significant difference between DSRC 802.11p and LTE-V2X PC5 for short-range communication under equivalent traffic conditions. Under certain conditions, LTE-V2X outperforms DSRC 802.11p in various metrics, primarily due to new mechanisms such as HARQ and Turbo Coding. Consistent test results from lab and field trials, as defined in the 5GAA test plan [8], confirm improved reliability and interference resistance of LTE-V2X in short-range communication. We recommend that since performance is scenario-dependent, ongoing observation and updates for emerging technologies are essential.
Development Roadmap
Even though DSRC has lost some momentum, it is far from dormant. DSRC and C-V2X are both consistently enhancing their standards. In the case of DSRC, ETSI is developing ITSG5 Release 2 while IEEE has introduced 802.11bd, a modernised version addressing some limitations of the original DSRC standards. Concurrently, 3GPP has crafted 5G-V2X specifications. The 802.11bd is fully backward compatible with 802.11p, and 5G-V2X is compatible with LTE-V2X for advanced layer applications. However, due to differences in access layer technology, LTE-V2X and 5G-V2X may face compatibility challenges during the transition from LTE-V2X's current 20 MHz spectrum to 5G-V2X's anticipated broader spectrum (40 MHz or more). A proposed solution for this compatibility issue is adjacent channel coexistence, which would allow both systems to operate concurrently on separate channels.
The rapid advancement of technology is driving the development of more and more use cases for the deployment of C-ITS. According to the design plan, the 5G-V2X technology promises substantial improvements in terms of increased throughput, enhanced reliability, and lower latencies, allowing for the creation of cutting-edge use cases. However, full implementation of 5G-V2X suites may still take 5-10 years. Meanwhile, the next-generation DSRC standard, IEEE 802.11bd, is working towards improving its performance and incorporating new modes of operation to enhance its capabilities. Two leading alliances in this field are 5GAA and C2C-CC. 5GAA, backed by major telcos and car manufacturers, champions the adoption of C-V2X technology. On the other hand, organisations like C2CCC, EU C-Roads, and Volkswagen lend their support to DSRC ITS-G5. Both alliances have published roadmaps for C-V2X and DSRC, outlining the potential for both current and future use cases. 5GAA's roadmap [9] provides a clear technical evolution of use cases, with a timeline that projects the full realisation of advanced use cases by 2029. This timeline has been agreed upon by 5GAA experts and stakeholders and is based on the current state of development. Both C-V2X and DSRC have the potential to support current and future use cases. As the debate on C-ITS standards continues, we anticipate that within the next 1-2 years, the conversation will become clearer and more consistent, allowing for greater clarity on the potential advantages and disadvantages of both DSRC and C-V2X technologies.
ITS Spectrum Requirements for Short-Range Communications
With the emergence of C-V2X technology, determining the allocation of spectrum in the 5.9 GHz band (5855 MHz to 5925 MHz) for short-range communications for ITS has become a vital consideration. The question of whether to implement a single technology or allow for the coexistence of DSRC and C-V2X in the ITS spectrum is at the forefront of this debate.
In the USA, the Federal Communications Commission (FCC) is progressively updating the ITS spectrum allocation policies, favouring C-V2X. The new change involves allocating 20 MHz for C-V2X while reserving 10 MHz for DSRC shared with C-V2X [10]. This is a noteworthy departure from past strategies favouring DSRC.
On the other hand, Europe, in its latest 2020 plan [11-13], has proposed a technologyneutral stance. 5GAA has recommended allocating the upper 20 MHz of the spectrum in the 5.9 GHz band to LTE-V2X. Additionally, 5GAA has suggested that 5G-V2X might require at least a 40 MHz bandwidth, though this requirement has not yet been finalised [14].
Meanwhile, in Australia, the spectrum allocated for ITS is currently regulated under the Radiocommunications (Intelligent Transport Systems) Class Licence 2017 (ITS Class License), issued by the Australian Communications and Media Authority (ACMA), with DSRC ITS-G5 defaulting to use 70 MHz [15-17]. To make Australia’s C-ITS system futurecompatible and equipped to address evolving road-user needs, we suggest a technologyneutral approach, akin to the one recommended by the Global System for Mobile Communications Association (GSMA) [18], referring to Europe’s 2020 plan for the ITS spectrum. As part of this transition, the allocation of the upper 20 MHz to LTE-V2X should be considered to encourage development and progress in Australia’s C-ITS industry. Ultimately, we anticipate the market, heavily influenced by automaker preferences, will guide the transition to the dominant technology. This strategy positions Australia favourably in line with global trends while maintaining flexibility for future innovations.
Future Use Cases of C-ITS: Connected and Automated Vehicles (CAVs)
How do these C-ITS technologies align with advancements in technology and its practical applications, including the management of roads and the potential for higher levels of automation in vehicles? C-ITS substantially improves the visual and perception abilities of autonomous vehicles, providing an ‘around-the-corner’ view and extending the range of obstacle detection through real-time V2I communication. Use cases including automated intersection crossing, cooperative lane merge, and remote automated driving improve road safety, especially during mixed traffic scenarios where understanding human drivers' actions is vital.
Four use cases identified by 5GAA representing Road Operators' expectations include local hazard and traffic information (LHTI), greenlight optimal speed advisory (GLOSA), highdefinition maps (HD maps) data collection and sharing, and probe vehicle data (PVD) [19]. One of the European leading car brands, Audi, has launched vehicles featuring autonomous driving and C-V2X technology in line with these use cases in China [20]. These use cases represent priorities for metropolitan traffic management and are closely aligned with the goals set in the Principles for a National Approach to C-ITS in Australia [1].
C-ITS is crucial for the progression of autonomous driving. Austroads emphasises the importance of investing in infrastructure for automated driving, particularly digital
infrastructures like AV/HD maps and C-ITS communications. These are essential for CAVs to detect physical structures when direct vision is obstructed.
Level 3 autonomous vehicles rely on C-ITS to achieve greater autonomy. In 2022, the German Federal Council and EU Commission introduced requirements for Level 4 autonomous vehicles, effective from July 2024 [21, 22]. These rules require safety assessments for these vehicles before they can enter the EU market. If there's a system failure or a near-accident, a remote operator can step in, provided it's safe. However, the Automated Driving System (ADS) oversees most driving functions, with remote intervention limited to speeds below 6km/h. We would anticipate that DSRC and C-V2X suit safetycritical applications, while advanced use cases demand the ultra-low latency of 5G networks. Increased automated vehicle penetration amplifies the need for C-ITS for object detection and traffic coordination. 5G integration would enhance vehicle data transmission, support various advanced use cases, and elevate traffic control and safety.
Security and Privacy
No matter the choice between C-V2X or DSRC communication technologies, prioritising data security and privacy is indispensable in the rollout of C-ITS. The devices involved in C-ITS will continuously share data with other unfamiliar devices, elevating the need for robust protection measures. The C-ITS Security Credential Management System (SCMS), with its focus on Public Key Infrastructure (PKI), is integral to this process. PKI utilises advanced encryption techniques and certificate management to enable secure and trusted communication. Currently, the European Union's C-ITS Security Credential Management System (EU SCMS) is recognised as a global front-runner, showcasing best practices for maintaining C-ITS security.
To effectively deploy C-ITS in Australia, it is crucial to focus on key strategic components that ensure security and privacy. The following points outline the core strategies that need to be addressed:
Prioritising PKI for C-ITS Deployment: Creating a secure PKI system is essential for a successful C-ITS platform. The PKI structure involves a trusted Certificate Authority issuing digital certificates and cryptographic keys to vehicles and infrastructure components, ensuring secure and authenticated communication within the C-ITS. Regardless of the underlying communication technology employed, whether it is DSRC or C-V2X, the performance of PKI remains unaffected. Initially, Australia could utilise the European SCMS PKI for testing or insights, but ultimately, it should develop its own PKI infrastructure, potentially managed by a third-party entity with strong cybersecurity capabilities. In the long run, the creation of a native PKI in Australia offers critical advantages in national security, data privacy, regulatory compliance, and customisation for local C-ITS needs, ensuring greater control and alignment with national interests compared to adopting a foreign system like the European SCMS.
Implementing an Across Jurisdictions Security Trust Model: A well-structured PKI promotes interoperability of C-ITS systems across jurisdictions, allowing smooth device communication regardless of the manufacturer or service provider. This interoperability can enhance the overall value and benefits of the C-ITS ecosystem.
Aiming for a centralised SCMS: A unified SCMS across all Australian states and territories ensures smooth interoperability and data management, providing better protection against cyber threats and addressing data privacy concerns.
Technology Extrinsic Factors
Global Support
In Europe, C-ITS deployment is largely driven by the DSRC ITS-G5 standard, heavily supported by consortiums such as C-Roads and C2C-CC. Transitioning to C-V2X poses substantial technical hurdles due to DSRC's historical dominance. DSRC-based C-ITS is highlighted by C-Roads and C2C-CC for its proven performance and the potential to combine short-range and cellular communication. The C-Roads project, focusing on ‘Day 1’ safety applications, validated C-ITS services across 18 EU nations, even amidst the Covid19 pandemic [23]. Furthermore, 37 cities have implemented C-ITS services to prioritise public transport and emergency services. The new DSRC 802.11bd standard also shows promise for automated driving. Emulating the European model, Australia's Ipswich Connected Vehicle Pilot in 2020 [24] effectively demonstrated a blend of short- and longrange communication for optimal coverage.
Established in 2016, the 5GAA is a cross-industry global organisation focusing on C-V2X technology development. As of late 2022, it has 119 members, including top automakers and telcos [25, 26]. The organisation has led successful LTE-V2X trials worldwide. China has made rapid strides in C-V2X development and implementation, adopting C-ITS aligned with 3GPP architectures. In 2018, China designated the 5.9 GHz band exclusively for C-V2X direct communications. Four regions have been sanctioned by the Chinese Ministry of Industry and Information Technology to create national-level C-V2X trial zones. A cooperation project between China and Germany led to the establishment of the largest C-V2X trial site in Chengdu City in 2022. Many mainstream car manufacturers have already made a de facto choice, to bet on the C-V2X camp. Ford China successfully deployed its C-V2X system in six Chinese cities at the end of 2022. The propensity of mainstream car manufacturers to embrace C-V2X, coupled with the national policies of the USA and China endorsing C-V2X as the exclusive C-ITS standard, implies a rapid forthcoming deployment of C-V2X technology.
As Australia predominantly imports vehicles, its future in C-ITS is influenced significantly by international car manufacturers, who typically prioritise the expansive Chinese market for their technological advancements. Notably, there's a fading commitment to DSRC, as most car manufacturers are members of the 5GAA, thereby gravitating towards C-V2X technology. This trend is particularly dominant in China, where vehicle testing has yielded a robust balance of short- and long-range communications. Car manufacturers prefer a unified C-ITS standard due to the costs and complexities of dual-mode transmission. The USA is moving towards C-V2X, with DSRC being phased out. Full adoption of 5G-V2X is expected around 2029. For Australia, these international trends should serve as crucial markers when deciding on its C-ITS standard.
Long-Range Communications in C-ITS
Telcos have evolved from passive network providers to active game players in C-ITS since the advent of C-V2X technology and the creation of 5GAA in 2016. The telco representatives we spoke to criticise DSRC for its limited range and reliance on roadside units, preferring the
wider coverage of 5G instead. They display reluctance to get involved in direct communication methods. Telcos assert that long-range communication allows for broader information distribution, benefiting everyone, especially mobile users. They regard shortrange communication, like DSRC or C-V2X PC5, as supplementary, reserved for particular scenarios, pointing out that alternative collision avoidance technologies currently already in vehicles, will provide similar functionality.
Verizon and Nissan have conducted successful C-ITS trials utilising Verizon's 5G Edge with Amazon Web Services (AWS) for real-time processing. Similarly, Audi and Verizon plan to integrate 5G Ultra-Wideband technology into certain Audi models from 2024. Vodafone has emerged as a leader in embedding C-ITS into Edge Computing using long-range 4G/5G networks. Vodafone’s Safer Transport for Europe Platform (STEP), operational in the UK and Germany, delivers basic safety applications and Day 2 use cases for Vulnerable Road Users (VRUs), using ETSI ITS message standards [27]. In contrast to Day 1 use cases, which primarily focus on critical road safety such as collision warning, Day 2 use cases explore more complex scenarios and typically demand advanced traffic management, cooperative manoeuvring, and, in some instances, vehicle automation (refer to Table 16 in Chapter 4 for details).
At present, RSUs (DSRC or C-V2X PC5) enable low-latency communication between vehicles and surrounding infrastructure through direct communication. To address latency issues of long-range communications, telcos are investing in ultra-low latency 5G communication and edge computing. In March 2022, Cisco and Verizon demonstrated how Verizon's LTE network and 5G Edge could support autonomous driving with minimal latency, without the need for physical RSUs. The test findings confirm that the virtual RSU on the mobile edge effectively meets latency needs for safety applications in V2I and essential V2V scenarios. Vodafone's STEP platform, leveraging edge computing, has shown promising performance with millisecond-level latency. Telcos emphasise that system efficiency, coverage, and accessibility of information outweigh latency concerns. Onboard sensors are favoured for critical V2V cases because collision avoidance remains a challenge for longrange communication.
In ITS America’s 2023 National V2X Deployment Plan [28], use cases involving VRUs are given priority. Spoke's VRU2X platform, supported by T-Mobile, Deutsche Telekom, and Audi, leverages C-V2X, LTE/5G, and camera/radar technology for improved safety of VRUs. Similarly, Vodafone's STEP platform uses mobile networks to enhance cyclist safety, indicating the potential of indirect communication for VRU safety. In 2020, the ETSI ITS Standard Release 2 introduced a VRU protection specification featuring the Vulnerable Road User Awareness Message (VAM) for different VRU profiles [29], while C2C-CC and the Connected Motorcycle Consortium (CMC) later recommended incorporating motorcycle VAM into CAM messages for improved path prediction [30]. This development highlights a growing emphasis on improving VRU safety, in line with the ongoing advancements in mobile technology.
Currently in Australia, the ITS Class Licence references the ETSI standard EN 302 571. EU ITS-G5 has been tested in several trials, including Australia’s largest C-ITS trial. C-V2X has also been trialled in Australia. However, due to global uncertainty around C-ITS standards, it may be advisable to defer commitment to EU ITS-G5. Instead, Australia should closely monitor global trends and industry reactions before finalising its own short-range C-ITS standards. During this period, C-ITS solutions based on both DSRC and C-V2X should aim
to fully utilise existing mobile network infrastructure. Austroads predicts a 16-75% uptake of C-ITS systems in vehicles by 2030 and a nearly 100% incorporation of mobile data connectivity by 2025 [31]. Consequently, the demand for vehicles offering C-ITS safety and enhanced services via mobile connectivity is expected to remain strong over the next 5-10 years. Due to advancements in onboard sensor technology and telematics, several automakers are hesitant to integrate short-range V2V communication in vehicles. This suggests that it might be more practical to initially focus on the advantages of long-range communication in C-ITS and view short-range communication as an added enhancement. Interviews with telco representatives highlight the value of mobile networks for accessible traffic information to all road users. Mobile networks enable road operators to contribute efficiently to a broader ecosystem by sharing crucial road data, as seen in popular apps like Live Traffic NSW and VIC Traffic. Therefore, regardless of the specific short-range communication technology chosen, maximising the potential of mobile networks is recommended for expediting the development of a C-ITS ecosystem.
Cost, Value Model and Governance
Short-range communication at the 5.9 GHz ITS band remains superior for critical V2V/V2I use cases despite advancements in 4G and 5G. Notably, road sections requiring critical, low-latency communication necessitate RSU deployment. The ITS America 2023 V2X National V2X Deployment Plan [28] recommends initiating V2X at signalised intersections, using existing infrastructure for quick deployment. Non-signalised areas could consider longrange communication or virtual RSUs. Intersections are increasingly being targeted for deployment as automakers and road operators focus on intersection-based V2I applications such as red-light alerts and pedestrian safety messages, particularly to ensure the safety of Vulnerable Road Users. ITS America underscores the need for government-installed C-V2X RSUs at signalised intersections and suggests prioritising messages for national interoperability and effective C-V2X deployment.
Some ITS experts argue that the private sector may not effectively provide traffic safety due to profit motives, thus government involvement is essential. Implementing and maintaining C-ITS infrastructure incurs deployment, operational, and connectivity costs. Despite using the class-licensed 5.9GHz ITS band for either DSRC or C-V2X PC5, data costs are unavoidable but can be reduced with telcos and C-ITS operators' collaboration. C-Roads infrastructure deployment costs fell from 59 million EUR in 2021 to a projected 42 million EUR in 2023 [23]. Early research by the United States Department of Transportation (USDOT) in 2014 claims that DSRC field infrastructure deployment costs can range from 13,000 USD to 21,000 USD per site, with the average cost estimated at 17,600 USD [32]. In the UK, each RSU installation costs 7,200 EUR and 735 EUR annually for operating [33]. The 5GAA also suggests that using existing infrastructure, such as cellular networks, can significantly reduce costs for C-ITS deployment.
Based on interviews conducted, representatives of telcos and car manufacturers are hesitant about investing in short-range infrastructure, due to concerns about low immediate financial returns. Although DSRC has been successful in delivering basic C-ITS use cases over the past 20 years, government funding has been the primary source of support for the deployment of DSRC RSUs at critical locations, such as intersections, which enabled lowlatency V2I applications. Should Australia opt for DSRC as the sole C-ITS standard, it could encourage self-sustaining infrastructure management. This scenario would likely shift a
greater share of the responsibility for C-ITS development onto the government, necessitating primary investment. A possible future scenario for C-ITS deployments might involve cellular communication enhanced by short-range capabilities. Recently, the C-V2X standard emerged to address the latency issues in cellular communication that DSRC proponents often criticise, by enabling short-range communication. If Australia were to adopt C-V2X which combines long-range with short-range communication, it might attract more investment from telcos, reduce government expenditure, and even allow for fees on noncritical services. Many automakers support the cellular-based C-V2X solution, which, with its proven benefits for VRU safety, could motivate ‘wait and see’ manufacturers to integrate it, enhancing alerts and Advanced Driver-Assistance System (ADAS) compatibility, and fostering a deeply invested ecosystem. It's worth considering that this approach might align with the interests of some telcos and car manufacturers.
The technology deployed should be easily upgradable, affordable, and should consider factors such as cost, licensing, and the complexity of updates. It should also have layered software for simple maintenance and modular hardware for easy troubleshooting and replacement. A suitable governance structure for C-ITS operations can help achieve these goals. The structure includes monitoring, change management, information management, security management, stakeholder support, and end-user support. If cooperative governance for C-ITS operations is to be established, the first step is to create a framework enabling partnerships between different stakeholders.
Envisioning Future C-ITS Adoption
Overall, enabled by C-ITS, a compelling vision emerges of a more sustainable, much safer transport ecosystem, including:
Adoption of mobile apps in connected cars and phones will provide users with realtime traffic updates, crossing safety, and hazard warnings. Edge computing and other technologies will increasingly improve the relevance, latency and reliability of warnings [26, 34].
A world where C-ITS technology not only facilitates low-latency interactions between vehicles and infrastructure but also seamlessly integrates with mobile devices like smartphones and smart helmets. C-V2X technology has the potential to redefine the future of a more diversely interconnected network, extending beyond traditional V2V/V2I communication. This integration will enable the rapid dissemination of crucial warnings and real-time traffic information directly to users. Moreover, the convergence of C-ITS with camera-based technologies and automated systems, whether factory-installed or aftermarket, holds the potential to significantly enhance collision prevention measures by providing a wider global view of traffic conditions. Specialised C-ITS devices, mobile phone applications, and wearables will be developed specifically for Vulnerable Road Users (VRUs) including pedestrians, cyclists, and individuals utilising assistive technologies. These custom-designed C-ITS devices will incorporate a variety of interfaces such as C-V2X direct communication, cellular communication, and cameras. This integration aims to enhance the detection of hazard safety warnings more effectively to VRUs [35, 36].
C-ITS devices or apps can offer various functionalities for a personalised journey experience based on user preferences. For instance, they can facilitate interaction
with traffic lights for the elderly and people with disabilities, monitor health and alertness, notify emergency services when needed, and adjust comfort settings during a CAV journey.
A diverse array of IoT technologies can provide warnings about challenging conditions such as icy patches, waterlogged areas, or pedestrian movements at crosswalks and real-time parking space availability can be monitored through Smart City IoT sensors, using Narrowband IoT (NB-IoT) technology [37].
Cellular connectivity will become increasingly indispensable and 5G is likely to become mandatory to support increasing levels of automation, to enable remote control, software updates, and emerging CAV regulatory compliance [21, 38].
MAIN REPORT
1 Background
Cooperative intelligent transport systems (C-ITS) enable real-time communication between vehicles (V2V), pedestrians (V2P), and infrastructure (V2I) to enhance road safety, efficiency, and comfort. This chapter explores key C-ITS concepts and compares the specifications of two primary C-ITS standards: Wi-Fi-based Dedicated Short-Range Communications (DSRC) and cellular-based Cellular Vehicle-toEverything (C-V2X). It examines the development and deployment of these technologies in different regions, highlighting the need for a decision on the preferred C-ITS standard. Successfully implementing C-ITS requires addressing challenges related to regulatory frameworks, market size, national platforms, security protocols, and infrastructure coverage. This chapter concludes with an outline of the research objectives.
1.1 Key C-ITS Concepts
C-ITS leverages V2V, V2I and V2P real-time communication to enhance road safety, efficiency, and comfort. This is achieved through the use of wireless communication technologies connecting various road traffic entities, such as vehicle On-Board Units (OBUs), Roadside Units (RSUs), traffic control systems, data platforms, and a credential security management system. An example of modern C-ITS is shown in Figure 2. The integration of cutting-edge technologies, such as the Internet of Things (IoT), edge computing, and Artificial Intelligence, further strengthens the capabilities of C-ITS [39].
C-ITS facilitates the seamless exchange of data between a diverse range of vehicles, including cars, trucks, buses, emergency vehicles, and specialised vehicles, as well as between the roadside and urban infrastructure, control and service centres, and other road users such as pedestrians and cyclists [40, 41]. To ensure the secure and effective management of communications and data, C-ITS integrates multiple access technologies and communication protocols through a unified approach.
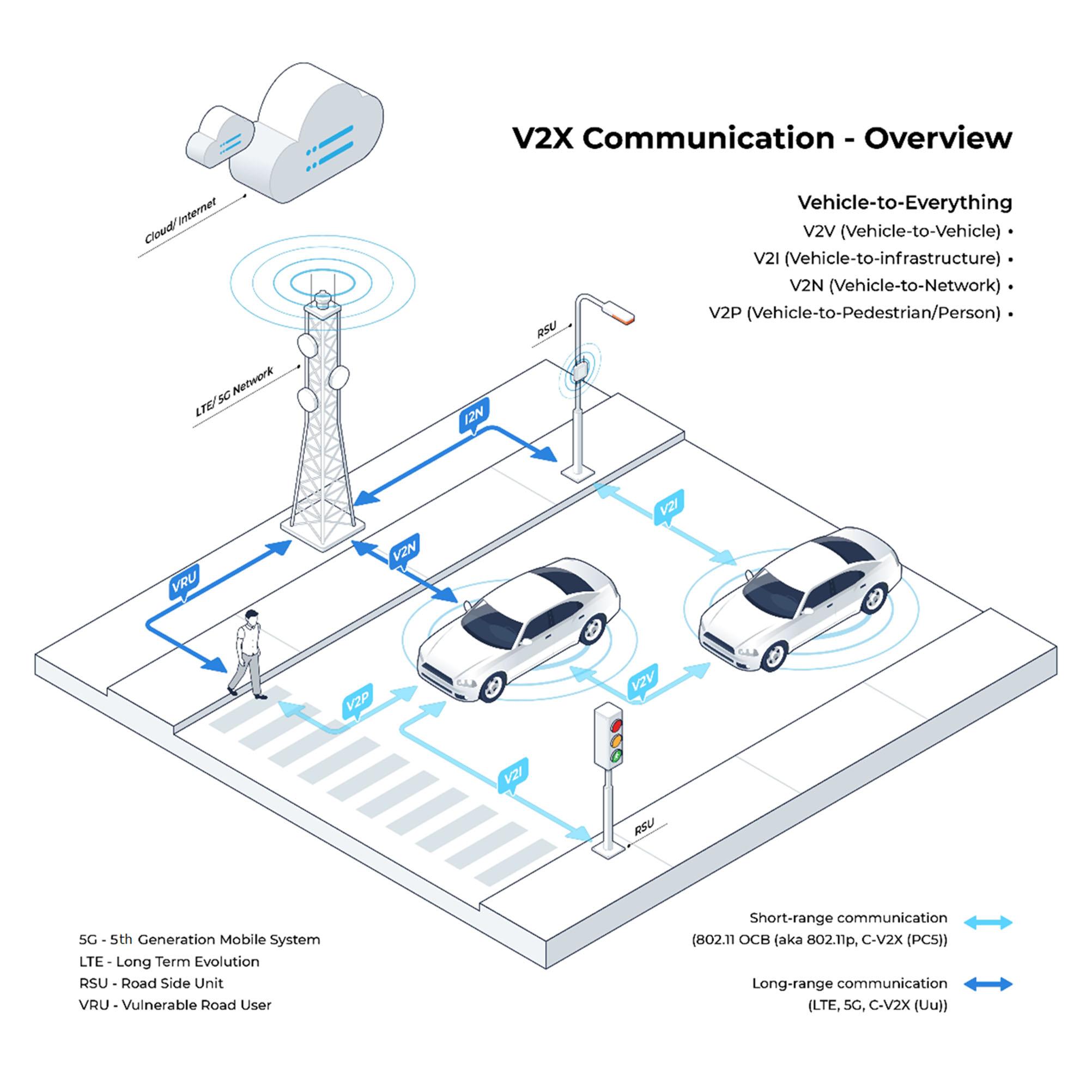
1.2 Competition of C-ITS Standards
C-ITS technologies offer both ‘long-range’ and ‘short-range’ communications. Long-range communications, e.g., mobile radio (4G LTE, in future 5G), are typically telecom-based data communication via the Internet. Short-range communications consist of hotspot installations on the road for direct communication with vehicles. At present, there are two main sets of C-ITS communication standards in the world, namely Dedicated Short-Range Communications (DSRC) and Cellular Vehicle-to-Everything (C-V2X). Other technologies are not yet ready for series production.
DSRC is a Wi-Fi derivative technology developed in the USA, i.e., vehicular networking communication technologies based on IEEE 802.11p and IEEE1609 suite standards. The term ‘dedicated’ refers to the designated frequency band allocated by the United States’
Figure 2 Examples of C-ITS communications (Source: La Trobe University)
Federal Communications Commission (FCC) for DSRC at 5.9 GHz, and short-range refers to a shorter communication distance than cellular communication, usually 100-400 meters. DSRC is predominantly a short-range communication technology as defined in the standards. It is designed to allow for highly secure, high-speed direct communication between vehicles (V2V), the surrounding infrastructure (V2I), and other road users (V2P) within its range.
Different from Wi-Fi-based DSRC standards, C-V2X uses cellular technology, which is the same as the 3G/4G/5G network we use daily on our mobile phones. The main difference is that C-V2X technology has both direct communication and non-direct communication modes. Direct communication means that the vehicle can directly communicate with other vehicles (V2V) or roadside units (V2I), which is consistent with the function of DSRC. Nondirect communication refers to the exchange of information with other traffic entities through the cellular network (V2N), which is not specifically defined in the DSRC 5.9 GHz communication standard. In a complex traffic environment, the non-direct communication function is very useful for a global view of traffic information in the area and proactive traffic management [42].
During past implementation trials, it was uncommon for DSRC networks to rely solely on 5.9 GHz direct communication in a decentralised manner. Instead, an Internet connection, such as a cellular network, is frequently used to connect DSRC networks to access additional information such as road traffic data. This practice is referred to as DSRC and cellular hybrid communication. Hybrid DSRC does not represent a specific standard but rather a technique for system execution. The implementation of hybrid DSRC does not need to adhere to mandatory standards, allowing for significant flexibility. The inclusion of cellular communication, along with Bluetooth, Wi-Fi, or even fibre, in DSRC deployments is not restricted. For instance, an ISO standard [43] provides guidelines for hybrid communications and supports functionalities for cooperative ITS services, which are widely applicable to C-ITS, including DSRC and cellular hybrid methods but are not limited to them.
DSRC and C-V2X technologies have predominantly been trialled by leading countries, including Europe, the USA, Japan, and China. Since the turn of the millennium, Europe and Japan have been primary adopters of DSRC technology. In contrast, over the past five years, C-V2X technology has seen significant growth, particularly in the USA and China.
For the past 20 years, the USA has shown a strong commitment to DSRC technology, designating 75 MHz of the 5.9 GHz band to DSRC. However, in the USA, DSRC has not moved significantly beyond large trials such as the Mcity Test Facility by the University of Michigan, and this critical mid-band spectrum has largely been unused for decades. In November 2020, the FCC adopted new rules for the 5.9 GHz band (5.850-5.925 GHz). The new band plan designates the lower 45 MHz (5.850-5.895 GHz) for unlicensed uses and the upper 30 MHz (5.895-5.925 GHz) for enhanced automobile safety using C-V2X technology [10]. The FCC believes that the transition away from DSRC will hasten the actual deployment of ITS services which will improve automotive safety [10].
From a global perspective, C-V2X is emerging as a leading technology, gaining support for its superior performance and clear evolution. The development of 5G-V2X as part of 3GPP Release 16 is rapidly advancing and attracting increased attention [44]. C-V2X has demonstrated a strong technical development momentum, aimed at supporting a range of intensive use cases, such as intersection warnings, green wave priority, VRU warnings, etc.
[45]. C-V2X has the support of mobile operators, leading mobile equipment makers and many automakers, including Audi, BMW, Mercedes-Benz, GM, Ford, Mitsubishi, Nissan, Volvo, Honda, Hyundai, SAIC, etc. [46]. Prominent members and partners of the 5G Automotive Association (5GAA) including Audi, Continental, Ericsson, Fiat Chrysler Automobiles, Harman Samsung, Marelli, Pirelli, Qualcomm, and Vodafone, have actively demonstrated the current state of C-V2X and the road to future, V2V, V2I, and V2N applications based on 4G LTE and 5G [47]. At the 2021 ITS World Congress (Hamburg), 5GAA demonstrated the latest C-V2X development which showed that 5G-V2X can have a significant impact by reducing accidents and saving millions of lives [48]. Future C-V2X use cases include cooperative navigation, autonomous vehicles, assisted border crossing, extended sensors with multi-access edge computing (MEC) [49], remote driving, and others [45].
However, in the current international C-ITS industry, DSRC still occupies a large share in the field of safety applications. Presently, the ITS Class Licence in Australia follows the European standards for C-ITS deployment by referencing the ITS-G5 standard defined by ETSI EN 302 663 [50]. One of the key factors contributing to the commercialisation of the DSRC-based C-ITS platform is its extensive history of research and validation. The existence of such history provides significant advantages for C-ITS technologies in general. In November 2016, the European Commission adopted the European Strategy on C-ITS for Cooperative, Connected and Automated Mobility (CCAM) and adopted a delegated regulation on specifications for the provision of C-ITS supported by an impact assessment in 2019 [51]. In Europe, the deployment of DSRC V2X technology in traffic infrastructure is becoming a reality. For example, the city of Ludwigsburg has equipped all of its traffic lights and intersections with Cohda Wireless DSRC V2X technology to allow fire trucks and rescue services to request priority passage at traffic lights and synchronise their movements with traffic signals, enabling them to reach accidents and emergency sites without delay. In Austria, the motorway operator ASFINAG is equipping its motorways nationwide with DSRC, providing reliable and low-latency communication between road infrastructure and vehicles. In Hamburg, the city's Port Authority has been working with NXP, Siemens, and Technolution to provide truck drivers with the ability to request priority passage at traffic crossings, as well as receive speed advice information and countdown information for the next green light [52]. From the perspective of manufacturers, Volkswagen, NXP Semiconductors, Israeli chip maker Autotalks, and Austrian ITS provider Kapsch continue to promote DSRC. In October 2019, Volkswagen announced the rollout of its new Golf equipped with NXP’s life-saving RoadLINK V2X communication solution, the first mainstream European car model equipped with DSRC technology [52].
1.3 The Choice of Short-Range Communications
C-ITS is a new and rapidly emerging industry that poses high demands for technology integration. The development of C-ITS can improve transport management efficiency and reduce traffic accidents, effectively decrease urban congestion and vehicle fuel consumption for environmental protection and enhance the driving experience and safety of consumers. Our interviews uncovered a number of challenges in the deployment of C-ITS in Australia:
The operating business model for C-ITS in Australia (and elsewhere) is unclear, and the final development direction has yet to be determined. Rather than concentrating solely on technology acquisition, the emphasis should be on formulating efficient
management strategies and operational models. For the successful deployment of C-ITS, the establishment and execution of a robust regulatory framework are of paramount importance. This framework should address key areas such as data privacy and security, interoperability, liability, and ethical considerations. Providing stakeholders with a clear set of guidelines and a degree of certainty is essential to stimulate action. Over time, this regulatory framework should be progressively refined and optimised.
The Australian market is relatively small – with only 1.08 million new car sales for 2022 [53], which is 1% of the world market [54]. Australia's relatively smaller market size means it has less influence on technology and business compared to larger markets. As a result, end-users, such as drivers, may not be eager to invest additional funds to access the advanced services offered by C-ITS. This is seen as one of the challenges to overcome.
Despite the absence of a nationwide C-ITS platform, some states have developed their own C-ITS systems. While these efforts demonstrate significant progress, the focus remains on pursuing a standardised approach and common security protocols to enhance uniformity and integration nationally. Especially, the absence of a nationwide Public Key Infrastructure (PKI) hinders the establishment of a common security protocol for managing C-ITS security, which is crucial for both short- and long-range communication regardless of the C-ITS technology used.
In addition to the aforementioned challenges, other potential hurdles could impact the successful implementation of C-ITS. One significant challenge is the limited coverage of the current infrastructure, including RSUs and cellular towers, which may not adequately support the desired level of information quality, particularly in terms of real-time accuracy.
1.4 Research Approach
Our research objective is primarily to review the standards suites of the two competing shortrange communication technologies, DSRC and C-V2X. We also provide an overview of the considerations that the Government should take into account with regard to a consistent nationwide approach to C-ITS short-range communications. The questions that we answer are as follows:
Which technology provides the preferable technical performance, and more suitable deployment practices?
Which technology provides the most support, has had the most use cases, the most field tests, and shows a promising future development?
Which other factors, not necessarily intrinsic to the technology, should the government take into account?
In this study, we compare the short-range communications technologies and their international standards suites, the current development state and future development directions. Our focus is on major countries, such as Europe, the USA, and China, to gain an understanding of the key markets. Based on this analysis, we provide recommendations on short-range communication deployment strategies, infrastructure networks, business models, data governance, data security, and their implications for autonomous vehicles. We
have gathered insights through in-depth interviews with 40 leading experts and executives in the field of C-ITS, from the following organisations:
Table 5 Interviewee list
Groups
Organisations
V2X Chip Manufacturers Qualcomm, Autotalks
C-ITS Equipment Manufacturers
Government Agencies
Telcos
Standard Institute Members
Consortiums
Car Manufacturers
Security and Technology
Cohda Wireless, Q-Free America, Kapsch USA., Kapsch Europe
Department of Transport and Main Roads, Queensland Government, Australia, Department for Transport UK, European Commission (Intelligent Transport)
Telstra AU, Vodafone UK, Vodafone Deutschland, Ericsson, Deutsche Telekom
ISO TC204 ITS WG, CEN/TC 278 PT1605, ETSI ES 200 674-1, ISO DIS 21218, CEN/TC278 PT1601
5GAA, European C-Roads, CAR 2 CAR Communication Consortium, ERTICO – ITS Europe, ITS Australia, ITS America, The Federal Chamber of Automotive Industries (FCAI)
Audi, Ford Australia, Stellantis, OHMIO, Toyota USA
ATOS (EU SCMS), Yogoko, Insurtech Australia
After analysing the results, we identified the key decision factors and recommendations, which we have summarised in this report.
2 C-ITS Standards
To understand the differences between DSRC and C-V2X, the first thing is to understand the standards that have been developed for each technology. This chapter provides an overview of Standards Development Organisations (SDOs), the architecture of the ITS, and a comparison between DSRC and C-V2X standards in the context of C-ITS.
2.1
Standards Development Organisations (SDOs)
Table 6 outlines the key SDOs, their primary roles, their main operating regions, and the CITS standards they have contributed to or maintained.
Table 6 Main C-ITS SDOs
SDO
International Organisation for Standardization (ISO)
European Committee for Standardization (CEN)
European Telecommunications Standards Institute (ETSI)
Standardisation of various industries, including ITS
Development of European standards, including ITS
Standardisation of ICT aspects of ITS
The 3rd Generation Partnership Project (3GPP) Mobile telecommunications standards
Society of Automotive Engineers (SAE)
Institute of Electrical and Electronics Engineers (IEEE)
International Telecommunication Union (ITU)
Development of engineering standards for automotive and aerospace systems
Electrical and electronic technology standards
Regulation and standardisation of
Global Various ITS standards by Technical Committees (TCs) 204 (ITS)
Europe Various ITS standards by TC 278
Europe European DSRC Standards: ITS-G5
C-V2X (4G and 5G)
Global SAE J2735 (DSRC Message Set Dictionary), SAE J2945 (Performance requirements for ITS V2X)
Global DSRC IEEE 802.11p and Wireless Access for Vehicular Environments (WAVE) IEEE 1609
Global ITS standards on vehicular communications,
SDO Name Main Scope of Work Main Country Relevant C-ITS Standards
global telecommunications
Internet Engineering Task Force (IETF)
Development and promotion of Internet protocols
Table 7 C-ITS Working Group activities
SDO Description WG
ISO/CEN More focused on the high-level definition, technologyagnostic and generically applicable functionalities (vehicles, roadside infrastructure, personal devices and control centres)
ISO/TC 204/WG 1
ISO/TC 204/WG 16
ISO/TC 204/WG 17
ISO/TC 204/WG 18
CEN/TC 278/WG 16
CEN/TC 278/WG 17
ETSI Focused on vehicle-centric technologies and services using ITS-G5 and its associated set of protocols
automation, telematics, and security
Global Networking and Transport Communication Protocol for C-ITS standards: IPv4, IPv6, GeoNetworking, Fast Networking & Transport Protocol (FNTP), etc.
Work Scope
Architectural issues and globally unique identifiers
Access technologies, communication protocols, and probe data
Personal devices
Cooperative ITS (applications and protocols)
Cooperative ITS (joint WG with ISO/TC 204/WG 18):
- Generic facilities messaging: vehicle navigation, coordination, real-time digital map
- Infrastructure-centric messaging: traffic light phases, intersection geometry
Mobility integration and urban ITS (joint WG with ISO/TC 204/WG 19)
ITS WG1 Application requirements and servicesdeveloping vehicle-centric localised messages (CAM, DENM, …) and other vehicle-centric facilities (vehicle-LDM, POTI)
ITS WG2 Architecture and cross-layers
ITS WG3 Transport and network
ITS WG4 Media and medium related: development of ITS-G5 (extension with IEEE 802.11bd) and LTE-V2X/5G-V2X (based on 3GPP Release 17) access layer specifications for Release 2
ITS WG5 Security
In the context of SDOs, relevant standardisation activities are carried out by specific Working Groups (WGs) to advance the development and implementation of the technology, as shown in Table 7. The C-ITS standards in the European Union are predominantly rooted in the ETSI standards, bolstered by complementary standards from ISO.
Currently, Release 1 is the ETSI ITS standard widely recognised by the industry. From the middle of 2023 to the present, the ETSI TC has been gradually releasing the Release 2 ITS standards. Compared to Release 1, a significant upgrade in Release 2 is the addition of new content of LTE-V2X and 5G-V2X, for ITS, such as access layer [55] and network layer [56] specifications. Thus, it's evident that C-V2X technology has significantly influenced ETSI ITS standards, owing to its rapid growth.
Simultaneously, CEN is chiefly devoted to formulating application standards. In recent years, ETSI has demonstrated a steadfast commitment to communication standards, actively pursuing the incorporation of pertinent ISO and CEN standards within its own framework.
2.2 Standard Architecture
A functional architecture – also referred to as ITS station architecture4 – is specifically designed to manage various aspects integral to C-ITS. This includes the oversight of security measures, the facilitation of communications, and the handling of data relevant to the provided C-ITS services. The ITS station architecture is specified in ISO 21217, prepared by ISO/TC 204 in a set of related standards, as shown in Figure 3. The architecture of the ITS station consists of three communication layers: the ITS station access layer, the ITS station networking and transport layer, and the ITS station facilities layer. These layers provide the foundation for the ITS Applications entity, which is situated on top of them, enabling the deployment of various ITS applications. Additional cross-layer entities in charge of the management activities (management of ITS station units, communications and security) support communications and applications [40].
A complete list of ITS standards can be found in Annex 1. The full ETSI ITS standards Release 1 are in Annex 2. ISO/CEN provides detailed guidelines on how to use C-ITS standards [57] and also provides the download link of the implementation Abstract Syntax Notation One (ASN.1) source code. Any C-ITS equipment manufacturer can access this document and download a series of ASN.1 source codes for ITS standard suites to be integrated into their products in a cross-platform way (e.g., Android, Apple, Linux, or Windows). This is advantageous for quickly developing new C-ITS products and ensuring standard compliance.
4 ‘ITS station’ is a term used in Intelligent Transportation Systems (ITS) standards. It refers to any entity that implements ITS services, including On-Board Units (OBUs) in vehicles, Roadside Units (RSUs), and servers. These stations are responsible for running various ITS applications as specified in the standards.
2.3 Basic Applications
In 2009, ETSI defined the basic set of application requirements for ITS, which are basic safety applications required for V2X systems (mainly DSRC at that time) to implement [58], as shown in Table 8. However, despite the definition of applications in the ETSI standard [58], previous trials of DSRC-based C-ITS have primarily focused on Active Road Safety and Cooperative Traffic Efficiency use cases. With evolving technology, it is crucial to explore new use cases that align with emerging needs and advancements, surpassing the limitations of the basic use cases outlined in this document to meet future requirements.
Figure 3 ITS station architecture [40]
Table 8 ITS basic use cases [58]
Applications Class Application
Active Road Safety
Driving assistanceCooperative awareness
Driving assistanceRoad Hazard Warning
Use case
Emergency vehicle warning
Slow vehicle indication
Intersection collision warning
Motorcycle approaching indication
Emergency electronic brake lights
Wrong way driving warning
Stationary vehicle - accident
Stationary vehicle - vehicle problem
Traffic condition warning
Signal violation warning
Roadwork warning
Collision risk warning
Decentralised floating car data
- Hazardous location
- Precipitation
- Road adhesion
- Visibility
- Wind
Cooperative Traffic Efficiency Speed management
Cooperative navigation
Cooperative Local Services Location-based services
Regulatory/contextual speed limits notification
Traffic light optimal speed advisory
Traffic information and recommended itinerary
Enhanced route guidance and navigation
Limited access warning and detour notification
In-vehicle signage
Point of Interest notification
Automatic access control and parking management
ITS local electronic commerce
Media downloading
Global Internet Services Community services
ITS station life cycle management
Insurance and financial services
Fleet management
Loading zone management
Vehicle software/data provisioning and update
Vehicle and RSU data calibration
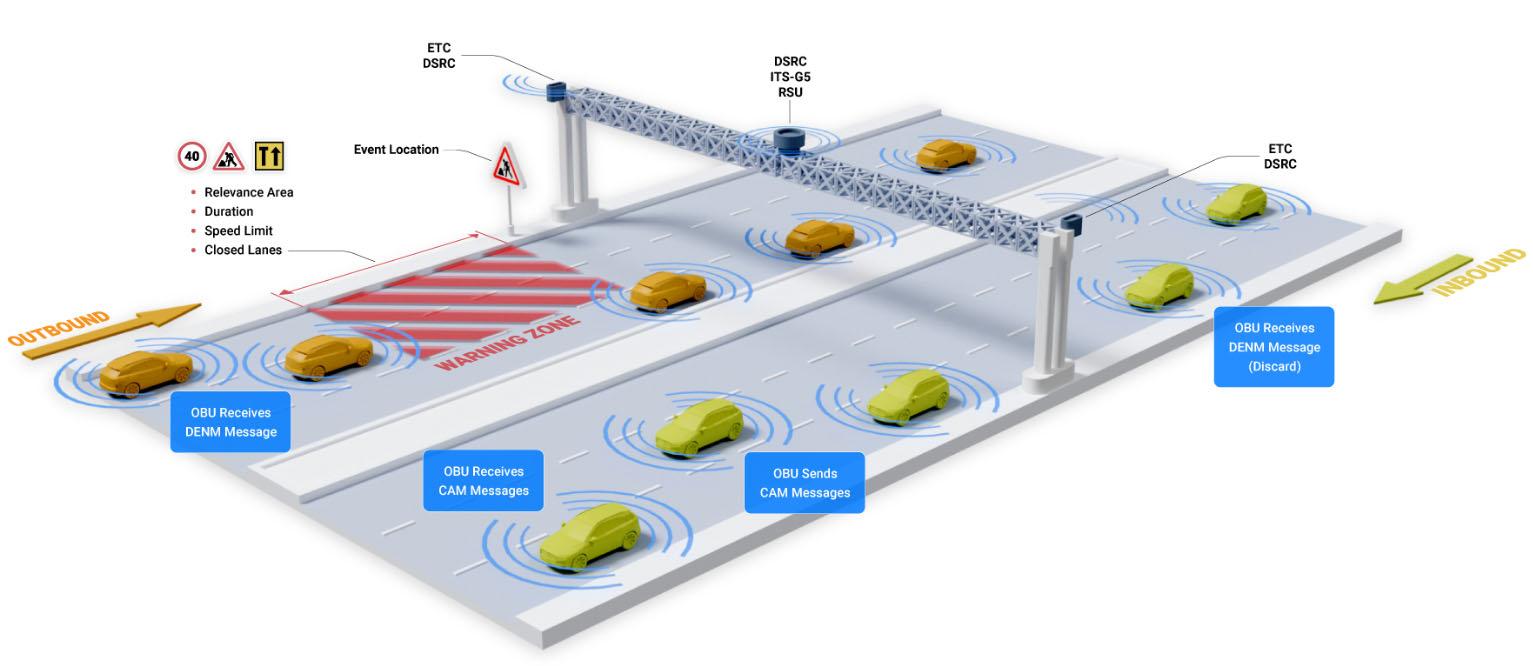
4 Implementation of basic application use cases in DSRC ITS-G5 (Source: La Trobe University)
Figure 4 provides a demonstration of basic use cases in DSRC-based C-ITS. The Melbourne EastLink field trial conducted between 2018 and 2019 involved testing the performance of DSRC-based V2V and V2I communication systems on highway gantries. DSRC RSUs broadcast Decentralised Environmental Notification Message (DENM) to provide drivers with vital information regarding roadworks, lane closures, relevance distances, and changes in speed limits (V2I). These messages are essential for drivers to make informed decisions within warning zones. Cooperative Awareness Message (CAM) is another type of message exchanged between vehicles (V2V), enabling the sharing of crucial details like position, speed, and direction, thereby facilitating collision avoidance. The seamless integration of V2V and V2I communication plays a pivotal role in the successful implementation of C-ITS.
2.4 DSRC and C-V2X Standards
Both DSRC and C-V2X are wireless communication technologies designed to meet ITS standard suites, aiming to enhance safety, efficiency, and mobility. They facilitate V2I and V2V communication, crucial for various ITS applications. DSRC, developed over two decades, primarily focuses on short-range V2V and V2I scenarios using the 802.11p standards. In contrast, C-V2X is a newer technology that aligns with core ITS standards and also utilises cellular network resources. Table 9 briefly compares the DSRC and C-V2X standards in different regions [59].
Dedicated Short-Range Communications (DSRC) standards include:
USA IEEE DSRC: IEEE 802.11p/WAVE (Wireless Access for Vehicular Environments) [2-4]. IEEE 802.11p provides access layer specification; the WAVE standard provides a broad range of features for implementing safety and non-safety applications in vehicular environments. The detailed protocol specification is in Annex 3.
Figure
ETSI ITS-G5: The European ETSI standard of IEEE 802.11p/WAVE standard [50]. The detailed protocol specification is shown in Annex 2.
The Cellular Vehicle-to-Everything (C-V2X) standard was first introduced in 3GPP Release 14 in 2017, as an extension of LTE’s Proximity Services (ProSe) used for the Device-toDevice (D2D) / Ad-hoc direct communication services [60]. C-V2X defines two Complimentary Transmission Modes [61]:
Direct communication mode (PC5): Direct low latency communication for Vehicleto-Vehicle (V2V), Vehicle-to-Infrastructure (V2I), and Vehicle-to-Person (V2P). In this mode, C-V2X works in the 5.9 GHz ITS spectrum, which is independent of cellular networks. Vehicles can directly communicate with each other in an area without any cellular coverage.5
Network communications mode (Uu): Vehicle-to-Network communication (V2N) operating in the mobile operator's licensed spectrum for complementary services such as receiving information about road conditions and traffic in the area [62].
Technology Region Key
Europe ETSI ITS-G5 suites
Japan ARIB STD-109
4G LTE-V2X Global 3GPP Release 14-15 [6]
3GPP TS 22.185 (Service requirements for V2X), TS 23.285 (Architecture enhancements for V2X and LTE), TS 23.286 (Application layer support for V2X), 3GPP 36 series (Radio access), TS 36.785-.788 (V2X radio transmission), TS 33.185 (Security for V2X)
5G-V2X
Global 3GPP Release 16-17 [63], 3GPP Release 18 5GAdvanced (started Q2-2022)
3GPP TS 22.186 (Service requirements for enhanced V2X), TS 23.287 (Architecture enhancements for 5G-V2X services), 3GPP 38 series (Radio access), TR 38.885-.886 (5G-V2X services), TS 33.185 (Security for V2X)
5 PC5 operates both in-coverage (Mode 3) and out-of-coverage (Mode 4) scenarios. Transmission Mode 3 is defined as when the network does the scheduling of resources for vehicles to communicate on. On the other hand, Transmission Mode 4, allows vehicles to autonomously select and use communication resources based on their sensing of the surrounding environment, without the involvement of the network in resource selections (source: "Cellular-V2X Technology Overview," Qualcomm, 2019)
Table 9 Comparison of DSRC and C-V2X standards globally
Characteristic
Safety applications
DSRC
Origin
Current standards
Next-generation standards
Short-range communication
Long-range communication
C-V2X
Both DSRC and C-V2X use ETSI ITS standards for safety-centric applications, which means that the syntax of the warnings – that is, the key information shared (referred to as CAM and DENM6 messages) – is the same. Although DSRC and C-V2X utilise different access layer technologies for message transmission, with DSRC employing Wi-Fi technology and C-V2X using cellular communication, the content of the messages, once received and processed by ITS safety applications, remains identical. This means that regardless of the transmission method, the critical safety information conveyed in these messages is consistent across both technologies.
Developed by IEEE in the USA in the late 1990s based on Wi-Fi 802.11 standards.
USA: IEEE 802.11p / Wireless Access in Vehicular Environments (WAVE)
Europe: ETSI ITS-G5
IEEE 802.11bd
DSRC is allocated a spectrum of either 70 MHz or 75 MHz in the 5.9 GHz ITS band, where each channel spans 10 MHz. This setup is akin to multiple lanes on a highway, with each 10 MHz channel acting as a separate lane within the broader 5.9 GHz frequency range.
In DSRC, the primary focus is on short-range, direct communication for low-latency and time-critical applications such as collision warnings. However, this is effectively complemented by long-range communication for non-critical information, like speed zone
Introduced by 3GPP in 2016-2017, based on cellular technology.
4G LTE-V2X (3GPP Release 14-15)
5G-V2X (3GPP Release 16-18), also known as 5G New Radio (NR) V2X
Typically, LTE-V2X PC5 mode utilises the upper 20 MHz band of the 5.9 GHz ITS band, ranging from 5.905 GHz to 5.925 GHz. As for 5GV2X, it is expected to require a broader spectrum, potentially 40 MHz or more, to accommodate its advanced capabilities, although this specification has not been definitively confirmed yet.
In C-V2X, long-range communication, such as 4G/5G, is inherently included in the C-V2X standard suites, referred to as network or Uu mode. This facilitates vehicle-to-network (V2N) communications, enhancing the reliability and coverage of shortrange communication. The latest 5G-
6 CAM (Cooperative Awareness Messages) and DENM (Decentralised Environmental Notification Messages) are two fundamental message types defined within C-ITS, which enable vehicles and infrastructure to communicate with each other and detect road hazard.
Table 10 Comparison of characteristics between DSRC and C-V2X technologies
Characteristic DSRC C-V2X
updates. This integration, known as DSRC-Cellular Hybrid, allows both short-range and long-range communications to operate in coordination, each within their respective coverage areas, ensuring a hybrid communication network for various vehicular use cases.
V2X specification incorporates Quality of Service in the network service, specifically optimising C-ITS message communication for longrange scenarios. For autonomous and connected vehicles, road operators often require the benefits of long-range communication, such as high bandwidth and low latency, to facilitate advanced applications like High-Definition (HD) Maps Data Collection and Sharing.
Modulation technique
Network resource efficiency
Orthogonal frequency division multiplexing (OFDM) is a method used in many wireless communications systems, including DSRC, to send many signals simultaneously over the same communication channel. It offers robust communication in a dense and dynamic environment. OFDM can be compared to a multi-lane freeway where each small chunk of data is like a car, and every car gets its lane (or frequency) to drive in. So, it is comparable to dispatching numerous small cars, each in their own lanes, all at the same time. OFDM is specifically designed for high-reliability and lowlatency data delivery.
Traditionally, this has not been the focus of DSRC as it assumes a dedicated channel.
Single-carrier frequency division multiplexing (SC-FDM) is used in digital communications to transmit large amounts of data. SC-FDM can be compared to a single, large truck carrying a big load (all packages) on a single lane (frequency) at once, instead of sending many small cars at once (as in OFDM). SC-FDM is designed to excel in network environments with less complex technical device requirements and less stringent power requirements.
As C-V2X has been designed with network resources in mind, it includes a comprehensive set of standards that features both Uu mode and PC5 mode. This aims to improve integration, compatibility, and network resource efficiency. For example, vehicles can seamlessly switch between Uu mode and PC5 mode.
Characteristic DSRC C-V2X
Message delivery verification
Error protection coding
DSRC does not verify if a sent message is received. It uses broadcast mode for highfrequency transmission in a dedicated channel, making the need for verification redundant.
Hybrid Automatic Repeat reQuest (HARQ) is a technique used in communication systems, particularly in wireless networks, to improve the reliability of data transmission. By using a combination of the original and previous data, as well as adding extra error correction information, the receiver has a better chance of correctly decoding the packet even if some parts were lost or corrupted during transmission. HARQ facilitates the retransmission of only those messages containing errors.
Data transmission
Convolutional coding is an error correction method of adding redundancy (extra information) to data before it is transmitted over a communication channel. This redundancy helps in detecting and correcting errors that might occur during transmission. It involves using a special mathematical operation called convolution to generate the redundant bits.
Convolutional coding is used in various communication systems, including satellite communication, wireless networks, and digital broadcasting, to improve the reliability of data transmission by detecting and correcting errors.
Carrier-Sense Multiple Access with Collision Avoidance (CSMA-CA) is a protocol used in wireless networks to manage how multiple devices share the same communication channel to avoid sending data simultaneously, which could lead to data collisions and loss. CSMA-CA helps wireless
Turbo coding is an advanced error correction technique that combines multiple convolutional codes to achieve even higher levels of error correction performance. It was developed in the 1990s and has proven to be highly effective in improving communication system reliability. Turbo coding is used in various modern communication standards, such as 3G, 4G LTE, and some digital broadcasting systems. Turbo codes play a crucial role in ensuring data integrity and reliability, especially in noisy or error-prone communication channels.
Semi-persistent scheduling is a way to manage wireless communication by dividing devices into priority categories and reserving resources at fixed, regular intervals. This can help improve overall system performance and meet the diverse communication needs of different devices.
Characteristic DSRC C-V2X
devices take turns using the communication channel to reduce the chance of data collisions and improve overall network efficiency.
Time synchronisation
Predictive quality of service (QoS)
DSRC employs a loose asynchronous timing, utilising the Global Navigation Satellite System (GNSS) for time synchronisation:
Loose Timing: DSRC communication may not rely on strict, synchronised timing between devices. Unlike some real-time communication systems where data must be transmitted and received at precise moments, DSRC may allow for some variation in the timing of messages.
Asynchronous Communication: DSRC devices can send and receive data independently without the need for strict coordination. Asynchronous communication means that devices don't have to wait for each other to finish transmitting before sending their own data.
While DSRC may not inherently provide predictive QoS features, it can still achieve reliable communication through techniques such as message repetition, error correction, and adaptive transmission power control.
Tight synchronous requirements, also utilising GNSS. When GNSS is unavailable, a device utilises other sources for synchronisation, including base stations, other vehicles, and roadside units. In the context of C-V2X, ‘tight synchronous requirements’ means that the communication between vehicles and infrastructure, or between vehicles themselves, is highly synchronised. In other words, there are strict timing standards that devices must adhere to when transmitting and receiving data.
Utilising the enhanced feature of predictive QoS, C-V2X can adaptively boost performance. For instance, during cooperative lane merges, if latency crosses the 20 milliseconds threshold, the QoS monitor will intervene and reallocate network resources to ensure lower latency and more secure lane merges.
C-V2X possesses both a direct short-range communication mode (PC5) and a network mode (Uu) that leverages the capacity of cellular networks. Its standards are tailored towards the seamless integration of these direct short-range and network communication modes. One distinct advantage of C-V2X is its built-in readiness for LTE integration, enabling it to pair with LTE cellular networks for advanced V2V and V2I communications. On the other hand, while DSRC is primarily designed for 5.9 GHz communication between vehicles and roadside units, in practice, it is often combined with a cellular modem to access expanded information sources, even though this is not explicitly defined in the DSRC standard.
C-V2X based on 3GPP Release 14 establishes the foundation for V2X communication for basic safety message exchange [6]. 3GPP Release 15 based on C-V2X, incorporates additional features like Transmit Diversity and 10 milliseconds Packet Delay Budget (PDB) which improve the range and reliability [6]. The 5G-V2X standard, based on the next 3GPP Release 16/17 standard, will offer much higher throughput, much higher reliability, and lower latencies, and enable possibilities of newer advanced use cases [61, 63]. Detailed C-V2X standards suites defined by the USDOT are in Annex 4. A detailed comparison of release use cases is in Annex 5.
Figure 5 illustrates the protocol architectures of DSRC and C-V2X. Both technologies, DSRC and C-V2X PC5, utilise the 5.9 GHz frequency band for direct radio communication. However, their access layers are not compatible. DSRC employs a contention-based MAC protocol, allowing multiple devices to transmit data simultaneously, with the receiver determining successful transmissions. In contrast, C-V2X utilises a scheduled-based MAC protocol, where transmissions are pre-scheduled, and devices are assigned specific time slots for data transmission. Nevertheless, the network layer, security layer, and application layer remain the same for both technologies. They use the same message set (SAE J2735 and J2945) and similar digital signatures to ensure secure and reliable message transmission. In simpler terms, DSRC and C-V2X can utilise common higher-layer ETSI and ISO standards and share security standards. This allows ITS road operators to upgrade C-ITS devices for specific communication technologies while still utilising existing application software and data structures. This compatibility simplifies the process for operators to adopt new communication technologies without significant changes to their existing systems.
Both DSRC and C-V2X are continuously evolving technologies. To address the performance disparities where C-V2X surpasses DSRC 802.11p, the IEEE Task Group 802.11bd (TGbd) was established in January 2019. Its objective is not only to bridge the performance gap but also to introduce additional operational modes and enhance the throughput of DSRC. The next-generation DSRC aims to double the throughput at the MAC level and extend communication ranges by reducing noise sensitivity [65]. The latest edition of 802.11bd was released in February 2023. 3GPP Release 18 content was finalised as of December 2021, and the study and work for 5G-Advanced Release 18 commenced in Q2-2022.
2.5 Conclusion
In summary, both DSRC and C-V2X serve as access layer communication technologies within the ITS architecture, facilitating over-the-air message transmission and reception. The primary distinctions between DSRC and C-V2X lie in physical layer aspects such as modulation and error protection. Many protocols can therefore be either shared or reused across these technologies because both C-V2X and DSRC use the same higher-layer applications based on ETSI standards.
The standards of both DSRC and C-V2X address basic use cases within their respective scopes. C-V2X standards have particularly focused on integrating short-range communication and long-range communication.
DSRC and C-V2X standardisation bodies remain highly active, as evidenced by their latest updates, including 802.11bd for DSRC and 5G-V2X Release 18 for C-V2X.
Figure 5 DSRC vs. C-V2X protocol architectures [64]
3 C-ITS Technology Readiness
This chapter provides a performance comparison of DSRC and C-V2X short-range communication technologies. DSRC has been in development for over 20 years and offers mature products, while C-V2X has seen rapid progress since 2017. The chapter discusses the latest advancements and research findings on technology readiness and performance.
3.1 Competing Standards in C-ITS
C-V2X and DSRC each offer distinct capabilities with the potential to revolutionise vehicular communication, yet they vary notably in their advantages and potential applications. DSRC, which originated in the USA in the late 1990s, is built on the 802.11p standard. This technology enables fast and efficient direct communication between vehicles, as well as between vehicles and infrastructure. Its effectiveness in supporting basic safety applications and improving traffic efficiency has been validated through extensive testing and standardisation efforts, particularly in regions such as Europe and the USA [5].
C-V2X, developed by 3GPP between 2016 and 2017, is an advanced feature of cellular communication designed to facilitate direct communication for V2V and V2I. While it serves a purpose similar to DSRC, C-V2X operates within cellular standards. C-V2X incorporates both the direct mode (PC5) and network mode (Uu) communication per the 3GPP standard. By leveraging network communication capabilities (V2N), C-V2X takes advantage of the bigger bandwidth provided by cellular infrastructure. This, within a unified cellular standard, enhances performance in non-safety applications such as HD maps sharing, automated driving, and cooperative driving.
DSRC advocates contend that it is a mature technology, outpacing the less intensive road trials of the newer C-V2X. They suggest DSRC may excel over C-V2X in delay-sensitive scenarios, like collision warnings, where ultra-low latency is crucial. Some researchers also highlight the relatively larger end-to-end communication delay of C-V2X, indicating this could constrain its effectiveness in such time-sensitive situations [45].
The competition between DSRC and C-V2X continues unabated. In February 2023, C2C-CC published a new white paper on Next-Generation V2X IEEE 802.11bd, presenting it as a fully backward-compatible evolution of DSRC 802.11p [5]. The latest release of DSRC 802.11bd introduces advanced features to support a wider range of use cases, highlighting the enhancements, as shown in Annex 3.
We believe that the vast expertise garnered from two decades of DSRC trials has the potential to accelerate the deployment of new C-ITS initiatives. Existing advanced applications of DSRC, such as the IEEE 1609 WAVE resource manager, network service, and security service, can be adapted for use across various C-ITS technologies, regardless of the specific underlying communication technologies. As a result, emerging C-ITS standards are likely to ‘repurpose’ well-established and mature elements originally developed for DSRC.
3.2 C-ITS Chip/Device Manufacturers
Table 11 Mainstream C-ITS equipment
Cohda (Now Danlaw) MK6
DSRC 2x NXP RoadLink® SAF5400 chipset
Q-Free Kapsch RIS9260 RSU
2 Channel IEEE 802.11p
5.850 – 5.925 GHz
C-V2X Qualcomm SA515 chipset supporting 3GPP Release15, LTE-V2X NA C-V2X 3GPP Release 14 5.855 – 5.925 GHz (LTE B47) 10 MHz channel spacing, PC5 sidelink
Cellular Cellular 5G with fallback support for 4G (LTE Cat 19)/3G/2G
a/b/g/n/ac
Standards
IEEE 802.11- 2012 IEEE 1609 - 2020
ETSI ES 202 663
SAE J2735 – 2020
SAE J3161/1, J3161/1A, J31612022 T/CSAE-2021 CERTIFICATION*
EU RED, FCC, OmniAir
voice and Internet
Commsi gnia ITS RS4
Danlaw RouteLink RSU
Autotalks / NXP /Marvell Chips
Qualcom m 9150 V2X
HARMAN Savari StreetWA VE
Yunex Traffic(Sie mens) RSU2X
(3GPP Release 14/15)
-ISO 21217/ETS I 320 665
-EN 302 636 series
-TS 103 097/102 940 series -CPOC
-ETSI ITSG5 -IEEE 1609
- IEEE 802.11p™/IEE E 802.11™
- C-V2X 3GPP Release 14
- SAE J2735 2016
- ETSI ITS-G5 standard set
- IEEE WAVE 2016 standard set
Complet e RealTime V2X Stack (ETSI, IEEE, SAE, ISO)
IEEE 802.11p, IEEE 1609.2, IEEE1609.3, IEEE 1069.4, SAE J2735, NTCIP, SNMP, USDOT v4.1 RSU specification, SAE J2735
Multiregion supports compliance with China, EU and US standards and regulations Supports all global V2X standards
DSRC has been developed and trialled for over 20 years, resulting in a wide range of products available in the market. C-V2X technology has experienced rapid progress since 2017. Currently, the prevailing products in the market are DSRC 802.11p and 4G LTE-V2X (3GPP Release 14-15). The newly released DSRC protocol, 802.11bd, became available in February 2023. To support autonomous driving, 5G-V2X has been introduced in Releases 16-18, coexisting with LTE-V2X. However, equipment supporting 802.11bd and 5G-V2X (Release 16-18) is not yet manufactured or available for purchase in the market at the time of writing this report.
In the V2X chip market, there are currently three major manufacturers: Qualcomm, Autotalks, and NXP. Qualcomm, as a member of the 5GAA, promotes its C-V2X chips as the primary product and has been working closely with 5GAA partners to support the global deployment of C-V2X. On 28th February 2023, Qualcomm announced the launch of its Snapdragon Auto 5G Modem-RF Gen 2-Release 16. This new addition to Qualcomm's connected car technology portfolio includes 4G LTE-V2X (Release 14/15) with a 20 MHz channel bandwidth, PC5 support, and 23 dBm power.
NXP, a Dutch semiconductor designer and manufacturer, is a leading producer of the DSRC single-mode modem for the 5.9 GHz and 760 MHz bands (SAF5100 series). In collaboration with Volkswagen, NXP is driving the production of Volkswagen's electric cars equipped with DSRC-based V2X technology (802.11p protocol).
Autotalks, an Israeli V2X chip company, has produced a low-cost dual-mode chip called CRATON2 [66] which is compatible with both DSRC and C-V2X protocols. CRATON2 is a dual-mode V2X chip that integrates mobility-optimised IEEE802.11p DSRC and C-V2X direct communications modems based on 3GPP Release 14 and 15. However, it does not support simultaneous transmission of C-V2X and DSRC messages, although it can be configured as a single mode at any given time. Although academic research suggests that the dual mode of simultaneous DSRC and C-V2X transmission offers higher reliability and lower delays [67], Autotalks chips are not designed to support this method. Despite this, CRATON2 remains a cost-effective solution for V2X communication and provides valuable options for vehicle manufacturers and infrastructure providers.
The leading C-ITS equipment manufacturers are shown in Table 11. CohdaWireless, QFree, and Kapsch are prominent competitors in the C-ITS market, commanding a significant market share. To broaden their user base and market penetration, these companies have adopted a strategy of offering dual-mode communication (DSRC 802.11p and LTE-V2X) on a single OBU/RSU device, catering to the evolving needs of the industry.
In China, C-V2X has become the dominant C-ITS standard, and leading companies in the Electronic Toll Collection (ETC) industry, such as WanJi (Vanjee) Technology and JinYi (Genvict) Technology, are actively involved in the C-ITS sector. As part of China's initiative to expand ETC smart parking services nationwide by the end of 2023, significant investments have been made in C-ITS and smart city technologies, with a strong emphasis on C-V2X and the integration of V2X with ETC systems. JinYi Technology has developed LTE-V2X OBU and RSU devices that comply with industry standards, while WanJi Technology offers a range of C-ITS products, including C-V2X OBU and RSU devices, as well as V2X testing instruments.
3.3 Technical Performance Comparison
The common performance metrics used by a C-ITS communication system are outlined in Table 12, primarily focusing on reliability, availability and Quality of Service (QoS) to ensure the quick and successful delivery of safety application messages.
Metrics Description
Reliability This criterion focuses on the system's reliability in high-traffic areas. It must maintain reliable communication despite obstacles and effectively manage interference from other systems or unauthorised devices.
Availability This pertains to the system’s operational continuity and coverage. It is imperative that the system maintains a broad area of coverage and exhibits resilience in promptly recovering from any potential disruptions.
Quality of Service (QoS)
This metric focuses on the efficiency of the system in terms of data transmission speed, task completion time, data handling capacity, seamless transition between tasks or locations, and the accuracy of location determination.
Typical Key Performance Indicators (KPIs)
Packet Error Rate (PER), Packet Reception Rate (PRR)
Receive Signal Strength Indicator (RSSI)
Communication Latency or End-ToEnd Delay in Applications
As of the writing of this report, products based on the latest C-ITS standards for C-V2X (5GV2X) and DSRC (802.11bd) are not yet commercially available. This makes it challenging to conduct a comprehensive evaluation to determine which technology outperforms the other based on these most recent standards. Most performance comparisons between DSRC and C-V2X in existing literature rely on IEEE 802.11p and LTE-V2X equipment, as these are the versions currently available on the market.
From March to September 2018, Ford and Qualcomm conducted systematic V2V tests, the reliability of which was further confirmed by an authoritative report from the 5G Automotive Association (5GAA). The report [8] evaluated the performance of both DSRC 802.11p and LTE-V2X radio in the ITS band ranging from 5.850 GHz to 5.925 GHz, based on key performance indicators outlined in Table 12. This analysis aids in comparing performance under different conditions in transmitting V2V safety messages, as shown in Table 13.
Table 13 Comparable performance of C-V2X and DSRC in 5GAA tests [8]
Metrics Tests
Reliability Lab Cabled Transmission and Receiving Tests
Field Line-of-Sight (LOS) Range Tests
Field Non-Line-of-Sight (NLOS) Range Tests
Interference Lab Cabled Test with Simulated Co-Channel Interference
Lab-Cabled Near-Far Test
Field Coexistence with Wi-Fi 80 MHz Bandwidth in UNII-3
Field Coexisting of V2X with Adjacent DSRC Carrier
Congestion Lab Cabled Congestion Control
Result
In a laboratory setting, when transmitting and receiving signals, C-V2X performed better.
When testing the range of the signal in a real-world environment where the transmitter and receiver can see each other, C-V2X performed better.
When the transmitter and receiver cannot see each other in a real-world environment, C-V2X performs better.
In a laboratory setting, when they simulated interference from two different transmitters using the same channel, C-V2X handled it better than DSRC.
When testing signal strength between close and distant receivers in the lab, both C-V2X and DSRC performed satisfactorily.
When testing how well the technologies work alongside Wi-Fi signals in the real world, C-V2X performed better.
When testing if DSRC can work in harmony with C-V2X in a real-world setting, both perform satisfactorily.
Both DSRC and C-V2X successfully handled a high volume of data congestion in the lab tests.
The reported performance summary is as follows [8]:
Both C-V2X and DSRC exhibited similar end-to-end application layer latencies under non-congested conditions, and both technologies met the latency requirements for the V2V safety applications defined in SAE J2945/1. In non-congested scenarios, DSRC demonstrates an average latency range of 16.2-16.6ms, whereas C-V2X achieves a latency of 14-16.8ms. For congested scenarios, the C-V2X latency budget is < 100 milliseconds.7
7 The term ‘latency budget’ means the maximum time that is allowed for a message or piece of data to travel from its source to its destination. ETSI TS 122 186 V16.2.0 (2020-11) “5G Service requirements for enhanced V2X scenarios” recommends 5ms latency for 5G NR V2X remote control use cases.
The test results indicate gains in terms of communication range for C-V2X compared to DSRC. The lab tests have shown significant link budget gain for C-V2X compared to DSRC. Under varying radio environment conditions (Line-of-Sight, Non-Line-ofSight and interference), the field tests show that C-V2X has a 1.3x-2.9x range advantage over DSRC. The LOS advantage was 1.7x, however, the improvements rose to 2.2x in more realistic NLOS conditions involving signal obstruction. With the interference in close proximity the improvement in range with U-NII-3 interferer was 1.7x while the improvement with the adjacent DSRC interferer was 2.9x.
C-V2X has shown performance gains over DSRC but both need to improve in interference scenarios. Above 800m for Static Vehicles and more than 1000m for moving vehicles, DSRC does not perform well against interference, compared to C-V2X. The net effect is elevated channel noise level at the V2X receiver. With the interference in close proximity, the improvement in range for C-V2X over DSRC with U-NII-3 interferer was 1.7x while the improvement for C-V2X over DSRC with the adjacent DSRC interferer was 2.9x [8].
In some tests, C-V2X displayed marginally improved reliability and resistance to interference. This enhanced performance can be attributed to its unique feature, the Hybrid Automatic Repeat reQuest (HARQ) mechanism.
Honda Research Institute USA conducted a trial to compare the direct communication performance of DSRC 802.11p and LTE-V2X in the Fowlerville Proving Ground in Michigan, from November 2019 to March 2020 [68]. Based on range analysis, LTE-V2X delivers a longer range for smaller packet sizes (around 200 bytes), while DSRC provides a longer range for larger packet sizes (around 1400 bytes). For Basic Safety Messages (BSM) with a full security profile (around 360 bytes), both technologies deliver similar performance. However, scenarios involving congestion or interference are beyond the scope of this research.
In our interviews, three primary manufacturers of C-ITS equipment asserted that DSRC outperforms or exhibits comparable performance to C-V2X (PC5). One of these manufacturers specifically highlighted that both technologies yielded similar performance outcomes when tested in a scenario involving 500 cars within a range of 300 meters. Overall, these manufacturers express greater confidence in DSRC devices, which have undergone trials for decades and have been deployed commercially numerous times. Moreover, a spokesperson from a leading car manufacturer pointed out that DSRC is more bandwidth-efficient than LTE-V2X. Specifically, a single 10 MHz DSRC control channel is sufficient to handle key safety features such as Basic Safety Messages (BSM), Signal Phase and Timing (SPaT), and MAP intersection messages. In contrast, the new FCC regulations allocated a 20 MHz channel to LTE-V2X to manage essentially the same set of applications—twice the bandwidth required by DSRC. Given the limited availability of the 5.9 GHz spectrum, which is even at risk in some areas, this less efficient use of bandwidth is unfavourable.
In 2019, Qualcomm performed tests to assess communication range and the safest maximum speeds across varied road conditions [69]. The findings, as shown in Table 14, indicate that C-V2X generally outperforms DSRC in communication range. Furthermore, vehicles with C-V2X can maintain higher safe speeds on both normal and icy roads.
In dense highway scenarios, one research [70, 71] presents a comparison between the performance of C-V2X and DSRC. It shows that while both technologies exhibit good performance at shorter distances, C-V2X performs better than DSRC at ranges greater than 100m with a similar bandwidth of 10 MHz. Moreover, with a larger bandwidth of 20 MHz, C-V2X further improves performance.
DSRC relies heavily on the Global Navigation Satellite System (GNSS) for timing synchronisation to send and receive safety messages. In contrast, C-V2X has flexible mechanisms for cost-effective synchronisation from various sources, even when GNSS is unavailable. 3GPP offers a detailed protocol that enables vehicles to synchronise using multiple alternative sources, such as other vehicles, base stations, and RSU timing [72].
3.4 Quality of Service
C-V2X has been designed with architectural improvements in 5G to enhance the QoS for V2X services as outlined in 3GPP TS 23.287 (Release 16) [73]. In the network mode (Uu), predictive QoS sustainability analytics are used, enabling the network to foresee if a desired QoS can be met in a designated area before launching the service. This empowers the C-V2X application layer to refine its operational strategy. Furthermore, the 3GPP is investigating the potential of Artificial Intelligence (AI) and Machine Learning (ML) to enhance and optimise C-V2X applications in the future.
The 2023 5GAA report [74] detailed that C-V2X can greatly enhance its performance through the application of predictive QoS. For example, in a scenario involving cooperative lane merging, the QoS predictive notification threshold is set at 20 milliseconds. If latency exceeds this 20 milliseconds threshold, the QoS Change Threshold activates to reallocate resources more optimally. This mechanism aids in significantly improving the latency performance, ensuring smoother and safer cooperative lane merges [74].
For the direct communication mode (PC5), V2X messages from applications are assigned QoS levels, with priority determined by the service type. Given varying use case intensities, such as cooperative lane changes, automated driving, collision avoidance, or sensor sharing, messages are designated with distinct priorities and delay allowances to optimise V2X services within C-V2X. In C-V2X, the integrated QoS management for both Uu and PC5 modes, particularly for V2X messages, represents a pioneering approach not found in the DSRC-cellular hybrid system. In the latter, DSRC messages transmitted over cellular
Table 14 Performance comparison of DSRC and C-V2X conducted by Qualcomm [69]
networks receive no special prioritisation or optimisation, being treated the same as other data messages.
3.5 Conclusion
In conclusion, based on current test results for 802.11p and LTE-V2X devices, it's clear that both technologies fulfil the requirements for C-ITS essential safety applications. DSRC standards have been extensively tested over the past 20 years. C-V2X standards have undergone some large-scale trials since 2017, and optimisation efforts are ongoing. Early comparison tests under specific conditions, such as dense highway scenarios and complex environments, indicate that C-V2X can outperform DSRC in reliability, communication range, and maximum allowable vehicle speed. DSRC meets performance metrics in standalone or independent systems where device diversity and interference are minimal. The main difference between C-V2X and DSRC is that C-V2X has focused on performance optimisation of the integration with the network, whereas DSRC has focused on optimising short-range communications only. It can be anticipated that C-V2X offers more robust performance in less-than-ideal conditions. With features like Turbo Coding, HARQ and predictive QoS mechanisms, C-V2X is designed to manage network congestion and latency efficiently.
We recommend that stakeholders continuously monitor development roadmaps and new performance reports for next-generation DSRC 802.11bd and 5G-V2X (3GPP Release 18), focusing on addressing specific use cases in the future.
4 C-ITS Technology Roadmaps
This chapter provides an overview of the roadmap for C-ITS technology. It focuses on the key stakeholders, consortiums, and technologies that are driving the global advancement of C-ITS. This chapter delves into the roadmaps for DSRC ITS-G5 and C-V2X technologies, highlighting their use cases, deployment phases, and anticipated timelines. It also explores the evolving road map of C-ITS, including the integration of advanced technologies, safety measures, and considerations for vulnerable road users.
4.1 Stakeholders and Consortiums
This section highlights key global and regional stakeholders and consortiums advancing C-ITS. These stakeholders facilitate C-ITS through research, standard setting, implementation, harmonisation, and policy advocacy, contributing to global traffic management, road safety, and transportation efficiency [75], as shown in Table 15.
Table 15 Major C-ITS stakeholders
Stakeholders
5G Automotive Association (5GAA)
C-Roads Platform
CEDR
CAR 2 CAR Communication Consortium
European Automotive and Telecoms Alliance (EATA)
C-ITS Deployment Group
EC sub-group on Cooperative Intelligent Transport systems
CCAM partnership
Description
The global consortium developing end-to-end connectivity solutions.
Joint initiative for piloting and deploying C-ITS services focusing on cross-border harmonisation.
Cooperation and promotion of improvements to the road system.
An industry-driven organisation promoting C-ITS.
Cooperation between Europe's automotive and telecom sectors.
Promotes C-ITS to increase road safety and decrease congestion.
Supports EU-wide cybersecurity infrastructures for C-ITS.
Prioritises and aligns European research and innovation for CCAM technologies.
Main Regions
Global
Europe
Europe
Europe
Europe
Europe
Europe
Europe
Stakeholders Description
European Data for Road Safety Initiative
EU ITS Platform
Maximises safety-related traffic information powered by vehicle and infrastructure data.
Fosters, accelerates and optimises current and future ITS deployments in Europe.
National Access Point Coordination Organisation for Europe Works towards harmonisation and interoperability of European ITS.
International Association of Public Transport
Platforms for Harmonising Data Exchange
USDOT and ITS JPO
Contributes to traffic management and ITS, including C-ITS in urban and public transport.
Facilitates C-ITS operations, focusing on harmonizing data exchange.
Guides research, development, and implementation of C-ITS in the United States.
ITS America Advocates for research and deployment of intelligent transportation systems.
OmniAir Consortium
Connected Vehicle Trade Association (CVTA)
Auto Alliance (Alliance for Automotive Innovation)
MLIT, Japan
Promotes interoperability and certification in ITS.
Advances the interests of entities in the vehicle communication environment.
Represents USA automakers working towards ITS and autonomous driving technologies.
Main Regions
Europe
Europe
Europe
Europe
Europe
The USA
The USA
The USA
The USA
The USA
Leads C-ITS implementation in Japan. Japan
ITS Japan Promotes research and development of Intelligent Transportation Systems.
National ITS Center, Korea
Promotes research and deployment of ITS.
Japan
South Korea
Stakeholders Description Main Regions
ITS Australia
iMOVE Cooperative Research Centre
Advocates for advanced technologies in transport across all modes.
Oversees research and implementation projects related to ITS.
Australia
Australia
ITS Canada Fosters the application of ITS. Canada
ITS Asia-Pacific
Ministry of Industry and Information Technology
4.2 DSRC ITS-G5 Roadmaps
The federation includes ITS interests from the Asia-Pacific region.
Set standards, promote research and development, and facilitate the deployment of these technologies.
Asia-Pacific
China
Emerging technologies in C-ITS deployment bring forth new use cases. For instance, future autonomous cars will need to convey real-time navigational, locational, and sensor data to the cloud through the network, generating significant data per second. The traditional use cases outlined in the ETSI standard [58] may not suffice for these upcoming trends.
Addressing this, the CAR 2 CAR Communication Consortium (C2C-CC) has introduced Day 1/2/3 use cases [39], depicted in Table 16. These represent the C-ITS innovation stages, beginning with Day 1's currently implemented basic information and warning services on European roads. The implementation of advanced services linked to the Day 2 and Day 3+ phases is contingent upon the broad adoption of automated driving technologies and the evolving demand of road operators to efficiently oversee a growing number of connected vehicles from a holistic, global viewpoint.
In July 2021, C2C-CC released a Day 2 and beyond roadmap [76], as shown in Figure 6. The C2C-CC has emerged as a significant influencer in the initial rollout and subsequent innovative stages of C-ITS, utilising ETSI ITS-G5 DSRC technology across Europe. They have drafted a V2X implementation roadmap that is divided into successive phases, each named after the time of deployment (Day 1, Day 2, and so forth up to Day N). This strategic plan has been formed in response to the current noticeable augmentation of automation features in production vehicles, and the anticipated growth in penetration of V2X-integrated systems. The initial phase, Day 1 (Awareness Driving), signifies the advent of C-ITS and is already underway. In this stage, vehicles and infrastructure systems broadcast information regarding their current status (for instance, vehicle dynamics for cars and powered twowheelers, and details about tolling stations from RSUs). They also provide data about unexpected events such as hazardous weather conditions or potentially dangerous situations. Moving on to the Day 2 phase (Sensing Driving), there are plans to further expand the V2X system to allow both vehicles and RSUs to not only transmit but also to
share information about objects detected via onboard sensors such as cameras, LiDAR or radars (at ISAD8 Level B).
Table 16 C2C-CC Day 1, Day 2 and Day 3 use cases [39]
Service Description
Day 1Awareness Driving
Day 2Sensing Driving
Cooperative V2X communication enables the exchange of status data, such as position, speed, driving direction, and incidents like vehicle defects. It provides information and warning services to support road users in driving proactively and becoming aware of potential risks that may not be visible to them yet.
Cooperative V2X-capable road users can also share sensor-based observations and advanced environmental information. This helps warn other traffic participants about dangers they cannot perceive and ensures the protection of noncommunicating road users in various traffic situations.
Use Case Examples
Intersection Collision Warning
Emergency Vehicle Warning
Dangerous Situation Warning
Stationary Vehicle Warning
Traffic Jam warning
Pre-/Post-crash Warning
Day 3Cooperative Driving
Cooperative V2X road users can provide intention data in addition to status and sensor data. This allows them to intelligently interact and coordinate their behaviour, even in complex traffic scenarios. Predicting the expected behaviour of all road users is crucial for achieving the longterm goal of highly automated and autonomous driving.
Overtaking Warning
Extended Intersection Collision Warning
Vulnerable Road User Warning
Cooperative Adaptive Cruise Control
Long-term Road Works Warning
Special Vehicle Prioritisation
Platooning (Static or dynamic) Area reservation
Cooperative Merging
Cooperative Lane Change
Cooperative Overtaking
Following the completion of the Day 2 deployment phase, the advent of the Day 3 phase, known as 'Cooperative Driving', is anticipated. This phase will capitalise on the roll-out of vehicles equipped with progressively advanced automated driving features, specifically those within SAE levels 3-4. The distinguishing aspect of these CAVs resides in their ability
8 Infrastructure Support for Automated Driving (ISAD Levels):
Level E: Conventional infrastructure without automation support.
Level D: Digital map with static regulatory information.
Level C: Full digitalization of infrastructure elements, including VMS and traffic lights.
Level B: Infrastructures perceive complete traffic situations using specialised sensors.
Level A: Infrastructure provides microscopic traffic management to automated and connected vehicles.
to plan and execute driving responsibilities. As a result, they have the capacity to convey their strategic intentions (such as preferred routes, maneuvers, or trajectories) as well as their presently implemented actions (including short- and long-term trajectories) to other entities within the traffic framework.
Day1 Awareness Driving
• Warning applications (e.g. collision avoidance)
• Information from infra (e.g. road/intersection signage)
• CAMS, DENMs (status, dynamics & notificatons)
• SPAT/MAPs,IVls (static & dinamic signage)
• Security support (use of EU CCMS)
Day2 Sensing Driving
• Advanced warnings
• VRU protection (e.g. pedestrian, PTW +Infra support)
• Semi-automated driving (e.g. Coop. emergency brake assistance, Coop. ACC
• Cooperation with traffic light controllers
• Extended CAMs, DENMs (e.g. for PTW, ASIL qualifiers)
• CPMs (detected objects)
• RTCMEM (position corrections)
• SSREMs, SSEMs (priority, preemption)
• Functional safety support (e.g. Certification of ASIL qualifiers for tx data)
• Misbehavior detection
• Improved positioning support (e.g. GP corrections from infra)
Day3 Cooperative Driving
• Cooperative automated driving (e.g. Advanced CACC-strings, cooperative lane merging)
• Cooperation with Infra for automated driving (e.g. intersection crossing, assisted transition of control, valet parking)
• Extended CAMS (e.g. supported AD level, planned maneuvers/routes,...)
• Extended IVls (e.g. signage for automated driving ,. ..)
• MCMs + extensions (planned/desired trajectories , infra-support) VAMs (dedicated VRUs awareness)
• Automated coordination (e.g. Objective Matching, AD coordination rules)
Support from risks & info dissemination to coordinated automation
Trustworthiness (for functional safety)
Accuracy (position and time)
Timeliness (update frequency)
Over two decades of rigorous testing and successful deployment have shown that Day 1 applications are robustly supported by DSRC 802.11p, and it is expected that the forthcoming 802.11bd will extend this success [5]. This technology has been critical in enhancing safety by supporting applications such as Forward Collision Avoidance (FCA), Cooperative Adaptive Cruise Control (CACC), Signal Phase and Timing (SPAT) Enabled Red Light Running (ERLR), and Railway Crossing (RC).
Since its inception in the 2016 C-Roads project, the DSRC ITS-G5-based system for road safety has seen significant deployment in Europe on both vehicular and infrastructure fronts. According to the C-Roads representative, by the end of 2022, about 20,000 km of European roads were equipped with DSRC-based Roadside units (RSUs), and nearly 1 million vehicles were fitted with DSRC OBUs compliant with the ETSI ITS-G5 standards. Since 2019, around 50 million EUR have been invested annually into DSRC trials as part of the European C-Roads Projects, as indicated in the 2021 annual report [23].
Annexes 6 and 7 contain a compilation of use cases that have been conducted in the European C-Roads DSRC trials. Given the current extent of DSRC technology deployment in Europe, transitioning to a new technology such as C-V2X could pose significant challenges. The potential waste and redundancy of private and public investment in DSRC
Figure 6 C2C-CC C-ITS roadmap [76]
technology may cause reluctance in its abandonment. It is therefore unsurprising that DSRC is projected to maintain a substantial share in Europe's technology market in the near term.
In March 2023, the C2C-CC released a new version of the use cases blueprint [77], aiming to shed light on new technological advancements and specific requirements, particularly emphasising Day 2+ use cases such as automated driving. For partial and high automation, the use cases range from Hazardous Location Notification for Vehicle Assistance Cooperative Adaptive Cruise Control (CACC), to Advanced Pre-crash Sensing, offering potential solutions to tackle the emerging complexities in automated vehicle operations. Moreover, advanced warning and information systems also garnered considerable attention. It emphasises innovative applications such as Advanced Slow Vehicle Warning (ASVW) and Advanced Intersection Collision Warning (AICW), along with dedicated use cases addressing the challenges related to motorcycle overtaking and filtering. Vulnerable Road Users (VRU), such as pedestrians and cyclists, were specifically mentioned, suggesting a growing focus on safety for all road users. Use cases in this category include VRU Presence Awareness, VRU Collision Warning, and VRU Brake or Steering Intervention, clearly demonstrating the efforts to mitigate the risks associated with VRUs in real-world scenarios.
Another notable aspect in this version is the introduction of a new category, agricultural use cases, signifying the extension of V2X communication technologies into non-traditional automotive sectors. With the advent of precision agriculture, use cases like Task Data Exchange, Geo-Referenced Data Exchange, Agricultural Platooning, In-Field Safety, and Agricultural Work Awareness are anticipated to contribute significantly to the efficiency and safety of agricultural operations.
This future-oriented approach embodies C2C-CC's commitment to fostering a safe and efficient road traffic environment, extending its scope from urban streets to agricultural fields. While this new use case document doesn't provide a specific timeline, it serves as an excellent guide for stakeholders supporting the DSRC ITS-G5 standard. It provides a robust framework to assist in the development of practical use cases, ultimately facilitating the successful rollout of products.
4.3 C-V2X Roadmaps
Inspired by the advent of high-speed 5G communication technology, C-V2X is considered capable of fulfilling advanced use case requirements for future C-ITS [78-80], as categorised in Table 17. Currently, 3GPP has published 27 use cases for LTE-V2X (3GPP TR 22.885) [81] and 30 for 5G-V2X (3GPP TR 22.886) [82], with an emphasis on future applications. The 27 use cases outlined for LTE-V2X in TR 22.885 mainly focus on assisted driving features, including active safety protocols like collision alerts and emergency braking, as well as functions for improving traffic flow, such as vehicle speed guidance and information services. Due to the current state of technological development and specific user needs, manufacturers of C-ITS equipment generally opt to integrate only the most immediately practical and relevant use cases into their products, rather than incorporating all the use cases specified in the standards. Annex 7 shows the list of use cases.
Table 17 C-V2X use cases [78-80]
Application Class Application
Safety
This group includes use cases that provide enhanced safety for vehicles and drivers. These use cases would in many apply equally to autonomous vehicles or to assist drivers.
Vehicle Operations Management
Convenience
This group includes use cases that provide operational and management value to the vehicle manufacturer.
This group includes use cases that provide value and convenience to the driver.
Use case
Cross Traffic Left Turn Assist
Intersection Movement Assist
Emergency Brake Warning
Traffic Jam Warning and Route Information
Real-Time Situational Awareness and High Definition Maps Hazardous Location Warning
Cooperative Lane Change of Automated Vehicles
Cooperative Traffic Gap
Interactive VRU Crossing
Software Update of Reconfigurable Radio System
Vehicle health monitoring
Remote support
Automated Valet Parking - Joint Authentication and Proof of Localisation
Automated Valet Parking (Wake Up)
Awareness Confirmation
Cooperative Curbside Management
Cooperative Lateral Parking
In-Vehicle Entertainment (IVE) - HighDefinition Content Delivery
Obstructed View Assist
Vehicle Decision Assist
Autonomous Driving
This use case group addresses use cases that are relevant for Autonomous/selfdriving vehicles (Society of Automotive Engineers (SAE) level 4 and 5).
Automated Intersection Crossing
Autonomous Vehicle Disengagement Report
Cooperative Lane Merge
Cooperative Maneuvers of Autonomous Vehicles for Emergency Situations
Coordinated, Cooperative Driving Maneuvers
High-Definition Map Collecting and Sharing
Infrastructure-Assisted Environment Perception
Infrastructure-Based Tele-Operated Driving
Application Class Application Use case
Remote Automated Driving Cancellation (RADC)
Tele-Operated Driving
Platooning
Traffic Efficiency and Environmental Friendliness
This use case group addresses use cases that are relevant for platooning, examples in this group are platoon management, which is of interest to transport companies and potentially by road operators/road traffic authorities since road infrastructure could be used more efficiently.
This group includes use cases that provide enhanced value to infrastructure or city providers, where the vehicles will be operating.
Society and Community This group includes use cases that are of value and interest to the society and public, with a business modelling point of view attractive to the public/private sector.
Tele-Operated Driving Support
Tele-Operated Driving for Automated Parking
Vehicle Collects Hazard and Road Event for AV
Collect and establish a platoon
Determine the position in the platoon
Dissolve a platoon
Manage distance within the platoon
Leave a platoon
Vehicles platooning in steady state
Bus Lane Sharing Request
Bus Lane Sharing Revocation
Continuous Traffic Flow via Green Lights Coordination
Group Start
Traffic Jam Information
Smart Routing Adisory
Accident /Crash Report
Patient Transport Monitoring
Vulnerable Road User (VRU) protection
Public services, such as ambulances, police, fire brigades, and emergency vehicles approaching
Traffic light priority
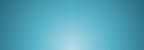
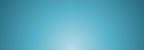
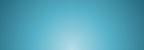
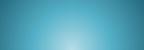
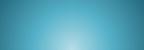
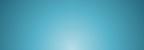
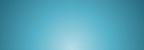
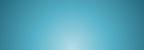
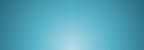
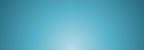
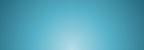
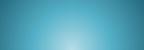
Figure 7 Expected timelines for mass deployment of C-V2X use cases [9]
In December 2022, 5GAA published a significant white paper [9] on the prospective C-V2X roadmap. This document emphasises advanced driving use cases, including automated driving, teleoperation, automated valet parking, and sensor sharing, as summarised in Table 18. These contribute to global safety, mobility, environmental sustainability, and transportation equity objectives. Figure 7 outlines the anticipated timelines for the widespread deployment of C-V2X use cases, which are validated by numerous 5GAA experts and stakeholders, based on their extensive industry experience. We believe that the outlined use cases and timelines are achievable within the current technological framework. Security, spectrum, and privacy regulations may continue to evolve, depending on the policies of individual countries.
The 5GAA's 2022 annual report [25] presents an evolved roadmap, demonstrating a focus on network-centric solutions aimed at safeguarding VRUs. It recognises that more complex use cases will require extended development periods. Concurrently, the automotive and telecom sectors are playing pivotal roles in standardisation efforts. Standardisation organisations like ETSI, SAE International, National Technical Committee of Automotive Standardisation (NTCAS), China Society of Automotive Engineers (C-SAE), and Association of Radio Industries and Businesses (ARIB) are working on upper-layer safety protocols, whereas the radio layer standardisation is primarily the remit of 3GPP, which is presently developing Release 18.
The 5GAA's strategic focus in the forthcoming years will be on advancing 5G technologies to facilitate new use cases, potentially transforming our road systems into digital ecosystems. This evolution will bring benefits for a wide array of stakeholders including vehicles, road users, road operators, and smart cities. The report also underscores the significant progression of several use cases since 2020, spurred primarily by region-specific safety regulations and incentives. Among these, Intersection Safety has emerged as a prioritised use case. It has undergone trials in various global regions and has proven to be a critically important application for safety [25].
Use Case
Local Hazard and Traffic Information
Hazard Information Collection Sharing
Basic Safety
Intersection Safety
VRU Collective Awareness
Group Start
Cooperative Adaptive Cruise Control
Cooperative Manoeuvres
VRU Complex Interactions
HD Maps Data Collection and Sharing
Data Sharing of Dynamic Objects
Non-analysed Sensor Signal Sharing
Dynamic Intersection Management
Dynamic Cooperative Traffic Flow
Automated Valet Parking (AVP)
Tele-operated Driving
Description
Host vehicle (HV) receives information on route events either from other vehicles or a backend/cloud service.
Vehicles gather hazard and road event data via sensors for use by AVs and V2X application servers.
Day-1 use cases focusing on hazard information and warnings.
Focuses on intersection safety, including Cross-Traffic Left-Turn Assist and Intersection Movement Assist.
Alerts host vehicles of approaching Vulnerable Road Users (VRUs) and warns of collision risks.
Self-driving or semi-automated vehicles form a group to start moving at a traffic light. No turns are permitted.
Extension of adaptive cruise control. It controls speed and distance with the preceding vehicle.
An AV coordinates with neighbouring AVs to undertake joint maneuvers in difficult situations.
VRUs share their intent to cross a street, and vehicles acknowledge, providing the VRU reassurance to cross safely.
Collects and shares data on static road objects and temporary conditions to create dynamic HD maps.
Collects and shares data on dynamic objects in or near the road based on sensor data, including metadata.
Shares raw sensor data to support the development of automated driving capabilities.
AV goes through or stops at an intersection based on traffic signal timing. Other traffic participants are coordinated dynamically.
A vehicle communicates its intent to perform a maneuver (e.g., lane change, U-turn). Informed participants either support or decline the action.
The vehicle parks itself through automated driving with the aid of a parking data centre.
The vehicle starts sharing video and/or sensor data when it needs remote support. The remote operator then provides trajectory or maneuver instructions.
4.4 Backward Compatibility
The DSRC 802.11bd standard is promoted as being fully backward compatible with its predecessor, 802.11p. From the inception of the IEEE 802.11bd definition, backward compatibility has been a pivotal requirement [5]. However, this view is not universally held, particularly among some representatives from the 5GAA. These individuals propose a different perspective, arguing that continuous technological development diminishes the significance of backward compatibility. For example, if the newly introduced 802.11bd is backward compatible with 802.11p, an 802.11bd-based device could potentially be downgraded to the lower version, namely 802.11p. This downgrade would cause the device to lose out on the inherent advantages of the newer technology. Thus, as the technological landscape evolves, it is expected that the older version will eventually be phased out of the market. This perspective will be incorporated into subsequent sections of the discussion. 5G-V2X and LTE-V2X are different in their access layer technologies and radio spectrum access protocols, which could lead to compatibility challenges during the transition from LTE-V2X to 5G-V2X. Presently, LTE-V2X operates using a 20 MHz spectrum at 5.9 GHz, primarily for V2V/V2I communications in support of Day 1 basic safety ITS services. In contrast, the forthcoming 5G-V2X is anticipated to leverage a broader spectrum range, potentially an additional 40 MHz or more at the same frequency, to accommodate more advanced driving applications, encompassing V2V, V2I, and V2P communications [14]. However, the exact specifications of this additional spectrum usage for 5G-V2X are not yet confirmed and are expected to be part of a longer-term development. One proposed solution to this challenge is the concept of adjacent channel coexistence [83, 84]. This approach involves concurrently running 5G-V2X and LTE-V2X systems on separate channels, thereby facilitating coexistence. In effect, this strategy addresses compatibility issues and provides users with the flexibility to choose between systems.
Despite the access layer incompatibility, Qualcomm posits that 5G-V2X maintains backward compatibility at the upper layers [7]. This suggests that the purpose of 5G-V2X is not to supersede LTE-V2X, but to augment C-V2X with advanced capabilities that LTE-V2X cannot support [84]. From the perspective of chip manufacturers, 5G-V2X compatibility can be resolved through certain chip design techniques. One such method involves integrating both 5G-V2X and LTE-V2X functionalities into a single chip, an approach that's seen as viable in the future. A representative from Qualcomm has also mentioned the potential for a single chip to support both LTE-V2X and 5G-V2X. However, despite this potential solution, we speculate that there may not be a strong motivation to pursue this strategy. Many stakeholders are keenly awaiting the maturity of 5G-V2X technology and show a preference for deploying the advanced 5G-V2X directly. They would rather bypass an intermediary version that might still grapple with backward compatibility issues in the future.
4.5 Conclusion
In summary, this chapter presents roadmaps indicating that both C-V2X and DSRC technologies are poised to manage a range of current and upcoming use cases in C-ITS, highlighting anticipated advancements in the future.
DSRC ensures full compatibility from 802.11bd to 802.11p. 5G-V2X may face backward compatibility challenges with 4G LTE-V2X, although this issue is still under discussion.
Looking forward, we found more published evidence that 5GAA focuses on future use cases such as interoperability, automated driving, and the safety of vulnerable road users.
Ultimately, the success of any technology will be judged by its ability to meet the transportation industry's needs in terms of safety, efficiency, and mobility.
5 Global Support
The global deployment of C-ITS relies on the collaboration of vital players such as car manufacturers, government bodies, and tech providers. Vehicle manufacturers are central to integrating C-ITS technology into their products, while governments back this venture with funding, policymaking, and supporting the deployment of innovative technologies and infrastructure. Governments further contribute by supplying crucial traffic, road network, and regulatory data, contributing to road safety and efficiency. Their commitment to ensuring this data is accessible plays a pivotal role in C-ITS functionality and the delivery of significant services for road users. Although the process may face hurdles, successful implementation will transform the transportation system, creating benefits for everyone.
5.1 DSRC ITS-G5 in Europe
Currently, the development of C-ITS in Europe involves several key considerations. These include the implementation of advanced driver-assistance systems (ADAS) and in-vehicle infotainment (IVI) systems, evaluating C-ITS through Field Operational Tests (FOTs), ensuring security and privacy in V2X communication systems, developing open platforms for mobility services across Europe, and improving energy efficiency in road transport with C-ITS.
In Europe, the dominant standard for actual C-ITS deployment is DSRC ITS-G5, with significant support and promotion from key consortiums such as C-Roads and C2C-CC. However, the technical challenges related to C-V2X interoperability are still being addressed in the early stages. This is primarily due to the historical context of the C-Roads project, which began in 2015 when DSRC equipment was the only mature C-ITS product available, while C-V2X equipment was not yet manufactured. The extensive deployment of DSRC RSUs and OBUs in Europe, coupled with the advanced state of the ITS-G5 standard, presents a formidable challenge for transitioning to C-V2X as it would necessitate starting from scratch and duplicating past investments.
The C-Roads project emphasises safety-sensitive applications, known as ‘Day 1’, across 18 EU nations. Using the technical protocols developed from 2017 onwards, the C-Roads pilot locations identified particular use cases and actively partnered to incorporate C-ITS systems. Equipment supporting ITS-G5 standards, such as DSRC RSUs and OBUs, were installed at various roadside points and on trial vehicles. Meanwhile, Kapsch provided crucial support services for the C-ITS central station, and Dynniq supplied the RSUs, OBUs, and the software for the C-ITS service application [23].
Despite the obstacles set by the COVID-19 pandemic, a C-ITS testing and validation campaign was launched, successfully validating the seamless operation of C-ITS services across 18 EU member states. Physical tests were also carried out, notably on the AustrianItalian and Spanish-Portuguese borders, demonstrating the flawless interoperability of crossborder operations. In certain border regions, 4G cellular networks serve as a means to achieve extensive communication coverage over long distances, complementing short-range communication methods.
Beyond highway installations, many C-Roads member states have introduced C-ITS services in city environments. This aims to prioritise public transport and emergency vehicles, enhancing traffic flow and safety. To date, 37 cities have joined the C-ITS rollout, employing various use cases spanning different C-ITS services, marking a significant step forward in traffic management and safety [23].
We categorise C-Roads field trial scales, use cases and partners in all countries in Annex 6.
According to representatives from C-Roads and C2C-CC, the DSRC-based C-ITS has demonstrated exceptional performance and successfully met the objectives for Day 1 use cases. The key advantages highlighted are as follows:
DSRC (IEEE 802.11p) is a highly mature and reliable V2X standard that operates independently of telecom infrastructure. It is specifically designed for V2X safety applications and boasts stringent performance specifications. The ITS-G5 standard, built on DSRC, has been extensively developed and proven efficient in long-term trials. C-Roads field trials have satisfactorily covered Day 1 use cases, including collision warnings, hazard warnings, emergency vehicle warnings, and effective implementation of VRU use cases.
Cellular communication can be used as a complementary solution for short-range communication, particularly in bridging communication gaps in border regions. In C-Roads trials, cellular communication has been successfully integrated with DSRC short-range communication. Additionally, fibre optic cables can be employed to connect RSUs along highways, establishing a highly reliable decentralised network without relying on cellular communication.
The newly released DSRC 802.11bd standard, which is fully backward compatible with 802.11p, offers enhanced performance specifically suited for automated driving applications, making it a promising next-generation DSRC standard.
Representatives from the C2C-CC argue that conducting minor trials of C-V2X will not significantly impact the existing systems, as they are expected to perform adequately. They believe that C-V2X still requires large-scale validation to demonstrate its reliability.
Overall, the feedback from C-Roads and C2C-CC emphasises the proven track record and advantages of DSRC-based C-ITS, while acknowledging the need for further evaluation and validation of C-V2X.
Using the ETSI ITS-G5 standards, Australia has conducted trials similar to the C-Roads projects. A prominent example is the Ipswich Connected Vehicle Pilot (ICVP) initiated in 2020. In this trial, up to 500 vehicles in Ipswich, South East Queensland, were equipped with Cohda’s MK5H OBUs [85]. Led by the Department of Transport and Main Roads (Queensland), the trial combined short-range DSRC and long-range cellular communication for six use cases. V2I use cases via short-range DSRC ITS-G5 included Advanced RedLight Warning (ARLW) and Turning Warning for Vulnerable Road Users (TWVR). V2N use cases via 4G cellular communication include In-Vehicle Speed (IVS), Back of Queue (BoQ), Road Work Warning (RWW), and Road Hazard Warning (RHW). These use cases do not require the ultra-low latency of critical V2V interactions, making cellular communication an ideal supplement to short-range communication. This combination ensures maximum
coverage at a minimal cost. Similarly, in the C-Roads project, cellular communication has been used to bridge communication gaps in border areas.
5.2 Fast-Paced C-V2X
Founded in September 2016, 5GAA is a global cross-industry organisation with a primary focus on advancing future mobility and transportation services through the development, verification, and promotion of C-V2X technology. As of the end of 2022, the organisation has grown to encompass 119 members, including 13 of the top 15 global automakers and telecommunications companies, as well as the top five global wireless infrastructure companies [25]. Over the past few years, 5GAA has taken the lead in conducting successful C-V2X trials, primarily based on LTE-V2X technology, in key regions such as North America, Europe, and China. In 2020, Telstra Australia partnered with Ericsson and Lexus and conducted a C-V2X trial in Australia, with life-saving use cases (Day 1) [86], which is the first C-V2X trial partnered with telcos in Australia. Detailed information regarding the scale of major field trials use cases, and partners can be found in Annex 7, where we categorise them for reference.
China stands as a notable example of swift C-V2X development and implementation. C-V2X has emerged as the sole C-ITS standard in China, and the country has adopted a C-ITS architecture closely aligned with 3GPP architectures. In 2018, China allocated the 5.9 GHz band specifically for LTE-V2X direct communication. Furthermore, China has established well-defined guidelines for C-ITS deployment within a national architecture framework. In recent years, China has made significant strides in developing urban interconnection ITS services across demo cities, aiming to enhance traffic efficiency, safety, and overall service experience. At the 2019 China-SAE Congress and Exhibition, Autotalks showcased its V2X chipset's compliance with Chinese C-V2X standards in a large-scale interoperability demonstration of multi-brand C-V2X communication, including five automakers: RenaultNissan, Brilliance Auto, FAW, Great Wall, and another major European OEM [87]. In April 2020, 13 Chinese automakers, including SAIC, GAC, Dongfeng, Changan, FAW, BAIC, JAC, Great Wall, Southeast, Zotye, Jiangling, BYD, and Yutong, announced plans to integrate C-V2X into mass-produced vehicles [88].
Until 2022, the Ministry of Industry and Information Technology of China has approved the support of 4 regions to create national-level C-V2X trial zones: Jiangsu Province (Wuxi City), Tianjin City (Xiqing), Hunan Province (Changsha City), and Chongqing City. For example, Wuxi City took the lead in launching the large-scale construction of C-ITS infrastructure and has deployed more than 600 intersection RSUs in highway, urban and rural areas covering more than 300 km2. Hunan (Changsha) built the leading Big Data Center, a 100-km smart highway, a 100 km2 smart city network, a 7.8-km smart bus demo line, a 5 km2 test field, and more than 2,000 LTE-V2X terminals, etc. Four highways, Jinghu, Shiyu, Yangchong and Jingxiong have been upgraded with C-V2X network coverage and smart RSUs [89]. Starting from 2023, there are plans within the national framework to carry out extensive installations of C-V2X RSUs at ETC toll stations, with the purpose of offering C-V2X services on a large scale.
China and Germany commenced cooperation in the field of Automated and Connected Driving in July 2018. In June 2022, the largest C-V2X trial site based on the China-Germany Cooperation Project on Intelligent Connected Vehicles, was settled in Chengdu City, Sichuan province. The total area was 6.29 km2, including 9 functional areas, 18.8 km of test
roads, and 266 test scenarios [90]. In September 2022, the China Academy of Information and Communications Technology (CAICT) published a white paper on the China-Germany Cooperation on Intelligent Connected Vehicles [91]. The report introduced the C-V2X trials conducted by GAC Group, BMW, and Audi. From 17 common use cases, the project team selected 5 use cases that are most prioritised for mass deployment, including Intersection Collision Warning, VRUs Collision Warning, Speed Limit Warning, Red Light Warning, and Green Wave Speed Guidance.
On 26th December 2022, Ford China made an official announcement regarding the deployment of its C-V2X system in Wuhan and Nanjing. This deployment marks a significant milestone as Ford's proprietary C-V2X system has now been implemented in a total of six Chinese cities, namely Wuxi, Changsha, Guangzhou, Xi'an, Wuhan, and Nanjing. The system is incorporated as a standard feature in six Ford vehicle models available in China, including the new Mondeo, the new F-150 Raptor, the EVOS, the Mustang Mach-E, the Edge PLUS, and the new Explorer. Ford's C-V2X system offers users a range of six key functions, including push notifications for traffic light signals, green light optimal speed advice, green light start reminders, red light warnings, road information broadcasts, and electronic road signs [92], which are Day 1 use cases. The successful deployment of the C-V2X system by commercial entities in China demonstrates its expected performance and commercial viability.
The 5GAA representatives anticipate that the market will deliver C-V2X Day 2 and 3 use cases outlined in the 2022 roadmap by 2029. With the widespread deployment of mobile communication technology, C-ITS stands to benefit from increased information availability and higher bandwidth. C-V2X solutions, which leverage cellular communication, offer more advantages compared to DSRC-based solutions. Global trials of C-V2X have demonstrated superior performance and greater market potential compared to DSRC. Following the FCC's new rules on the ITS band, the USA is strongly inclined towards adopting the C-V2X standard, and major automotive manufacturers are likely to accept it. The C-V2X community has made significant efforts to align with Chinese C-ITS standard-setting bodies, aiming to establish a standardised stack similar to the American approach. This coordination allows non-Chinese OEMs to supply their products to China while seamlessly transferring the same hardware and software to other markets, including the USA. This streamlined cross-market compatibility could serve as a catalyst, accelerating the future deployment and preferences of C-V2X in the USA.
5.3 Position of C-ITS Equipment Manufacturers
The representatives of three leading OEMs strongly recommend the use of DSRC and suggest that Australia aligns with the European ITS-G5 standards. Their recommendation is influenced by the fact that a majority of vehicles imported to Australia come from Europe. Additionally, the current Australian ETC standards and existing C-ITS standards follow European norms, ensuring consistency across the board. The representatives believe that DSRC generally performs better than or at least on par with C-V2X in most scenarios.
Focusing on decentralised systems, DSRC supports both asynchronisation and synchronisation mechanisms9 and is less sensitive to asynchronisation issues. Conversely, in certain situations like tunnels, C-V2X direct communication (PC5) may experience synchronisation losses due to stringent requirements and the loss of connectivity to a base station for timestamping, potentially impacting performance. However, it should be noted that Qualcomm has claimed that the 3GPP specified feature called SLSS (Sidelink Synchronisation Signals) [93] can be used for time and frequency synchronisation, which needs to be further addressed in upper-layer standards. With SLSS and multi-hop GNSS timing features, LTE, being a reservation-based system, can potentially overcome the synchronisation issue even in tunnels. Additionally, supplementary technology like in-tunnel base stations can improve C-V2X reliability by providing stable signals and timing in lowinterference areas.
The representative from a prominent V2X chip manufacturer maintains a neutral stance on the standards selection, as their V2X chips are highly adaptable and support both DSRC and C-V2X technologies. However, they express the opinion that having both technologies coexist is not an optimal choice. Instead, they recommend that Australia adopt a single standard and remain consistent with it, allowing OEMs and car manufacturers to align their strategies accordingly. It should be noted that both DSRC and C-V2X require infrastructure development, including the construction of RSUs, making the cost of electronic devices a minor portion of the overall expenses. In the broader context, one of the leading V2X chip manufacturer representatives emphasises that Australia should prioritise the construction of a nationwide C-ITS data and security platform. This prioritised investment in foundational infrastructure is crucial for the successful rollout of C-ITS in the country.
5.4 Position of Car Manufacturers
The future trajectory of C-ITS in Australia is heavily influenced by the stance of international car manufacturers who import their vehicles into the country. Australia, with a limited car manufacturing capability, only recorded 1.08 million new car sales in 2022 [53]. Given this, Australia tends to follow the C-ITS standards established by more prominent global players. International car manufacturers don't prioritise the Australian market when making decisions regarding the direction of their connected vehicle technology. In contrast, they often view the vast Chinese market as crucial for their future tech development, due to its massive potential.
When it comes to deciding between short-range communication technologies, such as C-V2X or DSRC, car manufacturers exhibit caution. It is improbable that the US government would mandate the installation of C-V2X in all cars. Consequently, the maturity of the technology and the market potential continue to be the primary factors influencing their selection of technology. Currently, most car manufacturers are prioritising long-range communication, while still determining the optimal timing for short-range communication decisions.
9 Synchronous communication is a mode of communication in which data is sent and received at the same time, or 'in sync'. In this mode, both the sender and receiver establish a communication by following a certain timing or sequence. Asynchronous communication enables devices to exchange information without strict synchronisation, which offers flexibility and adapts to changing transmission conditions.
Recent trends indicate a declining number of car manufacturers committing to the inclusion of DSRC technology in their new models. For instance, Volkswagen, with its Golf 8 launched in October 2019 and the more recent ID. electric series equipped with NXP DSRC 802.11p chips [94], is one of the few manufacturers still featuring DSRC in their models. However, Toyota, in response to the FCC's new regulation of the 5.9 GHz band, opted out of its plan to equip its vehicles with DSRC-supported connectivity in the USA.
Currently, the 5GAA counts most leading car manufacturers among its members, except Toyota. These 5GAA members are primarily committed to the 3GPP standards, focusing on both long- and short-range communication within their vehicle connectivity platforms. Compared to other regions where some uncertainty persists, car manufacturers exhibit heightened enthusiasm and confidence in promoting C-V2X-compatible vehicles in China. This is largely due to China's steadfast endorsement of C-V2X as the C-ITS standard, coupled with the huge profitability of the Chinese market. While China's C-V2X standard slightly deviates from the USA’s version, they are both essentially grounded in the 3GPP standards. Consequently, vehicles trialled in China could be introduced to the American market with minimal adjustments.
In the previous sections, we discussed successful cases of RSU deployments for LTE-V2X in six Chinese cities, and how international car manufacturers, like Ford, are fervently promoting C-V2X in China. Vehicles tested in China have demonstrated an efficient balance in short- and long-range communications for varied use cases, with the 20 MHz upper band allocation in the 5.9 GHz spectrum. In summary, China offers an ideal test site for C-V2X for automobile manufacturers. It serves as a vital preparatory step for future large-scale deployments of C-V2X in the USA once such programs commence. Industry insiders predict a key turning point in 2024, when they foresee all new vehicles being launched in the market to be equipped with V2X technology.
In various regions of the globe, it is evident that vehicles equipped with long-range V2X technology currently garner more preferences than those with short-range V2V communication capabilities. Car makers are using the attractiveness of Internet connectivity in vehicles as a significant selling point to enter the rapidly growing market of connected technologies. Conversely, vehicles with V2V capabilities are still being assessed for market acceptance.
Another critical point to consider is the reluctance of most car manufacturers to support dualmode (simultaneous transmission) DSRC and C-V2X messages, due to cost, technological complexity, and maintenance challenges. From our limited discussions with car manufacturer representatives, it appears that car manufacturers prefer that a vehicle supports a single, country-specific standard. They express hope for Australia to commit to one standard, enabling them to plan accordingly. Considering the incompatibility between DSRC and C-V2X, it's vital that Australia pays attention to the dominant C-ITS technology in the majority of vehicles. Moreover, it's crucial to stay abreast of the current trends in connected car technology when deciding on the adoption and investment in a specific standard. By aligning investments in C-ITS roadside infrastructure with a widely accepted CITS standard, this approach safeguards against the risk of the substantial infrastructure serving only a small portion of vehicles or even becoming obsolete. Therefore, we believe, Australia should refrain from hastily committing to a specific standard within the next one to two years. Instead, the country needs an evaluation of trends and prevalent technologies before making a well-informed decision.
At the current stage, vehicles truly equipped with 5G-V2X (3GPP Release 16+) do not yet exist, and all vehicles currently under testing are based on 4G LTE-V2X, mirroring the largescale deployment in China. China is leveraging the term 5G-V2X as a marketing strategy to highlight the presence of 5G-enabled mobile connectivity in vehicles. As predicted by 5GAA, it is anticipated that the full-scale adoption of 5G-V2X will not occur until at least 2029. On 28th February 2023, Qualcomm announced the launch of its Snapdragon Auto 5G ModemRF Gen 2 – R16 which supports 5G communication and 4G LTE-V2X (Release 14/15) operating a 20 MHz channel bandwidth and PC5 support, marking a significant advancement towards the goals set in the 5GAA roadmap. As of the time of this report, the definition of parameters and profiles for 5G-V2X intended for OEM manufacturing is still in progress and will take further time to solidify.
Car manufacturers, fully cognizant of the current status, are considering two key factors: the timeline for the 5G-V2X standard to mature for widespread market adoption and the clarification of C-V2X band regulations and market prospects in regions like Europe. With the FCC's approval of 20 MHz operation for C-V2X trials, stakeholders have initiated trials for swift deployment in the USA. We believe that the near future holds the promise of increased bandwidth allocation to 5G-V2X and the introduction of advanced vehicle models directly supporting 5G-V2X.
In this transition, many stakeholders have initiated the proceedings of deploying C-V2X in the USA in order to occupy the potential market in North America. The Departments of Transportation (DOTs) of the State of Florida and the State of Georgia, and the State of Maryland’s State Highway Administration, have requested a waiver of the FCC’s rules to operate RSUs with C-V2X-based technology in the upper 30 MHz (5.895-5.925 GHz). The car manufacturers, state DOTs and equipment manufacturers that have requested waivers, include Audi of America, Inc., Ford Motor Company, Jaguar Land Rover, the Utah Department of Transportation, the Virginia Department of Transportation, AAEON Technology Inc., Advantech Co., Ltd., Applied Information, Inc., Cohda Wireless Pty Ltd., Commsignia, Inc., Danlaw Inc., HARMAN International Industries, Inc., Kapsch TrafficCom USA Inc., and Panasonic Corporation of North America [95].
In a positive development, the FCC approved on 24th April 2023, to allow initial deployments of C-V2X technology in the 5.905-5.925 GHz frequency range using a 20 MHz channel [96]. This indicates that as DSRC is gradually phased out in America, the deployment of C-V2X is set to proceed more seamlessly. On 28th April 2023, ITS America published the National V2X Deployment Plan [28]. This plan underscores the critical need for the FCC's further action to outline key aspects like power limits, certification standards, and RSU registration for C-V2X. Additionally, it highlights the importance of the National Highway Traffic Safety Administration (NHTSA)’s endorsement of C-V2X within their safety ratings and promotion of V2X benefits to the public. Following the FCC's new order, on 15th June 2023, the City of Peachtree Corners in Georgia, USA, and Audi of America have embarked on a collaboration to enhance the development of C-V2X technology. Taking advantage of Peachtree Corner’s smart city ecosystem, the alliance strives to unify all facets of C-V2X, involving both autonomous vehicles and VRUs. The success of Audi's previous ventures in C-V2X, particularly in improving work zone safety and securing school areas, is expected to contribute significantly to this joint effort [37].
5.5 Conclusion
In summary, DSRC has made significant progress in Europe, supported by initiatives like the C2C-CC and C-Roads projects. In China, intensive testing, and commercial deployment of C-V2X technology have taken place. In the USA, trials of C-V2X have been approved following waivers to existing FCC ITS band regulations.
The scope of this research did not allow a final indication regarding the direction that car manufacturers will take with regard to short-range communications. It seems that many car manufacturers have implicitly chosen to align with the C-V2X camp. Car manufacturers focus more on long-range communications than short-range communications.
Some C-ITS equipment manufacturers suggest Australia should adopt the DSRC standard, citing alignment with existing European ITS-G5 norms already in use in Australia's C-ITS systems. Both equipment and chip manufacturers agree that adopting a single standard would be more efficient and they recommend against the simultaneous operation of both DSRC and C-V2X technologies.
6 Long-Range Communications
This project primarily focuses on short-range communication technologies. However, these short-range communications require integration with long-range or cellular communications. In the context of DSRC, this integration is known as DSRC Hybrid, while in the case of C-V2X, it is referred to as Uu mode. The question is to what extent long-range communications can deliver critical safety applications, with the implicit question: Do we need short-range communications?
This chapter explores the evolving role of telcos as C-V2X technology emerges alongside rapidly advancing 5G networks. Telcos are advocating for the integration of long-range communication with edge computing. This combination aims to reduce latency, achieving performance close to short-range communications, with an emphasis on VRUs.
6.1 Telcos' Transformation with C-V2X Emergence
Not until the creation of the 5GAA in 2016 has there been much enthusiasm in the telecommunications sector for involvement in C-ITS. Over the past 20 years, DSRC rollout in the USA has been limited, leaving most drivers yet to reap the benefits of C-ITS. In the DSRC era, telecommunication companies, or 'telcos', played more of a data communication provider role, rather than being key players actively driving technological innovation and investment in the C-ITS field. This has changed with the advent of C-V2X, an extension of cellular technology. Through their concerted efforts in recent years, telcos have introduced many ground-breaking innovations in C-ITS, turning imagination into reality. The ITS America's National V2X Deployment Plan published in April 2023, distinctly emphasises collaboration with USDOT, the National Telecommunications and Information Administration (NTIA), and the FCC to earmark at least an additional 40 MHz of spectrum for 5G-V2X technology and automated vehicle application deployments [28].
6.2 Telcos' Advocacy for Long-range Communication over DSRC
In April 2019, BMW CEO Harald Krueger and Deutsche Telekom's Tim Hoettges urged the German government to reject a European Commission proposal for a Wi-Fi-based standard (DSRC) in connected cars. They highlighted the need to consider 5G mobile networks as an alternative to avoid lagging behind China in future mobility [97]. This stance is indicative of a common viewpoint among telecom companies, showing a marked lack of interest in DSRCbased solutions. Telco representatives often criticise DSRC for its limited 500m coverage and reliance on RSUs, while they advocate for the expansive 10-30 km range of 5G networks. They worry that a heavy reliance on DSRC for C-ITS communication could lead to considerable information gaps in larger areas with an insufficient number of RSUs installed. This aligns with the insights gleaned from our interviews with telcos, demonstrating a clear reluctance to endorse direct communications, seemingly due to the uncertainty of profitable returns. They assert that focusing on long-range communications, with its wider reach, presents a more advantageous strategy to distribute information universally, benefiting all parties, especially mobile-equipped road users. Meanwhile, short-range communication technologies, such as DSRC or C-V2X PC5, are seen as additional features, designated for
localised, safety-critical coverage. For broader applications, 4G and 5G networks are typically more suitable for expansive, ubiquitous coverage.
6.3 Long-range Communication Potential
Table 19 Vodafone STEP platform use cases and services supported [27]
ETSI Standards Messages Descriptions
Cooperative Awareness Message (CAM)
Decentralised Environmental Notification Message (DENM)
In-Vehicle Information Messages (IVIM)
Signal Phase and Timing Extended Messages (SPATEM)
Shares basic presence information: vehicle type, position, heading, speed, acceleration, etc.
Warns drivers about different dangers on the road, including accidents, hazardous weather, traffic jams, road works, etc.
Includes the contents of electronic traffic signs.
Describes the traffic light status such as timing on traffic light changes, from red to green and vice versa, and additional information on closed lanes, detected pedestrian presence, etc.
Day 1
Day 1
Day 1
Day 1 & Day 2
MAP Extended Message (MAPEM)
VRU Awareness Message
Describes an intersection from a topology point of view: Lane direction, Lane type, Lane coordinates, Traffic Light ID linked to this lane, Maneuvering, Maximum speed, and Altitude.
Classifies Vulnerable Road users, like pedestrians, bicyclists, motorcyclists, and animals, based on position, profile, type, speed, direction, orientation, predicted trajectory, predicted velocity, heading changes, and braking indicators.
Day 1
Day 2
Verizon and Nissan North America have jointly conducted a proof-of-concept test, successfully demonstrating near real-time processing and communication of sensor data from vehicles and surrounding infrastructure at the edge of Verizon's network. The test utilised the C-V2X process in several scenarios, like pedestrian identification and detection of oncoming traffic from obscured sources, leveraging Verizon's 5G Edge with AWS Wavelength on Nissan’s Intelligent Shared World platform [26]. In February 2022, Audi of America and Verizon teamed up to embed 5G Ultra-Wideband technology into certain Audi vehicles, starting with the 2024 models. This partnership leverages mobile edge computing
with 5G cellular communication, ensuring minimal delay for vital C-V2X communications. This setup enables vehicles to interact in near real-time with other vehicles, surrounding infrastructure, and proximate devices [38].
In recent consultation with various telcos, Vodafone has distinctly surfaced as a leading advocate for embedding C-ITS into edge computing using long-range 4G/5G networks. Currently, they've successfully deployed a commercial V2X C-ITS service, termed the Safer Transport for Europe Platform (STEP), in both the UK and Germany, as shown in Figure 8. With an ambition to expand the STEP services across Europe, Vodafone aims to proficiently manage C-ITS's critical use cases via this scalable, low-latency framework. Tackling the challenge of data fragmentation, STEP methodically circulates data across numerous traffic and transport sectors in Europe, while ensuring that all exchanges through Vodafone's V2X SDK adhere to the ETSI ITS-G5 standard. Table 19 gives a summary of the use cases (Day 1 and Day 2) and services supported by the Vodafone STEP platform in alignment with ETSI standards. For Day 1, STEP backs Cooperative Awareness Messages (CAM) for basic vehicle information, Decentralised Environmental Notification Messages (DENM) for realtime hazard alerts, In-Vehicle Information Messages (IVIM) to broadcast electronic traffic sign data, and MAP Extended Messages (MAPEM) to depict intersection layouts. It also includes Signal Phase and Timing Extended Messages (SPATEM), which report traffic light statuses for both Day 1 and Day 2 use cases. Progressing to Day 2, VRU Awareness Messages are added for VRUs, thereby ensuring road safety across multiple deployment stages [27].
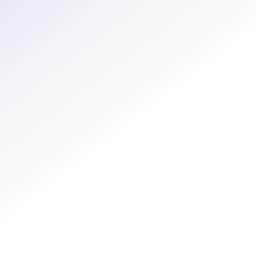
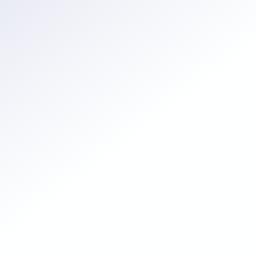
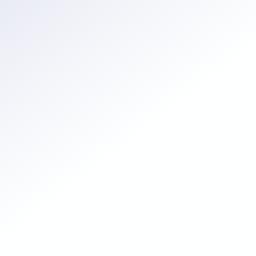
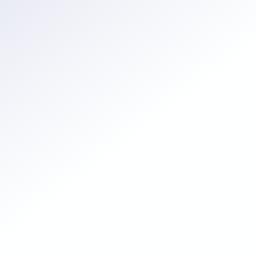
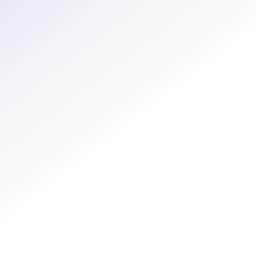
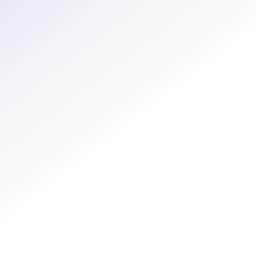
6.4
Still Need Short-Range Communications
Currently, the low-latency communication between vehicles and the surrounding connected infrastructure is achieved using RSUs (DSRC or C-V2X PC5), using direct communication,
Figure 8 Vodafone STEP C-ITS platform [27]
therefore, reducing communication time. However, the deployment of these RSUs is expensive and time-consuming.
To tackle latency challenges, telcos are focusing their investments on ultra-low latency 5G communication and edge computing solutions. In March 2022, Cisco and Verizon presented a demonstration in Las Vegas showcasing how Verizon's LTE network and public 5G Edge can enable autonomous driving solutions with minimal latency, eliminating the need for physical RSUs [98]. In Cisco's technical report [99], it is documented that Verizon has deployed a Virtual RSU software on the Mobile Edge. The report details the results of various tests, explicitly highlighting the performance of SPaT message conversations at intersections. Within a sample of 14 conversations recorded during the test period, the latency ranged from a minimum of 34.773 ms to a maximum of 62.272 ms. These metrics, along with the benchmarks for safety and non-safety applications, are presented in Table 20. The test findings confirm that the virtual RSU on the mobile edge effectively meets latency needs for safety applications in V2I and essential (not critical) V2V scenarios.
Virtual RSUs offer an affordable approach that delivers performance on par with direct communication methods. While automakers and telcos focus mainly on 5G and LTE, there may still be a need for physical RSUs at crucial intersections to enhance safety. For instance, ITS America sees RSU deployment as a key future task [28]. Despite telcos showing less interest in direct communication, their involvement can be valuable for rapid ecosystem development. Hence, a mix of virtual and physical RSUs could offer a swift rollout option.
Vodafone's STEP platform utilises edge computing and has undergone testing. Although no official report was received from Vodafone, their representatives have conveyed satisfaction with its performance during interviews. Telco representatives also claim that millisecondlevel latency is attainable and challenges the common perception that the speed of wireless transmission or latency in communication channels is the primary constraint for DSRC or long-range communication systems.
Telco representatives suggest that the efficiency of information processing systems carries greater significance. They contend that for safety applications, latency up to one second is acceptable and their STEP platform consistently meets this benchmark. They emphasise that the true determinant of performance is not latency but the coverage and accessibility of information.
In traffic light scenarios, they argue that the latency inherent in mobile networks suffices given the seconds-long switching cycle. For emergencies like abrupt braking, they assert that this falls on vehicle manufacturers to incorporate these critical V2V use cases into safety features. They propose that onboard sensors, such as LiDAR and cameras, offer more reliable and accurate data compared to V2V messages via short-range communication.
While we find validity in Vodafone's perspective, it is important to consider critical V2V use cases, like collision avoidance, as potential challenges to long-range communication. Based on the long-range testing conducted by Cisco and Verizon, and when compared to the metrics in Table 20, we can see that critical V2V applications still necessitate the use of short-range communication technologies for optimal performance.
6.5 Day 2+ Use Cases: Protecting VRUs
In ITS America's National V2X Deployment Plan, use cases involving VRUs are given priority. One of these pioneers is Spoke, a startup aiming to transform roadway safety and rider connectivity by delivering the first-ever connected IoT ecosystem for VRUs. Spoke's VRU2X system leverages three levels of connectivity within the mobility system. This includes C-V2X (powered by Qualcomm Chips) to provide direct and immediate communication between cars and VRUs, LTE/5G cellular communications for advanced contextual awareness alerts between cars and VRUs and a camera/radar system for vehicle identification and visualisation. Utilising these levels of connectivity, Spoke's system guarantees that vehicles can identify VRUs, such as pedestrians and cyclists, and also allows VRUs to become aware of approaching vehicles sooner than human sight permits. On 13th July 2022, T-Mobile and Deutsche Telekom announced their collaboration with Spoke to employ T IoT, a global IoT connectivity solution, to enhance road safety for cyclists and motorists by providing near real-time updates [35]. Moreover, Spoke collaborates extensively with high-end automaker Audi to pilot C-V2X implementations, focusing on developing bicycle-to-vehicle communication solutions in Peachtree Corners City [36].
Vodafone's STEP platform, designed to alert cyclists via long-range communication, capitalises on the ubiquity of smartphones among VRUs. Vodafone maintains that utilising DSRC or C-V2X PC5 for VRU scenarios can be problematic due to the currently limited accessibility of mobile devices equipped with the 5.9 GHz band. In the STEP trials, cyclist safety was enhanced by transmitting data from a smartphone app via mobile networks to the STEP platform. The platform relayed the cyclist's location every second to nearby vehicles, allowing them to assess collision risks and alert drivers as necessary. Vodafone proposes that an ecosystem prioritising indirect communication could be the optimal solution for all VRUs. They argue that developing a system similar to STEP is both cost and time-efficient, as it leverages existing mobile networks, eliminating the need for short-range communication infrastructure.
In September 2023, Bosch, in collaboration with Mercedes-Benz, Nokia, IT-Designers, InMach, Ulm University, and the University of Duisburg-Essen, showcased the findings of the LUKAS project. This project leverages edge servers and sensors on streetlights to aid cooperative behaviour in urban traffic. The preprocessed sensor information is relayed to an edge server, which is directly connected to the 5G network near the junction and provides data transmission with minimal delay times. The edge server utilises AI to contribute to a detailed environmental model, assist in cooperative maneuver planning, and incorporate a warning system for the safety of pedestrians and cyclists. The approach developed by LUKAS can help make automated driving in urban mixed traffic safer and more efficient for all traffic participants [100].
In November 2020, ETSI introduced a new technical specification ETSI TS 103 300-3 in its ITS Standard Release 2 [29] to enhance VRU protection. This update features the Vulnerable Road Users Awareness Message (VAM), a key element designed specifically for VRUs within the VRU basic service. This service is an integral part of the facilities layer in the ITS communication architecture, as defined in ETSI EN 302 665. The VAM message includes VRU profiles that are specifically designed for pedestrians, bicyclists, motorcyclists, and animals. On 23rd October 2023, C2C-CC and the Connected Motorcycle Consortium (CMC) released a white paper recommending the incorporation of the VAM motorcycle profile into CAM messages. This integration aims to optimally predict the motorcycle's path, thereby enhancing the effectiveness of collision avoidance scenarios specifically tailored for motorcycles [30]. Consequently, we can see a growing emphasis on VRU protection from both SDOs and consortiums, paralleled by advancements in mobile technology.
6.6 Aligning Mobile Networks with Rising Connected Car Adoptions
Leveraging the existing capabilities of mobile networks before fully investing in short-range communications is a smart move. This is because utilising the current mobile network infrastructure can significantly improve the safety and efficiency of transportation. Even if investment in short-range communication is made today, it will take several years, potentially up to 5 years, for all vehicles in Australia to be equipped with this technology.
Regarding vehicle connectivity forecasts, Austroads’ report underlines two crucial types of connectivity: C-ITS and mobile data [31]:
Forecast C1: The adoption of standards-based interoperable C-ITS systems in vehicles, regardless of specific communication standards (ETSI ITS-G5 DSRC or C-V2X). The projection suggests adoption starting in 2021, with a sales uptake of 1675% (average 40%) by 2030.
Forecast C2: Vehicles with built-in mobile data connectivity to the cloud, enabling various services such as live traffic info, over-the-air updates, crash alerts, and more. This requires connectivity to be incorporated within the vehicle, not reliant on smartphone pairing. The expected adoption continues to rise, with sales uptake increasing to nearly 100% by 2025 in the fast scenario, or only 26% by 2030 in a slower uptake scenario.
While these two forecasts are not interdependent, industry insight strongly suggests that vehicles equipped with C-ITS (C1) are likely to incorporate embedded mobile connectivity (C2) as well. Furthermore, it is important to note that the current mobile network infrastructure will continue to play a significant role in ensuring safety during the upcoming 5
to 10 years. Users will have the capability to leverage their mobile phones, equipped with safety applications, to seamlessly exchange data with C-ITS servers, thereby enhancing overall safety and efficiency in the transportation ecosystem. In the time-critical safety space, telcos are offering several services and capabilities through cellular networks. These offerings leverage the real-time communication capabilities of cellular technology to enable swift and efficient responses to safety-related incidents.
Moreover, according to BITRE estimates, it is projected that by 2028, 100% of new cars/SUVs and by 2026, 100% of new Light Commercial Vehicles (LCVs) will be equipped with Wi-Fi connectivity. Wi-Fi features in vehicles encompass both connectivity between vehicles and infrastructure (V2X) and connectivity for audio-visual devices unrelated to automation. Furthermore, new vehicles sold with in-vehicle telematics capability10 are forecast to reach 100% in 2035 and 2047 for cars/SUVs and LCVs, respectively [101].
Auto manufacturers exhibit reluctance towards adopting short-range V2V communication technology due to various factors. Both mobile networks and short-range communications face the challenges of promptly predicting accidents over minimal distances, a task currently managed predominantly by onboard sensors. Progress in sensor technology has facilitated swift responses in hazardous scenarios, lessening the appeal of V2V communication for some manufacturers. Further, the inclusion of telematics systems, which can offer details about proximate roadworks, enhancing navigation and infotainment capabilities, also makes V2V communication less of a priority for some car manufacturers. Overall, we anticipate that car manufacturers will initially focus on prioritising long-range communication in connected cars. Subsequently, they will explore ways to enhance performance through short-range communication technologies, such as DSRC or C-V2X.
6.7 Optimising Traffic Data Access via Mobile Networks
Consultations with telcos such as Vodafone and Deutsche Telekom have emphasised the importance of providing convenient access to traffic information for customers through mobile networks, which will deliver information to customers, regardless of whether they are drivers, passengers, motorcyclists, or pedestrians.
Mobile networks present an opportunity for road operators to contribute to a growing ecosystem. Road operators can share a digital model of road data, including traffic conditions, light timings, optimal speeds, and lane closures, using both short-range communication and mobile networks. This information will be available to service providers through a public interface, with a manageable latency of 100 milliseconds to 300 milliseconds achievable through mobile networks. This approach is evident in widely used apps such as Live Traffic NSW and VIC Traffic in Australia, which deliver real-time traffic data through this technology. The increasing adoption of API-based services like the NSW Open Data Traffic APIs highlights the potential growth and opportunities for road operators. Further, the system can prioritise public transportation or bicycle platoons at specific traffic
10 Telematics is a system with a vehicle tracking device that collects GPS data and various vehicle-specific information from sensors and engine diagnostics. The data is stored temporarily in a telematics device and transmitted via private cellular networks to a central server for interpretation.
lights for VRUs. Additionally, if the infrastructure exists, other applications such as priority for emergency vehicles can be implemented.
6.8 Conclusion
Given the current global uncertainty surrounding C-ITS standards, we recommend closely monitoring global trends and emerging standards for the next 1-2 years before making any long-term commitments. Moreover, the important role of mobile networks in C-ITS is evident. Research findings confirm that the virtual RSU on the mobile edge effectively meets latency needs for safety applications in V2I and essential V2V scenarios.
Furthermore, long-range communication is capable of supporting advanced use cases such as those involving Vulnerable Road Users (VRUs).
Nonetheless, critical safety scenarios still necessitate the use of short-range communication technologies.
7 Optimising the ITS Spectrum for Short-Range Communications
This chapter explores the 5.9 GHz ITS spectrum used globally for vehicle communication. We discuss regional differences, with a focus on the USA's shift towards C-V2X technology, and Europe's technology-neutral approach. The chapter also highlights spectrum requirements for C-V2X, challenges of DSRC and C-V2X coexistence, and proposes a tech-neutral strategy for Australia with a 20 MHz C-V2X allocation.
7.1 ITS Spectrum
The ITS spectrum is a specific range of frequencies used for communication in transportation systems. In leading markets, the ITS spectrum is 5.9 GHz free class license for ITS devices to communicate directly, for example, 5.855-5.925 GHz for Europe, and 5.850-5.925 GHz for the USA [102]. In 2017, the Australian Communications and Media Authority (ACMA) issued a free class license for the 5.9 GHz spectrum, aligning with the prevailing European standard [15]. Japan employs the 750 MHz spectrum for ITS safety applications and the 5.8 GHz band for non-safety purposes like Electronic Toll Collection. However, this case is beyond the scope of our research. China allocated the upper 20 MHz in the 5.9 GHz band for C-V2X, which is the only short-range communication to be officially used on roads for C-ITS use cases [103].
7.2 FCC Modernised ITS Spectrum for C-V2X
In the USA, 5.9 GHz has been designated for DSRC for over 20 years. However, according to the American Association of State Highway and Transportation Officials (AASHTO), there are only 57 DSRC projects in operation, including 6,182 DSRC roadside units (RSUs) and 15,506 vehicles equipped with DSRC on-board units (OBUs) [10]. 99.9% of the 274 million registered vehicles on the road in the United States still don’t have DSRC OBUs [104]. On 18th November 2020, the Federal Communications Commission (FCC) adopted new rules for the 5.9 GHz band (5.850-5.925 GHz) to make a new spectrum plan designating the lower 45 MHz (5.850-5.895 GHz) for unlicensed uses such as Wi-Fi, and the upper 30 MHz (5.895-5.925 GHz) for enhanced automobile safety using C-V2X technology (and 10 MHz can still be reserved for DSRC possibly), as shown in Figure 9. The FCC’s new change indicates that the USA has a strong will to choose C-V2X as the future pathway of C-ITS. In June 2022, the FCC published a public notice to remind ITS licensees that they will no longer be authorised to operate in the lower 45 MHz band, while they can continue to dedicate the upper 30 MHz (5.895-5.925 GHz) portion of the band for ITS operations [105]. The FCC proposed that the transition of ITS operations from DSRC-based technology to C-V2X-based technology would occur according to a timeline to be determined in a future Report and Order [105]. In the interim, ITS licensees that wish to operate in the upper 30 MHz using C-V2X-based technology may submit waivers requesting that the FCC waive the existing DSRC-based rules [106]. In April 2023, the FCC approved a Joint Waiver Request led by Audi of America, Ford, and Jaguar Land Rover, authorising the use of C-V2X
technology over a 20 MHz channel in the 5.905-5.925 GHz band. This indicates that the rollout of C-V2X in the USA is poised to advance more smoothly [96].
7.3 EU Designated ITS Spectrum on Technology Neutral Basis
European administrations have designated the bands 5.855-5.875 GHz and 5.8755.925 GHz, referred to as the 5.9 GHz band, for use by road ITS as specified in ECC Recommendation (08)01 [11] and ECC Decision (08)01 [12], respectively, both of which were approved by the ECC (CEPT) in March 2020 [13]. These are complemented by Decision 2020/1426 of the European Commission as adopted in October 2020. As is common practice in Europe, the spectrum is designated on a technology-neutral basis, as shown in Figure 10. Specifically, 5.855-5.875 GHz is designated for non-safety road ITS, whereas 5.875-5.935 GHz is designated for safety-related ITS. Furthermore, 5.875-5.915 GHz and 5.915-5.925 GHz are prioritised for road-ITS and rail-ITS applications, respectively, and with the frequency range 5.925-5.935 GHz available only for rail-ITS.
GSMA (GSM Association)11 recommends that regulators should adopt a technology-neutral approach to the spectrum that is set aside for safety-related ITS Automotive technology (e.g., the 5.9 GHz band) and does not take steps to pre-empt market forces by mandating or preferring one approach [18]. Regulators should adopt technology-neutral spectrum licenses to support network-connected vehicles and work with the mobile industry to support connected vehicles in future spectrum planning [18]. The EU's new plan aligns with this guide.
11
Figure 9 FCC Proposed ITS Band Plan in November 2020 [10]
Figure 10 Spectrum designations at 5.9 GHz in Europe [11] [12] [13]
7.4 Australia’s ITS Band
In ACMA’s five-year spectrum outlook 2023–28 [17], the interest in changes to the ITS class licence to accommodate C-V2X has been noticed, but further work on V2X channelisation needs to be prudent.
The current Radiocommunications (Intelligent Transport Systems) Class Licence 2017, i.e., ‘ITS Class Licence’ issued by ACMA [16], specifically references ETSI Standard EN 302 571. This standard is related to ITS and specifies radio equipment operating within the 5.855 GHz to 5.925 GHz frequency band - commonly used for DSRC. As such, before C-V2X came along, DSRC ITS-G5 was the default standard in Australia. Indeed, given that DSRC has been extensively trialled in Australia, it is the main user of this band. However, the ITS Class Licence, established during the emergence of C-V2X, should not impede the adoption of newer technologies, particularly considering that the USA has permitted C-V2X testing in the ITS band, and the EU's 2020 plan aimed to render the ITS band technology-neutral. This suggests that Australia may need to revise its ITS Class Licence to keep up with global trends, and potentially remove or modify this compliance in the foreseeable future.
7.5 Spectrum Requirements for C-V2X Technology
Table 21 Required spectrum for C-V2X [14]
Short-range communication (PC5 mode)
Network-based communications (Uu mode)
Day 1: V2V, V2I
Advanced use cases: V2V, V2I, V2P
Advanced automotive V2N services - Rural environments and affordable cost
Advanced automotive V2N services- Citywide and high-capacity
LTE-V2X 10 and 20 MHz of spectrum at 5.9 GHz
LTE-V2X and 5G-V2X
LTE-V2X and 5G-V2X
LTE-V2X and 5G-V2X
An additional 40 MHz or more of the spectrum at 5.9 GHz
At least 50 MHz of additional service-agnostic low-band (< 1 GHz)
At least 500 MHz of additional service-agnostic mid-band (1 to 7 GHz) spectrum
In reality, C-ITS technology for basic safety use cases does not need even half of the 70-75 MHz currently allocated for ITS spectrum at 5.9 GHz [107]. 5GAA classifies 40 use cases as Initial/ Day-1 and Advanced use cases. As shown in Table 21, 5GAA recommends 10-20 MHz for Day 1 use cases involving direct communications among road users or between road users and ITS roadside infrastructure (V2V, V2I), and an additional 40 MHz or more for advanced use cases (V2V, V2I, V2P). For V2N services based on network communication, depending on cost and capacity, 50 MHz at < 1 GHz or 500 MHz at 1-7 GHz is required [14]. More spectrum is a prerequisite for the advanced use cases supporting automated driving in the future.
In Europe, presently 5GAA seeks 20 MHz (5.905-5.925 GHz) for the provision of LTE-V2X Day 1 safety-related use cases (V2V/V2I) and attempts have been made to collaborate with 5GAA car manufacturers to agree on the usage of this band [108], as shown in Figure 11. 5GAA does not take a position on the use of 5.895-5.905 GHz by C-V2X because this is currently used by DSRC. Other 20 MHz (5.875-5.895 GHz) can be reserved for the future. In the current C-V2X trials in Europe, only 20 MHz in the upper ITS spectrum was used. The allocation of the 40 MHz band required for 5G-V2X in the future has not been finalised yet. 5GAA will determine the 5G-V2X spectrum allocation once the technology is more mature and the market conditions are clearer.
For Future Use For Future Use
The 5G-V2X technology is designed to support advanced autonomous driving services that demand a higher QoS and data flow. According to the advice of 5GAA, using 5G-V2X PC5 as a standalone technology without LTE-V2X is not a sustainable long-term evolution option. 5G-V2X PC5 requires an additional ITS spectrum as originally planned, but this spectrum is not yet allocated in the USA and China. Another alternative is to use both 5G-V2X and LTEV2X PC5 in the same channel. The 3GPP Release 18 standard for enabling cochannel coexistence was triggered when the USA decided to change the frequency band.
7.6 Coexistence of DSRC and C-V2X
The challenge of having both DSRC and C-V2X systems in connected vehicles is a key issue for ensuring vehicle communication regardless of the V2X radios used. As DSRC and C-V2X use different communication technologies, they cannot interact with each other, posing a significant challenge to car manufacturers and infrastructure developers. Running both DSRC and C-V2X in the same area is especially difficult due to the incompatibility of these two technologies.
For the best use of the resources available for short, medium and long-range, safety-critical communications, Communications Access for Land Mobiles (CALM) architecture was initiated by ISO TC 204/Working Group 16 [109], to define a set of wireless communication protocols and air interfaces for a variety of communication scenarios spanning multiple modes of communications and multiple methods of transmissions in ITS. The CALM architecture is based on an IPv6 convergence layer that decouples applications from the communication infrastructure [110]. However, the CALM protocol is not adopted by the industry for their C-ITS products, as commented by representatives of a leading chip manufacturer. The ISO TC204 WG16 CALM protocol is not commercially implemented in any region, and it is considered to be immature. The CALM in-vehicle ITS station architecture assumes some open interfaces, which many large OEMs prefer to keep closed. This protects their intellectual property, prevents unauthorised access, and enhances safety,
Figure 11 5GAA proposed deployment spectrum configuration in Europe [108]
particularly for critical functions like autonomous driving. Closed interfaces also simplify maintenance and reduce costs, as multiple interfaces can complicate quality control and provide limited benefits.
From industry consultations, most participants do not recommend the coexistence of DSRC and C-V2X. We agree with this conclusion that a single standard is a preferred direction for most OEMs and stakeholders.
7.7 Recommended Approach for Australia: A Tech-Neutral Plan with 20 MHz C-V2X Allocation
During the 20-year DSRC ITS-G5 trial, the primary use of the 10 MHz band (5.895-5.905 GHz) control channel (CCH 180) has been to broadcast safety-critical messages such as BSMs in the USA, and CAMs in ITS-G5. However, the remaining 60 MHz band, which comprises six service channels (SCHs), has largely been neglected. This significant underuse over two decades led the FCC to assert that the ITS 5.9 GHz band was not being optimally utilised. The FCC's updated proposal maintains a reservation of 10 MHz for DSRC (5.895-5.905 GHz), intended for the operation of the DSRC Control Channel, although this reservation may potentially be revoked in the future. In Australia, the ACMA has designated the ITS 5.9 GHz band as a free class license, with several operational DSRC-based C-ITS projects. However, despite the ITS band being 70 MHz, only 10 MHz is exploited, leading to 60 MHz of wasted band.
We recommend Australia adopt a technology-neutral strategy, similar to the European ITS spectrum 2020 plan, during this transition. This aligns with strategies already employed by GSMA and would be advantageous in maintaining technological neutrality and enhancing spectrum efficiency. However, while the goal is to ensure technology neutrality, it is vital to reach a consensus on band allocations for ITS-G5 and C-V2X to avoid device interference. If industry consensus can be reached, allocating 20 MHz (5.905-5.925 GHz) to C-V2X for initial trials would be a flexible and forward-thinking solution.
7.8 Conclusion
In conclusion, the coexistence of DSRC and C-V2X poses a concern for most stakeholders and represents the least desirable outcome. It is likely, but not a given, that the market will naturally dictate the dominant technology based on the majority of car manufacturers' choices for embedded V2X connectivity. During this transition, allocating 20 MHz to LTEV2X could position Australia favourably alongside leading countries.
8 C-ITS for Connected and Autonomous Vehicles
In this chapter, we explore the capabilities and limitations of current vehicular sensing systems and highlight the potential of C-ITS in enhancing the perception of vehicles, especially autonomous vehicles. In addition, we offer an overview of the latest technical legislation in Europe that pertains to fully autonomous vehicles. The chapter also examines the expectations and priorities of road operators concerning autonomous and connected vehicles. Lastly, we emphasise the role of digital infrastructure in supporting the functionalities and capabilities of autonomous vehicles.
8.1 C-ITS Extends the Vision of Vehicles
At present, autonomous vehicles operate decisions predicated on the data collected through sensor arrays, LiDAR, radar, and camera systems [111]. However, these line-of-sight sensing mechanisms have a limited field of view, which is a primary constraint impacting the safety of self-driving vehicles. Given these systems are vehicle-mounted, a ‘blind spot’ scenario is inevitable. No matter how advanced the onboard devices are, they cannot process the information they don't see. Moreover, managing multiple inputs from diverse sensors poses an additional challenge for AI in making accurate and final decisions. Therefore, enhancing driving safety is achievable by having the onboard systems collaborate with C-ITS. These systems could provide a broader outlook and even an around-the-corner view, expanding the range for detecting obstacles.
C-ITS can significantly enhance automated driving by enabling real-time communication between vehicles and infrastructure, thereby increasing situational awareness and safety. 5GAA discussed the inclusion of advanced use cases [79], especially for autonomous driving as shown in Table 22.
Table 22 5GAA Advanced use cases for autonomous driving
Use Case Category 5GAA Advanced Use Cases for Autonomous Driving
Intersection Assistance Automated Intersection Crossing
Cooperative Maneuvers
Cooperative Lane Merge, Cooperative Maneuvers of Autonomous Vehicles for Emergency Situations, Cooperative Driving Maneuvers
Map Sharing High-Definition Map Collecting and Sharing
Vehicle Control and Remote Driving
Environmental Hazard Detection
Remote Automated Driving Cancellation (RADC), Tele-Operated Driving, Tele-Operated Driving Support, Tele-Operated Driving for Automated Parking, Autonomous Vehicle Disengagement Report, Infrastructure-Based Tele-Operated Driving
Infrastructure-Assisted Environment Perception, Vehicles Collect Hazard and Road Event for Autonomous Vehicles
As connected vehicles enter the market before automated vehicles, C-ITS can improve road safety even in the short to midterm in line with the penetration of connected cars. In mixed traffic scenarios, autonomous vehicles face challenges in understanding and responding to the collaborative actions of human drivers, particularly during maneuvers like lane merging. Human drivers rely on visual cues for coordination, posing a hurdle for autonomous vehicles which lack this comprehension. This can lead to traffic congestion and disruptions. C-ITS technology enables coordination and communication between autonomous vehicles and other road users, allowing autonomous vehicles to receive and interpret information, including the intentions of nearby human drivers. This facilitates smoother coordination during complex manoeuvres, reducing congestion and improving traffic flow in mixed scenarios.
8.2 European Technical Legislation for Fully Driverless Vehicles
Ideally, an autonomous driving system should handle all hazard scenarios but there can be situations where the system might not be able to identify the obstacle and might need remote operator assistance for decision-making [112]. On 20th May 2022, the German Federal Council officially approved the draft regulation that complements the Act on Autonomous Driving from the previous year. This regulation outlines the technical and procedural requirements for operating autonomous vehicles at SAE/ISO level 4 on public roads [21]. Shortly after this, in July 2022, the EU Commission adopted technical legislation under regulation 2022/1426, specifically for fully autonomous vehicles operating at Level 4 of automation [22]. This regulation marks the first rule of its kind on a European Union and international scale. The technical rules set out via a delegated and implementing act establish an assessment of the safety and maturity of fully automated vehicles before they go onto the EU market, which is set to take effect in July 2024.
This new rule states the remote intervention operator where applicable. In the event of system failure or near-accident, people located outside the fully automated vehicle may remotely achieve the tasks of the onboard operator provided it is safe to do so. Automated Driving System (ADS) can be certified with remote intervention as a part of its safety strategy. However, the remote intervention operator will not drive the fully automated vehicle and the ADS will continue to perform the Dynamic Driving Task (DDT). The only caveat, however, is that remote driving is permissible at speeds not exceeding 6 km/h.
8.3 Expectations and Priorities of Road Operators
In January 2023, 5GAA work item BRIDGE released a recommendation report [113] to address the business priorities of the Road Operators (ROs) and how C-V2X services provided by telcos can support them directly. As shown in Table 23, the following four use cases and operational priorities were determined by 5GAA to be a good overall representation of the ROs’ expectations and they need higher data flow [19]:
Table 23 Road Operators' prioritised use cases
Use Cases Description
Local Hazard and Traffic Information (LHTI)
Greenlight Optimal Speed Advisory (GLOSA)
HD Maps Data Collection and Sharing (HD Maps)
Improve driving safety and efficiency by informing road users of known hazards in a specific location.
Provide speed advisory information for road users approaching signalised traffic intersections.
Uses HD maps for automated driving to enhance predictability and safety. Vehicles equipped with sensor technologies (e.g., LiDAR, radar, video) collect data about their surrounding road environment and share it with HD maps providers.
Operational Priority
Vision Zero (Aims to reduce road accidents and deaths)
CO2-free (energy/fuel consumption reduction)
Digital Twin (Supports road traffic modelling and planning)
Cooperative (Connected, and Automated Mobility)
Probe Vehicle Data (PVD)
Multiple vehicles send their information (speed, direction, type) to the RO C-ITS platform to be processed and visualised significantly. RO will have full visibility of the traffic conditions.
Urban Digital Twin (enhanced services for realtime support traffic monitoring and control in urban spaces)
As listed in Annex 8, in 2022, Audi introduced new models in China equipped with autonomous driving and C-V2X technology [20]. Meanwhile, Hyundai Motor Company is developing V2X technology to further develop the usability of renewable energy sources and reduce strain on local electricity networks. The company has undertaken two V2X pilot projects in the Netherlands and Germany. These projects, utilising the IONIQ 5, focus on two applications: Vehicle-to-Grid (V2G) and Vehicle-to-Home (V2H), to balance the energy grid and home systems [114]. These vehicles incorporated various use cases, such as safety sensing, traffic light information, energy transfer, and local hazard warnings, all of which are in line with the above expectations of ROs. The shift towards V2I collaboration offers a more cost-effective alternative for car manufacturers compared to relying solely on single-vehicle intelligence. This approach minimises the need for high-performance chips and costly onboard equipment, making it a more financially viable strategy.
Regarding this matter, our observations are as follows:
The ROs have prioritised four use cases that primarily focus on delivering road safety and traffic control information to vehicles. However, it is important to note that these prioritised use cases are not associated with safety-critical events that demand ultralow latency communication, akin to what C-V2X (PC5) or DSRC can provide. ROs perceive the implementation of such safety-critical systems as the domain of OEMs.
Present-day mobile networks, while not capable of providing ultra-low latency as short-range communication systems do, are still able to meet the requirements set by
the ROs. The latencies delivered by modern 4G networks, although not as low as short-range, are deemed sufficient for the ROs' prioritised use cases.
The main demands from ROs are for broad information coverage and availability. However, responsibility and liability for critical safety use cases requiring direct communication remain unclear among telcos, ROs, and OEMs.
8.4 C-ITS Digital Infrastructures for CAVs
Table 24 Complementary digital infrastructures [115]
Investment item
Likelihood infrastructure gaps are closed by digital infrastructure Complementary digital infrastructure
Line marking and signage Likely AV/HD maps
Work-zone signage Likely V2X
Traffic signals and school-zone signage Likely V2X and HD maps
Kerb and footpath management Unlikely N/A
Grade-separated unsignalised interchanges Likely V2X and HD maps
Safe-stopping zones Unlikely N/A
Bridges and pavements Likely V2X and HD maps
Dedicated AV transit lanes Likely V2X
Dynamic, dedicated AV freight lanes Likely V2X
Remote AV parking facilities and mobility hubs Unlikely N/A
Austroads outlines the minimum infrastructure investment required for automated driving. Such future automated vehicles are likely to interact digitally with their surrounding physical infrastructures during transit. The use of digital infrastructures, including audio-visual/highdefinition (AV/HD) maps and Vehicle-to-Everything (V2X) communication, is integral to achieving this interconnectedness [115]. Table 24 presents the relationship between the physical infrastructures identified and the role that digital infrastructure may play in closing the infrastructure gaps. Digital infrastructures can address physical infrastructure gaps by enabling real-time notifications of roadworks, traffic signal status, motorway safe-stopping areas and urban pick-up/drop-off zones capacities, emergency vehicle warnings, and coverage of poorly lit areas. Though digital infrastructure can serve as supplements, current regulations stipulate that physical infrastructure is the primary reference. At present, we foresee a concurrent existence of both physical and digital infrastructure, although this dynamic may change in the future.
8.5 5G is Critical to CAVs
Presently, Level 3 autonomous driving relies on onboard perception systems such as LiDAR and cameras. For advancing to superior autonomy levels like Level 4, incorporating C-ITS systems to broaden the detection range becomes more advantageous. Regarding immediate safety-critical applications, we consider both DSRC and C-V2X to be adequately equipped to fulfil these requirements. But with growing demand for advanced Day 2/3 use cases with larger data flows, the advent of 5G networks, with their ultra-low latency communication capabilities, is set to further propel autonomous vehicle development [116]. Vehicles incorporating C-V2X technology, harnessing the power of 5G communication, could enjoy significant advantages. Embracing 5G technology will be a critical factor in the successful fusion of CAVs into C-ITS systems, for instance:
The low-latency, real-time transmission of encrypted vehicle status and operational data, including high-resolution video from an autonomous vehicle (AV) to a control room, supports a broader range of use cases such as remote monitoring, TeleOperated Driving, platoon determination, and remote control.
The high-speed low-latency transmission of encrypted sensor data enables the realtime analysis and fusion of sensor data from multiple stations, including in-vehicle and roadside sensors, which will then be used to assist the cooperative driving, convenience, and traffic efficiency use cases.
Automated vehicle penetration: CAVs will operate more reliably with C-ITS, in particular for reliable object detection with rich data, traffic light indication, coordination between road users and the ability to detect obstructing objects. The greater the penetration of highly automated vehicles, the higher the need for V2X Day 2 and Day 3 deployment [117].
8.6 Conclusion
In conclusion, enhancing driving safety is achievable by having the onboard systems collaborate with C-ITS, to provide a broader around-the-corner view of obstacles. Australia's National Land Transport Technology Action Plan [118] highlights the safety benefits and expanded perception offered by C-ITS. Comparable to the advanced use cases for TeleOperated Driving and Support as detailed by 5GAA, the EU Commission and Germany have approved technical legislation governing the operation of autonomous vehicles at SAE/ISO level 4 on public roads. This legislation clarifies the role of remote intervention operators when required.
In terms of advanced driving, road operators have prioritised use cases like local hazard and traffic information (LHTI), greenlight optimal speed advisory (GLOSA), HD maps data collection and sharing, and probe vehicle data (PVD). These use cases are not associated with safety-critical events that demand ultra-low latency communication.
Road operators and car manufacturers are currently emphasising the importance of 5G communication. Digital infrastructures like AV/HD maps and V2X communication prove indispensable in bridging the gaps in physical infrastructure. High-speed 5G connectivity is increasingly vital for efficient data transmission and aligns well with road operators' priorities. We observe that car manufacturers are primarily focused on embedding cellular connectivity in CAVs, particularly since 5G is essential for advanced driving scenarios like Tele-Operated Driving. Audi has launched new models equipped with autonomous driving features and
4G/5G-enabled C-V2X technology. In this context, it implies that car manufacturers are currently prioritising cellular-based solutions for new vehicles, specifically leaning toward 5G technology. They seem to be favouring C-V2X as a subset of cellular communication technologies, viewing it as a more future-proof solution for short-range communication, compared to DSRC.
9 Cost, Value Model and Governance
While the associated costs are substantial, the significant improvements in public safety and efficiency make a compelling case for government investment in C-ITS infrastructure. This chapter provides a high-level review of cost considerations, deployment strategies, and potential challenges, drawing on a variety of sources including the 2023 National V2X Deployment Plan as presented by ITS America. This chapter explores the business model for advancing C-ITS, comparing DSRC and C-V2X standards. It highlights financial considerations, the roles of stakeholders, and the need for an effective governance structure.
9.1 Prioritising Intersections for V2X
Despite the significant progress made by long-range communication technologies such as 4G and 5G in minimising latency, the 5.9 GHz short-range communication still remains the fastest for V2I applications. Critical V2I scenarios require the use of Roadside Units (RSUs) for direct, high-speed communication with vehicles. While it is possible to deploy C-ITS using long-range virtual RSUs without the need for physical ones, this approach does not fully exploit the speed advantages of short-range communication. For locations of critical importance, the government must bear the responsibility and inevitable cost of RSU installation. Nonetheless, these costs are justified by the resultant public benefits: improvements in safety, efficiency, and the overall functioning of our transportation systems. In the 2023 National V2X Deployment Plan [28], ITS America suggests the initial deployment of C-ITS technology at signalised intersections due to their existing infrastructure (poles, power, electronics cabinets and backhaul communications), which facilitates rapid implementation. For non-signalised areas, it may be more appropriate to consider virtual RSUs or long-range communication technologies other than low-latency short-range communications. As automakers and road operators concentrate on intersection-specific V2I applications such as Red-Light Violation Warnings and Pedestrian Safety Messages, and with an increasing focus on Vulnerable Road Users (VRUs) safety, intersections have emerged as the primary deployment zone [28]. Approximately 330,000 signalised intersections exist within the USA. The task of equipping a substantial percentage of these intersections with C-V2X RSUs over several years falls on various levels of government, such as the Departments of Transportation (DOTs) and transportation agencies. For national interoperability, ITS America prioritises essential messages to drive robust C-V2X technology deployment, as shown in Table 25.
Messages Use Case Descriptions
Basic Safety Message (BSM)
Signal Phase and Timing (SPaT) and Map (MAP)
Radio Technical Commission for Maritime Services (RTCM)
Traveller Information Message (TIM)
Information about a vehicle’s state
Information about a traffic signal’s state, intersection geometry and lane attributes
Correction values for the GPS coordinates
Information about roadside advisories, work zones, road signs, etc.
Signal Request Message (SRM) Request for preferential treatment at a signalised intersection for certain vehicle types
Signal Status Message (SSM) Confirmation of a preferential treatment request by an SRM
Road Safety Message (RSM) Information about curve and work zone speeds, lane closures, etc.
9.2 Cost of Infrastructure Deployment
Many ITS SDO specialists view traffic safety as a societal benefit that the private sector cannot efficiently provide due to profit motives. They argue that the government is best suited to maintain transportation systems to societal standards and needs.
The following costs will be incurred when deploying and maintaining C-ITS infrastructure:
Deployment costs: capital expenditure for network assets and infrastructure.
Operation and maintenance costs: yearly expenses to operate and maintain the network infrastructure, e.g., fault detection and alert system, electric repair, and replacement.
Connectivity costs: correspond to fees related to connectivity services, depending on the subscriptions of the data plan [33]. Despite using short-range systems like DSRC or C-V2X PC5 on a free 5.9 GHz band, costs may still apply if long-range connectivity is needed. In C-ITS deployments, data costs are unavoidable but can be mitigated with the telcos' involvement and C-ITS operators' enterprise plans. Drivers cover their vehicle's data expenses.
Annex 6 presents the 2021 C-Roads infrastructure deployment costs [23]. The total expense for C-Roads trials across 19 European countries was 59 million EUR in 2021, dropping to 48 million EUR in 2022, and is projected to be reduced further to 42 million EUR in 2023.
Early research by the USA DOT in 2014 claims that DSRC field infrastructure deployment costs can range from 13,000 USD to 21,000 USD per site, with the average cost estimated at 17,600 USD [32]. Table 26 shows the average total direct cost per DSRC site.
Table 26 average total direct costs (USD) per DSRC site (Source: USDOT/AASHTO 2014) [32]
The cost of deploying RSUs can be determined by: a) calculating the average number of RSUs needed to cover each type of road, and b) estimating the annual Capital Expenditure (CAPEX) and Operating Expenditure (OPEX) costs, along with the deployment rate [33]. A British case is illustrated in Table 27, Table 28 and Table 29. Short-range communication performs better within a 300-500m range. Each RSU installation carries a CAPEX of 7200 EUR and an OPEX of 735 EUR. The expenses for network deployment and maintenance over 10 years, based on previous assumptions, are detailed in Table 29. Currently, an RSU device supports both DSRC and C-V2X, making their installation costs nearly identical.
Table 27 Dimensioning of RSU deployment (Great Britain) [33]
Table 28 RSU costs reference for CAPEX and OPEX [33]
ITS America's 2023 National V2X Deployment Plan [28] estimates the cost based on a scenario of deploying C-V2X PC5 RSUs over several years. The plan depends on joint V2I deployment and OBUs in vehicles transmitting key BSM data, vital for road operators to improve safety. As shown in Table 30, the short-term (5 years) and long-term (10 years) goals are outlined for equipping various community types with RSUs as part of the plan. Every state's Department of Transportation must create a deployment plan as part of the national plan, detailing deployment strategy and funding, to qualify for federal funds, with a focus on signalised intersections. The estimated infrastructure development costs are indicated in Table 31.
The assessment of utilising pre-existing infrastructure, such as RSUs or cellular towers, will hold even greater significance in terms of cost savings and expediting technology implementation. For C-V2X, 5GAA claims that using existing infrastructure does reduce cost [33]. 5GAA highlights the complementarity between cellular long-range technologies (mobile networks) using the cellular (Uu) interface and PC5 side link technologies. Leveraging existing cellular networks is beneficial because traffic signal status, variable road signs, etc. are already digitally connected and managed centrally. 5GAA shows that the public cost of using existing cellular networks to provide C-ITS services is significantly lower (even a hundredfold) compared to the widespread rollout of dedicated RSUs [33].
Table 29 Estimated cost evolution for RSUs [33]
RSU Installation
Large Communities (population > 400k)
Mid-size Communities (population 50k400k)
Rural Communities (population < 50k)
Long-term Goal (10 years)
250,000 intersections (75% of all signalised intersections)
Equip 85% of all signalised intersections
Equip 60% of all signalised intersections
Equip 20% of all signalised intersections, focusing on intersections with demonstrated safety challenges
Short-term Goal (5 years)
100,000 intersections (40% of long-term target)
Equip 35% of all signalised intersections
Equip 25% of all signalised intersections
CAPEX
Equip 8% of all signalised intersections
Initial setup cost for ‘ready’ intersection (per intersection)
Planning, engineering, and procurement cost (maximum per intersection)
Additional costs for ‘not ready’ intersection: New cabinet, signal controller, and other features
Additional costs for ‘not ready’ intersection: Nonintrusive detection systems (e.g., radar)
Annual agency-wide security license/management portal fee
Large-scale deployment (initial cost for 1700 intersections)
$7K - $15K
Up to $50K
$20K - $25K
$20K - $40K
$10K - $100K
$25M - $45M
Small-scale deployment (initial cost for 100 intersections) $1.5M - $2.7M
10-year national deployment goal (initial cost) $6.5B
Table 30 ITS America's 2023 National V2X Deployment Plan [28]
Table 31 Estimated Infrastructure Deployment Costs [28]
Expense Type Cost Category
OPEX Ongoing costs for maintenance, monitoring, replacement, compliance testing
Ongoing costs for data backhaul (transfer to server/cloud) Variable
Annual costs for security certificate updates Variable
9.3 Advancing C-ITS Requires Business Model
In the last two decades, DSRC has played a significant role as a short-range communication medium, forming decentralised, reliable, and simple C-ITS networks without business model dependencies. Over the past five years, the newly emerged C-ITS standard, C-V2X, has bridged the gap in cellular communication's disadvantages, specifically its low-latency direct communication, a point heavily criticised by DSRC proponents. As suggested by ITS America [28], we concur that long-range communication cannot entirely replace short-range communication. This is especially true in crucial areas such as signalised intersections, where low-latency communication is critical for use cases. The deployment of RSUs at crucial points such as intersections and highways facilitates swift, low-latency V2I applications, an initiative primarily funded by governments in the past. Additionally, key aspects like the SCMS security system, central stations, and road data uplift and management will likely require government investment in the C-ITS domain, exceeding the costs of RSU. Presently, mainstream RSU devices support both DSRC and C-V2X communications, thus the deployment costs for these specific short-range technologies are comparable. As we move forward, we anticipate a reduction in the cost of electronic devices, while human resources costs may rise. This shift will proportionately decrease the role of communication technology in the overall cost of ITS solutions.
During our discussions with telecom representatives, it became apparent that telcos are currently hesitant to invest in the deployment of short-range communication infrastructures, such as DSRC or C-V2X, due to the lack of immediate financial returns. They lean towards participating in the cellular-based ecosystem, deriving profits from backend services, with a preference for a virtual RSU coupled with edge computing to deliver ultra-low latency communication. Similarly, car manufacturers' representatives express caution regarding short-range communication, valuing safety as an essential factor rather than a revenuegenerating feature like in-car entertainment.
Currently, the DSRC network operating on the 5.9 GHz band is typically integrated into the broader C-ITS ecosystem. This is because nearly all C-ITS deployments require long-range communication to gather diverse data inputs, leading to a ‘hybrid’ mode that combines DSRC 5.9 GHz communication and cellular communication. Although this hybrid mode isn't explicitly defined in the DSRC standards, which primarily focus on 5.9 GHz band communication, it can be flexibly implemented through various means, including fibre, Wi-Fi, and Bluetooth, with cellular being the most preferred method in C-ITS projects. In the future, the most likely V2X deployment scenario could incorporate a short-range V2X device (either DSRC or C-V2X PC5) in combination with 4G/5G modems. Vehicles could be equipped with
aftermarket short-range communication devices, leveraging their existing connectivity to interface with an online message broker. For instance, in the DSRC trials conducted in Ipswich, Australia, 4 out of 6 use cases leveraged 4G cellular connections to deliver warnings directly from the Internet. The information regarding road hazards can also be distributed to a wide range of cellular commercial services through Application Programming Interfaces (APIs). This underscores the requirement for local governments to have a dual focus on delivering public services and recognising the value of commercially oriented services.
Looking ahead, if DSRC remains the sole C-ITS standard in Australia, its advantages include minimal reliance on telecommunications services for road operators and the capability to independently manage C-ITS infrastructure like traffic lights and automatic number plate recognition cameras. However, mirroring the unsuccessful large-scale DSRC rollout in the US over the past two decades, Australia's implementation of RSUs at signalised intersections is currently not widespread, and the pace of progress is sluggish. If Australian governments plan to persist with DSRC for safety use cases in the future, it will still necessitate significant government-led investment for RSU installations. Telcos appear less likely to engage if DSRC remains the mainstream standard. Australian governments would bear sole responsibility for installation, data management, and overall C-ITS system liability. A hybrid mode of DSRC and cellular communication is unlikely to draw in telcos. They will primarily serve as ISPs and not actively contribute to expanding the C-ITS ecosystem for rapid deployment as game players.
The C-V2X standard, leveraging the power of both short-range and cellular communications, seems to have more potential to draw investments from telcos and other stakeholders once it becomes effective in Australia. By integrating with cellular networks, telcos could be incentivised to participate in infrastructure investment and operations, including RSU deployments and other C-ITS services. Their involvement could potentially lower the government's CAPEX. Additionally, for non-critical use cases such as HD maps, guidance, road assistance, and trip planning, small fees could be levied on end-users. Presently, leading automakers, mostly members of 5GAA, are committed to cellular-based V2X solutions, initiating long-range 4G/5G modem communication. In China, for instance, trials of C-V2X direct communication are widespread. As outlined in the ITS America 2023 National V2X Deployment Plan [28], the key challenge in V2X deployments is the hockey stick value proposition where benefits of deployment materialise late in the cycle after a critical mass of deployed units is achieved. For example, V2X onboard units can be installed on bicycles more quickly than OEM vehicle development cycles. These units can support both C-V2X (short range) and Uu (cellular long range) technologies, offering a unique solution to VRUs safety. Devices transmitting short-range (C-V2X) direct messages could encourage vehicle manufacturers, who are currently undecided, to adopt modems with C-V2X capabilities. This technology would enable them to deliver superior alerts and warnings to drivers and eventually integrate these systems with Advanced Driver-Assistance Systems (ADAS). With the adoption of C-V2X, we envision a future where both automakers and telcos are more engaged and invested in the ecosystem.
While partnering with telecom companies could help the government lessen its load in managing and maintaining the C-ITS infrastructure, it is not without its risks. Potential issues include an over-reliance on these telecom networks and concerns about data security. Therefore, Australian governments need to proceed with caution, taking the time to carefully
assess the advantages and drawbacks before making a deployment decision. When it comes to advanced use cases such as Tele-Operated Driving, which fall under the Day 2 and Day 3 categories, additional expenditures for high-end hardware and software will likely be necessary. This report does not provide specific cost estimates for these scenarios. For a reliable estimate of minimum investment in Automated Driving use cases, reference [115] can be consulted. Please note that this report does not cover the costs of other crucial investments like data platforms and security PKI platforms.
Finally, we do not recommend a solution supporting both DSRC and C-V2X simultaneously, as both OEMs and car manufacturers prefer a single standard to reduce infrastructure complexity. Dual support will increase costs due to the development and maintenance of integrated systems. Governments, telcos, and road operators could face challenges in investment sharing, liability, and operating model coordination depending on their choice of C-ITS technology.
9.4 Governance Structures for C-ITS Operations
Table 32 Governance structure for C-ITS operations [75]
Elements Description
Organisational elements
Monitoring focuses on the supervision of the overall C-ITS service performance and the further development
Technical elements Change Management
Security system in place
Information on active C-ITS services
Information on data and services available
Regulatory aspects are considered
Update and authorisation of specifications and systems
Agreement on implementation plans for new releases
Set up and perform cross-tests, including validation
Roll-out of new versions/generation of PKI certificates
Elements fostering sustainable stakeholder engagement
Information Management
Security Management
Stakeholder Support
End-user support
Data sources used
The consistency of the C-ITS service chain
The operation of a central PKI infrastructure
Transport layer security
Handling of security issues
Newcomers should be provided with a central service
Usually managed by a single stakeholder for their customers
The technology to be deployed must exhibit ease of upgradeability and cost-effective maintenance:
Upgradability: The technology needs a well-defined path for future upgrades and developments, taking into account factors such as cost, licensing, and the intricacy of hardware and software updates. Such a clear path will simplify decision-making for Australian governments and users.
Maintenance: The technology should employ a clear, layered software architecture, facilitating simplified maintenance of applications at different levels. Moreover, hardware components should be modular to support effortless troubleshooting and replacement, without adversely affecting overall network performance.
To achieve the above aims, a possible governance structure for C-ITS operations is stated in [75] and is shown in Table 32. If a pan-European cooperative governance structure for C-ITS operations is to be established, the first consideration is to establish a framework that enables partnerships between different stakeholders. Therefore, an agreement on a common C-ITS vision, a common mission and cooperation agreements need to be finalised.
9.5 Conclusion
Successful deployment necessitates planning, investments, and a collaborative approach that fosters participation from a diverse set of stakeholders. Drawing from the insights gained through the consultation interviews and literature review, as we journey towards a future of advanced, interconnected, and intelligent transportation systems, we conclude:
5.9 GHz Communication for Signalised Intersections: Utilising 5.9 GHz shortrange communication, prioritised for critical areas, ensures the fastest speeds for V2I applications with the use of physical RSUs. ITS America's 2023 National V2X Deployment Plan, advocating the initiation of C-ITS at signalised intersections, while exploring alternative long-range technologies or virtual RSUs elsewhere, appears to be a justified and strategic approach.
Adoption of a Single C-ITS Technology: It is evident that OEMs and car manufacturers tend to favour a single standard technology, either DSRC or C-V2X. This preference is rooted in the desire to reduce infrastructure complexity and associated costs. Therefore, a singular technology adoption strategy is recommended, avoiding dual support that can lead to increased cost and complexity.
Encouraging Telcos’ Participation in C-ITS Large-Scale Deployment: Our study suggests that collaboration with telecom providers can serve as a cost-effective strategy that can alleviate some of the financial and operational burden from Australian governments in managing and maintaining the C-ITS infrastructure. The adoption of the C-V2X standard presents a more attractive opportunity for telcos to invest in C-ITS, potentially accelerating the deployment speed.
Prioritising C-ITS Data Platform: Regardless of the choice between DSRC or C-V2X technology, Australian governments must prioritise the establishment of a universal C-ITS data platform, possibly accompanied by a nationwide Public Key Infrastructure (PKI). Additionally, it goes without saying that it is vital for Road Operators (ROs) to actively cooperate in supplying the necessary ITS data sources.
Implementing a Robust Governance Structure: The chosen technology needs to exhibit ease of upgradability and cost-effective maintenance. This requirement can be fulfilled through a well-planned governance structure that emphasises straightforward upgrade paths and simplified maintenance of applications. While partnerships can provide significant benefits, it is equally important to be cognizant of the potential risks. Over-reliance on telecommunication networks and concerns around data security are two such challenges that need to be addressed proactively.
Fostering Collaboration Among Stakeholders: For successful C-ITS deployment, fostering an environment of collaboration and cooperation among various stakeholders, governments, OEMs, telecom providers, and ROs, is essential. The involvement of commercial capital from stakeholders can aid in establishing a nationwide, unified C-ITS ecosystem more quickly and serve a wider range of road users.
In summary, despite the unique set of challenges associated with C-ITS implementation, the potential benefits make it a significant and necessary step forward. With careful planning, effective partnerships, and a clear governance structure, we can successfully navigate the path towards a more efficient and safe transport ecosystem.
10 Security and Privacy
This chapter explores the critical aspects of C-ITS security and privacy. The focus is on the use of Public Key Infrastructure (PKI) to ensure secure communication and authentication. Notably, we highlight the European Union C-ITS Security Credential Management System (EU SCMS) as a leading and successful example. Additionally, the chapter suggests the specific priorities for enhancing C-ITS security in Australia, emphasising the importance of a robust PKI system, and promoting interoperability across different jurisdictions.
10.1 What is C-ITS Security and Privacy?
A C-ITS device will be constantly encountering and exchanging data with C-ITS devices with which it has no prior relationship, such as a car passing an unfamiliar infrastructure, and vice versa, hence trusts need to be established to enable high levels of interoperability and confidence in the content and security of messaging [121]. In these environments, messages are constantly exchanged, requiring both security and the assurance of user privacy. A security system for C-ITS will have the following well-known information security pillars:
Confidentiality – C-ITS governance framework and security policy to ensure that sensitive information remains protected and accessible only to authorised participants.
Integrity – authenticity and accuracy to record traceable logs to avoid abuse.
Availability – always on service to provide constant security and meet users’ requirements crossing multiple platforms and lower-layer communication technologies.
C-ITS and self-driving technology will gather and preserve data related to both vehicle usage and passenger information for operational and safety reasons. Some of this data will be categorised as personal or sensitive, making it subject to privacy and data protection regulations. The aim is to solve the dilemma of data privacy protection and circulation in the ITS system for management purposes [122]. ISO/IEC 15408-2 identifies 4 key attributes that relate to privacy [123]:
Anonymity: Anonymity is critical for preserving user privacy and fostering trust, as continuous data exchange happens between vehicles and infrastructure. However, it comes with limitations. Anonymity hinders accountability for actions such as traffic violations, complicates the monitoring essential for safety and efficiency, and restricts service personalisation based on user behaviour.
Pseudonymity: This is a privacy approach where an ITS user uses a pseudonym (or false name) to interact with the system, instead of their real identity. This ensures that an ITS station may use a resource or service without disclosing its identity but can still be accountable. Pseudonymity can offer a balance, providing privacy while maintaining necessary system functionality.
Unlink ability: Unlink ability ensures that an ITS station may make multiple uses of resources or services without others being able to link them together.
Unobservability: A user acts or uses a system resource without others being able to observe that the resource is being used.
10.2 C-ITS Security Credential Management System
SCMS uses a Public Key Infrastructure (PKI)-based approach that employs highly innovative methods of encryption and certificate management to facilitate trusted communication. Authorised system participants use digital certificates issued by an SCMS to authenticate and validate the safety and mobility messages [125]. In 2019, C-Roads reported [126] the successful implementation of the European C-ITS CP and SP, which involved the full lifecycle security of C-ITS stations, including the process steps of registration, start-up, operation (including updates), and decommissioning, as well as secure management of the certificate materials at the end of their lifetime. This serves as a valuable example of the effective application of C-ITS security in real-world projects.
At present, standardisation organisations such as ETSI, IEEE, and 3GPP have carried out standardisation work for V2X communication security. IEEE 1609.2 defines V2X-specific message formats and certificate formats, as well as selected cryptographic algorithms [3]. ETSI TS 102 940 defines the C-ITS security architecture [127]. ETSI TS 102 941 builds a PKI for C-ITS based on IEEE 1609.2 [123]. 3GPP SA WG3 - Security and Privacy, has carried out research and standard formulation work on LTE-V2X security, including TR 33.885 [128] and TS 33.185 [128].
The European Union C-ITS Security Credential Management System (EU SCMS) [129] is the leading security framework in Europe, and even in the world. The European Union has carried out the technical verification of the ETSI C-ITS security architecture through the PRESERVE project [130]. In the USA, the initial Security Credential Management System (SCMS) proof-of-concept (POC) was developed jointly through the USDOT and the Crash Avoidance Metrics Partnership (CAMP) [125]. The SCMS POC was formally closed in October 2018. So far, the nationwide security platform has not widely been deployed in the USA.
Figure 12 Why PKI is needed [124]
10.3 Public Key Infrastructure (PKI)
Public key infrastructure (PKI) allows for the encryption of digital keys and certificates, generally using X.509 certificate format. The certificate policy [131] of EU SCMS defines requirements for the management of public key certificates for C-ITS applications by issuing entities and their usage by end entities in Europe. The operational roles depicted in a trust model are shown in Table 33.
Table 33 Operation roles in the trust model
Operation Role Description
Root Certification Authority (RCA)
The RCA is the highest-level CA in the certification hierarchy. It provides EA and AA with proof that it may issue enrolment credentials, respectively authorisation tickets
Enrolment Authority (EA) The security management entity is responsible for the life cycle management of enrolment credentials. Authenticates an ITS-S and grants it access to ITS communications
Authorisation Authority (AA)
Sending C-ITS Station
Relaying C-ITS Station
Receiving C-ITS Station
The security management entity is responsible for issuing and monitoring the use of authorisation tickets. Provides an ITS-S with authoritative proof that it may use specific ITS services
Acquires rights to access ITS communications from the Enrolment Authority
Negotiates rights to invoke ITS services from Authorisation Authority
Sends single-hop and relayed broadcast messages
Receives broadcast messages from the sending C-ITS Station and forwards them to the receiving ITS-S if required
Receives broadcast messages from the sending or relaying C-ITS Station
Manufacturer / Operator Installs necessary information for security management in the C-ITS Station at production and provides updates during its operation
Figure 13 shows a PKI architecture used by EU SCMS. In a PKI, the root of the certificate hierarchy is the Root Certification Authority (RCA), which provides trust to the entire certificate chain. The digital certificates are issued by two sub-certification authorities (SCAs) under the RCA: the Enrolment Authority (EA) and the Authorisation Authority (AA). The EA is responsible for issuing long-term certificates, called Enrolment Certificates (ECs), which serve as unique identifications for individuals, similar to human IDs. The AA, on the other hand, issues short-term certificates called Authorisation Tickets (ATs) for temporary access to C-ITS communications, similar to bus tickets. Vehicles in C-ITS communications typically use ATs frequently, with an average usage of 60-100 ATs per week, while C-ITS RSUs use around 1 AT per week. To protect the privacy of individuals, ATs do not have unique names, which prevents the linking of ATs with ECs. Privacy is further ensured by dynamically changing the driver's identity using a Pseudonymous Certificate (PC) with an expiration date.
Each vehicle manages its own list of PCs, which are valid for a limited time. When a PC expires, the vehicle switches to a new one.
As shown in Figure 13, the middle blue zone is the EU RCA, Enrolment Authority and Authorisation Authority currently used by EU SCMS. Any station that receives this blue PKI digital certificate will trust other C-ITS stations. At the top of this architecture is the C-ITS Point of Contact (CPOC), European Certificate Trust List (ECTL) and Trust List Manager (TLM). ECTL can reduce the trait of the digital certificates of the RCA.
Security mechanisms do allow vehicles from other jurisdictions to be part of the local C-ITS system. The green zone indicates EAs and AAs that can share the EU RCA. For example, the French Government does not need to establish a separate RCA but can directly use the existing EU RCA to establish its independent external EA and AA. Digital certificates issued to the green zone can also be trusted in the blue zone.
An External RCA can be thought of as offering a PKI to car manufacturers. For instance, Toyota has its own External RCA, similar in structure to what's represented in the blue zone. By delivering the certificate to the Toyota cars, the vehicles in the yellow zone will usually only trust the digital certificate and the trust of the RCA in the yellow zone. Originally,
Figure 13 Architecture Schemes and Services [124]
vehicles in the yellow zone would not be able to trust messages signed by vehicles in the blue zone.
To allow mutual trust, the External RCA can process a registration to the CPOC to ensure equal trust in the blue zone. Then, the yellow zone will be able to trust the blue zone and vice versa. For example, a European RCA can trust Toyota RCA’s digital certificate.
L0
What? Testing & integration works
Less mature pilot projects
How? Low requirements
Easy registration process
When? Now
L0 ECTL published on 15 September 2023
L1
Stable pilot operation Production, not Certificate Policy (CP) and Security Policy (SP) certified
CP/SP with exceptions Intermediate registration process
Once L1 ECTL is published
L2
Full-scale EU CP/SPcertified production
CP/SP compliance & audit
Strict registration process
As soon as C-ITS stations can be SPcompliant
Presently, three levels of maturity and service are defined: L0, L1 and L2, as shown in Table 34. In Europe, L0 has been deployed; L1 is in progress; L2 is in full production which needs PKI to be 100% compliant with the certificate policy and audit it required. Currently, the L1 ECTL is not yet available, and a specific release date has not been provided. Once it is ready, the PKI platform for L1 will become accessible to the European Union.
While Australia can certainly gain valuable insights from the European SCMS PKI, the creation of a native PKI infrastructure offers significant advantages for several reasons:
National Security and Sovereignty: Managing its own PKI allows Australia to maintain control over its national security infrastructure. Relying on a foreign PKI, such as the European SCMS (Security Credential Management System), may pose risks in terms of dependence on external entities for critical infrastructure security.
Data Privacy Control: With its own PKI, Australia can ensure strict adherence to its data privacy laws and regulations. This aspect is particularly vital in the realm of CITS, where handling sensitive user information necessitates robust privacy and data protection measures.
Customisation for Domestic Requirements: Australia needs a custom PKI tailored to its unique regulatory and operational requirements. A domestically developed PKI would better meet local requirements and integrate more effectively with existing Australian C-ITS infrastructure, ensuring optimised compatibility.
Regulatory Compliance: Telecommunications and cybersecurity regulations can vary significantly from one country to another. By developing its own PKI, Australia ensures that it remains compliant with its regulatory framework, avoiding potential legal and operational conflicts that might arise from using an international system.
Table 34 EU C-ITS service levels
In summary, the creation of a native PKI infrastructure offers significant advantages in terms of security, privacy, control, customisation, and regulatory alignment, thereby serving national security and sovereignty more effectively.
10.4 Conclusion
With the rapid evolution of automotive technology, vehicle manufacturers need robust cybersecurity and software update management systems to protect against potential cyber threats. UN/ECE Regulations No. 155 and No. 156 serve as the legal framework for such systems, requiring security measures from the earliest stages of vehicle development. They enforce a Cybersecurity Management System (CSMS) for new vehicle type approvals, which should manage cyber risks and ensure secure software distribution. UN-R 156 establishes processes for secure and reliable software updates. Undeniably, guaranteeing the security of Australia's C-ITS system is critical. Since C-ITS heavily depends on secure vehicle communication, the cybersecurity protocols and software updating systems outlined in these regulations serve as a strong line of defence. For the construction of a solid and secure CITS system in Australia, we suggest prioritising the following actions:
Giving Priority to PKI for Safeguarding C-ITS Deployment: The creation of a resilient and secure PKI system is pivotal in the construction of a prosperous C-ITS platform in Australia. The choice of communication technology, be it DSRC or C-V2X, doesn't restrict PKI's versatility and standalone implementation. In the immediate future, Australia could link up with the European SCMS PKI to leverage its L0/L1 services for testing or gaining insights. However, a longer vision should aim for Australia to construct its own distinct PKI infrastructure, serving as a C-ITS backbone, similar to what Germany has done. By establishing a PKI system similar to the EU's SCMS, with a focus on stringent security measures, Australia stands to gain from agile solutions capable of swiftly addressing threats while maintaining alignment with the latest global security best practices and compliance standards.
Across Jurisdictions Security Trust Model: The PKI security framework is integral in fostering the interoperability of C-ITS systems across multiple jurisdictions. A wellstructured PKI enables vehicles from diverse regions such as the USA or European nations to integrate seamlessly into the C-ITS platform. It ensures every device in the network adheres to set standards and communicates smoothly with other devices, irrespective of the manufacturer or service provider. This is made possible when all External RCAs register with the CPOC, thereby establishing a mutual trust. For instance, in countries like Germany and France, the SCMS CPOC allows safe information exchange between C-ITS stations, promoting smooth communication and interoperability. Centralisation of this platform gives a common point of reference that guarantees data exchange integrity and security. By fostering interoperability among various C-ITS systems, the PKI security framework can enhance the overall value and benefits of the C-ITS ecosystem across jurisdictions and vehicle organisations. By enforcing uniform C-ITS security standards in Australia, the universal PKI will cater to varying vehicle organisations that may not be under Commonwealth management.
Aiming at Centralised SCMS: Establishing a unified SCMS across all Australian states and territories will ensure smooth interoperability and consistent data management, bolstering public confidence in C-ITS technologies. Centralised
security frameworks, compared to their distributed counterparts, offer enhanced protection against cyber threats, as they are easier to monitor and manage. It is vital to strategically address data privacy concerns. Any data held should be purged after a set duration, and regulations must precisely outline the permitted use of this data by enforcement agencies and telecom companies to avert misuse or unauthorised access. Meeting PKI standards can be a difficult task for vehicle organisations outside of Commonwealth governance. For these bodies, Australian governments should consider enacting rigorous legislation that mandates PKI implementation. The initiation of public-private partnerships will be essential, bridging the gap between public sector units and private vehicle organisations, and aiding the shift to a centralised system where PKI is effectively integrated. By spearheading these initiatives, Australia is positioning itself at the forefront of digital security and privacy protection.
To sum up, Australia's commitment to data security and privacy is evident in its push for widespread PKI application across diverse sectors. This commitment should be showcased via campaigns, regulatory blueprints, and financial motivators to encourage organisations to harness PKI to fortify C-ITS.
11 Envisioning Future C-ITS Devices for Road Users
Drawing upon the findings in this report, we envision a future for C-ITS devices that markedly enhance both safety and the overall driving experience for road users.
C-ITS Applications via Long-Range Communication for the General Public
In addition to vehicle-based technologies, we foresee the widespread adoption of mobile applications for long-range communication among the general public. These smartphone and mobile app-based C-ITS solutions are expected to feature applications that deliver realtime information about traffic conditions, crossing safety, and road hazard warnings to users, without requiring specific short-range communication hardware such as DSRC or C-V2X. Edge computing technologies are anticipated to support these applications, ensuring users receive essential warnings with satisfactory performance [34].
In-Vehicle Short-Range Communication Devices
A core component of this vision involves the integration of in-vehicle short-range communication devices. Whether installed by OEMs or as aftermarket onboard devices, these systems will enable vehicles to communicate with each other or directly with infrastructure elements, such as traffic lights and electronic signs. This communication is designed to occur at an extremely low latency, enabling the rapid conveyance of critical warnings to both drivers and automated systems.
Specialised C-ITS for Vulnerable Road Users (VRUs)
Furthermore, we anticipate the prominent emergence of C-ITS devices specifically designed for Vulnerable Road Users (VRUs), including pedestrians and cyclists. These devices, potentially resembling the size of mobile phones, such as Spoke devices, will likely include integrated C-V2X PC5, 4G/5G, and camera functionalities. These devices are forecasted to act as a significant driving force behind C-ITS adoption, thereby encouraging automakers that are currently undecided to integrate C-ITS technologies into their Advanced Driver Assistance Systems (ADAS) [35, 36].
Personalised Journey Experience Based on User Preferences
The integration of mobile devices and wearable technology is seen as paramount for refining the CAV user experience. Linking passenger smartphones to CAV systems will allow for customisation of settings and prioritisation of journey preferences. Additionally, integrating data from wearable devices could provide insights into a passenger’s health status, potentially leading CAVs to make adjustments for enhanced passenger comfort or in critical situations, to alert emergency services.
IoT Technology Integration
At the heart of this vision lies seamless interoperability between a diverse array of IoT technologies, grounded in the high-speed capabilities of C-ITS. Vehicles equipped with builtin IoT sensors will be able to communicate with complementary sensors positioned alongside roads, thus alerting drivers to challenging conditions, such as traffic jams, icy
patches, waterlogged areas, or obstructions. Additionally, IoT sensors at crosswalks could detect pedestrian movements and alert drivers, prompting appropriate vehicle responses through activating warning signals. Within urban environments, smart city Narrowband Internet of Things (NB-IoT) sensors in parking zones can offer real-time updates on space availability, significantly aiding CAV navigation [37].
5G Connectivity for Connected Vehicles
In this envisioned future, 5G connectivity is projected to be a requisite for connected vehicles. This essential upgrade will empower remote intervention capabilities and facilitate consistent software updates, thereby aligning vehicles with the evolving regulations for CAVs [21, 38].
In conclusion, to maintain the adaptability and diversity of C-ITS and to accommodate the varied needs and preferences of a broad range of end users, a multi-technology ecosystem is required.
References
[1] "Principles for a National Approach to Co-operative Intelligent Transport Systems (C-ITS) in Australia," DITDRCA, 2024. [Online]. Available: https://www.infrastructure.gov.au/infrastructure-transport-vehicles/transport-strategypolicy/office-future-transport-technology/principles-national-approach-co-operativeintelligent-transport-systems-c-its-australia
[2] IEEE Standard for Information technology-- Local and metropolitan area networks-- Specific requirements-- Part 11: Wireless LAN Medium Access Control (MAC) and Physical Layer (PHY) Specifications Amendment 6: Wireless Access in Vehicular Environments, IEEE, 2010. [Online]. Available: https://standards.ieee.org/ieee/802.11p/3953/
[3] IEEE 1609.2 Standard for Wireless Access in Vehicular Environments (WAVE)--Certificate Management Interfaces for End Entities, IEEE, 2020. [Online]. Available: https://standards.ieee.org/ieee/1609.2.1/10172/
[4] IEEE Guide for Wireless Access in Vehicular Environments (WAVE) - Architecture, IEEE, 2013. [Online]. Available: https://standards.ieee.org/ieee/1609.0/5660/
[5] "Next Generation V2X – IEEE 802.11bd as fully backward compatible evolution of IEEE 802.11p," C2C-CC, 2023. [Online]. Available: https://www.car-2car.org/fileadmin/documents/General_Documents/C2CCC_WP_2098_IEEE_802.11bd_TheV 2XEvolution_V1.0.pdf
[6] 3GPP Release 15, 3GPP, 2019. [Online]. Available: https://www.3gpp.org/release-15
[7] "Cellular-V2X Technology Overview," Qualcomm, 2019. [Online]. Available: https://www.qualcomm.com/content/dam/qcomm-martech/dm-assets/documents/cv2x_technology.pdf
[8] "V2X Functional and Performance Test Report; Test Procedures and Results," 5GAA, 2019. [Online]. Available: https://5gaa.org/content/uploads/2018/11/5GAA_P-190033_V2XFunctional-and-Performance-Test-Report_final-1.pdf
[9] "A visionary roadmap for advanced driving use cases, connectivity technologies, and radio spectrum needs - 2022 " 5GAA, 2022. [Online]. Available: https://5gaa.org/content/uploads/2022/11/5GAA_White-Paper_Roadmap_2022.pdf
[10] "FACT SHEET: Modernizing the 5.9 GHz Band," in "First Report and Order, Further Notice of Proposed Rulemaking, and Order of Proposed Modification –ET Docket No. 19-138," Federal Communications Commission, 2020.
[11] ECC Recommendation (08) 01 on the use of the band 5855-5875 MHz for Intelligent Transport Systems (ITS), E. E. C. Committee, 2020. [Online]. Available: https://docdb.cept.org/download/798c1836-20c6/REC0801.pdf
[12] ECC Decision (08) 01on the harmonised use of SafetyRelated Intelligent Transport Systems (ITS) in the 5875-5935 MHz frequency band, E. E. C. Committee, 2020. [Online]. Available: https://docdb.cept.org/download/b470d271-048b/ECCDEC0801.PDF
[13] Commission Implementing Decision (EU) 2020/ 1426 of 7 October 2020 on the harmonised use of radio spectrum in the 5 875-5 935 MHz fr equency band for safety-related applications of intelligent transport systems (ITS) and repealing Decision 2008/671/EC (notified under document C(2020) 6773), 2020. [Online]. Available: http://data.europa.eu/eli/dec_impl/2020/1426/oj
[14] "Study of spectrum needs for safety related intelligent transportation systems- day 1 and advanced use cases," 5GAA, 2021. [Online]. Available: https://5gaa.org/content/uploads/2021/10/5GAA_Day1_and_adv_Use_Cases_Spectrum_Ne eds_Study_V2.0.pdf
[15] "Five-year spectrum outlook 2022–27 and 2022–23 work program," ACMA 2022. [Online]. Available: https://www.acma.gov.au/sites/default/files/2022-03/Draft%20FYSO%20202227%20for%20consultation.pdf
[16] Radiocommunications (Intelligent Transport Systems) Class Licence 2017 made under section 132 of the Radiocommunications Act 1992, ACMA, 2021. [Online]. Available: https://www.legislation.gov.au/Details/F2021C01285
[17] "Five-year spectrum outlook 2023–28 and 2023–24 work program Draft for consultation," ACMA, 2023. [Online]. Available: https://www.acma.gov.au/consultations/2023-03/draftfive-year-spectrum-outlook-2023-28
[18] "Spectrum for Intelligent Transport Systems GSMA Public Policy Position," GSMA, 2017. [Online]. Available: https://www.gsma.com/spectrum/wpcontent/uploads/2018/12/Spectrum-for-Intelligent-Transport-Systems.pdf
[19] "Road Operator Use Case Modelling and Analysis," 5GAA, 2023. [Online]. Available: https://5gaa.org/road-operator-use-case-modelling-and-analysis/
[20] "Driverless test drives in China." Audi https://www.audi.com/en/innovation/autonomousdriving/driverless-test-drives-in-china.html (accessed 13 Feb., 2023).
[21] "German Federal Council clears the way for autonomous driving." https://www.jdsupra.com/legalnews/german-federal-council-clears-the-way-2860136/ (accessed 13 Sep. , 2023).
[22] Motor vehicle safety and automated/connected vehicles, 2022. [Online]. Available: https://single-market-economy.ec.europa.eu/sectors/automotive-industry/vehicle-safetyand-automatedconnected-vehicles_en
[23] "Annual pilot overview report 2021 " C-Roads, 2022. [Online]. Available: https://www.croads.eu/fileadmin/user_upload/media/Dokumente/M22_Annual_pilot_overview_report_2 021_v2.1_FINAL.pdf
[24] "Ipswich Connected Vehicle Pilot." https://www.qld.gov.au/transport/projects/cavi/ipswichconnected-vehicle-pilot (accessed 13 Feb., 2023).
[25] "ANNUAL REPORT 2022 5GAA - Connected Mobility For People, Vehicles and Transport Infrastructure," 5GAA 2022. [Online]. Available: https://5gaa.org/content/uploads/2023/06/5gaa-ar-2022.pdf
[26] "Verizon and Nissan test C-V2X proof of concept." https://www.autonomousvehicleinternational.com/news/v2x-news/verizon-and-nissantest-c-v2x-proof-of-concept.html (accessed 13 Feb, 2023).
[27] "Android V2X SDK User Guide." Vodafone https://github.com/Vodafone/HelloV2XWorldAndroid (accessed 14 May, 2023).
[28] "ITS America National V2X Deployment Plan: An Infrastructure & Automaker Collaboration " ITS America 2023.
[29] ETSI TS 103 300-3 V2.1.1 (2020-11) Intelligent Transport Systems (ITS); Vulnerable Road Users (VRU) awareness; Part 3: Specification of VRU awareness basic service; Release 2 ETSI, 2020. [Online]. Available:
https://www.etsi.org/deliver/etsi_ts/103300_103399/10330003/02.01.01_60/ts_10330003 v020101p.pdf
[30] "White Paper: Path Prediction for PTWs," C2C-CC, CMC, 2023. [Online]. Available: https://www.car-2car.org/fileadmin/documents/General_Documents/C2CCC_CMC_WP_2303_PTW_PathPredi ction_V1.1.pdf
[31] "Research Report AP-R654-21: Future Vehicles Forecasts Update 2031 Addendum to Future Vehicles 2030," Austroads, 2021. [Online]. Available: https://austroads.com.au/publications/connected-and-automated-vehicles/ap-r65421/media/AP-R654-21_Future_Vehicles_2031.pdf
[32] "Researchers identify that DSRC field infrastructure deployment costs." https://www.itskrs.its.dot.gov/its/benecost.nsf/ID/e3e596296c66702d85257d4f0069f496 (accessed 13 Feb. , 2023).
[33] "C-ITS Vehicle to Infrastructure Services: how C-V2X technology completely changes the cost equation for road operators white paper here.," 5GAA, 2019. [Online]. Available: https://5gaa.org/5gaa-releases-white-paper-on-the-benefits-of-using-existing-cellularnetworks-for-the-delivery-of-c-its/
[34] L. BUTCHER. "Verizon and Nissan test C-V2X proof of concept." https://www.autonomousvehicleinternational.com/news/v2x-news/verizon-and-nissantest-c-v2x-proof-of-concept.html (accessed 13 Feb., 2023).
[35] "Spoke Chooses T IoT from T Mobile and Deutsche Telekom to Pave the Way for Safer Cycling." https://www.t-mobile.com/news/business/spoke-chooses-t-iot-fromt-mobile-anddeutsche-telekomto-pave-the-way-for-safer-cycling (accessed 30 June, 2023).
[36] "Spoke Partners with Curiosity Lab to Test and Develop the First Vulnerable Road User and C-V2X Technologies to Improve Roadway Safety." https://www.prnewswire.com/newsreleases/spoke-partners-with-curiosity-lab-to-test-and-develop-the-first-vulnerable-roaduser-and-c-v2x-technologies-to-improve-roadway-safety-301803771.html (accessed 30 June, 2023).
[37] "Audi and Peachtree Corners collaborate on C-V2X." https://www.itsinternational.com/its7/news/audi-and-peachtree-corners-collaborate-c-v2x (accessed 30 June 2023).
[38] "Audi of America, Verizon partner to bring 5G to vehicle lineup " (accessed 13 Sep. , 2023).
[39] "C-ITS: Cooperative Intelligent Transport Systems and Services." Car-2-Car. https://www.car2-car.org/about-c-its (accessed 13 Feb., 2023).
[40] ISO 21217:2020(en) Intelligent transport systems — Station and communication architecture, ISO, 2020. [Online]. Available: https://www.iso.org/obp/ui/#iso:std:iso:21217:ed-3:v1:en
[41] "V2X in the Connected Car of the Future." QORVO. https://www.qorvo.com/designhub/blog/v2x-in-the-connected-car-of-the-future (accessed 13 Feb., 2023).
[42] "CONNECTED MOBILITY C-V2X explained." 5GAA (accessed 13 Feb., 2023).
[43] ISO/TR 21186-2:2021 Cooperative intelligent transport systems (C-ITS) — Guidelines on the usage of standards — Part 2: Hybrid communications, ISO, 2021. [Online]. Available: https://www.iso.org/standard/79948.html
[44] T. Inoue, "5G NR Release 16 and Millimeter Wave Integrated Access and Backhaul," in 2020 IEEE Radio and Wireless Symposium (RWS), 26-29 Jan. 2020 2020, pp. 56-59, doi: 10.1109/RWS45077.2020.9050108.
[45] "Service performance measurement methods over 5G experimental networks - ICT-19 performance KPIs," 5GPP, 2021. [Online]. Available: https://doi.org/10.5281/zenodo.4748385
[46] "Connecting Vehicles Today and in the 5G Era with C-V2X (Cellular Vehicle-to-Everything)," GSMA, 2019. [Online]. Available: https://www.gsma.com/iot/wpcontent/uploads/2019/08/Connecting-Vehicles-Today-and-in-the-5G-Era-with-C-V2X.pdf
[47] "5GAA LIVE DEMOS SHOW C-V2X AS A MARKET REALITY." https://iotautomotive.news/5gaa-live-demos-show-c-v2x-as-a-marketreality/#:~:text=Prominent%20members%20and%20partners%20of,Vehicle%2Dto%2DNetw ork%20(V2N (accessed 13 Feb., 2023).
[48] "5GAA shares latest C-V2X developments at ITS World Congress (Hamburg)." FOX29 WFLX. https://www.wflx.com/prnewswire/2021/10/14/5gaa-shares-latest-c-v2x-developments-itsworld-congress-hamburg-5g-will-have-most-revolutionary-impact-by-reducing-accidentssaving-millions-lives/?outputType=amp (accessed 13 Feb., 2023).
[49] J. Qin and J. Liu, "Multi-Access Edge Offloading Based on Physical Layer Security in C-V2X System," IEEE Transactions on Vehicular Technology, pp. 1-1, 2022, doi: 10.1109/TVT.2022.3164896.
[50] ETSI EN 302 663 V1.2.0 (2012-11) Intelligent Transport Systems (ITS); Access layer specification for Intelligent Transport Systems operating in the 5 GHz frequency band, ETSI, 2012. [Online]. Available: https://www.etsi.org/deliver/etsi_en/302600_302699/302663/01.02.00_20/en_302663v01 0200a.pdf
[51] "Cooperative, connected and automated mobility (CCAM)." https://transport.ec.europa.eu/transport-themes/intelligent-transportsystems/cooperative-connected-and-automated-mobility-ccam_en (accessed 13 Feb., 2023).
[52] P. Valerio. "NXP, Volkswagen, and several European projects bet on WiFi DSRC for V2X." https://iot.eetimes.com/nxp-volkswagen-and-several-european-projects-bet-on-wifi-dsrcfor-v2x/ (accessed 13 Feb., 2023).
[53] M. COSTELLO. "VFACTS: Australia's new car sales results for 2022." https://www.carexpert.com.au/car-news/vfacts-australias-new-car-sales-results-for-2022 (accessed 13 Feb., 2023).
[54] "FCAI releases new car sales figures for November 2022." https://www.fcai.com.au/news/index/view/news/786 (accessed 13 Feb., 2023).
[55] ETSI EN 303 798 V2.0.1 (2023-10) Intelligent Transport Systems (ITS); LTE-V2X and NR-V2X Access layer specification for Intelligent Transport Systems operating in the 5 GHz frequency band; Release 2 ETSI, 2023. [Online]. Available: https://www.etsi.org/deliver/etsi_en/303700_303799/303798/02.00.01_20/en_303798v02 0001a.pdf
[56] ETSI TS 103 836-4-3 V2.1.1 (2023-10) Intellig ent Transport Systems (ITS); Vehicular Communications; GeoNetworking; Part 4: Geographical addressing and forwarding for pointto-point and point-to-multipoint communications; Sub-part 3: Media-dependent functionalities for NR-V2X PC5 and LTE-V2X PC5; Release 2 ETSI, 2023. [Online]. Available:
https://www.etsi.org/deliver/etsi_ts/103800_103899/1038360403/02.01.01_60/ts_103836 0403v020101p.pdf
[57] "Cooperative intelligent transport systems (C-ITS) Guidelines on the usage of standards," ISO/CEN, 2020. [Online]. Available: https://www.itsstandards.eu/app/uploads/sites/14/2020/10/C-ITS-Brochure-2020FINAL.pdf
[58] Intelligent Transport Systems (ITS); Vehicular Communications; Basic Set of Applications; Definitions, ETSI, 2009. [Online]. Available: https://www.etsi.org/deliver/etsi_tr/102600_102699/102638/01.01.01_60/tr_102638v0101 01p.pdf
[59] "5G Americas White Paper: Cellular V2X Communications Towards 5G," 2018. [Online]. Available: https://www.5gamericas.org/wpcontent/uploads/2019/07/2018_5G_Americas_White_Paper_Cellular_V2X_Communications _Towards_5G__Final_for_Distribution.pdf
[60] "C-V2X technology." Autotalks. https://auto-talks.com/technology/c-v2x-technology/ (accessed 13 Feb., 2023).
[61] "Introduction to Cellular V2X," Qualcomm, 2019. [Online]. Available: https://www.qualcomm.com/content/dam/qcomm-martech/dm-assets/documents/cv2x_intro.pdf
[62] "Cellular Vehicle-to-Everything (C-V2X) Enabling Intelligent Transport " GSMA, 2017. [Online]. Available: https://www.gsma.com/iot/wp-content/uploads/2017/12/C-2VXEnabling-Intelligent-Transport_2.pdf
[63] 3GPP, "Technical Report 21.916 Release 16 Description; Summary of Rel-16 Work Items." [Online]. Available: https://portal.3gpp.org/desktopmodules/Specifica tions/SpecificationDetails.aspx?specificati onId=3493
[64] "ITS Stack," Qualcomm, 2019. [Online]. Available: https://www.qualcomm.com/content/dam/qcomm-martech/dm-assets/documents/cv2x_its_stack.pdf
[65] G. Naik, B. Choudhury, and J. M. Park, "IEEE 802.11bd & 5G NR V2X: Evolution of Radio Access Technologies for V2X Communications," IEEE Access, vol. 7, pp. 70169-70184, 2019, doi: 10.1109/ACCESS.2019.2919489.
[66] "Global V2X Communication Processor." Autotalks. https://autotalks.com/products/craton2/ (accessed 13 Feb., 2023).
[67] Z. H. Mir, J. Toutouh, F. Filali, and Y. B. Ko, "Enabling DSRC and C-V2X Integrated Hybrid Vehicular Networks: Architecture and Protocol," IEEE Access, vol. 8, pp. 180909-180927, 2020, doi: 10.1109/ACCESS.2020.3027074.
[68] E. Moradi-Pari, D. Tian, M. Bahramgiri, S. Rajab, and S. Bai, "DSRC Versus LTE-V2X: Empirical Performance Analysis of Direct Vehi cular Communication Technologies," IEEE Transactions on Intelligent Transportation Systems, vol. 24, no. 5, pp. 4889-4903, 2023, doi: 10.1109/TITS.2023.3247339.
[69] "C-V2X Technical Performance," Qualcomm Technologies, Inc. [Online]. Available: https://www.qualcomm.com/content/dam/qcomm-martech/dm-assets/documents/cv2x_technical_performance_faq.pdf
[70] H. I. Abbasi, R. Gholmieh, T. V. Nguyen, S. Patil, and J. Misener, "LTE-V2X (C-V2X) Performance in Congested Highway Scenarios," in ICC 2022 - IEEE International Conference on Communications, 16-20 May 2022 2022, pp. 303-308, doi: 10.1109/ICC45855.2022.9838706.
[71] "C-V2X performance in highly congested highway scenarios." Qualcomm. https://www.qualcomm.com/news/onq/2021/01/c-v2x-performance-highly-congestedhighway-scenarios (accessed 13 Feb., 2023).
[72] "Top 10 things you may not know about C-V2X." https://www.qualcomm.com/news/onq/2017/10/top-10-things-you-may-not-know-aboutc-v2x (accessed 13 Feb., 2023).
[73] 3GPP TS 23.287 V16.5.0 (2020-12) 3rd Generation Partnership Project; Technical Specification Group Services and System Aspects; Architecture enhancements for 5G System (5GS) to support Vehicle-to-Everything (V2X) services (Release 16), 3GPP, 2020. [Online]. Available: https://www.3gpp.org/ftp//Specs/archive/23_series/23.287/23287-g50.zip
[74] "Predictive QoS and V2X Service Adaption," 5GAA, 2023. [Online]. Available: https://5gaa.org/content/uploads/2023/01/5gaa-wi-presa-tr-predictive-qos-and-v2xservice-adaptation.pdf
[75] "Ecosystem for fully operational C-ITS service delivery-The infrastructure perspective " WG1 - C-ITS Organisation C-Roads, 2022.
[76] "Guidance for day 2 and beyond roadmap CAR 2 CAR Communication Consortium," 2021. [Online]. Available: https://www.car-2car.org/fileadmin/documents/General_Documents/C2CCC_WP_2072_RoadmapDay2AndBey ond_V1.2.pdf
[77] "Use Cases CAR 2 CAR Communication Consortium," CAR 2 CAR Communication Consortium, 2023. [Online]. Available: https://www.car-2car.org/fileadmin/documents/General_Documents/C2CCC_UC_2097_UseCases_V1.0.pdf
[78] "C-V2X Use Cases:Examples and Service Level Requirements White Paper " 5GAA, 2019. [Online]. Available: https://5gaa.org/content/uploads/2019/07/5GAA_191906_WP_CV2X_UCs_v1-3-1.pdf
[79] T. Linget, "C-V2X Use Cases Volume II:Examples and Service Level Requirements White Paper " 5GAA, 2020. [Online]. Available: https://5gaa.org/content/uploads/2020/10/5GAA_WhitePaper_C-V2X-Use-Cases-Volume-II.pdf
[80] "C-V2X Use Cases and Service Level Requirements Volume I," 5GAA 2020. [Online]. Available: https://5gaa.org/content/uploads/2020/12/5GAA_T-200111_TR_CV2X_Use_Cases_and_Service_Level_Requirements_Vol_I-V3.pdf
[81] 3GPP TR 22.885 V14.0.0 (2015-12) 3rd Generation Partnership Project; Technical Specification Group Services and System Aspects; Study on LTE support for Vehicle to Everything (V2X) services (Release 14), 3GPP, 2015. [Online]. Available: https://www.3gpp.org/ftp/Specs/archive/22_series/22.885/
[82] 3GPP TR 22.886 V16.2.0 (2018-12) 3rd Generation Partnership Project; Technical Specification Group Services and System Aspects; Study on enhancement of 3GPP Support for 5G V2X Services (Release 16), 3GPP, 2018. [Online]. Available: https://www.3gpp.org/ftp/Specs/archive/22_series/22.886/
[83] K. M. Andrew Turley, Alessio Filippi, Vincent Martinez, "C-ITS: Three observations on LTE-V2X and ETSI ITS-G5—A comparison ", 2018. [Online]. Available: https://www.nxp.com.cn/docs/en/white-paper/CITSCOMPWP.pdf
[84] Z. Ali, S. Lagén, L. Giupponi, and R. Rouil, "3GPP NR V2X Mode 2: Overview, Models and System-Level Evaluation," IEEE Access, vol. 9, pp. 89554-89579, 2021, doi: 10.1109/ACCESS.2021.3090855.
[85] "Australia’s Largest Connected Vehicle Trial A Model for Others to Follow." Cohda Wireless https://www.cohdawireless.com/australias-largest-connected-vehicle-trial-a-model-forothers-to-follow/ (accessed 30 June 2023).
[86] "Towards Zero: Creating safer roads with cellular-V2X in Australia," 2020. [Online]. Available: https://www.ericsson.com/4900e3/assets/local/cases/customercases/2020/towards_zero_safer_roads-with-cellular-v2x.pdf
[87] "Autotalks’ C-V2X Solution is Market-Ready for China, Following Successful Interoperability and Conformance Testing with Multiple OEMs, Tier1s and the Broader Ecosystem." https://auto-talks.com/autotalks-c-v2x-solution-is-market-ready-for-china-followingsuccessful-interoperability-and-conformance-testing-with-multiple-oems-tier1s-and-thebroader-ecosystem/ (accessed 10 Oct, 2023).
[88] "C-V2X global market momentum continues to accelerate." https://www.qualcomm.com/news/onq/2021/01/c-v2x-global-market-momentumcontinues-accelerate (accessed 10 Oct. , 2023).
[89] "Innovation ecological development of Internet of Vehi cles," CAICT, 2022. [Online]. Available: http://www.caict.ac.cn/kxyj/qwfb/ztbg/202201/P020220128564097792425.pdf
[90] "Sino-German Intelligent Connected Vehicle Sichuan Test Base Settled in Chengdu." http://zw.china.com.cn/2022-05/16/content_78220518.html?f=pad&a=true (accessed Feb 14, 2023).
[91] "China-Germany Internet of Vehicles (Intelligent Connected Vehicle) C-V2X Mass Production Research Report," CAICT, 2022. [Online]. Available: http://www.caict.ac.cn/kxyj/qwfb/ztbg/202209/t20220920_409171.htm
[92] Gabriella. "Ford China deploys C-V2X system in Wuhan, Nanjing." https://autonews.gasgoo.com/china_news/70021950.html (accessed 13 Feb., 2023).
[93] "Device to Device Communication in LTE Whitepaper." [Online]. Available: https://scdn.rohdeschwarz.com/ur/pws/dl_downloads/dl_application/application_notes/1ma264/1MA264_0e _D2DComm.pdf
[94] "NXP, Volkswagen and Partners Continue to Accelerate the V2X Rollout." https://www.nxp.com/company/blog/nxp-volkswagen-and-partners-continue-to-acceleratethe-v2x-rollout:BL-THE-V2X-ROLLOUT (accessed 13 Feb., 2023).
[95] "Recent Federal Communications Commission Public Notices on the 5.9 GHz Safety Band." U.S. DoT. https://www.its.dot.gov/press/2022/safetyband_fcc.htm (accessed Feb 14, 2023).
[96] "Before the Federal Communications Commission Washington, D.C. 20554- In the Matter of Request for Waiver of 5.9 GHz Band Rules to Permit Initial Deployment of Cellular VehicletoEverything Technology, ET Docket No. 19-138," FCC, 2023. [Online]. Available: https://docs.fcc.gov/public/attachments/DA-23-343A1.pdf
[97] R. Staff. "German bosses urge govt to stop EU proposal on connected cars " https://www.reuters.com/article/eu-autos-technology-idUKL3N21X36C (accessed 15 June 2023).
[98] P. G. Bob Banks. "Cars that can talk to ever ything? Our test shows the technology is ready to go." https://www.vodafone.com/business/news-and-insights/blog/gigabit-thinking/carsthat-can-talk-to-everything-our-test-shows-the-technology-is-ready-to-go (accessed 13 Feb., 2023).
[99] "Verizon-Cisco Collaboration Advances Autonomous Vehicle Tech with Streamlined Architecture." Cisco. https://newsroom.cisco.com/c/r/newsroom/en/us/a/y2022/m03/verizon-ciscocollaboration-advances-autonomous-vehicle-tech-with-streamlinedarchitecture.html?dtid=oblgzzz000659 (accessed 10 May, 2023).
[100] "How connectivity can make city traffic safer and more efficient." https://www.boschpresse.de/pressportal/de/en/how-connectivity-can-make-city-traffic-safer-and-moreefficient-258560.html (accessed 23 Oct., 2023).
[101] "Forecasting uptake of driver assistance technologies in Australia," BITRE, 2021. [Online]. Available: https://www.bitre.gov.au/sites/default/files/documents/research-report-153.pdf
[102] R. C. Hongyuan Zhang, Yan Zhang,et.al., "802.11 for Next Generation V2X Communication," IEEE, 2018. [Online]. Available: https://mentor.ieee.org/802.11/dcn/18/11-18-0513-020wng-802-11-for-next-generation-v2x-communication.pptx
[103] "UK National and International V2X Capabilities: Status and Evolution " ZENZIC, 2022. [Online]. Available: https://zenzic.io/content/uploads/2022/04/V2X-Capabilities-Report.pdf
[104] A. PAI, "STATEMENT OF CHAIRMAN AJIT PAI Re: Use of the 5.850-5.925 GHz Band, ET Docket No. 19-138," Federal Communications Commission, 2020.
[105] "Wireless Telecommunications Bureau and Public Safety and Homeland Security Bureau Remind 5.9 GHz Band ITS Licensees of The July 5, 2022, Deadline To Transition out of The 5850-5895 MHz Portion of The Band and The July 20, 2022, Deadline To Notify The Bureaus of The Transition ET Docket No. 19-138," FCC, 2022. [Online]. Available: https://www.fcc.gov/document/wtb-and-pshsb-remind-59-ghz-band-its-licenseestransition-deadline
[106] "Dedicated Short Range Communications (DSRC) Service." https://www.fcc.gov/wireless/bureau-divisions/mobility-division/dedicated-short-rangecommunications-dsrc-service (accessed 13 May, 2023).
[107] "Relocating V2X to Better Spectrum Will Benefit Consumers." https://www.newamerica.org/oti/reports/59-ghz-band/relocating-v2x-to-better-spectrumwill-benefit-consumers/ (accessed 13 Feb., 2023).
[108] "Deployment band configuration for C-V2X at 5.9 GHz in Europe," 5GAA, 2021. [Online]. Available: https://5gaa.org/deployment-band-configuration-for-c-v2x-at-5-9-ghz-in-europe/
[109] "ISO TC204 Intelligent Transport Systems." http://www.concepts-servicesconsulting.de/en/service-portfolio/iso-tc204-intelligent-transport-systems/ (accessed 30 May, 2023).
[110] ISO 13183 Intelligent transport systems — Communications access for land mobiles (CALM) — Using broadcast communications, ISO, 2012 [Online]. Available: https://www.enstandard.eu/iso-13183-intelligent-transport-systems-communications-access-for-landmobiles-calm-using-broadcast-communications/
[111] M. Hadded. "V2X is key to truly autonomous vehicles." https://insights.globalspec.com/article/16016/v2x-is-key-to-truly-autonomous-vehicles (accessed 13 Feb. , 2023).
[112] "Safety Treatment in Connected and Autonomous Driving Functions Report," 5GAA, 2019. [Online]. Available: https://5gaa.org/content/uploads/2021/03/5GAA_T210009_STiCAD_TR-V1-compressed_2.pdf
[113] "Conclusions and Recommendations for Communications Service Providers Supporting Road Operator Priorities and Expectations," 5GAA, 2023. [Online]. Available: https://5gaa.org/content/uploads/2023/01/5gaa-conclusions-and-recommendations-forcommunications-service-providers-supporting-road-operator-priorities-and-expectations2.pdf
[114] "Hyundai Motor broadens its energy solutions to include Vehicle-to-Everything technology " https://www.hyundai.news/eu/articles/press-releases/hyundai-broadens-its-energysolutions-to-include-vehicle-to-everythingtechnology.html#:~:text=,Germany%20that%20feature%20IONIQ%205 (accessed 13 Oct. , 2023).
[115] "Minimum Physical Infrastructure Standard for the Operation of Automated Driving Research Report AP-R665A-22," Austroads, 2022.
[116] N. N. Jessica Tong, Patricia Lavieri, Peter Sweatman, Majid Sarvi, Stacey Ryan, Susan Harris, "Putting the Connectivity in C-ITS - Investigating pathways to accelerate the uptake of road safety and efficiency technologies," 2020. [Online]. Available: https://cms.itsaustralia.com.au/assets/images/PDFs/1-Literature-Review.pdf
[117] "Functional Safety for Enabling Present and Future V2X Use-Cases," AutoTalks, 2021. [Online]. Available: https://auto-talks.com/wp-content/uploads/2021/07/Functional-Safetyfor-V2X-use-cases.pdf
[118] "NATIONAL LAND TRANSPORT TECHNOLOGY ACTION PLAN 2020-2023," Transport and Infrastructure Council Australia 2019. [Online]. Available: https://www.infrastructure.gov.au/sites/default/files/migrated/transport/land-transporttechnology/files/national_land_transport_technology_action_plan_2020-2023.pdf
[119] A. Mason, " Socio-Economic benefits of Cellular V2X," 5GAA, 2018. [Online]. Available: https://5gaa.org/content/uploads/2018/06/2.-Presentation-on-cellular-V2X-socioeconomic-benefit-study-08022018.pdf
[120] J. G. Wright, J. Kyle ; Hill, Christopher J., "National Connected Vehicle Field Infrastructure Footprint Analysis," 2014. [Online]. Available: https://rosap.ntl.bts.gov/view/dot/3440
[121] "Towards a national vision for a secure, connected future through Cooperative Intelligent Transport System (C-ITS)," Transport Certification Australia 2016. [Online]. Available: https://apo.org.au/sites/default/files/resource-files/2016-09/apo-nid67558.pdf
[122] "Policy paper: Regulating government access to C-ITS and automated vehicle data " National Transport Commission, 2019.
[123] ETSI TS 102 941 V1.4.1 (2021-01) Intelligent Transport Systems (ITS); Security; Trust and Privacy Management ETSI, 2021 [Online]. Available: https://www.etsi.org/deliver/etsi_ts/102900_102999/102941/01.04.01_60/ts_102941v010 401p.pdf
[124] A. SANDOT, "IDnomic C-ITS PKI Foundation of Security & Trust," ATOS, 2022.
[125] "SECURITY CREDENTIAL MANAGEMENT SYSTEM (SCMS)," US DOT. [Online]. Available: https://www.its.dot.gov/factsheets/pdf/CV_SCMS.pdf
[126] "Draft Report on European Security Mechanism," C-Roads, 2019. [Online]. Available: https://www.croads.eu/fileadmin/user_upload/media/Dokumente/20190125_TF1_Security_report_v1.4_f inal.pdf
[127] ETSI TS 102 940 V2.1.1 (2021-07) Intelligent Transport Systems (ITS); Security; ITS communications security architecture and security management; Release 2 ETSI, 2021 [Online]. Available: https://www.etsi.org/deliver/etsi_ts/102900_102999/102940/02.01.01_60/ts_102940v020 101p.pdf
[128] Specification #: 33.185 Security aspect for LTE support of Vehicle-to-Everything (V2X) services, 3GPP, 2017. [Online]. Available: https://portal.3gpp.org/desktopmodules/Specifica tions/SpecificationDetails.aspx?specificati onId=3141
[129] "C-ITS Point of Contact." European Commission. https://cpoc.jrc.ec.europa.eu/ (accessed 13 Feb., 2023).
[130] "PRESERVE Preparing secure V2X communication systems " https://www.preserveproject.eu/www.preserve-project.eu/index.html (accessed 13 Feb., 2023).
[131] "ANNEX to the Commission Delegated Regulation supplementing Directive 2010/40/EU of the European Parliament and of the Council with regard to the deployment and operational use of cooperative intelligent transport systems," EUROPEAN COMMISSION, 2019.
[132] ETSI TR 101 607 V1.2.1 (2020-02): Intelligent Transport Systems (ITS); Cooperative ITS (C-ITS); Release 1 2020. [Online]. Available: https://www.etsi.org/deliver/etsi_tr/101600_101699/101607/01.02.01_60/tr_101607v0102 01p.pdf
[133] ARC-IT databases, U. S. DoT. [Online]. Available: https://www.arcit.net/html/resources/databases.html
[134] 3GPP TR 22.885 V14.0.0 (2015-12) Technical Report 3rd Generation Partnership Project; Technical Specification Group Services and System Aspects; Study on LTE support for Vehicle to Everything (V2X) services (Release 14), 3GPP, 2015. [Online]. Available: https://portal.3gpp.org/desktopmodules/Specifica tions/SpecificationDetails.aspx?specificati onId=2898
[135] 3GPP TR 22.886 V16.2.0 (2018-12) Technical Report 3rd Generation Partnership Project; Technical Specification Group Services and System Aspects; Study on enhancement of 3GPP Support for 5G V2X Services (Release 16) , 3GPP, 2018. [Online]. Available: https://portal.3gpp.org/desktopmodules/Specifica tions/SpecificationDetails.aspx?specificati onId=3108
[136] "Enabling Public Transport to Vehicle Communication for Safety Applications in Melbourne, Victoria." https://eng.unimelb.edu.au/industry/aimes/news-and-events/enabling-publictransport-to-vehicle-communication-for-safety-applications-in-melbourne,-victoria (accessed 13 Feb., 2023).
[137] K. V. Katsaros, "5G for cooperative & connected automated MOBIlity on X-border corridorsD5.1 Evaluation methodology and plan," 5G MobiX-European Union’s Horizon 2020 research and innovation programme under grant agreement No [825496], 2020. [Online]. Available:
https://www.5g-mobix.com/assets/files/5G-MOBIX-D3.5-Report-on-the-evaluation-datamanagement-methodology-and-tools-v1.0_Final.pdf
[138] "5GAA partnered with ETSI and DEKRA to showcase C-V2X Interoperability and discuss deployment during the plugtests at the DEKRA Automobil Test Center in Klettwitz, Germany." https://5gaa.org/5gaa-partnered-with-etsi-and-dekra-to-showcase-c-v2xinteroperability-and-discuss-deployment-during-the-plugtests-at-the-dekra-automobil-testcenter-in-klettwitz-germany/ (accessed 13 Feb., 2023).
[139] "Live Trial of 5G Connected Car Concept To Launch in Turin, Italy." https://5gaa.org/live-trialof-5g-connected-car-concept-to-launch-in-turin-italy/ (accessed 13 Feb., 2023).
[140] "Live Trial of 5G Connected Car Concept Launches in Blacksburg, Virginia (VA)." https://5gaa.org/news/live-trial-of-5g-connected-car-concept-launches-in-blacksburgvirginia-va/ (accessed 13 Feb., 2023).
[141] "5GAA Demonstrated C-V2X Technology Applications and Safety Benefits for Road Users last October in Málaga, Spain " https://5gaa.org/5gaa-demonstrated-c-v2x-technologyapplications-and-safety-benefits-for-road-users-last-october-in-malaga-spain/ (accessed 13 Feb., 2023).
[142] M. Alleven. "Qualcomm to kick off C-V2X trials with AT&T, Nokia and Ford." https://www.fiercewireless.com/wireless/qualcomm-to-kick-off-c-v2x-trials-at-t-nokia-andford (accessed 13 Feb., 2023).
[143] "5G Harmonised Research and Trials for Service Evolution between EU and China," 5G-Drive, 2018. [Online]. Available: https://5g-drive.eu/wp-content/uploads/2021/08/5GDD4.4_Final-report-of-V2X-trials.pdf
[144] "C-V2X Trial in Japan," Qualcomm, 2018. [Online]. Available: https://www.rit.edu/wisplab/sites/rit.edu.wisplab/files/documents/misc/Qualcomm%20%20C-V2X%20Trial%20in%20Japan%20Results%20(Dec%202018).pdf
[145] "Report on C-V2X Technolo gy Trial for Autonomous Vehicle at Hong Kong International Airport," HKT 2020. [Online]. Available: https://www.ofca.gov.hk/filemanager/ofca/en/content_669/tr202011_03.pdf
Annex 1: C-ITS Standards List
Table 35 ISO standards [57]
ISO Standard
ISO 4426, Intelligent transport systems — Lower layer protocols for usage in the European digital tachograph
ISO/IEC 7498-1:1994, Information technology — Open Systems Interconnection — Basic Reference Model: The Basic Model
ISO 14812, Intelligent transport systems — Vocabulary
ISO 14813-1:2015, Intelligent transport systems — Reference model architecture(s) for the ITS sector — Part 1: ITS service domains, service groups and services
ISO 14816:2005, Road transport and traffic telematics — Automatic vehicle and equipment identification — Numbering and data structure
ISO 14823:2017, Intelligent transport systems — Graphic data dictionary
ISO 14906:2018 with FDAmd 1, Electronic fee collection — Application interface definition for dedicated short-range communication
ISO 15628:2013, Road transport and traffic telematics — Dedicated short-range communication (DSRC) — DSRC application layer
Note: A technically equivalent standard exists from CEN: EN 12834
ISO/TS 16460:2016, Intelligent transport systems — Communications access for land mobiles (CALM) — Communication protocol messages for global usage
Note 1: Specifies messages harmonized with IEEE 1609.3.
Note 2: Under revision and upgrade to ISO 16460.
ISO 16461:2018, Criteria for privacy and integrity protection in probe vehicle information systems
ISO 17419:2018, Intelligent Transport Systems — Cooperative Systems — Globally unique identification
Note: Under Vienna Agreement: EN 17419:2018
ISO 17423:2018, Intelligent Transport Systems — Cooperative Systems — Application requirements and objectives
Note: Under Vienna Agreement: EN 17423:2018
ISO/TS 17425:2016, Intelligent transport systems — Co-operative systems — Data exchange specification for the in-vehicle presentation of external road and traffic-related data
Note: Under Vienna Agreement: CEN TS 17425:2016
ISO/TS 17426:2016, Intelligent Transport Systems — Cooperative Systems — Contextual speeds
Note: Under Vienna Agreement: CEN TS 17426
ISO 17427, Intelligent Transport Systems — Cooperative Systems —
Part 1: Roles and responsibilities in the context of co-operative ITS based on architecture(s) for cooperative systems
Part 2: Framework overview
Part 3: Concept of operations (ConOps) for 'core' systems
Part 4: Minimum system requirements and behaviour for core systems
Part 5: void
ISO Standard
Part 6: 'Core system' risk assessment methodology
Part 7: Privacy aspects
Part 8: Liability aspects
Part 9: Compliance and enforcement aspects
Part 10: Driver distraction and information display
Note: Under Vienna Agreement
ISO/TS 17429:2017, Intelligent transport systems — Cooperative ITS — ITS station facilities for the transfer of information between ITS stations
Note 1: Under Vienna Agreement: CEN TS 17429
Note 2: Under revision with split into a multi-part document
Cooperative intelligent transport systems (C-ITS) — ITS station facility services
Part 1: Communication profile handler
Part 2: Facility services handler
Part 3: Content subscription handler
ISO 17465-3:2015, Intelligent transport systems — Cooperative ITS — Part 3: Release procedures for standards documents
ISO 17515-1:2015, Intelligent transport systems — Evolved-universal terrestrial radio access network — Part 1: General usage
ISO 17515-3:2019, Intelligent transport systems — Evolved-universal terrestrial radio access network — Part 3: LTE-V2X
ISO 18750:2018, Intelligent transport systems — Cooperative ITS — Local dynamic map
Note 1: Under Vienna Agreement: EN 18750
Note 2: Includes EN 302 895, and additionally provides PICS for conformance testing
ISO 19414:2019, Intelligent transport systems — Service architecture of probe vehicle systems
ISO/TS 19091:2017, Intelligent transport systems — Cooperative ITS — Using V2I and I2V communications for applications related to signalized intersections
Note: Under Vienna Agreement: CEN/TS 19091
ISO 19079:2016, Intelligent transport systems — Communications access for land mobiles (CALM) — 6LoWPAN networking
ISO 19080:2016, Intelligent transport systems — Communications access for land mobiles (CALM) — CoAP facility
ISO/TS 19321:2020, Intelligent transport systems — Cooperative ITS — Dictionary of in-vehicle information (IVI) data structures
Note 1: Under Vienna Agreement: CEN TS 19321
ISO/TS 20026:2017, Intelligent transport systems — Cooperative ITS — Test architecture
ISO 20922:2016, Information technology — Message Queuing Telemetry Transport (MQTT)
CEN/TS 21176:2020, Cooperative intelligent transport systems (C-ITS) — Position, velocity and time functionality in the ITS station
Note: Under Vienna Agreement: ISO/TS 21176
TS 21177:2019, Intelligent transport systems — ITS-station security services for secure session establishment and authentication between trusted devices
Note: Under Vienna Agreement: CEN/TS 21177
ISO Standard
CEN/TS 21184:2020, Cooperative intelligent transport systems (C-ITS) — Global transport data management (GTDM) framework
Note: Under Vienna Agreement: ISO/TS 21184
ISO/TS 21185:2019, Intelligent transport systems — Communication profiles for secure connections between trusted devices
Note: Vienna Agreement cancelled by CEN; almost identical TS at CEN published with a slightly different title: ISO/TS 21185:2019 Cooperative intelligent transport systems (C-ITS) — Communication profiles
ISO 21186-1:2020, Cooperative intelligent transport systems — Guidelines on the usage of standards — Part 1: Standardization landscape and releases
ISO 21186-2:2020, Cooperative intelligent transport systems — Guidelines on the usage of standards — Part 2: Hybrid communications
ISO 21186-3:2020, Cooperative intelligent transport systems — Guidelines on the usage of standards — Part 3: Security
Note: Further parts are under preparation:
Part 4: Usage of the service announcement protocol FSAP specified in EN 22418
Part 5: Dynamically extendable data and protocol parameters ("Information Object Classes" and "Information Object Sets"; based on ASN.1 type CLASS
Part 6: Usage of the GTDM framework specified in TS 21184
ISO 21210:2012 with Amd 1:2017, Intelligent transport systems — Communications access for land mobiles (CALM) — IPv6 Networking
Note: International Standard under revision with split into a multi-part document: Intelligent transport systems - IPv6 networking -
Part 1: Common terms, definitions and requirements
Part 2: Addressing and forwarding
Part 3: Mobility management
Part 4: ITS station management adaptation entity
ISO 21212:2008, Intelligent transport systems — Communications access for land mobiles (CALM) — 2G Cellular systems
ISO 21213:2008, Intelligent transport systems — Communications access for land mobiles (CALM) — 3G Cellular systems
ISO 21214:2015, Intelligent transport systems — Communications access for land mobiles (CALM) — Infra-red systems
ISO 21215:2018, Intelligent transport systems — Localized communications — ITS-M5
Note: Edition 2 (2018) includes EN 302 663 V1.2.1
ISO 21217:2014, Intelligent transport systems — Communications access for land mobiles (CALM) — Architecture
Note 1: Edition 2 (2014) includes EN 302 665
Note 2: Under revision with a new title: Intelligent transport systems — Station and communication architecture. The publication is expected in 2020.
ISO 21218:2018, Intelligent transport systems — Hybrid communications — Access technology support
ISO Standard
ISO 22418:2020, Intelligent transport systems — Fast service advertisement protocol (FSAP) for general purposes in ITS
Note: Under Vienna Agreement: EN / ISO 22418:2020.
ISO 22837:2009, Vehicle Probe Data for Wide Area Communication
ISO 24100:2010, Intelligent transport systems — Basic Principles for Personal Data Protection in Probe Vehicle Information Services
ISO 24102-1:2018, Intelligent transport systems — ITS station management — Part 1: Local management
ISO 24102-2:2018, Intelligent transport systems — ITS station management — Part 2: Remote management of ITS-SCUs
ISO 24102-3:2018, Intelligent transport systems — ITS station management — Part 3: Service access points
ISO 24102-4:2018, Intelligent transport systems — ITS station management — Part 4: Stationinternal management communications
ISO 24102-6:2018, Intelligent Transport Systems — ITS station management — Part 6: Path and flow management
ISO 24102-7, Intelligent transport systems — ITS station management — Part 7: ITS-S capabilities
Note: Under development
ISO 24102-8, Intelligent transport systems — ITS station management — Part 8: ITS-S application processes
Note: Under development
ISO 24102-9, Intelligent transport systems — ITS station management — Part 9: ITS-S managed entities
Note: Under development
ISO 24103:2009, Intelligent transport systems — Communications access for land mobiles (CALM) — Media adapted interface layer (MAIL)
ISO 24978:2009, Intelligent transport systems — ITS Safety and emergency messages using any available wireless media — Data registry procedures
ISO 25111:2009, Intelligent transport systems — Communications access for land mobiles (CALM) — General requirements for using public networks
ISO 25114:2010, Intelligent transport systems — Probe data reporting management (PDRM)
ISO 29281-1:2018, Intelligent transport systems — Localized communications — Part 1: Fast networking & transport layer protocol (FNTP)
ISO 29281-2:2019, Intelligent transport systems — Localized communications — Part 2: Legacy system support
ISO 29282:2011, Intelligent transport systems — Communications access for land mobiles (CALM) — Satellite networks
ISO 29284:2012, Intelligent transport systems — Event-based Probe Vehicle Data
36 CEN standards [57]
CEN Standards
EN 16157-1:2019, Intelligent transport systems — DATEX II data exchange specifications for traffic management and information — Part 1: Context and framework
EN 16157-2:2019, Intelligent transport systems — DATEX II data exchange specifications for traffic management and information — Part 2: Location referencing
EN 16157-3:2019, Intelligent transport systems — DATEX II data exchange specifications for traffic management and information — Part 3: Situation Publication
EN 16157-7:2019, Intelligent transport systems — DATEX II data exchange specifications for traffic management and information — Part 7: Common data elements
EN 12253:2005, Road transport and traffic telematics - Dedicated Short Range, Communication (DSRC) - Physical layer using the microwave at 5,8 GHz
EN 12795:2005, Road transport and traffic telematics — Dedicated Short Range Communication (DSRC) — DSRC data link layer: medium access and logical link control
EN 12834:2005, Road transport and traffic telematics - Dedicated Short Range Communication (DSRC) - DSRC application layer
CEN/TS 17182:2019, Intelligent transport systems — eSafety — eCall via an ITS-station
CEN/TS 17378:2019, Intelligent transport systems — Urban ITS — Air quality management in urban areas
CEN/TS 17380:2019, Intelligent transport systems — Urban ITS — 'Controlled Zone' management for UVARs using C-ITS
CEN/TS 17496:2020, Cooperative intelligent transport systems (C-ITS) — Communication profiles
Note: Vienna Agreement cancelled by CEN; almost identical TS at ISO published with a slightly different title: ISO/TS 21185:2019 Intelligent transport systems — Communication profiles for secure connections between trusted devices
CEN/TC 278 preliminary WI_00278472, Intelligent transport systems — Management for Electronic Traffic Regulations (METR) — Part 1: General concept and architecture
Table 37 ETSI standards [57]
ETSI Standards
EG 202 798 V1.1.1, 2011-01, Intelligent Transport Systems (ITS); Testing; Framework for conformance and interoperability testing
EN 301 893 V2.1.1, 2017-06, 5 GHz RLAN; Harmonised Standard covering the essential requirements of article 3.2 of Directive 2014/53/EU
Note: Included in ISO 21215 by reference
EN 302 571 V2.1.1, 2017-06, 5 GHz RLAN; Harmonised Standard covering the essential requirements of article 3.2 of Directive 2014/53/EU
Note: Included in ISO 21215 by reference
EN 302 890-1 V1.2.1, Intelligent Transport Systems (ITS); Facilities layer function; Part 1: Services Announcement (SA) specification
Table
ETSI Standards
EN 302 890-2, Intelligent Transport Systems (ITS); Facilities layer function; Part 2: Position and time management (PoTi); Release 2
EN 302 636-4-1 V1.3.1, 2017-08, Intelligent Transport Systems (ITS); Vehicular Communications; GeoNetworking; Part 4: Geographical addressing and forwarding for point-to-point and point-tomultipoint communications; Sub-part 1: Media-Independent Functionality
EN 302 636-5-1 V2.2.1, 2019-05, Intelligent Transport Systems (ITS); Vehicular Communications; GeoNetworking; Part 5: Transport Protocols; Sub-part 1: Basic Transport Protocol
EN 302 636-6-1 V1.2.1, 2014-05, Intelligent Transport Systems (ITS); Vehicular Communications; GeoNetworking; Part 6: Internet Integration; Sub-part 1: Transmission of IPv6 Packets over GeoNetworking Protocols
EN 302 637-2 V1.4.1, 2019-04, Intelligent Transport Systems (ITS); Vehicular Communications; Basic Set of Applications; Part 2: Specification of Cooperative Awareness Basic Service
EN 302 637-3 V1.3.1, 2019-04, Intelligent Transport Systems (ITS); Vehicular Communications; Basic Set of Applications; Part 3: Specifications of Decentralized Environmental Notification Basic Service
EN 302 663 V1.2.1, 2013-07, Intelligent Transport Systems (ITS); European profile standard for the physical and medium access control layer of Intelligent Transport Systems operating in the 5 GHz frequency band
EN 302 665 V1.1.1, 2010-09, Intelligent Transport Systems (ITS); Communications Architecture
Note: Included in ISO 21217
EN 302 895 V1.1.1, 2014-09, Intelligent Transport Systems (ITS); Vehicular Communications; Basic Set of Applications; Local Dynamic Map (LDM)
Note: Included in EN 18750
EN 302 931 V1.1.1, 2011-07, Intelligent Transport Systems (ITS); Vehicular Communications; Geographical Area Definition
TR 102 638 V1.1.1, 2009-06, Intelligent Transport Systems (ITS); Vehicular Communications; Basic Set of Applications; Definitions
TS 101 539-1 V1.1.1, 2013-08, Intelligent Transport Systems (ITS); V2X Application; Part 1: Road Hazard Signalling (RHS) application requirements specification
TS 101 539-2 V1.1.1, 2018-06, Intelligent Transport Systems (ITS); V2X Application; Part 2: Intersection Collision Risk Warning Specification
TS 101 539-3 V1.1.1, 2013-11, Intelligent Transport Systems (ITS); V2X Application; Part 3: Longitudinal Collision Risk Warning Specification
TS 101 556-1 V1.1.1, 2012-07, Intelligent Transport Systems (ITS); Infrastructure to Vehicle Communication; Part 1: Electric Vehicle Charging Spot Notification Specification
TS 101 556-2 V1.1.1, 2016-02, Intelligent Transport Systems (ITS); Infrastructure to Vehicle Communication; Part 2: Communication system spec ification to support application requirements for Tyre Information System (TIS) and Tyre Pressure Gauge (TPG) interoperability
TS 101 556-3 V1.1.1, 2014-10, Intelligent Transport Systems (ITS); Infrastructure to Vehicle Communication; Part 3: Communications system for the planning and reservation of EV energy supply using wireless networks
ETSI Standards
TR 102 893 V1.2.1, 2017-03, Intelligent Transport Systems (ITS); Security; Threat, Vulnerability and Risk Analysis (TVRA)
TS 302 636-4-2 V1.1.1, 2013-10, Intelligent Transport Systems (ITS); Vehicular Communications; GeoNetworking; Part 4: Geographical addressing and forwarding for point-to-point and point-tomultipoint communications; Sub-part 2: Media dependent functionalities for ITS-G5A media
TS 102 637-1 V1.1.1, 2010-09, Intelligent Transport Systems (ITS); Vehicular Communications; Basic Set of Applications; Part 1: Functional Requirements
TS 102 687 V1.2.1, 2018-04, Intelligent Transport Systems (ITS); Decentralized Congestion Control Mechanisms for Intelligent Transport Systems operating in the 5 GHz range; Access layer part
TS 102 724 V1.1.1, 2012-10, Intelligent Transport Systems (ITS); Harmonized Channel Specifications for Intelligent Transport Systems operating in the 5 GHz frequency band
TS 102 731 V1.1.1, 2010-09, Intelligent Transport Systems (ITS); Security; Security Services and Architecture
TS 102 792 V1.2.1, 2015-06, Intelligent Transport Systems (ITS); Mitigation techniques to avoid interference between European CEN Dedicated Short Range Communication (CEN DSRC) equipment and Intelligent Transport Systems (ITS) operating in the 5 GHz frequency range
TS 102 894-1 V1.1.1, 2013-08, Intelligent Transport System (ITS); Users and Applications requirements; Part 1: Facility layer structure, functional requirements and specifications
TS 102 894-2 V1.3.1, 2018-08, Intelligent Transport Systems (ITS); Users and applications requirements; Part 2: Applications and facilities layer common data dictionary
TS 102 940 V1.3.1, 2018-04, Intelligent Transport Systems (ITS); Security; ITS communications security architecture and security management
TS 102 941 V1.3.1, 2019-02, Intelligent Transport Systems (ITS); Security; Trust and Privacy Management
TS 102 942 V1.1.1, 2012-06, Intelligent Transport Systems (ITS); Security; Access Control
TS 102 943 V1.1.1, 2012-06, Intelligent Transport Systems (ITS); Security; Confidentiality services
TS 103 097 V1.3.1, 2017-10, Intelligent Transport Systems (ITS); Security; Security header and certificate formats
TS 103 157 V1.1.1, 2013-10, Intelligent Transport Systems (ITS); Cross Layer DCC Management Entity for operation in the ITS G5A and ITS G5B medium
TS 103 175 V1.1.1, 2015-06, Intelligent Transport Systems (ITS); Decentralized Congestion Control (DCC); Cross Layer DCC control entity
TS 103 248 V1.3.1, 2019-04, Intelligent Transport Systems (ITS); GeoNetworking; Port Numbers for the Basic Transport Protocol (BTP)
TS 103 301 V1.2.1, 2018-08, Intelligent Transport Systems (ITS); Vehicular Communications; Basic Set of Applications; Facilities layer protocols and communication requirements for infrastructure services
Table 38 IEEE standards [57]
IEEE Standards
IEEE Std 1609.0™-2019, Guide for Wireless Access in Vehicular Environments (WAVE) — Architecture
IEEE Std 1609.2™-2016, Standard for Wireless Access in Vehicular Environments (WAVE) — Security Services for Applications and Management Messages
IEEE Std 1609.3™-2016, IEEE Standard for Wireless Access in Vehicular Environments (WAVE) — Networking Services
IEEE Std 1609.4™-2016, IEEE Standard for Wireless Access in Vehicular Environments (WAVE) — Multi-channel Operation
IEEE Std 802™-2014, IEEE Standard for Local and Metropolitan Area Networks: Overview and Architecture
IEEE Std 802.11™-2016, IEEE Standard for Information technology—Telecommunications and information exchange between systems — Local and metropolitan area networks — Specific requirements — Part 11: Wireless LAN Medium Access Control (MAC) and Physical Layer (PHY) Specifications
IEEE Std 802.15.4™-2015, IEEE Standard for Information technology — Telecommunications and information exchange between systems — Local and metropolitan area networks — Specific requirements — Part 15.4: Wireless Medium Access Control (MAC) and Physical Layer (PHY) Specifications for Low Rate Wireless Personal Area Networks With amendments from 2017 and 2019
IEEE Std 802.15.5™-2009, IEEE Recommended Practice for Information technology — Telecommunications and information exchange between systems — Local and metropolitan area networks — Specific requirements Part 15.5: Mesh Topology Capability in Wireless Personal Area Networks (WPANs
Table 39 ITU standards [57]
ITU Standards
ITU-R M.1452-2: Millimetre-wave vehicular collision avoidance radars and radiocommunication systems for intelligent transport system applications
ITU-R M.1453-2: Dedicated Short Range Communications (DSRC) at 5.8GHz
ITU-R M.1890: ITS Guidelines and Objectives
SAE J2735:2016, Dedicated Short Range Communications (DSRC) Message Set Dictionary
SAE J2945/1:2016, On-Board System Requirements for V2V Safety Communications
Table 40 Project documents [57]
Project Documents
European Council Directive 1999/37/EC of 29 April 1999 on the registration documents for vehicles
European Commission Directive 2003/127/EC of 23 December 2003 amending Council Directive 1999/37/EC on the registration documents for vehicles
European Commission, Directive 2010/40/EU OF THE EUROPEAN PARLIAMENT AND OF THE COUNCIL of 7 July 2010 on the framework for the deployment of Intelligent Transport Systems in the field of road transport and for interfaces with other modes of transport
Project Documents
European Commission, M/453, 6. October 2009, Standardisation mandate addressed to CEN, CENELEC and ETSI in the field of Information and Communication Technologies to support the interoperability of Co-operative systems for Intelligent Transport in the European Community European Commission, 2019, ANNEX 2 to the Commission Delegated Regulation supplementing ITS Directive 2010/40/EU of the European Parliament and of the Council about the provision of cooperative intelligent transport systems
European Commission, M/546, 12. February 2016, Standardisation request to the European standardisation organisations as regards Intelligent Transport Systems (ITS) in urban areas in support of Directive 2010/40/EU of the European Parliament and of the Council of 7 July 2010 on the framework for the deployment of Intelligent Transport Systems in the field of road transport and for interfaces with other modes of transport
D.CVIS.3.3, Architecture and System Specifications, CVIS, WP3, Version 1.1, July 31st, 2007
CONVERGE project, Deliverable D6, Final Assessment, Version 1.0, 19.1.2016
C-Roads, The Platform of harmonised C-its deployment in Europe
HARTS, http://htg7.org/html/about/about.html
CEN/TC 278/PT 1605, Cooperative intelligent transport systems (C-ITS) — Guidelines on the usage of standards, https://www.itsstandards.eu/cits
Table 41 Conformance test specifications [57]
Conformance Test Specifications
EN 15876 – all parts, Electronic fee collection — Evaluation of on-board and roadside equipment for conformity to EN 15509
ISO/TS 20594, Intelligent transport systems — Cooperative ITS — Test requirements and protocol implementation conformance statements (PICS) pro forma for TS 17429
ISO/TS 20597, Intelligent transport systems — Cooperative ITS — Test requirements and protocol implementation conformance statements (PICS) pro forma for TS 19321
ISO/TS 20598, Intelligent transport systems — Cooperative ITS — Test requirements and protocol implementation conformance statements (PICS) pro forma for TS 19091
ISO/TS 21189, Intelligent transport systems — Cooperative ITS — Test requirements and Protocol Implementation Conformance Statement (PICS) pro forma for CEN ISO TS 17426
Note: Under Vienna Agreement: CEN/TS 21189
ETSI TS 102 797-2 V1.2.1, Intelligent Transport Systems (ITS); Communications Access for Land Mobiles (CALM); Test specifications for ITS station management (ISO 24102); Part 2: Test Suite Structure and Test Purposes (TSS & TP)
Note: To be revised
ETSI TS 102 797-3 V1.2.1, Intelligent Transport Systems (ITS); Communications Access for Land Mobiles (CALM); Test specifications for ITS station management (ISO 24102); Part 3: Abstract Test Suite (ATS) and partial PIXIT proforma
Note: To be revised
ETSI TS 102 859-1 V1.2.1, Intelligent Transport Systems (ITS); Testing; Conformance test specifications for Transmission of IP packets over GeoNetworking; Part 1: Test requirements and Protocol Implementation Conformance Statement (PICS) proforma
Conformance Test Specifications
ETSI TS 102 859-2 V1.2.1, Intelligent Transport Systems (ITS); Testing; Conformance test specifications for Transmission of IP packets over GeoNetworking; Part 2: Test Suite Structure and Test Purposes (TSS & TP)
ETSI TS 102 859-3 V1.2.1, Intelligent Transport Systems (ITS); Testing; Conformance test specifications for Transmission of IP packets over GeoNetworking; Part 3: Abstract Test Suite (ATS) and Protocol Implementation eXtra Information for Testing (PIXIT)
ETSI TS 102 868-1 V1.4.1, Intelligent Transport Systems (ITS); Testing; Conformance test specifications for Cooperative Awareness Basic Service (CA); Part 1: Test requirements and Protocol Implementation Conformance Statement (PICS) pro forma
ETSI TS 102 868-2 V1.4.1, Intelligent Transport Systems (ITS); Testing; Conformance test specifications for Cooperative Awareness Basic Service (CA); Part 2: Test Suite Structure and Test Purposes (TSS & TP)
ETSI TS 102 868-3 V1.4.1, Intelligent Transport Systems (ITS); Testing; Conformance test specifications for Cooperative Awareness Basic Service (CA); Part 3: Abstract Test Suite (ATS) and Protocol Implementation eXtra Information for Testing (PIXIT)
ETSI TS 102 869-1 V1.5.1, Intelligent Transport Systems (ITS); Testing; Conformance test specifications for Decentralized Environmental Notification Basic Service (DEN); Part 1: Test requirements and Protocol Implementation Conformance Statement (PICS) pro forma
ETSI TS 102 869-2 V1.5.1, Intelligent Transport Systems (ITS); Testing; Conformance test specifications for Decentralized Environmental Notification Basic Service (DEN); Intelligent Transport Systems (ITS); Part 2: Test Suite Structure and Test Purposes (TSS & TP)
ETSI TS 102 869-3 V1.5.1, Intelligent Transport Systems (ITS); Testing; Conformance test specifications for Decentralized Environmental Notification Basic Service (DEN); Part 3: Abstract Test Suite (ATS) and Protocol Implementation eXtra Information for Testing (PIXIT)
ETSI TS 102 870-1 V1.1.1, Intelligent Transport Systems (ITS); Testing; Conformance test specifications for GeoNetworking Basic Transport Protocol (BTP); Part 1: Test requirements and Protocol Implementation Conformance Statement (PICS) proforma
ETSI TS 102 870-2 V1.1.1, Intelligent Transport Systems (ITS); Testing; Conformance test specifications for GeoNetworking Basic Transport Protocol (BTP); Part 2: Test Suite Structure and Test Purposes (TSS&TP)
ETSI TS 102 870-3 V1.1.1, Intelligent Transport Systems (ITS); Testing; Conformance test specifications for GeoNetworking Basic Transport Protocol (BTP); Part 3: Abstract Test Suite (ATS) and Protocol Implementation eXtra Information for Testing (PIXIT)
ETSI TS 102 871-1 1.4.1, Intelligent Transport Systems (ITS); Testing; Conformance test specifications for GeoNetworking ITS-G5; Part 1: Test requirements and Protocol Implementation Conformance Statement (PICS) pro forma
ETSI TS 102 871-2 1.4.1, Intelligent Transport Systems (ITS); Testing; Conformance test specifications for GeoNetworking ITS-G5; Part 2: Test Suite Structure and Test Purposes (TSS & TP)
ETSI TS 102 871-3 1.4.1, Intelligent Transport Systems (ITS); Testing; Conformance test specifications for GeoNetworking ITS-G5; Part 3: Abstract Test Suite (ATS) and Protocol Implementation eXtra Information for Testing (PIXIT)
Conformance Test Specifications
ETSI TS 102 916-1 V1.1.3, Intelligent Transport Systems (ITS); Testing; Coexistence of Cooperative ITS-G5 with TTT DSRC; Part 1: Protocol Implementation Conformance Statement (PICS)
ETSI TS 102 916-2 V1.1.3, Intelligent Transport Systems (ITS); Testing; Coexistence of Cooperative ITS-G5 with TTT DSRC; Part 2: Test Suite Structure and Test Purposes (TSS & TP)
ETSI TS 102 916-3 V1.1.1, Intelligent Transport Systems (ITS); Testing; Coexistence of Cooperative ITS-G5 with TTT DSRC; Part 3: Abstract Test Suite (ATS) and partial Protocol Implementation eXtra Information for Testing (PIXIT)
ETSI TS 102 917-1 V1.1.1, Intelligent Transport Systems (ITS); Test specifications for the channel congestion control algorithms operating in the 5,9 GHz range; Part 1: Protocol Implementation Conformance Statement (PICS)
ETSI TS 102 917-2 V1.1.1, Intelligent Transport Systems (ITS); Test specifications for the channel congestion control algorithms operating in the 5,9 GHz range; Part 1: Protocol Implementation Conformance Statement (PICS)
ETSI TS 102 917-3 V1.1.1, Intelligent Transport Systems (ITS); Test specifications for the channel congestion control algorithms operating in the 5,9 GHz range; Part 1: Protocol Implementation Conformance Statement (PICS)
ETSI TS 102 985-2 V1.2.1, Intelligent Transport Systems (ITS); Communications Access for Land Mobiles (CALM); Test specifications for non-IP networking (ISO 29281); Part 2: Test Suite Structure and Test Purposes (TSS & TP)
Note: To be revised
ETSI TS 102 985-3 V1.2.1, Intelligent Transport Systems (ITS); Communications Access for Land Mobiles (CALM); Test specifications for non-IP networking (ISO 29281); Part 3: Abstract Test Suite (ATS) and partial PIXIT proforma
Note: To be revised
ETSI TS 103 096-1 V1.3.3, Intelligent Transport Systems (ITS); Testing; Conformance test specifications for ITS Security; Part 1: Protocol Implementation Conformance Statement (PICS)
ETSI TS 103 096-2 V1.3.3, Intelligent Transport Systems (ITS); Testing; Conformance test specifications for ITS Security; Part 2: Test Suite Structure and Test Purposes (TSS & TP)
ETSI TS 103 096-3 V1.3.2, Intelligent Transport Systems (ITS); Testing; Conformance test specifications for ITS Security; Part 3: Abstract Test Suite (ATS) and Protocol Implementation eXtra Information for Testing (PIXIT)
ETSI TS 103 191-1 V1.2.1, Intelligent Transport Systems (ITS); Testing; Conformance test specifications for Facilities layer protocols and communication requirements for infrastructure services; Part 1: Test requirements and Protocol Implementation Conformance Statement (PICS) pro forma
ETSI TS 103 191-2 V1.2.1, Intelligent Transport Systems (ITS); Testing; Conformance test specifications for Facilities layer protocols and communication requirements for infrastructure services; Part 2: Test Suite Structure and Test Purposes (TSS & TP)
[ETSI TS 103 191-3 V1.2.1, Intelligent Transport Systems (ITS); Testing; Conformance test specifications for Facilities layer protocols and communication requirements for infrastructure services; Part 3: Abstract Test Suite (ATS) and Protocol Implementation eXtra Information for Testing (PIXIT)
Annex 2: ETSI ITS Standards (Release 1)
Table 42 ETSI ITS standards (Release 1) [132]
Layers
ETSI C-ITS Release 1: 2019-2020 Standards
General Communications Architecture-ETSI EN 302 665 V1.1.1, Security Services and Architecture-ETSI TS 102 731 V1.1.1, Facility layer structure, functional requirements and specifications-ETSI TS 102 894-1 V1.1.1, Applications and facilities layer common data dictionary-ETSI TS 102 894-2 V1.1.1, Network architecture-ETSI EN 302 636-3 V1.2.1,
Framework for conformance and interoperability testing-ETSI EG 202 798 V1.1.1, Framework for Public Mobile Networks in Cooperative ITS (C-ITS)-ETSI TR 102 962 V1.1.1
Application requirements Road Hazard Signalling (RHS)-ETSI TS 101 539-1 V1.1.1,
Intersection Collision Risk Warning Specification-ETSI TS 101 539-2 V1.1.1, Longitudinal Collision Risk Warning Specification-ETSI TS 101 539-3 V1.1.1, Electric Vehicle Charging Spot Notification Specification-ETSI TS 101 556-1 V1.1, Communication system specification to support application requirements for Tyre Pressure Monitoring System (TPMS) (TPMS)-ETSI TS 101 556-2 V1.1.1, Communications system for the planning and reservation of EV energy supply using wireless networks-ETSI TS 101 556-3 V1.1.1, Basic Set of Applications; Definitions-ETSI TR 102 638 V1.1.1
Facilities Specification of Cooperative Awareness Basic Service-ETSI EN 302 637-2 V1.3.1, Specifications of Decentralized Environmental Notification Basic Service-ETSI EN 302 637-3 V1.2.1,
Local Dynamic Map (LDM) Specification-ETSI EN 302 895 V1.1.1, Position and time facility specification-ETSI TS 102 890-3 V1.1.1,
CAM: (PICS) proforma-ETSI TS 102 869-1 V1.1.1, CAM: (TSS&TP)-ETSI TS 102 868-2 V1.1.1, CAM: (ATS&PIXIT)-ETSI TS 102 868-3 V1.1.1, DENM: (PICS) proforma-ETSI TS 102 869-1 V1.1.1, DENM: (TSS&TP)-ETSI TS 102 869-2 V1.1.1, DENM: (ATS&PIXIT)-ETSI TS 102 869-3 V1.1.1, CAM Validation report-ETSI TR 103 061-1 V1.1.1, DENM Validation report-ETSI TR 103 061-2 V1.1.1
Network & Transport
GeoNetworking; Requirements -ETSI EN 302 636-1 V1.2.1, GeoNetworking; Scenarios -ETSI EN 302 636-2 V1.2.1, GeoNetworking; Geographical addressing and forwarding for point-to-point and point-to-multipoint communications; Media-Independent Functionality-ETSI EN 302636-4-1 V1.2.1,
GeoNetworking; Geographical addressing and forwarding for point-to-point and point-to-multipoint communications; Media dependent functionalities for ITS-G5A media-ETSI TS 102 636-4-2 V1.1.1, GeoNetworking; Transport Protocols; Basic Transport Protocol -ETSI EN 302 6365-1 V1.2.1,
GeoNetworking; Internet Integration; Transmission of IPv6 Packets over GeoNetworking Protocols -ETSI EN 302 636-6-1 V1.2.1
Layers ETSI C-ITS Release 1: 2019-2020 Standards
Access & Media Physical and MAC-ETSI EN 302 663 V1.2.1,
Access Layer-ETSI EN 302 663 V1.2.1,
PICS-ETSI TS 102 917-1 V1.1.1, TSS & TP-ETSI TS 102 917-2 V1.1.1, ATS & PIXIT-ETSI TS 102 917-3 V1.1.1
Management Architecture and addressing schemes-ETSI TS 102 723-1 V1.1.1, Management information base-ETSI TS 102 723-2 V1.1.1,
The interface between the management entity and access layer-ETSI TS 102 7233 V1.1.1,
Interface between management entity and networking & transport layer-ETSI TS 102 723-4 V1.1.1,
The interface between management entity and facilities layer-ETSI TS 102 723-5 V1.1.1,
The interface between the access layer and networking & transport layer-ETSI TS 102 723-10 V1.1.1
Security Threat, Vulnerability and Risk Analysis (TVRA) -ETSI TR 102 893 V1.1.1, Stage 3 mapping for IEEE 1609.2-ETSI TS 102 867 V1.1.1, Confidentiality services-ETSI TS 102 943 V1.1.1, Trust and Privacy Management-ETSI TS 102 941 V1.1.1,
Access Control-ETSI TS 102 942 V1.1.1, ITS communications security architecture and security management-ETSI TS 102 940 V1.1.1,
Security header and certificate formats-ETSI TS 103 097 V1.1.1
Layers Note
MAC/PHY Layer (ITS-G5, EN 302 663)
Use of IEEE 802.11p (MAC/PHY) over the European spectrum (ES 202 663
ITS European profile standard) for the physical and medium access control layer of Intelligent Transport Systems operating in the 5 GHz frequency band.
Decentralized Congestion Control (TS 102 687)
1. Motivation: Channel congestions can largely degrade communication performance. For safety-related applications, the network load has to be controlled by an additional DCC algorithm.
2. No need to switch between CCH and SCH
3. Cross-layer extension to IEEE 802.11 CSMA/CA plus EDCA for congestion control at
Access layer (Transmit power/rate control)
Network & Transport
Facilities
Management plays a central role.
4. DCC avoids channel overload by message rate control, additional transmit power control, and offloading data traffic to other channels.
5. The first implementation step will be done by a local CBR measurement and a message rate control.
6. To improve fairness and safety, information sharing between the ITS stations will be necessary later on.
7. A coordinated power control will be necessary when high penetration rates are reached.
Layers Note
8. Multi-channel DCC can be used.
Networking Layer
Transport Layer
Facilities Layer
GeoNetworking protocol (EN 302 636-4): provides single-hop and multi-hop packet delivery in an ad hoc network of vehicles and roadside stations. Specifically, it utilizes geographical positions carried in the packet headers for geographical addressing and forwarding of packets on the fly.
IPv4/v6
UDP, TCP Protocols
Basic Transport Protocol, BTP (ETSI EN 302 636-5-1 V1.2.0, 2013-10)) provides a UDP-like connectionless transport protocol service, on top of GeoNetworking. An enhanced Reliable BTP (RBTP) may be used.
Decentralized Environmental Notification Message (DENM): Standard (EN 302 637-3) specifies a protocol for dissemination of event-driven safety information in a geographical region, typically via multiple wireless hops.
Cooperative Awareness Messages (CAM) EN TS 102 637‐2: Periodic time‐triggered position messages 1‐10 Hz, packet length including security up to 800 bytes
Local Dynamic Map (LDM, ETSI TR 102 863 V1.1.1, 2011-06): Dynamic data received from other ITS stations through CAM and DENM to store in a database that combines lane-level map information with sensed objects.
Application Layer
The basic set of applications TR 102 638
Road safety (driving assistance) – Emergency vehicle, slow vehicle, wrong-way driving, traffic condition, roadwork, etc.
Traffic efficiency – Speed limits notification, enhanced route guidance
Cooperative local services and global Internet services – Applications in the cooperative local services and global Internet services classes advertise and provide on-demand information to passing vehicles on either a commercial or non-commercial basis. These services may include Infotainment, comfort and vehicle or service life cycle management. Cooperative local services are provided from within the ITS network infrastructure. Global Internet services are acquired from providers in the wider Internet.
Table 43
IEEE 802.11p and IEEE 1609 standards [2-4]
Layers
IEEE 802.11p PHY/MAC layer
Message sublayer
Network and Transport layers
Application layer
Description
IEEE 802.11ax-2021, IEEE Standard for Information Technology Telecommunications and Information Exchange between Systems Local and Metropolitan Area Network--Specific Requirements Part 11: Wireless LAN Medium Access Control (MAC) and Physical Layer (PHY) Specifications Amendment 1: Enhancements for High-Efficiency WLAN
5.850-5.925 GHz band divided into 7 channels (each 10 MHz)
1-400m (1000m max) range, high data rate 6-27 Mbps
Define SAE J2735 and Basic Safety Message (BSM) sets, and message formats for especially safety applications. Frequently broadcasted among vehicles
Meet V2V/V2I safety application requirements
TCP/UDP: IETF RFC 9293 / RFC 768
IPv4/v6: IETF RFC 8200
V2V
Each vehicle broadcasts its core state information in a “Basic Safety Message” (BSM) nominally 10 times/sec
BSM is sent in a 360o pattern using IEEE 802.11p technology
Upon receipt of BSM, the vehicle safety host builds a model of each neighbour’s trajectory, assesses the threat to the host vehicle, and warns the driver (or takes control) if the threat becomes acute
Forward Collision Avoidance (FCA)
Emergency Electronic Brake Lights (EEBL)
Blind Spot Warning (BSW)
Lane Change Assist (LCA)
Do Not Pass Warning (DNPW)
Intersection Collision Warning (ICA)
Wrong Way Driver Warning (WWDW)
Cooperative Adaptive Cruise Control (CACC)
V2I
Two intersection RSU messages enable a suite of intersectionrelated safety applications.
o SPaT messa–e - Signal Phase and Timing
Red Light Running (RLR)
Left Turn Assist (LTA)
Right Turn Assist (RTA)
Pedestrian Signal Assist (PED-SIG)
Emergency Vehicle Preempt (PREEMPT)
Layers Description
Transit Signal Priority (TSP)
Freight Signal Priority (FSP)
Rail Crossing (RCA)
o MAP message – Intersection geometry
Other RSUs can send Traveler Information messages on curve speed, height restriction, icy roads, etc.
IP data exchange with servers on the Internet – RSU acts as a forwarder
P1609.1 Resource Manager
P1609.2 Security Services for Applications and Management Messages
P1609.3 Networking Services
P1609.4 Multi-Channel Operations
Describe key components of WAVE system architecture and define data flows and resources
Define command message formats and data storage formats to specify the types of devices that may be supported by OBU
Define secure message formats and processing circumstances for using secure message exchanges
Define network and transport layer services, including addressing and routing, in support of secure WAVE data exchange
Define WAVE Short Messages (WSM), providing an efficient WAVE-specific alternative to IP that can be directly supported by applications. WSMP allows the applications to directly control the lower-layer parameters such as transmit power, data rate, channel number and receiver MAC addresses
Define MIB for WAVE protocol stack
Enhancement to 802.11 MAC to support WAVE multi-channel operations on top of IEEE 802.11p
Implement multi-channel operations by dividing the available access time between the Control Channel (CCH) and Service Channel (SCH) into intervals of 50 milliseconds
Table 44 Toolbox of technical advances of IEEE 802.11bd compared to IEEE 802.11p [5] IEEE 802.11p IEEE 802.11bd Benefits of IEEE 802.11bd
Modulation
Error correction
BPSK, QPSK, 16QAM, 64-QAM
BCC
BPSK-DCM, BPSK, QPSK, 16-QAM, 64QAM & 256-QAM
BCC & LDPC
Channel bandwidth 10 MHz or 20 MHz Interoperable 10 MHz and 20 MHz
Data subcarriers 48 48 & 52
MIMO N/A
Frequency bands 5.9 GHz
Adaptive Repetitions N/A
Localization N/A
DCM mode N/A
Up to 44% higher throughput (27 Mb/s versus 39 Mb/s)
2-3 dB better sensitivity
Improved robustness
Range extension
Improved interoperability
8% higher throughput
2x2 MIMO 2x higher throughput for unicast transmission
Improved robustness and range
5.9 GHz & 60 GHz New applications
1-3 repetitions, depending on CBR
Improved robustness
Range extension
Supported New applications
Supported 3 dB better sensitivity
Improved robustness
Range extension
Channel tracking Proprietary Proprietary & Midamble-based
Total Benefit:
Range extension
Throughput
Lower complexity receiver
DCM and LDPC, repetitions up to 3 times longer range
256 QAM and MIMO up to 3 times higher throughput
Annex 4: C-V2X R14 US-DOT Standards
Table 45 C-V2X R14 US-DOT standards [133]
Layer
Security Layer
Management Layer
Standard Name
IEEE 1609.2 Security Bundle, Transport Layer Security (TLS) Transport Model for the Simple Network Management Protocol (SNMP)
ETSI Security Architecture
SAE J3161, W3C WSDL 1.1, ETSI 302 890-1
Facilities LayerApplication Layer Presentation Layer
Session Layer
Network Layer and Transport Layer
ISO 15784-2, NTCIP AP-STMF
LTE-V2X WSMP, LTE-V2X TCP, LTE-V2X IPV6
IEEE 1609.3, IETF RFC 793TCP
Access Layer-Data Link Layer 3GPP R14 C-V2X (PC5)
Standard URL
https://tools.ietf.org/html/rfc5246 https://tools.ietf.org/html/rfc6347
Access Layer- Physical Layer 3GPP R14 C-V2X (PC5)
https://www.w3.org/TR/wsdl/
https://www.etsi.org/deliver/etsi_ ts/102800_102899/10289001/01 .01.01_60/ts_10289001v010101 p.pdf
https://www.sae.org/standards/c ontent/j3161/
http://www.ntcip.org/library/stand ards/default.asp?documents=ye s&qreport=no&standard=2301, https://www.iso.org/standard/55 233.html
http://standards.ieee.org/findstds /standard/1609.3-2016.html https://tools.ietf.org/html/rfc793
https://portal.3gpp.org/desktopm odules/Specifications/Specificati onDetails.aspx?specificationId= 3179
https://portal.3gpp.org/desktopm odules/Specifications/Specificati onDetails.aspx?specificationId= 3179
Annex 5: 3GPP C-V2X Use Cases
4G LTE-V2X primarily focuses on basic safety features, offering essential functionalities like collision warnings and emergency stop notifications. In contrast, 5G-V2X expands upon this by introducing more advanced use cases, particularly in the areas of vehicle platooning and advanced driving, thereby building on the capabilities established by 4G LTE-V2X.
Table 46
4G-V2X (Release 14) and 5G-V2X (Release 16) use case comparison [134] [135]
Use case group
3GPP TR 22.885 use cases: 4G
Vehicle to Everything (V2X), Release 14 [134]
Safety Forward Collision Warning
Control Loss Warning
V2V Emergency Stop Use Case
V2I Emergency Stop Use Case
Curve Speed Warning
Wrong way driving warning
Pre-crash Sensing Warning
Road safety service
V2X Road safety service via infrastructure
3GPP TR 22.886 enhanced use cases: 5G Vehicle to Everything (V2X), Release 16 [135]
Vehicle Operations Management
Convenience
Platooning
Remote diagnosis and just-in-time repair notification
V2X by UE-type RSU
eV2X support for Remote Driving
Communication between vehicles of different 3GPP RATs
V2X in areas outside network coverage Multi-PLMN environment
Use case for V2X access when roaming Use case on Multi-RAT
V2X message transfer under MNO control Use case out of 5G coverage
Dynamic Ride Sharing
Tethering via Vehicle
eV2X support for Vehicle
Platooning
Information exchange within a platoon
Use case group
3GPP TR 22.885 use cases: 4G Vehicle to Everything (V2X), Release 14 [134]
3GPP TR 22.886 enhanced use cases: 5G Vehicle to Everything (V2X), Release 16 [135]
Automated Cooperative Driving for Short distance Grouping
Information sharing for limited automated platooning
Information sharing for full automated platooning
Changing Driving-Mode
Traffic Efficiency and Environmental friendliness
Queue Warning
V2N Traffic Flow Optimisation
V2N Use Case to provide an overview to road traffic participants and interested parties
Mixed-Use Traffic Management
V2V Use case for emergency vehicle Warning
Society and Community Warning to Pedestrian against Pedestrian Collision
Vulnerable Road User (VRU) Safety
Pedestrian Road Safety via V2P awareness messages
Advanced and Automated Driving
Cooperative Adaptive Cruise Control Cooperative Collision Avoidance (CoCA)
Automated Parking System
Extended Sensor
Information sharing for limited automated driving
Information sharing for full automated driving
Emergency Trajectory Alignment
Intersection Safety Information Provisioning for Urban Driving
Cooperative lane change (CLC) of automated vehicles
3D video composition for V2X scenario
Teleoperated Support (TeSo)
Automotive: Sensor and State Map Sharing
Use case group
3GPP TR 22.885 use cases: 4G Vehicle to Everything (V2X), Release 14 [134]
Security and Privacy
Vehicle Quality of Service (QoS) support
Privacy in the V2V communication environment
V2X Minimum QoS
Enhancing Positional Precision for Traffic Participants
3GPP TR 22.886 enhanced use cases: 5G Vehicle to Everything (V2X), Release 16 [135]
Collective Perception of Environment
Video data sharing for Automated Driving
Proposal for secure software update for electronic control unit
QoS aspect of vehicle platooning
QoS aspects of advanced driving
QoS aspects of remote driving
QoS Aspect for extended sensor
Different QoS estimation for different V2X applications
Annex 6: C-Roads ITS-G5 Trials Overview
Table 47 C-Roads ITS-G5 trials [23]
Europe C-Roads platform The C-Roads Platform is a joint initiative of European Member States and road operators for testing and implementing CITS services in light of crossborder harmonisation and interoperability.
Table 48 Indicative budget overview C-Roads 1+2+3 (in k€) [23]
States committed to participating with their pilot sites
1 Austria 175 ITS-G5 RSU (DSRC)
525 RSU, 100 additional RSU till 2025. 2250 kilometres (2022 onwards)
2 Belgium/ Flanders 3G-4G/LTE mobile communication networks
HERE Location Cloud and the local Traffic Management Centre
Cities of Graz, Wien and Salzburgpublic transport services, signalized intersections as well as hazardous location notifications
ASFINAG
Siemens Mobility
Austria GmbH, now Yunex Traffic
Austria GmbH (Contractor)
Kapsch TrafficCom
AG (Contractor)
Stadt Graz
Land Salzburg
Stadt Wien
Wiener Linien
ASFINAG’ s road operator vehicles. 100 vehicles contract awarded in late 2020 to Kapsch TrafficCo m AG
3 Belgium/ Wallonia 5 DSRC ITS-G5 RSU for A602 junction
4G network
1000 test drivers to receive and potentially update selected Safety Related Traffic Information (SRTI) using low latency data exchange.
European Commission Delegated Regulation 886/2013
Approximately 427 km of Walloon motorways via the Coyote community
Flemish Department of Mobility
HERE ITS.be
Tractebel
Engineering
COYOTE (4G) Siemens (DSRC)
Wallonia
Infrastructure
SOFICO ITS.be
Tractebel
Engineering
Table 49 C-Roads trial overview [23]
4 Czech Republic
DSRC ITS-G5
DSRC and 4G Hybrid New Cellular LTE-V
230 km of motorways are covered by ITS-G5 technology, approximately 360 km of motorways are covered also by LTE technology, 3 cities were involved, 115 RSUs at all pilot sites and 25 urban intersections were equipped with RSU
DT1 Brno agglomeration
DT2 Brno city
DT3 – Motorways D1, D5, D11 and I/52 / D52
DT4 – Public transport deployment in the cities of Plzen and Ostrava
DT5 – Railway crossing pilot
DT6 – Cross-border testing
Road and motorway Directorate (RSD) of the Czech Republic
City of Brno (via. Brněnské komunikace)
Správa železnic, state organization (SŽDC)
City of Ostrava and Plzeň (via. their public transport companies)
AŽD Praha
O2 INTENS
Corporation
T-Mobile
Škoda Auto Czech Technical University in Prague, Faculty of Transportation Sciences (CTU)
240 vehicles and VMS warning trailers
5 Denmark scale up existing CITS services by supporting cloud-tocloud hybrid communication
6 Finland scale up existing CITS services by supporting cloud-tocloud hybrid communication
NordicWay
3:Demonstrates as a large-scale pilot the feasibility of Day 1 and Day 1.5 services using hybrid communication solutions
Close gaps regarding C-ITS messages and security in new pilots Cross border tests
Part of the NodicWay test: Pre-studies for the Tampere pilot for traffic light interoperability, in which a node will be developed which will publish traffic light signal status messages (SPATem and MAPem)
Emergency vehicle approaching pilot, delivered over cellular
The Danish Road Directorate
Traficom, Väylä, Finnish Transport Infrastructure Agency Fintraffic City of Tampere
VTT Technical Research Centre of Finland Ltd
Countries Current Deployment Use cases Partners Vehicles
communications. Demonstration of the use of 3D sensor and Lidar Edge AI edge intelligent solution for analysing traffic in intersections, and warning drivers about potential collisions with VRUs.
This proof of concept was performed in the Helsinki Jätkäsaari district.
Pre-study of interactive and cooperative traffic management in the Helsinki region. The different steps to deploy a data platform, which can be used for the publication of C-ITS services have been proposed.
Study on Automated driving on Motorways, addressing the readiness of the road network to support automated driving
Traficon
Ramboll Finland
LTD
Varala Engineering
Nodeon
Swarco
Dynniq
Normivalaistus Oy
7 France
DSRC ITS-G5
162 RSU Cellular networks
C-Roads France (CRoads 1): CITS
smartphone application supporting early I2V (infrastructure-tovehicle) services roll up and further scale up
InDiD (C-Roads 2): framework of the SCOOP, InterCor and C-ROADS projects, with a scope extended to urban and autonomous vehicles
A – Probe Vehicle Data (PVD)
B – Road Works Warning (RWW)
C-Roads France
InDiD
Road operators
Concessions companies and providers of road transport services
ASFA: The Association of French Motorway Companies
APRR:
ꞏ Société des autoroutes du Nord et de l'Est de la France
SANEF: French freeway
Renault PSA
Countries Current Deployment Use cases Partners Vehicles
C – Signage Applications
D – Hazardous Location Notifications (HLN)
E – Traffic Information and Smart Routing
F – Parking, Park and Ride, Multimodality
G – Intersection (SI)
H – Traffic management
I – Vulnerable users
J – Multimodal Cargo Transport Optimization
K - Level Crossing (Use cases SNCF - open level crossing)
L – Law Enforcement
M – Payment services
concession company
Vinci Autoroutes: French freeway concession company
ꞏ Interdepartmental Directorate of CentreEast Roads (deconcentrated state service)
Interdepartmental Directorate of north Roads (deconcentrated state service)
ꞏ Interdepartmental Directorate of Atlantic Roads (deconcentrated state service)
Concessions companies and providers of road transport services
ASFA: The Association of French Motorway Companies
APRR:
Société des autoroutes du Nord et de l'Est de la France
SANEF: French freeway concession company
Vinci Autoroutes: French freeway concession company
Cities / Public Entities
ꞏ Eurometropolis of Strasbourg (EMS) Bordeaux Metropole
Countries Current Deployment Use cases Partners Vehicles
City of Paris AixMarseille-Provence
Metropole
Département de l’Isère
Bordeaux
Metropole
European
Collectivity of Alsace (CEA)
Eurometropolis of Strasbourg (EMS)
Car manufacturers
Renault
PSA
Research institutes / Universities
CEREMA
Université
Gustave Eiffel
Université
Clermont-Auvergne
Université de Reims ChampagneArdennes
CEREMA
ꞏ Université
Gustave Eiffel
Université
Clermont Auvergne
Université de Reims ChampagneArdennes
ꞏ Bordeaux INP
Institut MinesTélécom
ꞏ Université
Polytechnique
Hauts de France
Eurecom
Security experts
ꞏ
IDNomic IDNomic
Mobility Labs /
Geography experts
Transpolis
Institut Vedecom
ꞏ Le LAB
IGN
Solution providers: C-ITS / Mobility /
8 Germany 168 DSRC ITS-G5
RSU
11 different C-ITS services, which will be deployed in 5 different pilot sites and harmonised by the Federal Highway Research Institute (BASt).
Pilot Hessen
Road Works Warning Service Deployment (RWW) (extension of the existing service for long-term roadworks)
Maintenance Vehicle Warning (MVW)
Emergency Vehicle Approaching Service Deployment (EVA)
ꞏ Traffic Jam Ahead Warning Service Deployment (TJW)
ꞏ Shockwave Damping Service Deployment (SWD)
Green Light Optimal Speed Advisory Service Deployment (GLOSA)
Probe Vehicle Data (PVD) Service Deployment (extension of the existing version to support the services of TJW and SWD)
DIGITAL Valeo
ꞏ TomTom Green
communication
Transdev
Autonomous
Transport Systems
ATC France
The Hessen pilot site is organised by the German motorway operator Autobahn GmbH.
For the Niedersachsen pilot site, the two companies NORDSYS and OECON Products & Services, which will be supported by the associated partner Deutsches Zentrum für Luft- und Raumfahrt (DLR), are responsible for local activities.
9 Greece 10 RSUs and 1 mobile unit have been installed on Attica Tollway. As far as Egnatia Odos Motorway is concerned, 25 RSUs and 1 mobile unit have already been procured and are planned to be installed by mid2022.
The selected Day 1 CITS services are the following:
Road Works Warning (RWW)
o Lane closure and other restrictions (RWW-LC)
ꞏ Hazardous Locations Notification (HLN)
o Stationary Vehicle (HLN-SV)
o Weather Condition
Hellenic Ministry of Infrastructure and Transport (MIT)
o The following implementing bodies are under MIT:
o Institute of Communication and Computer Systems, designated by MIT;
o the University of Patras, designated
Countries Current Deployment Use cases Partners Vehicles
Warning (HLN-WCW)
o Obstacle on the Road (HLN-OR)
In-vehicle signage (IVS)
o Embedded VMS “Free Text” (IVS-EVFT)
o Shockwave Damping (IVS-SWD)
ꞏ Probe Vehicle Data (PVD)
o Cam Aggregation (PVD-CA)
The only selected Day 1.5 C-ITS service for the Greek action is Smart Routing (SR)
by MIT; C-Roads annual pilot overview report 2021 53
o Centre for Research and Technology Hellas – Hellenic Institute of Transport, designated by MIT; o EGNATIA ODOS
S.A., designated by MIT
COSMOTE Mobile Telecommunication
s S.A. (Cosmote)
G4S Telematix
S.A. - G4S Private Company of Security Services, Trading And Provision of Advanced Telematic and Information Technology Services and Products (G4S Telematix) Telenavis S.A. (Telenavis) Hellenic Association of Toll Road NetworkHellastron (Hellastron) Attikes Diadromes
S.A. Operation and Maintenance Company for the Elefsina Stavros Spata Airport Free Motorway and Imitos Western Peripheral Motorway (Attikes)
INTRACOM S.A. TELECOM
10 Hungary
123 DSRC ITS-G5
RSU’s have been installed so far, and 23 are planned for installation in 2022
Future- hybrid cellular with DSRC
CROCODILE project: 8 junctions equipped with C-ITS applications
Day-1 and Day-1.5-CITS-services with the option to be upscaled to Day-2-C-ITS-services.
Beneficiary: Ministry for Innovation and Technology (formerly Ministry of National Development) Implementing body: Hungarian Public Roads
Other stakeholders: Budapest University of Technology and Economics (BUTE) Budapest Public Road Plc Automotive Proving Ground Zala Ltd (APZ)
ꞏ Municipality of Győr Municipality of Zalaegerszeg Commsignia Ltd Microsec Plc
ꞏ Transport Research Institute Non-profit Ltd. (KTI) – Mobility Platform (PKI issues) ITS Hungary Association (dissemination)
40 test vehicles equipped with OBUs
11 Ireland DSRC ITS-G5 Day 1 and Day 1.5 CITS services on both Transport Infrastructure Ireland’s road network (i.e. the TEN-T road network) and within the urban centre of Dublin using V2V, I2V and V2I communications.
In-vehicle signage
ꞏ Ireland’s DoT (Department of Transport) – the beneficiary of the Grant Agreement Dublin City Council – the urban centre where urban-based C-ITS services will be piloted Road Safety
Countries Current Deployment Use cases Partners Vehicles
Hazardous location notification
Road works warning
In-vehicle signage and probe vehicle data
12 Italy 84 DSRC RSUs installed along the 368Km
V2X technologies, for the following automated driving applications:
1. trucks Platooning
2. passenger cars
Highway Chauffeur
3. combined scenarios of trucks and passenger cars
Cross-border tests with Austria
a set of “Day 1” and “Day 1.5” C-ITS
Authority – Ireland’s primary road safety organisation and an official the statutory body charged with reducing the number of fatalities and injuries on Irish roads
Enterprise Ireland – a government organisation responsible for the development and growth of Irish enterprises
IDA – (Industrial Development Authority) a semistate organisation promoting overseas direct investment into Ireland
Kapsch – A partner to provide C-ITS central station support and services
ꞏ Dynniq - A partner to supply and install RSUs and OBUs together with the provision of C-ITS service application software
Italy 3: Autostrada del Brennero (A22)
Autostrade per l’Italia (ASPI)
ꞏ Concessioni
Autostradali Venete (CAV)
North Italy Communications (Nicom)
Roma Servizi per la Mobilità (RSM)
S.p.A. Autovie Venete
Countries Current Deployment Use cases Partners Vehicles
services, for example:
• Green Light Optimal Speed Advisory (GLOSA)
• Traffic signal priority request by designated vehicles
• Signal violation/Intersection safety
• On-street parking management & information
• Traffic Information and Smart Routing
13 Netherlands TESTFEST on services, using DSRC ITS-G5 communication
RWW (trailer-based portable solution)
IVS (fixed roadside units)
PVD
14 Norway same as NordicWay3 project Part of the NordicWay 3 project. Trondheim (RWW-RC, RWW-RM, SI-SPTI, SI-GLOSA)
Oslo (RWW-RC, SITLP)
C-ITS Day 1 services: Connected Traffic Signals including:
o Traffic signal priority request by designated vehicles (TSP)
o Green Light Optimal Speed Advisory (GLOSA)
o Signal Phase and Timing Information (SISPTI)
Roads works warning. C-ITS Day 1.5 services:
The project coordinator is Rijkswaterstaat, a part of the Dutch Ministry of Infrastructure and Water management. Furthermore the Province of NoordBrabant and the Province of Utrecht are involved.
The Norwegian Public Road Administration and the following public and private organisations:
ꞏ Combitech ꞏ SINTEF Volvo Car Corporation
ꞏ Aventi ꞏ City of Oslo Polestar
Bouvet Audi Aimsun
ꞏ Q-Free ꞏ BMW ꞏ COWI
Univrses Scania
CV AB ꞏ Vianova
BMW Volvo Trucks
Rijkswater staat test vehicle
Countries Current Deployment Use cases Partners Vehicles
Road pricing (not yet specified in C-Roads)
15 Portugal DSRC ITS-G5
5 C-ITS testbed Macro Pilot cases in the Atlantic Corridor in Portugal Day 1 services and, selected, Day 1.5 Day 1 C-ITS services: Emergency electronic brake light;
ꞏ Emergency vehicle approaching; Slow or stationary vehicles;
ꞏ Traffic Jam Ahead warning; Other hazardous location notification; C-Roads annual pilot overview report 2021 85
ꞏ Roads works warning; Weather conditions; In-vehicle signage;
ꞏ In-vehicle speed limits; Probe vehicle data; Shockwave damping. Day-1.5 C-ITS services: Off-street parking information; Park and Ride information; Information on alternative fuel vehicles and charging stations; Traffic information and smart routing; Zone access control for urban areas; Wrong way driving.
Instituto da Mobilidade e dos Transportes, I.P.;
ꞏ Infraestruturas de Portugal, I.P. IP Telecom, S.A.;
ꞏ Câmara Municipal de Lisboa; Câmara Municipal do Porto;
ꞏ STCP - Sociedade de Transportes Coletivos do Porto, S.A.; EMEL - Empresa Publica Municipal de Estacionamento de Lisboa, E.E.M.; FEUP - Faculdade de Engenharia da Universidade do Porto; Brisa Concessão Rodoviária, S.A.; Ascendi Beiras Litoral e Alta, Auto Estradas das Beiras Litoral e Alta, S.A.; Autoestradas Norte Litoral – Soc. ConcessionariaAENL, S.A.;
ꞏ Autoestrada do Algarve - Via do Infante – Soc. ConcessionariaAAVI, S.A.; CaetanoBUSFabricação Carroçarias, S.A.; Brisa Inovação e Tecnologia, S.A.;
ꞏ GMVIS Skysoft, S.A.; ARMIS, Sistemas
Countries Current Deployment Use cases Partners Vehicles de Informação, Lda.;
ꞏ Siemens Mobility Unipessoal Lda.; Vialivre, S.A.; DMS Displays & Mobility Solutions Lda.; ScutviasAutoestradas da Beira Interior, S.A.; LusoponteConcessionaria para a Travessia do Tejo, S.A.; BrisalAutoestradas do Litoral, S.A.; AEDLAutoestradas do Douro Litoral, S.A.; AEAAutoestradas do AtlânticoConcessões Rodoviárias de Portugal, S.A.; Ascendi Grande LisboaAutoestradas da Grande Lisboa, S.A.; Ascendi do Grande PortoAutoestradas do Grande Porto, S.A.; ꞏ Ascendi NorteAutoestradas do Norte, S.A.; Ascendi Costa de PrataAutoestradas da Costa de Prata. S.A.; Norscut Concessionaria de Autoestradas, S.A.; TIS.PT, consultores em Transportes,
Countries Current Deployment Use cases Partners Vehicles
lnovação e Sistemas. S.A.;
ꞏ Via Verde Serviços, S.A.;
16 Slovenia Phase 1: 10 DSRC
ITS-G5 RSU covers 24 km of TEN-T core network
Phase 2: 300 km on complete motorway A1, A3, H4, H5, H6 and at critical points of A2.
C-ITS services using 3g/4g/LTE cellular networks and hybrid solutions has been in progress in 2020 and concluded in 2021.
Day 1.0 services coexistence of the CITS system with the RTTT DSRC tolling system location and driving direction awareness is envisaged to deliver real-time traffic information and test V2I and I2V
C-Roads Slovenia 2 aims to provide a more pilot area including new critical motorway sections (e.g. tunnels)
Ministry of Infrastructure DARS d.d. (Motorway Company of the Republic of Slovenia)
3G/4G/LT E Cellular Connecte d Vehicle with the Cloud Informatio n Services w
17 Spain
DGT3.0: 12,270 Km
3G/4G/LTE
SISCOGA pilot: 151
DSRC RSU and 80 cars
Madrid “calle 30” pilot: 34 RSUs, 15 OBUs Cantabric Pilot: 31 RSU and 22 OBU
Mediterranean pilot: 20RSU, 25OBU
SISCOGA
• Stationary Vehicle
• Road Work Warning
• In-Vehicle Signage applications (IVS)
• Emergency Vehicle Priority
• Emergency Vehicle Approaching
• Traffic Jam Ahead
• In-vehicle speed limits
• Signal Phase and Timing information
• Emergency Brake Light
• Weather Conditions
• Embedded VMS “Free Text”
• Animal or person on the Road
• Vehicle Data Collection
MADRID
• Roads Work Warning (RWW)
• Hazardous Location Notification
Public authorities: o Dirección General de Tráfico (DGT) –Ministry of Interior o Dirección General de Carreteras (DGC) – Ministerio de Fomento o Madrid Calle 30 S.A.
ꞏ Associations: o Foro de Nuevas Tecnologías en el Transporte (ITS España) o Asociación Clúster de Movilidad y Logística de Euskadi (MLC ITS EUSKADI) o SEOPAN, Asociación de empresas constructoras y concesionarias de infrastructures
Countries Current Deployment Use cases Partners Vehicles
• In-Vehicle Signage applications
• Off-Street parking (Serrano Park)
• Traffic Information & Smart Routing
• Hybrid Services (events received from MC30 and DGT3.0 platform
CANTABRIC • Slow or Stationary Vehicle (Bizkaia)
• Traffic Jam Ahead (Asturias,Bizkaia,Galici a)
• Road Work Warning (Bizkaia)
• Weather Conditions (Bizkaia)
• Probe Vehicle Data (Bizkaia)
• Park & Ride information (Asturias)
• Traffic Information/ Smart Routing (Asturias)
• Emergency Brake Alert (Galicia)
MEDITERRANEAN
• Stationary vehicle (Catalonia, Andalusia)
• Shockwave Damping (Catalonia)
• Traffic Jam Ahead (Andalusia)
• Road works warning (Catalonia, Andalusia)
• Weather conditions (Catalonia, Andalusia)
• Probe vehicle data (Catalonia)
• In-vehicle signage (Catalonia, Andalusia)
• In-vehicle speed limits (Catalonia)
• Obstacle on the road (Andalusia)
Private companies: o Transport
Simulation Systems S.L. (TSS) o Equipos de Señalización y Control (ESYCSA) o Indra Sistemas S.A. (Indra) o Grupo Mecánica del Vuelo Sistemas
S.A.U. (GMV Sistemas) o Opus Remote Sensing Europe (OPUS RSE) o Gertek Sociedad de Gestiones y Servicios S.A. (Gertek) o ICEACSA Consultores, S.L.U. o Ingartek Consulting, S.L. o Abertis Autopistas España S.A. o Automóvil Club Asistencia S.A. (ACASA) o Ferrovial Corporación S.A. o Kapsch TrafficCom Transportation S.A.U.
Universities: o Universidad Politécnica de Madrid (UPM) o Universitat Politècnica de Catalunya (UPC) o Universitat de Valencia – Estudi General (UVEG)
Countries Current Deployment Use cases Partners Vehicles
• Smart Slip Road (Andalusia)
• Information on charging station (Catalonia)
18 Sweden aligning NordicWay 3 with C-Roads specifications for Hybrid communication
19 UK
DSRC ITS-G5
A2/M2 Connected Vehicle Corridor (A2/M2 CVC)
Part of the NordicWay 3, take part and verify cross border tests with other pilots in European countries who have implemented the CRoads hybrid communication specification C-ITS Day 1 services: Emergency vehicle approaching (EVA) Connected Traffic Signals including: o Traffic signal priority request by designated vehicles (TSP)
o Green Light Optimal Speed Advisory (GLOSA)
Roads works warning. C-ITS Day 1.5 services: Dynamic and semidynamic traffic restrictions
Cross-border harmonisation and interoperability of C-ITS deployments in four Member States (MS), Belgium, France, the The Netherlands and the United Kingdom, linking the different national initiatives towards a harmonised strategic roll-out, and common specifications
Ensure that future UK deployment of these services will be compatible and
SOS Alarm
Region Stockholm Carmenta AB Ciy of Uppsala
Monotch City of Gothenburg
Scania CV AB
Swarco Sverige AB
ꞏ Volvo Car Corporation dynniq
ꞏ VTI ꞏ EVAM
ACTIA ITXPT
Knowit RISE
Interactive Institute AB FourC Technolution
ꞏ City of Stockholm TTS
Department for Transport (DfT) Highways England (HE)
ꞏ Transport for London (TfL)
Kent County Council (KCC)
Countries Current Deployment Use cases
Vehicles interoperable with European deployment of the four services (RWW, IVS, PVD and GLOSA).
Annex 7: C-ITS Global Deployment Cases
Table 50 C-ITS global deployment cases
Standard Date /Location
DSRC C-ITS trial – European standard Sep 2020Sep 2021 QLD Australia
Use Cases Partners
In-vehicle speed (IVS)
Red light warning (ARLW)
Road hazard warning (RHW)
Back-of-queue (BoQ)
Turning warning for bicycle riders and pedestrians (TWVR)
Road Works Warning (RWW)
DSRC C-ITS trial – European standard Mid 2021Feb 2022 VIC Australia
C-V2X 8-10 Oct 2018, A2/M2 in Kent, UK
Tram to vehicle safety (Lexus RX Communicates With Trams and Traffic Lights in Melbourne)
TMR Australia, Toyota Lexus Telstra, QUT, etc. [24]
C-V2X 28 Mar - 1 Apr 2022
Klettwitz, Germany
Roadworks Warning (RWW) using DENM messages
In-vehicle Signage (IVS) using IVI messages
Probe Vehicle Data (PVD) (ITS-G5 only) using CAM and DENM messages
Green Light Optimised Speed Advice (GLOSA) using CAM, SPaT and MAP messages
Vodafone Safer Transport for Europe Platform (STEP)
Road Works Warning, Time To Green, In-Vehicle Signage, Traffic Jams Warning and Hazard On The Road Warning
Cohda MK6- Road Works Warning, Traffic Jams Warning and Hazard On The Road Warning
Commsignia- Intersection Collision Risk Warning and Longitudinal Collision Risk Warning
Toyota Lexus AIMES La Trobe Uni. YarraTrams [136]
Vodafone Group
InterCor Hybrid TESTFEST
DfT UK [137]
C-V2X 2 Dec 2021, Turin, Italy
NSA 5G networking capabilities and the Edge Cloud solution to deliver connected car use cases
Multi-MNO scenario: How can a vehicle, that has radio access to MNO
5GAA partnered with ETSI and DEKRA [138]
5GAA, Cisco, Telecom Italia (TIM), Telefonica [139]
Standard
C-V2X 24 Mar 2022
Virginia Smart Road
A, use an MEC application, which is operated by MNO B -> Interworking between MNOs (by NOT losing the benefits of low latency)
Global operational Availability: How can an OEM as the MEC application developer be sure, especially on a global basis, that a MEC application works in the same way if it’s operated by MNO A, or if it’s operated by MNO B
Multi-MNO with roaming scenario: Where the two operators can seamlessly transfer the V2X service from one operator to the other as the car OEM moves from one geo to the other in a roaming scenario. Typically, when an in-vehicle driver does a crossborder travel that involves two operators
Blacksburg VA As above
C-V2X 20 Oct 2022
Málaga, Spain
Protecting vulnerable road users and increasing the safety of cyclists on the road by alerting them of a possible collision
Enabling smart intersections to enhance VRU safety by sending awareness messages to C-V2Xenabled cyclists
Warning road users of traffic incidents between connected vehicles
5GAA, Virginia Tech Transportation Institute [140]
5GCroCo, Anritsu, Autotalks, Commsignia, Continental, Deutsche Telekom, Ericsson, Huawei, Intel, Keysight, Molex, Nokia, Rohde & Schwarz, Stellantis, Vodafone, Omniair [141]
Standard Date /Location
C-V2X Dec 2022
Wuhan, Nanjing China
C-V2X Oct 2017
The first announced C-V2X trials in the USA
Push notification of traffic light signals, green light optimal speed advisory, green light start reminder, red light warning, road information broadcasting, electronic road signs
V2V use cases, including do not pass warning, intersection movement assist, and left turn assist
Advanced vehicle communication capabilities for improved traffic efficiencies, such as real-time mapping updates and event notifications relayed using AT&T’s cellular network and Nokia Cloud Infrastructure
Ford China [92]
Qualcomm, AT&T, Nokia, Ford [142]
C-V2X Sep 2018Jun 2021 Europe & China
C-V2X Jul - Oct 2018
Japan
C-V2X May - Nov 2019
Hong Kong
Intersection safety (intelligent intersection with VRU crossing)
GLOSA (individual speed advice to C(A)V utilising LTE-V2X communication)
Overtaking, Car Following, Hazardous Location, Crossing, Pedestrian Scenarios
Early detection for autonomous vehicles before it enters the single-lane road
Early detection for autonomous vehicles after it enters the single-lane road
Autonomous Vehicle is out of RSU coverage
C-V2X Oct 2021 identifying vulnerable pedestrians and detecting oncoming traffic
European Commission, China Mobile, 17 partners from 11 countries [143]
Qualcomm, Continental, Ericsson, Nissan, NTT DOCOMO, OKI [144]
HKT, ASTRI and Hong Kong Airport Authority [145]
Verizon and Nissan North America [34]
Annex 8: Autonomous Vehicles C-ITS Trials
Table 51 Autonomous vehicle C-ITS trials
Automotive C-ITS
Audi China
CARIAD China 2022
China Wuxi IoT Smart City, team
Both 5G and CV2X (Cellular Vehicle-toEverything)
Hyundai - 2022 Hyundai conducted two V2X pilot projects in the Netherlands and Germany for use cases of Vehicle-to-Grid (V2G) and Vehicle-to-Home (V2H)
V2X technology
Audi Traffic Light Information (TLI) system
Audi Local Hazard Information (LHI) and Local Hazard Warning (LHW) functions
Green Light Optimised Speed Advisory (GLOSA) and time-to-green functions
Transfers energy stored in an electric car battery to an electricity network or grid
New production models for the Chinese market such as the Audi A7 L and Audi A6 L [20]
IONIQ 5 [114]