Computational and DataDriven Chemistry Using Artificial Intelligence Fundamentals, Methods and Applications
First Edition
Takashiro Akitsu
Department of Chemistry, Faculty of Science, Tokyo University of Science, Tokyo, Japan
Table of Contents
Cover image
Title page
Copyright
Contributors
About the editor
Preface
Chapter 1: Introductory chapter
Abstract
Acknowledgment
1: Chemistry using the “inductive” research style
2: Cyano-bridged copper complexes
3: Cyano-bridged 3d-4f complexes
4: Hybrid materials of copper complexes and photocatalysts
5: Hybrid materials of azobenzene and metal complexes
6: Hybrid materials of metal complexes and proteins
7: Conclusion
References
Chapter 2: Goal-directed generation of new molecules by AI methods
1: Introduction
2: Molecular representation
3: Guiding and evaluating generative methods
4: Generative methods
5: Conclusion
Chapter 3: Salen-type metal complexes based on structural database of X-ray crystallography
1: Background
2: Docking calculation of protein and salen complexes
3: Systematic structure analysis of 3d-4f salen complexes
4: Failure research on photoelectric conversion dyes
Chapter 4: Approaches using AI in medicinal chemistry
1: History of machine learning applications in medicinal chemistry
2: QSAR
3: De novo design
4: AI-assisted synthesis prediction
5: Closing the loop: Active learning and autonomous design
Chapter 5: Application of machine learning algorithms for use in material chemistry
2: ML algorithms
3: Connecting ML with HTE
4: Examples
5: Outlook
Chapter 6: Predicting conformers of flexible metal complexes using deep neural network
1: Tremendous Rubik’s snake versus polyhedral puzzle
2: Enumerating conformers of metal complexes
3: Machine learning and deep neural network
4: Tools for deep learning and related studies
5: Conformational analysis for [M(dmso) 6 ] ² + complexes
6: Time saving by AI in conformational search for [Mg(dmso) 6 ] ² +
7: Conformational prediction for [VO(dmso) 5 ] ² + without using AI
8: Conformational prediction for [VO(dmso) 5 ] ² + using DNN
9: Concluding remarks
Chapter 7: Predicting activity and activation factor of catalytic reactions using machine learning
Acknowledgments
2: Extraction of metal cluster catalytic activity factors using sparse modeling [11]
3: Activity prediction of heterogeneous catalyst using the surface adsorption calculation database [19]
4: Concluding remarks
Chapter 8: Convolutional neural networks for the design and analysis of nonfullerene acceptors
2: Models
3: Dataset
4: Results Index
Copyright
Elsevier
Radarweg 29, PO Box 211, 1000 AE Amsterdam, Netherlands
The Boulevard, Langford Lane, Kidlington, Oxford OX5 1GB, United Kingdom
50 Hampshire Street, 5th Floor, Cambridge, MA 02139, United States
Copyright © 2022 Elsevier Inc. All rights reserved.
No part of this publication may be reproduced or transmitted in any form or by any means, electronic or mechanical, including photocopying, recording, or any information storage and retrieval system, without permission in writing from the publisher. Details on how to seek permission, further information about the Publisher’s permissions policies and our arrangements with organizations such as the Copyright Clearance Center and the Copyright Licensing Agency, can be found at our website: www.elsevier.com/permissions .
This book and the individual contributions contained in it are protected under copyright by the Publisher (other than as may be noted herein).
Notices
Knowledge and best practice in this field are constantly changing. As new research and experience broaden our understanding, changes in research methods, professional practices, or medical treatment may become necessary.
Practitioners and researchers must always rely on their own experience and knowledge in evaluating and using any information, methods, compounds, or experiments described herein. In using such information or methods they should be mindful of their own safety and the safety of others, including parties for whom they have a professional responsibility.
To the fullest extent of the law, neither the Publisher nor the authors, contributors, or editors, assume any liability for any injury and/or damage to persons or property as a matter of products liability, negligence or otherwise, or from any use or operation of any methods, products, instructions, or ideas contained in the material herein.
Library of Congress Cataloging-in-Publication Data
A catalog record for this book is available from the Library of Congress
British Library Cataloguing-in-Publication Data
A catalogue record for this book is available from the British Library
ISBN: 9978-0-12-822249-2
For information on all Elsevier publications visit our website at https://www.elsevier.com/books-and-journals
Publisher: Susan Dennis
Acquisitions Editor: Anneka Hess
Editorial Project Manager: Lindsay Lawrence
Production Project Manager: Sruthi Satheesh
Cover Designer: Mark Rogers
Typeset by STRAIVE, India
Contributors
Takashiro Akitsu Department of Chemistry, Faculty of Science, Tokyo University of Science, Tokyo, Japan
Golnaz Bissadi University of Applied Sciences Niederrhein, Institute for Surface Technology, Krefeld, Germany
Thomas Cauchy Laboratoire MOLTECH-Anjou, UMR CNRS 6200, UNIV Angers, SFR MATRIX, Angers, France
Kevin Cremanns University of Applied Sciences Niederrhein, Institute of Modelling and High-Performance Computing, Krefeld, Germany
Béatrice Duval Laboratoire LERIA, UNIV Angers, SFR MathSTIC, Angers, France
Dea Gogishvili Medicinal Chemistry, Research and Early Development, Respiratory and Immunology (R&I), BioPharmaceuticals R&D, AstraZeneca, Gothenburg, Sweden
Junpei Iwama Department of Chemistry, Faculty of Science, Tokyo University of Science, Tokyo, Japan
Masato Kobayashi
Faculty of Science & WPI-ICReDD, Hokkaido University, Sapporo
ESICB, Kyoto University, Kyoto, Japan
Thierry Kogej Hit Discovery, Discovery Sciences, MolecularAI, R&D, AstraZeneca Gothenburg, Sweden
Jules Leguy Laboratoire LERIA, UNIV Angers, SFR MathSTIC, Angers, France
Benoit Da Mota Laboratoire LERIA, UNIV Angers, SFR MathSTIC, Angers, France
Eva Nittinger Medicinal Chemistry, Research and Early Development, Respiratory and Immunology (R&I), BioPharmaceuticals R&D,
AstraZeneca, Gothenburg, Sweden
Atanas Patronov Hit Discovery, Discovery Sciences, MolecularAI, R&D, AstraZeneca Gothenburg, Sweden
Shi-Ping Peng State Key Laboratory for Physical Chemistry of Solid Surfaces, iChEM, Fujian Provincial Key Lab of Theoretical and Computational Chemistry, and College of Chemistry and Chemical Engineering, Xiamen University, Xiamen, People’s Republic of China
Hiroshi Sakiyama Yamagata University, Yamagata, Japan
Christian Schmitz University of Applied Sciences Niederrhein, Institute for Surface and Coatings Chemistry, Krefeld, Germany
Christian Tyrchan Medicinal Chemistry, Research and Early Development, Respiratory and Immunology (R&I), BioPharmaceuticals R&D, AstraZeneca, Gothenburg, Sweden
Xin-Yu Yang State Key Laboratory for Physical Chemistry of Solid Surfaces, iChEM, Fujian Provincial Key Lab of Theoretical and Computational Chemistry, and College of Chemistry and Chemical Engineering, Xiamen University, Xiamen, People’s Republic of China
Yi Zhao State Key Laboratory for Physical Chemistry of Solid Surfaces, iChEM, Fujian Provincial Key Lab of Theoretical and Computational Chemistry, and College of Chemistry and Chemical Engineering, Xiamen University, Xiamen, People’s Republic of China
About the editor
Takashiro Akitsu is a full professor of chemistry at Tokyo University of Science. He completed his undergraduate school training (chemistry) at Osaka University, Japan and his graduate school training (physical and inorganic chemistry, especially coordination, crystal, and bioinorganic chemistry) at Osaka University (PhD 2000). Following positions at Keio University, Japan, and Stanford University, United States, he moved to his current affiliation in 2008. He has published almost 220 articles in peerreviewed journals and has presented multiple posters at international
exhibitions. Prof. Akitsu has been a peer reviewer of many journals and acted as an organizing committee of several international conferences.
Preface
With the practical application of the third-generation artificial intelligence (AI), the possibility of computational chemistry and chemical researches based on databases are increasing gradually. In a recent decade, indeed, with the development of AI, applications of data sciences for chemistry or materials science including drug design have been reported more and more. In this book, the editor is aiming at editing review chapters mentioning each field (computational, theoretical, and analytical chemistry; database; crystallography; spectroscopy) of recent works from chemistry and informatics aspects including tutorial explanation. Beyond conventional computational chemistry or use of databases, this area is a newly developed field and there are few books summarizing new studies or tutorial information at present. This book may be recommended to graduate students and upper researchers of chemistry, because this area is a newly developed field and there may be few competitors or challengers to use such methods so far. To tell the truth, the editor himself is interested in using it for chemistry, but he is not an expert. Therefore, the editor examined the possibility of using a new method in comparison with his conventional chemistry research style of searching suitable compounds (especially with the help of crystal structure databases and computational chemistry). Ideal goal that I think now is improving conventional (empirical) chemical researches to find the law inductively from systematic data, searching for compounds with desired structures and functions from systematic experiments, and narrowing down candidate compounds by prediction by databases and computational chemistry. While reviewing the style and past research of the authors, one will be introduced to examples of research on AI utilization that may be applicable. While learning about this new style of chemistry research and how it compares with an experiment-centered style, readers will also be provided with low-threshold chemical content and topics, with points of particular interest noted.
Chapter 1: Introductory chapter
Takashiro Akitsu Department of Chemistry, Faculty of Science, Tokyo
University of Science, Tokyo, Japan
Abstract
With practical application of third-generation artificial intelligence, the possibilities of computational chemistry and chemical research based on databases are increasing gradually. As a way of chemical research, which mainly consists of conventional experiments performed previously, researchers have proposed “inductive laws” based on the experimental data taken from systematic research or searching with testing. First, the author recaptures a “spectrochemical series,” which is one of the famous laws in the field of inorganic coordination chemistry, as an example of research using inductive laws based on many experimental data. Next, in the study of cyano-bridged metal complexes and their metal-organic frameworks (MOFs), the author introduces a case example of discovering specific phenomena by systematic experiments. Finally, in the research of hybrid functional materials including metal complexes, the author introduces some of his studies that have been experimentally (trial-anderror) and computationally performed for a combination of components, and shows the prospect of application of a data-driven style based on the latest papers.
Keywords
Inductive research; Data-driven chemistry; Coordination chemistry; Metalorganic framework; Organic-inorganic hybrid materials; Crystallography; Computational chemistry
Contents
1. Chemistry using the “inductive” research style
1.1 Theory and model in chemistry
1.2 Ligand field theory and spectrochemical series
1.3 Discovery of spectrochemical series from experimental data
2. Cyano-bridged copper complexes
2.1 Topics of cyano-bridged copper complexes
2.2 Systematic study for this system
2.3 Possibility of sophisticated research methods
3. Cyano-bridged 3d-4f complexes
3.1 Topics of cyano-bridged 3d-4f complexes
3.2 Systematic study for this system
3.3 Possibility of sophisticated research methods
4. Hybrid materials of copper complexes and photocatalysts
4.1 Topics of hybrid materials of copper complexes and photocatalysts
4.2 Systematic study for this system
4.3 Possibility of sophisticated research methods
5. Hybrid materials of azobenzene and metal complexes
5.1 Topics of hybrid materials of azobenzene and metal complexes
5.2 Systematic study for this system
5.3 Possibility of sophisticated research methods
6. Hybrid materials of metal complexes and proteins
6.1 Topics of hybrid materials of metal complexes and proteins
6.2 Systematic study for this system
6.3 Possibility of sophisticated research methods
7. Conclusion Acknowledgment
References
Acknowledgment
This work was partly supported by Japan Society for the Promotion of Science KAKENHI Grant Number 20H00336.
1: Chemistry using the “inductive” research style
1.1: Theory and model in chemistry
With the development of artificial intelligence technology, the possibility and applications of computational chemistry and chemical research based on data science are increasing more and more actively. Researchers who have been trained in ordinary (only experimental) chemistry research to adjust to such emerging fields will face a definitive manpower shortage. For changing their research style to the new era’s one smoothly and quickly, the first way is joint research with information scientists (artificial intelligence, machine learning, or data science), in which the difficulty or importance of communication with experts of other fields will be emphasized. The second way is that chemists should be trained to have a computer science background as well as chemistry, where reform of university (undergraduate) and graduate school education should be undertaken, and it must take a long time more than the first way. The third way is to review the “inductive” research style used by former chemists, too. Applying new methods in data science may be partly the same to extracting laws from empirical experimental data even for welleducated conventional chemists. It is important to improve the methodology of major scientific research.
From this point of view, in this chapter as a preface to this book, the spotlight from a new perspective is applied to the “spectrochemical series,” which is the law in the field of inorganic coordination chemistry, and also a typical inductive law based on experimental data. And the author will introduce some of his research on metal complexes by carrying out systematic experiments and accumulating data to discover only a few important experimental facts. Additionally, the possibility of applying the methods of chemical research based on artificial intelligence or data science will also be mentioned by leading researchers in recent years.
Generally speaking, when new facts found do not fit into the existing model or law, natural science really advances. For example, we look back on the progress of binding theory in metal complexes historically, or from the viewpoint of approximation and precision of theoretical treatments. (1) Valence bond method (including hybrid orbitals) can explain magnetism regardless of improper treatment of beautiful color of coordination compounds. (2) Crystal field theory was a model based on electrostatic theory essentially and could explain color and thermodynamic stability simply. However, it ignored π-bonds between metal and coordination atoms of ligands. (3) Ligand field theory could include covalent bonds σ- and π -bonding characters between metal and coordination atoms, which enabled to treat π-back donation or large splitting of d-orbital due to strong ligand field. However, it did not include all atoms of organic ligands, which sometimes play an important role in function of whole coordination compounds. (4) Molecular orbital theory could finally attain description including whole organic ligands as well as metal environments. Even with molecular orbital treatment, limited highsymmetry molecules can be treated in mathematical methods (group theory). (5) Nowadays, after semiempirical and ab initio methods, computational programs using density functional theory (DFT) are employed widely in recent studies of metal complexes [1] .
1.2: Ligand field theory and spectrochemical series
When an electrostatic interaction is exerted from around a positively charged metal ion from negatively charged ligands (also considering the unpaired electrons of the neutral ligand as a negative charge), the direction of the ligand is changed. According to crystal field theory the dorbitals of metal ions with broad lobes are repelled and destabilized energetically. The ligand field theory considers the contribution of covalent bonds (especially π-bonds).
In the case of a hexacoordinated octahedral complex (represented as “O h symmetry” by the point group symbol), a metal ion is on the origin and six ligands are located at both positive and negative points on the x , y , and z axes at the identical distances. For free metal ions, which can be thought of as an electron cloud distribution like a spherical soft ball, the five d-orbitals are degenerated. It can be inferred from the situation that pressure is applied to the soft ball from all directions, but first, as the
negative charge approaches, the entire five d-orbitals are destabilized (while degenerated still). It can be inferred from the situation that local pressure in the negative charge direction is applied to the soft ball, that is, the (negative) ligands are further approached, but the d x 2- y 2 or d z orbitals are compressed like a soft ball by the negative charged field from the direction of the x , y , z axes. The energy level of two d-orbitals becomes higher while being destabilized and double degenerate ( e g ). On the other hand, the d xy , d yz , and d zx orbitals that extend in the direction between the x , y , and z axes with no negative charge, that is, no ligand, are analogized from the bulge of the soft ball because the center of gravity is maintained. As described above, the three d-orbitals are relatively stabilized, and the energy level becomes low with triple degeneracy ( t 2 g ). In this way, Δ O (or 10Dq) denotes the ligand field splitting for the hexacoordinated octahedral ones ( Fig. 1 ).
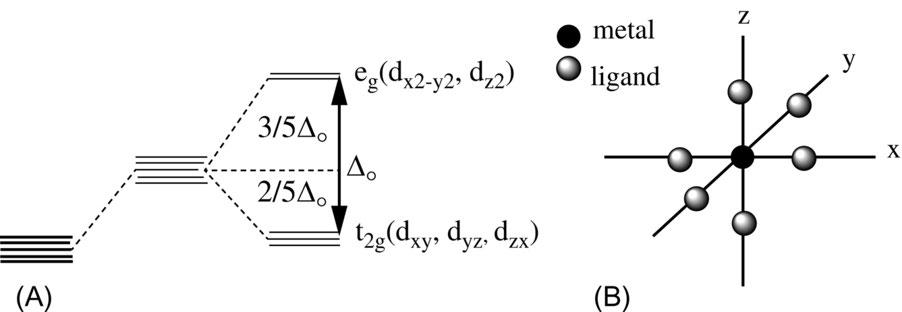
Fig. 1 (A) Ligand field splitting for O h symmetry. (B) Coordinates for a metal complex in O h symmetry.
Most metal complexes show various colors in the visible region. In the mechanism called d-d transition, from the orbitals with a low energy level to the orbitals with a high energy, a wavelength absorbing the light corresponds to the energy difference of Δ O . The complementary color of the absorbed light can be seen as the color of the compound for the human eye.
Consider a reddish purple hexacoordinated octahedral complex [Ti(H 2 O) 6 ] ³ + of d ¹ electron configuration. The low-energy t 2 g orbitals and the high energy e g orbitals are split by the effect of the ligand field due to six water ligands. Because of the light with the energy of Δ O = Δ E
= hν (where ν = Δ O / h is wavenumber of light and h is Planck’s constant) is absorbed, electrons are excited from t 2 g orbitals to e g orbitals. Namely the transition is and the electron configuration of the five d-orbitals changes before and after absorption of light.
The magnitude of ligand field splitting (Δ O for hexacoordinated octahedral type) varies depending on the type of ligands even under the same metal ion. For example, [Ni(en) 6 ] ² + of d ⁸ electron configuration absorbs light with a higher wavenumber (in other words, shorter wavelength) than [Ni(H 2 O) 6 ] ² + . This is confirmed by the shift of the peak wavelength of the UV-visible (electronic) spectra. Consequently, the ethylenediamine (en) ligand has a greater ability to split the ligand field than the water ligand ( Fig. 2 ). A series of ligands shown below (bold letters denote coordination atoms) arranged in the order of large ligand field splitting is called a “spectrochemical series.” The higher the ligand in the series, the “stronger” the ligand field is formed. From molecular orbital consideration, large ligand field splitting Δ O is ascribed to strong π-accepting property, and as a result, a low-spin complex tends to be formed for d ⁴ -d ⁷ electron configurations.

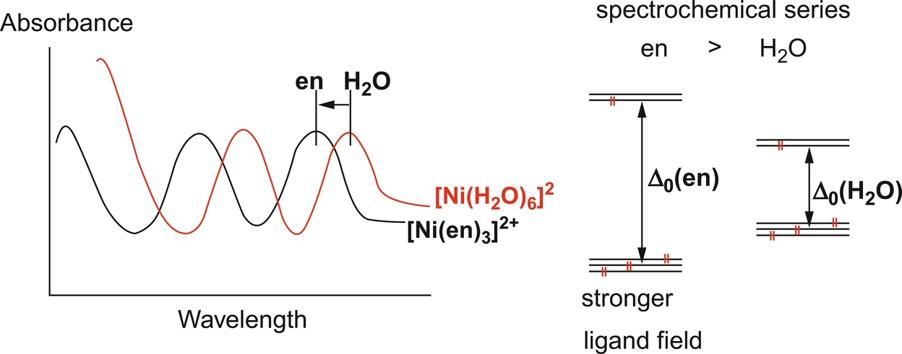
Fig. 2 [Left] Shift of electronic spectra of [Ni(en) 3 ] ² + and [Ni(H 2 O) 6 ] ² + . [Right] Corresponding ligand field strength for ethylenediamine (en) and water ligands.
1.3: Discovery of spectrochemical series from experimental data
Originally, Prof. Ryutaro Tsuchida (Osaka University, Japan) systematically investigated [Co(NH 3 ) 5 X] n + complexes by substituting X ligands and inductively proposed a spectrochemical series [2] . He systematically selected various series of metal complexes for experiments, focusing on Co(III) and Cr(III) complexes, and conducted thorough research based on the following results.
• A transition metal complex shows two absorption bands in electronic spectra. He also named the first and second absorption bands from the long wavelength side.
• In addition to the two absorption bands, a further absorption band may appear on the short wavelength side.
• A new absorption band is observed when two anions (Cl − , NO 2 − , etc.) are coordinated in trans -sites.
• When a special ligand (NCS − , etc.) is included in the complex group, a new absorption band peculiar to this ligand is also observed.
• The reason for the first absorption band was associated with inside the complex group, especially deep inside, not filled with central metal ions,
due to electronic transition in the transition shell (d-electron shell).
• The reason for the second absorption band was closely considered the relationship seen with the strength of the coordination bond between metal and ligand, which can be attributed to change in the energy states of coordination electrons.
• It was discovered that if the ligand (NH 3 ) was kept constant regarding types and numbers and only the ligand (X) was changed in [Co(NH 3 ) 5 X] n + , it would change in a fixed order. The order of the ligand is called as “spectrochemical series” later.
Their original paper [3] mentioned that systematic experimental data of electronic spectra for [Co(NH 3 ) 5 X] n + complexes in correlation order with “quantity P”, which associated differences of wavenumbers in the first and second absorption bands. Introducing the “ P ” values, reasonable rearrangement of the X ligands in the order of “ P ” values, he successfully came to the discovery of the order of ligands that shift the absorption bands. In later years, he and his colleagues developed spectrochemical series for the validation of other types of metal complexes using experimental data by others and more so their own experimental data [4 , 5] .
By the way, is it possible to apply a rule other than the set of data that guides the rules (theory or prediction)? Also, from the absorption spectra of many [Co III A 4 B 2 ] complexes in solutions, the relationship between the cis - and trans -type absorption bands is shown. The following conclusions were obtained. In the spectrochemical series:
• When A is behind B, the first absorption band of a trans -type complex appears on the shorter wavelength side than a cis -type one.
• When A is not behind B, the first absorption band of a cis -type complex appears on the shorter wavelength side than a trans -type one.
In this way, the spectrochemical series, which was an empirical rule deduced from systematic experimental data, may be origin and essence of the first and second absorption bands of a complex. A clear theoretical interpretation became possible after the introduction of ligand field theory
in the 1950s, namely after more than 20 years of the discovery of the spectrochemical series.
2: Cyano-bridged copper complexes
2.1: Topics of cyano-bridged copper complexes
As for cyano-bridged copper and other bimetallic complexes, we (the author and colleagues in those days) are interested in the direction of flexible Jahn- Teller strain of Cu(II) units and cyano-bridges in crystal, aiming at design or discovery of external field responsible MOFs (especially thermal and/or photoinduced phase transition). For many years, the crystal structure analysis of the mononuclear copper complexes as well as bimetallic complexes was also performed vigorously [ 6 – 21 ]. During this study by changes of substituents of ligands and central metals, we happened to find a quite long Cu
N axial bond (at least, semicoordination in a crystal). Once the author wrote the summary article entitled “Cu(II) complexes’ Jahn-Teller distortion and electronic function in proteins and molecular crystal environment” [22] . Furthermore, comparison with deuterium and light hydrogen (D/H) and nonlinear optical [17] effects were also dealt with after publishing this review article. In addition, we also developed hybrid or composite materials with inorganic materials and Cu(II) complexes and powder crystal analysis was carried out for them. After all, as mentioned later, only classification and consideration for characterized compounds can be carried out to our knowledge.
In 2010s, with the development of neutron crystallography, many compounds containing coordinated water and water of crystallization were focused on, in which an isotope-substitution effect may be expected for deuterium. As for cyano-bridged bimetallic complexes, in this way, we have studied the relationship between the changes in crystal lattice and coordination environment and their anisotropy. Thus, we began to investigate [CuL 2 ] 3 [M(CN) 6 ] 2 · n H 2 O complexes (L = diamine) to replace into deuterated water (D 2 O) for comparison of the difference in crystal structure during temperature change ( Fig. 3 ) [7 , 17] . Isotopesubstituted complexes were synthesized employing commercially available deuterated water for nuclear magnetic resonance (NMR) solvent in the
same manner as normal water. Single crystal X-ray structure analysis, synchrotron radiation powder X-ray diffraction (KEK-PF BL-8B), and infrared (IR) spectral measurements were performed to compare them.
Fig. 3 Crystal structure of [CuL 2 ] 3 [Co(CN) 6 ] 2 · n H 2 O (L = Nethylethylenediamine) marked with crystalline water for H/D substitution.
The complexes in Fig. 3 contained crystalline water only, without coordinated water. Although the crystal structures of H/D-forms were isomorphic, a large shift in the diffraction angle due to the temperature change was observed in deuterium. It is considered that this is because the deuterium substitution weakens the intermolecular hydrogen bonds, and the crystal lattice is significantly affected by the external temperature conditions.
On the other hand, the related chiral complexes incorporated L = (1 R ,2 R )-diaminocyclohexane contain both coordination water ligands of the mononuclear Cu(II) units (exhibiting large differences of axial bond distances in temperature dependence) and crystallization water molecules in the space of the cyano-bridges. The crystal structures of H/D-forms were isomorphic without significant difference during temperature changes. Of course, the H-form of L = trans -1,2-diaminocyclohexane complex (achiral diastereomers of the chiral ones) affords different crystal structure containing only water of crystallization. This is a very “rare” case in which the D-form exhibits weak intermolecular hydrogen bonds.
2.2: Systematic study for this system
Beside them, we have also systematically studied other cyano-bridged bimetallic complexes including a Cu(II) moiety with different diamine ligands for comparison of dimensional structures (such as 1D linear, 1D zigzag, 2D, 3D, 3D including guest mononuclear complex, and so on) of MOFs and Jahn-Teller distortion due to the Cu(II) moiety. Typical abbreviations of diamine ligands are en = ethylenediamine, N -Meen = N -methylethylenediamine, N , N -Me 2 en = N , Ndimethylethylenediamine, N , N ′-Me 2 en = N , Ndimethylethylenediamine, N -Eten = N -methylethylenediamine, chxn = cyclohexane-( 1R , 2R )-diamine, and so on [ 22 – 26 ]. In such a system in which the deformation is large and the direction of deformation is also limited, it can be seen that the initial Jahn-Teller strain is small and the tetragonal structural changes accompanying the temperature change become large. It is highly suggestive to examine the structural and chemical meanings from the viewpoint of the magnetic field, and it is also expected to be applied to the design guidelines for external fieldresponsive molecule-based magnets. For example, a chiral chxn complex with four-coordinated cyano units formed a 1D zigzag chain with zigzag molecular planes along the b axis direction, whose axial Cu
N(CN) bond is longer than conventional bond lengths known as semicoordination bonds in the classic structural chemistry of Cu(II) complexes. Because of characteristic crystal packing features, it can be interpreted that weakly electrostatic interaction tied anion (cyano complex) and cation [Cu(II) complex] moieties in a crystal. Such a crystal structure cannot be expected before crystal structure analysis and of course molecular design from the beginning [16] .
Generally, in the structural chemistry of Cu(II) complexes, the axial coordination of the hexacoordinated trans -[CuA 4 B 2 ] coordination is found frequently. The T value ( T = in-plane Cu-A average bond length/axis Cu
B average bond length) signifies the degree of tetragonal strain in the B
B direction. An ideal octahedron in O h symmetry exhibits T = 1, while large tetragonal distorted octahedron exhibits a smaller T value than 1. It may be natural that the steric hindrance of diamine ligands may act to elongate axial and equatorial bond distances around the Cu(II) ion. However, symmetry may also affect the coordination environment. For example, Cu(II) complexes in D 4h symmetry do not show Jahn-Teller distortion strictly. Due to pseudo-Jahn-Teller, in the actual D 4h systems, degree and direction of distortion of a coordination environment can be controlled considering with vibration modes [ 7 , 27 – 29 ].
Substitution of metal ions in a cyano-anion moiety has been also investigated by using variable temperature X-ray diffraction (XRD) patterns. As mentioned, a chiral [Cu(chxn) 2 ][Ni(CN) 4 ]·2H 2 O complex had a long axial Cu
N bond of 3.120(8) Å and also exhibited negative thermal elongation associated with this long Cu
N bond. In order to elucidate appropriate factors about crystal packing to observe lattice and Jahn-Teller distortion separately, we measured variable-temperature XRD patterns for some cases: (1) [Cu(chxn) 2 ] [M(CN) 4 ]·2H 2 O (M = Pd and Pt) to compare ion size; (2) [Cu( N Eten) 2 ][M(CN) 4 ]·2H 2 O for combinatorial preparations by using M = Ni, Pd, and Pt to test suitable ion size; (3) [Cu(Mechxn) 2 ] 3 [M(CN) 6 ] 2 · n H 2 O (Mechxn = ( 1R , 2R )- N , N ′-dimethyldiaminocyclohexane; M = Cr, Co, and Fe) by using different water of isotopes (H 2 O, D 2 O, and H 2 ¹⁸ O) for isotope effect on intermolecular hydrogen bonds.
Generally, slow diffusion of an aqueous solution (10 mL) of CuL 2 (H 2 O) 2 2 (0.1 mmol) onto an aqueous solution (10 mL) of K 2 [Pd(CN) 4 ] (0.1 mmol) gave rise to blue plate-like single crystals of [Cu(chxn) 2 ] [Pd(CN) 4 ] at 298 K. The rest of samples were prepared in a similar way by employing the corresponding ligands (L), cyanide precursors, and water of isotopes. Powder XRD patterns of the complexes were measured at BL-8B (8 keV, λ = 1.54 Å) at 100–300 K [18] .