Chemosphere
journal homepage: www.elsevier.com/locate/chemosphere
Ammonia volatilization mitigation in crop farming: A review of fertilizer amendment technologies and mechanisms
Tianling Li a, c , Zhengguo Wang a , Chenxu Wang a , Jiayu Huang a , Yanfang Feng b , Weishou Shen a , Ming Zhou c, * , Linzhang Yang b
a Collaborative Innovation Centre of Atmospheric Environment and Equipment Technology, Jiangsu Key Laboratory of Atmospheric Environment Monitoring and Pollution Control, School of Environmental Science and Engineering, Nanjing University of Information Science & Technology, Nanjing, Jiangsu, 210044, PR China
b Key Laboratory of Agro-Environment in Downstream of Yangtze Plain, Ministry of Agriculture and Rural Affairs, Jiangsu Key Laboratory for Food Quality and SafetyState Key Laboratory Cultivation Base, Ministry of Science and Technology, Institute of Agricultural Resources and Environment, Jiangsu Academy of Agricultural Sciences, Nanjing, 210014, PR China
c Centre for Clean Environment and Energy, Griffith University, Gold Coast campus, QLD, 4222, Australia
HIGHLIGHTS
• A vital step in reducing emissions is to inhibit the conversion of fertilizer to NH3
• Enhanced efficiency fertilizers technique can mitigate over 54% of NH3 emissions.
• Low environmental impact biofertilizers provide more sustainable NH3 control.
• Biochar-based fertilizers can either promote or inhibit NH3 volatilization.
• Membrane/film-based mulching methods achieve NH3 mitigation via barrier effect.
ARTICLE INFO
Handling Editor: Dr Y Yeomin Yoon
Keywords:
Ammonia volatilization
Mitigation technology
Agriculture
Nitrogen fertilizer
Farmland
* Corresponding author.
GRAPHICAL ABSTRACT
ABSTRACT
Good practices in controlling ammonia produced from the predominant agricultural contributor, crop farming, are the most direct yet effective approaches for mitigating ammonia emissions and further relieving air pollution. Of all the practices that have been investigated in recent decades, fertilizer amendment technologies are garnering increased attention as the low nitrogen use efficiency in most applied quick-acting fertilizers is the main cause of high ammonia emissions. This paper systematically reviews the fertilizer amendment technologies and associated mechanisms that have been developed for ammonia control, especially the technology development of inorganic additives-based complex fertilizers, coating-based enhanced efficiency fertilizers, organic waste-based resource fertilizers and microbial agent and algae-based biofertilizers, and their corresponding mechanisms in farmland properties shifting towards inhibiting ammonia volatilization and enhancing nitrogen use efficiency. The systematic analysis of the literature shows that both enhanced efficiency fertilizers technique and biofertilizers technique present outstanding ammonia inhibition performance with an average mitigation efficiency of 54% and 50.1%, respectively, which is mainly attributed to the slowing down in release and hydrolysis of nitrogen fertilizer, the enhancement in the adsorption and retention of NH4+/NH3 in soil, and the promotion in the microbial consumption of NH4+ in soil. Furthermore, a combined physical and chemical means,
E-mail address: ming.zhou@griffith.edu.au (M. Zhou).
https://doi.org/10.1016/j.chemosphere.2022.134944
Received 1 March 2022; Received in revised form 7 May 2022; Accepted 9 May 2022
Availableonline13May2022 0045-6535/©2022ElsevierLtd.Allrightsreserved.
namely membrane/film-based mulching technology, for ammonia volatilization inhibition is also evaluated, which is capable of increasing the resistance of ammonia volatilization. Finally, the review addresses the challenges of mitigating agricultural ammonia emissions with the aim of providing an outlook for future research.
1. Introduction
With the foreseeable growth of the global population in the years ahead, the reliance on fertilizer is predictably increasing to meet the growing demand for crops (Li et al., 2019b). Due to the necessity of nitrogen for crop growth, nitrogen fertilizer is generally adopted as the mainstay of agriculture; hence, it is the most crucial nutrient being used in farming (De Campos Bernardi et al., 2016). The world agricultural nitrogen fertilizer consumption increased from 2015 to 118.7 million tons in 2020, with an average annual growth of 1.5% (Martínez-Dalmau et al., 2021; Swarbreck et al., 2019). However, as much as 40–70% of nitrogen losses result from ammonia volatilization, denitrification, runoff, leaching and other ways (Chien et al., 2009; Li et al., 2019a; Timilsena et al., 2015), leading to the accumulation of reactive nitrogen (i.e., organic nitrogen and inorganic nitrogen compounds exclude N2) in the natural systems, which further contributes to a series of environmental issues (Minoli and Carozzi, 2015). Ammonia volatilization accounts for a considerable amount (average of 10–14%) of total nitrogen loss (Bouwman et al., 2001; Pan et al., 2016; Zhou et al., 2021a); it is, therefore, one of the main pathways of nitrogen loss. The volatile ammonia is not only an indispensable precursor of the atmospheric fine particulates (e.g., PM2.5) but also an incentive of water eutrophication and soil acidification after atmospheric deposition, causing detrimental effects on air, water and soil (Liu et al., 2020; Ti et al., 2019). Furthermore, some studies have revealed that ammonia volatilization is closely implicated with the emissions of greenhouse gases such as N2O emission from agriculture (David Ussiri, 2012; SN Behera et al., 2013), which is a crucial contributor to global warming. Therefore, many agricultural studies have taken ammonia volatilization as a necessary monitoring parameter for estimating agricultural systems, and the development of mitigation strategies has gained tremendous interest in both agronomic and environmental research.
The factors that can affect ammonia volatilization in agricultural
systems are the breakthroughs in developing ammonia control technologies. These factors could be natural factors, such as meteorological conditions (e.g., temperature, wind speed and water regime) (Gong et al., 2013; Liu et al., 2007; Peng et al., 2009), farmland properties (e.g., soil properties and crop features) (Corstanje et al., 2008; Griggs et al., 2007; Hayashi et al., 2006; Rochette et al., 2009), and factitious factors, such as fertilization and irrigation strategies (Barakat et al., 2016; Ding et al., 2021; Dong et al., 2012; Liu et al., 2015; Zhong et al., 2021), fertilizer amendments (De Campos Bernardi et al., 2016; Minoli and Carozzi, 2015; Shan et al., 2015; Timilsena et al., 2015), and other factors. Many studies have revealed that improved fertilizer technologyies can effectively inhibit the volatilization of ammonia on farmland, which have presented great development prospects and application potential. This review thus summarizes the recent advances in the mitigation strategies of ammonia volatilization through fertilizer amendments techniques, which is of the most critical significance for improving nitrogen fertilizer use efficiency and subsequently mitigating environmental pollution risks. Furthermore, this review also discusses the effects and mechanisms of the reuse of organic waste in agriculture on ammonia volatilization and the membrane/film-based mulching technology for ammonia volatilization inhibition and future development opportunities.
2. The mechanism of ammonia volatilization in farmland
Ammonia volatilization refers to the loss of nitrogen fertilizer to the atmosphere in the form of ammonia gas. After applying ammonium nitrogen fertilizer to the farmland, a series of biochemical and chemical processes related to ammonia formation occurs regardless in paddy field or dry field. As shown in Fig. 1, the hydrolysis of ammonium nitrogen fertilizer (e.g., urea) is a critical initial step. On the one hand, the hydrolyzed ammonium ion can dissolve in farmland surface water or soil water and further be converted into ammonia gas (i.e., NH3) and other
Fig. 1. The nitrogen cycle in the agriculture system.
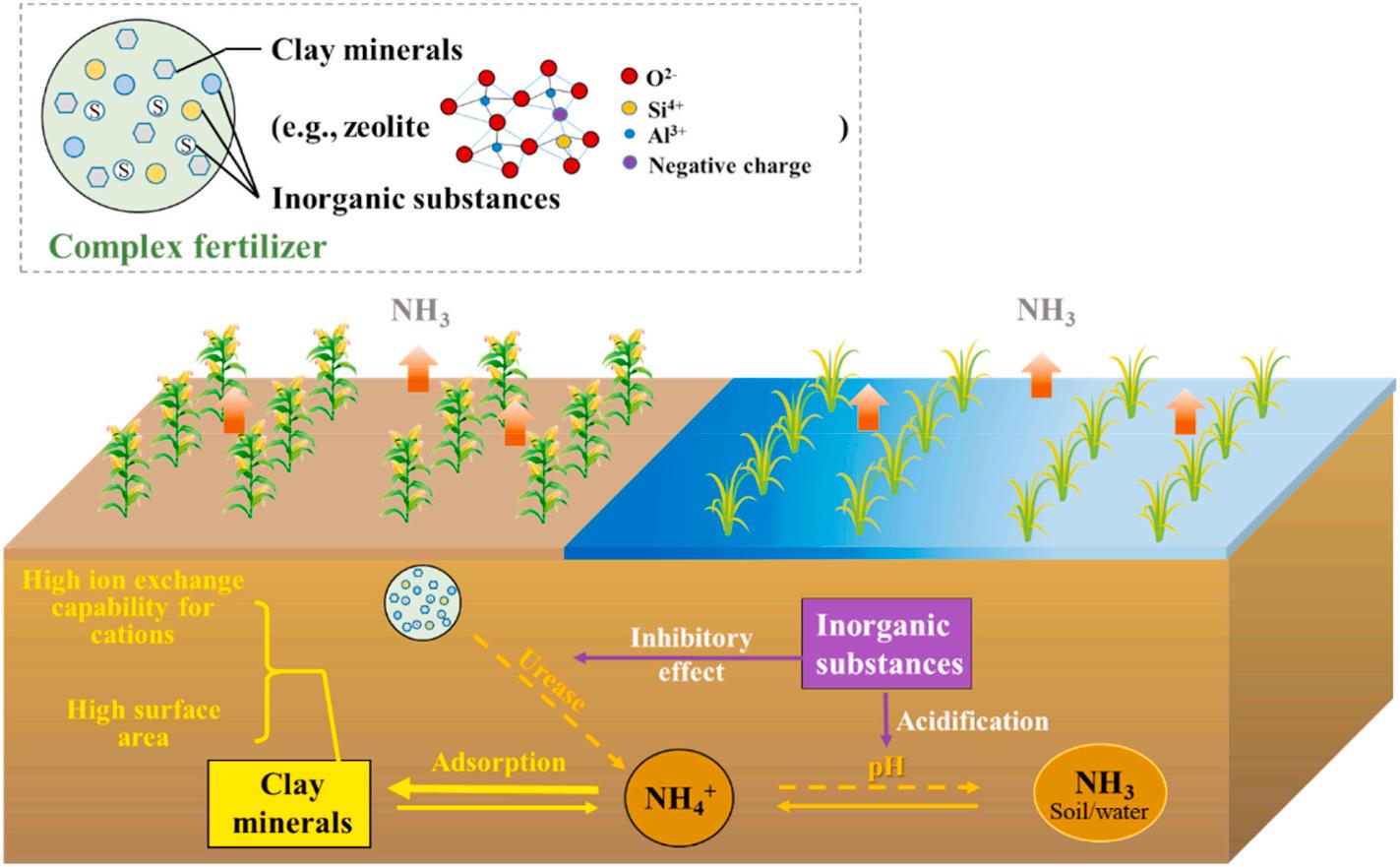
reactive nitrogen (e.g., NO and N2O) through nitrification and/or denitrification processes, ending up with volatilizing into the atmosphere. After that, part of the volatile ammonia returns to the farmland through atmospheric nitrogen deposition, and the rest reacts with atmospheric gaseous pollutants (such as VOCs, NOx, SO2, etc.) to accelerate the formation of PM2.5, threatening human health. On the other hand, chemical migration processes in soil allow part of hydrolyzed ammonium to leach into the surface and underground water, contributing to water eutrophication (Recio et al., 2018). As only nitrate and ammonium are “plant-available” , most of lost nitrogen fertilizers are no longer available to the crops, dwindling both fertilizer utilization efficiency and potential crops yields. Meanwhile, some ammoniums are retained in the soil through “exchange” (binding to soil particles or utilizing by microorganisms) that could be eventually available, indirectly reducing nitrogen loss.
The transformation of ammonium to ammonia is a reversible process, which strongly links to nitrogen fertilizer loss via volatilization; hence the conditions that stimulate the formation of ammonia promote the emission of ammonia. This insinuates that the emission of ammonia can be inhibited by restraint or control of ammonia volatilization promoting processes or conditions. Given this, this study reviews a variety of control technologies, mainly involving fertilizer amendments and barrier effects that are developed to suppress nitrogen fertilization loss through ammonia volatilization in various ammonia promotion related scenarios such as soil-water interface, water-air interface and soil-air interface. In addition, as the regional distribution pattern of ammonia emission also shows strong correspondence with local arable land areas and agronomic strategies that can be briefly sorted out into soil fertilization practices and irrigation practices (Barakat et al., 2016; SN Behera et al., 2013), agronomic strategies capable of reducing ammonia volatilization are systematically reviewed in the Supplementary Material.
3. Current fertilizer amendment technologies for mitigating ammonia volatilization
To date, nitrogen fertilizers used in the farmland are mostly quickacting fertilizers such as urea and ammonium bicarbonate. There are two potential problems with using this type of fertilizer. On the one hand, after fertilizer being applied to the soil, the concentration of soluble nitrogen ions (e.g., NH4+) in the soil will increase rapidly in a short time, thereby promoting the N loss through ammonia volatilization. On the other hand, the fertilizer release duration is also relatively short,
which cannot meet the nitrogen demand of crops throughout the whole growth period. Based on the above-mentioned problems, developing advanced fertilizer amendment technologies is an effective way to improve fertilizer utilization efficiency and reduce N loss through ammonia volatilization.
3.1. Clay mineral and inorganic additives-based complex fertilizer technology
As shown in Fig. 2, complex fertilizers are generally composed of soil additives and nitrogen fertilizer. Clay minerals and some inorganic substances are two major soil additives. The used clay minerals are usually crystalline hydrated aluminosilicates of alkali or alkaline-earth metals, structured in a three-dimensional rigid crystalline network with high-density pores, cavities and canals at the nanoscale. Such composition allows them to retain and release water content and exchange cations without changing the crystal structure (De Campos Bernardi et al., 2016). The high ion exchange capability and surface area of clay minerals (e.g., clinoptilolite zeolite and stilbite zeolite) for cations, such as ammonium, not only enhance fertilizer retention but also achieve slow-release to minimize the rate of converting ammonium to ammonia (Palanivell et al., 2015). Previous studies have shown that zeolite and bentonite can reduce ammonia volatilization by up to 50% and are beneficial to increase nitrogen fertilizer utilization and crop yields (Palanivell et al., 2016; Pratt et al., 2016; Sun et al., 2019d). Moreover, total ammonia volatilization and NH4+-N and NO3 -N leaching loss decreased with increasing rates of zeolite amendment (Latip et al., 2011; Sun et al., 2019d). A 25.3% of ammonia loss reduction was achieved by using clinoptilolite zeolite to extend the retention of NH4+ and NO3 in waterlogged condition soil (Palanivell et al., 2015). Also, the ammonia loss reduction ratio was achieved 40–50% when applying zeolite with sago wastewater irrigation under waterlogged soil, attributing to the combined effects of high absorption ability of zeolite and acidifying capacity of the irrigation wastewater (Omar et al., 2010). Additionally, some inorganic substances can also be incorporated with a nitrogen fertilizer to mitigate ammonia volatilization due to their accessibility and low cost. The added inorganic substances retards the hydrolysis of urea by reducing the urease activities and increases NH4+ retention by changing soil properties, such as acidifying the soil and enhancing soil cation exchange capacity. Using this principle, Soaud et al. employed S0 to amend alkaline and calcareous soils and investigated the varying rates of S0 application in sandy calcareous soils. The
Fig. 2. Schematic diagram of using complex fertilizer technology to mitigate ammonia volatilization.
Table 1
Mineral and inorganic additives for ammonia volatilization mitigation.
Type of additives Ammonia mitigation efficiency
Mitigation mechanisms
Clinoptilolite zeolite 25.3% Improve the retention of NH4+ and NO3 in soil.
Clinoptilolite zeolite
Cuban zeolite
Clinoptilolite zeolite
Andosols
33.2%
Increase the cation exchange capacity of the amended soil due to the inclus ion of zeolite can maintain more NH4+ in the soil.
33% Allow NH4+ to enter the porous structure of zeolites so is held to convert into NH3
26% Increase the affinity of clinoptilolite zeolite for NH4+
0.2% 1) Lower the soil pH;
2) Increase the effective cation exchange capacity; 3) Increase the nitrification potential for a high consumption rate of the applied ammonium.
Soil/crop type Reference
Typic paleudults (Bekenu series) soil
Palanivell et al. (2015)
Tipik tualemkuts (Bekenu series) soil (Latip et al., 2011)
Fertilizer pellets in aqueous solutions (Esp´ ecie Bueno et al. 2015)
Typic paleudults (Bekenu series) soil
Palanivell et al. (2016)
Volcanic ash soil with wheat Hayashi et al. (2011)
Vermiculite + bentonite 50% Improve the effectiveness of NH4+ adsorption/fixation to clays. Sandy sodosol soil Pratt et al. (2016)
Zeolite + sago wastewater 40–50% 1) Form channels in zeolites that effectively absorb ammonium ions and release them slowly; 2) Acidify the soil, and lower soil pH by introducing the sago wastewater, facilitating the formation of ammonium ions over ammonia.
Clinoptilolite zeolite + alternate wetting and drying irrigation 35–37%
Clinoptilolite + cellulose 85–96%
Zeolite + triple superphosphate 34–49%
Zeolite + triple superphosphate + humic acid >30%
Enhance soil adsorption capacity for NH4+ and more crystallized urea and urease being sequestered in the zeolite pores. Zeolite can retain NH4+ within a short time after N fertilization due to its high cation exchange capacity, which buffer the excessive N supply significantly and produce a better efficiency in reducing NH3 volatilization.
1) Increase NH4+ retention on cation-exchange sites; 2) Enhance microbial growth and activities.
Significantly increase soil-exchangeable Ca, K and Mg, and benefit the formation of NH4+ over NH3 compared with urea without additives.
1) Encourage the formation of NH4+ over NH3 via hydrolysis of superphosphate that can acidify the soil, lower soil pH; 2) Increase the retention of NH4+ on the humic acid and zeolite; 3) Promote the formation of metastable reaction products such as Ca (NH4)2(HPO4)2 would help to conserve NH4+
Gypsum 13% Decrease the pH.
Boric acid 11–16% Significantly slow down the rate of urea hydrolyzation, prolonging its half-life.
Calcium superphosphate
Urea + pyrite + CuSO4
Urea + KCl + CuSO4
39.2% Alleviate the negative effect of pH of the flood water.
sand
Sandy clay loam (Typic kandiudult)
Sandy clay loam (Typic kandiudult)
Haruna Ahmed et al. (2008)
Ahmed et al. (2006)
Coastal saline alluvial soil Zhu et al. (2020)
Chernozem and red soil Gao et al. (2021)
Yellow-brown soil with cabbage Shan et al. (2015)
24.6%
30.3% Induce a strong inhibitory effect on urease activity by using Cu2+ , and acidify alkaline urea microsites by using pyrite or KCl.
Urea + Cu + B 37% 1) Block the enzyme site and reduce its activity in the soil through the Cu2+ produced by the reaction of the ion with the sulfhydryl group from urease, thus promoting N maintenance in amidic form; 2) Reduce the pH around the granules by introducing B.
results showed that the neutralization effect between alkaline urea and H2SO4 generated from S0 suppresses the conversion of NH4+ to NH3 In this way, up to 55% ammonia loss has been reduced (Soaud et al., 2011).
A similar investigation focused on the effect of co-application of potassium chloride and copper sulfate on ammonia volatilization found that 25% of ammonia loss reduction was achieved due to the strong inhibitory effect of Cu2+ on urease activity and acidification of alkaline urea microsites by KCl (Damodar Reddy and Sharma, 2000). Moreover, there were other studies suggested that triple superphosphate and some acidic additives (such as boric acid and humic acid) possess good abilities for ammonia volatilization control and can be used as effective nitrogen fertilizer additives (Ahmed et al., 2006; He et al., 2002; Zhu et al., 2020).
Table 1 summarizes some common mineral and inorganic additives for mitigating ammonia volatilization. It can be seen that in the investigated studies, the inhibition effect of a single additive on ammonia volatilization (average inhibition efficiency ca. 23%) is inferior to the combined effects of two or more additives (average inhibition efficiency ca. 42.8%), and the total average inhibition efficiency is about 33.5%. To sum up, clay mineral and inorganic additives-based complex fertilizers have the advantage of being low-cost, most indigenously available from natural minerals or industrial by-products. However, excessive application of such additives can induce soil acidification and compaction or damage the soil structure, which may cause secondary environmental
Alfisol soil (Typic haplustalf) with sunflower (Damodar Reddy and Sharma, 2000)
Dystrophic red latosols with maize (Eduardo Lopes Cancellier et al., 2016)
pollution and rise potential environmental risks (De Campos Bernardi et al., 2016).
3.2. Coating and inhibition-based enhanced efficiency fertilizer technology
Several attempts have been made to develop means for ammonia evolution controlling during urea hydrolysis. A prevalent strategy is using enhanced efficiency fertilizers (EEF) with additional coating and urease/nitrification inhibitors. As demonstrated in Fig. 3, the added coating controls fertilizer nutrient release by suppressing one or more nitrogen transformation processes responsible for the N losses (Liu et al., 2020; Timilsena et al., 2015; Yang et al., 2020b).
The most used coated fertilizer, also known as controlled/slowrelease fertilizer, includes polymer coating, inorganic/organic additives coating, etc. The coating additives regulate the release rate of nitrogen fertilizer, thus maintaining a low level of ammonium nitrogen in the soil or field water and supporting the long-term supply of N for crop absorption, thereby reducing ammonia loss and boosting nitrogen utilization efficiency (Tang et al., 2018; Tian et al., 2017). In recent years, a wide variety of coated fertilizers have been developed. Compared with traditional urea, coated fertilizers have been proven to significantly reduce ammonia volatilization. The research conducted by Lam et al.
Mineral soil Omar et al. (2010)
Rice paddy soil Sun et al. (2019d)
Calcareous riviera fine
He et al. (2002)
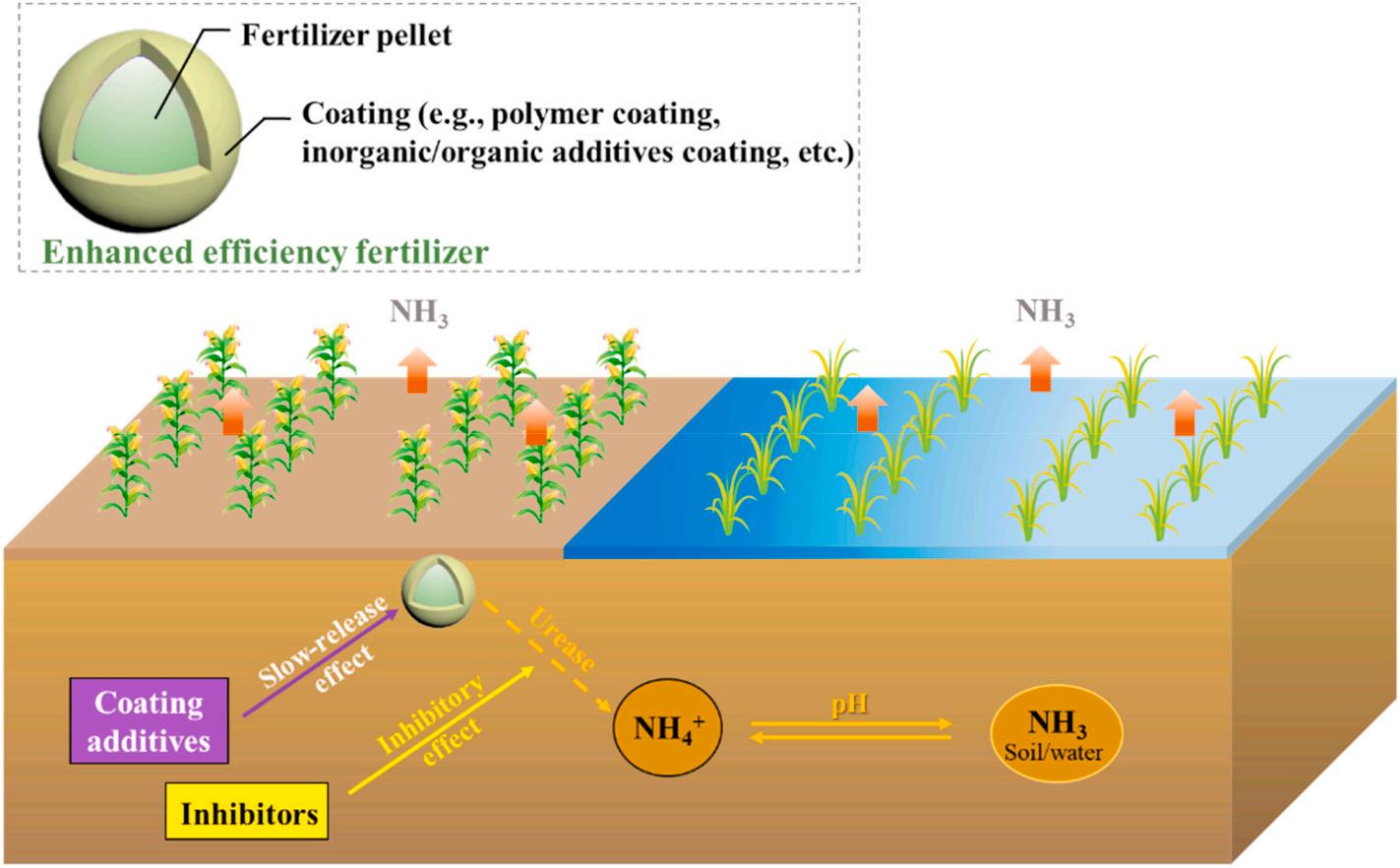
found that polymer-coated fertilizers can reduce ammonia volatilization by 80% in tenosol soil grown with perennial ryegrass (Lam et al., 2019). Later, Shan et al. suggested that sulfur-coated fertilizers can also reduce ammonia volatilization, with a reduction rate ranging from 60.7% to 68.8% in yellow-brown soil planted with cabbage (Shan et al., 2015). On this basis, Tian et al. collaboratively applied polymer-coated and sulfur-coated fertilizers to calcaric ochri-aquic cambosol soil planted with cotton, achieving an ammonia loss reduction of as much as 105% (Tian et al., 2017). Besides, the studies carried out by Sun et al. and Shan et al. revealed that bulk-blend controlled-release fertilizer performed different effects on ammonia volatilization inhibition under different farmland types, specifically, when being applied in hydroagric stagnic anthrosol planted with rice paddy and in yellow-brown soil planted with cabbage, this type of EEF can reduce ammonia loss by 22.8% and 77.7–83.1%, respectively (Shan et al., 2015; Sun et al., 2016). Lately, Pan et al. used meta-analysis to comprehensively analyze the effects of a variety of common coated fertilizers on ammonia volatilization. The analysis revealed that controlled/slow-release fertilizers can reduce ammonia volatilization by 68% on average. Among them, thermoplastic resin-coated urea, sulfur-coated urea, and polyfin-coated urea can mitigate ammonia loss by 82.7%, 78.4% and 69.4%, respectively (Pan et al., 2016).
Urease inhibitors are another important type of EEF for ammonia volatilization control. As a hydrolase that acts on amide bonds, urease can catalyze the conversion of urea and organic nitrogen, contributing to the formation of ammonium nitrogen. Since the urease activity can directly affect the ammonia volatilization process on the soil, urease inhibitors can be added to control the urease activity so that urea enters the deep soil before hydrolysis and forms an exchange complex with the soil, resulting in decreasing the NH4+ concentration produced by hydrolysis, and ultimately reducing the loss of ammonia volatilization (Sigurdarson et al., 2018; Silva et al., 2017). Many studies have suggested that the application of urease inhibitors has a critical effect on the reduction of ammonia volatilization in farmland. For example, N-(n-butyl) thiophosphoric triamide (NBPT) is recognized as a high-efficiency urease inhibitor, which has been studied and applied in a variety of farmland types (Cantarella et al., 2018). Awale’s research found that applying NBPT in sandy loam soil and silty clay soil can effectively reduce the ammonia volatilization loss by 32.3% and 71.4%, respectively (Awale and Chatterjee, 2017), while Singh et al. also revealed that, in temperate pasture soil, NBPT could reduce ammonia volatilization losses by 22–47% and increase nitrogen uptake to 7.61 g
m 2 compared with 1.90 g m 2 in the application of urea only (Singh et al., 2013). The study conducted by Ahmed et al. showed that an even higher ammonia volatilization reduction efficiency (up to 90%) could be achieved by the combined use of NBPT and dicyandiamide, which significantly improved the utilization of nitrogen fertilizer (Ahmed et al., 2018). In addition, other urease inhibitors, such as Benzoylthiourea-type urease inhibitor (RTB68), Benzimidazole-type urease inhibitor (BZI1) and Limus®, have also been proven to be capable of controlling ammonia volatilization, reaching 10%, 22% and 55–60% loss reduction, respectively (Barberena, 2019; Li et al., 2017b). Table 2 summarizes some coated fertilizers and urease inhibitors for mitigating ammonia volatilization. It is observed that this type of fertilizer amendment technique shows a high ammonia volatilization inhibition performance with an average inhibition efficiency of 54.3%, among which the coated fertilizers can effectively hinder ca. 63.6% ammonia volatilization while urease inhibitors mitigate ca. 45.6%, in the investigated 37 studies. In general, such coating and inhibition-based EEF realize ammonia reduction and nitrogen fertilization efficiency enhancement by hindering the hydrolysis of nitrogen fertilizer and coordinating nitrogen supply pattern so that they usually present the characteristics of “long-acting, slow-release, energy-saving, and environmental-friendly” However, there are also some drawbacks, such as expensive price, vulnerable coating material, potential damage to the soil structure, and the inability of nutrient release characteristics to fully match the nutrient requirements of the crop during the growth period. Therefore, the solutions to these existing problems will be the future development directions of such ammonia volatilization mitigation technology.
3.3. Microbial agent and algae-based biofertilizer technology
Another critical practice already in use to minimize ammonia emissions is the application of biofertilizers (Fig. 4). This type of fertilizer is considered the alternative to chemical fertilizers, which can directly or indirectly improve soil structure, restore soil fertility, maintain rhizosphere microflora balance, and degrade toxic substances. Differ from synthetic fertilizer, microbial agent-based biofertilizers are approved to alleviate ammonia volatilization mainly by reducing the amount of NH4+-N that remained in the soil, including both reducing the accumulation of NH4+-N by inhibiting the conversion of nitrogen fertilizers into NH4+-N and increasing the consumption of NH4+-N, that is, accelerating nitrification of NH4+-N, which present more economical and
Fig. 3. Schematic diagram of using enhanced efficiency fertilizer technology to mitigate ammonia volatilization.
Table 2
Coated fertilizers and inhibitors for ammonia volatilization mitigation.
Type of additives
Polyaspartic acid (PASP) with a molecular weight of 7568
Sulfur-coated urea
Ammonia mitigation efficiency
Mitigation mechanisms
47.5% Capture the NH4+ by the negative charge ions of PASP.
60.7–68.8% Prevent the direct contact of urea and soil water by the sulfur-coat and microcrystalline wax in this fertilizer, thus delaying the hydrolysis of urea.
Bulk-blend controlled-release fertilizer 77.7–83.1% Enhance the slow-release effect and promote N uptake to a greater extent.
Soil/crop type
Reference
Fluvo-aquic soil with maize Yang et al. (2019)
Yellow-brown soil with cabbage Shan et al. (2015)
Yellow-brown soil with cabbage Shan et al. (2015)
22.8% Delay the availability of nutrients. Hydroagric stagnic anthrosol with rice paddy Sun et al. (2016)
Cyclohexyl phosphoric triamide 86.6% Inhibit urease activity by slowing down and minimizing urea hydrolysis.
A meta-analysis of 824 observations Pan et al. (2016)
Methylene urea 87.5%
Thermoplastic resin-coated urea 82.7%
Sulfur-coated urea 78.4%
Polyfin-coated urea 69.4%
Polyurethane-coated urea; degradable polymer-coated urea; water-based polymer-coated urea
42.3–65%
Lower NH4+ concentration and pH in the floodwater.
Loam paddy soil with late rice Li
Polymer-coated urea 80% Slow urea release from the fertilizer granules. Tenosol soil with perennial ryegrass Lam et al. (2019)
Polyolefin-coated urea 59–91% Maintain lower surface water pH and NH4+ concentration. Ferralic cambisol soil with double-rice Xu et al. (2013)
Controlled release polymer-coated urea 84.2% in sandy loam
Slow urea release by the polymer-coating. Ulen sandy loam (sandy, mixed, frigid aeric calciaquoll)
Zinc sulfate (ZnSO4)-coated urea with Boron 58–81% Temporarily inhibit urease enzyme activity by boron compounds and thus slowing urea hydrolysis and NH3 volatilization.
Neem coated urea 27.5% Inhibit the urease producing microbial activities via the presence of alkaloids in the neem oil, resulting in a low urea hydrolysis rate that reduces the NH3–N loss.
Pine oleoresin (POR) coated urea 41.1% Change the antimicrobial properties and microsite pH through the POR-induced urea hydrolysis reduction by inhibiting ureaseproducing microbes.
A fertilizer mixture of sulfur-coated urea and polymer-coated urea
Polymer-coated urea (environmentally smart nitrogen [ESN] with methylene di-urea as conditioner, 44% N)
Oxamide
Phosphoric acid diamide-amended urea
65–105%
Prevent the direct contact of urea and soil moisture, so slow down the rate of urea hydrolysis.
5–6% Slow down the rate of urea hydrolysis by using polymer-coated urea and induce different influences on NH3 emissions according to different cropping systems and the source of polymer-coated urea.
38.3–62.7% Inhibit Ammonia volatilization via the slow hydrolysis of oxamide achieved by its slight water solubility.
50% Inhibit urease activity.
45–55% Inhibit urease activity and delay urease hydrolysis by the urease inhibitor, NBPT.
Crowley silt loam, mowata silt loam and kinder silt loam soil
Vertisol soil
Awale and Chatterjee (2017)
Adotey et al. (2017)
Jadon et al. (2018)
Calcaric ochri-aquic cambosol soil with cotton Tian et al. (2017)
Cancienne loam soil with cotton Tian et al. (2015)
Hydromorphic paddy soil with rice Tang et al. (2018)
Wheat Khalil (2011) Green UreaNV® (urea coated with the urease inhibitor N-(n-butyl) thiophosphoric triamide, NBPT)
SuperU® (urea containing urease and nitrification inhibitors)
Urease inhibitor N-(n-butyl) thiophosphoric triamide (NBPT)
44%
45% Delay the SuperU hydrolysis through the urease inhibitor.
58–63% Inhibit urea hydrolysis by using NBPT in different types of soil and cropping systems, and under different climate influences.
45% Weaken enzymatic activity to decrease the exchangeable NH4+ pool.
22–47% 1) Inhibit urea hydrolysis and the diffusion of non-ionic urea molecules in the presence of urea inhibitors; 2) Reduce diffusion of NH4+ ions in the absence of urea inhibitors.
32.3% in sandy loam and 71.4% in silty clay soil
Adsorb significant amounts of NH4+ and reduces NH4+ availability for NH3 production by high cation exchange capacity.
Tenosol soil with perennial ryegrass Lam et al. (2019)
Vertosol soil with bluegrass Lam et al. (2018)
Judith clay loam (fine–loamy, carbonatic, frigid typic calciustolls) (Keshavarz Afshar et al. 2018)
Cancienne loam soil with cotton Tian et al. (2015)
Calcaric fluvisol soil with sunflower crop Sanz-Cobena et al. (2008)
Temperate pasture soil Singh et al. (2013)
Temperate pasture soil ulen sandy loam (sandy, mixed, frigid aeric calciaquoll), and fargo silty clay (fine, smectitic, frigid typic epiaquert)
Awale and Chatterjee (2017)
52% Delay urease hydrolysis by using NBPT. A meta-analysis of 121 observations Silva et al. (2017)
55–60% Delay urease hydrolysis by using Limus® Li et al. (2017b) (continued on next page)
et al. (2017a)
Table 2 (continued ) Type
Limus® (a urease inhibitor consisting of 75% N-(n-butyl) thiophosphoric triamide (NBPT) and 25% N-(n-propyl) thiophosphoric triamide (NPPT))
77–88% Delay urease hydrolysis and increase fertilizer N retention by the urease inhibitor.
Fluvo-aquic soil and alluvial soil with maize
Agricultural soil with winter wheat Li et al. (2015)
Benzimidazole-type urease inhibitor (BZI1) 22% Slightly delay urease hydrolysis. Clayey yellow oxisol soil
Benzoylthiourea-type urease inhibitor (RTB68)
Urease inhibitor N-(n-butyl) thiophosphoric triamide (NBPT)
10%
81.9% Significantly delay urease hydrolysis. N-(n-butyl) thiophosphoric triamide (NBPT) + N-(n-propyl) thiophosphoric triamide (NPPT)
Urea coated with potassium sulfate with N-(n-butyl) thiophosphoric triamide (NBPT)
Urea coated with calcium sulfate with N(n-butyl) thiophosphoric triamide (NBPT).
6.3–24.5% Inhibit urease enzyme activity by using NBPT/ NPPT.
25.4–35.6% Inhibit urease hydrolysis by using NBPT.
24.1–35.1%
Soil samples from 79 agricultural fields from various cropping systems, tillage practices, landscape positions, and soil textures throughout the United States
Wheeling silt loam soil
diagram of using biofertilizer technology to mitigate ammonia volatilization.
beneficial in mitigating ammonia volatilization, increasing crop productivity, enhancing fertilizer utilization efficiency, and protecting the ecological environment (Vassilev et al., 2015). Studies have shown that microbial agents-based biofertilizers, such as Bacillus amyloliquefaciens biofertilizer and Bacillus subtilis biofertilizer, can effectively inhibit ammonia volatilization by 68% and 44%, respectively (Sun et al., 2020a; Xue et al., 2021). Similar results were also found in several later representative works. For example, Wang et al. have found that both viable Trichoderma viride (T. viride) and nonviable T. viride biofertilizer can control ammonia volatilization from alkaline soil, achieving ammonia loss reduction by 42.21% and 32.42%, respectively (Wang et al., 2018). These advantages of biofertilizers are achieved via a unique mechanism promoted by various compounds in the fertilizer. Firstly, it avoids the high soil pH that is usually induced while using conventional urea-based fertilizer (Wang et al., 2018). Meanwhile, it enhances soil nitrification capacity by increasing the abundance of nitrifying microorganisms, which promotes the conversion of NH4+-N to NO3 -N and reduces the proportion of nitrogen loss in the form of ammonia volatilization (Wang et al., 2018). Recently, another hidden
Barberena (2019)
Sunderlage and Cook (2018)
Frame et al. (2012)
mechanism of mitigating ammonia volatilization by using algae-based biofertilizers was revealed by Castro et al., who found out that the physical barrier forming on the paddy field water allowed it to hinder the ammonia exchange between water/soil and air phase, thus reducing volatilization (Castro et al., 2020). Free-floating algae in rice field has attracted researchers’ attention because, as a kind of nitrogen source to rice field, they can directly or indirectly change the physical, chemical and biological properties of soil and soil-water interface (Castro et al., 2020). In the study conducted by Castro et al., microalgal biofilm was confirmed to be capable of reducing ammonia volatilization by 75.6% through slow-release nitrogen from the degradation of microalgae organic matter (Castro et al., 2017). As a common hydrophyte, Azolla showed outstanding ammonia volatilization inhibition ability when applying to rice fields in both gleyi-stagnic anthrosolos and hydragric anthrosolos soils, contributing to the reduction of ammonia loss by 42–55.4% (Yang et al. 2020a, 2021; Yao et al. 2018a, 2018b). Another common alga in the paddy field, duckweed, was also validated for having ammonia volatilization mitigation capability (Li et al., 2009). When it is used in combination with sewage irrigation in rice fields, the
Fig. 4. Schematic
Table 3
Biofertilizers for ammonia volatilization mitigation.
Nonviable T. viride biofertilizer 32.4%
Viable Trichoderma viride (T. viride) biofertilizer 42.2% 1) Lower soil pH and NH4+-N concentration; 2) Promote the absorption of fertilizer nitrogen in sweet sorghum and increase the utilization rate of fertilizer; 3) Enhance nitrification by increasing the abundance of functional genes of ammonia-oxidizing archaea (AOA) and ammonia-oxidizing bacteria (AOB).
Bacillus amyloliquefaciens (BA) biofertilizer 68% Reduce the transformation of fertilizer nitrogen to NH4+-N, and simultaneously accelerate NH4+-N into the nitrification process.
Bacillus subtilis biofertilizer 44% Reduce the “source” and increase the “sink” of NH4+-N through the application of B. subtilis biofertilizer, contributing to reducing the retention of NH4+-N in alkaline soil and mitigating NH3 volatilization.
Azolla biofertilizer 42% Prevent the rapid increase of the floodwater temperature and uptake of NH4+-N by the Azolla physical barrier that suppresses the algal growth.
Microalgal biofilm 75.6% Reduce NH3 loss via the slow N release nature of algal biomass resulting from organic matter degradation.
Alkaline soil precultivated with two sorghum plants Wang et al. (2018)
Alkaline farmland soil with pakchoi Xue et al. (2021)
Alkaline fluvo-aquic soil with pakchoi Sun et al. (2020a)
Gleyi-stagnic anthrosol soil with rice
Yao et al. (2018a)
Dystrophic red-yellow latosol soil Castro et al. (2017)
Paddy Azolla 52.2–55.4% Lower the NH4+-N concentrations, pH, and temperature. Hydragric anthrosolos soil with rice Yang et al. (2020a)
Duckweed (Lemna minor L.) 20–53.7% The duckweed cover lowers the total ammoniacal nitrogen concentration, pH, and temperature of the floodwater.
Floating duckweed with irrigation with wastewater generated by livestock production
Paddy Azolla
Paddy Azolla combination with deep placement
55.2% Duckweed takes in N and decreases the floodwater pH and temperature or provides a physical barrier to hinder NH3 volatilization.
50.3% Lower surface water ammonia concentration and pH.
47% 1) Azolla cover can act as a physical barrier to trap liberated NH3; 2) More rice roots proliferate under the Azolla mat and even grow into the Azolla mat, which could promote the absorption of N released from Azolla
ammonia volatilization can be decreased by up to 55.2% (Sun et al., 2016). Some common biofertilizers for ammonia volatilization mitigation have been summarized in Table 3 The excellent average mitigation efficiency (ca. 50.1%) suggests it is a promising fertilizer amendment technique for ammonia inhibition. In recent years, biofertilizers development has emerged as a rising research direction for ammonia control technology. However, due to their strict requirements on environmental conditions, there is still a long way to go in developing biofertilizers with high-efficiency ammonia inhibition capabilities and fast adaptability to the environment. It is predicted to be the priority in the development of future ammonia volatilization control technology (Vassilev et al., 2015).
3.4. Organic waste-based resource fertilizer technology
As more and more innovative fertilizers have been developed to meet today’s requirement for low environmental impacts, using sustainable resources and introducing recycled waste as fertilizer sources are inevitable trends for resource conservation and sustainable development. The research on the reduction of ammonia emissions during the applications of these waste-based organic fertilizers has gained popularity (Erwiha et al., 2020). Organic fertilizer and organic waste-based fertilizers are usually processed from biological materials, animal and plant wastes, and plant residues. It plays a vital role in improving the physical and chemical properties and biological activity of the soil, providing comprehensive nutrition for crops and reducing the loss of N through runoff, ammonia volatilization and nitrogen leaching (Dai et al., 2021). It has been confirmed that the combined application of organic and inorganic fertilizers can slow down the loss of nitrogen by delaying the process of organic nitrogen mineralization, thereby effectively reducing ammonia volatilization by 8.8–12.7% (Shan et al., 2015). In addition, organic fertilizers incorporated with a low application rate or deep placement, instead of inorganic fertilizers, could reduce ammonia volatilization loss by 24.6% and 89%, respectively, while ensuring crop yields (Shang et al., 2014; Zhang et al., 2017). Win et al. applied anaerobically digested cattle slurry and wood vinegar to a rice field and
Clayey blue-purple paddy soil with rice Li et al. (2009)
Hydroagric stagnic anthrosol with rice paddy Sun et al. (2016)
Hydragric anthrosol with rice paddy Yang et al. (2021)
Gleyi-stagnic anthrosol with rice paddy Yao et al. (2018b)
found that compared with traditional fertilization, ammonia volatilization loss was reduced by 72–79%, mainly through the decrease of soil pH (Win et al. 2009, 2010). Moreover, Feng’s research also found that hydrothermal carbonization aqueous products (HCAP) could reduce ammonia loss by 7.6–11.2% by affecting the NH4+-N concentration in surface water, ammonia-oxidizing archaea (AOA) abundance, pH and soil urease activity in rice paddy soil (Feng et al., 2021). Table S1 summarizes some organic and organic waste-based fertilizers for ammonia mitigation. It can be seen that the organic waste-based fertilizers are usually applied together with other agronomic measures, and the investigated average mitigation efficiency of ammonia volatilization is about 45.6%. It is advocated to use organic fertilizers to replace chemical fertilizers from the perspective of crop growth and environmental protection. However, since the research on organic waste-based fertilizers is still relatively limited, although they can reduce ammonia volatilization to a certain extent, their long-term impacts on crop growth and soil properties need to be further studied.
It is worth mentioning that biochar is a typical organic waste-based fertilizer. This carbon-rich solid material is obtained from thermochemical conversion of biomass under oxygen-limited or anaerobic conditions (Fidel et al., 2018). According to the pyrolysis carbonization method and the hydrothermal carbonization method, it can be divided into pyrolysis carbon and hydrothermal carbon, named hydrochar (Chu et al., 2020a). Biochar usually possesses high porosity, large specific surface area, rich in negatively charged functional groups, and good adsorption performance. These superior features support its widely used in farmland ecosystems to deal with resource utilization, soil improvement, environmental pollution, and other issues (Spokas et al., 2012; Zhang et al., 2022). Attributing to biochar’s unique properties, it can change the physical and chemical properties of the soil after being applied to the soil, thereby directly or indirectly regulating the soil nitrogen cycle. However, agricultural practitioners should be careful when using biochar for reducing nitrogen loss via ammonia volatilization because it can either decrease or increase ammonia volatilization according to the biochar characteristics.
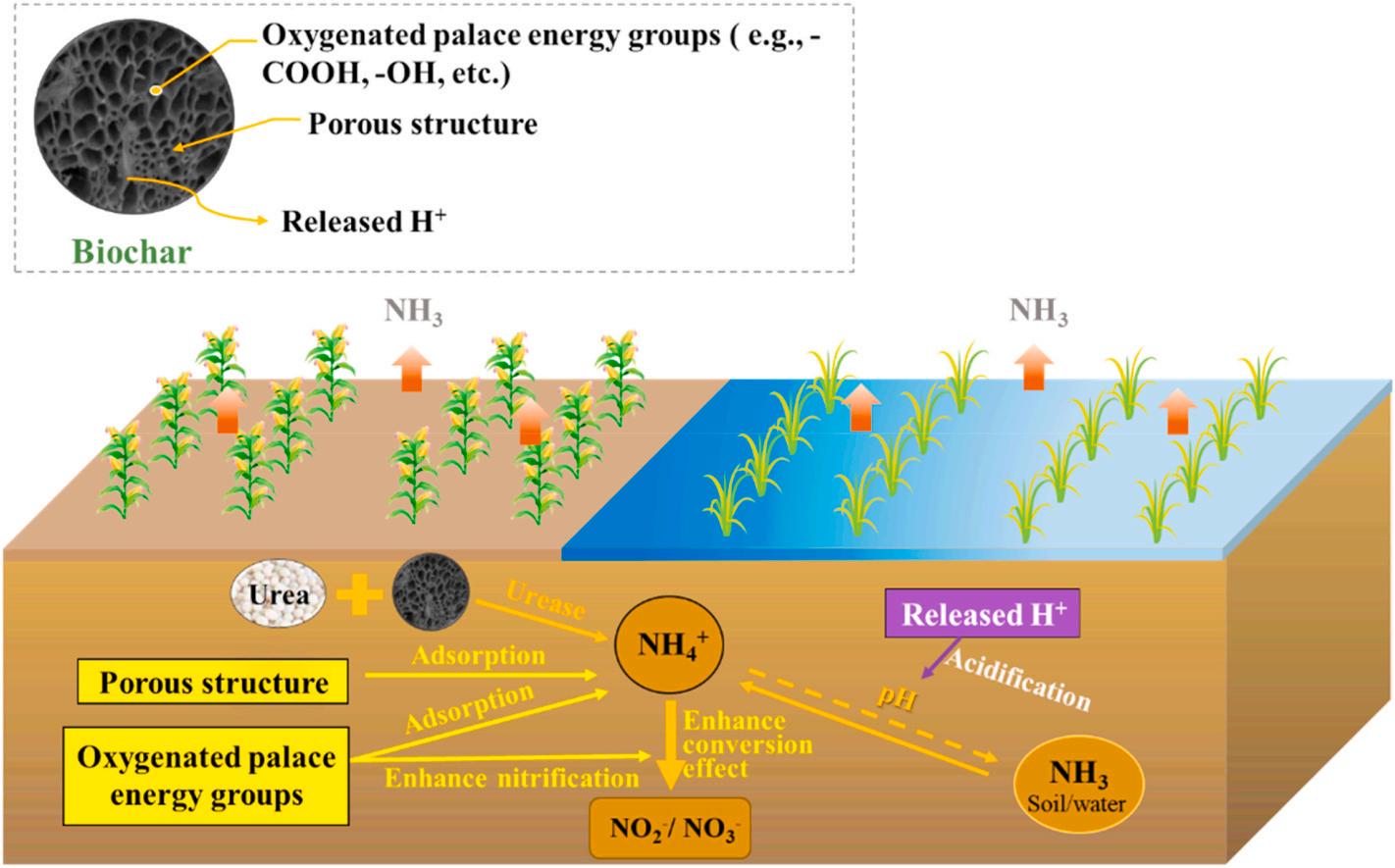
Fig. 5 demonstrates the mechanism of applying biochar for ammonia volatilization reduction. The main feature determining a biochar’s function in regulating ammonia release from farmland is its acid-base property. Specifically, neutral or acidic biochar can reduce ammonia volatilization. The mechanism is obvious. The low pH of neutral or acidic biochar can induce a neutralizing effect when applied to soil, which lowers the soil pH, thus inhibiting the conversion of NH4+ to NH3. In addition to this, studies have revealed other mechanisms that assist in cutting down nitrogen loss via ammonia volatilization: addition of biochar can i) promote nitrification process and then increases soil microbial nitrogen fixation capacity; ii) improve physical/chemical adsorption capacity through biochar’s large surface area and special functional groups; iii) enhance soil cation exchange capacity to increase soil nitrogen fixation capacity (Amin, 2020; Purakayastha et al., 2019; Sha et al., 2019). A representative work that was adapting these mechanisms in reducing ammonia volatilization was conducted by Mandal et al., who applied three kinds of biochar produced from 250 ◦ C to 700 ◦ C, they are, poultry manure biochar, green waste compost biochar and wheat straw biochar to calcareous soil and found all of them were capable of inhibiting ammonia volatilization, reducing ammonia loss by 53%, 38% and 35%, respectively (Mandal et al., 2018). Later, Amin et al. found that although applied to alkaline sandy soil, calotropis biochar, produced at 250 ◦ C with a low pH of 7.15, can still reduce ammonia volatilization by up to 71.5% by enhancing soil cation exchange capacity (Amin, 2020). In addition, many other studies also manifested that various biochar could increase crop yields while reducing ammonia volatilization (Chu et al., 2020c; Sun et al., 2019b). For example, poultry litter biochar, having a property of pH 8.66, surface area 12.8 m2 g 1 and pore volume 0.015 ± 0.15 cm3 g 1 , can not only reduce ammonia volatilization by 70.5% in wheat fields but also increase dry wheat weight by 24.24% and improves nitrogen uptake by 76.11% (Mandal et al., 2016). The work carried out by Sun et al. unveiled the effects of the combined use of wheat straw biochar and duckweed on ammonia volatilization, and the results suggested that ammonia volatilization could be reduced by 50.6–54.2%, while crop yield was increased by 0.1–0.3 t ha 1 , and the nitrogen utilization efficiency was promoted by 11.4–23.2% (Sun et al., 2019b). In the meanwhile, a more complex hydrochar derived from sewage sludge by Chu et al. was found to be capable of reducing the yield-scale ammonia loss by 20.3–41.2% in the rice field; While compared with the treatment without adding hydrochars, the dry grain weight was enhanced by 1.2–1.31 fold (Chu et al., 2020c).
On the other hand, some biochar can promote ammonia volatilization, alkaline biochar specifically, as it promotes a high soil pH, which favors the conversion of NH4+ to NH3 Other than this, alkaline biochar would also promote nitrogen loss via ammonia volatilization through different pathways: i) It inhibits the nitrification process, thereby increasing the accumulation of ammonium in soil; ii) It increases soil respiration, thereby increasing the exchange of soil gas (e.g., ammonia); iii) It enhances soil microbial activity, which accelerates the decomposition of soil organic nitrogen, thereby increasing the concentration of mineralized ammonium (Feng et al., 2017; Purakayastha et al., 2019; Sha et al., 2019). With these in mind, some studies have shown that applying fresh wheat straw biochar (pH 9.2, CEC 24.3 cmol kg 1 , and surface area 9.0 m2 g 1) to rice field can increase ammonia volatilization by 3.1–48% (Dong et al., 2019), and the amount of ammonia loss increases with the elevated temperature during the biochar pyrolysis due to a higher temperature leads to a higher biochar pH. The study revealed that rising the temperature from 500 ◦ C to 700 ◦ C led to ammonia volatilization loss increase from 40.8% to 70.9% (Feng et al., 2017). Interestingly, the study conducted by Wu et al. suggested that biochar produced from wheat straw with a pH of 9.42, total C content of 602 g kg 1 , total N content of 11.20 g kg 1 , and maximum NH+ 4 -N adsorption capacity of 2.68 g kg 1 , could increase ammonia volatilization (25.8%) while also increase rice yield by 26.5–35.3% (Wu et al., 2019). Similarly, Chu et al. added chlorella vulgaris-derived hydrochar made with water or citrate acid as the reaction medium to a rice field and found that they could increase ammonia volatilization by 53.8% and 72.9%, respectively, and also increased rice yield by 10.5–26.8% (Chu et al., 2020b). However, it is not always the case that hydrochar application improves crop yield. For example, a contrary finding was observed in Sun’s research, which demonstrated that biochar actually cut down crop yields by 12.6% and lower nitrogen utilization efficiency by 3.0–8.4%, along with increasing ammonia volatilization by 25.6–43.7% (Sun et al., 2019a). Due to the complex structure and properties of biochar produced from a variety of materials, its influence on farmland ammonia volatilization is related to many factors. Therefore, more studies are expected to provide insightful data to support scientific applications of biochar for reducing nitrogen loss and enhancing yields. Table 4 summarizes the effects of applying biochar on ammonia volatilization under different agricultural conditions. A quantitative analysis of nearly 20 studies on the inhibitory effect of biochar on ammonia volatilization realves that its extensive inhibitory efficiency (4.4%–82%) indicates that the inhibitory effect of biochar on ammonia volatilization is deeply
Fig. 5. Schematic diagram of using biochar technology to mitigate ammonia volatilization.
Table 4
Effects of adding biochar on ammonia volatilization.
Type of biochar
Wheat straw biochar
Wheat straw pyrolyzed at 500 ◦ C and 700 ◦ C
Sawdust biochar
Biochar-based fertilizer (BF)
Biochar
Calotropis biochar at 650 ◦ C
Effect on ammonia volatilization
Increase the NH3 loss by 25.6–53.6%.
Increase the NH3 losses by 25.8%.
NH3 loss increase with the biochar application rate in moderately saline soil. –
Increase the cumulative NH3 loss by 91.4–107% during the flooded rice season.
Increase the NH3 loss by 14.1% in the first rice season and decrease NH3 loss in the second rice growth season by 6.8%.
Increase the NH3 loss by 25.6–43.7%.
Increase the NH3 losses by 40.8–70.9%.
Increase the NH3 loss by 26.7–43.2%.
Increase the NH3 loss by 0.41%.
Increase the total NH3 loss by 8.6–17.9%.
Increase the cumulative NH3 loss by 73.3%. –
–
Effect on crop yield and nitrogen use efficiency (NUE)
Total N concentrations of soil are maintained at the same levels under with or without biochar treatments.
Increase rice yield by 26.5–35.3%.
Lower rice yield and reduce fertilizer 15N use efficiency by 32.6–76%.
Increase rice yield by 7.4–16.5% and NUE from 29.4% to 42.5%.
Decrease the grain yield by 12.6% and lower NUE by 3.0–8.4%.
–
Increase NH4+-N and total N content of top (0–15 cm) soil.
Significantly improve yield, plant N uptake, and NUE of water spinach and minimize N losses via leaching.
Increase rice yields by 4.2–5.2%.
Soil/crop type
Reference
Coastal saline soil Sun et al. (2017)
Rice paddy soil amendments with vermicompost
Wu et al. (2019)
Coastal saline alluvial soil Zhu et al. (2020)
Homestead anthrosol with paddy rice-wheat rotation Sun et al. (2019b)
Rice field
He et al. (2018)
Hydragric anthrosol with rice paddy Sun et al. (2019a)
Rice paddy
Paddy rice soil
Vegetable crops (water spinach, ipomoea aquatica)
Gleyi-stagnic anthrosol with a wheat-rice rotation system
Bauxite residue sand
Feng et al. (2017)
Feng et al. (2018)
Zhou et al. (2021b)
Wang et al. (2017)
Alkaline sandy soil Amin (2020) Greenwaste biochar applied in the soil with different pH (5, 7, 8, 9) NH3 loss increase with the pH increase, and with the additional increase of biochar rate at the same pH.
Chlorella vulgaris-derived hydrochar employ water as the reaction medium
Chlorella vulgaris-derived hydrochar employ citrate acid solution as the reaction medium
Fresh wheat straw biochar
Increase the total NH3 loss by 53.8%.
Increase the total NH3 loss by 72.9%.
Increase the grain yield by 13.5–26.8%. Hydroagric stahnic anthrosol soil with rice paddy
Increase the grain yield by 10.5–23.4%. Hydroagric stahnic anthrosol soil with rice paddy
Chen et al. (2013)
Chu et al. (2020b)
Chu et al. (2020b)
Rice-wheat rotation system Dong et al. (2019) Aged wheat straw biochar
Wheat straw biochar
Chicken litter biochar
Poultry litter biochar
Reduce the NH3 losses by 13.3–36.8%.
Increase the NH3 losses by 3.1–48%. Both field-aged and fresh biochar reapplication improve rice and wheat NUE but no significant increase in rice and wheat yields.
Reduce the NH3 loss by 17.6–29.4%.
Wood vinegar application alone or with biochar has no significant effect on total NH3 volatilization reduction, but yield-scale NH3 loss is reduced by 13.6%.
Reduce the NH3 losses by 6%. –
Reduce the NH3 loss up to 70.5%.
Increase the wheat plant biomass, grain yield, and total N uptake by 10–21%, 5–15%, and 11–25%, respectively.
Increase rice grain yields up to 11.2% and increase the NH4+-N contents of topsoil by 10.9–17.8% and 16.1–36.2%.
Silt clay loam soil with wheat Dawar et al. (2021)
Rice paddy soil amendment with wood vinegar Sun et al. (2020b)
Typic paleudults acid soil under waterlogged condition
Palanivell et al. (2017)
Wheat Mandal et al. (2016) Macadamia nutshell biochar
Calotropis biochar at 250 ◦ C
Reduce the NH3 loss by 64.1%.
Reduce the cumulative NH3 loss by 71.5%. –
Increase wheat dry weight and N uptake as high as by 24.2% and 76.1%, respectively.
Rice straw biochar Reduce the ammonia loss by 4.4%. Increase the grain yield from 6.6% to 32.5%.
Aged acidic Eucalyptus Pilularis biochar (collected after 44 years from wildfire occurred in 1961)
Eucalyptus wood biochar
Rice straw biochar
Poultry manure biochar
Reduce the NH3 losses by 82%. The acidic biochar treatment can retain about 73% of N, compared with <25% in alkaline biochar treatments.
Reduce the NH3 loss by 14%. –
Reduce the NH3 loss by 10.8%–20.9%. –
Reduce the NH3 loss by an average of 53%. –
Green waste compost biochar Reduce the NH3 loss by an average of 38%.
Wheat straw biochar Reduce the NH3 loss by an average of 35%.
Sewage sludge-derived hydrochars Reduce the yield-scale NH3 loss by 20.3–41.2%.
Clay hydrochar composites derived from poplar sawdust via hydrothermal carbonization (BHTC) mixed with bentonite
Alkaline sandy soil Amin (2020)
Rice field Sun et al. (2019c)
Alkaline bauxite residue sand
Agricultural clay soil cultivated with maize and sorghum
Deserted field of high salinity soil
Esfandbod et al. (2017)
Puga et al. (2020)
Liu et al. (2021)
Calcareous soil Mandal et al. (2018)
Enhance the dry grain weight by 1.20fold–1.31-fold.
Hydroagric stahnic anthrosol soil with paddy rice
Chu et al. (2020c)
Reduce the NH3 loss by 41.8%.
Improve the rice yield by 18.8% and plant NUE by 37.4%.
Paddy rice
Chu et al. (2020a)
(continued on next page)
Table 4 (continued )
Type of biochar Effect on ammonia volatilization Effect on crop yield and nitrogen use efficiency (NUE)
Biochar mixed with calcium superphosphate
Wheat straw biochar with duckweed
Wheat straw biochar with coapplication of biofertilizer
Reduce the NH3 loss by 39.2%. –
Reduce the NH3 loss by 50.6–54.2%.
Increase the grain yield by 0.1–0.3 t ha 1 and promote the NUE by 11.4–23.2%.
Reduce the NH3 loss by 12.3%. Biofertilizer and/or biochar increases rice grain yield by 16.5–38.3%.
Soil/crop type Reference
Rice paddy fields with Nrich wastewater generated by livestock irrigation Sun et al. (2016)
Hydragric anthrosol soil with rice paddy Sun et al. (2019a)
Rice paddy soil Sun et al. (2021)
affected by various factors such as biochar source type and soil type, with an average inhibitory efficiency of 36.6%.
3.5. Membrane/film-based barrier effect technology
Membrane/film-based mulching technology is a technique that uses membrane as a means to suppress ammonia volatilization from paddy fields. It (Fig. 6) inhibits ammonia loss through physical and chemical effects without changing the properties of nitrogen fertilizer and fertilization methods. Besides, the use of membrane/film can also reduce water evaporation, increase surface water temperature, inhibit the growth of algae, and improve nitrogen utilization efficiency, thereby saving fertilizer, water, and increasing yield. Table 5 summarizes some typical membrane/films for ammonia volatilization control that indicates the average mitigation efficiency of ammonia volatilization is ca. 29%.
To date, Polyethylene film and organic surface molecular membrane are two prominent ammonia suppression films commonly used for rice fields. Both Yang et al. and Li et al. have found that polyethylene film can control the ammonia volatilization rate by 50%, i.e., reduced ammonia loss of 1.09 kg N ha 1 on average, by restricting the exchange of ammonia between soil and the atmosphere (Li et al., 2021; Yang et al., 2015). There were also a few comparative researches have observed the same effect. A simulation experiment conducted by Yin et al. showed that compared with pure ammonium sulfate solution, the ammonia volatilization rate in the ammonium sulfate solution spraying with the octadecanol surface molecular membrane was greatly reduced, and the ammonia loss can be reduced by up to 90% (Yin et al., 1996). Meanwhile, Wang et al. compared the effects of three molecular membranes, i.e., Polylactic acid (PLA), Span60 and Zein, on ammonia volatilization
in a rice field, and the results demonstrated that the cumulative ammonia volatilization was reduced by 9.61%, 5.63% and 12.46%, respectively (Wang et al., 2019). Another comparative experiment on Polylactic acid (PLA) and Lecithin (LEC) also indicated that they could increase rice yields by 21% and 24.1% while reducing ammonia loss by 19.9% and 14.2% (Wang et al., 2020). It is expected that these membranes/films can be degraded naturally without causing secondary pollution, presenting good environmental and economic benefits. However, the existing membranes/films have problems such as unstable film formation, easy breakage, high cost and easy aggregation. Therefore, research on the property improvement and the appropriate application of these membrane/films will become one of the hotspots in future ammonia volatilization control technology research.
4. Conclusions and future outlooks
In general, all attempts that can inhibit the conversion of nitrogen fertilizers into ammonia can be breakthroughs in ammonia volatilization control. This study systematically reviews the inhibitory effects and inhibitory mechanisms of agricultural ammonia volatilization from the perspectives of fertilizer amendments. Specifically, various fertilizer improvement technologies can extend the process of nitrogen fertilizer release into the soil by changing soil structure and physicochemical properties, soil microbial activity, fertilizer properties, and hydrolysis rate so that more nitrogen fertilizers can be utilized by crops instead of loss via volatilization. Moreover, membrane/film-based mulching technology achieves ammonia volatilization mitigation by increasing the resistance of ammonia volatilization and reducing the gas exchange between the soil/water-air interface. In particular, among the studies investigated, enhanced efficiency fertilizer technology performs the
Fig. 6. Schematic diagram of using membrane mulching technology to mitigate ammonia volatilization.
Table 5
Ammonia volatilization mitigation based on membrane/film technology.
Type of membrane/film Application rate/ application method
Cetyl alcohol molecular membrane
Octadecanol dissolved in water with film-forming material
High carbon alkanol molecular membrane
A mixture of 16–18-octadecanols emulsified with sodium dodecyl sulfate and polyalkoxylated polyolols surface film-forming material (SFFM)
Spread on the water, average in 13.7 kg ha 1
Spread on the water.
Spread on the water, 3.5 g m 2
Spread on the water, 3.5–8 g m 2
Polylactic acid (PLA) molecular membrane Spread on the water, 8 g m 2
Span60 molecular membrane Spread on the water, 1 g m 2
Zein molecular membrane Spread on the water, 2 g m 2
Polylactic acid (PLA) molecular membrane Spread on the water, 8 g m 2 .
Lecithin (LEC) molecular membrane Spread on the water, 8 g m 2
Polyethylene film Film mulching
Polyethylene film Film mulching
Ammonia mitigation efficiency
Ammonia flux densities exceed 0.66 kg N ha 1 h 1 on the control area but never exceeded 0.34 kg N ha 1 h 1 on the area treated with cetyl alcohol.
Reduce the accumulative loss of ammonia by 90%.
Reduce ammonia loss by 30%.
Reduce cumulative NH3 loss by 12.8 kg ha 1 in the rice field.
Mitigation mechanisms Crop
Decrease the rate constant and increase the half-life by more than five-fold.
Decrease the volatilization rate constant.
Reduce the gas exchange between gas and liquid phases.
Change the resistance of NH3 volatilizing from liquid to air.
Flooded rice field
Water solution containing 50 g N m 3 in the form of ammonium sulfate
Rice paddy and enhanced rice yield by 10%.
Reference
Cai et al. (1988)
(Yin et al., 1996)
Shunyao (2002)
Rice filed Zhuang and Wang (2009)
Reduce ammonia loss by 9.6%. Reduce pH in surface water. Rice-wheat rotation system (Wang et al., 2019)
Reduce ammonia loss by 5.63%. Reduce NH4+ concentration in surface water.
Reduce ammonia loss by 12.46%. Reduce both pH and NH4+ concentration in surface water.
Reduce ammonia loss by 19.9%. 1) Form a physical barrier to reduce ammonia volatilization; 2) Inhibit the algae growth to reduce the water pH.
Reduce ammonia loss by 14.2%. 1) Reduce pH in surface water; 2) Increase NH4+ concentration in soil.
Reduce ammonia loss by 1.09 kg N ha 1 on average.
Reduce average fertilizer ammonia loss rates in two growth seasons by ca. 50%.
most effective inhibition capacity of ammonia volatilization (ca. 54.3%), followed by biofertilizer technology (ca. 50.1%). The organic and organic waste-based fertilizers technology are often applied with various agronomic measures with an average ammonia mitigation efficiency of 45.6%, and typical biochar addition technology can either increase or decrease ammonia volatilization deeply depending on the biochar source types and soil properties. Traditional complex fertilizer technology can inhibit ammonia volatilization by 33.5%, while current membrane/film-based control technology can alleviate ammonia volatilization by 29%.
Although a number of technologies have been developed and successfully applied to achieve ammonia volatilization control, as the agriculture system is an open and complex ecosystem, there are still some future development prospects, including i) Improve the pertinence of fertilizer amendment technologies. This involves the classification of crops depending on their fertilizer requirements and customization of new fertilizer so that an optimized fertilizer nutrients release pattern can be achieved during the specified crop growth period and congruously meet the needs of crops. ii) Enhance the environmental friendliness of fertilizer amendment-based technologies, especially in the selection of fertilizer additives, coating materials and membrane/film materials. Besides, further development in minimizing the interference of added materials on the natural soil and its original material circulation and investigations into both short-term and long-term effects on the agroecological system. iii) Strengthen field-scale rather than laboratory-scale research. Specifically, future research should focus more on in situ verification of the ammonia volatilization inhibition performance of any developed technologies by comprehensive assessments of soil ammonia
Restrict the gas flow between the soil and the atmosphere to decrease ammonia emissions.
Mitigate ammonia volatilization through a physical barrier or blocking, which enhances the topsoil water content and soil temperature, mineral nitrogen (NH4+-N and NO3 -N).
Rice-wheat rotation system (Wang et al., 2019)
Rice-wheat rotation system (Wang et al., 2019)
Rice-wheat rotation system and enhance rice yield by 21%.
(Wang et al., 2020)
Rice-wheat rotation system and enhanced rice yield by 24.1%. (Wang et al., 2020)
Wheat Yang et al. (2015)
Wheat Li et al. (2021)
volatilization loss in combination with crop yield and nitrogen utilization efficiency. iv) Comprehensively evaluate the cheapness, ease of operation, and feasibility of large-scale promotion of these developed fertilizer amendment-based technologies.
Overall, with continued developments and applications in tandem with emerging environmentally friendly materials and methods, the ammonia mitigation technology is developing in a more diverse, efficient and maintainable way. The advances in mitigation technologies of ammonia volatilization from agriculture not only contribute to reducing environmental pollution and maximizing agricultural yield but also generate new opportunities in nitrogen reuse, supporting resourcesaving sustainable agriculture development.
Credit author statement
Tianling Li: Conceptualization, Methodology, Resources, Formal analysis, Writing – original draft. Zhengguo Wang: Investigation, Data curation, Writing – editing. Chenxu Wang: Investigation, Data curation, Writing – editing. Jiayu Huang: Investigation, Data curation. Yanfang Feng: Investigation, Data curation. Weishou Shen: Investigation, Data curation. Ming Zhou and Linzhang Yang: Conceptualization, Methodology, Resources, Writing - review & editing, Supervision.
Declaration of competing interest
The authors declare that they have no known competing financial interests or personal relationships that could have appeared to influence the work reported in this paper.
Acknowledgement
This work was supported by the National Natural Science Foundation of China (No. 21806080); the Jiangsu Key Laboratory for Food Quality and Safety-State Key Laboratory Cultivation Base; Ministry of Science and Technology (No. 028074911709); and the Startup Foundation for Introducing Talent of NUIST (No. 2018r017).
Appendix A. Supplementary data
Supplementary data to this article can be found online at https://doi. org/10.1016/j.chemosphere.2022.134944
References
Adotey, N., Kongchum, M., Li, J., Whitehurst, G.B., Sucre, E., Harrell, D.L., 2017. Ammonia volatilization of zinc sulfate-coated and NBPT-treated urea fertilizers. Agron. J. 109 (6), 2918–2926. https://doi.org/10.2134/agronj2017.03.0153
Ahmed, O.H., Aminuddin, H., Husni, M.H.A., 2006. Reducing ammonia loss from urea and improving soil-exchangeable ammonium retention through mixing triple superphosphate, humic acid and zeolite. Soil Use Manag. 22 (3), 315–319. https:// doi.org/10.1111/j.1475-2743.2006.00040.x
Ahmed, M.Y.W., Lei, M., Raza, S., Zhou, J., 2018. Mitigation of ammonia volatilization with application of urease and nitrification inhibitors from summer maize at the Loess Plateau. Plant Soil Environ. 64 (4), 164–172. https://doi.org/10.17221/46/ 2018-PSE
Amin, A.E.-E.A.Z., 2020. Carbon sequestration, kinetics of ammonia volatilization and nutrient availability in alkaline sandy soil as a function on applying calotropis biochar produced at different pyrolysis temperatures. Sci. Total Environ. 726, 138489. https://doi.org/10.1016/j.scitotenv.2020.138489
Awale, R., Chatterjee, A., 2017. Enhanced efficiency nitrogen products influence ammonia volatilization and nitrous oxide emission from two contrasting soils. Agron. J. 109 (1), 47–57. https://doi.org/10.2134/agronj2016.04.0219.
Barakat, M., Cheviron, B., Angulo-Jaramillo, R., 2016. Influence of the irrigation technique and strategies on the nitrogen cycle and budget: a review. Agric. Water Manag. 178, 225–238. https://doi.org/10.1016/j.agwat.2016.09.027
Barberena, I.M., 2019. Use of urease inhibitors to reduce ammonia volatilization in Amazonian soils. Pesqui. Agropecu. Bras. 54, e00253 https://doi.org/10.1590/ S1678-3921.pab2019.v54.00253
Rome (Italy) Bouwman, A.F., 2001. Land and water development div. Eng. In: Boumans, L.J.M., Batjes, N.H., et al. (Eds.), Global Estimates of Gaseous Emissions of NH3, NO and N2O from Agricultural Land. International Fertilizer Industry Association
Cai, G., Freney, J., Humphreys, E., Denmead, O., Samson, M., Simpson, J., 1988. Use of surface films to reduce ammonia volatilization from flooded rice fields. Aust. J. Agric. Res. 39 (2), 177–186. https://doi.org/10.1071/AR9880177
Cantarella, H., Otto, R., Soares, J.R., Silva, AGdB., 2018. Agronomic efficiency of NBPT as a urease inhibitor: a review. J. Adv. Res. 13, 19–27. https://doi.org/10.1016/j. jare.2018.05.008
Castro, JdS., Calijuri, M.L., Assemany, P.P., Cecon, P.R., de Assis, I.R., Ribeiro, V.J., 2017. Microalgae biofilm in soil: greenhouse gas emissions, ammonia volatilization and plant growth. Sci. Total Environ. 574, 1640–1648. https://doi.org/10.1016/j. scitotenv.2016.08.205
Castro, JdS., Calijuri, M.L., Ferreira, J., Assemany, P.P., Ribeiro, V.J., 2020. Microalgae based biofertilizer: a life cycle approach. Sci. Total Environ. 724, 138138. https:// doi.org/10.1016/j.scitotenv.2020.138138
Chen, C.R., Phillips, I.R., Condron, L.M., Goloran, J., Xu, Z.H., Chan, K.Y., 2013. Impacts of greenwaste biochar on ammonia volatilisation from bauxite processing residue sand. Plant Soil 367 (1), 301–312. https://doi.org/10.1007/s11104-012-1468-0
Chien, S.H., Prochnow, L.I., Cantarella, H., 2009. Chapter 8 Recent developments of fertilizer production and use to improve nutrient efficiency and minimize environmental impacts. Adv. Agron. 102, 267–322. https://doi.org/10.1016/S00652113(09)01008-6 Academic Press.
Chu, Q., Xu, S., Xue, L., et al., 2020a. Bentonite hydrochar composites mitigate ammonia volatilization from paddy soil and improve nitrogen use efficiency. Sci. Total Environ. 718, 137301. https://doi.org/10.1016/j.scitotenv.2020.137301
Chu, Q., Xue, L., Cheng, Y., et al., 2020b. Microalgae-derived hydrochar application on rice paddy soil: higher rice yield but increased gaseous nitrogen loss. Sci. Total Environ. 717, 137127. https://doi.org/10.1016/j.scitotenv.2020.137127
Chu, Q., Xue, L., Singh, B.P., et al., 2020c. Sewage sludge-derived hydrochar that inhibits ammonia volatilization, improves soil nitrogen retention and rice nitrogen utilization. Chemosphere 245, 125558. https://doi.org/10.1016/j. chemosphere.2019.125558
Corstanje, R., Kirk, G.J.D., Pawlett, M., Read, R., Lark, R.M., 2008. Spatial variation of ammonia volatilization from soil and its scale-dependent correlation with soil properties. Eur. J. Soil Sci. 59 (6), 1260–1270. https://doi.org/10.1111/j.13652389.2008.01087.x
Dai, X., Guo, Q., Song, D., et al., 2021. Long-term mineral fertilizer substitution by organic fertilizer and the effect on the abundance and community structure of ammonia-oxidizing archaea and bacteria in paddy soil of south China. Eur. J. Soil Biol. 103, 103288. https://doi.org/10.1016/j.ejsobi.2021.103288.
Damodar Reddy, D., Sharma, K.L., 2000. Effect of amending urea fertilizer with chemical additives on ammonia volatilization loss and nitrogen-use efficiency. Biol. Fertil. Soils 32 (1), 24–27. https://doi.org/10.1007/s003740000208
David Ussiri, R.L., 2012. The role of fertilizer management in mitigating nitrous oxide emissions. Soil Emission of Nitrous Oxide and its Mitigation 315–346. https://doi. org/10.1007/978-94-007-5364-8_10
Dawar, K., Khan, H., Zaman, M., et al., 2021. The effect of biochar and nitrogen inhibitor on ammonia and nitrous oxide emissions and wheat productivity. J. Plant Growth Regul. 40 (6), 2465–2475. https://doi.org/10.1007/s00344-020-10283-1
De Campos Bernardi, A.C., Polidoro, J.C., de Melo Monte, M.B., Pereira, E.I., de Oliveira, C.R., Ramesh, K., 2016. Enhancing nutrient use efficiency using zeolites minerals a review. Adv. Chem. Eng. Sci. 6, 295–304. https://doi.org/10.4236/ aces.2016.64030
Ding, Z., Ali, E.F., Aldhumri, S.A., et al., 2021. Effect of amount of irrigation and type of P fertilizer on potato yield and NH3 volatilization from alkaline sandy soils. J. Soil Sci. Plant Nutr. 21 (2), 1565–1576. https://doi.org/10.1007/s42729-021-00462-8
Dong, N.M., Brandt, K.K., Sørensen, J., et al., 2012. Effects of alternating wetting and drying versus continuous flooding on fertilizer nitrogen fate in rice fields in the Mekong Delta, Vietnam. Soil Biol. Biochem. 47, 166–174. https://doi.org/10.1016/ j.soilbio.2011.12.028
Dong, Y., Wu, Z., Zhang, X., Feng, L., Xiong, Z., 2019. Dynamic responses of ammonia volatilization to different rates of fresh and field-aged biochar in a rice-wheat rotation system. Field Crop. Res. 241, 107568. https://doi.org/10.1016/j. fcr.2019.107568
Eduardo Lopes Cancellier, D.R.G.S., Faquin, Valdemar, Bruno, de Almeida, Gonçalves, Lopes Cancellier, Leandro, Roberto Spehar, Carlos, 2016. Ammonia volatilization from enhanced-efficiency urea on no-till maize in brazilian cerrado with improved soil fertility. Cienc. E Agrotecnol 40 (2), 133–144. https://doi.org/10.1590/141370542016402031115
Erwiha, G.M., Ham, J., Sukor, A., Wickham, A., Davis, J.G., 2020. Organic fertilizer source and application method impact ammonia volatilization. Commun. Soil Sci. Plant Anal. 51 (11), 1469–1482. https://doi.org/10.1080/00103624.2020.1784919
Esfandbod, M., Phillips, I.R., Miller, B., et al., 2017. Aged acidic biochar increases nitrogen retention and decreases ammonia volatilization in alkaline bauxite residue sand. Ecol. Eng. 98, 157–165. https://doi.org/10.1016/j.ecoleng.2016.10.077
Esp´ ecie Bueno, S.C., Filho, M.B., de Almeida, P.S.G., et al., 2015. Cuban zeolite as ammonium carrier in urea-based fertilizer pellets: photoacoustic-based sensor for monitoring N-ammonia losses by volatilization in aqueous solutions. Sensor. Actuator. B Chem. 212, 35–40. https://doi.org/10.1016/j.snb.2015.01.114.
Feng, Y., Sun, H., Xue, L., et al., 2017. Biochar applied at an appropriate rate can avoid increasing NH3 volatilization dramatically in rice paddy soil. Chemosphere 168, 1277–1284. https://doi.org/10.1016/j.chemosphere.2016.11.151
Feng, Y., Sun, H., Xue, L., et al., 2018. Sawdust biochar application to rice paddy field: reduced nitrogen loss in floodwater accompanied with increased NH3 volatilization. Environ. Sci. Pollut. Control Ser. 25 (9), 8388–8395. https://doi.org/10.1007/ s11356-017-1059-y
Feng, Y., He, H., Li, D., et al., 2021. Biowaste hydrothermal carbonization aqueous product application in rice paddy: focus on rice growth and ammonia volatilization. Chemosphere 277, 130233. https://doi.org/10.1016/j.chemosphere.2021.130233
Fidel, R.B., Laird, D.A., Spokas, K.A., 2018. Sorption of ammonium and nitrate to biochars is electrostatic and pH-dependent. Sci. Rep. 8 (1), 17627. https://doi.org/ 10.1038/s41598-018-35534-w
Frame, W.H., Alley, M.M., Whitehurst, G.B., Whitehurst, B.M., Campbell, R., 2012. In vitro evaluation of coatings to control ammonia volatilization from surface-applied urea. Agron. J. 104 (5), 1201–1207. https://doi.org/10.2134/agronj2012.0009
Gao, J., Luo, J., Lindsey, S., et al., 2021. Effects of boric acid on urea-N transformation and 3,4-dimethylpyrazole phosphate efficiency. J. Sci. Food Agric. 101 (3), 1091–1099. https://doi.org/10.1002/jsfa.10719
Gong, W., Zhang, Y., Huang, X., Luan, S., 2013. High-resolution measurement of ammonia emissions from fertilization of vegetable and rice crops in the Pearl River Delta Region, China. Atmos. Environ. 65, 1–10. https://doi.org/10.1016/j. atmosenv.2012.08.027.
Griggs, B.R., Norman, R.J., Wilson, C.E., Slaton, N.A., 2007. Ammonia vvolatilization and nitrogen uptake for conventional and conservation tilled dry-seeded, delayedflood rice. Soil Sci. Soc. Am. J. 71 (3), 745–751. https://doi.org/10.2136/ sssaj2006.0180
Haruna Ahmed, O., Husin, A., Husni Mohd Hanif, A., 2008. Ammonia volatilization and ammonium accumulation from urea mixed with zeolite and triple superphosphate. Acta Agric. Scand. Sect. B Soil Plant Sci 58 (2), 182–186. https://doi.org/10.1080/ 09064710701478271
Hayashi, K., Nishimura, S., Yagi, K., 2006. Ammonia volatilization from the surface of a Japanese paddy field during rice cultivation. Soil Sci. Plant Nutr. 52 (4), 545–555. https://doi.org/10.1111/j.1747-0765.2006.00053.x
Hayashi, K., Koga, N., Fueki, N., 2011. Limited ammonia volatilization loss from upland fields of Andosols following fertilizer applications. Agric. Ecosyst. Environ. 140 (3), 534–538. https://doi.org/10.1016/j.agee.2011.01.015
He, Z.L., Calvert, D.V., Alva, A.K., Li, Y.C., Banks, D.J., 2002. Clinoptilolite zeolite and cellulose amendments to reduce ammonia volatilization in a calcareous sandy soil. Plant Soil 247 (2), 253–260. https://doi.org/10.1023/A:1021584300322
He, T., Liu, D., Yuan, J., et al., 2018. A two years study on the combined effects of biochar and inhibitors on ammonia volatilization in an intensively managed rice field. Agric. Ecosyst. Environ. 264, 44–53. https://doi.org/10.1016/j. agee.2018.05.010
Jadon, P., Selladurai, R., Yadav, S.S., et al., 2018. Volatilization and leaching losses of nitrogen from different coated urea fertilizers. J. Soil Sci. Plant Nutr. 18 (4), 1036–1047. https://doi.org/10.4067/S0718-95162018005002903.
Keshavarz Afshar, R., Lin, R., Mohammed, Y.A., Chen, C., 2018. Agronomic effects of urease and nitrification inhibitors on ammonia volatilization and nitrogen utilization in a dryland farming system: field and laboratory investigation. J. Clean. Prod. 172, 4130–4139. https://doi.org/10.1016/j.jclepro.2017.01.105
Khalil, M.I., 2011. Physical and chemical manipulation of urea fertilizer to limit the emission of reactive nitrogen species understanding greenhouse gas emissions from agricultural management. ACS (Am. Chem. Soc.) Symp. Ser. 1072, 149–164. https:// doi.org/10.1021/bk-2011-1072.ch009 American Chemical Society.
Lam, S.K., Suter, H., Bai, M., et al., 2018. Using urease and nitrification inhibitors to decrease ammonia and nitrous oxide emissions and improve productivity in a subtropical pasture. Sci. Total Environ. 644, 1531–1535. https://doi.org/10.1016/j. scitotenv.2018.07.092
Lam, S.K., Suter, H., Bai, M., et al., 2019. Decreasing ammonia loss from an Australian pasture with the use of enhanced efficiency fertilizers. Agric. Ecosyst. Environ. 283, 106553. https://doi.org/10.1016/j.agee.2019.05.012.
Latip, B.N., Osumanu, H.A., Make, J., Franklin, R.K., 2011. Ammonia volatilization from urea at different levels of zeolite. Inernational Journal of Physical Sciences 6 (34), 7717–7720. https://doi.org/10.5897/IJPS11.592
Li, H., Liang, X., Lian, Y., Xu, L., Chen, Y., 2009. Reduction of ammonia volatilization from urea by a floating duckweed in flooded rice fields. Soil Sci. Soc. Am. J. 73 (6), 1890–1895. https://doi.org/10.2136/sssaj2008.0230
Li, Q., Yang, A., Wang, Z., et al., 2015. Effect of a new urease inhibitor on ammonia volatilization and nitrogen utilization in wheat in north and northwest China. Field Crop. Res. 175, 96–105. https://doi.org/10.1016/j.fcr.2015.02.005
Li, P., Lu, J., Hou, W., et al., 2017a. Reducing nitrogen losses through ammonia volatilization and surface runoff to improve apparent nitrogen recovery of double cropping of late rice using controlled release urea. Environ. Sci. Pollut. Res. 24 (12), 11722–11733. https://doi.org/10.1007/s11356-017-8825-8
Li, Q., Cui, X., Liu, X., et al., 2017b. A new urease-inhibiting formulation decreases ammonia volatilization and improves maize nitrogen utilization in North China Plain. Sci. Rep. 7 (1), 43853. https://doi.org/10.1038/srep43853
Li, T., Zhou, M., Fan, Z., et al., 2019a. Online conductimetric flow-through analyzer based on membrane diffusion for ammonia control in wastewater treatment process. ACS Sens. 4 (7), 1881–1888. https://doi.org/10.1021/acssensors.9b00768
Li, T., Zhou, M., Qiu, Y., et al., 2019b. Membrane-based conductivity probe for real-time in-situ monitoring rice field ammonia volatilization. Sensor. Actuator. B Chem. 286, 62–68. https://doi.org/10.1016/j.snb.2019.01.099
Li, H., Wang, L., Peng, Y., et al., 2021. Film mulching, residue retention and N fertilization affect ammonia volatilization through soil labile N and C pools. Agric. Ecosyst. Environ. 308, 107272. https://doi.org/10.1016/j.agee.2020.107272
Liu, G., Li, Y., Alva, A.K., 2007. High water regime can reduce ammonia volatilization from soils under potato production. Commun. Soil Sci. Plant Anal. 38 (9–10), 1203–1220. https://doi.org/10.1080/00103620701328289
Liu, T.Q., Fan, D.J., Zhang, X.X., Chen, J., Li, C.F., Cao, C.G., 2015. Deep placement of nitrogen fertilizers reduces ammonia volatilization and increases nitrogen utilization efficiency in no-tillage paddy fields in central China. Field Crop. Res. 184, 80–90. https://doi.org/10.1016/j.fcr.2015.09.011
Liu, X., Chen, L., Hua, Z., Mei, S., Wang, P., Wang, S., 2020. Comparing ammonia volatilization between conventional and slow-release nitrogen fertilizers in paddy fields in the Taihu Lake region. Environ. Sci. Pollut. Control Ser. 27 (8), 8386–8394. https://doi.org/10.1007/s11356-019-07536-2
Liu, S., Li, Y., Xu, J., et al., 2021. Biochar partially offset the increased ammonia volatilization from salt-affected soil. Arch. Agron Soil Sci. 67 (9), 1202–1216. https://doi.org/10.1080/03650340.2020.1786537
Mandal, S., Thangarajan, R., Bolan, N.S., et al., 2016. Biochar-induced concomitant decrease in ammonia volatilization and increase in nitrogen use efficiency by wheat. Chemosphere 142, 120–127. https://doi.org/10.1016/j.chemosphere.2015.04.086
Mandal, S., Donner, E., Vasileiadis, S., Skinner, W., Smith, E., Lombi, E., 2018. The effect of biochar feedstock, pyrolysis temperature, and application rate on the reduction of ammonia volatilisation from biochar-amended soil. Sci. Total Environ. 627, 942–950. https://doi.org/10.1016/j.scitotenv.2018.01.312
Martínez-Dalmau, J., Berbel, J., Ord ´ onez-Fern´ andez, R., 2021. Nitrogen fertilization. A review of the risks associated with the inefficiency of its use and policy responses. Sustainability 13 (10), 5625. https://doi.org/10.3390/su13105625
Minoli, S., Ma, Carozzi, M., 2015. NH3 emissions from land application of manures and N-fertilisers: a review of the Italian literature. Italian Journal of AgrometeorologyRivista Italiana di Agrometeorologia 20 (3), 5–24
Omar, O.L., Ahmed, O.H., Muhamad, A.M.N., 2010. Minimizing ammonia volatilization in waterlogged soils through mixing of urea with zeolite and sago waste water. Int. J. Phys. Sci. 5 (14), 2193–2197. https://doi.org/10.5897/IJPS.9000372
Palanivell, P., Ahmed, O.H., Susilawati, K., Ab Majid, N.M., 2015. Mitigating ammonia volatilization from urea in waterlogged condition using clinoptilolite zeolite. Int. J. Agric. Biol. 17 (1), 149–155. https://doi.org/10.1080/03650340.2015.1077229
Palanivell, P., Ahmed, O.H., Ab Majid, N.M., 2016. Minimizing ammonia volatilization from urea, improving lowland rice (cv. MR219) seed germination, plant growth variables, nutrient uptake, and nutrient recovery using clinoptilolite zeolite. Arch. Agron Soil Sci. 62 (5), 708–724. https://doi.org/10.1080/03650340.2015.1077229
Palanivell, P., Ahmed, O.H., Ab Majid, N.M., 2017. Minimizing ammonia volatilization from urea in waterlogged condition using chicken litter biochar. Commun. Soil Sci. Plant Anal. 48 (17), 2083–2092. https://doi.org/10.1080/00103624.2017.1406497
Pan, B., Lam, S.K., Mosier, A., Luo, Y., Chen, D., 2016. Ammonia volatilization from synthetic fertilizers and its mitigation strategies: a global synthesis. Agric. Ecosyst. Environ. 232, 283–289. https://doi.org/10.1016/j.agee.2016.08.019
Peng, S., Yang, S., Xu, J., 2009. Ammonia volatilization and its influence factors of paddy field under water-saving irrigation. Trans. Chin. Soc. Agric. Eng. 25 (8), 35–39. https://doi.org/10.3969/j.issn.1002-6819.2009.08.007.
Pratt, C., Redding, M., Hill, J., Brown, G., Westermann, M., 2016. Clays can decrease gaseous nutrient losses from soil-applied livestock manures. J. Environ. Qual. 45 (2), 638–645. https://doi.org/10.2134/jeq2015.11.0569
Puga, A.P., Queiroz, MCdA., Ligo, M.A.V., et al., 2020. Nitrogen availability and ammonia volatilization in biochar-based fertilizers. Arch. Agron Soil Sci. 66 (7), 992–1004. https://doi.org/10.1080/03650340.2019.1650916
Purakayastha, T.J., Bera, T., Bhaduri, D., et al., 2019. A review on biochar modulated soil condition improvements and nutrient dynamics concerning crop yields: pathways to climate change mitigation and global food security. Chemosphere 227, 345–365. https://doi.org/10.1016/j.chemosphere.2019.03.170
Recio, J., Vallejo, A., Le-Noe, J., et al., 2018. The effect of nitrification inhibitors on NH3 and N2O emissions in highly N fertilized irrigated Mediterranean cropping systems. Sci. Total Environ. 636, 427–436. https://doi.org/10.1016/j.scitotenv.2018.04.294
Rochette, P., Angers, D.A., Chantigny, M.H., MacDonald, J.D., Bissonnette, N., Bertrand, N., 2009. Ammonia volatilization following surface application of urea to tilled and no-till soils: a laboratory comparison. Soil Tillage Res. 103 (2), 310–315. https://doi.org/10.1016/j.still.2008.10.028
Sanz-Cobena, A., Misselbrook, T.H., Arce, A., Mingot, J.I., Diez, J.A., Vallejo, A., 2008. An inhibitor of urease activity effectively reduces ammonia emissions from soil treated with urea under Mediterranean conditions. Agric. Ecosyst. Environ. 126 (3–4), 243–249. https://doi.org/10.1016/j.agee.2008.02.001
Sha, Z., Li, Q., Lv, T., Misselbrook, T., Liu, X., 2019. Response of ammonia volatilization to biochar addition: a meta-analysis. Sci. Total Environ. 655, 1387–1396. https:// doi.org/10.1016/j.scitotenv.2018.11.316
Shan, L., He, Y., Chen, J., Huang, Q., Wang, H., 2015. Ammonia volatilization from a Chinese cabbage field under different nitrogen treatments in the Taihu Lake Basin, China. J. Environ. Sci. 38, 14–23. https://doi.org/10.1016/j.jes.2015.04.028
Shang, Q., Gao, C., Yang, X., et al., 2014. Ammonia volatilization in Chinese double ricecropping systems: a 3-year field measurement in long-term fertilizer experiments. Biol. Fertil. Soils 50 (5), 715–725. https://doi.org/10.1007/s00374-013-0891-6
Shunyao, z, 2002. Study on Logistic model of surface molecular membrane reducing ammonia volatilization. SolIandEnvironmentai Sciences 11 (1), 3
Sigurdarson, J.J., Svane, S., Karring, H., 2018. The molecular processes of urea hydrolysis in relation to ammonia emissions from agriculture. Rev. Environ. Sci. Biotechnol. 17 (2), 241–258. https://doi.org/10.1007/s11157-018-9466-1
Silva, A.G.B., Sequeira, C.H., Sermarini, R.A., Otto, R., 2017. Urease inhibitor NBPT on ammonia volatilization and crop productivity: a meta-Analysis. Agron. J. 109 (1), 1–13. https://doi.org/10.2134/agronj2016.04.0200.
Singh, J., Kunhikrishnan, A., Bolan, N.S., Saggar, S., 2013. Impact of urease inhibitor on ammonia and nitrous oxide emissions from temperate pasture soil cores receiving urea fertilizer and cattle urine. Sci. Total Environ. 465, 56–63. https://doi.org/ 10.1016/j.scitotenv.2013.02.018
Sn Behera, M.S., Aneja, V.P., Balasubramanian, R., 2013. Ammonia in the atmosphere: a review on emission sources, atmospheric chemistry and deposition on terrestrial bodies. Environ. Sci. Pollut. Control Ser. 20 (11), 8092–8131. https://doi.org/ 10.1007/s11356-013-2051-9
Soaud, A.A., Saleh, M.E., El-Tarabily, K.A., Sofian-Azirun, M., Rahman, M.M., 2011. Effect of elemental sulfur application on ammonia volatilization from surface applied urea fertilizer to calcareous sandy soils. Aust. J. Crop. Sci. 5 (5), 611–619. https://doi.org/10.3316/informit.280812805256218
Spokas, K.A., Novak, J.M., Venterea, R.T., 2012. Biochar s role as an alternative Nfertilizer: ammonia capture. Plant Soil 350 (1–2), 35–42. https://doi.org/10.1007/ s11104-011-0930-8
Sun, H., Zhang, H., Min, J., Feng, Y., Shi, W., 2016. Controlled-release fertilizer, floating duckweed, and biochar affect ammonia volatilization and nitrous oxide emission from rice paddy fields irrigated with nitrogen-rich wastewater. Paddy Water Environ. 14 (1), 105–111. https://doi.org/10.1007/s10333-015-0482-2
Sun, H., Lu, H., Chu, L., Shao, H., Shi, W., 2017. Biochar applied with appropriate rates can reduce N leaching, keep N retention and not increase NH3 volatilization in a coastal saline soil. Sci. Total Environ. 575, 820–825. https://doi.org/10.1016/j. scitotenv.2016.09.137
Sun, H., A D, Feng, Y., et al., 2019a. Floating duckweed mitigated ammonia volatilization and increased grain yield and nitrogen use efficiency of rice in biochar amended paddy soils. Chemosphere 237, 124532. https://doi.org/10.1016/j. chemosphere.2019.124532
Sun, H., Zhang, H., Xiao, H., et al., 2019b. Wheat straw biochar application increases ammonia volatilization from an urban compacted soil giving a short-term reduction in fertilizer nitrogen use efficiency. J. Soils Sediments 19 (4), 1624–1631. https:// doi.org/10.1007/s11368-018-2169-y
Sun, X., Zhong, T., Zhang, L., Zhang, K., Wu, W., 2019c. Reducing ammonia volatilization from paddy field with rice straw derived biochar. Sci. Total Environ. 660, 512–518. https://doi.org/10.1016/j.scitotenv.2018.12.450
Sun, Y., Xia, G., He, Z., et al., 2019d. Zeolite amendment coupled with alternate wetting and drying to reduce nitrogen loss and enhance rice production. Field Crop. Res. 235, 95–103. https://doi.org/10.1016/j.fcr.2019.03.004
Sun, B., Bai, Z., Bao, L., et al., 2020a. Bacillus subtilis biofertilizer mitigating agricultural ammonia emission and shifting soil nitrogen cycling microbiomes. Environ. Int. 144, 105989. https://doi.org/10.1016/j.envint.2020.105989
Sun, H., Feng, Y., Xue, L., et al., 2020b. Responses of ammonia volatilization from rice paddy soil to application of wood vinegar alone or combined with biochar. Chemosphere 242, 125247. https://doi.org/10.1016/j.chemosphere.2019.125247
Sun, H., Zhang, Y., Yang, Y., et al., 2021. Effect of biofertilizer and wheat straw biochar application on nitrous oxide emission and ammonia volatilization from paddy soil. Environ. Pollut. 275, 116640. https://doi.org/10.1016/j.envpol.2021.116640
Sunderlage, B., Cook, R.L., 2018. Soil property and fertilizer additive effects on ammonia volatilization from urea. Soil Sci. Soc. Am. J. 82 (1), 253–259. https://doi.org/ 10.2136/sssaj2017.05.0151
Swarbreck, S.M., Wang, M., Wang, Y., et al., 2019. A roadmap for lowering crop nitrogen requirement. Trends Plant Sci. 24 (10), 892–904. https://doi.org/10.1016/j. tplants.2019.06.006
Tang, Y., Li, X., Shen, W., Duan, Z., 2018. Effect of the slow-release nitrogen fertilizer oxamide on ammonia volatilization and nitrogen use efficiency in paddy soil. Agronomy-Basel 8 (4), 53. https://doi.org/10.3390/agronomy8040053
Ti, C., Xia, L., Chang, S.X., Yan, X., 2019. Potential for mitigating global agricultural ammonia emission: a meta-analysis. Environ. Pollut. 245, 141–148. https://doi.org/ 10.1016/j.envpol.2018.10.124
Tian, Z., Wang, J.J., Liu, S., Zhang, Z., Dodla, S.K., Myers, G., 2015. Application effects of coated urea and urease and nitrification inhibitors on ammonia and greenhouse gas emissions from a subtropical cotton field of the Mississippi delta region. Sci. Total Environ. 533, 329–338. https://doi.org/10.1016/j.scitotenv.2015.06.147
Tian, X., Geng, J., Guo, Y., Li, C., Zhang, M., Chen, J., 2017. Controlled-release urea decreased ammonia volatilization and increased nitrogen use efficiency of cotton. J. Plant Nutr. Soil Sci. 180 (6), 667–675. https://doi.org/10.1002/jpln.201600518
Timilsena, Y.P., Adhikari, R., Casey, P., Muster, T., Gill, H., Adhikari, B., 2015. Enhanced efficiency fertilisers: a review of formulation and nutrient release patterns. J. Sci. Food Agric. 95 (6), 1131–1142. https://doi.org/10.1002/jsfa.6812
Vassilev, N., Vassileva, M., Lopez, A., et al., 2015. Unexploited potential of some biotechnological techniques for biofertilizer production and formulation. Appl. Microbiol. Biotechnol. 99 (12), 4983–4996. https://doi.org/10.1007/s00253-0156656-4
Wang, S., Shan, J., Xia, Y., et al., 2017. Different effects of biochar and a nitrification inhibitor application on paddy soil denitrification: a field experiment over two consecutive rice-growing seasons. Sci. Total Environ. 593, 347–356. https://doi.org/ 10.1016/j.scitotenv.2017.03.159
Wang, X., Xu, S., Wu, S., et al., 2018. Effect of Trichoderma viride biofertilizer on ammonia volatilization from an alkaline soil in Northern China. J. Environ. Sci. 66, 199–207. https://doi.org/10.1016/j.jes.2017.05.016
Wang, M., Yu, Y., Yang, B., Xie, F., Hou, P., Yang, L., Xue, L., Sun, Q., 2019. Effect of different surface molecular membrane materials on inhibition of ammonia volatilization and the action pathways in paddy fields. Journal of Agro Environment Science 38 (8), 1685–1695. https://doi.org/10.11654/jaes.2019-0657
Wang, M., Yu, Y., Yang, B., Hou, P., Yang, L., Xue, L.*, Sun, Q., 2020. Effects of interface barrier materials on rice yield, nitrogen use efficiency, and NH3 volatilization. Chin. J. Eco-Agric. 28 (6), 803–812. https://doi.org/10.13930/j.cnki.cjea.200049
Win, K.T., Toyota, K., Motobayashi, T., Hosomi, M., 2009. Suppression of ammonia volatilization from a paddy soil fertilized with anaerobically digested cattle slurry by wood vinegar application and floodwater management. Soil Sci. Plant Nutr. 55 (1), 190–202. https://doi.org/10.1111/j.1747-0765.2008.00337.x
Win, K.T., Nonaka, R., Toyota, K., Motobayashi, T., Hosomi, M., 2010. Effects of option mitigating ammonia volatilization on CH4 and N2O emissions from a paddy field fertilized with anaerobically digested cattle slurry. Biol. Fertil. Soils 46 (6), 589–595. https://doi.org/10.1007/s00374-010-0465-9
Wu, D., Feng, Y., Xue, L., et al., 2019. Biochar combined with vermicompost increases crop production while reducing ammonia and nitrous oxide emissions from a paddy soil. Pedosphere 29 (1), 82–94. https://doi.org/10.1016/S1002-0160(18)60050-5
Xu, M., Li, D., Li, J., et al., 2013. Polyolefin-coated urea decreases ammonia volatilization in a double rice system of southern China. Agron. J. 105 (1), 277–284. https://doi. org/10.2134/agronj2012.0222
Xue, L., Sun, B., Yang, Y., et al., 2021. Efficiency and mechanism of reducing ammonia volatilization in alkaline farmland soil using Bacillus amyloliquefaciens biofertilizer. Environ. Res. 202, 111672. https://doi.org/10.1016/j.envres.2021.111672
Yang, Y., Zhou, C., Li, N., et al., 2015. Effects of conservation tillage practices on ammonia emissions from Loess Plateau rain-fed winter wheat fields. Atmos. Environ. 104, 59–68. https://doi.org/10.1016/j.atmosenv.2015.01.007
Yang, J., Liu, T., Liu, H., et al., 2019. Biodegradable PASP can effectively inhibit nitrification, moderate NH3 emission, and promote crop yield. Arch. Agron Soil Sci. 65 (9), 1273–1286. https://doi.org/10.1080/03650340.2018.1562275
Yang, G., Ji, H., Sheng, J., et al., 2020a. Combining Azolla and urease inhibitor to reduce ammonia volatilization and increase nitrogen use efficiency and grain yield of rice. Sci. Total Environ. 743, 140799. https://doi.org/10.1016/j.scitotenv.2020.140799
Yang, Y., Li, N., Ni, X., et al., 2020b. Combining deep flooding and slow-release urea to reduce ammonia emission from rice fields. J. Clean. Prod. 244, 118745. https://doi. org/10.1016/j.jclepro.2019.118745.
Yang, G., Ji, H., Liu, H., et al., 2021. Nitrogen fertilizer reduction in combination with Azolla cover for reducing ammonia volatilization and improving nitrogen use efficiency of rice. PeerJ 9, e11077. https://doi.org/10.7717/peerj.11077
Yao, Y., Zhang, M., Tian, Y., et al., 2018a. Azolla biofertilizer for improving low nitrogen use efficiency in an intensive rice cropping system. Field Crop. Res. 216, 158–164. https://doi.org/10.1016/j.fcr.2017.11.020
Yao, Y., Zhang, M., Tian, Y., et al., 2018b. Urea deep placement in combination with Azolla for reducing nitrogen loss and improving fertilizer nitrogen recovery in rice field. Field Crop. Res. 218, 141–149. https://doi.org/10.1016/j.fcr.2018.01.015
Yin, B., Shen, R.F., Zhu, Z.L., 1996. Use of new water soluble surface film-forming material to reduce ammonia loss from water solution. Pedosphere 6 (4), 329–334
Zhang, M., Yao, Y., Zhao, M., et al., 2017. Integration of urea deep placement and organic addition for improving yield and soil properties and decreasing N loss in paddy field. Agric. Ecosyst. Environ. 247, 236–245. https://doi.org/10.1016/j. agee.2017.07.001
Zhang, Y., Jeyakumar, P., Xia, C., Lam, S.S., Jiang, J., Sun, H., Shi, W., 2022. Being applied at rice or wheat season impacts biochar’s effect on gaseous nitrogen pollutatns from the wheat growth cycle. Environ. Pollut. 306 (2022), 119409. https://doi.org/10.1016/j.envpol.2022.119409
Zhong, X., Zhou, X., Fei, J., et al., 2021. Reducing ammonia volatilization and increasing nitrogen use efficiency in machine-transplanted rice with side-deep fertilization in a double-cropping rice system in Southern China. Agric. Ecosyst. Environ. 306, 107183. https://doi.org/10.1016/j.agee.2020.107183.
Zhou, M., Li, T., Liu, P., et al., 2021a. Real-time on-site monitoring of soil ammonia emissions using membrane permeation-based sensing probe. Environ. Pollut. 289, 117850. https://doi.org/10.1016/j.envpol.2021.117850
Zhou, M., Ying, S., Chen, J., Jiang, P., Teng, Y., 2021b. Effects of biochar-based fertilizer on nitrogen use efficiency and nitrogen losses via leaching and ammonia volatilization from an open vegetable field. Environ. Sci. Pollut. Control Ser. 28 (46), 65188–65199. https://doi.org/10.1007/s11356-021-15210-9
Zhu, H., Yang, J., Yao, R., et al., 2020. Interactive effects of soil amendments (biochar and gypsum) and salinity on ammonia volatilization in coastal saline soil. Catena 190, 104527. https://doi.org/10.1016/j.catena.2020.104527
Zhuang, S.Y., Wang, M.K., 2009. Model estimation of ammonia volatilization from a paddy rice field after application of a surface film-forming material. J. Agric. Sci. 148 (1), 95–100. https://doi.org/10.1017/S0021859609990293