School Energy Managers
Student Guide
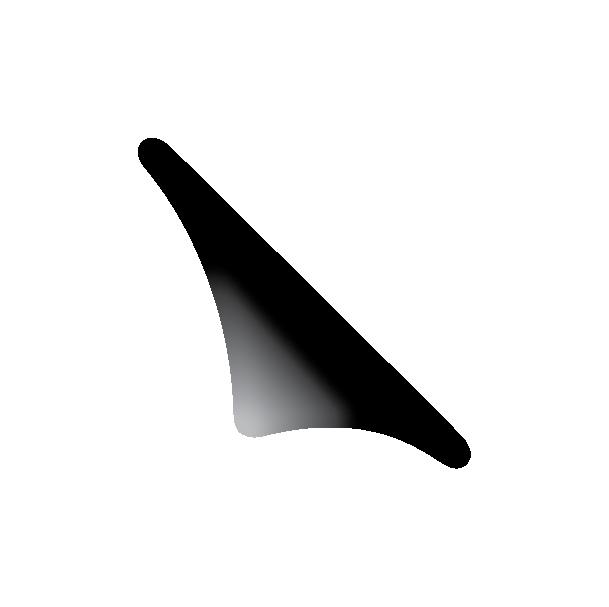
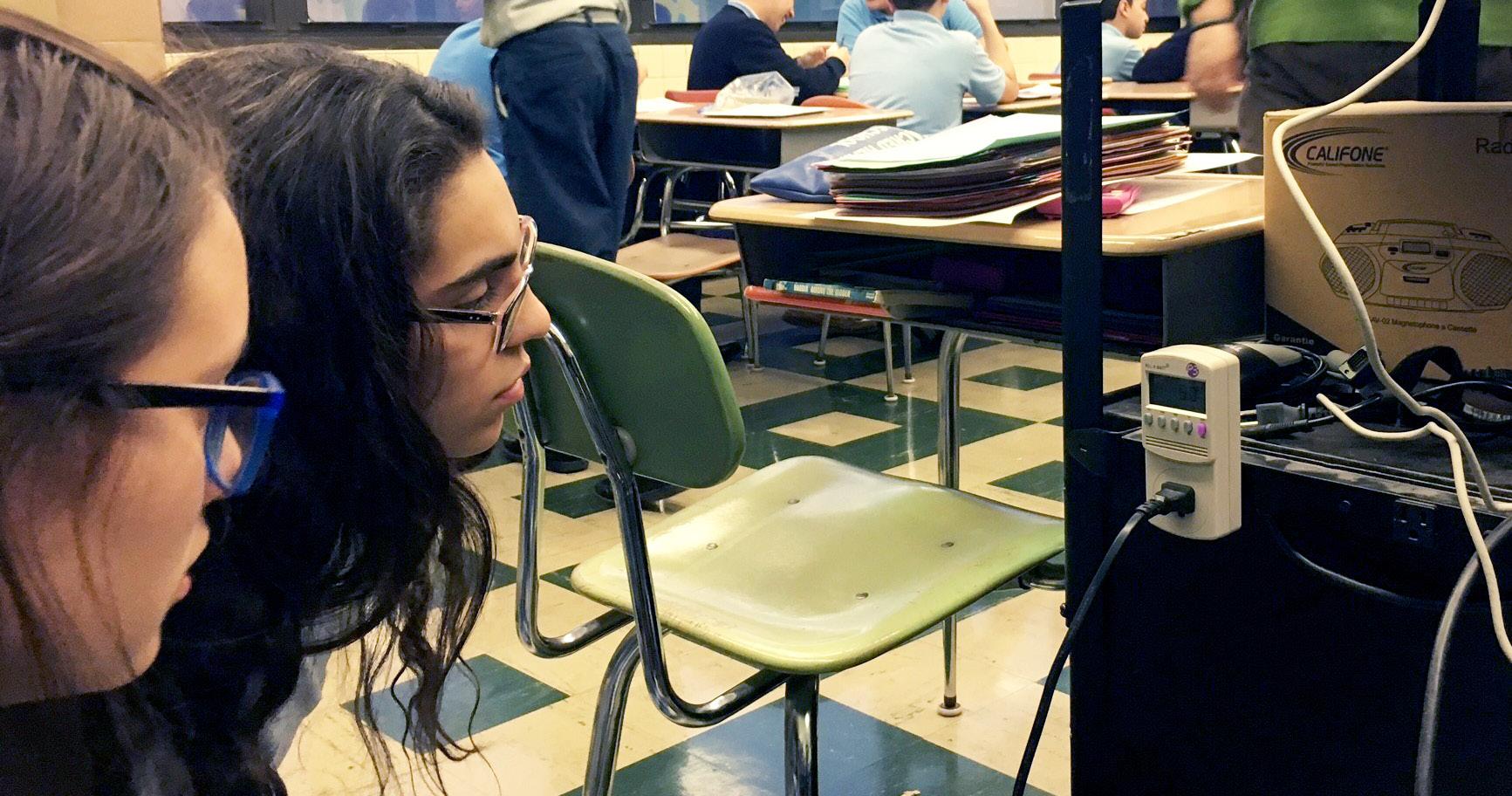

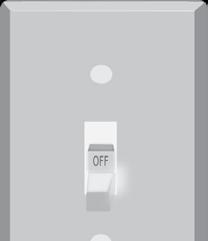
Energy has been a driving factor behind the advancement of our society. Until coal replaced wood as an energy source, technological developments occurred somewhat slowly. When energy sources with a higher energy density were discovered, the Industrial Revolution was launched. Since then, we have been using more and more energy as our population has grown and our technology has evolved. Today, people think a lot about energy – where to get it, how much of it they use, what sources they’re using for that energy – and it has become more important than ever to use energy wisely. Because most of the resources we use for energy are limited, using them wisely has become imperative. The text and activities in this guide are designed to help you think about the energy you use and learn to use less of it.
There are many forms of energy, but they all fall into two categories–potential or kinetic.
POTENTIAL ENERGY
Potential energy is stored energy and the energy of position, or gravitational potential energy. There are several forms of potential energy, including:
Chemical energy is energy stored in the bonds of atoms and molecules. It is the energy that holds these particles together. Foods we eat, biomass, petroleum, natural gas, and propane are examples of stored chemical energy.
During photosynthesis, sunlight gives plants the energy they need to build complex chemical compounds. When these compounds are later broken down, the stored chemical energy is released as heat, light, motion, and sound.
Elastic energy is energy stored in objects by the application of a force. Compressed springs and stretched rubber bands are examples of elastic energy.
Nuclear energy is energy stored in the nucleus of an atom— the energy that binds the nucleus together. The energy can be released when the nuclei are combined or split apart. Nuclear power plants split the nuclei of uranium atoms in a process called fission. The sun combines the nuclei of hydrogen atoms into helium atoms in a process called fusion. In both fission and fusion, mass is converted into energy, according to Einstein’s Theory, E = mc2.
Gravitational potential energy is the energy of position or place. A rock resting at the top of a hill contains gravitational potential energy because of its position. Hydropower, such as water in a reservoir behind a dam, is an example of gravitational potential energy.
Kinetic energy is motion—the motion of waves, electrons, atoms, molecules, substances, and objects.
Electrical energy is the movement of electrons. Everything is made of tiny particles called atoms. Atoms are made of even smaller particles called electrons, protons, and neutrons. Applying a force can make some of the electrons move. Electrons moving through a wire are called electricity. Lightning is another example of electrical energy.
Chemical Energy
Elastic Energy
Nuclear Energy
Gravitational Potential Energy
Electrical Energy
Radiant Energy
Thermal Energy
Motion Energy Sound Energy
Radiant energy is electromagnetic energy that travels in transverse waves. Radiant energy includes visible light, x-rays, gamma rays, and radio waves. Solar energy is an example of radiant energy.
Thermal energy, which is often described as heat, is the internal energy in substances—the vibration and movement of atoms and molecules within substances. The faster molecules and atoms vibrate and move within a substance, the more energy they possess and the hotter they become. Geothermal energy is an example of thermal energy.
Motion energy or mechanical energy is the movement of objects and substances from one place to another. According to Newton’s Laws of Motion, objects and substances move when an unbalanced force is applied. Wind is an example of motion energy.
Sound energy is the movement of energy through substances in longitudinal (compression/rarefaction) waves. Sound is produce when a force causes an object or substance to vibrate. The energy is transferred through the substance in a wave.
Your parents may tell you to conserve energy. “Turn off the lights,” they say. But to scientists, conservation of energy means something quite different. The Law of Conservation of Energy says energy is neither created nor destroyed.
When we use energy, we do not use it completely—we just change its form. That’s really what we mean when we say we are using energy. We change one form of energy into another. A car engine burns gasoline, converting the chemical energy in the gasoline into motion energy that makes the car move. Old-fashioned windmills changed the kinetic energy of the wind into motion energy to grind grain. Solar cells change radiant energy into electrical energy.
Energy can change form, but the total quantity of energy in the universe remains the same. The only exception to this law is when a small amount of matter is converted into energy during nuclear fusion and fission.
Energy efficiency is the amount of useful energy you can get out of a system. In theory, a 100 percent energy efficient machine would change all of the energy put in it into useful work. Converting one form of energy into another form always involves a loss of usable energy, usually in the form of thermal energy.
In fact, most energy transformations are not very efficient. The human body is no exception. Your body is like a machine, and the fuel for your “machine” is food. Food gives us the energy to move, breathe, and think. Your body is very inefficient at converting food into useful work. Most of the energy in your body is released as thermal energy.
A traditional incandescent light bulb isn’t efficient either. This type of light bulb converts only ten percent of the electrical energy into light and the rest (90 percent) is converted into thermal energy. That’s why these light bulbs are so hot to the touch. Their inefficiency is also why these bulbs are no longer sold for use in homes, and why many consumers use LEDs and CFLs for lighting.
Most electric power plants that use steam to spin turbines are about 35 percent efficient. Thus, it takes three units of fuel to make one unit of electricity. Most of the other energy is lost as waste heat. This heat dissipates into the environment where we can no longer use it as a practical source of energy.
When we are discussing how we use energy, efficiency refers to the equipment or machinery that we are using. For example, washing machines all clean our clothes. However, some are designed to do so using less water or electricity than other similar machines. Clothes come out clean from both machines, but one machine is more efficient in the way it cleans them.
Not to be confusing, but Conservation of Energy and energy conservation are not the same thing. One is a universal, governing law of science; the other is a term explaining behaviors we employ to use less energy.
When discussing how much energy we use, conservation refers to how we behave while using that energy. For example, the owners of the most efficiently built home can still waste energy if they leave the lights on and the refrigerator door open while pouring a glass of milk. People who have older, less efficient appliances can still save energy by using them wisely. Energy conservation refers to the things we choose to do that use less energy.
People have always used energy to do work for them. Thousands of years ago, early humans burned wood to provide light, heat their living spaces, and cook their food. Later, people used the wind to move their boats from place to place. A hundred years ago, people began using falling water to make electricity.
Today, people use more energy than ever from a variety of sources for a multitude of tasks and our lives are undoubtedly better for it. Our homes are comfortable and full of useful and entertaining electrical devices. We communicate instantaneously in many ways. We live longer, healthier lives. We travel the world, or at least see it on television and the internet.
The ten major energy sources we use today are classified into two broad groups—nonrenewable and renewable.
Nonrenewable energy sources include coal, petroleum, natural gas, propane, and uranium. They are used to generate electricity, to heat our homes, to move our cars, and to manufacture products from candy bars to cell phones.
These energy sources are called nonrenewable because they cannot be replenished in a short period of time. Petroleum, a fossil fuel, for example, was formed hundreds of millions of years ago, before dinosaurs existed. It was formed from the remains of ancient sea life, so it cannot be made quickly. We could run out of economically recoverable nonrenewable resources some day.
Renewable energy sources include biomass, geothermal, hydropower, solar, and wind. They are called renewable energy sources because their supplies are replenished in a short time. Day after day, the sun shines, the wind blows, and the rivers flow. We use renewable energy sources mainly to make electricity.
Is electricity a renewable or nonrenewable source of energy? The answer is neither. Electricity is different from the other energy sources because it is a secondary source of energy. That means we have to use another energy source to make it. In the United States, natural gas is the number one fuel for generating electricity.
NONRENEWABLE, 91.47%
RENEWABLE, 8.54%
Petroleum 37.29%
Uses: transportation, manufacturing - Includes Propane
Natural Gas 35.28%
Uses: electricity, heating, manufacturing - Includes Propane
Biomass 5.10%
Uses: electricity, heating, transportation
Wind 1.57%
Uses: electricity
Coal 10.40%
Uses: electricity, manufacturing
Hydropower 0.94%
Uses: electricity
Uranium 8.50%
Uses: electricity
*Propane consumption gures are reported as part of petroleum and natural gas totals.
Propane
Uses: heating, manufacturing
Data: Energy Information Administration
Solar 0.80%
Uses: electricity, heating
Geothermal 0.13%
Uses: electricity, heating
**Total may not equal 100% due to independent rounding.
Imagine how much energy you use every day. You wake up to an electric alarm clock and charge your cell phone. You take a shower with water warmed by a hot water heater using electricity or natural gas. You listen to music on your smart phone as you catch the bus to school. And that’s just some of the energy you use to get you through the first part of your day!
Every day, the average American uses about as much energy as is stored in a little less than seven gallons of gasoline. That’s every person, every day. Over a course of one year, the sum of this energy is equal to a little more than 2,300 gallons of gasoline per person. This use of energy is called energy consumption.
The U.S. Department of Energy uses categories to classify energy users—residential, commercial, industrial, electric power, and transportation. These categories are called the sectors of the economy.
Residences are people’s homes. Commercial buildings include office buildings, hospitals, stores, restaurants, and schools. Residential and commercial energy use are often lumped together because homes and businesses use energy in the same ways—for heating, air conditioning, water heating, lighting, and operating appliances.
The residential/commercial sector of the economy consumed 11.58 percent of the primary energy supply in 2022, with a total of 10.97 quads of energy. The residential sector consumed 6.41 quads and the commercial sector consumed 4.56 quads.
The industrial sector includes manufacturing, construction, mining, farming, fishing, and forestry. This sector consumed 22.80 quads of energy in 2022, which accounted for 24.08 percent of total consumption.
The electric power sector includes electricity generation facilities and power plants. All of the other sectors consume electricity generated by the electric power sector. The electric power sector consumed 34.95 percent of the total energy supply in 2022, more than any of the other sectors, with a total of 33.10 quads.
The transportation sector refers to energy consumption by cars, buses, trucks, trains, ships, and airplanes. In 2022, the U.S. consumed 27.68 quads of energy for transportation, which accounted for 29.22 percent of total consumption. 89.91 percent of this energy was from petroleum products such as gasoline, diesel, and jet fuel.
Several decades ago, in 1973, Americans faced a major oil price shock due to an oil embargo. People didn’t know how the country would react. How would Americans adjust to skyrocketing energy prices? How would manufacturers and industries respond? We didn’t know the answers.
Now we know that Americans tend to use less energy when energy prices are high. We have the statistics to prove it. When energy prices increased sharply in the early 1970s, energy use dropped, creating a gap between actual energy use and how much the experts had thought Americans would be using.
The same thing happened when energy prices shot up again in 1979, 1980, and more recently in 2008 and 2020—people used less energy. When prices started to drop, energy use began to increase.
We don’t want to simplify energy demand too much. The price of energy is not the only factor in the equation. Other factors that affect how much energy we use include the public’s concern for the environment and new technologies that can improve the efficiency and performance of automobiles and appliances.
Most reductions in energy consumption in recent years are the result of improved technologies in industry, vehicles, and appliances. Without these energy conservation and efficiency technologies, we would be using much more energy today.
In 2022, the United States used 25 percent more energy than it did in 1973. That might sound like a lot, but the population has increased by over 57 percent and the nation’s gross domestic product was 4.18 times that of 1973.
You may wonder why the 1970s are important—it was so long ago. However, the energy crisis during this decade taught us a valuable lesson. If every person in the United States today consumed energy at the rate we did in the 1970s, we would be using much more energy than we are—perhaps as much as double the amount. Energy efficiency technologies have made a huge impact on overall consumption since the energy crisis of 1973.
Speaking of energy consumption, did you know that the fresh water we use every day carries with it a high energy price tag? It’s true – extracting and purifying water for daily use requires a significant amount of energy. Leaving the faucet running is also a large energy liability.
Every step along the energy use pathway involves energy. Pumps are used to extract water from the ground or surface source and carry it to the treatment facility. More pumps and other electricallypowered equipment are used to move the water through as it is
cleaned and made suitable for home use. Getting that water to your home or school requires even more pumps and more energy. And then after you are done using the water, carrying it to wastewater treatment facilities also requires energy.
Different kinds of commercial buildings use water in different ways, but they all have domestic / restroom use in common. Except for restaurants, this is the largest water use in commercial buildings. In restaurants, it is the second largest use, after kitchen use.
All forms of energy fall under two categories:
Stored energy and the energy of position (gravitational).
CHEMICAL ENERGY is the energy stored in the bonds between atoms in molecules. Gasoline and a piece of pizza are examples.
NUCLEAR ENERGY is the energy stored in the nucleus or center of an atom – the energy that holds the nucleus together. The energy in the nucleus of a plutonium atom is an example.
ELASTIC ENERGY is energy stored in objects by the application of force. Compressed springs and stretched rubber bands are examples.
GRAVITATIONAL POTENTIAL
ENERGY is the energy of place or position. A child at the top of a slide is an example.
The motion of waves, electrons, atoms, molecules, and substances.
RADIANT ENERGY is electromagnetic energy that travels in transverse waves. Light and x-rays are examples.
THERMAL ENERGY or heat is the internal energy in substances – the vibration or movement of atoms and molecules in substances. The heat from a fire is an example.
MOTION ENERGY is the energy of the movement of a substance from one place to another. Wind and moving water are examples.
SOUND ENERGY is the movement of energy through substances in longitudinal waves. Echoes and music are examples.
ELECTRICAL ENERGY is the movement of electrons. Lightning and electricity are examples.
Btu: British thermal unit; a measure of thermal energy (heat); the amount of heat needed to raise the temperature of one pound of water by one degree Fahrenheit; one Btu is approximately the amount of energy released by the burning of one wooden kitchen match
Ccf: one hundred cubic feet; a unit used to measure natural gas usage
Current: the flow of electrons; the number of electrons flowing past a fixed point; measured in amperes — A
Energy: the ability to do work; work involves a change in movement, temperature, energy level, or electrical charge
Electricity: the energy of moving electrons; measured in kilowatt-hours — kWh
Force: a push or pull that gives energy to an object, causing it to start moving, stop moving, or change direction
kWh: kilowatt-hour; one kilowatt of electricity expended over one hour; one kilowatt-hour of electricity is the amount of energy it takes to burn a 100-watt light bulb for 10 hours; in 2022, the average cost of one kilowatt-hour of electricity for residential customers in the U.S. was about $0.150; the average cost for commercial customers, such as schools, was about $0.124 Mcf: one thousand cubic feet; a unit used to measure natural gas usage
MMBtu: 1,000,000 British thermal units (Btu)
Therm: a measure of thermal energy; one therm equals 100,000 Btu
Voltage: electric push or pressure; the energy available to move electrons; measured in volts — V
Watt: the measure of electric power; the number of electrons moving past a fixed point in one second multiplied by the pressure or push of the electrons; W = A x V
In 2022, the average heat content of natural gas for the residential, commercial, and industrial sectors was about 1,037 Btu per cubic foot.
1 cf = 1,037 Btu
1 Ccf = 103,700 Btu or 1.037 therms
1 Mcf = 1.037 MMBtu or 10.37 therms
1 kWh = 3,412 Btu
1 therm = 100,000 Btu
The cost of natural gas varies widely by sector of the economy. In 2022, one Mcf of natural gas cost $7.51 in the electric power generation sector, $7.66 in the industrial sector, $11.32 in the commercial sector, and $14.75 in the residential sector.
1. Choose a sector of the economy other than residential/ commercial. Create a fictitious scenario from that sector that describes good energy efficiency but bad energy conservation.
2. Choose a sector of the economy different from what you used in number 1, that is neither residential nor commercial. Create a fictitious scenario from that sector that describes bad energy efficiency but good energy conservation.
3. Re-read the two scenarios you created in numbers 1 and 2 above. Using evidence from the text, explain which scenario you believe would have the greatest impact (reduction or increase) on our total energy use as a nation if everyone in that sector employed it. Explain your reasoning well. GOOD ENERGY EFFICIENCY BUT BAD ENERGY CONSERVATION BAD ENERGY EFFICIENCY BUT GOOD ENERGY CONSERVATION
Using the form below, survey your classroom or other work area. Make a note of the devices using energy, any cold air (or warm air) currents you feel, any mysterious sounds that indicate something is running and not easily identified, and any similar items. The goal is to start paying detailed attention to the way you use energy every day while at school.
ITEMS YOU SEE THAT ARE USING ENERGY
ITEMS YOU HEAR THAT ARE USING ENERGY
ITEMS YOU FEEL THAT ARE USING ENERGY
Before you leave for the day, what is the first thing you think about with regard to the weather? If you said temperature, don’t be surprised. The temperature of the air around us dictates greatly what we will do. If the day is too hot, we will dress in lightweight clothes, and schedule our outdoor activities for the morning or evening to avoid overheating. If the day is too cold, we will bundle up with scarves, hats, gloves, heavy coats, and sometimes insulated pants and boots in order to stay warm. We wear very warm clothing in the winter so we don’t freeze to death, but we also wear the types of clothing we do each season to stay comfortable.
Keeping the interior of our homes comfortable is important, too. You may not think about it much, but the temperature indoors helps keep you healthy. If you’re comfortable, you don’t think at all about the heating system. However, if that heating system stops working properly and your house gets cold, you will probably think about it all the time.
The same can be said for schools. How difficult is it to concentrate on school work when your hands are cold? How easily can you stay awake if the room is too warm? The temperature of the room dictates our comfort level, and our comfort level greatly influences our ability to concentrate and be productive.
In Lesson 1, you read that most energy transformations also involve some “wasted” energy that is thrown off as thermal energy, and that energy is not typically useful. However, when it comes to keeping us comfortable, thermal energy is the most important form of energy.
Thermal energy is the energy that allows atoms and molecules to move. As you can imagine, solids have less thermal energy than gases; liquids fall somewhere in between. As long as you are comparing the solid, liquid, and gaseous state of the same substance, it is safe to say solids have the least amount of thermal energy and gases have the most. However, because different substances have the ability to retain different amounts of thermal energy, it is not always the case. For example, a gram of ice will have more thermal energy than a gram of oxygen gas. The amount of thermal energy a substance can retain is called its specific heat capacity, and it is different for each element or compound. Substances with a high specific heat capacity require a lot of thermal energy to increase in temperature, but they also can hold that energy for a longer time. Glass, water, and ceramics have high specific heat capacities. A substance with a lower specific heat capacity will heat up quickly and cool down quickly. Specific heat capacity factors into whether a substance is used to transfer thermal energy to heat a space. Thermal energy is transferred via three mechanisms: conduction, convection, and radiation. Conduction occurs when thermal energy is transferred from one object in direct contact with another object. In order for conduction to be feasible, the substance being used to transfer the energy must be a good conductor. In general, metals are good thermal conductors and non-metals are not. That is why cookware is often made of metals like copper, iron, and
stainless steel. Metals typically have low specific heat capacities, which is a desired quality in this case because the purpose is not to heat the cooking pot, but to transfer the heat from the burner to the food inside the pot.
Convection transfers thermal energy by heating a fluid, then circulating that fluid. The fluid must have a specific heat capacity large enough to make convection a worthwhile way to heat a space. Water has a very high specific heat capacity, and makes an excellent fluid for convection. The water is heated in a boiler, then circulated through pipes or tubes, releasing some of its energy and returning to the boiler at a lower temperature. Air does not have as high a specific heat capacity as water, but because it is easy to circulate air with fans, it is used in forced-air heating systems.
Radiation is the third mechanism for transferring thermal energy, and it does not require the assistance of a substance like steel or water. Rather, the thermal energy is emitted from the hot object in waves. You have experienced radiation if you’ve ever sat around a fire on a cool evening, toasting marshmallows or warming your hands. The thermal energy source is the combustion of the wood and paper on the fire, and you can feel the thermal energy radiating outward from the fire.
When it comes to transferring thermal energy to a specific object, conduction is the most efficient means to do so. However, conduction is not very efficient when it comes to heating entire rooms and it is impractical to have large metallic pieces radiating from a heat source into the rooms of a building. Therefore, convection and radiation are how spaces are usually heated. Small electric or kerosene space heaters use radiation. Forced-air furnaces use convection. Boiler systems with radiators use both convection and radiation.
The first schools were one small room, and had a wood stove in them that kept the room warm. The seats closest to the wood stove were quite warm, and those students seated farthest away from the stove might get cold. They relied on radiation to warm the school.
A century ago, schools were built with a large boiler that was fueled by coal. The boiler heated water, which was circulated through the school through radiators. The heat was either on all the time, or shut down entirely, and there was no control of the temperature from one room to the next. As a result, rooms on the upper floor of the school were often quite warm, while rooms on the lower floor or in the basement could be too cool to be comfortable.
Schools today often have boiler systems that circulate hot water or steam, but they are usually fueled by natural gas, and the water circulates into a device that blows air over the hot water piping system to heat the room. Some systems even have individual thermostats in each room that can open and close valves to control the temperature.
Buildings are designed to keep the indoor air separated from the outdoor air. Otherwise, the heating and cooling system is trying to heat or cool the air outside the building as well. That would be terribly inefficient. Buildings are constructed out of materials that insulate, or block thermal energy transfer. Insulating materials do not conduct thermal energy well.
The parts of the building that separate indoors from outdoors construct the building envelope. The walls, floor, roof, doors, and windows are parts of the building envelope system. Their purpose is to keep the interior of the building a comfortable, healthy place to work or learn while allowing access into and out of the building.
The space above the ceiling, below the floor, and within walls is filled with insulation, which prevents thermal energy transfer. Insulation is graded by its R-value, which is an abbreviation for its resistance to conduction. Different materials have different R-values, and the amount of insulation needed in total R-value varies according to the climate in which you live. Industry professionals calculate the R-value per inch thickness of different materials and builders install the appropriate thickness of locally-available materials to provide the proper amount of insulation. The materials used in buildings in your area depend on what is easily available and how many people are trained in their installation.
All of Alaska is in Zone 7 except for the following boroughs in Zone 8:
Bethel Northwest Arctic, Dellingham Southeast Fairbanks, Fairbanks N. Star Wade Hampton, Nome Yukon-Koyukuk, North Slope
Zone 1 includes Hawaii, Guam, Puerto Rico, and the Virgin Islands.
CATHEDRAL CEILING
R30 to R49
R30 to R60
R30 to R60
R38 to R60
R38 to R60
R49 to R60
R49 to R60
R49 to R60
Data: U.S. Department of Energy
R22 to R38
R22 to R38
R22 to R38
R30 to R38
R30 to R60
R30 to R60
R30 to R60
R30 to R60
CAVITY
R13 to R15
R13 to R15
R13 to R15
R13 to R15
R13 to R21
R13 to R21
R13 to R21
R13 to R21
INSULATION SHEATHING
None
None
R2.5 to R5
R2.5 to R6
R2.5 to R6
R5 to R6
R5 to R6
R5 to R6
FLOOR
R13
R13, R19 to R25
R25
R25 to R30
R25 to R30
R25 to R30
R25 to R30
R25 to R30
In order for the building envelope to do its job, air must not be allowed to flow into or out of the building. The most obvious way to avoid air infiltration is to keep doors and windows closed when the heating or cooling system is running. However, there are other ways air can find its way into or out of a building that are just as important to control. Doors and windows that leave gaps even when closed need a strip of weatherstripping to seal those spaces. The space between doors or windows and the walls that contain them also need to be sealed. Immediately after a building is completed, the doors and windows fit snug in their respective spaces. Over time, however, the building expands and contracts slightly as it heats and cools with the seasons, and the doors and windows may not be as tight a fit as they once were. Therefore it is important to inspect these junctions annually and fill any gaps with weathertight caulking.
Attic spaces are also frequent culprits in air infiltration. Any ventilation piping systems that exit the building, such as a flue from a boiler or a vent pipe for plumbing, have the potential to allow air to enter or exit the building. Because hot air is less dense than cold air, it rises, and can escape through the roof of a building if the attic is not well sealed.
Windows made of metallic frames were often used in schools built in the 1950s and 1960s. The aluminum in the frames is an excellent thermal conductor and will conduct the energy right through to the other side. As you can well imagine, this is not a great material for constructing window frames. Most windows today are made of vinyl, wood, or composite materials that are better insulators. The number of panes of glass in a window is important, too. Singlepane windows are not well insulated; double- or triple-pane glass has an insulating space between the glass that allows light in but keeps thermal energy from transferring across the window.
Most schools have two sets of doors at each entry point. This prevents large amounts of air from rushing in or out of the building as people enter and exit. To work properly, both sets of doors need to be kept closed when not being used.
Up until this point we have been considering heating or cooling indoor spaces under the assumption that moisture levels remain constant. The amount of water in air varies from day to day, according to climate, weather, and temperature, and is measured according to its relative humidity
Think of air as a moisture sponge. Warm air can hold more moisture because the atoms and molecules are moving faster and are more spread out. Warm air is like a bigger sponge. Cool air holds less moisture because the particles are spaced closer together. Cool air is like a smaller sponge. Imagine the sponge can increase or decrease in size as the temperature changes. If you add 100 mL of water to a small sponge, it might be completely saturated – it is holding all of the moisture it can hold. If that sponge expands, but no more moisture is added, it is no longer saturated, and is now holding a fraction of the water that it could potentially hold.
often has storm window, screen, or combination space between glass may be gas- lled glass may be low-E type with coatings
space between glass may be gas- lled glass may be low-E type with coatings
Improved frame materials
Wood composites, vinyl, and berglass frames reduce heat transfer and help insulate better.
Multiple panes
Two panes of glass, with an air- or gas- lled space in the middle, insulate much better than a single piece of glass. Some ENERGY STAR-quali ed windows include three or more panes for even greater energy e ency, increased impact resistance, and sound insulation.
Some energy e cient windows have argon, kyrpton, or other gases between their panes. These odorless, colorless, non-toxic gases insulate better than regular air.
Special coatings re ect infrared light, keeping heat inside in winter and outside in summer. They also re ect damaging ultraviolet light, which helps protect interior furnishings from fading.
Data: ENERGY STAR®
A spacer keeps a window’s glass panes the correct distance apart. Today’s warm edge spacers—made from steel, foam, berglass, or vinyl—reduce heat ow and prevent condensation.
Cool air is like a small sponge—it holds a small amount of water. Warm air is like a larger sponge—it holds more moisture. Warming the air increases the amount of water it can hold, but the relative humidity decreases because no additional moisture is added.
Air works the same way. Cool air, like the smaller sponge, holds a certain amount of water. If that air is warmed, but no more moisture is added, the relative humidity goes down because it is only holding a fraction of the water that the warm air could potentially hold. In winter, when the temperature is very cold, air holds little water. For the sake of argument, let’s say the cold air outdoors is at 50% relative humidity. That same air is warmed indoors, but no additional moisture is added. The warmer air is now at 18% relative humidity. The amount of moisture hasn’t changed, but the percent saturation – relative humidity – has changed because cold air holds less moisture than warm air. The amount of moisture in the air is very important. Air with too little moisture dries out skin, eyes, noses, and mouths and promotes static electricity buildup. Air with too little moisture also will not retain thermal energy as well, and the heating system needs to work harder to maintain a comfortable temperature. Too much moisture will cause the pages of books to curl and promotes the growth of mold and mildew, which are significant health hazards. During cooling season, air with too much moisture feels muggy and uncomfortable, and the cooling system will need to work harder to keep the room comfortable. The optimal relative humidity level for indoor spaces is 30-60%.
In schools, what is mixed in the air is a major concern because schools tend to be closed up tight all day, every day. Some classrooms may have an open window here and there, but for the most part, the doors and windows are kept closed.
Because schools have a lot of people in a smaller space than homes do, bringing fresh air in from outside is important. Each person in the building is a living, breathing, thermal energy and carbon dioxideproducing machine. In a sealed building, the carbon dioxide will build up, which creates a health hazard of its own. When carbon dioxide is added to possible mold growth, cleaning product fumes, cooking odors, and the smells of gym class, the need for fresh air becomes readily apparent.
Commercial buildings like schools have HVAC systems – heating, ventilation, and air conditioning. Your home has a heating system and might have an air conditioner, but ventilation is usually accomplished by opening and closing doors and windows. Because you have relatively few people per square foot in your home, adding fresh air is unnecessary. In commercial buildings, it is not only desirable, it is vital to keep the occupants healthy.
Ventilation is measured by the number of air changes per hour. One air change in one hour means enough fresh air is brought into the room such that in one hour all of the air is exchanged. Fresh air exchange is important not only to remove the carbon dioxide produced by the people, but also to keep moisture at a healthy level to prevent mold growth and to remove chemicals from activities like cooking, cleaning, science lab experiments, and art projects. Different buildings with different uses are required to exchange the air at different rates. Local building codes and regulations also vary. Therefore, what may be sufficient in one school may not meet regulated ventilation requirements in another school. You can check with your school’s maintenance supervisor or your local building code enforcement office to find out how many air changes per hour are needed.
You may be wondering at this point what a discussion of air quality has to do with thermal energy in a building’s energy use picture, especially if you live in a climate where you can have the doors or windows open almost all the time. However, in climate zones where winter temperatures get very cold or summer temperatures are very hot, the balance between fresh air and indoor comfort is difficult to maintain.
On the one hand, the building needs to be kept at a comfortable temperature, either by heating or cooling the air. This is achieved by using a heating or cooling system, and recirculating the air in the building to add or remove thermal energy from it.
On the other hand, the occupants of a building need fresh air to stay alert and healthy. If the air outside is very cold, bringing significant amounts of it into the building will place a heavy burden on the heating system. If the outdoor air is very warm, the cooling system must work hard. Both situations will require more energy. Moisture just adds to the complexity of the situation.
Maintenance supervisors need to maintain a balance in their buildings between what is comfortable and what is necessary for the occupants of the building to get the fresh air they need. A century ago, most people were not very concerned with indoor air quality except in extreme situations. Today, though, indoor air quality is a major factor in selecting the HVAC equipment for a commercial building. Fortunately, computer systems and other guides are available that help building managers keep that balance.
When constructing a building, a vapor barrier is often placed inside the wall. The purpose of the vapor barrier is to resist moisture diffusing through the wall. Its presence can minimize the amount of moisture that will build up inside the wall, which in turn prevents mold growth. Placing the moisture barrier properly ensures that the insulation does its job, and the inside air is kept separate from the outside air. It also prevents moisture as condensation from accumulating and adversely affecting indoor air quality.
? Question
Which materials are the best thermal insulators? What procedure should you follow to answer this question?
Materials
Your teacher will provide a list of suggested or required materials for this activity.
Hypothesis
In your science notebook, write a statement to address which types of materials you think make the best thermal insulators.
Procedure
1. Before you begin, as a class discuss how you are going to collect data to evaluate each insulation material. You will write the procedure you each will follow when testing your materials.
2. Design your data collection process and charts in the space provided below. Copy this into your science notebook, leaving plenty of room to record your data and observations.
3. Carry out the procedure you have developed as a class, recording your data and observations.
4. Clean up and return all materials according to the instructions given by your teacher.
Data
Use this space to develop your data collection plan and record observations.
Conclusion
Which material was the best insulator? Use the evidence you collected to support your answer.
You will be measuring air flow through your ventilation system in feet per minute using an anemometer. Multiplying the anemometer measurement by the area of the vent, in square feet, will provide a certain number of cubic feet per minute (CFM) that, when combined with the volume of your classroom, will determine how many air changes per hour are happening with your ventilation system. However, there are two important things to keep in mind when conducting this activity.
1. The minimum amount of air exchanged in a classroom according to ASHRAE standards is 2.5 changes per hour, but not all of the air coming through your vent is fresh air. Some of it is recirculated air, and in times of extreme temperature events, as seen in the coldest winters and hottest summers, the outside ventilation may be eliminated or severely restricted. This is because of the amount of energy needed to heat extremely cold or cool extremely hot air. In the interest of energy efficiency, sometimes outside ventilation is set aside to keep the indoor temperature and humidity at a safe and comfortable level.
2. This airflow measurement activity is not meant to be an official, inspection-level measurement. Ideally you will finish calculating the air flow into your classroom and arrive at an answer somewhere between 2.5 and 4.0 changes per hour. However, because of the nature of the instruments you are using and their accuracy, do not be alarmed if you arrive at exceptionally high or low air exchange rates with this activity. If you are concerned about the indoor air quality in your classroom, gather data on several different days, and discuss it with your administrator and building maintenance staff.
? Question
How quickly could the air in your classroom be refreshed?
Materials
Anemometer from kit
Measuring tape or laser measuring device
Calculator
Hypothesis
In most science classrooms, International System (SI) units are used, and you would expect to be measuring air volumes in liters rather than cubic feet. However, because American HVAC specialists and building codes work in Imperial units, this activity has you working with feet and inches rather than liters, meters, and centimeters.
Estimate how many times the air in your classroom could be exchanged with fresh air by the ventilation equipment present.
Procedure
1. Copy the first data table into your science notebook, once for each vent in your classroom. For example, if you have four vents, you will need to draw the table four times. Then copy the second data table into your notebook after the first.
2. Use the anemometer to measure the wind speed in feet per minute from your classroom’s ventilation system. If you have more than one vent, you will need to do this for each vent in your room.
3. Measure the cross-sectional area of the vent:
a. If the vent is rectangular in shape, measure the length and width in inches. Divide each number by 12, and multiply them together. This is the area in ft2.
b. If the vent is circular, measure the diameter in inches. Divide this by two to get the radius, then by 12 to convert inches to feet. Square this number, and multiply by π (pi), to get the area of the vent in ft2.
c. If the vent is neither rectangular nor circular, trace it onto a large sheet of paper. Using a ruler, divide the shape into regular shapes (semicircles, rectangles, triangles, etc.) and calculate the area of each shape, then add them together to get the area of the entire vent.
4. Multiply the airflow by the cross-sectional area to get cubic feet per minute (CFM).
5. Repeat steps 2-4 for the rest of the vents, if applicable. Add the airflow in cubic feet per minute for all of the vents in the room to get the total airflow in CFM for the entire room and enter this value into the second data table.
6. Multiply cubic feet per minute (CFM) by 60 to get cubic feet per hour.
7. Measure the length, width, and height of your classroom, in feet.
8. Calculate the volume of your classroom in cubic feet.
9. Divide the volume of your classroom in cubic feet by the airflow in cubic feet per hour, to get the number of room volumes per hour, or air changes per hour, that are moving through your classroom’s ventilation system.
Data
Copy the following table into your science notebook one time for each of the vents in your classroom.
Vent Location:
Airflow speed (from anemometer):
Vent Area
Total Airflow (CFM) Airflow speed (ft/min) × vent area (ft2) =
Copy the following table into your science notebook once:
inches ÷ 12 =
÷ 12 =
÷ 12 =
(CFM)
airflow (CFM) Sum of airflow of all vents =
Total hourly airflow (ft3/hr)
airflow (CFM) × 60 =
÷ Volume of room (ft3) = changes/hr
1. Most building codes require a bare minimum of 2.5 changes per hour of fresh air for classrooms (some are higher). Would your ventilation system be able to provide that if the vent is open all the way? What is the minimum percentage that your ventilation system can be open to achieve this requirement?
2. Identify sources of error in this activity. Using these error sources as a basis, explain why your calculations should not be used in an official capacity to determine compliance with building codes.
3. One of the most difficult tasks for building managers to work through is the balance between keeping a room at a comfortable temperature and ensuring enough fresh air is brought into the building. Write a paragraph or two describing why this is difficult. Include a discussion of scenarios that might favor a room temperature approach over fresh air, and other scenarios that might favor fresh air over a comfortable room temperature.
What is the first thing you think about when reading the word, “energy?” If you thought of electricity first, you’re not alone. Energy and electricity are frequently used interchangeably, although in Lesson 2 about thermal energy you learned that clearly energy is more.
In Lesson 1 you learned that the electric power generation sector uses the most energy. This is not surprising when you consider all of the things we do that require electricity. Chances are that while you are reading this, you are using artificial light powered by electricity to see. Stop and think about the first fifteen minutes of your day. How many of those minutes involved something electric?
Electricity is a little different from the other sources of energy that we talk about. Unlike coal, petroleum, or solar energy, electricity is a secondary source of energy. That means we must use other primary sources of energy, such as coal or wind, to make electricity. It also means we can’t classify electricity as a renewable or nonrenewable form of energy. The energy source we use to make electricity may be renewable or nonrenewable, but the electricity is neither.
Almost all electricity made in the United States is generated by large, central power plants. There are about 25,000 power plants in the U.S. Most power plants use natural gas, coal, nuclear fission, or other energy sources to superheat water into steam in a boiler. The very high pressure of the steam (it’s 75 to 100 times normal atmospheric pressure) turns the blades of a turbine. (A turbine turns the linear motion of the steam into circular motion.) The blades are connected to a generator, which houses a large magnet surrounded by coiled copper wire. The blades spin the magnet rapidly, rotating the magnet inside the coil producing an electric current.
The steam, which is still very hot but now at normal pressure, is piped to a condenser, where it is cooled into water by passing it through pipes circulating over a large body of water or cooling tower. The water then returns to the boiler to be used again. Power plants can capture some of the heat from the cooling steam. In old plants, the heat was simply wasted.
Not all power plants use thermal energy to generate electricity. Hydropower plants and wind farms use motion energy to turn turbines, turning a generator, which produces electricity. Photovoltaic plants use radiant energy to generate electricity directly.
Three basic types of power plants generate most of the electricity in the United States – fossil fuel, nuclear, and wind. There are also hydropower, geothermal, waste-to-energy, and solar power plants, but together they generate about 11.12 percent of the electricity produced in the United States in 2022.
Fossil fuel plants burn coal, natural gas, or petroleum. These plants use the chemical energy in fossil fuels to superheat water into steam, which drives a steam generator. Fossil fuel plants are sometimes called thermal power plants because they use heat to generate electricity. Natural gas and coal are the fossil fuels of choice for many generation companies across the U.S., providing 39.82 and 19.54 percent of total U.S. electricity respectively. Petroleum produces 0.55 percent of the electricity in the U.S.
Nuclear plants generate electricity much as fossil fuel plants do, except that the furnace is a reactor and the fuel is uranium. In a nuclear plant, a reactor splits uranium atoms into smaller elements, producing a great amount of thermal energy in the process. The heat is used to superheat water into highpressure steam, which drives a turbine generator. Like fossil fuel plants, nuclear power plants are thermal plants because they use heat to generate electricity. Nuclear energy produced 18.18 percent of the electricity in the U.S. in 2022.
Wind turbines use the energy of moving air, or wind, to push on blades attached to a hub, eventually driving a generator. Wind energy has been the fastest-growing renewable energy source in the last 20 years and is now the fourth largest electricity producer in the U.S. In 2022, wind energy accounted for 10.25 percent of electric power generation.
Power plant generates electricity
Transmission lines carry electricity long distances
Transformer steps up voltage for transmission
TRANSMISSION LINES
We are using more and more electricity every year. One reason that electricity is used by so many consumers is that it’s easy to move from one place to another. Electricity can be produced at a power plant and moved long distances before it is used. Let’s follow the path of electricity from a power plant to a light bulb in your home.
First, the electricity is generated at the power plant. Next, it goes by wire to a transformer that “steps up” the voltage. A transformer steps up the voltage of electricity from the 2,300 to 22,000 volts produced by a generator to as much as 765,000 volts (345,000 volts is typical). Power companies step up the voltage because less electricity is lost along the lines when the voltage is high. The electricity is then sent on a nationwide network of transmission lines made of aluminum. Transmission lines are the huge power lines you may see when you’re on a highway connected by tall power towers. The lines are interconnected, so should one line fail, another will take over the load. Step-down transformers located at substations along the lines reduce the voltage to 12,000 volts. Substations are small buildings in fenced in areas that contain the switches, transformers, and other electrical equipment. Electricity is then carried over distribution lines that bring electricity to your home. Distribution lines may either be overhead or underground. The overhead distribution lines are the electric lines that you see along streets.
Distribution lines carry electricity to houses
Step-down transformer reduces voltage (substation)
Neighborhood transformer on pole steps down voltage before entering house
Before electricity enters your house, the voltage is reduced again at another transformer, usually a large gray can mounted on an electric pole. This neighborhood transformer reduces the electricity to 240 and 120 volts, the amount needed to run the appliances in your home.
Electricity enters your house through a three-wire cable. The “live wires” are then brought from the circuit breaker or fuse box to power outlets and wall switches in your home. An electric meter measures how much electricity you use so the utility company can bill you. The time it takes for electricity to travel through these steps—from power plant to the light bulb in your home—is a tiny fraction of one second.
Everyone knows how important electricity is to our lives. All it takes is a power failure to remind us how much we depend on it. Life would be very different without electricity—no more instant light from flicking a switch, no more television, no more refrigerators, or stereos, or video games, or hundreds of other conveniences we take for granted. We depend on it, business depends on it, and industry depends on it. You could almost say the American economy runs on electricity. It is the responsibility of electric utility companies to make sure electricity is there when we need it. They must consider reliability, capacity, baseload, peak demand, and power pools
Reliability is the capability of a utility company to provide electricity to its customers 100 percent of the time. A reliable electric service is without blackouts or brownouts. To ensure uninterrupted service, laws require most utility companies to have 15 to 20 percent more capacity than they need to meet peak demand. This means a utility company whose peak demand is 12,000 megawatts (MW) must have 14,000 MW of installed electrical capacity. This ensures that there will be enough electricity to meet demand even if equipment were to break down on a hot summer afternoon.
Capacity is the total quantity of electricity a utility company has on-line and ready to deliver when people need it. A large utility company may operate several power plants to generate electricity for its customers. A utility company that has seven 1,000 MW plants, eight 500 MW plants, and 30 100 MW plants has a total capacity of 14,000 MW.
Baseload power is the electricity generated by utility companies around-the-clock, using the most inexpensive energy sources— usually coal, nuclear, and hydropower. Baseload power stations usually run at full or near capacity.
When many people want electricity at the same time, there is a peak demand. Power companies must be ready for peak demands so there is enough power for everyone. During the day’s peak, between 12:00 noon and 6:00 p.m., additional generators must be used to meet the demand. These peaking generators run on natural gas, diesel, or hydropower and can be put into operation in minutes because they require little start-up time. The more this equipment is used, the higher our utility bills. By managing the use of electricity during peak hours, we can help keep costs down.
The use of power pools is another way electric companies make their systems more reliable. Power pools link electric utilities together so they can share power as it is needed. A power failure in one system can be covered by a neighboring power company until the problem is corrected. There are eight regional power pool networks in North America. The key is to share power rather than lose it.
The reliability of U.S. electric service is excellent, usually better than 98 percent. In some countries, electric power may go out several times a day for several minutes or several hours at a time. Power outages in the United States are usually caused by such random occurrences as lightning, a tree limb falling on electric wires, or a fallen utility pole.
How much does electricity cost? The answer depends on the cost to generate the power (56 percent), the cost of transmission (13 percent), and local distribution (31 percent). The average cost of electricity is about 15.0 cents per kWh (15.04¢) for residential customers, twelve cents (12.41¢) for commercial customers, and a little more than eight cents (8.32¢) for industrial customers. A major key to cost is the fuel used to generate the power. Electricity produced from natural gas, for example, costs more than electricity produced from uranium or hydropower. Location plays a part in electricity costs. Hawaii and Alaska residents can pay up to 43 cents and 23 cents per kWh, respectively, while residents of Washington pay only 10.26 cents per kWh. Commercial customers in the U.S. follow the same patterns, but because they buy more electricity at once, their cost is lower, averaging at about 12 cents per kilowatt hour.
Another consideration is how much it costs to build a power plant. A plant may be very expensive to construct, but the cost of the fuel can make it competitive to other plants, or vice versa. Nuclear power plants, for example, are very expensive to build, but their fuel— uranium— is very cheap. Coal-fired plants, on the other hand, are much less expensive to build than nuclear plants, but their fuel— coal—is more expensive.
When calculating costs, a plant’s efficiency must also be considered. In theory, a 100 percent energy efficient machine would change all the energy put into the machine into useful work, not wasting a single unit of energy. But converting a primary energy source into electricity involves a loss of usable energy, usually in the form of thermal energy. In general, it takes three units of fuel to produce one unit of electricity from a thermal power plant.
Power is the rate (time) of doing work. A watt is a measure of the electric power an electrical device uses. Most electrical devices require a certain number of watts to work correctly. Light bulbs, for example, are rated by watts (13, 32, 60, 75, 100 watts), as are appliances, such as a 1500-watt hairdryer.
A kilowatt is 1,000 watts. A kilowatt-hour is the amount of electricity used in one hour at a rate of 1,000 watts. Visualize adding water to a pool. In this analogy, a kilowatt is the rate at which water is added to the pool; a kilowatt-hour is the amount of water added to the pool in a period of time.
Just as we buy gasoline in gallons or wood in cords, we buy electricity in kilowatt-hours. Utility companies charge us for the kilowatt-hours we use during a month. If an average family of four uses 899 kilowatt-hours in one month, and the utility company charges 15.0 cents per kilowatt-hour, the family will receive a bill for $135.21. (899 x $0.1504 = $135.21) Electric utilities use megawatts and gigawatts to measure large amounts of electricity. Power plant capacity is usually measured in megawatts. One megawatt is equal to one million watts or one thousand kilowatts.
Gigawatts are often used to measure the electricity produced in an entire state or in the United States. One gigawatt is equal to one billion watts, one million kilowatts, or one thousand megawatts.
In 1900, most power plants were only four percent efficient. That means they wasted 96 percent of the fuel used to generate electricity. Today’s thermal power plants are over eight times more efficient with efficiency ratings around 35 percent. Still, this means 65 percent of the initial thermal energy used to make electricity is lost. You can see this waste heat in the clouds of steam pouring out of giant cooling towers on newer power plants. A modern coal plant burns about 4,500 tons of coal each day, and about twothirds of the energy in this is lost when the chemical energy in coal is converted into thermal energy, then into electrical energy. A hydropower plant, on the other hand, is about 90 percent efficient at converting the kinetic energy of moving water into electricity. But that’s not all. Between three and eight percent of the electricity generated at a power plant must be used to run equipment. And then, even after the electricity is sent over electrical lines, another seven percent of the electrical energy is lost in transmission. Of course, consumers pay for all the electricity generated, lost or not.
The cost of electricity is affected by what time of day it is used. During a hot summer afternoon from noon to 6 p.m., there is a peak of usage when air-conditioners are working harder to keep buildings cool. Electric companies charge their industrial and commercial customers more for electricity during these peak load periods because they must turn to more expensive ways to generate power.
A school building contains many electrical devices, called plug loads, that contribute to the learning process and help occupants stay comfortable and safe. It is estimated that about 32 percent of the total electricity consumed by a school is used to power these electrical devices. Managing the use of such equipment can greatly reduce a school’s electricity consumption.
Look around any classroom and you will see many appliances. A quick survey of the typical classroom and school building reveals many kinds of electrical appliances, such as:
coffee makers
computers and monitors
fans
desk and table lamps
microwaves
refrigerators
televisions
laptops/tablets
DVD players/BluRay players
window air conditioners
vending machines
printers and scanners
fax machines
copiers
fish tanks
digital projectors
ranges and stoves
vocational equipment
clocks
drinking fountains
pencil sharpeners
Many of these devices are important to the learning environment. In addition, there are appliances that teachers and school staff bring from home that are not related to teaching, but are routine devices found in any office. Many electrical appliances, such as computers, printers, and copiers, waste energy when they are left on 24 hours a day. Often they are left on as a matter of convenience because they have a long warm–up time. Turning these machines off at the end of the day, and turning other machines off when they are not being used, can save a lot of energy.
Once students, teachers, and staff are educated about the impacts of energy consumption, they are often willing to reduce their use of these devices. By simply monitoring daily use of plug loads, students and staff can lower the school’s utility bills, saving the school system money.
Many computers, TVs, DVD players, and other electrical devices use electricity even when they are turned off. This type of electricity consumption is known as phantom load, because it can easily go unnoticed. Phantom loads are also known as standby power or leaking electricity. Phantom loads exist in many electronic or electrical devices found in schools. Equipment with electronic clocks, timers, or remote controls, portable equipment, and office equipment with wall cubes (small box-shaped plugs that plug into AC outlets to power appliances) all have phantom loads and can consume up to 40 watts when turned off, depending on the device. These appliances should be plugged into surge protectors so that all of the power can be turned off when they are not in use, or at the end of the day.
When shopping for a new appliance or lighting, look for the ENERGY STAR® label—your assurance that the product saves energy. ENERGY STAR® appliances have been identified by the U.S. Environmental Protection Agency and Department of Energy as the most energy efficient products in their classes. A list of these appliances and devices can be found on the ENERGY STAR® website at www.energystar.gov.
Another way to determine the efficiency of appliances is to compare energy usage using EnergyGuide labels. The Federal Government requires most appliances to display bright yellow and black EnergyGuide labels. Although these labels do not say which appliance is the most efficient, they provide the annual energy consumption and average operating cost of each appliance so you can compare them.
? Question
How much energy do all the plug-in devices in school use? How much does this electricity cost?
Materials
Computer with a spreadsheet program, like Microsoft Excel
Kill A Watt® meter
Stopwatches or timers (optional)
Hypothesis
In your science notebook, write a statement to address which types of appliances use the most energy and cost your school the most to operate.
1. In your science notebook, make a list of every single plug-in device in your classroom, regardless of whether it is currently being used. Do not include overhead lighting turned on with a wall switch. List duplicate devices only once, noting how many are present. For example, if there are three computers, just list “computer” and then note that there are three present.
2. Using the Kill A Watt® meter, determine the power load of the device when it is running. Also determine whether the device has a phantom load by observing the meter when the device is powered off. Phantom loads show as power used on the meter even when the device is off. If you are unable to plug the device into the meter, use the UL label to determine the maximum power the machine uses. The UL label is located on the back or in another inconspicuous area.
3. Estimate how many hours per day each device is used. You might do this in a small group or as a class, as instructed by your teacher. You can also time how long a device is used, and then estimate the number of times per day it is used and multiply by this time. For example, shredders do not run all the time, and are usually only used a few times a day. You would time how long it takes to shred a sheet of paper, the multiply by the number of times a sheet is shredded.
4. Most devices run all of the time, but some do not. For example, the compressor on a mini-fridge only runs about 1/3 the time (33%) and even though it is plugged in all day, every day, it only runs about 1/3 of the time. This is called its cycle time. Note the devices that have a cycle time of less than 100%. Develop a quick procedure for determining the cycle time of refrigerators, air conditioners, space heaters, etc. Devices that stay plugged in but only run intermittently, like pencil sharpeners, should only be calculated based on the actual number of minutes per day they are used. They do not have a cycle time.
5. Devices with multiple operating modes, like printers and copiers, should be checked with the meter in each mode, and the number of minutes the device runs in each mode should be estimated.
6. Determine the number of days per year that the devices are used. Many things in a school are turned off over weekends and breaks, but not all are. For example, refrigerators are usually left plugged in all day, every day. Computers might be in sleep mode overnight and on weekends, but are powered completely off over long breaks.
7. Create a spreadsheet or use the one your teacher provides to calculate the total kilowatt-hours (kWh) of electricity used by the device in one year, and to calculate the cost to run this device. If more than one is present, multiply by the number of devices of this type in your classroom.
If you are setting up a spreadsheet of your own, construct it in a way that makes sense to you but also makes the information your teacher is looking for easy to find. Make sure you include places to record or calculate the watts, kilowatt-hours, cycle time, mode, and cost to run for a year. Intermediate calculations that are helpful but not required are number of each device, watts to kilowatts, cost of operation for a day, number of days used in one year (up to 365), and annual kWh.
1. Which devices are the biggest energy users in your classroom? Which devices use the least amount of energy?
2. Were you surprised by any devices that used more or less energy than you originally expected?
3. Identify three action items your class can take to reduce energy consumption, and calculate how much money can be saved by implementing them.
How do you know which appliance is the best long-term investment?
Computer with a spreadsheet program, like Microsoft Excel
EnergyGuide labels
Procedure
1. Using the EnergyGuide labels provided by your teacher, build a spreadsheet that calculates the life cycle cost of two appliances that are similar, but that are not the same efficiency. Use the purchase price and operating cost per year to determine life cycle cost.
2. Determine the overall cost of each appliance each year for seven consecutive years.
3. Continue calculating the cost of each appliance until you have reached the “payback period”, or amount of time that the added energy savings makes up for the greater purchase price of a more efficient appliance.
Submit your spreadsheet file to your teacher electronically. If you are not able to do so, print a copy of it and staple it into your science notebook.
1. What was the type of appliance you studied? What was the purchase price and annual cost to operate of each?
2. How much did each of your two appliances cost overall after seven years?
3. What was the payback period of your appliance?
Lighting is a significant consumer of energy in a school system. An average school uses about 17 percent of the electricity (9% of the total energy) it consumes to light buildings and outside areas. Most schools are lit mainly with fluorescent lights.
A fluorescent lamp is a glass tube, whose inner surface has a powdered, phosphor coating. The tube is filled with argon gas and a small amount of mercury vapor. At the ends of the tubes are electrodes that emit electrons when heated by an electric current. When electrons strike the mercury vapor, the mercury atoms emit rays of ultraviolet (UV) light. When these invisible UV rays strike the phosphor coating, the phosphor atoms emit visible light. The conversion of one type of light into another is called fluorescence. Fluorescent lights have ballasts that regulate the electricity through the gas inside the bulb. Ballasts are electromagnets that produce a large voltage between the ends of the bulbs so the electricity will flow between them. There are two types of ballasts, magnetic and electronic. Magnetic ballasts produce a frequency of 60 Hertz (Hz), which means the light is flickering on and off 60 times a second. Electronic ballasts produce a frequency of at least 20,000 Hz. Fluorescent lights with electronic ballasts are more energy efficient than those with magnetic ballasts. Electronic ballasts use up to 30 percent less energy than magnetic ballasts. Electronic ballasts operate at a very high frequency that eliminates flickering and noise. Some electronic ballasts even allow you to operate the fluorescent lamp on a dimmer switch, which usually is not recommended with most fluorescents.
Although fluorescent tubes in ceiling fixtures are always more energy efficient than incandescents, there are new, even more efficient lamps that use better electrodes and coatings. They produce about the same amount of light with substantially lower wattage.
Most light fixtures in schools use four-foot long lamps, although three-foot lamps are common as well. Older fixtures often contain T12 lamps that are 1 1/2” in diameter and consume 34–40 watts. These lamps can be replaced with energy-saving T8 lamps that are 1” in diameter and typically consume 28–32 watts. Some newer systems are now using T5 lamps that are 5/8” in diameter and are even more efficient than the T8 lamps.
Incandescent lighting is only used in specific applications within schools, such as in stage lighting. Only 10 percent of the energy consumed by an incandescent bulb produces light; the rest is given off as heat. Legislation under the Energy Independence and Security Act of 2007 restricted how much energy light bulbs are allowed to use. Today, most general use incandescent bulbs have been replaced on store shelves by more efficient light emitting diode bulbs (LEDs). The transition from incandescent to LED lighting had two technologies that bridged from inefficient to very efficient lighting: Halogen light bulbs and compact fluorescent lights (CFLs).
Halogen light bulbs are sometimes referred to as energy-saving incandescent bulbs. They work much the same way as a traditional incandescent, but the filament is encapsulated and surrounded by
Mercury and inert gases
Phosphor coating
Base with bi-pin plug
In fluorescent tubes, a very small amount of mercury mixes with inert gases to conduct the electric current. This allows the phosphor coating on the glass tube to emit light.
In uorescent tubes, a very small amount of mercury mixes with inert gases to conduct the electric current. This allows the phosphor coating on the glass tube to emit light.
A T12 bulb consumes up to 40 watts of energy to produce a given amount of light. T8 and T5 bulbs use less energy to produce the same amount of light.
LEDs offer better light quality than incandescent bulbs and halogens, last 25 times as long, and use even less energy than CFLs. LEDs now have a wide array of uses because technology has improved and costs have decreased. CFLs are not as available in stores, and their use has drastically decreased, like the use of halogen bulbs. Some may still use these bulbs in their fixtures at home. They come in several shapes and sizes.
halogen gas, allowing it to last longer and be more efficient. Most halogen bulbs available on store shelves are specialty bulbs with pin-style bases. Halogen bulbs for general use are very difficult to find because most people have moved to entirely LED bulbs.
Fluorescent lights produce very little heat and are much more energy efficient than either type of incandescent bulb. CFLs use the same technology as overhead fluorescent lights, but they are designed to fit into lamps and other fixtures where incandescents are commonly used. All CFL bulbs have electronic ballasts.
Light emitting diode bulbs are even more efficient than CFL bulbs, last about 25 times longer than incandescent bulbs, and more than two and a half times longer than CFLs. One LED bulb has several tiny LEDs inside of it. LEDs contain semiconductors like solar panels and other diodes, however the difference is in the way the electrical energy is used by the LED. Three layers within the LED – p-type, n-type, and a depletion zone – combine to produce light. Basically, a minimum voltage is needed to energize electrons and they move from the n-layer to the p-layer. When the electrons move back to the n-layer again, they emit light that we see. Read more about this process in the graphic “How Light Emitting Diodes Work” below.
1. Diodes are made of semiconductors and conducting materials that need to be added to the semiconductor. In an LED the most common conductor added is aluminum-gallium-arsenide (AlGaAs). The AlGaAs is “doped” by adding small amounts of another material. One material will have more valence electrons than AlGaAs, and another doping material will have fewer electrons. The two doped materials are put together in a crystal. The material with more electrons is the “n-type” (n for negative) and the material with fewer electrons is the “p-type” (p for positive). When these materials are sandwiched together, the electrons move to balance themselves out. The area between the materials, called the p-n junction, is also called the “depletion zone.”
2. Connecting a power source to the diode, such as a battery, provides electric current that carries electrical energy. The electrons in the n-type are repelled by the electric current, and move through the depletion zone to the p-type. They are energized, and will want to return to their original, unenergized state in the n-type.
3. When the electrons move back through the depletion zone to the n-type, they release energy as light. This is the light that we see from the LED. This process continues over and over again–electrons absorbing energy, moving, then moving back and releasing the energy, until the power supply is disconnected or depleted.
4. Connecting the power supply in the wrong orientation does not allow the LED to work. Instead, it merely increases the size of the depletion zone. Therefore, it is important that LED’s be wired to their power supply in the correct orientation.
p-type layer
p-n type junction
n-type layer
CFL and LED bulbs were once significantly more expensive to purchase. As a result, some people were reluctant or unable to purchase them because they cost more. However, these bulbs are just as affordable as halogen bulbs are now, and incandescent bulbs are all but impossible to find. CFL bulbs have mostly been replaced by LEDs on shelves as well, due to their low cost and technology applications. Using an LED bulb where an incandescent bulb used to be can save up to $80 over the life of the bulb. They also reduce carbon dioxide emissions because so much less electricity is used to operate them. Efficient options are even available for stage lighting that are more affordable in the long run and that will not create the high temperatures for which stage lights have become infamous. There is almost no excuse to not use efficient lighting.
Lighting controls are devices that turn lights on and off or dim them. The simplest type is a standard snap switch. Other controls include photocells, timers, occupancy sensors, and dimmers. Snap switches, located in many convenient areas, make it easier for people in large, shared spaces to turn off lights in unused areas. Photocells turn lights on and off in response to natural light levels.
Photocells switch outdoor lights on at dusk and off at dawn, for example. Advanced designs gradually raise and lower fluorescent light levels with changing daylight levels.
Mechanical or electronic time clocks automatically turn indoor or outdoor lights on and off for security, safety, and tasks such as janitorial work. An occupancy sensor activates lights when a person is in the area and then turn off the lights after the person has left.
Dimmers reduce the wattage and output of incandescent and fluorescent lamps. Dimmers also significantly increase the service life of incandescent lamps; however, dimming incandescent lamps reduces their light output more than their wattage, making them less efficient as they are dimmed. Dimmers for fluorescents require special dimming ballasts, but do not reduce the efficiency of the lamps.
Even the best lighting system is not efficient if people do not use it wisely. In most schools, more light is used than needed and lights are often left on when no one is present. All lights that are not necessary for safety should be turned off when rooms are not in use. The same is true for outside lights. Using sunlight is a good idea whenever possible. Studies have shown that students learn better in natural light than in artificial light.
1. Insert the battery into the battery compartment in the back of the meter.
2. Slide the ON/OFF Switch to the ON position.
3. Slide the Range Switch to the B Position.
4. On the back of the meter, pull out the meter’s tilt stand and place the meter on a flat surface in the area you plan to measure.
5. Hold the Light Sensor so that the white lens faces the light source to be measured or place the Light Sensor on a flat surface facing the direction of the light source.
6. Read the measurement on the LCD Display.
7. If the reading is less than 200 fc, slide the Range Switch to the A position and measure again.
A lumen (lm) is a measure of the light output (or luminous flux) of a light source (bulb or tube). Light sources are labeled with output ratings in lumens. A T12 40-watt fluorescent tube light, for example, may have a rating of 3050 lumens.
A foot-candle (fc) is a measure of the quantity of light (illuminance) that actually reaches the work plane on which the light meter is placed. Foot-candles are work plane lumens per square foot. The light meter can measure the quantity of light from 0 to 1000 fc.
Another measure of light is its brightness or luminance. Brightness is a measure of the light that is reflected from a surface in a particular direction. Brightness is measured in footlamberts (fL).
PROCEDURE:
1.Place the incandescent bulb in one lamp and the compact fluorescent bulb in the other.
2.Place the lamps on a table about 40 cm apart facing a blank wall.
What is the difference in thermal energy output of different light bulbs?
3.Tape the thermometers to the wall so that the lamps shine directly on them, as shown in the diagram below.
What is the difference in light output of different light bulbs?
How do light bulbs compare in the amount of energy used?
Materials
2 Lamps
4.Record the thermometer readings in the chart below.
5.Turn on the lamps. Record the thermometer readings at 2-minute intervals for 10 minutes.
6.Calculate and record the change in temperature for each bulb. Compare. Δ Δ Δ Δ = change.
1 Light emitting diode bulb (LED) and its packaging
Incandescent bulb (provided by your teacher)
RESULTS:
2 Thermometers
Tape
Kill A Watt® meter
Light meter
Ruler or meter stick
Calculators
In your science notebook, write hypotheses stating which bulbs you think will be the hottest, brightest, and use the most energy.
Procedure
1. Copy the data table into your science notebook, if necessary, leaving plenty of room to record your observations.
CONCLUSION:
2. Place the incandescent bulb in one lamp and the LED bulb in the other lamp. If you do not have two lamps, conduct two trials, one for each bulb.
3. Place the lamps on a table about 20 cm away from a blank wall. The light should face the wall.
4. Tape the thermometers to the wall so the lamps shine directly on them, as shown in the diagram.
5. Record the thermometer readings every two minutes.
6. Calculate and record the change in temperature (ΔT) for each bulb.
7. Turn on the light meter and remove the cover from the sensor. Place the sensor on the wall in front of the thermometer and record the foot-candles for each bulb.
8. Turn off each lamp and unplug them. Plug one into the Kill A Watt® meter and plug the meter into the wall. Push the Watts button and turn on the lamp. Record the power used by the lamp. Repeat for the other lamp.
9. Answer the conclusion questions. PAGE 30 Learning & Conserving Student
Copy the following questions into your science notebook, leaving plenty of room for answers.
1. Compare the lumen rating on the light bulb packaging with the foot-candle measurements you took using the light meter. Is the brightest bulb the one with the higher lumen rating? Explain your answer using data from the investigation.
2. Each bulb uses a different method for emitting light. Based on your observations of temperature change, which bulb do you think is more efficient? Does this agree with the watts recorded on the Kill A Watt® meter? Justify your answer using data from the investigation.
The adults in your home probably remind you and any other kids you live with to please, turn off the lights when you leave the room. In fact, they might say it so much you can do a pretty good impression of them! That is because your family’s budget is probably limited, and the more money you have to spend on electricity, the less is available for something else.
Does anyone get after you at school to keep the lights off when they’re not needed? Probably not. You may have some conscientious individuals in school who are vigilant in keeping those lights off, but for the most part nobody really gives it much thought. However, lighting accounts for about 17 percent of electricity costs and almost 9 percent of total energy expenditures. That’s a significant amount of money! If an elementary school spends about $300,000 per year on utilities, lighting will be $27,000! And that does not include the cost of the lights themselves.
You cannot just turn the lights off everywhere. However, by taking advantage of any natural light coming through windows and keeping rooms lit properly, your school can save significant amounts of money. This activity will help you figure out how much your classroom’s lighting costs, how much it could cost if properly lit, and how much your school could save by switching to LEDs.
1. Look at the fixtures in the ceiling. What kind of lamp (“light bulb”) do they have? While most schools use fluorescent lighting, many schools have LED lighting. If you cannot easily tell, ask the person in charge of maintenance at your school.
2. If the lamps are fluorescent, determine the size, whether T5, T8, or T12. Use the information on page 25 to help you determine the size. Also, squint and peer through your eyelashes. If you see individual dots along the tube, they are LED replacement tubes.
How long are the lamps?
How many are in each fixture?
5. How many light fixtures are there in the classroom?
6. Use the table below to figure out how many watts each lamp uses.
7. Calculate how many watts, in total, are being used in the classroom when all the lights are on.
1. How many hours each school day are the lights on? Don’t forget about before school, after school, and any evening programs that happen regularly in your classroom.
2. Calculate the number of kilowatt-hours consumed each school day in the classroom when all the lights are on.
1. How well-lit are the work areas when all the lights are on? To determine this, you will need to use the light meter. Place the light meter sensor in the middle of a desk or work table. Pull out the stand from the back so the meter sits upright and stand back. If your meter does not have a stand in the back just prop it up on a couple of books so you can see it. Stay at least a meter away from the sensor and determine the number of foot-candles of light illuminating that surface. It is always a good idea to measure the illuminance at several points around the room and take an average. This will account for variations due to position under light fixtures or near a window.
2. Most classrooms require lighting to provide about 50 foot-candles. How does your classroom compare when all the lights are on?
Hold up one finger on one hand. Hold your other hand, palm facing the first, about a foot away from it. Imagine your finger is a candle, and your hand is a sheet of paper. If the candle were the only light source, the amount of light reaching the paper (your hand) from the candle (your finger) from a distance of one foot is one foot-candle. It is a very old unit of measurement but is still used throughout industry today.
3. Can you adjust the lights to alter the amount of light being produced, such as with multiple switches or by using dimmers? If so, how many fewer lamps are lit? Measure the illuminance at points around the room. If any work surface is below 30 foot-candles, it is not receiving enough light.
4. Does the reduction in light keep the room appropriately lit at 30-50 foot-candles? A variation of 5 foot-candles is acceptable.
5. How many kilowatt-hours are saved by having fewer lamps lit?
1. Ask your principal or custodian how much per kilowatt-hour your school pays for electricity. If they do not know or cannot tell you, use the 2022 national average of $0.124/kWh.
2. Calculate how much it costs to keep all the lights on for one school day.
3. Calculate how much it costs to keep all the lights on each school day for one school year.
4. How much money is saved by reducing the number of lamps that are lit to achieve 50 foot-candles?
The top two energy sources used to generate electricity in the United States are natural gas and coal, in order. When these energy sources are used, they are burned. Combustion of these fossil fuels releases carbon dioxide, a greenhouse gas. Climatologists have determined that fossil fuel combustion is driving climate change and its effects.
How much carbon dioxide is released into the atmosphere by using your classroom lights? You can look up your state’s average amount of carbon dioxide emitted by going to https://www.eia.gov/electricity/state/ and clicking on your state. Scroll down to find the number of pounds of CO2 per MWh, then divide by 1,000 to get the number of pounds CO2 / kWh. Or, you can use the national average of 0.855 pounds CO2/kWh. Keep in mind, though, that carbon dioxide emissions per kWh electricity varies widely depending on the energy sources used in each state.
1. Calculate the amount of carbon dioxide produced every school day when all the lights are on.
2. Calculate the amount of carbon dioxide produced by leaving all the lights on every school day for an entire school year.
3. Calculate the amount of carbon dioxide not produced in one day and also in one school year by reducing the number of lamps lit to achieve 50 foot-candles.
1. How many classrooms are in your school? Use a school map to get an accurate count.
2. Assume every classroom is lit the same as your own. Calculate the total number of kilowatt-hours, money, and carbon dioxide emitted by having all the lights in all the classrooms on all day long.
3. Send one person into the hallway to count the number of light fixtures in the hall. Use that number to estimate the total number of light fixtures in all hallways combined. Use the same process you just used for your classroom to determine how many watts are used by the lights in all the hallways combined.
4. Calculate the number of kilowatt-hours, money, and carbon dioxide emitted by lighting the hallways.
5. Add the total cost to light all classrooms and all hallways for one day, and for the whole school year.
6. What areas of the school are not included in the answer above? Estimate the additional electricity and cost needed to using these lights.
Understanding how much energy, and the impact it has on the environment and your school district’s budget, is one thing; using that information to improve your school is quite another. Complete the following items according to your teacher’s instructions.
1. Propose a plan for ensuring the school is properly lit.
2. Name three ways you can rally the entire school to reduce energy used for lighting.
3. If you have fluorescent lights, significantly less energy can be used to provide the same amount of light by switching to LED lighting. Re-do this exercise using data for a LED replacement tube in place of each fluorescent tube in your classroom. If your classroom has T5 or T12 tubes, the T8 replacement is close enough to be an acceptable substitute.
Below is a list of recommended illumination levels for school locations in foot-candles. These illumination levels align with the recommendations from the Illumination Engineering Society of North America.
Auditorium (Assembly)
Auditorium (Exhibitions) 15-25
Auditorium (Social Activities) 5-10
Cafeteria (Eating)
Data compiled from the Illuminating Engineering Society
When managing the systems of a school to minimize energy consumption, it’s important to maintain the health and comfort of the occupants. After all, the reason energy is being used in the first place is to provide a good learning environment. Human beings have specific requirements for temperature, relative humidity, and general air quality. They also have requirements for the quality and quantity of lighting. If light levels are too low, or of poor quality, they can cause eyestrain, headaches, and safety issues. Energy can be saved by turning off lights and lowering the heat in winter, but doing so thoughtlessly can cause unsafe or unhealthy conditions in the building. When the building is treated as a system, energy is saved while maintaining or improving the indoor environment. The school is not only a system in itself, but also a part of a global energy system that has finite energy resources.
While keeping students and staff comfortable and healthy in a school, the systems in the building need to work together. Up until this point we have discussed them as if they exist isolated one from another; however, as you are aware, they all are in the same building, working at the same time. Sometimes the function of one system will cause another system to work harder. For example, if the lighting system is producing a lot of heat, it will be working against the cooling system but assisting the heating system, according to the weather outdoors. The need for fresh air might be overridden by the need to heat the building on very cold days. Understanding how one system might affect another is key to keeping the building functioning efficiently and the occupants comfortable and healthy.
As few as twenty years ago, the systems of a school were monitored and maintained by walking from room to room to check temperatures, thermostat settings, the airflow of ventilation and heating systems, and the condition of the lighting system. Today, in new buildings and those that have been upgraded, an operations and maintenance employee can monitor all of those systems from a central computer, and often through an app on a tablet or smart phone. A century ago, the school’s boilers used coal as their fuel source, and someone had to stay at school overnight and on weekends and breaks to keep the fire in the boiler stoked and ready to heat the building. Today, a building manager can check the status of the school’s systems from the comfort of his or her home via the internet!
Managing your home is done by one of your parents or another adult in your family. That person controls the thermostat setting and reminds people to turn things off when not in use. Managing the energy use of a home is important, but not overly complicated. However, managing the energy systems in a school is an entirely different situation. People who oversee the maintenance and operation of commercial buildings like schools often have some level of post-secondary training, and often have an associate’s degree in building management. These programs train people in the operation and maintenance of HVAC, water, electrical, and lighting systems. Many community and junior colleges offer programs in building operations and maintenance, and some companies hire and train their own employees to manage their commercial buildings.
Operations engineers are often mechanical or electrical engineers who have taken specialized courses in the operation and maintenance of commercial buildings. In addition to the skills that a building maintenance supervisor would have, operations engineers also know how to upgrade systems and choose the equipment for new construction. Mechanical and electrical engineers might also work in the energy management field by working with construction managers to design systems from one manufacturer to meet the specifications in a building design. They choose the equipment, oversee its installation, and set up the control systems, testing them to make sure they are operational.
Certified Energy Managers are a specific group of people who have gone through training and passed a test certifying that they are qualified to conduct energy audits and manage the energy use of a building. Their responsibilities include maintaining all the energyusing systems in commercial and industrial buildings but can also include conducting formal energy audits for companies who do not employ energy managers full-time.
There are other careers in energy management that are important. Technical writers, accountants, and marketing and advertising people can apply their skills in energy management by working for a company that manages properties or seek employment in a large corporation that has its own energy management staff.
How does the placement of a vapor barrier affect indoor air quality and moisture in a building?
&Background
In most climates with cold winters and hot summers, vapor barriers are included in wall construction. A vapor barrier is a sealed material that is impermeable to water vapor. Most vapor barriers are made of polyethylene, but spray-foam insulation can also serve a dual role as a vapor barrier. The purpose of a vapor barrier is to prevent moisture buildup in the walls of the building, but its proper placement is vital to functioning correctly.
Large fish tank or transparent, watertight container with top
2 Zip-closed quart-sized plastic bags
2 Zip-close sandwich-sized bags
Cotton quilt batting
2 Thermometers
Hypothesis
Ice
Water
2 Handwarmers
Hygrometer
String, tape, meter stick (optional)
Write a hypothesis explaining how you think a plastic vapor barrier should be placed, inside or outside of the insulation, to minimize moisture buildup inside the wall.
Procedure
1. Your teacher will assemble the apparatus for you, because the amount of time needed for meaningful data is more than one class period. Inside the tank two bags are suspended. One has the insulation inside, which simulates an external placement of the vapor barrier; the other bag has the insulation outside, which simulates an internal placement of the vapor barrier. In both cases, the inner wall surface and outer wall cladding have been eliminated to simplify the activity.
2. One of two scenarios will be set up. Either the tank will have very warm water, with baggies of ice inside the insulated plastic bags, or the tank will have very cold ice water, with handwarmers inside the insulated plastic bags. Your teacher may decide to do one scenario on one day, and the other on another day.
3. Observe both bags. Write down the presence of any moisture you can see or feel, the temperature in the tank, the humidity in the tank, and the temperature inside both bags.
4. Add your observations to the collaborative document you are building with other classes. Be sure to include the observations from other class periods with your own in your notebook.
Data
In your science notebook, copy the data collected by earlier class periods. Then record the time of day and your measurements and observations. Add this data to the collaborative document your teacher is using for all class periods.
Conclusions
1. Based on the evidence you and your classmates collected during this activity, explain where a vapor barrier is installed, and why.
2. How do vapor barriers help contribute to better indoor air quality? Explain your answer.
Eisenhower High School is a fictitious school built in 1955 in Anytown, Nebraska. It is a two-story building with the original boilers that were converted to burn natural gas in 1975. The construction is brick exterior and concrete block on the interior of the building, and the single-pane windows have aluminum frames. Each classroom has an original thermostat that controls a pneumatic (compressed air switching) system that opens and closes the valve to heat the room. In 2005, most of the lights were replaced with T8 fluorescent fixtures with electronic ballasts, a project that paid for itself in energy savings in the first year. However, in some areas, the fixtures were not replaced, and the old magnetic fixtures remain. These areas include the ceiling above the boilers, two storage rooms, and the area around the loading dock. Furthermore, the lights in the two gyms kept their 400 W metal halide lights with magnetic ballasts. In the boiler room, an old trash incinerator still sits, with the flue pipe still leading out of the building. On very windy days, pieces of the incinerator flue rattle around. The school is not air conditioned.
Dr. Jacobs, the superintendent of Anytown Community Schools, would like to introduce a more balanced calendar to his district because it improves the amount of knowledge students retain over the summer from one year to the next. School would begin in late July, and longer breaks in the fall, at the holidays, and in the spring would make up the difference. School would dismiss for the year at the end of May, giving kids about seven weeks off each summer. However, Dr. Jacobs knows he will not get support for this schedule unless the old high school building is air conditioned.
Dr. Jacobs has a proposal he will present at the next school board meeting that requests funds for a new heating, cooling, and air conditioning system at Eisenhower High School. The cost to replace all the old, outdated equipment with new, efficient, computer-controlled machinery is one million dollars. Dr. Jacobs knows this is a lot for the district, but is going to request two million dollars, so that more upgrades and improvements can be made. Dr. Jacobs will be relying on the upgrades to provide enough energy savings to pay the school back within six years.
Which systems would you upgrade to save energy? Which would you leave as they are?
1. Re-read the description of Eisenhower High, taking note of the HVAC, lighting, electrical, and building envelope items that could use replacing or upgrading.
2. List your items from number 1 in order of most important to least important. List at least two reasons after each describing why they are important to change.
3. Use the list of potential upgrades and improvements on the next page to determine how you would spend the additional million dollars that Dr. Jacobs is requesting.
4. Use a weighted average and the payback period for each item on the list to calculate the average payback period for your entire list of upgrades. The weighted average must not exceed six years. The weight for the average will be the cost of the system. For example, an upgrade costing $100,000 will carry ten times the weight as a system costing $10,000.
5. When you have completed your upgrade selection from the list, write a short proposal describing each upgrade and justifying why you believe it is important to implement. Include a table that lists the cost and payback period of each item on your list. At the bottom, provide a total for the project, not to exceed $1 million, and provide the weighted average you calculated for the average payback period of the entire list. If your teacher shows you how to use a calculator to determine the actual payback period of your list, use that method instead of the weighted average method.
6. Prepare a presentation that you would use if you were going before the Anytown Community Schools board to convince them to fund your project.
Additional HVAC Options
Replace existing HVAC system with geothermal system
Remove incinerator and close hole for flue
Lighting
Replace T8 fluorescent bulbs with T5 bulbs
Replace T8 fluorescent bulbs with LED fixtures
Replace metal halide lights in two gyms with LED
Replace remaining magnetic fixtures with electronic fixtures
Install motion-activated light switches
Building Envelope
6 months
Replace all windows with insulated double-pane glass $750,000 7 years
Replace all exit doors with new, insulated doors $300,000 7 years
Add insulation to attic space above upper floor classrooms
Inject foam insulation in wall cavities of all exterior walls $250,000 3 years
* A geothermal system requires significant construction and equipment costs beyond a standard HVAC system. It is very expensive, but eliminates natural gas expenditures. Therefore, if you choose this upgrade for Eisenhower High School, your justification must include a significant discussion of the benefits of such an expensive installation. Dr. Jacobs would have to request a significant amount of money from the school board to install a geothermal system, so he needs to have a sound argument for it. It would be the only upgrade he would request at this time, because it includes a cooling system for summer-weather occupancy.
Everything you have learned has been leading up to Lesson 6 and describing energy audits. It’s time to put it all together, and audit the energy use in your building. An energy audit done by a professional energy manager is a formal, detailed walk-through of the entire building, room-by-room, measuring everything from temperature to relative humidity to airflow, and making detailed notes about what is running, plugged in, or drawing a phantom load. A professional energy audit also includes a review of the school’s utility bills and includes a list of recommendations to reduce energy consumption much as the list you assembled in Lesson 5.
The most important auditing tools are always with you – your ears and eyes. They will tell you more about how a building is using energy than any other tool. With them you can see and hear things that are running and can ask yourself why. You can see gaps around doors and windows, or hear the whistle of air being forced through a crack on a windy day. You can see lights left on in an empty room. You can see condensation building up, or in bad situations, mold or mildew that you can also smell. Do not discount the importance of good observation skills when you audit your work area.
The other tools you will use are a thermometer, hygrometer, light meter, and Kill A Watt® meter. These will give you objective measurements to use in evaluating the energy use in a room or building. You have already worked with the light meter and Kill A Watt® meter in previous lessons. Review the instructions for operating the other tools found on the next few pages, and if you have any questions be sure to ask your teacher for clarification.
You will work in groups of 2-4 students and evaluate a work area assigned to you. This may be a single room, or it may be several rooms or spaces such as hallways and stairways. When you enter a work area, first ask the teacher for permission to proceed. Remember to show courtesy and respect by working quickly and quietly and returning things where you found them.
The second side of the auditing form has an area for notes. This is where you will record unusual observations, such as a gap around a window, an unusually noisy HVAC unit, etc. If there are things plugged in and running, but you are unable to use the Kill A Watt® meter to measure them, make a note of it.
If you see something potentially dangerous, don’t wait to tell someone. Inform that teacher, your teacher, the principal, or a maintenance staff member about the problem right away.
If you spend all this time collecting data but then do nothing with it, you have just wasted a lot of time. The point of collecting data on an audit is to evaluate it and look for ways your school as a community can improve. As a class, look over all the data collected for each room and see if some trends emerge. Are a cluster of classrooms too hot or too cold? Is an older section of a building more air-tight than a newer section? These are the kinds of things you are looking for. You may also see something that stands out in stark contrast to the rest of the building. If most rooms are a comfortable 72 degrees, but one room is a chilly 66 degrees, something is not right with the HVAC system in that one room.
After you have found trends across the entire building, or anomalies within your data, the next step is to write recommendations. These will probably start as bullet point-type statements, but they should be formulated into complete sentences or paragraphs. State what you found, what it should be, and make a recommendation to amend it. Professional energy audits include recommendations for upgrades and improvements, their cost, and the amount of money saved through reduced energy use. You will not go this far, but do not hesitate to suggest efficiency upgrades where they would obviously make a significant impact on the energy used in school. For example, replacing metal halide lights with LED in the gym has a moderate up-front cost that will yield significant energy savings right away. Whatever recommendations you make, do so with respect, citing the data you collected on your audit. Do not be upset if the authorities in your school district do not take all of your recommendations immediately. If you get them thinking about what they can do to improve, your audit has been successful.
A digital thermometer measures the temperature of a substance and displays the temperature reading on its face. It has a battery for power. Sometimes they are waterproof for measuring the temperature of a liquid.
This digital thermometer can measure the temperature in Fahrenheit or Celsius. It shows the temperature range of the thermometer. It can read temperatures from -40° to 392°F and -40° to 200°C.
It has three buttons. The button on the bottom left is the ON/ OFF switch. If the thermometer is not used for a few minutes, it turns itself off.
The C/F button on the bottom right switches from the Celsius scale to the Fahrenheit scale. The face of the thermometer will show a C or an F to indicate which scale is being used.
The mode button on the top holds the temperature reading when it is pushed. If you need the exact temperature of a liquid, you push the hold button while the thermometer is in the liquid, then remove the thermometer to read it. This button will also allow you to view the maximum and minimum temperatures measured when pushed two or three times.
The metal stem of the thermometer can measure the temperature of the air or the temperature of a liquid. The stem should be placed about halfway into a liquid to measure the temperature.
Scientists measure the amount of water vapor in the air in terms of relative humidity—the amount of water vapor in the air relative to (compared to) the maximum amount it can hold at that temperature. Relative humidity changes as air temperature changes. The warmer the air is, the more water vapor it can hold.
Air acts like a sponge and absorbs water through the process of evaporation. Warm air is less dense and the molecules are further apart, allowing more moisture between them. Cooler air causes the air molecules to draw closer together, limiting the amount of water the air can hold.
It is important to control humidity in occupied spaces. Humidity levels that are too high can contribute to the growth and spread of unhealthy biological pollutants. This can lead to a variety of health effects, from common allergic reactions to asthma attacks and other health problems. Humidity levels that are too low can contribute to irritated mucous membranes, dry eyes, and sinus discomfort.
This digital humidity/temperature pen measures relative humidity and temperature and displays the readings on its face. It has a battery for power. It can display the temperature in Fahrenheit or Celsius. The reading shown on the right is 68.5°F. Devices that measure humidity are also called hygrometers. The hygrometer displays relative humidity in terms of percentage. The hygrometer shown reads 35%. This means that the air contains 35 percent of the water vapor it can hold at the given air temperature. When the air contains a lot of water vapor, the weather is described as humid. If the air cannot carry any more water vapor, the humidity is 100 percent. At this point, the water vapor condenses into liquid water. Maintaining relative humidity between 40 and 60 percent helps control mold. Maintaining relative humidity levels within recommended ranges is a way of ensuring that a building’s occupants are both comfortable and healthy. High humidity is uncomfortable for many people. It is difficult for the body to cool down in high humidity because sweat cannot evaporate into the air.
Press the ON/OFF key to turn the power on or off.
°F/°C
Press the °F/°C key to select the temperature unit you want to use, Fahrenheit or Celsius.
Press the MAX/MIN key once to display the stored maximum readings for temperature and humidity.
An up arrow will appear on the left side of the display to indicate the unit is in the maximum recording mode.
Press the MAX/MIN key a second time to display the stored minimum readings for temperature and humidity. A down arrow will appear on the left side of the display to indicate the unit is in the minimum recording mode.
Press the MAX/MIN key a third time to return to normal operation.
If an up or down arrow is displayed, press the CLEAR key until - - - appears on the display. The memory is cleared. New maximum or minimum values will be recorded within 3 seconds.
1. When was the school built?
2. What changes have been made since the school was built? When were they made?
3. What things use energy on the school grounds? Lighted fields? Outdoor lighting?
4. What fuels are used in the school? For heating, cooling, water heating, lighting, other?
5. How much does the school pay each year for energy? How much for electricity? How much for heat?
6. Are there other energy costs that the school pays for, like buses?
7. How many hours is the school in use each week?
8. Do other groups that use the school pay for the energy they use?
9. Who is in charge of controlling energy use in the school?
10. Who is in charge of maintaining energy-use equipment? Is there a maintenance schedule for all energy-using systems?
1. What is the building made of? Is it in good condition?
2. In which direction does the building face?
3. How many windows are on each side of the building? Are any windows cracked or broken?
4. Are the windows single or double-paned? Can they be opened? Do the windows have adjustable blinds?
5. How many outside doors are there? Are they insulated? Are there windows in the doors? Are any cracked or broken?
6. Does the building have insulation in the walls and ceiling?
7. Are inside stairwells open or enclosed?
8. Do windows and doors seal tightly, or do they leak air?
9. Are trees placed around the building to provide shade in warm months?
10. Are there awnings or overhangs over the windows to shade windows from the overhead direct sun in warm weather, yet allow the slanted rays in winter to enter?
1. What kind of heating system is used in the school? What fuel does it use?
2. How old is the heating system?
3. Does the heating system have a programmable thermostat to control temperature? What are the settings?
4. What kind of cooling system is used in the school? What fuel does it use?
5. How old is the cooling system?
6. Does the cooling system have a programmable thermostat to control temperature? What are the settings?
7. Is there an air exchange system to provide fresh air when the heating and cooling systems are not operating?
8. Are the boilers, pipes, and ducts sealed and insulated?
9. Are the heating and cooling systems maintained on a regular basis?
10. Does your school make use of passive solar heating?
1. What fuel is used to heat water in the school?
2. Is there more than one water heater? How many?
3. How old are they?
4. Do the water heaters have timers?
5. At what temperatures are the water heaters set?
6. Are the water heaters and water pipes insulated?
7. Are there leaks in the hot water system?
8. Are flow restrictions used?
1. What kind of lighting is used in the school? Outside the school? Exit lights?
2. Can the lights be controlled with dimmer switches? In which areas or rooms?
3. Does the school make use of skylights and natural lighting?
4. Are there timers for the outside lights so they go off automatically?
5. Are there automatic timers for any of the indoor lights?
Date: ___________________ Time: ______________________
Outdoor Temperature: ______________
Outdoor Relative Humidity: _______ Weather: _______________________________________
Is the heating system in use? yes no
Is the cooling system in use? yes no
Optional: Temperature of air exiting vent: __________________________________________________
Work Area Description: _________________________________________________________________
Who is in the room? _______________________________________________________________
Can you feel any air currents in the room? If so, describe where: _____________________________________________________________
Are there any vents that can be opened to the outdoors? yes no
If yes, are they currently open? yes no
Temperature of vent ______________
Number of Outside Windows: __________ Open __________ Closed
Results of Tissue Paper Test: __________________________________________________________________________________________
Indoor Temperature of Room: __________
Relative Humidity: _________
Thermostat setting: ________
Landscaping and surfaces outside of room ______________________________________________________________________________
Turn on the water, and start timing until hot water is delivered.
Hot Water Temperature: ___________
Length of Time for Hot Water: ___________
Are there any dripping faucets? _____________________
Lighting Types Present: _______________
Light Meter Reading: ______________
Can the lights be dimmed? yes no
Can some lights be turned on, and some left off? yes no
Were the lights on when you entered the room? yes no
Were the blinds closed when you entered the room? All Some None N/A
Are doors leading outside tightly closed? yes no N/A
Are doors leading inside tightly closed? yes no
Fill in the table below for every electrical device (plug-in) in the room:
Other notes and comments:
Engineers study building systems, then report the results of their investigations and recommend ways to use less energy. Use this form to organize the data you gathered on the Student Audit Recording Forms to prepare a presentation on your findings and recommendations. In your presentation, include an introduction and conclusion that explain your findings and recommendations.
What We Learned:
Our Recommendations To Save Energy:
What We Learned:
Our Recommendations To Save Energy:
What We Learned:
Our Recommendations To Save Energy:
What We Learned:
Our Recommendations To Save Energy:
What We Learned:
Our Recommendations To Save Energy:
What We Learned:
Our Recommendations To Save Energy:
baseload power the minimum amount of electricity a utility must provide to customers round–the–clock, using the least expensive sources available
blades the part of a wind turbine that is pushed by moving air
building envelope everything that separates the interior of a building from the outdoors
chemical energy energy stored in the chemical bonds of a substance and released during a chemical reaction such as burning wood, coal, or oil
commercial the part of the economy having to do with buying and selling of goods and services
compact fluorescent a light bulb consisting of a gas-filled tube and a magnetic or electronic ballast; electricity flows from the ballast through the gas, causing it to give off ultraviolet light; the ultraviolet light excites a white phosphor coating on the inside of the tube, which emits visible light; compact fluorescent light bulbs use less energy and produce less heat than a comparable incandescent bulb
conduction the transfer of thermal energy from one particle to another through vibrations in a solid
convection the transfer of thermal energy from one particle to another by movement in a fluid
efficiency the ratio of the energy delivered by a machine to the energy supplied for its operation; often refers to reducing energy consumption by using technologically advanced equipment without affecting the service provided
elastic energy energy stored through the application of a force to stretch or compress an item
electric power the rate at which electronical energy is transferred; measured in Watts; the part of the economy related to electricity generation
electrical energy the energy associated with electric charges and their movements
energy the ability to do work or make a change
energy conservation saving energy through behavior changes and installing energy efficient devices
energy density amount of energy that can be stored in a given mass
ENERGY STAR® a Federal G overnment program that recognizes the most energy efficient machines with a logo EnergyGuide label the label on an appliance that shows how much energy the appliance uses in comparison to similar appliances
hub the part of a wind turbine that connects the blades to the generator gravitational potential energy energy of position or place
incandescent a type of electric light in which light is produced by a filament heated by electric current; the most common example is the type you find in table and floor lamps
industrial the part of the economy having to do with the production of goods
insulation a material used to separate surfaces to prevent the transfer of electricity, heat, or sound
kinetic energy the energy of motion
Law of Conservation of Energy the law governing energy transformations and thermodynamics; energy may not be created or destroyed, it simply changes form, and thus the sum of all energies in the system remains constant
light emitting diode energy saving bulb that generates light through the use of a semiconductor lumen a measure of the amount of light produced by a bulb
motion energy the displacement of objects and substances from one place to another nonrenewable fuels that cannot be renewed or made again in a short period of time, such as petroleum, natural gas, coal, propane, and uranium nuclear energy energy stored in the nucleus of an atom that is released by the joining or splitting of the nuclei peak demand a period when many customers want electricity at the same time; may require additional generation by utility
phantom load a device that draws electric power when not in use or powered on potential energy the energy stored within a body power plants facility where power is generated power pool a group of utilities able to share power as needed radiant energy any form of energy radiating from a source in electromagnetic waves radiation high-speed transmission of energy in particles or waves reactor part of a nuclear power station where fission occurs relative humidity ratio of moisture in the air compared to what the air can hold at the temperature reliability the ability of a power provider to provide electricity to customers without disruption renewable fuels that can be made or used again in a short period of time, such as solar, wind, biomass, geothermal, and hydropower
residential the part of the economy having to do with homes and neighborhoods R-value a measure of a material’s resistance to heat flow in units of fahrenheit degrees x hours x square feet per Btu; the higher the R-value of a material, the greater its insulating capability sound energy energy that travels in longitudinal waves specific heat capacity the quantity of heat required to raise the temperature of one gram of a substance by one degree Celsius
steam generator a generator in which the turbines are powered by steam system substances or items directly involved that work together thermal energy the total potential and kinetic energy associated with the random motions of the atoms and molecules of a material; the more the molecules move and vibrate the more energy they possess transformations a change from one form of energy to another transportation the part of the economy dealing with the movement of people, goods, and services
AES
AES Clean Energy Development
American Electric Power Foundation
Appalachian Voices
Arizona Sustainability Alliance
Atlantic City Electric
Baltimore Gas & Electric
Berkshire Gas - Avangrid
BP America Inc.
Bob Moran Charitable Giving Fund
Cape Light Compact–Massachusetts
Celanese Foundation
Central Alabama Electric Cooperative
CITGO
The City of Cuyahoga Falls
Clean Virginia CLEAResult
ComEd
Con uence
ConocoPhillips
Constellation
Delmarva Power and Light
Department of Education and Early Childhood
Development - Government of New Brunswick, Canada
Dominion Energy, Inc.
Dominion Energy Charitable Foundation
DonorsChoose
East Baton Rouge Parish Schools
East Kentucky Power Cooperative
EcoCentricNow
EDP Renewables
EduCon Educational Consulting
Enel Green Power North America
ENGIE
Entergy
Equinix
Eversource
Exelon
Exelon Foundation
Foundation for Environmental Education
FPL
Generac
Georgia Power
Gerald Harrington, Geologist
Government of Thailand–Energy Ministry
Greater New Orleans STEM
GREEN Charter Schools
Green Power EMC
Guilford County Schools–North Carolina
Honeywell
Iowa Governor’s STEM Advisory Council -
Scale Up
Iowa Lakes Community College
Iowa State University
Illinois Clean Energy Community Foundation
Illinois International Brotherhood of Electrical
Workers Renewable Energy Fund
Independent Petroleum Association of New Mexico
Intuit
Iron Mountain Data Centers
Kansas Corporation Energy Commission
Kansas Energy Program – K-State Engineering
Extension
Katy Independent School District
Kentucky Environmental Education Council
Kentucky O ce of Energy Policy
Kentucky Power–An AEP Company
Liberty Utilities
Llano Land and Exploration
Louisiana State Energy O ce
Louisiana State University – Agricultural Center
LUMA
Marshall University
Mercedes Benz USA
Minneapolis Public Schools
Mississippi Development Authority–Energy Division
Motus Experiential
National Fuel
National Grid
National Hydropower Association
National Ocean Industries Association
National Renewable Energy Laboratory
NC Green Power
Nebraskans for Solar
NextEra Energy Resources
Nicor Gas
NCi – Northeast Construction
North Shore Gas
O shore Technology Conference
Ohio Energy Project
Oklahoma Gas and Electric Energy Corporation
Omaha Public Power District
Ormat
Paci c Gas and Electric Company
PECO
Peoples Gas
Pepco
Performance Services, Inc.
Permian Basin Petroleum Museum
Phillips 66
PowerSouth Energy Cooperative
PPG
Prince George’s County O ce of Human
Resource Management (MD)
Prince George’s County O ce of Sustainable Energy (MD)
Providence Public Schools
Public Service of Oklahoma - AEP
Quarto Publishing Group
The Rapha Foundation
Renewable Energy Alaska Project
Rhoades Energy
Rhode Island O ce of Energy Resources
Salal Foundation/Salal Credit Union
Salt River Project
Salt River Rural Electric Cooperative
Schneider Electric
C.T. Seaver Trust
Secure Solar Futures, LLC
Shell USA, Inc.
SMUD
Society of Petroleum Engineers
South Carolina Energy O ce
Southern Company Gas
Snohomish County PUD
SunTribe Solar
TXU Energy
United Way of Greater Philadelphia and Southern New Jersey
Unitil
University of Iowa
University of Louisville
University of North Carolina
University of Northern Iowa
University of Rhode Island
U.S. Department of Energy
U.S. Department of Energy–O ce of Energy
E ciency and Renewable Energy
U.S. Department of Energy - Solar Decathlon
U.S. Department of Energy - Water Power
Technologies O ce
U.S. Department of Energy–Wind for Schools
U.S. Energy Information Administration
United States Virgin Islands Energy O ce
Virginia Cooperative Extension
Vistra Energy
We Care Solar
West Virginia O ce of Energy
West Warwick Public Schools