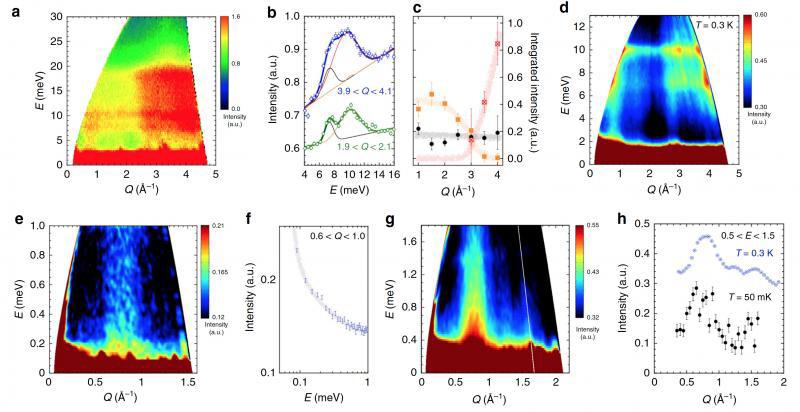
18 minute read
Breaking News
Unusual State of Matter in New Material Holds Promise for Transformative Quantum Technologies
ANSTO has provided supporting experimental evidence of a highly unusual quantum state, a quantum spin liquid (QSL), in a two-dimensional material as reported by an international collaboration led by Tokyo University of Science. Materials with quantum spin liquid states could be used in the development of spintronic devices, quantum computers and other transformative quantum technologies. In a quantum spin liquid, an elusive state of matter that is the subject of much investigation worldwide, the electron spins, in a magnetic material, never align, but continue to fluctuate even at the lowest temperatures. This phenomena has been described as a fluctuating liquid-like state. Low energy spin excitations, evidence of a QSL, were detected at a range of very low temperatures during experiments in Japan. Importantly, the expected spin ordering or freezing was not detected in the inelastic neutron scattering spectra. Scientists Dr Richard Mole and Dr Dehong Yu used inelastic neutron scattering, a spectroscopic technique to detect the vibrations of atoms. “When we analysed the Pelican data at 25K, 15K and 48mK, we could see the same spin excitations and they persisted to the lowest temperature, which is only slightly above absolute zero,” Dr Mole said. In order to create these low-temperature environments, a special type of cryostat, called a dilution insert, was optimised on the Pelican instrument. “A quantum spin liquid state possesses extensive many-body entanglements, a kind of correlation, or a link between all the spins. As an analogy, think of a bucket of water with several fishing floats on the surface. If you disturb one float, all the floats will also be disturbed,” Dr Yu explained.
Inelastic neutron scattering data of KCu6AlBiO4(SO4)5Cl. Pelican measurements plotted at h. Image courtesy of ANSTO.
Spin-Gapless Semiconductors Review: More Candidates for Next-Generation Low Energy and High Efficient Spintronics

The University of Wollongong recently published an extensive review of spin-gapless semiconductors (SGSs). SGSs are a new class of zero gap materials that have fully spin polarised electrons and holes. The study enhances the search for materials that would allow for ultra-fast, ultra-low energy spintronic electronics, with no wasted dissipation of energy from electrical conduction. The defining property of SGS materials relates to their ‘bandgap’ – the gap between the material’s valence and conduction bands – which defines their electronic properties. In general, one spin channel is semiconducting with a finite band gap, while the other spin channel has a closed (zero) band gap. The band structures of the SGSs can have two types of energymomentum dispersions: Dirac (linear) dispersion or parabolic dispersion. The new review investigates both Dirac and the three sub-types of parabolic SGSs in different material systems. For Dirac type SGS, the electron mobility is two to four orders of magnitude higher than in classical semiconductors. Very little energy is needed to excite electrons in an SGS, charge concentrations are very easily ‘tuneable’. The Dirac type spin gapless semiconductors exhibit fully spin polarised Dirac cones, and offer a platform for spintronics and low-energy consumption electronics through dissipation-less edge states, driven by the quantum anomalous Hall effect. In a spin-gapless semiconductor, conduction and valence band edges touch in one spin channel, and no threshold energy is required to move electrons from occupied (valence) states to empty (conduction) states. This property gives these materials unique properties, as their band structures are extremely sensitive to external influences such as pressure or magnetic field. Most SGS materials are all ferromagnetic materials with high Curie temperatures.
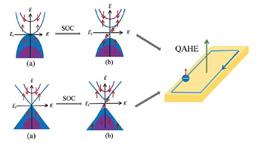
LEFT: The band structures of parabolic and Dirac type SGS materials with spin-orbital coupling, which leads to the quantum anomalous Hall effect. ABOVE: FLEET Chief Investigator Professor Xiaolin Wang
BREAKING NEWS
Through The Nanoscale Looking Glass: Fleet Researchers Determine Boson Peak Frequency in Ultra-Thin Alumina
Glasses, which are disordered materials with no long-range chemical order, have some mysterious properties that have remained enigmatic for several decades. Recent collaborative work between the Australian Research Council (ARC) Centre of Excellence in Future Low-Energy Electronics Technologies (FLEET) partners, the University of Wollongong, RMIT University and ANSTO has revealed the frequency of the boson-peak in the density of states of ultrathin alumina with thicknesses of two nanometres. However, many of the fundamental properties of alumina remain unknown owing to the fact it is thermodynamically unstable at the macroscale. The research team overcame this issue by focusing on nanoscale glasses, in the context of core-shell particles of an aluminium sphere wrapped in a thin skin of its native alumina oxide. Armed with the novel (and slightly explosive) samples, researchers deployed neutron spectroscopy, at ANSTO, to measure the lattice vibrations in the core shell particles. Using the small particles to enhance the surface contrast, the group revealed a THz-frequency feature for the feature, for the boson peak, that aligns with theoretical calculations. “I was excited to see the match between the molecular dynamics performed by the Cole group and our neutron experiment,” said lead author Dr David Cortie. “Our ability to predict the vibrational and electronic properties of ultra-thin materials and heterointerfaces is getting better year-on-year.” The new measurements are useful in identifying methods to control heat transfer through ultra-thin alumina. In addition to the FLEET experimentalists at the University of Wollongong and ANSTO, and theorists from RMIT, the study also represents a research partnership between two ARC Centres of Excellence in molecular dynamics modelling and exciton science.
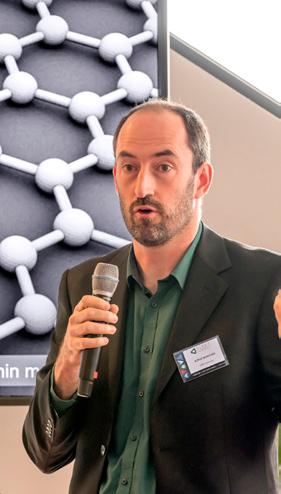
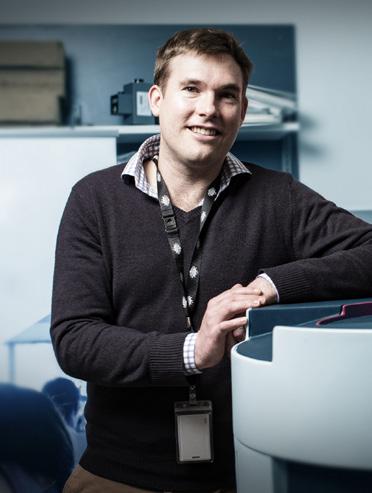
TOP LEFT: Co-author (experimental): FLEET AI Dr David Cortie (University of Wollongong). TOP RIGHT: Co-author (theoretical) FLEET CI Prof Jared Cole (RMIT). BOTTOM (L to R): Modelling nanoscale glass: Molecular dynamics model of 3-nmthick glass interface on a crystalline substrate. Experimental verification: neutron spectroscopy study of aluminium particle spheres wrapped in a thin alumina skin. Theoretical modelling and experiment verification combined: molecular dynamics model overlaid on TEM image of interface between crystalline core and oxide shell. (Image: Martin Cyster)
Scientists Discover How to Smash Quantum Limits
A team of Australian researchers has made a surprising discovery, working out how to break quantum limits. Scientists from The University of Western Australia’s (UWA) Centre of Excellence for Gravitational Wave Discovery (OzGrav) surpassed the limit in their quest to build better gravitational wave detectors using squeezed light technology on 40kg test masses in LIGO detectors. A quantum limit comes about from the interaction between light and a test mass, and breaking this limit, just like breaking the sound barrier, once seemed impossible. Gravitational wave detectors are the most precise measurement devices ever built, and the result shows they are now poised to see, and exploit, the effects of quantum physics, which governs the smallest objects in the universe, on human-sized objects such as their 40kg test masses. UWA physicist Dr Carl Blair, who was part of the team to make the discovery said discovering how to break quantum limits was significant for physics and science. “It’s amazing to think that sitting in the control room at LIGO, by manipulating some controls on a computer, you can manipulate the quantum noise of a 40kg mirror,” Dr Blair said. “We were able to break the limit doing something very mysterious – squeezing the quantum vacuum,” he continued. “In breaking this limit, we are now entering a world where quantum limits on measurements can be routinely surpassed.” Scientist Dr Xu Chen, also from UWA, said OzGrav and their collaborators were able to smash through the quantum noise barrier of gravitational-wave detectors. “At UWA, we aim to improve the sensitivity further with a white-light cavity. This works best at higher frequencies where we can see more binary neutron stars colliding,” Dr Chen said.
BREAKING NEWS
Supercharged Bandages to Revolutionise Chronic Wound Treatment
World-first plasma-coated bandages, with the power to attack infection and inflammation, could revolutionise the treatment of chronic wounds such as pressure, diabetic or vascular ulcers that do not heal on their own. Developed by the University of South Australia (UniSA), the novel coating comprises a special antioxidant that can be applied to any wound dressing to simultaneously reduce inflammation and break up infection to aid in wound repair. In Australia, nearly half a million people suffer from chronic wounds, costing the health system an estimated $3 billion each year. More than 5.7 million people suffer from chronic wounds in the United States. In the United Kingdom, more than two million people are currently living with chronic wounds at a cost of £5 billion per year. With growing rates of global obesity, diabetes and an ageing population, chronic wounds are increasingly affecting large proportions of the general population, yet few treatments have historically shown such positive results. Dr Thomas Michl, who leads the project at UniSA, said that upgrading current dressings with this state-of-the-art coating will promote effective healing on chronic wounds and reduce patient suffering. “Proper care for chronic wounds requires frequent changes of wound dressings, but currently, these wound dressings are passive actors in wound management,” Dr Michl said. “Our novel coatings change this, turning any wound dressing into an active participant in the healing process – not only covering and protecting the wound, but also knocking down excessive inflammation and infection.” The technology is highly scalable and sustainable, making it a viable option for broad application worldwide.
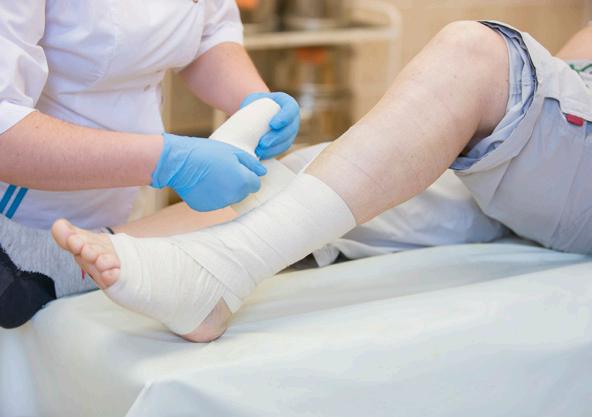
World-first plasma-coated bandages, with the power to attack infection and inflammation, could revolutionise the treatment of chronic wounds.
Publication: The Multi-Faceted MechanoBactericidal Mechanism of Nanostructured Surfaces
A publication from Surface Engineering for Advanced Materials (SEAM) on the bactericidal action delivered by rigid nanopillar arrays stems from the mechanical rupture of the bacterial cell membrane. Image courtesy of SEAM.
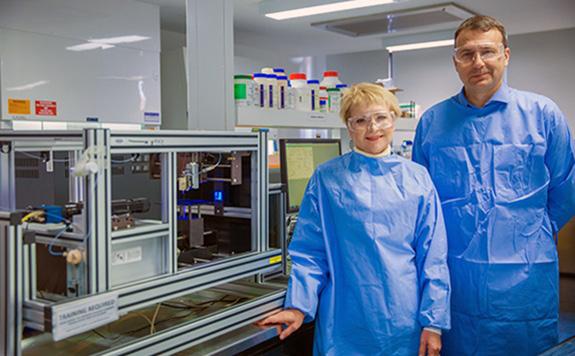
A publication from Surface Engineering for Advanced Materials (SEAM) on the bactericidal action delivered by rigid nanopillar arrays, stems from the mechanical rupture of the bacterial cell membrane; however, the precise mechanism may be unique to the individual nanopillar geometries. In this work, researchers presented a new model of mechanobactericidal action, which is specific to the elasticity of highly ordered arrays of symmetrical silicon nanopillars. Specific control over the height and spacing, simultaneously, was achieved using carefully controlled deep UV immersion lithography and plasma etching. At a certain aspect ratio, nanopillar elasticity contributes to the onset of pillar-to-pillar interactions upon bacterial adsorption to the surface. Previous mechano-bactericidal models have described the nanopillar surface as a ‘bed of nails’, which assumes complete rigidity in the surface structures. However, this work confirms the elastic pillar-pillar interactions to be reversible cluster formations, that are capable of delivering pillar-induced tension to the bacterial membrane due to their flexible bending and straightening motions. The enhanced rates of bactericidal activity observed in this study, for pillars of 360nm and 35nm diameters, can be attributed to the stress-induced deflection of the nanopillars upon bacterial membrane adsorption. This generates increased stretching of the membrane as the pillars deflect and revert to their original position, as opposed to the mechanistic action developed for rigid nanopillars. The Australian Research Council Industrial Transformation Training Centre in SEAM is Australia’s premier manufacturing research and development centre, which focuses on applied research with tangible outcomes.
BREAKING NEWS
Applying ‘Magic Angle’ Twistronics to Manipulate the Flow of Light
Monash University researchers, who are part of an international collaboration applying ‘twistronic’ concepts, are seeking to manipulate the flow of light in extreme ways. Twistronic concepts – the science of layering and twisting two-dimensional materials to control their electrical properties – can lead to advances in a variety of light-driven technologies, including nano-imaging devices; high-speed, low-energy optical computers; and biosensors. It is the first application of Moiré physics and twistronics to the light-based technologies, photonics and polaritonics, opening unique opportunities for extreme photonic dispersion engineering and robust control of polaritons on two-dimensional materials. The research team took inspiration from the recent discovery of superconductivity in a pair of stacked graphene layers that were rotated to the ‘magic twist angle’ of 1.1 degrees. Researchers discovered that an analogous principle can be applied to manipulate light in highly unusual ways. At a specific rotation angle between two ultrathin layers of molybdenumtrioxide, the researchers were able to prevent optical diffraction and enable robust light propagation in a tightly focused beam at desired wavelengths. “Our experiments were far beyond our expectations,” said Dr Qingdong Ou, who led the experimental component of the study at Monash. “By stacking ‘with a twist’ two thin slabs of a natural 2D material, we can manipulate infrared light propagation, most intriguingly, in a highly collimated style,” Dr Ou explained. In addition to support from the Australian Research Council, there were other stakeholders with a vested interest, including the US Air Force Office of Scientific Research; Singapore’s Agency for Science Technology and Research; and China’s National Natural Science Foundation.
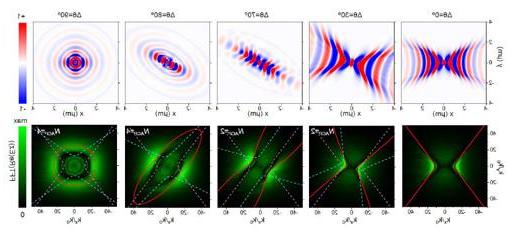
BELOW: Field distributions (top panel) and corresponding dispersion (bottom panel) at varying rotation angles. BOTTOM L-R: Twisted bi-layer (tBL) -MoO3. A bilayer of molybdenum trioxides supports highly collimated, directive, and diffractionless propagation of nano-light when the two layers are aligned at the photonic ‘magic angle’. Dr Qingdong Ou (Monash University) with s-SNOM facility at Melbourne Centre for Nanofabrication (MCN)
Towards Ultra-Sensitive Diagnostic Chips
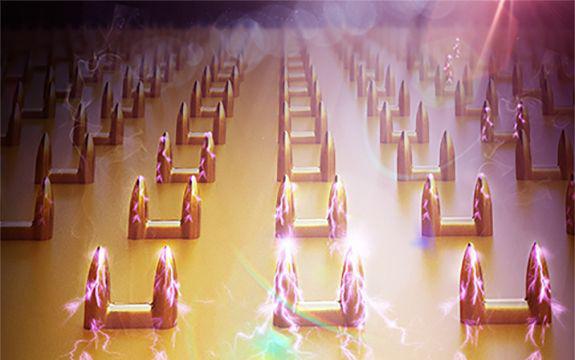
An ultra-thin nanostructure gold film – or metasurface – with the potential to revolutionise next-generation bio-sensing chips, has been developed by an international team, led by Swinburne University of Technology. Image courtesy of Swinburne University.
An ultra-thin nanostructure gold film – or metasurface – with the potential to revolutionise next-generation bio-sensing chips, has been developed by an international team, led by Swinburne University of Technology. The new metasurface could be used to create an extremely sensitive diagnostic chip to detect disease in small amounts of body fluids. The researchers include Founding Director of the Centre for Translational Atomaterials at Swinburne, Professor Baohua Jia and Distinguished Professor Yuri Kivshar from the Australian National University, who recently developed the metasurface, which is capable of strong light-matter interaction with higher sensitivity. The metasurface consists of an array of standing double-pillar meta-molecules that support strong dark mode resonances or electromagnetic configurations, that can ‘trap’ light energy and prevent it from escaping. Once the dark modes are excited, the structure ‘squeezes’ light into the tips of the pillars. “When the metasurface is illuminated by light at some specific oblique angles, dark modes can be excited and they can ‘trap’ all the energy of incident light, leading to the highest field enhancements at the tips of pillars,” said Swinburne PhD candidate Yao Liang, who also co-authored the paper. The strong light field enhancement in the infrared molecular fingerprint wavelength region has many applications. “For example, it could be applied to build an ultra-compact and extremely sensitive diagnostic chip that can detect disease in small samples of blood or saliva, helping people monitor their health in real-time,” said co-author Dr Fengchun Zhang from South China Normal University. The breakthrough shows great potential for other applications such as ultra-fast thermal imaging and quantum emitters.
BREAKING NEWS
Weird Science
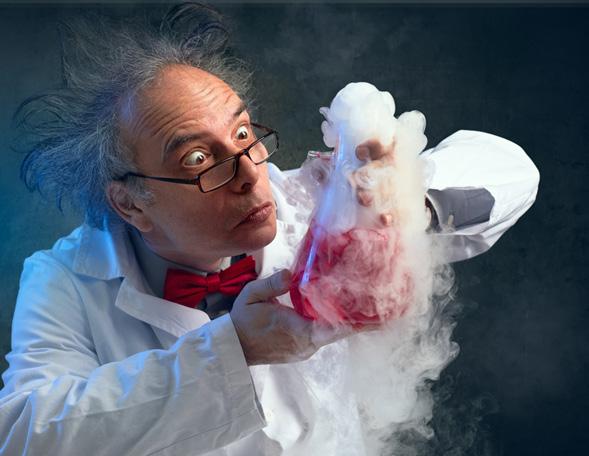
New research from the University of South Australia (UniSA) and the Australian Catholic University (ACU) shows how gender stereotypes influence young people’s perceptions of scientists.
White lab coats and dangerous experiments all epitomise the ‘mad scientist’, but beyond Hollywood, the stereotype lives on, and according to new research, it could influence the next generation of potential scientists. New research from the University of South Australia (UniSA) and the Australian Catholic University (ACU) shows how gender stereotypes influence young people’s perceptions of scientists. UniSA researcher Dr Garth Stahl, and Dr Laura Scholes from ACU, said understanding how stereotypes of science and scientists can influence children’s career aspirations – even at the primary school level – is important to address the skills shortage in science, technology, engineering and maths (STEM). “In the case of science, media often shows scientists to be eccentric men in white coats,” Dr Stahl said. “The problem with stereotypes is that they tend to stick, so what we’re seeing with primary school students is that their perceptions of science and scientists are influencing their ideas of future careers.” Forty-five year four primary school students, across six economically and geographically diverse schools, were interviewed about their future job ambitions; their interest in becoming a scientist; what kind of work a scientist did; and what a scientist might look like. The majority of students (55 per cent) had no aspirations to be a scientist; six were ambivalent; and 13 said they would strongly consider a job as a scientist. Nearly 40 per cent of students said they ‘did not like’ science, and that it was ‘boring’ or ‘weird’. Researchers found that most students did not see gender as a defining factor for a scientist, with only two students saying a scientist was ‘usually a man’.
New Partnership in Lightning Protection
A new partnership between Swinburne University of Technology and Lightning Protection International (LPI) will develop novel materials for LPI’s lightning protection devices, known as ‘air terminals’. The industry-led research project has been awarded $154,000 by the Australian Government, through the Innovative Manufacturing Cooperative Research Centre (IMCRC), matching LPI’s research and development investment. It will enable the launch of nextgeneration air terminals that focus on corona minimisation. The project will be led by Swinburne’s Dr Andrew Ang and Dr Rosalie Hocking, and LPI’s Dr Franco D’Alessandro. “One of the challenges with air terminals is that water droplets and air pollution deposits can impact their performance,” Dr Rosalie Hocking said. “The novel materials we are developing will overcome this issue,” Dr Franco D’Alessandro, Chief Technology Officer of LPI added. During a thunderstorm, all objects, especially sharp ones, can produce a charge in the air around their extremities that can be counterproductive for air terminals, whose job it is to capture the lightning strike. Air terminals intercept lightning strikes and safely pass their extremely high currents to ground through connected ‘downconductors’, which protect structures. The project aims to benefit manufacturing and other industry sectors, both in Australia and internationally. David Chuter, Managing Director and CEO of IMCRC, said the value of collaborative research and development will be highly beneficial. “This research collaboration is a great example of a manufacturing SME being ambitious, and strategically entering a partnership with an Australian university to solve real world problems and develop tangible and exportable technology. We are delighted to see Tasmania’s LPI work with Victoria’s Swinburne University,” Chuter said.
Image courtesy of LPI: LPI’s international air terminal installations (two terminals visible). These air terminals are protecting a hospital in India.
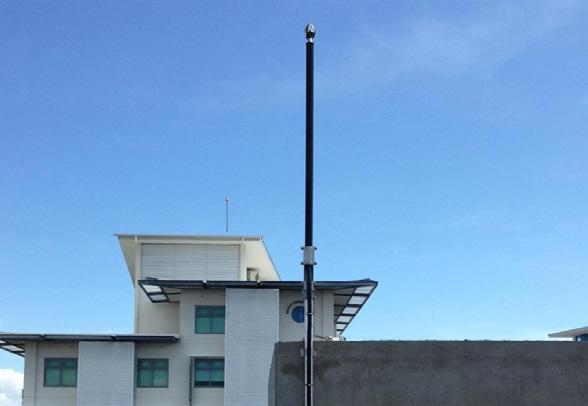
BREAKING NEWS
Now You See It, Now You Don’t: Adding Chameleon-Like Capabilities to Defence Drones
In conjunction with the Department of Defence, University of South Australia material scientists have developed a range of lightweight panels that can change colour on demand, allowing drones to match their appearance to the background colours of the sky. Ever since the French had the bright idea of using hydrogen balloons for military surveillance in the late 18th century, aviation capability has played a central role in intelligence, surveillance and reconnaissance (ISR) operations. Today unmanned aerial vehicles (UAVs), or drones, are a huge tiny Black Hornet – which is about the size of a whiteboard marker – to larger models with wide ranging surveillance capabilities. Despite their ubiquity and utility, all military UAVs are currently hindered by the same simple problem – the sky changes colour, but they do not. Given the huge importance of remaining undetected during ISR operations, the static colour of drones can be a significant problem, but now, thanks to researchers at The University of South
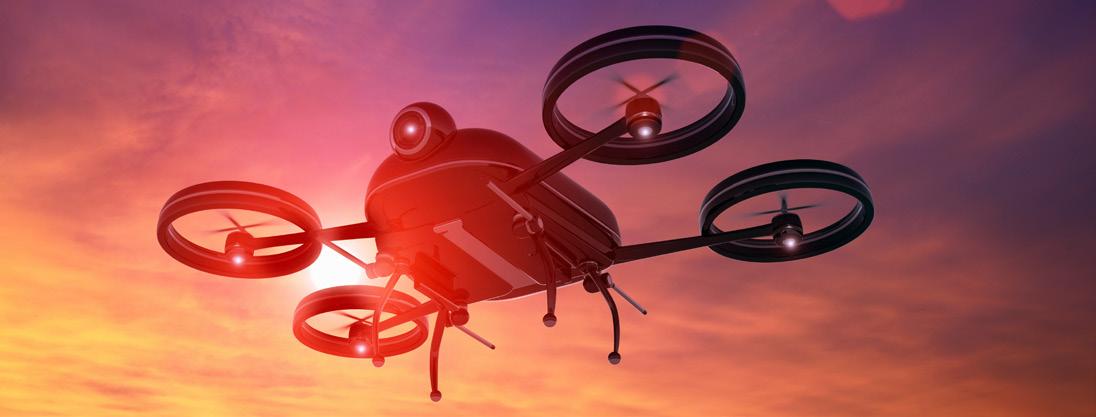
Australia’s Future Industries Institute (FII), the solution is at hand. In a collaboration with the Department of Defence, FII researchers, led by Dr Kamil Zuber, have developed a range of lightweight polymer panels that can change colour on demand. The polymers are what are known as electrochromic materials, meaning they change colour in response to an electric field, and the exact colours can be tuned to specific voltages. “Similar technology has been used in luxury cars, for dimming mirrors, and on the windows of the Boeing 787 Dreamliner,” Dr Zuber said. “But those applications are slow, require high power consumption to switch, and the electric flow must be maintained
asset for ISR. The Australian Army has drones ranging from the band (above the Fermi level), though there have been tantalising hints that the actual velocity of the electrons is much larger than to sustain the change state.” “Our panels, on the other hand, have switching speeds in the range of seconds and offer colour memory, which means they retain their switched colour without a continuously applied voltage. They also operate in the range from -1.5 to +1.5 volts, which means you only need to use an AA battery to activate the change.” In addition to their chameleon-like characteristics, the panels are inexpensive, lightweight and durable, and can be either rigid or flexible, making them ideal for use on drones of all sizes and specifications.
Unexpectedly-Fast Conduction Electrons in Na3Bi
An Australian-led study uses a scanningtunnelling microscope ‘trick’ to map electronic interference,” said lead author Dr Iolanda di
structure in Na3Bi, seeking an answer to that material’s extremely high electron mobility. In studying the topological Dirac semimetal, effects are crucial to electron speed, and therefore mobility, and thus, to the use of this exciting class of materials in future ultra-low energy electronics. To date, little has been known about the band dispersion of Na3Bi in the conduction theoretical predictions. “We grew thin films of Na3Bi and investigated their band structure via quasiparticle
the team found that exchange and correlation Bernardo. “Our calculations revealed that to understand the extremely high experimental velocities of the charge carriers, particularly in the conduction band, exchange and correlation effects are crucial.”
FLEET Research Fellow Dr Iolanda di Bernardo, School of Physics and Astronomy, Monash University.
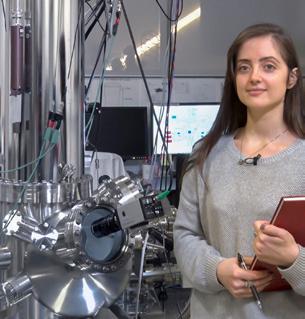