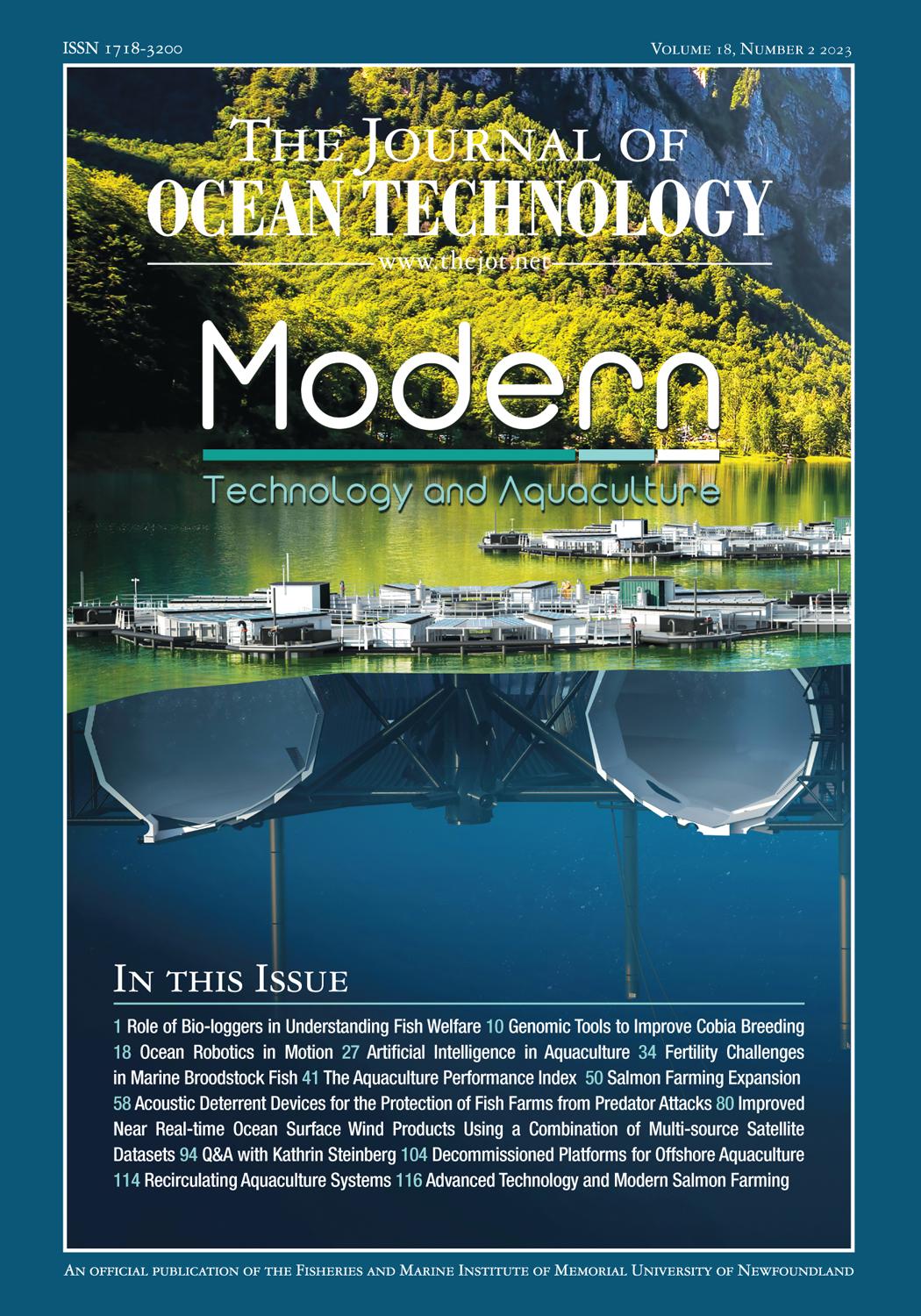
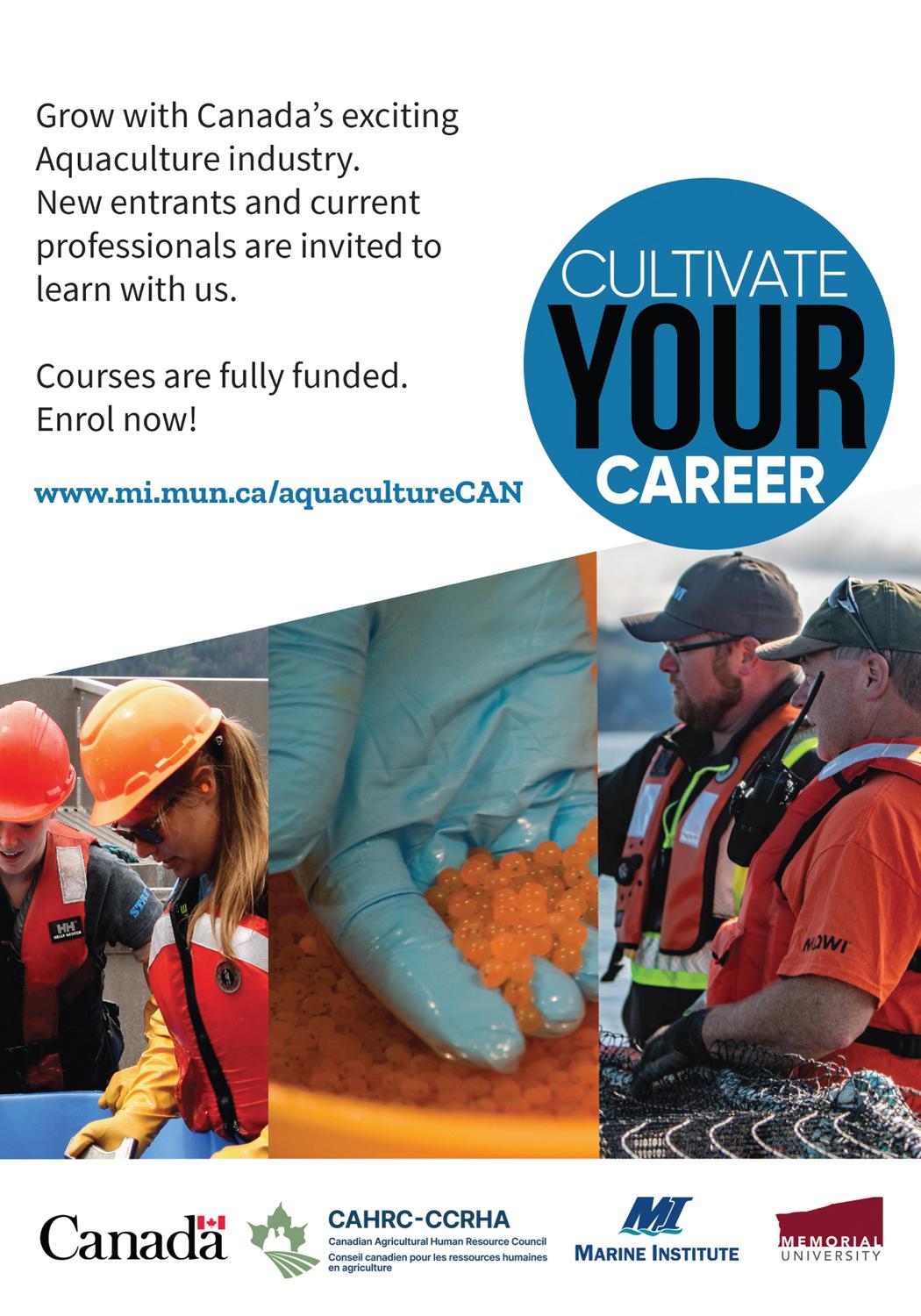
1 Emerging Technologies on the Road to Precision Aquaculture: The Role of Bio-loggers in Understanding Fish Welfare
Jennie Korus, Innovasea
10 Genomic Tools to Improve Cobia Breeding
Alcina Carvalho-Neta, José Torres-Júnior State University of Maranhão
Charles James McGuigan, Daniel Benetti University of Miami
18 Ocean Robotics in Motion: Applying BUGWRIGHT2 Top-down Regulatory-model to the Aquaculture Sector
Tafsir Matin Johansson, Dimitrios Dalaklis, Aspasia Pastra, World Maritime University
27 Old MacDonald had a Farm: AI. AI. O.
Cyr Couturier, Fisheries and Marine Institute
34 Overcoming Fertility Challenges in Tropical and Subtropical Marine Broodstock Fish
José Torres-Júnior, Alcina Carvalho-Neta State University of Maranhão
Carlos Tudela, Julio Camperio, Jorge Suarez, Daniel Benetti, University of Miami
41 The Aquaculture Performance Index Applied to Species Selection for Offshore Aquaculture
Daniel Benetti, Carlos Tudela, University of Miami
Fabio dos Santos Neto Universidade Federal de Sao Paulo
Tyler Sclodnick, Innovasea
Jenna Baggett, Florida Atlantic University
50 Supporting the Legitimacy of Salmon Farming Expansion
Carlos H. Carroza Meza, Daniel Benetti University of Miami
58 Acoustic Deterrent Devices for the Protection of Fish Farms from Predator Attacks: A Solution or Menace
Arnold Ebuka Irabor, Hardin Jrn Pierre
University of St. Andrews
Oghenebrorhie Obakanurhe, Ikechukwu Augustine Chukwurah, Augustine Onyemaechi Ozor, Jovita
Oghenenyerhovwo
Dennis Osadebay University
Francis Oster Nwachi, Jerimoth Kesena Ekelemu Delta State University Abraka
77 Lodestar Sindy Dove, Carlos Carroza Meza, Marianne Parent
80 Improved Near Real-time Ocean Surface Wind Products Using a Combination of Multi-source Satellite Datasets
Meisam Amani, Sahel Mahdavi, Terry Bullock, Steven Beale WSP Environment and Infrastructure Canada Limited
Dennis Jay
Yonathan
Lori Mercer, Wild Floss Designs
PUBLISHER
Bill Carter Tel. +001 (709) 778-0762
info@thejot.net
MANAGING EDITOR
Dawn Roche Tel. +001 (709) 778-0763
info@thejot.net
TECHNICAL CO-EDITORS
Dr. David Molyneux Director, Ocean Engineering Research Centre Faculty of Engineering and Applied Science Memorial University of Newfoundland
WEBSITE AND DATABASE
Scott Bruce
Dr. Keith Alverson USA
Dr. Randy Billard Virtual Marine Canada
Dr. Safak Nur Ertürk Bozkurtoglu Ocean Engineering Department Istanbul Technical University Turkey
Dr. Daniel F. Carlson Institute of Coastal Research Helmholtz-Zentrum Geesthacht Germany
Dr. Dimitrios Dalaklis World Maritime University Sweden
Randy Gillespie Windover Group Canada
Dr. Sebnem Helvacioglu
Dept. Naval Architecture and Marine Engineering
Istanbul Technical University Turkey
GRAPHIC DESIGN/SOCIAL MEDIA
Danielle Percy Tel. +001 (709) 778-0561
danielle.percy@mi.mun.ca
Dr. Katleen Robert Canada Research Chair, Ocean Mapping School of Ocean Technology Fisheries and Marine Institute
FINANCIAL ADMINISTRATION
Michelle Whelan
S.M. Asif Hossain National Parliament Secretariat Bangladesh
Dr. John Jamieson Dept. Earth Sciences Memorial University Canada
Paula Keener Global Ocean Visions USA
Richard Kelly Centre for Applied Ocean Technology Marine Institute Canada
Peter King University of Tasmania Australia
Dr. Sue Molloy Glas Ocean Engineering Canada
Dr. Kate Moran Ocean Networks Canada Canada
EDITORIAL ASSISTANCE
Paula Keener, Randy Gillespie, Bethany Randell
Kelly Moret Hampidjan Canada Ltd. Canada
Dr. Glenn Nolan Marine Institute Ireland
Dr. Emilio Notti Institute of Marine Sciences Italian National Research Council Italy
Nicolai von OppelnBronikowski Memorial University Canada
Dr. Malte Pedersen Aalborg University Denmark
Bethany Randell
Centre for Applied Ocean Technology Marine Institute Canada
Prof. Fiona Regan
School of Chemical Sciences
Dublin City University Ireland
Dr. Mike Smit School of Information Management Dalhousie University Canada
Dr. Timothy Sullivan
School of Biological, Earth, and Environmental Studies University College Cork Ireland
Dr. Jim Wyse Maridia Research Associates Canada
Jill Zande MATE Inspiration for Innovation USA
The Journal of Ocean Technology is a scholarly periodical with an extensive international editorial board comprising experts representing a broad range of scientific and technical disciplines. Editorial decisions for all reviews and papers are managed by Dr. David Molyneux, Memorial University of Newfoundland, and Dr. Katleen Robert, Fisheries and Marine Institute.
The Journal of Ocean Technology is indexed with Scopus, EBSCO, Elsevier, and Google Scholar. Such indexing allows us to further disseminate scholarly content to a larger market; helps authenticate the myriad of research activities taking place around the globe; and provides increased exposure to our authors and guest editors. All content in the JOT is available online in open access format. www.thejot.net
The Journal of Ocean Technology, ISSN 1718-3200, is protected under Canadian
Copyright Laws. Reproduction of any essay, article, paper or part thereof by any mechanical or electronic means without the express written permission of the JOT is strictly prohibited. Expressions of interest to reproduce any part of the JOT should be addressed in writing. Peer-reviewed papers appearing in the JOT and being referenced in another periodical or conference proceedings must be properly cited, including JOT volume, number and page(s). info@thejot.net
Bluegreen is a Norwegian company with leading expertise in thermoplastics, and has the world’s largest high density polyethylene structures on its track record. It designs and builds pioneering closed containment systems for the aquaculture industry, such as its own proprietary Marine Donut design, as well as other innovative solutions.
Marine Donut is a patented floating closed containment system for fish farming. It prevents escape and contamination, and minimizes the risk of disease, algae, and sea lice. The need for antibiotics and other medicines and preparations is significantly reduced. Breeders get full control over the fish and the environment through digitization and monitoring; and good control over the efficient feed factor, which further reduces costs.
Bluegreen’s mission is contributing to creating a sustainable and profitable future for fish farmers, worldwide. The company was established in 2020 by CEO Nils-Johan Tufte and two close colleagues and partners, Geir Andresen and Elg Ragnar Thunes. They saw an opportunity within thermoplastics and next generation aquaculture – and seized it. Bluegreen clearly hit a need in the market as its growth has been remarkable. It now employs about 40 engineers, technicians, and project managers locally in Norway, and also has a subsidiary in Canada that is led by Mark Lane.
Bluegreen’s core pillars are engineering and production of floating closed containment aquaculture systems and fusion of thermoplastic structures and infrastructure, including land-based fish farming facilities. https:// bluegreengroup.no/en/services/marine-donut
Copyright Journal of Ocean Technology 2023
The JOT production team invites the submission of technical papers, essays, and short articles based on upcoming themes. Technical papers describe cutting edge research and present the results of new research in ocean technology or engineering, and are no more than 7,500 words in length. Student papers are welcome. All papers are subjected to a rigorous peer-review process. Essays present well-informed observations and conclusions, and identify key issues for the ocean community in a concise manner. They are written at a level that would be understandable by a non-specialist. As essays are less formal than a technical paper, they do not include abstracts, listing of references, etc. Typical essay lengths are up to 3,000 words. Short articles are between 400 and 800 words and focus on how a technology works, evolution or advancement of a technology as well as viewpoint/commentary pieces. All content in the JOT is published in open access format, making each issue accessible to anyone, anywhere in the world. Submissions and inquiries should be forwarded to info@thejot.net.
All themes are approached from a Blue Economy perspective.
Fall 2023 Ocean vehicles: data pickers of the ocean
Winter 2023 Smart ships
Spring 2024 Remote operation centres: accessing the ocean
Summer 2024 Deep (ocean) learning
Fall 2024 Sensing the ocean: lights, camera, sensors
Winter 2024 Safety first: humans at sea
The challenge of feeding an estimated global human population of nine billion by 2050 is daunting. Approximately three billion people across the globe are dependent on fish as the principal source of animal protein. Aquaculture has become the fastest growing sector of food production and contributes more than half of the world’s seafood for human consumption. The latest report of the Food and Agriculture Organization of the United Nations predicts that two-thirds of the global seafood supply by 2030 will be farmed and that there will be shortages to the additional 30-40 million metric tons required to feed the world in the next three to four decades.
In this issue of the JOT, renowned experts have authored articles covering some of the most important technological advances as well as the greatest challenges faced by the expansion of modern aquaculture. The articles address topics ranging from genomics, breeding, and species selection to advances in cage and land-based systems engineering, environmental monitoring, feeding and biomass estimation, mortalities removal – all aiming at improving management strategies and the ecological and economic efficiencies of the operations.
The aquaculture production cycle encompasses several stages, each comprised of multiple variables that make the process rather lengthy and complex. It includes aspects such as genetics and molecular biology, research and technology development, engineering, biology, nutrition, hydrology, chemical and physical oceanography, modelling, bioenergetics, water quality, legal and regulatory framework, animal welfare, biosecurity, business, market and marketing strategies, traceability, and quality control. Environmental, socio-economic, and policy issues are equally important. In addition, in recent years, it has become evident that cellular aquaculture, or lab grown seafood, will play a significant role in producing seafood for human consumption.
Aquaculture starts at basic levels of site assessment and species selection. These obvious first steps often seem to be overlooked; it is not uncommon to see aquaculture operations being planned, designed, and implemented with unsuitable species for unsuitable sites. That lack of preliminary planning leads to failures. When the right species is selected, the appropriate site found, and the business and production plans properly developed, the likelihood of success increases dramatically.
Hatchery technology is of paramount importance since the success of any animal protein production activity will, to a great extent, depend on the quality of the seedstock. To that end, genomic tools and reproductive biology and physiology research aimed at improving fish breeding programs targeting specific approaches (e.g., pelagic
Dr. Daniel Benetti is a professor in the Department of Marine Biology and Ecology, Rosenstiel School of Marine, Atmospheric, and Earth Science (RSMAES), at the University of Miami. He has extensive international professional experience, having worked, consulted, and established research, commercial, and educational programs and projects for the private and government sectors in more than 20 countries throughout five continents over the last 40 years. He has over 200 scientific and technical publications and is internationally recognized for his contributions to the field of aquaculture. Dr. Benetti is the director of the Aquaculture Academic and Research Graduate Program at RSMAES and carries out interdisciplinary research and technology transfer for the development of sustainable aquaculture projects in the U.S., Australia, Brazil, Ecuador, Chile, Colombia, Panama, Mexico, the Bahamas, China, Turkey, Norway, and other Asian, European, and Latin American countries. Prior to joining the faculty of the University of Miami in 1998, Dr. Benetti was a research scientist at Harbor Branch Oceanographic Institution in Ft. Pierce, Florida.
species for offshore aquaculture) are gaining momentum. All hatchery stages are crucial components of the entire cycle.
Land-based recirculating aquaculture systems (RAS), integrated multi-trophic aquaculture, integrated agriculture and aquaculture, aquaponics – as well as traditional flow-through ponds, raceways, and tanks – are progressive and viable options. RAS are poised to make important contributions to production in areas close to the end market. However, large-scale industrial production will have to take place in the open ocean – where available areas, stronger currents, and greater depths increase the carrying capacity of the environment. Raising fish in exposed, high-energy areas offshore requires advanced technologies demanding high levels of investment and long-term commitment. These systems are complex and expensive to implement and run. Hence, fish produced offshore must be sold at high prices to compensate the high capital and operating costs required, limiting their demand in a highly competitive white fish market. To that end – as fish welfare became a key pillar to sustainability, affecting final consumers’ perceptions, acceptance, and price of the product in the marketplace – fish produced offshore present a marketing edge.
Offshore aquaculture continues to expand rapidly the world over. Tools for site assessment and environmental monitoring have been established. Hurdles still must be overcome before commercial viability can be secured. Some issues such as optimizing genetics, nutrition, and disease control are inherent to all forms of aquaculture – whereas, managing stocking, feeding, chemical treatments, net cleaning, predator avoidance, escapements, biomass estimates and crop management, mortalities collection, and harvesting operations are exacerbated in offshore systems. Full automation is progressing fast but still needs refinement. Modern operations are incorporating machine learning and artificial intelligence tools to control water quality, feeding levels, aeration, dissolved oxygen, ammonia, temperature, etc., aiming at optimizing productivity.
Despite serious challenges, technology continues to improve steadfastly, allowing the aquaculture industry to further explore opportunities in areas such as offshore and new species. However, with fishing resources at or above their maximum sustainable yields; competing coastal areas; and land, water, and other resources exhausted; the aquaculture industry can only continue expanding by advancing technology and improving ecological efficiency using fewer natural resources to produce more.
Science and technology are key to understanding what it takes for expanding an environmentally sustainable and economically viable industry. All indicators point to excellent opportunities. The market demand continues to grow, and there have been remarkable engineering and technological progress in the industry. The prospects are bright.
The role of Bio-loggers in Understanding Fish Welfare
by Jennie KorusThe 21st century has seen aquaculture become an integral source of global food production with fish farming accounting for almost half of all fish consumed globally and it shows no signs of slowing down. Farmed salmon, in particular, have mimicked intensive production growth strategies used by the agriculture industry to meet increased demand due to its affordability, high nutritional value, and sustainability compared to common land-based animal protein sources.
The sustainability and future of the industry are being challenged by several factors such as environmental changes, inefficient production systems, and disease outbreaks. Precision aquaculture has emerged as a promising approach to address some of these challenges by leveraging advanced sensor technologies, artificial intelligence, and the digitization of operations to gain insights, optimize production, and reduce risk. These tools allow farmers to monitor and control their environmental conditions at their farm, gain real-time insights into the health and welfare of their livestock, and improve inefficiencies in their production cycles.
Regulators can also benefit from technology advancements. For example, a new regulation in Norway has allowed the use of a camera system that automatically counts sea lice on fish. Not only does this eliminate the manual counting process, which is both time-intensive and stressful for fish, but this provides a more accurate estimate of sea lice abundance; digitizes this data, which can now be integrated into a farm management platform; and allows farms to take mitigation actions sooner, which can minimize the spread of sea lice to neighbouring farms. Machine vision algorithms have gained considerable traction within the industry since cameras are already widely used for essential operations like feeding and expanded to use for biomass estimation, pellet detection, fish satiation, and individual fish identification for health and welfare assessments.
Novel technology like bio-loggers or fish tags are another example of advanced sensor technology that can be investigated as a tool to monitor fish behaviour and optimize health and growth by providing real-time data on fish condition. Their use in aquaculture to date has been restricted to academic studies, but there is a case to be made for their inclusion in regular operations because of the data they can provide.
Unlike the earlier example where machine vision algorithms for sea lice counts are improving upon a dataset that was already being recorded, bio-loggers can provide new data streams that are currently unavailable to farmers. The capabilities of biological data loggers have significantly increased in recent years with new sensors, improved battery life, and storage space. Parameters like heart rate, acceleration, temperature, and depth can be monitored with tags that are commercially available and have been validated in studies that have produced valuable insights into both fish physiology and behaviour on aquaculture farms.
For example, studies have shown that heart rate becomes significantly elevated in response to common aquaculture practices such as crowding, grading, and vaccination procedures. Heart rate data can provide information on when fish have recovered from essential operations and help with farm management to ensure adequate spacing between these events. Other studies have examined preferential swimming depths and behaviours in response to key environmental drivers such as light, temperature, oxygen, and water currents, which are known to vary across farms and throughout the water column.
Aquaculture farms are complex environments with many confounding effects that all play a role in determining the welfare of an individual or the entire population. Welfare on farms is measured
by operational welfare indicators (OWIs; Figure 1) that are meant to provide a simple mechanism for farms to assess welfare. OWIs are measurements or observations that must be simple, quick, and repeatable in order to be adopted into standard operating procedures. However, welfare measurements are complex and often subjective in nature and there is not a single measurement that can be obtained to provide an absolute determination of an animal’s welfare status. Often, many different indicators must be considered and weighted appropriately based on their impact to an individual or group but applying this in practice can be complicated.
There are no standardized rules or regulations for fish welfare on all farms as most countries have their own set of regulations and, in most cases, they only specify that good welfare must be maintained without explicitly outlining how to achieve this. Eco-certification schemes such as Aquaculture Stewardship Council or Best
Aquaculture Practices are an additional set of voluntary standards that can help producers market their product as sustainable and ethical and can occasionally be required by retailers. From a welfare perspective, both certification standards require farms to maintain high water quality, manage disease and parasites in an environmentally responsible manner, and mandate fish health professionals to observe and report on fish welfare. However, there is no list of prescribed metrics or methodology that must be followed to comply with the standards. This leaves the onus on producers to decide which metrics to deploy to determine fish welfare.
There are many kinds of OWIs ranging from indirect environmental indicators such as oxygen, temperature, stocking density, current speed, and harmful plankton levels to direct measures of an individual’s health including gill/skin/fin condition, opercular movement, and lice levels. Other indicators are measured
at a group level and include mortality, appetite, swimming behaviour, and growth rate. There are an additional set of indicators known as laboratory-based welfare indicators (LABWIs) that can be sampled on the farm but need to be sent to a laboratory for analysis.
Indirect environmental indicators are some of the most straightforward OWIs as they can easily be measured in-situ with sensors but, as an indirect measurement, inferences must be made on the effect they have on fish. In addition, complex hydrodynamics and water stratification influence these variables over time and across small spatial scales and so a sensor in a single point location may not be representative of the whole farm environment.
Most observations of individual health require sampling a significant number of individuals to inform husbandry practices at the farm level and this is time consuming and stressful to fish that are handled during the process. Some observations can be accomplished through camera feeds, but they are difficult to quantify and limited by water clarity and the proximity of fish as they swim by the lens.
Group indicators are commonly used since many of them are used to track production cycle metrics, e.g., growth rate or mortality, but by the time they are calculated, it is too late to take any action and, inherently, most of these indicators are retroactive. Similarly, lab-based indicators are very insightful for fish health and identifying disease; however, there is a delay in receiving results. Both types of indicators are a necessary part of operations, but they do not provide immediate feedback to site staff, which makes them helpful for long-term husbandry management but not for day-to-day decisionmaking. There are some indicators such as sea lice counts that fall under their own regulatory framework but there are no standards on which indicators to use strictly for welfare assessments, and the responsibility falls on fish health teams to decide which are the most critical and how to weigh each indicator to report a holistic welfare metric.
There are many limitations of current OWIs and the lack of standard procedures across the industry make it difficult to determine the efficacy of their use on farms. Bio-loggers offer a unique perspective into the behaviour and physiological state of farmed fish as they experience regular operations and varying environmental conditions and have the potential to be used as modern, digital, and direct OWI. Typically, tags are surgically implanted (Figure 2) and can record a variety of physiological parameters like heart rate and temperature as well as behavioural information with acceleration and depth data.
When a fish experiences or perceives a potential threat, the biological primary stress response kicks into action – very similar to when a person perceives a threat, and the body prepares the fight or flight response (Figure 3). Hormones are released into the bloodstream, and this leads to many secondary responses including increased cardiorespiratory activity. Secondary responses have impacts on the whole animal, resulting in tertiary responses that can be identified by farmers and are currently used as OWIs unlike the primary and secondary responses. Tertiary effects are detectable but only after some period of delay (hours, days, or even weeks depending on the stressor and its severity) and these include growth, immune function, and swimming activity.
Fish heart rate varies in response to their physiological needs such as oxygen consumption or carbon dioxide release, but it is also influenced by numerous factors including (but not limited to) genetics, physical fitness, diet, environment, disease, and their interactions with each other. Heart rate is part of the secondary stress response so monitoring it in real time and in an unobtrusive manner makes it viable as a quantitative early indicator of stress.
Heart rate provides a measurement of fish response to any perceived stressor significantly earlier and can provide direct
feedback to operators that can help to inform management decisions in real time. This type of data would remove the need for farms to determine how to weigh confounding variables to determine the effect on individuals as they can now use heart rate as a direct measurement of stress. Studies have shown that following stressful operations like crowding, it can take as long as 24 hours for heart rate to return to normal levels. Other studies have looked at the effects of cumulative stressors and have identified that there is a diminished cardiac response indicating a maximum capacity of individuals to respond to consecutive stressors. These insights show how useful this data can be for developing standard operating procedures and farm management planning.
Another indicator that can be used is acceleration, which is measured using tri-axis accelerometers that describe changing velocity,
and this data can indicate erratic or lethargic behaviour. As part of normal feeding regimens, operators observe fish activity levels to determine how much feed to deliver and when to stop feeding. These decisions are based on the subjective opinion of the operator and their experience. Activity data could provide insights into activity levels during feeding and provide a quantitative measurement as the basis for this decision-making. These might seem like minor adjustments with little impact, but feed is the single greatest cost variable in a production cycle and one goal of precision aquaculture is making small efficiency gains by fine-tuning operations.
Temperature and depth are very simple measurements that are more straightforward to interpret. Depth data can be a useful tool to understand how fish are occupying the vertical space in a pen and whether they are
seeking out optimal environmental conditions or avoiding areas with deleterious water quality or where divers are carrying out operations. As ectotherms, the internal body temperature of fish is very similar to the temperature of their environment as they do not regulate it, but temperature is the main driver of all biological functions, so it is a critical factor to consider in fish welfare assessments.
There is work to be done in determining exactly how bio-logger data would be used on farms as OWIs but the data they provide are unique and insightful into a fish’s lived experience. Bio-loggers offer a new data stream to farmers that can assist in decisionmaking on farms; however, these data can be complex and, if implemented as an OWI, there must be some validation in the analysis and interpretation of this data. For example, heart rate in beats per minute is generally easy to interpret but thresholds for what defines stress can vary under different environments. Acceleration, on the other hand, can be complex to understand – especially when both erratic and lethargic behaviour can be indicators of stress. For this data to be used most efficiently, more studies are required to determine what parameters or combinations of parameters should be used and whether models can be developed that consider multiple factors. This is an area of active research as commercial bio-loggers are increasingly being used in aquaculture studies, but more research is needed before a robust tool can be provided to farmers that minimizes the amount of interpretation that site staff must do, instead providing inferences that can be acted upon.
Despite the novelty of bio-loggers and the benefits they can provide to farmers, there are some limitations that must be considered prior to their adoption on commercially operating farms. One major reason tags have been primarily used in research applications is that, in many cases, data are stored internally on the tag and so it must be retrieved to offload the data. This presents
two major challenges for commercial scale operations: how do you make use of the data for real-time operational decisions and how do you retrieve the tags during the harvest and processing phase to ensure they do not end up in packaged products.
Depending on its size, a single pen can hold up to 400,000 fish; an entire farm might have anywhere from six to 20 cages, and often processing centres are in a central location and will process fish from many different farms. It would be simpler if every fish had a tag as implementing a standard operating procedure to remove a tag from every individual would be simpler than attempting to locate the individuals with tags and removing them. However, tagging every individual is costprohibitive and excessive for data analysis but tagging only a representative population introduces a complication on a processing line that is built for efficiency.
The lack of real-time data is a barrier that can be overcome with different tag technologies. For example, acoustic tags can transmit data wirelessly to a cabled receiver that can send data to the cloud in real time. However, acoustic data transmission is limited in the amount of data that it can transmit and only some of the above-mentioned parameters are commercially available in acoustic tags. Real-time data are critical for operational decision-making and future development of tag technology may include a full suite of parameters that are transmissible in real time, but this has only been trialled in academic studies to date.
Another potential disadvantage of bio-loggers is that fish may be impacted by the presence of the tag. There are guidelines on tagging that recommend tags be no more than 2% of the body weight to minimize their impact on fish but some studies have shown that tags can affect buoyancy control in salmon, which can affect the depths they occupy within a pen (Figure 4). Tagging is inherently a stressful operation for fish and while most fish recover
fully, if not given a chance to recover postsurgery, tagging can have deleterious effects on the individual that may not be representative of the rest of the population.
Finally, from a technical point of view, there are always tradeoffs with sampling frequency and battery life of tags that must be considered. As sampling frequency increases, battery life diminishes faster and so the minimum sampling frequency that provides sufficient insights must be determined. With current battery life limitations, tags would not last a full production cycle and so this begs the question – at what life stage do you tag? Smaller size fish need smaller tags with smaller batteries and, therefore, have a shorter lifetime. Larger fish are of higher value and so perhaps tag data is more critical
closer to harvest. Tagging procedures are more easily carried out while fish are in land-based facilities, but acoustic tags are best suited to open water conditions. All these factors play a role in determining the usefulness of these tools on a commercial farm and there will always be tradeoffs with any technology but if the data are valuable enough then it is worth pursuing solutions to some of these challenges.
As with any novel technology, there are barriers that must be overcome before it is widely adopted by industry. In the current state of tag technology, more research is required to determine the value of bio-logger data and how the data can be leveraged in commercial aquaculture operations. There is
no question regarding the usefulness of the data but there are challenges with accessing data in real time and producing actionable insights from the data.
Interest in fish welfare has increased as production capacity continues to grow, and consumers become more aware and interested in the rearing conditions and source of their food. Bio-loggers can provide valuable data to help improve fish welfare and perhaps in the future there will be highly digitized operations with real-time data streams from bio-loggers providing live stress indicators to farmers but there are some obstacles to face before this becomes a reality. In the interim, scientific studies will continue to advance this technology and the insights we can derive from this data, and this helps push the industry forward. Aquaculture is a vital component of world food production, and cutting-edge
technology and the adoption of precision farming are critical steps in increasing production to meet growing demand and helping to reduce its environmental impact. u
Jennie Korus is an aquaculture scientist at Innovasea and part of the Aquaculture Intelligence team in Halifax, Nova Scotia. She holds an honours degree in marine biology and statistics from Dalhousie University and an advanced diploma in ocean technology from Nova Scotia Community College. She is currently working towards her master’s in oceanography at Dalhousie with a focus on using sensors and bio-loggers to monitor fish stress on aquaculture farms.
This is where it all starts. Without a thorough understanding and application of improved genetics in aquaculture – or any other form of farming or agriculture – the production efficiency throughout the entire cycle will be compromised and inevitably bound to fail. Here, we use a fast growing, energetically demanding fish species as a model for a case study exploring using genomic tools for improving breeding efficiency in aquaculture.
Marine aquaculture is the fastest growing food production sector and is rapidly becoming the main source of seafood for the human diet, serving as an essential supply to meet increasing animal protein demands.
Cobia ( Rachycentron canadum ) is a highly valued tropical and subtropical marine fish species exhibiting outstanding prospects for offshore aquaculture. The species features a number of key positive characteristics for production including broad adaptability, prolificacy, fast growth rates, and excellent meat quality.
As cobia aquaculture expands, new technologies that can result in quantitative and qualitative improvements in production continue to emerge. Although breeding technologies are well-established, more genetic information is necessary to guide biotechnology initiatives and/or genetic improvement of this and other aquacultured species. Genomic information, technological advances involving genetic tools, as well as the genomic databases currently available for this species are still quite limited when compared to highly-optimized genetic breeding programs of temperate fish such as salmonids.
These limitations are progressively being eliminated; more comprehensive genomic information for cobia has recently been described and genetic annotations are now available that can provide important insights about their physiology, nutrition, breeding, immune responses, and growout performance. These meaningful advances
should enable the application of novel genetic techniques to cobia aquaculture breeding programs and will strengthen genomic research in aquaculture as a whole.
The manipulation of chromosome sets, initiated decades ago, has progressed beyond the experimental field and is increasingly being used in aquaculture. Current approaches provide chromosomal data in fish that allow the implementation of ploidy management protocols, gynogenetic production, establishment of monosex cultures, and identification of quantitative trait loci for various marine and freshwater species. These techniques can produce multiple desirable effects including increased growth and/ or weight gain, improved understanding of genetic diversity and its impacts on conservation, as well as pathogen resistence and in-situ adaptability. Due to the growing economic importance of aquaculture and the increasingly sophisticated cultivation techniques, commercial breeding initiatives of cobia stocks from the Western Atlantic have been highly successful and are increasingly producing new useful characteristics for genomics that are leading to genetic improvement and biotechnological innovations.
Continued improvements in the efficiency and reliability of cobia production will require the application of modern genetic tools as they develop. Genotyping by sequencing approach, with a single nucleotide polymorphism (SNP) marker identified for genetic sex prediction, may provide the best tool for rapid sex identification in cobia, considering there is no phenotypic sexual dimorphism in the species. Additionally, a newly designed low-density panel (<200 SNPs) has been used for attributing progeny to parents, family classification, and genomic selection and application in breeding programs. The complete genome of cobia has not yet been described. However, led by the Center of Aquaculture Technologies (CAT), collaborative efforts towards this goal are being undertaken by James Cook University, Beijing Genomics
Institute, the University of Chile, Open Blue Sea Farms, and the University of Miami. Knowledge of this genetic panel will allow the expansion of studies already started with the existing transcriptomes and will help in the understanding of important characteristics such as disease resistance and improved genomic selection approaches.
These studies are already underway in cobia, and with the low and medium density panels (> 2,000 SNPs), commercial production is being optimized for genomic selection and obtaining better conversion of feed into biomass. Low and medium density snips can also be used for phenotypic distribution understanding; genealogy reconstruction from the knowledge of the genetic origin of each parent, from which pedigree information can be obtained; and estimation of heritability and prediction of genetic value.
Next-generation sequencing studies have become an essential molecular tool in aquaculture, aiding in the production of
several commercial fish species (Figure 1). De novo transcriptome assembly can be used in several different contexts, such as genomic analyses and gene expression studies, which can be applied in conservation genetics, selective breeding, reproductive biology, and nutrition. As applied to nutrition, the identification of genes associated with protein and lipid metabolism in the liver can help in the development of specific diets to improve the production chain of commercial aquaculture species, including cobia. Through the de novo platform, recent work has described the transcriptome of the cobia liver, providing descriptions of relevent gene sequences for this important commercial criteria. The Illumina sequencing process has provided substantial information about the genomic content of R. canadum
The information contained in this dataset contributes to the knowledge of important biological and physiological characteristics, establishing a solid basis for future studies involving especially nutrigenomics in this species. Various studies utilizing this
same platform of gene analysis have been conducted to improve the understanding of immune function and response in cobia.
Until the last decade, analysis of the cobia transcriptome used short reads of tissues isolated on limited platforms and did not produce meaningful advances in production. However, in the last five years, new transcripts have been discovered, including transposons, alternative splicing variants, and genomic annotations, which together compose the cobia genome. Third-generation sequencing platforms (TGS) have emerged as new genomic research tools due to the advent of high-throughput sequencing technology. Assembly of complete transcripts of a species and splicing variants allow for precise annotations of protein-coding transcriptomes, enabling the understanding of factors that directly affect fish nutrition and health in
commercial settings. Recently, researchers used the TGS platform to obtain complete transcripts from tissue sequencing at different life stages of cobia. That assay described comparative panels on homology with other species, and identified the KEGG pathways of cobia transcripts at different ages. These findings contribute to the knowledge of physiological properties, discovery of new genes, and understanding of mRNA structure in the species, but primarily serve to identify potential nutritional markers in cobia. These techniques can and have been used in other species.
Genome editing technology using CRISPR/ Cas9 is another genomic technique that has been analyzed for potential use in cobia aquaculture. However, its application has only been reported in several cold water and freshwater fish species, as well as oysters and other bivalves. Following CRISPR/Cas9
protocols developed in model organisms such as zebrafish (Danio rerio), aquaculture application typically relies on the injection of the CRISPR/ Cas9 complex into freshly fertilized eggs as close as possible to the unicellular stage of development. This technique allows the mRNA encoding the Cas9 protein to be injected with a (g)RNA guide, leading to the high editing efficiency demonstrated in several other species aimed at editing target genes controlling pigmentation, immunity, omega 3 metabolism, sterility, reproductive processes, and muscle development. Importantly, this CRISPR/Cas9 technology requires the description of highquality, well-annotated reference genomes for the effective design of target gRNAs with high specificity and minimal change from off-target editing. In particular, the metabolic events and unique physiological traits in several fish lineages create significant challenges for the use of gene editing tools.
Practically speaking, genome editing has such significant potential for research applications in aquaculture species because of the ease of access to many thousands of externally fertilized embryos, the large size of these embryos facilitating manual microinjection, and the potential for wide dissemination of improved germplasm for rapid impact through selective breeding (Figure 2). This demonstrates what a powerful tool CRISPR/ Cas9 can be in tropical marine fish production, including in cobia breeding programs.
Presently, the main focus in genome editing studies in aquaculture species are sterility, growth, and disease resistance. Sterility is desirable in aquaculture, especially in species raised in open sea cages, as a method of preventing escapements of farmed fish that may affect wild populations by transmitting edited alleles to wild stocks. Sterility achieved
through CRISPR/Cas9 may also avoid the negative energetic costs of premature maturation, thus allowing greater growth rates and biological efficiency.
It has been widely demonstrated that using genomic tools is of paramount importance for aquaculture – particularly in the most common method of cage aquaculture in open waters. Hence, genome editing technologies will certainly be used with greater frequency and specificity in commercial aquaculture production in the coming years. However, to achieve maximum benefit and minimum risk, this technology must be seamlessly integrated with well-managed selective breeding programs and careful management of genetic diversity to avoid potential inbreeding depression. The CRISPR/Cas9 technology to edit production animals or multipliers has emerged as a powerful tool capable of carrying desirable alleles in the germplasm in cobia broodstock, editing inducible characteristics related to sterility, induction of dysmorphisms for the production of neomales or neofemales, maturation, weight gain, and immunity, but all carried out within all the necessary ethical criteria in gene manipulation technologies. u
Dr. Alcina V.C. Carvalho-Neta, PhD, is a professor at the State University of Maranhão, Brazil, Department of Biology and Animal Sciences. She is a researcher and advisor of the master’s program in animal sciences and the doctoral program in biodiversity and biotechnology. Her areas of interest cover molecular pathology applied to animal health. Dr. Carvalho-Neta is currently a visiting researcher at the University of Miami Rosenstiel School of Marine, Atmospheric, and Earth Science, supported by Maranhão Research and Scientific and Technological Development Foundation (postdoc scholarship process BPD-05172/21).
Dr. José R.S. Torres-Júnior, PhD, is a professor at the Department of Oceanography and Limnology of the Federal University of Maranhão, Brazil. He is a researcher and advisor of the master’s program in oceanography and the doctoral program in biodiversity and biotechnology. His areas of interest cover fish reproduction and marine fish aquaculture. Dr. Torres-Júnior is currently a visiting researcher at the University of Miami Rosenstiel School of Marine, Atmospheric, and Earth Science, supported by Maranhão Research and Scientific and Technological Development Foundation (postdoc scholarship process BPD-05174/21).
Charles J. McGuigan, MS, is a PhD candidate at the University of Miami, U.S., Rosenstiel School of Marine, Atmospheric, and Earth Science. His doctoral research centres on the ecophysiology and ontogenic development of the northern red snapper, Lutjanus campechanus. He is particularly focused on the implementation of novel academic findings in established aquaculture facilities.
Dr. Daniel Benetti, PhD, is a professor and director of aquaculture at the University of Miami Rosenstiel School of Marine, Atmospheric, and Earth Sciences. He works extensively with the academic, government, and private sectors internationally, and has over 200 scientific and technical publications in the field of aquaculture. His areas of interest include technologies of marine fish hatcheries and growout aquaculture operations.
Innovation helps transcend human-centric boundaries. Its applied form found in a gamut of technologies are sound alternatives (to the human element) only if there is compliance with umbrella standards. Regulatory standards cannot be abrogated. When enforced through a top-down approach, regulatory standards do not limit the potential of technologies; rather, they help avoid major bottlenecks and complex challenges that may stall progressive development of a certain branch of technology. Technological applications involving the use of robotics – whether ocean observation, vessel inspection, or aquaculture survey – are still young. New and hybrid technologies in this era, often termed as Industry 4.0, will reshape special sectors that support fundamental benefits derived from marine ecosystem services. Drawing on critical findings from the European Horizon 2020 BUGWRIGHT2 project, this essay documents a sequence of important strands of influence for consideration so that remote inspection techniques could be effectively and efficiently integrated into aquaculture and its current manual driven survey and monitoring framework.
Historically, the fishery and associated activities are moored to the notion of food security. Evidently, continuing in this anthropocenic epoch, fishery remains an acknowledged source of nutrition and is supporting the livelihood of more than three billion people globally. The estimates provided by the United Nations Food and Agriculture Organization (FAO) adds credence to the above claim. Numbers crunched by FAO researchers note that fisheries and aquaculture production have already reached a record peak of 214 million tonnes in the year 2020 with a total of 20.2 kilogram per capita designated for human consumption. Unfortunately, fishery resources are plummeting. Recognizing the invaluable potentials of aquaculture to the staggeringly increasing population of the world, invested efforts seek to achieve sustainability in the fisheries domain.
At the outset, we note that innovation and sustainable fisheries are conjoined concepts.
A cascade of technological breakthroughs under the auspices of the fourth industrial revolution (Industry 4.0) has catalyzed a paradigm shift in maritime and ocean operations. Innovation bolsters support in sustainable movements and approaches – a concept that applies to natural resources.
Labelled as robotics and autonomous systems (RAS), a conflux of disruptive technologies, such as micro aerial vehicles, crawlers, and remotely operated vehicles (ROVs), has added meaning to the abstract concept of sustainability. Central in this innovationled environment is the intention to sustain the ability of the human element. In other words, integrating RAS and machine learning systems helps complete monitoring and inspection tasks that are otherwise dull, risky, and at times strenuous in nature.
Observably, a plethora of challenges are associated with manual inspection, especially when it comes to underwater monitoring that entails inspecting water salinity, temperature, and/or potential of hydrogen (pH) level, as well as oversight in feeding and breeding tasks. Moreover, real-time observation is required to contain corollary effects from untreated effluent discharges with heavy organic load and fish farming infrastructure development. Patently, qualitative assessments indicate that net/fish cages are prone to biofouling and other sources of stress caused by waterbody movements that could potentially lead to net/fish cage deformation. These are instances where RAS integration could act as an improved and perhaps safer method of completing the necessary monitoring and inspection tasks (Figure 1). The industry, as it appears, is slowly turning to service suppliers that are unleashing intervention tools replacing divers with the simultaneous objective of saving time and money, and mitigating environmental concerns.
It is correct to assume that the world of technology is standard reliant. The technology industry, similar to its counterparts, is
assessed based on performance built on safety and risk management systems, among other things. Today, safety and management systems are being integrated into standards. Systems and standards are collectively intertwined into a horizontal topic that necessitates the interaction between public and non-public norms. Private and industry developed standards, as well as corresponding norms and guidelines, serve as external sources of the wider regulatory regime. Adherence and
compliance are critical to the economic value of those industries, while safeguarding public interests. The latter is deemed as one of the principal mandates of governmental bodies that encourage regulators to orchestrate the use of industrial standards. This is done with a view to striking a balance between “hard” and “soft” elements embedded in the subject specific regulatory regime. Taking advantage of industry-based standards can help national regulators maintain a robust regulatory regime
and navigate in a complex landscape shared with other stakeholders to keep pace in a changing technological environment.
Markedly, there is no international regulatory guidance that underscores the “dosˮ from the “don’tsˮ with reference to aquaculture related underwater inspection and monitoring tasks. Notably, the 2018 FAO publication titled Guidance on Spatial Technologies for Disaster Risk Management in Aquaculture only proffers insights into opportunities from spatial technologies including remote sensing, geographical information system, and information and communication technologies while highlighting the need for policy support. Inspection and monitoring technologies remain outside the scope of the 2018 publication.
Deficits are conspicuous when deploying remote inspection techniques. Other than issues emanating from latent defects, usage of emerging technologies or technologies with emerging applications may sporadically give rise to collateral problems that require more than just rebooting the system to eradicate technical errors. In fact, one is faced with challenges for reconciling the tensions between human and robots simply because the techno-regulatory landscape is not fully autonomous despite autonomy being a term that is in common parlance. We notice a system that is marked by “human-in-the-loop” or “human-on-the-loop.”
In the context of human-robot interaction, BUGWRIGHT2: Autonomous Robotic Inspection and Maintenance on Ship Hulls and Storage Tanks (funded by the European Union’s Horizon 2020 research and innovation program under grant agreement No. 871260) is a project that deals with remote technologies applied in vessel hull inspection and maintenance that are statutory and classification in scope. Notably, project BUGWRIGHT2 has proceeded with the objective to bridge the gaps between current and desired potentials of selected remote technologies that are also deployed in aquaculture inspection and monitoring.
Three years into the project’s life cycle, academic partner World Maritime University has produced a state-of-the-art regulatory blueprint comprised of several strands of influence. Founded on state-of-the-art qualitative analysis and 33 interviews with organization representatives from the United States, Canada, China, Singapore, Netherlands, and Norway, the final outcome could serve as a tangible reference once dialogue and discussions commence at the International Maritime Organization (IMO) level pursuant to previous requests tabled by member states (MS) for amendments to the Harmonized System of Survey and Certification and to the Revised Guidelines on the implementation of selected IMO instruments.
It is important to note that BUGWRIGHT2 regulatory blueprint mirrors the need for “harmonization” of international rules and requirements that come into play taking into account the 1982 United Nations Convention on the Law of the Sea’s rule of reference through which MS could implement generally accepted international rules and standards. Cutting a long way short, “harmonization” requires the existence of several parallel stand-alone rules and requirements developed by individual international organizations. Unfortunately, the aquaculture domain, apparently, lacks common minimum standards developed by a specific mandated organization unlike the vessel survey and inspection regime where classification societies are seen as playing a proactive role. A brief overview of standards developed by the International Organization for Standardization (ISO) seems to indicate that ISO/TC 34, ISO/TC 94, and ISO/TC 207 do not necessarily provide insights into procedures for technology integration.
What are the noteworthy takeaways from BUGWRIGHT2 that could be applied to aquaculture technology? The starting point could be that the existing vacuum of procedural requirements should be viewed with a positive outlook since stakeholders have the opportunity to reap the benefits of lessons learned from trial
and errors from other sectors; move forward in unison from the start which would inevitably preclude the need for complex bureaucratic harmonization process (due to efforts being duplicated) in the long run; and establish a top-down platform comprised of organizations/ actors that could collectively reform/amend standards at any given stage. Opportunities are many. That being said, top-down efforts need to concentrate on determining the breadth and scope of specific provisions that could altogether serve as the main frame of reference.
When dissecting two primary regulatory standards, case in point the 1996 Guidelines for Use of Remote Inspection Techniques for Surveys and Unified Requirement Z17 titled Procedural Requirements for Service Suppliers, developed by the International Association of Classification Societies, we note elements for a common minimum standard blueprint. The above documents prescribe the types of permissible technologies and areas of application (under the heading titled general); specific conditions for technology deployment (under the heading titled condition); procedures concerning niche applications (under the heading titled procedures); and procedures related to the work of the human element/service suppliers and the service firm that is approved by the main company (under the heading titled procedural requirements for service suppliers).
Subsequently, we turn to the BUGWRIGHT2 strands of influence to take stock of the dispensable elements that require consideration for transition from manual to technology-based solutions, which would ameliorate and expedite technology integration into aquaculture inspection and monitoring activities. Axiomatically, the first of these strands call for research into cost-benefit assessments to observe whether the advantages of technology deployment outweigh the disadvantages, taking into account, e.g., duration of inspection, costs of deploying technology, and operational downtime. Such assessment could help
companies determine the economic feasibility of turning to remote inspection techniques and rationalized through evidence-based research.
The second strand considers the need for vetted, refined, and up-to-date definitions on each and every type of remote techniques. In tandem, there needs to be in place definitions of important terms, such as autonomy, robot, service robot, and mobile robot. Template definitions exist. Examples are ripe in documents such as ISO 8373:2021; ISO 19649: 2017; ISO/IEC 17000 (2020); Guidance Notes on the Use of Remote Inspection developed by the American Bureau of Shipping; and Guidelines for Use of Unmanned Aerial Vehicles developed by the China Classification Society. Whether template definitions are adapted or whether a completely new set of definitions are developed, it is important to benchmark the term autonomy through a clear and distinct overarching definition. Closely connected to this aspect is a strand that calls for the need to follow the degree of autonomy thread that currently guides the state of affairs for maritime autonomous surface ships (Table 1).
Identifying levels of autonomy and associating them with different classes of techniques could help keep track of the autonomy-paradigm trajectory. Again, the current system is not fully autonomous (i.e., systems that enable machines to interact with the environment (through built-in sensors) and respond/take decisions accordingly), and requires categorization so as to help review the extent of involvement of the human element. This, in turn, has an explicit nexus to what is known as a well-calibrated trustworthy ecosystem that requires a form of constructive balance between the human agency and autonomous modes. Until the human stays “in-the-loop” or “on-the-loop,” carving out the degrees of autonomy is a vital stepping stone to ascertaining, projecting, and designing effective and efficient human-robot teams for the conduct of survey and inspection. The next strand that would apply to the aquaculture profile is the need to carve out
operational and technical common minimum standards. Generally speaking, operational standards emanate from tests and risk assessments that help narrow down all potential risks associated with the deployment of each and individual categories of technologies. Risk information is relayed (by manufacturers) via product information notes for consideration by end users or service suppliers. Different remote inspection techniques are marked by operational and technical differences. It is worth noting that surveys using aerial drones, unlike crawler and ROVs, can easily be compromised due to humidity, lighting, and air turbulence. Furthermore, hybrid techniques that have the potential to conduct underwater biofouling cleaning, in addition to survey operations, require limiting all possible risks prior to deployment.
Risks on air or underwater tend to range from dropped object risks to collision risks (with other remote inspection technologies) to lost link risks (that originate from network compromise), which could be an issue once technologies reach the fourth degree, i.e., full autonomy. “Stealth technology” is a term often ascribed to autonomous underwater vehicles and the likes, and therefore, completion of tasks without disturbing underwater ecosystems is highly anticipated. Contingency
plans will, nevertheless, need to be developed taking into account operational standards so that solutions could be forged before the occurrence of environmental damages.
Another important strand of influence corresponds to a feature innate to technological devices applied in observational work and is aptly known as data management. Generally speaking, data acquisition lies at the heart of all technological interventions. Stakeholders involved in this process generally include non-human actors, e.g., technological tools and infrastructure, and human actors, i.e., service providers and companies (end users). The latter is coined as “human-in-the-loop” with supervisors, operators, and surveyors remaining engaged during data storage and verification of data collected through remote inspection technique-based inspections and surveys. In essence, the technological platform communicates data to “human-in-the-loop” via five independent layers: hardware, network, internet, infrastructure, and application. The last layer, i.e., application, mirrors implementation of decision.
Although the BUGWRIGHT2 regulatory model considers survey and inspection data as belonging to the asset, i.e., the ship, thus forming a part of the owner’s proprietary
rights, this very theory on ownership might not apply verbatim in the field of aquaculture. Notwithstanding, the following questions may still be subject to further consideration from an aquaculture perspective:
• Who should retain the copyright of data gathered from underwater remote technologies?
• What are the secured ways through which data could be shared between end users and stakeholders?
• To what extent do provisions on data control and security apply in the field of aquaculture survey and inspection?
• What is the duration of data preservation, and should there be any mechanisms to safeguard service providers against thirdparty liability?
The final strand is tied to a critical aspect: in-water environmental consideration. Depending on the location of aquaculture method and practice (freshwater, brackish water, and marine), risk assessments will need to be conducted at regular intervals to check for impacts and water conditions, especially if hybrid remote techniques will be used to clean pen nets and cages. This begs the question whether those techniques are properly equipped with storage systems for storing debris collected during clean-up operations. In this regard, the Baltic and International Maritime Council developed standards for in-water cleaning that could serve as a foundation for furthering control over impact of technology in-water. Although developed for in-water cleaning of a vessel’s hull and other niche areas, the ROV cleaning standards encapsulated in the section titled “operating requirements of the cleaning system” contains an important checklist comprised of postcleaning inspections (s. 9.3), post-cleaning safety and environmental requirements (s. 9.4), and service reports after cleaning (s. 9.5) that serve as model provisions for consideration by the industry. The checklist may very well be pertinent to remote inspection technique-based operations underwater.
At this juncture, it is essential to ask one final question: why are the strands of influence discussed relevant? Technology is a terraforming practice – one that could possibly shape and structure the environment. Yet technology, in parallel, is merely a product. Products can be defective. Defective products could give rise to unforeseen circumstances. Those are the circumstances that might inhibit end users from untethering the full potentials of Industry 4.0 byproducts that are able to add strength in projects that purport to support sustainable actions. Standards embedded in previous discussions are strands that could positively influence technology deployment in maritime projects. Strands such as liability and in-water environmental damage will certainly have a bearing on the technologies that will be deployed in aquaculture so that companies may derive good results from automated farming practices.
Fragmentation must be avoided should there be any intention to transfer technology to other parts of the world, namely developing and least developed countries where aquaculture production is relatively higher than other parts of the world. Despite current practices, self-regulation does not always help set robust standards that determine the strengths and limits of a certain technology. By the same token, selfregulation may not be a viable approach as different industries utilizing the same type of technology for different purposes contribute to the development of disparate standards. If the status quo is not rectified beforehand, collateral dormant problems will be transferred with the technology. Whatever pathways are explored, it is important to establish standard methodologies for technological platforms to buttress adherence and compliance. u
Dr. Tafsir Matin Johansson is an assistant professor at the World Maritime University-Sasakawa Global Ocean Institute (GOI) in Malmö, Sweden. Dr. Johansson is a techno-policy analyst with a PhD in maritime affairs from the World Maritime University. His duties at the GOI include ocean governance and policy research, teaching, and developing innovative policy models to better assess drivers and indicators relevant to ocean research agenda. He has published extensively on maritime and ocean issues including techno-regulatory dynamic governance, Arctic governance, vessels of concern, corporate social responsibility, marine pollution, climate change, conflict management and trust ecosystem, and Brexit and fisheries. He has worked on or led a number of multidisciplinary projects, including regulatory development projects funded by Transport Canada (Government of Canada) since 2014, as well as those funded under the Canadian Government’s Oceans Protection Plan covering numerous topics critical to the maritime and ocean domain. Currently, Dr. Johansson serves as a CO-PI in a European Union Horizon 2020 Programme funded project titled “Overcoming Regulatory Barriers for Service Robotics in an Ocean Industry Context.”
Dr. Dimitrios Dalaklis joined the World Maritime University in 2014, upon completion of a 26-year distinguished career with the Hellenic Navy. His expertise revolves around the interrelated maritime safety and security domains. He is an associate fellow of the Nautical Institute and a member of the International Association of Maritime Economists. With a bachelor’s degree from the Hellenic Naval Academy, his postgraduate studies took place in the Naval Postgraduate School of the United States (M.Sc. in information technology management, with distinction, and M.Sc. in defence analysis). He then conducted his PhD research at the University of the Aegean, Department of Shipping, Trade, and Transport. He is the author/co-author of many peer-reviewed articles, books, and studies in both Greek and English languages, with a strong research focus on issues related to the implementation of the SOLAS Convention and especially electronic equipment/ systems supporting the safety of navigation.
Dr. Aspasia Pastra has been appointed as a post-doc fellow and maritime policy analyst at the World Maritime UniversitySasakawa Global Ocean Institute in Malmö, Sweden. To date, she has been involved in a number of state-of-the-art regulatory projects in maritime policy, ocean technology, environmental protection, port governance, and gender diversity in the maritime sector. Dr. Pastra has published extensively in the field of maritime policy and governance, maritime robotics and techno-regulatory advancements, global environmental change, team dynamics, and leadership. She has been a lecturer in U.K. institutions in the field of business and maritime administration. She has extensive experience in shipping as she worked for many years in large shipping companies. She has also participated in the Marine Environment Protection Committee and Maritime Safety Committee of the International Maritime Organization, as a member of the Greek Delegation. Dr. Pastra holds a B.Sc. in public administration from Panteion University of Social and Political Sciences in Greece, an MBA from Cardiff University in the U.K., and an M.Sc. in maritime administration from the World Maritime University. She was awarded her PhD in the area of corporate governance from Brunel University in London.
A slightly reduced version of this essay is published in the Newfoundland and Labrador Aquaculture Industry Associationʼs Cold Harvester magazine, summer edition 2023.
The concept of artificial intelligence, or AI, was first described in the mid-20th century in a now famous article titled Computing Machinery and Intelligence (Turing, 1950). At the time it was thought that computers could enable humans and eventually machines to perform certain tasks to address production needs. However, it was expensive to use computers with limited computing capacity that were the size of a standard bungalow, and it often took hours, if not days, to do large computations. It was also difficult for computers to connect machines by any other means than a phone line, as cellular phones and the internet had not yet been widely invented (1960s for cells, 1990s for the internet, and 2000s for cloud computing). Fast forward to the present day with cloud computing, the internet, wireless communications (cell or satellite), even our handheld smartphones, are much more powerful and faster (almost real time) and allow humans to activate or enable certain tasks to be done on the farm remotely by machines. The definition of AI from the Oxford Dictionary is “the theory and development of computer systems able to perform tasks that normally require human intelligence, such as visual perception, speech recognition, decisionmaking, and translation between languages.” It has taken a while to get to this point since AI was first recognized almost 75 years ago.
When combined with machinery that serves a purpose, AI algorithms are capable of machine learning, whereby input data from a variety of sensors (visual, auditory, or other) are used repeatedly and, if offered by the farmer, decision-making on a course of action. AI systems do result in the recognition of individual animals and can take on a task suitable for that animal’s welfare. One only has to think about milking robots used in
the dairy industry today, all powered by AI and “learned” machine technology. In this case, the computer develops an algorithm (a mathematical model) that is constantly being updated millions of times over, which tells the robot which cow is entering the milking area, the size and shape of her udder and teats, her daily production of milk, and whether she is developing mastitis, among other health attributes. In other words, the machine (i.e., the robot) has learned to take care of the individual dairy cow, and relays that information to the farmer (on wireless or other communications) so they can take appropriate management action for the animal’s welfare.
These AI powered machine learning agriculture and agrifood operations are referred to nowadays as precision farming or smart farming. It has also been employed in terrestrial agriculture for well over a decade, from the dairy milking robots mentioned earlier, to spreading fertilizer and monitoring plant growth in field crops by using real-time indicators of water and nutrient levels in the soil. An additional benefit of these systems is that they allow farmers to lower greenhouse gas emissions to reduce costs of production and enhance yield and productivity in their crops. Some robots can even identify weeds and pests, and remove these mechanically or chemically, all using AI-powered algorithms.
Machine learning, of course, is only as good as the sensors, the input data they generate, the algorithms behind it all, and the communications available for decisionmaking. Communications in real time, via satellite, mobile phone, or other internet connection, is crucial for effective decisionmaking, and so are infrastructure needs in order for this to be of benefit to all farm producers, whether on land or sea.
AI is currently being used in aquaculture settings on land and at sea for a variety of purposes, including environmental monitoring, tracking from egg to plate, early disease and
parasite detection and animal health, growth and biomass estimation, processing, and feeding, among others. Some aquaculture machinery that is AI powered even serves to treat animals for health conditions.
One example of a fish health application, fit for purpose, is the Stingray delousing system, developed over a decade or so (Figure 1). The devices can count and size sea lice stages, determine infection rates, and destroy the parasites with the use of underwater lasers. This laser system has been trained to delouse a couple of salmon and cod parasites – all of this without further human intervention or decisionmaking. The system also uses biometric data to estimate biomass and growth in a given net pen of salmon. After years of development, the system appears to work well with over 800 units in operation in Norwegian and Nordic salmon farms at present, with a huge order backlog. Continuous development of both hardware and software over the years has improved the
system several times. All nodes are online and in a virtual neural network that secures overthe-air updates and frequent training of each detector. The overall system is now referred to as the Fish Health Hub from Stingray.
New upstart companies are also developing even smarter AI systems for fish health management, providing treatment options depending on the environmental conditions in which the farms are located. For example, AquaByte (Figure 2) highlights the following on its site: “Healthy fish at lower cost. Automatic lice counting, welfare scoring, and biomass control. Information for datadriven decision making to increase revenue.” One of the different attributes in this system is that real-time environmental data can be incorporated into the algorithm for more rapid development of patterns and treatment options. This has captured the attention of several large fish farming corporations in Europe, North America, and South America.
Another startup has planted its feet firmly on land-based fish farming systems. ReelData uses optical and water quality data in real time to optimize feeding of salmonids autonomously, while measuring growth, biomass, and stress in fish in real time in recirculating aquaculture systems (Figure 3). A number of these systems are in operation in North America, Europe, and South America.
Innovasea is an ocean technology leader using the best in-situ communications and environmental monitoring for fish growth, biomass, autonomous and remote feeding, and oxygenation in ocean pens, using specialized acoustic receivers to transmit data via satellite or cellular. All of this is in real time so rapid decisions can be made by the farmer on a course of action. The CageEye system from
Norway aims to accomplish the same in automated feeding for salmonids in particular.
On the plankton monitoring front, there are AI systems emerging to detect plankton that may provide much better predictive capacity and decision support for mitigating impacts on these types of concerns. The leader in this space is Blue Lion Labs, which uses machine learning to automatically identify and count plankton for dealing with toxic or harmful plankton concerns for aquaculture farms (Figure 4).
Wittaya Aqua has developed a digital ecosystem for aquaculture producers, feed manufacturers, and feed ingredient suppliers (Figure 5). Its AquaOp Farm and AquaOp Feed platforms allow companies to optimize aquafeed nutrition and improve farm production practices through the power of data. Wittaya’s software has been developed for most farmed species and is based on decades of cutting-edge research. The software is cloud-based and accessible anywhere in the world by subscription.
Optoscale and iFARM are yet other versions of smart fish farming in development at the moment, showing promise for real-time fish welfare and health tracking and management. These systems, as with others, are meant to operate in both net pens in the ocean and/or semienclosed containment systems for fish rearing.
Fish are not the only animals being used in aquaculture smart farming operations. The most valuable aquaculture output globally (in U.S. dollars) is the shrimp farming sector, ranging from small-scale “ mom and pop ˮ operations to intensive and hyperintensive systems operating on land. With increasing needs to monitor inputs and outputs of these farms for production, some operations are resorting to AI and machine learning for controlling the growout cycle. Similar to approaches used in finfish AI systems, various sensors, cameras, and probes are employed to monitor in real time the shrimps’ health and performance, and to control environmental parameters for optimal production.
Xpertsea is a fairly new startup from Canada for semi-intensive shrimp farms and touts itself as the future of sustainable shrimp farming globally. It is currently developing its machine learning and algorithms in southeast Asia and soon in central America.
Vertical Oceans is a commercial land-based vertically integrated shrimp farming company in Singapore whose claim to fame is fullcycle production efficiencies (zero-waste, 100% recirculating aquaculture system) using AI, machine learning, and various insitu sensors to manage production. In fact, shrimp harvests are distributed daily within
hours from its smart farm modular system, based on customer demand, so it is the freshest possible. The interesting part of this system is the broodstock, post-larvae, and growout systems in tanks as well as customer demands are integrated into the algorithms vertically so there is little wastage.
Last, but not least, autonomous delivery of feed by vessels to remote salmon farms has taken place in Norway recently, powered by AI and machine learning to deliver the food safely in open ocean conditions. These are but a sampling of a few types of AI-machine learning applications in aquaculture. Future endeavours in farm AI at sea and on land in aquaculture will almost certainly include robot mitigation of unfavourable environmental conditions such as plankton blooms, low oxygen levels, temperature anomalies, and other health and welfare challenges. At some point, entire farms will be autonomous production facilities, requiring only oversight by humans on the technical side of things.
Communications and sensor performance are essential for good AI solutions for fish farming. If reliable data cannot be transmitted in real time to the operator, they may not be able to manage or instruct robots on performing a stock-saving operation or an environmental mitigation instruction for the fish. These communication tools need to be rapid, precise, and use reliable sensors for a well-run operation, with animal welfare always first. This is often a constraint in many parts of the world where cell or internet services are unreliable, but perhaps satellite high-speed internet might be option at relatively low cost (e.g., Starlink).
Another possible constraint is that farmers will need access to data scientists or employ these folks to develop and understand their AI systems. These positions are increasingly in demand across economies of the world using large data inputs and analytics tools. Farmers will always want to own the data from their
own farms, so they need to be careful as to the terms of service and security provided by AImachine learning specialists and providers. Security of data and who uses it and for what purpose is always something a farmer will be concerned about.
One exciting trend in the AI-machine learning sphere is the use of real-time eDNA monitoring for pests, parasites, diseases, even fish presence and absence. Readers may be familiar with detection of infection loads from the COVID-19 virus and its variants in municipal wastewater, but systems are now available to detect organism presence or absence in aquatic systems in real time. Why not use them for predicting plankton, disease organisms, or pests like sea lice larvae, and other interactions among farmed and wild organisms in real time? There is plenty to be done on this in the near future.
The race is on now for optimizing food production both on land and at sea in the agriculture and agrifoods business. Improvements will be made at rapid pace, each year bringing new solutions for reducing environmental impacts of farming, improving animal health and welfare, and connecting with customers in real time. It is an exciting time to be in the seafood farming business with all of these innovations. u
Cyr Couturier is marine biologist, aquaculture scientist, and chair of the M.Sc. Sustainable Aquaculture program at the Fisheries and Marine Institute of Memorial University. He has 35+ years of experience in applied research and development, training, and education in aquaculture and fisheries. He is a board and executive member of several farming and development associations, including Canadian Aquaculture Industry Alliance (CAIA), Canadian Federation of Agriculture, Canadian Agricultural Human Resource Council, HORIZON TNL; and is a past president of Aquaculture Association of Canada, CAIA, and Newfoundland and Labrador Aquaculture Industry Association. He has worked in aquaculture and fisheries development in over 18 countries. The views expressed herein are his own. cyr@mi.mun.ca | Twitter @aquacanada
Tropical and subtropical marine finfish aquaculture, although incipient, has already demonstrated cases of success in largescale production, either on land or in offshore cages. At the University of Miami Experimental Hatchery (UMEH) facilities, we conduct research and technology development of commercially important tropical and subtropical marine fish species, particularly on reproduction and hatchery technologies. The focus is on practical and applied research to support the industry, in addition to training scientific, technical, and managerial staff as well as undergaduate and graduate students. Along with genetics and nutrition, effective broodstock management aimed at producing high quality offspring is one of the most important aspects for the success of the entire aquaculture production cycle – from eggs to market.
Cobia (Rachycentrum canadum), snappers (Lutjanidae spp.), Seriola (Seriola spp.), mahi-mahi (Coryphaena hippurus), groupers (Epinephelus spp.), red drum (Sciaenops ocellatus), and pompano (Trachinotus carolinus) are just a few of many challenging new species of marine finfish currently being produced at some level. Despite their potential for production, several challenges and biotic/abiotic factors can and do affect their broodstock fertility, including but not restricted to genetic and nutritional issues, seasonality, environmental and management stress, behavioural dynamics, and pathogens. Some of these issues are addressed and discussed in the following sections.
An important issue in broodstock (Figure 1) selection is to carry out genetic screening to increase genetic variability and detect genes that are important for reproduction and production. This topic falls under nutritional genomics, which can be divided into two disciplines: “nutrigenomics,” which study the effect of dietary ingredients on gene expression, and “nutrigenetics,” which study
how the genetic makeup reacts to a specific diet. Any errors in this process can lead to inappropriate broodstock, the occurrence of inbreeding depression, reproductive failures, and poor progeny performance. Parental diet and nutritional variations can manipulate offspring physiology and phenotype, a mechanism that is believed to be controlled by epigenetics. Additionally, digested and absorbed feed, ingredients, and additives influence gene activation and transcription, enzyme activities, protein expression, gut microbiota, and metabolism. Mating between genetically related individuals and increased homozygosity could mean an incalculable loss to the performance of offspring of exceptionally promising species such as cobia.
Genetic marker-assisted breeding programs and the production of offspring based on pedigree mating systems remain a new and unexplored method for tropical and subtropical marine finfish aquaculture. It is important to consider that the reproductive failures are not only attributed to changes in genetic characteristics, but also to multiple environmental, nutritional, social, and individually non-heritable factors.
The energy partition in finfish is linked to their genetically inherited potential and to environmental and external variations. Lack of essential nutrient reserves in broodstock compromises gonadal maturation in both males and females, egg composition, and the survival capacity of the larvae in the yolk-dependent phase. The reproductive performance of marine broodstock depends on a high-quality diet that contains rich and digestible proteins, energy, lipids, vitamins, and minerals. Our own experience has proven that satisfactory reproduction rates are achieved for several species of tropical and subtropical marine finfish using as a basis a strict feed regimen of high-quality sardine, squid, and shrimp – provided at satiation levels corresponding to 2-5% of the total tank biomass per day. Additionally, the broodstock of each and all species must receive a nutrient
supplementation with a customized premix maturation stimulator containing high levels of essential fatty acids, amino acids, carotenoids, vitamins, and minerals.
Fertility potential is controlled by genetic factors and strongly influenced by diet, the rearing system, and other intrinsic and extrinsic conditions. Environmental seasonality deserves to be highlighted as it is a key factor to stimulate or inhibit the reproductive pathways.
In addition, it is recognized that one of the biggest challenges in broodstock management of marine fish as well as penaeid shrimp worldwide continues to be replacing fresh or fresh-frozen feeds with dry feeds to reduce the risk of pathogens being transferred from the natural feeds to the broodstock. This represents a biosecurity breach that is often overlooked as the advantages of providing fresh/frozen natural diets to broodstock
outweigh the risks. Indeed, natural food items such as small pelagic fish, squid, and shrimp as well as bloodworms not only provide key nutrients but also have an important role in the feeding behaviour of the broodstock, spawning performance, fertilization, and egg and offspring quality.
Reproductive seasonality has been a challenging factor for controlling and maintaining yearly linear egg production on a commercial scale. Seasonality is not only restricted to temperature effects, but also to photoperiod and water characteristics that can also vary strongly with rainfall. In regions located at lower latitudes with seawater temperatures generally ranging from 20 to 30°C, several species can and do spawn volitionally throughout the year when properly managed. Proper management includes photoperiod and temperature
control, outstanding water quality parameters, adequate sex ratio, nutrition, as well as a deep knowledge of the biological and physiological characteristics and demands that must be met for each species. Large or abrupt variations in seawater temperature generally occur in tropical and subtropical regions. In tropical regions, where water temperature ranges from 18 to 32°C, for example, temperature drops below 22°C are common and known to cause stress, immediate volitional spawning disruption, and, quite often, lead to outbreaks of ectoparasitic diseases such as Cryptocarion spp. in tropical species.
In these regions, for most species, high spawning efficiency and egg viability can be achieved when water temperature is naturally or artificially maintained above 26°C. That being said, many breeding centres do not have the facilities or financial support to maintain temperature control using a heater/ chiller unit for an extended period of time. In these cases, an alternative would be the use of hormones such as human chorionic gonadotropin, gonadotropin-releasing hormone, and luteinizing releasing-hormone synthetic and analogues agonists or dopamine
inhibitors to induce and synchronize ovulation/ spermiation with relative success. In species that do not respond well to hormone treatments alone, success can be achieved by combining hormone injection and photothermal manipulation. Hence, in aquaculture, for difficult species to spawn, a combination of endocrine and environmental manipulation is used to control reproduction with a variety of products that act to stimulate the endocrine system either at the level of the hypothalamus, the pituitary, or the gonad.
Stressors affect captive broodstock reproduction in a variety of ways. Depending on the nature and severity of the stressor, the endocrine system that regulates reproductive development in fish – known as the hypothalamus-pituitary-gonadal axis – may be compromised and disrupted altogether. The domestication in restricted environments has contributed to many reproductive problems such as disruption of final maturation and failure of spontaneous spawning and unfertilized eggs, for example. In nature, the process is controlled by environmental factors such as temperature, photoperiod,
Low fertility Low hatchability
Low offspring resistance
Aggressive chasing
Economical losses
ENVIRONMENT No hormonal stimulus
No hormonal stimulus nor synthesis
No oocyte maturation No volitional spawns
MANAGEMENT Immunosuppression
Parasites/pathogens
Lesions/mortality
Mating inhibition
Spawn retention
Asynchronic spawns
Subfertility
Inbreeding depression
Phenotipic field selection
Low genetic variability
Natural behaviour
“unclear”
Inadequate temperature
Inadequate photoperiod stimulus
Low feeding activity
“unclear”
High biomass density/poor water quality
Stressful manipulation/drugs injection
Ineffective hormonal induction
Abnormal mating behaviour
Inadequate male:female ratio
Adaptation timing
Parasites/pathogens
“unclear”
nutrition, water quality, behavioural dynamics, migration patterns, etc. A lack of these maturational processes has been reported in wild caught species like Seriola spp. and Lutjanus spp. due to the fact that confinement stress may be the cause of inherent difficulties in maturation and spawning under culture conditions due to release of inhibiting hormones and elevated cortisol levels. However, researchers have managed to counteract specific challenges and, with proper management, achieved control of reproduction of Seriola spp. and Lutjanus spp. as well.
Conversely, cobia (Rachycentrum canadum) and mahi (Coryphaena hippurus) broodstock, for example, tend to adapt to tank conditions quickly and, when captured in their full reproductive period, respond to proper management by volitionally spawning within weeks to months – as long as optimal conditions of water temperature, biomass, stocking densities, and sex ratio as well as adequate nutrition and feeding are maintained. Combined with state-of-the-art maturation systems, conditioning and volitionally spawning of several commercially and ecologically important tropical and subtropical
Genetic sampling Analysis
Marker-assisted selection
Pedigree-based tagging
Rotational spawning program
Introducing wild broodstock
Photothermal manipulation
Water temperature ≥ 24 -26°C
Light photoperiod ≥ 12 h
Hormonal induction
Ideal biomass density/larger tanks
Ideal male:female ratio
Photothermal control
Hormone induction
Tank adaptation time
Monitoring mating/feeding behaviour
Biosecurity prophylactic procedures
Symbiotic cleaning stations
marine finfish species has been achieved, as reported in the literature and seen in practice with the commercial production of a number of these species by the private sector (Figure 2).
A main challenge is to provide management procedures that mitigate stressful conditions that can cause abnormalities in the mating behaviour of finfish. Behavioural dynamics can be extremely complex and are of paramount importance, particularly in reef species such as snappers (Lutjanidae spp.) and groupers (Epinephelus spp.). For example, aggressiveness in chasing females or other males can occasionally be responsible for serious injuries and even death. These events are common and occur in cobia, mahi-mahi, snappers, and groupers broodstock at the UMEH and other facilities around the world we collaborate with, such as the CEINER in Colombia. In any case, during the first days after mating groups are formed, it is recommended to diligently observe the broodstock behaviour, reduce the biomass, or replace fish within the tank, if necessary.
Regardless of species, broodstock living under stressful conditions become more
susceptible to diseases. High biomass can lead to fish aggression that, coupled with disruptive feeding behaviour, can cause high susceptibility to diseases. Prevention is the most viable disease management strategy in aquaculture and is primarily driven by strategies to avoid or minimize the stress.
Strict biosecurity protocols must be implemented to reduce stress and consequential disease outbreaks and broodstock management disruptions. The protocols include freshwater bath, thorough inspection and removal of parasites, formalin or hydrogen peroxide baths, followed by a one to two week quarantine period during which the newly introduced fish remain isolated. In addition, the broodstock should be kept in tanks under standard mechanical filtration procedure with sand filters, fine mesh bag filters, biofilters, protein skimmer, and UV sterilizer. Additionally, fish should be moved to clean tanks that have been disinfected. Eventually one can use cleaning stations in the broodstock tanks in which cleaner fish such as the neon goby (Elacatinus oceanops) are placed to symbiotically alleviate stress and reduce or eliminate ectoparasite infestation on the broodstock fish. Table 1 shows the main issues and recommendations for reproductive success in tropical and subtropical marine fish aquaculture. u
Dr. José R.S. Torres-Júnior, PhD, is a professor at the Department of Oceanography and Limnology of the Federal University of Maranhão, Brazil. He is a researcher and advisor of the master’s program in oceanography and the doctoral program in biodiversity and biotechnology. His areas of interest cover fish reproduction and marine fish aquaculture. Dr. Torres-Júnior is currently a visiting researcher at the University of Miami Rosenstiel School of Marine, Atmospheric, and Earth Science, supported by Maranhão Research and Scientific and Technological Development Foundation (postdoc scholarship process BPD-05174/21).
Dr. Alcina V.C. Carvalho-Neta, PhD, is a professor at the State University of Maranhão, Brazil, Department of Biology and Animal Sciences. She is a researcher and advisor of the master’s program in animal sciences and the doctoral program in
biodiversity and biotechnology. Her areas of interest cover molecular pathology applied to animal health. Dr. CarvalhoNeta is currently a visiting researcher at the University of Miami Rosenstiel School of Marine, Atmospheric, and Earth Science, supported by Maranhão Research and Scientific and Technological Development Foundation (postdoc scholarship process BPD-05172/21).
Carlos E. Tudela, MS, is the assistant manager at the University of Miami Experimental Hatchery (UMEH). He oversees all UMEH production operations: broodstock, larval rearing, shipments, equipment maintenance, fish health, data collection/ experiments, and personnel training. Mr. Tudela has over 10 years of aquaculture experience. His area of interest is in production technolgy of marine finfish.
Julio Camperio, MS, is an aquaculture nutrition PhD student at the University of Miami Rosenstiel School of Marine, Atmospheric, and Earth Science (RSMAES) working on cobia ( Rachycentron canadum ) in-vivo digestibility, endogenous digestibility markers, and bioenergetics. In 2020 he received a master of science from RSMAES, which focused on evaluating rabbitfish ( Siganus lineatus ) feeds where fishmeal had been completely replaced by plantbased meals in Palau, Micronesia.
Dr. Jorge Suarez, PhD, is an aquaculture nutritionist with a proven track record of excellence in research and development of aquafeeds. He is an accomplished and experienced scientist whose research focuses on the quality, cost, and performance of feeds for commercially important cultured species of fish and crustaceans. His investigations at the University of Miami are focused in developing practical diets for marine fish of high commercial value.
Dr. Daniel Benetti, PhD, is a professor and director of aquaculture at the University of Miami Rosenstiel School of Marine, Atmospheric, and Earth Sciences. He works extensively with the academic, government, and private sectors internationally, and has over 200 scientific and technical publications in the field of aquaculture. His areas of interest include technologies of marine fish hatcheries and growout aquaculture operations.
Biological, technological, and market criteria as well as feasibility levels must be evaluated to rank the suitability of marine fish species for commercial aquaculture. We developed a methodology termed Aquaculture Performance Index (APIX) considering all these parameters represented by a simple model to determine the prospects of any species for commercial aquaculture development in land-based, coastal, or offshore systems. Here, as a case study, we focus on tropical and subtropical marine fish species selection for offshore aquaculture in the Gulf of Mexico. The APIX equation considers the following basic criteria, each assigned different weights to calculate a respective final value/score: feed conversion rate, growth rate to market size, survival rate, stocking density, feed costs, fish processing yield, marketability (demand and price) as well as the feasibility levels.
APIX scores were calculated and assigned to each species. Red drum (Sciaenops ocellatus) was the top selected species using this methodology (Figure 1). Even though red drum has a lower market value when compared to some of the other species considered, it was found to be the most feasible since it thrives at wide temperature ranges (16 to 32°C), tolerates a much higher stocking density (>30 kg/m3), and has a lower feed cost since good growth and survival results can be obtained using a high percentage of soy protein as a substitute for fishmeal. In addition to that, there is a long history of red drum production in the southern U.S., which makes fingerlings and feed readily available as well as an established market. As a competitive advantage, red drum produced offshore will likely have an improved taste (no off-flavour) when compared to pondreared fish and, for this reason, has a potential of being marketed as premium. However, we suggest a thorough market research study to establish the price and support the economic analysis model for the species in offshore cages. It is also worth considering an innovative approach conducting trials combining the fish farming operation with
seaweeds and/or bivalves culture in an integrated multi-trophic aquaculture (IMTA) system. Some of the native species of mollusk bivalves with potential are the Lion’s paw scallop (Nodipecten nodosus), the Atlantic oyster (Crassostrea virginica), and the hard clam (Mercenaria mercenaria). Euchema spp., Gracilaria spp., and Hypnea spp. are likely suitable seaweed species to be recommended based upon their application for agar and carrageenan production among other potential uses (biostimulants, feed, cosmetics, etc.).
IMTA adds unfed aquaculture organisms –filter feeders (mollusks) and nutrient stripers (seaweeds) – to fed aquaculture (marine finfish) and has the potential of producing additional crops with relatively low inputs while improving water quality utilizing the organic and inorganic wastes of the fish. It then represents an interesting option and deserves serious consideration when implementing offshore aquaculture in the Gulf of Mexico and elsewhere.
With respect to feasibility levels, the broad criteria analyzed were combined to determine whether the species rank either at the Experimental , Technological , or Commercial feasibility level. These concepts are flexible and often overlap as the technology for producing a species from egg to market might exist and be prevalent but its commercial viability has not been yet proven. This is the case for several tropical marine species. Indeed, there are still no operations raising some species whose technology for full cycle production is available and turnkey projects with business and production plans are handy. Investors prefer less risky alternatives when deploying the extensive resources required to develop a commercial aquaculture operation. It is a long-term commitment requiring high investments and a thorough understanding of the business.
The following criteria related to the species to be cultured were considered for the final selection:
Market criteria: Market and marketing related criteria are crucial for species selection. Market demand and value are decisive for the business planning and implementation of the operation. This is where it all starts and how revenues are generated. Whether the species selected already has a high market demand and reasonable price are the most important considerations. At the very least, the species must have the potential to be turned into an appealing and high-quality product that will meet consumer expectations. Relevant considerations include acceptance by a large segment of the target population, which may include mass markets and/or specific niche markets such as white tablecloth restaurants, Japanese restaurants (sashimi grade), live products, etc. It is also important to understand the changes in the market and the market behaviour in response to certain predictors. Organoleptic characteristics such as flavour, taste, odour, etc. may be more important to certain market niches than others. Also, the appearance of the product (external and flesh: white flesh, red muscle tissue, marble colour, fat content, etc.) as well as its texture (firm, buttery, mushy, fatty, etc.) are key factors in the process. The level of processing (whole fish, gutted, fillet, frozen, fresh, fresh/flash-frozen, ready-to-eat portions, etc.) is also a key factor. Processing level is determined by the target market needs.
Technological criteria: The level of readily available technological development for the culture of the species from egg to market is also a crucial consideration. It is important to ensure that there are hatchery, nursery, and growout technologies available to raise the species throughout its entire life cycle, from egg all the way to harvest. Technological criteria encompass maturation, spawning, incubation, larval husbandry, fingerling production, nursery techniques, adequate feeds, growout equipment and methods (including cages, net cleaning/replacement), and harvesting techniques. Likewise, availability of feeds formulated to meet the nutritional requirements of the species at the various life stages is an important consideration.
Technology is available for all the pre-selected priority species in this case. In addition, recent developments in seaweed and bivalve cultures in offshore systems enables an IMTA approach, which can take advantage of the infrastructure, generate additional revenues, and minimize environmental impacts.
Socio-economic and regulatory criteria: It has become increasingly important to take social and economic considerations into account when selecting a species for commercial aquaculture development. Responsible aquaculture development must be socially accepted and viewed as a powerful means of generating high quality food/protein, jobs, and income, with a minimal environmental footprint. In essence, aquaculture should minimize competition with existing fisheries. Instead, aquaculture should be regarded as a harmonic complement to fisheries by diminishing the pressure upon declining and overfished stocks. It is also important to consider the regulatory situation and the role of government in establishing laws, rules, and regulations for open ocean aquaculture development. The process for obtaining the concession of an area may still be relatively complex, expensive, and time consuming, requiring expertise to navigate the system.
Ecological criteria: Whether the species is native, endemic, or exotic to the particular region in question is an important ecological consideration. The introduction of non-native species for aquaculture is not recommended since it is unlikely to be authorized. In addition, many non-governmental organizations and environmentalists are against introduction of non-native species and that can also create undesired public opposition to a project. These considerations are highly relevant for obtaining permits for new operations and must be considered when selecting a species to be raised in open environments. This is even more important when cages are to be used in exposed areas in the open ocean, considering risks of escapements that, even though mitigated, could potentially occur. For such reasons, only
native species to the Gulf of Mexico are being considered for this case study.
Biological criteria: In terms of priority, biological criteria are likely to be the most important for species selection because they determine the technical feasibility and express the aquaculture performance of any given species in terms of densities, survival, growth to market size, and feed conversion rates. These characteristics are recognized as the most important criteria for species selection for commercial aquaculture. The selected species need to have physiological characteristics that will enable it to thrive and grow to market size in the proposed site. Technically, an ideal species for aquaculture must be able to withstand wide ranges of temperature (eurythermal) and salinity (euryhaline) as well as other environmental parameters such as low dissolved oxygen levels, high ammonia, pH variations, etc. The precise environmental stressors likely to be encountered in the given environment and production method can usually be determined based on similar operations and are of chief concern. The open ocean environment considered in this case study is very unlikely to experience low dissolved oxygen or high ammonia levels, as well as other metabolic byproducts, whereas those are high concerns in recirculating systems. Another important biological aspect is regarding the fish diets. It is well known that marine fish species can be carnivorous, herbivorous, or omnivorous. While herbivorous and omnivorous species are less demanding when it comes to animal protein requirement in their diets – which represents the highest costs in the feeds – these species also tend to have a lower demand and a lower price in the market. On the other hand, carnivorous marine fish require higher levels of animal protein –mainly derived from fishmeal and fish oil – yet in turn present higher levels of omega-3 and invariably have a higher demand and higher prices in the market.
Other biological criteria are the species behaviour (schooling and gregarious species
are preferred over territorial and aggressive species) and cannibalism (especially in the hatchery/nursery stages). Specifically considering the high energy levels found in the open ocean, where greater depths and strong currents prevail, the right candidate species needs to exhibit the ability to cope with continuous swimming at velocities commensurate with the current velocities to which they would be exposed (Figure 2). This would require that the species exhibit exceptional physiological, biochemical, and anatomical adaptations – including respiratory, cardiovascular, and proportions of white and red muscle tissues – fit for enduring exercise. For example, the red drum (S. ocellatus) has been shown to be able to swim between 0.5 to 0.75 m/s, the equivalent of 1-1.5 knots. This is not surprising, since this species is associated with constant waves motion and rip and tidal currents that characterize most coastal waters where it inhabits. Cobia (C. canadum) can also swim
under high current conditions (>1 knot), although it routinely rests at the bottom if given the opportunity. From the results of previous research, and from the literature references available, these species can likely endure currents of 1 knot without fatigue. Indeed, in theory, except perhaps for a few strictly epipelagic species such as tunas, mahi, barracudas, and wahoo (to name a few), no fish species would fulfil all ideal criteria for being raised in a high energy environment with currents at or above 1 knot without incurring fatigue or negative physiological impact. Finally, it is important for the species to be hardy, being resistant to diseases (viral, parasitic, bacterial, and fungal) under environmental fluctuations. Generally, native species exhibit these characteristics when cultured within their respective habitat ranges.
The criteria used for establishing the feasibility of each species are determined by
the consistent and replicable success in the following basic aspects:
1. Maturation: broodstock availability and management; easy to obtain, handle, keep, and feed; resistant to diseases; adaptability to captivity; ease of domestication.
2. Spawning: volitional, natural, environmentally conditioned and/or hormone induced; controlled reproduction is of paramount importance.
3. Larval rearing: level of control of larval husbandry techniques.
4. Survival rates: during the larval rearing, nursery, and growout stages.
5. Feed conversion rates: feeds and feeding strategies mastered; basic information on nutritional requirements known and wellestablished.
6. Market and marketing: established, stable, good demand and value.
Experimental feasibility: Research level .
The species is generally hard to be raised, with little or no control over maturation, spawning, and larval rearing. Survival rates from fry to harvest size are low (between 0-1%). The species has been and can be experimentally raised but results cannot be consistently repeated. Research at this level is generally conducted at universities or research institutions and is funded by government grants.
Technological feasibility: Research and development level . High level of control over maturation, spawning, and larval rearing. Survival rates are generally low to medium (between 5-20% at the hatchery). The species has been and can be raised but not yet on a commercial basis. Results can be repeated consistently. R&D is generally conducted in private companies, universities, and research institutions using both private and government funds.
Commercial feasibility: Economic feasibility level. Total control is achieved over all stages of the production cycle (maturation,
spawning, larval rearing, nursery, and growout). Survival rates are generally medium to high (10-30% at the hatchery and 70-90% in the growout). The species has been and is being commercially cultured at a profit by the private sector.
Overview of Pre-Selected Candidate Species
Red drum (Sciaenops ocellatus), almaco jack, also known as Kampachi (Seriola rivoliana), pompano (Trachinotus carolinus), red snapper (Lutjanus campechanus), and cobia (Rachycentron canadum) were considered top candidate species for commercial offshore aquaculture development and were preselected for this evaluation.
The criteria and values assigned to rank the species are shown in Table 1. The maximum number of APIX points with the feasibility level multiplier is 90. No APIX was calculated for seaweeds and mollusks. The feasibility level of all species listed is considered commercial (3).
Using the criteria presented and discussed in Table 1, we developed a matrix to evaluate the viability of several pelagic, demersal, reef, and coastal species for open ocean aquaculture in the Gulf of Mexico. Table 2 presents a ranking of the species pre-selected for offshore aquaculture development in the region. We applied the APIX considering all criteria and respective weights assigned for each criterion and assigned each species a final score. These variables were assigned a weight of 1. Adding to that, we incorporated the level of feasibility, which was given a weight of 1, 2, or 3 depending on the level of species readiness for commercial aquaculture development. The APIX can be applied with different weights to each project at the users’ discretion to support specific project goals.
Our evaluation indicates that red drum, almaco jack (a.k.a. Kampachi), pompano, red snapper, and cobia can all be considered potential candidate species for commercial aquaculture development in subtropical marine locations.
FCR (Feed Conversion Rate)
1. Poor FCR; ranges from 2.5 and beyond
2. Average FCR; ranges from 1.5 to 2.5
3. Excellent FCR; ranges from 1.2 to 1.5
Survival rate (30 g to harvest)
1. Very poor survival (20-40%)
2. Poor survival (40-60%)
3. Acceptable survival (60-80%)
4. Excellent survival (>80%)
Fish yield
1. Poor fish yield (<20-30%)
2. Moderate fish yield (30-50%)
3. Good fish yield (50-75%)
4. Excellent fish yield (75-90%; whole)
Growth rate
1. Slow growth rate to market size (<500 g/yr)
2. Average growth rate (500-1,000 g/yr)
3. Fast growth rate (>2 kg/yr)
Feed costs
1. High feed costs (>60% of total production costs)
2. Average feed costs (50-60% of total production costs)
3. Low feed costs (<50% of total production costs)
Marketability (demand and consumer awareness)
1. Poor marketability (few people know the species)
2. Moderate marketability (carried by specialty fish markets)
3. Good marketability (commonly encountered on restaurant menus)
4. Excellent marketability (high demand at high prices by all sectors)
Market price
1. Low market price: at or below $5/lb
2. Average market price: $5-10/lb
3. High market price: ≥ $10/lb
Processing Level
1. Requires processing (fillets, steaks, smoking, canning, etc.)
2. Does not require processing (whole fish, head on and gutted)
Stocking density
1. Low stocking density (<10 kg/m3)
2. Average stocking density (10-25 kg/m3)
3. Excellent stocking density (> 25 kg/m3)
Feasibility level (multiplier)
1. Experimental (1)
2. Technological (2)
3. Commercial (3)
Specifically considering the high energy levels found in the open ocean, where greater depths and strong currents prevail, the right candidate species needs to exhibit the ability to cope with continuous swimming at velocities commensurate with the current velocities to which they will be exposed. Red drum is known for being a strong fish and cobia has shown the same, being produced successfully in open ocean conditions at Open Blue Sea Farms in Panama.
We selected tropical and subtropical native species and the offshore environment in the Gulf of Mexico for this case study. However, the APIX is directly related to the environmental conditions of the site and the method to be used, and can be adapted for any species, environment, location,
and method. For example, Atlantic salmon ( Salmo salar ) would certainly have a high APIX and, combined with its commercial feasibility level, rank very high for farming in temperate areas. Likewise, the Japanese or olive flounder ( Paralichthys olivaceus ) would rank exceedingly high for landbased aquaculture in flow-through or recirculating aquaculture systems where ideal temperatures of 18-24°C can be maintained. Both species have reached commercial feasibility level and have excellent aquaculture performance in terms of growth, survival, feed conversion rate, and marketability. The selected species ranked closely and some criteria might be deemed subjective, making it reasonable to consider some species as having no significant difference. However, in a data sparse field,
the process reflects the information, data, and knowledge available, and provides valuable guidance to interested parties outside the industry.
Red drum (Sciaenops ocellatus) was the top species selected using the APIX grade combined with feasibility level (Figure 3). Nonetheless, while it is technologically feasible to produce all species listed offshore, their respective aquaculture performance in terms of growth, survival, and feed conversion rates as well as the economic viability of their farming can only be proven in practice. u
Dr. Daniel Benetti, PhD, is a professor and director of aquaculture at the University of Miami Rosenstiel School of Marine, Atmospheric, and Earth Sciences. He works extensively with the academic, government, and private sectors internationally, and has over 200 scientific and technical publications in the field of aquaculture. His areas of interest include technologies of marine fish hatcheries and growout aquaculture operations.
Fabio Dos Santos Neto, MS, is a marine biologist with over 20 years of aquaculture experience both in the public and private sectors, as well as in non-governmental organizations. He has acquired professional experience through leading, developing, and managing R&D and commercial projects comprising the major aquaculture groups – algae, mollusks, shrimp, and finfish – in the various links of the value chain. His area of interest encompasses sustainable aquaculture systems and development.
Tyler Sclodnick, M.Sc., MBA, is the principal scientist at Innovasea, a designer and retailer of the most technologically advanced aquatic solutions for aquaculture and fish tracking. He has worked on projects in over 15 countries across five continents, helping new farms plan their business, obtain permits and financing, and work through the early struggles of producing fish.
Jenna K. Baggett, M.P.S., is a biological research technician for the Aquatic Animal Nutrition Laboratory at the Harbor Branch Oceanographic Institute of Florida Atlantic University. She oversees aquatic animal nutrition experiments and operations, data collection and analysis, husbandry, equipment maintenance, laboratory coordination, and water chemistry. Her areas of interest are marine finfish nutrition and sustainable aquaculture of marine finfish.
Carlos E. Tudela, MS, is the assistant manager at the University of Miami Experimental Hatchery (UMEH). He oversees all UMEH production operations: broodstock, larval rearing, shipments, equipment maintenance, fish health, data collection/ experiments, and personnel training. Mr. Tudela has over 10 years of aquaculture experience. His area of interest is in production technolgy of marine finfish.
To continue expanding, the salmon aquaculture industry must rely on science-based, solid data and information for legitimacy.
Introduction
Since the early 1980s, aquaculture has expanded to become the most successful food production sector worldwide. Over the last decade, it has overtaken wild catch fisheries production as a source of seafood for human consumption. It has surpassed beef production and continues to expand while arguably improving its socio-economic impact and ecological and economic efficiency.
Salmon farming is one of the most recognizable and controversial forms of aquaculture. However, according to the 2022 report on the State of Fisheries and Aquaculture of the Food and Agriculture Organization of the United Nations, salmon farming represents only 4% and ranks ninth among the major aquaculture finfish species produced worldwide. Norway, Chile, Scotland, and Canada lead the industry as the world’s largest producers. In these countries, aquaculture has become a well-established
industry and an important component of the economy (Figure 1). For example, in 2017, Norway’s aquaculture industry produced 1.31 million tonnes of fish with a value of Can$8.2 billion, employing approximately 8,000 people. In Chile, salmon farming, directly and indirectly, employs 71,000 people. In Scotland, the salmon industry supports about 10,000 jobs in rural and island communities, making it the largest employer in that country. In Canada, salmon farming contributes to 20% of total seafood production and employs over 14,000 full-time workers. These numbers are staggering and often unappreciated.
Despite the industry’s development in those four countries, the rapid growth of salmon aquaculture has not been without controversy. Environmental problems have triggered negative perceptions of the industry and have proved to be a significant barrier to growth. Further, society’s increased focus on the environment is more acute than ever due to the increased awareness of fish welfare, the marine ecosystems, and their interrelationships. In many instances, opposition groups have
successfully slowed or even stopped salmon aquaculture development. These conflicts demonstrate the importance of including social dimensions when designing and implementing salmon and any other aquaculture operation anywhere in the world. It is important to note, however, that these problems are unique to each country’s idiosyncrasies, including political, social, economic, cultural, and educational background.
In December 2022, the Norwegian government imposed a temporary halt of permit applications for new land-based fish farms until it can create new regulations to account for the increasing number of coastal projects. In January 2023, the deadline was further extended stating that “It is important that the committee does a thorough job of assessing how we can get a simpler and more holistic system.” In Chile, the government has pointed out that “the salmon industry has grown a lot and has had significant income.” The government demanded a higher standard of strict compliance with the law from the industry. Working conditions must be decent for all, and the link with the communities must be greater than the current levels. Following these statements, a bill was reactivated in February 2023 to suspend new aquaculture concessions for 10 years.
In Scotland, a coalition of animal welfare groups and members of the Scottish Parliament have recently called for a halt to the expansion of the salmon farming industry. That call comes after the number of fish deaths in 2022 almost doubled compared to 2021 and tripled compared to 2020. The coalition argues that the industry is not adequately prepared to handle the changing conditions caused by climate change and more fish are expected to die with detrimental consequences to the environment. The signatories of the letter, which was coordinated by animal protection non-governmental organizations (NGOs)
Animal Equality U.K. and One Kind, include Green and Labour members of the Scottish Parliament, as well as Conservative and Liberal
Democrat members of Parliament. They are asking for a moratorium to be imposed on the expansion of the industry, and for regulatory bodies to investigate the issue of mortality.
Finally, in Canada, Fisheries and Oceans Canada announced in February 2023 that it will not be renewing licenses for 15 net pen Atlantic salmon farms in the Discovery Islands (British Columbia, B.C.). This decision is in line with the initial decision in 2020 to avoid salmon farming in this region for a period of 18 months. According to the B.C. Salmon Farmers Association, the phased-out farms in the region of Discovery Islands shut down 24% of B.C. salmon production, put 1,500 jobs at risk, and forced the euthanasia of more than 10 million salmon.
These experiences are highlighting an important juncture in the history of aquaculture: the era of explosive growth in the salmon farming industry may be nearing its end. While this trend may be worrisome, three main approaches could be implemented to improve the legitimacy of this sector and could potentially alleviate the resistance the sector is currently facing in its main producing countries (Figure 2). The recommendations must be backed by solid data and information. These approaches are change the governance; increase environmental measures, monitoring, and control; and move farms far from the coast and away from their current places.
Governance is defined as the network of rules, institutions, and actors involved in the decision-making of a country. Good governance in the context of aquaculture is thus a supportive state whose regulations and policies provide security for stakeholders to prosper while maintaining social harmony and stewardship of the natural environment. This approach could be a valid option to improve the legitimacy of salmon farming. When food production systems lack proper regulatory standards, negative outcomes for the environment and industry can emerge,
and aquaculture is no exception. Indeed, Mikkelsen and colleagues pointed out that legitimate governance processes are fundamentally important, especially in the case of coastal zones where aquaculture takes place, as this domain includes a wide range of political processes with multiple actors and levels that are involved.
Improved governance could be achieved through changes to the legal framework, which increase the participation of local communities and local government in the decision-making process; ideally, local stakeholders should feel that they are involved in decisions that affect their territory.
Companies could potentially implement their own version of marine spatial planning, incorporating the input of local communities and areas of community sensitivity into their decision-making processes. For instance, in Chile, there are approximately 1,200 authorized sites for salmon farming. However, companies typically utilize only 400 to 600 sites per year, indicating that they own more sites than they actively operate. Presently, the criterion for site reuse is largely economic,
with preference given to sites that yield higher profits from salmon fattening, faster fish growth, or lower production costs, leading to subsequent cycles of use. To address conflicts and dissatisfaction within local communities, companies operating in a particular area could collaborate and coordinate their site usage. It may be worthwhile to introduce the concept of “social rest” – a period dedicated to reducing conflicts with local communities, similar to the concept of “sanitary rest,” which pertains to reducing pathogen load in a given area. Improvements in governance, however, are not all dependent on government actions. The private sector has the potential to create its own parallel governance system through the incorporation of private certifications. Aquaculture companies have used this approach to address socio-environmental and ethical sustainability challenges in their production processes in the past to some success. The Aquaculture Stewardship Council (ASC) and Best Aquaculture Practices (BAP) are two of the most successful private certifications available in terms of coverage within the salmon industry. These certifications provide a set of voluntary rules that cover a wide range of areas such as better environmental standards,
the use of pesticides and antibiotics, monitoring of sea mammals, fair income for employees, relations with local communities, greenhouse gas emissions, and other issues that help consumers ensure that they are purchasing a responsibly produced product along the entire supply chain. Despite the popularity of these certifications, there is still limited research on their actual impact on environmental and social outcomes at the local level. Nevertheless, studies have shown that consumers trust these standards to be an indicator of better practices and are, therefore, willing to pay more for products bearing the ASC or BAP seals.
Maybe the most relevant source of nonconformity with the salmon industry is the release of organic and inorganic wastes and chemicals into the environment. These impacts perturb ecosystems and change the biochemical composition of the benthic sediment at the sea bottom. These changes trigger a chain reaction that ultimately affect organisms such as mollusks, fish, and mammals, which then ultimately affects people who rely on the sea as their source of income, way of life, or a place of ancient beliefs. To gain a better understanding of the initial changes in the ecosystem, next-generation sequencing (NGS) offers an opportunity to detect microbial communities with a high level of taxonomic sensitivity. NGS analysis provides numerous advantages over classical microbial community analysis as it does not require microbiological cultivation, enabling the analysis of nonculturable bacteria that represent approximately 75% of the bacteria that inhabit the sediment below farming cages. Moreover, it allows the characterization of large volumes of samples in a short period of time. This approach provides a relatively unbiased view of the microbial diversity present in the system while providing data on the community structure and genetic basis of the environment. Improving knowledge on how the salmon industry affects the environment is essential to reduce the industry’s impact on ecosystems and minimize discomfort for local communities, and NGS might be one
of the best approaches to do so. This could be an innovative, proactive initiative of the private sector that would be highly appreciated by all stakeholders – from the government sector to the community, to NGOs, and consumers.
Closely related to the environmental approach, there is a substantial body of research that aims at relocating salmon farms away from their current locations into the open ocean, far away from shore. Countries such as Norway, China, Canada, the U.S., Japan, and Chile have been actively engaged in developing technologies that can withstand extreme weather conditions. In Norway, the primary objective is to move farms in order to reduce the incidence of sea lice infections and, consequently, decrease the usage of anti-parasitic treatments. In Chile, the purpose of relocating farms is to alleviate pressure on coastal ecosystems. Moving salmon farms offshore will have multiple benefits for the entire industry. The advantages and disadvantages must be carefully evaluated, yet the benefits will likely outweigh the increased costs and risks.
Operating farms in the open ocean will be more complex and costly, presenting higher risks. However, the high energy of the offshore environment, where stronger currents and greater depths increase the carrying capacity of the sites, will allow for higher stocking densities and benefit fish welfare, producing added-value fish that could be sold at premium in the market. Indeed, fish produced offshore must be recognized for their superior quality and sold at high prices to compensate for the high capital and operating costs required to raise them.
Offshore aquaculture presents both new opportunities and significant challenges. Currently, there are only a few companies that farm under offshore conditions in the Americas – one being Open Blue Sea Farms in Panama. The site is approximately 13 km offshore, with depths ranging from 55 to 65 m. The farm has been operating
continuously since 2010 and it is dedicated to the production of cobia (Rachycentron canadum). The company is a verticallyintegrated operation, encompassing all stages of the production cycle, from egg to market, including processing and rendering plants that use wastes and byproducts to produce fishmeal and fish oil – aiming at zero waste, hence making significant progress towards a circle economy and carbon neutrality. Blue Ocean Mariculture is the only offshore company operating in the U.S. using submersible cages producing Kampachi, a.k.a. almaco jack (Seriola rivoliana) in Kona, Hawaii. Both utilize the most advanced technologies and sustainable, certified practices to produce cobia and Kampachi from egg to market.
Offshore farming is a risky business, and although remarkable progress continues to be achieved, the technologies for operating at full automation are still being perfected. Stocking, feeding, chemical treatments, net cleaning, predator avoidance, escapements, biomass estimates and crop management, mortalities collection, and harvesting complexities are exacerbated in offshore systems.
Regarding offshore salmon farming, for example, there are two or three initiatives that are still mainly in the experimental and implementation stages. One is Ocean Farm 1, built by Ocean Farming AS, a subsidiary of the SalMar Group. The farm was designed to operate under the extreme conditions of the Frohavet Sea, but this experience was not exempt from problems. In 2018, it was reported that around 16,000 salmon escaped their cage when Ocean Farm 1 tilted after water entered an inspection hatch that had been accidentally left open. The top few metres of the net were submerged up to 18 cm underwater for a brief period, allowing fish to escape. A second escape, caused by a tear in a net, occurred in August 2020. The cage was withdrawn until a new design could be obtained, illustrating that further improvements are required for operating under offshore
conditions. As with any relatively new industry, hurdles must be overcome before large-scale operations can be successfully implemented and commercial viability can be secured.
Farming under offshore conditions is a new paradigm. Everything seems to indicate that offshore farms will be larger than the current farms, with fewer sea cages, but with higher water volume per cage. This transition could help move the salmon farms production away from traditional places and might provide the grounds for a new beginning. In order to sustain past successes and revitalize the growth of the industry, learning from past mistakes is crucial.
It is important to highlight that all three of these approaches are interconnected. For example, if the technology for offshore aquaculture is further developed and commercialized, it will be necessary to change the legal framework and adopt governance measures accordingly. Private certification could accelerate the adoption of new tools to measure environmental impact because changing their standards might take less time than changing the regulation. In the end, however, no matter which approach we choose, it is time to address past perceived or real mistakes and to work to improve the industry’s legitimacy to sustain growth and achieve sustainable development. u
Carlos Carroza Meza is a Chilean PhD student at the University of Miami with a bachelor’s degree in marine science and aquaculture engineering; a master’s degree in aquaculture; and an interest in institutional governance, environmental monitoring, and offshore aquaculture. Working as a research assistant at the Rosenstiel School of Marine, Atmospheric, and Earth Science, he is dedicated to enhancing the legitimacy of the salmon farming industry to benefit the environment, coastal ecosystems, local communities, and stakeholders involved.
Dr. Daniel Benetti, PhD, is a professor and director of aquaculture at the University of Miami Rosenstiel School of Marine, Atmospheric, and Earth Sciences. He works extensively with the academic, government, and private sectors internationally, and has over 200 scientific and technical publications in the field of aquaculture. His areas of interest include technologies of marine fish hatcheries and growout aquaculture operations.
Acoustic Deterrent Devices for the Protection of Fish Farms from Predator Attacks: A Solution or Menace Arnold Ebuka Irabor, Hardin Jrn Pierre, Oghenebrorhie Obakanurhe, Francis Oster Nwachi, Ikechukwu Augustine Chukwurah, Augustine Onyemaechi Ozor, Jovita Oghenenyerhovwo Sanubi, and Jerimoth Kesena Ekelemu
Improved Near Real-Time Ocean Surface Wind Products Using a Combination of Multi-Source Satellite Datasets Meisam Amani, Sahel Mahdavi, Terry Bullock, and Steven Beale
Who should read this paper?
This work is a review paper that dives into the use of acoustic deterrent devices (ADDs) for the protection of aquaculture species from attacks by aquatic mammals – the effectiveness, challenges, and possible remedies. This article is beneficial to those working in the aquaculture sector. The content of this review paper serves as a basis for policy-makers as well as researchers to guide them in issues around the use of acoustic deterrent devices and the relevant considerations to better the functionality while reducing the adverse effects of their use. It gives a deeper understanding of the impacts of the use of ADDs on target and non-target species as well as the environment at large. It also broadens the knowledge of cage aquaculture farmers on how best to curtail some of the challenges associated with ADDs such as the use of air bubble curtains.
Why is it important?
To tackle the challenges of ADDs, innovative modifications are suggested to bring about minimal danger to target and non-target species as well as the environment. Although the effectiveness of the devices is not in doubt, slight modifications on both aquaculture facilities and ADDs will give a better result. With the proposed adjustments, this technique of protecting aquaculture species and facilities from damage will be helpful.
Most aquaculture facilities and aquatic mammals in the context of this review paper are directly or indirectly related to the ocean environment. This automatically makes the paper a priority interest to the ocean community saddled with responsibilities such as conservation, sustainability, policy formation, and enforcement.
Irabor Arnold Ebuka is a researcher from Nigeria with interests spanning from sustainable aquaculture (fish production and sustainability), fish nutrition and feed formulation, fish gut health, and conservation of aquatic resources. He holds a master’s degree in sustainable aquaculture from the University of St. Andrews, Scotland; has successfully authored over 18 internationally published articles; and is a member of the renowned Fisheries Society of Nigeria.
Jrn Pierre Hardin is a PhD student of the Department of Aquaculture, Kagoshima University, Japan, with interests in fish nutrition and feed formulation and aquatic life conservation as reflected in his numerous publications. His contribution to aquatic life preservation is well known to St. Lucians via the Ministry of Agriculture, Fisheries Department. Presently, he is conducting research work on Japanese shrimp.
Dr. Obakanurhe Oghenebrorhie holds a PhD in animal nutrition (poultry) with knowledge spanning through various aspects of animal science and production as evident in most publications he has authored. He is a lecturer in the Department of Animal Science and Production, Dennis Osadebay University, Anwai, Asaba, Nigeria. Presently he is researching sweet potato leaf meal as supplement in poultry feed.
Dr. Nwachi Oster Francis is a lecturer in the Fisheries and Aquaculture Department at Delta State University, Abraka, Nigeria. He is an expert in fish genetics and biotechnology and has published over 25 articles in renowned international journals. Presently, he is working on a project on phenotypic responses of fingerlings obtained from a cross between albino and normal catfish (Clarias gariepinus).
Chukwurah Ikechukwu Augustine is a lecturer in the Department of Environmental Management and Toxicology, Dennis Osadebay University, Asaba, Delta State, Nigeria, with vast knowledge in environmental management. He is widely read and has authored a number of publications. He is presently working on research involving environmental toxicology with respect to the impact of industrial waste on the well-being of aquatic habitats.
Dr. Ozor Augustine Onyemaechi holds a PhD in agricultural extension from Delta State University, Abraka, Delta State, Nigeria. He is a lecturer in the Department of Agricultural Extension and Rural Development, Dennis Osadebay University, Anwai, Asaba, Delta State, Nigeria. His area of specialization is agricultural communication where he has carried out research and several publications.
Sanubi Jovita Oghenenyerhovwo is an aspiring animal geneticist with a bachelor’s degree in animal science from Delta State University, Abraka, Nigeria. She is currently working as a graduate assistant at Dennis Osadebay University, Asaba, Delta State, Nigeria. She is a book lover who has interest in research involving animal welfare.
Dr. Ekelemu Jerimoth Kesena is a renowned professor of fisheries and hydrobiology with specialty spanning through fish gears and crafts, aquatic life conservation and welfare, culture facility modification and population, and distribution and diversity of the aquatic ecosystem. He is a professor in the Department of Fisheries and Aquaculture, Delta State University, Abraka, Nigeria. He is also a respected fellow in the Fisheries Society of Nigeria.
Arnold Ebuka Irabor1,2, Hardin Jrn Pierre1, Oghenebrorhie Obakanurhe3, Francis Oster Nwachi4, Ikechukwu Augustine Chukwurah5, Augustine Onyemaechi Ozor6, Jovita Oghenenyerhovwo Sanubi3, and Jerimoth Kesena Ekelemu4
1Department of Sustainable Aquaculture, School of Biology, University of St. Andrews, Glasgow, Fife, Scotland; arnold.irabor@dou.edu.ng
2Department of Fisheries and Aquaculture, Dennis Osadebay University, Anwai, Asaba, Delta State, Nigeria
3Department of Animal Production, Dennis Osadebay University, Anwai, Asaba, Delta State, Nigeria
4Department of Fisheries and Aquaculture, Abraka, Delta State University Abraka, Delta State, Nigeria
5Department of Environmental Management and Toxicology, Dennis Osadebay University, Anwai, Asaba, Delta State, Nigeria
6Department of Agricultural Economics and Extension, Dennis Osadebay University Anwai, Asaba, Delta State, Nigeria
Sound-producing devices have been used in deterring predatory sea mammals from aquaculture zones thereby improving and sustaining fish production. However, some challenges have arisen concerning the use of these devices due to observed adverse effects on target and nontarget species and the aquatic ecosystem in general. To this end, a review of the use of these devices, especially the working techniques considered to have some deleterious effects on both the predators and prey, was the main objective of this study. Some of the identified negative impacts of the use of acoustic deterrent devices on these target and non-target species are erratic movements, masking communication, hearing disorder, physiological stress, mortality, and ecosystem alteration. It is, therefore, recommended that adequate modification (reenforcements) be carried out on aquaculture facilities to prevent predatory access. Furthermore, the use of hazardous sound-producing devices such as explosives should be handled with care or even prohibited. Designs of acoustic deterrent devices should be eco-friendly to minimize adverse effects, and proper impact evaluation must be carried out regularly to ascertain the best techniques to adopt. Also, the adoption of underwater noise-reducing equipment such as air bubble curtains is paramount. Lastly, extension service agents should ensure to communicate the harm of such devices to the locals while educating them on the suggested preferred techniques and devices.
Aquaculture zones; Ecosystem; Organisms; Pinniped; Sea mammals
The disturbance of carnivorous sea mammals in the marine environment posing a serious challenge to fish farming has been a major area of concern in the world, especially for fish farmers recording serious financial losses [Nash et al., 2000; Fakhru'l-Razi et al., 2020]. Consequently, a series of issues have been raised regarding how to curtail the activities of these mammals since the productivity and sustainability of aquaculture are adversely affected [Fakhru'l-Razi et al., 2020]. The use of devices that reduce the preying activities of sea mammals was, therefore, adopted. These devices include tensioned nets (i.e., stainless steel nets connected to high current generating panels used to bar predators from gaining entrance into the culture facility), acoustic deterrent devices (ADDs), and anti-predator nets (i.e., outer firmer nets placed around cages to prevent access to predators) [Würsig and Gailey, 2002; Gümpel et al., 2019; Espinosa-Miranda et al., 2020].
For decades, these devices have been used but, specifically, the acoustic deterrent device is mostly used to prevent sea mammals from approaching cage aquaculture facilities [Coram et al., 2014; Monaco et al., 2019]. These acoustic deterrent devices function by the sound principle whereby loud sound is emitted around the environment by installed ADDs. This sound helps to chase away sea mammals and other predators from the farm zones; this sound (within 1 to 20 kHz) causes a level of discomfort to the sea mammals. The aim is not only to keep these mammals away from preying on small fishes, but also to prevent them from being injured by some
of these culture facilities securing devices (e.g., tensioned nets).
Earlier investigations revealed that these devices are highly effective since they also help in preventing the destruction of bycatch of small cetaceans [Reeves et al., 2001; Erbe et al., 2019; Fanny et al., 2020]. Some researchers also reported that the use of these devices has been efficient, accomplishing expected results [Kraus, 1999; O’Neil, 2020]; however, major challenges have arisen which has made them more of a threat to the sea mammals, non-target species, and the surrounding ecosystem. This is attributed to the very high unpleasant sound emitted by the device, consequently producing sounds with detrimental effects on the physical and psychological well-being of both target and non-target species [Fanny et al., 2020].
Severe damages incurred from the use of these devices have been reported in numerous studies [Erbe et al., 2019; Fangqi et al., 2019; O’Neil, 2020; Schoeman et al., 2020]. Therefore, this study suggested possible solutions while reviewing the effects of sound-producing devices, especially acoustic deterrent devices, on the well-being of aquatic organisms and the marine ecosystem in general.
Several techniques have been identified in the interaction between sea mammals and preyed fishes [Würsig and Gailey 2002; de Oliveira et al., 2020]. These techniques are grouped into six: Harassment Technique, Aversive
Conditioning, Exclusion, Non-Lethal Removal, Lethal Removal, and Population Control. These techniques have been extensively described and evaluated by numerous researchers to ascertain their effectiveness and challenges. Although all are considered effective, the non-lethal removal (ADDs) has been reported to pose a minimal threat to the non-target and target species [Costa, 1986; Pemberton and Shaughnessy, 1993; Buck, 2000; Nash et al., 2000; Adele, 2018; Calamnius et al., 2018; Findlay et al., 2018; Hamilton and Baker, 2019; Cerqueira et al., 2020; Charla et al., 2020; Daniel et al., 2020; Schoeman et al., 2020; Nunny, 2020; Findlay et al., 2021; Hiley et al., 2021; Todd et al., 2021].
ADDs can be defined as a mechanism that uses sound as a measure to checkmate and control the preying activities of sea mammals in an aquatic ecosystem [Erbe et al., 2019; Fangqi et al., 2019]. They can also be referred to as devices with structures and principles built to deter large sea mammals from preying on small fishes and encroaching into the human environment using loud sounds [Erbe et al., 2019; Rowley, 2020].
Corbett [2019] posited that these devices have a very low intensity otherwise known as radiance (i.e., the ratio between the average power and the area through which the power passes). It also has a low-frequency level that allows for wider range coverage [Fangqi et al., 2019]. These devices have been ascribed many names, including acoustic mitigation device, acoustic harassment device, scrammers, scarers, pingers, etc. All these devices are in
use within the aquatic marine environment, which helps to mitigate predatory activities of large sea mammals.
According to Erbe et al. [2018], ADDs are known to have employed a principle of emitting continuous sound processes that leads to the formation of a sound field. Expanding on this principle, Rowley [2020] explained that for ADDs to function adequately the sound produced needs to be continuous and sufficiently audible to create the necessary sensitive impulse that affects the hearing range of aquatic mammals, thereby making the environment non-conducive for them. As an implication of this continuous sound strategy, the mammals are scared of the aquaculture zone.
Northridge et al. [2010] stated that most fish farmers leave the device continuously running on their farms; even during the perceived presence of prey, predators are forcefully deterred. This strategy is found to be effective since it does not only reduce the presence of the predator but also causes a level of discomfort thereby distracting them completely from the aquaculture zone. However, the continuous sound processes might cause damage to other aquatic organisms such as seahorses, octopuses, turtles, wild fishes, etc. constantly exposed to the sound emitted.
The second strategy as also observed by Northridge et al. [2010] is a reactive measure where the device is only switched on when the presence of sea mammals is noticed within a specified perimeter (the aquaculture zone) and switched off when the presence of the predator is no longer noticed within that area. In other words, it is a responsive measure used
only in reacting to the presence of large sea mammals [Erbe et al., 2018]. This method is observed to be more eco-friendly as there is limited hearing damage caused to non-target species. Also, modern ADDs being used are modified to minimize the sound produced at frequencies that would only deter cetaceans without causing harm. These devices function by particularly adapting signals to induce avoidance reflexes in seals at the low sound, significantly reducing the possibility of nontarget species being affected.
Furthermore, methods for reducing the sound output of existing ADDs have been investigated, such as a significant reduction of the emitted sound and duration per time, which implies that ADDs only transmit sound when these mammals are around the aquaculture zone. Nevertheless, there are still concerns about the effectiveness of these devices because seals may over time acclimatize to the regulation pattern of the device, which may pose further danger. Also, many marine mammals are considered endangered species, hence protected. The introduction of ADDs that may cause severe harm to them is yet another reason to assess the potential effects of ADDs on sea mammals before use.
Acoustic deterrent devices emit various sounds such as that of a killer whale or harsh cries transmitted between 12 and 17 kHz. The frequency and volume of acoustic deterrent devices are typically approximated to 10 kHz. This device has been tested to have an intensity level of up to 194 dB re 1uPa @ 1 m, and the sound may be heard up to a distance of 50 kilometres [de Oliveira et al., 2020; Schoeman et al., 2020]. These features make it very effective for deterring seals and other aquatic
mammals from culture facilities. Besides the working principles of these devices, there is further categorization based on the techniques of usage [Schoeman et al., 2020].
The effectiveness of ADDs using the aversive sound technique cannot be overemphasized. In line with this, most observations have been reported by several researchers. Brandt et al. [2013] observed that ADDs using aversive sound techniques have effectively helped to mitigate the potential damages that sea mammals cause both to themselves and small fish species. Monaco et al. [2019] also reported that with the effectiveness of the aversive sound technique in preventing the attacks of sea mammals, more benefits have been ascribed to the offshore area, including the renewal of the area and its dwellers.
Although the use of these devices has, over the years, received growing interest from researchers and practitioners, there also have arisen some misconceptions on the relationship between effectiveness and perceived damages caused by some techniques [Brandt et al., 2013]. Quick et al. [2004] argued that these devices using the sound alert system are not meant to harm the hearing capacity of sea mammals, but rather to alert them of potential risks and hazards that may be dangerous to their well-being or existence. This was supported by De La Croix [2008], who claimed these devices have a very low frequency, making them harmless to sea mammals but rather deters them from risky environments. Jefferson and Curry [1996] also posited that
these devices act only as a dinner bell or warning bell that reminds sea mammals of the presence of food in a particular location.
Zwicker and Fastl [2004] observed that just as humans feel a sense of unpleasantness anytime a disturbing sound comes close to the auditory system, the same principle applies to the use of these devices because the loudness, fluctuation of strength, and sharpness of the sound emitted are always perceived to be annoying to sea mammals, making them move away from the zone. However, McDermott and Hauser [2004] suggested that the unpleasantness that is perceived by humans as a result of sound may not apply to other non-human primates thereby posing a serious challenge to further adoption of these devices.
In the management of the aquatic environment concerning the control of sea mammals and their preying activities on smaller aquatic species, a lot of credit has been given to the use of ADDs since they have been observed to effectively deter sea mammals as well as prevent them from harm [Schoeman et al., 2020]. Despite the efficiency in protecting small fishes from sea mammals, some researchers have found fault with this operation in the field as it has led to the exposure of these sea mammals to predators [Thomas and Vincent, 2010; Coram et al., 2014; Ida et al., 2018].
organisms are the primary cause of irregular dislodgment, hearing disorder, physiological stress, masking, and subsequent mortality [Popper et al., 2014; Debusschere et al., 2016; Southall et al., 2019; Sarnocińska et al., 2020].
Irregular dislodgement of these sea mammals leads to numerous challenges that threaten the population of these species. During dislodgements, most young ones that cannot keep up with the pressure caused by the movement are left behind and this exposes them to predators while some die due to lack of parental care and physiological stress [Calamnius et al., 2018; Cattelan et al., 2020; Findlay et al., 2021]. Also, collisions during such chases lead to severe injuries and possible stampedes, especially on vulnerable young ones with fragile bodies [Heide, 2020; Todd et al., 2021]. The nesting and reproductive activities of such mammals are also altered due to constant dislodgements leading to significant depletion of the population of these species [Holmes et al., 2017; Weilgart, 2018; Hiley et al., 2021].
The adverse effects of the unpleasant sound emitted from ADDs and other sound-producing devices on sea mammals and other aquatic
Most aquatic organisms have over time become familiar with certain routes within the waterbody that are known to be dangerfree. However, with irregular dislodgements due to the sudden sound produced by ADDs, such routes might not be taken thereby exposing dislodged pinniped to life-threatening zones [Todd et al., 2019]. Mueller-Blenkle et al. [2010] and Corbett [2019] reported significant dislodgement (speedy movement) of Atlantic cod and Dover sole in net pens exposed to loud sounds from pile-driving in the sea. Also, repeated distortion in the movement was
observed in European seabass juveniles [Thomsen et al., 2020], and increased ventilation was a result of stress in Nile tilapia due to pile-driving sound effects [Kusku et al., 2020]. In some other organisms, such as European eels and Bluefin tuna, an unusually sluggish movement that made them more vulnerable to attacks from predators was reported [Sarà et al., 2007; Simpson et al., 2015].
Many sea mammals suffer from hearing disorders as a result of constant noise discharged from some of the sound-producing deterrent devices such as explosives. There are specific ultrasound frequency ranges (10 Hz to 200 kHz) as well as tolerance levels of these aquatic organisms and when such range is constantly exceeded, their auditory function is affected negatively [Erbe et al., 2018; Charla et al., 2020]. Although modern pingers have been modified to emit species specific sounds, most facilities still use pingers that are not programmed to be species specific. Aquatic organisms are affected at varying ranges, which will expose those with lower tolerance to noise quicker than even the target species [Staaterman et al., 2020]. Also, the preying sensitivity of these predators is automatically impelled since most species use sound waves to identify locations of prey [Wale et al., 2013; Pitman et al., 2018].
The constant emission of loud sound from these devices is known to travel beyond the aquaculture zone, which deprives sea mammals of rest and other health beneficial behavioural activities thereby subjecting them to levels of physiological stress [Radford et al., 2016; Poulton et al., 2016; Spiga et al.,
2017; Corbett, 2019]. The secretion of certain stress-related hormones has been observed to increase due to sound emission in an aquatic environment [Corbett, 2019]. In line with this, there has been increased and prolonged cortisol contained in fish blood when exposed to loud sounds. Such prolonged stress resultant hormones can be detrimental to fish health [Wysocki et al., 2006; Pottinger, 2008; Rolland et al., 2012]. Furthermore, an increased aeration and ventilation rate was reportedly observed in fish and crabs exposed to excessive sound [Simpson et al., 2015; Purser et al., 2016; Kusku, 2020; Kusku et al., 2023].
In addition, long exposure of sea mammals (target and non-target species) to these sounds emitted from ADDs results in injuries to the auditory system and swim bladder thus causing physiological stress [Putland and Mensinger, 2019]. Aside from physical injuries, noise has been attributed to increased metabolism and cardiovascular problems in fish and other aquatic organisms [de Vere et al., 2018].
Sound-making among some aquatic organisms is part of the steps toward reproduction; however, this means of communication between species suffers masking due to sound interference from ADDs [Radford et al., 2014; Corbett, 2019]. Such interferences may hinder the effectiveness and efficiency of the breeding period of these sea mammals in turn negatively affecting the population size. Some of the techniques (e.g., harassment) that use explosives can lead to mass mortality of non-target species as well as disrupt the balance of the ecosystem.
Kraus [1999] and Smith et al. [2020] observed that the use of these devices has adversely
affected aquatic and terrestrial environments through sound echoes such as the echolocation of cetaceans. Similarly, Todd et al. [2019], while comparing ADDs and pulse power devices, reported that the use of these devices and others such as predator noise, boats, and taste aversion in deterring seals and pinniped from the culture areas did not sustain the claimed effectiveness and suggested that further research be conducted concerning the modification and reconfiguration of the devices to address the observed negative impacts.
In the same vein, Iwama et al. [1997] opined that the devices should be ignored or neglected as a tool in handling sea mammals’ activities as they claimed the only benefit derived from such use has been short-term. It was also suggested that instead of using the ADDs as a tool, the fish should be physically relocated [Iwama et al., 1997]. In the same vein, Nash et al. [2000] refuted the use of the device claiming that it only provides a short-term solution that might even prompt the pinniped to acclimatize causing more damage to the aquatic system. These identified shortcomings of ADDs have necessitated some level of modifications on its working principles to ensure zero or minimal hazards impacted on target species (predator) as well as non-target species alongside optimal function.
this includes programming the device to become more species specific since studies have shown that in the cause of deterring the sea mammals another non-target aquatic organism is affected [Putland and Mensinger, 2019]. Götz and Janik [2013] stated that the device can be re-designed in such a way that only certain predatory species can be affected without having any effect on the cultured species such as salmon. Also, there is a need to program the sound system of the device to suit specific mammals’ auditory systems that might not cause harm to them and other species in the aquatic environment [Cerqueira et al., 2020; Nunny, 2020].
Importantly, there is a need to develop and design the device to suit different culture facilities [Schoeman et al., 2020]. Coram et al. [2014] noted that different devices with different specifications should be designed to fit culture facilities installed in rivers, the ocean, and stations as well as new zones depending on the presence of predators. It is a known fact that the device is designed to deter sea mammals far away from the culture facilities; therefore, there is a need to re-program the device to reduce the cases of injury with regard to contact with aquatic mammals [Orenstein et al., 2006].
The working principles of ADDs to a large extent need to be modified to better improve their adaptability. Some of these modifications have been suggested in some research and
Redesigning of ADDs has been proposed by numerous researchers as the solution to the adverse effects attributed to its use; however, some equipment such as air bubble curtains is also being suggested to minimize underwater noise caused by other noise-generating activities (e.g., percussive piling and drilling), in the water that can adversely affect these sea mammals [Simmonds and Dolman, 2016].
Air bubble curtains can be effective in reducing underwater noise generated by percussive piling and drilling activities, as well as adopted for reducing adverse effects resulting from ADDs usage. An air bubble curtain works by creating a barrier of bubbles around the area where the percussive piling or drilling is taking place. This barrier helps to absorb and scatter the sound waves, reducing the amount of noise that escapes into the surrounding water [Hatch et al., 2019; Kusel et al., 2019]. Also, there are some other impacts of air bubble curtains on the environment such as improving the level of dissolved oxygen (DO), temperature, pH, and other physicochemical parameters, steady water purification by dispersing solid particles, and reducing the occurrence of pollution resulting from biological waste (ammonia) generated from the cages and spills from barrels. Since bubbles are constantly generated by pumping oxygenated air into the water, the DO level of the water, as well as other physicochemical parameters, is optimized and this help to improve the well-being of the cultured species within the cages.
The effectiveness of an air bubble curtain depends on several factors, including the depth of the water, the distance between the source of the noise and the receiver, and the size and strength of the bubble curtain. Additionally, its design and construction contribute to the ability to withstand the forces of the water and the activities taking place [Jones et al., 2016; Carpine and Dumbser, 2017]. Air bubble curtains are produced by using specialized equipment called bubble diffusers, which
release streams of air into a body of water, creating a continuous stream of bubbles that rise to the surface. The equipment typically consists of a compressor, a diffuser, and a flexible hose or tubing that connects the two. The diffuser is placed at the bottom of the water body, and the compressor pumps air through the hose and into the diffuser, which then releases the air as a stream of bubbles. By adjusting the flow rate and placement of the diffusers, a continuous curtain of bubbles that rises to the surface is created, and this effectively blocks the spread of pollutants or thermal stratification [Hu and Kang, 2016; Sun et al., 2018; Jiang et al., 2019]. The size and spacing of the bubbles in the curtain can also be adjusted by varying the pore size of the diffuser, the air pressure, and the flow rate. This allows for customization of the curtain to the specific needs of the body of water (noise reduction) and the displacement of pollutants in the water body. This customization principle could also be adopted in the regulation of noise produced by ADDs.
Air bubble curtains have been successfully used in several construction projects to reduce the impact of underwater noise on marine life and thus proposed as a measure to curtail the adverse effects of the use of ADDs. However, they are not a perfect solution, and further research is needed to fully understand their potential limitations, and in turn, improve their effectiveness.
There are several other types of underwater noise-reducing equipment, each with unique characteristics and capabilities that makes them adoptable in this course. Some of them include acoustic curtains that are underwater
barriers made from materials that absorb or reflect sound waves, thereby helping to reduce noise levels that reach the target and non-target species within the specified areas. They can also be used to create quiet zones for research and other purposes that need some level of quietness [Lam et al., 2013; Liu et al., 2018; Shi et al., 2019]. Also, underwater silencers can be attached to underwater machinery, such as pumps or compressors, to reduce noise levels. They work by creating a path for the noise to travel that is longer than the direct path, allowing the noise to be absorbed or reflected before it reaches the surrounding water [Kim et al., 2018]. This principle can effectively channel the sound emitted from the ADDs or other noise-generating activities from the dwelling place of the non-target species.
Numerous principles and equipment have been suggested to help curtail the hazardous impact of noise-generating devices (ADDs) and other activities (percussive piling and drilling) on aquatic organisms and the environment; however, involvement of extension services remains an integral part. Most of the locals who have devised various means, including the use of ADDs and other noise-generating activities to deter predators from their aquaculture facilities, are not properly informed on some of the harms caused by these devices. Most of these fish farmers do not have opportunities to examine research conducted at various research-oriented institutions; this already creates a significant gap that can hinder the efforts to bring about the well-being of both aquatic resources (prey, predators, and the ecosystem) and the farmers. Extension agents, therefore, need to ensure regular and proper communication of findings to locals using various effective means.
Sound-producing devices such as acoustic deterrent devices have been used to keep sea mammals from specific installations or activities where their presence may result in damage to human interest and the mammals themselves. However, the present state of some of these devices has been reported to cause more damage to other aquatic organisms, sea mammals, and the ensonification of the surrounding environment.
These devices have been reported to have some negative impacts on the target or nontarget aquatic organisms. Therefore, it is recommended that there should be some level of modification in the design of specific installations to prevent sea mammals from causing havoc. Harmful deterrent measures such as the use of explosives should be prohibited while sound-producing deterrent devices such as ADDs are improved to reduce present challenges. Also, the need to assess the impact of these devices via an environmental impact assessment routine is paramount before adoption. Furthermore, regular evaluation of the potential effects of the use of acoustic deterrent devices on endangered species of sea mammals is necessary. This will help to better improve the acoustic deterrent devices and also minimize cases of sea mammal mortalities caused by injuries from the use of ADDs.
Acknowledgments: We wish to acknowledge the entire staff of the Sustainable Aquaculture Department, University of St. Andrews, Scotland, and Fisheries Department, Faculty
of Agriculture, Delta State University, Asaba Campus for their immeasurable support. Authors’ contributions: All authors contributed to the conceptualizing, drafting, proofreading, and approving of the submission of this manuscript. Availability of data and materials: The data and materials of this study are available from the corresponding author upon reasonable request.
Adele, R. [2018]. Impacts of ambient noise on minke whale (Balaenoptera acutorostrata) habitat use and behavior
Dissertation submitted in partial fulfillment of the requirements for the degree of Doctor of Philosophy at George Mason University.
Brandt, M.J.; Hoschle, C.; Diederichs, A.; Betke, K.; Matuschek, R.; and Nehls, G. [2013]. Seal scarers as a tool to deter harbor porpoises from offshore construction sites. Marine Ecology Progress Series, Vol. 475, pp. 291-302.
Buck, E.H. [2000]. Fishery, aquaculture, and marine legislation in the 106th congress. Congressional Research Service report. The Committee for the National Institute for the Environment, Washington, D.C.
Calamnius, L.; Lundin, M.; Fjälling, A.; and Königson, S. [2018]. Pontoon trap for salmon and trout equipped with a seal exclusion device catches larger salmons. Plos one, Vol. 13, No. 7, p.e0201164.
Carpine, C. and Dumbser, M. [2017].
Numerical simulation of air bubble curtains for the mitigation of underwater noise Marine Pollution Bulletin, Vol. 124, No. 2, pp. 915-925.
Cattelan, S.; James, H.; Paolo, P.; Alessandro,
D.; Matteo, G.; Andrea, P.; and Chiara, M. [2020]. Maternal predation risk increases offspring’s exploration but does not affect schooling behavior. Behavioral Ecology, Vol. 31, No. 5, pp. 1207-1217. https://doi.org/10.1093/beheco/araa071.
Cerqueira, M.; Millot, S.; Felix, A.; Silva, T.; Oliveira, G.A.; Oliveira, C.C.; Rey, S.; MacKenzie, S.; and Oliveira, R. [2020]. Cognitive appraisal in fish: stressor predictability modulates the physiological and neuro-behavioural stress response in sea bass. Proceedings of Royal Society B.28720192922. http://doi.org/10.1098/ rspb.2019.2922.
Charla, J.B.; Benno, W.; Charlotte, N.; and Marianne, H.R. [2020]. Behavioural responses of humpback whales (Megaptera novaeangliae) to two acoustic deterrent devices in a northern feeding round off Iceland. Aquatic Mammals, Vol. 46, No. 6, pp. 584-602. DOI 10.1578/AM.46.6.2020.584.
Coram, A.; Gordon, J.; Dave, T.; and Simon, N. [2014]. Evaluating and assessing the relative effectiveness of non-lethal measures, including acoustic deterrent device on marine mammals. Scottish Government.
Corbett, W.T. [2019]. The behavioural and physiological effects of pile-driving noise on marine species. Thesis submitted to the University of Exeter. http://hdl.handle. net/10871/36001.
Costa, D. [1986]. Physiological effects of prey aversion protocol using lithium chloride on Californian sea lions. U.S. Marine Mammal Commission, Washington, D.C.
Daniel, F.; Cardozo, P.; Lara, T.; Zane, C.N.; and Matthew, B.P. [2020]. A new look at the role of Mesoamygdaloid dopamine neurons in aversive conditioning. Journal
of Neuroscience Vol. 40, pp. 7590-7592. DOI: https://doi.org/10.1523/JNEUROSCI.
De La Croix, R. [2008]. Preventing damage to fish by seals and sea lions. https://silo. tips/download/preventing-damage-to-fishby-seals-and-sea-lions.
de Oliveira, L.R.; Ana, C.P.; Rodrigo, M.; Mônica, T.E.; Paulo, H.O.; Enrique, A.C.; and Silvio, M. [2020]. Assessing the economic impact caused by South American sea lions based on onboard check versus fishermen’s perception: the two sides of the same coin. Marine Policy, Vol. 121, 104193. doi.org/10.1016/j.marpol.2020.104193.
De Vere, A.J.; Lilley, M.K.; and Frick, E.E. [2018]. Anthropogenic impacts on the welfare of wild marine mammals. Aquatic Mammals, Vol. 44, No. 2, pp.150-180.
Debusschere, E.; Hostens, K.; Adriaens, D.; Ampe, B.; Botteldooren, D.; De Boeck, G.; De Muynck, A.; Sinha, A.K.; Vandendriessche, S.; Van Hoorebeke, L.; Vincx, M.; and Degraer, S. [2016]. Acoustic stress responses in juvenile sea bass Dicentrarchus labrax induced by offshore pile driving Environmental Pollution, Vol. 208, pp. 747757. DOI 10.1016/j.envpol.2015.10.055.
Erbe, C.; Dunlop, R.; and Dolman, S. [2018]. Effects of noise on marine mammals. In: Slabbekoorn H.; Dooling R.; Popper A.; Fay, R. (eds). Effects of Anthropogenic Noise on Animals. Springer Handbook of Auditory Research, Vol. 66. Springer, New York, NY. https://doi.org/10.1007/978-14939-8574-6_10.
Erbe, C.; Marley, S.A.; Schoeman, R.P.; Smith, J.N.; Trigg, L.E.; and Embling, C.B. [2019]. The effects of ship noise on marine mammals –a review. Frontiers in Marine Science, Vol. 6. DOI=10.3389/fmars.2019.00606
Espinosa-Miranda, C.; Caceres, B.; Blank, O.; Fuentes-Riquelme, M.; and Heinrich, S. [2020]. Entanglements and mortality of endemic Chilean dolphins (Cephalorhynchus eutropia) in salmon farms in southern Chile. Aquatic Mammals, Vol. 46, No. 4, pp. 337-343.
Fakhru'l-Razi, A.; Mohd, M.; Ridwan, W.; and Aini, M.S. [2020]. Consequences of the 2004 Indian Ocean tsunami in Malaysia. Safety Science, Vol. 121, pp. 619-631. doi. org/10.1016/j.ssci.2019.05.016.
Fangqi, C.; Yonghui, W.; Zhenyu, D.; Xin, X.; Shaoheng, L.; Haiwu, Z.; Chunli, D.; Gentian, Y.; and Yunlong, Z. [2019]. A novel triboelectric nanogenerator based on electrospun polyvinylidene fluoride nanofibers for effective acoustic energy harvesting and self-powered multifunctional sensing. Nano Energy, Vol. 56, pp. 241-251. doi.org/10.1016/j.nanoen.2018.11.041.
Fanny, B.; Josefa, E.; Steffi, M.; Sarah, B.M.; Kraak, H.; and Strehlow, V. [2020]. Boats don’t fish, people do – how fishers’ agency can inform fisheries-management on bycatch mitigation of marine mammals and sea birds. Marine Policy, Vol. 122, 104268. doi.org/10.1016/j.marpol.2020.104268.
Findlay, C.R.; Ripple, H.D.; Coomber, F.; Froud, K.; Harries, O.; van Geel, N.C.F.; Calderan, S.V.; Benjamins, S.; Risch, D.; and Wilson, B. [2018]. Mapping widespread and increasing underwater noise pollution from acoustic deterrent devices. Marine Pollution Bulletin, Vol. 135, pp. 1042-1050. doi.org/10.1016/j.marpolbul.2018.08.042.
Findlay, C.R.; Aleynik, D.; Farcas, A.; Merchant, N.D.; Risch, D.; and Wilson, B. [2021]. Auditory impairment from acoustic seal deterrents predicted for harbour
porpoises in a marine protected area Journal of Applied Ecology, Vol. 58, No. 8, pp.1631-1642.
Götz, T. and Janik, V.M. [2013]. Acoustic deterrent devices to prevent pinniped depredation: efficiency, conservation concerns and possible solutions. Marine Ecology Progress Series, Vol. 492, pp. 285302. https://doi.org/10.3354/meps10482.
Gumpel, P.; Hörtnagl, A.; and Sorg, M.; [2019]. High tensile stainless steel as a sustainable material for aquaculture. Procedia Manufacturing, Vol. 30, pp. 315-322.
Hamilton, S. and Baker, G.B. [2019]. Technical mitigation to reduce marine mammal bycatch and entanglement in commercial fishing gear: lessons learnt and future directions. Reviews in Fish Biology and Fisheries, Vol. 29, pp. 223-247. https://doi. org/10.1007/s11160-019-09550-6.
Hatch, L.T.; Clark, C.W.; and Van Parijs, S.M. [2019]. Anthropogenic noise in the ocean: measurement and mitigation. In: The Effects of Noise on Aquatic Life II. Springer, pp. 441-448.
Heide, T. [2020]. Assessing the sustainability of seal tourism at Duiker Island, Hout Bay Faculty of Science, Department of Biological Sciences. http://hdl.handle.net/ 11427/32722.
Hiley, H.M.; Janik, V.M.; and Götz, T. [2021]. Behavioural reactions of harbour porpoises Phocoenaphocoena to startle-eliciting stimuli: movement responses and practical applications. Marine Ecology Progress Series, Vol. 672, pp.223-241.
Holmes, L.J.; McWilliam, J.; Ferrari, M.C.O.; and McCormick, M.I. [2017]. Juvenile damselfish are affected but desensitize to small motorboat noise. Journal of
Experimental Marine Biology and Ecology, Vol. 494, pp. 63-68.
Hu, Z. and Kang, Y. [2016]. A study on the noise reduction effect of air bubble curtain in underwater blasting. Shock and Vibration Ecology, Vol. 494, pp. 63-68.
Ida, C.; Len, T.; Carlström, J.; Amundin, M.; Teilmann, J.; Tregenza, N.; Tougaard, J.; Koblitz, J.C.; Sveegaard, S.; Wennerberg, D.; Loisa, O.; Dähne, M.; Brundiers, K.; Kosecka, M.; Kyhn, L.A.; Ljungqvist, C.T.; Pawliczka, I.; Koza, R.; Arciszewski, B.; Galatius, A.; Jabbusch, M.; Laaksonlaita, J.; Niemi, J.; Lyytinen, S.; Gallus, A.; Benke, H.; Blankett, P.; Skóra, K.E.; and Acevedo-Gutiérrez, A. [2018]. Basin-scale distribution of harbour porpoises in the Baltic Sea provides basis for effective conservation actions. Biological Conservation, Vol. 226, pp. 42-53. doi.org/ 10.1016/j.biocon.2018.06.031.
Iwama, G.; Linda, N.; and John, F. [1997]. Aquatic mammals and other species. Salmon Aquaculture Review, Vol. 3. Technical Discussion Papers, part E. Jefferson, T. and Curry, B. [1996]. Acoustic methods of reducing or eliminating marine mammal-fishery interactions: do they work? Ocean Coastal Management, pp. 41-70.
Jiang, M.; Li, J.; Wang, Z.; and Zhang, J. [2019]. Experimental and numerical investigation of the noise reduction performance of an air bubble curtain in underwater piling Journal of Environmental Management, Vol. 236, pp. 644-651.
Jones, N.L.; Vu, H.Q.; and Kim, H. [2016]. Characteristics of air bubble curtains for underwater noise reduction. Journal of the Acoustical Society of America, Vol. 140, No. 5, EL464-EL469.
Kim, H.; Hwang, J.; Yoon, J.; Lee, J.; Lee, S.; Lee, J.; and Joo, K. [2018]. Underwater noise reduction using a novel compact silencer. Applied Acoustics, Vol. 138, pp. 36-45.
Kraus, S. [1999]. The once and future ping: challenges for the use of acoustic deterrents in fisheries. Maritime Technology Journal, Vol. 33, No. 2, pp. 90-93.
Kusel, E.T.; Bland, M.; and Hatch, L. [2019]. Underwater noise monitoring: a review of methods and applications. In: The Effects of Noise on Aquatic Life II. Springer, pp. 649-656.
Kusku, H. [2020]. Acoustic sound-induced stress response of Nile tilapia (Oreochromis niloticus) to long-term underwater sound transmissions of urban and shipping noises. Environmental Science and Pollution Research, Vol. 27, pp. 36857-36864. https:// doi.org/10.1007/s11356-020-09699-9.
Kusku, H.; Yigit, Ü.; Yilmaz, S.; Yigit, M.; and Ergün, S. [2020]. Acoustic effects of underwater drilling and piling noise on growth and physiological response of Nile tilapia (Oreochromis niloticus) Aquaculture Research, Vol. 51, pp. 31663174. https://doi.org/10.1111/are.14652.
Kusku, H.; Yigit, M.; Yilmaz, S.; Yigit, Ü.; and Ergün, S. [2023]. Multiple exposures to thunderstorm-sound in Nile tilapia (Oreochromis niloticus): physiological response and stress recovery. Annals of Animal Science. DOI: 10.2478/aoas-20220075.
Lam, Y.W.; Leung, K.M.; and Tang, S.K. [2013]. Design and optimization of air bubble curtains for underwater noise mitigation from impact pile driving. Applied Acoustics, Vol. 74, No. 4, pp. 517-527.
Liu, M.; Zhao, D.; Zhang, X.; and Ding, P. [2018]. Experimental investigation on noise reduction effect of air bubble curtains for underwater piling. Journal of Sound and Vibration, Vol. 413, pp. 383-392.
McDermott, J. and Hauser, M. [2004]. Are consonant intervals music to their ears? Spontaneous acoustic preferences in a nonhuman primate. Cognition, Vol. 94, No. 2, pp. B11-B21.
Monaco, C.; Cavallé, M.; and Peri, I. [2019]. Preliminary study on interaction between dolphins and small-scale fisheries in Sicily: learning mitigation strategies from agriculture. Acces la Success; Bucharest, Vol. 20, No. 2, pp. 400-407.
Mueller-Blenkle, C.; McGregor, P.K.; Gill, A.B.; Andersson, M.H.; Metcalfe, J.; Bendall, V.; Sigray, P.; Wood, D.T.; and Thomsen, F. [2010]. Effects of piledriving noise on the behaviour of marine fish. COWRIE Ref: Fish 06-08, Technical Report. https://dspace.lib.cranfield.ac.uk/ handle/1826/8235.
Nash, C.E.; Iwamoto, R.N.; and Mahnken, C.V. [2000]. Aquaculture risk management and marine mammal interactions in the Pacific Northwest. Aquaculture, pp. 307-323.
Northridge, S.; Gordon, J.; Booth, J.; Corderans, S.; Cargill, A.; Coram, A.; Gillespie, D.; Lonergan, M.; and Webb, A. [2010]. Assessment of the impact and utility of acoustic deterrent device. A report commissioned by SARF and prepared by SMRU.
Nunny, L. [2020]. Animal welfare in predator control: lessons from land and sea. How the management of terrestrial and marine mammals impacts wild animal welfare in human-wildlife conflict scenarios in Europe
Animals, Vol. 10, No. 2: 218. https://doi. org/10.3390/ani10020218.
O’Neil, D. [2020]. Reducing bat fatalities using ultrasonic acoustic deterrent technology: a potential mechanism for conservation at offshore wind energy sites. Master’s thesis, Harvard Extension School.
Orenstein, S.; Langstaff, L.; and Manning, L. [2006]. Acoustic committee on acoustic impacts on marine mammals. Sponsored by the Marine Mammal Commission, 63 pages.
Pemberton, D. and Shaughnessy, P.D. [1993]. Interaction between seals and marine fishfarms in Tasmania, and management of the problem. Aquatic Conservation: Marine and Freshwater Ecosystems, Vol. 3, pp. 149-158.
Pitman, R.L.; Fearnbach, H.; and Durban, J.W. [2018]. Abundance and population status of Ross Sea killer whales (Orcinus orca, type C) in McMurdo Sound, Antarctica: evidence for impact by commercial fishing? Polar Biology, Vol. 41, pp. 781-792. https://doi. org/10.1007/s00300-017-2239-4.
Popper, A.N.; Hawkins, A.D.; Fay, R.R.; Mann, D.A.; Bartol, S.; Carlson, T.J.; Coombs, S.; Ellison, W.T.; Gentry, R.L.; Halvorsen, M.B.; Løkkeborg, S.; Rogers, P.H.; Southall, B.L.; Zeddies, D.G.; and Tavolga, W.N. [2014]. ASA S3/SC1.4 TR-2014 Sound exposure guidelines for fishes and sea turtles: a technical report. Prepared by ANSI-Accredited Standards Committee S3/SC1 and Registered with ANSI American National Standards Institute. 10.1007/978-3-319-06659-2.
Pottinger, T.G. [2008]. The stress response in fish mechanisms, effects and measurement. Fish Welfare, Blackwell Science, pp. 32-48.
Poulton, D.A.; Porteus, C.S.; and Simpson, S.D. [2016]. Combined impacts of elevated
CO2 and anthropogenic noise on European sea bass (Dicentrarchuslabrax). ICES
Journal of Marine Science, Vol. 73, pp. 1-73.
Purser, J.; Bruintjes, R.; Simpson, S.D.; and Radford, A.N. [2016]. Condition dependent physiological and behavioural responses to anthropogenic noise. Physiology and Behavior, Vol. 155, pp. 157-161.
Putland, R.L. and Mensinger, A.F. [2019]. Acoustic deterrents to manage fish populations. Reviews in Fish Biology and Fisheries, Vol. 29, pp. 789-807. https://doi. org/10.1007/s11160-019-09583-x.
Quick, N.J.; Middlemas, S.J.; and Armstrong, J.D. [2004]. A survey of anti-predator controls at marine salmon farms in Scotland Aquaculture, pp. 169-180.
Radford, A.N.; Kerridge, E.; and Simpson, S.D. [2016]. Repeated exposure reduces the response to impulsive noise in European seabass. Global Change Biology, Vol. 22, No. 10, pp. 3349-3360.
Reeves, R.R.; Read, A.J.; and Notarbartolo, S. [2001]. Report of the workshop on interactions between dolphins and fisheries in the Mediterranean: evaluation of mitigation alternatives. Istituto Centrale per la RicercaScientifica e TecnologicaApplicata al Mare, Rome, Italy. 44.
Rolland, R.M.; Parks, S.E.; Hunt, K.E.; Castellote, M.; Corkeron, P.J.; Nowacek, D.P.; Wasser, S.K.; and Kraus, S.D. [2012]. Evidence that ship noise increases stress in right whales. Proceedings of the Royal Society B: Biological Sciences, Vol. 279, No. 1737, pp. 2363-8.
Rowley, K. [2020]. Aquaculture interactions with endangered species bibliography. National Oceanic and Atmospheric Administration, U. S. Department of
Commerce. https://doi.org/10.25923/x6mv8952.
Sarà, G.; Dean, J.M.; D’Amato, D.; Buscaino, G.; Oliveri, A.; Genovese, S.; Ferro, S.; Buffa, G.; Lo Martire, M.; and Mazzola, S. [2007]. Effect of boat noise on the behaviour of bluefin tuna Thunnus thynnus in the Mediterranean Sea. Marine Ecology Progress Series, Vol. 331, pp. 243-253.
Sarnocińska, J.; Teilmann, J.; Balle, J.D.; van Beest, F.M.; Delefosse, M.; and Tougaard, J. [2020]. Harbor porpoise (Phocoenaphocoena) reaction to a 3D seismic airgun survey in the North Sea Front. Marine Science, Vol. 6, No. 824.
Schoeman, R.P.; Patterson-Abrolat, C.; and Plön, S. [2020]. A global review of vessel collisions with marine animals. Frontiers in Marine Science, Vol. 7, No. 292. doi=10.3 389/fmars.2020.00292.
Shi, H.; Wu, X.; and Cheng, W. [2019]. Experimental study on the noise reduction of air bubble curtains in underwater pile driving. Applied Acoustics, Vol. 154, pp. 139-145.
Simmonds, M.P. and Dolman, S.J. [2016]. Noise from marine pile driving and its effects on fishes. Journal of the Marine Biological Association of the United Kingdom, Vol. 96, No. 1, pp. 87-97.
Simpson, S.D.; Radford, A.N.; Holles, S.; Ferarri, M.C.O.; Chivers, D.P.; McCormick, M.I.; and Meekan, M.G. [2015]. Smallboat noise impacts natural settlement behavior of coral reef fish larvae. Advances in Experimental Medicine and Biology, Vol. 875, pp. 1041-1048.
Smith, B.; Jaques, N.; Appleby, R.; Morris, S.; and Jordan, N. [2020]. Automated shepherds: responses of captive dingoes to
sound and an inflatable, moving effigy. Pacific Conservation Biology. https://doi. org/10.1071/PC20022.
Southall, B.L.; Finneran, J.J.; Reichmuth, C.; Nachtigall, P.E.; Ketten, D.R.; Bowles, A.E.; Ellison, W.T.; Nowacek, D.P.; and Tyack, P.L. [2019]. Marine mammal noise exposure criteria: updated scientific recommendations for residual hearing effects. Aquatic Mammals, Vol. 45, No. 2, pp. 125-232, 10.1578/AM.45.2.2019.125.
Spiga, I.; Aldred, N.; and Caldwell, G.S. [2017]. Anthropogenic noise compromises the antipredator behaviour of the European sea bass, Dicentrarchus labrax (L.). Marine Pollution Bulletin, Vol. 122, No. 1-2, pp. 297-305.
Staaterman E.; Gallagher, A.J.; Holder, P.E.; Reid, C.H.; Altieri, A.H.; Ogburn, M.B.; Rummer, J.L.; and Cooke, S.J. [2020]. Exposure to boat noise in the field yields minimal stress response in wild reef fish Aquatic Biology, Vol. 29, pp. 93-103. https://doi.org/10.3354/ab00728.
Sun, W.; Zhang, H.; and Zhou, Z. [2018]. Study on underwater noise attenuation effect of bubble curtain based on laboratory and field experiments. Ocean Engineering, Vol. 166, pp. 330-340.
Thomas, G. and Vincent, M.J. [2010]. Aversiveness of sound in Phocid seals: psycho-physiological factors, learning processes and motivation. Journal of Experimental Biology, Vol. 213, pp. 15361548.
Thomsen, F.; Lüdemann, K.; Kafemann, R.; and Piper, W. [2020]. Effects of offshore wind farm noise on marine mammals and fish. Biola, Hamburg, Germany, on behalf of COWRIE Ltd, 62 pages.
Todd, V.L.; Jiang, J.; and Ruffert, M. [2019]. Potential audibility of three acoustic harassment devices (AHDs) to marine mammals in Scotland, U.K. International Journal of Acoustics and Vibration, Vol. 24, No. 4, pp. 792-800.
Todd, V.L.; Williamson, L.D.; Jiang, J.; Cox, S.E.; Todd, I.B.; and Ruffert, M. [2021]. Prediction of marine mammal auditoryimpact risk from acoustic deterrent devices used in Scottish aquaculture. Marine Pollution Bulletin, Vol. 165, p. 112171.
Wale, M.A.; Simpson, S.D.; and Radford, A.N. [2013]. Noise negatively affects foraging and anti-predator behaviour in shore crabs. Animal Behaviour, Vol. 86, No. 1, pp. 111118.
Weilgart, L. [2018]. The impact of ocean noise pollution on fish and invertebrates. Ocean Care and Dalhousie University, Report for Ocean Care, Switzerland.
Würsig, B. and Gailey, G.A. [2002]. Marine mammals and aquaculture: conflicts and potential resolutions. In: Responsible Marine Aquaculture, eds. Stickney, R.R. and McVey, J.P. CAB International.
Wysocki, L.E.; Dittami, J.P.; and Ladich, F. [2006]. Ship noise and cortisol secretion in European freshwater fishes. Biological Conservation, Vol. 128, No. 4, pp. 501-508.
Zwicker, E. and Fastl, H. [2004]. Psychoacoustic: facts and models Springer-Verlag, Berlin and Heidelberg, 428.
Informative
Cutting Edge
Provocative
Challenging
Thought Provoking
International thejot.net
So far, the hatchery team has determined that oysters can successfully be grown in floating bags to avoid the parasite. As well, details about the biology of MSX, such as its preferred environment and thermal tolerances, have been discovered. So, what is next? Ms. Dove and her team are in the early stages of growing algae to feed the oysters in the hatchery to provide a more natural diet that will aid in the transition from hatchery to farm.
www.verschurencentre.ca
sdove@verschurencentre.ca
PHD STUDENT, ENVIRONMENTAL SCIENCE AND POLICY
UNIVERSITY OF MIAMI RESEARCH ASSISTANT
ROSENSTIEL SCHOOL OF MARINE, ATMOSPHERIC, AND EARTH SCIENCE
MIAMI, FLORIDA, U.S.
For more than a decade, Carlos Carroza Meza has been researching salmon aquaculture, a major economic activity in his native Chile. Over the course of his work, he has observed the positive impacts this industry has had on the lives of many people. Nevertheless, the industry continues to face staunch opposition due to institutional framework inadequacies and poor corporate practices. Mr. Meza is motivated to contribute to the creation of a novel relationship among the industry, local communities, and authorities. This can be achieved through modifications to institutional governance, environmental monitoring, and the relocation of current production sites to areas with greater energy, such as offshore aquaculture.
The main aim of Mr. Meza’s current research is to identify the incentives, protocols, tools, and
technologies that can effectively augment the legitimacy of salmon farming for the authorities, consumers, and local communities. It is pertinent to acknowledge that each stakeholder has its own unique priorities and concerns about the salmon farming industry, and understanding them is the first step towards tackling the issues at hand and devising solutions.
The primary advantage of the salmon farming industry would be the improvement of coastal ecosystems and the well-being of the local communities situated near the salmon farms. Ultimately, sustainable salmon farming will benefit all stakeholders directly and indirectly involved in the activity, from labourers to producers all the way to consumers.
Field activities such as collecting samples and interviewing individuals working in the salmon industry provide Mr. Meza with an opportunity to gain a deeper understanding of the reality of this sector, its relationship with the environment, and its importance for those who rely on it as a source of livelihood.
https://www.ere.earth.miami.edu/people/ carloscarozzameza/index.html
chc99@earth.miami.edu
DOCTOR OF PHILOSOPHY CANDIDAT E IN EPIDEMIOLOGY
ATLANTIC VETERINARY COLLEGE
UNIVERSITY OF PRINCE EDWARD ISLAND
CHARLOTTETOWN, P.E.I., CANADA
Dr. Marianne Parent hopes that those involved in the culture of Atlantic salmon might find the results from her research meaningful and useful. She currently works on a project looking at the dispersal of sea lice within and among the Atlantic salmon farms of the Bay of Fundy in New Brunswick, Canada.
Using a dataset of the counts of sea lice on fish, she creates complex models that consider the variability of the sea lice counts in relation to the environmental conditions and anti-lice treatments applied. Using these models, Dr. Parent estimates the effects that a count of sea lice a few weeks ago will have on the current count of sea lice at a salmon farm, and the influence that its neighbour has on its count of sea lice. Another model she constructed predicts the sea lice counts using historical counts, environmental data, and treatment information. The goal is to gain insight into
the dispersal of sea lice in the area because the management of sea lice poses a significant challenge on the farmers and managers.
She became interested in the project through the promise to work with a large dataset and the combination of this data with hydrological models. This research unites her skills in veterinary medicine and data analysis to address an important issue in salmon farming – sea lice management. The most satisfying part of the project, and veterinary epidemiology in general, is finding solutions to complex problems. Dr. Parent gets the satisfaction of solving a puzzle while helping animals.
https://pubmed.ncbi.nlm.nih.gov/34411315 marianne.i.parent@gmail.com
Who should read this paper?
Those who are interested in learning how satellite observations and advanced remote sensing techniques can be used for offshore and oceanographic applications will find this paper of value. It will be of interest to operators in the ocean environment and those who are looking for effective monitoring solutions for offshore installations.
Why is it important?
Combining datasets from different satellites to improve ocean surface wind vector maps enhances the spatial resolution, frequency, and latency of the current satellite-based ocean products. The generated ocean surface wind products can be used for various offshore activities, such as navigation, aviation, and commercial fisheries. They can also be used within meteorological and oceanographic models to map and forecast different met-ocean parameters, such as ocean fog, current, and waves. Finally, researchers can use these products in understanding ocean dynamics.
Dr. Meisam Amani is the remote sensing team lead at WSP Environment and Infrastructure Canada Limited, where he manages and leads various industrial, governmental, and academic projects related to remote sensing and geospatial technologies. Over the past 15 years, he has utilized various remote sensing datasets (e.g., UAV, optical, LiDAR, SAR, scatterometer, radiometer, and altimeter) along with different machine learning and big data processing algorithms to provide effective solutions for industrial challenges. A list of his research works, including over 100 peer-reviewed journal and conference papers, can be found at www. researchgate.net/profile/Meisam_Amani3.
Dr. Sahel Mahdavi is currently affiliated with the remote sensing team at WSP Environment and Infrastructure Canada Limited. She has almost 14 years of academic and industrial background in remote sensing and is familiar with a wide array of topics relevant to remote sensing and GIS and their applications in various environmental aspects. She has authored more than 60 peer-reviewed articles.
Terry Bullock is a senior physical environmental scientist with over 35 years’ operational and research experience in Canada and internationally. He worked as a consulting marine meteorologist since 1985 and specializes in on-site support for environmentally sensitive projects, such as heavy lift operations, wind energy resource monitoring and assessment, and regulatory compliance. Mr. Bullock’s research and development activities include wind boundary layer modelling, wind turbine energy potential and site assessment, marine and terrestrial climatological analysis, extreme event analysis, atmospheric mass and wind analysis, real-time data assimilation into analysis and forecasting systems, dynamical and statistical metocean-ice modelling, and weather-observation data processing and quality control.
Dr. Steven Beale is a data scientist/engineer developing and deploying predictive meteorological models at WSP Environment and Infrastructure Canada Limited. His current work is focused primarily on the design and development of data pipelines for meteorological forecast data and remote sensing data and the automation of predictive models using that pipeline.
Meisam Amani1, Sahel Mahdavi1, Terry Bullock2, and Steven Beale2
1WSP Environment & Infrastructure Canada Limited, Ottawa, O.N., Canada
2WSP Environment & Infrastructure Canada Limited, St. John’s, N.L., Canada
Accurate ocean surface wind vector (OSWV) products are important for various offshore operations. Currently, different types of satellites provide OSWV data, using one of which alone does not yield a high accuracy, reasonable revisit time, and full coverage over large ocean environments. Moreover, the current OSWV products that were generated by fusing different satellite-based wind datasets have their own limitations for operational applications (e.g., long latency). Therefore, the main objective of this study was to produce improved OSWV products using a spatiotemporal fusion algorithm applied to a combination of wind datasets collected by seven satellites: scatterometers (ScatSat-1, MetOp-A, MetOp-B, and MetOp-C), a nonpolarimetric radiometer (GCOM-W), and imaging synthetic aperture radar (SAR) systems (Sentinel-1A and Sentinel-1B). The spatial resolution, frequency, and latency for the final fused OSWV product were 1 km, six hours, and two to six hours, respectively. The comparison of the results with those derived from meteorological reanalysis models at different study areas showed the high potential of the proposed method for operational OSWV mapping and monitoring.
Ocean wind; Remote sensing; Synthetic aperture radar SAR; Scatterometer; Radiometer
Ocean surface wind vector (OSWV; i.e., both wind speed and direction) is an important parameter for various offshore activities, such as navigation, aviation, and commercial fisheries. OSWV is also required as an input parameter for various ocean dynamics modelling studies, such as ocean wave and ocean current estimation [Liu and Xie, 2006; Young and Donelan, 2018; Zou et al., 2017]. It also plays a key role in different forecasting models, such as fog nowcasting [Amani et al., 2020; Mahdavi et al., 2021; Wentz et al., 2017].
There are various methods for OSWV measurements, such as in-situ and remote sensing observations. Although in-situ measurements of OSWV are usually accurate, they have several limitations. For example, they are point-based and do not provide enough coverage over large oceanographic areas. On the other hand, remote sensing systems, such as satellites, have many advantages for measuring oceanographic variables, such as OSWV. For example, satellites cover large areas and can acquire near real-time data with a high frequency over any area in the ocean. Moreover, many satellites, such as scatterometers, radiometers, and synthetic aperture radar (SAR) systems, can work during both daytime and nighttime and in any weather conditions, which can be very helpful in continued monitoring of OSWV in any ocean environment. Finally, most satellite data for ocean applications are open access, which makes them suitable for operational ocean wind studies over a long period of time [Amani et al., 2022C; 2022A; 2022D; 2022B].
There are different satellite types, such as scatterometers, radiometers, and SARs, which can be used for OSWV estimation. Each of these satellites has its own advantages and, therefore, a combination of them is usually used to produce more accurate OSWV products with the required spatial and temporal resolutions for operational projects. For example, Zou et al. [2017] combined wind products generated from three scatterometers (i.e., ASCAT, OSCAT, and HSCAT), two nonpolarimetric radiometers (i.e., AMSR-2 and SSMI), and one polarimetric radiometer (i.e., Windsat) using a spatiotemporally weighted fusion algorithm. Their OSWV map had the spatial and temporal resolutions of 25 km and three hours, respectively. Moreover, Bentamy et al. [2007] used an optimum interpolation algorithm based on the kriging method to fuse the OSW products generated from QuickSCAT scatterometer, SSM/I radiometer, and the European Centre for Medium-Range Weather Forecasts (ECMWF) datasets. They produced improved OSW vector maps with a spatial resolution of approximately 25 km and frequencies of three hours and six hours over the Mediterranean Sea. Furthermore, the root mean square errors were respectively 1.2 m/s and 20.6˚ for the OSW speed and direction when compared to the in-situ data.
The Grand Banks area, located in Eastern Canada’s offshore, is rich in oil and gas and commercial fish and, thus, includes many aviation and navigation activities. Reliable and up-to-date information about the ocean, such as OSWV, is critical for these operations [Bullock et al., 2016; Mahdavi et al., 2021]. Although several met-ocean models have so far been developed and tested in this study area,
there is no satellite-based method to accurately estimate and predict OSWV. Moreover, although there are several satellite-based OSWV products produced worldwide, there are still several limitations for effectively using them in operational applications. The main shortcomings of current satellite-based OSWV products to be used in the Grand Banks and the main objectives of this study to address these disadvantages are provided below.
As mentioned, the OSWV map produced by only one satellite does not provide enough coverage and frequency over large ocean environments. Additionally, there are several OSWV products, produced by a combination of satellite datasets, that still do not have appropriate frequency (e.g., daily) for operational applications. Therefore, in this study, the OSWV products generated from seven satellites were fused to produce improved OSWV maps every six hours with full coverage of the Grand Banks.
Near real-time datasets are of considerable importance for offshore operations. However, the latency of the current OSWV products is usually more than six hours, which is not appropriate for operational purposes. Thus, an algorithm was developed in this study to produce OSWV with a latency between two to six hours.
Most ready-to-use OSWV datasets have been developed as global products and do not have enough accuracy for specific regions with its own met-ocean characteristics. Consequently, one of the main objectives of this study is developing an algorithm that can deal with the unique weather and oceanographic specifications of the Grand Banks, although it
was demonstrated that the proposed algorithm could provide reasonable accuracies in other offshore regions as well.
The fused OSWV products were mostly generated from a combination of datasets acquired by scatterometers and radiometers and, thus, have a coarse spatial resolution (e.g., 10 km). Therefore, the data collected by the most recent radiometers, scatterometers, and SAR systems were combined in this study to generate not only more accurate OSWV products, but also with a higher spatial resolution (i.e., 1 km).
The Grand Banks, located in Newfoundland’s offshore area in Canada (latitude = 40˚ N to 55˚ N, longitude = 45˚ W to 70˚ W), was selected as the study area for producing OSWV maps (see Figure 1). This area is a relatively shallow bathymetric feature and is important for various offshore operations, such as oil and gas activities. For instance, due to harsh weather in this area, OSWV can produce ocean wave heights of up to 20 metres, which can pose serious threats to both aviation and navigation operations. Consequently, many private and governmental organizations need accurate and frequent OSWV information about the Grand Banks area [Amani et al., 2020; Bullock et al., 2016; Mahdavi et al., 2021].
The OSW datasets from four sensor types on board seven satellites were used in this study. The specifications of these instruments are provided in Table 1 and discussed in more detail below.
ASCAT is a scatterometer sensor flying on board the European MetOp-A/B/C satellites, launched on October 19, 2006, September 17, 2012, and November 7, 2018, respectively. This sensor measures both wind speed and direction over the globe. In this study, the ASCAT Level-2 OSWV datasets provided by the EUropean organization for the exploitation of METeorological SATellites (EUMETSAT) were used [EUMETSAT, n.d.]. Specifically, the ASCAT Coastal Winds at 12.5 km Swath Grid – MetOp products with a spatial resolution of 12.5 km were employed.
OSCAT is a Ku-band scatterometer sensor flying on board the Indian ScatSat-1 satellite, launched on September 26, 2016, which measures both wind speed and direction over the globe. In this study, the OSCAT Level-2 OSWV datasets provided by the EUMETSAT were used [EUMETSAT, n.d.]. Specifically, the OSCAT Winds at 25 km Swath Grid – ScatSat products with a spatial resolution of 25 km were employed.
AMSR-2 is a radiometer carried on board the Japanese Global Change Observation MissionWater (GCOM-W) satellite, launched on May 18, 2012. Since AMSR-2 is a non-polarimetric radiometer, it only provides OSW speed. In this study, the Level-2 wind product provided by the National Oceanic and Atmospheric Administration were utilized [NOAA, n.d.]. Specifically, the GCOM-W Advanced Microwave Scanning Radiometer 2 (AMSR2) Level 2 (AMSR2_L2) products with different spatial resolutions varying between 10 km to 50 km were used.
which were launched on April 3, 2014, and April 25, 2016, respectively. Both OSW speed and direction can be derived from the backscattering coefficient collected by Sentinel-1A/B satellites over the ocean. In this study, the ocean wind field component of the Level-2 Ocean product of Sentinel-1A/B, provided by the European Space Agency [ESA, n.d.], were employed. Specifically, the OSWV products generated from the interferometric wide (IW) mode with a spatial resolution of 1 km were utilized.
The proposed satellite-based method for OSWV mapping had several steps as described below:
The Sentinel-1 data were initially downloaded by defining the boundary of the study areas. Afterwards, since the ASCAT, OSCAT, and AMSR-2 wind datasets are provided with global coverage, an algorithm was developed to select and clip the datasets based on the study areas.
Since the objective of this study was to produce OSWV maps with a six-hour interval (i.e., 00h, 06h, 12h, 18h UTC), an automatic algorithm was developed to create 6h-interval directories. Then, the datasets were inserted into the correct time interval directories based on the time of the acquisition of each satellite data.
C-SAR is an imaging microwave sensor on the European Sentinel-1A/B satellites,
Since the purpose of this study was producing OSWV maps with the spatial resolution of 1 km (i.e., equal to the spatial resolution of the Sentinel-1 data), a grid with a cell size of 1 km by 1 km was created for
each OSWV dataset. Considering the size and location of the Grand Banks study area (Figure 1), each grid contained 1,681 and 2,122 cells along the latitude and longitude directions, respectively. The initial values of the cells were set to Not a Number.
All the datasets, except for the Sentinel-1 data, were initially resampled to a spatial resolution of 1 km. Then, the location of pixels within the corresponding grid was determined through the georeferencing procedure. Subsequently, OSWV values of each cell was derived using the nearest neighbour interpolation. The nearest neighbour interpolation was utilized due to simplicity of this algorithm and having the least impact on the original wind values.
A temporal interpolation was also employed to apply weights based on the acquisition time of data. To this end, an inverse distance weighting interpolation algorithm (Equation (1)) was employed [Shepard, 1968]. In this algorithm, the parameter value can be estimated based on the weighted average of values within the time interval (i.e., each six hours in this study). The weights are inversely proportional to a power of time difference with the end of time interval, meaning that as the acquisition time of the OSWV data approaches the end of time interval, it is given higher weights.
value at the time of observation, which is within the time interval; ti is the time of observation; T is time interval in hours, which is six in our case; and N is the number of observations within the time interval.
After applying both spatial and temporal interpolations and calculating the OSWV values for each grid (each wind data had one grid), all the OSWV values derived from different satellite data for each cell were averaged to produce the OSWV map. The value of each cell was calculated based on the following criteria:
• If there is more than one OSWV data for a cell, the final wind value was calculated by averaging all the values.
• If there is only one OSWV data for a cell, that value was assigned to the cell.
• If there is no OSWV data for a cell, the value was filled out from the map produced in the previous six hours.
After producing the OSWV map, several refinements were applied to improve the visual representation of the final OSWV map. For example, there were no OSWV values near the coastline areas because most of the remote sensing systems do not operate over these areas. These areas were filled out using the OSWV values of the nearest cells.
in which OSWV(tE) is the OSWV value at the end of time interval; OSWVi is the OSWV
Figure 2 illustrates several examples of the produced OSWV map with a six-hours interval using a combination of different radiometer, scatterometer, and SAR datasets. It was visually observed that the produced
maps were reasonable and provided valuable information over large ocean regions. For example, these sample maps were produced for a time when there was a windstorm in the Grand Banks region. This fact can be clearly observed in the produced maps, which include a cyclone over the Grand Banks. This showed that the proposed method could be a valuable warning tool for many offshore operations in the Grand Banks.
The results of the proposed method were compared to the meteorological reanalysis models (i.e., ERA5 model outputs, [ECMWF, n.d.]), two examples of which are demonstrated in Figure 3. The results of the satellite-based OSWV algorithm corresponded well with those of reanalysis models. It should be noted that the maps produced using ERA5 data are not near real time and have several limitations for operational purposes.
p.m.)
19
a.m.),
However, the OSWV maps produced by the proposed method have a latency of two to six hours. Moreover, the spatial resolution of ERA5 model outputs was coarser than those produced through this project (ERA5: 30 km, proposed method: 1 km).
The proposed model was applied to the U.K. offshore area to show the high potential and robustness of the proposed remote sensing method for OSWV mapping in other study areas (see Figure 4). The wind maps generated from ERA5 data are also demonstrated for the same times to compare the results. The results showed
that both meteorological and the proposed methods provided similar results in terms of wind speed values (background colour). However, there were differences between wind speed values derived from two approaches (values of the legends). This was rooted in the fact that the spatial resolution of the maps produced by the proposed method in this study was finer than those of the ERA5 (1 km versus 30 km) and, therefore, the proposed model showed more details, especially over regions where Sentinel-1 SAR data were available (e.g., see the red areas near coastlines in the maps illustrated in Figure 4). In fact, the areas of high wind speed increased
dates/times of (a), (c), and (e), respectively.
the maximum wind speed values in the legend of the maps produced using satellite data. In terms of wind direction, both methods provided similar results, except for some regions where differences could be observed.
Although the produced OSWV maps could be a great help for different organizations operating in Canada’s eastern offshore area, they had multiple limitations that are described below. Several suggestions are
also provided to improve the accuracy of the maps in future studies.
As can be seen in Figures 2-4, there were some sudden changes in the wind vectors in some parts of the maps produced by the proposed method. The main reason was utilizing several satellite data without smoothing the results. To resolve this, a smoothing filter could be applied so that better visualization and a smoother look, like that of ERA5, is obtained.
The Canadian Radarsat Constellation Mission (RCM) satellites provide the best SAR data over Canada in terms of temporal resolution (e.g., daily data over the ocean). Future studies could include the data acquired by RCM within the proposed model to increase the accuracy and temporal resolution of the maps.
The accuracies of the produced maps in this study were only assessed by visual interpretation and by comparing with the ERA5 model outputs. However, it is suggested to conduct a more in-depth validation using in-situ data and the outputs of other meteorological models.
In this study, operational and improved near real-time OSWV products were generated by fusing datasets acquired by different scatterometers, radiometers, and SAR remote sensing systems. The accuracies of the products were also assessed over two different study areas using ERA5 data. The results showed the high potential of the proposed method in providing accurate information about OSWV and windstorms in offshore areas. Additionally, the proposed method produced better OSWV
maps compared to the current models in terms of latency, spatial, and temporal resolutions.
Amani, M.; Mahdavi, S.; Bullock, T.; and Beale, S. [2020]. Automatic nighttime sea fog detection using GOES-16 imagery Atmospheric Research, Vol. 238, 104712. https://doi.org/10.1016/j.atmosres.2019. 104712.
Amani, M.; Ghorbanian, A.; Asgarimehr, M.; Yekkehkhany, B.; Moghimi, A.; Jin, S.; Naboureh, A.; Mohseni, F.; Mahdavi, S.; and Layegh, N. [2022A]. Remote sensing systems for ocean: a review (part 1: passive systems). IEEE Journal of Selected Topics in Applied Earth Observations and Remote Sensing, Vol. 15, pp. 210-234. https://doi. org/10.1109/JSTARS.2021.3130789.
Amani, M.; Mehravar, S.; Asiyabi, R.M.; Moghimi, A.; Ghorbanian, A.; Ahmadi, S.A.; Ebrahimy, H.; Moghaddam, S.H.A.; Naboureh, A.; Ranjgar, B.; Mohseni, F.; Nazari, M.E.; Mahdavi, S.; Mirmazloumi, S.M.; Ojaghi, S.; and Jin, S. [2022B]. Ocean remote sensing techniques and applications: a review (part II). Water, Vol. 14, 3401. https://doi.org/10.3390/w1421 3401.
Amani, M.; Moghimi, A.; Mirmazloumi, S.M.; Ranjgar, B.; Ghorbanian, A.; Ojaghi, S.; Ebrahimy, H.; Naboureh, A.; Nazari, M.E.; Mahdavi, S.; Moghaddam, S.H.A.; Asiyabi, R.M.; Ahmadi, S.A.; Mehravar, S.; Mohseni, F.; and Jin, S. [2022C]. Ocean remote sensing techniques and applications: a review (part I). Water, Vol. 14, 3400. https://doi.org/10.3390/w14213400.
Amani, M.; Mohseni, F.; Layegh, N.F.; Nazari,
M.E.; Fatolazadeh, F.; Salehi, A.; Ahmadi, S.A.; Ebrahimy, H.; Ghorbanian, A.; Jin, S.; Mahdavi, S.; and Moghimi, A. [2022D]. Remote sensing systems for ocean: a review (part 2: active systems). IEEE Journal of Selected Topics in Applied Earth Observations and Remote Sensing, Vol. 15, pp. 1421-1453. https://doi.org/10.1109/ JSTARS.2022.3141980.
Bentamy, A.; Ayina, H.-L.; Queffeulou, P.; Croize-Fillon, D.; and Kerbaol, V. [2007]. Improved near real time surface wind resolution over the Mediterranean Sea Ocean Science, Vol. 3, pp. 259-271. https:// doi.org/10.5194/os-3-259-2007.
Bullock, T.; Isaac, G.A.; Beale, J.; and Hauser, T. [2016]. Improvement of visibility and severe sea state forecasting on the Grand Banks of Newfoundland and Labrador Proceedings: Arctic Technology Conference, OTC. https://doi.org/10.4043/27406-MS.
ECMWF [n.d.] European Centre for MediumRange Weather Forecasts ERA5. Retrieved from: www.ecmwf.int/en/forecasts/ datasets/reanalysis-datasets/era5.
ESA [n.d.] European Space Agency Copernicus Open Access Hub. Retrieved from: https:// scihub.copernicus.eu.
EUMETSAT [n.d.] EUropean organization for the exploitation of METeorological SATellites OSI SAF Ocean Wind Products. Retrieved from: www.eumetsat.int/osi-saf.
Liu, T. and Xie, X. [2006]. Measuring ocean surface wind from space. In: Remote Sensing of the Marine Environment, Manual of Remote Sensing, pp. 149-178. https://doi.org/https://doi.org/10.1023/A: 1015832919110.
Mahdavi, S.; Amani, M.; Bullock, T.; and Beale, S. [2021]. A probability-based
daytime algorithm for sea fog detection using GOES-16 imagery. IEEE Journal of Selected Topics in Applied Earth Observations and Remote Sensing, Vol. 14, pp. 1363-1373. https://doi.org/10.1109/ JSTARS.2020.3036815.
NOAA [n.d.] National Oceanic and Atmospheric Administration CLASS Products. Retrieved from: www.vtccorp. com/noaa-class.
Shepard, D. [1968]. A two-dimensional interpolation function for irregularlyspaced data. Proceedings, 23rd ACM National Conference. ACM Press, New York, N.Y., U.S., pp. 517-524. https://doi. org/10.1145/800186.810616.
Wentz, F.J.; Ricciardulli, L.; Rodriguez, E.; Stiles, B.W.; Bourassa, M.A.; Long, D.G.; Hoffman, R.N.; Stoffelen, A.; Verhoef, A.; O’Neill, L.W.; Farrar, J.T.; Vandemark, D.; Fore, A.G.; Hristova-Veleva, S.M.; Turk, F.J.; Gaston, R.; and Tyler, D. [2017]. Evaluating and extending the ocean wind climate data record. IEEE Journal of Selected Topics in Applied Earth Observations and Remote Sensing, Vol. 10, pp. 2165-2185. https://doi.org/10.1 109/JSTARS.2016.2643641.
Young, I.R. and Donelan, M.A. [2018]. On the determination of global ocean wind and wave climate from satellite observations. Remote Sensing of Environment, Vol. 215, pp. 228-241. https://doi.org/10.1016/j. rse.2018.06.006.
Zou, J.; Lin, M.; Zou, B.; Guo, M.; and Cui, S. [2017]. Fusion of sea surface wind vector data acquired by multi-source active and passive sensors in China Sea. International Journal of Remote Sensing, Vol. 38, pp. 6477-6491. https://doi.org/10.1080/0143116 1.2017.1356486.
Board member, European Aquaculture Society. Bachelor of Science, marine technologies. Master of Science, sustainable aquaculture. PhD, aquaculture. Volunteer swim instructor and lifeguard. Current head of research with the Aquaculture Stewardship Council, an independent not-for-profit organization founded in 2010 to manage global standards for responsible aquaculture.
Where were you born? Where is home today?
I was born in a small village close to Bremen in Northern Germany. I moved around a little bit for studying and working. I now live only 300 m away from the shore in a small fishing town in the far north of Germany.
What is your occupation?
I am head of research at the Aquaculture Stewardship Council (ASC), an impact organization running the world’s leading certification program for responsibly farmed seafood.
Why did you choose this occupation?
As a kid I wanted to study marine biology, but I was also very interested in maths and physics. When I found an engineering degree on marine technologies, I knew that was what I wanted to study – without a sense of what I would do with it afterwards. There were a few courses on aquaculture in the program, and I found the field interesting. I ended up doing a master’s degree in sustainable aquaculture and later a PhD. Working in ASC combines my interest in aquaculture with my strong sense of doing good and reducing impacts. And there is still so much to learn and improve, it certainly never gets boring.
Where has your career taken you?
My career has taken me to the laboratory for days as well as on stages, speaking to nearly 2,000 people. In the geographical sense, it has taken me to nearly all continents, except Antarctica. I started with an internship in Honduras and did another one in New Zealand. I had the privilege of doing my M.Sc. research in Bangladesh. I visited farms in Kenya, Chile, Canada, the U.S., and several countries in Europe; and I attended quite a few conferences around the world as well.
If you had to choose another career, what would it be?
I love teaching and enjoy every opportunity that I get to do that, either speaking to students about aquaculture and certification, or teaching kids to swim and adults how to teach swimming. If I had to change careers, I would probably become a high school teacher teaching sports, biology, and math.
What is sustainable aquaculture and why is it difficult to achieve?
The World Bank defines sustainable aquaculture as “a dynamic concept and the sustainability of an aquaculture system will vary with species, location, societal norms and the state of knowledge and technology.” There is no “one size fits all” solution for sustainable aquaculture and that makes it difficult to achieve but, at the same time, working towards it is so interesting and full of variety.
What hobbies do you enjoy?
I love the water, not only in my job. I volunteer as a swim instructor and lifeguard in our local lifeguard association, and I passionately play underwater hockey (yes, that is a thing!).
Where do you like to vacation?
I basically live at the beach so no need to go somewhere for the beach feelings, but we hardly get any snow here and I am always keen for a proper, snowy holiday in winter.
What has been the highlight of your career so far? One of the most important things in my career so far was and is to be part of the European Aquaculture Society (EAS). I started in the student group and have now been part of the EAS board for five years. Working alongside so many great and inspiring scientists and learning to have a voice in this group has certainly shaped me and my career more than I care to admit.
What do you like most about working in this field? The diversity, both of tasks and people. Okay, yes, the aquaculture research field is still very male dominated, but that is slowly and continuously changing. You meet people who taught themselves how to raise fish, others studied aquaculture like myself – people with various backgrounds from various countries but they all have this one passion in common: to expand and improve the current aquaculture practices.
What are some of the biggest challenges your job presents? The same thing that makes me love it so much – the variety and diversity. Getting people with diverse backgrounds on the same page can be tough. It is also a challenge that aquaculture is often used as this one overarching theme, as if raising salmon in
a cage in Norway can be easily compared to shrimp farming in a pond in Southeast Asia.
What technological advancements have you witnessed?
Land-based recirculating aquaculture systems (RAS) have grown significantly over the last decade, both in numbers but also in size. There are now RAS (planned) that could produce 100,000
tons of fish per year. Of course, these come with different environmental and social impacts that the ASC Standards must address.
What does the future hold for this industry?
It is still a very fast-growing industry, and it will continue to grow. But we do have to get better at addressing the main impacts, through certification, but also through consumer awareness. There are still many prejudices towards the industry, and we need to address those. Research will definitely be important for that and we at the ASC are working hard to fulfil our role in supporting, driving, and – hopefully also soon – funding research in the relevant fields.
What advice do you have for those just starting their careers?
My main advice would be to go for it! There are so many options and careers in the sector that I am sure that everybody will be able to find their passion. There are also a lot of different mentorship programs and networks, like the European Aquaculture Society, for example, that are worth joining. I also recommend a good dose of persistence, patience, and passion. After all, we are working with animals as well as people’s livelihoods, so things take time.
The European Aquaculture Society (EAS) is an independent non-profit association, created in 1976, that promotes contacts and disseminates information to stakeholders of European aquaculture. EAS has 1,000 members in more than 60 countries. Membership is mainly scientific/academic, but also includes producers, suppliers, and policy-makers. The EAS Secretariat is at Oostende in Belgium.
Principal activities:
• Provision of membership products and services
◦ Peer-reviewed scientific journal (Aquaculture International, AQUI); a dedicated aquaculture magazine (Aquaculture Europe, AE); and members’ newsletter
◦ Online member access to all publications, EAS webinars/podcasts, members’ directory, AE event abstracts, job offers, etc.)
• Organization of the annual Aquaculture Europe events, since 1981
◦ The major forum for multi-disciplinary aquaculture research and innovation
◦ Scientific conference, trade event, and industry/innovation forums
◦ Extensive networking activities for students
◦ More than 2,000 attendees
• Communication activities within the European Union research and innovation projects
◦ Short articles, social media posts, summaries of key public deliverables
◦ Connections and communication to key aquaculture stakeholders for project activity
◦ Outreach to 19,000 social media followers
EAS is, therefore, all about bringing people together; offering free membership for students and retirees; and providing individuals, institutes, and companies with various membership benefits. Our Aquaculture Europe events promote interdisciplinary research and provide a “helicopter” view of the status of aquaculture in Europe and beyond. Our communication activities reach out to the wider aquaculture community – in person, on paper, and online.
For more information:
www.aquaeas.eu
For over 27 years, the National Aquaculture Association (NAA) has been the unifying voice of the United States’ aquaculture sector. NAA remains committed to the growth and success of all American aquaculture farms through collaborations with state and federal governments that foster a positive business climate and cost-effective regulations and ensure environmental stewardship and sustainability. As a not-for-profit trade association, we fulfill our mission primarily through government advocacy, public outreach, and farm promotion.
Aquaculture represented 57% of global seafood production in 2020. An additional 13% increase in production is projected to occur by 2030. The U.S. currently imports 70-85% of the seafood we consume and is the world’s largest seafood importing nation. A variety of U.S. agencies and environmental groups recognize that offshore marine aquaculture in the United States’ exclusive economic zone holds tremendous potential for advancing the public health, food security, and economic interests of Americans, yet the nation has not seen significant marine aquaculture development since passing the National Aquaculture Act in 1980. Despite intense interest in domestic development by farmers for decades, U.S. farmers supply only 7% of domestic seafood demand and rank 18 th in the world for seafood production.
Many in the U.S. environmental community continue to frame potential, adverse effects based on outdated production technologies and standards. The NAA provided an in-depth
response to the common, speculative criticisms of offshore finfish production via a paper published in Reviews in Fisheries Science & Aquaculture. While critics of sustainable domestic production suggest additional regulation is necessary, the reality is that the U.S. is already a global leader in well-regulated, responsible aquaculture development, production, processing, and marketing.
Sufficient federal authority, unilaterally or in partnership with the states, already exists to protect the marine environment, prevent impacts to wild fisheries and protected species, require responsible drug and chemical use, prevent the introduction of non-native species and deleterious genetics, ensure safe navigation, and assure product food safety. Existing laws include, but are not limited to, the Animal Health Protection Act; Animal Medicinal Use Drug Clarification Act; Coastal Zone Management Act; Endangered Species Act; Federal Food Drug and Cosmetic Act; Federal Insecticide, Fungicide and Rodenticide Act; Federal Water Pollution Control Act (Clean Water Act); Lacey Act; Magnuson-Stevens Fishery Conservation and Management Act; Marine Mammal Protection Act; Migratory Bird Protection Act; National Environmental Policy Act; Outer Continental Shelf Lands Act; and Rivers and Harbors Act.
The U.S. Environmental Protection Agency, National Oceanic and Atmospheric Administration, U.S. Department of Agriculture, U.S. Army Corps of Engineers, U.S. Coast Guard, U.S. Department of Defense, Federal Aviation Administration, U.S.
Fish and Wildlife Service, Bureau of Ocean and Energy Management, and state agriculture, natural resources, and environmental protection agencies all have important regulatory roles relative to offshore aquaculture through rulemaking, judicial rulings, and an opportunity to comment on significant permitting by other agencies. Coastal states are also provided an opportunity to comment on proposed federal permits and leases associated with offshore marine aquaculture.
The sufficiency of U.S. laws and responsible environmental stewardship of commercial entities is clearly demonstrated by reviewing the historical and current offshore aquaculture sector. Commercial-scale net pen farms in the states of Maine, Washington, and Hawaii produce Atlantic salmon, steelhead, almaco jack; and thousands of shellfish farms in the state waters of Alabama, Alaska, California, Connecticut, Delaware, Florida, Georgia, Hawaii, Louisiana, Maine, New Hampshire, New Jersey, New York, Maryland, Massachusetts, North Carolina, Oregon, Rhode Island, Virginia, South Carolina, Texas, and Washington produce abalone, clams, oysters, mussels, or scallops. Marine aquaculture farms are sited and managed in compliance with a myriad of state and federal regulations and lease provisions. They are operated with regulatory transparency supported by environmental monitoring data, inspections, and public reporting as required by state and federal laws.
The U.S. is not a world leader in aquaculture production by volume or value, but we are a world leader in the thoughtful and rigorous development of regulatory and nonregulatory production practices, animal nutrition and health management, technological innovation, and the efficient processing and distribution of high-quality, wholesome seafood.
Global population and per capita protein consumption have been increasing and will continue to do so well into the mid-century.
Aquaculture will continue to have a larger role in the global food supply, and the U.S. is projected to further increase our reliance on imported seafood products.
The NAA has for decades championed a different pathway for America’s seafood sector that increases our domestic food security, provides new jobs to rural communities, and upholds strong environmental standards. Action from Congress is critically needed to provide security of tenure for offshore farmers as a bankable asset to obtain capital and insurance. Proud American farmers stand ready to fulfil this imperative. We invite you to learn more about this important issue by visiting our website or contacting us directly.
For more information:
https://thenaa.net
naa@thenaa.net
850-216-2400
See graphic on next page Sustainable Marine Aquaculture in the United States.
The rationale for considering reuses of hydrocarbon infrastructure in support of offshore aquaculture operations has been well documented, debated, and published –and still no operational examples currently exist in the United States. With nearly 1,600 structures planned for decommission in the Gulf of Mexico (GoM) alone, few are possibly aware that the oil and gas industry does not hold an exclusive market on available platforms for alternative use considerations, as the Department of Defense has a few in its inventory awaiting final decommissioning as well. The U.S. Air Force has approximately 14 communication towers scattered throughout the GoM approximately 30 nautical miles (NM) from shore. Similarly, the U.S. Navy (Beaufort) has eight offshore Tactical Aircrew Combat Training System towers located approximately 43 to 90 NM off the coast of Brunswick, Georgia. Avoiding the not-so-ill fate of a rigs-to-reef disposition, ample infrastructure appears to be available for alternative use considerations, particularly in support of offshore aquaculture operations.
This hopeful destination and outcome, however, then presumes a successful navigation through infrastructure liability release and overcoming regulatory constraints. Some entrepreneurs are attempting to seek
platform reuse permits directly from the U.S. Bureau of Ocean Energy Management and U.S. Bureau of Safety and Environmental Enforcement via a release of liability from platform owners. If successfully achieved, a concurrent and challenging pursuit is the application for, and permit awards of, a National Pollutant Discharge Elimination System permit from the Environmental Protection Agency and a Section 10/404 permit from the U.S. Army Corps of Engineers in order to operate and site an aquaculture facility in offshore federal waters of the GoM. Today, while both of these arduous actions must be pursued by each individual commercial aquaculture business, a grand opportunity and long overdue obligation resides with the U.S. federal government. While many wait for the federal government’s best intentions to come to fruition, environmental permitting initiatives by the aquaculture industry have been fraught with ill-guided opposition from their inception.
A U.S. regulatory framework is desperately needed to support and advance a sustainable offshore aquaculture industry in U.S. federal waters. Beginning in 2019, U.S. Sen. Roger Wicker, R-Mississippi, first convened a hearing titled, Feeding America: Making Sustainable Offshore Aquaculture a Reality, which finally led to the reintroduction of a bipartisan bill –Advancing the Quality and Understanding of American Aquaculture Act of 2021, to establish a regulatory system for sustainable offshore
aquaculture in the U.S. exclusive economic zone (EEZ). In early 2022, Stronger America Through Seafood sent a letter signed by more than 65 supporters of aquaculture (including yours truly) to Congress, requesting support for aquaculture expansion in the U.S. By the close of 2022, along comes yet another (parallel) bipartisan bill in support of sustainable aquaculture – the Science-based Equitable Aquaculture Food (SEAfood) Act, introduced by U.S. Rep. Alan Lowenthal, D-California. The SEAfood Act enters stage just on the heels of a report published in Marine Policy by the lead scientist of the Environmental Defense Fund (EDF). Member organizations (including EDF) of the Coalition for Sustainable Aquaculture are generally supportive of such initiatives as the SEAfood Act.
The EDF report focuses on the ecological risks, proposed remedies, and knowledge gaps, calling for more research to address the unanswered questions to create a regulatory framework that is science-based in order to protect the environment. All scientists are not necessarily in agreement that these initiatives require so much more research to solve the unresolved knowledge gaps that aquaculture industry data cannot provide. Most notably from the SEAfood Act language, with regard to this JOT article, is a directive for the National Oceanic and Atmospheric Administration to “solicit and accept applications for participation in the assessment program” to gather data from on-the-water “commercialscale and demonstration projects” that close said knowledge gaps. This effectively transitions the offshore aquaculture industry priorities from focusing purely on more science-based modelling research to targeted opportunities providing the science-based, documented monitoring data.
The environmental conditions and ecological communities associated with offshore hydrocarbon infrastructure within the EEZ have been some of the most heavily monitored and scrutinized operations. Collocating offshore aquaculture facilities and operations
with decommissioned platforms provides an opportunity for utilizing historical baseline data collected from these sites in conjunction with real-time operational science-based monitoring data. This scenario serves as an alternative for addressing the EDF report’s call for more research to fill the knowledge gaps, while satisfying the need for commercial and demonstration-scale project data set forth in the SEAfood Act. The final outcome, of course, is to finally establish a permitting pathway and science-based regulatory framework supporting sustainable offshore aquaculture within the EEZ.
Dennis Peters is president and CEO of Gulfstream Aquaculture, LLC, in Miramar Beach, Florida, which focuses on marine finfish research and development; offshore, coastal, and land-based siting analyses; demonstration and pilot farm operations; and commercial feasibility assessments for land-based and open ocean aquaculture.
Informative
Cutting Edge
Provocative
Challenging
Modelling the Effect of Ocean Acidification on Global Photosynthetic Activity: Analysis of Effect of pH on the Growth Rate of Tetraselmis striata
Eldar Isgandarov is a Grade 12 student at Holy Heart of Mary High School, St. John’s, Newfoundland and Labrador, Canada; and winner of the Science and Technology Marine Institute Award during the 2023 Regional Science Fair. Congratulations, Eldar!
what's new Turnings
NOVACAVI has supplied a special lan hybrid underwater cable for deployment on the experimental farm project, Nemo’s Garden. This world’s first underwater cultivation system of terrestrial plants located off the coast of Noli, southwest of Genoa, Italy, consists of an array of suspended, transparent, dome-shaped greenhouses called biospheres, anchored to the bottom of the sea. With all aspects monitored from land through cameras and sensors, this underwater cable will enhance the benefits of using the sea for farming. www.novacavi.it/news
Urchin harvesting in Newfoundland and Labrador is typically a late fall-winter fishery, as that is when roe yields are highest before spawn-out in AprilJune. That timeframe presents challenges for harvesting, mostly due to the poor weather and water conditions that divers must endure. Research partners tested the effectiveness of a C Disc harvesting tool developed by C Robotics in Norway. The unit is designed to increase the catch per unit effort while decreasing the time needed for a diver to be in the water. Learn more.
https://ccfi.ca | https://miawpukekhorizon.com | www.mi.mun.ca
TEMPERATURE
PHYTOPLANKTON SALINITY
INORGANIC CARBON
Now that aquaculture has become the fastest growing sector of agriculture globally, efforts are underway to ensure that it is efficient, cost effective, and environmentally responsible. Most modern, intensive finfish aquaculture is currently practiced by farming the fish in floating ocean cages, either in coastal water or offshore. Floating cages have often been criticized for their potential adverse biological and chemical effects on the marine (mainly coastal) environment. Additionally, fish farmed in ocean cages are often exposed to sub-optimal conditions, thereby compromising performance, as well as to pathogens, harmful algal blooms, and possible coastal pollutants. An alternative aquaculture farming practice that addresses these concerns is the rapidly emerging recirculating aquaculture systems (RAS) approach, which can be implemented either on land or in the ocean.
Recent advancements in the biology and engineering of land-based RAS technology enable a high degree of containment, minimizing any interactions with the environment. Cutting-edge platforms of modern biology, microbiology, and genomics have led to the development of optimal microbial communities, which are inoculated into the RAS biological filters and very efficiently remove dissolved waste products produced by the fish (mostly nitrogenous compounds), enabling the systems to recycle and re-use up to 99% of the tank water. As such, all environmental conditions in the fish tanks can be fully controlled and tailored to
the requirements of the species of interest, in order to drive optimal performances. These include water salinity (from fresh to full salinity water), temperature, and water quality. Species like Atlantic salmon, Mediterranean seabream, and European seabass have been shown to grow 50-100% faster from fry to harvest in RAS farms, compared to floating cages in the sea. Being able to adjust culture conditions in RAS operations also allows for species diversification and enables selecting the species to be farmed based on economic considerations, as opposed to geographic locations. RAS tanks are usually supplied with disinfected ground water, or even municipal water, thereby avoiding introduction of pathogens into the farms and enabling the establishment of RAS farms in any location, rural or urban. Solid organic waste generated by the fish is removed from the systems and, for freshwater farms, can be used as fertilizers for terrestrial crops or as a nutrient source for hydroponic production of plants in a practice referred to as aquaponics. Marine (or freshwater) RAS farms can use the process of anaerobic digestion, which has recently been optimized for saline solid wastes, to efficiently convert over 90% of the
organic waste to fuel-grade methane, a source of bioenergy that can offset up to 10% of the energy requirement of the farm. Therefore, all waste products are biologically captured, and the most advanced RAS farms generate near-zero discharge to the environment. Additionally, RAS farms are fully bio-secure and are the only aquaculture platforms that are authorized to produce non-native species and genetically engineered fish.
Land-based, partial-RAS operations have already been employed for many years, for instance in the salmon industry where smolts are produced inland before being transported to the sea cages. However, during the last five years, significant technology and engineering progress has enabled the scalability of highly contained, land-based RAS operations for the full life cycle of marine fish. Indeed, several large-scale RAS farms have recently started to operate, producing thousands of tons of high-value fish like salmon and kingfish (yellowtail). Additionally, billions of dollars are currently being invested in the planning and construction of RAS farms around the world for the above fish and additional high-value species like European seabass, Mediterranean
seabream, flounders, cod species, cobia, eels, and several others. Such farms are built in large warehouses of up to ~80,000 square metres, where fish are cultured in very large tanks of a few thousand cubic metres.
RAS technology is also applicable for floating cages and platforms in the ocean. Experimental and commercial projects are currently underway in Norway and other countries where floating cages or tanks are contained and RAS technology, such as biological filtration and anaerobic digestion, is implemented in these ocean farms to enable all the above-mentioned benefits of RAS in the sea.
Thus, it appears quite evident that the future of sustainable aquaculture will be the integration of in-the-ocean and land-based farming technologies that generate little environmental discharge, are contained, and optimize conditions for the most efficient performances.
The industry that puts your favourite seafood on the table is producing more food with more precision and safety than ever before. Salmon farming in Canada is led by science, featuring state-of-the-art technology to produce a healthy protein with a low-carbon footprint.
The technology is getting smarter – connected devices are improving modern fish farming and revolutionizing the way business is done. Technological solutions have vastly improved operational efficiency, which is good not only for farmers and companies, but also for the planet and for consumer safety.
These new technologies and innovations are being implemented at the hatchery, growout, and processing phases of the farm-raised salmon production cycle. Here are some examples of what salmon farming operations across the country are doing to improve their processes through the technology of connectivity.
Ocean-based farm-raised salmon containment systems are an essential part of modern farms that raise salmon in their natural environment.
Monitoring this ocean rearing environment is a key aspect of keeping the fish healthy. Changing water chemistry (dissolved oxygen and temperature) and harmful algal blooms
are just some of the risks to the health and welfare of the fish.
Canada’s seafood farmers rely on management platforms that provide real-time data on ocean environmental conditions. The platforms combine a complex system of marine data visualization tools layered with numerical ocean models and statistical analysis of local waterways to provide historical, current, and future-focused models of performance; the models allow farm managers to monitor current conditions, revisit past weather or mortality events, and plan for weather-related or environmental events that may take place in the future.
The platforms unify existing sensor networks on farms to collect information and provide a clear window into how salmon farms react to changing ocean conditions using both inpen data and publicly available data. These forecasting and mitigation technologies provide farms with the opportunity to reduce production impact of marine risks, such as harmful plankton blooms and sea lice outbreaks, as well as improve fish welfare and efficiency.
In addition to technology that captures environmental information, there is also imaging and sensors technology available to continuously survey stocks and monitor the health status of individual fish within a pen.
Sensors register fish size, number of sea lice, and possible signs of disease. Real-time data analysis increasingly enables farmers and company veterinarians to swiftly detect and respond to irregularities with the potential to divert individual fish requiring
treatment for treatment or harvest – without stressing the remaining fish.
The breeding process has also been revolutionized by technological innovation. Advancements made in DNA mapping and animal health diagnostic tools over the last few decades provide today’s salmon breeders with access to an abundance of data regarding the health and genetics of the fish under their care. These DNA tools allow for the selection of individual fish for breeding programs based on desirable traits identified in their existing genetic fingerprint.
Salmon breeding programs select stock that will thrive in a farmed environment to ensure the healthiest and strongest go on to create the next generation. Selection for
traits such as improved growth, flesh quality, and resistance to disease and parasites is important not only to animal welfare but also to improve husbandry.
From the farm to the table, modern technology is powering the evolution of the aquaculture sector. “Smart farming” is the way of the future, and as progress continues apace, the salmon farming sector will continue to adapt in the direction of better, safer, more sustainable production. This is critical at a time when the world is hungry for more high-quality and healthy seafood.
I have always been fascinated with ocean life, especially with jellyfish. Visually, they are stunning creatures with their vibrant colours and bioluminescent features. Even the way they move through the water is mesmerizing. When I thought about embroidering a jellyfish, I knew I wanted it to be more than just a visually appealing design. I wanted to create a sensory experience – a jellyfish you could actually touch. The bell of the jellyfish is handstitched using a stumpwork technique that allows me to create the 3D effect. The tentacles are crafted using beads, floss, and threads of varying shapes and sizes to bring texture and movement to the design. Add in a little sea breeze and this tactile jellyfish really comes to life! Instagram: @wildflossdesigns