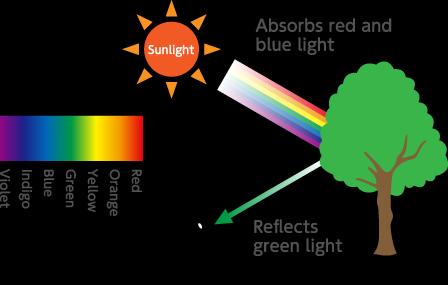
8 minute read
WHY IS GRASS GREEN?
Eleanor Baird, Madeleine Gallon and Lucia Macheta
Abstract
Grass owes its green colour to the pigment in chloroplasts called chlorophyll, specifically two types called chlorophyll A and B. Chlorophyll A absorbs light in the violet-blue and red part of the absorption spectrum, and chlorophyll B the blue and red-orange range. The light a chlorophyll molecule can absorb corresponds to energy gaps between the highest filled pi orbital and the lowest/second lowest empty pi orbital. It is because there are no energy gaps for the absorption of light in the green range that chlorophyll molecules reflect green light. It is this reflection of the green light that means we view grass as being green.
Introduction
The answer to the question ‘Why is grass green?’ can be summed up in one word: chlorophyll. Although this green pigment seems to provide an easy, succinct explanation, more complex processes need to be considered when establishing why chlorophyll itself appears green. The various types of chlorophyll and differences between them have gradually been understood since 1818, when Joseph Bienaimé Caventou and Pierre Joseph Pelletier first isolated and named chlorophyll.1 Nineteen years later, Hugo von Mohl gave the earliest description of a chloroplast, the organelle that contains chlorophyll. 2 In the early 20th century, the Russian plant physiologist and biochemist Mikhail Tsvet identified the two main chlorophyll pigments: chlorophyll A and B.
3 Other forms of chlorophyll do exist (chlorophylls C, D, E and F), but these are less common and are not found in grass. For example, chlorophyll D, found in red algae and cyanobacteria, absorbs far-red wavelengths of light and some wavelengths in the blue-green region. These other types of chlorophyll have been less-well researched as they have been discovered more recently than chlorophyll A and B. 4
What is chlorophyll and where is it found?
Grass cells contain cytoplasmic organelles called chloroplasts, which are best known as the site of photosynthesis, the process by which plants convert light energy into chemical energy stored as sugars. Chloroplasts also play a key role in the physiology and development of a plant, such as the synthesis of chlorophyll, carotenoids, and fatty acids. 5 It is the green pigment chlorophyll that gives grass, as well as all green plants, its distinctive colour.
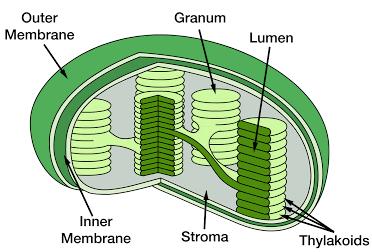
Figure 1: Structure of a chloroplast 6
Within each chloroplast, thylakoid membranes are arranged into stacks called grana, and all of the chlorophyll in the plant is situated in these thylakoid discs. Chlorophyll is the location of photosynthetic activity, absorbing sunlight and using the energy for photosynthesis. Other pigments, including carotenoids, act as accessory pigments, trapping solar energy and passing it on to chlorophyll.7,8 Chlorophyll does not absorb the green wavelength of light, only light in the red and blue regions of the visible light spectrum, and it is because the green light is reflected that we see grass as being green.9
Figure 2: How a human eye sees reflected green light 10
Role of Chlorophyll A and B
Chlorophyll A is the primary pigment of photosynthesis and the most abundant of all molecules, making up around 75% of the green pigment found in nature.1 All organisms that perform photosynthesis contain chlorophyll A, but not all have chlorophyll B.11 Chlorophyll A absorbs light from the orange-red and violet-blue areas of the electromagnetic spectrum, transfers this energy to the reaction centre and donates two excited electrons to the electron transport chain. Chlorophyll B is an accessory pigment because it is not necessary for photosynthesis to occur. Chlorophyll B’s central role is to expand the absorption spectrum of organisms. That way, organisms can absorb more energy from the higher frequency blue light part of the spectrum. The presence of chlorophyll B in cells helps organisms convert a wider range of the energy from the sun into chemical energy. Having more chlorophyll B in chloroplasts of cells is an adaptation to the shade. Plants that receive less sunlight have more chlorophyll B in their chloroplasts, as it allows the plant to absorb a broader range of wavelengths of light. Chlorophyll B transfers the extra energy it absorbs to chlorophyll A.
Structure of Chlorophyll
Chlorophyll molecules are complex organic compounds formed by derivatives of porphyrin.1 Porphyrins are a group of compounds containing four pyrrole rings, which are heterocyclic organic compounds with the formula C4H5N, connected by methine bridges, which consist of a carbon atom bound by two single bonds and one double bond, in a cyclic structure to which a variety of side chains are attached.12,13,14. Chlorophyll molecules have a central atom of magnesium as well as a propionic acid chain esterified with the alcohol phytol.1 Chlorophyll B differs from chlorophyll A by the presence of a CHO group instead of the CH3 group at C7 (Figure 3). Chlorophyll B is produced via the oxidation of the methyl group to aldehyde which is catalysed by the enzyme oxygenase.
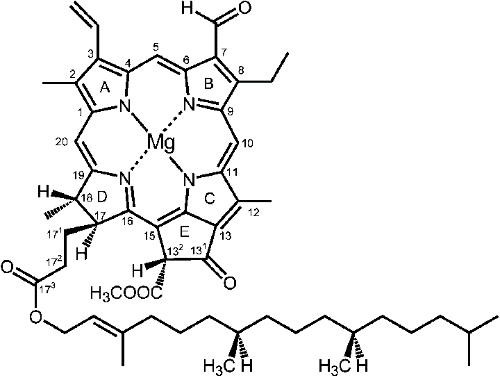
Chlorophyll A: R = CH3 Chlorophyll B: R = CHO
Figure 3: Structure of chlorophyll A and B15
Differences in Absorption
Chlorophyll A appears more bluish green whereas chlorophyll B gives a bright green colour. The differences in saturation of the pyrrolic rings alter the absorption spectrum (Figure 4), causing the variation in colour. Chlorophyll A gives absorption peaks at 430 nm and 662 nm, which are responsible for violet-blue and red colours respectively, while chlorophyll B gives peaks at 453 nm and 642 nm, which are responsible for blue and red-orange colours.16 This means there is a large spectral region, between around 500 and 600 nm, where very little light is absorbed. This light is in the green region of the spectrum, therefore green light is reflected into our eyes making the grass appear green.
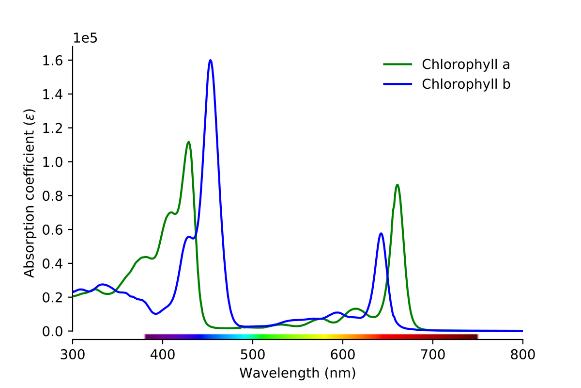
Figure 4: Absorption spectrum of chlorophyll A and B17
Role of Pi Orbitals in Absorption
Chlorophyll has 23 carbon atoms and 1 oxygen atom which make sigma bonds, and each atom has one remaining p orbital and one electron. This means there are 24 p orbitals in conjugation, which is the overlap of one p orbital with another across a σ bond.18 When atomic orbitals overlap, they form molecular orbitals: areas of space where electrons are shared between atoms. When two p orbitals overlap, two π molecular orbitals are formed.
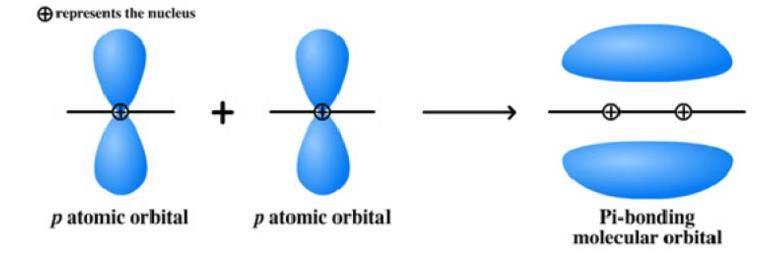
Figure 5: Formation of a pi bond19
Figure 6: Energy diagram for two pi molecular orbitals in a typical pi bond20
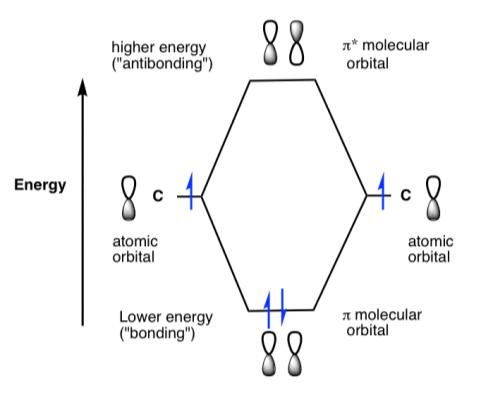
The lowest energy light (in the red region) that either chlorophyll molecule absorbs corresponds to the energy gap between the highest filled π orbital and the lowest empty π orbital. The next band absorbed by the chlorophyll molecules (in the blue region) corresponds to light with the same energy as the gap between the highest filled π orbital and the second lowest empty π orbital.21
Because there are no equivalent energy gaps for the absorption of green light, it is not absorbed by either chlorophyll molecule. However, there are also other chlorophyll molecules with slightly different structures that absorb light at marginally lower and higher bands.22 Taken together, the chlorophyll pigments sufficiently absorb all the red and blue light, leaving only the middle region of the spectrum to be reflected into our eyes as green.
Electronic Transitions
When a chlorophyll molecule absorbs light from the sun, the energy from the light excites an electron from its ground energy level to an excited energy level.23 This high-energy electron is very unstable and can have several different fates. For most lightabsorbing compounds, the electron simply returns to the ground state and the absorbed energy is converted into heat.
Figure 7: Excitation of an electron from its ground state to a higher energy level due to the absorption of light23
However, if a suitable electron acceptor is in close proximity, then the excited electron can move from the initial molecule to the acceptor. This process results in the formation of a positive charge on the initial molecule, and a negative charge on the acceptor, so is therefore referred to as photoinduced charge separation, and the site where the separational change occurs is called the reaction centre.20
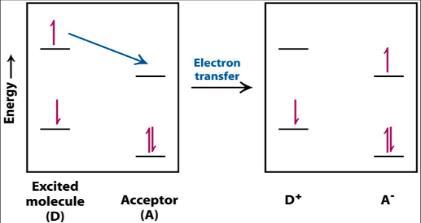
Figure 8: Transfer of an excited electron to an electron acceptor23
Reaction centres are key components of the photosystem used to carry out the primary photochemistry of photosynthesis. Found in the thylakoid membranes of plants, a photosystem consists of a light-harvesting complex and a reaction centre.22 Pigments in the light-harvesting complex pass light energy to two distinct chlorophyll A molecules within the reaction centre. The light excites an electron from the chlorophyll A pair, which passes to the primary electron acceptor.24 The excited electron must then be replaced, by either an electron produced from the reactions within photosystem I or II. In photosystem II the electron is released as a result of the splitting of water, which produces protons and electrons as well as releasing molecular oxygen as a waste product. This is known as the photolysis of water and can be represented by the equation25:
2H2O → O2 + 4H+ + 4e-
In photosystem I the electron comes from the chloroplast electron transport chain, known as cyclic electron transport.20 This process involves the transportation of electrons (via plastoquinone) from ferredoxin to a proton pump, cytochrome b6f, then they are returned (via plastocyanin) to the chlorophyll molecule.26
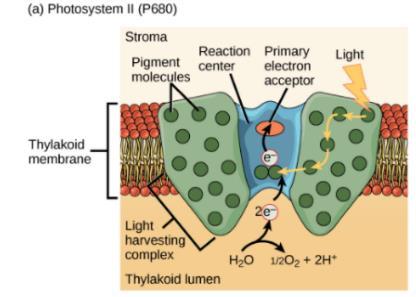
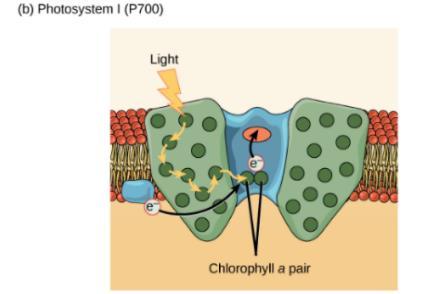
Figure 9: Replacement of excited electron via photosystem I and II 27 Although abstract scientific studies have long since disputed that the greenness of plants is due to the preferential absorption of blue and red light by chlorophyll, comparisons of the different absorption spectra of chlorophyll A and B have established that the green-colour we see is actually due to the reflectance of green light by the organic pigments. Owing to the lack of any energy gaps between the filled and unfilled π orbitals in the chlorophyll molecules, neither chlorophyll A nor B absorbs any green light. Although the reason why plants do not absorb green light still remains unanswered, it is clear that it is the green pigment chlorophyll that gives grass, as well as all green plants, its distinctive colour.