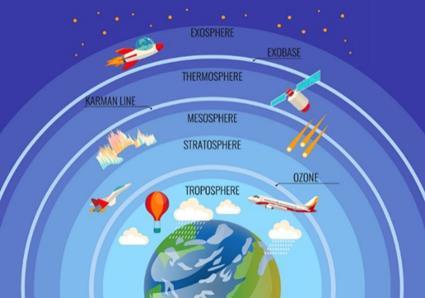
10 minute read
WHY IS OZONE LOSS MORE SEVERE IN ANTARTICA?
Why is ozone loss more severe in Antarctica and how is it affected by the seasons?
Serena Chohan, Victoria Liu and Abarna Sivanesan
Abstract
Ozone naturally depletes and forms at the same rate, leaving a constant layer of ozone in the earth’s stratosphere, which protects the earth from UV radiation. However, the ozone layer began to steadily deplete and in 1984, the ‘ozone hole’ appeared.
Introduction
The ozone layer is important as it absorbs some of the UV radiation from the sun, preventing it from reaching the earth. UV radiation is harmful as it can cause skin cancer, cataracts, damage DNA and weaken the immune system.1 However, in the past century, the ozone layer has depleted significantly due to certain industrial activities. The term ‘ozone depletion’ refers specifically to two related events: a steady decrease of around 4% of the total amount of ozone in the atmosphere, and the severe depletion of stratospheric ozone2 in the late winter and early spring in Antarctica (figure 1).
Figure 1: The different layers of the atmosphere.
Formation of ozone
Ozone is formed in the atmosphere by free radical reactions. UV light breaks the O=O bond in oxygen molecules, forming two oxygen radicals which react with more oxygen molecules to form ozone (O3).3
O2 →2●O●
●O● + O2 ⇌ O3
Discovery of the Antarctic ozone hole
In the 1980s, research bases in Antarctica discovered the depletion of the ozone using ground-based Dobson spectrophotometers. The observations showed that during the late winter to the early months of spring, there was a low total ozone in comparison to the observations made in the earlier years. Scientists observed that the depletion of ozone in the centre of the south pole increased over a bigger region during late winter to the early months of spring. The low level of ozone each spring formed the term, ‘ozone hole’. The ozone hole and its depletion are now researched about through the use of satellites, groundbased observations and balloon observations (figure 2).4
Figure 2: How the size of the ozone hole has increased from 1979-1996.
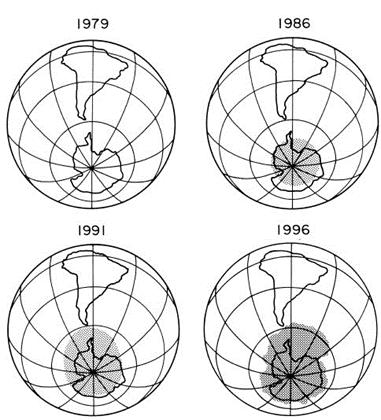
Decomposition of ozone Chlorine free radicals
Ozone can be decomposed by free radical substitution reactions of chlorine. It begins with photolysis (the decomposition or separation of molecules by the action of light). The ultraviolet light is simply a source of energy and is being used to break bonds. Chlorofluorocarbons (CFCs) can reach the atmosphere since they are very volatile. The UV light causes the C-Cl bond in CFCs to break. This is because the C-F bond is stronger than the C-Cl bond, so it is less likely to break. This produces chlorine radicals.3 CCl3F → ●CCl2F + ●Cl
The chlorine radicals released by CFC photolysis react in a catalytic chain reaction that decomposes ozone,
O3.
●Cl + O3 → ClO● + O2
ClO● + O3 → 2O2 + Cl●
Similarly, UV light has enough energy to break the CBr bond into two reactive radicals. It is easier to break C-Br bond in comparison to the C-Cl bond because it is weaker.
●Br + O3 → BrO● + O2
BrO● + O3 → 2O2 + Br●
Bromine and chlorine oxide
This cycle is initiated by the reaction between ClO and BrO under UV light. The reaction forms bromine and chlorine radicals in two different ways. It can either form a Br radical, Cl radical and O2 directly: ClO + BrO → Cl● + Br● + O2
Or it can form BrCl as an intermediate which decomposes into Br and Cl radicals under sunlight: ClO + BrO → BrCl + O2
BrCl → Cl● + Br●
The halogen oxide formed react with ozone and reduce it into oxygen molecules and halogen oxides radicals4: Cl● + O3 → ClO● + O2
Br● + O3 → BrO● + O2
Net: 2O3 → 3O2
Nitrogen monoxide
Nitrogen oxides are released into the air when fuels are burned (e.g., in car engines). When nitrogen dioxide is produced, it can decompose into a nitrogen monoxide radical.
NO2 → ●NO + 2●O●
The nitrogen monoxide radical then reacts with an ozone molecule and destroys it. ●NO + O3 → NO2 + O2
The oxygen radical can react with the nitrogen dioxide to produce another nitrogen monoxide radical.5 NO2 + ●O● → ●NO + O2 Nitrogen monoxide works as a catalyst, so it is able to repeat the process of destroying more ozone molecules without getting used up.6
How ozone decomposition can be prevented
There are ways to prevent chlorine radicals from reacting with ozone. Some of these ways are known as interference reactions.
ClO● + NO2 → ClNO3
Cl● + CH4 → HCl + CH3
The reaction products, ClNO3 and HCl, are known as reservoir molecules because they store chlorine radicals and stop them from reacting with ozone. These molecules might serve as condensation nuclei for cloud droplets (the small water drops that clouds are composed of) or might dissolve in the water in clouds. In both cases the chlorine radicals are prevented from reacting with ozone.2
Why ozone loss is more severe in Antarctica
There are a variety of factors that contribute to the significant depletion of ozone in Antarctica. Firstly, strong winds blowing around the continent form a polar vortex. This is a large, rotating low-pressure system which deteriorates during the winter and isolates the air in Antarctica from the rest of the world.7
There is also the formation of Polar Stratospheric Clouds (PSCs), also known as nacreous clouds. These clouds form in the winter polar stratosphere. PSCs contribute to ozone depletion over Antarctica, as reactions on the surfaces of solid and liquid PSCs can significantly increase the abundance of chlorine radicals.2
There is a significantly larger amount of ozone loss in Antarctica compared to the Arctic. This is due to the Antarctic winters having minimum daily temperatures, which are generally much lower and less variable than the Arctic winters. Antarctic temperatures remain below the PSC formation temperature for much longer periods during winter than Arctic regions (figure 3). The winter temperatures are low enough for PSCs to form in the Antarctic for nearly the entire winter whereas in the Arctic such temperatures only remain for 10–60 days4. This means there are more PSCs in Antarctica, which causes more severe ozone loss.
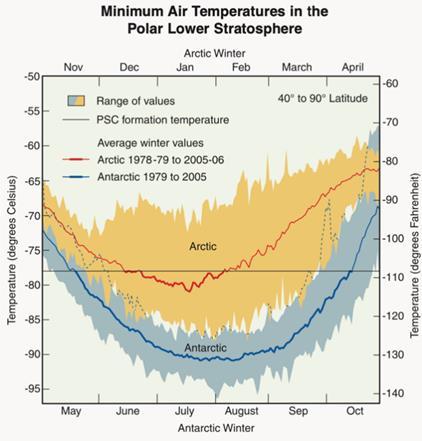
Figure 3: A graph showing the difference in temperatures in the Artic and Antarctica.
The polar vortex and Coriolis effect
The earth’s rotation creates a force called the ‘Coriolis force’ that deflects moving air to the right of its initial direction in the Northern Hemisphere and to the left of its initial direction in the Southern Hemisphere. This effect sets up a strong westerly circulation around the South Pole (polar vortex) (figure 4). The air in this vortex remains relatively isolated from the rest of the world so ozone-depleting substances build-up.7 It also helps to keep temperatures low which encourages the formation of Polar Stratospheric Clouds (figure 5).
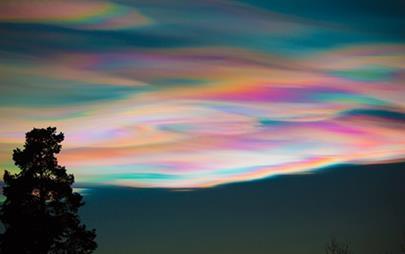
Figure 5: Polar Stratospheric Clouds formed in the lower stratosphere.
How PSCs cause more depletion of ozone
Polar stratospheric clouds form in the winter when temperatures in Antarctica fall below -80°C. Reservoir molecules from the interference reactions react on the frozen surface of PSCs to release ClNO2 and Cl2.
Reactions of reservoir molecules on surfaces of PSCs:
ClNO3 + HCl → Cl2 + HNO3
HCl + N2O5 → ClNO2 + HNO3 radicals which will go on to deplete ozone. PSCs can also react with and store nitrogen compounds like NO2 that could otherwise react with ClO radicals and prevent them from depleting ozone.2
Figure 4: Southern hemisphere total column ozone and potential vorticity (strength of polar vortex) on 22 August 2011. The white lines with arrows indicate the direction of wind flow.
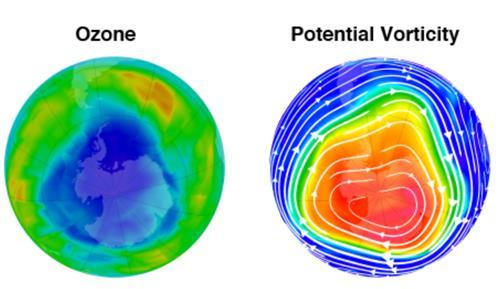
The anomalous 2002 Antarctic ozone hole
The 2002 Antarctic ozone hole showed features that surprised scientists (figure 6). They considered it anomalous at the time because the area of the hole was much smaller when viewed from space compared to the values from preceding years.8 The smaller area was unexpected because the conditions required to deplete ozone (low temperatures and reactive halogen gases) do not have large variations from year to year. The anomalous shape and size occurred because of specific atmospheric air motions that sometimes occur in polar regions. The Antarctic stratosphere was warmed by very strong, large-scale weather systems in 2002 that originated in the troposphere in late September.4 In late September, Antarctic temperatures are generally very low and ozone destruction rates are near their peak values. However, these tropospheric systems in 2002 disturbed circulation and warmed the lower stratosphere, causing less formation of PSCs and thus less depletion of ozone.
Figure 6: The anomalous 2002 ozone hole compared to the ozone hole in 2001 and 2003.
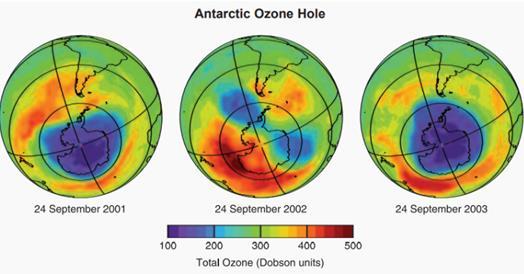
Effects of the ozone hole on the Earth’s climate
When it is intact, the ozone layer absorbs UV light, which warms the stratosphere. Therefore, when the ozone hole forms each spring, a strong springtime cooling trend in the lower polar stratosphere occurs. This springtime cooling intensifies the temperature difference between the poles and the equator. Models indicate that springtime cooling of the polar stratosphere due to ozone loss plays a large role in changes in atmospheric circulation across the Southern Hemisphere. These circulation changes, along with increased warming from the ozone hole, have had effects on climate around the world (figure 7).9 The prevailing mid-latitude westerly winds have strengthened and shifted closer to the pole, causing more rainfall and storms. More intense surface winds appear to have intensified the subtropical ocean gyres and increased the overturning circulation in the Southern Ocean.
In subtropical latitudes, summertime precipitation has increased. The Southern Ocean in late winter has warmed along with the summertime warming of the Antarctic Peninsula9 .
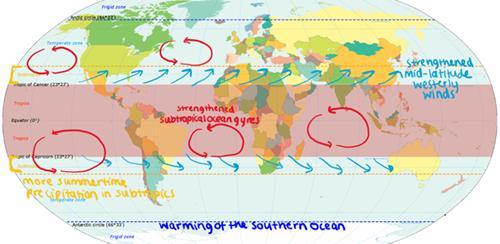
Figure 7: A diagram to show the effects of the ozone hole on the Earth’s climate.
How the ozone hole has recovered
The ozone layer is recovering, according to a new report published by the World Meteorological Organization and the UN. The ozone depletion, caused by human-produced chlorine and bromine compounds, is expected to gradually disappear by 2050s, as these compounds are slowly removed from the stratosphere by natural processes.10 The ozone layer has recovered by 1 to 3 percent per decade since 2000 and is forecasted to recover completely in the Northern Hemisphere and mid-latitude areas in the 2030s.11 A lot of this recovery is a result of the Montreal Protocol. In 1987, a global agreement, signed by many countries, was made to regulate the production and release of 100 ozone depleting substances into the environment and slow the depletion.10 The agreement has become the most successful in protecting the environment. The protocol has helped to remove 98%12 of the ozone depleting substances, and consequently protected two million people from getting skin cancer.13 In 2019, the smallest ozone hole was recorded.14 It is estimated that by 2060, the Antarctic ozone hole will have mostly recovered (figure 8). However, the future state of the ozone layer depends on factors other than just the stratospheric concentrations of chlorine and other halogens.15 It will also be affected to some extent by the changing atmospheric abundances of several other human-influenced constituents, such as greenhouse gases, nitrous oxides, sulfate particles16 and by the changing climate of the Earth. As a result, the ozone layer is unlikely to be identical to the ozone layer that existed before the 1980s17 .
Figure 8: How the ozone hole is predicted to recover.
Conclusion
Antarctica is prone to ozone depletion due to its geography; its circulation patterns, location and low temperatures make it susceptible to ozone loss as these factors increase the chance of the chemical reactions that destroy ozone. The reactants for these reactions enter the atmosphere via various human activities such as the use of CFCs in refrigerants. Therefore, ozone loss is known to be caused by humans, but is most severe in Antarctica due to Antarctica’s natural characteristics. Whilst the ozone hole over Antarctica is beginning to recover, it is uncertain of when, if ever it will return to its state prior to its first discovery.

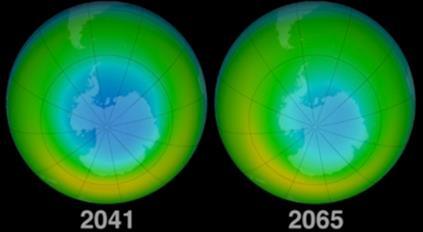