
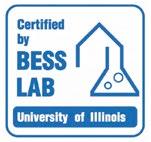
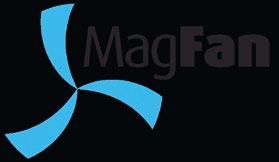
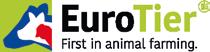
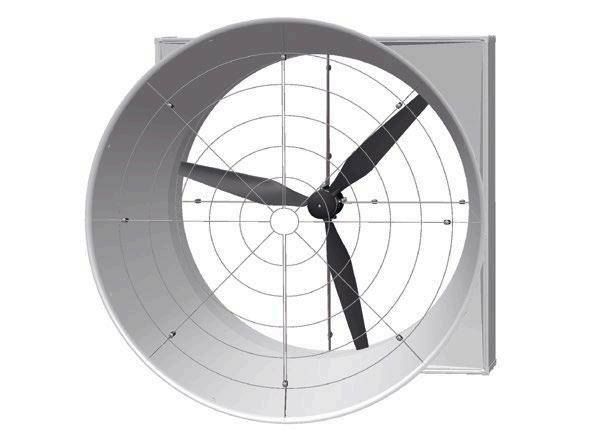
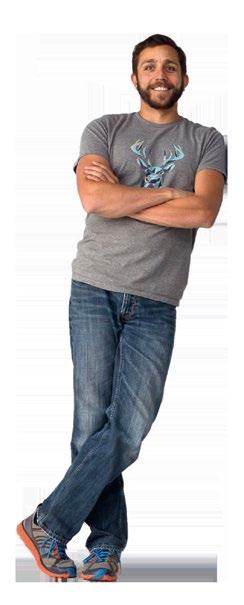
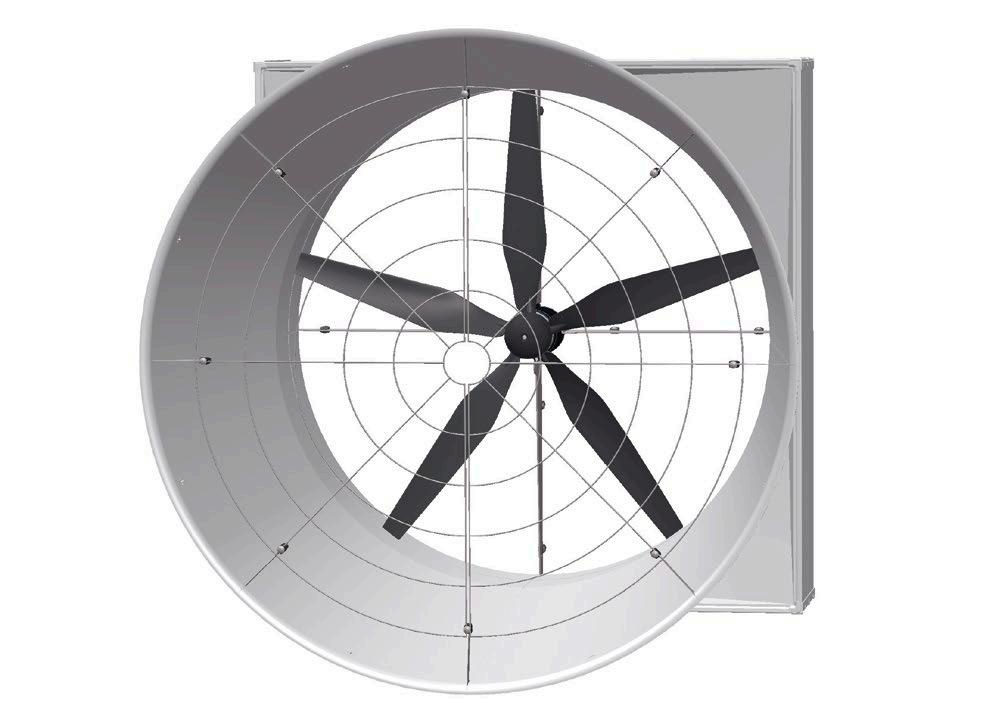
For this September issue, we look at the growing relationship of poultry farming with new technologies, such as Artificial Intelligence. The use of AI and machine learning technologies has further enhanced the accuracy and timeliness of predictive systems’ decision-making. Dra. Elein Hernández highlights in her article “Animal Welfare and Artificial Intelligence” key concepts in welfare assessment and point out some scientific advances for its evaluation by means of artificial intelligence.
Edgar Oviedo gives us his vision in an article entitled “Biosecurity Compliance: The Balance Between Culture, Personality, Experience, Education and Technology”. He states that technologies can help to overcome the challenges caused by the human factor in biosecurity.
In this opportunity we will learn about Applications of Saponins in Poultry Production. Saponins are natural compounds found in a large variety of plants that have been shown to produce a wide variety of biological effects.
We also take a look at The Quality Parameters of Soybean and Alternative Protein Feedstuffs in Poultry Nutrition. In the article “High Oleic Soybeans and Their Impact on Egg and Poultry Meat Quality” we will see that extruded full-fat soybeans with high oleic content can be included in feed for chickens and layers.
On the other hand, Guillermo Tellez-Isaias shows us the significant repercussions of chronic stress and intestinal inflammation on the health and performance of commercial birds.
Nestor Ledesma Martínez tells us in his article “Pathology of the Immune System in the Diagnosis of Immunosuppression in Poultry” that due to the multifactorial aetiology for immunosuppression, diagnosis is not always straightforward, requiring a clinical history, necropsies and complementary laboratory tests if necessary.
Respiratory diseases are a constant challenge for poultry producers and veterinarians since they do not show pathognomic signs, making diagnosis more complex. Edgar Oviedo teaches us that the Avian Metapneumovirus (aMPV) is an important pathogen in respiratory diseases but is frequently neglected.
For this edition, we had the privilege of interviewing Dr. Brian Fairchild, President of the PSA and Professor and Extension Poultry Specialist in the Department of Poultry Science at the University of Georgia, on the occasion of the 2024 PSA Annual Meeting, organized by the Poultry Science Association (PSA), which was held July 15-18, 2024. Enjoy reading it!
EDITOR
GRUPO DE COMUNICACIÓN AGRINEWS LLC
ADVERTISING
Luis Carrasco +34 605 09 05 13 lc@agrinews.es
Adoración Guerrero Guzmán +(34) 664 66 06 05 int@grupoagrinews.com
Nicole Carletti Ramírez +57 312 391 9330 avinewslatam@grupoagrinews.com
CUSTOMER SUPPORT
Mercé Soler
EDITORIAL STAFF
José Luis Valls
Osmayra Cabrera
María de los Angeles Gutiérrez
Nicole Carletti Ramírez Héctor David Corredor Martínez
TECHNICAL DIRECTION
Edgar Oviedo
Eduardo Cervantes
info@grupoagrinews.com avinews.com grupoagrinews.com Subscription
Biosecurity compliance: the balance between culture, personality, experience, education and technology
Edgar O. Oviedo-Rondón Prestage Department of Poultry Science, North Carolina State University. Raleigh, NC
The prevention and control of highly pathogenic avian influenza (HPAI), Newcastle disease, and all other diseases depend mainly on implementing complete biosecurity management programs.
Advancing poultry health: the role of predictive analytics in disease prevention 12
Talha Siddique
Institute of Animal and Dairy Sciences (IADS), University of Agriculture Faisalabad, Pakistan
Artificial intelligence (AI) is reshaping the future of various industries, and the poultry sector is no exception. Machine learning (ML) and predictive analytics are both segments of Artificial intelligence (AI). AI predictive analytics use machine learning (ML) techniques and models that acquire knowledge from data over time.
Animal welfare and artificial intelligence: a combination of the poultry present or future? 22
Dra. Elein Hernández Faculty of Veterinary Medicine of Higher Studies Cuautitlán UNAM, Mexico
The interest and evaluation of welfare has transcended theoretical concepts such as the five freedoms and has been applied in the development of new ones such as artificial intelligence.
Pathology of the immune system in the diagnosis of immunosuppression in poultry
Nestor Ledesma Martínez Department of Poultry Medicine and Zootechnics, Faculty of Veterinary Medicine and Zootechnics, Universidad Nacional Autónoma de México
Due to the multifactorial aetiology for immunosuppression, diagnosis is not always straightforward, requiring a clinical history, necropsies and complementary laboratory tests if necessary.
Controlling insects in poultry production
Gracieli Araujo Specialist in Animal Welfare, Cobb LatCan
Insects can cause physical damage including structural damage to the houses. Breeder operations can be impacted by many insect species but the primary issues are caused by flies, beetles, and ants.
In ovo vaccination with embrex® technology helps support earlier, more robust immune response in chicks
Zoetis Technical Team
Recombinant vaccines pair well with Embrex in ovo vaccination technology to help deliver strong early protection against viral challenges.
The quality parameters of soybean and alternative protein feedstuffs in poultry nutrition
Güner Gövenç
Bursa Uludag University, Faculty of Agriculture, Department of Animal Science, Nilüfer, Bursa TURKEY
In poultry nutrition, protein feeds are one of the most expensive and limiting ingredient in diet formulations and one of the most important protein sources for poultry is soybean.
Metapneumovirus epidemiology and seasonality
Edgar O. Oviedo-Rondón
Prestage Department of Poultry Science, North Carolina State University. Raleigh, NC
The Avian Metapneumovirus (aMPV) is an important pathogen in respiratory diseases but is frequently neglected. It can affect the respiratory and reproductive systems, facilitating the development of other diseases.
Effects of chronic stress and intestinal inflammation on commercial Poultry health & performance: Part I
Guillermo Tellez-Isaias
Department of Poultry Science, University of Arkansas Agricultural Experiment Station, Fayetteville, AR, USA
Currently, animal production systems require a constant search to reduce the effects of stress and chronic inflammation to improve energy use by producing animals.
Ken Bafundo Poultry Technical Consultant.
Among many natural strategies that have been studied and then applied commercially, saponins are gaining traction throughout the livestock and poultry industries.
High oleic soybeans and their impact on egg and poultry meat quality
Edgar O. Oviedo-Rondón
Prestage Department of Poultry Science, North Carolina State University. Raleigh, NC
High oleic soybean oil, a product of constant innovation in soy production, is rich in oleic acid, similar to olive oil. This high-oleic oil, with its 75% oleic acid content, is gaining popularity due to its numerous health benefits.
H&N Technical Team
One of the key parameters in commercial egg production is undoubtedly egg size. The production of high-weight eggs has challenges such as early degradation of shell quality, loss of accumulated eggs by a late start, deterioration of the conversion rate or the increased accumulated mortality.
Brian Fairchild interview
Brian Fairchild Professor/Extension Poultry Scientist - University of Georgia
On the occasion of the 2024 PSA Annual Meeting, organized by the Poultry Science Association (PSA), which was held July 15-18, 2024, AviNews International had the privilege of interviewing Dr. Brian Fairchild, President of the PSA and Professor and Extension Poultry Scientist in the Department of Poultry Science at the University of Georgia.
Edgar O. Oviedo-Rondón
Prestage Department of Poultry Science, North Carolina State University. Raleigh, NC
Endemic and emergent diseases remain a constant threat to poultry production, welfare, and the economy of the poultry industry worldwide. The prevention and control of highly pathogenic avian influenza (HPAI), Newcastle disease, and all other diseases depend mainly on implementing complete biosecurity management programs.
Biosecurity encompasses infrastructure, technologies, techniques, management, and basic hygiene practices.
While poultry facilities, fencing, and equipment locations should be designed to facilitate biosecurity practices, effective communication is always a crucial factor.
Convincing all involved in the production system and potential visitors to incorporate biosecurity practices into their routines is a necessity.
Biosecurity measurements have been discussed many times. Effective biosecurity depends on strict, constant, and daily compliance at the farm and regional levels. Compliance with biosecurity, such as consistently following sanitation protocols, changing footwear, or respecting areas before entering a production facility, has been reported to reduce diseases significantly.
Unfortunately, poor compliance with biosecurity is an endemic problem in all types of animal production systems worldwide, and it is the main reason biosecurity may not be effective.
In all cases, the human factor is critical.
Many experts in biosecurity have concluded that to be successful in this task, it is necessary to create a culture of biosecurity, address personality traits, provide experiences, and most importantly, ensure continuous education.
Applying new technologies to monitor, audit, and ensure biosecurity is also crucial.
However, it’s important to understand that no single intervention or corrective action can solve the problem of low biosecurity compliance.
Human behavioral factors are crucial to implementing effective biosecurity.
The lack of biosecurity compliance is so prevalent in the whole animal industry that it has raised the need for a new area of research.
A better understanding of the complex human decision-making process influencing workers’ willingness to comply with biosecurity protocols requires innovative research approaches and data-collection techniques.
Humans should participate in the strategy, tactics, and operations, which are guided by a long-term objective. Biosecurity issues at the strategic scale are often related to network interactions of people located in diverse facilities and service providers across production chains.
Everybody who works or provides a service in a poultry facility must understand and follow the rules and procedures.
Pressure to complete work efficiently with time constraints has created scenarios where farm workers find it unrealistically challenging to complete their jobs while complying with biosecurity standards.
At the tactical level, farm managers or owners decide whether or not to invest in and implement preventive biosecurity protocols. From a narrower, more localized perspective, operational-level biosecurity can be viewed as a continuous series of decisions made by animal production workers indicating their willingness to follow or comply with daily biosecurity protocols.
The operational, tactical, and strategic levels of biosecurity are interconnected.
For example, tactical decision-making influences operational-level protocols.
Moreover, while it is known that operational-level compliance with biosecurity will impact the implementation and efficacy of tactical and strategic biosecurity decisions, limited feedback describing operational-level behavior is currently available to inform tactical- and strategic-level decision-making.
Research has suggested that poultry farmers in the United States exhibit risk tolerance but may be more likely to implement or comply with biosecurity as their perceived risk of infection increases.
Indeed, as the actual infection risk increased, so did compliance with a biosecurity practice.
The certainty of infection risk information is also expected to affect worker biosecurity implementation and compliance.
However, the effect may change depending on the domain of interest, i.e., tactical vs. operational.
Worker decisions at the operational level have direct economic and sociological consequences when an outbreak occurs. Social cues, all nonverbal communication, and examples will result in different compliance strategies among individuals. The behavior of others, even if not directly impacting the individual, will impact their decision-making to comply with biosecurity measurements.
Then, social incentives and social norms play an essential role in maintaining compliance.
Workers in an animal facility may be exposed to various information about the consequences of an animal infection.
Still, they balance the cost of infection with complacency and a tendency to become lax in day-to-day activities.
It is generally perceived that short-term visits to the facilities are lower threats, and consequently, biosecurity procedures are forgotten in these cases, becoming the most common cause of outbreaks.
This relaxation of biosecurity effort has been examined from a temporal perspective, with evidence suggesting that people view the likelihood and impact of an animal disease event to be reduced as the time the event increases. Referred to as temporally based psychological distancing, the farther in the past an event occurs, the less likely and impactful such an event is perceived to be.
Studies have indicated that continuous training programs coupled with measure-specific training sessions based on observed needs should address issues related to visits by all poultry personnel and visitors.
The training should emphasize that biosecurity procedures must be applied with the same rigor regardless of the duration and moment of the visit.
Introducing new posters with the grower’s list of required biosecurity measures may enhance short-term compliance.
But constant rotation of information is necessary.
Training should also address the notion of threat perception when engaging in protective behavior. This means infusing the concept that animal diseases may affect them directly in their income and their own health.
Studies have demonstrated that being a member of a grower’s family improves biosecurity compliance. Nevertheless, a personality trait is always necessary to comply with biosecurity.
A study conducted by Racicot et al. (2012) identified that compliance was associated with people who showed responsibility, complexity, and action-oriented traits.
Recently, Racicot et al. (2022) evaluated two technologies based on radio-frequencyidentification (RFID) to monitor and continuously improve biosecurity compliance in poultry facilities.
Farm boots were equipped with RFID chips placed under the soles.
Three antennas in the barn entrance were connected to the RFID:
1
the first in the frame of the exterior door identifying the person entering the barn (access card already used by employees),
2
the second in the dirty area, allowing for the detection of chipped farm boots (indicating biosecurity non-compliance), and
3
the third in the clean area, connected to the hand sanitizer, detecting hand sanitization when entering and leaving the clean area as well as the RFID chips in farm boots (indicating compliance with changing boots).
Two pressure mats (one in the dirty area and one in the clean area) were also installed in the barn entrance to facilitate the detection of visitors, assess the direction of people (i.e., entering vs. leaving), and detect a lack of compliance if farm boots were recorded in the clean area (Figure 1).
Results confirmed the ability of the RFID system to monitor boot and hand sanitizing compliance. A continuous monitoring system seems to improve biosecurity compliance, with almost twice the compliance percentage compared to previous studies using hidden cameras.
Participants reacted each time the alarm sounded, often wondering what they may have done wrong.
Participants reported that the RFID system was helping increase daily compliance and recommended using it on a larger scale to improve overall compliance. They reported being comfortable with shoes being chipped and did not find the project intrusive.
Figure 1. Radio-frequencyidentification-based (RFID) real-time continuous automated monitoring system. (a) Soft chips inserted in work shoes used by personnel getting to the farm; (b) rigid chips inserted in the soles of farm boots; (c) RFID antenna; (d) Device (e-box) with data management program; (e) hand sanitizer device. Source: Racicot et al., 2022 Front. Vet. Sci. z
The same group led by Racicot and collaborators had evaluated in 2012 hidden cameras at Quebec poultry farms. They documented 44 biosecurity lapses workers and visitors made over four weeks.
The presence of a visible camera in a barn entrance enhanced overall visit compliance, specifically boot and area compliance.
However, six months after its installation, compliance with the area during the visit was the only biosecurity measure enhanced at the medium-term assessment.
Biosecurity compliance: The balance between culture, personality, experience, education and technology DOWNLOAD PDF
Compliance declined over time.
Audits of biosecurity did not have any impact on improving daily biosecurity compliance whenever another person was not present.
These results indicated that an automatic system for monitoring and recording each person’s activities could improve biosecurity compliance even more. More innovation is needed to implement this technology in other biosecurity practices. Technologies can help to overcome the challenges caused by the human factor in biosecurity compliance.
Talha Siddique
Institute of Animal and Dairy Sciences (IADS), University of Agriculture Faisalabad, Pakistan
Artificial intelligence (AI) is reshaping the future of various industries, and the poultry sector is no exception.
Machine learning (ML) and predictive analytics are both segments of Artificial intelligence (AI). AI predictive analytics use machine learning (ML) techniques and models that acquire knowledge from data over time.
These models undergo training using past data in order to recognize and analyze patterns and interactions (Ravi et al., 2018).
After being trained, the models are utilized to create predictions about future outcomes using new, previously unseen data (Figure 1).
Disease and inadequate hygiene are two of the many problems that confront the chicken production sector. Coccidiosis, Newcastle, Gumboro pullorum, and Salmonella are among the most prevalent diseases (Machuve et al., 2022).
The diagnostic testing for these diseases can be expensive, timeconsuming, and laborious.
1. Simple depiction of predictive analytics (Source: Ogirala et al., 2024).
This is where predictive analytics have provided a breakthrough solution for the poultry industry.
Farm managers can take appropriate measures even before the diseases manifest clinically, thanks to predictive analytics models that derive results from historical data and real-time inputs to forecast outbreaks.
The key to successful predictive analytics in poultry farming is the collection and management of different data.
The prominent data types on the farm include environmental factors like temperature, humidity, and air quality (key for poultry health).
Tracking them helps to forecast conditions that may favor a disease outbreak (Jung et al., 2021; Johansen et al., 2019).
loT Technology
Cameras
Microphones Wi-Fi, Sensors
Zigbee, GPRS
Ardunio, RFID
Bluetooth
Raspberry Pi
Wearable sensors
Biosensors
Air Quality
Feed consumption
DC Fan Heat Lamp Indoor Parameters
Moreover, sensors and video surveillance can help monitor bird behavior (feeding patterns, activity levels, and interactions with surroundings) (Figure 2).
Such behavioral indices can help identify distress or disease at earlier stages than traditional assessment alone (Reboiro-Jato et al., 2011).
Diseases
Avian In uenza
Newcastle, Infectious Bursal Bronchitis, Fowlpox
Salmonella, Aspergllloss Coccidlosis, E. coll
Behaviour
Walking
Eating
Posture
Drinking
Pecking
Rale sound
Resting
Running Temperature
Rel. Humidity
Light Intensity
Ventilation
Litter Moisture Content
Ammonla
Carbon dioxide
Data Types
Audio Images Videos
Text
Processing Types
Image Analysis
Vocalization Analysis
Data Processing
Classi cation
Regression
Clustering
Health status
Lameness, Weight, Growth, Heat Stress
Al ML Techniques
Support Vector Machines, Deep Learning, Linear Regression, Bayesian Network
Decision Trees, Neural Networks
Fuzzy Logic Control System
K-means, Logistic Regression
K-Nearest Neighbours
Random Forest, Association Rule
Principal Component Analysis
Lasso Regression, Least Squares Regression
Dempster-shafer, Genetic algorithm
Linear Discriminant, Quadratic Discriminant
Gradient Boosting Machines, C-Means
Figure 2. Overview of IoT and ML in poultry health management (Source: Ojo et al., 2022).
Health records, which include vaccination history, medication records, and previous medical diagnoses, are another important dataset.
This insight is essential for better risk disease modeling and understanding the temporal health issue patterns that might spread throughout an entire flock (Huang et al., 2019).
The scale and complexity of these data types require reliable systems to process, validate, and use all this information for real-time conclusions.
Internet of Things (IoT) devices and sensors primarily automate data collection, providing continuous input to integrated management systems.
State-of-the-art databases and cloud storage solutions store the massive amount of data.
Data analytics platforms utilize advanced algorithms and machine learning models to analyze the data, look for hidden relationships or patterns in the processed ways, and predict possible outcomes (LeCun et al., 2015) (Figure 3).
Figure 3. The general workflow of machine learning-based chicken monitoring systems (Source: Okinda et al., 2020).
ML-based techniques employ feature extractors to transform raw data, such as pixel values from photos, into feature vectors.
In addition, learning subsystems are employed to perform regression or identify patterns in the acquired features (LeCun et al., 2015).
Deep learning algorithms, derived from conventional machine learning techniques, have the ability to autonomously discover features or data representations from raw data, eliminating the need for knowledge in feature extraction engineering (LeCun et al., 2015).
Recurrent neural networks, autoencoders, convolutional neural networks, belief networks, generative adversarial networks, and reinforcement learning are all models that make up deep learning architectures.
To solve difficult problems, deep learning networks need highperformance processing systems, large datasets, optimization methods to keep data immutable, and complex topologies (Oyedele et al., 2021).
Convolutional neural networks are widely used in deep learning for the purpose of digital image processing (Zhuang & Zhang, 2019).
Most machine learning systems use past data as input to project future output values. Learning algorithms (e.g., ANN, SVR, random forest, CNN, GLM) and many learning models (e.g., classification, regression, clustering) define ML as either supervised or unsupervised (Milosevic et al., 2019). Supervised learning methods ensure the correctness of their classifications or predictions by means of labelled data.
Robertson and Yee created an automated data extraction and analysis pipeline using four keywords linked with poultry infection with AIV to examine Twitter tweets on AIV. Their model showed a positive relationship between avian influenza cases in poultry reported by the World Organization for Animal Health and Twitter posts linked to AIV between 2015 and 2016 (Robertson & Yee, 2016).
Belkhiria et al. (2018) created a disease distribution map to project California’s high pathogenicity avian influenza virus (HPAIV) risk. They combined several kinds of data, including the count of broiler farms in a given location, the distance to coastlines, and the historical low pathogenic avian influenza virus infection count of wild birds, to create their result. The map links areas of California with HPAIV infection risk, therefore enabling the use of suitable precautions should an outbreak strike.
One of the primary advantages is the significant reduction in bird mortality rates.
Early detection greatly reduces the reliance on antibiotics, traditionally used as a blanket preventive measure.
By preventing outbreaks, farms can avoid the substantial costs associated with mass culling and lost productivity.
Infrastructure problems, economic challenges and data governance concerns will be among the challenges facing the development of a standardized system to forecast the emergence of diseases in poultry.
There is insufficient internet access in rural farm areas, leading to a lack of connectivity. Additionally, the development of sensors and biosensors for diagnosing poultry infections is still in its early stages.
Therefore, further research is required to create technology that can effectively operate in a commercial poultry house environment.
The adoption of new technology on farms necessitates that farmers acquire knowledge and training in those areas. The problem of bias and noise in particular data sources is another obstacle that predictive algorithms will have to overcome.
As an example, assessments relying on web-based sources often end up with a lot of noise and useless data that needs to be deleted (Milinovich et al., 2014).
Farmers might have experienced data governance problems when considering harmonized prediction models with several stakeholders. While models should include information from as many farms as feasible to predict disease development, producers might not wish their data made public.
Data can be anonymised, but reidentification methods are improving (Ferris, 2017).
Improving data quality, building technical expertise, and reducing economic and operational barriers can effectively implement predictive analytics, leading to enhanced disease management and improved operational efficiency (Figure 4).
Improving Data Quality Automated Data Collection Data Veri cation Protocols
Building Technical Expertise Partnerships with Tech Companies Continued Education and Training
Reducing Economic and Operational Barriers Modular Implementation Government and Private Funding Cost-Bene t Analysis
Furthermore Ojo et al. (2022) proposed a strategy aimed at enhancing precision livestock farming for intelligent poultry health and welfare.
Their approach specifically tackles the obstacles encountered in current systems. This notion was formulated by a comprehensive examination of previous treatments conducted by other researchers in the field of poultry welfare management.
The framework possesses the qualities of scalability, resilience, extensibility, and security.
The framework consists of several fundamental components, namely the deep learning (DL) module, Digital Twin module, cloud edge computing (cloud-fog-based) module, communication module,
The future of predictive analytics in poultry farming is expected to improve with artificial intelligence (AI) and machine learning, as well as an integration into Internet of Things (IOT) devices and big data that would greatly enhance what these technologies can do.
To ensure commercial viability and applicability, poultry monitoring systems require further research and development.
Integrated approaches in livestock science and engineering must address these challenges to improve the overall performance of chicken monitoring in a PLF and increase its resilience (Figure 6).
Monitoring animal weight over development helps one assess slaughter time and feeding plans. Should the measured weight deviate from the expected growth curve, it suggests a condition such as disease or other vitality issues requiring counteractions. Live weight thus defines the welfare of animals.
The main difficulties for chicken weight estimate systems are variable ambient lighting and flock localization.
Flexible image sensors and illuminant invariant cameras for farms would address the changing light problem. Weight estimation makes advantage of IR-based depth cameras such as Microsoft Kinect (Mortensen et al. 2016).
Figure 6. Prospects
Arti cial Intelligence and Machine Learning: Enhancing predictive analytics with advanced technologies.
Health Status Classi cati on Systems:
Utilizing color and viscosity of droppings to diagnose health issues, with potential for expanding datasets and improving detection methods.
Integration with loT and Big Data:
Using loT devices and big data to collect and analyze extensive variables for real-time engagement.
Poultry Tracking Systems:
Implementing multi-object detection and non-visible light-based sensors for continuous behavior and health monitoring.
Live Weight Estimation Systems: Employing IR-based depth cameras and exible image sensors to monitor animal weight and welfare.
Challenges and Research Needs: Addressing the need for further research in sensor technology, data integration, and machine learning to improve the accuracy and applicability of predictive models in poultry farming.
Lameness Detection Systems: Tracking mobility to assess tness, detect potential diseases, and improve animal welfare.
Future Prospects: Anticipating improvements in predictive models through better algorithms that can process complex datasets quickly, identify early warning signs, and detect new disease strains.
Mobility is crucial for living organisms. Being mobile is associated with fitness and wellness.
Birds with trouble walking can starve, decreasing their feed conversion ratio, weight and growth, chest soiling, hock burns, and be vulnerable to predators.
According to wellbeing-Quality® (2009), the foregoing variables indicate poor animal wellbeing. Thus, tracking a bird’s mobility indicates its welfare.
Wang et al. (2019b) used dropping color and viscosity to diagnose digestive disorders in broilers.
Feed type can also affect falling color.
Water intake also affects decreasing viscosity.
Thus, this method would be difficult to use with free-range or diverse-fed hens. Additional research should be done to create a poultry dataset with particular categories such broiler, layers, chicks, etc., fit for usage in poultry detecting systems.
Still, the retraining process of new applications would make this laborintensive. Therefore, a lot of study should be directed on innovative learning strategies, like semisupervised learning and adaptive learning.
The evaluation of behavioral (types of activities) and physical (lameness and health) factors in poultry welfare depends critically on tracking of poultry.
Behavior phenotyping and welfare monitoring demand for constant recording of bird movement and behavior phenotyping.
Zhuang and Zhang (2019) have already implemented multi-object detection in unhealthy broiler detection, which has recently garnered significant attention.
Furthermore, additional research should be conducted on DL networks that employ non-visible light-based sensors to combat illumination variation issues and enable tracking to be conducted during nighttime hours (lighting regime).
When we consider the integration of IoT devices with big data, it revolutionizes predictive analytics in poultry farming by providing farmers with more detailed information.
By incorporating sensors, cameras, and the IoT in general, big data platforms are utilized to analyze a wide range of variables, from environmental factors to individual bird behavior, and then feed this data into a platform for real-time engagement (Nakrosis et al., 2023).
Adding AI functionality not only broadens the scope of data analysis but also expedites the process, enhancing the efficiency and promptness of decision-making.
We hope that the increase in accuracy and efficiency of predictive models will continue to trend this way as solutions combining bio-analytics, AI, and machine learning improve over time.
Better algorithms, which have the potential to process datasets more complex than ever before faster and far from perfect, are just beginning to emerge.
This will enable us to identify the rarer, smaller signals of impending outbreaks before full pathology emerges, and potentially identify new disease strains as they emerge.
Predictive modeling helps mine historical and real-time data to provide heads-up alerts on likely health outcomes, preventing diseases before they happen.
By moving from reactive to proactive management practices, we can optimize animal welfare and bring about considerable economic gains in the form of decreased mortality rates, reduced medication spending, or expedited turnover tracking.
The use of AI and machine learning technologies has further enhanced the accuracy and timeliness of these predictive systems’ decision-making.
In addition, the introduction of IoT devices and big data analytics has further evolved this landscape, providing advanced insights and monitoring support.
Advancing Poultry Health: The Role of Predictive Analytics in Disease Prevention
DOWNLOAD PDF
Dra. Elein Hernández Faculty of Veterinary Medicine of Higher Studies Cuautitlán UNAM, Mexico
Interest in animal welfare has increased over the years and is likely to remain on the agenda of producers and consumers of animal products.
The interest and evaluation of welfare has transcended theoretical concepts such as the five freedoms and has been applied in the development of new ones such as artificial intelligence.
The aim of this article is to highlight key concepts in welfare assessment and to point out some scientific advances for its evaluation by means of artificial intelligence.
The World Organization for Animal Health, OIE, defines animal welfare as:
The physical and mental state of an animal in relation to the conditions in which it lives and dies, and refers to the also known as the five freedoms:
Some of these challenges include the large number of animals, diverse housing types and species-specific characteristics.
Therefore, the use of objective and practical animal welfare indicators is recommended as a matter of routine practice.
Based on the animal(s): These are those that are evaluated directly on the animal and include physiological and behavioral changes and some other animal health indicators.
Resource-based: Includes direct observations of facilities and operations related to animal care and handling.
However, the use of the five freedoms as a means of animal welfare assessment may have some limitations when used in production animals such as poultry
Management-based: Includes documentary review of animal health and care procedures and records.
There are recommendations on the criteria to be evaluated within each of these indicators, but there may be national and international differences, as well as differences between national and private standards.
WOAH encourages private standards to develop transparency mechanisms and to work towards harmonization between public and private standards.
In general an animal welfare program should be governed by the following principles from Main et al (2014):
1
2 3
Dynamic animal welfare management program: The program should be proactive and be able to promote continuous improvement.
Progressive standards: Standards should at least meet the minimum stipulated by law and seek a relevant certification program in addition to promoting areas for improvement.
Have pre-defined evaluation guidelines and promote behavioral change: It is important to establish priorities and animal welfare concerns in advance, as well as to promote the interest of program participants to generate change.
Programs must offer technical advice, as well as have permissible or non-permissible criteria within evaluations.
4
Participation of external evaluations: Through evidence-based programs, evaluations can be developed and reviewers (external inspectors or auditors) can be invited to participate.
Modern poultry farming has been characterized by its continuous search for the optimization of resources in order to achieve productive efficiency, ensure optimum bird development and minimize mortality.
This has been possible thanks to the effort of diverse technologies and multidisciplinary developments.
Therefore, the inclusion of concepts such as “precision livestock” is not alien to poultry farming, since it focuses on the automated monitoring of animal consumption and behavior and information analysis using artificial intelligence tools such as machine learning or deep learning.
Identify the appropriate feeding of animals
Identify the appropriate feeding of animals
Reduce environmental impact
Reduce environmental impact
The main objectives of precision livestock farming are:
The main objectives of precision livestock farming are:
Ensure the safety and traceability of livestock products. Agricultural resource management
Ensure the safety and traceability of livestock products. Agricultural resource management
Improve animal health and welfare
Improve animal health and welfare
This holistic view on information capture and automated analysis for decision making in animal production has an important role to play in the fourth industrial revolution or also called Industry 4.0.
This digital transformation of the countryside includes tools such as: Machine learning which consists of the linear evaluation of information ranging from capturing information, processing it and presenting results.
However, there are more complex tools such as deep learning that use algorithms that allow the analysis of such information in a non-linear way.
The main advantages offered by this technology for animal assessment is that it is objective by minimizing the subjectivity of human judgment.
Currently, the evaluation of animal welfare depends largely on the experience and knowledge of the evaluator.
Another advantage is that it is noninvasive, since the collection of information such as images or data can be analyzed remotely
In other words, they use classification methods and data regressions similar to the pattern of learning and analysis of the human brain.
The scope of such tools for poultry welfare assessment remains to be seen, but current scientific research has focused on the use of artificial intelligence in animal- and resource-based indicators.
This represents an advantage in terms of biosecurity and stress or behavioral changes of birds in the presence of humans.
Real-time analysis is potentially another advantage, but depends largely on the equipment to be used.
Okinda and colleagues (2020) identified research with the use of artificial intelligence in poultry production focused on animal-based indicators such as:
Weight gain assessment
Lameness and locomotor problems
Identification of health problems
Monitoring systems for movement and behavioral changes
The methodology to perform these analyses differs among studies.
In general, in the case of weight gain evaluation, images of the birds are captured in an automated manner and the bird is assigned or coded a geometric figure according to the space occupied by its body in relation to the environment.
The analysis consists of evaluating the changes of the geometric figure or body of the animal in relation to the environment.
In theory, this processing seems straightforward, but it depends on several factors such as production type and image capture:
On a farm with birds on the floor, such as broiler chicken, it is simpler.
Birds in an aviary or cage where it is more complicated to identify the animal in isolation.
There are also other practical complications such as the effect of illumination on image quality and estimation errors as animal density changes.
In the case of locomotor problem analysis, artificial intelligence has advantages such as the automation of the evaluation.
Locomotor problems in chicken production can affect between 15-25% of the population and severely affect their welfare by preventing the development of expected behaviors such as eating and drinking.
For persistency that keeps going and going, choose Hy-Line. Reach more weeks over 90% production to a late age.
Contact your Hy-Line representative to learn more.
The conventional method of lameness assessment consists of a visual inspection of the chickens’ gait and scoring on a scale of 0 to 5, with 5 being the score that reflects the most difficulty in walking. This procedure requires experience, time and is performed individually per bird.
While the use of artificial intelligence requires the placement of marks or visual cues on the legs and joints so that by capturing images or videos these marks can be recognized and the system can measure the speed, acceleration and degree of angulation.
This provides an objective and more practical analysis of the chickens’ gait.
Research with this technology reports identifying birds with problems with better accuracy compared to the conventional method, which represents an advance in favor of animal welfare by being able to identify health problems in a timely manner and take corrective and preventive actions.
However, there are also technical limitations in the use of these tools, since images of lateral positions of the birds are required to evaluate their gait, while obtaining images from above or on top of the birds is less invasive.
Likewise, these investigations were carried out in controlled environments and the movement of the birds was temporarily restricted to facilitate the capture of individual information.
The assessment of health problems has been studied with various approaches using artificial intelligence.
Examples include the assessment of caloric stress using images captured with infrared technology and their subsequent analysis with machine learning.
Other applications of artificial intelligence for the assessment of animal welfare based on resource indicators are those used to measure changes in temperature, humidity and other environmental conditions on the poultry farm in an automated manner.
The goal of their use is better resource management to ensure poultry care and minimize environmental impact.
Behavioral changes in birds undergoing infectious processes and even changes in color and viscosity of excreta have also been evaluated as indicators of health, but the practical limitations are similar to those mentioned above.
In conclusion, the use of artificial intelligence tools poses several solutions to current challenges in poultry production.
However, its application still has limitations and is oriented to integrated systems or systems with a certain degree of technological advancement.
Nevertheless, there are benefits for poultry welfare if their integration into production sites is achieved.
It is important to mention that the use of this technology still requires the operator to make decisions and it is the responsibility of the producer, veterinarian and farm personnel to take care of the birds’ care and welfare.
Nestor Ledesma Martínez
Department of Poultry Medicine and Zootechnics
Faculty of Veterinary Medicine and Zootechnics
Universidad Nacional Autónoma de México
Modern poultry lines are characterised by high performance and specialisation. Advances in genetics, feeding, management, environment and breeding have significantly improved production parameters.
While today’s birds are more efficient and productive, they are also less hardy than in the past, making them more susceptible to disease.
Because of this increased susceptibility, modern production requires strict biosecurity measures and efficient vaccination schedules.
More and better vaccines are now available, however, the immune system of the birds needs to be in optimal working condition.
Physical agents such as sudden temperature changes, handling during vaccinations, sorting, regrouping.
The immune system is constantly subjected to immunosuppressive agents of various kinds;
Due to the multifactorial aetiology for immunosuppression, diagnosis is not always straightforward, requiring a clinical history, necropsies and complementary laboratory tests if necessary.
It is very important for the field clinician to be aware of the macroscopic changes in the immune system that can be observed during necropsy. This guides the diagnosis and facilitates sampling.
In the following, the most relevant aspects of immunosuppression-oriented pathology of the immune system are discussed.
Chemical agents such as mycotoxins and biogenic amines.
Biological agents such as viruses.
The avian immune system consists of primary and secondary lymphoid organs.
During the embryonic stage, undifferentiated cells migrate from the yolk sac to the bone marrow, thymus and bursa of Fabricius.
In these organs the cells differentiate into T- or B-lymphocytes where they express surface markers and by negative selection those lymphocytes that are not useful are eliminated.
They then migrate to secondary lymphoid organs such as the spleen, caecal tonsils, Harder’s gland, mucosa-associated lymphoid tissue, and germinal centres in connective tissue.
It is present only in birds, located in the dorsal part of the cloaca, connected to the intestine by a duct.
The bursa of Fabricius is the site of differentiation of B lymphocytes and captures antigens when the bird defecates, as the smooth muscle layer of the intestine continues into the bursa of Fabricius so that the contraction of the muscle makes the bursa function as a suction knob.
Inside the bursa of Fabricius there are major and minor folia, these folia are lined by a columnar epithelium and contain the lymphoid follicles supported by a matrix of connective tissue.
Each lymphoid follicle is made up of a cortex and medulla, separated by a layer of cortico-medullary cells that continue with the cells of the epithelium lining the folia.
The interpretation of lesions in lymphoid organs requires taking into account the age of the birds and vaccination schedule, as primary lymphoid organs atrophy when birds reach sexual maturity and most routine vaccines cause changes in the lymphoid organs.
The bursa of Fabricius, in the absence of infectious agents or immunosuppressants, should be present until 12 to 14 weeks of age, at which time it begins to involute, so that by 20 weeks only traces remain.
In production birds, the use of vaccines, mainly against infection of the bursa of Fabricius, causes atrophy before this time.
The involuted bursa of Fabricius appears macroscopically as a small, hard, yellowish-white nodule.
Microscopically, the folia are small, the follicles have lost the differentiation between cortex and medulla, the epithelium shows folds and cysts.
The thymus in birds is located along the neck and is composed of 6 to 7 lobes running parallel to the jugular veins and the vagus nerve.
T-lymphocytes differentiate in the thymus.
Macroscopically, the lobules of the thymus are seen with small lobules and on sectioning, a cortex and medulla can be distinguished.
In the absence of infectious or immunosuppressive agents, the thymus should remain until 15 to 17 weeks, after which time it begins to involute so that by 30 weeks only vestiges remain.
Histological examination of involuted thymus shows loss of the cortex and fibrosis of the medulla.
In 1-day-old chicks, clusters of heterophils are frequently found in the subepithelial tissue; these are foci of extramedullary granulopoiesis and are common in various tissues of the chick.
Only non-pneumatic bones such as the femur and tibiotarsus have bone marrow, it is considered a primary and secondary lymphoid organ as bone marrow is the source of:
Undifferentiated cells that migrate to the thymus and bursa of Fabricius in embryonic life and;
On the other hand, they are a source of these same cells in adult animals or in the repopulation of the thymus and bursa following severe damage with loss of lymphocytes.
The spleen is attached to the gizzard and proventriculus by its visceral side. It is a secondary lymphoid organ, made up of a capsule of connective tissue and trabeculae on which it is supported:
The germinal centres (white pulp) and arterioles.
The dendritic cells.
Red blood cells (red pulp).
The spleen in young chicks is a centre of granulopoiesis and in older birds a centre of antigen presentation.
In addition to the age-related involution of birds, there are many factors that cause lymphoid atrophy.
Birds are subjected during the production process to stress factors such as:
Heat. Cold.
Handling.
Vaccinations.
Feed restrictions.
Selection and regrouping among others.
Which trigger the secretion of glucocorticoids which cause apoptosis in lymphoid cells.
The process of apoptosis is normal in birds during negative selection processes of clones that are not useful. A histological slice of lymphoid organs shows apoptosis, which is described in texts as ‘starry sky’.
This is considered physiological unless the apoptosis is such that the size of the organ is decreased.
On the other hand, mycotoxins are considered to be immunosuppressive agents that cause atrophy of lymphoid organs, the mechanism of atrophy following two pathways:
They inhibit protein synthesis and nucleic acid replication, which are necessary for lymphoid cell differentiation and proliferation and;
Birds reject feed, which generates stress and glucocorticoid release.
If decreased lymphoid organ size is observed, mycotoxins should be considered in the differential diagnosis.
In the case of the thymus, atrophy due to abundant apoptosis occurs in cases of infection with infectious anaemia virus. This vertically transmitted virus targets T lymphocytes and bone marrow germ cells.
Birds under 5 weeks of age show severe atrophy of the thymus cortex, decreasing considerably the size of the thymus, sometimes at necropsy the thymus may go unnoticed.
In addition to apoptosis, necrosis also leads to atrophy of lymphoid organs, but in this case the inflammatory process may transiently increase the size of the organ.
In the case of the bursa of Fabricius, the best known agent is the bursa of Fabricius infection virus (IBF), also known as Gumboro disease The changes that the virus causes in the bursa of Fabricius have been well characterised.
Lymphoid cell necrosis with oedema between follicles and germinal epithelium, as well as heterophilic infiltration are the first changes.
When infection is caused by very virulent strains (hot strains) haemorrhages occur.
During the first 3 to 5 days, the bursa of Fabricius increases in size and after a week there is proliferation of cortico-medullary cells and fibrosis with a decrease in the size of the bursa, the epithelium is folded and cysts can be seen.
These changes are not exclusive to IBF, as other agents such as Marek’s disease virus (MDV), Reovirus and Newcastle disease virus (NDV) can also cause necrosis and inflammation.
For this reason, the diagnosis of IBF by histopathology must be accompanied by antibody determination by ELISA or virus serum neutralisation.
A particular case is IBF virus infection by variant strains, as they do not cause necrosis and inflammation and the bursa of Fabricius only atrophies, these viruses cause lymphoid cell death by apoptosis.
In the absence of secondary infections, the bursa of Fabricius may recover after 8 weeks, in which case areas of regeneration characterised by intense basophilic staining can be seen in the vicinity of depopulated follicles.
Regeneration depends on the absence of secondary infections, if there is a bacterial complication or cryptosporidium infestation the bursa of Fabricius has a fibrinous to fibrinocaseous exudate that may fill the bursa.
Necrosis and inflammation of the thymus is rare, usually associated with the application of emulsified vaccines, in which case a granulomatous reaction is observed.
On the other hand, necrosis of lymphoid cells and hyalinisation of arterioles can be observed in cases of NDV in its velogenic presentation or in Avian Influenza (AI), particularly with the H5N2 virus.
Most viral agents that cause immunosuppression with lymphoid cell necrosis or apoptosis result in spleen cell depletion, and when the immune system is constantly stimulated by other viruses and bacteria, lymphoid hyperplasia occurs.
With the naked eye, the cut surface shows white stippling, which microscopically corresponds to nodules of lymphoid hyperplasia. In cases of NDV or AI, areas of infarction with hyalinisation of arterioles are present.
In birds, Marek’s disease (MD) and Lymphoid Leukosis (LL) viruses cause lymphoid cell neoplasms (lymphomas) occurring in various organs.
Figure 3. Marek’s disease lymphoma in the heart and lung of a 12-week-old bird.
Marek’s disease has 5 presentations depending on the site of T-cell infiltration. With the exception of the cutaneous presentation, all other presentations are nonproductive transforming, that is, complete virions are not produced and the infected cell becomes neoplastic.
Clinical manifestations depend on the organ infiltrated, thus birds present:
Blindness at ocular presentation.
Paralysis or pendulous crop in the nervous presentation.
Various disorders including diarrhoea, dyspnoea, renal failure, ascites, etc.
The above depends on the organ infiltrate in the visceral presentation. In this case the organs present firm, white nodules in most cases. Muscular presentation is the rarest.
The interpretation of lesions in the lymphoid system, as well as the repercussions in the flock, requires a detailed clinical history, a correct selection of the birds and complementary serological tests are very helpful.
Waste or selection chickens are not always the most representative of the flock. Since in these birds the lymphoid organs generally show atrophy because their feed consumption is always lower.
Within the differential diagnosis with LL, it should be considered that LL is only visceral, so it is important:
The examination of nerves and bursa of Fabricius.
The appearance of the neoplastic cells.
The age of the birds.
Lymphoid leukosis presents intrafollicular infiltration (within the lymphoid follicles) in the bursa of Fabricius and the tumour is frequent. While in cases of MD the infiltration is interfollicular (outside the follicle, occupying the connective tissue between the epithelium and follicles) and on the other hand the presence of nodules is less frequent.
Also, since lesions in the lymphoid system can be caused by several agents, it is necessary for the clinician to be familiar with serological tests and the results obtained.
In recent years, molecular tests have provided valuable data in the diagnosis and epidemiology of immunosuppressive viral diseases, however, it should not be lost sight of the fact that a positive PCR test result does not always have diagnostic value.
For example: in the case of common infectious anaemia virus in six to seven week old broilers or 10 week old replacement pullets. At this age, virus infection does not cause immunosuppression, so the presence of virus genetic material in the sample alone is not necessarily the origin of the observed picture and other possibilities must be ruled out.
Pathology of the immune system in the diagnosis of immunosuppression in poultry DOWNLOAD PDF
Gracieli Araujo, Specialist in Animal Welfare, Cobb LatCan
Pests can cause significant losses to production by reducing productivity and transmitting diseases. Some insects can also cause physical damage including structural damage to the houses. Breeder operations can be impacted by many insect species but the primary issues are caused by flies, beetles, and ants.
Insects are attracted to poultry operations as food, water, and habitats are readily available
Chemical insecticides are not always effective as insects become more resistant to multiple pesticides.
Mechanical means of control (traps) are an option and should be part of an insect control program.
However, good management practices along with a prevention program are the most effective ways to prevent insect infestations.
Professional pest control companies have experts that can readily evaluate situations and help develop a good pest management program.
Once established, good record keeping is a valuable tool to identifying and mitigating pest issues before they become an infestation problem.
To prevent an insect infestation, it is crucial to control moisture. Insect eggs and larvae require moisture to hatch and survive, so it is important to keep the house environment dry. Likewise, eliminate potential food sources.
Consider the following points:
1
If darkling beetles are an issue, leave the litter in the house for at least one day and treat it with an effective insecticide. The beetles will come out in mass once the birds leave the house so control needs to take place quickly.
After removing the litter, cover it to keep the insects out of the litter and to contain others until it can be removed from the farm.
2
Ensure that the house drains well and is ventilated correctly to prevent wet litter. Check for and repair any water leaks immediately.
3
Ensure that water around the outside of the house drains away and that any drainage systems (ditches, piping) is not blocked with debris or vegetation.
Ensure that the birds have good quality drinking water. Poor quality water can induce episodes of diarrhea and flushing which will add moisture and manure to the litter.
Prevent high temperatures in the house which will cause the birds to drink more water.
4 5 6 7
Repair any structural damage as these areas can be used as burrows for insects, especially darkling beetles.
Allow at least 4 weeks of downtime between flocks. This will eliminate the food and water sources and give any applied insecticides time to work.
8
Quickly remove and dispose of any mortality.
9
Check for feed spills regularly and clean them immediately.
Image 1. Ensure that water drains away from the house. Standing water around the house can support insect survival and reproduction.
The choice of pesticide should be based on pest target, effectiveness, potential hazards (to humans and birds) as well as local regulations. A professional pest control consultant can provide more information about the right chemicals for your operation. Here is a bit of information about some common insecticides.
Carbaryl based products can be used to control multiple insect species. They block the nervous system by inhibiting the enzyme acetylcholine-esterase.
Pyrethrin based products are safe to use while birds are in the house.
These chemicals are effective against ants, flies, and beetles.
They cause temporary paralysis, but beetles can produce enzymes that detoxify the chemical.
Using pyrethrins synergistically with other insecticides can be more effective.
Insect growth regulators are also available which prevent formation of chitin so that the larvae cannot turn into an adult beetle.
If any ant trails are seen moving from mounds outside the house to inside the house, insecticides can be sprayed around the house perimeter. Any ant mounds can also be treated with chemical pesticides.
Several biological control agents are available for pest control. Be aware that not all these agents are suitable for use in all climates.
Boric acid can be applied as a pellet or crystal to control beetles and flies, but should only be applied between flocks because it can also injure animals.
Some fungi are available that infect and kill insect larvae.
Fly parasitoids are tiny wasps that can kill flies in the pupal stage. If using beneficial insects, be aware that many chemical pesticides can kill the pest and beneficial species.
Ants primarily prey on other insects. Controlling insect infestations can therefore help prevent ant infestations.
There are many types of traps available that include pheromone, sticky tape, and electrical based traps (bug zappers).
Flies will not move against the wind so fans can be used around doorways to prevent flies from entering.
Gracieli Araujo worked for Cobb as a contracted consultant for 5 years supporting our insect control program. In August 2019, she joined Cobb as an Animal Welfare
Analyst conducting training and audits in animal welfare and pest management.
Gracieli has bachelor’s and master’s degrees in Animal Biology with a specialization in insects from the Universidade Estadual Paulista.
Image 2. Mechanical traps can be used to trap flies, a leading pest in poultry production.
Controlling Insects in Poultry Production
DOWNLOAD PDF
Zoetis Technical Team
Recombinant vaccines pair well with Embrex in ovo vaccination technology to help deliver strong early protection against viral challenges.
At hatch, chicks can be exposed to health challenges, such as Marek’s disease virus (MDV), Newcastle disease (ND) and infectious bursal disease (IBD), and their immune systems may be too underdeveloped to protect against infection.
It can take one to two weeks for the newly hatched chick’s immune system to fully mature, creating a period of vulnerability. But there are ways to help protect chicks sooner.
Recombinant vaccines based on herpesvirus of turkeys (HVT) applied accurately through in ovo vaccination help hasten maturation of the chicken embryo immune system, shifting the onset of immunity closer to hatch and allowing the chick to respond to a disease challenge sooner.
Accurate site of injection during in ovo vaccination, in the amnion, is important to help maximize the full potential of vaccine investments and support an immune response.
In a study, chickens vaccinated against MDV at Day 17 of incubation had a lower incidence of lesions when challenged with MDV during the first five days after hatch compared with birds vaccinated at hatch, demonstrating that chicks vaccinated in ovo initiated a prehatch immune response (Figure 1)
Recombinant vaccines – such as Poulvac® Procerta® – that are based on HVT, are commonly used with in ovo vaccination systems.
The HVT virus – related to MDV and conferring protection against MDV – is a large virus, and that helps make it a good candidate for gene inserts from other viruses (particularly ND virus, IBD virus and infectious laryngotracheitis).
Research has shown that replication of recombinant HVT, or rHVT, is greater when administered in ovo than when administered subcutaneously at day of age.
Percentage of dead and MDV lesions after RB1B challenge
In ovo vaccination, 17 days Nonvaccinated control
vaccination at hatch
Figure 1. An MDV challenge study compared chicks vaccinated in ovo at Day 17 of incubation with chicks vaccinated subcutaneously at hatch against MDV. It showed that the in ovo-vaccinated group had a lower incidence of lesions at Days 1 to 5 after challenge compared with the subcutaneous-vaccinated group, demonstrating that an in ovo-vaccinated chick has protection up to five days earlier.
Since rHVT vaccines are cell-associated, they may overcome maternal antibodies and confer lifelong protection because they establish latency and periodically reactivate in long-lived birds.
In ovo vaccination not only helps with adaptive immunity through development of antibodies (humoral immunity) but also helps chickens develop cell-mediated immunity — an immune response that helps fight pathogens by destroying infected cells that display certain proteins known as antigens on their surface.
Research has demonstrated that in ovo vaccination with HVT also can hasten maturity of the chick embryo immune system. In ovo administration of HVT at Day 18 of incubation resulted in innate and cell-mediated immunity responses comparable to those of chicks 1 to 2 weeks of age..
Earlier development of the immune system via in ovo vaccination helps protect young birds against pathogens unrelated to those they’ve been vaccinated against.
In a study, a group of chicks were given an HVT in ovo vaccination.
A second group received a sham or diluent-only in ovo vaccination.
All the in ovo-vaccinated chicks were exposed to KLH, an unrelated antigen.
Unvaccinated 7- and 14-day-old chicks were also exposed to KLH.
When KLH antibodies were evaluated a week later,
100% of the HVT in ovo vaccinated chicks developed detectable antibodies to KLH, as well as 100% of the unvaccinated 7- and 14-day-old chicks.
Only 20% of the sham in ovo vaccinated chicks developed detectable KLH antibodies.
When all the chicks were exposed to KLH a second time, the antibody activity level for the HVT in ovo vaccinated chicks was much higher compared to the sham in ovo vaccinated group.
Comparing the HVT in ovo vaccinated chicks to the unvaccinated 7- and 14-dayold chicks, the HVT-in ovo vaccinated chicks had 61% of the KLH antibody activity compared to the 7-day-old chicks (46.7% compared with 76.4%).
This shows in ovo vaccination helps enhance maturation of the chicken immune system. Previously it was thought that chicks do not develop humoral immune response until 1 to 2 weeks of age.
The fast-acting line of Poulvac Procerta vector vaccines from Zoetis are designed to help protect against costly viral challenges.
The pairing of the accurate and reliable Embrex in ovo vaccination systems with Poulvac Procerta vaccines can enable great value and optimum results from a hatchery vaccine program.
Poulvac Procerta HVT-ND, offering protection against MDV and ND virus, showed 93% to 98% protection at 19 days of age when administered with an Embrex in ovo vaccination system on Day 18 of incubation.
To help protect against IBD, Poulvac Procerta HVT-IBD helps protect chicks fast and helps deliver strong overall protection to contemporary IBD virus challenges, demonstrating protection against IBD virus in chicks with high maternal antibody levels.
In a study comparing with a competitor, Poulvac Procerta HVT-IBD provided the earliest protection against a very virulent IBD challenge – Day 12 – when administered in ovo with Embrex technology.
Another study, comparing with a competitor, showed Poulvac Procerta HVT-IBD provided the highest protection against classic IBD challenges at Day 14 when administered in ovo with Embrex technology.
Poulvac Procerta HVT-IBD-ND, an advanced double insert vector vaccine, delivers powerful early protection against three diseases – MDV, IBD and ND – through a single-dose vaccine.
A study showed Poulvac Procerta HVT-IBD-ND provided protection against a very virulent IBD challenge at Day 18 when administered in ovo with Embrex technology.
Another study showed Poulvac Procerta HVT-IBD-ND provided early protection against a velogenic ND virus challenge at Day 21 when administered in ovo with Embrex technology.
Those five in ovo technology success factors include:
Adaptive egg location;
Maintains vaccine integrity. 1 / 2 / 3 / 4 / 5 /
Cconsistent shell penetration;
Thorough sanitation;
Accurate site of injection;
Embrex technology is backed by more than 30 years of combined poultry health and engineering experience, working with poultry producers to effectively immunize chickens and address disease challenges.
Zoetis is committed to delivering on the excellence in service, training, support and in ovo technology that the Embrex brand is consistently known for around the world.
Note: Product information, registration and availability may vary per country and may change without notice. Contact your local Zoetis representative for details.
All trademarks are the property of Zoetis Services LLC or a related company or a licensor unless otherwise noted. © 2024 Zoetis Services LLC. All rights reserved. MM-35236
References upon request from the author
In ovo vaccination with Embrex® technology helps support earlier, more robust immune response in chicks DOWNLOAD PDF
Zoetis is committed to helping you meet your poultry needs and achieve your success, no matter what challenges you face. From plans to products, we’re ready to work with you to customize programs that will exceed your flock health goals.
Learn how we can help with your success at zoetis.com
Güner GÖVENÇ
Bursa Uludag University, Faculty of Agriculture, Department of Animal Science, Nilüfer, Bursa TURKEY
The global population is increasing day by day, which results in a significant increment for global demand of food and feed (Parrini et al., 2023).
By 2050, it is estimated that the world population will be more than 9 billion people; subsequently agricultural production is expected to show an increment by 50% (Lombardi et al., 2021).
al., 2020) and one of the most important protein sources for poultry is soybean.
For this reason, the need for soybean is increasing day by day and soybean production is becoming more and more important especially in animal nutrition with a usage amount as 67% of the animal feed market (Pettigrew et al., 2002).
As seen in Figure 1, soybean production in the world constitutes an increasing graph. According to the U.S. Department of Agriculture data, 398.210 million metric tons of soybeans were produced worldwide in 2023.
As a result of increasing demands, soybean production is expected to increase even more every year. The majority of these high production capacities in soybean production are in 3 countries that are active in production.
As can be seen more clearly in Figure 2, the United States and Brazil account for more than half of total soybean production. The 3rd largest producer is Argentina, followed by China. Other countries account for a much smaller share of total production. The largest producers, Brazil, the United States and Argentina, account for about 70% of total production.
Soybean (Glycine max L.) is a high quality protein source due to its favorable attributes such as relatively high protein content and suitable amino acid profile except methionine, minimal variation in nutrient content, ready availability year-round, and relative freedom from intractable antinutritive factors if properly processed.
The most important quality criteria’s for soybean are crude protein, moisture, KOH and crude oil contents.
In poultry nutrition, soybeans cannot be used directly in feed formulation due to its high oil content, cellulose in the hull and anti-nutritional factors.
To avoid the negative effects of cellulose, soybeans are dehulled.
They are then cooked, which greatly reduces the anti-nutritional actors, and then the oil is separated to produce soybean meal.
Therefore, the by-products of soybean as soybean meal and soybean oil is used in poultry nutrition, with an average value of 30% of diet.
These criteria vary greatly depending on the origin of the soybean.
In addition to these analyses, physical analyses such as seed size, color and seed shape are also important in soybeans.
It has been reported that geographical location of soybean production, soybean variety, and processing methods affects the crude protein and amino acid composition of soybean meal (Parsons et al., 1991, 2000; de Coca-Sinova, 2008, 2010; Baker et al., 2011).
Soybean is a rich source of vegetable protein in terms of the variety and amount of amino acids it contains.
One of the features that makes soybeans stand out from other vegetable proteins in poultry nutrition is its high lysine content.
However, if soybeans are exposed to more heat than necessary in the heating applied to destroy the anti-nutritional factors in soybeans, some essential amino acids, especially lysine and arginine, are either destroyed or rendered unusable.
The aim of this article was to determine the variation of crude protein and crude fat values of soybeans according to origin and to observe the differences in nutritional value between producing countries.
A total of 227 soybean samples from six countries of origin (Ukraine, Argentina, Brazil, USA, Paraguay and Uruguay) were analyzed for crude protein and crude oil content.
The crude protein and crude fat content of the soybeans samples was determined according to AACC Method (46-11.02, 30-25.01, International, 2010a, 2010b).
The obtained data were analyzed using the GLM procedures of statistical software (Minitab, 2013). Significant differences among the means were compared using the Tukey test and were considered statistically different at a level of P <0.05.
The crude protein content of soybean samples from 6 countries of origin (Ukraine, Argentina, Brazil, USA, Paraguay and Uruguay) varied between 30.7% and 38.8% when compared by country of origin. The lowest average crude protein content was observed in Ukraine origin soybeans with 33.9%, while the highest crude protein content was observed in USA origin soybeans with 35.6%.
The crude oil content of soybean samples from 6 countries of origin varied between 17.7% and 23.0% when compared by country of origin. The highest and lowest crude oil contents were observed in soybeans produced in Paraguay (21.3%) and USA (19.2%, P<0.005).
Crude protein content of soybeans grown in different countries is shown in Figure 3. As shown in the figure, crude protein content was lower in soybeans originating from Argentina (34.3%) and Ukraine (33.9%) compared to other countries (P<0.001).
Crude oil content of soybeans grown in different countries is shown in Figure 4. As shown in the figure, the highest average oil content was observed in soybeans produced by Brazil (21.0%) and Paraguay (21.3%) (P<0.001).
The soybean meal is a primary source of protein in diets; therefore, any factors affecting the protein content of soybean could have major interest for feed industry.
On the other hand, fat content of soybean has also crucial importance to the industry because of its high economic value.
It is well known that the soybeans grown in different environmental conditions and agricultural practices cause huge variations in quality parameters.
Besides, the meal processing conditions, e.g. processing temperature, moisture and drying time, cause differences in both chemical composition and quality of soybean meal (Thakur and Hurburgh, 2007).
According to Zhang et al. (2024), soybeans with a moisture content of less than 13% and a crude fat content of more than 20% are accepted as high-oil soybeans.
In a previous study performed by Grieshop and Fahey (2001), soybeans from China had a higher crude protein (42.1%) and a lower fat content (17.3%) than those from Brazil (40.9% and 18.7%) and US (41.6% and 18.7%) on a dry matter basis.
Grieshop and Fahey (2001) reported that the fat content of soybeans from both Brazil (range between 18.0 and 19.8%) and the U.S. (17.89-19.65%) were quite stable, however fat content of soybeans from China showed many variations from 14.5% to 18.0%.
Nutritional Specialty Products
Vaccines
No matter the type of poultry operation, our portfolio provides the tools you need to help protect the health of your flock and optimize performance. With unmatched quality, many years of experience, and proven success in poultry health, Phibro Animal Health is the trusted partner for producers worldwide.
Learn more about our portfolio
12 to 15 November Hanover, Germany Visit us at EuroTier 2024 Hall 21 - Booth C12
Although the high nutritional value of soybean as a plant protein source provides an efficient usage in poultry nutrition, both the social and environmental impact of the soybean industry and genetically modified soybean production has led to a growth in demand for more sustainable alternative protein sources (Gkarane et al., 2020).
The production and supply of soybean are critical steps due to their environmental impact and feed/ food competition for land use.
Therefore, research is focusing on finding alternatives to replace soybean partially or totally.
However, alternative ingredients should ensure similar growth performance, carcass traits, and meat quality characteristics compared to conventional soybean-based diets (Silvia Parrini et al., 2023).
At that point, recent scientific studies have focused on the usage possibilities of Hermetia illucens (black soldier fly) larvae and the microalga spirulina (Arthrospira platensis) in nutrition (Schiavone et al., 2017; Park et al., 2018; Kawasaki et al., 2019; Smetana et al., 2019).
Among the most innovative feeds, microalgae have been gaining attention in the last few years thanks to their high protein content and the potential to cultivate them without using arable land.
Crude oil and crude protein analyses of 227 samples collected from 6 different countries show that;
As environmental conditions change in soybeans, large differences in nutritional values can occur.
Differences in nutritional value represent high economic values for the feed industry.
However, in some cases, despite the good nutritional value of soybeans, problems such as low crude protein, high levels of urease or low protein digestibility are encountered in the meal produced due to improper processing practices.
These criteria are of great importance for poultry nutrition.
Metal frame system for evaporative cooling pads with tank-gutter
Energy Efficient: Lower costs, optimal conditions
Made in Italy: Designed and produced in Italy
Easy Maintenance: Simplified installation and upkeep
Consistent Performance: For maximum productivity
Visit Us at next Eurotier 2024! 12-15 November in Hannover, Germany Hall16 Stand F16
Ken Bafundo
Ph.D. (Poultry Consultant – University of Georgia)
In the past 2 decades, the global poultry industry has faced dramatic changes in the feeding strategies, primarily due to the reduction of in-feed antibiotic (including ionophores) usage.
However, the need to maintain intestinal health and improve broiler performance has not changed, and in the absence of antibiotics, that need is actually greater.
In this non-antibiotic era, intestinal health disorders are ranked as the major challenges to the poultry industry worldwide.
Coccidiosis and necrotic enteritis (bacterial enteritis) have become the prominent diseases affecting broiler productivity and economic return (estimated USD 20 billion in yearly losses).
There are several benefits of Quillaja and Yucca saponins in animal feeding:
Ammonia reduction.
As a result, many specialists are anxious to learn of new non-antibiotic feed additives that may reduce the challenges produced by Eimeria spp. and Clostridium perfringens and improve poultry growth performance as well.
Among many natural strategies that have been studied and then applied commercially, saponins are gaining traction throughout the livestock and poultry industries.
Saponins are natural compounds found in a large variety of plants that have been shown to produce a wide variety of biological effects.
Quillaja saponaria, the Chilean soap bark tree, and Yucca schidigera, a plant of the arid regions of the American southwest, have become major sources of commercially used saponins.
Antiprotozoal and antimicrobial activity.
Stimulation of the immune response.
Anti-inflammatory and antioxidant effects.
Enhanced nutrient digestibility.
Image 1. Quillaja-saponaria-flower
Saponins have been widely used in US since 2000’s, and in the last 10 years it has been estimated that about 25% of American broilers receive a saponinbased product in feed (>2.5 billion broilers per year). In Brazil saponins are approved as natural growth promoters, and most recently, their use has been expanding in Europe and Asia.
Quillaja saponins (QS) have been used in both human and animal vaccines as adjuvant to increase immunogenicity of an antigen, resulting in increased antibodies and cytotoxic of T-lymphocytes.
In turn, vaccine efficacy is improved. Recently QS was used as adjuvant in a Covid-19 and malaria vaccine development.
In poultry, many studies suggested that in-feed supplementation with saponins, stimulates specific immune response (cellular & humoral) against different pathogens.
Bafundo et al, demonstrated that birds raised in a high challenge environment and fed a diet containing a combination of Quillaja and Yucca saponins, showed a significant reduction in numbers of Clostridium perfringens and Salmonella spp. incidence (Table 1).
As expected, significant improvements in bird performance occurred as well.
Table 1. The effects of graded levels of a Quillaja-Yucca saponins combination (QY) on Clostridium perfringens counts, percent Salmonella positive broilers and performance (Bafundo et al., EC Veterinary Science, 2021).
In the presence of high enteric disease challenge, or coccidiosis vaccination, birds fed with saponins show a significant improvement of intestinal villus height and reduction crypt depth.
In addition, up-regulation of tight junction proteins (reduced gut permeability) and improvements in the apparent intestinal uptake of dry matter, organic matter, fats and minerals, and N retention occurred (Table 2 and 3).
As a consequence, better feed conversion rate (FCR) and higher body weights (BW) were recorded in saponin-fed groups (Table 4).
Trial results and observations indicated that the positive effects in the gut integrity and nutrient digestibility may be associated with the reduction of the coccidia replication and changes in the bacteria populations in gut.
Tables 2 and 3. The effect of graded levels of Quillaja and Yucca saponins on percent apparent total tract digestibility (ATTD) and percent nitrogen retention of broilers measured from days 21 to 25 (Bafundo et al., Br. Poult. Sci. 2021).
Table 4. The effects of graded levels of saponins on performance, body weight gain, feed conversion and European Poultry Efficiency Factor (EPEF) – Straight correlation between gut integrity, digestibility and performance. (Bafundo et al., Br. Poult. Sci. 2021).
Since saponins have membranolytic properties, they can complex with cholesterol in protozoal (and bacterial) cell membranes, causing cell lysis.
Although it seems evident that the reduction in oocyst passage is a direct result of the anticoccidial effects of Quillaja and Yucca saponins, quillaja saponins are also known to improve antigenic recognition, and this effect may result in enhanced immune responsiveness of birds to the presence of coccidial infection and contribute to the reduced reproductive potential of the parasites.
OPG results - D28
Recent research has shown that saponins can significantly reduce the adverse effects of Eimeria infections in chickens, by reducing the oocyst per gram of feces (OPG) by 40% to 60%, decreasing lesion score (LS), and providing performance improvements (FCR, BW and Mortality).
Although saponins are not anticoccidials, these molecules, when used in combination with ionophores or chemicals anticoccidials, improve anticoccidial effects of these methods and provide better performance.
In birds vaccinated against coccidiosis, saponins enhance the immune responses, reduce the coccidia-vaccine intestinal damage and enhance performance parameters.
Adj. FCR - D28
NarasínNarasín+QS ClopidolClopidol+QS
Graph 1 and 2. Quillaja saponins (QS) reduce OPG (oocysts per gram of feces) and improve FCR (feed conversion rate) from all Anticoccidial methods used in this trial.
*IUC: Infected Untreated Control
Currently, there is not a lot of published data on the effects of saponins in broiler breeders, breeder pullets or in layers.
For the past few years however, commercial companies have evaluated saponin use in long-lived birds and are finding applications leading to better performance and productivity, such as improved uniformity and reduced mortality in pullets, as well as improved egg production and egg shell quality.
Considering previously mentioned effects in lowering intestinal pathogen loads, maintaining gut integrity, and improving digestibility of critical nutrients, saponins are likely to have a meaningful effect on long-lived birds.
Saponins are plant-derived products that exert numerous biological effects in broilers.
Both research trials and commercial use have shown that these products improve intestinal health by decreasing the occurrence of coccidial lesions, reducing the severity of intestinal bacterial infections, and promoting better intestinal immunity.
In addition, studies demonstrate that nutrient digestibility is enhanced when saponins are fed to growing broilers.
Saponins have been shown to compliment the activity of many anticoccidial programs and have been widely used to improve the responses of live coccidiosis vaccines.
They are compatible with feeds additives of all types.
Applications of Saponins in Poultry Production DOWNLOAD PDF
Edgar O. Oviedo-Rondon Prestage Department of Poultry Science, North Carolina State University. Raleigh, NC
The United States produces high quantities of soybeans since this oilseed provides more than 50% of all the vegetable oils used in this country.
The demand for this oil is increasing due to its application in generating biodiesel, and soybean meal is a co-product of this oil industry.
High oleic soybean oil, a product of constant innovation in soy production, is rich in oleic acid, similar to olive oil. This high-oleic oil, with its 75% oleic acid content, is gaining popularity due to its numerous health benefits.
It offers greater stability for food and processed food producers, functionality comparable to hydrogenated oils, but without the issues of trans fatty acids.
It also allows the elimination of chemical preservatives such as TBHQ and EDTA, making it a healthier choice for consumers.
High-oleic soybean oil stands out with its three times longer frying life than conventional soybean oil and an oxidative stability index exceeding 25 hours.
This reduces costs, consumption, and waste and minimizes polymer buildup during cooking.
Its neutral and light flavor enhances the taste of foods, and its melting properties make it suitable for solid and semi-solid soy butter with pastry applications.
Moreover, it has a higher smoke point than traditional high-oleic soybean, canola, or sunflower oil, as shown in Figure 1.
Figure 1. Comparison of fatty acid profiles of various oils, including those high in oleic acid. Source: United Soybean Board.
The production of high-oleic soybean oil is poised for significant growth in the coming years, presenting a promising future for the industry. Currently exclusive to the United States, these seeds are backed by a robust system that preserves their origin. The 12 most prominent suppliers of high oleic soybeans or soybean oil are currently using two varieties: the biotechnologically derived Plenish® and the non-genetically modified SOYLEIC® obtained through selection. Table 1 provides a detailed composition of these varieties per the companies that developed them.
Due to the properties of oleic acid products indicated above, it has been suggested that meat products and eggs could be enriched with this fatty acid by feeding chickens, hens, and fish whole soybean meal and other oilseeds.
In collaboration with the USDA-ARS, our research group at North Carolina State University developed a research project to evaluate the use of higholeic extruded whole soybeans.
A non-genetically modified variety similar to SOYLEIC® but from the USDA selection process in Raleigh, North Carolina, was used. The nutritional composition, energy value, and amino acid digestibility of extruded whole soybeans from high oleic soybeans were evaluated in several experiments.
The inclusion of extruded whole soybeans was evaluated in hen and chicken diets to observe the impact on bird performance, growth, egg production, and feed conversion.
Likewise, the impact on meat and eggs was evaluated, especially on the lipid profile of these products.
In these experiments, the results of high-oleic soybean meal were compared with diets that included traditional soybean products with average oleic content, such as soybean meal extracted by solvent and extruded full-fat soybeans.
The nutritional composition of the evaluated products is presented in Table 2.
Table 2. Nutritional composition of the soybean meal sources evaluated.
AVIARY VISION for LAYERS
AVIARY GREEN WAY for LAYERS
AVIARY GREEN START for REARING-BREEDING NEW WEBSITE
The research results indicated that extruded high-oleic full-fat soybeans have an energy content similar to traditional or normal-oleic full-fat soybeans (3,112 kcal/kg AMEn) and higher than solventextracted soybean meal. The digestibility of the two extruded full-fat soybeans is almost the same for all amino acids (80 to 87%) and consistently 3 to 10% points lower than that of solvent-extracted soybeans.
Including high-oleic extruded full-fat soybeans did not significantly affect weight, feed intake, feed conversion, egg production, or average egg weight (Table 3) compared to hens fed containing the other soybean sources evaluated.
The only parameter affected by the treatments on egg quality was yolk color (Table 4).
Extruded whole soybeans ended up with paler yolk colors.
However, the fatty acid profile of the soybeans was affected, increasing, as expected, the oleic acid and reducing the palmitic, linoleic, and linolenic acid when the extruded full-fat soybeans with high oleic content were included (Table 5).
The total fat content of the yolk was not affected by the treatments.
Table 4. Effects of inclusion of high oleic extruded full-fat soybean meal on egg quality.
Broiler chicken body weight gain and chicken feed conversion were lower for diets with normal oleic or high oleic extruded whole soybeans.
The higher levels of trypsin inhibitors from full-fat extruded soybeans probably affected performance.
Improving the process can overcome this issue.
Carcass yield was also reduced with high oleic extruded soybeans.
However, the soybean meal source affected the fatty acid profile of chicken meat (Table 6).
Diets with high oleic extruded whole soybeans increased oleic acid and reduced linoleic and linolenic acid.
A similar effect was observed in the meat of laying hens fed with these same soybean meal sources (Table 7).
A reduction in palmitic, palmitoleic, and arachidonic fatty acids and other saturated fatty acids was observed in poultry meat.
Table 5. Fatty acid profile of egg yolk from hens-fed diets containing high oleic extruded full-fat soybeans and other soybean sources with traditional oleic acid content.
Table 6. Effect of inclusion of high-oleic extruded full-fat soybean meal on the fatty acid profile in the pectoral muscles of 47-day-old broiler chickens.
Table 7. Effect of high-oleic extruded full-fat soybean meal on the fatty acid profile in the pectoral muscles of laying hens.
Extruded full-fat soybeans with high oleic content can be included in feed for chickens and layers.
In extruded soybeans, paying greater attention to the levels of trypsin inhibitors to avoid adverse effects on performance, especially for broiler chickens, is essential.
The energy value and amino acid digestibility of this soybean with higher oleic acid content is similar to that of traditional extruded full-fat soybeans.
The high oleic acid content is effectively transferred to eggs and meat, reducing linoleic and linolenic acids.
These poultry products are expected to have greater oxidation stability during storage.
High oleic soybeans and their impact on egg and poultry meat quality DOWNLOAD PDF
Optimal incubation takes 24 days
SetCare is the new HatchTech setter. Improve your performance with an incubation process of 24 days.
• 3% lower embryo mortality
• Overall improved chick quality
• Improved FCR and uniformity Don’t settle for less
Edgar O. Oviedo-Rondon Prestage Department of Poultry Science, North Carolina State University.
Respiratory diseases are a constant challenge for poultry producers and veterinarians since they do not show pathognomic signs, making diagnosis more complex.
The Avian Metapneumovirus (aMPV) is an important pathogen in respiratory diseases but is frequently neglected.
It can affect the respiratory and reproductive systems, facilitating the development of other diseases like colibacillosis, which is the most common co-infection in broilers, while in turkeys, it is Ornithobacterium rhinotracheale.
Mycoplasma gallisepticum infection can prolong the viral replication, but as a secondary infection, aMPV can delay M. gallisepticum infection.
The aMPV affects turkeys and chickens and can also be found in guinea fowl, ducks, and pheasants.
It is an enveloped negative-sense RNA virus included in the genus Metapneumovirus of the Pneumoviridae family.
The global impact of aMPV is significant. Six viral subtypes of aMPV are recognized worldwide, each with its unique distribution.
Subtypes A and B are found in Europe, Brazil, and the African continent, while subtype C has been identified in the United States, Canada, China, France, and South Korea.
The D subtype was only reported in France, and the two new subtypes are found in the United States and Canada in wild birds (black back gull and Monk parakeet chicks).
The genetic differences among subtypes are based mainly on the glycoprotein (G) variability that also affects replication in the target cell and, consequently, the pathogenicity in the host.
Schematic figure representing aMPV: (G) Glycoprotein, (F) Fusion protein, (SH) Small hydrophobic protein and other structural proteins, (M) Matrix protein, (N) Nucleocapsid protein, (P) Phosphoprotein, (L) RNA-dependent RNA polymerase and RNA strand.
Currently, subtype B is the most prevalent worldwide. However, subtypes A and B are causing outbreaks in several states in the USA, following a prolonged period without aMPV detection.
The recent introduction of aMPV-A and -B into the US has coincided with the increased spread of H5N1 HPAI by migratory waterfowl.
Whether these two occurrences are causal relationships or random effects remains to be determined.
The shift in the predominance of aMPV subtypes from A to B and the increased detection of aMPV-A in North America in recent years are significant and may reflect ongoing evolutionary dynamics and changing epidemiological patterns.
The presence of aMPV has been confirmed in wild migratory birds in several countries, indicating that this factor must be considered in epidemiological studies, as well as seasonality and biosecurity to prevent it. Horizontal transmission through aerosol is the most common route of infection, and no vertical transmission has been reported.
Metapneumovirus has a high tropism for the upper respiratory tract, infecting sinuses, larynx, and trachea.
It causes cessation of ciliary movements or ciliostasis and even complete loss of these cilia (desciliation).
These lesions cause mucus not to be removed and accumulate in the passages and cavities, causing the main sign of this disease, the swollen head.
It causes severe respiratory infection in turkeys, better known as Turkey Rhinotracheitis (TRT).
In laying hens can cause a drop in egg production, and it has been isolated from cockerels’s testes, causing reduced fertility together with the Infectious Bronchitis Virus.
Common symptoms include:
Sneezing.
Nasal and eye discharge.
Conjunctivitis.
Submandibular edema.
Infraorbital sinus swelling. Cracking. Rales.
In chickens, it causes swelling of the periorbital and infraorbital sinuses, torticollis, disorientation, and opisthotonos. The clinical manifestation may progress to reddening of the conjunctiva with edema of the lacrimal gland.
Between 12 and 24 hours, the birds show a subcutaneous swelling on the head, which starts around the eyes, increases under the entire head, and then descends to the submandibular tissue and back of the neck.
After three days, chickens may show neurological signs such as apathy and torticollis.
The permanence period of aMPV is only 4 to 7 days, which impairs virus detection for molecular diagnosis.
Vaccines from subtypes A and B are available, and both products provide good cross-protection.
Currently, no licensed vaccine is available for use in the USA.
A possible selection by vaccine pressure has been reported in the past two decades.
The heterologous protection capacity between vaccines of both subtypes is demonstrated, although it is susceptible to viral escapes.
Then, vaccination must always be paired with biosecurity and virus surveillance for strategic control.
There are contradictory reports regarding the evolution of aMPV.
Some studies report that aMPV is a relatively slow-evolving virus compared to other avian RNA viruses, and others estimate that its rate of viral evolution is within the normal range.
Nonetheless, viral evolution is based on both the pressure exerted by vaccine programs and the type of host and environment; consequently, multiple strains of the same subtype can phenotypically circulate in distinct regions of the globe.
The genetic diversity in the study sequences highlights the urgent need to carry out more whole genome sequencing of this virus to better understand the variants circulating in the field and the evolution of this virus over time.
The phylogenetic relationships among the aMPV-B strains, reconstructed using the maximum likelihood method in the Molecular evolutionary genetics analysis (MEGA X) software, showed that aMPV-B has evolved in Europe since its first appearance.
The 40% of aMPV-B viruses analyzed were identified as vaccine-derived strains, sharing phylogenetic similarities and displaying high nucleotide similarity with live commercial vaccine strains licensed in Europe.
The remaining 60% were considered field strains because they clustered separately and showed a low nucleotide identity with vaccines and vaccine-derived strains. Contrary to vaccine-derived strains, field strains tended to cluster based on their geographical origin and irrespective of the host species where the viruses had been detected.
All experts recommend monitoring birds by serology to detect antibodies by ELISA and detect the virus to identify the prevalence of the subtype and determine the best vaccine to use.
The following commercial ELISA kits are available:
Idexx Avian Pneumovirus antibody test kit to detect subtypes A, B, and C
BioChek Avian rhinotracheitis (ART) antibody test kit to detect subtypes A and V
AMPV subtypes A, B, C, and D can be detected by traditional real-time PCR or RT-qPCR. However, virus isolation and genome sequencing of the G gene region can be necessary to identify subtypes.
The molecular characterization of aMPV and the differentiation between vaccines and field strains through G gene sequence analysis can be valuable tools for correct diagnosis.
They should be routinely applied to better address the control strategies.
Common samples for analysis are oropharyngeal, nares, and sinus cavity swabs that should be submitted in transport media. For asymptomatic birds, the nares are the most reliable sample site for molecular diagnosis of aMPV, with the choanal cleft and trachea being secondary options. Symptomatic birds normally have detectable virus in the choanal cleft, nares, and trachea.
Maintaining a cold chain from the point of collection through transport to the diagnostic lab is critical.
If shipping may take more than 24 hours, samples should be frozen at—80 oC and shipped express on dry ice.
The control of aMPV requires correct identification of the agent, epidemiological surveillance, effective diagnosis, adequate immunoprophylactic programs, and constant biosecurity.
Figure 1. (A) Turkey showing swollen infraorbital sinuses, ocular discharge, and conjunctivitis (courtesy of Dr. Ashley Mason); (B) five-week-old chicken showing swollen head, swollen infraorbital sinuses, plugged nostrils, and opaque crusted ocular discharge (courtesy of Dr. William McRee).
Source: Luqman et al., 2024. Viruses 16 (4): 508.
Metapneumovirus epidemiology and seasonality
DOWNLOAD PDF
Guillermo Tellez-Isaias Department of Poultry Science,
University of Arkansas Agricultural Experiment Station, Fayetteville, AR, USA
Modern broiler chickens are most certainly the most visible manifestation of genetic advancements.
Genetic selection, food, health, and management measures carried out intensively have resulted in these successes.
However, it is essential for production to keep the gastrointestinal tract (GIT) in good condition.
As the growing period of broilers shortens and feed efficiency improves, the need for improved health and nutrition programs becomes more apparent.
Because the changes during intestinal growth are minute, they are often overlooked, yet gut health impacts general health and productivity.
Currently, animal production systems require a constant search to reduce the effects of stress and chronic inflammation to improve energy use by producing animals.
Although there is no a “silver bullet” to prevent multifactorial conditions associated with chronic stress, several studies show improvement in intestinal microbial balance, metabolism, and intestinal integrity through alternative products such as:
Probiotics
Prebiotics
Organic acids
Plant extracts
Essential oils
Trace minerals
This being an international scientific trend, due to the anti-inflammatory, antioxidant and immunomodulatory effects, as well as improvement in intestinal integrity.
Substitution of antibiotics in production systems with alternative products, improved management practices, strict biosecurity, quality ingredients, absence of diseases (Mycoplasma/Salmonella), and effective immunization programs are efficient strategies for health objectives and productivity.
In this work, we focus on reviewing the significant repercussions of chronic stress and intestinal inflammation on the health and performance of commercial birds.
In addition to absorbing and digesting water and food, the intestinal tract contains a diverse and complex microbial community (Celluzzi and Masotti, 2016), as well as an enteric nervous system (ENS) of metazoans considered the “second brain” of the organism (Schneider et al., 2019).
In addition to this complexity in the structure and microbial relationships, in chickens, the gut-associated lymphoid tissue (GALT) contains the highest concentration of immune cells in the organism, showing its relevance (Peralta et al., 2017; Casteleyn et al., 2010).
Furthermore, the digestive system has primary lymphoid organs such as the bursa of Fabricius, where B lymphocytes originate and multiply; this component of GALT in avian species is essential for protecting the digestive system (Bar-Shira et al., 2003).
The intestinal microbiome can influence host biology, nutrition, immunity, and the neuroendocrine system (Dimitrov, 2011). GIT function appears to be mediated by short-chain fatty acids (SCFA) generated by bacterial fermentation (Wu et al., 2017), communication between the microbiota and neurons (Megur et al., 2020), the endocrine system (Fukui et al., 2018), the immune system (Maslowski and Mackay, 2011) and modulation of the intestinal epithelial barrier (Sharma et al., 2010).
The ENS and endocrine gut network control the GIT motility and disruption in functional GIT disorders (Fukui et al., 2018).
Enteroendocrine cells (EECs) are found throughout the GIT epithelium and
Over 50 hormones or bioactive peptides have been identified, making the GIT the primary organ displaying endocrine, neuroendocrine, autocrine, or paracrine activities (Rao and Wang, 2010; Gribble and Reimann, 2017).
In metazoans, intestinal enterochromaffin cells, a subpopulation of numerous EECs, produce 90% of the neurotransmitter serotonin (5-hydroxytryptamine) (Lund et al., 2018).
The intestinal microbiota partially controls the secretion of serotonin, dopamine, oxytocin, and endorphins produced by EECs (Forsythe et al., 2010; Liang et al., 2014; Mayer et al., 2014). There is great wisdom in the old saying “gut feelings”.
Prolonged stress and inflammation negatively impact the microbiotabraingut axis, causing dysbacteriosis and disrupting the tight junction proteins with systemic translocation of bacteria and other antigens (Figure 2).
During chronic stress and, as a result, chronic intestinal inflammation, energy for growth and reproduction is diverted away from these functions to sustain the inflammatory response. Poultry is no exception to this rule.
The hypothalamic–pituitary–adrenal axis (HPA axis) is a complex network of direct influences and feedback interactions between three components:
In general, constant damage or the presence of pathogenic agents induces a stress process in the GIT, increasing inflammation and, therefore, oxidative stress (Federico et al., 2007).
Among the different possible pathogens in the GIT, we can include bacteria, and even protozoa, which infections can induce severe enteric damage, causing illness and high mortality rates.
In chickens, Eimeria tenella is a primary cecal pathogen that negatively affects growth, feed
Alternatives to control protozoa infections include feed additives and other immunomodulators, adjuvants, and recombinant vaccine development.
No commercial vaccines are available for H. meleagridis (Liebhart et al., 2017).
There is an undeniable need to identify more effective solutions to lessen the impact of chicken coccidiosis and histomonosis.
Yet, crucial resources still need to be included, making rapid progress difficult.
In murine models, Salmonella growth is aided, ironically, by acute inflammatory responses to pathogenic bacteria in the intestine, as there is increased migration of neutrophils and production of reactive oxygen species (ROS) and reactive nitrogen species (RNS) as a result of the Salmonella infection disrupting the balance of the microbiota (Winter et al., 2010a).
Bacterial infections are considered one of the main GIT-associated infections, which due to their infection process and presence, are inducers of acute or even chronic inflammatory processes (Yamamoto et al., 2013).
An increase in molecular oxygen in the lumen of the gut depletes important commensal anaerobes like Bacteroidetes and Clostridiales, which are essential butyric acid-producing bacteria (Rigottier-Gois, 2013).
The thiosulfate oxidation to tetrathionate is also a ROS byproduct (Winter et al., 2010b). In murine models, it has been shown that Salmonella uses tetrathionate to strengthen its development (Winter et al., 2010b); tetrathionate broth is a component of enriched media for the culture of Salmonella in diagnostic laboratories.
Figure 1. Interactions between the host (poultry species) and intrinsic or extrinsic factors that influence gut health (created with BioRender.com).
However, a recent study has found that this differs for chickens (Saraiva et al., 2021).
In contrast to murine models, in poultry, the ttrA and pduA genes do not appear to be significant virulence determinants in fecal excretion of invasiveness for Salmonella Enteritidis and Salmonella Typhimurium.
Interestingly, the deletion of both genes does not attenuate the pathogen but slightly decreases the numbers of Salmonella Enteritidis and Salmonella Typhimurium in caecum in poultry, reducing inflammation and allowing the bacteria to more easily invade gut epithelial cells and disseminate systemically, leading to severe clinical signs and higher mortality rates (Saraiva et al., 2021).
Avian species are unique because they lack a urinary bladder, and renal discharge accesses the GIT directly.
Both turkeys and chickens have a cloaca or common excretory pathway (Goldstein, 2006).
Unlike mammals, where the kidney regulates the extracellular fluid composition independently, the urine flows into the cloaca in birds by a reverse peristaltic action to the ceca (Karasawa and Duke, 1995; Duke, 1999).
Thus, the kidneys and lower GIT must regulate the extracellular fluid composition. Here, the avian ceca perform water reabsorption, fiber digestion (by bacterial fermentation), nitrogen recycling, microbial vitamin synthesis, and osmoregulation (Duke, 1982; Duke et al., 1983; Hall and Duke, 2000).
The dietary composition can affect the moisture in fecal droppings.
The water content of the feces directly impacts the litter moisture in poultry production systems, with moisture levels ranging from 15% to 44%.
Chronic Stress
Environment Physical Chemical Nutritional Biological Psychological HPA axis
Nutrients diverted to in ammatory response
Impaired nutrient uptake and water reabsorption
Poultry litter contains high levels of proteins and nitrogen (Kelleher et al., 2002). A significant issue with poultry litter is the loss of nitrogen, such as ammonia, during microbial fermentation of urea and uric acid (Nahm, 2003).
In chicken houses, ammonia volatilization is one of the most stressful gases to poultry that severely affects the birds’ welfare, health, and performance (Moore et al., 2011; van der Hoeven- Hangoor et al., 2014).
Epithelial permeability & bacterial entry
Lipoperoxidation
Cell & mitochondrial membrane dysfunction
Apoptosis & cellular necrosis
Figure 2. Chronic stress (regardless of its source) has a direct impact on the hypothalamic–pituitary–adrenal axis (HPA axis), the brainmicrobiota-gut axis (BMG axis), and the endocrine and immunological systems. Intestinal and chronic systemic inflammations originate from disruptions in the delicate balance and environment of the intestinal microbiota (dysbacteriosis) and alterations in tight junction proteins among enterocytes causing leaky gut. Prolonged oxidative stress induced by the inflammatory process causes phospholipid peroxidation in cell and mitochondrial membranes, leading to apoptosis, cellular necrosis, and multiple organ failure (created with BioRender.com).
Pathogen-associated molecular patterns (PAMPs) are elements of pathogenic bacteria that metazoans recognize.
PAMPs include lipopeptides, peptidoglycans, and teichoic acids (Salminen and Isolauri, 2006).
The endotoxin lipopolysaccharide (LPS), found in Gramnegative bacteria outer membranes, is a classic example (Kallapura et al., 2014).
Generally, infectious agents (bacterial, protozoal, viral, helminth) stimulate host pro-inflammatory responses. For instance, in domestic poultry, coccidiosis may cause necrosis and inflammation in the intestine, resulting in fever, depression, reduced performance, and death depending on the Eimeria spp.
Polymorphonuclear (PMN) leukocytes and macrophages fight pathogens as the first line of defense by producing reactive molecules capable of inducing oxidation or reduction reactions (Qureshi, 2003; Petrone-Garcia et al., 2021).
These include ROS, such as superoxide, hydrogen peroxide, and hydroxyl radical.
RNS comprise nitric oxide and peroxynitrite molecules.
Both ROS and RNS are highly toxic to fight against invaders.
They can penetrate the microbial cell wall easily, causing irreversible damage (Gostner et al., 2013).
Immune signaling only initiates the production of these ROS and RNS molecules to intercept and kill pathogens (Sun et al., 2020).
However, when ROS molecules overreact, they become immunotoxins capable of damaging the host cells and adjacent tissues, leading to severe local and systemic inflammation and multiple organ failure (Chen and Kevil, 2020).
Due to this, its control is key to avoiding the adverse effect due to its overproduction and its negative impact (Lian et al., 2020).
Effects of chronic stress and intestinal inflammation on commercial poultry health and performance: Part I DOWNLOAD PDF
One of the key parameters in commercial egg production is undoubtedly egg size. The production of high-weight eggs has challenges such as early degradation of shell quality, loss of accumulated eggs by a late start, deterioration of the conversion rate or the increased accumulated mortality.
However, even taking all of this into account, the production of high-weight eggs can be economically viable.
This is especially true in those countries where the market pays a very high differential price for these bigger eggs.
Egg size has a genetic component as it is a parameter with high heritability. However, changes in bird management and nutrition have an even greater impact than genetics.
The objective of this document is to clarify the key points to adapt egg production to the demand of markets with strong demand for high-caliber eggs. The egg size is based on three pillars:
Bird management.
Lighting program.
Nutrition.
The body weight of the bird at the age of sexual maturation has a direct correlation with the weight of the eggs produced. In a simple way, birds with weights suitable at this age have a better productive profile throughout their life and are better suited to the different production requirements in relation to the desired egg size.
It is estimated that for every 45 g above the standard at week 18 of life, there is an increase of 0.5g in the accumulated egg weight at the end of production.
However, if growth occurs in the final part of the rearing it is not effective, as the growth will be in form of fat deposition on the body of the bird.
The key period for the development of the bird’s carcass is well known to peak at 6th week of life (as shown in Figure 1
of production that the same batch will have during the period of 24-59 weeks of life. These results confirm the important relationship between chick body development and egg production. (See Table 1).
The only way to monitor the body development of the flock is through systematic weighing of a significant sample of birds.
The flock weighing should be started at the end of the first week of life, repeating it weekly.
In the case of caged hens, the same cages from different areas of the barn should always be weight for better follow-up.
In alternative systems, birds must be selected from different parts of the barn and weighed as regularly as in cage hens.
In addition to body weight, flock uniformity should also be calculated. This is equally important in the assessment of
Folck A Folck B
Folck A Folck B
Figure 2. Comparison of the body development during the rearing between flocks.
Stimulation of feed consumption should begin as soon as the birds start the rearing, considering that the body weight of the birds as early as the 6th week will be directly related to the weight of the eggs produced.
During the brooding period (and up to the 4th week of life), it is recommended to feed the chicks with crumb feed if the body weights aren’t achieved continuously. The crumble feed is well accepted by birds, improving consumption, and body and gut development.
One mistake that is usually made when we encounter flocks under the standard body weight and don’t have the correct feed intake is to increase the number of feed runs.
Force the feed intake of the fine particles of the feed, allowing a complete nutrition of the birds.
Help on the development of the bird’s digestive system and particularly the crop and gizzard.
The digestive tract must be developed so that the hen at the start of the production has the ability to ingest the amount of feed needed to ensure the growth of this period and the production of the first egg.
In addition to the above-mentioned management, it is necessary to avoid any factor capable of reducing feed intake, such as:
Stress.
Heat stress.
Hight bird density.
Diseases.
Poor feed quality.
Refer to the H&N Management Guide for more information on recommended flock densities for rearing and laying phases.
The housing density is a parameter that must be defined before the reception of the birds as it has a direct impact on: the body development at rearing and production period; flock uniformity; and daily feed intake.
Body weight, gr 1.397
Table 2. Impact of bird density on body development during rearing. (CAREY, J.B., (1986), Effects of Pullet-Stocking Density on Performance of Laying Hens)
Hens, like any other hot-blooded animals, have biological tools to regulate their body temperature.
However, this capacity is limited; the extended periods of high temperatures can directly affect the bird’s behavior. It could be shown as reduction of the feed intake, reduction of the production parameters, as well as increased of the stress.
The thermo neutral zone for a hen is between 18-25°C.
If the temperature goes above the high temperature, the biological heat loss mechanisms of the bird will start to maintain body temperature.
The high temperature of the barn, in addition, has a negative effect on feed intake during the rearing period (Figure 5).
This reduction in consumption has a negative impact on the body development and weight of the bird
%reduction in feed consumption
in the raring barn
Figure 5. Decrease in daily intake (in %) due to the variation of the average temperature (1°C) inside of the rearing barn. (Bell, D.D. and W.D. Weaver, Jr. (2002) Commercial Chicken Meat and Egg Production. 5th Edition. Kluwer Academic Publishers)
There are several management practices that can be applied to cope with these adverse conditions:
Group feed distributions in periods at periods of the day with lower temperature.
These are usually the first and last hours of the day. Likewise, during the production phase, the concentration of feed runs in the last hours of the day has an additional advantage: to make available to the hen nutrients just at the time of greatest need since the bird is in full formation of the next day egg.
We must make sure there’s a feed delivery in place two hours before the lights go out.
Additional feed delivery at night, in rearing and/or production.
It is about allowing birds to feed at night. The consumption during this period will be higher because temperatures are lower. To do this, the lighting system must be turned on and a feed run must be done at night.
During the production phase, the night light time can be between 60 to 120 minutes, but in any case, this period must be preceded and followed by a period of darkness of at least 3 hours as shown in the next chart.
6. 24 hour period.
The same strategy can also be applied during rearing, but if applied from week 12 of life, it can result in early flock stimulus.
Conceptually, hens activity could be reduced to transforming kilos of feed into kilos of egg mass. This egg mass is the product of the number of eggs laid by the weight of these and depends mainly on the genetics of the bird, as well as applying the correct feeding and management.
However, with a proper light stimulation, it is possible to guide the production of the bird towards one of the two parameters, number of eggs or size of egg.
Egg mass = Number of egss x weight of the eggs
of eggs
Light program is the key in the size of the eggs
of egg
The hens will start laying eggs when they achieve the mature body weight, when the photoperiod isn’t inhibitory, or they have lost the circadian cycle.
The photoperiods can be classified as:
Stimulanting:
They are those with a growing photoperiod, the light time of the day increases in duration. The hens exposed to this lighting tend to have an earlier start of production.
Decreasing or constant:
They are not stimulanting or promoting the start of production.
It is important to note that in commercial laying hens there is no refractory photo period as is the case in other bird species. This means that hens are sensitive to light stimulation from an early age.
It is necessary to work with specific parameters that allow us to make a measurable and repeatable approach when we want to make the optimum light stimulation. Among the most interesting for this purpose are the following:
It is the most used indicator to decide when to start the flock stimulation. Correlates well with weight at the start of light stimulation if the flock has a body weight close to the standard and is uniform.
Otherwise, it can lead to erroneous stimulations that can lead to unwanted production results.
It is a good predictive indicator of what batch production will look like and how the stimulation program has worked. It is very difficult to get the weight exactly in cage systems as it requires weighting the birds after collecting the eggs, but in alternative systems with the new systems on the farm can be easier.
Stimulation at 1.4kg
Body weight, in gr
Dely the stimulation until the body weight is achivieved
Flock body weight on target Flock body weight below target
7. Different weight on target and below target.
It is a good predictive data of how the production will be as log as the flock is at the standard body weight and it is uniform. It is more often used than the weight at 50% of production because it is very easy to calculate if the egg production is collected daily.
This data allows a review of the flocks which have produced a right egg size for the production needs and establish a control point for the light program. We can make decisions of which light program suits the production targets.
Treatment Age of simulation Weight at stimulation Sge at 50% of production
Number of egss 16 weeks 1400 gr 140-145 days
Balanced 17 weeks 1500 gr 145-150 days
Egg size 19-20 weeks 1600 gr 150-155 days
Table 3. Number of eggs
In the Table 3 there are the different stimulation programs to adjust the egg size to the market needs. These are based on the standard of the breed and should be taken only as an indication.
It should not be forgotten that in addition to light stimulation there are other parameters that influence egg size.
Bibliography on request from the author.
Egg size DOWNLOAD PDF
Egg size can be controlled by the nutrition of the birds, but it will not work if the rest of the key points explained in this text haven’t been completed before.
There are four nutritional concepts controlling the egg weight, but the energy will have a bigger impact in the hens in cage free systems than the ones in cage systems.
Energy needs are divided into three parts in cage production:
Maintenance needs account for 65% of total production needs and except at the beginning of production where bird growth is significant. In the rest of the production, the rest of the energy will be used for egg mass production.
The hen needs its maintenance needs covered before allocating resources to egg-sized production.
If you want to know more about the topic, visit: https://hn-int.com/
On the occasion of the 2024 PSA Annual Meeting, organized by the Poultry Science Association (PSA), which was held July 15-18, 2024, at The Galt House Hotel in Louisville, Kentucky (USA), AviNews International had the privilege of interviewing Dr. Brian Fairchild, President of the PSA and Professor and Extension Poultry Specialist in the Department of Poultry Science at the University of Georgia.
What is the Poultry Science Association (PSA) about?
To me PSA is several things.
1
First it is a society involved in research in the poultry sciences (physiology, management, nutrition, genetic, immunology, engineering, microbiology, etc.) and dissemination of that knowledge through publication of two journals and the annual meeting.
2
A second is an opportunity to network with members of the society that are involved in all the poultry sciences.
What can attendees expect from 2024 PSA Annual Meeting to be held in Louisville, USA?
An opportunity to learn about recently completed and ongoing research in the poultry sciences. In addition, it will be a chance to meet new people working in these areas as well as an opportunity to catch up with colleagues and learn what they are working on as well.
What was your year as PSA president been like?
It has been busy, educational, productive and enjoyable. Our PSA members serve on several committees and many of these committees have been productive this past year.
Our journals Poultry Science and Journal of Applied Poultry Research continue to do well and have both seen a decrease in time from submission to first decision as well as overall time to publication.
With the help of several committees PSA is set to offer our first professional development conference for our members later this year (October 28-29).
What is the vision of the PSA for the future of poultry farming in USA?
This is a tough one. I would say that, as a whole, the PSA vision is help poultry companies and farmers continue to raise healthy birds in an optimal environment so that we continue to provide economical and nutritious poultry meat and egg products for consumers.
and how is the association addressing these challenges?
This will vary depending on who you ask. There are a number of pressures from regulatory, food safety, bird health, bird welfare and financial points that the poultry industry is facing.
One that I see as a growing concern is education. With each generation more consumers are not familiar with how their poultry meat and egg products are produced.
Unfortunately, many go to the internet for information on this and the material they read is not always focused on the facts.
Unfortunately, they do not realize this.
Currently PSA handles this through published quality research in its two journals. Our members comprise poultry scientists that work in the poultry industry and in academia.
Together they work to provide basic poultry knowledge to students at all levels of education (preK through 12 and college) and industry, farmers and consumers.
association promoting to foster research and development in the field of poultry farming?
Continue to provide information on current research that can be implemented to improve bird health, growth, efficiency and food safety through better nutrition, management and protection from disease.
How does it collaborate with other organizations and institutions at the American and international level to promote the interests of poultry farming?
Joint meetings like the Latin American Scientific Meeting in Brazil in October 2024, World Poultry Congress in 2026, and the joint meeting with the AAAP in 2027.
Could you tell us about the new world trends in poultry environmental control?
Conserving energy (electricity and gas usage) while still maintaining optimal environmental conditions for housing and rearing the birds. Utilizing new technologies to do a better job of early identification when there are issues developing whether it be subpar environmental conditions or bird health related.
The UGA Poultry Housing lab has looked several aspects of air quality that include CO2, CO, NH3, dust and moisture in terms of air quality at methods as well as technology to reduce dust and lower ammonia concentrations.
I would say our primary focus is on moisture control. If the moisture load in a poultry house is maintained well all of the air quality parameters mentioned above will be in acceptable range.
We have tested numerous types of sensors including but not limited to ammonia, CO2, water usage, humidity and bird weight scales. Much of our work focuses on methodology/management methods to control moisture as economically as possible for farmers.
Recently our former PhD student, Garret Ashbranner, focus his work for his dissertation on looking at providing a dark period to chicks starting on the first day of placement at the farm.
Traditionally, chicks have been provided 24 hours of light with the thought that it would help get them off to a better start by finding feed, water and heat sources.
Our work demonstrates that broilers do just as well when provided a 4- or 6-hour dark period in the first week after being placed on the farm.
Pen trials suggested that there might be some weight and FCR benefits but this was not observed in our 3 field trials. However, there were no negative impacts on bird performance (weight, FCR, livability) in the field trials.
Not surprising is that the birds provided a dark period had higher melatonin levels than those in the control with 24 hours of light in the first week.
The interesting observation was that the melatonin levels in the birds with dark period remained higher than the controls even after the control birds were put on a dark period at Day 7.
While there were no differences in broiler performance, our data indicated that there was better livability and less lame birds at the end of the flock. This work shows that producers can provide chicks a dark period without any detrimental impacts on flock performance.
Have you worked on water quality issues? What new technologies exist today to ensure that our birds have the best water quality?
Yes. We have done several projects to collect data and share with producers on how to properly design and manage drinker systems. Metering pump technology continues to improve to deliver the right amount of product in the water.
I have done numerous presentations on water quality its impact on poultry house drinker and evaporative cooling equipment as well as bird performance.
We know that you have worked on important principles of evaporative cooling, what can you tell us about it?
First, this is that many producers overuse it. It is the air speed that is going to cool the birds. The evaporative cooling system enhances the ability of the air movement (tunnel ventilation) to cool the birds.
The thing that producers need to remember is that for every degree of cooling provided by the evaporative cooling system the relative humidity in the house is going to increase. This makes it more difficult for the birds to lose heat from respiration, a primary way that birds lose heat as they do not sweat.
The increased humidity can also lead to higher moisture loads in the house, which as we discussed in an earlier question is the root of many environmental, bird health/wellbeing and bird performance issues.
Please tell us about weight gain vs. body temperature in broilers.
Hot bird will not eat as much as birds with normal body temperature. We recently did a project that demonstrated that birds with body temperature of 110 oF (normal is 106 oF) did not gain any weight during the weeklong observation period of our field study on market age broilers.
Is it true that uneven bird distribution can prove costly?
Absolutely. The one assumption that is made in poultry house design and management is that the birds are evenly distributed down the length of the poultry house.
We continue to look at new options for migration fences to help keep birds evenly distributed throughout the flock.
Interview with Brian Fairchild DOWNLOAD PDF
Luis Carrasco Managing Director
lc@agrinews.es
+(34) 605 09 05 13
Adoración Guerrero
Guzmán
Key
Account Sales Manager
int@grupoagrinews.com
+(34) 664 66 06 05