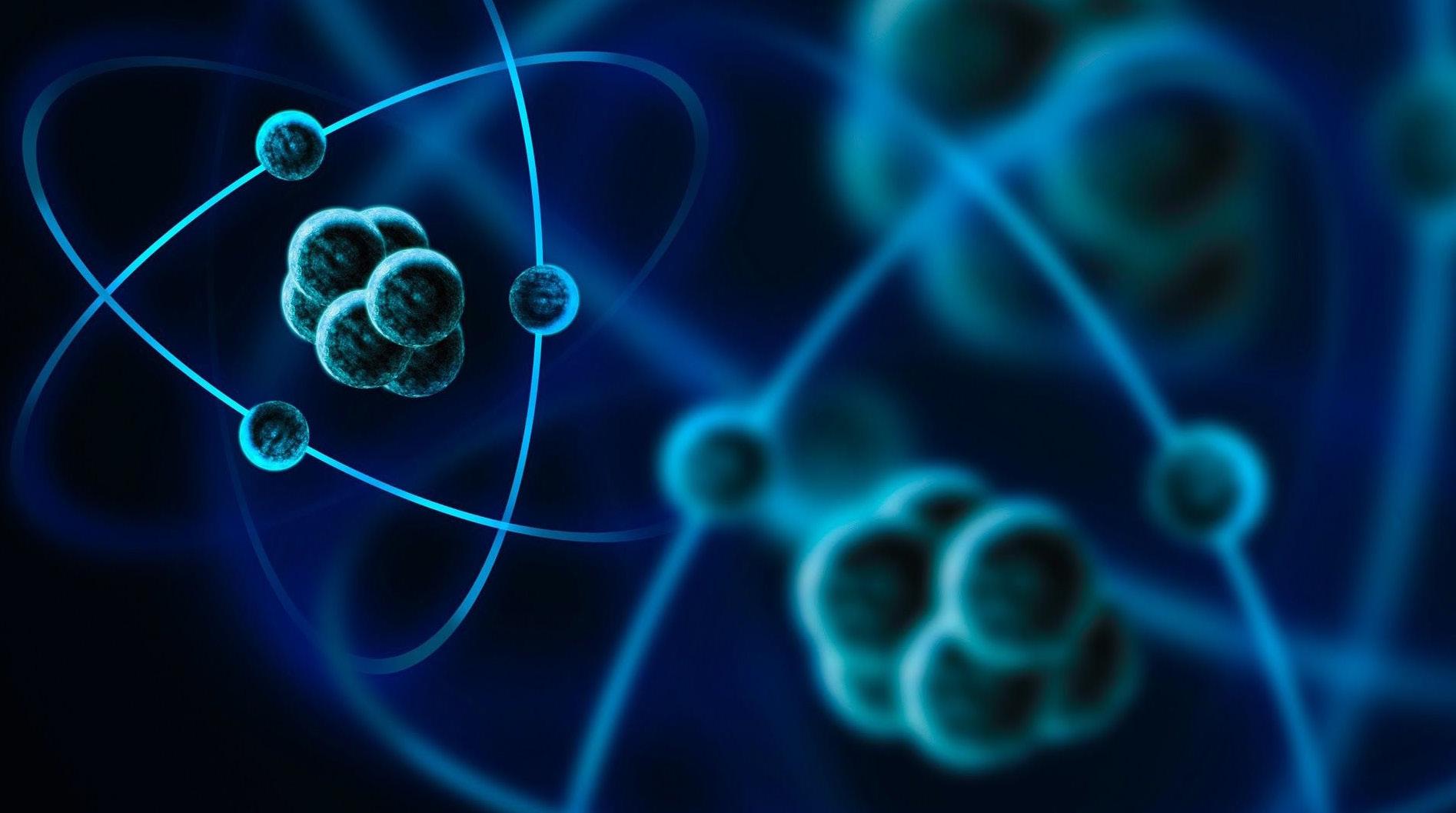
6 minute read
Quantum teleportation
from openME 52.2
by Simon Stevin
QUANTUM ENTANGLEMENT
One of the main rules of physics that we learn is that the maximum speed of an object is c, the speed of light, at 299.792.458 m/s. We don’t usually question this, and the exact reason why this is, would be a whole other article on its own.
Advertisement
WRITTEN BY ROEL HAZELHOF
From this constant, we are able to calculate things such as the wavelength of light, radiation, and much more – it is an absolute necessity when calculating and measuring things on the scale of the universe. It serves as proof that everything we can see and observe that carries weight, takes time to travel – just as light itself. This includes the waves, signals and radiation that carry information, which we use for almost everything on a daily basis. From your phone to a cell tower, to a satellite, and to a phone on the other side of the globe. On earth, these travel times are as good as negligible. However, if we zoom out to a more astronomical scope, these distances, and thus travel times, become immensely large. A message to the moon and back takes 2.5 seconds to travel, to the sun 16 minutes already and to Jupiter 90 minutes on average (depending on its orbit compared to ours). As you might imagine, this can cause communication problems in our future, when spaceflight becomes more prevalent. That is where quantum teleportation comes in. What if we could send information instantly, without delay? This is precisely what is on the forefront of physics research these days.
Beginnings of Quantum teleportation
How does this work? Well, the word ‘quantum’ may already spoil it a little bit: we are not dealing with regular physics. Quantum physics describes the physics that occur on an atomic level; on the level of the very building blocks of the world around us. This means that instead of looking at the physics of billions of small particles attached to each other, we look at how a single particle reacts to its surroundings – more fragile and prone to influences. An important aspect of quantum physics is the form in which a particle possesses energy; known as ‘spin’. This describes the rotation of a particle around its own axis, like an impulse moment. We theorize that the spin of a particle is not simply defined by a positive or negative spin (spin up or spin down), but that in reality it is in both states simultaneously
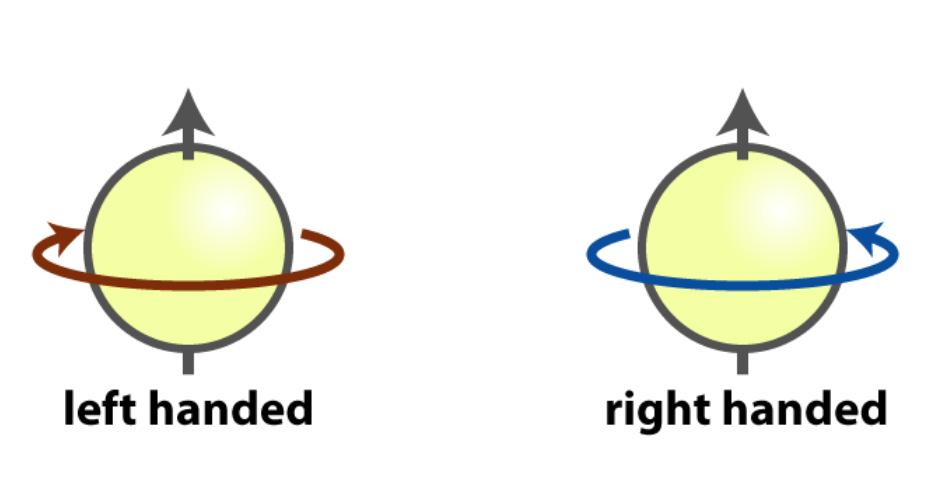
This is much like the wave-particle, which can display properties of a wave as well as a particle at the same time. We know that particles ‘dance’ between these states and can show different properties with new measurements.
(superposition). As soon as we measure it however, it takes either the value of a spin up or a spin down. Consequently, there is a 50/50 chance for a particle to display one of the two states. This superposition is one of the famous confusing aspects of quantum mechanics, but we will now hold it as true and clear. Particles contain weight and can spin. Therefore, they carry energy and have the possibility to influence the world and be influenced themselves. Some of the ‘traditional’ laws of physics still very much hold true in quantum mechanics. The most important and relevant one for this subject is the 1st law of thermodynamics (conservation of energy) – stating that the total amount of energy in the world cannot change. If this fundamental law would not hold, it would lead to the instability of the entire universe. This can also be seen in Newton’s third law; that for every action, there must be an equal and opposite reaction. This remains true on the atomic scale.
Now take the two subjects described in the previous paragraphs and apply them to this concept: if you have an electron and put a certain amount of energy in it, the electron reaches a higher energy state and becomes unstable. To get back to its initial ground state, the electron wants to release a photon that carries the added energy. However, we can also force the electron to keep the energy and add even more, causing it to reach an even higher energy state. Consequently, it will have to release two photons to be able to release the extra energy. With carefully chosen conditions and enough attempts, an electron can be forced to release these two photons just close enough to each other for the release to be considered ‘at the same time’.
The paradox
As you might imagine, these two new particles originate from a single particle. Following from the 1st law of thermodynamics and Newton’s third law, these two particles should then have an equal and opposite energy. This makes us able to more accurately predict what state a particle will be in when measured. When measuring two particles that are entangled at the same time, the second particle will have the opposite spin of the first particle around 75% of the time, unlike the 50% that should normally be the case. Imagine it like this: you buy a new pair of shoes at the store, but somehow the clerk didn’t put one of the shoes in the box. You know this, because you can feel only one shoe bouncing around, when shaking the box. You do not know which one you have (left or right) until you open the box, which puts the shoe in the box in a theoretical superposition - Schrödinger’s cat; the cat is both dead and alive, until you open the box. At home, you open the shoe box and find the left shoe in it. Within an instant, without there being any communication from the shoe left at the store, you know that the shoe at the store is the right shoe. The shoes are in an inter-dependent system and thus entangled.
Obstacles
As you might imagine, this entanglement and its result of instant knowledge about an entangled system, could theoretically allow for instant communication. However, we are not quite there yet. We are able to reliably produce (quantum) entangled photon pairs and test them over long distances, with the longest so far being more than 100 kilometres apart (from a Chinese satellite to the earth’s surface!). But there is a giant obstacle between communication through this quantum teleportation and reality.
Entanglements are extremely fragile and as soon as we measure a particle (forcing it into a certain state), the entanglement is broken. This means that in essence, we cannot encode information within a set of particles that are entangled with other particles in a different location. Aside from this problem, there are a lot of other problems as well: an example of this is that the accuracy of correctly predicting the state of quantum entangled particles, is a little bit more than 75%. Even if we were able to raise this percentage, these inaccuracies can ruin an entire batch of coded information and we wouldn’t be able to find out if a batch of information is corrupt. To find out if a batch is corrupt, we would have to use conventional methods of communication to compare the two quantum sets. And this would defeat the purpose of this new form of communication.
The future
Right now, research is focused on addressing the problematic areas: researchers at the TU Delft (QuTech) are working on ways to more reliably and quickly produce quantum entangled particles, improve the stability of these particles and the accuracy of predicting the state of the particles. Researchers in China are also working on the stabilization of entanglements, focusing on spanning the distance between earth and space. Hopefully this research will gain a lot of ground, so that we can experience a revolutionary way of communication in our lifetimes. Another example of entangling particles.
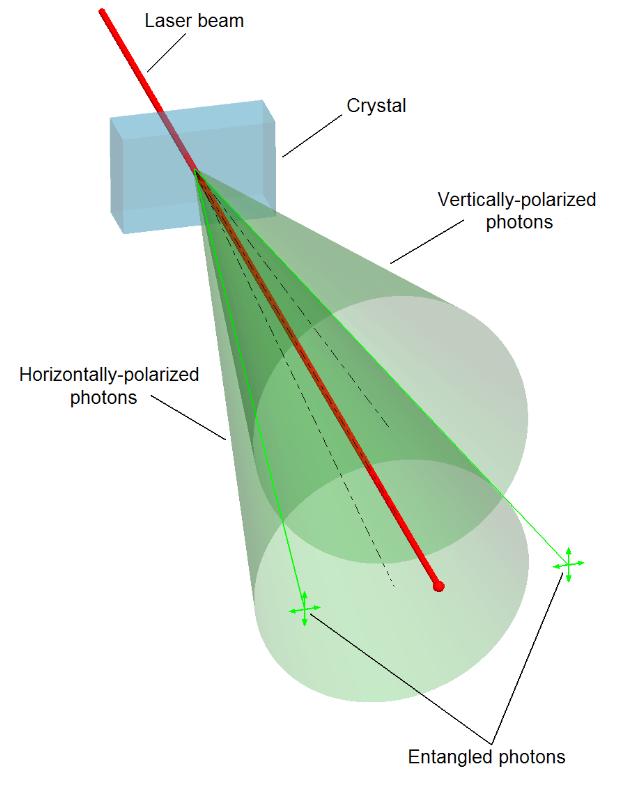