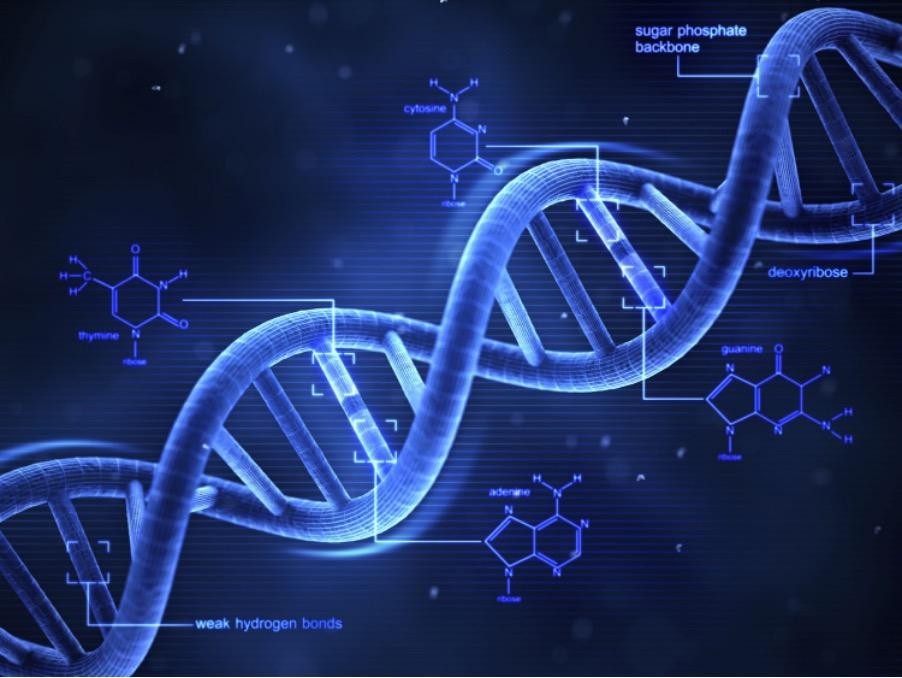
5 minute read
Junk DNA - Hamish Rimmer
from Serpentes Issue 6
‘Junk DNA’ may, at first glance, seem like a somewhat oxymoronic title – famously, upon discovering the double-helical shape of the DNA molecule in 1953, Francis Crick and James Watson walked into a pub in Cambridge and declared “we have found the secret to life” - surely to label any DNA as ‘Junk’ is heresy. ‘Junk DNA’, is in fact a term which refers to the sections of genetic code in an organism which do not code for proteins. The term first appeared in the 1960s, when it was mentioned only in passing in an academic paper. It was formalized by Susumu Ohno in 1972; since then, more and more discoveries have been made suggesting that so-called ‘Junk DNA’ is teeming with functions and vital roles. Hence, the term ‘Junk DNA’ has been questioned due to the provocation of a strong prior assumption of a lack of functionality – the term ‘non-coding DNA’ has been suggested to take its place. However, unfortunately, the name has stuck, and ‘Junk DNA’ remains widely used to refer to any genomic sequences which do not code for proteins. Nonetheless, by delving further into the dark matter of the genome, it is plain to see that ‘Junk DNA’ is far from useless.
Often, only a small percentage of a genome is responsible for coding for proteins in any particular organism. However, an increasing proportion of that which doesn’t is shown to have other functionality. It is estimated that almost 98% of the human genome is non-coding DNA, meaning only 2% codes for proteins. That 98% was assumed for a long time to be mostly, if not completely, useless. However, in 2011 the ENCODE Pro ject, a public research pro ject aiming to follow up from the Human Genome Pro ject in 2001, published results suggesting that over 80% of human DNA has biochemical functions; of course, there is still some debate surrounding the validity of this statistic as the criteria for what constitutes ‘functional DNA’ can vary.
Now that we have established that noncoding DNA contains a treasure trove of functionality, we can delve into these functions further. One function which has been determined for some non-coding DNA in general is called de novo gene birth, in which new genes are fashioned from noncoding DNA over time. This is because, due to a loss of selective pressure, pseudogenes (a sequence of DNA that once resembled a gene but has been rendered inactive through mutation) will accumulate mutations more rapidly than coding sequences. These pseudogenes could therefore serve as raw material for genetic evolution, resulting in new genes being formed. These pseudogenes were accordingly dubbed ‘potogenes’. On a more detailed scale, there are a range of types of non-coding DNA including: cis and trans-regulatory elements, introns, pseudogenes, repeat sequences, transposons, viral elements, and some non-coding DNA can be transcribed into functional non-coding RNA, however we don’t have time to unpack all that now. All these variations of non-coding DNA can serve separate purposes, ranging from ribozyme functionality to the control of the transcription of nearby genes. Due to the nature of much of non-coding DNA, a mutation on a regulatory section of ‘Junk DNA’ can cause a protein to be expressed in the wrong place, or at the wrong time, or can alter the expression of an important protein when it is needed, resulting in too little or too much. For example, mutations in noncoding DNA have been linked to developmental disorders such as isolated Pierre Robin sequence (a set of physical abnormalities of the face, such as a cleft palatte), which is caused by changes in enhancer elements that disrupt the activity of a gene called SOX9, altering the proteins
it produces. As such research into this section of Junk DNA suddenly becomes much more important as it could be linked with the prevention of this syndrome.
Although non-coding DNA hosts a myriad of functions, some organisms have significantly less non-coding DNA. The classic example is bacteria, whose genomes have traditionally been viewed as very compact with around 90% of the genome consisting of proteincoding genes. Generally, prokaryotes tend to have very little ‘Junk DNA’ (often between 6-14% compared to our 98%). Another example is the pufferfish, the genome of which is only around 13% of the size of that of a human, and yet they contain a similar number of genes to us. In experiments, (relatively) large sections of non-coding DNA have also been removed from mice before birth, this deletion of parts of their genome seemed to have little to no effect on their development, longevity, or reproduction. However, note the study doesn’t prove that Junk DNA has no function, just that removing a little over 1% of a mouse’s genome resulted in no abnormalities which they tested for in the laboratory. Clearly, it can be argued that Darwinian selection is a tougher test than surviving a single generation under laboratory conditions.
As mentioned previously, mutations in non-coding DNA can have just as serious implications in an individual as any mutation in the protein-coding DNA of a person might. As such, research into these sections of non-coding DNA could hold the key to several health problems, and even unlock a lengthier lifespan for humans. There are many inherited genetic disorders, such as fragile X syndrome or Friedreich’s Ataxia, and there are many health problems, such as various cancers, which can arise from random mutation in your genome, especially in long non-coding RNA sequences. For example, differences in non-coding genes within a population can influence susceptibility to Ewing sarcoma, a form of aggressive bone cancer. They can also play a role in infectious disease susceptibility, such as hepatitis C. ‘Junk DNA’ can additionally serve a purpose in a more physical way, without affecting other genes. For example, at the end of each of our chromosomes, we possess "caps” of non-coding DNA called telomeres. These sections of DNA act as a sort of insulation, wearing down over the lifetime of a cell. There is growing evidence that certain lifestyle factors may affect telomere length which, in turn, can affect the pace of aging and onset of age-associated diseases. This has led to research into reversing the effect of telomere shortening, and in the future may even hold the key to lengthening the lifespan of humans. This effect can already be observed in bats - the longest living mammals (relative to body size) - which keep their natural lives long by preserving their telomeres.
Clearly when you look past the name ‘Junk DNA’, you see that just because it does not code for proteins, does not mean noncoding DNA is useless. Far from it. It is clear then, that so called ‘Junk DNA’ will no doubt prove to be at the forefront of medical research influencing the scientific landscape of prominent fields such as oncology and genetics.