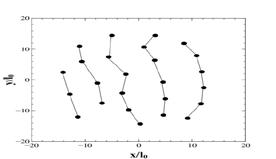
6 minute read
The Anisotropic Electronic States in the Fractional Quantum Hall Regime
Anisotropic electronic states in the fractional quantum Hall regime Isaac Berry and Orion Ciftja Department of Physics, Prairie View A&M University, Prairie View, Texas 77446, USA
Abstract Phases in the Lowest Landau Level Configuration of N=25 electrons
This study is concerned with the quantum Hall state at filling factor 1/6 of the lowest Landau level. This state is very close to the critical filling factor where the liquid-solid transition takes place. In this work we investigate whether an anisotropic Coulomb interaction potential is able to stabilize an anisotropic electronic liquid state at this filling factor. Monte Carlo calculations for small systems of electrons in disk geometry are implemented to obtain various quantities of interest . The results are consistent with the existence of an anisotropic liquid state of electrons in the lowest Landau level. 2D electronic systems in a perpendicular magnetic field form sets of discrete energy states called the Landau levels (LLs). An important characteristic parameter of such a system is the filling factor defined as the ratio of the total number of electrons relative to the capacity of each LL. The most robust fractional QHE state occurs in the lowest Landau level (LLL) at filling factors, υ=1/3 and 1/5. Such states are very well described by a wave function that is called Laughlin’s wave function. Wigner crystallization happens at filling factor υ=1/7 , thus the liquid-solid phase transition happens in the range of filling factors 1/7-1/5.
Fractional Quantum Hall Regime
We consider a two-dimensional (2D) system of fully spinpolarized electrons subject to a strong perpendicular magnetic field in the z direction. The magnetic field leads to the creation of discrete energy levels (Landau levels). Degeneracy of Landau levels is proportional to the value of the magnetic field. For large magnetic fields, electrons fill a fraction of the states in the lowest Landau level. This is the fractional quantum Hall regime. Under these conditions, the system manifests novel behavior such as the phenomenon of fractional quantum Hall effect (FQHE), a problem first explained by Laughlin.
Anisotropic Coulomb Potential

Energy Difference Per Electron
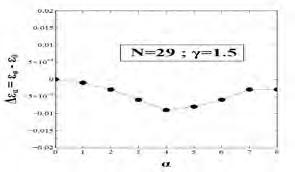
Laughlin’s State at Filling Factor 1/3
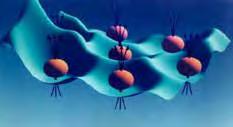
(From Bell Labs Picture Gallery)
Anisotropic Wave Function (ν=1/6)
Ψ=∏ j>k (z j -z k ) 4 (z j -z k +a)(z j -z k -a) exp[-(1/4)∑ j |z j | 2 ] Det[Plane waves]
The variables, z j are two-dimensional position vectors in complex notation (magnetic length is set to one). The parameter a breaks the rotational symmetry of the ground state wave function. Plane waves correspond to lowest-lying plane wave energy states of an ideal 2D spin-polarized Fermi gas.
Conclusions
We describe a possible anisotropic quantum Hall liquid state at filling factor 1/6 of the lowest Landau level stabilized by the presence of an anisotropic Coulomb interaction potential. The state is described by a wave function that contains a built-in anisotropy parameter that breaks rotational symmetry. Monte Carlo results for small systems of electrons indicate stability of liquid crystalline order in presence of an anisotropic interaction potential.
Acknowledgements R&I’s Office of Undergraduate Research (OUR and Physics Department,, Prairie View A&M University.
Introduction
The invention of novel nanofabrication techniques has made the realization of stable finite systems of almost perfectly confined particles in arrangements that can be easily controlled with external tools such as an electric or magnetic field. For example, it has been successfully demonstrated that electrons can be confined via a combination of electric and magnetic fields in an almost ideally two-dimensional layer. This project tried to gain some understanding of the behavior of anisotropic electronic states in the fractional quantum Hall regime, achieved when a two-dimensional system of electrons is subjected to a very high perpendicular magnetic field. The idea explored was to detect signs of anisotropy in the system due to some form of internal anisotropy.
Materials and Methods
The current research project was a theoretical study involving standard quantum calculation methods. The idea was to follow the theoretical calculations with numerical simulations done by the PI and his undergraduate student.
Results and Discussion
We investigated the theoretical possibility that an anisotropic Coulomb interaction potential leads to the stabilization of a liquid crystalline state of electrons with broken rotational symmetry. We describe such an anisotropic state by means of the liquid crystalline wave function, which can be adiabatically connected to the actual wave functions for the isotropic fractional quantum Hall effect states. We were able to perform all the theoretical calculations. The PI was able to do some quantum Monte Carlo simulations in standard disk geometry to gain a better understanding of the properties of this state. The following Figure shows a simulation snapshot of a system of 25 electrons. In PI’s view, this result provides some (very) preliminary evidence of layering behavior. Thus, we did observe signatures of anisotropic behavior in the system. However, the work was interrupted when the university closed. Numerical simulations of this nature cannot be done from home.
Conclusion(s) or Summary
Recent experiments raise many fundamental questions regarding the inner nature of various anisotropic electronic states observed in the fractional quantum Hall regime. The interplay between effective electron mass anisotropy and electron-electron correlation effects in a magnetic field can create a wide variety of possibilities. In particular, the electron mass anisotropy for a two-dimensional electron gas effectively leads to an anisotropic Coulomb interaction potential between electrons that may strongly influence the stability of various quantum phases, which are very close in energy. Since the electronic system’s overall stability is very sensitive to local order, several anisotropic electronic phases may emerge even in the lowest Landau level in regimes where one would not expect them. In this work, we study the lowest Landau level state with filling factor 1/6, a state which is very close to the critical filling factor where a liquid to Wigner solid transition takes place. The findings seem to indicate the stability of the liquid crystalline order. They are consistent with the existence of an anisotropic electronic state in the fractional quantum Hall regime. The PI may consider returning to this research after summer 2020 if normality returns.
References
1. O. Ciftja, Detailed solution of the problem of Landau states in asymmetric gauge, Eur. J. Phys. 41, 035404, (2020). https://doi.org/10.1088/1361-6404/ab78a7/ 2. O. Ciftja, Results for charged disks with different forms of surface charge density, Res. Phys. 16, 102962 (2020). https://doi.org/10.1016/j.rinp.2020.102962 3. O. Ciftja, Energy of a finite three-dimensional electron gas of spinless electrons, J. Phys. Chem. Solids 136, 109135 (2020). https://doi.org/10.1016/j.jpcs.2019.109135 4. O. Ciftja, Impact of an elliptical Fermi surface deformation on the energy of spinless twodimensional electron gas, Phys. Scr. 94, 105806, (2019). https://doi.org/10.1088/1402- 4896/ab23c6 5. O. Ciftja, Exact results for a quantum Hall state with broken rotational symmetry, J. Phys. Chem. Solids 130, 256, (2019). https://doi.org/10.1016/j.jpcs.2019.02.021 6. O. Ciftja and I. Berry, Interaction energy of a pair of identical coplanar uniformly charged nanodisks, AIP Adv. 8, 035209 (2018). https://doi.org/10.1063/1.5025336 7. O. Ciftja, Anisotropic magnetoresistance and piezoelectric effect in GaAs Hall samples, Phys. Rev. B 95, 075410 (2017). DOI: https://doi.org/10.1103/PhysRevB.95.075410
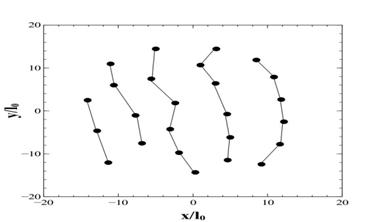
Isaac Berry is a senior, majoring in Mechanical Engineering.Dr. Orion Ciftja is a Professor of Physics with research interests in Theoretical Condensed Matter Physics, Nanotechnology and Materials Science.