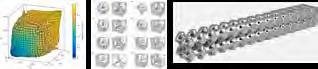
9 minute read
Geometric Stiffness Control Techniques for Metal-Based Implants
Prevailer Mba, and Jaejong Park, Ph.D.
Department of Mechanical Engineering, Prairie View A&M University, Prairie View, TX 77446
ABSTRACT
Bone defects can develop from various situations such as trauma, infections, tumor, surgery, etc. In some cases where the defect is large, making bone not being able to regenerate itself, the defect site needs to be replaced for functional requirements and aesthetic reasons. ant and that of the surrounding bones have to be close Bone grafting is one of such methods available for such purposes. This method includes repurposing another bone in the body (or using a substitute) by modifying it to fit into the affected area. Metal-based bone implants are another option. Titanium and its alloys are widely used in reconstruction surgeries. Since the design and fabrication are done using virtually Ex vivo, metal-based bone implants have geometric freedom compared to bone grafts. However, one of the main shortcomings of metal-based bone implants is their inherent strong mechanical properties. A substantial mismatch and discontinuity between the metal implant and the bone often lead to stress shielding, which may lead to a failure in the long run. To resolve this issue, the stiffness of the implant and that of the surrounding bones have to be close to each other, such that the bone starts to heal in the interference. This can be done in many different ways, utilizing less stiff materials, less material volume, modifying the structure geometry, etc. This study aims to focus on various techniques for stiffness control by changing the geometry of the overall structure confined in a design domain.
MOTIVATION
Physical trauma or bone cancer often requires the removal of damaged bone. In the case where the damage is significant and located in the midface (see Figure 1), proper reconstruction surgeries are crucial. It should address both appearance and functionality of missing bone. Inadequate treatment results in long-term disfigurement with devastating physical, psychological, social, and economic consequences for patients.
Figure 1. Patients with various craniofacial bone defects. Natural bone grafting including both autograft and allografts has long been a popular choice among reconstruction surgeons due to its biocompatibility. Fibula or iliac crest are commonly used for natural bone grafting but creating a required geometry for the substitution is not an easy task due to predefined geometry of those bones which limits the necessary revival of functional requirement as shown in Figure 2.
Figure 2. Limited geometric options using natural bone grafting (Asterick). With the surge of advancements in manufacturing methods, synthetic bone grafting is becoming important in reconstruction surgeries. Machined or 3D printed metal implants provides unlimited geometric freedom compared to natural bones [1]. However, its excellent mechanical properties arise another problem in the long-run, stress shielding.
METAL BASED BONE IMPLANTS
Metallic implants needs to provide important features to reliably serve its role for long time without causing any negative effect. An ideal metal implant should satisfy critical requirements such as biocompatibility, resistance to corrosion and wear, and ability to provide environment for new bone formation (osseointegration). Long interdisciplinary research efforts have found that metals, specifically, stainless steel, cobalt-chromium alloy, titanium alloys and noble metal-based alloys are good candidate materials for orthopedic, dentistry, and cardiovascular applications [2]. While abovementioned metal-alloys are biocompatible, and corrosion and wear resistance, their inherently strong mechanical properties creates unfavorable stress distribution. This discontinuity in mechanical property leads the neighboring bone to degrade overtime which potentially result in bone fracture, and implant failure. Figure 3 shows a significant bone loss near the metal femur implant two and 18 years after surgery accordingly.
Figure 3. Development of Grade 3 stress-shielding after total hip arthroplasties [3]
STIFFNESS CONTROL TECHNIQUES
• SHS Process Self – propagating high-temperature synthesis (SHS) is a type of combustion synthesis manufacturing process used in various industries to produce cheap, fast materials of high purity. It is a relatively cheap method, which makes it attractive for large scale manufacturing. Materials that a re produced by SHS are generally characterized by high porosity. An alternative to the conventional furnace technology, SHS can be used to control the properties of the alloy to be produced by controlling the composition of the reaction mixture. Figures 4 and 5 below show a metal alloy produced by SHS.
Fig 4. (a) Axial and (b) radial acroscopic morphology of TI-SI alloy compacts by SHS [4]. Here the heat of material leads to composition change as well as higher porosity.
Fig 5. SEM Micrographs of the fracture surface demonstrates different microscopic composition of TI-SI alloy from SHS[4]
• MIM Process Metal Injection Molding (MIM) is a type of manufacturing process that can be used to produce complex and intricate geometries. It combines two already established techniques to make manufacturing easier, and more cost efficient. The technologies are injection molding and powdered metallurgy. The properties of a metal produced by MIM can be controlled by changing different parameters during the sintering process. Lattice structures
The stiffness of a structure can be altered by inducing porosity to the structure. The size and configuration of the pores found to greatly affect the structural response. Challis et al [] numerically designed a family of meso-scale structures. Using an interpolation hexahedron in a cube consist of parameter vectors (�������� 1 ,�������� 2 , and �������� 3 ), each representing the Young’s modulus of principal directions, many microstructures with different anisotropic properties and volume fractions in a cubic domain. The microstructure library can be used to generate structures with a functionally graded structure which can be essential in bone implants.
Figure 6. Structural optimization for lattice structure design with a functionally graded mechanical properties. [5]. • Functional microstructures Similar to lattice structures, microstructures can be engineered based on the manufacturing process used. Powdered bed fusion techniques have been found to be very effective in controlling the structures of metals. From figure 7 below, we can see that the microstructures and mechanical differ with each type ofadditive manufacturing (AM) process. This means that coupling precise compositions ofpowdered metals with the right AM process could be a step in the right direction, towards desired mechanical properties.
Fig 7. Comparison of microstructures of Titanium alloys produced using different AM processes [6].
DISCUSSION & FUTURE RESEARCH
This literature review on techniques to control the stiffness of metal-based structures allowed us to divide primary methods into two categories: manufacturing processes and porosity control. A hybrid approach that considers both geometric configurations as well as the manufacturing process is being developed. The manufacturing method will be mathematically introduced into structural topology optimization methods to achieve this. This novel technique is expected to provide a much broader range of property controllability when compared to traditional schemes. This can potentially open up avenues to design metal-based implants with better stress shielding avoidance.
REFERENCES
[1] Wang, Xiaojian, et al. "Topological design and additive manufacturing of porous metals for bone scaffolds and orthopaedic implants: A review." Biomaterials 83 (2016): 127-141. [2] Manam, N. S., et al. "Study of corrosion in biocompatible metals for implants: A review." Journal ofAlloys and Compounds 701 (2017): 698-715. [3] Engh Jr, C. Anderson, et al. "Clinical consequences of stress shielding after porous-coated total hip arthroplasty." Clinical Orthopaedics and Related Research® 417 (2003): 157-163. [4] Ran, H., Niu, J., Song, B., Wang, X., Feng, P., Wang, J., … Farid, A. (2014). Microstructure and properties of Ti5Si3-based porous intermetallic compounds fabricated via combustion synthesis. Journal ofAlloys and Compounds, 612, 337–342. [5] Challis, V. J., A. D. Cramer, and A. P. Roberts. "An optimised family of anisotropic microstructures with application to functionally graded materials." International Journal ofSolids and Structures 171 (2019): 17-29. [6] Dutta, Bhaskar, and Francis H. Sam Froes. "The additive manufacturing (AM) of titanium alloys." Titanium powder metallurgy. Butterworth-Heinemann, 2015. 447 -468.
Introduction
Bone defects can develop from various situations such as trauma, infections, tumor, surgery, etc. In some cases where the defect is significant, making bone unable to regenerate itself, the defect site needs to be replaced for functional requirements and aesthetic reasons. Bone grafting is one of such methods available for such purposes. This method includes repurposing another bone in the body (or using a substitute) by modifying it to fit into the affected area. Metal-based bone implants are another option. Titanium and its alloys are widely used in reconstruction surgeries. Since the design and fabrication are done using virtually Ex vivo, metal-based bone implants have geometric freedom compared to bone grafts. However, one of the main shortcomings of metal-based bone implants is their inherent strong mechanical properties. A substantial mismatch in mechanical properties between the metal implant and the bone may lead to a failure in the long run. The property gap between the metal implant and neighboring bone needs to be closed to avoid stress shielding. This study aims to focus on various techniques for stiffness control by changing the geometry of the overall structure confined in a design domain. We are studying how to make the structures ‘porous’ to control the stiffness of the structures. While making structures porous intuitively reduce the stiffness of the structure, the study pursues explicitly algorithms where the stiffness can be systematically controlled. Various internal structuring techniques, such as lattice structures with different geometric configurations, architectural materials, and auxetics, are being investigated.
Materials and Methods
This work is intended to be a review of stiffness control. Therefore, we will be considering published manuscripts from trusted websites such as Google Scholar, using keywords such as tunable stiffness, stress shielding, e.t.c. Successful methods that align with our goal of systematically controlling the stiffness will be reviewed carefully. Inferences and results obtained by the authors of these published papers will inform our expected results. A family of journal articles was assessed to find the state-ofthe-art approaches for stiffness control of pure metal and metal alloy based structures.
Results and Discussion
We found that the significant techniques to control the stiffness of metal-based structures can mainly be divided into manufacturing and porosity control. Two different manufacturing methods have been identified that could be used to alter metal implants. They are Self-propagating High-temperature Synthesis (SHS), Metal Injection Molding (MIM) [1]. Special treatment and handling of the material leads to different material composition, thereby modifying its properties.
Another category is porosity control. Porosity refers to the voids in any structure, and the size, count, and configuration within the structure are found to be very useful in manipulating the structural response. Various optimization problems are formulated to design mesoscale structures for coveted macroscopic mechanical properties. Their study also showed it is possible to achieve a functionally graded property, which can be significantly advantageous for bone implant design applications. to develop a hybrid method that considers both functional design and manufacturing aspects, which can broaden the property controllability for the bone-implant design.
References
[1] Ran, H., Niu, J., Song, B., Wang, X., Feng, P., Wang, J., … Farid, A. (2014). Microstructure and properties of Ti5Si3- based porous intermetallic compounds fabricated via combustion synthesis. Journal of Alloys and Compounds, 612, 337–342 [2] Challis, V. J., A. D. Cramer and A. P. Roberts. “An optimized family of anisotropic microstructures with application to functionally graded materials.” International Journal of Solids and Structures 171 (2019): 17-29.
Prevailer Mba is a Senior, majoring in Mechanical Engineering student. Dr. Jaejong Park is a Professor of Mechanical Engineering with Research interests in the biomimetic structures, rehabilitation robots, and 3D/4D printing.
Figure 1. Mesoscale structural design for functionally graded mechanical properties [2].
Conclusion
This was the first phase of our project for developing a design tool for the stiffness matching bone implants. We are currently digging deeper into these possible methods for stiffness control that were found. The ultimate aim is