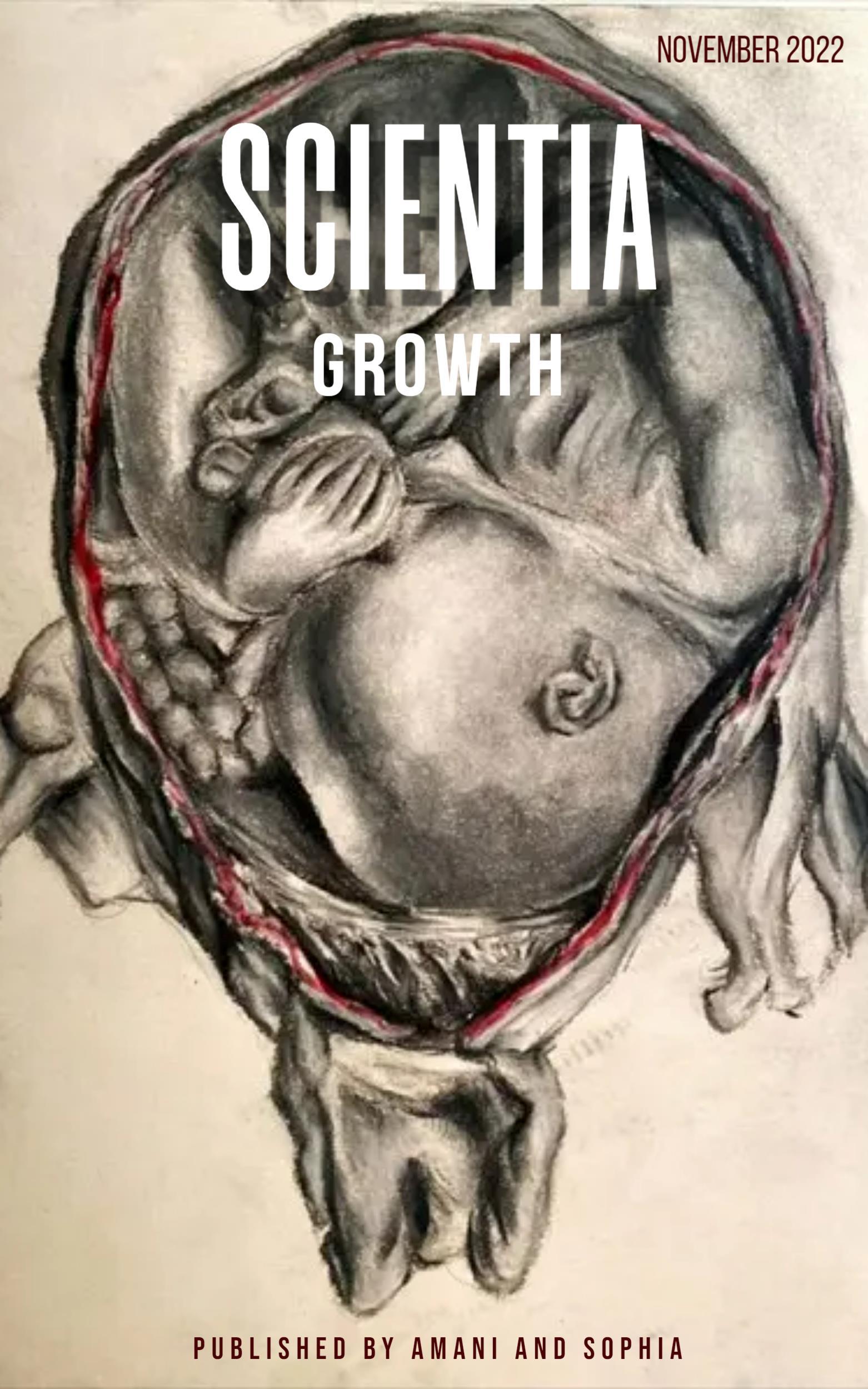
HEAD OF BIOLOGY Anagha
WRITERS Kate Camilla Kat Ghanage Tharunya Sara Trina
EDITORS-IN-CHIEF
Amani Sophia ILLUSTRATOR Anoushka
HEAD OF CHEMISTRY Solene
WRITERS Nadia Alice Jiya Erin
HEAD OF PHYSICS Diora
WRITERS Anantaa Anonymous
HEAD OF BIOLOGY Anagha
WRITERS Kate Camilla Kat Ghanage Tharunya Sara Trina
Amani Sophia ILLUSTRATOR Anoushka
HEAD OF CHEMISTRY Solene
WRITERS Nadia Alice Jiya Erin
HEAD OF PHYSICS Diora
WRITERS Anantaa Anonymous
The Future of Neurological Treatments: Mini brains ……………………….…4
Cancer Cell Metabolism: The Warburg Effect …………………… ……….….7
Adaptive Growth ……………………………………………………………………9
The increasing fitness of MRSA bacteria ……………………………………….10
Can we speed heal ourselves? …………………………………………………..13
Human Growth Hormones ……………………………………………………….15
King of Earth; Homo Sapiens…………………………………………………….17
The Past, Present and Future of mRNA vaccines ……………………………...19
Carbon Nanotubes and how they grow ……………………………………… .21
Bionic Photosynthesis: The future of fuel production? ...…………………….23
Growing Diamonds ……………………………………………………………….26
Quasicrystalline Products ………………………………………………………...30
The Growth of Quantum Physics ………………………………………………..32
The Growth of the Early Universe ……………………………………………….34
MORE Puzzles and Games (inc. an astrophysics wordsearch) .…..……………36 References ………………………………………………………………..…38
The human brain itself continues to remain as one of the largest mysteries in the world. The brain is the most complex structure in the universe with approximately 1×10¹¹ neurons interconnecting with each other in a series of over billions of networks, yet only weighs 1.5 kg in mass.[1] Neurons are specialised cells that are responsible for receiving sensory input from the external world, sending motor commands to our muscles and transmitting electrical signals between every step. Moreover, it is these interactions that defines us as people; our political beliefs, our preferences and our emotions the holy grail of human consciousness. [1]
Despite the fact that the brain has an incredible intellectual capacity, the vital organ is often affected by neurological diseases, many of which have been globally recognised as the major causes of death and disability.[2] Unfortunately for many of these diseases, such as Alzheimer’s and epilepsy, they are incurable and thus many patients on life long medications receive very little, effective treatment.
If we examine the anatomy of the brain, it is not only protected by the skull and cerebrospinal fluid, but also theblood barrier,whichhastheability to intercept any toxins that may be present within the blood and prevent the entry of pathogenic diseases.[3] The barrier is located between the brain’s capillaries and the tissues and
Fig 1: A diagram illustrating the structure of the brain blood barrier [3]
therefore, offers an endothelial tight junction. This means that endothelial cells line across the interior of the bloodvesselsandarecloselylinkedto eachother, formingtheso calledtight junctions.[3] As a result, the barrier tightlyregulatesthemovementofonly small ions, soluble fat molecules and specific gases to pass through, restricting pathogens and harmful bacteria to enter. [4] However, the blood barrier also prevents the entry of substances and drugs that are meant to help the brain, including chemotherapy drugs which are designed to kill cancer cells. [5] Scientists are trying to find ways to penetrate or trick the barrier to open in order to allow more effective treatments to work. However, once the blood barrier is open, other toxins
and bacteria can enter and harm or cause infection to the brain.
The study of the human brain is challengingandsomewhatrestricting, not only because of our lack of understanding, but also because of the ethical limitations in front of us. Scientistsoftenhavetoadheretostrict ethicalcodeandguidelinestoprevent any inhumane scientific practises like using live animals when it comes to researching the brain.
So, what if we grew a brain instead?
Recently researchers have been growing in vitro “mini brains,” otherwise known as brain organoids, which are essentially living samples of brain tissue. In vitro refers to enclosed test tubes, in which a combination of particulartypesofstemcellsgrowand form self organised, three dimensional tissue cultures under controlled conditions.[6] These are known as organoids as they can replicate some parts of the complex structure of an organ and in this case, the brain. Stem cells have the unique ability to divide indefinitely and produce different types of cells to from their progeny. To date, researchers have been producing organoids that have resembled the brain, the lung, the small intestines, the stomach, the liver, and the kidney. [6]
There are two types of stem cells which are involved in this process: adult stem cells and embryonic stem cells. Human induced pluripotent stem cells (iPSCs), which are a type of adult stem cells, are derived from skin or blood cells that have been reprogrammed back into an embryonic like pluripotent state. As a
result, this enables the development of an unlimited source of any type of human cell needed for therapeutic purposes, likewise to embryonic stem cells. [7]
[8]
In an interesting case study at the University of Cambridge, a sample of embryonic stem cells and iPSCs were taken from a patient suffering from frontotemporal dementia and was embedded into an extracellular matrix. It was then cultivated in a rotating bioreactor to allow tissue amplification and neural differentiation. While the cell culturewas beginning to develop, small molecules were supplemented to grow the brain organoid. As a result, mini brains had been produced. They were roughly the size of a pea and resembled parts of the human cerebral context. The brain organoid bore a similar structure towards the brain and cell to cell interactions were able to occur. The team was able to show that a drug, GSK2606414, was effective at relievingcommoncellularproblemsin frontotemporal dementia, including theaccumulationoftoxicproteins,cell stress and the loss of the nerve cells. [9] Therefore, they were able to block pathways that contributed to the
progression of the disease. Drugs formulated through such research are now more suitable as treatment and now can be approved and tested in clinical trials.
Scientists are now able to effectively test drugs on brain tissue ethically. Despite the organoids lacking a consciousness like a real brain, they can respond to drugs and show us the way to cure common brain diseases. One disease that could immensely benefit from this discovery is Alzheimer’s, a type of dementia that causes that brain to shrink and as a resultaffectsthememoryandthinking skills. There are only a few successful drugs that can slow down the progression rate of this debilitating condition. [10] By allowing pharmaceutical companies to test their compounds directly onto the
brain organoids, scientists will be able to formulate new drug compositions at a faster rate.
Additionally, mini brains have also enabled gene editing which can be used to fix the mutations in the DNA thatcarrythecondition.Theotherway is to grow mini brains from anonymous healthy people and then edit the gene that carries the disease. The advantage of this method is that it avoids some of the ethical and data protection issues that arise when dealing with specific patients and allows the mini brains to be used by pharmaceutical companies for research. Soon, there may be new drug formulations and revolutionary treatments from such pathbreaking research leading to potential cures for manyneurologicaldiseases.Professor Jurgen Knoblich of the Institute of Molecular Biotechnology of the Austrian Academy of Sciences, Vienna, Austria, says, “I’m convinced we are going to see a complete revolution that might actually end in a situation where biology and medicine are no longer separate disciplines. There is hope for new drugs and for new treatments.” [10]
-Trina
Cancer cells alter their metabolism to encourage growth, proliferation, and long term survival. A common feature of this altered metabolism is an increased uptake of glucose and fermentation of glucose to lactate, even in the presence of oxygen and functioning mitochondria. This phenomenon is called the Warburg Effect. Research on the Warburg Effect has advanced knowledge of the conditions for oncogenesis (the development and growth of tumours), but the function of the effect is unknown.
Cancerous cells have a higher demand for glucose and glutamine (an amino acid). In normal cells, pyruvate, which is formed from glucose in glycolysis, is converted to acetyl CoA and then enters the Krebs cycle in mitochondria. This then undergoes oxidative phosphorylation under aerobic conditions,resultinginthe production of 32 ATP molecules. It is only in anaerobic conditions that normal cells will produce large amounts of lactate instead of acetyl CoA. Cancer cells, despite having fully functioning mitochondria and a sufficient supply of oxygen, convert pyruvate to lactate, hence their metabolism often being referredtoas“aerobicglycolysis.”This is a specific hallmark of tumour cells. As a result, to meet the increased requirement for rapid tumour growth, an increased rate of glucose uptake is required. However, this is a less efficient method of energy production, and scientists aren't sure
why tumour cells use it. Otto Warburg originally hypothesized that cancer cells develop a mitochondrial defect that leads to a subsequent reliance on
glycolytic metabolism. However, later work has showed that mitochondrial functionisnotimpairedinmostcancer cells, suggesting an alternative explanation for aerobic glycolysis in cancercells.Wenowknowthatcancer cells exhibit aerobic glycolysis because of activation of oncogenes and loss of tumour suppressors.
Furthermore, the metabolism of cancer cells is altered from being catabolic (breaking down molecules) to anabolic (building molecules) to generate the extra protein, nucleic acids, and lipids required to maintain their increasing rate of proliferation. They absorb amino acids as well, with glutaminebeingespeciallysignificant. It is an essential source of nitrogen for thesynthesis ofamino acids,aswellas a source of carbon for replenishing Krebs cycle intermediates and producing more pyruvate. Some tumours are so dependent on glutamine that if they are deprived of it, they stop growing and die.
The Warburg Effect and Rapid ATP Synthesis. Aerobic glycolysis in cancer cells is an inefficient method of producing ATP per unit of glucose when compared to mitochondrial respiration. However, the rate of glucose uptake via aerobic glycolysis is faster, with lactate produced 10 100 times faster than full oxidation of glucose in the mitochondria in normal cells. As a result, a reasonable explanation for why cancer uses aerobic glycolysis should account for this inherent difference in the rate of glucose uptake. Although oxidative phosphorylation produces more ATP per mole of glucose, proliferative cells primarily rely on aerobic glycolysis as it generates ATP faster and provides substances to support cell proliferation and metastasis (the spreading of cancers). However, certain elements of this theory do not fully make sense as empirical calculations show that the amount of ATP a cancer cell releases is not even close to a tumour’s demand for ATP.
Warburg Effect and the tumour microenvironment. The Warburg Effect may provide an advantage for cellular metabolism in a multicellular environment as the acidification of the microenvironment and other metabolic features may enhance oncogenesis. Due to lactate secretion, increased glucose uptake lowers the pH in the microenvironment. Acidosis has a variety of potential advantages forcancercellsasonetheorysuggests that an acidic microenvironment would cause H+ ions to be secreted from cancer cells. These ions would then diffuse into the surrounding
environment, altering the tumorstroma (the structural components holding tumour tissues together) and causing penetration and spreading of cancer cells into neighbouring tissues. The availability of glucose is the result of direct competition between tumour and tumour infiltrating lymphocytes (immune cells that recognise and penetrate tumours). The high rates of glycolysis reduce the availability of glucose for tumour infiltrating lymphocytes and they require a high amount of glucose to perform their functions. Research shows that tageting aerobic glycolysis in proliferated cells will increase the amount of glucose for tumour infiltrating lymphocytes, thereby enhancing their main function, which is to eradicate tumour cells. The Warburg Effect is likely to provide an overall advantage that promotes a favourable microenvironment for tumoursthatleadstoproliferationand long term survival.
Warburg Effect and biosynthesis. The Warburg Effect could potentially promote a metabolic environment that allows for rapid biosynthesis to support growth and proliferation. Increased glucose consumption is utilized as a source of carbon for anabolic (promoting metabolism) processes.Thisextracarbonisusedto create lipids, nucleotides and protein from scratch which would help the tumour grow and proliferate. However, more research is needed to determine whether the Warburg Effect supports biosynthesis.
Research on the Warburg effect has been vital in helping scientists
understand the requirements of cancer cells to proliferate and survive. However, many elements of the WarburgEffectanditsfunctionarestill unclear but nonetheless this concept is highly significant for therapeutic advances to be made in treating
cancer. This can be done through intervention in a cancer cell’s metabolism through the immune system, especially in terms of glucose and glutamine.
-TharunyaWe humans, and other organisms, grow at an astonishing rate while still embryos and foetuses in the mother’s womb. Which means there should be a lot of opportunity for things to go wrong, yet most organisms leave the womb looking okay, in the sense that they are symmetrical. Why is this the case?
Alberto Roselló Díez, along with a few other scientists, carried out research to see what would happen when a mouse foetus’ growth in one leg was restricted. It was known that in fly larvae, injury to individual tissues wouldkickstartmechanismsthatacted both in the local tissue, and across the whole body to compensate for the injury. Ultimately, this allows the injured tissue to grow to become of a normal proportion compared to other tissues. However, catch up growth in vertebrates, which have a more complicated route of development, was still a foggy area.
Roselló Díez and his team used foetal micefortheir experimentsandcarried out experiments in which they triggered insult to the left limb during themouse’slastgestationalweek.This was done through the manipulation of a cell cycle suppressor p21, which they introduced exclusively to the cartilage that was driving the growth of the left hindlimb. During cell
division, the cells monitor and regulate the process, for which they require cell cycle checkpoints (CPs). One example is tumour suppressor genes, which inhibit cell division and thus, tumour development. At times, they can even trigger apoptosis, or programmed cell death. By introducing p21 to cartilage cells (chondrocytes), the chondrocytes were unable to divide, preventing the growth of the left limb. The right limb would serve as an internal control.
What Roselló Díez and his team found was that left right symmetry remained normalinthemouse,whichmeantthat some type of compensatory mechanism must have taken place during its development. Through further research they found that there were two main such mechanisms, one local and one systemic.
Locally, they found a hyperproliferation an abnormally high rate of cell division leading to a rapid increase in number of the unaffected left limb chondrocytes. This indicated that these cells must be responding to a signal, probably comingfromthetargetedcells.Firstly, extracellular matrix production is increased which results in a decrease in cell density. This means that the cartilage scaffolding is still being laid down as usual, and endochondral
ossification the process of replacing cartilage with bone takes place at an almost normal rate. Additionally, in cases where the cell density drops below a certain level, it was found that the compensatory proliferation that occurred in the remaining chondrocytes happened to an extent proportional to the number of affected chondrocytes. Therefore, it is thought that the spared chondrocytes are responding not to the cell density, but to stress signals released by the affected chondrocytes.
The systemic response involved the stunting of the growth of the right limb. This correlates with levels of placental insulin like growth factor (IGF) signalling, revealing that communication was taking place between the bone and the placenta. Insulin like growth factor (IGF) is a group of hormones which controls the effects of growth hormone (GH) in our body. They are called insulin like due to their similarity to insulin in their structure, and growth factor refers to any protein that can stimulate cell proliferation.
There are two proposed mechanisms for how the communication between the injured bone and placenta takes place.Thefirstisthatlocallyproduced stress signals are released into circulation and impair the functions of placental cells. In particular, if a subpopulation of natural killer cells in the placenta which are known to promote placental function and foetal growth are impaired, this could explain the reduction in growth.
The second mechanism is a size monitoring system which detects impaired long bone growth and causes system growth reduction. However, there is still little evidence for such a mechanism.
This new type of catch up growth, which takes place during insult to tissue, is known as adaptive growth. Althoughitisstillarelativelynewfield, further research could aid our understanding of human growth disorders and have applications in treating such disorders.
-SaraMRSA bacteria is a common bacterium that is resistant to many antibiotics. It is most known as the ‘superbug’, and the use of different antibiotics has led to mutations in genes, which has given rise to the growth of different strains. Antibiotic resistanceisbecominganincreasingly important issue, and this essay will explore the fitness of different strains
and potential solutions to reduce resistant bacteria.
Methicillin is an antibiotic which works by inhibiting penicillin binding proteins which synthesise peptidoglycan, a polymer that forms the cell walls of bacteria. Staphylococcus aureus has become resistant to methicillin through expressing foreign penicillin binding proteins (PBP) and can replicate the production of PBP’S. S. aureus also shows susceptibility to gentamicin, an example of an aminoglycoside antibiotic that is used as prophylaxis. In 1992, a new strain of MRSA emerged in France, and the incidence of this strain, with a new phenotype, has increased steadily. Instead of just looking at the evolution and growth of these strains from a molecular point of view, there has been a shift to looking at the ‘physiological characteristics’, which has led to the discoveries of
The study has shown that the GS MRSA A1 strain has a much faster growthratethanoftheGR MRSA,soit has increased fitness. Although both strains were inoculated at equal amountsatthebeginningofthestudy, the GS MRSA A1 strain accounted for 88 97% of the population 8 hours later.Thedifferenceingrowthratehas led to the GS MRSA A1 strain being a predominant issue and will eventually replace the old MRSA strain. For researchers, it is even more difficult to treatthesebacteriaastheyhavefound ways to mutate and adapt to their environment. Consequently, the increaseintheprevalenceofthisstrain has led to reduced consumption of gentamicin antibiotics.
maximal growth rate and generation times.
A possible solution can be to find agents that are able to reduce resistance to an existing antibiotic. Research has shown that there are other proteins in cell wall synthesis, so these are possible targets and antibiotics can be made to inhibit the production of these proteins. Compounds can also be formed to regulate methicillin resistance. In some cases, the only antimicrobial therapy available is the glycopeptide antibiotic, vancomycin, mainly used to treat infection in the intestines.
India,anLIC,doesnothavethemoney to spend on advancing medical facilities. This means that diagnoses are uncertain and often, MRSA infections are unrecognised thus the primary antibiotic that is prescribed is important. In many areas in Kolkata, the antibiotic that is normally prescribed is linezolid. Although, it is more costly, the uncertainty surrounding the type of infection the patients have, leads to precautions being taken immediately. A recent study showed that linezolid is a better option than vancomycin, as the rate of success was significantly higher and people who received this antibiotic stayed a shorter duration in hospital
andhadreducedintravenoustherapy. Therefore, these are two possible antibiotics that can be used as opposed to methicillin.
Quorum sensing is the process in which bacteria become pathogenic. Each bacterium sends out a chemical whilst also interpreting the other chemicals released by the bacteria surrounding them, so they can tell whether they can fight against the immune system now. Disruption of this process will treat the infection and natural selection is avoided as no selective pressures are applied to cause resistant bacteria to remain. However, this has not been fully investigated in humans but only seen to work in animals. The mechanics couldbeapotentialsiteofexploration and eventually, solutions can be devised to disrupt quorum sensing.
In conclusion, the growth of other strains, which are almost ‘cloned’ versions of the original MRSA, but have slight mutations that increase their fitness, is leading to increasing pressuretodiscovernewantibioticsto treatinfections.The discoveryofother proteinsinthecellwallsofthebacteria
is useful as they are targets, however, it is not possible to continue producing antibiotics as the bacteria will eventually become resistant. Therefore, other solutions, using
knowledge of quorum sensing, need to be devised to kill the bacteria without antibiotics.
-Ghanage
Many complex mechanisms work in our body to fix anything we throw at it from cuts and scrapes to broken bones, the body manages to restore itself to its former glory when given some time. How does it do this, and does anything affect the speed at which these processes takes place? Can we alter the body’s pace at which it heals?
In order to heal skin lacerations or abrasions, over a short period of time, blood clots over the opening, preventing further blood loss from thewoundandentryofpathogens.A scabforms,andoverthenextcouple of days the body’s immune system works to prevent infections from occurring at the site of the wound. White blood cells travel there, and fightinfectionandbloodvesselsdilate intheareatosupplymoreoxygenand nutrient rich blood to aid in the healing of the wound. As a few weeks go by, collagen is supplied by red blood cells and provides a strong, fibrous foundation for the new tissue to grow. This new tissue is called granulation tissue and on this new skin will start forming.
A diagram showing stages of a skin laceration healing.
In terms of fractures, bleeding occurs in the area and blood starts to clot (forming a hematoma), which fills the
gap between the fracture. The area becomes inflamed due to immune responses and stem cells migrate to the fracture to start the healing process. Bone forms near the edge of the fracture and soft cartilage grows between thebrokenends.Thisformationpeaks around 8 days after the incidence of the fracture. In a little less than a month, new bone starts to grow, but it can take several years for the bone to fully grow back.
Therearemanydifferentfactorswhich can control the speed at which our body heals after an injury. One of the main factors delaying the healing of bone fractures is smoking the nicotine found in cigarettes interferes with this. Concerning lacerations, wounds of individuals with diabetes often heal muchslower(ordonothealatall)than wounds of those without diabetes. This increases the likelihood of infections occurring; these can be life threateningif leftuntreated.Sincediabetes affects insulin production and therefore maintenance of blood glucose levels, if blood glucose levels remain high then white blood cells are unable to carry out their functions properly, therefore infection is not foughteffectively.Ifthediabetesisnot managed, blood circulation is also affected and blood also moves slower, affecting transport of nutrients to the site of injury. Diabetic neuropathy is a common condition amongst diabetics lack of a controlled blood glucose level affects nerves and therefore sensationofpain,leadingtonumbnessso in the case of a laceration, the person
may be unaware of the injury: the person will get no treatment which in some cases can deteriorate the in-
Image showing diabetic neuropathy of the feet.
There have been some developments to speed up healing of tissues. Recently, scientists have developed a new biodegradable plaster which is made of 3D printed skin. It is made of polyurethane a plastic and gelatine; analysis revealed that more than double the number of blood vessels and collagen were at the site of the wound treated with the 3D printed plaster than the control (commercially available) plasters. The wounds were also more than half the size after a month, showing the effectiveness of this treatment.
Anothertreatmentwhichhasbeendeveloped is wound dressings with electric fields. This doesn’t require any technical, expensive equipment and works by having four layers. The bottom layer, which rubs against the skin, producesstaticelectricitythroughfrictional forces. The layer above that is made of silicone and can conform to the curves of the skin. The next layer is a shape memory alloy which pushes the two edges of the wound together, sotheyaretouching, andthelastlayer
is a second layer of silicone. One improvement that needs to be made in the future with this technology is making it more applicable to irregular shaped wounds.
Anewbreakthroughinhealinginjured organsiswhatfascinatedmethemost, though. Recently, scientists at the University of Manitoba, Canada, have formulated a freeze dried powder which transforms into a gel upon touching blood. What drew my attention particularly was the fact that this gel was madefromavegetable okra.Inaddition to physically blocking wounds to prevent blood loss (which it does very effectively all bleeding from the dog and rabbit hearts and livers stopped within 1 minute), it also causes blood to clot very quickly. The mechanisms behind this are yet to be discovered but it is speculated that there is a carbohydrate in the gel which is responsible for this effect. The gel also dissolves in the body after 4 6 weeks and causes no further complications, which gives hope for this to be a feasible method of treating injured organs in the future.
Allthesetechnologieshavethepotential to change the future of those who are affected by medical conditions which affect blood circulation and clotting mechanisms. Now, diabetes contributes up to 90% of all amputationswithincertainpopulations.Thisis due to infection spreading from wounds to muscle and bone, which is called osteomyelitis. This develops into gangrene, leading to amputation. In the most severe cases, the person can develop sepsis which can be fatal. With quick healing technologies, a lot of lives would be improved and even saved, which would aid people in fulfilling more normal lives and having to take less precautions in order to care for a wound. As seen with the okra gel for organs, these can also be used in life or death situations this would have a huge positive impact on emergencyhealthcareifmethodsarefound to administer this treatment even more easily.
KatHuman growth hormone (HGH), also referredtoas‘somatropin’,isaprotein molecule of recombinant human growth hormone, comprised of a single chain of 191 amino acids. Although synthesised in a laboratory, it is biologically identical to ‘somatotropin’, a growth hormone naturally producedinthebody,secretedbytheanterior pituitary gland.
Each amino acid is encoded for by a different sequence of DNA, and these sequences are brought together until
the entire protein is encoded. Also, the manufacture of HGH uses recombinant DNA technology. rDNA is made artificially in a laboratory and combines genetic material from multiple origins; effectively, it isolates genes and attaches them to other sourcesofDNA.Itismadepossibleby the fact that the DNA in all living organisms is similar in chemical structure. After the protein is encoded, the recombinantDNAisinsertedintocells that mass produce the protein.
Therehavebeenvariousmethodsthat have successfully produced rHGH, the first of which being “inclusion body technology.” rHGH collects in small bodies in E. coli bacteria and is then segregated and purified. However, it was not 100% accurate, as the protein moleculesdifferedbyoneextraamino acid at the end. A newer technique, “protein secretion technology”, is used to manufacture most forms of rHGH currently available on the market. The main benefit is that the rHGH is secreted from the E. coli rather than accumulated in the inclusion body. Therefore, it is more easily purified, and ensures the production of rHGH identical to human growth hormone produced in the body.
It is important to maintain good growth hormone levels for both males and females, because lowering levels stops receptor cells in the body from performing their specific functions, which can cause a range of problems, includinglowenergy, reducedmuscle mass,andevenfeelingsofanxietyand depression; symptoms are not just physiological, they can also be psychological and emotional. Therefore, adults suffering from growth hormone deficiency require human growth hormone injections to supplement them with hormones needed for essential physiological functions, replacing the lack of natural growth hormone produced by the pituitary gland.
The use of HGH is only legal if approvedbyamedicalprofessionalfor a prescription medication; conditions include children with growth failure, adults with growth hormone defi-
ciency,cachexia, and short bowelsyndrome. It is not available as an over the counter medication to prevent misuse; for example, it has been popularlyusedforbodybuildingpurposes andtoimproveheavylifting,ortoslow ageing.
However, HGH therapy can also be used to treat other specific ailments. These consist of:
1. Prader Willi Syndrome: a rare genetic disorder that causes weakness in muscle tone, delayed development, and difficulties feeding
2. Noonan Syndrome: a genetic disorder that causes abnormal development in multiple parts of the body
3. Turner’s Syndrome: Women have partially or fully missing sex chromosomes, which resultsinunderdevelopedfemale characteristic
Generally, if the correct dosage is taken, HGH injections rarely have side effects; if they do occur, it is no more than some redness, swelling, or slight pain at the injection site. However, more serious complication can arise from abuse, misuse, or illegal use, as for a person with normal growth hormone levels, HGH injections will raise them too high. Side effects include insulin resistance, fluid retention, and carpel tunnel syndrome, but substanceabusecanevencausetype2diabetes or cancer; tumour growth could potentially be increased, since growthhormonestimulatescellreproduction.
-Kate
Human evolution has now led to the culture bearing, upright walking species that first lived about 315, 000 years ago in Africa. Homo sapiens arenowthemostdominantspecieson Earth, but why?
Since Charles Darwin first published his books ‘On the Origin of Species (1859)’ and ‘The Descent of Man (1871),’ where he claimed that we were descended from apes, the search for the history behind our species has continued. However, the fact wearedescendedfromapesstilldoes not answer how we have managed to become more sophisticated over our ancestral species. You may think that it’s just purely due to our resourcefulness and awareness of our environment and you are not completely wrong. However, I’m sure it would interest you to know that there are key anatomical differences betweenusandtheapeswhichmeans we are so superior. For example, the way we walk, our hand dexterity and even down to our brain mass. What first differentiates us from apes, isourabilitytomoveontwolegs.Otherwise known as bipedalism, we can stride unlike most other mammals. Walking involves one to tilt forward slightly and keep up with the displacedcentreofmassmuchliketrying to keep your bike stable as you pedal. It is shocking to think that this motion which we carry out subconsciously every day has led to notable anatomic differences between the pelvis and lower limbs of humans and the great apes. Differences that have led to our modern species.
As demonstrated in the diagram we have short ilia bones with a short, broad sacrum. Conversely, great ape hips have long ilia with a small sacrum which is long and narrow. This simple difference allows humans to facilitate swift and smooth movements unlike apes. It is a key reason behind our growth.Itfreedupourupperlimbsfor tool use and meant less body surface was exposed to damaging solar rays during midday tropical sunlight.
Images showing an ape pelvis (1) and human pelvis (2).
Another essential limb which has contributed to our growth as a species is the hand. Being primates, we use our hands continuously whether this be to feed ourselves, clean ourselves or even as a defence. They can be argued as the most important limb of the body but, what makes them so powerful?
The features of human hands are easily distinguishable from those of the great apes, its unique structure allows forexquisitemotorcontrol.Oneofthe most complex adaptations is the thumb, wherein a fully independent muscle aids a remarkable amount of strength and grip. Additionally, a spe-
cial saddle joint and associated ligament at the base of the thumb enable refined rotation. These differences had manipulatory capabilities, we were able to hold weapons steadily and throw them with increased precision and power. Itis what caused us to pose such a major threat to other species. Deposited hand bones in a cave in Africa from 2.8 2.5 million years ago evidence that even back then hands had developed for advanced tool use.
The advanced mammals that we are meansthatwemusthavemuchhigher cognitive abilities and therefore, bigger brains. This is true. But just how much bigger have our brains got? Scientistshavestudiedthebrainthroughout human evolution and have witnessed its continual expansion. From the average brain masses of 435g to modern day 1,400g which require 20% of our body’s energy. We now have a greater capacity to understand complex questions and abstract consequences such as the belief in a God, which the great apes cannot.
Our many distinguishable differences combined has meant we have been able to climb our way to the top of the food chain. We now sit comfortably in top spot because of our ancestors’ numerous evolutions and adaptations to their environment across millions of years.
-CamillaVaccines immunise the body against unwanted viruses and bacteria, limitinginfection.Mostvaccines(e.g.,inactivated vaccines or live attenuated vaccines) introduce a dose of a killed or weakened version of a pathogen into the body, resulting in an immune response. However, over the past several decades, mRNA vaccines have been developed by scientists. mRNA vaccines do not require the actual virus or bacteria to be injected. Scientists take a virus’s RNA blueprint and isolate the protein part of the virus from the RNA blueprint which is mainly responsible for infection. This RNA blueprint is used to create messenger RNA. Messenger RNA is put intovaccines,enterscellsandinstructs cells to build large quantities of the same protein from the virus. From here, the body recognises the foreign protein and creates antibodies; antibodies are kept in the body so that it can react quickly if exposed to the virus again. Compared to other vaccines, the chemistry behind mRNA vaccines is beneficial, as they do not cause illness and are also quicker to produce. However, it has taken over six decades for the first mRNA vaccine to be used in humans. This article will lookat howthe uncertaintiesof mRNA vaccines are being overcome, and the growth of mRNA usage in the future.
In 1961, Sydney Brenner, Francois Jacob, and Matthew Meselson founded the molecule mRNA, and discovered that it gains genetic information from thenucleusandgivesmRNAtranscript to the cell to create a specific protein. Once mRNA had been isolated in 1969, the chemical development of
liposomes used for drug and vaccine delivery continued, and by 1990, mRNA findings had significantly grown, as the novel liposome wrapped mRNA was given to mice. Mice were also used as a testing source for influenza and cancer mRNA vaccines between 1993 and 1995. However, during these several decades, humans were not used as a testing source, because uncertainties in the development of mRNA vaccines remained. The greatest uncertainty wastheeffectivenessofmRNAtranslation and its stability; mRNA was quickly degraded and taken up by the body before it could pass the RNA transcript to cells. This was soon solved from advances in nanotechnologyin1995 2005,involvingthedevelopment of lipid nanoparticles that enclosed mRNA, allowing mRNA entry into cells without degrading.
Recently, particularly during the Covid 19 pandemic years, there has been significant growth in the use of mRNA vaccines in humans. When the coronavirusarosein2019 2020,avaccine was key to settling the crisis. Vaccines not only needed to immunise people but had to be produced quickly. mRNA vaccines, which had been hidden in previous years, became a leading chemical resource in the SARS CoV 2 vaccine race. mRNA vaccines are quick to produce, as they do not require the chemical productionofaninfectiousvirus.Thevaccines are also easily adaptable and often only take a fortnight to make once scientists attain the virus’s RNA sequence. These discoveries about mRNA technology had already been made before the pandemic, thus the past provided a foundation for rapid growth of mRNA vaccines. Furthermore, from 2014 2019, researchers such as of the US National Institute of Health had worked specifically on coronavirus vaccine development, as the world had already experienced two variations of coronavirus: severe acute respiratory syndrome in 2003, and Middle East Respiratory Syndrome in 2012. Dr. Kizzmekia Corbett and others therefore explored some RNA sequences of covid variations and looked at the “spike protein” of coronavirusanditsrelevanceinmRNA covid vaccine growth. As Professor Shabaz Mohammed (University of Oxford) said, “this pre existent work allowed us to react rapidly at the beginning of the COVID 19 pandemic”. Pfizer’s COVID 19 vaccine was proven as extremely effective and safe, and it is the first mRNA vaccine with complete FDA approval in the U.S., an evident sign of growth in mRNA usage.
Since Covid 19, mRNA vaccines have become a promising and reliable prospect for the future in medicine advancement, primarily because of their easy adaptability and the speed with which they can be produced. These benefits mean that there is plenty of opportunity to grow and develop mRNAvaccinesbeyondCovid 19,and to tackle some of the biggest health problems in the world. For example, HIV is one of the Big Three Infectious Diseases, and the HVTN 302 study (National Institute of Health, March 2022) tests three HIV mRNA vaccines. The NIH’s threechemical formulae will aim to present the spike protein of HIV, encouraging an immune response from the body. A trial is expected to happen in July 2023. FollowingthesuccessandsafetyofCovid mRNA vaccines, chemists predict that mRNA vaccines for diseases like HIV and cancer, could also be safe if using similar methods when being made.
-JiyaIn chemistry lessons, most of us have learnt about structures formed by carbon that have different properties (allotropes), from diamond (one of the hardest materials in existence) to graphite (which can conduct electricity due to its carbon atoms only bonding with 3 other atoms, leaving mobile electrons that can carry charge). Carbon nanotubes (CNTs) are one of the structures carbon can take that we
do not learn much about, and so this articlehopestoexploretheproperties and applications of carbon nanotubes aswellastheprocessinwhichtheyare produced.
CNTs are extremely thin carbon structures and were first discovered by Iijima in 1993, and have similar properties to graphene in terms of electroconductivity and thermoconductivity, but are regarded as stronger ‘the stiffestandstrongestman madematerial known to date’ ( Sattar, R., Kausar, A., & Siddiq,M. 2015) than graphene due to their structure, easily explained using the analogy of how ‘a rolled piece of paper is stronger than the same paper as a flat sheet’ (Slomski. 2010). According to research conducted by the ACS, CNTs are ‘at least 117 times stronger than steel and 30 times stronger than Kevlar, the material used in bulletproof vests’ per unit of material (American Chemical Society.2010.). There may be some confusion caused as CNTs are referred toas ‘one dimensional conductors’, but this just means that they are ‘materials whose electrical conductivity is high only along one direction’ (Kagoshima, S., Nagasawa, H.& Sambongi, T. 1988).
This, in turn, proposes many possible applications of CNTs in our daily lives, including: Electron emitters (i.e. x ray machines) (Baughman R.H. 2010) as CNTs are electron emitters with low chemical reactivity; microchips, as CNTs can act as semi conductors and can cope with high temperatures as they can act as a ‘heat sink’; batteries, which can extremely lightweight and flexible, as well as highly performing throughamethodofapplyingCNTink onto paper (Hu, L. Et al. 2009); solar cells, whereby extremely thin CNTs are deposited onto a bacterial cellulose membrane template to create an electrically conductive and optically transparent layer (Jung, R. et al. 2008) that can replace transparent conducting films, etc.
As with most carbon based structures, CNTs have high thermal conductivity and electrical conductivity, like metals, but achieve this via the vibration of covalent bonds between the carbon atoms, enhanced by the ‘stiffness’ of the carbon bond, as described in Nanotechnology for Dummies (Boyson, Muir et al. 2011, p. 76). However, not all CNTs are the same, and their individual properties depend on the type of CNT they are. CNTs are categorized into 2 main categories:single wallcarbonnanotubes (SWCNTs) and multi walled carbon nanotubes (MWCNTs). SWCNTs are much smaller in diameter (typically ~1nm) (Faktri, N. et al. 2009) and also more curved compared to MWCNTs (typically ~50 150nm) (Nagai, H. Et al. 2011), which is rather self explanatory as they are ‘single
walled’. This means that they are more flexible and elastic, making them suitable in reinforcement of other materials such as plastics. As well as this, SWCNTs are useful for ‘exploiting the electronic properties which vary with their chirality’ (Jindal, V. 2012). Chirality indicates the structure of the CNT through the way by which the structure is ‘twisted’, and can be described as‘chiral’,‘zigzag’(whichcanmakethe CNT a semi conductor), or ‘armchair’ (which makes the CNT ‘metallic’ highly conducting). On the other hand, MWCNTs have, along with similar properties as SWCNTs, a higher tensile strength as it was found that ‘tensile strength of composite is proportional to the volume fraction of high modulus and low modulus fibres’ (Muzammil, S.B. et al. 2019). Other studies include the categories: double walled carbon nanotubes (DWCNTs)andfunctionalusedcarbon nanotubes (f CNTs) (Mehra N.K. and Palakurthi S. 2016 ), which have ‘an open end structure on both sides’ (Grumezescu, A.M. 2018).
Figure 1 (Kumar, M. 2010) a) tip growth model b) base growth model
To be able to incorporate the properties of CNTs, the most important thing to do is to understand how to create them efficiently. There are 3 commonly used methods of synthesizing (i.e.growing)CNTs:theArcDischarge Method, the Laser Ablation Method,
and Chemical Vapor Deposition (CVD). This article will look specifically into the CVD method, which is currently the most popular method of ‘growing’ CNTs as it has high yields, can produce at relatively low temperatures and can ‘control size by varying the size of catalyst particles’ (Purohit, R. Et al. 2014). Unlike Arc Discharge and Laser Ablation, which are based on heating graphite/graphene to produce ‘tangled nanotubes mixed randomly with byproducts’ (Dai,H. 2002, Pg.2), the CVD method involves heating a catalytic transition metal nano particle species supported on (typically) alumina in a patterned array and feeding a hydrocarbon gas through a tube reactor at between 700 900C, wherebythehydrocarbongasdecomposes into hydrogen and carbon (which are then deposited for CNTs to grow). According to the Ames Research Centre (Delzeit, L.D. 2004), the conditions of the formation of SWCNTs and MWCNTs differ, with SWCNTs preferring CH4 (which maintain thermal stability at night temperatures) at 900C and MWCNTs preferring C2H2/C2H4 at 750C. This demonstrates that SWCNTs have a higher energy of formation, which originates from their ‘characteristics of small diameter, large curvature, and high strain energy (Wang, X., Vinodgopal, K., Dai, G., 2019). The size of the CNTs depends on the size of the catalytic particle, which is shown in Figure 1.
The Vapour Liquid Solid (VLS) method by which the CNTs grow in CVD as ‘traditionally it is widely accepted that the growth of SWCNTs from metal catalysts follows the VLS mechanism’ (Liu, C. and Cheng, H.
2013) is also quite fascinating and almost mimics the growth of a plant shoot ‘as supersaturation occurs, carbon atoms precipitate out from the particle’, forming a nanotube that has a ‘dome shaped end’ (Dai, H. 2002). (Dai,2002) introduced 3 methods in which CNTs may align themselves: ‘self assembly by intratube van der Waals interactions’ of MWCNTs, ‘self assembly by van der Waals Interactions with substrates’ of ‘suspended’ SWCNTs and ‘electric filed directed nanotube growth’ of SWCNTs where CNTs were grown on silicon nanowires (SiNW) (Yoshida, H. et al. 2007)
Althoughthepotentialbenefitstosociety CNTs can offer are voluminous, therearereasonswhytheapplications suggestedabovehavenotbeenused. Some of these reasons include: the amount of CNTs, as “most mass produced CNTs are highly defective, and high qualityCNTsarehardtoproduce in large quantity” (Aron, J. 2016);purity of the CNTs, as ‘purification difficulties are great because of the insol-
ubility of CNT and the limitation of liquid chromatography’ (Jahanshahi, M. andKiadihi,A.S.2013); environmental risks, as CNTs have only been looked into for around 30 years, which means it is difficult to fully understand the impacts it can have of used in large amounts.
The idea of artificial growth of CNTs has been discussed, but there is also some research that does suggest the possibility of naturally made CNTs given the right conditions researchers have found ‘evidence of naturally occurring MWCNTs produced from Pinus oocarpa and Pinus pseudostrobus, following a forest wildfire’ as well as extractions of ice cores containing ‘carbon nanotubes and fullerene nano crystals’(Murr, L. E. et al. 2004, p.2). In the future, as we discover more evidenceandhaveadeeperunderstanding of the natural formation of CNTs, we may be able to develop sustainable new ways of creating CNTs that may be sufficient for more commonplace use in our daily lives.
-AliceFor over a century, scientists have been fascinated with artificially replicating the process of photosynthesis that takes place in living organisms. In 1912, Giacomo Ciamician, an Italian chemist wrote, “So far, human civilization has made use almost exclusively offossilsolarenergy…Woulditnotbe advantageous to make better use of radiant energy?” He went on to suggest that “Meshing plants with tech-
nology would turbocharge photosynthesis.” [1] It could be argued that Ciamician’s ambitions have since been achieved; we have discovered how to convert solar energy to electrical energy, through the use of solar panels. The typical consumer solar panel constructed from rigid silicon crystals generally has efficiency rates of between 15 to 20 per cent, althoughsomepanelssuchasthemonocrystalline solar panel, have even higher levels of efficiency. [2] This
completely outstrips the theoretical maximum amount of sunlight that plants can convert to biomass: 4.5 per cent, and the actual average of 1 per cent. [3]
However, it is still the case that we have not fully harnessed the potential of solar energy, because electricity cannot be constantly generated from photovoltaiccells(solarpanels),asthe sundoesnotcontinuallyshineonpanels. Electricity generated from solar energymustbestoredusingbatteries, so that it can be used later on. Storing electricity using batteries is inefficient and costly, not least because batteries degradeeverychargecycle.[3]Plants, on the other hand, have a much greater capacity to store energy as they store it in chemical bonds rather than charged particles in sugars such as starch and sucrose which are fuels. It is difficult to artificially produce fuels from solar energy in the same way and replicate photosynthesis, partly because of the light dependentreactionthatmusttakeplace, to allow this process to occur. In this reactionthephotolysis(breakdown)of water by light, catalysed by the oxygen evolving complex (a water splitting enzyme), forms protons (H+ ions), electrons and oxygen: H2O → 2H+ + 2e + ½O2
So far, the scientist who has most successfully replicated this reaction and converted sunlight and carbon dioxide into fuel is Daniel Nocera, of HarvardUniversity,throughhiscreationof the artificial leaf and bionic leaf. What is the Artificial leaf?
The artificial leaf, that Nocera originally pioneered at MIT between 2009 and 2010, is a thin wafer that has the power to split water. At its centre is a semiconducting silicon sheet, or a photovoltaic panel. When light shines
onthis,energyisconvertedintoaflow of wireless energy within the wafer, thus no external circuit is required for theleaf tooperate.[4]Thispowersthe electrolysis process that can be used to split water. In one of the first methods that Nocera developed, the current generated runs through an indiumtinoxideanode,whichhadbeen placed in a solution of cobalt and phosphate salts. Co2+ions are oxidated at the anode to form Co3+ , which then forms a precipitate with phosphate ions (cobalt phosphide). This precipitate forms a catalytic film overtheanode,andthefilmpullselectrons from the water, leaving behind protonsandoxygen atoms.Then,oxygenatomsarebroughttogetheratthe surface of the anode and the gas bubbles to the surface, whilst cobalt gains electrons and falls into solution as Co2+. At the same time, phosphate anions carry protons (H+ ions) to a platinum cathode where they are reduced, to form hydrogen gas. [5] In newer versions of the model, an alloy (NiMoZn) is used in place of platinum to generate hydrogen. [6] What is the bionic leaf?
In order to produce fuel with the hydrogen collected from the splitting of water molecules, Nocera partnered with colleague Pamela Silver, a bioengineer at Harvard, to create the bionic leaf (which also contains the technology of the artificial leaf) in 2015. The hydrogen produced by the artificial leaf is fed to pre starved bacteria, Ralstonia eutropha, which are placed in the same jar as the water splitting leaf. The R. eutropha have been modified to essentially switch from growth to panic mode resulting in the microbes consuming the hydrogen and carbon dioxide. Carbon dioxide is absorbed either from the atmosphere or pure
sources. [7] The bacteria can then synthesise biomass and fuels such as isopropanol,isobutanolandisopentanol.
[8] Overall, the bionic leaf fuses two distinct fields: inorganic chemistry, to split water, and biology to convert hydrogen and carbon dioxide to fuel. This is what sets it apart from similar projects and allows for it operate to at roughly 10 per cent efficiency at producing alcohol fuels, when provided with pure CO2. [9]
What are the benefits of this technology?
What is most advantageous about the bionic leaf is that it could provide a net zero method of producing combustible fuels. The carbon dioxide released during the combustion of fossil fuels can be re used by the bionic leaf to build renewable fuels, in a similar manner to plants. Crucially, the leaf is made of cheap and readily available materials, and operates at a neutral pH,undernospecialconditions.Itcan simply be placed into tap water, exposedtosunlightandwillproducehydrogen or other combustible fuels. Nocera believes that the technology of the bionic or artificial leaf could be easily adopted into everyday life, in many households. He envisions the technology bringing cleaner fuels to those disconnected from the energy
grid, in developing nations. "This scienceyoucandoinyourbackyard.You don't need a multi billion dollar massive infrastructure.” [7]
The bionic leaf promises to provide a feasible alternative to the use of fossil fuels, through the reversal of the process of combustion. This has been achieved by replicating photosynthesis; using solar energy and to split water and convert the hydrogen produced and atmospheric carbon dioxide into fuel. Despite this, it is unlikely that this technology will be implemented imminently because is in its early stages of development. At the moment, the leaf cannot produce large volumes of fuel in a short time period, and so will not compete with fossil fuel prices for some time. Still, Nocera and Silver’s innovation offers hope for the future and a potential solution to reverse excessive and lethal carbon dioxide emissions. [7,8]
Diamonds: the symbol of love and rarity but also the cause of many brutal civil wars in several African countries, including Sierra Leone, as depicted in the movie Blood Diamond. Additionally, they cause huge environmental destruction due to irresponsible mining techniques resulting in acid mine drainage, contaminant leaching, air pollution (with a median CO2 footprint of 108.5Kg per carat) (1), rerouting of rivers, deforestation and soil erosion which leaves large areas of land impossibletofarmandwithnowildlife (2) . However, the profitability of these minesdependsontheperceivedrarity of diamonds; a myth fashioned by DeBeers (the company which for a long time controlled the production of most of the world’s diamonds) with their signature slogan ‘A diamond is forever’ used since 1948, in order to increase their price by making them artificially scarce (3) .
The technology and process to produce synthetic diamonds has existed since the 1940s. Due to the start of the Second World War, however, the project pioneered by General Electric (codenamed “Project Superpressure”) was initially postponed, succeeding years later in December 1954 to produce the first diamonds synthesised in a lab (4). The 1950s represented the start of large scale diamond manufacture which, due to them not being as high quality as natural diamonds, were used for industrial purposes, including laser optics and healthcare. Gem quality diamonds were first synthesised by researchers at General
Electric (GE) in 1970, followed by other manufacturers in the mid 1980s (5) But can these lab grown diamonds really replace a mined stone, uprooting the established $82billion diamond industry?
Diamonds naturally form around 150 200km deep in the Earth’s mantle where temperatures average 900 1300oC and pressures range from 45 to60kilobars.Intheseconditions,carbon atoms are able to crystallise into regular arrays and form diamonds (6); a process that can take millions of years. A narrow vertical pipe of igneous rock called kimberlite, created by a highly explosive volcanic eruption, brings the diamonds closer to the surface where they can be found and mined. The kimberlite ore (which is then mechanically broken down, separating thediamondsfromtherock)hasavery low diamond content with roughly one part diamond per million parts rock requiring large amounts or ore to be processed and yielding large volumes of waste.
Natural diamonds form an octahedral shape, with the diamond growing outward from the centre resulting in growth on all the faces, giving a concentric square pattern to the crystal.
Infrared spectroscopy (a process with uses infrared light and measures absorption, reflection and emission when used on a molecule) is the primary way of identifying different types of diamonds. In the 1930s, scientists began recognising that certain kinds of diamonds displayed similar properties; for example, a blue diamond often exhibited electrical conductivity. Based on differences in transparency toultravioletradiationandthefactthat nitrogen was the principal chemical impurity in natural diamonds (which was discovered in 1959), they grouped diamonds into two main categories: Type 1 which contain nitrogen, and the less common Type 2 which does not. Both types have subcategories that further differ in their atomic structure. Type 1A and 1B vary in how the carbon and impurity atoms are arranged; the former has aggregated nitrogen atoms while the latter has a single nitrogen atom replacing a carbon atom. Type 2B diamonds contain a small amount of boron while Type 2A are chemically pure.
There are two main techniques for growing diamonds in the lab: the High Pressure, High Temperature (HPHT) method and the Chemical Vapour Deposition (CVD) method. The former was the initial process used by GEandworksbymimickingtheconditions that naturally occurring diamonds form under. The latter, a more modern and increasingly popular
technology, uses lower pressures and temperatures than HPHT as well as cheaper equipment. As shown in the table opposite, the technique used to produce lab grown diamonds influences their shapes and properties.
This process uses a small diamond fragment known as a seed crystal which acts as a diamond template and a special apparatus which is able to produce the very high pressures that diamonds need in order to form. The seed is placed in a capsule containing graphite or another suitable pure carbon alternative which is dissolved in a molten flux, made up of a metal catalyst such as iron, nickel or cobalt, lowering the temperature and pressure needed for diamond growth. As the seed is cooler, the carbon material migrates through the flux towards it and crystalizes on the surface forming a synthetic diamond crystal. This process is much faster than forming naturaldiamondsasitoccursoveraperiod of several days to weeks to grow one or several different crystals.
With more recent technological advancements in the HPHT method, scientists have now been able to produce colourless crystals up to a size of 10 carats and above.
In order to make sure that the diamondssynthesisedareofahigher purity, the growth time must be increased with greater control over pressure and temperature conditions, while nitrogen, which when included will make the diamonds a yellow colour, must be excluded from the growthenvironment.Ifbluediamonds are desired, boron is introduced whereas other colours, including pink and red, are obtained through post growth treatment involving radiation and heating.
HPHT synthetic diamonds are easily distinguishable from natural ones due to their differing internal growth patterns. While natural diamonds, as shown above, typically form octahedrons, the HPHT technology forms diamonds which have cubic faces. In some cases, the synthesised diamonds may have dark flux metal (i.e. a metal solvent) inclusions or may exhibit phosphorescence which means that the material, having been exposed to ultraviolet light, will continue to glow even when the light source has been removed. Furthermore, diamonds that are grown with the HPHT method often have unique colour distribution, fluorescent zoning and graining patterns due to their cross shapedgrowthstructurewhicharenot found in natural diamonds.
Similar to the HPHT process, chemical vapour deposition requires a diamond seed crystal. However, unlike the above method, the seed is placed in a vacuum chamber which is then filledwithahydrogenandcarboncontaining gas such as methane. A microwave beam, or another suitable source of energy, is used to break down the gas molecules in the chamber. The carbon atoms in the gas then diffuse towards the colder diamond seed. Over a period of weeks, crystallisation takes place resulting in the growth of a diamond. With this method, the exact number of diamonds grown can vary depending on the size of the chamber used and the number of initial seed plates placed in the chamber.
The diamonds synthesised by CVD have a tubular shape and are brown in colour (which is removed by a heat treatment known as HPHT annealing). Theyoftenhavearoughedgeofblack graphite that is then cut away.
In the same way as the HPHT growth process, the CVD method also continues to improve due to technological advancements, resulting in larger sized of crystals with improved colour and clarity.
Despite the fact that natural and synthetic diamonds have the same chemical composition, their unique growing conditions contribute to them havingspecificfeatureswhichallowscientists to identify by which method they came about. These are summarised in the following table and are described further below.
transmitted light, but have a metallic shine in reflected light, are often present in HPHT synthetic diamonds. This isaconsequenceofthemetalsusedas catalysts entering the diamond during growth; these fluxmetal alloys contain elements such as iron, nickel and cobalt which can result in the diamonds being able to be picked up with magnets if they contain a large amount of these metallic inclusions. In contrast, dark graphite or mineral inclusions can be found in CVD synthesised diamonds instead of metallic inclusions which mean they do not have a metallicshinetothem.Naturaldiamondson the other hand, have none of these inclusions.
Colour distribution/zoning - During a diamond’s formation, any change in temperature or uneven incorporation of trace elements results in an irregular distribution of colour within a diamond. Natural diamonds tend to not have any colour zoning, however, whentheydo,theyarenotofaregular pattern. Coloured diamonds formed by the HPHT method usually present with a geometric colour zoning pattern as a result of how elements which contributetothecolourconcentratein the diamond during the formation process. On the other hand, CVD diamonds commonly have an even internal colouration.
Metallic flux inclusions and dark pinpoint inclusions - Metallic flux inclusions appear black and opaque in
Strain patterns. Stress, due to erupting from deep in the Earth’s mantle to the surface, can result in natural diamonds exhibiting a crosshatched or mosaic pattern of interference (or ‘strain’)whichcanbe seenusingpolarising filters angled at 90 degrees to one another. Synthetic diamonds do not show the same pattern of interference while CVD diamonds can display banded interference patterns while HPHT diamonds have no strain patterns or weak banded ones due to them growing at nearly uniform pressure and are consequently not subjected to any stress.
Fluorescence - Synthetic diamonds differtotheir natural counterparts due to differing fluorescence colours and patterns. For example, natural diamond fluorescence is stronger at long wavelength ultraviolet light while weaker at shorter wavelengths while the contrary is true for synthetic diamonds which also have distinctive colour patterns not present in natural diamonds. (7)
In a survey, prior to 2021, of which around 1,500 US consumers responded with their response to the question “What comes to your mind when you think about lab grown diamonds?”, the terms “Artificial” and “Fake” were the most prevalent (8) . When you compare this image to that aroundrealdiamonds,whichareassociated with glamour and romance, it is difficultto imagine that lab grown diamonds will survive and expand in the luxury market and be sold by top brands. However, unlike real diamonds which need to be mined, diamonds grown in labs provide an ethically sourced alternative which are typically sold at two thirds the price. With continued improvements in the HTHP and CVD technologies, these prices can be expected to drop further. This opens up synthetic diamonds to a wider market of luxury goods consumers who are more price sensitive. They can also appeal to consumers who opt for products that causelessdamagetotheenvironment and raise less concerns over human
rights issues. From a regulatory perspective, the US Federal Trade Commissions expanded its definition of a diamondin2018toincludelab grown stones which could further stimulate their increase in sales (9)
The industrial applications of diamonds also continue to grow. Recently, diamonds have found application in the rapidly expanding field of quantum computing. Unlike natural diamonds, lab grown diamonds will be able to support this industry due to their fast growth time and cheaper prices.
The diamond industry has many facets.Itisclearthat labgrowndiamonds will be essential for existing and new industrial applications that require the unique features of diamonds. In contrast,theextentofentryofsyntheticdiamonds into the luxury goods market will depend more on social trends related to economics, sustainability and ethical considerations.
-NadiaA quasicrystal is an aperiodic crystal whose structure bothdisplays the precision of a crystal lattice as well as amorphouscharacteristics,inwhichits pattern is more subtle and nonrepeating. (1)
Before the discovery of quasicrystallinesolidsin1984,therewereonlytwo known forms of solids: crystalline and amorphous solids.(2) Quasicrystals were allegedly discovered, through the use of an advanced electron microscope, by Dan Shechtman (researcher from the Israel Institute of Technology), after the process of mixing aluminium with manganese in an
approximately six to one proportion caused a pericyclic reaction and resulted in an alloy of five fold symmetry (symmetry forbidden in crystallography and quasiperiodicity ) (3) This process involved the rapid cooling of this molten manganese and aluminium alloy through ‘melt spinning’. This discovery was such a phenomenon thatitgaverisetoalotofcontroversial thoughtsandscepticism,evenleading to Schechtman being dismissed from the lab by his boss who initially doubted his findings. (2) But what Shechtman’s boss didn’t know was thatShechmanhimselfwouldgoonto win the 2011 Nobel Prize in chemistry for his incredible discovery.
ing the axes) to be seen. (4) The reasoning behind why there is an irregularityinperiodicityis simplyduetothe idea that pentagons placed side by side will not fill the space like a sequence of squares would. This was demonstrated by Pat Theil’s (scientist at the US Energy Departments Ames Laboratory) bathroom tiling analogy. Therefore, additional atomic shapes are naturally added to fill in the gaps. (5)
Icosahedralquasicrystalswerethefirst reported quasicrystal. They exhibit long range order, meaning both symmetry and the regularity of particle arrangement are present within the structure, however unlike regular crystals, there is a lack of translational periodicity. This is implied by the five fold symmetry which can be identified usingelectrondiffraction,allowingthe ten concentric rings of spots (indicat-
Quasicrystals have been proven to have many favourable qualities such as being light absorbing as well as possessing beneficial electrical properties. However, suspicions were raised when miniscule cracks within thequasicrystalscalled‘grainboundaries’werediscovered.Thesecracksresulted in the quasicrystals becoming more susceptible to risks such as the onset of corrosion, making them ineffective substances for domestic use. Despite this set back, it was later
proven that the collision between two or more small quasicrystals results in the formation of a crystal which no longer possesses the grain boundary or the consequent imperfections. This processwasvirtuallyrecreatedinalab multiple times in order to obtain the precise conditions necessary for the combining of these quasicrystals. (6) With our knowledge of quasicrystals
increasingly growing, it is possible that quasicrystalline products will become more abundant and it may even become easier to find new types of them forming naturally beyond the walls of the labs.
-ErinQuantum Physics is arguably one of the most ground breaking branches of physics today. It has shown us a whole new side to the scientific realm: the absurd rules that govern the very tiny. Quantum physics plays a part in much of everyday life, for instance helping our mobile phones to work, by helping to design the silicone based elements of our phone circuits. Many concepts that are known today inquantumphysicsactuallygrewfrom discoveries about light and radiation made by physicists in the 18 and 19 hundreds.So,inthisarticleIwantedto enlighten you about some of these discoveries and how they led to the growth of this theory.
One of the first things that led to growth of quantum physics, was questions about the nature of light. This began with the renowned Isaac Newton, who had some very interesting ideas about particles. He held the belief that light was made up of many small particles called corpuscles. However, this belief was not held by some other scientists of the time. A Dutch scientist named
Christiaan Huygens believed that light was a wave, comparing it to the way that pebbles caused ripples in water. At first, Huygens and his theory about waves was not shared by most at the time.However,later,ascientistnamed Youngwasabletoprovethatlightwas a wave. He used the double slit experiment to show that light did followthepatternofthewave,aswhen light was filtered through a single slit and a double slit, it spread out in circles, behaving exactly as a wave should. However, we now know that light is actually a particle and a wave, depending on whether it is being observed or not.
Now these reflections on light were furthered by Gustav Kirchhoff’s discovery of blackbody radiation. This was essentially the spectrum of radiation emitted from a ‘blackbody’ or perfect emitter when it is heated. There is very little radiation emitted at short wavelengths, and long wavelengths, while a lot of radiation is emitted at middle wavelengths. This seemed confusing at the time, because it went against the classical
laws of physics. There should be far more radiation emitted at shorter wavelengths, as there should be an infinite amount of energy at these wavelengths. So, Kirchhoff’s discoveries seemed to disagree with traditional laws of physics when it came to shorter wavelengths, but fitted the pattern suggested by physics when it came to longer wavelengths. This was quite a conundrum, known as the ultraviolet catastrophe, however later developed by a man named Max Planck, who revolutionised quantum physics.
Planck was most interested in thermodynamics, the study of thermal energy. He worked for a long time on the ultraviolet catastrophe and in the end, merged two equations, to create one that successfully described the blackbody radiation However, Planck’s work was not finished. He then merged his blackbody radiation equation with some statistical equations, believing that energy emitted and absorbed by electrons in an atom must only be in a certain constant amount, called a quantum, and the energy of such a quantum must be related to its frequency where we get the name quantum physics.Theequationhecameupwith wasEnergy=hxfrequency,wherehis a new constant named Planck’s constant; a constant that today has become central to quantum physics, describing the quantum nature of energy. Planck’s work showed that there was a limitation to classical physics, and so much more to explore beyond that, through light and particles.
Now, Planck’s worth was furthered even more by none other than Albert Einstein. Einstein was very interested
in developing Planck’s ideas and finding the physical implications to his equation.AllhedidwasapplyPlanck’s ideas of quanta to electromagnetism. He said that light is not made up of waves, but little packets of lights, in essence, particles, which we now call photons. Photons each have the same frequency, explaining why the same amountofenergyisemittedeachtime by electrons, as said by Planck. Now Einstein used this to explain the electromagnetic attractions, suggesting that when photon hits an electron, it gives it energy, causing it to move. Different wavelengths of light simply mean that each photon is carrying a different amount of energy. Einstein was actually against the idea of quantum physics when it began to be theorised, believing it to be too absurd, and describing a central part of it, quantum entanglement to be “spooky action at a distance”. However, this work of his on photons endedupcontributingtothequantum theory of electrodynamics, which described electromagnetism at a quantum level, and it is this work that won him his famous Nobel Prize in 1922.
Now, these are just some of the ground breaking theories that led to the growth of quantum physics, however they hold great importance. Newton’s questions about light led to people drawing more attention to the science of light, and Kirchoff’s blackbody radiation eventually led to Planck theorising his constant and Einstein theorising photons. Quantum physics is one of the most interesting and vital theories in physics, so I hope through this article I have shed some light on how it grew.
-AnantaaScientists claim that the Universe started 13.8 billion years ago, with the Big Bang. But how did this happen? And what will happen in the future?
Theuniversebeganfromanindividual pointofhighdensityandtemperature. The forceful explosion of this small point was named the Big Bang. Scientistsbelievethattheexplosionsentenergy out at the speed of light (300,000,000 ms 1) and at 1000 trillion degrees Celsius. However, this explosion did not occur within something, like space, but rather, it was so powerful that scientists believe it created space itself.
After this initial explosion, scientists split time into 5 stages. These are as follows:
• The very early universe
• The early universe
• The Dark Ages
• The universe as it appears today
• The far future and ultimate fate
But what exactly happened in the very early universe?
And what awaits us in the future?
Physicists consider this stage to have lasted from 10 43 to 10 22 seconds after the Big Bang a very small amount of time. The main problems that physicists face, is explaining how the universecamefromnothing,becausethis goes against one of the fundamental laws of physics: conservation of energy. This law states that energy cannot be created or destroyed, only
transferred from one state to another. However,weknowwehavealotofenergy in the Universe now.
The question is, how did it get there?
The answer we don’t know yet.
The universe was at such a high temperature and density, that the modern laws of physics don’t seem to apply at all. This means that we can’t replicate or study the conditions at the time, makingitimpossibletocarryoutaccurate experiments. The best we can do with the current technology is make theoretical assumptions.
The first epoch, or period of time, was the Planck epoch. This epoch occurred less than 10−43 seconds after the Big Bang. Very little is known about this epoch, mainly because modern day physics falls apart; the 3 subatomic particles (protons, neutrons and electrons) and even the 4 fundamental forces (strong, weak, electromagnetic, gravitational) couldn’t form because of the conditions. Most of the assumptions we have about this epoch rely on the theory of general relativity by Einstein, but even this theory falls short at this level, due to the quantum effects.
To sum up, the beginning of the universe was very hot and very dense, andthistopiccontinuestoconfusescientists today, due to the lack of knowledge we have about how physics works in these conditions. As the universe begins to cool, the fundamental forces, as well as many particles we are now familiar with could also form. This means that we know quite well what happened during the
‘inflation’ or the period where the universe started expanding very rapidly.
The beginning, however, continues to elude us.
But first, let us briefly look at a theory which tries to explain the Big Bang. As we discussed before, one of the biggest questions we have is how something could come from nothing, and yet the universe continues to expand into supposed nothingness. One of the theories, which attempts to link Einstein’s theory of general relativity and quantum mechanics, relies on the notion of quantum fluctuations. The breakthrough occurred in 1960, when thephysicistsJohnWheelerandBryce DeWitt managed to combine the two ideas into what we now call, the Wheeler DeWitt equation. Scientists andmathematiciansaregettingcloser and closer to finding an answer as our technology and ideas progress. In 2014, mathematicians from the WuhanInstituteofPhysicsandMathematics in China managed to come up with a rigorous proof, helping to bring scientists closer to finding out what exactly happened all those years ago.
Now, let us go back to the future, and see what will happen to the universe as it continues to grow.
There are 3 main theories.
The first is the Big Freeze, or the Big Chill. This theory states that eventually, as all things do, stars and galaxies will exhaust their energy. Eventually, the Universe will reach a point, at which everything has stopped completely. Since nothing has energy, no changes will occur, and our Universe will remain cold and desolate forever.
This is quite a bleak outlook, but it is alsothemostpeacefuloutofthethree options that you will see here.
The second is the Big Rip. This theory statesthattheuniversewillbegintoincrease at a faster rate, and eventually the galaxies, stars and even atoms themselves are ripped apart.
The third one is the Big Crunch. This theory claims that gravity will eventually increase in strength and begin to pull the universe back into its initial condensed point, until everything is crunched back into a point.
Both the second and third theory rely on dark matter creating instability within the universe. However, as we know,allgoodthingsmustcometoan end.Wedoknowthatouruniversewill have an ending; scientists aren’t quite sure exactly what it will look like yet, though.
And, if what Wheeler and DeWitt believed is true, there might be other universes out there, which have long expired or which are yet to be born.
After reading this, you may have ended up with more questions than answers, but that is the beauty of science. No matter how much we learn and understand, there is still more to find out. And who knows what we will discover next? Eventually we might understand exactly the Universe began its story, or how it will end.
-Anonymous
[1]https://mindmatters.ai/2022/03/yes the human brain is the most complex thing in the universe/ [2]https://www.thelancet.com/journals/la neur/article/PIIS1474 4422(18)30499 X/fulltext
[3] https://www.rit.edu/spotlights/blood brain barrier
[4] https://qbi.uq.edu.au/brain/brain anatomy/what blood brain barrier
[5]https://www.ncbi.nlm.nih.gov/pmc/arti cles/PMC4292164/#:~:text=The%20bloo d%20vessels%20that%20vascularize,the %20blood%20and%20the%20brain
[6]https://www.smithsonianmag.com/sm art news/mini brains grown stem cells developed eyes can sense light 180978478/
[7] https://stemcell.ucla.edu/induced pluripotent stem cells#:~:text=iPSC%20are%20derived%2 0from%20skin,cell%20needed%20for%2 0therapeutic%20purposes
[8]https://scanberlin.com/2019/10/20/m eet the organoids/
[9]https://www.cam.ac.uk/research/news /lab grown mini brains hint at treatments for neurodegenerative diseases
[10] https://ec.europa.eu/research and innovation/en/horizon magazine/mini brains offer hope search new drugs brain disorders
[11]https://geneticliteracyproject.org/20 17/09/26/mini brains aid study dementia mental illness
https://www.ncbi.nlm.nih.gov/pmc/ar ticles/PMC4715268/
https://www.ncbi.nlm.nih.gov/pmc/articl es/PMC2849637/ https://www.ncbi.nlm.nih.gov/pmc/articl es/PMC4783224/ https://www.ncbi.nlm.nih.gov/pmc/articl es/PMC4928883/
[1] Fitness and competitive growth advantage of new gentamicin susceptible MRSA clones spreading in French hospitals | Journal of Antimicrobial Chemotherapy | Oxford Academic (oup.com)
[2] Using cheaper antibiotics to treat MRSA infection in India | The BMJ
[3] Methicillin resistance in Staphylococcus aureus PMC (nih.gov)
[4] Glycopeptides: Drug Class, Uses, Side Effects, Drug Names (rxlist.com)
[5] Efficacy and safety of linezolid versus vancomycin for the treatment of complicated skin and soft tissue infections proven to be caused by methicillin resistant Staphylococcus aureus PubMed (nih.gov)
[6] Fighting bacteria without antibiotics | Jody Druce | TEDxYouth@ISPrague
https://medlineplus.gov/ency/patientinst ructions/000741.htm#:~:text=Red%20bl ood%20cells%20help%20create,and%20 the%20wound%20gets%20smaller
https://www.medicalnewstoday.com/arti cles/318961#Cartilage and bone https://www.medicalnewstoday.com/arti cles/318961#Blood comes first https://www.newscientist.com/article/23 36913 wound dressing made of 3d printed skin could help people with diabetes/ https://www.newscientist.com/article/23
42040 gel made from okra stops bleeding in damaged hearts/
[1] Growth hormone injections: Uses and side effects (medicalnewstoday.com)
[2] Human Growth Hormone (HGH) Injections: Uses, Brands, Benefits (kingsbergmedical.com)
[3] Human Growth Hormone Injections for Adults | Types, Price, Comparison (hgha.com)
[4] HGH Injections | Benefits & Side Effects | Types & Cost (medzone.clinic)
[5] Growth Hormone Deficiency in Children: Testing, Symptoms, Causes & Treatment (emedicinehealth.com)
Carbon nanotubes & how they grow
American Chemical Society.2010 www.sciencedaily.com/releases/2010/09/100915140334.htm
Aron, J. 2016. Carbon nanotubes too weak to get a space elevator off the ground. https://www.newscientist.com/article/2093356 carbon nanotubes too weak to get a space elevator off the ground/ Baughman R.H. 2010. Carbon nanotubes The route toward applications. Science vol 297, pp.787 792. Boyson, Muir et al. 2011. Nanotechnology for Dummies, p. 76. Hoboken, New Jersey: Wiley Dai,H. 2002. Carbon Nanotubes: Synthesis, Integration, and Properties, Acc.Chem.Res 35(12), pp.1035 1044
Delzeit, L.D. 2004. Patterned growth of carbon nanotubes or nano fibers. https://www.techbriefs.com/component/content/article/tb/pub/briefs/materials/1663
Faktri, N. et al. 2009. Diameter dependent bending dynamics of single walled carbon nanotubes in liquids. Proceedings of the National Academy of Sciences 106
https://www.pnas.org/doi/10.1073/pnas. 0904148106
Grumezescu, A.M. 2018. Fullerens, Graphenes and Nanotubes, A Pharmaceutical Approach. Oxford: William Andrew, Elsevier Hu, L. Et al. 2009. Highly conductive paper for energy storage devices. Proceedings of the national Academy of Sciences [Online]106. Available at: https://www.pnas.org/doi/full/10.1073/p nas.0908858106
Jahanshahi, M. and Kiadihi, A.S. 2013. Fabrication, Purification and Characterisation of carbon nanotubes: Arc Discharge in Liquid Media (ADLM). In: Suzuki, S (ed.). 2013, Syntheses and Applications of Carbon Nanotubes and Their Composites, IntechOpen, London. Jindal, V. 2012. Re: What is the difference between a SWCNT and MWCNT and expecting your explanation in terms of its applications. https://www.researchgate.net/post/What_is_the_difference_between_a_SWCNT_a nd_MWCNT_and_expecting_your_explanation_in_terms_of_its_applications
Jung, R. et al. 2008. Electrically conductive transparent papers using multi walled carbon nanotubes. Polymer Physics vol 46(12), pp.1235 1242 Kagoshima, S., Nagasawa, H.& Sambongi, T. 1988. What Are One Dimensional Conductors? In: One Dimensional Conductors. Springer Series in Solid State Sciences, vol 72: Springer, Berlin, Heidelberg.
Kumar, M. 2010. Carbon nanotube synthesis and growth mechanism. In: Yellampalli, S (ed.). 2011, Carbon Nanotubes Synthesis, Characterization, Applications, IntechOpen, London. Chapter 3
Liu, C. and Cheng, H. 2013. Carbon nanotubes: controlled growth and application. Materials today 16 (1 2), pp. 19 28
Mehra N.K. and Palakurthi S. 2016. Interactions between carbon nanotubes and bio actives: a drug delivery perspective. Drug Discov Today 21(4), pp.585 97
Murr, L E et al. 2004. Chemistry and nanoparticulate compositions of a 10,000 year old ice core melt water. Water research 38(19), pp. 4282 96.
Muzammil, S.B. et al. 2019. Effect of MWCNT inclusions on tensile behavior of G CFRP and G KRFP hybrid nanocomposites. AIP Conference Proceedings [Online] 2057 (1) https://aip.scitation.org/doi/10.1063/1.5085613
Nagai, H. Et al. 2011. Diameter and rigidity of multi walled carbon nanotubes are critical factors in mesothelial injury and carcinogenesis. Proceedings of the National Academy of Sciences [Online]108 https://www.pnas.org/doi/10.1073/pnas. 1110013108
Purohit, R. Et al. 2014. Carbon Nanotubes and their growth methods. Procedia Material Science 6, pp. 716 28
Sattar, R., Kausar, A., & Siddiq, M. 2015. Advances in thermoplastic polyurethane composites reinforced with carbon nanotubes and carbon nanofibers: A review. Journal of Plastic Film & Sheeting, vol 31, pp186 224.
Slomski. 2010. Graphene and Carbon Nanotubes. https://medium.com/prime movers lab/graphene and carbon nanotubes part 1 2092776fc5bf
Wang, X., Vinodgopal, K., Dai, G., 2019, 'Synthesis of Carbon Nanotubes by Catalytic
Chemical Vapor Deposition', in H. E. Saleh, S. M. M. El Sheikh (eds.), Perspective of Carbon Nanotubes, IntechOpen, London. 10.5772/intechopen.86995. Yoshida, H. et al. 2007. Growth of single walled carbon nanotubes on silicon nanowires. Solid State Communications 141(11), pp.632 634
[1] Ciamician, G. (1912). The Photochemistry of the Future. Science, [online] 36(926), pp.385 394. Available at: https://www.jstor.org/stable/1637055#metadata_info_tab_contents [Accessed 30 Oct. 2022].
[2] www.projectsolaruk.com. (2021). How Efficient Are Solar Panels? | Project Solar
UK. [online] Available at: https://www.projectsolaruk.com/blog/how efficient are solar panels/ [Accessed 30 Oct. 2022].
[3] Azvolinsky, A. (2017). Make like a leaf: How copying photosynthesis can change society. [online] New Scientist. Available at: https://www.newscientist.com/article/mg23431210 400 make like a leaf how copying photosynthesis can change society/ [Accessed 30 Oct. 2022].
[4] L. Chandler, D. (2011). ‘Artificial leaf’ makes fuel from sunlight. [online] MIT News | Massachusetts Institute of Technology. Available at: https://news.mit.edu/2011/artificial leaf 0930 [Accessed 30 Oct. 2022].
[5] Bennett2008 07 31T15:10:00+01:00, H. (2008). Breakthrough catalyst for splitting water. [online] Chemistry World. Available at: https://www.chemistryworld.com/news/breakthrough catalyst for splitting water/3000627.article [Accessed 30 Oct. 2022].
[6] Nocera, D.G. (2012). The Artificial Leaf. Accounts of Chemical Research, 45(5), pp.767 776. doi:10.1021/ar2003013 [Accessed 30 Oct. 2022].
[7] Biello, D. (2015). ‘Bionic Leaf’ Makes Fuel from Sunlight. [online] Scientific American. Available at: https://www.scientificamerican.com/article/bionic leaf makes fuel from sunlight/ [Accessed 30 Oct. 2022].
[8] Biello, D. (2016). Bionic Leaf Makes Fuel from Sunlight, Water and Air [online] Scientific American. Available at: https://www.scientificamerican.com/article/bionic leaf makes fuel from sunlight water and air1/ [Accessed 30 Oct. 2022].
[9] Martin, R. (2016). A Big Leap for an Artificial Leaf. [online] MIT Technology Review. Available at: https://www.technologyreview.com/2016/06/07/159777/a big leap for an artificial leaf/ [Accessed 30 Oct. 2022].
[1] http://www.imperial consultants.co.uk/wp content/uploads/2021/02/Final report Environmental Impacts of Mined Diamonds.pdf [2] https://blueandgreentomorrow.com/environment/true environmental and social costs of mined diamonds/ [3] https://www.luxuryacademy.co.uk/diamond engagement ring/ [4] https://www.gemsociety.org/article/brief history of lab grown diamonds/ [5] https://www.gia.edu/gia news research/manmade diamonds questions answers [6] https://www.capetowndiamondmuseum.org/about diamonds/formation of diamonds/ [7] https://www.gia.edu/hpht and cvd diamond growth processes [8]https://www.forbes.com/sites/pamdan ziger/2021/02/14/lab grown or natural diamonds the choice is getting clearer for consumers and retailers/?sh=4cc918bb62f9 [9]https://www.youtube.com/watch?v=s1 h4gze_ktk