
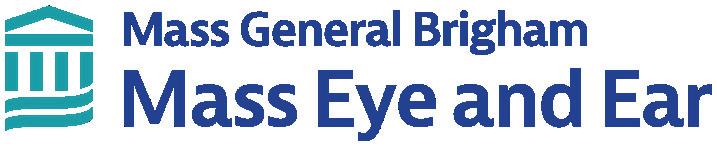
MASS EYE AND EAR I BOSTON, MA I JANUARY 24, 2025
Course Directors
Marta Stevanovic, MD, MSc and Joan W. Miller, MD
Resident Coordinators
Edward S. Lu, MD and Rachel Tandias, MD
2025 Claes H. Dohlman Visiting Professor in Ophthalmology
J. Fernando Arevalo, MD, PhD
Edmund F. and Virginia B. Ball Professor of Ophthalmology, Johns Hopkins School of Medicine Chief, Wilmer at Johns Hopkins Bayview Medical Center
The complete course book is available here:
Friday, January 24, 2025 - Mass Eye and Ear,
11:00 - 11:30 AM I REFRESHMENTS
11:3011:35 AM
11:3512:00 PM
12:0012:25 PM
12:2512:50 PM
12:501:15 PM
Welcome and Overview
Edward S. Lu, MD and Rachel Tandias, MD
Laser-based Refractive Surgery: A Review of Select Clinical Challenges and Future Directions
Dennis Akrobetu, MD and Roberto Pineda II, MD
Private Equity in Ophthalmology: An Innovation Here to Stay?
Darren A. Chen, MD and Jo-Ann Haney Tilton, MD
Expanded Field Swept-Source Optical Coherence Tomography Angiography in the Assessment of Proliferative Diabetic Retinopathy and Associated Complications: A Review
Edward S. Lu, MD and John B. Miller, MD
Refractory Macular Hole Surgery: A Review of Recent Surgical Innovations
Ryan S. Meshkin, MD and Dean Eliott, MD
1:15 - 2:00 PM I LUNCH
2:002:25 PM
2:252:50 PM
2:503:15 PM
3:153:40 PM
Innovation in Keratoprostheses: A Review of Modern Devices and Strategies for Preventing and Managing Complications
Rachel Tandias, MD and Thomas H. Dohlman, MD
Artificial Intelligence in Oculoplastic Surgery: Current Landscape
Hursuong Vongsachang, MD, MPH and Michael K. Yoon, MD
Innovations in Big Data and ‘Omics towards Phenome- and Genome-Wide Association Studies for Retinal Disease
Seyedeh Maryam Zekavat, MD, PhD and Elizabeth Rossin, MD, PhD
Gene Therapy in Age-Related Macular Degeneration
Henry W. Zhou MD, MS and Leo A. Kim, MD, PhD
3:40 - 4:00 PM I BREAK & CLASS PHOTOS
4:004:05 PM Introduction of Dr. J. Fernando Arevalo Demetrios Vavvas, MD, PhD
4:055:05 PM
2025 Claes H. Dohlman Visiting Professor Lecture “Retinal Prosthesis Update”
J. Fernando Arevalo, MD, PhD
5:055:10 PM Conclusion
Edward S. Lu, MD and Rachel Tandias, MD
6:00 - 9:00 PM I RECEPTION & DINNER (by invitation only)
J. Fernando Arevalo, MD, PhD
Edmund F. and Virginia B. Ball Professor of Ophthalmology, Johns Hopkins School of Medicine
Chief, Wilmer at Johns Hopkins Bayview Medical Center
Dr. Arevalo completed his medical and ophthalmology training in Caracas, Venezuela (his native country), before traveling to Bogota, Colombia for a two-year Retina and Vitreous fellowship. After this intensive training, he traveled to the United States for a two-year Retina and Vitreous/Uveitis and Intraocular Inflammation fellowship at UCSD. The following year, Dr. Arevalo went to Philadelphia for an Ocular Oncology fellowship at Wills Eye Hospital.
After a successful academic career in Venezuela for 15 years, Dr. Arevalo was invited by Johns Hopkins University in Baltimore to work as a Professor of Ophthalmology and the Chief of the Retina Division of the King Khaled Eye Hospital (KKESH) in Riyadh, Saudi Arabia for a four-year tenure, followed by an appointment at the Wilmer Eye Institute as the Edmund F. and Virginia B. Ball Professor of Ophthalmology in the Retina Division, and an appointment as chief of ophthalmology at Johns Hopkins Bayview Medical Center in 2015. As a clinical scientist, Dr. Arevalo has more than 900 scientific publications (400 in Pubmed), 18 books, and more than 1300 invited lecture presentations that have led to international recognition and awards.
Dennis Akrobetu, MD, Roberto Pineda II, MD
Abstract
Advances in laser-based corneal refractive surgery have allowed for a diverse array of surgical options available to patients seeking vision correction and contact lens/glasses-free independence. The most common laser-based corneal refractive surgeries include laser-assisted in situ keratomileusis (LASIK), photorefractive keratectomy (PRK), and small incision lenticule extraction (SMILE). Although advancements in the technology have been made, select clinical challenges such as higher-order aberrations, post-operative ectasia, diffuse lamellar keratitis (DLK)/central toxic keratopathy (CTK), and pressure-induced interlamellar stromal keratitis (PISK) remain as post-refractive issues to be addressed in laser-based corneal refractive surgery. Recent improvements in preoperative evaluation, the development of instrumentation to measure and treat higher-order optical aberrations, and new techniques for the treatment of presbyopia all serve as unique avenues for innovation in the field. Despite its many advances, laser-based corneal refractive surgery has specific clinical limitations which may be better addressed through lens-based refractive techniques.
Refractive error is one of the leading causes of visual deficit globally1-4. Myopia, which serves as the most common form of refractive error5,6, has increased in incidence around the world with billions of people projected to be affected over the next 50 years7. Given the high prevalence and increasing impact of refractive error across the globe, the popularity of refractive surgery as a means to achieve vision correction has also escalated. In addition, advances in technology have expanded the variety of refractive surgeries available to patients4,8. Focusing specifically on laser-based refractive surgeries, the most common procedures include LASIK, PRK, and SMILE. Although the efficacy of refractive surgery is very high, complications such as induced higher-order aberrations, post-operative ectasia, DLK, CTK and PISK remain as potential clinical challenges4,9-12. Recent advancements in the preoperative evaluation and instrumentation to measure higher-order optical aberrations coupled with refinements in excimer laser delivery have led to the development of wavefront-guided, wavefront-optimized, and topographyguided treatment4,13-17. In addition, the use of stromal lenticule implantation (acquired through SMILE) and refinements in laser software for the treatment of presbyopia serve as two areas of innovation in the field18. Despite its many breakthroughs, cornea refractive surgery has certain clinical limitations which may be better addressed through lens-based refractive techniques.
Overview
LASIK
LASIK surgery is a common refractive surgery technique that involves the creation of an anterior corneal lamellar flap (Fig. 1). The main advantages of LASIK are that it is associated with early visual recovery, less post-operative pain, reduced stromal inflammation, and a lower chance of post-operative corneal haze in comparison to PRK19,20 Careful patient selection with tomographic assessment is needed for proper preoperative evaluation of LASIK candidates given the small risk of post-operative ectasia4,21. Furthermore, there is a chance of degradation of dry eye syndrome after LASIK, highlighting the need for thorough evaluation for preexisting dry eye syndrome4,21,22
In PRK, unlike LASIK, no epithelial flap is created. Instead, the epithelium is removed through a variety of techniques, and a 193nm excimer laser is used to expose and ablate the corneal stroma4,23 (Fig. 2). After laser ablation, the epithelium is allowed to regenerate over the ablated corneal surface4,23 Since PRK does not rely on the creation of a flap, there is no chance for flap related complications associated with LASIK. Also, the absence of a flap in the cornea may result in a biomechanically stronger cornea, and there is less of a risk of worsening dry eye syndrome with PRK in comparison to LASIK4,24,25 Although the overall risk is low, there tends to be a greater incidence of post-operative haze in PRK when compared to LASIK26 Regeneration of the epithelium and corneal surface takes time so PRK can be associated with higher amounts of post-operative pain and a longer amount of time before the final visual outcome is achieved in comparison to LASIK27
Unlike LASIK and PRK, SMILE involves the use of a 1064nm femtosecond laser to shape a lenticule of the desired correction within the cornea which is then extracted through a small corneal incision28,29. Similar to PRK, SMILE also affords all the advantages of a flapless laser corrective surgery technique4. It is reported to lead to a lower incidence of post-operative dry eye syndrome in comparison to LASIK
surgery30,31. In addition, given the lower laser energy requirements, there is thought to be fewer induced higher-order aberrations and corneal inflammation32,33. However, in comparison to LASIK, most studies cite slower visual recovery34 Despite the many advantages of SMILE, given the fact that it involves manual lamellar dissection (Fig. 3a) within the cornea followed by efficient extraction of the corneal lenticule, it is a more technically challenging surgery in comparison to LASIK and PRK (Fig. 3 b and c) 4 As a result, a much steeper learning curve is required for refractive surgeons35,36. Novice refractive surgeons may be faced with a myriad of complications including unsuccessful lenticule extraction, retention of corneal lenticule fragments and stromal scarring, all of which can lead to worse vision in comparison to LASIK or PRK35,36 In addition, the use of a femtosecond laser in SMILE has also been linked to a slightly higher incidence of post-operative rainbow glare than LASIK37,38. Rainbow glare is a condition in which patients report seeing a spectrum of colored bands emanating from a white-light source in dark environments37,38 Overall, SMILE is a technology that is expected to advance significantly over the next few years with several companies expecting to enter the SMILE market.
Figure 3. a) Dissection of lenticule interface39. b and c) lenticule extraction4,39
Higher order aberrations
Optical aberration refers to the difference between a real image and an ideal image and is a direct result of the ability of light to be refracted via Snell’s Law40. Ideally, light should focus on one optical point within the eye. However, since the eye is not usually a perfect circle for focusing incoming light, an optical aberration can occur as a result of light focusing at multiple points within the eye40. Myopia, hyperopia, and astigmatism are considered lower-order aberrations and are readily addressed by many of the common laser-based corneal refractive surgery techniques41,42. Higher order aberrations (spherical, coma, trefoil, etc) also exist and often result in less defined visual symptoms such as haloes, glare, and ghost-images4,43,44 Furthermore, higher-order aberrations tend to increase in effect after correction of lower-order aberrations through refractive surgery40. The development of wavefront- and topographyguided technology (see below) have worked to reduce the incidence and symptoms of post-operative higher-order aberrations45,46. However, many limitations still exist in current laser platforms for addressing all higher-order aberrations, especially in particularly aberrated corneas47,48
Akrobetu, Dennis
Corneal-based refractive surgery often involves reshaping the cornea or the creation of a flap to achieve refractive outcomes. Rarely, this can lead to weakening of the biomechanical strength of the cornea which can then progress to corneal ectasia49. Post-operative ectasia is most commonly seen after LASIK surgery but has also been reported after SMILE4,49,50. Ectatic changes post LASIK/SMILE often results in progressive corneal steepening inferiorly or centrally which can eventually result in decreased quality of vision from severe irregular astigmatism49 Biomechanical instability of the cornea has been identified as the underlying mechanism for postoperative ectasia after corneal-based refractive surgery51-53 and serves as the basis for many of the predisposing risk factors for the complication including forme-fruste keratoconus, low residual stromal bed thickness (through high myopia, thin preoperative cornea, or thick LASIK flap) and irregular corneal topography50. Advances in preoperative management (discussed below) have allowed for improvement in early detection of these clinical risk factors.
DLK can occur after any lamellar-based surgery in which a corneal lamellar incision allows for an interface through stromal tissue54. Initially, it was described most commonly after LASIK but has since been observed in patients undergoing other lamellar corneal surgeries including SMILE and Descemet stripping automated endothelial keratoplasty (DSAEK)55-57. Clinically, it manifests as sterile inflammation of the cornea characterized by diffuse, multifocal infiltration at flap interfaces54 Early signs of DLK can present on post-operative day one, but, in some cases, signs may not be evident until several days after lamellar corneal surgery54 A 4-stage classification system is commonly used for accurate characterization of the location and severity of DLK58 Stage 1 is characterized by involvement of granular cells along the periphery of the lamellar flap (Fig. 4a)58. Stage 2 is characterized by central migration of previously peripheral cells (in Stage 1) towards the central visual axis (Fig. 4b)58. Stage 3 results when there is marked aggregation of white, coalesced cells in the central visual axis with peripheral clearing (Fig. 4c)58 Stage 4 is characterized by the addition of severe complications from keratitis including stromal melt, flap melt, and severely limited visual acuity (Fig. 4d)9,10 Although no one specific etiology has been decided as the driving factor for DLK, many different possible causes have been proposed including femtosecond flap creation, immune dysregulation, bacterial endotoxin and corneal epithelial injury52,53,59,60 Management of DLK can be challenging and most often consists of aggressive use of topical steroids58 . Mechanical irrigation of the flap interface in LASIK can also be employed in more severe cases of DLK58 Central toxic keratopathy (CTK) is a complication of corneal-based laser refractive surgery closely related to DLK but entirely separate. At one point, CTK was considered to be part of the DLK clinical spectrum. However, it was eventually categorized separately due to the absence of inflammatory cells and lack of response to topical steroids both of which serve as two key features of DLK12. CTK often presents within one week of corneal-based laser refractive surgery and is typically characterized by central striae and a focal, white-appearing central opacity without any associated inflammation or inflammatory cells61,62 Unlike DLK, the management of CTK involves observation only given that the condition is self-limiting and often resolves over a span of months63
4. Stages of diffuse lamellar keratitis58 (a) Stage 1 (b) Stage 2 (c) Stage 3 (d) Stage 4
Pressure-induced interlamellar stromal keratitis (PISK)
PISK is a rare sight-threatening complication of refractive surgery and is characterized by fluid collection at the flap interface64-66 It is most commonly observed after LASIK surgery but has been reported after SMILE and lamellar keratoplasty64. PISK occurs when elevated intra-ocular pressure leads to dysfunction of the endothelial pump system resulting in corneal edema as corneal cells imbibe with fluid64,65 However, since LASIK and SMILE lead to the creation a potential low-pressure space between the corneal flap and stromal bed, fluid can accumulate in this potential space leading to PISK64,67 In both PISK and DLK, examination reveals haze confined to the flap interface65. However, in sharp contrast with DLK, patients with PISK worsen with the treatment of topical steroids and improve only after reduction or discontinuation of steroids along with initiation of intraocular pressure lowering drops65
Innovation and Future Directions
Improved preoperative evaluation
Accurate preoperative assessment is critical to successful refractive surgery outcomes. Thorough evaluation allows for detection and exclusion of any potentially contraindicated corneal conditions such as subclinical corneal ectasia21. Generally, traditional preoperative evaluation includes the use of corneal tomography which provides insight into the corneal surface shape18. Recent advances in preoperative evaluation now allow for the addition of corneal biomechanics to corneal topography as a potential way for more accurate preoperative assessment of refractive surgery candidates18,68 One such example is the use of the corneal visualization tonometer which uses an ultra-high speed Scheimpflug camera that visualizes corneal changes during deformation to produce useful preoperative parameters68. In one study, biomechanical parameters obtained from the Corvis ST tonometer were integrated with Pentacam HR topography and analyzed using artificial intelligence, and the combination of the two preoperative strategies allowed for greater accuracy in determining subclinical keratoconus among eyes which were categorized as normal with topographic assessment alone69 Recent developments in Brillouin microscopy may also allow for improved evaluation of corneal biomechanics using quantum light scattering70-74 Optical coherence tomography (OCT) can also serve as a useful supplement to preoperative evaluation75,76. For example, high-resolution swept-source OCT imaging can provide detailed anterior segment imaging and measurements in one platform75,76. High frequency ultrasound
Akrobetu, Dennis
analysis of the cornea in combination with high-resolution OCT technology has allowed for the development of epithelium thickness maps which can also aid in identification of early corneal ectasia or focal areas of thinning with high granularity69
Wavefront-optimized and wavefront-guided treatments
Innovation in in excimer laser delivery as well as refinements in instrumentation to measure higher-order aberrations have allowed for the development of wavefront-optimized (WFO) and wavefront-guided (WFG) treatments in keratorefractive surgery18. As discussed earlier, outcomes of conventional cornealbased refractive surgery can be limited due to unwanted side-effects (glare, halos, starbursts) from higher-order aberrations4,43,44. In comparison to conventional ablations, wavefront ablations improve final visual outcomes for patients by minimizing the effects of higher-order aberrations77,78. In WFO treatments, individual ablation profiles are generated based on population averages of aberrometry data77,78. In WFG treatments, individual ablation profiles are generated based on preoperative measurements of each patient’s specific higher-order aberrations79-81 The treatment goal of WFG ablation is centered around reducing existing higher-order aberrations while minimizing creation of new higher-order aberrations after refractive surgery79-81. Both WFO and WFG treatment strategies have proven to be effective methods for minimizing incidence of post-refractive surgery higher-order aberrations18,77
Topography and tomography-guided lasers serve as another recent technological innovation in cornealrefractive surgery47,77. These lasers rely on the use of anterior (primarily) corneal topography rather than wavefront data47,77. Specifically, data obtained from a topolyzer, which integrates changes from the anterior corneal surface through placido-disc, and an oculyzer, which integrates both anterior and posterior corneal changes through pentacam, can be synchronized with the Alcon Wavelight excimer system to perform topography and tomography-based treatments that include treating higher-order aberrations82 In the United States, the Contoura (commercialized through Wavelight) is available for use and utilizes data obtained from the placido-disc based topolyzer 83 The Schwind Amaris84 is another commonly used laser platform outside the USA that has similar abilities as the Alcon Wavelight system In general, by employing corneal topography data, an elevation profile of the corneal surface is calculated and used as the basis for the generation of an “ideal” corneal surface47,77. The difference between this “ideal” corneal surface and the true, calculated corneal topography serves the topography-guided ablation profile47,77. Similar visual outcomes have been demonstrated between wavefront ablations and topography-guided ablations, but there are select instances when topography-guided ablations have resulted in superior visual outcomes in comparison to wavefront-guided ablations mainly, when ablations are being performed in highly aberrated corneas for which acquisition of wavefront data proves challenging47,77,85-87
lenticule implantation for the treatment of presbyopia
Corneal inlays have become a popular clinical strategy for the correction of presbyopia. However, given that inlays often employ synthetic material, various challenges may arise including improper biocompatibility and restriction of nutrient flow within the stromal bed18. With advancements in femtosecond laser precision, stromal lenticules obtained after SMILE are now being explored as biological inlays for the correction of presbyopia29,88. These inlays fall under standard eye banking regulatory guidelines89-91One advantage of stromal lenticule implantation for presbyopic correction is the relatively low risk of rejection 89-91 . A major limitation of SMILE inlays is the unpredictable nature, at times, of the refractive outcomes given that the final visual outcome after the procedure is contingent on proper stromal remodeling of both the biological inlay and the recipient stroma92,93. More studies and analysis of this technique will be needed to determine its effectiveness and predictability.
Modifying the Q-value or asphericity quotient to enhance depth of focus is not a new idea and has been explored on other platforms. However, the new technology from ZEISS has allowed for yet another innovation in presbyopic correction through Laser Blended Vision LASIK. Using a non-linear, aspheric ablation profile, PRESBYOND induces micro-anisometropia while leveraging a controlled amount of spherical aberration to increase depth of focus and allow for a “blend zone” of continuous clear vision over a range of distances94-97. In comparison to contact lens monovision (CLM), which serves as another popular alternative for presbyopic correction, PRESBYOND has been found to be tolerated by 95% of patients who receive it in contrast to only 59-67% of patients who tolerate CLM97,98
Laser-based corneal refractive surgery offers many advantages to patients seeking vision correction through refractive surgery. However, in comparison to lens-based approaches, laser refractive surgery has some limitations. The first major limitation of corneal-based laser approaches center around the fact that the final visual outcome (post-procedure) hinges on a proper healing response by the cornea after correction. Although a small risk, there is always a chance that certain patients may be prone to an unpredictable healing course which may limit the final visual outcome. Another major limitation of cornealbased laser refractive surgery is that the approach can be subject to certain anatomical limitations. For example, some patients may not be candidates for corneal-based approaches because they do not have sufficient corneal tissue required for a safe ablation, or that their refractive error falls outside the corrective range for corneal ablation. For these situations, a lens-based approach is often preferred. These include both phakic lens implants or refractive lens exchange. One lens-based approach that has gained popularity in these clinical situations is the EVO-ICL (Implantable Collamer Lens), a phakic lens implant. EVO-ICL allows for phakic-IOL implantation and has been approved for patients with very high myopia (-3.0D to -20.0D) and irregular and thin corneas99. It also has a lower incidence of post-operative dry eye syndrome, a condition that may be a contraindication for some patients interested in certain corneal-based approaches100
Significant advances have been made in laser-based corneal refractive surgery and a variety of surgical options are now available to patients, most of whom are very satisfied with their results Innovation in preoperative management, wavefront technology, and topography-guided treatments have allowed for further refinement and improvement in the efficacy of the laser-based corneal treatments. In addition, multiple advances in the area of presbyopic treatments have also been developed and continue to move the field forward. Despite these overall advances, there remains select clinical challenges and limitations unique to laser-based corneal refractive surgery, some of which can be more effectively addressed through lens-based approaches such as phakic-IOL implantation.
Akrobetu, Dennis
1. Lou L, Yao C, Jin Y, Perez V, Ye J. Global Patterns in Health Burden of Uncorrected Refractive Error. Invest Ophthalmol Vis Sci. Nov 1 2016;57(14):6271-6277. doi:10.1167/iovs.16-20242
2. Fricke TR, Holden BA, Wilson DA, et al. Global cost of correcting vision impairment from uncorrected refractive error. Bull World Health Organ. Oct 1 2012;90(10):728-38. doi:10.2471/BLT.12.104034
3. Naidoo KS, Leasher J, Bourne RR, et al. Global Vision Impairment and Blindness Due to Uncorrected Refractive Error, 1990-2010. Optom Vis Sci. Mar 2016;93(3):227-34. doi:10.1097/OPX.0000000000000796
4. Kim TI, Alio Del Barrio JL, Wilkins M, Cochener B, Ang M. Refractive surgery. Lancet. May 18 2019;393(10185):2085-2098. doi:10.1016/S0140-6736(18)33209-4
5. Morgan IG, Ohno-Matsui K, Saw SM. Myopia. Lancet. May 5 2012;379(9827):1739-48. doi:10.1016/S01406736(12)60272-4
6. Pan CW, Ramamurthy D, Saw SM. Worldwide prevalence and risk factors for myopia. Ophthalmic Physiol Opt. Jan 2012;32(1):3-16. doi:10.1111/j.1475-1313.2011.00884.x
7. Holden BA, Fricke TR, Wilson DA, et al. Global Prevalence of Myopia and High Myopia and Temporal Trends from 2000 through 2050. Ophthalmology. May 2016;123(5):1036-42. doi:10.1016/j.ophtha.2016.01.006
8. Sandoval HP, Donnenfeld ED, Kohnen T, et al. Modern laser in situ keratomileusis outcomes. J Cataract Refract Surg. Aug 2016;42(8):1224-34. doi:10.1016/j.jcrs.2016.07.012
9. Randleman JB, Shah RD. LASIK interface complications: etiology, management, and outcomes. J Refract Surg Aug 2012;28(8):575-86. doi:10.3928/1081597X-20120722-01
10. Segev F, Mimouni M, Sela T, Munzer G, Kaiserman I. Risk Factors for Sporadic Diffuse Lamellar Keratitis After Microkeratome Laser-Assisted In Situ Keratomileusis: A Retrospective Large Database Analysis. Cornea. Sep 2018;37(9):1124-1129. doi:10.1097/ICO.0000000000001674
11. Yesilirmak N, Chhadva P, Cabot F, Galor A, Yoo SH. Post-Laser In Situ Keratomileusis Epithelial Ingrowth: Treatment, Recurrence, and Long-Term Results. Cornea. Dec 2018;37(12):1517-1521. doi:10.1097/ICO.0000000000001760
12. Moshirfar M, Hazin R, Khalifa YM. Central toxic keratopathy. Curr Opin Ophthalmol. Jul 2010;21(4):274-9. doi:10.1097/ICU.0b013e32833a8cb2
13. Phusitphoykai N, Tungsiripat T, Siriboonkoom J, Vongthongsri A. Comparison of conventional versus wavefrontguided laser in situ keratomileusis in the same patient. J Refract Surg. Mar-Apr 2003;19(2 Suppl):S217-20. doi:10.3928/1081-597X-20030302-08
14. Schallhorn SC, Tanzer DJ, Kaupp SE, Brown M, Malady SE. Comparison of night driving performance after wavefront-guided and conventional LASIK for moderate myopia. Ophthalmology. Apr 2009;116(4):702-9. doi:10.1016/j.ophtha.2008.12.038
15. Kim TI, Yang SJ, Tchah H. Bilateral comparison of wavefront-guided versus conventional laser in situ keratomileusis with Bausch and Lomb Zyoptix. J Refract Surg. Sep-Oct 2004;20(5):432-8. doi:10.3928/1081-597X20040901-04
16. Jun I, Kang DS, Tan J, et al. Comparison of clinical outcomes between wavefront-optimized versus corneal wavefront-guided transepithelial photorefractive keratectomy for myopic astigmatism. J Cataract Refract Surg. Feb 2017;43(2):174-182. doi:10.1016/j.jcrs.2016.11.045
17. Lee WS, Manche EE. Comparison of simulated keratometric changes following wavefront-guided and wavefrontoptimized myopic laser-assisted in situ keratomileusis. Clin Ophthalmol. 2018;12:613-619. doi:10.2147/OPTH.S161387
18. Ang M, Gatinel D, Reinstein DZ, Mertens E, Alio Del Barrio JL, Alio JL. Refractive surgery beyond 2020. Eye (Lond). Feb 2021;35(2):362-382. doi:10.1038/s41433-020-1096-5
19. Hersh PS, Brint SF, Maloney RK, et al. Photorefractive keratectomy versus laser in situ keratomileusis for moderate to high myopia. A randomized prospective study. Ophthalmology. Aug 1998;105(8):1512-22, discussion 1522-3. doi:10.1016/S0161-6420(98)98038-1
20. Pallikaris IG, Siganos DS. Excimer laser in situ keratomileusis and photorefractive keratectomy for correction of high myopia. J Refract Corneal Surg. Sep-Oct 1994;10(5):498-510.
21. Chan C, Ang M, Saad A, et al. Validation of an Objective Scoring System for Forme Fruste Keratoconus Detection and Post-LASIK Ectasia Risk Assessment in Asian Eyes. Cornea. Sep 2015;34(9):996-1004. doi:10.1097/ICO.0000000000000529
22. Shtein RM. Post-LASIK dry eye. Expert Rev Ophthalmol. Oct 2011;6(5):575-582. doi:10.1586/eop.11.56
23. Munnerlyn CR, Koons SJ, Marshall J. Photorefractive keratectomy: a technique for laser refractive surgery. J Cataract Refract Surg. Jan 1988;14(1):46-52. doi:10.1016/s0886-3350(88)80063-4
24. Sanchez P, Moutsouris K, Pandolfi A. Biomechanical and optical behavior of human corneas before and after photorefractive keratectomy. J Cataract Refract Surg. Jun 2014;40(6):905-17. doi:10.1016/j.jcrs.2014.03.020
25. Nair S, Kaur M, Sharma N, Titiyal JS. Refractive surgery and dry eye - An update. Indian J Ophthalmol. Apr 2023;71(4):1105-1114. doi:10.4103/IJO.IJO_3406_22
26. Wachtlin J, Langenbeck K, Schrunder S, Zhang EP, Hoffmann F. Immunohistology of corneal wound healing after photorefractive keratectomy and laser in situ keratomileusis. wachtlin@ukbf.fu-berlin.de. J Refract Surg. Jul-Aug 1999;15(4):451-8. doi:10.3928/1081-597X-19990701-11
27. Faktorovich EG, Melwani K. Efficacy and safety of pain relief medications after photorefractive keratectomy: review of prospective randomized trials. J Cataract Refract Surg. Oct 2014;40(10):1716-30. doi:10.1016/j.jcrs.2014.08.001
28. Ang M, Mehta JS, Chan C, Htoon HM, Koh JC, Tan DT. Refractive lenticule extraction: transition and comparison of 3 surgical techniques. J Cataract Refract Surg. Sep 2014;40(9):1415-24. doi:10.1016/j.jcrs.2013.12.026
29. Ang M, Tan D, Mehta JS. Small incision lenticule extraction (SMILE) versus laser in-situ keratomileusis (LASIK): study protocol for a randomized, non-inferiority trial. Trials. May 31 2012;13:75. doi:10.1186/1745-6215-13-75
30. Vestergaard AH, Grauslund J, Ivarsen AR, Hjortdal JO. Efficacy, safety, predictability, contrast sensitivity, and aberrations after femtosecond laser lenticule extraction. J Cataract Refract Surg. Mar 2014;40(3):403-11. doi:10.1016/j.jcrs.2013.07.053
31. Moshirfar M, McCaughey MV, Reinstein DZ, Shah R, Santiago-Caban L, Fenzl CR. Small-incision lenticule extraction. J Cataract Refract Surg. Mar 2015;41(3):652-65. doi:10.1016/j.jcrs.2015.02.006
32. Riau AK, Angunawela RI, Chaurasia SS, Lee WS, Tan DT, Mehta JS. Early corneal wound healing and inflammatory responses after refractive lenticule extraction (ReLEx). Invest Ophthalmol Vis Sci. Aug 5 2011;52(9):6213-21. doi:10.1167/iovs.11-7439
33. Ganesh S, Gupta R. Comparison of visual and refractive outcomes following femtosecond laser- assisted lasik with smile in patients with myopia or myopic astigmatism. J Refract Surg. Sep 2014;30(9):590-6. doi:10.3928/1081597X20140814-02
34. Ji YW, Kim M, Kang DSY, et al. Lower Laser Energy Levels Lead to Better Visual Recovery After Small-Incision Lenticule Extraction: Prospective Randomized Clinical Trial. Am J Ophthalmol. Jul 2017;179:159-170. doi:10.1016/j.ajo.2017.05.005
35. Krueger RR, Meister CS. A review of small incision lenticule extraction complications. Curr Opin Ophthalmol. Jul 2018;29(4):292-298. doi:10.1097/ICU.0000000000000494
36. Titiyal JS, Kaur M, Rathi A, Falera R, Chaniyara M, Sharma N. Learning Curve of Small Incision Lenticule Extraction: Challenges and Complications. Cornea. Nov 2017;36(11):1377-1382. doi:10.1097/ICO.0000000000001323
37. Bamba S, Rocha KM, Ramos-Esteban JC, Krueger RR. Incidence of rainbow glare after laser in situ keratomileusis flap creation with a 60 kHz femtosecond laser. J Cataract Refract Surg. Jun 2009;35(6):1082-6. doi:10.1016/j.jcrs.2009.01.026
38. Zhang Y, Chen YG. High incidence of rainbow glare after femtosecond laser assisted-LASIK using the upgraded FS200 femtosecond laser. BMC Ophthalmol. Mar 5 2018;18(1):71. doi:10.1186/s12886-018-0734-1
39. Yesilirmak N, Davis Z, Yoo SH. Refractive Surgery (SMILE vs. LASIK vs. Phakic IOL). Int Ophthalmol Clin Summer 2016;56(3):137-47. doi:10.1097/IIO.0000000000000120
40. Zeng J, Lan G, Zhu M, et al. Factors associated with corneal high-order aberrations before and after femtosecond laser-assisted in situ keratomileusis. Ann Transl Med. Jun 2021;9(12):989. doi:10.21037/atm-21-2367
41. Sugar A, Rapuano CJ, Culbertson WW, et al. Laser in situ keratomileusis for myopia and astigmatism: safety and efficacy: a report by the American Academy of Ophthalmology. Ophthalmology. Jan 2002;109(1):175-87. doi:10.1016/s0161-6420(01)00966-6
Akrobetu, Dennis
42. Varley GA, Huang D, Rapuano CJ, et al. LASIK for hyperopia, hyperopic astigmatism, and mixed astigmatism: a report by the American Academy of Ophthalmology. Ophthalmology. Aug 2004;111(8):1604-17. doi:10.1016/j.ophtha.2004.05.016
43. Buhren J, Pesudovs K, Martin T, Strenger A, Yoon G, Kohnen T. Comparison of optical quality metrics to predict subjective quality of vision after laser in situ keratomileusis. J Cataract Refract Surg. May 2009;35(5):846-55. doi:10.1016/j.jcrs.2008.12.039
44. Pesudovs K. Wavefront aberration outcomes of LASIK for high myopia and high hyperopia. J Refract Surg. SepOct 2005;21(5):S508-12. doi:10.3928/1081-597X-20050901-18
45. Myrowitz EH, Chuck RS. A comparison of wavefront-optimized and wavefront-guided ablations. Curr Opin Ophthalmol. Jul 2009;20(4):247-50. doi:10.1097/icu.0b013e32832a2336
46. He L, Manche EE. Contralateral eye-to-eye comparison of wavefront-guided and wavefront-optimized photorefractive keratectomy: a randomized clinical trial. JAMA Ophthalmol. Jan 2015;133(1):51-9. doi:10.1001/jamaophthalmol.2014.3876
47. Holland S, Lin DT, Tan JC. Topography-guided laser refractive surgery. Curr Opin Ophthalmol. Jul 2013;24(4):3029. doi:10.1097/ICU.0b013e3283622a59
48. Lin DT, Holland SR, Rocha KM, Krueger RR. Method for optimizing topography-guided ablation of highly aberrated eyes with the ALLEGRETTO WAVE excimer laser. J Refract Surg. Apr 2008;24(4):S439-45. doi:10.3928/1081597X20080401-22
49. Bohac M, Koncarevic M, Pasalic A, et al. Incidence and Clinical Characteristics of Post LASIK Ectasia: A Review of over 30,000 LASIK Cases. Semin Ophthalmol. 2018;33(7-8):869-877. doi:10.1080/08820538.2018.1539183
50. Giri P, Azar DT. Risk profiles of ectasia after keratorefractive surgery. Curr Opin Ophthalmol. Jul 2017;28(4):337342. doi:10.1097/ICU.0000000000000383
51. Dawson DG, Randleman JB, Grossniklaus HE, et al. Corneal ectasia after excimer laser keratorefractive surgery: histopathology, ultrastructure, and pathophysiology. Ophthalmology. Dec 2008;115(12):2181-2191 e1. doi:10.1016/j.ophtha.2008.06.008
52. Roberts CJ, Dupps WJ, Jr. Biomechanics of corneal ectasia and biomechanical treatments. J Cataract Refract Surg. Jun 2014;40(6):991-8. doi:10.1016/j.jcrs.2014.04.013
53. Sinha Roy A, Dupps WJ, Jr. Effects of altered corneal stiffness on native and postoperative LASIK corneal biomechanical behavior: A whole-eye finite element analysis. J Refract Surg. Oct 2009;25(10):875-87. doi:10.3928/1081597X-20090917-09
54. Balestrazzi A, Balestrazzi A, Giannico MI, Michieletto P, Balestrazzi E. Diagnosis, Clinical Trend, and Treatment of Diffuse Lamellar Keratitis after Femtosecond Laser-Assisted in situ Keratomileusis: A Case Report. Case Rep Ophthalmol Sep-Dec 2018;9(3):457-464. doi:10.1159/000493338
55. Smith RJ, Maloney RK. Diffuse lamellar keratitis. A new syndrome in lamellar refractive surgery. Ophthalmology Sep 1998;105(9):1721-6. doi:10.1016/S0161-6420(98)99044-3
56. Reinstein DZ, Stuart AJ, Vida RS, Archer TJ, Carp GI. Incidence and Outcomes of Sterile Multifocal Inflammatory Keratitis and Diffuse Lamellar Keratitis After SMILE. J Refract Surg. Nov 1 2018;34(11):751-759. doi:10.3928/1081597X20181001-02
57. Maier P, Reinhard T, Cursiefen C. Descemet stripping endothelial keratoplasty rapid recovery of visual acuity. Dtsch Arztebl Int. May 2013;110(21):365-71. doi:10.3238/arztebl.2013.0365
58. Linebarger EJ, Hardten DR, Lindstrom RL. Diffuse lamellar keratitis: diagnosis and management. J Cataract Refract Surg. Jul 2000;26(7):1072-7. doi:10.1016/s0886-3350(00)00468-5
59. Lin HY, Ho WT. Diffuse lamellar keratitis as a rare complication of diamond burr superficial keratectomy for recurrent corneal erosion: a case report. BMC Ophthalmol. Sep 7 2022;22(1):362. doi:10.1186/s12886-022-02589-3
60. Peters NT, Iskander NG, Anderson Penno EE, Woods DE, Moore RA, Gimbel HV. Diffuse lamellar keratitis: isolation of endotoxin and demonstration of the inflammatory potential in a rabbit laser in situ keratomileusis model. J Cataract Refract Surg. Jun 2001;27(6):917-23. doi:10.1016/s0886-3350(00)00779-3
61. Koh K, Jun I, Kim TI, Kim EK, Seo KY. Central Toxic Keratopathy after Small Incision Lenticule Extraction. Korean J Ophthalmol. Jun 2020;34(3):254-255. doi:10.3341/kjo.2019.0122
62. Moshirfar M, Hall MN, West WB, Jr., et al. Five-Year Occurrence and Management of Central Toxic Keratopathy After Femtosecond Laser-Assisted LASIK. J Refract Surg. Jan 1 2021;37(1):25-31. doi:10.3928/1081597X-20201030-01
63. Yim CK, Zhu D. Central Toxic Keratopathy in Siblings After Laser-Assisted Keratomileusis: Case Report and Literature Review. Cornea. May 1 2022;41(5):640-643. doi:10.1097/ICO.0000000000002890
64. Moshirfar M, Somani AN, Vaidyanathan U, Ronquillo YC, Hoopes PC. Pressure-Induced Interlamellar Stromal Keratitis After Small-Incision Lenticule Extraction Procedure: A Case Report. Cornea. Feb 2020;39(2):254-257. doi:10.1097/ICO.0000000000002196
65. Lee V, Sulewski ME, Zaidi A, Nichols CW, Bunya VY. Elevated intraocular pressure-induced interlamellar stromal keratitis occurring 9 years after laser in situ keratomileusis. Cornea. Jan 2012;31(1):87-9. doi:10.1097/ICO.0b013e31821140fa
66. Lyle WA, Jin GJ. Interface fluid associated with diffuse lamellar keratitis and epithelial ingrowth after laser in situ keratomileusis. J Cataract Refract Surg. Jul 1999;25(7):1009-12. doi:10.1016/s0886-3350(99)00083-8
67. Ehlers N. Mechanical factors in the maintenance of normal corneal deturgescence. Acta Ophthalmol (Copenh) 1967;45(5):658-72. doi:10.1111/j.1755-3768.1967.tb06534.x
68. Roberts CJ, Mahmoud AM, Bons JP, et al. Introduction of Two Novel Stiffness Parameters and Interpretation of Air Puff-Induced Biomechanical Deformation Parameters With a Dynamic Scheimpflug Analyzer. J Refract Surg. Apr 1 2017;33(4):266-273. doi:10.3928/1081597X-20161221-03
69. Ambrosio R, Jr., Lopes BT, Faria-Correia F, et al. Integration of Scheimpflug-Based Corneal Tomography and Biomechanical Assessments for Enhancing Ectasia Detection. J Refract Surg. Jul 1 2017;33(7):434-443. doi:10.3928/1081597X-20170426-02
70. Webb JN, Zhang H, Sinha Roy A, Randleman JB, Scarcelli G. Detecting Mechanical Anisotropy of the Cornea Using Brillouin Microscopy. Transl Vis Sci Technol. Jun 2020;9(7):26. doi:10.1167/tvst.9.7.26
71. Vinciguerra R, Palladino S, Herber R, Romano MR, Vinciguerra P. The KERATO Biomechanics Study 1: A Comparative Evaluation Using Brillouin Microscopy and Dynamic Scheimpflug Imaging. J Refract Surg. Aug 2024;40(8):e569-e578. doi:10.3928/1081597X-20240701-02
72. Yun SH, Chernyak D. Brillouin microscopy: assessing ocular tissue biomechanics. Curr Opin Ophthalmol. Jul 2018;29(4):299-305. doi:10.1097/ICU.0000000000000489
73. Scarcelli G, Pineda R, Yun SH. Brillouin optical microscopy for corneal biomechanics. Invest Ophthalmol Vis Sci Jan 20 2012;53(1):185-90. doi:10.1167/iovs.11-8281
74. Loveless BA, Moin KA, Hoopes PC, Moshirfar M. The Utilization of Brillouin Microscopy in Corneal Diagnostics: A Systematic Review. Cureus. Jul 2024;16(7):e65769. doi:10.7759/cureus.65769
75. Ang M, Baskaran M, Werkmeister RM, et al. Anterior segment optical coherence tomography. Prog Retin Eye Res Sep 2018;66:132-156. doi:10.1016/j.preteyeres.2018.04.002
76. Ang M, Chong W, Huang H, et al. Comparison of anterior segment optical tomography parameters measured using a semi-automatic software to standard clinical instruments. PLoS One. 2013;8(6):e65559. doi:10.1371/journal.pone.0065559
77. Manche E, Roe J. Recent advances in wavefront-guided LASIK. Curr Opin Ophthalmol. Jul 2018;29(4):286-291. doi:10.1097/ICU.0000000000000488
78. Perez-Straziota CE, Randleman JB, Stulting RD. Objective and subjective preoperative refraction techniques for wavefront-optimized and wavefront-guided laser in situ keratomileusis. J Cataract Refract Surg. Feb 2009;35(2):256-9. doi:10.1016/j.jcrs.2008.10.047
79. He L, Liu A, Manche EE. Wavefront-guided versus wavefront-optimized laser in situ keratomileusis for patients with myopia: a prospective randomized contralateral eye study. Am J Ophthalmol. Jun 2014;157(6):1170-1178 e1. doi:10.1016/j.ajo.2014.02.037
80. Schallhorn SC, Farjo AA, Huang D, et al. Wavefront-guided LASIK for the correction of primary myopia and astigmatism a report by the American Academy of Ophthalmology. Ophthalmology. Jul 2008;115(7):1249-61. doi:10.1016/j.ophtha.2008.04.010
81. Kim A, Chuck RS. Wavefront-guided customized corneal ablation. Curr Opin Ophthalmol. Jul 2008;19(4):314-20. doi:10.1097/ICU.0b013e328302ccae
Akrobetu, Dennis
82. Cummings AB, Mascharka N. Outcomes after topography-based LASIK and LASEK with the wavelight oculyzer and topolyzer platforms. J Refract Surg. Jul 2010;26(7):478-85. doi:10.3928/1081597X-20090814-05
83. Wallerstein A, Gauvin M, Cohen M. WaveLight((R)) Contoura topography-guided planning: contribution of anterior corneal higher-order aberrations and posterior corneal astigmatism to manifest refractive astigmatism. Clin Ophthalmol. 2018;12:1423-1426. doi:10.2147/OPTH.S169812
84. Arbelaez MC, Arba Mosquera S. The SCHWIND AMARIS Total-Tech Laser as An All-Rounder in Refractive Surgery. Middle East Afr J Ophthalmol. Jan 2009;16(1):46-53. doi:10.4103/0974-9233.48868
85. Chen X, Stojanovic A, Zhou W, Utheim TP, Stojanovic F, Wang Q. Transepithelial, Topography-guided Ablation in the Treatment of Visual Disturbances in LASIK Flap or Interface Complications. J Refract Surg. Feb 2012;28(2):120-6. doi:10.3928/1081597X-20110926-01
86. Lin DT, Holland S, Tan JC, Moloney G. Clinical results of topography-based customized ablations in highly aberrated eyes and keratoconus/ectasia with cross-linking. J Refract Surg. Nov 2012;28(11 Suppl):S841-8. doi:10.3928/1081597X-20121005-06
87. Mysore N, Krueger R. Advances in Refractive Surgery: May 2013 to June 2014. Asia Pac J Ophthalmol (Phila) Mar-Apr 2015;4(2):112-20. doi:10.1097/APO.0000000000000117
88. Jacob S, Kumar DA, Agarwal A, Agarwal A, Aravind R, Saijimol AI. Preliminary Evidence of Successful Near Vision Enhancement With a New Technique: PrEsbyopic Allogenic Refractive Lenticule (PEARL) Corneal Inlay Using a SMILE Lenticule. J Refract Surg. Apr 1 2017;33(4):224-229. doi:10.3928/1081597X-20170111-03
89. Alio Del Barrio JL, El Zarif M, Azaar A, et al. Corneal Stroma Enhancement With Decellularized Stromal Laminas With or Without Stem Cell Recellularization for Advanced Keratoconus. Am J Ophthalmol. Feb 2018;186:47-58. doi:10.1016/j.ajo.2017.10.026
90. Liu YC, Teo EPW, Ang HP, et al. Biological corneal inlay for presbyopia derived from small incision lenticule extraction (SMILE). Sci Rep. Jan 30 2018;8(1):1831. doi:10.1038/s41598-018-20267-7
91. Li M, Li M, Sun L, Ni K, Zhou X. Predictive Formula for Refraction of Autologous Lenticule Implantation for Hyperopia Correction. J Refract Surg. Dec 1 2017;33(12):827-833. doi:10.3928/1081597X-20171016-01
92. Pradhan KR, Reinstein DZ, Carp GI, Archer TJ, Gobbe M, Gurung R. Femtosecond laser-assisted keyhole endokeratophakia: correction of hyperopia by implantation of an allogeneic lenticule obtained by SMILE from a myopic donor. J Refract Surg. Nov 2013;29(11):777-82. doi:10.3928/1081597X-20131021-07
93. Damgaard IB, Ivarsen A, Hjortdal J. Biological Lenticule Implantation for Correction of Hyperopia: An Ex Vivo Study in Human Corneas. J Refract Surg. Apr 1 2018;34(4):245-252. doi:10.3928/1081597X-20180206-01
94. Reinstein DZ, Ivory E, Chorley A, et al. PRESBYOND Laser Blended Vision LASIK in Commercial and Military Pilots Requiring Class 1 Medical Certification. J Refract Surg. Jan 2023;39(1):6-14. doi:10.3928/1081597X-20221129-02
95. Fu D, Aruma A, Xu Y, Han T, Xia F, Zhou XT. Refractive outcomes and optical quality of PRESBYOND laserblended vision for presbyopia correction. Int J Ophthalmol. 2022;15(10):1671-1675. doi:10.18240/ijo.2022.10.16
96. Reinstein DZ, Carp GI, Archer TJ, Gobbe M. LASIK for presbyopia correction in emmetropic patients using aspheric ablation profiles and a micro-monovision protocol with the Carl Zeiss Meditec MEL 80 and VisuMax. J Refract Surg. Aug 2012;28(8):531-41. doi:10.3928/1081597X-20120723-01
97. Reinstein DZ, Archer TJ, Gobbe M. LASIK for Myopic Astigmatism and Presbyopia Using Non-Linear Aspheric Micro-Monovision with the Carl Zeiss Meditec MEL 80 Platform. J Refract Surg. Jan 2011;27(1):23-37. doi:10.3928/1081597X-20100212-04
98. Evans BJ. Monovision: a review. Ophthalmic Physiol Opt. Sep 2007;27(5):417-39. doi:10.1111/j.14751313.2007.00488.x
99. Albo C, Nasser T, Szynkarski DT, et al. A Comprehensive Retrospective Analysis of EVO/EVO+ Implantable Collamer Lens: Evaluating Refractive Outcomes in the Largest Single Center Study of ICL Patients in the United States. Clin Ophthalmol. 2024;18:69-78. doi:10.2147/OPTH.S440578
100. Parkhurst GD, Psolka M, Kezirian GM. Phakic intraocular lens implantation in United States military warfighters: a retrospective analysis of early clinical outcomes of the Visian ICL. J Refract Surg. Jul 2011;27(7):473-81. doi:10.3928/1081597X-20110106-03
Darren A. Chen, MD and Jo-Ann Haney Tilton, MD
Private equity (PE) acquisition of medical practices has been a contentious topic in the past decade. These recent acquisitions represent another wave of private equity involvement in outpatient medicine, the first being the formation of physician practice management companies (PPM) that emerged in the 1990s. In recent years, dermatology, gastroenterology, and ophthalmology private practices have been the major targets for PE groups.1,2 The overarching goal of PE acquisition, regardless of field, is to increase the value of the acquired entity in order to sell the business for a multiple return. In the medical practice sector, the strategy employed by PE groups to increase value largely entails consolidating smaller practices, streamlining operations, removing inefficiencies, and negotiating for higher insurance or pharmaceutical reimbursement/rebates, among other revenue generating measures. From 2012-2019, an estimated 228 ophthalmology and optometry practices were acquired by PE groups in the United States.3 This change in practice management has led to questions regarding the quality and cost of ophthalmic care in PE owned practices. This paper will review the recent primary investigations regarding private equity acquisition of ophthalmology practices, current viewpoints, and future implications.
Private equity refers to ownership of stock in a company not listed on a public stock exchange. In common parlance, private equity oftentimes refers to firms that employ capital and debt to provide financial backing for private businesses.4 The overarching priority for any private equity firm is profit and return on investment (ROI); for this reason, ophthalmology has been targeted as an attractive acquisition. Ophthalmology private practices are often well-established, high-volume businesses encompassing both comprehensive medical and subspecialized surgical care. Experts cite high volume procedures, an aging populace, ancillary revenue streams, and cash pay elective surgeries as the principal reasons PE finds ophthalmology practices attractive.5 All of these components allow well run practices to achieve high revenues and subsequent profits.
The process prior to acquisition involves due diligence – that is, the comprehensive evaluation of a practice’s financial health and future viability. Typically, a practice’s health can be gauged roughly by its earnings before interest, taxes, depreciation, and amortization (EBITDA). The importance of this metric in the scheme of private equity acquisitions cannot be understated.
EBITDA is a generally accepted accounting principle (GAAP) that approximates a company’s profitability and financial performance; it can provide a convenient proxy for the valuation of a company when multiplied by an industry specific multiple, and this valuation can then be compared between similar businesses. In general, small independent ophthalmology practices, when acquired, have been estimated to trade in the 5x-9x EBITDA multiple.5,6 These primary acquisitions are commonly referred to as the “first sale” and represent far and away the majority of transactions thus far. The “second sale”, in which PE firms aim to resell the practice to another buyer have been few and far between, likely secondary to the current high interest rate environment, post-pandemic economy, and declining Center for Medicare Services (CMS) reimbursement rates.7 It will be interesting to see what percentage of these PE owned practices will successfully undergo a profitable second sale in the coming years.
Why is ophthalmology attractive for PE? The first reason revolves around a basic tenet of economics –supply and demand. The demand for eye care services is rapidly increasing and is projected to increase at least through the next one to two decades Simultaneously, the supply of ophthalmologists is projected
Chen, Darren
to be inadequate to care for a rapidly aging populace in the US. Berkowitz et al demonstrates that from 2020 until 2035, there will be a 12% decrease in full time ophthalmologists (2,650 full time ophthalmologists) and total demand will increase by 24% (5150 full time ophthalmologists).8 This also corresponds to the Association of American Medical Colleges report which predicts an 34.1% increase in the population aged 65 and older with a concurrent shortage of 86,000 physicians by 2036.9 The increasing prevalence of age-related eye diseases such as glaucoma and macular degeneration 10,11 will likely further exacerbate the supply and demand curve. Widespread use of diagnostic imaging such as ocular coherence tomography, high volume utilization of intravitreal medications, and adoption of surgical modalities such as minimally invasive glaucoma surgery (MIGS) are all byproducts of an aging populace, and demand for these services will surely increase.12,13 The inelasticity of both supply and demand from a revenue generating standpoint is clearly advantageous, and the consolidation of eyecare practices via private equity can exploit these trends. A second reason that ophthalmology is a target is the potential for high volume, outpatient surgical procedures with cash pay components such as refractive surgery, femtosecond laser-assisted cataract surgery (FLACS), premium intraocular lenses, and cosmetic surgery.14 Finally, ophthalmology private practices continue to be fragmented in the marketplace, with estimates for consolidated eye care still under 10%.6,15 This represents a tremendous opportunity for PE firms to consolidate a fragmented marketplace for financial profit.
Ophthalmologists may find working with private equity enticing for a myriad of reasons. For one, partnering with private equity may provide the influx of capital needed to expand the practice regionally Diffusing the financial burden of increasing overhead towards an outside entity allows for a safer degree of risk management. CMS reimbursements have also been shown to be steadily decreasing over the past few years for ophthalmology, with certain retina and oculoplastics procedures being essentially unprofitable in the current CMS reimbursement landscape 16,17 As such, partnering with private equity into a large physician practice management corporation can allow for strong collective bargaining power with insurance payors for more favorable reimbursements. Furthermore, practices also benefit from bulk pricing for high volume medications (i.e. anti-VEGF) from pharmaceutical companies. Finally, the upfront cash payment from a sale could prove particularly persuasive for physician owners especially if they are seeking an exit strategy and practice succession
PE groups in the 2010’s have been acquiring small practices and consolidating them into physician practice management (PPM) companies. Recent data estimate that there are 35 PPM in ophthalmology 7 From 2013-2016, a study by Zhu et al. noted a total of 355 private practices acquired by PE across all subspecialties with ophthalmology having 11 practices acquired representing 3.1% of acquisitions during this time frame and encompassing 134 ophthalmologists 18 In a more comprehensive time series analysis from 2012 to 2021 examining private equity acquisitions of ophthalmology and optometry practices, Chen et al found that there were 245 practices acquired by 30 PPMs.3 This represents a combination of 948 ophthalmologists and optometrists that were absorbed into a PE owned practice. In this study, the authors also found that the number of acquisitions peaked in 2018 with approximately 90 acquisitions. While acquisitions in the space have been accelerating in the past few years, what is unclear, and probably of greater interest to both retiring and early career ophthalmology attendings, are the valuations of these purchases. Investment information can often be found on platforms such as Pitchbook, AlphaSense, and CB Insights; however, most practices go through private acquisitions shrouded in nondisclosure agreements. Of the ones disclosed to the public on these platforms, one large “second sale” in 2021 was that of Cincinnati Eye Institute, which was initially bought by Revelstoke in 2018 Cincinnati Eye Institute was then sold to a PPM, EyePartners, for $600 million in 2021.19
One relevant research question relates to quality of care in a PE setting, which so heavily focuses on efficiency and profit In a systematic review, Borsa et al found that the results regarding quality were mixed across the health care spectrum.20 The authors found costs to patients and payers consistently increased in private equity owned practices, while health outcomes for patients showed equivocal results.
A primary investigation by Bruch et al examining 204 private equity-acquired hospitals compared to 532 similar hospitals not acquired by private equity found that those acquired by PE had larger increases in net income, charges, charge to cost ratios, and improvement in some quality measures for specific pathologies.21
Regarding ophthalmology specifically, there have been two primary investigations that examined changes in cost and quality in private equity acquired practices. In a study by Singh et al , the authors examined medicare spending in PE acquired retina practices compared to controls.22 They found that PE-owned groups increased the use of more expensive anti-vegf medications (aflibercept) and a higher frequency of injections – an increase of 6.5 injections per fiscal quarter from a baseline of 33 resulting in approximately $23,500 more in Medicare spending. In a more recent study published by Braun et al examining Medicare spending in PE run eyecare groups, the authors found the number of patients a single optometrist examined did increase, but no such change was seen in ophthalmologists. There was no significant increase in spending, but there was again, however, a shift towards the use of more expensive ranibizumab injections and concomitant decrease in less expensive bevacizumab injections;23 the authors speculate that this is perhaps due to specific pharmaceutical rebates that these private equity owned practices were able to access. Overall, the study suggested that PE-acquired practices, when examined from a Medicare spending perspective, had little or no overall effect on the total dollar cost.
The research in cost and quality of care for private equity practices is still limited. The main reason is that the methodology for such studies is inherently difficult. Convincing enough practices to open their books to compare spending and quality of outcomes both before and after private equity acquisitions would be an extremely complex task. Finding appropriate “control” practices for comparison would also be difficult given the nature of PE practices that tend to consolidate in geographic locales, thereby acting as a high barrier of entry for similar sized private practices to thrive in the same location.
In a recent qualitative study by O’Donnell et al , the authors conducted structured interviews with 35 different stakeholders ranging from PE directors to physician practice partners.24 Interviewees who had undergone acquisition by PE argued that there was minimal change in the day-to-day operations and governance of their clinical roles. Instead, overarching business development and business side operations changed and were no longer the responsibility of physicians Fortunate physicians also viewed the awarded stock options for the PE entity favorably. Opponents in the same study noted that PE firms have misaligned incentives, especially with using more expensive drugs – a fact that has been well established in the aforementioned studies above.
As far as the American Academy of Ophthalmology is concerned, it has not yet taken a firm stance once way or another. In a 2020 commentary, then president David Parke II, MD, noted that more research on cost and quality would need to be conducted before the Academy takes a firm stance and urged individual members to form their own opinion.25
Overall, it is still too soon to tell whether private equity acquisitions in ophthalmology will continue to accelerate leading to widespread consolidation or if market trends will reverse. A recent report, as of June 2024, produced by Stout LLC with data from Levin Associates is summarized in the figure below 26
Acquisitions peaked in 2021 with 100 deals according to this data; this contrasts with 2024, in which only 14 transactions have taken place up until June. They project that another 41 practices will close before the end of the fiscal year. On November 12, 2024, it was announced that Retina Consultants of America, the largest network of retina practices across the nation, currently owned by Webster Equity Partners, will undergo a second sale to Cencora for an estimated $4.6 billion in cash. Financial industry professionals seem to be anticipating continuing consolidation at least in the short term, especially if interest rates continue to fall.27,28 With regards to federal regulations , the Federal Trade Commission (FTC), Department of Justice, and Department of Health and Human Services launched a public inquiry into the private-equity healthcare sector in early 2024.29 It is unclear whether this inquiry will result in new legislation especially with a new administration coming into power after the election cycle.
Private equity acquisition of ophthalmology practices represents a significant shift in the delivery and management of eye care services. The growing interest in this field is driven by the high demand for ophthalmic care, the financial appeal of outpatient procedures, and the fragmented nature of the market. While PE acquisitions offer potential benefits, such as improved operational efficiency, expanded access to capital, and bargaining leverage, they also raise concerns about the alignment of profit motives with patient care quality and affordability.
Current evidence suggests mixed impacts of PE acquisitions on cost and quality, with some studies noting increased use of high-cost treatments and others indicating minimal changes in patient outcomes or spending. However, limitations in available research, including challenges in data transparency and control selection, make definitive conclusions difficult. Additionally, stakeholders continue to hold divergent views, with some emphasizing the operational support and financial opportunities provided by PE, while others criticize potential misaligned incentives.
Looking ahead, the trajectory of PE involvement in ophthalmology remains uncertain, influenced by factors such as market conditions, regulatory scrutiny, and broader trends in healthcare consolidation. The outcome of ongoing federal investigations and evolving interest rates will likely shape the future landscape. Ultimately, further comprehensive research is essential to fully understand the long-term implications of PE ownership on patient care, physician autonomy, and the broader ophthalmology sector.
1. Tan S, Seiger K, Renehan P, Mostaghimi A. Trends in Private Equity Acquisition of Dermatology Practices in the United States. JAMA Dermatol. 2019;155(9):1013-1021. doi:10.1001/jamadermatol.2019.1634
2. Konda S, Francis J, Motaparthi K, Grant-Kels JM. Future considerations for clinical dermatology in the setting of 21st century American policy reform: Corporatization and the rise of private equity in dermatology. J Am Acad Dermatol. 2019;81(1):287-296.e8. doi:10.1016/j.jaad.2018.09.052
3. Chen EM, Cox JT, Begaj T, Armstrong GW, Khurana RN, Parikh R. Private Equity in Ophthalmology and Optometry: Analysis of Acquisitions from 2012 through 2019 in the United States. Ophthalmology. 2020;127(4):445-455. doi:10.1016/j.ophtha.2020.01.007
4. Gilligan J, Wright M. Private Equity Demystified: An Explanatory Guide. Oxford University Press, USA; 2020.
5. Baker-Schena L. Private Equity and Ophthalmology. American Academy of Ophthalmology. November 1, 2019. Accessed November 17, 2024. https://www.aao.org/eyenet/article/private-equity-and-ophthalmology
6. Calcagnini. Eyes on Ophthalmology for M&A Opportunities. Stout. May 22, 2019. Accessed November 17, 2024. https://www.stout.com/en/insights/industry-update/eyes-ophthalmology-ma-opportunities
7. Yetter E. Ophthalmology – 2024 Update: Entering a Mature Stage. FOCUS. March 28, 2024. Accessed November 17, 2024. https://focusbankers.com/ophthalmology-2024-update-entering-a-mature-stage/
8. Berkowitz ST, Finn AP, Parikh R, Kuriyan AE, Patel S. Ophthalmology Workforce Projections in the United States, 2020 to 2035. Ophthalmology. 2024;131(2):133-139.
9. The Complexities of Physician Supply and Demand: Projections From 2021 to 2036. AAMC; 2024.
10. The Eye Diseases Prevalence Research Group*. Prevalence of Age-Related Macular Degeneration in the United States. Arch Ophthalmol. 2004;122(4):564-572. doi:10.1001/archopht.122.4.564
11. Wong WL, Su X, Li X, et al. Global prevalence of age-related macular degeneration and disease burden projection for 2020 and 2040: a systematic review and meta-analysis. Accessed November 17, 2024. https://www.thelancet.com/journals/lango/article/PIIS2214-109X(13)701451/fulltext?sm_guid=MTQ4NTAxfDExNjE0NjM5fC0xfGFubmZvbmZhQGFvbC5jb218ODcxMjMxfHwwfDB8MzM5MDE5NjV8O DM3fDB8MHw1
12. Desai S, Sekimitsu S, Rossin EJ, Zebardast N. Trends in Anti-Vascular Endothelial Growth Factor Original Medicare Part B Claims in the United States, 2014–2019. Ophthalmic Epidemiol. 2024;31(5):468-477. doi:10.1080/09286586.2024.2310854
13. Williams PJ, Hussain Z, Paauw M, et al. Glaucoma Surgery Shifts Among Medicare Beneficiaries After... : Journal of Glaucoma. J Glaucoma. 2024;33(1):59-64.
14. Leonard CY. How Practices Are Making Private Equity Work. Review of Ophthalmology. April 4, 2024. Accessed November 17, 2024. https://www.reviewofophthalmology.com/article/how-practices-are-making-private-equity-work
15. Smith JF, Hintze BC, Anderson ST, Tailor PD, Xu TT, Starr MR. Trends in Ophthalmology Practice Consolidation. Accessed November 17, 2024. https://www.aaojournal.org/article/S0161-6420(23)00311-1/abstract
16. Pan WW, Portney DS, Mian SI, Rao RC. The Cost of Standard and Complex Pars Plana Vitrectomy for Retinal Detachment Repair Exceeds Its Reimbursement. Ophthalmol Retina. 2023;7(11):948-953. doi:10.1016/j.oret.2023.06.021
17. Chen D, Azad A, Lin L, Yoon M. A cost analysis of enucleation and evisceration surgeries for treatment of blind, painful eyes. Ophthal Plast Reconstr Surg. Accepted.
18. Zhu JM, Hua LM, Polsky D. Private Equity Acquisitions of Physician Medical Groups Across Specialties, 20132016. JAMA. 2020;323(7):663-665. doi:10.1001/jama.2019.21844
19. US Ophthalmic Market: A Wave of Consolidation. Vector Medical Group; 2022:1-7.
20. Borsa A, Bejarano G, Ellen M, Bruch JD. Evaluating trends in private equity ownership and impacts on health outcomes, costs, and quality: systematic review. BMJ. 2023;382:e075244. doi:10.1136/bmj-2023-075244
Chen, Darren
21. Bruch JD, Gondi S, Song Z. Changes in Hospital Income, Use, and Quality Associated With Private Equity Acquisition. JAMA Intern Med. 2020;180(11):1428-1435. doi:10.1001/jamainternmed.2020.3552
22. Singh Y, Aderman CM, Song Z, Polsky D, Zhu JM. Increases in Medicare Spending and Use after Private Equity Acquisition of Retina Practices. Ophthalmology. 2024;131(2):150-158. doi:10.1016/j.ophtha.2023.07.031
23. Braun RT, Lelli GJ, Pandey A, Zhang M, Winebrake JP, Casalino LP. Association of Private Equity Firm Acquisition of Ophthalmology Practices with Medicare Spending and Use of Ophthalmology Services. Ophthalmology. 2024;131(3):360-369. doi:10.1016/j.ophtha.2023.09.029
24. O’Donnell EM, Lelli GJ, Bhidya S, Casalino LP. The Growth Of Private Equity Investment In Health Care: Perspectives From Ophthalmology. Health Aff (Millwood). 2020;39(6):1026-1031. doi:10.1377/hlthaff.2019.01419
25. Parke DW II. Corporatization in Ophthalmology. Ophthalmology. 2020;127(4):456-457. doi:10.1016/j.ophtha.2020.01.009
26. Forsythe L, Levin D, Janiga N. A Closer Look: LEye Care Indsutry Outlook 2024. Stout Risius Ross, LLC; 2024:120
27. Eye Care Add-On Opportunities Drive PE Investments in Physician Practices. Accessed November 18, 2024. https://www.stout.com/en/insights/commentary/eye-care-add-on-opportunities-drive-pe-investments-physician-practices
28. Sterrett T. Private Equity’s Uncertain Arc in Ophthalmology. CRSToday. Accessed November 18, 2024. https://crstoday.com/articles/aug-2024/private-equitys-uncertain-arc-in-ophthalmology
29. Federal Trade Commission, the Department of Justice and the Department of Health and Human Services Launch Cross-Government Inquiry on Impact of Corporate Greed in Health Care. Federal Trade Commission. March 4, 2024. Accessed November 18, 2024. https://www.ftc.gov/news-events/news/press-releases/2024/03/federal-trade-commissiondepartment-justice-department-health-human-services-launch-cross-government
Edward S. Lu, MD, Francesco Romano, MD, FEBO, Xinyi Ding, MD, and John B. Miller, MD
Abstract
Retinal imaging plays a critical role in the detection and management of proliferative diabetic retinopathy (PDR). Commonly used imaging modalities in the retina clinic include ultra-widefield fundus photography, ultra-widefield fluorescein angiography (FA), and optical coherence tomography (OCT). OCT angiography (OCTA) provides a non-invasive method to assess the retinal microvasculature and detect vascular alterations including retinal neovascularization (NV). More recently, the emergence of expanded field swept-source OCTA (SS-OCTA) has offered faster en-face and cross-sectional imaging of the retina with a broader field of view. Prior reviews have highlighted the role of SS-OCTA in assessing associated lesions of PDR including NV, microaneurysms, foveal avascular zone, intraretinal microvascular abnormalities, and capillary non-perfusion. In this review, we explore the clinical applications of expanded field SS-OCTA in characterizing NV morphology, comparing NV detection to FA, and offering potential imaging biomarkers associated with vision-threatening complications of PDR including vitreous hemorrhage, neovascular glaucoma, and tractional retinal detachment.
Introduction
Diabetic retinopathy (DR) is a common microvascular complication of diabetes mellitus (DM) and a leading cause of blindness among adults in the United States Approximately 9.6 million individuals are affected by DR, with a prevalence of 26% among people with DM.1 Proliferative DR (PDR), the most severe stage of DR, is defined by the presence of retinal neovascularization (NV) and is associated with significant psychological and physical morbidity.2
Historically, DR classification has relied on slit-lamp fundus examination; however, retinal imaging has become an invaluable tool for the detection and monitoring of PDR Commonly used retinal imaging modalities in the retina clinic include (ultra-)widefield fundus photography, ultra-widefield fluorescein angiography (FA), and optical coherence tomography (OCT). OCT angiography (OCTA), a functional extension of OCT, allows for non-invasive assessment of the retinal microvasculature and choroid, including epiretinal NV at both the optic disc and elsewhere. More recently, the emergence of expanded field swept-source OCTA (SS-OCTA)3,4 has allowed for faster, non-invasive en-face and cross-sectional imaging of the retina with a larger field of view.5,6 Prior work assessed the role of SS-OCTA in assessing associated lesions of PDR including NV, microaneurysms, foveal avascular zone changes, intraretinal microvascular abnormalities (IRMAs), and areas of capillary non-perfusion.7
Here, we discuss the clinical applications of expanded field SS-OCTA in characterizing NV morphology, detecting NV compared to FA, and providing imaging biomarkers associated with vision-threatening complications of PDR, including vitreous hemorrhage (VH), neovascular glaucoma (NVG), and tractional retinal detachment (TRD).
Compared to conventional spectral-domain OCTA, which is limited to imaging areas of 3x3 mm and 6x6 mm,8,9 the faster acquisition speed of swept-source technology significantly expanded the field of view achievable with OCTA. Single scans can now capture 12x12 mm and 15x15 mm images, with even larger areas possible through montage imaging.10 This greater field of view is particularly important to capture non-perfusion areas, NV, IRMAs, and other DR lesions that may occur outside the macula but have
significant clinical implications 10–13 Compared to FA, SS-OCTA has several advantages. First, SS-OCTA is non-invasive, eliminating the need for intravenous sodium fluorescein injection and thereby avoiding associated risks of patient discomfort (e.g., nausea) and allergic reactions. Additionally, SS-OCTA can be repeated in the clinic more frequently than FA due to its fast acquisition time and non-invasive nature.
SS-OCTA is faster and more efficient, and prior work has demonstrated the 12x12 mm scan protocol may be optimal to efficiently identify DR lesions.10 SS-OCTA allows for depth-resolved assessment of retinal vascular plexuses, thus allowing clinicians to distinguish lesions such as NV and IRMAs, and detect subclinical NVs not seen on clinical examination of fundus photographs 14,15 Additionally, SS-OCTA is not affected by leakage but can better show capillary loss and early retinal NV 8 In addition to NV, prior work has highlighted the use of SS-OCTA in assessing non-perfusion areas, which may be a valuable biomarker for monitoring DR severity and predicting complications.11,16 Given the relatively low predictive value of standard fundus examinations, non-invasive imaging biomarkers may be useful to personalize patient care and better predict outcomes, especially in diseases like DR since the poor compliance of these patients is well-established.
OCTA provides en face, cross sectional, and, with appropriate post-processing, volumetric images of the retina, thus offering additional insights into NV morphology. Prior studies have proposed novel classification of NV morphology based on OCTA images (Table 1). Ishibazawa et al. defined exuberant vascular proliferation (EVP) as irregular proliferation of fine, smaller-caliber new vessels on en face imaging, in contrast to pruned NV observed as fibrotic changes or inactive leakage on FA.17 EVP was predominantly seen in treatment-naïve PDR eyes and was notably reduced in eyes treated with PRP, supporting EVP as a sign of active NV. Using en face and B-scan images, Pan et al. demonstrated the utility of OCTA in the identification of the origin and morphology of NV. 18 Type 1 NV originated from veins in a tree-like shape, Type 2 NV originated from capillary networks and had an octopus-like appearance, and Type 3 NV originated from IRMAs and had a sea-fan configuration.
Vaz-Pereira et al. used cross-sectional OCT images to reclassify NV morphology 19 Flat NV were confined to the posterior hyaloid face, forward NV traversed the posterior hyaloid face, and tabletop NV were displaced anteriorly by vitreous traction and tethered by vascular “pegs.” Prior work used this crosssectional morphological classification system to assess NV detection rates using the vitreoretinal interface (VRI) slab on OCTA, which includes the region 10 to 300 microns above the internal limiting membrane 20 Our group found that the VRI slab detects NV with high sensitivity (99.1%); however, flat NV had lower rates of detection due to segmentation errors.20 By characterizing NV morphology, SS-OCTA provides valuable insights into NV activity, response to treatment, and its anatomical relationship to the vitreous. Russell et al. showed that SS-OCTA compared to FA showed similar progression or regression of NV after panretinal photocoagulation, suggesting SS-OCTA may be a reasonable alternative to FA for the longitudinal evaluation of NV.21 Parrulli et al. assessed NV changes in PDR eyes after 3 monthly intravitreal injections of ranibizumab and found that the tabletop cross-sectional morphology, higher vessel density, and the presence of focal vitreous adhesions overlying NV were imaging biomarkers associated with increased chance of regression of NV after treatment 22
Determining the presence or absence of NV is critical for identifying the proliferative stage of DR While ultra-widefield FA has progressively replaced fundus examination and photography as the gold standard for detecting NV, due to its ability to detect leakage, recent studies using different SS-OCTA scan protocols have demonstrated comparable efficacy (Table 2). Sawada et al. reported high sensitivity (100%) and specificity (97%) of SS-OCTA compared to FA in a cohort of 58 eyes using the 12x12 mm scan protocol.23 In a large cohort of 651 eyes with PDR, Russell et al. reported a similarly high sensitivity of 98.3%.24 Hirano et al. used 15x15 mm montage images and reported a lower sensitivity of 73% that improved to 84% after correction of segmentation errors.25 In a cohort of 152 patients including 75 with PDR, our group previously reported high sensitivity (97%) and specificity (95%) using the Montage 15x15
mm scan protocol after correction of segmentation errors.26 Yang et al used 12x12mm images at 5 fixation points and reported a 100% sensitivity and 86% specificity of NV detection compared to FA.27 More recently, Hamada et al. used a wider field of view (23x20 mm) and reported high sensitivity (95%) and specificity (88%) without correction of segmentation errors in a real clinical setting.28
Several studies including residents and ophthalmologists across training levels demonstrated similar detection rates of NV using SS-OCTA compared to FA, further suggesting that SS-OCTA may be an alternative to FA in diagnosing diabetic NV.29,30 Other studies have reported a high sensitivity of NV detection using SS-OCTA using OCT B-scans, biomicroscopy, and color fundus photographs as the gold standard.31–33,15 Taken together, SS-OCTA emerges as a promising non-invasive alternative to FA in the detection of NV in PDR.
Expanded field SS-OCTA provides valuable insights into the development of severe ocular complications of PDR including VH, NVG, and TRD and aids retina specialists in identifying patients at the highest risk for these complications Cui et al. prospectively assessed SS-OCTA metrics that may predict the development of diabetic VH in 55 eyes with PDR Their findings indicated that the presence of NV with forward morphology on cross-sectional B-scan and extensive NV (total area >4 disc diameters) were associated with the development of VH.34 Prior work explored SS-OCTA biomarkers associated with the presence of NVG in PDR eyes 16 Our group found that a greater ischemia index (non-perfusion area/total retinal area) and lower best-corrected visual acuity were associated with the presence of NVG. Russell et al. assessed 31 eyes of 21 patients with diabetic TRDs using SS-OCTA before and after surgical repair.35 The authors reported that SS-OCTA revealed all clinically salient features of TRDs at baseline and longitudinally after vitrectomy and suggested that SS-OCTA could serve as a standalone imaging modality to diagnose and monitor diabetic TRDs. Thus, SS-OCTA may provide clinically important biomarkers associated with severe complications of PDR and be useful to monitor complications longitudinally.
Expanded field SS-OCTA presents several limitations and challenges for clinical practice. First, SS-OCTA devices are expensive, with most currently approved for research purposes only. As the technology improves and costs decrease, SS-OCTA may become more widespread and accessible. In addition, quantitative metrics may not be consistent across OCTA devices, with limited comparative studies to establish standardization.36 These quantitative metrics are generally not available in device review software, which hinders clinical implementation. Obtaining expanded field SS-OCTA images with larger field of view requires longer acquisition time, operator expertise, and patient cooperation. However, devices with faster scanning speeds will continue to reduce acquisition times. Patients with poor vision have difficulty fixating and may be unable to acquire high-quality images. Furthermore, imaging artifacts and segmentation errors by the device often require manual correction that is not feasible in a busy retina clinic.37 Unlike FA, SS-OCTA does not detect leakage. While SS-OCTA can locate the depth of microaneurysms, it exhibits lower sensitivity compared to FA.38 Finally, the scan range of expanded field SS-OCTA is still limited compared to ultra-widefield fundus photography and FA.
However, advancements in ultra-wide field SS-OCTA not only provide a single scan range of 21x26-mm, but also the creation of five-field montage images offering a field of view comparable to ultra-widefield FA.39 Future work with artificial intelligence (AI) may help identify clinically useful SS-OCTA biomarkers and aid the retina specialist in interpreting retinal images in a busy clinic. AI and deep learning algorithms may improve segmentation issues, predict disease progression, and make SS-OCTA biomarkers more clinician-friendly.40–42
Expanded field SS-OCTA provides value in the assessment of NV morphology and detection compared to FA and may offer non-invasive biomarkers associated with vision-threatening complications including VH, NVG, and TRD. Taken together, SS-OCTA may serve as a valuable tool in the retina clinic in addition to fundus photography and ultra-widefield FA.
1. Lundeen EA, Burke-Conte Z, Rein DB, et al. Prevalence of Diabetic Retinopathy in the US in 2021. JAMA Ophthalmol. 2023;141(8):747-754. doi:10.1001/jamaophthalmol.2023.2289
2. Nagda D, Mitchell W, Zebardast N. The functional burden of diabetic retinopathy in the United States. Graefes Arch Clin Exp Ophthalmol. 2021;259(10):2977-2986. doi:10.1007/s00417-021-05210-3
3. Romano F, Ding X, Miller JB. Spotlight on Expanded Field Imaging Findings in Proliferative Sickle Cell Retinopathy. Ophthalmic Surg Lasers Imaging Retina. Published online September 1, 2024:1. doi:10.3928/2325816020240906-03
4. Romano F, Ding X, Miller JB. Expanded field: filling the gap between macula and widefield. Eye (Lond). 2024;38(9):1612-1613. doi:10.1038/s41433-024-02978-6
5. Waheed NK, Rosen RB, Jia Y, et al. Optical coherence tomography angiography in diabetic retinopathy. Prog Retin Eye Res. 2023;97:101206. doi:10.1016/j.preteyeres.2023.101206
6. Laíns I, Wang JC, Cui Y, et al. Retinal applications of swept source optical coherence tomography (OCT) and optical coherence tomography angiography (OCTA). Prog Retin Eye Res. 2021;84:100951. doi:10.1016/j.preteyeres.2021.100951
7. Wang M, Garg I, Miller JB. Wide Field Swept Source Optical Coherence Tomography Angiography for the Evaluation of Proliferative Diabetic Retinopathy and Associated Lesions: A Review. Semin Ophthalmol. 2021;36(4):162-167. doi:10.1080/08820538.2021.1887901
8. Hwang TS, Jia Y, Gao SS, et al. OPTICAL COHERENCE TOMOGRAPHY ANGIOGRAPHY FEATURES OF DIABETIC RETINOPATHY. Retina. 2015;35(11):2371-2376. doi:10.1097/IAE.0000000000000716
9. Jia Y, Bailey ST, Hwang TS, et al. Quantitative optical coherence tomography angiography of vascular abnormalities in the living human eye. Proc Natl Acad Sci U S A. 2015;112(18):E2395-2402. doi:10.1073/pnas.1500185112
10. Zhu Y, Cui Y, Wang JC, et al. Different Scan Protocols Affect the Detection Rates of Diabetic Retinopathy Lesions by Wide-Field Swept-Source Optical Coherence Tomography Angiography. Am J Ophthalmol. 2020;215:72-80. doi:10.1016/j.ajo.2020.03.004
11. Garg I, Uwakwe C, Le R, et al. Nonperfusion Area and Other Vascular Metrics by Wider Field Swept-Source OCT Angiography as Biomarkers of Diabetic Retinopathy Severity. Ophthalmol Sci. 2022;2(2):100144. doi:10.1016/j.xops.2022.100144
12. Le R, Cui Y, Lu ES, et al. Prevalence of venous loops and association with retinal ischemia in diabetic retinopathy using widefield swept-source OCT angiography. Graefes Arch Clin Exp Ophthalmol. 2023;261(7):1861-1870. doi:10.1007/s00417-022-05957-3
13. Ding X, Romano F, Garg I, et al. Expanded Field OCT Angiography Biomarkers for Predicting Clinically Significant Outcomes in Non-Proliferative Diabetic Retinopathy. Am J Ophthalmol. Published online October 25, 2024:S00029394(24)00485-9. doi:10.1016/j.ajo.2024.10.016
14. Arya M, Sorour O, Chaudhri J, et al. DISTINGUISHING INTRARETINAL MICROVASCULAR ABNORMALITIES FROM RETINAL NEOVASCULARIZATION USING OPTICAL COHERENCE TOMOGRAPHY ANGIOGRAPHY. Retina. 2020;40(9):1686-1695. doi:10.1097/IAE.0000000000002671
15. You QS, Guo Y, Wang J, et al. DETECTION OF CLINICALLY UNSUSPECTED RETINAL NEOVASCULARIZATION WITH WIDE-FIELD OPTICAL COHERENCE TOMOGRAPHY ANGIOGRAPHY. Retina. 2020;40(5):891-897. doi:10.1097/IAE.0000000000002487
16. Lu ES, Cui Y, Le R, et al. Widefield swept-source optical coherence tomography angiography metrics associated with neovascular glaucoma in patients with proliferative diabetic retinopathy. Graefes Arch Clin Exp Ophthalmol. 2024;262(4):1111-1120. doi:10.1007/s00417-023-06290-z
17. Ishibazawa A, Nagaoka T, Yokota H, et al. Characteristics of Retinal Neovascularization in Proliferative Diabetic Retinopathy Imaged by Optical Coherence Tomography Angiography. Invest Ophthalmol Vis Sci. 2016;57(14):6247-6255. doi:10.1167/iovs.16-20210
Lu, Edward
18. Pan J, Chen D, Yang X, et al. Characteristics of Neovascularization in Early Stages of Proliferative Diabetic Retinopathy by Optical Coherence Tomography Angiography. Am J Ophthalmol. 2018;192:146-156. doi:10.1016/j.ajo.2018.05.018
19. Vaz-Pereira S, Dansingani KK, Chen KC, Cooney MJ, Klancnik JM, Engelbert M. TOMOGRAPHIC RELATIONSHIPS BETWEEN RETINAL NEOVASCULARIZATION AND THE POSTERIOR VITREOUS IN PROLIFERATIVE DIABETIC RETINOPATHY. Retina (Philadelphia, Pa). 2017;37(7):1287-1296. doi:10.1097/IAE.0000000000001336
20. Lu ES, Cui Y, Le R, et al. Detection of neovascularisation in the vitreoretinal interface slab using widefield sweptsource optical coherence tomography angiography in diabetic retinopathy. Br J Ophthalmol. 2022;106(4):534-539. doi:10.1136/bjophthalmol-2020-317983
21. Russell JF, Shi Y, Hinkle JW, et al. Longitudinal Wide Field Swept Source OCT Angiography of Neovascularization in Proliferative Diabetic Retinopathy After Panretinal Photocoagulation. Ophthalmol Retina. 2019;3(4):350-361. doi:10.1016/j.oret.2018.11.008
22. Parrulli S, Airaldi M, Folegani V, et al. Multimodal imaging features of retinal neovascularization in response to 3 anti-VEGF injections. Can J Ophthalmol. Published online November 30, 2024:S0008-4182(24)00341-7. doi:10.1016/j.jcjo.2024.11.001
23. Sawada O, Ichiyama Y, Obata S, et al. Comparison between wide-angle OCT angiography and ultra-wide field fluorescein angiography for detecting non-perfusion areas and retinal neovascularization in eyes with diabetic retinopathy. Graefes Arch Clin Exp Ophthalmol. 2018;256(7):1275-1280. doi:10.1007/s00417-018-3992-y
24. Russell JF, Flynn HW, Sridhar J, et al. Distribution of Diabetic Neovascularization on Ultra-Widefield Fluorescein Angiography and on Simulated Widefield OCT Angiography. Am J Ophthalmol. 2019;207:110-120. doi:10.1016/j.ajo.2019.05.031
25. Hirano T, Hoshiyama K, Hirabayashi K, et al. Vitreoretinal Interface Slab in OCT Angiography for Detecting Diabetic Retinal Neovascularization. Ophthalmol Retina. 2020;4(6):588-594. doi:10.1016/j.oret.2020.01.004
26. Cui Y, Zhu Y, Wang JC, et al. Comparison of widefield swept-source optical coherence tomography angiography with ultra-widefield colour fundus photography and fluorescein angiography for detection of lesions in diabetic retinopathy. Br J Ophthalmol. 2021;105(4):577-581. doi:10.1136/bjophthalmol-2020-316245
27. Yang Y, Li F, Liu T, Jiao W, Zhao B. Comparison of widefield swept-source optical coherence tomographic angiography and fluorescein fundus angiography for detection of retinal neovascularization with diabetic retinopathy. BMC Ophthalmol. 2023;23(1):315. doi:10.1186/s12886-023-03073-2
28. Hamada M, Hirai K, Wakabayashi T, et al. Practical Utility of Widefield OCT Angiography to Detect Retinal Neovascularization in Eyes with Proliferative Diabetic Retinopathy. Ophthalmol Retina. 2024;8(5):481-489. doi:10.1016/j.oret.2023.11.009
29. Al-Khersan H, Russell JF, Lazzarini TA, et al. Comparison Between Graders in Detection of Diabetic Neovascularization With Swept Source Optical Coherence Tomography Angiography and Fluorescein Angiography. Am J Ophthalmol. 2021;224:292-300. doi:10.1016/j.ajo.2020.11.020
30. Wai KM, Moon JY, Garg I, et al. Comparison of Neovascularization Detection in Proliferative Diabetic Retinopathy Using Widefield Swept-Source Optical Coherence Tomography Angiography and Fluorescein Angiography Among Ophthalmology Residents at a Single Institution. Ophthalmic Surg Lasers Imaging Retina. Published online November 1, 2024:1-6. doi:10.3928/23258160-20241114-01
31. Pichi F, Smith SD, Abboud EB, et al. Wide-field optical coherence tomography angiography for the detection of proliferative diabetic retinopathy. Graefes Arch Clin Exp Ophthalmol. 2020;258(9):1901-1909. doi:10.1007/s00417-02004773-x
32. Khalid H, Schwartz R, Nicholson L, et al. Widefield optical coherence tomography angiography for early detection and objective evaluation of proliferative diabetic retinopathy. Br J Ophthalmol. 2021;105(1):118-123. doi:10.1136/bjophthalmol-2019-315365
33. Hirano T, Hoshiyama K, Takahashi Y, Murata T. Wide-field swept-source OCT angiography (23 × 20 mm) for detecting retinal neovascularization in eyes with proliferative diabetic retinopathy. Graefes Arch Clin Exp Ophthalmol. 2023;261(2):339-344. doi:10.1007/s00417-022-05878-1
34. Cui Y, Zhu Y, Lu ES, et al. Widefield Swept-Source OCT Angiography Metrics Associated with the Development of Diabetic Vitreous Hemorrhage: A Prospective Study. Ophthalmology. 2021;128(9):1312-1324. doi:10.1016/j.ophtha.2021.02.020
35. Russell JF, Scott NL, Townsend JH, et al. Widefield Swept Source OCT Angiography of Diabetic Tractional Retinal Detachments Before and After Surgical Repair. Retina (Philadelphia, Pa). 2021;41(8):1587. doi:10.1097/IAE.0000000000003146
36. Lu Y, Wang JC, Zeng R, et al. Quantitative Comparison Of Microvascular Metrics On Three Optical Coherence Tomography Angiography Devices In Chorioretinal Disease. Clin Ophthalmol. 2019;13:2063-2069. doi:10.2147/OPTH.S215322
37. Cui Y, Zhu Y, Wang JC, et al. Imaging Artifacts and Segmentation Errors With Wide-Field Swept-Source Optical Coherence Tomography Angiography in Diabetic Retinopathy. Transl Vis Sci Technol. 2019;8(6):18. doi:10.1167/tvst.8.6.18
38. Salz DA, de Carlo TE, Adhi M, et al. Select Features of Diabetic Retinopathy on Swept-Source Optical Coherence Tomographic Angiography Compared With Fluorescein Angiography and Normal Eyes. JAMA Ophthalmol. 2016;134(6):644-650. doi:10.1001/jamaophthalmol.2016.0600
39. Kim A, Ding X, Miller JB. Ultra-widefield Swept-Source OCT Angiography vs. Ultra-widefield Fluorescein Angiography in Diabetic Retinopathy. Am J Ophthalmol. 2024;268:e3-e4. doi:10.1016/j.ajo.2024.09.002
40. Wang RY, Zhu SY, Hu XY, Sun L, Zhang SC, Yang WH. Artificial intelligence applications in ophthalmic optical coherence tomography: a 12-year bibliometric analysis. Int J Ophthalmol. 2024;17(12):2295-2307. doi:10.18240/ijo.2024.12.19
41. Wongchaisuwat N, Wang J, White ES, Hwang TS, Jia Y, Bailey ST. Detection of Macular Neovascularization in Eyes Presenting with Macular Edema using OCT Angiography and a Deep Learning Model. Ophthalmol Retina. Published online October 24, 2024:S2468-6530(24)00488-3. doi:10.1016/j.oret.2024.10.017
42. Heinke A, Zhang H, Broniarek K, et al. Cross-instrument optical coherence tomography-angiography (OCTA)based prediction of age-related macular degeneration (AMD) disease activity using artificial intelligence. Sci Rep. 2024;14(1):27085. doi:10.1038/s41598-024-78327-0
Table 1. Neovascularization morphology on OCT/OCTA
Author/Year N (eyes) OCTA device Scan protocol NV classification (en face vs. B-scan)
Ishibazawa (2016) 40 RTVue XR
Avanti, Optivue
3x3, 4.5x4.5 En face
Vaz-Pereira (2017) 51 Spectralis OCT B-scan B-scan
NV morphology Findings
Exuberant vascular proliferation (EVP): irregular proliferation of fine, smallercaliber new vessel (vs. pruned NVD/NVE without EVP)
- Flat NV: confined to the posterior hyaloid face
- Forward NV: traversed posterior hyaloid face
- Tabletop NV: anterior displacement by vitreous traction, tethered by vascular “pegs” with the bulk of the neovascular tissue growing along the posterior hyaloid face
EVP is a sign of active NV
- Most neovascularization complexes arise and grow along the posterior hyaloid face
- Vitreoschisis is more prevalent than previously found in ultrasound studies
- Tabletop configuration does not seem to result from invasion of the bursa as previously suggested
Pan (2018) 35 RTVue XR
Avanti, Optivue
3x3, 4.5x4.5 En face, B scan
Type 1: originated from venous side, in a tree-like shape
Type 2: originated from capillary networks, octopuslike appearance
Type 3: originated from IRMAs, sea fan shape
Lu (2022) 142 Plex Elite, Zeiss 12x12 B-scan Forward, flat, tabletop as defined by VazPereira et al. (2017)
OCTA allows for identification of origins and morphology of NV
- VRI slab detects NV with high sensitivity
- Flat NV had lower rates of detection due to segmentation error
Table 2. Studies comparing SS-OCTA to FA in the detection of diabetic neovascularization
Author /Year N (eyes) OCTA device Scan protocol Sensitivity Specific
Sawad a (2018) 58 (31 NPDR, 27 PDR) Plex Elite, Zeiss 12x12 (5 fixation points)
Russell (2019) 651 (PDR) Plex Elite, Zeiss 12x12 (5 fixation points; Simulated field of view )
(eyes)
Hirano (2020) 42 (PDR) Plex Elite, Zeiss 15x15 73% (NV), 84% after correcting segmentatio n errors N/A Yes FA
Pichi (2020) 82 (PDR) Plex Elite, Zeiss 12x12 (5 fixation points)
Cui (2021) 152 (75 PDR, 57 NPDR, 20 no DR) Plex Elite, Zeiss
15x15
(NV)
Hirano (2023) 64 (PDR) Xephili o OCTS1 (Cano n) 23x20 96% (NV; fovea centered), 99% (disc centered), 100% (both) N/A No biomicroscopy, color fundus photography, FA Yang (2023) 53 VG200 , SVisio n Imagin g 12x12 (5 fixation points)
Hamad a (2024) 108 (PDR) S1, Canon 23x20 67% (NV); 95% (eyes)
Ryan S. Meshkin, MD and Dean Eliott, MD
Abstract
Following the first successful macular hole surgery performed by Kelly and Wendel in 1990, advances in surgical techniques and instrumentation have established pars plana vitrectomy with internal limiting membrane (ILM) peeling and gas tamponade as the gold standard for macular hole treatment. Closure rates are widely regarded as over 90% with this approach. In cases of persistent or recurrent fullthickness macular holes (FTMHs), new surgical techniques have been pioneered over the past decade for holes with worse prognosis related to size, chronicity, myopia, prior unsuccessful surgery, or other factors. This review aims to summarize the surgical developments, outcomes, and rationale for three techniques: the inverted ILM flap, amniotic membrane grafting, and autologous retinal transplantation.
Introduction
Macular hole was first described by Knapp in 1869 in a case of trauma 1 A full-thickness macular hole (FTMH) is a round, complete break in the foveal center spanning all retinal layers from the internal limiting membrane (ILM) to the retinal pigment epithelium (RPE). FTMHs can present with sudden vision loss, a central scotoma, and metamorphopsia 2 They can occur secondary to high myopia and trauma, although the vast majority are due to vitreous traction, termed ‘primary’ or ‘idiopathic’. Primary FTMHs have a prevalence of 0.02% to 0.8% 2–6 in the general population and more commonly affect individuals over 60 years old with an incidence of 7.8 new cases per 100,000 population per year.2 There is a female-male preponderance of 2.2-3.3:1. 2,5,7 The pathophysiology of primary FTMHs is thought to involve an abnormal vitreoretinal interface resulting in traction transmitted in both antero-posterior and tangential axes 8 These forces disrupt the retinal layers and contribute to macular hole formation. Additionally, degeneration of foveal inner retinal layers has been proposed as a complementary hypothesis, thereby predisposing those tractional forces to progress to FTMH 9
A complete characterization of macular holes is necessary for appropriate intervention. Descriptive characteristics include etiology (idiopathic or secondary to myopia, trauma, or inflammation), association with concurrent retinal detachment (RD) or epiretinal membrane (ERM), chronicity, size, status of the posterior hyaloid, whether the hole is primary or refractory (and if refractory, whether it is persistent or recurrent), as well as the nature of prior surgical interventions, such as whether prior ILM peeling has been performed. In addition to helping further elucidate the pathophysiology of FTMHs, the advent of optical coherence tomography (OCT) has helped refine its staging system. In 2013, the International Vitreomacular Traction Study Group released an updated anatomic classification system based on the extent of perifoveal vitreous separation, the presence of anatomic foveal distortion, the diameter of vitreous attachment to the macular surface, as well as the size of the hole. Hole size subclassification was reported as: small (<250 microns), medium (250-400 microns), and large (>400 microns). 10 Later, the Manchester Large Macular Hole Study noted more significant changes in hole closure prognosis when hole size exceeded 650 microns.11
Discussion of various treatment approaches must needs begin with characterization of the outcomes sought when treating FTMHs. A successful anatomic result is obtained when the hole undergoes closure with reconstitution and realignment of the inner and outer retinal layers. Beyond hole closure, a functional outcome, namely an improvement in visual acuity (VA) is also sought. Lastly, the number of surgical interventions required to achieve the desired anatomic and functional result is also considered.
The first successful surgery for FTMHs was performed and reported by Kelly and Wendel in 1990. They reported 52 cases with a success rate of 58% 12 Their approach incorporated vitrectomy, posterior
vitreous detachment (PVD), peeling of any co-existing ERM, fluid-gas exchange, and face-down postoperative positioning. The widely accepted gold standard surgical approach utilized most often today for treatment of primary FTMH involves pars plana vitrectomy (PPV), peeling of ILM, and utilization of gas tamponade. PPV and PVD induction alleviate antero-posterior traction while ILM and ERM peeling address the tangential tractional forces. OCT has demonstrated that the posterior vitreous is attached to the edge of the macular hole in a small minority of cases 13 It is felt, therefore, that the main benefit of macular hole surgery derives from addressing the persistent tangential traction with peeling techniques and by creating space for gas tamponade. Various explanations for the role of gas tamponade in macular hole repair include dehydrating the edges of the hole and facilitating healing by reducing fluid currents within the eye 14 The use of gas tamponade is not always necessary as studies have shown hole closure with vitreolysis alone. One series studying the vitreolysis agent ocriplasmin, reported nearly 60% closure in holes less then 250 microns, and this rate increased substantially when gas tamponade was utilized 15,16 OCT studies have demonstrated that hole edges begin to flatten with a reduction in hole size noted on the day after surgery and hole closure typically occurs by post-operative day 3 to 7 depending on hole size 17
Nowadays, the closure rate exceeds 95% following a single intervention.18–20 Visual outcomes have similarly improved such that after cataract surgery, mean postoperative VA tends to be better than 20/40 (in the absence of co-existing retinal pathology) and improves over time. 21,22 In a large multi-center prospective series of 2455 eyes in whom 95.6% achieved hole closure, the mean improvement in VA at 12 months was 19.2 letters. 20 Microperimetry has enabled detection of relative or absolute microscotomas post-operatively in some patients which may be attributed to microtrauma during membrane peeling.21
Despite the significantly improved success rate since Kelly and Wendell in 1990, there are instances when FTMHs do not close after a single procedure. Factors associated with risk of hole closure failure include large hole size, traumatic etiology, duration greater than 6 months, high myopia, previous failed macular hole surgery, flat hole edge configuration, RD-associated macular holes, inadequate ILM peeling, and inability to position appropriately post-operatively 23,24 There still lacks consensus defining what constitutes a “large” hole with poor prognosis, however, Ch’ng et al. demonstrated good anatomic outcomes with conventional surgery in FTMHs up to 650 microns 11 Patients with small holes have been shown to have a better final VA when compared to patients with large holes 25 Studies comparing ILM peeling and non-peeling have shown closure rates of 77-89% with non-peeling as compared to 92-97% with ILM peeling 26,27 Tadayoni et al. showed that the effect of ILM peeling on successful FTMH closure is more apparent in large holes (>400 microns) than small holes (<400 microns) 28 There are mixed results when it comes to assessing the benefit of post-operative positioning on hole closure. A randomized prospective trial comparing face-down and seated positioning showed superior closure rate in the facedown positioning cohort, although this difference did not persist for hole size smaller than 400 microns 29 Alternatively, a different randomized trial failed to show benefit in closure rate with face-down positioning for holes larger than 400 microns 30 Ultimately, it appears that while post-operative face-down positioning is not always necessary, the general consensus is that prolonged gas bubble-macula contact may support healing in holes with worse closure prognosis.31 Results from the American Society of Retina Specialists (ASRS) Preferences and Trends (PAT) Survey in 2020 showed that 46% of U S retina specialists recommend 5-7 days of face-down positioning after macular hole repair 32
Over the last decade, new and innovative surgical approaches have emerged for the treatment of refractory FTMHs. The aim of this review is to summarize the evolution of three of these surgical approaches, their underlying principles, and briefly, their reported outcomes.
The inverted ILM flap technique was first reported by Michalewska et al. in 2010 33 The technique, as originally described, involved peeling the peripheral macular ILM while keeping the ILM attached at the edges of the macular hole. The edges of the detached ILM would then be inverted into the hole. The authors state that the principle is to not completely remove the peeled ILM but to leave a small remnant at
Meshkin, Ryan
the surface of the hole to serve as a hinge. In doing so, the remnant is inverted such that the side that was previously oriented to the vitreous is made to face the RPE, and by extension, the side previously touching the underlying retinal layers is made to face the vitreous cavity. The initial series reported a 98% hole closure rate as compared to 88% with the conventional ILM peeling approach as well as improved VA outcomes. This technique was among the first to report an improvement in closure rate and visual function for large macular holes (> 400 microns)33. Thereafter, several larger multi-center studies further demonstrated the utility of the inverted ILM flap technique. Naranyan et al. reported an 89% closure rate when compared to conventional peeling, which had a 78% closure rate, for holes >800 microns, with superior post-operative visual acuity 34 Rizzo et al. achieved a 96% closure rate with inverted ILM flap vs. 79% with conventional peeling in a series of >400 micron FTMHs 35 In a retrospective Japanese study with 165 eyes comparing outcomes between the inverted ILM technique and conventional peeling, Yamashita et al. reported a closure rate of 100% (19/19) and 95.2%, respectively, for medium-large (400550 micron) holes.36 This trend also applied to extra-large (>500 micron) holes with conventional ILM peeling achieving 88.4% (38/43) closure and inverted ILM peeling achieving 100% (41/41) closure. These findings were extended to myopic holes and macular hole-associated RDs. 37–39 The superb outcomes summarized here may explain why over 99% of U.S. retina surgeons chose to treat a 1-month old 400 micron FTMH with PPV plus ILM peeling or ILM flap technique on the 2020 ASRS PAT Survey.32 Moreover, 89% of US retina surgeons similarly opted to treat a 1000 micron chronic macular hole with PPV plus ILM peeling or ILM flap.
Since Michalewska’s original inverted ILM flap technique was reported, numerous variations have also been utilized. In the temporal inverted ILM flap technique, ILM is peeled temporally up to the macular hole, with the edges of the ILM kept attached to the hole. The temporal ILM flap is then draped over the macular hole (Figures 1 and 2) 40 This technique was found to be associated with fewer cases of dissociated optic nerve fiber layer (DONFL). DONFL is defined as retina with striae along the optic nerve fibers corresponding to discontinuities in the inner retinal layers that are visible on OCT. The ‘texas taco’ technique involves peeling nasal ILM just beyond the temporal edges of the hole, such that it is undermined 360-degrees off the macular hole circumference The fold of the taco is beyond (more temporal than) the FTMH. Once fluid–air exchange is performed, the flap is drawn nasally to completely cover the hole 41 Another variation that is useful in situations when prior complete ILM peeling has been performed is the ILM free flap technique. As the name suggests, a complete circular area of ILM is harvested from another area of the retina and brought to, and typically inserted within, the FTMH 42 The original series reported by Morizane et al. in 2014 found a 90% closure rate in 10 eyes with refractory FTMH 42 Multiple other series have substantiated these findings 43–46
Additional variations of the ILM flap technique including the pedicle ILM flap47, the temporal/nasal hemiflaps48, the double ILM flap insertion technique49, the ILM retracting door technique50, and most recently, the superior wide-base ILM flap transposition (SWIFT) technique51 , have all been reported with similarly favorable outcomes. In the SWIFT technique, a ring of ILM has been previously removed during prior surgery. A flap of ILM is harvested superiorly and draped over a horizontal base inferiorly to cover the macular hole. It is proposed that the SWIFT flap may be more stable given the wide inferior flap base as well as the superior-to-inferior drape directionality, which, aided by gravity, helps maintain the ILM’s position and reduce microtrauma associated with techniques that tuck the ILM into the hole. In a series of 17 total eyes, 6 had chronic holes, 4 had high myopia, and 3 had prior macular hole surgery with ILM peeling. The mean inner hole diameter was 516.1 microns, of which 6 eyes had an inner diameter >650 microns. The authors report a closure rate of 94% with improvement in baseline mean VA from 0.88 logMAR to 0.54 logMAR using the SWIFT technique.51
The inverted ILM flap technique and the associated variations are thought to facilitate hole closure by providing a tissue scaffold. The ILM has been suggested to serve as an interface along which Müller cells are guided to migrate and proliferate to bridge the hole defect 42 This hypothesis has been supported by immunohistochemical studies on FTMH treated with the inverted ILM flap technique in primates which showed GFAP-positive proliferating cells along the ILM flap. Various authors have hypothesized that ILM may additionally contain Müller cell fragments which can further induce gliosis 52,53 Various known promoters of Müller cell proliferation in ILM, including type IV collagen and fibronectin, have been shown in vitro to support Müller cell activity.54 Various neurotrophic factors contained within ILM extracellular
matrix have similarly been expressed by Müller cells in vitro55 , and which, by virtue of their capacity to prevent neuronal cell apoptosis, may help facilitate hole closure 56,57
Human amniotic membrane is derived from the inner layer of human placenta. It is composed of three layers: a single-layered epithelium overlying a thick basement membrane and a stromal layer. Its original use was identified in treating anterior segment disorders, specifically in corneal ulcers and corneal chemical injuries 58 Building on experimental studies by Rosenfeld et al. showing that human amniotic membrane could be placed subretinally in rabbit eyes without transplant rejection, Rizzo and colleagues reported the first series of patients undergoing amniotic membrane transplantation. 59 The surgical technique can be performed with cryopreserved, lyophilized, or dehydrated human amniotic membrane graft 60 The graft is cut to the approximate appropriate size necessary to overlap the hole diameter, typically 1-2mm, which can be further fine-tuned with vitreoretinal scissors 61 Studies show that the graft should be roughly 500 microns larger in diameter than the hole itself 62 A four-port PPV utilizing chandelier endo-illumination has been shown helpful in allowing for the delicate bimanual approach 59,61 Forceps are used to approximate the edges of the graft and insert it through one of the ports. Within the vitreous cavity, care is taken to check the directionality of the graft. The stromal side of the graft can be identified as it tends to stick to the forceps. The stromal side of the graft is placed facing the RPE. The ultimate positioning of the graft can either be within or overlying the FTMH. If placing within the hole, Caporossi et al. have recommended ensuring the edges of the hole are elevated off the RPE, either by massaging the hole edges or by infusing balanced salt solution (BSS) with a soft-tip instrument to raise the hole edges 61 The membrane can be manipulated under perfluorocarbon liquid (PFCL) to assist with control and handling of the graft. Following fluid-air exchange, gas tamponade is typically administered to assist with graft adherence to the RPE 63
The surgical outcomes for FTMHs treated with human amniotic membrane grafting vary. Typically, this procedure is used in cases when the ILM peeling/flap technique has proven unsuccessful resulting in a persistent or recurrent FTMH. The initial series published by Rizzo et al. using this technique reported 8 patients experiencing a 100% hole closure rate and mean improvement in VA from 20/800 to 20/100 at 3 months post-operatively 59 All patients had previously undergone PPV with ILM peeling and gas tamponade. OCT showed retinal adhesion over the amniotic membrane transplant during the first postoperative week. Retinal ingrowth on the hole margins over the amniotic graft was seen by post-operative months 2 through 3. The investigators opted to implant the amniotic membrane in the subretinal space to maximize interaction with the RPE. The thin retinal substrate overlying the amniotic membrane provided evidence for the membrane’s ability to stimulate tissue proliferation. Normal retinal layer organization was appreciated on post-operative OCT on the overlying neuroretina including in the outer retinal layers such as the external limiting membrane (ELM) and ellipsoid zone (EZ) They surmise that this reconstitution of the outer retinal layers is the likely explanation for the improvement in visual acuity and foveal sensitivity after the procedure, which reached its peak at six months after surgery. To the contrary, there have been reports of persistent absence and disorganization of the ELM and EZ even in holes that close 60 In a series of 7 refractory FTMHs, hole re-opening occurred in 28% of the eyes that were treated with amniotic membrane placed epiretinally, suggesting that a subretinal placement may be superior 64
Following Rizzo’s initial series, Caporossi et al. presented two similarly successful case series of the amniotic membrane transplantation technique. In both series, the amniotic membrane plug was inserted between the macular hole edges and RPE. The first included 20 eyes with persistent FTMHs with 100% hole closure and mean VA improvement from 20/320 to 20/63 63 They subsequently showed in 16 eyes with high myopia experiencing recurrent FTMH, that 15 eyes had hole closure with mean VA improvement from 20/200 to 20/100 61 The most commonly reported complication following amniotic membrane transplantation is graft dislocation. The rate of graft dislocation varies widely from 5.9% to 28.6% 60–63 Graft contracture, parafoveal atrophy, and iatrogenic trauma associated with subretinal graft placement have also been reported.60,62 In further analyzing performance of various graft preservation
Meshkin, Ryan
techniques for use in treating refractory FTMHs, Zhang et al. reported that cryopreserved grafts with a thicker stroma tended to have better closure rates than dehydrated grafts 65
The rationale behind using human amniotic membrane transplantation is multifactorial. Animal studies have shown amniotic membrane to be non-toxic to the retina suggesting that it promotes wound healing and serves as a scaffold for glial cell proliferation 66,67 The membrane itself is avascular and contains type IV collagen, fibronectin, and laminin, much like the ILM itself 68,69 The success of amniotic membrane grafting for FTMH closure has been demonstrated when placed over the hole and subretinally. In instances of subretinal placement, it is theorized to release growth factors that encourage hole closure. When positioned on top of the hole, the scaffold hypothesis is invoked whereby the membrane helps retinal tissue to approximate the hole. Prior to its adoption and subretinal utilization in patients, Kiilgard and Ohno-Matsui had shown subretinal placement to be well tolerated and to support RPE proliferation 70,71 OCT-angiography has shown similar deep capillary plexus density in eyes treated with human amniotic membrane for refractory FTMHs between the treated and fellow eye. This supports that the membrane may assist with deep capillary plexus reconstruction 59,61 Parafoveal segment vascularization has also been related to VA improvement in cases of hole closure with amniotic membrane transplantation in non-myopic eyes.61
Autologous retinal transplantation was first introduced in 2016 when Grewal and colleagues reported a new technique involving a retinal free flap for eyes with refractory FTMHs after prior failed ILM peeling/flap surgery.72 Their patient with -15 diopter myopia had a persistent 1100 micron macular hole with VA 20/200. Intraoperatively, there was poor staining with indocyanine green in the staphyloma, but ILM remnants in the macula confirmed adequate ILM peeling had already been performed. They were unable to harvest additional ILM to use in a free flap technique. As such, they proceeded to harvest neurosensory retina from just superior to the supero-temporal arcade. Endolaser barricade was applied around a 2-disc diameter area of retina which was subsequently diathermized. Chandelier illumination enabled utilization of a bimanual approach. The graft edge was held with forceps and cut with vertical scissors. The diathermy marks served to maintain correct orientation of the free flap. PFCL was used to hold the flap in place and flatten the flap edges over the full extent of the hole. A direct PFCL to silicone oil exchange was carried out and the patient was instructed to maintain facedown positioning for one week. OCT post-operatively showed hole closure. The patient reported subjective improvement in distortion and decreased scotoma size. Her VA improved gradually to 20/160 at post-operative week one and then to 20/80 by three months. The harvest site was stable. Microperimetry showed retinal sensitivity improvement from 7.5 dB at 1 week to 12.3 dB at 3 months after surgery. The utility of this novel approach was initially considered to be in cases with significant myopia where ILM peeling/flap creation is challenging due to poor ILM staining 42,73 An advantage of the autologous retinal transplant, is that the flap is thicker and can be successfully positioned over the macular hole rather than needing to be inserted subretinally, thereby reducing potential trauma.
Grewal and colleagues’ subsequent case series included large (mean diameter 825 microns), myopic macular holes in which a peripheral autologous retinal transplant was placed into the macular hole with either gas, oil, or short-term PFCL. Of the 41 eyes, 88% experienced hole closure with over one third of patients experiencing 3+ lines of VA improvement 74 In 2021, a large international multicenter, retrospective, consecutive case series of 130 patients undergoing autologous retinal transplantation for primary (n=35) and refractory (n=76) FTMHs, as well as for macular hole-associated retinal detachments (MH-RD) (n=19), was performed.75 Thirty-three surgeons contributed cases. They reported mean maximum and minimum macular hole diameters of 1470 and 840 microns, respectively. 89% of FTMHs closed, and 95% of MH-RDs closed. The authors did, however, consider patients who had a residual small eccentric defect as having achieved closure. In terms of functional outcomes, 43% of patients experienced a 3-line improvement in VA, 29% had a 5-line improvement in VA, and 12% were left with 20/50 or better VA. They found EZ reconstitution and alignment of neurosensory layers on OCT to be associated with better final VA. There were 5 cases of graft dislocation (3.8%). In subgroup analysis focused only on the refractory FTMHs, mean pre- operative and post-operative VAs were 20/362 and 20/231, respectively. Of the 76 patients in this subgroup, OCT analysis was available for 39 eyes and
showed EZ and ELM reconstitution in two-thirds of eyes and alignment of neurosensory layers in one-fifth of eyes. No significant association was found between tamponade agent, PPV gauge, graft size, or type of grant and closure rate or final VA OCT angiography was obtained for 11 cases, descriptive analyses suggested some vascularization into the region of the graft in the superficial and deep capillary plexuses. Vessel growth, however, was grossly limited and scattered with many areas of avascularity on long-term follow-up. Microperimetry data in 12 cases showed a very mild increase in fixation in the graft area. The authors reported a mean response of 6.7 dB. Three cases with multifocal electroretinography indicated slow but measurable B-wave amplitudes in the region of the transplant.
The most recent systematic review of autologous retinal transplant surgery carried out by Patel et al. included 10 series with a minimum of five eyes in each study. The weighted mean FTMH closure rate was 88% (range: 66.7% - 100%) 76 Many case series reported some improvement in visual acuity. Weighted mean pre-operative visual acuity improved from 1.35 logMAR (~20/450) to 1.02 logMAR (~20/210). Reported complications with this technique include intraoperative hemorrhage (3.1%), graft slippage (2.3%), undersized graft (1.5%), and subfoveal RPE damage (0.8%) 77 Other rare complications that have been published include post-operative RD and cystoid macular edema.78,79
Several additional considerations and best-practices associated with this technique bear keeping in mind. Adequate graft size is vital with some surgeons recommending a flap 0.5 disc diameters larger than the hole diameter to allow for sufficient overlap, easier handling, and hole coverage even in the case of mild flap decentration. The original technique suggests oversizing the graft by 1.2-1.5 times the original defect size due to shrinkage when the graft integrates into the adjacent retinal tissue 72,74 Moysidis and colleagues concluded in their large multicenter series that because there was no significant difference in closure rate and VA between transplanted tissue placed subretinally and epiretinally, it may be favorable to proceed with epiretinal placement to minimize iatrogenic trauma associated with raising the hole edges. Since introduction of this approach, modifications to the technique have been reported such as covering the transplant with autologous blood80 or dispersive viscoelastic material81 once the graft is in place to assist with stabilization in a glue-like function. The use of autologous blood had previously been proposed as an adjuvant to augment healing in conjunction with the ILM flap technique.73 Chang and colleagues reported good outcomes with placing autologous blood or viscoelastic within the hole prior to autologous retinal graft insertion 81
Although the choice of tamponade agent did not appear to affect outcomes, it is well-established that silicone oil blocks oxygen transfer between the anterior and posterior segments after vitrectomy82, so perhaps oxygen diffusion to the graft may be better served with short-term PFCL. Various approaches exist for tamponade agent administration including short-term PFCL with a staged removal approach after two weeks which allows for verification of graft position with OCT and the ability to further adjust graft position during subsequent PFCL removal. A direct PFCL-gas exchange can be performed, although it can result in graft slippage or outright dislocation. With regards to positioning, Grewal et al. in their original description of the technique recommended positioning face-down for one week or in a supine position if short-term PFCL was used (typically for 3 weeks prior to removal).74 In terms of graft harvest site, the most common sites were superior (45%), inferonasal (17%), superotemporal (11%), although Moysidis et al. did not report any significant impact on outcomes based on harvest site.75 Theoretically, a superior graft site has a lower risk for post-operative proliferative vitreoretinopathy from the iatrogenic retinal break creation. In instances of subretinal graft placement, the induction of a small focal retinal detachment with a subretinal BSS injection was typically achieved with a 41-gauge cannula, although this approach does presumably increase the risk of subretinal PFCL migration. Focal retinal detachment induction may generate shearing forces that could in turn damage photoreceptors, which is another reason why surgeons might opt for epiretinal graft placement.
Autologous retinal transplants have been theorized to replace retinal tissue and integrate within existing healthy photoreceptors by a variety of mechanisms. Grewal and colleagues showed post-autologous retinal transplant OCT images with progressive flattening of macular hole edges and migration of surrounding retina with graft integration 72,74 By serving a plug-like function, the retinal graft acts as a barrier between the vitreous and subretinal space allowing the RPE to remove subretinal fluid and achieve hole closure. In addition to the scaffold function enabling glial cell proliferation that was attributed
Meshkin, Ryan
to amniotic membrane grafts and ILM flaps, supporters of the autologous retinal transplant technique have proposed that this approach carries with it cell rehabilitation advantages. These claims are based around prior animal model studies which demonstrated synaptic plasticity after photoreceptor-bipolar cell dissociation.83–85 It is thought that transplanted retinal photoreceptors can directly synapse with bipolar cells through a process called ectopic synaptogenesis. In this way, healthy photoreceptors could promote cell-cell communication with adjacent damaged photoreceptors, exerting a rehabilitation effect. One example of this phenomenon stems from retinitis pigmentosa models that show reactivation of dormant cones after healthy rod photoreceptor transplantation, as evidenced by restoration of glucose transport and cone outer segment synthesis 86
Autologous retinal transplants may also have cell rehabilitation properties because they contain retinal progenitor cells (RPCs). Karl et al. showed that RPCs from the anterior retina near the ciliary margin showed significant regenerative capabilities in fish and amphibians 87 To further support this, proponents cite studies showing Müller cells from cadaveric human retina demonstrating pluripotency in vitro with expression of neural stem cell markers 88,89 Perhaps these harvested Müller cells could differentiate into rod photoreceptors and ganglion cells and integrate with outer and inner retinal layers as seen in retinal transplants carried out in animal models.90,91 Mahato et al. showed that integrated precursor cells have led to functional improvements, specifically in the pupillary reflex and with scotopic tasks in mouse models of rod degeneration.92 The injured retina’s improved incorporation of RPCs when compared to normal retina may stem from relative hypoxia in the extracellular matrix that increases RPC pluripotency 90,93 Retinal degeneration models have similarly shown that RPCs may rehabilitate surrounding retinal tissue through a mechanism referred to as material transfer. Proteins and RNA are capable of being transferred to photoreceptors away from the site of local subretinal RPC injection and have been theorized through this mechanism to augment cell functions previously lost in degenerative retina models 94
Despite the cited experiments supporting various cellular properties that could support functioning of grafted retinal tissue, further studies are needed before concluding that grafted tissue is viable. Authors claim that the increased retinal sensitivity and VA are proof that the autologous retinal flap can restore retinal function. They noted gradual restoration of the nasal ELM and EZ and suggest that perhaps there is potential for the outer retina to be restored 75 Authors have concluded that grafted retina may serve as a bridge where it is placed as evidenced by partial outer retinal layer reconstitution and signs of graft reperfusion 74,75,95 With regards to vascular perfusion, it is theorized that the graft depends on oxygen diffusion from the choroid for survival in the early postoperative period before new vessels anastomose with old vessels around the graft and that areas of vascular leakage at the graft-host junction may positively suggest neovascularization 95
Critics of this technique raise various counter-points. It is important to consider that in the peripheral retina, rods outnumber cones 30:1, making it difficult to believe that such sparsely cone-dense retina could manifest meaningful retinal function 96 Furthermore, in the process of transplanting the tissue, the retinal fibers are transected with those at the margins being subjected to diathermy and laser photocoagulation. Their neural and vascular connections are entirely interrupted prior to further manipulation involved with graft fixation over the defect. It seems challenging to accept that such adulterated tissue is capable of retinal function. It is conceivable that some of the reported VA gains are secondary to eccentric fixation. Tests of retinal sensitivity with microperimetry, for example, are subject to misinterpretation if there is not exact correspondence between the points being tested. Furthermore, postoperative OCT analysis in several studies claimed reconstitution of various retinal layers, however, many of the published images only show partial reconstitution at the graft margins but not within the graft. Nevertheless, this surgical approach certainly carries with it various advantages in achieving hole closure. Donor tissue is always available to be harvested in cases where ILM flaps or peeling is not feasible. Autologous retinal tissue can be customized in terms of size dimensions to allow for easier surgical handling due to its greater thickness than ILM. Although the ability to close large, chronic FTMHs with autologous retinal transplantation has made surgery possible for patients who could not be treated previously, questions remain around how graft survival can be supported metabolically, how peripheral retina transplanted to the fovea can function, and how the tissue can integrate with the rest of the macula
The inverted ILM flap, amniotic membrane graft, and autologous retinal transplant each have their unique advantages and drawbacks. These approaches represent only three among many techniques that have been developed for the treatment of refractory FTMHs. Although there is no established superior technique, specific patient characteristics and surgeon preference may lend themselves to a particular approach. The surgical armamentarium has substantially grown over the past decade, and we look forward to future studies to further improve both anatomic and functional outcomes.
Meshkin, Ryan
1. Knapp H. Uber isolirte zerreissungen der aderhaut in folge von traumen auf dem augopfel. Arch Augenhetlkd 1869;1:6-29.
2. McCannel CA, Ensminger JL, Diehl NN, Hodge DN. Population-based incidence of macular holes. Ophthalmology 2009;116(7):1366-1369. doi:10.1016/j.ophtha.2009.01.052
3. Mitchell P, Smith W, Chey T, Wang JJ, Chang A. Prevalence and associations of epiretinal membranes. The Blue Mountains Eye Study, Australia. Ophthalmology. 1997;104(6):1033-1040. doi:10.1016/s0161-6420(97)30190-0
4. Rahmani B, Tielsch JM, Katz J, et al. The cause-specific prevalence of visual impairment in an urban population. The Baltimore Eye Survey. Ophthalmology. 1996;103(11):1721-1726. doi:10.1016/s0161-6420(96)30435-1
5. Sen P, Bhargava A, Vijaya L, George R. Prevalence of idiopathic macular hole in adult rural and urban south Indian population. Clin Experiment Ophthalmol. 2008;36(3):257-260. doi:10.1111/j.1442-9071.2008.01715.x
6. Wang S, Xu L, Jonas JB. Prevalence of full-thickness macular holes in urban and rural adult Chinese: the Beijing Eye Study. Am J Ophthalmol. 2006;141(3):589-591. doi:10.1016/j.ajo.2005.10.021
7. Ezra E. Idiopathic full thickness macular hole: natural history and pathogenesis. Br J Ophthalmol. 2001;85(1):102. doi:10.1136/bjo.85.1.102
8. Schumann RG, Eibl KH, Zhao F, et al. Immunocytochemical and ultrastructural evidence of glial cells and hyalocytes in internal limiting membrane specimens of idiopathic macular holes. Invest Ophthalmol Vis Sci. 2011;52(11):78227834. doi:10.1167/iovs.11-7514
9. Smiddy WE, Flynn HW. Pathogenesis of macular holes and therapeutic implications. Am J Ophthalmol 2004;137(3):525-537. doi:10.1016/j.ajo.2003.12.011
10. Duker JS, Kaiser PK, Binder S, et al. The International Vitreomacular Traction Study Group classification of vitreomacular adhesion, traction, and macular hole. Ophthalmology. 2013;120(12):2611-2619. doi:10.1016/j.ophtha.2013.07.042
11. Ch’ng SW, Patton N, Ahmed M, et al. The Manchester Large Macular Hole Study: Is it Time to Reclassify Large Macular Holes? Am J Ophthalmol. 2018;195:36-42. doi:10.1016/j.ajo.2018.07.027
12. Kelly NE, Wendel RT. Vitreous surgery for idiopathic macular holes. Results of a pilot study. Arch Ophthalmol Chic Ill 1960. 1991;109(5):654-659. doi:10.1001/archopht.1991.01080050068031
13. Philippakis E, Amouyal F, Couturier A, Boulanger-Scemama E, Gaudric A, Tadayoni R. Size and vitreomacular attachment of primary full-thickness macular holes. Br J Ophthalmol. 2017;101(7):951-954. doi:10.1136/bjophthalmol2016-309212
14. Berger JW, Brucker AJ. The magnitude of the bubble buoyant pressure: implications for macular hole surgery. Retina Phila Pa. 1998;18(1):84-86; author reply 86-88. doi:10.1097/00006982-199801000-00020
15. Dugel PU, Group MTS. A Single Injection Of Ocriplasmin For The Treatment Of Symptomatic Vitreomacular Adhesion (sVMA): Results Of The Phase III MIVI-TRUST Program. Invest Ophthalmol Vis Sci. 2011;52(14):6628.
16. Jackson TL, Verstraeten T, Duchateau L, Lescrauwaet B. Visual function response to ocriplasmin for the treatment of vitreomacular traction and macular hole. Acta Ophthalmol (Copenh). 2017;95(8):e740-e745. doi:10.1111/aos.13369
17. Kikushima W, Imai A, Toriyama Y, Hirano T, Murata T, Ishibashi T. Dynamics of Macular Hole Closure in Gas-Filled Eyes within 24 h of Surgery Observed with Swept Source Optical Coherence Tomography. Ophthalmic Res 2014;53(1):48-54. doi:10.1159/000368437
18. Kumagai T, Shoji T, Yoshikawa Y, et al. Comparison of central visual sensitivity between monocular and binocular testing in advanced glaucoma patients using imo perimetry. Br J Ophthalmol. Published online March 9, 2020. doi:10.1136/bjophthalmol-2019-315251
19. Sheidow TG, Blinder KJ, Holekamp N, et al. Outcome results in macular hole surgery: an evaluation of internal limiting membrane peeling with and without indocyanine green. Ophthalmology. 2003;110(9):1697-1701. doi:10.1016/S0161-6420(03)00562-1
20. Essex RW, Hunyor AP, Moreno-Betancur M, et al. The Visual Outcomes of Macular Hole Surgery: A Registry-Based Study by the Australian and New Zealand Society of Retinal Specialists. Ophthalmol Retina. 2018;2(11):1143-1151. doi:10.1016/j.oret.2018.04.022
21. Haritoglou C, Gass CA, Schaumberger M, Gandorfer A, Ulbig MW, Kampik A. Long-term follow-up after macular hole surgery with internal limiting membrane peeling. Am J Ophthalmol. 2002;134(5):661-666. doi:10.1016/s00029394(02)01751-8
22. Bonnabel A, Bron AM, Isaico R, Dugas B, Nicot F, Creuzot-Garcher C. Long-term anatomical and functional outcomes of idiopathic macular hole surgery. The yield of spectral-domain OCT combined with microperimetry. Graefes Arch Clin Exp Ophthalmol 2013;251(11):2505-2511. doi:10.1007/s00417-013-2339-y
23. Steel DH, Donachie PHJ, Aylward GW, Laidlaw DA, Williamson TH, Yorston D. Factors affecting anatomical and visual outcome after macular hole surgery: findings from a large prospective UK cohort. Eye. 2021;35(1):316-325. doi:10.1038/s41433-020-0844-x
24. Michalewska Z, Michalewski J, Dulczewska-Cichecka K, Nawrocki J. Inverted internal limiting membrane flap technique for surgical repair of myopic macular holes. Retina Phila Pa. 2014;34(4):664-669. doi:10.1097/IAE.0000000000000042
25. Liu PK, Chang YC, Wu WC. Management of refractory macular hole with blood and gas-assisted autologous neurosensory retinal free flap transplantation: a case report. BMC Ophthalmol. 2018;18(1):230. doi:10.1186/s12886018-0909-9
26. Tognetto D, Grandin R, Sanguinetti G, et al. Internal limiting membrane removal during macular hole surgery: results of a multicenter retrospective study. Ophthalmology. 2006;113(8):1401-1410. doi:10.1016/j.ophtha.2006.02.061
27. Kumagai K, Furukawa M, Ogino N, Uemura A, Demizu S, Larson E. Vitreous surgery with and without internal limiting membrane peeling for macular hole repair. Retina Phila Pa. 2004;24(5):721-727. doi:10.1097/00006982-20041000000006
28. Tadayoni R, Gaudric A, Haouchine B, Massin P. Relationship between macular hole size and the potential benefit of internal limiting membrane peeling. Br J Ophthalmol. 2006;90(10):1239-1241. doi:10.1136/bjo.2006.091777
29. Guillaubey A, Malvitte L, Lafontaine PO, et al. Comparison of face-down and seated position after idiopathic macular hole surgery: a randomized clinical trial. Am J Ophthalmol. 2008;146(1):128-134. doi:10.1016/j.ajo.2008.02.029
30. Pasu S, Bell L, Zenasni Z, et al. Facedown Positioning Following Surgery for Large Full-Thickness Macular Hole: A Multicenter Randomized Clinical Trial. JAMA Ophthalmol. 2020;138(7):725-730. doi:10.1001/jamaophthalmol.2020.0987
31. Yamashita T, Sakamoto T, Yamashita T, et al. Individualized, spectral domain-optical coherence tomography-guided facedown posturing after macular hole surgery: minimizing treatment burden and maximizing outcome. Retina Phila Pa. 2014;34(7):1367-1375. doi:10.1097/IAE.0000000000000087
32. American Society of Retina Specialists (ASRS). Preferences and Trends (PAT) Survey.; 2020. Accessed December 12, 2024. https://www.asrs.org/content/documents/_2022-pat-survey-us-and-intl-for-website.pdf
33. Michalewska Z, Michalewski J, Adelman RA, Nawrocki J. Inverted Internal Limiting Membrane Flap Technique for Large Macular Holes. Ophthalmology. 2010;117(10):2018-2025. doi:10.1016/j.ophtha.2010.02.011
34. Narayanan R, Singh SR, Taylor S, et al. SURGICAL OUTCOMES AFTER INVERTED INTERNAL LIMITING MEMBRANE FLAP VERSUS CONVENTIONAL PEELING FOR VERY LARGE MACULAR HOLES. Retina Phila Pa 2019;39(8):1465-1469. doi:10.1097/IAE.0000000000002186
35. Rizzo S, Tartaro R, Barca F, Caporossi T, Bacherini D, Giansanti F. INTERNAL LIMITING MEMBRANE PEELING VERSUS INVERTED FLAP TECHNIQUE FOR TREATMENT OF FULL-THICKNESS MACULAR HOLES: A
Meshkin, Ryan
COMPARATIVE STUDY IN A LARGE SERIES OF PATIENTS. Retina Phila Pa. 2018;38 Suppl 1:S73-S78. doi:10.1097/IAE.0000000000001985
36. Yamashita T, Sakamoto T, Terasaki H, et al. Best surgical technique and outcomes for large macular holes: retrospective multicentre study in Japan. Acta Ophthalmol (Copenh). 2018;96(8):e904-e910. doi:10.1111/aos.13795
37. Shen Y, Lin X, Zhang L, Wu M. Comparative efficacy evaluation of inverted internal limiting membrane flap technique and internal limiting membrane peeling in large macular holes: a systematic review and meta-analysis. BMC Ophthalmol. 2020;20(1):14. doi:10.1186/s12886-019-1271-2
38. Marques RE, Sousa DC, Leal I, Faria MY, Marques-Neves C. Complete ILM Peeling Versus Inverted Flap Technique for Macular Hole Surgery: A Meta-Analysis. Ophthalmic Surg Lasers Imaging Retina. 2020;51(3):187-A2. doi:10.3928/23258160-20200228-08
39. Matsumae H, Morizane Y, Yamane S, et al. Inverted Internal Limiting Membrane Flap versus Internal Limiting Membrane Peeling for Macular Hole Retinal Detachment in High Myopia. Ophthalmol Retina. 2020;4(9):919-926. doi:10.1016/j.oret.2020.03.021
40. Michalewska Z, Michalewski J, Dulczewska-Cichecka K, Adelman RA, Nawrocki J. TEMPORAL INVERTED INTERNAL LIMITING MEMBRANE FLAP TECHNIQUE VERSUS CLASSIC INVERTED INTERNAL LIMITING MEMBRANE FLAP TECHNIQUE: A Comparative Study. Retina Phila Pa. 2015;35(9):1844-1850. doi:10.1097/IAE.0000000000000555
41. Major JC, Lampen SIR, Wykoff CC, et al. THE TEXAS TACO TECHNIQUE FOR INTERNAL LIMITING MEMBRANE FLAP IN LARGE FULL-THICKNESS MACULAR HOLES: A Short-Term Pilot Study. Retina Phila Pa. 2020;40(3):552556. doi:10.1097/IAE.0000000000002431
42. Morizane Y, Shiraga F, Kimura S, et al. Autologous Transplantation of the Internal Limiting Membrane for Refractory Macular Holes. Am J Ophthalmol. 2014;157(4):861-869.e1. doi:10.1016/j.ajo.2013.12.028
43. De Novelli FJ, Preti RC, Ribeiro Monteiro ML, Pelayes DE, Junqueira Nóbrega M, Takahashi WY. Autologous Internal Limiting Membrane Fragment Transplantation for Large, Chronic, and Refractory Macular Holes. Ophthalmic Res 2015;55(1):45-52. doi:10.1159/000440767
44. Pires J, Nadal J, Gomes NL. Internal limiting membrane translocation for refractory macular holes. Br J Ophthalmol 2017;101(3):377-382. doi:10.1136/bjophthalmol-2015-308299
45. Ozdek S, Baskaran P, Karabas L, Neves PP. A Modified Perfluoro-n-octane-Assisted Autologous Internal Limiting Membrane Transplant for Failed Macular Hole Reintervention: A Case Series. Ophthalmic Surg Lasers Imaging Retina. 2017;48(5):416-420. doi:10.3928/23258160-20170428-08
46. Primavera V, Centoducati T, Agea L, Zucchiatti I, Querques L, Querques G. Pilot Evaluation of a New Surgical Technique for Persistent or Recurrent Large Macular Holes. Ophthalmic Surg Lasers Imaging Retina 2018;49(4):266-268. doi:10.3928/23258160-20180329-09
47. Gekka T, Watanabe A, Ohkuma Y, et al. Pedicle Internal Limiting Membrane Transposition Flap Technique for Refractory Macular Hole. Ophthalmic Surg Lasers Imaging Retina. 2015;46(10):1045-1046. doi:10.3928/2325816020151027-10
48. Chou HD, Chong YJ, Teh WM, et al. Nasal or Temporal Internal Limiting Membrane Flap Assisted by SubPerfluorocarbon Viscoelastic Injection for Macular Hole Repair. Am J Ophthalmol. 2021;223:296-305. doi:10.1016/j.ajo.2020.09.023
49. Chen SN, Yang CM. Double Internal Limiting Membrane Insertion for Macular Hole-Associated Retinal Detachment. J Ophthalmol. 2017;2017:3236516. doi:10.1155/2017/3236516
50. Finn AP, Mahmoud TH. Internal Limiting Membrane Retracting Door for Myopic Macular Holes. Retina Phila Pa 2019;39 Suppl 1:S92-S94. doi:10.1097/IAE.0000000000001787
51. Tabandeh H, Morozov A, Rezaei KA, Boyer DS. Superior Wide-Base Internal Limiting Membrane Flap Transposition for Macular Holes: Flap Status and Outcomes. Ophthalmol Retina. 2021;5(4):317-323. doi:10.1016/j.oret.2020.12.003
52. Astir S, Shroff D, Gupta C, Shroff C. Inverted flap technique for a large traumatic macular hole with choroidal rupture and subretinal hemorrhage. Oman J Ophthalmol. 2018;11(1):68. doi:10.4103/0974-620X.226348
53. Mahalingam P, Sambhav K. Surgical outcomes of inverted internal limiting membrane flap technique for large macular hole. Indian J Ophthalmol. 2013;61(10):601. doi:10.4103/0301-4738.121090
54. Wu AL, Liu YT, Chou HD, et al. Role of growth factors and internal limiting membrane constituents in müller cell migration. Exp Eye Res. 2021;202:108352. doi:10.1016/j.exer.2020.108352
55. Shiode Y, Morizane Y, Matoba R, et al. The Role of Inverted Internal Limiting Membrane Flap in Macular Hole Closure. Invest Ophthalmol Vis Sci. 2017;58(11):4847-4855. doi:10.1167/iovs.17-21756
56. Di Polo A, Aigner LJ, Dunn RJ, Bray GM, Aguayo AJ. Prolonged delivery of brain-derived neurotrophic factor by adenovirus-infected Müller cells temporarily rescues injured retinal ganglion cells. Proc Natl Acad Sci 1998;95(7):3978-3983. doi:10.1073/pnas.95.7.3978
57. Frasson M, Picaud S, Léveillard T, et al. Glial Cell Line–Derived Neurotrophic Factor Induces Histologic and Functional Protection of Rod Photoreceptors in the rd/rd Mouse. Invest Ophthalmol Vis Sci. 1999;40(11):2724-2734.
58. Cursiefen C, Rummelt C, Beckmann MW, Kruse FE. Amniotic membrane-covered bio-onlays for treatment of ocular surface disease. Br J Ophthalmol. 2007;91(6):841-842. doi:10.1136/bjo.2006.108134
59. Rizzo S, Caporossi T, Tartaro R, et al. A Human Amniotic Membrane Plug to Promote Retinal Breaks Repair and Recurrent Macular Hole Closure. Retina Phila Pa. 2019;39 Suppl 1:S95-S103. doi:10.1097/IAE.0000000000002320
60. Huang YH, Tsai DC, Wang LC, Chen SJ. Comparison between Cryopreserved and Dehydrated Human Amniotic Membrane Graft in Treating Challenging Cases with Macular Hole and Macular Hole Retinal Detachment. J Ophthalmol. 2020;2020:9157518. doi:10.1155/2020/9157518
61. Caporossi T, Pacini B, De Angelis L, Barca F, Peiretti E, Rizzo S. HUMAN AMNIOTIC MEMBRANE TO CLOSE RECURRENT, HIGH MYOPIC MACULAR HOLES IN PATHOLOGIC MYOPIA WITH AXIAL LENGTH OF ≥30 mm. Retina Phila Pa. 2020;40(10):1946-1954. doi:10.1097/IAE.0000000000002699
62. Tsai DC, Huang YH, Chen SJ. Parafoveal atrophy after human amniotic membrane graft for macular hole in patients with high myopia. Br J Ophthalmol. 2021;105(7):1002-1010. doi:10.1136/bjophthalmol-2019-315603
63. Caporossi T, Tartaro R, Finocchio L, et al. HUMAN AMNIOTIC MEMBRANE TO TREAT MACULAR HOLES THAT FAILED TO CLOSE, SULFUR HEXAFLUORIDE ENDOTAMPONADE VERSUS AIR ENDOTAMPONADE: A Prospective Comparative Study. RETINA. 2021;41(4):735. doi:10.1097/IAE.0000000000002913
64. Szurman P, Wakili P, Stanzel BV, Siegel R, Boden KT, Rickmann A. Persistent macular holes - what is the best strategy for revision? Graefes Arch Clin Exp Ophthalmol Albrecht Von Graefes Arch Klin Exp Ophthalmol 2021;259(7):1781-1790. doi:10.1007/s00417-021-05252-7
65. Zhang H, Li Y, Chen G, Han F, Jiang W. Human amniotic membrane graft for refractory macular hole: A single-arm meta-analysis and systematic review. J Fr Ophtalmol. 2023;46(3):276-286. doi:10.1016/j.jfo.2022.07.001
66. Rosenfeld P, Merritt J, Hernandez E, Mollor D, Rosa R, Tsong S. Subretinal implantation of human amniotic membrane:A rabbit model for the replacement of Bruch’s membrane during submacular surgery. Invest Ophthalmol Vis Sci. 1999;40(206).
67. Tseng SCG, Espana EM, Kawakita T, et al. How does amniotic membrane work? Ocul Surf. 2004;2(3):177-187. doi:10.1016/s1542-0124(12)70059-9
68. Fukuda K, Chikama T ichiro, Nakamura M, Nishida T. Differential Distribution of Subchains of the Basement Membrane Components Type IV Collagen and Laminin Among the Amniotic Membrane, Cornea, and Conjunctiva. Cornea. 1999;18(1):73.
69. Jirsova K, Jones GLA. Amniotic membrane in ophthalmology: properties, preparation, storage and indications for grafting-a review. Cell Tissue Bank. 2017;18(2):193-204. doi:10.1007/s10561-017-9618-5
Meshkin, Ryan
70. Kiilgaard JF, Scherfig E, Prause JU, la Cour M. Transplantation of Amniotic Membrane to the Subretinal Space in Pigs. Stem Cells Int. 2012;2012(1):716968. doi:10.1155/2012/716968
71. Ohno-Matsui K, Ichinose S, Nakahama K ichi, et al. The effects of amniotic membrane on retinal pigment epithelial cell differentiation. Mol Vis. 2005;11:1-10.
72. Grewal DS, Mahmoud TH. Autologous Neurosensory Retinal Free Flap for Closure of Refractory Myopic Macular Holes. JAMA Ophthalmol. 2016;134(2):229-230. doi:10.1001/jamaophthalmol.2015.5237
73. Lai CC, Chen YP, Wang NK, et al. Vitrectomy with Internal Limiting Membrane Repositioning and Autologous Blood for Macular Hole Retinal Detachment in Highly Myopic Eyes. Ophthalmology. 2015;122(9):1889-1898. doi:10.1016/j.ophtha.2015.05.040
74. Grewal DS, Charles S, Parolini B, Kadonosono K, Mahmoud TH. Autologous Retinal Transplant for Refractory Macular Holes: Multicenter International Collaborative Study Group. Ophthalmology. 2019;126(10):1399-1408. doi:10.1016/j.ophtha.2019.01.027
75. Moysidis SN, Koulisis N, Adrean SD, et al. Autologous Retinal Transplantation for Primary and Refractory Macular Holes and Macular Hole Retinal Detachments: The Global Consortium. Ophthalmology. 2021;128(5):672-685. doi:10.1016/j.ophtha.2020.10.007
76. Patel SN, Mahmoud TH, Kazahaya M, Todorich B. AUTOLOGOUS NEUROSENSORY RETINAL TRANSPLANTATION: Bridging the Gap. Retina Phila Pa. 2021;41(12):2417-2423. doi:10.1097/IAE.0000000000003210
77. Takeuchi J, Kataoka K, Shimizu H, et al. INTRAOPERATIVE AND POSTOPERATIVE MONITORING OF AUTOLOGOUS NEUROSENSORY RETINAL FLAP TRANSPLANTATION FOR A REFRACTORY MACULAR HOLE ASSOCIATED WITH HIGH MYOPIA. Retina Phila Pa. 2021;41(5):921-930. doi:10.1097/IAE.0000000000003000
78. Tanaka S, Inoue M, Inoue T, et al. AUTOLOGOUS RETINAL TRANSPLANTATION AS A PRIMARY TREATMENT FOR LARGE CHRONIC MACULAR HOLES. Retina Phila Pa. 2020;40(10):1938-1945. doi:10.1097/IAE.0000000000002693
79. Sonmez K. Autologous neurosensory retinal transplantation for large refractory idiopathic macular hole. Int Ophthalmol. 2021;41(4):1415-1425. doi:10.1007/s10792-021-01716-1
80. Wu AL, Chuang LH, Wang NK, et al. Refractory macular hole repaired by autologous retinal graft and blood clot. BMC Ophthalmol. 2018;18(1):213. doi:10.1186/s12886-018-0898-8
81. Chang YC, Liu PK, Kao TE, et al. MANAGEMENT OF REFRACTORY LARGE MACULAR HOLE WITH AUTOLOGOUS NEUROSENSORY RETINAL FREE FLAP TRANSPLANTATION. RETINA. 2020;40(11):2134. doi:10.1097/IAE.0000000000002734
82. Mccuen BWI, De Juan EJ, Machemer R. SILICONE OIL IN VITREORETINAL SURGERY PART 1: SURGICAL TECHNIQUES. RETINA. 1985;5(4):189.
83. Peng YW, Hao Y, Petters RM, Wong F. Ectopic synaptogenesis in the mammalian retina caused by rod photoreceptor-specific mutations. Nat Neurosci. 2000;3(11):1121-1127. doi:10.1038/80639
84. Lewis GP, Linberg KA, Fisher SK. Neurite outgrowth from bipolar and horizontal cells after experimental retinal detachment. Invest Ophthalmol Vis Sci. 1998;39(2):424-434.
85. Haverkamp S, Michalakis S, Claes E, et al. Synaptic plasticity in CNGA3(-/-) mice: cone bipolar cells react on the missing cone input and form ectopic synapses with rods. J Neurosci Off J Soc Neurosci. 2006;26(19):5248-5255. doi:10.1523/JNEUROSCI.4483-05.2006
86. Wang W, Lee SJ, Scott PA, et al. Two-Step Reactivation of Dormant Cones in Retinitis Pigmentosa. Cell Rep 2016;15(2):372. doi:10.1016/j.celrep.2016.03.022
87. Karl MO, Reh TA. Regenerative medicine for retinal diseases: activating endogenous repair mechanisms. Trends Mol Med. 2010;16(4):193-202. doi:10.1016/j.molmed.2010.02.003
88. Klassen H, Ziaeian B, Kirov II, Young MJ, Schwartz PH. Isolation of retinal progenitor cells from post-mortem human tissue and comparison with autologous brain progenitors. J Neurosci Res. 2004;77(3):334-343. doi:10.1002/jnr.20183
89. Lawrence JM, Singhal S, Bhatia B, et al. MIO-M1 Cells and Similar Müller Glial Cell Lines Derived from Adult Human Retina Exhibit Neural Stem Cell Characteristics. Stem Cells. 2007;25(8):2033-2043. doi:10.1634/stemcells.2006-0724
90. Giannelli SG, Demontis GC, Pertile G, Rama P, Broccoli V. Adult human Müller glia cells are a highly efficient source of rod photoreceptors. Stem Cells Dayt Ohio. 2011;29(2):344-356. doi:10.1002/stem.579
91. Singhal S, Bhatia B, Jayaram H, et al. Human Müller glia with stem cell characteristics differentiate into retinal ganglion cell (RGC) precursors in vitro and partially restore RGC function in vivo following transplantation. Stem Cells Transl Med. 2012;1(3):188-199. doi:10.5966/sctm.2011-0005
92. Mahato B, Kaya KD, Fan Y, et al. Pharmacologic fibroblast reprogramming into photoreceptors restores vision. Nature. 2020;581(7806):83-88. doi:10.1038/s41586-020-2201-4
93. Baranov PY, Tucker BA, Young MJ. Low-oxygen culture conditions extend the multipotent properties of human retinal progenitor cells. Tissue Eng Part A. 2014;20(9-10):1465-1475. doi:10.1089/ten.TEA.2013.0361
94. Pearson RA, Gonzalez-Cordero A, West EL, et al. Donor and host photoreceptors engage in material transfer following transplantation of post-mitotic photoreceptor precursors. Nat Commun. 2016;7(1):13029. doi:10.1038/ncomms13029
95. Tabandeh H. Vascularization and Reperfusion of Autologous Retinal Transplant for Giant Macular Holes. JAMA Ophthalmol. 2020;138(3):305-309. doi:10.1001/jamaophthalmol.2019.5733
96. Curcio CA, Allen KA, Sloan KR, et al. Distribution and morphology of human cone photoreceptors stained with antiblue opsin. J Comp Neurol. 1991;312(4):610-624. doi:10.1002/cne.903120411
Figure 1 – A) Pre-operative OCT of the right eye with full-thickness macular hole possibly secondary to trauma ~7 months earlier with 3 months of visual symptoms (presenting VA 20/100). B) Post-operative week 3 following 25-gauge pars-plana vitrectomy, ILM peel/temporal inverted flap with 20% SF6 gas tamponade demonstrating hole closure, ILM flap overlying foveal contour, and reconstitution of ellipsoid zone and external limiting membrane (VA 20/50). C) Post-operative month 4 with mild nasal dissociated optic nerve fiber layer and residual ILM bridge (VA 20/40).
Figure 2 – Drawing of the temporal inverted ILM flap technique. A semicircular flap of ILM is peeled from the temporal to nasal direction (blue arrow) up to the temporal edge of the macular hole (red circle) and inverted over the complete area of the macular hole.
Tandias, Rachel
Rachel Tandias, MD, Leyla Yavuz-Saricay, MD, and Thomas H. Dohlman, MD
Introduction
Corneal blindness is a major cause of ocular morbidity that affects approximately 4.5 million people worldwide.1 Keratoprosthesis (KPro), an artificial cornea, is an effective therapeutic option for many patients with severe corneal diseases that are not amenable to conventional treatments or corneal transplantation. Keratoprostheses also offer a viable solution for corneal blindness in areas of the world with limited supply of corneal donor tissue. Collaborations between scientists and surgeons have led to the development of various new and modified KPros designed to improve outcomes, reduce costs, and increase global accessibility. This review summarizes the evolution and clinical applications of modern KPro devices, with a primary focus on the Boston Keratoprosthesis Type I, and explores innovative solutions for common KPro-associated complications.
The Boston Keratoprosthesis Type 1 (B-KPro Type I, Massachusetts Eye and Ear, Boston, MA) is the most widely used keratoprosthesis globally, with nearly 20,000 devices implanted to date.2 Initially introduced by Dr. Claes Dohlman in the 1970s, the B-KPro was approved by the U.S. Food and Drug Administration (FDA) in 1992 for use in patients with multiple failed corneal grafts.3 Advancements and innovation in B-KPro design have resulted in improved outcomes and expanded indications over time.
The B-KPro Type I has a collar-button design featuring a front plate made of polymethylmethacrylate (PMMA) with a 5-mm diameter and a central optical stem measuring 3.35 mm in diameter, which engages securely into a backplate composed either of PMMA or titanium. A donor corneal graft is placed between the front and back plates, serving as a carrier to facilitate implantation and integration into the host tissue. The back plate was originally designed to be screwed onto the stem intraoperatively.4 To address postoperative loosening and minimize donor cornea damage during assembly, a titanium posterior locking ring was added in place of the screw threads.5 This was further refined with the introduction of a ‘click-on’ version with a backplate that snaps directly into a groove on the posterior stem. Early versions of the B-KPro backplate were composed of solid PMMA and were associated with a high incidence of sterile keratolysis. The introduction of fenestrated backplates with round holes to enhance aqueous flow and nutrient delivery to the donor cornea significantly reduced rates of keratolysis and tissue melt around the KPro stem.6 Backplates are fabricated in two sizes: 8.5-mm diameter for adults and 7-mm for pediatric eyes, respectively, and are available in original PMMA and a newer titanium model. Titanium has become the standard choice for most surgeons given its superior biocompatibility.7,8 The B-KPro is available in a standard pseudophakic plano power or customized aphakic powers based on axial length. Postoperative management requires continuous wear of a soft contact lens to prevent dehydration of the corneal tissue, stromal melt, and dellen formation. The contact lens can also be used for additional refractive correction.9
The B-KPro Type I has demonstrated favorable outcomes, including through extended (10 year) follow up 10 Results of the largest multi-center trial showed a retention rate of 95% with best-corrected visual acuity (BCVA) of ≥20/200 in 57% of cases and ≥20/40 in 19% at an average follow-up of 8.5 months 11 A meta-analysis of 406 studies reported that 60% of patients attained 6/60 vision or better at two years postoperatively and 51% at five years.12 The combined retention rate was 88% at two years and 74% at five years. The prognosis following B-KPro Type I implantation is most strongly linked to the patient’s degree of prior pathology and category of corneal disease The best outcomes are observed in patients with non-cicatrizing conditions such as corneal dystrophies, bacterial or viral infections, and aniridia. In
contrast, autoimmune and cicatrizing diseases like Stevens-Johnson syndrome (SJS) and mucous membrane pemphigoid (MMP) are associated with poorer prognoses, likely due to chronic inflammation that predisposes to corneal melting, necrosis, leakage, and infection.13 Outcomes have improved with prophylactic postoperative measures, such as daily topical antibiotics, anti-inflammatory treatments, protective soft contact lenses, and frequent screening and management of common postoperative complications, including glaucoma and vitreoretinal complications.
Lucia Keratoprosthesis
The Lucia keratoprosthesis (Lucia KPro) is a variant of the B-KPro Type I that is produced at a reduced cost with a simplified manufacturing process.14,15 Like the B-KPro, the Lucia KPro has a two-piece design with a PMMA front plate and a titanium backplate. However, the backplate of the Lucia KPro is photoetched from medical-grade titanium sheets and curved over a mold, in contrast to the B-KPro backplate which is lathed from a titanium rod. The 7.75 mm backplate diameter is suited for both adult and pediatric eyes. Cosmetic enhancements to the backplate include petal-shaped rather than round holes and the use of anodized titanium to allow for a more natural appearing brown or blue tint.
The Lucia KPro was approved by the FDA in 2019, and clinical trials to assess visual and safety outcomes are currently underway. A recent study in Mexico City showed a 96% device retention rate in 48 patients over an average follow-up of 20.5 months (range: 3-40), with 71% of recipients experiencing improved visual acuity.16 At least one complication occurred in 75% of cases, and the most common complication was development of retroprosthetic membrane, observed in 35% of eyes. Despite these complications, 63% of patients achieved functional success at the final follow-up, and 23% achieved a BCVA of 20/40 or better.
Aurolab Keratoprosthesis
Efforts have been made to expand accessibility of the Boston KPro to developing countries, where the burden of corneal blindness and need for keratoprosthesis are high. As part of these efforts, Massachusetts Eye and Ear shared the Boston KPro design with an Indian manufacturer for production and distribution within India of a lower-cost version called the Aurolab keratoprosthesis (AuroKPro, Aurolab, Madurai, India).17 The AuroKPro is based on the B-KPro’s snap-on design, featuring a PMMA optic and backplate and a titanium locking ring, and is produced in two versions for either pseudophakic or aphakic eyes.
A retrospective study comparing the 5-year outcomes in 78 B-KPro eyes and 56 AuroKPro eyes revealed slightly higher rates of retention and functional recovery in the B-KPro group, though these differences were not statistically significant.17 The AuroKPro had a higher rate of complications, including intraoperative device breakage and postoperative extrusion, but rates of glaucoma and infection were similar between groups. The cost of implantation was significantly lower for the AuroKPro ($326) compared to the B-KPro ($1,113), though the average cost of follow-up surgical procedures over the course of the follow-up period was higher in the AuroKPro group ($346/patient versus $266/patient in the B-KPro group). Another retrospective case series comparing 99 B-KPro eyes and 62 AuroKPro eyes with a mean follow-up of 1.8 ± 1.7 years similarly showed no significant difference in anatomical retention between the two groups.18 No statistically significant differences between B-KPro and AuroKPro eyes were observed in rates of pre-existing and post-operative glaucoma, glaucoma surgeries performed before and after KPro implantation, or rate of glaucoma progression within two years post-KPro. These promising results support the use of the AuroKPro as an affordable and reasonable alternative to the BKPro in India
The minimally invasive Boston KPro (mi-KPro) is a partial-thickness keratoprosthesis developed at Massachusetts Eye and Ear with the aim of reducing complications associated with traditional, fullthickness B-KPro devices. The device features an ultra-thin, titanium-nickel backplate that is implanted anterior to Descemet’s membrane, and only the optic penetrates the anterior chamber.19 The reduced invasiveness of implantation and flexibility of the backplate help to decrease postoperative inflammation ,
Tandias, Rachel
which helps preserve angle anatomy and reduce posterior segment complications, specifically optic nerve axon loss
A preliminary study in rabbits comparing mi-KPro to penetrating keratoplasty (PKP) revealed that mi-KPro eyes had excellent anatomic retention after 12 months with no evidence of ocular hypertension, retroprosthetic membrane (RPM) formation or infection, and a significant reduction in retinal thinning and optic nerve axon loss.19 Further evaluation is underway in preparation for human clinical trials.
Pre-assembled Boston Keratoprosthesis
In addition to financial considerations, logistical challenges restrict the accessibility of B-KPro in many parts of the world. The implantation process necessitates the separate procurement of an ethylene oxide (ETO)-sterilized BKPro and a donor corneal graft. These components must be assembled in the operating room at the time of surgery. ETO sterilization is expensive, time-intensive, and requires use of a toxic and flammable gas.20 Donor cornea shortages, complex storage and transport requirements, and the surgical expertise required for KPro assembly further compound these challenges.
Gamma and electron beam (E-beam) irradiation have been studied as sterilization techniques with the goal of enabling long-term, room-temperature storage of KPros preassembled with carrier corneal tissue.20-22 Both methods were found to have minimal effects on the biocompatibility, mechanical, or optical properties of the KPro device. Unlike other methods of prolonging the viability of corneal tissue, such as cryopreservation, glycerol-preservation, and freeze-drying, irradiated corneal grafts are sterile and can be immediately used without the need for reconstitution. In comparison to gamma irradiation, Ebeam irradiation is more rapid, less prone to cause yellowing of the PMMA component, and can be delivered at higher dosing rates, which reduces exposure time and potential degradation of the irradiated tissue. E-beam irradiated devices pre-assembled with corneal tissue were stable for 6 months at roomtemperature.22 Production of an off-the-shelf, pre-assembled KPro is a promising innovation that would improve logistical challenges associated with transportation and storage, increase access in areas where donor corneal tissue is limited, and simplify the B-KPro implantation procedure.
CorNeat Keratoprosthesis
The CorNeat Keratoprosthesis (CorNeat Vision Ltd, Israel) offers several potential advantages to prior KPro devices, including a fully synthetic design, an optical component that closely resembles the natural cornea, and a simplified, one-stage implantation process that utilizes a novel approach of subconjunctival integration.23,24 Surrounding the device’s central PMMA optic is a thin, flexible skirt composed of electrospun carbonated polyurethane fibers designed to integrate into the host conjunctiva. The device has a fixed optical power of 40.8 diopters and can be used in phakic and pseudophakic eyes. The lens has a spherical surface of 10 mm diameter with a wide optical zone of 6.5 mm diameter. The rim of the optical component features three pairs of holes for suturing the implant to the cornea and four access ports into the anterior chamber, including a 2.6 mm IOL injection port, to allow postoperative surgical interventions. After trephination of the host cornea, a custom spatula tool is used to manipulate the corneal edge into the posterior groove of the optic, and the conjunctiva is repositioned to cover the skirt of the device. The design aims to minimize the risk of device extrusion and bypasses the host cornea interface, eliminating the need for carrier tissue.
The first human implantation of the CorNeat KPro in a single patient was reported in 2023 with promising short-term results.23 Visual acuity was improved at 12 months with no significant complications. The keratoprosthesis was properly positioned and tactile intraocular pressure was assessed as normal. A multicenter clinical trial in the United States, Canada, France, Israel and the Netherlands is currently ongoing (ClinicalTrials.gov Identifier: NCT04485858) and results are pending. The company anticipates securing initial marketing approval for the CorNeat KPro late in 2025.
The GORE synthetic cornea, in development by W.L. Gore & Associates and Johns Hopkins University, is
a full-thickness, single-piece and fully synthetic keratoprosthesis that consists of a flexible central optic and surrounding skirt.25 The device is made of a proprietary perfluoroalkoxy alkane that is flexible, suturable, and optically clear. The optic and skirt are lined with porous expanded polytetrafluoroethylene (ePTFE) to enhance bio-integration with host corneal tissue. The optic is fitted into a central corneal trephination, and the skirt is inserted into a circumferential intrastromal lamellar pocket and secured with 16 permanent sutures.26 The optic has a diameter of 4.60-mm anteriorly and 4.28-mm posteriorly and provides a refractive power of 43.5 diopters, which is suitable for pseudophakic eyes.
In addition to eliminating the need for donor tissue, the flexibility of the GORE device helps to minimize the effect of shear forces at the optic-device junction, which is thought to enhance tissue integration and reduce inflammation and tissue loss. Studies in animal models have shown promising results, with early signs of successful bio-integration within six weeks of implantation.26,27 An early human feasibility study is currently underway.
The AlphaCor KPro was developed at the Lions Eye Institute in Australia and was approved by the FDA in 2002.28 The device was composed of a transparent central optic core made of gel PHEMA (poly 2hydroxyethyl methacrylate) and an outer skirt made of opaque, porous PHEMA. The high water content and porous structure of the skirt were thought to allow for better biointegration by cellular ingrowth and collagen deposition.29 The AlphaCor KPro was available in two powers, one for aphakic patients and another for phakic or pseudophakic patients, and was implanted in a two-stage procedure. It was placed within a lamellar corneal pocket with tissue posterior to the optic being removed at the time of the first stage of surgery and the tissue anterior to the optic being removed in the second stage. Despite initially promising outcomes, the AlphaCor KPro was found to have severe mid- and long-term complications, including stromal melt, optic deposition, and retroprosthetic membrane formation, and is no longer available on the market.14
KeraKlear Keratoprosthesis
The KeraKlear keratoprosthesis (KeraMed Inc., Sunnyvale, California) is a single-piece device designed to be used as an artificial anterior lamellar graft. The device is made from a flexible, proprietary acrylic material and can be folded or injected through a 3.5-mm incision into an 8 mm intra-stromal pocket created manually or by femtosecond laser.30,31 It is secured to the corneal stroma with sutures passed through peripheral holes in the device, which eventually allow stromal growth and integration for permanent fixation. The KeraKlear KPro is 7 mm in diameter with a 4- mm central optic and is produced in two powers, 44 diopters for phakic/pseudophakic eyes and 60 diopters for aphakic eyes.
The KeraKlear KPro is advantageous in that it can be implanted in a one-stage procedure and does not require a donor cornea. The partial-thickness implantation technique reduces the risk of complications associated with penetrating surgery, such as intraocular infection and hemorrhage, and is appropriate for corneal opacities involving the anterior cornea. The KeraKlear has received CE mark approval in Europe but has not been FDA-approved for use in the U.S. Small in-human studies have shown promising results, though a multi-center clinical trial in the US was terminated due to the slow pace of recruitment and insufficient financial resources.
Boston Keratoprosthesis Type II
The B-KPro Type II was designed to enable implantation through surgically closed eyelids and features an optical stem that is 2 mm longer than the Type I design. The B-KPro Type II is typically reserved for patients with severe autoimmune conditions, such as Stevens–Johnson syndrome or mucous membrane pemphigoid, or patients with chemical injuries. It is intended for use in cases with significant symblepharon or ankyloblepharon, impaired eyelid function and/or ocular surface keratinization The BKPro Type II is more readily available than the modified osteo-odonto-keratoprosthesis (MOOKP) and can be implanted in a single surgical procedure. It can be used in edentulous patients or those unable to undergo MOOKP implantation due to the risks associated with general anesthesia.
Tandias, Rachel
The MOOKP features a PMMA optical cylinder supported by a biological haptic composed of the patient’s tooth and alveolar bone. It is used primarily in patients with bilateral end-stage ocular surface disease, including Stevens-Johnson Syndrome, ocular mucous membrane pemphigoid, and chemical injuries. Originally designed by Strampelli in 1963 and later modified by Falcinelli, the MOOKP procedure involves replacing the ocular surface with a full-thickness oral mucosa graft that provides vascular and structural support to the patient’s tooth and bone graft.32 Use of an autograft enhances biointegration and durability, and it eliminates the need for a donor cornea. Eligibility for MOOKP requires at least one healthy canine or premolar tooth, minimal gum disease, and intact retinal and optic nerve function. Allograft teeth or tibial bone may be used as alternatives for edentulous patients, though these options generally yield less favorable outcomes.33
MOOKP implantation is a complex, two-stage procedure that requires technical expertise and a multidisciplinary team approach.34 In the first stage, the ocular surface is prepared by removing keratinized scar tissue and corneal epithelium. Buccal mucosa is harvested and grafted onto the ocular surface. A canine tooth and a small segment of the mandible is removed and trimmed to for m an osteoodonto-acrylic lamina. A hole is drilled perpendicularly into the lamina to accommodate the PMMA optical cylinder. The completed osteo-odonto-acrylic lamina is then inserted below the lower orbital rim into a subcutaneous pouch to promote fibrovascular investment and vascularization. The second stage is performed 10–16 weeks later and involves retrieving the lamina from the lower orbital rim. The mucosal graft is partially detached to expose the cornea, which is trephined and excised to accommodate the optical cylinder. A 360-degree iridectomy is performed followed by cryo-extraction of the lens. The PMMA optic is inserted into the lamina, which is positioned through the corneal opening and sutured to the sclera. The remaining cornea is covered by the flap of the oral mucosa. After the second stage, patients are hospitalized for at least one week and receive broad-spectrum antibiotics and oral acetazolamide to reduce the risk of subchoroidal hemorrhage. Close follow-up is essential in the weeks following surgery to assess implant viability and monitor for complications such as ulcers, thinning, or infections.35
MOOKP implantation typically achieves excellent long-term outcomes and offers the highest long-term anatomical and functional success rates among all KPro options for patients with severe ocular surface disease.35 However, complications such as mucosa-related issues and laminar resorption pose significant challenges. Few specialized centers across the world offer MOOKP, underscoring its complexity and limited availability.36
The LV Prasad keratoprosthesis (LVP KPro) is a modified version of the B-KPro I that was developed for use in cases in which implanting the modified osteo-odonto-keratoprosthesis (MOOKP) would be difficult or contraindicated, such as children, edentulous patients, and those at high risk of mucosal complications. The LVP KPro consists of an AuroKPro implanted under a pre-placed oral mucous membrane graft, a design inspired by the AlphaCor device to prevent desiccation of the donor corneal graft.37,38 To help prevent mucosal overgrowth, the optical stem was made to be 0.75 mm longer than the AuroKPro, which allows the mucous membrane graft to be tucked under the front plate. Implantation is a two-stage procedure: the first stage involves harvesting and suturing a mucous membrane graft over the ocular surface. The second stage includes assembly of the LVP KPro using a donor corneal graft, removal of the crystalline or intraocular lens, and implantation of the device into the patient’s trephined cornea.
Compared to the MOOKP and Boston Type II KPro, the LVP KPro allows for relatively more straightforward management of posterior segment complications. Intravitreal injections can be performed and vitrectomy ports can be placed directly through the mucosal graft, and eye drops can be administered into the inferior fornix. A 2018 prospective case series of 58 LVP KPro eyes of 56 patients (12 children and 44 adults) showed a retention rate of 91% at 1 year, 80% at 2 years, and 77% at 3–5 years.38 At final follow-up, 78% had greater than two-line improvement in BCVA from the baseline, with 61.5% maintaining 20/400 or better at 1 year. Complications included retroprosthetic membrane formation (43%), glaucoma (26%), vitritis (22%), and endophthalmitis (12%), though mucosal issues were
significantly lower (19%) compared to previous rates reported with MOOKP. Long-term outcomes of the LVP KPro are yet to be reported.
Lux Keratoprosthesis
The Lux keratoprosthesis was developed at Massachusetts Eye and Ear for patients with severe cicatricial keratoconjunctivitis who are not candidates for the Boston KPro Type II or MOOKP due to inadequate eyelid tissue, unsuitable teeth, or previous failed B-KPro Type II or MOOKP surgery. The Lux KPro is a 3-piece device with a cone-shaped PMMA cylinder, a titanium sleeve, and a titanium back plate.39 It is assembled in a corneal donor graft like the B-KPro Type II and is inserted through a preplaced mucous membrane graft, similar to MOOKP. The titanium sleeve surrounding the PMMA stem is aimed at improving bioadhesion and prevents direct contact between PMMA and ocular tissues, reducing the risk of mucosal retraction around the device. The Lux and B-KPro I Lucia designs use the same 7.8mm-diameter back plate, which allows for a better fit than the larger 8.5-mm backplate of the B-KPro II in eyes with smaller corneal diameters after chemical burn or other failed corneal procedures.
Short-term results from a case series of nine patients were promising, with a 100% retention rate at a mean follow-up of 18.7 months (range 7-28 months) and all patients achieving at least ambulatory vision.39 The rate of retroprosthetic membrane (RPM) formation was comparable to that of the B-KI, with only one case of visually significant RPM.
MIRO Cornea UR
The MIRO® Cornea UR (MIRO GmbH, München, Germany) was developed as a synthetic alternative to the MOOKP. It consists of a flexible, single-piece keratoprosthesis made of hydrophobic acrylic polymer with an 8-mm diameter and a 3-mm central optic.40 Its flexible haptic reduces shear stress between the device and ocular tissues and is coated with engineered fibronectin to promote tissue integration. Implantation occurs via a 2-stage procedure that starts with covering the eye with buccal mucous membrane graft. The optical cylinder is placed in a central corneal trephination, and the haptic is inserted into a pocket between the globe and the mucosa layer and secured by a skirt of donor sclera or a collagen membrane.
The MIRO offers a less complex surgical alternative to the OOKP that does not require tooth extraction. An initial human trial published in 2014 showed that three of the four eyes had successful adherence between the MIRO haptic and the surrounding tissue within months of implantation.40 However the MIRO was explanted and replaced by an OOKP in one of these three eyes after 42 months due to dense deposits on the back surface of the optic.
A persistent challenge with keratoprostheses is adhesion of the corneal graft to the PMMA stem. PMMA is an inert and hydrophobic material that promotes fibrous tissue encapsulation but lacks the bioactivity needed for true integration with adjacent tissue. Poor bioadhesion between the device and surrounding tissue can lead to complications such as graft detachment, corneal melting, and infection. Various surface modification techniques have been explored to enhance adhesion between the corneal tissue and the PMMA surface of the device without altering its bulk characteristics. Titanium has been shown to be a superior substrate for cell growth in comparison to PMMA.7,41,42 Animal studies further validate these findings, showing that titanium-coated stems significantly enhance corneal tissue adhesion compared to uncoated PMMA.43 The use of magnetron sputtering for nanometer-thickness titanium deposition on the PMMA stem of the B-KPro showed improved biocompatibility in vitro, with increased cell adhesion, proliferation, and collagen deposition using the titanium coated stem.44 Leveraging titanium’s biocompatibility, the Lux keratoprosthesis incorporates a titanium sleeve around the device stem.39
In addition to titanium, several other surface modifications have been explored, including titanium dioxide (TiO2) over a layer of polydopamine, dopamine deposition followed by calcium phosphate coating, TiO2 nanoparticle dip coating, covalent modification of PMMA with L-DOPA (3,4-dihydroxy-L-phenylalanine),
Tandias, Rachel
and hydroxyapatite (HAp).43,45-48 HAp coating is a common surface modification that has been used for medical implants to mitigate inflammation and reduce fibrosis, promoting biointegration. While HAp coating is well established for use in dental and orthopedic implants, its broader clinical application remains limited. A recent study investigated the use of nanohydroxyapatite (nHAp) coating applied to the optical cylinder of the AuroKPro using a dip-coating strategy.49 nHAp coated devices were found to have improved tissue adhesion, reduced fibrosis, and reduced tear protein secretion in response to stimuli of injury compared to unmodified PMMA devices when tested in rabbit models, suggesting that the technique improved biocompatibility of PMMA.
Reliance on human donor corneas as carriers for all Boston KPro devices remains a limitation of the procedure. Given the global shortage of donor corneas, there is growing interest in exploring alternatives to fresh human donor corneas. Cryopreservation, glycerol-preservation, and gamma- and electron beam (E-beam) irradiation are different approaches aimed at allowing long-term tissue storage, though these methods require human tissue.22,50-53
Xenografts, or tissues obtained from non-human animal species, represent a promising alternative for use as KPro carriers. Several methods for xenogeneic corneal decellularization have been developed.54,55 Decellularization involves the removal of cellular and genetic material from the donor tissue to reduce immunogenicity while preserving its biocompatibility, structural integrity, and bioactive components. This process is critical to minimizing the risk of immune rejection and disease transmission. Decellularization techniques utilize physical, chemical, or enzymatic processes, either independently or in combination, with chemical detergents being the most commonly employed due to their effectiveness and accessibility.55
Although corneas of several different animal species have been successfully used for corneal decellularization to construct tissue-engineered corneal scaffolds, porcine corneas are the most commonly used due to their accessibility, structural similarity to human corneas, and low immunogenicity. Porcine corneas can undergo chemical decellularization, followed by sterilization and recellularization with human corneal cells. Acellular porcine corneas (APCs) have been used as partial-thickness corneal treatments for various corneal diseases.56-59 One challenge of decellularization is that it weakens the collagen fibers and disrupts the extracellular matrix (ECM) microstructure, making the tissue less mechanically stable and more susceptible to collagenase degradation. To address this, crosslinking with ultraviolet A (UVA)/riboflavin has been used to improve stability and biocompatibility of decellularized APC.60 The crosslinked APC was used as a carrier for B-KPro in rabbit models with promising short-term results.
Recent efforts have focused on developing partial and full-thickness corneal implants from synthetic, semi-synthetic, and natural polymers and peptides, as well as hydrogels (crosslinked polymer networks that can absorb fluid) and biomaterials produced by cell-based technologies.61-63 Advances in 3D bioprinting have enabled the creation of corneal scaffolds using a range of materials and cellular bioinks.64,65 Advances in biofabrication techniques have the potential to produce viable alternatives to human donor tissue for KPro implantation 66
Glaucoma
Despite advancements in surgical techniques and post-operative care, glaucoma remains a major cause of irreversible vision loss following KPro implantation and can progress rapidly. Glaucoma is present in up to 76% of eyes prior to KPro surgery and can also develop de novo postoperatively.67 Risk factors for preexisting glaucoma include underlying ocular conditions like aniridia, chemical burns, and autoimmune diseases as well as prior intraocular surgeries The mechanism of secondary glaucoma is multifactorial. The formation of iridocorneal adhesions can occur with progressive angle closure due to contact between the back plate and iris. Mechanical distortion of the iridocorneal angle during KPro implantation and postoperative corticosteroid use and inflammation further exacerbate the risk of secondary glaucoma.
Surveillance is also challenging given difficulties accurately measuring intraocular pressure (IOP) and obtaining reliable results using other testing modalities in KPro eyes. Early surgical intervention is often necessary given the rapid nature of glaucoma progression. Implantation of a glaucoma drainage device (GDD), particularly the Ahmed glaucoma implant (AGI), is recommended before or concurrently with the B-KPro for patients without a pre-existing GDD, especially those already on glaucoma medications or with significant risk factors.68,69
Reliable IOP measurement in eyes after KPro implantation is challenging due to the rigid surface of traditional KPro implants and altered biomechanics of the scleral wall. Although digital palpation is the most common and preferred method of IOP measurement post-KPro, other more objective tonometry methods are under active investigation. Implantable IOP sensors have been studied for several years as a potential solution for the challenge of IOP monitoring in patients with KPros.70 Hui and colleagues investigated the integration of a fiber-optic pressure sensor with the B-KPro's optical stem.71 The sensor consisted of a lead-in multimode fiber (MMF) adhered to a Fabry-Perot sensing element and insulated by a polyimide tubing. Preimplantation testing in vitro demonstrated stable pressure readings with minimal baseline drift for over 4.5 years. IOP measurements obtained with the sensor implanted in rabbits agreed with intracameral IOP measurements initially but started to deviate from the second week of measurement onward. IOP drift in vivo was attributed to retroprosthetic membrane (RPM) formation on the sensor secondary to surgical inflammation, which the authors note to be more severe in rabbit models compared to humans. Future design improvements, including methods of detecting and inhibiting RPM growth on the sensing membrane, will be necessary to optimize in vivo performance prior to human trials.
The Eyemate-IO (Implandata Ophthalmic Products GmbH, Hannover, Germany) is another implantable device designed to enable continuous telemetric IOP monitoring.72,73 The telemetric IOP sensor ring consists of a microelectromechanical system and is paired with a hand-held reading device. A multicenter clinical trial reported one-year outcomes of 12 eyes that underwent combined implantation of the B-KPro I and Eyemate-IO.73 Prior to B-KPro insertion, the Eyemate-IO was placed by an ‘open sky approach’ in the sulcus or sutured to the sclera if capsular support was insufficient. Telemetric IOP readings showed strong correlation with IOP measurements obtained with intracameral manometry (correlation r = 0.68; If 3 events of presumed measurement errors were excluded, r = 0.87). Safety outcomes and adverse events aligned with the expected rate of complications after B-KPro I implantation. The most frequent complications were RPM formation and insufficiently controlled IOP.
The Eyemate device has undergone iterative development and demonstrated safety and reliability in animal models and human trials. However, ciliary sulcus placement can cause issues such as iris chafing and pigment dispersion and was unsuitable for phakic patients. To address these issues, a miniaturized version of the device, called the Eyemate- SC, was developed for suprachoroidal implantation.74 This version can be implanted in phakic eyes and showed good biocompatibility and agreement with direct intracameral IOP measurements in animal studies. The first in-human clinical trial assessed the EyemateSC in patients with primary open-angle glaucoma undergoing nonpenetrating glaucoma surgery.74 The device was found to be safe and well-tolerated and allowed accurate, continuous IOP monitoring over 12 months of follow-up.
Regular monitoring for the development and progression of glaucoma is critical to mitigating vision loss in KPro patients. Goldmann visual field (GVF) testing is commonly used in KPro eyes, and Humphrey 10–2 visual field testing has also been shown to provide repeatable and reliable results in these patients.75 One group described the use of digital planimetric quantification of GVF as a novel method of analyzing visual field changes in KPro patients. Three-dimensional spectral domain optical coherence tomography (SDOCT) volume scans can provide useful, quantitative measurements of the optic nerve head and peripapillary region in selective B-KPro eyes.76,77 Anterior segment OCT can be used to visualize the anterior chamber angle in KPro patients.78,79 Ultra-widefield imaging provides visualization of the posterior segment after KPro implantation,80 though cup-to-disc ratio using color photographs can be highly variable between graders.75
Tandias, Rachel
TNF-α Inhibition
Recent insights into the pathophysiology of secondary glaucoma highlight a shift toward investigating the existence of an inflammatory, IOP-independent mechanism of secondary glaucomatous damage after acute events in the eye, including corneal surgery. Earlier work showing the efficacy of anti-TNF-α antibodies in preventing corneal melt around KPros in high-risk patients with autoimmune disorders led to further research on the pathophysiology of such treatment.81,82 Studies in animal models of corneal alkali burns and corneal surgery revealed that TNF-α is upregulated in the anterior segment after injury to the eye and can rapidly diffuse to the retina, where it can cause ganglion cell apoptosis and optic nerve axon degeneration.83,84 These processes may contribute to the development of secondary glaucoma
A number of studies have suggested that early administration of anti-TNF-α antibodies following corneal injuries and surgery might be beneficial in reducing inflammation and the risk of developing secondary glaucoma.83,85,86 Monoclonal antibodies targeting TNF-α, including etanercept, infliximab, and adalimumab, have shown significant neuroprotective effects on the retina and optic nerve in animal models when administered systemically soon after injury. Sustained delivery of infliximab via subconjunctival implantation of a polymer-based drug delivery system (DDS) has been studied in animal models of corneal alkali burn.87 Eyes treated with the DDS showed significantly reduced retinal cell apoptosis, with measurable biological effects lasting for over a month.
Subconjunctival injection of 4.0 mg adalimumab provided nearly full protection against retinal cell apoptosis 3 days following corneal alkali burn in rabbit models, and 100 mg infliximab was only slightly less effective.81 Neuroprotective effects were also observed at 90 days post-injury. Both biologics were well-tolerated at doses of up to 4.0 mg of adalimumab and 100 mg of infliximab, but high-dose adalimumab (40 mg) showed retinal toxicity. These findings and results of prior animal studies have paved the way for promising opportunities in anti-inflammatory treatments for prevention of secondary glaucoma after ocular trauma or surgery
Retroprosthetic Membrane
Retroprosthetic membrane (RPM) development is the most common complication of KPro implantation and occurs in up to 65% of KPro cases.88 Growth of fibrovascular tissue behind the device can obstruct the visual axis and block the holes on the B-KPro back plate, which may cause melting of the carrier cornea due to impaired nutrient delivery.89 RPM-induced traction on the iris, anterior chamber angle, ciliary body, and retina can result in secondary complications such as angle-closure glaucoma, hypotony, and retinal detachment.88 Visually significant RPMs can be treated with neodymium-doped yttrium aluminum garnet (Nd:YAG) laser membranotomy. Severe cases may require surgical membranectomy or KPro exchange.
RPM formation is thought to result from cellular downgrowth of keratocytes from the host stroma at the graft-host junction, with contributions from donor keratocytes and epithelial cells in some cases.90,91 Activated keratocytes differentiate into myofibroblasts that produce the extracellular membranous components that form the RPM. Myofibroblasts also release cytokines and matrix metalloproteinases (MMPs), enzymes that amplify TGF-β1 activity and further myofibroblast proliferation.92 MMP-9 levels in the aqueous humor of rabbit eyes with corneal alkali burns have been shown to be significantly higher in KPro eyes that developed RPM compared to those that did not develop RPM.93 Targeted therapies inhibiting fibrocytes, MMP-9, or TGF-β1 may help mitigate RPM formation. Antimetabolite therapies are also promising therapeutic targets for preventing RPM growth. Intravitreal methotrexate is an established treatment for intraocular lymphoma and has been investigated as a treatment for epithelial downgrowth and proliferative vitreoretinopathy 94,95 Additional investigation of the pathophysiology of RPM formation is needed to develop innovative treatment strategies, such as targeted anti-inflammatory therapies.
Factors associated with an increased incidence of RPM include history of silicone oil placement prior to KPro implantation, GDD placement after KPro implantation, and poor preoperative visual acuity.96,97 Pars plana vitrectomy (PPV) with shaving of the vitreous base at the time of KPro surgery has been associated with reduced RPM development as compared to eyes that underwent anterior or partial vitrectomies.98 PPV reduces potential scaffolding for membrane formation and clears pro-inflammatory material from the vitreous. The iris and capsular bag may also act as scaffolds for RPM formation. Intraocular lens
extraction at the time of KPro implantation has been negatively correlated with RPM development.99 Iris explantation has also been proposed as an approach to decrease the rate of RPM formation, as OOKP eyes undergo total iridectomy and have a lower incidence of RPM.88
The use of titanium backplates has been suggested to reduce RPM formation, but studies comparing the rate of RPM development in eyes with titanium and PMMA backplates have shown mixed results.8,100 Enlargement of the titanium backplate diameter has also been investigated with the aim of providing better wound apposition at the posterior graft-host junction and impeding the downgrowth of keratocytes. This approach was associated with a lower rate of primary RPM formation, but a higher number of retinal detachments were observed in these patients.101
The incomplete bioadhesion of hardware in KPro eyes poses a significant risk of infectious endophthalmitis, a severe complication that often leads to substantial vision loss. The overall incidence of endophthalmitis has been reported as 2.7% per patient-year, with rates significantly reduced through lifelong daily topical antibiotic prophylaxis. Common postoperative antibiotic regimens include a topical fourth-generation fluoroquinolone (moxifloxacin or gatifloxacin) alone or combined with topical vancomycin. Increased cases of gram-positive endophthalmitis prompted the inclusion of topical vancomycin in some prophylaxis regimens. Vancomycin preserved with 0.005% benzalkonium chloride allows room-temperature storage for up to 60 days, reducing costs and improving patient compliance. Most surgeons recommend dual-agent coverage with a fluoroquinolone and vancomycin during the initial postoperative period, with longer treatment duration in patients with autoimmune conditions or monocular patients. For lower-risk patients, long-term prophylaxis with a single-agent fluoroquinolone or polymyxin B/trimethoprim 2–3 times is recommended. Some surgeons also advocate for antibiotic cycling and periodic anti-fungal administration to prevent microbial resistance and fungal infection, respectively Adherence to lifelong antibiotic prophylaxis is essential to mitigate the risk of infection. Even with strict adherence to hygiene and medication protocols, infection can still occur and is challenging to treat. Recent advancements have focused on incorporating antibacterial properties into KPro devices
Surface antimicrobial modifications represent a promising strategy for improving prophylaxis for KProassociated infections. A recent study explored the feasibility of improving the biocompatibility and antimicrobial properties of the B-KPro titanium backplate through functionalization of titanium surfaces with a dual peptide platform (PTF).102 The PTF was a molecule with a branched structure linking the celladhesive RGD peptide in its cyclic version (cRGD) and the antimicrobial peptide (AMP) LF1–11, which is composed of the first 11 residues of lactoferrin. Functionalized surfaces demonstrated antibacterial activity against Pseudomonas aeruginosa and improved the long-term proliferation of human corneal keratocytes (HCKs) on both smooth and rough titanium surfaces.
Others have attempted to leverage the antimicrobial properties of silver to prevent KPro-related infections. Constant pulse electrodeposition, an innovative electrochemical anodizing process, was used to deposit silver particles onto rough titanium surface.103 The silver-treated surfaces demonstrated significant antibacterial activity against P. aeruginosa and S. epidermidis, thought to be a result of increased production of reactive oxygen species (ROS), despite minimal Ag+ ion release. The technology produced rough titanium surfaces with firmly anchored silver particles that exhibited strong antibacterial efficacy while remaining compatible with HCKs. No cytotoxic effects were observed, as the silver-treated surfaces supported the adhesion and proliferation of HCKs. The findings highlight the potential of this technique to prevent early infections associated with B-KPro implantation. Ongoing research aims to assess the antibacterial performance of silver-treated surfaces in in vivo models.
Electrospinning technology has been utilized to create a soluble borneol-based antibacterial coating for the B-KPro, demonstrating effective antibacterial activity against E. coli and S. aureus while maintaining negligible cytotoxicity toward epithelial cells.104 This approach leverages borneol, a natural antibiotic with known antibacterial and anti-inflammatory properties, addressing its low water solubility by incorporating it into a polyvinylpyrrolidone (PVP) electrospun coating. The borneol/PVP coating allows rapid release of borneol, with 100% release achieved within 125 minutes, and exhibits good biocompatibility with minimal
Tandias, Rachel
cytotoxic effects. In vitro studies confirmed its bactericidal effects and significant reduction in bacterial adhesion on the KPro surface. Rapid release of antibacterial treatment during the initial hours postimplantation may be critical for preventing infection. Further research is needed to evaluate the in vivo performance of these soluble antibacterial coatings to ensure sustained efficacy and biocompatibility.
Numerous strategies for improving ocular drug delivery have been investigated, including nanocarriers, hydrogels, contact lenses, and implants. Among these, contact lenses hold significant promise due to their ability to provide sustained drug release and high drug-loading capacity. Antibiotic-releasing contact lenses have previously been proposed for use in KPro eyes.105,106 However, challenges persist, including the initial burst release during drug loading, which results in short release times and fluctuating drug concentrations, and the risk of drug leakage during storage.107 Addressing these issues, a novel drugreleasing contact lens was designed to minimize storage-phase drug release while ensuring consistent and gradual drug delivery. This lens leverages ROS, which are elevated during ocular inflammation, to trigger the release of levofloxacin and diclofenac, combining antibacterial and anti-inflammatory effects.108 In vitro and in vivo studies in rabbit models demonstrated that the lens sustained drug release for seven days, significantly reducing dosing frequency and stabilizing drug concentrations. Additionally, the lens protected epithelial cells from mechanical damage caused by blinking and eyelid movement. These findings highlight the lens' potential as a powerful tool for treating ocular surface diseases associated with ROS production, offering adaptive, real-time therapeutic responses for improved patient outcomes.
Corneal melt, or sterile necrosis of the corneal stroma, is one of the most severe complications of KPro implantation and the leading cause of device failure.11 The reported incidence of corneal melt ranges from 2% to 30% and increases with the duration of follow up.109,110 The pathophysiology is thought to involve excessive matrix metalloproteinases production which interrupts the normal healing process.111 Risk factors include impaired nutrient delivery due to RPM formation, infectious keratitis, persistent epithelial defects, and the presence of autoimmune disease.112 Mild cases can be managed medically with highoxygen transmission bandage contact lenses and matrix metalloproteinase inhibitors such as oral tetracyclines or topical medroxyprogesterone. Severe cases may require gluing, lamellar patch graft, or KPro exchange.
A study of 19 patients with corneal melt reported no significant difference in visual acuity improvement or complication rates between those managed with KPro repair (n=13) and repeat KPro (n=6).113 The rate of melt recurrence was higher in the repair group, suggesting that KPro replacement may be a more definitive treatment. Cross-linking the carrier corneal has been investigated as a method of increasing resistance to enzymatic degradation, with promising results in preclinical research and small case series.114,115 However, a randomized, prospective, multi-center trial did not demonstrate that crosslinking of the carrier cornea improved device retention or decreased the rate of sterile keratolysis following BKPro implantation in eyes at high risk for sterile keratolysis.116
The development of modern keratoprostheses is a testament to the dynamic nature of medical innovation in response to complex and challenging clinical needs. The evolution in technology from the original Boston Keratoprosthesis to current variants and novel devices illustrates a continuous mission to optimize visual restoration while mitigating associated risks. Emerging devices such as the Lucia and minimallyinvasive KPros, as well as novel strategies such as TNFα inhibition to reduce complications demonstrate a commitment to expanding accessibility, enhancing procedural simplicity and improving outcomes Future advancements are expected to focus on refining these devices to extend their applicability and efficacy further. With ongoing research geared towards improving these prostheses, the prospect of restoring vision in patients with previously untreatable corneal diseases is increasingly attainable.
1. Mathews PM, Lindsley K, Aldave AJ, Akpek EK. Etiology of Global Corneal Blindness and Current Practices of Corneal Transplantation: A Focused Review. Cornea. 2018;37(9):1198-1203.
2. Dohlman C. The Boston Keratoprosthesis-The First 50 Years: Some Reminiscences. Annu Rev Vis Sci. 2022;8:132.
3. Salvador-Culla B, Kolovou PE. Keratoprosthesis: A Review of Recent Advances in the Field. J Funct Biomater. 2016;7(2).
4. Dohlman CH, Harissi-Dagher M, Khan BF, Sippel K, Aquavella JV, Graney JM. Introduction to the use of the Boston keratoprosthesis. Expert review of ophthalmology. 2006;1(1):41-48.
5. Dohlman CH, Harissi-Dagher M, Graney J. The Boston keratoprosthesis: a new threadless design. Digit J Ophthalmol. 2007;13(3):1-5.
6. Harissi-Dagher M, Khan BF, Schaumberg DA, Dohlman CH. Importance of Nutrition to Corneal Grafts When Used as a Carrier of the Boston Keratoprosthesis. Cornea. 2007;26(5).
7. Ament JD, Spurr-Michaud SJ, Dohlman CH, Gipson IK. The Boston Keratoprosthesis: comparing corneal epithelial cell compatibility with titanium and PMMA. Cornea. 2009;28(7):808-811.
8. Todani A, Ciolino JB, Ament JD, et al. Titanium back plate for a PMMA keratoprosthesis: clinical outcomes. Graefes Arch Clin Exp Ophthalmol. 2011;249(10):1515-1518.
9. Kammerdiener LL, Speiser JL, Aquavella JV, et al. Protective effect of soft contact lenses after Boston keratoprosthesis. Br J Ophthalmol. 2016;100(4):549-552.
10. Tsou BC, Koseoglu ND, Akpek EK, Liu TYA. Ten-Year Outcome of Boston Type I Keratoprosthesis Surgery at a Tertiary Care Center. Cornea. 2024;43(8):982-988.
11. Ciolino JB, Belin MW, Todani A, Al-Arfaj K, Rudnisky CJ. Retention of the Boston keratoprosthesis type 1: multicenter study results. Ophthalmology. 2013;120(6):1195-1200.
12. Priddy J, Bardan AS, Tawfik HS, Liu C. Systematic Review and Meta-Analysis of the Medium- and Long-Term Outcomes of the Boston Type 1 Keratoprosthesis. Cornea. 2019;38(11):1465-1473.
13. Yaghouti F, Nouri M, Abad JC, Power WJ, Doane MG, Dohlman CH. Keratoprosthesis: preoperative prognostic categories. Cornea. 2001;20(1):19-23.
14. Moshirfar M, Moody JJ, Barke MR, et al. The historical development and an overview of contemporary keratoprostheses. Surv Ophthalmol. 2022;67(4):1175-1199.
15. Bakshi SK, Paschalis EI, Graney J, Chodosh J. Lucia and Beyond: Development of an Affordable Keratoprosthesis. Cornea. 2019;38(4):492-497.
16. Ortiz-Morales G, Vera-Duarte GR, Jimenez-Collado D, et al. Results of Lucia Keratoprosthesis Implantation in Severe Corneal Disease. Am J Ophthalmol. 2024;268:388-394.
17. Basu S, Serna-Ojeda JC, Senthil S, Pappuru RR, Bagga B, Sangwan V. The Aurolab Keratoprosthesis (KPro) versus the Boston Type I Kpro: 5-year Clinical Outcomes in 134 Cases of Bilateral Corneal Blindness. Am J Ophthalmol. 2019;205:175-183.
18. Senthil S, Mohamed A, Shanbhag SS, et al. Glaucoma Evaluation and Management in Eyes With Boston Type 1 and Aurolab Keratoprostheses in an Indian Cohort. Cornea. 2022;41(5):552-561.
19. Sharma J, Dohlman TH, Zhou C, et al. Minimally invasive keratoprosthesis (mi-KPro) for vision restoration after severe chemical injury. Investigative ophthalmology & visual science. 2023;64(8):5136-5136.
20. Gonzalez-Andrades M, Sharifi R, Islam MM, et al. Improving the practicality and safety of artificial corneas: Preassembly and gamma-rays sterilization of the Boston Keratoprosthesis. Ocul Surf. 2018;16(3):322-330.
21. Fadlallah A, Atallah M, Cherfan G, Awwad ST, Syed ZA, Melki SA. Gamma-irradiated corneas as carriers for the Boston type 1 keratoprosthesis: advantages and outcomes in a surgical mission setting. Cornea. 2014;33(3):235239.
22. Sharifi S, Sharifi H, Guild C, et al. Toward electron-beam sterilization of a pre-assembled Boston keratoprosthesis. Ocul Surf. 2021;20:176-184.
Tandias, Rachel
23. Bahar I, Reitblat O, Livny E, Litvin G. The first-in-human implantation of the CorNeat keratoprosthesis. Eye (Lond). 2023;37(7):1331-1335.
24. Litvin G, Klein I, Litvin Y, Klaiman G, Nyska A. CorNeat KPro: Ocular Implantation Study in Rabbits. Cornea. 2021;40(9):1165-1174.
25. Li G, Aldave AJ, Amescua G, et al. Design and Biocompatibility of a Novel, Flexible Artificial Cornea. Transl Vis Sci Technol. 2024;13(5):19.
26. Akpek EK, Aldave AJ, Amescua G, et al. Twelve-Month Clinical and Histopathological Performance of a Novel Synthetic Cornea Device in Rabbit Model. Transl Vis Sci Technol. 2023;12(8):9.
27. Akpek EK, Van Court S, Glass S, Schmiedel T, Troutman M. Short-Term Clinical Outcomes of a Novel Corneal Prosthetic Device in a Rabbit Model. Cornea. 2020;39(6):706-712.
28. Holland G, Pandit A, Sánchez-Abella L, et al. Artificial Cornea: Past, Current, and Future Directions. Front Med (Lausanne). 2021;8:770780.
29. Jirásková N, Rozsival P, Burova M, Kalfertova M. AlphaCor artificial cornea: clinical outcome. Eye (Lond). 2011;25(9):1138-1146.
30. Kilian R, Lammer J, Roberts P, et al. Nonpenetrating Foldable Intrastromal Keratoprosthesis: A Review of the Literature. Cornea. 2024;43(7):909-915.
31. Pineda R. The keraklear artificial cornea. In: Keratoprostheses and Artificial Corneas: Fundamentals and Surgical Applications. Springer; 2014:213-219.
32. Falcinelli G, Falsini B, Taloni M, Colliardo P, Falcinelli G. Modified osteo-odonto-keratoprosthesis for treatment of corneal blindness: long-term anatomical and functional outcomes in 181 cases. Arch Ophthalmol. 2005;123(10):1319-1329.
33. Charoenrook V, Michael R, de la Paz MF, Ding A, Barraquer RI, Temprano J. Osteokeratoprosthesis Using Tibial Bone: Surgical Technique and Outcomes. Ocul Surf. 2016;14(4):495-506.
34. Hille K, Grabner G, Liu C, et al. Standards for modified osteoodontokeratoprosthesis (OOKP) surgery according to Strampelli and Falcinelli: the Rome-Vienna Protocol. Cornea. 2005;24(8):895-908.
35. Ortiz-Morales G, Loya-Garcia D, Colorado-Zavala MF, et al. The evolution of the modified osteo-odontokeratoprosthesis, its reliability, and long-term visual rehabilitation prognosis: An analytical review. Ocul Surf. 2022;24:129-144.
36. Falcinelli G, Colliardo P, Falcinelli G, Taloni A, Taloni M. Modified Osteo-odonto-keratoprosthesis (MOOKP): Indications, Contraindications, and Surgical Technique. In: Cortina MS, de la Cruz J, eds. Keratoprostheses and Artificial Corneas: Fundamentals and Surgical Applications. Berlin, Heidelberg: Springer Berlin Heidelberg; 2015:191-204.
37. Basu S, Sureka S, Shukla R, Sangwan V. Boston type 1 based keratoprosthesis (Auro Kpro) and its modification (LVP Kpro) in chronic Stevens Johnson syndrome. BMJ Case Rep. 2014;2014.
38. Basu S, Nagpal R, Serna-Ojeda JC, Bhalekar S, Bagga B, Sangwan V. LVP keratoprosthesis: anatomical and functional outcomes in bilateral end-stage corneal blindness. Br J Ophthalmol. 2018.
39. Bakshi SK, Graney J, Paschalis EI, et al. Design and Outcomes of a Novel Keratoprosthesis: Addressing Unmet Needs in End-Stage Cicatricial Corneal Blindness. Cornea. 2020;39(4):484-490.
40. Duncker GI, Storsberg J, Müller-Lierheim WG. The fully synthetic, bio-coated MIRO® CORNEA UR keratoprosthesis: development, preclinical testing, and first clinical results. Spektrum der Augenheilkunde. 2014;6(28):250-260.
41. Paschalis EI, Chodosh J, Spurr-Michaud S, et al. In vitro and in vivo assessment of titanium surface modification for coloring the backplate of the Boston keratoprosthesis. Investigative ophthalmology & visual science. 2013;54(6):3863-3873.
42. Zhou C, Lei F, Chodosh J, Paschalis EI. The Role of Titanium Surface Microtopography on Adhesion, Proliferation, Transformation, and Matrix Deposition of Corneal Cells. Investigative ophthalmology & visual science. 2016;57(4):1927-1938.
43. Salvador-Culla B, Jeong KJ, Kolovou PE, et al. Titanium Coating of the Boston Keratoprosthesis. Transl Vis Sci Technol. 2016;5(2):17.
44. Sharifi S, Islam MM, Sharifi H, et al. Sputter Deposition of Titanium on Poly(Methyl Methacrylate) Enhances Corneal Biocompatibility. Transl Vis Sci Technol. 2020;9(13):41.
45. Riau AK, Mondal D, Yam GH, et al. Surface Modification of PMMA to Improve Adhesion to Corneal Substitutes in a Synthetic Core-Skirt Keratoprosthesis. ACS Appl Mater Interfaces. 2015;7(39):21690-21702.
46. Riau AK, Mondal D, Setiawan M, et al. Functionalization of the Polymeric Surface with Bioceramic Nanoparticles via a Novel, Nonthermal Dip Coating Method. ACS Appl Mater Interfaces. 2016;8(51):35565-35577.
47. Sharifi R, Mahmoudzadeh S, Islam MM, et al. Covalent Functionalization of PMMA Surface with L-3,4Dihydroxyphenylalanine (L-DOPA) to Enhance its Biocompatibility and Adhesion to Corneal Tissue. Advanced Materials Interfaces. 2020;7(1):1900767.
48. Wang L, Jeong KJ, Chiang HH, et al. Hydroxyapatite for keratoprosthesis biointegration. Investigative ophthalmology & visual science. 2011;52(10):7392-7399.
49. Thirunavukarasu AJ, Morales-Wong F, Halim N, et al. Nanohydroxyapatite Coating Attenuates Fibrotic and Immune Responses to Promote Keratoprosthesis Biointegration in Advanced Ocular Surface Disorders. ACS Appl Mater Interfaces. 2024;16(20):25892-25908.
50. Sharifi S, Sharifi H, Akbari A, et al. Critical media attributes in E-beam sterilization of corneal tissue. Acta Biomater. 2022;138:218-227.
51. Sabeti S, Daoud R, Robert MC, Harissi-Dagher M. Frozen versus fresh corneal graft carriers in Boston keratoprosthesis surgery: 10-year outcomes. Can J Ophthalmol. 2022;57(2):127-133.
52. Feilmeier MR, Tabin GC, Williams L, Oliva M. The use of glycerol-preserved corneas in the developing world. Middle East Afr J Ophthalmol. 2010;17(1):38-43.
53. Gupta N, Upadhyay P. Use of glycerol-preserved corneas for corneal transplants. Indian J Ophthalmol. 2017;65(7):569-573.
54. Fernández-Pérez J, Ahearne M. Decellularization and recellularization of cornea: Progress towards a donor alternative. Methods. 2020;171:86-96.
55. Procházková A, Poláchová M, Dítě J, Netuková M, Studený P. Chemical, Physical, and Biological Corneal Decellularization Methods: A Review of Literature. J Ophthalmol. 2024;2024:1191462.
56. Zhang Y, Hu Z, Qu J, et al. Tissue-Engineered Corneal Endothelial Sheets Using Ultrathin Acellular Porcine Corneal Stroma Substrates for Endothelial Keratoplasty. ACS Biomater Sci Eng. 2022;8(3):1301-1311.
57. Li S, Li M, Gu L, et al. Risk factors influencing survival of acellular porcine corneal stroma in infectious keratitis: a prospective clinical study. J Transl Med. 2019;17(1):434.
58. Liang T, Wang X, Wu J, Cheng Y. Small-diameter acellular porcine corneal stroma for peripheral corneal ulceration treatment. Int J Ophthalmol. 2024;17(5):831-837.
59. El-Massry A, Ibrahim O, Abdalla M, Osman I, Mahmoud S. Safety and Indicative Effectiveness of Porcine Corneal Lenticular Implants in Patients with Advanced Keratoconus and Post Lasik Ectasia: A Retrospective Clinical Study. Clin Ophthalmol. 2021;15:3165-3171.
60. Li Q, Zhao H, Wang H, Zhao G. Properties of the acellular porcine cornea crosslinked with UVA/riboflavin as scaffolds for Boston Keratoprosthesis. Biomater Adv. 2022;137:212822.
61. Lohchab M, Gour A, Vohra M, Sangwan VS. Bridging the gap: The promise of corneal bioengineering and regeneration. Indian J Ophthalmol. 2024;72(4):483-488.
62. Bonato P, Bagno A. Replace or Regenerate? Diverse Approaches to Biomaterials for Treating Corneal Lesions. Biomimetics (Basel). 2024;9(4).
63. Mancha Sánchez E, Gómez-Blanco JC, López Nieto E, et al. Hydrogels for Bioprinting: A Systematic Review of Hydrogels Synthesis, Bioprinting Parameters, and Bioprinted Structures Behavior. Front Bioeng Biotechnol. 2020;8:776.
64. Fuest M, Yam GH, Mehta JS, Duarte Campos DF. Prospects and Challenges of Translational Corneal Bioprinting. Bioengineering (Basel). 2020;7(3).
65. Mirsky NA, Ehlen QT, Greenfield JA, et al. Three-Dimensional Bioprinting: A Comprehensive Review for Applications in Tissue Engineering and Regenerative Medicine. Bioengineering (Basel). 2024;11(8).
Tandias, Rachel
66. Jia S, Bu Y, Lau DA, et al. Advances in 3D bioprinting technology for functional corneal reconstruction and regeneration. Front Bioeng Biotechnol. 2022;10:1065460.
67. Crnej A, Paschalis EI, Salvador-Culla B, et al. Glaucoma progression and role of glaucoma surgery in patients with Boston keratoprosthesis. Cornea. 2014;33(4):349-354.
68. Geoffrion D, Hassanaly SI, Marchand M, Daoud R, Agoumi Y, Harissi-Dagher M. Assessment of the Role and Timing of Glaucoma Surgery in Boston Keratoprosthesis Type 1 Patients. Am J Ophthalmol. 2022;235:249-257.
69. Sun JA, Manz SN, Shen LQ. Glaucoma management in patients with penetrating keratoplasty or keratoprosthesis. Curr Opin Ophthalmol. 2023;34(2):95-102.
70. Wu KY, Mina M, Carbonneau M, Marchand M, Tran SD. Advancements in Wearable and Implantable Intraocular Pressure Biosensors for Ophthalmology: A Comprehensive Review. Micromachines (Basel). 2023;14(10).
71. Hui PC, Shtyrkova K, Zhou C, et al. Implantable self-aligning fiber-optic optomechanical devices for in vivo intraocular pressure-sensing in artificial cornea. J Biophotonics. 2020;13(7):e202000031.
72. Enders P, Hall J, Bornhauser M, et al. Telemetric Intraocular Pressure Monitoring after Boston Keratoprosthesis Surgery Using the Eyemate-IO Sensor: Dynamics in the First Year. Am J Ophthalmol. 2019;206:256-263.
73. Enders P, Cursiefen C. Device profile of the EYEMATE-IO™ system for intraocular pressure monitoring: overview of its safety and efficacy. Expert Rev Med Devices. 2020;17(6):491-497.
74. Szurman P, Gillmann K, Seuthe AM, et al. EYEMATE-SC Trial: Twelve-Month Safety, Performance, and Accuracy of a Suprachoroidal Sensor for Telemetric Measurement of Intraocular Pressure. Ophthalmology. 2023;130(3):304312.
75. Akpek EK, Karakus S, Yohannan J, et al. Reliability of Several Glaucoma Tests in Patients With Boston Type 1 Keratoprosthesis. Cornea. 2022;41(3):310-316.
76. Castillejos AG, Devlin J, Saini C, et al. Artifacts in OCT Retinal Nerve Fiber Layer Imaging in Patients with Boston Keratoprosthesis Type 1. Ophthalmol Glaucoma. 2024;7(2):206-215.
77. Khoueir Z, Jassim F, Braaf B, et al. Three-Dimensional Optical Coherence Tomography Imaging For Glaucoma Associated With Boston Keratoprosthesis Type I and II. J Glaucoma. 2019;28(8):718-726.
78. Silva LD, Santos A, Sousa LB, Allemann N, Oliveira LA. Anterior segment optical coherence tomography findings in type I Boston keratoprosthesis. Arq Bras Oftalmol. 2018;81(1):42-46.
79. Nascimento ESR, Taniguchi EV, Cruzat A, et al. Angle Anatomy and Glaucoma in Patients With Boston Keratoprosthesis. Cornea. 2020;39(6):713-719.
80. Bloom WR, Karl MD, Smith SB, et al. Ultra-wide field imaging to assess the optic nerve and retina in Boston type I and II keratoprosthesis patients. Eye Vis (Lond). 2022;9(1):18.
81. Paschalis EI, Zhou C, Sharma J, et al. The prophylactic value of TNF-α inhibitors against retinal cell apoptosis and optic nerve axon loss after corneal surgery or trauma. Acta Ophthalmol. 2024;102(3):e381-e394.
82. Dohlman CH, Zhou C, Lei F, et al. Glaucoma After Corneal Trauma or Surgery-A Rapid, Inflammatory, IOPIndependent Pathway. Cornea. 2019;38(12):1589-1594.
83. Paschalis EI, Zhou C, Lei F, et al. Mechanisms of Retinal Damage after Ocular Alkali Burns. Am J Pathol. 2017;187(6):1327-1342.
84. Chen X, Lei F, Zhou C, et al. Glaucoma after Ocular Surgery or Trauma: The Role of Infiltrating Monocytes and Their Response to Cytokine Inhibitors. Am J Pathol. 2020;190(10):2056-2066.
85. Cade F, Paschalis EI, Regatieri CV, Vavvas DG, Dana R, Dohlman CH. Alkali burn to the eye: protection using TNF-α inhibition. Cornea. 2014;33(4):382-389.
86. Roh M, Zhang Y, Murakami Y, et al. Etanercept, a widely used inhibitor of tumor necrosis factor-α (TNF-α), prevents retinal ganglion cell loss in a rat model of glaucoma. PLoS One. 2012;7(7):e40065.
87. Zhou C, Lei F, Sharma J, et al. Sustained Inhibition of VEGF and TNF-α Achieves Multi-Ocular Protection and Prevents Formation of Blood Vessels after Severe Ocular Trauma. Pharmaceutics. 2023;15(8).
88. Park J, Phrueksaudomchai P, Cortina MS. Retroprosthetic membrane: A complication of keratoprosthesis with broad consequences. Ocul Surf. 2020;18(4):893-900.
89. Sivaraman KR, Hou JH, Allemann N, de la Cruz J, Cortina MS. Retroprosthetic membrane and risk of sterile keratolysis in patients with type I Boston Keratoprosthesis. Am J Ophthalmol. 2013;155(5):814-822.
90. Stacy RC, Jakobiec FA, Michaud NA, Dohlman CH, Colby KA. Characterization of retrokeratoprosthetic membranes in the Boston type 1 keratoprosthesis. Arch Ophthalmol. 2011;129(3):310-316.
91. Hou JH, Sivaraman KR, de la Cruz J, Lin AY, Cortina MS. Histopathological and immunohistochemical analysis of melt-associated retroprosthetic membranes in the Boston type 1 keratoprosthesis. JAMA Ophthalmol. 2014;132(9):1133-1136.
92. de Oliveira RC, Wilson SE. Fibrocytes, Wound Healing, and Corneal Fibrosis. Investigative ophthalmology & visual science. 2020;61(2):28-28.
93. Gao M, Sang W, Liu F, et al. High MMP-9 Expression May Contribute to Retroprosthetic Membrane Formation after KPro Implantation in Rabbit Corneal Alkali Burn Model. Journal of Ophthalmology. 2016;2016(1):1094279.
94. Hébert M, Kyrillos R, Snyder ME, et al. Intraocular methotrexate for epithelial downgrowth: long-term outcomes in a multicentre case series. Br J Ophthalmol. 2023;107(9):1383-1389.
95. McAllister MA, Moore SM, Bullock B, Christoforidis JB. Intraocular Methotrexate for the Treatment and Prevention of Proliferative Vitreoretinopathy: A Review. J Vitreoretin Dis. 2023;7(2):144-153.
96. Iyer G, Srinivasan B, Agarwal S, et al. Keratoprostheses in silicone oil-filled eyes: long-term outcomes. Br J Ophthalmol. 2019;103(6):781-788.
97. Gabriel BS, Robbins CB, Wisely CE, Gabriel RS, Daluvoy MB, Fekrat S. Incidence, risk factors, and treatment of retroprosthetic membranes following Boston keratoprosthesis eyes and the impact of glaucoma surgery. Graefes Arch Clin Exp Ophthalmol. 2024;262(8):2561-2567.
98. Perez VL, Leung EH, Berrocal AM, et al. Impact of Total Pars Plana Vitrectomy on Postoperative Complications in Aphakic, Snap-On, Type 1 Boston Keratoprosthesis. Ophthalmology. 2017;124(10):1504-1509.
99. Khair D, Daoud R, Harissi-Dagher M. Retroprosthetic Membrane Formation in Boston Keratoprosthesis Type 1: Incidence, Predisposing Factors, Complications, and Treatment. Cornea. 2022;41(6):751-756.
100. Talati RK, Hallak JA, Karas FI, de la Cruz J, Cortina MS. Retroprosthetic Membrane Formation in Boston Keratoprosthesis: A Case-Control-Matched Comparison of Titanium Versus PMMA Backplate. Cornea. 2018;37(2):145-150.
101. Kaufman AR, Cruzat A, Colby KA. Clinical Outcomes Using Oversized Back Plates in Type I Boston Keratoprosthesis. Eye Contact Lens. 2018;44(6):399-404.
102. Gómez SG, Guillem-Marti J, Martín-Gómez H, et al. Titanium Boston keratoprosthesis with corneal cell adhesive and bactericidal dual coating. Biomater Adv. 2023;154:213654.
103. Gómez SG, Ginebra MP, Gil FJ, Barraquer RI, Manero JM. Antibacterial and cytocompatible silver coating for titanium Boston Keratoprosthesis. Front Bioeng Biotechnol. 2024;12:1421706.
104. Luo Y, Wang T, Yang K, Liu T, Bai X. Electrospun borneol/PVP antibacterial coating for postoperative management of keratoprosthesis implantation. Materials Technology. 2024;39(1):2309596.
105. Carreira AS, Ferreira P, Ribeiro MP, et al. New drug-eluting lenses to be applied as bandages after keratoprosthesis implantation. Int J Pharm. 2014;477(1-2):218-226.
106. Ciolino JB, Stefanescu CF, Ross AE, et al. In vivo performance of a drug-eluting contact lens to treat glaucoma for a month. Biomaterials. 2014;35(1):432-439.
107. Lanier OL, Christopher KG, Macoon RM, Yu Y, Sekar P, Chauhan A. Commercialization challenges for drug eluting contact lenses. Expert Opin Drug Deliv. 2020;17(8):1133-1149.
108. Sun R, Zhang J, Chen X, et al. An adaptive drug-releasing contact lens for personalized treatment of ocular infections and injuries. J Control Release. 2024;369:114-127.
109. Zerbe BL, Belin MW, Ciolino JB. Results from the multicenter Boston Type 1 Keratoprosthesis Study. Ophthalmology. 2006;113(10):1779.e1771-1777.
110. Sejpal K, Yu F, Aldave AJ. The Boston keratoprosthesis in the management of corneal limbal stem cell deficiency. Cornea. 2011;30(11):1187-1194.
Tandias, Rachel
111. Robert MC, Dohlman CH. A review of corneal melting after Boston Keratoprosthesis. Semin Ophthalmol. 2014;29(5-6):349-357.
112. Bouhout S, Robert MC, Deli S, Harissi-Dagher M. Corneal Melt after Boston Keratoprosthesis: Clinical Presentation, Management, Outcomes and Risk Factor Analysis. Ocul Immunol Inflamm. 2018;26(5):693-699.
113. Daoud R, Sabeti S, Harissi-Dagher M. Management of corneal melt in patients with Boston Keratoprosthesis Type 1: Repair versus repeat. Ocul Surf. 2020;18(4):713-717.
114. Robert M-C, Arafat SN, Ciolino JB. Collagen Cross-Linking of the Boston Keratoprosthesis Donor Carrier to Prevent Corneal Melting in High-Risk Patients. Eye & Contact Lens. 2014;40(6).
115. Kanellopoulos AJ, Asimellis G. Long-Term Safety and Efficacy of High-Fluence Collagen Crosslinking of the Vehicle Cornea in Boston Keratoprosthesis Type 1. Cornea. 2014;33(9).
116. De Arrigunaga S, Akpek EK, Aldave AJ, Mian SI, Zurakowski D, Ciolino JB. Prospective, Randomized, Multicenter, Double-Masked, Clinical Trial of Corneal Cross-Linking for Boston Keratoprosthesis Carrier Tissue. Am J Ophthalmol. 2023;249:39-48.
Hursuong Vongsachang, MD, MPH, and Michael K. Yoon, MD
Introduction
Artificial intelligence, the development and manifestation of automated intelligent behavior, has become a rapidly growing field within ophthalmology in recent years, as evidenced by the dramatic rise in research in this area and the increasing focus on this topic by professional societies internationally.1,2 In brief, within artificial intelligence, machine learning refers to the training and validation of algorithms through data input, which can be labelled or unlabeled, supervised or unsupervised by human expertise.3 Ideally, the data inputted should be high quality, diverse, and representative of the intended population to which the program will be applied. Deep learning is a further advancement of machine learning whereby output generated at a certain level of input is then propagated forwards and backwards through multiple layers and orders of “neurons.” Of particular interest in deep learning are convolutional neural networks, a form of deep learning that primarily utilizes images as the data source. In ophthalmology, these processes can ultimately manifest as the ability to automate screening of retinal diseases from fundus photos or the diagnosis of anterior segment pathology from corneal topography, for example. Test characteristics such as sensitivity, specificity, false positives, false negatives, and area under the receiver operator characteristic curve are primary study outcomes to understand the performance of an artificial intelligence model.
Similar to other fields in ophthalmology, oculoplastic surgery is a field with ample opportunity for application of artificial intelligence given the high utilization of images such as external photos and radiography. The present article provides a broad overview of the current landscape of artificial intelligence across several conditions in oculoplastic surgery
Patients may present with various benign or malignant eyelid lesions to the general practitioner, comprehensive ophthalmologist, or oculoplastic surgeon Suspicious lesions may be biopsied for definitive histopathologic diagnosis. The most frequently encountered malignant lesions include basal cell carcinoma, squamous cell carcinoma, melanoma, and sebaceous carcinoma. Deep learning has shown promise in not only identifying the presence of eyelid neoplasms in photographs, but further, differentiating malignant from benign lesions.4–7 In some studies, these models can perform at a level equal, if not superior to, ophthalmologists. For example, one of the models by Lee et al achieved over 90% mean accuracy and sensitivity in categorizing malignant and benign lesions, which was comparable to the performance of three oculoplastic surgeons but better in sensitivity than three general ophthalmologists (mean 64.2%) and three residents (mean 73.8%).4
In a similar vein, deep learning has been used to identify basal cell carcinoma, sebaceous carcinoma, and melanoma on whole slide images of eyelid tissue with excellent results 8–10 In fact, the study by Luo et al demonstrated that their deep learning model had significantly higher accuracy (0.983) differentiating between basal cell and sebaceous carcinoma on whole slide images than two recently graduated pathologists (0.644 and 0.729) and one seasoned pathologist (0.831).8 Besides using artificial intelligence directly for automatic diagnosis, using output from the models, such as images with characteristic diagnostic areas highlighted, as an adjunct increased the accuracy of the three pathologists between 1030% compared to their accuracy without these diagnostic aids 8
Blepharoptosis
Blepharoptosis, abnormally low eyelid position that may obstruct the visual axis, is a common complaint evaluated in the oculoplastic surgery clinic. Standard practice during a consultation for blepharoptosis
requires a handheld ruler to take eyelid measurements such as margin reflex distance, as well as external photography. Convolutional neural networks can take these clinical photos and automate several eyelid measurements with excellent performance metrics.11–19 The basis of these models involve identifying the eye region in facial photographs, then segmenting landmarks such as the cornea, pupils, and brows for calculations of eyelid parameters While most utilize a single photo in primary gaze, more dynamic input such as a series of photos in different gazes or frames from a video recording of a blink cycle can help assess other pertinent measures like levator function and fluctuations in margin reflex distance 17,18 This then translates into the ability to detect blepharoptosis and further, evaluate post-operative appearance in various areas such as eyelid height, contour, and symmetry, although it is worthwhile to note that not all models generate or use these measurements 20–28
Moreover, these tools could potentially be used outside of the oculoplastic clinic by general ophthalmologists and non-ophthalmology physicians whose patients may have eyelid changes and complaints When compared to a family medicine physician, emergency medicine physician, and a neurologist, the model studied by Hung et al demonstrated higher accuracy (90% versus mean 77.33%), sensitivity (92% versus mean 72%), and specificity (88% versus mean 82.67%) in identifying ptosis and ptosis mimickers (e.g. dermatochalasis) on a test of 50 images.29 Overall, by analyzing patient photographs, artificial intelligence has potential to help non-oculoplastic physicians identify suitable candidates for oculoplastic evaluation of ptosis and possibly other concerns of eyelid malposition, standardize and automate measurements in the clinic, and promote non-synchronous visits.
The diagnosis of thyroid eye disease is based on several clinical parameters including symptoms and physical examination along with ancillary imaging and laboratory values. Some of the earliest efforts in applying artificial intelligence towards thyroid eye disease date back to 2002, where Salvi et al input clinical variables into an artificial neural network to differentiate patients with inactive or no thyroid eye disease from those with active disease requiring subsequent clinic visits for monitoring.30 Since then, deep learning models in this field have largely shifted to image- based data as the training and validation datasets. These deep learning models use computed tomography (CT), magnetic resonance imaging (MRI), and external photographs to not only detect, but also classify the severity of thyroid eye disease and whether the disease is active.20,31–38 In terms of disease monitoring, most of the literature have focused on developing deep neural networks to identify individual Clinical Activity Score (CAS) items and composite CAS scores based on photos taken in the clinic and even smartphones for potential at-home monitoring.39–41 One advantage of utilizing artificial intelligence is the potential to diagnose and classify thyroid eye disease based on a single image alone without other clinical data. However, incorporating multimodal data formats, such as radiography, subjective symptoms, and slit lamp photographs, can enhance a model’s ability to accurately detect certain thyroid eye disease features, such as ocular motility deficits and conjunctival chemosis, for example.36,42 Finally, there is hope that MRI-based machine learning algorithms can eventually predict which patients with active and moderate-to-severe thyroid eye disease will respond to intravenous glucocorticoid therapy.43,44
The bones around the orbit can be commonly fractured during trauma owing to their thin nature. Computed tomography (CT) is critical for the diagnosis and management of orbital fractures and typically necessitates trained personnel to annotate and measure fracture areas and volumes with digital calipers in the imaging software. Naturally, artificial intelligence researchers have used CT images to train convolutional neural networks to identify and segment orbital fractures, automating a traditionally timeconsuming and operator-dependent manual process while preserving accuracy.45–49 For instance, the system used by Bao et al achieved high accuracy of 0.9693 in fracture identification as well as high similarity with manual methods in fracture area segmentation with intersection over union and Dice coefficients greater than 0.80 46 Additionally, in the study by Chepurnyi et al, there was no significant difference between the calculated changes in pre- and post-operative orbital volumes, defect areas, and prolapsed orbital soft tissue volumes performed by manual versus automatic processes 49 Hou et al even
used the segmentation output generated by their models to produce 3D models for surgical planning with favorable results 47
Imaging, particularly MRI, is a mainstay in the diagnosis of orbital pathology, which include neoplastic, inflammatory, infectious, and vascular etiologies. Deep learning can increase the efficiency and quality of image segmentation and even reconstruction.47,50–53 Convolutional neural networks can be trained on radiographic images to detect the presence of specific orbital pathology, differentiate benign from malignant features, or distinguish between two specific entities, sometimes even better than experienced neuroradiologists.54–60 Current examples in the literature include distinguishing cavernous venous malformation from solid tumor and lymphoma from non-specific inflammation.54,56,57 Investigators have explored how to further optimize these models with the addition of clinical data and qualitative and quantitative data extracted from radiographic images.54–56,59 Beyond diagnosis, there is also research on using deep learning to prognosticate and manage orbital disease, for example using deep learning measurements of abscess size to predict need for surgical intervention of pediatric subperiosteal abscess and helping identify orbital invasion of sinonasal tumors.61,62 Notably, most deep learning work with regards to orbital lesions has relied on MRI and less frequently on CT. Finally, deep learning can also be trained on histopathology slides of orbital tissue to aid in diagnosis.63
In the realm of oculoplastic conditions, there is relatively less research on applying artificial intelligence to lacrimal system disorders. The few studies available currently have suggested the use of deep learning to screen for nasolacrimal dysfunction based on anterior segment imaging and other measures of the ocular surface 64,65 Kim et al tested a model to detect and classify the location of nasolacrimal duct obstruction based on dacryocystography with good results.66
Large language models (LLM) are trained on the vast amount of text data available on the web to provide conversational, intelligible responses to human questions. Open-source models have propelled the popularity and widespread use of LLM, prompting research into its clinical applicability, including within oculoplastic surgery When tested on hundreds of simulated clinical scenarios and patient queries across various oculoplastic domains, LLM can often produce highly satisfactory answers but ultimately are still limited by occasional inaccuracies and other practical considerations, like readability and complexity of answers.67–72 Switching to text-to-image generation, Balas et al demonstrated that publicly available artificial intelligence tools can process text-based prompts to produce predictive images of a patient’s expected post-operative outcome based on a patient’s own clinical photo for patients considering eyelid surgery.73 These machine learning photos were similar to the patients’ actual post-surgical photos.
The rise in artificial intelligence is an exciting time in oculoplastic surgery, garnering a rush of interest and research that promise practice-changing clinical applications in the future, as summarized in the present review However, it remains to be known how or when artificial intelligence will reach the standards to be incorporated clinically. Maximizing accuracy, reliability, generalizability, clinical utility, accessibility, feasibility, and security are just some of the paramount criteria that must be met before such technology can be deployed into real practice settings.3 Furthermore, it is unknown how artificial intelligence tools will change the role of the oculoplastic surgeon, what the relationship between the physician and these tools will look like, and the response of physicians and patients to this technology potentially taking part in their care. Nonetheless, as artificial intelligence continues to become more advanced and ubiquitous, changes to the field of oculoplastic surgery and medicine overall are inevitable. Understanding and embracing this budding technology offers the oculoplastics community a unique opportunity to better patient care and outcomes.
1. Maleki A, Anesi SD, Look-Why S, Manhapra A, Foster CS. Pediatric uveitis: A comprehensive review. Surv 1. Deng J, Qin Y. Current Status, Hotspots, and Prospects of Artificial Intelligence in Ophthalmology: A Bibliometric Analysis (2003-2023). Ophthalmic Epidemiol. Published online August 15, 2024:1-14. doi:10.1080/09286586.2024.2373956
2. Artificial Intelligence. Accessed November 16, 2024. https://www.aao.org/education/artificial-intelligence
3. Kang D, Wu H, Yuan L, Shi Y, Jin K, Grzybowski A. A Beginner’s Guide to Artificial Intelligence for Ophthalmologists. Ophthalmol Ther. 2024;13(7):1841-1855. doi:10.1007/s40123-024-00958-3
4. Lee MJ, Yang MK, Khwarg SI, et al. Differentiating malignant and benign eyelid lesions using deep learning. Sci Rep 2023;13(1):4103. doi:10.1038/s41598-023-30699-5
5. Li Z, Qiang W, Chen H, et al. Artificial intelligence to detect malignant eyelid tumors from photographic images. NPJ Digit Med. 2022;5(1):23. doi:10.1038/s41746-022-00571-3
6. Adamopoulos A, Chatzopoulos EG, Anastassopoulos G, Detorakis E. Eyelid basal cell carcinoma classification using shallow and deep learning artificial neural networks. Evol Syst. 2021;12(3):583-590. doi:10.1007/s12530-021-09383-4
7. Jiang J, Liu H, He L, et al. HM_ADET: a hybrid model for automatic detection of eyelid tumors based on photographic images. Biomed Eng OnLine. 2024;23(1):25. doi:10.1186/s12938-024-01221-3
8. Luo Y, Zhang J, Yang Y, et al. Deep learning-based fully automated differential diagnosis of eyelid basal cell and sebaceous carcinoma using whole slide images. Quant Imaging Med Surg. 2022;12(8):4166-4175. doi:10.21037/qims-22-98
9. Wang L, Jiang Z, Shao A, et al. Self-supervised learning mechanism for identification of eyelid malignant melanoma in pathologic slides with limited annotation. Front Med. 2022;9:976467. doi:10.3389/fmed.2022.976467
10. Wang L, Ding L, Liu Z, et al. Automated identification of malignancy in whole-slide pathological images: identification of eyelid malignant melanoma in gigapixel pathological slides using deep learning. Br J Ophthalmol. 2020;104(3):318323. doi:10.1136/bjophthalmol-2018-313706
11. Cao J, Lou L, You K, et al. A Novel Automatic Morphologic Analysis of Eyelids Based on Deep Learning Methods. Curr Eye Res. 2021;46(10):1495-1502. doi:10.1080/02713683.2021.1908569
12. Thomas PBM, Gunasekera CD, Kang S, Baltrusaitis T. An Artificial Intelligence Approach to the Assessment of Abnormal Lid Position. Plast Reconstr Surg Glob Open. 2020;8(10):e3089. doi:10.1097/GOX.0000000000003089
13. Rana K, Beecher M, Caltabiano C, et al. Artificial intelligence to automate assessment of ocular and periocular measurements. Eur J Ophthalmol. Published online May 6, 2024:11206721241249773. doi:10.1177/11206721241249773
14. Shao J, Cao J, Wang C, Xu P, Lou L, Ye J. Automatic Measurement and Comparison of Normal Eyelid Contour by Age and Gender Using Image-Based Deep Learning. Ophthalmol Sci. 2024;4(5):100518. doi:10.1016/j.xops.2024.100518
15. Nam Y, Song T, Lee J, Lee JK. Development of a neural network-based automated eyelid measurement system. Sci Rep. 2024;14(1):1202. doi:10.1038/s41598-024-51838-6
16. Song B, Kwon H, Kim S, Ha Y, Oh SH, Song SH. Introduction of Deep Learning-Based Infrared Image Analysis to Marginal Reflex Distance1 Measurement Method to Simultaneously Capture Images and Compute Results: Clinical Validation Study. J Clin Med. 2023;12(23):7466. doi:10.3390/jcm12237466
17. Van Brummen A, Owen JP, Spaide T, et al. PeriorbitAI: Artificial Intelligence Automation of Eyelid and Periorbital Measurements. Am J Ophthalmol. 2021;230:285-296. doi:10.1016/j.ajo.2021.05.007
18. Chen HC, Tzeng SS, Hsiao YC, Chen RF, Hung EC, Lee OK. Smartphone-Based Artificial Intelligence-Assisted Prediction for Eyelid Measurements: Algorithm Development and Observational Validation Study. JMIR MHealth UHealth. 2021;9(10):e32444. doi:10.2196/32444
19. Choi CJ, Lefebvre DR, Yoon MK. Validation of the facial assessment by computer evaluation (FACE) program for software-aided eyelid measurements. Orbit Amst Neth. 2016;35(3):117-120. doi:10.3109/01676830.2016.1139595
20. Hanai K, Tabuchi H, Nagasato D, et al. Automated detection of enlarged extraocular muscle in Graves’ ophthalmopathy with computed tomography and deep neural network. Sci Rep. 2022;12(1):16036. doi:10.1038/s41598-022-20279-4
21. Song X, Tong W, Lei C, et al. A clinical decision model based on machine learning for ptosis. BMC Ophthalmol 2021;21(1):169. doi:10.1186/s12886-021-01923-5
22. Hung JY, Perera C, Chen KW, et al. A deep learning approach to identify blepharoptosis by convolutional neural networks. Int J Med Inf. 2021;148:104402. doi:10.1016/j.ijmedinf.2021.104402
23. Lou L, Yang L, Ye X, et al. A Novel Approach for Automated Eyelid Measurements in Blepharoptosis Using Digital Image Analysis. Curr Eye Res. 2019;44(10):1075-1079. doi:10.1080/02713683.2019.1619779
24. Bahçeci Şimşek İ, Şirolu C. Analysis of surgical outcome after upper eyelid surgery by computer vision algorithm using face and facial landmark detection. Graefes Arch Clin Exp Ophthalmol Albrecht Von Graefes Arch Klin Exp Ophthalmol. 2021;259(10):3119-3125. doi:10.1007/s00417-021-05219-8
25. Lou L, Cao J, Wang Y, et al. Deep learning-based image analysis for automated measurement of eyelid morphology before and after blepharoptosis surgery. Ann Med. 2021;53(1):2278-2285. doi:10.1080/07853890.2021.2009127
26. Tabuchi H, Nagasato D, Masumoto H, et al. Developing an iOS application that uses machine learning for the automated diagnosis of blepharoptosis. Graefes Arch Clin Exp Ophthalmol Albrecht Von Graefes Arch Klin Exp Ophthalmol. 2022;260(4):1329-1335. doi:10.1007/s00417-021-05475-8
27. Abascal Azanza C, Barrio-Barrio J, Ramos Cejudo J, Ybarra Arróspide B, Devoto MH. Development and validation of a convolutional neural network to identify blepharoptosis. Sci Rep. 2023;13(1):17585. doi:10.1038/s41598-02344686-3
28. Lee S, Song T, Lee JK, Lee J. Effective encoder-decoder network for pupil light reflex segmentation in facial photographs of ptosis patients. Sci Rep. 2024;14(1):26220. doi:10.1038/s41598-024-77001-9
29. Hung JY, Chen KW, Perera C, et al. An Outperforming Artificial Intelligence Model to Identify Referable Blepharoptosis for General Practitioners. J Pers Med. 2022;12(2):283. doi:10.3390/jpm12020283
30. Salvi M, Dazzi D, Pellistri I, Neri F, Wall JR. Classification and prediction of the progression of thyroid-associated ophthalmopathy by an artificial neural network. Ophthalmology. 2002;109(9):1703-1708. doi:10.1016/s01616420(02)01127-2
31. Lin LY, Zhou P, Shi M, et al. A Deep Learning Model for Screening Computed Tomography Imaging for Thyroid Eye Disease and Compressive Optic Neuropathy. Ophthalmol Sci. 2024;4(1):100412. doi:10.1016/j.xops.2023.100412
32. Lin C, Song X, Li L, et al. Detection of active and inactive phases of thyroid-associated ophthalmopathy using deep convolutional neural network. BMC Ophthalmol. 2021;21(1):39. doi:10.1186/s12886-020-01783-5
33. Alkhadrawi AM, Lin LY, Langarica SA, et al. Deep-Learning Based Automated Segmentation and Quantitative Volumetric Analysis of Orbital Muscle and Fat for Diagnosis of Thyroid Eye Disease. Invest Ophthalmol Vis Sci 2024;65(5):6. doi:10.1167/iovs.65.5.6
34. Song X, Liu Z, Li L, et al. Artificial intelligence CT screening model for thyroid-associated ophthalmopathy and tests under clinical conditions. Int J Comput Assist Radiol Surg. 2021;16(2):323-330. doi:10.1007/s11548-020-02281-1
35. Karlin J, Gai L, LaPierre N, et al. Ensemble neural network model for detecting thyroid eye disease using external photographs. Br J Ophthalmol. 2023;107(11):1722-1729. doi:10.1136/bjo-2022-321833
36. Liu Z, Tan K, Zhang H, et al. CT-based artificial intelligence prediction model for ocular motility score of thyroid eye disease. Endocrine. 2024;86(3):1055-1064. doi:10.1007/s12020-024-03906-0
37. Lee J, Seo W, Park J, et al. Neural network-based method for diagnosis and severity assessment of Graves’ orbitopathy using orbital computed tomography. Sci Rep. 2022;12(1):12071. doi:10.1038/s41598-022-16217-z
38. Lee J, Lee S, Lee WJ, Moon NJ, Lee JK. Neural network application for assessing thyroid-associated orbitopathy activity using orbital computed tomography. Sci Rep. 2023;13(1):13018. doi:10.1038/s41598-023-40331-1
39. Moon JH, Shin K, Lee GM, et al. Machine learning-assisted system using digital facial images to predict the clinical activity score in thyroid-associated orbitopathy. Sci Rep. 2022;12(1):22085. doi:10.1038/s41598-022-25887-8
40. Huang X, Ju L, Li J, et al. An Intelligent Diagnostic System for Thyroid-Associated Ophthalmopathy Based on Facial Images. Front Med. 2022;9:920716. doi:10.3389/fmed.2022.920716
41. Shin K, Choung H, Lee MJ, et al. A Preliminary Evaluation of the Diagnostic Performance of a Smartphone-Based Machine Learning-Assisted System for Evaluation of Clinical Activity Score in Digital Images of Thyroid-Associated Orbitopathy. Thyroid Off J Am Thyroid Assoc. 2024;34(6):744-752. doi:10.1089/thy.2023.0621
42. Yan C, Zhang Z, Zhang G, et al. An ensemble deep learning diagnostic system for determining Clinical Activity Scores in thyroid-associated ophthalmopathy: integrating multi-view multimodal images from anterior segment slitlamp photographs and facial images. Front Endocrinol. 2024;15:1365350. doi:10.3389/fendo.2024.1365350
43. Hu H, Chen L, Zhang JL, et al. T2 -Weighted MR Imaging-Derived Radiomics for Pretreatment Determination of Therapeutic Response to Glucocorticoid in Patients With Thyroid-Associated Ophthalmopathy: Comparison With Semiquantitative Evaluation. J Magn Reson Imaging JMRI. 2022;56(3):862-872. doi:10.1002/jmri.28088
44. Zhang H, Jiang M, Chan HC, et al. Whole-orbit radiomics: machine learning-based multi- and fused- region radiomics signatures for intravenous glucocorticoid response prediction in thyroid eye disease. J Transl Med. 2024;22(1):56. doi:10.1186/s12967-023-04792-2
45. Morita D, Kawarazaki A, Soufi M, Otake Y, Sato Y, Numajiri T. Automatic detection of midfacial fractures in facial bone CT images using deep learning-based object detection models. J Stomatol Oral Maxillofac Surg. 2024;125(5S2):101914. doi:10.1016/j.jormas.2024.101914
46. Bao XL, Zhan X, Wang L, Zhu Q, Fan B, Li GY. Automatic Identification and Segmentation of Orbital Blowout Fractures Based on Artificial Intelligence. Transl Vis Sci Technol. 2023;12(4):7. doi:10.1167/tvst.12.4.7
47. Morita D, Kawarazaki A, Koimizu J, et al. Automatic orbital segmentation using deep learning-based 2D U-net and accuracy evaluation: A retrospective study. J Cranio-Maxillo-fac Surg Off Publ Eur Assoc Cranio-Maxillo-fac Surg 2023;51(10):609-613. doi:10.1016/j.jcms.2023.09.003
48. Kang D. Evaluating the Accuracy and Reliability of Blowout Fracture Area Measurement Methods: A Review and the Potential Role of Artificial Intelligence. J Craniofac Surg. 2023;34(6):1834-1836. doi:10.1097/SCS.0000000000009486
49. Chepurnyi Y, Chernohorskyi D, Prykhodko D, Poutala A, Kopchak A. Reliability of orbital volume measurements based on computed tomography segmentation: Validation of different algorithms in orbital trauma patients. J CranioMaxillo-fac Surg Off Publ Eur Assoc Cranio-Maxillo-fac Surg. 2020;48(6):574-581. doi:10.1016/j.jcms.2020.03.007
50. Hamwood J, Schmutz B, Collins MJ, Allenby MC, Alonso-Caneiro D. A deep learning method for automatic segmentation of the bony orbit in MRI and CT images. Sci Rep. 2021;11(1):13693. doi:10.1038/s41598-021-93227-3
51. Estler A, Zerweck L, Brunnée M, et al. Deep learning-accelerated image reconstruction in MRI of the orbit to shorten acquisition time and enhance image quality. J Neuroimaging Off J Am Soc Neuroimaging. 2024;34(2):232-240. doi:10.1111/jon.13187
52. Wang G, Yang B, Qu X, et al. Fully automated segmentation and volumetric measurement of ocular adnexal lymphoma by deep learning-based self-configuring nnU-net on multi-sequence MRI: a multi-center study. Neuroradiology. 2024;66(10):1781-1791. doi:10.1007/s00234-024-03429-5
53. Fu R, Leader JK, Pradeep T, et al. Automated delineation of orbital abscess depicted on CT scan using deep learning. Med Phys. 2021;48(7):3721-3729. doi:10.1002/mp.14907
54. Xie X, Yang L, Zhao F, et al. A deep learning model combining multimodal radiomics, clinical and imaging features for differentiating ocular adnexal lymphoma from idiopathic orbital inflammation. Eur Radiol. 2022;32(10):6922-6932. doi:10.1007/s00330-022-08857-6
55. Duron L, Heraud A, Charbonneau F, et al. A Magnetic Resonance Imaging Radiomics Signature to Distinguish Benign From Malignant Orbital Lesions. Invest Radiol. 2021;56(3):173-180. doi:10.1097/RLI.0000000000000722
56. Hou Y, Xie X, Chen J, et al. Bag-of-features-based radiomics for differentiation of ocular adnexal lymphoma and idiopathic orbital inflammation from contrast-enhanced MRI. Eur Radiol. 2021;31(1):24-33. doi:10.1007/s00330-02007110-2
57. Bi S, Chen R, Zhang K, et al. Differentiate cavernous hemangioma from schwannoma with artificial intelligence (AI). Ann Transl Med. 2020;8(11):710. doi:10.21037/atm.2020.03.150
58. Han Q, Du L, Mo Y, Huang C, Yuan Q. Machine Learning Based Non-Enhanced CT Radiomics for the Identification of Orbital Cavernous Venous Malformations: An Innovative Tool. J Craniofac Surg. 2022;33(3):814-820. doi:10.1097/SCS.0000000000008446
59. Ren J, Yuan Y, Qi M, Tao X. MRI-based radiomics nomogram for distinguishing solitary fibrous tumor from schwannoma in the orbit: a two-center study. Eur Radiol. 2024;34(1):560-568. doi:10.1007/s00330-023-10031-5
60. O’Shaughnessy E, Senicourt L, Mambour N, Savatovsky J, Duron L, Lecler A. Toward Precision Diagnosis: Machine Learning in Identifying Malignant Orbital Tumors With Multiparametric 3 T MRI. Invest Radiol. 2024;59(10):737-745. doi:10.1097/RLI.0000000000001076
61. Fu R, Bandos A, Leader JK, et al. Artificial Intelligence Automation of Proptosis Measurement: An Indicator for Pediatric Orbital Abscess Surgery. Ophthalmol Ther. 2023;12(5):2479-2491. doi:10.1007/s40123-023-00754-5
62. Nakagawa J, Fujima N, Hirata K, et al. Utility of the deep learning technique for the diagnosis of orbital invasion on CT in patients with a nasal or sinonasal tumor. Cancer Imaging Off Publ Int Cancer Imaging Soc. 2022;22(1):52. doi:10.1186/s40644-022-00492-0
63. Tagami M, Nishio M, Yoshikawa A, et al. Artificial intelligence-based differential diagnosis of orbital MALT lymphoma and IgG4 related ophthalmic disease using hematoxylin-eosin images. Graefes Arch Clin Exp Ophthalmol Albrecht Von Graefes Arch Klin Exp Ophthalmol. 2024;262(10):3355-3366. doi:10.1007/s00417-024-06501-1
64. Imamura H, Tabuchi H, Nagasato D, et al. Automatic screening of tear meniscus from lacrimal duct obstructions using anterior segment optical coherence tomography images by deep learning. Graefes Arch Clin Exp Ophthalmol Albrecht Von Graefes Arch Klin Exp Ophthalmol. 2021;259(6):1569-1577. doi:10.1007/s00417-021-05078-3
65. Song X, Li L, Han F, Liao S, Xiao C. Noninvasive Machine Learning Screening Model for Dacryocystitis Based on Ocular Surface Indicators. J Craniofac Surg. 2022;33(1):e23-e28. doi:10.1097/SCS.0000000000007863
66. Kim S, Lee H, Roh HG, Shin HJ. Using Artificial Intelligence to Diagnose Lacrimal Passage Obstructions Based on Dacryocystography Images. J Craniofac Surg. Published online November 6, 2024. doi:10.1097/SCS.0000000000010829
67. Parikh AO, Oca MC, Conger JR, McCoy A, Chang J, Zhang-Nunes S. Accuracy and Bias in Artificial Intelligence Chatbot Recommendations for Oculoplastic Surgeons. Cureus. 2024;16(4):e57611. doi:10.7759/cureus.57611
68. Gernandt S, Aymon R, Scolozzi P. Assessing the accuracy of artificial intelligence in the diagnosis and management of orbital fractures: Is this the future of surgical decision-making? JPRAS Open. 2024;42:275-283. doi:10.1016/j.jpra.2024.09.014
69. Ali MJ. ChatGPT and Lacrimal Drainage Disorders: Performance and Scope of Improvement. Ophthal Plast Reconstr Surg. 2023;39(3):221-225. doi:10.1097/IOP.0000000000002418
70. Balas M, Janic A, Daigle P, et al. Evaluating ChatGPT on Orbital and Oculofacial Disorders: Accuracy and Readability Insights. Ophthal Plast Reconstr Surg. 2024;40(2):217-222. doi:10.1097/IOP.0000000000002552
71. Al-Sharif EM, Penteado RC, Dib El Jalbout N, et al. Evaluating the Accuracy of ChatGPT and Google BARD in Fielding Oculoplastic Patient Queries: A Comparative Study on Artificial versus Human Intelligence. Ophthal Plast Reconstr Surg. 2024;40(3):303-311. doi:10.1097/IOP.0000000000002567
72. Rajabi MT, Rafizadeh SM, Ghahvehchian H. Exploring the Use of ChatGPT in Delivering Evidence-Based Information to Patients with Thyroid Eye Disease. Ophthal Plast Reconstr Surg. 2024;40(1):113-115. doi:10.1097/IOP.0000000000002468
73. Balas M, Micieli JA, Wulc A, Ing EB. Text-to-image artificial intelligence models for preoperative counselling in oculoplastics. Can J Ophthalmol J Can Ophtalmol. 2024;59(1):e75-e76. doi:10.1016/j.jcjo.2023.09.006
Seyedeh Maryam Zekavat, MD, PhD, and Elizabeth J. Rossin, MD, PhD
Abstract
The human retina is a multi-layered tissue with diverse embryological origins and is therefore a window for assessment of ocular, neurological, vascular, and other systemic conditions. Innovations in big data, particularly involving large population biobanks, have enabled improved datasets that facilitate a better understanding of connections between the retina and systemic health. Retinal fundus and optical coherence tomography imaging data provide rich resources for describing interpretable imaging endophenotypes and evaluating their utility as biomarkers for future ocular and systemic conditions. Furthermore, the addition of genomic data to retinal and ophthalmic information has enabled unbiased discovery of the biological mechanisms driving changes in the retina. Assessment of inherited common and rare variants influencing ocular phenotypes through genome-wide association studies, in silico analyses for gene prioritization, pathway enrichment analysis, and experimental validation may enable identification of biological targets for therapeutic modulation of retinal microvascular indices, neuronal health, and other imaging biomarkers. Further development of polygenic risk scores for retinal phenotypes enables a personalized medicine approach to quantifying inherited disease risk for an individual. While interpretation of causality from observational associations is difficult, Mendelian randomization (MR) analyses utilizing genomics can help improve understanding of the causal relationship between different phenotypes. Given the routine, non-invasive nature of retinal imaging, the findings from retinal cross-phenotype and genome-wide analysis have the direct potential for application clinically including in diagnosis, monitoring and prevention, and in treatment of both ocular and systemic conditions.
Introduction
The human retina is a complex multi-layered tissue combining neuronal, microvascular, and epithelial cells, which may provide insights not only to ocular health but also to brain, cardiovascular, and systemic health. Innovations in multi-omic big data across large ethnically diverse population biobanks, have enabled improved understanding of links between retinal and systemic health. The connection of retinal phenotypes with electronic health record data, other clinical traits, blood cell counts, metabolomics, and other medical imaging data, has improved our understanding of interactions between systemic disease and retinal health. Furthermore, analysis of genomic data has enabled unbiased discovery of the inherited biological mechanisms driving changes in the retina. This review article highlights innovations in population biobanks, retinal phenotyping, and cross-phenotype and genome-wide association analyses that together have enabled improved clinical and biological understanding of retinal physiology and pathophysiology (Figure 1).
The current era of big data has resulted in an explosion of datasets with rich phenotypic and genotypic data that have led to an increase in multi-omic analyses enabling improved understanding of human physiology and pathophysiology. This review will focus on several key biobanks that have driven multiomic analyses of retinal disease and retinal image-based phenotypes with other systemic phenotypes and also with genomics, including the UK Biobank1, the Million Veteran’s Program2, FinnGen3, and the Mass General Brigham Biobank. While the focus of this review paper is specifically on biobanks with both phenotypic and genotypic data, we acknowledge that there are also many rich datasets available with ophthalmologic phenotypic data and retinal imaging available (such as the Moorfields Eye Hospital Database4 and the IRIS registry5).
The UK Biobank1 is a population biobank of 500,000 individuals ages 40-70 years at baseline visit in 2010 from the UK who have >10 years of follow-up data. Deep phenotypic data is available, including electronic health record (EHR) information through ICD-9 and ICD-10 codes, quantitative clinical traits (ex: blood pressure, heart rate, body mass index, pulmonary function tests, etc), blood cell traits, metabolomics, and medical imaging (brain MRI, cardiac MRI, whole-body MRI, carotid ultrasound images, full body DXA scans, and retinal imaging). In particular, approximately 88,000 individuals also have retinal fundus and OCT images available. The Million Veteran’s Program (MVP)2 has also conducted extensive genomic analyses across >2,000 EHR-derived disease phenotypes amongst 635,969 participants from diverse backgrounds. The FinnGen3 study is a large-scale project amongst the “bottlenecked” Finnish population
which also integrates genetic data with EHR and has thus far also performed genomic analyses across nearly 2,000 conditions. Given their rich nature, meta- analyses of EHR-derived disease GWAS across the UK Biobank, MVP, and FinnGen across 330 aligned disease endpoints have recently been performed (available here: https://mvp-ukbb.finngen.fi), including many ophthalmic and retinal conditions. Additionally, multiple hospital-specific biobanks are also in creation, such as the Mass-General-Brigham Biobank (MGBB), which currently has 53,000 genotyped individuals with complete EHR data and a variety of imaging modalities.
These population databases have been used in a multitude of ways towards retinal phenotyping for downstream epidemiologic and genomic analyses. For example, studies have utilized a combination of ICD-9 and ICD-10 codes to define ophthalmic disease phenotypes for genomic analyses. Separately, other work has utilized retinal imaging to extract various features of the retina for downstream analyses. For example, deep learning of retinal fundus photos has been used to study the biology surrounding variation in different features of the retinal vasculature6, 7 and optic nerve. Retinal OCT images have been used towards genetic analyses of retinal layer thicknesses8, and towards machine-learning-derived predictions of disease. Multimodal use of both fundus and OCT images have been used towards predictions of retinal age for downstream epidemiologic and genetic analyses
Machine learning (ML) is a branch of artificial intelligence that can learn patterns and make predictions or decisions based on data. By harnessing ML techniques, researchers can uncover complex associations between retinal imaging features and genomic data, advancing our understanding of the genetic basis of retinal diseases and creating opportunities for personalized medicine approaches.
One of the main areas of focus for ML in retinal imaging has been on feature extraction, enabling the identification of complex imaging traits and their subsequent connection to genetic variants associated with retinal diseases. Different retinal imaging techniques, such as color fundus photography and OCT, produce extensive datasets with complex features that are often challenging to analyze using traditional methods. ML algorithms, particularly convolutional neural networks (CNNs), offer the computational capability to extract these subtle imaging features and correlate them with genomic data, effectively bridging the phenotype-genotype divide. CNNs can be used to segment out different features and thereby extract quantitative imaging features from fundus photos. For example, CNNs have enabled segmentation of the retinal vasculature from retinal fundus photos for quantification of features such as vascular density7, tortuosity, and cup-to-disc ratio9. Similarly, when applied to OCT photos, CNNs can segment out different layers of the retina and quantify retinal thickness10, calculate drusen volume, and assess for different retinal pathologies including the presence of choroidal neovascularization, macular edema, macular hole, central serous retinopathy, vitreomacular traction, geographic atrophy, and epiretinal membranes4. These extracted features enable improved unbiased analyses of population-wide OCT images, without the need to rely on possible inaccuracies of ICD codes or perform manual chart review. These retinal image-derived features serve as phenotypes in GWAS, enabling the discovery of genetic associations with retinal phenotypes.
Multimodal data integration across multiple retinal and other imaging modalities has enabled understanding of the biologic connections between the retina and other systemic diseases. ML excels in integrating retinal imaging data with genomics, clinical records, and demographic information, forming a comprehensive framework for identifying multivariate genetic associations. An example is combining retinal imaging with brain imaging modalities like MRI and genetic data to uncover novel phenotypic and genetic links between eye and brain traits. In recent work11, researchers have analyzed a total of 156 retinal imaging traits, including ML-derived features from utilized retinal imaging (OCT and fundus photos) alongside structural and functional brain MRI data from the UK Biobank to identify genetic pleiotropy across 65 genomic loci shared between retinal and brain traits. This approach revealed insights into links between retinal layer thicknesses and the volumes of multiple brain cortical and subcortical structures including the pericalcarine, thalamus, pallidum, and putamin. Associations between features of the retina and neuronal conditions such as stroke, Alzheimer’s disease, and schizophrenia were also established, highlighting the retina as a noninvasive proxy for studying brain health. Such multimodal imaging
frameworks can enhance the understanding of complex genetic and phenotypic connections linking retinal biomarkers with systemic health
Population-based analyses of retinal image-derived phenotypes in and of themselves have enabled new insights into basic findings about the retina. For example, in comparing features between right versus left eyes, right eye vascular density tends to be significantly higher than left eye vascular density across the population7. Likewise, thicknesses of multiple retinal layers, including the photoreceptor layer, are significantly higher in right eyes than in left 8 (Figure 2). Furthermore, age-related changes across these retinal features had previously not been characterized across a large population. As may be expected, aging is associated with decline in retinal vascular density7 and thinning of nearly all retinal layers except for the combination of the retinal pigment epithelium plus Bruch’s membrane, which together increase in thickness at around age 60 across the population; this increased thickness likely signifies drusen development, which on average occurs over a decade after photoreceptor thinning accelerates in the general population (at age ~48y)12
Epidemiologically
Once a retinal phenotype is developed for study, there are multiple forms of downstream analyses that may be carried out. One of the first analyses that is epidemiologically of interest is to see what systemic and ocular traits are linked with that retinal phenotype. Such analyses are possible in an unbiased fashion with the use of hierarchical ICD-based PheCode definitions that group ICD-9 and ICD-10 codes into different diseases. Further analyses may be performed looking at other available phenotypes such as
blood cell traits, plasma metabolites, associations with other socioeconomical, demographic, lifestyle, and clinical traits, and links with medical image-derived phenotypes (ex: from MRI, CT, or ultrasound). Such analyses, which we call cross-phenotype association analyses, enable improved understanding of epidemiological links between the retina and systemic health. Below, we discuss several discoveries from cross-phenotype association studies.
Recent work has derived various retinal imaging features to assess together with ocular and systemic health in the UK Biobank.
In particular, machine learning of retinal fundus photos has been utilized to segment out the retinal vasculature and extract various microvascular features such as retinal vascular density and fractal dimension7, a measure of branching complexity, as well as tortuosity6 Phenome-wide analyses of retinal vascular traits across 1,866 incident, and separately, prevalent phenotypes, as well as 88 quantitative biomarkers in multivariate models identified significant links with both systemic and ocular traits. Retinal vascular density and fractal dimension are associated with an increased prevalence of, and separately increased risk for, cardiometabolic (hypertension, diabetes, renal failure, elevated HbA1c, and BMI), pulmonary (sleep apnea and abnormal pulmonary function tests), and hematopoietic (anemia) conditions. Such systemic links may reflect changes in the microvasculature as a result of cellular injury and stress. Additionally, significant associations were identified between these microvascular indices and incident ocular conditions influencing both the posterior segment of the eye (retinal detachment, diabetic retinopathy, macular degeneration, and vitreous hemorrhage), as well as the anterior segment of the eye (glaucoma and cataracts), which together contribute towards a significant association between these retinal microvascular indices and impaired visual acuity7 (Figure 3)
Moreover, analyses of retinal OCT imaging data has enabled associations of retinal layer thicknesses with systemic health8. Significant phenotypic associations have been detected between thinner retinal layers and ocular, neuropsychiatric, cardiometabolic, and pulmonary conditions. In particular, each standard deviation of retinal layer thinning for the respective layers listed has been associated with increased risk for development of future ocular (retinal nerve fiber layer, RNFL- glaucoma hazard ratio, HR 1.77; photoreceptor segment, PS- AMD HR 1.39), neuropsychiatric (ganglion cell layer, GCL-multiple sclerosis HR 2.87, epilepsy HR 1.55; PS-schizophrenia HR 1.90), cardiac (PS-myocardial infarction HR 1.17, hypertension HR 1.09; choroid layer, CSI-hypertrophic cardiomyopathy HR 2.05), metabolic (PSType 2 diabetes HR 1.28, diabetic polyneuropathy HR 1.67), and pulmonary disease (PS-COPD HR 1.31, chronic bronchitis HR 1.52), as well as end-organ failure (PS-respiratory failure HR 1.46, heart failure HR 1.21, Non-alcoholic steatohepatitis HR 1.53) and mortality (PS HR 1.16; GCC HR 1.12)8 (Figure 3). A more focused analysis of the relationship between PS thinning and RPE+BM thickening, which denotes
drusen development, showed that photoreceptor thinning precedes RPE+BM thickening in the general population, and has significant association with increased risk of future AMD (PS: HR 1.34, 95% CI 1.241.45, P=7.4x10-14) Further comparison of inner versus outer PS thickness has shown that outer PS thinning is specifically and independently associated with future AMD risk. This risk is independent of inner PS thickness, age, age2, sex, smoking status, and the first ten principal components of genetic ancestry. There is no association between inner PS thinning and AMD13 (Figure 4). Further analyses with genomics showed a concordant association between inherited genomic markers associated with PS thinning and AMD risk, suggesting a possible causal link between the two12
Genome-wide association studies assess the association of genetic variants with disease, typically after adjusting for sex, age, smoking status, and principal components of genetic ancestry. One of the first common variant genome-wide association studies (GWAS) performed was in the field of ophthalmology in 2005 and identified the CFH locus for AMD14, 15. Later in 2016, a larger seminal AMD GWAS was performed16 across >12 million variants among 16,144 advanced AMD patients and 17,832 controls from the International AMD Genomics Consortium. This study identified 52 independently associated common and rare variants (P < 5×10-8) distributed across 34 loci. These loci included putative genes involved in inflammation (ex: CFH, C3, PILRB, VTN, CFI), angiogenesis (VEGFA), lipid metabolism (ex: CETP, APOE, APOA1, LIPC), and matrix metalloproteases ( MMP19, MMP9), amongst others.
Additional GWAS studies have been performed on other ICD-based retinal traits including central serous chorioretinopathy (5 loci)17, epiretinal membrane18 (48 loci), and multiple retinal image-based phenotypes including retinal vascular density7 (13 loci), fractal dimension7 (7 loci), tortuosity6 (175 loci), retinal layer thicknesses8 (259 loci across 9 retinal layers), and image-derived optic nerve cup-to-disc ratio19 (145 loci).
Further downstream analyses may be performed to help prioritize genes at each GWAS locus through algorithms such as PoPs20, which integrates GWAS summary statistics with gene expression, biological pathways, and predicted protein-protein interaction data to identify likely causal genes at each genomewide significant locus. Enrichment analyses are often performed on these prioritized genes to look for biological pathways that are enriched in genes from associated regions. This has resulted in interesting findings in the most recent retinal image-derived GWAS analyses. For example, the prior retinal vascular
density GWAS identified genetic links between genes involved in angiogenesis, cancer, pigmentation, and inflammation. In the retinal vascular GWAS, among genes prioritized by the PoPs algorithm, there was a significant genetic enrichment in pathways related to angiogenesis (VEGF, PDGF, Angiopoietin), which are pathways therapeutically targeted to inhibit neovascularization in diabetic retinopathy, advanced age-related macular degeneration, and many cancers. Interestingly, a significant genetic correlation between retinal vascular density with skin color was also observed, and several of the finemapped top variants are strongly associated with skin neoplasms and lighter skin color (at the PoPsprioritized genes IRF4, SLC45A2, OCA2, DUSP22, ACTG1). Indeed, OCA2 encodes the oculocutaneous albinism 2 protein that is known to result in lighter skin color and predisposes to skin cancers21 Additionally identified on both the common variant GWAS and the rare variant association analyses were predicted deleterious variants in MITF, a transcription factor necessary for normal melanocyte differentiation. MITF genetic variants have been linked to Waardenburg syndrome, characterized by pigmentation anomalies of eyes, hair, and skin22-24. Prior work has also identified that MITF labelling in human tumor samples is strong around the vessels25, and that Mitf knock-out mice have a significant increase in retinal arterial and venular diameter26. Inflammatory and chemokine pathways were also significantly enriched in the retinal vascular density GWAS study, with contributing genes prioritized by PoPS including IL2RA, IL23A, IL1R2, IL2, IL7R, IL6, MEF2A, PDGFRA, HLA markers, and others. Interleukins are known modulators of angiogenesis and anti-angiogenesis in tumors27, and inhibition of interleukins has been found to suppress VEGF expression in tumors. Thus, study of genetic contributors to microvascular indices not only provides insight onto biological contributors to the microvasculature but also on possible therapeutic targets that may be leveraged towards treating retinopathy, cancer, and microvascular disease in other tissues.
Polygenic risk scores (PRS) may be used in downstream analyses after a GWAS has been performed to as quantify the summative burden of disease-associated genetic variants in a given individual. There are many different PRS calculation methods, but the most basic PRS is created as a summative score across the regression betas of genome-wide significant independent variants carried by an individual for a given phenotype. These PRS may be developed as such: ∑ ���������������� × �������������������� ���� ���� =1 , where ���������������� is the weight for each of the N independent genome-wide significant variants in the GWAS, and �������������������� is the number of alleles (i.e., 0, 1, or 2) for ���������������� carried by individual ����. The purpose of defining an individuals’ polygenic risk for a phenotype is to quantify an individuals’ inherited risk for future disease. PRS analyses may also be used to develop a genetic marker of disease risk for analyses with other phenotypes amongst a population, classically called a phenome-wide association analysis (or PRS-PheWAS). Performed across many phenotypic outcomes, this enables comparison of individual-level epidemiologic cross-phenotype analyses with genetic PRS-PheWAS for different retinal traits.
For example, OCT-based measurements of RNFL thickness are used clinically to monitor risk of glaucoma and to monitor glaucoma progression. An RNFL thickness GWAS in the UK Biobank identified 43 independent genetic loci linked to RNFL thickness8. Each 1 SD decrease in RNFL PRS was significantly associated with glaucoma (OR 1.12, P=4.2x10-5), independent of age, sex, smoking, myopia, spherical equivalent, height, weight, and principal components of genetic ancestry8. Similarly, photoreceptor thinning has been significantly associated with incident AMD phenotypically (Figure 6a) The connection between PS thinning and AMD development was also observed genetically, with each 1 SD decrease in genetic PS thickness (across individuals not included within the GWAS study) being associated with increased risk of AMD in the UK Biobank (OR 1.1, P=2.9x10-12)8 This finding indicates that genetic variants linked to a thinner PS are also connected to AMD development, suggesting a possible causal link between PS atrophy and risk of AMD8 (Figure 6b).
While interpretation of causality from observational associations is difficult, Mendelian randomization (MR) analyses can help improve understanding of the causal relationship between different phenotypes. MR can be thought of as a genetic version of a clinical randomized control trial (RCT). While an RCT randomizes individuals to drug versus placebo, MR uses human genetics for causal inference by leveraging the random assortment of genetic variants during meiosis at conception, which diminishes susceptibility to confounding or reverse causality28. MR analyses assess whether there are concordant significant associations between independent, genome-wide significant variants for an exposure phenotype (ex: low density lipoprotein (LDL) levels) on outcome phenotype (ex: myocardial infarction). By assessing whether randomly assorted genetic variants influencing a certain risk factor are also significantly affected with an outcome, one can infer that the exposure is causally linked to the outcome. There are three main assumptions in MR analyses: 1) the genetic variant is associated with the risk factor, 2) the genetic variant is not associated with confounders, and 3) the genetic variant influences the outcome only through the risk factor. The first assumption can be assessed by examining the strength of the instrumental variable or the group of genetic variants associated with the risk factor. The second and third assumptions can be assessed through the performance of sensitivity analyses, but are more difficult to prove
One classic use of MR has been in the field of cardiology. Genetic variants associated with low density lipoproteins (LDL) are also strongly associated with coronary artery disease, but genetic variants associated with high density lipoproteins (HDL) are not. These findings correlate with the success of LDLlowering drugs such as statins and the failure HDL modulating CETP inhibitors in reducing the risk of coronary artery disease29. Similarly, MR can be applied towards the field of ophthalmology to help understand what systemic risk factors may be causal towards a particular ophthalmic disease in order to help guide therapeutics.
Across retinal phenotypes, MR has been used to link systolic blood pressure with glaucoma and with thinner RNFL and GCC layer30. Separately, MR has linked smoking and alcohol consumption with AMD31, as well as photoreceptor degeneration with AMD8, 12 As previously discussed, the AMD GWAS studies have identified multiple lipid loci linked to AMD, which appear to also be involved in plasma HDL metabolism. Prior MR analysis has found that while genetic variants associated with HDL are also significantly associated with AMD, this association is specific to the CETP locus and is no longer significant after excluding CETP32; these findings suggest a possible local role of CETP in AMD pathogenesis Further systematic MR analyses between possible risk factors and retinal disease may enable improved understanding of causal mediators of retinal disease for prevention.
Innovations in big data have improved our understanding of observational and genetic links between the retina and systemic health. The onset of large population biobanks with retinal imaging data and genomic data has enabled understanding of the phenotypic links with retinal features and has enabled unbiased discovery of the genetic architecture of retinal phenotypes As a result, new connections between the retina and cardiometabolic, brain, pulmonary, brain, and ocular health have been identified. Machine learning of retinal imaging has enabled feature extraction of different retinal phenotypes across large populations for downstream analyses of the retinal vasculature6 7, optic nerve19, and retinal layer thicknesses8, among others. This has resulted in many new discoveries, including a new imaging biomarker, outer photoreceptor segment thinning, linked to risk of future age-related macular degeneration (AMD) 8 12 13, the leading cause of blindness in the US among older adults. It has led to improved understanding of the role of genes linked to angiogenesis, inflammation, and melanocyte function in the retinal microvasculature. We have developed a user-interface for all of the UK Biobank retinal layer thickness cross-phenotype and GWAS data and further downstream analyses that other users can perform on a webplatform called the "Ocular knowledge portal" detailed here:https://ocular.hugeamp.org/dinspector.html?dataset=Zekavat2021_RetinalLayerThickness_EU . Further comparison of genome-wide association results across a wide array of ocular phenotypes and systemic phenotypes may also be performed on the Ocular knowledge portal (https://ocular.hugeamp.org). Together these studies highlight how retinal phenotypes and retinal imaging may be integrated with electronic health records, genomic data, and other biomarkers, to advance our understanding of disease mechanisms and help inform risk prediction and risk modification strategies. Continued advances in population datasets with retinal imaging and other omic data, in combination with advances in machine learning methods for retinal image-based phenotyping, will help further our knowledge of the retina.
Abbreviations
RNFL = retinal nerve fiber layer, GCL = ganglion cell layer, IPL = inner plexiform layer, PS = photoreceptor segment, RPE = retinal pigment epithelium, BM = Bruch’s membrane, CSI = choroid layer, AMD = age-related macular degeneration, GWAS = genome-wide association study, MR = Mendelian randomization, PRS = polygenic risk score, OCT = optical coherence tomography
1. Bycroft C, Freeman C, Petkova D, et al. The UK Biobank resource with deep phenotyping and genomic data. Nature 2018;562(7726):203-9.
2. Verma A, Huffman JE, Rodriguez A, et al. Diversity and scale: Genetic architecture of 2068 traits in the VA Million Veteran Program. Science 2024;385(6706):eadj1182.
3. Kurki MI, Karjalainen J, Palta P, et al. Author Correction: FinnGen provides genetic insights from a wellphenotyped isolated population. Nature 2023;615(7952):E19.
4. De Fauw J, Ledsam JR, Romera-Paredes B, et al. Clinically applicable deep learning for diagnosis and referral in retinal disease. Nat Med 2018;24(9):1342-50.
5. Lee CS, Blazes M, Lorch A, et al. American Academy of Ophthalmology Intelligent Research in Sight (IRIS(R)) Registry and the IRIS Registry Analytic Center Consortium. Ophthalmol Sci 2022;2(1):100112.
6. Tomasoni M, Beyeler MJ, Vela SO, et al. Genome-wide Association Studies of Retinal Vessel Tortuosity Identify Numerous Novel Loci Revealing Genes and Pathways Associated With Ocular and Cardiometabolic Diseases. Ophthalmol Sci 2023;3(3):100288.
7. Zekavat SM, Raghu VK, Trinder M, et al. Deep learning of the retina enables phenome-and genome-wide analyses of the microvasculature. Circulation 2022;145(2):134-50.
8. Zekavat SM, Jorshery SD, Rauscher FG, et al. Connecting ocular and systemic health: Phenome-and genomewide analyses of retinal layer thicknesses from optical coherence tomography imaging across> 50k participants. Investigative Ophthalmology & Visual Science 2024;65(7):1014-
9. Alipanahi B, Hormozdiari F, Behsaz B, et al. Large-scale machine-learning-based phenotyping significantly improves genomic discovery for optic nerve head morphology. Am J Hum Genet 2021;108(7):1217-30.
10. Wilson M, Chopra R, Wilson MZ, et al. Validation and Clinical Applicability of Whole-Volume Automated Segmentation of Optical Coherence Tomography in Retinal Disease Using Deep Learning. JAMA Ophthalmol 2021;139(9):964-73.
11. Zhao B, Li Y, Fan Z, et al. Eye-brain connections revealed by multimodal retinal and brain imaging genetics. Nat Commun 2024;15(1):6064.
12. Zekavat SM, Sekimitsu S, Ye Y, et al. Photoreceptor layer thinning is an early biomarker for age-related macular degeneration: epidemiologic and genetic evidence from UK Biobank OCT data. Ophthalmology 2022;129(6):694-707.
13. Zekavat SM, Sekimitsu S, Doroodgar Jorshery S, et al. Reply. Ophthalmology 2023;130(4):e15-e6.
14. Klein RJ, Zeiss C, Chew EY, et al. Complement factor H polymorphism in age-related macular degeneration. Science 2005;308(5720):385-9.
15. Haines JL, Hauser MA, Schmidt S, et al. Complement factor H variant increases the risk of age-related macular degeneration. Science 2005;308(5720):419-21.
16. Fritsche LG, Igl W, Bailey JN, et al. A large genome-wide association study of age-related macular degeneration highlights contributions of rare and common variants. Nat Genet 2016;48(2):134-43.
17. Ramo JT, Abner E, van Dijk EHC, et al. Overlap of Genetic Loci for Central Serous Chorioretinopathy With AgeRelated Macular Degeneration. JAMA Ophthalmol 2023;141(5):449-57.
18. Gelernter J, Levey DF, Galimberti M, et al. Genome-wide association study of the common retinal disorder epiretinal membrane: Significant risk loci in each of three American populations. Cell Genom 2024;4(6):100582.
19. Han X, Steven K, Qassim A, et al. Automated AI labeling of optic nerve head enables insights into cross-ancestry glaucoma risk and genetic discovery in >280,000 images from UKB and CLSA. Am J Hum Genet 2021;108(7):1204-16.
20. Weeks EM, Ulirsch JC, Cheng NY, et al. Leveraging polygenic enrichments of gene features to predict genes underlying complex traits and diseases. Nat Genet 2023;55(8):1267-76.
21. Rayner JE, Duffy DL, Smit DJ, et al. Germline and somatic albinism variants in amelanotic/hypomelanotic melanoma: Increased carriage of TYR and OCA2 variants. PLoS One 2020;15(9):e0238529.
22. Tachibana M. Evidence to suggest that expression of MITF induces melanocyte differentiation and haploinsufficiency of MITF causes Waardenburg syndrome type 2A. Pigment Cell Res 1997;10(1-2):25-33.
23. Tassabehji M, Newton VE, Read AP. Waardenburg syndrome type 2 caused by mutations in the human microphthalmia (MITF) gene. Nat Genet 1994;8(3):251-5.
24. Wang XP, Liu YL, Mei LY, et al. Wnt signaling pathway involvement in genotypic and phenotypic variations in Waardenburg syndrome type 2 with MITF mutations. J Hum Genet 2018;63(5):639-46.
25. Cheli Y, Giuliano S, Fenouille N, et al. Hypoxia and MITF control metastatic behaviour in mouse and human melanoma cells. Oncogene 2012;31(19):2461-70.
26. Eysteinsson T, Daníelsson SB, García Llorca A, et al. Mutations in the microphthalmia transcription factor (Mitf) gene affect retinal vessel diameter. Investigative Ophthalmology & Visual Science 2018;59(9):5854-
27. Ribatti D. Interleukins as modulators of angiogenesis and anti-angiogenesis in tumors. Cytokine 2019;118:3-7.
28. Smith GD, Ebrahim S. 'Mendelian randomization': can genetic epidemiology contribute to understanding environmental determinants of disease? Int J Epidemiol 2003;32(1):1-22.
29. Emdin CA, Khera AV, Kathiresan S. Mendelian Randomization. JAMA 2017;318(19):1925-6.
30. Rajasundaram S, Segre AV, Gill D, et al. Independent Effects of Blood Pressure on Intraocular Pressure and Retinal Ganglion Cell Degeneration: A Mendelian Randomization Study. Invest Ophthalmol Vis Sci 2024;65(8):35.
31. Kuan V, Warwick A, Hingorani A, et al. Association of Smoking, Alcohol Consumption, Blood Pressure, Body Mass Index, and Glycemic Risk Factors With Age-Related Macular Degeneration: A Mendelian Randomization Study. JAMA Ophthalmol 2021;139(12):1299-306
32. Liu DJ, Peloso GM, Yu H, et al. Exome-wide association study of plasma lipids in >300,000 individuals. Nat Genet 2017;49(12):1758-66.
Henry W Zhou MD, MS, and Leo A Kim, MD, PhD
As described by Friedmann and Roblin in 1972, gene therapy was traditionally conceived as a therapeutic option for inherited human genetic diseases.1 In this classical framework, exogenous therapeutics (via isolated DNA segments or viral vectors) delivered to the proper tissues can sustain endogenous production of proteins and/or enzymes that are absent, non-functional, or otherwise aberrant in the individual with an inherited disease-causing mutation.1,2 The allure of curative therapy from a single treatment, along with the development of gene delivery vectors such as re-engineered retroviruses and adeno-associated viruses,3–5 led to the first human trials of gene therapy in the 1990s 4 The first successful gene therapy treatment was performed in children with mutations in adenosine deaminase (ADA) causing severe combined immunodeficiency (SCID) 4 Despite the innovations made in the field for the treatment of monogenic diseases, our most prevalent human diseases are more complex, often polygenic, and prone to environmental influence 6 One such example in ophthalmology is age-related macular degeneration (AMD) 7
Despite its modern-day ubiquity in retina practices across the United States, AMD is a disease of modernity. In 1911, Ernst Fuchs dedicated only a single sentence of his textbook “Text-book of Ophthalmology” to AMD, which he described as “senile changes.”8,9 Today, AMD is a leading cause of blindness in the United States and is the number one cause of blindness among White persons.10 Recent estimates suggest that nearly 20 million individuals in the US age 40 years or older carry a diagnosis of AMD. Of those, nearly 1.5 million individuals are living with late-stage AMD.11
The genetic and molecular pathogenesis of AMD is multifactorial yet has a strong genetic predisposition Two loci have been found to be most strongly linked with the development of AMD: complement factor H (CFH), on chromosome 1q, and high-temperature requirement protein A1 (HTRA1), on chromosome 10q. Together, these loci account for approximately half of the heritability of AMD.12–16 Subsequent genomewide association studies have further highlighted the contribution of diverse systems in the pathogenesis of AMD, including complement activation, collagen synthesis, lipid metabolism and cholesterol transport, receptor-mediated endocytosis, endodermal cell differentiation, and extracellular matrix organization 17
At first glance, the absence of a single deficient or dysfunctional gene product makes AMD an unlikely target for ophthalmic gene therapy. The first FDA-approved in vivo ophthalmic gene therapy, voretigene neparvovec-rzyl,18 was designed to deliver a functional copy of the gene RPE65 to patients with biallelic RPE65-related Leber congenital amaurosis type 2 via an AAV vector.18,19 RPE65-related LCA is a prime example of a monogenic disease and a canonical target of traditional gene therapy. However, recent advances in gene therapy and salient features of the current AMD therapeutic landscape have led to increased interest in applying gene therapy approaches to AMD.
This review will discuss approaches to drug administration, viral and non-viral delivery vectors, and current trials in gene therapy for both wet and dry AMD.
Subretinal injection allows for direct contact between medications and the photoreceptors and RPE, which are primarily affected in AMD. The subretinal space is considered an immune privileged space that would reduce the immunogenicity of any delivered therapeutic, given proper surgical delivery and a relatively intact blood-retina barrier 20–22 Additionally, the ability to bypass the internal limiting membrane (ILM) can improve transduction efficiency of gene therapies, particularly for early-generation AAVs 23
The most common technique for subretinal injection, which was employed and validated in the voretigene neparvovec-rzyl trials,24 involves creating a localized retinal detachment, typically in the macular region, to administer the medication in a subretinal “bleb” (Figure 1a).23–25 With this technique, the patient is put under general anesthesia before the vitreoretinal surgeon performs a pars plana vitrectomy to clear the posterior cortical vitreous and allow access for creation of a retinotomy 19,25 An injection of medication is performed in the subretinal fluid, generating a dome-shaped localized retinal detachment. A fluid-air exchange is frequently performed prior to closure of the trocar incisions.19
Limitations of the subretinal approach to drug delivery include the need for a vitreoretinal surgeon experienced with the technique, the need for an operating room setting to perform pars plana vitrectomy, a relatively small area of treated retina in direct or close contact with the subretinal bleb, and the risk of forming a macular hole or persistent retinal detachment as a result of iatrogenic retinal detachment.26,27 Of note, some patients undergoing subretinal injection of voretigene neparvovec-rzyl later developed progressive perifoveal chorioretinal atrophy, albeit it is currently not yet clear whether the atrophy was instigated by ocular, surgical delivery, or vector-related factors.28
Intravitreal injection of medications is the most widely used and best studied method of pharmacologic administration into the intraocular and vitreoretinal space.29,30 Although the technique differs slightly between practitioners, the general principle is relatively straightforward.31 Patients are anesthetized, either with topical or subconjunctival local anesthesia,32 antisepsis is placed (typically povidone-iodine, provided the patient does not have an allergy) on the ocular surface,33 eyelids are kept away from the planned site of injection often via a speculum,34 and injection of the drug of choice is performed via a small-gauge needle, often a 30-gauge but no larger than 27-gauge (Figure 1b) 35 The point of injection differs between phakic and pseudophakic patients, typically 3.5mm from the limbus in pseudophakic (or aphakic) patients and 4.0mm from the limbus in phakic patients.31 The needle is directed either toward the optic nerve or the center of the globe, to avoid damage to the lens and/or retina.35 Finally, the physician may assess for central retinal artery perfusion in a variety of manners, such as via visual acuity assessment, tonometry, or direct visualization of the optic nerve head.36
Complications of intravitreal injections are well-studied and include hemorrhage, retinal detachment, and endophthalmitis.35 Specific to the goals of chorioretinal gene therapy, intravitreal injections pose several limitations that helped catalyze the development of other approaches to administration, as discussed in this review. One key limitation is the internal limiting membrane (ILM), which serves as a barrier to efficient viral transduction of gene therapies by irreversibly binding adeno-associated virus (AAV) virions.37 Direct disruption of the ILM improves viral transduction during intravitreal injections,37 which may be achieved by a direct ILM peel in the operating room, but this approach would negate many of the putative benefits of intravitreal injections (e.g. decreased cost, in-office vs operating room, reduced anesthesia usage, and higher risk of infection or other surgical complications). One promising approach involves the use of engineered capsid-mutated AAV vectors to minimize ILM binding, reduce in vivo cellular turnover, and improve rates of photoreceptor transduction.38–40
Administration of medications via a suprachoroidal route is an emerging area of interest for gene therapies and traditional therapies alike (Figure 1c) 25,41,42 The suprachoroidal space is a potential space that lies between the sclera and choroid that allows for aqueous drainage via the uveoscleral pathway43 and for sliding during accommodation 44 The space contains collagen fibers, elastic fibers, melanocytes, fibrocytes, ganglion cells, and nerve plexi.42 There has been growing interest in use of the suprachoroidal space for drug administration as medications administered here have been shown to remain in the posterior segment with relative sparing of anterior structures such as the lens and cornea.45,46 This delivery profile is particularly favorable for medications such as corticosteroids, which have adverse anterior segment side effects. Agents delivered via the suprachoroidal space distributes within the sclera, choroid, RPE, and retina.41 The suprachoroidal approach has already been validated in a Phase 3 clinical trial (PEACHTREE) assessing safety and efficacy of suprachoroidally-delivered triamcinolone acetonide formulation for patients with macular edema secondary to noninfectious uveitis.47 Suprachoroidal
Zhou, Henry
injections of AAV have been shown to result in widespread expression of transgene payload in ocular tissue, highlighting the possibility of using suprachoroidal injections in gene therapy drug delivery.48
One alternative approach employs a hybrid between the subretinal and suprachoroidal approach.49,50 This system, being developed by Gyroscope Therapeutics and named the ORBIT system, uses a flexible cannula threaded through the suprachoroidal space. Once the cannula is placed under the desired region, a needle is advanced through the choroid and directed at the subretinal space where a localized detachment can be generated via administration of medication.49,50 This approach circumvents the need for vitrectomy and generation of a retinotomy, but further work is necessary to assess if this approach is viable for clinical use.
Viral vectors
Adeno-associated virus (AAV) vectors are currently the most commonly used and investigated gene delivery vectors for ophthalmic applications 23 Originally identified in 1965 as a contaminant of adenovirus preparations,51,52 AAVs have gained a significant adoption lead over other viral vectors such as adenovirus and retrovirus/lentivirus. The first use of an AAV vector in humans was performed in 1995, where a patient with cystic fibrosis was treated with an AAV carrying the cystic fibrosis transmembrane regulator (CFTR) gene.53 Since then, investigation and use of AAVs for clinical applications has exploded. Many advances have been made in service of improving AAVs’ clinical applications, for example depleting the vector genome of CpG dinucleotides54 or insertion of cell type-specific microRNA to reduce innate immunity effects.55,56 Capsid engineering is another area of intense research, as the AAV capsid is the site of tissue/cell tropism for drug delivery as well as the epitope for host immunity recognition.57 Rational AAV capsid design has been limited by the capacity to isolate and modulate phenotypes while retaining capsid integrity and desirable functions of the viral particle. One novel approach to AAV capsid engineering employs ancestral sequence reconstruction methods to predict ancestral AAV capsid monomers and generated a novel viral-like, AAV-like particle.58,59 Particularly important for gene therapy applications, ancestral particles potentially have reduced levels of B-cell and T-cell recognition, although further confirmational studies are required.58
Non-AAV vectors confer some benefit over AAVs. One major consideration is the increased carrying capacity afforded by non-AAV vectors.60 AAV typically have carrying capacities of only 4.7kb, whereas lentiviruses can carry up to 9kb and third-generation, high-capacity adenoviruses can accommodate up to 36kb of genetic payload.61–63 Nevertheless, the host immune response to adenovirus largely results in diminished transgene expression. Lentiviruses carry other safety concerns such as unintended generation of replication-competent provirus (herpes simplex virus 1, in many applications), although significant work has gone into generating third-generation lentivirus vectors capable of self-inactivation 63 Given the drawbacks and risks of adenovirus and lentivirus vectors, AAV remains the most popular vector in ophthalmic applications, though each vector has strengths and weaknesses 64 Viral vector selection for specific use cases, and vector development to reduce weaknesses such as immunogenicity and uncontrolled replication, will continue to be key issues as ophthalmic gene therapy evolves and matures.
Although viral vectors comprise the lion’s share of current therapeutic delivery vectors, non-viral vectors are appealing due to their potential for lower immunogenicity and ease of large-scale production compared to viral vectors 65 Lipid-based nanoparticles (Figure 2a) have shown promise in efficient delivery of genetic material.66,67 Lipid nanoparticles can induce genetic changes by encapsulation of DNA, RNA, or CRISPR-Cas9 protein/nucleic acid components,68 facilitating their delivery to the desired retinal cell population Lipid-based nanoparticle surfaces can be modified (e.g. PEGylation to for PEG-lipids)69 to enhance cell-specific targeting and transfection efficiency
The other significant class of nonviral vectors is polymer-based vectors (Figure 2b), such as polyethylenimine (PEI), chitosan, and dendrimers (e.g. polyamidoamine, PAMAM).70 These vectors can form complexes with nucleic acids through electrostatic interactions, protecting the genetic material from degradation and aiding in cellular uptake. In contrast to lipid nanoparticles, polymer-based vectors do not
encapsulate their genetic payload within an organized complex (e.g. such as the double-layered organization of liposomes, an early subtype of lipid nanoparticles).71 Instead, polymer-based vectors have been described as “spaghetti and meatballs” type complexes, where the genetic payload (the “meatballs”) are entangled within the elongated polymer (the “spaghetti”).72,73
There are two experimental gene therapies currently in clinical studies for the treatment of wet AMD Vascular endothelial growth factor (VEGF) is an initiator of angiogenesis in hypoxic conditions74 and has been identified as the key factor driving neovascular (wet) AMD.75 Inhibition of VEGF was demonstrated to inhibit and reverse neovascular eye disease.76,77 Several anti-VEGF therapeutics have since been brought to market and are now widely available for clinical use in patients with wet AMD.78 However, realworld treatment schedules and adherence to frequent intravitreal injections often results in significant psychosocial and logistical burdens,79 and failure to adhere to consistent treatment schedules can lead to undertreatment.80,81 Three years following the Comparison of Age-Related Macular Degeneration Treatment Trial, which was a highly structured, multicenter, single-blind noninferiority trial designed to assess the relative efficacy and safety of ranibizumab to bevacizumab and sought to compare an asneeded injection regimen with a monthly regimen,78 patients resumed standard clinical care and were found to have mean best corrected visual acuity (BCVA) decreased by 11 letters from the 2-year study timepoint.82 Such data supports the need for improved durability of anti-VEGF agents. One approach for this is via gene therapy for continuous production of anti-VEGF using endogenous protein production machinery. Two such therapeutics are in the early stages of clinical trials
ADVM-022 (Advernum Biotechnologies), now called ixoberogene soroparvovec or ixo-vec, is a novel recombinant AAV-based intravitreal injection for treatment of wet AMD.83 The AAV capsid of ixo-vec was engineered via directed evolution for efficient retinal transduction via invtravitreal administration. The capsid carries an expression cassette with a codon-optimized cDNA of aflibercept.83 Sustained expression of aflibercept via ixo-vec is designed to reduce the treatment burden of frequent aflibercept intravitreal injections. Non-human primate studies found that single injections of ixo-vec resulted in sustained expression for 30 months.84 There were no measurable abnormalities in retinal structure or function at 2.5 years, as measured by OCT, ERG, and histology. Inflammatory responses were found to peak at 1 month post-treatment, with eyes demonstrating mild aqueous cell infiltrates, mild vitreous cell infiltrates, mild to moderate keratic precipitates, and incidental mild lens capsule deposits that trended toward self-resolution without anti-inflammatory treatment.84 Intravitreal injection of ixo-vec 13 months prior to laser-induced choroidal neovascularization in a primate model demonstrated equivalent efficacy to an intravitreal bolus of aflibercept delivered at the time of laser.
The OPTIC phase I trial is the first human trial for use of ixo-vec in patients with wet AMD.85 The OPTIC trial is a two-year, open-label, prospective, multicenter phase I study that recruited 30 patients between November 14, 2018 and June 30, 2020 across nine study sites in the US. Subjects were randomized to two different vector genome (vg) doses and two different steroid regimens (oral vs topical). The study’s primary endpoint was type, severity, and incidence of ocular and systemic adverse events. Secondary endpoints were mean change in BCVA, mean change in central subfield thickness and macular volume, mean number of supplemental anti-VEGF injections, and percent of participants requiring supplemental anti-VEGF. A subset of patients underwent aqueous humor analysis for detection of ixo-vec expression. Vision and central subfield thickness were stable through the two-year study period. Annualized antiVEGF utilization was decreased in both dose groups; the cohort receiving 2x1011 vector genomes per eye (vg/eye) reduced annual anti-VEGF use by 80% (from 10.0 mean annualized injections to 1.9 mean annualized injections), and the 6x1011 vg/eye cohort reduced annual anti-VEGF use by 98% (from 9.8 mean annualized injections to 0.2 mean annualized injections). Two serious adverse events were reported and deemed likely secondary to ixo-vec: a case of dry AMD and a case of recurrent uveitis. The most common adverse event was anterior chamber cell and was seen in 18 total subjects. Vitreous cell was found in eleven subjects. Rates of ocular inflammation appeared to be dose- dependent. Notably, all patients in the 2x1011 vg/eye group were able to discontinue topical corticosteroids by the end of the study period. In the higher-dose group, seven out of 15 required ongoing topical corticosteroid therapy.85
Zhou, Henry
RGX-314 (REGENXBIO Inc.) is another novel recombinant AAV-based subretinal therapy for treatment of wet AMD. RGX-314 expresses a monoclonal fragment similar to ranibizumab, a humanized monoclonal VEGF antibody targeted to VEGF-A. Investigators conducted a two-year, open-label, prospective, multicenter phase I/IIa dose escalation study that recruited 42 patients between May 12, 2017 and May 21, 2019 across eight study sites in the US.86 The primary outcome was safety of RGX-314 up to week 26. Key secondary outcomes included RGX-314 protein concentration in aqueous fluid, BCVA, central subfield thickness, and need for supplemental anti-VEGF injections up to week 106. Subjects were randomized into five different cohorts, receiving either 3x109, 1x1010, 6x1010, 1.6x1011, or 2.5x1011 vg/eye (with the last two cohorts being added later).
There was stable or improved BCVA and central subfield thickness in most subjects. There were no meaningful mean changes in choroidal neovascular lesion size in any cohort during the study. Thirteen patients experienced serious adverse events, and most were experienced in cohorts receiving 6x1010 or greater vg/eye. There were seven instances (17%) of retinal degeneration that occurred around 6-12 months following therapy, such as ellipsoid zone changes (n=4), retinal thinning over bleb (n=2), and RPE clumping (n=1). Most patients showed RPE changes (69%), most of which were observed in higher dose cohorts. Most events of reduced visual acuity occurred in cohorts receiving fewer vg/eye.
Both RGX-314 and ixo-vec show promise as gene therapies for treatment of wet AMD. Ixo-vec side effects appear to be primarily inflammatory, while the adverse effects of RGX-314 are predominantly related to its subretinal delivery technique.
There are two experimental gene therapies currently in clinical studies for the treatment of dry AMD. In comparison to wet AMD, dry AMD has relatively fewer treatment options for patients. Neovascularization is not a characteristic of dry AMD, and therefore the use of anti-VEGF agents is not effective in treatment of dry AMD. Advanced dry AMD, also known as geographic atrophy, is characterized by loss of RPE cells and dysfunction/loss of overlying retinal photoreceptors. The complement system has been implicated as a key driver of geographic atrophy pathogenesis.87 The complement system includes the classical and lectin pathways, triggered by apoptotic cells, and the alternative pathway, perpetually active and triggered by spontaneous hydrolysis, both of which converge with the generation of C3b from C3.88 The first FDAapproved medication for treatment of geographic atrophy, pegcetacoplan, inhibits complement system activation by selectively targeting C3 and C3b.89,90 Avacincaptad pegol is the second FDA-approved therapy for geographic atrophy and selectively inhibits C5.91,92
The experimental drug GT005 (Gyroscope Therapeutics) is a novel recombinant, AAV-based, subretinal therapy for treatment of dry AMD.93 GT005 expresses complement factor I (CFI), which has been shown to cleave C3b and inhibit activation of the alternative pathway activation.94 Preclinical studies demonstrated that GT005 was able to drive expression of CFI specifically in RPE and retinal tissue. Attempted codon optimization resulted in reduced transcription and expression levels compared to wildtype CFI.93 Data from preclinical studies were used for the FOCUS trial, an open-label, prospective, multicenter phase I/II study of GT005 administered via subretinal injection for patients with macular atrophy due to dry AMD. The study sought to recruit 75 patients in total. Some patients were assigned to undergo subretinal delivery using a suprachoroidal approach. According to the ClinicalTrials.gov entry for the FOCUS trial, the study was terminated in the summer of 2024 due to interim analyses demonstrating a lack of treatment efficacy.95 Similarly, the phase II trials EXPLORE and HORIZON were also terminated due to lack of treatment efficacy.96,97
The experimental drug JNJ -81201887 (aka JNJ-1887, Janssen Pharmaceuticals), formerly known as HMR59 from Hemera Biosciences and later acquired by Janssen, is a novel AAV-based, intravitreal gene therapy for treatment of dry AMD.98 JNJ-1887 expresses a soluble form of CD59, a membrane-bound protein that exhibits the final step of the complement cascade, membrane attack complex (MAC) formation, on cell surfaces. Patients with dry AMD demonstrate decreased rates of CD59 expression in areas affected by drusen and geographic atrophy,99 suggesting that CD59 may play a key role in advanced dry AMD pathogenesis and may serve as a useful therapeutic target. The first in human study of JNJ-1887 was a two-year, open-label, single-center, prospective Phase I study in patients with foveal
center-involving geographic atrophy.98 Seventeen subjects were recruited and injected intravitreally with one of three possible doses of JNJ-1887: 3.56x1010 vg/eye, 1.071x1011 vg/eye, or 3.56x1011 vg/eye. There was no prophylactic corticosteroid therapy. The primary endpoint of the study was to assess safety and tolerability of JNJ-1887. Secondary objectives included change in area and rate of growth of GA between treatment arms, rate of conversion to wet AMD, and protein expression of soluble CD59 in the aqueous humor.
Of the seventeen individuals that underwent treatment with JNJ-1887, sixteen completed the full two years of follow-up. No serious adverse events were considered related to treatment, nor did related adverse events lead to discontinuation in the study. One subject in the high-dose group (3.56x1011 vg/eye) died of leukemia, which was deemed unrelated to the study intervention. Five subjects (29.4%) experienced ocular inflammation that resolved with either observation (n=2) or topical corticosteroid therapy (n=2). Two patients experienced increased cup-to-disc ratios with onset between 240-300 days following treatment; one was deemed possibly related to study treatment, and the other was deemed unrelated to study treatment. Overall, GA growth from baseline was similar in all three treatment arms. Growth rates tended to decrease over the study period. Aqueous humor levels of CD59 were detected in highly variable amounts among 5 patients from the high-dose group (3.56x1011 vg/eye).
In summary, the early phase I/II trial for GT005 was discontinued for lack of treatment efficacy, but a small phase I study of JNJ-1887 demonstrated an acceptable safety profile despite the relatively smaller sample size. JNJ-1887 is currently being studied in a follow-up multi-national, multi-center, doublemasked, dose-ranging, randomized sham-controlled trial.
Gene therapy for AMD is an area of active bench and clinical research ripe with opportunities for innovation. Several viable delivery options exist, although current therapeutics in the pipeline focus on intravitreal and subretinal methods of drug delivery. AAV-based viral vectors currently dominate the ophthalmic gene therapy landscape, but basic and translational work in non-viral vectors such as lipid nanoparticles and polymer-based vectors are rapidly improving and may prove useful in clinical applications of the future. Four early-stage AMD gene therapies, two each for wet and dry AMD, are actively being studied for safety and efficacy. Trial results for each are on the horizon and will provide valuable data on the still-developing field of AMD gene therapy.
FIGURE 1: Routes of gene therapy administration
Depiction of current routes of administration for intraocular gene therapies. A. Subretinal injection following pars plana vitrectomy. B. Intravitreal injection. C. Suprachoroidal injection.
FIGURE 2: Nonviral vectors for gene therapy delivery
A. Lipid nanoparticle-based vectors encapsulating genetic material, with the outer shell demonstrating PEGylated lipids and cholesterol molecules. B. Polymer-based vectors demonstrating a “spaghetti-andmeatballs” configuration for delivery of genetic material.
1. Friedmann T, Roblin R. Gene therapy for human genetic disease? Science 1972;175(4025):949–55.
2. Dunbar CE, High KA, Joung JK, Kohn DB, Ozawa K, Sadelain M. Gene therapy comes of age. Science 2018;359(6372):eaan4672.
3. High KA, Anguela XM. Adeno-associated viral vectors for the treatment of hemophilia. Hum Mol Genet 2015;25(R1):R36.
4. Blaese RM, Culver KW, Miller AD, et al. T lymphocyte-directed gene therapy for ADA- SCID: initial trial results after 4 years. Science 1995;270(5235):475–80.
5. Manno CS, Pierce GF, Arruda VR, et al. Successful transduction of liver in hemophilia by AAV-Factor IX and limitations imposed by the host immune response. Nat Med 2006;12(3):342–7.
6. Visscher PM, Yengo L, Cox NJ, Wray NR. Discovery and implications of polygenicity of common disease. Science 2021;373(6562):1468.
7. Wąsowska A, Teper S, Matczyńska E, et al. Polygenic Risk Score Impact on Susceptibility to Age-Related Macular Degeneration in Polish Patients. J Clin Med 2022;12(1):295.
8. Fuchs, Ernst. Text-book of ophthalmology. 4th edition. Philadelphia, London: JB Lippincott Company; 1911.
9. Guymer RH, Campbell TG. Age-related macular degeneration. Lancet Lond Engl 2023;401(10386):1459–72.
10. The Eye Diseases Prevalence Research Group*. Causes and Prevalence of Visual Impairment Among Adults in the United States. Arch Ophthalmol 2004;122(4):477–85.
11. Rein DB, Wittenborn JS, Burke-Conte Z, et al. Prevalence of Age-Related Macular Degeneration in the US in 2019. JAMA Ophthalmol 2022;140(12):1202–8.
12. Edwards AO, Ritter R, Abel KJ, Manning A, Panhuysen C, Farrer LA. Complement factor H polymorphism and agerelated macular degeneration. Science 2005;308(5720):421–4.
13. Haines JL, Hauser MA, Schmidt S, et al. Complement factor H variant increases the risk of age-related macular degeneration. Science 2005;308(5720):419–21.
14. Klein RJ, Zeiss C, Chew EY, et al. Complement factor H polymorphism in age-related macular degeneration. Science 2005;308(5720):385–9.
15. DeWan A, Liu M, Hartman S, et al. HTRA1 Promoter Polymorphism in Wet Age-Related Macular Degeneration. Science 2006;314(5801):989–92.
16. Yang Z, Camp NJ, Sun H, et al. A Variant of the HTRA1 Gene Increases Susceptibility to Age-Related Macular Degeneration. Science 2006;314(5801):992–3.
17. DeAngelis MM, Owen LA, Morrison MA, et al. Genetics of age-related macular degeneration (AMD). Hum Mol Genet 2017;26(R1):R45–50.
18. Russell S, Bennett J, Wellman JA, et al. Efficacy and safety of voretigene neparvovec (AAV2-hRPE65v2) in patients with RPE65-mediated inherited retinal dystrophy: a randomised, controlled, open-label, phase 3 trial. Lancet Lond Engl 2017;390(10097):849–60.
19. Maguire AM, Simonelli F, Pierce EA, et al. Safety and Efficacy of Gene Transfer for Leber’s Congenital Amaurosis. N Engl J Med 2008;358(21):2240–8.
20. Wenkel H, Streilein JW. Analysis of immune deviation elicited by antigens injected into the subretinal space. Invest Ophthalmol Vis Sci 1998;39(10):1823–34.
Zhou, Henry
21. Al-Amro S, Tang L, Kaplan HJ. Limitations in the Study of Immune Privilege in the Subretinal Space of the Rodent. Invest Ophthalmol Vis Sci 1999;40(12):3067–9.
22. Zhang X, Bok D. Transplantation of retinal pigment epithelial cells and immune response in the subretinal space. Invest Ophthalmol Vis Sci 1998;39(6):1021–7.
23. Kovacs KD, Ciulla TA, Kiss S. Advancements in ocular gene therapy delivery: vectors and subretinal, intravitreal, and suprachoroidal techniques. Expert Opin Biol Ther 2022;22(9):1193–208.
24. Sengillo JD, Gregori NZ, Sisk RA, et al. Visual Acuity, Retinal Morphology, and Patients’ Perceptions after Voretigene Neparovec-rzyl Therapy for RPE65-Associated Retinal Disease. Ophthalmol Retina 2022;6(4):273–83.
25. Scherbakova I, Ragi SD, Sharma T. Ocular Injection Techniques for Retinitis Pigmentosa: Intravitreal, Subretinal, and Suprachoroidal [Internet]. In: Tsang SH, Quinn PMJ, editors. Retinitis Pigmentosa. New York, NY: Springer US; 2023 [cited 2024 Nov 16]. p. 375–92.Available from: https://doi.org/10.1007/978-1-0716-2651-1_34
26. Irigoyen C, Amenabar Alonso A, Sanchez-Molina J, Rodríguez-Hidalgo M, Lara-López A, Ruiz-Ederra J. Subretinal Injection Techniques for Retinal Disease: A Review. J Clin Med 2022;11(16):4717.
27. Dhurandhar D, Sahoo NK, Mariappan I, Narayanan R. Gene therapy in retinal diseases: A review. Indian J Ophthalmol 2021;69(9):2257–65.
28. Gange WS, Sisk RA, Besirli CG, et al. Perifoveal Chorioretinal Atrophy following Subretinal Voretigene Neparvovecrzyl for RPE65-mediated Leber Congenital Amaurosis. Ophthalmol Retina 2022;6(1):58–64.
29. Baum J, Peyman GA, Barza M. Intravitreal administration of antibiotic in the treatment of bacterial endophthalmitis. III. Consensus. Surv Ophthalmol 1982;26(4):204–6.
30. Peyman GA. Antibiotic administration in the treatment of bacterial endophthalmitis. II. Intravitreal injections. Surv Ophthalmol 1977;21(4):332, 339–46.
31. Doshi RR, Bakri SJ, Fung AE. Intravitreal injection technique. Semin Ophthalmol 2011;26(3):104–13.
32. Cintra LP, Lucena LR, Da Silva JA, Costa RA, Scott IU, Jorge R. Comparative study of analgesic effectiveness using three different anesthetic techniques for intravitreal injection of bevacizumab. Ophthalmic Surg Lasers Imaging Off J Int Soc Imaging Eye 2009;40(1):13–8.
33. Speaker MG, Menikoff JA. Prophylaxis of endophthalmitis with topical povidone-iodine. Ophthalmology 1991;98(12):1769–75.
34. Aiello LP, Brucker AJ, Chang S, et al. Evolving guidelines for intravitreous injections. Retina Phila Pa 2004;24(5 Suppl):S3-19.
35. Jager RD, Aiello LP, Patel SC, Cunningham ET. Risks of intravitreous injection: a comprehensive review. Retina Phila Pa 2004;24(5):676–98.
36. Kim JE, Mantravadi AV, Hur EY, Covert DJ. Short-term intraocular pressure changes immediately after intravitreal injections of anti-vascular endothelial growth factor agents. Am J Ophthalmol 2008;146(6):930-934.e1.
37. Do JL, Pedroarena-Leal N, Louie M, et al. Mechanical Disruption of the Inner Limiting Membrane In Vivo Enhances Targeting to the Inner Retina. Invest Ophthalmol Vis Sci 2023;64(15):25.
38. Ross M, Obolensky A, Averbukh E, et al. Evaluation of Photoreceptor Transduction Efficacy of Capsid-Modified Adeno-Associated Viral Vectors Following Intravitreal and Subretinal Delivery in Sheep. Hum Gene Ther 2020;31(13–14):719–29.
39. Kay CN, Ryals RC, Aslanidi GV, et al. Targeting photoreceptors via intravitreal delivery using novel, capsid-mutated AAV vectors. PloS One 2013;8(4):e62097.
40. Reid CA, Ertel KJ, Lipinski DM. Improvement of Photoreceptor Targeting via Intravitreal Delivery in Mouse and Human Retina Using Combinatory rAAV2 Capsid Mutant Vectors. Invest Ophthalmol Vis Sci 2017;58(14):6429–39.
41. Olsen TW, Feng X, Wabner K, et al. Cannulation of the suprachoroidal space: a novel drug delivery methodology to the posterior segment. Am J Ophthalmol 2006;142(5):777–87.
42. Rai UDJP, Young SA, Thrimawithana TR, et al. The suprachoroidal pathway: a new drug delivery route to the back of the eye. Drug Discov Today 2015;20(4):491–5.
43. Krohn J, Bertelsen T. Corrosion casts of the suprachoroidal space and uveoscleral drainage routes in the human eye. Acta Ophthalmol Scand 1997;75(1):32–5.
44. Chiang B, Jung J, Prausnitz M. The suprachoroidal space as a route of administration to the posterior segment of the eye. Adv Drug Deliv Rev 2018;126:58.
45. Goldstein DA, Do D, Noronha G, Kissner JM, Srivastava SK, Nguyen QD. Suprachoroidal Corticosteroid Administration: A Novel Route for Local Treatment of Noninfectious Uveitis. Transl Vis Sci Technol 2016;5(6):14.
46. Gu B, Liu J, Li X, Ma Q, Shen M, Cheng L. Real-Time Monitoring of Suprachoroidal Space (SCS) Following SCS Injection Using Ultra-High Resolution Optical Coherence Tomography in Guinea Pig Eyes. Invest Ophthalmol Vis Sci 2015;56(6):3623–34.
47. Yeh S, Khurana RN, Shah M, et al. Efficacy and Safety of Suprachoroidal CLS-TA for Macular Edema Secondary to Noninfectious Uveitis: Phase 3 Randomized Trial. Ophthalmology 2020;127(7):948–55.
48. Ding K, Shen J, Hafiz Z, et al. AAV8-vectored suprachoroidal gene transfer produces widespread ocular transgene expression. J Clin Invest 2019;129(11):4901–11.
49. de Smet MD, Lynch JL, Dejneka NS, Keane M, Khan IJ. A Subretinal Cell Delivery Method via Suprachoroidal Access in Minipigs: Safety and Surgical Outcomes. Invest Ophthalmol Vis Sci 2018;59(1):311–20.
50. Gray AP, Sato Y, Meyer T, Stoner K, Beltran WA. Surgical Procedure and Applicability of the Orbit Subretinal Delivery System (SDS)TM in the Normal Adult Canine Eye. Invest Ophthalmol Vis Sci 2022;63(7):4118-F0355.
51. Atchison RW, Casto BC, Hammon WM. ADENOVIRUS-ASSOCIATED DEFECTIVE VIRUS PARTICLES. Science 1965;149(3685):754–6.
52. Rose JA, Hoggan MD, Shatkin AJ. Nucleic acid from an adeno-associated virus: chemical and physical studies. Proc Natl Acad Sci U S A 1966;56(1):86–92.
53. Flotte T, Carter B, Conrad C, et al. A phase I study of an adeno-associated virus-CFTR gene vector in adult CF patients with mild lung disease. Hum Gene Ther 1996;7(9):1145–59.
54. Faust SM, Bell P, Cutler BJ, et al. CpG-depleted adeno-associated virus vectors evade immune detection. J Clin Invest 2013;123(7):2994–3001.
55. Boisgerault F, Gross D-A, Ferrand M, et al. Prolonged gene expression in muscle is achieved without active immune tolerance using microrRNA 142.3p-regulated rAAV gene transfer. Hum Gene Ther 2013;24(4):393–405.
56. Majowicz A, Maczuga P, Kwikkers KL, et al. Mir-142-3p target sequences reduce transgene-directed immunogenicity following intramuscular adeno-associated virus 1 vector-mediated gene delivery. J Gene Med 2013;15(6–7):219–32.
57. Mingozzi F, High KA. Immune responses to AAV vectors: overcoming barriers to successful gene therapy. Blood 2013;122(1):23–36.
58. Zinn E, Pacouret S, Khaychuk V, et al. In silico reconstruction of the viral evolutionary lineage yields a potent gene therapy vector. Cell Rep 2015;12(6):1056–68.
59. Zolotukhin S, Vandenberghe LH. AAV capsid design: A Goldilocks challenge. Trends Mol Med 2022;28(3):183–93.
60. Planul A, Dalkara D. Vectors and Gene Delivery to the Retina. Annu Rev Vis Sci 2017;3:121–40.
61. Naldini L, Blömer U, Gallay P, et al. In Vivo Gene Delivery and Stable Transduction of Nondividing Cells by a Lentiviral Vector. Science 1996;272(5259):263–7.
Zhou, Henry
62. Alba R, Bosch A, Chillon M. Gutless adenovirus: last-generation adenovirus for gene therapy. Gene Ther 2005;12(1):S18–27.
63. Bulcha JT, Wang Y, Ma H, Tai PWL, Gao G. Viral vector platforms within the gene therapy landscape. Signal Transduct Target Ther 2021;6(1):1–24.
64. Reichel MB, Ali RR, Thrasher AJ, Hunt DM, Bhattacharya SS, Baker D. Immune responses limit adenovirally mediated gene expression in the adult mouse eye. Gene Ther 1998;5(8):1038–46.
65. Koirala A, Conley SM, Naash MI. A review of therapeutic prospects of non-viral gene therapy in the retinal pigment epithelium. Biomaterials 2013;34(29):7158–67.
66. Masuda I, Matsuo T, Yasuda T, Matsuo N. Gene transfer with liposomes to the intraocular tissues by different routes of administration. Invest Ophthalmol Vis Sci 1996;37(9):1914–20.
67. Hangai M, Kaneda Y, Tanihara H, Honda Y. In vivo gene transfer into the retina mediated by a novel liposome system. Invest Ophthalmol Vis Sci 1996;37(13):2678–85.
68. O’Hare M, Miller WP, Arevalo-Alquichire S, et al. An mRNA-encoded dominant-negative inhibitor of transcription factor RUNX1 suppresses vitreoretinal disease in experimental models. Sci Transl Med 2024;16(775):eadh0994.
69. Chen S, Deng Z, Ji D. Advances in the development of lipid nanoparticles for ophthalmic therapeutics. Biomed Pharmacother 2024;178:117108.
70. Dehshahri A, Alhashemi SH, Jamshidzadeh A, et al. Comparison of the effectiveness of polyethylenimine, polyamidoamine and chitosan in transferring plasmid encoding interleukin-12 gene into hepatocytes. Macromol Res 2013;21(12):1322–30.
71. Tenchov R, Bird R, Curtze AE, Zhou Q. Lipid Nanoparticles─From Liposomes to mRNA Vaccine Delivery, a Landscape of Research Diversity and Advancement. ACS Nano 2021;15(11):16982–7015.
72. Kneuer C, Sameti M, Bakowsky U, et al. A Nonviral DNA Delivery System Based on Surface Modified SilicaNanoparticles Can Efficiently Transfect Cells in Vitro. Bioconjug Chem 2000;11(6):926–32.
73. Casper J, Schenk SH, Parhizkar E, Detampel P, Dehshahri A, Huwyler J. Polyethylenimine (PEI) in gene therapy: Current status and clinical applications. J Controlled Release 2023;362:667–91.
74. Leung DW, Cachianes G, Kuang WJ, Goeddel DV, Ferrara N. Vascular endothelial growth factor is a secreted angiogenic mitogen. Science 1989;246(4935):1306–9.
75. Adamis AP, Miller JW, Bernal MT, et al. Increased vascular endothelial growth factor levels in the vitreous of eyes with proliferative diabetic retinopathy. Am J Ophthalmol 1994;118(4):445–50.
76. Aiello LP, Pierce EA, Foley ED, et al. Suppression of retinal neovascularization in vivo by inhibition of vascular endothelial growth factor (VEGF) using soluble VEGF-receptor chimeric proteins. Proc Natl Acad Sci U S A 1995;92(23):10457–61.
77. Adamis AP, Shima DT, Tolentino MJ, et al. Inhibition of vascular endothelial growth factor prevents retinal ischemiaassociated iris neovascularization in a nonhuman primate. Arch Ophthalmol Chic Ill 1960 1996;114(1):66–71.
78. The CATT Research Group. Ranibizumab and Bevacizumab for Neovascular Age-Related Macular Degeneration. N Engl J Med 2011;364(20):1897–908.
79. Boyle J, Vukicevic M, Koklanis K, Itsiopoulos C, Rees G. Experiences of patients undergoing repeated intravitreal anti-vascular endothelial growth factor injections for neovascular age-related macular degeneration. Psychol Health Med 2018;23(2):127–40
80. Droege KM, Muether PS, Hermann MM, et al. Adherence to ranibizumab treatment for neovascular age-related macular degeneration in real life. Graefes Arch Clin Exp Ophthalmol Albrecht Von Graefes Arch Klin Exp Ophthalmol 2013;251(5):1281–4.
81. Boulanger-Scemama E, Querques G, About F, et al. Ranibizumab for exudative age-related macular degeneration: A five year study of adherence to follow-up in a real-life setting. J Fr Ophtalmol 2015;38(7):620–7.
82. Comparison of Age-related Macular Degeneration Treatments Trials (CATT) Research Group, Maguire MG, Martin DF, et al. Five-Year Outcomes with Anti-Vascular Endothelial Growth Factor Treatment of Neovascular Age-Related Macular Degeneration: The Comparison of Age-Related Macular Degeneration Treatments Trials. Ophthalmology 2016;123(8):1751–61.
83. Grishanin R, Vuillemenot B, Sharma P, et al. Preclinical Evaluation of ADVM-022, a Novel Gene Therapy Approach to Treating Wet Age-Related Macular Degeneration. Mol Ther J Am Soc Gene Ther 2019;27(1):118–29.
84. Kiss S, Oresic Bender K, Grishanin RN, et al. Long-Term Safety Evaluation of Continuous Intraocular Delivery of Aflibercept by the Intravitreal Gene Therapy Candidate ADVM-022 in Nonhuman Primates. Transl Vis Sci Technol 2021;10(1):34.
85. Khanani AM, Boyer DS, Wykoff CC, et al. Safety and efficacy of ixoberogene soroparvovec in neovascular agerelated macular degeneration in the United States (OPTIC): a prospective, two-year, multicentre phase 1 study. eClinicalMedicine [Internet] 2024 [cited 2024 Dec 1];67. Available from: https://www.thelancet.com/journals/eclinm/article/PIIS2589-5370(23)00571-0/fulltext
86. Campochiaro PA, Avery R, Brown DM, et al. Gene therapy for neovascular age-related macular degeneration by subretinal delivery of RGX-314: a phase 1/2a dose-escalation study. Lancet Lond Engl 2024;403(10436):1563–73.
87. Boyer DS, Schmidt-Erfurth U, van Lookeren Campagne M, Henry EC, Brittain C. THE PATHOPHYSIOLOGY OF GEOGRAPHIC ATROPHY SECONDARY TO AGE-RELATED MACULAR DEGENERATION AND THE COMPLEMENT PATHWAY AS A THERAPEUTIC TARGET. Retina Phila Pa 2017;37(5):819–35.
88. Merle NS, Church SE, Fremeaux-Bacchi V, Roumenina LT. Complement System Part I - Molecular Mechanisms of Activation and Regulation. Front Immunol 2015;6:262.
89. Liao DS, Grossi FV, El Mehdi D, et al. Complement C3 Inhibitor Pegcetacoplan for Geographic Atrophy Secondary to Age-Related Macular Degeneration: A Randomized Phase 2 Trial. Ophthalmology 2020;127(2):186–95.
90. Heier JS, Lad EM, Holz FG, et al. Pegcetacoplan for the treatment of geographic atrophy secondary to age-related macular degeneration (OAKS and DERBY): two multicentre, randomised, double-masked, sham-controlled, phase 3 trials. Lancet Lond Engl 2023;402(10411):1434–48.
91. Jaffe GJ, Westby K, Csaky KG, et al. C5 Inhibitor Avacincaptad Pegol for Geographic Atrophy Due to Age-Related Macular Degeneration: A Randomized Pivotal Phase 2/3 Trial. Ophthalmology 2021;128(4):576–86.
92. Khanani AM, Patel SS, Staurenghi G, et al. Efficacy and safety of avacincaptad pegol in patients with geographic atrophy (GATHER2): 12-month results from a randomised, double-masked, phase 3 trial. The Lancet 2023;402(10411):1449–58.
93. Dreismann AK, McClements ME, Barnard AR, et al. Functional expression of complement factor I following AAVmediated gene delivery in the retina of mice and human cells. Gene Ther 2021;28(5):265–76.
94. Lachmann PJ, Halbwachs L. The influence of C3b inactivator (KAF) concentration on the ability of serum to support complement activation. Clin Exp Immunol 1975;21(1):109–14.
95. Gyroscope Therapeutics Limited. FOCUS: An Open Label First in Human Phase I/II Multicentre Study to Evaluate the Safety, Dose Response and Efficacy of GT005 Administered as a Single Subretinal Injection in Subjects With Macular Atrophy Due to AMD [Internet]. clinicaltrials.gov; 2024 [cited 2024 Dec 4]. Available from: https://clinicaltrials.gov/study/NCT03846193
96. Gyroscope Therapeutics Limited. EXPLORE: A Phase II, Outcomes Assessor-masked, Multicentre, Randomised Study to Evaluate the Safety and Efficacy of Two Doses of GT005 Administered as a Single Subretinal Injection in Subjects With Geographic Atrophy Secondary to Age-related Macular Degeneration [Internet]. clinicaltrials.gov; 2024 [cited 2024 Dec 4]. Available from: https://clinicaltrials.gov/study/NCT04437368
97. Gyroscope Therapeutics Limited. HORIZON: A Phase II, Open-label, Outcomes-assessor Masked, Multicentre, Randomised, Controlled Study to Evaluate the Safety and Efficacy of Two Doses of GT005 Administered as a Single
Zhou, Henry
Subretinal Injection in Subjects With Geographic Atrophy Secondary to Dry Age-related Macular Degeneration [Internet]. clinicaltrials.gov; 2024 [cited 2024 Dec 4]. Available from: https://clinicaltrials.gov/study/NCT04566445
98. Heier JS, Cohen MN, Chao DL, et al. Phase 1 Study of JNJ-81201887 Gene Therapy in Geographic Atrophy Secondary to Age-Related Macular Degeneration. Ophthalmology 2024;131(12):1377–88.
99. Ebrahimi KB, Fijalkowski N, Cano M, Handa JT. Decreased membrane complement regulators in the retinal pigmented epithelium contributes to age-related macular degeneration. J Pathol 2013;229(5):729–42.