Buildings as carbon banks Master MASSMASTERThesisINTIMBER DESIGN 2021/22The role of buildings in the decarbonization of the built environment
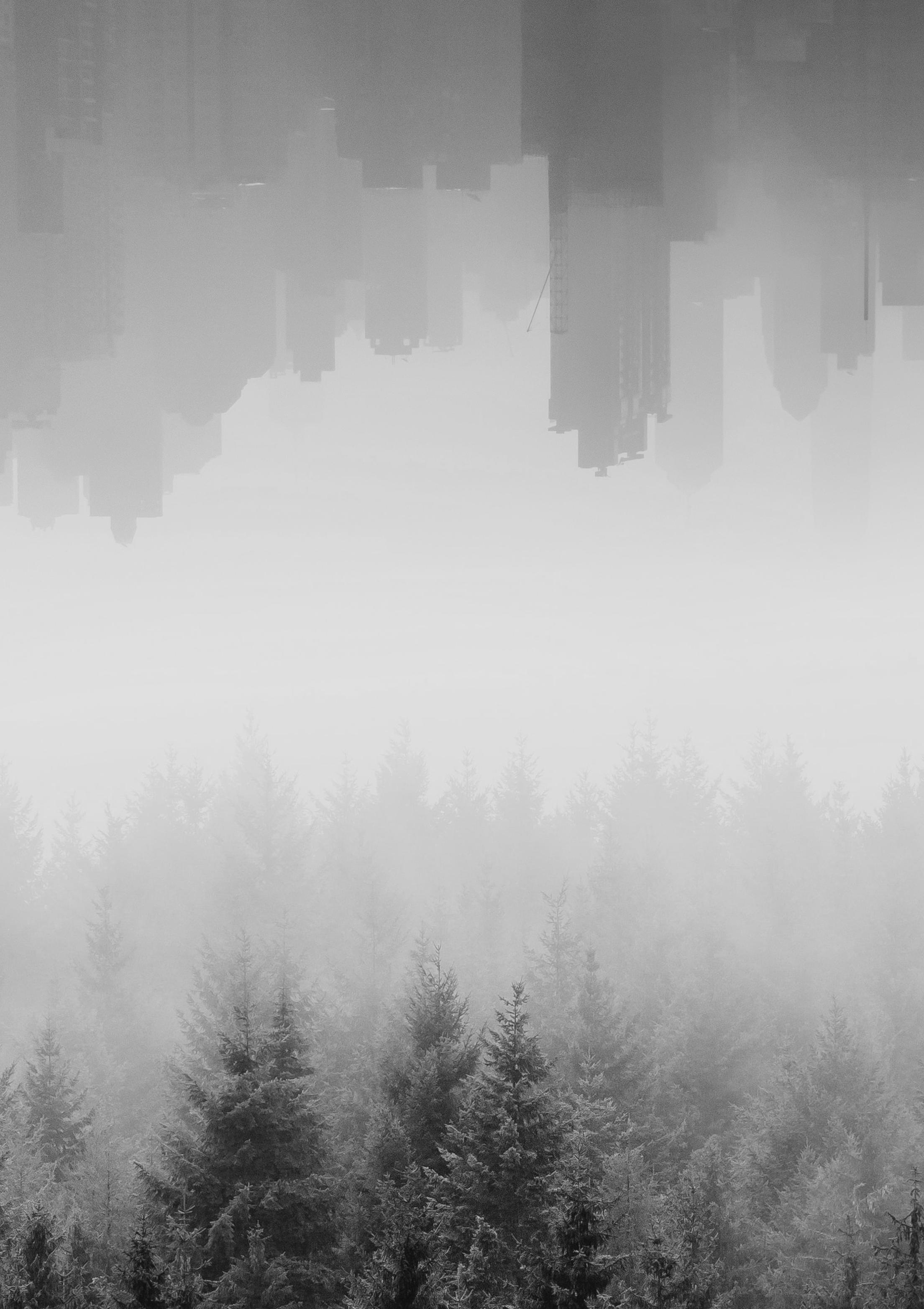
2
3
The role of buildings in the decarbonization of the built environment
Faculty: Rebecca Sawcer Student: Maria Cotela Dalmau Thesis
Master
MASTER IN MASS TIMBER DESIGN
Buildings as carbon banks
4
5 01THESISCARBON01 | 1 CARBON EMISSIONS 01 | 2 CARBON IN WOOD PRODUCTS 01 | 3 CARBON IN THE FORESTS 01 | 4 THE SUBSTITUTION EFFECT 02 BUILDINGS AS CARBON BANKS 03 | 1 SPEKULATUS BY ULTRAMODERNE 03 | 2 BANK OF NORRLAND BY ANDERS BERENSSON ARCHITECTS 03 | 3 TIMBER CITY BY GRAY ORGANSCHI ARCHITECTURE 03 CASE STUDY: NEW BULL RING MARKET IN BIRMINGHAM 04 | 1 METHODOLOGY 04 | 2 EQUIVALENCIES 04 | 3 COMPARISON 04 CONCLUSIONS 05 INDEXACKNOWLEDGEMENTSBIBLIOGRAPHY
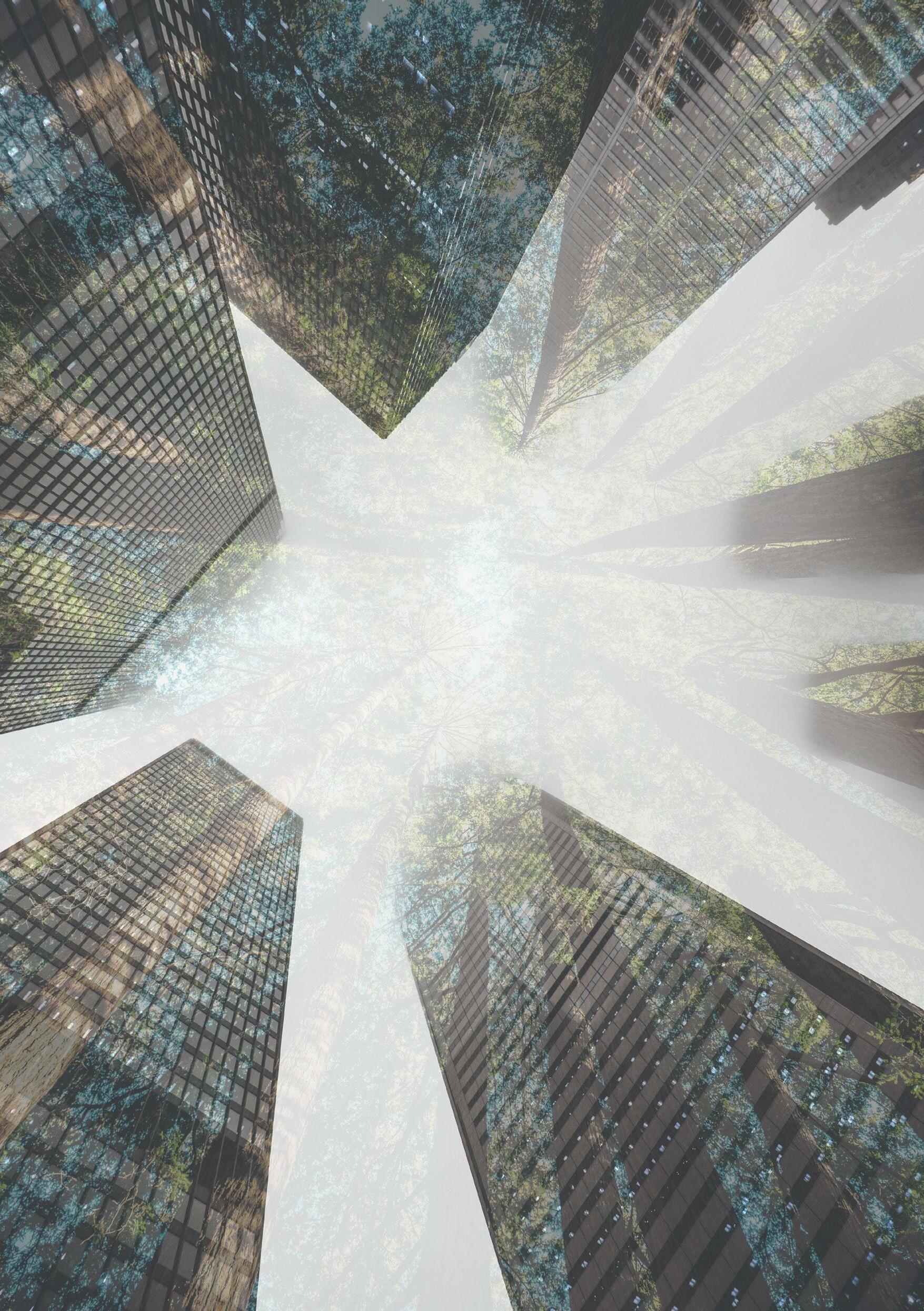
“Architecture,aspresentlytaughtandpracticed,isapervasiveformofclimatechangedenial”-KielMoe
07
Thesis
timber buildings give architects the opportunity to address both issues and transform buildings from carbon sources to carbon banks. Engineered wood pro ducts (EWPs) in mass timber, such as glulam and CLT, have the structural capacity to build at height in urban areas, and at the same time act as carbon sinks. Thanks to the process of photosynthesis, trees capture CO2 from the atmosphere and lock it inside their structure. This sequestered carbon stays inside the material for as long as it is in use, and will only be released when the timber is burned or allowed to decay, at the end of its lifecycle. When building with mass timber, carbon is stored into buildings for deca des, contributing to the decarbonization of the atmosphere. This carbon-storing quality has already been embraced by many architects around the globe, and in recent years we have seen a growing interest for mass timber buildings with more of them rising in diffe rent countries.Butjust as architecture has been blind to the consequences that fabricating carbon-intensive materials can have on our natural landscapes, forests must be taken into consideration when building with mass timber. Questions about forest management, mass timber production and the lifespan of timber products, which can be longer if de signing for disassembly and reuse, are vital to fully understand the real potential of this material.
The building industry is facing two of the most pressing challenges of our time: that of climate change and that of a growing population. Estimated to be responsible for about 40 percent of global greenhouse gas emissions, the building industry needs to drastically lower its environmental impact. At the same time, since the Earth’s population is expected to increase, urban environments will need to be densified to host their new inhabitants.Mass
08 This thesis looks further into the concept of timber buildings as carbon banks and tries to tackle some of these questions. Mass timber construction presents an in credible opportunity to both the decarbonization of the built environment and the de velopment of our landscapes of production, in this case the forests, when considering the whole value chain of this construction material. Understanding the mutually beneficial relationship between forests and the densification of urban areas with mass timber buil dings is key to shift towards a more sustainable building industry.
the
carbon
Key concepts related with emissions, timber products and built
environment
09 CARBON# 01
Source: Chris LeBoutillier @chrisleboutillier via Unsplash.
Release of combustion gases into the atmosphere
10
FIG 1. keeling curve showing the atmospheric concentration of co2 during last decades.
Source: Britannica. https://www.britannica.com/science/greenhouse-gas
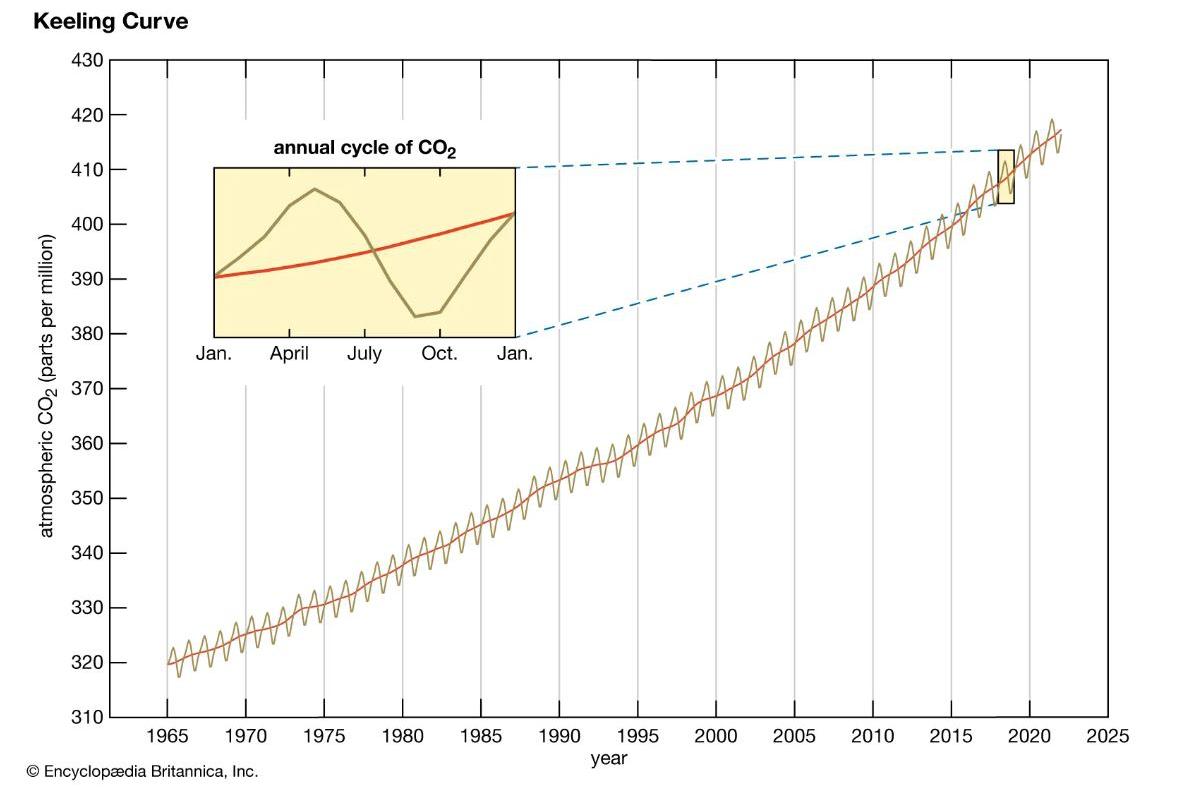
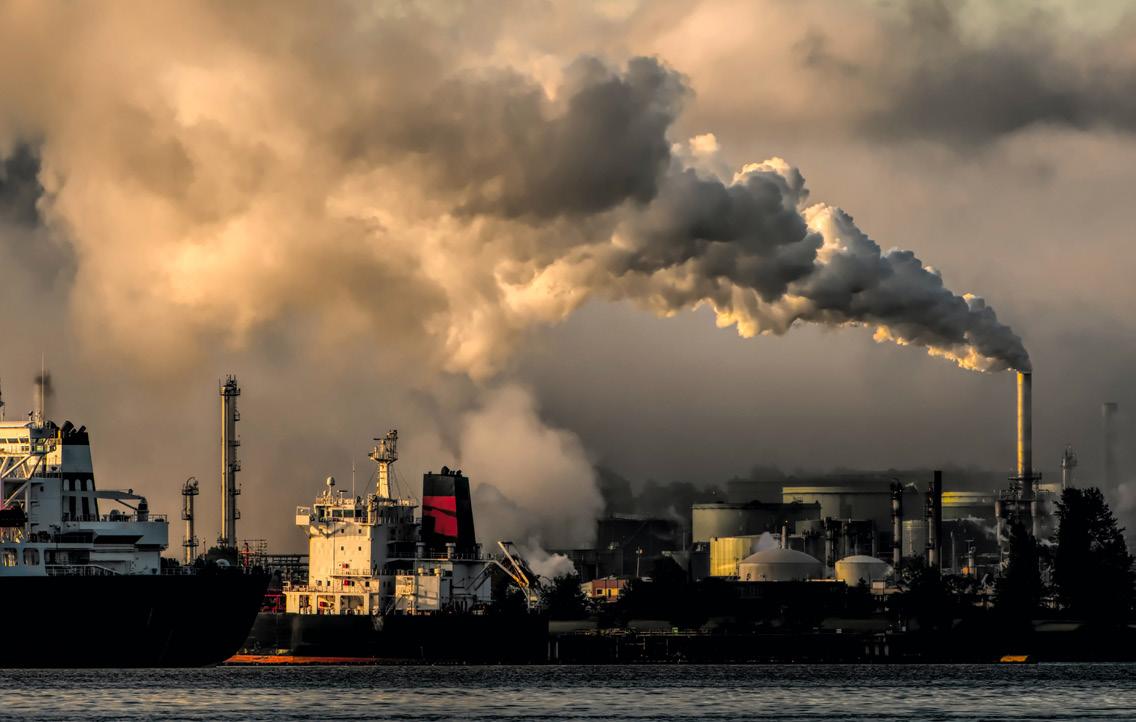
Greenhouse gasses are naturally present in the atmosphere. They trap heat from solar radiation to the Earth’s surface and radiate part of this heat back to space as infrared light. Their presence helps keep the planet at temperatures that allow human and animal life. Over the last centuries, an increase of greenhouse gasses has elevated atmospheric temperatures to previously unseen levels, breaking the equilibrium of the natural carbon cycle, and triggering climate change. This increase of greenhouse gasses is due to human activities, mainly the burning of fossil fuels for energy that started with the Industrial Revolution and continues until today. It is estimated that the Earth’s aver age temperature has increased by one degree Celsius between preindustrial times and now and will continue to rise1. This will have devastating effects for life on Earth that can already be witnessed, such as extreme weather events, sea level rise, and the alteration of ecosystems and natural habitats.
Carbon emissions and climate change
11 01 I 1
The main gasses responsible for the greenhouse effect are carbon dioxide, methane, nitrous oxide, and water vapor, the latter being a consequence of temperature variation. Carbon dioxide accounts for 76 percent of the total human-caused emissions across the globe, making it the most significant greenhouse gas. Human activities such as the burning of fossil fuels for transportation and energy production, deforestation and the burning of forests release as much as 7 gigatons of carbon into the atmosphere, exceeding by far the capacity of absorption of natural carbon sinks, such as forests, oce ans, and soils2. The concentration of carbon dioxide in the atmosphere has increased uniformly from 280ppm (parts per million) before the Industrial Revolution to 410ppm in 2018 (FIG 1) and could reach 560ppm by the middle of the 21st century if continuing with the current rates of fossil-fuel use3
The Architectural Engineering and Construction (AEC) industry is estima ted to be responsible for about 40 percent of the global greenhouse gas emissions4, which puts the decarbonization of the building sector at the top of the architec tural agenda in order to reach the Sustainable Development Goals (SDGs) set by the Paris Agreement in 2015. On the other hand, demographic prospects estimate that the world’s population will grow from the current 7.6 billion to 8.6 billion by 2030,
The rise of the global population will mostly affect urban areas
triggering12 the urban development of cities. Urban areas are expected to grow from 56.2 percent in 2020 to 60.4 percent by 2030 to accommodate a growing population that will mostly live in cities5. If continuing with the status quo, using fossil fuels for power, and concrete and steel as default construction materials, the necessary urbanization of cities will release enormous amounts of CO2 to the atmosphere. But if turning to renewable materials such as engineered timber products, and integrating the carbon cycle to buil ding desgin, cities can become an ally to decarbonize the atmosphere and act as active carbon sinks thanks to the natural properties of wood.
Source: Ryoji Iwata @ryoji__iwata via Unsplash.
Concrete building under construction Source: C Dustin @dianamia via Unsplash
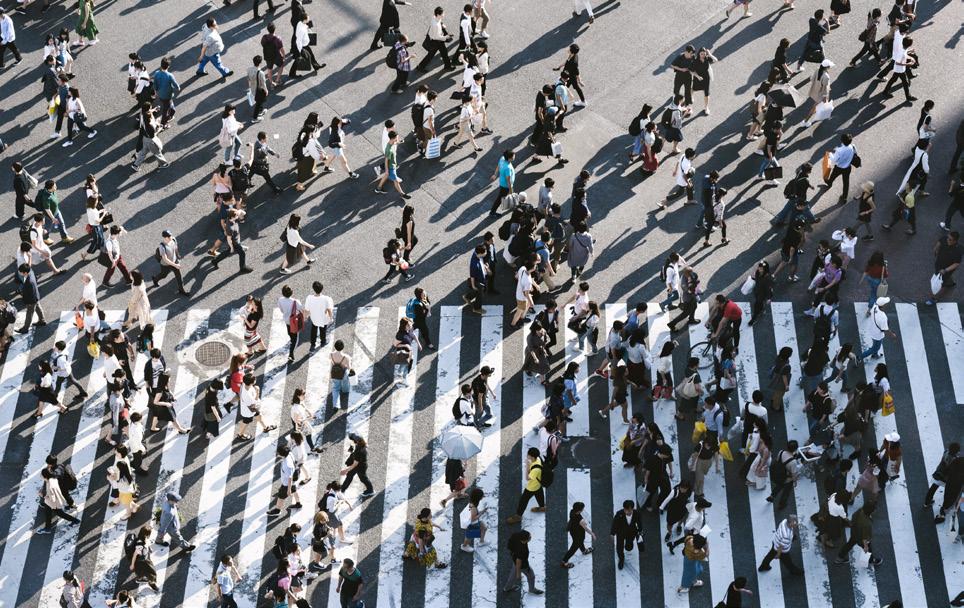
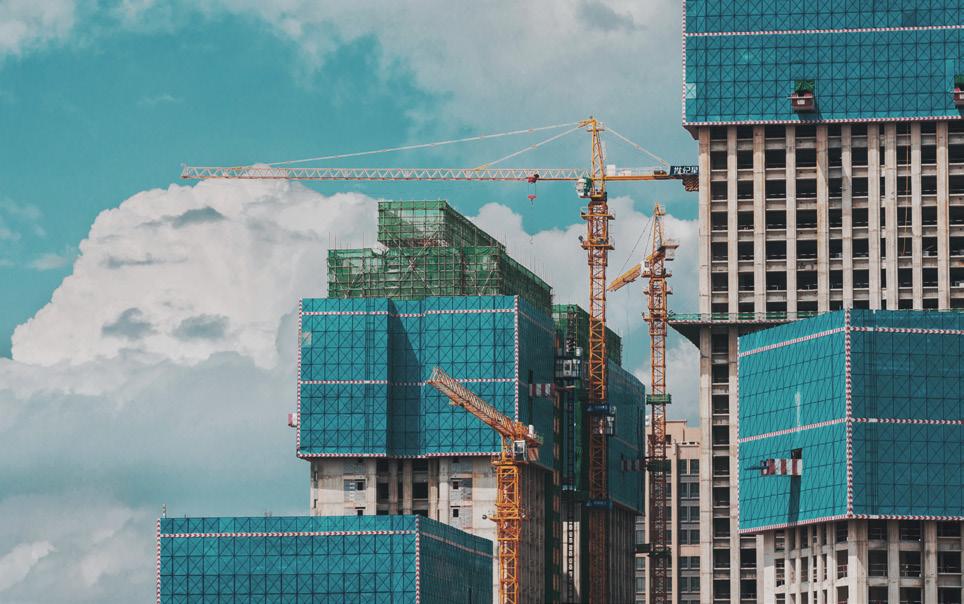
13 ATMOSPHERIC CARBON AND GREENHOUSE GASES CARBON STORED INTO TIMBER BUILDINGS EMISSIONS BIOGENIC CARBON FOSSIL FUELS ASSIMILATIONBYPHYTOPLANKTON COMBUSTIONOFFOSSILFUELS EXTRACTIONOFFOSSILFUELS OCEANS RESPIRATION(ORGANICDECOMPOSITION) PHOTOSYNTHESIS DECOMPOSITION DIFFUSION FIG 2: Carbon cycle and greenhouse gases. Cities can become carbon sinks if building with mass timber Source: by the author
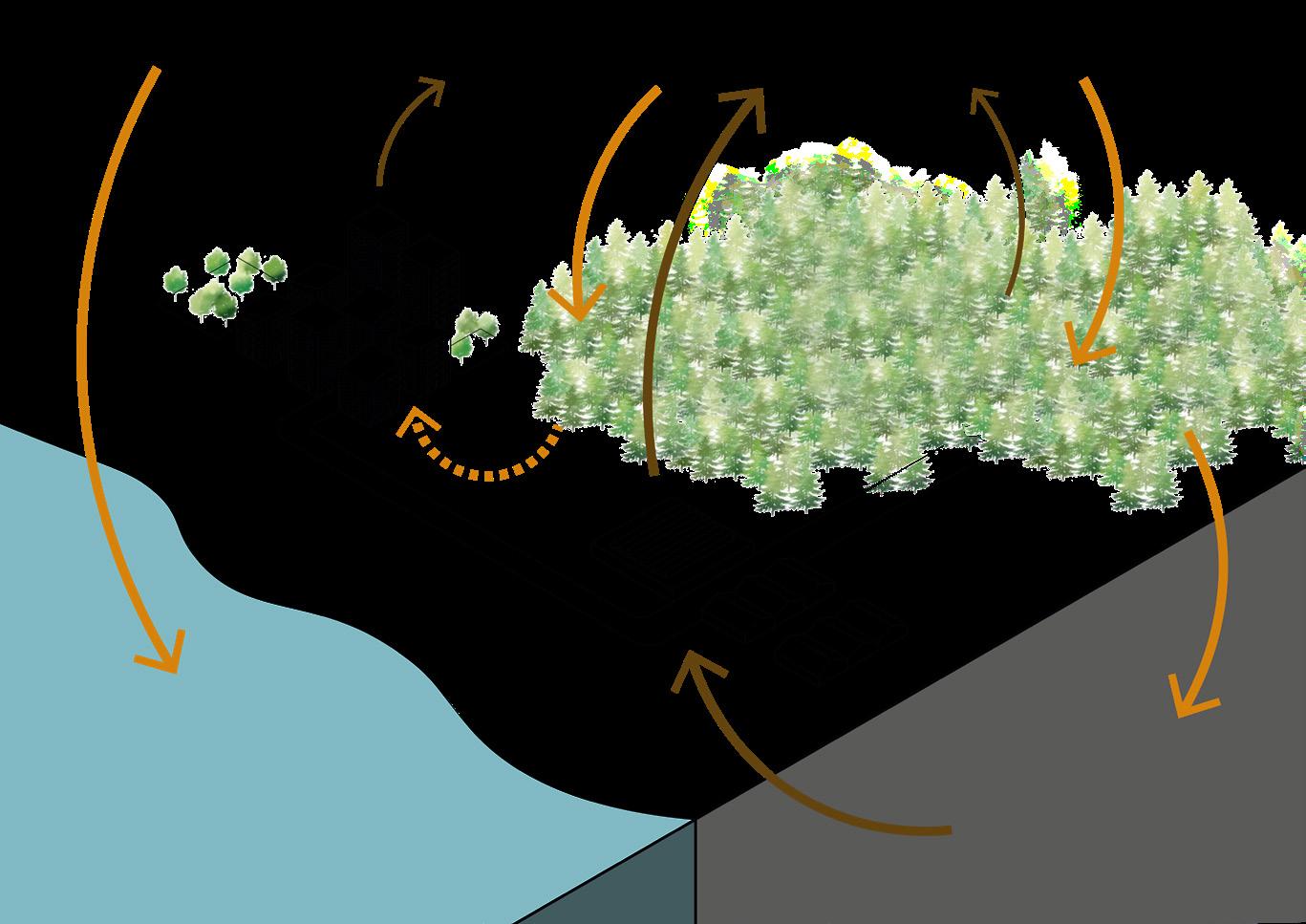
14 01 I 2
With only sunlight, water and air, trees bind carbon dioxide through the natural process of photosynthesis. This biogenic carbon will stay within the tissues of the tree for the rest of its life, until it dies and decays, when the carbon dioxide will be released back to the atmosphere.Whenlogging, this biogenic carbon is taken from the forest and “stored” in the form of timber products, let it be engineered wood products, wall panels or furniture. About 50 percent of the dry weight of wood is carbon6, and when building with timber, this carbon stays locked out of the atmosphere for as long as the building is in use, while new trees are allowed to grow in the forest, absorbing more carbon dioxide and thus making the timber product carbon neutral. That’s why both time and forest management are of the essence when we are talking about timber products. To consider a product as carbon negative, its embodied carbon (that is, the carbon emitted to produce, transport and erect it) must be lower than its sequestered carbon. In engineered wood products, this is usually the case. But that alone does not guarantee that a timber structure will automatically contribute to the decarbonization of the built environment. To do that, we need to consider the whole value chain of the product to ensure that the sequestered carbon can be considered as a net positive contribution to the global carbon stores.
First, to consider the product carbon neutral, the stored carbon taken from the forest by cutting down the trees needs to be reabsorbed again within the forest so that the carbon within the product can be seen as a “new” or additional store, and not merely displaced stored carbon from the forests. To ensure this, the wood must come from sus tainably managed forests that guarantee a regular harvest while maintaining the forest’s biodiversity and a constant if not increasing forest stand or volume. Currently, the most common labels for sustainable forest management are FSC (Forest Stewardship Council) and PEFC (Program for the Endorsement of Forest Certification). This carbon neutrality also requires the timber product to remain in use for at least the period of regrowth for the trees, but ideally as long as possible. To lengthen the building, and its structure’s, lifespan, considerations about building for disassembly, reuse and repurpose must be made. Trees used for mass timber manufacturing take around 60 years to regrow to a size
Carbon in wood products
End
15 101000 654321000000020 30 40 50 60 70 80 90 100 110 120 50 sequestered sequestrationCO2 years Seedling stage. Harvested areas release emissions to the atmosphere 6543210000000 FIG 4. Carbon Storage And Emissions In Timber Buildings Source: by the author carbon storage in timber products kTCO2sequestered carbon emissions through time Manufacturing and construction account for most of the building emissions
of life. Disassem bly, recycling, landfill Manufacturing,constructiontransportation, Operational phase (min 60 years, as long as possible)
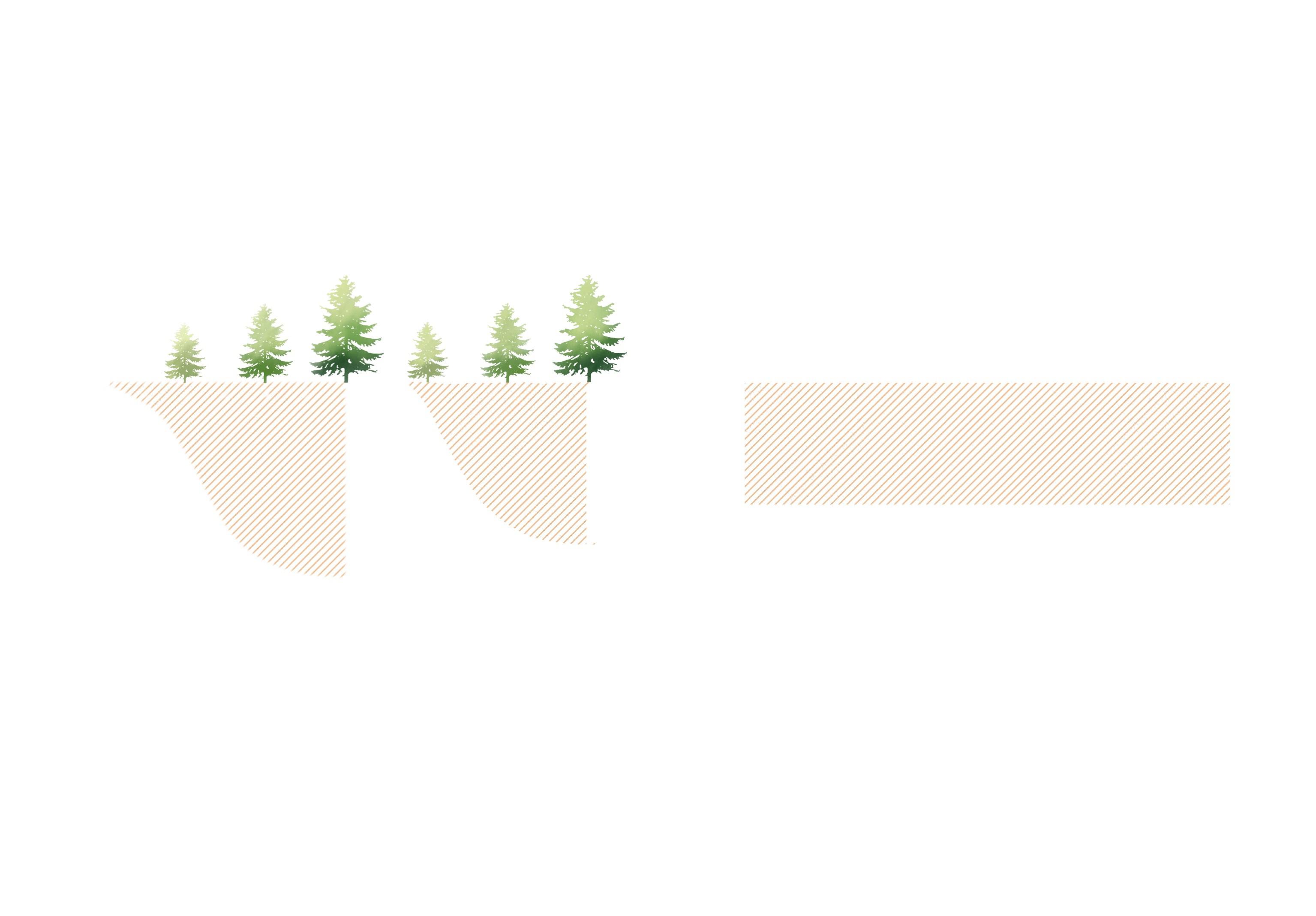
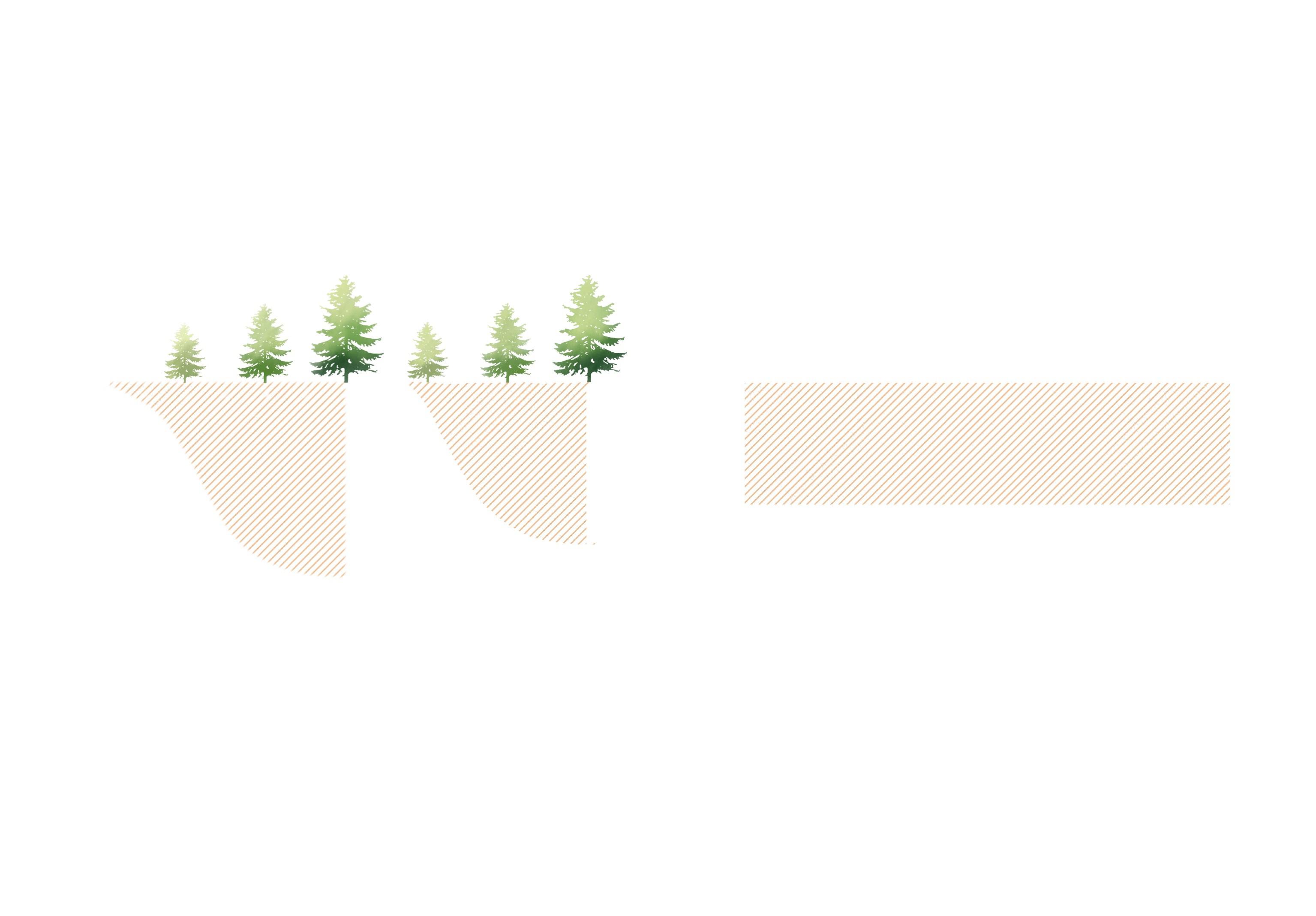
Biomass power / Biomass fuel and animal bedding releaseCO2 60% of sawn wood -7% during kiln drying -15% through cutting and planing transportation to manufacturing plant absorptionCO2 distribution
FIG 5. CLT Manufacturing Source: by the author. Adaptation from the publication 100 Projects UK CLT by Think Wood, p.16 40% to wood chips and sawdust
In addition, it is important to note that during the manufacturing phase of timber products, some wood will be lost in the process. For CLT for example, about 60 percent of the sawn wood volume is lost during cutting, kiln drying and planing. The discarded wood is commonly used for biomass or animal bedding, which means its lifespan is far shorter than if it was part of the CLT7. Using this discarded timber for biomass is currently the more sustainable option of these two as it is able to substitute energy generation from fossil fuels, however as grids across Europe decarbonize through transitioning to rene wable energy sources such as wind and solar, this aspect of the wood value chain will be under greater scrutiny and efficiencies that we can make in manufacture to reduce this ‘wasted’ material or use it in more long lasting products will become essential.
suitable16 for harvesting, which must therefore be the minimum lifespan of timber buildings.
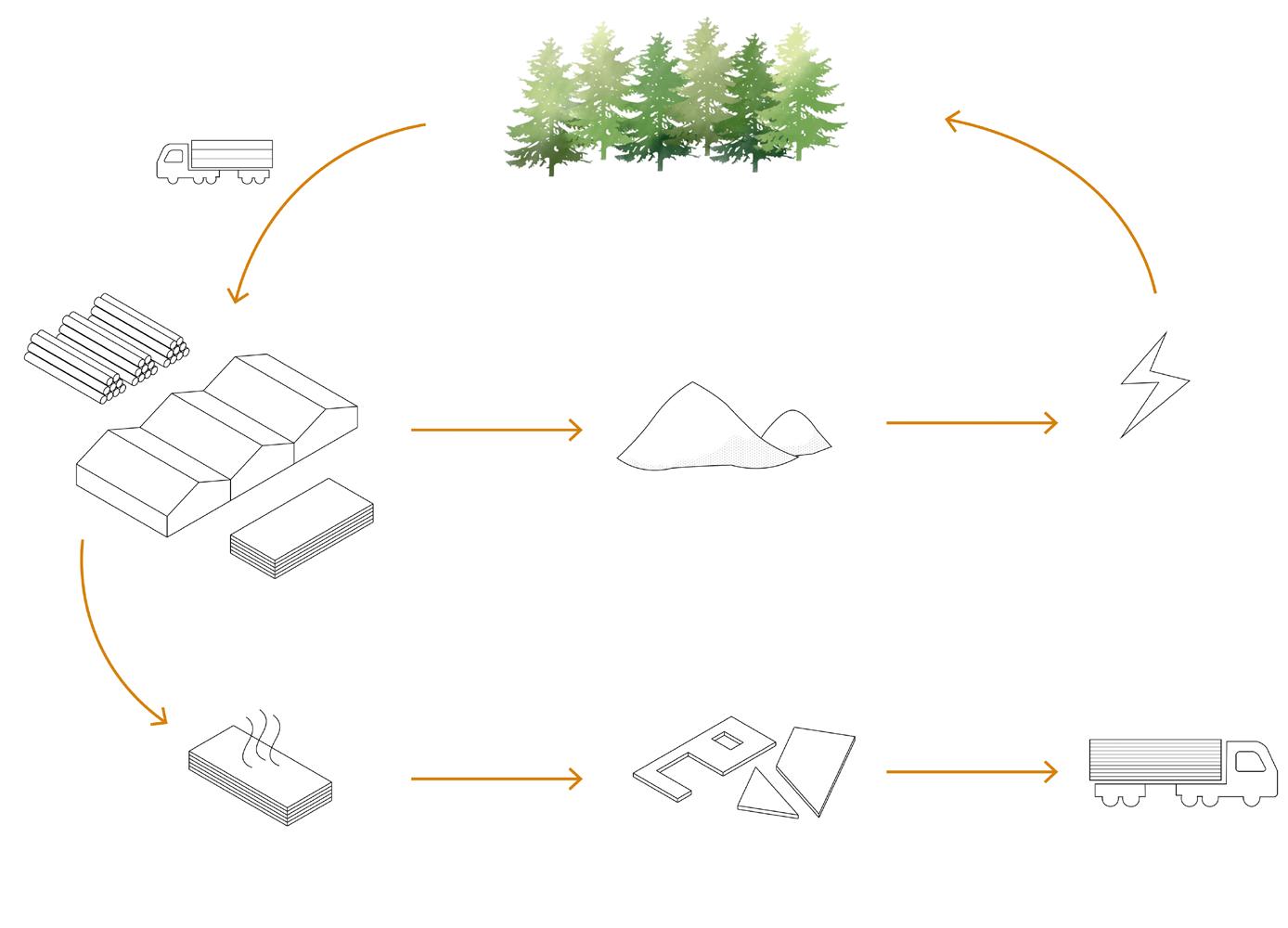
17 01 I 3
Carbon in the forest
Since forests are natural carbon sinks that store carbon for hundreds of years, some question the benefits of building with wood as opposed to leaving the forests as they are. But the capacity of trees to absorb carbon changes over time8. Younger trees will take in more carbon while they are growing, and after some decades their carbon intake stabilizes. If trees are harvested when they have reached their carbon sequest ration peak, transformed into timber products, and storage in buildings, while new trees are planted to replace them, over time the volume of stored carbon will be higher than if the forest remained untouched9. Moreover, the current high risk of wildfires derived from climate change makes forests less reliable environments to store carbon. While some fo rests should be protected and preserved for their natural value, most forests, if managed in a sustainable way, can guarantee a stable harvest while preserving biodiversity and generating spaces where both animals and humans can thrive. Moreover, if there is an increase in the demand for timber products, more sustainably managed forests could be planned and planted, generating an increase of forested areas and new carbon sinks. This mutually beneficial connection between the use of mass timber and forest development is key to understand the full potential of mass timber construction.
TCO2sequestered
Source: by the author harvest sequestrationCO2 carbon sequestration if the forest is not harvested years
18 FIG 6. Carbon sequestration cycle in sustainably harvested forest. Estimated harvest every 60 years
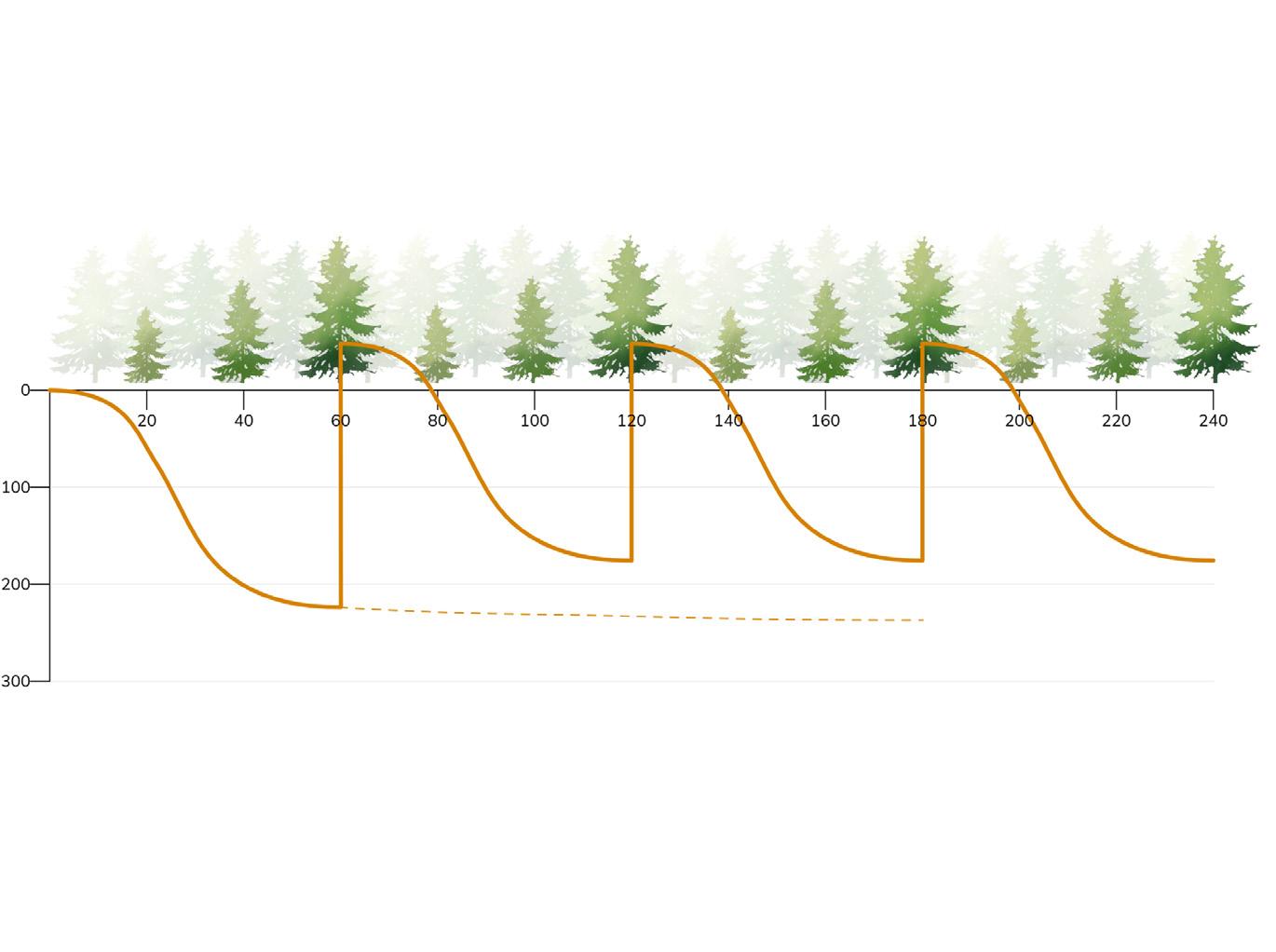
19 FIG 7. City densification and carbon storage Source: by the author increasing accumulated carbon storage with mass timber buildings kTCO2sequestered
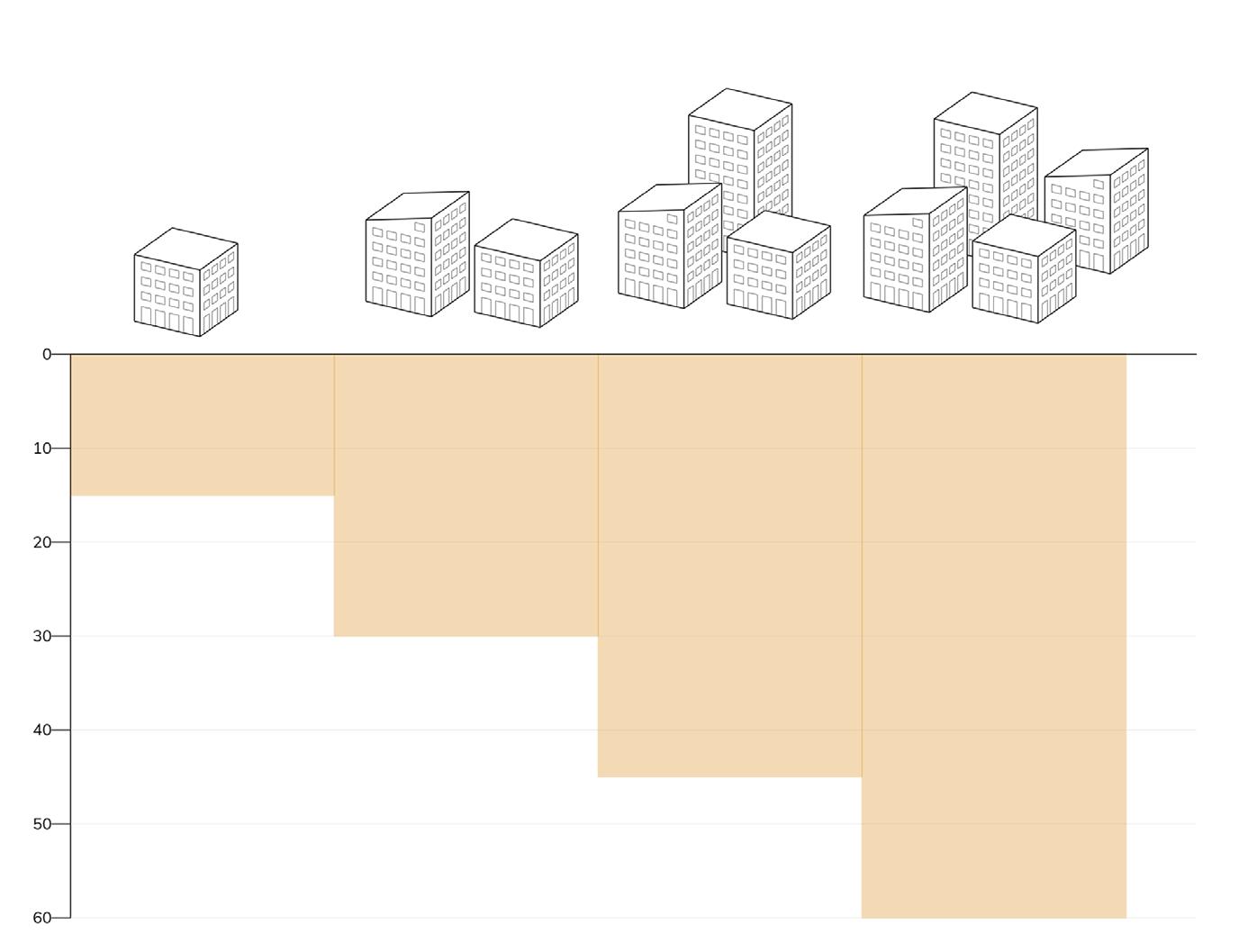
20 01 I 4 Substitution effect
important to remember that all materials have a carbon cost, including timber, and the most sustainable option is not to build. But when building, the material optimization as well as specification of re-used/recycled and lower embodied carbon materials for every part of a building must be considered if it is to have any hope to truly claim carbon neutrality.
One of the most important and often overlooked benefits of using engineered wood products for building construction is the substitution effect, in other words the emissions that are avoided by not using other carbon-intensive materials, such as con crete and steel. Usually, the substitution effect accounts for the greatest carbon saving when building with EWPs or timber products. In fact, about 80 percent of the energy associated with modern building processes is used in the production of raw matter th roughout the building’s life cycle, from the extraction, manufacturing, installation, main tenance, replacement and disposal10.
If the construction sector would substitute carbon-intensive materials with re newable, carbon-storing ones, and avoid the use of fossil fuels, it could potentially affect between 14 to 31 percent of the world’s carbon emissions, and 12 to 19 percent of its fuel consumption11. Instead of carbon-generating cities, we can have carbon-storing urban developments.Itisalso
21 -0.50-0.250.751.00.500.250 CEMENT STEEL TIMBER1T material 0.29 T max 0.16 T mean 0.05 T min 0.12 T max 0.52 T max 0.48 T mean 0.44 T min 0.05 T min 0.12 T mean 0.20 T max 1.04 T max 0.37 T min 0.54 T mean T Carbon emissions T Carbon storage FIG 8. Carbon emissions and carbon storage for producing 1 ton of material Source: copy of Fig.2|Physicaldimensions,carbonemissionsandcarbonstorage capacityof1tofcement, steel and timber materials in Churkina, G., Organschi, A., Reyer, C.P.O. et al. Buildings as a global carbon sink. Nat Sustain 3, 269–276 (2020). https://doi.org/10.1038/s41893019-0462-4
22 PROJECT EXAMPLES# 02 Critical analysis of three speculative projects
TIMBER
BANK OF NORRLAND - ANDERS BERENSSON
norrland/https://andersberenssonarchitects.com/work/bank-of-ARCHITECTS
- ULTRAMODERNE
23
SPEKULATUS Source: https://www.ultramoderne.net/buildings.php
http://timbercity.org/
The concept of timber buildings as carbon banks has been explored in theoreti cal projects that depict a near-future where carbon storage has been given an economic or social value. We will go through three of such projects, from smaller to bigger scale. All of them propose buildings that visualize and take advantage of the capacities of mass timber buildings as carbon sinks.
CITY - GRAY ORGANSCHI ARCHITECTURE
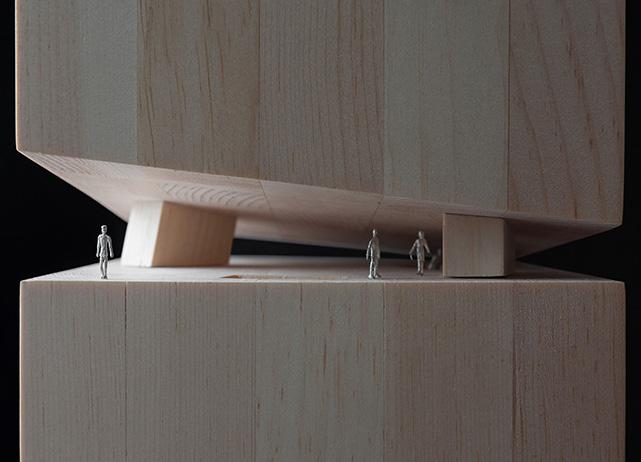
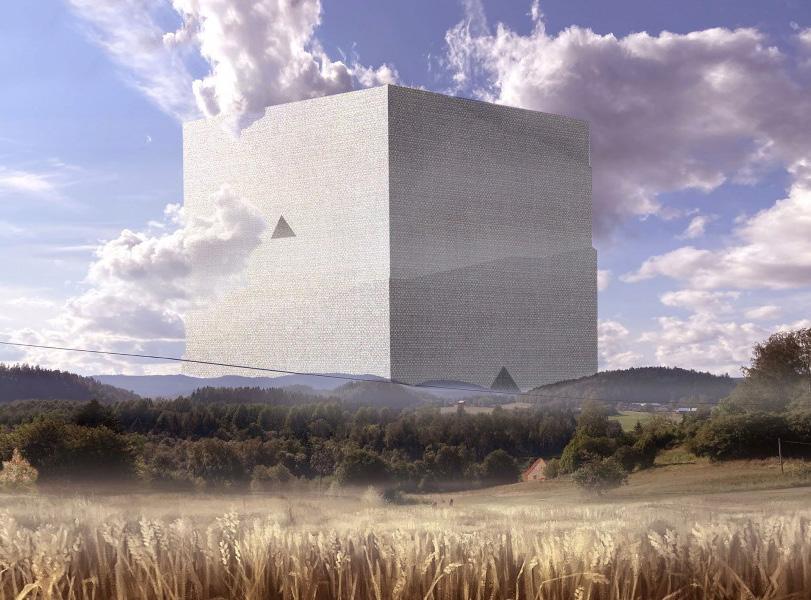
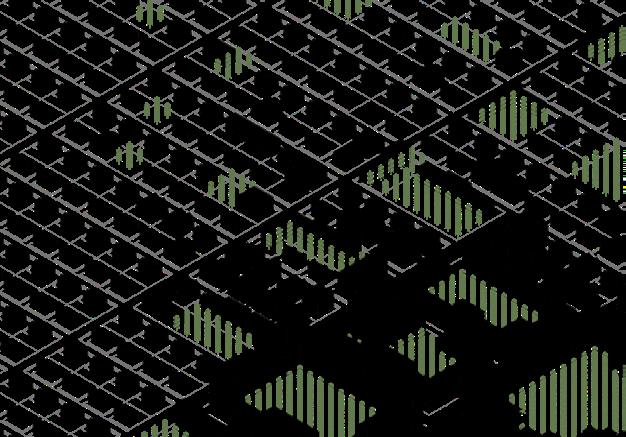
In this context, Spekulatus rises as a response to both situations. As long as the condos of the solid timber tower stay uninhabited, they will continue to store carbon and won’t lose their investment value. The skyscraper’s volume is transversely cut to create accessible terraces, including its top. This way, the project draws a parallel between the value of real estate goods and the value of carbon stocks, which might increase following the current climate emergency. It also presents the capacity of timber to create new public spaces and new forms of public architecture through its terraces. On the other hand, it ignores the fact that spaces for living will be needed, since the world population is growing, and proposes a solid tower without inhabitable spaces. The tower becomes more like a sculpture to carbon sequestration than a skyscraper. Finally, the project does not consider the forest role within the project or the development of new urban areas. In a satirical way, this project points out how the indiscriminate construction of high-standing apartments in towers for economic benefit is leading us to increased car bon emissions and far from the Paris Agreement (which the Trump administration pulled out of) and is completely ignoring the need for more economically accessible housing.
Spekulatus by American architecture firm Ultramoderne is a massive timber tower where the volume of the building is divided into condos sold as carbon shares. The fiction takes place in 2024, after two terms of the Trump-Sanders administration (the project was published before the elections of 2016) which have resulted in increased social inequality and more severe regulations for carbon emissions. Hence, carbon-emis sion costs outpace investment returns in high-rise developments. At the same time, due to social inequality, the general population has less and less access to public space.
24 02 I 1
Spekulatus by Ultramoderne
25
https://www.ultramoderne.net/buildings.phpSource:
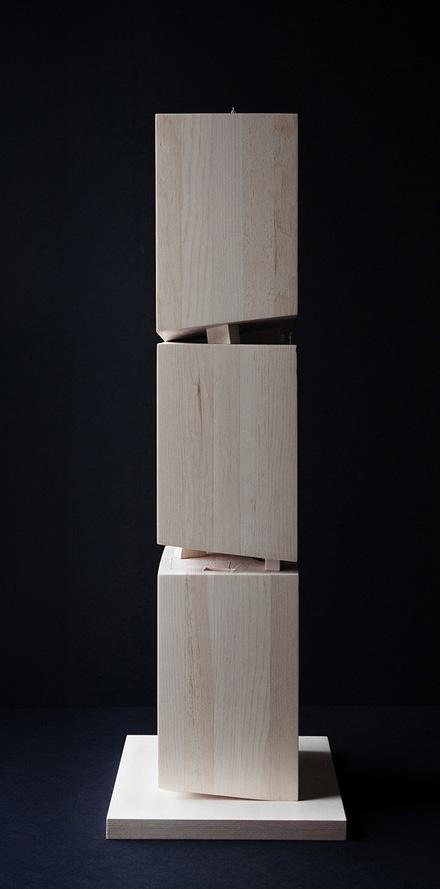
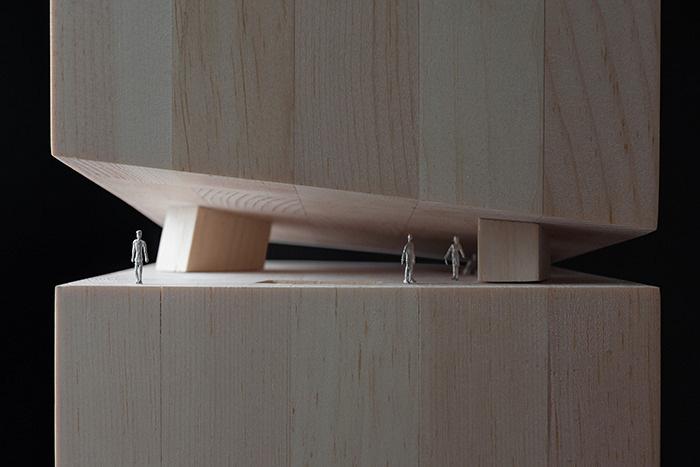
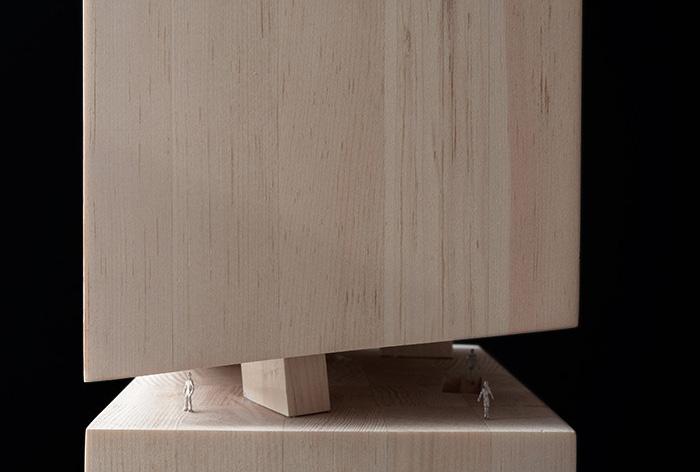
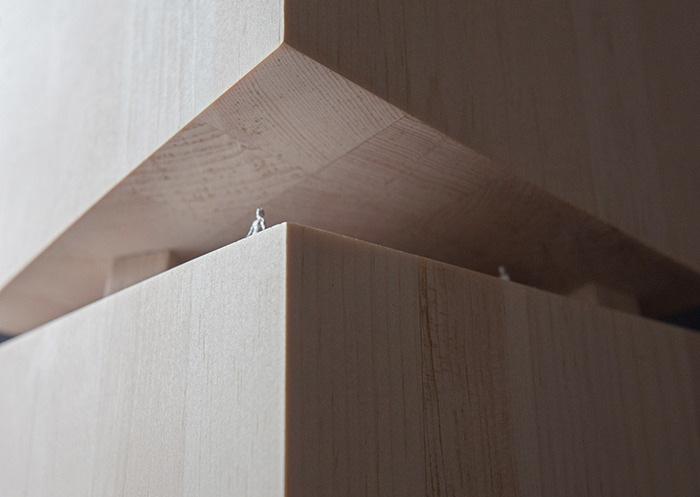
26 02 I 2 Bank of Norrland by Anders Berensson Architects Bank of Norrland by Anders Berensson Architects is a science-fiction project that proposes a giant timber structure of one cubic kilometer to address the “green” carbon emissions derived from the Swedish forest industries.
Under the Paris Agreement, Sweden committed to reduce its carbon emissi ons to 55 percent of 1990 levels by 2030 and to 95 percent of 1990 levels by 2050. Today, most of the logged wood in Sweden is used to make pulp, paper products and biofuel, which accounts for 33 percent of Sweden’s total carbon emissions. Moreover, the production of pulp and paper generates a byproduct called black liquor, that is usually burned and accounts for 15 percent of the country’s total emissions. Since all emissions that come from burning wood are classified as “renewable”, they are counted as carbon neutral and therefore ignored. In addition, in recent years Sweden‘s forest area has suf fered natural disasters such as fires and storms, that have felled trees which are then usually used for biofuel. To address this real situation, Anders Berensson imagines the Bank of Norrland, a structure made of logged trees with the main purpose to store carbon dioxide. Trees felled by storms or other natural disasters are also stored here. The logs are placed to dry in interlocking rows one on top of the other to form a giant cube of 1 km3, becoming the world’s largest timber structure. At its full capacity, the Bank can contain 900 million logs, which is the equivalent of around 330 million cubic meters of wood. All wood in the bank is used for wood construction or to produce wood products with a lifespan of at least 50 years. In this fiction, Sweden has committed to become a country free of all forms of combustion, getting rid of the “green” half of the country’s carbon emissions, those produced by the forest industry. Instead, timber products will only be used to purposes that can store carbon for decades.
Although it is unlikely that humanity can stop burning wood for energy alto gether, especially during the transition from fossil fuels to less carbon-intensive energies, this project is denouncing and addressing the problem of carbon emissions inside the forestry industry itself and positioning using wood for construction as the most effective way to store carbon from the atmosphere for a long time.
Source:CompositionSource:Entranceshttps://andersberenssonarchitects.com/work/bank-of-norrland/totheBankofNorrlandhttps://andersberenssonarchitects.com/work/bank-of-norrland/oftheBank’sstructurehttps://andersberenssonarchitects.com/work/bank-of-norrland/
27
timber
Bank of Norrland seen from afar
Images illustrating facts about and storage into
Source:
emis sions
Source:
carbon
chitects.com/work/bank-of-norrland/Source:pulp,Thechitects.com/work/bank-of-norrland/https://andersberenssonarmajorityofsawnwoodgoesintopaperproductsandbiofuelhttps://andersberenssonar
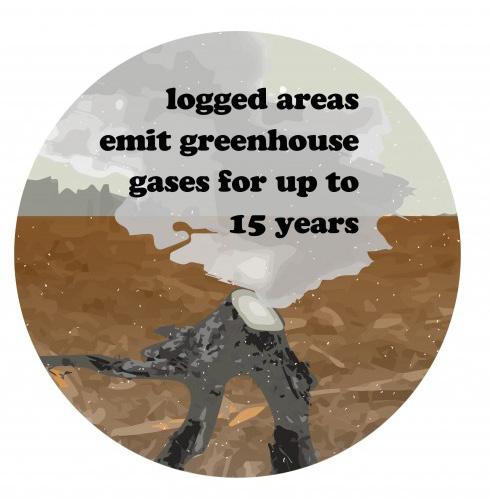
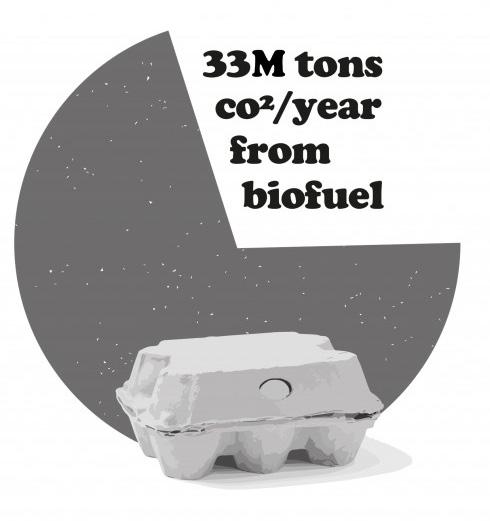
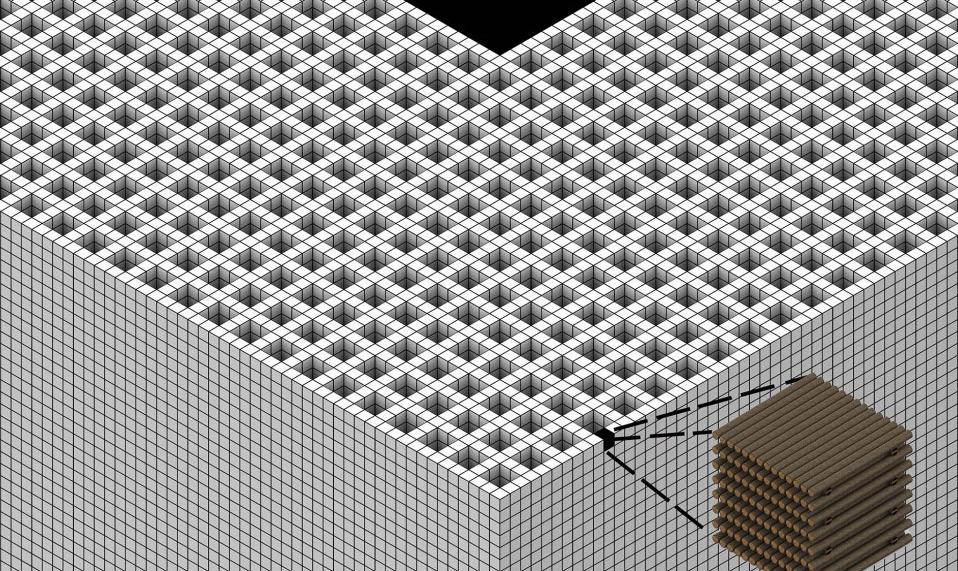
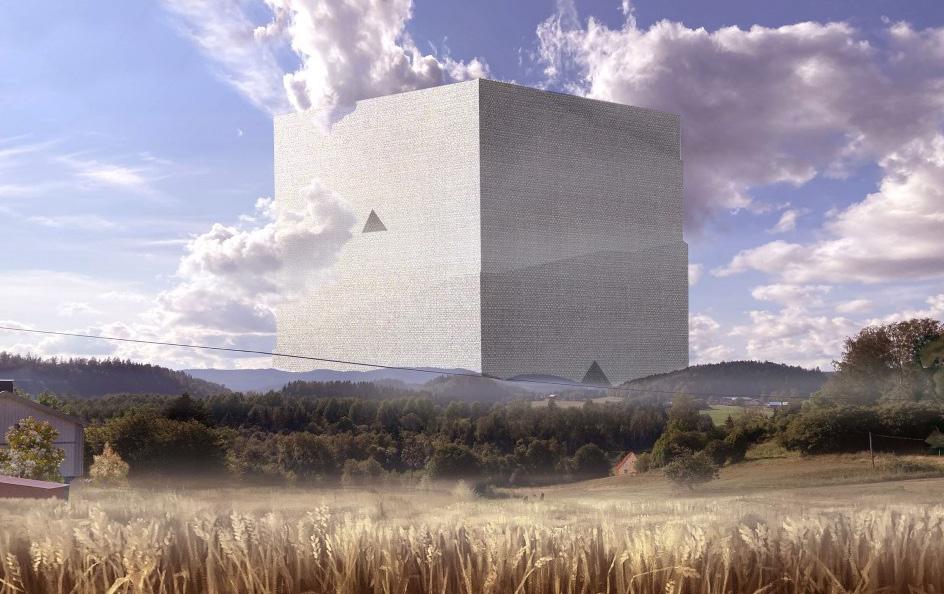
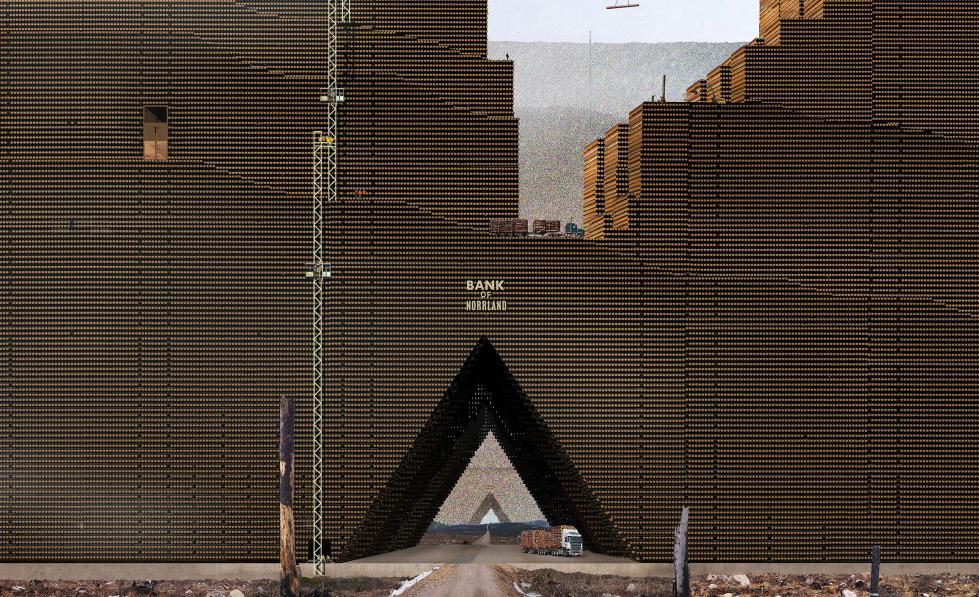
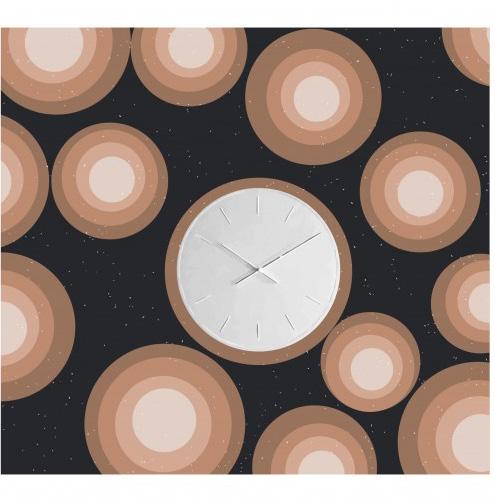
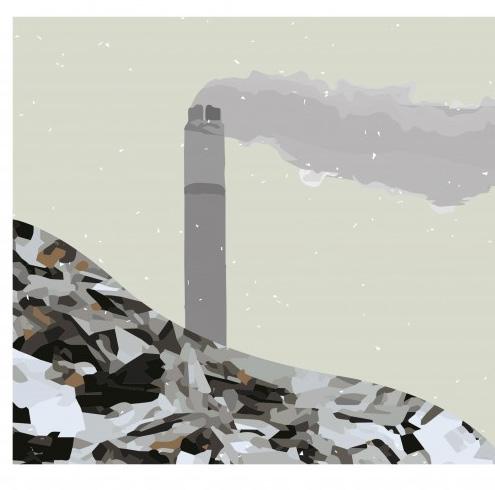
Timber City by Gray Organschi Timber City is an ongoing research project by Gray Organschi Architecture supported by the Hines Research Fund for Advanced Sustainability at Yale University. It proposes to densify North American cities using mass timber instead of carbon-intensi ve materials. The project aims to manage the whole value chain of mass timber, from tim ber harvest in sustainably managed forests, the production in sawmills and manufacturing plants and the distribution at a regional level. The project is a response to the issue of a growing population in a context of a climate emergency. By 2050, 6.3 billion people will live in urban areas, and cities will need to grow to accommodate 2.9 billion people. Specifically for the US, this could mean a population increase of 120 million people in only 36 years. If continuing with the current state of affairs, the densification of cities will release an enormous amount of carbon emissions to the atmosphere. Timber City underlies the intrinsic connection that can exist between forests and urban areas, offering the possibility of turning cities into carbon sinks while developing the regional forests at the same time. This way, cities and forests can grow together, in a mutually beneficial dynamic that can help to mitigate climate ch ange and boost the region’s economy.
28 02 I 3
From carbon source of emissions to carbon sink Source: http://timbercity.org/
Architecture
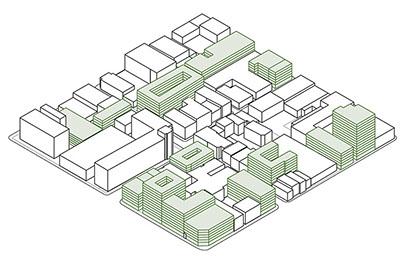
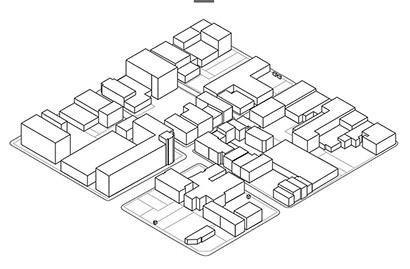
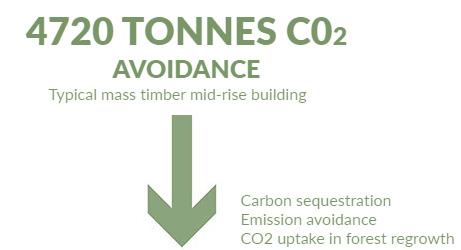
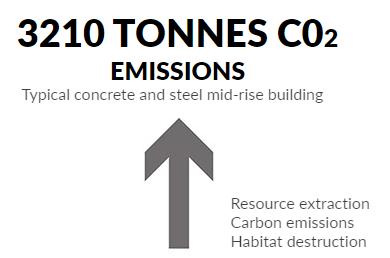
29 http://timbercity.org/Source: This project is probably the most realistic of all three projects presented here, and although it is specific to regions with forest areas nearby, it proposes a solution with a territorial vision that fulfills the whole potential of building with mass timber.
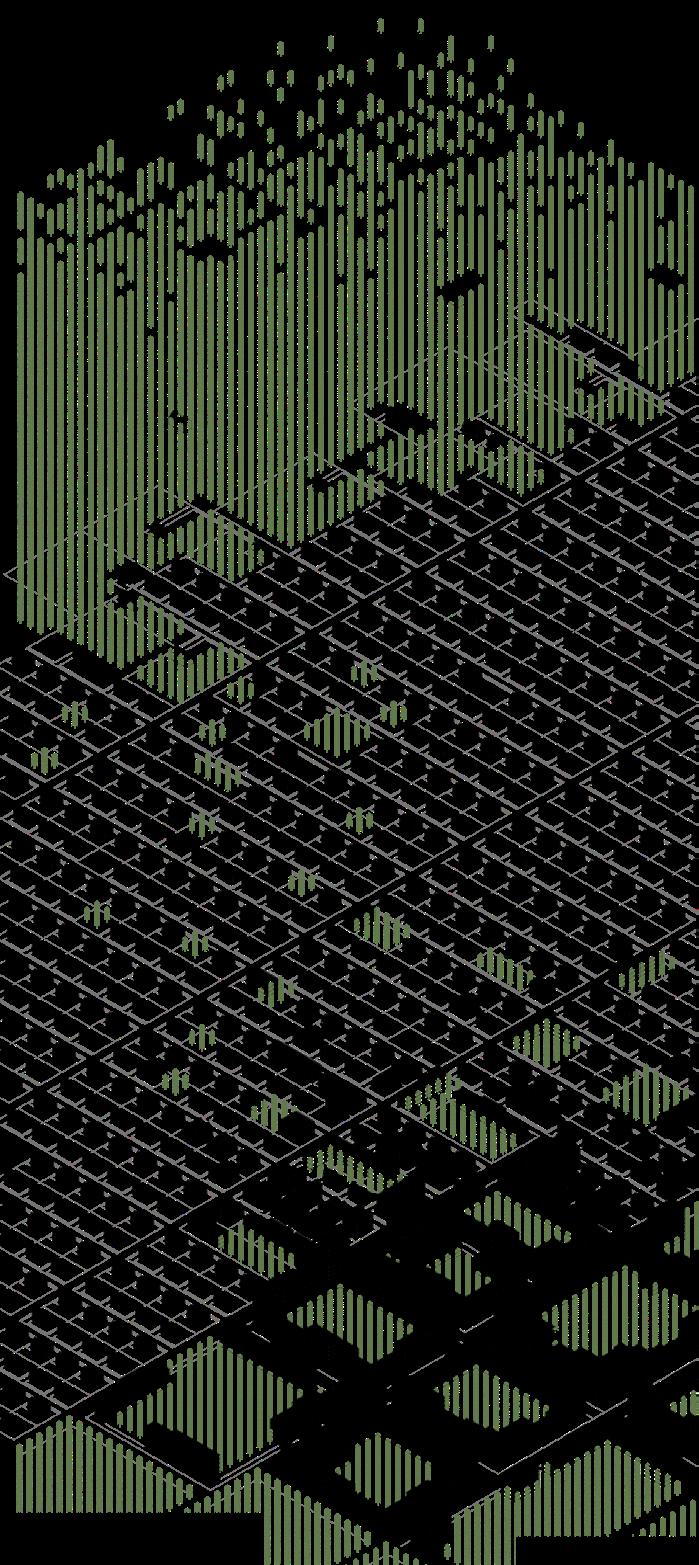

30 CASE STUDY# 03
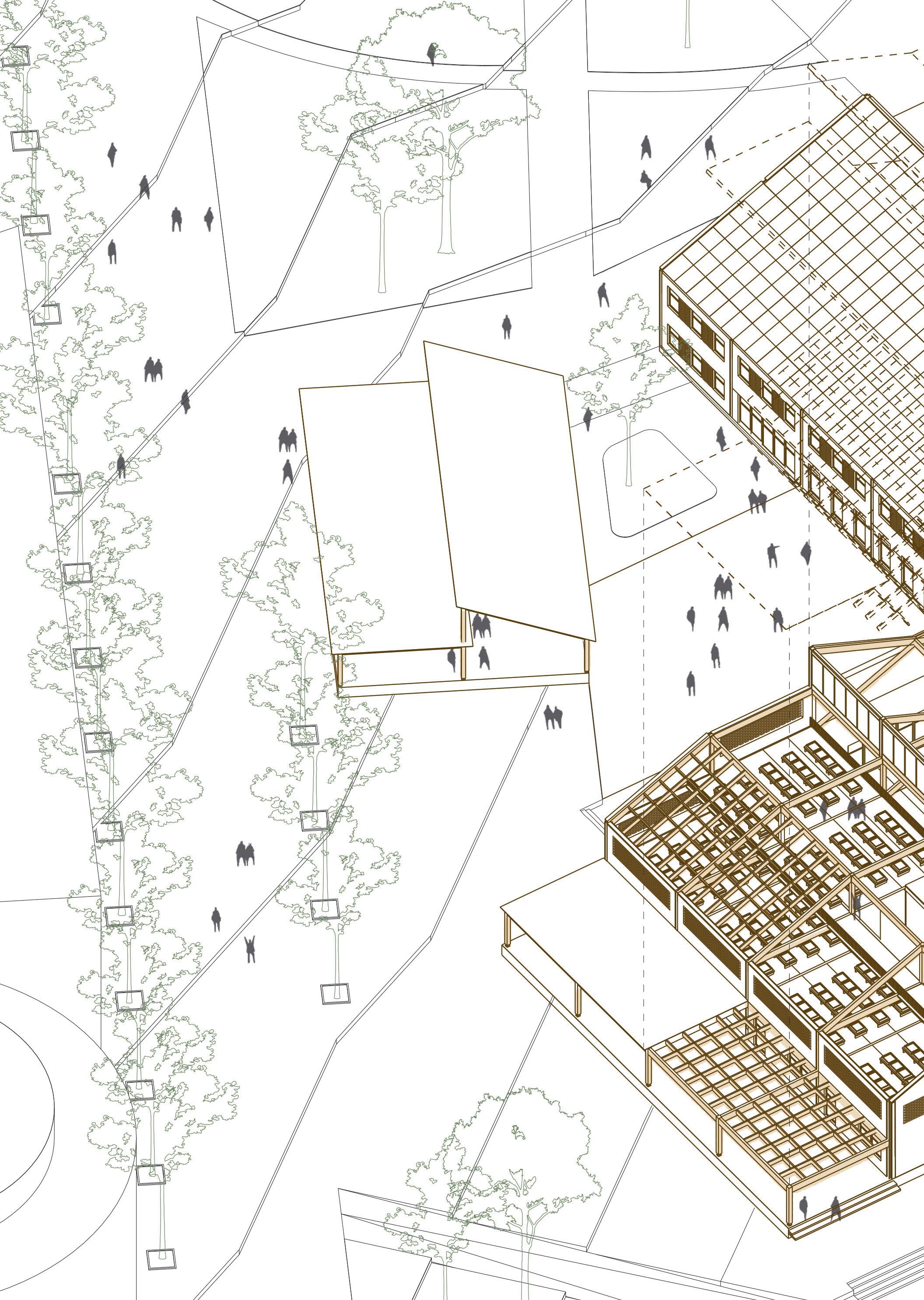
31 New Bull Ring Market in Birmingham
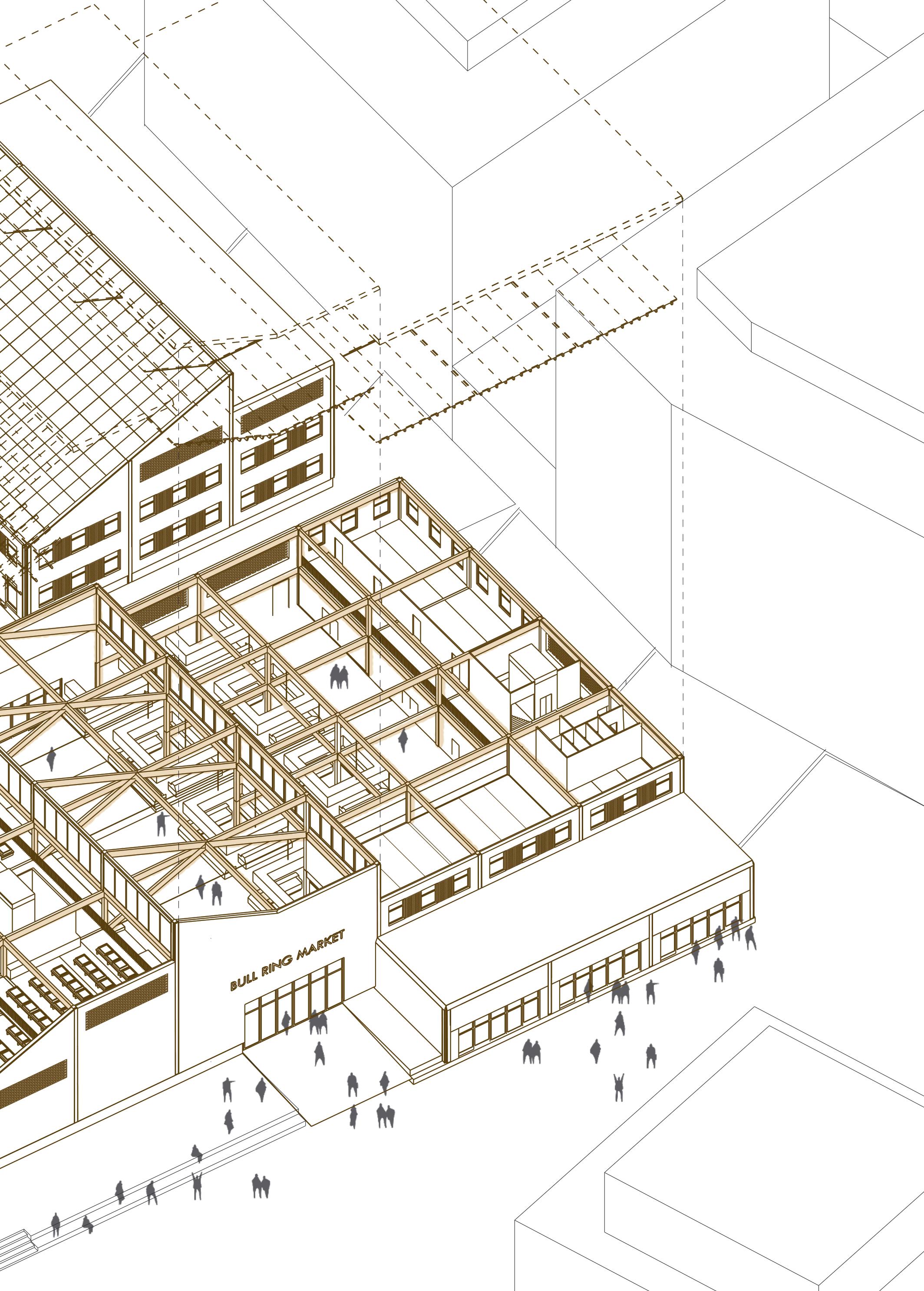
32
Despite the carbon-storage potential of mass timber, it won’t be the only para meter driving architectural design. Other aspects such as transportation, the duration of the construction process, material efficiency and budget among others will also be con sidered. In addition, the impacts on the forest should also be examined. To illustrate these different variables, I developed three alternative structures for the last term’s project of the Master in Mass Timber Design.
The project is a large-span timber structure in Birmingham, UK, hosting a cover ed permanent market, a temporary market, greenhouses, and a research center with of fice space. The different programs are organized in three different volumes. The building’s structure is a 9 by 9 meters square grid, and it was conceived with design for disassembly in mind to lengthen the lifespan of its timber components.
Interior of the market hall. Source: by the author
Multi-purpose office and retail buildings will also be needed in future urban developments, and the possibilities of variation of the structure offered by the project grid were thought to be more suitable for this exercise than other projects developed in previous terms.
03 I Case Study: New Bull Ring Market in Birmingham
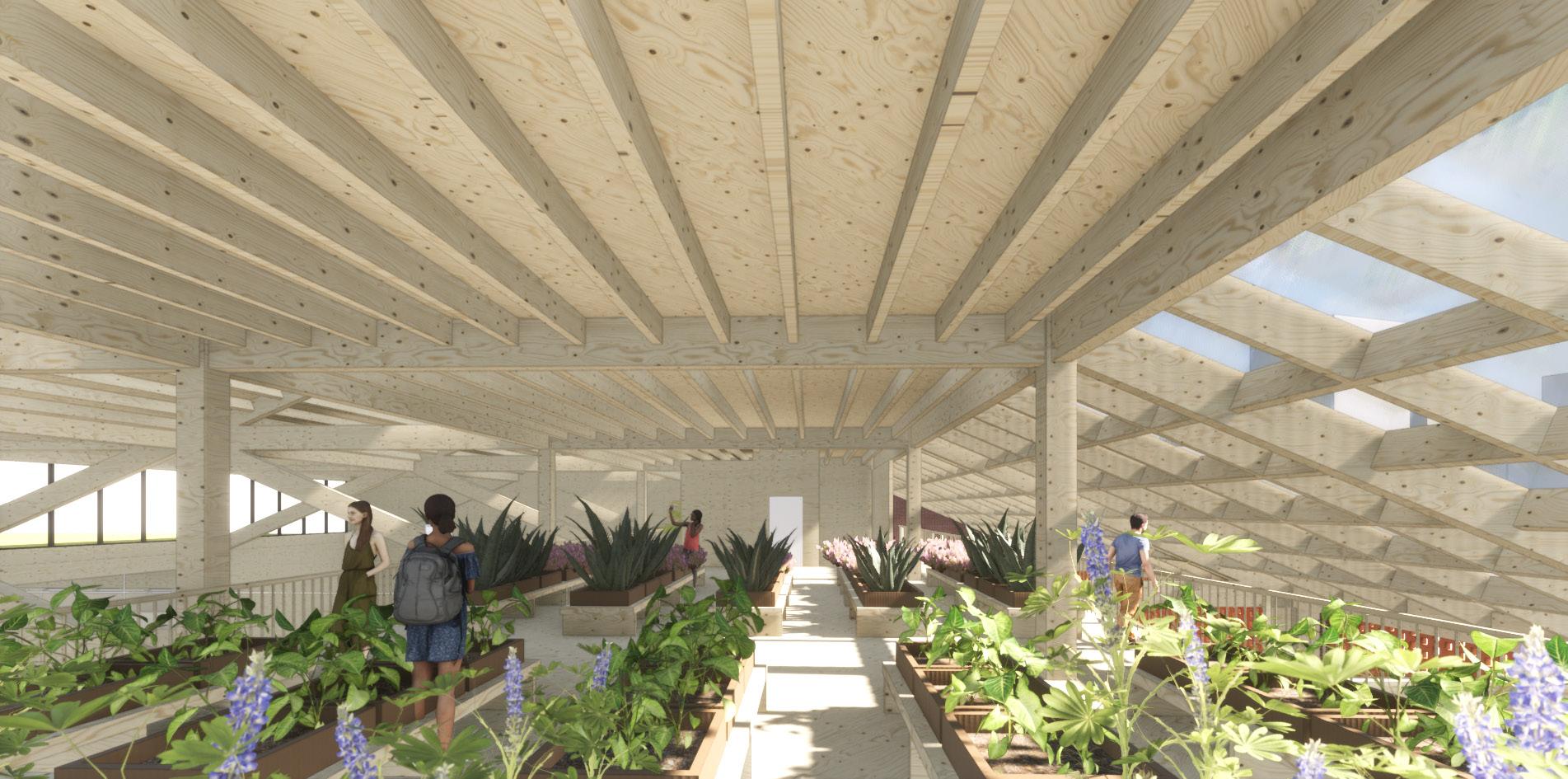
perforated brick bond facade brick opaque facade triangular brick bond facade
Concealed metal profile fixed with screws to the timber beams. Factory production and transportation to site in three parts. Assembly of parts on site
1 32
33
3. EXPOSED COLUMN TO CONCRETE FLOOR Metal post base fixed to floor and con nected with screws and metal profile to timber column. Example rothoblaas highly-resistant post base s50.
photovoltaic glass office facade ground floor office facade
1. GIRDER GRID JOIN
Concealed hook timber-to-timber Connector fixed with screws to both Column and Reinforcementbeam.with diagonal screws Example rothoblaas lock T connector In-situ assembly
2. COLUMN TO BEAM JOIN
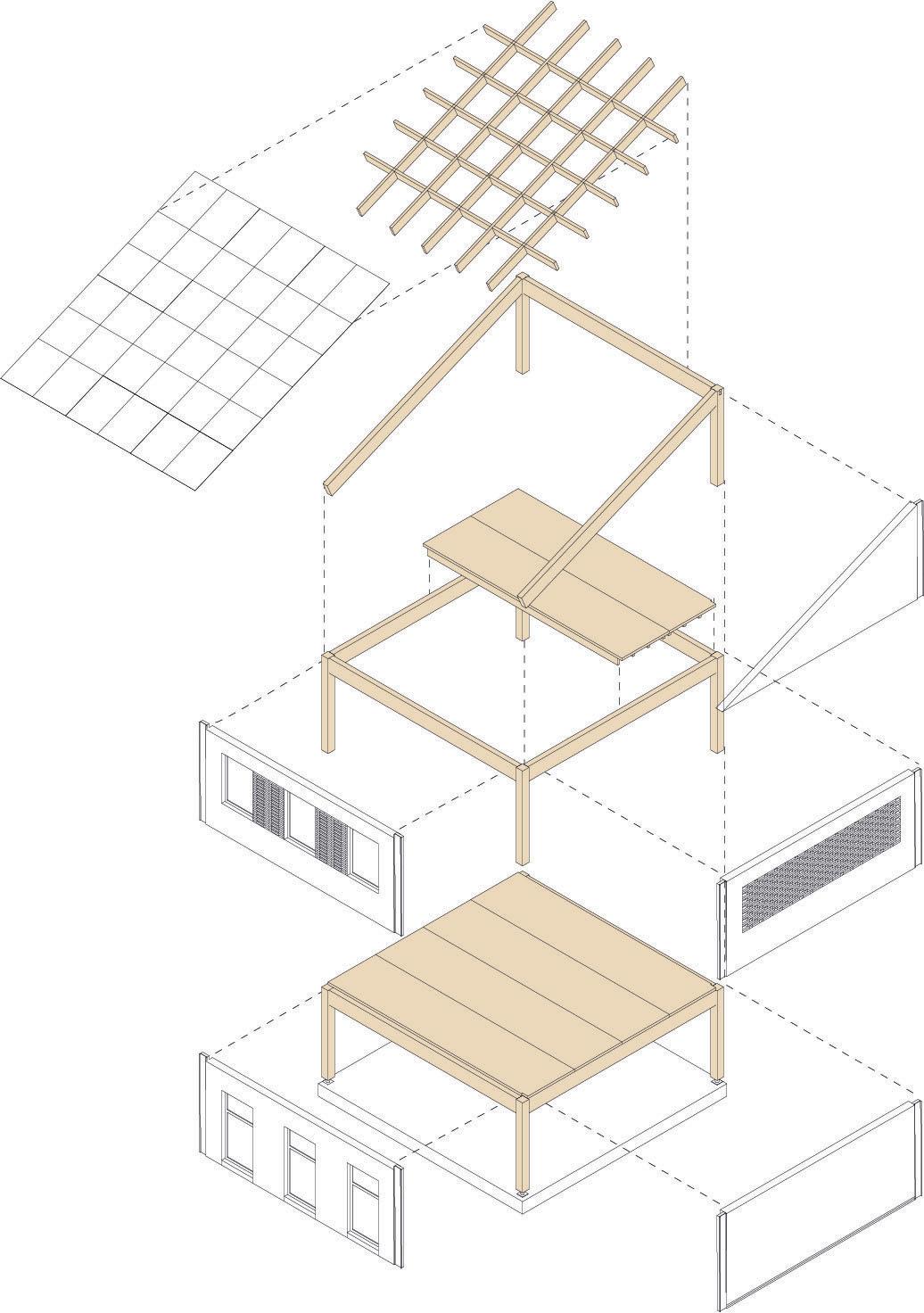
To perform the different calculations, some previous assumptions were made. Since the UK is not a timber-producing country, the raw material would probably come from somewhere else. Due to previous personal research about the Swedish forest in dustries and the fact that UK is the biggest importer of Swedish sawn timber products, it has been assumed that the timber will come from Sweden for this exercise. This Scandi navian country has a forest area that accounts for 70 percent of the total land area and is the fifth global producer of wood products and the third exporter in the world (wood products include pulp, paper, and sawn wood). Sweden produced 18.4 million cubic me ters of sawn timber in 2020 and almost 19 million in 2021. Its forest area increases about 120 million cubic meters per year, and it has doubled during the last 100 years. Most of Sweden’s managed forests are FSC or PEFC-certified12
The most common species to produce mass timber products found in the Swedish forest are Scots Pine and Norway Spruce. Scots Pine is a fast-growing conifer tree usually reaching 30 to 40 meters of height. With a lifespan of 150 to 300 years, the oldest specimen in Sweden is 760 years old. Norway Spruce is an evergreen conifer tree that can reach up to 60 meters of height and has a usual lifespan of 40 to 400 years. Ne vertheless, the oldest specimen in Sweden is 9550 years old, and is found in the province of Dalarna. Both trees are usually harvested once they reach around 50 to 60 years of age, and not later than 100 years old. The minimal waiting time for logging a tree in Swe dish forests is 45 years13.
Exports of Swedish sawn timber. UK is the biggest importer of Swedish timber Source: Swedish Forest Industries (Skogsindustrierna)
34 03 I 1 Methodology and limitations
Production of Swedish sawn timber Sweden produced 18,4 million m3 sawn timber in 2020 and nearly 19 million m3 in 2021
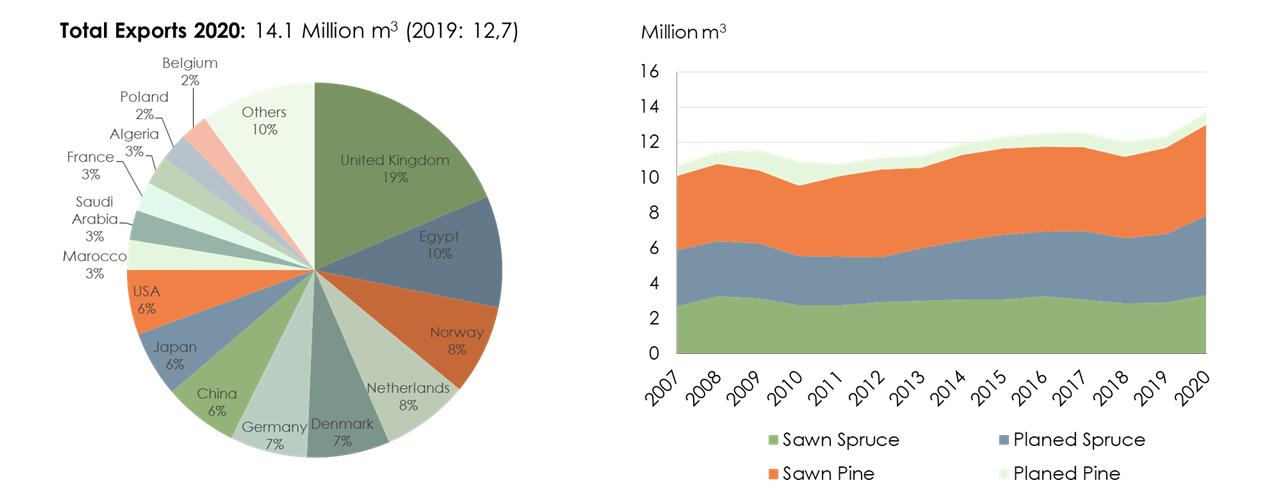
SCOTS PINE Height: 30-40m Lifespan: 150-300 years Logging age: 45 - 100 years
Image source: Modification of an image from Imageum=android_app&utm_source=sharelhi3hh/the_topography_of_sweden/?utm_medihttps://www.reddit.com/r/MapPorn/comments/below:Bytheauthor
NORWAY SPRUCE Height: 30-60m Lifespan: 150-400 years Logging age: 45 - 100 years
35 6543210000000
For the carbon storage calculations, I have used the FCBS Carbon Calculator, a free online tool de veloped by British architecture firm Feilden Clegg Brad ley Studios that calculates embodied and sequestered carbon for the different elements of a building project. The tool consists of various Excel Sheets that generate easy-to-read graphics to visualize the carbon emissions of the building throughout its lifespan, which in the tool is 60 years for the building structure. For this exercise, we will only consider the volu me of the structure of the building, without façade, floor, or roof assemblies. This should be enough to illustrate the purpose of the exercise. There are other limits to the calculation for this thesis that are worth mentioning, like the fact that emissions derived from the transportation of the timber from Sweden to England were not added to the tool’s results. Finally, for the timber volume, since the tool assumes a fixed grid size of 7.5 meters, calculations for the volume of each alternative were made before hand and the numbers were then adjusted accordingly within the tool.
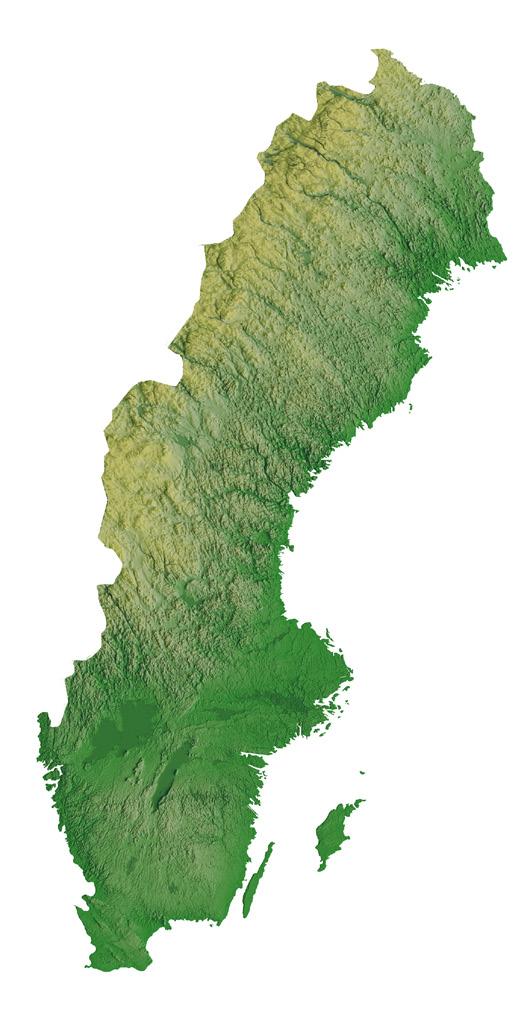
36 03 I 2 Alternatives
Case 1 consists of a post-and-beam structure with girder grid decks throug hout. This would be the most efficient approach in terms of timber use, but the 9 by 9 meters girder grid would probably be more difficult to transport and install than the rib decks. It would also probably need more metal connections than the other two alterna tives. As for the carbon calculations, this structure would sequester 703 tons of CO2, the equivalent of the energy consumption of 272 homes for one year. However, due to the large concrete slab at ground floor, its carbon balance would not be negative, meaning that the structure produces more emissions than it sequesters. The volume of timber used for this structure represents 0.03 percent of the total Swedish sawn timber impor ted to the UK, and it could be built 1444 times with the imported timber. The structure is equivalent to 730 harvested trees, and it would claim around 2.2ha of forest to offset its carbon emissions14.Case2presents a post-and-beam structure with both girder grids and rib pa nels, depending on the use of the space below. This structure would sequester 1212 tons of carbon dioxide, the annual energy consumption of 236 homes. Its timber volume re presents a 0.045 percent of the total sawn timber imported from Sweden, which in its turn could be used to build this structure 889 times. It is the equivalent of 1186 trees, and 2.6 ha of forest would be necessary to offset its carbon emissions. The carbon balance of this alternative is negative, -57 tons of CO2.
All options have a continuous reinforced concrete slab at ground floor. Case 1 will be the one with a minimal timber structure, case 2 the structure corresponding to the original proposal, and case 3 will maximize the use of mass timber products.
Three different alternatives for the building structure are proposed correspon ding to a mini-midi-maxi approach in terms of timber volume, and derive from the pro posal that was made during the third term of the Master in Mass Timber Design. Because of the squared proportions of the grid and its big dimensions (9x9m), two structural al ternatives were used for the decks: an LVL girder grid and LVL rib decks with a CLT plate.
37 Carbon calculation with FCBS Carbon Tool for Case 1 Carbon conversion source: https://www.epa.gov/energy/greenhouse-gas-equivalencies-calcu lator. Source: FCBS Carbon Tool Non-domesticRIBA 2030 Challenge 2030 2025 2020 Pre - 2020 Sequestered carbon Embodied carbon over the lifecycle TOTAL Embodied Carbon: 136 kgCO2e/m2 TOTAL Including Sequestration: 38 kgCO2e/m2 500 650 800 1100 LIFECYCLE EMBODIEDCARBON-98 91 45 -150-100-50 0 50 100 150 InternalSuperstructureSubstructureUpperfloorsRoofExternalwallsWindowsInternalwallsfinishesServices kgCO2e/m2 Axonometry of Case 1 Source: FCBS Carbon Tool
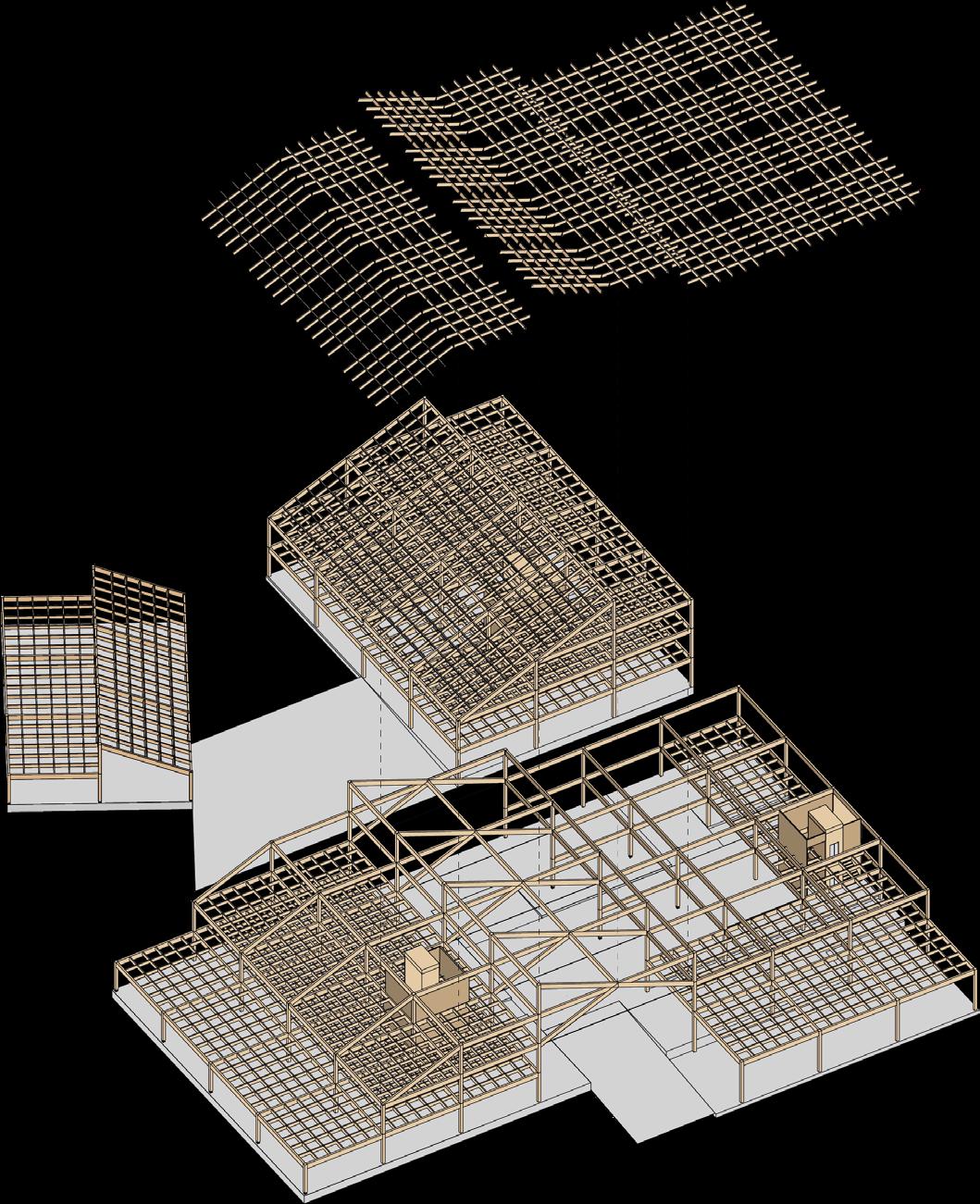
38 Non-domesticRIBA 2030 Challenge 2030 2025 2020 Pre - 2020 Sequestered carbon Embodied carbon over the lifecycle TOTAL Embodied Carbon: 162 kgCO2e/m2 TOTAL Including Sequestration: -8 kgCO2e/m2 500 650 800 1100 LIFECYCLE EMBODIEDCARBON-144 -25 91 61 InternalSuperstructureSubstructure-200-150-100-500501001509UpperfloorsRoofExternalwallsWindowsInternalwallsfinishesServiceskgCO2e/m2 Carbon calculation with FCBS Carbon Tool for Case 2 Carbon conversion source: https://www.epa.gov/energy/greenhouse-gas-equivalencies-calcu lator. Source: FCBS Carbon Tool Axonometry of Case 2 Source: FCBS Carbon Tool
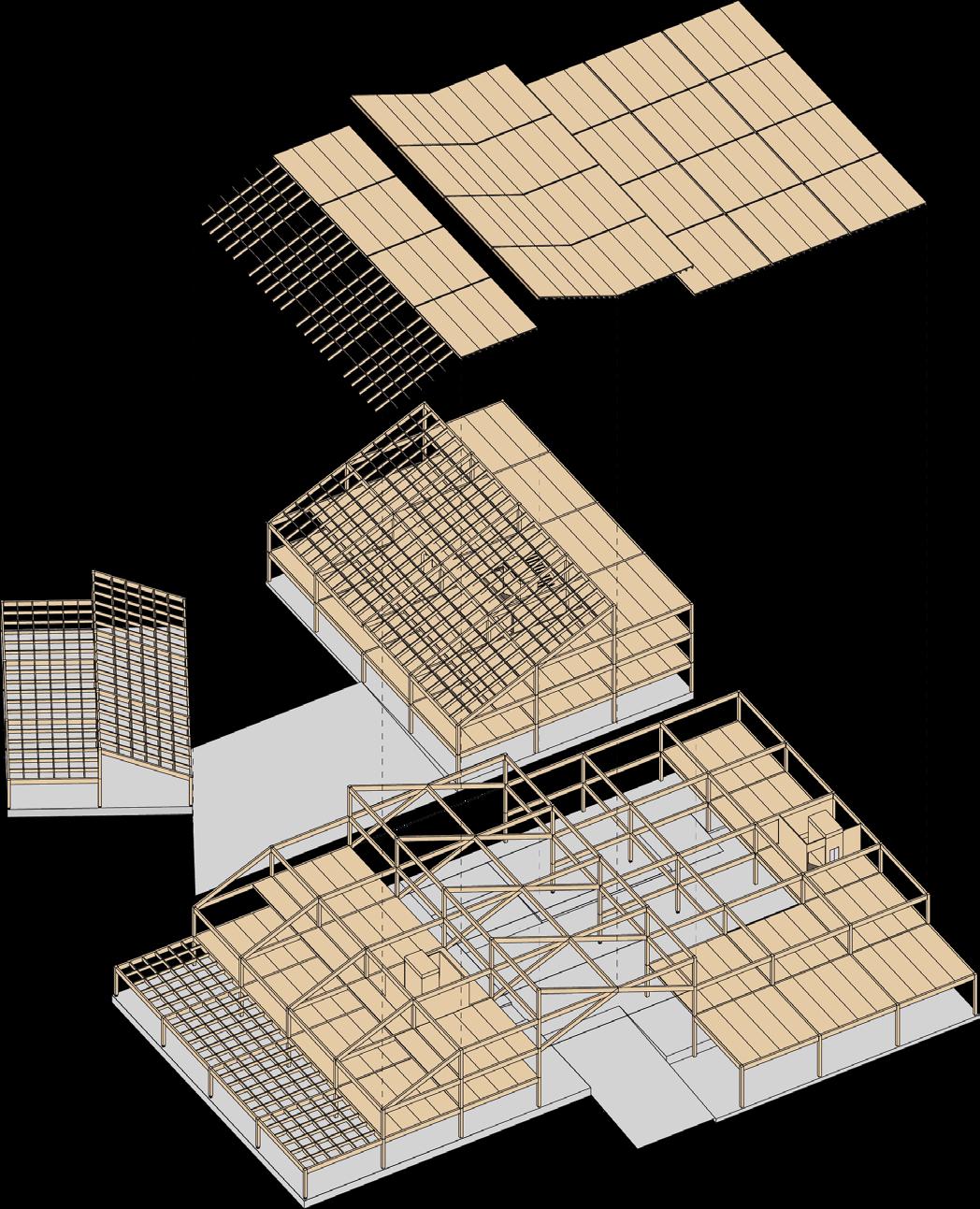
39 Non-domesticRIBA 2030 Challenge 2030 2025 2020 Pre - 2020 Sequestered carbon Embodied carbon over the lifecycle TOTAL Embodied Carbon: 162 kgCO2e/m2 TOTAL Including Sequestration: -8 kgCO2e/m2 500 650 800 1100 LIFECYCLE EMBODIEDCARBON-144 -25 91 61 InternalSuperstructureSubstructure-200-150-100-500501001509UpperfloorsRoofExternalwallsWindowsInternalwallsfinishesServiceskgCO2e/m2 Carbon calculation with FCBS Carbon Tool for Case 3 Carbon conversion source: https://www.epa.gov/energy/greenhouse-gas-equivalencies-calcu lator. Source: FCBS Carbon Tool Axonometry of Case 3 Source: FCBS Carbon Tool
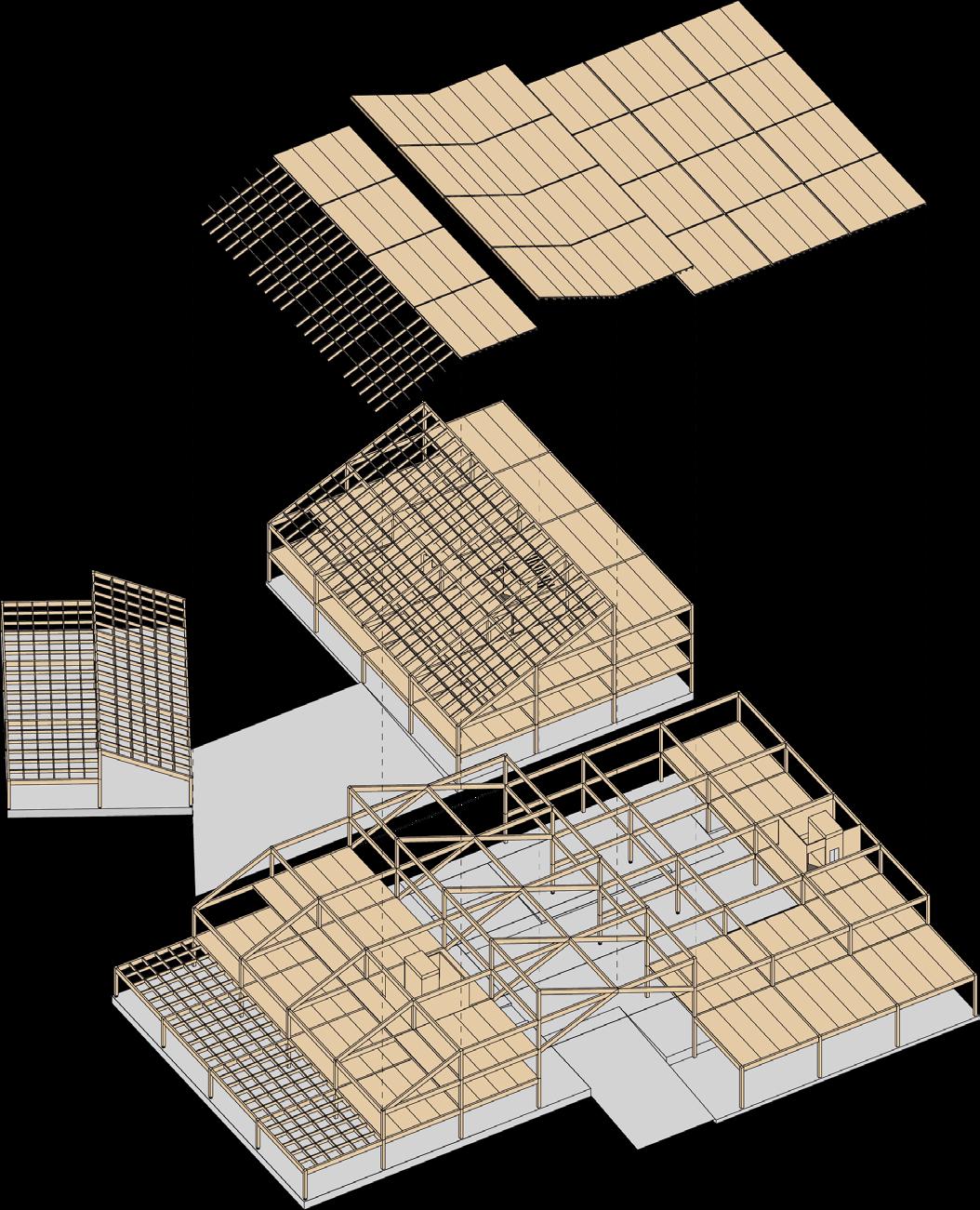
Case 3 maximizes the use of timber by adding CLT panels to the façade struc ture and using exclusively rib panels for the decks instead of a girder grid. This structure sequesters the equivalent to the annual energy consumption of 262 households, with a negative carbon balance of -122 tons of carbon emissions. Its timber volume represents 0.05 percent of the total Swedish sawn timber imported to the UK, and with that sawn timber the structure could be built about 798 times. Its timber volume equals about 1321 logged trees, and 2.7 forest hectares would be necessary to offset the structure’s carbon emissions. Source:by
CASE 3 TIMBER TRANSPORTATIONVOLUME TO SITE BUILDING SEQUESTEREDTIME CARBON CARBON BALANCE POSITIVE NEGATIVE NEGATIVE CASE 2CASE 1 Diagramatic comparison of all three alternatives
40
the author
41 03 I 3 Comparative
In terms of carbon sequestration, case 3 would be the best since it represents the maximum amount of carbon storage within the three possibilities. However, it clearly uses more material than is needed, generating avoidable embodied carbon emissions, and using more timber that could otherwise be used elsewhere. In addition, more material means also more transportation and more trucks from the manufacturing plant to the building site, generating more emissions and increasing the project budget. The budget of the project would also be increased by the larger volume of unnecessary timber itself.
Consequently, case 2 would be the most suitable once everything considered. It is a carbon negative structure that uses the necessary timber for its program structural demands and optimizes connections and construction times. Its consequences in the forest are measured, allowing for a controlled harvest and forest regrow.
It is also important to note that given that UK’s population is expected to grow from 67.2 inhabitants to 69.2 in 203015, and nowadays the retail space rate per inhabitant is 1,1 m2, this building would claim only 0,3 percent of the needed retail space increase16.
Finally, maybe the most important thing to note is the substitution effect: by using mass timber instead of carbon-intensive materials, each of these structures is avoiding a thousand tons of carbon emissions that a concrete building would generate. That is the equivalent of 200 households’ electricity consumption for one year. By using the moderate solution which is optimized to the structural demands and other require-
For case 1, the complexity of the dimensions of the girder grid would claim more working hours and more transportation, since it would probably be necessary to divide the grid into parts that would be later assembled on site. This would also mean more steel connections. In addition, in this case the structure is not carbon neutral, due to the slender dimensions of the timber elements in comparison to the extensive concrete slab. Finally, it would present more challenges than the other two alternatives in case of fire, most likely requiring more gypsum or cement based linings for floor and wall assemblies which have high embodied carbon.
42 EQUIVALENCIES CASE 1 CASE 2 CASE 3 TIMBER VOLUME (m3) 1000 1625 1810 EMBODIED CARBON* (TCO2e) 975 1155 1226 CARBON SEQUESTRATION*(TCO2e) 703 1212 1348 EQUIVALENT CO2E** 137 homes236 homes262 homes in yearly home energy concumption CARBON BALANCE (TCO2e) 272 -57 -122 SHARE OF IMPORTED TIMBER 0,03 0,045 0,05 TIMES THE STRUCTURE COULD BE BUILT WITH THE 1444 889 798 IMPORTED TIMBER NUMBER OF TREES 730 1186 1321 FOREST HA NECESSARY TO OFFSET THE STRUCTURE'S 2,2 2,6 2,7 EMISSIONS (ha)* Data summary total Swedish production total 2021 19000000 m3 imported timber UK 19% 3610000 m3 production yield 40% of sawn wood 1444000 m3 tree factor 1,37factor used in Cases course retail space rate UK (m2/person) 1,1 building surface 7170 m2 estimated future retail (m2) 22000002 million population increase UK * Data from the FCBS Carbon Tool results **Data from https://www.epa.gov/energy/greenhouse-gas-equivalencies-calculator FIG 9. Comparative table with results for all three cases Source: by the author requirements mentioned, the available timber can be used on as many construction pro jects as possible, providing the greatest substitution benefit that is achievable.
43 CONCLUSIONS# 04
The different case scenarios presented serve to illustrate that a building is not just a finite product without further consequences. It is carbon emissions; it is new landscapes of production that are created. In the case of mass timber, it is also seques tered carbon, it is felled trees in the forest and, if it is a sustainably managed forest, new trees that will grow, expand the forest area and sequester new carbon from the atmo sphere. It is imperative that architects stop ignoring the consequences that construc tion has to the environment and assume our share of responsibility for carbon emissions. A circular approach to building, first refurbishing and reusing when possible, and building sustainably when needed, accounting for the whole value chain of the building process, should be the aim of architectural practices around the globe. With mass timber pro ducts, we have the possibility to integrate our landscapes of production to our urban landscapes in a mutually beneficial relationship that can help us reconnect not only with nature but also with other disciplines within the construction sector.
44 04 I Conclusions
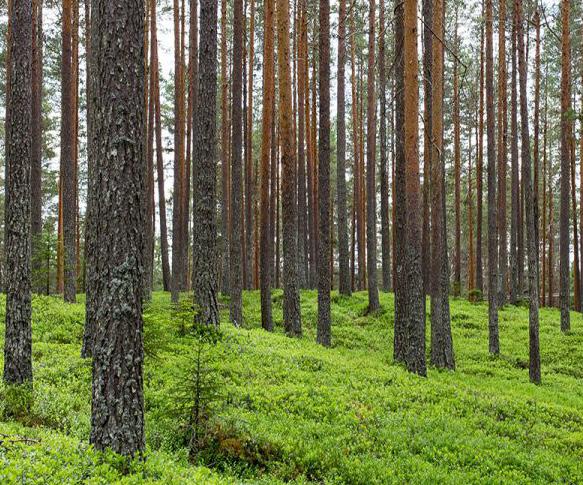
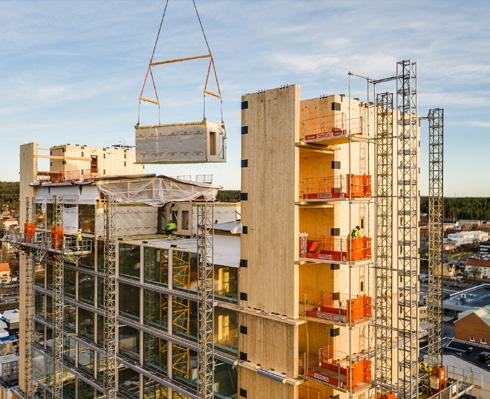
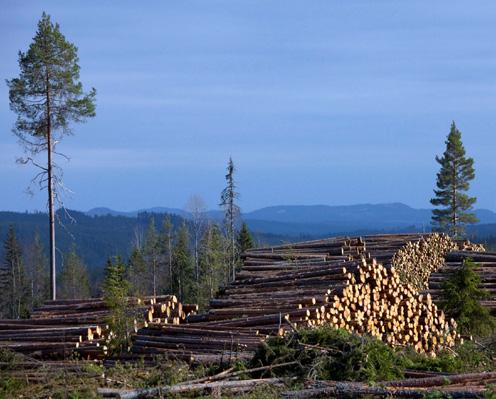
45 FIG 10. SUSTAINABLE FOREST MANAGEMENT CYCLES INTEGRATED IN URBAN DEVELOPMENT
Source: by the author Mass timber construction is being embraced by many architects and real estate compa nies around the world. Its benefits as a lightweight, renewable building material with high structural performance, easy prefabrication, shorter construction times, and the capacity of timber to store carbon throughout its life are frequently touted. But we also need to start talking about the forest and the beneficial exchange that timber construction can be for cities and forests, to deploy the full potential of this necessary shift of the building industry and help with the decarbonization of the built environment.
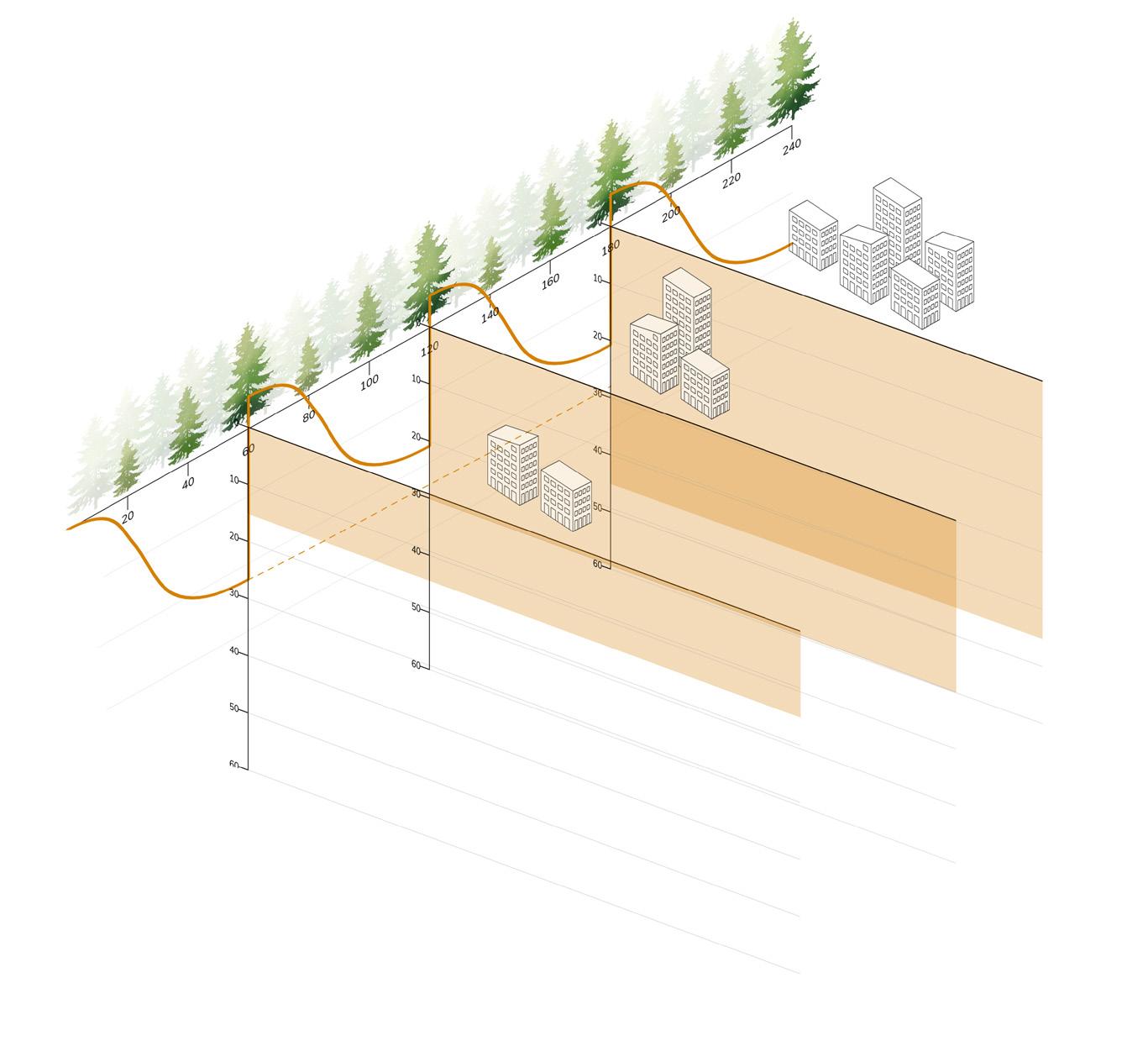
46 BIBLIOGRAPHY# 05
12. Swedish Forest Industries. https://www.forestindustries.se/siteassets/dokument/fakta-nyckeltal/fact-and-key-figu res-2022-.pdf. Accessed July 2022 13. Skogsstyrelsen. https://www.skogsstyrelsen.se/lag-och-tillsyn/skogsvardslagen/. Accessed July 2022; and also htt ps://www.skogskunskap.se/skota-barrskog/slutavverka/slutavverkningens-grunder/lagen-och-slutavverkning/ , acces sed July 2022 14. See Links to calculation tools below (6.9%25%20increase). Accessed July 2022 15. Office for National Statistics website. https://www.ons.gov.uk/peoplepopulationandcommunity/populationand to%20Euromonitor%2C%20the%20UK,European%20average%20of%201.14m2.16.lation,million%20(6.9%25%20increase).migration/populationprojections/bulletins/nationalpopulationprojections/2020basedinterim#:~:text=3.-,UK%20popuAccessedJuly2022PatelMiller.https://www.patelmiller.com/single-post/2017/04/04/is-uk-retail-over-spaced#:~:text=According%20AccessedJuly2022
47 Cited Quote from Kiel Moe in conference Climate change, architecture change: 1.https://www.youtube.com/watch?v=iomFhd3Mmj8NRCD(NaturalResourcesDefenseCouncil) website. 11.Jane10.Jane9.diversity8.7.Kiel6.events/pdf/other/21/21June_FINAL%20PRESS%20RELEASE_WPP17.pdf.5.sed4.3.2.text=The%20main%20gases%20responsible%20for,gases%20(which%20are%20synthetic),https://www.nrdc.org/stories/greenhouse-effect-101#:~:accessedJuly2022IdemBritannica.https://www.britannica.com/science/greenhouse-gas.AccessedJuly2022Archdaily.https://www.archdaily.com/974165/steps-taken-in-2021-towards-decarbonizing-the-aec-sector.AccesJuly2022UNwebsite–SustainableDevelopmentGoalsPressRelease.https://www.un.org/en/development/desa/population/AccessedJuly2022MattiKuittinen,“Carbon”,inWoodUrbanism.FromtheMoleculartotheTerritorial,ed.DanielIbáñez,JaneHuttonandMoe(ActarPublishers2019)92-97WaughThistletonArchitectsthroughThinkWood,100ProjectsUKCLT,2018ChadwickDearingOliver,NedalT.,Nassar,BruceR.Lippke&JamesB.McCarter(2014)Carbon,FossilFues,andBioMitigationWithWoodandForests,JournalofSustainableForestry,33:3,248-275AlanOrganschi,“TheCarbonTransect”ininWoodUrbanism.FromtheMoleculartotheTerritorial,ed.DanielIbáñez,HuttonandKielMoe(ActarPublishers2019)112-127KielMoe,“CarbonandTimberBuilding”,inWoodUrbanism.FromtheMoleculartotheTerritorial,ed.DanielIbáñez,HuttonandKielMoe(ActarPublishers2019)84-89Idem
https://www.architectmagazine.com/design/firm-profile/ultramoderne_o
https://www.epa.gov/energy/greenhouse-gas-equivalencies-calculatorhttps://fcbstudios.com/fcbscarbon
Links to reference projects Spekulatus
48
Also consulted Churkina, G., Organschi, A., Reyer, C.P.O. et al. Buildings as a global carbon sink. Nat Sustain 3, 269–276 (2020). https:// Hurmekoski,doi.org/10.1038/s41893-019-0462-4Elias,“Howcanwoodconstruction
reduce environmental degradation?” European Forest Institute, 2017 Timber Development UK, “Assessing the carbon-related impacts and benefits of timber in construction products and buildings”, Technical Paper, November 2021
https://vimeo.com/189798419Ultramoderne
https://grayorganschi.com/research/initiatives/timber_cityhttp://timbercity.org/Timberhttps://andersberenssonarchitects.com/work/bank-of-norrland/Bankus-is-solid-timber-tower/10156585611437706/https://nenp.facebook.com/architecturemodels/posts/ultramodernespekulatiusultramodernedescription-spekulatiofNorrlandCity(GrayOrganschiArchitecture)
Links to calculation tools
I would like to express my gratitude to all faculty members of the first edition of the Master in Mass Timber Design, and special thanks to Rebecca Sawcer, associate architect at Waugh Thistleton Architects, for her help as thesis mentor.
49
Acknowledgements
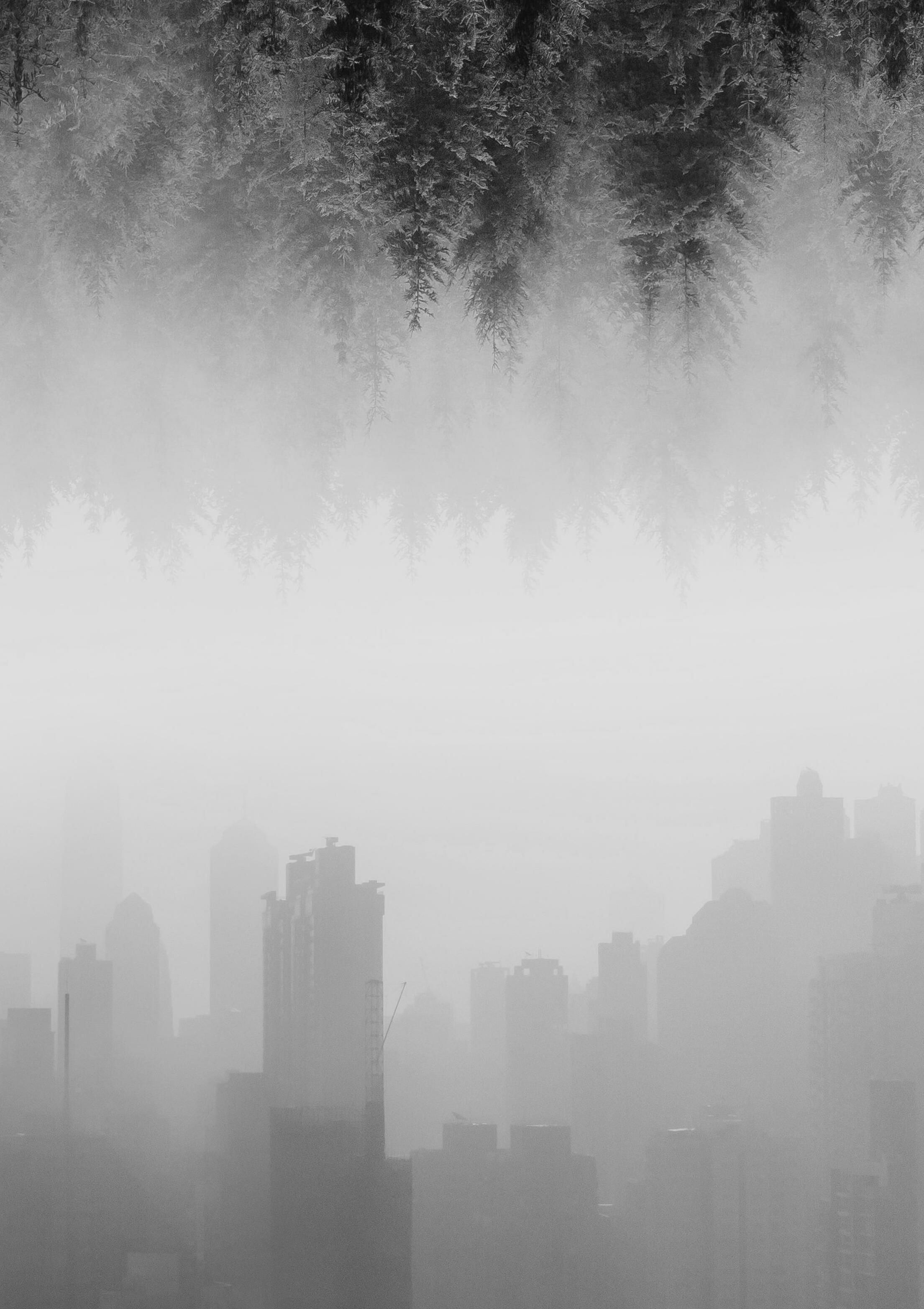