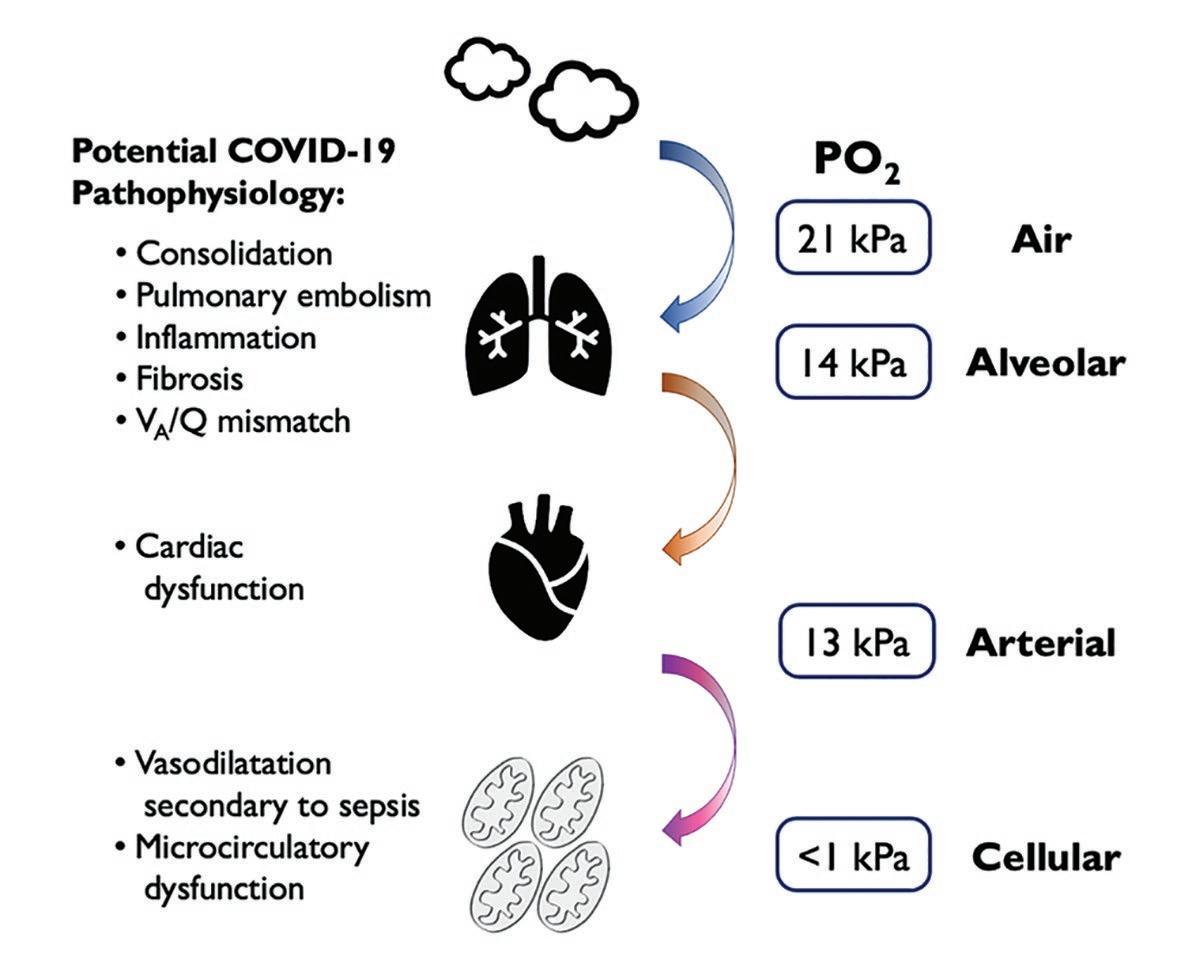
13 minute read
The Role of Blood Gas Analysis in the Management
from Improving the Diagnosis and Monitoring of Critically ill Patients and the Role of Blood Gas Testing
The Role of Blood Gas Analysis in the Management of Patients with Severe COVID-19
Professor Daniel Martin OBE, Intensive Care Consultant, Royal Free Hospital, London, UK
Advanced blood gas analysers can support the monitoring and management of COVID-19 patients.
Introduction
Airborne infectious diseases are a constant threat to society. Transmissible via the air in droplet or aerosol form, and through contact with contaminated surfaces, they primarily affect the respiratory tract leading to a range of illnesses with varying severity. In 2019, a novel coronavirus named severe acute respiratory syndrome coronavirus 2 (SARS-CoV-2) emerged, spreading rapidly to cause coronavirus disease 2019 (COVID-19) and eventually resulted in a pandemic that changed the world. To date, 14.8 million people have tested positive for the virus, and over 600,000 are known to have died from COVID-19 38 . These figures are likely to be underestimates of the total disease burden and will have increased by the time this report is published. Approximately 80% of people who are affected by COVID-19 develop mild symptoms (most commonly a fever, persistent cough, and shortness of breath), which requires no specific treatment. Around 15% of those infected with the virus will develop severe acute respiratory failure with breathlessness and hypoxaemia, requiring hospital admission for the administration of oxygen 1 . If giving oxygen by simple measures such as a nasal cannula or face mask fails to correct the hypoxaemia then admission to an intensive care unit (ICU) may be required. This occurs in around 5% of those infected, and most will go on to require mechanical ventilation via an endotracheal tube 2-4 . Unfortunately, the mortality of those admitted to the ICU is approximately 50% 5,6 . Older people, males, and those with diabetes, obesity, and hypertension appear to be prone to a more severe form of the infection. In the absence of a definitive treatment for COVID-19, supporting respiratory function is the cornerstone of the current strategy to promote survival in patients with COVID-19. Optimum application of all of the various modalities available to improve oxygenation in hospitalised patients relies on rapid, accurate, and reliable measurement of arterial oxygenation by point-of-care test (POCT) arterial blood gas (ABG) analysis to titrate to the required physiological target.
COVID-19 and Oxygenation
At the cellular level, oxygen is essential for the preservation of energy production via oxidative phosphorylation. When oxygen is in short supply, normal cellular function deteriorates and ultimately fails altogether. A continuous supply of oxygen must be maintained along a pathway known as the oxygen cascade (Figure 1). The cascade describes the journey taken by oxygen from the air, along the respiratory tract, into the blood, and then via the circulatory system to the tissues that use it. Oxygen transport can be impaired by pathology at any point along this pathway. COVID-19 primarily affects gas exchange at the interface between the respiratory and cardiovascular systems, where gaseous oxygen and carbon dioxide are exchanged at the alveolar membrane. Of the five commonly described physiological causes of hypoxaemia (Table 1), ventilation-perfusion (VA/Q) mismatching is likely to be the dominant factor in COVID-19. Commonly, patients present to the hospital with rapid, shallow breathing, but often without the other classic signs of respiratory distress, such as increased work of breathing. The typical picture is a type-1 respiratory failure with severe hypoxaemia but a normal (or low) arterial partial pressure of carbon dioxide (PaCO 2 ). Chest X-ray and computed tomography (CT) scanning of the lungs tends to show patchy areas of consolidation throughout both lungs and widespread groundApproximately 80% of people who are affected by COVID-19 develop mild symptoms (most commonly a fever, persistent cough, and shortness of breath), which requires no specific treatment
Table 1. The relationship between alveolar–arterial oxygen partial pressure difference and physiological causes of hypoxaemia
(Patho-)physiology
Hypoventilation Low inspired oxygen tension Right-to-left shunt (pulmonary or cardiac) Ventilation–perfusion mismatch Impaired diffusion
A-aPO 2 : Alveolar-arterial partial pressure difference
glass opacities 7 . Perfused alveolar units that are consolidated or contain pulmonary oedema reduce the VA/Q ratio, resulting in a right to left intra-pulmonary shunt. In addition to this, a high incidence of pulmonary embolism has been noted in patients with COVID-19. This further exacerbates hypoxaemia by creating areas in which ventilated alveolar units are not perfused, increasing the physiological dead space, resulting in a high VA/Q ratio. Thus, in COVID-19, different pathophysiological processes are interacting to create severe and sustained hypoxaemia and progressive hypercapnia that can be challenging to treat (Figure 1).
Assessment and Monitoring of the Hypoxaemic Critically Ill Patient
While pulse oximetry is the mainstay of monitoring systemic oxygenation in mild to moderate disease, as hypoxaemia worsens, ABG analysis becomes a critical component of patient management and decision-making. It is also useful to remember that oximetry saturation (SpO 2 ) is error-prone and may differ from the arterial oxygen saturation
A-aPO 2
(SaO 2 ) measured by the co-oximeter of an ABG system 8 . ABG analysis is essential in patients with respiratory failure to assess the severity of their condition, determine its cause, and monitor the effectiveness of treatments. One of the first things to determine in a hypoxaemic patient is whether they have type-1 (hypoxaemic) or type-2 (hypercapnic) respiratory failure, which requires measurement of PaCO 2 levels. In the majority of patients with early COVID-19 related respiratory failure, PaCO 2 is either normal or low (type-1), the latter likely due to the hypoxic ventilatory response triggering hyperventilation.
Knowledge of the PaO 2 value allows clinicians to make a more comprehensive assessment of the severity of hypoxaemia than can be made from SpO 2 alone. The term hypoxaemia has no strict definition other than a level of arterial oxygen that is lower than ‘normal’. The normal PaO 2 in a healthy individual is generally accepted to be between 10.7-13.3 kPa. Quantification of the severity of respiratory failure needs to take into account the concentration of oxygen a patient is breathing, and this is usually expressed as the P/F (PaO 2 :F i O 2 ) ratio. P/F ratio is used as part of the diagnostic criteria for acute respiratory distress syndrome (ARDS), with 300 mmHg (40 kPa) being the current threshold for diagnosis (Table 2) 9 . The alveolar-arterial (A–a) oxygen partial pressure difference can also be calculated using ABG data. This may help in elucidating the cause of hypoxaemia (Table 1); however, the alveolar gas equation must be used to estimate the alveolar partial pressure of oxygen (PaO 2 ) as it cannot be easily measured (Equation 1).
Table 2. Severity of acute respiratory distress syndrome as determined by P/F ratio 9
ARDS Severity
Mild
Moderate
Severe
PaO 2 /F i O 2
200-300 mmHg 100-200 mmHg < 100 mmHg
Values are relevant for patients with a PEEP of ≥ 5 cmH 2 O PaO 2 /F i O 2 : ratio of arterial partial pressure of oxygen to fractional inspired oxygen concentration; PEEP: positive end-expiratory pressure
Equation 1. Calculation of the alveolar partial pressure of oxygen using the alveolar gas equation
PaO 2 = P i O 2 - PaCO 2 x (F i O 2 /RQ) P i O 2 = F i O 2 (PB - PH 2 O)
PaO 2 : P i O 2 : PaCO 2 : F i O 2 : RQ :
PB : alveolar partial pressure of oxygen Inspired partial pressure of oxygen arterial partial pressure of carbon dioxide Fractional inspired oxygen concentration respiratory quotient (0.8 in healthy individuals) barometric pressure
Respiratory Support for Patients with COVID-19
Correct interpretation of ABG data facilitates the appropriate selection of treatment modality for an individual patient with COVID-19. Mild hypoxaemia may be managed with the administration of oxygen via simple nasal cannula, while a higher requirement for oxygen will require either a variable or fixed performance oxygen mask. If these modalities prove inadequate, non-invasive ventilation can be considered in one of two formats. The first is continuous positive airway pressure (CPAP) ventilation; this may be of benefit in early type-1 respiratory failure as the maintenance of positive pressure at the end of expiration acts to prevent alveolar collapse. This, in turn, reduces VA/Q mismatching, improves lung compliance, and reduces the work of breathing. The second is bilevel positive airway pressure (BiPAP); it is appropriate for patients with type-2 respiratory failure, where the delivery of additional pressure during inspiration should increase minute ventilation and reduce PaCO 2 . These non-invasive modes of ventilation may allow the correction of hypoxaemia without the need for escalation to intubation and mechanical ventilation. CPAP, in particular, has been used extensively in patients with COVID-19, and strategies for its use have been outlined 10 . Alongside clinical signs, ABG analysis can help to signal when non-invasive ventilation is failing, and intubation and mechanical ventilation are required. The PaCO 2 will begin to rise, and PaO 2 will fall further as patient fatigue and respiratory failure worsens.
The decision to intubate is a complex and multi-factorial one based on ABG results, clinical signs, disease trajectory, and the likelihood of a long-term successful outcome. Once intubated, frequent ABG analysis is essential for the titration of positive end-airway pressure (PEEP), respiratory rate, and F i O 2 . Traditionally in ARDS, PEEP was set according to F i O 2 , as outlined in the ARDSnet guidelines 11 . However, it has been noted that such high levels of PEEP may be disadvantageous in patients with COVID-19, as lung compliance appears to be better than was expected 12 . This means that a more individualised approach to setting PEEP is required, taking into account lung compliance measurements and the gas exchange response to a range of levels of PEEP. ABG analysis also helps to determine the mode of ventilation best suited for an individual patient. Airway pressure release ventilation (APRV), an inverse ratio pressurecontrolled mode of ventilation that permits unrestricted spontaneous breathing, is thought to promote alveolar recruitment and therefore improve oxygenation 13 . It does, however, require careful titration of its settings against ABGs to avoid excessive hypercapnia and assess the effectiveness of the recruitment process.
After the acute phase of illness has subsided, active weaning from mechanical ventilation will be required. This can be a lengthy process if pulmonary inflammation develops into fibrosis, if patients develop critical illness polyneuropathy (a form of severe weakness that follows critical illness) or if there is severe cardiac dysfunction. In those patients for whom extubation has not been possible after approximately two weeks, the formation of a tracheostomy will be necessary to aid ventilatory weaning. Complete separation from mechanical ventilation may take many weeks for some patients, and the risk of recurrent bacterial ventilator acquired pneumonia is high. Throughout this time, weaning plans will need to be adjusted according to the patient’s process, and ABG analysis is a key component in the judgement of daily success.
Blood Gas Systems: More Than Just Blood Gases
Modern blood gas analysers provide much more than just pH and gas exchange measurements. Also, they have an ever-increasing array of additional biochemical information (Figure 2). Two of the most frequent and arguably most important of these during the COVID-19 pandemic were glucose and lactate. With almost 20% of hospitalised COVID-19 patients having a diagnosis of diabetes 14 , frequent monitoring of blood glucose became a key component of their ICU management. Presentation with diabetic ketoacidosis (DKA) and hyperosmolar hyperglycaemic syndrome (HHS) was also
Figure 2. POC RAPIDPoint 500e System Touchscreen
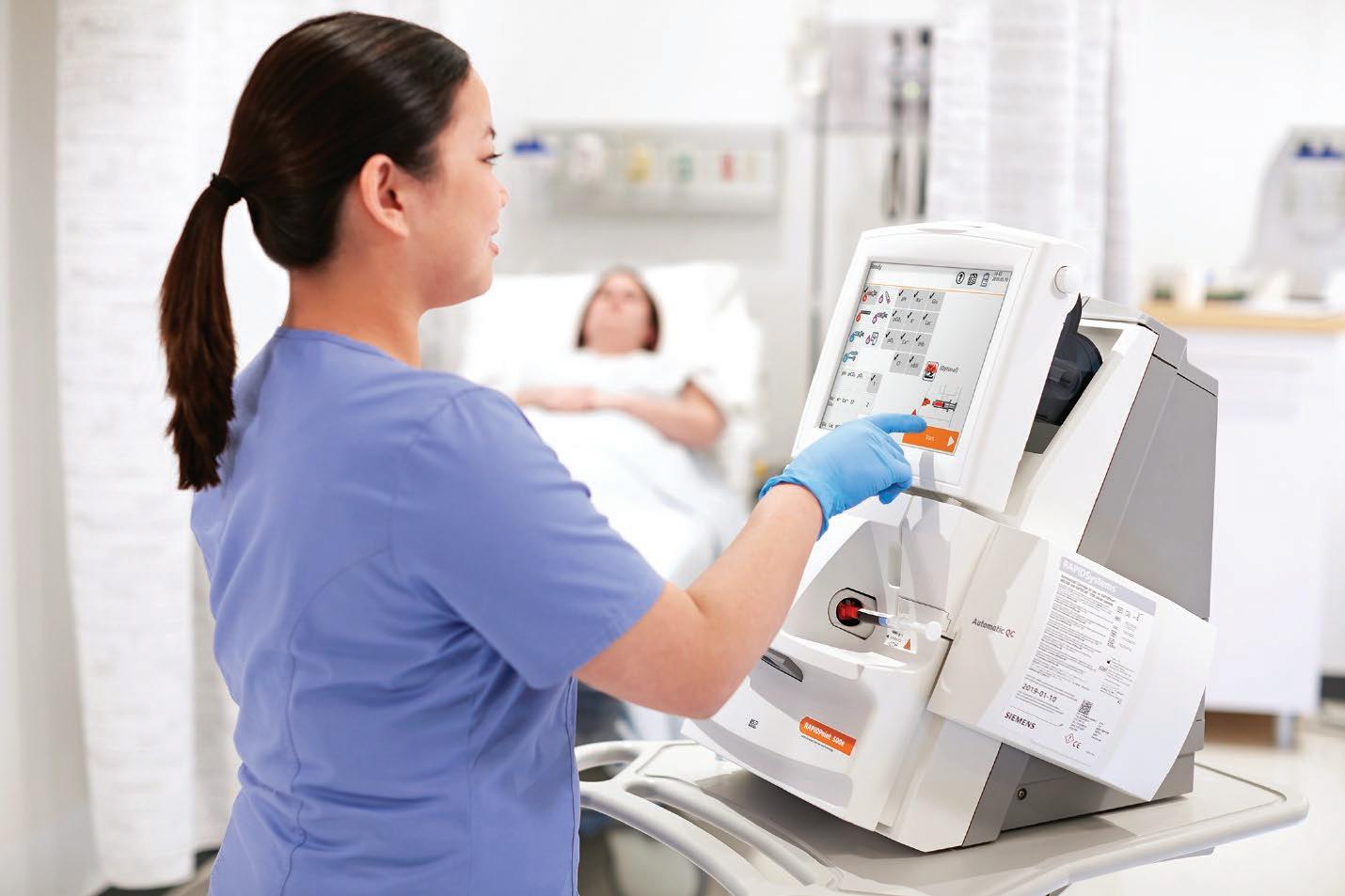
frequently observed, both of which require frequent blood gas analysis to avoid harm. The calculation of base deficit and anion gap help differentiate the causes of acidaemia. The measurement of blood lactate has become an integral component of the assessment of patients with sepsis, particularly in the critically ill 15 . In patients with COVID-19, a rising lactate is likely to signify organ hypoperfusion due to low cardiac output, meaning the need for intravenous fluid resuscitation and potentially the need for vasopressor or inotropic support. Other measurements key to the successful management of critically ill patients include haemoglobin concentration, sodium, potassium, magnesium, and creatinine. POCT analysis of all of these additional measurements will speed up diagnoses and facilitate a more rapid implementation of life-saving interventions.
Point of Care Testing During a Pandemic
The delivery of precision care for critically ill patients with COVID-19 would be extremely challenging without access to POCT blood gas analysis. Every hospital must decide the POCT strategy that works for their specific setting and consider the strengths and weaknesses of different devices available on the market. Key criteria for devices that can deliver POCT blood gas analysis are summarised in Table 3. All of these factors need to be considered when selecting instruments for different areas of a hospital, along with the training required for staff who will use them. A blood gas instrument should no longer be thought of as a stand-alone device. If integrated into a hospital’s information technology network, it can transform patient care. Minimising variation of the systems across a hospital will
Table 3. Properties of an ideal point of care blood gas analysis system
Property
Accuracy
Accessibility
Reliability
Robustness
Security and connectivity
Support
Features
Sample integrity must be core to the design of the system to ensure test results must reflect those produced in a standard laboratory setting. The system needs to be able to perform meticulous quality checks in the background, at every stage of analysis.
The system must have operational simplicity. Devices must be close to the bedside and in sufficient numbers to reflect the workload of the area.
The system must be able to cope with high numbers of samples without affecting performance.
Systems need to be able to withstand the rigorous environments found in some clinical settings.
There is a need for heightened cyber security and data connectivity in modern data enabled healthcare services. Barcode reading sensors save time and reduce errors*. Integration with hospital information technology systems is essential.
Servicing, repairs, assistance and supplies are crucial components to a well-functioning system.
Figure 3. POC RAPIDPoint 500e Barcode Scanning of Syringe
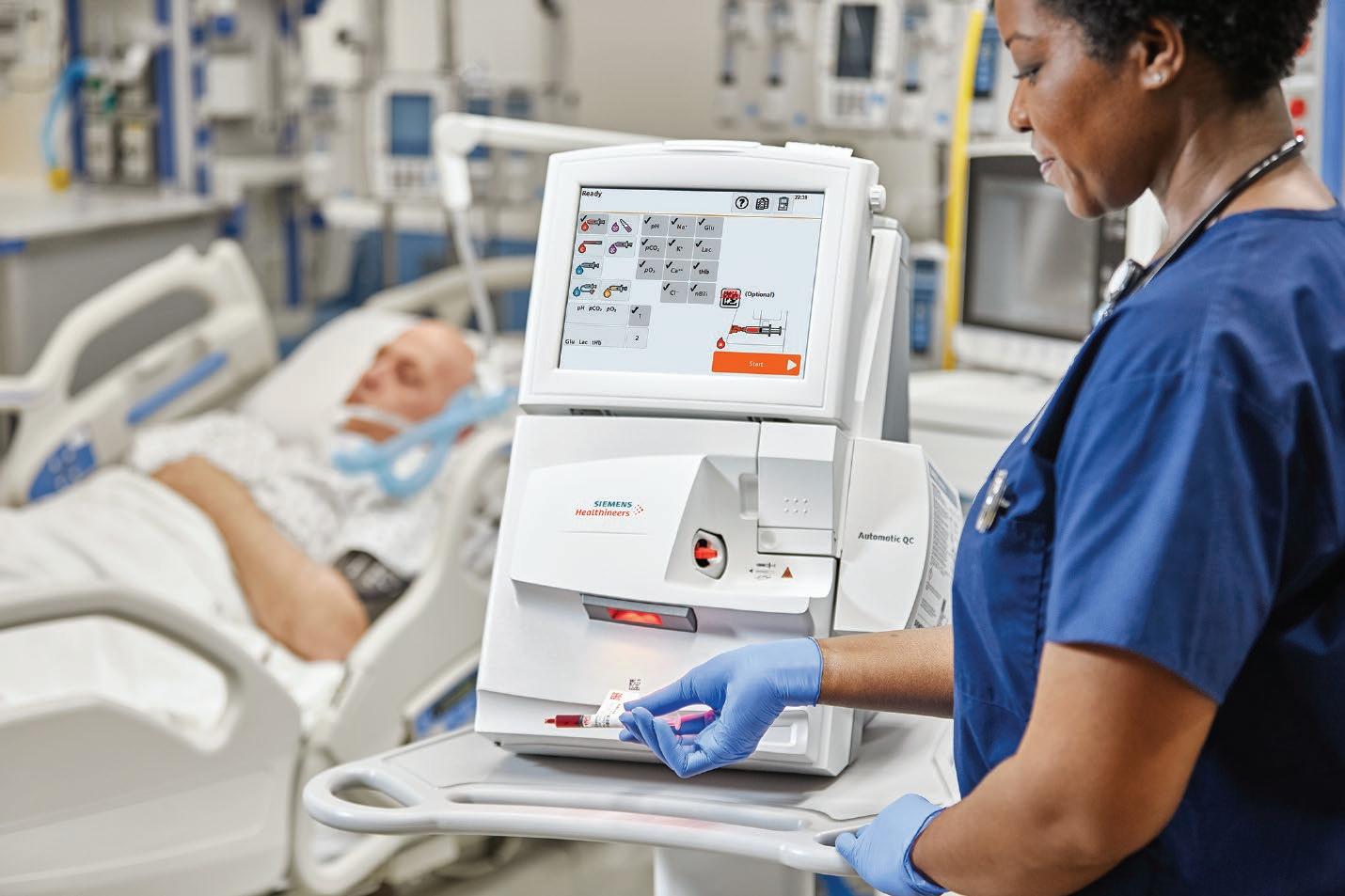
Table 4. Potential advantages to using hand-held POCT blood gas analysers in a pandemic
Immediate bedside access to testing saves vital time in life-threatening situations Devices are easily and quickly decontaminated due to their size Simplicity of use as a result of cartridge-based technology Cartridges ensure no blood comes into contact with the internal workings of the device Multiple cartridge options provide a broad array of POCTs
help maintain a high level of competence and confidence amongst staff, particularly in times when teams may be working in unfamiliar environments. Many blood gas instruments now feature cartridge-based technology, reducing the need for high-level technical support. An increased capacity for POCT blood gas analysis may be required in certain areas of a hospital during a pandemic, so there needs to be a plan of which devices are moved into key areas when patient numbers begin to rise. One strategy adopted by some hospitals is to use hand-held POCT systems (Figure 4); the potential advantages are summarised in Table 4. Portable analysers offer flexibility that cannot be matched by larger benchtop systems, so they should be considered as part of any expansion plan during a pandemic. Also, by bringing the analysis device directly to the bedside, hand-held systems provide a solution to the infection-control issues surrounding the transportation of samples around a unit or hospital.
Conclusions
Over the last few decades, POCT analysis has revolutionised intensive care medicine and allowed clinicians to optimise patient care in real-time. COVID-19 has taught us many lessons
Figure 4. An example of a cartridge-based hand held blood gas analyser
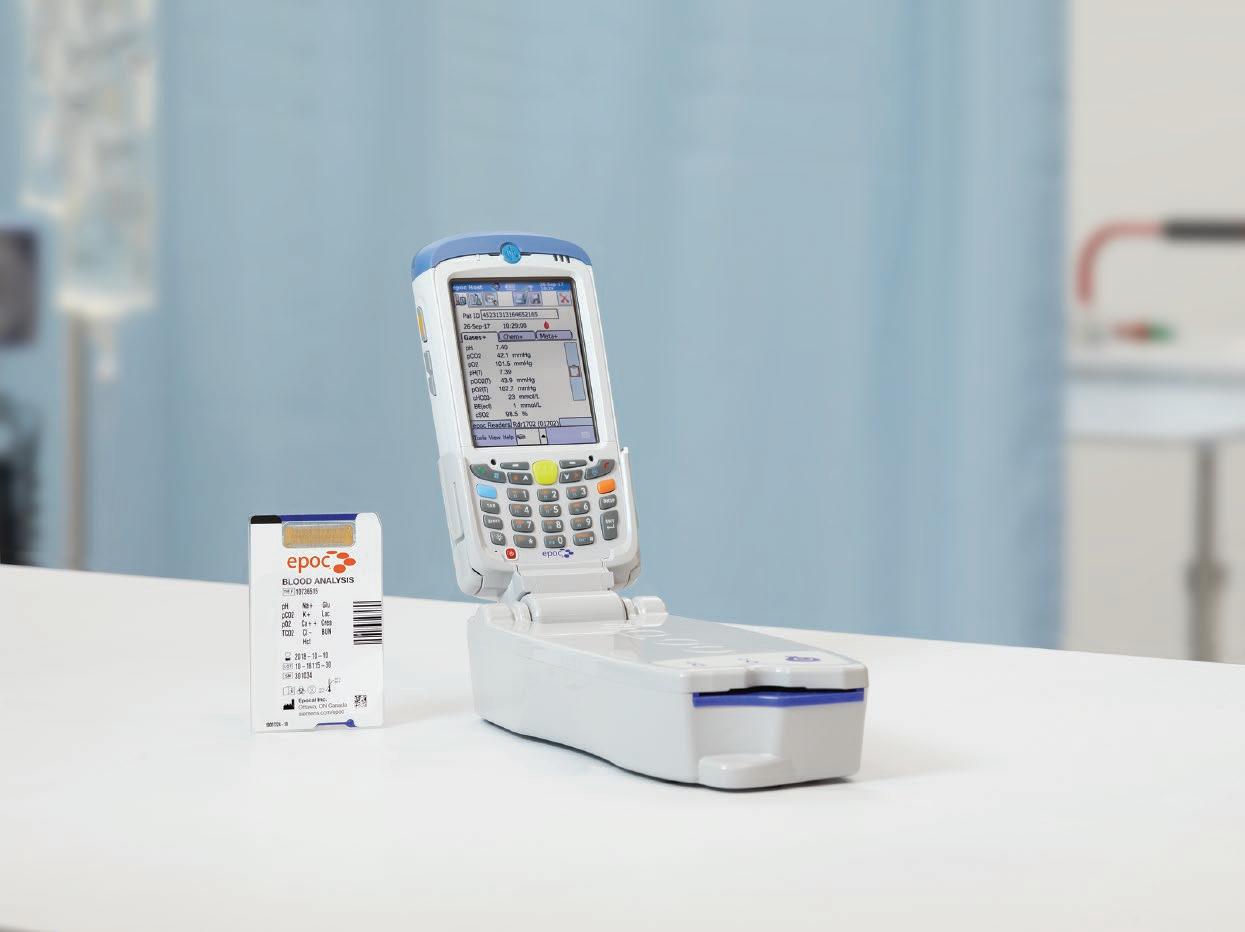
about pathophysiology and strategic planning, which will undoubtedly shape healthcare in the future. POCT blood gas analysis has proven to be an essential part of the treatment of hospitalised patients with COVID-19 and must be central to future pandemic planning. A blood gas instrument should no longer be thought of as a stand-alone device. If integrated into a hospital’s information technology network, it can transform patient care