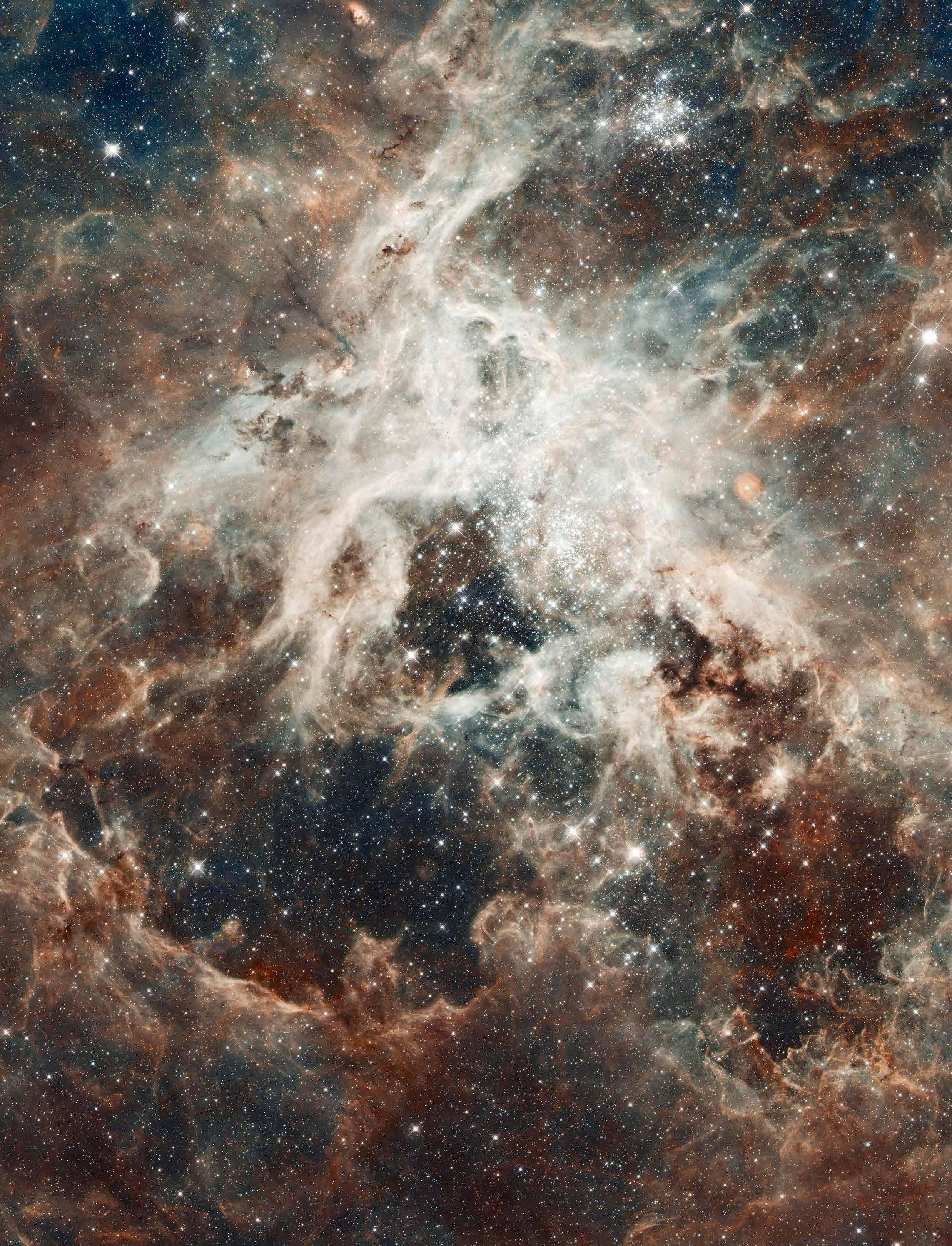
10 minute read
MAGALOPS
The magnetism of the Milky Way
The Galactic magnetic field exerts a strong influence on the formation of stars, motions of gas clouds, and the way cosmic rays travel through the Milky Way, yet much remains to be learned about its nature and extent. We spoke to Professor Marijke Haverkorn about the work of the MAGALOPS project in developing a next generation model of the Galactic magnetic field.
The Galactic magnetic field is by nature very difficult to observe, and the information that has been gathered is typically partial, relating just to specific components. While it has been established that the Galactic magnetic field has a largescale uniform component and a smallscale turbulence component, there are still many unanswered questions in this area. “We lack details on the characteristics of the components. What is the situation nearer to the Galactic centre? What does the Galactic magnetic field look like in the Milky Way’s halo?” asks Marijke Haverkorn, Associate Professor of Astrophysics at Radboud University in the Netherlands. This is a complex area of research, as magnetic fields don’t radiate light like stars, but rather influence whatever light comes through. “If a star emits light, then under certain circumstances that light is altered a little bit as it propagates by the Milky Way’s magnetic field,” explains Professor Haverkorn. “By studying changes in the polarisation of that light, we can gain new insights into how the magnetic field has influenced it.”
MAGALOPS project
This is a topic central to Professor Haverkorn’s work as the Principal Investigator of the MAGALOPS project, an ERC-backed initiative in which researchers aim to develop a nextgeneration model of the Galactic magnetic field. This involves the analysis of data from several sources. “We need very different types of measurements and different kinds of instruments to study the magnetic field of the Milky Way. The Milky Way is all around us, so ideally we would want observations over the whole sky,” outlines Professor Haverkorn. A survey of selected regions of the sky has already been conducted and the data is being analyzed, with plans for a survey of the whole sky, while Professor Haverkorn and her colleagues are also using distance data gathered from the Gaia satellite. “With the release of data from the Gaia satellite, we now know the distances to over a billion stars,” she explains. “By combining our polarimetry data with the distance data that the Gaia satellite gives us, we can learn more about the location of these stars and the interstellar medium between these stars and earth.”
From this point, it’s possible to identify which specific parts of the Milky Way that light has travelled through before it reaches
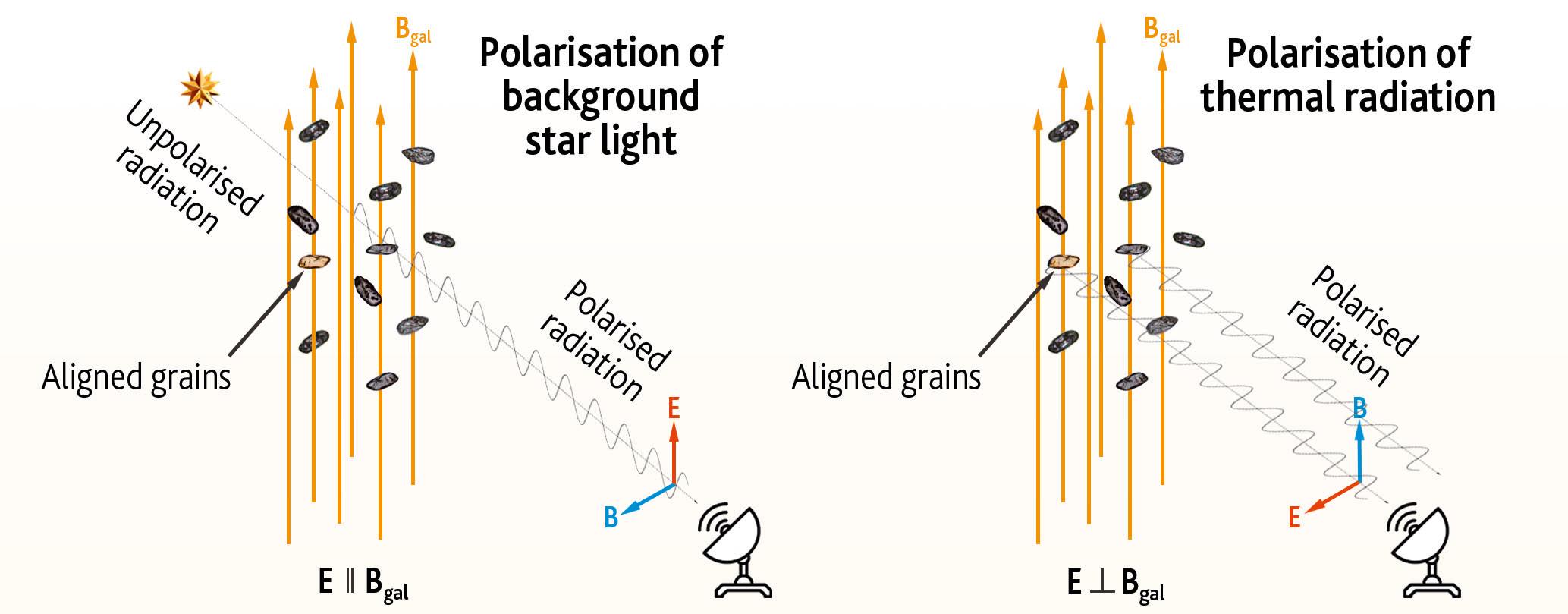
us on earth. By measuring the polarisation of relatively nearby stars, and comparing that to the polarisation of more distant stars, Professor Haverkorn hopes to gain new insights into the magnetic field in the space inbetween. “This is the innovative thing about this large data set. Traditionally, galactic magnetic fields are measured with radio polarisation,” she says. This meant that the observed polarised radiation represented an
integration of all the gas along the line-ofsight. “You would see polarisation and gas, but you wouldn’t know where along the line of sight it came from,” explains Professor Haverkorn. “This has always been a problem, but I think this data set will change that, in the sense that we now know the distances to the sources of this polarisation. So we will get a kind of 3-d image of the Galactic magnetic field for the first time.”
The polarisation evident from these data is largely inter-stellar, so caused by the Galactic magnetic field, yet there are also cases where polarisation is due to a star itself. Some
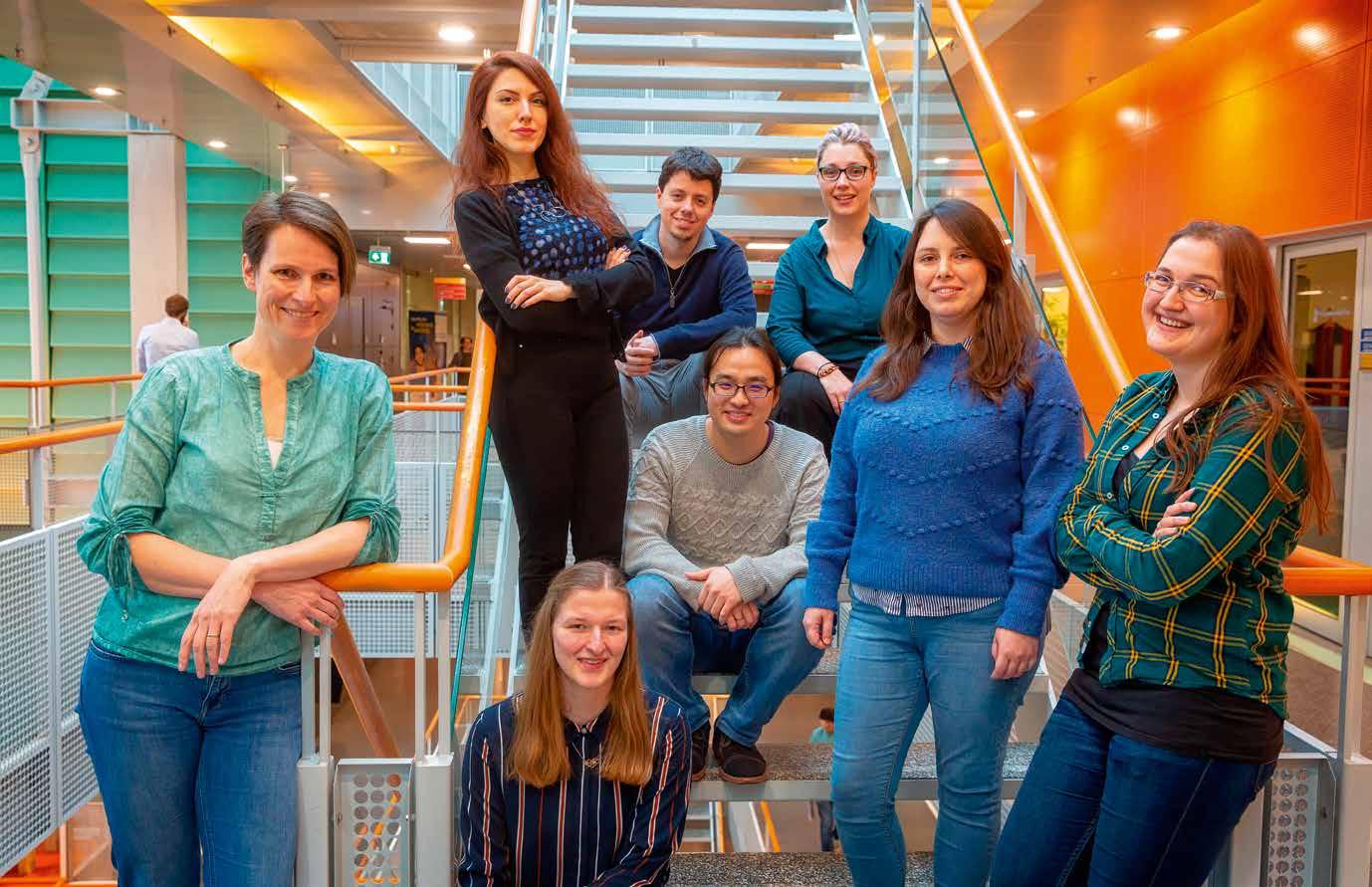
massive stars do have intrinsic polarisation, something which researchers are taking into account. “We expect a few of those stars in our data-set, and we have to be careful to distinguish them,” acknowledges Professor Haverkorn. The presence of dust grains in the Milky Way is another important consideration in the project’s research. “These dust grains are elongated and they spin. Their spin will automatically align with the magnetic field, so they will mostly spin in the same direction,” explains Professor Haverkorn. “If they all spin in the same direction, they will absorb more light polarised in one direction than light polarised in the other. This effect causes the polarisation from which we can measure the magnetic field. However, it means that the distribution and properties of the dust also influence the polarisation. Therefore, in order to measure the magnetic field, we also need to know how the dust is distributed. ”
A more detailed picture of dust distribution is correspondingly important to the wider goal of building a new model of the Galactic magnetic field. Data from the Gaia satellite is again invaluable in this respect, says Professor Haverkorn. “Much better models are available nowadays. The presence of dust leads to stars appearing much redder than they would intrinsically be, which can be observed. By combining these observations together with distance data from Gaia, people have tried to trace the 3-d dust distribution in the galaxy,” she explains. This approach gives a much more detailed picture of dust distributions than previously possible. “Dust distribution is fairly clumpy. Hot gas has been spewed out by supernovae explosions in some parts of the Milky Way for instance, and in that hot gas almost all the dust is destroyed. We cannot really see what is going on in the hot gas, because there is no dust,” continues Professor Haverkorn. “There’s also colder gas in the Milky Way. The very coldest bits of gas are the molecular clouds, where stars form.”
The Galactic magnetic field is of course enormously complex, and so it’s not realistic to expect that the available data can accurately determine every specific parameter in a model. Researchers in this project are using Bayesian methods to build
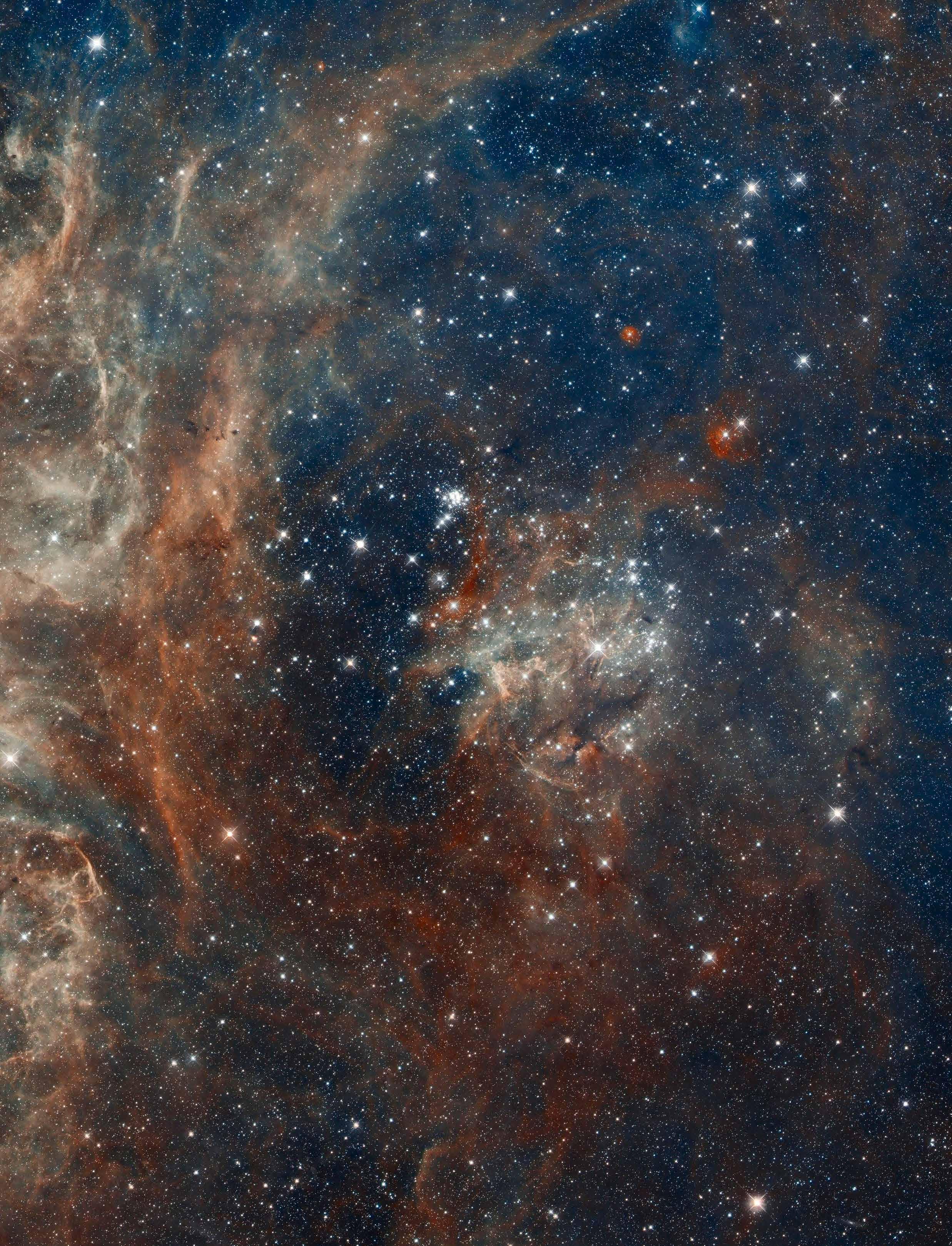
models, assess their quality and identify the most relevant parameters. “We can make a model of the Galactic magnetic field, and a model of a certain dust distribution, then calculate what kind of observables that would produce. If we had a star at a certain location in a model, what kind of polarisation would that star have? We can then re-tune the model,” outlines Professor Haverkorn. There are many different parameters to consider here; Professor Haverkorn says Bayesian methods play an important role in this respect. “Bayesian methods give you a kind of quantitative measure of not only what are the best-fit parameters, but also the uncertainty ranges involved,” she says. “For instance, if I put a Galactic magnetic field strength of say 3 μG (microGauss) into a model, then Bayesian methods will also tell you the impact if you change that to 3.5 μG.”
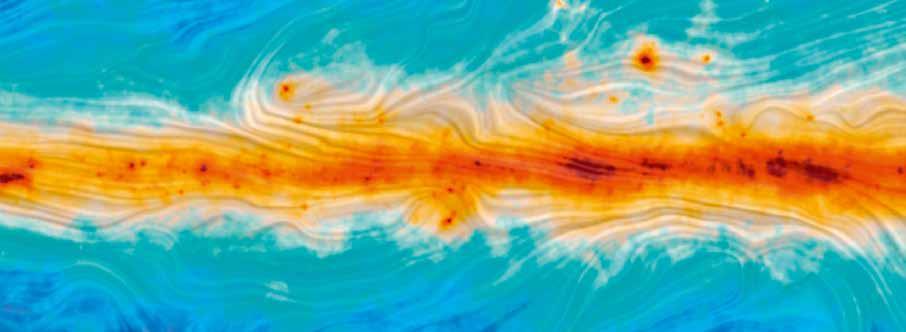
MAGALOPS The magnetic field of the Galaxy, from optical starlight polarization Project Objectives
This research will result in a next-generation Galactic magnetic field model that includes all observational tracers and can include current knowledge on this magnetic field as prior information. It will allow mapping out interstellar magnetized turbulence in the Galaxy, instead of providing averaged parameters only, and understanding the interplay between the local interstellar magnetic field, gas and dust. Its legacy is a 1000x increased stellar polarization catalog, an all-sky 3D dust model, a bayesian sampler for Galactic magnetic field models, and a preferred Galactic magnetic field model for use in cosmic ray modeling or foreground subtraction.
Project Funding
Funded under an ERC Consolidator Grant (ERC-2017-COG nr 772663) H2020-EU.1.1. - EXCELLENT SCIENCE - European Research Council (ERC)
Project Partners
* IAG/University of Saõ Paulo, Prof. Dr.
Antonio-Mario Magalhães * Instituto Nacional de Pesquisas Espaciais,
Dr. Claudia Vilega Rodrigues * IMAGINE collaboration
Contact Details
Marijke Haverkorn Department of Astrophysics Institute for Mathematics, Astrophysics and Particle Physics Radboud University PO Box 9010 6500 GL Nijmegen The Netherlands T: +31 24 365 2809 E: m.haverkorn@astro.ru.nl W: http://www.astro.ru.nl/~haverkorn/magalops/
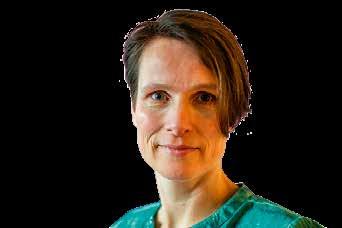
Professor Marijke Haverkorn
Marijke Haverkorn is an Associate Professor in the Department of Astrophysics at Radboud University, Nijmegen, a position she has held since July 2017. She is Chair of the Netherlands LOFAR For Astronomy Consortium, and has been a board member of the internetional LOFAR telescope since October 2016.

Distribution of known stellar polarizations over the sky (Heiles 2000). The circles show the fields in the conducted observations of selected regions, with each circle representing hundreds of polarized stars. Image credit José Versteeg-Veltkamp.
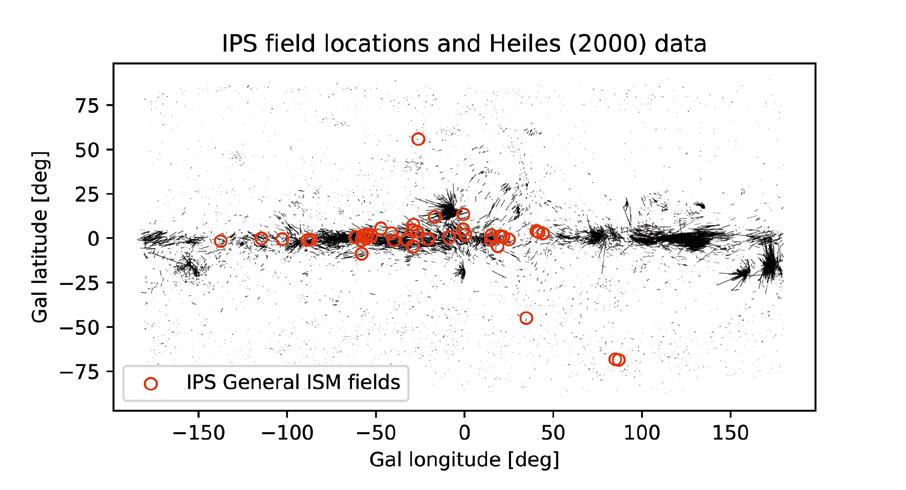
Stellar polarization orientations in one selected region, where every line segment represents a polarized star. The background color indicates magnetic field orientation as measured by the Planck satellite. Image credit: Marijke Haverkorn; data credit Antonio-Mario Magalhães/José Versteeg-Veltkamp; background credit ESA/Planck Collaboration.
IMAGINE consortium
This research is closely related to Professor Haverkorn’s work in the IMAGINE consortium, which brings together collaborators from several different fields to build a deeper understanding of interstellar magnetic fields. While there have been many studies on the Galactic magnetic field, Professor Haverkorn says these research efforts have historically been separate from each other. “The new thing about the IMAGINE consortium is that we want to combine all these efforts together,” she explains. Bayesian modelling is at the core of the consortium’s work. “We are developing a big software package, where you can essentially input any module that you want,” continues Professor Haverkorn. “For instance, I could input a module that includes radio polarisation or optical polarisation data, or maybe a dust distribution model. Groups around the world are working on different parts, and we want to bring them together.”
The wider aim in this research is to build a deeper understanding of the Galactic magnetic field, a topic which holds intrinsic interest for Professor Haverkorn, while it’s also essential to several other areas of study. For instance, researchers who study the propagation of cosmic rays need to take the Galactic magnetic field into account. “Cosmic rays are very high velocity particles, they move at close to the speed of light. The fastest cosmic rays are very rare particles, they’re the most energetic particles in the universe. This means they can only be produced in very energetic sources, close to supermassive black holes,” outlines Professor Haverkorn. It’s very difficult to identify the source of these cosmic rays, as they are deflected during their journey. “You need to know about the Galactic magnetic field to calculate that deflection,” says Professor Haverkorn. “Another area in which the Galactic magnetic field is important is in investigating cosmic microwave background (CMB) polarisation.”
This provides evidence of the early history of the universe, so is of great interest to cosmologists, however it’s again important to consider the Galactic magnetic field. The CMB signal is very weakly polarised, and Professor Haverkorn says the Galactic magnetic field effectively gets in the way. “The Galactic magnetic field also produces a polarisation foreground which is much stronger than the CMB signal. So if you’re looking for the polarised signal of the CMB you have to know the Galactic foreground,” she explains. This underlines the wider relevance of the project’s research. “It is imperative to know about the Galactic magnetic field when you’re looking to make these very sensitive measurements and detect evidence of the early universe. A number of research communities are interested in the magnetic field, so that they can draw the correct insights from their own measurements,” says Professor Haverkorn.
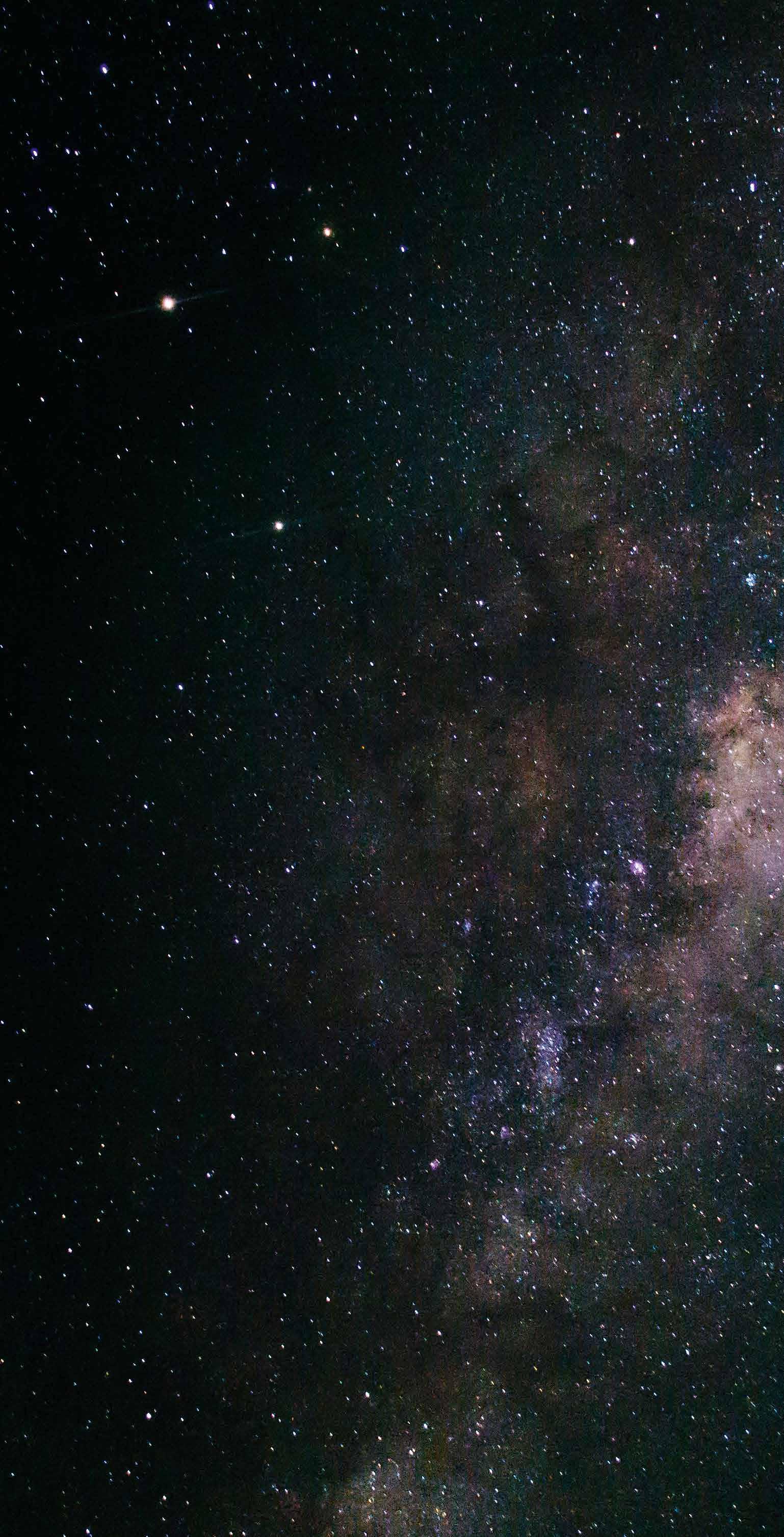
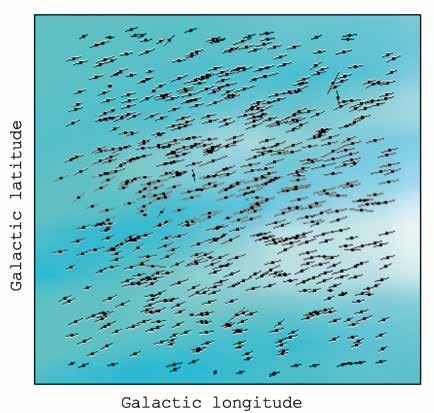