Ogden at 20
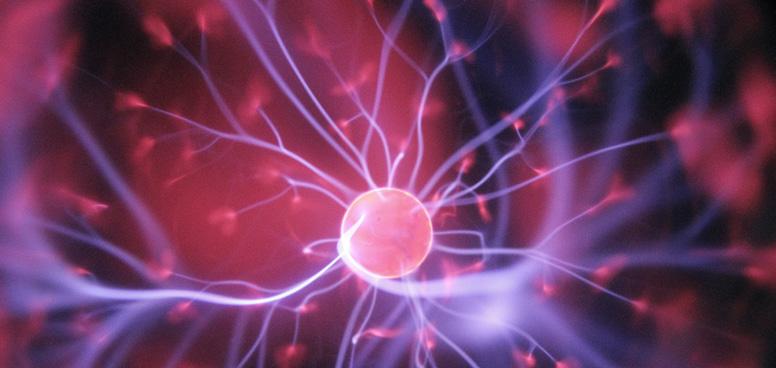
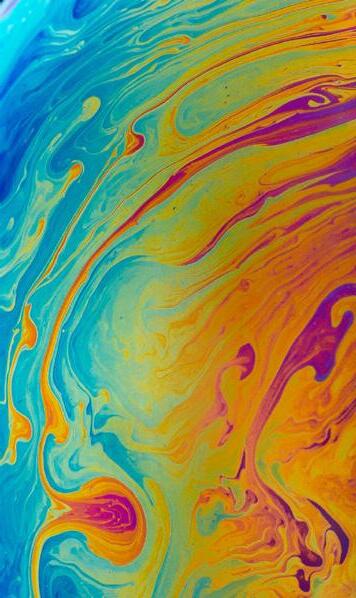
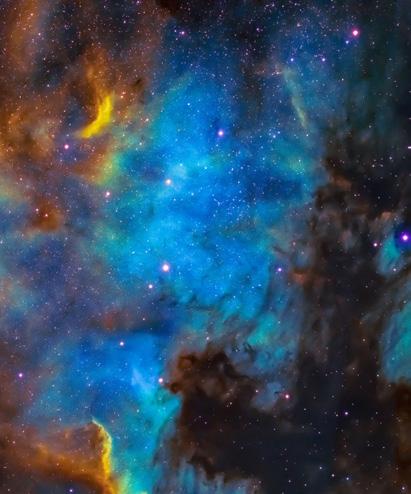
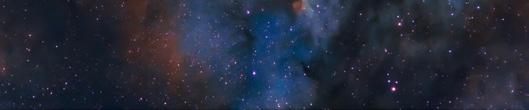

Celebrating 20 years of infinite possibilities
Celebrating 20 years of infinite possibilities
The Ogden Centre for Fundamental Physics is an international centre of excellence dedicated to research into the structure and constituents of our Universe. It is made up of the Institute for Computational Cosmology (ICC) and the Institute for Particle Physics Phenomenology (IPPP).
Our researchers confront the oldest and most fundamental questions in science. How and when did our Universe begin? What is it made of? How did it acquire its current appearance? To tackle them, we use some of the most sophisticated techniques and machines ever produced, including advanced mathematics, supercomputers that perform hundreds of billions of arithmetic operations per second, and particle accelerators that reproduce the conditions in the Universe a millionth of a second after the Big Bang. There has been tremendous progress in the past two decades towards answering some of these questions. Observations have uncovered a surprising mix of cosmic constituents, with ordinary atoms making up only 5% of the Universe, dark matter 20%, and dark energy the rest. Gigantic surveys of galaxies are revealing how the Universe is structured. Large supercomputer simulations recreate the evolution of the Universe, from the Big Bang to the present. Data from the Large Hadron Collider are being analysed to test the Standard Model of Particle Physics and explore possible extensions. Ogden Centre researchers are playing a central role in these developments.
The immediate future is full of promise. We already know that the Standard Model of Particle Physics is incompatible with the existence of dark matter and with the standard “cold dark matter’’ model of cosmology, to whose development the ICC has greatly contributed. The Ogden Centre is bound to be at the forefront of the search for a new physical formulation of our world.
new route for Moon formation
Precision Simulations for Particle Collider Experiments
Precision calculations for high-energy physics and the structure of the proton
Deciphering the nature and properties of the Higgs boson
The Millennium Simulation was the first supercomputer simulation to follow the dark matter evolution in a representative patch of the Universe with enough detail to resolve the dark matter clumps or “halos” in which galaxies form.
Cosmic structure originates from tiny ripples imprinted in the Universe a fraction of a second after the Big Bang during “cosmic inflation”. This phase lasted a very short time during which the Universe grew exponentially. Quantum fluctuations associated with inflation were stretched to macroscopic scales by this expansion seeding the Universe with ripples in its energy density.
The primordial ripples were subsequently amplified by the gravity of dark matter, which makes up about 25% of the Universe. Although yet to be discovered in the laboratory, it is thought to consist of fundamental particles created in the young Universe and collectively known as “cold dark matter”.
To simulate the evolution of the Universe, its contents must be specified, and the computer programmed to solve the relevant equations of physics: Einstein’s theory of relativity and Newton’s law of gravity. The gravity of dark matter, acting over 13.7 billion years of cosmic evolution, amplifies the primordial ripples causing clumps of matter to collapse into galactic halos. On large scales the dark matter ends up distributed in an intricate pattern of filamentary structures known as the cosmic web.
The Millennium Simulation was a collaboration between the ICC and the Max Planck Institute for Astrophysics in Munich. The data have been used in over 1,100 scientific papers worldwide and is one of the most influential cosmology programmes ever.
Accurately measuring the expansion history of the Universe is greatly helped by having a standard cosmic ruler that can be seen at different times. Nature provides exactly this: the “Baryonic Acoustic Oscillations” (BAO).
These are sound waves that propagate in the hot plasma of the early Universe but stop 380 million years after the Big Bang. At this point, these waves left a small imprint in the matter irregularities that would go on to form the galaxies and large-scale structure of our Universe. The scale of this imprint, the BAO scale, is the distance sound travelled between the Big Bang and the time when the gas recombined. This standard ruler is about 500 million light years in length!
The first detection of the BAO imprint in the large-scale galaxy distribution was simultaneously made with the Anglo-Australian “2dF Galaxy redshift survey” (2dFGRS, top image) and the US-led “Sloane Digital Sky Survey” (SDSS). The imprint is manifest as a slight excess in the number of pairs of galaxies at this separation compared to neighbouring scales (see top image). The 2014 Shaw Prize was awarded jointly to Shaun Cole (Durham), Daniel Eisenstein (Harvard), and John Peacock (Edinburgh) for the discovery.
The BAO scale is now used in new generations of galaxy redshift survey, such as DESI, in which Durham is a partner, and the space mission Euclid. These surveys will measure the expansion history of the Universe with exquisite accuracy, which will help determine properties of the dark energy that is driving the Universe’s accelerating expansion.
Galaxies form when cosmic gas is gravitationally driven into “halos” of dark matter, where it cools, settles into a spinning disc and fragments into stars. The halos and their attendant galaxies form in a hierarchical way (see “Millennium Simulation”, page 4), with small fragments forming first and then, attracted by the force of gravity, colliding and merging together making larger and larger structures.
Although their origin is not fully understood, black holes form at the centre of these fragments. Mergers of large proto-galactic units funnel gas towards the centre where it is swallowed by the black hole, reaching masses of hundreds of billions of suns. The huge amounts of energy injected by mergers can destroy a disc and turn it into an elliptical galaxy.
The most massive stars explode as supernovae, injecting energy and heating the cooling gas. Gas accreting into a supermassive black hole releases its gravitational energy just before it falls in, heating the surrounding gas. These feedback processes give rise to huge outflows of gas in the form of galactic winds.
In 2005, the ICC, alongside several groups around the world, achieved a breakthrough in the ability to simulate the complex processes involved in galaxy formation. It became possible to make realistic galaxies in a supercomputer based only on the known equations of physics.
The Cold Dark Matter (CDM) model has become the standard model of cosmology. ICC researchers have greatly contributed to this for four decades. Its most striking prediction is that the Universe should be teaming with dark matter halos of masses ranging over 20 orders of magnitude, from that of the Earth to that of a galaxy cluster. Most are too small for a galaxy to have formed within them, so they remain completely dark.
ICC researchers and collaborators carried out the first simulations in the 1980s and 1990s that established the number and internal structure of halos. In 2008, they completed the Aquarius project, the largest and most detailed halo simulations to date, culminating in 2020 with the definitive study of halos over the entire mass range. All these projects were reported in the journal Nature
The predicted number of dark matter halos offers a definitive test of CDM. This is being pursued at the ICC and elsewhere using gravitational lensing to detect dark, galaxy-less halos. A further test is provided by the number and structure of the faintest galaxies, known as “dwarfs”, which can only be seen in our local neighbourhood as satellites of the Milky Way. To predict their observable properties, ICC researchers and colleagues carried out the influential “Apostle” project, which showed that CDM provides an excellent match to current data. The most stringent test will come from lensing analyses of forthcoming James Webb Space Telescope data.
The Moon is thought to have arisen from the debris produced during a giant impact into the early Earth by a Mars-sized body called Theia, because of similarities between lunar rocks returned by the Apollo missions and samples from Earth’s mantle.
Computer simulations of such a collision have typically yielded a disk of diffuse debris, with the majority originating in Theia. A molten Moon would accrete material from this disk over decades, eventually cooling and solidifying.
Our recent, more detailed, simulations have led to a qualitatively different understanding, whereby a Moonsized satellite is formed in the immediate aftermath of the giant impact. This proto-Moon has a centre almost exclusively made from Theia and outer layers that are more like the early Earth. This might help explain the isotopic similarity of Moon and Earth rocks and predict a less molten Moon that will have a thinner crust after it solidifies, in alignment with recent crustal thickness measurements by NASA’s GRAIL mission. This immediate Moon-sized satellite could be placed onto an inclined orbit around Earth, helping address the mystery of why the Moon’s orbital plane differs from that of Earth’s equator.
When particles collide at high energies, for example the protons at the Large Hadron Collider (LHC) at CERN, the released energies show themselves as showers of elementary particles that will eventually hit the detectors and reveal the elementary degrees of freedom and its most fundamental dynamics.
To simulate such events in their entirety, a profound knowledge of this underlying dynamics is necessary. At the shortest time scales, probabilities for the production of a handful of highly energetic and possibly massive particles, quarks and gluons, weak gauge or Higgs bosons, photons and leptons, can be calculated based on first-principles methods that can be systematically improved, another important IPPP activity.
These particles, and in particular the quarks and gluons, may radiate many secondary particles. Finally, the quarks and gluons will transform into ultimately observable hadrons, and unstable particles will decay. For this part of the simulation, often only a qualitative description is available, and the resulting models must be fitted to existing data.
IPPP hosts and continues to further develop and improve two of the three multi-purpose simulation programmes, also known as Monte Carlo event generators, which aim at a complete description of these aspects of collision events, and which are used by most experimental analyses at the LHC and beyond. These essential tools are supported by a host of more specialised programmes, which target aspects of the overall simulation with tailored methods and algorithms.
The Large Hadron Collider smashes protons together at unprecedented energies and intensities, producing final states governed predominantly by Quantum Chromodynamics (QCD). The concept of QCD factorisation allows us to understand the complex dynamics at different energy scales and to separate the non-perturbative from the perturbative.
The non-perturbative dynamics of the quarks and gluons that make up the proton are described by parton distribution functions (PDFs), which encode the momentum distributions of partons inside the proton. Since 1987, Durham has led global analyses of high-energy scattering data to determine the PDFs, which are used in every single prediction for the LHC and future hadron colliders.
Quantitative predictions, which are crucial for making new discoveries, are obtained using perturbation theory, which expresses physical quantities as a series in the strong coupling. More accurate predictions are obtained by including higher orders in the perturbative expansion. The leading order (LO) captures only the gross features of an observable, inclusion of next-to-leading order (NLO) corrections is required to predict the overall normalisation. Even higher orders are mandatory for describing detailed event properties or when percent level precision is needed. IPPP scientists were instrumental in both the NLO (2008-11) and NNLO (2013-2018) revolutions and are now pushing the boundary to include N3LO effects.
Since the foundation of the IPPP in 2000, its researchers made significant contributions in preparing for the Higgs boson’s impending discovery at the LHC. They proposed search channels for its discovery, provided precision calculations for its production cross sections, and provided guidance on the Higgs boson’s mass by evaluating its impact on electroweak precision observables. Since the discovery of the Higgs boson in 2012, the IPPP has worked at the forefront in proposing ways to measure the properties of the Higgs boson precisely, for example, its coupling to other Standard Model particles, to itself or the Higgs boson’s lifetime.
While the Higgs boson completes the particle content of the Standard Model of particle physics, the Standard Model cannot account for many observations in nature, such as the dark matter content of the Universe, why the Higgs boson mass is so much smaller than the Planck mass or why we observe more matter than antimatter in the Universe. It is plausible that the Higgs field and its potential can provide a window to improving our understanding of these major outstanding questions in modern physics. Thus, scientists at the IPPP study multi-particle production of Higgs bosons at very high energies, non-perturbative instanton-induced scattering processes and (non-supersymmetric) string theories to uncover the global structure of the electroweak potential.
Neutrinos are ultralight particles whose properties may hold the key to discovering new fundamental physics. Over the past 20 years, the IPPP has pioneered several research directions in neutrino physics. IPPP physicists first demonstrated that neutrino physics could generate the imbalance between matter and antimatter observed throughout the Universe. They also instigated the search for heavy sterile neutrinos at the Large Hadron Collider.
The observation of gravitational waves in 2015 was a revolutionary event for fundamental physics. Recently, the IPPP has dived into gravitational wave science with studies of particle physics in compact astrophysical objects, data analysis of gravitational wave experiments and membership of key collaborations. IPPP researchers recently highlighted the unique synergy between neutrino and gravitational wave experiments as a probe of unified theories of particle physics. Astrophysical observations provide a unique opportunity to uncover the identity of the dark matter that makes up 85% of matter in the Universe. IPPP physicists have proposed novel candidates and search strategies for dark matter, including showing that dark matter could consist of very light particles and providing a framework to characterise dark matter searches at both particle colliders and dark matter detection experiments. The IPPP is also training the next generation of astroparticle physicists.
Over the past 20 years, IPPP researchers have been at the forefront of studying flavour-changing processes of elementary particles, to improve our understanding of elementary interactions at the smallest distances.
They achieved this by separating effects that are well understood, but hard to compute due to the strong interaction, from the potential new effects by forces beyond our current understanding. IPPP’s flavour researchers have contributed to the global theory effort to achieve this separation and explain nature’s behaviour at the smallest scales. They have helped to define the length scales at which potential new interactions become relevant. Their phenomenological analyses have become increasingly important due to the so-called flavour anomalies: persistent and significant tensions between theory predictions and experimental measurements.
Examples of their research include hadronic form factors needed for the interpretation of semileptonic and rare B-meson decays as well as hadronic matrix elements needed to extract B-meson mixing parameters.
Our cosmologists have shown how the present pattern of galaxies in the cosmos grew from tiny fluctuations in the density of matter just after the Big Bang. The 2sFGRS (2-degree Field Galaxy Redshift Survey) provided the missing link that directly relates modern galaxies like our own Milky Way to the Big Bang that created our Universe 14 thousand million years ago.
Featured media includes: BBC News, Science Daily, Phys.org, The Northern Echo, The Chronicle.
Dark matter’s “cosmic scaffold” mapped
We helped to map the cosmic “scaffold” of dark matter upon which stars and galaxies are assembled. Researchers used 1,000 hours of Hubble Space Telescope observations to show that the distribution of galaxies follows the distribution of dark matter.
Featured media includes: BBC News.
Working with our partners in the international Virgo Consortium, we used a massive computer simulation showing the evolution of a galaxy like the Milky Way to “see” gammarays given off by dark matter.
The Aquarius Project showed how halos – structures surrounding galaxies which contain a trillion times the mass of the Sun – grew through a series of violent collisions and mergers between smaller clumps of dark matter that came from the Big Bang.
Featured media includes: Reuters, NBC, BD News 24, Space.com, Daily Mail.
We used a computer simulation to predict what the very early Universe would have appeared like 500 million years after the Big Bang. The images produced show the “Cosmic Dawn” – the formation of the first big galaxies in the Universe.
Working with the Virgo Consortium, we produced data suggesting that our understanding of the composition of the Universe may be incomplete. The team created computer simulations to visualise how the dwarf galaxies formed using their assumptions about Cold Dark Matter, and found that the final results of these simulations did not match what we observe as well as expected. A different assumption about the identity of the dark matter may be required.
Featured media includes: BBC News.
Our particle physicists are providing the theory and analysis behind a number of experiments at the Large Hadron Collider where the Higgs boson was discovered in 2012. Evidence that the Higgs boson –which helps explain why elementary particles (the smallest building blocks of nature) have mass – exists is seen as a “triumph” for particle physics.
Featured media includes: The Guardian, Daily Mail, MyScience, ITV, The Northern Echo, Phys.org, The Conversation.
To learn more, please visit durham.ac.uk/ogdenat20/media
We have used supercomputer simulations to see how the Moon might have formed following a huge collision involving the early Earth 4.5 billion years ago.
Featured media includes: Daily Mail, LBC, Daily Express, AOL, Metro, Sky News, The Hindu, The Guardian, UKRI, Space.com, New Scientist, Astronomy Magazine, India Today.
You might not think that studying the Universe could benefit research into serious illnesses like cancer, but our researchers have joined forces with cancer researchers to improve the diagnosis and treatment of patients using advanced statistical techniques developed for cosmology.
Featured media includes: The Times, Palatinate.
We are part of an international team building a new kind of telescope. It weighs as much as a car and is transported to the edge of space by a helium balloon the size of a football stadium. The Superpressure balloon-Borne Imaging Telescope (SuperBIT) will be used to measure the properties of dark matter.
Featured media includes: The European Times, BBC Newsround, Universe Today, The Guardian, Science.
We are part of an international team that has helped create the most detailed ever 3-D map of the Universe. Durham is a key partner in the Dark Energy Spectroscopic Instrument (DESI), helped design and build the new telescope instrument, and is using supercomputer simulations to help interpret the data.
Featured media includes: Daily Mail, ITV, BBC, The Independent, Yahoo!, Cosmos Magazine, Morning Express (India), Persia Digest, New Scientist, The Northern Echo.
We have helped produce the largest and most accurate supercomputer simulation to date of our local patch of the Universe. SIBELIUS-DARK is part of the “Simulations Beyond the Local Universe” (SIBELIUS) project and is the largest and most comprehensive ‘constrained realisation’ simulation to date –covering a volume up to 600 million lightyears from Earth.
Featured media includes: Sky News, Yahoo!, Metro, Daily Mail, Universe Today, Evening Standard, The Independent, Bloomberg, Royal Astronomical Society, WIRED.
The James Webb Space Telescope (JWST) – which Durham helped to develop and engineer – has captured its first images. Our scientists will be among the first to observe the cosmos using the JWST as they hunt for dark matter and investigate early galaxy formation.
Featured media includes: New Scientist, The Times, Space.com, The Northern Echo, Kauai Now.
It is wonderful that our University community is able to count on supportive alumni passing on their good fortune either during their lifetime or after they are gone – or both. This is the case of one of our alumni who graduated with a Physics degree in the early 1960s from Grey College who is currently a donor and plans to continue to support students in the future.
He explains the importance of his time at Durham and his motivation to give back.
“My legacy is a way of expressing my appreciation for the qualifications and experience that seven years in Durham gave me which served as a fine springboard to my subsequent career, all provided at no cost to me or my parents. Such an education and the associated benefits of a ‘Durham experience’ are no longer possible unless a student is willing to take on a substantial debt or has independent financial means, and I see the financial hurdles to be surmounted in getting a university education only getting higher in the coming years. I left Durham with both a trained mind and, to my great good fortune, a lifetime partner and I hope that my legacy will enable others to have the same start in their lives as I have had.”
Under the donor’s current scholarship, he receives regular reports which give an idea of the impact of his gift. One of the recipients describes the impact of his award as follows. “I am incredibly thankful for all the opportunities this scholarship has provided me with. Undoubtedly the most valuable experience has been the chance to work with, learn from, and be part of an incredible community. This scholarship has been a fantastic experience and has opened up many avenues for future careers that I could not have considered before.”
We would be delighted to hear from anyone considering making a donation or leaving a gift in the future from their will or their pension. We would be pleased to illustrate the concept and explain the impact it is going to have.
For further information, please visit dunelm.org.uk/legacies
“
My legacy is a way of expressing my appreciation”Current Ogden Centre donor
During the Ogden at 20 celebrations, we also take the opportunity to celebrate the life of a great supporter of Physics at Durham and University College alumnus, Arthur Browne, 1932-2022. We are grateful for his contributions to our work and for his legacy.
Through a range of award-winning activities we stimulate interest in science among young people, their teachers and the wider community.
We enthuse, engage and inspire, showcasing Durham’s world-class scientific research and teaching, by developing collaborative programmes that involve our local community in the excitement of science.
We work in partnership with local communities, schools and other organisations, and many programmes include visits and on-site workshops and sessions, supporting and involving our researchers and students.
SOE contribute to, and participate in, many regional networks and groups including teacher networks, Science Learning Partnerships, business-education alliances, and cultural organisations.
Full details of many of our past and current activities can be found on the SOE webpages.
To
More than 66,000 7-11 year-olds and their families have come along to Celebrate Science since our first festival in 2010.
13-15 year-old pupils and their teachers from 42 local secondary schools have attended the last five Durham Schools’ Science Festivals.
Since 2006, over 500 third year undergraduate students have participated in the Science into Schools Module working with more than 130 local schools.
Over 2,500 young people from 145 schools and community organisations across the North East have been trained on our Science Ambassadors programme.
The Ogden Trust North East schools’ partnerships involve over 150 schools across the region, many in areas identified as facing multiple indices of deprivation.
For 2018-19 (the last full year of in-person sessions), the 38 County Durham primary Science Ambassador teams alone were estimated to have engaged over 4,500 other young people, not including families and others at out of school events.
Over 290 Year 10 and Year 12 students from schools across the North East have received awards at our annual Schools’ Physicist of the Year event.
Each year since 2009, SOE have organised a training day for local secondary school science teachers and their technicians. Both online events in 2020 and 2021 attracted audiences of around 70 teachers from around the UK.
In 2018, our Primary Physics Professional Learning Day was attended by 66 teachers from 59 primary schools across the North East. 85 local primary school teachers attended the event in 2019.
Since 2012, we have engaged well over 5,000 members of the public through our programme of talks to community groups, u3a, The Women’s Institute, Café Scientifiques, etc.
It is estimated that our ‘Gravity, Gas and Stardust’ DVD has been viewed by over 500,000 Key Stage 4 secondary school students in the UK alone.
We are grateful to Sir Peter Ogden for the generous gifts that made possible the creation of the Ogden Centre and the two buildings in which it is housed, and to the Wolfson Foundation for their contribution to the second building. We also thank Damon de Laszlo for his gift of the sculptures that adorn the two buildings.
We remember our colleagues, James Stirling and Mike Pennington, who played a key role in the establishment of the Institute for Particle Physics Phenomenology.
Visit durham.ac.uk/ogdenat20 for more information.
Durham University and Durham University logo are registered Trade Marks of the University of Durham. Unless otherwise stated, all material in this publication is copyright of the University of Durham. The University makes every effort to ensure that the information contained here is accurate. Please note that the University’s website is the most up-to-date source of information and we strongly recommend that you always visit the website before making any commitments.