Introduction 8
1. Multiple Tree Origins
What is a tree? 10
The tree life-form 12
Non-seed trees of the first forest 14
Devonian trees 16
Hardwoods and softwoods 18
Developing woodiness on islands 20
2 Patterns of Tree Diversity
Global diversity 22
Tropical rainforests 24
Why are there so many species in the tropics? 26
Tree distributions in biomes 28
Diversity anomalies 30
Evolution and diversity patterns 32
3. Tree Leaves
Morphology 34
Leaf characteristics 36
Types of trees 38
Photosynthesis 40
Photosynthesis, CO2, and H2O 42
Photosynthesis and climate change 44
4. Tree Trunks
Global patterns of height 46
Mechanics of growing tall trees 48
Trunk strength trade-offs 50
Apical dominance 52
How to be a hyper-tall tree 54
Non-monopodial large “trees” 56
5. The Architecture of Trees
Crown shyness 58
Hallé/Oldeman models 60
Crown geometry 62
Adaptive crown geometry 64
Shinozaki pipe model theory 66
Forest plantations 68
6. Tree Bark
Bark variation 70
Jigsaw-puzzle bark 72
Bark anatomy 74
Bark variation in eucalypts 76
Bark as an adaptation to fire 78
Uses of bark 80
7. Tree Roots
Unique root macro-structures 82
The metabolic costs of tree roots 84
Mycorrhizae 86
The rhizosphere 88
Nitrogen fixation 90
Root-feeding insects 92
8. Seeds and Life History
Life history 94
Seeds and seed crops 96
Seed dispersal 98
Seed dormancy 100
Devil's walking stick 102
Growth rate and life stages 104
9. Ecology and Forest Dynamics
Primary and secondary succession 106
Gap replacement 108
The forest as a dynamic mosaic 110
Ponderosa fire recovery 112
Fir waves 114
Forest area and dynamic mosaics 116
10. Conservation
Conservation challenges 118
Habitat loss and fragmentation 120
Species invasions 122
Changes in ecological processes 124
Pollution and climate change 126
Future forests 128
11. Myths and Folklore
The sacred Bodhi tree 130
Heartwood, sapwood, and the British longbow 132
The Kula Ring 134
Indigenous knowledge and biological heritage 136
The talking tree 138
The didgeridoo 140
12. Curious Facts
Largest and oldest living beings 142
Seeds in search of safe sites 144
Living with fire 146
Drought and the baobab 148
Living with salt and storms 150
Playing defense 152
Glossary 154
Further reading 156
Index 158
Acknowledgments 160
1. Multiple Tree Origins
WHAT IS A TREE?
Atree is a tall, woody, perennial plant with an elongated stem or trunk. The trunk typically supports woody branches, twigs, and leaves. “Tree” is not a taxonomic grouping; it is a life-form of plants with tree attributes. Some trees are evolutionarily related with shared ancestors. However, other trees have independent origins, even though they seem similar. One might expect the nearest relatives of a tree to be other trees, but this is not always so. There are many plant genera with tree, shrub, and/or vine life-forms that are strongly related from an evolutionary point of view. DNA analyses provide multiple examples of trees with kindred species of alternative plant lifeforms. An extreme case is the large plane trees of the genus Platanus, which are most related to the aquatic sacred lotus (Nelumbo nucifera) and vice versa. Plane trees are also the lotus’s nearest kin. Their last common ancestor dates from 60 Mya (Mya = million years ago).
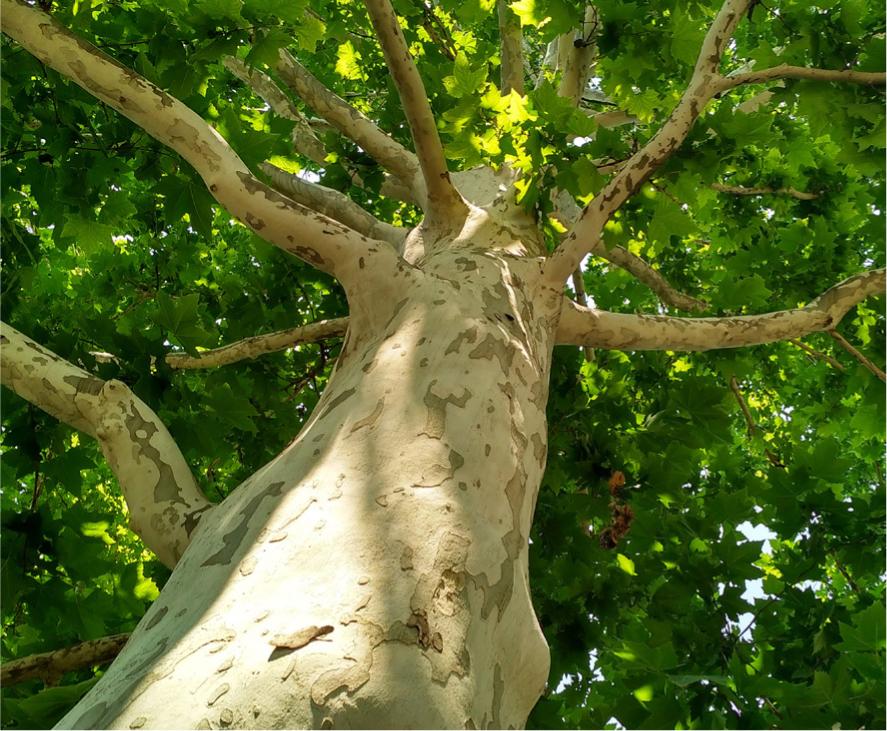
The Oriental plane tree (Platanus orientalis). The nearest living relative to plane trees (
).
COMPETITION BETWEEN PLANTS
A tree’s height gives it preemptive access to incoming light, a winning strategy when other resources (water, soil nutrients) are in ample supply. Light competition is oneway: the taller plants get sunlight first and the smaller plants can only access the overhead light that has streamed past the taller plants above. In contrast, competition between individual plants for nutrients and/or water is two-way—when a tree’s roots capture nutrients or
Platanus) is the aquatic and herbaceous sacred lotus (Nelumbo
water, these are then made unavailable to other plants, regardless of their sizes.
ADVANTAGES AND DISADVANTAGES OF HEIGHT
There are many advantages to height among plants competing for resources, but there are also challenges in being a tall plant. Transporting water and nutrients to leaves high in the air requires roots to supply water transport systems in the trunks of trees. In general, plants require some sort of woody fiber to attain height. In most trees, this involves two different modes of growth: primary growth (extending roots and branches) and secondary growth (thickening the trunk, branches, and roots). Along with primary and secondary growth, the evolution of tree-like plants has also involved solutions to several structural problems—forming self-supporting structures, monopodial trunks, and fluid transport in tall plants—all of which are discussed in this chapter.
THE TREE LIFE-FORM
Evolutionary relatedness of plants traditionally involved determining fossil dates and comparing morphologies, particularly of flowers. Modern DNA analysis is revealing surprising relations among plants. Flowering plants (angiosperms), originally thought to have arisen from a single ancestor, may actually be a collection of species with five different origins. The “clock” provided by the accumulation of DNA mutations has pushed separations between taxa back as much as 60 million years. Microscopic detection of fossil pollen has displaced the origins of different seed plants by a similar degree.
The tree life-form developed in different evolutionary pathways as solutions to the problems of building plants with tall, stable trunks. The trunks of trees can be formed in several different ways, depending on the structural challenges involved in building taller plants (wood macrogeometry problem); growing the wooden parts of trees to adjust for increasing the tree’s height and mass (secondary-growth problem); and lifting water to the treetops (hydraulic problem).
Female cones of the monkey puzzle tree (Araucaria araucana) are round, 4½–8 in (12–20 cm) across, and hold about 200 seeds.
Male (pollen) cones are initially 1½ in (4 cm) long. They expand to 3–4½ in (8–12 cm) long by 2–2½in (5–6 cm) wide at pollen release.
Araucaria araucana trees growing in Conguillío National Park, in the Lakes District of Chile. Monkey puzzle trees share a common ancestry with trees from the time when Australia, Antarctica (fossil plants), and South America were linked by land in the supercontinent known as Gondwanaland.
NON-SEED TREES OF THE FIRST FOREST
Trees and forests originated 393–383 Mya in the Middle Devonian Period. In 2020, Professor William Stein and colleagues, of Binghampton University in New York state, excavated the oldest such intact forest in the Catskill region near Cairo, New York. They uncovered and mapped a buried ancient soil and fossilized tree roots across an area measuring ¾ acre (3,000 m2). This ancient forest existed at the transition of the Earth from a planet virtually devoid of forests to one covered by them. Professor Stein and his colleagues’ detailed mapping of this area allows us to visualize a forest that is now lost deep in time.
EARLY FOREST PLANTS
The trees of early forests reproduced through spores, not seeds. The roots and the size of the trees at the Catskill site help us imagine what this incredibly ancient forest must have looked like. There were a few Stigmaria, the roots of lycopsids—the so-called “giant club mosses” that could reach heights of 98 ft (30 m). The forest was also home to tree-fern-like trees called cladoxylopsids, which have no living relatives but are related to ferns and horsetails (Equisetum). At full size, these stood up to 40 ft (12 m) tall and had no leaves, but they did develop a canopy of photosynthesizing twigs and branches. Unusually detailed fossil cladoxylopsids from China indicate that they were hollow, and as the plant grew larger, the resulting strain would tear the trunk. These rips and tears would repair themselves over time to produce diameter growth—a violent, Procrustean solution to the secondarygrowth challenge of increasing the diameter of the trunk as a tree grows taller.
THE ARCHAEOPTERIS GENUS
At the New York site, protogymnosperms of the genus Archaeopteris, distant ancestors of modern gymnosperms, were abundant. These trees could grow up to 100 ft (30 m) tall and had base diameters of almost 5 ft (1.5 m). The name Archaeopteris derives from the Greek archaīos (ancient) and ptéris (fern), and the plants were initially classified as ferns. They had leafy, fern-
like foliage arranged in umbrellas of fronds. The resultant canopy captured light efficiently and the leaves may have been shed seasonally, as in modern deciduous trees. The roots resembled those of today’s trees in that they were both spatially extensive and deep. These then-novel roots provided the buried organic carbon compounds that could change the chemistry of soils.
Archaeopteris trees were the first to evolve deep and extensive root systems like those of today’s trees, leading to the building of soils and generating planetary chemical changes.
The resounding success of Archaeopteris covered the planet with a soilbuilding, biomass-accumulating forest unlike anything that had come before. Storing carbon in trees and forest soils, it contributed to the chemistry of the planetary atmosphere and the oceans, much like modern forests.
ARCHAEOPTERIS DOMINATION
Archaeopteris trees came to dominate the Earth and eventually composed 90 percent of the planetary forests. The genus remained until the Lower Carboniferous, about 50 million years after the time of the early forests mapped near Cairo, New York. Archaeopteris fossils have been reported from North and South America, Europe, and Asia. One species, Archaeopteris notosaria, grew within the then Antarctic Circle and fossilized Archaeopteris fronds have also been found on Bear Island above the Arctic Circle in the Norwegian Svalbard archipelago.
DEVONIAN TREES
About 372 Mya, the Devonian Epoch ended with a massive and complex extinction, particularly of the remarkably diverse coastal reefs, primarily in tropical, shallow water, which had been evolving and diversifying until that time. This extinction was one of the “Big Five” such occurrences in the Earth’s history.
The Late Devonian extinction appears to be the result of several different events. The first of these, 372 Mya, was the Kellwasser Event, a massive extinction pulse of marine invertebrates. Evidence of this event can be seen in the rock strata in the Kellwasser Valley in Lower Saxony, Germany. In these strata, the rocks contain two distinct shale layers, which implies that there were two occurrences of ocean anoxia (lack of oxygen). The Hangenberg Event, which occurred 13 million years later and was named for strata from the German Hangenberg Black Shale, documents an extinction event associated with a disappearance of reefs and many fish genera.
There is little debate over whether the collective events of the Late Devonian were anything but horrific. But there are several explanations and considerable debate as to the complex cause(s) of the events of these Late Devonian extinctions. Collectively, they eliminated about 80 percent of the planet’s animal species, mostly from the diverse array of marine species of the shallow tropical seas.
PLANETARY EFFECTS OF FORESTS
Although the causes of the Late Devonian extinctions are complex, with competing potential reasons, initial forestation across Earth might have played a role in massive planetary change. Since we are now in a time of planetary deforestation, appreciating the global effects of forests is central to understanding how Earth functions.
Fossil trilobites from the Devonian Epoch in Ontario, Canada. Trilobites were a diverse, abundant, and dominant animal species in Devonian coastal environments. They were greatly diminished by the end of the Devonian.
WHAT CAUSED THE LATE DEVONIAN EXTINCTIONS?
One theory regarding the possible causes behind these extinctions indicts the success of Archaeopteris roots in weathering soils, increasing runoff, and generating global changes in tropical ocean chemistry. Professors MeyerBerthaud, Scheckler, and Wendt have conjectured that the primeval, early success of Archaeopteris contributed to a large decrease in carbon dioxide in the atmosphere (from 10 to 1 percent) and an increase in atmospheric oxygen (from 5 to 20 percent)—conditions more like those of today. These chemical changes could have induced the much cooler temperatures involved in the Late Devonian Mass Extinction.
HARDWOODS AND SOFTWOODS
Foresters classify trees according to the features of the wood they produce. For practical foresters, trees are either softwoods, which are more easily sawn and nailed, or hardwoods, which are more difficult to saw and join. This utilitarian classification is clouded by the additional formal classification used in forestry that uses “hardwoods” to describe tree-form angiosperms (flowering plants) and “softwoods” for tree-form conifer trees (in the gymnosperm division, Pinophyta).
In combination, these definitions produce glaring paradoxes when comparing hardwoods and softwoods. For example, balsa (Ochroma pyramidale) is a hardwood tree in the sense that it is an angiosperm, but it has the softest commercially available wood. The opposite also occurs and some softwoods (conifers) can have very hard wood.
TYPES OF TREE TISSUE
Macroscopically, angiosperm and gymnosperm trees have similar arrangements of plant tissues, seen as rings in cross section, which allow for secondary growth. Both angiosperm and gymnosperm trees are typically covered by bark, which varies greatly from a fleshy, smooth, almost skinlike covering to a very hard and thickened, rough covering. Bark is produced by a thin layer of bark cambium tissue that grows outward to produce bark and repairs the outer protective bark layer.
Inside the bark is a layer of phloem tissue, which primarily transports the sugars made by photosynthesis. Phloem tissues may also contain specialized cells that provide mechanical support, flexibility, storage, and protection from herbivores. Phloem is composed of living tissue in contrast to the dead cells in most of the xylem tissue. Inside the ring of phloem tissue is another ring of living tissue called the vascular cambium. This has two kinds of cells. Some are long and oriented along the growth axes of the tree (fusiform initials) and eventually produce the xylem tubes that transport water to the tree’s leaves. Other cambial cells are smaller and produce rays that conduct water outward from the tree’s center.
XYLEM IN ANGIOSPERMS AND CONIFERS
Angiosperms and conifers converge at the macro level in forming stronger, tougher, more supportive heartwood over time in the water-conducting sapwood. But they diverge microscopically in how the xylem cells solve the strength versus water-conduction trade-off. In conifers, the xylem cells are tracheids, which transport water upward and provide the mechanical strength for building the tall, wooden tree structure. Thick-walled tracheids provide strength, while thinner-walled tracheids, which are larger in cross-sectional diameter, transport more water, faster. In angiosperms, the xylem is more complex, since the tissues have specialized wood fibers to give the xylem wood mechanical strength. Water-conducting pit vessels are collections of tracheids and other structures that form a wider cross section and allow for greater volume in the water transport system and faster velocities of water moving upward.
The innermost part of the tree consists of xylem tissue produced by the vascular cambium. This tissue is involved in the transport of water and nutrients and, unlike the sap-transporting phloem, is composed of dead cells. Typically, in both angiosperms and conifers, the outer xylem wood is lighter in color and weight and is called sapwood. In most trees, the older xylem found in the tree’s center is chemically transformed to form heartwood, which is often darker, heavier, more decay-resistant, and stronger than the younger sapwood surrounding it.
Cross section of a tree trunk showing the key structures, as follows: (A) annual ring, (B) rays, (C) pith, (D) xylem, (E) heartwood, (F) sapwood, (G) vascular cambium, (H) phloem, (I) bark.
DEVELOPING WOODINESS ON ISLANDS
Tall plants reaching heights of tens of meters require wood fibers for strength. A conspicuous feature of the plants growing on remote islands is a high proportion of woody plants that evolved from herbaceous continental ancestors. Charles Darwin (1809–1882) noted this tendency during his fiveyear journey on HMS Beagle, and later, his 1859 book On the Origin of Species attributed this to natural selection. The phenomenon of insular woodiness can also occur on continents and is referred to as secondary woodiness.
Professor Frederic Lens and colleagues, of Leiden University, tabulated the occurrences of woodiness in plants of herbaceous origins on the Canary Islands. There, they found that 220 native flowering plants represent 34 genera from 15 plant families—this diversity originated from 38 events in the development of woodiness in herbaceous lineages. While there are clearly advantages in the height granted by woodiness over herbaceous competitors, insular woodiness is also associated with drier conditions and drought resistance.
Cretan viper’s bugloss (Echium creticum) in Spain. It grows to a height of 9–36 in (25–90 cm).
of jewels (Echium simplex) is a closely related Canary Island species. It grows to 10 ft (3 m) in height.
Tower
The dragon tree (Dracaena draco) is native to the Canary Islands. A member of the Asparagus family, it has secondary woody tissue and can attain heights (and widths) of 50 ft (15 m) in nearly frost-free climates.
acid deposition 126
acorns 97
alpine ash 64
American beech 120
angiosperms 18–19, 143 ants 153
apical dominance 52
Archaeopteris 14–15, 17, 85 ash 111
balsa 18, 24, 104
banyan 56, 143 baobab 143
drought adaptation 148–9
bark 70–1
adaptation to fire 76, 78–9
bark anatomy 74–5
bark variation in eucalypts 76–7
jigsaw-puzzle bark 72–3
lenticels for gas exchange 71 uses of bark 80–1
beech 33, 71, 111
bigleaf magnolia 30
bio-piracy 136–7
biodiversity 27
biological heritage 136–7
biomes 28–9
black gum tree 30
black locust 91
black mangrove 82
black spruce 64–5
Bodhi tree 130–1
bristlecone pine 143
bunya pine 63
Cecropia 104, 153
climate change 44–5, 126, 129
climatic shifts 32–3
clonal trees 57, 142, 143
INDEX
coast redwood 54–5, 142 competition between plants 11 conifers 18–19
conservation challenges 118–19
continental distributions 29
continental drift 32
cork oak 80–1
crown geometry 62–4
crown shyness 58
date palm 100–1
dawn redwood 30–1, 33 defense strategies 152–3 chemical tree attack 153 defensive mutualisms 153
devil’s walking stick 102–3, 104
Devonian extinctions 16–17
didgeridoos 140–1
dipterocarp trees 46–7 diversity 22, 26
counting the world’s trees 22 diversity anomalies 30 elevation and tree diversity 23 evolution and diversity patterns 32–3
latitude and diversity 29 why are there so many species in the tropics? 26–7 dragon tree 20–1
eastern hemlock 109 ebony tree 24, 104
Echium spp. 20 ecosystem management 129 energy–diversity theory 26
Eucalyptus 76–7, 79, 140 evolution 12, 30 convergent and divergent evolution 37 evolution and diversity patterns 32–3 extinctions 118, 120
ivory-billed woodpecker 117
Late Devonian 16–17
figs 29
fir waves 114–15 fires 54–5, 76, 116, 124 bark as an adaptation to fire 78–9
living with fire 146
Ponderosa fire recovery 112–13
floods 124 forests 14
Archaeopteris 14–15
change in ecological processes 124–5 differing compositions and structures 111 early forest plants 14 forest regularity 67 future forests 128–9 ghost forests 123
interaction of forest processes and patterns 110–11 managed forests and the site index 68–9 planetary effects of forests 16 plant and animal diversity 116–17
regeneration in an old forest 108 self-thinning 68 fragmentation 120–1
giant sequoia 49, 142, 143, 145, 146
Gingko biloba 33, 38 girdling 75
Gleiwitz Radio Tower, Poland 46
gray birch 120
Grime’s triangular model 105 growth rate 104–5
gymnosperms 18, 143, 146
habitat loss 120–1
Hallé/Oldeman models 60–1 hardwoods 18–19 heartwood 132 height 48
advantages and disadvantages 11 global patterns of height 46 what restricts tree height? 49 withstanding structural damage 48–9 world’s tallest trees 54–5
Indaba tree 138–9 indigenous knowledge 136–7 inducible defenses 152 insular woodiness 20 invasive species 122, 129 pests and diseases 123
kauri 57
Kula Ring 134–5
leather tanning 81 leaves 34–6 boundary layer 43
convergent and divergent evolution 37 deciduous leaves 39 effect of climatic differences 36–7
environmental leaf morphology 36 stomata 42 sun-leaves and shade-leaves 62, 63 types of trees 38–9
Leeuwenberg’s model 61
life history 94, 104–5 longbows 132–3
Magnolia 33 mangroves 150–1 maple 33 masting 97
monkey puzzle 12–13 mono- and multi-layered trees 62–3
Montezuma cypress 142–3 mountain ash 54–5 mycorrhizae 86–7
narrow endemics 119 nitrogen fixation 90–1 non-seed trees 14–15
oak 94–5, 97
Osage orange 99, 133 Ötzi 132–3 outrigger canoes 134–5
Pandanus 82–3, 134, 135 phenology 45 phloem 43 photosynthesis 40–3 climate change 44–5 pin cherry 100–1, 144 plane trees 10, 11, 72–3 pollution 126
Ponderosa pine 112–13 primary succession 106
quaking aspen 57, 119, 143 quinine 137
r-K selection 105 red oak 46 rhizosphere 88–9 roots 82
effect on acidity levels 85
metabolic costs 84–5
root-grafted trees 57
root interaction with the rhizosphere 88
root-feeding insects 92
tree root systems 84
rubber tree 136–7
sacred fig 130–1
sandbox tree 98–9
sapwood 132
sea levels 126
secondary succession 106
Ponderosa fire recovery 112–13
seeds 96
saving seed 128
seed dispersal 98–9
seed dormancy 100–1
seed numbers 96
seed sizes 96–7
surviving the odds 144–5
serotiny 146
shepherd’s tree 82
Shinozaki Pipe Model 66–7
Siberian larch 46 softwoods 18–19
southern beech 29, 32
species invasions 122–3, 129
stringybark 79 succession 106
gap replacement 108–9
sumac 61
table mountain pine 146–7
time-stability theory 27
tree distributions 28–9
tree models 60–1
trees 8–9
largest and oldest trees 142–3
tree life-form 12
types of tree tissue 18–19
what is a tree? 10–11
trophic cascades 124, 125
tropical rainforests 22, 24–5
trunk strength 50
water flow 51
wood density 50
vegetative regeneration 101
Wollemi pine 118–19
wood density 50, 104
woodpecker, ivory-billed 117
woody plant development on islands 20
xylem 18–19, 43
yew 132–3