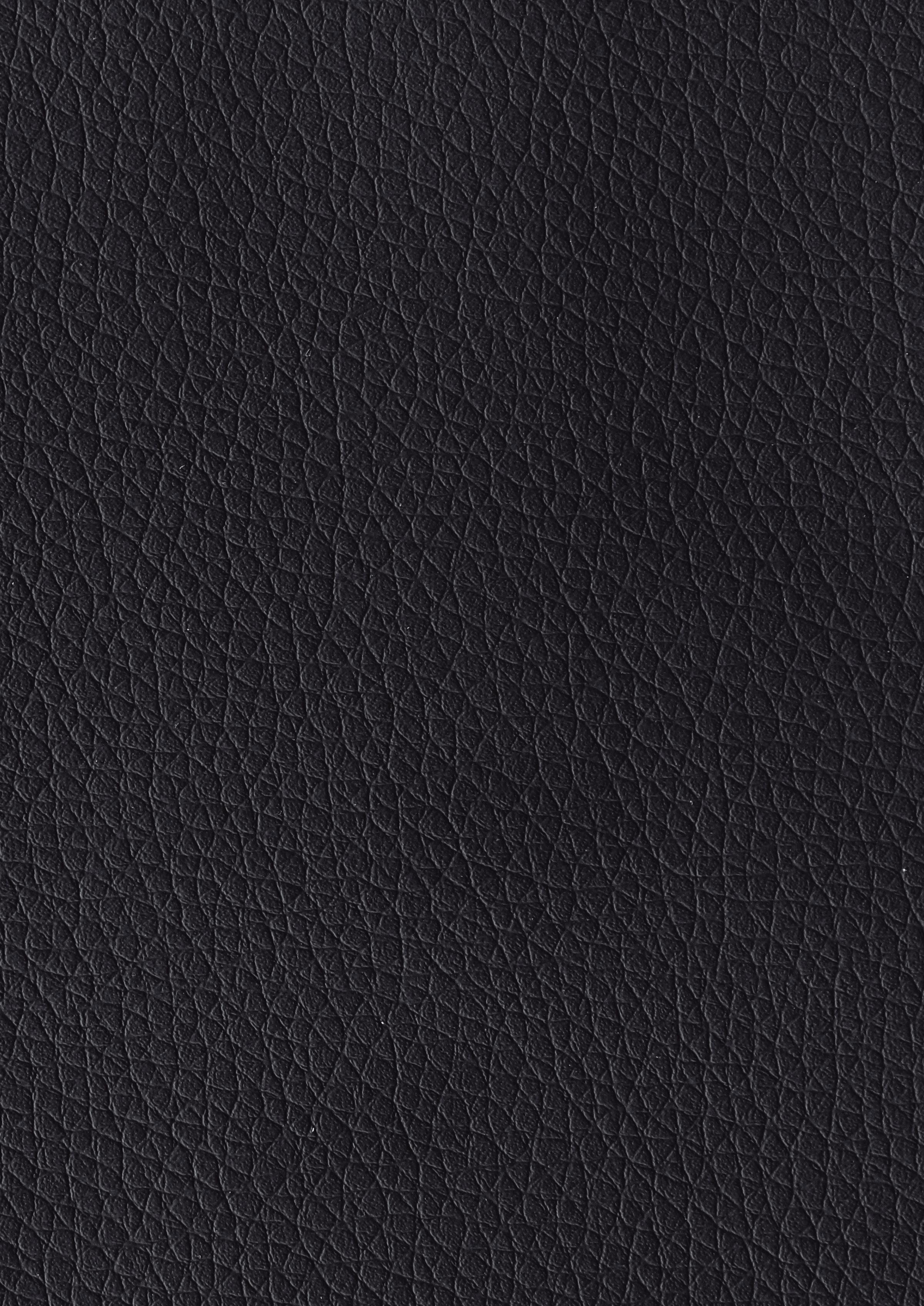
Editorial
Dr. Edwin Cevallos Arellano
Oncologist / Scientific Creator of BIRM President, BIRM Verónica Peñaherrera Marketing Director, BIRM
Dra. Samantha Vasco Yánez Editor
GIRO Agency Design and layout
Dr. Edwin Cevallos Arellano
Oncologist / Scientific Creator of BIRM President, BIRM Verónica Peñaherrera Marketing Director, BIRM
Dra. Samantha Vasco Yánez Editor
GIRO Agency Design and layout
A world in constant evolution needs professionals committed to healthcare and disease prevention. We have set ourselves new goals trying to treat highly aggressive diseases. It is the duty of health professionals to research innovative therapies for the future. We seek to find new medical discoveries that will provide positive results. Decades after the unveiling of BIRM, our commitment to improved health endures.
A decade after the beginning of BIRM’s successful legacy, we have decided to compile all the scientific research carried out worldwide on a product with a permanent commitment to humanity, a product that contributes to disease prevention and recovery from chronic pathologies. BIRM is a natural product that has helped thousands of Ecuadorian families throughout its history. It is as an adjuvant immunomodulator for all types of diseases. We have seen such good results that we continue to innovate day by day, seeking new discoveries for humanity’s benefit.
BIRM –an acronym for Biological Immune Response Modulator– is a product with proven scientific efficacy, which can be administered from children to seniors, with high levels of effectiveness and with zero toxicity.
The purpose of the following scientific review is the timely dissemination of information and the promotion of the credibility and sustainability of the product. We hope this will promote access to the product’s benefits, as the only adjuvant that acts on the immune system as a potent immunomodulator. BIRM acts at the cellular level, producing apoptosis in cancer cells, or even remission of acute, chronic and serious diseases. BIRM also aids in the prevention and control of autoimmune and infectious disease s.
After rigorous analysis and verification, the United States Patent and Trademark Office granted BIRM patent No. US 7,250,180 B2, which recognizes the product’s originality and authorizes its medicinal use in the prevention and cure of various diseases. This certificate guarantees BIRM’s quality and protects it from any attempts at counterfeiting.
University of Miami School of Medicine, Coral Gables, FL, United States
Patients with malignant diseases commonly consume poorly characterized medicine or dietary supplements. Extensive use of untested formulations, provide both opportunities and danger. BIRM, an Ecuadorian oral solution, is a dietary supplement developed from the Andean variety of Solanum dulcamara L, which is widely consumed in the Americas for a variety of diseases, including prostate and breast cancers, without any noted toxicity. Initial laboratory studies of BIRM on prostate cancer models showed strong cytotoxic antitumor activity, including induction of apoptosis, cell-cycle arrest, reduction in androgen receptor levels and down-regulation of pro-survival genes. Oral dosing of BIRM in a rat Dunning MAT LyLu model and in human prostate cancer xenografts showed chemopreventive and antimetastatic activities of BIRM. It decreased tumor incidence (75%), tumor growth (50%) and metastasis (63%). These observations provide the basis for further investigation into the use of BIRM as a chemopreventive dietary supplement. The main hypothesis, to be tested in this project, is that BIRM is a non-toxic, chemopreventive, natural product with the potential to retard tumor growth, progression and recurrence. The main objective of this application is to establish the chemopreventive and antimetastatic activity of BIRM in transgenic models of prostate cancer with the goal of establishing its safety and efficacy for use in controlled clinical trials.
In Aim 1, the minimum effective dose, optimum effective dose and maximum tolerated dose of BIRM will be established in the TRAMP (transgenic adenocarcinoma of the mouse prostate) model. In addition, the chemopreventive and antitumor activities of BIRM will be investigated using two distinct transgenic models that develop prostate tumor by either an androgen-independent (GvT-15 model) mechanism or by the conditional knock-out of a tumor suppressor gene (PTEN) in the prostate (PTEN loxp/loxpPBCre-4).
In Aim 2, the molecular mechanism of BIRM induced apoptosis, cell cycle arrest and androgen receptor degradation will be investigated by delineating the alterations in the molecular signatures of respective signaling pathways.
In Aim 3, the efficacy of BIRM either alone, or in combination with standard chemotherapy, will be evaluated for preventing the emergence of hormone-refractory prostate cancer in two human prostate cancer orthotopic xenograft models, LNCaP and LAPC-4, which demonstrate distinct molecular forms of androgen receptor. Relevance: This study will establish safety and toxicity profiles of BIRM and determine whether BIRM, a complex natural product, has 1. a proven chemopreventive efficacy against prostate cancer and 2. if the efficacy of proven cancer therapies are enhanced or significantly compromised by BIRM. This study should also provide a rationale for further development (if any), of this chemopreventive agent, i.e., clinical trials for prostate cancer and other malignant cancers.
Agency Institute Type Project # Application # Study Section Program Officer Project Start Project End Budget Start Budget End Support Year Fiscal Year Total Cost Indirect Cost
National Institute of Health (NIH)
National Center for Complementary & Alternative Medicine (NCCAM) Research Project (R01) 1R01AT003544-01A1 7197505
Chemo/Dietary Prevention Study Section (CDP) Sorkin, Barbara C 2007-08-01 2011-07-31 2007-08-01 2008-07-31 1 2007 $302,940
Institution
Name Department Type DUNS # City State Country Zip Code
University of Miami School of Medicine Urology Schools of Medicine 052780918
Coral Gables FL United States 33146
Pg. 2
NIH 2010 R01 AT
Antitumor and chemopreventive activity of the Ecuadorian plant extract BIRM Lokeshwar, Bal L. / University of Miami School of Medicine $293,913
NIH 2009 R01 AT
Antitumor and chemopreventive activity of the Ecuadorian plant extract BIRM Lokeshwar, Bal L. / University of Miami School of Medicine $296,881
NIH 2008 R01 AT
Antitumor and chemopreventive activity of the Ecuadorian plant extract BIRM Lokeshwar, Bal L. / University of Miami School of Medicine $296,881
NIH 2007 R01 AT
Antitumor and chemopreventive activity of the Ecuadorian plant extract BIRM Lokeshwar, Bal L. / University of Miami School of Medicine $302,940
Shamaladevi, Nagarajarao; Araki, Shinako; Lyn, Dominic A et al. (2016)
The andean anticancer herbal product BIRM causes destabilization of androgen receptor and induces caspase-8 mediated-apoptosis in prostate cancer.
Oncotarget 7:84201-84213
Salazar, Nicole; Castellan, Miguel; Shirodkar, Samir S et al. (2013)
Chemokines and chemokine receptors as promoters of prostate cancer growth and progression.
Crit Rev Eukaryot Gene Expr 23:77-91
Shamaladevi, Nagarajarao; Lyn, Dominic A; Shaaban, Khaled A et al. (2013)
Ericifolin: a novel antitumor compound from allspice that silences androgen receptor in prostate cancer.
Carcinogenesis 34:1822-32
Zhang, Lei; Lokeshwar, Bal L (2012)
Medicinal properties of the Jamaican pepper plant Pimenta dioica and Allspice.
Curr Drug Targets 13:1900-6
Singh, Rajendra Kumar; Lokeshwar, Bal L (2011)
The IL-8-regulated chemokine receptor CXCR7 stimulates EGFR signaling to promote prostate cancer growth.
Cancer Res 71:3268-77
Lokeshwar, Bal L (2011) Chemically modified non-antimicrobial tetracyclines are multifunctional drugs against advanced cancers.
Pharmacol Res 63:146-50
Shamaladevi, Nagarajarao; Lyn, Dominic A; Escudero, Diogo O et al. (2009)
CXC receptor-1 silencing inhibits androgen-independent prostate cancer.
Cancer Res 69:8265-74
Singh, Rajendra K; Lokeshwar, Bal L (2009)
Depletion of intrinsic expression of Interleukin-8 in prostate cancer cells causes cell cycle arrest, sponta neous apoptosis and increases the efficacy of chemotherapeutic drugs.
Mol Cancer 8:57
1. Southern Research Institute, Results Of Evaluation, 1990.
2. Tenth International Conference On AIDS Yokohama, Japan; 1994.
3. An Orally Active Amazonian Plant Extract (BIRM) Inhibits Prostate Cancer Growth And Metastasis, 2003.
4. Amazonian Plant Extract BIRM Reverses Chronic Neuropathic Pain In Rat Sciatic Nerve Chronic Constriction Injury Model, 2015.
5. The Andean Anticancer Herbal Product BIRM Causes Destabilization
Of Androgen Receptor And Induces Caspase-8 Mediated-Apoptosis In Prostate Cancer, 2016.
6. Antioxidant Activity Study of the “BIRM” Immunomodulator Product, 2018
7. GT198 is a target of oncology drugs and anticancer herbs, 2021.
The BIRM® ECA 10-142 in vitro, reports non-cytotoxic even at the highest concentration tested, and moderate anti-HIV activity with % reduction in syncytia-formation of 59%; (Southern Research Institute, Birmingham Alabama, U.S.A.; July 1991)
Results:
PB0294 BINATIONAL EXPERIENCE IN THE TREATMENT OF AIDS WITH A LOW MOLECULAR WEIGHT NATURAL CARBOHIDRATE (ECA-10-142), AS STIMULATE OF THE INMUNOLOGY SISTEM.
Cevallos Arellano Edwin, Head of the Oncology Clinic at the Metropolitan Hospital, Quito, Ecuador; Ardila Ardila, Henry, Director of the Liga Colombiana de Lucha Contra el SIDA, Santa fé de Bogotá D.C., Colombia.
To evaluate the stimulant properties of the immunological system of the (ECA-10-142) a low weight natural origen carbohydrate, in 70 IV CDC stage AIDS patients. Methods: 70 patients with AIDS confirmed diagnosis were selected, 30 Colombians and 40 Ecuadorian, for oral treatment with (ECA10-142). In a period of 9 months. In the evaluations were considered clinical symptoms and signs, total percentage of linfocites, CD4 and CD8 cells percentage and its relationships. In In vitro studies was demonstrated also that this carbohydrate stimulates the immunological system in the AIDS patients.
The obtain results in the 9 months demonstrated a clinical improvement as to signs and symptoms in 64 (91.4%) patients, immunological recuperations of the CD4 levels in 66 (94.3%) patients, clinical and immunological improvement were presented in 64 (91.4%) patients, 4 (5.7%) patients died due to their advanced state of the sickness. They only received treatment during one month and had their CD4 levels under 100.
Discussion and Conclusion:
The (ECA 10-142) has demonstrated to be useful vitro and in vivo stimulated of the immunological system as inhibits the formation of sincitiales masses in a 59% and is effective as the clinical and immunological improvement of AIDS patients. Kinetics studies are required and observations in a longer time in order to conclude its imunostimulating activity in AIDS patients.
Cevallos A. Edwin Dr., Chief of Oncology and Radiotherapy Services of Metropolitan Hospital, Quito, Ecuador.
The BIRM® ECA 10-142 in vitro, reports non-cytotoxic even at the highest concentration tested, and moderated and its HIV activity with % reduction in syncytia-formation of 59%; (Southern Research Institute, Birmingham Alabama, U.S.A.; July 1991) In vivo double-blind reported an elevation in the number of CD4 cells. The Chemical analysis report in Nuclear Magnetic Resonance stablished the empirical formula C3H502, or a multiple thereof. Methods: Twenty patients in state IV of CDC, were chosen, and survived from January 1989 to April 1993. In every patient the CD4 cells were elevated notoriously. For example: 149 CD4 cells to 480 CD4 cells, in 3 months of treatment with BIRM® .
Results:
Discussion and Conclusion:
The results were evaluated based on the number of CD4, CD8 and quotient CD4/CD8; clinical evolution of the patient in his signs and symptoms. Patients with CD4 as low as 1%, survived for 15 months, and in the second group the patients are still alive after 22 months of treatment.
The BIRM® ECA 10-142 has demonstrated been effective in all the states of HIV/AIDS, BIRM® has been evaluated “in-vitro*”and “in-vivo”; and its composition has demonstrated to be an carbohydrate of low molecular weight, which performs at the level of cellular adhesion and reduces in 59% the syncytia formation. The elevation in the number of CD4 cells indefinitely in all the patients treated, shows that we are in front of a modulator and stimulator substance of the Immune System, a substance of enormous application in this kind of patients.
Authors:
Abstract:
Devendra S. Dandekar Æ Vinata B. Lokeshwar Edwin CevallosArellano, Mark S. Soloway Balakrishna L. LokeshwarPoor efficacy of conventional chemo-therapeutic drugs against metastatic hormone-refractory prostate cancer (CaP) drives patients to try ‘‘alternative medicine’’. The antitumor activity of one such agent, ‘‘BIRM’’ (biological immune response modulator; ‘‘Simple Ecuadorian Oral Solution: an extract of an Amazonian plant’’), was characterized in vitro and in vivo using established CaP cell lines and a tumor model. Methods: The cytotoxicity of BIRM in four human and one rat CaP cell line was evaluated using cell proliferation inhibition and clonogenic survival assays. BIRMinduced apoptosis, alterations in cell cycle phase progression and inhibition of the extracellular matrix-degrading enzyme hyaluronidase were also investigated in these cells. The in vivo efficacy of BIRM was evaluated in rats with subcutaneous tumor implants of Dunning EGFP-MAT LyLu cells. The active species in BIRM were characterized by gel filtration chromatography. Results: BIRM inhibited cell proliferation and clonogenic growth of the CaP cells (IC50 about 8.0 ll/ ml). It increased cell accumulation in the G0/G1 phase by 33.8% and decreased the proportion of cells in S phase by 54.6%. Apoptotic cell death in BIRM-treated cells was associated with activation of cell death-associated caspases. BIRM inhibited the activity of hyaluronidase, a hyaluronic acid-degrading enzyme, at 1 ll/ml. Treatment of MAT LyLu tumor-bearing rats with BIRM by oral gavage resulted in a significant decrease in tumor incidence (50%), tumor growth rate (18.6±1.3 days for 1 cc tumor growth in control rats and 25.7±2.6 days in BIRM-treated rats), and only one out of six BIRM-treated rats versus four out of six in the control group developed lung metastasis. Three active ingredients in BIRM with a relative molecular mass (Mr) of ‡3500 were identified by ultracentrifugation and gel filtration chromatography and were found to be resistant to proteinase and heat (100LC). Conclusion: The plant extract BIRM contains antitumor compounds of Mr ‡3500 with potent antiproliferative activity in vitro and in vivo against prostate cancer cells.
Keywords:
Natural herbal anticancer products, Prostate cancer, Invasion and metastasis Chemoprevention, Apoptosis.
CaP Prostate cancer/cancer of the prostate
EGFP Enhanced green fluorescence protein Æ HA Hyaluronic acid
HAase Hyaluronidase Æ MTT Methyl thiazolyl tetrazolium bromide [(3-[4,5-demethylthiozol-2-y]-2,5-diphenyl tetrazolium bromide]
Cancer of the prostate (CaP) is the most frequently diagnosed malignant cancer in American men with an estimated 189,000 new cases in 2002 [13]. The majority of CaP-related deaths, estimated to be 30,200 in 2002, are likely the result of failure of all currently available conventional treatments. Besides undergoing conventional therapy, CaP patients often seek treatment by unproven therapeutic approaches [12]. It is estimated that 30–40% of men with CaP experiment with one or more complementary therapies which include high-dose vitamins and minerals, herbal preparations and supplements of soy, saw palmetto etc. [14]. Moreover, there is a dramatic increase in the number of patients moving towards complementary and alternative medicine and consuming plant extracts from ‘‘folklore medicine’’ [26]. We have come across one such natural herbal medicine ‘‘BIRM’’ (biological immune response modulator; ‘‘Simple Ecuadorian Oral Solution: an extract of an Amazonian plant’’) formulated by a physician (E.C.-A.), promoted in South America, and based on the local folklore of the Ecuadorian native population. The formulation is dispensed as a natural remedy for a variety of maladies including HIV-1 infection and cancer [1, 3, 4]. Very little systematic information is currently available on BIRM, and no studies have been undertaken to investigate the structure-function correlations in the ingredients of BIRM. Therefore, we decided to evaluate the efficacy and antiproliferative effects of BIRM in a CaP model.
BIRM was a gift from BIRM Inc. (Quito, Ecuador). BIRM is an aqueous extract of dried roots of a plant of the genus Dulcamara (family Solanaceae) grown in Ecuador, and marketed as a greenish-brown suspension with a mild bittersweet smell. The inactive ingredients in BIRM comprise 16% solid particles, likely root fibers, and the remainder, a lipid-free liquid. BIRM is prepared by aqueous extraction of dried roots followed by oxidation/reduction of the extract. During this process, the number of roots and the timing of oxidation/reduction are carefully controlled to minimize batch-to-batch variation. Prior to initiation of this project, the efficacy of BIRM samples from five different batches were selected randomly and tested in two different cell lines (PC3ML and LNCaP) by the MTT assay to determine the degree of interpatch variation. We found no interpatch variation in the potency of BIRM for induction of cytotoxicity. In the present study, BIRM samples from lot number 011-2000 were used. For all the studies reported here, BIRM clarified by centrifugation at 10,000 g was used.
Established human CaP cell lines (LNCaP and DU-145) were ob-tained from the ATCC (Rockville, Md.). A recently established bone metastatic PSA+ CaP line (VCaP) was generously provided by Drs. Pienta and Cooper (Karmanos Cancer Center, University of Michigan, Ann Arbor, Mich.) [11, 17, 23]. A metastatic variant of a PC-3 cell line, PC-3ML, was a gift from Dr. M.E. Stearns (Allegheny University Hospitals, Philadelphia, Pa.) [15, 27]. All cultures were maintained in a complete medium containing RPMI-1640 basal medium, 10% fetal bovine serum (Atlanta Biologicals, Atlanta, Ga.),
and 10 lg/ml gentamicin. The EGFP-MAT LyLu cell line was generated by stable transfection of Dunning MAT LyLu rat CaP cells with pEGFP-1 plasmid DNA (Clontech, Palo Alto, Calif.) and was maintained in complete medium with 250 nM dexamethasone as described previously [19, 28].
A 3H-thymidine incorporation assay was performed as described previously [8]. Following incubation in medium containing BIRM or without BIRM, the cells were pulselabeled with 3H-thymidine (1 lCi/ml) for 2 h. Incorporation of 3H-thymidine into cellular DNA was stopped by the addition of 10% trichloroacetic acid and the acid-precipitable radioactivity was determined by liquid scintillation counting [8]. Clonogenic survival of CaP cells exposed to BIRM for 24 h was assayed by the colony assay as described previously [8].
BIRM-induced apoptosis was assayed using a cell death ELISA kit (Cell Death ELISA-Plus kit; Roche Molecular Biochemicals, Mannheim, Germany). The assay measured the number of free nucleosomes in cell lysate resulting from programmed cell death [9]. The relative number of free nucleosomes present in cell lysates from cultures incubated with BIRM for 4 h or 24 h was estimated according to the supplier’s instructions.
CaP cells (1•105) were cultured in 60-mm culture dishes. After an overnight culture, the cells were treated with 10 or 25 ll/ml of BIRM for 24 h. BIRM-treated and untreated cells were harvested and stained with 50 lg/ml propidium iodide. The amount of propidium iodide bound to DNA was profiled in an EPICS XL flow cytometer as described previously [19]. The fraction of dead cells at the time of harvesting was about 16% as determined by trypan blue exclusion. The majority of these cells were floating, so were discarded at the time of washing. The remaining dead cells were gated out using the forward angle light scatter and side scatter gating’s during flow cytometry. About 20,000 propidium iodide-stained cells were analyzed in the flow cytometer from each sample. The MODFIT LT program (Verity Software House, Topsham, Me.) was used for the cell cycle phase analysis [29].
Caspase activation in CaP cells treated with BIRM was determined using a kit (Homogeneous Caspases Assay, fluorometric; Roche) which determined collectively activated caspases nos. 2, 3, 6, 7, 8, 9 and 10. The assay measured the free rhodamine 110 (R110) resulting from the cleavage of a common caspase substrate, DEVD, conjugated with R110. The amount of free R110 was determined fluorometrically at an excitation wavelength of 499 nm and an emission wavelength of 528 nm, and is expressed as relative fluorescence units (RFU) [25].
We tested whether BIRM affects HAase activity secreted in DU-145 culture-conditioned medium and partially purified
HYAL1 using a HAase activity ELISA-like assay [20, 22]. HYAL1-type HAase was partially purified from the urine of patients with high-grade bladder cancer as described previously [20]. The assay was performed in a 96-well microtiter plate coated with HA (200 lg/ml, ICN Biomedicals). Wells were incubated with various concentrations of BIRM or column fractions from a Sephadex G-50 gel-filtration column (see below) in a HAase assay buffer at 37LC for 15 h [20]. Following incubation, HA degraded by HAase was washed off and the HA remaining in the microtiter wells was estimated using a biotinylated bovine nasal cartilage HA-binding protein, and an avidin-biotin detection system (Vector Laboratories, Burlingame, Calif.) [21].
To study heat inactivation, BIRM was heated at 100LC for 5 min in a water bath. BIRM was digested with proteinase K (10 U/ml) at 37LC for 18 h. For size fractionation studies, clarified BIRM was loaded into ultrafiltration mini-Centriprep tubes (Millipore, Bed-ford, Mass.) with membrane barriers with different molecular weight cut-off points (i.e. about 3.5, 10 and 30 kDa). Following three cycles of centrifugation and separation of the low molecular weight fractions, both the filtrate and the retentate were assayed for cytotoxic activity. BIRM solution was also treated with charcoal-dextran (50 mg/ml) at 4LC for 12 h to remove lipids and steroids (if any).
Following the various treatments, BIRM was centrifuged and various concentrations of the supernatant were added to PC3ML cells cultured in 24-well plates (2•104 cells/well). BIRMinduced cytotoxicity was estimated using the MTT reduction assay following a 24-h treatment [19].
Particle-free BIRM was loaded onto a G-50 Sephadex column (1.5•120 cm) equilibrated with 20 mM Tris-HCl, pH 7.4, containing 150 mM NaCl buffer (Tris/NaCl buffer) [20]. The column was eluted in Tris/NaCl buffer at 7 ml/h and 3-ml fractions were collected. Each fraction was assayed for protein (BCA-Bio Rad), uronate [2], inhibition of cell growth (MTT assay) [19] and inhibition of HAase activity (HAase ELISA-like assay).
This experiment was performed according to a protocol approved by the University of Miami Animal Care and Use Committee and as stipulated in the NIH Guide to the Humane Care and Use of Laboratory Animals. A suspension (1•105 cells, 0.5 ml) of growing EGFP-MAT LyLu cells was implanted subcutaneously into the dorsal flank of adult (about 250 g) male Copenhagen rats (Harlan Sprague Dawley, Indianapolis, Ind.) under mild anesthesia [19]. The rats were housed in a room under a 12-h light/12-h dark cycle and provided with food and water ad libitum throughout the experiment. Following implantation, the rats were randomly divided into two groups of six animals and gavage with 1 ml of either distilled water (vehicle control, group 1) or
BIRM (group 2) using a 3-inch stainless-steel intubation cannula on day 1 of tumor implantation and then daily for 30 days. Tumor growth was examined by palpating the skin around the site of injection. After the tumors became palpable (about day 5), they were measured three times a week using calipers, and the volumes calculated assuming approximation to an ellipsoid (length height•-width•0.524). Animals were euthanized when the tumor volume was about 10 cc or the tumor became significantly necrotic. At necropsy, lungs were collected and viewed under a Nikon stereo-microscope with a fluorescence attachment (SMZ 1500) to examine the presence of fluorescent metastatic tumor foci.
Triplicate samples were assayed in all in vitro experiments. Statistical analysis was performed using parametric and nonparametric Student’s t-tests.
BIRM inhibited cell proliferation in all the CaP cell lines tested in a dose-dependent manner (Fig. 1). The concentration of BIRM causing 50% growth inhibition.
Fig. 1 Cytotoxicity of BIRM against prostate tumor cell lines. CaP cells cultured in growth medium (1•104 cells/well, 48-well plates) were exposed to various concentrations of BIRM. Following incubation for 24 h, DNA synthesis was determined by measuring 3H-thymidine incorporation in the proliferating cells (vertical bars means ± SEM from three independent assays)
(IC50) was 8 ll/ml (i.e. 0.8% v/v). Furthermore, the inhibitory activity of BIRM was comparable among all CaP cell lines regardless of their androgen sensitivity (androgen-sensitive LNCaP and VCaP cells versus androgen-resistant PC-3ML and DU-145 cells). Similar results were obtained by cell counting and Trypan blue exclusion assays (data not shown). The results presented in Fig. 1 and similar observation from other assays suggested that BIRM-induced inhibition of cell proliferation led to either cell death (cytotoxicity) or arrest of cell proliferation (cytostasis). To distinguish between these mechanisms, we investigated the colony-forming efficiency of CaP cells treated with BIRM. The clonogenic assay revealed a dose-dependent inhibition of colony formation in BIRM-treated CaP cells. Neither cell colonies nor cell clusters were observed in cultures exposed to BIRM at doses
of 10 ll/ml and above for 24 h (Fig. 2A). The IC50 of BIRM for inhibiting clonogenic survival was also 8 ll/ml, the same as the value obtained in the 3H-thymidine assay (Fig. 2B).
As shown in Table 1, the proportion of cells in G0/G1 phase increased significantly from 56.4±0.9% in control to 75.5±2.2% in cultures treated with BIRM at 25 ll/ ml. The increase in the G0/G1 phase fraction in the BIRM-treated cells was contrasted with a decrease in the S-phase fraction. The S-phase fraction in BIRM-treated cells was 13.1±2.9% compared to 28.9±2.1% in the control. A small decrease of 15–22% in the G2/M fraction was also observed in BIRMtreated cells.
We did not observe a significant difference in the levels of free nucleosomes in BIRM-treated cells during 4 h of treatment, but after 24 h of treatment the intracellular levels of free nucleosomes showed a two- to threefold dose-dependent increase (Fig. 3).
As shown in Fig. 4, the activities of one or more of caspases 2, 3, 7, 8, 9 and 10 were increased significantly in BIRM-treated cells as compared to the activities in control cells. We initially detected a time-dependent increase in combined caspase activity, beginning at 4 h of exposure to BIRM and peaking at 18 h. The dose-dependent increase in caspase activities showed a 50% increase in cells treated at 5 ll/ml BIRM over the activity in control cells following incubation for 18 h or longer (Fig. 4).
As shown in Fig. 5, following tumor implantation, oral administration of BIRM (4 ml/kg body weight) to rats resulted in slow tumor growth. While the tumor incidence was 100% in the control group, only four out of six BIRM-treated animals (67%) developed tumors. The tumor growth rate estimated using non-linear regression analysis of tumor volumes over time for each animal confirmed a decreased growth rate in BIRMtreated animals. The time taken for tumors to reach 1 cc was 18.6±1.3 days in control animals and 25.7±2.6 days in BIRMtreated animals (mean ± SE from four animals). A 38% delay in tumor growth was observed in BIRM-treated animals compared with control animals. The difference in the growth rate between control and BIRM-treated animals was statistically significant (unpaired t-ctest: P=0.03, t=2.773, df=6, 95% CI 0.835–13.36). Fluorescence imaging of the lungs at necropsy revealed that only one out of six BIRM-treated animals had metastatic lung foci, whereas five out of six control animals had tumor metastasis to the lungs. Furthermore, the tumor foci in the lungs of the BIRMtreated animal were significantly smaller than those in control animals (Fig. 6). These results indicate that ingredients in BIRM either delay or block spontaneous lung metastasis.
Fig. 2a, b Effect of BIRM on the growth of CaP cells. Cells cultured at low density in 60-mm culture dishes were exposed to BIRM for 24 h. Surviving cells at the end of incubation were allowed to form adherent cell colonies during the next 7–10 days. Cell colonies stained with 0.1% crystal violet and colonies containing >50 cells were counted manually using a hand-held electronic counter in a blinded fashion. a Colonies of surviving PC3-ML cells exposed to BIRM for 24 h. b Clonogenic survival of CaP cells cultured with BIRM. The results are presented as means ± SEM from three independent experiments.
Investigation of inhibition of matrix metalloproteinase activity by BIRM using a 3H-labeled collagen degradation assay [18] showed no changes in matrix metalloproteinase activity following BIRM treatment (data not shown). We next examined whether BIRM could inhibit the activity of HYAL1-type HAase. We have previously shown that HYAL1 is the major HAase expressed in cancers of the prostate and bladder [21, 22]. Furthermore, invasive tumor cells express high levels of HYAL1 [5, 21, 22]. As shown in Fig. 7, BIRM potently inhibited HAase activity. BIRM inhibited the HAase activity present in the culture-conditioned medium of DU-145 cells (a good source of HYAL-1 [21]) and the activity of partially purified HYAL1, in a dose dependent manner (IC50 0.25 ll/ml).
Fig. 3 BIRM kills tumor cells by induction of apoptosis. PC-3ML, DU-145 and Mat Lylu CaP cells cultured in growth medium (1•104 cells/well; 48-well plates) with or without BIRM at various concentrations for 24 h were analyzed for apoptotic activity using the Cell Death ELISA Plus assay kit, which allowed measurement of soluble nucleosomes by spectrophotometry. The data presented as means ± SEM from three independent experiments.
Fig. 4 BIRM induced increases in caspase activity. CaP cells were treated with BIRM for 4 to 24 h and the total activities of cell death-associated caspases were measured using a kit that employed a rhodamine110-conjugated peptide substrate common to all cell death-related caspases. The results are presented as means ± SEM from three independent experiments in each of which cell lysates incubated with BIRM for 24 h were used for caspase activity determination. Caspase activity in untreated control cultures was detectable, but was typically ten times less than the activity detected in the positive controls provided with the assay kit
BIRM retained its full cytotoxic activity after boiling for 5 min or digestion with proteinase K, indicating that reactive species present in BIRM most likely are heat-stable proteinase-resistant compounds. Similarly, char-coal-dextran extraction also did not result in any loss in the cytotoxic activity associated with BIRM, suggesting that the active species present in BIRM are not lipid-soluble compounds such as alkaloids or steroids. No loss in cytotoxic activity was found upon ultrafiltration through a 3.5-kDa membrane barrier. However, a 40% loss in activity was observed after ultrafiltration through 10-kDa and 30-kDa membranes (Fig. 8). These results indicate that BIRM contains at least two species with different molecular mass, i.e. one with a molecular mass between 3500 and 10,000 and a second with a molecular mass of ‡30,000, with cytotoxic activity against CaP cells. The possibility of the growth-inhibitory activity being associated with carbohydrate derivatives was investigated using the Bitter and Muir modified carbazole assay to measure glycosaminoglycans and proteo-glycans containing D-glucuronic acid (i.e. uronate) [23]. The results showed that BIRM is rich in urinate containing carbohydrates (19.5 mg/ml). Gel filtration chromatography on a Sephadex G-50 column also showed two active fractions (fraction nos. 28 and 42, Fig. 9).
Fig. 5 Effect of daily dosing of BIRM on growth and metastasis of Dunning MAT LyLu tumors in rats. Tumor growth in rats with subcutaneous tumor implant with EGFP-MAT Lylu cells (1•105 cells/animal). The data are presented as means ± SEM of each treatment group over time. Data from the control group include measurements from six tumor bearing rats, whereas the BIRM-treated group had only four animals; the other two animals in this group did not develop tumors.
Fig. 6 A, B EGFP-MAT LyLu rat prostate tumors metastatic to lungs. The figure shows rat lungs with fluorescent tumor foci (arrows). A Control animal (•40); B a typical tumor metastatic to the lung in a BIRM-treated animal (•20). Tumor foci in the lungs of BIRM-treated animals were typically ten times smaller or absent.
Fig. 7 BIRM inhibits tumor-derived HAase. Effect of BIRM on the HAase activity present in DU-145 cell-conditioned medium and partially purified preparation of HYAL1 was carried out using an HAase ELISA-like assay as described in Materials and methods. The data shown are from a typical experiment. Similar results were obtained in three other experiments using LNCaP cells (data not shown)
A systematic investigation of promising plant products has led to the discovery and development of antineoplastic agents with unique modes of action and striking efficacy (e.g. paclitaxel, vinblastine, etoposide, etc.) [7]. In this report, we present evidence of the antineoplastic activity of a plant-derived nutritional supplement, BIRM. The medicinal value of BIRM is believed to be associated only with the Amazonian variety of the plant Dulcamara, where the micronutrients present in the soil of the Upper Amazon basin promote the synthesis of medicinal compounds in the plant. BIRM inhibited cell proliferation and clonogenic survival (Figs. 1 and 2) and caused apoptotic cell death via the caspase activation pathway (Figs. 3 and 4). In vivo studies on the growth and metastasis of Dunning MAT LyLu tumors suggested that oral dosing with BIRM resulted in lower tumor incidence, slower tumor growth and reduced spontaneous metastasis to the lungs (Figs. 5 and 6). A preliminary biochemical characterization and size-exclusion chromatography suggested that there were at least four active species present in BIRM, three with cytotoxic activity and one with HAase inhibitory activity. We have not yet determined whether the three cytotoxic species present in BIRM have the same chemical composition but different polymer length. Nonetheless, all four active ingredients were heat-stable and unlikely to be proteins or lipid-soluble compounds.
Fig. 8 Cytotoxicity of modified BIRM as assayed by MTT assay and compared with unmodified BIRM. BIRM solution was treated with proteinases (proteinase K), heated for 5 min in a bath of boiling water or subjected to ultrafiltration as described in Materials and methods. Following treatment, untreated and treated BIRM solutions were tested for cytotoxic activity in PC3-ML cells by MTT assay, as described in Materials and methods (vertical bars means ± SEM from four independent assays).
Fig. 9 Fractionation of antineoplastic ingredients present in BIRM by gel filtration chromatography. Clarified BIRM (5 ml) was fractionated on a Sephadex G-50 column. The column fractions were assayed for protein (A280 nm), uronate concentration (Bitter and Muir assay), HAase activity (HAase activity ELISA) and cytotoxicity (MTT assay). A single protein peak was detected in fraction no. 20, which had neither cytotoxic activity nor HAase-inhibitory activity.
Inhibition of tumor growth in the rat CaP model following the oral administration of BIRM clearly suggests that the active ingredient(s) of BIRM are absorbed in the gastrointestinal tract. The reduction in tumor incidence (33%) and the number of tumor foci in the lungs (>80%) in BIRM-treated animals suggest that BIRM may exert both antiproliferative and antimetastatic activities. It is estimated that 20–40% of patients initially diagnosed with local CaP have either locally advanced disease (stage C) or metastatic disease (stage D) [10, 16], and the cure of metastatic disease still re-mains a challenge. Our observation that CaP cell cultures treated with BIRM showed a significant reduction in cell proliferation and undergo apoptosis (Figs. 1, 2, and 3) indicates that the active ingredients present in BIRM have potential for use in controlling advanced hormone-refractory prostate cancer. We investigated whether the BIRM-induced cytotoxic effect was due to inhibition of mitotic spindle separation. This would lead to mitotic inhibition and arrest of cells in the G2/M phase. Contrary to our expectation, incubation with BIRM arrested CaP cells in G0/G1, which was compensated for by a significant decrease (56%) in the proportion of cells in S phase and a modest decrease in G2/M, indicating lack of mitotic arrest or cytokinesis (Table 1). This finding is novel in the sense that most plant-derived compounds used in cancer therapy interfere with tubulin polymerization (e.g. vinblastine and vincristine) or depolymerization (paclitaxel), or inhibit topoisomerase I activity (e.g. irinotecan, topotecan, 9-aminocamptothecin and 9-nitrocamptothecin) [6] or topoisomerase II activity, leading to cell cycle arrest in the G2/M phase (e.g. paclitaxel, etoposide and teniposide) [24]. Apoptotic cell death may be one of the mechanisms involved in BIRM-induced cytotoxicity. BIRM in-creased the apoptosis in three CaP cell lines (Fig. 3). Furthermore,
induction of apoptosis in BIRM-treated CaP cells was coincident with activation of cell-death caspases (Fig. 4). In addition to its cytotoxic effects, BIRM appeared to be a potent inhibitor of metastasis. Although, the mechanism by which it may inhibit metastasis is unknown at present, our results suggest that BIRM is a potent inhibitor of HAase, a class of matrix-degrading enzymes whose levels have been shown to correlate with CaP progression [20, 22].
The recommended minimum dose of BIRM for human consumption is 4 ml/day (as indicted on the bottle label), a significantly lower dose than that used in the current study. We based the dosage to rats on the observed efficacy in vitro. We found no observable systemic toxicity in rats at a dose of 4 ml/kg. Given its effect on tumor growth and metastasis and no systemic toxicity, inclusion of BIRM as an adjuvant to standard therapy has potential to reduce/halt disease progression.
In summary, our study demonstrated that BIRM shows cytotoxic activity against both androgen dependent and androgen-independent CaP cells in vitro. More importantly, it reduced tumor incidence, delayed tumor growth and caused a significant reduction in metastasis in an experimental model of late-stage CaP. Further-more, no systemic toxicity was seen following continuous administration of BIRM in an in vivo rat model. These useful properties of BIRM indicate that further investigation of its mechanism of action and clinical trials involving its use in advanced CaP are warranted.
Acknowledgements: The authors dedicate this work to Mr. Christian DeGetau von Forckenbeck (late) for introducing us to BIRM. The authors thank Rita Moure Latos, Monica Lopez and Dr. Tie Yan Shang for technical assistance and Dr. A. Krishan for the use of his flow cytometry facility
1. BIRM Carbohydrate of low molecular weight ECA10-142 controls AIDS (1994) Tenth International Conference on AIDS (Yokohama, Japan). Abstracts, vol 2 (abstract no. 0291)
2. Bitter T, Muir H (1966) Mucopolysaccharides of whole human spleens in generalized amyloidosis. J Clin Invest 45:963–975
3. Cevallos EA (1994) Binational experience in the treatment of AIDS with a low molecular weight natural carbohydrate (ECA-10-142), as a stimulant of the immune system. Tenth International Conference on AIDS (Yokohama, Japan). Abstracts, vol 1 (abstract no. 0294)
4. Cevallos EA (1996) ‘‘BIRM: La estrategia terapéutica del futuro’’. Abstract of Congresso Mundial de SIDA en Vancouver
5. Delpech B, Girard N, Bertrand P (1997) Hyaluronan: fundamental principles and applications in cancer. J Intern Med 242:41–48
6. Dennis RAM, Adriana BN, Rocha DA, Gilberto S (2000) Anti-cancer drug discovery and development in Brazil: targeted plant collection as a rational strategy to acquire candidate anticancer compound. Oncologist 5:185–198
7. Donehower RC, Rowinsky EK (1993) Anticancer drugs de-rived from plants. In: DeVita VT Jr, Hellman S, Rosenberg SA (eds) Principles and practice of oncology, 4th edn. Lippincott, New York
8. Dudak SD, Lopez A, Block NL, Lokeshwar BL (1996) Enhancement of radiation response of prostatic carcinoma by lonidamine. Anticancer Res 16:3665–3671
9. Eisel D, Fertig G, Fischer B, Manzow S, Schmelig K (eds) (2000) Guide to cell proliferation and apoptosis methods, 2nd edn (technical manual). Roche Applied Science, Mannheim, Germany
10. Harris K, Reese DM (2001) Treatment options in hormone-refractory prostate cancer: current and future approaches. Drugs 61:2177–2192
11. Horoszewicz JS, Leong SS, Kawinski E, Karr JP, Rosenthal H, Chu TM, Mirand EA, Murphy GP (1983) LNCaP model of human prostatic carcinoma. Cancer Res 43:1809–1818
12. Jacobson JS, Chetty AP (2001) Complementary and alternative medicine in prostate cancer. Curr Oncol Rep 3:448–452
13. Jamel A, Thomas A, Murray T, Thun M (2002) Cancer statistics 2000. CA Cancer J Clin 52:23–47
14. Jones HA, Metz JM, Devine P, Hahn SM, Whittington R (2002) Rates of unconventional medical therapy use in patients with prostate cancer: standard history versus directed questions. Urology 59:272–276
15. Kaighn ME, Narayan KS, Ohnuki Y, Lechner JF, Jones LW (1979) Establishment and characterization of a human prostatic carcinoma cell line (PC-3). Invest Urol 17:16–23
16. Kojima M, Troncoso P, Babaian RJ (1995) Use of prostate-specific antigen and tumor volume in predicting needle biopsy grading error. Urology 45:807–812
17. Korenchuk S, Lehr JE, Mclean L, Lee YG, Whitney S, Vessella R, Lin DL, Pienta KJ (2001) VCaP, a cellbased model system of human prostate cancer. In Vivo 15:163–168
18. Lokeshwar BL, Selzer MG, Block NL, Gunja-Smith Z (1993) Secretion of matrix metalloproteinases and their inhibitors (tissue inhibitor of metalloproteinases) by human prostate in explant cultures: reduced tissue inhibitor of metalloproteinase secretion by malignant tissues. Cancer Res 53:4493–4498
19. Lokeshwar BL, Selzer MG, Zhu BQ, Block NL, Golub LM (2002) Inhibition of cell proliferation, invasion, tumor growth and metastasis by an oral non-antimicrobial tetracycline analog (COL-3) in a metastatic prostate cancer model. Int J Cancer 98:297–309
20. Lokeshwar VB, Lokeshwar BL, Pham HT, Block NL (1996) Association of elevated levels of hyaluronidase, a matrix-degrading enzyme, with prostate cancer progression. Cancer Res 56:651–657
21. Lokeshwar VB, Young MJ, Goudarzi G, Iida N, Yudin AI, Cherr GN, Selzer MG (1999) Identification of bladder tumor-derived hyaluronidase: its similarity to HYAL1. Cancer Res 59:4464–4470
22. Lokeshwar VB, Rubinowicz D, Schroeder GL, Forgacs E, Minna JD, Block NL, Nadji M, Lokeshwar BL (2001) Stromal and epithelial expression of tumor markers hyaluronic acid and hyaluronidase in prostate cancer. J Biol Chem 276:11922–11932
23. Mickey DD, Stone KR, Wunderli H, Mickey H, Paulson DF (1980) Characterization of a human prostate adenocarcinoma cell line (DU 145) as a monolayer culture and as a solid tumor in athymic mice. Prog Clin Biol Res 37:67–84
24. Pienta KJ, Naik HN, Jeffrey EL (1996) Effect of estramustine, etoposide and taxol on prostate cancer cell growth in vitro and in vivo. Urology 48:164–170
25. Roche Applied Science (2002) Homogeneous caspases assay, fluorometric (pack insert/product instruction). Roche Applied Science, Mannheim Germany
26. Smith M, Mills EJ (2001) Select complementary/ alternative therapies for prostate cancer: the benefits and risks. Cancer Pract 9:253–255
27. Wang M, Stearns ME (1991) Isolation and characterization of PC-3 human prostatic sublines, which preferentially metastasize to select organs in S.C.I.D. mice. Differentiation 48:115– 125
28. Wenger AS, Mickey DD, Hall M, Silverman LM, Mickey GH, Fried A (1984) In vitro characterization of MAT LyLu: a Dunning rat prostate adenocarcinoma tumor subline. J Urol 131:1232–1236
29. Yamamura Y, Rodriguez N, Schwartz A, Eylar E, Bagwell B, Yano N (1995) A new flow cytometric method for quantitative assessment of lymphocyte mitogenic potentials. Cell Mol Biol (Noisy-le-grand) 41:121–132
Authors:
1Department of Zoology, The M. S. University of Baroda, Vadodara 390 002, Gujarat, India
2Division of Biology, GVK Biosciences Pvt. Ltd., Hyderabad 500 076, Telangana, India
3BIRM Inc, Quito, Ecuador
Abstract:
Neuropathic pain condition remains poorly managed by currently available therapeutics. There is therefore a dire need for development of efficacious therapeutics with minimal side effects. BIRM (Biological Immune Response Modulator), an extract of Amazonian plant Solanum Dulcamara, consumed as a dietary supplement by natives in Ecuador, is considered as a natural remedy for a number of ailments (AIDS and Cancer, among others). The aim of the current study was to test the efficacy of BIRM in in vivo neuropathic pain model to elucidate its anti-neuroinflammatory potential. Rats subjected to chronic constriction injury (CCI) were divided into CCI-control, CCI-Gabapentin and CCI-BIRM groups along with a normal control group. BIRM was administered orally (4 ml/kg, daily) to animals of CCI-BIRM group from day 14 post-surgery till day 28. Repeated oral administration of BIRM inhibited CCI-induced mechanical allodynia and thermal hyperalgesia. It also inhibited CCI-induced activation of microglial cells and upregulation of COX-2 and TNF-α in the dorsal horn of the lumbar spinal cord. These data indicate that the marketed formulation BIRM, has anti-neuroinflammatory and anti-nociceptive properties in neuropathic rats and can serve as an adjuvant to standard therapy or as a stand-alone therapeutic agent for the treatment of neuropathic pain disorders.neuropathic pain model at preclinical stage, BIRM has potential to improve pain condition or reduce the disease progression as a standalone therapeutic or adjuvant to standard therapy.
Neuropathic pain is a chronic pain condition and is heterogeneous in nature. It is considered to arise from damage to nerves due to tumors, diabetic neuropathy, herpes zoster, complex regional pain syndrome, AIDS, sclerosis multiplex, hypoxia or stroke [1] and occurs worldwide. It greatly impairs quality of life, and has a high economic impact on society. The Institute of Medicine reports that at least 116 million American adults suffer from chronic pain and estimates for people suffering from neuropathic pain are as high as 17.9% [2]. Symptoms of neuropathic pain are often severely debilitating such as allodynia, hyperalgesia, spontaneous pain, as well as behavioral disabilities. Several animal models mimicking peripheral nerve injury have been developed to study neuropathic pain. Most widely used animal models are chronic constriction injury (CCI) of sciatic nerve [3], partial ligation of the sciatic nerve (PNL) [4] and ligation of one or more of the spinal nerves (SNL). Studies carried out using these animal models in last decade provides evidence of interactions between neurons, inflammatory immune and immune like glia cells, inflammatory cytokines and chemokines. Peripheral nerve injury provokes a reaction from the immune system and has been observed at various
anatomical locations including the injured nerve, the dorsal root ganglia (DRG), the spinal cord and supraspinal sites associated with pain pathways [5]. Emerging lines of evidence have revealed that changes also occur in spinal microglia, the immune cells of the central nervous system [6]. Activation of microglia is a major feature of neuropathic pain and growing evidence suggests that microglia have a causal role in pathogenesis of persistent neuropathic pain and hence a detailed study of the microglial cells and its exact role in central pain seems necessary. Until today, Neuropathic pain remains a poorly managed pathological condition by currently available therapeutics. Current treatments available for neuropathic pain indicate general insensitivity to nonsteroidal anti-inflammatory drugs and relative resistance to opioids. These treatments have untoward side effects when given at higher doses to obtain adequate analgesia [7]. Researchers around the globe are looking for alternate treatment which can offer adequate analgesia devoid of severe side effects. Under these circumstances, BIRM (Biological Immune Response Modulator) seems to be promising herbal formulation. BIRM is an oral solution which has been formulated from extracts of Amazonian plant Solanum Dulcamara by a physician E. Cevallos-Arellano,
native of Ecuador. This formulation is considered as a natural remedy for number of ailments (AIDS, cancer) and is consumed as a dietary supplement by Ecuadorian native population [8]. Earlier studies using BIRM have shown anti-metastasis properties in in vivo prostate cancer model [9] and inhibition of PGE2production by COX1and COX2 [10]. Recently, studies performed using BIRM in preclinical animal models of pain and inflammation exhibit its analgesic and anti-inflammatory properties [11]. Based on these data available, we found it good enough to explore undiscovered benefits of BIRM and study it systematically for assessing its anti-nociceptive effect in neuropathic pain conditions.
Animals and housing conditions Male Wistar rats (180-210 g) were procured from CPCSEA and AAALAC approved Vivarium Facility at GVK Biosciences Pvt. Ltd., Hyderabad, India. They were allowed to acclimatize for a minimum duration of one week prior to surgical intervention. They were housed in groups of three in polypropylene cages under ambient conditions prior to surgery and housed individually post-surgery. Room temperature and humidity were maintained at 22–25°C and 65-70%, respectively. 12 h light/dark cycle was maintained. Standard laboratory rodent diet and portable drinking water were provided ad libitum. To prevent wound infection after a surgical procedure, the surgical area was dusted with streptomycin before suturing the incision in all the animals. Experimental protocols were approved by IAEC (Institutional Animal Ethics Committee) of GVK Biosciences Pvt. Ltd. according to CPCSEA (Committee for the purpose of Control and Supervision of Experiments of Animals), India[1125/PO/c/ CPCSEA/019(2012)] All animal procedures were performed in accordance with guidelines of CPCSEA.
The method described by Bennett and Xie [2] was generally followed. Rats were anesthetized with gaseous anesthesia Isoflurane (Baxter, Germany). The right common sciatic nerve was exposed at the level of the mid-thigh by blunt dissection through the biceps femoris. Proximal to the sciatica’s trifurcation, about 12 mm of nerve was freed of adhering tissue and four ligatures (chromic catgut, Johnson & Johnson) were tied loosely around it with an interval of about 1 mm among ligatures. The length of nerve thus affected was 6-8 mm long. Great care was taken to tie the ligatures such that the diameter of the nerve was just barely constricted. The desired degree of constriction retarded, but did not arrest, circulation through the superficial epineural vasculature. The incision was closed in layers. Test compound and treatment regimen BIRM was a gift from BIRM Inc. (Quito, Ecuador). It is an aqueous extract of dried roots of a plant S. Dulcamara (family Solanaceae) grown in Ecuador, and marketed as a greenish-brown suspension with a mild bittersweet smell. In the present study, BIRM samples from same lot number were used and it was clarified by centrifugation at 10,000 g prior to usage as described by Dandek are et al. [9]. Gabapentin (Sigma Aldrich, USA) was used as a standard reference. Though the recommended minimum dose of BIRM for human consumption, as per the container label, is 4 ml/day, we used BIRM at 4 ml/kg dose based on dose range finding
study in in vivo experiments (acute inflammatory models). The commercially available formulation was directly administered without further dilution at 4 ml/kgb.wt. BIRM when administered orally at 4 ml/kg dose level was found to be well tolerated and found no observable systemic toxicity in rodents. Experiment was performed in two sets (n=4 per group in each set). Data obtained from both sets was collated and is represented hereby as one experiment. Surgically operated CCI rats were randomly selected after assessment of mechanical allodynia and divided into four groups (n=8 per group). Drug testing was initiated on day 14 post-surgery. Animals from Normal Control (NC) and CCI operated Control (VC-CCI) were orally administered distilled water throughout the study duration. Animals from BIRM –CCI operated group (BIRM-CCI) were administered BIRM daily at 4 ml/kg dose volume (day 14-day 28 post-surgery) through oral outer. Gabapentin (30 mg/kg, p.o.) was administered once on day 14,21 and 28 post-surgery Neuropathic pain measurements Mechanical Allodynia Mechanical allodynia was assessed by modified Dixon’s Up and Down method using a set of von-Frey filaments (0.4, 0.6, 1.0, 2.0, 4.0, 6.0, 8.0 and15.0 g). It was assessed at 3hr post first dose on day 14,21 and day 28 post-surgery. The pattern of positive and negative responses was tabulate dosing the convention, X = withdrawal; O = no withdrawal and the 50% response threshold was interpolated using the method followed by Chaplanet al [12]. Thermal Hyperalgesia Thermal response was determined by measuring hind paw withdrawal latency of affected paw employing Hargreaves’ plantar test. Sample Preparation On day 28 post-surgery (14 days post treatment), the rats from NC, CCI-VC and CCI-BIRM were deeply anesthetized with isoflurane 5% and immediately perfused intracardially with 400-500 ml of cold phosphate buffered saline (0.01 M, pH -7.4) followed by 2% paraformaldehyde in 0.01 M phosphate buffer(pH-7.4) through the ascending aorta. Then their lumbar spinal cords (L4-L6 region) were quickly removed. From another set of four animals from each group, the lumbar spinal cord tissues for RT-PCR and western blot analysis were collected and immediately stored at -80°C until analysis. Western blot –expression of Iba-1 protein –microglia cell marker Protein was resolved on 15% polyacrylamide gel followed by transfer on to nitrocellulose membrane. Immunoprobing of Iba-1 protein was by Anti-Iba-1(Ab Cam, ab5076) used at 1:1000 dilution. For staining, Horseradish peroxidase coupled with ECL detection reagent (GE Healthcare-Amersham, USA) was used. Anti-β-actin was used as the loading control antibody. Densitometric analysis was carried out using Alpha Ease FC software, version 4.0.034(Alpha In no tech, USA) and results were normalized to loading control. Real time PCR Total RNA from the lumbar spinal cord was extracted using the standard phenol/chloroform extraction with TRIzol Reagent (Invitrogen) according to the manufacturer’s guidelines. Samples were treated with DNase (Invitrogen) to remove any contaminating DNA. Total RNA was reverse transcribed into cDNA using Revert Aid First Strandc DNA Synthesis Kit (Thermo Scientific, USA). All cDNA samples were stored at -80°C until real-time PCR (qPCR) was performed. The primers for TNF-α,IL10, COX-2 and GAPDH were
F: 5 ‘–GATGGGCTGTACCTTATCTACTCCCAGG3’,R5’ CCTTAGGGCAAGGGCTCTTGATGGC; 5’ –TAAGGGTTACTTGGGTTGCCAAGCC-3’, reverse 5’–GCAGCTGTATCCAGAGGGTCTTCAGC - 3’; 5’ –CAGTATCAGAACCGCATTGCCTCTG -3’, reverse 5’– GTGAGCAAGTCCGTGTTCAAGGAGG - 3’; 5’ –CAAGGTCATCCATGACAACTTTGGC - 3’, reverse 5’–CAAGGTCATCCATGACAACTTTGGC - 3’, respectively.
Amplification of the cDNA was performed, in a blinded procedure, using SYBR® Select Master Mix kit (Life Technologies/ABI) in MicroAmp® 96 well plate (Life Technologies/ABI) on Step-one Real Time PCR machine (Applied Biosystems). Each sample was measured in triplicate. The reactions were initiated with a hot start at 94ºC for 5 min, followed by 40 cycles of 5 s at 94ºC (denaturation), 10 sat55ºC (annealing), and 10 sat 72ºC (extension). Melt curve analyses were conducted. The comparative cycle threshold (Ct) method was used for relative quantification of gene expression. The amount of mRNA, normalized to the endogenous control (GAPDH) and relative to a calibrator, was given by 2-Δ(ΔCt). Immunohistochemistry Spinal cord tissues collected after transcardial perfusion were washed with phosphate buffer and cryopreserved in sucrose at 4°C, till the tissues settled at bottom. Frozen tissue was embedded in OCT (Tissue-Tek, Sakura Finetek, USA) and sections were taken at 12 µm thickness with cryotome (Reichert-Jung, Cryocut E Cryostat). Tissues were immunolabeled using standard immuno-histochemistry methods for microglial localization and Iba1 expression using goat polyclonal antiIba1antibody (1:300; Abcam, overnight incubation at4°C). The ABC-DAB system was used for immunostaining. From each animal’s spinal cord, four to five sections within the L4L5 region were included in the analysis. The sections were observed under Leica DM2500 microscope and the images were captured using EC3 camera utilizing Leica LAS EZ (V 1.6.0) software. Colour intensity was quantified using Doc ItLS software (Genei, Bangalore, India) by an observer unaware of experimental conditions. The pixel measurement was used for counting the density-slicing area in the image of the positive area of the dorsal horn of the spinal cord. Then, the fold change in the staining density between NC, CCIVC and CCI-BIRM was calculated. The criteria for resting and activated microglia were as described previously [13]. All samples from all groups were numbered randomly and blinded observation was carried out to prevent bias.
One-way ANOVA followed by Tukey’s multiple comparison test was applied for 50% PWT analysis and 50% PWL analysis. Unpaired Student’s t-test was used for analysis of data generated from gene expression studies. p ≤0.05 was considered statistically significant. For ease of reading, the basic statistical values are shown in the text while the more extensive statistical information can be found in the figure legends. Results The effect of BIRM on CCI -induced changes in behavioral and neuropathic pain measurements
After CCI surgery, the rats gradually showed the typical signs of allodynia and hyperalgesia such as toe closing, foot eversion and paw licking. There was nonsignificant difference observed in ipsilateral 50% paw withdrawal threshold (PWT)
and paw withdrawal latency (PWL) among the groups (p>0.05)in animals subjected to CCI surgery (CCI-VC, CCIBIRM and CCI-Gabapentin) after characterization of CCI on day 13(Table 1 and 2; Figure 1 and 2) .Mechanical Allodynia
With respect to mechanical allodynia, CCI-vehicle control group showed significant decrease in 50%PWT as compared to normal control group throughout the study duration post CCI induction on days 13,14,21 and 28 (P ≤0.001). Repeated oral treatment with BIRM (4 ml/kg) as a single dose daily for 14 days showed a mean protection of 35% and38%at 3 h post BIRM administration on day 14 and day 21, respectively. The increase in 50% PWT was significantly higher than CCI-vehicle control group (CCI-VC) on day 14 (p ≤0.05) but not on day 21 analyzed by One Way ANOVA followed by Tukey’s multiple comparison test. BIRM showed mean protection of 70%on day 28. This increase in 50%PWT was significantly higher than CCI - vehicle control group (p ≤0.001) as analyzed by One Way ANOVA followed by Tukey’s multiple comparison test (Table 1; Figure 1). Treatment with Gabapentin at 30mg/kg showed a mean protection of 82%-83%at all assessment time i.e., on day 14,21 and 28. The increase in 50% PWT was significantly higher (P ≤0.001) than CCI - vehicle control group as analyzed by One Way ANOVA followed by Tukey’s multiple comparison test (Table 1; Figure 1).Thermal hyperalgesia With respect to thermal hyperalgesia, CCI-Vehicle control group (CCI-VC) showed significant decrease in50% PWL throughout the study duration post CCI induction i.e., on days 14,21 and 28 (p ≤0.001). But treatment with BIRM (4 ml/kg/day) showed a significant increase in PWLs at 3h post administration on day 14 (p ≤0.05), day 21 (p ≤0.001) and day 28(p ≤0.001). This increase in PWLs was significantly higher than CCI - vehicle control group as analyzed by Oneway ANOVA followed by Tukey’s multiple comparison test (Figure 2).Treatment with Gabapentin showed an average increase in PWLs at 3hr post administration on day14,21 and 28. The increase in PWLs was significantly higher than CCI - vehicle control group as analyzed by One-way ANOVA followed by Tukey’s multiple comparison test (Table 2; Figure2).The effect of BIRM on CCI-induced Iba-1 protein expression Quantitative analysis of Western blots showed thatIba-1 protein level had significantly increased in lumbar spinal cord tissue on day 28 post CCI surgery (p<0.001). Significant reduction in Iba-1 protein level was observed in the CCI group treated with BIRM (p≤0.01) (Figure 3). These findings in western blot experiment suggest neuroprotective effect of repeated oral treatment of BIRM (4 ml/kg/day) on microglia activation in lumbar spinal cord tissue. The effect of BIRM on CCI –induced COX-2, TNF-α,IL-10 expression in spinal cord Mean fold increase was observed in mRNA levels of TNF-α(0.022 times) and COX-2 (0.141 times) in lumbar tissue (L4-L5) in CCI-VC animals as compared to normal control (NC) animals. Mean fold change of 0.008 times was observed in mRNA levels of IL-10 in animals of CCI-VC group. But treatment with BIRM was able to bring significant reduction in mean fold change in mRNA levels of TNF-α(0.007 times)(p ≤0.01) and COX-2 (0.032times) (p ≤0.001)in lumbar tissue (L4-L5) in CCI-BIRM animals as compared to CCI-VC group. Similarly, significant increase in fold change in mRNA levels of IL-10 (0.029) (p ≤0.01)
in CCI-BIRM group was observed as compared to CCI-VC group. Results were analyzed using Unpaired Student’s test (Figure 4). The effect of BIRM on CCI-induced changes in spinal microglia cells Immunohistochemistry was performed using the Iba-1 antibody which is known to selectively label activated microglia in nervous tissue. In the normal control sections, a few Iba-1 positive cells could be seen (Figure 5A) as compared to higher number of Iba-1 positive cells in CCI-VC and CCI-BIRM sections (Figure 5B and 5C). The morphology of microglia cells in normal control sections exhibited the resting type shape to some extent, which has small compacts Mata bearing long, thin, ramified processes (Figure5A, arrow marked). Although the thin, ramified processes are not clearly visible in our sections, soma diameter is found to be much smaller and compact compared to activated microglial cells in CCI-VC and CCIBIRM groups. Microglia exhibited an activated phenotype, showing hypertrophy and retraction of cytoplasmic processes in the sections of CCI rats. Compared to normal control rats, a significant shift from resting to activated morphology was found in CCI rats. Chronic treatment of BIRM (4ml/kg) for 14 days was found to reduce the proportion of the activated phenotype in microglial cells (Figure 5C). Quantification of Iba-1immunoreactivity in lumbar dorsal horn shows significant increase in Iba-1 immunoreactivity in CCIVC group as compared to naïve/normal rats(p≤0.001) but repeated oral treatment of BIRM significantly inhibits CCIinduced upregulation of Iba-1 immunoreactivity as compared to CCI-VC group(Figure 5D) Discussion The present study demonstrates that microglia cells are the useful tool for evaluating the effects of anti-neuroinflammatory effects of novel compounds. Repeated oral administration of BIRM, an aqueous extract of dried roots of a plant of the species Dulcamara, has significantly inhibited thermal hyperalgesia and mechanical allodynia in animal model of CCI-induced neuropathic pain. The immunohistochemical results have shown the CCI-induced microglia activation, which is evident from their morphologies in CCI-VC group. The western blot results showed increased expression of Iba-1 protein in lumbar spinal cord in CCI induced neuropathic pain which supports the immunohistochemical data. Increased expression of Iba-1 protein under neuropathic condition indicates activation of microglia cells in the spinal cord. Repeated administration of BIRM orally, improved pathological conditions in animal model of neuropathic pain in the spinal cord by reducing the expression of Iba1protein and the proportion of activated microglia cells along with significant inhibition of neuropathic pain symptom, thermal hyperalgesia and mechanical allodynia. Microglial cells are the resident immune cells of the central nervous system (CNS). They act as the main form of active immune defense in the CNS and upon getting activated following any insult to the nervous tissue, they become the main source of inflammatory mediators (e.g.: IL-1β, IL-6, TNF-α, PGE2, NO, BDNF etc.) in the nervous system [14].
Microglia, once activated, gets engaged in phagocytosis and also participates in the adaptive immunity by presenting antigens to T cells. Apart from their role in inflammatory processes and regulation of cell survival, they are also capable
of detecting specific aspects of normal and pathological levels of activity in brain, and its repercussions. Nerve injury induces extensive proliferation of spinal microglia and related gene expression. They become activated and adopt the immunological functions of the tissue following the damage [1]. The increased presence of Iba-1positive cells in L4-L5 region of spinal cord in present study following induction of neuropathic pain is in line with the earlier report on the L4-L5spinal cord dorsal and ventral horn following sciatic nerve injury [15]. Moreover Patro et al. [16] have also reported activation of microglia and increased expression of Iba-1 in the proximity of the sensory and motor neurons in the L4-L5 spinal cord of threats subjected to nerve injury. This data suggests importance of the role being assayed by Iba-1protein in regulation of activated microglia functions. Using Iba-1 as microglia marker, Tawfik etal. [17] and RomeroSandoval et al. [18] has also shown the importance of role being played by microglia in the maintenance of neuropathic pain for longer duration. The microglial activation (presence of Iba1 positive cells) in the L4-L5 region of spinal cord following induction of neuropathic pain through CCI reported in this article supports the above findings. Repeated administration of BIRM to CCI rats helps in restoring microglia cells to its resting stage from the activated stage (Figure5C). Further, the gene expression analysis of COX-2 and pro-inflammatory cytokines (TNF-α) showed fold increase in their mRNA levels in lumbar spinal cord tissue of rats from CCI-induced vehicle control group as compared to normal control group. Repeated oral administration of BIRM not only inhibited the neuropathic pain symptom namely thermal hyperalgesia and mechanical allodynia but also prevented CCI-induced changes in spinal cord and significantly reduced fold increase of inflammatory mediators like COX-2 and TNF-α in lumbar spinal cord tissue. At the same time, we were able to observe fold increase in mRNA levels of anti-inflammatory cytokine (IL-10) in lumbar spinal cord tissue of CCI-BIRM treated rats. It is well documented that COX exists in two isoforms: COX-1and COX-2. COX-1 is constitutively expressed in most cells under physiological conditions whereas COX-2 is highly inducible in response to cytokines, growth factors, or other inflammatory stimuli and lasts for several months or even several years [10,19]. These enzymes catalyze the rate limiting steps of prostaglandin and thromboxane synthesis. Prostaglandins play a crucial role in nociceptive transmission at peripheral sites and in the spinal cord [20,21]. The present gene expression studies show the mRNA levels of COX-2 in lumbar spinal cord of naïve rats (Figure 4). COX-2, being an inducible enzyme, increases in the peripheral and central nervous system post injury or inflammation [22,23]and plays an important role in neuropathology. Jeanet al. [24] observed overexpression of COX-2 uninjured nerve in rats following CCI, partial sciatic nerve ligation, spinal nerve ligation and complete sciatic nerve transaction intervention. Supporting this observation, the present gene expression study shows a significant increase in COX-2 mRNA levels in the lumbar spinal cord in CCI rats as compared to normal/naïve rats (Figure 4). Matsunaga et al. [25] showed that inhibition of COX-2 by selective inhibitors attenuates hyperalgesia in neuropathic rats. This increase in COX-2 mRNA levels was inhibited by repeated oral treatment of BIRM in CCI-BIRM treated rats. These results suggest that BIRM produces an analgesic effect on neuropathy via inhibition of the expression of COX-2 mRNA levels in the spinal cord.
The current results support the earlier findings reported by Jaggi et al. [10] demonstrating inhibitory effect of mother tincture Solanum Dulcamara on PGE2 production via COX-1and COX-2 in vitro. In addition, central neuroimmune activation and neuro-inflammation have also been postulated to mediate and/or modulate the pathogenesis of persistent pain states. Proinflammatory cytokines (IL-1β, IL-6 and TNF-α), signaling proteins are uniquely powerful and have been associated with cell proliferation, differentiation and changes in gene expression and synthesis of matrix proteins important to cell growth and tissue repair [26]. They induce a long-term alteration of synaptic transmission in the CNS and play a critical role in the development and maintenance of neuropathic pain [27,28], but on the other hand they are also essential in fighting infection and responding to injuries. Each of these pro-inflammatory cytokines has been observed in spinal cord under pathological conditions implicating its role in pain facilitation. These cytokines activate neurons as wells as glia via specific receptors. In the CNS, the major contributors of cytokine release are glia. Microglia can produce cytokines on activation [29]. Nerve injury or peripheral inflammation has-been reported to activate glial cells and increase the pro-inflammatory cytokine levels in the CNS [30]. Also, as per the previous findings, TNF-α, IL-1 and/or IL-6 mRNA expression is elevated in spinal cordin response to peripheral nerve injury [31], spinal nerve injury [32], each of which elevates pain responses (hyperalgesia and allodynia). In line with this, the present study showed fold increase in mRNA levels of TNF-α in the lumbar spinal cord of rats following the sciatic nerve ligation (CCI) (Figure4). Repeated oral administration of BIRM to CCI-rats lowered the fold increase in mRNA levels of pro-inflammatory cytokine (TNF-α) (Figure 4) and these could be due to its direct interaction with immune cells of the CNS. There are reports showing increase in TNF-α, IL-1 and/or IL-6 protein levels in spinal cord following peripheral nerve injury [32,33].IL-10, being a suppressor of macrophages, is considered as an antiinflammatory cytokine. It potently down-regulates production and release of pro-inflammatory cytokines like TNF-α, IL1βand IL-6 [34]. Although the precise functions of IL-10 in the CNS require further clarification, it is well known as an important negative regulator of pro-inflammatory gene expression. It can down-regulate the expression of receptors for pro-inflammatory cytokines [35,36]. In our present study we observed significant fold increase in IL-10 mRNA levels in the lumbar spinal cord of CCI-rats treated with BIRM as compared to CCI-vehicle treated rats (Figure 4). Ledeboer et al. [37] have reported that IL-10, when injected in a region of the spinal cord where activated glial cells were present, drastically reduced the pain symptoms in animal models of chronic pain. The latter record consolidates our current finding of BIRM induced heightened expression of IL-10 with concomitant reduction in the expression of TNF-α. This together with the observed reduction in the expression of COX-2 and the attended decline in prostanoid synthesis explain the reasons for the effective amelioration of neuropathic pain observed in BIRM treated rats. In summary, our study with BIRM shows inhibition of microglia activation in the CNS and downregulates pro-inflammatory cytokines andCOX-2. As demonstrated in this study, BIRM attenuates the development of hyperalgesia and allodynia
in the rat model of neuropathic pain. Overall, this study not only demonstrates the effectiveness of BIRM in improving pathological conditions of nerve injury induced neuropathic pain but also showed the important role played by microglia in regulating the induction of a chronic pain state induced by peripheral nerve ligation. Based on the results obtained using BIRM as a therapeutic agent in neuropathic pain model at preclinical stage, BIRM has potential to improve pain condition or reduce the disease progression as a standalone therapeutic or adjuvant to standard therapy.
There is no conflict of interest.
We are thankful to GVK Biosciences Pvt. Ltd. for providing infrastructure assistance in carrying out these studies. We are also thankful to Dr. Amit Sharma for his assistance in surgical procedures and to Dr. Chetan Sharma and Dr. Mukesh Gandhari for technical assistance. PB is grateful to the Council of Scientific & Industrial Research, New Delhi, India for financial assistance as fellowship.
1. Mika, J., Modulation of microglia can attenuate neuropathic pain symptoms and enhance morphine effectiveness. Pharmacol Rep 2008; 60:297-307.
2. von Hehn, C.A., Baron, R., Woolf, C.J., Deconstructing the neuropathic pain phenotype to reveal neural mechanisms. Neuron 2012; 73:638-652.
3. Bennett, G.J., Xie, Y.K., A peripheral mononeuropathy in rat that produces disorders of pain sensation like those seen Inman. Pain 1988; 33:87-107.
4. Seltzer, Z., Dubner, R., Shir, Y., A novel behavioral model of neuropathic pain disorders produced in rats by partial sciatic nerve injury. Pain 1990; 43:205-218.
5. Austin, P.J., Moalem-Taylor, G., The neuro-immune balance in neuropathic pain: involvement of inflammatory immune cells, immune-like glial cells and cytokines. J Neuroimmunol2010; 229:26-50.
6. Inoue, K., Tsuda, M., Microglia and neuropathic pain. Glia2009; 57:1469-1479.
7. Dray, A., Neuropathic Pain: Emerging treatments. Br JAnesth 2008; 101:48.
8. Cevallos-Arellano, E., Binational experience in the treatment of AIDS with a low molecular weight natural carbohydrate (ECA-10-142) as a stimulant of the immune system. Tenth International Conference on AIDS 1994 (Yokohama, Japan).
9. Dandekar, D.S., Lokeshwar, V.B., Cevallos-Arellano, E., et al., An orally active Amazonian plant extract (BIRM) inhibits prostate cancer growth and metastasis,
Cancer Chemother Pharmacol 2003;52:59-66.
10. Jaggi, R., Wurgler, U., Grandjean, F., et al., Dual inhibition of5-lipoxygenase/cyclooxygenase by a reconstituted homeopathic remedy; possible explanation for clinical efficacy and favourable gastrointestinal tolerability. Inflamm Res 2004; 53:150-157.
11. Ravalji M, Cevallos-Arellano E, Balakrishnan S. Investigation of centrally and peripherally acting analgesic and anti-inflammatory activity of biological immune response modulator (an Amazonian plant extract) in animal models of pain and inflammation. Int J Basic Clin Pharmacol 2015; 4:342-348.
12. Chaplan, S.R., Bach, F.W., Pogrel, J.W., et al., Quantitative assessment of tactile allodynia in the rat paw. J Neurosci Methods 1994; 53: 55-63.
13. Hains, B.C., Waxman, S.G., Activated microglia contribute o the maintenance of chronic pain after spinal cord injury. J Neurosci 2006; 26: 4308.
14. Hung, J., Chansard, M., Ousman, S.S., et al., Activtion of microglia by neuronal activity: results from a new in vitro paradigm based on neuronal-silicon interfacing technology. Brain Behav Immun 2009; 24(1): 31-40.
15. Saxena, K., Patro, N., Patro, I., FK506 protects neurons following peripheral nerve injury via immunosuppression. Cell Mol Neurobiol 2007; 27: 1049-1057.
16. Patro, N., Nagayach, A., Patro, I.K., Iba1 expressing microglia in the dorsal root ganglia become activated following peripheral nerve injury in rats. Indian J Exp Biol 2010; 48(2): 110-6.
17. Tawfik, V.L., Nutile-McMenemy, N., Lacroix-Fralish, M.L., et al., Efficacy of propentofylline, a glial modulating agent on existing mechanical allodynia following peripheral nerve injury. Brain Behave Immune 2007; 21(2): 238-46.
18. Romero-Sandoval, E.A., Horvath, R.J., DeLeo, J.A., Neuroimmune interactions and pain: focus on glial modulating targets. Curr Opin Investig Drugs 2008; 9(7): 726-34.
19. Zhu, X.Y., Huang, C.S., Li, Q., et al., p300 exerts an epigenetic role in chronic neuropathic pain through its acetyltransferase activity in rats following chronic constriction injury (CCI). Mol Pain 2012; 8: 84.
20. Yamamoto, T., Nozaki-Taguchi, N., The role of cyclooxygenase-1 and -2 in the rat formalin test. Anesth Analg 2002; 94: 962.
21. Jean, Y.H., Chen, W.F., Duh, C.Y., et al., Inducible nitric oxide synthase and cyclooxygenase-2 participate in antiinflammatory and analgesic effects of the natural marine compound lemnalol from Formosan soft coral Lemnalia
cervicorni. Eur J Pharmacol 2008; 578: 323-331.
22. Seibert, K., Zhang, Y., Leahy, K., et al., Pharmacological and biochemical demonstration of the role of cyclooxygenase 2 in inflammation and pain. Proc Natl Acad Sci 1994; 91: 12013-12017.
23. Zhao, Z., Chen, S.R., Eisenach, J.C., et al., Spinal cyclooxygenase-2 is involved in development of allodynia after nerve injury in rats. Neurosci 2000; 97: 743-748.
24. Jean, Y.H., Chen, W.F., Sung, C.S., et al., Capnellane, a natural marine compound derived from soft coral, attenuates chronic constriction injury-induced neuropathic pain in rats. Br J Pharmacol 2009; 158: 713-725.
25. Matsunaga, A., Kawamoto, M., Shiraishi, S., et al., Intrathecally administered COX-2 but not COX-1 or COX-3 inhibitors attenuate streptozotocin-induced mechanical hyperalgesia in rats. Eur J Pharmacol 2007; 554: 12-17.
26. Wieseler-Frank, J., Maier, S.F., Watkins, L.R., Central proinflammatory cytokines and pain enhancement. Neuro signals 2005; 14: 166-174.
27. Sweitzer, S.M., Arruda, J.L., DeLeo, J.A., The cytokine challenge: methods for the detection of central cytokines in rodent models of persistent pain. In Kruger L ed. Methods in Pain Research, CRC, New York. 2001: 109.
28. Raghavendra, V., Rutkowski, M.D., DeLeo, J.A., The role of spinal neuroimmune activation in morphine tolerance/hyperalgesia in neuropathic and shamoperated rats. J Neurosci 2002; 22: 9980-9989
29. Dong, Y., Benveniste, E.N., Immune function of astrocytes. Glia 2001; 36: 180.
30. DeLeo, J.A., Yezierski, R.P., The role of neuroinflammation and neuroimmune activation in persistent pain. Pain 2001; 90: 1-6.
31. Arruda, J.L., Colburn, R.W., Rickman, A.J., et al., Increase of interleukin-6 mRNA in the spinal cord following peripheral nerve injury in the rat: potential role of IL-6 in neuropathic pain. Brain Res Mol Brain Res 1998; 62: 228-235.
32. Winkelstein, B.A., Rutkowski, M.D., Sweitzer, S.M., et al., Nerve injury proximal or distal to the DRG induces similar spinal glial activation and selective cytokine expression but differential behavioral responses to pharmacologic treatment. J Comp Neurol 2001; 439: 127-139.
33. DeLeo, J.A., Colburn, R.W., Nichols, M., et al., Interleukin-6- mediated hyperalgesia/allodynia and increased spinal IL-6 expression in a rat mononeuropathy model. J Interferon Cytokine Res 1996; 16: 695-700.
34. Moore, K.W., O’Garra, A., Malefyt, R.W., et al., Interleukin10. Annu Rev Immunol 1993; 11: 165-190.
35. Hamilton, T.A., Ohmori, Y., Tebo, J.M., et al., Regulation of macrophage gene expression by pro- and anti-inflammatory cytokines. Pathobiology 1999; 67: 241-244.
36. Sawada, M., Suzumura, A., Hosoya, H., et al., Interleukin-10 inhibits both production of cytokines and expression of cytokine receptors in microglia. J Neurochem 1999; 72:1466-1477.
37. Ledeboer, A., Jekich, B.M., Sloane, E.M., et al., Intrathecal interleukin-10 gene therapy attenuates paclitaxel-induced mechanical allodynia and proinflammatory cytokine expression in dorsal root ganglia in rats. Brain Behav Immun 2007; 21: 686-698.
Authors:
Abstract:
Nagarajarao Shamaladevi1,*, Shinako Araki1, 6,*, Dominic A. Lyn1, Rajnikanth Ayyathurai2, Jie Gao3, Vinata B. Lokeshwar4, Hugo Navarrete5, Bal L. Lokeshwar3 University of Miami, 2016.
BIRM is an anticancer herbal formulation from Ecuador. Previous study established its antitumor and antimetastatic activity against prostate cancer models. The activity of BIRM against human prostate cancer (PCa) cells was investigated to uncover its mechanism of antitumor activity. In androgen receptor (AR)expressing PCa cells BIRM was 2.5-fold (250%) more cytotoxic in presence of androgen (DHT) compared to cells grown in the absence of DHT. In AR-positive cells (LAPC-4 and LNCaP) BIRM caused a dose and time-dependent down-regulation of AR and increased apoptosis. Exposing cells to BIRM did not affect the synthesis of AR and AR promoter activity but increased degradation of AR via proteasome-pathway. BIRM caused destabilization of HSP90-AR association in LAPC-4 cells. It induced apoptosis in PCa cells by activation of caspase-8 via death receptor and FADD-mediated pathways. A synthetic inhibitor of Caspase-8 cleavage (IETD-CHO) aborted BIRM–induced apoptosis. The effect of BIRM on AKT-mediated survival pathway in both AR+ and AR- negative (PC-3 and DU145) showed decreased levels of p-AKTser 473 in all PCa cell lines. BIRM dosed by oral gavage in mice bearing PC-3ML tumors showed selective efficacy on tumor growth; before tumors are established but limited efficacy when treated on existing tumors. Moreover, BIRM inhibited the LNCaP tumor generated by orthotropic implantation into dorsal prostate of nude mice. Partial purification of BIRM by liquid-liquid extraction and further fractionation by HPLC showed 4-fold increased specific activity on PCa cells. These results demonstrate a mechanistic basis of anti-tumor activity of the herbal extract BIRM.
Systematic investigations of promising plant products have led to the discovery and development of several anticancer agents with unique modes of action (e.g., paclitaxel, vinblastine, camptothesin, etc.) [1]. Prostate cancer strikes the elderly men (median age > 69) many of whom already have co-morbid conditions such as diabetes and heart disease, which make them less tolerant to aggressive treatments [2–4] including chemotherapy, radiation and even hormonal therapies. It is estimated that both in Europe and the United States, over 50% of the men with PCa experiment with one or more complementary therapies. These treatments include high dose vitamins and minerals, herbal preparations and supplement of soy, saw palmetto, and other supplements of less defined efficacy [5–8]. In addition, some of the recent products which have been shown to slow the development and progression of cancers of the prostate, breast, colon and
lung include lycopene from tomatoes, curcumin from turmeric roots, phenethyl isothiocyanate and indole-3-carbinol from cruciferous vegetables [9–17]. Interestingly, many of these products are active ingredients in dietary supplements and herbal formulations in indigenous medicines. However, recommending the efficacy of complementary treatments without preclinical evaluation is not only inexact but also dangerous.
BIRM is an aqueous extract of dried roots of an Ecuadorian medicinal plant, Kalanchoi gastonis bonnieri. BIRM is extensively used in Western Hemisphere mainly as Herbal immune booster, as by the manufacturer (EcuaBIRM Inc., Quito, EC and on their website [WWW.EcuaBIRM.Com]. In addition, as per the manufacturer of BIRM, this product has been dispensed as a complementary medicine for ailments such as AIDS, lupus, arthritis related psoriasis and various treatment-refractory cancers. However, the mechanism of
BIRM action and whether it is a cancer chemo preventative is unknown. We previously reported anti-tumor activity of BIRM against prostate cancer models, and have shown that it inhibits proliferation of all PCa cells, induces apoptosis and inhibits extracellular matrix (ECM) degrading enzyme, hyaluronidase [18]. Further, we showed that BIRM inhibits tumor growth and metastasis of Dunning MAT LyLu rat prostate tumor with no observable adverse effect. The mechanism of BIRM inhibitory activity against PCa, both in vitro and in vivo, has opened a new avenue for us to investigate the mechanism of anti-tumor activities of BIRM.
In this report we present evidence that BIRM inhibits growth of human PCa in subcutaneous and orthotopic models by oral administration. We further provide evidence that it inhibits AR mediated growth signaling by destabilizing AR-HSP-90 association and accelerated AR degradation. In addition, we demonstrate the mechanism by which BIRM induces apoptosis by the extrinsic cell death-induction pathway.
Previously, we reported dose-dependent inhibition of proliferation by BIRM in established PCa cell lines. The 50% growth inhibition determined by DNA synthesis activity (IC50) was 3 μl/ml (0.3% v/v) for LNCaP, and 8 μl/ml (0.8% v/v) for DU145 and PC-3ML cells [18]. This observation prompted us to inquire whether cytotoxicity of BIRM is dependent on the AR-mediated growth signaling in androgen responsive LNCaP and LAPC-4 cells. As shown in Figure 1A, BIRM at 1 μl/ml caused 45% and 69% decrease in DNA synthesis activity in LNCaP and LAPC4 respectively, in the absence of DHT whereas 75% and 85% inhibition were observed in cells cultured with DHT, respectively. Therefore, we tested whether BIRM-induced growth inhibition is mediated through DHT-dependent AR growth signaling in androgen responsive PCa cells.
Indeed, as shown in Figure 1B, BIRM significantly inhibited the PSA promoter activity by 50% (18 h) and 90% (48 h). Consistent with PSA promoter activity, PSA protein level also was decreased by ≥ 80% in cells treated with BIRM (Figure 1B, Insert). Since AR signaling (PSA promoter activity) was blocked by BIRM treatment, we next explored the effect of BIRM on AR protein expression levels in AR expressing cells. We found a reduction of AR protein expression in both LNCaP and LAPC-4 cells from as early as 3 h following BIRM treatment (Figure 1C) and further, dose dependent AR decrease was also observed in LAPC-4 with concomitant decrease in the phosphorylated-AR (Figure 1C). Furthermore, we examined whether BIRM affects AR translocation to the nucleus. LAPC4 cells were treated with 5 μl/ml BIRM for 24 h and AR levels in cytosol and nucleus were determined by first isolating the two fractions as described in methods or using a kit (NEPER Nuclear and Cytoplasmic extraction reagents, ThermoScientific, Cat#78833) then by western blotting of respective fractions. We found a significant decrease in AR levels in both cytosol and nucleus as found in whole cell extract, suggesting that BIRM did not affect AR translocation, but reduced the AR protein levels (Figure 1D).
Since AR protein expression was diminished in BIRM-treated cells, we next investigated whether AR down-regulation was by inhibition of AR synthesis or degradation. The effect of BIRM on AR synthesis was determined by qPCR in LAPC-4 cells. BIRM did not affect the AR mRNA significantly as confirmed by semi-quantitative PCR [Figure 2A (i)], qPCR [Figure 2A (ii)] and AR promoter activity (data not shown). BIRM did not alter the AR mRNA levels even under androgen deprivation condition suggesting that de novo AR synthesis was not suppressed by BIRM treatment.
We next examined whether BIRM affects AR stability, specifically whether BIRM increases the rate of AR degradation. AR is degraded via ubiquitin/proteasome pathway activated by PI3KAkt signaling pathway [19]. Therefore, LAPC-4 and LNCaP cells (with DHT) were treated with combination of BIRM, proteasome inhibitor MG132 and PI3K inhibitor LY294002. AR levels were determined by immunoblotting. As shown in Figure 2B, AR levels were low without DHT but were not affected by BIRM, or MG132. They were stabilized in cells treated with LY294002. However, BIRM treatment decreased the level of AR protein with or without LY294002 suggesting, AR stability in dormant state is independent of phosphorylation by AKT. However, in the presence of DHT, AR levels in BIRM treated cells were significantly lower (≥ 50%) than that in untreated cells, and further, co-treatment with S26 proteasome inhibitor, MG132 prevented this depletion. This rescue by MG132 was similar in both cell lines expressing AR (LNCaP and LAPC-4). We also observed similar rescue by another proteasome inhibitor, epoxomicin (an inhibitor of 22S proteasome; data not shown).
To further substantiate BIRM-mediated accelerated AR degradation is through ubiquitination, we performed coimmunoprecipitation of AR and ubiquitinated AR from LAPC4 cells with or without pre-incubation with BIRM. We found BIRM mediated ubiquitination was dose dependent (Figure 2C), comparable to protein treated with emodin (Figure 2C), a drug that was shown previously, to increase AR-ubiquitination and accelerated degradation [33–34]. Furthermore, co-treatment with BIRM and MG132, an inhibitor of S26 proteasome inhibitor that slows degradation of ubiquitinated-AR, showed abolition of ARdegradation, indicating ubiquitination and subsequent degradation of AR, is the major cause of AR down-regulation by BIRM.
Ligand-free AR, along with other co-factors, forms a heteromeric complex with Hsp90 that stabilizes ligand-free AR [20–25]. Previous studies have shown that a kinase inhibitor (i.e. Emodin) and Hsp90 inhibitor (i.e., Geldanamycin) disrupt the association between AR and Hsp90 [24, 25]. Therefore, we next examined the effect of BIRM on AR-Hsp90 formation. As shown in Figure 2D (i), BIRM exposure significantly disrupted the association between AR and Hsp90 in LAPC-4 cells. BIRM (5 µl/ml, 4 h) abolished AR-Hsp90 interaction by > 90% (Figure 2D, top panel), comparable to that by Geldanamycin (GA), an HSP90 inhibitor. Hyperacetylation of Hsp90 has been shown to inactivate Hsp90 and relieve bound protein off this chaperone [25, 26]. This prompted us to examine acetylation levels of Hsp90 after BIRM treatment.
Figure 1: BIRM mediated AR down regulation. (A) DNA synthesis inhibition in LAPC-4 and LNCaP cells treated with indicated dose of BIRM for 48 h with or without DHT were measured by [3H] Thymidine incorporation assay. (B) LAPC-4 cells were transiently transfected with PSA-luc and TK-Renilla. 24 h later, cells were exposed to 10 μl/ml BIRM in medium with 1 nM DHT for indicated time points and promoter activity was monitored by Dual-luciferase assay. Luciferase activity was normalized by Renilla activity and% promoter activity was measured compared to untreated (left panel). LAPC-4 cells were treated with 10 μl/ml BIRM in medium with or without DHT for 48 h and PSA production was monitored by ELISA (Inset). *p = 0.002; (C) Androgen dependent LAPC-4 and LNCaP cells cultured in growth medium were exposed to 10 μl/ml BIRM for indicated time point (left panel) or treated with various concentrations of BIRM for 24 h. Cells were harvested after incubation time and AR levels were analyzed by Immunoblotting. Actin was used as loading control. (D) LAPC-4 cells were treated with 10 μl/ml BIRM for 24 h and cytosol and nuclear fractions were prepared for immunoblotting to examine the effect of BIRM on AR localization. C: cytoplasmic AR, N: Nuclear AR in (A) and (C), the data presented as means ± SEM from two or more independent experiments in triplicate.
We found that treating cells with BIRM caused hyper acetylation of Hsp90 [Figure 2D (ii)] as shown by probing the western blot with anti-N-acetyl lysine antibody. This observation indicated that BIRM caused hyper acetylation and inactivation of Hsp90, resulted in disruption of AR-Hsp90 interaction, and further degradation of AR.
Since AKT regulates several survival pathways in normal and tumor cells, we examined whether BIRM treatment alters AKT activity in PCa cells. Since AKT mediated survival pathway is common among all PCa cell lines, we examined the levels of phosphorylated AKT (pAKT) in both androgen-responsive and castration resistant PCa cell lines (LNCaP, LAPC-4, DU 145 and PC-3). As shown in Figure 3A, BIRM treatment decreased p-Aktser473 levels in a dose-dependent manner.
We hypothesized that hypo-phosphorylation of Akt by BIRM contributes G1 cell cycle arrest. To elucidate that, we transiently transfected the LAPC-4 cells with a plasmid coding for kinase-dead Akt (KD-Akt) [27]. Transfected cells were incubated with BIRM for 24 h and p-Rb levels were monitored. As expected, BIRM treatment caused dephosphorylation of Rb protein (Figure 3B). Transient expression of KD-Akt decreased p-Rb levels, compared to that of control (Figure 3B). Furthermore, combination of BIRM and KD-Akt caused further decrease in p-Rb levels, suggesting that dephosphorylation of Akt by BIRM treatment is correlated with BIRM mediated cell cycle arrest at G1 [18]. We used SH5, a specific inhibitor of Akt, to further corroborate our observation that BIRM induced cell cycle blockade at G1 is mediated by inhibition of AKT activation [27, 28]. We obtained same results with or without BIRM treatment as with KD-Akt which, further confers that Akt involvement is correlated with BIRM mediated cell cycle arrest at G1 phase.
Figure 2: BIRM induced decrease in AR is due to increased proteasomal degradation of AR. (A) Total RNA was purified from LAPC-4 treated with 10 μl/ml BIRM with or without 1 nM DHT for 48 h and semi-quantitative RT-PCR (i) was performed. GAPDH was amplified as an internal control. (ii) Quantitative real time PCR was performed on LAPC-4 cells treated with BIRM with 1nM DHT, the graph shows a relative AR mRNA expression normalized against a β-actin mRNA. (B) LAPC-4 and LNCaP cells incubated with or without BIRM (20 μl/ml) for 48 h, were pretreated with 10 μM LY294002 (PI-3Kinase inhibitor) for 1 h, or co-treatment with MG132 (proteasome inhibitor) for 4 h at the end of treatment. Decrease AR levels were monitored by Immunoblotting. (C) LAPC-4 cells were treated with indicated doses of BIRM for 48 h with or without MG132 treatment as described above. Immunoprecipitation was performed using anti-AR antibody and Western blotting was performed with anti-Ubiquitin antibody. Emodin treatment was performed for positive control of AR ubiquitination. (D) LAPC-4 cells were treated with various doses of BIRM for 48 h or 5 μg ml geldanamycin (GA) for 18 h and harvested. Cell lysate was used for Immunoprecipitation with a-Hsp90 antibody, separated by SDS-PAGE and probed with a-AR or a-acetyl lysine antibody. GA treated samples was used as a positive control of decrease interaction between AR and Hsp90. Due to high background in control band (0 µl/ml BIRM) in lane1, accurate densitometry of band intensity was difficult. However, there was > 50% decrease in the Hsp90-bound AR at 5 µl/ml BIRM. BIRM induced strong acetylation of Hsp90 at 5µl/ml. The disintegrated band at higher BIRM exposure might be associated with cells undergoing apoptosis. GA: Geldanamycin treated cell lysates, IgG: normal mouse IgG as negative control in IP.
We reported previously that BIRM caused executioner caspase (e.g., caspase-3) activation in all PCa cells [18]. To further elucidate whether apoptosis induction by BIRM is mediated through extrinsic (death receptor mediated caspase-8 activation) or intrinsic (mitochondria depolarization) pathway, we measured the critical cellular determinants of extrinsic (caspase-8 activation) versus intrinsic (permeabilization of mitochondria) apoptosis pathways activated by BIRM. In all cell lines, FADD and active caspase-8 (cleaved caspase-8) were increased after BIRM treatment, resulted in increased level of cleaved PARP (Figure 4A). We also attempted to detect potential activation of Caspase 9, an initiator caspase that is activated by cytochrome-c release and formation of apoptosome via adapter protein AFAF-1 [29]. However, BIRM did not affect the caspase 9 activity in DU145 cells (Figure 4B) that implied, BIRM mediated apoptosis in PCa cells is unlikely due to “intrinsic” mechanism. Furthermore, LAPC-4 cells treated with BIRM resulted in significant increase in active caspase-8 (Figure 4C) that in turn activates caspase-3 (data not shown) and increased PARP cleavage. Moreover, induction of caspase-8 activation by BIRM was confirmed, by the absence
of apoptosis when a specific inhibitor of caspase-8, (AcIETD-CHO) was co-incubated with BIRM. This inhibitor also suppressed BIRM induced increase in downstream apoptotic event, cleaved PARP (Figure 4C). The cytotoxic effect of BIRM on LAPC-4 cells was suppressed (31%) by Caspase-8 inhibitor when compared to vehicle control (Figure 4D).
Gavaging mice with BIRM for 42-days had no detectable effect on body weight or other forms of toxicity. As shown in Figure 5A Table, tumors were evident in 21 days where 21 of the 24 mice injected with PC-3ML tumor cells had palpable tumors. Mice gavage with BIRM on the same day as of tumor cell injection showed significant decrease in tumor growth and terminal tumor weight (Group B) as compared to the mice in Group A where they were gavage with saline alone (p = 0.016, t-test). Further, more significantly, only 2 out of 8 mice in Group B developed tumors beyond palpability. This result suggest BIRM has strong antitumor activity. However, in mice allowed to develop palpable tumor (14/16 mice) by 21 days after injection, gavage with BIRM from day 21 to 42 showed some small difference in tumor weight (321 ± 87 mm3 vs 460 ± 122 mm3, p = 0.37) but not significant, suggesting BIRM is unlikely to have significant effect on established tumor in this castration resistant tumor model.
We observed a low incidence of LNCaP tumors in the prostate (dorsal, ventral or lateral) gland of athymic mice. The incidence was about 36% in control (3/11; given normal drinking water)
and 0% (0/11) in Group 2, where the animals were given 2% BIRM in drinking water continuously from the day tumor cells were injected. In the group of mice given BIRM, 3 weeks after tumor cell injection, the tumor incidence was 40% (2/5), similar to that observed in untreated control (Figure 5B).
Figure 3: Effect of BIRM in phosphorylation of Akt. (A) LNCaP, LAPC-4, PC-3 and DU145 cells were treated with various doses of BIRM for 48 h and p-Akt levels were monitored by Immunoblotting. Relative levels of p-Akt against t-Akt were calculated compared to untreated samples. (B) LAPC-4 cells were transiently transfected with either KDAkt or PPCDNA3 (control). Then, cells were treated with 10 μl/ml BIRM for 24 h. KDAkt: Kinase-Dead domain containing AKT transfectants.
Figure 4: BIRM induces apoptosis by extrinsic (DISC) pathway. (A) FADD, Cleaved caspase-8 and cleaved PARP levels were monitored in LAPC-4 and LNCaP cells treated with indicated doses of BIRM for 48 h (left panel), and DU145 and PC-3 cells treated with 10 μl/ml BIRM for indicated time points (right panel) by Immunoblotting. (B) DU145 cells were treated with BIRM for 48 h or staurosporine (STS) for 18 h and indicated protein levels were monitored by Immunoblotting. (C) LAPC-4 cells were pretreated with 50 μM caspase-8 inhibitor (Ac-IETD-CHO) for 1 h, followed by 50 μl/ml BIRM for 48 h. Then, cells were harvested for Immunoblotting to detect cleaved caspase-8 and PARP. (D) DU145 cells were pretreated with 1 μM CHO for 1 h, followed by BIRM for 48 h. Cytotoxicity of BIRM was monitored by MTT assay. The data presented as means ± SEM from two or more independent experiments in triplicate. FADD: Fas-Associated via Death Domain. PARP: Poly-ADP-Ribose Polymerase; C-PARP: Cleaved PARP; UT: Untreated; C-Casp 8: Cleaved (activated) caspase 8; C-casp9: Cleaved (activated) caspase 9; STS: Staurosporin. CHO: Inhibitor of Caspase 8 activation.
Figure 5: BIRM reduced incidence of PC-3 and LNCaP tumors. (A) Summary of PC-3ML tumor growth in athymic mice. *Only two mice in BIRM treated group from day of tumor cell injection were palpable out of 8 mice. Comparison was made on average tumor weight of two mice vs, that from untreated mice (7 animals). (B) Tumor generated by orthotopic injection of LNCaP-EGFP cells. Three groups of athymic mice were injected with LNCaP-EGFP cells intraprostatically to dorsolateral prostate. Tumor incidence (9/30) control, and BIRM treated by providing in drinking water from second day of tumor implant (group 2: 12/15) and Group 3: BIRM was given 3 weeks after tumor implant (4/15) was noted in each of the three groups. No significant fluorescence was detected in GU organs of Group 2. Fluorescence was not uniform in any of the groups. Animals with tumors were euthanized after 95 to 124 days. The results presented are from one experiment. A significant number of animals died in all groups or were euthanized due to loss of body weight (not shown). As shown in the figure, at the time of necropsy, genitourinary system except the seminal vesicles was dissected and weighed to estimate the total weight of the GU apparatus. Error bars represent ± SD, n = 4 to 12.
About 16% (Vol/Vol) of BIRM, as extracted from ground roots, is insoluble fibrous matter. After centrifugation, the liquid BIRM with soluble fiber had a dry mass of ~ 40 mg/mL equivalent. For fractionation of active principle, BIRM was first extracted with methanol where most of the solid matter composed of aqueous-precipitable and methanol-soluble material (70%) were precipitated. The methanol soluble extract was evaporated using a Rota-evaporator, dried sample was reconstituted with water and extracted sequentially with different solvents: hexane, chloroform, ethyl acetate (EA) and n-butanol [29] by liquid-liquid extraction method. A complete description of the extraction process is summarized in the flow chart (Figure 6A). The yield of BIRM from various extracts were: 1% (F1), 3% (F2) 5% (F3) and 14% (F4) from hexane, chloroform, ethyl acetate and n-butanol, respectively. The highest yield (% of total weight) acquired from BIRM liquid-liquid extraction method was the water extract 77% (F5). The cytotoxic activities of these solvent extracts were tested on DU145 cells by MTT reduction assay. The fractions F1, F2 and F3, except F4 and F5 showed cytotoxicity on DU145 cells at ≥ 500 μg/ml. However, the EA fraction showed 4-fold increase in specific activity (IC50 = 120 μg/ml) compared to BIRM (Figure 6B).
The fractions extracted from the solvents were subjected to LC-Mass spectrometry. The chromatogram profile showed a common peak in hexane, chloroform and EA fractions (Figure 6C). Further spectroscopic characterization of the common peak by proton NMR analyses showed a compound as benzoic acid, however it showed no specific activity in PCa cells and did not inhibit AR levels when tested on LNCaP cells (data not shown). Therefore, we reasoned, benzoic acid is unlikely to be an active anti-tumor agent in BIRM. Moreover, the LCMS chromatogram profile of BIRM EA (F3) fraction showed multiple maxima other than benzoic acid. A sub-fractionation of EA by HPLC resulted in 8 peaks (F3A-F3H). The MTT cytotoxicity assay on these peaks showed an enhanced specific activity on F3F and F3G peaks compared to BIRM (Figure 7A). Furthermore, the AR protein level was also reduced on peaks F3F and F3G [Figure 7B (i & ii)]. The induction of apoptosis by the peaks F3F and F3G on DU145 cells was more than 75% [Figure 7C (Ii & ii) and Figure 7D (i & ii)]. These results suggest that there are chemically closely related multiple active compounds in BIRM which attributes to its antiproliferative and anti-AR activities.
Figure 6: Fractionation of BIRM. (A) Schematic representation of solid-liquid extraction of BIRM using different organic solvents and their yield. (B) Cytotoxicity BIRM fractions (F1-F5) extracted from different solvent in DU145 cells. (C) LC/MS chromatogram of all the fractions from F1-F5. (D) Uronic acid estimation in Fractions, note all fractions contained uronic acid containing GAG-like compounds. All three peaks that showed anti-proliferative activity against PCa cells were assayed for uronic acid content for identifying chemical nature of the MS-LC peaks. All the fractions had uronic acid content that varied from 25 ug to 80 ug. Further, we also analyzed these peak fractions for potential to inhibit HYAL1, a tumor specific hyaluronidase that was inhibited by BIRM [18]. These results suggested, the cytotoxic activity in BIRM is very likely correlated with the presence of HA-like compounds.
These studies were conducted to demonstrate mechanistic basis of anti-tumor activity of BIRM specific to PCa. Our previous reports [18] presented the evidence of anti-neoplastic activity of BIRM on PCa cells. The activities of BIRM included inhibition of cell proliferation (arrested at G0/G1 in PC-3ML and LNCaP), inhibition of clonogenic growth of both androgen dependent (LNCaP) and castration–resistant PCa cells (DU145 and PC-3) and induction of apoptosis. More importantly, BIRM reduced incidence, delayed tumor growth and caused a significant decrease of metastasis of Dunning EGFP-MAT Lylu tumors (18). However, the molecular target of BIRM and its mechanism of therapeutic action on prostate cancer was not investigated at that time.
Our present study showed the mechanistic action of BIRM inhibition of cell growth in both androgen dependent and independent PCa cells. LAPC-4 cells were more sensitive to BIRM mediated cell growth inhibition in the presence of DHTmediated growth when compared to that in androgen deprived LAPC-4 cells (Figure 1A). BIRM caused an inhibition of PSA production in LAPC-4 cells with DHT (Figure 1B), suggesting that BIRM suppressed AR signaling even at the basal signaling level. A repressed AR signaling in androgen dependent PCa cells was due to BIRM mediated AR degradation (Figure 1C) via the S-26 proteasome mediated pathway (Figure 2B). Furthermore, treatment with BIRM caused neither inhibition of AR synthesis (Figure 2A) nor AR translocation (Figure 1D).
Figure 7: Biological activities of F3 sub-fractions. Column fractions of F3 were assayed for both for cytotoxic activity and anti-AR activities. (A) Cytotoxicity of F3AF3H on DU 145 cells at 50 and 100 µg/ml. (B) Anti-AR activities of BIRM fraction F3A-H. Several subfractions (F3F and F3G, and F2 and F3 had strong anti-AR activity when LNCaP cells were exposed to these fractions for 24 h. (Bii): densitometry trace of AR levels. (C) Apoptotic activities of BIRM sub-fractions. DU 145 cell cultures were exposed to F3 sub fractions and levels of cleaved-PARP was detected in cell lysates by Western blot. Only F3F and F3G had both pro-apoptotic and antiAR activities. (D) Densitometry trace (estimate) of C-PARP (D.i) and AR (D.ii) are shown.
The inhibition of Hsp90 function results in degradation of targeted proteins, such as hormone receptors and cell cycle related protein. Hsp90 has been also reported that it is involved in AR folding for its maturation and stabilization [29]. As similar to Hsp90 inhibitor, geldanamycin, BIRM caused disruption of Hsp90-AR complex formation, and resulted in reduced level of AR due to its degradation (Figure 2Di). Furthermore, BIRM caused hyper acetylation of Hsp90 in PCa cells (Figure 2Dii), suggesting that BIRM mediated acetylation of Hsp90 may cause inhibition of Hsp90 function, which is consistent with previous report [22, 24, 25], resulted in AR degradation. This result gives us the hypothesis that BIRM may down-regulate not only AR, but also other Hsp90 targeted protein, such as ER and EGFR, via inhibition of Hsp90 functions, which are not investigated for this report.
We observed that BIRM reduced the levels of phosphorylation ofAkt at ser 473 (Figure 4A) which is linked to survival pathway through activation of transcription of anti-apoptotic genes [27]. Interestingly, Zhang et al. [28] have reported that p-Akt phosphorylates and activates the winged helix family of transcription factors, FKHRL1, FKHR and AFX. This resulted in retaining FKHRL1 and FKHR in the cytoplasm. Further, inhibitors of mTOR, a downstream effector of the phosphatidylinositol 3-kinase (PI3K)/Akt signaling pathway, prevent cyclin dependent kinase (CDK) activation, inhibit Rb protein phosphorylation (at pRbs780), and accelerate the turnover of cyclin D1, leading to G1-phase arrest [28]. Moreover, it has been shown that FOXO fork head transcription factors down regulate cyclin D1 in a p27kip1-independent mechanism [27, 30]. Thus,
we conjecture that decreased phosphorylation of Akt levels by BIRM results in G1 cell cycle arrest via downregulation of cyclin D1 and concomitant de-phosphorylation of Rb. In addition to acceleration of AR degradation, BIRM also showed cytotoxicity in both AR dependent and independent PCa cells. Apoptosis is tightly regulated by prosurvival and proapoptotic molecules, including proteins of the caspase family, and can be mediated by several different pathways. It is imperative to elucidate the mechanisms by which potential chemotherapeutic/ chemo preventive drugs cause apoptosis. We showed that BIRM caused apoptosis in both AR positive and AR negative cell lines [18]. BIRM caused increase FADD, cleaved caspase-8 and PARP in all PCa cell lines examined (Figure 4A). Furthermore, BIRM caused cleaved caspase 8 but not caspase 9 (Figure 4B) The specific caspase inhibitor (AcIETD-CHO) reverted the action of BIRM induced apoptosis on LAPC-4 cells (Figure 4C) and a synthetic inhibitor of caspase-8 (Ac-IETD-CHO) rescued the BIRM induced cytotoxicity (Figure 4D) suggesting that BIRM induced apoptosis is through caspase-8 activated death-inducing signaling complex (DISC) pathway [31].
Previously, we showed that BIRM contains at least three molecular species of different mass that have cytotoxicity [18]. In this investigation, we further characterize the BIRM. The active compounds in BIRM are rich in uronic acid sugar containing poly- and oligosaccharides (Figure 6). Our data showed that BIRM contains several compounds, chondroitin sulfate-like, heparansulfate-like or hyaluronic acid oligosaccharide containing 10 sugar residues with some modification. Further investigations to
identify the exact molecule contained in BIRM and to explore the mechanism of each component are underway.
These results suggest potential chemo preventive function of BIRM in both androgen-independent and androgen-sensitive PCa xenografts. These observations showed that BIRM has substantial stronger antitumor activity when given before the tumors are established, as shown by the lack of tumor incidence (75%–80% reduction) and slower growth of incident tumors (71% decrease in tumor volume, Figure 5. We have shown previously in the Dunning MAT Ly Lu rat tumor model, BIRM also significantly inhibits tumor metastasis [18]. Therefore, BIRM may be a potential candidate as an effective chemo preventive agent, for preventing the growth of primary and metastatic tumors.
BIRM was a gift from EcuaBIRM Inc. (Quito, Ecuador). BIRM was prepared by extracting the powdered roots of a medicinal plant widely referred to as “Dulcamara” or “Life Root” in Ecuador. The botanical identification of Dulcamara was performed at the Catholic University of Quito. The reference specimen from the identified plant, Kalanchoe gastonisbonnieri [formerly known as Bryophyllum gastonis-bonnieri (RayamHamet & H Perrier)] is deposited and maintained at the QCA Herbarium, Pontific a Catholic University of Quito, Ecuador.
BIRM was prepared by organoleptic method of the Government of Ecuador certified contractor, Renase CIA LTDA following authentication of the starting material by QCA Herbarium. The roots were dried at 65°C in an indoor oven and the liquid extraction of BIRM was processed as per the EcuaBIRM established proprietary standards; each batch was certified by the Department of Sanitation, Government of Ecuador. Before use, BIRM was clarified by centrifugation at 10, 000 g for 10 min, supernatant that contained active principle and solubilized fiber were separated from insoluble fiber and used for all in vitro experiments. BIRM used in oral gavage to test its anti-tumor activity in vivo was not separated from insoluble fibers before. Further standardization of the product used in the present study is described under Results (in section: molecular characterization of BIRM).
Cell lines LNCaP and DU-145 cells (ATCC, Manassas VA) and PC-3ML (a gift from Dr. M. E. Stearns, Drexel University, Philadelphia, PA) [32] were maintained in RPMI-1640 medium with 10% fetal bovine serum (FBS, Mediatech, Inc. Herndon, VA) and 10 mg/ml gentamicin (Invitrogen, Inc., NY). AR dependent LAPC-4 cells were obtained from Dr. Charles Sawyer’s laboratory in UCLA (Los Angeles, CA) and cultured in Iscove’s media (Invitrogen) containing 1nM DHT (Sigma, St. Louis, MO), 7.5% FBS and 10 mg /ml gentamicin. The cell lines used in the study were authenticated for their origin by Genetica DNA laboratories Inc. Cincinnati, OH).
LY294002 (PI3 kinase inhibitor), IETD CHO (Caspase-8 inhibitor), MG132 and epoxomicin (proteasome inhibitors) were purchased from Calbiochem (San Diego, CA). Geldanamycin was purchased from Stressgen (Victoria, BC, Canada). Staurosporin was purchased from Cell Signaling (Beverly, MA).
Total RNA was isolated from untreated and BIRM treated LAPC4 cells with RNeasy Mini kit (Qiagen. com). RNA was reverse transcribed and PCR-amplified, expressions of AR and PSA mRNA were analyzed by semi-quantitative RT-PCR and real time quantitative PCR (qPCR) using Biorad icycler IQ as described before [33, 34]
We used both colorimetric (MTT) and [3H-Methyl] deoxythymidine [3H-TdR] incorporation assays to determine cell viability and DNA synthesis activity of PCa cell cultures treated with BIRM (0–20 ml/ml). The cytotoxicity of BIRM on PCa cell cultures was measured using Thiozoyl Blue (3(4, 5-dimethylthiazol-2-y)-2, 5-diphenyltetrazolium bromide (MTT) reduction assay [33].
Relative levels of various cellular proteins were determined by immunoblotting using a routine procedure described before [33]. After BIRM treatment, whole cell extracts were prepared in ice-cold NP-40 buffer (50 mM Tris pH8.0, 1% NP-40, 150 mM NaCl, 2 mM EGTA, 2 mM EDTA, 50 mM NaF, 0.1 mM NaVO4 and Protease inhibitor cocktail). Protein samples were dissolved in 2X loading buffer, separated by 8–16% gradient SDS-PAGE gel (LifeGel, Frenchs Forest, NSW, Australia) or 10% SDS-PAGE gel and transferred onto Immunobilon-P (Millipore, Bedford, MA). Indicated proteins were probed with specific antibodies. Antibodies to AR (Ab-1) and cyclin B1 (Ab-3) were purchased from LAB VISION (Fremont, CA). Anti-phospho-AR (Ser213/210) IgG was purchased from IMAGENEX (San Diego, CA). Anti-cleaved PARP (Asp214), FADD, phopho-FADD (Ser194), p-Akt (Ser 473), p-RB and cleaved caspase-8 (Asp374) were purchased from Cell signaling. Antibodies to β-actin (actin), and Akt were purchased from BD Pharmingen (San Diego, CA). Antibody to Hsp-90 was from Stressgen (Victoria, BC, Canada). The immunoblots were visualized by an enhanced chemiluminescence kit (ECL; Amersham, Piscataway, NJ). For Immunoprecipitation, cells were lysed in NP-40 buffer, mixed incubated with either anti-AR Antibody (LAB VISION) or anti-Hsp 90 IgG and incubated for 2 h in cold room with rotation. Then, Protein G Agarose Beads (Pierce, Rockford, IL) was added to the mixture and incubated for overnight at 4ºC. Immunocomplexes were washed with a washing buffer (0.3% FBS, 1% NP-40 in PBS) four times, and dissolved in 2 X SDS-sample buffer. SDS-Solubilized sample aliquots were fractionated on SDS-Polyacrylamide gel electrophoresis (SDS PAGE). Gels were blotted onto PCDmembrane and the resulting blot was sequentially incubated with blocking buffer (5% non-fat milk in PBS with 0.05% Tween 20) anti-polyubiquitin antibody (Zymed, South San Francisco, CA) to detect ubiquitinated AR or anti-AR antibody (LAB VISION) to examine the interaction between Hsp90 and AR. Co-Immunoprecipitation followed by western blotting was used to determine AR-HSP90 association with a technique as described before [33]. Band density was normalized to β-actin by re-probing the blot with anti-β actin antibody (Santa Cruz Technology Inc., Dallas, TX).
Activation of AR and PSA promoters [34, 35] were assayed in cells transfected with an AR or PSA promoter luciferase reporter constructs as described before [36, 37]. Briefly, LAPC-4 (1 × 104) cells were co-transfected with PSA-luc along with pRL-TK Renilla using Effectene reagent (QIAGEN, Inc., Valencia, CA). Twentyfour hours after transfection, cells were treated with indicated doses of BIRM for several time points before measuring the luciferase activity using the Duo Glo luciferase kit and the Glomax Plate reader (Promega Corporation, Madison, WI). Relative Light Units (activity, RLU) were normalized to TK-Renilla activity.
Cell fractionation was performed to detect AR levels in nuclear and cytoplasmic fractions. Briefly, the procedure involved: after treatment with BIRM, cells were trypsin zed and washed with cold PBS once. Then, cells were lysed in ice-cold CSK buffer (10 mM PIPES pH 6.8, 100 mM NaCl, 300 mM sucrose, 3 mM MgCl2, 1 mM EGTA, 0.5% Triton X-100 with protease inhibitor cocktail) for 10 min on ice. The cytosolic fractions as supernatant was collected by centrifugation. The nuclear fraction as pellet was washed once with CSK buffer, resuspended in buffer C (20 mM HEPES, pH 7.9, 0.4 M NaCl, 1 mM EDTA, 1 mM EGTA, 1 mM DTT and protease inhibitor cocktail) and kept on ice for 30 min, then, collected by centrifugation. Both fractions were boiled in 2X SDS-Gel sample buffer for 3 min and stored at 4ºC awaiting anlysis by Western blotting. Cytoplasmic AR was normalized against β-actin and nuclear AR was normalized using PARP antibody respectively.
(i): We used FOXN1 inbred athymic male mice were purchased from Charles River Laboratories (Durham, NC) for all studies reported here. A power analysis was performed for determining sample size for each of the experiments. The power analysis showed that for PC-3ML tumors, with take rate of > 90%, we needed 7–8 animals per group to get 80% confidence [38]. We injected 6-week old mice with 2 × 10 6 cells/site/ per animal in 50% Matrigel on the dorsal flank. Mice were treated with BIRM by oral gavage (0.2 ml/mouse/day; for up to 21 days) as described in Figure 5A. Tumor growth over time was evaluated by tumor volume measurement followed by tumor weight estimation following euthanasia on day 42. (ii): Orthotopic implantation and treatment: We injected LNCaP cells over expressing Enhanced Green Fluorescent Protein (EGFP-LNCaP) in the dorsolateral prostate of FOX N1 nude mice as described before using the procedure adapted from Stephenson et al [39]. Briefly, we performed orthotopic implant of EGFP-LNCaP cells on 10 mice for each control and experimental groups. Mice aged 6 to 8 weeks were anesthetized using isoflurane in a ventilated facility. Anesthetized mice were placed on the stage of a dissection microscope in the sterile hood to perform survival surgery. Abdomen was opened with a midline incision to expose prostate and 0.02 ml suspension of 2 × 106 EGFP-LNCaP cells was injected into the posterior prostatic lobe with a Hamilton push-button syringe fitted with a # 30 needle. A distinct blob of cells in the prostate indicated proper injection. Animals were sutured in two layers with internal chromic gut sutures and outside with silk sutures. Animals were allowed to recover under warming light until they
regained movement after which they were injected with 0.01 ml of Buprenorphine every 12 hours for three days. Animals were examined under a Fluorescence in vivo imaging devise (Light Tools Research, Encinitas, CA) every two weeks, until the animals were euthanized at the end of 12 weeks. Although tumors could not be palpated reliably with fingers, fluorescence signal from EGFP-LNCaP cells was detectable percutaneously in 6 weeks and beyond.
Group 1, the control group, received normal autoclaved drinking water given to athymic mice. Animals in Group 2 were given 0.2% BIRM in drinking water from the day of tumor cell injection, and Group 3 were given 2.0% BIRM starting 3 weeks after tumor cell injection. The BIRM containing water was replaced two times a week.
Tumor cell injected mice were anesthetized and imaged every two weeks using the In vivo imaging device [that captures fluorescence in a cooled-CCD video camera] and digital images were captured using the software provided by the devise vendor. All animals were euthanized at the end of 12 weeks, when two animals in control group had developed large tumors. At necropsy, the skin was removed to expose the tumors and fluorescence was imaged using the CCD camera. Following imaging, the entire genitourinary apparatus (bladder, and all prostate lobes), minus the seminal vesicle was removed and its weight was recorded. A part of the tumor was fixed and a histology was performed. Quantification of fluorescence image to estimate tumor size was not accurate due to poor distribution of fluorescent intensity along the curvature of the tumor, therefore are not presented. However, tumor weight from site of injection was quantified at the time of necropsy.
Three separate batches of BIRM, each manufactured by EcuaBIRM INC, were analyzed for chemical composition that included, elemental analysis, analysis of organic components, and macromolecular composition, MS and 1H and C13-NMR. These analyses were done at the Mikroanalytisches Labor Pascher, Remagen-Bandorf, Germany as reported previously [40]. Elemental analysis for relative abundance of C, H, and N, was determined by oxidative micro-combustion followed by microtitration. Percent oxygen in BIRM was determined by the hot gas extraction followed by analysis on an infrared-analyzer. Sulfur and metal analyses were also performed using Inductively Coupled Plasma Atomic Emission Spectroscopy. Other chemicals, such as alkaloid by TLC [41], polyphenol using the Folin-Ciacalteu method [42] and tannins by ferric chloride protein precipitation assay [29] and uronic acid content in BIRM and its fractions were also conducted [43, 44].
AR: Androgen receptor, BIRM: Trademark name from EcuaBIRM Inc. C-PARP: Cleaved (~85KD) fragment of Poly ADP-RibosePolymerase-1 (PARP); DHT: Dihydrotestosterone; EGFP: Enhanced green fluorescent protein; GA: Geldanamycin; MTT: (3(4, 5-dimethylthiazol-2-y)-2, 5-diphenyltetrazolium bromide; PCa: Prostate cancer; ECM: extracellular matrix; RT-PCR, Reverse
transcriptase-PCR; Q-PCR: Quantitative RT-PCR; C-Casp9: Cleaved Caspase-3, STS: Staurosporine.
The authors are grateful to a large number of individuals who helped with this project. The authors are especially grateful to the owners and employees of EcuaBIRM Inc., Quito, Ecuador and Mr. Luis E Lopez (University of Miami), Jurgen Rohr, Khaled Shaaban at the School of Pharmacy, University of Kentucky. Lexington KY (for initial fractionation of BIRM). This work was supported by funds from: NIH 5R01AT3544, NIH 1R01CA156776-1 and Florida Department of Health Bankhead-Coley Biomedical Research Grant No. 06BCBG1-12.
The authors declare no conflicts of interest.
1. Gioti K, Tenta R. Bioactive Natural Products against Prostate Cancer: Mechanism of Action and Autophagic/Apoptotic Molecular Pathways. Planta Med. 2015; 81:543–62.
2. LeMasters TJ, Madhavan SS, Sambamoorthi U, Kurian S. Health behaviors among breast, prostate, and colorectal cancer survivors: a US population-based case-control study, with comparisons by cancer type and gender. J Cancer Surviv. 2014; 8:336–48.
3. Gavin AT, Drummond FJ, Donnelly C, O’Leary E, Sharp L, Kinnear HR. Patient-reported ‘ever had’ and ‘current’ longterm physical symptoms after prostate cancer treatments. BJU Int. 2015.
4. Bock PR, Friedel WE, Hanisch J, et al. Retrolective, comparative, epidemiological cohort study with parallel groups design for evaluation of efficacy and safety of drugs with „well-established use“. Forsch Complementarmed Klass Naturheilkd. 2004; 11:23–9.
5. Nelson PS, Montgomery B. Unconventional therapy for prostate cancer: good, bad or questionable? Nat Rev Cancer. 2003; 3:845–58.
6. Lucarelli G, Rutigliano M, Galleggiante V, Giglio A, Palazzo S, Ferro M, Simone C, Bettocchi C, Battaglia M, Ditonno P. Metabolomics profiling for the identification of novel diagnostic markers in prostate cancer. Expert Rev Mol Diagn. 2015; 1-14.
7. Eng J, Ramsum D, Verhoef M, et al. A population-based survey of complementary and alternative medicine use in men recently diagnosed with prostate cancer. Integr Cancer Ther. 2003; 2:212-6.
8. Singh H, Maskarinec G, Shumay DM. Understanding the motivation for conventional and complementary/ alternative medicine use among men with prostate cancer. Integr Cancer Ther. 2005; 4:187-94.
9. Powolny AA, Bommareddy A, Hahm ER, Normolle DP, Beumer JH, Nelson JB, Singh SV. Chemopreventative potential of the cruciferous vegetable constituent phenethyl isothiocyanate in a mouse model of prostate cancer. J Natl Cancer Inst. 2011; 103:571-84.
10. Kim MJ, Kim H. Anticancer Effect of Lycopene in Gastric Carcinogenesis. J Cancer Prev. 2015; 20:92-6.
11. Song JM, Qian X, Molla K, Teferi F, Upadhyaya P, O Sullivan G, Luo X, Kassie F. Combinations of índole 3-carbinol and silibinin suppress inflammation-driven mouse lung tumorigenesis by modulating critical cell cycle regulators. Carcinogenesis. 2015; 36:666-75.
12. Mohapatra P, Satapathy SR, Siddharth S, Das D, Kundu CN. Resveratrol and curcumin synergistically induce apoptosis in cigarette smoke condensate transformed breast epithelial cells through a p21 Waf1/Cip1 mediated inhibition of Hh-Gli signaling. Int J Biochem Cell Biol. 2015.
13. Scarpa ES, Ninfali P. Phytochemicals as Innovative Therapeutic Tools against Cancer Stem Cells. Int J Mol Sci. 2015; 16:15727-42.
14. Donehower RC, Rowinsky EK. Anticancer drugs derived from plants. In: DeVita VT Jr., Hellman S, Rosenberg SA (Ed). Principles and practice of oncology, 9th N. American Edition, Lippincott. 2011.
15. Yan J, Wang Y, Zhang X, Liu S, Tian C, Wang H. Targeted nanomedicine for prostate cancer therapy: docetaxel and curcumin co-encapsulated lipid-polymer hybrid nanoparticles for the enhanced anti-tumor activity in vitro and in vivo. Drug Deliv. 2015; 1-6
16. Liu X, Zhang DY, Zhang W, Zhao X, Yuan C, Ye F.
17. The effect of green tea extract and EGCG on the signaling network in squamous cell carcinoma. Nutr Cancer. 2011; 63:466-75.
18. Afaq F, Adhami VM, Ahmad N, et al. Botanical antioxidants for chemoprevention of photocarcinogenesis. Front Biosci. 2002; 7:d784-92.
19. Dandekar DS, Lokeshwar VB, Cevallos-Arellano E, Soloway MS, Lokeshwar BL. An orally active Amazonian plant extract (BIRM) inhibits prostate cancer growth and metastasis. Cancer Chemother Pharmacol. 2003; 52:59-66.
20. Qi J, Tripathi M, Mishra R, Sahgal N, Fazli L, Ettinger S, Placzek WJ, Claps G, Chung LW, Bowtell D, Gleave M, Bhowmick N, Ronai ZA. The E3 ubiquitin ligase Siah2 contributes to castration-resistant prostate cancer by regulation of androgen receptor transcriptional activity. Cancer Cell. 2013; 23:332-46.
21. Gan L, Chen S, Wang Y, Watahiki A, Bohrer L, Sun Z, Wang Y, Huang H. Inhibition of the androgen receptor as a novel
mechanism of taxol chemotherapy in prostate cancer. Cancer Res. 2009; 69:8386-94.
22. Cha TL, Qiu L, Chen CT, et al. Emodin down-regulates androgen receptor and inhibits prostate cancer cell growth. Cancer Res. 2005; 65:2287-95.
23. Burnstein KL. Regulation of androgen receptor levels: implications for prostate cancer progression and therapy. J Cell Biochem. 2005; 95:657-69.
24. Fang Y, Fliss AE, Robins DM, Caplan AJ. Hsp90 regulates androgen receptor hormone binding affinity in vivo. J Biol Chem. 1996; 271:28697-702.
25. Segnitz B, Gehring U. The function of steroid hormone receptors is inhibited by the hsp90-specific compound geldanamycin. J Biol Chem. 1997; 272:18694-701.
26. Solit DB, Zheng FF, Drobnjak M, Münster PN, Higgins B, Verbel D, Heller G, Tong W, Cordon-Cardo C, Agus DB, Scher HI, Rosen N. 17-Allylamino-17-demethoxygeldanamycin induces the degradation of androgen receptor and HER-2/neu and inhibits the growth of prostate cancer xenografts. Clin Cancer Res. 2002; 8:986-93.
27. Thomas M, Dadgar N, Aphale A, Harrell JM, Kunkel R, Pratt WB, Lieberman AP. Androgen receptor acetylation site mutations cause trafficking defects, misfolding, and aggregation similar to expanded glutamine tracts. J Biol Chem. 2004; 279:8389-95.
28. Brunet A, Bonni A, Zigmond MJ, Lin MZ, Juo P, Hu LS, Anderson MJ, Arden KC, Blenis J, Greenberg ME. Akt promotes cell survival by phosphorylating and inhibiting a Forkhead transcription factor. Cell. 1999; 96:857-68.
29. Zhang HS, Cao EH, Qin JF. Homocysteine induces cell cycle G1 arrest in endothelial cells through the PI3K/Akt/
30. FOXO signaling pathway. Pharmacology. 2005; 74:57-64.
31. Sarkar, S. D., Z. Latif, and A. I. Gray. “Natural product isolation.” 2005; 323-351. 2006 Humana press 2nd Edition. 27-46.
32. Gao N, Zhang Z, Jiang B-H, Shi X. Role of PI3AKT/mTOR signaling in cell cycle progression of human prostate cancer. BBRC 2003; 310:1124-1132.
33. Shamaladevi N1, Lyn DA, Shaaban KA, Zhang L, Villate S, Rohr J, Lokeshwar BL. Ericifolin: a novel antitumor compound from allspice that silences androgen receptor in prostate cancer. Carcinogenesis. 2013; 34:1822-32.
34. Dolloff NG, Shulby SS, Nelson AV, Stearns ME, Johannes GJ, Thomas JD, Meucci O, Fatatis A. Bone-metastatic potential of human prostate cancer cells correlates with
Akt/PKB activation by alpha platelet-derived growth factor receptor. Oncogene. 2005; 24:6848-54.
35. Araki S, Omori Y, Lyn D, Singh RK, Meinbach DM, Sandman Y, Lokeshwar VB, Lokeshwar BL. Interleukin-8 is a molecular determinant of androgen independence and progression in prostate cancer. Cancer Res. 2007; 67:6854-62.
36. Wang LG, Ossowski L, Ferrari AC. Androgen receptor level controlled by a suppressor complex lost in an androgen-independent prostate cancer cell line. Oncogene. 2004; 23: 5175-84.
37. Perez-Stable CM, A de las Pozas, BA Roos. A Role for GATA Transcription Factors in the Androgen Regulation of the Prostate-Specific Antigen Gene Enhancer. Mol CellEndocrin. 2000;167: 43-53.
38. Dandekar DS, Lokeshwar BL. Inhibition of cyclooxygenase (COX)-2 expression by Tet-inducible COX-2 antisense cDNA in hormone-refractory prostate cancer significantly slows tumor growth and improves efficacy of chemotherapeutic drugs. Clin Cancer Res. 2004;10: 8037-47.
39. Salazar N, Muñoz D, Kallifatidis G, Singh RK, Jordà M, Lokeshwar BL1. The chemokine receptor CXCR7 interacts with EGFR to promote breast cancer cell proliferation. Mol Cancer. 2014; 13:198-205.
40. Dupont WD, Plummer WD. Power and sample size calculations: a review and computer program. Controlled Clin. Trials.1990; 11:116-128.
41. Stephenson RA, Dinney CP, Gohji K, Ordóñez NG, Killion JJ, Fidler IJ. Metastatic model for human prostate cancer using orthotopic implantation in nude mice. J Natl Cancer Inst. 1992; 84:951-7.
42. Lokeshwar VB, Selzer MG, Cerwinka WH, et al. Urinary uronate and sulfated glycosaminoglycan levels: markers for interstitial cystitis severity. J Urol. 2005; 174:344-9.
43. Klyushnichenko VE, Yakimov SA, Tuzova, TP, et al. Determination of indole alkaloids from R. serpentina and R. vomitoria by high-performance liquid chromatography and high-performance thin-layer chromatography. Journal of Chromatography A. 1995; 704:357-362.
44. Price ML, Butler LG. Rapid Visual Estimation and Spectrophotometric Determination of Tannin Content of Sorghum Grain J. Agric. Food Chem. 1977; 25:1268-1273.
45. BITTER T, MUIR HM. A modified uronic acid carbazole reaction. Anal Biochem. 1962; 4:330-4.
46. Lokeshwar VB, Selzer MG, Cerwinka WH, Gomez MF, Kester RR, Bejany DE, Gousse AE. Urinary uronate and sulfated glycosaminoglycan levels: markers for interstitial cystitis severity. J Urol. 2005; 174:344-9.
Ecuador is one of the eight originating centers of native plants, since the influence of geographic and ecological factors grants it a wide diversity of flora. It is estimated that Ecuador is one of the countries with the widest genetic diversity per surface unit, with 20,000 to 25,000 species of vascular plants being reported. Many of the Ecuadorian species are cataloged as plant genetic resources within agriculture and food. They have local and global importance, and have an incalculable potential value.
Phenolic compounds in particular are considered as one of the most important classes of natural antioxidants. One or more aromatic rings with one or more hydroxyl groups make up its molecules. Chemically, polyphenols can be divided into several classes, such as phenolic acids, flavonoids, anthocyanins, isoflavones, stilbenes, lignans and tannins (Machu et.al., 2015).
Polyphenols are directly related to the antioxidant activity of a particular food, plant or product, since they inhibit the oxidative degradation of organic materials, including a large number of aerobic biological organisms and commercial products (Rappoport, 2004).
The present study focused on determining the total phenolic content, the antioxidant capacity and proposing an alternative to reduce the bitter taste present in BIRM. This study underwent the following stages:
1. Review of information on BIRM, antioxidant capacity, and total phenolic content.
2. Preparation of each of all the necessary resources, including reagents, materials, equipment, etc.
3. Experimental development of the analyses to determine the total content of phenolic compounds and the antioxidant capacity in BIRM.
STUDY OF ANTIOXIDANT ACTIVITY 0F THE “BIRM” IMMUNOMODULATOR PRODUCT, SOUTHBOROUGH, MA; 2018.
4. Analysis and interpretation of the experimental results and report of final conclusions.
This research paper presents the determination of the antioxidant capacity of Ecuadorian natural resources, a capacity that is directly related to the total phenolic content, proposing the analysis of the antioxidant capacity of BIRM, an Ecuadorian product of great interest due to the properties that it possesses and the use of dulcamara (Ecuadorian plant) in its formulation. This study provides new scientific information for the BIRM product, which lays the groundwork for continuing the study of alternatives based on natural resources and the development of future research that will allow to add greater value to existing resources in our country.
From a legal point of view, this study is important because:
• Article 385 verse 3 of the 2008 constitution of the Republic of Ecuador, which states “To develop technologies and innovations that boost national productivity, increase efficiency and productivity, improve quality of life and contribute to the realization of good living ”(Constituent Assembly, 2008).
From the point of view of the National Plan for Good Living, this study is important because it contributes to the achievement of several of its objectives:
• To promote reciprocal interaction between education, the productive sector and scientific and technological research, for the transformation of the productive matrix and the satisfaction of needs.
• To articulate scientific and technological research, and higher education with the productive sector, for a constant improvement of productivity and systemic competitiveness, within the framework of current and future needs of the productive sector and the development of new knowledge.
• To promote and transfer, development and technological innovation, in order to boost national quality production with high added value, with emphasis on prioritized sectors.
• To diversify and generate greater added value in national production.
• To consolidate the productive transformation of the industrial and manufacturing priority sectors, with processes of incorporation of added value that maximize the national component and strengthen the capacity for innovation and collective learning.
• To consolidate existing external markets, with emphasis on the region’s countries and foster the promotion of non-traditional products, with added value and those relating to the transformation of the productive matrix.
• To promote, facilitate and technify the promotion, protection, preservation and dissemination of nationally created intellectual property rights.
• To promote a dialogue of knowledge between communities and academia, in the research and documentation of social memory, cultural heritage and diverse knowledge.
• Study the antioxidant activity and propose an alternative for flavor improvement in the immunomodulator product BIRM.
• Determine the amount of total phenolic compounds of BIRM using the Folin-Ciocalteau method, using gallic acid as a benchmark.
• Determine the antioxidant activity of BIRM using the ABTS method, using Trolox as the benchmark.
The medical product BIRM (Biological Immune Response Modulator) is a completely natural brown viscous liquid, made with the Amazonian root extract from Solanum dulcamara, which is used as food by native communities in Ecuador. In vivo studies on rats which had induced neuropathic problems show anti-neuroinflammatory and antinociceptive properties. Additionally it can be used as an auxiliary to standard therapy or as a therapeutic agent for the treatment of neuropathic disorders (Ravalji et.at ., 2015).
BIRM is a product approved by the Ministry of Public Health of Ecuador, known for the ability to prevent the development of prostate cancer and even prevent metastasis. Several scientific studies have been carried out inside and outside the country. This liquid is recommended for treating cancer, lupus, HIV and different diseases caused by the poor health of the immune system. It has great applications in chemotherapy or radiotherapy treatments, where BIRM® syrup serves as a complement (BIRM, 2013). BIRM contains antitumor compounds with proven activity on prostate cancer cells, according to studies conducted in vivo and in vitro (Dandekar et.al., 2003).
The product is of interest because of its high percentage of efficiency against cancer. In countless cases a very rapid effective response (from 60 to 90 days) has appeared so that the malignant cancerous cells begin to be eliminated from the body due to the capacity for apoptosis that this product possesses. In healthy people, ingesting this product does not cause any negative effects; on the contrary, it helps prevention, keeping the immune system balanced and strengthening the body’s defenses against any disease. As Dr. Edwin Cevallos has mentioned, the action of BIRM syrup is aimed at boosting the immune system and in this way the body is able to fight effectively against external agents or alterations that may occur, as well as to sweep the cancer cells off an organism (BIRM, 2013).
The benefits of BIRM syrup in cancer cells are detailed below:
• It affects the cell membrane and phospholipids present in cancer cells, which prevents these cells from feeding.
• It decreases the energy capacity that these cells have to reproduce, because this medicine alters the electrical charge of the mitochondria, which is the cell’s engine.
• It keeps cancer cells in a resting phase.
• It causes apoptosis or the programmed death of malignant cells.
• It prevents the spread of the disease to different parts of the body by preventing cancer cells from creating hyaluronidase, which causes metastasis.
The most active components in BIRM are Oligosaccharides rich in glycosaminoglycans (GAG). BIRM is orally bioavailable without systemic toxicity. Androgen receptor (AR) levels and activities are critical for prostate cancer (PCa) progression. Negative regulation of AR can slow or stop the progression of PCa (Cevallos, 2007).
The concentration of total phenolic compounds was determined using the Folin-Ciocalteu method. A stock solution of gallic acid (AG) of 100 ppm was prepared. Suitable aliquots are taken from this solution to prepare a calibration curve with gallic acid between 0 and 10 ppm. For this, the aliquot taken is placed in a 25 ml volumetric balloon, 1 ml of Folin-Ciocalteau reagent is added and allowed to stand in the dark for 15 minutes. Then, 5 ml of 7.5% sodium carbonate is added, the final volume is shaken with water and allowed to stand in the dark for 2 hours. The above mixture is placed in a cuvette and the absorbance at 765 nm is measured. The sample for analysis is prepared in the same way as the standards, starting from an aliquot of 25 uL of BIRM. The measurements were made in triplicate. The calibration curves can be seen in Fig. 1.
Fig. 1. Calibration curves with gallic acid. The absorbance results of the standards and samples are indicated in Table 1, where the corresponding calculations are included:
The results indicate that the BIRM product has a concentration of total phenolic compounds of 891.62 mg of gallic acid per 100 ml of product. If this value is compared with the concentration data of total phenolic
compounds found in fruits (Vasco, et.al., 2008) and vegetables (Číž, M. et.al., 2010), it can be established that BIRM is a product that has a very high concentration of total phenolic compounds.
The determination of the antioxidant capacity was based on the application of the method of discoloration of the ABTS radical. +, using Trolox as a reference standard for antioxidant compound. A stock solution of Trolox, concentration 700 µM was prepared. Aliquots are taken from this solution to prepare a calibration curve between 0 and 700 µM. To do this, the aliquot taken is placed in a 10 ml volumetric balloon and is added to the final volume with phosphate buffer pH 7.5. On the other hand, a solution of 7.4 mM ABTS in pH 7.5 phosphate buffer and another solution of 2.6 mM potassium peroxydisulfate in water was prepared. In a 25 ml balloon, 0.5 ml of both solutions A and B are mixed and allowed to react for two and a half hours so that the ABTS free radical is formed, then reflux with pH 7.5 phosphate buffer. 2100 uL of the ABTS free radical solution is taken and placed in a 1 cm spectrophotometric cuvette. 100 uL of standard solution is added, homogenized and absorbance is measured at 735 nm. The sample is analyzed in the same way as the standards, using 100 uL of previously diluted BIRM in 0.5: 10 ratio with pH 7.5 phosphate buffer. The measurements were made in triplicate. The calibration curves can be seen in Fig. 2.
The absorbance results of the standards and samples are indicated in Table 2, where the corresponding calculations are included:
The results indicate that the BIRM product has an antioxidant activity of 67.82 umol of trolox per ml of product. If this value is compared with antioxidant capacity data found in fruits (Vasco, et.al., 2008) and vegetables (Číž, M. et.al., 2010), it can be established that BIRM is a product that has a very high antioxidant action.
BIRM is a product that has a high content of phenolic compounds and antioxidant capacity, properties that corroborate its functionality and properties already found in previous studies, since there is a direct correlation between the content of phenolic compounds and the antioxidant capacity with respect to the uptake of free radicals, very reactive and oxidizing agents, responsible for aging.
The incorporation of the two flavor enhancing agents into the BIRM formula does not affect its functionality at all and, above all, keeps its manufacturing process intact from beginning to end.
• Cevallos, E. (2007). U.S. Patent No. 7,250,180. Washington, DC: U.S. Patent - Trademark Office. BIRM. (2013) desde http://jarabebirm.blogspot.com/
• Číž, M., Čížová, H., Denev, P., Kratchanova, M., Slavov, A., & Lojek, A. (2010). Different methods for control and comparison of the antioxidant properties of vegetables. Food Control, 21(4), 518-523.
• Constituyente, E. A. (2008). Constitución de la República del Ecuador.
• Dandekar, D. S., Lokeshwar, V. B., Cevallos-Arellano, E., Soloway, M. S., & Lokeshwar, B. L. (2003). An orally active Amazonian plant extract (BIRM) inhibits prostate cancer growth and metastasis. Cancer chemotherapy and pharmacology, 52(1), 59-66.
• Machu, L., Misurcova, L., Vavra Ambrozova, J., Orsavova, J., Mlcek, J., Sochor, J., & Jurikova, T. (2015). Phenolic content and antioxidant capacity in algal food products. Molecules, 20(1), 1118-1133.
• Rappoport, Z. (2004). The Chemistry of Phenols, 2 Volume Set: John Wiley & Sons.
• Ravalji, M., Buch, P., Uggini, G. K., Awasthi, A., CevallosArellano, E., & Balakrishnan, S. (2015). Amazonian plant extract birm reverses chronic neuropathic pain in rat sciatic nerve chronic constriction injury model. Pharmacology online, 3, 33-43.
• Ravalji, M., Cevallos-Arellano, E., & Balakrishnan, S. (2015). Investigation of centrally and peripherally acting analgesic and anti-infl ammatory activity of biological immune response modulator (an Amazonian plant extract) in animal models of pain and infl ammation. International Journal of Basic & Clinical Pharmacology | Vol 4 | Issue 2 Page 342-348.
• Vasco, C., Ruales, J., & Kamal-Eldin, A. (2008). Total phenolic compounds and antioxidant capacities of major fruits from Ecuador. Food chemistry, 111(4), 816-823.
Authors:
Abstract:
Front Oral Health. 2021; 2: 679460.
Published online 2021 Jun 11. do: 10.3389/froh.2021.679460
PMCID: PMC8409151
NIHMSID: NIHMS1717758
PMID: 34476412
Authors: Junfeng Pang, 1 , † Jie Gao, 2 Liyong Zhang, 3 , † Nahid F. Mivechi, 1 , † and Lan Ko 1 , 4 , * , †.
Tumor angiogenesis is a hallmark of cancer. Therapeutic drug inhibitors targeting angiogenesis are clinically effective. We have previously identified GT198 (gene symbol PSMC3IP, also known as Hop2) as an oncoprotein that induces tumor angiogenesis in human cancers, including oral cancer. In this study, we show that the GT198 protein is a direct drug target of more than a dozen oncology drugs and several clinically successful anticancer herbs. GT198 is a DNA repair protein that binds to DNA. Using an in vitro DNA-binding assay, we tested the approved oncology drug set VII from the National Cancer Institute containing 129 oncology drugs. Identified GT198 inhibitors include but are not limited to mitoxantrone, doxorubicin, paclitaxel, etoposide, dactinomycin, and imatinib. Paclitaxel and etoposide have higher binding affinities, whereas doxorubicin has higher binding efficacy due to competitive inhibition. GT198 shares protein sequence homology with DNA topoisomerases, which are known drug targets, so that GT198 is likely a new drug target previously unrecognized. To seek more powerful GT198 inhibitors, we further tested several anticancer herbal extracts. The positive anticancer herbs with high affinity and high efficacy are all clinically successful ones, including allspice from Jamaica, Gleditsia sinensis or honey locust from China, and BIRM from Ecuador. Partial purification of allspice using an organic chemical approach demonstrated great feasibility of natural product purification, when the activity is monitored by the in vitro DNA-binding assay using GT198 as a target. Together, our study reveals GT198 as a new targeting mechanism for existing oncology drugs. The study also delivers an excellent drug target suitable for compound identification and natural product purification. In particular, this study opens an opportunity to rapidly identify drugs with high efficacy and low toxicity from nature.
KEYWORDS: tumor angiogenesis, oncology drug target, anticancer herbs, GT198, oral cancer
Angiogenesis is a hallmark of many types of human solid tumors [1, 2]. Drugs that target tumor angiogenesis are proven to have great therapeutic value [3–5]. Mounting evidence indicates that angiogenic blood vessels in tumor tissues are not normal counterparts; rather, they serve as the epicenters of tumor development [6–9]. In tumor tissues, angiogenic blood vessels could be malignant themselves [10].
Central to the control of angiogenesis is the pericyte [11]. Pericytes are perivascular cells initiating angiogenesis in response to stimuli [12]. Pericytes are stem or progenitor cells capable of differentiating into various cell lineages [13]. Normal pericytes may differentiate into new tissues during embryonic development or adult tissue repair [11–13]. However, this process is hijacked in tumors in which pericytes are angiogenic and malignant [14], and their differentiation is jeopardized. The angiogenic pericytes detach, migrate, and overgrow into undifferentiated tumor cells [10]. Tumor cells with migratory pericyte behavior have been previously observed [15]. Pericyte-derived tumor cells surrounding
blood vessels are also described as vascular mimicry [6, 16, 17]. Multiple lines of evidence support that sustained tumor cell growth is replenished by cancerous stem cells [13], which would include angiogenic pericytes [11, 18].
To target tumor angiogenesis, a number of angiogenesis drug inhibitors have been developed [19, 20]. Many are kinase inhibitors or VEGF pathway inhibitors [21, 22], acting on blood vessel formations. Drug resistance may occur due to tumor vascular mimicry lacking endothelial cells [16], so that targeting to angiogenic pericytes could overcome drug resistance and suppress tumor angiogenesis more specifically. We and others have previously identified a DNA repair oncoprotein called GT198 (gene symbol PSMC3IP, alias Hop2) [10, 23–25]. The GT198 protein is overexpressed in angiogenic pericytes in tumors but not in quiescent normal pericytes [10]. In this study, we show that GT198 protein is an excellent drug target, inhibited by a panel of approved oncology chemotherapeutic drugs as well as several anticancer herbs known to be effective in human cancer treatment.
The GT198 protein is originally characterized as a transcriptional coactivator [23, 26]. The human GT198 gene carries germline mutations in early-onset breast and ovarian cancer families [27, 28], in familial ovarian disease [29], and insufficiencies [30–32]. Somatic mutations in GT198 are prevalent and recurrent in the tumor microenvironment of sporadic cancers [27, 33]. Importantly, GT198 mutations lead to its protein overexpression, which can be detected in angiogenic pericytes and their descendent vascular smooth muscle cell lineage, such as myoepithelial cells and adipocytes in breast cancer [34], the cells in ovarian cancer [35], and myofibroblasts in prostate and bladder cancers [36]. GT198-expressing pericytes have also been found in oral cancers as well as in multiple other solid tumors [10] and in mouse tumors [37]. Abnormal angiogenic pericytes in the microenvironment appear to be the origin of human cancer development.
The role of GT198 in pericytes may be associated with its expression and function in stem cells [10, 33]. Overall GT198 expression resembles cancer-testis antigens with high levels in the embryo, testis, cancer, and with low levels in normal adult tissues [10]. Normal stem cell differentiation requires a switch from functionally counteracting the GT198 splice variant to its wild type [33]. In human tumors, identified somatic mutations generate variants similar to the normal splice variants so that stem cell differentiation could be jeopardized [33]. Indeed, the angiogenic pericytes in oral tumor stroma produce undifferentiated cells [10].
GT198 is a small DNA-binding protein dimer, containing 217 amino acids in its monomer [23, 33]. The GT198 protein includes an N-terminal domain, a leucine zipper dimerization domain, a DNA-binding domain capable of binding to either single- or double-stranded DNA [24, 33], and a C-terminal auto-inhibitory domain [33]. Many biochemical studies published using a GT198 alias name Hop2 demonstrate that mammalian GT198 is a critical DNA repair factor stimulating homologous DNA recombination and regulating meiosis [24, 38, 39]. The mounting evidence collectively puts GT198 as a master nuclear controller with central importance. The processes of transcriptional activation, recombination in DNA repair, and pairing of homologous chromosomes in meiosis, all require the opening and binding of DNA strands. Thus, the DNA-binding is a critical activity of GT198. Through detecting its DNA-binding activity, GT198 inhibitors can be tested.
In this report, we extend our previous observations [10], and further show angiogenic pericytes expressing GT198 in human oral cancer. To test whether GT198 could be a target of angiogenesis inhibitors, we screened 129 clinical oncology drugs derived from the National Cancer Institute (NCI) using an in vitro DNA-binding assay of GT198. Surprisingly, we found that a panel of chemotherapeutic drugs, including angiogenesis inhibitors, such as doxorubicin, mitoxantrone, paclitaxel, etoposide, and gleevec, are also GT198 inhibitors. GT198 is likely a hidden drug target previously unrecognized. To further seek powerful GT198 inhibitors, we tested a number of anticancer herbs with historical success in human cancer treatment. We have identified several positive herbs,
including Jamaican allspice, Chinese Gleditsia Sinensis, and Ecuadorean BIRM, which directly inhibit GT198 with high affinity and efficacy. Partial purification of allspice using methods in organic chemistry confirmed great feasibility of natural product purification monitored by inhibiting of GT198 activity. Together, our finding reveals that GT198 is a hidden target of many existing oncology drugs and anticancer herbs. GT198 can be served as a target for future chemical compound drug identification and also natural product purification because a target is now available.
We have previously identified GT198-expressing angiogenic blood vessels in precursor lesions of various human solid tumors, including oral cancer [10, 34–36]. In agreement with our previous finding in human oral cancers, GT198 protein is a clear marker to reveal angiogenic blood vessels in the tumor microenvironment (Figures 1A,B). When oral cancer pathology slides are examined by immunohistochemistry of GT198, we observed that the earliest lesions are GT198+ pericytes, which appear to differentiate into multiple types of descendent cells. Initially, GT198+ pericytes are present in small clusters of angiogenic vessels while the surrounding quiescent vessels have GT198− pericytes (Figure 1B). Next, the cytoplasmic volume of GT198+ pericytes increases so that the capillary wall abnormally thickens (Figure 1B). Further, GT198+ pericytes detach from vessels becoming nodules in tissue stroma. At this stage, GT198+ fibroblasts in stroma also increase. Then, the pericyte nodule proliferates into a tumor mass often enclosing a blood vessel at its center (Figure 1B). This type of blood vessel in a tumor is still functional with red blood cells in its cavity, which is consistent with the observations from others that the tumor produces its own vessels [6, 16]. It is important to note that classically described tumor development from epithelial basal layers can also be observed. However, in that case, the affected epithelia are always surrounded by GT198+ angiogenic blood vessels, which carry the vascular smooth muscle marker αSMA (Figure 1C). The affected epithelial cells are also GT198+ compared with nearby normal epithelia, which are GT198− (Figure 1C). Because pericyte stem cells may replenish progenitor cells in the basal layer of the epithelium, it is possible that epithelium- and stromal nodule–derived oral tumors are both originated from angiogenic pericytes. The concepts of tumor initiation from epithelia vs. from angiogenic vessels may now be reconciled when using GT198 protein as a pericyte marker. Tumor initiation from vessels is further supported by scattered GT198+αSMA+ cells found within the tumors that enclose vessels (Figure 1D and Supplementary Figure 1). Angiogenic vessels appear to develop into early stage tumors and later disintegrate when tumors mature or advance. In agreement with our previous study [10], the evidence collectively suggests that angiogenic pericytes give rise to tumors in human oral cancer.
Figure 1
GT198+ angiogenic blood vessels in human oral cancers. (A) A hypothetic model comparing pericytes in quiescent vessel in green and in angiogenic and tumor enclosed vessels in orange. (B) Immunohistochemical staining of GT198 in human oral cancer. Pericytes in quiescent vessel are GT198−. Angiogenic pericytes, detached pericytes, and pericytes in a tumor are GT198+. The tumor enclosed vessel is functional with red blood cells inside. Boxed areas are enlarged below. (C) In an epithelial oral cancer, multiple GT198+ angiogenic vessels are present around the GT198+ epithelia in tumor development. An adjacent section is immunofluorescent double-stained with GT198 in red and αSMA in green and counterstained with DAPI in blue. (D) An oral tumor enclosing a vessel from the same patient is double-stained with GT198 and αSMA showing a vessel at tumor center. Boxed areas are enlarged at the right. Extended view is shown in Supplementary Figure 1. Arrows indicate GT198+ pericytes. Immunohistochemistry sections are counterstained with hematoxylin. Scale bars = 100 μm..
GT198 is a specific marker for tumor angiogenesis. To test the idea that GT198 inhibitors would have an anticancer effect, we first selected clinically successful oncology drugs for analysis. In order to measure GT198 activity in vitro for direct inhibition, a DNA-binding assay was developed in which recombinant GT198 proteins are coated on 96-well plates and assayed for the binding of biotin-labeled DNA in the presence of drug inhibitors. Bound biotinylated DNA can be detected by streptavidin-horseradish peroxidase (HRP) conjugate and chemiluminescence (Figures 2A,B). The binding affinity between DNA and GT198 was first tested to be EC50 = 43 nM using BSA as a negative control (Figure 2C). This result revealed a DNA concentration before the binding saturation as approximately 150 nM (Figure 2C), an optimal DNA concentration to allow both maximal signal detection and efficient drug competition in subsequent analyses.
GT198 is a target of oncology drugs. (A) A model of allosteric or competitive drug inhibition of the DNA binding of GT198 dimer. (B) A flowchart of the DNA-binding assay. (C) The increasing concentrations of biotinylated DNA bind to GT198 at EC50 = 43 nM using BSA as a negative control. (D) Inhibition of DNA binding to GT198 by increasing concentrations of clinical drugs as indicated. IC50s are measured by sigmoidal curve fit. Data represent mean ± s.e.m of duplicate experiments. (E) A graph model comparing binding affinity and efficacy.
A number of chemotherapy drugs were then tested for GT198 inhibition by measuring the IC50, half maximal inhibitory concentration under serial diluted concentrations of drugs and in the presence of 150 nM DNA. Surprisingly, we identified many positive GT198 inhibitors. Doxorubicin has low affinity (IC50 = 341 nM) but high efficacy for inhibition, and paclitaxel (IC50= 5.0 nM) and etoposide (IC50=24.2 nM) have high affinities and poor efficacies (Figures 2D,E). Camptothecin has poor affinity (IC50= 2.06 μM), and carboplatin does not inhibit GT198 (Figure 2D). This is the first time to cross-compare binding characteristics of these drugs in vitro using their common target. The finding may be clinically relevant because higher dose tolerance with low toxicity in paclitaxel or etoposide reflects their high affinities [40, 41], potent doxorubicin reflects its high efficacy albeit toxicity [42], and the clinical weakness of camptothecin in contrast to highly successful paclitaxel may be due to its very poor affinity [43, 44].
GT198 being a genuine drug target became more evident when the similarities among drug analogs were found. Among doxorubicin analogs, mitoxantrone (IC50 = 187.4 nM) and daunorubicin (IC50 = 149.9 nM) showed better affinities than idarubicin (IC50 =362.4 nM), epirubicin (IC50 = 749.6 nM), and valrubicin (IC50 = 973.3 nM) (Figure 3A). Mitoxantrone has the highest efficacy leading to an almost complete inhibition. However, their binding affinities are all within the hundred nanomolar range. In contrast, camptothecin and its analogs irinotecan and topotecan have very poor affinities or fail to inhibit GT198 (Figure 3B).
Figure 3
Competitive inhibition of GT198 by doxorubicin. (A,B) Inhibition of the DNA binding to GT198 under increasing concentrations of doxorubicin analogs and camptothecin analogs. Their IC50s are as indicated. (C) Increasing concentrations of biotinylated DNA bind to GT198 in the presence (blue) or absence (red) of 100 nM doxorubicin. Doxorubicin as a competitor shifts the binding curve to the right. (D) Double reciprocal plot using the means of highconcentration data points (37, 111, 333, 1,000 nM) from the panel C. The constant Vmax and increased Km with doxorubicin indicate competitive inhibition. (E) Primary sequence homology among human GT198 and DNA topoisomerase I and IIB (Clustal Omega). Asterisks denote identical residues and dots denote homologous residues. Fulllength alignment is shown in Supplementary Figure 2.
We further found that doxorubicin is a competitive inhibitor of GT198 (Figures 3C,D). The binding of GT198 by doxorubicin competed with increasing concentrations of DNA (Figure 3C). Double-reciprocal plot analysis of the same data revealed competitive binding by DNA and doxorubicin (Figure 3D). The constant Vmax with increased Km in the presence of 100 nM doxorubicin vs. 0 nM control suggested that doxorubicin binds to the same site on GT198 as DNA binds. Thus, it directly blocks the DNA-binding site of GT198 (model in Figure 2A). As a competitive inhibitor, doxorubicin exerts high efficacy (model in Figure 2E). In contrast, paclitaxel was found to be an allosteric inhibitor or noncompetitive inhibitor in a separate study [45]. The allosteric inhibition may lead to rather incomplete inhibition, resulting poorer binding efficacy (Figures 2A–D). Our results provide explanations that doxorubicin is a more potent drug due to high efficacy, and paclitaxel is less potent but more sensitive due to higher affinity. Higher affinity is linked to low toxicity because lower drug concentration is sufficient.
It was initially unexpected but later no longer surprising for many drugs found to be GT198 inhibitors because GT198 shares protein sequence homology with DNA topoisomerases
I and II (Figure 3E and Supplementary Figure 2). DNA topoisomerases are previously known targets of analogs of doxorubicin, etoposide, and camptothecin [46–48]. Like GT198, DNA topoisomerases are DNA-binding proteins participating in transcription and DNA recombination. Thus, the sequence homology further validates GT198 as a previously unrecognized target of topoisomerases inhibitors.
The above findings prompted us to further test additional oncology drugs as GT198 inhibitors. Using the DNAbinding assay above, we screened 129 oncology drugs from the Approved Oncology Drugs Set VII derived from the NCI (Table 1 and Supplementary Table 1). The selected 40 drugs are shown for comparison (Figure 4), and IC50 values and efficacies were only analyzed in a number of positive inhibitors (Table 1). Identified GT198 inhibitors include doxorubicin family analogs, paclitaxel and docetaxel; etoposide and teniposide. In addition, positive inhibitors also include dactinomycin, carfilzomib, sirolimus (rapamycin), imatinib (Gleevec), sunitinib, trifluridine, and aminolevulinic acid (Figure 4 and Table 1). Celastrol, which was not from the NCI drug collection, was also found to be positive (Table 1). Many drugs are negatives, including platinum inhibitors, methotrexate, and vincristine. Of all the drugs tested as GT198 inhibitors, mitoxantrone has the highest efficacy, and paclitaxel has the best affinity (Table 1) [45].
Table 1
Clinical oncology drugs inhibit GT198. Clinical oncology drugs were tested by the DNA binding assay. The drug activities of GT198 inhibition are shown as the relative reciprocal amounts of DNA binding in a bar graph. Black arrows indicate doxorubicin analogs. White arrows indicate paclitaxel analogs. Stars indicate statistically significant activities. Selected 40 oncology drugs are shown, and the complete list is in Supplementary Table 1.
It is important to note that many drugs are well-characterized with other mechanism of actions in the history. For example, Gleevec is an Abl tyrosine kinase inhibitor. However, during traditional drug development, there are multiple selection procedures from hit to lead and further to candidate. Often, the later steps require cell and animal testing whereas the GT198 protein is a prominent cytotoxic target inducing apoptosis [33] and is also overexpressed in many mouse tumor models [10, 37]. Therefore, Gleevec may inhibit both Abl tyrosine kinase and GT198 in vivo, but this was not revealed until this study. Similarly, some other GT198 inhibitors identified here may also have more than one in vivo target.
To seek more potent GT198 inhibitors with both high affinity and high efficacy, we looked into herbs having historical success in the treatment of human cancers. Detailed rationales for each herb selection are described in the Discussion section. The two most promising herbs with a long history of cancer treatment, allspice (Pimenta dioica) native to Jamaica and honey locust thorns (Gleditsia sinensis L., GSL) native to China, were extracted by ethanol and tested by the DNAbinding assay. Both allspice (IC50 = 1.77 ng/μl, efficacy = 86%) and GSL (IC50 = 0.54 ng/μl, efficacy = 92%) showed excellent affinities and efficacies compared with licorice root, which was used as a negative control (Figures 5A,B). Using silica gel chromatography to analyze eluting profiles for polarity, we found that the active ingredients from two plants have distinct polarity (Figure 5C). The active ingredient in GSL is more polar than that in allspice, suggesting the presence of two distinct drug inhibitors that can later be purified. When the GSL extract was tested by a TUNEL assay for apoptosis on HeLa cells, significant apoptotic activity was detected (Figure 5D). This result confirmed the presence of cytotoxic ingredient in the GSL extract. Allspice has been previously shown to be apoptotic [49].
Anticancer herbs inhibit GT198. (A) The thorn needles of GSL and berries of allspice extracts inhibit the DNAbinding activity of GT198. Licorice is a negative control. IC50s are as indicated. (B) Photographs of the plants and their corresponding herbs used in panel A. (C) Silica gel chromatography of GSL and allspice. Arrows indicate their most active constituents with different polarities when eluted from nonpolar to polar solvents. (D) TUNEL assay for apoptotic activity of GSL in HeLa cells. Quantitative representation of positive cell numbers in microscopic views is shown on the right. **P < 0.01.
We also tested a set of anticancer herbs originating from Taiwan containing branches of four trees (Figures 6A,B). The mix of four crude herbs is an anticancer remedy in Taiwan with extensive cancer treatment testimonies. These trees are mulberry (Morus australis), walnut (Juglans regia L.), island mahonia (Mahonia oiwakensis), and rosewood (Dalbergia odorifera). When individually tested, we found that all four extracts positively inhibit GT198 although their activities are much lower than allspice as a positive control under the same concentrations (Figure 6A). All four herbs are commonly used in Asia, and their combinations may exert higher potency albeit low activity in each herb.
Figure 6
Commercial herbal medicines inhibit GT198. (A) Ethanol extracts from four tree branches inhibit the DNA-binding of GT198. The four trees are mulberry, walnut, island mahonia,
and rosewood from a Taiwanese anticancer remedy. Allspice is a positive control. (B) Photographs of the four plants, their corresponding herbs, and ethanol extracts assayed in panel A. (C) BIRM, an Ecuador product of dulcamara root extract, inhibits the DNA-binding activity of GT198. Allspice as a positive control. IC50s are indicated. (D) Photographs of dulcamara plant. *P < 0.05 and **P < 0.01
Another tested herb is a commercially available online health product from Ecuador called BIRM. BIRM stands for biological immune response modulator. BIRM is an aqueous extract of dried roots of the Ecuadorian plant dulcamara (Kalanchoe gastonis-bonnieri Raym). The BIRM extract showed high efficacy as well as high affinity (IC50 = 7.12 ng/μl) in inhibiting GT198 (Figures 6C,D). In addition to human cancer treatment testimonies, research studies have previously shown that BIRM is effective for prostate cancer by regulating androgen receptor in cellular and animal models [50, 51]. The current study first pinpoints that GT198 is a direct target of BIRM, while GT198 is also an androgen receptor coactivator [23].
Very interestingly, most identified anti-GT198 anticancer herbs are also herbs known to be anti-infection (Table 2). Online testimonies in the treatment of viral and bacterial infections by allspice and BIRM are extensive. GSL has also been shown to be anti-HIV [52] and was used for COVID-19 in the 2020 pandemic in Asia. Because angiogenesis is activated by both acute inflammation in infection and chronic inflammation in cancer, GT198 inhibitors suppressing angiogenesis could be a subset of dual effective anticancer and anti-infective drugs.
Table 2
Efficacies of GT198 inhibition are measured with 120 ng/μl of herbal ethanol extracts. Clinical evidence of anticancer and anti-infection is indicated as: +++, extensive; ++, significant; +, evidence present; -, evidence absent.
Natural product purification using organic chemical methods requires an efficient in vitro assay to monitor constituent activities in each purification step. The highly sensitive DNAbinding assay described above is found to be ideal to monitor purifications of herbs. Allspice extract was first fractionated using silica gel chromatography to obtain an active constituent of fraction 8 (Figure 7A), which was further fractioned by preparative reverse-phase HPLC to obtain an active peak 3 (Figure 7B). The peak 3 was then purified by mono-Q anion exchange chromatography. The most active fraction was eluted at 60 mM NaCl (Figure 7C). The 60-mM fraction was further analyzed by reverse-phase HPLC, and the constituent fraction 3 with the highest activity corresponded to the peak (Figure 7D). Based on further analysis, it appeared that the fraction 3 was not yet pure, and further purification is needed to obtain a single compound.
Figure 7
Partial purification of allspice. (A) Ethyl acetate extract of allspice was purified by silica gel chromatography to obtain an active fraction 8. Colors of each fraction are shown below.
(B) Fraction 8 was purified by reverse-phase HPLC to obtain an active peak 3. (C) The peak 3 was further purified by mono-Q anion exchange chromatography with active fraction in 60 mM NaCl. (D) The 60 mM fraction was analyzed by reverse-phase HPLC with each fraction assayed for activities.
These results demonstrate great feasibility of natural product purification when constituent activity is monitored by the DNA-binding binding assay using GT198 as the drug target. Because the assay is fast and highly sensitive, we expect that many positive herbs could be purified to obtain chemical drugs or partially purified to remove toxic components to become safer herbal medicines.
GT198 cDNA was first reported in 1995 in an effort to find breast cancer genes in the chromosome 17q21 locus [53]. GT198 has now emerged out of the shadow of BRCA1 as an ever-important cancer gene. The original gene symbol at NCBI was HUMGT198A, and much later, it was renamed PSMC3IP. The full-length human GT198 was initially reported as a transcriptional coactivator [23], and its mouse homolog as a TBP-interacting protein [54]. Subsequently, it
was published using the alias name Hop2 in meiosis [55] and in DNA repair [24, 39] due to its functional similarities to yeast Hop2 protein. Today, the alias names in the literature include GT198 in cancer studies, Hop2 or TBPIP in biochemical studies, and PSMC3IP in genetic studies.
The divergent research focuses reflect highly complex functions of GT198, which are hard to reconcile during early discoveries. As evidence accumulates, the roles of GT198 become more unified. Regarding the nuclear biochemical activities, GT198 binds to DNA so that it can stimulate transcription, recombination, DNA repair, and meiosis [56]. Many nuclear proteins interact with GT198, including steroid hormone receptors [23] and DNA repair factors [56]. From a cancer biology prospective, GT198 regulates stem cells, stimulates angiogenesis [34, 36], and induces apoptosis [33]. From a genetic prospective, the GT198 gene carries germline and somatic mutations in cancers [27, 28, 34] and in ovarian diseases [29]. Our current study extends the role of GT198 into a new dimension as a multidrug target, providing further support for GT198 in oncogenesis [57].
The finding of multiple clinical successful oncology drugs targeting GT198 (Table 1), invites a revisit of previous mechanisms of the action of many drugs. We found that much evidence in the past is indeed consistent with a mechanism targeting to GT198. The GT198 protein is homologous to DNA topoisomerases I and II (Figure 3E), providing a direct explanation of doxorubicin, etoposide, and camptothecin and their homologs as GT198 inhibitors. Consistently, suppression of angiogenesis has also been shown in doxorubicin [58], etoposide [59], and camptothecin [60]. The clinical cardiovascular side effects of doxorubicin may also be correlated with the inhibition of blood vessel pericytes.
Dactinomycin is cytotoxic and regulates DNA functions [61], which can be explained as a GT198 inhibitor. In addition, the mechanism of paclitaxel has been linked to mitotic arrest, apoptosis, and angiogenesis [62], and paclitaxel’s clinical side effects are correlated with the normal expression of GT198 [10, 45]. Consistent with roles as angiogenesis inhibitors, paclitaxel and docetaxel are common chemotherapy drugs used for human oral cancer. Inhibition of angiogenesis is also shown as an activity in Gleevec [63], aminolevulinic acid [64], and celastrol [65]. Platinum DNA inhibitors did not inhibit GT198 directly (Figure 2D and Table 1), possibly due to their DNA crosslinking rather than DNA intercalating property. The above evidence collectively supports GT198 as a master oncoprotein unmatched by many others characterized to date. Potentially, more GT198 inhibitors could be found in the future because many drugs have not been tested.
Herbs have been a principal form of medicine since ancient times and are still used in most developing countries. Mounting sophisticated uses of herbs have been described in numerous medicinal books, and around 70,000 plant species have been used as medicines throughout history [66]. Because humans co-evolved together with plants in the environment, many natural ingredients have been adapted by humans with less toxicity when compared to chemically synthesized nonnatural compounds. Various successful clinical oncology
drugs are originally derived from plants, such as paclitaxel from the Pacific yew tree and etoposide from Mayapple.
After our discovery of GT198 inhibitors among existing oncology drugs and realizing that none of them are perfect in both GT198 binding affinity and efficacy, we speculated that better GT198 inhibitors potentially exist in anticancer herbs. This judgment was based on the fact that GT198 plays an important role in cancer initiation; GT198 protein is a druggable target, and mounting historical testimonies are present in anticancer herbs. We then selected and tested herbal medicines with successful human cancer treatment.
GSL (Gleditsia sinensis L.) was selected from a Chinese medicinal book named Ben Cao Gang Mu, a UNESCO registered heritage, in which the texts were adapted from an ancient herbal scientist Li Shi-Zhen (1518–1593) [67]. Among more than 1200 herbs described, only GSL stands out to have an impact on all sex organs, including breast, ovary, testis, and uterus, which link to functional characteristics of GT198. GSL is described by the book as super effective to treat women’s abdominal tumor masses. Today, GSL is widely used as an anticancer herb in Asia with the tree massively planted for therapeutic interests. Approximately 100 references in PubMed describe the studies of Gleditsia sinensis, including anticancer activities in breast and prostate cancers [68, 69] and in tumor angiogenesis [70, 71]. GSL biological activity is also previously summarized [72].
The selection of allspice and BIRM was due to their historical treatments of human prostate cancers in South America. Allspice is well-known for its health benefits, including antiinfection, antioxidant, and enhancing immune properties. Allspice is often used by dentists for oral antiseptic healthcare. Researchers from our own institute showed that active ingredients exist in allspice extract [49, 73, 74], and in BIRM [50, 51] with apoptotic and anticancer activities in mouse models. Both herbs affect androgen receptor–mediated functions in prostate cancer. Because GT198 is an androgen receptor coactivator, we tested and confirmed GT198 as a direct target (Figures 5A, ,6C6C).
An herbal remedy from Taiwan containing branches of four trees was selected to test because this medicine has extensive testimonies in the treatment of human colon cancer. Mulberry and walnut have been described by medicinal books with various health benefits, and island mahonia and rosewood are well-known anticancer herbs with a long history. Although they showed less activity than allspice in inhibition of GT198 (Figure 6A), the combination of the four may have a synergistic anticancer effect. The advantage is that all four tree herbs are less toxic.
In addition, we concurrently tested many other herbal extracts as negative controls (Table 2 and data not shown) to ensure detected activities are not nonspecific due to isolating from plants. The highest sensitivity was observed in GSL extract, which can be detected at 0.2 ng/μl. Given more than hundreds of different molecules that are normally present in the crude extracts, the active compound affinity would predictably be extremely high (IC50 <1 nM) if GSL were purified. When
toxicity is considered, allspice may be a best medicine without complete purification because it is a common organic spice with plenty of health benefits.
After anti-GT198 activity is found in herbal medicines, an interesting phenomenon has emerged that all positive anticancer herbs are also anti-infection (Table 2). In fact, allspice and BIRM are more prominent for their antiviral and antibacterial activities than for anticancer activity. GSL and rosewood have been used in combating the COVID-19 pandemic in 2020. This is not surprising if considering the fact that acute inflammation in infection and chronic inflammation in cancer share the same angiogenic pericytes, in both cases overexpressing GT198. Inflammatory signals normally aim to activate pericyte stem cells for subsequent growth and inflammatory responses except that chronic and persistent pericyte activation leads to cancer. Thus, a subset of drugs as GT198 inhibitors will likely be both anti-infective and anticancer. The reason most oncology drugs have limited uses is because the drugs are only approved within the scope of conducted clinical trials in a subset of cancer patients. Our current finding helps to expand the usage of approved oncology drugs (Table 1) in angiogenic cancers, including oral cancer as well as in infectious diseases. The effort may accelerate combating future pandemics, not only using herbal medicines, but also using approved chemical drugs. GT198 is essential for the development of dual-effective anticancer and anti-infective drugs in the future.
In summary, we extend the previous observations and further showed that angiogenic pericytes express GT198 in human oral cancer. We identified a panel of existing oncology drugs, including mitoxantrone, doxorubicin, paclitaxel, etoposide, dactinomycin, and imatinib, as direct GT198 inhibitors. We further found a number of anticancer herbs with historical success in human cancer treatment as GT198 inhibitors. We confirmed the feasibility of natural product purification in organic chemistry monitored by the GT198 target. Together, this study reveals GT198 as a new targeting mechanism for many existing oncology drugs. GT198 is an excellent drug target suitable for compound identification and natural product purification. This study may also accelerate identification of high-efficacy and low-toxicity drugs to combat both cancer and infection.
Polyclonal rabbit antibody against GT198 was affinity purified and previously described [23, 34]. Formalin-fixed paraffin-embedded (FFPE) sections were deparaffinized and dehydrated through xylene and ethanol series, followed by antigen retrieval in 10 mM sodium citrate buffer, pH 6.0, containing 0.05% Triton at 90°C for 20 min. Anti-GT198 (1:200) was incubated at 4°C overnight. Antibody binding was detected using biotinylated secondary antibody followed by detecting reagents (Abcam). Sections were counterstained with hematoxylin.
Human oral tumor FFPE sections were obtained from
the Head and Neck Cancer SPORE at the University of Pittsburgh Cancer Institute. Samples were obtained following institutional IRB guidelines using de-identified human cancer specimens that cannot be traced back to the subject. Clinical staging of five oral cancer specimens used in this study were (1) male, age 56, normal oral mucosa, Figure 1B, quiescent vessel; (2) male, age 50, T4N3M0, Figure 1B, angiogenic vessel; (3) male, age 53, T3N2M0, Figure 1B, detached pericytes; (4) male, age 65, T2N1M0, Figure 1B, vessel in tumor; and (5) male, age 51, T3N1M0, adjacent tissue in Figure 1C; tumor tissue in Figure 1D and in Supplementary Figure 1..
The Approved Oncology Drugs Set VII containing 129 drugs (plates 4,845 and 4,846) were derived from NCI, Division of Cancer Treatment and Diagnosis (DCTD), and Developmental Therapeutics Program (DTP). Chemical structures of each drug compound can be found in the NCI’s website https://dtp. cancer.gov/dtpstandard/platemap/index.jsp, and the drugs are also listed in Supplemental Table 1. Each well in the 96-well plates contained 20 μl of 10 mM drugs in DMSO. In addition to 129 NCI drugs, a number of clinical oncology drugs were obtained from the Augusta University pharmacy.
The GSL herb with the Chinese name ZaoJiaoCi is the thorn needles of Gleditsia sinensis L. tree from Yunnan province in China. Its ethanol extract dry powder was provided by the Drug Research Institute of KPC Pharmaceuticals Inc. in Yunnan. Allspice with an origin of Jamaica was obtained from the World Spice Merchants, WA, USA. The dry powder of BIRM extract originated from Ecuador and was provided by Dr. Bal Lokeshwar at Augusta University. The branches of four trees in an anticancer herbal mix containing mulberry, walnut, island mahonia, and rosewood were from an herbal drug store in Taiwan. Two negative control herbs, licorice and garden mum, were obtained from a TongRenTang herbal retail store in China (Table 2). Ethanol extracts of herbs were used in the DNA-binding assays to determine activities. Methanol extracts of GSL and allspice were used in chromatography purifications. The extracts were dried overnight, weighted using an analytical balance, dissolved in DMSO at 30 mg/ml, and stored at −80°C.
The full-length GT198 protein has 217 amino acids [23]. The N-terminus and the DNA-binding domain are essential for its dimerization and DNA binding. However, we have previously reported that its C-terminal tail (aa 181–217) reduces its own DNA-binding activity and serves as an auto-inhibitory domain [33]. In vivo GT198 activity might be tightly guarded until regulated. For in vitro assays, the C-terminal removal will enable maximal DNA binding and signal detection. Thus, this study used a C-terminal truncated version of GT198 (aa
1–180) to ensure high sensitivity of the assay. The potential drawback of the C-terminal removal is that certain drugs may not be detectable if the C terminus is required. N-terminal His-tagged recombinant human GT198 protein without its C-terminus (aa 1–180) were expressed in E. coli BL21 (DE3) pLysS and purified through Ni-NTA-agarose (Qiagen) as previously described [33]. Proteins were eluted by 200 mM imidazole, desalted, concentrated using Amicon YM-10 spin columns, and stored at −80°C before use. Protein concentrations were determined using protein assay dye reagent concentrate solution (Bio-Rad) and 2 mg/ml bovine serum albumin (BSA) standard (Sigma).
In the in vitro 96-well plate binding assay, the binding of biotinylated DNA to GT198 was detected by chemiluminescence. A single-stranded 25-mer biotinoligonucleotide (Biotin)-cctggggttgctgaggtcctggcag was used in the assay because it is sufficient to bind one GT198 dimer. White MicroLiteTM 2+ 96-well plates (Thermo Scientific, #7572) were coated by drying overnight at 37°C containing a solution of 400 ng/well of recombinant Histagged GT198 proteins together with 5 ug/well of purified BSA (NEB) in a volume of 50 ul. BSA alone was included as a control for background. No subtraction of background was needed due to very low background. Duplicated wells were used for each experimental point. Each experiment was repeated three times. The GT198-coated plates were blocked with 5% BSA in a solution containing PBS with 0.1% Triton X-100 (TPBS) for 1 h. The binding was carried out for 4 h to overnight at 4°C using 150 nM Biotin-DNA and serial diluted drugs (0.128, 0.64, 3.2, 16, 80, 400, 2,000, 10,000 nM) or herb extracts (0, 0.0384, 0.192, 0.96, 4.8, 24, 120, 600 ng/μl) in the binding buffer (20 mM TrisHCl, pH 7.5, 50 mM NaCl, 75 mM KCl, 0.5 mM MgCl2, 0.05% Triton X-100, 10% glycerol, 1 mM dithiothreitol). After binding, the plates were washed three times with TPBS solution for total 45 min. The plates were then incubated with streptavidinconjugated HRP (Roche Molecular Biochemicals, #1089153) at 1 U/ml in TPBS for 1 h at 4°C and further washed three times with TPBS for total 30 min. Bound biotinylated DNA were detected by chemiluminescence with 50 μl/well ECL detection reagents (Amersham Pharmacia Biotech) using a luminometer. Reading of plates was repeated three times within 45 min, and usually the second reading yielded the most consistent results. The finished plates were stained with Bio-Rad protein assay dye reagent to evaluate the bound GT198 protein on the plates. MicroLiteTM 2+ plates have excellent binding capacity without losing coated GT198 protein after repeated washing steps.
The IC50 value is the drug concentration of 50% inhibition of the DNA binding. The IC50 values were calculated through Kaleidagraph software (Synergy Software) using nonlinear regression sigmoidal dose-response curve fit. The equation for calculation is y = m1 + (m2 – m1)/[1 + (x /m3)m4], where m1 is the minimum, m2 is the maximum, m3 is the IC50 value
calculated by Kaleidagraph, and m4 is the slope at midpoint of the curve. The analysis of Vmax and Km in competitive binding assays were carried out in double-reciprocal plots, using means of four high-concentration data points, omitting points at lower concentrations with reciprocal values offscale. See a reference as examples [45]. Double-reciprocal plots reveal competitive binding as constant Vmax with increased Km in the presence of drugs or allosteric binding with reduced Vmax and constant Km in the presence of drugs. In general, competitive inhibitors directly bind to the DNA-binding surface of the target and kick out the DNA. By contrast, allosteric inhibitors bind to a site nearby and induce protein conformational changes to reduce the DNA-binding to the target. Competitive inhibitors may have higher binding efficacy.
Silica gel is highly polar and can interact strongly with compounds. Using a gradient of nonpolar to polar solvents, compounds with different polarity can be separately eluted from silica gel in constituents. The 5 ml methanol extracts containing 60 mg of GSL or allspice were mixed with 1.5 g of high-purity grade, pore size 60 Å, 70–230 mesh silica gel (Sigma #288624), and air-dried overnight. The herb-bound silica gel was loaded on to a chromatography column (BioRad, 3 ml gel in 14 ml column) and batch eluted by 6 ml of the following solvents with polar index in parentheses. Hexane (0.1), 1:1 hexane and ethyl acetate (2.2), 1:2 hexane and ethyl acetate (3.0), ethyl acetate (4.4), 1:1 ethyl acetate and methanol (4.7), methanol (5.1), and 1:1 methanol and water (7.6). The eluted fractions were vacuum dried, weighted, and tested for activity in the DNA-binding assays.
Allspice berries (50 g) were ground into powder and extracted by 1,000 ml methanol overnight at room temperature. The methanol-soluble fraction was liquid–liquid extracted using an equal volume of ethyl acetate. The ethyl acetate fraction was further dried for subsequent chromatography. The extracted allspice (1.5 g) was bound to silica gel and loaded onto a silica gel column (3 × 30 cm), which was first eluted by hexane-chloroform gradient and then by ethyl acetate-10% methanol in ethyl acetate gradient. A total of 10 fractions (50 ml each) were collected, and the highest activity was in fraction 8 (Figure 7A). This fraction was concentrated and further purified by preparative reverse-phase HPLC (C18, 4.6 × 150 mm, flow rate 0.5 ml/min), using 5% methanol in water to 100% methanol gradient to obtain an active peak 3 (Figure 7B). The peak three was dried (600 μg) and further purified by mono-Q anion exchange chromatography (BioRad HiTrap Q HP column, 1 ml). The column was step-eluted by 1.2 ml of increasing concentration of NaCl (0–360 mM) in a buffer containing 95% methanol, 50 mM NH4HCO3, pH 8.5. All fractions were measured for absorbance at A254 and assayed for activity. The most active fraction was eluted at 60 mM NaCl (Figure 7C). The 60 mM fraction was then analyzed by reverse-phase HPLC (Waters, C18, 4.6 × 75 mm, flowrate 0.5 ml/min), yielding a fraction 3 with highest
activity (Figure 7D). In each step, the testing samples were dried and dissolved in DMSO or in the binding butter for the DNA-binding assays.
HeLa cells (ATCC, CCL-2) were maintained in chamber slides in DMEM supplemented with 10% fetal bovine serum, 100 U/ml penicillin, and 0.1 μg/μl streptomycin. Cells were incubated in 5% CO2 at 37°C. Cells were treated with 200ng/ μl GSL extract overnight and fixed with100%methanol for 10 min at −20°C. Cells were then made permeable using 0.1% Triton in 10 mM sodium citrate pH 6.0 for 2 min on ice. TUNEL staining was carried out using the In Situ Cell Death Detection Kit (Roche) following the manufacturer’s protocol. Briefly, prepared cells in a chamber side were labeled in the dark for 1 h at 37°C with TMR-red labeled dUTP and terminal transferase solution, which labels DNA fragments produced in apoptotic cells. After washing with PBS, slides were counterstained with DAPI before visualization by fluorescence microscopy.
Protein sequence alignment was carried out using the Multiple Sequence Alignment tool of Clustal Omega at EMBLEBI (https://www.ebi.ac.uk/Tools/msa/clustalo/). Protein sequences of human GT198, human DNA topoisomerase I, and IIB were entered for alignment using default settings in the program. Asterisks denote identical amino acid residues, and dots denote homologous residues (Supplementary Figure 2). Selected section of results was shown in Figure 3E.
Statistical analyses were carried out using GraphPad Prism software. Bar graphs were derived from data points in duplicates and are presented for the DNA-binding assays. P-values were calculated using unpaired two-tailed t-test. *P < 0.05, **P < 0.01, ***P < 0.001; NS, not significant. A P-value of < 0.05 is considered statistically significant.
The original contributions presented in the study are included in the article / supplementary material, further inquiries can be directed to the corresponding authors.
LK and JG conceived and designed experiments. LK, JP, JG, and LZ performed experiments. JP, LZ, and NM contributed reagents. LK wrote manuscript. All authors contributed to the article and approved the submitted version.
LK is a founder of OnkoTarget LLC, and is the inventor of GT198 patents. The remaining authors declare that the research was conducted in the absence of any commercial or financial relationships that could be construed as a potential conflict of interest.
We thank the National Cancer Institute (NCI), Division of Cancer Treatment and Diagnosis (DCTD), and Developmental Therapeutics Program (DTP) for providing oncology drug plates (4845 and 4846) in the Approved Oncology Drugs Set VII. We thank the Head and Neck Cancer SPORE at University of Pittsburgh for human oral tumor samples. We thank Dr. Wei Zhang and Dr. Jianwen Zhang at KPC Pharmaceuticals Inc, China for GSL extracts. We thank Augusta University faculty Dr. Dorothy Tuan for herbal materials, Dr. Bal Lokeshwar for BIRM, and Dr. Ahmed Chadli for drug materials. We thank the following photographers for their images of herbal plants: Forest Starr and Kim Starr, Maui, Hawaii, USA for allspice plant (Pimenta dioica, Figure 5B); Michael Wolf, Niedersteinbach, Germany for licorice plant (Glycyrrhiza uralensis, Figure 5B); Li Yekting, Zoological and Botanical Gardens, Central, Hong Kong for island mahonia plant (Mahonia oiwakensis, Figure 6B); Hong Kong Herbarium, Agriculture, Fisheries and Conservation Department, Kowloon, Hong Kong for rosewood plant (Dalbergia odorifera, Figure 6B); Jordi Bosch Janer and David J. Stang at Wikimedia Commons for dulcamara plant (Kalanchoe gastonis-bonnieri, Figure 6D).
1. Folkman J. Tumor angiogenesis: therapeutic implications. N Engl J Med. (1971) 285:1182–6. 10.1056/NEJM197111182852108 [PubMed] [CrossRef] [Google Scholar]
2. Carmeliet P, Jain RK. Molecular mechanisms and clinical applications of angiogenesis. Nature. (2011) 473:298–307. 10.1038/nature10144 [PMC free article] [PubMed] [CrossRef] [Google Scholar]
3. Plate KH, Scholz A, Dumont DJ. Tumor angiogenesis and anti-angiogenic therapy in malignant gliomas revisited. Acta Neuropathol. (2012) 124:763–75. 10.1007/s00401012-1066-5 [PMC free article] [PubMed] [CrossRef] [Google Scholar]
4. Sennino B, McDonald DM. Controlling escape from angiogenesis inhibitors. Nat Rev Cancer. (2012) 12:699–709. 10.1038/nrc3366 [PMC free article] [PubMed] [CrossRef] [Google Scholar]
5. Sandler A, Gray R, Perry MC, Brahmer J, Schiller JH, Dowlati A, et al.. Paclitaxel-carboplatin alone or with bevacizumab for non-small-cell lung cancer. N Engl J Med. (2006) 355:2542–50. 10.1056/NEJMoa061884 [PubMed] [CrossRef] [Google Scholar]
6. Leslie M. Tumors’ do-it-yourself blood vessels. Science. (2016) 352:1381–3. 10.1126/science.352.6292.1381 [PubMed] [CrossRef] [Google Scholar]
7. Jain RK, Carmeliet P. SnapShot: Tumor angiogenesis. Cell. (2012) 149:1408 e1401. 10.1016/j.cell.2012.05.025 [PubMed] [CrossRef] [Google Scholar]
8. Takano S. Glioblastoma angiogenesis: VEGF resistance solutions and new strategies based on molecular mechanisms of tumor vessel formation. Brain Tumor Pathol. (2012) 29:73–86. 10.1007/s10014-011-0077-6 [PubMed] [CrossRef] [Google Scholar]
9. Lugano R, Ramachandran M, Dimberg A. Tumor angiogenesis: causes, consequences, challenges and opportunities. Cell Mol Life Sci. (2020) 77:1745–70. 10.1007/s00018-019-03351-7 [PMC free article] [PubMed] [CrossRef] [Google Scholar]
10. Zhang L, Wang Y, Rashid MH, Liu M, Angara K, Mivechi NF, et al.. Malignant pericytes expressing GT198 give rise to tumor cells through angiogenesis. Oncotarget. (2017) 8:51591–607. 10.18632/oncotarget.18196 [PMC free article] [PubMed] [CrossRef] [Google Scholar]
11. Armulik A, Genove G, Betsholtz C. Pericytes: developmental, physiological, and pathological perspectives, problems, and promises. Dev Cell. (2011) 21:193–215. 10.1016/j.devcel.2011.07.001 [PubMed] [CrossRef] [Google Scholar]
12. Papetti M, Herman IM. Mechanisms of normal and tumor-derived angiogenesis. Am J Physiol Cell Physiol. (2002) 282:C947–970. 10.1152/ajpcell.00389.2001 [PubMed] [CrossRef] [Google Scholar]
13. Bautch VL. Stem cells and the vasculature. Nat Med. (2011) 17:1437–43. 10.1038/nm.2539 [PubMed] [CrossRef] [Google Scholar]
14. Weller M. Angiogenesis in glioblastoma: just another moving target? Brain J Neurol. (2010) 133:955–6. 10.1093/brain/awq063 [PubMed] [CrossRef] [Google Scholar]
15. Farin A, Suzuki SO, Weiker M, Goldman JE, Bruce JN, Canoll P. Transplanted glioma cells migrate and proliferate on host brain vasculature: a dynamic analysis. Glia. (2006) 53:799–808. 10.1002/glia.20334 [PubMed] [CrossRef] [Google Scholar]
16. Andonegui-Elguera MA, Alfaro-Mora Y, CaceresGutierrez R, Caro-Sanchez CHS, Herrera LA, DiazChavez J. An overview of vasculogenic mimicry in breast cancer. Front Oncol. (2020) 10:220. 10.3389/ fonc.2020.00220 [PMC free article] [PubMed] [CrossRef] [Google Scholar]
17. Dunleavey JM, Dudley AC. Vascular mimicry: concepts and implications for anti-angiogenic therapy. CurrAngiog. (2012) 1:133–8. 10.2174/2211552811201020133 [PMC free article] [PubMed] [CrossRef] [Google Scholar]
18. Valle IB, Schuch LF, da Silva JM, Gala-Garcia A, Diniz IMA, Birbrair A, et al.. Pericyte in oral squamous cell carcinoma: a systematic review. Head Neck Pathol. (2020) 14:1080–91. 10.1007/s12105-020-011882 [PMC free article] [PubMed] [CrossRef] [Google Scholar]
19. Ribatti D. The history of angiogenesis inhibitors. Leukemia. (2007) 21:1606–9. 10.1038/sj.leu.2404756 [PubMed] [CrossRef] [Google Scholar]
20. El-Kenawi AE, El-Remessy AB. Angiogenesis inhibitors in cancer therapy: mechanistic perspective on classification and treatment rationales. Bri J Pharmacol. (2013) 170:712–29. 10.1111/bph.12344 [PMC free article] [PubMed] [CrossRef] [Google Scholar]
21. Zirlik K, Duyster J. Anti-Angiogenics: current situation and future perspectives. Oncol Res Treat. (2018) 41:166–71. 10.1159/000488087 [PubMed] [CrossRef] [Google Scholar]
22. Cook KM, Figg WD. Angiogenesis inhibitors: current strategies and future prospects. Can J Clin. (2010) 60:222–43. 10.3322/caac.20075 [PMC free article] [PubMed] [CrossRef] [Google Scholar]
23. Ko L, Cardona GR, Henrion-Caude A, Chin WW. Identification and characterization of a tissue-specific coactivator, GT198, that interacts with the DNA-binding domains of nuclear receptors. Mol Cell Biol. (2002) 22:357–69. 10.1128/MCB.22.1.357-369.2002 [PMC free article] [PubMed] [CrossRef] [Google Scholar]
24. Enomoto R, Kinebuchi T, Sato M, Yagi H, Shibata T, Kurumizaka H, et al.. Positive role of the mammalian TBPIP/HOP2 protein in DMC1-mediated homologous pairing. J Biol Chem. (2004) 279:35263–72. 10.1074/ jbc.M402481200 [PubMed] [CrossRef] [Google Scholar]
25. Pezza RJ, Voloshin ON, Vanevski F, Camerini-Otero RD. Hop2/Mnd1 acts on two critical steps in Dmc1promoted homologous pairing. Genes Dev. (2007) 21:1758–66. 10.1101/gad.1562907 [PMC free article] [PubMed] [CrossRef] [Google Scholar]
26. Satoh T, Ishizuka T, Tomaru T, Yoshino S, Nakajima Y, Hashimoto K, et al.. Tat-binding protein-1 (TBP1), an ATPase of 19S regulatory particles of the 26S proteasome, enhances androgen receptor function in cooperation with TBP-1-interacting protein/Hop2.
Endocrinology. (2009) 150:3283–90. 10.1210/en.20081122 [PMC free article] [PubMed] [CrossRef] [Google Scholar]
27. Peng M, Bakker JL, DiCioccio RA, Gille JJP, Zhao H, Odunsi K, et al.. Inactivating mutations in GT198 in familial and early-onset breast and ovarian cancers. Genes Can. (2013) 4:15–25. 10.1177/1947601913486344 [PMC free article] [PubMed] [CrossRef] [Google Scholar]
28. Schubert S, Ripperger T, Rood M, Petkidis A, Hofmann W, Frye-Boukhriss H, et al.. GT198 (PSMC3IP) germline variants in early-onset breast cancer patients from hereditary breast and ovarian cancer families. Genes Can. (2017) 8:472–83. 10.18632/genesandcancer.132 [PMC free article] [PubMed] [CrossRef] [Google Scholar]
29. Zangen D, Kaufman Y, Zeligson S, Perlberg S, Fridman H, Kanaan M, et al.. XX ovarian dysgenesis is caused by a PSMC3IP/HOP2 mutation that abolishes coactivation of estrogen-driven transcription. Am J Hum Genet. (2011) 89:572–9. 10.1016/j.ajhg.2011.09.006 [PMC free article] [PubMed] [CrossRef] [Google Scholar]
30. Yang X, Touraine P, Desai S, Humphreys G, Jiang H, Yatsenko A, et al.. Gene variants identified by wholeexome sequencing in 33 French women with premature ovarian insufficiency. J Assis Reprod Genet. (2019) 36:39–45. 10.1007/s10815-018-1349-4 [PMC free article] [PubMed] [CrossRef] [Google Scholar]
31. Mei L, Huang L, Huang Y, Wu X, He H, He X, et al.. Two novel biallelic mutations in PSMC3IP in a Chinese patient affected by primary ovarian insufficiency. Res Squ Prep. (2020). 10.21203/rs.3.rs-33852/v1 [PubMed] [CrossRef] [Google Scholar]
32. Al-Agha AE, Ahmed IA, Nuebel E, Moriwaki M, Moore B, Peacock KA, et al.. Primary ovarian insufficiency and azoospermia in carriers of a homozygous PSMC3IP stop gain mutation. J Clin Endocrinol Metab. (2018) 103:555–63. 10.1210/jc.2017-01966 [PMC free article] [PubMed] [CrossRef] [Google Scholar]
33. Peng M, Yang Z, Zhang H, Jaafar L, Wang G, Liu M, et al.. GT198 splice variants display dominant negative activities and are induced by inactivating mutations. Genes Cancer. (2013) 4:26–38. 10.1177/1947601913486345 [PMC free article] [PubMed] [CrossRef] [Google Scholar]
34. Yang Z, Peng M, Cheng L, Jones K, Maihle NJ, Mivechi NF, et al.. GT198 expression defines mutant tumor stroma in human breast cancer. Am J Pathol. (2016) 186:1340–50. 10.1016/j.ajpath.2016.01.006 [PMC free article] [PubMed] [CrossRef] [Google Scholar]
35. Peng M, Zhang H, Jaafar L, Risinger JI, Huang S, Mivechi NF, et al.. Human ovarian cancer stroma contains luteinized theca cells harboring tumor suppressor gene GT198 mutations. J Biol Chem. (2013) 288:33387–97. 10.1074/jbc.M113.485581 [PMC free article] [PubMed] [CrossRef] [Google Scholar]
36. Zhang L, Liu Y, Cheng L, Zhao C, Ko L. Mutant GT198 in angiogenesis as a common origin of human prostate and bladder. BioRxiv. (2019). 10.1101/726679 [CrossRef] [Google Scholar]
37. Achyut BR, Zhang H, Angara K, Mivechi NF, Arbab
AS, Ko L. Oncoprotein GT198 vaccination delays tumor growth in MMTV-PyMT mice. Cancer Lett. (2020) 476:57–66. 10.1016/j.canlet.2020.02.005 [PMC free article] [PubMed] [CrossRef] [Google Scholar]
38. Cho NW, Dilley RL, Lampson MA, Greenberg RA. Interchromosomal homology searches drive directional ALT telomere movement and synapsis. Cell. (2014) 159:108–21. 10.1016/j.cell.2014.08.030 [PMC free article] [PubMed] [CrossRef] [Google Scholar]
39. Petukhova GV, Pezza RJ, Vanevski F, Ploquin M, Masson JY, Camerini-Otero RD. The Hop2 and Mnd1 proteins act in concert with Rad51 and Dmc1 in meiotic recombination. Nat Struct Mol Biol. (2005) 12:449–53. 10.1038/nsmb923 [PubMed] [CrossRef] [Google Scholar]
40. Kelly K, Pan Z, Wood ME, Murphy J, Bunn PA, Jr. A phase I study of paclitaxel, etoposide, and cisplatin in extensive stage small cell lung cancer. Clin Cancer Res. (1999) 5:3419–24. [PubMed] [Google Scholar]
41. Fisherman JS, McCabe M, Noone M, Ognibene FP, Goldspiel B, Venzon DJ, et al.. Phase I study of Taxol, doxorubicin, plus granulocyte-colony stimulating factor in patients with metastatic breast cancer. J Natl Cancer Inst. (1993) 15:189–94. [PubMed] [Google Scholar]
42. Chatterjee K, Zhang J, Honbo N, Karliner JS. Doxorubicin cardiomyopathy. Cardiology. (2010) 115:155–62. 10.1159/000265166 [PMC free article] [PubMed] [CrossRef] [Google Scholar]
43. Li F, Jiang T, Li Q, Ling X. Camptothecin (CPT) and its derivatives are known to target topoisomerase I (Top1) as their mechanism of action: did we miss something in CPT analogue molecular targets for treating human disease such as cancer? Am J Can Res. (2017) 7:2350–94. [PMC free article] [PubMed] [Google Scholar]
44. Posey JA, 3rd, Saif MW, Carlisle R, Goetz A, Rizzo J, Stevenson S, et al.. Phase 1 study of weekly polyethylene glycol-camptothecin in patients with advanced solid tumors and lymphomas. Clin Cancer Res. (2005) 11:7866–71. 10.1158/1078-0432.CCR-050783 [PubMed] [CrossRef] [Google Scholar]
45. Yang Z, Gurvich VJ, Gupta ML, Mivechi NF, Ko L. Oncoprotein GT198 is a direct target of taxol. BioRxiv. (2019). 10.1101/675579 [CrossRef] [Google Scholar]
46. Tewey KM, Rowe TC, Yang L, Halligan BD, Liu LF. Adriamycin-induced DNA damage mediated by mammalian DNA topoisomerase II. Science. (1984) 226:466–8. 10.1126/science.6093249 [PubMed] [CrossRef] [Google Scholar]
47. Pommier Y. Topoisomerase I inhibitors: camptothecins and beyond. Nat Rev Cancer. (2006) 6:789–802. 10.1038/nrc1977 [PubMed] [CrossRef] [Google Scholar]
48. Wu CC, Li TK, Farh L, Lin LY, Lin TS, Yu YJ, et al.. Structural basis of type II topoisomerase inhibition by the anticancer drug etoposide. Science. (2011) 333:459–62. 10.1126/science.1204117 [PubMed] [CrossRef] [Google Scholar]
49. Zhang L, Shamaladevi N, Jayaprakasha GK, Patil BS, Lokeshwar BL. Polyphenol-rich extract of Pimenta dioica berries (Allspice) kills breast cancer cells by autophagy and delays growth of triple negative breast
cancer in athymic mice. Oncotarget. (2015) 6:16379–95. 10.18632/oncotarget.3834 [PMC free article] [PubMed] [CrossRef] [Google Scholar]
50. Dandekar DS, Lokeshwar VB, Cevallos-Arellano E, Soloway MS, Lokeshwar BL. An orally active Amazonian plant extract (BIRM) inhibits prostate cancer growth and metastasis. Can Chemother Pharmacol. (2003) 52:59–66. 10.1007/s00280-0030612-1 [PubMed] [CrossRef] [Google Scholar]
51. Shamaladevi N, Araki S, Lyn DA, Ayyathurai R, Gao J, Lokeshwar VB, et al.. The andean anticancer herbal product BIRM causes destabilization of androgen receptor and induces caspase-8 mediated-apoptosis in prostate cancer. Oncotarget. (2016) 7:84201–13. 10.18632/oncotarget.12393 [PMC free article]
[PubMed] [CrossRef] [Google Scholar]
52. Li WH, Zhang XM, Tian RR, Zheng YT, Zhao WM, Qiu MH. A new anti-HIV lupane acid from Gleditsia sinensis Lam. J Asian Nat Prod Res. (2007) 9:551–5. 10.1080/10286020600883419 [PubMed] [CrossRef] [Google Scholar]
53. Rommens JM, Durocher F, McArthur J, Tonin P, LeBlanc JF, Allen T, et al.. Generation of a transcription map at the HSD17B locus centromeric to BRCA1 at 17q21. Genomics. (1995) 28:530–42. 10.1006/geno.1995.1185 [PubMed] [CrossRef] [Google Scholar]
54. Ijichi H, Tanaka T, Nakamura T, Yagi H, Hakuba A, Sato M. Molecular cloning and characterization of a human homologue of TBPIP, a BRCA1 locus-related gene. Gene. (2000) 248:99–107. 10.1016/S03781119(00)00141-4 [PubMed] [CrossRef] [Google Scholar]
55. Petukhova GV, Romanienko PJ, Camerini-Otero RD. The Hop2 protein has a direct role in promoting interhomolog interactions during mouse meiosis. Dev Cell. (2003) 5:927–36. 10.1016/S1534-5807(03)003691 [PubMed] [CrossRef] [Google Scholar]
56. Sansam CL, Pezza RJ. Connecting by breaking and repairing: mechanisms of DNA strand exchange in meiotic recombination. FEBS J. (2015) 282:2444–57. 10.1111/febs.13317 [PMC free article] [PubMed] [CrossRef] [Google Scholar]
57. Ko L. Human solid cancer decoded. Zenodo Prep. (2019). [Google Scholar]
58. Lennernas B, Albertsson P, Lennernas H, Norrby K. Chemotherapy and antiangiogenesis–drugspecific, dose-related effects. Acta oncologica. (2003) 42:294–303. 10.1080/02841860310001835 [PubMed] [CrossRef] [Google Scholar]
59. Panigrahy D, Kaipainen A, Butterfield CE, Chaponis DM, Laforme AM, Folkman J, et al.. Inhibition of tumor angiogenesis by oral etoposide. Exp Ther Med. (2010) 1:739–46. 10.3892/etm.2010.127 [PMC free article] [PubMed] [CrossRef] [Google Scholar]
60. Clements MK, Jones CB, Cumming M, Daoud SS. Antiangiogenic potential of camptothecin and topotecan. Can Chemother Pharmacol. (1999) 44:411–6. 10.1007/ s002800050997 [PubMed] [CrossRef] [Google Scholar]
61. Sobell HM. Actinomycin and DNA transcription. Proc Natl Acad Sci USA. (1985) 82:5328–31. 10.1073/ pnas.82.16.5328 [PMC free article] [PubMed]
[CrossRef] [Google Scholar]
62. Wang J, Lou P, Lesniewski R, Henkin J. Paclitaxel at ultra low concentrations inhibits angiogenesis without affecting cellular microtubule assembly. Anti Drugs. (2003) 14:13–9. 10.1097/00001813-200301000-00003 [PubMed] [CrossRef] [Google Scholar]
63. Kumar K, Ghanghas P, Sanyal SN. Chemopreventive action of Imatinib, a tyrosine kinase inhibitor in the regulation of angiogenesis and apoptosis in rat model of lung cancer. Mol Cell Biochem. (2018) 447:47–61. 10.1007/s11010-018-3292-1 [PubMed] [CrossRef] [Google Scholar]
64. Mercea PA, Mischkulnig M, Kiesel B, Wadiura LI, Roetzer T, Prihoda R, et al.. Prognostic Value of 5-ALA fluorescence, tumor cell infiltration and angiogenesis in the peritumoral brain tissue of brain metastases. Cancers. (2021) 13:603. 10.3390/cancers13040603 [PMC free article] [PubMed] [CrossRef] [Google Scholar]
65. Gao Y, Zhou S, Pang L, Yang J, Li HJ, Huo X, et al.. Celastrol suppresses nitric oxide synthases and the angiogenesis pathway in colorectal cancer. Free Rad Res. (2019) 53:324–34. 10.1080/10715762.2019.1575512 [PMC free article] [PubMed] [CrossRef] [Google Scholar]
66. Chevallier A. Encyclopedia of Herbal Medicine. London, UK: Dorling Kindersley Limited; (2016). [Google Scholar]
67. Zheng J. [Preliminary textual research on Zhijintang Sanctum re-revised version of Jinling edition of Ben cao gang mu (Compendium of Materia Medica)]. Zhonghua Yi Shi Za Zhi. (2014) 44:106–10. [PubMed] [Google Scholar]
68. Shoemaker M, Hamilton B, Dairkee SH, Cohen I, Campbell MJ. In vitro anticancer activity of twelve Chinese medicinal herbs. Phytother Res. (2005) 19:649–51. 10.1002/ptr.1702 [PubMed] [CrossRef] [Google Scholar]
69. Ryu S, Park KM, Lee SH. Gleditsia sinensis thorn attenuates the collagen-based migration of PC3 prostate cancer cells through the suppression of alpha2beta1 integrin expression. Int J Mol Sci. (2016) 17:328. 10.3390/ijms17030328 [PMC free article] [PubMed] [CrossRef] [Google Scholar]
70. Lee J, Yi JM, Kim H, Lee YJ, Park JS, Bang OS, et al.. Cytochalasin H, an active anti-angiogenic constituent of the ethanol extract of Gleditsia sinensis thorns. Biol Pharm Bull. (2014) 37:6–12. 10.1248/bpb.b13-00318 [PubMed] [CrossRef] [Google Scholar]
71. Yi JM, Park JS, Oh SM, Lee J, Kim J, Oh DS, et al.. Ethanol extract of Gleditsia sinensis thorn suppresses angiogenesis in vitro and in vivo. BMC Complement Altern Med. (2012) 12:243. 10.1186/1472-6882-12243 [PMC free article] [PubMed] [CrossRef] [Google Scholar]
72. Gao J, Yang X, Yin W. From traditional usage to pharmacological evidence: a systematic mini-review of spina gleditsiae. Evid Based Compl Alternat Med. (2016) 2016:3898957 10.1155/2016/3898957 [PMC free article] [PubMed] [CrossRef] [Google Scholar]
73. Shamaladevi N, Lyn DA, Shaaban KA, Zhang L, Villate S, Rohr J, et al.. Ericifolin: a novel antitumor compound from allspice that silences androgen receptor in prostate cancer. Carcinogenesis. (2013) 34:1822–32. 10.1093/ carcin/bgt123 [PMC free article] [PubMed] [CrossRef] [Google Scholar]
74. Zhang L, Lokeshwar BL. Medicinal properties of the Jamaican pepper plant Pimenta dioica and Allspice. Curr Drug Targ. (2012) 13:1900–6. 10.2174/138945012804545641 [PMC free article] [PubMed] [CrossRef] [Google Scholar]
Annexes:
1. Histological Report, Zacatecas Autonomous University, 1994.
2. Appointment as a researcher at the Autonomous University of Madrid of Immunomodulators and Antitumor Agents of Natural Origin, 2000.
3. Report in Discovery Salud Medical Magazine, Birm Effective Product against Cancer, 2005.
4. Histological Report Center for Biological Research and Evaluation (CEIBEB), University of Havana, 2006.
5. Miami Herald newspaper, article “Mysterious Cure for Prostate Cancer” by Fred Tasker. October 16TH, 2007.
6. Sponsor of the University of Miami Symposium entitled “Cancer Prevention and Treatment” organized by the University of Miami, FL-USA, January 24TH, 2014.
7. Phytochemical Report, University of Antioquia, 2017.
8. Award to the Leader in Research and Health Sciences for The Benefit Of Mankind, 2018.
9. Certificate of International Health Coach, SIISDET, 2018.
10. Acknowledgement. Cartagena, International Medical Scientific Congress and Business Development in Health (SIISDET), Award of the Best Research Work “BIRM , Biological Modulator of the Immune Response, Prevention and Treatment in Cancer”, 2018.
11. International Society for Research, Health, Business Development and Technologies, SIISDET, 2018.
12. Harvard Medical School, Office of Online Learning, External Education HMX Fundaments – Immunology, 2019.
13. Acknowledgement and honorary title “Honoris Causa Doctor in Medical Sciences” granted by SIISDET to Dr. Edwin Cevallos A., MD from Ecuador. Held in University of Miami Miller School of Medicine. Miami, FL - USA. October 26, 2019.
14. Presentation of BIRM® in the 2nd Emirates Congress on Integrative and Complementary Medicine 2019. Dubai, UAE. November 22, 2019.
15. Acknowledgement of Scientific Merit to the Creator of BIRM, the first ecuadorian product in prevention and treatment of cancer, autoimmune and degenerative diseases conferred by María José Carrión, Assemblywoman for the Province of Pichincha. November 27, 2019.
16. Master’s Degree in Education in the Teaching and Research Area, awarded by the University of Santander. Cancun, Mexico. February 18, 2020.
17. Prize for Philanthropy in Education and Research awarded by the SIISDET, at the VI International Congress on Medical Science and Business Development in Health - “United for Health”. Bogota Colombia. June 24, 25 and 26, 2021
18. Honorary Doctorate in Health, the highest honorary degree awarded by SIISDET, at the VII International Congress on Medical Science and Business Development– SIISDET, Reunion of Two Continents- “Unifying Concepts”. Santander – Spain, from June 03 to 05, 2022.
ZACATECAS AUTONOMOUS UNIVERSITY HISTOLOGICAL REPORT, 1994. APPOINTMENT AS A RESEARCHER AT THE AUTONOMOUS UNIVERSITY OF MADRID OF IMMUNOMODULATORS AND ANTITUMOR AGENTS OF NATURAL ORIGIN, 2000.
Acknowledgements:
In May 2005, Dr. Edwin Cevallos gave a conference on BIRM® syrup at the “First World Cancer Conference with Complementary Treatments” held in Madrid - Spain and organized by Discovery Salud from Spain.
AS ANTITUMORAL AGENT AND IMMUNOMODULATOR OF PLANT ORIGIN, IN THE AUTONOMOUS UNIVERSITY OF MADRID “UAM”.
AS ANTIRETROVIRAL AGENT AND IMMUNOMODULATOR BY THE WORLD FOUNDATION DIRECTED BY MR. DR. LUC MONTAGNIER.
From left to right: PH.D. Balakrishna Lokeshwar, PH.D.Vinata Lokeshwar, Dr. Mark Soloway, Dr. Edwin Cevallos, Decano Dr. Pascal Goldschmidt, Director de Sylvester Cancer Center Dr. Stephen Nimer. en Universi ty of Miami Miller School of Medicine.
SPONSOR OF THE UNIVERSITY OF MIAMI SYMPOSIUM ENTITLED “CANCER PREVENTION AND TREATMENT” ORGANIZED BY THE UNIVERSITY OF MIAMI, FL-USA, JANUARY 24TH, 2014.
“AWARD TO THE LEADER IN RESEARCH AND HEALTH SCIENCES FOR THE BENEFIT OF MANKIND 2018” which is awarded by the Steering Committee, evaluators, national and international of the International Society for Research, Health, Business Development and Technologies, SIISDET
For participating in the III International Medical Scientific Congress
CERTIFICATE OF INTERNATIONAL HEALTH COACH - SIISDET, 2018.
Award:
PRIZE TO THE LEADER IN RESEARCH AND HEALTH SCIENCES FOR THE BENEFIT OF MANKIND, 2018.
Scientists from many countries met with the endorsement of the University of Cartagena, among them: United States, Spain, Mexico, Argentina, Paraguay, Nicaragua, Panama, Honduras, Colombia, Peru, Chile, Puerto Rico and Ecuador. There, Doctor Edwin Cevallos received the following acknowledgement:
ACKNOWLEDGEMENT AND HONORARY TITLE “HONORIS CAUSA DOCTOR IN MEDICAL SCIENCES” GRANTED BY SIISDET TO DR. EDWIN CEVALLOS A., MD FROM ECUADOR. HELD IN UNIVERSITY OF MIAMI MILLER SCHOOL OF MEDICINE. MIAMI, FLUSA. OCTOBER 26TH, 2019.
PRESENTATION OF BIRM® IN THE 2ND EMIRATES CONGRESS ON INTEGRATIVE AND COMPLEMENTARY MEDICINE 2019. DUBAI, UAE. NOVEMBER 22TH, 2019.
ACKNOWLEDGEMENT OF SCIENTIFIC MERIT TO THE CREATOR OF BIRM, THE FIRST ECUADORIAN PRODUCT IN PREVENTION AND TREATMENT OF CANCER, AUTOIMMUNE AND DEGENERATIVEDISEASES CONFERRED BY MARÍA JOSÉ CARRIÓN, ASSEMBLYWOMAN FOR THE PROVINCE OF PICHINCHA. NOVEMBER 27TH, 2019.
PRESENTATION OF BIRM® AT PANAMERICAN MEDICAL ASOCIATION - PAMA, A NONPROFIT MEDICAL AND SCIENTIFIC ORGANIZATION, WILL HOLD THE CONGRESS OF SURGICAL AND ULTRASOUND MEDICINE, NUMBER62 THAT WILL BE NAMED AFTER DR. TEÓFILO LAMA, A PRESTIGIOUSPHYSICIANFROMGUAYAQUILANDFOUNDER OF THE KENNEDY CLINIC IN GUAYAQUIL, ECUADOR. NOVEMBER 15TH, 2019.
HONORARY MEMBER OF THE PAN AMERICAN MEDICAL ASSOCIATION (PAMA) FOR HIS LAUDABLE SCIENTIFIC AND ACADEMIC MERITS. GUAYAQUIL, ECUADOR. DECEMBER 5TH, 2019.
MASTER’S DEGREE IN EDUCATION IN THE TEACHING AND RESEARCH AREA, AWARDED BY THE UNIVERSITY OF SANTANDER. CANCUN, MEXICO. FEBRUARY 18, 2020.
PRIZE FOR PHILANTHROPY IN EDUCATION AND RESEARCH AWARDED BY THE SIISDET, AT THE VI INTERNATIONAL CONGRESS ON MEDICAL SCIENCE AND BUSINESS DEVELOPMENT IN HEALTH - “UNITED FOR HEALTH”. BOGOTA COLOMBIA. JUNE 24, 25 AND 26, 2021
HONORARY DOCTORATE IN HEALTH, THE HIGHEST HONORARY DEGREE AWARDED BY SIISDET, AT THE VII INTERNATIONAL CONGRESS ON MEDICAL SCIENCE AND BUSINESS DEVELOPMENT– SIISDET, REUNION OF TWO CONTINENTS- “UNIFYING CONCEPTS”. SANTANDER –SPAIN, FROM JUNE 03 TO 05, 2022.
During more than 45 years, BIRM has been the most tested and studied immunomodulator. Laboratories and Universities around the world, have examined its healing properties with incredible results:
VISIT US: www.birm.com
CONTACT US IN ECUADOR: consultas@birm.com.ec
CONTACT US IN COLOMBIA: ventasbirmcolombia@greenterra.com.co www.birm.com.co
CONTACT US IN THE UNITED STATES: info@birmproducts.com www.birmproducts.com
CONTACT US IN SPAIN: ventas@greenmedilab.com
CONTACT US IN PERU: pfranco@grupofs.com
Quito - Ecuador Manuel Iturrey N2805 y Bello Horizonte Edificio Artes, 2005, Piso 2 PBX: (+593) 2 2992910 / Tel.: (+593) 2 2509861 / (+593) 2 2562995 / (+593) 2 2563918 Fax: (+593) 2 2564374
Bogota - Colombia GREEN TERRA LIFE S.A.S Carrera 11 No. 86-32 Tel: (+57) 3182854042
Morgan Hill - U. S. A. 17100 Monterey Rd., Suite 1A, Ca 95037 Tel.: +1 (669) 888-3005
Santiago del Surco - Peru Av. del Pinar 110 oficina 405 Tel.: (+51) 939 343 332
FOLLOW US: