Gernot Minke
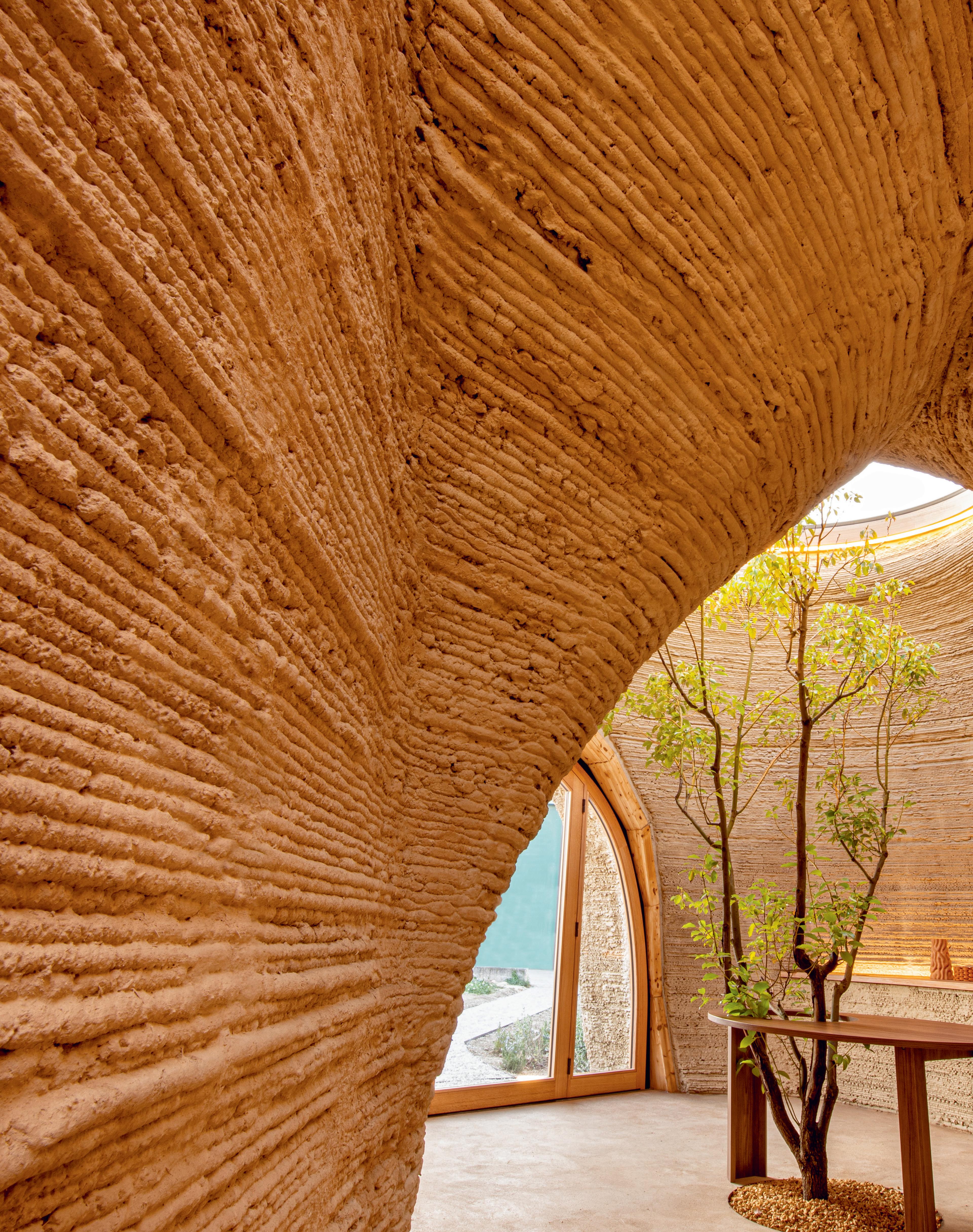
Gernot Minke
Design and Technology of a Sustainable Architecture
Fifth and revised edition
Birkhäuser
Gernot Minke
Design and Technology of a Sustainable Architecture
Fifth and revised edition
Birkhäuser
Basel
Preface 7
1 Introduction 9
History 9
Earth as a building material: the essentials 11
Improving indoor climate 13
Prejudices against earth as a building material 16
2 The properties of earth as a building material 17
Composition 17
Tests used to analyse the composition of loam 19
Effects of water 22
Effects of vapour 26
Influence of heat 29
Strength 30
pH-value 32
Radioactivity 33
Shelter against high-frequency electromagnetic radiation 33
3 Preparing of loam 34
Soaking, crushing and mixing 34
Sieving 36
Mechanical slurrying 36
Water curing 36
Thinning 36
4 Improving the characteristics of loam by special treatment or additives 37
Reduction of shrinkage cracks 37
Stabilisation against water erosion 38
Enhancement of binding force 40
Increasing compressive strength 40
Strength against abrasion 45
Increasing thermal insulation 45
Lightweight loams 46
5 Rammed earthworks 50
Formwork 51
Tools 52
Method of construction 53
Shaping of openings 53
Wall construction techniques 54
Rammed earth domes 59
Drying 59
Labour input 59
Thermal insulation 59
Surface treatment 59
6 Working with earth blocks 60
History 60
Production of earth blocks 61
Material composition 64
Laying earth blocks 64
Surface treatment 65
Fixing fasteners to walls 65
Lightweight earth blocks 65
Special acoustic green bricks and adobes 66
7 Large blocks and panels 67
Large blocks 67
Earth-filled wall panels 67
Clay panels 69
Heating panels 71
Floor slabs 71
Floor tiles 72
Extruded loam slabs 72
8 Direct forming with wet loam 73
Traditional wet loam techniques 73
The “Dünne loam loaf” technique 75
The stranglehm technique 75
9 Wet loam infill in skeleton structures 80
Thrown loam 80
Sprayed loam 80
Rolls and bottles of straw loam 81
Lightweight loam infill 81
Infill with stranglehm and earth-filled hoses 82
Sprayed loam in steel-reinforced walls and ceilings 82
10 Tamped, poured or pumped lightweight loam 85
Formwork 85
Tamped lightweight straw loam walls 85
Tamped lightweight wood loam walls 87
Tamped, poured or pumped lightweight mineral loam walls 87
Pumped lightweight mineral loam floors 88
Loam-filled hollow blocks 88
Loam-filled hoses 90
11 Loam plasters 92
Preparation of substrate 92
Composition of loam plaster 92
Guidelines for plastering earth walls 94
Sprayed lightweight plaster 94
Lightweight mineral loam plaster 95
12
Thrown plaster 95
Plastered straw bale houses 95
Wet formed plaster 97
Protection of corners 97
Stabilised loam plasters 97
Characteristics of different loam plasters 97
Weather resistance, coatings and coverings 100
Consolidating the sur face 100
Paints 100
Making sur faces water-repellent 103
Lime plasters 104
Shingles, planks and other covers 105
Structural methods 105
13 Repair of loam structures 106
Occurrence of damage 106
Repair of cracks and joints with loam fillers 106
Repair of cracks and joints with other fillers 107
Repairing larger damaged areas 107
Retrofitting thermal insulation with lightweight loam 107
Residences
Vineyard residence, Mornington Peninsula, Victoria, Australia 148
Residence cum office, Kassel, Germany 150
Residence, Merrijig, Victoria, Australia 152
Residence, Helensville, New Zealand 154
Residence, Palo Alto, California, USA 156
Weekend house, Ajijic, Mexico 158
Desert Outpost residence, Paradise Valley, Arizona, USA 160
Artist’s residence, Boulder, Colorado, USA 162
Casa Martha, La Misión, Baja California, Mexico 164
Condominiums Los Maitenes, Peñalolén, Santiago de Chile, Chile 166
Residence at Valle Tucán, Emboscada, Paraguay 170
Barrel-vaulted residential buildings at Valle Tucán, Emboscada, Paraguay 172
Cultural, educational and sacred buildings
National Environment Centre at Thurgoona Campus, Albury, New South Wales, Australia 176
14
Customised design solutions 109
Joints 109
Special wall constructions 111
Intermediate floors 113
Rammed earth floorings 113
Inclined roofs filled with lightweight loam 115
Ear th-covered roofs 115
Ear th block vaults and domes 117
Ear then storage wall in winter gardens 129
Loam in bathrooms 129
Built-in furniture and sanitar y objects from loam 131
Passive solar wall heating system 132
15 Earthquake-resistant building 133
Structural measures 134
Openings for doors and windows 136
Bamboo-reinforced rammed earth walls 139
Steel-reinforced sprayed loam walls 139
Steel-reinforced adobe wall system 141
Domes 141
Vaults 141
Textile walls with loam infill 144
Steel-reinforced earth walls 146
School, Rudrapur, Bangladesh 178
Chapel of Reconciliation, Berlin, Germany 180
WISE Centre for Alternative Technology, Machynleth, Wales, UK 182
Primary school, Tanouan Ibi, Mali 184 Cemetery, Bushey, Hertfordshire, UK 188
The Village Nursery, Bellingdon, Buckinghamshire, UK 192
Centre for People with Disabilities and Dipdii Textiles Studio, Rudrapur, Bangladesh 196
Adobe Dome Music Space, Aiguá, Uruguay 200
Burkina Institute of Technology, Koudougou, Burkina Faso 204
Maison pour tous, Four, Isère, France 208
Commercial, hospitality and health buildings
Rivergreen Centre, Aykley Heads, Durham, UK 212
High Country Visitor Information Centre, Mansfield, Victoria, Australia 214
Mii Amo Spa, Sedona, Arizona, USA 216
Bayalpata Hospital, Acham, Nepal 218
Office pavilion Earthbox, Asunción, Paraguay 222
Alnatura Office Building, Darmstadt, Germany 226
Prototype building TECLA, Massa Lombarda, Italy 230
Dior Pop-up Store, Jumeirah, Dubai 232
Bibliography 236
About the author 239
Illustration credits 239
Subject index 240
This handbook was written in response to an increasing worldwide interest in building with earth. While in Europe and North America earthen architecture may never play the dominant role as in warmer regions, we nevertheless find an increasing tendency to build with loam also in cooler climate zones. One reason for this is the growing environmental awareness as well as the desire to live in a balanced and healthy indoor environment. Thus this fifth edition is timely and should encourage architects to explore the potential of this sustainable building material further. The publication provides a survey of all applications and construction techniques of earth as a building material, including the relevant physical data, while explaining its specific qualities and the possibilities of optimising them. On many accounts, earth can offer an interesting alternative to industrialised building materials. The data and expert knowledge contained in this volume may be used as guidelines for a variety of construction processes and possible applications by engineers, architects, builders and clients who seek to use humanity’s oldest building material for their purposes.
Earth as a building material comes in many different compositions, and can be variously processed. Loam, or clayey soil, as it is referred to scientifically, has different names when used in various applications, for instance rammed earth, soil blocks, mud bricks or adobe. This book documents the results of experiments and research conducted at the Preface
Forschungslabor für Experimentelles Bauen (Building Research Institute – BRI) at the University of Kassel in Germany from 1978 to 2011. Moreover, the specialised techniques that the author developed and the practical experience he gathered in the course of designing earth buildings in a number of countries have also found their way into this book.
This volume is loosely based on the German publication Handbuch Lehmbau (Publisher: Ökobuch Verlag, Staufen), first published in 1994 and now in its nineth edition (2017). Besides this English edition, the publication was translated into numerous languages such as Spanish, Russian, Japanese, Czech, Farsi, Arabic and Rumanian.
While this is first and foremost a technical book, the introductory chapter also provides the reader with a short survey on the history of earth architecture. In this fifth English edition, the relatively recent technology of prefabricated rammed earth elements is introduced and the growing segment of clay panels is documented. The book’s final chapter depicts a number of attractive earth buildings from various regions of the world. These constructions demonstrate the impressive versatility of earth architecture and the many different uses of the building material earth. Within this chapter, several older built examples were replaced by seven new projects.
Kassel, March 2025
Gernot Minke
In nearly all hot-arid and temperate climates, earth has always been the most prevalent building material. Even today, one third of the human population resides in earthen houses; in developing countries this figure is more than one half. It has proven impossible to fulfil the immense requirements for shelter in the developing countries with industrial building materials, i.e. brick, concrete and steel, nor with industrialised construction techniques. Worldwide, no region is endowed with the productive capacity or financial resources needed to satisfy this demand. In the developing countries, requirements for shelter can be met only by using local building materials and relying on do-it-yourself construction techniques. Earth
is the most important natural building material, and it is available in most regions of the world. It is frequently obtained directly from the building site when excavating foundations or basements. In the industrialised countries, careless exploitation of resources and centralised capital combined with energy-intensive production is not only wasteful; it also pollutes the environment and increases unemployment. In these countries, earth is being revived as a building material. Increasingly, people when building homes demand energy- and cost-effective buildings that emphasise a healthy, balanced indoor climate. They are coming to realise that mud, as a natural building material, is superior to industrial building materials such as concrete, brick and lime-sandstone. Newly developed, advanced earth building techniques demonstrate the value of earth not only in do-it-yourself construction, but also for industrialised construction involving contractors. This handbook presents the basic theoretical data concerning this material, and it provides the necessary guidelines, based on scientific research and practical experience, for applying it in a variety of contexts.
Earth construction techniques have been known for over 9000 years. Mud brick (adobe) houses dating from 8000 to 6000 BC have been discovered in Russian Turkestan (Pumpelly, 1908). Rammed earth foundations dating from ca. 5000 BC have been
3 Loam saves energy and reduces environmental pollution
The preparation, transport and handling of loam on site requires only ca. 1% of the energy needed for the production, transport and handling of baked bricks or reinforced concrete. Loam, then, produces virtually no environmental pollution.
4 Loam is always reusable
Unbaked loam can be recycled an indefinite number of times over an extremely long period. Old dry loam can be reused after soaking in water, so loam never becomes a waste material that harms the environment.
5 Loam saves material and transportation costs
Clayey soil is often found on site, so that the soil excavated for foundations can then be used for earth construction. If the soil contains too little clay, then clayey soil must be added, whereas if too much clay is present, sand is added. The use of excavated soil means greatly reduced costs in comparison with other building materials. Even if this soil is transported from other construction sites, it is usually much cheaper than industrial building materials.
6 Loam is ideal for do-it-yourself construction
Provided the building process is supervised by an experienced individual, earth construction techniques can usually be executed by non-professionals. Since the processes involved are labour-intensive and require only inexpensive tools and machines, they are ideal for do-it-yourself building.
7 Loam preserves timber and other organic materials
Owing to its low equilibrium moisture content of 0.4% to 6% by weight and its high capillarity, loam conserves the timber elements that remain in contact with it by keeping them dry. Normally, fungi or insects will not damage such wood, since insects need a minimum of 14% to 18% humidity to maintain life, and fungi more than 20% (Volz, 2004, p.
60). Similarly, loam can preserve small quantities of straw that are mixed into it. However, if lightweight straw loam with a density of less than 500 to 600 kg/m3 is used, then the loam may lose its preservative capacity due to the high capillarity of the straw when used in such high proportions. In such cases, the straw may rot when remaining wet over long periods (see 10.3, p. 86).
8 Loam absorbs pollutants
It is often maintained that earth walls help to clean polluted indoor air, but this has yet to be proven scientifically. It is a fact that earth walls can absorb pollutants dissolved in water. For instance, a demonstration plant exists in Ruhleben, Berlin, which uses clayey soil to remove phosphates from 600 m3 of sewage daily. The phosphates are bound by the clay minerals and extracted from the sewage. The advantage of this procedure is that since no foreign substances remain in the water, the phosphates are converted into calcium phosphate for reuse as a fertiliser.
In moderate to cold climates, people usually spend about 90% of their time in enclosed spaces, so indoor climate is a crucial factor in well-being. Comfort depends upon the temperature, movement, humidity, radiation to and from surrounding objects, and pollution content of the air contained in a given room. Although occupants immediately become aware when room temperatures are too high or too low, the negative impacts of excessively elevated or reduced humidity levels are not common knowledge. Air humidity in contained spaces has a significant impact on the health of inhabitants, and earth has the ability to balance indoor humidity like no other building material. This fact, only recently investigated, is described in detail later in this section.
Research performed by Grandjean (1973) and Beckert (1986) has shown that a relative humidity of less than 40% over a long period may dry out the mucous membrane, which
can decrease resistance to colds and related diseases. This is so because normally the mucous membrane of the epithelial tissue within the trachea absorbs dust, bacteria, viruses etc. and returns them to the mouth by the wavelike movement of the epithelial hair. If this absorption and transportation system is disturbed by drying, then foreign bodies can reach the lungs and may cause health problems (1.16). A high relative humidity of up to 70% has many positive consequences: it reduces the fine dust content of the air, activates the protection mechanisms of the skin against microbes, reduces the life of many bacteria and viruses, and reduces odour and static charge on the surfaces of objects in the room.
A relative humidity of more than 70% is normally experienced as unpleasant, probably because of the reduction of oxygen intake by the blood in warm-humid conditions. Increasing rheumatic pains are observed in cold humid air. Fungus formation increases significantly in closed rooms when the humidity rises above 70% or 80%. Fungus spores in large quantities can lead to various kinds of pain and allergies. From these considerations, it follows that the humidity content in a room should be a minimum of 40%, but not more than 70%.
In moderate and cold climates, when the outside temperatures are much lower than inside temperatures, the greater degree of fresh air exchange may make indoor air so dry that negative health effects can result. For example, if outside air with a temperature of 0°C and 60% relative humidity enters a room and is heated to 20°C, its relative humidity decreases to less than 20%. Even if the outside air (temperature 0°C) had 100% humidity level and was warmed up to 20°C, its relative humidity would still drop to less than 30%. In both cases, it becomes necessary to raise the humidity as soon as possible in order to attain healthy and comfortable conditions. This can be done by regulating the humidity that is released by walls, ceilings, floors and furniture (1.17).
Porous materials have the capacity to absorb humidity from the ambient air and to desorb humidity into the air, thereby achieving humidity balance in indoor climates. The equilibrium moisture content depends on the temperature and humidity of the ambient air (see 2.29, p. 28). The effectiveness of this balancing process also depends upon the speed of the absorption or desorption.
Vineyard residence
Mornington Peninsula, Victoria, Australia
Architect: John Wardle Architects, Melbourne, Australia
Completion: 2002
Wall system: Rammed earth Floor area: 400 m²
The predominant elements of this residence, which is situated in a large vineyard, are the rammed earth walls. The living area extends out to the north veranda, the kitchen to an informal terrace area. The study opens up to the garden.
The principal bedroom, with its walls angling outward, evokes the impression of continuing into the landscape. The entrance screen reduces western sun into the living area. Cross ventilation is achieved throughout all areas.
Architect: Alfredo Navarro Tiznado
Design team: Kenia Esmeralda García Rosas, Hanna Appel Hernández, Giancarlo Reyes Olguin
Completion: 2021
Wall system: Rammed earth walls
Floor area: 310 m²
Casa Martha, the retirement home for an archaeologist couple, Martha and Bill, is located between the municipalities of Ensenada and Rosarito, in the urban area of La Misión. The plot has a size of 15 × 40 metres, on a terrain of rugged topography oriented towards the Pacific Ocean.
The house comprises four levels in a terraced arrangement. The ground floor is the access level. The first floor is divided into two areas: a visitor’s area with two rooms and a study space that doubles as a painting and carpentry workshop or as a garage. There are also two small interior gardens. The second floor accommodates the core of the house – the entertainment room, dining room, and kitchen – with large windows and porches. Woven salt pine shutters provide protection from the prevailing winds while maintaining the privacy of the interior. They also create a play of light and shadow on the interior walls. At the back of the social area, a staircase leads to the third floor, where the master bedroom is located. This uppermost level has panoramic views onto the desert landscape.
Concept and design: Anna Heringer
Project management: Stefano Mori
Consultants: Martin Rauch (earth), Andreas Guetling (roof construction), Emmanuel Heringer (bamboo roof)
Contractor: Montu Ram Shaw
Completion: 2020
Wall system: Cob
Floor area: 174 m² (rooms), 180 m² (ramp and veranda)
The two-storey-building accommodates a centre for people with disabilities combined with a small studio for the production of textiles, allowing women to find work in their village. It is called “Anandaloy” which can be translated as “place of profound joy”. The name strikes a positive note in a culture that traditionally shuns people with disabilities as punished by God. A large ramp leads to the first floor in order to be accessible for wheel chairs. The foundation was made of burnt bricks while the walls consist of locally sourced rammed earth using the cob technique that needs no formwork. Cob is an ancient building technology that is both simple and sturdy. Clay subsoil, sand, straw and water are mixed together to make a malleable mud that is then formed into monolithic walls. When the cob dries it becomes hard which results in very durable walls and columns. The columns, ceilings and the roof construction are bamboo. The ventilated roof has a lower layer of straw and is covered by metal sheeting. A special feature inside the building are the “caves”, spaces for self-learning and relaxing formed by hand from clayey soil lumps (also built in cob technique). Because the Anandaloy project mainly uses earth available nearby and bamboo from local farmers, the biggest part of the budget was invested in local crafts(wo)men. Thus, the building became a real catalyst for local development. Because of its sustainable and holistic concept, the centre received the Obel Award 2020.
Built examples
Aiguá, Uruguay
Design and supervision dome: Gernot Minke, Kassel, Germany
Organisation and supervision: Macarena Albarracin, Santiago Escarrá, Salta, Argentina
Completion: 2020
Vault system: Adobe masonry without formwork
Floor area: 38.5 m²
At a farm on the outskirts of the small town of Aiguá, a dome was built from adobe, designed for relaxation, meditation and music-making for up to 50 people. Due to its special acoustics, the building also serves as a recording studio for experimental music. The inner diameter of the dome is 7 m, the clear height in the middle is 5.40 m. The foundation and plinth as well as the circular entrance are made of locally available natural stones. The entrance has a diameter of 1.60 m, so the room has to be entered in a stooped position and its height is only experienced once inside. The floor slopes 20 cm towards the centre, so that no horizontal level is perceptible. This creates a special spatial impression, resulting in a sense of security and well-being. The top has an opening which is covered by an octagonal, pyramidical skylight, exuding a sense of being in touch with the cosmos.
The dome was built during a two-week workshop directed by the author with the assis-
tance of Macarena Albarracin and Santiago Escarrá. It was built up utilising the “acoustic adobes” developed by the author. These have rounded edges to diffuse the sound reflection and are walled up at an angle of approximately 20° to the outside to reflect part of the sound upwards, thus reducing the focusing of the reflected sound towards the centre of the room. In addition, the joints have been recessed to improve sound absorption. In order to achieve the statically optimal cross-section of the dome, the rotational guide developed by the “Research Laboratory for Experimental Building” at the University of Kassel was used, which defines the correct position for each adobe. On the
outside, the dome was first covered with a two-layer earth plaster and then sealed with a fleece-reinforced acrylic paint to make it weatherproof. The last layer of the transparent paint was mixed with sand and clay powder to achieve a clay-like appearance and to provide additional protection against ultraviolet radiation.
The floor is made of rammed earth, its surface is divided into eight fields and a central octagon by wooden strips. The top layer of the floor consists of an approximately 2-cmthick earth render, which has been stabilised with some lime and cement.
Architect: Kéré Architecture, Jaime Herraiz
Completion: 2020
Wall system: Cement-stabilised rammed earth
Floor area: 1000 m²
The Burkina Institute of Technology is based on a system of repeated modules, housing classrooms and auxiliary functions, arranged orthogonally to define a rectangular courtyard. The orthogonal layout of modules allows the campus to be expanded incrementally according to its needs. The modules are staggered, allowing air to flow through the central void, creating a cool space where students can relax and interact.
The walls are made of cement-stabilised local lateritic clayey soil poured into the onestorey-high formwork and then rammed by a special vibrator. Due to the special mixture, which contains a high content of large particles, it was sufficient to use for stabilisation only 4.5% of cement by mass, which corresponds by volume to 100 kg per m³. The basic mixture contains per volume 57% of soil, 29% of crushed granite 05/15 and 14% of coarse sand. The formwork was removed after 4 weeks.
The roof profiles, repeated regularly, create a dynamic rhythm and form a chimney at the back of each module where warm air can be released. Suspended ceilings, made of local eucalyptus wood, brighten the interior spaces.
During the rainy season, water is channeled into a large underground tank and stored there. It is later used to irrigate the extensive mango plantations on the campus.
Office pavilion Earthbox
Asunción, Paraguay
Architects: Equipo de Arquitectura (Horacio Cherniavsky and Viviana Pozzoli)
Structural engineer: Emilio Richer
Rammed earth consultant: Yago GarcíaEnríquez
Completion: 2018
Wall system: Rammed earth walls
Floor area: 45 m²
This pavilion was built by Equipo de Arquitectura for themselves. A single continuous wall wraps around a basic square plan. It opens up to create the entrance to the office and bends around a little restroom next to it. A pre-existing guavirà tree was integrated into the design, rises from the patio, and creates a division between working spaces and kitchen. Outside, a flame tree screens the earthbox from the sun.
The 30-cm rammed earth walls hold the weight of the roof, resting on only 20 cm of their width and thus exploiting the structural qualities of this material. The remaining 10 cm on the perimeter hide the ceiling slab when seen from the outside.
All of the furniture and doors are made of recycled wooden formwork that was used to cast the concrete slab that covers the office. The library shelves are detached from the walls and suspended.
Earth, in common use for architectural construction for thousands of years, has in recent years attracted new attention as a healthy, environment-friendly and economical building material. An impressive number of buildings has been realized not just in hot and dry regions but also in the colder climates of Europe and North America. Technical innovations such as prefabricated rammed earth components, clay panels or earth walls from a 3D printer facilitate the use of this sustainable material.
This manual, now in its fifth and revised editon, describes the building technology of rammed earth. The physical properties and characteristic values are explained in a hands-on manner: With proper moisture protection, earth buildings are very durable, and in particular the combination with wood or straw allows a wide spectrum of design options. Thirty international built examples demonstrate the range of applications for this fully recyclable material.
ISBN 978-3-0356-2766-4