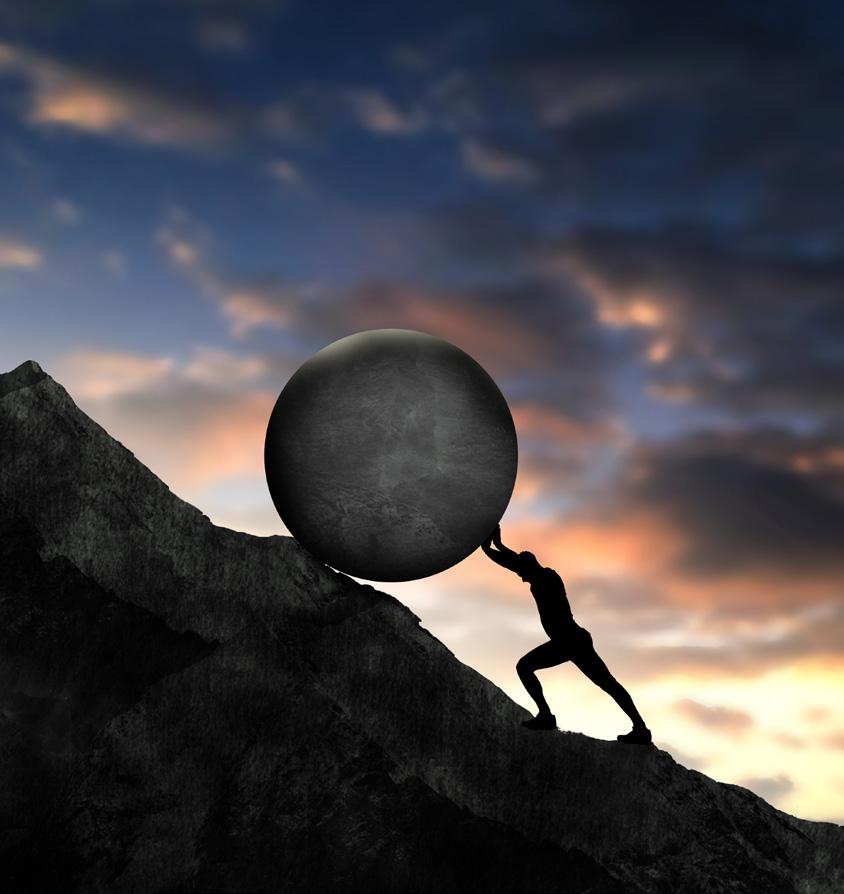
BIOBASED TRANSITIONS: AN UPHILL BATTLE?!
Martijn Zieverink Inaugural lecture
April 5, 2024
Martijn Zieverink Inaugural lecture
April 5, 2024
The content of this book is based on the inaugural lecture held on Friday April 5th 2024, with the title Biobased Transitions, an uphill battle?! Here I have made an attempt to bring together some of the ideas and concepts that I have been developing in the past year.
Chapter 1 gives the briefest of introductions into the fossil age in which we now live. Chapter 2 gives a short overview of the history of climate change research. Although not necessary to the rest of the story it does form a large part of the Why and therefore I decided to include it here. Chapter 3 outlines why we have petrochemicals and what they can be used for. Chapter 4 introduces the concept of biobased or renewable carbon and the role it can play in de-fossilizing materials. Some of the different routes that are available are laid out. Chapter 5 considers transitions: what needs to be done versus what can be done and how this can be overcome. Chapter 6 introduces the ambition of the group and suggests three research lines. Finally, Chapter 7 asks the question ’do we want all this?’, are all the scenarios that consider growth and business as usual as inevitable as they now appear to many.
I would like to thank my colleagues Bas Koebrugge, Marlies Schets and Wendy van Rijsbergen for their assistance in preparing the manuscript. Thanks to Mijke Kleisterlee for all the administrative support. Thanks also to the people at PeelPioneers who allowed me to pursue this new opportunity. Special thanks to my recently joined group members Philippa, Maddalena and Willem for their support in the past few months. I look forward to our future collaboration! Their contributions, ideas and suggestions are already scattered throughout the text. Any omissions, mistakes or problems are of course my own.
A final word of thanks to my family without whom none of this would be remotely possible.
Large scale and systematic use of fossil fuel started with coal in the 18th century. The earliest steam engines were hungry beasts requiring wood to burn at an ever expanding scale. The industrial revolution that started in Great Britain would have come to a grinding halt if it had not been accompanied by the discovery of large amounts of coal in Scotland and Wales. Despite this transition the UK now has some of the lowest amounts of remaining intact forests in Europe.
The advent of liquid fossil fuels started with the discovery of crude oil in the United States. Standard Oil, one of the first multinationals and one of the largest companies ever was broken up in 1911. It became the predecessor of companies such as Chevron, Exxon, BP and Conoco Phillips. Because of higher efficiency, the British navy decided to retool the boilers of their warships from coal to oil, giving them a tactical edge over Germany at the start of the first world war.
The use of fossil fuels did not stop at energy. The 19th century discovery that coal tar extracts could be used to make synthetic dyes led to the making of the German chemical industry with companies such as BASF and IG Farben. Germany’s chemical head start would last until the second world war [18].
The amount of fossil material that is consumed world wide on an annual basis is staggering. In total about 16 billion tons of oil, coal and gas is used, equal to a cube with sides of roughly 2.5 km! Approximately 90% is burned for energy, releasing some 34 billion tons of CO2 every and the remainder is converted into materials in the form of chemicals and plastics.
Making materials also costs energy: even if only 10% of all fossil carbon is turned into materials, an additional 7% is used as energy to drive the processes to make them [29].
Another way of looking at these huge volumes is value: at the beginning of 2024 the website www.companiesmarketcap.com listed 161 chemical companies with a total value of 1600 billion US$. In comparison, the oil and gas companies total a staggering 7000 billion US$. There is some overlap between the categories but it shows that fossil fuels create more value in absolute terms than petrochemicals, roughly in line with the amounts used.
Everything fossil that is extracted from the earth was once part of a living creature. Hundreds of millions of years and geological pressure converted primeval forests into underground seas of oil, gas and coal. (Coal miners had first hand knowledge of fossils; they encountered the prints of strange plants and animals in the coal they dug up. It is quite possible that because of this some silently doubted the religious dogma of a young, recently created world).
This process of carbonization stopped a long time ago. The amounts of fossil carbon are therefore finite and could theoretically at some point run out. Unfortunately, ’reserves’ are so large that they offer us the opportunity to dramatically and irreversibly change our climate.
Figure 1.3: prediction made in 1984 of expansion of world wide oil production due to new discoveries and technologies [4]. Figure taken from [36]. The author was conservative, in 2021 total oil production was already 90 million barrels per day
Not that long ago the question was not ’should we stop burning oil/coal/gas’ but rather ’Are we not running out?!’ In 1985 Peter Baxendell, then chairman of the Royal Dutch/Shell group, projected that by the development of new technologies and finds, the production of oil could be maintained far into the 21st century [4]. If the longer term consequences to the climate were known to him (and it is likely that they were) this did not enter into the consideration.
Figure 1.3 is of course nearly 40 years old and therefore misses several boom and bust cycles, including the recent shale gas boom in the US. More recent versions can be found in for example [42, 6] or on the website of the International Energy Agency.
The message however remains the same: there need not be a shortage of oil (or gas, or coal) in the foreseeable future. In fact large parts of the currently known ’reserves’ need to remain unexploited to have some chance of limiting the impact of climate change. According to McGlade and Ekins [32] if global temperature rise is to be limited to 2oC, a third of all oil reserves, half of the available gas and over 80% of coal should not be used.
An alternative title for this chapter could have been the age of shareholder capitalism. One of the distinguishing features of this system is that it seeks to optimize profit. Since the negative consequences of doing business remain hidden or are postponed to some future date that profit can be much higher than it should be. By taking the negative environmental effects of products and services into account (so-called true pricing) what is now considered quite profitable may at a single stroke become the opposite. Petrochemical industries are perhaps the best example of this; while creating billions in revenue the environmental consequences much have a greater global cost.
It is beyond the scope of this document but it seems clear to me that only waiting for the market to solve these problems will not work. Legislation and/or regulation is needed and that requires politicians, who are dependent on voters who are hesitant to vote for measures that they perceive to lower their standard of living.
About half of all emitted CO2 ends up in the ocean, the rest remains in the atmosphere. As a result the amount of atmospheric CO2 is increasing steadily. Starting in 1958 Charles Keeling was the first to measure this on a regular basis and was able to show the long term increase in the amount of CO2 present in the atmosphere [25]. At the same time dissolving CO2 slowly increases the acidity of the oceans. This has a negative impact on coral health and the ocean’s ecosystems as a whole [13]. The rising global temperature only excarbates this effect.
Another source of CO2 emissions is the slashing and burning of plants and forests. There happens to be a small difference in the amount of natural radioactivity of CO2 coming from living material versus CO2 from fossil sources (after millions of years underground, fossil carbon is a bit less radioactive than carbon in living materials which is constantly being bombarded by radiation from the sun). Based on these differences, scientists have been able to show which part of atmospheric CO2 is caused by burning of forests. This correlates well with what is known about the area of forest that is lost each year [26].
Fossil based materials and especially plastics tend to be around for a very long time. Every ocean now contains a garbage patch, huge areas where circulating streams tend to accumulate floating debris. A recent conservative estimate puts the amount of plastic floating on the surface at more than 3 Mton, to which 500 kton is added each year [24]. Trillions pieces of microplastic are now present in the oceans with an increasing impact on marine ecosystems and human health [28]. Eriksen et al [15] speak of a growing plastig smog, afloat in the world’s oceans.
The idea that the world’s climate is changeable by man’s action is relatively new and still controversial to some. An argument that can still be heard today goes along the lines I don’t understand how we tiny humans can make changes to this vast planet or 350 ppm CO2 that is less than 0.1%! How can that matter? Unfortunately we now know better (or at least should know better). If you are interested in a little refresher, read on! If not or if the topic depresses you feel free to move on to the next chapter.
In the following I would like to give an introduction to the history of the concept of climate change. This is not the place for a deep dive but since the existence of climate change is an important driver for the new Biobased Transitions group a short introduction seems to be in order. The interested reader may want to pick up the recent books by Alice Bell [5] or Spencer Weart [53]. Both offer excellent overviews of the history of climate science.
Before we get started: many of the early researchers did not consider climate change to be bad! They might consider that an increase in temperature and CO2 levels could lead to better harvests. Especially those living in frigid climates actually relished the idea of higher ambient temperature! So with that in mind lets cast ourselves back to the year 1856.
On Saturday the 23rd of August 1856 the American Association for the Advancement of Science held its annual meeting. As was customary, research papers were presented and discussed. The paper Circumstances affecting the Heat in the Sun’s Rays [16] was not read by its author, Eunice Newton Foote but instead by a man. Her work was overseen or ignored by later researchers, possibly because of a combination of her gender and the relative obscurity of the journal in which she published. Only recently has her priority in the field of climate change research been established [21].
Foote reported the results of a simple but effective experiment: glass containers containing different gases were placed in the sun. The increase in temperature was measured. Her results showed that moist air heats more than dry air and CO2 enriched air even more. In a single paragraph she came to a far reaching conclusion:
“An
atmosphere of that gas would give to our earth a high temperature; and if as some suppose, at one period of its history the air had mixed with it a larger proportion than at present, an increased temperature from its own action as well as from increased weight must have necessarily resulted”
Although Foote’s findings were largely ignored the investigation of different gases and their properties continued apace. Already in 1860 the Irishman John Tyndall measured the radiation absorption of CO2 gas, laying the ground floor for quantitative research into the effect of the composition of the atmosphere on climate [46].
In 1896 the Swede Svente Arrhenius published the groundbreaking article On the influence of carbonic acid in the air upon the temperature on the ground [3]. After long and complex calculations he concluded that a doubling of the amount of CO2 in the atmosphere would lead the earth’s temperature to increase by 5-6oC. However, based on the industrial activity in his time he estimated that this doubling might take about three thousand years (!) Little did he know it would only take about a century. Maybe it is a result of the frigid Swedish weather but Arrhenius mainly saw advantages in a warmer climate [1].
Just then as now, it is unrealistic to expect the ordinary reader to be up to date on the ongoing scientific debate. However even at the beginning of the twentieth century popularizing articles were written about the possible correlation between CO2 emissions and climate. An often quoted example is an article in a 1912 issue of the magazine Popular Mechanics [33]. The author shows that the effect of burning coal will be noticeable within a few centuries, already much faster than Arrhenius’ prediction. They did not yet take into account exponential population growth and the greatly increasing use of oil and gas.
The furnaces of the world are now burning about 2,000,000,000 tons of coal a year. When this is burned, uniting with oxygen, it adds about 7,000,000,000 tons of carbon dioxide to the atmosphere yearly. This tends to make the air a more effective blanket for the earth and to raise its temperature. The effect may be considerable in a few centuries
As an estimate of the order of magnitude a few centuries is not that wide of the mark. This was written about a century before the effects of CO2 emissions on climate became noticeable to all. The author also mentions the capacity of the oceans to buffer some of this CO2 and suggest that this capacity will have its limits. Since then both concepts have been proven both by theory and experiment.
Already in 1938 the first scientific study is made linking actual CO2 emissions to climate change [9]. After reviewing thousands of data points collected around the world Guy Stewart Callendar showed that between 1888-1938 the average global temperature increased by 0.25oC. He concluded that this was due to the 8% increase in CO2 levels in the atmosphere since the start of the industrial revolution. He also showed that this amount is roughly equivalent to the estimated total amount of coal that has been burned until that moment.
Just like Arrhenius before him he also sees advantages to this trend: higher temperatures and CO2 concentration will cause crops to grow faster. Also that there is less need to worry about the start of a new ice age...
Other researchers start taking the potential consequenses more seriously: in 1956
Gilbert Plass calculated that based on the then known fossil reserves a global temperature rise of 7-13oC could be expected [37]. Even then, he underestimated the total reserves. He foresaw serious consequenses but still assumed these would take hundreds of years to manifest themselves. He also predicted that removing CO2 from the air will be extremely costly (the modern term for this is Direct Air Capture or DAC), something that is true to this day.
In fact the temperature rise from this cause may be so large in several centuries that it will present a serious problem to future generations. The removal of vast quantities of carbon dioxide from the atmosphere would be an extremely costly operation
At the same time Roger Revelle and Hans Suess investigated the uptake of CO2 into the ocean. Their article contains a paragraph which gave rise to the expression ’the Great Experiment’ [41]. This is the concept that by changing the planet’s atmosphere, mankind is carrying out an experiment of unique scale and proportion. Fossil materials that were created and stored for millions of years are now being released as CO2 with the span of a little bit more than a century. For better or for worse, this experiment can only be carried out once. . .
Human beings are now carrying out a large scale geophysical experiment of a kind that could not have happened in the past nor be reproduced in the future. Within a few centuries we are returning to the atmosphere and oceans the concentrated organic carbon stored in sedimentary rocks over hundreds of millions of years
These discussions are not limited to the academic world. In 1962 the Committee on Natural Resources of the American National Academy of Sciences delivers a report with the title Energy Resources [20]. This report contains the following paragraph:
There is evidence that the greatly increasing use of the fossil fuels [...] is seriously contaminating the earth’s atmosphere with CO2. Analyses indicate that the CO2 content of the atmosphere since 1900 has increased 10 per cent. [...] it is possible that it is already producing a secular climatic change in the direction of higher average temperatures. This could have profound effects both on the weather and on the ecological balances
Main author of the report: Marion King Hubbert, geologist, employed by the Shell Development Company. Ironically, even part of the remedy is described:
In view of the dangers of atmospheric contamination by both the waste gases of the fossil fuels and the radioactive contaminates from nuclear power plants, Professor Hutchinson urges serious consideration of the maximum utilization of solar energy
This professor George Evelyn Hutchinson is himself well acquainted with Callendar’s previous work, thus closing the circle. This shows that already in 1962 at least one Shell employee was aware of the risk of continued use of fossil fuel. Recent research has shown that on an institutional level, companies like Shell and Exxon already knew in the 1970s of the long term damage their products were doing to the world’s climate [43, 2].
As of writing this there are several lawsuits worldwide trying to determine what companies knew and when they knew it. Whether the public was intentionally misled and whether there should be consequences will be for the courts to decide.
Where do all these years of research into climate change leave us? The 2023 Intergovernmental Panel on Climate Change (IPCC) report [30] states the following:
“The cumulative scientific evidence is unequivocal: climate change is a threat to human well-being and planetary health. Any further delay in concerted anticipatory global action on adaptation and mitigation will miss a brief and rapidly closing window of opportunity to secure a liveable and sustainable future for all”
Climate change is a game of chance: as Table 2.1 shows, we have a 66% chance of keeping the average temperature rise below 1.5oC if emissions stop by the year 2043. Accepting a fifty-fifty chance increases the amount of time left somewhat. One might ask themselves how much they would be willing to stake on a single coin throw?
Table 2.1: total CO2 budget and the chance that average temperature change is limited to 1.5oC, time at which net zero must be reached
Since actual emissions are usually larger than what is projected it is quite possible that the amount of time left to prevent destructive warming is less than the estimates given in the table.
Most people will agree that the use of fossil feeds for energy and materials needs to be phased out as soon as possible. In the European context this means no later than 2050. Fast expansion of wind and solar energy is necessary to cover the gap but will also lead to plenty of challenges, including the hunt for scarce metals. This is where the materials and energy transition overlap and can come into conflict.
The production of materials from fossil feeds is closely connected to fuel refining. Most materials are made from side-products of the production of fuels. Only 10-15% of each barrel of oil is turned into something tangible, the rest is turned into fuels. Both routes are coupled: less fuel production means less feed to make materials out of.
The long term effect of a succesful energy transition will therefore be less fossil feedstock available for making materials (chemicals, plastics). However as we’ll see in the next chapter the medium term outlook is quite the opposite! In the medium term there even may be less need for the use of renewable or biobased feedstocks.
Petrochemicals have dramatically changed the world we live in. There is of course the matter of pollution and climate change but we can also not overlook the fact that these materials have had a huge positive impact on the quality of life. Without ammonia based fertilizers feeding seven billion people would be next to impossible even as ammonia can also lead to excess nitrification and loss of biodiversity. Packaged food can be more hygienic and pre-packaged sterilized medical tools and bandages make for better healthcare at the cost of more waste.
The majority of all petrochemicals are derived from seven building blocks. Their combined production accounts for the lion’s share of the industry’s energy and materials consumption. Ammonia is the starting compound for nitrogen-based fertilizers. Most of the demand for ammonia is driven by the agricultural sector. Although it does not contain carbon, lots of (fossil) carbon is involved in the manufacture of ammonia. Methanol is the simplest possible alcohol. Highly toxic to humans, it is used to make resins, plastics and other kinds of materials. Ethylene and propylene, also referred to as olefins, are mainly used in the production of plastics. Benzene, toluene and xylenes, collectively also known as BTX or aromatics, are converted into plastics, solvents and many other high value added materials.
Many alternative (or at least reduced in fossil carbon) production routes to these primary building blocks are available on a technical level but are not (yet) economical. The requirement of additional renewable energy is another example of the intersection of the energy and materials transition. Biobased chemical production is currently considered cost prohibitive. However, the statement bio-based product or process XYZ is too expensive or not economical could perhaps better be rephrased to considering its impact, fossil based product or process XYZ is too cheap.
However, the medium term expectation is that fossil feedstocks will become more available and possibly even cheaper! The ongoing energy transition leads to reduced demand for lighter refinery fractions which would otherwise be sold as transportation fuels. In order to retain profitability, producers may decide to change the ratio of fuel to chemicals production [29]. An unwelcome outcome of the energy transition could therefore be that the introduction of renewable base chemicals becomes more difficult instead of less so.
Table 3.1 shows some examples of alternative routes to chemical building blocks and companies involved in those routes. This list shows only a fraction of what is currently going on.
Table 3.1: examples of alternative routes to the major chemical building blocks and a random sampling of some of the companies involved
building block alternative route company
ammonia
methanol
olefins
BTX
green hydrogen by electrolysis Stamicarbon
Yara
CO2 combined with green hydrogen Carbon Recycling
biomass torrefaction
biomass (steam)cracking
Int. torrgas
chemical recycling of PET plastics Ionica
biomass pyrolysis BioBTX
Table 3.2 shows the major mass flows in the global chemical industry for the year 2013 [31]. A combined 677 Mtons of fossil feed is used, together with 142 Mton nitrogen to make 275 Mton fertilizer and 546 Mton chemicals, plastics, solvents and additives. Carbon dioxide is used as a feedstock for the production of urea but is also released, resulting in a net production of around 135 Mton.
Table 3.2: major mass flows in the chemical sector expressed in millions of tons in the year 2013. Adapted from [31]
The industry requires another estimated 400+ Mton of fossil energy, resulting in 1.31.5 gigaton additional CO2 emissions. Because a large amount of fossil feed ends up in products, the sector achieves the seemingly contradictory feat of being both the largest industrial energy consumer and yet only the third-largest industrial carbon dioxide emitter [35]
So what do all these large numbers in the previous section amount to in terms of money? The 2022 world chemical sales totalled 5400 billion euro. After China’s 2390 billion euro, Europe is the second-largest chemical producer in the world with total sales of 760 billion euro still just ahead of the US. The Netherlands has a relatively large chemical industry with a 87 billion euro turnover. However only 20% of Dutch total production is meant for the internal market: 64% is exported within Europe and the remainder to outside Europe. The Dutch chemical industry represents slightly more than 2% of the national gross product and employs some 45000 people [44, 52].
Figure 3.2: Green House Gas intensity expressed in kg GHG-equivalent per euro added value. Different industries compared to the average of total Dutch industry and the total Dutch economy. Figure adapted from [8]
A recent TNO report [8] argues that with 4.2% the R&D intensity of the Dutch chemical industry is low compared to the 10-22% of national high-tech industries (R&D intensity is defined as the R&D expenditure as percentage of added value). It is also low compared to Germany’s chemical industry’s 8%. The same report states that Dutch industry in general is facing a strong challenge in the form of increasing costs for the emission of carbon dioxide. Especially the petrochemical industry has very high CO2 emissions per unit of value added, which combined with relatively low R&D expenditure leaves it vulnerable [8].
With these challenges in mind let us now look at the opportunities for making chemicals and materials from biobased feedstocks.
Everything alive consists of a combination of carbon, oxygen and nitrogen, with some other elements sprinkled into the mix for flavor. Plants grow by way of photosynthesis, combining water and CO2 to form biomass. Animals eat plants and some animals eat other animals. Biomass is a catch-all name for any living material that is also renewable. By this definition animal products such as lard are also biomass but here we will focus on plant based biomass only
Table 4.1: examples of residual biomass resulting from a production crop
production crop residual streams
primary use food non-food
secondary use non-food food
examples wheat wheat straw maize maize stover palm oil empty fruit bunches
There are two main classes of (plant based) biomass: residual streams and production crops. A production crop’s main use is for human or animal consumption. Processing production crops inevitably leads to residual streams, often rich in lignocellulose but poor in nutritional value. Traditionally these residual streams are burned or left to rot on the land, ideally also replenishing the soil. An illustration of a production crop (sunflower plants for oil) and its residual streams and potential use is shown in Figure 4.1.
Most production crops can be used in different ways. Grain can be baked into bread but also fermented to bio-ethanol. This potential for multiple usage has lead to undesired consequenses: when the EU required the addition of bio-diesel to normal diesel demand naturally increased. As a result forests were burned all over SouthEast Asia to make room for new palm plantations, the palm oil to be converted into bio-diesel. The CO2 emissions as a result of forest burning and released from the soil, not to mention the loss of biodiversity makes the net effect of palm-based biodiesel very disputable. On the other hand, developing economies such as Indonesia consider palm oil a valuable commodity and resent being told where it can and cannot be used [47].
A similar debate will no doubt occur when the focus shifts towards chemicals, biodiesel or SAF (’Sustainable’ Aviation Fuel) based on vegetable oils produced in Europe. In the long term however this disctinction will have to become moot: as we will see later on, production crops are much better suited for transformation into materials than residual streams. This is a message that legislators need to hear more often.
Over the course of millions of years, evolution has developed materials that are just right: sugars to store energy in, lignocellulose to give strength with hundreds if not thousands of more examples of molecules that are fit for purpose. Of course, plant based biomass will always contain a mixture of materials. Some plants have been engineered by humans to increase the yield of a certain component.
We speak of functionality: carbon atoms combine with oxygen and nitrogen to form complex structures that fulfil a certain purpose. Carbon in biomass is always functionalized to some extend. For someone wanting to use biomass to make (chemical) products this can be either a blessing or a curse.
An advantage of a fossil feedstock such as naphtha is the low amount of oxidation / functionalization. Each carbon is only bound to a hydrogen or to another carbon atom. This means that these atoms have a high willingness to combine with oxygen or nitrogen into a new product. Additionally, reactions can be relatively easily steered by conditions and/or catalysts.
The concept of functionality is further illustrated in Figure 4.2 which shows the different routes that can be taken towards existing or new products (chemicals, materials, plastics). Each individual arrow symbolizes a transformation, not shown are the concomitant side and waste streams. The only exception is the addition of the arrow from biomass to water, to emphasize the importance of dealing with the water inherently present in any natural feedstock.
Figure 4.2: different routes. Black arrows: existing products from fossil feedstock. Blue arrows: defunctionalization route, orange arrows: drop-in route, green arrows: new product route. What is not shown here are the enthalpy ’humps’, the additional energy needed to drive the process
The height on the y-axis symbolizes the energy content. Going up requires energy input and usually results in CO2 emissions. This figure focusses on oxygen rich materials but the same principle applies for nitrogen rich materials. The classical route starts with crude oil and natural gas1, which after refining is split into naphtha and methane, ethane and propane. These fractions are then converted into about a dozen so-called base chemicals, which are the basis of all chemicals2
In contrast there are at least three biobased routes. The first, which I shall call the de-functionalization route, takes biomass and removes most of the functionality, mainly by removing most of the oxygen. What is left is a stream that mimics a fossil feed and can go into existing processes (blue arrows) making existing products. The second route (orange arrows) makes smart use of the functionality already present in the biomass and converts into existing products or into products that are slightly different but still comparable (so-called drop-ins). The third route (green arrow) leaves existing functionality mostly intact and moves towards new products with also new properties.
Each route has its own problems and challenges. It is also good to remind ourselves of the scale represented by the black arrows, symbolizing the existing petrochemical industries as given in Table 3.2. For example, in Europe alone, ethylene production averages somewhere between 15 and 20 Mton each year. In comparison, the only commercial facility in the world that produces bio-ethylene from ethanol has recently been expanded to 260 kton [40] with a second one announced only recently [39].
On a global scale there may be enough biomass to replace all fossil feedstock. Elbersen et al [14] estimate that each year around 3.5 billion ton of (dry) residual lignocellulosic biomass is produced, excluding wood. A study for the continental United States estimated an annual total of 1.3 billion ton [45]. These numbers are in large excess compared to the amounts shown in Table 3.2. This of course conveniently ignores the reality of cost, location, transportation, seasonal availability and many other factors. There is also the question of nutrient depletion to consider if large amounts of biomass are taken out of the natural order.
1 the term ’natural’ gas is a misnomer: a product of millions of years that cannot be replaced, there is little still natural about it. I shall still use the term to avoid confusion
2 a highly detailed overview of these routes can be found on www.petrochemistry.eu
Table
Adapted from [14]
A major part of any biobased transition will be logistics. Production will have to consider the connection between available streams and products. As we will see in the next section a regional connection is desired but not always possible. Just as oil, gas and coal can be transported over thousands of kilometers the same may have to be done with biomass. A major challenge to a succesful transition is the lack of one or more standardized biobased commodities that can be made from as many feeds as possible.
The previous section sketched in broad strokes the availability of biomass (residues) on a global scale. Looking a bit closer to home, Avans University of Applied Science is headquartered in Breda and considers the Dutch province of North Brabant its hinterland.
The province North Brabant currently has about 2.5 million inhabitants. Its total area is a bit less than six thousand square kilometers, of which 62% is used for agricultural purposes [51]. The total agricultural production comes to almost 6000 kton, that is, gross weight including water (Table 4.3).
Located in the municipality of Moerdijk is a large petrochemical complex fed by the output of single large naphtha cracker. This cracker produces mainly ethylene which is transported by pipeline or converted in situ into products such as ethylene glycol and ethylene oxide. Other products include propylene, benzene and butadiene [54]
crop area (km2) gross weight (kton)
As shown in Table 4.4 the Moerdijk complex consumes approximately 3000 kton naphtha on an annual basis [54]. Compare this to the estimated gross agricultural production of 5700 kton for the whole province (Table 4.3). When correcting for water content, the current agricultural sector produces some 1500-3000 kton of organic dry matter. Leaving aside carbon to oxygen ratios (naphtha is mainly carbon, biomass contains lots of oxygen) most of the agricultural output is already earmarked for human and/or animal consumption. It seems obvious that it will be impossible to run this refinery on only locally sourced biobased feedstock, based on current production and consumption levels. There simply is not enough biomass available in the province itself.
Table 4.4: the Moerdijk complex in numbers compared to North Brabant as a whole (annual basis). Data taken from [27] and [54]
whole province Moerdijk complex
The above example is of course flawed in many ways: regional boundaries are arbitrary and much of the output of the Moerdijk complex will be transported to elsewhere. It does however illustrate the point that simply replacing naphtha with regional biomass will be difficult. Unless alternative ways of production are implemented, large streams of biomass will have to be transported.
For a country such as the Netherlands with a relatively large petrochemical sector this will mean importing some form of (pre-treated) biomass. In a sense this is not much different from the current situation where the fossil crude has to be imported. In fact, most bio-ethanol currently produced and used in the Netherlands is made from imported biomass.
Table 4.4 also shows how large a percentage of total energy use and emissions this complex has compared to the province as a whole. Humorists have suggested that slightly redrawing the province boundaries would result in meeting most provincial climate targets at a single stroke. . .
On February 20, 2024, representatives of twenty industrial sectors (including the petrochemical industry) signed the Antwerp Declaration for a European Industrial Deal [17]. Mention is made of competition by the US and its generous Inflation Reduction Act and Chinese overcapacity, both increasing pressure on the European market. The declaration calls on the European Commission for a comprehensive action plan for a stronger business:
“We need our industry for their innovation capacity. To come up with tomorrow’s climate solutions. That is why Europe should not only be a continent of industrial innovation, but should remain a continent of industrial production. . . The question in front of us is: how do we continue to grow our European industry? The answer is: with a European Industrial Deal at the same level of a European Green Deal. Not in opposition to each other but to reinforce each other”
The European Green Deal is of course the strategy by which the EU aims to reduce its greenhouse gas emissions at least 55% by 2030 compared to 1990 and become climate neutral by 2050. The recent Fit for 55 package is a set of proposed changes and amendments to EU law to make this a reality.
In addition to the Antwerp declaration, Martin Brudermüller, president of the European Chemical Industry Council said the following [12]:
“Basic industries in Europe are grappling with historical challenges: demand is declining, investments in the continent are stalling, production has dropped significantly, and sites are threatened. We want to drive the transformation of our companies. For this, we urgently need decisive action to create the conditions for a stronger business case in Europe”
The same Martin Brudermüller is also the CEO of BASF, one of the largest chemical producers in the world. BASF is of course investing 10 billion euro in Zhajiang, China, making it their third-largest site. This is a perfect illustration of conflicting messaging: on the one hand calling for a stronger European industry but on the other hand investing heavily outside Europe.
1 Trillion euro...
A recent report commisioned by the European Chemical Industry Council outlined what is needed to make the European chemical industry part of climate neutrality in 2050 [48]. The report states that it will require 400 to 600 billion euro in capital expenditures for new equipment and modification of existing facilities, combined with 200-300 billion in standstill cost and 250-300 billion in additional research, coming to a grand total of 1 trillion euro.
Compared to funds already allocated this leads to a reported gap of 365 billion euro (Figure 5.2). Unfortunately the underlying study and assessment of 236 European plants remains confidential, making it difficult to fully assess these numbers. A recurring theme is that the availability of renewable energy at a competitive price level is necessary to achieve climate neutrality. Whatever the actual numbers are it is clear that the amount of money involved in this transition is staggering.
Where the previous section gives a snapshot of the cost and scale of transition of the existing industry there are also national and European efforts to build a new industry almost from the ground up.
The 20 billion euro Dutch Nationale Groeifonds exists to finance projects that increase the future earning power of the Netherlands. Specifically relevant for the chemical transition are Circular Plastics NL [34] and Biobased Circular [10]. In short, both projects aim to reduce emissions by increased plastics recycling and
the production of plant based materials and building blocks. Biobased Circular is expected to lead to 3500 new jobs and an eventual annual reduction in CO2 emissions of 2.5 Mton [19]. To put this into perspective: this is the same amount as emitted by the single refinery complex mentioned in Table 4.4.
The total amount of subsidies involved in both projects is on the order of magnitude of half a billion euro. This is a substantial amount. However, most of these funds will not go to the building of actual production capacity but rather to research and development. Also, circumstances can change quickly: the original Biobased Circular proposal included a provision for the building of a factory for the production of polylactic acid (PLA) which has since been pulled. One of the current nine project lines still involves the production of bio-ethylene glycol (EG) [11] while the only Dutch company currently involved in (pilot)scale bio-EG recently announced a stop to these activities, firing or re-allocating the personnel involved [38].
There are also European initiatives such as the Circular Bio-Based Europe Joint Undertaking [49] which co-funds plants at the demonstration scale. Reportedly each euro invested leads to another five in private investment.
However there is a tendency towards optimism as to the actual impact projects will have. A 2020 report commissioned by the Circular Biobased Delta mentions 21 high potential projects in the so-called Delta provinces (Zeeland, North Brabant and South Holland) [22]. Together these required a 3.2 billion euro investment and would lead to 5 Mton annual reduction in CO2 emissions. In reality as of March 2024 the top five projects were either shelved or disbanded, equivalent to 60% of the hoped for impact.
When it comes to providing (venture) capital for start-ups and scale-ups, Europe is running behind the US and the Netherlands is not a European frontrunner. The Dutch Regional Development Agencies or ROMs are well financed but are not set up to finance multiple multi-million scale outs. Recently the North Brabant ROM announced a funding gap of 2 to 4 billion euro (figure 5.3). Admittedly not all this money is supposed to go to companies that support the biobased transition.
New companies might make it to the ton pilot scale but struggle to get funding for their first ’real’ kton (or beyond) scale factory. At the moment there are several companies such as Relement and Susphos that are being lionized by both press and politicians for their innovative technology and potential climate saving impact, yet struggle for funding.
To illustrate the amount of capital required consider Avantium’s first 5 kton/yr plant for the production of 2,5-furandicarboxylic acid (FDCA), a biobased replacement for terephthalic acid. Construction of this so-called demonstrator plant will reportedly cost in excess of 255 MEuro [38]. It is to be hoped that after successful demonstration, the investment cost per ton production capacity for a second and larger plant will be much lower.
Table 5.1 lists a number of potential differences between scale-ups vs existing industries when it comes to making the Biobased Transition a reality. These are mainly still a set of hypotheses which the new Biobased Transitions group intends to investigate further. If however the existing industry expects to have to spend 1 trillion euro in the next 30 years, the question remains if start-ups and scale-ups can ever reach the same scale within the limited time left.
Table 5.1: comparing the building of new industries and the change of existing industries
start-ups / scale-ups
limited techno-economical expertise
’re-invent the wheel’
existing industries
techno-economical expertise
entrepreneurial: taking risks by default entrepreneurial without risk taking may have to build new value chain operates within existing value chains can it be scaled in time? can it be re-tooled in time? regulation seen as an enabler regulation seen as a blocker nothing to lose?!
everything to lose?!
Given the challenges set out in Chapters 4 and 5 I would like to formulate the following ambition for the new Biobased Transitions group:
“Understand what it takes to increase the production of biobased materials and chemicals and decrease the use of fossil feeds by both existing and new industries.”
This is obviously a huge task! To insert a bit of realism I therefore propose the following three research lines:
1. Quantify change Put the rate of change into numbers. What investments are made, what subsidies are being given, how much biobased or renewable feedstock is used, leading to actual product volumes. There have been several private-public partnerships in the past, how many kilotons of production capacity do these represent
2. Understanding blockers/enablers What is the influence of legislative frameworks, subsidies. What is the role of individual decision makers. The role and availability (or absence) of different types of capital. What can we learn from both succesful and failed projects
3. Qualify Given the above, what course of action is the most likely to speed the biobased transition, where should (political) influence be exerted
Each of these three lines can be extended towards both the existing petrochemical industry and the nascent start-up / scale-up culture. In the former case we will need to look at the EU as a whole, in the latter we will start with the Netherlands at first. In practice it will of course be impossible to look at all product groups or value chains so we will have to make choices.
It is clear to me that Qualify contains a value judgement and can therefore not be a true research line. Though dispassionate research remains important, I have come the conclusion that today’s global challenges also require a more activist role.
Table 6.1: proposed research lines
industries existing new EU (Netherlands) Netherlands (EU) quantify change investments made subsidies received feedstock and product volumes revenues generated understanding role of legislation blockers/enablers role of subsidies impact of decision makers capital (un)availability qualify influence who/what/where to enhance the transition
This inaugural lecture has the title Biobased transitions: an uphill battle?! combining both an exclamation mark and a question mark. The exclamation mark symbolizes the excitement that goes along with doing and making new things. It shows the huge challenges that are happily being tackled by many new and even some existing companies. The question mark represents the majority of existing industries that see change as a risk instead of something that has to be done regardless of risk.
The picture on the front page shows Sisyphus, doomed to forever roll a large boulder up a hill. In this particular context, forever is of course too long a time. For a process of change that will take many decades if not a lifetime to complete it surely is an apt metaphor.
The topic of Biobased Transitions offers a multitude of teaching opportunities. Students need to understand what the current petrochemical landscape looks like before they can start thinking of changing it. There is technology to be studied, value chains, products, process economics, (raw) materials, the list goes on and on.
In one of our first teaching projects we invited students to look back from the year 2073 and visualize what a large biobased chemical company would look like. Not only that, they were asked to imagine the chain of events that led to such a company, beginning with the founding of a humble start-up.
Consider the following graph taken from a report by the Nova Institute and the Renewable Carbon Initiative [50]. It is deceptively simple: it shows the type of carbon used in chemicals now and a projection of the type of carbon that will be used in 2050.
Several things are obvious: the role of fossil carbon is reduced to nearly zero, recycling takes up half of the feedstock, CO2 and biobased feed make up the remainder in almost equal measure. This distribution is of course up for debate. For example, nearly 300 Mton of CO2-based carbon equals more than a gigaton of CO2. The current capacity for CO2 capture is a fraction of this. The amount of green energy required to capture and convert this CO2 will be staggering and as always will compete with other demands. This is not to say it can’t be done but the relative contributions may very well turn out to be different in the end.
The authors are at pains to explain that the transition shown in this graph is not a foregone conclusion but rather an (optimistic) scenario of how things might proceed. There is however one other assumption that needs addressing: the column to the right assumes a doubling of the amount of carbon-based materials used in 2050 compared to 2020. No doubt this was reached at assuming a certain amount of yearly economic ’growth’ and of course the continuing increase of the world’s population. This begs the question: do we need all these chemicals and materials?
Another doubt in my mind is that we are trying to replace a production system based on shareholder capitalism with another system, but still using the same underlying economical mechanisms. Perhaps the startups / scaleups of the Biobased Transition should not be financed by venture capital looking for high returns but some other way? If European companies start shipping cheap biomass from across the world for processing in Europe instead of creating value locally would we not be doing the same as we did with oil and gas? How do we ensure that value is distributed (more) evenly throughout the chain?
There is a famous quote attributed to Isaac Newton about being able to see further, because of standing on the shoulders of giants. I should like to counter that the same holds true without using the word giants and that ordinary shoulders will do.
Everyone alive today is standing on the shoulders of previous generations. Most of these were ordinary people, doing ordinary things. Each generation of parents and caregivers strives to make the lives of their children a bit better. Until recently the larger impact this had on the planet and our environment was unknown or at least overlooked. (Notwithstanding those cultures who did manage to find some sort of balance with the natural world).
It is all too easy to blame today’s problems on previous generations. ’If only they stopped pumping oil earlier, if only they decided that single-use plastics were a bad idea’ - the list is endless. While one can always point to individuals who willfully made wrong decisions I do not think this should be generalized. Not only did most people not have access to the insights we now have they often also lacked the agency to affect change. Then as now, most of us are part of larger systems that are very difficult to escape from, assuming that we even know or understand that we are part of one.
Just as we are standing on top of other people’s shoulders, next generations will be standing on ours. These future generations will no longer be able to absolve us: we now know too much for that. Wether we will have acted in time remains to be seen.
[1] Thomas R. Anderson, Ed Hawkins, and Philip D. Jones. CO2, the greenhouse effect and global warming: from the pioneering work of Arrhenius and Callendar to today’s earth system models. Endeavour, 40(3):178, 2016
[2] Leah Aronowsky. Gas guzzling gaia, or: A prehistory of climate change denialism. Critical Inquiry, 47(2):306–327, 2021. 11
[3] Svante Arrhenius. On the influence of carbonic acid in the air upon the temperature of the ground. The London, Edinburgh, and Dublin Philosophical Magazine and Journal of Science, 41(251):237–276, 1896
[4] Peter Baxendell. Enhancing Oil Recovery - Making the Most of What We’ve Got. Transactions of Mining and Metallugy, 94A:A84–A89, April 1985.
[5] Alice Bell. Our biggest experiment: A history of the climate crisis. Bloomsbury Publishing, 2021
[6] R. W. Bentley. Global oil & gas depletion: an overview. Energy policy, 30(3):189–205, February 1, 2002
[7] BP BP. Statistical review of world energy 2022. Technical report, BP London, UK, 2022
[8] Thijmen van Bree, Ton Bastein, Joris Vierhout, and Wimar Bolhuis. Toekomst van de Nederlandse industrie. Technical report, TNO Vector, October 2023
[9] G. S. Callendar. The artificial production of carbon dioxide and its influence on temperature. Quarterly Journal of the Royal Meteorological Society, 64(275):223–240, 1938
[10] Biobased Circular. https://www.biobasedcircular.com/.
[11] Biobased Circular. https://www.biobasedcircular.com/programma/industrieleglycolproductie/
[12] The European Chemical Industry Council. https://cefic.org/mediacorner/newsroom/ antwerp-declaration-for-a-european-industrial-deal/
[13] Scott C. Doney, Victoria J. Fabry, Richard A. Feely, and Joan A. Kleypas. Ocean Acidification: The Other CO2 Problem. Annual Review of Marine Science, 1(1):169–192, January 2009
[14] Wolter Elbersen, Iris Vural Gursel, Juliën Voogt, and Koen Meesters. To be or not to be a biobased commodity. Assessing requirements and candidates for lignocellulosic based commodities. Technical report, Wageningen University & Research, 2022. Report 2242, WFBR project number 6224088700
[15] Marcus Eriksen, Win Cowger, Lisa M. Erdle, Scott Coffin, Patricia VillarrubiaGómez, Charles J. Moore, Edward J. Carpenter, Robert H. Day, Martin Thiel, and Chris Wilcox. A growing plastic smog, now estimated to be over 170 trillion plastic particles afloat in the world’s oceans—urgent solutions required. PLOS ONE, 18(3), 2023
[16] Eunice Foote. Circumstances affecting the heat of the sun’s rays. The American Journal of Science and Arts, 22(56):382–383, 1856
[17] Antwerp Declaration for a European Industrial Deal. https://antwerpdeclaration.eu/.
[18] Michael Freemantle. The chemists’ war: 1914-1918. Royal Society of Chemistry, 2015
[19] Nationaal Groeifonds. www.nationaalgroeifonds.nl/overzicht-lopende-projecten/ thema-sleuteltechnologieen-en-valorisatie/biobased-circular
[20] M. K. Hubbert. Energy resources: a report to the committee on natural resources of the national academy of sciences–national research council. Technical report, National Academy of Sciences - National Research Council, Washington, D.C. (USA), 1962
[21] Roland Jackson. Eunice Foote, John Tyndall and a question of priority. Notes and Records: the Royal Society Journal of the History of Science, 74(1):105, 2019
[22] Chris Jongsma, Isabel Nieuwenhuijse, Bettina Kampman, Martijn Broeren, and Diederik Jaspers. CO2 - reductie met de circular biobased delta. Technical report, CE Delft, December 2020. publicatienummer 20.190303.147
[23] Jan Bart Jutte, Iris Pronk, and Guido Israels. Circulaire Atlas Noord-Brabant. Technical report, Royal HaskoningDHV, April 21, 2020. Projectnummer BG6246-101-100
[24] Mikael L. A. Kaandorp, Delphine Lobelle, Christian Kehl, Henk A. Dijkstra, and Erik van Sebille. Global mass of buoyant marine plastics dominated by large long-lived debris. Nature Geoscience, 16(8):689, 2023
[25] Charles D. Keeling. The concentration and isotopic abundances of carbon dioxide in the atmosphere. Tellus A: Dynamic Meteorology and Oceanography, 12(2):200–203, 1960
[26] Ralph F. Keeling, Stephen C. Piper, and Martin Heimann. Global and hemispheric CO2 sinks deduced from changes in atmospheric O2 concentration. Nature, 381(6579):218–221, 1996
[27] Regionale Klimaatmonitor. https://klimaatmonitor.databank.nl/home
[28] G. Lamichhane, A. Acharya, R. Marahatha, B. Modi, R. Paudel, A. Adhikari, B. K. Raut, S. Aryal, and N. Parajuli. Microplastics in environment: global concern, challenges, and controlling measures. International Journal of Environmental Science and Technology, 20(4):4673, 2022
[29] J.P. Lange. Towards circular carbo-chemicals - the metamorphosis of petrochemicals. Energy & environmental science, 14(8):4358–4376, 2021
[30] Hoesung Lee, Katherine Calvin, Dipak Dasgupta, Gerhard Krinner, Aditi Mukherji, Peter Thorne, Christopher Trisos, José Romero, Paulina Aldunce, and Ko Barret. IPCC, 2023: Climate Change 2023: Synthesis report, summary for policymakers. contribution of working groups i, ii and iii to the sixth assessment report of the intergovernmental panel on climate change [core writing team, H. Lee and J. Romero (eds.)]. IPCC, Geneva, Switzerland. 2023
[31] Peter G. Levi and Jonathan M. Cullen. Mapping global flows of chemicals: From fossil fuel feedstocks to chemical products. Environmental science & technology, 52(4):1725–1734, 2018
an Uphill Battle?!
[32] Christophe McGlade and Paul Ekins. The geographical distribution of fossil fuels unused when limiting global warming to 2oC. Nature, 517(7533):187–190, 2015
[33] Francis Molena. Remarkable weather of 1911. The effect of the combustion of coal on the climate - what scientists predict for the future. Popular Mechanics, March 1912
[34] Circular Plastics NL. https://circularplasticsnl.org/
[35] Araceli Fernandez Pales and Peter Levi. The future of petrochemicals. Technical report, International Energy Agency, 2018
[36] Max Perutz. Is science necessary? Essays on science and scientists. Barrie & Jenkins Ltd, 1989
[37] Gilbert N. Plass. Carbon dioxide and the climate. American Scientist, 44(3):302–316, 1956
[38] Avantium Press Release, December 13, 2023. Avantium focuses on maximising the potential of its FDCA and PEF technology
[39] Braskem Press Release, August 17, 2023. Braskem and SCG Chemicals join forces to advance in the bio-based Ethylene project in Thailand
[40] Braskem Press Release, July 24, 2023. Braskem expands its biopolymer production by 30% following an investment of US$ 87 million
[41] Roger Revelle and Hans E. Suess. Carbon dioxide exchange between atmosphere and ocean and the question of an increase of atmospheric CO2 during the past decades. Tellus, 9(1):18–27, 1957
[42] James Speight and M. R. Islam. Peak Energy. Peak energy: myth or reality. 1st edition, 2016
[43] Geoffrey Supran and Naomi Oreskes. Addendum to ‘Assessing Exxon-Mobil’s climate change communications (1977–2014)’ Supran and Oreskes (2017 environ. res. lett. 12 084019). Environmental Research Letters, 15(11), 2020
[44] the European Chemical Industry Counsil CEFIC, December 5, 2023. The European Chemical industry. Facts and Figures 2023
[45] Anthony Turhollow, Robert Perlack, Laurence Eaton, Matthew Langholtz, Craig Brandt, Mark Downing, Lynn Wright, Kenneth Skog, Chad Hellwinckel, Bryce Stokes, and Patricia Lebow. The updated billion-ton resource assessment. Biomass and Bioenergy, 70:149–164, November 2014
[46] John Tyndall. On the absorption and radiation of heat by gases and vapours, and on the physical connexion of radiation, absorption, and conduction.—the Bakerian lecture. The London, Edinburgh, and Dublin Philosophical Magazine and Journal of Science, 22(146):169–194, 1861
[47] Adam Tyson and Eugenia Meganingtyas. The status of palm oil under the European Union’s renewable energy directive: Sustainability or protectionism? Bulletin of Indonesian Economic Studies, 58(1):31–54, 2022
[48] Michael Ulbrich, Eike Christian Eschenröder, Bernd Elser, Mais Haddadin Finn, Nuno Faísca, Richard Sleep, and Alistair Hensman. The chemical industry’s road to net zero. Costs and opportunities of the EU Green Deal. Technical report, Accenture and NexantECA, 2022
[49] Circular Bio-Based Europe Joint Undertaking. https://www.cbe.europa.eu/
[50] Christopher vom Berg and Michael Carus. Renewable carbon as a guiding principle for sustainable carbon cycles. Technical report, 2023. Renewable Carbon Initiative.
[51] Centraal Bureau voor de Statistiek. https://opendata.cbs.nl/statline
[52] Vereniging voor Nederlandse Chemische Industrie VNCI. https://www.vnci.nl/ chemie-in-cijfers
[53] Spencer Weart. The Discovery Of Global Warming. Harvard University Press, 2008
[54] Louise Wong and Ton van Dril. Decarbonisation options for large volume organic chemicals production, Shell Moerdijk. Technical report, 2020. TNO project no. 060.33956 / TNO 2020 P11258
The Biobased Transitions group (Dutch ’lectoraat’) is part of the Centre of Expertise MNEXT, which itself is a joint venture between Avans University of Applied Science and Hogeschool Zeeland.
The group was started in March 2023 with the appointment of Martijn Zieverink as lector. Recently we welcomed new members (in Dutch called ’kenniskringleden’) Philippa, Willem and Maddalena. Together they represent a wonderful mix of experience and backgrounds.
Philippa Roots is an advocate for realizing climate change management strategies on local and systemic levels. She studied sustainability at McGill University and received a masters from the Western Norway University of Applied Sciences, both studies encompassing a broad range of social and physical sciences.
Philippa comes from western Canada, where she began her career working in regenerative agriculture through grassroots organizations, as both a farmhand and project coordinator. While maintaining involvement in the farming scene she also worked in the energy sector, gaining an appreciation of the challenges facing decarbonization in the industry. She continued recognizing the role of soil in climate change while writing her masters thesis, where she studied how carbon storage in peatlands responds to land use change.
During her masters Philippa co-founded and led a student organization that facilitated small scale climate action and circular economy initiatives in the local community. Returning again to industry, she held a short-term research position with Den Norske Veritas investigating untapped energy potential in the maritime space.
In many of her roles, Philippa has taken responsibility for maintaining an overview and ensuring that multiple aspects of sustainability are considered. She is a strong proponent of nature-based solutions and believes that in pursuing Biobased Transitions many of today’s other challenges can be addressed. Philippa has recently moved to the Netherlands and begun her role as a Biobased Transition Researcher with Avans University of Applied Science.
Willem van Liemt started his career studying photosynthesis in Leiden University. After his PhD he joined Unilever, developing new ingredients and formulations for laundry applications. In his first project leader role he introduced high-throughput methodology and robotics in the research of fastmovingconsumer-goods. He subsequently moved to Nova Chemicals/Ineos Styrenics where he led the development of new insulation products for the European market. Joining
Eastman Chemicals in 2012, Willem took commercial responsibility for the European Adhesives and Plasticisers Business, after which he returned to technology, leading the global technical team for the Care Chemicals in Eastman.
Work at Unilever and Nova Chemicals has led to multiple patents and has been recognized by a ’Unilever Research Team’ award and the ’Nova Chemicals Presidents’ award.
Having been part of Corporate R&D for most of his working life, Willem has witnessed how difficult it can be to successfully drive a sustainability agenda. Being part of the Biobased Transitions group at MNEXT is therefore a great opportunity for him to jointly investigate and understand such barriers in corporations and society, and to try to resolve these in order to secure a durable future for society.
Outside of MNEXT, Willem supports technical teams, companies and projects in the circular transition. To focus more fully on this he started his company TechPilot Innovation Consultancy in September 2023.
Maddalena Eloisa Logrieco was born in Bari and later relocated to Turin to study Chemical Engineering. During her master’s degree she spent a semester at the University of Queensland collaborating with the bioelectrochemical system team on the development of a microbial fuel cell combining urine treatment and fertilizer production.
Following her graduation, she entered industry as an EHS intern for GE Aviation with high hopes for sustainability. However, she soon realized that her department’s role resembled that of Jiminy Cricket – offering guidance that often went unheeded. She then moved to Lisbon to join the European agency focused on maritime safety (EMSA). There, she initially worked in the Remotely Piloted Aircraft Systems team, later extending her responsibilities to include the Earth Observation team. During her experience at EMSA, she primarily focused on emission monitoring and CleanSeaNet services, utilizing cutting-edge technologies for environmental protection.
She then shifted into consultancy as a PMO for R&D projects in electric mobility while still enjoying her passion for surfing in Lisbon. A significant shift occurred when she spent a year in Kansas, pursuing a Graduate Certificate in Applied
Statistics. During this period, she conducted part-time research on bioleaching of feedstock used in thermochemical conversion.
The common thread between all these experiences is a commitment to making a positive impact and being guided by integrity.
Martijn Zieverink studied chemical engineering and has a PhD in catalysis from the Delft University of Technology. He was born in Sittard, his (great)grandfathers were employed by the Dutch State Mines.
He started his career working in a refinery for edible oils and fats, leading a team of product developers and process engineers. He joined Avantium’s contract research division, improving existing and developing new catalysts for a wide array of clients, ranging from start ups to some of the world’s largest companies. In this role he saw at first hand the opportunities and challenges for newcomers to the world of chemicals and materials. While at Avantium, Martijn also played a small role in the development of the FDCA process. He spent the last two years setting up the new electrochemistry group ‘Volta’. After a short stint developing biobased resins at a startup Martijn joined PeelPioneers in his home town of ‘s-Hertogenbosch. He developed PeelPioneers patent portfolio and is still involved in trouble shooting and process development.
Martijn has always been interested in science and technology and he is listed as (co)inventor on eleven patents. However lately he has come to the conclusion that while important, the major challenges of our times can not (only) be overcome by technology. The technology needed for Biobased Transitions by and large exists, it is in the implementation, the creation of new value chains and possibly the creations of whole new industries that challenges now arise.
Martijn is married and has two children of primary school age. It is also with their future in mind that he accepted his new role of Lector Biobased Transitions at the Avans University of Applied Science.
New lectores within Avans University of Applied Science are given a so-called chain of office or ’lectorale keten’. As this is still a relatively new tradition there is room for innovation and doing things a bit different.
This particular chain of office was designed and made by Marlies Schets. As a symbol of the Lectoraat Biobased Transitions we decided on a chain not made from metal but combining non-traditional materials such as wood, biobased plastics and fossil based plastics. Each material signifies a different stage of the transition.
This is not meant to be an unbreakable chain but rather a changeable object. Individual chain links can be exchanged, symbolizing change and progress. When not in use, the chain can be dismantled. The links fit into each other and can be stored in a handsome box.
This is an edition of Avans University of Applied Sciences
Issued on the occasion of the inaugural lecture by Martijn Zieverink, delivered on April 5, 2024
ISBN 9789081309172
NUR 615 Boekwetenschappen algemeen
Editing and coordination
MNEXT
Printing and design De Studio, powered by Avans & Canon
Contact
MNEXT secretariaat.mnext@avans.nl www.mnext.nl
Lovensdijkstraat 63 Breda
Correspondence Address Postbus 90116 4800 RA Breda