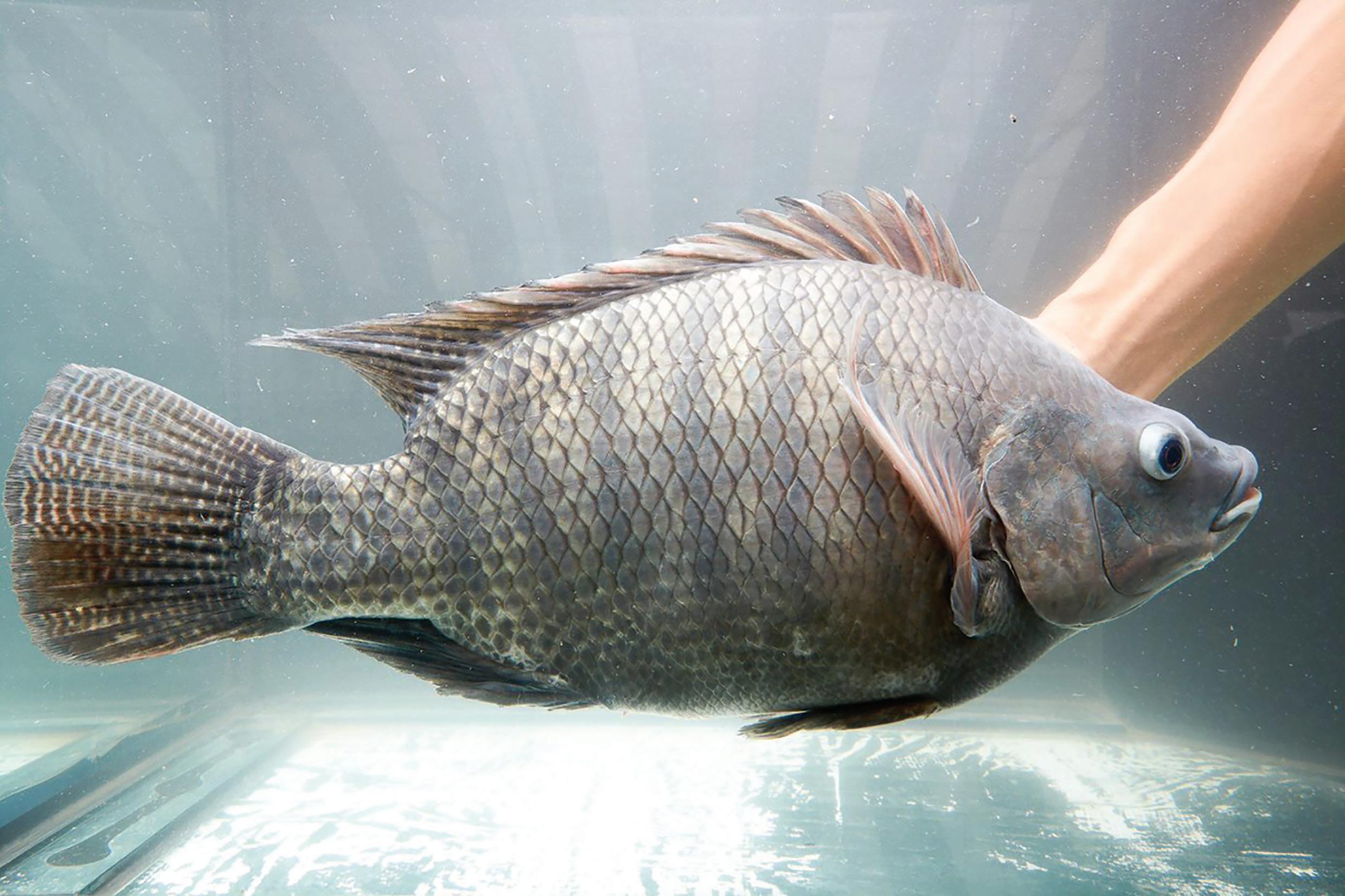
21 minute read
A mix of 17 free amino acids enhances performance of Nile tilapia
Pierrick Kersanté, BCF Life Sciences, Eakapol Wangkahart, Mahasarakham University, Guillaume Le Reste, Halieutica
To date, more than 30 studies have been conducted by BCF Life Sciences in partnership with research centers, universities and numerous studies directly on farms (60 studies in 2021) that underline the positive effects of Kera-Stim®50, a natural mix of free amino acids (MFAA), on zootechnical parameters for shrimp.
On this strong basis and with the objective to identify MFAA effects on tilapia farming performances, we have recently conducted a trial in partnership with Mahasarakham University, Thailand. In this trial, 450 healthy tilapia juveniles, 4.7g of initial body weight (IBW), were allocated in cages, at 30 fish per cage and 3 repetitions per treatment. Animals were fed five diets (control, control+0.25%, control+0.50%, control+0.75%, control+1.00%, MFAA) for 8 weeks with application of the MFAA into the pellet mix. Results underline the positive effects of MFAA on a wide range of parameters (Wangkahart et al., 2022).
MFAA supplementation of the feed influenced zootechnical performances, with an increase in growth parameters, final body weight (FWB) and FCR reduction. In relation to these observations, positive effects on feed utilization and body condition indices with significant improvements in protein efficiency
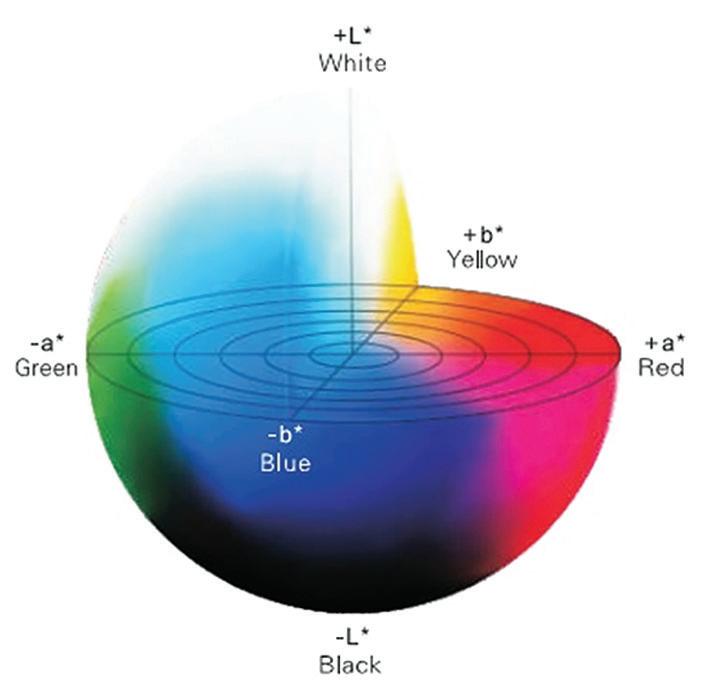
Figure 1. Lab color measure system.
ratio, viscerosomatic index (VSI) and carcass yield were observed. MFAA addition also influenced fillet composition and color.
Animal husbandry and feeding protocol
Four hundred and fifty healthy juvenile tilapia (O. niloticus), 4.76 ± 0.05 g IBW, were allocated in 15 cages (30 fish per cage and 3 repetitions per treatment) for a 56-day trial. High-quality feed was formulated (32% protein, 4.2% fat, 2.8% fiber, 9.7% ash) and used as a basal diet. Four different concentrations of KeraStim®50 were supplemented and tested: 2.5 g/kg of feed, 0.25%, 5 g/kg of feed 0.50%, 7.5 g/kg of feed, 0.75% and 10 g/kg of feed, 1.00%. The MFAA was included in the pellet mix, before pelletizing.
Fish were fed twice a day to apparent satiation (8:00, 16:00). The feeding rate applied was 5% of the biomass and was adjusted every 2 weeks, according to fish growth.
Evaluated parameters Feed utilization and body condition indices
Growth parameters were measured including final weight, weight gain (WG; g); specific growth rate (SGR; % day-1) and feed conversion ratio (FCR). Additionally, the following indices were calculated: Protein efficiency ratio (PER; %) = 100 × (total final body weight – total initial body weight) / (dry feed intake × protein content in the feed) and VSI (%) = 100 × visceral wet weight/ body wet weight).
Digestive enzymes activity
Amylase activity was measured according to Nater et al. (2006). The determination of proteinase activity was determined using the azocasein hydrolysis assay according to the method of Ivergen and JØrgensen (1995). The activity of lipase was determined following the method of Pencreac'h and Baratti (1996).
Analysis of fillet composition and color
At the end of the trial, 45 fish were randomly sampled and 9 additional fish (3 fish/tank x 3 tank/group) were collected from control and all treatment groups. These fish were filleted resulting in butterfly fillets. Fillet color was measured with a CR400 chroma meter (Minolta, Japan) where the redness index or a* (red-green intensity), yellowness or b* (yellow-blue intensity), and lightness or L* (dark to light) (Fig. 1).
Table 1. Growth performance, amylase activity, body condition indices and fillet composition of Nile tilapia fed experimental diets for 8 weeks.
Parameters MFAA0 MFAA0.25 MFAA0.50 MFAA0.75 MFAA1.0 P-value
WG (g) SGR ADG FCR PER
48.19 ± 0.02b 49.37 ± 0.38ab 56.97 ± 0.37a 60.56 ± 0.08a 63.39 ± 2.60a 0.024 4.03 ± 0.01b 4.02 ± 0.04ab 4.20 ± 0.08a 4.35 ± 0.02a 4.39 ± 0.06a 0.047 0.80 ± 0.04b 0.82 ± 0.01ab 0.95 ± 0.03a 1.01 ± 0.01a 1.06 ± 0.02a 0.033 1.92 ± 0.07a 1.85 ± 0.02ab 1.62 ± 0.10b 1.53 ± 0.10b 1.46 ± 0.14b 0.020 1.54 ± 0.00d 1.55 ± 0.00d 1.78 ± 0.01c 1.84 ± 0.00b 1.99 ± 0.01a 0.000
VSI (%)
10.04 ± 0.18a 8.12 ± 0.57b 8.60 ± 0.48b 8.53 ± 0.42b 8.08 ± 0.45b 0.019
Carcass yield (%)
58.0 ± 0.90b 59.8 ± 1.84ab 61.7 ± 1.56ab 62.6 ± 1.13a 63.4 ± 0.68a 0.018 Amylase (U/mg) 4.36 ± 0.19b 4.34 ± 0.23b 4.90 ± 0.28b 5.88 ± 0.25a 5.30 ± 0.42ab 0.010 Crude lipid (fillet composition) 2.74 ± 0.6b 2.88 ± 0.4b 4.44±0.5a 5.15 ± 0.1a 4.47 ± 0.4a 0.001 L value (fillet dorsal region) 61.5 ± 2.1b 62.0 ± 1.8ab 65.6±1.6ab 65.9 ± 0.1a 66.7 ± 0.8a 0.048
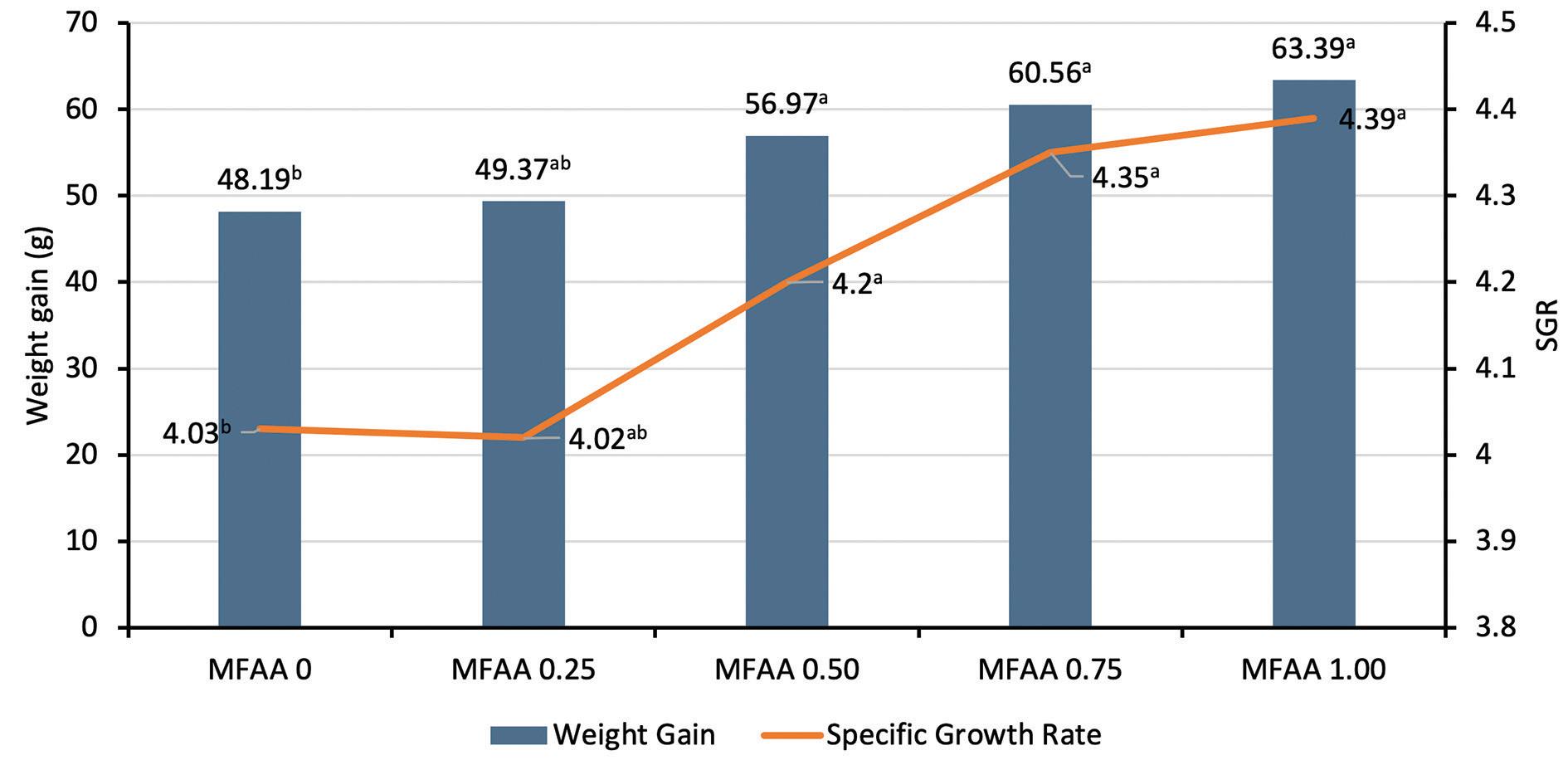
Figure 2. Weight gain and SGR in tilapia fed on different MFAA treatments.
Data were submitted to ANOVA and in case of significance (P≤0.05), a Duncan test was performed. Statistical analyses were made with the SPSS software.
Results
Interestingly, growth parameters underlined better zootechnical performances for animals fed with MFAA, with significant gains in biomass evolution. More precisely, MFAA included at 1.0% (equivalent to 10 kg/ton of feed), generated the best results with improvements in the final weight (+28.9%), WG (31.5%), SGR (8.9%) and an FCR reduction of 24% after 8 weeks (Table 1).
In addition, feed efficiency and body condition indices underlined better zootechnical performances for MFAA treatments, with significant gains in protein efficiency ratios, carcass yields and a reduction of the VSI (i.e. more muscle per kg of fish). All MFAA treatments induced significant improvements in those parameters. A dose effect was noteworthy observed. MFAA included at 1.00%, 10kg/ton of feed improved PER by 29.2% and carcass yield by 9.3%. It also reduced the VSI by 19.5%. We can hypothesize that this last parameter is directly related to better utilization of the feed and energy assimilation ensuring less fat deposition around the viscera.
The digestive enzyme activities, amylase, lipase and proteinase, were significantly increased with treatments including 0.75%, and 1.00% of MFAA, respectively from 34.9%, 10.4% and +9.9%, in relation to the improved feed utilization.
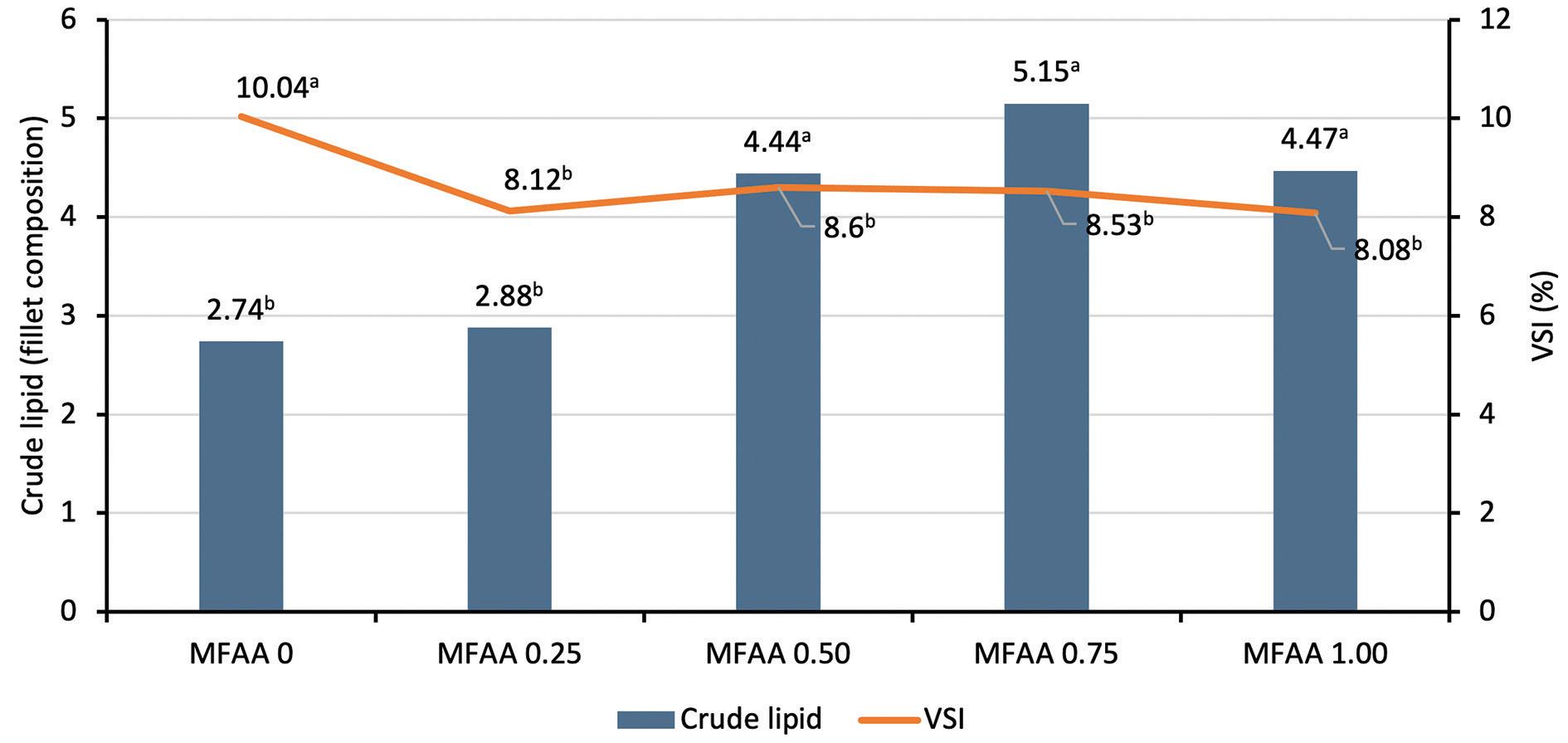
Figure 3. Crude lipid fillet composition and VSI in tilapia fed on different MFAA treatments.
The fillet composition analysis underlined a significant influence of MFAA with increased lipid composition in the fillet (+88% with 0.75% MFAA addition). According to these observations, the fillet color measured in ventral and dorsal regions was also significantly influenced by a higher value of L*, in relation to the lightness and white color of the fillet (respectively +13.7% and +7.2% for the L* value of the fillet ventral and dorsal regions with 0.75% MFAA addition). According to these observations, we can underline that there was no difference in the b* value (yellowness) for the fillet color.
Conclusion
During this study, we underlined particularly interesting effects of MFAA when applied to the feed for Nile tilapia fingerling. Firstly, regarding growth parameters with positive effects on biomass and feed utilization. Interestingly, strong improvements in body conditions indices, such as VSI, fillet composition and fillet color, were also observed. All those results suggest better feed assimilation and better use of the energy by the digestive system. In addition, we can hypothesize that the more pronounced white color of the fillet is probably in relation to a reduction of fat oxidation.
Interestingly, the reduction of the VSI can be linked with higher amylase activity generating a better utilization of the polysaccharides inducing less fat deposition around the viscera and more lipid content into the fillet. The strong effects of Kera-Stim®50 on digestive enzymatic parameters open new development possibilities to improve fish feed utilization, farming performances and tilapia meat quality. Mixes of free amino acids obtained from poultry keratin extensive hydrolysis are sustainable and efficient ingredients to improve fish farming performances.
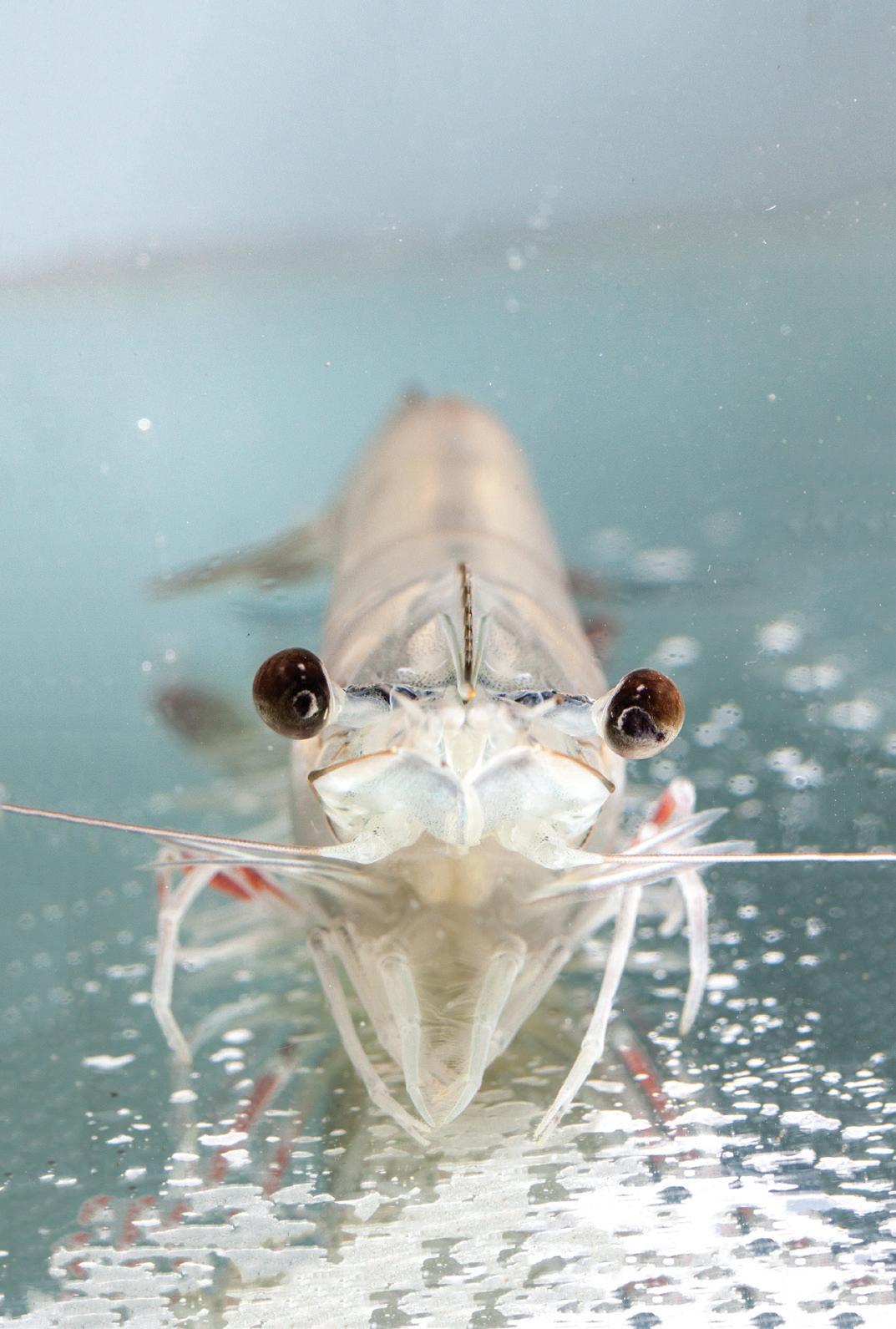
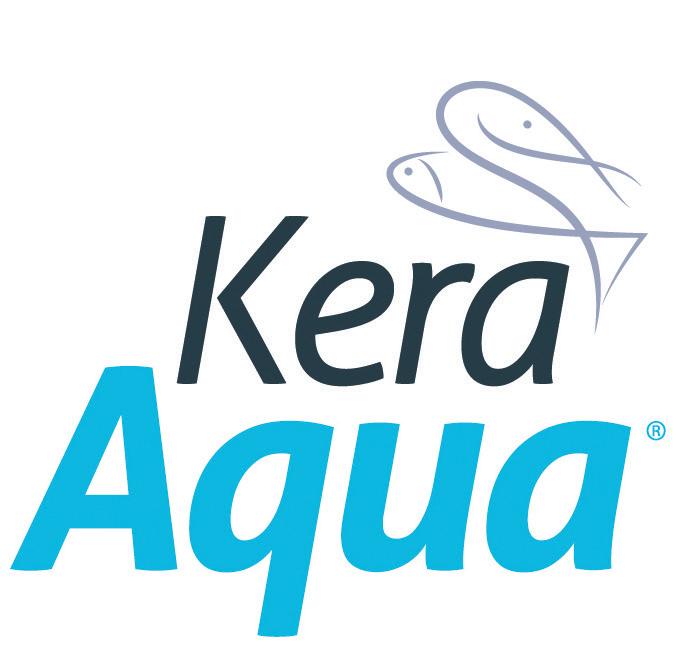
Free Amino Acid mixes for a SUSTAINABLE
More information: Pierrick Kersanté
R&D Aquaculture Manager BCF Life Sciences E: pkersante@bcf-lifesciences.com
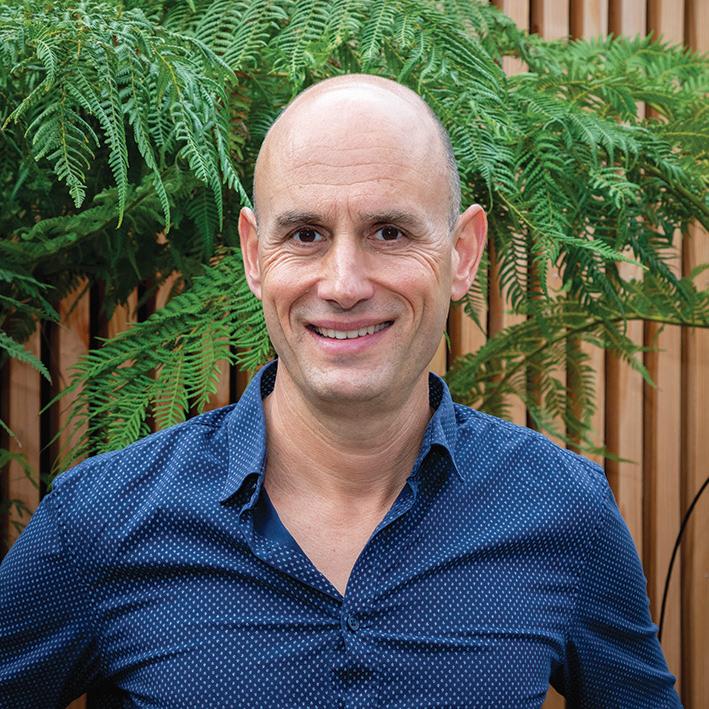
AQUACULTURE
FABRIQUÉ EN FRANCE
FABRIQUÉ EN FRANCE
Scan me to access the brochure
© Com d’Happy Création :
History of fish nutrition
Part I: 1900-1958
Ronald W. Hardy, Distinguished Professor Emeritus, Aquaculture Research Institute, University of Idaho
This is the first of a series of articles titled "History of fish nutrition".
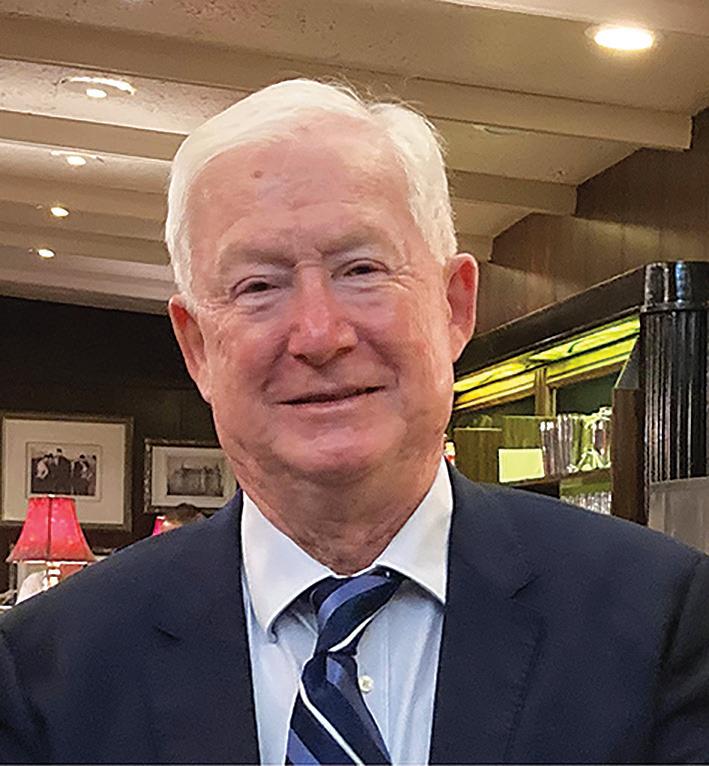
Fish nutrition is a relatively new field of study in the realm of animal and human nutrition. Fish nutrition research evolved by exploiting discoveries in animal and human nutrition over the past 100 years. These discoveries, in turn, built upon the foundation of information obtained over the previous century to characterize the nutrient and energy contents foods and animal matter, measure the metabolism of dietary energy and protein and identify the relationship between certain foods and human and animal diseases.
Discoveries from animal nutrition
At first, the main function of food was assumed to provide fuel for the body, similar to fuel for a furnace. Obviously, animals do not literally ‘burn’ food, but they were shown to extract energy from food for heat and activity by a similar but unknown mechanism. Dietary protein was assumed to be incorporated more-or-less directly from foods into tissue protein without much change (Carpenter, 2003a).
Animals were found to be useful subjects to study human nutrition. Dogs were especially popular because they would consume diets comprised of a single ingredient. One scholar wrote in 1816 that “Everyone knows that dogs can live very well on bread alone” (Magendie, cited by Carpenter, 2003a). However, when this statement was put to the test, dogs fed bread alone did not survive more than 50 days, suggesting that it lacked some essential materials to support life. Fresh animal meat and bones supported sustained growth and health of dogs, but refined animal products, such as gelatin extracted from bones or meat extracted with water, did not. Magendie suggested that water extraction of meat removed materials that were essential in the diet, possibly iron or other salts, fatty material or lactic acid.
Despite this scientific insight, more than 75 years passed before scientists returned to studying the constituents of meats and other foods that were necessary for feeds containing purified ingredients to support animal growth. One of the reasons for this was the publication of an influential book by Liebig in 1842 entitled Animal Chemistry or Organic Chemistry in its Application to Physiology and Pathology. Leibig argued that muscle tissue only contained protein and therefore muscle contraction came from an energy-yielding breakdown of protein molecules that powered muscles and produced urea. Therefore, he reasoned, protein was the only true nutrient (Carpenter, 2003a).
Nutrition through diseases
Nutrition also advanced through studies of several disease conditions prevalent in populations deprived of specific foods. Since ancient times, it was known that night blindness and scurvy were cured by feeding certain foods, i.e. carrots for night blindness and limes/lemons for scurvy, a condition that plagued sailors at sea.
In the late 19th century, rickets, a deformity of leg bones in children, became a widespread and serious problem in industrialized countries in Northern Europe as people moved from farms to polluted cities. The cause was unknown. Rickets was not associated with calcium intake but was cured by supplementing childrens’ diets with cod liver oil (Carpenter, 2003b). The finding that cod liver oil cured rickets supported
the concept that minor constituents of foods were essential in the diet.
In Asia, beriberi, a polyneuritis condition, afflicted prisoners in some prisons in Indonesia but not in other prisons. A Dutch physician, Conrad Ejikman, was tasked with identifying the cause of beriberi. It was noted that chickens grown in prisons where beriberi was prevalent in prisoners developed a similar condition. Chickens were fed left-over food that was mainly cooked rice. In prisons where unpolished (brown) rice was fed, neither prisoners nor chickens developed polyneuritis, whereas, in prisons where polished (white) rice was fed, beriberi was present in both prisoners and chickens. Many years later, the active, anti-beriberi substance in rice polishings was found to be thiamin, the first B vitamin to be identified.
Sailors deprived of fresh food on long sea voyages developed a disease condition called scurvy. Although it was known for centuries that consuming fresh citrus fruits cured scurvy, the mechanisms involved were unknown. One theory was that sea air clogged skin pores preventing the escape of poisons and that pores were unclogged by eating lemons and limes. Many decades later, the active material in limes and lemons that prevented scurvy was identified as ascorbic acid, named Vitamin C.
The next stages of nutrition research led to the discovery of essential dietary nutrients and influenced fish, animal and human nutritional concepts to this day. Foods that cured nutritional diseases were subjected to various processes to characterize active fractions, such as assessing sensitivity to heat treatment or whether the activity was reduced after extraction with water or other solvents. This approach produced concentrated extractions fractions that were added to diets containing purified ingredients to determine if they contained nutritionally essential components. Further fractionation and refinement showed that some fractions contained several compounds that were necessary to support animal growth and health. This highly productive and intellectually challenging period in nutritional science is summarized in a wonderful series of review articles by Carpenter (2003a; 2003b) that contain interesting details and historical contexts for their interpretation. These articles should be essential reading for any aspiring fish, animal or human nutritionist.
First aquaculture nutrition studies
Although aquaculture is an ancient practice, writings about fish feeds only began to appear in the second half of the 19th century associated with trout and carp farming in North America and Europe (Stickney, 1996). Farm-made feeds were produced using locally available materials, such as organ meats from animal slaughterhouses, milk curd, fresh chopped fish and chicken eggs (Rumsey, 1994). Feeds were generally combinations of wet and dry ingredients, so the aim was to combine ingredients that increased the water stability of feed particles. Commercial pelleting practices had not yet been adopted, so it was important that feed particles stuck together long enough to be consumed by fish.
One concept that influenced feed formulations for trout was based on the observation that wild trout were carnivores, consuming insects, aquatic invertebrates and small fish. An early study of the natural diet of trout involved analysis of the stomach contents of wild fish (Embody & Gordon, 1924). They found that the diet of wild trout was 49% crude protein, 15-16% fat, 8% crude fiber, and 10% ash, expressed on a dry weight basis. Therefore, the thinking went, trout feeds should only contain animal-based ingredients that were formulated to have a similar proximate composition.
It was several decades before fish culturists changed their thinking and began to accept the concept that fish feeds should be formulated to supply essential nutrients (Page, 1985, cited by Rumsey, 1994). Even then, it was essential to supplement trout fed early feeds with fresh animal liver to support fish growth and prevent mortality.
At first, two active fractions extracted from foods were prepared and called fat-soluble A and watersoluble B. The unknown compounds in the extracts were named vitamins by the pioneering nutrition researcher, Dr. Casimir Funk, because they were thought to be derived from ammonia and were thus “vital amines” (Funk, 1912). Each extract was thought to contain a single compound essential to life, but subsequent refinement of extracts showed
that each contained multiple active components. Fat-soluble A was found to contain four vitamins, A, D, E and K, known as the fat-soluble vitamins. The water-soluble B extract contained a number of vitamins that were named in order of discovery, i.e., B1 (thiamin), B2 (riboflavin), B3 (niacin), B5 (pantothenic acid), B6 (pyridoxine) and B7 (biotin), B9 (folic acid) and B12 (cobalamin).
It took decades of research to identify and characterize all of the B vitamins, with the structure of the last one, B12, only being described in 1948. Each vitamin appeared to have one or more specific and distinct functions in metabolism that were essential for normal growth and health in animals, poultry and humans. When any of these vitamins was omitted from the diet, clinical deficiency signs appeared that could be cured by restoring the vitamin to the diet.
A major development in fish nutrition was the establishment of the research partnership between Cornell University, the New York Department of Conservation and the U.S. Bureau of Fisheries. A fish nutrition research program was located at the Cortland National Hatchery in New York and began in 1932. Cornell University was a leader in poultry nutrition research, and this influenced the direction of fish nutrition research. Scientists at the Cortland Hatchery adopted the rigorous approaches and new technology in used in animal and poultry nutrition at Cornell. However, fish proved to be challenging research subjects because they are poikilothermic and are thus sensitive to water temperature as well as other environmental conditions, such as photoperiod and water quality. Also, due to their aquatic existence, fish excrete ammonia rather than urea from protein metabolism. Further, they obtain some essential minerals directly from rearing water and must maintain internal electrolyte homeostasis in either hypotonic or, for marine fish, hypertonic conditions. These factors made it necessary to modify methods and approaches used in nutrition research with chickens, rats or other laboratory animals. Another potential complication with fish nutrition research was that fish cannot be fed ad libitum like rats or chickens. Researchers must decide how much and how often to feed fish, and differences in these experimental factors complicate the interpretation of fish nutrition studies, especially between different laboratories.
Development of practical fish feeds
Up until the late 1950s, feeds for hatchery-reared trout and Pacific salmon were made on-site at federal, state and private hatcheries using locally available ingredients. Since the nutritional requirements of fish were not yet known, except in broad terms, feed formulations were developed by trial and error by state and federal fisheries agencies, and university researchers. Successful feed formulations supported fish growth, maintained rearing water quality and prevented clinical signs of nutritional deficiencies. As mentioned, fresh byproducts of animal slaughter, mainly beef liver, were considered essential dietary constituents to prevent nutritional deficiencies.
Feed formulations were developed empirically, rather than on the basis of meeting nutritional requirements which were, at the time, unknown. For example, in the first feed evaluation trial conducted at the Cortland Hatchery, these four diet formulations were tested: • Diet 1: 50 parts cottonseed meal, 50 parts raw beef liver and 50 parts dried skim milk • Diet 2: 50 parts cottonseed meal, 50 parts raw beef liver and 50 parts dried buttermilk • Diet 3: 50 parts cottonseed meal, 50 parts raw beef heart and 50 parts dried buttermilk • Diet 4: 50 parts cottonseed meal, 50 parts raw beef spleen and 50 parts dried buttermilk
The next round of trials was conducted using similar formulations but containing skim milk from different suppliers, substituting peanut meal for cottonseed meal and replacing beef parts with sheep plucks (viscera) or with dried meat scraps. From this work, researchers concluded that skim milk and buttermilk were similar in value as ingredients. However, trout health could not be maintained longer than eight weeks unless feeds were supplemented with raw animal liver or heart (Cortland Hatchery Report Number 1, 1932). Over the next 10 years, 809 feed ingredients were tested in trout feeds at the Cortland Hatchery to develop formulations that hatchery personnel could use to prepare economical diets from commonly available ingredients. However, no matter what combination of dry ingredients was used, raw beef liver or other organ meat had to be included in the diet to prevent early mortality of the trout.
Nutritional requirements
Concurrently with studies to develop feed formulations for hatcheries, research was being conducted to explore the nutritional requirements of trout. For example, Tunison and McCay (1935) investigated the calcium requirement of trout, showing that the calcium content in the whole body of trout was higher than could be accounted for by the calcium content of the feed they consumed. They concluded that trout could obtain calcium directly from rearing water. A major research topic was anemia, a widespread problem in hatchery-reared trout and salmon that was prevented by feeding beef liver. Liver was thought to contain an anti-anemia component that McCay named Factor H. Six B vitamins (thiamin, riboflavin, pyridoxine, pantothenic acid, niacin and biotin) were hypothesized to comprise Factor H. However, supplementing these vitamins in a trout diet, either alone or in various combinations, did not prevent anemia (Cortland Hatchery Report, No 13, 1944).
At that time, folic acid, an essential vitamin, had not yet been identified. Beef liver is a rich source of folic acid and was therefore hypothesized to be Factor H but later studies in which folic acid was supplemented with semi-purified diets did not prevent anemia in trout. It was only after vitamin B12 was shown to be the missing nutrient associated with Factor H that anemia could be prevented without supplementing trout diets with the fresh liver.
The 1920s and 1930s were a period of rapid progress in the discovery and characterization of vitamins, facilitated by using rats and mice as experimental animals (Carpenter, 2003c). Researchers used semipurified diets to determine requirements for individual vitamins and, in the late 1940s, fish nutritionists adopted this approach, first using semi-purified diet formulations that were used in rat nutrition studies. However, rat semi-purified diets consisting of casein and sucrose with vitamin and mineral supplements proved unsuitable for trout unless the fish received dried beef liver (McLaren et al., 1946). After much trial and error, a semi-purified diet that supported trout growth and health in short-term studies was developed (McLaren et al., 1947). Using this diet, B vitamins and ascorbic acid were omitted one-by-one from the vitamin mixture and added back in increments to the experimental diets, enabling scientists to make broad estimates of dietary requirements of trout for thiamin (1-10 mg/kg), riboflavin (5-15 mg/kg), pyridoxine (1-10 mg/kg), pantothenic acid (10-100 mg/kg), nicotinic acid (1-5 mg/kg), choline (50-100 mg/kg), inositol (250-500 mg/kg), biotin (0.05-0.25 mg/kg), folic acid (1-5 mg/kg) and ascorbic acid (250-500 mg/kg).
To estimate the vitamin requirements of trout more accurately, semi-purified diets had to be vitamin free. The semi-purified diets used in the McLaren et al. studies were not totally vitamin free because they contained casein and crab meal; both contained low levels of vitamins. An improved semi-purified diet was developed that contained casein, gelatin, lard, cooked potato starch, cellulose flour and minerals (Wolf, 1951). This diet supported trout with reasonable growth rates over a 25-week period equivalent to contemporary practical diets that contained beef liver. Commercial casein still contained small amounts of B vitamins, but when vitamin-free casein, made by extracting casein with solvents, was substituted for commercial casein, trout refused to eat the diet.
Regarding the need for vitamin supplementation, Wolf (1951) reported that deletion of pantothenic acid resulted in cessation of growth and gill disease after eight weeks of feeding. Likewise, deletion of folic acid slowed fish growth and resulted in anemia, and deletion of vitamin B12 resulted in fish mortality and anemia.
While the Cortland Hatchery was focusing on trout nutrition, salmon nutrition became a focus of study in the western USA at the University of Washington, Oregon State University and at regional state and federal fisheries agencies, notably the US Fish and Wildlife Service. Starting in the 1930s, dams for irrigation and hydroelectric power generation were constructed on major rivers in the western USA, especially in the Columbia River system. The dams blocked a large proportion of Pacific salmon migratory corridors to upstream spawning and juvenile nursery areas.
To mitigate the loss of spawning and salmon nursery habitats, many hatcheries were constructed, creating a need to provide suitable feeds to rear juvenile Pacific salmon for release as smolts to maintain salmon fisheries. Chinook (Oncorhynchus tshawytscha) and coho (O. kisutch) salmon were the main species raised in hatcheries, although sockeye salmon (O. nerka),
Check out worldwide industry job openings at Aquafeed.com Job Center
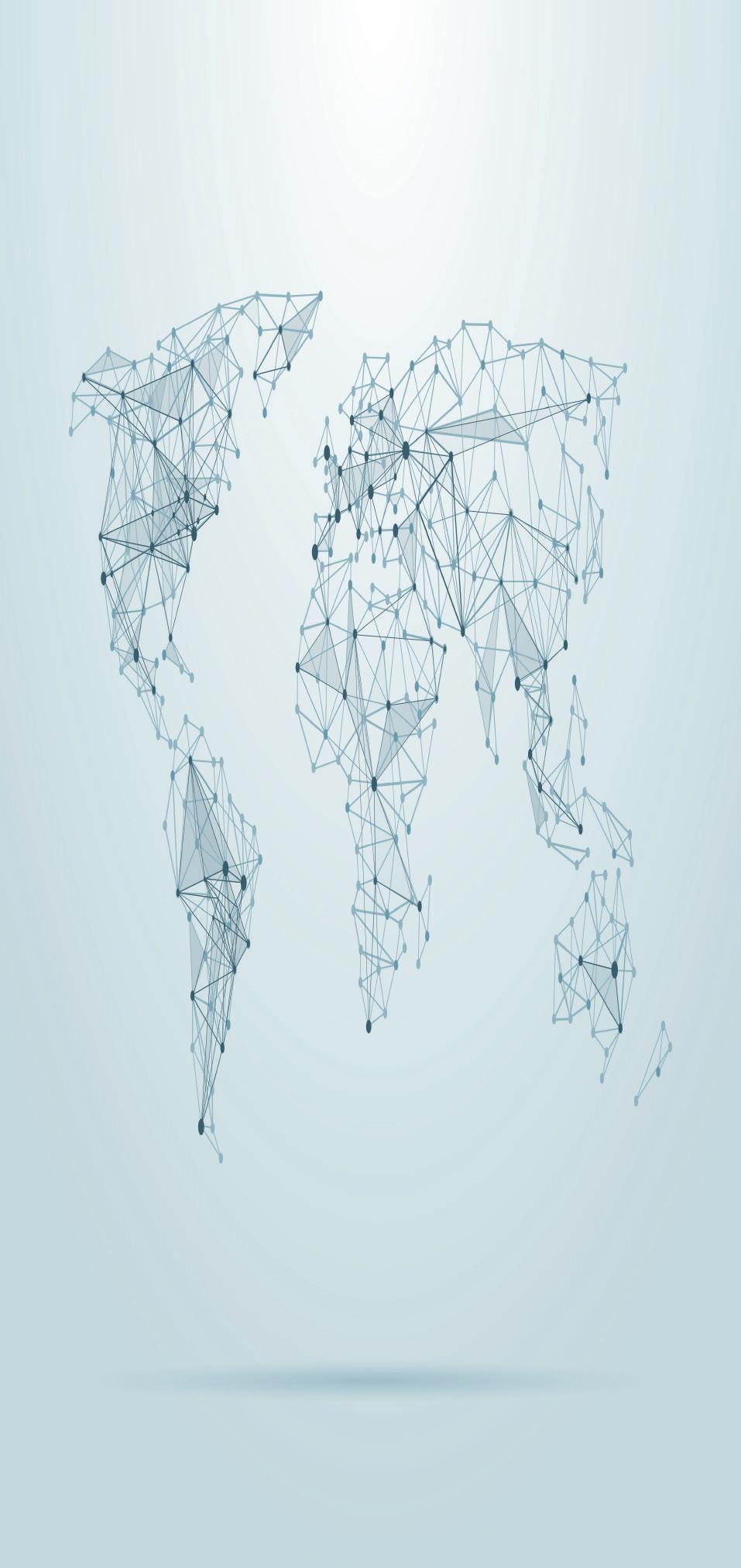
www.aquafeed.com
chum salmon (O. keta) and pink salmon (O. gorbuscha) were also raised. After spending several years at sea, salmon migrate to coastal areas where they enter commercial, tribal and recreational fisheries. A high smolt to adult return ratio was the primary goal of hatchery programs, and as a result, the focus of fish nutrition research was on developing feed formulations to produce healthy, robust juveniles likely to survive after hatchery release. The cost of feed was secondary to the goal of producing smolts that contributed to fisheries. Feeds cannot be precisely formulated without information on the nutritional requirement, so research focused on identifying the dietary nutritional requirements of juvenile salmon.
The substantial investments made in research capacity led to the development of standards for fish nutrition research worldwide during the second half of the 20th century, providing the foundation for the development of the global aquaculture industry decades later.
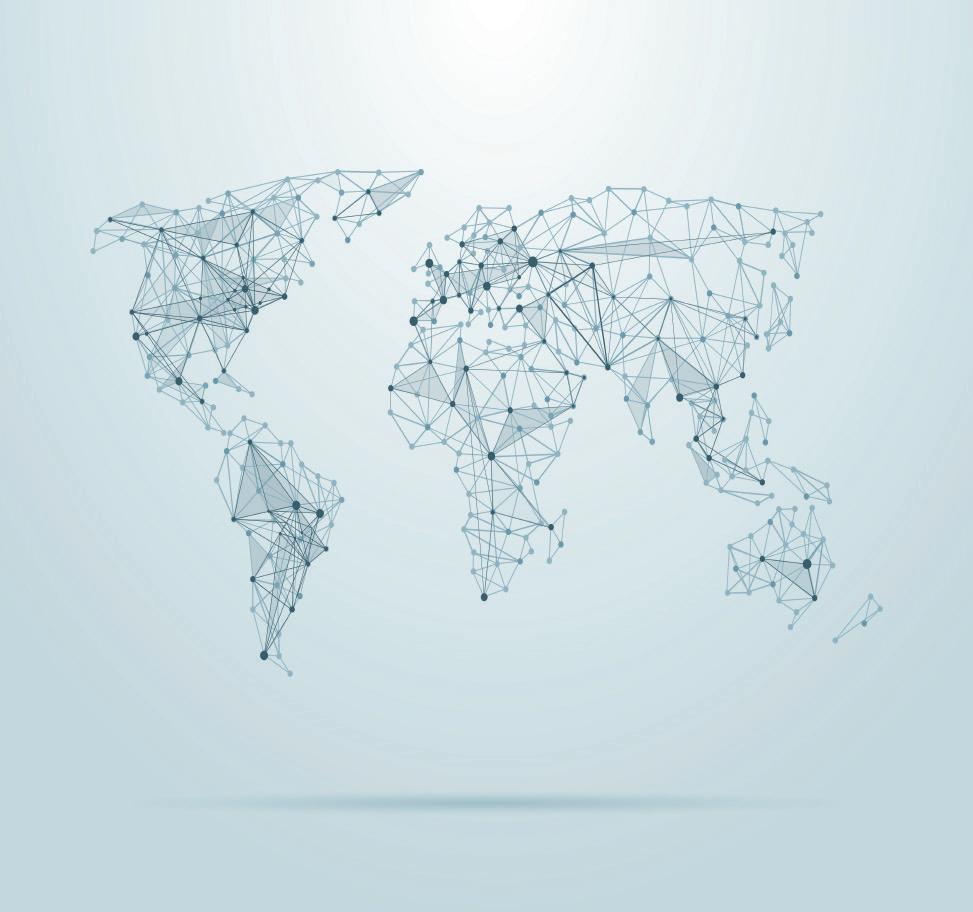
References available on request.
XX INTERNATIONAL SYMPOSIUM ON FISH NUTRITION AND FEEDING TOWARDS PRECISION FISH NUTRITION AND FEEDING
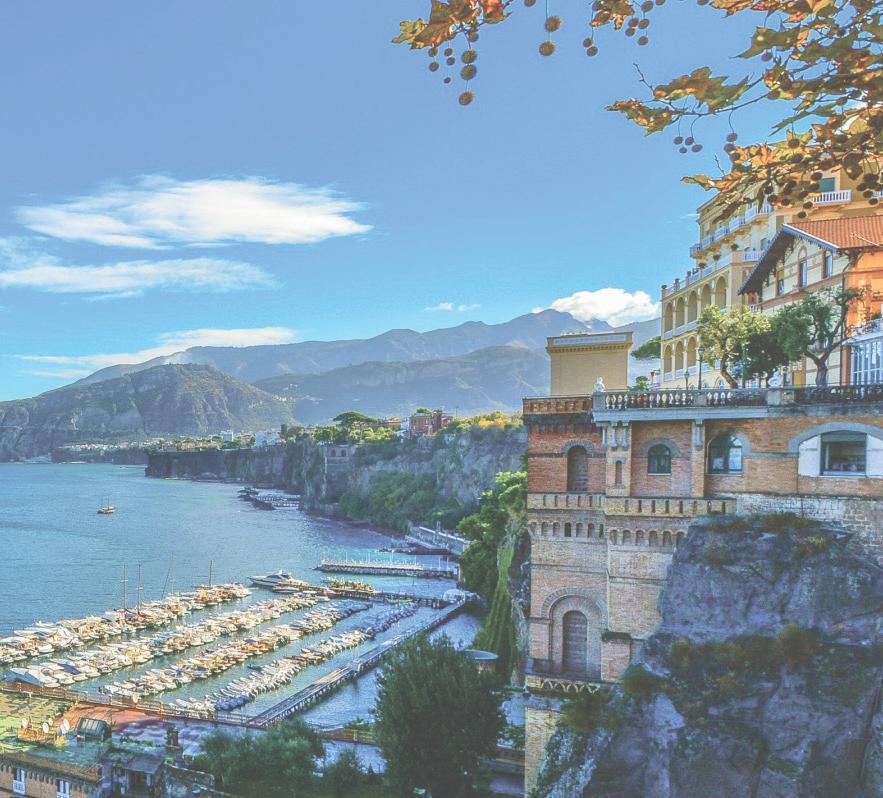
5th - 9th June 2022 | Sorrento, Italy
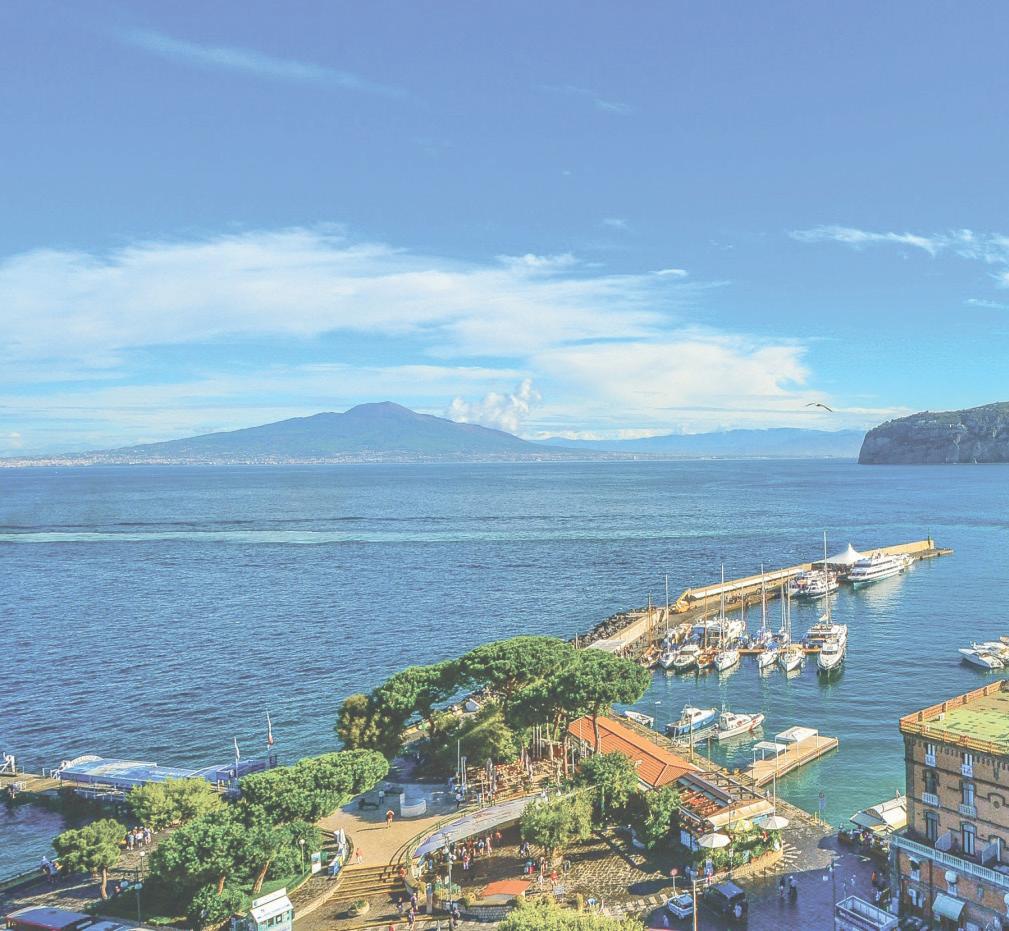
Chair: Alessio Bonaldo, University of Bologna
ORGANIZING SECRETARIAT
VET INTERNATIONAL SRL FRANCESCA.MAZZUCCHELLI@VETINTERNATIONAL.EU
www.isfnf2022.org
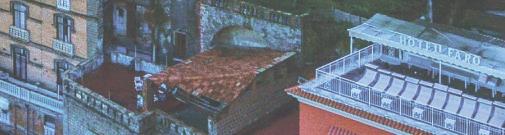