BREEDER MANAGEMENT AND NUTRITION
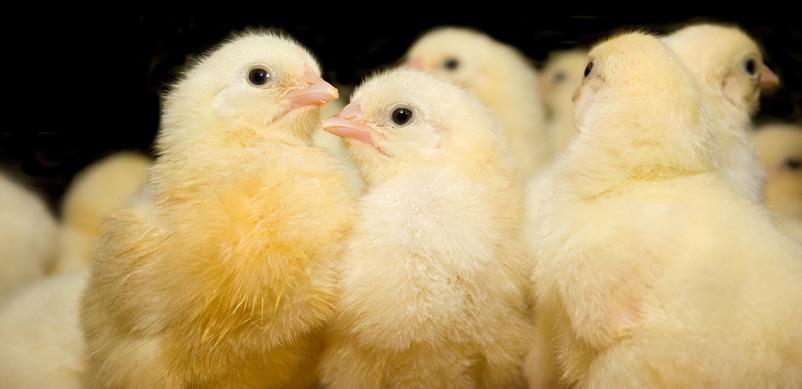
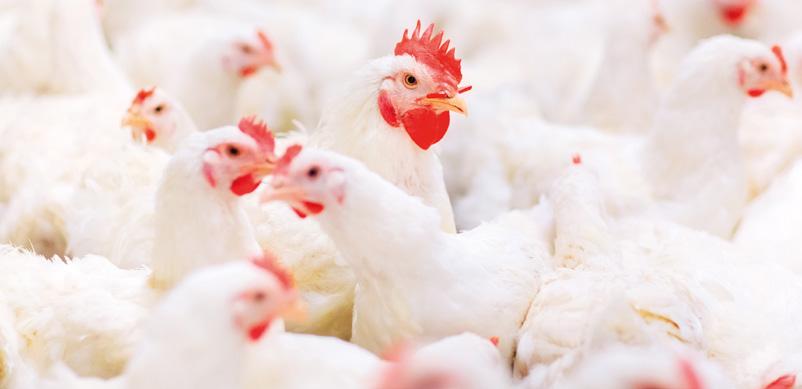
Edit: Novus International, Inc
Design: Grupo Editorial Agricola Española Henar Comunicación
ISBN: 978-84-17884-25-3
Deposit: M-19690-2022
The views and opinions expressed by the third-party authors in this book are those of the authors and do not necessarily represent those of Novus International, Inc.
The idea for this book on broiler breeders was launched soon after the Novus Key Opinion Leaders Breeder Workshop held in February 2019 where several experts addressed fundamental questions such as, “Have we already reached the full potential of broiler breeder production?” and “Are there multi- and trans generational ways to create profitable broilers?”. It was also clear during the event that parent breeder populations play a pivotal role in the poultry sector.
The workshop was a success notwithstanding the fact that the group con cluded that not all issues in broiler breeder production have been solved. Hence, the necessity to summarize relevant information in a reference book that could also serve as the basis for continuing the discussions regarding further optimizations of breeder reproduction and offspring performance.
This book is perhaps needed now more than ever as we see the escalating increase in meat protein production and consumption worldwide. This in crease in meat production and consumption is happening at an even faster pace than increases in the global human population. It has also become evident that the production of poultry meat has overtaken that of pork meat and, surely, of red meat. To meet this demand for poultry meat (main ly broiler), it is obvious that the pillars of the poultry industry, including companies providing breeding and genetics, nutrition, climate control and housing (including precision livestock farming), disease control and pre vention, and finally parent reproduction performance and incubation must act together in the effort of providing safe, healthy, affordable meat to the market. In addition, attention must be paid to the economic and societal impacts, animal welfare and ethics, and, perhaps most importantly, to the overall sustainability of the poultry sector.
It was decided at the start of creating this book that the contributions of international experts from the academic world, the industry, and breeding
companies be represented in a balanced way. With respect to the latter, we are extremely pleased to have the collaboration of some of the major broiler breeder and light breeder companies explaining their particular viewpoints and perspectives.
Due to the complexity of our sector, it was important to provide a sound structure of the topics addressed. You’ll see a clear distinction was made be tween quantitative and qualitative feeding strategies for breeder populations during rearing as well as during the laying period and providing details on their distinct effects on breeder reproductive performance, behaviour, and welfare. Addressing the importance of the male breeder, breeder diseases and control, recent developments in precision feeding, and breeder macroand micronutrient requirements were also considered paramount.
The avian world has recently shown more interest in the concept of epi genetics. Is it possible to alter the performance, immunity, physiology, be haviour, and welfare of the next generation (multigenerational) or of genera tions (transgenerational) of broilers by manipulating the parent population in one way or another? This question is considered in detail in this reference book.
The scientific coordinators acknowledge the Centre for Agriculture and Bioscience International (CABI) for allowing us to include the chapter compiled by Aviagen experts previously published in a CABI book as part of this Novus reference book unaltered.
Along with Novus International, the scientific coordinators are much in debted to all the experts from different fields for their valuable contribu tions. Without their contributions, it would not have been possible to com pile this reference book titled “Breeder management and nutrition: Moving the industry forward”.
Special thanks to the communications team who participated in the cre ation, organization and supervision of the contents that made the launch of this book possible, Susana Candel, Elizabeth Davis, Megan Hayes, Raquel Vázquez and other Novus colleagues.
Enjoy your reading!
Prof. Johan Buyse, KU Leuven Belgium Sandrine Durox, Novus International, Inc Dr. Silvia Peris, Novus International, Inc
1/ Global (poultry) meat production: a holistic view.
Eddy Decuypere, PhD
2/ Quantitative feed restriction and reproductive performance.
Aitor Arrazola, PhD
3/ Effects of breeder feed restriction on behaviour, welfare, reproduction, and health.
R.A. (Rick) van Emous, PhD, and A.J.W. (Annemarie) Mens, MSc
4/ The effects of qualitative feed restriction on performance, behaviour and welfare of broiler breeders.
Henk Enting, PhD
5/ Trace mineral nutrition in breeder hens.
Stanislaw Budnik, PhD, and Silvia Peris, PhD
6/ Macrominerals: calcium, phosphorus, and electrolytes.
Hugo Romero-Sanchez, PhD, and Dinabandhu Joardar, PhD
7/ Housing, equipment and management of broiler breeders.
R.A. (Rick) van Emous, PhD
8/ Management and nutrition of the male breeder.
Edgar Orlando Oviedo Rondón, PhD, and Hugo Romero-Sanchez, PhD
9/ Maternal programming of the offspring.
Rebecca Forder, PhD
10/ Harnessing epigenetics to improve chick quality with trace mineral zinc.
Juxing Chen, PhD, and Mercedes Vázquez-Añón, PhD
11/ Opportunities for multi- and transgenerational feeding strategies for sustainable broiler breeder management. Johan Buyse, PhD
12/ Poultry breeding for sustainability. Anne-Marie Neeteson, Santiago Avendaño, PhD, and Alfons Koerhuis, PhD
13/ Breeder management during rearing: a Cobb perspective. Juan Carlos Abad and Robin Jarquin, PhD
14/ Layer breeding: past, present and future perspectives. David Cavero Pintado, PhD, and Xabier Arbe Ugalde, PhD
Conclusion:
Johan Buyse, PhD, KU Leuven Belgium Sandrine Durox, Novus International, Inc Silvia Peris, PhD, Novus International, Inc Hugo Romero-Sanchez, PhD, Novus International, Inc
EDDY DECUYPERE, PhD., is the professor emeritus of the Physiology of Domestic Animals at KU Leuven (Belgium) and holds the title of extraordinary professor at several universities globally. Attending university at KU Leuven, Dr. Decuypere obtained his master’s degree in Zoology in 1969, his master’s in Agronomy in 1972, and his doctorate degree in Agricultural Sciences in 1979. From 1974 to 1977, he worked in Rwanda, Africa where he was responsible for agricultural practice and applied research in a developmental project. Dr. Decuypere spent his post-doc (1979-1980) at Rutgers University (United States) and Arizona State University (United States). From 1979 onwards, he was a research fellow of the Belgian National Fund for Scientific Research (NFWO), and then served as Research Director at NFWO until 1988 when he returned to KU Leuven. Throughout his career, Dr. Decuypere has supervised 45 doctoral students and led 35 scientific projects, primarily in poultry research. He has published more than 850 papers in international peer-reviewed journals and many book chapters and review papers. He is the recipient of the Tom Newman Memorial Award, the Gordon Memorial Award, Doctor Honoris Causa at the Veterinary University of Budapest, Fellow of the Japanese Society for the Promotion of Science, the Belgian Francqui Chair, and the Farner Medal for Avian Endocrinology.
Within the boundaries of the planet Earth, agriculture plays an essential role in the production of renewable resources for the needs and desires of the growing human population.
This increasing demand of agricultural output has to match with a finite agroproduction input such as soil, water and nutrients, which is the core of the concept of sustainability. Therefore, ethical dilemmas in the future will be the competition between different uses made of agricultural biomass production. The shift in consumption pattern towards an increased proportion of animal products, and in spite of the world population growth, is mainly triggered by increasing per capita income invariably followed by increasing consumption of animal products. Hence, a thorough discussion about the efficiency of animal production, also from an energetic point of view, is of great and crucial importance for further evaluation of the sustainability of animal production. For the latter, the entire feed and food chain should be taken into account, not only from farm to fork but also from fodder to farm.
Efforts can and have been made in many aspects of poultry production, such as improving lifetime performance, reducing feed loss and waste, increasing efficient use of natural resources and by-products, reducing emissions, etc. All of these attempts contribute to a relative uncoupling of growth from the use of resources, but sustainability should remain aware of the broader, holistic perspective of sustainable development as well as of the limited character of ecological resources.
Demand and offer. Challenges for the future context of the animal production.
Within the boundaries of planet Earth, agriculture plays an essential role in the production of renewable resources for the desires and needs of the growing human population.
Agriculture played, and still plays, an important role in the history and evolution
of humanity. In a world of hunter-gatherers only about a couple of hundred million people could be fed (Mayozer and Roudart, 2006), but agriculture made it possible to change the ecological equilibrium between the human species and the systems they relied on, allowing the human population to grow to more than 7.5 billion people and increasing daily. Agriculture did not only influence the total number of people but also changed their way of living, mostly as a consequence of sedentarity, following the initiation and
development of agriculture. The still-growing human population leads to increasing demand for food, animal products, energy, raw materials for construction, clothing, chemical industry, etc. Although awareness about caring for our planet is rising, increasing income and wealth makes it possible to not only fulfill our needs but also encourages consumers to create unnecessary but desired demands (e.g., Fresco, 2008). The question then arises, how can this increasing demand on agricultural output match the finite agroproduction input, such as soil, water, and nutrients?
Inevitably this leads to the question of sustainability, its definition, and the consequences for choosing to use agricultural products (including sylviculture). Indeed, although food production was the main goal, and still is the most important one, human society has long since learned that biomass (plants, animals, and forestry) could be used for other purposes than food production. A good overview of the use of agriculture biomass, including sylviculture, cereals, vegetables, sugar culture, tuberales, and root products, fruits and oil plants was published by Morrison (2016).
Apart from use as an energy source (mainly forestry products, sugar plants, and cereals for biofuel) about two-thirds of the biomass (in millions of tonnes) of all other agriculture products are used for food is for direct human consumption, while one-third is for feed use as basic agriculture commodities produced to feed animals. In 2011 worldwide, this meant a production of 3.340 million tonnes of biomass for food and 1.510 million tonnes for feed (Morrison, 2016). However,
taking into account that land can be used for agriculture, one-third is arable and two-thirds is available for pasture use only, and one-third of total biomass produced on arable land is for animal feed, the impact of animal production in terms of sustainable choices may be even greater than expected. Therefore, as discussed by Boonen et al. (2012a,b) and Boonen (2015), animal production can and may play an essential role in producing food in so far as it can maximally exploit “useless” land or convert “useless” energy or proteins.
Although during the last decades agriculture production strongly increased, still more than 800 million people suffer from hunger. With a global population that will go up to 9 or 10 billion people by 2050, focusing on increasing food production alone will not solve the hunger problem. One of the important ethical dilemmas of the future will be the competition among the different functions or uses of agricultural biomass production. There is no scientific solution for the question of how these functions or uses have to be balanced since this strongly depends on personal values, desires, and needs influenced by one’s sociocultural background and worldview. Therefore, this discussion has to be placed in a broader, philosophical-ethical perspective.
When observing the change in human populations as well as the changing consumption pattern of food over a sufficiently long period (40 to 50 years), world meat consumption is increasing faster than the global population increase, indicating a shift in consumption pattern to an increased
proportion of animal products in the diet worldwide. This is reflected in the worldwide per capita consumption of cereals and meat over the period 1960 to 2000. In 1960, the per capita cereal production was 280 kg and meat about 20 kg, while in 2000 the per capita cereal and meat production was around 340 kg and 38 to 40 kg, an increase of respectively 20% and 100%.
This shift in consumption pattern towards an increased proportion of animal products, and in spite of word population growth, is mainly triggered by increasing per capita income invariably followed by increasing consumption of animal products. This phenomenon can be repeatedly observed, in different historical periods in different parts of the world. How can this trend - a shift in consumption pattern towards more animal products - be reconciled with the fact that 800 million or close to 1 billion people are chronically underfed? It indicates at least that the problem is not just production, but efficient production which can and will help, especially where land use for feed/ food production is concerned.
There are many opinions regarding the supply of food for the human population. In the scientific-technological realm, it is said that we have to produce more food without areal expansion in order to save the natural environments left. So, solving the hunger problem has to be realized by applying modern technologies. On the other hand, many organizations (many nongovernmental organizations (NGOs) to a more or less extent) reject the so called “green revolution” and other modern technologies.
According to those who advocate rejection of these technologies, these are not sustainable, they increase the gap between the poor and the rich, and, moreover, there is too much animal production (e.g., Fresco, 2008).
Although there are conflicting ideas on how to address the problem of world hunger and the increasing demand for food worldwide, most people agree on the following important strategies:
- Limit the extension of agricultural land at the expense of forest, especially in tropical areas
- Increasing food supply for all by reducing waste and through more equitable distribution
- Increasing the efficiency of water use and nutrient use for crop production
- Increasing total production as well as production efficiency of crops via optimization by using the genetic potential of crops as well as by increasing this genetic potential
This leads us to the question: What about animal production and its efficiency within this broader framework? The increasing demand (including animal products) but limited production capacity, limited water, fertilizer, soil, and ecosystem of Earth, exacerbates the questions about animal production, its efficiency and legitimacy.
From a fundamental biological-ecological point of view, it is well known that in
terrestrial food pyramids about 10% of the energy of one level is incorporated in the next level. This means that from all vegetation used by herbivores about 10% is transformed in their body into energy, and roughly the same is true for carnivores eating the herbivores. When calculated for the efficiency of different animal husbandry species, at population level (including all young and adult, males and females, and replacement breeders, just as in natural populations) the following efficiency ratios for energy and protein were reached (Table 1. Van Es, 1975).
So, it can be argued that if humans behave more as herbivores, 5 to 10 times greater human population could be fed. However, this is an oversimplification that was elegantly pointed out by Van Es (1975) and contradicted by his calculated efficiency ratios for animal production.
Van Es (1975) stated that digestibility as well as the metabolisability of feedstuffs in the different species of our farm animals is not identical, compared to each other as well as with humans when they eat the feed. Therefore, it’s not the total energy content has to be taken into account, but the true metabolisable energy (ME) for the different levels in the food pyramid. Only through this
way can an estimation be made as to if passing the feed through the animal production chains means a loss or gain, and to what amount in the different animal husbandry species. Of course, this has to be done at the population level, including pregnancy, lactation, youngsters for slaughter or replacement, etc.
The ratio for this estimation is available ME for humans in the animals or animal products (numerator) divided by the available ME for humans if they can and will consume the feedstuffs given to animals (denumerator).
For these calculations some assumptions were made:
- Humans cannot nor will use fodder such as grass, hay, and straw as a source of energy for themselves for the simple reason that it is not digestible for monogastrics.
- Using concentrates for pigs and poultry, it is estimated that about 60% respectively 75% could be used by humans, while in cows this is only 50%, taking into account the less digestible (for monogastrics, including humans) components used for ruminants. Of course, using milk products for intensive veal production is 100% compatible with direct human use of these products.
Table 2. Available ME for human food as a ratio: ME from animal products /ME directly available to humans from feed but given to animals (Van Es, 1975; Boonen, 2015)
Food shortage
Full supply
Boonen (2015)
supply
Pig
Broiler
Layer
Dairy cow
Intensive meat cattle
Extensive meat cattle
- Using the ME ratios to calculate the digestibility coefficients for different feedstuffs in the case of direct human use were those calculated for pigs considering their comparable digestive system, hence also in digestibility.
- What humans can and especially are willing to eat can vary greatly over time and geographical distribution and will depend also or mainly on supply or shortage (e.g., war, famine).
Van Es (1975) calculated for each of the rations of available ME energy and digestible protein two ratios, one in the event of food shortage and one for full food supply. These calculations were reevaluated by Boonen (2015) taking into account new data about animal productivity and reproduction capacity, new feed sources, and feeding management types. Also, the influence of what we consider “edible” was taken into account but a thorough discussion of this aspect is out of the scope of this chapter. It may be noticed that in his calculations as presented here compared with the older data from Van Es (1975), the use of soybean meal has not been considered as
competitive for direct human food (although it could be in principle).
Based on data on the ecological energetic efficiency as calculated for husbandry species, monogastrics are more efficient than large ruminants. This is largely to do with reproductive efficiency. Based on these figures, one could agree it is more sustainable to use pig and poultry production for meat instead of cows or sheep, and this is beside the higher greenhouse gas emission for the latter per kg of meat produced. However, taking into account Van Es’s (1975) logic behind the efficiency of ME-use for human food, by directly eating plant production or indirectly after conversion to animal products, it is clear that ruminant meat consumption is far more energetically efficient, and in some cases may even constitute a gain of ME-use for human food consumption.
Focusing on a sustainable poultry production, two statements can be made for which there is now a universal agreement:
1. The livestock sector poses severe pressure on the environment (air, water, soil) and competes for scarce resources (land, water, …) for the production of feed.
2. The production and utilization of feed are the dominant factors determining environmental impacts of pig and poultry production, e.g., crop productivity and animal productivity.
An endless (economic and production) growth on a finite planet with limited resources seems to be therefore a “contradictio in terminis”. To escape this dilemma, an uncoupling of growth from the use of resources is advocated. A relative uncoupling is realized by increasing the efficiency of production. However, if the increase in growth is bigger than the increase in efficiency, then there is no absolute uncoupling at all and no sustainability of growth in the long term. The latter is the case for most domains of economic activity, including poultry production (considering the rapid increase in poultry and egg production worldwide).
Many efforts have been and are made in many aspects of livestock production e.g.:
- Increased land-use efficiency, which is important to reduce land-use requirements.
- By-products from arable production or from the food processing industry are important to reduce the environmental impact of livestock production. The feed industry should collaborate with the food- and biofuel industries to optimize the allocation of biomass streams between sectors. Efficient livestock production has to take the whole
feed/ food chain into account, not only from farm to fork but also from fodder to farm.
(Hendriks et al., 2019).
- Improvement of livestock production through genetic improvements, through more appropriate nutritional requirements, through elimination of mycotoxins, better sanitary conditions, etc.
In view of the competitiveness of poultry production with human food security, its environmental impact, as well as welfare and product quality issues, sustainable poultry production is under increased scrutiny from the value chain, policymakers, and associated stakeholders. This means that continuing to operate as we have done in the past is not an option.
The challenge we are facing requires new technologies, new business models, and especially new thinking in order to create a more sustainable industry including poultry production, protecting the planet, economically viable and socially responsible.
Many initiatives and actions can be listed, based on new approaches to business, greater collaboration through the value chain, and greater use of technologies.
Nickell and Saviani (2021) listed 6 key sustainability platforms in their recent contribution to sustainable animal production. Herein I include some concrete possible examples for each of these platforms for poultry production:
Laying hen selection to achieve a longer laying cycle is a good example; often it is said to increase the “longevity” of laying hens, but since the biological lifetime of chickens can be 6-10 years, we still make suboptimal use of this potential. The fact that laying hens are depreciated after only 1 year (more or less) of lay due to the current business model and requires new thinking.
Even in situations of high feed costs and low egg prices where the choice for a second and eventually third laying cycle is obvious (and can be calculated as beneficial for the farmers if the rejuvenation technique of artificial molting is done correctly), the contracts between farmers, pullet producers, hatcheries, etc., do not allow it.
In the aftermath of mad cow disease (bovine spongiform encephalopathy or BSE), a disequilibrium between our concept of food/ feed safety and food security has invaded the minds of consumers and many policymakers. Blood, feather meal, and slaughter waste could be excellent sources of animal proteins in feed, at least when properly treated. Moreover, these can be used as a substitute for fish meal and soybean meal. The cry, “No cannibalism!” in this context is a false paradigm.
A wealth of information on reducing nitrogen emissions is available. Techniques for reducing ammonia emission in the ventilation air from poultry houses by washing the air is just an example of a social responsibility of poultry farms.
Considering the shortage of phosphate resources in the world, and the way phosphates are overloading fields and surface waters in production areas densely populated with poultry (and swine), the use of phytase enzyme could greatly reduce the need for additional phosphate in the rations of poultry, especially of laying hens.
4. Making efficient use of natural resources
For example, new and greater use of byproducts of other agricultural speculations (e.g., byproducts of bioethanol or biofuel production) as well as algae production and/ or insect production for high-quality poultry feed supplements.
5. Reducing our reliance on marine resources
This is related to point 2 (Reducing food loss and waste). Make use of these waste products as a source of high-quality animal protein by substituting e.g., fish meal in poultry rations. Also, aquaculture as a source of DHA (docosahexaenoic acid) or EPA (eicosapentaenoic acid) for producing enriched eggs/meat in omega-3 or -6 fatty acids, should be replaced by marine algae production of these ingredients.
6. Helping tackle antimicrobial resistance
Smart nutrition leading to eubiotic solutions can possibly minimize or eradicate the subtherapeutic use of antibiotics. A wealth of recent poultry research in Africa, Asia, and Latin America is focusing on new ingredients, and natural plant products as alternatives for antibiotics, anticoccidials, anthelminthica (e.g., papaya-seeds, moringa oleifera leaves, powder or extracts, etc.).
All these examples, and many more, contribute to a relative uncoupling (and increased efficiency), but not necessarily to the long-term sustainability of production if the overall growth in poultry production overrides the growth in efficiency.
Sustainability from a holistic perspective, including sustainable poultry production worldwide, has to take into account many fundamental aspects listed as the 17 sustainable development goals of the United Nations (UN, 2020). Livestock production is at least included in 6 or 7 of these goals. The relative importance of several of these goals in relation to livestock production is shifting in time and according to the worldview of individual persons or communities. This could be observed already in the shift in livestock policy objectives in relation to economic development as published by the Food and Agriculture Organization in 2006 (FAO, 2006). In a 4-factor analysis, 4 aspects were taken into account for their importance in 4 different societal development conditions. These factors are, 1) food supply, 2) social/ poverty concerns, 3) food safety, and 4) environmental impact. While in low development conditions with a large number of smallholder farms, food supply, and social/poverty concerns are by far the most pressing factors, this concern shifts during a beginning or rapid industrialization phase to food safety and environmental impact. In a post-industrial society, food supply and social/poverty concerns are becoming of very minor importance while the most important factor for livestock policy is the environment (including animal welfare).
As a conclusion of such a broader perspective, it was estimated that if development in/for the world means the estimated 9 to 10 billion people in 2050 have to reach a way of life and consumption considered normal for the OESO-countries, then the economy in 2050 has to be 15 times the level it was in 2010. Of course, there are increases in efficiency, changes in technology, often unpredictable, and in consumption patterns perhaps. Awareness of the limited character of ecological resources and the limited character of growth of the world population is urgently needed. The UN’s 17 sustainable development goals are clear: our societies or our world society in the future will or will not be sustainable.
Boonen, R. 2015. How to feed and not eat our world? PhD-thesis, KU Leuven, 164 pp. Boonen, R., Aerts, S., Meganck, M., De Tavernier, J. and E. Decuypere. 2012a. Feed efficiencies in animal production: a non-numerical analysis. In: Climate Change in Sustainable Development. Potthast and Meisch (Eds.). Wageningen Academic Publishers, Wageningen, pp. 196-201. Boonen, R., Aerts, S. and J. De Tavernier. 2012b. Which sustainability suits you? In: Climate Change in Sustainable Development. Potthast and Meisch (Eds.). Wageningen Academic Publishers, Wageningen, pp. 43-48. Hendriks, W.H., Verstegen, M.W.A. and L. Babinszky. 2019. Poultry and pig nutrition: challenges of the 21st century. Wageningen Academic Publishers, 428 pp.
FAO, 2006. Livestock’s Long Shadow: environmental issues and options. Rome, 390 pp. Fresco, L.O. 2008. Challenges for food system
Breeder Management and Nutrition: Moving the industry forward
adaptation today and tomorrow. Environmental Science and Policy 12: 378-385
Morrison, B. 2016. La graine et le navet. Courrier International, nr 1324, p 41.
Mayozer, M. and L. Roudart. 2006. A history of world agriculture: from the Neolithic age to the current crisis. Monthly Review Press, New York, 528 pp. Nickell, D. and C.M. Saviani. 2021. Improving the sustainability of animal farming. Misset. Special
issue on sustainability and welfare, October 2021. pp. 42-43.
UN, 2020. www.undp.org/context/undp/en/home/ sustainable-development-goals.html.
Van Es, A.J.H. 1975. Heterotrofe productie bij dieren. In: Productiviteit in biologische systemen. Biologische Raad Reeks, Wageningen, pp 105-156.
AITOR ARRAZOLA, PhD., is a biologist interested in studying and understanding animal behaviour and how to apply this knowledge to improve animal welfare through research and find solutions to on-farm behavioural and welfare problems. He is currently a postdoctoral researcher with Purdue University (Indiana). His research experience focuses particularly on addressing animal welfare concerns in livestock and developing reliable behavioural and physiological welfare indicators to assess the wellbeing of animals under commercial conditions and management. He received his doctorate through the Department of Animal Biosciences at the University of Guelph (Canada).
Intense selection for meat production and efficiency in modern broilers has impaired the reproduction performance of their parent stock. In breeder hens and roosters, fast growth rate and heavy body weight result in low laying rate and poor hatchability under ad libitum feeding. Recent research has highlighted that excessive weight and poor body condition before photostimulation are determinant factors of asynchronous onset of lay and poor laying persistency. Therefore, quantitative feed restriction throughout rearing and lay is necessary to slow down growth rate and achieve optimal reproductive performance in broiler breeders.
Feed restriction during rearing aims to provide enough nutrients for skeletal development and sexual maturation while maintaining healthy body composition (having enough protein content at low-fat accumulation). Optimal body composition at the end of rearing is essential for proper ovary functioning and regulation during lay and to avoid problems associated with excessive fat deposition. However, feed restriction also leads to poor body weight uniformity and chronic stress due to feeding competition and frustrated feeding, respectively. Poor body weight uniformity and chronic stress are linked with reduced reproduction performance in broiler breeders, and finding solutions to these problems can particularly maximize laying rate and persistency in broiler breeder hens. This chapter covers into more detail the need for feed restriction and the rationale behind commercial feed restriction programs for broiler breeders. Then, practical solutions to prevent poor body weight and alleviate chronic feed restriction are discussed to, ultimately, improve the well-being and reproductive performance of broiler breeder under commercial conditions. Concluding remarks and future trends about feed restriction and the reproductive performance of broiler breeders are summarized at the end, including public awareness and welfare concerns about broiler breeder management.
The genetic selection of fast-growing broiler chickens is linked with limited reproductive performance and breeding problems when broiler breeders are fed to satiety (Mench, 2002). Broiler breeders share the same
genetic potential for a fast growth rate with their progeny (Havenstein et al., 2003), but selection traits that optimize broiler performance have negative consequences on the reproductive performance of modern broiler breeders fed ad libitum (Mench, 2002). Consequently, feed restriction in
conventional broiler breeders is a common on-farm practice used to avoid high mortality, health problems, and poor reproductive performance during lay (D’Eath et al., 2009).
The growth rate of broiler breeder hens and roosters is controlled using feed restriction throughout the entire life cycle. Under commercial practice, feed restriction provides enough nutrients to meet metabolic demands and support skeletal development at a slow growth rate without the negative consequences of overfeeding. Controlled growth rate prevents health and reproductive problems related to high body weight and excessive fat content in conventional broiler breeders fed ad libitum (Mench, 2002). However, chronic feed restriction also raises welfare concerns and production challenges (D’Eath et al., 2009; de Jong and Guémené, 2011).
Intensive genetic selection in pedigree lines and grandparent stock has resulted in broilers with a fast growth rate, capable of achieving their target body weight in less than five weeks. This remarkable growth rate in broilers is attributed to high feed intake and improved feed efficiency (Havenstein et al., 2003). Over time, broilers are becoming more feed efficient, grow faster per unit of time, and achieve a market body weight at a younger age (Tesseraud et al., 2000). This selection for fast growth has dramatically changed the metabolism and behaviour of modern broilers and, therefore, their parent stock (Tesseraud et al., 2000; Bokkers and Koene, 2003; Havenstein et al., 2003).
Broiler breeders are the parent stock of broilers and consequently share same strong predisposition for feeding motivation and feed efficiency as broilers. Excessive feed intake (overfeeding) and the fast growth rate in broiler breeders ultimately lead to metabolic disorders and pathologies related to overweight and obesity. Indeed, excessive body weight is associated with cardiovascular problems, skeletal and metabolic disorders, leg weakness, pododermatitis and hock burn, low immunocompetence, and sudden death syndrome (see review by Julian, 2005). As broiler breeders stay in production for over a year, excessive body weight gain and fat accumulation result in elevated mortality, the aforementioned pathologies, and poor reproductive performance when hens and roosters are fed to satiety (Walzem and Chen, 2014).
Research from the early 2000s concluded that conventional broiler breeders (hens and roosters) need feed restriction to achieve optimal performance during lay due to their propensity for a fast growth rate and to be overweight (Bruggeman et al., 1999; Heck et al., 2004). During rearing, fast growth rate and body composition before the onset of lay are determining factors of subsequent laying rate (onset, peak, and persistency) during rearing. During lay, excessive body weight and elevated fat accumulation are risk factors for poor reproductive performance (Ramachandran, 2014; Walzem and Chen, 2014).
Excessive body weight is ultimately responsible for a low laying rate of hens and
a low fertility rate in roosters (Chen et al., 2006). In hens, being overweight is linked with dysfunctional ovaries that, in the end, result in a poor laying rate. Excessive body fat contributes to a high number of mature yellow follicles, heavier residual ovary, and multiple follicle hierarchies that interfere with optimal follicle regulation. Problems establishing follicle hierarchy can result in ovulation abnormalities, such as higher incidence of double-yolks eggs, follicle dysregulation, poor follicle recruitment, and a low laying rate (Renema et al., 2001a,b). In roosters, high body weight is associated with low fertility and hatchability related to low breeding activity and poor sperm quality (Hocking and Bernard, 2000). Poor sperm quality directly compromises fertility, and low breeding activity in heavier roosters can be explained by the high prevalence and severity of foot problems and lameness. However, the link between excessive body weight in roosters and a low percentage of hatched or fertile eggs is unclear.
Problems related to poor reproductive performance in overweight hens often refers to a low laying rate, but heavy body weight in hens is also a risk factor for low fertility, embryo mortality, and reduced percentage of hatched or fertile eggs ( Walzem and Chen, 2014). The causal factors behind this relationship are not well-understood. The negative effect of excess body weight on embryo mortality is hypothesized to be mediated by an indirect effect of hens’ body condition and age on egg composition (van Emous et al., 2015). Hatching eggs with relatively heavier yolk laid by older hens can be at risk for embryo mortality, particularly in male embryos, due
to excessive endogenous heat production from lipid oxidation (Arrazola et al., 2019). However, the negative consequences of excessive fat content in breeder hens on embryo development are yet to be determined.
Feed restriction during rearing and lay mitigates the severity and incidence of disorders associated with poor liability, health problems, and impaired reproductive performance. To avoid these problems, a controlled growth rate in broiler breeder pullets and cockerels is designed to achieve the target body weight, optimal body condition, and proper frame size before the development of the reproductive organs (Vignale et al., 2016). Therefore, conventional broiler breeders are quantitatively feed restricted throughout the entire production following slow growth curves.
Quantitative feed restriction refers to limited weekly feed allotment to slow body weight gain and reach age-specific target body weight using standard broiler breeder diets (D’Eath et al., 2009; Zuidhof et al., 2015). Weekly feed allotment for broiler breeders slightly increases with age to support steady body weight gain as feed restriction is not intended to result in body weight loss or growth checks. For this reason, limited calorie intake aims to satisfy the nutritional requirements needed for a healthy growth rate while avoiding the negative consequences of overfeeding and being overweight. In other words, quantitative feed restriction provides enough nutrients to promote skeletal
Breeder Management and Nutrition: Moving the industry forward
development and sexual maturation during rearing, support egg production during lay, and limit lifelong fat accumulation (Chen et al., 2006; Vignale et al., 2016).
Commercial feed restriction programs
Feed restriction management during early development defines growth rate, body composition, and skeletal development leading to long-term consequences during lay (de Beer and Coon, 2009). Starting during the first week(s) of age, feed restriction limits the growth rate of both female and male broiler breeders throughout the entire production cycle. Under commercial feed restriction programs, the level of feed restriction varies throughout
the production cycle taking into account the developmental stage and sexual maturation of male and female breeders (Figure 1). In pullets, feed restriction level increases with age until mid-rearing and decreases before the development of sexual organs and during lay (Figure 1). Sexual maturity and the onset of lay are delayed under feed restriction, and rearing management must ensure that pullets achieve sexual maturity simultaneously before photostimulation (Zuidhof et al., 2015). Cockerels and roosters remain under chronic feed restriction following slow but steady growth curves during rearing and lay, respectively. Due to differential feed restriction levels, hens and roosters need separate-sex feeding and female feeders must be equipped with male-exclusion grills to avoid roosters feeding on hens’ feeders.
As artificial selection continuously accelerates growth rate and improves feed efficiency in broilers, broiler breeders need more severe feed restriction over time to maintain healthy body weight gain and body composition (de Jong and Guémené, 2011). For example, Figure 1 illustrates previous and current commercial feed restriction levels in broiler breeders relative to voluntary feed intake of broilers for the same body weight according to breeding company performance outcomes for Ross 308 females in the last decade. This figure shows that, compared to previous feed restriction management, broiler breeder pullets undergo greater feed restriction during early and late rearing in current commercial feed restriction programs.
Before photostimulation, pullets must maintain optimal body condition and develop proper frame size during rearing. Both are relevant to maintaining ovary functionality and regulation to sustain egg production during lay. As indicated in Figure 1, high feed restriction before the development of sexual organs is critical to optimizing egg production and laying persistency during lay (Bruggeman et al., 1999; de Beer and Coon, 2009). Pullets must keep lean body composition before photostimulation with just enough fat to support reproductive development. Chronic feed restriction during this phase limits development of adipose tissue which, in turn, upholds optimal laying rate, laying persistency, and high cumulative egg production by the end of the production cycle.
Feed restriction (around 70-80% of ad libitum feed intake) during the second or
third weeks of age is effective to the reduce development of adipose tissue later in life. However, early rearing is also a sensitive period for skeletal growth and frame size development (de Beer and Coon, 2009). Indeed, nutritional stress (e.g., non-daily quantitative feed restriction) during early rearing can compromise skeletal development and frame size resulting in smaller eggs during lay due to physical limitations (de Beer and Coon, 2009; Arrazola et al., 2019). Therefore, although limiting fat accumulation is key, optimal skeletal development and frame size in pullets also play a crucial role in musculature development that sustains egg production during lay.
Research from the last decades has wellestablished that conventional broiler breeders need controlled growth rate starting no later than two months of age to meet reproductive objectives (de Beer and Coon, 2009). Further understanding the role of body composition on reproductive performance highlights that excessive fat accumulation and development of adipose tissue are responsible for poor laying rate and persistency. In conventional strains of broiler breeders, excessive fat accumulation is a risk factor associated with dysfunctional ovaries and dysregulation of the hypothalamus-pituitary-gonadal axis (HPG) (Renema et al., 2001a,b; Heck et al., 2004; Chen et al., 2006). Experimental studies looking at the relationship between ovary morphology and overfeeding indicated that higher feed intake in conventional broiler breeders led to greater fat accumulation and
altered ovary morphology. This results in a higher frequency of abnormal ovaries, a higher number of hierarchical follicles, a lower count of small yellow follicles, and a higher count of atretic follicles (Chen et al., 2006). In return, these obesity-related problems due to overfeeding translate to poor laying rate in broiler breeder hens (Chen et al., 2006).
The incidence and severity of obesity-related problems on reproductive performance is, interestingly, driven by individual susceptibility to lipotoxicity (Chen et al., 2006; Walzem and Chen, 2014). The term lipotoxicity refers to the negative consequences of excessive fat accumulation in non-adipose tissues, such as the reproduction system (reviewed by Walzem and Chen, 2014). Understanding the relationship between excessive adipose tissue and ovary dysfunction (as well as other related obesity problems) in broiler breeder hens is necessary to address problems in broiler breeder reproduction. In addition to specific target growth curves, optimal body composition (enough protein content at lowfat content) during late rearing is a determinant to uniformly stimulating and maintaining laying rate (Renema et al., 2001a; Vignale et al., 2016). For this reason, feed restriction management in broiler breeders aims to uniformly sustain healthy body weight and optimal body composition without excessive fat accumulation to, ultimately, maximize reproductive performance.
Feed restriction is essential to control body weight gain and to prevent high mortality
and health problems (Dawkins and Layton, 2012), but feed restriction on its own is also associated with challenges to the reproductive performance of broiler breeder hens and roosters. From a behavioural standpoint, chronic hunger and unsatisfying feeding behaviour often result in competitive feeding, aggressive behaviour, and oral-redirected behaviour to drinkers and conspecifics in broiler breeders. These behavioural problems can cause additional issues in broiler breeder performance such as poor body weight uniformity, injurious pecking, and feather coverage loss (Arrazola et al., 2019).
Achieving a uniform, mature body weight and body conformation before photostimulation is essential to synchronize laying onset and laying rate. However, feed restriction leads to poor body weight uniformity due to competition of the limited feed allotment (Zuidhof et al., 2015). Under chronic feed restriction, dominant birds compete for feeder space and a larger feed ration while subordinate birds may not receive enough nutrients to meet metabolic requirements. At the flock level, this unequal feed allocation attributed to feeding competition results in poor body weight uniformity and suboptimal body composition in overfed dominant pullets and underfed submissive pullets. Therefore, poor body weight uniformity raises welfare and productivity concerns as dominant birds exceed target body weight while subordinate birds below target body weight can be undernourished.
Poor body weight uniformity at the end of rearing negatively impacts flock performance due to asynchronous laying rate and differential
nutrient requirements between sexually mature and immature pullets (Zuidhof et al., 2015). At the end of rearing, photostimulation activates the HPG axis and signals the onset of lay in sexually mature pullets while sexually immature pullets remain unresponsive. Consequently, flocks with poor body weight uniformity before photostimulation are unlikely to reach the expected peak of lay. Body weight uniformity is therefore critical to ensure that most hens come into lay simultaneously, reach peak of lay at the same time, and uphold laying persistency (de Beer and Coon, 2009; Zuidhof et al., 2015).
Body weight uniformity should be monitored regularly during rearing, and problems with body weight uniformity must be corrected as soon as possible. Under poor body weight uniformity, the growth rate of overweight birds must be adjusted (lower body weight gain) to ensure pullets remain within target body weight and optimal body composition before photostimulation. On the other hand, underweight pullets must receive additional feed intake to compensate for light body weight. Grading flock by body weight is a common on-farm practice to prevent and correct poor body weight uniformity under commercial conditions (Aviagen, 2018). During grading, pullets are individually weighed every month and divided into three categories based on body weight: underweight, on-target, and overweight. Feed restriction is then managed independently for the three separated groups of pullets to follow the target body weight.
Understanding the relationship between stress and reproductive performance in
feed-restricted broiler breeders is difficult due to the physical and psychological stress imposed by feed restriction. In laying hens, stressful experiences during lay can adversely impact egg production (Deviche et al., 2017). Fundamental studies have indeed demonstrated that elevated activation of the stress response (by infusion of adrenocorticotropic hormone) resulted in atresia in all treated hens, inhibition of laying rate, and lowered oviduct weight compared to control laying hens (Odihambo Mumma et al., 2006). These results suggest that activation of the hypothalamuspituitary-adrenal axis can interfere with the regulation of the HPG axis and activation of the physiological stress response can directly impair ovary regulation and functioning (Deviche et al., 2017).
Research concerning broiler breeder welfare has clearly demonstrated that quantitative feed restriction is a physical and psychological stressor that activates the physiological stress response (de Jong and Guémené, 2011). However, the extent to which reproductive performance of broiler breeder hens is compromised due to stress associated with feed restriction is unknown. Management practices that reduce stress during lay are hypothesized to improve egg production in broiler breeder hens.
Chronic stress during sexual maturation can also have detrimental carry-over effects during lay (Odihambo Mumma et al., 2006; Deviche et al., 2017), although the long-term effect of chronic stress during rearing on the reproductive performance is unclear. Recent evidence suggests that welfare-friendly
feeding strategies during rearing can yield improved laying rate, better hatchability, and high cumulative egg production at the end of lay (Arrazola et al., 2019). Therefore, understanding the effect of chronic stress (either during rearing, lay, or both) on reproduction can provide further venues to improve the performance of broiler breeders.
Finding practical solutions to challenges associated with feed restriction and reproductive performance of broiler breeders requires an interdisciplinary approach, balancing welfare concerns and production outcomes. In addition, decision-making processes regarding the management of broiler breeders must also consider ethical concerns about the well-being and health of hens, roosters, and their progeny.
Modern broilers and broiler breeders are highly predisposed to high feed intake that exceeds their nutritional requirements, mainly because their feeding motivation is largely driven by satiety mechanisms instead of hunger mechanisms (Bokkers and Koene, 2003). In other words, broilers (and broiler breeders if they are fed ad libitum) feed continuously until they are full even if they are not hungry. Under commercial conditions, feed restriction is therefore severely stressful for broiler breeders due to unsatisfied and frustrated feeding behaviour. To reduce the distress associated with chronic feed restriction, welfare-friendly feeding strategies for broiler breeders must consider means to satisfy feeding motivation
while maintaining the slow growth rate (D’Eath et al., 2009). The development of alternative feeding restriction programs focuses on strategies that increase feed intake and provide opportunities to engage in feeding behaviour without higher calorie intake (D’Eath et al., 2009).
Qualitative feed restriction refers to the reduction in dietary nutrient and calorie density using low-quality diets or alternative diets (D’Eath et al., 2009). Alternative diets limit nutrient intake by reducing dietary nutrient density (including insoluble fibrous ingredients like oat hulls and soybean hulls), reducing voluntary feed intake (using appetitive suppressants such as calcium propionate), or a combination of both. Under commercial feed restriction, the feed intake of broiler breeders fed alternative diets stills needs to be rationed to control growth rate (Sandilands et al., 2005). However, due to dietary dilution, pullets under qualitative feed restriction are fed a larger feed allotment than those under quantitative feed restriction (D’Eath et al., 2009).
Rationed alternative diets during rearing can reduce signs of chronic feed restriction and appear to enhance reproductive performance during lay (de Jong and Guémené, 2011; van Emous et al., 2015; Arrazola et al., 2019). Indeed, pullets under rationed qualitative feed restriction can uphold higher laying persistency after 30 weeks of age (van Emous et al., 2015). However, switching diets before laying can be stressful and delay the onset of lay (Arrazola et al., 2019). Pullets
reared on alternative diets would benefit from a transition period (switching diets progressively) a few weeks before lay, allowing hens to habituate to a layer diet gradually and minimize dietary stress (Sandilands et al., 2005). Nevertheless, hens can compensate throughout lay for delayed onset of lay due to higher laying rate and persistency (Renema et al., 2001b; Arrazola et al., 2019).
Non-daily feeding schedules are common feeding strategies used in North America for feed restriction management in broiler breeders. Non-daily feeding schedules reduce the number of feeding days per week in exchange for providing larger feed allotments during feeding days (de Beer and Coon, 2009; Arrazola et al., 2019). Common nondaily feeding schedules include feeding every other day (skip-a-day), four feeding days per week (4/3 schedule), and five feeding days per week (5/2 schedule). Due to the larger feed allotment on feeding days, non-daily feeding schedules are expected to improve body weight uniformity, reduce feeding competition, and promote satiety on feeding days (Zuidhof et al., 2015; Arrazola et al., 2019).
Compared to daily feed restriction, nondaily quantitative feed restriction can reduce behavioural signs of hunger and physiological indicators of chronic stress during rearing (Arrazola et al., 2019). From a performance point of view, non-daily feeding is less feedefficient than daily feeding due to the energy storage and mobilization during feeding and non-feeding days, respectively (Zuidhof
et al., 2015). However, broiler breeders show compensatory body weight gain when they switch from non-daily to daily feeding frequency (de Beer and Coon, 2009; Arrazola et al., 2019). The implications of this compensatory body weight gain on laying rate may depend on the pullets’ age and body weight. Broiler breeder pullets within target body weight can become overweight after switching from non-daily to daily feeding frequency at the end of rearing, resulting in poor laying performance (de Beer and Coon et al., 2009). However, switching from non-daily to daily feeding frequency two weeks before photostimulation can plausibly improve laying rate persistency due to leaner body composition at the end of rearing (Arrazola et al., 2019).
Non-daily feeding schedules during rearing can have metabolic programming consequences that impact body function and reproduction during lay. Compared to daily feed restriction, broiler breeder pullets reared under non-daily feeding have higher hepatic lipogenesis and fat accumulation by the end of lay (de Beer and Coon, 2009; Vignale et al., 2016). This suggests that conventional broiler breeder hens reared under non-daily feed restriction can be more susceptible to lipotoxicity if body weight slightly exceeds the target growth rate during lay (Arrazola et al., 2019). This hypothesis can explain why research looking at the effect of non-daily feed restriction during rearing found contradictory results on laying rate. Information about non-daily feed restriction before sexual maturation is scarce, and little is known about how to adjust for the compensatory growth rate when pullets switch from non-
daily to daily feeding frequency or about the consequences of lipogenic predisposition on their reproductive performance.
Environment enrichments that promote feeding behaviour in broiler breeders can alleviate the distress associated with chronic feed restriction. Indeed, recent studies have evaluated the effect of feeding enrichments in broiler breeder pullets under daily quantitative feed restriction (Tahamtani et al., 2020) and non-daily feed restriction (Aranibar et al., 2020). Providing a small amount of corn silage reduced signs of distress in feed-restricted pullets although performance outcomes were not reported in this paper. In non-daily feeding schedules, eliminating feeding days is a major ethical concern and is perceived as an insult to animal welfare (Lindholm et al., 2018). To solve this problem, Aranibar and colleagues (2020) evaluated the effect of providing a small ration of soybean hulls during nonfeeding days. Results from this research suggested that body weight uniformity and laying rate during peak lay was improved compared to control (skip-a-day schedule). These results suggest that providing feeding enrichments to broiler breeders can improve welfare and production outcomes.
Due to larger feed allotment, alternative feeding strategies (qualitative and non-daily quantitative feed restriction) are meant to improve welfare and reduce the negative consequences of chronic feed restriction (signs of hunger and distress, feeding competition, low body weight uniformity, feather coverage loss, and polydipsia/high water usage).
However, alternative feeding strategies do not eliminate signs of chronic hunger and distress in broiler breeders under simulated commercial conditions (Arrazola et al., 2019). Similarly, conventional broiler breeders still display signs of chronic hunger and distress when given feeding enrichments (Arrazola et al., 2019; Aranibar et al., 2020; Tahamtani et al., 2020). From a performance standpoint, alternative feeding strategies do not seem to have clear improvements in body weight uniformity at the end of rearing (Zuidhof et al., 2015; Arrazola et al., 2019). Some alternative feeding strategies are also ethically questionable, like using non-daily feeding schedules (Lindholm et al., 2018). Consequently, feed restriction in broiler breeders is still a major welfare concern and raises sustainability issues about using conventional strains of fast-growing broiler breeders.
Broiler breeders lay hatching eggs that satisfy the need for broiler chicks raised for meat production. However, the reproductive performance of conventional broiler breeders is still low despite the controlled growth rate and efforts to maintain hens and roosters within healthy body conformation. In conventional broiler breeders (e.g., Ross 308 and 708, Cobb 500), laying persistency decreases noticeably soon after hens reach the peak of egg production as well as hatchability in settable eggs laid by hens older than 45 weeks.
Limited reproductive performance and welfare concerns in conventional broiler
breeders can be mitigated by using broiler breeder strains with a low growth rate or that are less susceptible to obesity-related problems. Recent research exploring the use of alternative strains of broiler breeder roosters and hens suggests that slower and intermediate growing broiler breeders can achieve optimal laying rate and high cumulative egg production during lay (Heck et al., 2004; Gebhardt et al., 2018; Arrazola et al., 2022). In their study, Gebhardt and colleagues noted that slower-growing broiler breeders (Sasso SA31A hens with T44 roosters) had 40% higher egg production and 55% higher chick production than conventional broiler breeders (Ross 308 hens with 308 roosters). Looking at the reproduction performance of alternative strains in more detail, susceptibility to obesity-related disorders on laying rate seems to be strain-dependent (Heck et al., 2004; Arrazola et al., 2022).
Broiler breeder strains with lower growth rates can be a welfare-friendly solution to chronic feed restrictions for conventional broiler breeders (Dawkins and Layton, 2012). Indeed, alternative strains of broiler breeders are commercially available (e.g., Ranger Premium by Aviagen, JA57&JA57K by Hubbard, and SA51 by Sasso) and require a lower level of feed restriction to maintain a healthy body weight and slow growth rate (Heck et al., 2004; Jones et al., 2004). According to previous breeding company guidelines, the performance objectives for slower-growing broiler breeders indicate that hens under moderate feed restriction can achieve high cumulative egg production at the end of lay due to early onset of lay and
persistent laying rate after peak. Hence, using slower-growing broiler breeders can alleviate or remove welfare concerns linked with chronic feed restriction in broiler breeder production and achieve higher cumulative hatching egg production than conventional broiler breeders.
Heavy body weight in broiler breeders is associated with health problems and high mortality, but susceptibility to excessive adipose tissue is the main risk factor behind poor reproductive performance in conventional broiler breeder hens. Certainly, susceptibility to problems associated with excessive fat accumulation can vary among individuals and strains (Heck et al., 2004; Chen et al., 2006; Arrazola et al., 2022s). The mechanisms behind ovary sensitivity to lipotoxicity are complex, including individual differences in lipid metabolism and altered hormonal regulation of the HPG axis, and there are those yet to be determined (Chen et al., 2006). Understanding ovary function, hormonal profile, and lipid metabolism in hens less susceptible to overfeeding and high fat accumulation is key to comprehending and overcoming poor reproductive performance in broiler breeder hens. Meanwhile, selecting broiler breeders that are less susceptible to the negative consequences of excessive fat accumulation or those capable of maintaining low-fat content are plausible solutions to current challenges associated with lipotoxicity and poor reproductive performance in conventional strains. Indeed, alternative strains with these traits are already commercially available and can provide practical improvements to broiler breeder welfare and performance.
Most attention and concerns around broiler breeder welfare and performance focus on hens, while roosters remain chronically feed restricted during the entire production cycle. This chronic feed restriction throughout production results in high aggressive behaviour, feeding competition, and severe pecking that roosters redirect to hens and other males. From a welfare perspective, excessive maleto-female aggressive behaviour and forced matings account for elevated feather coverage loss, body lesions, and bone fractures in hens that need to be euthanized if the damage is severe. From a productive perspective, fertility rate and breeding activity drops in males around 25 weeks into lay. To avoid negative consequences associated with poor rooster performance, spiking flocks is a recommended on-farm practice to reduce the drop in fertility, hatchability and hatch of fertile during this period.This practice consists of replacing poorquality, 45-week-old males (sexually inactive and those exceptionally above or below target body weight) with sexually mature, 25-weekold roosters (Arrazola et al., 2019). In return, fertility and hatchability increase after spiking until late lay, but this practice raises biosecurity and ethical concerns. After removing the overweight, dominant males, old and young roosters fight severely to re-establish hierarchy causing elevated mortality and low breeding activity during the first week after replacement (Hocking and Bernard, 2000).
Means to improve the welfare and performance of roosters have received relatively little attention. However, research with broiler
breeder hens suggests that alternative feeding strategies, such as feeding diluted diets or adding non-nutritious feeding enrichments, can be used in roosters to alleviate their lifelong chronic feed restriction and, ultimately, reduce distress and improve their well-being. Enhancements in rooster welfare are also expected to improve production outcomes such as body weight uniformity and breeding activity. Additionally, alternative rooster strains, such as slower or intermediate growing roosters, can be a practical solution to previous welfare concerns and production challenges (Arrazola and Torrey, 2021). Due to the slower growth rate, alternative roosters are expected to require moderate feed restriction levels, have better reproductive performance, and have fewer behavioural problems such as high aggression.
Future trends and concluding remarks
Solutions to poor body weight uniformity and elevated stress in broiler breeders are likely to provide short-term, moderate improvements in the reproductive performance of hens. This is particularly because the current selection traits for high performance in broilers impair reproductive traits and liveability liability of conventional broiler breeder strains. Although the fast growth rate may not be the problem on its own, understanding the effects of lipotoxicity on ovary function and regulation provides avenues to select hens with lower fat accumulation or that are less susceptible to negative consequences of excessive fat content.
Due to previous challenges in conventional broiler breeder hens and roosters, improvement in the reproductive performance and welfare
of broiler breeders may require using alternative strains (Dawkins and Layton, 2012). Public pressure and consumer concerns about health and welfare in conventional broilers are pushing the poultry meat industry toward using alternative strains of hens and roosters with a slower growth rate and better welfare. Increasing interest in using slowergrowing broiler strains requires the use of slower-growing broiler breeder strains, and information about the performance and welfare of the parent stock of slower-growing broilers is very scarce. From a production standpoint, slower-growing broiler breeders can achieve optimal reproductive performance under acute/moderate feed restriction throughout the production cycle (Heck et al., 2004; Jones et al., 2004; Arrazola and Torrey, 2021). Some strains can certainly maintain a slow growth rate within a healthy body weight threshold and yield high cumulative egg production without feed restriction (Heck et al., 2004). More research is still needed to assess the feasibility of using slower-growing broiler breeders under commercial conditions and develop strain-specific management recommendations, nutrient requirements, and housing recommendations, during rearing and lay, in order to maximize the reproductive performance and well-being of these alternative strains.
Aranibar, C.D., Chen, C., Davis, A.J., Daley, W.I., Dunkley, C., Kim, W.K., Usher, C., Webster, A.B. and J.L. Wilson. 2020. Impact of an alternate feeding program on broiler breeder pullet behavior, performance, and plasma corticosterone. Poultry Science 99: 829-838.
Arrazola, A., Widowski, T.M., Guerin, M.T., Kiarie, E.G. and S. Torrey. 2019. The effect of alternative feeding strategies for broiler breeder pullets: 2. Welfare and performance during lay. Poultry Science 98: 6205-6216.
Arrazola, A. and S. Torrey. 2021. Welfare and performance of slower growing broiler breeders during rearing. Poultry Science 100: 101434.
Arrazola, A., Widowski, T.M., and S. Torrey. The effect of alternative feeding strategies for broiler breeder pullets: 2. Welfare and performance during lay. Poultry Science (Arrazola et al., 2022).
Aviagen. 2018. Ross Parent Stock: Management Handbook. Broiler Performance Objectives. Aviagen, Ltd., Huntsville, AL.
Bokkers, E. and P. Koene. 2003. Eating behaviour, and preprandial and postprandial correlations in male broiler and layer chickens. British Poultry Science 44: 538-544.
Bruggeman, V., Onagbesan, O., D’Hondt, E., Buys, N., Safi, M., Vanmontfort, M., Berghman, L., Vandesande, F. and E. Decuypere. 1999. Effects of timing and duration of feed restriction during rearing on reproductive characteristics in broiler breeder females. Poultry Science 78: 1424-1434.
Chen, S., McMurtry, J. and R. Walzem. 2006. Overfeeding-induced ovarian dysfunction in broiler breeder hens is associated with lipotoxicity. Poultry Science 85: 70-81.
D’Eath, R. B., Tolkamp, B. J., Kyriazakis, I. and A. B. Lawrence. 2009. ‘Freedom from hunger’ and preventing obesity: the animal welfare implications of reducing food quantity or quality. Animal Behaviour 77: 275-288.
Dawkins, M. and R. Layton. 2012. Breeding for better welfare: genetic goals for broiler chickens and their parents. Animal Welfare 21: 147-155. de Beer, M. and C. Coon. 2009. The effect of feed restriction programs and growth curves on reproductive performance, in vitro lipogenesis and
heterophil to lymphocyte ratios in broiler breeder hens. International Journal of Poultry Science 8: 373-388.
de Jong, I. and D. Guémené. 2011. Major welfare issues in broiler breeders. World’s Poultry Science Journal 67: 73-82.
Deviche, P., Bittner, S., Gao, S. and S. Valle. 2017. Roles and mechanistic bases of glucocorticoid regulation of avian reproduction. Integrative and Comparative Biology 57: 1184-1193. Gebhardt-Henrich, S.G., Toscano, M.J. and H. Würbel. 2018. Use of aerial perches and perches on aviary tiers by broiler breeders. Applied Animal Behaviour Science 203: 24-33. Havenstein, G. B., Ferket, P. R. and M. A. Qureshi. 2003. Growth, livability, and feed conversion of 1957 versus 2001 broilers when fed representative 1957 and 2001 broiler diets. Poultry Science 82: 1500-1508.
Heck, A., Onagbesan, O., Tona, K., Metayer, S., Putterflam, J., Jego, Y., Trevidy, J., Decuypere, E., Williams, J. and M. Picard. 2004. Effects of ad libitum feeding on performance of different strains of broiler breeders. British Poultry Science 45: 695703.
Hocking, P. and R. Bernard. 2000. Effects of the age of male and female broiler breeders on sexual behaviour, fertility and hatchability of eggs. British Poultry Science 41: 370-376.
Jones, E.K.M., Zaczek, V., MacLeod, M. and P.M. Hocking. 2004. Genotype, dietary manipulation and food allocation affect indices of welfare in broiler breeders. British Poultry Science 45: 725-737.
Julian, R. J. 2005. Production and growth related disorders and other metabolic diseases of poultry–a review. The Veterinary Journal 169: 350-369. Lindholm, C., Johansson, A., Middelkoop, A., Lees, J.J., Yngwe, N., Berndtson, E., Cooper, G. and J. Altimiras. 2018. The quest for welfare-friendly feeding of broiler breeders: effects of daily vs. 5: 2
feed restriction schedules. Poultry Science 97: 368377.
Mench, J. A. 2002. Broiler breeders: feed restriction and welfare. Worlds Poultry Science Journal 58: 23-29.
Odihambo Mumma, J., Thaxton, J., Vizzier-Thaxton, Y. and W. Dodson. 2006. Physiological stress in laying hens. Poultry Science 85:761-769.
Ramachandran, R. 2014. Current and future reproductive technologies for avian species. In Current and Future Reproductive Technologies and World Food Production. G.C. Lamb and N. DiLorenzo (eds). Springer, New York, pp. 23-31. Renema, R., Robinson, F. and P. Goerzen. 2001a. Effects of altering growth curve and age at photostimulation in female broiler breeders. 1. Reproductive development. Canadian Journal of Animal Science 81: 467-476. Renema, R., Robinson, F., Goerzen, P. and M. Zuidhof. 2001b. Effects of altering growth curve and age at photostimulation in female broiler breeders. 2. Egg production parameters. Canadian Journal of Animal Science 81: 477-486. Sandilands, V., Tolkamp, B. J. and I. Kyriazakis. 2005. Behaviour of food restricted broilers during rearing and lay - effects of an alternative feeding method. Physiology & Behavior 85: 115-123.
Tahamtani, F.M., Moradi, H. and A.B. Riber. 2020. Effect of qualitative feed restriction in broiler breeder pullets on stress and clinical welfare indicators. Frontiers in Veterinary Science 7: 316. Tesseraud, S., Chagneau, A.M. and J. Grizard. 2000. Muscle protein turnover during early development in chickens divergently selected for growth rate. Poultry Science 79: 1465-1471. van Emous, R., Kwakkel, R., Van Krimpen, M. and W. Hendriks. 2015. Effects of dietary protein levels during rearing and dietary energy levels during lay on body composition and reproduction in broiler breeder females. Poultry Science 94: 1030-1042.
Vignale, K., Caldas, J.V., England, J.A., Boonsinchai, N., Sodsee, P., Putsakum, M., Pollock, E. D., Dridi, S. and C.N. Coon. 2016. The effect of four different feeding regimens from rearing period to sexual maturity on breast muscle protein turnover in broiler breeder parent stock. Poultry Science 96: 1219-1227.
Zuidhof, M., Holm, D., Renema, R., Jalal, M. and F. Robinson. 2015. Effects of broiler breeder
management on pullet body weight and carcass uniformity. Poultry Science 94: 1389-1397.
Walzem, R.L. and S.E. Chen. 2014. Obesity-induced dysfunctions in female reproduction: lessons from birds and mammals. Advances in Nutrition 5: 199206.
R. A. (RICK) VAN EMOUS, PhD., is a Senior Researcher in Animal Nutrition at Wageningen Livestock Research in the Netherlands. He received his bachelor’s degree in Animal Production from HAS Dronten (Netherlands). Dr. van Emous started his career as a research assistant at COVP-DLO Centre for Poultry Research in Beekbergen. He joined Cobb-Europe as a Technical-Commercial Advisor before returning to COVP-DLO where he worked intensively studying how laying hens respond to different housing systems. He earned his doctorate from the Wageningen Institute of Animal Sciences (Netherlands) in 2015 with a focus on the feeding behaviour and practices of modern broiler breeders.
A.J.W. (ANNEMARIE) MENS, MSc., is a researcher in poultry and swine nutrition at Wageningen Livestock Research (Netherlands). In her research, Mens focuses on using nutrition as a tool, or connector, to improve the welfare, behaviour, physiology, reproduction, and health of both poultry and swine. For example, she studies how stress and nutrition during the early life of rearing hens can influence the development of (ab)normal behaviour. She also studies the underlying physiological mechanisms, the so-called gut-brain axis, and the microbiome in the gastrointestinal tract. As a PhD. candidate, Mens is researching early nutrition and the behaviour of laying hens at Wageningen University (Netherlands) where she earned her master’s degree in Animal Science.
This chapter starts with an introduction on the occurrence of feed restriction in broiler breeders and goes on to describe the level of feed restriction, followed by the effects of feed restriction on physiological parameters, behaviour, reproduction/health, and body composition. Finally, different methods to minimize the effects of feed restriction are discussed, complemented with future possibilities for research opportunities.
The modern broiler breeder has high genetic growth potential but its main goal is to produce hatching eggs. Providing broiler breeders with an ad libitum feed allowance supports fast growth and causes too high of a body weight with detrimental effects on health and reproduction. This implies that these birds should receive a limited amount of feed, especially during the rearing phase, to prevent excessive growth and their associated health and reproduction problems. According to the literature, feed restriction level during rearing is estimated at 70%, whereas feed restriction in this chapter based on energy intake is estimated to be 47%. In literature, there is no consensus on the effects of feed restriction on physiological parameters. However, there are numerous studies demonstrating that feed-restricted broiler breeders show abnormal stereotypical pecking behaviour towards equipment, which can be indicative of frustration, boredom, and hunger. Also, stereotypical tail feather licking or pecking can be an indication of frustration or boredom. Over-drinking (polydipsia) was also mentioned as a sign of abnormal behaviour caused by feed restriction, however, in modern pullets, overdrinking is no longer considered a problem. Pacing before feeding is often mentioned as an indication of stress or frustration, however, recent research questions if this could be normal behaviour since most animals (also less or not restricted) show this behaviour before feeding. To alleviate the negative effects of feed restriction on pullet behaviour, different feeding goals and strategies are investigated: body weight (BW) profile, diet composition, feeding frequency, and scattering feeding. In general, most of these methods, or combinations of these methods, had only small effects on abnormal behaviour and physiological parameters. Further research is necessary to diminish the negative effects of feed restriction on bird behaviour and welfare, and the combination of different feeding methods and strategies seems to be the most promising direction toward a solution.
Poultry meat is considered a good value and efficient protein source in human diets. The
high growth potential of broilers, with low feed conversion ratio and a short production period, makes broiler farming profitable all over the world. Despite the increasing interest during
the last decade for slow-growing broilers, the majority (>95%) of broilers, and thus broilers breeders, are still of the fast-growing breed. During the last six decades, broilers have been successfully selected for maximum growth and feed efficiency (Havenstein et al., 2003a,b; Zuidhof et al., 2014) and fast-growing broilers reach a body weight of 2.0 to 2.5 kg in 35 to 45 days (Augère-Granier, 2019). In a study of different strains representing broilers of 1957 or 1978 compared to a broiler strain from 2005 (Zuidhof et al., 2014), the 2005 strain showed, over a 56-day growth period, 4.6 times higher growth rate and 50% lower feed conversion ratio. The high growth rate and efficiency is advantageous in broilers but may lead to welfare and reproduction problems in parent stock during the rearing and laying periods. Feeding breeder pullets ad libitum, as is done in broilers, results in a much higher body weight with negative effects on reproductive performance and health (Katanbaf et al., 1989; Savory et al., 1993; Heck et al., 2004). In general, broiler breeders produce until 60 to 65 weeks of age (WOA), meaning that high mortality, poor reproduction, and reduced efficiency have a major impact on bird welfare and economic profitability (Decuypere et al., 2010).To prevent these detrimental effects on reproduction and health, feed restriction is necessary to promote breeder welfare and for health reasons, whereas feed restriction simultaneously creates another welfare problem (D’Eath et al., 2009). Breeders show abnormal behaviour like stereotypical pecking of objects such as walls, empty feed systems, empty water systems, and other parts in the house, sometimes in combination with excessive water intake (spoiling) (Hocking et al., 1996, 2001; Savory and Kostal, 1996; de Jong et al., 2002). There are (mostly indirect)
indications that these abnormal behaviours can be indicative of hunger and frustration and that broiler breeders experience hunger (Mench, 2002). The discrepancy between a healthy and reproductive life and impaired behaviour and welfare is known as ‘the broiler breeder paradox’ (Decuypere et al., 2010). In other words, feeding broiler breeders ad libitum has negative consequences on health, welfare, and production, but on the other hand, feed restriction has negative effects on behaviour and welfare. The European Food Safety Authority (EFSA, 2010), however, states that insufficient research has been done in this field to conclude whether feed restriction impaired the welfare of broiler breeders.
Despite the discrepancy previously explained, feed restriction is common practice during the rearing period of broiler breeders. In practice, it is common to feed pullets ad libitum for the first two weeks of age (WOA), whereafter feed restriction starts (de Jong and van Emous, 2017). The literature continuously emphasizes that feed intake in breeder pullets is restricted from 25% to 33% of the feed intake of ad libitum fed birds during the rearing period (Savory et al., 1993; Savory and Kostal, 1996; de Jong et al., 2002). This means a total feed reduction between 67% and 75% compared to the maximum possible intake. Furthermore, the literature describes that the period with the most severe feed restriction is between 7-8 and 15-16 WOA (Savory et al., 1993; de Jong and Jones, 2006). During the laying period, feed allowance is between 45% and 80% of the intake by ad libitum-fed breeders
of the same age (Bruggeman et al., 1999), resulting in a feed restriction level between 20% and 55%.
The level of feed restriction can be important for the effect it has on the birds. The determination of the restriction level is, however, arguable and might not be as simple as comparing the feed intake of ad libitumfed birds of the same age. It seems that feed restriction levels have been overestimated for many years. In the 1990s, the age comparison was already questioned by Savory et al. (1993). They postulated that determining feed restriction levels in breeder pullets at the same age is not a valid comparison because of the (large) body weight differences in controlled and ad libitum-fed pullets at the same age. Feed requirement is dependent on body weight because of the energy needed for maintenance, thus a heavier bird has a higher energy requirement. Surprisingly, in almost all studies performed on behaviour and welfare in breeders feed restriction level based on age is mentioned, ignoring the nuance of Savory et al. (1993). They observed feed intake of restricted and ad libitum fed pullets at the same age and found feed allowance of restricted birds was 44% at 2 WOA, around 25% between 7 to 15 WOA, and 51% at 20 WOA compared to ad libitum-fed birds. When Savory et al. (1993) compared the same body weight (and thus different age), they found a feed intake of 40% to 48% for restricted pullets compared to the ad libitum-fed pullets, resulting in a 52% to 60% feed restriction level. This is lower than the 67% to 75% of feed intake mentioned by Savory et al. (1993), Savory and Kostal (1996), and de Jong et al. (2002).
More recently, Arrazola (2018) introduced a novel method to estimate feed restriction levels by comparing the target feed intake of modern broiler breeders with the actual feed intake of modern broilers at the same body weight. He divided the rearing period into three periods: early (up to 7 WOA), middle (from 7 to 15 WOA), and late rearing (from 15 to 22 WOA). According to estimations, feed restriction level during the early rearing period (0 to 7 WOA) was between 0% (start rearing) and 50% (7 WOA). In the middle part of rearing period, feed restriction level was relatively constant between 50% and 55%. Feed restriction level decreased in the late rearing period from 55% to 35%. Feed restriction level during the laying period was between 35% (start of lay) with the lowest feed restriction level (approx. 20%) around 30 WOA, which increased to 30% around 65 WOA.
Calculating feed restriction based on body weight rather than age is an improvement toward more realistic representations of feed restriction levels. An even more realistic estimation of feed restriction levels is through the implementation of nutrients (especially energy) provided daily as proposed by van Emous (2021). Feeding pullets low energy diets will result in a higher feed allowance to achieve the same target weight at the end of the rearing period (Enting et al., 2007; de los Mozos et al., 2017). Broiler diets have a higher energy content (3,000-3,200 AMEn kcal/kg; Aviagen, 2019b) than broiler breeder diets (2,600-2,800 AMEn kcal/kg; Aviagen, 2016a,b). Calculations have been performed in our lab to determine the daily energy intake using feed intake targets and dietary energy
content at different phases of life for broiler females (Aviagen, 2019a,b) and breeder females (Aviagen, 2016a,b). The calculations showed that an energy restriction level between 7 and 16 WOA was 56%, and 47% for the entire rearing (Figure 1). This is lower than the 66% to 75% mentioned in the literature (Savory et al., 1993; Savory and Kostal, 1996; de Jong et al., 2002). For the laying period, feed restriction level is calculated to be 35%, which is comparable with the 37.5% for the laying period as mentioned by Bruggeman et al. (1999).
In this section, effects of feed restriction on physiology, behaviour, reproduction/health, and body composition are described.
Feed-restricted broiler breeders may show physiological signs of stress (van Krimpen and de Jong, 2014; D’Eath et al. , 2009). Various studies have reported physiological indicators of (chronic) stress, such as increased plasma corticosterone levels and increased heterophil to lymphocyte ratio in the blood in feed-restricted pullets (Hocking, 1993; Hocking et al. , 1996; Savory and Mann, 1997; de Jong et al. , 2003). In the last two decades, however, it has been discussed if these physiological indicators indeed reflect stress, frustration, and/ or hunger in the birds or if they merely reflect the metabolic state of the birds (de Jong et al. , 2003; D’Eath et al. , 2009).
Since then, experiments were performed to find more reliable methods to measure hunger and motivation for appetitive behaviour in broiler breeders. Different tests were developed, such as the Y-maze paradigm, novel food test, and water runway, but until now no reliable behavioural test to assess hunger is available for broiler breeders (Buckley et al., 2011a,b, 2015; Nielsen et al., 2011; Dixon et al., 2014). In general, all methods to measure hunger are indirect, which implies this may not reflect the real physiological state of the birds (D’Eath et al., 2009). Moreover, in studies where different methods to measure hunger were tested, contradictory results were found (de Jong et al., 2005). Besides the before-mentioned doubts and lack of proper methods to study the birds’ state, quantitative feed-restricted pullets probably experience hunger, which negatively affects the welfare of the bird (D’Eath et al., 2009).
As is commonly suggested, feed restriction leads to hunger or at least a disturbed metabolic state. The ratio of glucose to nonesterified fatty acids in the blood seems to be a good indicator of the metabolic state, which increases when restriction increases (de Jong et al., 2003; van der Wal et al., 1999; Scheurink et al., 1996). Regulation of hunger and feed intake is a complex interaction of hormones, gut filling, and metabolites. Understanding the endocrine regulation of chickens’ energy balance and, thus, avian homeostasis could optimise breeders’ welfare by taking the action where it is needed. However, the energy balance mechanisms in birds are less studied when compared to other vertebrates. In most vertebrates, the brain uses information
received from peripheral hormones regarding satiety or appetite to regulate food intake (reviewed by Honda et al., 2016). Adiposity hormones (leptin and insulin) suppress food intake, while gut hormones (such as gastrin, ghrelin, glucagon-like peptide-1, peptide YY (PYY), and cholecystokinin (CCK)) regulate appetite and digestive organ activity. In birds, the adiposity hormones are low and might not be primarily involved in food intake since body mass fat should be low for flying. Therefore, the appetite-regulating role of gut hormones might be more important in birds than in mammals (Honda et al., 2016). Furthermore, CCK might have other functions in the brain that are related to behaviour (Ballaz, 2017). The function of gastrin, CCK, and peptides in birds has been scarcely studied, especially in a feed-restricted state. Chronic feed restriction of growing broiler chickens is associated with increased hypothalamic neuropeptide Y (NPY) (Boswell et al., 1999). Furthermore, both a short-term and longterm nutritive state influence the expression of pancreatic PYY, while intestinal PYY does not fluctuate (Reid et al., 2017). As is shown by Reid and Dunn (2018) with NOVOgen BROWN birds, CCK expression does not respond to short-term satiety. Their results suggest a different expression under longterm nutritional challenges. Furthermore, the authors reported a paradoxical effect of gastrin, which was low when the birds reached satiety and low when in acute hunger. It was hypothesized that this was due to conditioning to ad libitum feeding conditions earlier in life, giving a heightened expression in anticipation of meal consumption as well as being accustomed to feed availability (Reid and Dunn, 2018). The melanocortin system
is responsible for monitoring and controlling appetite, as was reviewed by Hanlon et al. (2020), indicating the importance of agouti-related peptide (AgRP) and NPY for reproduction as well. Chronically restricted red junglefowl chicks with a lower body fat are hungrier according to AgRP and proopiomelanocortin augmentation (Lees et al., 2017). Another study has shown that AgRP (which induces obesity when over-expressed and stimulates food intake) and NPY gene expression provide an indication of chronic feed restriction, and AgRP potentially provides an integrated measure for recent satiety (Dunn et al., 2013), which has been confirmed by other studies (reviewed by Hanlon et al., 2020). The expression of AgRP could be a marker when studying feeding strategies to lift the negative impact of feed restriction on breeder welfare (Dunn et al., 2013). There are still many questions left considering the endocrinology behind hunger and the impact of chronic feed restriction but understanding the mechanisms could provide us with methods to test new strategies or innovative ideas to improve breeder welfare with or without restriction.
The ancestors of modern breeds like the red jungle fowl are omnivores that eat seeds and herbs as well as small insects (worms and insects) for energy and protein requirements (Savory et al., 1978). The eating behaviour profile of chickens is distinct between a feedseeking phase (or foraging) and a feed-eating phase (Kruijt, 1964). While foraging, chickens typically scrape their feet across the ground and
then peck for edible ingredients (foraging and scratching). Research conducted in (semi-) natural conditions showed that chickens spend 60% to 90% of their time during the day foraging and eating (Wood-Gush et al., 1978; Keeling, 2002; Appleby et al., 2004). In commercial circumstances, layers spent between 25% and 45% of the active time foraging (Appleby et al., 1989, 2004).
Chickens (thus also broiler breeders) use their beaks for a large part of the day performing eating-related and other behaviour (Arrazola et al., 2020). The beak replaces the function of a hand and is the main tool to explore and perform certain behaviours. Furthermore, chickens have taste and nutrient sensors in their beaks to guide their food choice (Cho, 2019). This potentially allows them to select feed not only based on taste but also on the type of nutrient (carbohydrates, fat, protein, and minerals). Chickens primarily peck to ingest food but the beak is also used for foraging, object and environment exploration, plumage maintenance and dust bathing. Increasing the feed restriction level reduces the time spent on feed intake, resulting in more time spent foraging (food-seeking and feeding behaviour) and reduces unwanted stereotypes such as object pecking (Hocking et al., 1996; van Emous et al., 2013, 2015a).
Studies have shown that feed restriction in breeder pullets results in abnormal behaviour that can be indicative of hunger and frustration (de Jong and Jones, 2006; D’Eath et al., 2009). Under chronic restrictions, this behaviour can develop into stereotypical behaviour (Mellor et al., 2018). Stereotypical behaviour is defined as repetitive behaviour, unchanging
in form, with no apparent purpose or function (Mason, 1991; Mason et al., 2006). It is a form of abnormal behaviour and is widely regarded as an indicator of decreased well-being (de Jong and Jones, 2006; D’Eath et al., 2009). In broiler breeders this manifests itself in pecking objects and high water intake (Kostal et al., 1992; Savory et al., 1993; Hocking et al., 1996, 2001Stereotypical object pecking is mostly observed as pecking at empty feeding systems, empty drinking systems, walls, and other equipment (Hocking et al., 1996, 2001; Savory en Kostal, 1996; de Jong et al., 2002). Behavioural scientists assume that feed restriction is frustrating and stressful for pullets, which manifests itself in stereotypical pecking behaviour. For example, in a study with restricted-fed pullets of 8 WOA, it was found that these birds pecked at the empty feeder about 40% during the light period (de Jong et al., 2002). A study by van Emous et al. (2015a) found an increase in stereotypical pecking behaviour (particularly towards empty feeding pans) from 15% to 30% during the day in pullets fed a standard diet. In contrast, they observed a lower level and a smaller increase (from 1% to 10%) of stereotypical pecking when pullets were fed a low crude protein diet. The authors hypothesized that the birds received a higher feed allowance resulting in a longer feed intake time leaving less time for unwanted behaviour.
The cause of stereotypical pecking of an empty feeding system can, in addition to redirected foraging behaviour, also be caused by small feed remains in the system (R.A. van Emous, 2020, personal observation). A small amount of feed may remain in the auger or pan system, resulting in increased
or continuous pecking on the pans or auger to get the remains. Several incentives could cause an increase in pecking. The birds could be motivated by hunger, causing strong feed intake motivation to peck as much as possible for all small feed residues (Buckley et al., 2011a,b). However, since the breeders’ excessive pecking is rewarded with a small amount of feed, they might become conditioned to continue this behaviour due to the dopamine reward system.
During the highest feed allowance in the laying period (between 165 and 170 g/bird/ day), breeders spend up to four hours per day eating. At the end of the production phase (up to 60 WOA) this is approximately three hours per day (de Jong and van Emous, 2017). At the onset of the laying period stereotypical object pecking and pecking at the litter rapidly decreases due to the reduced feed restriction (Sandilands et al., 2005). Van Emous et al. (2015a) observed a seven-fold lower level of stereotypical object pecking during the laying phase compared to the rearing phase. The primary behavioural and welfare challenges for broiler breeders therefore is mainly concentrated in the rearing phase (Arrazola, 2018).
In broiler breeders, gentle feather pecking and tail feather licking are regularly observed, especially between 15 and 25 WOA. These behaviours are relatively harmless however, they could indicate abnormal behaviour (Dixon et al., 2008). Gentle feather pecking is defined as pecking at feathers of conspecifics without removing the feathers or causing a reaction from the victim (Hocking et al., 2005;
Newberry et al., 2007; Dixon et al., 2008; de Jong et al., 2013). In contrast to layer flocks, the incidence of gentle feather pecking and severe feather pecking, in which the feather is pulled out of the plumage, is low in broiler parent stock (de Jong and Guémené, 2011). Feather pecking is mostly aimed at the tail and back feathers, while aggressive pecking aims at the head. Gentle feather pecking could also have a function in normal social interactions (allopreening) but could become problematic when it turns into stereotypical gentle feather pecking. Stereotypical gentle feather pecking behaviour that targets the plumage of conspecifics or of a bird’s own feathers can result in poor feathering (Morrissey et al., 2014a; Arrazola et al., 2019) and can occasionally result in severe feather pecking (Savory et al., 1993). In the study by van Emous et al. (2014, 2015a), the proportion of feather pecking (and tail feather licking) to other pullets during the rearing phase in the first and second experiment was 5% and 0.5%, respectively, while it was negligible during the laying phase.
Stereotypical gentle feather pecking in breeder pullets is also described as feather (or tail feather) licking (Nielsen et al., 2011; van Emous et al., 2015a). As a result of this abnormal behaviour, feathers may be damaged but are not removed. This stereotypical feather licking is mainly observed in the period between 15 and 25 WOA (Nielsen et al., 2011). This is roughly after the period (7 to 16 WOA) when pullets are most severely feed restricted (Savory et al., 1993; de Jong and Jones, 2006). This behaviour is primarily recognizable by the activity itself but also by the somewhat darker and dirtier feathers on
the tail (Nielsen et al., 2011). In experiments and in practice, this behaviour is not observed in all flocks, nor to the same extent, and as a result hardly any causes are mentioned in the (scientific) literature.
Tail feather licking could also have a nonstereotypical incentive. It is suggested that birds display this behaviour because they have a shortage of energy from the feed. Research by Sandilands et al. (2004 a,b) has shown that the preen gland, which is close to the tail, releases various types of fatty acids and esters. These substances are used to waterproof the plumage, maintain feather condition (Thompson, 1964), and act as an antimicrobial agent (Stevens, 1996). Furthermore, it might be possible that salts in the oils from the preen gland are a trigger to start tail feather licking since deficiency in salt can result in feather pecking (Hughes and Whitehead, 1979). Tail feather licking is regularly observed as group behaviour, which means that it is probably copying behaviour. However, when breeders cannot benefit from the energy or salt anymore, thereby losing apparent function but continue tail feather licking, this behaviour could become stereotypical.
Stereotypical pecking at the drinkers can lead to excessive drinking (Savory et al., 1993; Hocking, 1993; Hocking et al., 2001) and water spillage (Sandilands et al., 2005). The only scientific paper to investigate water restriction in breeder pullets found no evidence of physiological stress in these birds (Hocking, 1993). In that experiment, executed with feed-restricted Cobb and Ross pullets, a water-to-feed ratio of 4.7 and 2.4,
respectively, was used for free and limited access to water. Moreover, they observed that pullets with two to three hours of access to water in the morning did not appear to be particularly thirsty in the afternoon. It was therefore concluded that breeding hens do not need constant access of water.
The water system itself seems to be connected to over-drinking and spillage. In most experiments that report excessive drinking and water spillage, open water systems were used (Kostal et al., 1992; Savory et al., 1993; Hocking et al., 1996, 2001). In these systems, the water intake for breeder pullets is much easier than from modern nipple drinkers resulting in a higher water intake. In addition, open water systems led to more water spillage compared to nipple drinkers, which undesirably increased water intake and likely was also the cause of wet litter problems that were mentioned in all four experiments. Another explanation for the excessive drinking could be the much longer (14 vs. 8 hours) day length used by Kostal et al. (1992) and Savory et al. (1993). This offers the breeder pullets a longer and, therefore, higher opportunity to drink water. Providing unlimited water for a longer period, especially in combination with an open water system, can lead to compulsive drinking as a remedy for boredom resulting in excessive water use.
In a recent experiment by van Emous et al. (2021), it was observed that there was no difference in water intake in rearing pullets that were fed either a basal or 16% diluted diet with unlimited access to water during the light period. They assumed that water
intake for pullets was maximized due to the physical capacity of the crop and that the water saturation level was not influenced by feed restriction. Overall, the average waterto-feed ratio was higher (2.3 ml water to 1 g feed) than is normally advised under commercial conditions (Aviagen-EPI, 2017).
Despite the higher water-to-feed ratio, the litter quality was not affected. This was also observed in several experiments performed by Aviagen-EPI in recent years wherein unrestricted water was provided to pullets. During these experiments, a water-to-feed ratio between 2.15 and 2.30 was observed without problems with litter quality. Between 5 and 7 WOA, litter quality decreased because the pullets showed more drinking behaviour and water spillage. When water intake was restricted at that age, obsessive drinking behaviour actually increased (J. Lesuisse, 2020, personal communication). Around that critical age, it is important to regularly process the bedding so that it dries again to prevent litter problems. It is postulated that the issue of over-drinking can be summarized by the expression: “Drinkers are reared and not born.”
When pullets are feed-restricted they show increased activity, which is reflected in more walking behaviour (sometimes in a stereotypical form, so-called ‘pacing’), specifically before feeding. Pacing connected to feeding is mentioned by many studies as an indicator of hunger in broiler breeders or hunger and frustration due to a deficiency of feed (Hocking, 1993; Hocking et al., 1996; Savory and Maros, 1993; Savory and Kostal, 1996; Savory and Mann, 1997; de
Jong et al., 2002). Moreover, stereotypical walking is positively correlated with feed restriction level (Savory and Maros, 1993; Hocking et al., 1996). In recent years, however, the conclusion that walking before feeding is indicative of hunger in broiler breeders has been questioned. In a recent study, rearing pullets were fed once or twice a day in combination with a control and diluted diet (Mens et al., submitted). The pullets were all fed in the morning, and the second meal was provided in the afternoon. Since all treatments were housed within the same room, pullets that were only fed in the morning saw and heard the activity from both the caretakers and the pullets in the other pens (vocalizations, walking, running, and flying) that were receiving the second meal and did not respond by displaying (similar) active behaviour. They observed more sitting (resting) behaviour in once-aday fed pullets between 12:00 h and 15:00 h when twice-a-day fed pullets in adjacent pens were fed the second proportion of feed. The difference in behaviour during the day was also visible around 15 minutes before the second feeding. Pullets fed once-a-day showed less walking or pacing behaviour, which is known as stereotypical behaviour, directly before the second meal in the other pens. This indicates a certain anticipation of a meal since the pullets that would receive the second meal were getting ready to eat, while the pullets that would not receive another meal remained inactive. According to the authors, these observations could be explained in two ways. On the one hand, it is possible both nutritional strategies and the combination of both are not sufficient to overcome the negative effects of feed
restriction. Two meals might not be enough to fulfill their behavioural needs since chickens in natural environments eat several meals throughout the day (Dawkins, 1989). Furthermore, the dilution of the diet was not aligned with the feed restriction, which could still result in hunger and frustration. On the other hand, it is postulated that walking behaviour prior to feeding could be considered a normal behaviour that is also observed in other animals. Under the influence of habituation, animals know that they will be fed (biological clock, certain sounds and behaviours of the caretaker) and they respond by becoming active, walking back and forth in anticipation of the feed. The question is whether the walking behaviour before feeding is an indication of hunger or whether it is a normal behaviour that corresponds to the expectation that the animals will receive feed. This behaviour is also observed in captive wild animals under little or no feed restriction (McPhee and Carlstead, 2010).
It is commonly accepted that feed restriction results in a higher motivation to consume feed. In an experiment by Savory and Lariviere (2000), a positive correlation was found between higher feed restriction and higher feed intake motivation. This was confirmed in research by Sandilands et al. (2005) and Tolkamp et al. (2005) who fed broiler breeders ad libitum diets diluted with oat hulls (with additional calcium propionate, an appetite inhibitor). Furthermore, it was found that stereotypical pecking behaviour was virtually absent and that the time birds spend sitting increased significantly. Moreover, it was
observed that breeder pullets of 10 WOA ran faster to the feed with severe feed restriction (Buckley et al., 2011a). They also indicated that maybe due to the severe feed restriction, the pullets may not be able to learn certain tasks. Subjecting broiler breeder pullets to a novel feed test showed high motivations to feed with short latencies to reach and consume from a novel feeder with novel feed (Mens et al., personal communication).
One study tested the relationship between feed motivation and the incidence of floor eggs in broiler breeders (Sheppard and Duncan, 2011). It was shown that the motivation to consume a limited amount of feed during the production period, where the restriction level is lower compared to the rearing phase, was still high. They observed that females left the nest boxes during oviposition when feed was supplied, which resulted in an increase in floor eggs.
Feed restriction is associated with an improvement in egg production and health compared to ad libitum-fed broiler breeders. When broiler breeder pullets receive ad libitum feed, as in broilers, it results in excessive body weight development (two and a half times higher at onset of lay) with adverse effects on reproductive performance (Katanbaf et al., 1989; Savory et al., 1993; Heck et al., 2004). In the latter study, the authors found that ad libitum-fed pullets showed five times higher mortality, 34% lower egg production, and 74% higher feed conversion ratio between 32 and 40 WOA, in comparison to restrictedfed birds (Heck et al., 2004). Feed restriction
in broiler breeder pullets also prevents multiovulations that result in double yolks and regulate a normal follicle hierarchy (Mench, 2002). Fewer double yolk eggs result in less mortality due to reduction in the possibility of prolapse (bulge of the cloaca) during the first 10 weeks of the laying period (A. Steentjes, 2019, personal communication). Feed restrictions in breeders provides lower body weight, improved mobility and male stability, which results in more successful matings (Mench, 2002).
Both restricted and ad libitum feeding could affect bird health. Research suggests that adrenocorticotropin (ACTH) and glucocorticoids (such as corticosterone), which are commonly elevated in feed-restricted birds due to stress, can have a negative effect on the immune system (Montiel, 2016). Higher levels of ACTH and corticosterone can suppress both innate and induced immunity (Shini and Kaiser, 2009; Moe et al., 2010). The harmful effect of increased stress on the innate immune system results in a decreased efficacy of pathogen control programs in birds causing higher infection rates and, eventually, mortality (Latshaw, 1991; Montiel, 2016). It is postulated that transient stress situations in layers aid the transmission of Salmonella enteritidis from birds to consumption eggs, which is the main source of infection in humans during paratyphoid outbreaks in the past 20 years (Humphrey, 2006).
Body composition of adult breeders is important to ensure healthy and efficient production (van Emous, 2015). It was
concluded that higher abdominal fat pad weight and lower breast muscle content at the end of the rearing period positively affected reproduction (van Emous, 2015). Breeders with a higher body fat content are probably more able to mobilize energy reserves in periods of a negative energy balance (Renema et al., 2013), which probably prevents them from moulting. Lower muscle content may decrease the daily energy requirement for maintenance and increase the amount of energy available for egg production (Ekmay et al., 2013).
Applying different protein diets during the rearing period influences pullet body composition by increasing body fat content (Mba et al., 2010; van Emous et al., 2013,
2015b). In the studies of van Emous et al. (2013, 2015b), no effects of dietary protein on abdominal fat percentage were found at 15 WOA, while this was present at 10 and 20 WOA. Furthermore, Mba et al. (2010) observed a difference in abdominal fat percentage at 12 WOA that was influenced by differences in dietary protein, while this had disappeared at 19 WOA. It seems that the development of abdominal fat and total fat content of the body follows a specific pattern during the rearing period (Figure 2). This pattern in body composition was previously reported by Bennet and Leeson (1990) who found reduced total fat levels between 2 and 14 WOA, but increased fat levels between 14 and 24 WOA.
In this section, the different possibilities to reduce the effects of feed restriction are described.
Feed restriction is becoming a larger topic in societal discussion. By omitting restrictions altogether, the negative effects are most effectively solved but this leads to the problems indicated before in the broiler breeder paradox. In literature, using dwarf parents is a suggested solution to mitigate the negative effects of feed restriction because these birds have a relatively good reproduction rate when fed ad libitum (Decuypere et al., 2006, 2010). The offspring of dwarf breeders are still relatively fast-growing broilers if the hen is crossed with a fast-growing male that is feed restricted. However, in the study of Heck et al. (2004), it was found that egg production of dwarf breeders was lower than that of regular fastgrowing restricted-fed females. Research has shown that replacing regular males with dwarf males can improve welfare. This is mainly due to the lack of feed restriction and results in beneficial positive changes in behaviour, such as less stereotypical pecking behaviour (Jones et al., 2004). It is noted in literature that size difference between the female and male could cause problems in mating behaviour, leading to necessary artificial insemination (Decuypere et al., 2010). However, in practice, this is not observed and maybe even fewer problems are observed since males are not really mounting but are more standing over the females (I.C. de Jong, personal communication). In addition,
dwarf breeders are aimed at a specific market segment so using these birds is often not desirable for the poultry farmer for economic reasons (de Jong and Guémené, 2011).
Another mentioned solution is to use more slow-growing breeds and formulate a broader breeding goal with welfare implemented (Decuypere et al., 2010). However, similar to the situation of dwarf hens, slow-growing breeds are often for a specific market (de Jong and Guémené, 2011). It should be noted that replacing regular, fast-growing broilers with slow-growing broilers has consequences with regard to the environment, sustainability (e.g., higher feed conversion, higher land use, higher NH3 and fine dust emissions), feed efficiency, and other non-welfarerelated aspects. A desk study showed that the environmental impact of parent stock of slow-growing broilers is lower than that of parent stock of regular broilers due to the higher production and lower feed intake (Rougoor and van der Schans, 2019).
A higher body weight profile during rearing resulted in decreased feed restriction levels in birds with higher body fat mass at 20 WOA. Only two studies include behavioural observations connecting the influence on breeders’ welfare (van Emous et al., 2013; de los Mozos et al., 2017). Van Emous et al. (2013) studied the effects of three dietary protein levels and two different growth profiles (2,400 vs. 2,200 g at 20 WOA).
Although pullet feed intake maintained at 9%, higher body weight profile was increased (6.5%) resulting in an extended feeding time.
Stereotypical object pecking and bird pecking was not affected. In the study by de los Mozos et al. (2017), pullets were kept at a 15% higher body weight. The pullets kept according to the higher body weight profile spent 19 minutes longer consuming the feed. This study also showed no effects on stereotypical pecking behaviour.
Adjusting diet composition is another possibility frequently investigated as a method to reduce the effects of feed restriction. For example, by offering more feed with a lower level of all or some nutrients (energy and protein/amino acids: diluted diets), the feed restriction level could be limited. Another example is adjusting the energy-to-protein ratio in the feed (Sandilands et al., 2006, van Emous et al., 2014, 2015a). The studies by van Emous et al. (2014, 2015a) examined the effects of three different dietary protein levels and different body weight profiles (2,400 g versus 2,200 g at 20 WOA) on indicators of welfare during the rearing and production phase. Partly due to the higher amount of feed, a positive effect on welfare indicators was found for the low-protein diets during the rearing period. Stereotypical object pecking in the rearing phase was reduced in the low protein diet pullets compared to the high protein diet pullets.
The conclusions within the scientific community about alternative diets are contradictory to date and more research will have to show what the actual influences of these strategies are on bird welfare (D’Eath et al., 2009). Some works suggest
that alternative feeding strategies result in improved bird welfare by responding to more natural feeding behaviour and/or a feeling of fullness (Zuidhof et al., 1995; de Jong et al., 2005; Sandilands et al., 2006). Others, however, conclude that this method does not eliminate ‘metabolic hunger’ (de Jong et al., 2005; D’Eath et al., 2009). In addition, there are studies that found no or limited positive effects on behaviour and welfare as a result of adjusted diets (Savory and Lariviere, 2000; Hocking et al., 2001; Tahamtani et al., 2020; Mens et al., personal communication). For example, Decuypere et al. (2006) concluded that the provision of feed with high levels of crude fibre, resulting in a higher feed allowance with less nutritional value, gave negligible results on bird welfare.
Appetite suppressants (e.g., calcium propionate) in combination with ad libitum oat hulls as an alternative to feed restriction seem to have a positive effect on improving the stereotypical pecking behaviour and thus the welfare of parent stock (Tolkamp et al., 2005; Sandilands et al., 2005, 2006; Morrissey et al., 2014b; Arrazola et al., 2019). This alternative feeding strategy ensures that the desired growth pattern is followed despite ad libitum feeding. Object pecking is almost non-existent (<1%) in diets including calcium propionate compared to standard diets (about 50%). However, there are indications that the birds may feel ill from the appetite suppressants, which reduces bird welfare (Sandilands et al., 2005, 2006; Morrissey et al., 2014b; Arrazola et al., 2019). In addition, other behavioural and physiological parameters (such as plasma corticosterone levels) did not differ compared
to restricted birds (Sandilands et al., 2006), indicating there are no effects on the birds’ stress levels.
Studies with different feeding strategies during the rearing phase that focus on production performance are very limited. However, it has been shown that pullets fed diluted diets need a higher feed allowance to compensate for lower nutrient concentration to obtain the same body weight target at end of rear (Enting et al., 2007; Zuidhof et al., 2015; de los Mozos et al., 2017; van Emous et al., 2021). Breeders fed low-density diets during the laying phase showed increased egg production and egg weight compared to control diets (Enting et al., 2007). In a recent 2 x 2 factorial study, pullets were fed once or twice a day standard or 16% diluted diets (van Emous et al., 2021). The pullets fed the diluted diets received a higher feed allowance in combination with similar water intake, which resulted in a lower water/feed ratio compared to the control pullets, and thus in higher dry matter content in the litter and improved litter quality. The higher feed allowance resulted in a 20% higher total manure production at 20 WOA.
In addition to composition of the feed, it seems that the method of feeding could also influence breeder pullet behaviour and welfare. Pullets are often fed on a feeding schedule that provides them with one meal per day (most parts of Europe), or one meal every other day, or other weekly schedules (United States). The adjustment in feeding
frequency (twice compared to once a day) during the rearing phase has been investigated by van der Haar and van Voorst (2001) in combination with feeding via feeder pans or spin feeders. Pullets fed twice a day spent less time scratching, walking, and standing than pullets fed once a day. It was remarkable that the concentration of corticosterone in blood plasma at 12 WOA was the highest in pullets fed twice compared to those fed once a day. In addition, uniformity was lowest at 13 WOA in hens fed twice a day from feeder pans. de Jong et al. (2005) also investigated stress and hunger as effects of feeding twice versus once a day in addition to scattering feed in the litter (spin feeder) or feeder pans during the rearing period. They found no effects on behaviour or physiological indicators of stress.
A 2 x 2 factorial experiment of diet density and feeding frequency in rearing pullets was performed in our lab, focusing on both behaviour and performance. Although not scientifically properly recorded, it was noted by the animal caretakers that breeders of the twice-a-day fed groups reacted differently to entering the compartments. When pullets in the pens received the second proportion of feed, they showed pacing, running, fluttering, and made more noise. The pullets that were not fed at that time continued performing scratching, sitting, standing, and comfort behaviour almost without reaction to the animal keeper. It seems likely that the birds were conditioned to the pattern of feeding, causing them to react in this way. The pullets fed in the afternoon connected entry of the caretakers with feed, while the pullets that didn’t receive feed in the afternoon did not have that linkage. Thus, it might be that
behaviour around feeding is initiated due to habituation and expectation of feeding and maybe less with hunger. However, this cannot be said with certainty without properly recording the behaviour and measuring physiological parameters.
In addition to the behavioural observations, twice a day feeding improved flock body weight uniformity (11.5% vs. 14.2% BW CV) at 10 WOA (van Emous et al., 2021). However, at 20 WOA this difference had disappeared. This was the opposite to the results of van der Haar and van Voorst (2001) who found poorer body weight uniformity at 13 WOA when hens were fed twice a day. This discrepancy between the studies can be explained by the differences in feed structure (meal and pellets) and the different breeds (Ross 508 and Ross 308) used. In the same study of van Emous et al. (2021), pullets fed twice a day tended to reach earlier onset of lay, higher total egg production at 30 WOA, and better egg fertility.
From the previous sections describing different methods to minimize the effects of feed restriction it can be concluded that the researched feeding strategies are not giving sufficient effects on welfare. Therefore, new feeding strategies and combinations of different and/or more extreme strategies should be developed.
For example, it is possible that increasing feeding frequency to more than twice a day has effects on behaviour, welfare, and production. A precision feeding system
for breeder pullets was developed to use real-time body weight measurement to feed individual birds. They observed high variability in feeding behaviour patterns between the pullets (Zuidhof, 2018). The average number of visits to the precision feeding system ranged from 28 to 138 times per bird, per day (average 61 times/bird/ day), which clearly showed differences in the individual character of the pullets.
It is postulated that a combination of measures to increase feed intake time and reduce the consequences of feed restriction seems to generate the strongest effect. A multifactorial solution includes feeding several times a day, different methods of feed distribution, and dilution of the diet. Because it is currently difficult under practical circumstances to distribute a small amount of feed, the use of a spin feeder (distributing feed over the litter) is a good option. In addition, even more innovative solutions can be developed for quick distribution of the feed over the flock and/or offering it in a different form (e.g., large particles).
More exotic feed ingredients could also improve breeder welfare. Feeding pullets living insects in addition to regular rearing diets could be an interesting option. Providing living insects with pullets gives them the opportunity to express their natural feeding behaviour (Carr, 2016). This might be pleasurable for pullets and can therefore improve poultry welfare (Widowski and Duncan, 2000). Insects are not only an important energy and protein source but also a moving stimulus to activate chickens (Paul et al., 2016a,b, 2017; Clara et al., 2009). Under natural circumstances,
small animals and insects are an important part of the diet of the red junglefowl (Collias and Collias, 1967; Arshad et al., 2000) who are the ancestors of modern birds. Arshad et al. (2000) analysed crops of 80 individual Red jungle fowl birds and found 26 different invertebrates, with termites and ants predominant. In layer research, improvements in welfare are already found. An experiment with laying hens with intact beaks with a provision of live black soldier fly larvae saw a positive effect on feather cover (Star et al., 2020). When feeding novel feedstuffs such as insects, the risks for both breeder and products need to be closely monitored. Feeding insects to chickens must not increase microbiological and chemical contamination risks (EFSA, 2015).
In addition to the mentioned different feeding strategies, it is postulated that unrestricted access to water could decrease stress due to feed restrictions. The ancestors of modern chickens are known as nonobligatory drinkers since they fulfill their water requirement by ingestioning watercontaining arthropods, leeches, and tender leaves (Arshad et al., 2000). In commercial houses, however, breeder pullets are only fed diets that contain a low proportion of water (approximately 10%). Under commercial circumstances daily access to water is limited to a certain period (e.g., 2 to 3 h) of time or a maximum amount of water based on water-to-feed ratio (e.g., 1.8) (Hocking, 1993; de Jong et al., 2016). This means that pullets have no access to water during approximately 70% of the light period (2.5 h of 8 h light), initiating the discussion about water restriction and the effects on welfare.
The modern broiler breeder has high genetic growth potential but its main goal is to produce hatching eggs. Providing broiler breeders with an ad libitum feed allowance would support fast growth and causes too high body weight with detrimental effects on health and reproduction. This implies that these birds should receive a limited amount of feed, especially during the rearing phase, to prevent excessive growth and thereby health and reproduction problems. According to the literature, feed restriction level during rearing is estimated to be around 70%, whereas feed restriction, in this chapter, based on energy intake, is estimated to be 47%. In literature, there is no consensus on the effects of feed restriction on physiological parameters. However, there are numerous studies that have shown that feed-restricted broiler breeders show abnormal stereotypical pecking behaviour towards equipment, which can be indicative of frustration, boredom, and hunger. Also, stereotypical tail feather licking or pecking can be an indication of frustration or boredom. Over-drinking was also mentioned as a sign of abnormal behaviour caused by feed restriction, however, in modern pullets, over-drinking is no longer considered a problem. Pacing before feeding is often mentioned as an indication of stress or frustration, however, recent research questions if this could be normal behaviour since most animals (also less or not restricted) show this behaviour before feeding. To alleviate the negative effects of feed restriction on pullet behaviour, different feeding goals and strategies are investigated: body weight profile, diet composition,
feeding frequency, and scattering feeding. In general, most of these methods, or combinations of these methods, had only small effects on abnormal behaviour and physiological parameters. Further research is necessary to diminish the negative effects of feed restriction on bird behaviour and improve welfare and the combination of different feeding methods and strategies seems to be the most promising direction to a solution.
Appleby, M.C., Hughes, B.O. and G.S. Hogarth. 1989. Behaviour of laying hens in a deep litter house. British Poultry Science 30: 545-553. Appleby, M.C. Mench, J.A. and B.O. Hughes. 2004. Poultry Behaviour and Welfare. CABI Publishing, Wallingford, Oxfordshire, UK.
Arrazola, A. 2018. The effect of alternative feeding strategies for broiler breeder pullets throughout the production cycle. PhD Diss. The University of Guelph, Guelph, Ontario, Canada.
Arrazola, A., Mosco, E., Widowski, T.M., Guerin, M.T., Kiarie, E.G. and S. Torrey. 2019. The effect of alternative feeding strategies for broiler breeder pullets: 1. Welfare and performance during rearing. Poultry Science 98: 3370-3390.
Arrazola, A., Mosco, E., Widowski, T.M., Guerin, M.T. and E.G. Kiarie. 2020. The effect of alternative feeding strategies during rearing on the behaviour of broiler breeder pullets. Applied Animal Behaviour Science 224: 1-8.
Arshad, M.I., Zakaria, M., Sajap, A.S. and A. Ismail. 2000. Food and feeding habits of Red Junglefowl. Pakistan Journal of Biological Sciences 3: 10241026.
Augère-Granier, M.L. 2019. The EU poultry meat and egg sector. https://www.europarl.europa.eu/
thinktank/en/document.html?reference=EPRS_ IDA(2019)644195.
Aviagen. 2016a. European Parent Stock. Ross 308 Parent Stock: Performance Objectives. Aviagen, Ltd., Huntsville, Alabama, USA.
Aviagen. 2016b. Parent Stock. Ross 308 Parent Stock: Nutrition specifications. Aviagen, Ltd., Huntsville, Alabama, USA.
Aviagen-EPI, 2017. Management guide Ross 308 parent stock (in Dutch). Roermond, the Nederlands. Aviagen. 2019a. Broiler. Ross 308/Ross 308 FF Broiler: Performance objectives. Aviagen, Ltd., Huntsville, Alabama, USA. Aviagen. 2019b. Broiler. Ross Broiler: Nutrition specifications (all plan protein-based feeds). Aviagen, Ltd., Huntsville, Alabama, USA. Ballaz, S. 2017. The unappreciated roles of the cholecystokinin receptor CCK (1) in brain functioning. Reviews in the Neurosciences 28: 573585.
Bennett, C.D. and S. Leeson. 1990. Body composition of the broiler-breeder pullet. Poultry Science 69: 715-720.
Boswell, T., Dunn, I.C. and S.A. Corr. Hypothalamic neuropeptide Y mRNA is increased after feed restriction in growing broilers. Poultry Science 78: 1203-1207.
Bruggeman, V., Onagbesan, O., D’Hondt, E., Buys, N., Safi, M., Vanmontfort, D., Berghman, L., Vandesande, F. and E. Decuypere. 1999. Effects of timing and duration of feed restriction during rearing on reproductive characteristics in broiler breeder females. Poultry Science 78: 1424-1434.
Buckley, L.A., McMillan, L.M., Sandilands, V., Tolkamp, B.J., Hocking, P.M. and R.B. D’Eath. 2011a. Too hungry to learn? Hungry broiler breeders fail to learn a Y-maze food quantity discrimination task. Animal Welfare 20: 469-481.
Buckley, L.A., Sandilands, V., Tolkamp, B.J. and R.B. D’Eath. 2011b. Quantifying hungry broiler breeder
Breeder Management and Nutrition: Moving the industry forward
dietary preferences using a closed economy T-maze task. Applied Animal Behaviour Science 133: 216-227. Buckley, L.A., Sandilands, V., Hocking, P.M., Tolkamp, B.J. and R.B. D’Eath. 2015. Feedrestricted broiler breeders: State-dependent learning as a novel welfare assessment tool to evaluate their hunger state? Applied Animal Behaviour Science 165: 124-132.
Carr, N. 2016. Domestic Animals and Leisure. Springer, Berlin, Germany. Cho, S. 2019. Nutrient specific appetites and feather pecking behaviour in laying hens. PhD Diss. The University of Queensland, Queensland, Australia. Clara, E., Regolin, L., Vallortigara, G. and L.J. Rogers. 2009. Chicks prefer to peck at insect-like elongated stimuli moving in a direction orthogonal to their longer axis. Animal Cognition 12: 755-765. Collias, N.E. and E.C. Collias. 1967. A field study of the Red Jungle fowl in North-Central India. The Condor 69: 360-386.
Dawkins, M.S. 1989. Time budgets in Red Jungle Fowl as a baseline for the assessment of welfare in domestic fowl. Applied Animal Behaviour Science 24: 77-80.
D’Eath, R.B., Tolkamp, B.J., Kyriazakis, I. and A.B. Lawrence. 2009. ‘Freedom from hunger’ and preventing obesity: the animal welfare implications of reducing foot quantity or food quality. Animal Behaviour 77: 275-288.
Decuypere, E., Hocking, P.M., Tona, K., Onagbesan, O., Bruggeman, V., Jones, E.K.M., Cassy, S., Rideau, N., Metayer, S., Jego, Y., Putterflam, J., Tesseraud, S., Collin, A., Duclos, M. Trevidy, J.J. and J. Williams. 2006. Broiler breeder paradox: a project report. World’s Poultry Science Journal 62: 443-53.
Decuypere, E., Bruggeman, V., Everaert, N. Yue Li, Boonen, R., de Tavernier, J., Janssens, S. and N. Buys. 2010. The Broiler Breeder Paradox: ethical, genetic and physiological perspectives, and suggestions for
solutions. British Poultry Science 51: 569-579. de Jong, I.C., van Voorst, S., Ehlhardt, D.A. and H.J. Blokhuis. 2002. Effects of restricted feeding on physiological stress parameters in growing broiler breeders. British Poultry Science 43: 157-168. de Jong, I.C., van Voorst, A.S. and H.J. Blokhuis. 2003. Parameters for quantification of hunger in broiler breeders. Physiology & Behavior 78: 773-783. de Jong, I.C., Fillerup, M. and H.J. Blokhuis. 2005. Effect of scattered feeding and feeding twice a day during rearing on parameters of hunger and frustration in broiler breeders. Applied Animal Behaviour Science 92: 61-76. de Jong, I.C. and B. Jones. 2006. Feed restriction and welfare in domestic birds. In Feeding in Domestic Vertebrates. From Structure to Behavior. V. Bels (ed). CABI Publishing, Wallingford, UK. pp. 120-135. de Jong, I.C. and D. Guémené. 2011. Major welfare issues in broiler breeders. World’s Poultry Science Journal 67: 73–81. de Jong, I.C., Reuvekamp, B.F.J. and H. Gunnink. 2013. Can substrate in early rearing prevent feather pecking in adult laying hens? Animal Welfare 22: 305-314.
de Jong, I.C., van Harn, J., Koene, P., Ellen, H., van Emous, R.A., Rommers, J.M. and H. van den Brand. 2016. Risk assessment of water supply to broilers and broiler breeders (in Dutch). Report 989. Wageningen Livestock Research, Wageningen, the Netherlands. de Jong, I.C. and R.A. van Emous. 2017. Broiler breeding flocks: management and animal welfare. In Achieving sustainable production of poultry meat. T. Applegate (ed). Burleigh Dodds Science Publishing Limited, UK. pp 211-230. de los Mozos, J., García-Ruiz, A.I., den Hartog, L.A. and M.J. Villamide. 2017. Growth curve and diet density affect eating motivation, behavior, and body composition of broiler breeders during rearing. Poultry Science 96: 2708-2717
Dixon, L., Duncan, I. and G. Mason. 2008. What’s in a peck? Using fixed action pattern morphology to identify the motivational basis of abnormal featherpecking behaviour. Animal Behaviour 76: 1035-1042.
Dixon, L.M., Brocklehurst, S., Sandilands, V., Bateson, M., Tolkamp, B.J. and R.B. D’Eath. 2014. Measuring motivation for appetitive behaviour: food-restricted broiler breeder chickens cross a water barrier to forage in an area of wood shavings without food. Plos ONE 9. doi 10.1371/ journal. pone.0102322.
Dunn, I.C., Wilson, P.W., Smulders, T.V., Sandilands, V., D’eath, R.B. and T. Boswell. 2013. Hypothalamic agouti‐related protein expression is affected by both acute and chronic experience of food restriction and re‐feeding in chickens. Journal of neuroendocrinology 25: 920-928. EFSA. 2010. Scientific Opinion on welfare aspects of the management and housing of the grandparent and parent stocks raised and kept for breeding purposes. The EFSA Journal 8: 81. EFSA. 2015. Risk profile related to production and consumption of insects as food and feed. The EFSA Journal 13: 4257.
Ekmay, R. D., De Beer, M., Mei, S. J., Manangi, M. and C. N. Coon. 2013. Amino acid requirements of broiler breeders at peak production for egg mass, body weight, and fertility. Poultry Science 92: 9921006.
Enting, H., Boersma, W.J.A., Cornelissen, J., van Winden, S.C.L., Verstegen, M.W.A. and P.J. van der Aar. 2007. The effect of low-density broiler breeder diets on performance and immune status of their offspring. Poultry Science 86: 282-290.
Hanlon, C., Ramachandran, R., Zuidhof, M.J. and G.Y. Bédécarrats. 2020. Should I lay or should I grow: photoperiodic versus metabolic cues in chickens. Frontiers in physiology 11: 707.
Havenstein, G.B., Ferket, P.R. and M.A. Qureshi. 2003a. Growth, livability, and feed conversion of
1957 versus 2001 broilers when fed representative 1957 and 2001 broiler diets. Poultry Science 82: 1500-1508.
Havenstein, G.B., Ferket, P.R. and M.A. Qureshi 2003b. Carcass composition and yield of 1957 versus 2001 broilers when fed representative 1957 and 2001 broiler diets. Poultry Science 82: 15091518.
Heck, A., Onagbesan, O., Tona, K., Metayer, S., Putterflam, J., Jego, Y., Trevidy, J.J., Decuypere, E., Williams, J., Picard, M. and V. Bruggeman. 2004. Effects of ad libitum feeding on performance of different strains of broiler breeders. British Poultry Science 45: 695-703.
Hocking, P.M. 1993. Welfare of broiler breeder and layer females subjected to food and water control during rearing: Quantifying the degree of restriction. British Poultry Science 343: 53-64.
Hocking, P.M., Maxwell, M.H. and M.A. Mitchell. 1996. Relationships between the degree of food restriction and welfare indices in broiler breeder females. British Poultry Science 37: 263-278.
Hocking, P.M., Maxwell, M.H., Robertson, G.W. and M.A. Mitchell. 2001. Welfare assessment of modified rearing programs for broiler breeders. British Poultry Science 42: 424-432.
Hocking, P.M., Jones, E. and M. Picard. 2005. Assessing the welfare consequences of providing litter for feed-restricted broiler breeders. British Poultry Science 46: 545-552.
Honda, K., Saneyasu, T. and H. Kamisoyma. 2016. Gut hormones and regulation of food intake in birds. The Journal of Poultry Science 54: 103-110
Hughes, B.O. and C.C. Whitehead. 1979. Behavioural changes associated with the feeding of low sodium diets to laying hens. Applied Animal Ethology 5: 255-266.
Humphrey, T. 2006. Are happy chickens safer chickens? Poultry welfare and disease susceptibility. British Poultry Science 47: 379-391.
Jones, E.K.M., Zaczek, V., Macleod, M. and P.M. Hocking. 2004. Genotype, dietary manipulation and food allocation affect indices of welfare in broiler breeders. British Poultry Science 45: 725-737.
Katanbaf, M., Dunnington, E. and P. Siegel 1989. Restricted feeding in early and late-feathering chickens. 1. Growth and physiological responses. Poultry Science 68: 344-351.
Keeling, L.J. 2002. Behaviour of fowl and other domesticated birds. In The ethology of Domestic. An Introductory Text. Animals. Jensen, P. (ed). CABI International, Wallingford, UK, pp 101-119.
Kostal, L., Savory, C.J and B.O. Hughes. 1992. Diurnal and individual variation in behaviour of restricted-fed broiler breeders. Applied Animal Behaviour Science 32: 361-374.
Kruijt, J.P. 1964. Ontogeny of social behaviour in the Burmese red junglefowl (Gallus gallus spadiceus). PhD Thesis, University of Groningen. Latshaw, J. D. 1991. Nutrition-mechanisms of immunosuppression. Veterinary Immunology and Immunopathology. 30: 111-120.
Lees, J.J., Lindholm, C., Batakis, P., Busscher, M. and J. Altimiras. 2017. The physiological and neuroendocrine correlates of hunger in the Red Junglefowl (Gallus gallus). Scientific reports 7: 1-11.
Mason, G.J. 1991. Stereotypies and suffering. Behavioural Processes 25: 103-115.
Mason, G., Clubb, R., Latham, N. and S. Vickery. 2006. Why and how should we use environmental enrichment to tackle stereotypic behavior? Applied Animal Behaviour Science 102: 163-188.
Mba, E., Renema, R.A., Pishnamazi, A. and M.J. Zuidhof. 2010. Do dietary protein:energy ratios modify growth and frame size of young broiler breeder females? Poultry Science 89(Suppl. 1):122. (Abstr.)
McPhee, M.E. and K. Carlstead. 2010. The importance of maintaining natural behaviors in
captive mammals. In Wild Mammals in Captivity. D.G. Kleiman, K.V. Thompson, C.K. Baer (eds). The University of Chicago Press. Chicago, USA. pp. 303-313.
Mellor, E., Brilot, B. and S. Collins. 2018. Abnormal repetitive behaviours in captive birds: a Tinbergian review. Applied Animal Behaviour Science 198: 109-120.
Mench, J.A. 2002. Broiler breeders: feed restriction and welfare. World’s Poultry Science Journal 58: 23-30.
Moe, R., Guémené, D., Bakken, M., Larsen, H., Shini, S., Lervik, S. and R. Tauson. 2010. Effects of housing conditions during the rearing and laying period on adrenal reactivity, immune response and heterophil to lymphocyte (H/L) ratios in laying hens. Animal 4: 1709-1715.
Montiel, E.R. 2016. Influence of feeding programs on innate and adaptive immunity in broiler breeders. PhD Diss. The University of Georgia, Athens, Georgia, USA.
Morrissey, K.L.H., Widowski, T., Leeson, S., Sandilands, V., Arnone, A. and S. Torrey. 2014a. The effect of dietary alterations during rearing on feather condition in broiler breeder females. Poultry Science 93: 1636-1643.
Morrissey, K.L.H., Widowski, T., Leeson, S., Sandilands, V., Arnone, A. and S. Torrey. 2014b. The effect of dietary alterations during rearing on growth, productivity, and behaviour in broiler breeder females. Poultry Science 93: 285-295.
Newberry, R.C., Keeling, L.J., Estevez, I. and B. Bilčík. 2007. Behaviour when young as a predictor of severe feather pecking in adult laying hens: the redirected foraging hypothesis revisited. Applied Animal Behaviour Science 107: 262-274.
Nielsen, B.L., Thodberg, K., Malmkvist, J. and S. Steenfeldt. 2011. Proportion of insoluble fibre in the diet affects behavior and hunger in broiler breeders growing at similar rates. Animal 5: 1247-1258.
Paul, A., Frederich, M., Uyttenbroeck, R., Hatt, S., Malik, P., Lebecque, S., Hamaidia, M., Miazek, K., Goffin, D., Willems, L., Deleu, M., Fauconnier, M.L., Richel, A., De Pauw, E., Blecker, C., Monty, A., Francis, F., Haubruge, E. and S. Danthine. 2016a. Grasshoppers as a food source? A review. Biotechnology, Agronomy and Society and Environment 20: 337-352.
Paul, A., Frederich, M., Uyttenbroeck, R., Malik, P., Filocco, S., Richel, A., Heuskin, S., Alabi, T., Megido, R.C., Franck, T., Bindelle, J., Maesen, P., Francis, F., Lognay, G., Blecker, C., Haubruge, E. and S. Danthine. 2016b. Nutritional composition and rearing potential of the meadow grasshopper (Chorthippus parallelus Zetterstedt). Journal of Asia-Pacific Entomology 19: 1111-1116.
Paul, A., Frederich, M., Megido, R.C., Alabi, T., Malik, P., Uyttenbroeck, R., Francis, F., Blecker, C., Haubruge, E., Lognay, G. and S. Danthine. 2017.
Insect fatty acids: A comparison of lipids from three Orthopterans and Tenebrio molitor L. larvae. Journal of Asia-Pacific Entomology 20: 337-340. Reid, A.M., Wilson, P.W., Caughey, S.D., Dixon, L.M., D’Eath, R.B., Sandilands, V., Boswell, T. and I.C. Dunn. 2017. Pancreatic PYY but not PPY expression is responsive to short-term nutritional state and the pancreas constitutes the major site of PYY mRNA expression in chickens. General and Comparative Endocrinology 252: 226-235. Reid, A.M. and I.C. Dunn. 2018. Gastrointestinal distribution of chicken gastrin-cholecystokinin family transcript expression and response to shortterm nutritive state. General and comparative endocrinology 255: 64-70.
Renema, R.A., Moraes, T.G.V. and M.J. Zuidhof. 2013. Effects of broiler breeder nutrition on chick quality and broiler growth. In Proceedings 2nd International Poultry Meat Congress, Antalya, Turkey, pp 212-222. Robinson, F.E., Zuidhof, M.J. and R.A. Renema.
2007. Reproductive efficiency and metabolism of female broiler breeders as affected by genotype, feed allocation, and age at photostimulation. 1. Pullet growth and development. Poultry Science 86: 2256-2266.
Rougoor, C. and F. van der Schans. 2019. Comparison of environmental effects of broiler concepts (in Dutch). CLM report 982. Culemborg, the Netherlands.
Sandilands, V., Savory C.J. and K. Powell. 2004a. Preen gland function in layer fowls: factors affecting morphology and feather lipid levels. Comparative Biochemistry and Physiology 137: 217-225.
Sandilands, V., Powell, K., Keeling, L.J. and C.J. Savory. 2004b. Preen gland function in layer fowls: factors affecting preen oil fatty acid composition. British Poultry Science 45 :109-115.
Sandilands, V., Tolkamp, B.J. and I. Kyriazakis. 2005. Behaviour of food restricted broilers during rearing and lay - effects of an alternative feeding method. Physiology & Behavior. 85: 115-123.
Sandilands, V., Tolkamp, B.J., Savory, C.J. and I. Kyriazakis. 2006. Behaviour and welfare of broiler breeders fed qualitatively restricted diets during rearing: Are there viable alternatives to quantitative restriction? Applied Animal Behaviour Science 96: 53-67.
Savory, C.J., Wood-Gush, D.G.M. and I.J.H. Duncan. 1978. Feeding behaviour in a population of domestic fowls in the wild. Applied Animal Ethology 4: 13-27.
Savory, C.J. and K. Maros. 1993. Influence of degree of food restriction, age and time of day on behaviour of broiler breeder chickens. Behavioural Processes 29: 179-190.
Savory, C.J., Maros, K. and S.M. Rutter. 1993. Assessment of hunger in growing broiler breeders in relation to a commercial restricted feeding programme. Animal Welfare 2: 131-152.
Savory, C.J. and L. Kostal. 1996. Temporal patterning
Breeder Management and Nutrition: Moving the industry forward
of oral stereotypies in restricted-fed fowls: 1. investigations with a single daily meal. International Journal of Comparative Psychology 9: 117-139. Savory, C.J. and J.S. Mann. 1997. Is there a role for corticosterone in expression of abnormal behaviour in restricted-fed fowls? Physiology & Behavior 62: 7-13.
Savory, C.J. and J.M. Lariviere. 2000. Effects of qualitative and quantitative food restriction treatments on feeding motivational state and general activity level of growing broiler breeders. Applied Animal Behaviour Science 69: 135-147. Scheurink, A.W., Balkan, B., Strubbe, J.H., van Dijk, G.J. and A.B. Steffens. 1996. Overfeeding, autonomic regulation and metabolic consequences. Cardiovascular Drugs and Therapy 10: 263-274. Sheppard, K.C. and I.J.H. Duncan. 2011. Feeding motivation on the incidence of floor eggs and extraneously calcified eggs laid by broiler breeder hens. British Poultry Science 52: 20-29. Shini, S. and P. Kaiser. 2009. Effects of stress, mimicked by administration of corticosterone in drinking water, on the expression of chicken cytokine and chemokine genes in lymphocytes. Stress 12: 388-399.
Star, L., Arsiwalla, T., Molist, F., Leushuis, R., Dalim, M. and A. Paul. 2020. Gradual provision of live black soldier fly (Hermetia illucens) larvae to older laying hens: Effect on production performance, egg quality, feather condition and behavior. Animals 10, 216; doi:10.3390/ani10020216
Stevens, L. 1996. Lipids and their metabolism. In Avian Biochemistry and Molecular Biology. Cambridge, Cambridge University Press, Cambridge, UK. pp. 46-64.
Sun, J.M., Richards, M.P., Rosebrough, R.W., Ashwell, C.M., McMurtry, J.P. and C.N. Coon. 2006. The relationship of body composition, feed intake, and metabolic hormones for broiler breeder females. Poultry Science 85: 1173-1184.
Tahamtani, F.M., Hengameh, M. and A.B. Riber. 2020. Effect of qualitative feed restriction in broiler breeder pullets on stress and clinical welfare indicators. Frontiers in Veterinary Science 7: 1-11. Thompson, A.L. 1964. Oil gland. In A New Dictionary of Birds. A.L. Thompson (ed). Thomas Nelson and Sons Ltd, Edinburgh, pp. 551-552. Tolkamp, B.J., Sandilands, V. and I. Kyriazakis. 2005. Effects of qualitative feed restriction during rearing on the performance of broiler breeders during rearing and lay. Poultry Science 84: 1286-1293. van der Haar, J. and S. van Voorst. 2001. Welfare of breeder pullets fed twice a day (in Dutch). Praktijkonderzoek Veehouderij - Pluimvee. Lelystad, the Netherlands. pp: 21-25. van der Wal, P.G., Reimert, H.G.M., Goedhart, H.A., Engel, B. and T.G. Uijttenboogaart. 1999. The effect of feed withdrawal on broiler blood glucose and nonesterified fatty acid levels, postmortem liver pH values, and carcass yield. Poultry Science 78: 569-573.
van Emous, R.A., Kwakkel, R.P., van Krimpen, M.M. and W.H. Hendriks. 2013. Effects of growth pattern and dietary protein levels during rearing on body composition and performance in broiler breeder females during the rearing and laying period. Poultry Science 92: 2091-2100.
van Emous, R.A., Kwakkel, R.P., van Krimpen, M.M. and W.H. Hendriks. 2014. Effects of growth pattern and dietary protein level during rearing on feed intake, eating time, eating rate, behavior, plasma corticosterone concentration, and feather cover in broiler breeder females during the rearing and laying period. Applied Animal Behaviour Science 150: 44-54.
van Emous, R.A. 2015. Body composition and reproduction in broiler breeders: impact of feeding strategies. PhD Diss. Wageningen University, Wageningen, the Netherlands.
van Emous, R.A., Kwakkel, R.P., van Krimpen, M.M.
and W.H. Hendriks. 2015a. Effects of different dietary protein levels during rearing and different dietary energy levels during lay on behaviour and feather cover in broiler breeder females. Applied Animal Behaviour Science 168: 45-55.
van Emous, R.A., Kwakkel, R.P., van Krimpen, M.M. and W.H. Hendriks. 2015b. Effects of dietary protein levels during rearing and different dietary energy levels during lay on body composition and reproduction in broiler breeder females. Poultry Science 94: 1030-1042.
van Emous, R.A. 2021. Literature study feed control broiler breeders (in Dutch). Report 1291. Wageningen Livestock Research, Wageningen, the Netherlands.
van Emous, R.A., Mens, A.J.W. and A. Winkel. 2021. Effects of diet density and feeding frequency during the rearing period on broiler breeder performance. British Poultry Science 62: 686-694.
van Krimpen, M. and I.C. de Jong. 2014. Impact of nutrition on welfare aspects of broiler breeder flocks. World’s Poultry Science Journal 70: 139150.
Widowski, T.M. and I.J.M. Duncan. 2000. Working for a dustbath: Are hens increasing pleasure rather than reducing suffering? Applied Animal Behaviour Science 68: 39-53.
Wood-Gush, D.G.M., Duncan, I.J.H. and C.J. Savory. 1978. Observations on the social behaviour of domestic fowl in the wild. Biology of Behavior 3: 193-205.
Zuidhof, M.J., Robinson, F.E., Feddes, J.J.R. and R.T. Hardin. 1995. The effects of nutrient dilution on the wellbeing and performance of female broiler breeders. Poultry Science 74: 441-456.
Zuidhof, M.J., Schneider, B.L., Carney, V.L., Korver, D.R. and F.E. Robinson. 2014. Growth, efficiency, and yield of commercial broilers from 1957, 1978, and 2005. Poultry Science 93: 2970-2982.
Zuidhof, M.J., Holm, D.E., Renema, R.A., Jalal, M.A. and F.E. Robinson. 2015. Effects of broiler breeder management on pullet body weight and carcass uniformity. Poultry Science 94: 1389-1397.
Zuidhof, M.J. 2018. Lifetime productivity of conventionally and precision-fed broiler breeders. Poultry Science 97: 3921-3937.
HENK ENTING, PhD., is the Senior Poultry Technology Lead for Cargill Animal Nutrition. In this role, he is responsible for developing and implementing poultry technology within Cargill Animal Nutrition globally. He also contributes to the company’s innovation project portfolio. Dr. Enting graduated with distinction from Wageningen University (Netherlands) with a master’s degree in animal breeding, nutrition and agricultural economics and a doctorate thesis on the effect of low-density diets on broiler breeders and their offspring. After receiving his master’s degree, he worked as a poultry nutrition researcher and poultry cluster coordinator at Schothorst Feed Research in Lelystad (Netherlands). With his doctorate degree completed, Dr. Enting started as a poultry researcher at the Nutreco Poultry Research Center in Casarrubios del Monte (Spain). He returned to the Netherlands to join Trouw Nutrition as a global poultry technical manager and then to Nutreco as a poultry application and solution specialist before moving to Cargill.
Current feed intake restriction levels in broiler breeders can have a negative effect on bird welfare. In order to overcome part of this negative effect, qualitative feed restriction may help. This can include dilution of feeds with inert or low digestible nutrients, providing diets that change body composition and nutrient requirements, and the use of appetite reducing ingredients. Both pre-prandial factors that are associated with the satiety mechanism and post-prandial factors that relate to the hunger mechanism are important in studying effects of qualitative restriction.
Results of trials indicate it might be difficult to obtain a substantial reduction in hunger during the rearing period by applying qualitative feed restriction. A reduction in diet density of 20-30% with a certain but unknown level of soluble fiber seems to be most promising, and the provision of roughage also can help to reduce hunger-related stress parameters. Moving to a higher body weight target that allows for higher feed intake might also alleviate hunger feeling. It is not clear to what extend changes in body composition, that can translate into higher feed allowances in order to maintain body weight and performance targets, may alleviate hunger. Further work on qualitative feed restriction may include a wide range of endocrine and physiological parameters and body composition and should focus on both satiety and hunger mechanisms.
Quantitative feed restriction is less severe during the laying period compared to rearing. A high level of feed dilution can even have a negative effect on stress-related parameters. Diluted diets (10-15%) appear to have a positive effect on laying performance and at least part of this effect is originating from changes in the rearing period.
The amount of feed broiler breeders is provided to broiler breeders is lower than the ad libitum feed intake capacity (quantitative feed intake restriction) to control body weight and improve egg production. In ad libitum-fed broiler breeders, egg production is significantly lower than in restrictedly fed birds (Katanbaf et al., 1986; Decuypere et al., 1999). Due to the high feed intake capacity
and feed intake motivation of broiler breeders, feed restriction levels can exceed 50% of the ad libitum feed intake during the rearing period (de Jong et al., 2002). Feed intake restriction is generally less severe during the laying period (de Jong et al., 2002).
A high level of quantitative feed intake restriction can have a negative effect on broiler breeder welfare; both feed and water deprivation are included in the 2020 Five
Domains Model (Mellor et al., 2020). Signs of a negative impact include changes in behaviour, such as stereotypic behaviourand increased feed intake motivation; and stress physiology, such as increased plasma corticosterone levels and heterophil-to lymphocyte-ratio (Hocking et al., 1996; de Jong et al., 2002; Mench, 2002). An increase in plasma corticosterone levels can have a negative impact on concentrations of reproductive hormones like luteinizing hormone (Etches et al., 1984). Elevated plasma corticosterone levels can also be immunosuppressive (Griffin, 1989) and may result in a decrease in antibody production which might lead to lower maternal antibody concentrations in eggs (Knorr, 1991). Beside the negative effects on bird welfare, feed restriction may also have a negative effect on uniformity and offspring performance (Zuidhof et al., 1995; Enting et al., 2007c). Hocking et al. (1996) concluded that feed restriction in the range of 50-85% of the ad libitum intake might provide optimum welfare for broiler breeders based on behaviour and physiological indices.
Instead of quantitative feed restriction, qualitative feed restriction may help to overcome part of the negative effects of quantitative feed restriction by increasing satiety and reducing hunger feeling. Qualitative feed restriction can include diluting the with inert or low digestible feed ingredients, feeding diets with low levels of some nutrients such as amino acids that require a higher feed allowance in order to maintain performance, and providing appetite suppressants. de Jong and Guémené (2011) indicated that using both a fiber-rich feed and an appetite suppressant can be the most successful. In this chapter, an overview
is provided of changes that can be made in the feed that may help to reduce hunger and increase satiety in broiler breeders, while maintaining egg production performance.
Behaviour and stress indicators in quantitatively restrictedly fed broiler breeders show that birds are motivated to consume more feed than they are offered (Savory et al., 1993; de Jong et al., 2002). Pre-prandial and post-prandial factors can be identified to describe feed intake motivation. Pre-prandial factors are mainly associated with the satiety mechanism and post-prandial factors are associated with the hunger mechanism, according to Bokkers and Koene (2003).
Forbes (1999) identified three main factors in the regulation of feed intake: oropharyngeal, gastrointestinal, and postabsorptive. Oropharyngeal factors relate to the smell and taste of the feed or the form in which the feed is presented. Feed particle size for example can affect feed intake (Portella et al., 1988; Amerah et al., 2007) and has the potential to change eating behaviour but may not affect satiety or hunger. Gastrointestinal factors relate to feed intake as it is induced by the digestive tract emptying. Information from mechano- and chemoreceptors is transferred to the central nervous system (Mei, 1985) and is mediated by gastrointestinal hormones like cholecystokinin, glucagon-like peptide-1, and peptide YY (Honda, 2016; Honda et al., 2017). The release of these hormones can slow the feed passage rate (Cuche et al., 2000) resulting in a higher satiety and reduced feed intake (Baile et al., 1986; Kuenzel, 1994). Post-
absorptive factors include levels of glucose and non-esterified fatty acids in blood plasma; glucose plays a central role in the regulation of feed intake as low glucose drives feed intake motivation (Oomura, 1983; Forbes, 1999). Changes in nutrient levels in the liver and other body tissues may signal that feed has to be consumed in order to maintain nutrient levels (Forbes, 1995). Signals can originate from changes in deposition or mobilization of nutrients from the tissues, from metabolites produced from that, and include hormones that are involved in the regulation of body and blood plasma nutrient levels (Forbes, 1988; Denbow, 1994). Therefore, body composition and digestion kinetics may potentially play a role in the post-prandial factors that affect feed intake motivation. It remains unclear whether feed intake motivation in broiler breeders might be more controlled by physical satiety mechanisms rather than by hunger mechanisms; in most publications feed intake motivation and feed intake regulation parameters have not been measured consistently. Burkhart et al. (1983) concluded that genetic selection for an increased growth rate in broiler chickens has resulted in a reduction in satiety due to lower nutrient levels in the blood. This has led to feed intake levels that are close to the capacity of the digestive tract and may indicate that current feed intake capacity of broiler breeders might have become a limiting factor to reaching satiety.
Feed intake motivation –gastrointestinal and oropharyncheal factors
Broiler chickens may consume feed to
their maximum intake capacity to meet their high nutrient requirements for body weight gain (Bokkers and Koene, 2003). Savory et al. (1981) indicated that feed intake in broiler chickens and broiler breeders is more determined by maximum gastrointestinal capacity than nutrient levels in the blood. An increase in gastrointestinal contents by the inclusion of inert or fiber-rich raw materials in the diet and an increased feed allowance may increase satiety due to increased gut filling and might help to reduce the feeling of hunger in broiler breeders.
Initial studies in broiler breeders fed fiberrich or diluted diets showed conflicting results for potential changes in satiety and hunger feeling in the rearing period (Zuidhof et al., 1995; Savory et al., 1996; Savory and Lariviere, 2000; Hocking et al., 2004). Zuidhof et al. (1995) observed changes in behaviour and a reduction in the heterophilto-lymphocyte-ratio as a physiological indicator of stress in broiler breeders when diets were diluted by 15 or 30% of oat hulls. Hocking et al. (2004) observed a reduction in spot pecking when broiler breeders were provided a diet with 5% sugar beet pulp and 20% oat hulls as bulk ingredients compared to a control diet. They proposed that diets with sugar beet pulp could improve satiety and welfare.
Enting (2005) performed a study with broiler breeders in which feeds were diluted by reducing the energy content by 300 or 600 kcal/kg in the rearing and laying period by the inclusion of low-density byproducts. Heterophil-tolymphocyte-ratios were reduced at 9 weeks of age and feed consumption time increased
with lower energy levels. Amino acid levels were reduced proportionally to the reduced energy levels, and feed allowance was increased to keep the total nutrient intake the same per day for all treatments. Since offspring showed a significantly higher initial growth rate and a significantly lower mortality rate when breeders were provided the low-density diets, it was hypothesized that changes in hunger feeling and chronic stress in breeders could affect offspring performance.
In a follow-up study, the same diets were provided as in the first study. Behavioral data and results from the feed intake motivation test (Figure 1) indicated that the lowestdensity diet may result in less frustration and hunger during rearing as compared to the breeders from the other treatments.
However, measured physiological indicators of hunger and stress (i.e., blood plasma corticosterone, heterophil-to-lymphocyteratio, glucose, and non-esterified fatty acids) did not support these observations (de Jong et al., 2005). Buyse et al. (2000) found that corticosterone-induced hyperglycemia in feed-deprived birds and therefore plasma glucose levels could also indicate hunger. This may suggest that low-density diets affect satiety through feed intake motivation more than through hunger mechanisms.
Feed form may play a role in the variation in response of broiler breeders to lowdensity diets in the rearing period since pelleted feeds can result in a higher feed intake rate compared to mash diets (Gous and Danisman, 2016). Hocking (2006), who
included 20 or 40% of oat hulls, and de los Mozos et al. (2017), who used a 15% lower diet density, used pelleted or crumbled diets in their studies and did not find important differences in behaviour and physiological responses between diets in the rearing period. Enting (2005) and de Jong et al. (2005) used mash diets in their studies and observed more pronounced effects of low-density diets. However, de los Mozos et al. (2017) observed 30% lower feed consumption in a feeding rate test in broiler breeders when providing a low-density diet. This may still indicate a lower feeding motivation with low-density diets, also in pelleted and crumbled diets.
Jojoba meal was shown to be successful in reducing feed intake and controlling body weight in ad libitum-fed broiler breeders during the rearing period, but inhibited normal follicle development and maturation (Vermaut et al., 1998) and therefore was unfit for application in practice. It appeared that jojoba meal reduced feed intake by its taste rather than by increasing satiety (Vermaut et al., 1997).
Fiber type may influence the responses to low-density diets. Insoluble fiber can increase the rate of feed passage in the intestinal tract in broiler breeders (Enting et al., 2007a) and may therefore limit effects on gut filling. Soluble fibers may decrease the feed passage rate (van der Klis and van Voorst, 1993) along with coarse insoluble fiber (Amerah et al., 2009). In sows, the inclusion of fibrous raw materials rich in soluble fiber can help to reduce hunger feeling as measured by reduced stereotypic behaviour and reduced
feed intake motivation (Bergeron et al., 2000; de Leeuw et al., 2004). This positive effect is linked to more stable glucose and insulin levels in blood plasma. This may indicate that the inclusion of some soluble fiber may provide better responses than inert fiber in broiler breeders.
Nielsen et al. (2011) compared increased fiber levels from oat hulls and wheat bran (insoluble fiber) and from oat hulls, sugar beet pulp, potato pulp, and some wheat bran (increased soluble fiber) in the rearing feed of broiler breeders. Both fiber sources reduced compensatory feed intake, indicating a reduction in the level of hunger experienced by the birds. Broiler breeders fed high insoluble fiber showed less tail pecking and more dust bathing and comfort behaviour compared to the control group, while signs of discomfort were observed in broiler breeders fed the high soluble fiber diet due to poor litter quality. Tahamtani et al. (2020) had similar observations when they included sugar beet pulp in a soluble plus insoluble fiber diet during the rearing period. However, although the soluble plus insoluble fiber diets had the same nutrient density as the insoluble fiber diets used in this study, feed allowance increased less with the soluble plus insoluble fiber diets to maintain body weight (9.1% vs. 15.4%). Using the same diets, Tahamtani and Riber (2020) concluded that breeders fed the soluble plus insoluble fiber diet were in a hungrier state than breeders reared on insoluble fiber diets and that there was a negative impact on bird welfare (Riber et al., 2021). This indicates that the effects of soluble fiber on behaviour in broiler breeders are different from effects reported in swine and do
not contribute to more stable plasma glucose levels; the soluble fiber used in these studies may have caused too much fermentation in broiler breeders. de Jong et al. (2005) did not observe clear differences in behaviour between low-density rearing diets with a difference in composition; one of the diets included 15% of sugar beet pulp. Strict control of the water intake may have prevented excessive water intake in the high soluble fiber group in this study. However, water restrictions may also impair animal welfare (Mellor et al., 2020).
The use of a control feed plus maize silage showed improved welfare based on feather condition in the work of Tahamtani et al. (2020), but plasma corticosterone levels were not affected by the diet. Maize silage also showed a positive effect on welfare in nonbeak trimmed laying hens, where feather condition was improved and mortality due to cannibalism was strongly reduced (Steenfeldt et al., 2007). It is not clear if the effect of maize silage is from changes in behaviour due to increased foraging or if more stable glucose levels may prevent hunger feeling and an increase in activity as has been observed with sugar beet pulp in restricted fed sows (de Leeuw et al., 2004).
In a number of studies, soybean hulls were used to reduce diet density; compared to oat hulls, soybean hulls contain a higher soluble fiber level but less than sugar beet pulp. In most of the studies, soybean hulls were combined with the use of calcium propionate as an appetite suppressor. Morrissey et al. (2014) concluded that this combination was able to reduce hunger-related behaviour and that feather condition was improved, which
may be an indication of reduced stereotyped feather pecking. The authors suggested that soybean hulls combined with calcium propionate increased satiety compared with the control diet. Also, Arrazola et al. (2019) indicated that the combination of soybean hulls and calcium propionate can reduce signs of chronic hunger as measured through a reduced feed intake motivation and a delayed increase in basophil-to-lymphocyteratio. Based on treatments in which soybean hulls or calcium propionate were fed separately, Arrazola et al. (2020) concluded that the effect of calcium propionate alone was not clear. Earlier work of Pinchasov et al. (1993) indicated that dilution of rearing diets with barley and calcium propionate was sufficient to control body weight in ad libitum-fed birds. Dilution of the diet with barley alone was however not effective in controlling body weight.
Sandilands et al. (2006) combined different levels of oat hulls with different levels of calcium propionate during the rearing period. Breeders provided with oat hulls and calcium propionate showed almost no object pecking in contrast to the control treatment. Moreover, they had a lower feed intake compared to the control treatment in a feed intake motivation test. The authors concluded that the low-density diets may be beneficial for welfare based on behaviour, but that physiological indices of stress as heterophil-to-lymphocyte-ratio and plasma corticosterone levels did not differ from the control diet. Based on this work, it appears soybean hulls may be more effective in changing behaviour and physiological parameters than oat hulls.
Aranibar et al. (2020) provided soybean hulls on days without feed in a skip-aday program during the rearing period. Their results suggested that soybean hulls provided some satiety on days without feed; the behaviour on those days was more like that of days with feed. There was however no diet effect on corticosterone levels during the rearing period. Arrazola et al. (2019) and Aranibar et al. (2020) concluded that the degree of feed restriction and the period of time without feed between feeding times had the largest effect on plasma corticosterone concentrations. This is in line with de Jong et al. (2003) who found the largest increase in plasma corticosterone levels at feed restriction levels that exceeded 50% of the ad libitum feed intake. These results indicate that the amount of soybean hulls provided during days without feed was not enough to decrease the level of metabolic stress in feed-restricted birds during the rearing period.
It is not clear to what extent genetic selection has changed responses in physiology to quantitative and qualitative feed restriction. When feed intake levels are close to the capacity of the intestinal tract (Burkhart et al., 1983), hunger feeling might have become stronger when nutrient requirements have become higher over time. On the other hand, earlier performed trials tend to show more clear differences in plasma corticosterone levels than more recent work. Slower-growing breeds may still need a certain level of restriction to control body weight, but these breeds show fewer signs of hunger compared to fast-growing breeds (Arrazola and Torrey, 2021).
Since feed restriction levels during the laying period are less severe than in the rearing period (de Jong et al., 2005), the effects of low-density diets may be different. In contrast to the rearing period, they observed a very long feed intake time during the laying period when the dietary nutrient density was reduced by 21% compared to the control diet. Plasma corticosterone levels went up with this level of diet dilution, which may indicate a negative effect on bird welfare (de Jong et al., 2005). An intermediate lowdensity diet (10.7% lower density) provided a significantly better laying percentage compared to the control group (Enting et al., 2007b). Zuidhof et al. (1995) also observed a significantly better laying percentage with the dilution of 15% oat hulls, while no such effect was observed with the 30% oat hulls group. Using insoluble fiber (cellulose or wheat bran) in a 10% lower energy diet that was provided from 24-38 weeks of age significantly improved laying percentage compared to a control group and a 5% diluted diet using soluble fiber (pectin; Moradi et al., 2013). The insoluble fiber diets significantly decreased plasma corticosterone levels. Moradi et al. (2013) also measured ovarian morphology but found no relation with laying percentage. The inclusion of 3% inulin or 3% cellulose improved laying percentage in the second half of the laying period; with cellulose, this improvement was significant (Mohiti-Asli et al., 2012). Aranibar et al. (2020) found that laying performance was improved by providing soybean hulls on days without feed, which most likely was due to a difference in flock uniformity as better body weight uniformity can lead to
Figure 2. Effect of different amino acid-to-energy
the rearing period compared to standard levels on relative breast meat
weeks of age; body weight was the same for all treatments. CP:ME ratios were 6.6, 5.8, and 5.30 per 1000 kcal
fat pad weights
standard group for starter, grower, and developer phases, respectively.
from
higher egg production (Abbas et al., 2010). Although low-density diets may not show a clear effect on welfare parameters during the laying period and may be even negative at high levels of dilution, a moderate dilution of the diets (10%) can have a positive effect on laying performance. Part of this effect may originate from changes during the rearing period when providing diluted diets (Enting et al., 2007b).
Initial work in this field focused on the chemical regulation of feed intake to control the body weight of broiler breeders. The application of this work was limited due to feed regulation restrictions. Oyawoye and Krueger (1990) tested phenylpropanolamine hydrochloride and sodium monensin as appetite control agents. They found that 200
mg and 300 mg/kg of monensin sodium were more potent in suppressing feed intake and growth than phenylpropanolamine hydrochloride and hence could have an application in ad libitum-fed broiler breeders. Feeding high levels of monensin (400 mg/kg) resulted in a lower uniformity at 21 weeks of age and feeding 400 mg/kg monensin in combination with low protein resulted in a significant reduction in laying percentage and eggshell quality (Oyawoye and Krueger, 1986).
There are a limited number of studies that may indicate that qualitative feed restriction by reducing levels of certain nutrients that change body composition can contribute to changes in feed intake motivation. Kershaw and Flier (2004) and Ceciliani et al. (2018) indicated that cell-signaling molecules produced by adipose tissue, adipokines, can influence processes such as feed intake
Table 1. Effect of different amino acid to energy ratios during the rearing period on feed allowance during the laying period in order to keep body weight at target (week 22-64). Body weight was 2675 g on average at 22 weeks of age and 3937 g at 64 weeks of age with no differences between treatments; the tendency for a quadratic response in laying percentage was due to a significant quadratic effect in the period from week 52-64. Adapted from Soumeh et al. (2018)
CP:AA ratio
Feed intake, g/day
and reproduction. One of these adipokines, chemerin, showed a negative correlation with body fat condition and hatchability of fertile eggs in broiler breeders (Mellouk et al., 2018) and its gene expression was influenced by feed restriction levels. Changing body fat levels might therefore have an effect on feed intake motivation.
Soumeh et al. (2018) observed differences in body composition at the end of the rearing period by providing different crude proteinto-energy ratios (CP:ME), from -20% up to +5% compared to recommended levels (Figure 2). Broiler breeders fed higher levels of CP:ME during rearing linearly increased relative breast weight and reduced relative fat pad weights in week 22. The differences in body composition at the end of the rearing period translated into differences in the amount of feed that was required during the laying period to maintain body weight at the target level: higher breast meat and lower fat pad weights required more feed. Differences in daily feed allowance amounted to 12 g/ day (Table 1). These results are in line with those from Hocking and Whitehead (1990), who found evidence that optimal feed allowance for egg production was higher in lean compared to fat broiler genetic selection
P-value linear P-value quadratic
<0.001
lines. No physiological indicators of hunger and no feed motivation were measured in this study and further work is required to study the relationship between body composition and hunger.
When dietary amino acid levels are low, an increase in the incidence of abnormal behaviour, such as aggressive pecking, has been observed in laying hens (Ambrosen and Petersen, 1997). van Emous et al. (2014) reported that more feed was needed with low protein diets to reach the target body weight and that less stereotypic object pecking was observed. However, there were no differences in plasma corticosterone concentrations. Low dietary protein resulted in less good feathering, which may have had an origin other than feather pulling or pecking. A substantial increase in tryptophan levels may help to reduce aggressive pecking during the rearing period (Shea et al., 1990). Tryptophan is a precursor of indolamines (e.g., serotonin), which is a group of neurotransmitters that are involved in feed intake regulation (Denbow, 1989). Intubation of tryptophan in broilers and laying hens inhibited feed intake (Lacy et al., 1986). Mench (1991) found that the increase in plasma corticosterone levels due to feed restriction is less pronounced by dietary tryptophan. A level of 0.38% tryptophan was
effective in decreasing pecking behaviour in male birds compared to the control level of 0.19% tryptophan.
Another example of how a reduction in nutrient levels may increase feed allowance to maintain performance comes from an unpublished Cargill Animal Nutrition experiment with Ross 308 broiler breeders in which dietary crude protein was gradually decreased from 14% until 35 weeks of age to 10.5% from 53-58 weeks of age compared to a control group that received 14% until the end of lay. The reduction in dietary crude protein required a significant increase in feed allowance to maintain performance (Figure 3). No feed intake motivation test was done in this study, so it remains unclear to what extent body weight, body composition, and changes in feed allowance to maintain
body weight and performance could have contributed to a difference in satiety and hunger feeling. Based on the work of Forbes and Shariatmadari (1994), there might have been an effect on satiety. de los Mozos et al. (2017) found the lowest body fat percentage in hens that were provided low-density diets following the standard breeder body weight targets. The lower body fat may have impacted the lack of response to low-density diets in this study. Higher body fat and a higher feed allowance might both contribute to increased satiety.
The results of trials indicate that it might be difficult to obtain a substantial reduction in hunger by applying qualitative feed restriction in the rearing period. Diets with 20-30%
lower density with a certain but unknown low level of soluble fiber that can increase feed transit time may be most promising; a combination of soybean hulls with propionic acids seems to provide the most consistent changes in behaviour and feed intake motivation. Provision of roughage (5 g/bird/ day in the starter phase increasing to 15 g/ bird/day during the grower phase) also seems to be beneficial in reducing hunger-related stress parameters in broiler breeders. High soluble fiber coming from raw materials such as sugar beet pulp can have a negative effect on welfare due to an increase in water intake and wet litter.
Due to genetic selection for an increased growth rate and a constant pull for nutrients, it is hypothesized that current broilers consume feed to their maximum feed intake. Dilution of the diet may not change nutrient levels in the blood and can therefore have limited effects on hunger feeling. It looks like hunger mechanisms are not clearly affected by diet dilution and can overrule effects on satiety mechanisms that are related to gut fill. Furthermore, a reduction in feed transit time with low-density diets rich in insoluble fiber may partially offset the potential positive effect of these diets.
It is not clear to what extent changes in body composition, which may translate to a higher feed allowance in order to maintain body weight targets, alleviate hunger feeling. This effect might both come from the change in body composition and cell-signaling molecules produced by adipose tissue and from a higher feed allowance as such. Moving to higher body weight targets by providing more feed might also alleviate hunger feeling. Further work on
body composition and feed intake motivation and regulation can provide further direction on how to improve welfare in broiler breeders.
One missing piece is how responses to quantitative feed restriction have changed due to genetic selection. On the one hand, feed intake capacity and growth potential have increased over time. On the other hand, physiological responses may have changed as well. Earlier trials tend to show more clear differences in plasma corticosterone levels than more recent work, but this can also be due to increased hunger feeling due to the higher nutrient requirements that make the changes in feed allowance with diluted diets translate into too small effects. New work may indicate if optimal feed restriction levels need to change given current genetic potential and if this is still somewhere in the range of 0.50-0.85 of the ad libitum intake as earlier work suggested.
For further work, it seems to be important to combine behaviour studies with a wide range of endocrine and physiological parameters to assess stress and hunger and to focus both on satiety and hunger, that is to separate pre- and post-absorptive factors. Body composition can also be important to measure. In the case where gastrointestinal tract capacity is driving feed intake motivation more than blood nutrient levels, it might be difficult to reduce hunger feeling.
During the laying period, quantitative feed restriction levels are less severe than in the rearing period. Besides potential effects on welfare, it appears that low-density diets can improve laying performance in broiler
breeders. It is not clear what is driving this effect. At least part of this effect is originating from changes during the rearing period. The uniformity of birds may be involved in this but does not seem to be consistently improved in trials. The effects on laying performance may need further work.
Abbas, S. A., Gasm Elsig A.A. and M. K. Ahmed. 2010. Effect of body weight uniformity on the productivity of broiler breeder hens. International Journal of Poultry Science 9: 225–230. Ambrosen, L. and V.E. Petersen. 1997. The influence of protein level in the diet on cannibalism and quality of plumage of layers. Poultry Science 76: 559-563. Amerah, A.M., Ravindran, V., Lentle, R.G. and D.G. Thomas. 2007. Feed particle size: Implications on the digestion and performance of poultry. World’s Poultry Science Journal 63: 439-455. Amerah, A.M., Ravindran, V. and R.G. Lentle. 2009. Influence of insoluble fibre and whole wheat inclusion on the performance, digestive tract development and ileal microbiota profile of bro375.iler chickens. British Poultry Science 50: 366.
Aranibar, C.D., Chen, C., Davis, A.J., Daley, W.I., Dunkley, C., Kim, W.K., Usher, C., Webster, A.B. and J.L. Wilson, 2020. Impact of an alternate feeding program on broiler breeder pullet behaviour, performance, and plasma corticosterone. Poultry Science 99: 829-838.
Arrazola, A. and S. Torrey. 2021. Welfare and performance of slower growing broiler breeders during rearing. Poultry Science 100: 1-9.
Arrazola, A., Mosco, E., Widowski, T.M., Guerin, M.T., Kiarie, E.G. and S. Torrey. 2019. The effect of alternative feeding strategies for broiler breeder pullets: 1. Welfare and performance during rearing. Poultry Science 98: 3377-3390.
Arrazola, A., Widowski, T.M., Guerin, M.T., Kiairie, E.G. and S. Torrey. 2020. The effect of alternative feeding strategies on the feeding motivation of broiler breeder pullets. Animal 14: 2150-2158.
Baile, C.A., McLaughlin, C.A. and M.A. Della-Fera. 1986. Role of cholecystokinin and opioid peptides in control of food intake. Physiological Reviews 66: 172-234.
Bergeron, R., Bolduc, J., Ramonet, Y., Menier-Salaün, M.C. and S. Robert. 2000. Feeding motivation and stereotypies in pregnant sows fed increasing levels of fibre and/or food. Applied Animal Behaviour Science 70: 27-40.
Bokkers, E.A.M. and P. Koene. 2003. Eating behaviour, and pre-prandial and postprandial correlations in male broiler and layer chickens. British Poultry Science 44: 538-544.
Burkhart, C.A., Cherry, J.A., van Krey, H.P. and P.B. Siegel. 1983. Genetic selection for growth rate alters hypothalamic satiety mechanisms in chickens. Behaviour Genetics 13: 295-300.
Buyse, J., Decuypere, E., Darras, V.M., Vleurick, L.M., Kuhn, E.R. and J.D. Veldhuis. 2000. Food deprivation and feeding of broiler chickens is associated with rapid and interdependent changes in the somatotrophic and thyrotrophic axes. British Poultry Science 41: 107-116.
Ceciliani, F., Lecchi, C., Bazile, J., and M. Bonnet. 2018. Proteomics research in the adipose tissue. In: Proteomics in domestic animals: from farm to systems biology. Springer. p. 233-254.
Cuche, G., Cuber, J.C. and C.H. Malbert. 2000. Ileal short-chain fatty acids inhibit gastric motility by a humoral pathway. American Journal of Physiology 279: G925-G930.
Decuypere, E., Buys, N. and O. Onagbesan. 1999. A mechanistic approach for understanding nutritional and selection effects on reproductive functioning. Production diseases in farm animals: 10th International Conference 1998, Wageningen Pers, Wageningen, The Netherlands.
de Jong, I.C. and D. Guémené. 2011. Major welfare issues in broiler breeders. World’s Poultry Science Journal 67: 73-82.
de Jong, I.C., van Voorst, S., Ehlhardt, D.A. and H.J. Blokhuis. 2002. Effects of restricted feeding on physiological stress parameters in growing broiler breeders. British Poultry Science 43: 157-168. de Jong, I.C., van Voorst, S. and H.J. Blokhuis. 2003. Parameters for quantification of hunger in broiler breeders. Physiology & Behaviour 78: 773-783. de Jong, I.C., Enting, H., van Voorst, S. and H. J. Blokhuis. 2005a. Do low-density diets improve broiler breeder welfare during rearing and laying? Poultry Science 84: 194–203. de Leeuw, J.A., Jongbloed, A.W. and M.W.A. Verstegen. 2004. Dietary fiber stabilized blood glucose and insuline levels and reduces physical activity in sows (Sus scrofa). Journal of Nutrition 134: 1481-1486.
de los Mozos, J., García-Ruiz, A.I., Den Hartog, L.A. and M. J. Villamide. 2017. Growth curve and diet density affect eating motivation, behaviour, and body composition of broiler breeders during rearing. Poultry Science 96: 2708–2717
Denbow, D.M. 1989. Peripheral and central control of food intake. Poultry Science 68: 938–947.
Denbow, D.M., 1994. Appetite and its control. Poultry Science Reviews 5: 209-229.
Enting, H. 2005. Effect of low-density diets on broiler breeder and offspring performance. PhD. Thesis, Wageningen Institute of Animal Sciences, Wageningen University, Wageningen, The Netherlands.
Enting, H., Veldman, A., Verstegen, M.W.A. and P. J. van der Aar. 2007a. The effect of low-density diets on broiler breeder development and nutrient digestibility during the rearing period. Poultry Science 86: 720–726.
Enting, H., Kruip, T.A.M., Verstegen, M.W.A. and P. J. van der Aar. 2007b. The effect of low-density diets
on broiler breeder performance during the laying period and on embryonic development of their offspring. Poultry Science 86: 850–856.
Enting, H., Boersma, W.J.A., Cornelissen, J.B.W.J, van Winden, S.C.L., Verstegen, M.W.A. and P.J. van der Aar. 2007c. The Effect of low-density broiler breeder diets on performance and immune status of their offspring. Poultry Science 86: 282-290.
Etches, R.J., Williams, J.B. and J. Rzasa. 1984. Effects of corticosterone and dietary changes in the hen on ovarian function, plasma LH and steroids and the response to exogenous LHRH. Journal of Reproduction and Fertility 70: 121-130.
Forbes, J.M. 1988. Metabolic aspects of the regulation of voluntary food intake and appetite. Nutrition Research Reviews 1: 145-168.
Forbes, J.M. and F. Shariatmadari. 1994. Diet selection for protein by poultry. World’s Poultry Science Journal 50: 7-24.
Forbes, J.M. 1995. Voluntary food intake and diet selection in farm animals. Wallingford: CAB International.
Forbes J.M. 1999. Natural feeding behaviour and feed selection. In Regulation of feed intake. D. van der Heide, E.A. Huisman, E. Kanis, J.W.M. Osse, and M.W.A. Verstegen (Eds). CAB International, Wallingford, Oxon, United Kingdom.
Gous, R.M. and R. Danisman. 2016. Age, lighting treatment, feed allocation and feed form influence broiler breeder feeding time. South African Journal of Animal Science 46: 29-34.
Griffin, J.F.T. 1989. Stress and immunity: A unifying concept. Veterinary Immunology and Immunopathology 20: 263-312.
Hocking, P.M. and C.C. Whitehead. 1990. Relationship between body fatness, ovarian structure and reproduction in mature females from lines of genetically lean or fat broilers given different food allowances. British Poultry Science 31: 319–330.
Hocking, P.M., Maxwell, M.H. and M.A. Mitchell. 1996. Relationships between the degree of food restriction and welfare indices in broiler breeder females. British Poultry Science 37: 263-278.
Hocking, P.M., Zaczek,, V., Jones, E.K.M. and M.G. MacLeod. 2004. Different concentrations and sources of dietary fibre may improve the welfare of female broiler breeders. British Poultry Science 45: 9-19.
Hocking. P.M. 2006. High-fibre pelleted rations decrease water intake but do not improve physiological indexes of welfare in food-restricted female broiler breeders. British Poultry Science 47: 19-23.
Honda K. 2016. Glucagon-related peptides and the regulation of food intake in chickens. Animal Science Journal, 87: 1090-1098.
Honda, K., Saneyasu, T. and H. Kamisoyama, 2017. Gut hormones and regulation of food intake in birds. Journal of Poultry Science, 54: 103-110.
Katanbaf, M. N., Dunnington, E.A. and P. B. Siegel. 1986. Restricted feeding in early and late feathering chickens. 2. Reproductive Responses. Poultry Science 68: 352–358.
Kershaw, E. E. and J. S. Flier. 2004. Adipose tissue as an endocrine organ. The Journal of Clinical Endocrinology & Metabolism 89: 2548-2556.
Knorr, R. 1991. Qualitätsbeurteilung von Eiprodukten. Hohenheimer Arbeiten, Ulmer Verlag, Stuttgart. Kuenzel W.J. 1994. Central neuroanatomical systems involved in the regulation of food intake in birds and mammals. Journal of Nutrition 124: 1355S-1370S.
Lacy, M.P., van Krey, H.P., Skewes, P.A. and D.M. Denbow. 1986. Tryptophan’s influence on feeding and body temperature in the Fowl. Poultry Science 65: 1193-1196.
Mei, N. 1985. Intestinal chemosensitivity. Physiology Reviews 65: 211-237.
Mellor, D.J., Beausoleil, N.J., Littlewood, K.E., McLean, A.N., McGreevy, P.D., Jones, B. and
C. Wilkins. 2020. The 2020 five domains model: including human-animal interactions in assessment of animal welfare. Animals 10: 1870-1893.
Mellouk, N., Ramé, C., Marchand, M., Staub, C., Touzé, J.-L., Venturi, É., Mercerand, F., Travel, A., Chartrin, P., Lecompte, F., Ma, L., Froment, P. and J. Dupont. 2018. Effect of different levels of feed restriction and fish oil fatty acid supplementation on fat deposition by using different techniques, plasma levels and mRNA expression of several adipokines in broiler breeder hens. PLOS ONE 13: e0191121
Mench, J.A. 1991. Research note: Feed restriction in broiler breeders causes a persistent elevation in corticosterone secretion that is modulated by dietary tryptophan. Poultry Science 70: 2547–2550.
Mench, J., 2002. Broiler breeders: Feed restriction and welfare. World’s Poultry Science Journal, 58: 2329.
Mohiti-Asli, M., Shivazad, M., Zaghari, M., Rezaian, M., Aminzadeh, S. and G.G. Mateos. 2012. Effects of feeding regimen, fiber inclusion, and crude protein content of the diet on performance and egg quality and hatchability of eggs of broiler breeder hens. Poultry Science 91: 3097-3106.
Moradi, S., Zaghari, M., Shivazad, M., Osfoori, R. and M. Mardi. 2013. Response of female broiler breeders to qualitative feed restriction with inclusion of soluble and insoluble fiber sources. Journal of Applied Poultry Research 22 :370–381
Morrissey, K.L.H., Widowski, T., Leeson, S., Sandilands, V., Arnone, A. and S. Torrey. 2014. The effect of dietary alterations during rearing on feather condition in broiler breeder females. Poultry Science 93: 1636-1643.
Nielsen, B.L., Thodberg, K., Malmkvist, J. and S. Steenfeldt. 2011. Proportion of insoluble fibre in the diet affects behaviour and hunger in broiler breeders growing at similar rates. Animal 5: 1247-1258.
Oomura Y. 1983. Glucose as a regulator of neuronal
Breeder Management and Nutrition: Moving the industry forward
activity. Advances in Metabolic Disorders 10: 31-65.
Oyawoye, E.O. and W.F. Krueger. 1986. Effect of high levels of monensin during the growing period on subsequent breeding performance of adult broiler breeders. Poultry Science 65: 2246-2252.
Oyawoye, E.O. and W.F. Krueger. 1990. Potential of chemical regulation of food intake and body weight of broiler breeder chicks. British Poultry Science 31: 735-742.
Pinchasov, Y., Galili, D., Yonash, N. and H. Klandorf. 1993. Effect of feed restriction using self-restricting diets on subsequent performance of broiler breeder females. Poultry Science 72: 613–619.
Portella, F.J., Caston, L.J. and S. Leeson. 1988. Apparent feed particle size preference by laying hens. Canadian Journal of Animal Science 68: 915922.
Riber, A.B., Tahamtani, F.M. and S. Steenfeldt. 2021. Effects of qualitative feed restriction in broiler breeder pullets on behaviour in the home environment. Applied Animal Behaviour Science 235: 1-9.
Sandilands, V., Tolkamp, B.J., Savory, C.J. and I. Kyriazakis. 2006. Behaviour and welfare of broiler breeders fed qualitatively restricted diets during rearing: are there viable alternatives to quantitative restriction? Applied Animal Behaviour Science 96: 53-67.
Savory, C.J., Duke, G.E. and R.W. Bertoy. 1981. Influence of intravenous injections of cholecystokinin on gastrointestinal motility in turkeys and domestic-fowls. Comparative Biochemistry and Physiology A -Physiology 70: 179-189.
Savory, C.J., Maros, K. and S.M. Rutter. 1993. Assessment of hunger in growing broiler breeders in relation to a commercial restricted feeding programme. Animal Welfare 2: 131-152. Savory, C.J., Hocking, P.M., Mann, J.S. and M.H.
Maxwell. 1996. Is broiler breeder welfare improved by using qualitative rather than quantitative food restriction to limit growth rate? Animal Welfare 5: 105-127.
Savory, C.J., and J.M. Lariviere. 2000. Effects of qualitative and quantitative food restriction treatments on feeding motivational state and general activity level of growing broiler breeders.
Applied Animal Behaviour Science 69: 135-147. Shea, M.M., Mench, J.A. and O.P. Thomas. 1990. The effect of dietary tryptophan on aggressive behaviour in developing and mature broiler breeder males. Poultry Science 69: 1664-1669. Soumeh, E.A., Lamot, D.M., Enting, H., Koedijk, R. and S. Powell. 2018. Effects of dietary protein levels during rearing of broiler breeders on egg production and fertility during production, and offspring growth performance. In Proceedings 29th Australian Poultry Science Symposium, Sydney, Australia. pp. 47-50.
Steenfeldt, S., Kjaer, J.B. and R.M. Engberg. 2007. Effect of feeding silages or carrots as supplements to laying hens on production performance, nutrient digestibility, gut structure, gut microflora and feather pecking behaviour. British Poultry Science 48: 454-468.
Tahamtani, F.M. and A.B. Riber. 2020. The effect of qualitative feed restriction in broiler breeder pullets on fear and motivation to explore. Applied Animal Behaviour Science 228: 100509.
Tahamtani, F.M., Moradi, H. and A.B. Riber. 2020. Effect of qualitative feed restriction in broiler breeder pullets on stress and clinical welfare indicators. Frontiers in Veterinary Science 7: 316. van der Klis, J.D. and A. van Voorst. 1993. The effect of carboxy methyl cellulose (a soluble polysaccharide) on the rate of marker excretion from the gastrointestinal tract of boilers. Poultry Science 72: 503-512.
van Emous, R.A., Kwakkel., R.P., van Krimpen, M.
Breeder Management and Nutrition: Moving the industry forward
and W. Hendriks. 2014. Effects of growth pattern and dietary protein level during rearing on feed intake, eating time, eating rate, behaviour, plasma corticosterone concentration, and feather cover in broiler breeder females during the rearing and laying period. Applied Animal Behaviour Science 150: 4454.
Vermaut, S., de Coninck, K., Flo, G., Cokelaere, M., Onagbesan, M. and E. Decuypere. 1997. Effect of Deoiled Jojoba Meal on Feed Intake in Chickens: Satiating or Taste Effect? Journal of Agricultural and Food Chemistry 45: 3158-3163.
Vermaut, S., Onagbesan, O., Bruggeman, V., Verhoeven, G., Berghman, L., Flo, G., Cokelaere, M. and E. Decuypere. 1998. Unidentified factors in jojoba meal prevent oviduct development in broiler breeder females. Journal of Agricultural and Food Chemistry 46: 194-201. Zuidhof, M.J., Robinson, F.E., Feddes, J.J.R., Hardin, R.T., Wilson, J.L., McKay, R.I. and M. Newcombe. 1995. The effects of nutrient dilution on the wellbeing and performance of female broiler breeders. Poultry Science 74: 441-456.
STANISLAW BUDNIK, PhD., is the Senior Poultry Technical Services Manager for Northern Europe at Novus International where his focus is poultry production, farm management and feeding strategies. He has a career in product development, research and development, marketing and sales, and customer service in the animal nutrition industry. Dr. Budnik graduated from the University of Technology and Agriculture in Bydgoszcz (Poland), with a specialty in agricultural chemistry and finished his pedagogical course in parallel. His post-graduate studies in poultry production were completed at the Agricultural University in Wroclaw (Poland). He received his doctorate in agriculture, specializing in zootechnical sciences, from the University of Technology and Life Sciences in Bydgoszcz (Poland). Dr. Budnik has completed numerous technical courses in the field of poultry production in the Netherlands and the United States, and in team management in Poland and Switzerland. Dr. Budnik is (co)author of more than 60 scientific and popular publications and has participated in various international conferences on animal nutrition and production. He is currently vicepresident of the World’s Poultry Science Association Poland branch.
SILVIA PERIS MIRAS, DVM, PhD., is the Executive Regional Technical Services Manager for Europe, Africa & Middle East at Novus International. Prior to joining Novus, she led the team of Technical Documentation and Registers before becoming Technical Director at Industrial Técnica Pecuaria (ITPSA), Spain. Dr. Peris graduated with a degree in veterinary medicine from Autonomous University of Barcelona (Spain) where she went on to earn her doctorate in veterinary medicine with a focus in small ruminants, lactation physiology, and milk production. She completed her post-doctoral fellowship at the Hannah Research Institute (Scotland) before returning to Spain where she was named associate professor in the Department of Animal Production at the Autonomous University of Barcelona. Dr. Peris has (co)authored various papers and publications and spoken at many international industry meetings and activities during her career.
Trace minerals are essential cofactors for hundreds of cellular enzymes and transcription factors in breeder hens, as in all animal species, and thus participate in a wide variety of biochemical processes. The focus of the chapter is on zinc, copper and manganese trace minerals.
There is controversy between trace mineral requirements and the trace mineral recommendation levels that the breeder hen feeding industry uses in its formulations, not only to cover those requirements but to optimize hen productivity. Clearly, deficiencies in trace minerals can lead to deficits in any of the processes they participate, such as tissue and bone development and integrity, immune development and response, or protection against oxidative stress, among others, which will lead to reductions in performance. As such, breeder hen diets are supplemented with inorganic and/or organic forms of trace minerals, adding a safety margin and, therefore, going beyond the levels needed to simply overcome the deficiency symptoms reported in the literature. It is important here to mention that most of the research addressing deficiency studies are relatively old, while the current commercial poultry breeds have undergone a significant genetic improvement over the last decades. Finally, deficiency studies do not lead to a broader understanding of the requirements of an animal in a certain period, nor do they address the effects of marginal deficiency or excess.
The source of a trace mineral, either inorganic or organic, has also an impact on the performance of breeder hens, as different molecules have different properties, making the effects in the organism different. In this regard, the article describes how the molecular structure of different sources affect its stability under adverse conditions, such as the low pH conditions in the upper gastrointestinal tract, making the minerals more or less prone to antagonisms with other compounds in the digesta once inside the gastrointestinal tract and during the digestion process. Ultimately, the extent of trace element dissociation from their ligands will determine the occurrence of more or less antagonism; if more antagonism occurs, less mineral will be available at the small intestine for absorption, resulting in decreased bioavailability.
If a trace mineral source is capable of remaining associated with its ligand under low pH conditions in the gizzard and proventriculus, the compound will arrive at the small intestine where the specific mineral receptors are located for the absorption to take place, as described in the chapter. Again, this will signify more mineral availability to the animal. As a consequence, breeder hen performance will be the same or even improved when using lower levels of this particularly bioavailable mineral source in the diets, as described in the chapter, with the additional benefits in the environment, as lower levels of the trace mineral will be excreted through the faeces.
Poultry nutrition is aimed at optimizing the balance of nutrients in the diet to reach animal requirements while justifying the cost of final feed and considering the birds’ wel fare at the same time. In parallel, reducing the negative impact of animal production on the environment, like carbon dioxide emis sion and heavy metal pollution, is becoming more and more important. The specific com petition for food resources between humans and animals impacts the availability and price of raw materials used to manufacture compound feed for poultry and livestock. As a consequence, nutritionists are obliged to use alternative raw materials and also identi fy new sources of different nutrients to take into account today’s challenges.
The main groups of nutrients are carbohy drates, fats, proteins and amino acids, fibers, vitamins, and minerals. Within minerals, two groups are usually distinguished: macroand microminerals, also called trace minerals or trace elements.
Definition for macrominerals englobes those minerals present in the diet in greater amount than 100 ppm; their levels are ex pressed in % or g/kg. This group is comprised of calcium, chlorine, magnesium, phospho rus, potassium, sodium, and sulfur (Ewing and Charlton, 2007).
Conversely, microminerals include those existing in the diet at less than 100 ppm, and their levels are expressed in ppm or mg/ kg. This group is comprised of chromium, cobalt, copper, iodine, iron, manganese, mo
lybdenum, selenium, and zinc (Ewing and Charlton, 2007). Nutritionists in the feed industry currently optimize the levels of io dine, iron, selenium, manganese, copper, and zinc through supplementation.
The availability of minerals in the diets for poultry production is extremely important to assure the required mineral levels for the currently high efficiency poultry breeds. Ul timately the number of high-quality day-old chicks (DOC) obtained from each breeder hen depends on her health status and the egg quality (Araujo et al., 2019), where minerals have a big role.
This chapter is focused on the require ments of zinc (Zn), copper (Cu) and man ganese (Mn) for breeders and the benefits of supplementing their diets with these trace elements from different sources available in the market.
Biological functions of trace minerals (zinc, copper, and manganese)
Zinc (Zn) is a constituent or activator of about 400 different enzymes needed for growth, synthesis of DNA and two import ant protein types: collagen and keratin (Un derwood and Suttle, 2001). The development of the skeleton and internal tissues depends on collagen, whereas keratin is the structural protein of skin and skin elements like feath ers, beaks, and nails.
Zn finger proteins are involved in the reg ulation of DNA transcription (Luscombe et al., 2000) which controls differentiation of many cell types, including T-lymphocytes
and myeloid precursor cells (Powell et al., 2019; Shivdanasi, 2001; Staal et al., 2001). In fact, an engineered Zn finger protein has been used to cause differentiation of embry onic stem cells (Bartsevich et al., 2003). Thus, Zn is literally essential for the embryonic de velopment of all species, including poultry. After hatch, Zn finger proteins continue to bind to DNA and regulate its transcription in all tissues.
In addition, Zn-containing proteins are the receptors for steroid and thyroid hor mones (Evans, 1988; Luscombe et al., 2000). After binding with the appropriate ligand, these receptors move to the nucleus where they regulate the DNA transcription of hor mone response elements (Freedman and Lu isi, 1993).
Finally, Zn is the most common mineral constituent in metalloenzymes (Underwood and Suttle, 2001). These range in function from protection against oxidation by decom position of superoxide radicals (Bannister et al., 1971; Cook-Mills and Fraker, 1993) to the facilitation of cellular migration by digestion of basement membranes (Goodsell, 2000).
In poultry breeder diets, Zn is recognized as a factor involved in immunity, antioxidant ability, and reproductive (shell quality, hatch ability) and epigenetic processes (Huang et. al., 2019).
Copper (Cu) plays an important role in biological processes as a component or ac tivator of many enzymes, such as superoxide dismutase, cytochrome oxidase, tyrosinase, hydroxylase, and lysine oxidase (Leeson,
2009; Surai, 2016; Kara et al., 2021). As a component of antioxidant enzymes, it par ticipates in antioxidant processes. The pres ence of Cu ions determines the proper func tion of many hormones and vitamins. Cu is also involved in hematopoiesis (formation of the hemoglobin porphyrin ring; Chen et al., 2006; Leeson, 2009) and the metabo lism of connective tissue (collagen synthe sis), playing an important role in the proper cross-linking of collagen and elastin (Rucker and Murray, 1978; Opsahl et al., 1982).
Cu is essential for reproduction; it has been demonstrated that this mineral plays an important role in the activity of dopamine β-monooxygenase, which catalyzes the hy droxylation of dopamine to norepinephrine needed for the production of the gonadotro pin-releasing hormone (Roychoudhury et al., 2016). Hazum (1983) reported the induction of ovulation by Cu in female rats. Finally, it is important to consider the impact of Cu in breeder diets on the number and quality of day-old chicks, mainly due to its influence on shell membrane development (Rucker and Murray, 1978), as well as the benefits on embryo development. Indeed, the yolk is the largest deposit of Cu in the egg, there fore, the main source of the embryo during incubation (Richards, 1997). In this sense, it has been demonstrated that Cu deficiency negatively affects embryo development re sulting in gross structural and biochemical abnormalities (Roychoudhury et al., 2016) linked to its function in collagen synthesis and bone development. Cu deficiency also affects the occurrence of anemia due to its influence on iron transport and mobilization (Dibner, 2005).
Manganese (Mn) is essential for animal growth and fertility (Gallup and Norris, 1939). It plays a critical role in bone formation as well as in the activation of many different kinds of enzymes, such as glycosyltransferase, which is involved in the formation of proteoglycans. Therefore, it is essential for the formation of eggshell membranes (Lilburn, 2021). Interac tions of Mn with several enzymes have also been demonstrated, mainly as a component of metalloenzymes such as superoxide dismutase (Surai, 2016). This is critical for embryonic and postnatal development of bones during the growing phase. Mn deficiency in poultry is as sociated with structural and physiological dis orders, such as cartilage and skeletal malforma tions, as well as weakening of the antioxidant defense system (Xiao et.al, 2014; Noetzold et.al, 2020; Lilburn, 2021).
Trace mineral nutrition for breeder hens, besides supporting the hen’s requirements in terms of fertile egg development and hatch ability, should supply minerals for embryo development. Due to the low costs of supple menting inorganic forms of trace elements and their reduced inclusion rate in feed compared to other nutrients, little attention has been paid to in-depth research into trace element absorp tion and interactions among them and, final ly, their impact on optimizing animal perfor mance, until the beginning of the 21st century when alternative forms - organic trace mineral sources - appeared on the market.
Research focused on trace minerals for poultry and livestock is scarce. The cita
tions typically referenced in scientific liter ature on trace mineral supplementation in the diet refers to works from the 1960s or even earlier. Moreover, in relation to broiler breeders, only a few recent research studies exist (Kidd et al., 1992a, 1992b; Virden et al., 2003; Hudson et al., 2004a, 2004b; Dibner and Richards, 2006; Favero et al., 2013; Ber wanger et al., 2018; Torres and Korver, 2018; Güz et al., 2022, among few others). Most of these studies compare different trace mineral sources while others look only at the effects on the breeder’s progeny. However, with the exception of the work of Berwanger et al. (2018) in which they conclude providing an estimation of the Cu requirements according to production objectives, the rest have not been designed to determine mineral require ments and focus on describing the benefits of the trace minerals and sources. Therefore, due to the lack of supporting literature, rec ommendations for trace minerals supple mentation presented in the most popular re ferred tables of requirements for these hens are either nonexistent (NRC, 1994) or based on scientific studies considering minimum levels to prevent typical deficiency symptoms and adding safety margins to avoid subclin ical problems under standard management conditions (Rostagno et al., 2017; FEDNA, 2018; Cobb, 2019; Aviagen, 2021), as shown in Table 1. However, these recommendations do not consider extreme situations, such as stress, which may increase the need for ad ditional trace minerals related to the status of the immune system and antioxidant pro cesses. On the other hand, deficiency studies do not lead to a broader understanding of the requirements of an animal in a certain period, nor do they address the effect of mar
Table
FEDNA, 2018
Rostagno, 2017
Cobb500 Fast Feather Breeder, 2019
Aviagen Ross 308 Parent Stock, 2021
Aviagen Ranger Premium PS, 2018
legislation – Maximum content in compound feed for poultry (ppm complete feed)
ginal deficiency or excess. Finally, it should be noted that the requirements provided by these tables have not significantly changed in recent decades while constant improve ments in productivity have been occurring over time.
Rostagno et al. (2017) is the only source providing recommended levels for organic and inorganic trace mineral sources sepa rately. Others refer to the higher biological availability of organic sources versus inor ganic sources, therefore suggesting the ad justment of trace mineral levels in feed when using these organic sources (Aviagen, 2018; FEDNA, 2018).
The interrelationships of minerals, some of them well known, creating antagonism among different macro and trace minerals (Figure 1) deserve further attention. In this regard, a relative overestimation of one min eral can severely affect the availability of an other, leading to an antagonistic relationship. For example, high levels of Zn reduce the availability of Cu, and the opposite is also
(80-110)
true (O’Dell, 1989; Leeson and Summers, 2001; Zhao et al., 2008). Other compounds present in the feed matrix also interact with minerals, macro and trace minerals, antago nizing them and reducing their absorption and therefore bioavailability for the animal. In relation to this, phytic acid is able to form complexes with trace minerals that are very stable and highly insoluble, rendering the minerals unavailable for absorption (Ober leas et al., 1966; Leeson and Summers, 2001). The phytic acid-mediated antagonism is am plified in the presence of calcium (Oberleas et al., 1966).
Trace minerals used in poultry diets most ly consist of an inorganic form, such as ox ide (ZnO, CuO, MnO) or sulphate salts (ZnSO₄, CuSO₄); however, organic sources of these minerals are also available today on the market. Inorganic salts tend to dissoci ate in the low pH environment of the upper gastrointestinal tract, leaving the minerals susceptible to various nutrient and ingredi ent antagonisms that impair absorption (and thus reduce bioavailability; Underwood and
P - Phosporus
Cr - Chromium
Co - Cobalt
PB - Lead
Fe - Iron
Se - Selenium
Na - Sodium
Ca - Calcium
Ag - Silver
Cd - Cadmium
Hg - Mercury
Al - Aliminium
Cu - Copper
Mn - Manganese
K - Potassium
Mo - Molybdenum
I - Iodine
Mg - Magnesium
Zn - Zinc
Si - Silica
As - Arsenic
F - Florine
S - Sulfur
Direction of arrows denotes interference.
Arrows aimed at each other denote mineral synergy.
Arrows aimed away f rom each other denote mutual mineral interference or antagonism
Figure 1. Some of the one-way or two-way antagonisms between macro and microminerals (Mulder’s Chart (Nutritional Wheel) Journal of Environmental & Agricultural Sciences – JEAS (10 July 2017))
Suttle, 1999). An advantage of chelated trace minerals is that the binding of the organic ligand(s) to the mineral provides stability to the complex in the upper gastrointestinal system, thereby minimizing mineral loss to antagonists and allowing the complex to be delivered to the absorptive epithelium of the small intestine for mineral uptake (Leeson and Summers, 2001). It should be noted that different organic trace minerals are not equally stable at low pH and therefore will not necessarily increase the bioavailability of a given mineral to the same extent (Brown and Zeringue, 1994; Cao et al., 2000; Guo et al., 2001).
Nowadays and under practical conditions, several questions are becoming more and more important:
- Do the levels and source of trace elements supplemented in the feed optimize the ef ficiency of current poultry lines?
- What are the optimal levels and sources of trace minerals in commercial conditions?
- Can we reduce the mineral levels to protect the environment without adversely affect ing production results?
Currently, the industry uses several sources of information to optimize trace mineral lev els and the form of supplementation:
1. Legal regulations determining the maxi mum level of minerals in feed. In the Eu ropean Union, concerns for environmental pollution have been leading to regulations, setting the maximum total level of Zn to 120 ppm, Cu to 25 ppm, and Mn to 150 ppm in complete feed for poultry.
2. Nutritional institute/council publications for poultry species, publish tables of re quirements for the different nutrients.
3. Genetic companies’ recommendations for the different poultry commercial breeds.
4. Mineral producers, especially related to organic trace mineral producers.
In vertebrates, trace minerals are absorbed in the gut through the apical surface of the enterocytes (Cousins, 1985). The molecular mechanisms involved in the transepithelial transport of the minerals are currently not fully understood.
Zn uptake happens at the intestinal brush border membrane where it is transported from the lumen into the absorptive cells of the epithelium: the enterocytes (Maares and Haase, 2020). According to Goff (2018) and Maares and Haase (2020), intestinal Zn ab sorption is mainly mediated by different spe cific transporters regulated by the organism, described as such: A Zn transporting protein (ZIP4) in the apical membrane transports Zn ions into the cell. The amount of ZIP4 on the apical membrane is upregulated when the an imal needs Zn and downregulated when the animal is Zn-replete (Mao et al., 2007). Other ZIP transporters have been identified (ZIP11 and ZIP14) that may also play a minor role in
bringing Zn across the apical membrane. Zn can also use divalent metal transporter 1 pro tein (DMT1) to cross the apical membrane, though it must compete with iron and Mn for binding sites on this transporter; how ever, this is considered a minor pathway for Zn absorption across the apical membrane. The discovery of intestinal Zn transporters and elucidation of the role of Zn-binding metallothionein in maintaining enterocyte Zn homeostasis contributed to an increased understanding of the regulatory parameters of intestinal Zn absorption (Maares and Haase, 2020). Paracellular absorption has also been described but it is not the primary pathway, requiring higher dietary Zn levels to achieve the higher lumen-soluble Zn concentra tion needed to drive this secondary pathway (Condomina et al., 2002).
In relation to Cu, this element uses a spe cific high-affinity cuprous (Cu+) transport protein, CRT1, to cross the apical mem brane and enter the enterocyte; this process is highly efficient (Goff, 2018). Although CTR1 seems to be the most important path used to cross the apical membrane, there is evidence that DMT1 is also a minor path way for Cu movement across the apical membrane (Lutsenko et al., 2007). CTR1 appears to be the protein acting as the reg ulator for Cu absorption, but not DMT1 (Hill and Link, 2009).
Finally, as for Mn, the mechanisms under lying this trace mineral absorption remain mainly unknown, but it is thought that regu lation of whole-body Mn homeostasis occurs at least partially at the intestinal absorption level. The main transporter that has been de
scribed in the scientific literature concerning Mn absorption is DMT1 (Goff, 2018; Liao et al., 2019), but ZIP14 and ZnT10 trans porters have also been shown to play a role (Felber et al., 2019), as well as FPN1 (Yin et al., 2010).
In relation to the different mineral sourc es, there have been assumptions that organ ic trace minerals could be intactly absorbed through an amino acid or peptide transport er. However, these statements have been based on in vitro models. Nevertheless, even among this type of work, there is data using everted gut models suggesting that amino acid complexes (in this case Zn methionate or Zn lysinate) are not absorbed intact (Hill et al., 1987). More recently, Liao et al. (2019) demonstrated in an in vivo study using com mercial broilers and different Mn sources (sulphate, and 3 different Mn organic sourc es) that both DMT1 and FPN1 transport ers facilitated Mn absorption. The results of their work suggest that amino acid and peptide transporters might not be involved in the transport of organic Mn in the small intestine of broilers.
To be absorbed, minerals must overcome substantial challenges to reach the site of absorption intact so the metal can then be trapped by the transporter proteins locat ed in the enterocytes of the small intestine. For this to happen, minerals must make it through strong challenging conditions, such as those in the gizzard and proventriculus. These conditions will trigger dissociation of
the mineral from its ligand or ligands to a lower or larger extent depending on the mo lecular structure of the compound, which will determine its stability. In this sense, it has been shown that the most stable com plexes are those containing 2 ligands per each metal atom (ratio 2:1) with a structure of five member rings created between each of the ligands and the mineral (Predieri et al., 2005). This structure is named bis-chelate, as there are 2 five-member rings securing the mineral, in the middle of which confers neu tral charge to the molecule, in opposition to those of simple complexes containing 1 ligand per mineral (ratio 1:1) that remain positively charged (Predieri et al., 2005; Bel trami, 2008). The neutral charge achieved with the bis-chelated structure makes it less reactive to antagonisms in the environment, feed, and upper gastrointestinal tract, there by ensuring the mineral reaches the site of absorption.
In this regard, in an acidic pH environ ment like the gizzard and proventriculus of the bird, more protons are present, creating competition between molecules. This com petition can result in the dissociation of the desired complex. The resulting anions and cations, such as the minerals, will react with other charged substances present in the di gesta (i.e. phytic acid), creating complex structures that will precipitate and will be excreted through faeces. With the bis-che lated neutral structures, because the bond is more secure, the changes in pH that occur throughout the digestion process have less effect on the bond structure and dissociation of the mineral from ligands is greatly reduced (Ballarini and Predieri, 2007), allowing more
Zinc amino acid complex
Zinc bis-chelate of hydroxy analogue of methionien
(Adapted from Richards et al., 2008)
for Zn,
minerals to reach the site of absorption, at which time the specific transporter can grab the mineral because the affinity of the trans porter for the mineral is stronger than the forces holding the ligand and the mineral together.
Evidence suggests more mineral from Zn bis-chelates of methionine hydroxy ana logue (HMTBa) is absorbed compared to Zn from other sources, not only from inor ganic (Richards et al., 2015) but also from organic sources (Richards et al., 2008), by measuring the level of metallothionein expression in the jejunal mucosa of broil ers after being fed the same amount of Zn from different sources (Figure 2). Metallo thionein is a biomarker whose expression is regulated by Zn status (Davis and Cousins, 2000). Its mRNA and protein expression increases when more Zn is taken up by the
enterocytes and decreases when less Zn is taken up. As such, MT mRNA or protein expression is widely used as an indicator of the Zn status of humans and animals, and to evaluate the bioavailability of different Zn sources (McCormick et al., 1981; Martinez et al., 2004). Similarly, in recent works using the technique of stable isotopes as markers for Zn, which allows to tract the mineral to the different tissues within the animal, it has been demonstrated in dairy calves that Zn bis-chelates of hydroxy analogue of me thionine show greater tissue enrichment, therefore higher bioavailability, compared to inorganic (Zn oxide) and organic (gly cinate mineral complexes) sources (Tucker et al., 2016; Tucker and Provin, 2020). As a consequence of the higher bioavailabili ty over corresponding sources, bis-chelates can be used at a lower mineral inclusion rate without compromising performance
and therefore achieve a reduction of trace mineral excretion into the environment.
Poultry breeder nutrition and feeding strat egies during the periods of rearing and egg production have a strong impact on the liv ability and productivity of these birds. In the case of parent stock flocks, the main produc tion goal is the number of high-quality dayold chicks obtained from each hen housed. Therefore, in the end, we can say that we feed the eggs that would end up in the incubator.
Over the last years, various studies and field observations have been carried out in layer and broiler breeders comparing the benefits of bis-chelated trace minerals (MMHAC, me thionine hydroxy analogue chelates) on their
health, performance, egg quality, hatchabili ty, and progeny versus inorganic sources or a combination of inorganic and organic sources. MMHAC is an innovative Zn, Cu and Mn organic source in which the mineral is chelat ed to 2 molecules of methionine hydroxy ana logue, explaining the bis-chelated appellation.
In these trials, the standard trace miner al sources and levels (either inorganic or a combination of inorganic and organic sourc es), acting as the control group, were entire ly replaced with lower levels of MMHAC bis-chelates, which conformed the treatment group. This strategy is referenced as the RE DUCE AND REPLACE™ (R&R) strat egy, that is, lower supplementation of Zn, Cu and Mn trace minerals in the feed as MMHAC, entirely replacing the standard trace mineral sources. It should be noted that the methionine contribution coming from the MMHAC was always taken into account in
Table 2. Results on performance in different studies with broiler or layer breeders when supplementing the diets with methionine hydroxy analogue chelates of Zn, Cu and Mn (MMHAC) following the REDUCE AND REPLACE™ (R&R) strategy versus inorganic trace mineral sources (ITMs) or a combination of inorganic and other organic (OTMs) sources. Results expressed as improvement of MMHAC versus control (Control = 100%)
Breed (country) & Reference
Ross 308 (BE) Novus Int., 2016
Control vs Treatment (ppm Zn : Cu : Mn su pplemented in feed)
: 15 : 100 ITMs
: 10 : 65 MMHAC
Hubbard GGP male line (FR) Peris et al., 2015 100 : 10 : 100 ITMs vs 56 : 14 : 56 MMHAC
NOVOgen White (NL) Borren, 2017
Hy-line Brown (RU) Novus Int., 2017
treatments
: 13+2 : 58+12
vs 50 : 10 : 65 MMHAC
83+20 : 19+5 : 83+30
vs
: 10 : 60 MMHAC
a
Trial period (hen’s age in weeks)
Parameters (Control = 100%)
trial Hen mortality Nº day-old pullets FCR / day-old pullet
to 60 43 000 100.0 100.8 95.5
to 53 3
to
to
84.4 107.8 92.2
105.3 96.7
100.0 106.8 96.3
mineral
levels of each source
ppm (organic),
the amino acid balance of the diets in all trials as a source of methionine. In this regard, Yi et al. (2007) demonstrated that the methionine hydroxy analogue ligands from MMHAC were fully available as a methionine source. An overview of some of these trials and their outcomes are presented in Table 2.
In the study carried out with NOVOgen White layer breeder flocks (Borren, 2017), referred in Table 2, great emphasis was placed on the effect of the mineral sources and levels on the total number of hatched chicks in relation to the storage period of the eggs, short (7 to 9 d) versus long (17 to 19 d). The assessment was done twice during the duration of the study with a total of 2,400 eggs collected at 10 and 28 weeks after the start of the trial (corresponding to 27 and
Mn:
ppm
45 weeks of hen’s age, respectively). Animals were fed identical diets with the exception of the mineral sources and levels (50 ppm Zn, 10 ppm Cu, and 65 ppm Mn for the MMHAC group; 75 ppm Zn (64 ppm in organic + 11 ppm organic), 15 ppm Cu (13 ppm inorganic + 2 ppm organic), and 70 ppm Mn (58 ppm inorganic + 12 ppm organic) for the group combining ITMs and OTMs, respectively). During incubation, the eggs were candled to identify clear versus dead embryos. Higher fertility and lower embryo mortality were noticed in the MMHAC group versus those from the combination of inorganic and organic trace mineral sourc es. The benefit on embryo mortality became especially visible when the eggs were stored for a longer time (up to 35% lower embryo mortality), which finally resulted in a high
er hatchability percentage for the eggs de rived from MMHAC-fed hens (Figure 3). It was suggested that MMHAC improved egg fertilization and reduced losses due to em bryo defects during incubation, particular ly during the long storage time, suggesting a higher bioavailability of MMHAC trace minerals since the levels supplemented were lower than in the group combining ITMs and OTMs.
The impact of feeding breeder hens MMHAC at reduced levels (R&R) on final chick quality was also assessed in some stud ies (Peris et al., 2015; Novus International, 2016). The hen deposits minerals in the egg via 2 routes: the ovary to the yolk and the oviduct to the albumen, shell, and shell mem brane (Richards and Packard, 1996). Because
these routes provide the only mineral source for the embryo during incubation, proper development of the broiler embryo depends on the correct deposition of minerals by the hen into the egg (Wilson, 1997; Yair and Uni, 2011). However, studies have shown that increasing the concentration (feeding in excess) of most minerals in the diet has little or no effect on mineral concentrations in the egg (Naber, 1979; Angel, 2007). On the con trary, a study performed by Novus Interna tional (2011) using 19,000 Lohmann Brown breeders from 17 to 80 weeks of age showed that higher bioavailable minerals in breeder diets can also result in higher levels avail able for their chick development. This study demonstrated that MMHAC (50:10:65 ppm for MMHAC Zn, Cu and Mn, respec tively) resulted in higher Zn and Cu content
Figure 5. Increase in percentage of ash on tibia (dry matter basis) in day-old chicks resulting from MMHAC R&R strategy fed to breeder hens, compared to a diet supplemented with inorganic trace mineral sources
in the egg yolk in comparison to a diet based on 100 ppm Zn, 10 ppm Cu, and 100 ppm Mn from inorganic sources (Figure 4).
Different work performed in heavy broil er breeders (Hubbard GGP male line) in France evaluated the impact of trace min eral source on the level of mineralization of the tibia bones of their day-old chicks based on crude ash and Zn content (Peris et al., 2015). The significantly higher level of ash found in the tibia of chicks originating from MMHAC-fed hens in comparison to hens supplemented with inorganic trace mineral sources suggests a stronger skeleton, which is associated with fewer locomotion problems in broiler production (Yair et al., 2013). The results of this study are shown in Figures 5 and 6. Other benefits obtained in this study with MMHAC are summarized in Table 2.
In this same direction, Novus Interna tional (2016) conducted a large-scale com
Figure 6. Increase of Zn level (ppm) in tibia (dry matter basis) in day-old chicks resulting from MMHAC R&R strategy fed to breeder hens, compared to a diet supplemented with inorganic trace mineral sources
mercial study with 43,000 Ross 308 breeder hens from 20 weeks to 60 weeks of age in the Netherlands in cooperation with a lead ing hatchery organization in the European Union, BHV (Belgium). Among other pa rameters, early mortality at 7 days of age of progeny from supplemented hens was evalu ated as an indicator of chick health. Hens re ceived MMHAC during the testing period at the levels of 50 ppm Zn, 10 ppm Cu, and 65 ppm Mn. Hen and progeny performance were compared to the same parameters ob tained in the previous 5 years wherein the animals were supplemented with inorganic sources at levels of 100 ppm Zn, 15 ppm Cu, and 100 ppm Mn. Among other benefits de scribed in Table 2 the hens fed MMHAC during the period saw a higher number of viable chicks compared to the previous 5 years when diets contained inorganic trace mineral sources at significantly higher levels
MMHAC supplemented levels were 50 ppm Zn, 10 ppm Cu, and 65 ppm Mn from 20 weeks up to 60 weeks of age. Results were compared with the average of the previous 5 years for the same laying period during which hens were supplemented with 100 ppm Zn, 15 ppm Cu, and 100 ppm Mn from inorganic sources.
These findings demonstrate that support ing breeder hens with a highly bioavailable source of Zn, Cu, and Mn not only ensures better performance of the hens but also posi tively impact their progeny’s health and qual ity for a better start in the broiler production that follows. In addition, studies show pro viding bis-chelated mineral sources that are more bioavailable allows supplementing at lower levels in feed without a negative im pact on the performance, maximizing the animal’s use of the minerals and therefore
minimizing mineral excretion into the envi ronment (results not shown).
The importance of Zn, Cu and Mn trace minerals in animal agriculture is widely rec ognized and virtually all diets are supple mented with these minerals. Well-designed experiments that investigate the relative bio availability of trace mineral sources demon strate that certain organic trace minerals can more effectively satisfy the requirements of the current high-producing breeder hens, ei ther heavy or light genetic lines. Providing a more bioavailable source of minerals such as bis-chelated methionine hydroxy analogue trace minerals, has shown to exert a variety of positive effects, ending with higher per formance from these animals together with better progeny quality. Finally, due to the
Breeder Management and Nutrition: Moving the industry forward
higher bioavailability of bis-chelates, the mineral requirement can be met at lower levels of inclusion in the diet, resulting in re duced excretion into the environment.
Angel, R. 2007. Metabolic disorders: Limitations to growth of and mineral deposition into the broiler skeleton after hatch and potential implications for leg problems. Journal of Applied Poultry Research 16: 138–149.
Araujo, C.S.S., Hermes, R.G., Bittencourt, L.C., Silva, C.C., Araujo, L.F., Granghelli, C.A., Pelissari, P.H., Roque, F.A., and Leite, B.G.S. 2019. Different di etary trace mineral sources for broiler breeders and their progenies, Poultry Science 98: 4716–4721.
Aviagen. 2018. Ranger Premium Parent Stock Nutri tion Specifications. www.en.aviagen.com.
Aviagen. 2021. Parent Stock Ross 308 Nutrition Specifications. www.Aviagen.com.
Ballarini, G., and Predieri, G. 2007. I chelate metal lici nella nutrizione. Struttura, funzioni e metodi d’analisi. Progress in Nutrition 9: 1-11.
Bannister, J., Bannister, W., and Wood, E. 1971. Bo vine erythrocyte cupro-zinc protein. European Journal of Biochemistry 18: 178-186.
Bartsevich, V.V., Miller, J.C., Case, C.C., and Pabo, C.O. 2003. Engineered Zinc Finger Proteins for Controlling Stem Cell Fate. Stem Cells 21: 632-637. Beltrami, D. 2008. Development of new metal che lates for animal nutrition and of analytical meth ods for their quantitative determination and quality control. Dissertation. Parma University. 118 pp. Berwanger, E., Vieira, S.L., Angel, C.R., Kindlein, L., Mayer, A.N., Ebbing, M.A., and Lopes, M. 2018. Copper requirements of broiler breeder hens. Poul try Science 97: 2785–2797. Borren, H. 2017. The effect of organic chelated trace minerals on the technical performance of parent
stock. Dissertation. Van Hall Larenstein, University of Applied Sciences, The Netherlands.
Brown, T.F., and Zeringue, L.K. 1994. Laboratory Evaluations of solubility and structural integrity of complexed and chelated trace mineral supplements.
Journal of Dairy Science 77: 181-189.
Cao, J., Henry, P.R., Guo, R., Holwerda, R.A., Toth, J.P., Littell, R.C., Miles, R.D., and Ammerman, C.B. 2000. Chemical characteristics and relative bioavailability of supplemental organic zinc sources for poultry and ruminants. Journal of Animal Sci ence 78: 2039-2054.
Chen, H., Huang, G., Su, T., Gao, H., Attieh, Z.K., McKie, A.T., Anderson, G.J., and Vulpe, C.D. 2006. Decreased hephaestin activity in the intestine of copper-deficient mice causes systemic iron defi ciency. Journal of Nutrition 136: 1236–1241.
Cobb. 2019. Cobb500 Breeder Management Supple ment. Female Fast Feather. www.cobb-vantress.com. Combs, G. F., Jr. 1994. Clinical implications of sele nium and vitamin E in poultry nutrition. Veterinary Clinical Nutrition 1: 133–140.
Condomina, J., Zornoza-Sabina, T., Granero, L. and Polache, A. 2002. Kinetics of zinc transport in vitro in rat small intestine and colon: Interaction with copper. European Journal of Pharmaceutical Sci ences 16: 289–295.
Cook-Mills, J., and Fraker, P. 1993. The role of met als in the production of toxic oxygen metabolites by mononuclear phagocytes. Pages 127-140. S. Con ningham-Rundles, ed. Nutrient Moldulation of the Immune Response. Marcel Dekker, Inc, New York. Cousins, R.J. 1985. Absorption, transport, and hepat ic metabolism of copper and zinc: special reference to metallothionein and ceruloplasmin. Physiologi cal Reviews 65: 238-309.
Davis, S.R. and Cousins, R.J. 2000. Metallothionein expression in animals: A physiological perspective on function. The Journal of Nutrition 130: 10851088.
Dibner, J. 2005. Early nutrition of zinc and copper in chicks and poults: Impact on growth and immune function, Proceedings of the 3rd Mid-At lantic Nutrition Conference.
Dibner, J.J., and Richards, J.D. 2006. Mineral metab olism and chelated minerals for hatchlings. Chapter 4 in: Recent Advances in Animal Nutrition 2005, Nottingham University Press.
Evans, R.M. 1988. The steroid and thyroid hormone receptor superfamily. Science 240: 889–895. Ewing, W.N. and Charlton, S.J. (2007): The minerals directory, 2nd Ed. Leicestershire, Context Products Ltd.
Favero, A., Vieira, S.L., Angel, C.R., Bess, F., Cemin, H.S., and Ward, T.L. 2013. Reproductive perfor mance of Cobb 500 breeder hens fed diets supple mented with zinc, manganese, and copper from in organic and amino acid-complexed sources. Journal of Applied Poultry Research 22: 80–91.
FEDNA, 2018. Necesidades nutricionales para Avi cultura: Normas FEDNA, 2n Ed., Universidad Politécnica de Madrid, Madrid, Spain.
Felber, D.M., Wu, Y. and Zhao, N. 2019. Regulation of the metal transporters ZIP14 and ZnT10 by manganese intake in mice. Nutrients 11, 2099. Freedman, L., and Luisi, B. 1993. On the mechanism of DNA-binding by nuclear hormone receptorsa structural and functional perspective. Journal of Cell Biochemistry 51: 140-150.
Gallup, W.D., and Norris, L.C. 1939. The effect of a deficiency of manganese in the diet of the hen. Poultry Science 18: 83–88.
Goff, J.P. 2018. Invited review: Mineral absorption mechanisms, mineral interactions that affect acid–base and antioxidant status, and diet considerations to improve mineral status. Journal of Dairy Science 101: 1–51.
Goodsell, D.S. 2000. The molecular perspective: Ma trix metalloproteinase 2. Stem Cells 18: 73-75. Guo, R., Henry, P.R., Holwerda, R.A., Cao, J., Lit
tell, R.C., Miles, R.D., and Ammerman, C.B. 2001. Chemical characteristics and relative bioavailability of supplemental organic copper sources for poultry. Journal of Animal Science 79: 1132-1141.
Güz, B.C., de Jong, I.C., Bol, U.E., Kemp, B., van Krimpen, M., Molenaar, R., and van den Brand, H. 2022. Effects of organic macro and trace minerals in fast and slower growing broiler breeders’ diet on offspring growth performance and tibia character istics. Poultry Science 101: 101647.
Hazum, E. 1983. Copper and thiol regulation of go nadotropin releasing hormone binding and lutein izing hormone release. Biochemical and Biophysi cal Research Communications 1: 306-312.
Hill, D.A., Peo, E.R. Jr. and Lewis, A.J. 1987. Influ ence of picolinic acid on the uptake of zinc-amino acid complexes by the everted rat gut. Journal of Animal Science 65: 173-178.
Hill, G.M. and Link, J.E. 2009. Transporters in the absorption and utilization of zinc and copper. Jour nal of Animal Science 87: E85-E89.
Huang, L., Li, X., Wang, W., Yang, L., Zhu, Y. 2019: The Role of Zinc in Poultry Breeder and Hen Nu trition: an Update. Biological Trace Element Re search 192: 308-318.
Hudson, B.P., Dozier III, W.A., Wilson, J.L., Fair child, B.D., and Buhr, R.J. 2004a. Breeder age and zinc source in broiler breeder hen diets on proge ny characteristics at hatching. Journal of Applied Poultry Research 13: 55–64.
Hudson, B.P., Dozier III, W.A., Wilson, J.L., Sander, J.E., and Ward, T.L. 2004b. Reproductive perfor mance and immune status of caged broiler breeder hens provided diets supplemented with either in organic or organic source of zinc from hatching to 65 wk of age. Journal of Applied Poultry Research 13: 349–359
Kara, K., Kocaoglu Guclu, B., Senturk, M., Eren, M., and Baytok, E. 2021. Effects of catechin and copper or their combination in diet on productive perfor
mance, egg quality, egg shelf-life, plasma 8-OHdG concentrations and oxidative status in laying quail (Coturnix coturnix japonica). Journal of Applied Animal Research 49: 97-103.
Kidd, M.T., Anthony, N.B., Johnson, Z., and Lee, S.R. 1992a. Effect of zinc methionine supplementation on the performance of mature broiler breeders. Journal of Applied Poultry Research 1: 207–211.
Kidd, M.T., Anthony, N.B., and Lee, S.R. 1992b. Progeny performance when dams and chicks are fed supplemental zinc. Poultry Science 71: 1201–1206.
Leeson, S., and Summers, J.D. 2001. Scott’s Nutrition of the Chicken. 4th Ed. University Books, Guelph, Ontario.
Leeson, S. 2009. Copper metabolism and dietary needs. World’s Poultry Science Journal 65: 353-366. Liao, X.D., Wang, G., Lu, L., Zhang, L.Y., Lan, L.Y., Li, S.F. and Luo, X.G. 2019. Effect of manganese source on manganese absorption and expression of related transporters in the small intestine of broil ers. Poultry Science 98: 4994-5004.
Lilburn, M.S. 2021. Centennial Review: Trace miner al research with an emphasis on manganese. Poultry Science 100: 101222.
Luscombe, N.M., Austin, S.E., Berman, H.M., and Thornton, J.M. 2000. Review: An overview of the structures of protein-DNA complexes. Genome Biology 1: 001.1-001.10.
Lutsenko, S., Barnes, N.L., Bartee, M.Y. and Dmi triev, O.Y. 2007. Function and regulation of human copper-transporting ATPases. Physiology Reviews 87: 1011-1046.
Maares, M. and Haase, H. 2020. A guide to human zinc absorption: General overview and recent ad vances of in vitro intestinal models. Nutrients 12: 762.
Mao, X., Kim, B.E., Wang, F., Eide, D.J. and Pe tris, M.J. 2007. A histidine-rich cluster mediates the ubiquitination and degradation of the human zinc transporter, hZIP4, and protects against zinc
cytotoxicity. Journal of Biological Chemistry 282: 6992–7000.
Martinez, M.M., Hill, G.M., Link, J.E., Raney, N.E., Tempelman, R.J. and Ernst, C.W. 2004. Pharmaco logical zinc and phytase supplementation enhance metallothionein mRNA abundance and protein concentration in newly weaned pigs. Journal of Nu trition 134: 538-544.
McCormick, C. C., Menard, M. P. and Cousins, R. J. 1981. Induction of hepatic metallothionein by feeding zinc to rats of depleted zinc status. Ameri can Journal of Physiology 240: E414-E421.
Naber, E.C. 1979. The effect of nutrition on the com position of the egg. Poultry Science 58: 518–528. Noetzold, T.L., Vieira, S.L., Favero, A., Horn, R.M., Silva, C.M., and Martins, G.B. 2020. Manganese requirements of broiler breeder hens. Poultry Science 99: 5814-5826.
Novus International. 2011. Efficacy of MINTREX® Reduce & Replace strategy in Lohmann Brown layer breeders. Internal Technical Report. Novus International. 2016. New large-scale trial con firms breeder performance, egg shell structure and progeny development depend on the right trace mineral source in the diet. Internal Technical Report. Novus International. 2017. Applying MINTREX® Reduce & Replace strategy supports commercial lay er breeder performance. Internal Technical Report. NRC (National Research Council). 1994. Nutrient Requirements of poultry. 9th Ed. National Acade my Press, Washington D.C., USA.
O’Dell, B.L. 1989. Mineral interactions relevant to nutrient requirements. Journal of Nutrition 119: 1832-1838.
Oberleas, D., Muhrer, M.E., and O’Dell, B.L. 1966. Dietary metal complexing agents and zinc avail ability in the rat. Journal of Nutrition 90: 56-62.
Opsahl, W., Zeronian, H., Ellison, M., Lewis, D., Rucker, R.B., and Riggins, R.S. 1982. Role of cop per in collagen crosslinking and its influence on
Breeder Management and Nutrition: Moving the industry forward
selected mechanical properties of chick bone and tendon. Journal of Nutrition 112: 708–716.
Peris, S., Alleno, C., Michard, J., and Manangi, M. 2015. Effect of a reduced supply of Zn, Cu, Mn as chelate of methionine analogue on hatchability in broiler breeders and bone quality of progeny. Eu ropean Symposium on Poultry Nutrition, Prague, Czech Republic, 24-27 August 2015.
Powell, M.D., Read, K.A., Sreekumar, B.K., and Oes treich, K.J. 2019. Ikaros Zinc Finger Transcription Factors: Regulators of Cytokine Signaling Path ways and CD4+ T Helper Cell Differentiation. Frontiers in Immunology 10: 1299
Predieri, G., Elviri, L., Tegoni, M., Zagnoni, I., Cinti, E., Biagi, G., Ferruzza, S. and Leonardi, G. 2005. Metal chelates of 2-hydroxy-4-methylthiobutanoic acid in animal feeding. Part 2: Further characteriza tions, in vitro and in vivo investigations. Journal of Inorganic Biochemistry 99: 627–636.
Richards, J.D., Dibner, J.J., Winkelbauer, P., Atwell, C.A., Quiroz, M.A., York, T., Zhao, J., Shirley, R., Giesen, A.F. and Knight, C.D. 2008. Organic trace minerals for poultry enhance mineral bioavailabil ity and can improve performance and reduce en vironmental impact. Paper presented at: XXXIII World’s Poultry Conference (Brisbane, Australia).
Richards, J.D., Fisher, P.M., Evans, J.L. and Wede kind, K.J. 2015. Greater bioavailability of chelated compared with inorganic zinc in broiler chicks in the presence or absence of elevated calcium and phosphorus. Open Access Animal Physiology 7: 97-110.
Richards, M.P., and Packard, M.J. 1996. Mineral me tabolism in avian embryos. Poultry and Avian Biol ogy Reviews 7: 143–161.
Richards, M. P. 1997. Trace mineral metabolism in the avian embryo. Poultry Science 76: 152–164. Rostagno, H.S., Teixeira Albino, L.F., Hannas, M.I., Lopes Donzele, J., Kazue Sakomura, N., Perazzo, F.G., Saraiva, A., Teixeira de Abreu, M.L., Borges
Rodrigues, P., de Oliveira, R.F., de Toledo Barreto, S.L., and Oliveira Brito, C. 2017. Brazilian Tables for Poultry and Swine. Feedstuff Composition and Nu tritional Requirements. 4th Ed. Federal University of Viçosa-Department of Animal Science, Brazil.
Roychoudhury, S., Nath, S., Massanyi, P., Stawarz, R., Kacaniova, M., and Kolesarova, A. 2016. Cop per-induced changes in reproductive functions: In vivo and vitro effects. Physiological Research 65: 11–22.
Rucker, R.B., and Murray, J. 1978. Cross-linking ami no acids in collagen and elastin. American Journal of Clinical Nutrition 31: 1221-1236.
Shivdasani, R.A. 2001. Molecular and transcriptional regulation of megakaryocyte differentiation. Stem Cells 19: 397-407.
Staal, F.J.T., Weerkamp, F., Langerak, A.W., Hen driks, R.W., and Clevers, H.S. 2001. Transcription al control of T lymphocyte differentiation. Stem Cells 19: 165-179.
Stahl, J.L., Cook, M.E. and Sunde, M.L. 1986. Zinc supplementation: its effect on egg production, feed conversion, fertility and hatchability. Poultry Sci ence 65: 2104-2109
Surai, P.F. 2016. Antioxidant Systems in Poultry Bi ology: Superoxide Dismutase. Journal of Animal Research and Nutrition 1: 8.
Torres, C.A., and Korver, D.R. 2018. Influences of trace mineral nutrition and maternal flock age on broiler embryo bone development. Poultry Science 97: 2996–3003.
Tucker, H.A., Foran, C.K., Bettis, S., Fisher, P., Xue, J., Wedekind, K.J. and Vazquez-Anon, M. 2016. Bioavailability of different sources of zinc sing stable isotopes in male Holstein calves. Journal of Dairy Science 99: 704.
Tucker, H. and Provin, A. 2020. Benefit of zinc me thionine hydroxy analog chelate to increasing tissue enrichment with dietary antagonism in Holstein calves. Journal of Dairy Science 103: 290.
Breeder Management and Nutrition: Moving the industry forward
Underwood, E., and Suttle, N. 2001. The Mineral Nutrition of Livestock. CABI Publishing, London, UK.
Virden, W.S., Yeatman, J.B., Barber, S.J., Zumwalt, C.D., Ward, T.L., Johnson, A.B., and Kidd, M.T. 2003. Hen mineral nutrition impacts progeny liv ability. Journal of Applied Poultry Research 12: 411–416.
Wilson, H.R. 1997. Effects of maternal nutrition on hatchability. Poultry Science 76: 134–143.
Xiao, J.F., Zhang, Y.N., Wu, S.G., Zhang, H.J., Yue, H.Y., and Qi, G.H. 2014. Manganese supplemen tation enhances the synthesis of glycosaminogly can in eggshell membrane: A strategy to improve eggshell quality in laying hens. Poultry Science 93: 380–38.
Yair, R., and Uni, Z. 2011. Content and uptake of minerals in the yolk of broiler embryos during in cubation and effect of nutrient enrichment. Poultry Science 90: 1523–1531.
Yair, R., Shahar, R., and Uni, Z. 2013. Prenatal nu tritional manipulation by in ovo enrichment influ
ences bone structure, composition and mechanical properties. Journal of Animal Science 91: 2784–2793.
Yi, G.F., Atwell, C.A., Hume, J.A., Dibner, J.J., Knight, C.D., and Richards, J.D. 2007. Determin ing the Methionine Activity of Mintrex Organic Trace Minerals in Broiler Chicks by Using Radio label Tracing or Growth Assay. Poultry Science 86: 877-887.
Yin, Z., Jiang, H., Lee, E.S.Y., Ni, M., Erikson, K.M., Milatovic, D., Bowman, A.B. and Aschner, M. 2010. Ferroportin is a manganese-responsive pro tein that decreases manganese cytotoxicity and ac cumulation. Journal of Neurochemistry 112: 11901198.
Zhao, J., Shirley, R.B., Hampton, T.R., Richards, J.D., Harrell, R.J., Dibner, J.J., Knight, C.D., and Vazquez-Anon, M. 2008. Benefits of an organic trace mineral on performance with dietary Cu an tagonism in broilers. Poultry Science Association 97th Annual Meeting, July 20-23, 2008, Niagara Falls, Canada.
HUGO ROMERO-SANCHEZ, PhD., is the Executive Global Poultry Solutions Manager at Novus International, where he leads a cross-functional team to identify and improve practical nutritional solutions for poultry customers. Prior to joining Novus he worked with Trouw Nutrition, serving as a poultry nutritionist and technical manager in South America and the United States, and working in the global division where he supported poultry integrations through feed formulation, modeling, and farm management. Dr. Romero-Sanchez received his bachelor of science degree in Zootechnics from La Salle University (Colombia) before completing his master of science degree in Animal Health and Animal Production at the National University of Colombia where he researched energy and protein metabolism in broilers raised at high altitude. He was the recipient of an international scholarship to study at the University of Jerusalem (Israel) and was later appointed assistant professor at the University of Antioquia in Colombia. Selected for a prestigious Fulbright scholarship, he traveled to the United States to earn his doctorate at North Carolina State University. There he studied the effects of male broiler breeder feeding programmes on fertility and broiler performance. Dr. Romero-Sanchez has worked in academia in Colombia for 10 years, and in 2021 joined the Poultry Science Prestage Department at North Carolina State University as an adjunct professor.
DINABANDHU JOARDAR, P hD., is a poultry nutritionist with Cargill Animal Nutrition – North America, providing nutrition and technical services to Cargill feed mills and independent egg producers, and poultry meat producers in the United States and Canada. Dr. Joardar received his bachelor of Veterinary Science degree from the University of Agricultural Sciences in Bangalore (India) before earning his master’s degree in Applied Poultry Science from the University of Glasgow (Scotland). His doctorate degrees in Nutrition, and Animal and Poultry Science are from North Carolina State University in Raleigh (North Carolina). Dr. Joardar has published three research papers and over 20 peer-reviewed abstracts and is a reviewer for the Poultry Science Journal, the official journal of the Poultry Science Association. He has been invited to present at various scientific meetings and industry symposiums during his career.
Dietary calcium (Ca) and phosphorus (P) are intricately linked to one another and influenced by dietary electrolytes (Na+, Cl-, and K+), therefore, during the onset of the laying phase, which is characterized by an increase in Ca uptake and retention, and following the lay period, these minerals play an important role in broiler breeder micromineral nutrition. These minerals influence fecal moisture output and eggshell quality. The quantitative relationship between Ca and P is a result of chemical association and solubility thus, feeding a portion of Ca requirement in larger limestone particles is beneficial for eggshell quality. During eggshell calcification, both hydrogen (H+) and carbonate ions are generated, creating transitory acidosis. When bone Ca is mobilized the excess P is primarily excreted via urine. Therefore, adjusting dietary P and K+ for alkaline effects is beneficial in managing excreta fecal moisture.
In breeder diets, macromineral nutrition focuses on skeletal development during the rearing phase and egg production; eggshell quality and embryo development during the adult phase; with specific challenges during the transition phase (pre-lay) between rearing and onset of production (Joardar et al., 2017).
Most of the research in macrominerals has been conducted in commercial layers, and this data can be applied to the layer breeder and with some differences for broiler breeders. In broiler breeders, mineral concentration of the diet along with the feed restriction program allows better estimation of the daily mineral intake. However, there are differences in mineral recommendations when genetic lines are compared with some of the most recent updates (Table 1). This review will focus on the macromineral requirements of the broiler breeder female in pre-lay and production phases.
Calcium (Ca) and phosphorus (P) are macrominerals that are important for skeletal development, eggshell quality, reproductive performance, and diverse metabolic functions. In general, dietary Ca tends to be fed at higher levels than required. However, feeding large particle limestone increases the retention time in the gizzard, increasing Ca availability for subsequent intestinal absorption during the period of shell formation. With mash diets, it is easier to supply larger Ca particles, and due to the continuous Ca absorption, there may be a reduction in the mobilization of Ca from bone (less bone resorption). In addition, a bigger particle size stimulates gizzard functionality and prolongs feeding time, which is beneficial for gut health and welfare. Using non-colostomized breeders, Ekmay and Coon (2009) showed that larger particle limestone tends to reduce P excretion along with a significant increase in
percent tibia ash and the specific gravity of the egg. This study also indicated that the particle size (smaller or larger) of Ca source may not have an influence on urinary excretion of Ca and P but may influence retention. In the 6-wk breeder study, the P excretion was not different but there was a numerical improvement of 2.09% P retention and 3.69% Ca retention when breeders were fed larger size limestone particles compared to the breeders fed smaller particles. Previous data on commercial laying hens revealed a significant decrease in P excretion with increasing dietary levels of NPP when hens were fed large limestone particles compared to small particle limestone (Manangi et al., 2018). They also found that feeding larger particle size limestone significantly increased the specific gravity of the eggs compared to feeding smaller particle limestone (1.087 vs. 1.085). The increase of dietary Ca from 2.25% to 3.25% with 0.25% NPP gradually improved eggshell quality, while 0.4% dietary NPP did not affect the eggshell quality. Similar observations were reported by Narvaez-Solarte et al. (2006) who observed an improvement in eggshell quality when dietary Ca was increased from 2.6% to 4.2%, with a quadratic response, showing the best shell weight at 3.56% Ca; however, the authors used 0.35% NPP in the diet and the feed intake of laying hens was much lower than that of broiler breeders even if they are restricted fed.
The National Research Council (NRC, 1994) suggests that broiler breeders housed on litter only need 3.91 g of Ca per day (Wilson et al., 1980), and this value can be obtained with diets containing between 2.3% and 2.6% Ca (assuming a daily feed intake between 150g to 170g, from peak to the end of egg production, respectively). However, only the Brazilian
tables (Rostagno et al., 2017) suggest similar levels, while most commercial references are higher (2.8%-3.6%). Research diets from North Carolina State University (NCSU) are intermediate, ranging from 2.7% (RomeroSanchez., 2006; Joardar, 2019) to 3.0% (Walsh and Brake, 1999), when one or two breeder diets are implemented under controlled and commercial conditions, respectively, and this without affecting eggshell quality (specific gravity and broken eggs).
During bone resorption, hydroxyapatite is released from bone supplying Ca and P for shell calcification, thereby also balancing P excretion (Leeson and Summers, 2001; Manangi et al., 2018L). If excessive Ca is supplied in the diet, the bone mineralization decreases. As a consequence, less P is released into the bloodstream and, with less P for kidney acidbase homeostasis, more H+ will be excreted as water, increasing litter moisture (Joardar, 2019) and the occurrence of dirty eggs.
Some attempts to improve eggshell quality in breeders have studied the effects of feeding during the afternoon, however, eggs with thicker eggshells might reduce hatchability, or the birds become distracted from the mating activity. However, adding only the additional Ca (as big particles) in the afternoon seems ideal in improving eggshell quality, especially in brown layers where 65% of the Ca should be in particles of 2 mm to 4 mm (ISA, 2021). This also encourages hens to move from slats to the litter floor, improving the mating activity.
Since egg size increases and feed intake and eggshell quality decrease with age there is an advantage to phase feeding Ca and P. Ca
levels can be increased over time by 0.2% with the addition of extra limestone at 5 kg/MT to the diet between 45 weeks and 55 weeks of age. One reason for a decline in shell quality over time is the gradual loss in Ca deposition efficiency and withdrawal from medullary bone. Although the medullary bone reserve is essential for shell formation regardless of diet, its role is somewhat reduced if the bird has some Ca absorbed from the digestive tract during the night. This situation supports the idea of afternoon feeding of Ca to provide a Ca source in the digestive tract that can slowly be released during the night, supporting eggshell formation. Ideally, a mixture of fine and coarse particles should be used because this gives both rapid and slow release of Ca for metabolic needs.
Ca tetany is poorly defined in broiler breeder hens but results from acute hypocalcemia. It is characterized by impaired mobility, increased mortality, and a lack of any gross lesions that would explain the impaired mobility (Martin et al., 2011). It is unlikely to see this problem due to low Ca levels in the breeder diet. However, there are risk factors that affect proper Ca supply, such as poor uniformity, feed space, early photostimulation triggering production too quickly, small particle sized Ca (Turner and de Beer, 2009), and even leaving the prelay diet with a medium Ca level for too long before changing to production levels. Typical post-mortem findings include an active but congested ovary, a partially or fully formed egg in the oviduct (Martin et al., 2010), and possibly damage to the back
of the bird from male abuse (Turner and de Beer, 2009). Treatment of Ca tetany is through Ca supplementation in the form of oyster shell or large particle limestone at 2-5 g/bird/day for three consecutive days followed by three days of rest. This treatment should continue until mortality is under control. Nutritional knowledge suggests that amendment of vitamin D and bicarbonate levels are recommended as these compounds increase Ca absorption and improve dietary electrolyte balance.
The P needs of broiler breeder hens for production and progeny performance have not been well investigated. The NRC (1994) recommends 350 mg of NPP; however, the industry usually supplies around 600640 mg NPP/d (150-160 g/d x 0.4% avP). Previous studies have shown evidence that increasing P intake above 0.4% available P confers no additional benefit to production. Triyuwanta et al. (1992) showed that increasing available P to amounts as high as 1% in the diet in dwarf broiler breeders did not improve egg production, egg weight, or shell quality. Only marginal improvements were seen in fertility, hatchability, and hatching weight, and no improvements were seen in two-week progeny body weight or seven-week-progeny body weight. On the other hand, very little research has been conducted on lower NPP in broiler breeder diets. Chandramoni et al. (1998) showed that egg production, shell weight per unit surface area (SWUSA), and egg content were not improved with a total P above 0.43% in caged layers; while Keshavarz (2000) found
Breeder Management and Nutrition: Moving the industry forward no differences in egg production in layer hens fed low levels of NPP (0.15-0.10%) and phytase. Similar results were reported by Sodsee (2013), who did not find significant differences in egg production and shell quality for breeder hens fed either 0.25% or 0.4% NPP (with daily intake ranging from 0.34 to 0.55g NPP at peak feed intake) and with 3.25% Ca in the diet.
The study by Triyuwanta et al. (1992) showed that the current practice of feeding 600 mg NPP/d to breeders may not be necessary. The industry starts around those values and then decreases to 480-500 mg NPP/d (150-156 g/d/hen x 0.32% NPP). The Brazilian tables (Table 1) suggest 400 mg NPP/d, which is slightly higher than the 338 mg/d (0.41% total dietary P (718 mg/bird/d)) found by Wilson et al. (1980) for egg production. To reach 400 mg NPP for feed intake from 153 g/d to 167 g/d the diets should have 0.26% to 0.24% NPP, which is lower than the commercial recommendation for genetic broiler lines.
The P requirement is also fulfilled in phase feeding, but in contrast to Ca, available P is normally reduced in the production phase (Table 1). Only the Brazilian tables suggest a slight increment in the concentration (0.02%) to counteract the normal reduction in daily feed allocation that is used in broiler breeders. Ekmay and Coon (2010, 2011) evaluated the effect of particle size on Ca and P excretion and found that Ca excretion significantly increased with higher P intake. Feeding breeders large particle limestone did not improve shell quality or tibia bone strength but this trial was only conducted for 6 weeks. However, the authors concluded that
NPP can be fed at 0.2% (288 mg/d), without impacting performance and at 0.25% NNP (360 mg/d) to ensure bone integrity.
Plumstead et al. (2007) conducted floor pen feeding studies with breeders housed on slats to determine the water-soluble excretion while feeding corn/soybean/phytase diets (Table 2). The researchers fed corn-soy diets and added 0.10% AvP made available from phytase to the breeder diets and suggested that 0.37% AvP was needed for maximum fertility if pullets were reared from 10 weeks of age on corn/soybean and phytase diets. However, eggs and chicks per hen housed were numerically diminished when hens were fed 0.37% NPP.
Potassium (K+) is the primary cation in the intracellular fluid (ICF), while sodium (Na+) and chloride (Cl-) are the primary ions in the extracellular fluid (ECF). Each of these ions is involved in the regulation of acidbase balance via extracellular and intracellular buffering (Carlson, 1997). In the diet, the electrolyte balance between the cation, Na+, K+, and the anion Cl- can be expressed as a formula Na++K+-Cl -mEq/kg, which is known as dietary electrolyte balance (DEB) (Leeson and Summers, 2001). These electrolytes play a major role in osmotic regulation, cell physiology, animal metabolism, and body fluid acid-base balance (Leeson and Summers, 2001). Endogenous acid production and rates of renal clearance (Leeson et al., 1995) can be affected by supplying the diet with these electrolytes. In most situations 250 mEq/kg is
optimum for normal physiological functions (Mongin 1981; Leeson et al., 1995). However, the individual elements need to be carefully revised since it is possible to reach 250 mEq/ kg with very extreme levels of Na+ and Cl-.
Low dietary electrolyte balance (dEB) (<180 mEq/kg) values are considered acidotic while high values (>300 mEq/kg) are considered alkalotic (Mongin, 1981). The eggshell carbonate (CO32-) is derived from metabolic CO2 in the shell gland. Considerable amounts of hydrogen ions are produced during this process, which results in an altered acid-base balance. Excess H+ ions are often compensated by panting. This compensation induces a moderate respiratory alkalosis that causes a reduction in blood bicarbonate (HCO3-) that may lead to eggshell problems during heat stress when panting is exacerbated. Although the addition of NaHCO3 to feed or water will not correct respiratory alkalosis, it may mitigate eggshell problems (Leeson et al, 1995; Leeson and Summers, 2001).
Daily Na+ requirements for egg production, feed efficiency, egg weight, fertility, and hatchability of meat-type breeder hens have been established at 150 mg/d (NRC, 1994; Larbier and Leclerq, 1994), whereas sodium intake in excess of 320 mg/d were shown to reduce fertility (Damron et al., 1983). Although Harms et al. (1995) did not find effects on egg production and egg mass with Na+ levels above 114 and 105 mg, respectively, the Brazilian tables (Table 1) recommend higher levels (250 mg/d) similar to the minimum recommendations established by the genetic companies (calculated
>240 mg/d). This difference might be due to trial conditions with a low number of hens per pen vs. commercial conditions. High sodium levels are related to increased litter moisture; however, water restriction of broiler breeders is applied to avoid excessive water intake (Romero-Sanchez et al., 2007, 2008; Leksrisompong, 2014).
Although Harms and Wilson (1984) reported that 254 mg of chloride (Cl-) per hen daily resulted in the best overall performance of broiler hens as measured by egg production and hatchability, the NRC (1994) recommends 185 mg/d, which is 20% higher than Na+. In contrast, the Brazilian tables (Rostagno et al., 2017; Table 1) recommend 220 mg/d, which is 12% higher than Na+. High Cl- levels (0.4-0.5%) can exacerbate Ca excretion and deficiency (Austic, 1984; Leeson and Summers, 2001). Also, high Cl- concentrations in the blood can negatively affect the transport of HCO3- (Jonchère et al., 2012), which may further affect eggshell quality. By reducing chloride in the diet there is an increment in bicarbonate reabsorption from the kidney that benefits the eggshell formation process. However, Nys (1999) found that chloride levels below 0.2% were detrimental to eggshell quality.
Potassium (K+) is primarily within the cells, with similar functions to Na+ in the plasma and interstitial fluid, such as acid-base balance, osmotic pressure, and membrane potential regulation. K+ also activates a number of intracellular enzymes and it is required for normal heart activity. Replacing Na+ with K+
has shown to maintain dEB while reducing ascites mortality in broilers raised at high altitudes (Betancourt et al., 2004).
The NRC (1994) suggests a K+ requirement of 0.30% for broilers. In contrast, Oliveira et al. (2005) reported that the optimum body weight gain was achieved with K+ levels of 0.63% from 8-21 d of age, 0.71% from 2242 d of age, and 0.80% from 43-53 d of age. To maintain optimum live performance in broilers, practical diets are formulated with K+ levels that are two to three times higher than those suggested by the NRC (1994).
The role of K+ in the regulation of the acidbase balance has been investigated by many researchers, especially in relation to heat stress conditions in broilers (e.g., Teeter and Belay, 1996). Belay et al. (1992) reported that heat-stressed broilers exhibited an increased urinary excretion of K+. The respiratory alkalosis from heat stress-induced panting has been associated with a negative balance of K+ due to increased excretion of K+. Borges et al. (2007) reported the onset of respiratory alkalosis in broilers when supplemented K+ was supplemented as potassium bicarbonate (KHCO3). Smith and Teeter (1987) observed improved thermotolerance of broilers when supplemented with K salts (KCl) in drinking water. Rao et al. (2002) reported a K+ intake of 1.8 to 2.3 g/d for optimum body weight gain in broilers under heat stress conditions. However, Ait-Boulahsen et al. (1995) observed increased body weight gain and feed intake when dietary K+ was supplemented as 0.6% KCl in heat-stressed birds, together with a reduction in blood pH, resulting in less severe alkalosis.
Similarly, Ahmad et al. (2008) noticed that 0.6% KCl supplementation in drinking water reduced the blood pH to 7.31 of broilers of 42 d of age. The possible reasons for the changes in blood pH may have been due to the acidogenic effects of Clions. More recent studies (Joardar et al., 2020) demonstrated that during heat stress in broilers the blood Ca was correlated with K+, P, pH, and pCO2, and before and after heat stress blood pH was positively correlated with plasma K+.
In laying hens, Smith et al. (2000) demonstrated that increased dietary P resulted in a greater percent of excreta moisture in laying hens. Ziaei et al. (2008) recorded that reduced dietary P increased the dry matter of excreta, which resulted from increased retention of P. In broiler breeder hens, Joardar et al. (2019) reported an interaction between dietary AvP and K+ on the percent of excreta fecal moisture. The supplemental addition of dietary 0.2% K (as potassium carbonate) to 0.3% AvP breeder diets resulted in reduction of percent fecal moisture in breeder hens when compared with 0.2% K addition to 0.5% AvP-fed breeders. The data suggests that the effect of AvP on fecal moisture output seems to be manifested in presence of K+. Therefore, the addition of 0.2% K had a transitory effect on the reduction of the fecal moisture with 0.3% AvP in breeder diets, during the onset of lay. The dietary treatments did not influence the breeder egg characteristics (Joardar et al., 2017) measured over time, although lower fecal moisture might reduce dirty eggs.
Breeder Management and Nutrition: Moving the industry forward
Table 1. Macromineral requirements in broiler breeders in rearing and production. Range indicates values between 4 to 24 weeks of age for rearing and between first egg and 64 weeks of age.
Ross1 Cobb2 FEDNA3 CVB4 Brazilian Tables5
Calcium, g/kg
Available P, g/kg
Rearing 9-15 9.5-12 9-12 8.8-17
Production 30-34 30- 32 30-35 29-33 25-27
Rearing 4.5-4.0 4.2 3.8 4.1
Production 3.6-3.2 4.2-3.2 3.5-3.3 2.4-2.6
Retained P CVB, g/kg Rearing 3.2
Production 2.8-2.7 2.5-2.3
Sodium, g/kg Rearing 1.8-2.3 1.5-2.4 1.6-1.7 1.4 -1.8 1.8-1.7
Production 1.8-2.3 1.5-2.4 1.6-1.8 1.5-1.64
Chloride, g/kg Rearing 1.8-2.3 1.5-2.5 1.5-2.9 1.6-1.5
Production 1.8-2.3 1.5-2.4 1.5-2.6 1.7-1.8
Potassium, g/kg Rearing 6.0-9.0 6.0-9.0 5-5.5 6.0 – 9.0 5.5
Production 6.0-9.0 6.0 4.5-5.5 4.2-4.6
1 (Aviagen, 2021), 2 (Cobb Vantress, 2019), 3 (Santoma and Mateos, 2018), 4 CVB, (2017) 5 (Rostagno et al., 2017)
Table 2. Effect of varying dietary non-phytate P (NPP) levels with or without added phytase enzyme on performance variables of broiler breeders from 29 to 64 weeks of age. Adapted from Plumstead et al. (2007).
Item NPP (%) Added phytase (FTU/kg)
Treatment
Eggs per hen hou sed (n)
Feed per dozen eggs (g)
Hen-day egg production (%) Fertility* (%) Chicks per hen housed (n)
A 0.37 158.0 2,999xy 62.09b 97.5A 143.6
B 0.27 500 164.5 3,038x 62.12b 95.0B 145.2
C 0.19 163.9 3,068x 61.24b 95.5B 145.3
D 0.09 500 170.8 2,875y 65.18a 95.3B 152.4
SEM 3.74 46.7 0.893 3.26
P Value 0.178 0.059 0.041 <0.001 0.269
a,b Means within the same column with no common superscript differ (P≤0.05).
A,B Means within the same column with no common superscript differ (P≤0.01). x,y Means within the same column with no common superscript differ (P≤0.10).
* The SE for fertility was not available because of the categorical nature of the data
There are differences in macromineral requirements during the onset of lay and peak laying period in breeder hens. Both limestone particle size and solubility are important
considerations for maintaining eggshell quality throughout the laying cycle. Dietary phosphorus and potassium have been shown to affect fecal moisture during the onset of lay. Therefore, taken together, the dietary macromineral and dietary electrolytes are
Breeder Management and Nutrition: Moving the industry forward
important considerations in breeder nutrition to optimize laying performance.
Ahmad, T., Khalid, T., Mushtaq, T., Mirza, M.A, Nadeem, A., Babar, M.E. and G. Ahmad. 2008. Effect of potassium chloride supplementation in drinking water on broiler performance under heat stress conditions. Poultry Science 87: 1276-1280. Ait-Boulahsen, A., Garlich, J.D. and F.W. Edens. 1995. Potassium chloride improves the thermotolerance of chickens exposed to acute heat stress. Poultry Science 74: 75-87.
Aviagen, 2021. Parent stock nutrition specifications. Huntsville, AL.
Angel, R. and T. Applegate. 2001. Phytase use -what do we know? In: Proceedings of the 62nd Minnesota Nutrition Conference and Minnesota Corn Growers Association Technical Symposium, Bloomington, MN. pp.250-263.
Apke, M.P., Waibel, P.E., Larntz, K., Metz, L., Noll, S.L. and M. Walser. 1987. Phosphorus availability bioassay using bone ash and bone densitometry as response criteria. Poultry Science 66:713-720. Austic, R. 1984. Excess dietary chloride depresses eggshell quality. Poultry Science 63: 1773-1777.
Belay, T., Wiernusz C.J. and R.G. Teeter. 1992. Mineral balance and urinary and fecal mineral excretion profile of broilers housed in thermoneutral and heat-distressed environments. Poultry Science 71:1043-1047. Betancourt, L., Romero-Sanchez, H., Afanador G. and J. Brake. 2004. The effect of feed restriction and water alkalization on blood pH, hematological variables and pulmonary hypertension in broilers at high altitude. In proceedings of World’s Poultry Congress, Istanbul, Turkey. pp.312. Borges, S.A., Fischer, A.V. and A. Maiorka. 2007. Acid-base balance in broilers. World’s Poultry Science Journal 63: 73-81.
Carlson, G.P. 1997. Fluid, electrolyte, and acid-base balance. In Clinical Biochemistry of Domestic Animals. J.J. Kaneko (ed). Academic Press, London. pp.543-575. Cobb Vantress, 2019. Breeder Management Guide. Siloam Springs, AR.
CVB Table Booklet Feeding of Poultry 2018. Feeding standards, feeding advices and nutritional values of feed ingredients for poultry. Federatie Nederlandse Diervoederketen. www.cvbdiervoeding.nl/bestand/10563/ Chandramoni, S., Jadhao, B. and R.P. Sinha. 1998. Effect of dietary calcium and phosphorus concentrations on retention of these nutrients by caged layers. British Poultry Science 39: 544-548. Damron, B. L., Wilson, H.R. and R.H. Harms. 1983. Sodium chloride for broiler breeders. Poultry Science 62: 480-482.
Ekmay, R.D. and C.N. Coon. 2009. The effects of reduced NPP levels and limestone particle size on production, skeletal integrity, progeny quality, and P balance in broiler breeder hens. In: Proceedings and abstracts of 17th European Symposium on Poultry Nutrition; Edinburgh, Scotland. pp.189. Ekmay, R.D. and C.N. Coon. 2010. An examination of the P requirements of broiler breeders for performance, progeny quality and P balance 1. non-phytate phosphorus. International Journal of Poultry Science 9: 1043-1049.
Ekmay, R.D. and C.N. Coon, 2011. An examination of the P requirements of broiler breeders for performance, progeny quality and P balance 2. Ca Particle Size. International Journal of Poultry Science 10: 760-765. Harms, R.H. and H.R. Wilson. 1984. The chloride requirement of the broiler breeder hen. Poultry Science 63: 835-837.
Harms, R.H., Kuchinski, K.K., Sloan, D.R. and G.B. Russell. 1995. Sodium requirement for broiler breeder hens. Poultry Science 8: 1311-1316.
ISA 2021. Hendrix Genetics Parent Stock Management Guide. https://www.isa-poultry.
Breeder Management and Nutrition: Moving the industry forward
com/documents/608/Parent_Stock_management_ guide.pdf.
Joardar, D., Livingston, K. A., Edens, F.W., Nusairat, B., Qudsieh, R., Livingston M..L. and J. Brake. 2020. Effect of limestone particle size and potassium supplementation on live performance, blood physiology and breast muscle myopathy of male broiler chickens. Frontiers in Veterinary Science 7: 603284.
Joardar, D. 2019. Effect of dietary phosphorus and potassium in broiler breeders during onset of lay and effect of dietary limestone particle size, potassium and phytase in broilers. PhD dissertation. http:// www.lib.ncsu.edu/resolver/1840.20/36316.
Joardar, D., J. Brake, and C. T. Caraway. 2017. Effect of available phosphorus in growing and laying diets of broiler breeders on egg production and egg characteristics at the onset of lay. Poultry Science 96(E-suppl. 1): 136.
Jonchère, V., Brionne, A., Gautron, J. and Y. Nys. 2012. Identification of uterine ion transporters for mineralisation precursors of the avian eggshell. BMC Physiology 12:10.
Keshavarz, K. 2000. Nonphytate phosphorus requirement of laying hens with and without phytase on a phase feeding program. Poultry Science 79: 748-63.
Larbier, M. and B. Leclercq. 1994. In: Nutrition and Feeding of Poultry (J. Wiseman. (ed). Nottingham University Press, Loughborough. pp.305-319.
Leeson, S., Summers, J.D. 2001. Scott’s Nutrition of the chicken, 4th edition. University Books. Guelph, Canada. N1.H6N8.
Leeson, S., Summers, J.D. and G.J. Diaz, G.J. Poultry metabolic disorders and mycotoxins. 1995. 1st edition. University Books. Guelph, Canada. N1.H6N8.
Leksrisompong, N., Romero-Sanchez, H., OviedoRondón, E.O. and J. Brake. 2014. Effect of feeder space during the growing and laying periods and the rate of feed increase at the onset of lay on broiler
breeder female reproductive function. Poultry Science 7: 1599-1607.
Martin, M.P., Wineland, M., Fletcher, O.J. and H.J. Barnes. 2011. Selected blood chemistry values in mobility impaired broiler breeder hens with suspected calcium tetany using the i-STAT® handheld clinical analyzer. Avian Diseases 55: 340-345.
Martin, M.P., Wineland, M. and H.J. Barnes. 2010. Selected blood chemistry and gas reference ranges for broiler breeders using the i-STAT handheld clinical analyzer. Avian Diseases 54: 1016-1020.
Manangi, M. K., Maharjan, P. and C.N. Coon. 2018. Calcium particle size effects on plasma, excreta, and urinary Ca and P changes in broiler breeder hens. Poultry Science 97: 2798-2806.
Mongin, P. 1981. Recent advances in dietary cationanion balance: applications in poultry. In: Proceedings of the nutrition society 40:285–294. Published online by Cambridge University Press: 28 February 2007.
Narvaez-Solarte, W., Rostagno H.S., Soares, P.R., Uribe-Velasquez, L.F. and M.A. Silva. 2006. Nutritional requirement of calcium in white laying hens from 46 to 62 wk of age. International Journal of Poultry Science 5: 181-184.
Nys, Y. 1999. Nutritional factors affecting eggshell quality. Czech Journal of Animal Science 44: 135-143. NRC. 1994. Nutrient Requirement of Poultry. National Research Council, Washington, DC.
Oliveira, J. E., Albino, L.F.T., Rostagno, H.S., Paez, L.E. and D.C.O. Carvalho. 2005. Dietary levels of potassium for broiler chickens. Brazilian Journal of Poultry Science 7: 33-37.
Plumstead, P.W., Romero-Sanchez, H., Maguire, R.O., Gernat, A.G. and J. Brake. 2007. Effects of phosphorus level and phytase in broiler breeder rearing and laying diets on live performance and phosphorus excretion. Poultry Science 86: 225-231.
Rama Rao, S.V., Nagalakshmi, D. and V.R. Reddy. 2002. Feeding to minimize heat stress. International Journal of Poultry Science 41: 30-33.
Romero-Sanchez, H. 2006. Effect of male broiler breeder feeding programs on growth, reproductive performance, and broiler progeny (PhD. Dissertation). https://repository.lib.ncsu.edu/handle/1840.16/3358
Romero-Sanchez, H., Plumstead, P.W., Leksrisompong, N. and J. Brake. 2007. Feeding broiler breeder males.2. Effect of cumulative rearing nutrition on body weight, shank length, comb height, and fertility. Poultry Science 86: 175-181.
Romero-Sanchez, H., Plumstead, P.W., Leksrisompong, N.,Brannan K.E. and J. Brake. 2008. Feeding broiler breeder males.4. Deficient feed allocation reduces fertility and broiler progeny body weight. Poultry Science 87: 805-811. Rostagno, H.S., Teixeira Albino, L.F., Hannas, M.I., Lopes Donzele, J., Sakomura, N.K., Perazzo, F.G., Saraiva, A., Teixeira, M.L., Borges Rodrigues, P., De Oliveira, R.F., De Toledo, S.K., and C. De Oliveira. 2017. Tabelas Brasileiras para Aves e Suínos. Composição de Alimentos e Exigências Nutricionais. Santoma, G. and Mateos. 2018. Necesidades Nutricionales en Avicultura. Normas FEDNA. 2ª edición. Fundación Española para el Desarrollo de la Nutrición Animal. Madrid. España. Smith, M.O., and R.G. Teeter. 1987. Effect of ammonium chloride and potassium chloride on survival of broiler chickens. Nutrition Research 7: 677-681.
Smith, A., Rose, S.P., Wells, R.G. and V. Pirgozliev. 2000. Effect of excess dietary sodium, potassium, calcium and phosphorus on excreta moisture of laying hens. British Poultry Science 41: 598-607. Soares, J.H., Jr. 1995. Phosphorus Bioavailability. Pages 257-294 in: Bioavailability of nutrients for animals: amino acids, minerals, and vitamins. Eds. C. B. Ammerman, D. H. Baker, A. J. Lewis, Academic Press, London, UK.
Sodsee, P. 2013. The effect of calcium and nonphytate phosphorus on chicken genetic line, egg production, shell quality, bone homeostasis, and progeny bone quality. Dissertation University of Arkansas, Fayetteville
Teeter, R.G., and T. Belay.1996. Broiler management during acute heat stress. Animal Feed Science and Technology 58: 127-142.
Triyuwanta Leterrier, C. and Y. Nys. 1992. Dietary phosphorus and food allowance of dwarf breeders affect reproductive performance of hens and bone development of their progeny. British Poultry Science 33: 363-379.
Turner, B. and M. de Beer. 2009. Calcium Tetany in Broiler Breeder Hens: In UPDATE. Aviagen 2009. AviagenBrief_CalciumTetany_Apr09.pdf Walsh, T. and J. Brake. 1999. Effects of feeding program and crude protein intake during rearing on fertility of broiler breeder females. Poultry Science 78: 827–832.
Wilson, H.R., Miller, E.R., Harms, R.H. and B.L. Damron. 1980. Hatchability of chicken eggs as affected by dietary phosphorus and calcium Poultry Science 59:1284-1289.
Ziaei, N., Guy, J.H., Edwards, S.A., Blanchard, P.J., Ward, J. and D. Feuerstein. 2008. Effect of reducing dietary mineral content on growth performance, water intake, excreta dry matter content and blood parameters of broilers. British Poultry Science 49: 195-201.
R. A. (RICK) VAN EMOUS, P hD., is a Senior Researcher in Animal Nutrition at Wageningen Livestock Research in the Netherlands. He received his bachelor’s degree in Animal Production from HAS Dronten (Netherlands). Dr. van Emous started his career as a research assistant at COVP-DLO Centre for Poultry Research in Beekbergen. He joined Cobb-Europe as a Technical-Commercial Advisor before returning to COVP-DLO where he worked intensively studying how laying hens respond to different housing systems. He earned his doctorate from the Wageningen Institute of Animal Sciences (Netherlands) in 2015 with a focus on the feeding behaviour and practices of modern broiler breeders.
This chapter discusses the housing, equipment, and management of broiler breeders (or parent stock) of broiler chickens. After a description of the layout of broiler breeder houses (rearing and laying), different topics about the equipment are addressed. Research on house layout, different equipment and management in broiler breeders is scarce. Most of the information in this chapter is from the few scientific papers available, popular magazines, breeder management books, and breeder management guides in combination with expert knowledge.
Broiler breeder housing systems are very similar, and the birds are mostly kept in climatecontrolled houses. Pullet houses have a total litter floor with few elevated platforms. Production houses have a combination of litter and slatted floors. Males and females are reared separately until approximately 20 weeks of age and then transferred to the production farm where they are housed together until 60 to 65 weeks of age. Pullets and breeders receive feed via trough or pan feeders, and water via bell drinkers, nipple drinkers or drink cups, whereas spin feeders are only used during the rearing period. Feeder space, feeder time, feeding frequency and diet composition are important management measures to optimize egg production. Male-to-female ratio and (intra) spiking are key factors to maximizing egg fertility and hatchability. Breeder houses are equipped with different light systems, climate control systems, and elevated areas to support behaviour and reproduction. State-of-the-art nest boxes are necessary to maintain the highest number of first-class eggs (not dirty, broken or on the floor).
The main objective of broiler breeder production is to produce a maximum number of first-grade fertile eggs and firstclass healthy chicks. This implies that the breeder house layout is focusing on optimal production circumstances, floor eggs prevention, and optimal sexual interaction between the males and females (de Jong and Swalander, 2013). In the past, these issues
were the basis for the development of a very uniform housing system for broiler breeders.
Globally, two different production systems are used today: day-old-to-death and separated specialized rearing and laying farms. In the first system, birds are kept in the same house between day-old and death (between 60 and 65 weeks of age). In the second system, pullets are reared until 18 to 21 weeks of age, transferred to the production farms, and kept until 60 to 65 weeks of age (de
Jong and van Emous, 2017). In the day-oldto-death system, birds should have access to laying nests at oviposition by introducing nest boxes or by removing covering material over the nest boxes. The advantages of the day-old-to-death system are avoiding longdistance transport from the rearing to the laying farm, and the absence of differences in feeder and water system between the rearing and laying house. On the other hand, the cost of housing, labour, and the investment of young birds in the same house are relatively high (van Emous et al., 2020). The mean advantages of the separated rearing and laying farm system are more efficient, specialized management, and they are less costly.
Despite climate differences, legislation, and labour costs, only small variations in the layout of rearing houses for breeder pullets are found globally (de Jong and van Emous, 2017). The majority of breeder pullets are kept on a fully littered floor, and litter material varies between regions due to local availability. In Europe, wood shavings, straw, and peat are mostly used whereas rice hulls are often used in Asia. The use of cage systems during the rearing period is very scarce (de Jong and Swalander, 2013; EFSA, 2010). In the last decade, the use of aviary systems during the rearing phase is emerging, due to the increased use of slow-growing breeds (Hubbard) (H. Bel, 2020, personal communication). The slow-growing Hubbard breeders are more mobile and rearing in aviary systems can be beneficial for leg health and floor eggs prevention.
During the laying phase, the majority of the breeders are housed on floor systems consisting of both litter and slatted floors (de Jong and van Emous, 2017). Breeder houses with outdoor access are not used because of the higher likelihood of bacterial contaminations (de Jong and Swalander, 2013). Only a small part of broiler breeder houses are equipped with aviary systems and the use of furnished cages for both females and males is very scarce. These cages are equipped with nest boxes, perches, and a small litter area (de Jong and Swalander, 2013). In some regions, cage systems (about 0.5% of the breeders), in combination with artificial insemination, for breeders are used (Leeson and Summers, 2009).
The importance of optimal housing, equipment, and management is clearly demonstrated in an inventory of breeder farms by van Emous (2018). An assessment was conducted at 14 breeder farms with 46 houses with an average 27,500 broiler breeders per farm. The most important criteria for the selection of the breeder farms was that management and/or equipment was significantly adjusted in the previous five years with positive effects on reproduction and mortality. The study showed that through a combination of adjustments to management and equipment at the investigated farms, the mortality rate was almost halved (from 12.9% to 6.6%) and the number of chicks per female increased by 13 (from 133 to 146 chicks) during the last five years. Adjustments were done on a combination of the water system, feed system, climate control, and light system.
In general, two different floor systems are used worldwide for broiler breeders: 1) central slatted floor with mechanical egg collection and litter floors on both sides, and 2) central litter floor with slatted floors on both sides with manual or mechanical egg collection. Besides these two major breeder house systems, sometimes fully littered floors with manual egg collection are used (van Emous et al., 2020). The use of different layouts is regionally dependent and the proportion of litter floor versus raised slatted floor differs between regions and countries (de Jong and van Emous, 2017). The central slatted floor system is common in Europe and less common in other parts of the world whereas the central litter floor system is common in Asia, North and South America and not used in Europe.
No good comparable research has been done on the two major breeder house systems, however, breeder experts appoint some important advantages of central slatted floor breeder houses. The first advantage is the presence of two separated litter areas which is the preferred place for males during the day. The second advantage is the higher proportion of litter in central slatted floor houses compared to central litter houses (50% vs . 33%), which results in lower male density and therefore less aggressive interactions between males. The third advantage is the lower number of floor and slat eggs, which results in less eggs lost and more saleable eggs. Central slatted floor breeder houses result in higher fertility due to the above-mentioned separated litter areas and lower density of the males on the litter (van Emous et al .,
2020). This is because the majority (90%) of matings are performed on the litter area. More space and fewer interactions result in more successful matings (van Emous, 2010). On the other hand, females in a breeder house with a central litter floor have more opportunities to hide on the slats behind the nest boxes and avoid contact with the males, which can result in lower fertility (Fontana et al ., 1992).
Better litter quality (drier and more friable) could have beneficial side effects on leg health, such as the incidence of footpad dermatitis (Kaukonen et al., 2016). This is of the utmost importance for males because they spend most time of the day on the litter and therefore they are more sensitive to impaired foot health.
During the rearing and laying phase, stocking density varies between countries, depending on climatic conditions and/ or legislation (de Jong and van Emous, 2017). Some countries in Europe (Sweden, Norway and The Netherlands) have legislation on maximum stocking density for the laying phase. Breeder companies recommend stocking densities during rear between 7 and 10 birds/m2 for females and 4 to 8 birds/m2 for males (de Jong and Swalander, 2013). Lower stocking densities are usually applied in open-sided houses (EFSA, 2010). For alternative breeds (dwarf parental females) recommended stocking densities are 9-10 birds/m2 but stocking densities may be higher (15 birds/m2) in practice (EFSA, 2010).
Studies on the effects of stocking density on production performance and behaviour of pullets and breeders are very scarce. An assessment at commercial Dutch breeder farms was carried out in 2007 to determine the effect of stocking density on egg fertility (van Emous, unpublished data). Flocks were divided in low (>7.3 birds/m2), standard (7.3-7.7 birds/m2), and high (>7.7 birds/ m2) stocking density. The hatchability of the flocks at the low, standard, and high stocking density was 81.7%, 82.2%, and 78.0%, respectively (Figure 1).
In a study of de Jong et al. (2011), half of the female pullets were housed at a low stocking density (7.3 birds/m2) and the other half at a high density (14.5 birds/m2). Half of the male pullets were housed at a low density (5.5 birds/m2) and the other half at
a high stocking density (11.0 birds/m2). The experiment showed that a lower stocking density in rear resulted in more foraging and less standing of females. More aggressive interactions between males were observed at 3, 9, and 12 weeks of age. Less feather and skin damage was found during rear for males and females housed at reduced compared to standard stocking density.
Stocking density during the laying phase varies between 4.5 and 7.7 birds (females + males)/m2 depending on legislation and region (de Jong and van Emous, 2017). In Europe, stocking density varies between 5 and 8 birds/m2, whereas in other countries it varies between 4.5 and 6.5 birds/m2.
Data analysed on the effect of stocking density in commercial two-thirds slat houses
in the United States suggests that the threshold for impaired production is about 6 birds/m2 (Brake, 1998). In the previously mentioned study of de Jong et al. (2011), breeders were also followed during the laying phase and allotted to two different densities: low: 5.2 birds/m2 and high: 8.8 birds/m2. The lower stocking density had a positive effect on the quality of mating behaviour (more courtship behaviour and more successful matings). This resulted in higher fertility, and in combination with higher egg production, a higher number of chicks per hen housed (de Jong et al., 2011). Due to the much lower density, the chick production per square meter was, however, 34% lower, which negatively affected profitability.
Feeding systems - rearing phase Feed is provided in the rearing phase via pan feeders, chain feeders or spin feeders (where feed is distributed over the litter). Feed in pan and chain feeders is normally in mash or crumb form, and pellets are used in combination with spin feeders (de Jong and van Emous, 2017). For a good, uniform flock, and certainly when pullets are fed daily, a rapid (within three minutes) and equal distribution of the feed over all the birds is very important (Abad, 2020). However, for the modern broiler breeders, the general guideline is: ‘every individual pullet must receive their feed within three seconds’ (R.A. van Emous, 2021, personal communication).
Chain feeders have been used in pullet houses for six decades (Ketelaars, 1992), however, the modern variants are much
faster (up to 30 m/min) to prevent pullets from eating while the system is running (Brake, 1992). Besides the increased speed of the chain feeder, feeding systems are often winchable. Before feed distribution, the chain feeder is winched up (sometimes in the dark), filled with feed, and brought to bird height after filling so all birds can eat at the same time. Four decades ago, pan feeders were introduced as a novel feeding system for poultry and are, after adjustments and improvements, still used in pullet houses. Advantages of this system are the increased feeding space and the short and equal feed distribution time.
The distribution of feed on the litter has been adopted in several countries as a more equal and natural feed distribution method (de Jong et al., 2005; Zuidhof et al., 2015; Montiel, 2016). Early studies in the 1970s showed that pullets fed on litter (twice a day) had less abdominal fat at 26 to 27 weeks of age and produced heavier eggs than pullets fed via pan feeders (Chu, 1979). A longer shank length (13.1 vs. 12.5 cm) was observed at 20 weeks of age when male pullets were fed via a spin feeder compared to male pullets fed in feeding pans, suggesting that these birds had better-developed legs due to scratching activity (van Emous et al., 2004).
An improved innate immune response was found in broiler breeder pullets when they were fed on the floor (Montiel, 2016). Montiel (2016) observed that everyday fed pullets on the litter cleared E.coli faster than birds that were skip-a-day fed and everyday fed in feeder pans, and exhibited lower Salmonella enteritis colonization rates
towards the end of the restriction period (1722 weeks of age). This together suggests that feeding programmes including off-feed days have a negative impact on innate immune responses. They postulated this is possibly derived from feed competition and available feeding space in the skip-a-day fed or everyday fed in feeder pans programmes, and perhaps due to fasting during off-feed days in the skip-a-day programme or insufficient feed amounts delivered daily in the everyday fed in feeder pans birds.
Transferring pullets from a spin feeder system to a laying house with a trough or pan feeder, however, may sometimes result in adaption problems of the new feeder system, which has detrimental effects on flock startup and uniformity.
In general, a spin feeder system shows good performance results, less mortality, fewer leg problems, improved litter condition, and more uniform body weight (Aviagen, 2018).
In the rearing phase, feed is provided daily or via different feeding programmes with feed-less days (EFSA, 2010). Feeding programmes can be in the form of skipa-day (feed day followed by feed less day), 6/1, 5/2, or 4/3 feeding programmes (1, 2, or 3 days without feed each week and a larger portion feed on the feeding days). In Europe, legislation does not allow feeding programmes with feed-less days and most farmers apply daily feeding systems, whereas in North America feeding programmes with feed-less days are still applied (de Jong and van Emous, 2017). The reason for applying
feeding programmes was (and is) to obtain bodyweight uniformity in feed-restricted pullets (Leeson and Summers, 2009). Pullets are fed between 25 to 33% the amount of feed compared to pullets fed ad libitum (de Jong et al., 2002), and the most severe feed restriction period is between 6 and 16 weeks of age (de Jong and Jones, 2006). This results in a feed clean-up time in daily-fed birds between 15 and 30 minutes, leading to increased competition and aggressive behaviour around feeding time (de Jong et al., 2002). This was and is the case when pullets are reared in houses with insufficient feeder space and slow feed distribution (de Jong and Swalander, 2013). Modern pullet houses are equipped with enough feeder space and fast chain feeders (up to 30 m per minute) or pan feeders that guarantee an equal feed distribution within three minutes in the entire rearing house (Aviagen, 2018; Cobb, 2018). Elevating the feeder system during filling and/or feed system filling in darkness are additional management measures to improve feed distribution (Abad, 2020).
Everyday fed pullets are shown to experience less stress than skip-a-day fed pullets, evidenced by lower plasma corticosterone and insulin-like growth factor levels (Ekmay et al., 2010). Moreover, daily feeding is 5 to 10% more efficient in terms of feed intake to obtain target weight at the end of rear compared to feeding programmes (Vaughters et al., 1987; Bennett and Leeson, 1989; de Beer and Coon, 2007; Montiel, 2016). The reason for the difference in efficiency is that birds fed feeding programmes must store nutrients (fat and protein) on feed days that must be utilized for maintenance and
growth on the feed less day (de Beer and Coon, 2007; Leeson and Summers, 2009). This physiological process of deposition and re-utilization of nutrients is not 100% efficient resulting in a decreased efficiency and higher feed requirement. de Beer and Coon (2007) compared four different feeding programmes (skip-a-day, 4/3, 5/2, and every day) and found that breeder pullets fed every day during rearing consumed 8% less feed to reach the same bodyweight with comparable BW uniformity. Improved feed efficiency and comparable uniformity was seen when applying everyday feeding (Zuidhof et al.,2015). de Beer and Coon (2007) found during the laying phase, earlier peak egg production and higher settable egg production in everyday fed pullets compared to feeding programmes. Also, Montiel (2016) found a 9% decreased feed allowance during rear when applying everyday feeding compared to skip-a-day feeding.
Contrary to feeding programmes with feed-less days, van Emous et al. (2021) applied feeding once or twice a day in combination with control and diluted diets. The twice-a-day fed pullets showed a lower BW CV (is a higher BW uniformity) at 10 weeks of age, however, no effect was found at 20 weeks of age. Moreover, pullets fed twice a day tended to have an earlier onset of lay, higher total egg production at 30 weeks of age, and better fertility.
Breeders are conditioned to a quick feed clean-up time (15 to 30 min) during the rearing phase due to feed restrictions (de Jong et al., 2002; Leeson and Summers, 2005).
After transfer to the breeder house, they still show this aggressive eating behaviour during the first 5 weeks of the laying period, which can lead to unequal feed distribution, worse uniformity, and feather loss (van Emous and Mens, 2020). This implies that a rapid and equal feed distribution over the flock is also very important during the initial laying phase (Aviagen, 2018; Cobb, 2018). All feed should be distributed within 3 minutes, which is possible by a rapid chain feeder (30 m/min), additional feeder bins in the house (Cobb, 2018), feeder bins in the centre of the house, and/or feeding with lights off (van Emous, 2018).
Feeding systems in breeder houses are installed on the litter areas, or both on the litter and slat areas (EFSA, 2010). During the laying phase, female feed is distributed over the birds in different forms (mash, pellet, or crumb) via chain or pan feeders with a mail exclusion system. Different male and female target weights have been recommended for many years in breeding company management guides. Worldwide in the broiler breeder industry, males are reared separately from the females so that growth and development can be controlled (Leeson and Summers, 2009). The technique for feeding sexes separately during the laying phase was introduced by McDaniel (1986). An experiment showed that using separated sex feeding during the laying phase resulted in a better-controlled bodyweight of the males and, as a consequence, improved fertility in the last part of the laying phase (Stappers and Vahl, 1991).
Differences in relative heights and head sizes of males and females, age at mating,
and feeder types have led to much practical refinement. The most effective method of preventing male access to the female feeding system (chain or pan feeder) is using grills with a width between 45 and 47 mm and a height between 60 and 70 mm (Aviagen, 2018; Cobb 2018). Head width of males increases from approximately 44 mm at 20 weeks of age to 55 mm at 60 weeks of age whereas head width of females stays below 46.5 mm (Aviagen, 2018). Some feeding troughs are equipped with roller bars and the height between trough and roller bar can be adjusted while breeders age; between 43 mm at the start of lay, 47 mm at 30 weeks of age, and 50 mm at 50 weeks of age (Aviagen, 2018; Cobb, 2018). Males are fed via troughs or pan feeders located near the walls of the house at a height (min. 50 cm) that is unreachable for the females (de Jong and van Emous, 2017).
In the last five years this minimum height has increased to 52 cm due to the increased bodyweight and length of the females (R. van Oosten, 2021, personal communication). Field studies show that male feeders located at the walls resulted in roughly 60% lower number of floor eggs (Aviagen, 2015). When the male feeder system is installed close to the slats, the male and the system can function as barriers for the females to reach the nest boxes on the slats.
A new housing system for broiler breeders called the Quality Time (QT) was developed to improve sexual behaviour and reproduction (van Emous, 2010). Males are separated from females five hours a day using a separate feeding system and a moving fence which implies optimal separated sex feeding. The special male compartment(s) can also
be used during the first three weeks of the laying phase for 100% separated sex feeding.
Feeder space during the rearing and laying phase is important for an equal distribution of the feed over all birds with less mutual competition (de Jong and van Emous, 2017).
A shortness in feeder space negatively affects uniformity (Aviagen, 2018; Cobb, 2018) and thereby reproduction performance (Abbas et al., 2010). Besides this, the shortage in feeder space results in impaired feather cover due to competitive eating behaviour around the feeding system (van Emous and Mens, 2020).
In a study of Leksrisompong et al. (2014), however, no effect of less feeder space (5.3 vs. 7.0 cm/female) during the rearing phase was found on reproductive performance during the laying phase.
The recommended feeder trough space for females and males during the laying phase is 15 and 20 cm per bird (Leeson and Summers, 2009; Aviagen, 2108). The effect of feeder space on hatchability was investigated in 2007 via a commercial breeder farms assessment (van Emous, unpublished data). Flocks were divided into three categories: low (<12 cm/female), medium (between 12 and 13 cm/female), and high feeder space (>13 cm/female). Hatchability for low, medium, and high feeder space was 79.0%, 80.8%, and 82.2%, respectively (Figure 2).
In a recent on-farm experiment, four comparable houses each containing each approximately 6,000 breeders were used to compare feeder spaces (standard: 13.6 cm/female and high: 15.9 cm/female) (van
Emous and Mens, 2020). Feather cover quality of females housed at the high feeder space was significantly improved compared to the females housed at the low feeder space. Due to the severe feed restrictions during the rearing period (de Jong et al., 2002), breeders are accustomed to rapid feed intake which is still present during the first weeks of the laying period. This results around feeding time in more aggressive and competitive behaviour with more feather cover damage. Studies show that when breeders have more feeder space, there is relatively less aggression and competition around feeding, resulting in better feathering.
Broiler breeders are fed a limited amount of feed once a day with a feed clean-up time ranging from two to six hours (Roland and Farmer, 1984). Clean-up time is affected by
different factors such as diet energy level, feed structure, health, amount of feed, and most importantly ambient temperature (Leeson and Summers, 2005).
Feed distribution time during the laying phase is determined by the time frame when most eggs are laid. Research shows that 80% of the eggs are laid in the morning between two and seven hours after lightson (Zakaria et al., 2005; Zakaria and Omar, 2013). When breeders are fed in this period a certain proportion of the females are disturbed during nesting behaviour resulting in more floor eggs (Aviagen, 2018; Cobb 2018). It is therefore recommended to feed the birds before or after the majority of the eggs are laid (Aviagen-EPI, 2017; Cobb, 2018).
In the last decades, early morning (directly after lights-on) feeding has become a more
and more common practice in breeder houses in Europe due to reliable feed equipment. Feeding directly after lights-on in the early morning has some major advantages:
1) Feed distribution at the coolest part of the day. During hot climate conditions feed consumption can be extended when birds are fed later in the day, which sometimes results in underfeeding (Leeson and Summers, 2009). An experiment showed that 80% of the total amount of feed is consumed within two hours in a cooler house (10-25°C) compared to six to seven hours in a hotter house (21-39°C) (Samara et al., 1996).
2) Breeders are habituated during the rearing phase to receive feed directly when lightson. During the rearing phase, broiler breeders are conditioned that feed takes place directly available after lights-on. The standard daylight period in rearing is from 08.00 to 16.00 h, and when breeders are photo-stimulated during initial lay from 8 to 10 or 11 hours (between 21 and 23 weeks of age), lights are switched on at 05.00 or 06.00 h with feed distribution at 08.00 h. This timing is because farmers want to observe the birds’ eating behaviour. This implies that the birds have no access to feed and water for several hours, which affects daily behaviour patterns and results in more stress (van Emous et al., 2020).
3) Breeders are fed without disturbing egg laying behaviour. As mentioned before, the majority of eggs are laid between two and seven hours after lights-on (Zakaria et al., 2005; Zakaria and Omar, 2013; Akli and Zakaria, 2015). Feeding before
this period of the day helps to minimize floor and slat eggs. Feeding before this oviposition window becomes even more important as breeders age because older flocks show a broader distribution of oviposition time (Zakaria et al., 2005; Zakaria and Omar, 2013).
A disadvantage of feeding directly after lights-on is that feeding occurs during the night when the farmer is not present to observe feeding behaviour, bird distribution, and/or malfunctions of the feeding system (Leeson and Summers, 2009). Furthermore, the chance of spoiling the nest boxes is greater when feed is administrated before oviposition because the excretion of faeces already starts during nesting behaviour (Farmer et al., 1983a,b).
Twice-a-day-feeding / split feeding Despite increasing calcium levels in the breeder diet and/or applying additional calcium sources (oyster shell or large limestone), eggshell quality has decreased (Leeson and Summers, 2009). This is caused by the feeding practice applied in breeders: a limited feed allowance in the morning which is consumed within 2 to 6 hours (Ronald and Farmer, 1984; Backhouse and Gous, 2005). This practice does not support optimal nutrient requirements for egg production (e.g., Backhouse and Gous, 2006). The majority of females lay eggs in the morning between two and seven hours after lights-on (Zakaria et al ., 2005; Zakaria and Omar, 2013). After ovulation, albumen formation takes place during the next six hours, requiring protein,
followed by eggshell formation during the next 18 hours, which mostly requires calcium (Leeson and Summers, 2005). Feeding breeders twice a day can improve the availability of nutrients in egg and eggshell formation, maybe resulting in improved eggshell quality (Backhouse and Gous, 2006). Different studies also demonstrate that broiler breeder hens fed twice a day produced more eggs compared to breeders fed once a day (Spradley et al ., 2008; Taherkhani et al ., 2010; Moradi et al ., 2013; Soltanmoradi et al. , 2014). However, other authors did not observe such an effect (de Avila et al ., 2003; Backhouse and Gous, 2005; Londero et al ., 2015, 2016).
Feeding specially formulated morning and afternoon diets (so called ‘split-feeding’) can help to provide the different nutritional requirements during egg formation in layers (de Los Mozos and Sanchez, 2014; Molnár et al., 2018) and breeders (Trouw, 2021; van Emous and Mens, 2021).
De Los Mozos and Sanchez (2014) investigated split-feeding’ in old laying hens (between 95 and 98 weeks of age) and observed a higher eggshell weight, thicker eggshell and 30% less cracked and shell-less eggs. A study of Trouw (2021), saw higher chick production (+1.9 DOC/hh) in breeders fed via split-feeding, 9% lower feed costs, and improved feather cover due to less pecking.. In the study of van Emous and Mens (2021) it was concluded that twice-a-day feeding (same diets) improved behaviour while split feeding’ improved both egg production and behaviour in broiler breeders. However, no
effects were observed on eggshell quality and incubation traits.
The use of special male diets is globally still not a common practice which means males receive more nutrients (especially calcium and protein) than required (Leeson and Summers, 2005, 2009). Overall, there seems to be a positive effect of lower protein levels for males, both in the rearing and the laying phases. A study by Romero-Sanchez et al. (2007) showed that feeding male pullets low dietary protein diets (120 vs. 170 g/kg CP) during the rearing phase improved egg fertility. Early studies of the effect of dietary protein levels in male diets demonstrated that semen quality and fertility are improved when low-protein diets are used (McDaniel, 1986). Subsequently, several papers have investigated the effect of crude protein on semen production and fertility in broiler breeder males (Wilson et al., 1987a,b; Hocking, 1989; Hocking and Bernard, 1997; Zhang et al., 1999; Borges et al., 2006; Silveira et al., 2014). In a study, breeder males were fed five different daily amounts of crude protein: 12.0, 14.2, 16.4, 18.6, and 20.8 g per bird (Borges et al., 2006). They observed quadratic effects of daily crude protein intake on semen volume, spermatic concentration, motility, spermatozoa vigour and fertility, and concluded that 16.9 g crude protein per male per day meets requirements. In a more recent study of Silveira et al. (2014) an on-farm study with 26 houses with each approximately having 7,000 females and 840 males were fed a standard breeder diet (2,830 kcal ME/kg and 15.0% CP) or a special male diet (2,750 kcal ME/kg and 13.5% CP). At 65 weeks of age, bodyweight of males in houses
with the special male diet was 6% lower and egg fertility and hatchability was 1.5 and 4.2 percentage points higher, respectively.
Although the observed effects in the different studies are not universal, the general conclusion from this research corroborates the work of McDaniel (1986) showing that low crude protein diets have an advantage in semen production over high crude protein diets and can improve egg fertility and hatchability.
In both rearing and laying houses water is provided via bell drinkers, nipple drinkers or drink cups (de Jong and van Emous, 2017). The systems have different advantages and disadvantages. Open water systems are easily discoverable and accessible for one-day-old chicks and for pullets after transfer from the rearing to the laying house (van Emous et al., 2020). On the other hand, open water systems like bell drinkers, and to a lesser extent drink cups, are more sensitive for manure and bacterial contamination and therefore regular and frequent cleaning, especially with young chicks, is necessary (Aviagen, 2018; Cobb, 2018). Water spillage is easier with open water systems with a possible negative effect on litter quality and thus footpad health (van Emous et al., 2020). Nipple drinkers are less sensitive for manure and bacterial contamination and water spillage. The investment for nipple drinkers are higher than bell drinkers, and nipple drinker lines can function as an obstacle to the nest boxes, resulting in more slat and floor eggs (van Emous et al., 2020).
In an on-farm study at a commercial farm with four similar breeder houses, two houses were equipped with special male nipple drinker lines close to the male feeders (A. Spangberg, 2021, personal communication). After transfer, the males get used more easily to the new house which resulted in approximately 8% lower mortality and grading.
In breeder production with separated rearing and laying houses, it is important to avoid differences in water system between both phases (Aviagen, 2018). It is common practice that rearing houses are equipped with both bell and nipple drinkers to prevent water intake problems after transfer (J. Lemmens, 2020, personal communication). Crop fill assessment of 50 females and males over the entire house at 1 h and 24 h after transfer from the rearing to the laying farm is good practice to check water intake per individual hen and proper function of the system (Aviagen, 2018; Cobb, 2018).
Unrestricted access to water can lead to overdrinking in restricted fed broiler breeders, which may result in wet litter and, in severe cases, in polydipsia (Hocking et al., 1993). Maintaining good quality litter is essential for good foot health in pullets and breeders (Kaukonen et al., 2016) and for preventing nest and egg contamination (EFSA, 2010). Therefore, water restriction is applied to most broiler breeders (rearing and breeding farms) by a fixed water-to-feed ratio or a fixed number of hours of water during the day (de Jong et al., 2016). A water-to-feed ratio of around 1.8 is recommended under commercial conditions, dependent on house
temperature (Aviagen-EPI, 2017; Cobb, 2018; Hubbard, 2015). When using the fixed-hours-of-water method, farmers use the guideline that access to water is closed two hours after clean-up time (R. van Oosten, 2020, personal communication). During hot days (over 25°C) most breeder farmers give birds access to an extra period (between 30 min and 1 h) of water in the late afternoon. With both methods of water restriction, daily crop assessment a few hours after feeding on firmness of at least 20 birds per house during the entire lifecycle is recommended (Aviagen, 2018; Cobb, 2018).
Experts indicate that there may be a negative effect of water restriction on the welfare of breeders (de Jong et al., 2016). The only experiment carried out with water restriction in breeders, however, did not observe any effects on welfare indicators (Hocking et al., 1993). In a study of van Emous et al. (2021), breeder pullets had access to water 7¼ h of the day and waterto-feed ratio was on average 2.3. Stocking density in the experiment was somewhat lower (8 vs. 10 birds/m2) than what is common in practice. The results of the study, however, show that the higher water intake does not necessarily result in wet litter, which was confirmed in several studies of Aviagen at their research facilities in the Netherlands (J. Lesuisse, 2020, personal communication). In previous studies with over-drinking and litter problems, open water systems were used (Kostal et al., 1992; Savory et al., 1993; Hocking et al., 1996, 2001) sometimes in combination with much longer access (14 h) to the water system due to the longer day length (Kostal et al.,
1992; Savory et al., 1993). Over-drinking behaviour was not observed in the study of van Emous et al. (2021) and therefore they postulated that this was caused by the drinking system (nipple drinkers) and the shorter period of water access.
In the aforementioned study of van Emous et al. (2021) half of the pullets were fed control or 16% (on average) diluted diets. Against expectations, no difference in water intake was found between the two treatments resulting in lower water-tofeed ratio for pullets fed diluted diets. The authors speculated that water intake might be maximized by the capacity of the crop (Wehner and Harrold, 1982) and not per se by the amount of feed. Overall, it is concluded that severe water restriction is not necessary when pullets have longer access to water and perform calmer water intake behaviour. It is previously postulated that ‘over-drinkers’ are not born in that way but raised (O. van Tuijl, 2018, personal communication).
It is important to note that the water intake pattern can change during the first 10 weeks of lay due to decreased feed restriction severity (J. Lemmens, 2020, personal communication). Birds under practical conditions are used to eating first as quickly as possible and after feed clean-up, drinking as quickly as possible to prevent running out of feed and water, respectively. This behaviour is also observed in the production house during roughly the first five weeks, whereafter the feed allowance is increasing rapidly to approximately 90% of the maximum daily amount of feed (AviagenEPI, 2017). This results in a peak water intake after one to two hours after feed distribution,
which implies that the water capacity of the system must be calculated at that water peak. If not, water availability at the rear of the breeder house will be insufficient with an unequal water distribution over the flock as a result.
Globally, different broiler breeder house layouts exist and vary between fully climate-controlled (totally closed) without daylight entrance or with windows, or open-sided with mesh curtains. The layout of the house is dependent on the region, climate, labour costs, and legislation (de Jong and van Emous, 2017). Totally closed and controlled houses (or black-out housing) are windowless and rely totally on mechanical ventilation to remove heat and moisture from the building, and to provide air exchange (Leeson and Summers, 2009). For these houses, the most important part of the farm is an alternate electricity source (power generator) because, after electricity failure, birds usually start dying within one to four hours depending upon age, temperature, and stocking density. With a reliable energy source, totally closed houses are suited for all kinds of regions around the globe with environmental temperatures between -25 and 40°C. In regions with temperatures below -5°C, a well-insulated house is necessary. When environmental temperature increases above 30°C, a cooling system (pat cooling or fogging system in or outside the house) is necessary to prevent heat stress. Heat stress in breeders can easily arise because of their high bodyweight in combination with good feather cover, which, during heat stress, results in lower
reproduction and mortality (van Emous et al., 2020). This is shown in an experiment where half of the breeders kept on a low and high bodyweight profile between 22 (2,007 and 2,434 g, respectively) and 62 weeks of age (3,935 and 4,323 g, respectively) (van Emous et al., 2004). Around 46 weeks of age the birds experienced eight days of high maximum ambient temperatures (above 30°C) resulting in higher mortality (14.1% vs. 5.0%), lower total hatching eggs production (171.8 vs. 175.2), and lower average hatchability (90.1% vs. 91.5%). The effect on hatchability can be explained by the negative correlation between male body temperature and sperm quality (McDaniel et al., 1995; Karaca et al., 2002).
In higher temperatures, it has been observed that laying hens also experience lower egg production and higher mortality (Mashaly et al., 2004).
An increased temperature is not the only factor that causes discomfort for the birds, but the combination of temperature and humidity (Aviagen, 2014). To estimate the level of heat stress, the heat stress index (HI) was developed, which is the sum of the ambient temperature and the relative humidity (RH). The rule of thumb The general rule is that heat stress occurs when HI is above 100 (e.g., 30°C and 70% RH), and HI above 115 results in dead birds. The main indicator of heat stress is prolonged panting and if this is not rectified, it will lead to bird death (Lara and Rostagno, 2013). Besides dead birds, a certain number of birds will almost die. It is said that when one bird dies, approximately ten birds almost die due
to heat stress resulting in lower reproductive performance (van Emous et al., 2020).
Breeder companies recommend starting the production period with 8 to 11% males (Aviagen, 2018, Cobb, 2018). Moreover, it is recommended by Cobb (2018) to use a male-to-female ratio of 8 to 9% in houses with slats, and 9 to 10% in houses without slats. Male percentage, however, decreases during the laying phase due to grading (e.g., leg problems, BW loss) and mortality (de Jong and van Emous, 2017). Around 24 weeks of age mostly 9% good developed and active males are necessary for good initial fertility, resulting in 8 to 8.5% first-grade males around 30 weeks of age (Aviagen, 2018, Cobb, 2018). When males show aggressive and dominant behaviour, male-to-female ratio must be reduced to 5% and the 3 to 4% placed in a separated male compartment (van Emous, 2007; Cobb, 2018). About 15 to 25% of the males are graded during the laying phase and replaced by so called ‘spiking’ males (Casanovas, 2000, 2002). From 40 to 45 weeks of age, spiking of mature young males (around 25 weeks of age) is often applied. Spiking is replacing approximately 1 to 2% inactive males with mature young males, which is commonly applied in practice to maintain the fertility of hatching eggs (Leeson and Summers, 2009). Spiking males, however, involves the risk of introducing pathogens and may be stressful to the birds because male aggression may increase (EFSA, 2010). Sometimes intraspiking (swapping older males at the same farm from one house to the next) is used as a method to increase male activity and thus
fertility, which has a much lower biosecurity risk (Casanovas, 2000).
In the last decade, research in the Netherlands focussed on adjusting male and female management to influence and improve mating behaviour in breeders (van Emous, 2010; van Emous and Gunnink, 2011; van Emous and Mens, 2020). A decade ago, an innovative housing system for broiler breeders, called the Quality Time, was developed (van Emous, 2010). After a successful pilot experiment, two on-farm experiments were carried out in a commercial broiler breeder house with 15,000 birds. Males were separated from females for five hours per day using a sexseparate feeding system and a vertical moving fence running lengthwise the house. In the QT compartments, more voluntary and successful matings were observed, which resulted in an improved feather cover between 37 and 48 weeks of age (van Emous and Gunnink, 2011). Separating males from females did not increase aggressive behaviour between the males (van Emous, 2010). In the first flock no effect on fertility was found, however in the second flock average fertility was improved with 1.5%. The first version (QT 1.0) was followed by an on-farm experiment (QT 2.0) with 9,900 breeders with a separated male compartment at the rear of the house (R.A. van Emous, unpublished data). It was concluded that this system results in very good separation of the females (less than 0.1% females in the male compartment) and increased hatchability by approximately 2%. An additional advantage of the QT house is that males can be easily locked up in the male pen during the first two to three weeks after transfer for excellent separated sex feeding. Besides this, grading of low-quality
males is much easier when sexes are separated and depletion at end of lay is also easier (van Emous, 2010).
An experiment was carried out to study the effects of the Quality Time 3.0 or so called ‘skip-a-day male-female contact’ system (van Emous and Mens, 2020). In this system, males and females were mixed every other day followed by a day without mixing. The study showed that skip-a-day male-female contact has hardly any effect on reproduction results and behaviour. However, when this system is applicable in practice, it gives a 5 to 10% higher economical result due to the higher number of females and lower number of males.
Despite spiking, the overall male percentage declined roughly from 9% at the start to 7% males at the end of the laying phase (van Emous, 2010). From an objective perspective, this is a surprising method because the physical and physiological characteristics of the breeder males were also decreasing (van Emous, 2010). Research showed that mating activity (Duncan et al., 1990; Cassonovas, 2000), percentage of successful matings (Duncan et al., 1990), ejaculate quality (e.g., Casanovas, 2002; Zhang et al., 1999), sperm penetration ability (e.g., Bramwell et al., 1996; Barna et al., 2009) and testicular size (Sarabia Fragoso et al., 2013) has decreased as males age.
Therefore, adjusted male-to-female ratio management is suggested by van Emous (2007) and Renema (2011). Starting with a low male-to-female ratio (6-7%) and adding extra males at several moments during the laying period (e.g., +1% around 30, 40, and 50
weeks of age), resulting in 7.5 to 8.5% males around 50 weeks of age (Renema, 2011). This was earlier mentioned by van Emous (2007), who suggested starting with an even lower male-to-female ratio (4-5%) and increasing this by 3 times spiking to around 8% at 50 weeks of age. He advised to transfer 8 to 9% of the male-to-female ratio to the breeder farm, place 4% in a separated male house and use these males as reserve males, placing them in a four-to-five-week period in the main flock after 30 weeks of age. Recently, an on-farm experiment in a Veranda cage system was begun to investigate the effect of two different male-to-female ratios. In two cages, breeders were kept with a decreasing male-to-female ratio (from 7.2% to 6.4%), and in two cages the experiment started with 4% males which increased three times: 5% at 30, 6% at 40, and 7% at 50 weeks of age by adding spiking males. The preliminary results showed no difference in general behaviour, however, feather cover and mating behaviour were improved in cages with the adjusted male-to-female ratio (R.A. van Emous, unpublished data). Moreover, fertility and hatchability were approximately 3% higher from 49 weeks of age onwards in the cages with an increased male-to-female ratio.
Standard production houses in Northern Europe are windowless and mechanically ventilated (de Jong and van Emous, 2017). However, in Sweden and France windows are often present in the houses, although these may be covered with curtains in case they may conflict with the lighting schedule
or cause increased feather pecking (EFSA, 2010). Open-sided houses can be found in Italy, France, Spain, and Eastern Europe (EFSA, 2010). Due to welfare legislation in the last decade, more houses are equipped with windows for natural light (de Jong and van Emous, 2017). In Germany, in new buildings, additional daylight must be provided, whereby the light must fall evenly into the floor space. The incidence of light via wall or roof windows must be at least 3% of the house floor surface (QS, 2021).
In general, artificial light can be provided via conventional light bulbs (or incandescent lamps), fluorescent light (TL-tubes), highpressure sodium lamps, and more recently LED (Leeson and Summers, 2009). The different light systems have advantages and disadvantages in efficiency, lifespan, investment costs, light spectrum, and dimmability. For example, bulbs are less efficient than TL-tubes, sodium and LED and have a shorter lifespan (roughly 1,000 vs. 10,000 h). On the other hand, investment costs are the lowest for bulbs. Jones et al. (2001) found that a UVA-enriched light systems increased matings by 28%, locomotion by 43% and fertility by 2%.
During the first two days chicks are reared at a photoperiod of 24 h light (40 lx), which is gradually reduced to 8 h light and 5 to 10 lx. Due to injurious pecking or to reduce birds’ activity, sometimes lower light intensities are applied (EFSA, 2010). Age at photostimulation and light intensity (between 25 lx and 80 lx) varies globally and may affect reproduction performance
of broiler breeders (van Emous et al ., 2018). In some regions, breeders are photo-stimulated around 21 weeks of age while in other regions breeders are photostimulated around 23 weeks of age. This difference is mainly due to differences in threshold for minimal hatching egg size (50 or 52 g). Later photo-stimulated breeders generally compensate the extended age at sexual maturity with an higher egg production (Yuan et al ., 1994; Robinson et al ., 1996; Renema et al ., 2001; Joseph et al ., 2002). No effects of age at photostimulation on incubation traits were found in studies of Robinson et al . (1996) and Renema et al . (2001). In the study of Robinson et al. (1996), however, they found a higher chick production when birds were photo-stimulated at 140, 150, or 160 d compared to 120 and 130 d.
A study with a 2 x 2 factorial arrangement was conducted to determine the effects of two dietary CP levels (high and low) and 2 ages at photostimulation (early: 21 weeks of age and late: 23 weeks of age) (van Emous et al., 2018). Early stimulated birds showed an advanced age at sexual maturity and age at peak production of 4.6 and 5.3 d, respectively, resulting in 2.5 more total eggs and almost 5% increased fertility during the first phase (22 to 34 weeks of age). Chick production in the first phase was higher for early stimulated birds resulting in a tendency to higher overall chick production of almost 8 chicks over the entire laying phase.
The effects of different light schedules in breeders was comprehensively investigated
in South Africa by Lewis and co-workers (Lewis et al., 2007, 2010; Lewis, 2016, 2019). In an experiment, Ross 308 broiler breeder females were reared from 2 d of age on 8 h photoperiod and transferred abruptly to an 11, 12, 13, or 14 h photoperiod at 20 weeks of age (Lewis et al., 2010). Age at 50% egg production and egg production itself was progressively advanced between the 11 and 13 h groups but were similar for the 13 and 14 h birds. More floor and slat eggs were found, and consequently, more cracked and dirty eggs were produced by breeders on 11 and 12 h photoperiod compared to 13 and 14 h. Birds exposed to 11 and 12 h photoperiods had a higher BW at 60 weeks of age than birds exposed to 13 or 14 h photoperiods. Researchers concluded that a maximum day length of 13 h optimized egg production in broiler breeder females.
It is hypothesized that a shorter day length also affects mating behaviour and thereby egg fertility (van Emous, 2010). Previously in broiler breeder houses applying 16 h daylength, ‘open spots’ on the litter area at the end of the light period were observed due to the fact that females were resting on the slats. It is well known that the majority of matings (90%) are performed on the litter area (van Emous, 2010) during the last three to five hours of the day (e.g., Harris et al., 1980; Bilcik and Estevez, 2005). When sexes aren’t mixed frequently, mating frequency can decrease with detrimental effects on fertility and hatchability (van Emous, 2010). Because of the advantages on reproductive performance, daylength in northwest Europe decreased from 16-17 h to 13-14 h (van Emous, 2018). Since the transition to a
shorter daylength, ‘open spots’ at the end of the day are hardly seen anymore (R.A. van Emous, 2021, personal observation).
The ancestors of the modern chicken breeds were prey animals and roosted during the night on branches in trees (WoodGush et al., 1978; Blokhuis et al., 1984; Newberry et al., 2001). This anti-predator behaviour reduces the risk of being caught by a ground predator(Newberry et al., 2001; Wood-Gush and Duncan, 1976). Despite decades of selection, this ancestor behaviour is still observed in modern chickens (e.g., Olsson and Keeling, 2000; Schrader and Müller, 2009; Donaldson et al., 2012). The use of elevated places in breeder pullet and production houses is to fulfillthe natural roosting behaviour and also to help prevent floor eggs (Brake, 1987). In two consecutive studies, Brake observed a 40% decrease in the number of floor eggs during the laying phase when breeder pullets had access to perches. Therefore, it is recommended by the breeder companies (Aviagen, 2018; Cobb, 2018; Hubbard, 2015) to provide at least 1 m2 elevated platforms (with slats) per 500 pullets around 50 cm above the floor from about 6 weeks of age onwards. For additional vertical movement stimulation, a drinking water system is often installed above the elevated platforms (de Jong and van Emous, 2017; Riber et al., 2017). This training in vertical direction during the rearing phase promotes the movement towards the nest boxes during the laying phase resulting in a lower number of floor and slat eggs (Aviagen, 2018; Cobb, 2018; Hubbard, 2015).
In a study during the rearing phase, 7.2% and 3.4% of the breeder pullets were found on perches (5 cm per bird) during day and night, respectively (van Middelkoop and van Harn, 2003). In a more recent study, under practical conditions, 10% and 24% of the pullets were found on the perches during day and night, respectively (von Wachenfelt and Berndtson, 2014).
Studies with small groups of birds showed an even higher use (between 35% and 70%) of elevated areas (perches, A-frames or aviaries) by fast- and slow-growing breeders (Gebhardt-Henrich et al., 2017, 2018). Brandes et al. (2017) observed in commercial breeder houses that 23% (slow-growing) to 27% (fast-growing) of the birds used a perch at night and that the use of the perches increased for both breeds while aging. In general, hardly any research has been done on broiler breeder preferences for roosting on different types of elevated places. A pilot study was carried out in a pullet house (9,000 birds) under commercial conditions with elevated platforms (240 x 60 cm, 50 cm above the litter) equipped with plastic slats or three metal (2.5 cm die) round perches (van Emous et al., 2017). During the light period, 21 and 12 birds (per platform) were observed on the elevated resting areas with slats and perches, respectively (van Emous et al., 2017). The preference for an elevated slatted floor compared to perches was confirmed in a following study with broiler breeders during lay. A relative preference experiment was conducted between 40 and 60 weeks of age in 24 pens with 26 females and 3 males at the start (Mens and van Emous, in press). The birds could choose to roost on elevated
wooden slats (30% floor surface) and on two plastic rectangle perches with rounded edges (total 4 m), both 50 cm above the floor. Pens were further equipped with a littered floor area, a drinking line above the slats, and a nest box. The majority of the birds roosted on the slats (51.5 ± 1.4%), followed by the perches (23.9 ± 1.2%), drinking line (11.2 ± 0.7%), nest box (9.2 ± 0.7%), and litter (4.2 ± 0.8%). More and severe breast blisters were found in birds roosting on drinking lines and perches, respectively, compared to the slats. The higher prevalence of breast blisters was previously observed under commercial conditions by von Wachenfelt and Berndtson (2014).
A recent study investigated the effects of a raised slatted area on leg health, mating behaviour, and floor laying behaviour (van den Oever et al., 2021). They found lower floor eggs percentage and less mating behaviour in pens with raised slats compared to pens without raised slats.
Floor eggs
Eggs laid on the floor or on the slats are detrimental for a production due to labour costs for manual collection, bacterial contamination, lower hatchability, and decreased chick quality (Aviagen, 2016; van den Brand et al., 2016). Hence, breeder farmers spend an important part of the day during initial lay on floor egg prevention and one of the most important components of breeder houses are well-designed nest boxes. Prevention of floor eggs is extensively described in special floor egg guides and
general management guides from breeder companies (Aviagen, 2015, 2018; Cobb, 2018, 2019; Hubbard, 2015). Good practice in floor eggs prevention is based on three pillars: 1) Training: birds must use the nests, 2) Environment: keep the birds in the nests, and 3) Diminish alternative nest places (Aviagen, 2015). Within these three pillars a number of management measures can be learned from: elevated platforms during rear, feeding time lay, light intensity, avoiding draughts in nests, good laying nests, stocking density, collecting at least 10 times a day floor eggs, etc.
In the past, broiler breeder houses were equipped with individual nest boxes with litter material (Ketelaars, 1992). These nest boxes were cheap, but labour-intensive with low hygienic conditions for eggs. During the 1980s, mechanical colony nest boxes were developed as a result of the growth in farm size, labour costs, and hygienic standards (Ketelaars, 1992). The first prototypes of colony nest boxes were relatively unsuccessful and it cost many years of research and development to design modern nest boxes to produce less than 1% floor eggs (R.A. van Emous, 2021, personal communication). Early research showed that broiler breeders preferred smaller metal nests over larger wooden nests, with unpainted rather than black painted walls (Brake, 1985). A comparison of different colony nest with individual nest boxes in the Netherlands, however, showed that the improved Jansen colony nest (Jansen Poultry Equipment, Barneveld, the Netherlands) was comparable or better for egg production and reducing floor eggs than individual nest boxes (van der Haar, 1991, 1992; van der Haar and Meijerhof,
1994). Due to the negative correlation between floor eggs and egg production, the authors suggested that a high number of floor eggs probably also results in a high number of lost eggs (van der Haar and Meijerhof, 1994). It is postulated that the majority of the eggs laid on the slats break and are lost under the slats, which is expressed by the estimate: ‘5% total floor eggs result in at least 2.5% production loss’ (van Emous et al., 2020). Recently a study was performed to investigate the relative preference of four different nest designs (van den Oever et al., 2019). The following types of nests were tested: a plastic control nest, a plastic nest with a partition to divide the nest into two areas, a plastic nest with a ventilator underneath to create airflow inside the nest, and a wooden nest. They found a clear preference with the number of eggs in the wooden nest (69.3% compared to the control nest (15.1%), partition nest (10.2%), and the ventilator nest (5.4%). They also observed an increased time spent sitting, together with fewer nest inspections and visits per egg laid in the wooden nest.
Broiler breeder houses (rearing and laying) are very similar and housed in climatecontrolled environments. Pullet houses have a total litter floor with few elevated platforms, and production houses have a combination of litter and slatted floors. Males and females are reared separately until approximately 20 weeks of age and then transferred to the production farm where they are housed together until 6065 weeks of age. Pullets and breeders receive feed via trough or pan feeders and water via bell drinkers, nipple drinkers or drink cups, whereas spin feeders are only used during
the rearing period. Feeder space, feeder time, feeding frequency and diet composition are important management measures to optimize egg production. Male-to-female ratio and (intra) spiking are key factors to maximizing egg fertility and hatchability. Breeder houses are equipped with different light systems, climate control systems, and elevated areas to support behaviour and reproduction. State-of-the-art nest boxes are necessary to maintain the highest number of first-class eggs (not dirty, broken, or on the floor).
Abad, J.C. 2020. Management of males in broiler breeder operations. https://avicultura.info/en/ management-of-males-in-broiler-breederoperations/ Abbas, S.A., Gasm Elseid, A.A. and M-K.A. Ahmed. 2010. Effect of body weight uniformity on the productivity of broiler breeder hens. International Journal of Poultry Science 9: 225.230. Akil, R. and A.H. Zakaria. 2015. Egg laying characteristics, egg weight, embryo development, hatching weight and post-hatch growth in relation to oviposition time of broiler breeders. Animal Reproduction Science 156: 103-110. Aviagen. 2014. Hot season management of broiler breeders in open-sided houses. Arbor Acres service bulletin.
Aviagen. 2015. Best practice in the breeder house. Preventing floor eggs. http://en.aviagen.com/ assets/Tech_Center/Ross_Tech_Articles/RossBestPractice-Floor-Eggs-2015-EN.pdf
Aviagen-EPI. 2017. Management guide for Ross broiler breeders (in Dutch). Roermond, The Netherlands.
Aviagen. 2018. Ross parentstock management handbook. https://en.aviagen.com/assets/Tech_
Center/Ross_PS/RossPSHandBook2018.pdf
Backhouse, D. and R. M. Gous. 2005. The effect of feeding time on shell quality and oviposition time in broiler breeders. British Poultry Science 46: 255-259. Backhouse, D. and R. M. Gous. 2006. Responses of adult broiler breeders to feeding time. World´s Poultry Science Journal 62: 269-281.
Barna, J., Végi, B., Váradi, É., Szőke, Zs. and P. Péczely.
2009. Studies related to fertility in broiler breeders. XXI. International Poultry Symposium PB WPSA, Wroclaw-Szklarska Poreba, Poland. pp. 18-23.
Bennett, C.D. and S. Leeson. 1989. Research note: Growth of broiler breeder pullets with skip-a-day versus daily feeding. Poultry Science 68: 836-838. Blokhuis, H.J. 1984. Rest in poultry. Applied Animal Behaviour Science 12: 289-303.
Brake, J. 1985. Comparison of two nesting materials for broiler breeders. Poultry Science 64: 2263-2266. Brake, J. 1987. Influence of presence of perches during rearing on incidence of floor laying in broiler breeders. Poultry Science 66: 1587-1589.
Brake, J. 1998. Equipment design for breeding flocks. Poultry Science 77: 1833-1841.
Bramwell, R.K., McDaniel, C.D., Wilson, J.L. and B. Howarth. 1996, Age effect of male and female broiler breeders on sperm penetration of the perivitelline layer overlying the germinal disc. Poultry Science 75: 755-762.
Borges, C.A.Q., Rostagno, H.S., da Silva, J.H.V., Albino, L.F.T., Torres, C.A.A., Filho, J.J. and M.L.G. Ribeiro. 2006. Protein requirements and carcass composition of male broiler breeders from 27 to 61 weeks of age (in Portuguese). Revista Brasileira de Zootecnia 35: 1971-1977.
Brandes, A., Gierberg, M.F., Kemper, N. and B. Spindler. 2017. Gegenwärtiger Einsatz von Sitzstangen in der Masthühnerelterntierhaltung sowie deren Eignung hinsichtlich Nutzung und Tiergesundheit (Status quo Erhebung). Institut für Tierhygiene, Tierschutz und Nutztierethologie
Breeder Management and Nutrition: Moving the industry forward
Hannover.
Casanovas, P. 2000. Management techniques to improve male mating activity and compensate for the age-related decline in broiler breeder fertility: Intra-Spiking. Technical News, Quarterly Publication of Cobb-Vantress, Incorporated, USA. Casanovas, P. 2002. Management techniques to improve male mating activity and compensate for the age-related decline in broiler breeder fertility: intra-spiking. The Poultry Informed Professional 63: 1-13.
Chu, S.S.W. 1979. The effect of feeding management during the growing and laying periods on productivity and the characteristics of adipose tissue of the broiler breeders. Master thesis. University of British Columbia, Canada. Cobb. 2018. Cobb breeder management guide. CobbVantress, Siloam Springs, Arkansas, USA. Cobb. 2019. Broiler breeder management to minimize floor egg production. Cobb-Vantress, Siloam Springs, Arkansas, USA. de Avila, V.S., Penz Jr., A.M., de Brum, P.A.R., Rosa, P.S., Guidoni, A.L. and É.A.P. de Figueiredo. 2003. Performance of female broiler breeders submitted to different feeding schedules. Brazilian Journal of Poultry Science 5: 197-201. de Beer, M. and C.N. Coon. 2007. The effect of different feed restriction programs on reproductive performance, efficiency, frame size, and uniformity in broiler breeder hens. Poultry Science 86: 1927-1939. de Jong, I.C., van Voorst, S., Ehlhardt, D.A. and H.J. Blokhuis. 2002. Effects of restricted feeding on physiological stress parameters in growing broiler breeders. Poultry Science 43: 157-168. de Jong, I.C., Fillerup, M. and H.J. Blokhuis. 2005. Effect of scattered feeding and feeding twice a day during rearing on parameters of hunger and frustration in broiler breeders. Applied Animal Behaviour Science 92: 61-76.
de Jong, I.C. and B. Jones. 2006. Feed restriction and welfare in domestic birds. In Feeding in Domestic Vertebrates. From Structure to Behavior. V. Bels (ed). CABI Publishing, Wallingford, UK. pp. 120-135
de Jong, I.C., Lourens, A., Gunnink, H. and R.A. van Emous. 2011. Effect of stocking density on (the development of) sexual behaviour and technical performance in broiler breeders (in Dutch). 61 p. Wageningen UR Livestock Research, Lelystad, Report 457. de Jong, I.C. and M. Swalander. 2013. Housing and management of broiler breeders and turkey breeders. In Alternative Systems for Poultry: Health, Welfare and Productivity. V. Sandilands and P.M. Hocking (eds). CABI publishing, Wallingford, UK. pp. 225-249. de Jong, I.C., van Harn, J., Koene, P., Ellen, H., van Emous, R.A., Rommers, J.M. and H. van den Brand. 2016. Risk assessment of water provision for broilers and broiler breeders (in Dutch). Wageningen Livestock Research, report 989, Wageningen, the Netherlands. de Jong, I.C. and R.A. van Emous. 2017. Broiler breeding flocks: management and animal welfare. In Achieving Sustainable Production of Poultry Meat. T. Applegate (ed). Burleigh Dodds Science Publishing Limited, London, UK. pp. 1-19. de Los Mozos, J. and F. Sanchez. 2014. Split feeding meets layers’ specific needs. http://www. thepoultrysite.com/articles/3091/split-feedingsystem-for-more-sustainable-egg-production. Donaldson, C.J., Ball, M.E.E. and N.E. O’Connell. 2012. Aerial perches and free-range laying hens. The effect of access to aerial perches and of individual bird parameters on keel bone injuries in commercial free-range laying hens. Poultry Science 91: 304-315.
Duncan, I.J.H., Hocking, P.M. and E. Seawright. 1990. Sexual behavior and fertility in broiler breeder domestic fowl. Applied Animal Behaviour Science 26: 201-213.
EFSA. 2010. Scientific Opinion on welfare aspects of the management and housing of the grandparent and parent stocks raised and kept for breeding purposes. The EFSA Journal 8:81. doi:10.2903/j. efsa.2010.1667.
Ekmay, R.D., de Beer, M., Rosebrough, R., Richards, M.P., McMurtry, J.P. and C.N. Coon. 2010. The role of feeding regimens in regulating metabolism of sexually mature broiler breeders. Poultry Science 89: 1171-1181.
Farmer, M., Roland Sr., D.A., Brake, J. and M.K. Eckman. 1983a. Calcium metabolism in broiler breeder hens. 1. Calcium status of the digestive tract of broiler breeders throughout a 24 hour period. Poultry Science 62: 459-464.
Farmer, M., Roland Sr., D.A. and M.K. Eckman. 1983b. Calcium metabolism in broiler breeder hens.
2. The influence of the time of feeding on calcium status of the digestive system and egg shell quality in broiler breeders. Poultry Science 62: 465-471.
Gebhardt-Henrich, S.G., Toscano, M.J.H. and H. Würbel. 2017. Perch use by broiler breeders and its implication on health and production. Poultry Science 96: 3539-3549.
Gebhardt-Henrich, S.G., Toscano, M.J. and H. Würbel. 2018. Use of aerial perches and perches on aviary tiers by broiler breeders. Applied Animal Behaviour Science 203: 24-33.
Hocking, P.M. 1989. Effect of dietary crude protein concentration on semen yield and quality in male broiler breeder fowls. British Poultry Science 30: 935-945.
Hocking, P.M., Maxwell, M.H. and M.A. Mitchell. 1993. Welfare assessment of broiler breeder and layer females subjected to food restriction and limited access to water during rearing. British Poultry Science 34: 443-58.
Hocking, P.M., Maxwell, M.H. and M.A. Mitchell. 1996. Relationships between the degree of food restriction and welfare indices in broiler breeder
females. British Poultry Science 37: 263-278.
Hocking, P.M. and R. Bernard. 1997. Effects of dietary crude protein content and food intake on the production of semen in two lines of broiler breeder males. British Poultry Science 38: 199-202.
Hocking, P.M., Maxwell, M.H., Robertson, G.W. and M.A. Mitchell. 2001. Welfare assessment of modified rearing programmes for broiler breeders. British Poultry Science 42: 424-432.
Hubbard. 2015. Breeder management manual. Quintin, France.
Jones, E.K.M., Prescott, N.B., Cook, P., White R.P. and C.M. Wathes. 2001. Ultraviolet light and mating behaviour in domestic broiler breeders. British Poultry Science 42: 23-32.
Joseph, N.S., Dulaney, A.A.J., Robinson, F.E., Renema, R.A. and M.J. Zuidhof. 2002. The effects of age at photostimulation and dietary protein intake on reproductive efficiency in three strains of broiler breeders varying in breast yield. Poultry Science 81: 597-607.
Karaca, A.G., Parker, H.M., Yeatman, J.B. and C.D. McDaniel. 2002. Role of seminal plasma in heat stress infertility of broiler breeder males. Poultry Science 81:1904-1909.
Ketelaars, E.H. 1992. History of the Dutch poultry husbandry (in Dutch). BDU, Barneveld, the Netherlands.
Kostal, L., Savory, C.J. and B.O. Hughes. 1992. Diurnal and individual variation in behaviour of restricted-fed broiler breeders. Applied Animal Behaviour Science 32: 361-374.
Lara, L.J. and M.H. Rostagno. 2013. Impact of heat stress on poultry production. Animals 3: 356-369.
Laughlin, K.F. 2009. Breeder management: How did we get here? In Biology of Breeding Poultry. P.M. Hocking (ed). CABI. Wallingford, UK. pp. 10-25.
Leeson, S. and J.D. Summers. 2005. Commercial poultry nutrition. University Books, Guelph, Canada. Leeson, S. and J.D. Summers. 2009. Broiler breeder
production. University Books, Guelph, Canada. Leksrisompong, N. Romero-Sanchez, H., OviedoRondón, E.O. and J. Brake. 2014. Effects of feeder space allocations during rearing, female strain, and feed increase rate from photostimulation to peak egg production on broiler breeder female performance. Poultry Science 93: 1045-1052.
Lewis, P.D., Danisman, R. and R.M. Gous. 2010. Photoperiods for broiler breeder females during the laying period. Poultry Science 89: 108-114.
Londero, A., Rosa, A.P., Giacomini, C.B.S., Vivas, C.E.B., Orso, C., de Freitas, H.M., Gressler, L.T. and A.C. Vargas. 2015. Effect of different feeding schedules on reproductive parameters and egg quality of broiler breeders. Animal Feed Science and Technology 210: 165-171.
Londero, A., Rosa, A.P., Vivas, C.E.B., Orso, C., Fernandes, M.O., Paixão, S.J., Giacomini, C.B.S., Andrade, C.M. and H.E. Palma. 2016. The effect of different feeding schedules on egg quality, blood and bone parameters in broiler breeders. Animal Reproduction 13: 14-20.
Mashaly, M.M., Hendricks (3rd), G.L., Kalama, M.A., Gehad, A.E., Abbas, A.O. and P.H. Patterson. 2004. Effect of heat stress on production parameters and immune responses of commercial laying hens. Poultry Science 83: 889-894.
McDaniel, G.R. and J.C. Wilson, 1986. Feeding breeder males. Pages 66–74 in: Proceedings Carolina Nutrition Conference, Charlotte, NC.
McDaniel, C.D., Bramwell, R.K., Wilson, J.L. and B. Howarth, Jr. 1995. Fertility of male and female broiler breeders following exposure to elevated ambient temperatures. Poultry Science 74: 10291038.
Montiel, E.R. 2016. Influence of feeding programs on innate and adaptive immunity in broiler breeders. Thesis University of Georgia, Athens, Georgia, USA.
Molnár, A., Kempen, I., Sleeckx, N., Zoons, J., Maertens, L., Ampe, B., Buyse, J. and E. Delezie.
2018. Effects of split feeding on performance, egg quality, and bone strength in brown laying hens in aviary system. Journal of Applied Poultry Research 27: 401-415.
Moradi, S., Zaghari, M., Shivazad, M., Osfoori, R. and M. Mardi. 2013. The effect of increasing feeding frequency on performance, plasma hormones and metabolites, and hepatic lipid metabolism of broiler breeder hens. Poultry Science 92: 1227-1237.
Newberry, R.C., Estevez, I. and L.J. Keeling 2001. Group size and perching behaviour in young domestic fowl. Applied Animal Behaviour Science 73: 117-129.
Olsson, I.A. and L.J. Keeling. 2000. Night-time roosting in laying hens and the effect of thwarting access to perches. Applied Animal Behaviour Science 68: 243-256.
QS. 2021. https://www.qs.de/services/files/down loadcenter/4_leitfaeden/landwirtschaft/2021/ Leitfaden_Landwirtschaft_Elterntierhaltung_Ge fl%C3%BCgel_01.01.2021.pdf
Renema, R.A., Robinson, F.E., Goerzen, P.R. and M.J. Zuidhof. 2001. Effects of altering growth curve and age at photostimulation in female broiler breeders. 2. Egg production parameters. Canadian Journal of Animal Science 81: 477-486.
Riber, A.B., de Jong, I.C., van de Weerd, H.A. and S. Steenfeldt. 2017. Environmental enrichment for broiler breeders: an undeveloped field. Frontiers in Veterinary Science 4:1-6.
Robinson, F.E., Zuidhof, M.J. and R.A. Renema. 2007. Reproductive efficiency and metabolism of female broiler breeders as affected by genotype, feed allocation, and age at photostimulation.1. Pullet growth and development. Poultry Science 86: 2256-2266.
Roland Sr., D.A. and M. Farmer. 1984. Egg shell quality II: importance of time of calcium intake with emphasis on broiler breeders. Worlds Poultry Science Journal 40: 255-260.
Romero-Sanchez, H., Plumstead, P.W. and J. Brake. 2007. Feeding broiler breeder males. 1. Effect of feeding program and dietary crude protein during rearing on body weight and fertility of broiler breeder males. Poultry Science 86: 168-174.
Sarabia Fragoso, J., Pizarro Diaz, M., Abad Moreno, J.C., Casanovas Infesta, P., Rodriguez Bertos, A. and K. Barger. 2013. Relationships between fertility and some parameters in male broiler breeders (body and testicular weight, histology and immunohistochemistry of testes, spermatogenesis and hormonal levels). Reproduction in Domestic Animal 48: 345-352.
Savory, C.J., Maros, K. and S.M. Rutter. 1993. Assessment of hunger in growing broiler breeders in relation to a commercial restricted feeding programme. Animal Welfare 2: 131-152. Schrader, L. and B Müller. 2009. Night-time roosting in the domestic fowl - the height matters. Applied AnimAl Behaviour Science 121: 179-183.
Silveira, M.M. de Freitas, A.G., Moraes, C.A., Gomes, F.S., Litz, F.H., Martins, J.M.S., Fagundes, N.S. and E.A. Fernandes. 2014. Feeding management strategy for male broiler breeders and its effects on body weight, hatchability and fertility. Brazilian Journal of Poultry Science 16: 397-402.
Soltanmoradi, M.G., Seidavi, A., Dadashbeiki, M., Laudadio, V., Centoducati, G. and V. Tufarelli. 2014. Influence of feeding frequency and timetable on egg parameters and reproductive performance in broiler breeder hens. Avian Biology Research 7: 153-159. Spradley, J.M., Freeman, M.E., Wilson, J.L. and A.J. Davis. 2008. The influence of a twice-a-day feeding regimen after photostimulation on the reproductive performance of broiler breeder hens. Poultry Science 87: 561-568.
Stappers, H.P. and H.A. Vahl. 1991. Separate feeding of parent stock (in Dutch). Experiment report 310. De Schothorst, Lelystad, the Netherlands.
Taherkhani, R., Zaghari, M., Shivazad, M. and
A. Zare Shahneh. 2010. A twice-a-day feeding regimen optimizes performance in broiler breeder hens. Poultry Science 89: 1692-1702. Trouw. 2021. Innovative split-feeding system for broiler breeders. International Hatchery Practice 35: 7-9. van den Brand, H., Sosef., M.P., Lourens, A. and J. van Harn. 2016. Effects of floor eggs on hatchability and later life performance in broiler chickens. Poultry Science 95: 1025-1032. van den Oever, A.C.M., Rodenburg, T.B., Bolhuis, J.E., van de Ven, L.J.F., Hasand, Md.K., van Aerle, S.M.W. and B. Kemp. 2020. Relative preference for wooden nests affects nesting behaviour of broiler breeders. Applied Animal Behaviour Science 222: 104883.
van den Oever, A.C.M., Candelotto, L., Kemp, B., Rodenburg, T.B., Bolhuis, J.E., Graat, E.A.M., van de Ven, L.J.F., Guggisberg, D. and M.J. Toscano. 2021. Influence of a raised slatted area in front of the nest on leg health, mating behaviour and floor eggs in broiler breeders. Animal 15: 100109. van der Haar, J.W. 1991. An automated laying nest can give good results (in Dutch). Praktijkonderzoek Pluimveehouderij, Beekbergen, the Netherlands. van der Haar, J.W. 1991. Last round of laying nest research in Delden (in Dutch). Praktijkonderzoek Pluimveehouderij, Beekbergen, the Netherlands. van der Haar, J.W. and R. Meijerhof, 1994. Correct barn equipment results in less floor eggs (in Dutch). Pluimveehouderij 24: 14-15. van Emous, R.A., Lourens, A. and J. van Harn. 2004. Vitality of broiler breeders and ammonia measurements (in Dutch with English summary). PraktijkRapport Pluimvee 13. Animal Sciences Group, Praktijkonderzoek Veehouderij, Lelystad, the Netherlands. van Emous, R.A. 2010. Innovative housing in broiler breeder production. Pages 37-44 in Proc. 2nd Int. Symp.: Highlights in nutrition and welfare in poultry production, Wageningen, The Netherlands.
Animal Science Group, Lelystad, The Netherlands. van Emous, R.A. and H. Gunnink. 2011. Innovative broiler breeder housing system: ‘Quality Time’ house (in Dutch). Report 470. Wageningen UR Livestock Research, Lelystad, the Netherlands. van Emous, R.A., Gunnink, H. and G.P. Binnendijk. 2017. The effect of elevated resting place on use and behavior of breeder pullets (in Dutch). Wageningen Livestock Research, Report 1047. van Emous, R.A. 2018. More chicks due to less unrest (in Dutch). Pluimveehouderij 48: 14-15.
van Emous, R.A., de la Cruz, C.E. and V.D. Naranjo. 2018. Effects of dietary protein level and age at photo stimulation on reproduction traits of broiler breeders and progeny performance. Poultry Science 97: 1968-1979.
van Emous, R.A. Holleman, J. and T. van Schie. 2020. Breeder Signals. A practical guide to optimal management of broiler breeders. Roodbont Publishers B.V., Zutphen, the Netherlands. van Emous, R.A. and A.J.W Mens. 2020. Longer feeders lead to better plumage. Poultry world. https://www.poultryworld.net/Nutrition/ Articles/2020/10/Longer-feeders-lead-to-betterplumage-655456E/ van Emous, R.A., Mens, A.J.W. and A. Winkel. 2021. Effects of diet density and feeding frequency during the rearing period on broiler breeder performance. British Poultry Science 62: 686-694. van Middelkoop, J.A. and J. van Harn. 2003. Broiler breeder needs 5 cm perch length (in Dutch). Pluimveehouderij 33: 14-15. Vaughters, P.D., Pesti, G.M. and B.J. Howarth. 1987. Effects of feed consumption and feeding schedule on growth and development of broiler breeder males. Poultry Science 66: 134-146. von Wachenfelt, E. and E. Berndtson. 2014. Usage of perches in meat fowls. Sveriges lantbruksuniversitet Fakulteten för landskapsarkitektur, trädgårds- och växtproduktionsvetenskap. Report 2014:3.
Wehner, G. and R. Harrold. 1982. Crop volume of chickens as affected by body size, sex, and breed. Poultry Science 61: 598-600.
Wilson, J.L., McDaniel, C.D. and C.D. Sutton. 1987a. Dietary protein levels for broiler breeder males. Poultry Science 66: 237-242.
Wilson, J.L., McDaniel, C.D., Sutton, C.D. and J.A. Renden. 1987b. Semen and carcass evaluation of broiler breeder males fed low protein diets. Poultry Science 66: 1535-1540. Wood-Gush, D.G.M. and I.J.H. Duncan. 1976. Some behavioural observations on domestic fowl in the wild. Applied Animal Ethology 2: 255-260. Wood-Gush, D.G.M., Duncan, I.J.H. and C.J. Savory. 1978. Observations on the social behaviour of domestic fowl in the wild. Biology of Behaviour 3: 193-205.
Yuan, T., Lien, R.J. and G.R. McDaniel. 1994. Effects of increased rearing period body weights and early photostimulation on broiler breeder egg production. Poultry Science 73: 792-800.
Zhang, X., Berry, W.D., McDaniel, G.R., Roland, D.A., Liu, P., Calvert, C. and R. Wilhites. 1999. Body weight and semen production of broiler breeder males as influenced by crude protein levels and feeding regimes during rearing. Poultry Science 78: 190-196.
Zakaria, A.H., Plumstead, P.W., Romero-Sanchez, H., Leksrisompong, N., Osborne, J. and J. Brake. 2005. Oviposition pattern, egg weight, fertility, and hatchability of young and old broiler breeders. Poultry Science 84: 1505-1509.
Zakaria, A.H. and O.H. Omar. 2013. Egg laying pattern, egg weight, body weight at hatch and sex ratio bias relative to oviposition time of young- and mid-age broiler breeders. Animal Reproduction Science 141: 80-85.
Zuidhof, M.J., Holm, D.E., Renema, R.A., Jalal, M.A. and F.E. Robinson. 2015. Effects of broiler breeder management on pullet body weight and carcass uniformity. Poultry Science 94: 1389-1397.
EDGAR O. OVIEDO-RONDÓN, PhD., is a Professor and Extension Specialist in the Prestage Department of Poultry Science at North Carolina State University (United States).
In these roles, his responsibilities include research in broiler and broiler breeder nutrition and management, incubation, and data analytics. His research activities are related to feedstuff quality and processing, evaluation of feed additives - especially enzymes, mineral metabolism, intestinal health, avian bone and muscle development and its impacts on locomotion and meat quality, incubation effects, as well as the application of data analytics and computer modeling.
Dr. Ovideo earned his veterinary degree from the University of Tolima (Colombia) and his master of science in Animal Nutrition and Production from the State University of Maringa (Brazil). His doctorate in Poultry Science was earned at the University of Arkansas (United States) and he recently earned a master’s degree in business administration from North Carolina State University (United States). Dr. Oviedo is the author/co-author of over 100 peer-review publications in scientific journals, has participated in over 300 proceedings at international meetings, nearly 300 abstracts at national and international scientific meetings, and written over 90 press articles, and 10 book chapters.
HUGO ROMERO-SANCHEZ, PhD., is the Executive Global Poultry Solutions Manager at Novus International, where he leads a cross-functional team to identify and improve practical nutritional solutions for poultry customers. Prior to joining Novus he worked with Trouw Nutrition, serving as a poultry nutritionist and technical manager in South America and the United States, and working in the global division where he supported poultry integrations through feed formulation, modeling, and farm management. Dr. Romero-Sanchez received his bachelor of science degree in Zootechnics from La Salle University (Colombia) before completing his master of science degree in Animal Health and Animal Production at the National University of Colombia where he researched energy and protein metabolism in broilers raised at high altitude. He was the recipient of an international scholarship to study at the University of Jerusalem (Israel) and was later appointed assistant professor at the University of Antioquia in Colombia. Selected for a prestigious Fulbright scholarship, he traveled to the United States to earn his doctorate at North Carolina State University. There he studied the effects of male broiler breeder feeding programmes on fertility and broiler performance. Dr. Romero-Sanchez has worked in academia in Colombia for 10 years, and in 2021 joined the Poultry Science Prestage Department at North Carolina State University as an adjunct professor.
Roosters are critical for the reproductive success of broiler breeder flocks. This chapter addresses the main nutritional and management factors that affect male fertility. Fertility is not only related to sperm quality. Male reproductive success requires adequate body weight, physical condition, testis development, testosterone production, and libido. Aging, stress, diseases, and toxicosis can cause oxidative stress and higher corticosterone levels that decrease semen quality, and regress testicles, reducing testosterone levels and libido. Optimum feed amounts during rearing and production provide the energy and protein intake necessary for better rooster development, maintenance, and reproduction. Feed amounts dictate the feeding programme and growth curves that may impact reproductive performance differently. In this chapter, some effects of these growth curves will be discussed, including the impact on broiler progeny. To enhance or recover male fertility, specific nutrients and metabolites can be supplemented as feed ingredients or additives. Among the nutrients for better rooster fertility, we will discuss the effects of trace minerals like zinc, omega-3 PUFA levels, dietary antioxidants like vitamin C, E, CoQ10, and selenium, a variety of phytochemical antioxidants, probiotics, functional amino acids and their metabolites, and other additives. This chapter describes common management practices like photoperiods, conditions at the time of mixing and mating with hens, the stocking density during the laying phase, and the mating ratios. Finally, we included a brief discussion on spiking and intraspiking; management practices to overcome male fertility issues. This information aims to contribute to a comprehensive overview of nutritional and management methods to achieve optimum reproduction in broiler breeders.
The importance of males in the reproductive success of broiler breeder flocks is obvious since one male could be responsible for more than 1,000 fertilized eggs per year (Hocking, 1990; McGary et al., 2002, 2003; Bilcik and Estevez, 2005; Lagares et al., 2017; Wu et al., 2017). It is assumed that if the hen produced an egg, the fertility depended on the presence of spermatozoa in the oviduct. However, broiler breeder hens tend to mate with multiple males; thus, sperm competition through the increase
of motility within the female oviduct may contribute to a differential male’s reproductive success (Bilcik and Estevez, 2005). The female can still influence fertility due to spermatozoa storage in the oviduct (Hocking, 1990; Breque et al., 2003), but the male plays a major role in fertility through mating frequency, mating success, sperm quality, and forward-motility (Bilcik and Estevez, 2005). In natural mating settings, male reproductive fitness is associated with physical condition, size of secondary sexual characters (particularly comb size), appropriate body weight (BW), which should
be synchronic with female BW at mating time; musculoskeletal conformation, body fat composition, testis size, testosterone levels, libido, and sperm quality (Fouad et al., 2020).
Failures of fertilization as measured by fertility in embryodiagnosis through fertility tests frequently occur in broiler breeder flocks (Figure 1) during the initial phase of reproduction and generally after 40 or 45 weeks of age (Avital-Cohen et al., 2013; Sarabia et al., 2013; Lagares et al., 2017). Occasionally, infectious diseases (Villareal et al., 2007; Crespo et al., 2010), heat stress (McDaniel et al., 2004; Ebeid 2009, 2012), and some toxicosis (Vodela et al., 1997; Brake et al., 2000; Ortatatli et al., 2002) can impair male fertility. In contrast, Yegani et al
(2006) reported that Fusarium mycotoxins (6.4 mg of deoxynivalenol/kg, 0.3 mg of zearalenone/kg, and 0.3 mg of 15-acetyldeoxynivalenol/kg of diet) did not affect reproductive parameters of males but can make them more susceptible to diseases like infectious bronchitis that may impair male fertility (Villareal et al., 2007).
The most common fertility issues are related to increased plasma corticosterone levels due to stress and aggression, lower initial testicular weight or testicular regression, and reduced plasma testosterone levels (Hocking and Bernard, 2000; McGary et al., 2003; Sarabia et al., 2013). The semen volume, sperm concentration, characteristics, and composition of the sperm-like viability and
forward motility are important parameters. The polyunsaturated fatty acid (PUFA) and antioxidant concentrations in sperm fluid also decrease as roosters age. Fertility deteriorates because sperm membrane functionality, sperm mitochondrial activity, and sperm-egg penetration are impaired (Bazyar et al., 2019).
Nutrition, feeding control, and management during rearing are necessary to achieve BW targets, appropriate body conformation, testicular development, and maturity development synchronic with females at photostimulation and mating time (van Emous et al., 2020). During the breeding phase, feeding and BW control, management of male : female ratios, lighting control, adequate energy allotments, and optimum energy, protein, amino acid (AA), vitamin, and mineral levels are necessary to minimize the detrimental effects of aging, stress, and male-to-male competition on reproductive organs and, finally, infertility (Silveira et al., 2014; van Emous et al., 2020). Additionally, supplementation of antioxidants, phytochemicals, functional AA, and other sources of fatty acids can modulate physiological mechanisms to mitigate those negative effects in reproduction (Fouad et al., 2020).
Nutrients and feed additives at specific intake levels can exert their effects through activation or inhibition of hormone response, the supply of essential components or cofactors for reproductive function (Scanes and Harvey, 1982). Nutrients and energy levels may directly affect Luteinizing hormone (LH) secretion at the pituitary level or indirectly by decreasing LH-releasing hormone (LHRH) release from the median eminence (Buonomo
et al., 1982). The feed can also contain harmful contaminants such as mycotoxins (Brake et al., 2000; Ortatatli et al., 2002) and heavy metals, which can also come from drinking water (Vodela et al., 1997). This chapter will briefly discuss current and promising interventions to promote reproductive success in broiler breeders.
As in the female, restricted feeding regimes during rearing and breeding have been routinely used in the broiler breeder male to control BW, lengthen reproductive lifespan, and optimise reproduction potential (Hocking, 1990, van Emous et al., 2020). However, if the nutrient intake is insufficient, reproductive performance declines (RomeroSanchez et al., 2007a,b,c, 2008a,b) by affecting libido, semen volume and quality, fertility, and hatchability (Branwell et al., 1996), and there is evidence that this could negatively influence the slaughter BW of the broiler progeny (Attia et al., 1995; Romero-Sanchez et al., 2008a).
Determining the optimum level of daily or weekly feed allocation for each flock is still a challenge because energy and nutrient needs may vary depending on nutrition during rearing, environmental conditions, immunological challenges, and health status, among other management factors (Sakomura et al., 2003; Romero et al., 2009; Reyes et al., 2012; Darmani Kuhi et al., 2011, 2019). Feed energy content is always estimated and may vary greatly depending on feed ingredient quality and processing. The apparent metabolizable energy (ME) values for adult chickens have
been determined with White Leghorn cockerels, and further research is needed for male breeders (Pishnamazi et al., 2005). Consequently, monitoring bird responses to feed by collecting BW, egg numbers, and weight data are still crucial to guide breeder managers in determining those optimum feed amounts every week (van Emous et al., 2020). With the shortage of hand labor in the agricultural sector worldwide, estimating BW with automatic electronic scales and machine vision becomes more important in broiler breeders (Liu et al., 2021).
The current nutritional recommendations from genetic companies are used worldwide as guidelines to feed parent stock. During the rearing phase, males are commonly fed similar diets to those recommended for females with 2,800 or 2,850 kcal of ME/ kg, 19% crude protein (CP) and 0.93-0.95% digestible lysine (dLys) for starter diets, and 14-15% CP and 0.60-0.66% dLys for grower and developer diets. Consequently, feed allocations should be different for males to obtain different target weights at each age according to the growth curves (GC) that are expected to be optimum or ideal. The most recent energy and nutrient recommendations from genetic companies during the breeding phase are summarized in Table 1. The lower need for ME, CP, calcium (Ca), and AA in males justifies a different diet (Silveira et al., 2014) from the females.
Specific ME requirements for pullets and hen breeders have been established for
maintenance, growth, and egg production. Studies with serial analyses of carcass and egg composition (Sakomura et al., 2003; Reyes et al., 2012; Rostagno et al., 2017) and modeling (Romero et al., 2009; Darmani Kuhi et al., 2011, 2019) have been conducted to determine these values. However, energy and CP requirements for growth during the rearing and mating phases to achieve optimum male reproductive performance in natural mating has not been established. The feed allocation values used currently are based on limited research conducted more than 20 years ago, prediction equations, and mainly on experiences within each selection company to maintain the desired BW related to the best fertility observed.
Many studies have reported that limiting nutrient intake, and therefore growth rate during rearing delays the onset of puberty in many species (Nolan et al., 1990; RomeroSanchez et al., 2007 a,b). The effects of decreased nutrient intake related to puberty have been examined primarily at the testicular level in cockerels. When broiler breeder males received low ME diets during the rearing phase, they could maintain their BW with little or no surplus ME for BW gain.
In this scenario, the spermatozoal maturation process and motility might be affected. Few spermatozoa would reach the uterovaginal spermatozoal storage sites resulting in a lower mating frequency and affecting polyspermy (Attia et al., 1995). Although the testicular weights could
Nutrients Unit Hubbard, 2019 Cobb, 2020 Ross, 2021*
Energy kcal 2,800 2,700 2,800
Crude protein % 11.6 - 12.2 13.0 12.0
Lysine (dig) % 0.42 0.50 0.35
Methionine (dig) % 0.23 0.28 0.33
Methionine + Cystine (dig) % 0.40 0.48 0.58
Threonine (dig) % 0.32 0.44 0.43
Valine (dig) % 0.35 0.38 0.47
Tryptophan (dig) % 0.10 0.12 0.15
Arginine (dig) % 0.49 0.55 0.68
Leucine (dig) % 0.66
Isoleucine (dig) % 0.34 0.40 0.41
Histidine (dig) % 0.16
Crude fiber % 4.00 - 6.50
Crude fat % 3.00 - 6.50
Calcium % 0.92 - 0.98 0.95 0.70
Available phosphorus % 0.39 - 0.42 0.42 0.35
Sodium % 0.15 - 0.21 0.15 - 0.24 0.18 - 0.20
Chloride % 0.17 - 0.22 0.15 - 0.24 0.20 - 0.23
Potassium % 0.56 - 0.73 0.60 0.60 - 0.75
Copper mg 10 10 - 15 16
Iodine mg 2 1.5 2
Iron mg 50 20 - 50 40
Manganese mg 100 100 120
Selenium mg 0.30 - 0.40 0.30 0.3
Zinc mg 100 120
Vitamin A IU 12,000 10,000 13,000
Vitamin D3 IU 3,000 3,500 4,000
Vitamin E mg 40-100 100 100
Vitamin K mg 3 3 6
Vitamin B1 mg 3 2.75 5
Vitamin B2 mg 12 8 15
Vitamin B6 mg 4.5 3 5
Vitamin B12 mg 0.03 0.025 0.05
Niacin mg 55 40 50
D-Pantothenic acid mg 14 15 20
Folic acid mg 2 2 3
Biotin mg 0.25 0.25 0.3
Choline mg 750 1400
Linoleic Acid % 0.59 - 2.10 1.25
be adversely affected by a low ME diet, researchers have not consistently observed a clear relationship between testes weights, semen production or quality, libido, and persistence of semen production as the roosters age (Brown and McCartney, 1986; Wilson et al., 1987; McGary et al., 2002, 2003; Sarabia et al., 2013).
Experiments have not been conducted to test the amounts of ME and CP required to feed each day during rearing to achieve the best male reproductive performance during natural mating. But, every breeder farm can obtain growth curve of roosters with good reproductive performance and review the feed intake these roosters had during rearing. Using that information, it is possible to apply modeling methodologies described by Darmani Kuhi et al. (2011) and Darmani Kuhi et al. (2019) to estimate the ME and CP for maintenance (MEm and CPm), net energy, and protein for growth (NEg and CPg). These values can help breeder managers to determine the feed allocation for broiler breeder males more accurately. In the most recent evaluation, Darmani Kuhi et al. (2019) used Aviagen (2017) data of weekly BW, feed intake, and common dietary energy and nutrient concentrations to estimate ME and CP needs using modeling. They applied the monomolecular equation estimating the MEm in 385 kJ/d per kg BW (91.96 kcal/d/ kg BW) and between 10.85 and 12.65 kJ/g BW gain (2.59 -3.02 kcal/g BW). The CPm needed for males was estimated to be 3.74 g/d/kg BW, and the protein requirements for growth were between 0.243 and 0.279 g CP/g BW. This modeling technique proved that maintenance requirements are similar
for both sexes, but cockerel breeders are 1118% more efficient for growth than pullet breeders. The values estimated were very close to those obtained with factorial partitioning models that are more difficult to acquire due to the need for the chemical composition of carcasses (Sakomura et al., 2003; Reyes et al., 2012; Rostagno et al., 2017).
On the other hand, it has been suggested that a cumulative ME requirement previous to photostimulation exists for male broiler breeders. This requirement has been suggested to be between 29.5 and 32 Mcal for Ross 308 males (Romero-Sanchez et al., 2007b; Brake and Peak, 1999). Cumulative nutrient intake, regardless of the feeding programme during the rearing period, should be 29.6 Mcal of ME and 1,470 g of CP to obtain a Ross rooster broiler breeder with adequate BW, frame, and physical attributes at 21 weeks of age able to maintain optimum fertility and produce broiler progeny with the potential for rapid growth throughout the production period (Romero-Sanchez et al., 2007c).
Males seem to need less CP, but the excessive restriction of CP intake from late rearing can delay sexual development and impair fertility. Protein restriction (with isocaloric diets) decreased the circulating concentration of LH in young growing birds (Bruggeman et al., 1998). This effect is more severe in males and accounts for a decreased gonadal weight. However, in an early evaluation by Wilson et al. (1987) with CP levels between 9-15% fed from 7 to 50 weeks of age, no significant effects on semen quality and testes weight were observed. A positive linear impact on male BW was
observed as the CP increased. Reducing CP to 9% decreased male BW, increased carcass fat but had no adverse effects on semen production. Additionally, the percentage of males that produced semen was higher than in the groups fed diets with 12% and 15% CP up to 50 weeks of age. Zhang et al. (1999) also observed reproductive advantages in feeding males of two genetic strains with diets containing 12% CP instead of 16% CP from 4 to 28 weeks of age.
More recently, with new genetic strains more efficient in protein deposition, it was found that males fed 17% CP diet during the rearing period were heavier than those receiving 12% CP diets, but these differences tended to disappear after sexual maturity; however, the reduction in fertility as roosters aged (36-40 weeks) was worse in males fed 17% CP (Romero-Sanchez et al., 2007a). Behura et al. (2014) evaluated cockerel grower diets (6 to 20 weeks) with 2,750 kcal ME/kg and 12%, 14%, and 16% CP. This research group concluded that semen volume, spermatozoa concentration, and the number of spermatozoa per ejaculation were higher in the males fed 12% CP than those of 14% and 16% CP. Only cockerels provided diets with 16% CP were heavier than the other two groups. In an experiment with similar grower diets with 8%, 10%, 12%, and 14% CP (Talukdar et al., 2015), only the 8% CP had 0.5 mL lower semen volume up to 35 weeks and lower semen concentration up to 50 weeks.
In conclusion, feeding female diets with 1415% CP during grower or developer phases is unlikely to have protein or AA deficiencies
in males before photostimulation. More than 15% CP during rearing (6 to 20-22 weeks) can lead to overweight roosters, reduced fat reserves for mating activity, and potentially lower fertility at an advanced age. The minimum dietary concentration of CP for growing cockerels could be close to 10%; this is difficult to observe with current grower and developer pullet breeder diets.
During the mating period, various maintenance ME requirement values have been estimated. Attia (1993) suggested 81-95 kcal ME/kg of BW per d and this value was in agreement with traditional values obtained using different maintenance equations. These traditional maintenance values must have included activity as they were higher than those suggested by Sexton et al. (1989), who estimated the requirement for males in cages to be only 56 kcal ME/kg of BW per d. Although no requirements for sexual activity have been established, Peak (2001), with her prediction model, suggested 7 kcal/kg of BW per d to account for sexual activity during peak fertility. Therefore, a good percentage of fertility from males with different carcass composition is obtained if those males received enough nutrients to meet maintenance and reproductive requirements, assuming that they grew within a normal BW range (Romero-Sanchez et al., 2007a,c).
Several studies have demonstrated the deleterious effects of overfeeding in male broiler breeders (Cerolini et al., 1995; Romero-Sanchez et al., 2007b, 2008a,b).
These negative impacts were generally attributed to excess BW as the major factor negatively affecting fertility (Lopez and Leeson, 1994). However, many data suggest that excessive ME intake can directly affect spermatogenesis irrespective of BW (Robinson et al., 1982; Borges et al., 2006). Overfeeding male Leghorn breeders resulted in a decline in total spermatozoa production with an associated increase in dead spermatozoa. Spermatozoa motility depends on energy intake (Froman et al., 2003), and consequently, ME intake below 320 kcal/d is detrimental. Branwell et al. (1996) had observed a linear decrease in mean sperm penetration holes in the
germinal disc perivitelline layer when feeding between 290 and 370 kcal ME/d. At the end of the experiment, the rooster BW, testis weights, fertility, and hatchability were linearly reduced due to lower ME intake, and the best parameters were observed in roosters fed 370 kcal/d (Table 2). As roosters aged from 38 to 46 weeks of age (Table 3), the number of males producing semen, the ejaculate volume, and total live sperm count tended to decrease (Table 2), especially in roosters fed 290 kcal ME/d.
Ceroline et al. (1995) showed that the highest mean percentage of males producing semen was recorded with a moderate
Table 2. Bodyweight, testis weight, fertility, and hatchability of roosters fed different energy allotments.
from Branwell et al., 1996)
Dietary energy (kcal/d)
Body weight (g) Testis weight
wk
370 5,912 5,889 25.9
(%)
of set
wk 36 wk 44 wk
75.5 78.6 62.0 67.1
330 5,939 5,213 11.7 0.22 83.1 70.3 69.7 62.0
290 5,937 4,620 8.9 0.20 73.9 64.5 62.9 59.2
SEM 103 120 4.31 0.07 4.91 8.96 5.67 8.96
Regression 0.87 0.001 0.01 0.03 0.65 0.001 0.84 0.02
Table 3. Semen traits of broiler breeder
Dietary energy (kcal/d) Age male (wk)
fed
energy allotments. (Adapted from Branwell et al., 1996)
Semen production1 (%) Ejaculate volume (mL) Total live sperm count (x 109/mL)
38 42 46 38 42 46 38 42 46
370 100.0 100.0a 81.8a 0.42a 0.33a 0.26b 3.93b 4.10a 4.77a
330 100.0 72.7b 63.6b 0.37b 0.33a 0.28a 4.38a 3.61b 3.54b
290 100.0 54.5c 36.3c 0.30c 0.27b 0.22c 3.94b 2.86c 2.50c
Regression
0.01 0.01 0.01 0.05 0.05 0.05 0.01 0.01
1Percentage of males producing semen
Means with different superscripts are
Not
Chick age
Hatch 1
AME intake
P- value* 300 kcal/d 340 kcal/d 380 kcal/d
Day 1 39.6 40.5 40.3
Day 21 619 602 609
Day 42 1,937 1,958 1,986 <0.05
Hatch 2
Day 1 43.1 43.4 43.4 Day 21 684 706 706
Day 42 2,063 2,115 2,170 <0.001
Hatch 3
Day 1 45.7 45.8 46.0 Day 21 811 818 834 <0.05 Day 42 2,213 2,221 2,311 <0.001
*Probability that the linear regression coefficients relating chick BW to sire energy allotment were different than zero
restriction of 357 kcal ME/male/d (130g/d) compared with ad libitum programmes or groups that had been more severely restricted to 302-330 kcal ME/male/d. Attia et al (1995) evaluated in two experiments with individually caged roosters the effects of four levels of daily allotments between 280 and 380 ME/d from 24 and 57 weeks of age. These authors did not observe significant effects of ME intake on fertility, hatchability, semen, and plasma testosterone concentrations. However, they observed that BW and testes weight was increased with higher ME intake. Additionally, the ME intake of broiler breeder males showed a significant impact on the BW of the progeny (Attia et al., 1995) from three different hatches (Table 4). In contrast, Branwell et al. (1996) did not detect this sire ME intake on offspring BW up to six weeks, when feeding either 290, 330, or 370 kcal/ rooster/d. Nevertheless, Romero-Sanchez
et al. (2008a) concluded that optimum feed allocation improved fertility, and better fertility is always associated with heavier progeny at 42 days of age and better feed conversion ratio.
Borges et al. (2006) also observed a positive linear effect in rooster BW and body fat as ME intake increased between 290 and 370 kcal/rooster/d. Those experiments were conducted in floor pens with natural mating, and spermatozoa motility, vigor, and fertility were quadratically influenced by ME intake. Consequently, this research group was able to estimate that 346 kcal ME/d was the optimum level for Cobb 500 roosters between 26 and 61 weeks of age. This value corresponds to male diets with 2,660 kcal ME/kg of feed with an average feed intake of 130 grams. These findings and the more recent evaluation of Silveira et al. (2014) support the application
of separate male feeding programme systems that have resulted in better fertility in broiler breeders apparently due to the better control of feed intake in roosters with lower dietary ME content.
Based on previous research and commercial practice, it has been commonly accepted that broiler breeder males could be fed low CP diets during the breeding phase and maintain good fertility as conventional dietary concentrations for females, in the range of 14-16% CP, had deleterious effects on spermatogenesis (Wilson et al., 1987; Hocking, 1989; Tyler and Bekker, 2012; Silveira et al., 2014). The negative impact of high CP diets could be explained by the elevated plasma uric acid concentrations associated with nitrogen excretion from excess protein catabolism. Separate sex feeding during the laying period presents an additional advantage to feeding low CP diets (11.7% to 13%) for roosters (Tyler and Bekker, 2012; Silveira et al., 2014; Graff et al., 2018).
Currently, all nutritional guidelines for parent stock from genetic lines suggest dietary CP concentration between 11.6% and 13% for males during the production phase or approximately 16 g/d (Table 1). However, some poultry companies still feed the same breeder hen diet to both males and females, increasing the daily protein intake to ~20 g/d using 130 g/b/d and 15% CP. This experience is also common in broiler breeder research, and still, good fertility data is observed with small groups of roosters. Male competition and mating activity under natural mating can cause differences in responses to CP concentration under specific environmental
conditions (Bilcik and Estevez, 2005; Saraiba et al., 2013). Most of the results linking CP with fertility have been attributed to either individual performance or increased mortality in some treatments. However, it is important to mention that the level of CP can affect individuals differently. Thus the observed effects may have been due to an altered proportion of males producing semen rather than an effect on semen production and quality itself (Hocking and Duff, 1989; Bilcik and Estevez, 2005; Romero-Sanchez et al., 2007a).
Feeding programmes are essential for flock reproductive success. While small differences in dietary CP intake have been found to produce only slight differences in BW and fertility (Tyler and Bekker, 2012; Graff et al., 2018), small differences in caloric intake affect BW more profoundly (Vaughters et al., 1987; Romero-Sanchez et al., 2007a,b,c). Additionally, the weekly increments of the feed programme may affect growth rate, the growth curve, and body composition during the rearing period, but especially during the pre-lay and early production periods (Buzala and Janicki, 2016).
Male and female breeders can be fed every day or with any of the every other day programme options —skip-a-day, 4/3, 5/2, or 6/1— during rearing. However, each programme may alter breeder nutrient metabolism, hormonal control, uniformity, and reproductive organ development in
2,800
Male Broiler BW at 42 d (g
Figure 2. Effect of the rearing broiler breeder male diet on fertility during the production period and its effects on progeny. Low diet (29,580 kcal ME and 1,470 g CP) and high diet (33,500 of ME and 1,730 g CP), cumulative nutrients at 21 weeks of age. Adapted from Romero-Sanchez et al. (2007b)
pullets (De Beer and Coon, 2007), and similar responses could be expected in males. More than 30 years ago, it was observed that a limited everyday feed restriction programme during the rearing period had been shown to decrease testes weight, semen volume, and spermatozoa concentration when compared to an every-other-day programme (Sexton and Renden, 1988), probably because with the daily programme the efficiency of ME utilization was higher (Leeson and Summers, 1997, 1999),therefore, the roosters received less cumulative ME and CP during the rearing period.
Romero-Sanchez et al. (2007b) compared a low density diet (LoDiet) that provided a cumulative intake of 29,580 kcal of ME and 1,470 g of CP at 21 weeks of age with a high-density diet (HiDiet) with a total intake of 33,500 kcal of ME and 1,730 g of CP. Romero-Sanchez and coworkers (2007b) observed (Figure 2) that the LoDiet increased
fertility (4.5%) during the overall production phase, 28-64 weeks (P<0.01), with a higher difference in the last eight weeks of production. Interestingly, the HiDiet increased BW at 16, 20, and 24 weeks of age, but the difference disappeared after 28 weeks. Males reared on a HiDiet failed to sustain fertility after 36 or 46 weeks of age, and overweight of 200 grams could cause a significant difference in fertility. When the males were classified by BW, the heaviest 50% exhibited the poorest fertility when reared on the HiDiet programme but exhibited the best fertility when reared on the LoDiet programme. Therefore, the data suggested that males could be easily “overrestricted” with mating activity affected due to a ME deficiency (Romero-Sanchez et al., 2007b).
Rearing or production feeding programmes that produced males with higher fertility tended to result in improved broiler progeny performance. This indicated that the males
most probably adversely affected by less than adequate nutrient intake and ME were the heavier males that could produce better performing progeny, i.e., higher BW and better FCR. On the other hand, when there was no difference in fertility, there was no difference in broiler performance.
In another experiment, Romero-Sanchez et al. (2007c) evaluated slow, medium, and fast feed increases (from 84 to 110 g/ bird/d) from 16 to 23, 25, and 26 weeks of age, respectively, with photostimulation at 22 weekss of age (Figure 3A). No significant differences were observed in BW, other than an increased male BW for the slow feed increments. It was observed that the differences in feeding programmes played an important role in subsequent fertility (Figure 3B). The results showed that increasing the male feed allocation programme too rapidly during the period
around photostimulation caused the BW of these birds to reach a plateau at 36 weeks of age, indicative of inadequate nutrient intake to support further BW gains. The detrimental effect that this plateau in BW had on the fertility of birds in the fast and medium groups was evident (Romero-Sanchez et al., 2007c). During the production period, an increase in the male feed allocation of 5 g/male/d for all treatments at 55 weeks of age (RomeroSanchez et al., 2007c; 2020) restored fertility and caused the differences due to either feed allocation programme from 16 to 26 weeks of age or dietary antioxidants during the production phase to disappear. In adequate feed restricted roosters there is a decline in fertility that dietary antioxidants can attenuate; however, when feed allocation is increased, the fertility is restored, showing the benefits of antioxidants on fertility and the bigger effect of the total nutrient intake.
Figure
Male feeding programme
weeks
age,
feeding programmes
was increased by 5 g/male/d. Adapted from Romero-Sanchez
the same amount of
al. (2007a)
Growth can be described mathematically using various linear or non-linear models (Darmanhi Kuhi et al., 2011, 2019). These models have been useful for predicting nutritional requirements and body deposition of nutrients (Peak, 2001, Sterling et al., 2005; Darmanhi Kuhi et al., 2011, 2019). Although most of these GC are sigmoidal, the GC can also be simplistically described as linear, concave, or convex (Leeson and Summers, 1997; Buzala and Janicki, 2016). We can deduce that birds growing on a concave pattern have more efficient feed utilization following this approach. Sigmoid curves can have different interpretations depending upon how body composition was affected.
Although most of this type of research has been conducted in broilers, some data shows male breeders growing in a concave
pattern have a more predictable BW due to greater cumulative intake of CP and ME and lower mortality (Buzala and Janicki, 2016). On the other hand, broiler breeder males growing on a linear or sigmoid curve presented greater BW at an early age, with higher maintenance requirements and lower BW gain (Peak, 2001). The GC shape may determine how the bird deposits ME in lipids and protein, and how the different tissues contribute to heat production (Spratt et al., 1990; Buzala and Janicki, 2016). The avian breast muscle is high in white-type fibers with higher glycolytic potential, higher amounts of glycogen, and lower oxidative metabolism than red fibers. Since the breast muscle can represent a high proportion of the broiler breeder BW it may contribute an important proportion to the bird’s overall heat production and even survival during
stress.
In one experiment, Romero-Sanchez et al. (2007a) evaluated a concave and sigmoid feeding programme (Figure 4) together with two levels of CP during rearing. The data suggested that BW during the early rearing period did not have very obvious effects on fertility (Figure 5A), but that heavy males towards the end of the rearing period from the concave or 17% CP rearing treatments (Figure 5B) had higher maintenance requirements for ME and were unable to sustain fertility after 40 weeks of age without an appropriate increase in the allocation of feed (ME). To prove this point, when the male feed allocation was slowly increased 5 g/ wk, supplying ~15 kcal of ME/male/d during the production period (Romero-Sanchez et al., 2008), there was an improvement in fertility and a favorable impact on progeny performance (Figure 6).
The failure to gain BW consistently and appropriately was most likely the cause for
the lower fertility of the high BW males and constant treatments. Fertility is largely a function of the number of males mating in a flock. In the case of the high BW males, the larger males were most likely not mating, as evidenced by a failure to gain BW, and this resulted in lower broiler progeny BW at 42 days of age (Figure 6).
During the egg production period or breeding phase, feeding programmes should be designed to maintain BW gain rather than attaining some fixed BW. The largest males in a flock would be more subject to nutrient deficiencies (primarily ME) than smaller males and would be the first to reduce semen production and mating activity. In the experiments conducted by Romero-Sanchez et al . (2008b), when there were differences between male breeder treatments there were also differences in the live performance of broiler progeny.
Fertility (%) Broiler BW at 42 d (g
1g/3wks
Indeed, the treatments that produced the best fertility also had the heaviest broiler progeny at 42-d (Figure 2, Figure 6) and the best FCR. Although broiler breeders are supposed to exhibit the same genetic potential, the most plausible interpretation of this data is that there was a reduction in the mating frequency of the heaviest males with the greatest potential to produce heavy BW progeny. Similar results have been previously reported by Attia et al. (1993, 1995). Therefore, there might be an additional benefit of providing sufficient nutrients to maintain mating behaviour and libido of males, increasing flock fertility, and ameliorating growth and feed efficiency of the broiler progeny.
In conclusion, providing sufficient nutrients through a programmed increase in male feed allocation as they age maintained the males’ mating activity and libido as evidenced by
increased fertility of the flock and the positive effects of increased genetic potential for growth and feed efficiency of the broiler progeny.
Trace minerals are key cofactors of many enzymes, especially for cells like spermatozoids with high metabolism rates and nucleic acid replication. Generally, organic forms have better bioavailability and have been evaluated in reproduction (Shan et al., 2017). Zinc (Zn) supplementation has been one of the most investigated for male broiler breeders (Amen and AlDaraji, 2011; Abd et al., 2017). Organic Zn supplementation (100-110 mg Zn/kg diet) increased gonadal maturation, enhanced IGF and testosterone, testicular histology, improved semen quality, morphology
Breeder Management and Nutrition: Moving the industry forward and motility, and consequently fertility (Shanmugam et al., 2015; Jafari et al., 2021).
The presence of high levels of PUFAs omega-6 (n-6), especially arachidonic acid (C20:4 n-6) and docosatetraenoic acid (C22:4 n-6), in avian spermatozoa and seminal plasma provides conditions for dietary incorporation of PUFAs and different antioxidants like vitamin E, ascorbic acid, coenzyme Q10 (CoQ10), phytochemicals, and selenium (Se) to improve fertility (Fouad et al., 2020).
The supplementation of omega-3 PUFAs with fish oils like 4% salmon, or 3% tuna, or 2% menhaden (Blesbois et al., 1997; Surai and Sparks, 2000; Hudson and Wilson, 2003; Surai et al., 2000a, Cerolini et al., 2005) or 2-6% linseed (flaxseed) oil (Abbaspour et al., 2019; Qi et al., 2019; Zanussi et al., 2019) have improved fertility in roosters of different genetic lines. These effects could be due to higher levels of eicosapentaenoic (EPA; C20:5 n3) and docosahexaenoic (DHA, C22:6 n-3), improved energy production by the activation of betaoxidation in the spermatozoa that increases their forward-motility, and enhanced testosterone synthesis by the testicular Leydig cells, semen volume, sperm viability, and total sperm count. However, there are differences in the responses in rooster fertility to inclusion levels of these oils because avian species and genetic lines of poultry differ on fatty acid desaturation and elongation processes and, consequently, the generation of EPA and DHA (Fouad et al., 2020).
Vitamin E deficiency has been shown to negatively affect avian male fertility (Surai, 1999, 2000, 2002) and the whole antioxidant defense system in poultry (Surai et al., 2019). Low vitamin E levels decreased testosterone hormone synthesis in the Leydig cells, thus increasing the feedback mechanism control of LH secretion (Akazawa et al., 1987) and consequently is required to maintain normal testis development (Hooda et al., 2007) and spermatogenesis. It has been suggested that vitamin E plays a role as a natural stabilizer of the spermatozoal plasma membrane (Surai 1999, 2002, Surai et al., 2001, 2019; Zanussi et al., 2019), making spermatozoa more resistant to the stresses incurred during artificial insemination, short term storage, and cryopreservation (Surai, 2002). In experiments with turkeys, the best results were obtained when dietary vitamin E supplementation was increased from 20 to 80 mg/kg. However, supplementations of breeding toms with a 90 mg/kg diet have been observed under commercial conditions (Surai, 1999). The dietary supplementation (100-200 mg Vit E/kg feed) in the alpha-tocopherol content of semen was found to result in a significant reduction in the susceptibility of the semen to lipid peroxidation and improved male fertility (Danikowski et al., 2002; Lin et al., 2005; Min et al., 2018; Zanussi et al., 2019). The susceptibility of semen to peroxidation displayed a very high negative correlation (r = -0.998) with the alpha-tocopherol content of the semen. The vulnerability of testes homogenates to in vitro peroxidation was also reduced by dietary supplementation with alpha-tocopherol (Surai et al., 1998c; Surai et al., 2019). CoQ10 helps the inner
mitochondrial membrane move electrons and protons, generate ATP, and regenerate vitamin E and other antioxidants in the same line of functions as vitamin E. In older roosters, 300 mg CoQ10/kg of feed increased sperm production, motility, membrane integrity, and fertility percentage due to enhanced seminiferous tubule diameter, germinal cell layer thickness, and seminal antioxidant status (Sharideh et al., 2020).
However, different studies have shown contrasting results regarding the additive effects of vitamin E and ascorbic acid on male fertility. For example, dietary supplementation with low doses of vitamins C and E did not show any impact on spermatozoal quality; however, high amounts (300 mg/kg Vit C and 150-200 mg/kg Vit E) of these vitamins decreased the number of spermatozoa/mg of the epididymis and increased the percentage of spermatozoa with misshapen heads (Ten et al., 1997; Min et al., 2018). Regarding ascorbic acid, it has been shown that supplementation with 500 mg/kg of diet in cockerels housed under hot and humid tropical conditions improved semen volume, motile spermatozoa per ejaculate, and spermatozoal numbers per ejaculate (Monsi and Onitchi, 1991). Positive results have been obtained by supplementing another antioxidant like trimethylglycine, better known as betaine, at 1,000 mg/kg (Attia et al., 2019).
In testes from selenium-deficient roosters and toms, fewer Sertoli cells and hierarchies of spermatogonia were committed to spermatid formation than those fed Se (0.3
mg of Se/kg). Furthermore, Leydig cell formations appeared to be less developed in the basal-fed birds, negatively affecting testosterone production and secretion, ejaculate volume, and spermatozoal concentrations (Edens, 2002; Surai and Fisinin, 2014; Gao et al., 2017).
In an attempt to improve broiler breeder male fertility, several antioxidants have been used, and three were evaluated in experiments conducted by Romero-Sanchez et al. (2008b). Organic selenium (SeMe) and antioxidant vitamins (Vitamins C and E) appeared to have an energy-sparing effect when the daily ME allocations were marginal relative to BW. Antioxidants protected against infertility when feed allocations were marginal, but when feedwas increased, the antioxidant benefit disappeared, supporting the supposition that an energy sparing effect was present only when feed amounts were marginal. On the other hand, the data showed contradictory effects of Se source on fertility, embryonic mortality, and fertile hatchability during the production period. SeMe and other sources of Se (sodium selenite Na2SeO3 or nanoSe) are beneficial in semen quality and testis development for older (>55 weeks old) roosters (AlKaabi et al., 2021) or under injection of dexamethasone (Khalil-Khalili et al., 2021), heat stress (Ebeid, 2009, 2012; Shamiah et al., 2017), and cadmium intoxication (Marttová et al., 2013), but not always mitigate infertility and, on occasion, high doses (>0.4 mg/kg) have been shown to be detrimental in some experiments (Surai, 2018). SeMe is incorporated more efficiently in many proteins.
Research studies have shown positive antioxidant effects of several phytochemicals to improve semen traits and fertility in roosters, toms, and quails (Fouad et al., 2020). The supplements evaluated with positive results include curcumin from turmeric (Curcuma longa) at 0.8 mg/kg diet or 30 mg curcumin/rooster/d, gingerol and gingerdione from ginger (Zingiber officinale) at 15 g of ginger root powder/kg diet or 100 mL of ginger essential oil/kg BW, lycopene from tomatoes and fruits at 5 g/L or 0.05 mg/ mL of drinking water, 6 mg of canthaxanthin/ kg diet, 50 mg of chrysin (5,7-dihydroxyflavone a flavonoid and polyphenol) per day, 5 mg of soybean isoflavones per kg of diet, rosemary leaves at 5 g/kg diet, 0.25 mg of cinnamon bark oil/kg diet, and 20% inclusion of dried apple pomace (Fouad et al., 2020).
Probiotics from Saccharomyces cerevisiae, Bacillus subtilis (KATMIRA 1933), Bacillus amyloliquefaciens (B-1895) have been evaluated in aged rooster diets and shown to improve semen quality and fertility. Dietary probiotics boost absorption and utilization of nutrients by improving gut health, antioxidant status elevating plasma concentrations of Vit E and A, and modulating microflora (Fouad et al., 2020). Alpha-lipoic acid has also been evaluated as an antioxidant for aged breeder roosters and was found to improve semen quality (Ye et al., 2021).
The supplementation of L-arginine (Ahangar et al., 2017; Abbaspour et al., 2019), and
endogenous AA or metabolites like D-aspartic acid (Giacone et al., 2017; Ansari et al., 2018), L-carnitine (Elokil et al., 2019), and guanidino acetic acid (Tapeh et al., 2017; Nasirikhah et al., 2019) is demonstrated to improve the fertility of aged roosters (> 55-week-old). L-arginine has been supplemented at 0.14% of the diet, D-aspartic acid at 200 mg/kg BW, L-carnitine at 150 mg/kg diet, and GAA at 1,200 mg/kg feed with positive effects on libido, testicular development, and semen quality. Mechanisms include enhancements in testosterone plasma levels, development of the testes; Sertoli, Leydig, and spermatogonia cells; semen quality characteristics like sperm concentration, membrane integrity, forward motility, and sperm penetration. D-aspartic acid and L-carnitine are involved in mechanisms to suppress lipid peroxidation. L-carnitine also participates in lipid metabolism by transporting long-chain fatty acids into the mitochondria to initiate fatty acid β-oxidation. L-arginine produces GAA and nitric oxide, involved in fatty acid oxidation. GAA is required to make creatine and in its phosphorylated form, phosphocreatine, generates energy for the spermatozoid.
The pubertal phase of testicular development can be hastened by photostimulation from 16 to 18 weeks of age, but if it is not synchronic with BW and development this practice has generally led to the reduced persistency of testicular development (de Reviers, 1977,1980; Tyler and Gous, 2009; Floyd and
Housing
Tyler, 2011; Shi et al., 2021). Higher peak testes weights have been associated with more rapid testicular regression in heavy BW males (de Reviers, 1980). Photostimulation of parent stock males in advance of their female counterparts has been recommended (Etches, 1996) but has not been generally practiced due to the intermingling of sexes in many commercial facilities during the rearing period. Brake (1990), however, showed that when males were reared together with females, an increase in photoperiod from 8L:16D to 10L:14D at 18 weeks of age increased fertility compared to an 8L:16D control group with all birds shifted to 14L:10D at 20 weeks. Some aspects of mating itself may be involved in these results as these males did not differ in BW between treatments. Also, work done with turkey breeders indicated that moving from a rearing light programme with 8h/d of light to a 14h/d at photostimulation can induce a quick onset of sperm production. However, this is followed by a progressive reduction in testes weight and sperm concentration (Noiralt and Brillard, 2000).
From practical experiences (Table 5), it has been noticed that broiler breeder males growing in two housing conditions during
rearing with higher light intensity (open house 650-1200 lux) than the traditional dark house (close house 3-10 lux) for females developed better sexual traits such as comb size and fertility due to more mating activity (Romero-Sanchez et al., 2008b). An optimal day length of 8 hours might increase the photo-stimulatory effect seen on longer days (10 to a maximum of 13 hours) while minimizing the photorefractory decline in sperm production as the rooster ages (Robinson et al., 2003; Lewis, 2006; Tyler and Gous, 2009; Floyd and Tyler, 2011). Several studies have concluded that the male requirement during the production phase daylength should not exceed 13 hours for proper stimulation of hormones and to minimize testes regression (Robinson et al., 2003; Lewis, 2006; Tyler and Gous, 2009; Floyd and Tyler, 2011).
Transfer, mixing, and initial mating are especially stressful for males. In general, males have difficulties finding feed and water and becoming accustomed to the slats;
a time when they also have to become
dominant in the group of males and over the females. Therefore, placing the males several days before the females to familiarize them with the new housing arrangement might be advisable depending on the genetic line. The transfer should not be done after the females, making it more difficult for the males to become dominant. An additional feed increment of 5 to 10 g/d helps stimulate activity, as well as develop and maintain good condition during this transition. Good males stand upright, with their heads higher than those of the females. Good working males are strong with straight legs and toes, good muscle tone, fleshing and body condition. Roosters should be well feathered but display normal wearing due to mating near the vent. Comb and wattles of good size and color should be correlated with evidence of mating activity (Leeson and Summers, 1999; van Emous et al., 2020). When people enter the house, males should pay attention and move towards them. If not, there is a good chance that males are not feeling dominant for proper mating activity. However, the males should not be over-active and overaggressive towards the female or each other (Bilcik and Estevez, 2005).
Stocking density and mating ratio during the breeding phase plays an important role in male-male interactions and reproductive success (Leeson and Summers, 1999; Bilcik and Estevez, 2005; van Emous et al., 2020). Some evidence (Mtileni et al., 2007) indicated that as stocking density of breeders increases from 5 to 8.33 birds/m2, voluntary feed intake decreases along with egg production. Closer male-male interactions (Bilcik and Estevez, 2005) can augment
stress and plasma corticosterone levels, causing shrinkage of testicles and reducing plasma testosterone levels, sperm production, and fertility (Saraiba et al., 2013).
Male-to-female ratio are also a key aspect of management and should be planned depending on the maturity and quality of the males at mixing and reviewed weekly to minimize aggression among males and from males to females (Leeson and Summers, 1999; van Emous et al., 2020). Male to female aggression and overmating can cause feathering problems, injuries, skin scratches, and a reduction in mating and fertility. Nowadays, the optimum initial mating ratio at 22 weeks is close to 9.5 good males per 100 females, and it will be reduced to 8.0 or 9.0 after 50 weeks due to mortality and culling and the rise of more dominant males. Research results (Hazary et al., 2001) indicated that 9.5 males per 100 females increase only 1% flock fertility compared to having nine males/100 females. The removal of excessive males or non-working males from the flock should be a gradual, continuous process. When the number of good active males is reduced below critical limits causing infertility, management practices like spiking andintra-spikingcan be used (Leeson and Summers, 1999; van Emous et al., 2020).
To reach the target BW, males are normally raised separately from females with the same diet but different feeding programmes. However, the male feeding programme becomes a challenge at mating age since
they can access higher amounts of feed both male and female feed. Several practices can be implemented to avoid this situation, like nose bones or the more common grill in the female feeder, or male restaurants for separate feeding before delivering female feed. The male development should mature fast enough to grow bigger heads that cannot access the female feeder (Leeson and Summers, 1999; van Emous et al., 2020).
Despite these practices, some flocks decline in male fertility as roosters age (40 to 50 weeks), and some roosters become overweight. The most common management practices to improve fertility is spiking and intra-spiking. Spiking replaces overweight, non-fitted, worn-out males with males from other younger flocks, normally younger males. Intra-spiking implies moving groups of males inside the breeding house to other areas of the same breeder house or among houses of the same breeder flock (Leeson and Summers, 1999; van Emous et al., 2020). Spiking also helps to overcome mortality issues and can be done with same-age males, but is generally performed with roosters from younger flocks. However, spiking always becomes a biosecurity concern because new males may bring pathogens or viruses from vaccine strains different from those the original flock has been managing. Additionally, spiking implies that an extra number of males should be raised with additional costs to improve the fertility rate. Intra-spiking minimizes these biosecurity concerns but requires more males to be moved. Intra-spiking might be costly and impractical in big flocks due to labor requirements.
The competition between existing males in the house, pen or area, and the new males increases mating activity and increases mortality usually (0.10% to 0.45%). When fertility declines, these practices are shown to work better after 40 to 45 weeks. Spiking requires that 30-35% of the males are replaced (Seyed Sharifi et al., 2018), whileintra-spiking needs to move between 25% and 50% of the roosters. Mphepya et al. (2019) showed that shifting 45% Cobb 500 males in a doubleintraspiking at 40 and 48 weeks worked better to improve fertility and reduce mortality than intra-spiking 25% or 35% of the males.
Males play a key role in reproduction. Nutrition and management are essential in developing cockerels that can produce enough sperm with good forward-motility and maintain semen production and mating behaviour until the end of the production cycle. Energy and crude protein levels should be controlled by means of well-planned feeding programmes that help support growth rates that can produce fitted males for reproduction under each condition. By monitoring BW, body condition, and fertility breeder managers can regulate the responses to the amount and quality of feed offered when determining the next feed allocations within a planned feeding programme. Several antioxidant feed additives, organic trace minerals, functional amino acids, and fatty acids could be used to prevent the negative effects of aging and stress seen in male reproduction. Management control of lighting and mating ratio is necessary to optimise conditions for reproductive success.
Abbaspour, B., S. D. Sharifi, S. Ghazanfari, A. Mo hammadi-Sangcheshmeh, and S. Honarbakhsh. 2019. The effect of l-arginine and flaxseed on plas ma testosterone concentration, semen quality, and some testicular histology parameters in old broiler breeder roosters. Theriogenology, 128: 101-109.
Abd El-Hack M. E., Alagawany M., Arif M., Chaudhry M. T., Emam M., and A. Patra. 2017. Organic or inorganic zinc in poultry nutrition: a re view. World’s Poult Sci J. 73: 904–915.
Ahangar, M., S. Asadzadeh, V. Rezaeipour, and A. Z.Shahneh. 2017. Effects of L-Arginine supple mentation on semen quality, testosterone concen tration and testes histological parameters of Ross 308 breeder roosters. Asian Pac. J. Reprod., 6: 133135.
AlKaabi, A.A.H. and Ali, E.A., 2021. Effect of dos ing of broiler breeder roosters (Ross) with different levels of nano-selenium particles and organic se lenium on reproductive traits A Thesis Submitted. Ann. Romanian Soc. Cell Biol., 25: 3718-3726.
Amen M., and H. J. Al-Daraji. 2011. Effect of dietary zinc on semen quality of Cobb 500 broiler breeder males. Poult Sci., 10: 447–482.
Ansari, M., M. Zhandi, H. Kohram, M. Zaghari, M. Sadeghi, M. Gholami, H. Deldar, M. M. Di Fiore, and A. P. Benson. 2018. D-Aspartate amends re productive performance of aged roosters by chang ing gene expression and testicular histology. Re prod. Fertil. Dev., 30: 1038-1048.
Attia, Y. A., El-Naggar, A. S., Abou-Shehema, B. M. and A. A. Abdella. 2019. Effect of supplementation with trimethylglycine (betaine) and/or vitamins on semen quality, fertility, antioxidant status, DNA re pair and welfare of roosters exposed to chronic heat stress. Animals, 9(8): 547.
Attia, Y. A., K. A. Yamani, and W. H. Burke. 1993. Daily energy allotment and reproductive perfor
mance of broiler breeder males. Poult. Sci. 72:4250.
Attia, Y. A., W. H. Burke, K. A. Yamani, and L. S. Jensen. 1995. Daily energy allotments and per formance of broiler breeders. 1. Males. Poult. Sci. 74:247-260.
Avital-Cohen, N., R. Heiblum, N. Argov-Argaman, A. Rosenstrauch, Y. Chaiseha, N. Mobarkey, and I. Rozenboim. 2013. Age-related changes in gonad al and serotonergic axes of broiler breeder roosters. Domest. Anim. Endocrinol., 44: 145-150.
Aviagen, 2021. Parent stock nutrition specifications. Huntsville, AL. Bazyar, M. Sharafi, A. Shahverdi. 2019. Changes in seminal parameters and hormonal profile with use of aromatase inhibitor in management of aging broiler breeder roosters. Poult. Sci., 98: 6100-6107.
Behura, N. C., Mohanta, K. C., Biswal, C., Kumar, F., Singh, M. K., Mishra, S. K. and G. D. Nayak. 2014. Effects of dietary protein intake on growth and se men quality in broiler breeder males. Anim. Nutr. Feed. Technol. 14(3):617-623.
Bilcik, B. and I. Estevez. 2005. Impact of male–male competition and morphological traits on mating strategies and reproductive success in broiler breed ers. Appl. Anim. Behav. Sci., 92(4): 307-323.
Borges, C. A., H. S. Rostagno, J. H. V. da Silva, L. F. Teixeira Albino, C. Al. Alves Torres, J. J. Filho, M. L. Gomes Ribeiro. 2006. Energy requirements and carcass composition of male broiler breeders as a function of energy intake during the reproduc tion period. R. Bras. Zootec. 35: 1978-1984 doi. org/10.1590/S1516-35982006000700014
Brake, J. 1990. The effect of a 2-hour increase in pho toperiod at 18 week of age on broiler breeder per formance. Poult. Sci. 69:910-914.
Brake, J. 2002. Feeding and management of broiler breeder males.45-67 in: Proc. N.C Broiler Breeder and Hatchery Management Conference, States ville, N.C.
Brake, J., and G. R. Baughman. 1989. A comparison of lighting regimens during growth on subsequent seasonal reproductive performance of broiler breed er. Poult. Sci. 68:79-85.
Brake, J., and S. D. Peak. 1999. Feeding programs for broiler breeders.48-56 in: Proc. N.C Broiler Breed er and Hatchery Management Conference, States ville, NC.
Brake, J., Hamilton, P. B., and R. S. Kittrell. 2000. Effects of the trichothecene mycotoxin diacetoxy scirpenol on feed consumption, body weight, and oral lesions of broiler breeders. Poult. Sci., 79(6): 856-863.
Brake, J., J. D. Garlich, and G. R. Baughman. 1989. Effects of lighting program during the growing period and dietary fat during the laying period on broiler breeder performance. Poult. Sci. 68:11851192.
Breque, C., P. F. Surai, and J. P. Brillard. 2003. Roles of antioxidants on prolonged storage of avian sper matozoa in vivo and in vitro. Mol. Reprod. Dev. 66:314-323.
Bruggeman, V., E. D’Hondt, L. Berghman, O. Onag besan, D. Vanmontfort, F. Vandesande, and E. De cuypere. 1998. The effect of food intake from 2 to 24 weeks of age on LHRH-I content in the medi an eminence and gonadotrophin levels in pituitary and plasma in female broiler breeder chickens. Gen. Comp. Endocrinol. 112:200-209.
Buonomo, F. C., P. Griminder, and C. G. Scanes. 1982. Effects of gradation in protein-calorie re striction on the hypothalo-pituitary-gonadal axis in young domestic fowl. Poult. Sci. 61:800-803.
Buzala, M., and B. Janicki. 2016. Effects of different growth rates in broiler breeder and layer hens on some productive traits. Poult. Sci., 95(9): 21512159.
Cerolini, S., C. Mantovani, F. Bellagamba, M. G. Mangiagalli, L. G. Cavalchini, and R. Reniero. 1995. Effect of restricted and ad libitum feeding
on semen production and fertility in broiler breeder males. Br. Poult. Sci. 36:677-682.
Cobb Vantress, 2020. Breeder Management Guide. Siloam Springs, AR.
Crespo, R., and H. L. Shivaprasad. 2010. Decrease of fertility in a broiler breeder flock due to testicular atrophy. Avian Dis., 54(1): 142-145.
Danikowski, S., H. P. Sallmann, I. Halle, and G. Fla chowsky. 2002. Influence of high levels of vitamin E on semen parameters of cocks. J. Anim. Physiol. Anim. Nutr., 86: 376-382.
Darmani Kuhi, H., Rezaee, F., Faridi, A., France, J., Mottaghitalab, M. and E. Kebreab, 2011. Applica tion of the law of diminishing returns for partition ing metabolizable energy and crude protein intake between maintenance and growth in growing male and female broiler breeder pullets. J. Agric. Sci., 149: 385-394.
Darmani Kuhi, H., López, S., Shabanpour, A., Mohit, A., Falahi, S. and France, J., 2019. Application of sinusoidal equations to partitioning crude protein and metabolizable energy intake between mainte nance and growth in parent stock of broiler chick ens. Iran. J. Appl. Anim. Sci., 9: 299-308. de Reviers, M. 1977. Le development testiculaire chez le coq. V: Action de variations de la duree quotidi enne d’ eclairement. Ann. Biol. Anim. Bioch. Bio phys. 17:179-186. de Reviers, M. 1980. Photoperiodism, testis develop ment and sperm production in the fowl.515-526 in: 9th Int’l. Cong. Animal. Reprod. Artif. Insem, Madrid. de Reviers, M., and F. Seigneurin. 1990. Control of Fertility in Domestic Birds. Pages 220-231 in: In teractions between light regimes and feed restric tions on semen output in two meat-type strains of cockerels. (Les colloques de L’INRA, No. 54) ed. INRA, Tours, France. de Reviers, M., and J. B. Wiliams. 1984. Testis de velopment and production of spermatozoa in the
Breeder Management and Nutrition: Moving the industry forward
cockerel (Gallus domesticus). Pages 183-202 in: Reproductive Biology of Poultry. F. J. Cunningham, P. E. Lake, and D. Hewitt, eds. Brit. Poultry Sci. Ltd (Longman Group), Harlow, UK. de Reviers, M., and J. Williams. 1981. Predicting the adult daily sperm output after the first ejaculates in cockerels
Ebeid, T. A., 2009. Organic selenium enhances the antioxidative status and quality of cockerel semen under high ambient temperature. Br. Poult. Sci., 50: 641-647.
Ebeid, T.A., 2012. Vitamin E and organic selenium enhances the antioxidative status and quality of chicken semen under high ambient temperature. Br. Poult. Sci., 53(5): 708-714.
Edens, F. W. 2002. Practical aplications of selenome thionine:broiler breeder reproduction. Pages 349376 in: Nutritional Biotechnology in the Feed and Food Industry. Proceedings of Alltech’s 18th An nual Symposium. T. P. Lyons and K. A. Jacques, eds. Nottingham University Press, Nottingham. Elokil, A. A., A. A. Bhuiyan, H. Z. Liu, M. N. Hus sein, H. I. Ahmed, S. A. Azmal, L. Yang, and S. Li. 2019. The capability of L-carnitine-mediated an tioxidant on cock during aging: evidence for the improved semen quality and enhanced testicular expressions of GnRH1, GnRHR, and melatonin receptors MT ½. Poult. Sci., 98: 4172-4181.
Etches, R. J. 1996. Reproduction in Poultry. CAB In ternational, Cambridge.
Fouad, A. M., El-Senousey, H. K., Ruan, D., Xia, W., Chen, W., Wang, S., and C. Zheng. 2020. Nutri tional modulation of fertility in male poultry. Poult. Sci. 99:5637-5646.
Floyd, M. H. and N. C. Tyler. 2011. Photostimulation of male broiler breeders to different photoperiods. South African Journal of Animal Science, 41: 146155.
Froman, D.P., Bowling, E.R., Wilson, J.L. 2003. Sperm mobility phenotype not determined by
sperm quality index. Poultry Science, 82: 496-502. Gao, Y., J. Zhang, X. Huang, and G. Zhang. 2017. Glutathione peroxidase 1, selenoprotein K, and selenoprotein H may play important roles in chick en testes in response to selenium deficiency. Biol. Trace Elem. Res., 179: 271-276.
Giacone, F., R. A. Condorelli, L. M. Mongioì, V. Bullara, S. La Vignera, and A. E. Calogero. 2017. In vitro effects of zinc, D-aspartic acid, and coen zyme-Q10 on sperm function. Endocrine 56: 408415.
Graaf, B., Ciacciariello, M., and N. C. Tyler. 2018. The effect of crude protein intake on sperm quality of young and old male broiler breeders. S. Afr. J. Anim. Sci., 48(6).
Hazary, R. C., Staines, H. J., and G. J. Wishart. 2001. Assessing the effect of mating ratio on broiler breeder performance by quantifying sperm: egg in teraction. J. Appl. Poult. Res., 10:1-4.
Hocking, P. M. 1990. Influence of the male on the decline of fertility with age in broiler breeder flocks. Pages 213-218 in: Control of fertility in domestics birds, Les Colloques de I’INRA. Vol. 54. J. P. Bril lard, ed, Paris.
Hocking, P. M., A. B. Gilbert, M. Walker, and D. Waddington. 1987. Ovarian follicular structure of White Leghorns fed ad libitum and dwarf and normal broiler breeders fed ad libitum or restricted until point of lay. Br. Poult. Sci. 28:493-506.
Hocking, P. M., and G. W. Robertson. 2000. Ovarian follicular dynamics in selected and control (relaxed selection) male- and female-lines of broiler breed ers fed ad libitum or on restricted allocations of food. Br. Poult. Sci. 41:229-234.
Hocking, P. M., and S. R. Duff. 1989. Musculo-skel etal lesions in adult male broiler breeder fowls and their relationships with body weight and fertility at 60 weeks of age. Br. Poult. Sci. 30:777-784.
Hocking, P. M., D. Waddington, M. A. Walker, and A. B. Gilbert. 1989. Control of the development of
Breeder Management and Nutrition: Moving the industry forward
the ovarian follicular hierarchy in broiler breeder pullets by food restriction during rearing. Br. Poult. Sci. 30:161-173.
Hooda, S., P. K. Tyagi, J. Mohan, A. B. Mandal, A. V. Elangovan, and K. Tyagi Pramod. 2007. Effects of supplemental vitamin E in diet of Japanese quail on male reproduction, fertility and hatchability. Br. Poult. Sci., 48: 104-110.
Jafari, M., Irani, M. and V. Rezaeipour. 2021. Effect of different dietary zinc sources on the semen quality, testicular histology and sex hormone concentra tion in broiler breeder roosters. Ital. J. Anim. Sci. 20:489-496.
Jones, J. E., B. L. Hughes, and K. A. Wall. 1977. Effect of light intensity and source on tom reproduction. Poult. Sci. 56:1417-1420.
Khalil-Khalili, A. A., Zhandi, M., Zaghari, M., Meh rabani-Yeganeh, H., Yousefi, A. R., and M. Tava koli-Alamooti. 2021. The effect of dietary organic selenium on reproductive performance of broiler breeder roosters under dexamethasone induced stress. Theriogenology, 161, pp.16-25.
Lagares, M. A., R. Ecco, N. R. S. Martins, L. J. C. Lara, J. S. R. Rocha, D. A. R. Vilela, V. M. Barbosa, P. F. Mantovani, J. F. V. Braga, I. S. Preis, and V. A. Gheller. 2017. Detecting reproductive system ab normalities of broiler breeder roosters at different ages. Reprod. Domest. Anim., 52: 67-75.
Leeson, S., and J. D. Summers. 1997. Commercial Poultry Nutrition. University Books, Guelph, On tario, Canada.
Leeson, S., and J. D. Summers. 1999. Broiler Breed er Production. University Books, Guelph, Ontario, Canada.
Leeson, S., and J. D. Summers. 2001. Nutrition of the Chicken. 4th ed. University Books, Guelph, Ontar io, Canada.
Lewis, P. D. 2006. A review of lighting for broiler breeders. British Poultry Science, 47: 393-404.
Lin, Y. F., S. J. Chang, J. R. Yang, Y. P. Lee, and A.
L. Hsu. 2005.Effects of supplemental vitamin E during the mature period on the reproduction per formance of Taiwan native chicken cockerels. Br. Poult. Sci., 46: 366-373.
Liu, D., Vranken, E., Van Den Berg, G., Carpentier, L., Fernández, A.P., He, D. and T. Norton. 2021. Sepa rate weighing of male and female broiler breeders by electronic platform weigher using camera technolo gies. Comput. Electron. Agric., 182: 106009. Lopez, G., and S. Leeson. 1994. Nutrition and broiler breeder performance. J. Appl. Poult. Res. 3:303-312. Lopez, G., and S. Leeson. 1995. Response of broiler breeders to low-protein diets. 1. Adult breeder per formance. Poult. Sci. 74:685-695.
Marettová, E., Maretta, M., and J. Legáth. 2013. Ef fect of Cd with or without Se supplementation on spermatogenesis and semen quality in the rooster (Gallus gallus). Avian Biol. Res., 6(4): 275-280.
McDaniel, C. D., Hood, J. E., and H. M. Parker. 2004. An attempt at alleviating heat stress infertility in male broiler breeder chickens with dietary ascorbic acid. Int. J. Poult. Sci., 3(9): 593-602.
McGary, S., I. Estevez, and M. R. Bakst. 2003. Poten tial relationships between physical traits and male broiler breeder fertility. Poult. Sci. 82:328-337.
McGary, S., I. Estevez, M. R. Bakst, and D. L. Pol lock. 2002. Phenotypic traits as reliable indica tors of fertility in male broiler breeders. Poult. Sci. 81:102-111.
Min, Y. N., Z. Y. Niu, T. T. Sun, Z. P. Wang, P. X. Jiao, B. B. Zi, P. P. Chen, D. L. Tian, and F. Z. Liu. 2018. Vitamin E and vitamin C supplementation improves antioxidant status and immune function in oxidative-stressed breeder roosters by up-regu lating expression of GSH-Px gene. Poult. Sci., 97: 1238-1244.
Mphepya, L. C., van Rensburg, W. J., Mpofu, T. J., Mtileni, B. J. and K. A. Nephawe. 2019. Influence of male–male competition on reproductive perfor mance and mortality of broiler breeders following
intra-spiking. Poult Sci., 98: 4549-4554.
Mtileni, B. J., Nephawe, K. A., Nesamvuni, A. E., and K. Benyi. 2007. The influence of stocking density on body weight, egg weight, and feed intake of adult broiler breeder hens. Poult. Sci., 86: 1615-1619.
Nasirikhah, A., M. Zhandi, M. Shakeri, M. Sade ghi, M. Ansari, H. Deldar, and A. R. Yousefi. 2019. Dietary Guanidinoacetic acid modulates testicu lar histology and expression of c-Kit and STRA8 genes in roosters. Theriogenology, 130: 140-145. Nolan, C. J., D. A. Neuendorff, R. W. Godfrey, P. G. Harms, T. H. Welsh, Jr, N. H. McArthur, and R. D. Randel. 1990. Influence of dietary energy intake on prepubertal development of Brahman bulls. J. Anim. Sci. 68:1087-1096.
NRC. 1994. Nutrient Requirement of Poultry. Na tional Research Council, Washington, DC. Orunmuyi, M., Akanwa, C. L., and N. B. Ifeanyi. 2013. Semen quality characteristics and effect of mating ratio on reproductive performance of Hub bard broiler breeders. J. Ag. Sci. (Toronto), 5:154159.
Peak, S. D. 2001. Development of a bioenergetic growth model to determine the effect of feed allo cation program on male broiler breeder growth and performance. PhD Dissertation, North Carolina State Universiy, Raleigh, NC.
Pishnamazi, A., Pourreza, J., Edriss, M.A., and Samie, A.H., 2005. Influence of broiler breeder and laying hen breed on the apparent metabolizable energy of se lected feed ingredients. Int. J. Poult. Sci, 4(3): 163-166.
Reyes, M. E., Salas, C. and C. N. Coon. 2012. Metab olizable energy requirements for broiler breeder in different environmental temperatures. Int. J. Poult. Sci, 11(7): 453-461.
Robinson, F.E., G.M Fasenko., R.A Renema. 2003. Optimizing Chick Production in Broiler Breeders. Spotted Cow Press. Edmonton, Alberta, Canada.
Romero, L. F., Zuidhof, M. J., Renema, R. A., Robin son, F. E., and A. Naeima. 2009. Nonlinear mixed
models to study metabolizable energy utilization in broiler breeder hens. Poult. Sci., 88(6):1310-1320.
Romero-Sanchez, H., P.W. Plumstead, and J. Brake. 2007a. Feeding broiler breeder males.1. Effect of feeding program and dietary crude protein during rearing on body weight and fertility of broiler breeder males. Poult. Sci. 86:168-174.
Romero-Sanchez, H., P.W. Plumstead, and J. Brake. 2007b. Feeding broiler breeder males.3. Effect of feed allocation program from sixteen to twenty-six weeks and subsequent feed increments during the production period on body weight and fertility. Poult. Sci. 86:775-781.
Romero-Sanchez, H., P.W. Plumstead, N. Leks risompong, and J. Brake. 2007c. Feeding broiler breeder males.2. Effect of cumulative rearing nu trition on body weight, shank length, comb height, and fertility. Poult. Sci. 86:175-181.
Romero-Sanchez, H., P.W. Plumstead, N. Leks risompong, K.E., Brannan and J. Brake. 2008a. Feeding broiler breeder males.4. Deficient feed al location reduces fertility and broiler progeny body weight. Poult. Sci. 87: 805-811.
Romero-Sanchez, H, Freitas, R, and J. Brake, 2008b. Male broiler breeder management and nutrition: from the experimental research to the field expe rience. Proc. World’s Poultry Congress XXIII Ab stracts: 312. Brisbane, Australia.
Sakomura, N. K., Silva, R., Couto, H. P., C. Coon, and C. R. Pacheco. 2003. Modeling metabolizable energy utilization in broiler breeder pullets. Poult. Sci., 82(3): 419-427.
Sarabia, F. J., M. Pizarro Diaz, J. C. Abad Moreno, P. Casanovas Infesta, A. Rodriguez-Bertos, and K. Barger. 2013. Relationships between fertility and some parameters in male broiler breeders (body and testicular weight, histology and immunohisto chemistry of testes, spermatogenesis and hormonal levels). Reprod. Domest. Anim., 48: 45-352.
Sexton, K. J., and J. A. Renden. 1988. Effects of feed
ing regimen during early development on body composition, gastrointestinal tract size, and semen quality of broiler breeder cockerels after matura tion. Poult. Sci. 67:835-841.
Sexton, K. J., J. A. Renden, D. N. Marple, and R. J. Kempainen. 1989. Effects of dietary energy on se men production, fertility, plasma testosterone, and carcass composition of broiler-breeder males in cages. Poult. Sci. 68:1688-1694.
Seyed Sharifi, R., Mahdavi, D., Hedayat Evrigh, N., Seifdavati, J. and Sahraei, M., 2018. Investigating the effects of intra spiking flocks on fertility pro ductivity in economic viewpoint. J. Anim. Environ. Sci., 10: 73-78.
Shamiah, S. M., El-Karim, A., Ragaa, E., Eshera, A. A., Fouda, S. F., and H. K. Zaghloul. 2017. Effects of dietary selenomethionine supplementation on semen quality, fertility and antioxidant status of cockerels. EJNF, 20: 227-236.
Sharideh, H., Zeinoaldini, S., Zhandi, M., Zaghari, M., Sadeghi, M., Akhlaghi, A. and E. D. Peebles. 2020. Use of supplemental dietary coenzyme Q10 to improve testicular function and fertilization ca pacity in aged broiler breeder roosters. Theriogenol ogy 142: 355-362. Shi, L., Li, Y., Yuan, J., Ma, H., Wang, P., Ni, A., Ge, P., Chen, C., Li, D., Sun, Y. and J. Chen. 2021. Ef fects of age at photostimulation on sexual maturity and reproductive performance in rooster breeders. Poult. Sci., 100: 101011.
Silveira, M. M., A. G. de Freitas, C. A. Moraes, F. S. Gomes, F. H. Litz, J. M. S. Martins, N. S. Fagundes, E. A. Fernandes. 2014. Feeding management strategy for male broiler breeders and its effects on body weight, hatchability and fertility. Brazil. J. Poultry Sci., 16(4): 397-402. 10.1590/1516-635x1604397-402
Spratt, R. S., B. W. McBride, H. S. Bayley, and S. Lee son. 1990. Energy metabolism of broiler breeder hens. 2. Contribution of tissues to total heat produc tion in fed and fasted hens. Poult. Sci. 69:1348-1356.
Sterling, K. G., D. V. Vedenov, G. M. Pesti, and R. I. Bakalli. 2005. Economically optimal dietary crude protein and lysine levels for starting broiler chicks. Poult. Sci. 84:29-36.
Surai, P. F. 1999. Vitamin E in avian reproduction. Poult. Avian. Biol. Rev. 10:1-60.
Surai, P. F. 2000. Effect of the selenium and Vitamin E content of the maternal diet on the antioxidant system of the yolk and the developing chick. Br. J. Nutr. 41:235-243.
Surai, P. F. 2002. Natural Antioxidants in Avian Nu trition and Reproduction. First ed. Nottingham University Press, Nottingham, UK.
Surai, P. F., B. K. Speake, R. C. Noble, and N. H. Sparks. 1999. Tissue-specific antioxidant profiles and susceptibility to lipid peroxidation of the newly hatched chick. Biol. Trace Elem. Res. 68:63-78.
Surai, P. F., E. Blesbois, I. Grasseau, T. Chalah, J. P. Brillard, G. J. Wishart, S. Cerolini, and N. H. Sparks. 1998a. Fatty acid composition, glutathione peroxidase and superoxide dismutase activity and total antioxidant activity of avian semen. Comp. Biochem. Physiol. B. Biochem. Mol. Biol. 120:527533.
Surai, P. F., I. I. Kochish, M. N. Romanov, and D. K. Griffin. 2019. Nutritional modulation of the anti oxidant capacities in poultry: the case of vitamin E. Poult. Sci. 98: 4030-4041.
Surai, P. F., I. Kostjuk, G. Wishart, A. Macpherson, B. Speake, R. Noble, I. Ionov, and E. Kutz. 1998c. Effect of Vitamin E and selenium supplementation of cockerel diets on glutathione peroxidase activ ity and lipid peroxidation susceptibility in sperm, testes, and liver. Biol. Trace Elem. Res. 64:119-132.
Surai, P. F., R. C. Noble, and B. K. Speake. 2001. Re lationship between Vitamin E content and suscep tibility to lipid peroxidation in tissues of the newly hatched chick. Br. J. Nutr. 40:406-410.
Surai, P. F., S. Cerolini, G. J. Wishart, B. K. Speake, R. C. Nobel, and N. H. C. Sparks. 1998b. Lipid and
Breeder Management and Nutrition: Moving the industry forward
antioxidant composition of chicken semen and its susceptibility to peroxidation. Poult. Avian. Biol. Rev. 9:11-23.
Surai, P.F. and V. I. Fisinin. 2014. Selenium in poultry breeder nutrition: An update. Animal Feed Science and Technology, 191, pp.1-15.
Talukdar, D., Behura, N. C., Sethy, K., Mishra, P. C., Nayak, G. D., Mishra, S. K., Behera, K., and C. Mishra. 2015. Effects of different levels of dietary crude protein on growth and reproductive perfor mance of broiler breeder birds. Int. J. Bio-resour. Stress Manag. 6(6): 667-672.
Tapeh, R. S., M. Zhandi, M. Zaghari, and A. Akh laghi. 2017. Effects of guanidinoacetic acid diet supplementation on semen quality and fertility of broiler breeder roosters. Theriogenology, 89: 178182.
Tyler, N. C. and R. M. Gous. 2009. The effect of pho toperiod on testis weight and comb area of male broiler breeders. Br. Poult. Sci, 52: 517-522.
Tyler, N. C., and H. A. Bekker. 2012. The effect of dietary crude protein on the fertility of male broiler breeders. S. Afr. J. Anim. Sci., 42(3): 304-309.
Tyler, N.C. and R. M. Gous. 2009. The effect of age at photostimulation of male broiler breeders on tes tes growth and the attainment of sexual maturity. S. Afr. J. Anim. Sci., 39: 169-175.
Van Emous, R., J. Holleman, and T. van Schie. 2020. Breeder signals: A practical guide to optimal man agement of broiler breeders. 187 pp. Roodbont Publishers. ISBN 9789087403324.
Villarreal, L. Y. B., Brandão, P. E., Chacón, J. L., As sayag, M. S., Maiorka, P. C., Raffi, P., Saidenberg, A. B. S., Jones, R. C., and A. J. P. Ferreira. 2007. Orchi tis in roosters with reduced fertility associated with avian infectious bronchitis virus and avian metap neumovirus infections. Avian Dis., 51(4): 900-904.
Vodela, J.K., Lenz, S.D., Renden, J.A., McElhenney, W.H. and Kemppainen, B.W., 1997. Drinking water contaminants (arsenic, cadmium, lead, benzene, and trichloroethylene). 2. Effects on reproductive perfor mance, egg quality, and embryo toxicity in broiler breeders. Poultry Science, 76(11), pp.1493-1500.
Walsh, T. J., and J. Brake. 1997. The effect of nutrient intake during rearing of broiler breeder females on subsequent fertility. Poult. Sci. 76:297-305.
Walsh, T. J., and J. Brake. 1999. Effects of feeding program and crude protein intake during rearing on fertility of broiler breeder females. Poult. Sci. 78:827-832.
Ye, N., Lv, Z., Dai, H., Huang, Z., and F. Shi. 2021. Dietary alpha-lipoic acid supplementation im proves spermatogenesis and semen quality via an tioxidant and anti-apoptotic effects in aged breeder roosters. Theriogenology, 159, pp.20-27.
Yegani, M., Smith, T. K., Leeson, S. and H. J. Boer mans. 2006. Effects of feeding grains naturally con taminated with Fusarium mycotoxins on perfor mance and metabolism of broiler breeders. Poult. Sci., 85(9): 1541-1549.
Zanussi, H. P., F. Shariatmadari, M. Sharafi, and H. Ahmadi. 2019. Dietary supplementation with flax seed oil as source of omega-3 fatty acids improves seminal quality and reproductive performance in aged broiler breeder roosters. Theriogenology, 130: 41-48.
Zhang, X., Berry, W. D., McDaniel, G. R., Roland, D. A., Liu, P., Calvert, C., and R. Wilhite. 1999. Body weight and semen production of broiler breed er males as influenced by crude protein levels and feeding regimens during rearing. Poult. Sci., 78(2): 190-196.
REBECCA FORDER, PhD., is a Senior Lecturer at the School of Animal and Veterinary Sciences at the University of Adelaide (Australia) where she teaches physiology and poultry production. Her expertise in gastrointestinal physiology has complemented and strengthened projects aiming to develop novel dietary strategies to enhance gastrointestinal development and improve the lifelong productivity of animals in intensive farming systems. Through invaluable mentorship by Professor Philip Hynd (University of Adelaide), Dr. Forder’s interests have expanded into the area of developmental programming and how the maternal environment influences offspring growth and performance as a means to optimise commercial breeder management.
Environmental conditions during gestation/egg formation, primarily involving stress and nutrition, have the capacity to contribute, enhance or disrupt the programming of physio logical mechanisms that regulate progeny growth, health, behaviour, and production. This phenomenon, known as maternal or developmental programming can be affected by en vironmental stressors that compromise the maternal environment experienced by devel oping offspring, resulting in permanent physiological alterations to offspring phenotype, further impacting their health and productive performance. The link between maternal stress and developmental programming is becoming increasingly evident in both mam malian and avian literature, and such investigations are highly translatable to commercial breeder hens given the demand for high egg production and feed restriction practices. Mechanisms mediating the effects of maternal stress on offspring phenotype have been previously investigated in both mammalian and avian species; these include endocrine axes, epigenetic modification, and immune and intestinal microbiota modulation. Taking advantage of the potential transgenerational maternal effects within the breeding system pyramid may enable a targeted alternative approach regarding nutrition or other sup plemental interventions at significant physiological time points. Optimising health and reproductive output through the implementation of novel breeder management strategies can positively influence all facets of production, including improved behaviour, immunity, growth, and efficiency of subsequent generations.
Developmental programming is defined as “alterations in the foetal or in ovo environment, induced by the maternal environment, resulting in developmental adaptations that permanently change the structure, physiology, metabolism, health and production of the offspring” (Wu et al., 2004). Such adaptations can be induced by several environmental factors, including maternal nutrition and stress, which compromises the maternal environment provided during gestation/egg formation resulting in permanent physiological
alterations to offspring phenotype impacting their growth, health, behaviour, and productive performance (Reynolds et al., 2010; Hynd et al., 2016; Figure 1).
Physiological alterations can be induced directly through altered organ development and disrupted endocrine axes development (Henriksen et al., 2011b), which may be mediated through epigenetic effects with potential transgenerational impacts (Chango and Pogribny, 2015). Additionally, the microbial environment housed by the mother generates enormous interest through
Altered in ovo environment
Altered embryonic development
Altered offspring phenotype
Figure 1. Developmental programming. External stressors (health-related, nutritional, or environmental) experienced by the breeder hen can potentially alter the development of her offspring
its potential to contribute to environmental factors influencing the maternal environment via the brain-gut-microbiota axis and its potential influence on developmental programming mechanisms.
Pioneering work by Ho et al. (2011), utilising a yolk cross-fostering technique, elegantly demonstrated that a complex synergistic effect of breed-specific genotype and yolk environment exists very early in embryo development. This study has paved the way for further investigations into how both the maternal and egg environment can alter chick growth and performance. Recent findings from our group have demonstrated that maternal stress and nutrition in broilers can have significant negative effects on progeny body weight, stress-linked behaviour, immune response (Hynd et al., 2016; Bowling et al., 2018), and body composition (Angove et al., 2020). Considering commercial chicken meat birds now spend a third of their life inovo, the influence of current breeder hen practices on progeny development and links to maternal stress may provide a pathway
to improve production and management. Thus, through maternal programming mechanisms, there is substantial opportunity to optimise breeder hen health, reproductive capacity, and welfare standards. This chapter will focus primarily on maternal stress and the potential mechanisms responsible for embryonic reprogramming and consequent influence on offspring phenotype.
Maternal stress is well documented to influence progeny growth rate in various species and is a pressing issue in the broiler breeder industry due to current feed restriction practises (Tahamtani et al., 2020). Feed restriction allows hens to maintain optimum body weight and reproductive capacity, however, may result in hens experiencing chronic stress due to prolonged hunger (de Jong and Guemene, 2011). Several studies have shown that hens subjected to feed restriction exhibit elevated stress biomarkers, including plasma corticosterone levels and heterophil:lymphocyte ratios (de Jong et al., 2002; Najafi et al., 2015).
Gestational stress in mammalian species is linked with reductions in birth weight (Sloboda et al., 2005), permanent hypertension (O’Regan et al., 2001; Nuyt, 2008), hyperglycaemia/hyperinsulinemia (O’Regan et al., 2001), behavioural alterations, reduced immunocompetence, and endocrine axes disruption (Hyatt et al., 2007; Henriksen et al., 2011a). Similar physiological impacts have been noted in avian species in response to feed restrictions, malnourishment, and adverse environmental conditions, such as cognitive disruption, anxiety and aggressive behaviours (Lindqvist et al., 2007; Ahmed et al., 2014a); delayed sexual maturation (Henriksen et al., 2011a), compromised T-cell- and B-cellmediated immunit (Love et al., 2005), and elevated baseline plasma testosterone concentrations (Henriksen et al., 2013).
The ramifications of maternal stressors and their ability to influence the hormonal and nutritional composition of the egg are complex (Groothuis et al., 2019). Corticosterone depositions in the yolk of the egg have been identified, providing a link between maternal stress and progeny development in egg-laying species, similar to that of placental transfer in mammals (Seckl 2004; Henriksen et al., 2011b). However, the mechanisms as to how this affects the progeny are still elusive (Angove et al., 2020). It has been hypothesized that the initial exposure of glucocorticoids within the in ovo environment may influence embryonic developmental processes, including that of endocrine pathways, such as the hypothalamic–pituitary–adrenal (HPA) axis (Ahmed et al., 2014b; Vassallo et al., 2019).
Figure 2. The hypothalamo-pituitary (HPA) axis in response to stress. Hypothalamus (H); Pituitary Gland (P); Adrenal Glands (A); corticotropin-releasing hormone (CRH); adrenocorticotrophic hormone (ACTH); corticosterone (CORT)
The regulation of the HPA axis and its role in the stress response is well-reviewed in literature both for mammals (Sheng et al., 2020) and birds (Smulders, 2021). During a stressful event, neurons in the paraventricular nucleus (PVN) of the hypothalamus are stimulated to emit a corticotropin-releasing hormone (CRH), which results in increased adrenocorticotrophic hormone (ACTH) from the anterior pituitary, which acts on the adrenal cortex (zona fasciculata), elevating plasma corticosteroids (e.g., cortisol and corticosterone; Herman et al., 2012).
The HPA axis and blood corticosterone concentrations are maintained homeostatically via a negative-feedback loop (Figure 2). When blood corticosterone levels are elevated, they act on the glucocorticoid receptors (GR) within the PVN of the hypothalamus and anterior pituitary, reducing CRH and ACTH release, respectively, and therefore, corticosterone (Herman et al., 2012). When this feedback mechanism is unable to maintain this balance, in the case of chronic stress, corticosterone levels remain elevated. In mammals, overexposure to cortisol during pregnancy can affect this feedback loop by reducing the sensitivity of the GR and elevating cortisol (Seckl and Holmes, 2007). When this occurs in avian species, it can result in increased corticosterone deposition within the egg and, as a consequence, increased corticosterone exposure to the embryo (Hayward et al., 2006; Royo et al., 2008; Henriksen et al., 2013).
The mechanisms behind endocrine axis reprogramming in response to maternal stress continue to be investigated. One area believed to be influenced is the glucocorticoid receptor (GR), expressed predominately in the PVN of the hypothalamus (Talge et al., 2007) and highly expressed in the brain during development (Seckl and Holmes, 2007). It is thought that as the plasma levels of corticosterone increase, the sensitivity of these receptors is decreased, reducing negative feedback and increasing circulating corticosterone levels (Seckl and Holmes, 2007).
Alternatively, expression of the GR gene may be altered due to methylation of the promoter region of the gene (Parade et al., 2016). In rodents and humans, such
methylation events are responsive to many forms of prenatal and maternal stressors (Szyf and Bick, 2013; Lillycrop and Burdge, 2015). In rodents, decreased nurturing behaviour by the dam resulted in increased methylation of the hippocampal GR gene of the offspring and, as methylation blocks transcription, resulted in decreased GR expression (Weaver et al., 2004). A recent study in zebra finches also reported a link between methylation of the GR gene and hatchling stress, demonstrating that similar mechanisms exist in birds (Jimeno et al., 2019). Decreased GR gene expression could therefore lead to reduced negative feedback to glucocorticoids, and increased circulating corticosterone.
Corticosterone is also known to interact with other important endocrine axes, such as growth hormone/insulin-like growth factor-I (GH/IGF-I) axis through the promotion of hypothalamic somatostatin release, an inhibitor of GH. HPA axis disruption has the potential to interfere with the GH/IGF-I axis and the hypothalamic-pituitary-thyroid axis (De Groef et al., 2008), both of which are potent stimulators of growth and metabolism in avian species (Xiao et al., 2017). The functionality of the GH/IGF-I axis can thus have the potential to be influenced by maternal stressors either through neuroendocrine or epigenetic reprogramming, reducing the occurrence of growth-promoting events (Álvarez-Nava and Lanes, 2017). The importance of maternal reprogramming of endocrine axes during development and subsequent influence on performance parameters of chicken meat birds has been recently reviewed (Angove and Forder, 2020).
Endocrine axes are also intimately linked to the immune system. This extensive network with both the endocrine and nervous systems has been termed the neuroendocrine-immune (NEI) interplay (Ashley and Demas, 2017). A primary component of this network is communication via bioregulatory mediators including neurotransmitters, hormones, and cytokines (Ashley and Demas, 2017). Key hormones of the HPA axis have been demonstrated to modulate immune function with downregulation of cellmediated responses (T-cells and cytokines) and upregulation of humoral responses (antibodies/immunoglobulins; Murray et al., 2001; Giayetto et al., 2020). Many wild and domestic avian studies have discussed immune modulation from exposure to environmental stressors having a major influence on health status, fitness, and survival, including heat stress (Giayetto et al., 2020; Videla et al., 2020), nutrition stress (Najafi et al., 2015; Bowling et al., 2018), and immune challenge studies (Henriksen et al., 2013; Videla et al., 2020).
Transgenerational modulation with altered immune-related responses in progeny, such as elevated inflammatory response have shown reduced antibody production and smaller lymphoid organs have also been seen (Henriksen et al., 2013; Bowling et al., 2018; Giayetto et al., 2020; Videla et al., 2020). A study by our group in 2018, found that chicks hatched from commercial broiler breeder hens with feed restricted to maintain lower bodyweights to mimic hunger stress, had decreased infectious bronchitis virus titres compared to hens maintained at higher body
weight (less feed restricted). The increased antibody titres in progeny of a higher body weight, and less stressed hens suggest an enhanced immune response to the vaccination (Bowling et al., 2018). Such disruption to NEI homeostasis can thus increase susceptibility to disease as well as divert important energy reserves towards fighting disease and away from growth and other performance parameters.
As mentioned previously, the hen can modulate and transfer endocrine and immune constituents into their eggs (Henriksen et al., 2011a; Merrill and Grindstaff, 2014; Ruuskanen, 2015). Other studies have also demonstrated that the link between maternal immunity and stress, and subsequent offspring immune response may not be directly linked to in ovo maternal antibody transfer (Burness et al., 2018), outlining the complexity of neuroendocrineimmune responses in maternal programming. Recently, epigenetic mechanisms have been suggested to mediate the parental regulation of offspring phenotype.
Maternal factors such as stress and nutrition can lead to epigenetic changes which may affect transgenerational phenotypic traits. Epigenetic reprogramming is of great significance when considering breeder hen management due to the enormous transgenerational potential of broiler breeders, in regard to time to sexual maturity, rate of egg production, and fast embryonic development.
For birds, the maternal environment influences the physiological status of the
embryo during egg formation through DNA methylation, histone modification, and microRNA transfer (Wade et al., 2016; Perez and Lehner, 2019). In wild birds, maternal care, early life and nutrition stress have been linked to epigenetic modification of genes associated with growth, metabolic and behavioural traits (Mathot et al., 2017; Sepers et al., 2021). Using quail as a model, Leroux et al. (2017) studied epigenetic modification in response to a methylation modifier (genistein) as an environmental stressor. Interestingly, they found phenotypic alterations in adult body weight, sexual maturity, and social behaviour across multiple generations, highlighting that not only is the maternal environment important for inducing potential epigenetic change, but so is the environment of the grandparents and great-grandparents.
Epigenetic regulation of gene expression in birds is currently a hot topic in literature (Perini et al., 2021; Raddatz et al., 2021). Bednarczyk et al (2021) have recently reviewed the research to date investigating epigenetic changes during chicken embryonic development. Studies utilising in ovo models have identified epigenetic mechanisms responsible for transgenerational modification of traits associated with thermal tolerance, metabolism, growth, and disease susceptibility. Transgenerational epigenetic inheritance has immense implications in regard to breeder and hatchery management and warrants further investigation into the identification of new markers, molecular mechanisms and improved selection of such traits (Bednarczyk et al., 2021).
Progeny exposure to maternal stress during early development can change an organism’s microbiota composition, and the microbiota can alter the organism’s ability to respond to stress after birth (Jasarevic et al., 2017; Hechler et al., 2019). The extent to which alterations to the intestinal environment, including microbiota, affects the programming of gutbrain signaling pathways of both the hen and her progeny is an area of great interest.
The intimate interaction between the central nervous system (CNS), the gastrointestinal tract and the residing microbiota –coined the brain-gut-microbiota axis– has been extensively reviewed in the literature (Morais et al., 2021). Bi-directional communication exists between the gastrointestinal tract and the CNS not only ensures the proper maintenance of gastrointestinal homeostasis and digestion but also is likely to have multiple effects on higher cognitive functions (Mayer, 2011). Studies on germfree animals have demonstrated that the gut microbiota can modulate brain development, function and behaviour (Cryan and Dinan, 2012; Stilling et al., 2014; Kelly et al., 2016), and that brain function or behaviour can affect the microbiota composition and gastrointestinal function (Demaude et al., 2006; Park et al., 2009; Galley et al., 2014). Evidence supporting such modulation in chickens, however, is elusive. A study by Calefi et al. (2016), demonstrated that C. perfringens infection was able to evoke behavioural changes in chickens; increasing the frequency of sleep-like behaviour and decreasing feeding, walking, feather pecking,
and standing behaviours, when exposed to heat stress. Their results demonstrated a direct relationship between heat stress and C. perfringens-induced effects on gut inflammation, serum corticosterone levels, and immune-reactive neurons, which are related to HPA-axis activity, providing some insight into the importance of gutbrain interactions in maintaining intestinal homeostasis in birds.
Communication between the brain and gut, including microbiota, occurs via complex neural (CNS, autonomic and enteric nervous system), endocrine (specifically the HPA axis), and immune signaling pathways. (Figure 3; Fung et al., 2017; Powell et al., 2017). Disturbances to this axis, have been implicated in a wide range of human disorders, including functional and inflammatory gastrointestinal disorders or dysbiosis, such as inflammatory bowel disease and inflammatory bowel syndrome (Jones et al., 2006), as well as extra-intestinal disorders such as allergy, obesity, cardiovascular disease and neurological disorders, such as anxiety and depression. (Carding et al., 2015; Kelly et al., 2016). Dysbiosis or dysbacteriosis has been characterised in broiler chickens with non-infectious factors, including nutritional and management stressors being key causes (Teirlynck et al., 2011).
Of growing interest is the relationship between stressor-induced alterations to the maternal brain-gut-microbiota axis and the impact on progeny development. Primary mediators of the stress response, including corticosterone (HPA axis) and the sympathetic nervous system (SNS), can regulate multiple
Enteric to Autonomic NS
Immune Signaling Pathways
Bacteria Metabolites
Figure 3. The brain-gut-microbiota axis. Bidirectional communication exists between the gastrointestinal tract (GIT), intestinal microbiota and central nervous system (CNS) via endocrine and immune signaling pathways
aspects of immune function. In return, immune mediators trigger the stress response and modulate its effects on the gastrointestinal tract, including the growth and type of commensal and pathogenic organisms (Galley et al., 2014; Gur and Bailey, 2016; Fung et al., 2017; Powell et al., 2017). Such axes modulation via stress factors can cause alteration to intestinal permeability, motility, and changes in mucus production, causing an inflammatory response (Rubio and Huang, 1992; Powell et al., 2017). Consequently, increased permeability of the intestinal barrier and microbial-driven inflammation can then exert influence back on the HPA-axis. Continuous stress-induced impairment of the intestinal barrier creates a positive feedback-like situation whereby inflammatory cytokines persistently activate the SNS and HPA-axis resulting in barrier disruption, increased endotoxin translocation, and a low-grade inflammatory state (de Punder
Breeder Management and Nutrition: Moving the industry forward and Pruimboom, 2015). Interestingly, this continual low-grade chronic inflammation has been reported to affect fertility and subsequent embryo development in rodent models of obesity. Investigators were able to draw correlations between obesity-mediated gut dysbiosis and markers of ovarian inflammatory responses and oocyte gene expression (Xie et al., 2016). Further investigations into linking stress-mediated gut dysbiosis to reproductive performance through such mechanisms would be of great interest not only in clinical application but also for commercial poultry production where the health and performance of breeding stock are paramount.
While it is becoming increasingly evident that stressor-induced alterations in the microbiota of animals can significantly impact host physiology and associated disorders, there is also evidence to suggest that the consequences of these alternations can have long-term negative implications in regard to both pre-natal and post-natal development of their progeny (Gur and Bailey, 2016; VeigaFernandes and Pachnis, 2017). There are two possible mechanisms: as discussed, microbial communities have been implicated in altering the neuroendocrine axis, therefore changing the maternal profile of hormones and other signaling molecules that the developing foetus and chick are exposed to (Sudo et al., 2004). The second possibility is the well-established concept that a newborn is inoculated with bacteria from the maternal microbiota, either through the placenta, uterus and vagina or, in the case of poultry, discover that bacteria can be vertically transferred during egg development via colonisation of the oviduct (Ding et al., 2017). This initial colonisation of
the gut by maternally-derived microbes, can ultimately “reset” neuroendocrine axes and have a profound influence on growth, health, and development of progeny (Al-Asmakh et al., 2012).
Despite an increasing body of work focusing on the effects of avian maternal stress much remains to be elucidated. Decreased progeny growth rate and hatchling mass, altered cognition, and social and foraging behaviours have been reported in response to elevated maternal corticosterone levels (Henriksen et al., 2013; Schoenle et al., 2017), or in ovo administration of synthetic glucocorticoids to mimic the effects of maternal stress, although with limited consistency (Hayward and Wingfield 2004; Love et al., 2005; Boogert et al., 2013; Ahmed et al., 2014b). Differences between studies are likely to be influenced by species and breed. Indeed, given that maternal yolk hormone concentrations are vastly different between both commercial layers and broilers (Cogburn, 1991; Ho et al., 2011), it is not surprising that genotype is an important factor when investigating maternal programming and offspring performance and fitness (Henriksen et al., 2011b; Peixoto et al., 2020). Interestingly, these studies have reported mixed results when investigating correlations with maternal corticosterone, yolk corticosterone, and progeny plasma corticosterone concentrations.
Recent literature exploring the metabolism of maternally derived hormones within yolk has suggested that only a fraction of yolk corticosterone ends up in the embryo, with the
remaining metabolised by enzymes in the extraembryonic membranes of the egg (Kumar et al., 2019). This extra embryonic metabolism has been suggested as a protective mechanism to improve the fitness and survival of the chick (Carter et al., 2018). It remains unclear, however, what conditions or concentration of embryonic corticosterone (either maternal or de novoderived) are required to alter or “reprogram” embryonic development (Vassallo et al., 2019). Another crucial factor is at what point in time during embryonic development this programming occurs in order to permanently alter phenotype. Such alterations may include the progeny’s ability to cope with stress and/or changes in performance traits.
To add to the complexity, animal studies altering the maternal environment, either through nutritional or environmental factors, have highlighted significant phenotypic variations between male and female progeny. In ovo manipulation has been shown to alter stress response (HPA activity), body composition, hormonal profiles, muscle mass, growth and behaviour in a sex-dependant manner (Love et al., 2005; Hayward et al., 2006; Leroux et al., 2017; Bowling et al., 2018; Angove et al., 2020). Thus, maternal stress has implications for a wide variety of physiological functions for both male and female progeny which is immensely important when considering mixed flock performance and flock uniformity, especially in commercial chicken meat production.
Evidence from both mammalian and avian ecology literature regarding maternal stress
and developmental programming highlights the need for further investigation into the current management of commercial breeder hens. Taking advantage of the potential transgenerational maternal effects within the breeding system pyramid may enable a targeted alternative approach regarding nutritional or other supplement interventions at significant physiological time points. Optimising health and reproductive output through the implementation of novel breeder management strategies will positively influence all facets of production, including improved behaviour, immunity, growth, and efficiency of subsequent generations. However, more research is needed to disentangle the mechanisms underpinning maternal stress and developmental programming in commercial poultry production.
Ahmed, A. A., Ma, W., Ni, Y., Wang, S. and R. Zhao. 2014a. Corticosterone in ovo modifies aggressive behaviors and reproductive performances through alterations of the hypothalamic-pituitary-gonadal axis in the chicken. Animal Reproduction Science 146: 193-201.
Ahmed, A. A., Ma, W., Ni, Y., Zhou, Q. and R. Zhao. 2014b. Embryonic exposure to corticosterone mod ifies aggressive behavior through alterations of the hypothalamic pituitary adrenal axis and the seroto nergic system in the chicken. Hormones and Be haviour 65: 97-105.
Al-Asmakh, M., Anuar, F., Zadjali, F., Rafter, J. and S. Pettersson. 2012. Gut microbial communities modulating brain development and function. Gut Microbes 3: 366-73.
Álvarez-Nava, F. and R. Lanes. 2017. GH/IGF-1 Signaling and Current Knowledge of Epigenetics; a Review and Considerations on Possible Thera
Breeder Management and Nutrition: Moving the industry forward
peutic Options. International Journal of Molecular Sciences 18: 1624.
Angove, J. L., and R. E. A. Forder. 2020. The avian maternal environment: exploring the physiological mechanisms driving progeny performance. World’s Poultry Science Journal 76: 100-118.
Angove, J. L., Willson, N. L., Cadogan, D. J. and R. E. A Forder. 2020. In ovo corticosterone adminis tration alters body composition irrespective of argi nine supplementation in 35-day-old female chick en meat birds. Animal Production Science 61: 8-16.
Ashley, N. T. and G. E. Demas. 2017. Neuroendo crine-immune circuits, phenotypes, and interac tions. Hormones and Behavior 87: 25-34.
Bednarczyk, M., Dunislawska, A., Stadnicka, K. and E. Grochowska. 2021. Chicken embryo as a model in epigenetic research. Poultry Science 100: 101164. Boogert, N. J., Zimmer, C. and K. A. Spencer. 2013. Pre- and post-natal stress have opposing effects on social information use. Biology Letters 9: 20121088. Bowling, M., Forder, R., Hughes, R. J., Weaver, S. and P. I. Hynd. 2018. Effect of restricted feed intake in broiler breeder hens on their stress levels and the growth and immunology of their offspring. Trans lational Animal Science 2: 263-271.
Burness, G., Moher, D., Ben-Ezra, N., Kelly, R. J., Hasselquist, D., Chin, E. H. 2018. Maternal im munization increases nestling energy expenditure, immune function, and fledging success in a passer ine bird. Biology Open 7: bio028803.
Calefi, A. S., da Silva Fonseca, J. G., Cohn, D. W. H., Honda, B. T. B., Costola-de-Souza, C., Tsugiyama, L. E., Quinteiro-Filho, W. M., Piantino Ferreira, A. J. and J. Palermo-Neto. 2016. The gut-brain axis interactions during heat stress and avian necrotic enteritis. Poultry Science 95: 1005-1014. Carding, S., Verbeke, K., Vipond, D. T., Corfe, B. M. and L. J. Owen. 2015. Dysbiosis of the gut micro biota in disease. Microbial Ecology in Health and Disease 26: 26191.
Carter, A. W., Bowden, R. M. and R. T. Paitz. 2018. Evidence of embryonic regulation of maternally derived yolk corticosterone. Journal of Experimen tal Biology 221: jeb182600
Chango, A. and I. P. Pogribny. 2015. Considering ma ternal dietary modulators for epigenetic regulation and programming of the fetal epigenome. Nutri ents 7: 2748-2770.
Cogburn, L. A. 1991. Endocrine manipulation of body composition in broiler chickens. Critical Re views in Poultry Biology 3: 283-305.
Cryan, J. F. and T. G. Dinan. 2012. Mind-altering mi croorganisms: the impact of the gut microbiota on brain and behaviour. Nature Reviews Neuroscience 13: 701-12.
De Groef, B., Grommen, S. V. H. and V. M. Darras. 2008. The chicken embryo as a model for develop mental endocrinology: Development of the thyro tropic, corticotropic, and somatotropic axes. Molec ular and Cellular Endocrinology 293: 17-24. de Jong, I. C. and D. Guemene. 2011. Major welfare issues in broiler breeders. Worlds Poultry Science Journal 67: 73-81. de Jong, I. C., van Voorst, S., Ehlhardt, D. A. and H. J. Blokhuis. 2002. Effects of restricted feeding on physiological stress parameters in growing broiler breeders. British Poultry Science 43: 157-68. de Punder, K. and L. Pruimboom. 2015. Stress in duces endotoxemia and low-grade inflammation by increasing barrier permeability. Frontiers in Immu nology 6:223.
Demaude, J., Fioramonti, J., Ferrier, L. and L. Bue no. 2006. LPS from the commensal microflora participates to stress-induced colonic epithelial barrier dysfunction in mice. Gastroenterology 130: A239-A239.
Ding, J., Dai, R., Yang, L., He, C., Xu, K., Liu, S., Zhao, W., Xiao, L., Luo, L., Zhang, Y. and H. Meng. 2017. Inheritance and Establishment of Gut Microbiota in Chickens. Frontiers in Microbiology 8: 1967.
Fung, T. C., Olson, C. A. and E. Y. Hsiao. 2017. In teractions between the microbiota, immune and nervous systems in health and disease. Nature Neu roscience 20: 145.
Galley, J. D., Nelson, M. C., Yu, Z., Dowd, S. E., Wal ter, J., Kumar, P. S., Lyte, M. and M. T. Bailey. 2014. Exposure to a social stressor disrupts the commu nity structure of the colonic mucosa-associated mi crobiota. BMC Microbiology 14: 189. Giayetto, O., Videla, E., Chacana, P., Jaime, C., Marin, R. and F. Nazar. 2020. Modulating offspring responses: concerted effects of stress and immuno logic challenge in the parental generation. Journal of Experimental Biology 223: jeb219386. Groothuis, T. G. G., Hsu, B. Y., Kumar, N. and B. Tschirren. 2019. Revisiting mechanisms and func tions of prenatal hormone-mediated maternal ef fects using avian species as a model. Philosophical Transactions of the Royal Society B Biological Sci ences 374: 20180115.
Gur, T. L. and M. T. Bailey. 2016. Effects of stress on commensal microbes and immune system activity. Advances in Experimental Medicine and Biology. Springer New York LLC, 874: 289-300.
Hayward, L. S., Richardson, J. B., Grogan, M. N. and J. C. Wingfield. 2006. Sex differences in the orga nizational effects of corticosterone in the egg yolk of quail. General and Comparative Endocrinology 146: 144-148.
Hayward, L. S. and J. C. Wingfield. 2004. Maternal corticosterone is transferred to avian yolk and may alter offspring growth and adult phenotype. Gener al and Comparative Endocrinology. 135: 365-371.
Hechler, C., Borewicz, K., Beijers, R., Saccenti, E., Riksen-Walraven, M., Smidt, H. and C. de Weerth. 2019. Association between Psychosocial Stress and Fecal Microbiota in Pregnant Women. Scientific Reports 9: 4463.
Henriksen, R., Groothuis, T. G. and S. Rettenbacher. 2011a. Elevated Plasma Corticosterone Decreases
Yolk Testosterone and Progesterone in Chickens: Linking Maternal Stress and Hormone-Mediated Maternal Effects. PLoS ONE 6: e23824.
Henriksen, R., Rettenbacher, S. and T. G. G. Groo thuis. 2011b. Prenatal stress in birds: Pathways, ef fects, function and perspectives. Neuroscience and Biobehavioral Reviews 35: 1484-1501.
Henriksen, R., Rettenbacher, S. and T, G. Groothuis. 2013. Maternal corticosterone elevation during egg formation in chickens Gallus gallus domesticus. in fluences offspring traits, partly via prenatal under nutrition. General and Comparative Endocrinolo gy 191: 83-91.
Herman, J. P., McKlveen, J. M., Solomon, M. B., Car valho-Netto, E. and B. Myers. 2012. Neural regula tion of the stress response: glucocorticoid feedback mechanisms. Brazilian Journal of Medical and Bio logical Research 45: 292-298.
Ho, D. H., Reed, W. L. and W. W. Burggren. 2011. Egg yolk environment differentially influences physiological and morphological development of broiler and layer chicken embryos. Journal of Ex perimental Biology 214: 619-28.
Hyatt, M. A., Gopalakrishnan, G. S., Bispham, J., Gentili, S., McMillen, I. C., Rhind, S. M., Rae, M. T., Kyle, C. E., Brooks, A. N., Jones, C., Budge, H., Walker, D., Stephenson, T. and M. E. Symonds. 2007. Maternal nutrient restriction in early preg nancy programs hepatic mRNA expression of growth-related genes and liver size in adult male sheep. Journal of Endocrinology 192: 87-97.
Hynd, P. I., Weaver, S., Edwards, N. M., Heberle, N. D. and M. Bowling. 2016. Developmental pro gramming: a new frontier for the poultry industry?
Animal Production Science 56: 1233-1238.
Jasarevic, E., Howard, C. D., Misic, A. M., Beiting, D. P. and T. L. Bale. 2017. Stress during pregnancy al ters temporal and spatial dynamics of the maternal and offspring microbiome in a sex-specific manner. Scientific Reports 7: 44182.
Jimeno, B., Hau, M., Gómez-Díaz, E. and S. Verhulst. 2019. Developmental conditions modulate DNA methylation at the glucocorticoid receptor gene with cascading effects on expression and corticosterone levels in zebra finches. Scientific Reports 9: 15869.
Jones, M. P., Dilley, J. B., Drossman, D. and M. D. Crowell. 2006. Brain-gut connections in functional GI disorders: anatomic and physiologic relationships. Neurogastroenterology and Motility 18: 91-103. Kelly, J. R., Borre, Y., C, O. B., Patterson, E., El Aidy, S., Deane, J., Kennedy, P. J., Beers, S., Scott, K., Moloney, G., Hoban, A. E., Scott, L., Fitzgerald, P., Ross, P., Stanton, C., Clarke, G., Cryan, J. F. and T. G. Dinan. 2016. Transferring the blues: Depres sion-associated gut microbiota induces neurobe havioural changes in the rat. Journal of Psychiatric Research 82: 109-18.
Kumar, N., Lohrentz, A., Gahr, M. and T. G. G. Groothuis. 2019. Steroid receptors and their regu lation in avian extraembryonic membranes provide a novel substrate for hormone mediated maternal effects. Scientific Reports 9: 11501. Leroux, S., Gourichon, D., Leterrier, C., Labrune, Y., Coustham, V., Riviere, S., Zerjal, T., Coville, J. L., Morisson, M., Minvielle, F. and F. Pitel. 2017. Em bryonic environment and transgenerational effects in quail. Genetics Selection Evolution 49: 14. Lillycrop, K. A. and G. C. Burdge. 2015. Maternal diet as a modifier of offspring epigenetics. Journal of De velopmental Origins of Health and Disease 6: 88-95. Lindqvist, C., Janczak, A. M., Natt, D., Baranowska, I., Lindqvist, N., Wichman, A., Lundeberg, J., Lind berg, J., Torjesen, P. A. and P. Jensen. 2007. Trans mission of stress-induced learning impairment and associated brain gene expression from parents to off spring in chickens. PLoS ONE 2: e364. Love, O. P., Chin, E. H., Wynne-Edwards, K. E. and T. D. Williams. 2005. Stress hormones: A link be tween maternal condition and sex-biased reproduc tive investment. American Naturalist 166: 751-766.
Mathot, K. J., Olsen, A. L., Mutzel, A., Araya-Ajoy, Y. G., Nicolaus, M., Westneat, D. F., Wright, J., Kempenaers, B. and N. J. Dingemanse. 2017. Provi sioning tactics of great tits Parus major. in response to long-term brood size manipulations differ across years. Behavioral Ecology 28: 1402-1413.
Merrill, L. and J. L. Grindstaff. 2014. Maternal an tibody transfer can lead to suppression of humoral immunity in developing zebra finches Taeniopygia guttata. Physiological and Biochemical Zoology 87: 740-51.
Morais, L. H., Schreiber, H. L. and S. K. Mazmanian. 2021. The gut microbiota–brain axis in behaviour and brain disorders. Nature Reviews Microbiology 19: 241-255.
Murray, S. E., Lallman, H. R., Heard, A. D., Rit tenberg, M. B. and M. P. Stenzel-Poore. 2001. A genetic model of stress displays decreased lympho cytes and impaired antibody responses without al tered susceptibility to Streptococcus pneumoniae. Journal of Immunology 167: 691-8.
Najafi, P., Zulkifli, I., Soleimani, A. F. and P. Kashiani,. 2015. The effect of different degrees of feed restric tion on heat shock protein 70, acute phase proteins, and other blood parameters in female broiler breed ers. Poultry Science 94: 2322-9.
Nuyt, A. M. 2008. Mechanisms underlying devel opmental programming of elevated blood pressure and vascular dysfunction: evidence from human studies and experimental animal models. Clinical Science 114: 1-17.
O’Regan, D., Welberg, L. L., Holmes, M. C. and J. R. Seckl. 2001. Glucocorticoid programming of pituitary-adrenal function: mechanisms and phys iological consequences. Seminars in neonatology. 6: 319-329.
Parade, S. H., Ridout, K. K., Seifer, R., Armstrong, D. A., Marsit, C. J., McWilliams, M. A. and A. R. Tyrka. 2016. Methylation of the Glucocorticoid Receptor Gene Promoter in Preschoolers: Links
With Internalizing Behavior Problems. Child De velopment 87: 86-97.
Park, A. J., Blennerhassett, P., Denou, E., Bercik, P. and S. M. Collins. 2009. Examination of the Depressed Gut Reveals Changes in Microbiota and Serotonin Levels. Gastroenterology 136: A575-A575.
Peixoto, M. R. L. V., Karrow, N. A., Newman, A. and T. M. Widowski. 2020. Effects of Maternal Stress on Measures of Anxiety and Fearfulness in Differ ent Strains of Laying Hens. Frontiers in Veterinary Science 7: 128.
Perez, M. F. and B. Lehner. 2019. Intergenerational and transgenerational epigenetic inheritance in an imals. Nature Cell Biology 21: 143-151.
Perini, F., Cendron, F., Rovelli, G., Castellini, C., Cassandro, M. and E. Lasagna. 2021. Emerging Genetic Tools to Investigate Molecular Pathways Related to Heat Stress in Chickens: A Review. An imals 11: 46.
Powell, N., Walker, M. M. and N. J. Talley. 2017. The mucosal immune system: master regulator of bidi rectional gut–brain communications. Nature Re views Gastroenterology and Hepatology 14: 143. Raddatz, G., Arsenault, R. J., Aylward, B., Whelan, R., Böhl, F. and F. Lyko. 2021. A chicken DNA methylation clock for the prediction of broiler health. Communications Biology 4: 76.
Reynolds, L. P., Borowicz, P. P., Caton, J. S., Von nahme, K. A., Luther, J. S., Hammer, C. J., Mad dock Carlin, K. R., Grazul-Bilska, A. T. and D. A. Redmer. 2010. Developmental programming: the concept, large animal models, and the key role of uteroplacental vascular development. Journal of Animal Science 88: E61-72.
Royo, F., Mayo, S., Carlsson, H. E. and J. Hau. 2008. Egg corticosterone: a noninvasive measure of stress in egg-laying birds. Journal of Avian Medicine and Surgery 22: 310-4.
Rubio, C. A. and C. B. Huang. 1992. Quantification of the sulphomucin-producing cell population of
the colonic mucosa during protracted stress in rats. In Vivo 6: 81-4.
Ruuskanen, S. 2015. Hormonally-mediated maternal effects in birds: Lessons from the flycatcher model system. General and Comparative Endocrinology 224: 283-293.
Schoenle, L. A., Dudek, A. M., Moore, I. T. and F. Bonier. 2017. Red-winged blackbirds Agelaius phoeniceus. with higher baseline glucocorticoids also invest less in incubation and clutch mass. Hor mones and Behaviour 90: 1-7.
Seckl, J. R. 2004. Prenatal glucocorticoids and longterm programming. European Journal of Endocri nology 151: 49-62.
Seckl, J. R. and M. C. Holmes. 2007. Mechanisms of disease: glucocorticoids, their placental metabolism and fetal ‘programming’ of adult pathophysiology. Nature Clinical Practice: Endocrinology & Metab olism 3: 479-88.
Sepers, B., Erven, J. A. M., Gawehns, F., Laine, V. N. and K. van Oers. 2021. Epigenetics and Early Life Stress: Experimental Brood Size Affects DNA Methylation in Great Tits Parus major.. Frontiers in Ecology and Evolution 9:
Sheng, J. A., Bales, N. J., Myers, S. A., Bautista, A. I., Roueinfar, M., Hale, T. M. and R. J. Handa. 2020. The Hypothalamic-Pituitary-Adrenal Axis: Devel opment, Programming Actions of Hormones, and Maternal-Fetal Interactions. Frontiers in Behavior al Neuroscience 14: 601939.
Sloboda, D., Challis, J., Moss, T. and J. Newnham. 2005. Synthetic glucocorticoids: antenatal adminis tration and long-term implications. Current phar maceutical design 11: 1459-1472.
Smulders, T. V. 2021. Telencephalic regulation of the HPA axis in birds. Neurobiology of Stress 15: 100351. Stilling, R. M., Dinan, T. G. and J. F. Cryan. 2014. Microbial genes, brain & behaviour - epigenetic regulation of the gut-brain axis. Genes, Brain and Behaviour 13: 69-86.
Breeder Management and Nutrition: Moving the industry forward
Sudo, N., Chida, Y., Aiba, Y., Sonoda, J., Oyama, N., Yu, X. N., Kubo, C. and Y. Koga. 2004. Postnatal microbial colonization programs the hypothalam ic-pituitary-adrenal system for stress response in mice. Journal of Physiology 558: 263-75.
Szyf, M. and J Bick. 2013. DNA methylation: a mechanism for embedding early life experiences in the genome. Child Development 84: 49-57.
Tahamtani, F. M., Moradi, H. and A. B. Riber. 2020. Effect of Qualitative Feed Restriction in Broiler Breeder Pullets on Stress and Clinical Welfare In dicators. Frontiers in Veterinary Science 7: 316. Talge, N. M., Neal, C. and V. Glover. 2007. Ear ly Stress, Translational Research and Prevention Science Network: Fetal and neonatal experience on child and adolescent mental health. Antenatal maternal stress and long-term effects on child neu rodevelopment: How and why? Journal of Child Psychology and Psychiatry 48: 245-61. Teirlynck, E., Gussem, M. D., Dewulf, J., Haese brouck, F., Ducatelle, R. and F. Van Immerseel. 2011. Morphometric evaluation of “dysbacteriosis” in broilers. Avian Pathology 40: 139-44.
Vassallo, B. G., Litwa, H. P., Haussmann, M. F. and R. T. Paitz. 2019. In ovo metabolism and yolk glu cocorticoid concentration interact to influence em bryonic glucocorticoid exposure patterns. General and Comparative Endocrinology 272: 57-62. Veiga-Fernandes, H. and V. Pachnis. 2017. Neuroim mune regulation during intestinal development and
homeostasis. Nature Immunology 18: 116.
Videla, E. A., Giayetto, O., Fernandez, M. E., Chacana, P. A., Marin, R. H. and F. N. Nazar. 2020. Immediate and transgenerational effects of thymol supplemen tation, inactivated Salmonella and chronic heat stress on representative immune variables of Japanese quail. Scientific Reports 10: 18152.
Wade, B., Cummins, M., Keyburn, A. and T. M. Crowley. 2016. Isolation and detection of microR NA from the egg of chickens. BMC Research Notes 9: 283.
Weaver, I. C. G., Cervoni, N., Champagne, F. A., D’Alessio, A. C., Sharma, S., Seckl, J. R., Dymov, S., Szyf, M. and M. J. Meaney. 2004. Epigenetic programming by maternal behavior. Nature Neuro science 7: 847-854.
Wu, G., Bazer, F. W., Cudd, T. A., Meininger, C. J. and T. E. Spencer. 2004. Maternal nutrition and fe tal development. Journal of Nutrition 134: 2169-72. Xiao, Y., Wu, C., Li, K., Gui, G., Zhang, G. and H. Yang. 2017. Association of growth rate with hor mone levels and myogenic gene expression profile in broilers. Journal of Animal Science and Biotech nology 8: 43d.
Xie, F., Anderson, C. L., Timme, K. R., Kurz, S. G., Fernando, S. C. and J. R. Wood. 2016. Obesity-De pendent Increases in Oocyte mRNAs Are Associ ated With Increases in Proinflammatory Signaling and Gut Microbial Abundance of Lachnospiraceae in Female Mice. Endocrinology 157: 1630-43.
Breeder Management and Nutrition: Moving the industry forward
JUXING CHEN, P hD., is the Senior Manager of Structure and Functional Biology at Novus International. Since joining Novus in 2012 Dr. Chen’s research has included maternal feeding/epigenetic modification, gut health, structural integrity, and soybean meal quality. Dr. Chen’s research in maternal feeding mainly focuses on delineating the mode of action of maternal supplementation of organic trace minerals on the growth and development of progeny in poultry, swine and ruminants. Her research findings have been published in the book, Nutrition of Hyperprolific Sows; the Journal of Animal Science, and various industry publications.
MERCEDES VÁZQUEZ-AÑÓN, PhD., is the Senior Director of Strategic Initiatives and Account Collaboration at Novus International. She received her bachelor’s degree in Agriculture Engineer and Animal Science from Universidad Politécnica- Madrid, Spain. Her master’s degree was earned at Penn State University in the U.S. Her doctorate degree in Nutritional Science is from the University of Wisconsin-Madison in the U.S. Dr. VázquezAñón joined Novus in 1996 as a research manager where she developed the application of several Novus products across species, became a Fellow Scientist in 2006, the director of animal nutrition research in 2007, and in 2012 became responsible for global animal research and facilities. Her research expertise encompasses amino acid, trace mineral, antioxidant, enzyme, and lipid nutrition and metabolism in ruminants and monogastrics, holds patents on different technologies, has published more than 40 refereed scientific publications and numerous conference proceedings. She is bilingual in English and Spanish.
The poultry industry seeks ways to improve efficiency and reduce the cost of production. One way to improve efficiency is to optimize the nutrition and management of the breeder hen by optimizing its reproduction and the performance of the progeny. Historically, more attention has been on the nutrition of the hen to improve reproduction and the progeny was an afterthought.. However, new research has provided evidence showing how some micronutrients in the breeder hen diet can boost the immune system of the progeny, providing new opportunities to help the chick develop its immune system, particularly in the face of an antibiotic-free environment. Of the trace minerals, zinc (Zn) and especially in its chelated form when fed to the breeder hen can improve the livability of the young chick by modulating the cellular and humoral immune response to pathogenic challenges. With recent advances in epigenetics, the underlying mechanism by which maternal Zn can modulate gene expression of the chick in vivo and improve immune development are better understood. Maternal Zn is stored in the egg yolk, yolk sac, and albumen prior to its delivery to the embryo’s liver from where it is distributed to the developing organs as needed during embryo development and after hatch. Increasing level of Zn in the breeder diet - and in the form of chelates – is shown to improve storage of Zn in the egg yolk and albumen, so more Zn is available for embryo development. In the gut, Zn improves gut barrier function, mucosal and systemic immunity, and reduces gut inflammation of the progeny chicks by epigenetic modification of anti-inflammatory proteins.
In conclusion, feeding Zn, especially in the chelated form, to broiler breeders improves the chance for better livability of the chicks due to a more developed immune system, and provides a way to better prepare the chick for optimal performance in an antibiotic-free environment.
The poultry industry is always looking for ways to improve efficiency and reduce the cost of production. In broiler production, the cost of hatching the egg or a day-old chick is very small compared to the cost of kilograms of live bird (Callini and Sirri, 2007). These low-cost periods provide opportunities to optimize the nutrition and management of the breeder hen to improve its performance and that of the progeny.
However, most of the attention until now has been on broiler breeder nutrition and research to improve productivity in terms of saleable chicks produced, egg fertility, and hatchability and less attention has been given to the effect of maternal nutrition on the offspring broiler performance.
Kidd (2003) and Callini and Sirri (2007) summarized the breeder nutrition studies
published in the literature. The concept of feeding the hen to improve progeny performance is not new but sometimes the goals of breeder nutrition - maximizing reproductive performance - are at odds with improving progeny development. In spite of limited information in the literature, there are two aspects of progeny development that can be improved via maternal nutrition. One is growth performance and carcass yield, and the other is the health and immunity of the progeny (Callini and Sirri, 2007). Reducing dietary protein levels in a breeder’s diet can negatively affect the size of the egg, but does not appear to affect progeny performance (Kidd, 2003). Optimizing the level and type of energy in the breeder diet improves the carcass yield of the progeny. Brake et al. (2003) reported that progeny performance, especially in males, was improved when increasing protein and energy in the breeder diet. Lesson and Summer (2001), and Peebles et al. (1999, 2002a) showed that feeding fat sources with linoleic and linolenic acid content, such as corn oil, provided better progeny performance, carcass yield, and reduced embryo mortality than feeding poultry fat or lard. This effect was not observed when fed to younger breeders (Peebles et al., 2002 b). Later, Kidd et al. (2005) reported that L-carnitine supplementation in high energy hen diets improved performance and carcass yield, especially in males, suggesting carnitine helps with the utilization of energy in the hen and embryo to support rapid growth in the male offspring. It has been postulated that hen nutritional programmes that increase yolk weight over egg weight provide more energy and protein to the chick posthatch and support faster growth of the offspring, especially of male broilers.
Supplementation of vitamins and minerals in breeder hen diets has been found to be more effective than other nutrients in improving liveability and immunity of the progeny (Callini and Sirri, 2007). Because of the high content of polyunsaturated fatty acids in egg yolk, supplementation with selenium and high levels of vitamin E in breeder diets has been reported to increase their content in yolk, protecting the unsaturated fatty acids from oxidation and improve the antioxidant and humoral immune status of the progeny (Surai, 2000). As an example, maternal supplementation of 25-hydroxy vitamin D3 increased hatchability and in vitro chick innate immunity towards E. coli (Saunders-Blades and Korver, 2015). Adequate levels of calcium (Ca) and phosphorus (P) were reported to improve skeleton ossification of the embryo and progeny at hatch (Triyuwanta et al., 1992), and the addition of vitamin D improved calcification of the eggshell (Plaimast et al., 2015). Supplementation of Zn has been shown to improve humoral and cellular immunity and to enhancethe progeny response to pathogenic challenges (Kidd, 1992, 1993).The combination of Zn and Mn improved the livability of the progeny (Virden et al., 2003).
More recent work compiled by Dixon et al. (2016) identified factors in the prelay period and incubation environment that can have lasting effects on how the offspring responds to its environment, beyond genetics. During the prelay period, hen age, nutrition, and management can impact the performance of the offspring. It is well known that progeny
DNA methylation
DNA-histone compaction
Histone acetylation
DNA-histone relaxation
DNMT:
DNA methylation
DNA-histone compaction
Acetylation
DNA-histone relaxation
from middle-aged breeders have higher performance than those from young or older breeders. As mentioned earlier, energy type and the content of the hen diet can impact the performance of the offspring, whereas vitamins and minerals can affect progeny health. Developing the offspring’s immunity can be achieved by exposing the breeder to disease-associated pathogens through vaccination of attenuated live vaccines to enhance transmission of immunity via antibodies in the egg yolk (Hassan and Curtis, 1996).
New advances in epigenetics have led us to better understand the mechanism by which maternal nutrition and management can impact progeny development. Epigenetics is an important regulatory mechanism of gene expression independent of the DNA sequence. It can be viewed as a life-course strategy for the offspring to help it meet the
demands of the predicted environment in later life (Dixon et al., 2016). Changing this life course is possible through epigenetics. DNA methylation, which causes DNAhistone compaction and represses gene expression (Figure 1A), and acetylation of lysine 9 on histone protein 3 (H3K9), which causes DNA-histone relaxation and activates gene expression (Figure 1B, are two common ways of epigenetic modification (Moresi et al., 2015).
Recent studies have focused on understanding the mechanisms by which maternal nutrition could impact the health of progeny via epigenetic modification(s) (Berghof et al., 2013). In chickens, the adaptive immune system is functionally immature and newly hatched birds are dependent on maternal antibodies transferred through the egg yolk. In addition, the intestine is
Treatments
Hens
Chick Treatments
Low Zn: 20 ppm ZnSO4
Normal Zn: 70 ppm ZnSO4
the largest immune system of the body that plays a major role in resistance to infection. However, the functional development of gut-associated lymphoid tissues in neonatal chickens is slow and the functional maturation occurs in the second week of life (Bar-Shira and Friedman, 2006). Zinc (Zn) has been associated with different aspects of the immune system and maternal Zn can improve the development of the immune system of the offspring. Kidd et al. (1992, 1993) showed supplementation of organic Zn in the breeder diet increased embryonic bone weight and enhanced cellular immune response and primary antibody titers to
Salmonella pullorum antigen. Virden et al. (2003) fed breeders with a combination of Zn and Mn in organic and inorganic forms and found improvements in progeny livability with higher response from the organic form. More recent work has focused on the mechanisms by which maternal Zn supplementation modulates the immune system of the offspring. Zhang et al. (2012) documented how Zn prevented Salmonella enteric-induced loss of intestinal mucosal barrier function by increasing expression of the main proteins responsible for maintaining gut integrity, including occludin and claudin-1. Zhu et al. (2017) showed
Figure
chickens
ppm
that maternal dietary Zn supplementation, regardless of inorganic or organic source, enhanced the antioxidant ability of chick embryos from maternal normal and high temperatures. This was reflected in increased mRNA and protein expressions of metallothionein IV in the embryonic liver by
reducing DNA methylation and increasing H3K9 acetylation of the metallothionein IV promoter. Li et al. (2015) evaluated the role of maternal Zn on intestinal immunity of the offspring and the underlying epigenetic mechanisms in broiler chickens. In this study, broiler breeder hens (45 weeks old) were
first provided a Zn-deficient diet (0 ppm supplemental Zn) for 2 weeks to exhaust maternal Zn and then fed commercial (50 ppm) and high (300 ppm) levels of two sources of Zn, chelated (Zn methionine hydroxy analogue chelate, MHAC-Zn) and inorganic (ZnSO4); or 0 ppm supplemental Zn for 6 weeks (Figure 2). The F1 progeny chicks from those hens were fed either low (20 ppm) or normal (70 ppm) levels of inorganic Zn for 6 weeks.
Zn content in the egg yolk and albumen gradually increased with supplementation and was higher in the yolk than albumen in the chelated Zn treatments, suggesting more delivery of Zn to the progeny by the chelated Zn (Figure 3). Zn must first be stored in the yolk before it can be delivered to the embryo. The hen delivers Zn to the yolk via the vitellogenin transport protein, where it is stored and bound to lipovitellin and
phosphovitin. The stored Zn is transferred to the yolk sac for temporary storage before it´s delivered to the liver of the embryo where it is distributed to the rest of the organs when needed via metallothionine protein (Richards, 1997). The levels of Zn in the yolk and yolk sac are gradually depleted during the incubation period as they are transferred to the embryo (Richards, 1997).
Zinc and modulation of intestinal epithelium immunity
Li et al. (2015) investigated several aspects of maternal Zn supplementation on gut immunity in offspring broilers. The authors measured jejunal secretory IgA (sIgA) production as the first line of defense in protecting the intestinal epithelium from enteric pathogens, jejunal mucin 2 (MUC2) gene expression as the main intestinal mucin of the mucus layer that protects the gut epithelial cells, and serum immunoglobulin
expression
A20 gene expression (d35)
Figure 7. Zn supplementation in broiler breeder hens and offspring chickens increased A20 gene expression and reduced NF-κB and IL6 gene expression in the jejunum of offspring chickens at 35 d of age hatched from breeder hens supplemented with 0, 50, 300 ppm ZnSO4 or 50, 300 ppm Zn-MHAC. Treatments with different letters were significantly different (P < 0.05). (Adapted from Li et al., 2015)
methylation
promoter
acetylation at A20 promoter region
Figure 8. Zn supplementation in broiler breeder hens and offspring birds reduced DNA methylation at A20 promoter region and increased H3K9 acetylation at A20 promoter region in the jejunum of offspring birds at 35 d of age hatched from breeder hens supplemented with 0, 300 ppm ZnSO4 or 300 ppm Zn-MHAC. Treatments with different letters were significantly different (P < 0.05). (Adapted from Li et al., 2015)
Y (IgY) and antibody titers responses against Newcastle disease virus (NDV) and infectious bursal disease virus (IBDV). Compared to Zn-deficient or inorganic Zn treatments, chelated Zn supplementation in breeder hens 1) enhanced mucosal immunity by increasing sIgA production in progeny chickens at 35 days of age fed either a low or normal Zn diet
(Figure 4A); 2) improved gut barrier function by increasing MUC2 gene expression (Figure 4B); and 3) enhanced systemic immunity in progeny chicks by increasing concentration of IgY in the offspring at 1 d of age (Figure 5A), and antibody titer of NDV (Figure 5B) and IBDV (Figure 5C) in offspring chickens at 28 d of age. These results suggest that Zn
supplementation in the breeder diet improves gut barrier function, mucosal immunity and systemic immunity of progeny chicks and that Zn in the chelated form is more effective than in an inorganic form.
Zinc and modulation of inflammatory signaling pathway via epigenetics
Li et al. (2015) also studied the underlying molecular epigenetic mechanism by which maternal Zn modulates gut immunity of the progeny. Evidence in human literature postulated that Zn suppresses inflammation via induction of A20-mediated inhibition of nuclear factor-κB (NF-κB) signaling pathway (Prasad et al., 2011). Zn finger protein A20 can negatively regulate the inflammatory response by deubiquiting ubiquitin-dependent factors of NF-κB signaling cascades (Ma and Malynn, 2012; Catrysse et al., 2014). NF-κB signaling pathway is one of the major pathways to regulate inflammation induced by external stimuli such as bacteria, infection, and inflammation (Figure 6, modified from Neurath et al., 1998). A20 blocks the phosphorylation and activation of NF-κB, thereby inhibiting translocation of NFκB and suppressing inflammation cascade. Therefore, up-regulation of A20 would down-regulate NF-κB pathway and reduce inflammation (Figure 6).
Li and colleagues (2015) measured the expression of A20, NF-κB and IL6 genes, DNA methylation and histone acetylation at A20 promoter region. Chelated Zn supplementation to breeder hens showed a greater effect than ZnSO4 in 1) reducing gene expression of NF-κB p65 (Figure 7A)
as well as its downstream inflammatory cytokines such as IL6 (Figure 7B); and 2) increasing A20 gene expression in the jejunum of progeny (Figure 7C). As A20 negatively regulates NF-κB pathway, increase of A20 is consistent with reduction of NF-κB p65 gene expression. These results suggest that Zn supplementation in breeder diets reduced gut inflammation in progeny birds by up-regulation of A20 expression.
To determine the molecular mechanism by which Zn regulates A20 gene expression, DNA methylation and histone H3K9 acetylation at A20 promoter region was measured in the jejunum of progeny birds at 35 d of age. Both chelated Zn and ZnSO4 significantly reduced DNA methylation at A20 promoter (Figure 8A). Chelated Zn but not ZnSO4 significantly increased histone H3K9 acetylation at A20 promoter (Figure 8B). Because methylation represses gene expression and histone acetylation activates gene expression, lower DNA methylation and greater histone H3K9 acetylation at A20 promoter region suggests that breeder Zn supplementation activates A20 gene expression. This is consistent with the increase of A20 mRNA levels shown in Figure 7C.
In summary, Zn supplementation in breeder hens improved gut barrier function, mucosal immunity and systemic immunity, and reduced gut inflammation of progeny chicks by epigenetic modification of A20 promoter. The higher levels of Zn tested as a chelated source appeared to have the most consistent effect in epigenetic modulation of A20 promoter.
Based on new research, the impact of breeder nutrition, management, and environment on progeny development becomes more evident. The nutrition of breeder hens provide a tool to prepare the immune system of the chicken post-hatching, especially under an antibioticfree environment. Zn supplementation in the chelated form in breeder hen diets is an example of how maternal nutrition can modulate several aspects of the immune system of the progeny to help the chick to overcome pathogenic insults. The mechanism by which Zn improves the immune system of the chick has been elucidated thanks to the new developments in epigenetics. Maternal Zn transferred to the embryo epigenetically modulates A20 promoter, which upregulates A20 gene expression thereby down-regulating NF-kB gene expression, hence reducing gut inflammation, and improving gut barrier function, mucosal immunity, and systemic immunity of progeny chicks. More work in this area would allow for breeder hen nutrition to be used to further prepare the chick for optimal growth performance and meat yield.
Bar-Shira, E. and A. Friedman. 2006. A development and adaptations of innate immunity in the gastrointestinal tract of the newly hatched chick. Developmental and Comparative Immunology 30: 930-941.
Berghof, T.V., Parmentier, H.K. and A., Lammers. 2013. Transgenerational epigenetic effects on innate immunity in broilers: An underestimated field to be explored? Poultry Science 92: 2904-2913. Brake, J., Jenfestey, B.A. and P.W. Plumstead. 2003.
Broiler performance to 21 days as affected by cumulative broiler breeder pullet nutrition during rearing. Poultry Science 14: 81-85.
Calini, F. and F. Sirri. 2007. Breeder nutrition and offspring performance. Brazilian Journal of Poultry Science 9: 77-83.
Catrysse, L., Vereecke, L., Beyaert, R. and G. van Loo. 2014. A20 in inflammation and autoimmunity. Trends in Immunology 35: 22-31.
Dixon, L.M., Sparks, N.H. and K.M. Rutherford 2016. Early experiences matter: a review of the effects of prenatal environment on offspring characteristics in poultry. Poultry Science 95: 489-499.
Hassan J. O. and R. Curtiss. 1996. Effect of vaccination of hens with an avirulent strain of salmonella typhimurium on immunity of progeny challenged with wild-type salmonella strains. Infection and Immunity 64: 938-944
Kidd, M.T., Anthony, N.B. and S.R. LEE,. 1992. Progeny performance when dams and chicks are fed supplemental zinc. Poultry Science 71: 1201-1206.
Kidd M.T., Anthony, N.B., Newberry, L.A. and S.R. Lee. 1993. Effect of supplemental zinc in either a corn-soybean or a milo and corn-soybean meal diet on the performance of young broiler breeders and their progeny. Poultry Science 72: 1492-1499.
Kidd M.T. 2003. A treatise on chicken dam nutrition that impacts on Progeny. World’s Poultry Science Journal 59: 475-494
Kidd, M.T., McDaniel, C.D., Peebles, E.D., Barber, S.J., Corzo, A., Branton, S.L., Woodworth, J.C. 2005. Breeder hen dietary L-carnitine affects progeny carcase traits. British Poultry Science 46: 97-103.
Leeson, S. and J.D. Summers. 2001. Nutrition of the Chicken University Books, Guelph, Ontario, Canada.
Li., C, Guo, S., Gao, J., Guo, Y., Du, E., Lv, Z. and B. Zhang. 2015. Maternal high-zinc diet attenuates intestinal inflammation by reducing DNA
methylation and elevating H3K9 acetlylation in the A20 promoter of offspring chicks. Journal of Nutritional Biochemistry 26: 173-183
Ma, A. and B.A. Malynn. 2012. A20: linking a complex regulator of ubiquitylation to immunity and human disease. Nature Reviews Immunology 12:774-785.
Moresi, V., Marroncelli, N., Coletti, D. and S. Adamo. 2015. Regulation of skeletal muscle development and homeostasis by gene imprinting, histone acetylation and microRNA. Biochimica et Biophysica Acta 1849: 309-316.
Neurath, M.F., Becker, C. and K. Barbulescu. 1998. Role of NF-kappaB in immune and inflammatory responses in the gut. Gut. 43: 856-860
Peebles, E.D., Doyle, S.M., Pansky, T., Gerard, P.D., Latour, M.A., Boyle, C.R. and T.W. Smith.1999. Effects of breeder age and dietary fat on subsequent broiler performance. 2. Poultry Science 78: 512515.
Peebles, E.D., Zumwalt, C.D., Gerard, P.D., Latour, M.A. and T.W. Smith. 2002. Market age live weight, carcass yield and liver characteristics of broiler offspring from breeder hens fed diets differing in fat and energy contents. Poultry Science 81: 23-29. Peeble, E.D., Zumwalt, C.D., Smith, T.W., Gerard, P.D. and M.A. Latour. 2002b. Poultry fat and corn oil may be used to adjust energy in the diets of young breeder hens without affecting embryogenesis and subsequent broiler growout performance. Journal of Applied Poultry Research 11: 146-154. Plaimast, H., Kijparkorn S. and P. Ittitanawong. 2015. Effects of Vitamin D3 and Calcium on Productive Performance, Egg quality and Vitamin D3 Content in Egg of Second Production Cycle Hens. The Thai Journal of Veterinary Medicine. 45: 189-195.
Prasad, A. S, Bao, B., Beck, F.W. and F.H. Sarkar. 2011. Zinc-suppressed inflammatory cytokines by induction of A20-mediated inhibition of nuclear factor-kappaB. Nutrition 27: 816-823
Richards, M.P. 1997. Trace mineral metabolism in the avian embryo. Poultry Science 76:152-164
Saunders-Blades, J.L. and D.R. Korver. 2015. Effect of hen age and maternal vitamin D source on performance, hatchability, bone mineral density, and progeny in vitro early innate immune function. Poultry Science 94: 1233-1246.
Surai, P.F. 2000. Effect of selenium and vitamin E content of the maternal diet on the antioxidant system of the yolk and the developing chick. British Poultry Science 41: 235-243.
Triyuwanta, C.L., Leterrier, C. and Y. Nys. 1992. Dietary phosphorus and food allowance of dwarf breeders affect reproductive performance of hens and bone development of their progeny. British Poultry Science 33: 363-379.
Virden, W.S., Yeatman, J.B., Barber, S.J., Zumwalt, C.D., Ward, T.L., Johnson, A.B. and M.T. Kidd. 2003. Hen mineral nutrition impacts progeny livability. Journal Applied Poultry Research 12: 411416.
Zhang, B., Shao, Y., Liu, D., Yin, P., Guo, Y. and J. Yuan. 2012. Zinc prevents Salmonella enterica serovar typhimurium-induced loss of intestinal mucosal barrier function in broiler chickens. Avian Pathology 41: 361367.
Zhu, Y., Liao, X., Lu, L., Li, W., Zhang, L., Ji, C., Lin, X., Liu, H.C., Odle, J. and X. Luo. 2017. Maternal dietary zinc supplementation enhances the epigenetic-activated antioxidant ability of chick embryos from maternal normal and high temperatures. Oncotarget 8: 19814-19824.
JOHAN BUYSE, PhD., obtained his master’s in Bioscience Engineering with a specialty in animal production at the Katholieke Universiteit Leuven (Belgium) before beginning his doctoral research at the same university on the influence of intermittent lighting on broiler performance and underlying physiological working mechanisms at the Laboratory of Livestock Physiology (promotor prof. E. Decuypere). Since then, Dr. Buyse has served as a full professor at the Faculty of Bioscience Engineering (KU Leuven) before he was named the head of the Laboratory of Livestock Physiology there. In his current role, Dr. Buyse is responsible for several courses such as Animal Physiology, Animal Production and Welfare, Poultry Production and Husbandry, and Poultry Nutrition. During this research career, he was a laureate of the Royal Academy for Sciences, Letters and Fine Arts of Belgium for his contributions to agricultural research. He has (co)authored over 320 international, peer-reviewed papers, and several book chapters. He has also served as a member on the editorial board of several international journals.
Since the last two decades, a lot of attempts have been made to find appropriate solutions for the so-called broiler breeder paradox. This paradox states that is rather impossible to combine a fast muscle growth potential with an acceptable reproduction capacity and good health and welfare status in a feed- and body weight-controlled breeder hen.
The majority of attempts to solve this paradox are aiming on the breeder generation but no satisfactory answers could be postulated yet. More recent trials are now focusing on the carry-over effects of the imposed treatment on the parent generation on performance of the F1, F2 (multigenerational studies) up to the F3 generation (transgenerational studies). This chapter summarizes the current knowledge regarding multi- and transgenerational effects of in particular the quality (composition) of the breeder diet. It was clear that the quality of the (grand)maternal diet not only affects their own reproductive performance, body composition, physiology, gene expression, behaviour, etc. but also has an impact on several traits of subsequent offspring generations in a multi- or even transgenerational epigenetic way. The affected traits were in many cases gender-specific. Results so far are promising. Lastly, a selection of results regarding our unique transgenerational broiler breeder study spanning over three generations is presented. This project is focusing on the epigenetic inheritance effects of a reduced dietary crude protein content for F0 and F1 broiler breeders. This study identified multi- and true transgenerational inheritance effects for several traits. It is concluded that it must be possible to positively influence the performance of production animals in a sustainable and animal-friendly way by targeted changes in the environment (in casu dietary quality) of the (great) (grand)parent stock.
Poultry meat production (mainly broiler meat) continues to increase rapidly during the last decades. This increase is made possible thanks to ongoing improvements regarding the four pillars of the poultry sector: 1) genetics and selection (by yet a diminishing number of selection companies), 2) optimizing nutrition, 3) ameliorating housing and climate management, and 4) disease prevention and control.
As a consequence, the gain in body weight for the age of the breeder population stock has increased as well. The pure line and (great) grandparent and parent breeders must carry the genes for e.g., rapid growth and low feed conversion ratio (FCR) and pass these traits on to their (broiler) offspring. Next to their poor reproductive performance (in terms of settable eggs and day-old chick production), ad libitum-fed breeders are overweight and obese, are prone to develop metabolic diseases, and leg
problems, display high morbidity and mortality rates, and hence their health and welfare are compromised (Hocking, 1993; Decuypere et al., 2006, 2010; de Jong and Guémené, 2011; Mohiti-Asli et al., 2012). Consequently, a certain degree of controlled feeding (especially during the rearing period) must be imposed as this management strategy is shown to improve the reproductive performance of the breeders as well as their condition and health status considerably. However, feed-controlled breeders may have a hunger feeling and may display abnormal behaviour (e.g., frustration, boredom, object and bird pecking), creating new welfare issues. In order to solve the first ‘reproduction and health’ versus ‘fast muscle growth’ paradox (under ad libitum conditions) by controlling the appetite and body weight (gain) of the breeders, a new paradox, namely the ‘acceptable reproduction and good health’ versus ‘impaired welfare and hunger stress’ paradox, is created. This apparent deadlock is known as the ‘broiler breeder paradox’ (Decuypere et al., 2010).
Several efforts have been made to improve the welfare status related to the controlled feed allocation of parent stock. These attempts can be categorized as quantitative (amount of daily feed allocation, daily or skip-a-day feeding, spin feeding, etc.) or as qualitative (fiber addition, low-energy or low-protein levels, appetite-suppressive supplements, etc.) feeding strategies. These strategies at the level of the parent stock are discussed in other chapters of this volume and will not be repeated here.
This chapter is aimed to review the available literature as well as my own research in order to
seek or propose acceptable solutions to solve – at least to some extent – this paradox. In particular, the focus here is on multi- and transgenerational epigenetic inheritance effects of quality factors of breeder diets on offspring phenotypes.
It is clear that management interventions at the level of the breeders have an impact on an array of traits such as reproductive performance, health, welfare, behaviour, and physiology of the breeders themselves. It is consequently wondered if these treatments of the parent stock would also affect whatever traits of their offspring.
According to Skinner (2008), the term multigenerational refers to situations in which the reproductive-active maternal population (F0) is exposed to an exogenous (including nutrition) stressor(s) and how this affects traits of the F1 and F2 offspring. It follows that true transgenerational epigenetic effects are observable only on the F3 generation, as this is the first generation that is not directly exposed to the environmental challenge exerted on the F0 population. With respect to the paternal as well as the non-reproductive maternal side, a multigenerational effect is considered from the F0 father or nonreproductive mother to the F1 offspring whereas a true transgenerational effect is from the F0 father or non-reproductive mother to the F2 grand-offspring (e.g., Skinner, 2008; Thompson et al., 2020).
In mammals, it is now generally recognized that a wide variety of environmental (including
nutrition) factors imposed on the F0 generation can affect traits of the F1 and F2 offspring (multigenerational) or even the F3 generation (true transgenerational effects) by means of epigenetic mechanisms (e.g., Zambrano et al., 2005; Roseboom et al., 2006; Thompson et al., 2020). But what about non-mammalian species, in the case of avian species?
The impact of diverse quantitative feed restriction protocols applied to the F0 breeder population on F1 breeder and broiler offspring performance and welfare traits are explored in detail elsewhere in this volume. Just as an example, van der Waaij et al. (2011) showed that quantitative feed restriction of adult breeders for 5 weeks affected the growth trajectory and abdominal fat weight of their F1 broiler offspring albeit in a gender-specific way (see below). Next to the impact of quantitative feeding strategies, manipulations of the quality aspects of the breeder diet such as energy density and crude protein (CP) content can also influence the offspring phenotype in one way or another.
Indeed, F1 offspring of broiler breeder hens fed low-density diets (-21% reduction in nutrient density compared to a standard breeder diet) from 25 to 60 weeks of age showed a better embryonic development as a result of increased egg weight due to a higher egg white:yolk ratio (Enting et al., 2007a). In another study by Enting and co-workers (2007b), breeders were provided low-density diets (varying reduction in nutrient density between 11% and 23%) both during the rearing and layer period. It was observed that feeding low nutrient diets to breeders can have positive effects on day-old chick
weight, their postnatal growth rate, and humoral immune status, yet this is strongly dependent on breeder age and egg weight.
Next to nutrient density, the maternal dietary protein content can also alter F0 offspring performance. Rao et al. (2009) demonstrated that a maternal low protein diet for breeders can program the offspring as reflected in a lower hatch weight but higher post-hatch growth up to 4 weeks of age. In addition, changes in a whole array of physiological parameters such as plasma hormone levels and expression of some genes in the yolk sac membrane, muscle, and hypothalamus of the offspring of proteinrestricted dams were measured.
van Emous et al. (2015) studied the effects of breeder growth patterns and the CP content of their diet on the development of next-generation F1 embryos and their posthatch performance. Rearing the breeder pullets to a higher adult body weight at 20 weeks of age induced significant positive effects on embryonic parameters (better fertility and hatchability of set eggs and reduced early embryonic mortality) of eggs collected from 29-week-old breeders as well as a strong tendency towards better offspring performance. The CP content (low, medium, or high CP) of the phased rearing diets had apparently only a few effects on these parameters, except maybe for a higher breast percentage of only the male offspring from breeders fed the low CP diet. In a subsequent study, van Emous et al. (2018) investigated the effects of a factorial combination of two CP levels of phased layer diets (average difference of 15
g CP/kg) and two ages (early: 21 weeks, or late: 23 weeks) at photostimulation on egg production, hatchability, and F0 broiler offspring performance. There was a tendency that older (>46 weeks of age) hens reared on a reduced CP diet produced somewhat fewer eggs and with reduced hatchability. Advancing the age at photostimulation led to an overall increase in the number of chicks per hen and improved cumulative feed efficiency of their offspring. F0 progeny performance and slaughter yields were not significantly affected by the maternal dietary CP content.
Manipulation of the incubating avian egg and exploring its effects on embryo development and subsequent performance of the hatched phenotypes can be considered a special case of multigenerational transmission. As an example, partial removal of albumen (3 ml) of layer strain eggs before the initiation of incubation impaired the growth rate of layer pullets, their egg production (both number and weight of eggs), and reduced proportional albumen weight (Willems et al., 2015). In addition, offspring originating from dams that were albumen-restricted during their proper embryonic growth, displayed a temporary reduction in growth rate as well as an increased sensitivity to insulin. Moreover, changes in the hepatic transcriptome of these F1 day-old chicks originating from albuminrestricted hens including in pathways related to embryonic development, carbohydrate and amino acid metabolism, protein synthesis, and DNA methylation were identified (Willems et al., 2016). Hence, by using an avian model, it can be concluded that prenatal protein undernutrition induces long-term alterations
in the production characteristics of the parent generation and also exerts multigenerational carry-over effects on several traits of the F1 generations.
For mammals including humans, a mismatch between the poor nutritional state of parents and abundantly fed offspring can lead to a higher occurrence of metabolic diseases and other dysfunctions in the offspring (e.g., Zambrano et al., 2005; Roseboom et al., 2006; Langley-Evans, 2015). In mammals, there is a rather close in utero contact between the mother and the developing embryo/fetus, making the distinction between strict maternalimposed nutritional effects and the mediating interference of the dam difficult. This is not the case for avian species as the embryo develops in the incubating egg, hence independently from the mother hen. According to van der Waaij et al. (2011), avian models are therefore extremely well-suited to study the pure effects of the maternal nutritional conditions on offspring development and performance and to identify maternal-offspring match or mismatch situations. In their experiment, 60-week-old pure line Cobb hens were either fed more (near to ad libitum) or less (-41% compared to near ad libitum-fed counterparts) than their normal diet for 5 weeks. F1 broilers were either fed ad libitum or feed restricted (-30% less feed), resulting in four treatments (2 dietary treatments of hens x 2 dietary treatments of offspring). In brief, chicks from ad libitum fed breeders hatched earlier than those of restricted hens (male hatchlings only), were heavier (both males and female hatchlings), and had a better navel quality (female hatchlings only). Body weight of ad libitum fed male (not females) chicks originating from restricted breeders
remained significantly lower until 2 weeks of age, but this difference nearly disappeared (difference of -38.5g) by 6 weeks of age. At week 1 and week 6, ad libitum-fed male broiler chickens originating from ad libitum-fed breeders grew faster than their ad libitum-fed counterparts coming from restricted hens. For females, ad libitum-fed females from restricted breeders grew somewhat faster than those from ad libitum-fed hens (although only statistically discernable for week 3), resulting in a slightly higher body weight (difference of +18.8g) at 6 weeks of age. The authors suggested that these changes in growth trajectory up to commercial slaughter age due to the maternal nutritional state could be attributed to preprogrammed changes in muscle mass. Moreover, the accelerated growth of ad libitum-fed offspring of restricted dams could be due to a higher feed intake than ‘programmed’ because of the restriction of the dams. With respect to abdominal fat weight, the only significant difference was found for females, as ad libitum fed female broilers originating from restricted breeder hens were characterized by a higher abdominal fat weight compared to that of their ad libitum-fed counterparts coming from ad libitum-fed hens. Hence, a mismatch between maternal poor and offspring rich nutrition is disadvantageous in terms of growth and fat deposition, although gender-dependent. The authors concluded that a mismatch in the maternal-offspring nutritional environment must be taken into account in broiler breeder management if optimal dam reproduction and offspring performance is to be achieved.
One of the few (maternal) multigenerational studies spanning over 3 generations (F0 to F2) with an avian species, in this case Muscovy
ducks, was performed by Brun et al. (2015). In brief, a limited number of female Muscovy ducks were either provided a standard control diet or a methionine-deficient diet (-38% methionine reduction compared to the control diet). Methionine is an important supplier of methyl units that are required for DNA methylation and hence most likely to be involved in epigenetic effects. Representative F1 offspring drakes were mated to Muscovy or mule duck females to produce the F2 progeny. The reader is referred to the paper by Brun et al. (2015) for detailed information regarding the overall and generation-specific mating strategies. The F2 mule and Muscovy ducks of both sexes were subjected to forcefeeding for 2 weeks (between 12 and 14 weeks of age) after a period of ad libitum feeding and preparation for force-feeding. It was found that the grandmaternal diet elicited multigenerational effects on several traits recorded on the F2 offspring, which must have occurred through the male line. Notably, body weight of F2 mule ducks originating from methionine-deficient grandmothers was reduced compared to that of their F2 counterparts descending from grandmothers fed a normal diet. However, the former F2 mule ducks displayed a higher weight gain and lower feed conversion ratio during the force-feeding period. With respect to carcass traits, only absolute and proportional abdominal fat weights were increased due to the grandmother’s methionine-deficient diet. For F2 Muscovy ducks, body weights of ducks originating from methionine-deficient grandmothers were reduced up to 12 weeks of age, although only for male ducks. Feeding the grandmothers a methioninedeficient diet enhanced lipid metabolism
and deposition. Interestingly, the augmented lipid deposition was genotype-dependent; in mule ducks the abdominal fat was targeted whereas in Muscovy ducks liver steatosis was encouraged. It is also worthy to note that some traits such as body weight gain of Muscovy ducks (but not of mule ducks) were affected in a sex-specific way (grandmother diet by sex interaction). Brun and coworkers (2015) concluded that the diet of the grandmothers can influence some traits of their grand-offspring (surely in Muscovy ducks) by means of their sons. At that time, these authors stated it needs to be established if this transmission through the duck fathers is epigenetic in origin.
It can be inferred that there is already a lot of information available regarding
multigenerational effects in mammals and to some extent also in avian species. However, in contrast to these multigenerational studies, information about true transgenerational (F0 up to the F3 generation) epigenetic inheritance studies in avian species are sparse.
Besides the transgenerational study with broiler breeders mentioned earlier, we are aware of only one true transgenerational experiment with an avian species, in this case quail, performed by Leroux and coworkers (2017). These researchers established two ‘epilines’ of quail by injecting genistein, a natural isoflavone from soy with methylationmodifying properties, into fertilized quail eggs (Epi+ line). The control group (Epiline) remained untreated. The hatchlings were raised to adulthood (F0 generation)
F0 generation
Control (C)
Reduced Protein (RP, -25%)
F1 generation
Broilers
F2 generation
F3 generation
Broilers Broilers
C/C
C/RP RP/C RP/RP
C/C/C C/RP/C RP/C/C RP/RP/C
BroilersBroilers Broilers
Figure 1. C = control; RP = reduced balanced protein. Two treatments for F0 generation: C group fed with standard C diets and RP group fed with RP diets. Female F0 progeny of each group was subdivided into two dietary (C or RP) treatments, resulting in four treatments for the F1 generation: C/C, C/RP, RP/C, and RP/RP (letters indicating the breeder diet in F0/F1 generation). Female F1-progeny of each group were all fed with the standard C diet resulting in four treatment groups for the F2 generation: C/C/C, C/RP/C, RP/C/C, and RP/RP/C (letters indicating the breeder diet in F0/F1/F2 generation). For each generation, several trials with sexed broiler offspring were also performed
and two subsequent generations (F1 and F2) were produced according to a strict mirrored single-pair mating protocol for each line and generation in order to minimize the initial genetic variability between the lines (see Leroux et al. (2017) for detailed information on the mating strategies). Standard-reared F3 quail of both sexes were generated for the production trait studies. These latter studies clearly showed that genistein injections in F0 eggs affected body weight of 3-week-old F3 quail, albeit in an opposite way according to the sex. Indeed, body weight of Epi+ males was increased whereas body weight of Epi+ females was decreased compared to that of their Epi- counterparts. Genderdependent multi- and transgenerational inheritance effects on some particular traits were also observed as in other studies with avian species (e.g., Brun et al., 2015; Lesuisse et al., 2017) and this recurrent phenomenon surely merits further investigations. At slaughter age (27 weeks of age), Epi+ quail were significantly lighter than Epi- quail (regardless of gender) but contained more abdominal fat (only measured in females). With regard to reproductive traits of adult F3 individuals, Epi+ females produced fewer eggs, mainly due to a delayed onset of laying. As F3 individuals were not directly exposed to the stressor genistein, it can be inferred that the observed epiline differences could have occurred at least to some extent through epigenetic mechanisms, although according to these authors some genetic line variability cannot be totally excluded. Maybe surprisingly, there were no differences between the epilines in whole blood DNA methylation obtained from 23-week-old F0 and F3 individuals. However, Leroux
and coworkers (2017) acknowledged that besides tissue-specific DNA methylation profiles, other epigenetic marks could be involved in their observed transgenerational inherited effects. Indeed, at the molecular level and besides DNA methylations, hydroxymethylation of CG dinucleotides, posttranslational or chemical histone modifications, non-coding RNA expression, posttranslational RNA modifications, and chromatin condensation modes could all be involved. The reader is referred to the review paper of David et al. (2017) for more information on the molecular aspects of the epitome (of chickens).
In view of the lack of proper knowledge about true transgenerational inheritance in avian species, we have performed a (maternal) transgenerational study over three generations with broiler breeders and their offspring at the Laboratory of Livestock Physiology (KU Leuven, Belgium). The focus was on the effects of a reduced, yet balanced CP content (-25% CP) for the breeders (F0 and F1 generations) provided during both the rearing and laying period. The main research hypothesis was that feeding breeders a reduced balanced protein diet would induce maternal programming effects in such a way that the performance (e.g., growth, FCR, fat deposition) of the offspring should be better than that of offspring originating from control-fed breeders. Furthermore, it was also hypothesized that broiler offspring from protein-restricted (grand)parents would be programmed to cope better with lower CP
diets (match between maternal poor and offspring poor nutrition). Indeed, based on mammalian studies and sparse studies with avian species (e.g., van der Waaij et al., 2011), it was wondered what the consequences would be of direct (between two consecutive generations) maternal-offspring nutritional matches or mismatches. As there are many such combinations in this vast project, the focus in this book chapter is limited to maternal poor protein and adequate offspring protein nutrition combinations. However, would these direct relationships be changed by the grandmother’s diet?
In summary, the F0 generation was established with 160 pure-line A breeder hens that were provided with either a standard diet (control C diet) or a reduced balanced protein (RP) diet (Figure 1). Compared to the C diet, the iso-energetic RP diet was formulated to
have a 25% reduction in CP and also in amino acids (for details regarding diet formulations and breeder management, see Lesuisse et al., 2017; 2018a). The RP hens received on average 10% more feed than the C hens to achieve a similar body weight gain trajectory. It is important to note that the RP diets were provided both during the rearing (22 days to 154 days of age) and laying (>155 days) periods. Hens were artificially inseminated with pooled serum from standard-reared roosters (to avoid paternal interference) and eggs were incubated in a forced draft incubator under standard incubation conditions (Lesuisse et al., 2017). Day-old chicks were reserved for establishing the next F1 breeder generation or were raised as broilers (F1 broilers or F0 broiler offspring). For the F1 generation, female chicks of both F0 groups (C and RP group) were further subdivided into a C and RP group, resulting in four F1 breeder groups: C/C, C/RP, RP/C, and RP/RP (the first and second letter refer to the type of breeder diet in the F0 and F1
Table 1. Average body weight (BW), feed conversion ratio (FCR) and total tract nitrogen retention of male broiler offspring of C/C, C/RP, RP/C, and RP/RP breeders (letters indicating the breeder diet in the F0 and F1 generations, respectively; C= Control diet, RP= Reduced Protein diet)
on a control or low protein (LP) diet (-15% reduction in crude protein)
C/RP RP/C RP/RP
BW
BW
FCR
a,bDifferent superscripts
row
from Lesuisse et al. (2018b)
Breeder Management and Nutrition: Moving the industry forward
generation, respectively). Next, an F2 breeder generation was created, wherein all received the C diet: C/C/C, C/RP/C, RP/C/C, RP/ RP/C (the third letter refers to the type of diet (control C diet for all groups) for the F2 breeders). Within each generation, several broiler offspring trials were conducted with sexed broilers reared on isocaloric diets with varying protein levels. Recall that true transgenerational effects can only be observed in the broiler offspring of the F2 breeder hens as the female germ cells for generating the F2 breeder generation are already present in the F0 generation (Skinner, 2008).
For the F0 and F1 generations, RP breeders were characterized by higher proportional body fat and liver content and lower proportional breast weight compared to that of C breeders (Lesuisse et al., 2017, 2018a; Schroyen et al., 2021). The effect of the type of diet (C or RP) in the F1 generation was more determinative compared to the diet type provided in the F0 generation. RP breeders from the F0 and F1 generations had the poorest laying performance and produced the lightest eggs (Lesuisse et al., 2017, 2018a). In contrast, F2 hens from the RP/RP/C group realized the best laying performance, but egg weight and day-old chick weight were still lower compared to that of C/C/C hens, which remained the producers of the highest egg weights and day-old chick weights. Hence, providing a RP diet to F0 and surely to F1 breeder hens still reduced the average weight of eggs produced by F2 hens, notwithstanding the fact that all F2 hens were fed the C control
diet. This observation can be considered as a maternal multigenerational inheritance effect as the impact of the RP diet provided to the previous generation(s) on egg weight remained present. Control-fed F2 hens originating from CP-restricted F1 hens produced eggs with the lowest absolute and proportional albumen weights, and the highest proportional yolk weights, resulting in eggs with the lowest albumen to yolk ratio of all four F2 breeder groups.
Sexed F0 offspring chicks (F1 broilers) were reared on a standard broiler diet program and housing conditions. Male F0 broiler offspring originating from RP breeders realized a higher 5-week-old body weight and proportional breast weight, and a strong tendency (P=0.052) for an improved FCR compared to their male counterparts originating from F0 controlfed breeders (Lesuisse et al., 2017). Female F0 offspring from RP dams displayed a significantly improved FCR but no differences in slaughter weight and proportional breast muscle weight compared to their female counterparts originating from F0 control-fed breeders, illustrating again the gender-specific multigenerational inheritance according to the trait. In contrast, van Emous et al. (2015) did not observe a meaningful effect of maternal CP diet on offspring performance, but this is most probably related to the period of CP restriction (only rearing versus the entire life cycle period). In our study, proportional liver and abdominal fat pad weights of male and female F0 offspring were not affected by maternal dietary CP content.
Here, a mismatch between the undernutrition of the breeders and the adequate diet of the offspring – at least in terms of protein – did result in positive consequences. In contrast, apart from ageand gender-dependent differences in weekly growth rates (see above), van der Waaij et al. (2011) did not observe statistically significant differences in average 6-weekold body weight between ad libitum-fed male and female broilers originating from feed-restricted dams with that of their sexmatched counterparts originating from ad libitum-fed hens. However, the ad libitum fed female (not male) broilers from restricted dams had a significantly higher abdominal fat weight. Hence, in the latter study of van der Waaij et al. (2011), the nutritional maternal-offspring mismatch did not produce significantly favourable effects and even enhanced abdominal fat deposition.
Lesuisse et al. (2018b) reported that male F1 offspring (F2 broilers) from C/RP and RP/RP hens fed on a standard control broiler diet realized a higher 42-day slaughter weight compared to that of offspring from C/C dams, whereas RP/C offspring had intermediate body weights (Table 1). The differences in FCR between the four groups were not statistically discernible at the 5% level. Interestingly, compared to their C/C offspring counterparts, standard-fed male F1 offspring of breeders that received the RP diet in the F0 and/or F1 generation displayed a tendency (P=0.067) toward better nitrogen retention, which might contribute to their better growth rate during the finisher phase.
When focussing solely on the consequences of a specifically selected direct generationto-generation (mis)match case between a poor maternal and adequate offspring diet, the performance of standard-fed F2 broilers originating from control-fed F1 breeders (match) should be compared with their counterparts coming from RP-fed F1 hens (mismatch). Again - as already demonstrated for F0 breeders and F1 broilers - mismatch situations (restricted- fed F1 breeders versus standard-fed F2 broilers) enhanced performance in terms of slaughter weight, FCR, and N retention efficiency compared to that of broilers from a matched situation (control-fed F1 breeders versus standardfed F2 broilers). It is also worth noting that the positive outcomes of this mismatch were in the same line, irrespective of the type (C or RP) of F0 grandmother diet. Hence, adequate protein-fed offspring from CPrestricted mothers are programmed to be more efficient with dietary protein, which might be due to the epigenetic inheritance of a more efficient protein metabolism from their reproductive RP dams (Lesuisse et al., 2018a).
Is this also the case when the F1 broiler offspring is reared on a low protein (LP) diet? Are the broiler offspring from mothers confronted with a protein restriction (C/RP group) able to cope better with a low protein diet than their counterparts originating from parents that never experienced a protein restriction (C/C group)? The answer is clearly yes. Indeed, when fed a LP broiler diet (15% reduction in CP compared to the standard control broiler diet), the male C/RP offspring attained significantly higher body weights
at 42 d and realised a significant better FCR compared to that of offspring from C/C hens (no differences in proportional abdominal fat content). A nutritional match (maternal poor – offspring poor) situation is apparently also advantageous. Male offspring from grandmothers that received the RP diet (RP/C group) also performed better on the LP diet than the C/C offspring. Hence, it is clear that when confronting the (grand) parent generations with a RP diet program, the offspring perform better on an adequate, as well as on an inadequate protein diet. This observation confirms again that the acquired more efficient protein metabolism of RP-fed breeders is passed on to their offspring. Does it make a difference if only the mothers or if only the grandmothers were provided with the RP diet? There is a tendency that both standard-fed and LP-fed offspring from C/ RP breeders performed somewhat better than those originating from RP/C breeders. So, the answer is yes: it matters. Would it be extra beneficial to subject both the mothers and grandmothers to the RP program? To answer this, one should compare LP-fed F1 offspring from RP/C and C/RP mothers with offspring from RP/RP mothers. Clearly, the RP/RP offspring performed significantly poorer in terms of slaughter weight and FCR compared to RP/C and C/RP offspring, and their performance leveled off to that of the LP-fed C/C offspring (Table 1). Therefore, the answer is no: not beneficial but rather to the contrary. In contrast to the strong tendency (p=0.067) for group differences in total tract N retention between F1 broilers fed the standard broiler diet, there was no such tendency for F1 broilers reared on the LP diet. The overall group average for N
retention of control-fed broilers was 61.5% whereas this value increased to 65.1% for LP-fed broilers (+5.8% improvement). As observed previously (Buyse et al., 1992), providing inadequate protein diets to broilers improves their N retention efficiency. Hence, it is hypothesized that the LP diet already maximized N retention, leaving little space for additional amelioration by multigenerational inheritance.
In the next step, several broiler trials were carried out with sexed F2 offspring (F3 broilers) that were fed ad libitum either on low (-10% or -15%), standard, or high (+10% or +15%) CP diets. If effects between these broiler groups would be observed, these could only be caused by the breeder diet type in the F0 and/or F1 generations as their F2 mothers all received the same control breeder diet program and were raised in the same conditions.
True transgenerational maternal (F0–F3) inheritance effects can only be identified if there are trait differences between F3 broilers (F2 broiler offspring) due to different challenges (in this case CP provision) in the F0 generation. In our study, this boils down to trait differences between F3 broilers originating from C/C/C breeders and RP/ C/C breeders. Next to recording the technical performance (growth, FCR, mortality), proportional organ and tissue (liver, pancreas, abdominal fat, breast muscle, hypothalamus) weights were determined and tissue samples stored, blood samples were collected, and plasma stored for posterior determination of
hormones and metabolites, and age-related changes in body composition were measured by dual-energy x-ray absorptiometry. To date, tissue and plasma samples are being analyzed and data statistically processed. Not all data is available but is it already clear that true transgenerational inheritance effects are indeed present. As an example, when fed a LP broiler diet, female broiler offspring from RP/C/C breeders were characterized by a significantly lower proportional abdominal fat pad weight compared to that of offspring from C/C/C breeders. It is to be determined if more transgenerational effects for other traits and parameters will be identified.
Following studies on breeders and their broiler offspring performance, welfarerelated parameters were investigated (for details see Li et al., 2018, 2019a,b). Clearly, the litter moisture content of pens housing C breeders was much higher compared to that of RP breeder pens, resulting in an associated increase in footpad dermatitis incidence. In agreement with the observations of van Emous et al. (2014), providing the RP diet to breeder hens (both F0 and F1 generation) resulted in a poorer feather condition. Surprisingly, the RP/RP/C hens had the best feather condition compared to the other three groups. The nutritional status of breeder hens did not have an impact on learning ability or memory retention, but RP hens displayed more frustration-related and ‘craving for protein’ behaviour. F2 hens descending from RP/C or RP/RP hens however were characterized by reduced frustration behaviour and polydipsia. In
conclusion, multigenerational effects of the maternal dietary CP content on feather condition, polydipsia, and frustrationrelated behaviour were observed. The results clearly indicate that it is possible to program offspring performance as well as behavioural characteristics by altering the diet of the parent and even the grandparent populations.
The liver transcriptome of 44-weekold hens from the F1 generation (C/C; C/RP; RP/C and RP/RP groups) was studied by RNA sequencing in order to 1) investigate multigenerational effects on hepatic gene expression, and 2) to screen for the consequences of match and mismatch situations (Schroyen et al., 2021). Independently from the maternal F0 diet, it was clear that providing the RP diet in the F1 generation directly downregulated amino acid metabolism, as reflected in reduced transaminase activity corresponding with decreased amino acid degradation and uric acid synthesis. Moreover, compared to control hens (C/C hens), RP-fed F1 breeder hens originating from control-fed F0 dams were characterized by an activated hepatic fatty acid metabolism as reflected in increased gene expression of fatty acid desaturase 1, fatty acid desaturase 2, and elongation of very long-chain fatty acids protein 2. Intriguingly, F1 hens originating from protein-restricted F0 hens (but not from control F0 hens) that were also provided with the RP diet (RP/ RP group) were characterized by an altered glucose metabolism (more specifically upregulation of fructose and mannose metabolism) compared to that of RP/C
hens. It seems therefore that the redirection of the fat and carbohydrate metabolism of RP-fed F1 breeder hens is determined by the maternal F0 diet, representing a multigenerational inheritance effect at the level of the hepatic transcriptome.
This project has also environmental and bio-economical perspectives. Concerning the broilers, next to better technical performance, higher efficiency of dietary N retention also lowers the nitrogen waste output and pollution, leading to reduced eutrophication (fewer nitrates) of surface and groundwater and soil. In addition, it is assumed that this will also lead to curtailed emissions of ammonia and greenhouse gasses (such as nitrous oxide) and hence slow down global warming (environmental sustainability). A third major advantage of the enhanced N efficiency is that South American soy production (at the expense of the rainforest) and transport (greenhouse carbon dioxide production) can be scaled back as less protein is needed in feed formulations of feeds for parent stock but above all for broilers, which also definitely will contribute to environmental sustainability. Finally, broiler diets with reduced CP content will also be less costly as the CP fraction is the most expensive macronutrient in poultry diets.
The broiler breeder paradox is not completely solved yet, but solutions are underway. Feeding RP diets to (grand)
mothers showed clearly positive effects on a broiler level by reducing FCR, increasing daily weight gain and improving nitrogen retention, and also ameliorating the welfare status of the breeder generation. However, breeder reproductive performance was negatively influenced by the RP diet, leaving challenges to improve breeder reproductive performance by optimizing nutritional formulation for these RP diets.
Based on literature data and my own research it can be inferred that the quality of the (grand)mother’s diet does not only have an impact on their own reproductive performance, body composition, physiology, gene expression, and behaviour but also affects several traits of consecutive generations in a multi- or even transgenerational epigenetic way. It is really intriguing to realize that it is possible to steer - to some extent - the phenotype of the (great) (grand)offspring by changing the environment in a broad sense of the (great) (grand)parents. A common thread was also that for some traits the inheritance was gender-specific. This knowledge certainly opens perspectives for improving the performance, welfare, and adaptation capabilities of production animals in a sustainable way. It seems also that a mismatch between a maternal CP poor and adequate CP offspring diet, as well as a match between maternal CP poor and offspring CP poor diet, both result in enhanced offspring performance.
It is now undoubtedly established that phenotypic variation is not solely dependent on genetics, but that epigenetics are also involved, although the real contribution of
Breeder Management and Nutrition: Moving the industry forward
epigenetics needs to be assessed (GuerreroBosagna et al., 2018). Environmental and nutritional factors can induce tissue-specific epigenetic changes (without changes in DNA sequence) and the identification of the involved molecular epigenetic markers is a logical next step in explaining the observed results.
Buyse, J., Decuypere, E., Berghman, L., Kühn, E.R. and F. Vandesande, 1992. The effect of dietary protein content on episodic growth hormone secretion and on heat production of male broilers. British Poultry Science 33: 1101-1109. Brun, J-M., Bernadet, M-D., Cornuez, A., Leroux, S., Bodin, L., Basso, B., Davail, S., Jaglin, M., Lessire, M., Martin, X., Sellier, N., Morrison, M. and F. Pitel. 2015. Influence of grand-mother diet on offspring performances through the male line in Muscovy ducks. BMC Genetics 16: 145. David, S-A., Mersch, M., Foissac, S., Collin, A., Pitel, F. and V. Coustham. 2017. Genome-wide epigenetic studies in chicken: a review. Epigenomes 1: 20.
de Jong, I.C. and D. Guémené. 2011. Major welfare issues in broiler breeders. World’s Poultry Science Journal 67: 73-82.
Decuypere, E., Hocking, P.M., Tona, K., Onagbesan, O., Bruggeman, V., Jones, E.K.M., Cassy, S., Rideau, N., Métayer, S., Jego, Y. and J. Putterflam. 2006. Broiler breeder paradox: a project report. World’s Poultry Science Journal 62: 443-453.
Decuypere, E., Bruggeman, V., Everaert, N., Li, Y., Boonen, R., De Tavernier, J., Janssens, S. and N. Buys. 2010. The Broiler Breeder Paradox: ethical, genetic and physiological perspectives, and suggestions for solutions. British Poultry Science 51: 569-579.
Enting, Kruip, T.M., Verstegen, M.W.A. and P.J. Van der Aar. 2007a. The effect of low-density diets on broiler breeder performance during the laying period and on embryonic development of their offspring. Poultry Science 86: 850-856.
Enting, H., Boersma, W.J.H., Cornelissen, B.J.W., Van Winden, S.C.L., Verstegen, M.W.A. and P.J. Van der Aar. 2007b.The effect of low-density broiler breeder Diets on performance and immune status of their offspring. Poultry Science 86: 282-290. 285 Guerrero-Bosagna, C., Morisson, M., Liaubet, L., Rodenburg, T. B., de Haas, E. N., Košťál, L. and F. Pitel. 2018. Transgenerational epigenetic inheritance in birds. Environmental Epigenetics 1-8. Hocking, P.M. 1993. Welfare of broiler breeder and layer females subjected to food and water control during rearing: Quantifying the degree of restriction. British Poultry Science 34: 53-64. Langley-Evans, S.C. 2015. Nutrition in early life and the programming of adult disease: a review. Journal of Human Nutrition and Dietetics 28, Suppl 1, 1-14.
Leroux, S., Gourichon, D., Leterrier, C., Labrune, Y., Coustham, V., Rivière, S., Zerjal, T., Coville, J_L., Morisson, M., Minvielle, F. and F. Pitel. 2017. Embryonic environment and transgenerational effects in quail. Genetics, Selection and Evolution 49: 14.
Lesuisse, J., Li, C., Schallier, S., Leblois, J., Everaert, N. and J. Buyse. 2017. Feeding broiler breeders a reduced balanced protein diet during the rearing and laying period impairs reproductive performance but enhances broiler offspring performance. Poultry Science 96: 3949-3959.
Lesuisse, J., Li, C., Schallier, S., Clímaco, W.L.S., Bautil, A., Everaert, N. and J. Buyse. 2018a. Multigenerational effects of a reduced balanced protein diet during the rearing and laying period of broiler breeders. 1. Performance of the F1 breeder generation. Poultry Science 97: 1651-1665.
Lesuisse, J., Schallier, S., Li, C., Bautil, A., Li, B., Leblois, J., Buyse, J. and N. Everaert. 2018b. Multigenerational effects of a reduced balanced protein diet during the rearing and laying period of broiler breeders. 2. Zootechnical performance of the F1 broiler offspring. Poultry Science 97: 16661676.
Li, C., Lesuisse, J., Schallier, S., Climaco, W., Wang, Y., Bautil, A., Everaert, N. and J. Buyse. 2018. The effects of a reduced balanced protein diet on litter moisture, pododermatitis and feather condition of female broiler breeders over three generations. Animal 12: 1493-1500.
Li, C., Lesuisse, J., Schallier, S., Lamberigts, C., Wang, Y., Driessen, B., Everaert, N. and J. Buyse. 2019a. The learning ability and memory retention of broiler breeders: 1 effects of reduced balanced protein diet on reward based learning. Animal 13: 1252-1259.
Li, C., Schallier, S., Lesuisse, J., Lamberigts, C., Driessen, B., Everaert, N. and J. Buyse. 2019b. The learning ability and memory retention of broiler breeders: 2 transgenerational effects of reduced balanced protein diet on reward-based learning. Animal 13: 1260-1268.
Mohiti-Asli, M., Shivazadi, M., Zaghari, M., Rezaian, M., Aminzadeh, S. and G.C. Mateos. 2012. Effects of feeding regimen, fiber inclusion, and crude protein content of the diet on performance and egg quality and hatchability of eggs of broiler breeder hens. Poultry Science 91: 3097-3106.
Rao, K., Xie, J., Yang, X., Chen, L., Grossmann, R. and R. Zhao. 2009. Maternal low-protein diet programmes offspring growth in association with alterations in yolk leptin deposition and gene expression in yolk-sac membrane, hypothalamus and muscle of developing Langshan chicken embryos. British Journal of Nutrition 102: 848-857. Roseboom, T., De Rooij, S. and R. Painter. 2006. The Dutch famine and its long-term consequences
for adult health. Early Human Development 82: 485-491.
Schroyen, M., Lesuisse, J., Lamberigts, C., Schallier, S., Li, C., Buyse, J. and N. Everaert. 2021. Direct and maternal reduced balanced protein diet influences the liver transcriptome in chickens. British Journal of Nutrition 126: 337 – 344. Skinner, M.K. 2008. What is an epigenetic transgenerational phenotype? F3 or F2. Reproductive Toxicology 25: 2-6. Thompson, R.P., Nilson, E. and M.K. Skinner. 2020. Environmental epigenetics and epigenetic inheritance in domestic farm animals. Animal Reproduction Science 220: 106316. van der Waaij, E.H., van den Brand, H., van Arendonk, J.A.M., and B. Kemp. 2011. Effect of match or mismatch of maternal-offspring nutritional environment on the development of offspring in broiler chickens. Animal 5: 741-748. van Emous, R.A., Kwakkel, R., van Krimpen, M. and W. Hendriks. 2014. Effects of growth pattern and dietary protein level during rearing on feed intake, eating time, eating rate, behavior, plasma corticosterone concentration, and feather cover in broiler breeder females during the rearing and laying period. Applied Animal Behaviour Science 150: 44-55.
van Emous, R.A., Kwakkel, R.P., van Krimpen, M.M., van den Brand, H. and W.H. Hendriks. 2015. Effects of growth patterns and dietary protein levels during rearing of broiler breeders on fertility, hatchability, embryonic mortality, and offspring performance. Poultry Science 94: 681-691.
van Emous, R.A., de la Cruz, C.E. and V.D. Naranjo. 2018. Effects of dietary protein level and age at photo stimulation on reproductive traits of broiler breeders and progeny performance. Poultry Science 97: 1968-1979.
Willems, E., Koppenol, A., Deketelare, B., Wang, Y., Franssens, L., Buyse, J., Decuypere, E. and N.
Everaert. 2015. Effects of nutritional programing on growth and metabolism caused by albumen removal in an avian model. Journal of Endocrinology 225: 89-100.
Willems, E., Guerrero-Bosagna, C., Decuypere, E., Janssens, S., Buyse, J., Buys, N., Jensen, P. and N. Everaert. 2016. Differential expression of genes and DNA methylation associated with prenatal protein undernutrition by albumen removal in an avian model; Scientific Reports 6: 20837. Zambrano, E., Martinez-Samayoa, P.M., Bautista, C.J., Deas, M., Guillen, L., Rodriguez-Gonzales, C., Guzman, G.L., Larrea, F. and P.W. Nathanielsz. 2005. Sex differences in transgenerational alterations of growth and metabolism in progeny (F2) of female offspring (F1) of rats fed a low protein diet during pregnancy and lactation. Journal of Physiology 566: 225-236.
ANNE-MARIE NEETESON-VAN NIEUWENHOVEN, MSc, is the Global Vice President of Welfare and Compliance of Aviagen Group – she leads the International Poultry Council Environment and Sustainability Working Group, is a board member of the International Poultry Welfare Alliance and the U.S. Round Table for Sustainable Poultry and Eggs and active in various poultry working groups globally.
DR. SANTIAGO AVENDAÑO is originally from Uruguay and joined Aviagen in 2003 after graduating from Edinburgh University with a PhD. in Quantitative Genetics and Genome Analysis. He is currently Global Director of Genetics for the Aviagen Group with responsibility for the development, evaluation and implementation of new technologies within Aviagen’s breeding programmes. Previous to joining Aviagen, Santiago was a researcher and technical advisor in beef cattle and sheep breeding in pasture-based production systems in Latin America.
DR. ALFONS KOERHUIS is the Chief Technical Officer of Aviagen Group, responsible for the research and development of Aviagen chickens and turkeys. He has an MSc degree in Animal Breeding from Wageningen University and a PhD from the Edinburgh University.
Animal breeding for welfare and sustainability requires improving and optimising environmental impact, productivity and robustness and welfare. Breeding is a long term exercise at the start of the food chain with permanent cumulative outcomes, disseminated widely. This chapter explains, with a focus on poultry, breeding programme design and how broadening breeding goals and managing trait antagonism results in balanced breeding and more robust animal populations. Breeding progress in skeleton and skin health, physiology and body composition and behaviour is addressed. The economic impact of welfare and environmental improvements is worked out, and the ethical and societal aspects of genetic improvement are put in perspective. The consideration of feedbacks of all stakeholders, including customers and the wider society, is crucial. For each crossbreed, breeders will continue to improve overall welfare, health, productivity and environmental impact, but between the crossbreeds there will be clear differences answering specific demands of concepts and brands.
Animal breeding and genetics are situated at the start of the food supply chain, with a gene flow originating from the breeding programme and disseminating permanent and cumulative genetic change through the production base. We will address the global framework of which animal breeding, economics and welfare are part, and the technical and organisational details of breeding for sustainable poultry meat production. We will focus on poultry using examples from broiler and turkey breeding.
Population will grow to over 9 Billion people by 2050 with long-term increases in meat demand, limited availability of natural resources such as agricultural land and water,
and concerns about environmental impact of livestock production (e.g., Foresight, 2011; Herrero and Thornton, 2013; Herrero et al., 2013, LEAP 2015; United Nations (UN), 2015; Bryden, 2016).
At the same time, with societies becoming more affluent, alternative production systems with various schemes targeting thresholds for growth rates lower than 50 g/day and/ or specific requirements regarding welfare attributes, coloured feathering of birds and/ or ranging behaviour are gaining momentum (Neeteson-van Nieuwenhoven et al., 2016b; Avendaño et al., 2017). They require alternative production systems or new suitable crossbreeds (e.g., ‘Kip van Morgen’ (CBL, 2013) and ‘Beter Leven’ (beterleven.dierenbescherming. nl) in the Netherlands, ‘Für mehr Tierschutz’ (tierschutzlabel.info) in Germany, and
‘RSPCA Assured’(rspcaassured.org.uk) in the UK). In addition to these consumer requirements, also consumer choice, responsible use of antibiotics, dietary health and sustainability are items influencing food purchases in urban and prosperous societies.
Economy, welfare and breeding are an integral part of sustainability. The official definition of sustainability is “meeting the needs of the present without compromising the ability of future generations to meet their own needs” (Brundtland report, 1987). Sustainability is mostly referred to via the three Sustainability Pillars (UN general assembly, 2005): environment, society and economy. Later ‘society’ also got referred to as ‘ethics’. Animal welfare is an integral part of the ethics/society pillar; economy occupies a pillar of its own. Breeding contributes to sustainability by delivering genetic potential to match both environmental (through lower resource utilisation and carbon footprint), economic (through increased profitability of the wider industry) and welfare requirements (through meeting societal requirements).
The framework of sustainability in animal breeding was coined in 2003. Motivated by the apparent distance between animal production in its wider sense and consumers - breeders, scientists, welfare organisations, ethicists, sociologists and economists developed the concept of sustainability in animal breeding in European Commission (EC) funded efforts (Neeteson-van Nieuwenhoven et al., 2006).
As a first step, the ethical, legal and society implications of farm animal breeding were
described (Neeteson-van Nieuwenhoven et al., 1999). Breeders were guided by ethicist professor Peter Sandøe who proposed that they must investigate and decide where animal breeding can make a difference. In the second phase, socio-economic studies explored cultural differences, animal welfare, ethics and global economy in relation to animal breeding. At the same time, socioeconomic specialists provided a critical forum for the specialists in ruminant, pig, poultry and aquaculture breeding who independently identified similar areas across species where animal breeding can play a role: welfare and health, food safety, genetic diversity, efficiency, environment, and product quality. The end results were a definition of and scenarios for sustainable farm animal breeding and reproduction (Liinamo and Neeteson-van Nieuwenhoven, 2003; FABRE TP Annex II, 2008). In a third step, this was formalised in a Code of Good Practice for Sustainable Farm Animal Breeding and Reproduction (CodeEFABAR; responsiblebreeding.eu; EFFAB, 2018a, b) which is updated every 3 years and adopted by many breeding organisations. “The Code” is a pledge by the breeding companies with which they show how “selection for genetic potential in production is balanced by appropriate attention to reproduction, health and welfare-related traits” (Besbes and Neeteson-van Nieuwenhoven, 2006). Poultry breeding companies now take this Code a step further by adding an auditing component with Code-EFABAR Poultry Assured (CEPA; EFFAB, 2018c).
Animal production sectors have articulated their sustainability actions for
Breeder Management and Nutrition: Moving the industry forward
their respective species through several forums. In beef, there is an active Global Round Table for Sustainable Beef (grsbeef. org). The International Poultry Council has adopted the Poultry Sustainability Pillars, and has linked these to the UN Sustainable Development Goals (SDGs) (Declaration of São Paulo; IPC, 2017 and 2019). The International Poultry Council has adopted the Poultry Sustainability Pillars, and links these to the UN Sustainable Development Goals (SDGs) (IPC, 2017). The International Egg Commission announces it will work out how the egg sector contributes to the UN SDGs (IEC, 2018). Recently stakeholder platforms like the International Poultry Welfare Alliance (IPWA) and US Round Table for Sustainable Poultry and Eggs (US-RSPE) were launched (USPEA, 2018).
For a poultry breeder, welfare can be defined as developing suitable birds for a range of production systems via balanced breeding from large gene pools, handling birds with care and respect, ensuring health, welfare and productivity. At the same time it should support producers with the latest management and technical advice, and demonstrate corporate responsibility while meeting global food demand. This principle is described as the commitments to 1) health, food safety and food security by ensuring a safe and secure supply of healthy birds to help feed a growing global population; 2) biodiversity by providing a sustainable supply of breeding stock from pedigree programmes with large diverse gene pools; 3) balanced breeding programmes, in which simultaneous selection takes place for a large number of production, health and
welfare traits; 4) tailored management and stockmanship via extensive management support as management factors contribute significantly to the health, welfare and performance of a flock, and 5) transparency, communication and engagement: engaging with stakeholders through active, transparent communication and close cooperation with customers, associations and wider society (Aviagen Group, 2017).
2.1. The domestication and breeding of animals
2.1.1. Domestication
When hunter-gatherer populations became farmers, domestication of farm animals started, e.g., domesticated chickens originate from the Red Jungle Fowl (Gallus gallus; 25002100 B.C.; Laughlin, 2007). Domestication gradually evolved into animal breeding, which is the selective mating of animals with desirable genetic traits, to maintain or enhance these traits in future generations (FABRE TP, 2008; Nature, 2018).
Figure 1 shows two entangled pyramids, representing the broiler gene flow structure. The bottom pyramid contains requirements to develop and maintain a breeding programme – a gene pool including pure lines contributing to commercial broilers or turkeys and non-commercial lines (e.g., experimental and control lines), and the considerable support systems of modern genetics. Poultry breeders keep a wide range of pedigree lines (for instance in Aviagen > 30 in broilers, > 40 in turkeys; Defra, 2010).
Lobby groups
Health & Genes
years
Figure 1. Diversity of breeding stock, multiplication pyramid and feedback mechanism of Aviagen breeding programmes. GGP = Great Grandparent Stock. From: Laughlin, 2007
The inverted pyramid shows how genetic improvements made in poultry pedigree lines are disseminated to the rest of the chain through a series of multiplying generations into a variety of crossbreeds at parent and broiler/commercial turkey level to meet the needs of different markets. A crossbreed is typically made up of four different types of pedigree lines (Laughlin, 2007; Aviagen Group, 2016).
Animal breeding is the optimization of internal and external factors: maximizing the breeding objective(s) taking account of the opportunities and challenges from
the outside world (economy, environment, society), and the intrinsic opportunities and limitations from animal populations, structures and opportunities to correctly measure and weigh the genetic component of the desired characteristics. Successful breeding requires accurate prediction of breeding values with data recording, novel trait development, optimal breeding programme structure and design, the use of latest knowledge in analytics applied to genetics and genomics, and the ability to manage huge data sets at high throughput. In addition, the size of the breeding populations matters as genetic progress is a direct function of selection intensity.
Animal breeding is a long-term exercise. Although economic evaluation is useful to indicate the broad direction, the breeding programme needs must have a broad and long term focus. McKay et al. (2000) predicted that future selection progress also will include closer monitoring of physiological systems to ensure an integrated response to multiple traits. Neeteson-van Nieuwenhoven et al. (2013) queried animal breeding experts worldwide about the relative importance of trait groups in breeding goals in 1950, 1990, 2015 and 2025, showing that the complexity of breeding goals increases over time: the relative focus on productivity decreases and objectives such as efficiency (productive and environmental) and animal robustness increase. Breeding decisions require a long term view which at least should include a 10 year perspective – the consideration of feedbacks of all the industry stakeholders on a continual basis is crucial (EPB/EFFAB, 2009). Figure 1 shows the feedback from customers and the wider society via an arrow left from the pyramids.
An important aspect of animal multiplication is healthy propagation of breeding stock – in cattle and pig artificial insemination was introduced to eliminate venereal diseases – in most animal breeding programmes the biosecurity level is very high. Biosecurity is a condition sine qua non in global poultry breeding. Any breeding programme must commit to deliver genetic stock free of transmissible diseases including Mycoplasmas, Salmonellas and Avian Influenza (AI). Strict biosecurity procedures must be in place for disease prevention and thousands of serology and bacteriology samples are analysed annually to ensure
freedom from Salmonella and Mycoplasma. Avian Influenza represents the single most significant disease challenge, risk and threat to the poultry industry globally which in recent years had serious consequences to poultry trade and the poultry industry leading to the destruction of millions of domestic birds across the world (OIE, 2017). The arrows on the right illustrate the biosecurity of the pyramid, and its timeframe of 4 years from pedigree to poultry meat production.
The optimal design of the breeding programme is crucial for its sustainability. Maximising genetic gain while controlling inbreeding rates is an approach that ensures long-term sustainability of the breeding programme. A sustainable breeding programme requires continuous improvement of selection accuracy, novel recording technology, and understanding the genetic and, where possible, physiological relationships between traits of interest across the variety of lines in the gene pool (e.g., both conventional and slow grow genotypes) - as any product arising from these has to be both competitive and sustainable (EPB/EFFAB, 2009; Hill et al., 2016; Avendaño et al., 2017).
Genetic improvement is highly dependent on sustained accurate data collection which is paramount for reliable predictions of genetic merit. Data are gathered from pedigree animals and their relatives (ancestors, siblings, including information on traits which cannot be measured on the animal itself, e.g., egg production of a cockerel). In poultry breeding, a full pedigree structure of populations dates back to the late 1970s. Information recorded on the selection
candidates and its relatives are included to predict genetic merit for all the traits in the breeding goal. In poultry breeding, the recording of health and welfare traits dates back to before the 1970s (Aviagen Group, 2016).
In a multi-trait breeding goal there is a wide range of traits with different levels of complexity. There are some traits that are relatively easy to measure and they represent the final outcome of a biological process, for example, live weights, physical examination of broilers and turkeys for leg and skeletal defects, egg production or fertility. On the other hand there are traits that require significant technological developments because they underlie complex biological processes, for example feed efficiency and behaviour, immune response, and gut function. Transponder technology has allowed the recording of feed intake and feeding behaviour in large groups (e.g., Howie et al., 2011 in chickens). X-ray technology has contributed to the improvement of leg health in broilers through the detection of the clinical and sub-clinical incidence of Tibial Dyschondroplasia (TD). The recent introduction of 3D imaging technology in broiler breeding is going to provide unprecedented opportunities to manage trade-offs between traits and will benefit in particular welfare, processing and meat quality traits and balance of the breeding programme in general (Howie et al., 2011; Kapell et al., 2012b; Avendaño et al., 2017). Clearly, as more traits related to biological functions are included in the breeding goal, there will be a need to develop novel ways to measure underlying traits in the selection
candidate through live indicators (e.g., biomarkers) or imaging technology.
Traditionally, genetic evaluation is based on phenotypic records, which incorporate genetic information through the family relationship and estimates of the relationships (genetic variances, covariances) among the traits. The information on the animal, its parents, sibs and earlier generations are each combined optimally taking account of all these aspects including each trait’s heritability. With specifically designed models and analyses (e.g., Best Linear Unbiased Prediction, BLUP) this wealth of information is used to predict breeding values (Hill et al., 2016).
2.2.4 Genomics
Hill et al. (2016) explain that, when assessing individuals without own or progeny records (in particular unproven males for sex limited traits and for post mortem recorded traits), information from the ancestors does not include the effect of which unique sample of DNA an individual gets from the father or from the mother (Mendelian sampling). Genomics information allows predicting the Mendelian Sampling term in the absence of own phenotypic record hence allowing differentiating candidates from the same male and female parent which otherwise would have the same breeding value. Simultaneous estimation of marker associated effects across the whole genome creates genome wide breeding value estimates, an approach developed by Meuwissen et al. (2001; ‘genomic selection’). Genomic selection is implemented
Breeder Management and Nutrition: Moving the industry forward
in many dairy breeding programmes, and is routine in pig and poultry (e.g., Forni et al., 2011; Avendaño et al., 2012). Genomic selection combines pedigree, molecular and phenotypic data within the classical BLUP structure to maximise the accuracy of choosing selection candidates, allowing comparison of predicted breeding values of each individual directly despite being based on very different amounts and types of data, as with BLUP based on production data alone. Adding genomic prediction to traditional quantitative genetics evaluation in poultry increases the selection accuracy of 10 - 50% depending on the trait. The introduction of genomics into breeding programmes has thus increased the possibilities to select optimally balanced animals and the accuracy of selection for all traits related to welfare (Aviagen Group, 2016; Hill et al., 2016).
A breeding goal defines the desired change in an animal population in terms of the traits of any kind of interest and their relative importance (Neeteson-van Nieuwenhoven et al., 2013).
Breeding goals must be long term to achieve steady improvements over time, focus and improve the individual components, and weigh the optimum. Breeding goals also have to be adapted regularly, because the relative value of traits changes over time when there are changes in production conditions, market requirements, and societal developments.
Better insights into the biological background of traits and of technology development
lead to novel options for selection so that improving novel traits becomes feasible. Broiler breeding goals have expanded vastly in the last three decades, combining productivity and biological efficiency with liveability, robustness, adaptability and reproduction (Neeteson-van Nieuwenhoven et al.; 2013; Aviagen Group, 2016). Welfare-related traits represented 18% to 33% of the relative weight in the breeding goal in broiler breeding (Hiemstra and Ten Napel, 2013), including traits on skeletal integrity (e.g., leg strength, walking ability, TD), contact dermatitis, heart and lung function and liveability. The process of breeding goal expansion is not expected to stop but to continue even further.
Whether livestock breeding goals are sustainable into the future depends on the ability of breeding organizations to balance the increasingly complex demands for overall sustainability: food security and economic survival, environmental load, population diversity, food safety, and health, welfare, and ethical considerations. As explained, the further development of technology makes it increasingly feasible to aim for genetic improvement of productivity, product quality and animal adaptability simultaneously.
Hill et al. (2016) illustrate the principle of multi-trait genetic selection in the presence of trait antagonism in meat chicken breeding and demonstrate that when traits are included in a broad breeding goal and balanced selection is applied, the desired direction in each trait can be and has been achieved. We will explain the major decisive intrinsic components of a balanced breeding strategy: sufficient and
Improved FCR but decreased
Breeding
sustainable management of antagonistic traits and of genotype by environment interaction to improve robustness.
In complex breeding programmes including traits related to production, health, reproductive fitness, welfare, robustness and adaptability there will be often traits that are antagonistically related. A classical example often quoted is that improvements in production traits led to undesirable health‐related consequences (e.g., Rauw et al., 1998; Dawkins and Layton, 2012; Hocking, 2014). In the past, in broilers, increases in incidence of ascites and skeletal abnormalities were reported “in birds intensively selected for growth rate” (Chambers, 1990). The way to handle this antagonisms is through estimating the genetic correlations between trait groups
Improved Hatch %
Improved Hatch and FCR simultaneously
in the breeding goal (e.g., between biological performance and welfare or product quality) allowing improvements of antagonistically related traits simultaneously.
2.4.1 Antagonism in genetic terms
Avendaño et al. (2017] explained that, from a breeding point of view, the key parameter controlling the extent of the genetic antagonism between traits in a breeding goal is their genetic correlation (GC): “the GC measures the extent to which two traits are controlled by the same genes. A favourable GC means that the genes controlling both traits have the same effect on each trait while an unfavourable or antagonistic correlation means that the effect is the opposite in each trait.”
With an example from their breeding
programme, Avendaño et al., (2017) illustrate the genetic antagonism between feed conversion rate (FCR) and hatch%. Figure 2 shows the estimated breeding values (EBVs) for 1385 birds from a pedigree batch as deviations from the population mean. In this dataset, the GC between hatch% and FCR is 0.27, typical for the antagonism between broiler and breeder performance traits. The dotted double arrowed trend line indicates that as we move further to the left we will find birds with better FCR (i.e., lower) but worse hatch% while to the right we find birds with improved hatch but poorer FCR (i.e., higher). In this example FCR would get 0.012 (i.e., 12 g of feed per kg of live weight) worse per % improvement of hatch.
The solution to deal with this dilemma is to include both traits in the breeding goal and to select for birds which are good for both traits at the same time. The birds with breeding values in the right bottom box are the birds of this pedigree batch with better EBVs than the population average for both traits at the same time.
The current improved ways of recording both performance traits (e.g., body weight, breast yield%, FCR) and welfare-related traits (e.g., leg health and contact dermatitis) allow inclusion of all these traits in the breeding goal, and to improve them simultaneously in the long term (management of antagonisms or trade-offs in animal breeding). This is illustrated by e.g., Kapell et al. (2012a, b) and confirms that “continued genetic improvement for production efficiency and minimisation of demand on resources is feasible without sacrificing animal health and welfare in a multi-
trait selection set up including both fitness and production traits” (Hill, 2016).
Current and future animal populations will have to perform with increasing efficiency in a wide range of environments, characterized by varying management practices, feed quality (form and density) and gut and immune challenges. In these environments substantial genotype × environment (G×E) interactions will exist. (Neeteson van Nieuwenhoven et al., 2013; Hill et al., 2016)
The robustness component of genetic potential is particularly relevant when meat production expands globally in a wide range of production regimes (OECD/FAO, 2018). The pedigree populations are normally kept at high standards of management, health and biosecurity. Thus, it is crucial to include elements of differential production to the pedigree programme to minimise the GxE interaction and ensure the expression of the genetic potential in many varying local environments.
One approach is to base selection at the pedigree level on records gathered in a commercial production environment. Pedigree populations can best express their genetic potential for productivity in high-input conditions. As highlighted before, animals raised in bio-secure conditions are important to fulfill the other important role of breeding: dissemination of animals with a high health status. Methods used are combined crossbred and purebred selection (CCPS; Wei and Van der Steen, 1991) and commercial sibling
testing (CST; Kapell et al., 2012a). With CST, selecting on data from contrasting (pedigree and commercial) environments improves robustness. Sibs of selection candidates are grown in nonbio-secure commercial conditions, assessing gut health, digestive, and immune function along with liveability, growth, and uniformity. The result is improved productivity in both environments, increased animal adaptability to the wider range of management circumstances they may encounter in the field, and more robust populations with higher liveability and uniformity.
For reasons of practicality, we will first concentrate on welfare aspects where genetics
is the major component: balanced breeding programmes. In 10.5 we will also address other societal and ethical aspects influencing animal breeding.
For decades, breeding goals have been broad and included traits related to welfare. Apart from the overall balance in a broadly defined breeding goal, breeding programmes include individual traits related to welfare: healthrelated traits like leg, feett and gut health, stress susceptibility, muscle metabolism; liveability in general; and adaptability traits such as susceptibility to behavioural deprivation (stereotypies, lethargy), dominance, aggression, feather pecking, tail biting and piglet savaging
Figure
term relationships
body
strength (%), liveability (%), oxygen saturation in blood and crooked toes
coloured
represents the relationship between breeding values for each trait within a year. The broken arrow represents the joint direction of the average breeding value for each trait involved in the trade off.
Avendaño et al.
(after Rodenburg and Turner, 2012). FAWC (2012) confirms that indeed farm animal breeding programmes have met many of the concerns raised in the FAWC (2004) report. As described in 10.2.4 – 10.2.5, the knowledge and experience of the genetic basis of welfarerelated traits in modern breeding companies is such that these conditions can be, have been and will continue to be met.
Avendaño et al. (2017) describe how the concept illustrated in Figure 2 also is applied to manage the genetic antagonism between biological performance and welfare-related traits. Figure 3 shows the range of GC (across a number of pedigree broiler breeding lines) between body weight (BWT) and breast
yield (BY, %) and a range of welfare-related traits: leg bone deformities (LD, %), gait score (GS), TD (%), FPD (%), crooked toes (CT, %), liveability (LIV, %) and oxygen saturation level in blood (OXI, %). Clearly, all the correlations are, in absolute terms, below 0.35 indicating the extent of the antagonism is not extreme. In some cases the antagonism is insignificant (bars close to 0) or there is no genetic antagonism at all, e.g., between BWT and FPD, BY% and TD, FPD, CT and in some cases with OXI.
These GC ranges are essential – it means there are ample opportunities to improve biological performance and welfare-related traits simultaneously, even in the presence of genetic antagonisms, when both groups of traits are included in a broad and balanced
breeding goal (Avendaño et al., 2017). However, it also shows that the extent to which selection can be effective depends on: 1. records can be taken in a timely manner and, if these are proxies for the trait in question, are sufficiently closely correlated to it; 2. there is significant genetic variation in that trait to enable its improvement; 3. the trait is not so unfavourably correlated with other production, health and welfare traits that selection on to jointly improve overall productivity, efficiency and welfare would be unfeasible, and 4. whether the breeding programme can be appropriately structured to enable such selection to take place.
We will show the achievements in broiler breeding for welfare traits using some illustrative examples. Figure 4 (Avendaño et al., 2017) illustrates that growth rate and leg strength have been improved simultaneously over the long term while the antagonistic genetic relationship between both traits holds within a year. The data, based on Aviagen breeding programme data, describe the joint trajectory between BWT and OXI, Liveability, LD and CT over 22 years from 1996 to 2017. Each coloured line shows the relationship between the traits estimated breeding values (EBVs) for selection candidates hatched in a specific year. The broken arrow represents the joint direction of the average breeding value for each trait involved in the tradeoff. The relationships between traits remains antagonistic within each year but there is a favourable trajectory for each trait because of simultaneous selection, that is, as BWT increases, cardiovascular function, liveability,
leg strength increases while CT decreases. In addition, it can be seen that the worst EBVs for OXI, LIV, LD and CT in 2017 are far better than the maximum of the 1996 birds.
These examples show the principle of multi-trait genetic selection in the presence of trait antagonism and demonstrate that when traits are included in a broad breeding goal and balanced selection is applied, the desired direction in each trait can be and has been achieved.
3.3.1. Leg and toe integrity and heart and lung function
Improvements in leg, toe integrity and cardiovascular function trends are confirmed by independent data of Agriculture and AgriFood Canada (AAFC, 2018), peer reviewed literature (e.g., leg strengths and toes in Kapell et al., 2012a), the FAWC (2012) report and the EC broiler breeding study (Hiemstra & Ten Napel, 2013). Figure 10.5 shows the Canadian improvements of leg and toe integrity and heart and lung function from 1995-2017.
Ascites levels as measured by AAFC have fallen correspondingly from about 36 per 10 000 birds slaughtered (1995) to about 8 (2008), and continue to stay low. Although ascites and SDS are now generally considered to be historical problems, companies continue monitoring and selecting against them. Understanding of fundamental biological bases for the pulmonary hypertension/ascites syndrome in broilers and novel indicator traits may come from immunological and genomics research (Wideman et al., 2013; Hill et al., 2016). In turkeys similar trends can be seen when the same selection tools are used.
3.3.2. Liveability
Reported liveability increases within lines and crossbreeds vary from 0.2-1.0% per year (Hiemstra and Ten Napel, 2013).
During last decades welfare research on contact dermatitis shifted gradually from hock burn (measured and selected against
since the 1970’s), to FPD. Kapell et al. (2012b) showed how genetic selection can be effective against FPD in contrasting environments – this is effectively applied since 2008 with decreased levels at the production level as a result.
addition to sustained improvements
in biological performance, the modern broiler has shown improvements in body composition. Compared to unselected genotypes, as a function of live weight, the modern broiler has greater leg strength (measured as tibia breaking force) and additional digestive capacity (e.g., greater small intestine surface area, larger pancreas and liver), with no evidence of negative impacts on cardiovascular function, indicating the modern broiler has a support system suited to handle higher metabolic demands from and express the genetic potential for higher biological efficiency and development (Fancher, 2014; Avendaño et al., 2017).
In poultry breeding, recording individual meals during the rearing phase of birds housed in large groups has taken place since 2004, allowing measurements of individual bird intake and feeding behaviour in competition with other birds. These data have shown that there is a significant genetic component in feeding behaviour with heritabilities ranging from 0.24 to 0.57 (Howie et al. 2011). Feeding behaviour in broilers is governed by non-random bouts consistent with periods of hunger and satiety which are common not only among a wide range of chicken lines but also across selected and unselected species (Tolkamp et al., 2011) meaning that the underlying mechanisms of short-term feeding behaviour are conserved in broiler birds. In addition, short-term feeding behaviour is heritable but independent from selection for FCR as shown by Howie et al. (2011) who found low genetic correlations with
feed intake ranging from -0.20 to 0.25. Common feeding behaviour patterns are conserved across chicken lines with widely varying growth rates, and across chickens, turkeys and ducks (Howie et al., 2009, 2010, 2011; Aviagen Group, 2016; Hill et al., 2016).
3.3.6. Water intake behaviour
The individual feed intake technology has been expanded to measure individual water intake in both turkeys and broilers. This allows breeding for both feed and water efficiency jointly. Identifying and rejecting birds with very high levels of water consumption for a given level of feed intake will have a positive impact on gut health and the incidence of contact dermatitis through an improvement of the quality of the litter. (Aviagen Group, 2016, 2018).
3.3.7. Feed intake management of broiler breeders and satiety
An additional item raised is feed intake management of broiler breeders during puberty. The genetic correlation between early and later growth, and similarly between early and late appetite, are very high (Hill et al., 2016). It is possible to manage the chicken growth curve by selection (Ricard, 1975) but at the cost of very large sacrifices in early gain, because of the high genetic correlation with late gain. Dawkins and Layton (2012) argue that by changing selection goals, sampling other populations and incorporating appropriate QTL from non-pedigree populations the antagonism could be addressed. Cross-bred combinations of fast growing male lines with slower growing female lines have indeed
addressed an important part of the issue, with the slower growing females being fed closer to ad-libitum levels in both rear and lay without elevated levels of mortality or multiple ovulation.
Currently, management changes, e.g., feed intake control during puberty, are also a practical and effective approach to manage the antagonism. EFSA (2009) concluded that research in this area is limited and more research was needed to draw firm conclusions about feeding programmes in relation to bird welfare – increased research efforts on alternative feeding systems and their impact on feeding amounts, behaviour and stress indicators (e.g., Dunn et al.; 2013; Dixon et al., 2014; Van Emous et al., 2014; Van Emous, 2015; Chang et al.,2016; Cherrie et al., 2016; Vanderhoydonck, 2016; Lesuisse et al., 2017; Li, 2018; Cherrie et al., 2018) have shown promising results – more research on further fine-tuning and better understanding of the various processes is on-going and essential to lead to meaningful change.
An intrinsic part of the sustainability of the poultry sector is its viability in economic terms, and its ability to continue to provide farms and farming for the next generation. Important for this is the resilience of farmers and of the rest of the poultry chain in the short and longer term (Neeteson et al., 2016). Economic evaluation can give broad direction to breeding strategy but cannot be applied within the selection decisions (Laughlin, 2007).
Paragraph 3.3. has addressed how welfare and production have improved simultaneously over the last 2 decades, and Figure 5 shows the improvement of valgus/varus and ascites in approximately the same timespan (19952018) from AAAF – the AAAF website has similar data on other health traits. Comparisons between selected and unselected or heritage broiler lines provide annual improvements estimates over the last 50 years of around 50g bodyweight, feed conversion rate improvements of 15 to 25 g feed/kg of bodyweight, and around 0.2% higher breast meat yield (Havenstein et al., 1994, 2003a, b; Fleming et al., 2007; Mussini, 2012; Zuidhof et al., 2014). Longterm industry data are a reflection of both management practices and genetics – they show improvements of 25 to 30g live weight and 16 to 20g reduction in feed consumed per kg of live weight thus indicating sustained improvements in feed efficiency (Laughlin, 2007; Avendaño et al.; 2017; Leinonen et al., 2016; NCC, 2018). The economic impact of this is huge as around 70% of total poultry production costs can be attributed to animal feed costs. Low production costs, leanness of the meat and favourable affordability have contributed to making poultry the most used meat by both producers and consumers in the developing world. Developing countries will account for 76% of the output growth, with poultry seeing the most rapid expansion (OECD/FAO, 2018).
As global meat production is expected to increase, the environmental impact of livestock
Table 1. Biological performance1
Genotype
seven
Avendaño and Corzo, 2018]
%
Ross 308 65 38.5 1.62 73.2 22.7 96.5
Ross 708 63 39.7 1.63 74.1 24.2 97.0
Ranger Classic 49 50.0 1.83 72.2 20.8 97.5
Ranger Premium 50 51.0 1.83 72.7 21.6 97.5
Ranger Gold 47 55.0 1.90 71.4 19.8 97.8
Rowan Ranger 44 62.5 1.99 70.7 18.7 98.0
Rambler Ranger 34 74.6 2.15 69.2 17.0 98.5
ADG = Average Daily Gain;
production systems are a key component of sustainable production. The OECD/ FAO (2018) forecasts that global meat production is projected to be 15% higher in 2027 relative to the base period (2015-2017). Animal breeding improves environmental impact of animal production and sustainable agricultural land use. Poultry is in a favourable position compared to other meats with values of CO2 equivalents per unit of edible carcass ranging from around 20-60 for ruminants, 7-20 for pork and 3.7-5 for poultry. Poultry is expected to have a pre-eminent role in satisfying this increasing global demand for meat products with a contribution of 44% of total meat production growth (Williams et al., 2006, Leip et al., 2013, Herrero et al., 2013; Avendaño et al., 2017).
Based on 2010 FAO global broiler consumption numbers (86.5 million tons, equivalent to 123.6 million tons live weight; faostat.fao.org), and annual global commercial field FCR improvements of 0.015 kg/kg (a conservative estimate), cumulative annual savings are 0.015 × 123.6 = 1.85 million tons of feed. The main chicken
Breast%=Breast
meat producing countries realized a 2010 harvest yield of 466 tons of wheat per km2the above FCR improvement frees up 1.85 million/466 = about 4000 km2 of arable land, an area 1.5 times the size of Luxemburg or 3.3 times the size of New York City. This is a cumulative figure, realized every year (Neeteson van Nieuwenhoven et al., 2013).
Avendaño (Avendaño et al., 2017; Avendaño and Corzo, 2018) has calculated the environmental impact of a range of crossbreeds with different average daily gain and FCR using data from crossbreeds in their portfolio. Ross 308 and 708 are globally established commercial broiler crossbreeds; Rowan Range broiler types are slower growing crossbreeds (eu.aviagen.com/ brands/rowan-range). The Ranger genotypes in Table 10.1 fall under the requirements of current EU accreditation schemes like ‘Kip van Morgen’ and ‘Beter Leven’ in the Netherlands, ‘RSPCA Assured’ in the UK, and ‘Für mehr Tierschutz’ in Germany.
Biological performance to 2.5kg BWT difference between the fastest (Ross 308)
Breeder Management and Nutrition: Moving the industry forward and slowest (Rambler Ranger) crossbreed is 31.5g per day for daily gain (ADG), 36.1 days to achieve target weight, 0.53 kg feed per kg BWT, 3.8% eviscerated yield and 5.8% breast yield. Whereas production differences are large, liveability between the fastest and slowest crossbreed differs more modestly (2%). The reason is balanced breeding goals combining biological performance and liveability and welfare-related traits (Kapell et al., 2012a,b; Neeteson- van Nieuwenhoven et al., 2013; Avendaño et al., 2017), as is also shown in 10.3.3.
This has huge impacts on what is required to produce a kg of broiler meat. Annual requirements for feed, agricultural land, water and housing for an integration processing 1,000,000 birds per week have increased resource need when moving from standard to slower growing crossbreeds. Compared to Ross 308, the Rambler Ranger requires 32.7% more feed. Water and land increases by 32.7% which is in direct relationship with the FCR increase (Table 10.1) from 1.62 to 2.15. Housing requirements are three times as high, including the different stocking densities (s.d.) and number of annual cycles which are a function of the number of days to achieve the target weight. S.d.’s used were 42 kg/m2 for Ross 308 and 708, 38 kg/m2 for Rowan Classic and Rowan Premium (i.e., conforming with the ‘Kip van Morgen’ requirement), and 25 kg/m2 for Ranger Gold, Rowan Ranger and Rambler Ranger (i.e., conforming with Beter Leven and RSPCA Assured requirements). Increased resource requirements will impact cost of production, hence, to be profitable, the use of slow grow options will likely need to be accompanied by
capturing premium product prices exceeding the increased resource requirement and cost to remain profitable. In addition, a significant shift towards slow grow genotypes globally will likely mean an increased pressure on the ingredients market due to poorer FCR, with related higher prices for corn, soya and wheat, hence further driving up the production cost of meat.
With a Life Cycle Assessment (LCA) tool developed by Cranfield and Newcastle University (Poultry LCA Model Version 1.0) Avendaño et al. (2017) compared the environmental impact of the broiler crossbreeds in Table 10.1. The ‘cradle to farm gate’ LCA approach accounted for all inputs and outputs of a production system as described by Leinonen et al. (2012). FCR differences between crossbreeds stem from feed amount differences to reach the 2.5kg target weight at the corresponding age for their target daily gain - not from different feed densities. Input requirements for each system (electricity, heating, water, bedding) were modelled based on Leinonen et al. (2012) to 39d and adjusted to the corresponding age to reach 2.5kg, meaning input requirements increased linearly with bird age. In this example we have we estimated the real environmental impact of breeds and the systems in which they are used. The method can be used for other combinations of breeds and management systems and calculate their environmental impact outcomes.
In their paper, Avendaño et al. (2017) show biological efficiency is a major driver for environmental impact differences. As average daily gain and breast% increase,
kg bodyweight (Ross 308 is the base
global warming potential (GWP) decreases linearly - the opposite trend is seen for FCR. The more biologically efficient crossbreeds have the lowest environmental impact in terms of pollutant emissions and other contributes in LCA. These results are consistent with the findings by Leinonen et al. (2012): a free range and an organic production system had a predicted higher GWP of 16% and 28%, respectively over a standard production system. Acidification potential (AP), eutrophication potential (EP) and primary energy use (PEU) and biological performance show similar linear relationships (results not shown). This is in line with Herrero et al. (2013), concluding feed efficiency is a key driver of productivity, resource use and greenhouse gas emissions.
Figure 10.5 visualises relative GWP, AP, EP and PEU levels with Ross 308 as base reference level (1.0) for the seven crossbreeds as evaluated by Avendaño et
al . (2017). Ross 308 and 708 showed the lowest predicted environmental impactpredicted increased environmental impact of slow grow crossbreeds range from around 10% to 30% for GWP and 12% to 45% for AP, EP and PEU.
Like with GWP these results are in line with Leinonen et al. (2012): a standard production system had lower EP, AP and PEU than a free range and an organic production system. Leinonen et al. (2012) showed organic production system had environmental impacts with 40% higher EP, 96% higher AP and 59% higher PEU compared to standard production system. Leinonen et al. (2012) also illustrates how biological efficiency, in terms of feed requirements and length of the production cycle, are linked to environmental impacts of different production system inputs. Feed and water usage had the highest environmental impacts on GWP and
PEU (including production, processing and transport) to about 70% of GWP and 65-80% of PEU depending on production system. Farm gas and oil had second highest impact in PEU from 12-25% followed by farm electricity (ventilation, feeding and lighting). The use of gas, oil and electricity is generally lower in free-range and organic systems but the lower usage of these inputs does not compensate for the greater FCR and production cycle length.
Summarising, the impact of crossbreeds with lower biological efficiency on resource utilisation and environmental burdens is 30-40% higher compared to conventional genotypes. On the other hand, more broadly, the suitability of a crossbreed to a production system or market segment will depend not only on biological performance but also on consumer preference, product price and other product attributes including the perceived importance between performance and welfare (Avendaño et al., 2017).
Higher production levels in agriculture have allowed people to take up other activities than food production. Animal based food has become safer, healthy, and affordable. Food security has improved. And poultry production in particular has contributed to the viability of many poorer regions across the globe (LEAP, 2015).
On the one hand, consumers are more distant from animal food production, as fewer people produce food themselves, or
have family members who are farming. At the same time, people are more interested where their food comes from, and social media can bring the final consumer very close to the food product, the food production system and even the breeding programme as the customer is more interested and inquisitive where meat comes from and under which circumstances it was produced. Farm animal welfare is a major item in the public debate, particularly in affluent regions.
6.1. Definitions of animal welfare
A generally used means to describe animal welfare centers around the Five Freedoms, which were developed by FAWC (1979) as “the ideal state to strive for”, and the Three Essentials of Stockmanship (knowledge and skills on animal husbandry, and personal qualities: affinity and empathy with animals, dedication and patience; FAWC, 2007). “This provides a working definition for practical animal breeding centering around the animal’s homeostatic balance between i) its intrinsic potential and requirements and ii) its production environment, which includes nutrition, housing, health, the social environment, and stockmanship (each to support one of the Five Freedoms of the Farm Animal Welfare Council). Based on this, breeding goals can be designed to develop appropriate animals for various production systems supported by appropriate management” (Neetesonvan Nieuwenhoven et al., 2013).
People do not all have the same concept of animal welfare, and in addition to physical
aspects, people may put more emphasis on mental aspects and on naturalness. However, welfare perception sometimes is related to how a human would perceive a situation or condition. According to Dawkins (2012), it is dangerous to determine animal welfare in terms of anthropomorphism: ”a lot of people think that good welfare is when animals are allowed to perform natural behaviour, and you can judge welfare by how natural it is. … Animals in the wild are regularly chased by predators, and that would be natural”. She indicates we are ignorant about consciousness – it is better to face this than to pretend we have solved what is consciousness and use that as a basis for animal welfare. Dawkins defines animal welfare as animals that are healthy and have what they want (Rollin, 1993; Fraser et al., 1997; Neeteson et al., 2016b).
In addition to ‘naturalness’, also other items relevant for public awareness can become part of a general ‘food and goodness box’, such as dietary health (e.g., consumption of lean animal protein), indoor versus outdoor farming, farm size, use of machinery and technology, feed type (e.g., with or without animal protein, whether or not organic, whether or not genetically modified) and bird health (e.g., use of prophylactic or curative antibiotics) (e.g., Sonntag et al., 2018). In this general ‘good feeling food box, often the separate attributes are not recognised or distinguished: ‘animal welfare’, ‘natural’, ‘healthy’, ‘clean’, ‘whole’, ‘organic’, ‘unprocessed’ , ‘freshly made’, ‘preservative free’, ‘foods avoiding artificial ingredients and highly processed foods’,
and regularly also relate to different types of elimination diets, such as ‘vegan’ , ‘dairyfree’ , or ‘gluten-free ‘(Mantucci, 2018).
This is in line with the findings of a study by Deloitte (Ringquist et al., 2015) in the U.S., identifying a shifting of consumer value drivers. While historically consumers made decisions based on ‘Traditional Drivers’ (price, convenience and taste), a new set of ‘Evolving Drivers’ including Health and Wellness, Safety, Social Impact, Experience and Transparency are now influencing consumer purchase decisions. This study shows that about 50% of the purchase decisions were influenced by Evolving Drivers, and the corresponding broader purchase consideration arising from these new drivers was independent from region, age and income level. In addition, the consideration of Evolving Drivers in purchase decisions was important in both elaborated products (e.g., fresh prepared meals 66%) and non-elaborated products (e.g., meat, fish and poultry 49% and dairy 42%). Clearly some of the Evolving Drivers will be more related to retail aspects (e.g., safety), but social impact and health and wellness, have a direct link with breeding goals through sustainability, animal welfare and reduced usage of antimicrobials (Avendaño et al., 2017). Spiller et al. (2016) highlight that communication based on shared values, i.e. producing food to answer consumer needs, might be a good way forward for animal production as the basic attitude toward farmers in society is positive (Liebert, 2010). The same may be true for the development of products, and crossbreeds.
In addition, more affluent consumers appreciate if they can have an influence on and can make a choice from products available to them. These developments partly are the basis for the development of Speciality Production Brands in retail and food service, but also for breeds suited for their various requirements, e.g., colour variants, growth rate, outdoor access appreciative birds. In this way welfare as perceived by humans, often as part of a “food perception box”, becomes part of breeding goals for speciality types of production.
There may be intrinsic conflicts in the various aspects the public would appreciate. Slower growing chickens (perceived to have better welfare) have a higher environmental impact. Outside production systems have a higher disease risk which is in contradiction with food safety and human health. People prefer birds not to be beak trimmed for welfare reasons, but layers injuring each other by pecking seriously impacts their welfare, even fatally. The transparency role of the breeding sector is then to provide fact based information about the various characteristics of crossbreeds under certain production circumstances and to improve crossbreeds for the various markets such that a variety of options are available. A Dutch project ‘Greenwell’ is currently developing a model to combine sustainability, including welfare and environmental impact.
For each crossbreed, breeders will continue to improve overall welfare, health, productivity and environmental impact, but
between the crossbreeds there will be clear differences answering specific demands of concepts and brands. The environmental impact comparison of different growth rate crossbreeds as highlighted in more detail in 10.5 is an example of such information. Other examples are bird colour, or eagerness to go outside. Such information allows industry stakeholders (producers, food service, retail) to develop breed/management packages under labels that appeal to consumers in a factual way.
The above mentioned analysis of consumer drivers means from a breeding perspective the following (after Avendaño et al., 2017]:
Continued investment in R&D to elucidate the genetic basis of novel traits and emerging genetic correlations and trade-offs between new and existing traits in the breeding goal. This includes investing in novel recording and selection techniques for the improvement of genetic lines contributing to conventional and slow grow products;
A continual expansion of breeding goals with the ability to change and adapt as a response to current and future changes in global trends. This, in plain words just means more balanced progress across more traits;
An expanded genotype portfolio to be able to address the whole spectrum of industry requirements, from covering the sheer scaling up of broiler meat production and demand globally in a sustainable way to providing options to emerging niche markets (e.g., free range and organic). Thus, the need for large genetic pools suitable to generate broiler
products aligned with current and future market needs;
Continued investments in a) dialogue and cooperation with the wide stakeholder field, and its developments, so that the direction of the day after tomorrow and of tomorrow can be designed and implemented b) proof of breeding progress in meaningful terms for stakeholders, c) provision of management support to serve the changing markets.
In a growing and evolving marketplace, there will be room for both conventional, coloured and slower growing genotypes. While it is difficult to predict the relative representation of each type of product globally or regionally, clearly the focus from the primary breeder will be to offer genetic potential suited to all market segments while fulfilling sustainability requirements from economical, biological, welfare and environmental considerations.
AAFC (Agriculture and Agri-Food Canada) (2018) Available at: http://www.agr.gc.ca/ eng/industry-markets-and-trade/marketinformation-by-sector/poultry-and-eggs/ poultry-and-egg-market-information/ condemnations/?id=1384971854399#chicken (accessed 4 October 2018).
Avendaño, S., Watson, K.A. and Kranis, A. (2010) Genomics in poultry breeding: from Utopia to deliverables. In: Proceedings of the 9th World Congress on Genetics Applied to Livestock Production. Leipzig, Germany, Session 07-01. Avendaño, S., Watson, K.A. and Kranis, A. (2012) Genomics in poultry breeding—into consolidation
phases. In: Proceedings 24th World’s Poultry Congress, Salvador, Bahia, Brazil. Available at: http://www. facta.org.br/wpc2012-cd/pdfs/plenary/Santiago_ Avendano.pdf (accessed 14 October 2018).
Avendaño, S., Neeteson, A. and Fancher, B. (2017) Broiler Breeding for Sustainability and Welfare –are there Trade Offs? In: Proceedings Poultry Beyond 2023 Conference, Queenstown, New Zealand. 17pp. Avendaño, S. and Corzo, A. (2018) Selección de Pollos de Engorda - Sostenibilidad y Bienestar Animal.
avi News A. Latina Diciembre: 2-10.
Aviagen Group (2016) Decades of Welfare and Sustainability Selection at Aviagen. Chickens and Turkeys. Eds.: Neeteson, A.M., Swalander, M., Ralph, J. and Koerhuis, A. 11pp.
Aviagen Group (2017) Top 5 Commitments. Chicken Breeding. . Available at: http://eu.aviagen.com/ assets/Headers/Product-Page-Headers/AboutUs/Welfare-Top5Infographic-18-EN.pdf. Turkey Breeding. Available at: http://www.aviagenturkeys. com/uploads/2017/10/10/GEO5_V1_Aviagen%20 Turkeys%20Top%205%20Commitment%20 Infographic.pdf (accessed: 20 August, 2018).
Aviagen Group (2018) Water utilization in broilers. Aviagen brief. 5pp. Available at: http://eu.aviagen. com/tech-center/download/1256/AviagenBriefWaterUtilizationInBroilers2018-EN.pdf (accessed: 31 October 2018).
Bellamy, K. and Bogdan, E. (2016) Dairy and the Sustainable Development Goals. Rabobank Industry Note #574. October 2016, 8pp. Besbes, B. and Neeteson-van Nieuwenhoven, A.M. (2006) Code of Good Practice for Farm Animal Breeding Organisations. European Poultry Conference. In: EPC 2006: 12th European Poultry Conference, Verona, Italy. unpaginated World’s Poultry Science Association, Beekbergen, The Netherlands. 12pp. Brundtland report (1987). Our Common Future. World
Breeder Management and Nutrition: Moving the industry forward
Commission on Environment and Development.
Oxford: Oxford University Press, Oxford, UK.
Bryden, W.L. (2016) Water, energy and feed: the trifecta for food security. In: Proceedings Australian Poultry Science Symposium. World’s Poultry Science Association and Enhancing Poultry Performance. Camperdown, Australia, 27,7.
CBL (Centraal Bureau Levensmiddelenhandel) (2013) Factsheet Kip van Morgen. Available at: http://www.cbl.nl/fileadmin/user_upload/ formulieren__factsheets_etc/Factsheet_Kip_van_ morgen.pdf (accessed 14 October 2018).
Chambers, J. R. (1990) Genetics of growth and meat production in chickens. Pages 599–643 In: Poultry Breeding and Genetics. R. D. Crawford, ed. Elsevier Science Publishing Co., New York, NY.
Chang, A, Halley, J. and Silva, M. (2016). Can feeding the broiler breeder improve chick quality and offspring performance? Animal Production Science. 56, 8, 1254-1262.
Cherrie, G., Avendaño, S., Van Tuijl, O., Dunn, I.C., D’Eath, R.B. and Dixon, L. (2016) The effect of different feeding strategies on broiler breeder satiety. Abstract. UK World’s Poultry Science Association. Chester, UK. 1pp.
Cherrie, G., Avendaño, S., Dunn, I.C., D’Eath, R.B. and Dixon, L. (2018) The effect of different feeding strategies on female broiler breeder satiety International Society for Applied Ethology, Bristol UK. 1pp.
Dawkins, M. (2012) What do animals want? A conversation with Marian Stamp Dawkins. The Reality Club. Available at: https://www.edge.org/ conversation/marian_stamp_dawkins-what-doanimals-want (accessed 14 October 2018).
Dawkins, M.S. and Layton, R. (2012) Breeding for better welfare: genetic goals for broiler chickens and their parents. Animal Welfare. 21: 147-155. Defra (Department for Environment, Food and Rural Affairs) (2010) Poultry in the United Kingdom. The
Genetic Resources of the National Flocks. Available at: https://assets.publishing.service.gov.uk/ government/uploads/system/uploads/attachment_ data/file/69294/pb13451-uk-poultry-faw-101209. pdf (accessed 14 October 2018).
Dixon, L.M., Brocklehurst, S., Sandilands, V., Bateson, M., Tolkamp, B.J. and D’Eath, R.B. (2014) Measuring Motivation for Appetitive Behaviour: Food-Restricted Broiler Breeder Chickens Cross a Water Barrier to Forage in an Area of Wood Shavings without Food .PlosOne. Volume 9 | Issue 7 | e102322.
Dunn, I.C., Meddle, S.L., Wilson, P.W., Wardle, C.A., Law, A.S., Bishop, V.R., Hindar, C., Robertson, G.W., Burt, D.W., Ellison, S.J.H., Morrice, D.M. and Hocking, P.M. (2013) Decreased expression of the satiety signal receptor CCKAR is responsible for increased growth and body weight during the domestication of chickens American Journal of Physiology-Endocrinology and Metabolism 304 E909–E921.
EFFAB (European Forum of Farm Animal Breeders) (2018a) Code of Good Practice for Sustainable Farm Animal Breeding and Reproduction. Available at: www.responsiblebreeding.eu (accessed 14 August 2018).
EFFAB (European Forum of Farm Animal Breeders) (2018b) Code-EFABAR, the Commitment to Responsible Breeding. and The Code of Good Practice for Farm Animal Breeding and Reproduction Organisations. General Brochure. Available at: http://www.responsiblebreeding. eu/uploads/2/3/1/3/23133976/brochure_code_ efabar_2014-2016.pdf (accessed 14 October 2018).
EFFAB (European Forum of Farm Animal Breeders) (2018c) Code-EFABAR Poultry Assured. Available at: http://www.responsiblebreeding.eu/ uploads/2/3/1/3/23133976/code_efabar_cepa_ folder.pdf (accessed: 14 October 2018).
EFSA (European Food Safety Authority) Panel on
Animal Health and Welfare (AHAW) (2009)
Scientific Opinion on welfare aspects of the management and housing of the grand-parent and parent stocks raised and kept for breeding purposes.
EFSA Journal. 8, 1667.
EPB/EFFAB (European Poultry Breeders / European Forum of Farm Animal Breeders) (2009) The Context of Broiler Breeding. 7pp.
FABRE TP (Sustainable Farm Animal Breeding and Reproduction Technology Platform) (2008)
Strategic Research Agenda. 32pp. and Annex II: Horizontal Issues . 109pp.
Fancher, B. (2014) What is the Upper Limit to Commercially Relevant Body Weight in Modern Broilers? Proceedings of the New Zealand Poultry Beyond 2020 Conference. Queenstown, New Zealand. 20pp.
FAWC (Farm Animal Welfare Council) (1979)
FAWC Report on Stockmanship and Farm Animal Welfare, London, UK. Available at: https://www. gov.uk/government/uploads/system/uploads/ attachment_data/file/325176/FAWC_report_ on_stockmanship_and_farm_animal_welfare.pdf (accessed 14 October 2018).
FAWC (Farm Animal Welfare Council) (2004) FAWC report on the welfare implications of animal breeding and breeding technologies in commercial agriculture. Available at: https://assets.publishing.service.gov.uk/ government/uploads/system/uploads/attachment_ data/file/325222/FAWC_report_on_the_ welfare_implications_of_breeding_and_breeding_ technologies.pdf (accessed 14 October 2018).
FAWC (Farm Animal Welfare Council) (2011) Five Freedoms. Available at: http://webarchive. nationalarchives.gov.uk/20121007104210/http:// www.fawc.org.uk/freedoms.htm (accessed 29 November 2016).
FAWC (Farm Animal Welfare Committee) (2012) Opinion on the Welfare Implications of Breeding and Breeding Technologies in Commercial Livestock
Agriculture. Department for Environment, Food and Rural Affairs, London, UK. Available at: https:// www.gov.uk/government/uploads/system/uploads/ attachment_data/file/324658/FAWC_opinion_ on_the_welfare_implications_of_breeding_and_ breeding_technologies_in_commercial_livestock_ agriculture.pdf (accessed 14 October 2018).
Fleming, E.C., Fisher, C. and McAdam, J. (2007) Genetic progress in broiler traits – implications for welfare. Proceedings of the British Society of Animal Science. Southport, UK. p.50.
Foresight (2011) The Future of Food and Farming, Final project report. The Government Office for Science, London, UK.
Forni, S., Aguilar, I. and Misztal, I. (2011) Different genomic relationship matrices for single-step analysis using phenotypic, pedigree and genomic information. Genetics Selection Evolution. 43: 1. Fraser, D., Weary, D.M., Pajor, E.A. and Milligan, B.N. (1997) A scientific conception of animal welfare that reflects ethical concerns. Animal Welfare. 6, 187-205.
Havenstein, G.B., Ferket, P.R., Scheideler, S.E. and Larson, B.T. (1994) Growth, liveability, and feed conversion of 1957 vs. 1991 broilers when fed typical 1957 and 1991 broiler diets. Poultry Science. 73, 1785-1794.
Havenstein, G. B., Ferket, P.R. and Qureshi, M.A. (2003a) Growth, livability, and feed conversion of 1957 versus 2001 broilers when fed representative 1957 and 2001 broiler diets. Poultry Science. 82: 1500‐1508.
Havenstein, G.B., Ferket, P.R. and Qureshi, M.A. (2003b) Carcass Composition and Yield of 1957 Versus 2001 Broilers When Fed Representative 1957 and 2001 Broiler Diets. Poultry Science. 82:1509–1518.
Herrero, M. and Thornton, P.K. (2013) Livestock and global change: Emerging issues for sustainable food systems. PNAS. 110, 52, 20879- 20881.
Herrero, M., Havlík, P., Valin, H., Notenbaert, A., Rufino, M.C., Thornton, P.K., Blümmel, M., Weiss, F., Grace, D.and Obersteiner, M. (2013) Biomass use, production, feed efficiencies, and greenhouse gas emissions from global livestock systems PNAS. 110, 52, 20888–20893.
Hiemstra, S.J. and Ten Napel, J. (2013) Study of the impact of genetic selection on the welfare of chickens bred and kept for meat production, DG SANCO 2011/12254. IBF International Consulting, Brussels, Belgium. Available at: https://ec.europa. eu/food/sites/food/files/animals/docs/aw_ practice_farm_broilers_653020_final-report_ en.pdf (accessed 14 October 2018).
Hill, W.G. (2016) Is continued genetic improvement of livestock sustainable? Genetics. 202, 887-881. Hill, W.G., Wolc, A., O’Sullivan, N.P. and Avendaño, S. (2016) Chapter 11. Breeding for sustainability: Maintaining and enhancing multi-trait genetic improvement. In: Burton, E., Gatcliffe, J., Masey O’Neill, H. and Scholey, D. (eds.). Poultry Science Symposium Series Vol. 31. UK WPSA symposium September 2014 Chester. CABI Publishers, Oxforshire, UK. 193-213.
Hocking, P. M. and McCorquodale, C. C. (2008) Similar improvements in reproductive performance of male line, female line and parent stock broiler breeders genetically selected in the UK or in South America. British Poultry Science. 49, 282-289. Hocking, P.M. (2014) Unexpected consequences of genetic selection in broilers and turkeys: problems and solutions. British Poultry Science. 55:1, 1-12, DOI: 10.1080/00071668.2014.877692.
Howie , J.A., Tolkamp , B.J., Avendano, S. and Kyriazakis, I. (2009) The structure of feeding behavior in commercial broiler lines selected for different growth rates Poultry Science. 88,:1143–1150.
Howie, J.A., Tolkamp, B.J., Bley, T. and Kyriazakis, I. (2010) Short-term feeding behaviour has a similar
structure in broilers, turkeys and ducks British Poultry Science. 51, 6, 714-724.
Howie, J.A., Avendano, S , Tolkamp, B.J. and Kyriazakis, I. (2011) Genetic parameters of feeding behavior traits and their relationship with live performance traits in modern broiler lines. Poultry Science. 90, 1197-1205.
IEC (International Egg Commission) (2018) Egg Industry Announces Commitment to United Nation’s Sustainable Development Goals. Available at: https://www.internationalegg.com/ egg-industry-announces-commitment-to-unitednations-sustainable-development-goals/ (Accessed 26 September 2018).
IPC (International Poultry Council) (2017) IPC adopts Sustainability Principles. Available at: www. internationalpoultrycouncil.org (accessed 20-82018).
IPC (International Poultry Council) (2019) Global poultry leaders sign Sustainable Development Goals commitment with FAO. Available at: www. internationalpoultrycouncil.org (accessed 21-82019)
Kapell, D.N.R.G., Hill, W.G., Neeteson, A.M., McAdam, J., Koerhuis, A.N.M. and Avendaño, S. (2012a) Genetic parameters of foot-pad dermatitis and body weight in purebred broiler lines in 2 contrasting environments. Poultry Science. 91, 565–574.
Kapell, D. N. R. G., Hill, W. G., Neeteson, A. M., Mc Adam, J., Koerhuis, A. N. M. and Avendaño, S. (2012b) Twenty-five years of selection for improved leg health in purebred broiler lines and underlying genetic parameters. Poultry Science. 91, 3032-3043. Laughlin, K.F. (2007) The Evolution of Genetics, Breeding and Production, Temperton Fellowship. 15. Harper Adams University, Newport, UK.
LEAP (Livestock Environmental Assessment and Performance Partnership) (2015) Environmental performance of poultry supply chains. Version 1. Food
Breeder Management and Nutrition: Moving the industry forward and Agriculture Organisation, Rome, Italy.
Leinonen, I., Williams, A.G., Wiseman, J., Guy, J. and Kyriazakis, I. (2012) Predicting the environmental impacts of chicken systems in the United Kingdom through a life cycle assessment: Broiler production systems. Poultry. Science. 91, 8-25.
Leinonen, I., Williams, A.G. and Kyriazakis, I. (2016) Potential environmental benefits of prospective genetic changes in broiler traits. Poultry Science. 95, 228-236.
Leip, A., Weiss, F., Lesschen, J.P. and Westhoek, H. (2013) The nitrogen footprint of food products in the European Union. Nitrogen workshop special issue paper. Journal of Agricultural Science. Page 1-14. Cambridge University. DOI: 10.1017/ S0021859613000786.
Lesuisse, J., Li, C.,Schallier, S., Leblois, J., Everaert, N. and Buyse,J. (2017) Feeding broiler breeders a reduced balanced protein diet during the rearing and laying period impairs reproductive performance but enhances broiler offspring performance. Poultry Science. 0, 1–11.
Li, C. (2018) Transgenerational effects of controlled feed allocation and reduced balanced protein diet on the welfare, cognition and behaviour of broiler breeders. Doctoraatsproefschrift nr. 1494 aan de faculteit Bioingenieurswetenschappen van de KU Leuven. 155 pp. Liebert, T. (2010): Das Image der Landwirtschaft: Ist und Wege zum Soll.Systematische Differenzierungen und kommunikationsstrategische Ableitungen aus empirischen Befunden. In: Böhm, J., Albersmeier, F., Spiller, A. (Hrsg.): Die Ernährungswirtschaft im Scheinwerferlicht der Öffentlichkeit, Lohmar-Köln, 25-46.
Liinamo, A.E., and Neeteson-van Nieuwenhoven, A.M. (2003) Sustainable European Farm Animal Breeding and Reproduction (SEFABAR). Proceedings. EC ELSA Project 5th Framework Programme for RTD QLG7-CT-2000-01368. 123pp.
Mantucci, F. (2018) Is ‘clean’ the new healthy? Mintel blog. Available at: http://www.mintel.com/blog/ food-market-news/is-clean-the-new-healthy (accessed: 14 October 2018).
McKay, J.C., Barton, N.F., Koerhuis, A.M.N. and McAdam, J. (2000) The challenge of genetic change in the broiler chicken. In The Challenge of Genetic Change in Animal Production. (W.G. Hill et al., eds.) Occasional Publication British Society of Animal Science. 27, 1-7.
Meuwissen, T.H.E., Hayes, B.J. and Goddard, M.E. (2001) Prediction of total genetic value using genome-wide dense marker maps. Genetics. 157, 1819–1829.
Mussini, F. J. (2012) Comparative response of different broiler genotypes to dietary nutrient levels. Dissertation, University of Arkansas.
NCC (National Chicken Council) (2018) U.S. broiler performance. Available at: http://www. nationalchickencouncil.org/about-the-industry/ statistics/u-s-broiler-performance/ (accessed:14 October 2018).
Nature (2018) Animal Breeding. Available at: https://www.nature.com/subjects/animal-breeding (accessed 14 August 2018).
Neeteson, A.-M., Merks, J., Bagnato, A., Finnocchiaro, R., Sandøe, P. Christiansen, S., Noiville, C. and van Genderen, A. (1999) The future developments in farm animal breeding and reproduction and their ethical, legal and consumer implications. Report EC ELSA project 4th Framework Programme for RTD BIO4-CT98-0055.128pp.
Neeteson-van Nieuwenhoven, A.M., Merks, J., Bagnato, A. and Liinamo, A.E. (2006) Sustainable Transparent Farm Animal Breeding and Reproduction. Livestock Science. 103, 282-291.
Neeteson-van Nieuwenhoven, A.M., Knap, P. and Avendaño, S. (2013) The Role of Commercial Pig and Poultry Breeding for Food Security. Animal Frontiers. 3, 1, 52-57. Available at: http://www.
Breeder Management and Nutrition: Moving the industry forward
animalfrontiers.org/content/3/1/52.full.pdf+html (accessed: 14 October 2018).
Neeteson, A.M., Appleby, M. and Hogarth, G. (2016a) Making a resilient poultry industry in Europe. Chapter 1. In: Sustainable Poultry Production in Europe. In: Eds. Burton, E., Gatcliffe, J., Masey O’Neill, H. and Scholey, D. (eds.). Poultry Science Symposium Series Vol. 31. UK WPSA symposium September 2014 Chester. CABI Publishers, Oxforshire, UK. 3-24.
Neeteson, A.M. and Avendaño, S. (2016b) Sustainability and Productivity. Poultry Meat Production. In: Proceedings FACTA conference 2016, Expo Dom Pedro, Campinas, SP, Brazil. APINCO Foundation for Poultry Science and Technology. 12pp.
OECD/FAO (Organisation for Economic Cooperation and Development; Food and Agriculture Organisation). (2018) Agricultural Outlook 20182027, OECD Publishing, Paris. Available at: http:// www.fao.org/publications/oecd-fao-agriculturaloutlook/2018-2027/en/ (accessed: 31 October 2018).
OIE (World Organisation for Animal Health) (2017) OIE Situation Report Avian Influenza. http://www. oie.int/fileadmin/Home/eng/Animal_Health_in_ the_World/docs/pdf/OIE_AI_situation_report/ OIE_SituationReport_AI__6_8May2017.pdf (accessed 26 November 2018).
Rauw, W.M., Kanis, E., Noordhuizen-Stassen, E.N. and Grommers, F.J. (1998) Undesirable side effects of selection for high production efficiency in farm animals: A review. Livestock Production Science. 56, 15–33.
Ricard, F. H. (1975) A trial of selecting chickens on their growth curve pattern experimental design and 1st general results. Annales de Génétique et de Séléction Animale. 7, 427-444.
Ringquist, J, Phillips, T., Renner, B., Sides, R., Stuart, K., Baum, M. and Flannery, J. (2015) Capitalizing
on the shifting consumer food value equation. Deloitte Rodenburg,T.B. and Turner, S.P. (2012) The role of breeding and genetics in the welfare of farm animals. Animal Frontiers. 2, 3, 16-21.
Rollin, B.E. (1993) Animal production and the new social ethic for animals, In: Baumgardt, B. and Gray, H.G. (eds.) Food Animal Well-Being. Purdue University, West Lafayette, USA. 3-13.
Sonntag, W.I., Spiller, A. and Von Meyer-Höfer, M. (2018) Discussing modern poultry farming systems—insights into citizen’s lay theories. Poultry Science. 0, 1–8.
Spiller, A.; Von Meyer-Höfer, M. and Sonntag, W. (2016) Gibt es eine Zukunft für die moderne konventionelle Tierhaltung in Nordwesteuropa? Diskussionspapiere, Department für Agrarökonomie und Rurale Entwicklung, No. 1608 Econstor. 40pp.
Tolkamp, B.J., Allcroft, D.J., Barrio, J.P., Bley, T.A., Howie, J.A., Jacobsen, T.B., Morgan, C.A., Schweitzer, D.P. Wilkinson, S., Yeates, M.P. and Kyriazakis, I. (2011) The temporal structure of feeding behavior. American Journal of Physiology. Regulatory, Integrative and Comparative Physiology. 301, 2, 378-93.
United Nations General Assembly (2005) Resolution adopted by the General Assembly 60/1 10. World Summit Outcome 1. Values and Principles. United Nations, New York USA. United Nations. (2015) Transforming our world: the 2030 Agenda for Sustainable Development. General Assembly. 17th Session Agenda Items 15 and 116. A/RES/70/1. Available at: http:// www.un.org/ga/search/view_doc.asp?symbol=A/ RES/70/1&Lang=E (accessed: 14 August 2018).
USPEA (US Poultry and Egg Association) (2018) Ryan Bennett to lead US-RSPE and IPWA. Available at: http://www.uspoultry.org/ mediacenter/docs/2018_USRSPE_IPWA_ RBennett.pdf (accessed 14 October 2018).
Vanderhoydonck, C. (2016) Transgenerationele
Breeder Management and Nutrition: Moving the industry forward
beïnvloeding van de performantie van vleeskuikenmoederdieren en vleeskuikens als gevolg van een aangepaste voederstrategie bij de vleeskuiken(groot)moederdieren (Transgenerational effect on the performance of breeders and broilers as a result of an adapted feeding strategy of their (grand) parents). KU Leuven Bio-ingenieurswetenschappen. Master proef. 134 pp. Van Emous, R.A., Kwakkel, R., Van Krimpen, M. and Hendriks, W. (2014) Effects of growth pattern and dietary protein level during rearing on feed intake, eating time, eating rate, behavior, plasma corticosterone concentration, and feather cover in broiler breeder females during the rearing and laying period. Applied Animal Behaviour Science. 150, 44-54.
Van Emous, R.A. (2015) Body Composition and Reproduction in Broiler Breeders: Impact of Feeding Strategies. Thesis. Wageningen UniversityGraduate School of Wageningen Institute of Animal Sciences (WIAS). 173pp.
Wei, M., Van der Steen, H,A.M., Van der Werf, J.H.J. and Brascamp, E.W. (1999) Relationship between purebred and crossbred parameters II Variances and covariances under the one‐locus model. Journal of Animal Breeding and Genetics. 108, 253-261. Wideman, R., Rhoads, D., Erf, G. and Anthony, N. (2013) Pulmonary arterial hypertension ascites syndrome in broilers: a review. Poultry Science. 92, 64-83.
Williams, A.G., Audsley, E. and Sandars. D.L. (2006) Determining the environmental burdens and resource use in the production of agricultural and horticultural commodities. Main Report. Defra Research Project IS0205. Cranfield University, Bedford, UK. Zuidhof, M.J., Schneider B. L., Carney V. L., Korver D. R. and Robinson F. E. (2014) Growth, efficiency, and yield of commercial broilers from 1957, 1978, and 2005. Poultry Science. 93, 12, 2970–2982. Available at: https://doi.org/10.3382/ps.201404291 (accessed: 14 August 2018).
JUAN CARLOS ABAD, DVM., Technical Manager with Cobb-Spain. When not assisting customers he serves on the Facultad de Veterinaria at Universidad Complutense Madrid (Spain). Dr. Juan Carlos earned his degree at Universidad Complutense. His research in broiler breeders includes performance in antibiotic-free environments, pathogen response in traditional and ABF settings, and follicular development. Dr. Juan Carlos is a member of the Security Committee of Spanish Veterinary Drugs Agency, and the Poultry Veterinary Study Group of the European Union; secretary of the Spanish Branch.
ROBIN JARQUIN, PhD., is the Director of World Technical Services at Cobb-Vantress. He joined Cobb in 2005 and has held positions as a microbiologist, lab supervisor, and commercial manager for Mexico, Central America, and the Caribbean. He started his career at ConAgra Foods as a HACCP coordinator and quality assurance supervisor. Dr. Jarquin received his first bachelor’s degree at Zamorano - Escuela Agricola Panamericana (Honduras) before attending the University of Arkansas (United States) where he earned a bachelor’s in Poultry Science, master’s degrees in Agriculture Economics and Poultry Science, and doctorate degree in Poultry Science.
Each phase of rearing has specific management objectives and goals. The starter and accelerated growth phases emphasize weight gain, while maintenance phases are focused on maintaining uniformity. Achieving the objectives of each phase will produce a flock with optimal body condition that will be highly productive.
Collecting accurate data is critical to make any adjustments that are necessary to keep the flock on target. This includes using correct weighing techniques and assessing body conditioning consistently as well as sampling a representitive portion of the flock.
During rearing, applying a feed restriction programme will help to prevent issues and mortality during production. Each feeding system has advantages and disadvantages that can support an effective feed restriction programme. The feeding equipment should be chosen based on functionality, benefits, experience, and management and feeding programmes. The right feeding system and programme with good management can allow uniform feeding and reduce the stress of competition at the feeder.
Success in the rearing phase will provide a solid foundation for production performance. However, the production phase still requires good management. After transfer to the production farm, continue good management practices, correctly photo-stimulate the flock and apply an effective feeding programme.
Genetic improvements in broiler breeders have required rearing management programmes to adapt over time. Likewise, equipment and infrastructure have also changed with the goal of improving production performance and the health and welfare of the birds. Given that a proper start in rearing will support good performance in production, it is important to understand how management programmes, the infrastructure, and available equipment are choices that
producers need to consider to optimize their programmes. This chapter will focus on the highlights of broiler breeder rearing management, feeder, and feed management during the rearing period.
Body weight curve and body condition in rearing phases
In the first weeks of life, the goal is to have good growth to promote skeletal development and, in turn, good uniformity. Establishing
good uniformity early will make maintaining uniformity through rearing easier. In addition, adequate intake of high-quality feed, especially protein, is important for feathering and feather retention over the bird’s life.
Although birds are fed ad libitum during the 1st week, feed should be weighed to determine how much they are eating. Keep in mind that extra feed supplied may be wasted in the litter or scratch area. After the 1st week, when birds start consuming more feed, they may find leftover feed leading to overweight pullets.
Good skeletal development must continue to be supported, but feed restriction may cause some competition for feed. Crop checks after feeding can be performed to determine if all birds are securing feed. A rearing feeding programme and protocol should be designed and applied during this phase.
To maintain good uniformity in rearing, it is necessary to have a good grading system based on weight. Early gradings (4 weeks) will allow recovery of any small birds and limit the amount of feed for any overweight birds because they can be sorted, penned, and fed different allocations. Of the different weight groups, the smallest group should get the most attention because they are more susceptible to skeletal issues, poor feathering and feather quality, and tendon ruptures beginning at lay.
During the maintenance period, birds are feed-restricted but are given small weekly feed increases. If birds are above the target
body weight, small feed increases should continue. Excessive feed restrictions will make the birds nervous and manifest abnormal behaviours such as feather pecking and excessive water consumption. They may also be prone to intestinal issues and lameness associated with Staphylococcal infections.
At 12 weeks of age, 90% of skeletal development is complete. At this time, the birds accumulate mass, first as lean tissue and then as fat. To assess fat accumulation, pass a finger over the pelvic bone to determine if there is sufficient fat for photostimulation. Feed increases are provided to increase body weight and ensure the correct body conformation.
In phase 5, consistent weight gains are needed. Weight gains allow the females to develop the desired fleshing and sexual uniformity necessary to maximize peak egg production and maintain post-peak persistency. Although consistent weight gain is important during this phase, pullet fleshing scores are more important. The objective of the accelerated growth phase is to gain enough fleshing and fat reserves to last the hen through peak production.
The term “turn up” is used often during this period as a focus on how much body weight percentage was gained from 16 to 20 weeks of age. This is a great indicator of whether or not feed and feeding were managed correctly to achieve what the flock requires to perform in the production period. Typically, a minimum of 36% turn up in body weight from 16 to
20 weeks is the goal. More than 36% (within reason) is not detrimental to the flock, but under the 36% threshold will often lead to poor performance.
Feed is usually changed at 15 to 16 weeks of age from the grower to the developer (pre-breeder) ration. The developer feed is continued until the first egg or 5% production. The energy from the developer feed helps deposit fat and some flesh conformation giving the pullet reserve energy to reduce the stress of photostimulation, improve the onset of lay, and, most importantly, for persistency of lay after 40 weeks. The developer feed also provides slightly more calcium. It is important to note that underfeeding from 16 to 23 weeks can produce smaller chicks up to 27 weeks.
Body weight refers to the actual mass of each bird. However, it is challenging to control body weight in broiler breeders because they have been genetically selected to efficiently convert feed into muscle. It is necessary to restrict feed amounts in broiler breeders, because, without feed restriction, broiler breeders will grow like broilers and fail in reproductive performance. Specifically, overweight pullets will become overweight hens that are vulnerable to increased mortality caused by prolapse, yolk peritonitis, sudden death syndrome, and heat stress.
Body conditioning refers to the correct physiological condition prior to receiving photostimulation. Feeding programmes,
allocations, and formulations, are designed so that both the correct body weight and body condition are achieved. With the correct physiological condition, pullets can reach optimal development of reproductive organs to maximize peak egg production and persistency of lay. The criteria used to evaluate body condition include body weight, breast fleshing score, and fat deposition. Pullets have, in the rearing period, different fleshing conformation over time (Figure 1). Until four weeks of age, pullets should have a more rounded shape of breast; a fleshing number 3 on a scale of 5. In the maintenance period, the body conformation should change to fleshing number 2. During this period the breast muscle should not be prominent. From 12 weeks onwards the fleshing on the birds should gradually increase so they have a fleshing of 2 to 3 at 16 weeks, and 3 to 4 at 20 weeks.
Establishing flock uniformity requires the collection of accurate weight data using consistent sampling methods. The goal is to weigh enough birds to accurately represent the average body weight of the flock. Birds should be individually weighed at placement and 7 and 14 days. It is important that no less than 2% of the flock be weighed in order to correctly estimate average body weight and calculate flock uniformity. Uniformity is a measure of the variability of bird weight in a flock and is measured by weight +/- 10% of the average body weight and/or by the coefficient of variation (CV).
Birds are usually measured using a “dry” body weight when birds have not been fed
or were fed 6 to 8 hours prior to weighing. However, birds can also be weighed “wet,” meaning directly after eating. Regardless, it is important to weigh the birds at a consistent hour each time they are weighed. Since the weights are used to calculate the following week’s feed allocation, biasing the data with inconsistent weighing methods can cause a flock to divert from the body weight target.
Once the flock reaches 3 weeks of age, it is recommended to increase the sample size to 3% to 5% of the population. Weigh birds from three different locations (front, middle, back) of each house to make the sample more representative of the flock.
To increase the accuracy of the sampling, use a catching pen, do not randomly pick up and weigh birds, and do not force birds into the pen. Forcing birds into the pen or selectively catching birds and placing them in a pen can result in a non-representative flock sample for weighing. Instead, the catch frame should be placed to allow the birds to freely enter the pen. Weekly sample weights must be representative of the whole house. Do not place a catch frame near the main hopper area because birds in this area tend to weigh above average from eating feed from the hopper.
Weigh every bird individually inside the catch pen, including small birds, and do not reject any weights, except for sexing errors. After each bird is weighed, release the bird into the house. Continue weighing until the pen is empty. Record each weight and calculate the average weight as well as the flock weight distribution.
The scales used to measure body weight must have a maximum capacity of 5 kg (11.02 lb) and be accurate to +/- 20 g (0.05 lb). Scales must be calibrated on a regular basis. It is advantageous to use electronic scales with a printout capability. This will reduce human error and assist the weighing team.
Record each weight and calculate the average weight as well as the flock weight distribution. Plot the average body weight on the appropriate chart and use this data to calculate the feed amount for the following days. It is also important to determine if the feeding programme is working and keeping the birds close to the standard body weight.
Automatic scales are becoming more popular with improved weighing equipment and software. They can be used daily and over a specific time period each day. Typically, measurements are taken for 1 to 2 hours prior to feeding. As an example: if the lights turn on at 7 a.m., then scales operate from 7 a.m. to 8 a.m. or 9 a.m. with feeding starting at either 8:01 a.m. or 9:01 a.m.
On average, 100 females will step on the scale per hour. The automatic scales are either a suspended or fixed platform, but there is no clear advantage for either. The scale range is normally set between +/- 25 to 30% of the population’s mean body weight.
It is very important to have the flock at the correct weight and uniformity by 4 weeks
with a maximum of 5% above or below the standard. Correct weights and high uniformity at 4 weeks of age will prevent severe feed restrictions or increasing feed amounts during the maintenance period. An important tool to improve uniformity is grading. Grading is the process of sorting individual birds into categories based on body weight (super light, light, average, heavy). Birds within each category are then separated into pens and can be managed back to standard accordingly. Sorting birds into categories can be done after taking individual weights and calculating uniformity, CV and standard deviations.
Males follow the same grading concept as females and, in general, should be 5% higher in uniformity than females.
Weighing and grading by hand
Digital hanging scales are available for grading by hand. Many digital scales can record and store weights to the nearest gram, calculate an average weight, and display histograms of the weight distributions.
The cutoff values for sorting the flock into groups can be programmed to make weighing and sorting faster and easier. Finally, digital scales can also count the number of birds that were weighed and the final number of birds in each group can be displayed on the scale. It is important to note that, if the number of birds in each group is not accurate, then the feed allocations cannot be accurately calculated.
A range of models are available. A larger model is available and is suitable for
companies with flock sizes greater than 300,000. This machine can vaccinate pullets but is not easy to move. In contrast, smaller models are readily mobile and constructed of stainless steel for easy cleaning. These smaller models are limited to grading chicks and birds up to 20 weeks of age. In general, grading with machines is recommended for 2 gradings at 3 to 4 and 10 to 12 weeks of age to maintain average uniformity in rearing above 75% (i.e. 75% of the birds are within a certain body weight with a +/- 10% of the average range).
Advantages of automatic grading include:
• Can be faster than hand grading and typically requires less personnel
• Capacity-based models can grade from 1,500 to 3,000 birds per hour
• Sorts birds with precise weight ranges and no mistakes with counting birds that are sorted into groups (a main issue of hand grading)
After each weekly weighing, plot and analyze the data as soon as possible. Plotting the data into weight and uniformity graphs can help
track the development of the flock. If the flock body weight and uniformity are not according to the standard, investigate immediately to determine if there are any issues. Possible causes can include disease, high density, lack of feeder space, and feed and water problems. Finally, if the weighing results are inconsistent or not according to the expected results, it is better to repeat the weighing process before deciding on feed allowances and adjustments.
In rearing, uniformity is crucial to help maximize production results. Uniform flocks reach peak egg production earlier, peak higher, and persist longer than non-uniform flocks. High variation in sexual maturity, associated with underweight and heavy females, will delay the onset of production or females may come into production but persistence is drastically shortened.
In order to achieve a uniform flock, feeding uniformity is required. The goal is for each bird to have an equal opportunity to eat and an equal amount of feed at the same time. Although there are a number of systems available, no system
Table 1. Advantages and disadvantages of oval pan feeders compared to chain and spin feeders
Feed will fill all pans at the same time Cannot be used with fine mash feed; only good crumble and pellets
Less stress for the birds - not having to “chase” the feed around the feed line
System can be winched up providing more space for the birds, allowing birds to work litter
Less feed spillage
Birds are less active, do not jump, causing some issues with acces sing slats in production -requires training slats in rearing
Cleaning is difficult when disease challenge is present
Feeding time can be extended by reducing feed flow
Without beak conditioning, birds will poke holes in the bottom of pans; pans not an option where beak conditioning is prohibited
Table 2. Advantages and disadvantages of chain pan feeders compared to oval pan and spin feeders
Easy to see feed distribution
Durable system
Can use with mash, pellet, or crumble
Birds learn to hop over chain feeder, training them to jump onto slats in production and strengthens leg tendons
Can be thoroughly cleaned compared to oval pans
is fully automatic and all require maintenance and observation for mechanical performance.
Tubular feeders are being phased out and round pans have been replaced by ovals. The two most common feeder types used in broiler breeder systems are chain and oval pan feeders. Spin feeders are also popular in some regions of the world, such as western European countries. The choice of system is based on a number of factors including available labor, animal welfare practices and legislation restrictions, feed form, distribution abilities, production design, and economics. Consider the advantages and disadvantages of some of the most commonly used feeders:
In the following section, feed space per bird, stocking densities, and equipment will
Feeder is an obstacle for workers (vaccinations, grading)
Less feeder space per bird available than oval pans
Some feed loss to the litter with fine mash feeds
be considered and compared between oval pan and chain feeders.
Beginning at placement, a progressive feed space plan can be used to configure the system for the correct number of birds per pan or chain length at different ages and to obtain good feed and bird distribution. Feeder space increases should be gradual -based on bird age and the amount of feed needed for even distribution. For pan feeders, additional lines of pans can be dropped or individual pans can be switched “on” as needed. For chain feeders, additional loops of chain feeders can be added, or a loop can be extended to add extra feeding space.
Table 3. Advantages and disadvantages of spin feeders compared to oval pan and chain feeders
Provides more floor area with feeder suspended
Very easy to clean
Birds are very active so litter is turned well; provides good leg strength
Cannot be used in the first 4 weeks - feed trays or supplemental feeders required
More supervision is required
Uniformity can be an issue making grading and supporting slow-eating birds important
Light intensity must be high enough for birds to find pellets
Good pellet quality is required, durable enough to resist breakage during transport and distribution with low percentage of fines
Change to different feeding system in production required, which can cause issues
Feeder space calculations allow 15 cm for each full-grown female. For chain feeders, measure linear chain feeder space and divide by 15 cm to determine the number of females that can be accommodated. For example, 15 meters (1,500 cm) of chain length will accommodate 100 females per side.
With a round pan feeder, the linear feeder space is calculated by using the circumference of the circle and the formula 2πr. Since the widest part of the bird is actually 10 cm away from the feeder, add 20 cm to the pan diameter to account for the actual distance between hens feeding.
In Figure 2, the diameter of the pan is 40 cm (on left - blue dashed line). However, the widest part of the female is 10 cm away from the feeder. Therefore, to get the diameter of the feeding circle, add 20 cm. The actual diameter in this example is then 60 cm (on right - red dashed line).
Next, determine the linear feeding space around the pan feeder by calculating the circle circumference (2πr). Figure 2 illustrates that the linear feed space would be (2 X 3.14 X 30) = 188 linear cm. Dividing by 15 cm (per female), the pan feeder in Figure 2 will accommodate about 12 females.
Calculating the linear feeding space of oval feeders is a bit more complicated. Ovals have 2 axis (diameters). These 2 numbers can be averaged together and used as the diameter. However, be aware that a small error in calculation can be magnified in a very large house using many feeders.
For feeder space between lines, 60 cm leaves no space for hens to pass between the feeders. Place feeders at least 75 cm apart for one bird to pass and 90 cm apart to allow enough space for 2 birds to pass.
Breeder Management and Nutrition: Moving the industry forward
Table 4. Recommended progressive feeder space for females based on age and feeder type
Age (weeks) Chain* Round Pan (30 cm diameter)*
Oval Pan Manual Tubular (30 cm diameter) *
0-4 5 cm 20-25 23-28 20-25 1.9 in
5-8 9 cm 16-18 18-20 16-18 3.54 in
9-12 13 cm 14-16 16-18 14-16 5.12 in
13-21 15 cm 10-12 13-15 10-12 5.90 in 22-65 15 cm 10-12 13-15 10-12 5.90 in
* Calculation based on diameter and circumference of pan
Table 5. Recommended progressive feeder space for males based on age and feeder type
Recommended Progressive Feeder Space for Males
Male feeder type - Rearing
Age (weeks) Chain Male Round Pan (30 cm diameter)*
Male Oval Pan Male Manual Tubular (30 cm diameter) *
0-4 6 cm 20-23 23-25 20-23 2.36 in
5-8 10 cm 14-16 16-18 14-16 3.94 in
9-12 14 cm 12-14 14-16 12-14 5.51 in
13-21 18 cm 8-10 10-12 8-10 7.08 in
22-65 20 cm 8-10 10-12 8-10 7.87 in
* Calculation based on diameter and circumference of pan
In general, stocking densities with oval pans can be higher than with chain feeders because there is more feeder space per linear
meter with oval pans than with chain feeders. Roughly, 3 chain feeder lines are equal to approximately 2 oval pan feeder lines.
However, there are a number of factors besides space availability that must be considered:
• Pans in rearing are less suitable than chain feeders if using selection pens (pens used to manage different weight groups of birds) and a separate feeding programme is applied to each pen. Oval pans are better for large houses without pens or divisions.
• Using the same feeding system in rearing and production is ideal. However, it is not recommended to use pans in rearing and switch to chains in production, especially if no training slats were used in rearing. When birds are fed with pan feeders in rearing without training slats, they tend to have weaker leg tendons and have not learned how to jump up onto the slats in production. Additionally, they will have to learn how to jump over the chain feeders in production. These issues can cause a significant increase in mortality and floor eggs.
• Oval pans do not work with fine mash feed; only good crumble and pellets. Chain feeders can accommodate any type of feed.
• Consult your local regulations for feeding programmes, stocking densities, and beak conditioning. In areas where beak conditioning is prohibited, chain feeders are a better option. Some countries regulate feeding programmes and do not allow “skip-a-day” programmes. With everyday feeding programmes, it can be challenging to obtain good feed distribution with small amounts of feed depending on the feeding system and feed presentation.
• If using a 100% floor system in production, it is recommended to use pans in rearing and production with training slats, beak conditioning is required. When using slats in production and mechanical community nests, chain feeders are recommended.
Feed distribution is one of the keys to achieving good flock uniformity. The right feeding programme is essential for good feed distribution. When choosing a feeding programme, consider local legislation regarding any programme that involves feed restriction.
Depending on the producer and local legislation, some breeder farmers adopt everyday feeding programmes, while others apply alternate feeding days. However, with everyday feeding programmes, it can be difficult to distribute small amounts of feed in a uniform way so that all birds can eat. Feeding on alternate days allows a greater quantity of feed, which can be distributed more evenly around the feeder. The birds then have improved satiety, longer feed clean-up time, and are less competitive.
Alternate feeding programmes can be used with any feeders, but are most commonly used with chain feeders. The most common feeding programme is a 5/2, where birds fast for 2 non-consecutive days. One day is usually left for weighing and the other is a weekend day. Programmes 6/1 and 4/3 or skip-a-day are also used. An issue with skipa-day is that feed is supplied for 4 days one week and only 3 days the following week.
Birds that are fed every other day have larger crops and gizzards and lower nutritional efficiency, increasing the amount of feed they consume (2 to 5% more than birds fed everyday) in rearing to achieve the recommended weight.
Feed restriction in broiler breeders is necessary to achieve good production and prevent high mortality due to metabolic stress during laying. Broiler breeders are selectively bred for growth and feed efficiency. Without restricting feed, mating and production would not be possible because the birds would be too overweight.
Feed restriction can cause hunger and stress which are the primary welfare concerns. Optimizing feed delivery systems can decrease flock anxiety and reduce competition at the feeders. Feed distribution should be completed in less than 3 minutes with the same schedule, time and procedure.
There are three primary feed distribution methods used:
Regardless of the type of feeder used, it is recommended to distribute feed in the dark to prevent birds from crowding around the feed hopper or outlet. After turning on the light and once the birds are well distributed, the light is turned off while feed is distributed. The light then is turned back on during eating.
In the case of winched feeders, the feeders can be lowered after turning on the light, which helps the birds position themselves. The light is turned off, the feed distributed, and the lights are turned back on. Distributing the feed before lowering the feeders can result in nervous birds and increased anxiety. Do not lower feeders with the lights off as there is a risk of injuring birds.
The birds relate feeding time to routines and signals on the farm. For that reason, a light can be used as a signal for feeding time. The light signal will reduce birds’ stress during feed distribution and nervousness on “off feed” days (if using an alternative feed programme). The birds will learn to associate the arrival of feed with a light on. This method helps to uniformly distribute the birds (especially in trough feeders) and has four different phases:
1. The farmer enters with the lights on to inspect the house.
2. The lights are turned off and the signal light is turned on.
3. The feed is distributed.
4. The lights are turned back on.
If feed is distributed in the dark and the birds are well distributed around the feeder, the birds begin to eat before the light is turned on. If darkness is prolonged, the majority of the feed will be consumed. While feeding in the dark, the birds are not as aware of competition and are only concerned with finding the feed. The aim of this method is to extend the consumption time and reduce competition and nervousness.
Birds must be trained for dark feeding at least a week before beginning this method. This can be done in two ways depending on whether the light intensity can be reduced or not. If light intensity can be reduced, do it a week before feeding 100% in the dark and gradually reduce the intensity every day by 10 to 20% until reaching total darkness in approximately 7 days. If light intensity cannot be reduced, distribute feed in the
dark the first day, and leave the light off for 5 minutes. Each day increase the time the lights are off by 5 minutes until reaching 20 to 30 total minutes of darkness.
The objective of dark-out feeding is for 80% of the feed to be eaten in the dark, without competition from other birds, and the remaining feed be consumed when the light is on. This method requires that crops are checked to confirm that all birds have consumed feed.
The average rate of mortality on a broiler breeder farm during rearing is 3 to 5%. The highest mortality occurs in the first week of rearing, generally due to bacterial infections, most frequently colibacillosis.
Infection in the first week is usually caused by the colonization of E. coli during hatching and the first days of life. The main route of infection is through the lungs and digestive tract, although the yolk sac can also become infected when the navel does not properly close. Factors that make chicks more susceptible to colibacillosis depend on the housing conditions, such as cold stress, difficult access to water and feed, the microbiological quality of the water, or poor ventilation.
Usually, 1% of the breeders are culled in the rearing phase due to some type of lameness. Among the lameness of infectious origin, staphylococci are the most frequent. This infection usually presents as joint swelling with heat, redness, and pain, with a more or less liquid gaseous exudate observed post-mortem.
The appearance of this infection is related to wounds in the skin or gastrointestinal mucosal lining, as well as stress situations, related to feed restriction and weight profile, feed distribution, feeder space and density. This appears more in birds fed via chain feeders than those fed from spin feeders and is also influenced by nutritional aspects related to the skin integrity.
Impacted gizzards due to litter consumption can produce high mortality in rearing at 2 to 4 weeks of age. Birds with impacted gizzards become dehydrated, and have empty intestines and a gizzard filled with a greenish fibrinous mass due to secretions of the proventricular glands. To mitigate this, it is recommended to avoid using very fine shavings or sawdust as litter and limit spills of starter feed from the feeders that could promote litter pecking and consumption.
In some cases, mortality can be caused by birds piling. To prevent birds from piling, walk slowly through the house, do not make loud or sudden sounds, and work in low light intensity, especially on fasting days if using an alternative feeding programme.
Invaginations or prolapses can occur in the rearing phase due to stressors such as high light intensity, excess heat or high densities. Mortality due to colacal prolapse may occur on day-off feed on alternate feeding days. This can be aggravated by pecking, especially when there is high intensity of light. In this case, it is recommended to lower the light intensity to 5 lux (where allowed by local regulations) and return to an everyday feeding programme.
Transfer can cause stress because birds must adapt to a new environment, new feeders, and drinkers. On the day of the transfer, the birds have to fast at the rearing farm but must be fed upon arrival at the production farm. Increase the ration for females and males by 20 to 25% for 3 to 4 days after the transfer to facilitate a better distribution of feed and compensate for the stress involved in the transfer. The increase in feed can vary. Consider factors including the time of year, the duration of the transfer, and if vaccinations are done at the same time as the transfer.
It is recommended to transfer males first as it is more difficult for them to adapt to the change of feeder and drinker systems. When the males are transferred first in winter, the houses must be preheated to prevent cold stress. If houses are not preheated, females and males must be transferred at the same time.
If using slats on the production farm and the birds have not been trained in rearing to jump, birds may have difficulties jumping on the slats to drink causing some mortality. Birds that refuse to jump onto the slats can be seen sticking their beaks through the slats or eating fresh feces. They may have dirty beaks and poor feather quality. To prevent this, it is recommended to unload the birds directly on the slats so they can drink immediately. A step or ramp can also be installed, helping the birds access the slats easily.
The birds should be photo-stimulated only when they have sufficient body
condition and fat reserves, which is normally between 147 and 154 days of age. Any delay in production can reduce total egg numbers. However, photo-stimulating a flock that is not ready will reduce peak lay, can cause poor production persistence, increase double yolks and floor eggs, cause egg peritonitis, and increase mortality. The lighting programme is dependent on housing configuration i.e., using darkout or natural daylight in rearing and using dark-out, brown-out, or natural daylight in production. The following is an example of dark-out rearing to darkout production.
For photostimulation, both the duration and intensity of the photoperiod are gradually increased. Although many different programmes can be used, the most used is an increase from 8 hours per day to 11 or 12 hours and an increase in intensity from 50 to 100 lux (5 to 10 fc) all at once followed by an increase one hour weekly up to 15 hour/ day of light.
When the birds are slightly above the recommended weight, flock uniformity is ≥ 70%, and the birds have the correct fleshing and conformation, hens can be photo-stimulated at 144 to 147 days without risking overstimulating the birds.
When the flock is underweight, has poor uniformity, and poor body conformation, it is recommended to delay photostimulation until 157 to 160 days. Moreover, use smaller increases in light increments (2 to 3 hours in one step) to prevent overstimulation.
During the 5th rearing phase,the feed increments allow pullets to acquire fleshing and abdominal fat necessary to respond to photostimulation. After photostimulation, the feed increments should be small. Pullets can be overstimulated by both high increases in light intensity and duration as well as with large increases in feed.
Overstimulation with light can occur when birds are transferred to open houses mainly in the summer period (over 50% increase in light duration) or when transferred to dark houses with more than 100 lux and white light over 4000 Kelvin. Therefore, in production, it is recommended to use a color temperature of 2700 to 3000 Kelvin.
The feed increase will depend on the weight and body condition of the birds. Typically, increases are 2 to 4 g per week. Larger increases in feed and high increases in light duration and/or intensity can cause overstimulation of ovarian development, more double yolks, and increased mortality due to prolapses and egg peritonitis.
If pullets received a high feed allocation in rearing but weight and body condition are adequate, it is possible to use the same feed amount per bird the following week (no feed increase) without risking a delay in the beginning of production. This is especially important during the turn-up period because if feed allocations are insufficient, birds will only use the nutrients for metabolic maintenance. They will not have extra feed to allocate for fat storage, which is needed for good egg production.
The change from developer to breeder feed is done at the first egg. However, if weekly mortality is high at the start of lay, the change to breeder feed may be delayed until daily production is between 20 and 30%.
When hens reach 5% of daily production, the feed increases should be made according to the percentage of daily laying, increasing feed for every 10% increase in laying. Typically, 40% of the feed increase is when daily production is between 5% to 45%. The remaining 60% of the feed increases are given when production is between 45 and 80%.
Achieving high flock uniformity and good production performance during laying can be attributed to the technical work done during the rearing phase. Plan, organize and monitor important factors including body weight and feed consumption. It is important to monitor these key indicators and respond early to issues. Collect data accurately and routinely and analyze the data in a timely manner to support good management practices. Finally, for feeding programmes, consider animal welfare, equipment choices, and feeding management practices.
Arrazola, A., Mosco, E., Widowski, T.M., Guerin, M.T., Kiarie, E.G. and S. Torrey. 2019. The effect of alternative feeding strategies for broiler breeder pullets: 1. Welfare and performance during rearing. Poultry Science 98: 3377–3390
Cobb Breeder Management Guide. 2020. Cobb 500 Breeder Mahagment Suplement. 2020.
DAVID CAVERO PINTADO, P hD., is the Chief Geneticist at H&N International in Cuxhaven (Germany), one of the world’s leading layer breeding companies. He also leads the layer breeding program at H&N. His fields of expertise are comprised of animal breeding and husbandry with a special focus on poultry genetics including genomic selection. A native of Spain, Dr. Cavero graduated from the University Politécnica of Madrid (Spain) with a degree in Agricultural Engineering. He earned his doctorate from the Christian-Albrecht University in Kiel (Germany).
XABIER ARBE UGALDE, P hD., is the Managing Director for Technical Service and Nutrition at H&N International in Cuxhaven (Germany). After graduating with a veterinary degree from the University of Zaragoza (Spain), Dr. Arbe worked as a field veterinarian and led the research and development team at Granjas Cantos Blancos, the largest egg producer in Spain. From there he moved to Production General Manager and Nutritionist before joining Novus International as the Technical Manager for Spain and Portugal. He also served as the poultry and feed hygiene specialist for Novus Europe. From there he joined Novus’ s team in Southeast Asia Pacific where he worked as the Regional Technical Manager before being named Head of Technical Services for Novus Southeast Asia and South Asia Pacific. Prior to his current role, Dr. Arbe served as the Global Nutritionist for H&N International GmbH based in Bangkok supporting customers around the world.
Layer breeding companies have played an important role in the development of the egg production sector. They keep improving the performance of the hens through genetic selection of their purebred lines at the breeding farms. At the same time crossbred birds with known pedigree are tested in commercial farms under different conditions worldwide to select birds with a good adaptability to different field conditions.
The breeding values estimation has improved significantly by using molecular information. A dense set of markers distributed over the whole genome is linked with the phenotypic information increasing the accuracy of selection. Further improvements are expected by expanding the scope of phenotypes available, which will be recorded in the future with the help of sensors and cameras and transformed into valuable information using artificial intelligence.
Increasing the number of saleable eggs per hen housed remains the cornerstone of the selection process. Huge improvements have been made in terms of high peak and persistent rate of lay, quality of the eggs and liveability which has extended the productive life of the commercial flocks. As part of the sustainable goals, feed efficiency has been always in the focus. The strategy has been to select birds with higher egg mass output and keeping the feed intake at an appropriate level according to production.
There is an increasing interest of traits related with more environmentally friendly production and animal welfare which play a major role in the selection goals. Non-cage housing systems are gaining momentum, traits such as general livability, nesting behaviour, feather cover or bone stability are gaining importance and are stronger weighted in the selection index.
The layer selection is evolving based on the demands of the markets and is routinely incorporating new traits to make the egg production an affordable and sustainable source of food in a socially responsible way worldwide.
In the past, the consumption of eggs as a valuable source of protein for human nutrition was a privilege of the wealthier human population. The development of
high-producing genetic lines, disease control, modern housing systems, and the use of balanced compound feed has led to a dramatic reduction in cost and market price for poultry eggs. Furthermore, these improvements in productivity and feed
efficiency have contributed to a decrease in the environmental impact per egg produced. Not only are resources such as feed, water, and energy better used, but also the reduction in the number of animals required to produce one kilogram of protein has decreased the quantity of emissions and residues, consequently having a positive effect on the environmental footprint of the egg production. The sustainable production of animal protein to meet the requirements of a healthy diet for a growing world population is a great challenge. In this chapter, we give an overview of the egg industry and the changes that have been carried out in recent decades. We present the work that breeding companies have conducted to adapt layers to fit changing production environments, market demands, and legislations. Finally, future perspectives and challenges of the egg layer sector are discussed.
World egg production has substantially increased during the last 20 years, reaching 83.48 million metric tonnes (Mt) in 2019,
which means an average annual growth of 3.4% (Table 1). However, as can be seen in Table 1, the development has been very heterogeneous in the different regions. Asia is the dominating continent as it shares around 60% of the global population and contributed to almost 62% of the world’s egg production in 2019 (Table 2). Thus, most of the growth in the last two decades has taken place in Asia, which accounts for 70% of the global growth in egg production (23.70 Mt in Asia from 33.85 Mt in the world). This production growth has been mainly driven by actions in China, India, and Indonesia, which together represent 47.2% of the world’s egg production. Conversely, Europe’s continuous loss of importance in the global market, with its mature market and stable production, is quite remarkable.
Despite having great potential due to its big population, the egg production volume is low in Africa (Table 2). A possible explanations include low egg consumption, or a lack of financial assets, infrastructures, education, effective veterinary systems, and knowhow; all frequently exacerbated by unstable political situations (Windhorst, 2016).
World 49.63 (100.0)
Africa 1.95 (3.9)
Americas 10.11 (20.4)
Asia 27.97 (56.4)
Europe 9.38 (18.9)
Oceania
(0.4)
(4.0)
(20.4)
(58.8)
(16.3)
(100.0)
(4.1)
(20.7)
(61.9)
(12.9)
(0.4)
hens
production
Africa
It is also interesting to mention the difference between the shares in laying hens and in egg production presented in Table 2, which hints to the adoption of technical methods and new technologies, management skills, and production efficiency in Europe and the Americas (especially North America) in contrast to Asia and Africa.
China is the biggest egg producer worldwide, reaching 28.9 Mt of eggs in 2019, which represents 34.6% of the world’s
production. The 10 top egg producers worldwide are presented in Figure 1, accounting for 71.3% of the total production in 2019. The development of the different countries in the last 20 years hasn’t followed the same dynamics.
World egg trading is very limited due to the difficulty of transporting shell eggs and the short life of the product. According to the FAO (2021), in 2019 international egg trading accounted only for 3.3% of total egg
production. This volume is distributed in 2.32 Mt for shell eggs, 0.08 Mt for egg powder and 0.38 Mt for liquid eggs. The European Union was the leading trader of shell eggs in 2020 with a share in exports of 54.4% and 46,3% in imports, followed by Asia with 28.9% in exports and 33.3% in imports (FAO, 2021). The three leading egg exporting countries in 2020 are the Netherlands (19.2%), Turkey (10.1%), and Poland (9.2%). Whereas the three leading importing countries are Germany (16.3%), the Netherlands (10.5%), and China (8.4%). It can be concluded that most eggs are produced for the domestic market and only a small number of eggs are traded, mainly between bordering countries.
The average yearly egg consumption per person in the world was 10.57 kg in 2018 (FAO, 2021), which roughly accounts for 169 eggs. However, egg consumption per capita differs substantially from country to country. There are countries with more than 300 eggs per capita like Mexico and Japan and other countries, like India, do not even reach 100 eggs per capita.
In the early part of the 20th century, almost every small farm possessed some poultry (including chickens, waterfowl and pigeons). Many of these small flocks were selfreproducing, productivity was not recorded and was probably extremely low (Hunton, 1990). The trend of intensification and concentration was accelerated through discoveries in the fields of genetics, nutrition, housing systems, and disease control. Following World War II, poultry production developed from a small-
scale, local enterprise to an economically important branch of agriculture (Fröhlich et al., 2012). For centuries, reproduction and egg production in latitudes outside of tropic zones was seasonal. The introduction of artificial lighting in laying houses was an important first step in increasing egg production during winter months, and once the influence of changing day length on sexual development and molting was understood and applied in practice, fresh eggs could be produced year-round. Breeding chickens for egg production started during the first half of the 20th century, based on trapnesting in single sire pens. Poultry were first selected as purebreds, however around 1940 crossbreeding began to be used, since these hybrid birds had an advantage in production and viability traits compared to the parental lines, called heterosis. An additional advantage of crossbreeding was the possibility to use sex-linked genes for sexing day-old chicks. However, the transition to systematic breeding through crossbreeding took place slowly, even when the superiority of crossbreeds was clear (Hartmann, 2000). Most of the breeds and varieties that exist today were developed in the 19th century and only some of them are used in the commercial layer breeding, such as White Leghorn for white eggs and crosses of initially dual-purpose breeds such as Plymouth Rock, New Hampshire, Rhode Island Red, and Australorp, which are used for brown eggs (Crawford, 1990). Additionally, the use of conventional cages under commercial conditions started in the 1930s. Here automatization could be implemented and not only could economies of scale be applied by increasing flock size, but the hygienic conditions and health status of the birds could also be improved. Decreases in production
costs were mainly thanks to genetic progress, prevention of diseases, and adequate feeding of birds under the specialized housing systems. These developments have improved not only the productivity, liveability and efficiency of the birds but also the eco-footprints, such as the emission of greenhouse gases and ammonia, as well as improved the utilization of raw materials, energy, and water (Pelletier et al., 2014). Although public awareness about the environmental impact of commercial poultry farming and climate change has grown in the last decade, these authors revealed already drastic reductions of 54–63% in the total environmental footprint of egg production in the United States from 1960 to 2010.
Structural changes in the poultry breeding sector during the last century have led to
a tight concentration of providers as many genetic companies disappeared or were merged with others. Consequently, nowadays the worldwide demand for layers is supplied by a handful of breeding companies. As all these companies are active globally, they need to adjust breeding goals according to the different regional requirements and changing consumer preferences. Market demands are mainly differentiated by the requirements for adaptability of the birds to different local conditions, as well as preferences in terms of egg size and eggshell colour. Increasingly, animal welfare and environmental impact requirements are part of the local conditions to which birds need to be adapted.
Moreover, breeding companies cannot be unaware of the reality and the environment that surround them. A great effort has been done to develop well-dimensioned
and internationally distributed production hubs. This production network is critical to ensure the supply of breeding stock anytime and anywhere in the world, in the event of a possible crisis that restricts or hinders trading between countries (e.g., outbreaks of Avian Influenza).
Genetic improvement is realized by breeding companies through selection. Geneticists identify the individuals that carry the best combinations of genes to meet the selection goals and consequently prepare the birds to meet the current and future market requirements. In this way, generation after generation there is an accumulation of genes with a positive effect on the traits of interest within the population. These improvements are flowing through the multiplication process, making efficient use of a relatively small elite nucleus to achieve substantial improvements at the commercial level (Figure 2). The commercial layer is a cross of four lines, which makes the maximum use of the heterosis effect. The breeding work, performance testing, and selection take place in a few breeding farms, which are geographically distributed worldwide for hygienic and risk management reasons. International export is only possible if the breeding animals are kept under strict biosecurity conditions to guarantee that the delivered genetic material is free from any kind of disease.
The selection process considers many traits and new traits are permanently added to fulfill the requirements of the egg producers, consumers, legislation, and society. The breeding values of different traits are
weighted depending on their importance and quantified in a total merit index which allows the geneticists to rank the birds and make a balanced selection. Performance testing must therefore be designed to be particularly broad and comprehensive. Moreover, the information collected at breeding farms is supplemented by data from crossbred birds with known pedigree tested under practical conditions (climate, housing, etc.) at commercial farms
As various simulation studies with laboratory animals have shown, the breeding progress in closed populations decreases at some point from generation to generation if the same breeding goal is always pursued. To guarantee sustainable genetic progress, it is crucial that breeding companies maintain enough genetic variability within and across genetic lines. Layer breeding companies have heavily invested to increase their testing capacities and optimise the family structures of their purebred lines. The consequent minimization of inbreeding and the dynamic adjustment of the selection targets, including many different traits, reduce the risk of losses in the genetic variability. Furthermore, the scope of their lines has been expanded with broad breeding goals combining many traits. Additionally, breeding companies have expanded the number of pure lines to offer a wide portfolio of layers based on different crosses of these pure lines to fit better the local needs and be able to react to new market requirements.
Egg production has always been the cornerstone of the selection goal. Different
ways to analyse egg production have been proposed in the literature. One convenient way is to dissect the production curve in different parts records: start, peak and persistency of production. The heritability is high at the start of lay with heritability ranging between 0.3-0.5. Heritability goes down at peak of production to 0.10-0.15 and increases again at the end of production to 0.2-0.3. Each hen’s individual daily egg production is recorded on the breeding farms during its whole production life. This information allows for increasing the number of eggs per bird through genetic selection, which has been remarkably successful in the last decades.
In the past, selection was based on eggs laid until a specific age, putting special pressure on early sexual maturity. A significant decrease in age at 50% production from about 170 to 140 days of age is reported in the literature (Anderson et al., 2013). However, since early production and persistency tend to be negatively correlated, there is little interest to push too much further for an earlier start of lay. The goal of genetic selection for egg production is sexual maturity at an optimal age, rapid increase in egg weight, a high peak, and persistent rate of lay at the end of production. Due to the long history of selection for persistent egg production, today’s hybrid layers can lay one egg per day for several months, without a pause. Analyses of the clutch length of pure lines have shown that more than 50% of the hens in a flock lay at least 100 eggs in a sequence without a pause. In fact, the average rate of lay in flocks at the middle of the production cycle is so high and the difference between birds
so small that there is not much potential for improvement in this phase. The biological limit is one egg with a good shell in 24 hours, and this limit has almost been achieved at the peak of production. Therefore, selection for lifetime egg production is focused on persistency, increasing the rate of lay at the end of production, and expanding the production life of the layers on commercial farms. Today’s commercial layers produce 320-340 eggs during the first year of production and stay in production until 100 weeks of age or more without molting.
Only eggs with intact shells, which conform to the weight requirements of the market, can be classified as saleable. Eggshell strength is of special interest at the end of the laying period because shell quality tends to decrease after peak production and eventually determines the end of the productive period.
In the case of egg weight, it is a high heritable trait with h² ~ 0.5-0.7, which allows the genetic selection for an easy increase or decrease of this trait. However, the attempt is to hold it at the current level with a slightly upward selection to counteract the natural selection to reduce egg weight, since smaller eggs show better hatchability. Moreover, breeders are trying to modify the natural egg weight curve through selection. The goal is to have a fast increase in egg weight at the start of lay to reach the optimum level as fast as possible and to slow this growth and keep a flat curve at the end to avoid increased eggshell quality issues due to high egg size at the end of production. Breeding companies offer a range of layers
with different egg weight profiles to fulfill the egg size requirements of the markets. Thus, we can have a flock producing a high number of eggs, but if those eggs don’t satisfy the egg size that the market demands, the egg price will be low and, consequently, the profitability will be negatively impacted.
Liveability is one of the most important selection goals. First, to enhance or sustain animal welfare and second as an important contributing factor to the number of saleable eggs produced per hen housed together with the rate of lay. A high incidence of mortality will have a negative impact on the number of eggs per hen housed. Mortality and loss of productivity due to infectious diseases have been reduced in commercial settings through a combination of genetic selection, well-design health, and biosecurity programs as well as good management practices. Significant genetic variability for immune traits including responses to infections has been amply demonstrated through successful selection experiments (Pinard-van der Laan et al., 2020). However, as these authors further argued all these immune-related responses might be under different genetic control with many QTLs (quantitative trait loci) of small effects. Breeding programs are carried out under optimal standardised conditions to find the difference between birds with a low incidence of “noise” in the data. Under these conditions the incidence of mortality is very low, the heritability of liveability is close to zero and genetic improvement of this trait is almost impossible. Therefore, relative
hens (half-siblings) with known pedigree are tested under different field conditions with a history of health challenges to improve general liveability. Although the heritability of this trait is low, normally in the range of 0.05 to 0.10, giving liveability enough weight in the selection goal will have substantial cumulative improvements in this trait in the long run.
Successful egg production from an economic point of view has been basically driven by two key parameters: the egg mass produced per hen housed and feed intake. Feed conversion ratio (FCR), expressed as the ratio of a kilogram of feed per kilogram of egg produced, was from 1960 to 2010 improved from 3.41 to 1.98 in United States according to Pelletier et al. (2014). An optimum feed efficiency simultaneously improves economic and environmental criteria. In the last 20 years feed conversion has improved by at least 0.45 to 0.50 kg of feed per kg egg mass produced (Preisinger, 2021).
Higher body weight also means higher maintenance requirements and, consequently, negative impacts on feed efficiency. Since it is a trait that can be easily recorded and has a high heritability (h² ~ 0.6 to 0.8), it is not a surprise that body weight of layers was reduced during the 20th century. However, it has not been further reduced in the ...last two decades/once the optimum body weight within each line was achieved. Further lowering body weight could negatively impact egg production, liveability, and egg weight. Individual feed intake measurement
is needed to select more efficient birds that produce at the same level of others and, at the same time, need less feed or those that produce more with the same amount of feed. Feed intake is routinely measured at the peak of production when nutrient requirements are highest, and it shows a moderate heritability (h² ~ 0.3 to 0.5).
Feed efficiency is one of the major economic traits in the poultry industry since feed cost represents over two-thirds of the total egg production costs in laying hens. For this reason egg producers as the managers of an economic activity pay careful attention to feed consumption and FCR. However, FCR is probably the key performance indicator that may hurt most of the egg industry when it is not correctly interpreted within a context. Maintaining a low feed intake or restricting the feed intake can have a negative effect on the production of the flock. FCR can be improved by increasing egg mass or by reducing feed intake. The egg mass production per hen housed, which is calculated by multiplying the number of eggs per hen housed by the average egg weight, can be compared to the breed standard and provide clear and objective information about the performance of the flock. On the contrary, feed intake can easily be manipulated since laying hens adapt nutrient intake to their requirements, i.e., feed intake can be reduced by making a highly concentrated feed (Pérez-Bonilla et al., 2012). However, concentrated diets could have collateral problems related to gut health and higher metabolic stress for the birds. In addition, a low FCR might not be the most interesting option from an economic
point of view. Highly concentrated diets are only doable/affordable in countries where high-energy raw materials are available and affordable.
FCR has the disadvantage of depending on the age or the developmental phase of the bird, therefore residual feed intake (RFI) has been proposed to counteract this drawback (Emmerson, 1997). RFI is defined as the difference between observed and expected feed intake based on metabolic body weight (BW0.75), body weight gain, and egg mass production. Hence a bird with a lower RFI is considered more efficient. Heritability of RFI and FCR are moderate and very similar, ranging from 0.3-0.5. Moreover, the genetic correlation between both traits is high (above 0.8), thus it seems that RFI does not bring too much additional information for selection over FCR when egg mass output and daily feed intake are already included in the selection index.
The best way to evaluate feed efficiency is to measure the net profit of the production; egg income over feed cost per hen. This parameter allows a better comparison of the birds’ nutrient intake efficiency. The income of the eggs, contrary to egg mass, considers that not all eggs might have the same value in the market. Thus, income can be calculated based on the kg egg price, but also on the number of eggs produced in the different gradings with their respective prices, depending on the local mode of payment. Only eggs that are saleable and maintain an intact shell until they reach the consumer or retailer will have a positive impact on egg income. Therefore, the genetic improvement is focused on
Figure
Heritability of different
Albumen Height,
increasing the number of saleable eggs per hen housed, selecting birds with higher egg mass output, and keeping the feed intake at an appropriate level according to production. Selection of birds with a good feed intake capacity also has the additional advantage that the birds do not need a special highconcentrated diet. This opens the possibility to use local raw materials and different byproducts. The use of local raw materials that can avoid long transport is important to reduce carbon footprint, reduce feed cost, and reduce the dependency on imports from other countries.
The quality of eggs determines egg income for the producer, the profit margins of the egg industry and overall consumer satisfaction. Several criteria of external and
Weight,
Shell Strength, Kdyn: Dynamic Stiffness, ES: Egg Shape,
internal egg quality are routinely measured and subjectively assessed in breeding programs. The heritability of most of these traits is moderate, an overview is shown in Figure 3. Egg consumers prefer eggs with a perfect “oval” shape, a sound shell that is free of defect, as well as an attractive and uniform shell colour. However, it must be realized that eggs are not always easy to differentiate at a glance. Moreover, some attributes of its nutritional value cannot be assessed only through visual inspection. Consumers make few choices of egg buying just based on their visual examination. Frequently they use labels or additional information to support their purchase decision.
Wardy et al. (2014) found freshness, shell cleanness, absence of cracks, USDA-certified farm eggs, a label stating “packing/bestbefore date,” egg grade, secure packaging, and availability as “must-be attributes”. These
could produce absolute dissatisfaction from the consumer if absent and does not increase satisfaction when present. Furthermore, these authors found that sale price and egg size were classified as attractive attributes, which have a linear response of increasing consumer satisfaction with greater attribute fulfillment and are mostly demanded by consumers.
Cracked or damaged eggshells not only cause financial loss but constitute a risk in terms of food safety due to the easier ingress of microorganisms (Bain et al., 2006). These authors concluded that the dynamic stiffness measurement (Kdyn) can predict the probability of an egg cracking in the field with high precision. The heritability of Kdyn is high (0.35-0.55) and is combined with the conventional breaking strength measurement in layer breeding programmes to improve the stability of the eggshell. It should be pointed out that the main supply of calcium for eggshell formation is provided by the feed and the rest is derived from the mobilization of bone reserves, mostly coming from the medullary bones. Therefore, it is critical to deliver calcium in the feed at the right time, amount and granulometry to fulfill the requirements of the hen.
Apart from cleanness and absence of cracks, the consumer’s first choice starts with the eggshell colour. Even when there are significant differences between continents, the proportion of white and brown eggs consumed in the world is roughly 50:50. There are some markets where creamy eggs are quite popular, even green or chocolate eggshell colour from Araucana or Marans
hens can be found in small niche markets. A good shell colour improves the presentation of the eggs at point of sale. Eggshell colour has a high heritability that ranges between 0.4 to 0.6, which allows the geneticist to make good improvements in this trait (Cavero et al., 2012). The genetic correlations between measurements at different ages are high, meaning that hens with a nice colour at start of lay will also tend to lay nice eggs at the end of production.
Another important and very common criteria is the egg size. Each market has different preferences and most of them will stipulate different prices based on egg weight grading.
The next factor is based on the economic status of the consumer. Higher income customers tend to focus on regionality, production system, and nutritional information, whereas the low-income consumer might base their decision mainly driven by price.
Consumers have different approaches based on habits, culture, practices, and other factors. It is difficult to make one single assessment for the entire world, since consumers are very heterogeneous across and even within countries, but in all reviewed studies we can see freshness, food security, healthiness, and price as the most important criteria. In relation to healthiness, in recent years research on eggs has expanded beyond cardiovascular diseases and blood cholesterol studies that previously gave eggs an undeserved bad reputation. Many studies have shown the
positive effects on health when including eggs in the diet (Rains and Kanter, 2017).
Albumen height is associated with the “freshness” of eggs. In breeding programs, albumen height is measured several days after oviposition and has a heritability ranging between 0.25 and 0.35 (Figure 3), which allows genetic improvement of this trait. Despite this, it is crucial that eggs are cool stored and that the cold chain is maintained to preserve the albumen quality.
Once the consumer breaks the egg the visual journey of the customer continues. Egg yolk colour will be the first point of attention. Colour of preference is driven by cultural background, social memory, or regional marketing by the egg industry. This characteristic is dependent on the nutrition of the hen and the supplementation of carotenoids in the diet.
The absence of blood and meat spots is an important trait for product acceptance for the consumers. In some cultures, because of religious laws, the consumption of bloodspotted eggs is unacceptable (Johnston et al., 2011). Estimated frequency of blood and meat inclusions in brown layers is about 18% whereas it is just 0.5% in white egg layers (Honkatukia et al., 2011). Apart from genetics, low levels of vitamin A or K, mould or mycotoxins in the feed, stress, or diseases may increase the incidence of inclusions. The heritability of this trait is low ~0.05 (Figure 3), therefore it is difficult to achieve big improvements on this trait or eliminate completely the incidence of inclusions. The selection is focused on eliminating extreme
values and keeping the incidence of inclusions at a low level. Table egg processors and egg breaker plants use various forms of candling technology to detect and remove blood spot eggs before packaging or further processing. Regarding sensory perception, there are no reports showing significant differences between eggs from different production systems. The composition of the feed and the raw materials used will determine the taste and the nutritional content of the egg.
Unfortunately, eggs have become a commodity in many countries and are frequently offered by retailers at lower prices in advertising and promotional campaigns. Nevertheless, those consumers, having more awareness of nutrition and health or animal welfare, show more willingness to accept a premium price.
The environment in which birds are housed is defined by several factors: management, climate, housing system, nutrition, biosecurity, and health status. This environment interacts with the birds and has an impact on their performance. If birds are only tested under optimal conditions with excellent feed at the breeding farm, there is a risk that the best birds selected under these optimal conditions will perform below average in an environment with suboptimal conditions. This effect is known as genotypeenvironment interaction (GxE). This effect is of great relevance for breeding programs, although it might not be of much practical concern if special attention is paid to ensure good, or at least not extreme, environmental
conditions (Hull and Gowe, 1962). In some cases, it is possible to invest in equipment and housing to adjust the environmental conditions to the requirements of the desired genotype and reduce GxE effect. However, in many cases, such adjustments are either not affordable or simply not cost-effective.
In order to breed birds with good adaptability to different environments and that can produce well under harsh conditions, breeding companies not only use the information of birds housed under optimal conditions on the breeding farms. Crossbred birds with known pedigree are tested under commercial farms in different conditions worldwide, including different locations in hot climate areas. The information delivered from these field tests flows in the selection process and is combined with the information collected in the pure-line birds. This strategy allows to select birds that not only have good performance under optimal conditions but also are robust and have a good resilience to cope with the different challenges they might find at the commercial level. In addition, commercial layers are fourway crossbred, which exploits the effect of heterosis. In other words, they have a greater tolerance and resistance to environmental stress compared with their parents.
The thermoneutral zone for adult layers is 18-24°C, where the needs of energy for maintenance are at a minimum. As temperature increases above this comfort zone, birds will start to use part of their energy to lose heat; they will consume more water and less feed, which can have a negative impact on their performance. When
temperatures are below 18°C, there will be an increased feed intake to maintain body temperature. However, if the birds have good feather cover this increase in feed intake will be lower. In addition to direct improvements in adaptability, the selection for good feather cover and good feed intake capacity creates supplementary tools the bird can use to cope with unfavourable climate conditions.
The adaptation of the birds to diverse density diets with different granulometry and raw materials is one of the key parameters that we can expect from genetic selection. All available raw materials have potentially positive effects, for instance on the development of the digestive system, however, due to their composition they may also have negative effects that must be carefully monitored (Frikha et al., 2009). A commonly used cereal, such as wheat, or the main source of protein, such as soybean meal, in layer diets have antinutritional factors that can negatively affect performance (McDonald et al., 2002). Therefore, a good understanding of the limitation of raw materials is necessary. Moreover, understanding feed intake limitations under specific environments and applying the right technology to maximize the use of different local raw materials will have a huge impact on the sustainability of the egg production.
Sustainability is a complex and quite abstract term. The concept of sustainable development was described by the United Nations Brundtland Commission Report (Brundtland, 1987) as “meeting the needs of
current generations without compromising the ability of future generations to meet their own needs”.
The Food and Agriculture Organization of the United Nations (FAO) defines a sustainable food system (Nguyen, 2018) as a food system that delivers food security and nutrition for all in such a way that the economic, social and environmental bases to generate food security and nutrition for future generations are not compromised. This means that the system is profitable throughout (economic sustainability); it has broad-based benefits for society (social sustainability); and it has a positive or neutral impact on the natural environment (environmental sustainability).
Breeding companies are working towards more sustainable egg production and traits related to more environmentally-friendly production and animal welfare are gaining weight and importance in the selection goal in the last decades. Environmental health is one of the biggest impacts of genetic improvements. The extended life of commercial hens and layer parent stock is reducing resource need. It is expected that the production cycles will be additionally extended by 5 weeks in the next 10 years, reducing further the natural resources needed for egg production. These extended production cycles will make the practices of molting obsolete and no longer profitable, with a positive impact on the birds’ welfare.
Saving in natural resources due to genetic improvement of production efficiency is a key accomplishment of the layer breeding industry. Sometimes some of the aspects and
perspectives that define sustainability might not be align or even confronted. Van Asselt et al. (2015) assessed the sustainability of enriched cage, barn, free-range, and organic egg production systems analysing the social, environmental, and economic dimensions. These authors found that for the Dutch situation, enriched cage egg production was most sustainable, having the highest score on the environmental dimension, whereas freerange egg production gave the highest score in the social dimension (covering food safety, animal welfare, and human welfare). In the economic dimension both enriched cage egg and organic egg production had the highest sustainability score.
The movement to cage-free housing systems during the last years in many countries, especially in Europe, United States, Canada and Australia, to enhance animal welfare and promote more natural behaviours create an additional challenge for breeders that have to select birds that adapt well to these systems. Birds that have access to the range will enjoy natural sunlight, walk through the outside area, and can scratch and take sand baths. However, there are some risks associated since they are in contact with wild birds, which might carry diseases, or they can be hunted by predators, which have a negative impact on the health and the well-being of the birds. In addition, the higher activity of the birds will increase the resources needed for production. The access to the range will require 5-10% more feed energy for having the same production (Brainer et al., 2015).
The global population is continuously growing, and it is predicted to achieve 9.8
billion by 2050. Breeding companies are challenged to produce enough food of a good quality at a reasonable price in an environmentally responsible way and with the best health and well-being for the birds. Breeding programs have acknowledged these priorities and have broadened their breeding goals to include these traits to select birds with the best health and welfare, keeping at the same time excellent egg production and feed efficiency. New traits have been included and, through a well-balanced selection, it is possible to obtain robust birds with the best performance in order to enhance the sustainability of the egg production industry.
Animal welfare has become a major issue in poultry production in industrialized/ developed countries. Government authorities, non-governmental organizations (NGOs), retailers, and consumers are paying more attention to intensive livestock production systems, and also those used for egg production. The transition from extensive, small family flocks to big cage systems that allowed a high automatization of egg production and reduce the workload for the farmers started in the middle of the last century. The view of the traditional free-range system, however, persisted in the memory of the urban population and has been often idealised. The main points of concern of conventional cages are the restricted space and lack of structural elements, such as litter, nests, and perches to perform natural behaviours (Blokhuis et al., 2007). In Europe, United States, Canada, and Australia among others, there is a strong
movement to replace cages with barns or aviaries with or without access to winter garden or free-range. Windhorst (2021) reported that in 2020 cage-free systems were already dominating in the European Union with 51.9% of the hens being housed in alternative systems. Large groups and the behaviour of free-moving hens in alternative systems that can search for feed everywhere and that are in contact with faeces from other birds represent some challenges for egg producers and poultry breeders. Good nesting behaviour is required to reduce the number of floor eggs. Fear and sudden outbreaks of nervousness in large groups in cage-free systems not only lead to reduced performance but also increase mortality through suffocation of hens in the corners of the litter area. Management must adapt accordingly. Pullets must be reared in systems that are similar to the system that birds will find in production. Birds need to learn to fly and to move appropriately in the system to find water and feed. More labour and time are required to monitor the bird behaviour and to take appropriate actions if needed. Eggs laid outside the nests represent a microbiological risk since the eggs are in contact with litter. Minimizing floor eggs in commercial flocks requires special management procedures, such as early transfer of pullets to the layer facilities, frequent collection of floor eggs at the start of egg-laying, avoiding dark areas in the litter, facilitation of nest access, and attractive and sufficient nest space.
From a breeding point of view, in addition to conventional selection criteria such as egg production, feed conversion and egg quality, traits related to animal welfare received more
specific weight in the selection index in the last decades. Special testing performance under alternative systems has been developed to collect this information on an individual bird basis (Icken et al., 2012).. Primary poultry breeders have incorporated nesting behaviour in their selection goals to adapt layers to non-cage systems and contribute to reduce the number of floor eggs in commercial flocks. Nevertheless, welldesigned systems, appropriate management, and trained staff are required to accompany genetic selection to successfully produce in non-cage environments.
Feather cover allows birds to control their body temperature. The deterioration of feather cover disbalances this mechanism and limits the birds’ adaptability to their environment. It is described that layers, having lost a substantial part of their feathers, have an increase in feed intake of up to 10% (Leeson and Morrison, 1978). Furthermore, animal welfare legislation as well as retailers and consumers in developed countries are paying more attention to the birds’ feather cover status at the farm as an indicator of animal well-being. Damages caused by feather pecking can be exacerbated in large groups and improper feather cover represents not only an economic problem but a serious welfare concern. Many factors may contribute to the observed variation of incidence under commercial conditions. Nutrient deficiencies, lack of foraging materials, high light intensity, inadequate lighting system, group size, stocking density, and other risk factors have been identified (Nicol et al., 2013). Beak treatment does not avoid feather pecking
but is a preventive measure that reduces its damage. However, beak treatment can cause pain and has therefore been criticized by welfare organizations. In some countries, beak treatment is banned or not practiced at all. To control damages in intact-beak flocks more intense attention from the farmers is required to detect and react before pecking behaviour starts in the flock. Scoring feather conditions and recording mortality and incidence of feather pecking from birds with known pedigree housed in family cages has been used to select calmer birds that show less flightiness, better feather cover, less incidence of feather pecking, and lower mortality. Additionally, for several years genetic selection for a blunt beak has been incorporated in breeding programs to reduce the negative impact of feather pecking (Icken et al., 2017). Future technologies that can automatically capture and track individual behavioural information within a group of birds in a cage-free environment could substantially help to improve these traits. The geneticists have worked on selecting calm birds that do not express aggressive behaviours and maintain a good feather cover. However, improper management or decisions at the farm level like slow-moving feeders, high stocking density, lack of feeder space, or non-uniform flocks could trigger feather pecking and cannibalism as well as bad feather cover (Tablante et al., 2000).
Bone strength of laying hens is better in non-cage systems and there is a reduction in fractures associated with osteoporosis due to their higher activity (Lister and van Nijhuis, 2012). However, keel bone fractures appear to be more frequent in aviary systems, causing
pain and reducing the welfare of the birds. Hardin et al. (2019) reported 30 to 95% keel bone damage in flocks housed in non-cage systems, compared to 15 to 55% in furnished cages. The variation in the prevalence of keel bone fractures reflects differences in nutrition, management, housing system design, and genetic origin. Possible conflicts between bone mineralization and shell quality were discussed by Whitehead et al. (2003), whereas Jansen et al. (2020) did not find any association between total eggshell production and bone stability. Furthermore, there is also a fear that extending the productive cycle of laying hens might be associated with a greater risk of poor bone quality at advanced ages. In disagreement with this theory, Dunn et al. (2021) found that selection for increased persistency of egg production does not adversely affect bone quality. However, these authors alert that the onset of puberty and mineralization should be taken with care to ensure adequate bone development occurs before the hens start to lay. Studies to find suitable methods for measuring bone quality on live animals on a large scale in a cost-effective way are ongoing, simple methods such as keel bone palpation or ultrasound measurements as proposed by Andersson et al. (2017) with a moderate heritability (h² ranging from 0.17 to 0.30) can be useful to select birds with better bones.
Another welfare issue in layers is induced molting of hens by using feed restriction or alternative non-fasting methods. Since breeding companies are selecting for persistent egg production and stronger eggshell quality at an advanced age that
allows extending the productive life of the birds, molting layers may become unattractive and obsolete in most situations.
Due to the antagonism between laying rate and growth rate, males of layer lines show extremely slow growth and poor feed conversion compared to modern broilers. There is strong opposition in some countries against culling day-old male chicks. Rearing male chicks leads to high production costs and consumer prices, and the odd conformation of breast and thighs is not generally accepted by consumers (König et al., 2012). Males of dual-purpose breeds have a higher growth rate than males of conventional layer hybrid strains, but neither are they competitive with commercial broilers, nor are their sisters competitive with females of specialized layer strains. Because of the extended duration of the growing period and the poor feed conversion rate, the ecological footprints are inferior to specialized breeds (Damme et al., 2015). Krautwald-Junghanns et al. (2018) reviewed the different methods for sex determination in ovo: determination of the hormonal levels (estrone sulphate) in the allantoid fluid after nine days of incubation, molecular sexing assay of the allatonic fluid, optical-imaging methods, such as reflectance spectroscopy and hyperspectral imaging, infrared spectroscopy, Raman spectroscopy, magnetic resonance imaging and genetic engineering. Various methods have shown promising results in the laboratory. Currently, only a few companies are offering chicks from in ovo sex determination from day nine to day thirteen of incubation for the European market (Preisinger, 2021). Other projects continue their investigations to implement
in ovo sexing determination at an earlier age under commercial hatchery conditions.
Since the 1980s considerable efforts have been put into identifying regions or genes associated with quantitative traits of economic interest that later could be used in marker-assisted selection to improve the accuracy of selection (Dekkers, 2004). Abasht et al. (2006) reported almost 700 quantitative trait loci (QTLs) affecting different traits in poultry, approximately one-third of them being significant. However, only a few of these markers were integrated into commercial selection schemes. Genomewide association studies (GWAS) have identified mostly markers with small effects distributed all over the genome, confirming the assumption that most traits of interest in layers are inherited in a polygenic manner. The best-known example of applied markerassisted selection in layers was identifying the locus of the FMO3 gene (Honkatukia et al., 2005) and the subsequent elimination of brown-egg layers that may lay eggs with a fishy taint depending on the composition of the diet. Since then, increasing complexity and computationally demanding statistical approaches have been implemented to assess the breeding value of individual males and females, using phenotypic and pedigree information. The routine application of molecular information should further increase genetic improvement. In a pioneering paper, Meuwissen et al. (2001) proposed methods that could be used when marker information becomes available. The idea is to use a dense
set of markers distributed over the whole genome independent of their effect and link these markers with phenotypic information to estimate breeding values more accurately.
DNA can be analysed in both sexes, which is particularly helpful for sex-limited traits like egg production. Furthermore, the DNA can be analysed early in life, which helps to shorten the generation interval. Both factors have a positive effect on genetic progress.
Advances in molecular biology have contributed to the development of new and more powerful techniques for selection. Single Nucleotide Polymorphism (SNP) marker panels with varying densities were developed and used for large-scale genotyping in poultry (Kranis et al., 2013). Using several thousands of genetic markers distributed over the whole genome, individuals with the highest genetic merit can be identified early in life with greater precision and, thus, accelerate genetic improvement of the elite lines at an acceptable cost. Genomic selection is a recent paradigm shift for animal and plant breeding, a science with exponential advancement in recent years. Currently, custom-designed medium density SNP-chips are being used to shorten the generational interval and estimate breeding values more accurately compared to pedigreebased analysis, particularly in traits with moderate to high heritability. The molecular information also allows selection between full-sib males, which used to have identical breeding values, based on female relatives. Genomic selection provides tools that can be applied for the selection of birds during the growing period before their full- and halfsisters generate new information.
The current rate of genetic progress in lifetime egg production is even greater than 20 years ago. An improved structure and increased size of breeding populations, the application of new testing and recording technologies, and more powerful computer systems for breeding value estimation have contributed to more efficient use of existing genetic variation. The application of new technologies will play an even greater role in improving the genetic potential of hybrid layers.
In addition, recent developments in the field of gene editing have put back GWAS studies and the identification of candidate genes and causal mutations in a completely new perspective: if one wants to apply gene editing one needs to know what to edit (Bovenhuis et al., 2020). Furthermore, the availability of less costly sequencing technologies increases the power of GWAS, which together with better annotation information, will increase the resolution of mapping QTLs or find causative variants (Kranis and Maniatis, 2020). The geneediting tool CRISPR is very promising, however, the commercial application of this technique requires not only to have candidate genes to be edited but also it has to answer several questions: if these changes might negatively impact other traits if it is a cost-effective solution, and lastly and most importantly if the consumer will accept it (Fulton, 2018).
For the last two decades, genotyping and sequencing technologies have outpaced the most optimistic predictions, allowing industry-scale application of genomic
selection (Pérez-Enciso and Steibel, 2021). However, simulation and empirical results have shown that only marginal or no gains in genetic prediction accuracy can be expected by genome sequencing data instead of high-density genotyping data (Pérez-Enciso et al., 2015; van Binsbergen et al., 2015; Ni et al., 2017).
The layer breeding industry has gone through significant changes during the past decades and has a remarkable record to cope with new challenges. Increased egg production, improved feed efficiency, and adaptation of egg quality to consumer preferences contributed significantly to the success of the poultry industry. These genetic improvements and corresponding improvements in nutrition, disease control, and general farm management enabled the poultry industry to achieve its current position in the global food market.
In the future, while maximizing the genetic potential to produce high-quality protein at an affordable price remains a top priority, ethical concerns and demands of society have to be taken into account: (1) environmental impact of egg production, (2) animal welfare and well-being of the birds, (3) requirements of the egg processing industry and consumer preferences, (4) adaptability to modern management systems and resilience to environmental challenges wherever chickens are kept.
Furthermore, most farms still collect their data with pencil and paper. Implementation of digital data recording, where data is reliably
recorded, easily presented and available in realtime for the whole staff of a company can help to make better decisions and at the right time. Artificial intelligence can learn from different sources of data, not only given by the farmer but automatically recorded by sensors and cameras. These systems cannot only include warnings for current events on the farms but also are capable of producing predictions that can be automatically readjusted when new data is coming, which helps in decision making.
The improvement of fitness, robustness, and the behaviour of the birds have not been easy in the past to some extent due to a lack of easy-to-measure phenotypes that could be integrated into breeding programs. However, there is an opportunity in the future to develop novel health and behavioural phenotypes using new data from precision livestock farming and novel biomarkers.
Breeders look at the world market for a specific product to define breeding goals and to determine priorities. Genetic improvement is changing the business of parent stock layer suppliers and those selling day-old chicks or pullets. Genetic selection has extended the productive life of commercial layers to 100 weeks or more and this trend will continue in the future. This extension of the productive life of commercial hens reduce the need for yearly day-old chick production and it will influence the yearly needs of the parent stock. Fortunately, the market is growing due to the population growth and the increase in egg consumption per capita.
However, the expectation is that the amount of parent stock in developed countries will remain stable or even decrease, whereas the number of layers, and consequently of parent stock in North America and Asia, will grow.
The extended longevity of modern layers will lead to farmers having to manage older birds at the farm. Maintaining the health of birds will become critical to achieving their extended longevity. Understanding the health of later-aged birds will require extensive research. New trial modeling and investment will be needed to make the right evaluations regarding the needs of the birds as they age. Breeding companies are already testing their birds and recording information for extended cycles of more than 100 weeks.
The welfare regulations are affecting egg production at this moment, especially in Europe, United States, and Australia. Different states in the U.S. are applying different welfare regulations and the food industry there is requiring new welfare and housing system standards. In Europe, the first regulation in some countries regarding the ban on culling males in the laying sector is impacting hatcheries in Northern Europe, which could extend to the rest of Europe.
In the last decades, there have been great developments in the egg industry with breeding as an important driver of those improvements. There are a lot of new improvements, and breakthrough technologies coming that could be applied to collect more and better information about the birds and that will allow breeding companies to achieve further improvements.
Breeding companies will continue to adjust their breeding programs, selection tools, and breeding goals as well as continue to contribute to the sustainable and flourishing poultry industry.
Finally, all breeding efforts must be accompanied by appropriate feed formulation, vaccination, and biosecurity programmes together with good management practices to optimise the sustainability of egg production.
Abasht, B., Dekkers, J.C.M. and S.J. Lamont. 2006. Review of quantitative trait loci identified in the chicken. Poultry Science 85: 2079-2096. Anderson, K.E., Havenstein, G.B., Jenkins, P.K. and J. Osborne. 2013. Changes in commercial laying stock performance, 1958-2011: thirty-seven flocks of the North Carolina random sample and sequential layer performance and management test. World’s Poultry Science Journal 69: 489-514. Andersson, B., Icken, W., Cavero, D., Kaufmann, F. and M. Schmutz. 2017. Different methodologies to genetically improve the robustness of bones in layers. 10th European Symposium on Poultry Genetics. Bain, M.M., Dunn, I.C., Wilson, P.W., Joseph, N., De Ketelaer, E, B., De Baerdemaeker, J. and D. Waddington. 2006. Probability of an egg cracking during packing can be predicted using a simple non-destructive acoustic test. British Poultry Science 44: 462-469.
Blokhuis, H. J., van Fiks Niekerk, T., Bessei, W., Elson, A., Guémené, D., Kjaer, J. B. et al. 2007. The LayWel project. Welfare implications of changes in production systems for laying hens. World’s Poultry Science Journal 63: 101–114.
Bovenhuis, H., Farnir, F. and P. Le Roy. 2020. Theory of genome wide association for QTL detection.
Chapter 11. In: Advances in poultry genetics and genomics. S. Aggrey, H. Zhou, M. Tixier-Boichard, D. Rhoads (Eds). Burleigh Dodds Science Publishing Limited., Cambridge, UK. Pp: 327-342. Brainer, M.M.A., Rabello, C.B.V., Santos, M.J.B., Lopes, C.C., Ludke, J.V., Silva, J.H.V. and R.A. Lima. 2016. Prediction of the metabolizable energy requirements of free-range laying hens. Journal of Animal Science 94: 117-124.
Brundtland, G.H. 1987. Our Common Future: Report of the World Commission on Environment and Development. Geneva, UN-Document A/42/427. Cavero, D., Schmutz, M., Icken, W. and R. Preisinger. 2012. Atractive eggshell color as a breeding goal. Lohmann Information 47: 15-21.
Crawford, R.D. 1990. Poultry genetic resources: evolution, diversity and conservation. Chapter 2. In: Poultry Breeding and Genetics. R. D. Crawford (Ed). Elsevier, Amsterdam, The Netherlands. Pp 43-60. Damme, K., Urselmans, S. and E. Schmidt. 2015. Economics of Dual-Purpose Breeds. Lohmann Information 49: 4-9.
Dekkers, J.C.M. 2004. Commercial application of marker- and gene-assisted selection in livestock: strategies and lessons. Journal of Animal Science 82 (E-Suppl): E313-328.
Dunn, I., De Koning, D-J., McCormack, H., Fleming, R., Wilson, P., Andersson, B., Schmutz, M., Benavides, C., Dominguez-Gasca, N., Sanchez-Rodriguez, E. and A. Rodriguez-Navarro. 2021. No evidence that selection for egg production persistency causes loss of bone quality in laying hens. Genetics Selection Evolution 53: 11.
Emmerson, D.A. 1997. Commercial approaches to genetic selection for growth and feed conversion in domestic poultry. Poultry Science 76: 994-1002. FAO (2021). FAO database: www.faostat.org. Accessed on 5th August 2021.
Frikha, M., Safaa, H.M, Serrano, M.P., Arbe, X. and G.G. Mateos. 2009. Influence of the main cereal
Breeder Management and Nutrition: Moving the industry forward
and feed form of the diet on performance and digestive tract traits of brown-egg laying pullets. Poultry Science 88: 994-1002. Fröhlich, E.K.F., Niebuhr, K., Schrader, L. and H. Oester. 2012. What are alternative systems for poultry? Chapter 1. In: Alternative Systems for Poultry – Health, Welfare and Productivity. V. Sandilands, and P. Hocking (Eds). CABI Publishing, Wallingford, UK. Pp 1-22.
Fulton, J. 2018. Complementary: application of gene editing in the commercial poultry production industry. Poultry Science 97: 3007-3008.
Hardin, E., Castro, F. and W. Kim. 2019. Keel bone injury in laying hens: The prevalence of injuries in relation to different housing systems, implications, and potential solutions. World’s Poultry Science Journal 75: 285-292.
Hartmann, W. 2020. From Mendel to multi-national in poultry breeding. Archiv für Geflügelkunde 64: 189-203.
Honkatukia, M., Reese, K., Preisinger, R., TuiskulaHaavisto, M., Weigend, S., Roito, J., Mäki-Tanila, A. and J. Vilkki. 2005. Fishy taint in chicken eggs is associated with a substitution within a conserved motif of the FMO3 gene. Genomics 86: 225-232. Honkatukia, M., Tuiskula-Haavisto, M., Ahola, V., Uimari, P., Schmutz, M., Preisinger, R., Cavero, D., Vennerström, P., Arango, J., O’Sullivan, N., Fulton, J. and J. Vilkki. 2011. Mapping of QTL affecting incidence of blood and meat inclusions in egg layers. BMC Genetics 12: 55.
Hull, P. and R.S. Gowe. 1962. The importance of interactions detected between genotype and environmental factors for characters of economic significance in poultry. Genetics 47: 143-159. Hunton, P. 1990. Industrial breeding and selection. Chapter 40. In: Poultry Breeding and Genetics. R. D. Crawford (Ed). Elsevier, Amsterdam, The Netherlands. Pp 985-1028
Icken, W., Cavero, D., Schmutz, M. and R. Preisinger.
2012. New phenotypes for new breeding goals in layers. World’s Poultry Science Journal 68: 387-399.
Icken, W., Cavero, D. and M. Schmutz. 2017. Selection on beak shape to reduce feather pecking in laying hens. Lohmann Information 51: 22-27.
Jansen, S., Baulain, U., Habig, C., Weigend, A., Halle, I., Scholz, A.M., Simianer, H., Sharifi, A.R. and S. Weigend. 2020. Relationship between bone stability and egg production in genetically divergent chicken layer lines. Animals 10: 850.
Johnston, N.P., Jefferies, L. K., Rodriguez, B. and D. E. Johnston. 2011. Acceptance of brown-shelled eggs in a white-shelled egg market. Poultry Science 90: 1074-1079.
König, M., Hahn, G., Damme, K. and M. Schmutz. 2012. Untersuchungen zur Mastleistungund Schlachtkörper zusammensetzung von Stubenküken aus Masthybriden und verschiedenen Legehybridherkünften. Züchtung skunde 84: 511-522.
Kranis, A., Gheyas, A.A., Boschiero C., Turner, F., Yu, L., Smith, S., Talbot, R, et al. 2013. Development of a high density 600K SNP genotyping array for chicken. BMC Genomics 14: 59.
Kranis, A. and G. Maniatis. 2020. Application of genomic selection (GS) in breeding commercial meat-type chickens. Chapter 14. In: Advances in poultry genetics and genomics. S. Aggrey, H. Zhou, M. Tixier-Boichard, D. Rhoads (Eds). Burleigh Dodds Science Publishing Limited., Cambridge, UK. Pp: 385-402.
Krautwald-Junghanns, M-E, Cramer, K., Fischer, B., Förster, A., Galli, R., Kremer, F. et al. 2018. Current approaches to avoid the culling of day-old male chicks in the layer industry, with special reference to spectroscopic methods. Poultry Science 97: 749–757.
Lister, S. and B. Van Nijhuis. 2012. The effects of Alternative Systems on disease and health of Poultry. Chapter 4. In: Alternative systems for poultry: health, welfare and productivity. Ed. by V. Sandilands and P.M. Hocking. CABI Publishing, Wallingford, UK. Pp: 62-76.
Leeson, S. and W.D. Morrison. 1978. Effect of feather cover on feed efficiency in laying birds. Poultry Science 57: 1094-1096.
McDonald, P., Edwards, R.A., Greenhalgh, J.D.F. and C. A Morgan. 2010. Protein Concentrates. Chapter 23. In: Animal Nutrition (7th Ed). Prentice Hall, Harlow. Pp 563-593.
Meuwissen, T.H.E., Hayes, B.J. and M.E. Goddard. 2001. Prediction of total genetic value using genome-wide dense marker maps. Genetics 157: 1819-1829.
Nguyen, H. 2018. Sustainable food systems concept and framework. FAO. (http://www.fao.org/3/ ca2079en/CA2079EN.pdf).
Ni, G., Cavero, D., Fangmann, A., Erbe, M. and H. Simianer. 2017. Whole genome sequence based genomic prediction in laying chickens with different genomic relationship matrices to account for genetic architecture. Genetics Selection Evolution 49: 8. Nicol, C.J., Bestman, M., Gilani, A.M., De Haas, E.N., De Jong, I.C., Lambton, S., Wagenaar, J.P., Weeks, C.A. and T.B. Rodenburg. 2013. The prevention and control of feather pecking. Application to commercial systems. World’s Poultry Science Journal 69: 775-788.
Pelletier, N., Ibarburu, M and H. Xin. 2014. Comparison of the environmental footprint of the egg industry in the United States in 1960 and 2020. Poultry Science 93: 241-255.
Pérez-Bonilla, A., Novoa, S., García, J., Mohiti-Asli, M., Frikha, M. and G.G. Mateos. 2012. Effects of energy concentration of the diet on productive performance and egg quality of brown egg-laying hens differing in initial body weight. Poultry Science 91 :3156-3166.
Pérez-Enciso, M, Rincón, J.C. and A. Legarra. 2015. Sequence- vs. chip-assisted genomic selection: accurate biological information is advised. Genetics Selection Evolution 47: 43.
Pérez-Enciso M. and J.P. Steibel (2021). Phenomes:
the current frontier in animal breeding. Genetics Selection Evolution 53: 22.
Pinard-van der Laan, M.H., Kaufman, J., Psifidi, A., Zhou, H. and M. Fife. 2020. Genetics and genomics of immunity and disease traits in poultry species. Chapter 9. In: Advances in poultry genetics and genomics. S. Aggrey, H. Zhou, M. TixierBoichard, D. Rhoads (Eds). Burleigh Dodds Science Publishing Limited., Cambridge, UK. Pp: 263-305.
Preisinger, R. 2021. Commercial layer breeding: Review and forecast. Züchtungskunde 93: 210-228. Rains, T.M. and M. Kanter. 2017. The nutritional role of eggs. Chapter 14. In: Achieving sustainable production of eggs, Vol 1: Safety and Quality. J. Roberts (Ed.). Burleigh dodds, Cambridge, UK. Pp: 319-335.
Tablante, N.L, Vaillancourt, J.-P., Martin, S.W., Shoukri, M. and I. Estevez. 2000. Spatial Distribution of Cannibalism Mortalities in Commercial Laying Hens. Poultry science 79: 705-708.
van Asselt, E.D., van Bussel, L.G.J., van Horne, P., van der Voet, H., van der Heijden, G.W.A.M. and H.J. van der Fels-Klerx. 2015. Assessing the sustainability of egg production systems in The Netherlands. Poultry Science 94: 1742-1750. van Binsbergen, R., Calus, M.P.L., Bink, M.C.A.M., van Eeuwijk, F.A., Schrooten C. and R.F. Veerkamp. 2015. Genomic prediction using imputed wholegenome sequence data in Holstein Friesian cattle. Genetics Selection Evolution 47: 71. Wardy, W., Mena, B., Nongtaodum, S., No, H.K. and W. Prinyawiwatkul. 2014. Exploring the drivers of purchase intent and consumer satisfaction of chicken eggs using principal component analysis and the kano model. Journal of Sensory Studies 29: 463-473. Whitehead, C., Fleming, R., Julian, R. and P. Sorensen. 2003. Skeletal problems associated with selection for increased production. Chapter 3. In: Poultry
Genetics, Breeding and Biotechnology. Muir and Aggrey (Eds.). CABI Publishing, Wallingford, UK. Pp 29-52. Windhorst H.-W. (2016). The role of developing and newly industrialised countries in global egg production. Lohmann-Information 50: 24-29.
Windhorst H.-W. (2021).Patterns and dynamics of the EU poultry industry: a status report. Part 1 –Laying hen husbandry, egg production and egg trade. Zootecnica International 43 (No 12): 22-26.
You have come to the end of our journey: navigating through some of today’s very latest scientific insights that are working to move forward tomorrow’s management and nutrition systems making them more efficient, more sustainable, and more welfare-friendly.
It was inspiring, working with each of the contributing authors of this volume. Every chapter that we received during this almost year-long collaboration brought the same passion regardless of its origin from the industry or the academic world. We are also astonished to see the boundaries being pushed in every new hypothesis and perspective. Our Novus team could never express enough gratitude to all the contributors for having shared such a level of expertise. Amongst the contributors, we want to deeply acknowledge the precious and huge support given by Professor Johan Buyse, the scientific coordinator of this book. Without his generosity, patience, and precision, this book could not have emerged.
Now, what are the conclusions? There is no doubt that poultry meat production continues to grow at impressive rate year over year, fueled by consumer demands, who demand inexpensive, high-quality, and versatile protein sources. The poultry industry can already supply high-quality protein at affordable prices thanks to the continued genetic improvement on growth rate, feed conversion ratio, and egg production. However, genetic improvement in broiler and egg traits has repercussions provoking more challenges in the breeder hens.
This volume carries in it the major parameters of breeder reproductive performance: Fertility, hatchability, and hence the number of day-old chicks per breeder. A reduction in fertility can be related to nutritional factors, flock
management, or diseases. Hatchability is also affected by nutrition, several egg traits, hen age, and incubation conditions. Additionally, the quality of the day-old chick, which can be affected by maternal and paternal nutrition, and incubation conditions, might impact its potential growth performance. Traditionally, breeder managers have given more attention to the female birds. However, the male is responsible for 50% of the genetic potential and nutrition and management play important roles in cockerel semen production and mating behaviour until the end of the production cycle.
Proper nutrition, feeding control, and management during rearing are necessary to achieve weight targets, body conformation, reproductive development, and synchronized sexual maturity at the age of photostimulation. During the reproductive phase, feeding and body weight control, management of male-to-female ratios, lighting control, and optimum energy, protein, amino acid, vitamin, and mineral levels are necessary to optimize breeder reproductive performance as well as offspring performance. Several feed additives, such as organic trace minerals, antioxidants, functional amino acids, and fatty acids can be used to prevent the negative effects of aging and stress from breeder reproduction; and the selection of additives must be based on their efficacy during different climate and management conditions.
With increasing consumer awareness about sustainability and animal health and welfare, the traditional restrictive feeding programs (both quantitative and qualitative) come under scrutiny. The poultry industry is now developing and adopting new technologies to ameliorate these programs while also maximizing the number of day-old chicks with highperformance potential and finding ways to minimize nutrient excretion.
Following newly developed high-tech, day-by-day breeder management and feeding protocols during both the rearing and laying periods, the concept of epigenetics finally found its way to the poultry sector. Direct developmental programming of the growing embryo due to external factors (e.g., maternal nutrition) does occur in chickens and can have an impact on offspring performance. Furthermore, a few multigenerational and even
transgenerational poultry studies spanning over several generations were recently performed. Based on these studies, it can be concluded that it must be feasible to improve offspring performance in a sustainable and animalfriendly manner by considering (nutritional) interventions at the (great) (grand) parent level. Concomitantly, there is increasing interest from the academic world to investigate the underlying physiological, neuroendocrine, immunological, and molecular epigenetic mechanisms in avian species.
As stated in the preface, one of the aims of compiling this book was to seek solutions for industry problems and, at the same time, identify new opportunities for the breeder industry. We are convinced that, through this book, we succeeded in helping to move the poultry industry a tremendous step forward, and sincerely hope you have the same conviction after reading this volume.
Prof. Johan Buyse, KU Leuven Belgium Sandrine Durox, Novus International, Inc Dr. Silvia Peris, Novus International, Inc Dr. Hugo Romero-Sanchez, Novus International, Inc
Poultry is the most widely consumed meat in the world due to its availability, wholesomeness and affordability. Demand for safe and high-quality meat and eggs continues to grow, and so do regulatory requirements and consumer expectations.
Since 1991, Novus International, Inc., has offered the global poultry industry scientifically created and masterfully manufactured health and nutrition solutions that positively impact animal performance as well as farm profitability and sustainability.
From the moment a chick is hatched, a bird’s gut is susceptible to many factors that can impact performance. Novus offers solutions to support gut health through better use of raw feed, promoting stress reduction, supporting disease response and pathogen control.
The goal of any breeder program is to produce high-quality/low-cost chicks. Because chickens in particular “are what they eat,” research shows that optimal maternal nutrition is crucial for both hen and progeny performance. Novus solutions show greater egg production and hatchability in both layers and broiler breeders along with improved chick uniformity and development.
Feed is the top cost for any animal protein operation. Managing those costs without compromising development is an ongoing struggle. Novus’s feed cost reduction program can help producers achieve greater flexibility in formulations while optimizing diets for yield and cost efficiency without sacrificing performance.
When combined with strong management practices, Novus solutions can help with bone development and promote stronger skin and tissues, which contributes to improved animal welfare.
High quality meat is in high demand. Poorly developed breast, wing and leg meat, and myopathies like wooden breast and white striping are costly challenges. Novus solutions can help support improved meat yield and quality that meet consumer expectations while realizing a greater return on investment.
What does sustainability mean? Is it less minerals excreted in soil? Reducing nitrogen emissions? Is it getting the most from lowquality raw ingredients? Increasing the amount phosphorus captured from feed? Or is it about return on investment, the longevity of your operation, the viability of our industry? Any way you think about it, sustainability is what all producers are striving for. Talk to a Novus representative about how our products can help.
The ultimate challenge for the egg industry is increasing the overall quantity and quality of salable eggs. High-quality eggs start with a well-structured layer nutrition program. The proper balance of the right kind of minerals can, for instance, strengthen the shell and promote structural integrity of the egg.
As laying hens age, mineral nutrition is increasingly important in maintaining the overall well-being of the hen and the continued production of plentiful, highquality eggs.
Novus offers programs aimed at enhancing eggshell strength, reducing cracks and breaks and supporting hen productivity.
ALIMET® feed supplement is an active liquid source of methionine. In addition to proven performance, ALIMET® delivers an organic acid effect, reduces nitrogen excretions1 and supports performance during heat stress.2
MHA® feed supplement is a dry, active source of HMTBa methionine. Because of its granular powder form, MHA® is ideal for use in premixes and farm feed mills. MHA® delivers organic calcium and is shown to reduce nitrogen excretion1 and provide better performance during heat stress.2
CIBENZA® DP100 protease feed additive is a heat-stable, potent protease enzyme shown to improve dietary protein digestibility for optimal animal performance.4 This solution can reduce costs by allowing producers to lower protein levels in feed up to 10% without sacrificing animal performance, also helping to mitigate the negative effects of anti-nutritional factors.
CIBENZA® EP150 feed additive is an advanced, dual-component product containing Bacillus licheniformis spores and its protease derivative that optimizes health and performance. This solution is shown to decrease the incidence and impact of pathogen outbreaks as well as boost animal performance especially when nontraditional feed ingredients are used.5
MINTREX® bis-chelated trace minerals combine the HMTBa molecule found in methionine source ALIMET® with an essential trace mineral (zinc, copper or manganese) in a unique bis-chelate structure. The result is greater stability in the digestive tract and superior bioavailability. MINTREX® delivers improved performance parameters beyond what is traditionally expected from other sources of trace minerals.3
ACIDOMATRIX™ feed additives are a select blend of organic acids aimed at improving gut health. The solutions in this product family stabilize intestinal microflora to bolster the ability to control pathogens, especially in antibiotic-free programs.7
AVIMATRIX® feed supplement is a protected blend of benzoic acid, calcium formate and fumaric acid shown to have an antimicrobial affect.8 It specifically acts on the lower gut environment through a targeted release of its active ingredients. This mode of action is shown to have positive impacts on bird perfomance and welfare.
NEXT ENHANCE® feed additive is a 1:1 combination of thymol and carvacrol that supports optimal gut health in broilers, improving immune status, gut integrity,9 oxidative balance10 and gut microbiota.11
This solution is also shown to offer support during coccidiosis challenges.12
ACTIVATE® nutritional feed acid solutions are blends of organic acids and HMTBa, a highly bioavailable source of methionine. It is available in both liquid and dry form depending on location. ACTIVATE® is an important component of Salmonella reduction programs, in vaccination-free flocks and withdrawal diets, as well as help to optimize nutrient utilization.
7 Yan et al., 2017
8 Keller and Parker, 2013
9 Du et al., 2016
10 Hashemipour et al., 2013
11 Yin et al., 2017
12 Yan et al., 2017
13 Castillo et al., Spanish field trial, 2019
PROVENIA® feed supplement is a blend of benzoic and fumaric acid in an embedded matrix technology to ensure targeted release in the hind gut of birds where antimicrobial action happens. This mode of action is shown to reduce pathogenic bacteria and result to a positive impact in gut health and performance of the birds.13
Not all solutions are available in all areas. Contact your local Novus representative or visit novusint.com to learn how Novus can work with you.
NOTICE: While the information contained herein (“Information”) is presented in good faith and believed to be correct as of the date hereof, Novus International, Inc. does not guarantee satisfactory results from reliance upon such Information, disclaims all liability for any loss or damage arising out of any use of this information or the products to which said Information refers and MAKES NO REPRESENTATIONS OR WARRANTIES, EITHER EXPRESS OR IMPLIED, OF MERCHANTABILITY, FITNESS FOR A PARTICULAR PURPOSE OR OF ANY OTHER NATURE WITH RESPECT TO THE INFORMATION OR PRODUCTS, except as set forth in Novus’s standard conditions of sale. Nothing contained herein is to be construed as a recommendation to use any product or process in conflict with any patent, and Novus International, Inc. makes no representation or warranty, express or implied, that the use thereof will not infringe any patent.
Products not available in all countries.
® ACIDOMATRIX, ACTIVATE, ALIMET, AVIMATRIX, CIBENZA, MHA, MINTREX, PROVENIA, and NEXT ENHANCE are trademarks of Novus International, Inc., and are registered in the United States and other countries.
TM is a trademark of Novus International, Inc.
© 2022 Novus International, Inc. All rights reserved.