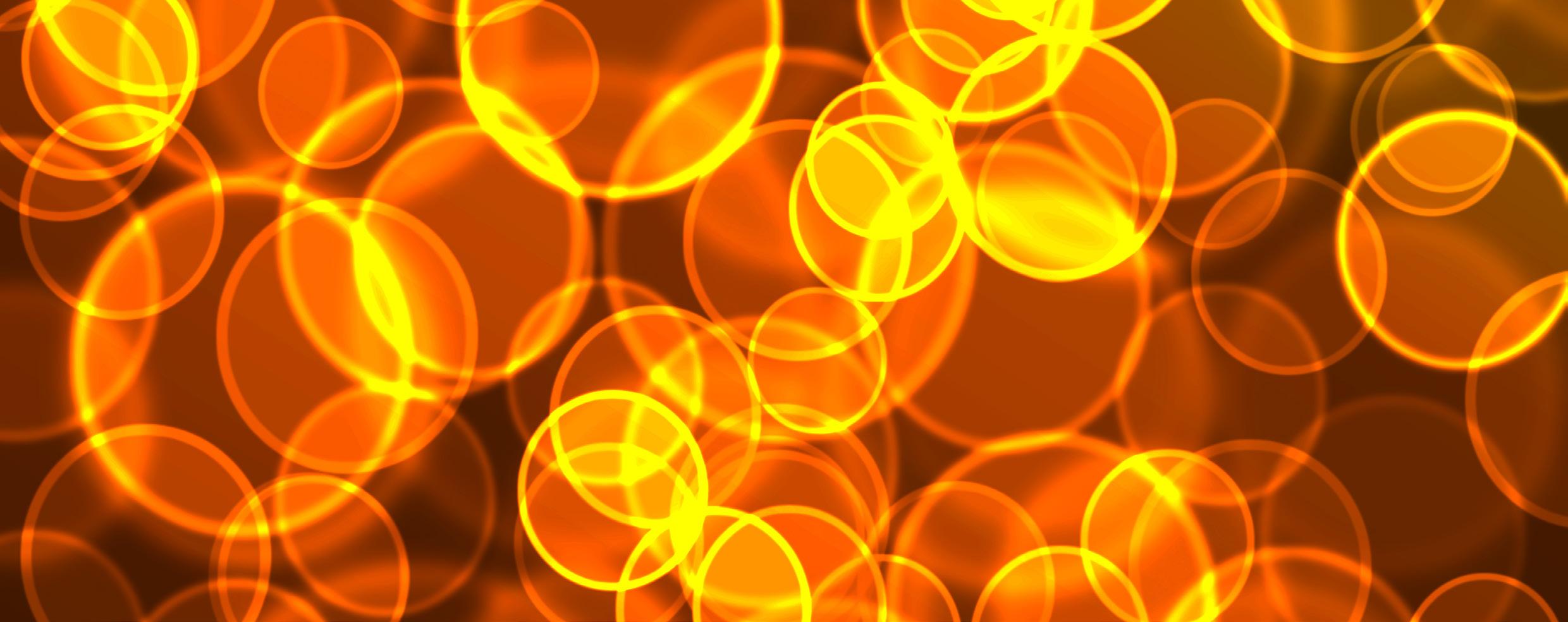
24 minute read
Optimizing Heat distribution in Grease Manufacturing Process - A critical parameter for Quality Assurance
Dr. Navendu Bhatnagar, Dr. Anoop Kumar and Bill Mallory Royal Mfg. Co. LP
Abstract
Lubricating greases are NonNewtonian fluids and nonconducting in nature which makes manufacturing of lubricating greases a complex process. As lubricating greases predominantly consists of base oil (75-85 %), thickener (1015 %) and balance additives, high temperatures adversely affect the quality of grease resulting in rapid thermal oxidation or carbonization though dependent upon mixing profile. Due to semi-solid nature of lubricating greases results in slow dissipation of heat compared to that in case of lubricating oils. This fact holds importance in the grease manufacturing process where large quantities of grease ( 5000-20000 lbs ) is heated by circulating thermic fluids at temperatures ranging from 400 °F – 480 °F [204°C – 249°C]. Imparting desired quality and performance characteristics to the grease product depends on ensuring proper control of the heat transfer from thermal exchange systems in a manner that temperatures stay well within the defined range as per the formulation process. A thermal shock can occur if temperature at the heat source is not regulated properly or reacting mass is not mixing in proper fashion resulting in adverse effects on grease quality. Proper control of heat during exothermic/endothermic reactions is needed to maintain the reaction kinetics.
This paper will focus on understanding the factors (such as mixing profiles) that affect the heat transfer in grease manufacturing process. Role of Heat transfer coefficients will be discussed from a commercial industry standpoint. Comparison in heat transfer profiles for different greases (for example lithium based vs. calcium sulfonate) will also be studied and analyzed. Another aspect that will be studied is the difference in heating profiles between a contactor and an open kettle cook. The conclusion of this paper will focus on identifying proper methods for ensuring uniform and controlled heat distribution during the grease manufacturing process.
INTRODUCTION
Lubricating grease has been defined in ASTM terms as dispersion of thickening agent in a liquid lubricant where the dispersion is a solid to semi-fluid product. Three basic components that form are oil, thickener and various additives as required [1, 2].
Over the years, a prominent debate has been the choice of oil or grease for lubrication purposes. Strong points for grease include better start-stop performance, ability to stick to the surface offering better retain characteristics, application as a sealant thereby serving as a protective coating and ability to disperse solid additives that may impart certain unique properties in operation. With such strong attributes on offer, it is imperative that manufactured grease must be high on quality standards and should be able to deliver the promises that are expected theoretically in a particular application.
Grease manufacturing however, is not a simple process, when compared to the case of lubricating
oils which involves mixing the different base oils and liquid additives for small period of time at room or slightly elevated temperatures. One of the main challenges for grease is the presence of different phases [3, 4] during the manufacture process which starts in a liquid phase but transforms into a semi-liquid to a semisolid phase during the course of chemical reaction and addition of additives, in many cases. This multi-phase operation requires a significantly more robust control of parameters to achieve a better quality product.
Scale-up of grease manufacturing process from lab to commercial level is challenging and requires a careful consideration of factors that can significantly affect the replication of product quality achieved at small scale [5]. Some of the notable factors are, a) Temperature control – Saponification reaction, like other reactions is known to accelerate with the rise in temperature [6]. However, there is no linear relationship between the kinetics and heat supplied. b) Mixing profiles – Even though a single uniform mixing profile can be employed for both reaction and finishing stages, mostly it is beneficial to provide a two stage mixing for process optimization. c) Exposure to open environment – Whether or not the saponification reaction is carried out under pressure changes the reactions times as well as grease formulations which plays an important role in commercial grease manufacture process.
Manufacturing can be performed in a batch or a continuous manner, however a batch process is the more popular one as it provides more flexibility in grease cooking and finishing compared to the continuous operation. Batch process may employ an open kettle cook where grease is manufactured at atmospheric pressure and complete process is finished in one kettle in contrast to closed kettle cook where saponification reaction is carried out under pressure in a contactor followed by finishing in a second kettle [6].
Main focus of this study is to understand the parameters and factors that play an important part in temperature control during the grease manufacturing process. Base oils are known to get oxidized at elevated temperatures and since they volumetrically contribute 75 – 85 % in grease formation, pose a danger of causing thermal oxidation of grease at temperatures above the recommended range. This oxidation results in darkening of grease and generation of unwanted oxidation products which may have an adverse effect on the thickener causing softening, oil bleeding followed by leakage when exposed to certain applications. Also, chain oxidation causes carbonization which results in crust formations. All these unwanted occurrences can be reduced or even eliminated by marking a better control on heat transfer to and from the reaction mixture and final grease product.
Overall Heat Transfer Coefficient (U)
One of the parameters that is used to study and measure the efficacy of heat transfer is the overall heat transfer coefficient which is defined as the ability of a certain medium or series of mediums to conduct heat.
The rate with which heat is removed or transferred from the surface can be defined by the following equation [8],
where Q is the heat removal rate, U is the overall heat transfer coefficient, A is the surface area of heat transfer and ΔT is the difference in temperature of solid surface and fluid in contact.
For systems involving coils or jackets, overall heat transfer coefficient can be calculated using the standard equation [8],
where α and αs are the process and service side heat transfer coefficients and 1/αF is the service side fouling resistance.
A higher overall heat transfer coefficient is an indication of better heat conduction through the material which is desirable. For grease manufacture, the cooking kettles and jackets are generally constructed from carbon steel or stainless steel material. This brings the typical value of ‘U’ equal to 400 W/m2-K (70.45 BTU/hr.ft2.°F) when heating the product [8]. In some cases cast iron may also be used. U can be calculated for a specific case by getting process and service side heat transfer coefficients using the equations between Nusselt and Reynolds number. This parameter cannot be varied on a daily basis and holds importance only when designing or modifying a grease manufacturing unit.
a) Type of heat transfer fluid. b) Method of heat transfer c) Flow rate. d) Distance from heat source. e) Mixing profiles and efficiency. f) Properties of reaction fluid g) Heat losses
Type of Heat Transfer medium
Old techniques employed exposing the grease kettle to a direct fire where a flame would be in touch with the outside kettle wall. This approach had obvious disadvantages as temperature control was a big concern and due to lack of surface area, heating times were increased. If mixing was not fast and effective, this type of heating could lead to burning or scorching of final product [7]. Also, direct fire increases the fire hazard, especially when dealing with oils of low volatility and flash points. Added precautions and considerations are required in plant designing when installing direct fire equipment [6]. Modern grease kettles are built with jackets where a heat transfer (HT) fluid is circulated to heat the reaction mixture. For low temperature cooking where reaction mixtures are to be heated up to 150 deg C, steam is a viable option. High pressure steam (operating @ 100-150 psi) can attain such temperature range. However, recent advances in grease formulations have enabled the manufacturers to make better quality greases if cooked at higher temperatures in the range of 380-410 deg F which cannot be provided by steam. One of the most common heat transfer fluids used in the current grease manufacturing industry is heat transfer oil. The formulation can be Group I or Group II oil based with either a naphthenic or paraffinic content in it depending on the application. These oils can provide working temperatures in the range of 100-600°F. However, since source is set at high temperature, better control of heat transfer is required. If not controlled properly, this can cause the temperatures to go out of the recommended range and affect grease in an adverse manner. Also, heat losses to the environment can lower the efficiency of the heat transfer if proper measure are not followed. These include insulation of transfer lines, kettle jackets and minimum distance between the source and kettle. Another factor to consider is the thermal degradation and oxidation of heat transfer (HT) oil. - 34 VOLUME 80, NUMBER 4
Oxidation occurs when HT oil comes in contact with air at high temperatures (>200°F [93°C]) resulting in formation of sludge that eventually deposits in the hot oil circuit thereby reducing the heat transfer efficiency. Thermal degradation of petroleum based fluids refers to the breakage of carbon-carbon bonds when exposed to temperatures in excess of recommended bulk operation temperature of oil. This increases the acid number of the oil resulting in slow corrosion of hot oil circuit. This results in chipping of solid wall material in contact with hot oil which may deposit on the heat transfer surface adversely affecting the heat transfer efficiency.
Method of heat transfer
Heat transfer in agitated vessels is carried out using the external jackets or internal coils or a combination of both [8]. External jackets can be conventional in design using spiral baffles welded to the wall of kettle or it can be dimpled jacket. In some cases, a half pipe (limpet coils) jacket can also be used but with a disadvantage that it covers less surface area and requires a higher extent of welding which may cause mechanical stability concerns when thermal shocks are encountered [9].
Figure 1[8]: External jackets; (a) spiral baffle, (b) dimpled, (c) half-pipe
In addition to external jackets, certain agitated kettles also employ internal coils which may be fully helical in shape or a series of smaller ringlet coils.
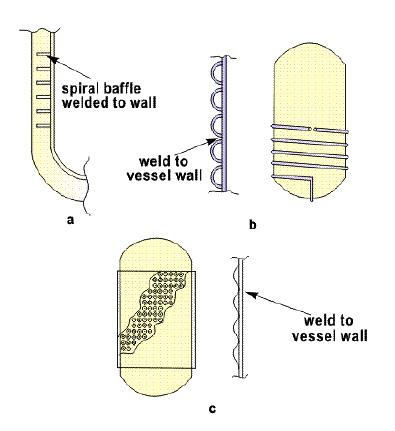
Figure 2[8]: Internal coil jackets; (a) Full helical, (b) ringlet coils
As it is evident, full helical coil offers an advantage of providing more surface area than the ringlet coils but requires the kettle body to be two-piece increasing the overall initial cost of fabrication. Ringlet coils can be inserted in a single piece vessel but may generate temperature gradient in the reaction mixture if mixing is not sufficient. In context to grease manufacturing, a conventional style spiral baffle jacket is more advantageous than other external jackets as it offers a higher surface area for heat transfer. This however is true when considering the open kettle cooks. One of the foremost innovations in grease manufacturing has been the advent of contactor [10] reactor which allows reactions to take place in closed, highly pressurized environments thereby increasing the kinetics of reactions by a multifold. This unit employs an internal double walled circulation tube which is an extension of a full helical coil in conjunction with an external heating jacket. This increases the heat transfer surface area almost by a factor of three when compared to an external jacket open kettle as shown in Figure 3.
Figure 3: Kettle TOP view; (a) External jacket only, (b) External jacket + internal coils
To summarize, a combination of external jacket system and internal coil network should be utilized to achieve a higher degree of heat transfer in grease manufacturing processes. Rate of heat transfer from the heating medium to reaction fluid depends on various factors such as type of heat transfer fluid, impeller speed, vessel geometry, area of heat transfer and also the temperature difference between the source and reaction fluid. This relationship is mathematically expressed as [8],
Where q is the rate of heat transfer, U is the overall heat transfer coefficient, A is the area of transfer and TH / TF are temperatures of heating fluid and reaction mixture respectively.
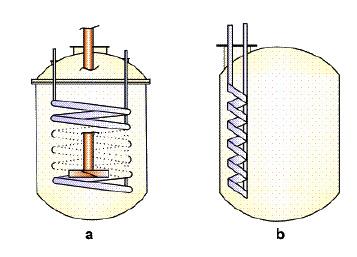
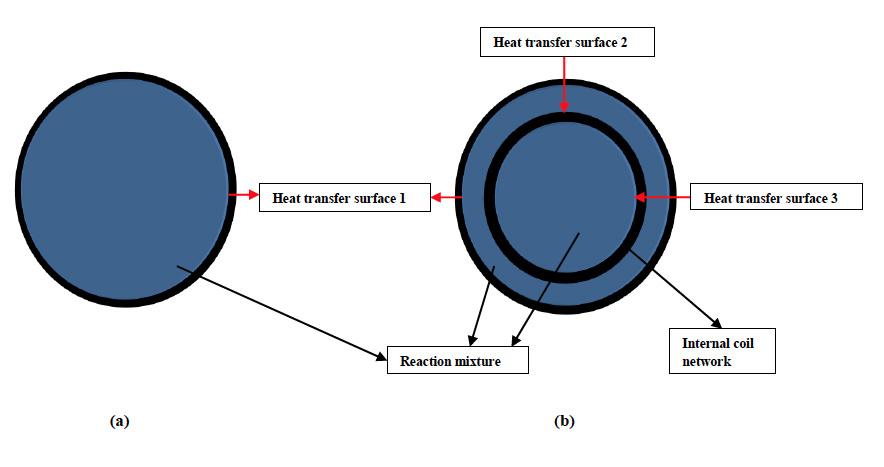
Energy balance involved in this step can be expressed as follows,
where qin is the heat input to the system, qout is the heat released from the system and qloss is the heat loss from the system.For simplification purposes considering the case of transient heat transfer in a batch manufacturing process with good insulation and minimal losses the above equation can be expressed as [11],
where mw is weight of mixture and C is the specific heat.
This equation is integrated to yield,
This equation provides a simplified method to estimate the overall heat transfer coefficients for a batch process. However, other factors such as mixing profiles (Reynolds number, Prandtl number) need to be considered for a more realistic depiction which is out of scope for this current study. Authors studied the temperature profile of grease mixture cooked in an open kettle and also in the contactor. Grease in contactor was lithium based and sodium based in the open kettle. Greases were of similar NLGI grade to avoid the effect of thickness on recorded data. Heating was started from same temperature and was continued till the desired temperature was achieved. Heat transfer fluid flow rate was also maintained at an equal level between the open kettle and contactor to achieve comparable conditions. Source temperature was maintained at 410°F [210°C].
Figure 4: Comparing heating profile for grease cooked in (a) contactor vs. (b) open kettle
As can be observed in the above figure, contactor unit utilizing an open jacket in conjunction with a closed internal coil network takes approximately two to three hours less than an open kettle (with just one external jacket) to heat up a grease reaction mixture. Contactors (or any pressurized kettle) are used where water is required to be present in the reaction mixture at a temperature higher than the boiling point [6]. It is important to mention here that pressure is also generated in the closed kettle when heating the reaction mixture.
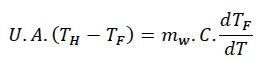
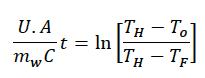
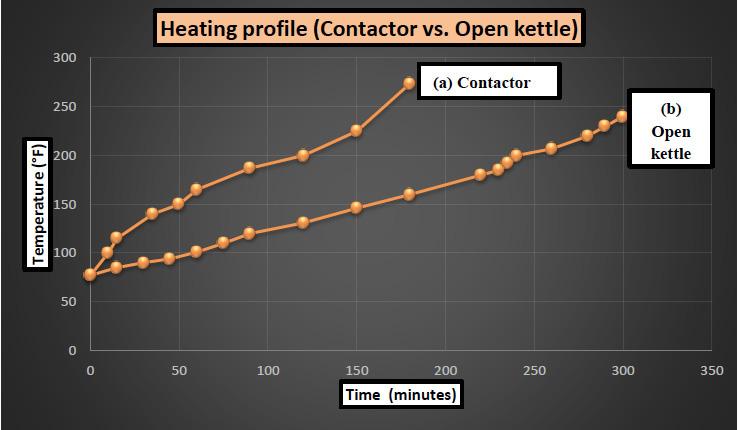
Flow rate of heating fluid (heat transfer oil in the current study) directly affects the rate of temperature rise which can be critical when the reaction mixture is being heated to the required range. If the reaction mixture is heated too fast then it may cause problems of a varied nature. In some cases, saponification reaction may not complete thereby lowering the overall yield and in some cases it may affect the quality of manufactured soap. In one of the studies performed, authors looked at two different cases for Lithium 12-hydroxystearicacid (12HSA) thickener based grease where the HT oil flow rate was increased by a factor of two and the effect on grease was evaluated. In the first case, temperature increased with a rate of 1.5°F/minute and comparatively in the second case, flow rate was increased by a factor of two which allowed the temperature to rise with a rate of 3.0°F/minute. After the reaction completed and soap was transferred into the finishing kettle, base penetrations and drop points were measured and overall grease appearance was compared. One of the striking results was the difference in appearance of soap. While the soap cooked with slower flow rate had a uniform texture, black particles were found to be uniformly distributed in the soap with an increased flow rate and a steep rise in temperature. This occurrence is attributed to the burnt solid powdered ingredient particles which did not get enough time to dissolve and an extremely fast heating rate scorched the powder resting on the inner heating coils. A slow heating rate allows enough time to mix and dissolve the solid raw materials thus avoiding such occurrence. In addition to this, penetration for case 1was lower than for case 2 which means that soap cooked with a slow uniform heating was thicker than the one cooked with a fast heating rate. This allows the yield to be higher for case 1. Drop points were also higher for case 1 than for case 2 which is a direct indication of better grease quality.
Figure 5: Lithium grease cooked with (a) low HT oil flow rate; (b) high HT oil flow rate
One of the major factors that plays a role in ensuring proper heat transfer in grease manufacture specifically is the type of mixing involved. Grease cooks pass through several stages during the manufacture. As mentioned earlier, it is a multiphase reaction where initial stage have more of a liquid phase with base oil acting as the dissolving medium for solid raw materials and liquid additives. During the course of reaction and subsequent soap formation, mixture thickens up to a semi-solid phase which is then thinned out in the finishing stages to a semi-liquid phase depending on the grease grade required. It is imperative that mixing needs to be vigorous in the initial stages of grease manufacture to improve the reaction kinetics and reduce the time taken for reaction completion. However, in the later stages when soap network is formed, mixing needs to be slow but effective. In terms of heat transfer, if mixing is not vigorous enough, it will generate a temperature gradient in the reaction mixture allowing pockets of low and high temperature regions. Another concern arises from the fact that, if mixing is poor, heat removal rate from the jacket wall (maintained at a temperature of around 400°F [204°C]) will be low which may end up scorching the product. A typical temperature gradient plot in agitated reaction vessels is shown below,
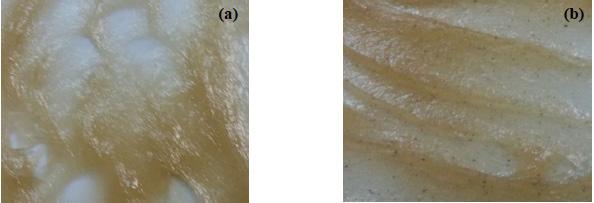
Figure 6: Schematic of a typical temperature gradient in an agitated vessel.
Authors carried out experiments where two grease manufacturing kettles with different mixing profiles were considered. Kettle 1 was equipped with a counter rotation
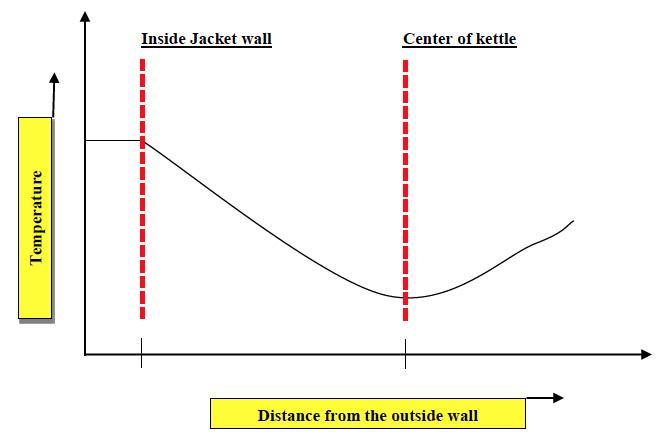
agitator (480V, 40 H.P.) with an RPM of 30. Kettle 2 was equipped with a single rotation agitator (480V, 30 H.P.) with an RPM of 22. Authors cooked similar greases in both kettles and monitored the temperatures along the central kettle axis. Temperatures were taken in similar working conditions when the source temperature / jacket temperature was stable at 240°F. A plot was generated with the data as shown below,
Figure 7: Temperature gradient for kettles with fast counter rotation (a) and slow single rotation mixing (b)
It can be clearly observed from the resulting plot that kettles having a vigorous mixing profile has a less temperature gradient (shown by red arrow) compared to the cased where mixing profile is relatively slow. The difference in the current study was about 30°F at the center of kettle when the source temperature was at 240°F [116°C]. This result is qualitative in nature and the temperature difference may change based on the source temperature, mixing profile or type of grease.
Another parameter that assists in evaluating the mixing needs for a particular mixture is tip speed which is defined as distance travelled by the outermost point of the mixer blade in a given amount of time. This is mathematically expressed as [12],
Where D is the diameter of mixer blade in ft. and N is the mixer rotation speed in rpm.
During scale-up calculations, the aim should be to attain a constant tip speed with varying diameter and rotational speeds. This mixing factor holds importance in the grease industry as grease matrix is known to deform under high shear conditions. One example is that of aluminum soap based greases which possibly are extremely sensitive to shear and are known to break down mechanically losing both their consistency and lubricating effectiveness [13]. A simple rule of thumb is that lower tip speeds are preferred for mixtures with higher viscosities and higher tip speeds for thin fluidic mixtures. This holds true for grease manufacturing as well where tip speeds in contactor are higher than in the finishing kettle almost by a factor of 10 thereby producing high shear rates. However, high tip speeds in the finishing kettles with viscous grease mixtures is not advisable as it may lead to grease break down.
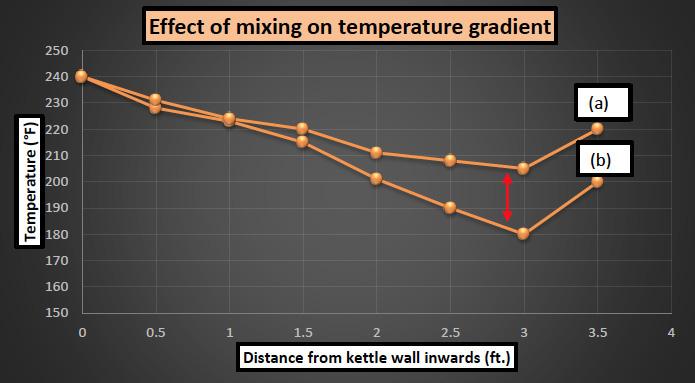
Heat transfer dependence on grease composition / reaction fluid
Grease composition may also be a factor affecting the rate of heat transfer through the reaction fluid and also once the grease is put under application. One reason for this is the difference in thermal conductivities of base oils. Base oil is the name given to lubrication grade oils initially produced from refining crude oil (mineral base oil) or through chemical synthesis (synthetic base oil). Base oil is typically defined as oil with a boiling point range between 550 and 1050 F, consisting of hydrocarbons with 18 to 40 carbon atoms. One of the studies shows that group V base oils have a higher thermal conductivity than group II base oils [14]. Base oils can be paraffinic or naphthenic based and another study shows that paraffin based oil have a better thermal conductivity than naphthenic base oil [15]. Since grease is ~ 80% base oil, thermal conductivity of grease reaction mixture is dominated by base oil properties.
A study was carried out where Lithium 12-hydroxystearicacid (HSA) thickener based grease samples with different base oils were heated with a constant heat supply rate. A temperature vs. time reading data was accumulated (Table 1)
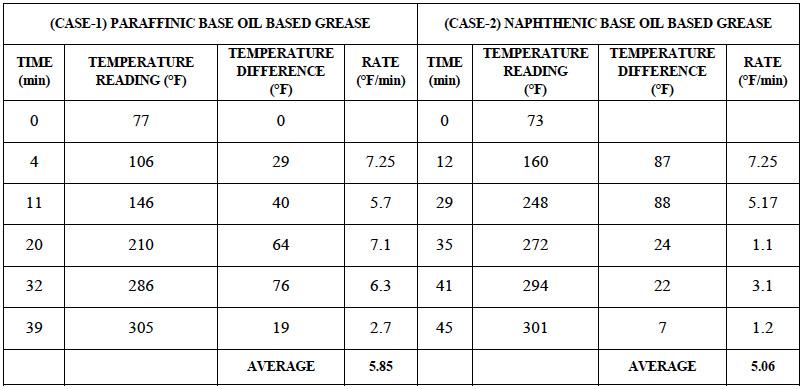
Table 1: Rate of heat transfer for grease composed of Paraffinic base oil (CASE-1) and Naphthenic base oil (CASE-2)
The data above shows that rate of heat transfer is higher for case-1 with an average heat transfer rate of 5.85°F/min compared to 5.06°F/min for case-2. This result indicates a higher thermal conductivity for greases cooked in paraffinic base oils compared to those cooked in naphthenic base oils.
Table 2: Rate of heat transfer for lithium complex structure grease (CASE-1) and standard lithium grease (CASE-2)
Another experiment with a similar set up was performed to test the effect of complex formation on the grease ability to conduct heat. A lithium soap based and a lithium complex soap based grease were heated at a constant rate to ~ 300°F [149°C] and the temperature vs time data was collected (Table 2). Results show that lithium complex grease provides more resistance to heat transfer than the regular
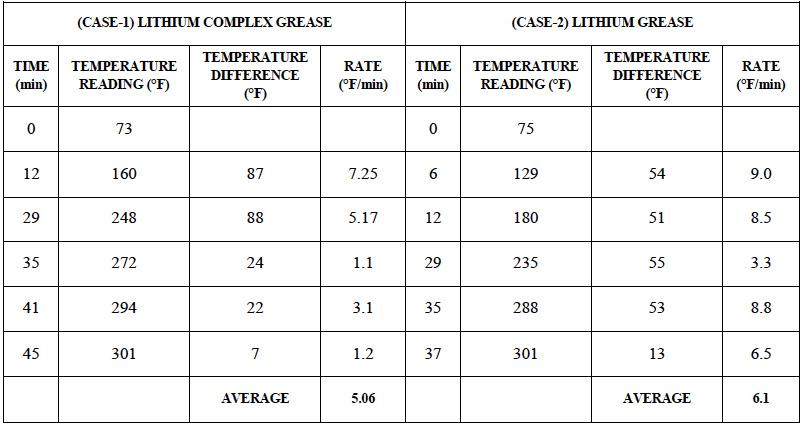
lithium greases. This probably is due to the microstructure of complex greases where more energy is required to break the complex structure than that for a simple lithium grease. Test samples were additive inclusive finished greases. These data are mostly qualitative and indicative of the effect of grease composition and structure on the ability of grease to transfer or conduct heat.
Effect of heat absorption capacity
Another study was performed to check the effect of grease composition on heat absorption capacity and subsequent rate of temperature rise. Heat capacity of a material is defined as the ratio of heat energy transferred to an object and the resulting increase in temperature of that material. This is represented by the following equation [16],
or it can also be expressed as,
where Q is the heat transferred to a material, m is the mass of material and c is the specific heat of the material. Grease 1 was lithium based and grease 2 was poly urea based and both were in the same penetration range of 300 – 305. 1000 gm of grease sample was put in an open mixer exposed to a heating coil bath. Heat source was set at a constant heating rate with the maximum attainable temperature of 180°F [82°C]. Heating was started from room temperature and data was recorded till a constant temperature was attained for both samples. Results for grease 1 and grease 2 were plotted together and are shown in Figure 8.
Figure 8: Effect of composition on heat absorption rate of lithium based (a) and poly urea based (b) grease.
Since the temperature difference between bath and grease mixture is higher in the beginning, the rate of heat absorption and temperature rise is higher initially for both grease samples and as it reaches close to the maximum temperature of bath (~180°F [83°C]), the rate decreases significantly towards the end. One clear difference between the two grease samples is that lithium
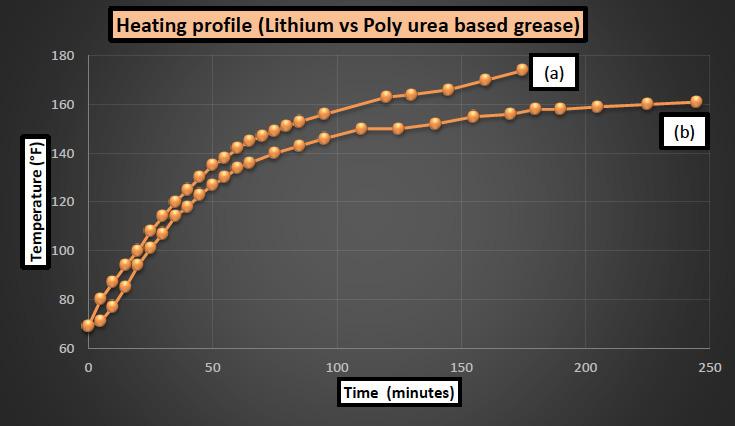
based grease attains a temperature of 174°F [79°C] after almost 3 hours of mixing whereas poly urea grease is at 158°F [70°C] after the same amount of time. This is an indication that lithium based grease has a higher heat capacity compared to poly urea based grease. Rate of temperature is comparable for both greases till 130°F [54°C] mark after which it slows down significantly for poly urea base grease compared to lithium base grease. Result of this nature suggests that during the manufacture of poly urea grease, more heat should be provided to reach a certain temperature compared to the case of lithium base grease.
SUMMARY / CONCLUSION
This study focused on significance of heat transfer and also the role of efficient mixing in controlling grease manufacturing operations at a commercial level. There is a constant objective to improve and optimize the manufacturing process by reducing the batch cycle time while improving the product quality in conjunction. Proper temperature control is a necessity to achieve this goal as shown in the above study. Various factors that affect heat transfer in batch operations were discussed with specific focus on grease manufacturing. Difference in temperature control between a contactor and open kettle were studied and it was established that heat transfer is more effective in a contactor owing to more heat transfer surface area and vigorous mixing profiles. Flow rate of heat transfer media is directly proportional to achieving heat transfer efficiency; however, proper control of the same is of paramount importance. Role of overall heat transfer coefficient (U) was discussed (with the defining mathematical relations) in deciding the materials for grease kettle construction. A higher value of ‘U’ indicates better capability of the material for heat transfer. Mixing has a direct effect on circulation of heat by reducing temperature gradients in the grease mixture. Studies indicated that counter rotating mixers were more effective than single rotation mixers in achieving a uniform temperature control. Grease is a broad term and based on the type of soap used for formation of multiphase grease network determines various grease properties. On similar lines, heat transfer may also be different for different greases and experimental results indicate that lithium based grease has a lesser resistance to heat transfer than a lithium complex grease. Also, results show that choice of base oil also affects the heat transfer properties. Overall, grease manufacture, just like any other chemical reaction is sensitive to methods of heat transfer and based on various factors (such as mixing, type of grease and so on) encountered in real life manufacturing processes, it is important to control and optimize the heat transfer mechanism to ensure reduction of batch cycle time while maintaining high quality standards.
ACKNOWLEGEMENT
Authors acknowledge the help of grease cooks in generating and documenting the data used in this study. Authors are also thankful to lab personnel especially Daniel Cruz for preparing grease samples and running some designed experiments relevant to the current study.
BIBLIOGRAPHY
1. ASTM D-288; www.astm.org 2. Wright, J., “Grease Basics” Machinery Lubrication
Issue 5, 2008 3. Couronne, I. et al. Tribology transactions, Vol. 46 (2003), 1, 31-36 4. Christiernsson, A. White Paper, Lubrisense, 04, 01 5. Euzen, J. P., Tramboze, P., Wauquier, J. P. “Scale up
Methodology for Chemical Processes”, Editions
TECHNIP, 1993. 6. Boner, C. J., “Manufacturing and Applications of
Lubricating Greases” Reinhold Publishing Corp., 430
Park Ave. NY, 1954 7. NLGI. “Lubricating Grease Guide” Published in
Kansas City, Missouri, 2006, pp. 11. 8. Carpenter, K. J. “Agitated Vessel Heat Transfer”,
Thermopedia (2011) 9. Markovitz, R. E. “Picking the Best Vessel Jacket”,
Chemical Engineering (1973) 10. STRATCO Inc.; www.stratcoinc.com 11. Nassar, N. N. et al. Education for Chemical
Engineers, 6 e83-e89 (2011) 12. Stuart, M.C. “Air propeller performance and design by the specific-speed method” Practical Engineer and Engineers Gazette. Volume 58, p 148-149 (1918) 13. “Thickeners in Grease Matrix” Lubrisense (2005) 02. 14. Scott, W. et al. Proceedings of World Tribology
Congress 64316 (2005) 15. Kraweic, S. et al. International Doble Conference
LUB-2403E (08.11) 16. http://en.wikipedia.org/wiki/Heat_capacity