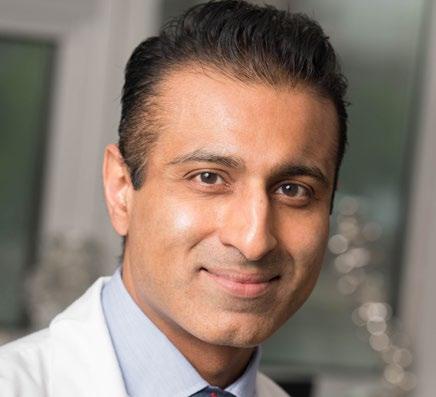
10 minute read
Robotic Spine Surgery
Current State and Future Outlook
Minimally invasive spine surgery (MISS) is associated with inadequate visualization and palpation of anatomical landmarks and hence is reliant upon intraoperative imaging.[1] Traditional fluoroscopy has been associated with high radiation exposure and low pedicle screw accuracy, resulting in the evolution of newer imaging modalities such as navigation and robotics over the past 2 decades.[2] Spinal robots were initially marred by limitations of lack of real-time visual feedback, difficulty with instrument localization, and unreliable depth gauging. However, with advancements of integrated 3-dimensional (3D) navigation, real-time visual feedback, better accuracy of registration, features to mitigate skiving, and rigid end-effectors, robotic platforms in spine surgery have seen a resurgence and are increasingly being adopted.[3] Recent studies have shown that the use of robotics in spine surgery leads to a decrease in intraoperative radiation exposure and an increase in pedicle screw accuracy.[4,5] Although robotic platforms are mostly used for placing pedicle screws, they are now also being used for decompression, disc space preparation, and interbody placement.[3] This article aims to provide an overview of the emergence of robotics in spine surgery, the current platforms being used, and the possible future advancements.
Current Stance: Where Are We at Present?
The current field of spine surgery is prospering with advancements in robotic techniques. Robotics in spine surgery has shown superior safety in various aspects of pedicle screw placement in spinal surgery. According to a recent meta-analysis,[6] robotic navigation was found to have 2.3 times greater accuracy than conventional freehand techniques. Additionally, robotic screw placement was ranked as the most effective technique in terms of optimal placement, thereby reducing overall implant-related complications.[6] Before the advent of robotics and navigation technologies, patients, surgeons, and operating room personnel were frequently exposed to high doses of intraoperative radiation to attain technical safety.[7] However, robotic navigation has the potential to reduce radiation exposure as well as provide high levels of safety during MISS. The skin-anchored spinal navigation system reduced intraoperative radiation exposure from 63.1 mGy with fluoroscopy to 28.3 mGy during a minimally invasive transforaminal lumbar interbody fusion.[8] Furthermore, a more recent study demonstrated that even when compared to skin-anchored navigation, robotic screw placement appears to be secure and efficient at reducing fluoroscopy time.[4] This technique has thus opened the possibility of performing MISS with reduced radiation exposure.
Currently, the U.S. Food and Drug Administration (FDA) has approved 5 robotic platforms for spinal surgery[3]: (1) Mazor X (Medtronic Spine, Memphis, TN, USA, approved in 2016) with Stealth Navigation (Medtronic Navigation Louisville, CO, USA), (2) ExcelsiusGPS (Globus Medical, Inc., Audubon, PA, USA, approved in 2017), (3) ROSA Spine (Zimmer Biomet, Montpellier, France, approved in 2019), (4) TiRobot (TINAVI Medical Technologies, Beijing, China, approved in 2020), and (5) Cirq with Loop-X (Brainlab, Munich, Germany, approved in 2021). Despite the benefits of increasing pedicle screw accuracy and reducing radiation exposure, these platforms share some drawbacks as well. One of the major issues is cost-effectiveness and affordability. Often, high installation costs, lack of affordability, and insufficient evidence make it difficult to justify the use of this technology. However, a study focusing on economic factors found that robotic spine surgery may be cost-effective based on factors such as a lower revision surgery rate, shorter hospital stays, and shorter operative times.[9] Additionally, robotics in spine surgery is not free from surgical pitfalls, wherein a technical guide might be required for all surgeons.10 Lack of haptic feedback and thereby skiving is a common issue when using navi- gation-guided drills during surgery. This may require a learning curve to achieve a high level of safety even in robotic spine surgery. Previous research has suggested that it may take approximately 20 cases for a surgeon to become proficient in robotic spine surgery.[11,12] To address the learning curve and improve safety, some platforms have added features such as an end effector that can detect excessive lateral forces generated by instrument skiving. Although newer robotic platforms are being developed to address the technical and economic challenges of current systems, they are still lacking sufficient clinical evidence. More platforms are expected to become available offering improved performance and cost-effectiveness. This opens the realm of future clinical studies in this ever-changing field of hardware and software.
Visionary Imagination: How the Future Unfolds?
The past few years have been instrumental in shaping the present and future of robotic spine surgery. Envisioning the successful use of robotics in spine will require a high volume of evidence-based studies reporting improved patient outcomes, patient satisfaction, surgeon expertise, and cost-effectiveness in the long run.[2,3] In addition, robust studies reporting reduced complications, morbidity, radiation, carbon footprint, and operative time are needed.[20]
In the future, it is essential to explore the safety and efficiency of robotics in applications other than pedicle screw placement. The application of robotic technology in surgeries of decompression, decortication, rod place- ment, deformity correction, and vertebral tumor resection is anticipated in the next few years.[14] Although in infancy, the new idea suggests modification of the robotic software to include vertebral tumor classification systems to help with precise tumor resection, in turn helping with the reconstruction of the fibrosed parts following chemotherapy.[15] Similarly, robotic-guided endoscopic spine surgery is an upcoming technology that requires higher quality evidence regarding puncture accuracy and fluoroscopy time.[13] Hence, the future goal will be to tap into the potential of robotic navigation in treating a wide range of pathologies of spine.[15]
Furthermore, additional evidence and research are required in the field of ergonomic efficacy and efficiency of robotics in the spine, especially cervical, thoracic, and craniovertebral junction surgical procedures.[20] Recently, Ueda et al have addressed the problem of autonomous collision in robotic spine surgery while working in closed spaces. Additionally, robotic surgery comes with the drawback of indirect visualization and a limited degree of freedom, which might pose a hazard in the field of MISS when the working spaces are narrow and deep. This often results in inevitable collision between the long arms of the robot with other instruments or soft tissues. With continued efforts and work on the robotic simulator and software, autonomous collisions can be avoided in the future using virtual fixtures and a well-thought artificial trajectory.[19]
In addition, the incorporation of virtual reality and augmented reality (AR) is expected to improve the learning curve and overall performance of surgeons in training.[17] The new school of thought builds on the idea of a semi-independent robot along with a wearable AR headset where a spine surgeon can visualize screw trajectories, review imaging, and operate with voice commands.18 One essential aspect of AR is projection overlay, which might be able to track the position and orientation of the operating field and personnel. This in turn will construct an accurate 3D picture and build communication between the operating surgeon and the machine. With the progress of digital optics, spatial measurements can be projected in the operation field along with the overlaying of high-resolution operative images. Hence, this will lend a helping hand to the surgeon performing spine surgery.16 Similarly, the addition of sensor gloves while operating with robotic spine surgery will help with natural hand motions and improved maneuverability, leading to a better sensory experience. Akin to this, the implementation of tactile stimulation can assist with outof-sight collisions. For the development of better haptic feedback, it is quintessential to improve the proprioception component of robotic navigation along with integration into the software to prevent any time lag in making decisions while performing life-saving procedures.[18]
The inclusion of artificial intelligence and the Internet of Skills in the field of robotics can revolutionize the way spine surgery is performed. With the combination of portability and machine learning, the Internet of Skills has the potential to utilize 5G connectivity with low latency in predicting surgical movements and allowing a surgeon to operate remotely with precision.[16] This will also help with real-time feedback to the surgeon when there is some evidence of deflection from a preplanned trajectory in a surgical procedure.
Conclusion
In a constant attempt to improve techniques in spine surgery, human efforts in the future will strive to achieve maximum precision along with high-functioning software in robotic systems. Though our imagination can uncover only a part of future innovation in robotics, what matters the most is our relentless dedication to providing our patients with the best surgical treatment for all their needs. Hence, the futuristic model of robotics in spine surgery will comprise the current elements with a paradigm shift toward more independent, affordable, accessible, and optimizable software and machines.
References
1. Virk S, Qureshi S. Navigation in minimally invasive spine surgery. J Spine Surg. 2019;5(S1):S25-S30.
2. Vaishnav AS, Othman YA, Virk SS, Gang CH, Qureshi SA. Current state of minimally invasive spine surgery. J Spine Surg. 2019;5(S1):S2-S10.
3. Kiran Alluri R, Sivaganesan A, Vaishnav AS, Qureshi SA. Robotic guided minimally invasive spine surgery. In: Perez-Cruet M, ed. Minimally Invasive Spine Surgery: Advances and Innovations. IntechOpen; 2022. Accessed January 11, 2023. http://dx.doi. org/10.5772/intechopen.97599
4. Shahi P, Vaishnav A, Araghi K, et al. Robotics reduces radiation exposure in minimally invasive lumbar fusion compared with navigation. Spine. 2022;47(18):1279-1286.
5. Fan Y, Du JP, Liu JJ, et al. Accuracy of pedicle screw placement comparing robot-assisted technology and the free-hand with fluoroscopy-guided method in spine surgery. Medicine. 2018;97(22):e10970.
6. Naik A, Smith AD, Shaffer A, et al. Evaluating robotic pedicle screw placement against conventional modalities: a systematic review and network meta-analysis. Neurosurg Focus. 2022;52(1):E10.
7. Rampersaud YR, Foley KT, Shen AC, Williams S, Solomito M. Radiation exposure to the spine surgeon during fluoroscopically assisted pedicle screw insertion. Spine. 2000;25(20):2637-2645.
8. Vaishnav AS, Merrill RK, Sandhu H, et al. A review of techniques, time demand, radiation exposure, and outcomes of skin-anchored intraoperative 3D navigation in minimally invasive lumbar spinal surgery. Spine. 2020;45(8):E465-E476.
9. Menger RP, Savardekar AR, Farokhi F, Sin A. A cost-effectiveness analysis of the integration of robotic spine technology in spine surgery. Neurospine. 2018;15(3):216-224.
10. Torii Y, Ueno J, Iinuma M, Yoshida A, Niki H, Akazawa T. Accuracy of robotic-assisted pedicle screw placement comparing junior surgeons with expert surgeons: can junior surgeons place pedicle screws as accurately as expert surgeons? [advance online publication July 18, 2022]. J Orthop Sci. https:// doi.org/10.1016/j.jos.2022.06.012
11. Hu X, Lieberman IH. What is the learning curve for robotic-assisted pedicle screw placement in spine surgery? Clin Orthop Relat Res. 2014;472(6):1839-1844.
12. Khan A, Meyers JE, Siasios I, Pollina J. Next-generation robotic spine surgery: first report on feasibility, safety, and learning curve. Oper Neurosurg. 2018;17(1):61-69.
13. Liounakos JI, Basil GW, Urakawa H, Wang MY. Intraoperative image guidance for endoscopic spine surgery. Ann Transl Med. 2021;9(1):92.
14. Shafi KA, Pompeu YA, Vaishnav AS, et al. Does robot-assisted navigation influence pedicle screw selection and accuracy in minimally invasive spine surgery? Neurosurg Focus. 2022;52(1):E4.
15. Perfetti DC, Kisinde S, Rogers-LaVanne MP, Satin AM, Lieberman IH. Robotic spine surgery: past, present, and future. Spine. 2022;47(13):909-921.
16. D’Souza M, Gendreau J, Feng A, Kim LH, Ho AL, Veeravagu A. Robotic-assisted spine surgery: history, efficacy, cost, and future trends. Robot Surg. 2019;6:9-23.
17. Yoon JW, Chen RE, Kim EJ, et al. Augmented reality for the surgeon: systematic review. Int J Med Robot. 2018;14(4):e1914.
18. Madhavan K, Kolcun JPG, Chieng LO, Wang MY. Augmented-reality integrated robotics in neurosurgery: are we there yet? Neurosurgl Focus. 2017;42(5):E3.
19. Ueda H, Suzuki R, Nakazawa A, et al. Toward autonomous collision avoidance for robotic neurosurgery in deep and narrow spaces in the brain. Procedia CIRP. 2017;65:110-114.
20. Huang M, Tetreault TA, Vaishnav A, York PJ, Staub BN. The current state of navigation in robotic spine surgery. Annal Transl Med. 9(1):86-86.