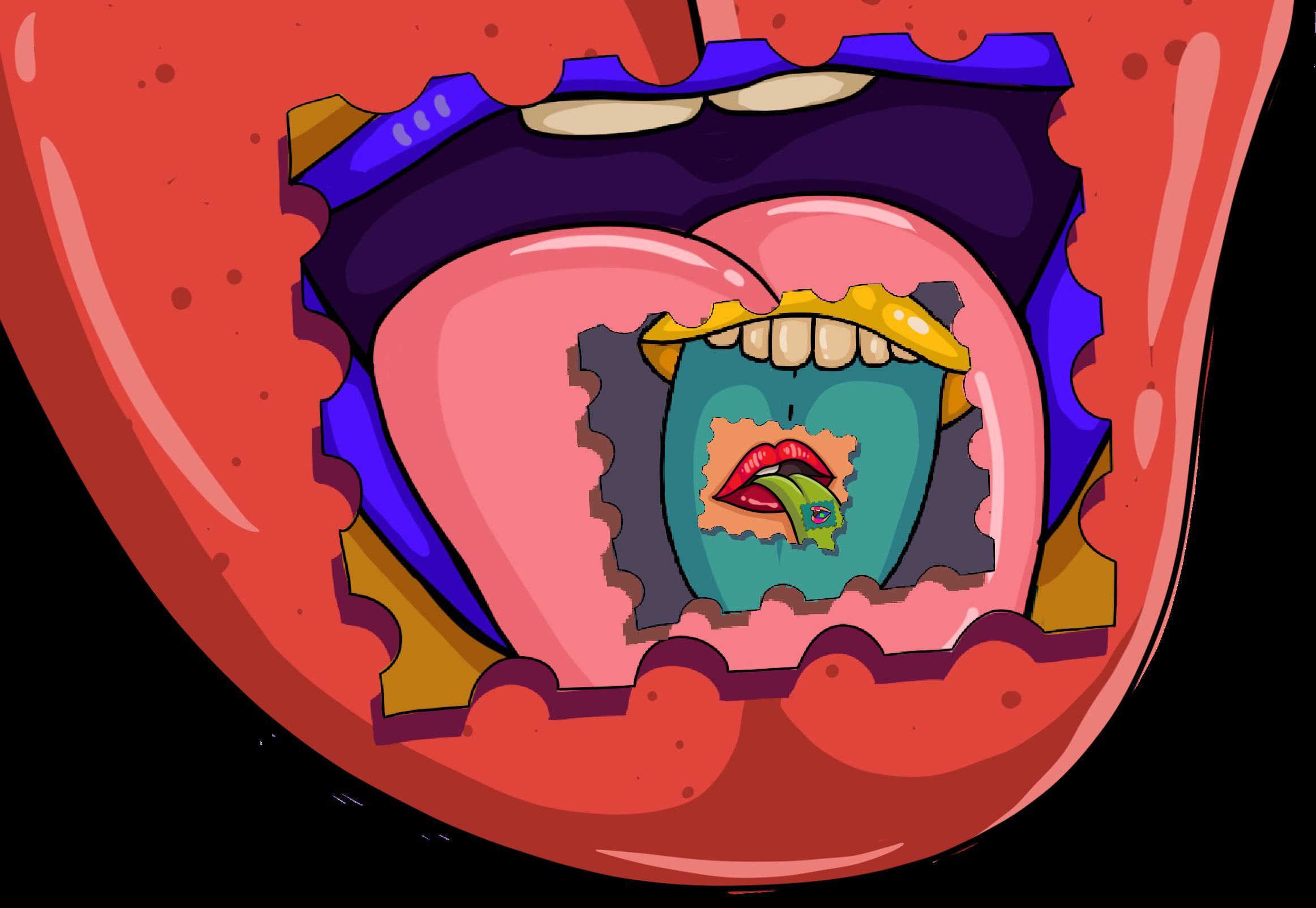
71 minute read
DEFYING THE “WAR ON DRUGS”: THE REBIRTH
DEFYING THE “WAR ON DRUGS”: THE REBIRTH OF PSYCHEDELIC MEDICINE &
RESEARCH by Nick Beebe / art by Cherrie Chang
Advertisement
Widely recognized by U.S. historians as igniting the “Summer of Love” (1967), San Francisco’s “Human Be-In” gathering made for anything but a typical day; more than 30,000 hippies flooded the Golden Gate Park in what ultimately became a defining moment for America’s counterculture movement. United by support of nonviolence and primary disdain for the U.S.’ role in the Vietnam War, hippies resulted during the 1960s as predominantly young Whites (ages 15–25) fully ‘dropping out’ of their everyday lives. One participant of the demonstration himself, Dr. Timothy Leary, psychologist and prominent LSD advocate, first vocalized the phrase “tune in, turn on, drop out.” In his signature soft-yet-monotone voice, this simple saying would soon blossom into an iconic saying amongst psychedelics users and the collective counterculture movement [1].
To the delight of its everyday supporters alongside hopeful scientists, including the late Dr. Leary, psychedelics are experiencing an enthusiastic rebirth across various healthcare systems. In the past, psychedelics (also known as hallucinogens) had stood as a promising “North Star’’ within U.S. medicine; however, the eclectic array of wavy chemicals saw its luster vanish just as the Nixon administration (1969–1974) anchored itself to a fervent anti-drug attitude. With the government’s “War on Drugs” surging ahead, the Nixon administration illegalized drugs to thwart the anti-establishment mentality sweeping the country [2,3].
As evidenced, the inexplicable allure of psychedelics is enough to instigate its use amongst humanity— from recreational use to scientific investigation. Most notably, their current return results from an entirely new psychedelic-based practice: microdosing. Even in just partial dosage (a microdose), their ‘rebirth’seeking clientele spreads far—from the innovative architects of Silicon Valley to the ceremonial yogis leading spiritual retreats [4–6]. Until recently, few researchers were permitted to revisit the past studies that had halted following psychedelic criminalization. Of the few psychedelic trials allowed to occur from the early 1970s until the late 2000s, most centered on rodent test subjects in preclinical contexts [3,7,8].
In stark contrast, studies of psychedelics — including psilocybin (the key compound of magic mushrooms, or “shrooms”), MDMA, and LSD — have finally entered into the clinical stages. Emerging evidence continues to highlight the drug class as game-changing for the treatment of various conditions, many of which are neuropsychological [9,10]. Despite the legal suppression psychedelics have faced, they continue to mesmerize and modify users’ minds. Recent studies of psychedelics have even helped researchers pinpoint some cognitive processes to specific brain regions [11]. Ultimately, current psychedelics research aims to alter and expand the scope of emerging medicine [12]. But is the world really ready for psychedelic-infused healthcare?
Psychedelics: Beyond Woodstock & Tame Impala
As is the case with most drugs, psychedelics have a complex history shaped by numerous sociocultural pressures. In regards to their origin(s), lies persisting across mainstream society still allow some to deem the emergence of psychedelics as a spontaneous “flower child” of the counterculture age. In reality, however, the first humans to interact with psychedelics were Indigenous populations, who have incorporated entheogens (plant-based psychedelics) into religious and ceremonial practices for centuries (and counting) [4,5]. Additionally, in the medical sector, psychedelics were incorrectly classified as “psychotomimetic,” implying that their use would invoke psychosis, or the alteration of the brain’s connection to reality. Naturally, this was able to overwhelmingly instill fear, panic, and consequent objection throughout the American public. However, as recent research refutes, any continuing association between psychedelics and psychosis stems from baseless assumptions [13,14]. In other words, psychedelics pose nearly none of their once-expected threat — provided that they are used under appropriate professional supervision.
Continued research has demonstrated that the toxicity of psychedelics is relatively low, meaning that these drugs may not be associated with oncefeared adverse health conditions (e.g., organ damage, overdose fatality, and cancer instigation) [15]. The success behind psychedelic administration also lies in the compiled testimonials that confirm these drugs promote unmatched changes in brain function and personality. In particular, improvements have best been demonstrated amongst individuals with major depressive disorder and post-traumatic stress disorder (PTSD) [2]. Regardless of the success of short-term clinical studies, much still remains uncertain about the long-term effects of psychedelics. As such, the gap spanning between possible and known information has been deeply perpetuated by widespread restrictions on access to psychedelics for the purpose of performing knowledge-expanding research.
In recent years, there has been a significant uptick in “biohacking,” or the process of developing one’s optimal self through the use of particular drugs, vitamins, and exercises [6]. One of the various “biohacking” practices gaining traction is the act of microdosing. Microdosers take psychedelic drugs, often several times a week, in small amounts to prevent a “trip.” Typically, this dosage level falls between 10–20% of any particular drug’s “normal” dose [16]. Similarities between user reports collectively suggest that microdosing can elevate cognitive performance, creativity, and productivity [16]. However, more clinical research is needed to explore these claims empirically.
One recent survey-based study with over 2,400 microdosing participants determined that those identifying as male were nearly twice as likely to microdose than females—and that tech industry workers and retired people were most likely to partake [17]. With the bounds of technology rapidly expanding, employment within this sector might overwhelm one’s creative abilities and cause them to microdose—though it is not entirely certain why retirees also prominently microdose. Furthermore, of 393 microdosers, nearly half reported using LSD, followed by psilocybin (~25%) and MDMA (~12%). Many in this pool also reported improvements to depression (~72%), sociability (~67%), increased focus (~59%), and decreased anxiety (~57%) following microdosing [17]. However, these rates are self-reported, meaning they only represent participants’ perceptions of their own experiences. Aside from these initial reports, very little is known about microdosing — particularly its effects, both during and after the duration of dosage [18,4].
One recent source shares that a participant’s optimism regarding microdosing treatment may impact its outcome, demonstrating one of the many variables relevant to developing effective psychedelic treatments [18]. Nevertheless, it has proven exceedingly difficult to decode the neurobiological mechanisms responsible for psychedelics’ beneficial impact on cognition with certainty. Due to the abundant ambiguity, some argue that these drugs are veering towards gaining the status of a panacea — a hypothetical remedy for all diseases and difficulties — despite the lack of scientific support for this position [19]. However, due to legal constraints, research into psychedelic drugs remains far from comprehensive. Researchers can rarely investigate beyond the most common drugs and have visibly failed to study the newest psychedelics of all: new psychoactive substances (NPS) [20,21]. Regardless of one’s stance, further research must be conducted on the use of psychedelics and NPS, particularly through microdosing, in order to assess their medicinal properties. To develop a consistent understanding of the potential benefit held within these drugs, additional supporting research is needed to decode them — most urgently being the substances most commonly used by microdosers: LSD, psilocybin, and MDMA (sometimes incorrectly referred to as “ecstasy”).
LSD
In an attempt to mirror the positive effects of plant-based ergotamine—once widely used to treat migraines—practicing drug manufacturers of the early 1900s began searching for synthetic molecules to act as superior medicines [22]. Swiss chemist Albert Hofmann was able to extract one portion of ergotamine’s dense structure: lysergic acid [1,8]. Years later, Hofmann sought to mix the lysergic acid he had produced with other organic molecules to create new drugs. During his twenty-fifth trial, Hofmann was able to fuse lysergic acid with nikethamide—known commercially as Coramine, a circulatory stimulant. However, this produced an array of unexpected behaviors in test rats and was subsequently ignored [8,1]. Years later, still captivated by his creation, Hofmann unknowingly consumed an indefinite amount of LSD while handling it in the lab [9,1]. When he first realized something was abnormal, Hofmann reported “a remarkable restlessness combined with a slight dizziness” in his notebook. Shortly after, he found himself exposed to “fantastic pictures, extraordinary shapes, with intense kaleidoscopic plays of color” [1]. Much later, Hofmann was unable to even handle pen and paper to record his experienced sensations.
We now know that LSD has the potential to decrease brain connectivity in ways that can last for years, potentially even impacting one’s personality (“sense of self” network, formally named the default mode network) [23,24]. This network is responsible for one’s capacity for mental time travel (the ability to think in past or future tense), self-reflection, and autobiographical memory. When these functions are disturbed, it becomes difficult for an individual to maintain their personal identity and connection with the physical world. So, should LSD temporarily skew the framework of the default mode network, a user may struggle to process the world around them, leading to
the experience of ego dissolution (disintegration from one’s self-identity) [24,25]. This comes as no surprise, given that immediately after consumption, LSD is known to distort one’s perception of time, identity, depth, size, and shape [26].
Following its accidental creation by Hofmann, LSD would soon be used to combat addiction, anxiety, and depression in over 40,000 patients up until the mid-1960s [1]. LSD has also demonstrated success in the treatment of alcoholism and other substance addictions [15,25]. The performed studies of LSD (on participants) have ultimately permitted researchers to further understand brain systems previously regarded as entirely unrelated to each other—one example being the brain’s visual processing component; LSD increases activity in brain regions tagged responsible for creating intense and dreamlike visions [24]. Ultimately, since LSD research was restricted during the War on Drugs, we have much more to learn about the fascinating compound. The resurrection of LSD, like other psychedelics undergoing clinical trials, has been astonishing — though it is clear that future trials and literature must weigh its potential negative effects with efficacy in changing the mind for the better [24,25].
PSILOCYBIN
In 1958, psilocybin was initially identified by Albert Hofmann, following its extraction from mushrooms [27]; in comparison to Hofmann’s other psychedelic ‘child’ (LSD), psilocybin is roughly 100x less potent [28]. However, the first clinical study involving psilocybin only concluded six years ago, resulting in 10 participants’ complete recovery from alcohol use disorder [9]. Today, the FDA has remarkably referred to psilocybin as a “breakthrough therapy” for the treatment of major depressive disorder, including treatment-resistant depression [29]. Most of the 30 registered trials currently studying psychedelic drugs center around psilocybin, examining its uses for treating anorexia, obsessive-compulsive disorder (OCD), addiction and substance use disorders, and depression [30,10,31]. As of 2018, similar trials also conducted with psilocybin were seeking to demonstrate its efficacy in alleviating alcoholism, tobacco addiction, and cocaine addiction [7]. In terms of tobacco addiction, two-thirds of 15 participants in a 2014–2016 study reported sustained abstinence from tobacco use in the year following initial treatment of psilocybin-aided psychotherapy [32,33]. While the U.S. has prioritized substance abuse and depression treatment in regards to psilocybin, there are several other conditions that psilocybin may treat—including Alzheimer’s and OCD [34–36]. For example, a recent case study found that a 38-year-old male subject with OCD reported a significant decrease in intrusive thoughts following psilocybin administration, despite initially finding the drug’s immediate effects unpleasant [36]. Though this source represents just one individual’s experience, it highlights one of the many circumstances where psilocybin can assist in healing [37]. Nevertheless, many newer studies have begun to further explore the role of psilocybin for individuals facing OCD [38].
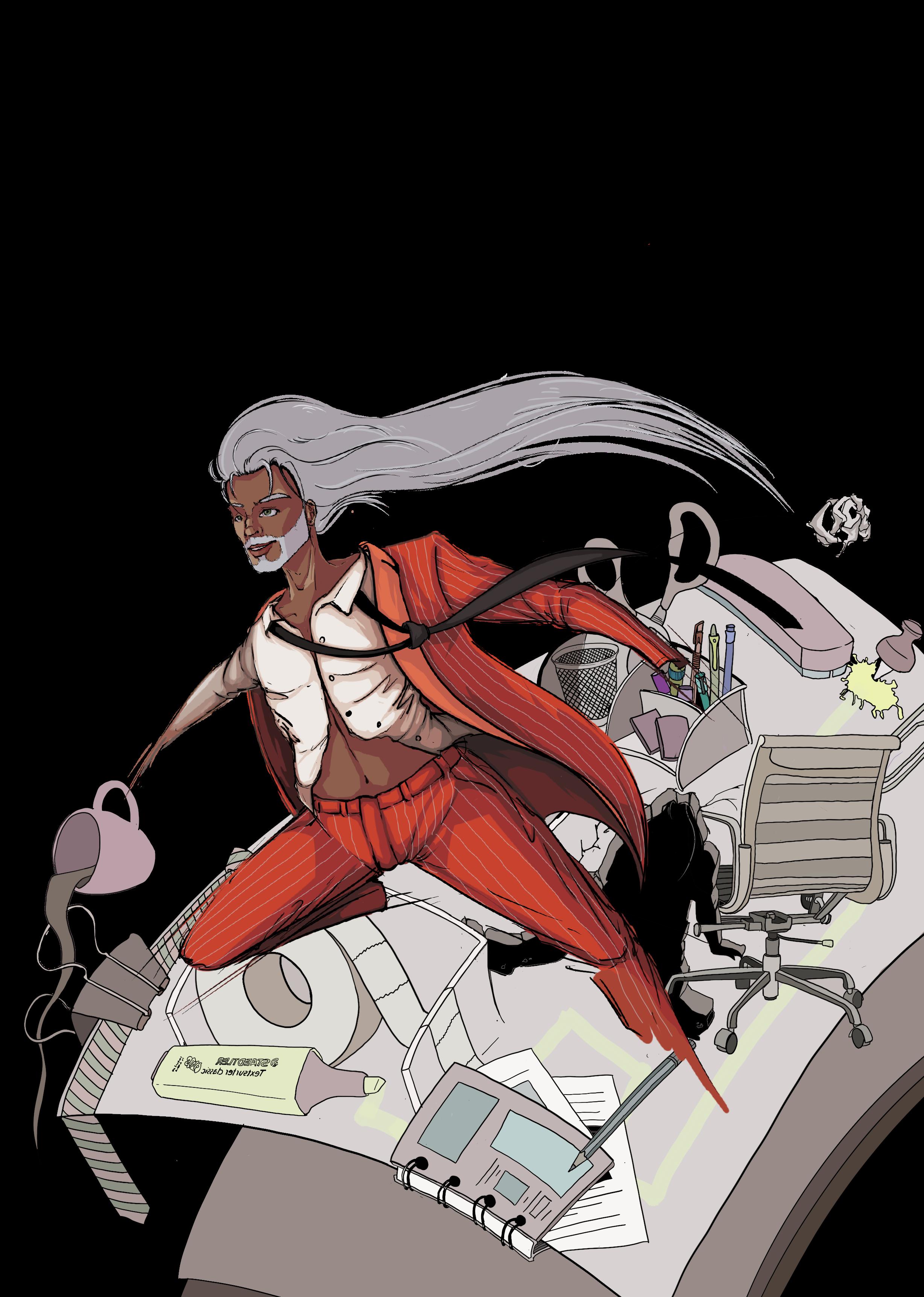
Furthermore, psilocybin has been praised for its ability to improve the quality of life of patients facing advanced or terminal cancer [39,9]. A recent study exploring this type of psilocybin use confirmed that even years following initial treatments, over 80% of participants maintained improved spirituality, positive behavior, and satisfaction. However, since most of these participants were White cancer patients, these findings cannot be generalized to individuals outside of these categories. While psilocybin shows
promise in treating numerous conditions such as addiction and depression, additional studies are needed to determine how the drug could affect larger populations [39].
MDMA
MDMA, alongside LSD and psilocybin, is another example of a psychedelic with gleaming value for medicine [40]. Initially designed by pharmaceutical company Merck to control bleeding, MDMA improves one’s overall mood by decreasing anxiety, while enhancing relaxation and sociality [41,42]. However, MDMA’s legacy is tainted by its association with ecstasy. While many people believe these drugs to be synonymous, ecstasy can actually contain several other substances apart from MDMA — though this fact remains widely unknown due to the prevailing public uncertainty surrounding most things psychedelicrelated [42]. Early research in MDMA’s effect of PTSD found that over half of participants experienced relief so significant that they no longer matched the criteria for a definitive PTSD diagnosis [4]. However, the study’s participant pool contained very little diversity, especially biogeographic ancestry — a common issue within clinical research. As briefly noted above, while some forms of psychedelic therapy might work for a White person, the same methods might not work for someone of differing biogeographic ancestry [4].
MDMA actively stimulates the release of neurotransmitters and hormones known to enhance prosocial behaviors, such as serotonin, oxytocin, and vasopressin. Psychiatrists have found numerous positive effects linked to MDMA-aided psychotherapy for patients with PTSD, including sensory intensification, increased emotional awareness, slight ego dissolution, and changes in interpersonal relationships [43]. Traumatic events are often followed by a critical period, a brief time span where the brain is more plastic (or subject to potential change[s]). Irreversible neuronal changes can occur during this period, however, meaning MDMA’s therapeutic success (for PTSD) may decrease with the passage of time. However, study participants receiving MDMA during the critical period reported elevated feelings of closeness and trust [41]. This means that MDMA, even when administered during one’s critical period, can still assist PTSD treatment. Closeness and trust, particularly, can prove vital to treatment success as they enhance patient-therapist bonding [41].
As such, MDMA-aided PTSD psychotherapy may help patients overcome the complex mental barriers that commonly already challenge PTSD treatment. Individuals with PTSD construct internal boundaries to protect themselves from experiencing the intense negative emotions that typically accompany the memory of traumatic events—and MDMA works to help unravel them. Undoubtedly, dissolving these boundaries with MDMA treatment offers an incredibly novel clinical approach to PTSD therapy [41]. Of a study devoted to MDMA-aided psychotherapy with participants of color, one participant memorably remarked that MDMA might be the best catalyst for those with PTSD to “push things along [and] enjoy their lives again” [4]. Given these positive clinical trials, approval of MDMA for medical use is likely to occur around in the next year or so, depending on the pandemic’s impact on research execution [42].
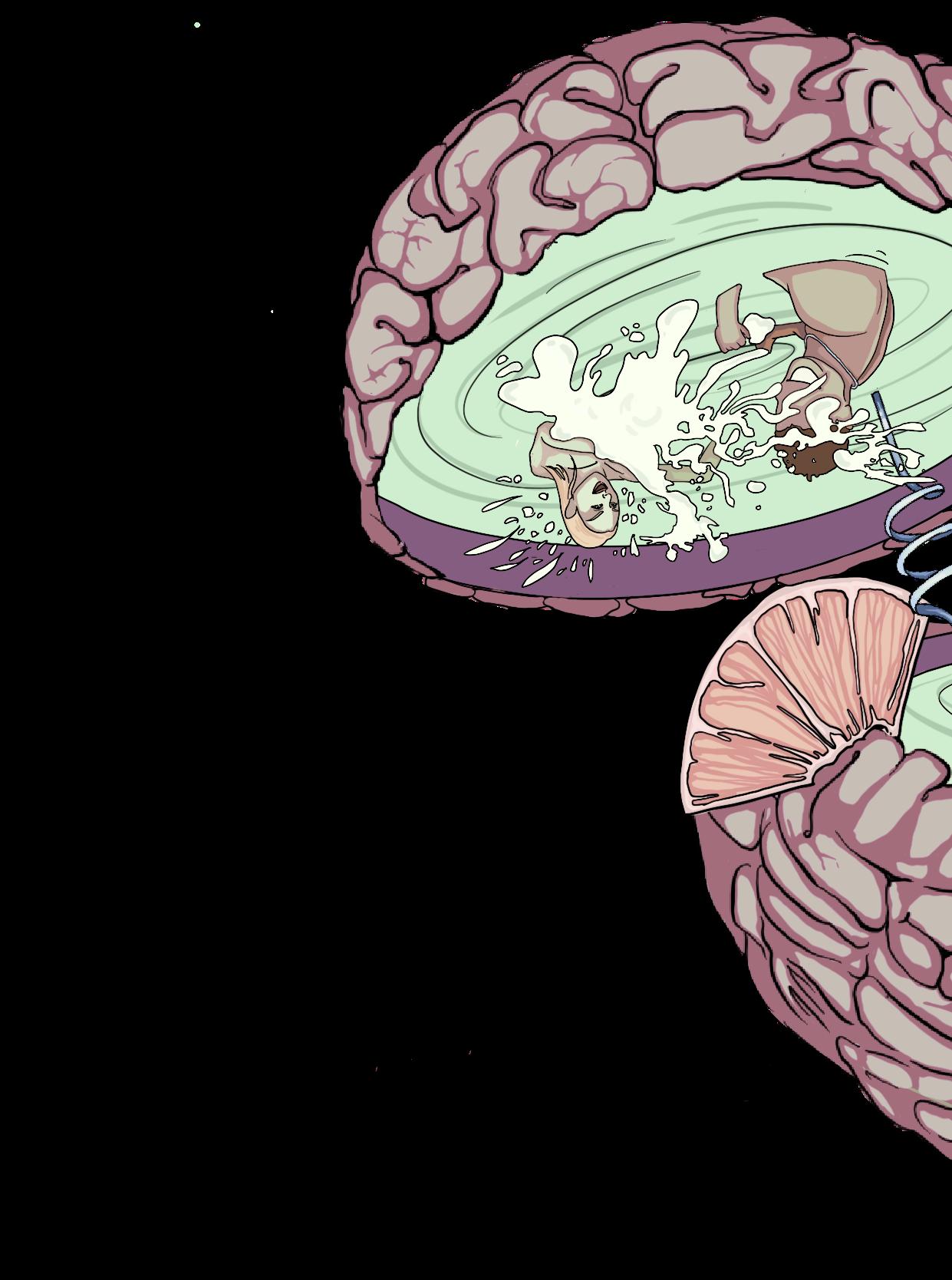
HOW WILL WE NAVIGATE PSYCHEDELIC MEDICINE?
Supported by the results of pertinent past work, the future of psychedelic medicine appears promising, though its trajectory remains vastly uncertain. Given the resistance that cannabis legalization has faced in the past decade, pushback is almost expected [17]. Along those lines, a collaborative recentering of mainstream attitudes towards potentially drugs with potentially restorative benefits is desperately needed. As psychologist Dr. Jamilah George, of the Multidisciplinary Association for Psychedelic Studies (MAPS), admits: “I’ve always seen drugs as dangerous, leading to violence and incarcerations[—n]ever something… as a means [of] healing” [43]. The intensive and holistic studies of psychedelics available infer that the body’s psyche wants to recover from traumatic experiences, much like how one’s immune system
tends to a physical wound. However, the complex nature of the human psyche does not succinctly signal where one most needs a neurobiological bandage; this is the basis for psychotherapy which, in the presence of psychedelics, displays deeper and swifter strives.
As with any drug, it is imperative to examine potential risks before choosing to experiment. Nearly one in twenty individuals (~5%) reported self-directed psychedelic use as a means of treatment reported visits to the
emergency department [44]. Though not a significant portion of the population, this statistic still exhibits that a “trip” (from any psychedelic) can force a user to hail medical attention. Since the outcome of a “trip” relies on a multitude of variables (e.g. biochemical, neurocognitive), it might be impossible to weave common threads through this small subgroup of users. In this regard, researchers may opt to rely on mechanisms, like scales, to derive numeric values from qualitative, intangible elements (e.g. personality, upbringing). However, one variable to certainly account for in this context is a user’s dependency on their psychedelic(s) of choice.
The Substance Abuse and Mental Health Services Administration recently concluded that although some psychedelic addiction exists in younger users (particularly those just entering adulthood), addiction in people over 25 is very rare. Considering the near-doubled rate of psychedelic use in America’s young(est) adults (ages 18–25), it is essential to understand what factors are motivating any use of psychedelics—from sporadic microdosing to intense tripping [28]. With clinical steps being made towards FDA approval, addiction rates may vary [20,7]. Though relatively rare, a hallucinogen use disorder (HUD) arises with one’s extensive use and experienced addiction to any psychedelic(s); even if addiction does not occur, prolonged psychedelic use may pose other severe neural impacts. For example, hallucinogen persisting perception disorder (HPPD) results when someone who has used psychedelics prior experiences extended visual hallucinations, similar to the distorted visual effects brought about by one’s use of psychedelics. The condition is most exaggerated for users of LSD and can be bolstered by stress, anxiety, exercise, or the use of other drugs [12,7]. With all of this in mind, it is imperative to continue clinical research to grow the understanding of psychedelics to create guidelines for all use to be safe.
An important thing to remember is that the clinical use of psychedelics is more often than not done alongside psychotherapy. That said, findings surrounding unassisted, self-administration of psychedelics have not yet reflected positive psychological benefits [45]. Leaving the administration of psychedelics to users could easily prompt abuse. Instead, the drugs have been successfully used to enhance physiological exploration within controlled therapy settings. With the country’s first legal psychedelic product coming in the form of fruity seltzers (and on sale now), it is clear that this drug class will likely remain prominent in the coming years, in various forms. Thus, the question still remains: are we ready for a psychedelic future? While we have much to learn about these distinctive drugs, the literature to-date significantly speculates an encompassed role of psychedelics within future medicine—though there is certainly no telling what that may look like.
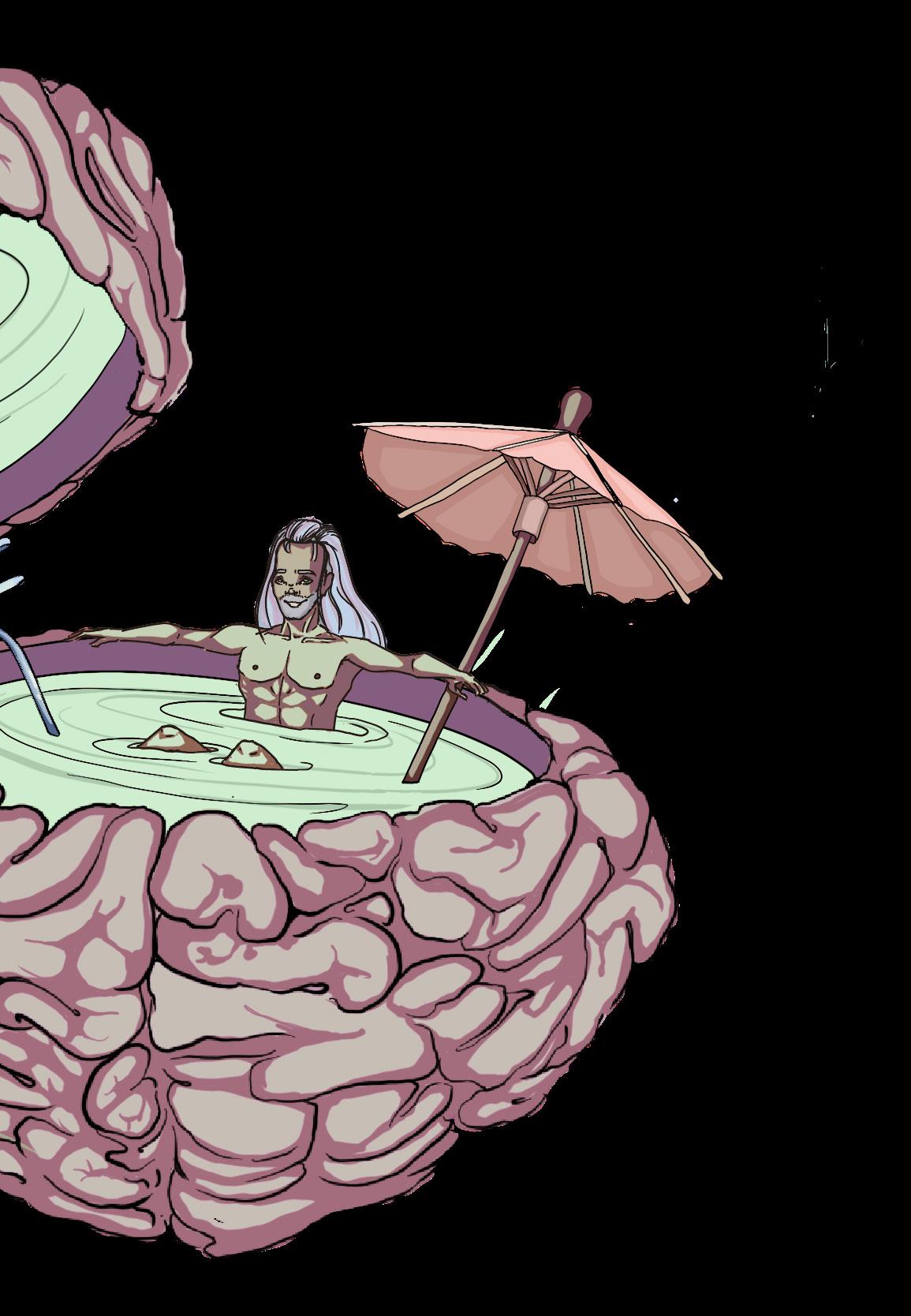
DEPRESSION AND ANXIETY: THE FRENEMIES NO COLLEGE STUDENT WANTS TO MEET
by Kaiya Bhatia / art by Ayane Garrison
Frenemies. We all know Jim and Dwight from The Office, or Perry the Platypus and Dr. Doofenshmirtz from Phineas and Ferb. Each side of these pairs has opposing agendas, but like all good frenemies, they occasionally work together to help one another out. College students, however, deal with a different kind of frenemy relationship: one that lives inside their heads.
The most common and insidious pair of frenemies present in the lives of college students is anxiety and depression, a difficult and sometimes deadly duo. The energetic volatility of anxiety versus the dull monotony of depression ignites a fierce rivalry within the host’s mind. And yet, each condition makes us more vulnerable for the other’s attack on our neural systems. For example, imagine you get anxious about failing your upcoming test, making you question if you’re prepared enough or how you might fare. In turn, you may begin to fear that you have no chance of passing the exam at all, leading you to feel hopeless and lost. These swells of depression may then render you unmotivated to study, compounding your initial anxiety of failure [1]. This cycle repeats itself over and over, enabling anxiety and depression to feed off of each other, ultimately leaving you paralyzed. It is a selfdefeating, self-fulfilling prophecy — one that can lead to fatal consequences if misunderstood.
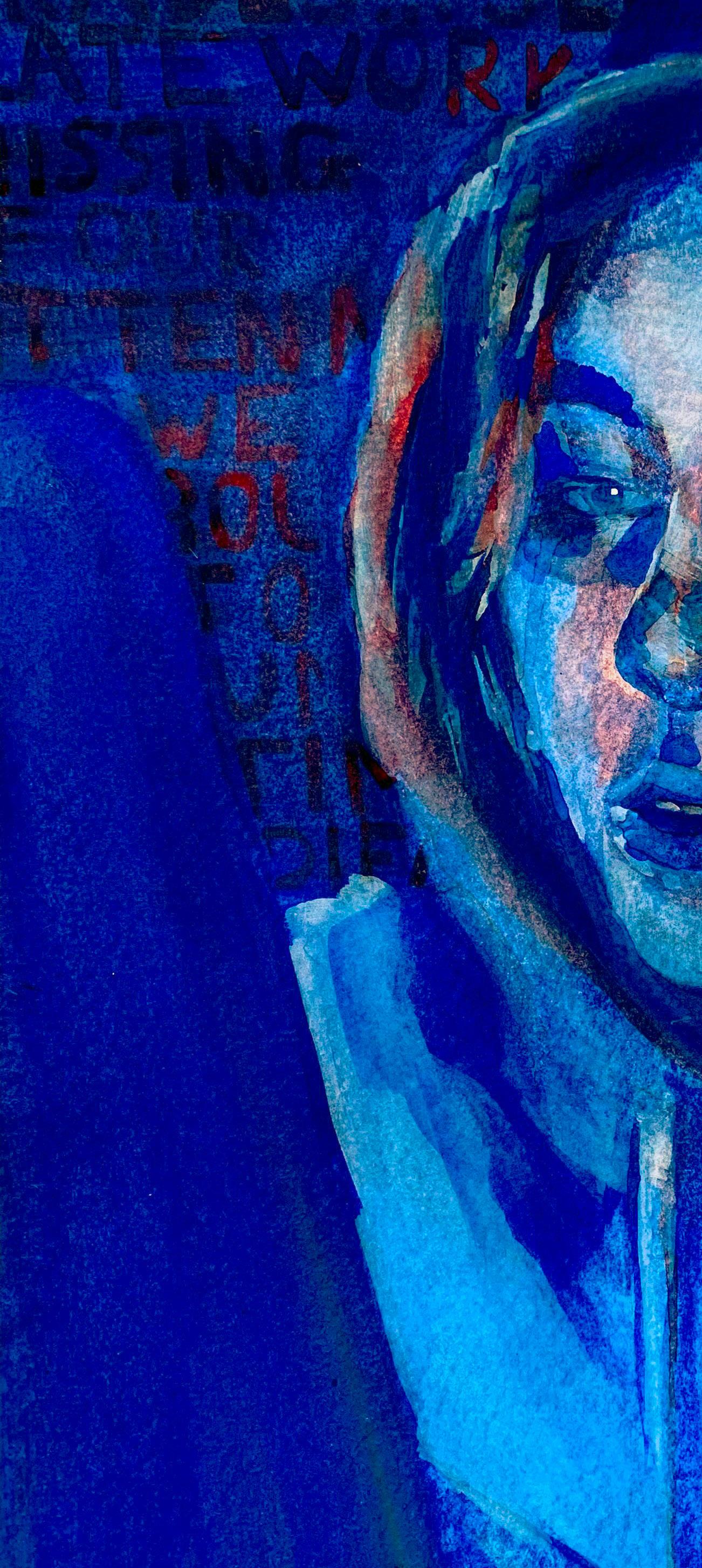
THE DIAGNOSES
While depression and anxiety exist in many different forms, college students are most commonly diagnosed with Generalized Anxiety Disorder (GAD) and/or Major Depressive Disorder (MDD). GAD is characterized by persistent and excessive worrying that is difficult to control, usually without apparent or rational cause for concern [2]. Experiencing these symptoms over a period of six months warrants an official diagnosis of GAD. MDD, on the other hand, is characterized by a period of at least two weeks of depressed mood or loss of interest. Related symptoms of MDD include issues with sleep, eating, energy, or concentration [3]. The “frenemy” relationship between GAD and MDD is clinically referred to as a comorbid relationship, which
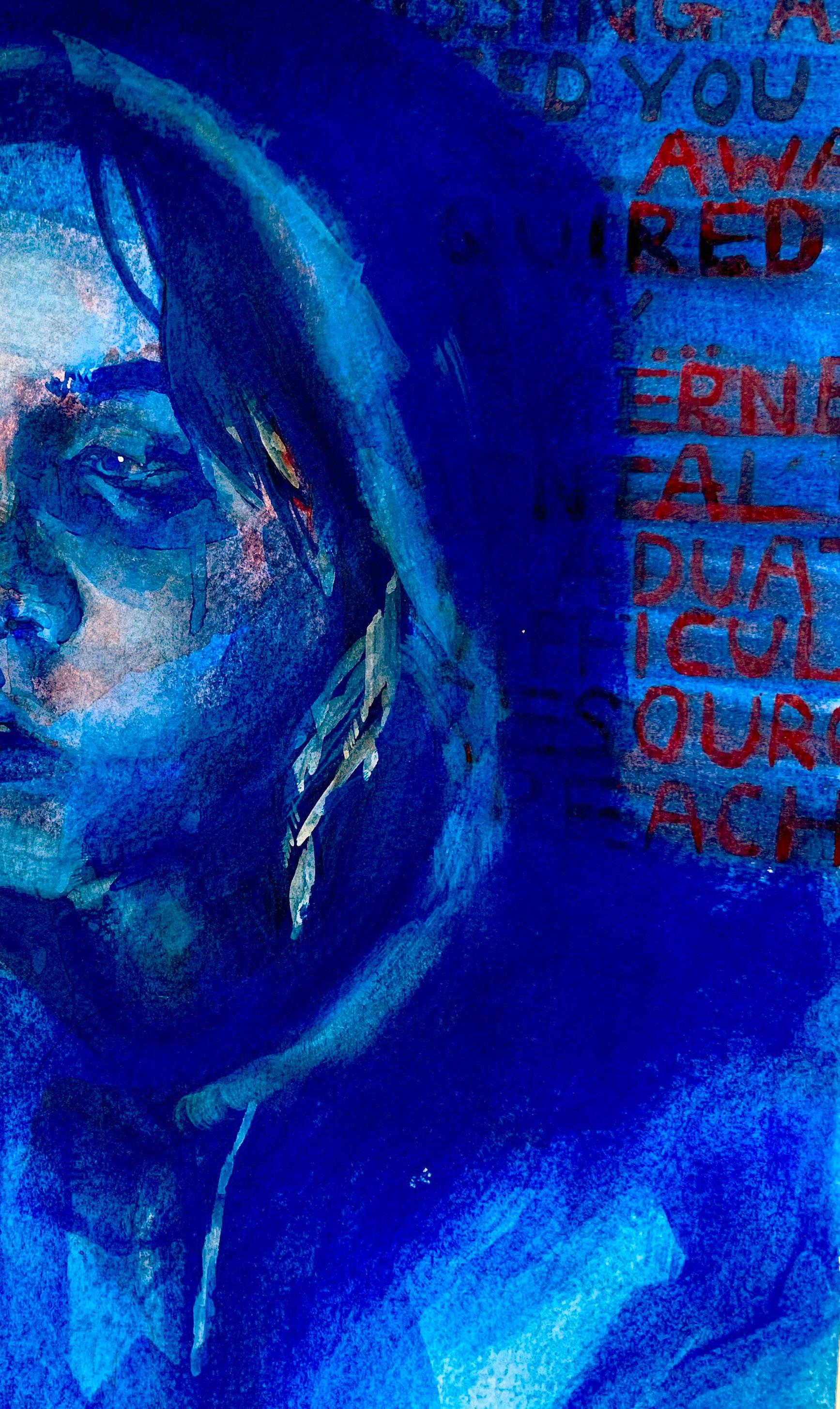
describes one or more additional conditions that exist with a primary condition; in this case, the primary condition can be either MDD or GAD.
THE COLLEGIATE MENTAL HEALTH CRISIS
GAD and MDD are the two most common mental health issues reported in college students, affecting 41.6% and 36.4% of students, respectively [5]. In fact, their presence is much more prevalent on college campuses than anywhere else in the country — a mere 2.7% of adults in the United States are estimated to have GAD, and only 7.1% are diagnosed with MDD [2, 3]. Interestingly, even with the overwhelming prevalence of both disorders in college students, this comorbidity is often overlooked in the investigation of mental health on college campuses [6]. However, this relationship actually plays a significant role in the college mental health crisis, as a recent study found an astronomical 29.8% of college females and 13.9% of college males are diagnosed with both GAD and MDD [6]. As comorbid GAD and MDD diagnoses are associated with much greater impairment compared to that of just one of the conditions alone, identifying and exploring this comorbidity is essential to providing better treatment to those affected.
A recent study has sought to address this lack of research attention on comorbidity, delving into the impact of comorbid GAD and MDD on college students’ wellbeing [6]. Researchers found that those who screened positive for both of the two psychiatric disorders showed a significant decrease in quality of life as compared to those diagnosed with either one [6]. One of the main explanations for this phenomenon lies in the cognitive impairment associated with comorbid MDD and GAD. The combination of MDD and GAD makes individuals generally more inclined to express negativity towards day-to-day problems. The overthinking and self-doubt characteristic of GAD are compounded, causing the individual to overassess a problem, leading to increased anxiety [7]. All the while, MDD stirs up feelings of hopelessness towards the issue at hand [7]. And, even beyond these affective symptoms, researchers also found that college students with comorbid MDD and GAD show cognitive impairments, despite their constant need to harness cognitive skills. These impairments often cause students to struggle with school work and other daily tasks, likely leading to an increase in stress. This build-up of stress significantly worsens the patient’s primary condition and quality of life.
While this combination of symptoms would make it difficult for anyone to deal with arising problems, college students can become especially frustrated and burnt out, as their academic and social environments push them to confront urgent and intellectually complex issues every day. As such, to deal with this emotional distress, college students with GAD and MDD often also develop a tendency for cognitive avoidance — the process of avoiding or eliminating negative thoughts — leading to high levels of overall thought suppression [7]. This is cause for concern, as college students are expected to reflect on highly conceptual material both in and out of the classroom. The college experience of comorbid GAD and MDD is presenting major barriers to academic success and emotional well-being.
GAD & MDD BRAIN CHEMISTRY
Before we consider how to treat these comorbid disorders, we first need to understand the key players involved. Brain chemistry refers to the activity of neurotransmitters and their effects on behavior or brain functioning. Neurons are a type of brain cell that communicate using neurotransmitters — chemical messengers that each serve a distinct purpose. These molecules are released by one neuron, the presynaptic neuron, and attach to the receptor of another, the postsynaptic neuron, resulting in either an inhibitory or excitatory response. This process is like receiving a letter in the mail, containing information regarding your future plans. The news could be disappointing, making you less likely to go through with your plans, or it could be exciting, preparing you to move forward. In this analogy, the letter is a neurotransmitter; inhibitory neurotransmitters render the postsynaptic neuron less likely to send a signal after the neurotransmitter binds to its receptor, while excitatory neurotransmitters make the neuron more likely to do so. Gammaaminobutyric acid (GABA) and serotonin are the two major players in the brain chemistry of anxiety and depression.
A new focus of research regarding GAD and MDD treatment centers around GABA. Recent studies have found that increased presence of GABA can have both antidepressant and anti-anxiety effects [8]. To achieve an anxiolytic effect, GABA binds to receptors on neurons in the amygdala, a brain region responsible for our fear and stress responses, inhibiting signals of fear and stress [9]. However, patients with anxiety have a decreased abundance of GABA receptors, meaning that they are less likely to get this antianxiety effect in their system [10]. Benzodiazepines are one of the most common treatments for anxiety, working to correct this imbalance by helping GABA neurotransmitters inhibit the postsynaptic neuron. By doing this, the benzodiazepines work to reduce anxiety effects within an individual. This medication has been used for 50 years to treat anxiety, and, while effective, it also raises concerns for long-term dependence and sedative effects [9].
In contrast, our understanding of GABA’s role in MDD
has been much more recent. Unlike in anxiety disorders, we do not see a change in GABA receptor abundance in patients suffering from MDD. However, this does not mean GABA has no role in MDD. On the contrary,
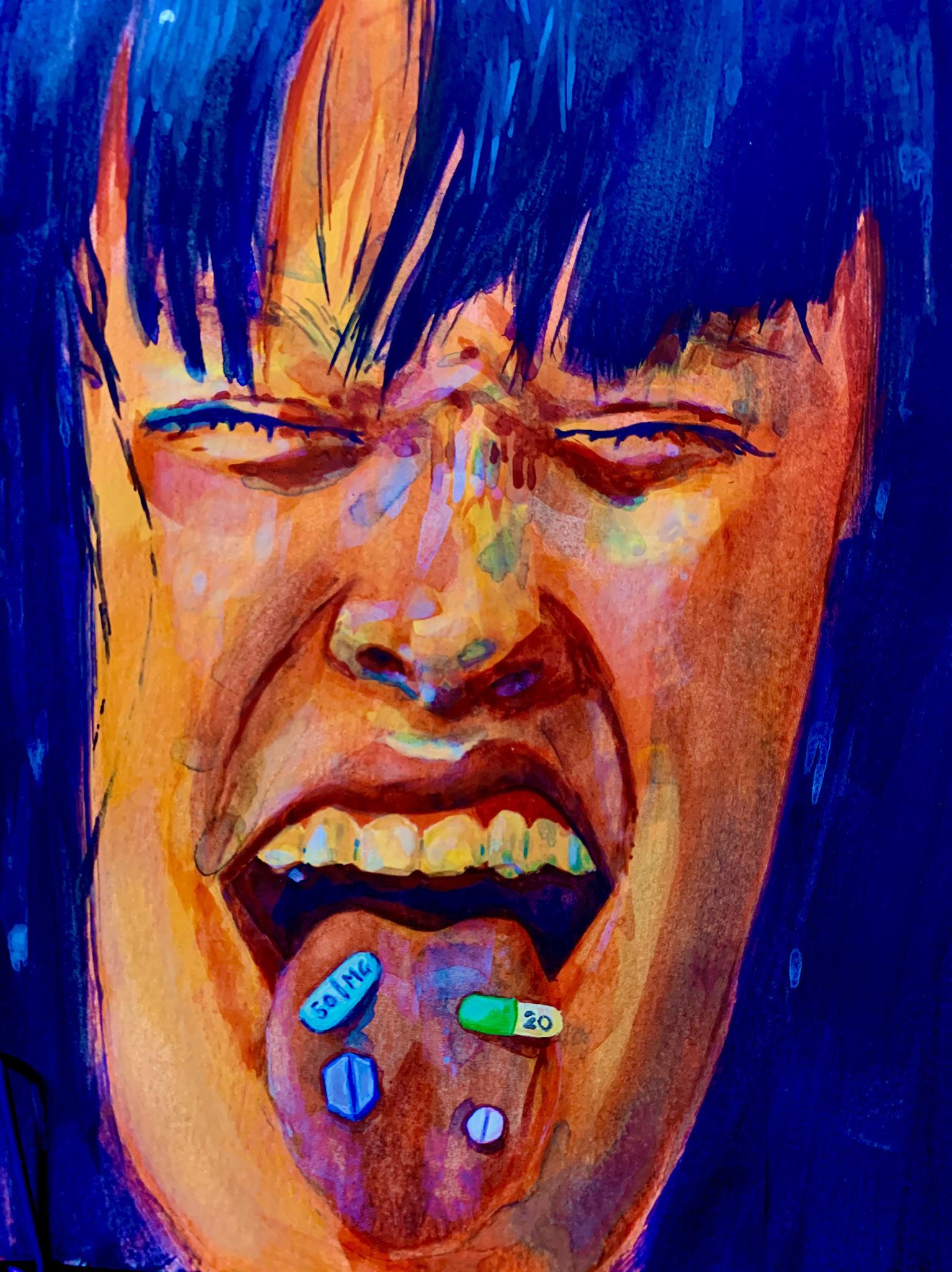
there is significant evidence showing patients with MDD to have overall reduced levels of GABA in brain regions such as the amygdala [10]. Furthermore, there is evidence that those with MDD may have an altered gene expression for GABA receptors as well [10]. Our current treatments for depression do not target the GABA system, but still increase inhibitory signals [11]. Researchers have proposed that targeting GABA receptors will help reduce depression, as the inhibitory nature of GABA may help slow down the cognitive processes involved with depressive thoughts [11]. While the specific mechanisms involved with GABA in MDD are not completely understood, related research still conveys the need for future antidepressants to correct neurological chemical imbalances rather than the symptoms themselves [10].
Serotonin’s role in MDD, on the other hand, is much clearer. Serotonin is important for regulating the mood, well-being and cognition of an individual. This inhibitory neurotransmitter has been consistently implicated as a cause for MDD but has not yet been strongly linked to GAD. Despite these differences in links, selective serotonin reuptake inhibitors (SSRIs) are still the starting point for the treatment of comorbid GAD and MDD. SSRIs work to influence the concentration of serotonin in the brain [12]. When neurotransmitters are released, SSRIs enter into the space between the presynaptic and postsynaptic neuron, known as the synaptic cleft. After some time, the neurotransmitters are absorbed back into the presynaptic neuron to be recycled. SSRIs work to prevent this reuptake so that more serotonin is present in the synaptic cleft, increasing the neurotransmitter’s effectiveness in the postsynaptic neuron.
However, as mentioned earlier, despite the success SSRIs have had with treating GAD, serotonin’s role in the disorder is not very well understood. Our best inferences on the role of serotonin in GAD come from looking at how SSRIs work to treat GAD. For example, a recent study found that the use of SSRIs in anxiety and depression treatment normalizes amygdala activity in the brain, as well as a decrease in activity in the limbic system (i.e., the region responsible for emotion) [13]. These changes were seen to cause a reduction in anxiety symptoms experienced by individuals. This suggests that reduction in activity is a potential mechanism of anxiety reduction. However, that the observed effective treatment of GAD with SSRIs is more of an accidental action than an intentional action of the drugs, further understanding of the mechanisms and serotonin’s role is still needed. Serotonin is arguably the most famous of the neurotransmitters implicated in MDD. The serotonin hypothesis was first proposed half a century ago, stating that decreased activity of serotonin pathways has a causal role in the pathophysiology of depression [14]. Like many hypotheses developed in psychiatry, the study claimed that the condition is caused by serotonin because we see that a drug involving serotonin is an effective treatment. While we cannot outright refute this hypothesis, as it does hold some truth, we need to acknowledge that MDD is not likely an issue due to the activity of a single neurotransmitter. The reason this can be said is because SSRIs do not work for everyone, suggesting that there are other neurotransmitters at play here (e.g. GABA).
COMORBID MDD & GAD TREATMENT
One of the biggest issues we face in the treatment of depression and anxiety is the lack of knowledge surrounding the nature of their comorbidity. Generally, researchers believe that the high prevalence of both GAD and MDD suggests there are commonalities in their causes. However, our current pharmaceutical tactic for treating GAD and MDD is to throw different drugs at the symptoms and hope that one sticks (without making any symptoms worse). The intention of this treatment is to reduce symptoms to allow the individual to improve functionality. However, the presence of one disorder often reduces the efficacy of treatment for the other, creating a tricky situation [15]. Thus, the first step towards effective treatment is being able to recognize the presence of a comorbidity, which increases chronicity and recurrence, and typically requires long-term pharmaceutical treatments [16]. Incomplete diagnoses, likely due to the symptom overlap associated with the disorders, may result in incomplete treatment. As such, the recognition of these conditions is essential in guiding treatment plans.
When considering medication to treat comorbid anxiety and depression, experts flock to SSRIs as their primary treatment option [16]. However, as we have discussed, SSRIs do not work for everyone. Furthermore, we see antidepressants improving symptoms for each disorder alone, but are such results enough to ensure that antidepressants will effectively treat both illnesses at the same time? It’s a difficult question to answer conclusively, yet it certainly highlights the need for more research concerning MDD and GAD treatment. As of now, treatment research for comorbidity is lacking, and there is no existing medication that
works to treat comorbid anxiety and depression [16]. Our current void in research suggests that our attempt at treatment is a patchwork of guesses with hints of understanding. Therefore, furthering our understanding of the similarities between GAD and MDD, as well as their differences, may help us improve treatment availability for the future.
LOOKING AHEAD
With so much uncertainty in our current understanding of comorbid depression and anxiety, it’s no wonder we are struggling to treat patients. Our tunnel-vision, symptom-based approach to treatment has blinded us to the significance of comorbidity, especially in the case of comorbid MDD and GAD. Yet, it’s also true that there is no easy fix. The collegiate mental health crisis won’t magically resolve itself with a few days off for student wellness or an email encouraging selfcare. A solution relies on recognizing the importance of comorbid conditions and redefining our focus, not just pharmaceutically. There is a crisis that is leaving a significant portion of college students emotionally and cognitively impaired, and we can only alleviate the stress of their conditions by addressing the issue headon. It may be unrealistic to implore every institution to divert resources towards funding treatment for comorbid GAD and MDD. However, it is necessary to ask schools to reconsider how they help students manage their symptoms, so that they may improve their emotional and academic well-being, and steer clear of these frenemies for good.

BRAIN BUSTERS
by Ally Thayer / art by Sophie Sieckmann
In 2013, the Obama Administration launched the BRAIN Initiative: a research effort aimed towards understanding how the human brain functions. When he spoke at its unveiling, President Obama pointed out the largest paradox of neuroscience: “As humans, we can identify galaxies light-years away and we can study particles smaller than an atom, but we still haven’t unlocked the mystery of the 3 pounds of matter that sits between our ears.”
This “mystery,” otherwise known as the brain, has intrigued humanity for millenia, as human beings have always possessed an innate curiosity and desire to understand ourselves. Many fields, such as philosophy, sociology, psychology and anthropology, have stemmed from this fascination, attempting to answer the fundamental questions of what makes us human. Neuroscience addresses these questions head on, investigating the human mind by exploring neural connections in the brain. Generally, neuroscience strives to answer: What is consciousness? How do we experience dreams and emotions? What neural factors are involved in cognition? None of these questions have a simple answer; hence, our everpresent interest in the workings of the mind.
This innate human curiosity has also led to the everincreasing prominence of neuroscience in pop culture and movies. However, filmmakers often inaccurately represent the science behind different neural phenomena. For example, the movie 50 First Dates (2004) tells the story of a woman who suffers from “Goldfield’s syndrome” after an accident. Each day her memory completely resets. Goldfield’s Syndrome is a fictional condition, but it is loosely based on a combination of several real types of memory loss. The critically acclaimed film Inception (2010) is also, neurologically speaking, inaccurate. The central premise of the movie is that while one sleeps, the dreamer can access several parallel realities and travel to other people’s dreams. Although fascinating, the events are not based on factual science. Film portrayals of complex neuroscience topics, such as dreams and memory have changed, disregarded, and even fabricated science. Because most viewers of these hit films do not have neuroscience backgrounds, they may be more inclined to believe this scientific misinformation. As a result, oversimplifications of complex neurological phenomena evolve into commonly believed myths that are eventually regarded as facts. In order to distinguish fact from fiction, I surveyed the neuroscience faculty at Vassar College, asking which popular neuroscience myths frustrate them the most. Here are the most common answers:
MYTH ONE: “WE ONLY USE 10% OF OUR BRAIN.”
The idea that we don’t utilize our full brains is one of the most commonly believed neuroscience myths. Several surveys have demonstrated that 65% of
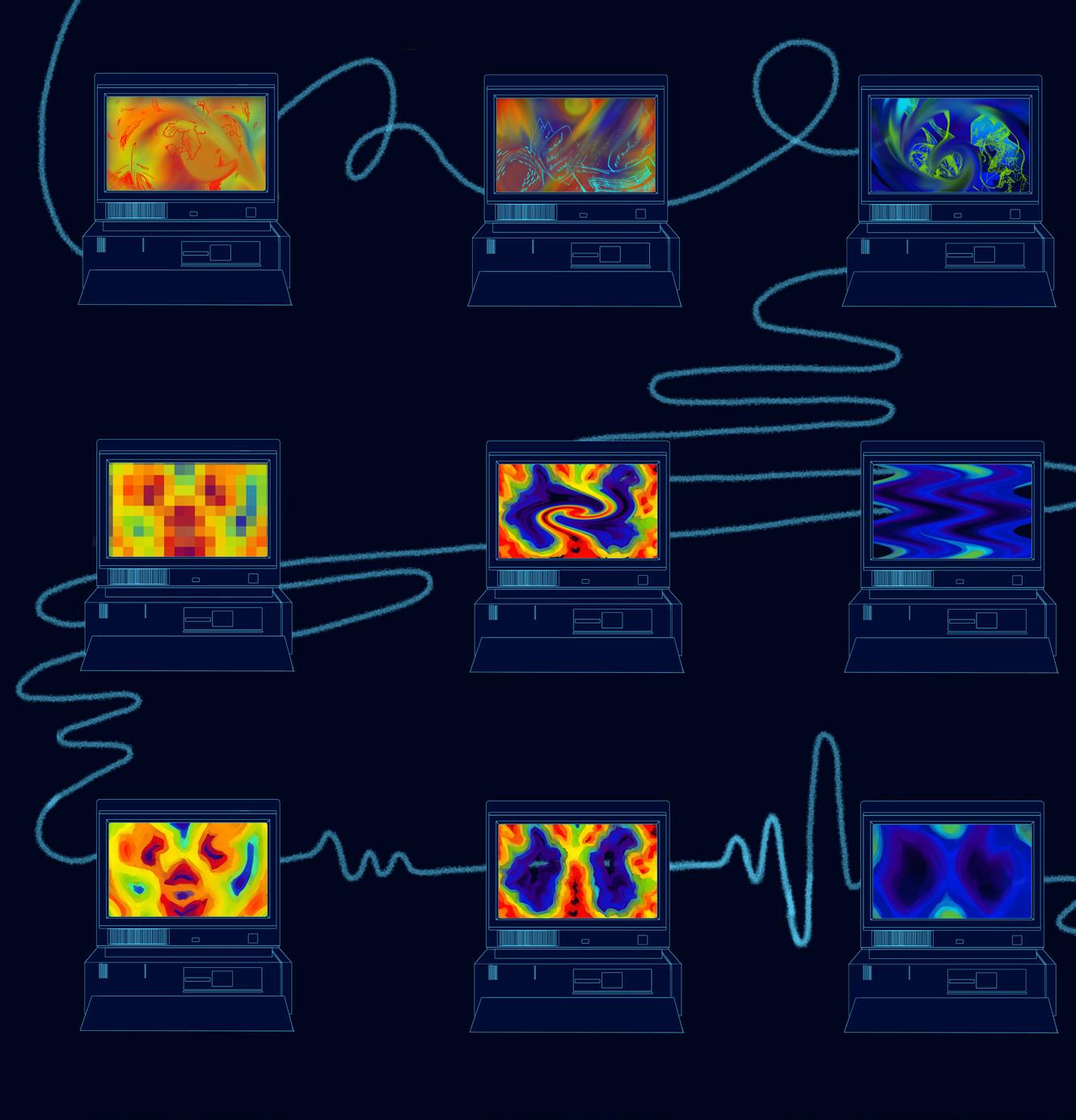
Americans, 1 in 3 people with a bachelor’s degree in psychology, and more than 1 in 20 neuroscientists agree with this claim [12, 10, 9]. The idea still lingers in modern-day pop culture, as movies like Lucy (2014), Defending Your Life (1991), and Limitless (2011) use plotlines emphasizing the “untapped superpower” residing in the supposed remaining 90% of the brain. However, despite how common this belief is, it is entirely false.
Many trace this myth back to Albert Einstein and William James, often referred to as the father of American psychology. Einstein has been misquoted as arguing that his above-average intelligence resulted from the ability to use more of his brain. In reality, though, Einstein never said this; but, it is very common to see quotes misattributed to him. Einstein’s brain was extensively studied after his death and it was actually found to be smaller than average;, however, he was reported to have higher-than-average numbers of a specific type of brain cell called glia [8]. William James did propose a theory that most of our brain and cognitive potential goes untapped; however, he never specified a percentage of brain use [11]. So, how did this myth get linked to James? Dale Carnegie’s best-selling book, How to Win Friends and Influence People, includes a misinterpretation of James’ work and implies we do not use all of our brain power [6]. This book was one of the first self-help books written and is considered by TIME magazine to be the 19th most influential nonfiction book written in English since 1923, meaning that many readers of Carnegie’s work likely took his misrepresentation of James’ theory as fact [18].
This myth may have also come from a misinterpretation of neurological research in the early twentieth century regarding glial cells. Glial cells are one of the two main cell types within the brain, lesser known to the general public than the other main cell type: neurons. While neurons fire to transmit neural information, glial cells act as the brain’s structural support, protecting and nourishing neurons. Several studies from the 1960s to the 2000s report a 10:1 glia-to-neuron ratio in the brain [19]. Because glial cells were thought to have no neural utility, researchers inferred that only 10% of the cells in the brain had a function. Thus, the idea that we only use 10% of our brain was born.
Neuroscientist Dr. Barry Beyerstein has provided several pieces of evidence to dispute this myth. First, Beyerstein reasons that if it were true that only 10% of the brain was in use, most neural damage would have no impact on normal functioning [5]. If 90% of the brain is inactive, most brain injuries would miss essential brain tissue and there would be no adverse effects. However, damage to virtually any part of the brain may cause personality changes, paralysis, sensory dysfunction, or the loss of language abilities. Brain imaging such as PET and fMRI (functional MRI) scans also show neural activity in all brain regions, no matter what the individual is doing [5]. This means that whether you are meditating, sleeping, or taking an exam, your brain is fully engaged. Finally, Beyerstein also makes an efficiency argument, explaining that the human brain spends 20% of the body’s total energy despite making up only 2% of the human body weight. If only a tenth of the brain is in use, devoting this much energy to the body part would be wasteful, and natural selection would have eliminated this inefficiency over the course of human evolution [5].
MYTH TWO: “DOPAMINE MAKES US HAPPY”
The media often refers to dopamine as the “feel-good chemical.” However, happiness is a complex emotion and neurological topic, and there is no single chemical or brain region responsible for one’s happiness. Dopamine is a neurotransmitter, or a specialized chemical that allows two neurons to communicate. Two communicating neurons do not physically touch one another; rather, a small space, called the synaptic cleft, separates them. When one neuron is activated, it releases a neurotransmitter into the synaptic cleft. This neurotransmitter makes its way to the second neuron to activate it, and the message is passed along.
The “feel-good chemical” myth arose from a 1950s study in rats by Dr. James Olds. Olds studied neurons that communicated via dopamine in rat brains; when these dopamine-releasing neurons were stimulated, Olds found that the rats were prompted to repeat the same activity over and over again, and he concluded that this repetition was due to the rats enjoying the activity [15]. It is also well known in the psychiatric community that individuals with depression have lower levels of dopamine and therefore, it is logical to connect dopamine to lower mood [3]. These theories have been disproven because animals still experience pleasure even if their dopamine neurons are killed [4]. In the late 1980s, Dr. Kent Berridge eliminated the dopamine neurons in rat brains and tested their responses to a sugar solution. He discovered that these rats, despite not having dopamine neurons, still showed signs of enjoyment and pleasure similar to the rats who had intact dopamine neurons [4].
If dopamine is not responsible for happiness, then what exactly is its role in the brain? Today, dopamine is thought to be involved in motivational behaviors [1, 7]. Because motivation can appear similar to pleasure and happiness, it can be easy to confuse them. But neurons that use dopamine aren’t actually telling us that we like performing an activity or task; rather, they are
communicating that we should do or pursue that activity again. This is essentially the difference between wanting something and liking something, and it is especially clear in individuals with depression. Depressed people are often less likely to pursue social interactions, but this is not because they do not enjoy the company of others. In fact, they might still appreciate time with friends, but the effort needed to engage in social situations might prevent them from joining in. Similarly, people and animals with low levels of dopamine are less likely to work for a reward. Therefore, this behavior is more of an internal debate on how to spend one’s time and energy than a reflection of personal enjoyment.
Interestingly, dopamine also plays a large role in drug addiction, as many addictive drugs cause dopamine levels in the brain to increase by promoting more dopamine release from neurons [20]. You may have heard the term “dopamine rush” and associated it with a rush of happiness. However, a dopamine rush is actually a rapid increase in dopamine that instructs your brain to seek out whatever caused the surge more frequently, motivating the brain to overcome boundaries to access the source of the rush. In the case of drugs, the powerful motivational force of an extreme dopamine rush can compel a person to pursue a substance no matter how serious the obstacles and consequences are . For some, this can mean life or death — especially those most vulnerable to developing substance use disorders or addictive behavior patterns. So, no: dopamine may motivate us, but it certainly does not always make us happy.
MYTH THREE: “RIGHT VS. LEFT-BRAINED PEOPLE”
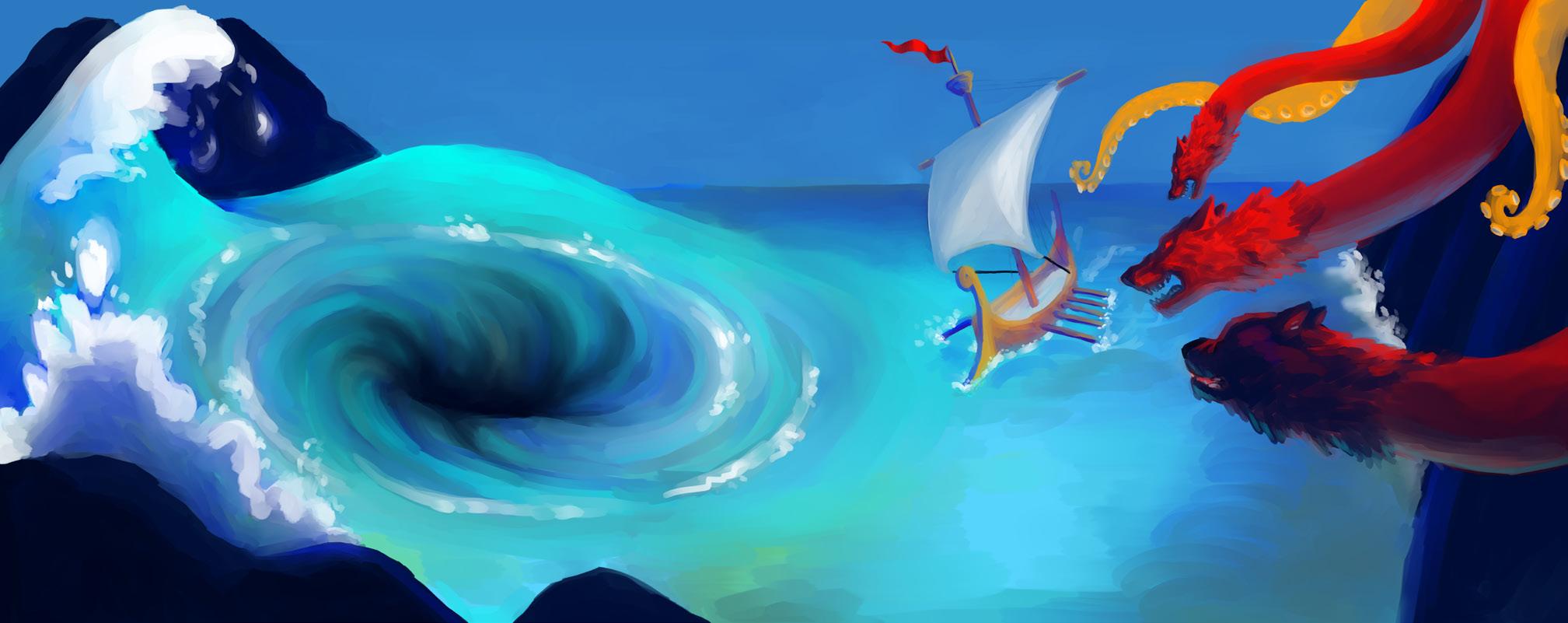
Another common neuroscience myth exists in the classification of people as either left-brained and right-brained. This idea is based on the brain’s two hemispheres and posits that people who think more logically, linearly, and sequentially (often excelling in science and math) have a dominant left hemisphere, while people who think more visually, imaginatively, and holistically (often excelling in the arts) have a dominant right hemisphere.
This myth originated from the work of neurologist Dr. Roger Sperry, winner of the 1982 Nobel Prize in Physiology or Medicine for research on the brain’s two hemispheres [17]. Sperry aimed to investigate the role of the corpus callosum, the bundle of neurons that connects the two hemispheres of the brain. Because surgery cutting the corpus callosum was one of the earliest treatments for epilepsy, Sperry studied the abilities of patients who had undergone this procedure and pioneered studying the functional differences between brain hemispheres. In his experiments, Sperry would present a word to either the participant’s left or right eye for only a moment and then would ask them what they saw [17]. At the time, Sperry knew that the right hemisphere of the brain exclusively responded to the image seen in the left eye, and vice versa. When he placed the word in front of individuals’ right eye, they were able to tell him what word they saw. However, when the word was presented to their left eye, participants could not tell Sperry what they
saw. From this, Sperry concluded that the left brain is necessary for language articulation and understanding. Next, he asked the same participants to draw the word that was presented only to their left eye with their left hand, knowing that, similar to visual control, muscular control is also governed by the opposite brain hemisphere. These individuals were able to draw the word and recognize their drawing as a word
but could not say it aloud. Sperry concluded that the right hemisphere can identify words as shapes, but can not articulate them. This contributed to the false notion that the combination of the two hemispheres is necessary for normal language functioning.
This study provides evidence for differences in hemispheres, and, therefore, evidence for the rightbrained left-brained myth, right? There is no dispute that there is functional lateralization within the brain; the two hemispheres are not identical, and there are functions that one primarily holds over the other. For example, the majority of language processing is governed by the left hemisphere, as Sperry suggested [13]. The left hemisphere is home to the brain regions Broca’s area and Wernicke’s area, which are responsible for language production and comprehension, respectively. This myth, however, incorrectly conflates the idea of functional differences with personality. Human beings love to classify each other into personality groups, as shown by the Myers-Briggs personality test, astrological signs, and Hogwarts houses. While yes, everyone’s brain has functional differences that vary by hemisphere, these differences are not necessarily related to personality. A 2013 study investigated whether or not brain activity in each hemisphere differs from person to person, looking at just over 1,000 individuals and analyzing over 7,200 cortical regions via fMRI. The results showed some variability in brain activity by region, but those differences were consistent across all individuals [14]. The researchers found no separation of the cohort into “left-brained” and “right-brained” individuals, thus disproving the common myth.
So what could explain individual differences in creative and logical thinking capabilities? In 2018, a group of researchers sought out to answer this question by using fMRI data to observe connectivity between brain regions of study participants after they had completed creative thinking tasks [2]. The study found that connectivity patterns and creativity scores were
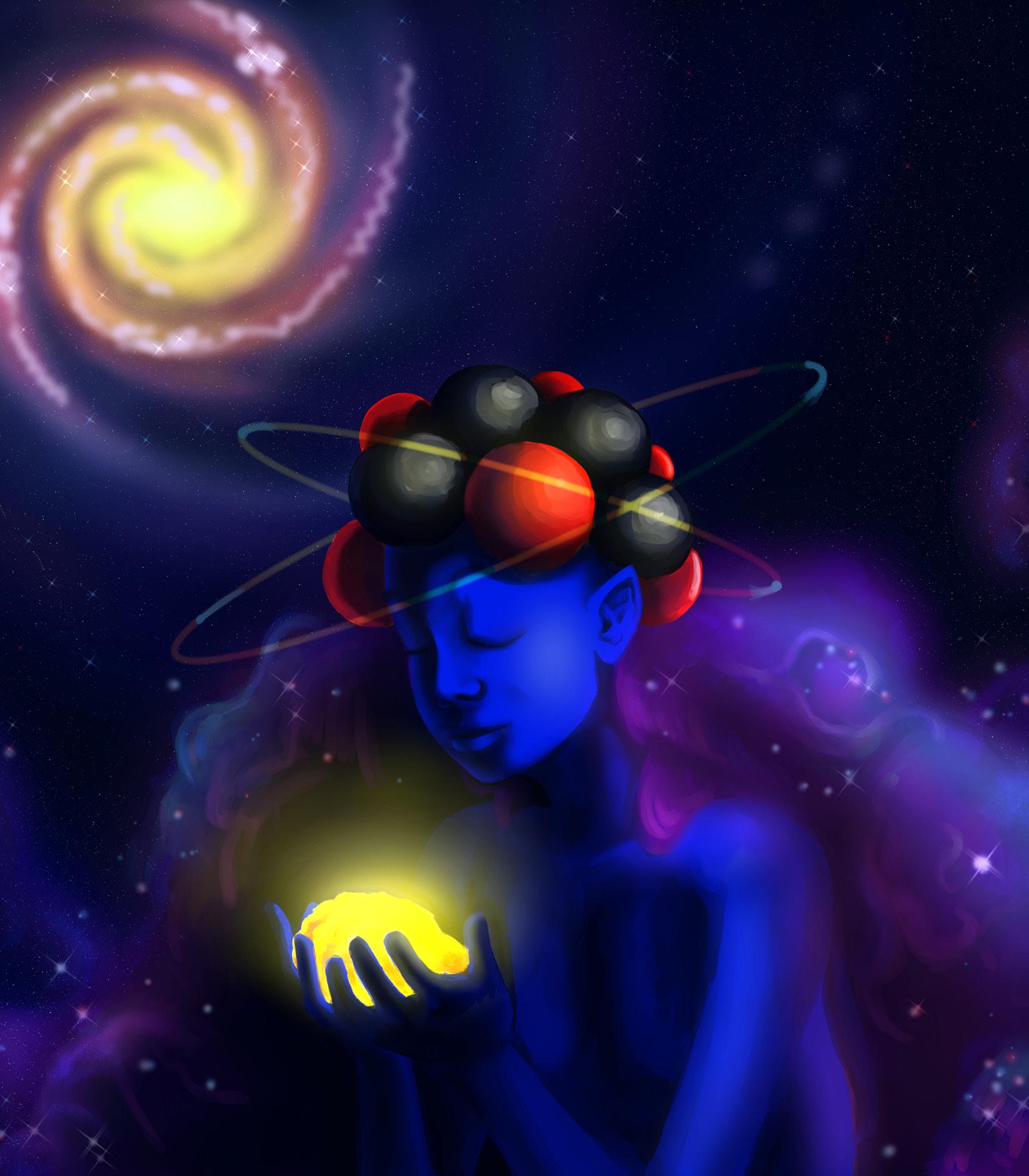
so strongly correlated that they could predict the creativity of a participant’s response by looking at their brain scan. They concluded that a person’s creative ability is based on the connectivity of three prominent brain networks: the default, salience, and executive networks. The default network includes regions in the cortex, the cerebellum, and striatum, which are best known for roles in thinking, balance, and movement respectively. These regions are activated when people are brainstorming or imagining. The executive network resides primarily in the frontal cortex and is responsible for evaluating if the brainstormed idea will actually work. Finally, the salience network is composed of the cingulate cortex and insula, which are brain regions necessary for emotion regulation, maintaining homeostasis, and switching between the two other networks.
In other words, the default network puts the mind into dreamer mode, the executive network puts the mind into realist mode, and the salience network mediates when each mode is necessary. Interestingly, while these three networks are rarely all activated at the same time, this study suggests the more creative a person is, the better able they are to co-activate the networks [2]. These results are also consistent with another fMRI study of jazz musicians as they improvise melodies [16]. If you are trying to understand why, neurologically speaking, one person is more creative than another, resist the urge to categorize people into left and right brained individuals. As with every topic in neuroscience, the true explanations are very complex.
Billions of brain cells work together to govern the human body and construct the human mind. One can devote their entire life to studying the brain and only scratch the surface of its inner workings. Every brain region has subregions. Every subregion has several different types of cells. Each of these cells have multiple, unique functions, which can also vary based on the types of cells that surround them. It’s easy to see why neuroscience can be so complicated to understand. Even neuroscientists find themselves subspecializing within the field because it is impossible to study it all. When neuroscientific findings are published, they can be difficult to interpret, even by someone with a science background; therefore, misinterpretations are inevitable. These misinterpretations manifest in popular culture as facts even if there is no intent to mislead society. When we are confronted with a neurological phenomenon in our daily lives, it is important that we question its scientific accuracy before blindly accepting it as true.
REFERENCES
REWIRING THE BRAIN: HOW THE NERVOUS SYSTEM HEALS ITSELF
1. Schiltz, N. K., & Fernandez-Baca Vaca, G. (2018). Epidemiologist’s view: Addressing the epilepsy surgery treatment gap with minimally-invasive techniques. Epilepsy Research, 142, 179–181. doi: 10.1016/j.eplepsyres.2018.01.013
2. Rogawski, M. A., Löscher, W., & Rho, J. M. (2016). Mechanisms of action of antiseizure drugs and the ketogenic diet. Cold Spring Harbor Perspectives in Medicine, 6(5). doi:10.1101/cshperspect.a022780
3. Lin, Y., Harris, D. A., Curry, D. J., & Lam, S. (2015). Trends in outcomes, complications, and hospitalization costs for hemispherectomy in the United States for the years 2000-2009. Epilepsia, 56(1), 139–146. doi: 10.1111/epi.12869
4. Armstrong-Wells, J., & Ferriero, D. M. (2014). Diagnosis and acute management of perinatal arterial ischemic stroke. Neurol Clin Pract, 4(5), 378–385. doi: 10.1212/CPJ.0000000000000077
5. Fountas, K. N., Smith, J. R., Robinson, J. S., Tamburrini, G., Pietrini, D., & Di Rocco, C. (2006). Anatomical hemispherectomy. Child’s Nervous System: ChNS: Official Journal of the International Society for Pediatric Neurosurgery, 22(8), 982–991. doi:10.1007/s00381-006-0135-2
6. Miller, J. W., & Hakimian, S. (2013). Surgical treatment of epilepsy. Continuum (Minneapolis, Minn.), 19(3 Epilepsy), 730–742. doi:10.1212/01. CON.0000431398.69594.97
7. Deransart, C., Vercueil, L., Marescaux, C., & Depaulis, A. (1998). The role of basal ganglia in the control of generalized absence seizures. Epilepsy Research, 32(1–2), 213–223. doi:10.1016/s09201211(98)00053-9 8. Villemure, J.-G., & Daniel, R. T. (2006). Periinsular hemispherotomy in paediatric epilepsy. Child’s Nervous System: ChNS: Official Journal of the International Society for Pediatric Neurosurgery, 22(8), 967–981. doi:10.1007/s00381-006-0135-2
9. Kim, J.-S., Park, E.-K., Shim, K.-W., & Kim, D. S. (2018). Hemispherotomy and functional hemispherectomy: Indications and outcomes. Journal of Epilepsy Research, 8(1), 1–5.
10. Campbell, J., & Sharma, A. (2016). Visual crossmodal re-organization in children with cochlear implants. PLOS ONE, 11(1), e0147793. doi:10.14581/ jer.18001
11. Shaffer, J. (2016). Neuroplasticity and clinical practice: Building brain power for health. Frontiers in Psychology, 7, 1118. doi:10.1371/journal.pone.0147793
12. Morris, R. G. M. (2003). Long-term potentiation and memory. Philosophical Transactions of the Royal Society of London. Series B, Biological Sciences, 358(1432), 643–647. doi:10.3389/fpsyg.2016.01118
13. Huttenlocher, P. R., & Dabholkar, A. S. (1997). Regional differences in synaptogenesis in human cerebral cortex. The Journal of Comparative Neurology, 387(2), 167–178. doi:10.1098/rstb.2002.1230
14. Klein, D., Mok, K., Chen, J.-K., & Watkins, K. E. (2014). Age of language learning shapes brain structure: a cortical thickness study of bilingual and monolingual individuals. Brain and Language, 131, 20–24. DOI:10.1002/(sici)10969861(19971020)387:2<167::aid-cne1>3.0.co;2-z
15. Ismail, F. Y., Fatemi, A., & Johnston, M. V. (2017). Cerebral plasticity: Windows of opportunity in the developing brain. European Journal of Paediatric Neurology: EJPN: Official Journal of the European Paediatric Neurology Society, 21(1), 23–48. doi:10.1016/j.bandl.2013.05.014
16. Sussman, D., Leung, R. C., Chakravarty, M. M., Lerch, J. P., & Taylor, M. J. (2016). The developing human brain: age-related changes in cortical, subcortical, and cerebellar anatomy. Brain and Behavior, 6(4), e00457. doi:10.1016/j.ejpn.2016.07.007
17. Abdul-Kareem, I. A., Stancak, A., Parkes, L. M., & Sluming, V. (2011). Increased gray matter volume of left pars opercularis in male orchestral musicians correlate positively with years of musical performance. Journal of Magnetic Resonance Imaging, 33(1), 24–32. doi:10.1002/brb3.457 doi:10.1002/jmri.22391
18. Schnack, H. G., van Haren, N. E. M., Brouwer, R. M., Evans, A., Durston, S., Boomsma D. I., , Khan, R. S., & Hulshoff Pol, H. E. (2015). Changes in thickness and surface area of the human cortex and their relationship with intelligence. Cerebral Cortex (New York, N.Y.: 1991), 25(6), 1608–1617. doi: 10.1093/cercor/ bht357
19. Kinsbourne, M. (2013). Somatic twist: a model for the evolution of decussation. Neuropsychology, 27(5), 511–515. doi:10.1093/cercor/bht357
20. Graveline, C. J., Mikulis, D. J., Crawley, A. P., & Hwang, P. A. (1998). Regionalized sensorimotor plasticity after hemispherectomy fMRI evaluation. Pediatric Neurology, 19(5), 337–342. doi:10.1037/ a0033662
21. Benecke, R., Meyer, B. U., & Freund, H. J. (1991). Reorganisation of descending motor pathways in patients after hemispherectomy and severe hemispheric lesions demonstrated by magnetic brain stimulation. Experimental Brain Research, 83(2), 419–426. doi:10.1016/S0887-8994(98)00082-4
22. Corballis, M. C. (2014). Left brain, right brain: facts and fantasies. PLOS Biology, 12(1), e1001767. doi:10.1007/BF00231167
23. Liégeois, F., Connelly, A., Baldeweg, T., & VarghaKhadem, F. (2008). Speaking with a single cerebral hemisphere: fMRI language organization after hemispherectomy in childhood. Brain and Language, 106(3), 195–203. doi:10.1371/journal.pbio.1001767
24. Ivanova, A., Zaidel, E., Salamon, N., Bookheimer, S., Uddin, L. Q., & de Bode, S. (2017). Intrinsic functional organization of putative language networks in the brain following left cerebral hemispherectomy. Brain Structure & Function, 222(8), 3795–3805. doi:10.1016/j.bandl.2008.01.010 25. Björklund, A., & Lindvall, O. (2000). Self-repair in the brain: Neurobiology. Nature, 405(6789), 892–893, 895. doi:10.1007/s00429-017-1434-y
SEX DIFFERENCES AND DEPRESSION: THE MALE-CENTRIC RESEARCH MODEL’S HARMFUL EFFECTS ON FEMALES
1. National Institute of Mental Health. (2019). Major depression. Retrieved April 05, 2021, from https://www.nimh.nih.gov/health/statistics/majordepression.shtml
2. Cassano, P., & Fava, M. (2002). Depression and public health: an overview. Journal of psychosomatic research, 53(4), 849–857. doi:10.1016/s00223999(02)00304-5
3. Tolentino, J. C., & Schmidt, S. L. (2018). DSM-5 Criteria and Depression Severity: Implications for Clinical Practice. Frontiers in psychiatry, 9, 450. doi:10.3389/fpsyt.2018.00450
4. LeGates, T. A., Kvarta, M. D., & Thompson, S. M. (2019). Sex differences in antidepressant efficacy. Neuropsychopharmacology, 44(1), 140–154. doi:10.1038/s41386-018-0156-z
5. Altemus, M., Sarvaiya, N., & Neill Epperson, C. (2014). Sex differences in anxiety and depression clinical perspectives. Frontiers in Neuroendocrinology, 35(3), 320–330. doi:10.1016/j. yfrne.2014.05.004
6. Sramek, J. J., Murphy, M. F., & Cutler, N. R. (2016). Sex differences in the psychopharmacological treatment of depression. Dialogues in clinical neuroscience, 18(4), 447–457. doi:10.31887/ DCNS.2016.18.4/ncutler
7. Schiebinger, L. (2003). Women’s health and clinical trials. Journal of Clinical Investigation, 112(7), 973977. doi:10.1172/jci200319993
8. McGregor, A. J. (2020). Sex Matters: how malecentric medicine endangers women’s health and what we can do about it. QUERCUS PUBLISHING.
JUST ANOTHER FACE IN THE CROWD: THE EVOLUTION AND MECHANISMS OF PRIMATE FACIAL PROCESSING
1. South Palomares, J. K., & Young, A. W. (2018). Facial first impressions of partner preference traits: Trustworthiness, status, and attractiveness. Social Psychological and Personality Science, 9(8), 990–1000. doi: 10.1177/1948550617732388
2. Oosterhof, N., & Todorov, A. (2008). The functional basis of face evaluation. Proceedings of the National Academy of Sciences, 105(32), 11087–11092. doi:10.1073/pnas.0805664105
3. Burrows, A. M. (2008). The facial expression musculature in primates and its evolutionary significance. BioEssays, 30(3), 212–225. doi: 10.1002/ bies.20719
4. Waller, B. M., Julle-Daniere, E., & Micheletta, J. (2020). Measuring the evolution of facial ‘expression’ using multi-species FACS. Neuroscience & Biobehavioral Reviews, 113, 1–11. doi: 10.1016/j. neubiorev.2020.02.031
5. Costa, M., Gomez, A., Barat, E., Lio, G., Duhamel, J.R., & Sirigu, A. (2018). Implicit preference for human trustworthy faces in macaque monkeys. Nature Communications, 9(1), 4529. doi:10.1038/s41467-01806987-4
6. Haselhuhn, M. P., Ormiston, M. E., & Wong, E. M. (2015). Men’s facial width-to-height ratio predicts aggression: a meta-analysis. PloS one, 10(4), e0122637. https://doi.org/10.1371/journal.pone.0122637
7. Deska, J. C., Lloyd, E. P., & Hugenberg, K. (2018). The face of fear and anger: Facial width-to-height ratio biases recognition of angry and fearful expressions. Emotion, 18(3), 453–464. doi: 10.1037/ emo0000328
8. Gore J. C. (2003). Principles and practice of functional MRI of the human brain. The Journal of Clinical Investigation, 112(1), 4–9. doi: 10.1172/JCI19010
9. Kanwisher, N., McDermott, J., & Chun, M. M. (1997). The fusiform face area: A module in human extrastriate cortex specialized for face perception. doi:10.1523/JNEUROSCI.17-11-04302.1997 10. Tsao, D. Y. (2006). A cortical region consisting entirely of face-selective cells. Science, 311(5761), 670–674. doi: 10.1126/science.1119983
11. Ratan Murty, N. A., Teng, S., Beeler, D., Mynick, A., Oliva, A., & Kanwisher, N. (2020). Visual experience is not necessary for the development of face-selectivity in the lateral fusiform gyrus. Proceedings of the National Academy of Sciences, 117(37), 23011–23020. doi:10.1073/pnas.2004607117
12. Mäkelä, P., Näsänen, R., Rovamo, J., & Melmoth, D. (2001). Identification of facial images in peripheral vision. Vision Research, 41(5), 599–610. doi:10.1016/ s0042-6989(00)00259-5
13. Kosslyn, S. M., Hamilton, S. E., & Bernstein, J. H. (1995). The perception of curvature can be selectively disrupted in prosopagnosia. Brain and Cognition, 27(1), 36–58. doi:10.1006/brcg.1995.1003
14. Parvizi, J., Jacques, C., Foster, B. L., Withoft, N., Rangarajan, V., Weiner, K. S., & Grill-Spector, K. (2012). Electrical stimulation of human fusiform faceselective regions distorts face perception. Journal of Neuroscience, 32(43), 14915-14920. doi:10.1523/ JNEUROSCI.2609-12.2012
15. Voss, J. L., Federmeier, K. D., & Paller, K. A. (2012). The potato chip really does look like Elvis! Neural hallmarks of conceptual processing associated with finding novel shapes subjectively meaningful. Cerebral Cortex, 22(10), 2354–2364. doi:10.1093/ cercor/bhr315
16. Sternberg, R. J., Sternberg, K., & Mio, J. S. (2012). Cognitive Psychology. Wadsworth/Cengage Learning.
17. Taubert, J., Weldon, K.B. & Parr, L.A. (2017). Robust representations of individual faces in chimpanzees (Pan troglodytes) but not monkeys (Macaca mulatta). Anim Cogn 20, 321–329. doi: 10.1007/s10071-016-10546
18. Wilmer, J. B., Germine, L., Chabris, C. F., Chatterjee, G., Williams, M., Loken, E., Nakayama, K., & Duchaine, B. (2010). Human face recognition ability is specific and highly heritable. Proceedings of the National Academy of Sciences, 107(11), 5238–5241. doi: 10.1073/pnas.0913053107
19. Biotti, F., & Cook, R. (2016). Impaired perception of facial emotion in developmental prosopagnosia. Cortex, 81, 126–136. doi: 10.1016/j.cortex.2016.04.008
20. Gainotti, G., & Marra, C. (2011). Differential contribution of right and left temporo-occipital and anterior temporal lesions to face recognition disorders. Frontiers in Human Neuroscience, 5, 55. doi: 10.3389/fnhum.2011.00055
21. Corrow, S. L., Dalrymple, K. A., & Barton, J. J. (2016). Prosopagnosia: Current perspectives. Eye and Brain, 8, 165–175. doi: 10.2147/EB.S92838
FAKING IT ‘TILL YOU MAKE IT: WHY WE SHOULD ALL SMILE MORE
1. Kraft, T. L., & Pressman, S. D. (2012). Grin and bear it: The influence of manipulated facial expression on the stress response. Psychological Science, 23(11), 1372-1378. doi:10.1177/0956797612445312
2. Ekman, P., Davidson, R. J., & Friesen, W. V. (1990). The Duchenne smile: Emotional expression and brain physiology: II. Journal of Personality and Social Psychology, 58(2), 342–353. doi: 10.1037/00223514.58.2.342
3. Gothard, K. M. (2014). The amygdalo-motor pathways and the control of facial expressions. Frontiers in Neuroscience, 8(43). doi: 10.3389/ fnins.2014.00043
4. Baxter, M. G., & Croxson, P. L. (2012). Facing the role of the amygdala in emotional information processing. Proceedings of the National Academy of Sciences, 109(52), 21,180-21,181. doi: 10.1073/ pnas.1219167110
5. Farhud, D. D., Malmir, M., & Khanahmadi, M. (2014). Happiness and health: The biological factors- Systematic review article. Iranian Journal of Public Health, 43(11), 1468-1477. PMID: 26060713
6. Meeten, F., Ivak, P., Dash, S. R., Knowles, S., Duka, T., Scott, R., Kaiser, J., & Davey, G. C. L. (2015). The effect of facial expressions on the evaluation of ambiguous statements. Experimental Psychology, 6(3), 253-263. doi:10.5127/jep.039613
7. Marmolejo-Ramos, F., Murata, A., Sasaki, K., Yamada, Y., Ikeda, A., Hinojosa, J. A., Watanabe, K., Parzuchowski, M., Tirado, C., & Ospina, R. (2020). Your face and moves seem happier when I smile. Facial action influences the perception of emotional faces and biological motion stimuli. Experimental Psychology, 67 (1), 14-22. doi:10.31234/osf.io/4uvdq 8. Davis, J. I., Senghas, A., & Ochsner, K. N., (2009). How does facial feedback modulate emotional experience. Journal of Research in Personality, 43(5), 822-829. doi:10.1016/j.jrp.2009.06.005
9. Ehrenfeld, T. (2013, February 23). Does botox blunt emotions? Psychology Today. Retrieved from https://www.psychologytoday.com/us/blog/opengently/201302/does-botox-blunt-emotions
10. Dutta, S. R., Passi, D., Singh, M., Singh, P., Sharma, S., & Sharma, A. (2016). Botulinum toxin the poison that heals: A brief review. National Journal of Maxillofacial Surgery, 7(1), 10-16. doi:10.4103/09755950.196133
11. Davis, J. I., Senghas, A., Brandt, F., & Ochsner, K. N. (2010). The effects of BOTOX® injections on emotional experience. Emotion, 10(3), 433-440. doi:10.1037/a0018690
12. Ulrich-Lai, Y. M., & Herman, J. P. (2014). Neural regulation of endocrine and autonomic stress responses. Nature Reviews Neuroscience, 10(6), 397409. doi: 10.1038/nrn2647
13. Harvard Health Publishing (2020, July 6). Understanding the stress response. Harvard Medical School. Retrieved from https://www.health.harvard. edu/staying-healthy/understanding-the-stressresponse
14. Zander-Schellenberg, T., Collins, I. M., Miché, M., Guttmann, C., Lieb, R., & Wahl, K. (2020) Does laughing have a stress-buffering effect in daily life? An intensive longitudinal study. PLOS ONE, 15(7),1-11. doi:10.1371/journal.pone.0235851
15. Okley, J. A., Weiss, A., & Gale C. R. (2017). The interaction between stress and positive affect in predicting mortality. Journal of Psychosomatic Research, 100, 53-60. doi:10.1016/j. jpsychores.2017.07.005
ENCOUNTERS WITH THE THIRD KIND: THE MEMORY TWILIGHT ZONE
1. Friedman & Marden. (2007). Captured! The Betty and Barney Hill UFO Experience. Weiser.
2. Winthrop, J. (1996). The Journal of John Winthrop, 1630-1649 (R. S. Dunn, J. Savage, L. Yeandle, Eds.). Harvard University Press. (Original work published 1630-1649).
3. Queensland Brain Institute. (2018). Where are memories stored in the brain?. Queensland Brain Institute. Retrieved from https://qbi.uq.edu.au/brainbasics/memory/where-are-memories-stored
4. Hellenthal, M. V., Knott, L. M., Howe, M. L., Wilkinson, S., & Shah, D. (2019). The effects of arousal and attention on emotional false memory formation. Journal of Memory and Language, 107, 54-68. doi:10.1016/j.jml.2019.03.010.
5. Williamson, A. (2019). What is hypnosis and how might it work? Palliative Care: Research and Treatment, 12. doi:10.1177/1178224219826581.
6. June, C. (2016). Sleep deprivation increases formation of false memories. Journal of Sleep Research, 25, 673-682. doi: 10.1111/jsr.12436.
7. Hessen-Kayfitz, K. & Scoboria, A. (2012). False memory is in the details: Photographic details differentially predict false memory formation. Applied Cognitive Psychology, 26, 333-341. doi: 10.1002/ acp.1839
8. Williamson, A. (2019). What is hypnosis and how might it work? Palliative Care: Research and Treatment, 12.
9. McNally, R. & Clancy, S. (2005). Sleep paralysis in adults reporting repressed, recovered, or continuous memories of childhood sexual abuse. Journal of Anxiety Disorders, 19, 595-602. doi: 10.1016/j. janxdis.2004.05.003.
10. Otgaar, H., Howe, M., Patihis, L., Merckelbach, H., Lynn, S., Lilienfeld, S., Loftus, E. (2019). The return of the repressed: The long persistent and problematic claims of long-forgotten trauma. Perspectives on Psychological Science, 14, 1072-1095. doi: 10.1177/174569161862306.
BEWARE THE POST-VACCINE BLUES
1. Al-Harbi, K. S. (2012). Treatment-resistant depression: Therapeutic trends, challenges, and future directions. Patient Preference and Adherence, 6, 369–388. doi:10.2147/PPA.S29716 2. Derry, H. M., Padin, A. C., Kuo, J. L., Hughes, S., & Kiecolt-Glaser, J. K. (2015). Sex differences in depression: Does inflammation play a role? Current Psychiatry Reports, 17(10). doi:10.1007/s11920-0150618-5
3. Fournier, J. C., DeRubeis, R. J., Hollon, S. D., Dimidjian, S., Amsterdam, J. D., Shelton, R. C., & Fawcett, J. (2010). Antidepressant drug effects and depression severity: A patient-level meta-analysis. JAMA - Journal of the American Medical Association, 303(1), 47–53. doi:10.1001/jama.2009.1943
4. Haapakoski, R., Mathieu, J., Ebmeier, K. P., Alenius, H., & Kivimäki, M. (2015). Cumulative meta-analysis of interleukins 6 and 1β, tumour necrosis factor and C-reactive protein in patients with major depressive disorder. Brain, Behavior, and Immunity, 49, 206–215. doi:10.1016/j.bbi.2015.06.001
5. Kessler, R. C., Berglund, P., Demler, O., Jin, R., Koretz, D., Merikangas, K. R., Rush, A. J., Walters, E. E., Wang, A., Rovner, B., & Casten, R. (2003). The epidemiology of major depressive disorder. EvidenceBased Eye Care, 4(4), 186–187. doi:10.1097/00132578200310000-00002
6. Kim, Y. K., Na, K. S., Myint, A. M., & Leonard, B. E. (2016). The role of pro-inflammatory cytokines in neuroinflammation, neurogenesis and the neuroendocrine system in major depression. Progress in Neuro-Psychopharmacology and Biological Psychiatry, 64, 277–284. https://doi.org/10.1016/j. pnpbp.2015.06.008
7. Miller, G. E., & Cole, S. W. (2012). Clustering of depression and inflammation in adolescents previously exposed to childhood adversity. Biological Psychiatry, 72(1), 34–40. doi:10.1016/j. biopsych.2012.02.034
8. Maes, M. (1995). Evidence for an immune response in major depression: A review and hypothesis. Progress in Neuropsychopharmacology and Biological Psychiatry, 19(1), 11–38.doi:10.1016/02785846(94)00101-M
9. Raison, C. L., Dantzer, R., Ph, D., Kelley, K. W., Marcus, A., Woolwine, B. J., Vogt, G., Spivey, J. R., Saito, K., & Miller, A. H. (2010). CSF concentrations of brain tryptophan and kynurenines during immune stimulation with IFN-alpha: relationship to CNS immune responses and depression. Molecular Psychiatry, 15(4), 393–403. doi:10.1038/mp.2009.116. CSF
10. Raison, C. L., Rutherford, R. E., Woolwine, B. J., Shuo, C., Schettler, P., Drake, D. F., Haroon, E., & Miller, A. H. (2013). A randomized controlled trial of the tumor necrosis factor antagonist infliximab for treatment-resistant depression: The role of baseline inflammatory biomarkers. Archives of General Psychiatry, 70(1), 31–41. doi:10.1001/2013. jamapsychiatry.4
11. Tsatsoulis, A., & Fountoulakis, S. (2006). The protective role of exercise on stress system dysregulation and comorbidities. Annals of the New York Academy of Sciences, 1083: 196-213. doi:10.1196/ annals.1367.020
12. Vollmer-Conna, U., Fazou, C., Cameron, B., Li, H., Brennan, C., Luck, L., Davenport, T., Wakefield, D., Hickie, I., & Lloyd, A. (2004). Production of pro-inflammatory cytokines correlates with the symptoms of acute sickness behaviour in humans. Psychological Medicine, 34(7), 1289–1297. doi:10.1017/ S0033291704001953
13. Wirtz, P. H., & von Känel, R. (2017). Psychological stress, inflammation, and coronary heart disease. Current Cardiology Reports, 19(11). doi:10.1007/s11886017-0919-x
14. Yirmiya, R., Rimmerman, N., & Reshef, R. (2015). Depression as a microglial disease. Trends in Neurosciences, 38(10), 637–658. doi:10.1016/j. tins.2015.08.001
RNA: A NEW FACE IN THE FIGHT AGAINST NEURODEGENERATION
1. Gilbert SF. Developmental Biology. 6th edition. Sunderland (MA): Sinauer Associates; 2000. The Cell Death Pathways. Available from: https://www.ncbi. nlm.nih.gov/books/NBK10103/ 2. Ghavami, S., Shojaei, S., Yeganeh, B., Ande, S. R., Jangamreddy, J. R., Mehrpour, M., Christofferson, J., Chaabane, W., Moghadam, A., R., Kashani, H., H., Hashemi, M., Owji, A., A., & Łos, M. J. (2014). Autophagy and apoptosis dysfunction in neurodegenerative disorders. Progress in Neurobiology, 112, 24–49. doi:10.1016/j. pneurobio.2013.10.004.
3. Harvard Medical School. (n.d.). The challenge of neurodegenerative diseases. Harvard NeuroDiscovery Center. Retrieved from https://neurodiscovery. harvard.edu/challenge
4. U.S. Department of Health and Human Services. (n.d.). What are the signs of Alzheimer’s Disease? National Institute on Aging. Retrieved from https:// www.nia.nih.gov/health/what-are-signs-alzheimersdisease.
5. Durães, F., Pinto, M., & Sousa, E. (2018). Old drugs as new treatments for neurodegenerative diseases. Pharmaceuticals, 11(2), 44. doi:10.3390/ph11020044
6. Liu, E. Y., Cali, C. P. &, Lee. E. B. RNA metabolism in neurodegenerative disease. Dis Model Mech. 2017 May 1;10(5):509-518. doi:10.1242/dmm.028613.
7. Ferry, G. (2019). The structure of DNA. Science, 575, 35-36. doi:10.1038/d41586-019-02554-z
8. Clancy, S. (2008) Chemical RNA structure. Nature Education, 7(1):60. Retrieved from https://www. nature.com/scitable/topicpage/chemical-structureof-rna-348/
9. Chan, J. J., & Tay, Y. (2018). Noncoding RNA: RNA regulatory networks in cancer. International Journal of Molecular Sciences, 19(5), 1310. doi:10.3390/ ijms19051310
10. Qureshi, A., Tantray, V. G., Kirmani, A. R., & Ahangar, A. G. (2018). A review on current status of antiviral siRNA. Reviews in Medical Virology, 28(4), e1976. doi:10.1002/rmv.1976
11. Belzil, V. V., Gendron, T. F., & Petrucelli, L. (2013). RNA-mediated toxicity in neurodegenerative disease. Molecular and Cellular Neurosciences, 56, 406–419. doi:10.1016/j.mcn.2012.12.006
12. Nussbacher, J. K., Tabet, R., Yeo, G. W., & LagierTourenne, C. (2019). Disruption of RNA metabolism in neurological diseases and emerging therapeutic interventions. Neuron, 102(2), 294–320. doi:10.1016/j. neuron.2019.03.01
13. Ramaswami, M., Taylor, J. P., & Parker, R. (2013). Altered ribostasis: RNA-protein granules in degenerative disorders. Cell, 154(4), 727-736. doi:10.1016/j.cell.2013.07.038.
14. Riva, P., Ratti, A., & Venturin, M. (2016). The Long Non-Coding RNAs in Neurodegenerative Diseases: Novel Mechanisms of Pathogenesis. Current Alzheimer Research, 13(11), 1219–1231. doi:10.2174/1567 205013666160622112234
15. Johnson R. (2012). Long non-coding RNAs in Huntington’s disease neurodegeneration. Neurobiology of disease, 46(2), 245–254. https://doi. org/10.1016/j.nbd.2011.12.006
16. Idda, M. L., Munk, R., Abdelmohsen, K., & Gorospe, M. (2018). Noncoding RNAs in Alzheimer’s disease. Wiley interdisciplinary reviews. RNA, 9(2), 10.1002/ wrna.1463. https://doi.org/10.1002/wrna.1463
17. Drew, L. (2019). Why rare genetic diseases are a logical focus for RNA therapies. Nature Outlook, 574, S16-S18. doi 10.1038/d41586-019-03075-5
18. Arraiano, M.C., Andrade, J.M., Domingues, S., Guinote, I.B., Malecki, M., Matos, R.G., Moreira, R.N., Pobre, V., Reis, F.P., Saramago, M., Silva, I.J., & Viegas, S.C. (2010). The critical role of RNA processing and degradation in the control of gene expression. FEMS Microbiology Reviews, 34(5), 883–923. doi:10.1111/ j.1574-6976.2010.00242.x
19. Katella, K. (2021, April 13). Comparing the COVID-19 vaccines: How are they different? Retrieved from https://www.yalemedicine.org/news/covid-19vaccine-comparison.
20. Centers for Disease Control and Prevention. (2021, March 4). Understanding mRNA COVID-19 Vaccines. Centers for Disease Control and Prevention. Retrieved from https://www.cdc.gov/coronavirus/2019-ncov/ vaccines/different-vaccines/mrna.html. 21. Wang, H., Zhang, Y., Huang, B., Deng, W., Quan, Y., Wang, W., Xu, W., Zhao, Y., Li, N., Zhang, J., Linag, H., Bao, L., Xu, Y., Ding, L., Zhou, W., Gao, H., Liu, J., Niu, P., Zhao, L., Zhen, W., Fu, H., Yu, S., Zhang, Z., Xu, G., Li, C., Lou, Z., Xu, M., Qin, C., Wu, G., Gao, G., F., Tan, W., & Yang, X. (2020). Development of an inactivated vaccine candidate, BBIBP-CorV, with potent protection against SARS-CoV-2. Cell, 182(3), 713–721. doi:10.1016/j.cell.2020.06.008
22. Immunisation Advisory Centre. (2020, January). A brief history of vaccination. Immunisation Advisory Centre. Retrieved from https://www.immune.org. nz/vaccines/vaccine-development/brief-historyvaccination
A HOT ISSUE: TEMPERATURE-DEPENDENT TOXICITY IN HERBIVOROUS MAMMALS
1. Parry, M. L. (2007). Climate Change 2007: Impacts, Adaptation and Vulnerability: Contribution of Working Group II to the Fourth Assessment Report of the Intergovernmental Panel on Climate Change. Cambridge: Cambridge University Press.
2. Dearing, M., Forbey, J., McLister, J., & Santos, L. (2008). Ambient temperature influences diet selection and physiology of an herbivorous mammal, Neotoma albigula. Physiological and Biochemical Zoology: Ecological and Evolutionary Approaches, 81(6), 891-897. doi:10.1086/588490
3. Waller, J. (2009). A forgotten plague: Making sense of dancing mania. The Lancet, 373(9664), 624-625. doi:10.1016/s0140-6736(09)60386-x
4. Guerre P. (2015). Ergot alkaloids produced by endophytic fungi of the genus Epichloë. Toxins, 7(3), 773–790. doi: 10.3390/toxins7030773
5. Florea, S., Panaccione, D. G., & Schardl, C. L. (2017). Ergot alkaloids of the family Clavicipitaceae. Phytopathology, 107(5), 504–518. doi:10.1094/PHYTO12-16-0435-RVW
6. Bobkova, N.V., Uteshev, V.K., Medvinskaya, N.I., Nesterova, I. V., & Arinbasarov, M. U. (2002). Mechanisms of amnestic effect of ergot alkaloid agroclavin. Bulletin of Experimental Biology and Medicine, 133, 366–368. doi: 10.1023/A:1016298005058
7. Eisemann, J. H., Huntington, G. B., Williamson, M., Hanna, M., & Poore, M. (2014, October 15). Physiological responses to known intake of ergot alkaloids by steers at environmental temperatures within or greater than their thermoneutral zone. Frontiers in Chemistry, 2. doi: 10.3389/ fchem.2014.00096
8. Lawler I. R., Stapley J., Foley W. J., & Eschler, B. M. (1999). Ecological example of conditioned flavor aversion in plant-herbivore interactions: Effect of terpenes of Eucalyptus leaves on feeding by common ringtail and brushtail possums. Journal of Chemical Ecology, 25, 401–415. doi:10.1023/A:1020863216892
9. Mclean, S., Brandon, S., Davies, N. W., Foley, W. J., & Muller, H. K. (2004). Jensenone: Biological reactivity of a marsupial antifeedant from eucalyptus. Journal of Chemical Ecology, 30(1), 19-36. doi:10.1023/ b:joec.0000013180.46747.07
10. Cubeddu, L., Hoffmann, I., Fuenmayor, N., & Malave, J. (1992). Changes in serotonin metabolism in cancer patients: Its relationship to nausea and vomiting induced by chemotherapeutic drugs. British Journal of Cancer, 66(1), 198-203. doi:10.1038/bjc. 1992.242
11. Marsh, K. J., Wallis, I. R., & Foley, W. J. (2005). Detoxification rates constrain feeding in common brushtail possums (Trichosurus vulpecula). Ecology, 86(11), 2946-2954. doi:10.1890/05-0303
12. Nersesian, C. L., Banks, P. B., & Mcarthur, C. (2011). Titrating the cost of plant toxins against predators: Determining the tipping point for foraging herbivores. Journal of Animal Ecology, 80(4), 753-760. doi:10.1111/ j.1365-2656.2011.01822.x
13. Kirmani, S. N., Banks, P. B., & McArthur, C. (2010). Integrating the costs of plant toxins and predation risk in foraging decisions of a mammalian herbivore. Oecologia, 164(2), 349–356. doi: 10.1007/s00442-0101717-y
14. Moore, B. D., Wiggins, N. L., Marsh, K. J., Dearing, M. D., & Foley, W. J. (2015). Translating physiological signals to changes in feeding behaviour in mammals and the future effects of global climate change. Animal Production Science, 55(3), 272. doi:10.1071/ AN14487 15. Marsh, K. J., Wallis, I. R., Mclean, S., Sorensen, J. S., & Foley, W. J. (2006). Conflicting demands on detoxification pathways influence how common brushtail possums choose their diets. Ecology, 87(8), 2103-2112. doi:10.1890/0012-9658(2006)87[2103:cdodp i]2.0.co;2
16. Moffett, D. B., Mumtaz, M. M., Sullivan, D. W., & Fowler, B. A. (2015). General considerations of doseeffect and dose-response relationships. In Handbook on the Toxicology of Metals (pp. 197–212). Elsevier. doi:10.1016/B978-0-444-59453-2.00010-X
17. Torregrossa, A. M., Azzara, A. V., & Dearing, M. D. (2012). Testing the diet-breadth trade-off hypothesis: Differential regulation of novel plant secondary compounds by a specialist and a generalist herbivore. Oecologia, 168(3), 711–718. doi:10.1007/ s00442-011-2121-y
DEFYING THE “WAR ON DRUGS”: THE REBIRTH OF PSYCHEDELIC MEDICINE & RESEARCH
1. History.com Editors. (2018, August 21). LSD. A&E Television Networks. Retrieved from history.com/ topics/crime/history-of-lsd.
2. Aixalà, M., dos Santos, R., Hallak, J., & Bouso, J.C. (2018). Psychedelics and personality. ACS Chemical Neuroscience, 9(10), 2304–2306. doi:10.1021/ acschemneuro. 8b00237.
3. Lewis, T. (2020, January 16). Johns Hopkins scientists give psychedelics the serious treatment. Scientific American. Retrieved from scientificamerican.com/article/johns-hopkinsscientists-give-psychedelics-the-serious-treatment.
4. Joiner, W. (2020, September 21). Who will benefit from psychedelic medicine? Washington Post. Retrieved from washingtonpost.com/ magazine/2020/09/21/psychedelic-medicine-will- itbe-accessible-to-all.
5. Walsh, Z., Hendricks, P., Smith, S., Kosson, D., Thiessen, M., Lucas, P., & Swogger, M. (2016). Hallucinogen use and intimate partner violence: Prospective evidence consistent with protective effects among men with histories of problematic substance use. Journal of Psychopharmacology, 30(7), 601–607. doi:10.1177/0269881116642538.
6. Kuchler, H. (2017, August 10). How Silicon Valley rediscovered LSD. Financial Times. Retrieved from ft.com/content/0a5a4404-7c8e-11e7-ab01a13271d1ee9c.
7. Tust, A. (2019, February 7). Would you consider trying psychedelics to take your practice to another level? Yoga Journal. Retrieved from yogajournal. com/lifestyle/yoga-trends/yoga-meditation- andpsychedelics.
8. Hagenbach, D., & Werthmüller, L. (2013, May 17). Turn on, tune in, drop out--and accidentally discover LSD [Excerpt]. Scientific American. Retrieved from scientificamerican.com/article/lsd-finds-itsdiscoverer.
9. Bogenschutz, M. P., & Ross, S. (2018). Therapeutic applications of classic hallucinogens. Current topics in behavioral neurosciences, 36, 361–391. doi:10.1007/7854_2016_464
10. Cormier, Z. (2016, April 11). Brain scans reveal how LSD affects consciousness. Springer Nature. Retrieved from www.nature.com/news/brain-scansreveal-how- lsd-affects-consciousness-1.19727.
11. Neelkantan, N., Mikhaylova, A., Stewart, A.M., Arnold, R., Gjeloshi, V., Kondaveeti, D., Poudel, M., & Kalueff, A. (2013). Perspectives on zebrafish models of hallucinogenic drugs and related psychotropic compounds. ACS Chemical Neuroscience, 4(8), 1137–1150. doi:10.1021/cn400090q.
12. Johns Hopkins Medicine. (2020, November 4). Psychedelic treatment with psilocybin relieves major depression, study shows. Johns Hopkins Medicine. Retrieved from https://www.hopkinsmedicine. org/news/newsroom/news-releases/psychedelictreatment-with-psilocybin-relieves-majordepression-study-shows.
13. De Gregorio, D., Enns, J., Nuñez, N., Posa, L., & Gobbi, G. (2018). d-Lysergic acid diethylamide, psilocybin, and other classic hallucinogens: Mechanism of action and potential therapeutic applications in mood disorders. Progress in Brain Research, 242, 69–96. doi:10.1016/bs.pbr.2018.07.008.
14. Cormier, Z. (2015, May 26). No link found between psychedelics and psychosis. Springer Nature. Retrieved from nature.com/news/no-link-foundbetween-psychedelics-and-psychosis-1.16968. 15. Krebs, T., & Johansen, P-Ø. (2012). Lysergic acid diethylamide (LSD) for alcoholism: Metaanalysis of randomized controlled trials. Journal of Psychopharmacology, 26(7), 994–1002. doi:10.1177/0269881112439253.
16. Carhart-Harris, R. L., Muthukumaraswamy, S., Roseman, L., Kaelen, M., Droog, W., Murphy, K., Tagliazucchi, E., Schenberg, E. E., Nest, T., Orban, C., Leech, R., Williams, L. T., Williams, T. M., Bolstridge, M., Sessa, B., McGonigle, J., Sereno, M. I., Nichols, D., Hellyer, P. J., … Nutt, D. J. (2016). Neural correlates of the LSD experience revealed by multimodal neuroimaging. Proceedings of the National Academy of Sciences, 113(17), 4853–4858. doi:10.1073/ pnas.1518377113.
17. Yaden, D. B., Yaden, M. E., & Griffiths, R. R. (2020). Psychedelics in psychiatry—Keeping the renaissance from going off the rails. JAMA Psychiatry. doi:10.1001/ jamapsychiatry.2020.3672.
18. New Scientist. (2021). Microdosing may be all in the mind. New Scientist, 249(3325). doi:10.1016/ s0262-4079(21)00406-1.
19. Cameron, L. P., Nazarian, A., & Olson, D. E. (2020). Psychedelic microdosing: Prevalence and subjective effects. Journal of Psychoactive Drugs. doi:10.1080/02 791072.2020.1718250.
20. Rougemont-Bücking, A., Jungaberle, H., Scheidegger, M., Merlo, M.C.G., Grazioli, V., Daeppen, J-B., Gmel, G., & Studer, J. (2019). Comparing mental health across distinct groups of users of psychedelics, MDMA, psychostimulants, and cannabis. Journal of Psychoactive Drugs. doi:10.1080/ 02791072.2019.1571258.
21. Kaertner, L., Steinborn, M., Kettner, H., Spriggs, M., Roseman, L., Buchborn, T., Balaet, M., Timmermann, C., Erritzoe, D., & Carhart-Harris, R. (2021). Positive expectations predict improved mental-health outcomes linked to psychedelic microdosing. Scientific Reports, 11(1). doi:10.1038/s41598-02181446-7.
22. Lieberman, J. A. (2021). Back to the future — The therapeutic potential of psychedelic drugs. New England Journal of Medicine, 384(15), 1460–1461. doi:10.1056/ nejme2102835.
23. Sutherland, R., Peacock, A., Whittaker, E., Roxburgh, A., Lenton, S., Matthews, A., Butler, K., Nelson, M., Burns, L., & Bruno, R. (2016). New psychoactive substance use among regular psychostimulant users in Australia, 2010–2015. Drug and Alcohol Dependence, 161, 110–118. doi:10.1016/j. drugalcdep.2016.01.024.
24. Fernández-Calderón, F., Cleland, C., & Palamar, J. (2018). Polysubstance use profiles among electronic dance music party attendees in New York City and their relation to use of new psychoactive substances. Addictive Behaviors, 78, 85–93. doi:10.1016/j.addbeh. 2017.11.004.
25. Tupper, K., Wood, E., Yensen, R., & Johnson, M. (2015). Psychedelic medicine: A re-emerging therapeutic paradigm. Canadian Medical Association Journal, 187(14), 1054–1059. doi:10.1503/cmaj.141124.
26. Davis, A., Barrett, F., May, D., Cosimano, M., Sepeda, N., Johnson, M., Finan, P., Griffiths, R. (2020). Effects of psilocybin-assisted therapy on major depressive disorder. JAMA Psychiatry. doi:10.1001/ jamapsychiatry.2020.3285.
27. National Center for Biotechnology Information (2021). PubChem compound summary for CID 8223, Ergotamine. NCBI. Retrieved from pubchem.ncbi.nlm. nih.gov/compound/Ergotamine.
28. Magness, J. (2018, March 4). LSD is “harmonizing” for the brain — and can change your personality for years, studies find. Miami Herald. Retrieved from miamiherald.com/news/nation-world/ article203341684.html.
29. Fuentes, J. J., Fonseca, F., Elices, M., Farré, M., & Torrens, M. (2020). Therapeutic use of LSD in psychiatry: A systematic review of randomizedcontrolled clinical trials. Frontiers in Psychiatry, 10. doi:10.3389/fpsyt.2019.00943.
30. Nichols, D., Johnson, M., & Nichols, C. (2016). Psychedelics as medicines: An emerging new paradigm. Clinical Pharmacology & Therapeutics, 101(2), 209–219. doi:10.1002/ cpt.557.
31. Anderson, A. (2016, April 13). LSD may chip away at the brain’s “sense of self” network. Scientific American. Retrieved from scientificamerican.com/ article/lsd-may-chip-away-at-the-brain-s-sense-ofself-network. 32. American Chemical Society. (2017, October 2). Psilocybin. American Chemical Society. Retrieved from acs.org/content/acs/en/molecule-of-the-week/ archive/p/psilocybin.html.
33. Mammoser, G. (2019, February 12). Mushrooms as medicine? Psychedelics may be next breakthrough treatment. Healthline. Retrieved from healthline.com/ health-news/benefits-of-medical-mushrooms.
34. Nichols, D. (2020). Psilocybin: From ancient magic to modern medicine. The Journal of Antibiotics, 73(10), 679–686. doi:10.1038/s41429-020-0311-8.
35. Nutt, D., & Carhart-Harris, R. (2021). The current status of psychedelics in psychiatry. JAMA Psychiatry, 78(2), 121–122. doi:10.1001/jamapsychiatry.2020.2171.
36. Daniel, J., & Haberman, M. (2017). Clinical potential of psilocybin as a treatment for mental health conditions. Mental Health Clinician, 7(1), 24–28. doi:10.9740/ mhc.2017.01.024.
37. Agin-Liebes, G., Malone, T., Yalch, M., Mennenga, S., Ponté, K.L., Guss, J., Bossis, A., Grigsby, J., Fischer, S., & Ross, S. (2020). Long-term follow-up of psilocybin-assisted psychotherapy for psychiatric and existential distress in patients with lifethreatening cancer. Journal of Psychopharmacology, 34(2), 155–166. doi:10.1177/0269881119897615.
38. Johnson, M., Garcia-Romeu, A., Cosimano, M., & Griffiths, R. (2014). Pilot study of the 5-HT2AR agonist psilocybin in the treatment of tobacco addiction. Journal of Psychopharmacology, 28(11), 983–992. doi:10.1177/0269881114548296.
39. Johnson, M., Garcia-Romeu, A., & Griffiths, R. (2016). Long-term follow-up of psilocybin-facilitated smoking cessation. The American Journal of Drug and Alcohol Abuse, 43(1), 55–60. doi:10.3109/00952990.20 16.1170135.
40. Vann Jones, S. A., & O’Kelly, A. (2020). Psychedelics as a treatment for Alzheimer’s disease dementia. Frontiers in Synaptic Neuroscience, 12. doi:10.3389/fnsyn.2020.00034.
41. Sessa, B., & Nutt, D. (2015). Making a medicine out of MDMA. British Journal of Psychiatry, 206(1), 4–6. doi:10.1192/bjp.bp.114.152751.
42. Wilcox, J. (2014). Psilocybin and obsessive compulsive disorder. Journal of Psychoactive Drugs, 46(5), 393–395. doi:10.1080/02791072.2014.963754.
43. Atasoy, S., Roseman, L., Kaelen, M., Kringelbach, M., Deco, G., & Carthart-Harris, R. (2017). Connectome-harmonic decomposition of human brain activity reveals dynamical repertoire reorganization under LSD. Scientific Reports, 7(1), 17661. doi:10.1038/s41598-017-17546-0.
44. Lugo-Radillo, A., MD, PhD, & Cortes-Lopez, J. L., MD (2020). Long-term Amelioration of OCD Symptoms in a Patient with Chronic Consumption of Psilocybin-containing Mushrooms. Journal of psychoactive drugs, 1–3. doi:10.1080/02791072.2020.1 849879.
45. Weiss, S. (2018, May 24). Here’s what MDMA did for my anxiety that meds couldn’t. Vice. Retrieved from vice.com/en/article/8xeqp4/psychedelicsmdma-versus-medicine-anxiety.
46. Weiss, S. (2021, January 14). How mixing mushrooms and MDMA affects your body and brain. Vice. Retrieved from vice.com/en/article/5dpkk8/ what-happens-when-you-mix-mdma-andmushrooms.
47. Lopez, G. (2016, November 30). Ecstasy could be available for medical use by 2021. Vox. Retrieved from vox.com/science-and-health/2016/11/30/13792958/ mdma-ecstasy-molly-study-fda.
48. Alcohol and Drug Foundation (Australia). (2020). MDMA-assisted therapy for PTSD edges closer. Alcohol and Drug Foundation. Retrieved from adf.org. au/insights/mdma-ptsd.
49. Bedi, G. (2018, March 28). Is psychiatry ready for medical MDMA?. The Conversation. Retrieved from theconversation.com/is-psychiatry-ready-formedical-mdma-94105.
50. Amoroso, T. (2015). The psychopharmacology of ±3,4 methylenedioxymethamphetamine and its role in the treatment of posttraumatic stress disorder. Journal of Psychoactive Drugs, 47(5). doi:10.1080/027 91072.2015.1094156. 51. Butler, G. (2021, March 5). Study reveals more people are using psychedelics to self-treat mental health. Vice. Retrieved from vice.com/en/article/ g5b7x9/study-reveals-more-people-are-usingpsychedelics-to-self-treat-mental-health.
52. Lamkin, M. (2019, July 31). Psychedelic medicine is coming. The law isn’t ready. Scientific American. Retrieved from blogs.scientificamerican.com/ observations/psychedelic-medicine-is-coming-thelaw-isnt-ready.
DEPRESSION AND ANXIETY: THE FRENEMIES NO COLLEGE STUDENT WANTS TO MEET
1. Tjornehoj, T. (2016). The relationship between anxiety and depression. Hartgrove Behavioral Health Systems. http://www.hartgrovehospital.com/ relationship-anxiety-depression/
2. National Institute of Mental Health (2017) Generalised Anxiety Disorder https://www.nimh.nih. gov/health/statistics/generalized-anxiety-disorder. shtml
3. National Institute of Mental Health. (2019). Major depression. https://www.nimh.nih.gov/health/ statistics/major-depression.shtml
4. Zhou, Y., Cao, Z., Yang, M., Xi, X., Guo, Y., Fang, M., Cheng, L., & Du, Y. (2017). Comorbid generalized anxiety disorder and its association with quality of life in patients with major depressive disorder. Scientific reports, 7(1), 1-8. doi:10.1038/srep40511
5. American Psychological Association. (2013). College students’ mental health is a growing concern, survey finds. APA, 44(6), 13. https://www.apa.org/ monitor/2013/06/college-students
6. Jenkins, P. E., Ducker, I., Gooding, R., James, M. & RutterEley, E. (2020) Anxiety and depression in a sample of UK college students: a study of prevalence, comorbidity, and quality of life. Journal of American College Health. 1940- 3208 doi: doi:10.10 80/07448481.2019.1709474
7. Dupuy, J. & Ladouceur, R. (2008). Cognitive processes of generalized anxiety disorder in comorbid generalized anxiety disorder and major depressive disorder. Journal of Anxiety Disorders, 22(3), 505-514. doi:10.1016/j.janxdis.2007.05.010
8. Kalueff, A. V., & Nutt, D. J. (2007). Role of GABA in anxiety and depression. Depression and Anxiety, 24(7), 495-517. doi:10.1002/da.20262
9. Nuss P. (2015). Anxiety disorders and GABA neurotransmission: a disturbance of modulation. Neuropsychiatric disease and treatment, 11, 165–175. doi:10.2147/NDT.S58841
10. Luscher, B., Shen, Q. & Sahir, N. The GABAergic deficit hypothesis of major depressive disorder. Mol Psychiatry 16, 383–406 (2011). doi:10.1038/mp.2010.120
11. Schmitz, T.W., Correia, M., Ferreira, C., Prescot, A., & Anderson, M.C. (2017). Hippocampal GABA enables inhibitory control over unwanted thoughts. Nature communications, 8(1), 1-12. doi:10.1038/s41467-01700956-z
12. Coplan, J. D., Aaronson, C. J., Panthangi, V. & Kim, Y. (2015). Treating comorbid anxiety and depression: Psychosocial and pharmacological approaches. World journal of psychiatry, 5(4), 366–378. doi:10.5498/wjp. v5.i4.366
13. Gorka, S. M., Young, C. B., Klumpp, H., Kennedy, A. E., Francis, J., Ajilore, O., Langenecker, S. A., Shankman, S. A., Craske, M. G., Stein, M. B., & Phan, K. L. (2019). Emotion-based brain mechanisms and predictors for SSRI and CBT treatment of anxiety and depression: a randomized trial. Neuropsychopharmacology : official publication of the American College of Neuropsychopharmacology, 44(9), 1639–1648. doi:10.1038/s41386-019-0407-7
14. Cowen, P. J., & Browning, M. (2015). What has serotonin to do with depression?. World Psychiatry, 14(2), 158. doi:10.1002/wps.20229
15. Garber, J., & Weersing, V. R. (2010). Comorbidity of anxiety and depression in youth: Implications for treatment and prevention. Clinical Psychology: Science and Practice, 17(4), 293-306. doi:10.1111/ j.1468-2850.2010.01221.x
16. Hirschfeld R. M. (2001). The Comorbidity of Major Depression and Anxiety Disorders: Recognition and Management in Primary Care. Journal of clinical psychiatry, 3(6), 244–254. doi:10.4088/pcc.v03n0609
BRAIN BUSTERS
1. Baik, J. (2013). Dopamine signaling in rewardrelated behaviors. Frontiers in Neural Circuits, 7(152). doi:10.3389/fncir.2013.00152
2. Beaty, R. E., Kenett, Y. N., Christensen, A. P., Rosenberg, M. D., Benedek, M., Chen, Q., Fink, A., Qiu, J., Kwapil, T. R., Kane, M. J., & Silvia, P. J. (2018). Robust prediction of individual creative ability from brain functional connectivity. Proceedings of the National Academy of Sciences of the United States of America, 115(5), 1087–1092. https://doi.org/10.1073/ pnas.1713532115
3. Belujon, P., & Grace, A. A. (2017). Dopamine system dysregulation in major depressive disorders. The International Journal of Neuropsychopharmacology, 20(12), 1036–1046. doi:10.1093/ijnp/pyx056.
4. Berridge, K.C., Venier, I.L., & Robinson, T.E. (1989). Taste reactivity analysis of 6-hydroxydopamineinduced aphagia: implications for arousal and anhedonia hypotheses of dopamine function. Behav Neurosci, 103(1), 36-45. doi:10.1037/0735-7044.103.1.36.
5. Beyerstein, B.L. (1999). “Whence cometh the myth that we only use ten percent of our brains?” In, S. Della Sala (Ed.), Mind Myths: Exploring Popular Assumptions About the Mind and Brain (pp. 1-24). John Wiley and Sons., Ltd.
6. Carnegie, D. (1936). How to win friends and influence people. Simon & Schuster.
7. Cools R. (2008). Role of dopamine in the motivational and cognitive control of behavior. Neuroscientist, 14(4):381-95. doi: 10.1177/1073858408317009.
8. Diamond, M.C., Scheibel, A.B., Murphy Jr., G.M., & Harvey, T. (1985). On the brain of a scientist: Albert Einstein. Experimental Neurology, 88(1), 198-204. doi:10.1016/0014-4886(85)90123-2.
9. Herculano-Houzel S. (2002). Do you know your brain? A survey on public neuroscience literacy at the closing of the decade of the brain. Neuroscientist, 8(2), 98-110. doi:10.1177/107385840200800206.
10. Higbee, K.L., & Clay, S.L. (1998). College students’ beliefs in the ten-percent myth. Journal of Psychology, 132, 469-476, 471. doi:10.1080/00223989809599280
11. James, W. (1907). The energies of men. The Philosophical Review, 16(1), 1-20. doi:10.2307/2177575.
12. Lilienfeld, S. O., Lynn, S. J., Ruscio, J., & Beyerstein, B. L. (2010). The top ten myths of popular psychology. Skeptic [Altadena, CA], 15(3), 36+. Retrieved from https:// link.gale.com/apps/doc/A220057908/ AONE?u=bron88970&sid=AONE&xid=2bc454c6.
13. Manning, L., & Thomas-Antérion, C. (2011). Marc Dax and the discovery of the lateralisation of language in the left cerebral hemisphere. Rev Neurol (Paris). 167(12), 868-72. doi:10.1016/j.neurol.2010.10.017. PMID: 21640366.
14. Nielsen, J. A., Zielinski, B. A., Ferguson, M. A., Lainhart, J. E., & Anderson, J. S. (2013). An evaluation of the left-brain vs. right-brain hypothesis with resting state functional connectivity magnetic resonance imaging. PLOS ONE, 8(8). doi:10.1371/ journal.pone.0071275.
15. Olds, J., & Milner, P. (1954). Positive reinforcement produced by electrical stimulation of septal area and other regions of rat brain. Journal of Comparative and Physiological Psychology, 47(6), 419-427. doi:10.1037/h0058775.
16. Pinho, A. L., Ullén, F., Castelo-Branco, M., Fransson, P., & De Manzano, Ö. (2015). Addressing a paradox: dual strategies for creative performance in introspective and extrospective networks. Cerebral Cortex, 26(7), 3052-3063. doi:10.1093/cercor/bhv130.
17. Sperry, R. (1982). Some effects of disconnecting the cerebral hemispheres. Science, 217(4566), 12231226.
18. Sun, F. (2011). All-TIME Nonfiction Books. TIME Magazine.
19. von Bartheld, C. S., Bahney, J., & HerculanoHouzel, S. (2016). The search for true numbers of neurons and glial cells in the human brain: A review of 150 years of cell counting. The Journal of Comparative Neurology, 524(18), 3865–3895. doi:10.1002/cne.24040. 20. Wise, R.A. & Robble, M.A. (2020). Dopamine and addiction. Annual Review of Psychology, 71:79-106. doi:10.1146/annurev-psych-010418-103337.
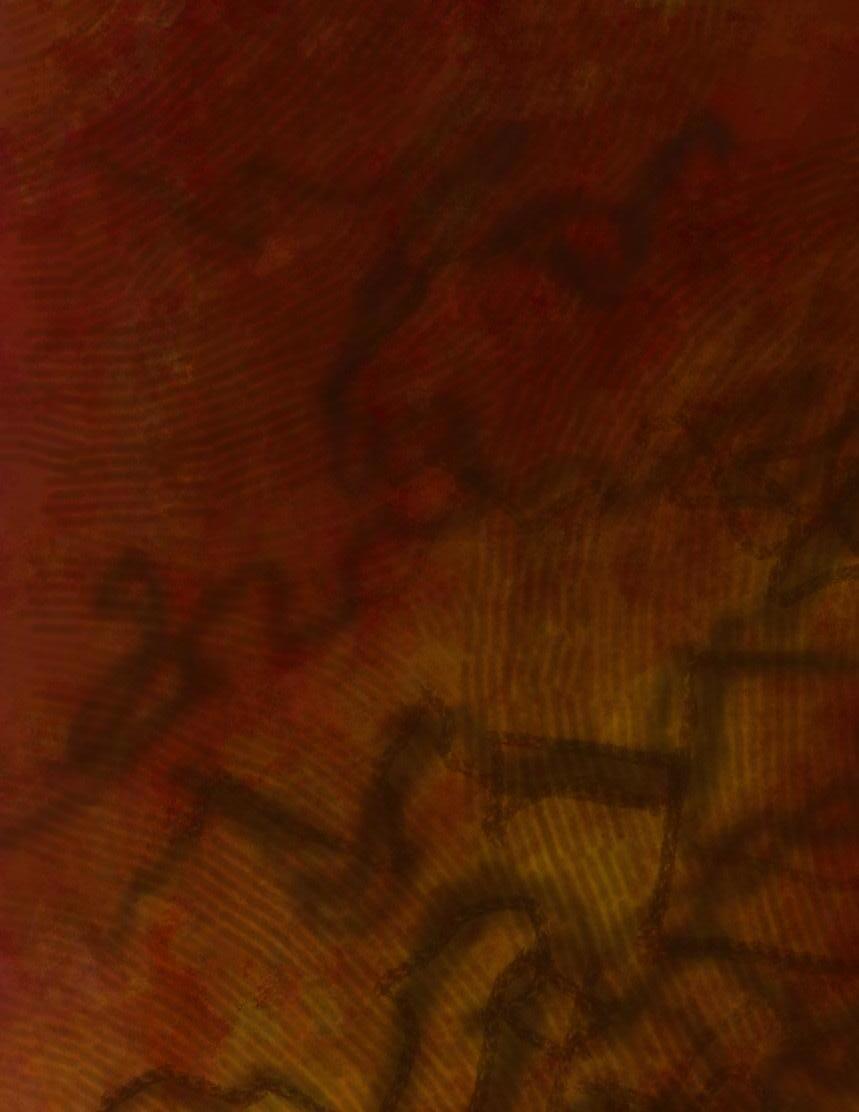
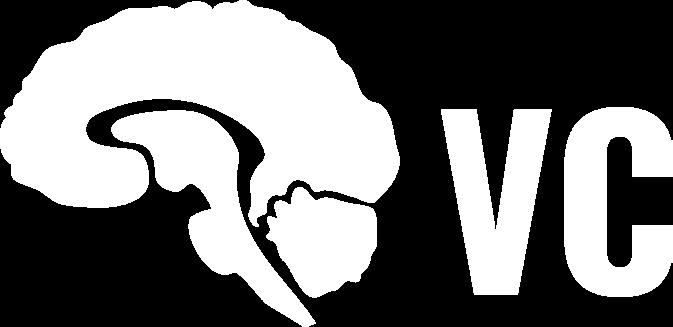