5 minute read
Drug Discovery for Space Radiation Using iPSCs and Clinical Trials
in Dish
[Xu Cao (Stanford Cardiovascular Inst., Stanford University School of Medicine, CA, USA and Dept. of Medicine, Division of Cardiology, Stanford University School of Medicine, CA, USA) , Michael M. Weil (Dept. of Environmental and Radiological Health Sciences, Colorado State University, CO, USA), Joseph C. Wu (Stanford Cardiovascular Inst., Stanford University School of Medicine, CA, USA / Dept. of Medicine, Division of Cardiology, Stanford University School of Medicine, CA, USA / Dept. of Radiology, Stanford University School of Medicine, CA, USA)]
Introduction
Space radiation exposure presents a significant challenge for astronauts during long-duration missions. The risks include increased cancer risk, cardiovascular dysfunction, central nervous system impairment, and other health problems, which are primarily due to high-energy, high charge (HZE) ions that can easily penetrate spacecraft shielding (Chancellor et al., 2014). Understanding the health effects of HZE ions is an important goal of space radiobiology as they create dense ionization tracks in living tissue known as high-linear energy transfer (LET). Health risks of space radiation remain poorly understood, with most insight based on studies of the effects of ground-based space radiation analogs and limited flight research. Drugs that can protect astronauts from both rare high-dose solar particle event (SPE) radiation and constant lowdose galactic cosmic rays (GCRs) are urgently needed. To understand the effects of space radiation on human health, it is important to study individual susceptibility and radio-sensitivity of different tissue types, which can be challenging to achieve through existing animal models. The advent of human induced pluripotent stem cells (iPSCs) and their use in preclinical drug development, known as «clinical trials in dish» (CTD), offers a new opportunity to study radiation biology and develop radioprotective countermeasures for extended space travel (Figure 1)
Human iPSCs as ideal cell source for CTD
The cost of developing a new FDA-approved drug is high, with 80% of the cost being spent on clinical trials and FDA approvals (DiMasi et al., 2016). CTD provides a tool to test candidate drugs on patient-specific cells before moving to clinical trials and can be valuable for assessing individual susceptibility to radiation exposure and designing personalized medicine. CTD requires high-quality patient-specific cell types, which has become more feasible with iPSCs. Human iPSCs have the ability to provide an unlimited source of autologous cells for cellular therapies, generate human genetic disease models using genome edi- ting techniques, provide patient-specific cells for drug screening, and predict individual disease risks and drug responses, making them a valuable tool for drug development (Sayed et al., 2016). More than 80% of drugs fail in clinical trials due to toxicity or lack of efficacy, highlighting the need for more efficient systems to better predict patients’ drug responses. Immortalized cell lines and animal models have limited success due to poor relevance to human physiology and pathophysiology. Progress in developing well-validated and standardized protocols for generating various cell types from iPSCs is expected to lead to the increased use of iPSCs derived cells and engineered tissues in preclinical drug development. Patient-specific iPSCs, which carry the same genetic information as donors, can be used to evaluate personalized countermeasures and reduce the cost and improve the efficiency of clinical trials by categorizing patients as drug responders and non-responders (Inoue et al., 2014). Additionally, the use of expression quantitative trait locus (eQTL) analysis can identify drug response-related genes and single nucleotide polymorphisms (SNPs) as biomarkers to predict individual risks and responses (Rong et al., 2021).
Space radiation effects: lessons learned from conventional cell models
In vitro and ex vivo studies in human cells and tissues are necessary for extrapolating risks of radiation injuries from animal models to humans and understanding inter-individual heterogeneity in radiation responses. Despite lacking genetic diversity and physiologically relevant environment, primary cell types like blood, endothelial cells, and fibroblasts provide a cost-effective platform for understanding human responses to radiation and screening candidate countermeasures, although low-LET radiation (e.g., x-rays and gamma rays) used in most studies compromise their translational value to space radiation.
Delineating radiation effects and developing countermeasures with CTD
Primary and immortalized cell lines are suitable for in vitro and ex vivo radiation assays at the initial stage of drug development but lack the genetic diversity of patient populations and the physiological complexity of tissues. Cells and engineered tissues derived from iPSCs are promising platforms for preclinical drug development that can reduce time and cost. The ability of iPSCs to differentiate into desired lineages allows the study of radiation effects on various cell types from diverse patients, which could be used for developing individualized radiation countermeasures. However, most studies using iPSC models have primarily concentrated on simulating human exposure to radiation rather than conducting drug screening or discovery. More work is needed to translate iPSC radiation models into high-throughput drug screening assays and to study genetic contributions to radiation-induced damage.
Moving toward 3D tissue engineering
The translation of preclinical studies using CTD into clinical applications depends on the development of iPSC-derived models with radiation-related phenotypes that can replicate cell-cell interactions and 3D environments in human tissues/organs, which can be achieved with recent advances in co-culture systems, 3D tissue engineering, organoids, and microphysiological systems (MPS) (Cho et al., 2021; Kim et al., 2022; Thomas et al., 2021). Although there are a limited number of radiation studies published using engineered human tissues derived from iPSCs, more studies have been published using 3D tissue models made from human primary or immortalized cells. Recent advancements of radiation studies using tissue engineering and their potential applications in space radiation countermeasure discovery are summarized in this section (Figure 2).
Conclusions
CTD provides a unique opportunity to discover space radiation countermeasures based on human systems, since iPSCs can be obtained from different individuals with diverse genetic backgrounds to predict individual drug responses within a large population. CTD allows for the study of radiation responses of human cells in 2D and 3D models, with 2D models being ideal for cost-effective assays at the initial stage of drug discovery and 3D models better resembling the in vivo physiological environment in a lower throughput secondary screens. The application of CTD in radiation drug discovery is still in its early stages. However, a number of studies have been conducted to understand human-specific radiation injuries and test specific drug candidates using various human primary cell types or iPSC-derivatives, providing evidence of the great value of human in vitro cell models for space radiation drug discovery (Figure 2). Last but not least, efforts are needed to simulate simultaneous exposure to microgravity and radiation using random positioning or clinostat within a radiation environment, potentially using 2D/3D cell models adapted for CTD. The ultimate goal is the development of countermeasures for the effects induced by simultaneous exposure to both factors.
Acknowledgment
This work was supported by National Institutes of Health (NIH) R01 HL123968, R01 HL146690, UH3 TR002588, and P01 HL141084 (JCW), and The Translational Research Institute for Space Health (TRISH) NNX16AO69A (MMW).
References
Chancellor, J., Scott, G., Sutton, J., 2014. Space Radiation: The Number One Risk to Astronaut Health beyond Low Earth Orbit. Life 4, 491–510. https://doi. org/10.3390/life4030491
Cho, S., Lee, C., Skylar-Scott, M.A., Heilshorn, S.C., Wu, J.C., 2021. Reconstructing the heart using iPSCs: Engineering strategies and applications. J Mol Cell Cardiol 157, 56–65. https://doi.org/10.1016/j. yjmcc.2021.04.006
DiMasi, J.A., Grabowski, H.G., Hansen, R.W., 2016. Innovation in the pharmaceutical industry: New estimates of R&D costs. J Health Econ 47, 20–33. https:// doi.org/10.1016/j.jhealeco.2016.01.012
Inoue, H., Nagata, N., Kurokawa, H., Yamanaka, S., 2014. iPS cells: a game changer for future medicine. Embo J 33, 409–17. https://doi.org/10.1002/embj.201387098 Kim, H., Kamm, R.D., Vunjak-Novakovic, G., Wu, J.C., 2022. Progress in multicellular human cardiac organoids for clinical applications. Cell Stem Cell 29, 503–514. https://doi.org/10.1016/j.stem.2022.03.012
Rong, Y., Dong, S.-S., Hu, W.-X., Guo, Y., Chen, Y.-X., Chen, J.-B., Zhu, D.-L., Chen, H., Yang, T.-L., 2021. DDRS: Detection of drug response SNPs specifically in patients receiving drug treatment. Comput Struct Biotechnology J 19, 3650–3657. https://doi.org/10.1016/j. csbj.2021.06.026
Sayed, N., Liu, C., Wu, J.C., 2016. Translation of Human-Induced Pluripotent Stem Cells: From Clinical Trial in a Dish to Precision Medicine. J Am Coll Cardiol 67, 2161–76. https://doi.org/10.1016/j.jacc.2016.01.083
Thomas, D., Kim, H., Lopez, N., Wu, J.C., 2021. Fabrication of 3D Cardiac Microtissue Arrays using Human iPSC-Derived Cardiomyocytes, Cardiac Fibroblasts, and Endothelial Cells. J Vis Exp Jove. https://doi. org/10.3791/61879
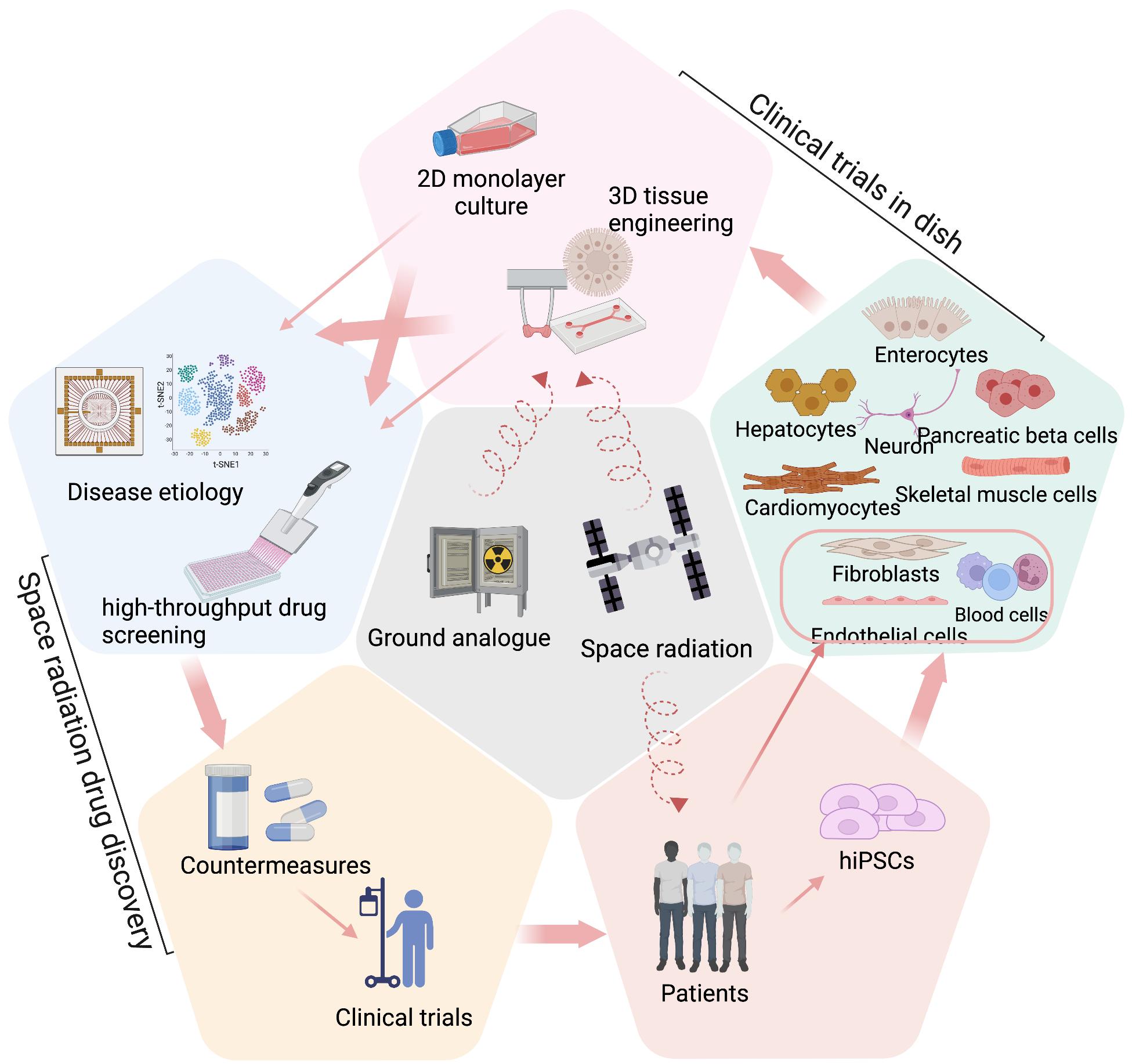