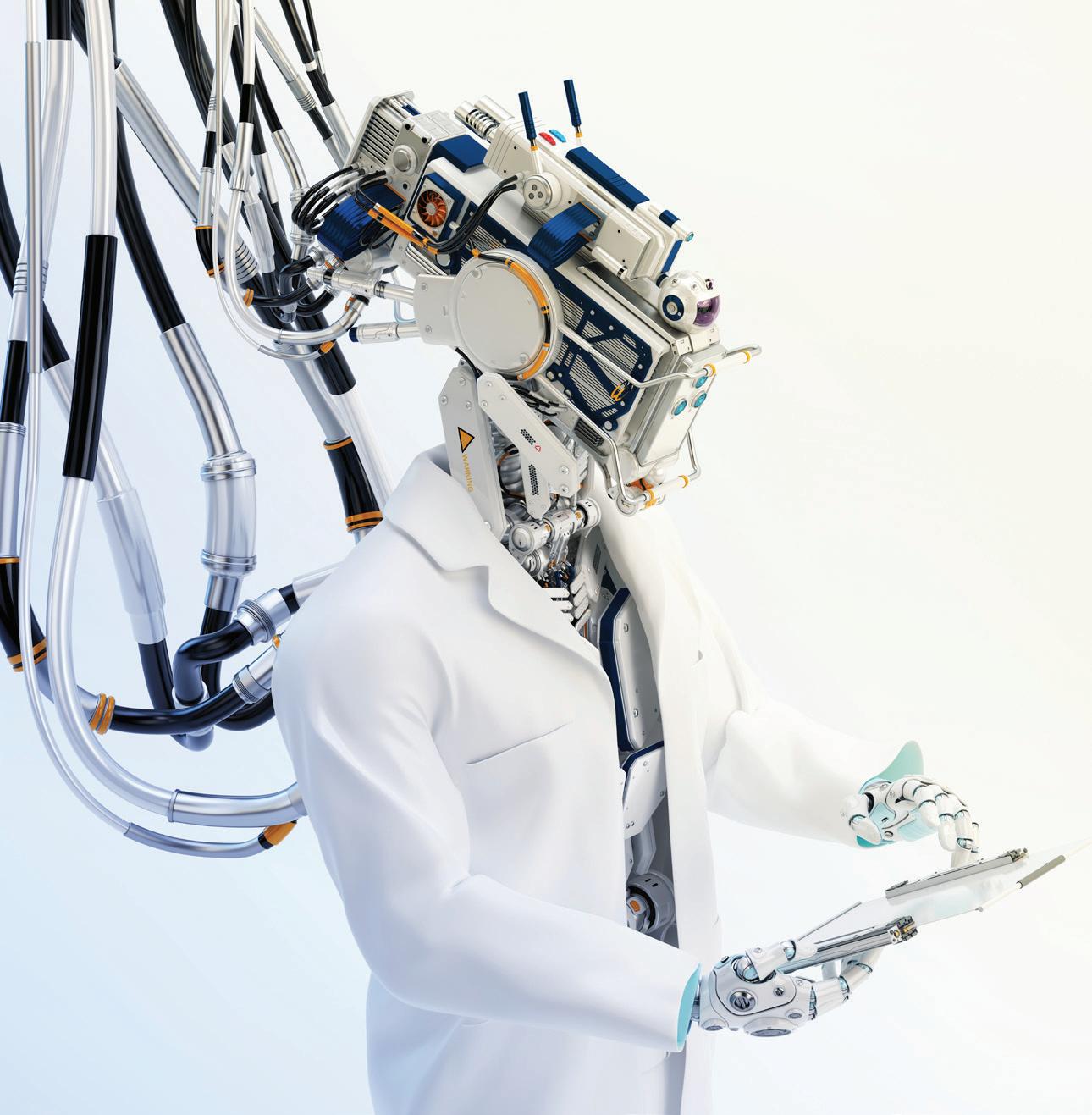
14 minute read
RESEARCH IN MEDICAL ROBOTICS
What is a robot?
The word “robot” was used by the Czech novelist Karel Čapek in his 1920 play entitled R.U.R. (Rossum’s Universal Robot). “Robot” in Czech is a word for a worker or servant.
Advertisement
“A robot is a reprogrammable, multifunctional manipulator designed to move material, parts, tools, or specialized devices through variable programmed motions for the performance of a variety of tasks.” – Robot Institute of America, 1979.
“Such a definition leaves out tools with a single task (e.g., a stapler), anything that cannot move (e.g., image analysis algorithms), and all nonprogrammable mechanisms (e.g., purely manual laparoscopic tools). As a result, robots are generally indicated for tasks requiring programmable motions, particularly where those motions are to be quick, strong, precise, accurate, untiring, and/or made via complex articulations.” (Beasley, 2012)
We also have the idea that a robot looks like a human being, but this kind of robot is better known as an android, a robot with a human appearance. Androids are among the few robots available to help humans with their daily activities.
robotics:
The field of robotics stands at the intersection of science, engineering, and the technology that produces machines, called robots, that can act as substitutes for (or replicate) human actions.
As technology progresses, so too does the scope of what may be considered robotics. In 2005, 90% of all robots could be found assembling cars in automotive factories. These robots consisted mainly of mechanical arms tasked with welding or screwing on certain parts of a car. Today, we have seen an evolved and expanded definition of robotics, which includes the development, creation, and use of “bots” that can explore the earth’s harshest environments, of robots that assist lawenforcement, and even of robots that assist in almost every facet of healthcare.
The laws of robotics
In his book I, Robot, Isaac Asimov proposed three “Laws of Robotics ” and later added the “zeroth law” (Asimov, 1950).
+ Law 0: A robot may not injure humanity or, through inaction, allow humanity to come to harm.
+ Law 1: A robot may not injure a human being or, through inaction, allow a human being to come to harm, unless this would violate a law of a higher order.
+ Law 2: A robot must obey orders given to it by human beings, except where such orders would conflict with a law of a higher order. + Law 3: A robot must protect its own existence, as long as such protection does not conflict with a law of a higher order.
The “laws” described above are fiction and are to be taken as such, but they may be useful and should perhaps be taken into account when we begin to think about the artificial intelligence (AI) that is being
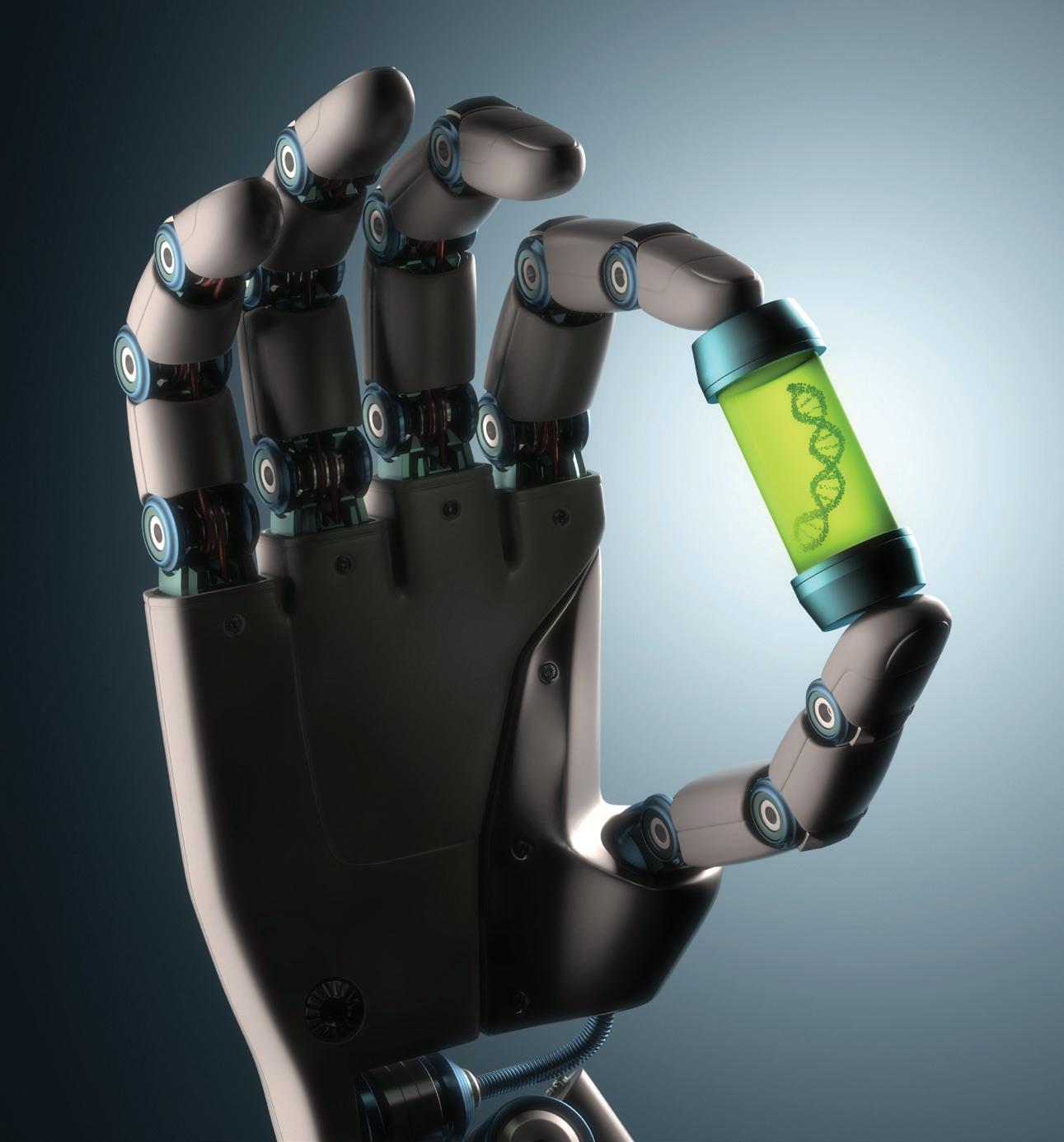
Fuente: https://upload.wikimedia.org/wikipedia/en/d/ d5/I_robot.jpg
developed as a tool to help human beings in their daily activities.
Bearing this in mind, we can devise countless useful activities where robots could help human beings, as described below (adapted from Mendes, 2014):
•Dirty tasks. In the manufacturing sector, many factory jobs are messy or dirty. Dirty tasks may include welding, grinding, molding, and casting. When robots are used to perform these tasks, they enable human workers to partake in more meaningful and creative pursuits.
•Repetitive tasks. Robots are reliable workers. They do not feel emotions and therefore do not feel worthless when performing menial tasks. For most people, the repetitive tasks performed in much industrial work are considered very dull or boring. For example, a robot’s only task may be to pick up an object from a conveyor belt and place it in a box. The robot can perform this task all day, every day, without getting “bored.” Other examples are packaging, lifting heavy objects, and installing parts in manufacturing.
•Dangerous tasks. In manufacturing, robots often perform tasks which are very dangerous for human beings. For example, using robots for tasks involving extreme temperatures reduces the risk of workplace accidents. Apart from these dangerous tasks in manufacturing, robots are also used to carry out important but perilous activities such as clearing out landmines, helping in rescue missions, or mopping up toxic leaks. Police robots are used to defuse and eliminate explosive devices. Sometimes, the police may have to detonate a device on site. Some robots are so tough that they can survive multiple blasts. •Impossible tasks. Working deep under water, exploring live volcanoes up close, or travelling to faraway planets are tasks that are simply impossible for humans to execute. Robots are often called upon to perform underwater salvage missions in order to find sunken ships or planes. In 1985, a team of researchers and a robot called Jason Junior were able to locate the wreck of the Titanic. Underwater robots operating a kilometer and a half under the ocean’s surface played a vital role in the fight to stop oil gushing into the Gulf of Mexico.
•Assistive and caregiving tasks. The world’s most sophisticated robots are now designed to support our surging population of elderly and disabled citizens. There is a range of household robots that can understand verbal instructions and help with any household chore. They can also assist disabled people with the use and control of artificial limbs. Finally, robots can help in the medical profession, assisting surgeons to perform operations.
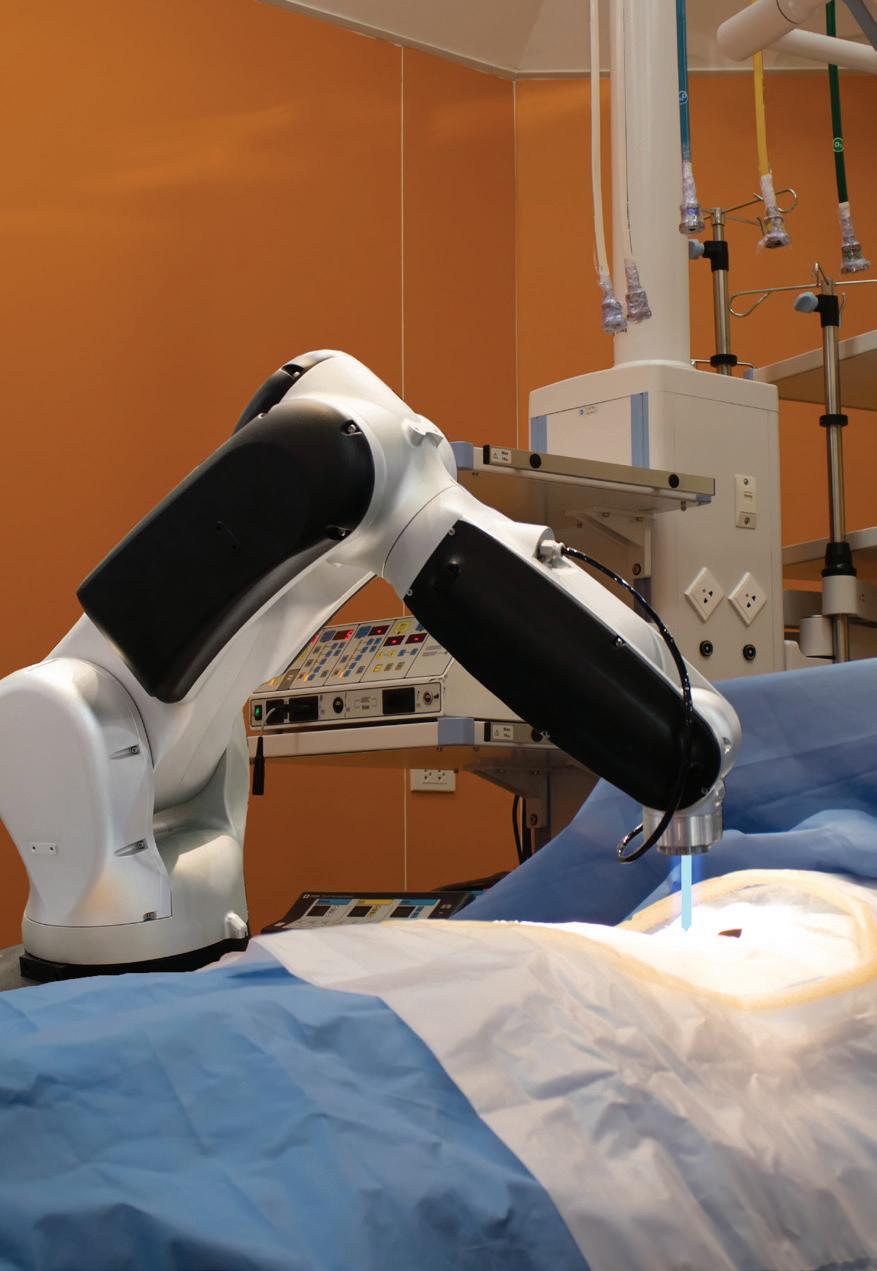
Why is robotics important?
As we have seen above, robotics is an emerging field with applications in many facets of our lives. It is important for all members of society to have an understanding of the technology that surrounds us. Robotics, however, is important for more than that reason. Robotics provides a unique combination of the pillars of STEM: science, technology, engineering, and math. When taught in schools, it allows students to explore a truly interdisciplinary field while studying an exciting cutting-edge subject. At the same time, the esthetics that go into the design and creation of robots allow students to experiment with their artistic sides, even as they work through technical principles. This combination rewards participants on a plethora of different learning levels.
robots in medicine
According to a recent report by Credence Research, the global medical robotics market was valued at US$7.24 billion in 2015 and is expected to grow to US$20 billion by 2023. A key driver for this growth is demand for using robots in minimally invasive surgeries, especially for neurologic, orthopedic, and laparoscopic procedures. As a result, wide ranges of robots are being developed to serve in a variety of roles within the medical environment. Robots specializing in human treatment include surgical robots and rehabilitation robots. The field of assistive and therapeutic robotic devices is also expanding rapidly. These include robots that help patients rehabilitate from serious conditions such as strokes, empathic robots that assist in
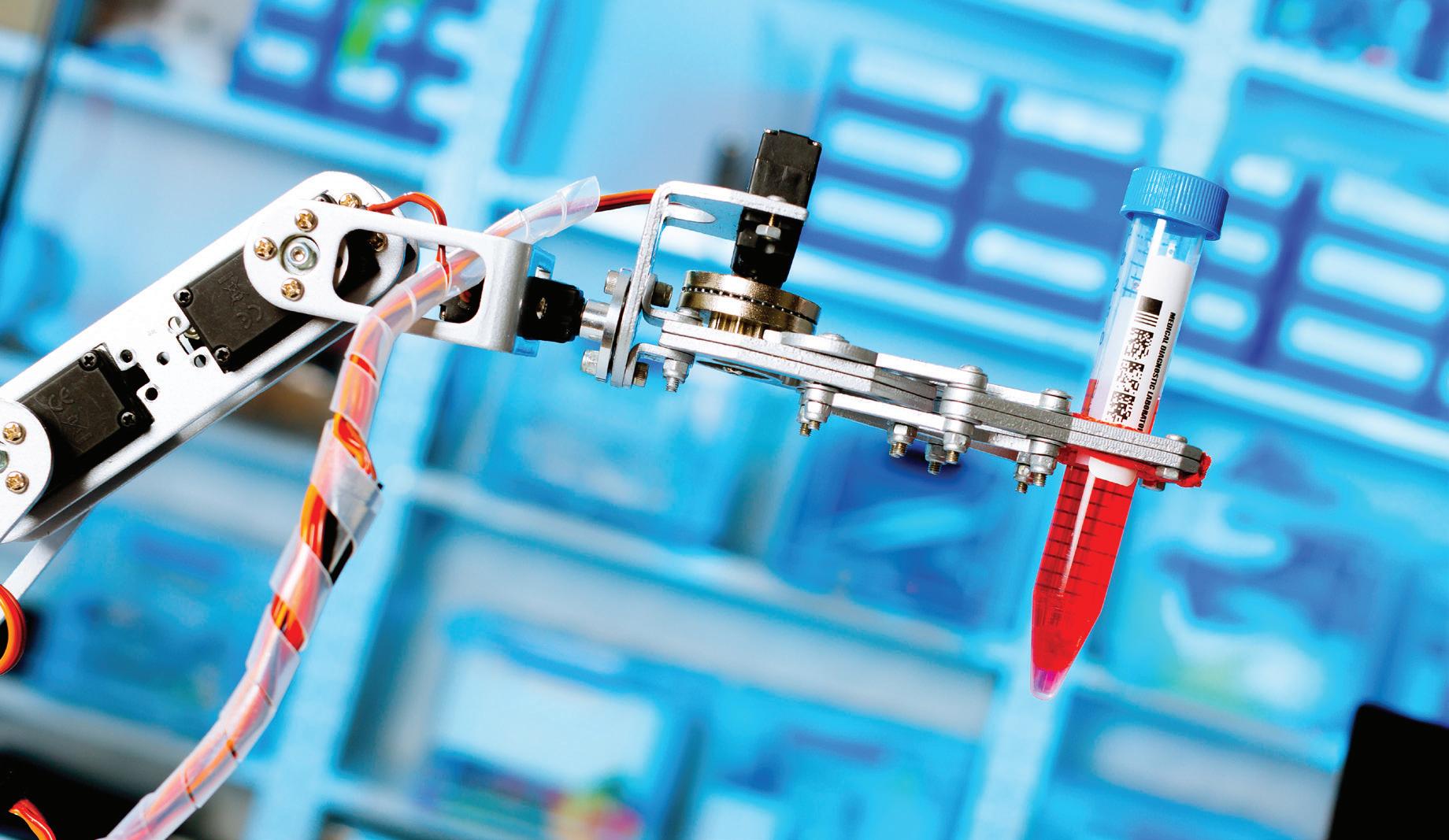
the care of older or physically/mentallychallenged individuals, and industrial robots that take on a variety of routine tasks, such as sterilizing rooms and delivering medical supplies and equipment, including medications (Crawford, 2016; Credence Research, 2016).
Medical robots have been reviewed in various papers since the 1990s. Many such reviews are domain-specific, focusing, for example, on surgical robots, urological robots, spine robots, and so on. We shall attempt to make a comprehensive review of those already available, according to therapeutic area, in order to provide an overview of these technologies and their uses in treating patients.
• Neurological
Brain surgery involves accessing a buried target surrounded by delicate tissue, a task that benefits from the ability of robots to make precise and accurate motions based on medical images. Thus, the first published account of the use of a robot in human surgery was in 1985. The robot was used for a brain biopsy using a computed tomography (CT) image and a stereotactic frame. In 1991, the Minerva robot (University of Lausanne, Switzerland) was designed to direct tools into the brain under real-time CT guidance.
Another robotic system, Pathfinder (Prosurgics, formerly Armstrong Healthcare Ltd.) allows the surgeon to specify a target and trajectory on a pre-operative medical image, while the robot guides the instrument into position with submillimeter accuracy. Reported uses of the system include guiding needles for biopsies and guiding drills to make burr holes.
•Orthopedics
The expected benefit of robot assistance in orthopedics is accurate and precise bone resection. Through good bone resection, robotic systems can improve the alignment of implant with bone and increase the contact area between implant and bone, both of which may improve functional outcomes and implant longevity. Orthopedic robots have so far targeted the hip and knee for replacements or resurfacing. Initial systems required the bones to be fixed in place, and all systems use bone screws or pins to localize the surgical site.
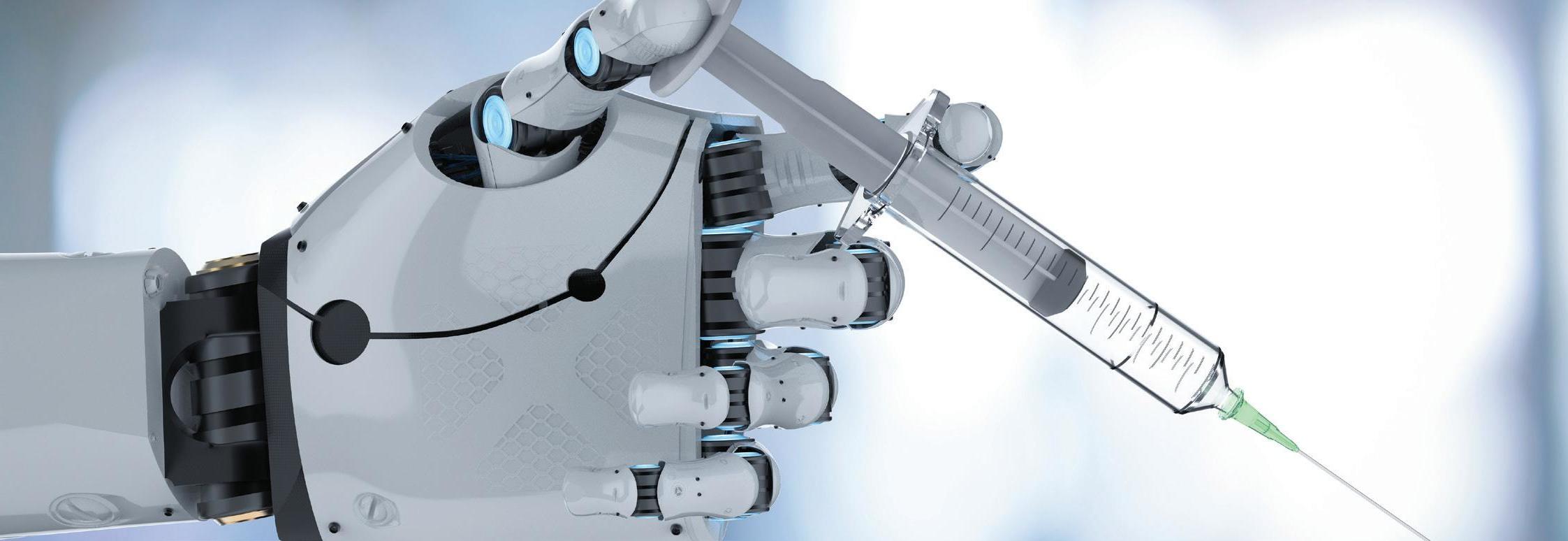
•General Laparoscopic
Robot assistance for soft-tissue surgery was first done in 1988, using an industrial robot to actively remove soft tissue during transurethral resection of the prostate. As with neurosurgery, the researchers deemed use of an industrial robot in the operating room to be unsafe. The experience provided the impetus for a research system, Probot, with the same purpose.
In 1995, Computer Motion combined two tool-holding robot arms with Aesop to create the Zeus system (since discontinued). The Zeus’s tool arms were teleoperated, following motions the surgeon made with instrument controls (a.k.a. “master” arms or joysticks) at the surgeon console. Technically, the Zeus is not a robot, because it does not follow programmable motions, but rather a remote computer-assisted telemanipulator with interactive robotic arms. To improve precision in tool motion, the Zeus filters out hand tremor, and can also scale large hand motions by the surgeon down to short and precise motions by the tool.
Meanwhile, Intuitive Surgical Inc. was developing the da Vinci. Like the Zeus, the da Vinci is a teleoperated system, with the surgeon manipulating instrument controls at a console and the robot arms following those motions with motion scaling and tremor reduction. Like the Zeus, the da Vinci was initially designed with three arms and therefore able to hold two tools and an endoscope, mounted on a single bedside cart.
•Steerable Catheters
Vascular catheterization is used to diagnose and treat various cardiac and vasculature diseases, including direct pressure measurements, biopsy, ablation for atrial fibrillation, and angioplasty for obstructed blood vessels. The catheter is inserted into a blood vessel and the portion external to the patient is manipulated to move the catheter tip to the surgical site, while fluoroscopy provides image guidance. Owing to the supporting tissue, catheters typically require only three degrees of freedom: tip flexion, tip rotation, and insertion depth. The potential benefits of robot-steered catheters are shorter procedures, reduced forces exerted on the vasculature by the catheter tip, increased accuracy in catheter positioning, and teleoperation (which reduces the exposure of the physician to radiation).
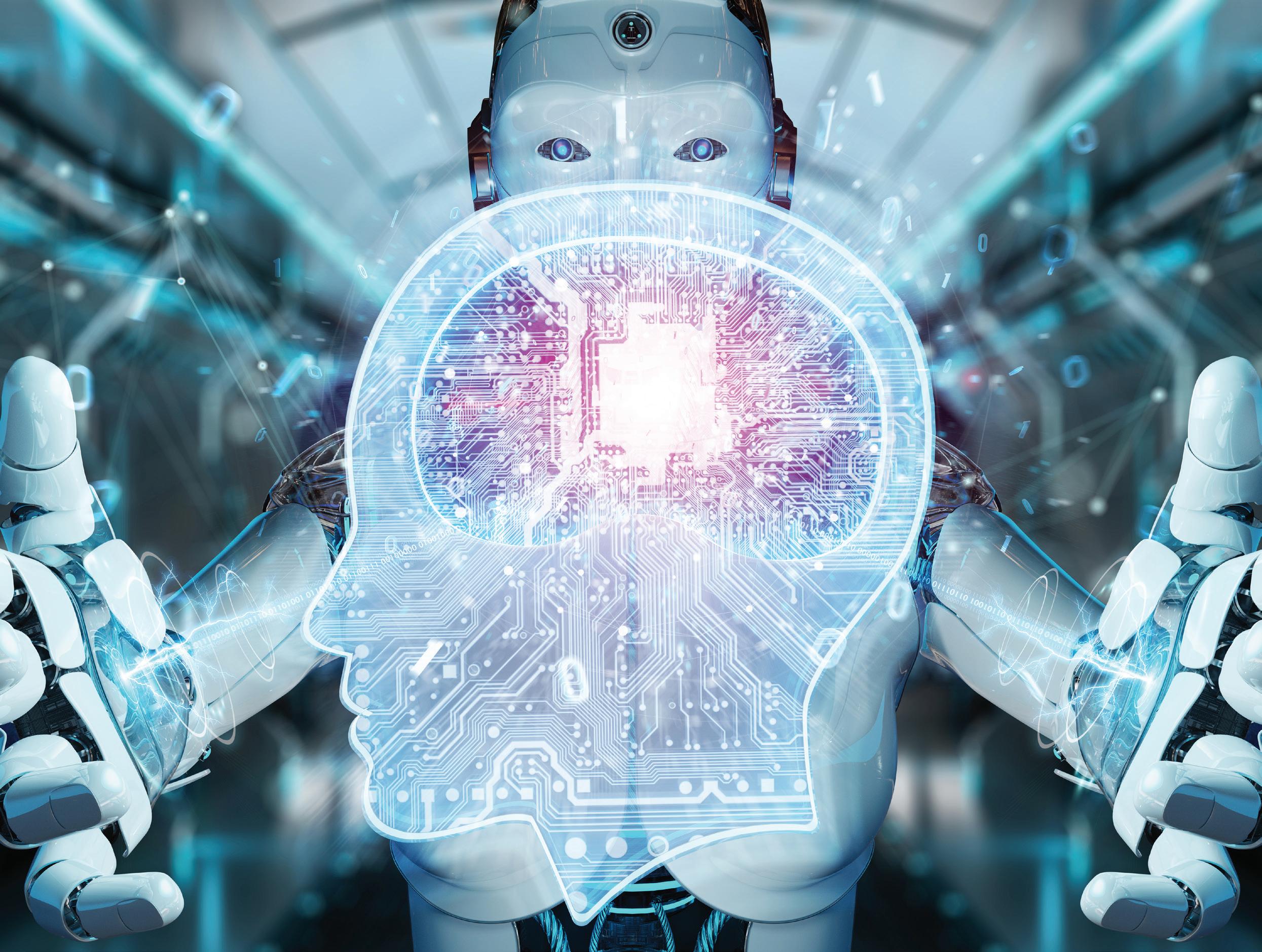
•Radiosurgery
Radiosurgery is a treatment (not a surgery), in which focused beams of ionizing radiation are directed at the patient, primarily to treat tumors. By directing the beam through the tumor at various orientations, high-dose radiation is delivered to the tumor while the surrounding tissue receives significantly less radiation. Prior to real time tissue tracking, radiosurgery was practically limited to treating the brain, using stereotactic frames mounted to the skull with bone screws. Now that real-time tissue tracking is feasible, systems are commercially available.
•Emergency Response
Few medical robot systems are suitable for use outside of the operating room, despite significant research funding on medical devices for disaster response and battlefield medicine. Typical goals for such research include improved ex-
traction of patients from dangerous environments, rapid diagnosis of injuries, and the semiautonomous delivery of lifesaving interventions. Current emergency response robots are little more than single-motor systems, but those systems can be controlled by health monitors to minimize the necessary attention by emergency responders. Such a feedback control makes it more likely that such systems will be autonomous, as for example automated external defibrillators.
•Prosthetics and Exoskeletons
Microprocessor-controlled prosthetics have been available since 1993, specifically the Intelligent Prosthesis knee (Chas. A. Blatchford & Sons, Ltd.). Several more microprocessor-controlled prosthetics exist today, predominantly for knee prosthetics, hand prosthetics, and exoskeletons.
• Assistive and Rehabilitation Systems
Assistive robotic systems are designed to allow people with disabilities more autonomy, covering a wide range of everyday tasks.
Current Research and Development in Medical Robotics
Medical robotics is a young and relatively unexplored field, made possible by technical improvements over the past couple of decades. Currently available systems have been available for too short a time to allow long-term studies. Nor are the benefits potentially provided by medical robots fully understood. Medical robots have only passed through a few technological generations, and the technology continues to change and leap into new areas. Yet by looking at the current market and representative research systems, educated guesses can be made about the impacts of robots on near-future medicine. Moreover, many more medical robots are currently being researched, and such research will lead to the new capabilities of future commercial systems.
Swallowable capsules take patient trauma reduction to an extreme, but current systems are limited to diagnostic uses. Core temperature measurement has been FDA-cleared since 1990, by CorTemp (HQ Inc., formerly HTI Technologies). More recently, capsule endoscopy systems, consisting of a forward-looking wide-angle camera taking regularly timed pictures, a battery, and lights, all contained in a capsule, utilize multiple sensors to measure pressure, pH level, gastric emptying time, and bowel emptying time. Sayaka (RF Co Ltd.) is a novel design, not yet FDA-cleared, which uses a lateral-facing camera that rotates inside the capsule to image the entire tract and is designed without a battery, relying instead on an externally applied magnetic field for inductive power supply. For the future, many enhancements have been proposed, including biopsy, real-time localization of the capsule, drug delivery, ultrasonic imaging, increasing motility by electrically inducing peristalsis, and utilizing an active locomotion system involving treads or legs.
In a more dramatic approach to in vivo robotics, micro/nanotechnology is a multibillion dollar area of research, including investigation for various medical robotic uses such as inexpensive directable drug delivery vehicles, radio-controlled biomolecules, tissue micromanipulation platforms, artificial mechanical white blood cells, and many other therapeutic approaches that may benefit
from robots working at the cellular level. Construction of functional systems is an ongoing area of research, particularly with respect to generating and powering motion. Many current prototypes are propelled and guided via magnetic fields, though some utilize external electrical energy sources. As far as we know, no clinical trials have been performed for any medical micro/nanorobot. In surgical robotics, there has been a trend away from autonomous or even semiautonomous motions toward synergistic manipulation and virtual fixtures. Thus, the robot acts as a guidance tool, providing information (and possibly a physical nudge) to keep the surgeon on target. Such use requires accurate localization of the tissues in the surgical site, even as the tissues are manipulated during surgery. Improved imaging systems (e.g., Explorer, an intraoperative soft tissue tracker by Pathfinder Therapeutics, or robot compatibility with MRI or CT will provide that loca-
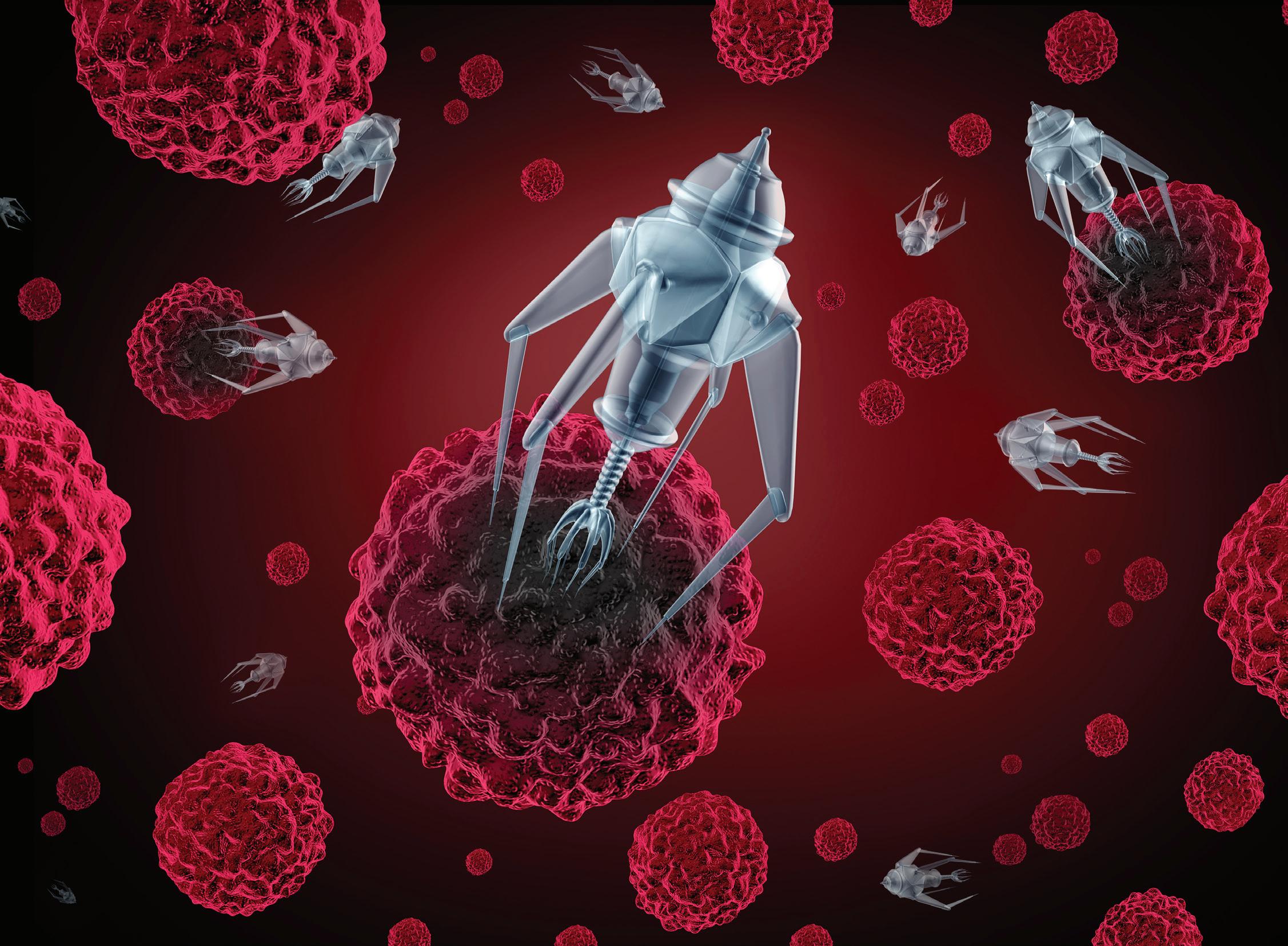
lization. In particular, MRI-guided robots will benefit from intraoperative 3D images with excellent soft tissue contrast and accurate registration between the tool and the tissue, thus allowing precise virtual fixtures, such as “snap-to” and “stand-off” behaviors. Furthermore, such imaging will allow modeling and rapid prototyping of patient-specific templates/jigs/implants. The physical designs for medical robots will continue to improve, reducing expense and size, while minimizing or compensating for non-idealities such as flexion, as in the case, for example, of the CRIGOS robot. With better physical designs, semiautonomous behavior will likely become more useful. “Macros” may become commonplace, wherein the surgeon presses a button and the robot performs a preprogrammed motion, such as passing a suture needle between graspers, or the Sensei’s auto-retract feature. Robots will see more use for medical training purposes, bolstered by improved tissue-modeling capabilities, by the increasing objectivity in healthcare assessment, by advances in computer simulations, and as a result of increased data mining, arising naturally from improved data connectivity between devices and between institutions. Some such systems are already available, such as the aforementioned da Vinci Skills Simulator, the Virtual I.V. Simulator by Laerdal, and the EndoscopyVR Surgical
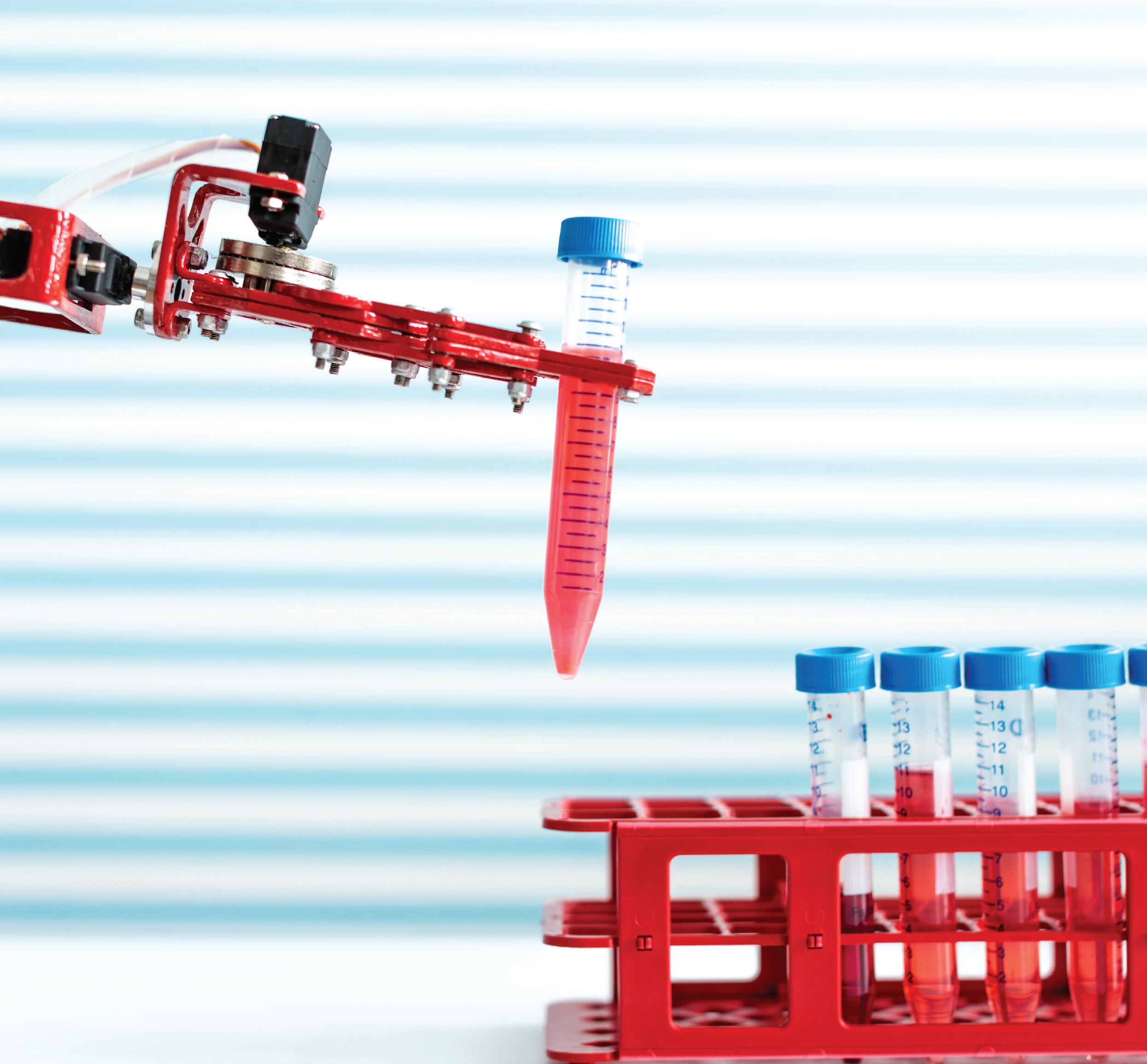
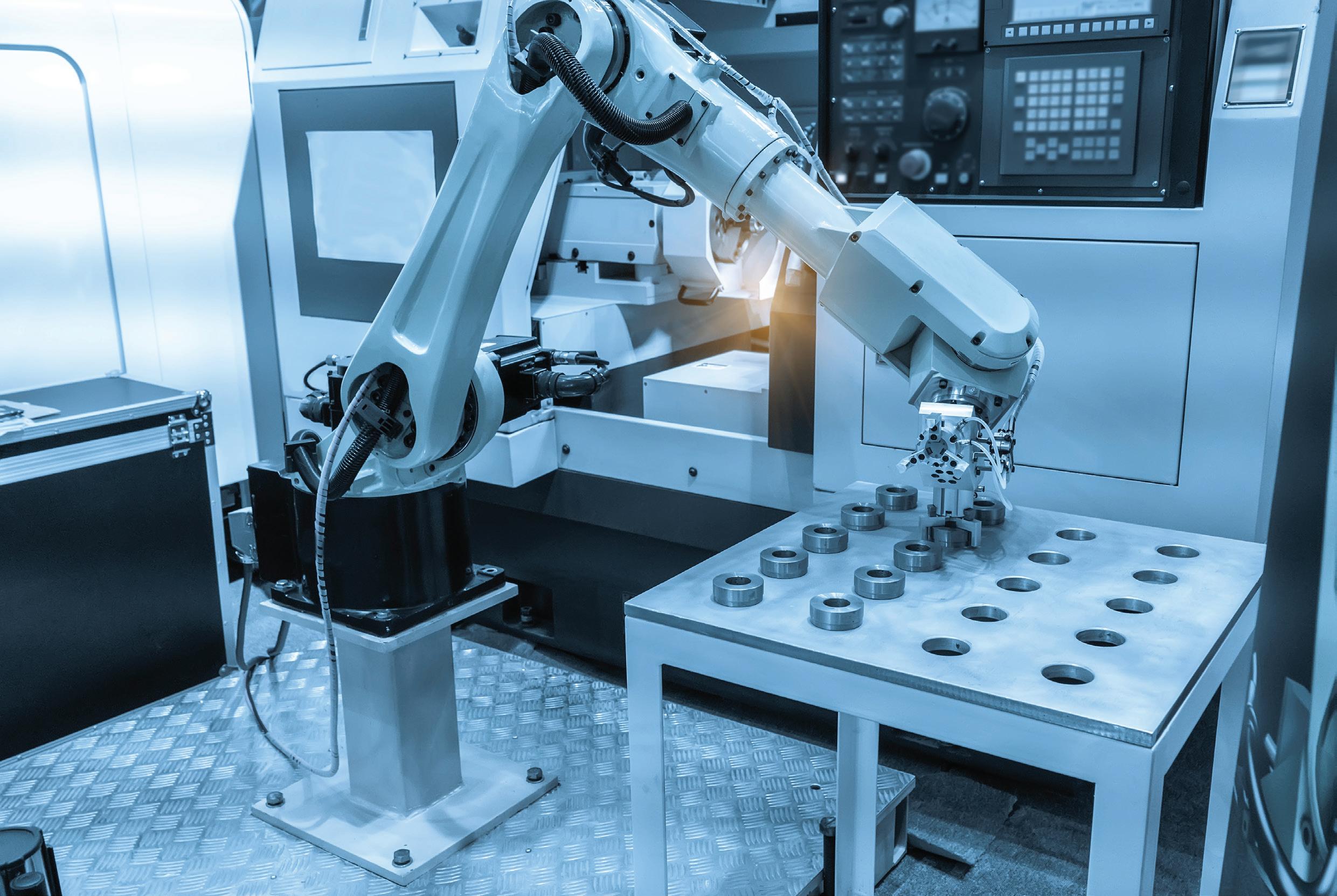
Simulator by CAE. For the same reasons, robotics will continue to make possible new medical procedures and treatments, such as new Single-Port Access procedures. Even as robots are developed for new medical areas, other tools may encroach on medical needs currently filled by robots. Medical robots must develop a firm basis in improved medical outcomes, or risk being displaced by pharmaceuticals, tissue engineering, gene therapy, and rapid innovation in manual tools (e.g., the SPIDER Surgical System by TransEnterix, and the EndoStitch by Covidien). To that end, improvements in medical robotics must address and solve real problems in healthcare, ultimately providing a clear improvement in quality of life when compared with the alternatives.
References 1.Beasley, Ryan A. Journal of Robotics. Vol 2012, Article ID 401613. 2.Asimov, Isaac. I Robot. Gnome Press. December 2, 1950. 3.Erika Mendes. https://prezi.com/uatrfrr1rrmc/differentpurposes-of-robots/ 4.Crawford, Mark. ASME.org. Sep 14th, 2016. https://www. asme.org/topics-resources/content/top-6-roboticapplications-in-medicine 5.https://www.credenceresearch.com/press/globalmedical-robotics-market

Guillermo Caletti, Ph.D.
Chief of Clinical Operations at Boehringer Ingelheim for Mexico and Central America.