thE BALANCE® MEthodoLogY
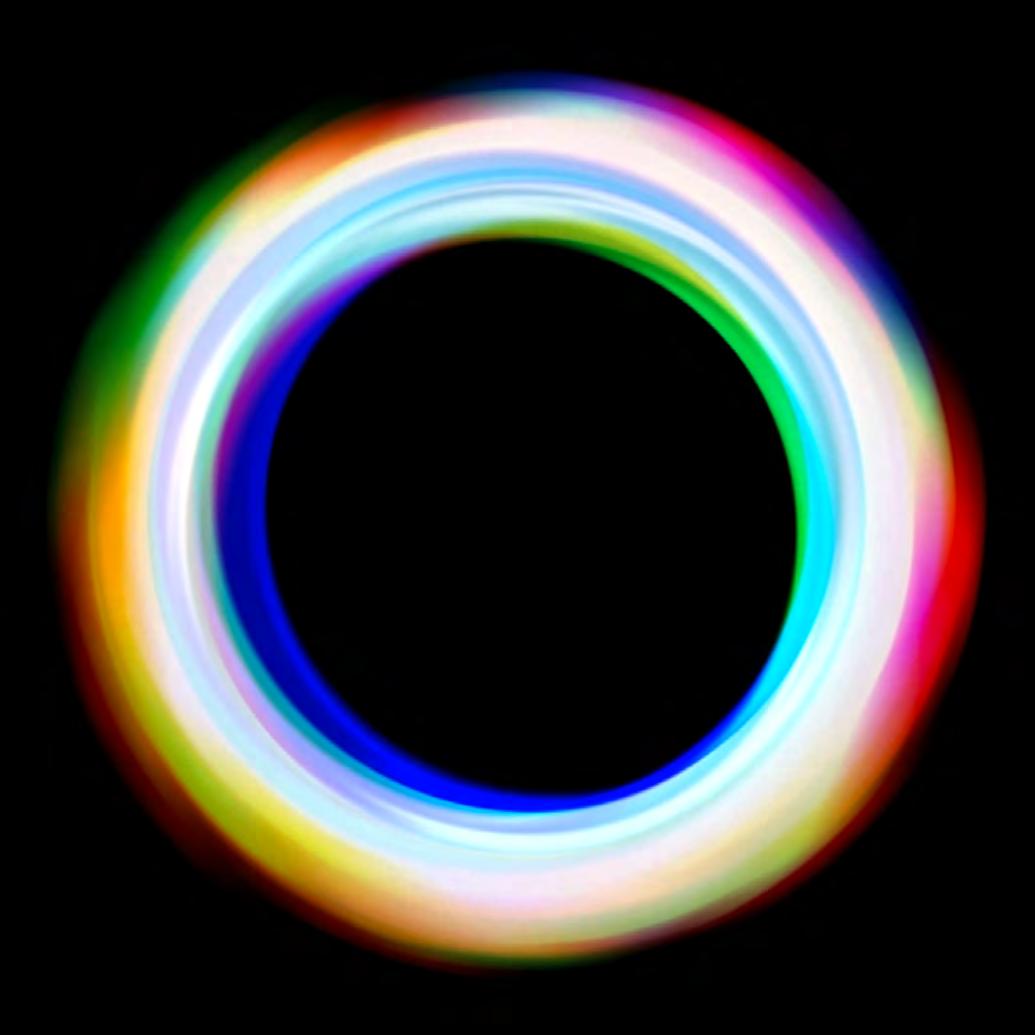
CoNvErtiNg CArBoN fiNANCE
iNto BiodivErsitY
A how-to MANuAL
Authors: Prof. Felix Dodds, Daniel B.J. Morrell and James Cameron
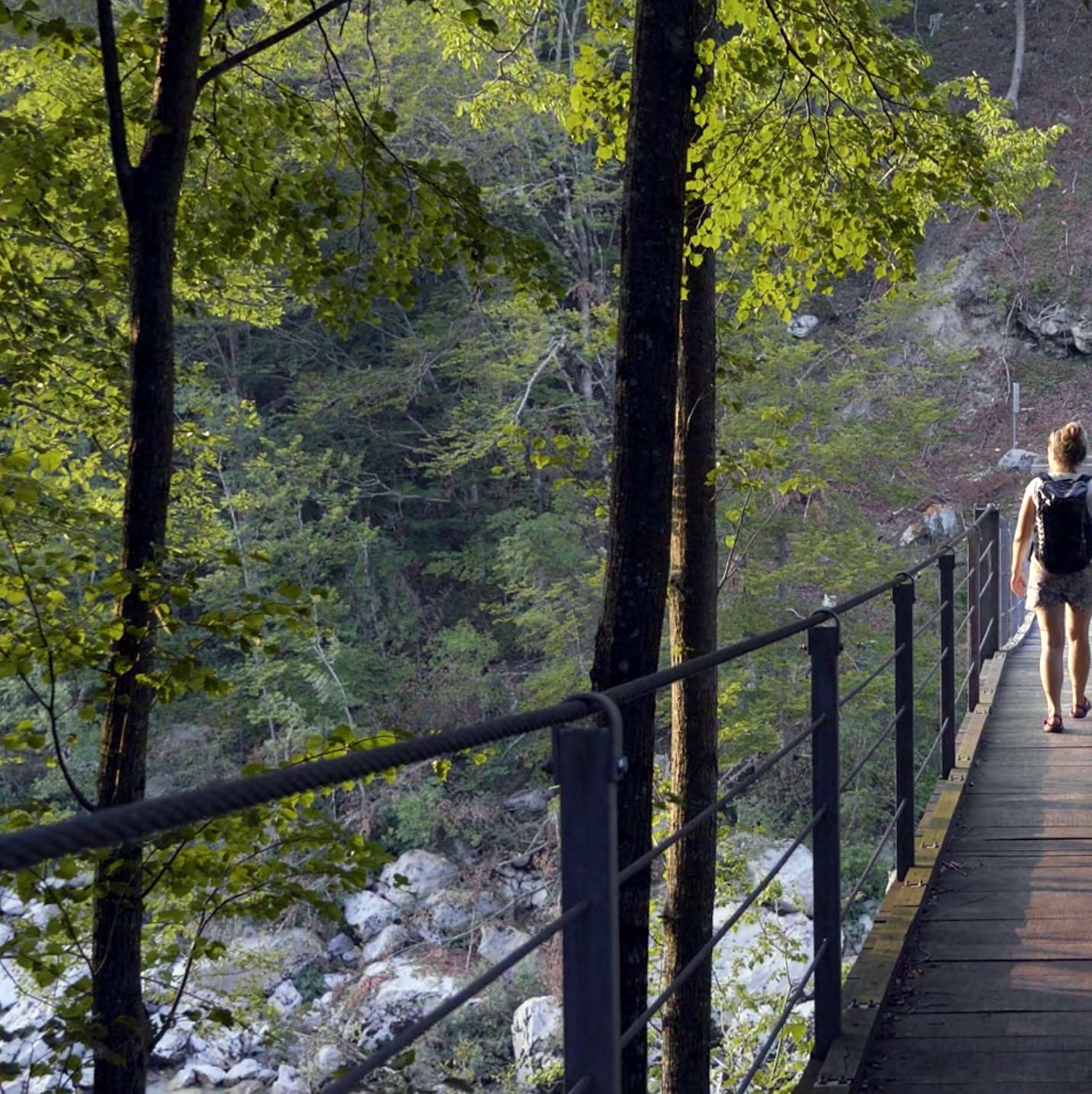
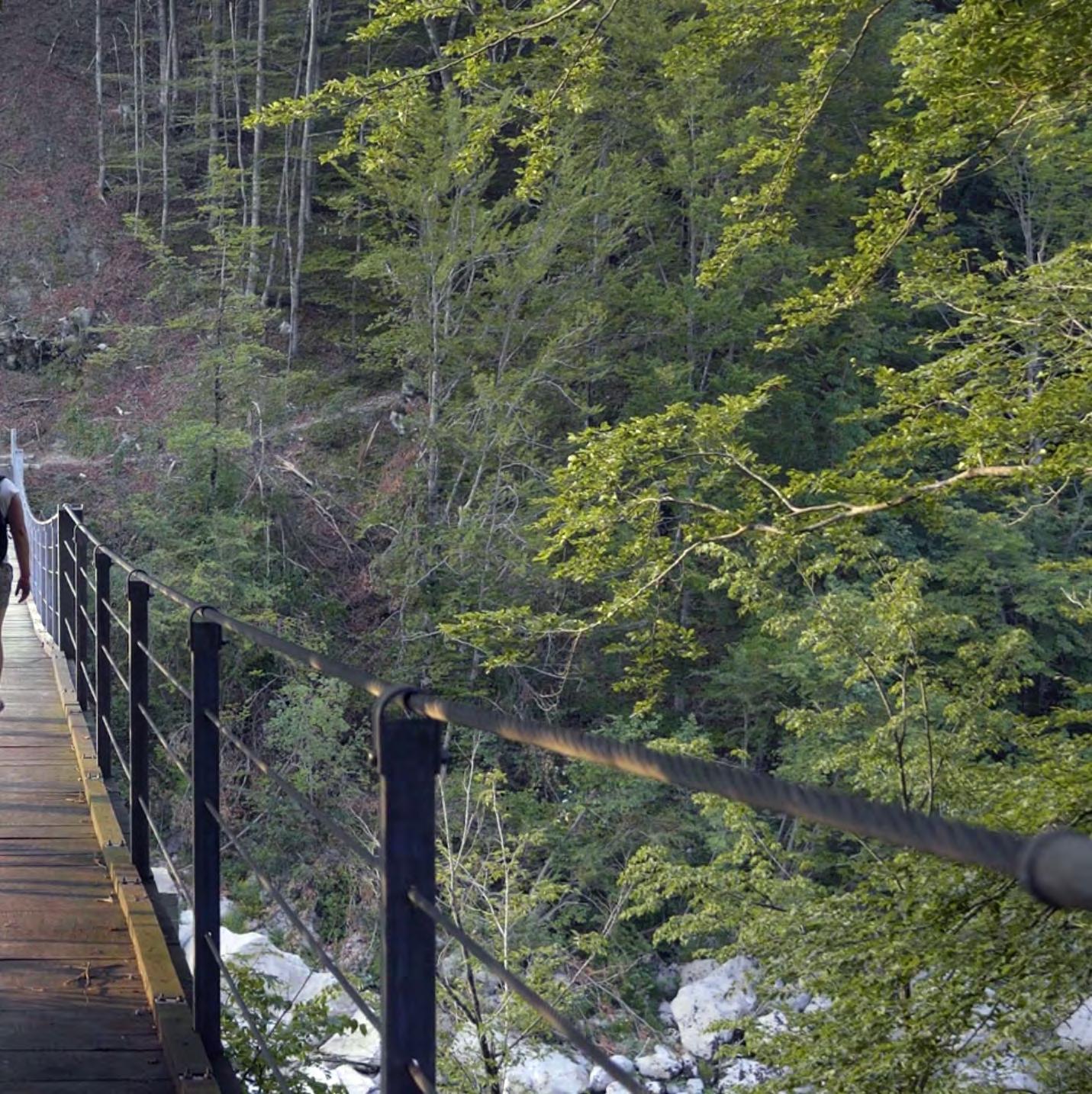
CoNvErtiNg CArBoN fiNANCE
iNto BiodivErsitY
A how-to MANuAL
Authors: Prof. Felix Dodds, Daniel B.J. Morrell and James Cameron
BALANCE® represents a new type of carbon offset initiative for contributing to global climate mitigation efforts. It synthesizes lessons learned from past carbon offsetting and NbS (Nature-Based Solutions), with the latest scientific evidence to capitalize on widespread support for biodiversity and ecosystem creation. By considering the ecosystem as a whole, the BALANCE® methodology accrues numerous benefits, including carbon sequestration and long-term sustainability and biodiversity creation..
As the core of this methodology, ‘BALANCE® Planting Principles’ establish prescriptive guidelines with which selected planting partners must comply, as detailed on our Planting Partner Contract. These obligations combine to form guarantees that support planting and protecting diverse, sustainable and efficient carbon-sequestering forests. BALANCE® methodology accounts for soil and climate context, topography, land use history, species diversity, disease and pest mitigation, forest resilience, and sustainability. The BALANCE® Planting Principles provide a framework for all future aforestation-based carbon offset providers. An overview of the benefits of biodiversity follows the Planting Principles. Finally, this article presents two short case studies, the first on the current state of forestry in the UK, and the second on the Forest of Marston Vale, which is a planting partner of BALANCE®
Evidence in this paper derives from a variety of sources found through a comprehensive literature review.
Methodology Reviewed by:
Prof Peter Cox, Exeter University
Dr Thomas Elliott, Crowther Lab Zürich
Dr Bertil Abbing, Ecometrica
Dr Vicky West, Woodlands Carbon Code
Research Assistant: Gabriel Ware
1. iNtroduCtioN P8
2. BALANCE® ANd whAt it is P24
3. BALANCE® PLANtiNg PArtNEr oBLigAtioNs P36
4. CAsE studiEs P68
5. CoNCLusioN: thE futurE of BALANCE® P92
6. dAtA AvAiLABiLitY stAtEMENt P94
Voluntary carbon credits can create a number of benefits, provided they adhere to a sound methodology. These benefits include biodiversity protection, emissions reduction, physical and mental health improvements in the general public, new jobs, and a variety of ecosystem services including ancillary incomes from permaculture, agroforestry, beekeeping, biochar, production and water catchment enhancement.
To achieve long-term emissions reductions in line with the Sustainable Development Goal 15 of the 2030 Agenda for Sustainable Development, BALANCE® repurposes biodiversity as the key differentiator in addressing the global biodiversity and climate crises.
‘BALANCE®’ is shorthand for a state of harmony with the natural environment. This is not achieved only through offsetting emissions, but also through fostering biodiversity in productive ecosystems by means of optimised emissions reductions and a commitment to environmental protection. The BALANCE® philosophy dictates more wide-ranging considerations than past carbon reduction initiatives did because it involves more than planting trees. BALANCE® also ensures that environmental protection does not burden consumers. As a trademarked term, BALANCE® represents an evolution from carbon-neutral to carbon-neutral 2.0. It shows consumers how their investments promote the long-term resilience of forest ecosystems. A BALANCED company seeks efficiency within its operations, and then BALANCE®s its unavoidable emissions, and reaches out to its suppliers to do the same.
humanity.
BALANCE® represents the synthesis of carbon and biodiversity. A tonne of carbon, produced in the BALANCE® process, does not solely represent a particular quantity of trees; it’s also the land mass required for the biodiverse forest to store a tonne of carbon, a quantity which varies depending upon the land’s capability to store carbon both in its soil and the planted biomass.
Our BALANCE® UNIT is a blend of a biodiversity and carbon credit to create a way of valuing nature and its benefits to humanity. Through BALANCE® we aim to develop a new marketplace for BALANCE® UNITS. In this hypothetical marketplace, the BALANCE® UNIT as a carbon-denominated instrument will have a multiplier attached to it due to its additional values, which include higher quality, integrity, and the creation of natural capital. BALANCE® UNITs fit with the evolving understanding of biodiversity credits that commercial entities purchase as part of their commitment to sustainable environmental practice.
Each tonne of carbon is allocated to a BALANCE® UNIT. In the UK, through our affiliation with the Woodland Carbon Code, we assign Woodland Carbon Units (WCUs) to clients. Each WCU represents a tonne of CO2e which has been sequestered in a WCC-verified woodland. The WCU has been independently verified, is guaranteed to be there, and can be used by companies to report against UK-based emissions assessments or to support claims of carbon neutrality or compliance with Net Zero emissions goals. WCUs are acquired upon the verification of a project, after Pending Issuance Units (PIUs) have vested over a 10-to-30-year interval. There is a one-to-one relationship between PIUs and WCUs because a PIU is effectively a promise to deliver a Woodland Carbon Unit in the future based on the predicted level of carbon sequestration performed when the involved trees have matured. PIUs help demonstrate the volume of potential future sequestration at the outset of the project. As planning tools and data points, PIUs can be assigned to buyers but not used or retired until they are transferred into WCUs, which can be used as full carbon credits1
Full details of the purchase, assignment and retiring process as per Woodland Carbon Code regulations are outlined comprehensively on p70.
BALANCE®
clients create biodiversity and natural mechanisms for carbon sequestration.
BALANCE® clients create biodiversity and natural mechanisms for carbon sequestration. The BALANCE® methodology mitigates a year’s worth of emissions by ensuring that carbon sequestration over 20 or 30 years will effectively negate the impact of those emissions. While tree growth determines the 10 to 30-year window over which PIUs convert to WCUs in the United Kingdom and delivered PIUs are eligible for conversion into WCUs after 10 years, it’s also important to note that BALANCE® Eco Limited and its planting partners hold land under contract for 99 years. This longer interval significantly increases the carbon sequestration capacity of the woodlands involved. The 99-year protection in BALANCE® contracts also guarantees that sequestration calculations underestimate the restorative impact of employing the BALANCE® methodology. 99 years is in harmony with the 100 year radiative forcing lifetime cycle of CO2 and gives time for biodiversity enhancement, such as deadwood for insects. Professor Peter Cox, Professor of Climate System Dynamics at the University of Exeter (in Exeter, Devon, United Kingdom) helped quantify and validate the positive impact of the 99-year timeframe that BALANCE® contracts support, which is a net gain of more than 10 percent.
The lack of retirement which has long been a factor of the compliance market attracted negative attention for permitting excess emissions elsewhere, or “carbon leakage.” Retirement of offset credits avoids double counting and non-additional credits being resold on the carbon market.
Moreover, prioritisation of indigenous species, and the planting of a variety of species adapted to the local abiotic context is a factor in methodology. Established forests in accordance with the UKFS (UK Forestry Standard), are verified through the UK Woodland Carbon Registry, the public registry of the Woodland Carbon Code, and validated by a certification body accredited by the UK Accreditation Service.
1.2. thE PLANtiNg PArtNEr CoNtrACt
thE BALANCE® PLANtiNg PArtNEr CoNtrACt CoNtAiNs sEvEN KEY oBLigAtioNs
Before contacting potential partners, BALANCE® ensures that a number of planting obligations are met. These include the following:
1.2.1 sPECiEs divErsitY
The right trees in the right places, including an understanding of the land’s historical context, and planting exclusively on previously forested land.
The prioritisation of native species from local genetic stock.
Consideration of soil type and soil organic matter when planting, and planning for carbon sequestration.
The formation of a varied stand with genetic diversity, a healthy understorey, and the use of relevant agement cycles to enhance biodiversity.
Inclusivity and equality in social and economic benefits where necessary.
N ecessary considerations in tree planting for adaptation to climate change
Documented consideration of how to mitigate threats of pests and diseases
Reduction of dependence on invasive species.
An understanding of the importance of forest structure, connectivity between forest ecosystems and tree size.
Commitment to the production of data and the frequent review of management and planting strategies based upon findings.
BALANCE®
commits to ethical land use as a part of existing human rights agreements, standards and principles in the international system.
Creation of natural forest cover with a minimum of 99 year protection and carbon sequestration capacity independently monitored to promote the longevity of created carbon sequestration ecosystems. This time frame reflects the radiative forcing cycle of carbon dioxide in the atmosphere, and the necessity for trees to die and create deadwood to optimise biodiversity and additional carbon sequestration benefits.
1.2.3
The public may visit forest areas which they have chosen to create. Planted trees also help create green spaces. Planting partners provide regular biodiversity enhancement information as it happens, which we then send back to our clients so they can see the benefits and pass on to their stakeholders and customers.
If the funding has not arrived, purchased trees cannot be planted, thus ensuring additionality of BALANCE® planting projects. Additionality protocols for UK-based projects are set out in the Woodland Carbon Code (WCC) verification system in the UK and the Gold Standard and REDD+ internationally. BALANCE® also monitors other reputable verification standards worldwide.
Force Majeure ensures compensation in case of unforeseen events, including but not limited to an epizootic or a plant disease affecting part or all of the Woodland, road construction, compulsory purchase, unforeseen building construction, or a severe natural disaster.
Human rights are at the core of the BALANCE® methodology. Climate action should be consistent with human rights obligations as it affects the human right to food, water, and a healthy environment (Humphreys, Robinson, 2009). BALANCE® commits to ethical land use as a part of existing human rights agreements, standards and principles in the international system. BALANCE® asks our project developers to ensure indigenous–local people will not be removed from land for carbon farming. BALANCE® works directly with local communities to receive stakeholder input and with local planting partners where appropriate. BALANCE® aims to encourage indigenous–local people to generate capital from the forests beyond remuneration and recreation, through enhanced commitments in our planting partner contract, by encouraging the use of co-benefits such as biochar, permaculture and agroforestry techniques to harness socio- economic benefits from the forest.
For each tonne of BALANCE®, an additional tree is twinned and planted in a noncarbon forest. This can be close to the client’s business, even in urban areas, and is linked to the carbon tonne through BALANCE®’s public database. This is unique amongst offset initiatives and designed to increase biodiversity benefits and create wildlife corridors in urban and rural environments. It also allows us to supply funding in locations that would not otherwise receive carbon-financed tree planting benefits.
These key points, in tandem with our selection process for trees and forest composition, ensure that our forests are planted to optimise additionality and accessibility, while promoting biodiversity, longevity, resilience and sustainability. Our planting process incorporates predictive climate modelling to ensure that selected species are able to survive local and regional climate change in the near future. Varying sapling quantities are planted depending on the location, because some locations require greater density of trees in order to store one tonne of carbon. BALANCE® tonnage generated by the planting of trees is found in the BALANCE® inventory, which is sold with a location reference. All sales are listed on the BALANCE® website (https://BALANCE.eco) to ensure transparency and prevent repeat selling. Once sold, inventory is publicly retired, along with the WCU (Woodland Carbon Credit) allocated to each tonne of carbon.
In the voluntary carbon market, BALANCE® monitors and incorporates various review and best practice processes, including the Voluntary Carbon Markets Integrity Initiative (VCMI) and its Provisional Claims Code of Practice. BALANCE® calculations scale to fit with the size of small, medium, and large companies. In other words, promotion of biodiversity and reduction in greenhouse gas (GHG) emissions via carbon sequestration as ratified by PIU to WCC conversion over time can be thought of as achievement in gold, silver, and bronze tiers relative to the VCMI standard.
Another initiative, the Taskforce for Scaling Voluntary Carbon Markets (TSVCM), an oversight board for carbon offsetting headed by Mark Carney, is dedicated to developing new methodologies for the voluntary carbon market. BALANCE® monitors these processes closely. Although it is impossible to guarantee that the current form of the BALANCE® Methodology will function for all time, BALANCE® will incorporate the most recent research in this methodology annually so as to deliver optimal environmental outcomes.
BALANCE® began as the product of BALANCE® Founder and CEO Daniel B J Morrell’s vision to reconsider offsetting in its current form as an endeavour which has failed to fulfil its potential. Morrell wanted to modernise approaches to ‘carbonneutral’ outcomes. The term ‘carbon neutral’ was co-coined by Morrell (and became the Oxford English Dictionary’s ‘word of the year’ in 2006) because ‘carbon sequestration’ did not effectively communicate the outcome of offsetting carbon emissions. The first carbon trade was to Maureen, who ran the Castlecary Pet Shop in Somerset in 1988. Shortly afterwards, Morrell met Rodney Bickerstaffe, leader of the trade union Unison, who was taken with carbon neutrality as an idea and promised to share it with his union members. A few weeks later Morrell received a cheque from a doctor in Hull, asking him to plant a tree for him to offset his carbon footprint. More cheques arrived. When the stream turned into a flood, Morrell established the Reforestation Britain Campaign, arguably the first business to address the consequences of global warming.
Morrell went on to found Future Forests in 1992, providing a way for businesses to ‘go green’. His partner in this was marketing consultant Sue Welland, with the science around carbon sequestration provided by Dr. Richard Tipper, who has worked as a science and policy advisor on climate change issues for major businesses, international organisations and governments, a lead author on IPCC reports, and CEO at Resilience Constellation and Chairman at Ecometrica.
Although many people found some of the issues around climate change and carbon offsetting confusing, the idea of planting trees to help the environment was a relatively easy one to get behind. This effort was helped by the prominent support of many musicians and artists, beginning with Joe Strummer of the Clash after a meeting with Morrell at Glastonbury in 1995. Strummer instantly committed to becoming the first carbon-neutral citizen, and the spontaneous support of such a respected figure proved a tipping point. Artists such as The Rolling Stones, Pink Floyd, and Leonardo Di Caprio also committed to becoming carbon neutral. This eventually resulted in over 100 million CDs being produced on a carbon neutral basis.
In 2006, Morrell began a campaign to inspire a billion people to act against climate change. If that many people could reduce their own emissions by just a ton a year, a significant blow would be struck in the battle against irreversible global climate change. Hence the name of the organisation – Global Cool – and its rallying cry of ‘One By One, Tonne By Tonne.’ A personal CO2 calculator was developed so people could easily quantify their own environmental impact, and participate in carbon offsetting through tree planting. The initiative was funded by companies such as Vodafone, Man Group, Logica, and The Body Shop, and endorsed by leaders in politics and culture.
Thanks to Global Cool, Morrell gained other opportunities to drive action on climate change, becoming a panelist at the Accounting for Sustainability project supported by Prince Charles. At the 2012 Doha Climate Change Conference (COP 18), following a chance conversation in a hotel lobby with eminent economist Lord Nicholas Stern, Morrell was asked to stand up before an audience including the Saudi and Qatari royal families to open COP 18 by explaining his part in the world’s first carbon trade. This was Morrell’s second interaction with the UN after an unscripted appearance in front of the General Assembly in New York in 1999.
Morrell went on to form the Carbon Advisory Service in 2007. The company refurbished existing buildings and designed efficient new builds, using new technology within an existing budget rather than simply renewing dated designs
Opening another front in the climate battle, Morrell (along with Green & Black’s founder Craig Sams) started Carbon Gold in 2008 in order to realise the carbonreducing potential of the earth beneath our feet. Carbon Gold is the first modern biochar company, broadening the appeal of a practice known for thousands of years which not only improves soil fertility but actually removes harmful CO2 from the atmosphere.
While the original ‘carbon-neutral’ idea was sufficient at a time when the climate crisis received far less attention than today, BALANCE® acknowledges that basic carbon neutrality is not enough, because forests created to offset carbon emissions need to be sustainable.
A true commitment to biodiversity encourages the symbiotic chains on which nature depends, such as leaving dead wood for bugs that can in turn be enjoyed by woodpeckers. BALANCE® supports natural regeneration and re-wilding projects which can help with this process.
BALANCE® in Business is an easy-to-use system for Small and Mid-Sized Enterprises (SMEs) and larger companies that wish to act in an environmentally responsible way, but find existing processes time-consuming and expensive. BALANCE® in Business offers a simple top-down carbon calculator to companies located within the UK, which provides a robust estimate of emissions using readily available data to arrive at results BALANCE® by sector.
The BALANCE® Calculator allows greater accessibility to a wider range of carbon emitting companies or individuals. The BALANCE® CO2 Estimation Methodology (2021) outlines the calculations through which estimates of required carbon offsets are achieved. Historically, the concept of generating an effective general calculator for measuring GHG has been hindered by complexity and inaccuracies. To address this problem, researchers introduced sector specific calculators. Sector specific calculators, for example, have been used to model a city’s emissions (Lin et al., 2014) and predict the future emission levels of an entire country (Nieves et al., 2019).
BALANCE® has created a calculator which uses sector-based analysis based upon national GDP, estimating the share of GHG for businesses based on their specific sector/sub-sector and their current GDP proportion to the sector/sub-sector GDP. This provides streamlined estimation more simply than other methods. BALANCE® simplifies the CO2 equivalent assessment process by using the consumer’s household income (if individual), or the company’s income, without regard for expenditure. The calculator compares client company turnover with government GDP data and DEFRA data on emissions broken down by 720 sectors; it can estimate a client company’s emissions by pro-rating the emissions on the basis of their share of sector GDP, thus establishing how much their footprint is within the sector proportionate to their turnover. The client company need only provide annual turnover and its industry sector (SIC) code, which is included in the company’s registration number via a simple, searchable interface. A country’s GDP and emission factors are typically in the public domain, and published by the IPCC.
The BALANCE® Calculator estimates the value of Sector Turnover based on the GDP Fraction. This is a conservative estimate used to calculate the proportion of GHG for a company based on company turnover. Subsequently, the sector turnover estimation uses a curve-fitting process to predict Sector Turnover (ST) based on the GDP Fraction (GDP). In this process, 98 different sectors with known GDP-F help develop the equation. The methodology of equation development is as follows:
1. I dentifying the conversion rate (CR) based on CR =
2. Based on the value of CR, sectors are grouped (G) as displayed in figure 1:
Figure 1. In this categorisation the main concept is to assign a category for every few CR values in the same range. The gap between ranges is not considered here, but has been taken care of in step 4. Also, the adjustment process has been done later in step 4 to modify categories for higher accuracy. The number of categories provided is based on the heuristic of performing a test on several scenarios, but in general, increasing the number of categories increases the accuracy in the equation.
BALANCE® in Business is an easy-to-use system for Small and Mid-Sized Enterprises (SMEs) and larger companies that wish to act in an environmentally responsible way, but find existing processes time-consuming and expensive.
3. In the next step, a simple linear progression based on the G and GDP is used to calculate CR.
4. The category value of sectors has been modified to increase the accuracy of curve fitting. The first and final values of categories are listed in figure 2.
5. The final accuracy review shows the average accuracy of 97.78 percent, with the standard deviation of 1.90 percent. This is the accuracy of predicting Sector Turnover based on the GDP Fraction.
6. The final step calculates the proportion of GHG for a company based on the Sector Turnover (ST) and the Company Turnover (CT) to measure the BALANCE® value, using the BALANCE® Equations:
sectorCO2 x 1000 1000
BALANCE = orgTurnover x ukGDP x sectorWeight
BALANCE = x sectorCO2e x 1000 orgTurnover sectorTurnover
The sector-based calculator accounts for in country scope 1 and 2 emissions only, not scope 3, and is review to be 97.8% accurate in all instances to Government data. If everybody in a company’s supply chain were balanced, scope 3 emissions would go away. BALANCE® encourages sector base calculator clients to ask that their supply chain become BALANCED to spread the cost of scope 3.
The GHG emissions quantity is translated into the amount of carbon required to offset emissions through our metric system, which equates one BALANCE® UNIT to one tonne of CO2e, allowing participants to comprehend their quantifiable contribution to biodiversity easily, and compensating for their emissions in a way that creates lasting benefits. The purchase of a BALANCE® Tonne guarantees that at least a tonne of carbon will be taken out of the atmosphere as a direct result of its purchase. Because all ‘additionality’ is mitigated, a BALANCE® Tonne results in genuine carbon reduction rather than being attached to planting that might have happened anyway.
Consumers using our calculator can work out how many tonnes of CO2e they generate. Afterward, the turnover and sector-based calculations translate this into the tonnage of carbon required to offset emissions. Once the carbon tonnage is calculated, the client is presented with a choice of forest to subsidise, and receives a certificate displaying the location of the woods2.
Statistics Reviewed by: Professor Hassan Chizari Gloucestershire University.
2. The sector-based calculator accounts for in-country scope 1 and 2 emissions only, not scope 3, and is review to be 97.8% accurate in all instances to Government data. If everybody in a company’s supply chain were balanced, scope 3 emissions would go away. BALANCE® encourages sector base calculator clients to ask that their supply chain become Balanced to spread the cost of scope 3.
1. ATMOSPHERIC EMISSIONS: GREENHOUSE GASES BY INDUSTRY AND GAS
https://www.ons.gov.uk/economy/environmentalaccounts/datasets/ukenvironmentalaccountsatmosphericemissionsgreenhousegasemissionsbyeconomicsectorandgasunitedkingdom
NOTES: The emissions of carbon dioxide, methane, nitrous oxide, hydro-fluorocarbons, perfluorocarbons, sulphur hexafluoride, nitrogen trifluoride and total greenhouse gas emissions, by industry (SIC 2007 group - around 130 categories), UK, 1990 to 2018 and provisional 2019.
2. PUBLIC AND PRIVATE SECTOR EMPLOYEES’ CONTRIBUTION TO GDP
https://www.ons.gov.uk/aboutus/transparencyandgovernance/freedomofinformationfoi/publicandprivatesectoremployeescontributiontogdp
NOTES: National Accounts: Blue Book 20
3. GDP(O) SOURCE CATALOGUE (BLUE BOOK)
https://www.ons.gov.uk/economy/grossdomesticproductgdp/datasets/gdpodatasourcescatalogue
NOTES: A breakdown of all the data sources that feed into the output measure of Gross Domestic Product. BB20 has a reference year of 2018 and the industry weights represent the relative contribution of each industry to the whole economy in 2018. The weights are calculated on a parts per thousand basis and are used within the GDP(O) processing system. However, due to rounding the GDP(O) weights sum to 1000.0001 for this publication.
4. ANNUAL TURNOVER BY INDUSTRY FOR ALL (SIC) INDUSTRIES
https://www.ons.gov.uk/aboutus/transparencyandgovernance/freedomofinformationfoi/annualturnoverbyindustryforallsicindustriesfrom1998to2015
NOTES: ONS Dataset providing accurate company turnover by sector and industry. Using this dataset, we can help provide a more accurate company to sector ratio aligning with GDP. This also helps to simplify the equation and allows us to use the results of this equation to obtain an accurate estimate of CO2 emissions.
Notes: 1. When using this methodology no improvement in results will be seen due to sustainability performance improvements you make yourself because the results are based on the industry average. 2. To track actual results, trends and improvements requires a more in-depth GHG assessment of your operations.
Beyond offset credits, BALANCE® provides a range of services through its consultations with client companies. Before considering offsetting greenhouse gas emissions through BALANCE®, potential clients need to complete at least the following:
MEASURE: Quantify their carbon or carbon dioxide equivalent (CO2e) output.
AVOID: Take steps to prevent avoidable emissions.
REDUCE: Reduce remaining emissions where possible.
BALANCE® offers advice on efficiencies and reductions on our website that can be implemented within a business. This process takes the user through a step-by-step process of understanding climate impacts from square footage of property, energy usage in heating and cooling, car mileage, number of staff, and flights and other travel. It is imperative to BALANCE® that each client reduces emissions throughout their supply chain, and that only excess emissions are considered for offsetting.
The BALANCE® Eco Foundation charity directly supports forest planting and protection. It receives 20 percent of the revenue from sales of the CHANT with BALANCE® music and art app, the remainder of which is largely used to pay music rights holders and artists fairly. Operating costs of the BALANCE® Eco Foundation are paid by BALANCE® Eco Limited, removing overheads so that all the money coming into the Foundation can be used for forest planting and protection. The Foundation also pays the direct costs of tree planting wherever possible, so that the absolute maximum from contributions goes directly into tree planting rather than administrative costs. BALANCE® Eco Foundation is registered with the charity commission (No. 1162529). Its trustees are David Taylor, Craig Sams, and Daniel Morrell.
The BALANCE® forest programme, operated by associate planting partners, supports long-term biodiversity, and is fully transparent and verifiable, with exact carbon sequestration amounts attached and the location of planting made visible. For every tonne of carbon identified through BALANCE®, BALANCE® plants an extratree in a twinned non-carbon planting registered on our database. This enables us to create migration corridors from urban to rural areas and planted trees are a visible icon near our customers. The single extra tree planted by BALANCE® becomes an integral part of biodiversity creating adjunct to the resilient and effective carbon-storing forest.
BALANCE® is focused on ensuring the correct trees are planted in the correct places, with prioritisation of locally-sourced indigenous tree species and the simultaneous acknowledgement of species from relevant geographical areas (for example, in the UK context, from within 2 degrees south) to mitigate future climate change and its impact on susceptible tree species. This process makes stand composition in associated forests varied and non-uniform, with many different sapling amounts planted depending on location. BALANCE® also ensures that the consumer is physically engaged in the process so that information concerning all trees is accessible to the consumer on our database. Similarly, the forests which BALANCE® plants are accessible to the public.
All BALANCE® forests will be monitored and verified by reputable, globally accredited third parties, including the Woodlands Carbon Code in the UK and REDD+ internationally. BALANCE® meets and exceeds REDD+ standards by following the Gold Standard approach to reforestation and carbon offsetting enhanced by the Balance six-point planting partner contract Planting Obligations. BALANCE® also partners with various verification bodies, depending on project location, so that the planting and protection activities are evident as has not always been the case with historical carbon offsetting.
All trees are planted with a 99-year maintenance contract, providing insurance for investors and clients. In this way, Balancing your business offers benefits over the length of the contract. On a broader scale, BALANCE® advocates Governmental guarantees, combining marketbased insurance with a stopgap on voluntary carbon market contracts and transactions, which does not exist yet. Although the WCC and REDD+ have buffer stocks, these often require careful management, and there is always a risk that markets will avoid them.
Offset credits in the UK are subsequently retired following the Woodland Carbon Code, meaning they cannot be re-circulated, eradicating the risk of double counting while reducing overall excess emissions, which arrive through reselling credits on the voluntary market. The principles by which BALANCE® and its partners operate in planting forests are discussed below.
oBLigAtioNs
BALANCE® guidelines are split into two sections: Forest Creation (3.1), and Resilience and Longevity (3.2), including obligations for consideration during the initial planting phase and the medium- and long term future of the forest.
The principles involved are as outlined previously in the section about the Planting Partner Contract, and explained below:
Forestry of all types has employed mixed and native species in line with national commitments to forest and landscape restoration, yet there are still many projects which fall short in terms of biodiversity, resilience and ecosystem services provision.
The BALANCE® ideology integrates the lessons learned from past carbon offsetting, using current evidence of the most effective and sustainable carbon offset projects through the lens of NbS. Each of the following points are included within the first motion of the five-point Planting Partner Contract. It is important to note that while these principles are relevant to current and future afforestation strategies, they are particularly relevant to carbon offset reforestation initiatives.
BALANCE® Eco-Ltd funds Carbon Removal projects only through a) afforestation, b) reforestation, c) natural regeneration.
Stressed here is the necessity to consider the exact types of trees for the location and geographical context of the project, and the importance of planting indigenous species while considering forest composition, while allowing the inclusion of exotic species for certain benefits, though in the right context and quantity to minimise negative ecological consequences.
Reforestation planting areas, comprising afforestation or reforestation efforts aimed at carbon offsetting, account for 7 percent, which is equivalent to 264 million hectares, of the global forest cover. The area of such reforestation is expanding quickly as plantations become increasingly relied upon for wood-based products, carbon management, soil and water conservation, and the rehabilitation and diversification of impoverished landscapes. This has collectively led to a significant anthropogenic influence on the composition of forests (Barsoum et al. 2016, p. 4, FAO, 2010, Pawson et al., 2013). It is also important to note that afforestation might not be a viable long-term adaptation solution in some regions. Tree planting can be especially problematic in native non-forest ecosystems (Veldman et al., 2015), that are often overlooked by restoration and conservation policies. BALANCE® therefore does not encourage planting trees in non-forest ecosystems.
In some areas, scientific research increasingly suggests that allowing forest regeneration to occur naturally can deliver a wider range of climate change adaptation services with fewer trade-offs than plantations. Several of these “rewilding” projects have led to the return of open woody habitats once grazing pressure from domestic livestock is reduced (Tree, 2018), creating some notable successes in restoring rare wildlife. Naturally-established forests can also be more cost and resource efficient because they rely on less costly and labour-intensive human interventions. They can also accumulate carbon rapidly once sufficient trees have colonised a site and tend to be more biodiverse than plantations (Cook-Patton et al. 2020).
Forestry plantations have often come at the expense of naturally occurring ecosystems, such as natural grasslands and peatlands (Veldman, et al., 2015), which may be more resilient to climate change impacts and may lack diversity entirely. In the BALANCE® process, it is important to define whether a target area was historically covered by forests, as this can be a key indicator of the suitability of the land for reforestation. Planted areas, generally, are beneficial where natural biodiversity is low, and where the land is suitable for forest regrowth, particularly on lands which were previously forested, as non-forested lands like grasslands or wetlands already contribute to carbon capture, so should be avoided. Selecting an area that is already in use for agriculture could result in further deforestation elsewhere, resulting in carbon leakage and potential loss to biodiversity. Finally, the number of trees planted in a given plantation area should be as close to the maximum as possible.
BALANCE® declares, as an obligation, that native species should be the first consideration in any tree planting project. A few key species of local provenance are picked to enhance local biodiversity development and ecosystem services, while space for exotic species should not be discarded at the outset.
In Europe, according to one study (Barsoum, 2016), 29 percent of forests are composed of a single tree species, and many of these are plantations composed of a single non-native species, with serious implications for biodiversity. It is important to note that, given the current warming scenario, the selection of ‘indigenous’ species may be reconsidered with a view to the future, so that species derived from warmer conditions, but as close to the location of the project as possible (from where the species would be migrating), are included.
Large-scale reforestation initiatives increasingly utilise multidisciplinary studies such as remote sensing and mapping of soils, topography, tree and forest cover, as well as other biophysical variables, to prioritise and choose species for specific planting locations, and to decide whether tree-planting is the correct solution for local conditions.
The characteristics of soils, including their fertility, texture, colour, depth, acidity, and water retention capacity, exhibit significant variation. Different soil types develop unique horizons (or layers), resulting in a distinctive soil profile. In contrast to agricultural soils, which are frequently disturbed by ploughing, forest soils are typically undisturbed, allowing them to retain their natural profiles. Soil horizons exhibit variation in properties such as “colour, quantity of organic matter (i.e., dead flora and fauna), size and proportions of soil particles, acidity, and nutrient availability for plant growth” vary in (Greenbelt Consulting, N.A.). Amongst the six basic soil types (clay, sandy, silty, loamy, chalky and peaty), each are favoured by a number of common tree species. Many species are capable of growing with a variety of variable soil types.
Soil is also a significant carbon sink. While this is not yet specifically accounted for in our calculation methodology, the selection and growth of diverse species is certainly beneficial to carbon sequestration in soils, thus elevating the potential benefits of planting based upon soil types, and further promoting the benefits of biodiversity-based planting. The provision of semi-permanent land cover, for example, creates physical shelter to minimise soil disturbance and thus reduces erosion. It also aids in the reduction of soil contamination by avoiding the high inputs of fertilisers and pesticides associated with many forms of agriculture, and restores organic content to soils through organic decomposition and heightened presence of biotic matter. Similarly, woodlands indirectly aid in reducing the incidence of landslides through the shelter of soils and the reduction of grazing pressures.
The ability of forests to deliver ecosystem services and the various co-benefits depends on biodiversity and necessary focus on the ecosystem as a whole, not just the trees. In fact, trees represent less than a third of the plant species across a range of forest types (Spicer, Mellor, & Carson, 2020). Forests host a diverse range of plants, animals, fungi and microbes that form symbiotic relationships that are critical to forest recovery. The explicit consideration of the natural composition of locally-situated ancient forests is therefore particularly useful, taking into account stand diversity and composition, as well as understorey vegetation such as shrubs and herbs.
Current forestry policy advocates a diversification of forest stands to achieve both more productivity and sustainability, favouring mixed age structures and polycultures over single-aged monocultures. Canopy phenology, defined as the timing of recurring the biological regrowth of the canopy, relies on biotic and abiotic forces and the interrelation among phases of the same or different species. It is also important to widen forest species diversity due to the diversification of traits between species (Barsoum et al., 2016).
The creation of forests with ‘contrasting’ traits can ‘neutralise’ the influence of specific tree identities on the composition of understorey vegetation, which allows for the proliferation of ground plant species that are often suppressed or excluded in a monoculture plantation due to the presence of a limited number of species identity influences. Monoculture stands generally have poor nutrient retention. Mixed stands thus reduce the abundance of species that would otherwise tend to dominate and limit the growth of ground vegetation communities in a monoculture.
Understorey vegetation impacts nutrient cycling and disturbance mediation; it also plays an important role in the provisioning of habitat and foraging material (e.g. pollen, nectar, foliage) for many species. Ground vegetation, in turn, is strongly influenced by the composition and structure of the overstorey, responding to differences in temperatures and the availability of light, water and soil nutrients at the forest floor level.
Also important are forest management cycles which allow healthy growth of selected species, whereby trees are planted, allowed to grow, then certain trees are ‘thinned’ (a proportion cut and harvested), allowing space for the remaining trees to grow larger and more effectively and for new growth to be accelerated through cyclical procedure. This process takes decades, which makes effective and thorough planning of the management cycles for biodiversity development and native species essential.
The creation of woodlands also plays a role in natural flood management, particularly with reforestation of hill slopes and gullies and the restoration of wetlands, floodplains and river channel meanders. Woodland can increase hydraulic “roughness” by slowing down and reducing run-off as water storage is increased and drainage to streams is delayed, which can help to desynchronise multiple flood peaks within a catchment and decrease the overall geographic scale, depth and frequency of floods (Scottish Forestry Commission, 2020).
As part of our due diligence, BALANCE® examines every partner project to ensure that issues of inclusivity, equality, and economic benefits are accounted for, particularly in locations where local communities are relatively disadvantaged.
Resilience is the capability of a forest to withstand external pressures and, in time, to recover from disturbances, which may involve returning to its pre-disturbance state so as to retain the same function, structure, identity and feedbacks (Cavers, Cottrell, 2015). It is the extent of perturbation that a system can experience before it undergoes a shift to an alternative state; in other words, a system’s ability to maintain elasticity and avoid reaching a state of malleability.
“When viewed over an appropriate time span, a resilient forest ecosystem is able to maintain its ‘identity’ in terms of taxonomic composition, structure, ecological functions, and process rates (Secretariat, CBD., p. 28) Based on current scientific evidence, it can be firmly asserted that forest resilience is significantly influenced by biodiversity at various levels, and that maintaining or restoring biodiversity in forests is pivotal to promoting their resilience to different types of external pressure. Biodiversity, crucially, should be considered at all scales, from ecosystem level to individual stands or trees, and in terms of all elements, whether that be genes, communities or species (Thompson et al., 2009).
The high level of uncertainty regarding whether locations that are currently climatically suitable for forests will remain so in the future is a major concern. “Climate change may threaten woodlands by increasing the frequency of disturbance events that kill trees. Unlike other sectors, adaptive measures for forestry need to account for long time lags between tree establishment and maturity” (Dunn et al, 2021, p. 28). The importance of biodiverse forests is paramount in the context of climate change and more extreme climate events. Regional impacts of events such as droughts, fires, and hurricanes might be sufficient to overcome the resilience of even some large areas of primary forests, pushing them into a permanently changed state. BALANCE® therefore requires planting partners to display their consideration of the impacts of climate change upon forest locations, and the necessary alterations to the considered tree species for planting.
Knowing exactly how the climate will change in the near future is impossible, but projects should work under the assumption that we will continue to experience a warming scenario, and choose species accordingly. The inclusion of species which are more resilient to higher temperatures, even if not native to the specific locale in which a project is located, seems worthwhile, as long as exotic species do not become dominant species.
The Forestry Commission in the UK recommends including species and provenances with more southerly origins (DEFRA, 2014). FC England, similarly, advises that at least one-third of the plant material should be local, but consideration should be given to including plants from southern areas of origin, especially from 2 to 5 latitudes further south (Cavers & Cottrell, 2015, p. 22) than the project location, with the exception of Eastern European sources in the UK due to a lack of suitability. Although provenances from farther south may be well adapted to the warmer weather expected by climate change, they are not always well suited to other conditions at British locations. As a consequence, this may merely lead to the substitution of one sort of maladaptation risk for another (Cavers & Cottrell, 2015, p. 22). It is necessary, then, to display to BALANCE® that the project has carried out careful planning for any inclusion of exotic species and their potential risks even in cases where they might be potentially suited to climatic conditions in the near future.
Significant barriers to forest adaptation and resilience include widespread tree mortality from pests and diseases, with risks increasing due to reliance on imported plants and the planting of monoculture forests. With limited genetic diversity, any pest or disease which targets the majority species of a monoculture forest threatens a large proportion of the forest’s trees. Using the UK as an example, About 300 pest and disease species that cause significant harm to trees are presently listed on the UK Plant Health Risk Register. These pests and diseases pose a more pressing and immediate threat to woodlands compared to the impacts of climate change. To mitigate the risks, various resistance strategies can be employed, including: implementing integrated pest management techniques, producing more planting stock within the UK, prioritising natural establishment where feasible, enhancing biosecurity measures to impede the movement of contaminated water and soil, and intensifying surveillance efforts to promptly detect outbreaks and minimise their occurrence (Dunn, et al, 2021). Increasing the biodiversity of a forest is also proven to increase resilience to pests and diseases, as monocultures are widely reported to be susceptible to disease and insect attack (Pichancourt, 2014).
BALANCE® requires planting partners to display an understanding and inclusion of such preventative measures.
Invasive species can introduce fatal diseases which prove disastrous to native species. Nevertheless, it must be recognised that certain degraded forests, especially those with invasive alien species, may be stable and resilient, and these forests can become serious management challenges if attempts are made to reestablish the natural ecosystem to recover original ecosystem services. This presents a complex problem in the reestablishment of native, diverse forests for their variety of benefits, though it can be mitigated by efforts against excess introduction of exotic species, particularly through the reduction of reliance on non-native tree crop species for plantation or reforestation projects. Promising strategies with potential value for improving woodland regeneration include population management tactics targeting deer and uncontrolled livestock, which impede regrowth by consuming seedlings and are no longer subject to natural predator control in UK woodlands. Additional measures include the eradication of invasive species such as Rhododendron ponticum to restore natural regeneration processes, and the introduction of ground flora and epiphytes to support recovery efforts (Dunn et al, 2021). BALANCE® therefore requires partner projects to minimise invasive or exotic species in planting phases.
ANd trEE sizE
New woodlands can be established with diverse canopy and forest structure planned from the outset, to increase resilience to hazards. Connecting or expanding reforested sites to an existing forest would help the new forest to regenerate naturally and expand the size of the existing forest, thus benefiting biodiversity and forest resilience. This can be achieved by maintaining connectivity across forest landscapes by reducing fragmentation, expanding protected area networks and establishing ecological corridors. BALANCE® therefore prioritises projects that incorporate forest connectivity and stand variety in planting phases.
Some species thrive in selectively-logged woodlands, but felling large, old trees and clearing deadwood is harmful to birds, bats, lichens, invertebrates and fungi that are woodland specialists, so these measures should be avoided (British Ecological Society, 2021). Deadwood stands are also important carbon stores. Old trees and deadwood should be retained in managed woodlands, as they are immensely valuable for woodland specialist species (Kirby et al., 2017) and are nationally uncommon (Forest Research, 2021). Ancient woodlands are particularly valuable sites for veteran trees, deadwood and woodland specialists, but occupy just 2.2 percent of the UK (Rackham, 2020). As it takes many decades to accumulate woodland specialists in secondary woodlands, BALANCE® avoids unnecessary harvesting of any veteran trees.
To assist with decisions such as species selection, existing scientific and local knowledge should be consulted prior to beginning a reforestation project. Before applying large-scale techniques, it is best to conduct smaller trials. Regular monitoring of success indicators, such as the recovery of an endangered species, is necessary to assess the state of an ecosystem’s recovery and permit project managers to adjust accordingly (Brewer, 2021). Logistical and managementbased principles, though not included here, should also be paramount, and might include planning for the most cost-effective ways to achieve the project’s goals, testing of methods prior to scaling up, adherence to regulations, distributing responsibilities for planting, caring for and monitoring the trees, site preparation measures, implementing a variety of restoration strategies, the engagement and inclusion of local people and stakeholders in decision making processes and in implementation, and the necessary training and education on planting methodology and appropriate practices specific to the project. BALANCE® therefore investigates all partner projects to ensure that the relevant scientific research has been studied.
Furthermore, projects should produce quantifiable datasets via consistent planning and monitoring (i.e. who will be responsible for monitoring, how will the data be used and by whom). As projects are carried out, any unintended consequences of tree planting should be observed and, if possible, corrective actions should be taken, though, of course, undesirable outcomes are to be expected. BALANCE® will not become involved in a project in which quantifiable data is not produced.
“Species-rich ecosystems are typically more resilient because different species respond differently to stressors, thereby buffering the system as a whole”
(Dunn et al. 2021, Jucker et al. 2014, Isbell et al. 2015)
“Species-rich ecosystems are typically more resilient because different species respond differently to stressors, thereby buffering the system as a whole” (Dunn et al. 2021, Jucker et al. 2014, Isbell et al. 2015). The cultivation of native tree species in environments that meet their climatic and abiotic requirements fosters intra-species genetic diversity, thereby enhancing prospects for climate adaptation and bolstering resilience. Accordingly, future woodland creation initiatives geared towards conservation of biodiversity would do well to prioritise the inclusion of natural regeneration and locally sourced seed as fundamental components. (Dunn et al. 2021, Ennos et al., 2019). Practices which select only certain types of trees for harvesting should be avoided in all cases.
Depending on whether ecosystem resilience or species resilience is being considered, stability may depend on either diversity of species throughout an ecosystem or intra-specific genetic diversity, respectively, and the processes governing their maintenance. Studies show that the level of genetic diversity within an individual species is important for delivering the potential for ‘evolutionary rescue’; that is, to adapt and change to different abiotic circumstances.
The mechanism of evolutionary rescue involves initial population decline followed by recovery as genotypes adapted to the new conditions prosper via natural selection. Trees, generally, maintain high levels of genetic diversity and are typically effective at gene dispersal, particularly in northern temperate forests. Many tree species are also capable of adapting genetically to local environments, although the degree and geographic scale over which they are distributed may vary depending on the heterogeneity of the landscape conditions. With the threats presented to forests now and in the future, individual tree species with optimised resilience through variants of genes or gene combinations, as well as particular forest compositions typically conferred by high biodiversity, will prove most resistant.
Generally, the larger and less fragmented a forest is, the better. Also, large tree species are important ‘drivers’ in an ecosystem, particularly in northern temperate forests such as the UK which are typically species-poor. The ideal circumstance is that intended large tree species are suitably resilient to potential threats. Thus, planning for optimal resilience of the primary tree species in any forest is essential. To do this, forest planting projects should plant with varying compositions based upon a variety of species, while prioritising one or two main tree species with intra-specific genetic diversity. The mode and scale of dispersal of species, as well as particular threats to certain species, must be accounted for.
Clearer insights might be achieved if the multidimensional outcomes and factors of biodiversity are condensed and made uniform so that a single robust indicator is used, and the same is done with carbon sequestration.
Many studies have explored the trade-offs of planning for biodiversity and carbon sequestration but have failed to provide any detailed guidelines on what to plant and how to manage these plantings, and many more studies which have found positive correlations in the past have been conducted in non-forest landscapes such as grasslands. Addressing this issue requires deciphering to what extent forest biodiversity and carbon sequestration influence each other. Few empirical or modelling studies address the trade-offs and synergies that can occur between biodiversity and carbon sequestration in forests, and even fewer have identified any potential mechanisms which facilitate that relationship.
Another stumbling block has been the heterogeneity and lack of regulation (as well as ambiguous categorisation) of carbon sequestration monitoring and quantification. This is because outcomes strongly depend on how the boundaries of the analysis are drawn and which aspects are incorporated, i.e. developments solely within the forest ecosystem itself, or inclusive of wood products and emission substitution effects. Clearer insights might be achieved if the multidimensional outcomes and factors of biodiversity are condensed and made uniform so that a single robust indicator is used, and the same is done with carbon sequestration.
Carbon is sequestered in forests through a mix of several ecosystem processes, of which photosynthesis is key, though respiration and decomposition subsequently play their part. Carbon can be stored as soil organic carbon (SOC), and as carbonates, the latter of which are created over thousands of years when carbon dioxide dissolves in water and percolates the soil, combining with calcium and magnesium minerals, forming “caliche” in desert and arid soil. Temperature and moisture levels in the soil are the most important climatic factors that influence photosynthesis, decomposition, and respiration rates. For instance, high levels of SOC occur when photosynthesis outpaces decomposition in cold, wet northern latitudes (Habumuremyi, 2019, p. 9; Todd & Schulte, 2012), while temperate ecosystems, the likes of which exist in the UK, are likely to have high productivity in the summer with high temperature and sufficient moisture levels.
One method by which soil can be optimised for carbon sequestration and health is through continuous improvement by a type of charcoal which we now know as ‘biochar’, a rich, black, fertile earth which can be made from all sorts of organic material including agricultural waste, tree trimmings, manure, and rice husks.
Because soil organic matter (SOM) is composed of compounds that are highly enriched in carbon, high levels of SOM typically allow for increased sequestration of carbon (SOC) for several decades. SOM is made up of a heterogeneous mixture of materials that range in stage of decomposition and include soil microbes like bacteria and fungi, decaying material from once-living organisms like plant and animal tissues, faecal material, and products formed from their decomposition. SOC levels do not change in any way when carbon inputs and outputs in a forest are in equilibrium. SOC levels rise over time when photosynthesis adds more carbon than it takes out. (Habumuremyi, 2019, p.8). This, of course, is achieved in planting projects, but can be optimised amongst those which directly benefit biodiversity.
One method by which soil can be optimised for carbon sequestration and health is through continuous improvement by a type of charcoal which we now know as ‘biochar’, a rich, black, fertile earth which can be made from all sorts of organic material including agricultural waste, tree trimmings, manure, and rice husks. It offers many agricultural benefits, improving fertility without the need for synthetic fertilisers, supporting healthy soil by providing a home for armies of microbes and mycorrhizal fungi, and increasing the soil’s ability to hold water, mitigating the effects of flooding and improving drought resistance. But biochar’s biggest benefit is enduring carbon sequestration, removing carbon from the atmosphere and holding it as an inert substance over the very long term
In all soil types, the benefits of biodiversity can work to ensure that SOM is more abundant and that this process occurs to store carbon at greater capacities and for longer periods. Similarly, with biodiversity enhancing the resilience of forests, carbon is stored for longer and the release of carbon into the atmosphere when the plants die is less frequent.
Primary native forests, particularly in the tropics, are most effective in storing carbon, as they hold the largest carbon pools of any forest habitats in the world. However, a great variation exists in the carbon stock estimates for different types of forests, and can even vary widely among the same forest types.
More recently, synergies between species richness and diversity and carbon storage have been found among various forest types all over the world. For example, Biber et al. (2020) argue that, though difficult to analyse, win-win situations for carbon sequestration and biodiversity and forest landscapes across Europe have been shown. Vayreda et al. (2012) found that species richness and structural richness variables are better predictors of C accumulation than climatic and local site variables in Western Mediterranean region. Poorter et al. found that diversity of species is strongly related to carbon storage at smaller scales, while structural attributes of forests are more related to carbon storage at larger scales, though are relevant at all scales. Liu et al. (2018) found that tree species richness enhances ecosystem-level C storage in the subtropical forests of China.
The Planting Principles ensure that any projects with which BALANCE® becomes associated consider and implement biodiversity with the same urgency with which it is required.
Kothandaraman et al. (2020) used tropical forests in India as an example of forests rich in biodiversity proving hugely beneficial for carbon storage by estimating ecosystem-level carbon stock with data from 70 forest plots in three major forest types. Considerable quantities of carbon per hectare were found in the more biodiverse tropical evergreen forests, with an average of 336. Mg C/ha, of which just under a third of the carbon was stored in understorey, litter, deadwood and soil respectively (with the majority stored in trees). Among the forest types, the tropical evergreen forest type, the most biodiverse of the studied forest types, had the highest average carbon stocks when compared to semievergreen forests and dry deciduous forests.
Within the tropical evergreen forests, 14.5 percent of carbon was stored in the understorey, raising the overall carbon sequestration per unit area, while the average contribution of understorey over every other forest type is only 2.2 percent. Pichancourt et al. (2014,) found that the type of forest, the landscape context and climate are all significant. Therefore, since there is no ‘one size fits all’ solution, the best management solutions must include complex planning.
In addition to their role in enhancing biodiversity, larger trees should also be prioritised for their carbon sequestering capabilities. Lutz e al. (2018) reported that large-sized trees account for nearly 41 percent of carbon storage in forests on a global level. Tree species richness is widely known to increase tree size inequality among and within species, creating varied and diverse structures with a larger number of large trees, thus enhancing overall carbon stocks in a forest. Tree species evenness, monoculture planting or excessive logging for timber, on the other hand, is known to have a negative effect on average large tree size, structural diversity and overall carbon storage (Kothandaraman et al. 2020).
The extent to which soil carbon storage is influenced by either species diversity or specific species identity is another crucial factor in being able to analyse how to approach planting for biodiversity.
Historically, offsetting and conservation initiatives that have been mainly focused on carbon storage failed to protect many species that exist in species-rich, biodiverse forests, and positive relationships between diversity and carbon sequestration have not been well captured by global carbon models. Today, forests are often planted to maximise a single prime objective, whether that be biodiversity or carbon sequestration, often using just a single metric to determine success, rather than maximising the two objectives concurrently. Ironically, this has damaged the stocks of carbon found in the whole ecosystem, in favour of fast-growing, monoculture tree species, as well as the long-term ability of many projects to sequester carbon by reducing the forests’ resilience. The potential tonnage of carbon available for sequestration if intensive agriculture is converted into agroforestry, therefore, is enormous.
The Planting Principles ensure that any projects with which BALANCE® becomes associated consider and implement biodiversity with the same urgency with which it is required.
Rewilding and natural regeneration, of course, is also performed by an increasing number of projects, and BALANCE® recognises the necessity for such projects in mitigating the biodiversity crisis.
Biodiversity faces significant pressures stemming from various sources such as agricultural practices, climate change, pollution, urbanisation, woodland management, land use conversion, and the proliferation of non-native species (Dunn et al, 2021). With the current decade addressed as the UN Decade on Ecosystem Restoration as a primary target for all UN nations, it is timely to consider how carbon offsetting projects can contribute to the establishment and conservation of biodiversity.
Forests provide vital habitat for biodiversity and essential ecosystem services (Biber et al. 2020). Whilst some species are able to persist or adapt to climate change, others have and will continue to go extinct (Pearce-Higgins et al. 2017, Hickling et al. 2006). Protecting existing habitats is also of vital importance, as is introducing stricter regulations against land-use change in natural high-biodiversity regions and protection from harmful human activities such as logging and poaching. Rewilding and natural regeneration, of course, is also performed by an increasing number of projects, and BALANCE® recognises the necessity for such projects in mitigating the biodiversity crisis.
A mature oak tree, for example, transpires more than 400 litres of water on one hot summers’ day, and each gram of water transformed into water vapour removes heat. If one imagines this process carried out amongst every tree and plant across an entire forest, it causes a significant cooling impact on the surrounding area.
The benefits of afforestation are well-researched, and shall be described briefly here. When water is absorbed by trees predominately through osmosis, it moves vertically upwards through the inner bark’s xylem through capillary action (enabled by the phenomenon of surface tension against the force of gravity). Once water reaches the leaves, it evaporates into water vapour through the stomata (pores), and if this process occurs more than the rate at which water vapour returns to a liquid state on the surface of the leaves, there is net evaporation (Kumagai, 2011). In this context, large quantities of water vapour can be emitted by trees, and larger trees, of course, incur more transpiration. This evaporation causes a decreasing effect on immediate surrounding temperature, as considerable energy is required to vaporise each gram of water. A mature oak tree, for example, transpires more than 400 litres of water on one hot summers’ day, and each gram of water transformed into water vapour removes heat. If one imagines this process carried out amongst every tree and plant across an entire forest, it causes a significant cooling impact on the surrounding area. Water also evaporates from soil, so the total output of water vapour into the air from a forest or any ecosystem is called evapotranspiration.
The benefits of transpiration are not limited to the cooling effect on temperatures, they also cause humidifying benefits across a widespread system of air currents, preventing desertification. The evapotranspiration from rainforests, other forests, fields, and yards adds water to the atmosphere in the form of clouds and general humidity. Most of these clouds release the water right back onto the local areas. In simplified but strongly evidenced terms, forests are barriers to desiccation, a process which can spiral into a cycle of aridification and forest destruction whereby both phenomena positively influence each other, while also promoting susceptibility to pests and diseases. Stressed trees, in drier atmospheres and higher temperatures, are also more susceptible to fire, which adds to the cyclical process of destruction. In the Amazon rainforest, for example, “flying rivers”, air currents which carry the precipitation from the forest, are critical for the transport of moisture across the continent of South America, and even beyond. Widespread deforestation, through its destructive impacts upon evapotranspiration, contributes significantly to potentially irreversible tipping points.
The current land-cover of forests in the UK (13 percent) is small compared to that of other European countries. It is, however, larger than the forest land cover which existed at the end of the First World War in 1918, when, it is thought, it was only 5 percent. Existing forests at the time were a combination of native woodlands and commercial forests of mostly native species, though a new regime of forest creation through afforestation created intensively managed monoculture forests, typically involving exotic species. Historically, clear-cutting practices were widespread, involving the harvesting of exotic tree species at economic intervals of between 40 and 70 years, after which replanting of comparable exotic species ensued. Subsequently, governmental administrations incentivized the afforestation of non-indigenous conifers, with Sitka spruce and select other conifer species being acknowledged as capable of yielding significantly larger volumes of merchantable timber than native woodlands. This facilitated the development of a rural industry that currently employs more than 43,000 individuals in forest management and primary wood processing, providing the country with a domestic supply of timber and wood products, thereby decreasing the nation’s reliance on imports (Dunn et al. 2021). Levels of woodland creation across the UK are generally low at present, however, with woodland creation targets of 30,000 hectares per year to aid in meeting the Net Zero by 2050 mission proving particularly challenging.
practice in the UK has increasingly come to acknowledge the necessity for native species.
Many tree planting initiatives have consisted of monoculture forests or mixed forests prioritising exotic species under the guise of tree planting as environmentally and economically beneficial. This has been a longstanding issue; in the 1980s, peatlands, bogs and moorlands were planted with conifer, with environmentally damaging results, because planting trees on peatland dries out the soil, whereas peat in its natural state can act as a powerful carbon sink. The promise of planting native broadleaf species and mixed composition forests in place of the more typical monoculture conifer species of past forests is considerable. In terms of adaptive capacity, native tree populations in the UK typically show a degree of adaptation to environmental and topographical conditions in Britain to a far greater degree than those from mainland Europe. In provenance trials with Scots pine, silver birch, sessile oak and alder, for instance, British provenances outperformed continental European ones in terms of growth and survival in 90% of cases (Cavers & Cottrell, 2015, p. 19; Gerber et al. 2014). Also, native mixed-species planting in the UK which leads to oaks dominance also results in more durable carbon stores than achievable by conifer forests. Local provenances are typically, therefore, best adapted to current climatic and abiotic conditions in Britain, and thus should be prioritised in tree planting programmes in the UK.
Planting practice in the UK has increasingly come to acknowledge the necessity for native species. Multiple guidelines within the UK today recommend the use of local provenances for planting of primarily native species, yet monoculture forests with tree species or exotic origins are still selected, particularly for forests intended for timber production.
Today, according to Cavers and Cottrell (2014), British forests consist of roughly half conifer, 32 percent broadleaf and 8 percent broadleaf/conifer mixtures. Most of the conifer species are of exotic provenance; Scots pine, which is endemic to the area, Sitka spruce and Lodgepole pine (both USA/Canada), Larch (Central Europe or Japan), North-Central European Norway spruce, Southern European Corsican pine, and American/Canadian Douglas fir are the main species grown (Cavers & Cottrell, 2015, p. 14). Sitka spruce is the most abundant species, and covers half of the area of commercial conifer forests. The only native conifer species, Scots pine, represents only 18 percent of planted species. Oak, beech, sycamore, ash, and birch are the most common broadleaf species. Of all the broadleaf species in Britain, oak and ash account for 30% and 14% of the standing volume, respectively (Cavers & Cottrell, 2015; Anon, 2013). Unfortunately, in most cases, studies of genetic diversity in British tree species are few, despite the role that this would play in understanding how forest resilience may be increased. This is because most British species have distributions that extend to mainland Europe and so studies have been carried out across a broad geographic scale with little consideration of the British context.
The UK Forestry Standard serves as a framework “for more sustainable forestry, discouraging geometric plantings of single species in large even-aged blocks in favour of mixed systems including native species (at least five per cent).”
(Dunn et al, 2021, p.31).
The UK’s forests are under particular threat from new pests and diseases of exotic provenance. At least 28 known pests and diseases are thought to have the potential to significantly harm British trees if they entered the country. There are numerous instances in which introduced pathogens have resulted in the widespread extinction of tree species elsewhere in the world, such as in North America with chestnut blight and white pine blister rust (Cavers & Cottrell, 2015. p. 13).
Current recommendations advise that large-scale tree planting programs should steer clear of ecologically important regions, such as peatlands, highly productive agricultural lands, and habitats with elevated conservation value. Instead, emphasis should be placed on repurposing underutilised grazing lands, given their abundant availability and potential to satisfy governmental commitments for afforestation. It is cautioned that afforestation of high-quality arable land must be avoided, unless accompanied by strategic compensation measures, as it would further impede the UK’s capacity to produce food, culminating in greater reliance on imported food products, which are often associated with tropical deforestation. Moreover, it is critical to note that open habitats of high conservation value or “priority habitats”, including lowland heathlands and species-rich grasslands, are susceptible to being compromised by the establishment of woodlands, and should be avoided (Dunn, et al, 2021).
Planting on peat that is deeper than 50 cm is now outlawed under the UK Forestry Standard, but planting on shallow peat continues, supported by evidence that these forests can sequester carbon over the production cycle if the productivity is high enough. However, modelling suggests that peats should be avoided altogether to avoid damaging the soil, and new forests should be created in low-grade agricultural land instead. In all, policies regarding the establishment of woodlands on carbon-rich soils need further refinement if evidence emerges of adverse effects on the large stocks of carbon held below ground.
In order to maximise the potential for large-scale afforestation in the UK, forests ought to be planted on lands with low species diversity, particularly grasslands. The Forestry Commission has identified a vast expanse of “low risk” land, measuring five million hectares, that could potentially be utilised for this purpose. However, Friends of the Earth have excluded grasslands that are species-rich and have been designated as priority habitat for conservation, narrowing their estimate to 1.4 million hectares (Dunn et al, 2021; Friends of the Earth, 2020).
Encouragingly, political frameworks have increasingly begun to incorporate the concepts of forest resilience, native species, and biodiversity. The UK Forestry Standard serves as a framework “for more sustainable forestry, discouraging geometric plantings of single species in large even-aged blocks in favour of mixed systems including native species (at least five per cent)” (Dunn et al, 2021, p.31).
Scotland is considered the most advanced in its modernisation of forestry techniques and renewing targets set for forest creation with incorporation of biodiversity and native species restoration. Although regimented monoculture forests of conifer, which are managed primarily for timber, can still be seen on a large scale in Scotland, recent efforts have been made to redress this issue by building a vision for Scottish woodlands in line with modern consensus on the necessary ecological, environmental, social and economic concerns when planting forests. Forests in Scotland, on the whole, are now far more diverse in structure, with forest certification and political readdressing of forestry in Scotland as one of the most important drivers for this change in approach.
Habitat Action Plan Targets for native woods in Scotlands were revised as early as 2006 along with the rest of the UK with the wide review of UK Biodiversity Action Plan targets. At that time, conifer constituted 39,741 hectares of the total 59,057, or 67 percent, of planted woodland sites in Scotland. Targeted for their replacement were ancient broadleaved woods and Scots pine in areas suitable to their proliferation, with ambitious targets of 51 percent potential expansion area for 1km woodland networks, and 43 percent for 250m woodland networks, citing that these native species should be able to disperse freely, and attract more investment as success is evidenced.
Unfortunately, in the decade since this report, such progress has been limited. As outlined by the Forestry Commission Scotland (2020), a report aimed to provide government advice to planning authorities on planning for forestry and woodlands, much more still needs to be done to expand native woodland cover. The major drivers for native reforestation outlined in the report are to aid Scotland in mitigating climate change, to heighten Scotland’s timber productivity, support sustainable economic growth, support community development, improve quality of life and wellbeing, improve health through greater access to woodlands, conserve and enhance Scotland’s biodiversity, and protect ecosystem services. Those ecosystem services include improving water quality, reduction of wind erosion and sedimentation of water courses, reduction of run-off, increased entry of rainwater into soil, maintaining soil health, minimising soil disturbance, managing floods, and providing shelter for farmland and riparian habitats. The development and enhancement of native woodlands is also supported for its help in developing forest habitat networks, as part of integrated habitat networks, to enhance habitat interconnectivity and resilience to climate pressures.
The vision set out in Scotland is to increase woodland cover to 25 percent of land area by 2050
The vision set out in Scotland is to increase woodland cover to 25 percent of land area by 2050, focusing on native species and the maximisation of the delivery of multiple benefits from Scottish woodlands, including the restoration of lost habitats and climate mitigation through the increased effectiveness of carbon sequestration in native forests. Scotland’s native woodlands support a disproportionately high proportion (36 percent) of threatened species in Scotland, as well as 7 UK priority habitat types, and are a key element of Scotland’s landscape and cultural heritage; conserving this is central to the Scottish Government’s new Scottish Biodiversity Strategy. Nevertheless, despite the promising nature of the suggested outcomes of their renewed forestry strategies, the Scottish government has not entirely shifted their priorities to native, mixed forests, but has instead included four main types of woodland envisaged as the future of Scotland’s forests: Native Woodlands; Mixed Woodlands; Softwood Forests; Energy Forests.
Native woodlands are thus positioned on equal terms of importance as “energy forests”, which are explicitly monoculture forests for the primary purpose of energy generation, and, tangentially, timber production. As long as “energy forests” are still included in long-term plans for national forestry strategies, the positive impacts of biodiverse forests cannot be optimised on a scale that would see widespread impacts.
Afforestation-centred carbon offset initiatives are limited in number and scope within the UK context, because many are located in developing regions of the Global South. Nevertheless, promising examples of successful forests aligned with the principles established in this methodology do exist, one of which is the Forest of Marston Vale, one of the forests through which BALANCE® operates.
The Forest of Marston Vale operates through the Woodland Carbon Code (WCC), a voluntary standard for woodland creation projects in the UK, based on an early carbon methodology established by Daniel Morrell and Richard Tipper in the 1990s, which today offers offsetting projects the greatest opportunity for tangible verification and validity based upon a number of integrated considerations. Other voluntary carbon standards, such as the UK Peatland Code, Verra’s Verified Carbon Standard, the Gold Standard, and Plan Vivo all operate for organisations within the UK, yet the WCC has seen endorsement and promotion accelerate under governmental decree in recent years when compared with the other standards.
In the most basic sense, the WCC encourages a much-needed consistency and uniformity among approaches to woodland offsetting projects, while providing validation and independent verification to projects based upon sustainable management to national standards, reliable estimation and monitoring of the amount of carbon sequestered, and adherence to transparent criteria and standards to ensure that benefits are delivered. To meet the requirements of the code, projects must demonstrate additionality, scalability and guaranteed maintenance for their duration, use standardised methods for estimating sequestered carbon, and have integrated long-term management plans to ensure project sustainability. The code accounts for most types of forest growth or regeneration and associated carbon sequestration and emissions reductions, including woodland created by planting and natural regeneration, multiple types of management regimes from frequent clear felling to minimum intervention woodland, and even emissions outside the woodland boundary which result from the project. The code does not, however, account for additional carbon sequestration due to changes to the management of existing woodland, or carbon stored in forest products.
The WCC ensures comprehensive planning and managing, including an outline of the necessary inputs and resources including a full financial analysis, a summary of operational techniques, consideration of species selection for current and future benefits, consideration of longevity and resilience in created forests, maps of the areas being planted, and a chronological plan of all key project operations to be established at the outset of the project.
The WCC ensures comprehensive planning and managing, including an outline of the necessary inputs and resources including a full financial analysis, a summary of operational techniques, consideration of species selection for current and future benefits, consideration of longevity and resilience in created forests, maps of the areas being planted, and a chronological plan of all key project operations to be established at the outset of the project. The code ensures that the management plan is updated regularly with renewed longer-term management targets and intentions beyond the project duration.
The WCC operates by allocating WCUs to organisations and individuals based on projects within the UK. WCUs are voluntary offset units and thus cannot be used in the compliance market, and cannot currently be used for emissions made outside the UK, thus are restricted purely to the voluntary market for organisations based primarily within the UK. They are acquired upon the verification of a project, but before that the units are called Pending Issuance Units (PIUs), the purpose of which is to demonstrate the quantity of potential future sequestration at the outset of the project, which can be assigned to buyers but cannot yet be used or retired until they are transferred into WCUs, which can be used as full carbon credits. All units are assigned a vintage, which is the period in which their delivery is anticipated, as determined by monitoring and verification schedules.
When the vintage has ended, the quantity of PIUs assigned to it is automatically converted into WCUs, and only verified WCUs can be used or retired to help compensate for an organisation’s emissions. All retirements are shown on the public view of the UK Land Carbon Registry, prior to using WCUs in any reports. This process helps to ensure the validity of all carbon units sold, with each unit designed to represent real and tangible carbon sequestration, and that carbon units cannot be resold to create further carbon leakage through excess emissions which are ‘compensated for’ elsewhere.
The designation of WCUs is achieved through determining the project’s net carbon sequestration, which is the total amount of carbon sequestered by the project which can be converted into carbon units. These are divided between the proportion that will contribute to the shared WCC buffer and the claimable carbon sequestration which is the amount the project can sell or claim. The net carbon sequestration is derived from the simple, REDD+ compliant, equation:
NEt CArBoN sEquEstrAtioN = ProJECt CArBoN + LEAKAgE – BAsELiNE
By this, the predicted number of carbon units is identified in accordance with the project’s verification schedule, and will then be divided into the claimable carbon sequestration units, WCUs, and the contribution to the WCC buffer. Offset value is only delivered when the offsetting has actually taken place. Monitoring of the carbon sequestered at 5 years into the project is based on the projected carbon sequestration established at the outset of the project, but from year 15 onwards (at intervals of 10 years), it is based upon field survey measurements.
Also significant is that all units are expressly publicly visible in regard to their current status and owner, providing clarity and transparency of carbon owners and claims that are made, and avoiding duplicity or ‘double counting’.
Developers are required to describe the original condition of the location, including details about vegetation cover, soil type and carbon content, through which they are to estimate the baseline for the carbon quantities at the site for the duration of the project in the hypothetical absence of the project’s activities. The WCC’s conservative approach to baseline construction means project activities must be more effective to accrue additional benefits, so only the most efficient carbon sequestering woodlands create a significant number of WCUs. Included in the baseline are carbon pools made up from tree biomass, soil, non-tree biomass and litter and deadwood, and measuring can be achieved with reference to any photographs, maps, field survey results or remotely-sensed images which indicate the condition of the vegetation and soil before project commencement. For tree biomass in the baseline scenario, which is most often the largest carbon pool prior to the start of a project, assessment can be done by determining the density of the trees and their current age, converting this to an equivalent area of woodland of a given age and using Carbon Lookup Tables to estimate the likely changes to that stock over time. The inclusion of non-tree biomass and litter and deadwood in the baseline scenario encourages the growth of biodiverse forests which facilitate understorey development as well as mixed stand structure and tree age, which, as discussed, enhances the resilience of the forest and its capability to sequester carbon. Also significant is that all units are expressly publicly visible in regard to their current status and owner, providing clarity and transparency of carbon owners and claims that are made, and avoiding duplicity or ‘double counting’.
Considerations of carbon leakage are taken into account by the WCC; small projects (of equal to or less than 5 hectares in planting area) are assumed to produce no leakage due to UK legislation which is designed to protect semi-natural habitats from threats such as deforestation, while standard projects (of 5 hectares or more in planting area) should account for any significant GHG emissions through land use changes in other areas over the project duration.
Additionality is also accounted for by the WCC. In order to assess additionally, the project must pass legal and carbon finance tests. If both are passed, an investment test is used to confirm a project’s additionality, but if that is not passed, a barrier test may be used.
Additionality is also accounted for by the WCC. In order to assess additionally, the project must pass legal and carbon finance tests. If both are passed, an investment test is used to confirm a project’s additionality, but if that is not passed, a barrier test may be used. The legal test is the assessment that there are no laws, regulations, orders, agreement or any legally binding agreement which requires the implementation of the project, and the carbon finance test is used to show the significance of income from carbon units; projects must demonstrate that income from the sale of carbon units over the project lifetime, equates to at least 15 percent of their planting and establishment costs up to and including year 10. The investment test is used to prove that carbon finance is crucial to making woodland creation economically attractive in the given circumstances, i.e. the net value of woodland creation is positive only with the support of investment. If the investment test is not passed, proof of other economic, environmental or social barriers which stop a project from going ahead with the absence of carbon finance is sufficient to demonstrate additionality.
Through the comprehensive list of WCC regulations, any project operating through the code is far more likely to provide the benefits which carbon offset projects are supposed to supply, while ensuring that invalid initiatives do not slip through the cracks. This makes the WCC a valuable contributor to a global effort to reinvent carbon offsetting and ensure that it contributes to climate mitigation as efficiently as possible, and aligns with the BALANCE® ethos as established in this methodology.
The Forest of Marston Vale is a group project consisting of ten sites, covering 61 square miles between Milton Keynes and Bedford in Bedford Borough and central Bedfordshire, providing a home for newly-grown forest ecosystems. The Forest is one of BALANCE®’s major planting partners. The project began in 1991 when the Government designated this area as one of 12 Community Forests across England, and the Forest of Marston Vale Trust was created by the founding partnership of Natural England, the Forestry Commission and local authorities. The individual planting projects in the scheme are managed by the Trust. Historically, the site upon which a considerable portion of the Forest has been planted had been damaged by industrial processes such as brick making and refuse sites over many decades. At the start of the project, the Forest area had just 3 percent tree cover.
Today, Marston Vale is home to an expanding range of forests which have increased overall tree cover to 15 percent, and have incorporated the key concepts of biodiversity, forest resilience and carbon sequestration to considerable success, with a key target of increasing tree cover to over 30 percent playing a central role in driving the project. Millions of trees have been planted with the participation of local communities and businesses, boosting the local economy and aiding in potential future growth. The site is home to some pre-existing semi-natural ancient woodlands, which help to connect and buffer the created forests.
An academic report published by the Forest of Marston Vale highlights the various achievements of the project over its 20 year lifespan, with an effort to quantify the social, environmental and economic impacts of the project. Thus far, tree cover has increased from just 3.6 percent in 1995 to more than 15 percent in 2015, and has created over 1,150 hectares of new woodland, more than trebling woodland cover. Overall economic benefits are valued annually at £UK12.83 million, which equates to benefits with a net present value of £UK339 million, and, according to the study, £UK11 of social, economic and environmental benefits for every £UK1. In terms of employment, the report estimates that the project has supported an additional 167 jobs per year for local residents, which includes both direct employment in the project, to service industry positions and contractors. The local economy has been especially supported by the preferential use of local goods and services by the project. In turn, these local businesses provide local jobs and boost local incomes.
Investment has been fruitful, with an average of 15 partnerships with public and private sector organisations per year, which have helped to secure a total inward investment so far of over £UK22.8m. The recreational benefits of creating the forest has helped the visitor economy in the area, bringing in an estimated £UK6.91 million per year from elsewhere.
The advantages to health and wellbeing of the forest, for example, are numerous; the majority of the new woodlands are close to residential areas, which enhances access and recreational opportunities, and public footpaths cross many of the sites.
Economic benefits, though useful for attracting investment, are only a small part of the story, however. The advantages to health and wellbeing of the forest, for example, are numerous; the majority of the new woodlands are close to residential areas, which enhances access and recreational opportunities, and public footpaths cross many of the sites. Using both visitor data and research on how physical activity outdoors can reduce hospital visits and increase life expectancy, the value of the physical health benefits through the provision of recreational space for exercise and contact with nature provided by the Forest was estimated at £UK4.95 per annum. This does not include the benefits to mental health and wellbeing, which, although not quantified, if comprehensive studies from around the world on forests and mental health issues such as stress and anxiety are to be believed, are almost certainly significant. Less quantifiable social impacts, such as the facilitation and fostering of social cohesion and a new sense of place, as well as the benefits derived from increased understanding of natural and ecological processes, are also important.
The Forest has long sold the carbon stored in the woodlands as a voluntary carbon offset initiative, with buyers allocated carbon offset units on the Markit Registry. Today, the Forest operates by the Woodland Carbon Code, and the overall benefits to carbon sequestration, forest resilience and biodiversity as transferred through the various requirements for WCC verification are embodied by the nature of the planting process. Total carbon sequestration of the involved trees has been estimated at 4,917 tonnes of CO2 annually, and has potential for creating an even larger carbon pool with the planting of more trees. Biomass carbon, including below ground root mass, and SOC, are considered in carbon sequestration models.
Woodlands vary between the different sites of the Forest, but generally they include plant protection (with fencing or the use of tree shelters), ground cultivation, and sward establishment using a ‘pollen and nectar’ grass and wildflower mix to form the understorey. Trees are planted with higher spatial density than found in ancient forests in the same region. Whereas the typical ancient woodland would have trees 8m apart, the Forest of Marston Vale project plants trees at 2m apart, in an attempt to acquire the same benefits from ecosystem services and carbon sequestration as found in ancient woodlands. This is because trees today are considered light-dependant; that is, they need to grow higher quicker to get the light they need to survive, and thus need to be closer together. Importantly, roughly three quarters of the landscape is planted with native species found on site or locally, creating a largely unhindered ancient woodland character comprised of oak, pine and maple woods, with particular consideration for resilience in the context of climate change by the inclusion of oak and hornbeam tree species which are projected to fare better with local temperature rises and climatic extremes in the near future.
The provenance and sourcing of seedlings is most often exclusive to the UK, from nurseries and contractors located in the UK, thus avoiding importation while selecting species most suited to the heavy clay soil found at the site’s location in Bedfordshire.
The provenance and sourcing of seedlings is most often exclusive to the UK, from nurseries and contractors located in the UK, thus avoiding importation while selecting species most suited to the heavy clay soil found at the site’s location in Bedfordshire. Amongst all the woodlands created in the project, forest composition normally incorporates an intermix of dominant UK native species and fewer exotic species which comprise a core of 2 or 3 species, as well as minor native species, which work to simultaneously create functioning biodiverse ecosystems while preparing the forests for future threats. Similarly, the inclusion of species from between 2 to 5 degrees south to suit projected climate shifts, as recommended by forestry experts, is in line with the Forestry Commission and the Forest of Marston Vale’s targets.
Mitigation of the threat of diseases is also considered as part of the project with the explicit avoidance of creating monoculture forests, which are more likely to be seriously impacted by diseases. The focus on native species and creating forest ecosystems which resemble ancient forests that exist on the site increases overall biodiversity, which elevates the forest’s overall resilience to pests and diseases. If any one of the species succumbs wholly to disease, the woodland should be able to repair itself.
Environmental benefits and ecosystem services of the project besides the storing of carbon have been numerous. Air pollution, for example, has been significantly reduced; it is estimated that the new woodland thus far created has been able to absorb 0.65 tonnes of sulphur dioxide (SO2 ) and 65 tonnes of particulate matter. While agriculture remains the primary land use within the Forest area, the shift in land use to woodlands has resulted in reduction of impacts such as GHG emissions from fertilisers and machinery, which are common with modern intensive agriculture, and has significantly benefited soil health and reduced soil erosion. To date, it is estimated, the creation of the Forest has reduced agricultural GHG emissions by 1,747 tonnes CO2e per annum, which is additional to the increased size of the carbon pool as a result of forest creation. Water quality has also been improved, and flood risk reduced, due to the slowing of the flow of water; the quantity of woodland created in the Forest so far is estimated to reduce peak flood flows by 5 percent.
The core target of increasing tree cover up to 30 percent from today requires the planting of around 5 million more trees by 2031. With the adoption of the Woodland Carbon Code, the future of the Forest of Marston Vale is extremely promising, as is its potential for use by BALANCE®
The Forest of Marston Vale is just one example of the type of reforestation which BALANCE® facilitates through its focus upon biodiversity and ecosystem creation. Going forward, BALANCE® plans to expand to planting forests internationally, with consideration in each location for appropriate forest composition, local topography and climate in order to enhance the resilience of the forest and thus more efficiently and sustainably sequester carbon. Planting shall always occur on previously lowdiversity lands, and avoid peatlands or high diversity grasslands or other productive biomes, in order to avoid carbon leakage and negative impacts on biodiversity. Selected planting partners, as a matter of both priority and necessity, shall strictly follow the planting principle obligations set out in this methodology.
Biodiversity is the key factor in implementing BALANCE® through forest creation. When focusing on biodiversity in selecting and planting species, a positive feedback loop encourages both tree and non-tree species diversity. This in turn promotes forest resilience, carbon sequestration and ecosystem services, as well as the various social, economic and environmental benefits associated with biodiverse forests.
Ultimately, BALANCE® targets the creation of large, connected forest ecosystems, with varying stand age and size, complimented by the prioritisation of large native tree species. The mitigation of the threat of diseases and serious climate related threats is simultaneously achieved through the creation of biodiversity and avoidance of monoculture planting.
Through BALANCE® in Business and the BALANCE® Calculator, a simple summation of carbon equivalent emissions is offered to the consumer, offering the share of GHG for businesses based on their specific sector/sub-sector and their current GDP proportion to the sector/sub-sector GDP, which works to provide an estimation in a much simpler way compared to other methods.
The data that support the findings of this study are available in BALANCE® Methodology at https://osf.io/ak3y8/?view_only=6adcbf7661c242728628f06ce1d2b263.
These data were derived from the following resources available in the public domain:
https://www.ons.gov.uk/economy/environmentalaccounts/datasets/ukenvironmentalaccountsatmosphericemissionsgreenhousegasemissionsbyeconomicsectorandgasunitedkingdom/current
https://www.ons.gov.uk/aboutus/transparencyandgovernance/freedomofinformationfoi/publicandprivatesectoremployeescontributiontogdp
https://www.ons.gov.uk/economy/grossdomesticproductgdp/datasets/gdpodatasourcescatalogue
https://www.ons.gov.uk/aboutus/transparencyandgovernance/freedomofinformationfoi/annualturnoverbyindustryforallsicindustriesfrom1998to2015
[total manuscript word count: approximately 14,820 references on the following 12 pages not included]
-Advantages and Disadvantages of Afforestation. Sciencing (2018). Available online at: <https:// sciencing.com/ advantages-disadvantages-clearcutting-8481691.html>
-Aerts, R., Honnay, O., & Van Nieuwenhuyse, A. (2018). Biodiversity and human health: Mechanisms and evidence of the positive health effects of diversity in nature and green spaces. British Medical Bulletin, 127(1), 5–22
-Alvarez, S. A., Gibbs, S. J., Bown, P. R., Kim, H., Sheward, R. M., & Ridgwell, A. (2019). Diversity decoupled from ecosystem function and resilience during mass extinction recovery. Nature, 574, 242– 245
-Anon. (2013). NFI preliminary estimates of quantities of broadleaved species in British woodlands, with special focus on ash. InNational Forest Inventory, Forestry Commission, 117, 13-26.
- Brewer, Grace. (2021). 10 golden rules for restoring forests, Learn how reforestation can tackle the climate and biodiversity crises. (Web article). https://www.kew. org/read-and-watch/10-golden-rules-for-reforestation
-Barsoum, N., Coote, L., Eycott, A. E., Fuller, L., Kiewitt, A., & Davies, R. G. (2016). Diversity, functional structure and functional redundancy of woodland plant communities: How do mixed tree species plantations compare with monocultures?. Forest Ecology and Management, 382, 244-256.
-Beechener G. et al (2021). Environmental Agency. Achieving Net Zero: A review of the evidence behind potential carbon offsetting approaches. Version 7.
-Behera, S. K. et al. (2017) Aboveground biomass and carbon stock assessment in Indian tropical deciduous forest and relationship with stand structural attributes. Ecol. Eng.99, 513–524. Available online at: <https:// doi.org/10.1016/j.ecoleng.2016.11.046>
-Bender, S. F., & van der Heijden, M. G. (2015). Soil biota enhance agricultural sustainability by improving crop yield, nutrient uptake and reducing nitrogen leaching losses. Journal of Applied Ecology, 52, 228–239
-Bennett, B., & Kruger, F. (2015). 1965 to 1995: Fluctuating fortunes and final dividends. Forestry and water conservation in South Africa, history: Science and policy (pp. 219– 242). ANU Press
-Biber P. et al. Forest Biodiversity, Carbon Sequestration, and Wood Production: Modeling Synergies and Trade-Offs for Ten Forest Landscapes Across Europe. Front. Ecol. Evol. (2020)
-Brancalion, P. H. S., & Holl, K. D. (2020). Guidance for successful tree planting initiatives. JOURNAL OF APPLIED ECOLOGY, 57(12), 2349–2361.
-Broadmeadow M. Matthews R. (2003) Forests, Carbon and Climate Change: the UK Contribution. Forestry Commission
-Bronson W. Griscom, Justin Adams, Peter W. Ellis, Richard A. Houghton, Guy Lomax, Daniela A. Miteva, William H. Schlesinger, David Shoch, Juha V. Siikamäki, Pete Smith, Peter Woodbury, Chris Zganjar, Allen Blackman, João Campari, Richard T. Conant, Christopher Delgado, Patricia Elias, Trisha Gopalakrishna, Marisa R. Hamsik, Mario Herrero, Joseph Kiesecker, Emily Landis, Lars Laestadius, Sara M. Leavitt, Susan Minnemeyer, Stephen Polasky, Peter Potapov, Francis E. Putz, Jonathan Sanderman, Marcel Silvius, Eva Wollenberg, Joseph Fargione. Natural climate solutions (2017). Proceedings of the National Academy of Sciences114 (44) 11645-11650; Available online at: <10.1073/pnas.1710465114>
-Browder, G., Ozment, S., Rehberger Bescos, I., Gartner, T., & Lange G.-M. (2019). Integrating Green and Gray: Creating Next Generation Infrastructure. Washington, DC: World Bank and World Resources Institute
-Brown, D. R., Dettmann, P., Rinaudo, T., Tefera, H. & Tofu, A. (2011). Poverty alleviation and environmental restoration using the clean development mechanism: a case study from Humbo, Ethiopia. Environmental Management, 48(2), 322–333
-Busch, J., Engelmann, J., Cook‐Patton, S. C., Griscom, B. W., Kroeger, T., Possingham, H., & Shyamsundar, P. (2019). Potential for low‐cost carbon dioxide removal through tropical reforestation. Nature Climate Change, 9, 463– 466
-Calvin, K.; Rose, S.; Wise, M.; McJeon, H.; Clarke, L. & Edmonds, J. (2015). Global climate, energy, and economic implications of international energy offsets programs. Climatic Change, 133(4), pp. 583–596.
-Cames M. et al. (2016) Öko Institut, How additional is the Clean Development Mechanism? Analysis of the application of current tools and proposed alternatives
-Cao, S., Zhang, J., Chen, L. & Zhao, T. (2016). Ecosystem water imbalances created during ecological restoration by afforestation in China, and lessons for other developing countries. Journal of Environmental Management, 183, 843–849
-Carbon Neutral.com. (2021) The Carbon Neutral Protocol: The global standard for carbon neutral programmes
-Cardinale, B. J., Duffy, J. E., Gonzalez, A., Hooper, D. U., Perrings, C., Venail, P., Narwani, A., Mace, G. M., Tilman, D., Wardle, D. A., Kinzig, A. P., Daily, G. C., Loreau, M., Grace, J. B., Larigauderie, A., Srivastava, D. S., & Naeem, S. (2012). Biodiversity loss and its impact on humanity. Nature, 486, 59– 67
-Cavers, S., & Cottrell, J. E. (2015). The basis of resilience in forest tree species and its use in adaptive forest management in Britain. Forestry: An International Journal of Forest Research, 88(1), 13-26. https://academic.oup. com/forestry/article/88/1/13/2756020
-Ceballos, G., Ehrlich, P. R., & Dirzo, R. (2017). Biological annihilation via the ongoing sixth mass extinction signaled by vertebrate population losses and declines. Proceedings of the National Academy of Sciences, 114(30)
-Chausson, A., Turner, B., Seddon, D., Chabaneix, N., Girardin, C. A. J., Kapos, V., Key, I., Roe, D., Smith, A., Woroniecki, S., & Seddon, N. (2020). Mapping the effectiveness of nature-based solutions for climate change adaptation. GLOBAL CHANGE BIOLOGY, 26(11), 6134–6155.
-Committee on Climate Change (2019) Net Zero The UK’s contribution to stopping global warming. UK Government. [online] Available at: <https://www.theccc. org.uk/wpcontent/uploads/2019/05/Net Zero-The-UKscontributionto-stopping-global-warming.pdf>
-Committee on Climate Change (2016). UK Climate action following the Paris Agreement
-Committee on Climate Change (2019). Net Zero Technical report. UK Government. Available online at: <https:// www.theccc.org.uk/publication/net-zerotechnical-report/>
-Committee on Climate Change (2019). Net Zero: The UK’s Contribution to stopping global warming. UK Government.
-Committee on Climate Change. (2020) Reducing UK emissions: Progress Report to Parliament. Committee on Climate Change, London
-Cook-Patton, S.C., Leavitt, S.M., Gibbs, D., Harris, N.L., Lister, K., Anderson-Teixeira, K.J., Briggs, R.D., Chazdon, R.L. Crowther, T.W. Ellis, P.W., Griscom, H.P., Herrmann, V., Holl, K.D., Houghton, R.A., Larrosa, C., Lomax, G., Lucas, R., Madsen, P., Malhi, Y., Paquette, A., Parker, J.D., Paul, K., Routh, D., Roxburgh, S., Saatchi, S., van den Hoogen, J., Walker, W.S., Wheeler, C.E., Wood, S.A., Xu, L. and Griscom, B.W. (2020). Mapping carbon accumulation potential from global natural forest regrowth. Nature, 585, 545–550.
-Dave, R., Maginnis, S., & Crouzeilles, R. (2019). Forests: Many benefits of the Bonn Challenge. Nature, 570, 164
-de Lamo, X., Jung, M., Visconti, P., Schmidt‐Traub, G., Miles, L., & Kapos, V. (2020). Strengthening synergies to achieve biodiversity goals. UNEP‐WCMC
-DEFRA (2014). Tree Health Management Plan. Available online at: <https://assets.publishing.service.gov.uk/ government/uploads/system/uploads/attachment_data/ file/ 307299/pb14167-tree-health-management-plan.pdf>
-Davies, C., Chen, W. Y., Sanesi, G., & Lafortezza, R. (2021). The European Union roadmap for implementing nature-based solutions: A review. Environmental Science and Policy, 121, 49–67.
-Defra. Clean Growth Strategy: executive summary (2019)
-Dhanda K. Kath, & Hartman Laura P. (2011). The Ethics of Carbon Neutrality: A Critical Examination of Voluntary Carbon Offset Providers. Journal of Business Ethics, 100(1), 119–149.
-Dhanda, K. K., & Murphy, P. J. (2011). The New Wild West Is Green: Carbon Offset Markets,Transactions, and Providers. Academy of Management Perspectives, 25(4), 37–49
-Dieler, J., Uhl, E., Biber, P., Müller, J., Rötzer, T. and Pretzsch, H. (2017). Effect of forest stand management on species composition, structural diversity, and productivity in the temperate zone of Europe. European Journal of Forest Research, 136, 739–766.
-Donadieu. P. (2019) Tree-planting to offset carbon emissions: no cure-all. phys.org. Available online at: <https://phys.org/news/2019-09-tree-planting-offsetcarbon-emissions-cure-all.html>
-Donofrio S. Maguire P. Zwick S. Merry W. (2020) Voluntary Carbon and the Post-Pandemic Recovery. Ecosystem Marketplace Insights Brief. A Special Climate Week NYC 2020 Installment of Ecosystem Marketplace’s State of Voluntary Carbon Markets 2020 Report.
-Drinan, T. J., Graham, C. T., O’Halloran, J., & Harrison, S. S. C. (2013). The impact of catchment conifer plantation forestry on the hydrochemistry of peatland lakes. Science of the Total Environment, 443, 608–620
-Dunn, C., Burden, A., Chamberlain, B., Danek, S., Evans, C., Freeman, C., Harvey, R., Proctor,S., & Walker, J. (2021). Nature-based solutions for climate change in the UK - Peatlands. In Nature-based solutions for climate change in the UK British Ecological Society, 28.
-Ennos, R., Cottrell, J., Hall, J. and O’Brien D. (2019). Is the introduction of novel exotic forest tree species a rational response to rapid environmental change? – A British perspective. Forest Ecology and Management, 432, 718–728.
-Environment Agency. (2020) Net Zero: Biological carbon sequestration. Research - Internal Evidence Review
-Forest Research. (2021). What our woodlands, and tree cover outside woodlands, are like today; NFI inventory reports and woodland map reports. Available online at: <https:// www.forestresearch.gov.uk/tools-andresources/national-forest-inventory/what-our-woodlandsand- tree-cover-outside-woodlands-are-like-today-8211nfi-inventory-reports-and-woodland-map- reports/>
-Forestry Commission Scotland. (n.d.) The right tree in the right place. Planning for forestry & woodlands. Available at <https://forestry.gov.scot/publications/96the-right-tree-in-the-right-place- planning-for-forestryand-woodlands>
-Fraser LH, et al. (2015) Plant ecology. Worldwide evidence of a unimodal relationship between productivity and plant species richness. Science 349:302–305.
-Friends of the Earth (n.d.). Finding land to double tree cover. [online] Available at: https://policy. friendsoftheearth.uk/insight/finding-land-doubletreecover
-García, C., Espelta, J.M. and Hampe, A. (2020). Managing forest regeneration and expansion at atime of unprecedented global change. Journal of Applied Ecology, 57, 2310–2315.
-Gerber, S., Chadœf, J., Gugerli, F., Lascoux, M., Buiteveld, J. and Cottrell, J. et al. (2014) High rates of gene flow by pollen and seed in oak populations across Europe. PlosOne. 9, e85130.
-Ghazoul, J., Bugalho, M., & Keenan, R. (2019). Plantations take economic pressure off natural forests. Nature, 570, 307
-Girardin, C. A. J., Jenkins, S. R., Seddon, N., Allen, M., Lewis, S. L., Wheeler, C. E., Griscom, B. W., & Malhi, Y. (in press). Nature-based Solutions for climate change mitigation: Contribution to peak warming and long-term global cooling.
-Global Commission on Adaptation (2019). Adapt now: a global call for leadership on climate resilience
-Gómez Martín, E., Giordano, R., Pagano, A., van der Keur, P., & Máñez Costa, M. (2020). Using asystem thinking approach to assess the contribution of nature based solutions to sustainable development goals. Science of the Total Environment, 738.
-Greenbelt Consulting. Shoreline Management And Stabilization Using Vegetation. Accessed March 2023. (Web Article). Link: http://www.greenbeltconsulting. com/ctp.html
-Habumuremyi, D. (2019). Assessment of Soil Carbon Storage in Different Land Use Managements: Kinoni Watershed, Musanze District-Rwanda. Éditions universitaires européennes. https://documents.pub/ document/donatienfinal-submission-of-researchproject-5th-year-2011-2013.html?page=1
-Hamilton et al. (2011). Back to the Future: State of the Voluntary Carbon Market 2011. Ecosystem Marketplace, Bloomberg New Energy Finance. www.ecosystemmarketplace.com/reports/ SOVCM2011
-Herr, D., Blum, J., Himes-Cornell, A., & Sutton-Grier, A. (2019). An analysis of the potential positive and negative livelihood impacts of coastal carbon offset projects. Journal of Environmental Management, 235, 463–479. Available online at: <https://doi-org.elib.tcd. ie/10.1016/j.jenvman.2019.01.067>
-Hickling, R., Roy, D. B., Hill, J. K., Fox, R. and Thomas, C. D. (2006). The distributions of a wide range of taxonomic groups are expanding polewards. Glob. Change Biol. 12, pp.450–455
-Houghton, R.A., Byers, B. and Nassikas, A.A. (2015). A role for tropical forests in stabilizing atmospheric CO2. Nature Climate Change, 5, 1022–1023.
-Hua, F., Wang, X., Zheng, X., Fisher, B., Wang, L., Zhu, J.Wilcove, D. S. (2016). Opportunities for biodiversity gains under the world’s largest reforestation programme. Nature Communications, 7(1), 12717
-Huang, L., Shao, Q. & Liu, J. (2012). Forest restoration to achieve both ecological and economic progress, Poyang Lake basin, China. Ecological Engineering, 44, 53–60
-IEMA (2020). Pathways to Net zero: Using he IEMA GHG Management Hierarchy-IGES (2014). CDM Project Database, as of 01/01/2014. Available online at <http://enviroscope.iges.or.jp/ modules/envirolib/view. php?docid=968.>
-IPBES (2019) Summary for Policymakers of the Global Assessment Report on Biodiversity and Ecosystem Services of the Intergovernmental Science-Policy Platform on Biodiversity and Ecosystem Services. IPBES Secretariat
-IPBES (2019): Summary for policymakers of the global assessment report on biodiversity and ecosystem services of the Intergovernmental Science-Policy Platform on Biodiversity and Ecosystem Services.
-IPCC (2019) Climate and Land: An IPCC Special Report on Climate Change, Desertification, Land Degradation, Sustainable Land Management, Food Security, and Greenhouse gas fluxes in Terrestrial Ecosystems
-IPCC, Summary for Policymakers of IPCC Special Report on Global Warming of 1.5ºC approved by governments, 2018
-IUCN. (2020). Guidance for using the IUCN global standard for nature‐based solutions (1st ed.)
-Isbell F, et al. (2015) Biodiversity increases the resistance of ecosystem productivity to climate extremes. Nature 526:574–577
-Jenkins M. Schaap B. (2018) Forest Ecosystem Services. Background Analytics Study 1. Background study prepared for the thirteenth session of the United Nations Forum on Forests.
-Jia, X., Zhu, Y. & Luo, Y. (2017). Soil moisture decline due to afforestation across the Loess Plateau, China. Journal of Hydrology, 546, 113–122
-Jucker, T., Bouriaud, O., Avacaritei, D. and Coomes, D.A. (2014). Stabilizing effects of diversity on aboveground wood production in forest ecosystems: linking patterns and processes. Ecology Letters, 17, 1560–1569
-Keith, H., Vardon, M., Obst, C., Young, V., Houghton, R. A., Mackey, B. (2021). Evaluating nature- based solutions for climate mitigation and conservation requires comprehensive carbon accounting. Science of the Total Environment, 769.
-Kendon, M., McCarthy, M., Jevrejeva, S., Matthews, A. and Legg, T. (2019). State of the UK climate 2018. Int. J. Climatol., 39, pp.1–55
-Kirby, K.J., Buckley, G.P. and Mills, J. (2017). Biodiversity implications of coppice decline, transformations to high forest and coppice restoration in British woodland. Folia Geobotanica. 52, 5–13.
-Kollmuss, A.; Füssler, J.; Spors, F.; Kennedy, P. & Guigon, P. (2015a). Overview of Carbon Offset ProgramsSimilarities and Differences: PMR (Partnership for Market Readyness, Technical Note 6.
-Kollmuss, A.; Schneider, L. & Zhezherin, V. (2015b). Has Joint Implementation reduced GHG emissions? Lessons learned for the design of carbon market mechanisms.
-Kothandaraman S. et al. (2020) Ecosystem-level carbon storage and its links to diversity, structural and environmental drivers in tropical forests of Western Ghats, India. Scientific Reports 10, Article number: 13444
-Kovats, R. S. and Osburn, D. (2016). UK Climate Change Risk Assessment Evidence Report: Chapter 5, People and the built environment. Available online at: <https://www. theccc.org.uk/ wp-content/uploads/ 2016/07/UK-CCRA2017-Chapter5-People-and-the-built-environment.pdf>
-Lal, R. (2004 )Soil carbon sequestration impact on global climate change and food security. Science 304, 1623-1627.
-Lal, R. (2005) Forest soils and carbon sequestration. For. Ecol. Manage.220, 242–258. Available online at: <https:// doi.org/ 10.1016/j.foreco.2005.08.015>
-Lamb, A., Green, R., Bateman, I., Broadmeadow, M., Bruce, T., Burney, J., Carey, P., Chadwick, D., Crane, E., Field, R., Goulding, K., Griffiths, H., Hastings, A., Kasoar, T., Kindred, D., Phalan, B,, Pickett, J., Smith, P., Wall, E., zu Ermgassen, E.K.H.J. and Balmford, A. (2016). The potential for land sparing to offset greenhouse gas emissions from agriculture. Nature Climate Change, 6, 488– 492.
-Lewis, S. L., Wheeler, C. E., Mitchard, E. T. & Koch, A. (2019). Restoring natural forests is the best way to remove atmospheric carbon. Nature, 568, 25–2
-Liang J, et al. (2016) Positive biodiversity-productivity relationship predominant in global forests.Science 354:aaf8957
-Lin, J., Jacoby, J., Cui, S., Liu, Y. and Lin, T. (2014). A model for developing a target integrated low carbon city indicator system: The case of Xiamen, China. Ecological indicators, 40, p.51-57
-Liu, J., Li, S., Ouyang, Z., Tam, C. & Chen, X. (2008). Ecological and socioeconomic effects of China’s policies for ecosystem services. Proceedings of the National Academy of Sciences of the United States of America, 105(28), 9477–9482
-Liu, X. et al. (2018) Tree species richness increases ecosystem carbon storage in subtropical forests. Proc. R. Soc. B Biol. Sci.285, 20181240. Available online at: <https://doi.org/10.1098/rspb.2018.1240>
-Liu, X., & Cui, Q. (2017). Baseline manipulation in voluntary carbon offset programs. Energy Policy, 111, 9–17. Available online at: <https://doi-org.elib.tcd.ie/10.1016/j. enpol.2017.09.014>
-Lutz, J. A. et al. Global importance of large-diameter trees. Glob. Ecol. Biogeogr.27, 849–864. (2018). Available online at: <https://doi.org/10.1111/geb.12747>
-Luyssaert, S., Schulze, E-D., Börner, A., Knohl, A., Hessenmöller, D., Law, B.E., Ciais, P. and Grace, J. (2008). Old-growth forests as global carbon sinks. Nature, 455, 213–215.
-MONAST, J. J., MURRAY, B. C., & WIENER, J. B. (2017). On Morals, Markets, and Climate Change: Exploring Pope Francis’ Challenge. Law & Contemporary Problems, 80(1), 135–162.
-Marando, F., Salvatori, E., Sebastiani, A., Fusaro, L., & Manes, F. (2019). Regulating ecosystem services and green infrastructure: Assessment of Urban Heat Island effect mitigation in the municipality of Rome, Italy. Ecological Modelling, 392, 92– 102. Available online at: <https://doiorg.elib.tcd.ie/10.1016/ j.ecolmodel.2018.11.011>
-McKinsey Report: Why investing in nature is key to climate mitigation (2021). Available online at: <https:// www.mckinsey.com/business-functions/sustainability/ourinsights/why-investing-in-nature- is-key-to-climate-mitigation>
-McKinsey Report: Why investing in nature is key to climate mitigation (January 25, 2021). doi <https://www.mckinsey. com/business-functions/sustainability/our-insights/whyinvesting-in-nature- is-key-to-climate-mitigation>
-Met Office, Latest Climate Projections. UKCP18 Marine. Available online at: <https://www.metoffice.gov.uk/ research/ approach/ collaboration/ukcp/index> [accessed May 2021]
-Morison, J., Matthews, R., Miller, G., Perks, M., Randle, T., Vanguelova, E., White, M. and Yamulki, S. (2012).
Understanding the carbon and greenhouse gas balance of forests in Britain. Forestry Commission Research Report. Forestry Commission, Edinburgh. i-vi, Pages 1-149. Available online at: <https://www.forestresearch.gov.uk/ research/understanding-the-carbon-and- greenhousegasbalance-of-forests-in-britain/>
-NaturalCapitalPartners.com. Forest Solutions. Available online at: <https://www.naturalcapitalpartners.com/ solutions/solution/forests>
-Nature-Based Solutions Handbook. Thinknature. (2019)
-Nieves, J.A., Aristizábal, A.J., Dyner, I., Báez, O. and Ospina, D.H. (2019) Energy demand and greenhouse gas emissions analysis in Colombia: A LEAP model application. Energy, 169, pp. 380-397.
-One Earth. (2020) Below 1.5ºC: a breakthrough roadmap to solve the climate crisis. Available online at: <https:// www.oneearth.org/below-1-5-c-a-breakthrough-roadmapto-solve-the-climate-crisis/>
-Ontl, T. A. & Schulte, L. A. (2012) Soil Carbon Storage. Nature Education Knowledge 3(10):35
-Osuri, A. M., Gopal, A., Raman, T. R. S., Defries, R., Cook‐Patton, S. C., & Naeem, S. (2020). Greater stability of carbon capture in species‐rich natural forests compared to species‐poor plantations. Environmental Research Letters, 15
-Pearce-Higgins, J.W., Beale, C.M., Oliver, T.H., August, T.A., Carroll, M., Massimino, D., Ockendona, N., Savaged, J., Wheatley, C.J., Ausden, M.A., Bradbury, R.B., Duffield, S.J., Macgregorh, N.A., McClean, C.J., Morecroft, M.D., Thomas, C.D., Watts, O., Beckmann, B.C., Fox, R., Royd, H.E., Sutton Walker, K.J. and Crick, H.Q.P., (2017). A national-scale assessment of climate change impacts on species: Assessing the balance of risks and opportunities for multiple taxa. Biological Conservation, 213, 124-134.
-Pichancourt J-P. Firn J. Chadès I. Martin T.G. (2014) Growing biodiverse carbon-rich forests. Global Change Biology 20, 282-393. <Available online at: 10.1111/ gcb.12345>
-Polonsky, M. J., Grau, S. L., & Garma, R. (2010). The New Greenwash? Potential Marketing Problems with Carbon Offsets. International Journal of Business Studies, 18(1), 49–54.
-Poorter, L. et al. (2015) Diversity enhances carbon storage in tropical forests. Glob. Ecol. Biogeogr.24, 1314–1328. Available online at: <https://doi.org/10.1111/geb.12364>
-Rackham O. (2020). The History of the Countryside: The Classic History of Britain’s Landscape,Flora and Fauna. Phoenix Press.
-Refinitiv, Carbon Market Survey (2020). Available online at: <https://www.refinitiv.com/en/resources/specialreport/ global-carbon-market-report>
-Schneider et al. (2012). Standardized Baselines for the CDM. Available online at <http://seius.org Publications_PDF/Policy-paper-2012-Standardizedbaselines-CDM.pdf>
- Secretariat, C. B. D. (2011). REDD-plus and Biodiversity. CBD Technical Series, (59). Link: https:// www.cbd.int/doc/publications/cbd-ts-59-en.pdf
- Seddon, N., Daniels, E., Davis, R., Chausson, A., Harris, R., Hou-Jones, X., . . . Wicander, S. (2020). Global recognition of the importance of nature-based solutions to the impacts of climate change. Global Sustainability, 3, E15.
-Seddon, N., Mace, G. M., Naeem, S., Tobias, J. A., Pigot, A. L., Cavanagh, R., Mouillot, D., Vause, J., & Walpole, M. (2016). Biodiversity in the Anthropocene: Prospects and policy. Proceedings of the Royal Society B‐Biological Sciences, 283, 20162094. Available online at: <https://doi-org.elib.tcd.ie/10.1098/rspb.>
-Seddon, N., Smith, A., Smith, P., Key, I., Chausson, A., Girardin, C., House, J., Srivastava, S., & Turner, B. (2021). Getting the message right on nature-based solutions to climate change. Global Change Biology, 27(8), 1518–1546.
-Seres, S. & Tewari, R. (2012). Assessing the impact of the Clean Development Mechanism. Available online at: <http:// www.cdmpolicydialogue.org/research/1030_ impact.pdf.>
-Siikamäki, P., Kangas, K., Paasivaara, A., & Schroderus, S. (2015). Biodiversity attracts visitors to national parks. Biodiversity and Conservation, 24, 2521– 2534
-Sing L. Patterson. G. (2008) Native Woodland Targets and Forest Habitat Networks in Scotland. Forestry Commission Scotland.
-Smith, A. C., Harrison, P. A., Pérez Soba, M., Archaux, F., Blicharska, M., Egoh, B. N., … Wyllie de Echeverria, V. (2017). How natural capital delivers ecosystem services: A typology derived from a systematic review. Ecosystem Services, 26, 111–126.
-Song L. ProPublica. An Even More Inconvenient Truth: Why Carbon Credits For Forest Preservation May Be Worse Than Nothing (2019). Available online at: <https://features.propublica.org/brazil- carbonoffsets/inconvenient-truth-carbon-credits-dont-workdeforestation-redd-acre-cambodia/ >
-Spalding-Fecher, R. (2013). National policies and the CDM rules. Available online at: <http://www. energimyndigheten.se/Global/Internationellt/ Carbon%20Limits%20-%20National%20Policies%20 and%20CDM.pdf>
-Spalding-Fecher, R.; Achanta, A. N.; Erickson, P.; Haites, E.; Lazarus, M.; Pahuja, N.; Pandey, N.;
-Stafford, R., Chamberlain, B., Clavey, L., Gillingham, P.K., McKain, S., Morecroft, M.D., Morrison- Bell, C. and Watts, O. (Eds.) (2021). Nature-based Solutions for Climate Change in the UK: A Report by the British Ecological Society. London, UK. Available online at: <www. britishecologicalsociety.org/nature-basedsolutions>
-State of Finance for Nature, Tripling investments in nature-based solutions by 2030. UN Environmental Programme (2021)
-Stephens, P.A., Mason, L.R., Green, R.E., Gregory, R.D., Sauer, J.R., Alison, J., Aunins, A.,Brotons, L., Butchart, S., Campedelli, T., Chodkiewicz, T., Chylarecki, P., Crowe, O., Elts, J., Escandell, V., Foppen, R., Heldbjerg, H., Herrando, S., Husby, M., Jiguet, F., Lehikoinen, A., Lindström, Å., Noble, D.G., Paquet, J., Reif, J., Sattler, T., Szép, T., Teufelbauer, N., Trautmann, S., van Strien, A., van Turnhout, C.A.M., Vorisek, P. and Willi, S.G. (2016). Consistent response of bird populations to climate change on two continents. Science 352, 84–87.
-Stott, P. A., Stone, D. A. and Allen, M. R. (2004). Human contribution to the European heatwave of 2003. Nature, 432, pp.610–614.
-Sullivan, T. P., Sullivan, D. S. & Lindgren, P. M. F. (2001) Influence of variable retention harvests on forest ecosystems. I. Diversity of stand structure. J. Appl. Ecol.38, 1221–1233. Available online at <https://doi.org/10.1046/j. 00218901.2001.00671.x>
-Tan, L., Ge, Z., Zhou, X., Li, S., Li, X., & Tang, J. (2020). Conversion of coastal wetlands, riparian wetlands, and peatlands increases greenhouse gas emissions: A global meta‐analysis. Global Change Biology, 26, 1638–1653.
-The Guardian. Greenhouse Gas Emissions: Trials to suck carbon dioxide from the air to start across the UK. (2021) <https://www.theguardian.com/environment/2021/may/24/ trials-to-suck- carbon-dioxide-from-the-air-to-start-across-theuk></https:>
-The Woodland Trust (2018). The current state of ancient woodland restoration. [online] Available at: https://www. woodlandtrust.org.uk/publications/2018/07/current-stateofancient-woodland- restoration/
-Thompson I. Mackey B. McNulty S. Mosseler A. (2009) UNEP. Forest Resilience, Biodiversity and Climate Change: A Synthesis of the Biodiversity/Resilience/Stability Relationship in Forest Ecosystems
-Todd & Schulte (2012).Soil Carbon Storage Department of Natural Resource Ecology and Management, Nature Education, Iowa State University, Ames, IA.
-Tozer, L., Hörschelmann, K., Anguelovski, I., Bulkeley, H., & Lazova, Y. (2020). Whose city? Whose nature? Towards inclusive nature-based solution governance. Cities, 107.
-Tree, I. (2018). Wilding: The Return of Nature to a British Farm. Pan Macmillan
-Tucker, Will. (2019). “Debunked: Eight Myths About Carbon Offsetting,” Ecosystem Marketplace, 19 September. Available online at: <https://www.ecosystemmarketplace.com/articles/ debunked-eight- myths-carbon offsetting>
-UNFCCC (2006a). Decision 3/CMP.1 Modalities and procedures for a clean development mechanism as defined in Article 12 of the Kyoto Protocol. Available at http://cdm.unfccc. int/ Reference/COPMOP/08a01.pdf.
-UNFCCC (2014). CDM database (31/07/2014). Bonn.
-UTS. Sectoral Pathways to Net Zero Emissions: Institute for Sustainable Futures. OECM Sector Pathways Report, 2020
-Vayreda, J. et al. (2012) Spatial patterns and predictors of forest carbon stocks in Western Mediterranean. Ecosystems15, 1258–1270. Available online at: <https://doi.org/10.1007/ s10021-012-9582-7>
-Veldman J.W. et al. (2015) Where Tree Planting and Forest Expansion are Bad for Biodiversity and Ecosystem Services. BioScience 65. 1011-1018.
-Warnecke, C.; Day, T. & Klein, N. (2015). Analysing the status quo of CDM project: Status and prospects. Cologne, Germany
-Wen-Hsien Tsai. (2020). Carbon Emission Reduction— Carbon Tax, Carbon Trading, and Carbon Offset. Energies, 13(6128), 6128.
-Wheeler, C. E., Lewis, S. K., Mitchard, E. T. A., & Koch, A. (2019). Restoring natural forests is the best way to remove atmospheric carbon. Nature, 568, 25–28. Available online at: <https://doi.org/10.1038/d41586-019-01026-8>
-Wilson, K. (2011). Access to Justice for Victims of the International Carbon Offset Industry. Ecology Law Quarterly, 38(4), 967-1031.
-World Meteorological Organisation. New climate predictions increase likelihood of temporarilyreaching 1.5 °C in next 5 years (2021). Available online at: <https://public.wmo.int/ en/media/press-release/new- climate-predictions-increaselikelihood-of-temporarily-reaching-15-%C2%B0c-next-5>
-edie. Carbon offsetting: How are businesses avoiding greenwashing on the road to net-zero? (2020). Available online at:<https://www.edie.net/registration/regwall.asp? mid =153477&origin=https%3A%2F%2Fwww%2Eedie%2Enet%2 Flibrary%2FCarbon-offsetting-- How-are-businesses-avoidinggreenwash-on-the-road-to-net-zero>
-ethical. net. Carbon Offsetting Companies Compared (2019). Available online at: <https://ethical.net/climate-crisis/ carbonoffsetting-companies-compared/ >
-Whitehouse Statement: FACT SHEET: President Biden’s Leaders Summit on Climate, April 23 2021
-Woodland Trust, Which Species to Plant. Available online at: <https://www.woodlandtrust.org.uk/planttrees/advice/choose/>