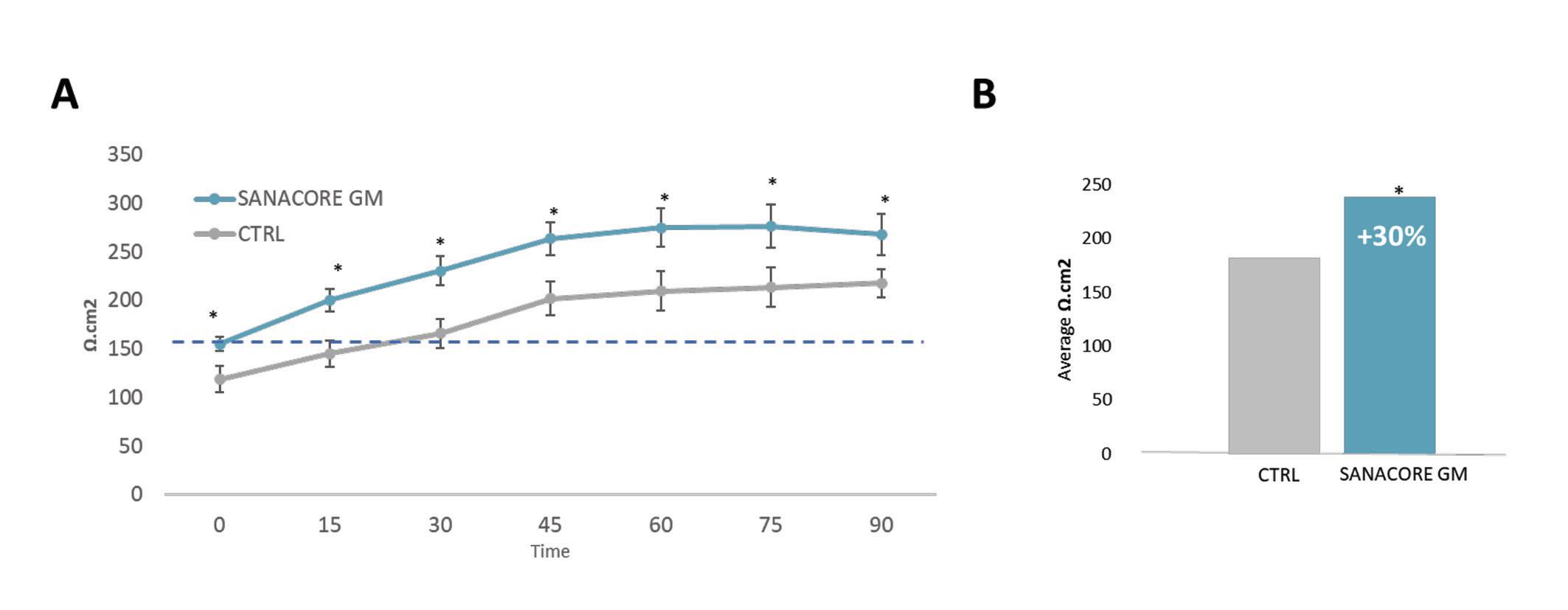
35 minute read
FEED ADDITIVE PROTECTS AGAINST INTESTINAL ENDOPARASITE
Functional feed additive for gut integrity and protection against the intestinal myxosporean endoparasite Enteromyxum leei
Panos G. Kalatzis, Maria Mercè Isern-Subich, Waldo G. Nuez-Ortín, Adisseo
The importance of gut in fish health and performance
A healthy alimentary canal is a fundamental element for the optimal growth and performance of all cultured fish. Apart from nutrient absorption and digestion, which are its main functions, the gut plays also a major role in sustaining fish health (Kristen et al., 2012; Tarnecki et al., 2017). However, there are several, both non-infectious and infectious factors, that may cause imbalance among the major components of the gut: microbiota, epithelium and intraepithelial leukocytes. The amount of scientific research on gut microbiota has been growing exponentially over the past few years (Egerton et al., 2018; Bozzi et al., 2021), however, the complexity of gut microbiome and the potential interventions that may lead to better fish health and performance has only scratched the surface (Montalban et al., 2015). The clinical picture of such an imbalanced gut is called enteritis and occurs in the form of generalized intestinal inflammation, triggered by the activation of the intraepithelial immune cells such as macrophages.
The inflammatory response of the intestine is the defensive mechanism of the organism due to an infection or an injury. Although it is rather beneficial in the short term, if prolonged, it can lead to chronic inflammation which undermines both nutrient absorption and immunity functions of the gut. The combination of nutritional factors, such as the use of plant-based ingredients in the feed and gut pathogens, for instance the myxosporean parasite Enteromyxum leei, can further cause severe disruption of the gut integrity, undermining the growth and performance of
Figure 1. (A) Transepothelial resistance of anterior intestine with and without SANACORE GM® supplementation (0.5%). Tissue resistance in the intestine of healthy seabream juveniles of 100g is expected to be >150 Ω cm2, therefore a threshold has been set with dotted line. (B) A statistically significant difference of 30% higher tissue resistance is reported under SANACORE GM® supplementation (0.5%). Statistical significance is stated by *.
Figure 2. (A) Internal:External perimeter ratio. On the left section (SANACORE GM®) the ratio is higher by 7% compared to the right (CONTROL) and the intestine is presented intact and well-developed with many well-shaped villi. (B) External:Internal diameter ratio. On the left section (SANACORE GM®) the ratio is higher by 11% compared to the right (CONTROL) because the development of the villi is more consistent, leading to a smaller lumen space.
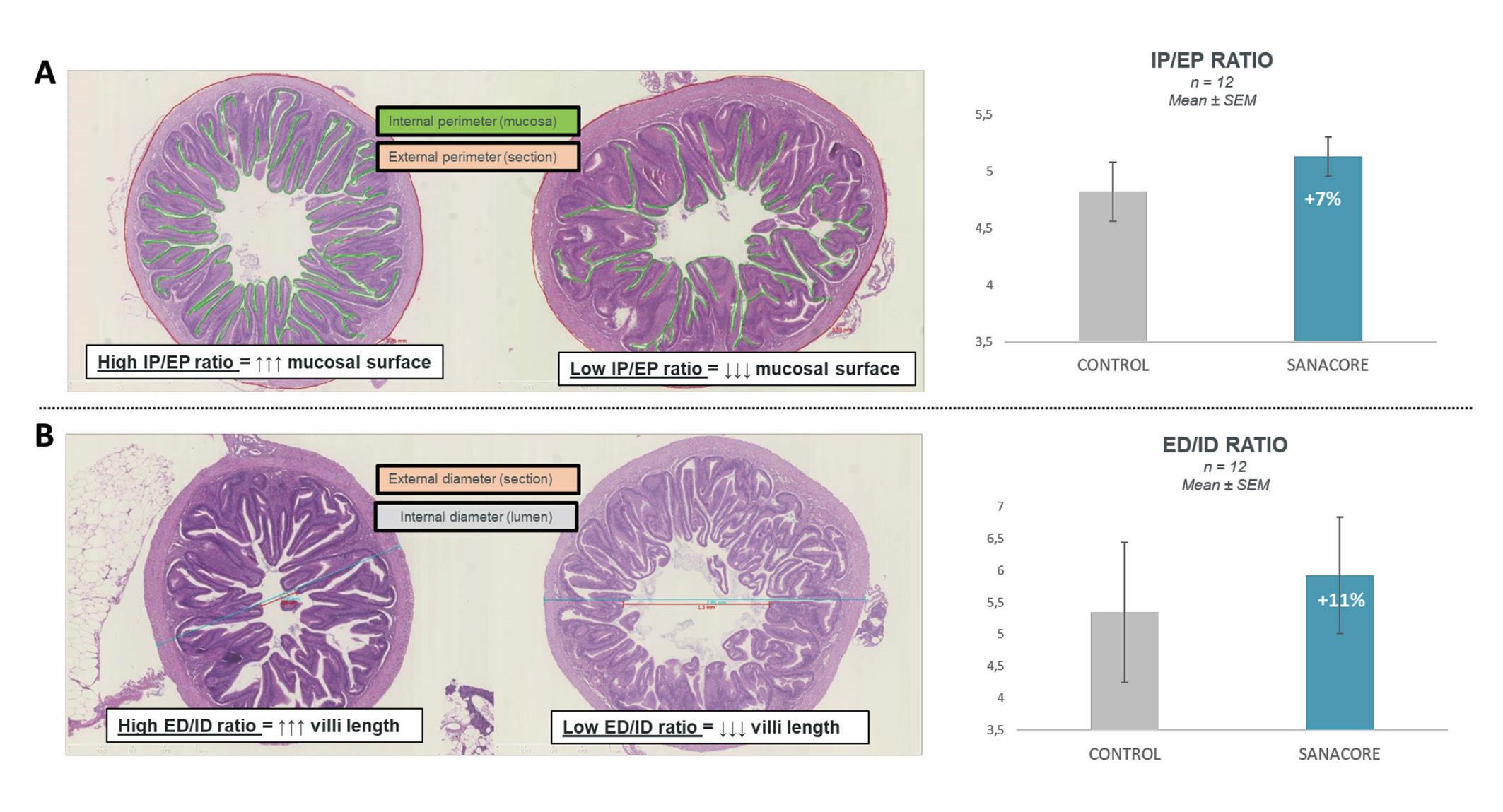
the fish (Sitjà-Bobadilla et al., 2019; Piazzon et al., 2022). It should also be noted that a well-functioning gut is a prerequisite for the avoidance of the subclinical picture of the fish, which may not show direct consequences at once but will do so as soon as any abrupt or steep changes eg. suboptimal temperature, high stocking densities, opportunistic pathogens, vaccination or transfer stress, etc. appear in the environment. Therefore, functional feed additives which are able to enforce and protect gut health may claim today a prominent role in the formula.
The objective of the present article is to assess and present the impact that the functional feed additive under the commercial name SANACORE GM® can render on the host’s gut. The documented beneficial effects on gut integrity, villi formation, antiinflammatory response and consequent protective activity against the myxosporean parasite E. leei draw the picture of a healthier gut which not only generates better performance indices but also makes it harder for pathogens to infiltrate and damage the tissue.
SANACORE GM® promotes gut integrity and protects against E. leei infestations
SANACORE GM® by Adisseo is a broad-spectrum, health-promoting additive based on components that deliver gut integrity and anti-inflammatory action. The positive impact of SANACORE GM® on gut integrity and health promotion has been extensively documented in the literature and reported by EU aquaculture projects such as ParaFishControl.
The functionality of the intestine for both digestion and health performance requires gut integrity to be intact. The transepithelial electrical resistance (TER) of the intestine, as an indicator of gut integrity, has been measured in gilthead seabream, Sparus aurata, fed a 10% fishmeal diet supplemented with SANACORE GM®. TER was 30% significantly higher under supplementation and in relation to the control group (Fig. 1). The tighter the junctions among the cells, the more difficult for the electrical current to pass, hence, a tangible improvement of gut integrity is capitalized to better nutrient absorption, improved growth performance, and more robust fish health.
Morphometrical studies on the seabream’s gut, corroborate the previous result by focusing on the length and surface of intestinal villi. The villi from the hindgut, which is the most sensitive to inflammation part of the gut, indicate that internal:external gut perimeter and external:internal gut diameter fractions are higher by 7% and 11%, respectively, under SANACORE GM® supplementation (Fig. 2). The biological
Figure 3. Resilience to inflammation following a cytomix stimulus. The ELISA results showed that in all 4 different concentrations (0.1x, 0.2x, 0.4x and 1x) and both time points (2h and 16h), SANACORE GM® was the most efficient additive in maintaining the inflammatory status below threshold.
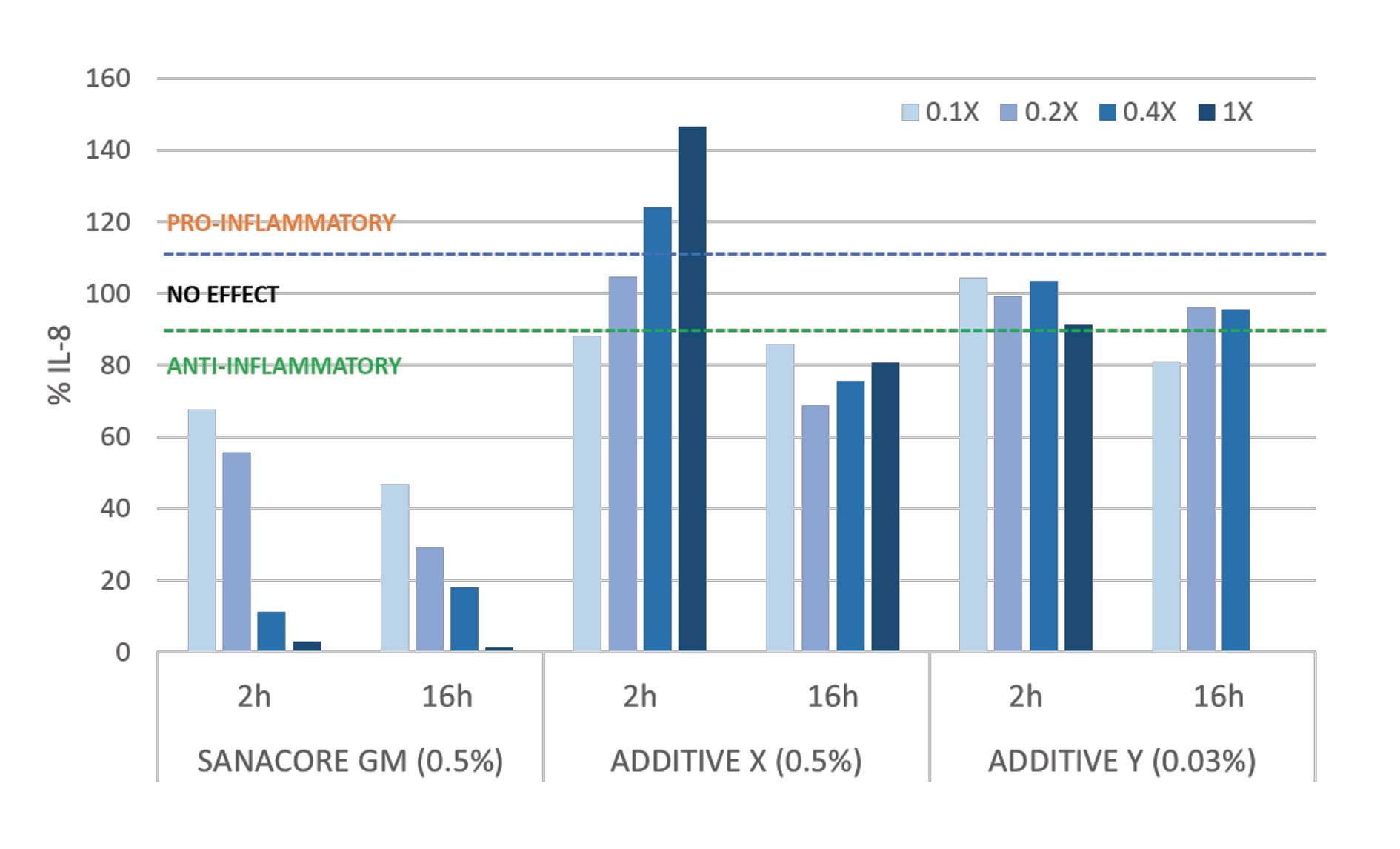
meaning of these numbers is translated into more consistently developed villi, less inflamed villi, larger lumen space, bigger mucosal surface and consequently improved gut integrity.
The inflammatory process is mediated by the secretion of proinflammatory cytokines including interleukins (IL). IL-8 has been particularly studied and extensively used in research as a biomarker for the inflammatory cascade acting as a neutrophil leukocyte chemoattractant or activator (Kim et al., 2019; Laing et al., 2002; Laing et al., 2004). The in vitro model using the caco-2 cell line (van de Walle et al., 2010), which originates from epithelial cells mimicking a functional intestine barrier, was used in order to assess the inflammatory response to SANACORE GM® and two other commercial feed additives. The cells were exposed to a mixture of proinflammatory cytokines (cytomix) and it was shown that supplementation with SANACORE GM® was the most efficient in maintaining the IL-8 levels below the anti-inflammatory effect threshold of 90%, whereas the other tested additives generated either no effect (90-110%) or proinflammatory effect (>110%) for most concentrations and time points (Fig. 3).
Supporting gut integrity and intestinal health is directly reflected in the successful application of SANACORE GM® as a preventive strategy against pathogenic infestations. E. leei is a myxosporean endoparasite that colonizes the gut and occasionally can also be found in the bile. Enteromyxosis in gilthead seabream is a more chronic, rather than acute, condition since instead of causing immediate mortality, it gradually causes anorexia, weight loss, muscle reduction and eventually death. However, when illustrated in more sensitive fish, such as the sharpsnout seabream (Diplodus puntazzo) and the red seabream (Pagrus major) mortality can be induced much faster (Palenzuela et al., 2020; Henry et al., 2020). The route of infection for the parasite passes through the already loose junctions among the epithelial cells and further exacerbates the clinical picture of enteritis. The disruption of gut integrity compromises the host’s health while rendering it vulnerable to secondary infections as well. E. leei is a case in point for the Mediterranean aquaculture, particularly for gilthead and sharpsnout seabream (Palenzuela et al., 2020; Henry et al., 2020). In the former case, SANACORE GM® could mitigate the SGR decrease caused by the infection by up to 15% versus the untreated infected control. A functional feed could also lower the prevalence of the infestation by 30% whereas the mean parasitic abundance per fish was significantly lower compared to untreated control (Palenzuela et al., 2020). In the case of sharpsnout seabream, SANACORE GM® had an
immune-promoting effect on the antibacterial, antiprotease and ceruloplasmin activity compared to the untreated positive control (Henry et al., 2020).
Efforts for sustainable aquaculture and high prices that accompany the ingredients of marine origin have led the industry to constantly increase the inclusion of plantbased components and encourage the development of alternative nutrient sources in aquaculture diets. A recent study has proved that reinforcing a zero fishmeal (FM) diet with SANACORE GM® to support fish in dealing with E. leei infection is as effective as a 20% FM in avoiding the increased intestinal susceptibility to the parasite (Piazzon et al., 2022).
Conclusion
The economic losses accompanied by hampered productivity along with the increasing research for plant-origin ingredients coupled with the stressors and pathogens to which culture fish are exposed, highlight the importance and necessity of functional feed additives in the feed formula. Under these circumstances, the beneficial effects SANACORE GM® on gut health and fish performance complemented by the protective impact against E. leei, enforce its role as a core functional feed additive for the Mediterranean aquaculture.
References available on request.
More information: Panos G. Kalatzis
Regional Manager Aquaculture Europe Adisseo E: panos.kalatzis@adisseo.com
CUSTOM AQUATIC FEED SOLUTIONS ARE WAITING
Surround Yourself with Unparalleled Resources for Extrusion and Drying Systems
Do you know what aquatic feed you need to produce – but unsure of the next steps? Wenger will put unsurpassed technology and process expertise to work, developing flexible solutions specific to your operation. We expertly evaluate and guide the development and processing applications for floating, sinking, shrimp and micro aquatic feeds. Every one of our systems is customized, serving you best by going beyond a standardized approach. Let us help you solve production challenges now. Email us at info@wenger.com today.
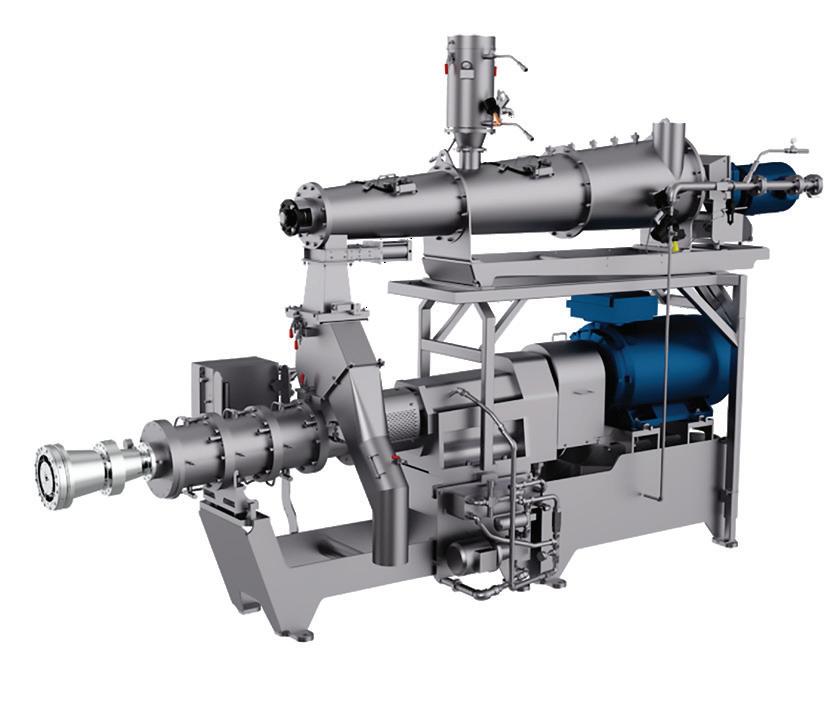
FLOATING
SINKING
SHRIMP
PHONE: 785.284.2133 | EMAIL: INFO@WENGER.COM | WENGER.COM USA | BELGIUM | TAIWAN | BRASIL | CHINA
SALMON MICRO
Enhancing gut health in tilapia with yeast-based probiotic for better feed efficiency and growth
Otavio Castro, Nadège Richard, Alban Caratis, Phileo by Lesaffre
Suboptimal water quality conditions, pathogens, feed composition and quality, or pollutants are all factors that can lead to dysbiosis and gut inflammation in fish, ultimately reducing growth, feed efficiency and overall health status. The use of probiotics in aquaculture has taken a prominent place in preventively addressing these challenges while simultaneously providing an alternative to the systematic use of chemical therapeutics.
Leveraging more than 160 years of expertise in the fermentation of yeasts, Phileo® by Lesaffre developed ActiSaf®, a yeast-based probiotic that improves gut health and thus, feed efficiency and growth performance of farmed tilapia.
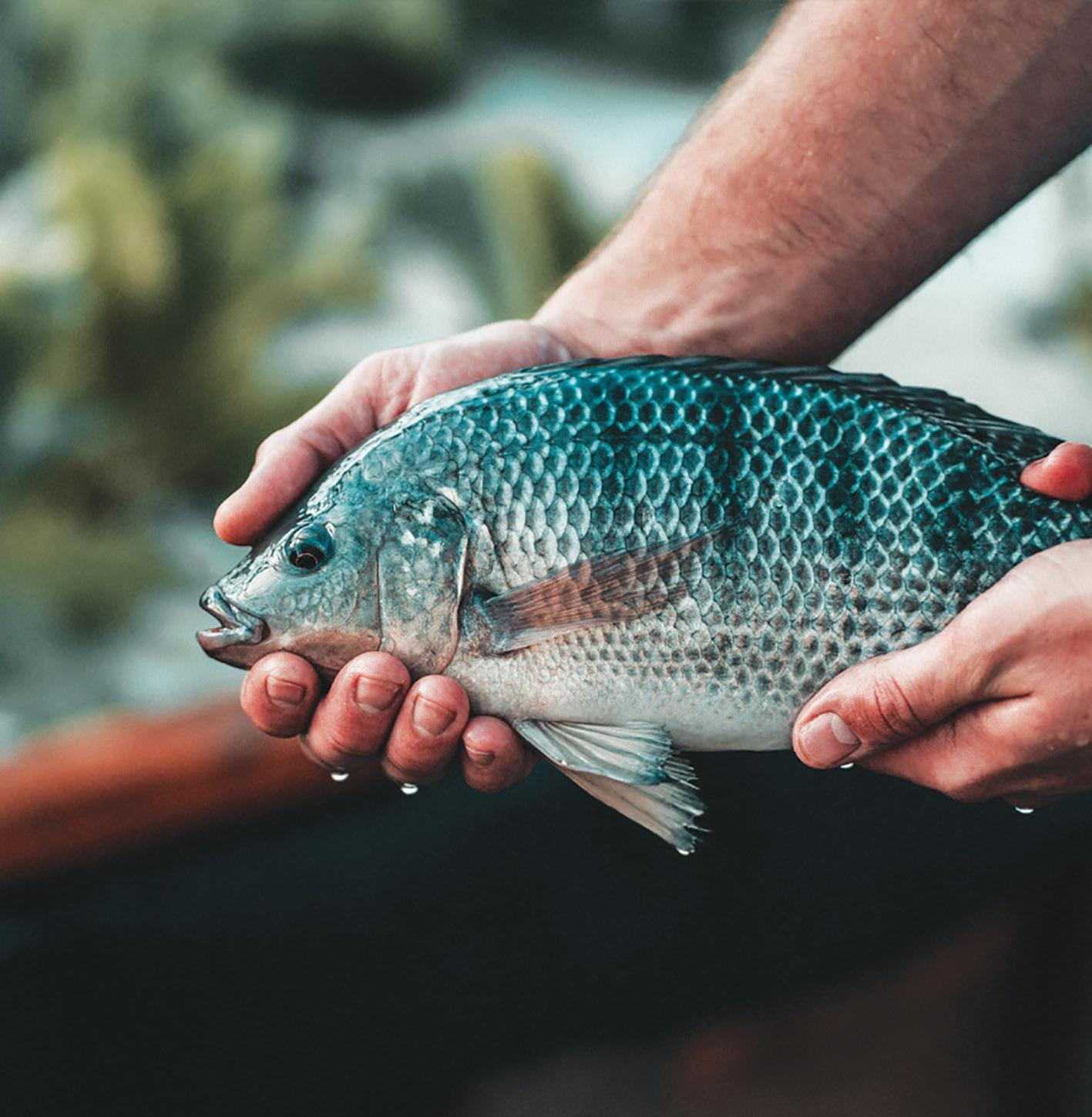
What gut health is and why it is important
The gut has the dual role of secreting digestive enzymes and triggering some immune functions in fish. A healthy gut with a balanced microbiota means fish can process
Group
Diet 1 Diet 1 + ActiSaf® Diet 2 Diet 2 + ActiSaf®
Midgut microvilli length (µm2)
0.93 ± 0.02a
1.02 ± 0.02b
1.05 ± 0.03b
1.17 ± 0.02c
Figure 1. ActiSaf® supplementation improves midgut microvilli length and reduces inflammatory expression in Nile tilapia fingerlings.
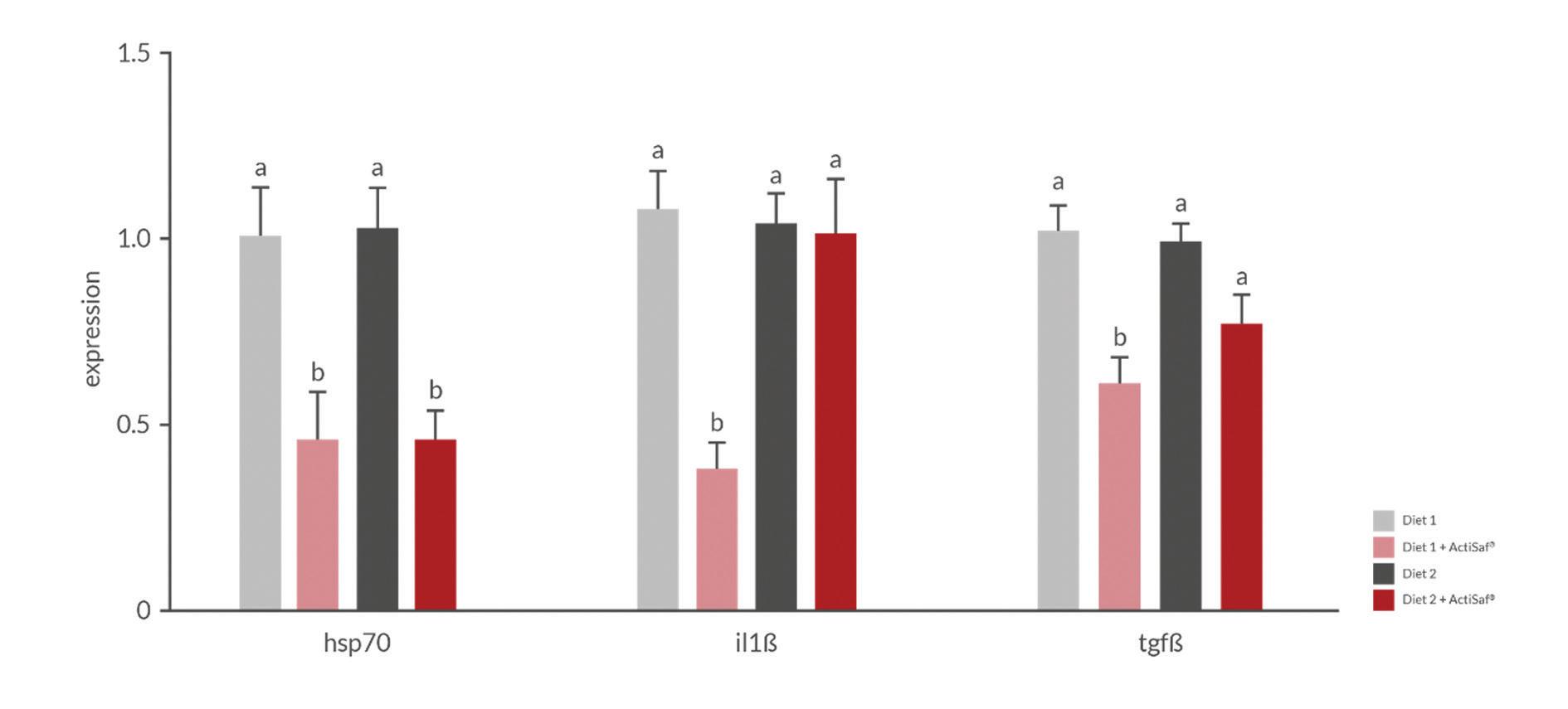
Figure 2. ActiSaf® supplementation improves survival, growth performance, protein efficiency ratio, and apparent protein digestibility in Nile tilapia reared under low-density (LD) and high-density (HD) conditions.
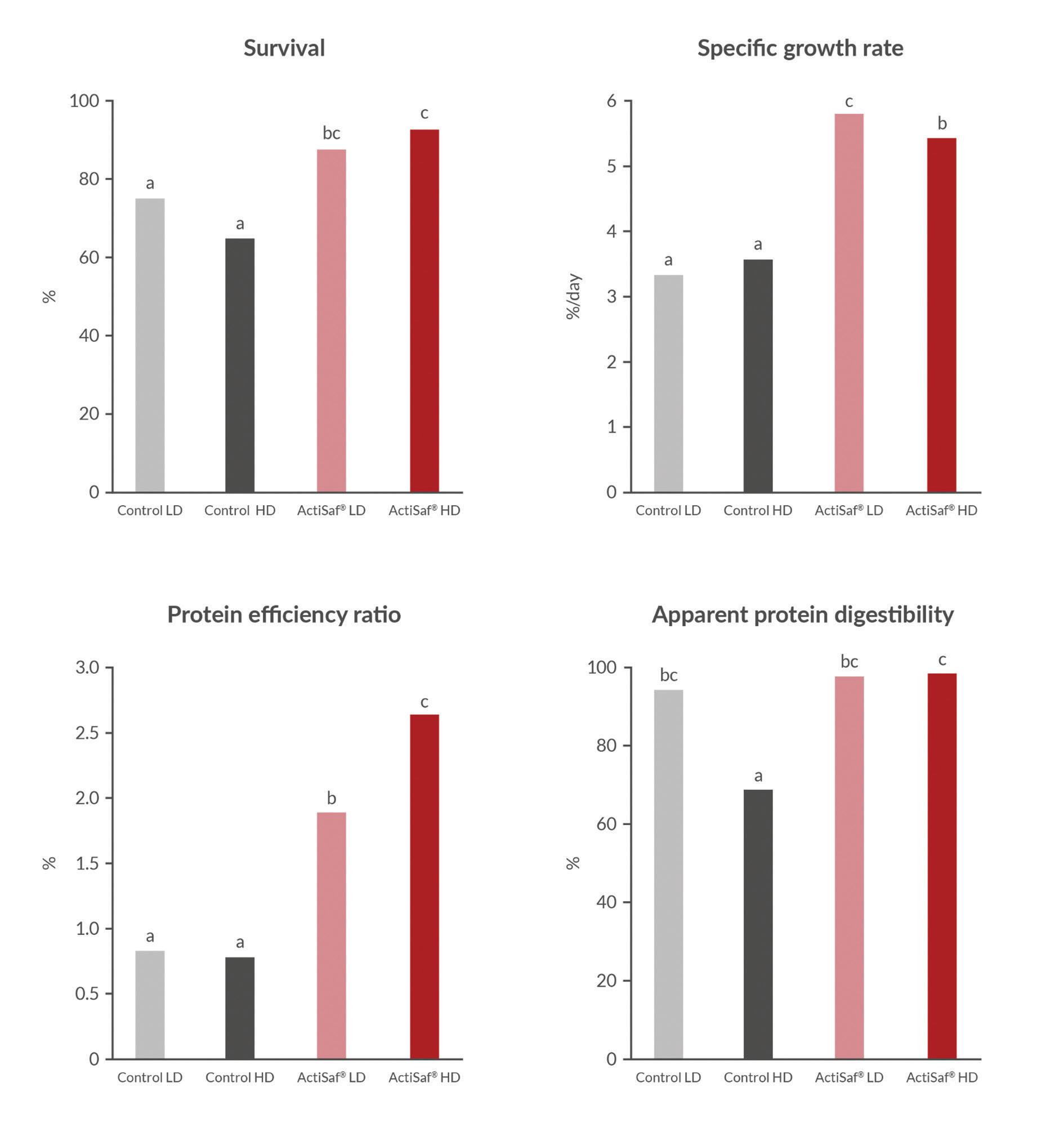
feed more efficiently, have a better immune system and are less susceptible to the pathogens present in their environment. On the other hand, without a stable microbiota, they tend to be more susceptible to stress and are more likely to develop some conditions.
The stability of the gut microbiome, as in any living organism, is a fragile balance that can be disturbed by the conditions and the variety of stress factors that fish encounter throughout production. For instance, the increasing price of commodities and the pressure to reduce feed formula costs have necessitated the utilization of more low-value vegetable ingredients in aquafeeds. These are also known sources of anti-nutritional factors, such as mycotoxins, enzymatic activity inhibitors and nonstarch polysaccharides, that can cause chronic gut inflammation and change the gut microbiome.
The gut health status of tilapia can also be disrupted by pathogen-related dysbiosis. This process happens when harmful toxins are produced by host-colonizing
Figure 3. Feed conversion performances of tilapia fed with Actisaf® supplementation. Source: Schwarz et al. (2016).
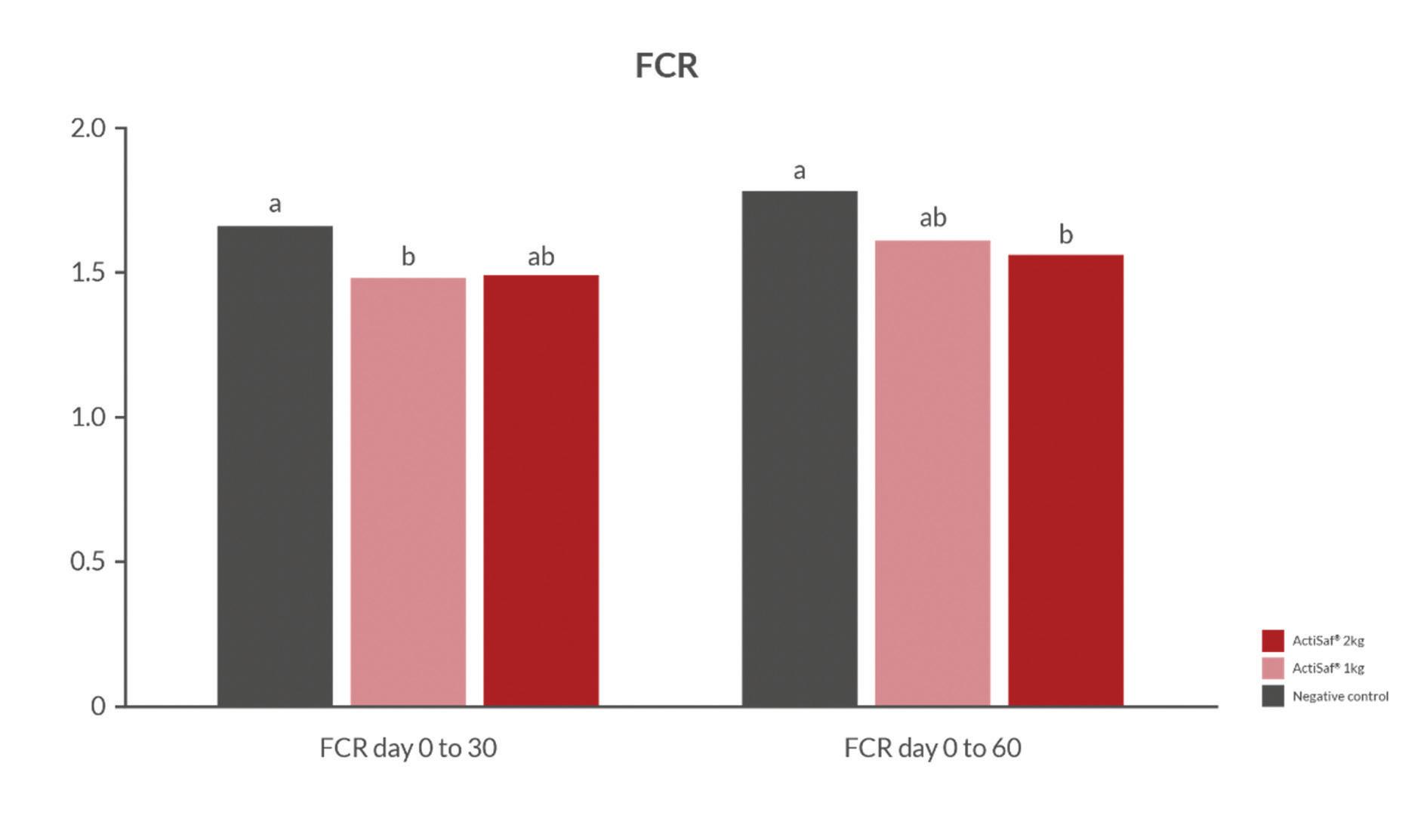
pathogens which subsequently damage the mucosa, resulting in an inflammatory response that affects intestinal morphology. This diminishes the fish’s nutrient absorption capacity and growth, thus making them more vulnerable to infections. Also, infections and other disease outbreaks in fish farming have a long history of being tackled using antibiotics. In addition to the sustainability issues that are tied to the use of antibiotics, such as resistance development and food safety concerns, their administration can significantly reduce intestinal bacterial diversity and suppress the fish’s immune response (He et al., 2011; Zhu et al., 2018). Gut health is therefore strongly connected to farm productivity and may lead to increased production costs.
Gut health management practices in aquaculture
The use of probiotics in aquaculture has grown along with the necessity of managing fish gut microbiome during production in order to optimize performances at harvest while minimizing the use of therapeutics. Probiotics are living micro-organism additives that are widely used in aquaculture to enhance fish production as they help improve fish microbiota and thus contribute to immune response enhancement and disease prevention (Islam, Rohani, and Shahjahan, 2021).
Although probiotics were initially used for controlling pathogens' pressure in the water, their use in aquaculture has extended to improving fish growth and feed conversion through feed application. In this context, the supplementation of live yeast-based probiotics in aquafeeds is gaining interest and their benefit on gut health has already been assessed in different trials (Ran, Huang, Hu et al., 2016; Ran et al., 2015), with some promising results reported in tilapia.
Maintaining gut health for higher production performances with ActiSaf®
ActiSaf® is a yeast probiotic that is produced by Phileo® from a selected proprietary strain of Saccharomyces cerevisiae that is exclusively dedicated to the animal production industry.
Ran et al. (2015, 2016) showed that ActiSaf® supplementation in the diet of Nile tilapia fingerlings (at the optimized ratio of 1 kg/ton of feed) increased midgut microvilli length, thus supporting higher nutrient absorptive capacity and final body weight. ActiSaf® also reduced the expression of inflammatory and stressrelated cytokines such as IL1β and TGFβ in the gut mucosa (Fig. 1).
ActiSaf® also proved to be helpful in both high and low fish density conditions, increasing microvilli length, improving trypsin activity in the intestine (a
Table 1. Strategies for the application of Actisaf® for the improvement of gut health and feed efficiency in tilapia, per production stage.
Fry Fingerling Growth I Growth II Finishing 0.1-2g 2-20g 20-50g 150-400g 400g-harvest
Gut health & feed efficiency support ActiSaf®
C C C C C
1 kg/ton 1 kg/ton 1-2 kg/ton 1-2 kg/ton 1-2 kg/ton
C = Continuous application. P = pulsed application with minimum of 14-21 days of supplementation before challenge peak.
digestive enzyme), and reducing hsp70 expression (thus decreasing crowding-induced stress) and alkaline phosphatase activity (Ran, Huang, Liu et al., 2016). Furthermore, ActiSaf® showed a beneficial impact on tilapia microbiota by enriching beneficial bacteria Lactococcus spp. in allochthonous microbiota (Ran et al., 2015). Additionally, Lara-Flores et al. (2003) established the outperformance of yeast probiotics over bacterial probiotics (Streptococcus faecium and Lactobacillus acidophilus) in the improvement of feed efficiency and growth performance characteristics in tilapia. Results from this study also demonstrated that Actisaf® can improve tilapia survival and specific growth rate independently of the density (high-density HD, and low-density LD), and support feed digestion under HD and more stressful conditions (Fig. 2).
By improving gut microbiota and nutrient absorption capacity, ActiSaf® significantly reduces FCR and generates significant savings on feed costs (Fig. 3). Consequently, the growth performance and overall health status of tilapia are also improved.
Conclusion
The shift of aquaculture practices towards more intensive systems and the necessity to optimize feed costs are two major root causes of gut issues in tilapia farming. In some areas, these issues are likely to grow in complexity due to the continued development of production, the demand for affordable products, the emergence of antimicrobial-resistant pathogens and climate change. Therefore, the use of probiotics for maintaining gut health in aquaculture will be increasingly required. As demonstrated earlier, ActiSaf® offers producers the possibility to prevent problems related to gut inflammation and dysbiosis, thus optimizing production performances at harvest while maintaining low feed costs.
Based on the results obtained in the lab and commercial trials reported here, we provide a variety of ActiSaf® recommendations ranging from 1 to 2 kg per tonne of feed depending on the extent of the gut issues and your own production objectives (Table 1).
For additional information, visit Aquasaf tilapia program.
References available on request.
More information: Otavio Serino Castro
Global Species Manager Aquaculture Phileo by Lesaffre E: o.castro@phileo.lesaffre.com
Nadège Richard
Research & Development Manager Aquaculture Phileo by Lesaffre E: n.richard@phileo.lesaffre.com
Alban Caratis
Global Program Manager Aquaculture Phileo by Lesaffre E: a.caratis@phileo.lesaffre.com
Botanical compounds for fish gut health: Case of soybean meal-induced enteritis in Atlantic salmon
Siméon Fagnon, Phytosynthese
Gut health is defined by complex homeostasis between gut immunity and microbiota. Considering gut health is key for fish health and performance, its management has been the focus of much recent research for feed strategies. At the same time, fish farming environment could be the source of many stresses which challenge gut heath.
In carnivorous species, the replacement of fish ingredients is needed for sustainable production. Soybean meal is currently one of the prominent alternatives for fishmeal replacement in aquafeed. Although its nutritious value has been demonstrated as an excellent protein and almost balanced amino acid complex, the high-level inclusion of this ingredient in aquafeed remains challenging (Wu et al., 2021). Soybean meal contains some antinutritional factors especially saponins which cause negative performances and degradation of intestinal health in some carnivorous fish species (Miao et al., 2018). In salmonids, the main effects of feed containing high antinutrients are gut inflammation induction, loss of important digestive functions and diarrhea, as well as reduced nutrient digestibility and subsequently growth.
Botanicals for intestinal health
Many strategies have been developed to overcome this issue. Natural botanical compounds have recently been identified as a potential option. Plants produce uncountable secondary metabolites, some of which have been used for millenniums by humans and wild animals to improve environmental resilience. Over recent years, botanical compounds were evaluated in the aquaculture sector for anti-inflammation, immunomodulation, antioxidant, microbiota balance and growth improvement (Fagnon et al., 2020). Regarding these well-documented properties in intestinal tissues, natural botanical compounds might contribute to alleviating the inflammatory reactions caused by standard soybean meal and subsequent consequences on overall health.
Phytosynthese is an independent French company specialized in sourcing and analytical chemistry of plants for 25 years. Its feed supplements provide high and constant concentration of botanical secondary metabolites. Recently developed by the company, Phyto AquaMeric is a combination of different flavonoids and terpenoids from botanicals and Brazilian green propolis. The formula was specifically designed
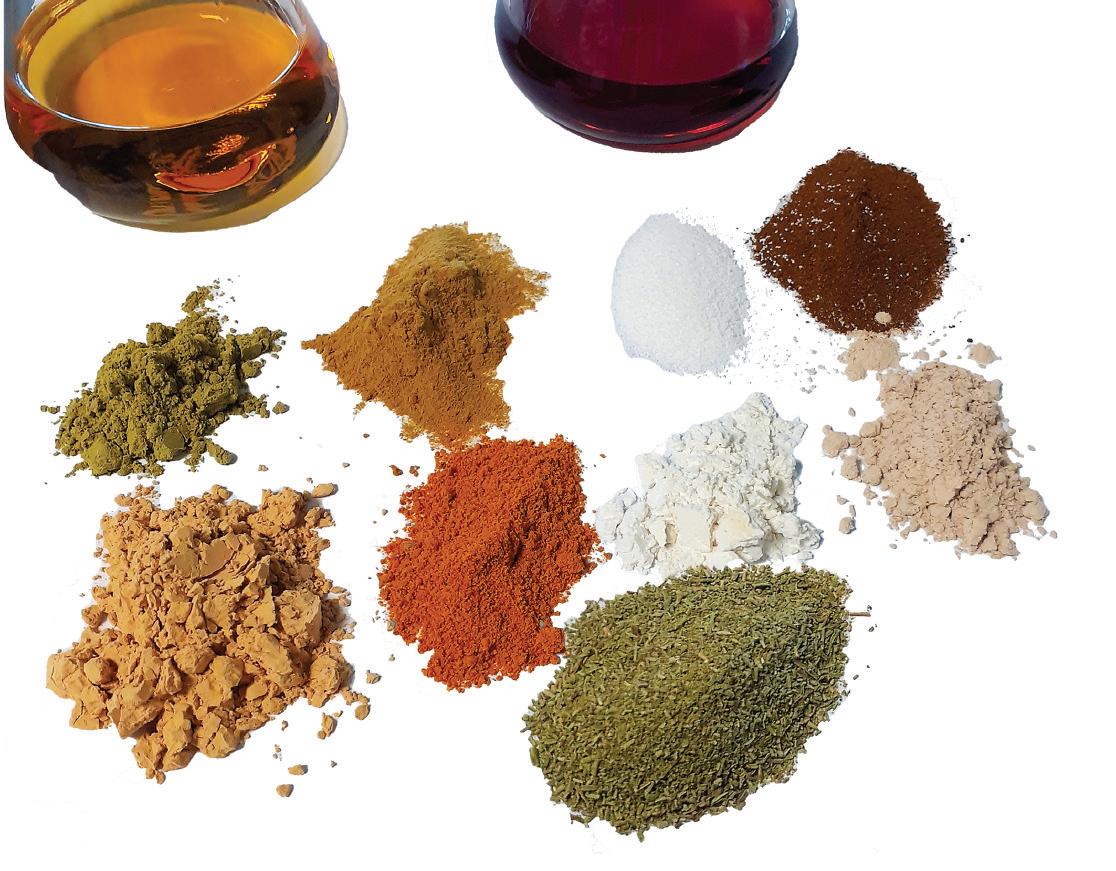
Table 1. Diet composition.
Ingredients
Soybean meal Wheat gluten Faba bean dehulled Soy protein concentrate Pea Protein Conc. 50 Lucatin pink, 10% Fish oil NA Fish meal NA Rapeseed oil Wheat Mineral and Vitamines MCP Ytrium premix DL methionine Ca-carbonate
Soy-based feed
25 20.74 5 12 5 0.01 9.3 5 8.6 6 0.5 2 0.1 0.3 0.45
to reduce intestinal inflammation and ensure overall health when faced with high-level inclusion of soybean in carnivorous fish.
Materials and methods
The trial was conducted at Nofima AS’ research station at Sunndalsøra (Norway). Post salmon of average weight of 234 grams were fed 10% in excess based on weighed daily rations and anticipated growth over 56 days. Thirty-five fish were stocked per tank, in 6 cylindrical, 1000 L, fiberglass tanks supplied with saltwater and maintained at 7.8°C. Each diet was fed to fish in three replicate tanks per feed, using automatic belt feeders.
Fish were fed with the following diets: a reference feed (CTR) as described in Table 1 and a supplemented reference diet with 0.05% of Phyto AquaMeric. The diets were formulated to fulfill the requirements of fish and contained 44% protein and 21% lipid.
At the end of the feeding period, six fish were randomly taken from each tank and euthanized. The weight of individual fish was recorded. Blood and plasma were collected from the caudal vein. The intact intestinal tract of the fish was removed, and divided into 3 regions: proximal, mid and distal intestines. Tissue samples were collected from all three segments and gene expression analyses were performed on distal intestine. Samples were stored for histology and gene expression according to Sahlmann et al. (2013).
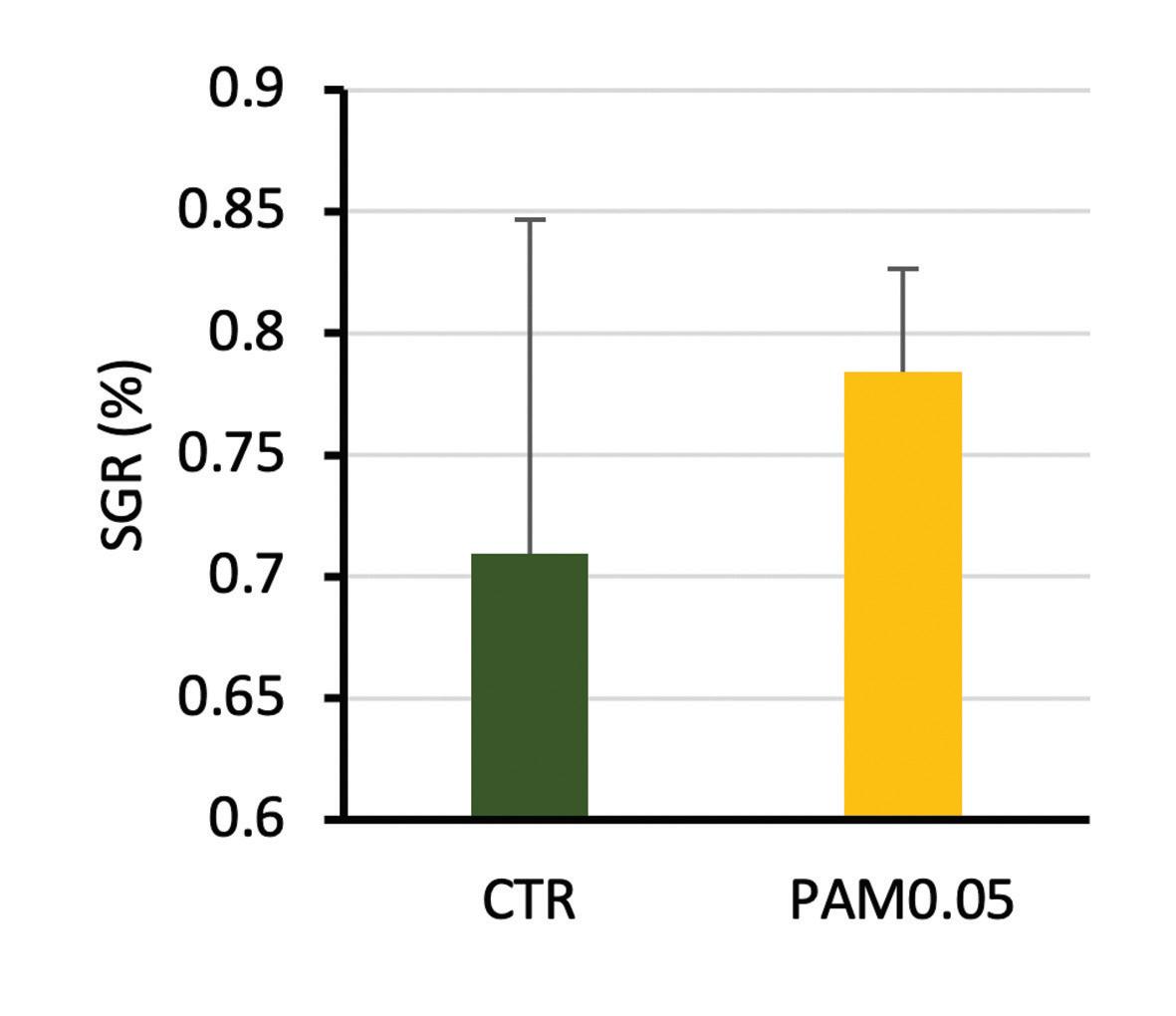
Growth performance
On the triplicate, no significant effect on specific growth rate (SGR) was observed in the group supplemented with Phyto AquaMeric (p>0.05). However, Phyto AquaMeric group showed the highest value of SGR (+9.5%) compared to the reference diet. The same tendency was found for the thermal growth coefficient (TGC).
Histology
An histology improvement was found in the group supplemented with Phyto AquaMeric compared to the reference diet. In the proximal submucosa, the percentage of normal fish in the Phyto AquaMeric group was higher than the control with the same benefit for pyloric steatosis (Fig. 2). Phyto AquaMeric protected more individuals from inflammatory changes.
In mid intestine submucosa, a significant reduction of inflammatory changes was also observed in Phyto AquaMeric group with no fish showing severe steatosis. No visual differences were observed in the distal intestine related to the high feed challenge.
Figure 1. Specific growth rate (SGR) for the reference feed (0%) (CTR) and 0.05% Phyto AquaMeric (PAM0.05).
Gene expression
In the distal intestine, gene expression revealed a positive effect of PhytoAquaMeric (Fig. 3). For example, interleukin (IL) 17α showed a down-regulation expression in Phyto AquaMeric group. The opposite was found in many inflammation regulatory markers
Figure 2. Number of mid intestine tissue sections that were scored “normal”, “moderate”, “marked” or “severe” for (A) enterocyte steatosis or hyper-vacuolization, and (B) increases in the width and inflammatory cell infiltration in the submucosa.
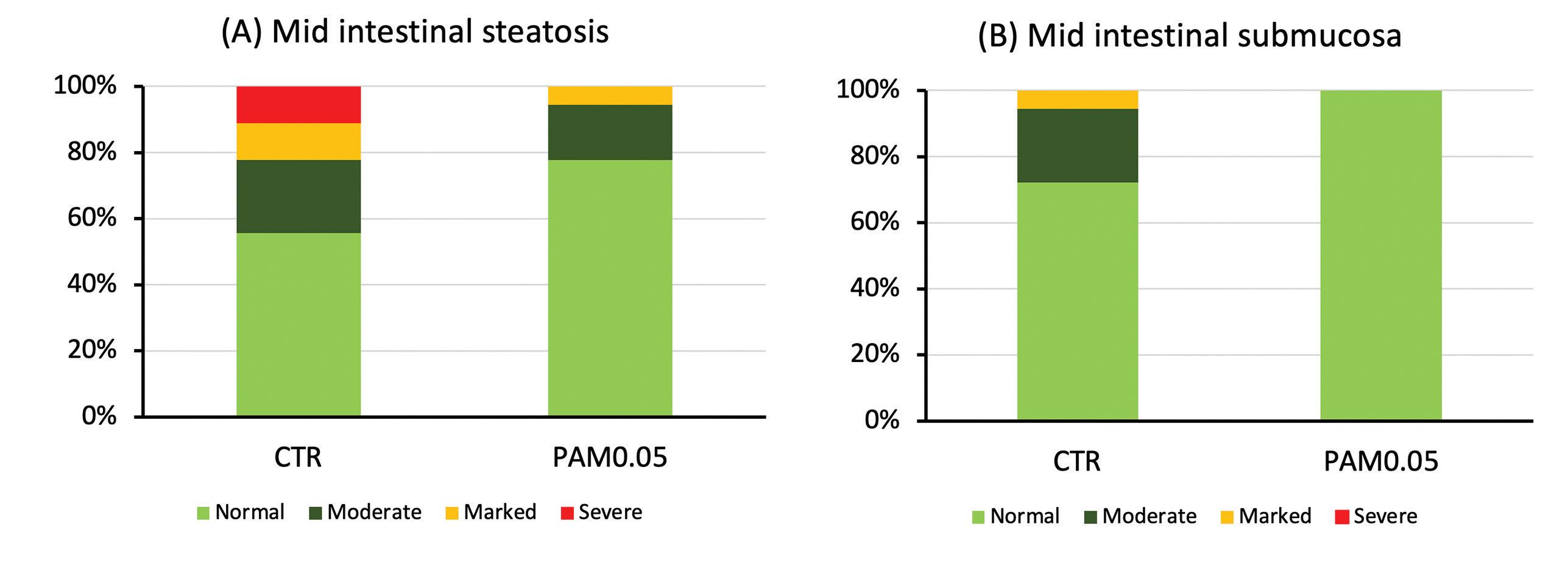
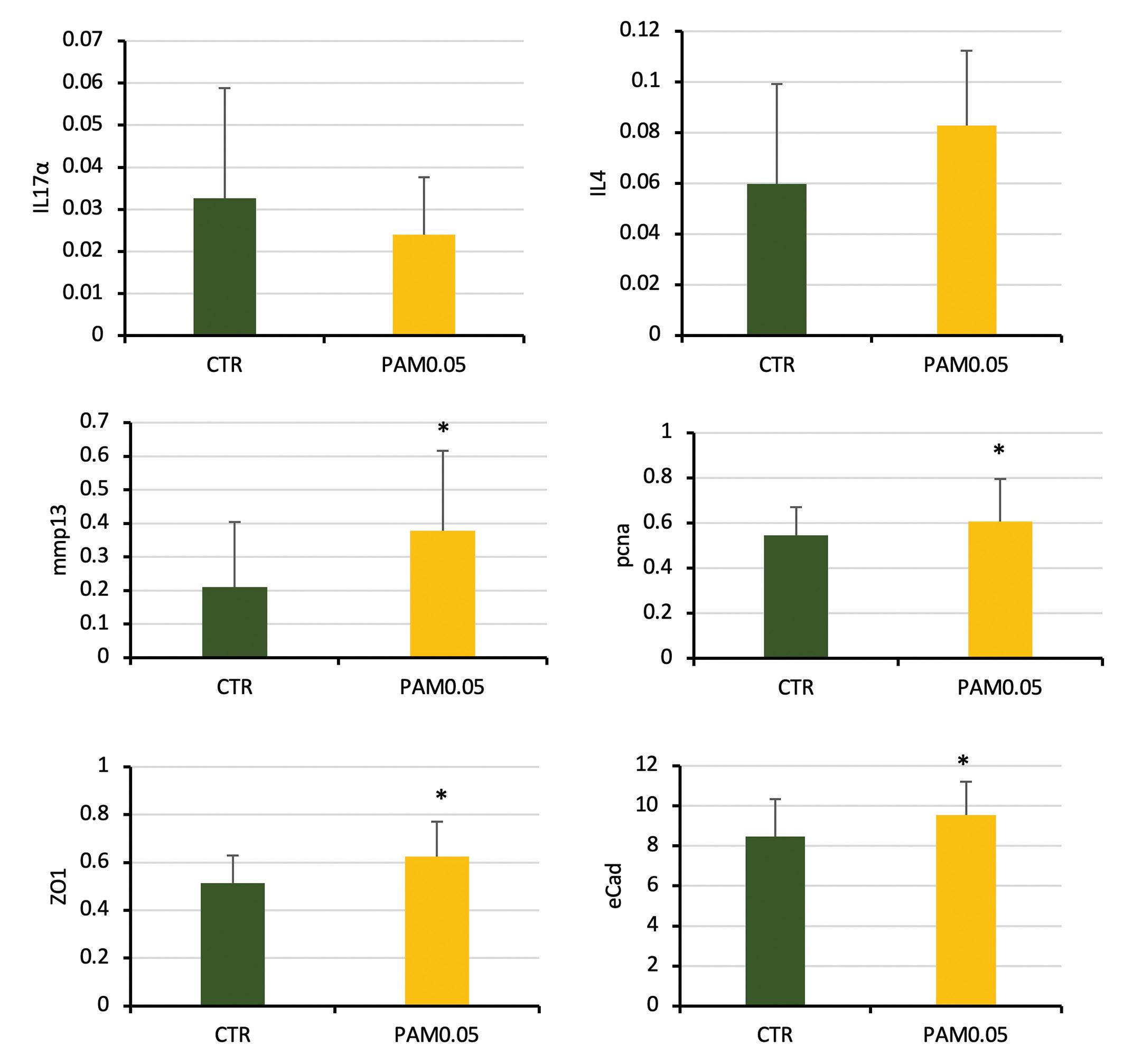
Figure 3. Relative expression levels in distal intestine of pro-inflammatory cytokine IL17α; anti-inflammatory markers IL4; immunomodulation markers (mmp13 & pcna); barrier function genes; plotted as mean tank levels (n=3) +/-SEM.
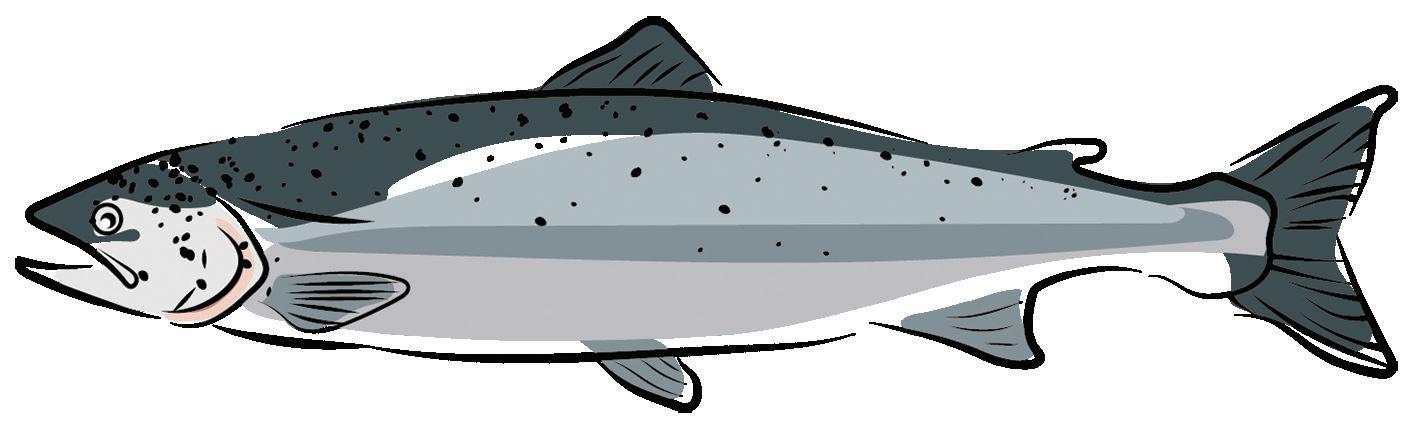
such as iIL4 and TGF-β. Other immune-related genes that revealed an up-regulation expression were extra-cellular matrix modulator collagenase (mmp13) and proliferating cell nuclear antigen (pcna). This observation might indicate a change in epithelial tissue remodeling or reorganization as an effect of the dietary supplement. Moreover, gene coding for tight-junction proteins like E-Cadherin (Ecad) or Zonula occludens 1 (ZO1) were up-regulated. These results indicate Phyto AquaMeric might contribute to restoring the integrity of intestinal barriers through the regulation of such genes.
Conclusion
In a highly challenging trial, Phyto AquaMeric improved growth performances. Results revealed alleviation of enteritis at proximal and mid intestines. This effect of Phyto AquaMeric on the first segments of intestines could be explained by immunomodulation activity expressed by the gene expression in the distal part of the intestine. Selected botanical compounds could contribute to reducing the consequences of intestinal inflammation in fish. The reproducibility of these results should be evaluated on a larger scale.
Botanical ingredient quality was shown essential to answering aquafeed industry needs.
References available on request.
More information: Siméon Fagnon
Innovation Product Manager Phytosynthese E: simeon.fagnon@phytosynbthese.fr
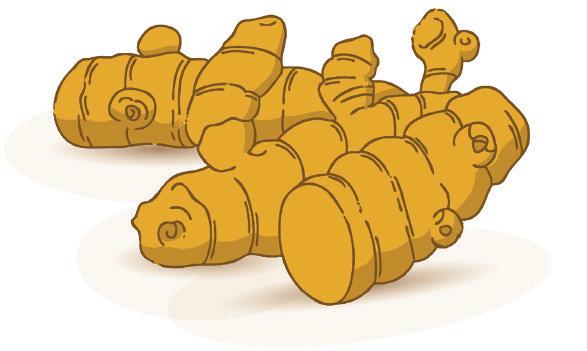
Black soldier fly improves the health status of rainbow trout increasing their high gut microbial richness
Alex Diana, Andrew Richardson, Innovafeed
As it has been widely demonstrated in human health, the microbiota affects many vital functions. It contributes to the regulation of the immune system, digestion of food, production of vitamins, and much more. Recent research demonstrates the impact of insect ingredients on fish species' microbiome.
The stomach is often called the second brain of the human body. It represents 100 million nerve cells that form the enteric nervous system (ENS) and gut microbes may stimulate immune cells, which then signal to the brain, meaning your gut’s health can have a very realworld impact on your well-being. Indeed, by modulating gut microbiome composition through proper nutrition and probiotics, we also help decrease anxiety and depression in humans. This is because Lactobacilli and inflammation are also recognized to affect the brain pathway and when an imbalance occurs, mood disorders develop.
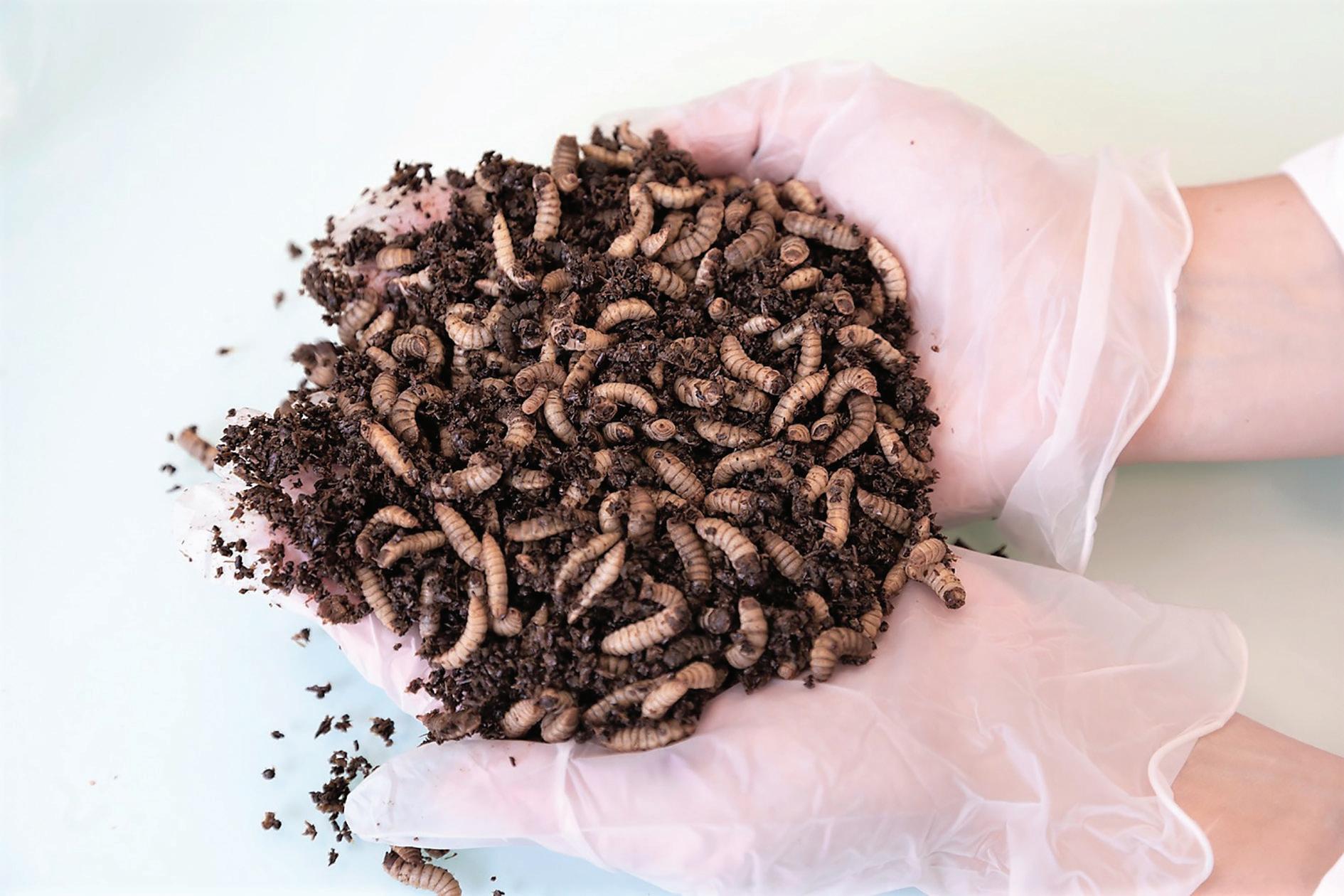
Figure 1. Relative abundance within the two diets.
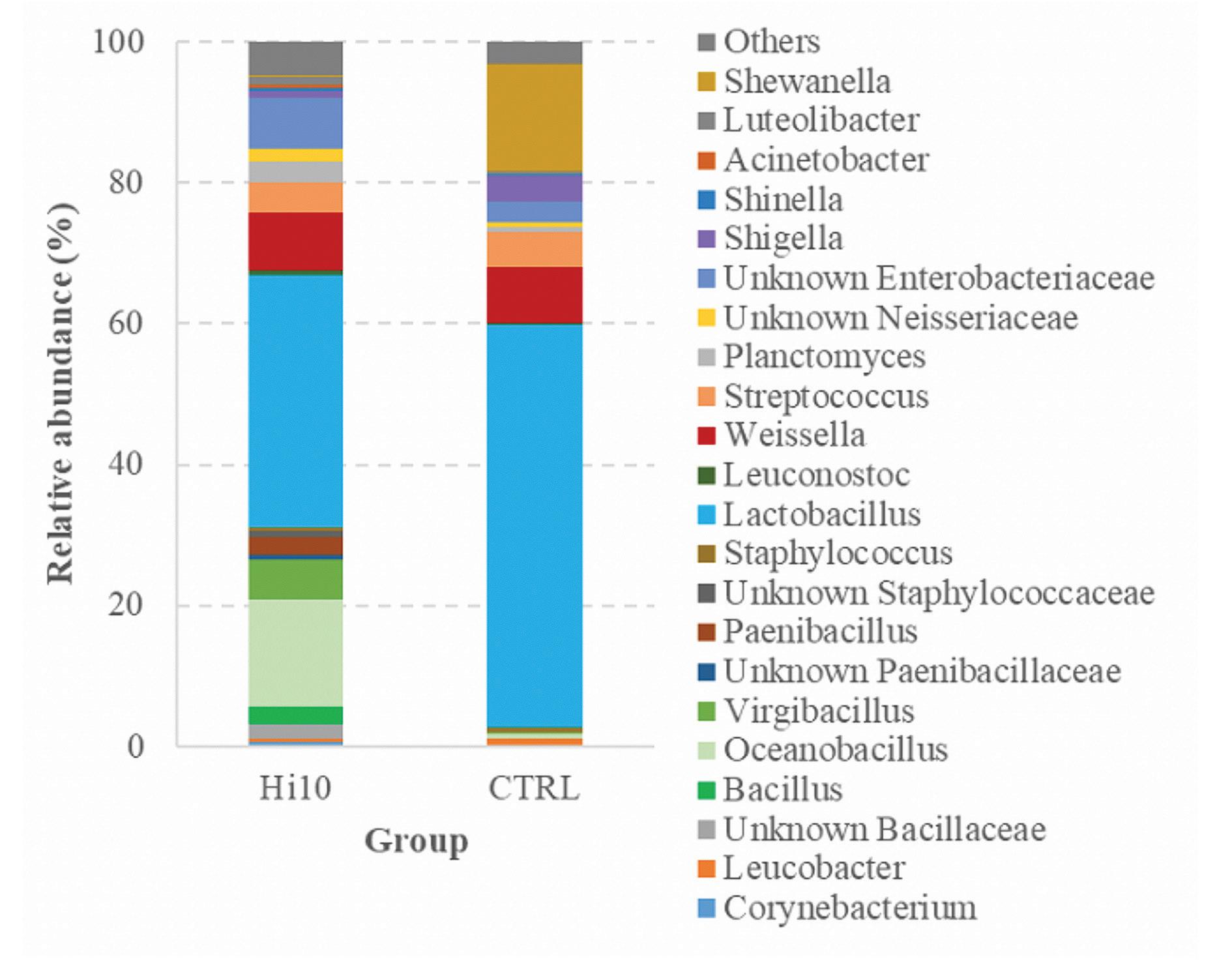
Aquaculture growth comes with important challenges that nutritionists, farmers, and formulators have to face daily, of which maintaining survival rate and strengthening immune development are the main priorities. These problems have been addressed primarily by advancements in automated feeding machines, reduced reliance on fishmeal-based feeds, and even disease control through commercial adoption of vaccine development along with biological control of parasite infection using cleaner fish.
Gut communities in aquaculture species
In aquatic animals, gut microbial communities shift with host development and living environments. Understanding the mechanism by which the environment impacts the gut microbial communities of aquatic animals is crucial for assessing and managing aquatic ecosystem health. Initially, fish embryos develop in a relatively constant bacteria-free environment (i.e., within the egg), although some environmental microbes quickly colonize the egg surface from the surrounding water after spawning. After hatching, environmental microbes colonize the gut of larvae through the ingestion of water. After the first feeding, new microbial communities are introduced into the gut with the diet, increasing microbial diversity. The gut microbiota further shifts with host development and with changes in diet. At an early stage, the gut microbiota is influenced mainly by the introduction of environmental microbes with water and diet. However, as the immune system and nutrition metabolism develop, gut microbes are selected and enriched gradually and can be altered with nutritional elements.
For this reason, the research project aimed to explore the impact of novel ingredients on these gut communities using metabarcoding analysis of feed- and gut-associated microbial communities. The feeding trial was set at a recirculation aquaculture system (RAS) with a growth phase of 87 days. A control diet containing only fish and plant proteins (CTRL) and an insect diet containing 10% of Hermetia illucens meal (Hi10) were tested in rainbow trout (Oncorhynchus mykiss) were used to source comprehensive samples of the gut.
Results
In agreement with the majority of studies on the partial substitution of fishmeal with insect meal, the experimental diet Hi10 had an important effect in modulating the intestinal microbial communities of trout. In particular, the Hi10 diet increased significantly gut microbial richness and numerically the diversity (Shannon diversity index p=0.05) as compared to control fish (Fig. 1).
In general, high gut microbial richness and diversity are considered desired features because they are usually associated with the health status of the host. Dietary inclusion of insect meal influenced the microbial intestinal profiles of trout both qualitatively and quantitatively and allowed a dominance of Firmicutes. Indeed, multivariate analysis of bacterial communities revealed a significant relationship between diet and microbiota associated with fish intestines.
The analysis of microbiota showed a significant increase in Bacillales in the intestine of trout fed with Hi meal. In this case, we can exclude the influence of feed’s microbiota on trout’s gut microbiota since the relative abundance of Bacilli was very similar between the two feeds. A possible explanation could be that chitin, contained in insect meal, may have acted as a substrate to increase the proliferation of gut chitinolytic bacteria, which include many Bacillus species.
Several studies in fish have shown that dietary Bacillus subtilis administration enhances immune responses and disease resistance in salmonids. Moreover, other genera of bacilli have been reported to produce high levels of lipase and therefore can help to break down fats from the feed diet, and therefore they can be better absorbed in the intestine.
Conclusion
Our results indicate that dietary inclusion of Hi meal at 10% modified trout gut microbiota by increasing its richness and therefore improving the health status of the fish and its microbiome. This is an important step when guaranteeing the animal welfare of the fish in a comprehensive approach with other good practices.
More information: Alex Diana
Product Manager Aquaculture Innovafeed E: alex.diana@innovafeed.com
Andrew Richardson
Product and Account Manager Innovafeed
History of fish nutrition
Part II (1957-2000)
Ronald W. Hardy, Distinguished Professor Emeritus, Aquaculture Research Institute, University of Idaho
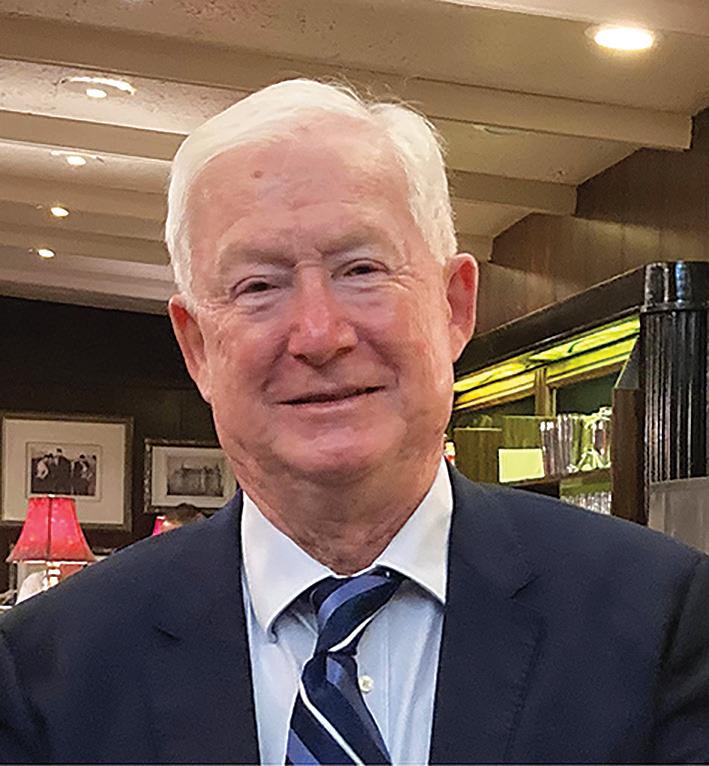
During the middle of the 20th century, a confluence of factors initiated a new era in fish nutrition research that led to the identification of essential nutrient requirements for a number of important farmed fish species. In just a few decades, the field of fish nutrition advanced more than it had in the previous century. New research approaches yielded reliable nutritional requirement information that made it possible to formulate feeds to match the nutrient requirements of selected fish species. As a result, a number of fish feed manufacturing companies became global providers of high-quality fish feeds that fueled the emergence of industrial fish and shrimp farming across the globe.
Halver’s foundational studies
The first factor was the development of a vitaminfree, semi-purified experimental diet that supported reasonable fish growth. Researchers first used this diet to determine the vitamin requirements of juvenile salmon and later to determine the requirements of salmon and other farmed fish and shrimp for vitamins, amino acids, minerals and, eventually, essential fatty acids. The approach in these studies was similar to that used in nutritional studies of livestock, poultry and lab animals; one vitamin (or other nutrient) was deleted from an otherwise nutritionally complete diet and added back to experimental diets at incremental levels. The dietary level above which no further growth response (or other dependent variable such as tissue level, enzyme activity or other health metric) was measured was considered the nutritional requirement.
The semi-purified diet that changed the course of fish nutrition research was developed at the University of Washington in Seattle. A graduate student in the Department of Biochemistry was assigned by the department chair to help a professor in the College of Fisheries investigate the persistent problem of anemia in chinook salmon fingerlings fed standard hatchery diets. The graduate student, John Halver, was an WWII army veteran whose experiences in post-war Germany with malnutrition inspired him to study human nutrition. His assignment to investigate potential solutions to salmon anemia was supposed to be a minor detour in his studies. Vitamin B12 had just been synthesized and the Department of Biochemistry had secured a few milligrams that Halver injected along with folic acid into anemic juvenile salmon. The fish recovered in 10 days.
Halver’s career goal changed; he switched to fish nutrition and undertook the challenge of developing a vitamin-free, semi-purified diet for fish as his Ph.D. research project. After a few attempts, Halver developed a semi-purified diet formulation that worked (Halver & Coates, 1957). He then used this diet to show that several B vitamins were essential in the diets of juvenile chinook salmon (Halver, 1957). Over time, the formulation of this diet changed as new information about the nutritional requirements of fish became available, but the general concept of the diet remained the same.
A second factor was the decision by the US Fish & Wildlife Service to build a second fish nutrition research laboratory in the western USA to complement the Cortland Hatchery in New York. Halver became the first director of this laboratory, located in Washington State along the Columbia River. The research focused
on the quantitative vitamin and later amino acid requirements of chinook salmon, coho salmon and rainbow trout fingerlings (Halver, 1972) (NRC, 1973). Halver’s training was in nutritional biochemistry, and he employed rigorous experimental approaches at the new laboratory that were subsequently used by researchers in the USA, Europe and Japan in the 1960s and 1970s to identify dietary requirements of salmonids, channel catfish, carp and other farmed fish. In the USA, catfish farmers were shifting to intensive production that depended on the use of nutritionally complete feeds. Research conducted by university and government researchers led to the development of high-quality and economical catfish feeds, resulting in a tremendous growth of the US catfish industry in the 1970s and 1980s. An excellent historical review of catfish nutrition research was recently published (Robinson & Li, 2019). By the 1970s, most of the quantitative nutrient requirements of juvenile salmon and catfish were known (NRC, 1973).
Fish nutrition research around the world
Aquaculture has a long history in Japan and in the second half of the 20th century, researchers in Japan made substantial contributions to fish nutrition, although many of their studies were published in Japanese with English abstracts. This limited recognition of their work by fish nutritionists in western countries. Examples of early fish nutrition research by Japanese scientists included amino acid composition of hatchery diets (Ogino, 1957), digestibility (Nose & Toyama, 1966), nutritional requirements of Penaeus japonicus, Japanese prawn (Kanazawa et al., 1971), vitamin requirements of trout (Kitamura et al., 1965; 1967), and dietary fatty acid requirements (Toyomizu et al., 1963; Toyomizu et al., 1963; Kayama, 1964; Higashi et al., 1966; Kaneko et al., 1966. By the late 1970s, Japanese researchers had estimated the dietary requirements for amino acids, fatty acids, vitamins and minerals for trout, carp, shrimp and other farmed species. Tokyo University of Fisheries and Kagoshima University under the leadership of T. Watanabe and S. Kanazawa established strong research programs that attracted and trained many international students who returned to their home countries to lead fish nutrition programs. Fish nutrition research also took hold in European countries in the 1970s, particularly in the UK, France, Germany, Italy, Norway, Greece, Poland and Hungary. In the UK, the Unit for Research into Fish Nutrition was established as a part of the National Institute for Research into Dairying at the University of Reading by C. Cowey, who subsequently joined the Institute of Marine Biochemistry in Aberdeen, Scotland, and led a team of researchers who approached fish nutrition from a biochemical perspective. Cowey organized the first International Symposium on Fish Nutrition and Feeding in Aberdeen, Scotland in 1985. This symposium became a biannual event rotating from Europe, the Americas and Asia. The most recent symposium was held in June 2022 in Sorrento, Italy. Germany hosted the first World Symposium on Finfish Nutrition and Fishfeed Technology in Hamburg, Germany in 1979 under the umbrella of the European Inland Fisheries Advisory Committee (EIFAC) of the FAO (Halver & Tiews, 1979). EIFAC also organized a dedicated workshop on Methodology for Determination of Nutrient Requirements in Fish in 1993 in Eichenhau, Germany.
Other government and academic centers in Europe added fish nutrition to their research portfolios. The Institute of Aquaculture, University of Stirling, Scotland, became a center of expertise in lipid nutrition and metabolism. In Norway, the Institute of Nutrition in Bergen added fish nutrition to its programs on animal and human nutrition in the late 1970s. Basic and applied fish nutrition research continues to this day at the Institute of Marine Research in Bergen.
In the 1970s, with the rapid development of Atlantic salmon farming in Norway, another major group dedicated to fish nutrition, Akvaforsk, now NOFIMA, was created to conduct research and development in fish nutrition and feeding. In France, Luquet established the first laboratory dedicated to fish nutrition as part of animal nutrition group in the National Institute for Agricultural Research (INRA) in Jouy en Josas. This laboratory then moved to the southwest of France in Saint Pee sur Nivelle in 1976, where the group became a leading laboratory conducting research on fish nutrition and metabolism. Currently, in almost all European countries, research teams as part of universities or public research institutes deal with dedicated work on various aspects of nutrition of different species of farmed fish at such locations as the Hellenic Centre for
Marine Research, Athens, Greece, the University of Las Palmas, Spain, the University of Porto, Portugal, and the University of Udine in Italy, just to mention a few.
China accounts for over 62% of global farmed fish and crustacea production but it was a relative latecomer to the study of fish nutrition. Throughout China's more than 3000-year history of aquaculture, the production relied on natural food in ponds to support fish growth. In the early 1950's, China was the first to succeed in the artificial propagation of four major fishes (black carp, grass carp, silver carp and bighead carp). Artificially propagated fingerings replaced wild sources to stock ponds and production depended on natural food, often enhanced by adding agricultural by-products to ponds to increase natural food production. Research on quantitative nutrient requirements of fish did not start until after 1980. In fact, in the mid-1980s, not a single feed company in China exclusively produced fish feeds. However, the situation changed quickly and within a few decades, China had over 12,000 fish feed factories. Availability of high-quality fish feeds in China enabled rapid intensification of freshwater finfish production through intensification and rapid expansion of marine finfish aquaculture production, including intensive production of many other species with pelleted feeds, including turtles and frogs.
First aquafeeds
In the early days of Pacific salmon hatchery operations, feeds were made on-site using frozen, adult salmon carcasses as a feed component. However, feeds containing salmon carcasses were discovered to be a vector for fish tuberculosis, a disease caused by Mycobacterium chelonei (Wood & Ordahl, 1958). This disease was a significant problem for the Pacific salmon hatchery programs because the main clinical manifestation of chronic infection was incomplete gonadal development in maturing fish. Hatchery programs were sustained by collecting and fertilizing eggs from salmon that returned to hatcheries and fish tuberculosis prevented hatcheries from meeting production goals due to a lack of viable eggs (Wood & Ordahl, 1958). Research showed the pathogen was not affected by freezing salmon carcasses, but heat treatment (pasteurization) killed it. Subsequently, pasteurized fish carcasses and byproducts from fish processing were used in an open-formula, semi-moist salmon feed called the Oregon Moist Pellet (OMP) that became the standard feed used in hatchery programs (Hublou, 1963). The OMP was produced in central locations by commercial companies, frozen and shipped to hatcheries. Hatchery-made feeds were dropped. The adoption of the OMP eliminated fish tuberculosis, improved hatchery water quality and supported consistent and predictable juvenile salmon growth at hatcheries.
The OMP had one major drawback; it was a highmoisture feed (~28%) and therefore had to remain frozen until it was fed. It was also relatively expensive. Research by the US Fish & Wildlife Service at the Cortland Hatchery and the Abernathy Fish Technology Center in Washington State led to develop open formulas for dry, compression-pelleted trout and salmon feeds (Phillips et al., 1964; Fowler & Burrows, 1971). The open-formula feeds were produced by commercial feed companies who bid to supply state, federal and tribal salmon hatcheries, and commercial trout farmers. The formulations were later changed by feed companies and became proprietary, closedformula feeds. The most important advantage of compressed pellets compared to moist feeds was that pellets did not require frozen storage. Another advantage was that feed formulations for compressed could include a greater number of common feed ingredients than moist feeds because moist feeds required a precise combination of feed ingredients with binding properties to hold pellets together.
Compressed pellets have several disadvantages as aquaculture feeds. First, they are dense and rapidly sink in water. Second, the water stability of compressed pellets is limited. Third, they can fracture if not handled carefully during shipping and storage, creating dust (fines) that are essentially wasted feed. Finally, their high density limits the amount of fat or oil that can be applied by top-dressed pellets to a maximum of 18-20% total lipid.
Extrusion technology
Using cooking-extrusion technology to produce fish feeds overcame the disadvantages of compressed pellets and propelled the aquaculture industry to much higher and efficient fish production.
The first major application of extrusion pelleting in aquaculture was by the US catfish industry. The
density of extruded pellets can be controlled by the feed formulation and conditions of pelleting to produce floating, slowly sinking or sinking pellets. Catfish are primarily farmed in large ponds and using floating feeds allowed farmers to see feeding activity and adjust feeding levels accordingly. However, when extrusion was first used to produce trout feeds by a major USA feed company, the results were disastrous. Feeding extruded pellets to rainbow trout caused high mortality from fatty liver disease. Trout farmers blamed the extrusion process and did not use extruded feeds for decades, even after extruded feeds were widely and successfully used in Europe by trout and salmon farmers.
Fatty liver disease in trout was, of course, not caused by the extrusion process per se but rather by using feed formulations designed for compressed pellets. These formulations specified relatively high starch levels. Cooking-extrusion processing greatly increased the degree of starch gelatinization, thereby increasing starch digestibility and raising blood glucose levels. Trout have limited ability to metabolize glucose, so excess glucose was metabolized into fat and stored in the liver, causing fatty liver disease. When starch levels were reduced in high-lipid, high-energy, extruded feed formulations, the level of digestible starch was also reduced to levels that salmon and trout could tolerate.
The adoption of extruded feeds by salmon and trout farmers improved feed efficiency, reduced environmental pollution from lost feed and reduced production costs. Control of feed density allowed feed producers to control feed buoyancy and increase lipid levels of feeds beyond 30%. The adoption of highenergy feeds allowed feed protein levels to be reduced, leading to lower feed conversion ratios, around 1.0 to 1.2 for commercial salmon farms. All of this improved the economics of salmon and trout aquaculture, making salmon the second-most valuable farmed fish species in the world, behind shrimp.
The period between 1958 and 2000 looks, in retrospect, to have been a golden age for fish nutrition research. New information on the nutritional requirements for a wide range of fish and shrimp species, combined with improvements in feed manufacturing processes, provided the foundation for tremendous increases in the amount and efficiency of global aquaculture production of fed aquaculture species. However, the rapid growth of aquaculture production created new challenges for the industry, notably bringing sustainability issues to the forefront of fish nutrition research. This will be the subject of the final installment of this series of columns.
Protecting your dreams
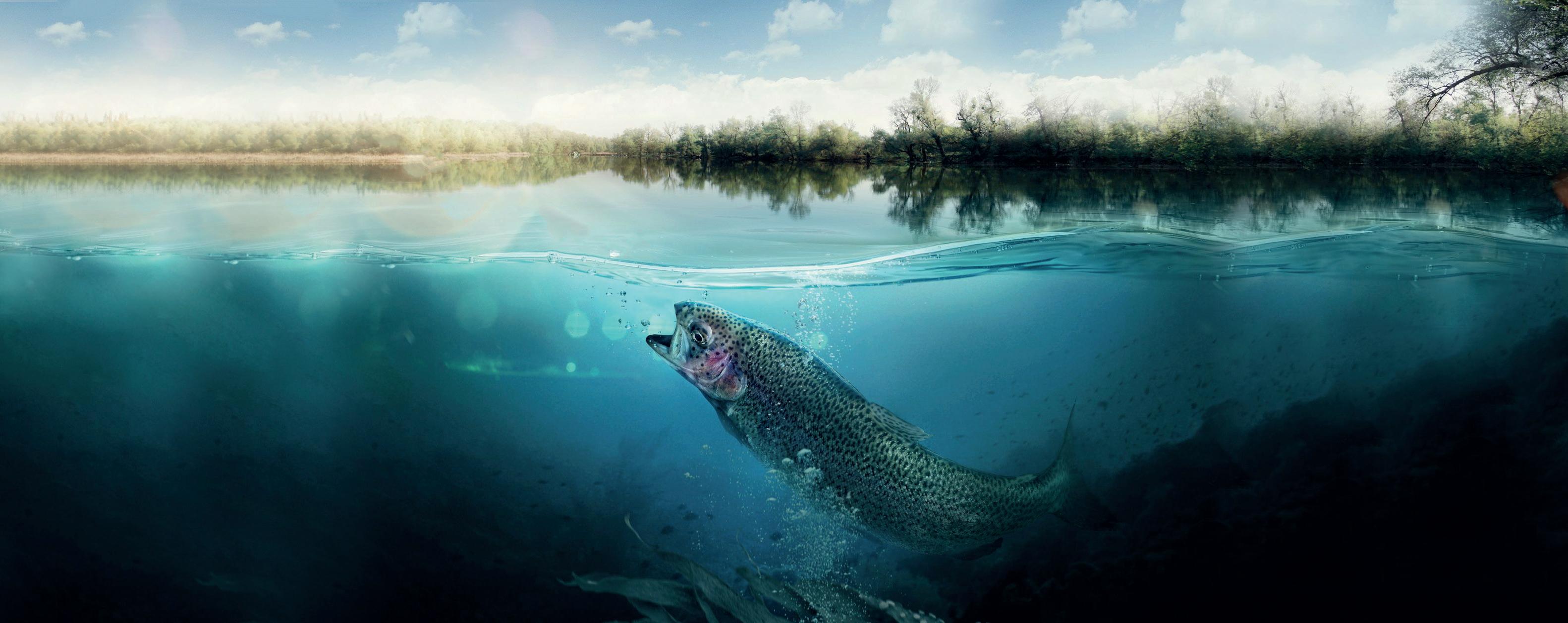
EXPERTS IN ANTIOXIDANTS
IONOL
BHT, BHA, PROPYL GALLATE, CITRIC ACID NATUROL
TOCOPHEROL, ROSEMARY EXTRACT, GALLIC ACID