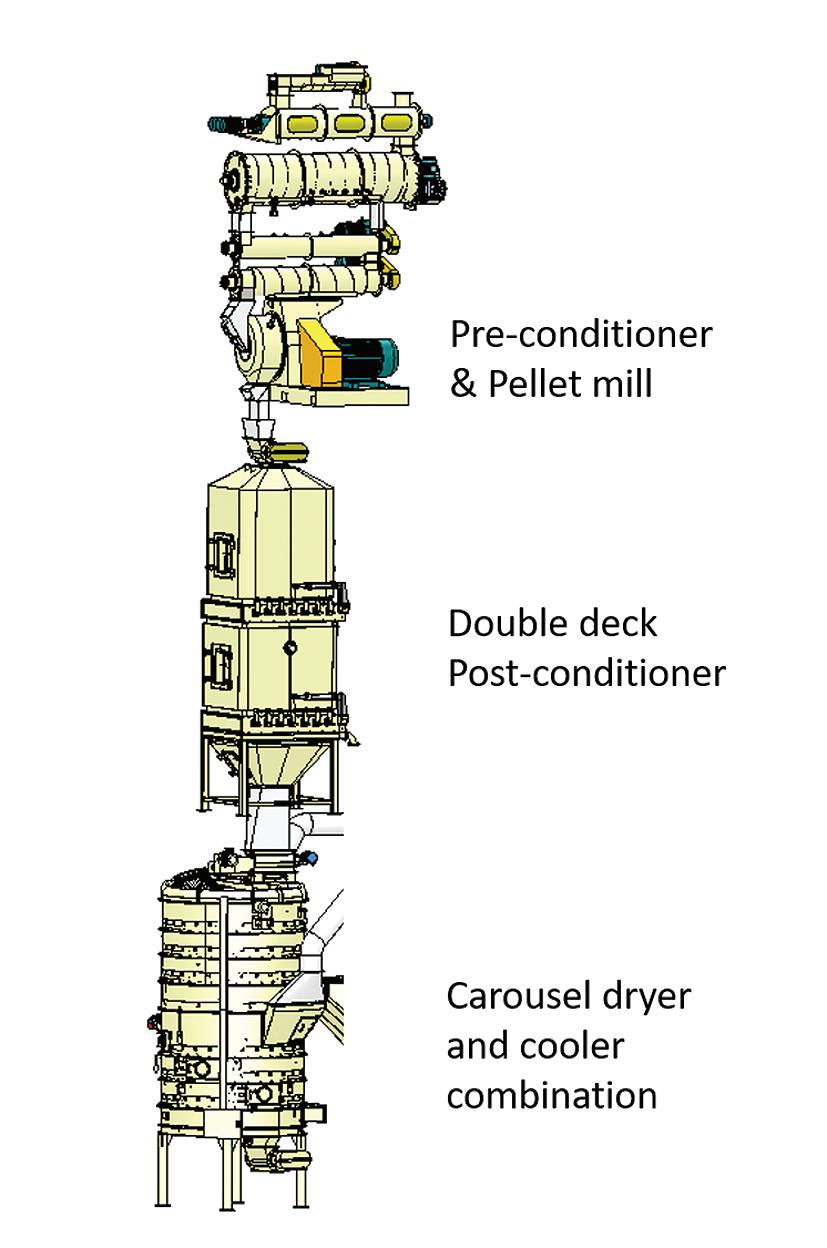
42 minute read
DRYING AND COOLING PROCESSES FOR PELLETED SHRIMP FEED
Albert Wang, IDAH Co., Ltd.
Development of shrimp feed
A few decades ago, a Japanese scientist Dr. Motosaku Fujinaga (also known as Dr. Hudinaga), became a pioneer in the technology of shrimp spawning and larval rearing. This technology development is an important milestone that marks the start of in-land shrimp cultivation. In the 1970s, commercial shrimp feed was sold to the public. These pellets are fast sinking and water-stable (Chamberlain, 2010).
Pelleted feed production in the early days
Early day formulation of shrimp feed incorporated a high percentage of an aquatic animal protein source, such as fishmeals or squid meal, which was readily digestible for the shrimp digestive tract (higher FCR) (Yun et al., 2017). The early pelleting process only incorporated a small amount of moisture and no significant drying process was needed.
The limitation and rising price of the animal-based feed source promoted the usage of a reasonable amount of plant-based protein, such as soybean meal as a protein source substitute (Hasan, 2001; Suárez et al., 2009). The incorporation of plant-based meals induced the need for a better ingredient cooking process because the cooking process can denaturate these plant proteins and promote starch cooking.
The first-generation (G1) of shrimp feed production machine, popular from the 1980s until the 2010s, consisted of a pellet mill, a post-conditioner, and a cooler. This machine setup can prolong the mash cooking while taking out a small amount of moisture. The pre-conditioner system with direct steam spraying incorporated into the pelleting system in the 1980s helped increase the moisture content of the mash by around 2-3% (wb). The higher moisture improvement can increase the feed stability in water. The following post-conditioning and cooling process will remove approximately 1-2% moisture from the feed, resulting in the finished product with a moisture content of 10-11%, a shelf-stable pelleted feed moisture content during storage.
Incorporation of the carousel dryer to shrimp feed pelleting lines
Even though the G1 technology is still a popular option in many Asian countries, there is a growing demand to increase the incorporation of sustainable plant-based protein while keeping the water stability (Bae et al., 2020). One way to improve the water stability of plantbased protein is by adding moisture to the mash. The benefits of increasing the moisture content during mash cooking are the increase of starch cooking and
Figure 1. The third-generation (G3) shrimp feed pelleting system development offers total moisture control and saves drying energy. (IDAH, Taiwan)
the lubricating effect of the mash when passing the pelleting hole.
Carousel dryer was first incorporated into the shrimp pelleting line back in 2013 in a large Indian shrimp feed manufacturing company. This started the secondgeneration (G2) pelleting system. This dryer can fulfill all conditions needed to dry the shrimp feed properly and produce high-quality shrimp feed. Nowadays, the G2 system has become popular and utilized in many different pelleting lines in Asia. The incorporation of the carousel dryer after the postconditioning process in the production line opens up the possibility of increasing the mash moisture before the pelleting process, due to the ability of the dryer to take out 4-5% of moisture content from the feed. The significant improvement of G2, when compared with the G1 in shrimp feed drying, are: • Optimum drying moisture control. The carousel dryer can dry material with ±0.5% moisture variance, much less than the G1 (± 1%). Having adequate moisture control means the water content in pellets can be precisely controlled and indirectly reduce the loss of raw materials. • The dryer optimally utilizes the drying air, thus minimizing energy use. • The G2 technology can reduce steam usage for the post-conditioning system by up to 15%.
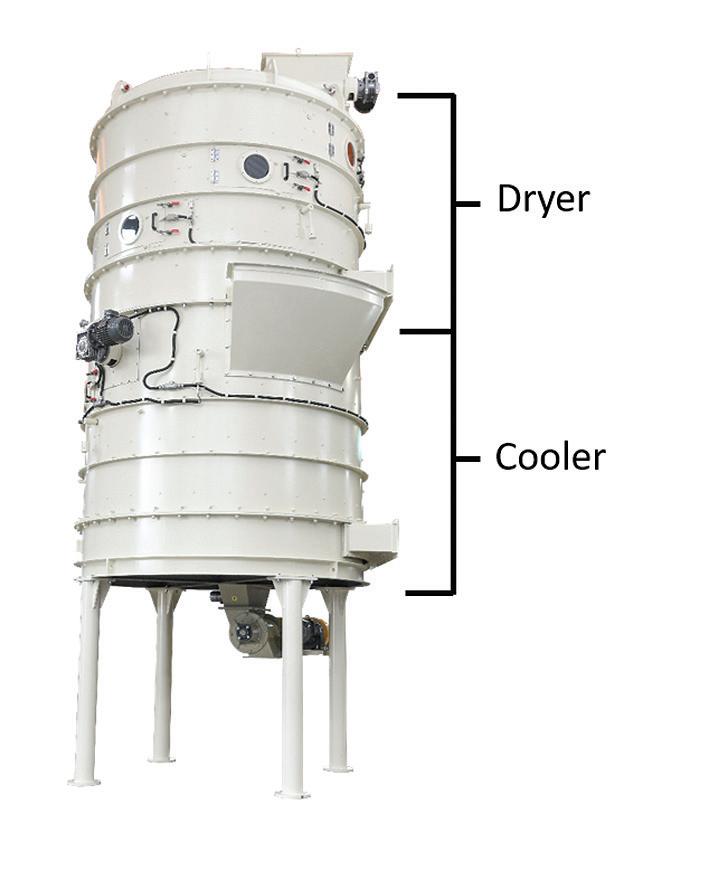
So what is the latest technology in drying and cooling shrimp feed? We considered all the requirements and the experience in the field and have developed a Generation 3 (G3) pelleting system that incorporates the dryer and cooler (TK-series) in one machine (Fig. 1).
IDAH sold the TK-series machine to China and India back in 2021 (Fig. 2). The two benefits of this G3 system when compared to G2 system are: • Total moisture control: easy and fast. The TK-series can produce feed with ±0.5% moisture variance in both dryer and cooler. This combination solved the high moisture variance problem that usually occurs when applying the conventional box cooler. This
TK-series machine lessens the point to control in the production flow. • Saving drying energy. In a carousel dryer, the counterflow drying airflow will support efficient heat transfer
Figure 2. The ±0.5% moisture variance in the carousel dryer-cooler lowers the amount of production flow control point in the shrimp feed pelleting production line. (IDAH, Taiwan)
and give a low exhaust air temperature. The system utilizes the air exhaust from the cooler as makeup air for the dryer. The dust-free makeup air discharged from the cyclone (or jet filter) still contains some amount of heat and can lower the energy use (1530%) and reduce the amount of air emission (30-50%) and decrease odor problems.
Conclusion
In the past 40 years, there have been breakthroughs in shrimp feed production technology. Along with the changes in the formulation from animal-based to become plant-based, we also utilized new technologies to enable the production of high-quality shrimp feed. The main changes were, first, the moisture level increase during the pre-pelleting process, which improved the cooking process and water stability; second, the post-conditioning system prolonged the cooking of the pellets; and lastly, the incorporation of carousel dryers, which gave total moisture control (+/- 0.5%), energy-saving, and lower dust/odor exhaust that benefited feed producers.
References available on request.
More information: Albert Wang, M.Sc.
Marketing Manager (IDAH Co., Ltd.) E: albert.wang@idah.com
Instant insights into aquafeed and ingredients with portable on-site analyzers
Chris Larkin, NeoSpectra by Si-Ware
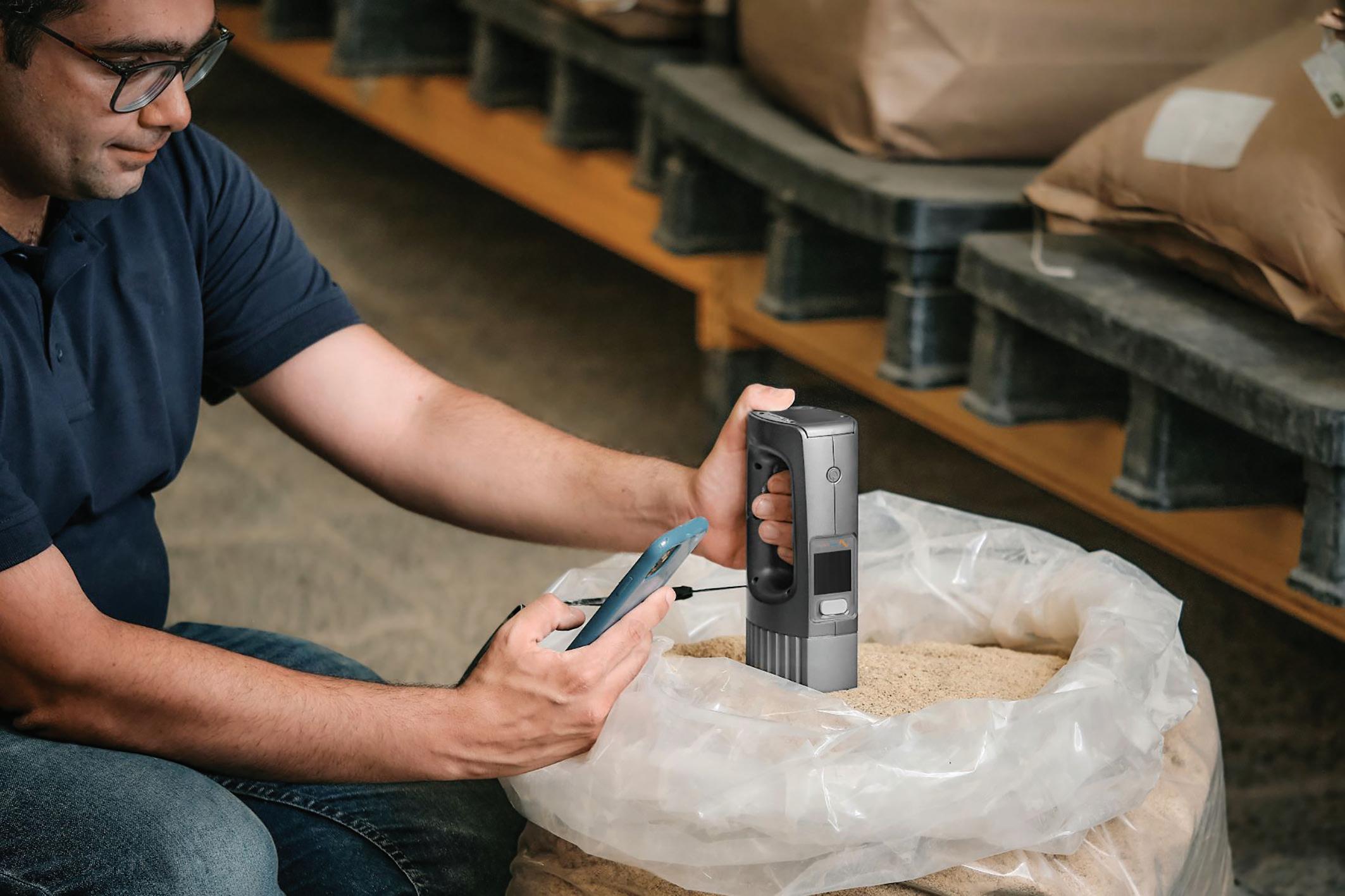
Aquaculture production is projected to increase globally by 8-15% over the next decade. Meeting the increased demand for seafood will require aquafeeds to meet nutritional and environmental sustainability standards. But new pressures imposed on aquafeed manufacturers pose a challenge to meeting increased demand. Factors like unstable global markets for core commodity ingredients, new aquafeed ingredient sources, and market and end-use changes threaten to obstruct industry production.
Like other livestock feed producers, aquaculture feed manufacturers are challenged to create recipes for a multitude of aquaculture feeds utilizing many different ingredients, often sourced globally, to balance the palatability and proper nutritional profile at the lowest price point.
Formulating feed recipes on a least-cost basis is not a simple task. On the quality side of the equation, aquaculture feed ingredients are natural products with inherent variability in composition based on growing environments, variety, processing, and storage conditions. Wheat bran can vary from 14-17% protein, as an example, while fishmeals can range from 60-75% protein depending on type and season.
The cost side of the equation is equally complex. From production and supply chain factors in the last two years to the Russian aggression in Ukraine in 2022, the global commodity market has seen record prices in 2022 for many aquaculture feed ingredients. Wheat, for example, hit record prices above USD 1200/bu in March and May of 2022 before declining to around USD 800/ bu, still an 8-year high. Soybeans have shown typical trends, reaching prices of over USD 1700/bu in June of 2022, almost double the pre-pandemic price in the USD 900/bu range.
But it’s not all bad news. Necessity and conflict often elicit a reaction from innovators. Accurate and frequent analysis of feed ingredients can provide the insight needed to optimize the production of nutritionally complete aquafeed at reduced costs.
NIR to optimize production costs of aquafeeds
Many aquafeed manufacturers are already familiar with the benefits of using NIR instrumentation technology to test ingredients for nutritional composition. This technology is commonly used in laboratories via benchtop instruments, but these instruments tend to be expensive and require skilled operators.
In terms of cost, NIR analysis is less expensive and faster than wet chemistry methods in general, with similar or better precision. For example, the cost of routine proximate analysis is only about one-third of the cost of wet chemistry, and the analysis is typically completed in less than a minute.
The benefits of frequent analysis can be seen throughout the aquafeed value chain. Incoming raw ingredients can be inspected to ensure they meet contract claims, providing evidence for claims where the quality is below specification and valuable data to take advantage of when quality is higher. Aquafeed producers can use this information to adjust formulations based on commodity or ingredient prices and still maintain proper nutritional profiles for the various final compound feeds.
Final compound aquafeed can be monitored at production to ensure labeled specifications and at intake at fish farms to validate each batch of incoming feed. Industry leaders are creating calibrations for new ingredients, such as insect proteins, so feed manufacturers can properly incorporate novel new materials into formulations. However, the actual value of frequent NIR testing can only be realized by
Table 1. Calibration statistics from a soy meal calibration created on a current benchtop FT-NIR and a NeoSpectra by Si-Ware Scanner analyzer. Reprinted with permission from Klevtech Consulting.
Protein
Fat
Fiber
N SECV
R2
Range
N SECV
R2
Range
N SECV
R2
Range
Leading Benchtop FT-NIR NeoSpectra Scanner Portable FT-NIR
138 0.58 0.91 39.7 - 49.8
137 0.54 0.91 39.7 – 49.6
135 0.298 0.93 0.47 – 8.1%
138 0.3 0.75 2.49 – 7.67%
145 0.32 0.95 0.4 – 8.7%
143 0.29 0.78 2.49 – 7.67%
Moisture
N SECV
R2
Range 95 0.19 0.85 10.2 – 11.7%
95 0.19 0.83 9.5 - 11.5%
N = number of samples in the calibration set SECV = standard error of cross-validation in the calibration set R2 = coefficient of determination for the cross-validation in the calibration set Range = minimum and maximum reference values in the calibration set
easy, on-site testing throughout the product chain. As technology has advanced, NIR has left the confines of the laboratory and become portable and accessible to feed manufacturers in their facilities. NIR is about putting more information into the hands of the feed manufacturer and livestock farms.
Portable NIR
With the cost of bench-top instrumentation ranging from 40 to 100K dollars and a concurrent requirement for a clean and stable environment and well-trained operators, many feed manufacturers are looking for reliable instrumentation that is portable, rugged, and adaptable to a wide range of sample types, feeding operations, and ways of handling feed. Additional requirements for the next-generation solutions are that they must also operate at every point in the manufacturing process (raw material through final product) at an affordable price point and still provide the benefits of improved efficiency and lowering costs.
Portable NIR devices have been on the market for some time, but many have not delivered the performance, reliability, and ease of use required for intuitive on-site analysis.
With the earliest examples of handheld NIR technology, the biggest issue was the poor transferability of calibrations from robust benchtop instruments to the portable platform. With nextgeneration spectrometers, such as those implementing MEMS technology, it is possible to eliminate these issues and transfer calibrations reliably. FT-based MEMs spectrometers also deliver accuracy and performance comparable to much more expensive benchtop units, even when faced with challenging conditions.
One recent study examined the performance of a portable MEMS-based NIR analyzer from NeoSpectra by Si-Ware with an industry-standard benchtop FT-NIR analyzer for soy meal analysis. Table 1 shows that the portable handheld NeoSpectra scanner performs as well as a benchtop instrument for protein, oil, fiber, and moisture analysis.
As the data above show, new portable FT-NIR analyzers can provide the insight required by aquafeed manufacturers to standardize consistent feed quality while minimizing raw material costs in an end-to-end quality program from ingredient to aquaculture farm. Cloud-based Intuitive mobile apps bring smart-phone ease of use to the operator, and cloud-based software aggregates and summarizes reported data for powerful insights into the operation.
The next-generation portable NIR instruments are ushering in a new era of aquafeed analysis with affordable on-site analysis in easy-to-use handheld devices.
More information: Chris Larkin
Senior Applications Engineer NeoSpectra by Si-Ware E: Chris.larkin@si-ware.com
AQUA FEED
PREMIUM PETFOOD
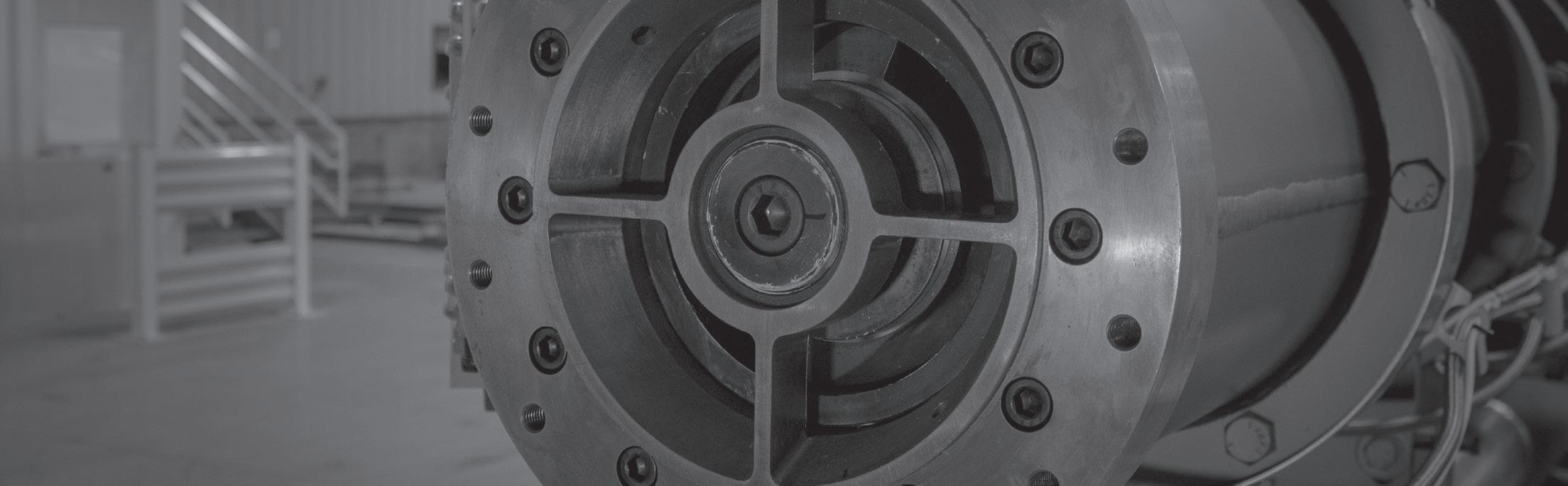
PETFOOD SNACKS
SINGLE SCREW MULTIPLE MARKETS
SINGLE SCREW EXTRUSION SYSTEM OPTIMIZES
Optimize the return on your capital investment FLEXIBILITY AND MARKET OPPORTUNITIES with a single screw extrusion system. Buy one system and cost-effectively deliver product to multiple market opportunities. Contact Extru-Tech today
at 785-284-2153 or visit us
• • One system capable of economy up to super premium fresh meat petfood Aquatic feeds that range from floating to sinking shrimp feed online at extru-techinc.com • Capitalize on high margin petfood treat opportunities • Significantly lower operating cost per ton versus competitive systems An Extru-Tech Single Screw Extrusion System provides all the flexibility and production efficiencies at around half the cost of competitive extrusion systems with high operating costs.
P.O. Box 8 100 Airport Road Sabetha, KS 66534, USA Phone: 785-284-2153 Fax: 785-284-3143 extru-techinc@extru-techinc.com www.extru-techinc.com
ET-337C.indd 1 1/28/21 8:32 AM
Managing moisture in aquafeed production
John Williamson, Devenish
Extruding aquafeed requires high moisture addition during processing. Moisture is added at various stages of production: in the mixer, in the conditioner and the extruder. The moisture content of the raw materials during production is pushed to levels up to 25% to ensure good cooking, particle adhesion and starch gelatinization. Aquafeed raw materials need to absorb this high moisture in a very short period of time. This is a challenge as raw materials used in producing aquafeed are generally hydrophobic and do not easily absorb moisture.
SmartMoisture, a new patented solution from Devenish, will accelerate moisture acceptance in feed particles during the various processes of extruding aquafeed. It is a novel patented technology that contains a combination of propionic acid esterified to glycerol, buffered organic acids, and surfactants.
The rate at which molds and bacteria grow after feed processing depends on factors such as pH, temperature, oxygen availability, and most importantly, water activity of the feed. The decision on what the final moisture content will be is based on risk. Lower moisture content in the final feed is targeted considering the volume of moisture used in the extrusion process and the hydrophobic nature of aquafeed raw materials to avoid free water or high-water activity. SmartMoisture technology is very effective in reducing water activity in aquafeed thereby reducing risk and allowing manufacturers to produce feed with higher moisture.
Stabilizing propionic acid
SmartMoisture contains unique glycerol esters of propionic acid and surfactants. Glycerol esters of propionic acid, are covalent bonds that stabilize
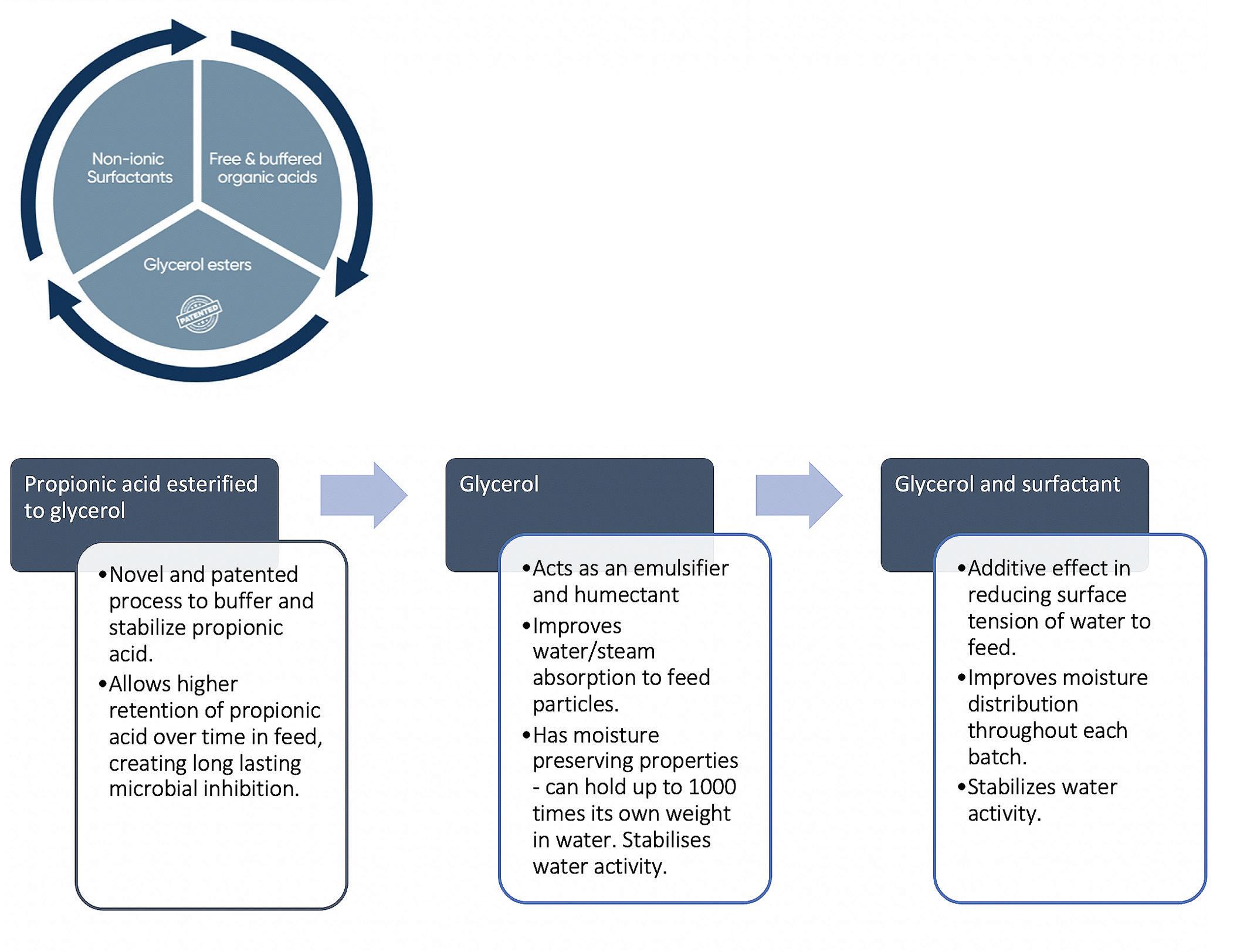
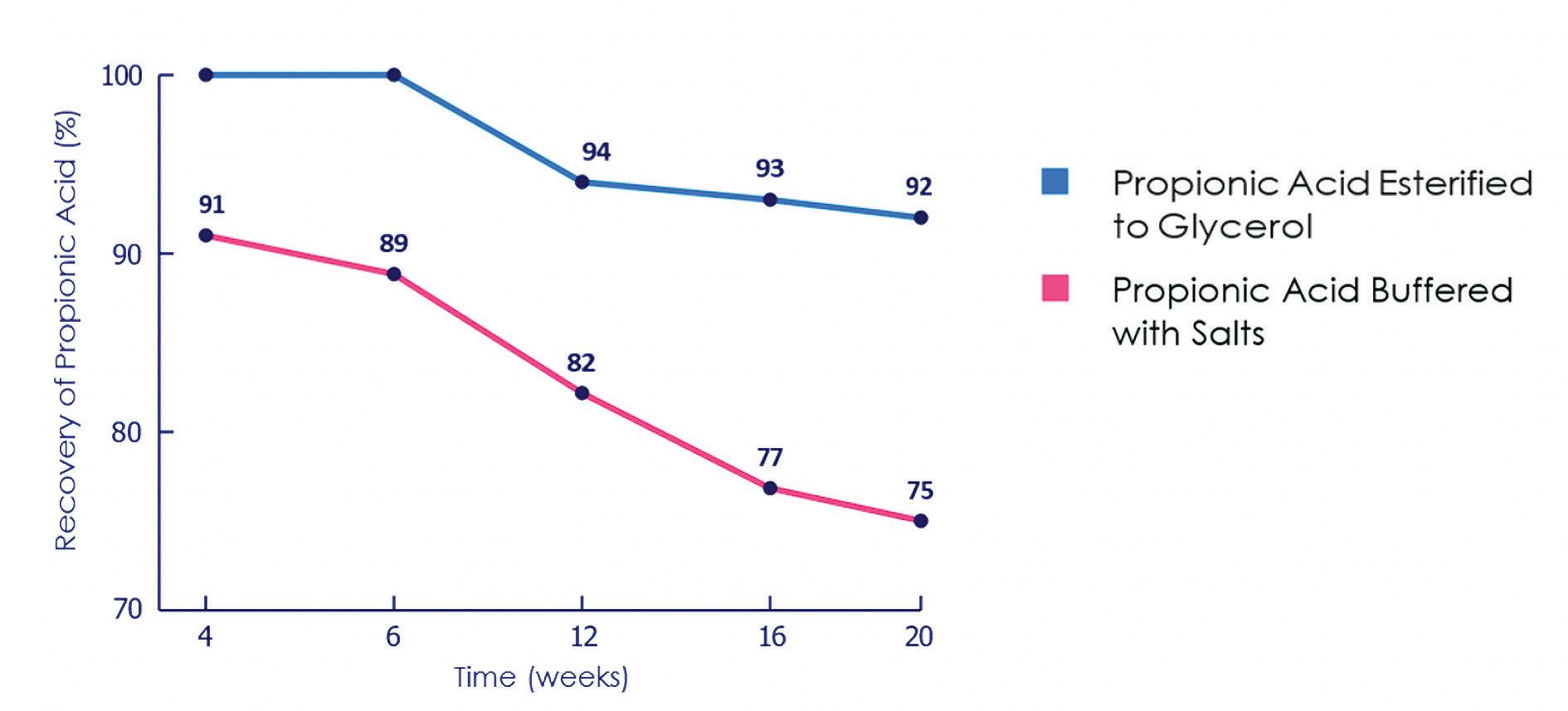
Figure 1. Stability of propionic acid from SmartMoisture.
propionic acid, allowing for a higher concentration and longer retention in aquafeed. The bond increases the retention time of propionic acid in feed ensuring longerlasting microbial inhibition (Fig. 1).
In addition, glycerol can hold up to 1,000 times its own weight in water. It works in synergy with surfactants. Surfactants reduce the water’s surface tension, improving moisture distribution. At the same time, the glycerol acts as a humectant drawing the moisture inside the feed particles and locking it inside. The moisture is now locked into feed particles and water activity is reduced. The propionic acid attached to the glycerol is absorbed by the feed particles.
Aquafeed trial
A trial was conducted by a commercial aquafeed producer who exports aquaculture globally. Due to challenges associated with shipping products to tropical regions, the company reduces its feed moisture to
Table 1. Water activity (aW) of aquafeeds treated and not treated with SmartMoisture.
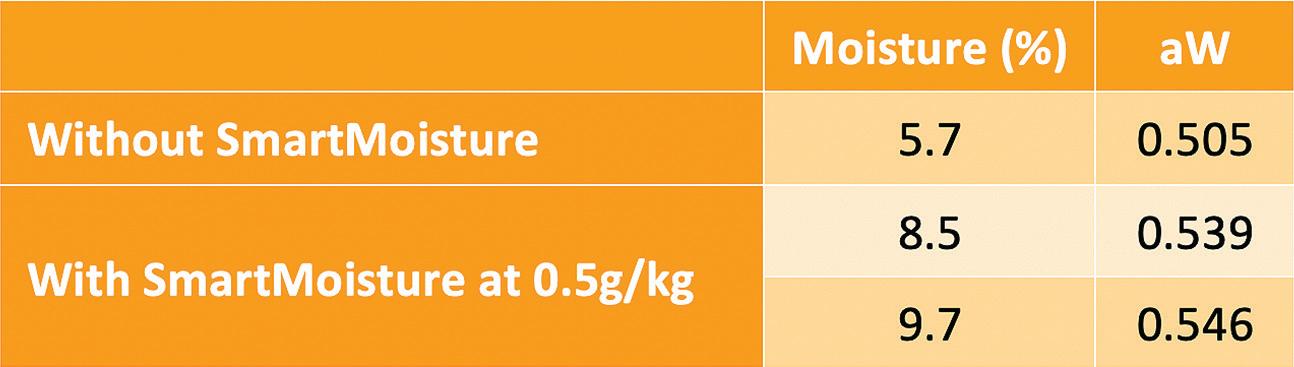
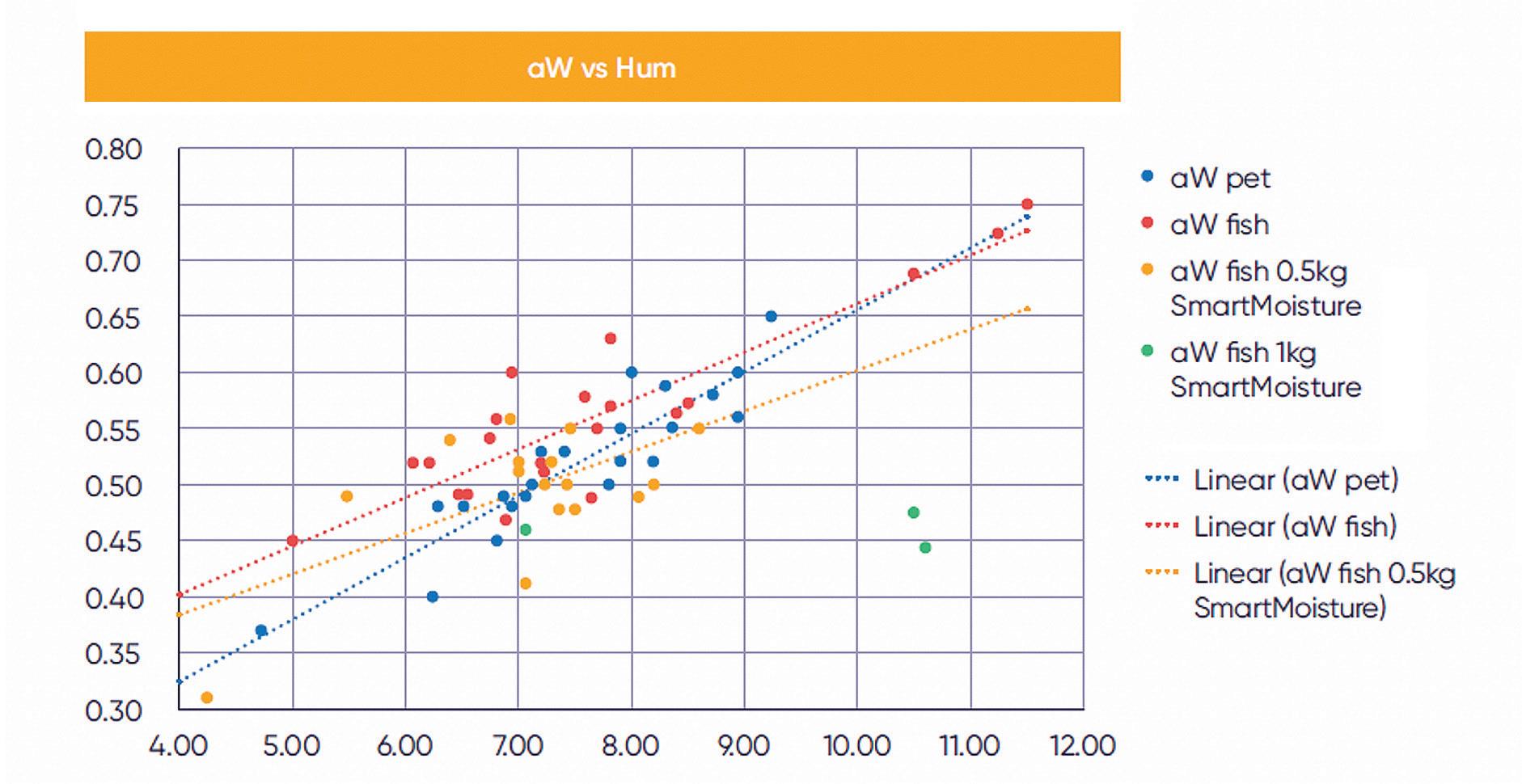
Figure 2. Higher SmartMoisture inclusion rates lead to higher aquafeed moisture.
below 6% in order to keep water activity below their rejection value of 0.6 aW. The trial assessed three treatments: • Control: No SmartMoisture with moisture level reduced to 5.7% . • Treatment 1: 0.5g/kg SmartMoisture with moisture level reduced to 8.5%. • Treatment 2: 0.5g/kg SmartMoisture with moisture level reduced to 9.7%. SmartMoisture was added to the mixer with all the usual liquid additions. Water activity was measured for all three treatments.
The humectant properties of SmartMoisture at the inclusion of 500g per ton stabilized the water activity when the aquafeed was left at a higher moisture content and even at a 4% moisture increase over the control, SmartMoisture achieved water activity below 0.55 aW (Table 1).
More tests were done and SmartMoisture inclusion was increased to 1 kg pushing moisture to 10.5 (Fig. 2). SmartMoisture at higher inclusion allowed for lower water activity at higher moisture content and gave this customer confidence to ship their aquafeed with higher moisture content.
The benefits of using SmartMoisture in aquafeed
SmartMoisture’s novel technology allows aquafeed manufacturers to: • Manage moisture levels. • Keep water activity low and stable. • Improve steam absorption. • Protect feed against microbial and mold contamination. • Improve starch gelatinization. • Save energy by reducing drying and cooling. • Increase yield.
More information: Janus Fouche
SmartMoisture Product Manager Devenish E: janus.fouche@devenish.com
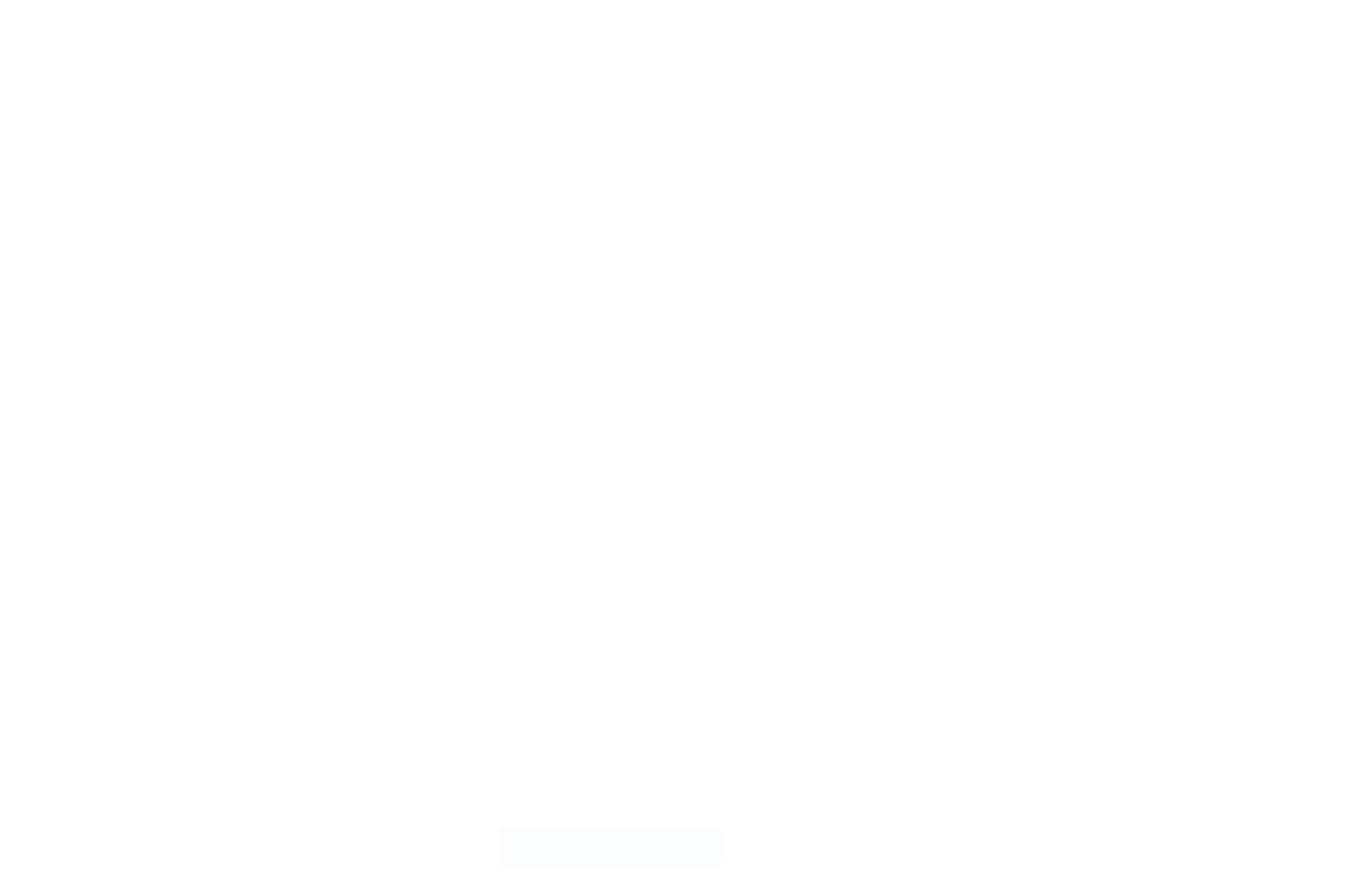
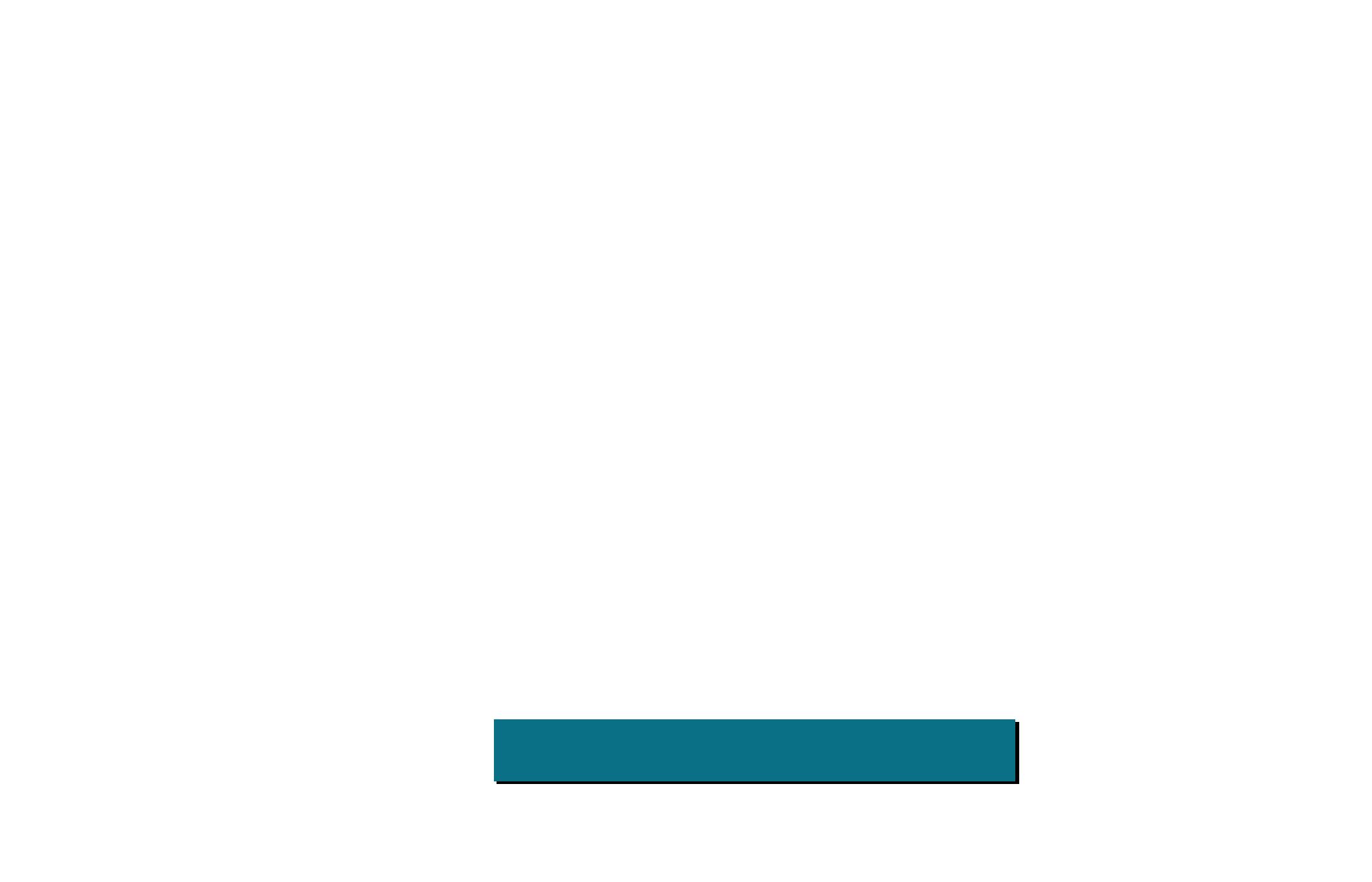
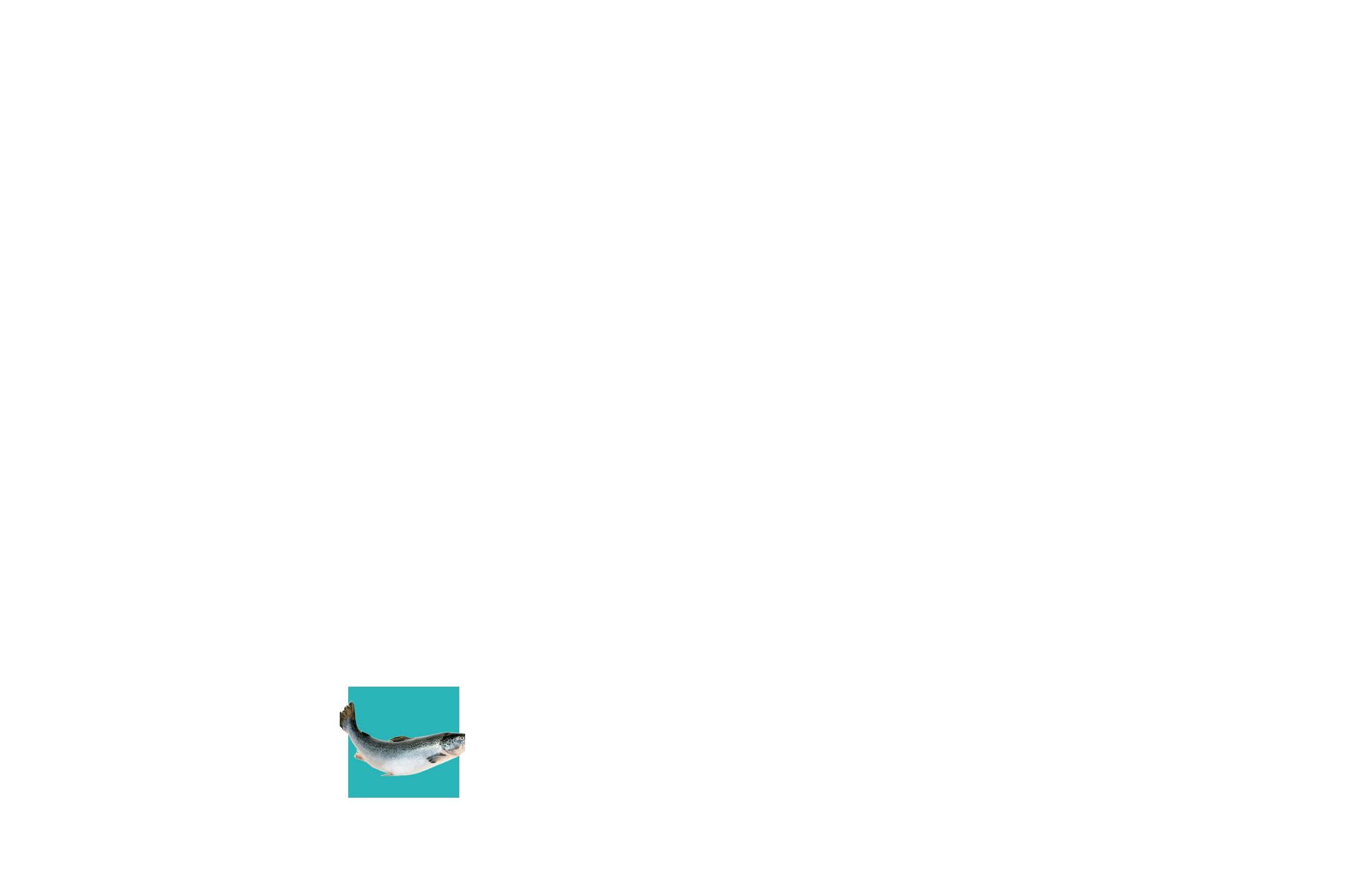
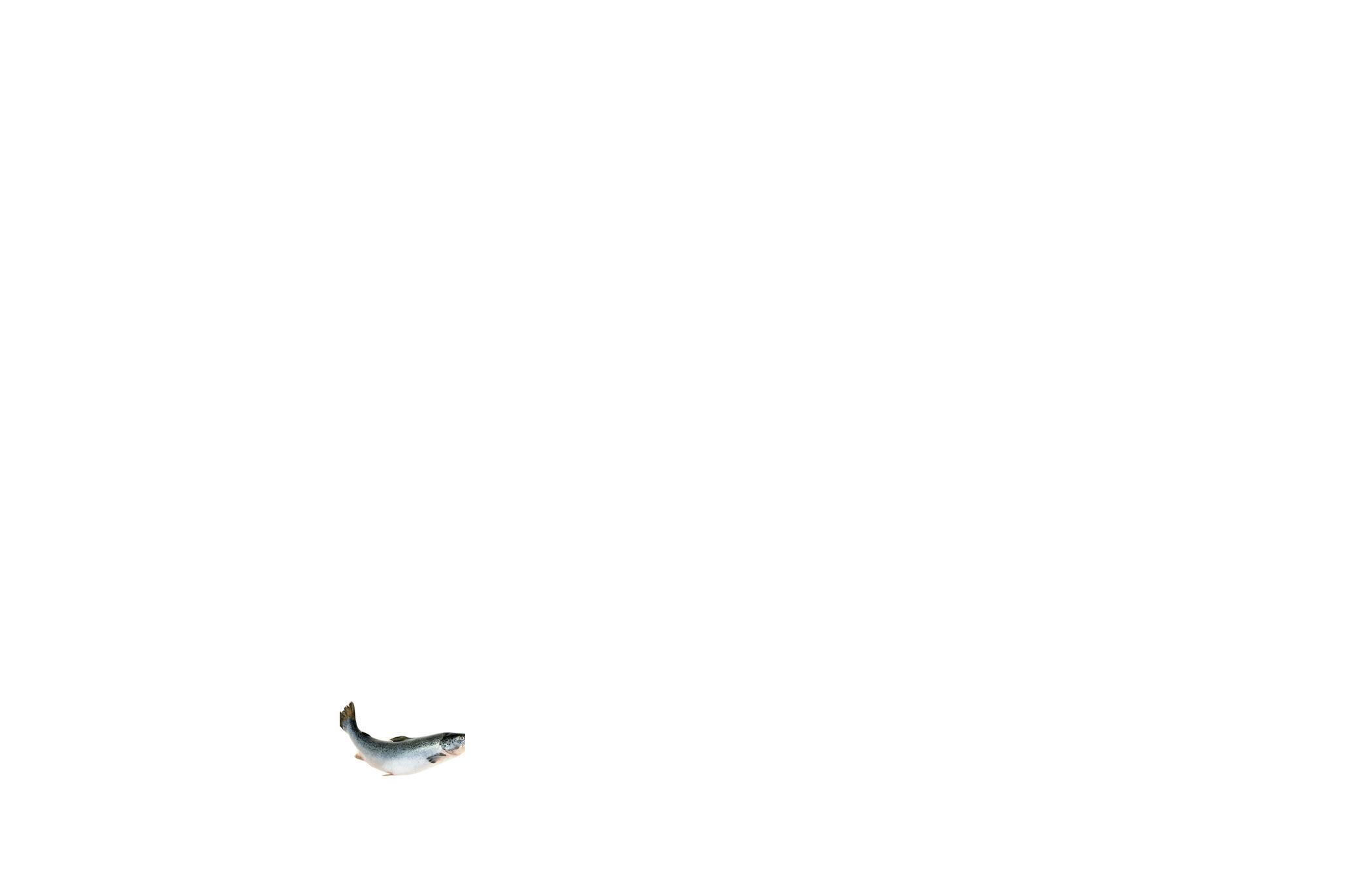
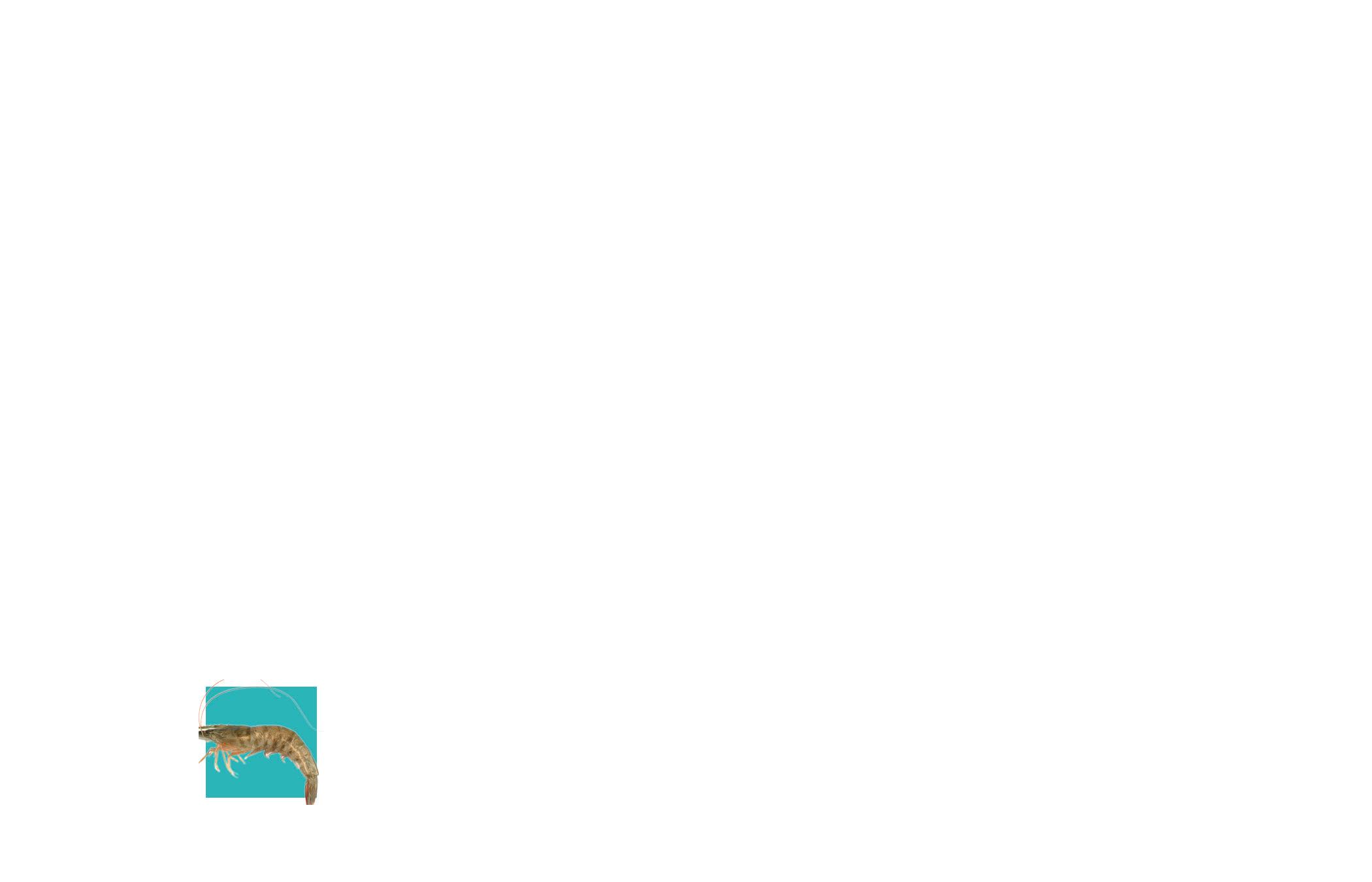
SUPPORT OF NATURAL DEFENSES AGAINST PATHOGENS
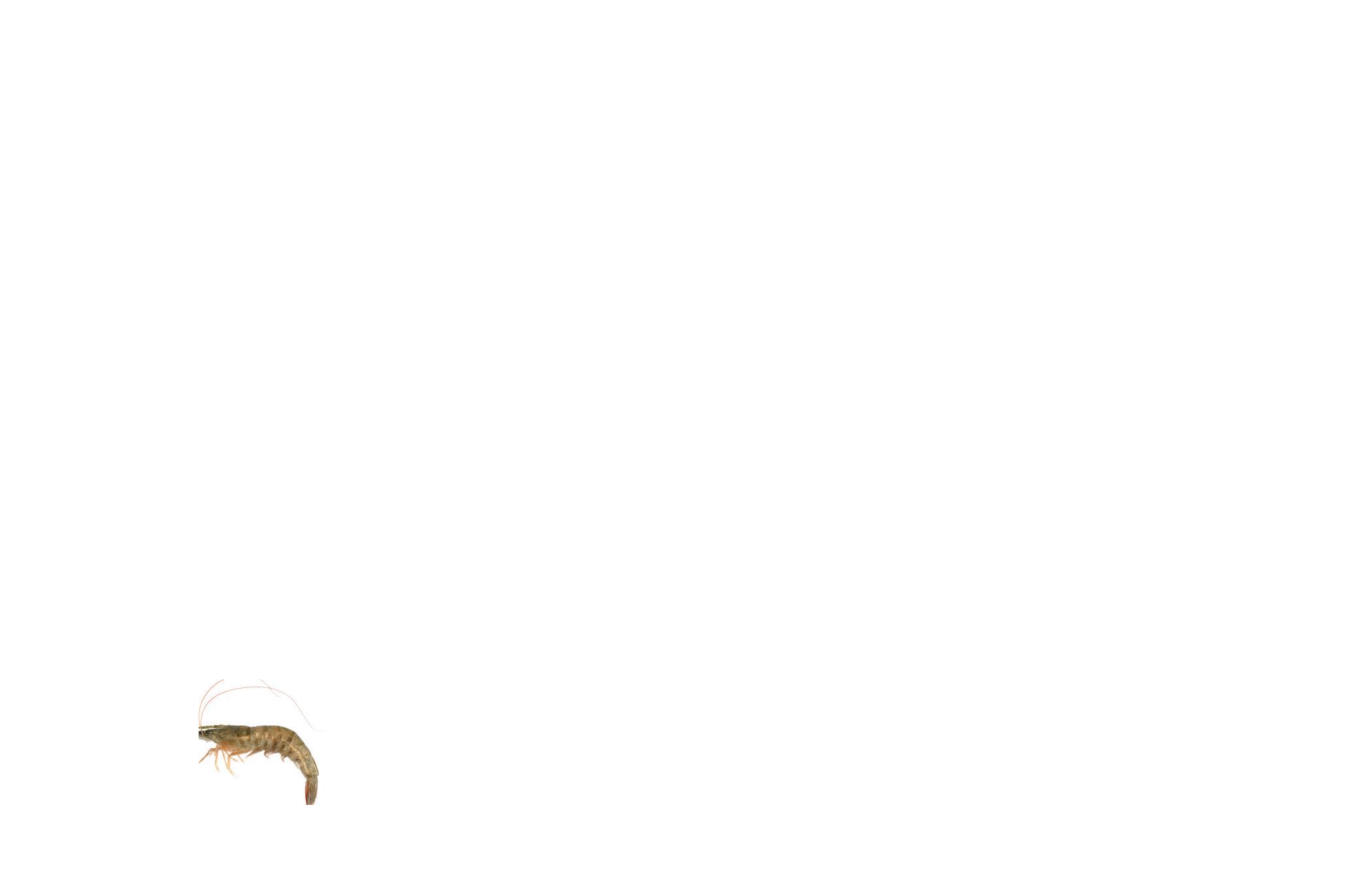
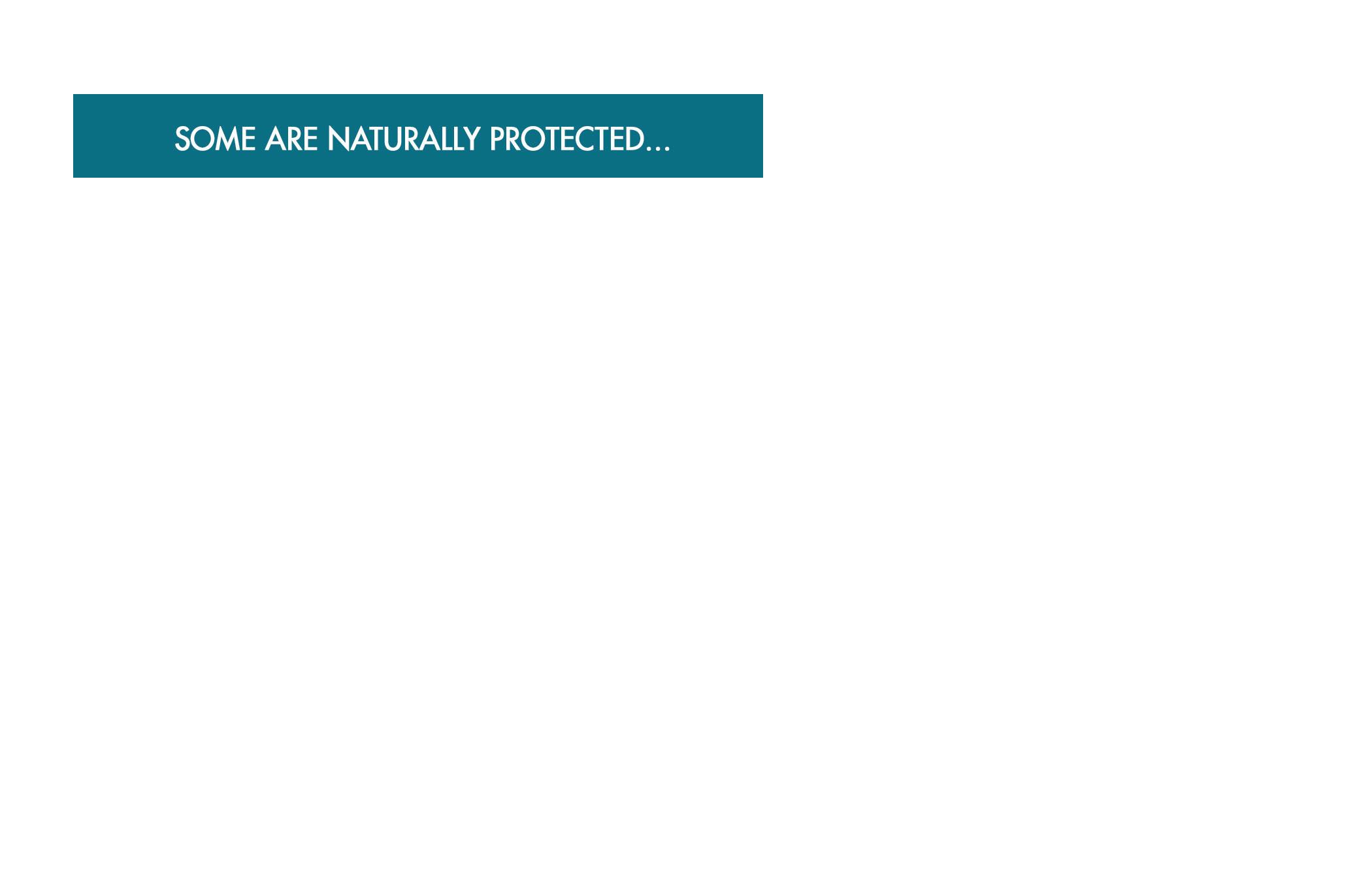
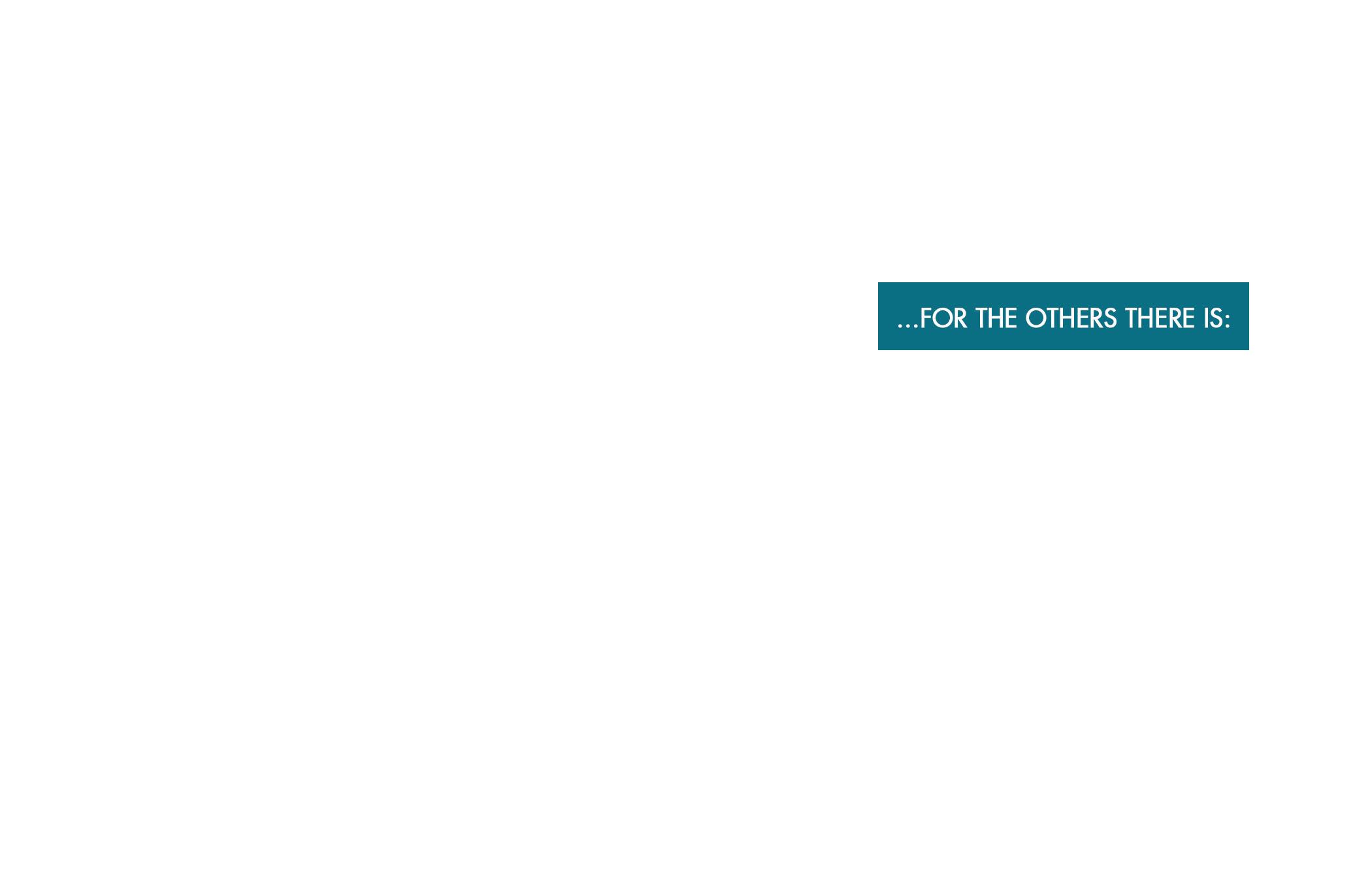
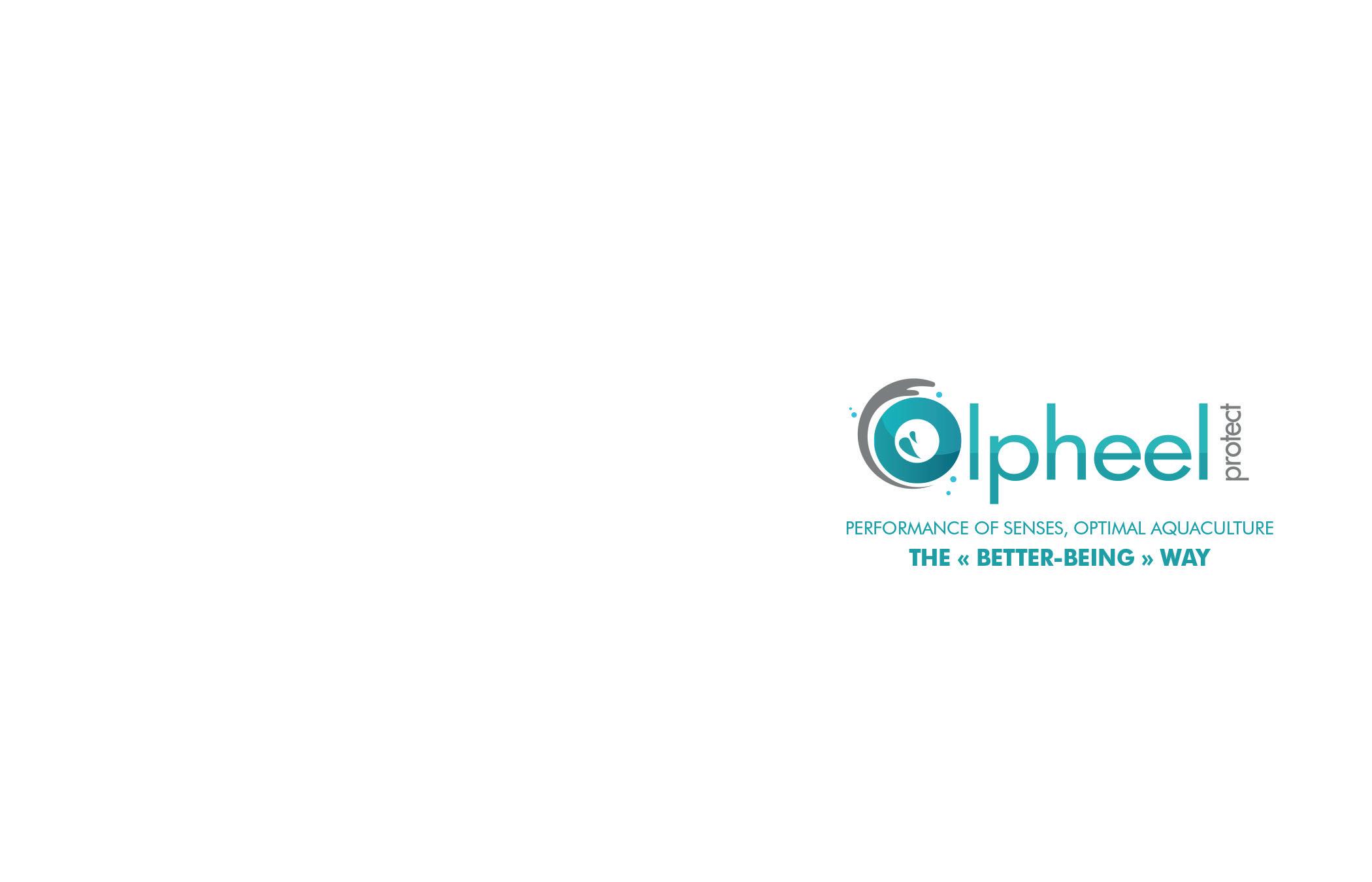
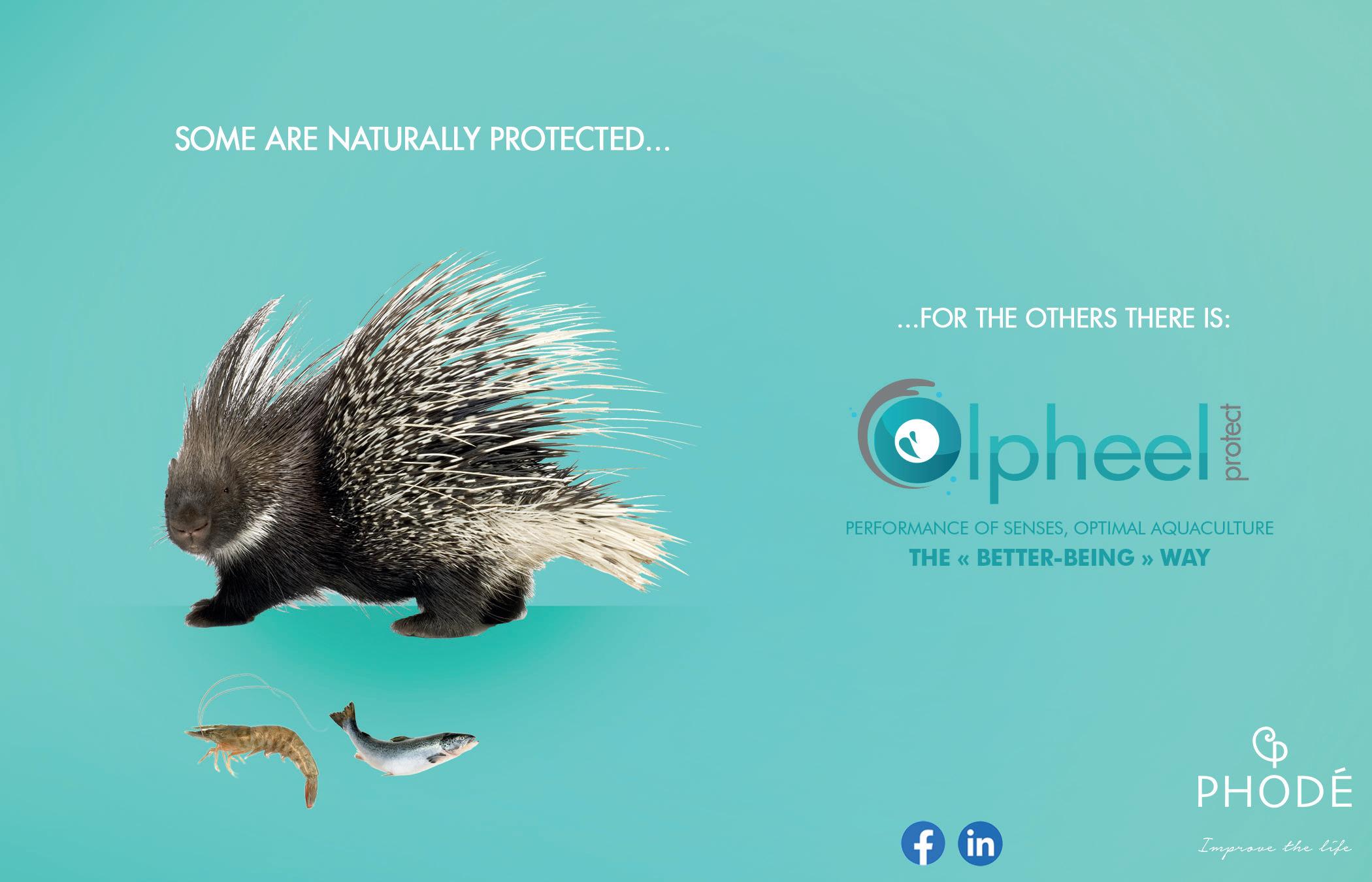
www.phode.com / Follow us
Development of aquafeeds: Reflections and future perspectives II.
The transformational years: 1980-2019
Louis R. D’Abramo, Professor Emeritus, Department of Wildlife, Fisheries and Aquaculture, Mississippi State University Thomas R. Zeigler, Senior Technical Advisor, Past President and Chairman, Zeigler Bros., Inc.

During the foundational years of 1940 -1979, basic knowledge of aquatic animal nutrition provoked the early development of feed manufacturing techniques. This period was followed by an arbitrarily defined period of 40 years (1980-2019) when information about specific nutritional requirements began to be systematically generated. The accumulation of this information ultimately led to efforts of synthesis whereby noteworthy publications were produced to serve as sources of knowledge and guidance. For the manufacturing of aquafeeds, a notable transition was realized. The choice of feed ingredients in formulations was moving from a domain based on an indiscriminate and rather arbitrary choice to selection guided by scientifically-based knowledge that specifically targeted the nutrition and physiology of a species. The aquafeed manufacturing sector resourcefully introduced technological improvements designed to achieve the efficient provision of nutrients to yield good survival and a reduction in the amount of feed consumed per increase in body weight (feed conversion ratio, FCR). These goals translated into an operational reduction in feed costs and the detrimental effects of feed waste on water quality. The aquafeed marketplace further compelled the industry to develop improved technologies of manufacture to address distinct needs. As a result, the global aquaculture enterprise entered a period that was truly transformational with the revolutionary development and use of aquafeeds.
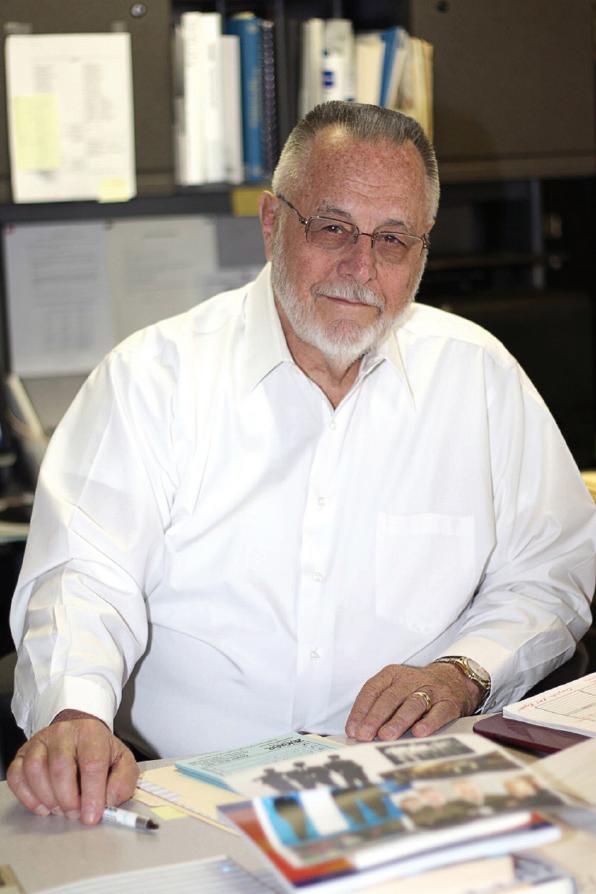
Synthesis of information about nutrient requirements of fish and crustacean species
Many efforts to synthesize the growing knowledge of nutrition of aquaculture species began to occur resulting in published books, manuals, and handbooks (Fig. 1). A chronology of some of the most notable publications that appeared from 1981 through 2011 follows. In 1987, the Food and Agricultural Organization (FAO) published three “field documents” that were inspired by the utilitarian need to produce training manuals to promote an understanding of the nutrition and feeding of farmed fish and shrimp. Three publications that carried the same title, The Nutrition and Feeding of Farmed Fish and Shrimp with different subtitles, the essential nutrients (Field Document 2), nutrient sources and composition (Field Document 5) and feeding methods (Field Document 7), were prepared by Dr. Albert G. J. Tacon. The manuals were derived from Tacon’s notes that he used to instruct trainees enrolled in the Fourth Senior Aquaculturists Training Course in Brazil during a period of approximately two weeks in 1986.
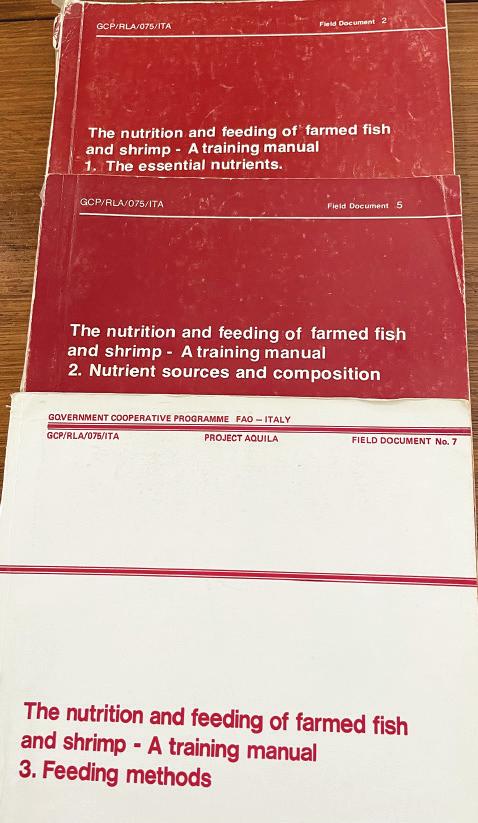
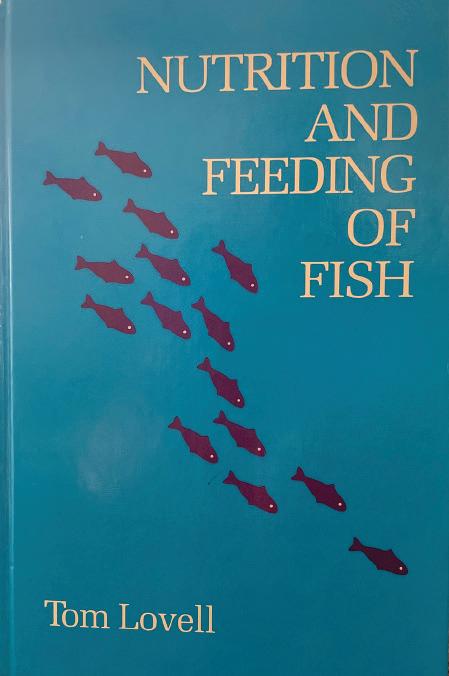
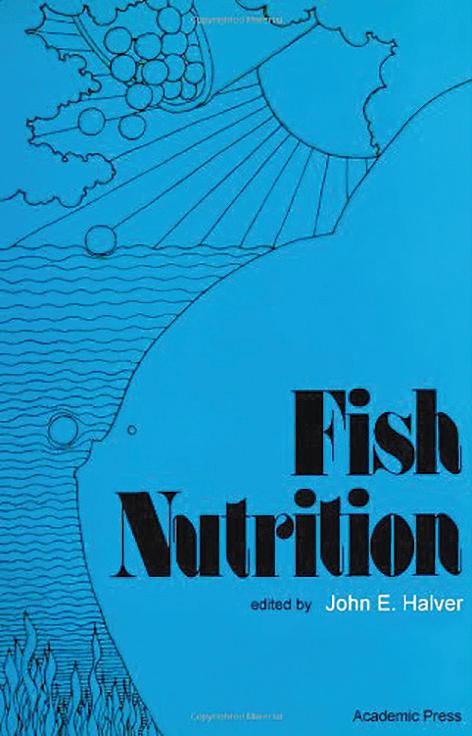
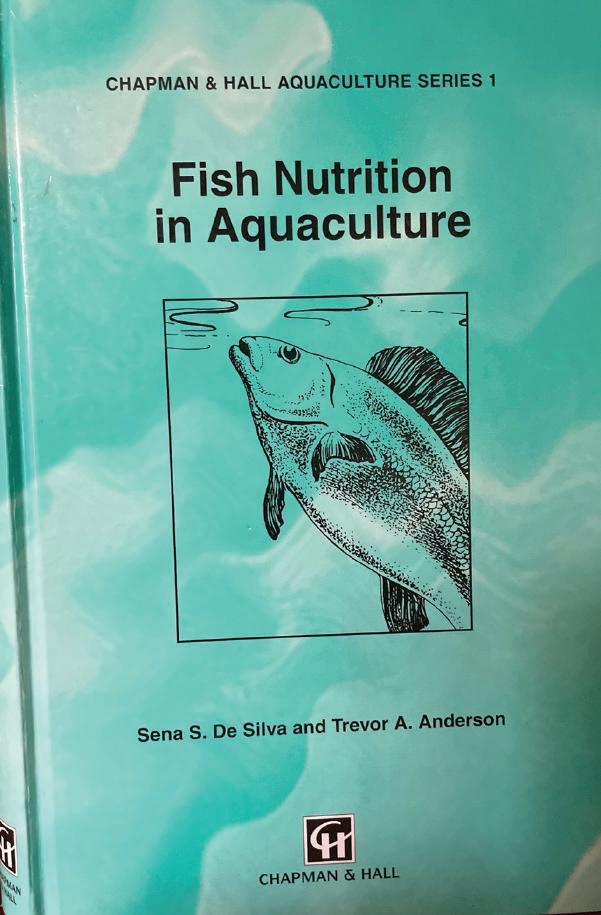
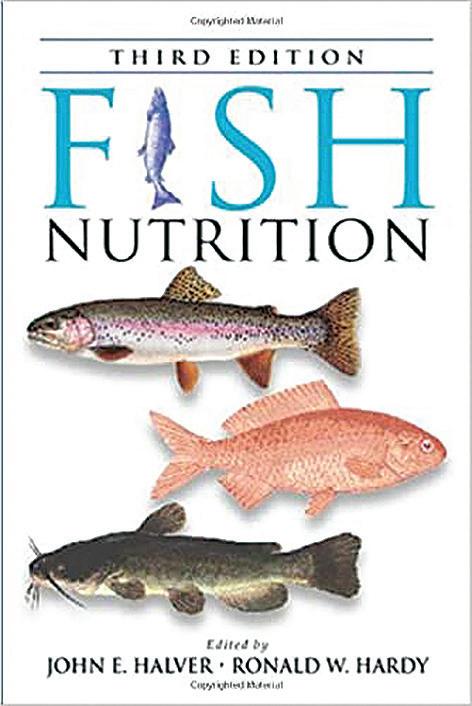
Figure 1. Some notable texts were published from 1981 to 2011 during the transformational years. They provided nutrient requirements and feeding information that contributed to advances in aquafeed development.
Preceding these publications was a 1981 publication produced by the National Research Council (NRC) of the National Academy of Sciences (NAS) titled Nutrient Requirements of Cold Water Fishes. In 1988, the book Nutrition and Feeding of Fish was published. The author, Tom Lovell of Auburn University, focused on channel catfish nutrition and feeding, including a chapter on feed formulation and processing. A group of researchers contributed additional chapters that addressed practical feeding of tilapia, penaeid shrimp, eels, salmon, trout, and crawfish. In 1993, the NRC followed with a publication titled Nutrient Requirements of Fish and Shrimp. This publication was updated by a select group of international aquatic animal nutritionists and published by NRC in 2011. The pioneering publication, Fish Nutrition (1st edition), edited by Dr. John Halver, became available in 1979 and was followed by 2nd and 3rd editions edited by Halver and Dr. Ron Hardy, and published in 1989 and 2002, respectively. In 1997, the first publication that offered a comprehensive synthesis of crustacean nutrition, Crustacean Nutrition, edited by Drs. Lou D’Abramo, Douglas Conklin, and Dean Akiyama, was published by the World Aquaculture Society as part of its book series.
The fate of proposed standardized reference diets for nutrient requirement research
As more information was being generated, a noteworthy effort, designed to promote a wellorganized generation of knowledge of crustacean nutrition, was collaboratively initiated by a 1985 publication by Dr. John Castell and colleagues in Canada and the United States. In the genre of Halver’s early work using a specific test diet to determine vitamin requirements of salmonids, they proposed a rather revolutionary approach designed to improve the value of crustacean nutrition studies. It was founded on the desire to reduce confusion arising from the lack of control of a very important experimental variable, the ingredient composition of the diet, used in the performance of nutritional experiments. The goal was to establish a universally available, standardized reference diet that would ideally be used by a majority of researchers in their nutritional requirement studies. Accordingly, the knowledge of nutrient requirements of crustacean species that was being increasingly generated could take on a greater significance and application among research laboratories.
The ingredient compositions of two proposed “standard reference diets” were presented and the results of comparative testing with different crustacean
species were reported. However, concerns arose about the ability to reliably provide the standard reference diet(s) to meet the research-based demand, and the possible inability to control inconsistencies in the nutrient composition of some ingredients and therefore different batches. Additionally, the lack of sufficient standardization of other experimental variables combined with differences in the feeding habits and habitat of a wide array of crustacean species introduced potentially confounding rather than elucidating information about nutrient requirements among species.
Clarification of the protein requirement of aquatic animal species
The interest in the protein content of aquafeeds was fueled by its integral role in the growth and its proportionally higher contribution to the overall ingredient cost. Good relationships between the ratio of an essential amino acid relative to total essential amino acids (A/E ratio) of muscle or whole-body protein and those of the dietary requirements of the ten essential amino acids were identified. The application of this relationship proved to be an effective way to estimate dietary requirements of essential amino acids without the need to conduct time-consuming, doseresponse experiments for each essential amino acid. This method was reviewed by Toshio Akiyama, Ichiro Oohara and Takeshi Yamamoto in a 1997 publication and they alluded to a specificity of essential amino acid requirements among fish species or families.
The publication, Dietary protein requirements of fishes: a reassessment, authored by Stephen Bowen in 1987, provided insight into the protein requirements of aquatic vs. terrestrial animals. His analysis of protein requirements found in published research results revealed how the median values of absolute protein requirements, defined as protein intake per gram of body weight consumed per day, were, for the most part, similar among aquatic and terrestrial species. In contrast, the relative dietary protein requirements, expressed as a percent of dietary protein, were found to be higher for aquatic species. This unstandardized difference is actually a consequence of reduced consumption of food by poikilotherms (fish, crustaceans and other invertebrate organisms) to meet the need for dietary energy in comparison to homeotherms (terrestrial vertebrates) that have higher energy needs. Consequently, the amount of feed consumed per increase in body weight (feed conversion ratio), an index of farm performance, was lower for fish and crustaceans. Other superior performance outcomes associated with aquatic species, such as edible protein yield and protein retention, were not addressed. However, this paradigm of comparative protein/energy requirements of aquatic versus terrestrial production species was foundational to the understanding of preferences in qualitative and quantitative dietary energy sources among different species of fish and crustaceans.
The rising consciousness of the roles of dietary protein and energy ultimately led to the recognition of the importance of dietary protein: energy ratios in the formulation of aquafeeds. Ratios were uniquely associated with physiological differences specific to life-history stages. Additionally, efficient utilization of dietary energy provided as lipid or carbohydrate was also species-dependent, principally associated with the feeding habits of the species. As a result, choice of feed ingredients used in the formulation of feeds was guided by the relative ability to use carbohydrates, lipids, or a combination as these sources of energy. This effort was aligned with the goal of minimizing the use of protein derived from comparatively high-cost ingredients as a source of energy, a focus termed “protein sparing”.
Specific requirements of lipid in addition to an energy source
Lipid serves as a source of not only energy but also essential fatty acids, polyunsaturated fatty acids (PUFA) as well as long-chain polyunsaturated fatty acids (LC-PUFA). Choice of sources of dietary lipid would be guided by the provision and availability of these nutrients that are essential for growth and other physiological processes. For larval and juvenile forms of crustaceans, unique requirements for lecithin, often composed of an array of phospholipids have been identified. Those phospholipids with the greatest activity were determined to be phosphatidylcholine and phosphatidylinositol. For crustaceans, a dietary requirement for cholesterol has been consistently reported. Plant-derived sterols (phytosterols) have not conferred the same growth performance when substituted for cholesterol on a 1:1 basis. However,
there is some evidence of the sparing of the cholesterol requirement using a comparatively small proportional amount of phytosterols.
Addressing particle size and feed stability in aquafeed manufacturing
With the increased knowledge of nutrient requirements, the objective of effective provision of nutrients via stability and availability of manufactured aquafeed became an important area of investigation. The resulting improvements contributed to meeting overarching goals of environmental and economic sustainability of aquafeed.
Stability encompasses a variety of characteristics that include reduced rate of loss of activity of nutrients, maintenance of physical integrity when handled, ability to retain size and shape, and reduction of loss due to leaching. Availability refers to the size and enhancement of encounters for consumption. The increase in the number of farmed species of fish and crustaceans and respective life history stages introduced many challenges within the aquafeed industry. Most manufacturing goals were driven by the need to produce feed to meet size and feeding behavior requirements whereby efficient consumption and delivery of essential nutrients were achieved.
For example, animals produced in hatcheries and nurseries increased the demand for smaller feed particles for consumption. This specific need was effectively addressed by improvements in the ability to grind ingredients to finer particle sizes so that a homogenous mixture was maintained in the formulations. Grinding of feed ingredients to finer particles also helped enhance water stability and improved the digestibility of feeds.
Water stable feeds were especially critical to the success of the ever-increasing shrimp aquaculture enterprise globally. Maintenance of the physical integrity of the feed was important because shrimp were protracted feeders that commonly used their mouthparts to break up feed into smaller particles prior to consumption. The increased surface area and duration of exposure to water exacerbated the leaching of water-soluble nutrients. Specific dietary ingredients were added to formulations to confer stability in conjunction with the feed manufacture process and evaluated. Urea-formaldehyde was identified as a highly successful binder, but the use of this and other effective chemical compounds was short-lived, eventually being prohibited in the European Union and by the Food and Drug Association (FDA) within the United States. In response to these policies, focus turned to the use of specific carbohydrates that would convey the desired level of feed stability.
A demand for larger feed size, from 1.27 cm to 2.54 cm, or even 3 to 4 cm in diameter, to satisfy needs for the farming of larger marine species also introduced challenges specifically related to manufacturing by extrusion. The larger pellets needed to be thoroughly dried to avoid deleterious mold growth and loss of nutrient activity within the core. However, utmost caution needed to be exercised because short-term drying at too extreme a temperature would risk denaturation of dietary protein and reduction of levels of activity of nutrients located on or close to the surface of the pellet. With the desire to increase the efficiency of production and economic sustainability, the salmon cage farming industry sought slow-sink feeds to maximize the time of encounter and consumption. Through combined manufacturing modifications in extruders and forced hot air dryers and changes in proportional amounts of carbohydrate ingredients, successful operational protocols were established for the production of a slow sink, floating, and large pellet feeds.
Maximizing availability of dietary nutrients
The objective of maximizing the availability of essential nutrients in aquafeeds has evolved and improved over time and is essentially founded on four strategies, use of highly digestible ingredients, supplementation to existing levels, protection/preservation of heatsensitive, highly degradable types, and provision of specific additives. Knowledge of the ability of particular aquaculture species to digest particular feedstuffs is essential in reducing waste and efficiently providing required dietary nutrients. The increased digestibility of dietary carbohydrates in a gelatinized form, a byproduct of feed manufacturing by extrusion, increased the amount of available dietary energy. New grinding equipment, designed to reduce the particle size of feedstuffs, contributed to increased digestion and was instrumental in the manufacture of small feed particles of homogeneous composition for juvenile
and larval stages. Variable speed and temperature dryers associated with the manufacturing of extruded feeds were introduced with the objective of effectively reducing nutrient loss.
Use of feed additives to maximize performance, health and associated economic benefits
The positive benefits of the inclusion of specific additives in formulated aquafeeds are well established. New or improved additives in aquafeeds will first be based on the additional cost for effective inclusion followed by that cost passed on to the consumer relative to the performance realized. As a feed additive, dietary ascorbic acid in crystalline form is highly watersoluble and subject to degradation during relatively short durations (~ 30 days) of feed storage. Fish that consumed feed that contained reduced ascorbic acid activity due to prolonged storage were afflicted with a vitamin C deficiency called lordosis which is an inward curvature of the spine. Required dietary levels of vitamin C, provided in crystalline form, for crustaceans were reported to be 10-20x those of fish. The presumed higher dietary requirements were misleading and actually reflective of a significant loss due to leaching into the water due to a protracted duration of feeding and exposure of smaller particles (higher surface area) derived from the consumptive behavior of crustaceans.
A process developed by Seib and Liao to produce ascorbic tripolyphosphate, a compound composed of a mixture of mono, di, and tri phosphorylated esters of ascorbic acid, served to launch a major breakthrough for the aquafeed industry. Adding this compound to aquafeed extended the durations of feed storage by 90 to 120 days with a gradual loss of activity as influenced by temperature, moisture and sunlight. Products providing these ester forms of ascorbic acid are also comparatively less water-soluble. Dietary additives of ascorbic acid in the form of phosphorylated esters (Stay-C) are preferably used in aquafeeds to meet the vitamin C requirement and contain approximately 35% active ascorbic acid.
Plant-derived ingredients, commonly found in aquafeed formulations as grains and oil seeds, contain a compound called phytate which is able to effectively sequester minerals and thereby make them metabolically unavailable. To remove the “antinutritional” effects of phytate, an enzyme additive, phytase, has been included as an aquafeed additive. The presence of active phytase allows important dietary elements (minerals) that would otherwise be lost to the environment to become physiologically available for absorption. Like all enzymes, phytase is a protein that is heat sensitive and subject to denaturation during the process of manufacturing by extrusion unless precautionary measures are taken.
During the last decade of the 20th century, the physiological role of both probiotics and prebiotics and the advantage of inclusion in aquafeeds to promote disease resistance and growth through their influential role in the composition of the gut microbiome were revealed. This recognition has paralleled what occurred in the 1970s in the poultry and swine production industries. However, one of the major challenges confronting the application of probiotics and prebiotics as additives in aquafeeds is the myriad of aquatic species that are currently farmed globally. Species specificity would seem to be a prime determinant of success and accordingly, the concept of “one size fits all” for application in the aquaculture industry does not appear to be likely. The benefits of particular probiotics would most probably be influenced by such factors as the trophic level of species, phylogenetic family, and production system. Additionally, caution needs to be exercised based on the acknowledgment of the possible confounding interactive effects of both quantity and quality of these additives within commercially produced aquafeeds. Specific feed manufacturing precautions are needed to preserve desired activity. For the aquafeed industry to meet the anticipated demand of the marketplace, these additives must be characterized by predictability and consistency. Dietary supplementation of nucleotides has also been shown to enhance immunocompetence and stress tolerance of fish.
Loss and waste of dietary nutrients due to heat sensitivity are protected by either of two approaches, encapsulation or top coating. Encapsulation produces a barrier that prevents steam and high temperature of the extrusion process from diminishing or eliminating the level of activity of a nutrient.
Top coating was an innovation introduced by the aquafeed equipment industry. Quantities of lipid, alone or containing a heat-sensitive ingredient, are introduced into a special mixer wherein a vacuum is created. The lipid is then sprayed onto the recently
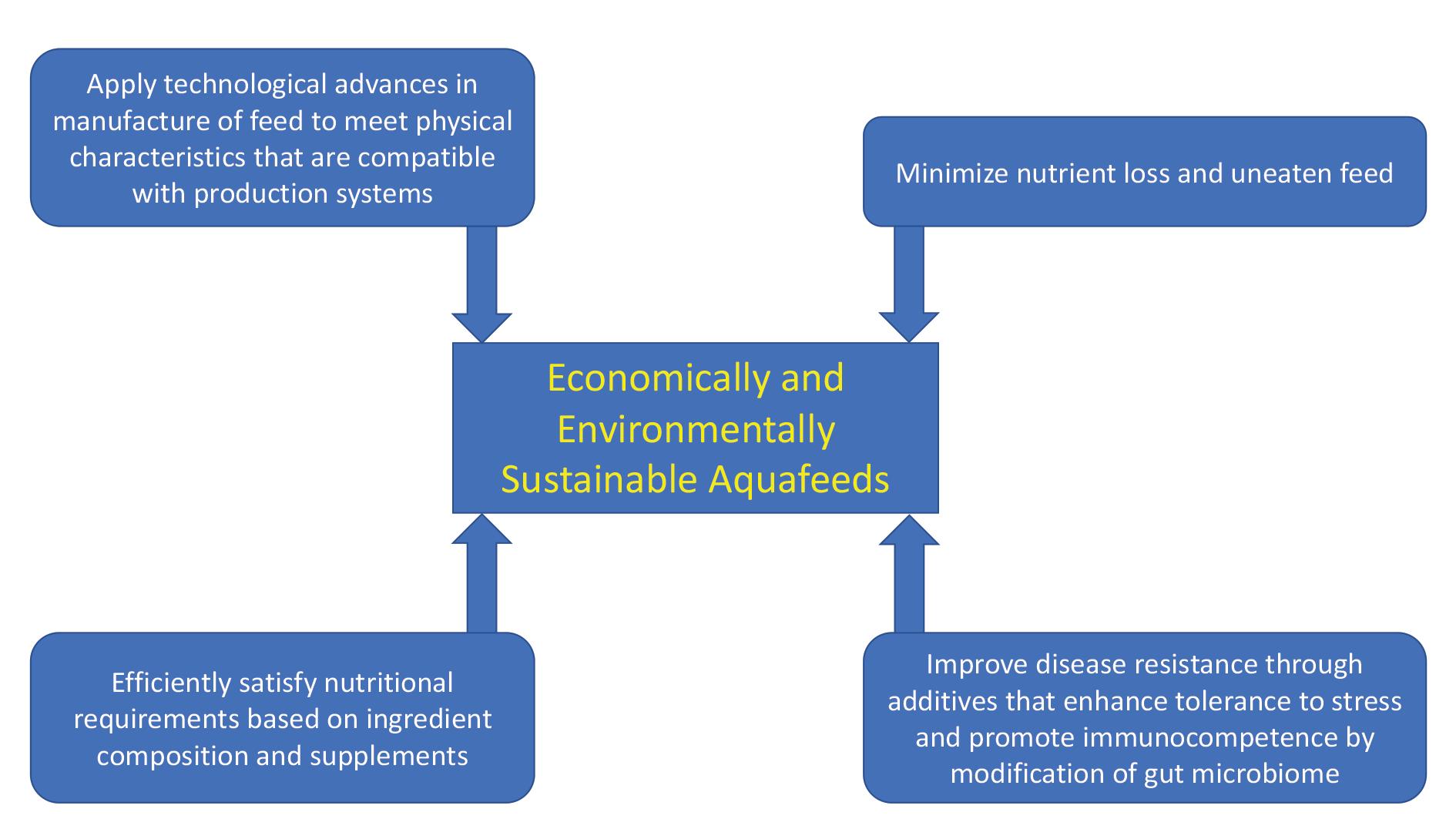
Figure 2. The four directives collectively contribute to the production of economically and environmentally sustainable aquafeeds as part of a dynamic and ongoing process of development.
produced extruded feed while it is still warm, and is drawn into the feed when released at atmospheric pressure. Incorporation of this technique into the manufacturing process also serves to attain dietary lipid levels that are not possible under normal conditions of extruded feed manufacture. Feeds containing levels of lipid of up to 35% are possible. Additional lipid provides a greater source of energy than that available from equivalent levels of carbohydrate. These higher levels of dietary lipid can also be effective in sparing the use of dietary protein as a source of energy that will correspondingly reduce the excretion of nitrogen into the culture environment.
The feed manufacturing industry has also introduced vitamin and mineral premixes and crystalline sources of first limiting essential amino acids, commonly methionine, lysine, and threonine for fish. Arginine is often a limiting amino acid in crustacean feed formulations and commonly will require supplementation. Taurine, a product of the metabolism of sulfur-containing amino acids, does not contain the characteristic carboxyl group of amino acids and has been characterized as a conditionally required metabolic nutrient. It is added in powder form to feeds formulated for marine and freshwater fish and shrimp species to compensate for deficiencies when plantbased sources of dietary protein proportionally exceed those that are animal-based. If needed, the level of supplementation appears to be influenced by species, life history stage, and process of feed manufacture. For shrimp feeds, the efficacy of crystalline amino acids and taurine as additives to meet dietary requirements is problematic based on solubility in water. Protracted periods of leaching into the aquatic medium occur due to characteristic delays in consumption of feed that is broken into smaller sizes. A possible option may be the inclusion of chelates of 2-hydroxy-4-methylthiobutyrate which are significantly less soluble in water, However, cost considerations vs. performance level must be considered. Reduction of activities of certain vitamins and mineral availability occurs due to manufacturing conditions and leaching. Accordingly, the dietary levels of these required nutrients that are added compensate for these losses.
Sustainable ingredient substitutes in feeds
For decades, marine-derived meals and oils have been the mainstay ingredients in aquafeeds produced for fish and crustacean aquaculture. It has become clear that these particular ingredients themselves are not critical, but rather the essential nutrients they contain. Thus, an ongoing objective was launched to produce cost-effective feeds using highly available plant-derived alternatives to these ingredients, such as soybean meal,
processed soybean meal, high protein corn gluten meal and corm distillers dried grains. These feedstuffs are commonly consistent in nutrient composition and not subject to vagaries in availability; however, as singular substitutes, they have inherent nutrient deficiencies. Mixtures of these sources appear to be a plausible solution. Other possible substitutes that potentially have merit are single-cell protein sources and insect meal as demonstrated by success in laboratory-based research. These potential feed ingredients also have the added advantage of consistency in a composition achieved through highly controlled production parameters. The process of the manufacturing of aquafeed has embraced the circular bioeconomy approach by using feed ingredients that are recycling products. In addition, byproducts (waste) of the rendering of terrestrial animal production offer highly acceptable and efficient substitutes with the added feature of their reduced carbon footprint. The search for oil sources that can potentially serve as effective substitutes for marinederived oils as sources of essential PUFAs and LC-PUFAs in sustainable aquafeeds remains an ongoing challenge.
Conclusion
At this point in our historical journey, we offer what we believe to be overarching directives that have evolved in concert with transformational changes fueled by advances in knowledge of aquatic animal nutrition and the ensuing market demands for manufacture of high performance aquafeed (Fig. 2). As established, these “directives”, are reflective of the quintessential goal of producing environmentally and economically sustainable aquafeed. Future research endeavors that yield more knowledge about the nutritional physiology of farmed aquatic species and will be complemented by dynamic innovation in feed formulation and methodology of the manufacturing of aquafeed. The greatest challenge resides in the variety of fed species being cultured globally. Collectively, some of the contributions based on each of the four overarching directives will take on a species-specific quality. Aquafeeds will move to a level of being characteristically “designed” to meet the needs of different species or arrays of species and their respective production systems. In the next issue of Aquafeed Magazine, the third and final part of the history of the development of aquafeeds will be presented as Development of Aquafeeds: Reflections and Future Perspectives III. The Sustainable Future. We will take on the challenge of addressing the fate of the most current trends. We will engage in speculating novel strategies that may prove to be rewarding in meeting the need to produce sustainable aquafeeds as an essential component of sustainable aquaculture enterprise globally.
Applications of phytogenics in shrimp farming
Ruby Yu, Teresa M. Agulles Teixidó, PlusVet Animal Health
For the past two decades, the farming industry is moving towards a reduction in the use of antibiotics to prevent the emergence of antibiotic-resistant strains in humans. Feeds without antibiotic growth promoters (AGPs) are a reality in many countries, while others are looking into ways to restrict them. Different types of feed additives can be used to replace antimicrobial growth promoters, always combined with other types of interventions such as changes in feed formulation and improvements in biosafety and management practices.
Since prehistoric times, humans have used plants to cure their own diseases and those of their animals. In the past 150 years, the use of medicinal plants has been shown to have a scientific basis: plants contain an abundance of chemicals, called “secondary compounds” or “phytochemicals”, that enable them to perform metabolic and ecological functions, such as resisting attacks by microorganisms and insects, and have been proved to exert useful health-promoting effects in human beings and animals.
In the world of animal nutrition, plant derivatives are called “phytogenics” or “botanicals”. The group includes plant extracts, essential oils, purified natural compounds such as saponins, flavonoids, and tannins, and synthetic compounds that are identical to natural substances.
Mechanisms of action of phytogenics
Since antibiotic growth promoters were banned in Europe in 2006, phytogenics have emerged as an effective alternative and their use is becoming widespread. When they began to be used on commercial farms, their mechanism of action was poorly understood, which made it difficult for nutritionists to include phytogenics in their feeds. However, in recent years, research work has multiplied, and it has uncovered that the mechanism of action of plant derivatives as AGPs replacers is complex and multifunctional. Such complexity represents an advantage over other types of feed additives.
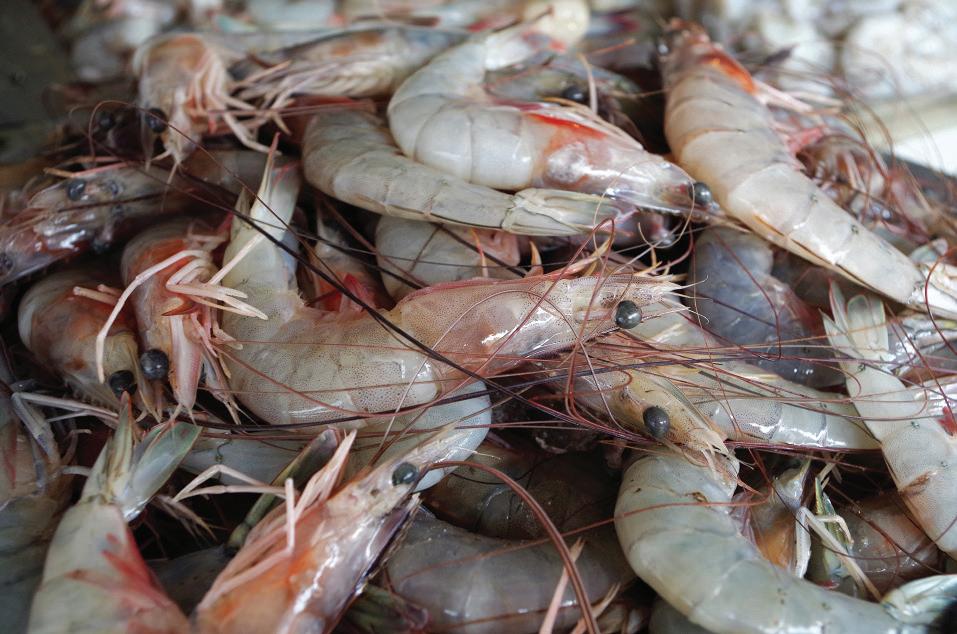
Antimicrobial activity
Many phytogenics are microbiocidal against Grampositive and Gram-negative bacteria, yeasts and fungi. As the microbiocidal activity of phytogenics arouses interest as substitutes for antibiotics, a multitude of research works has been carried out trying to determine their mechanism of action.
It has been determined that their antibacterial activity takes place through different mechanisms: • Alteration of quorum sensing. When the number of bacteria within a population is large enough, each individual secretes and receives small signal molecules that allow the whole colony to act as a group. This communication system is called quorum sensing.
Thanks to quorum sensing, bacterial colonies can form biofilms, become more virulent and secrete toxins, multiply faster and cross the intestinal barrier to enter the bloodstream. Since quorum sensing is responsible for pathogen virulence, finding active principles that can inhibit this communication system is a promising strategy to control pathogenic bacteria.
Many phytogenics have been proven to disrupt bacterial quorum sensing at concentrations much lower than those needed to kill bacteria.
• Direct effect on the bacterial cell. Toxicity to bacteria can occur in different ways, but the best known is the disruption of the bacterial wall. Thanks to the lipophilic nature of many phytochemicals, they bind to the bacterial wall and increase its permeability, which ultimately results in the death of the microorganism. Gram-positive bacteria are more sensitive to phytogenics, as their plasma membrane is covered by a cell wall layer consisting of peptidoglycans, which are lipophilic. On the other hand, the outermost layer of Gram-negatives, called the outer membrane, is made of hydrophilic lipopolysaccharides, making it more difficult for phytogenics and other hydrophobic antimicrobials to penetrate. When formulating products based on plant-derived compounds, it is possible to weaken the outer membrane of the Gram-negative bacteria by adding permeabilizes, substances that act by disintegrating the outer membrane. A little-known aspect of the use of plant derivatives is that, when antibiotic therapy is necessary, phytogenics and antibiotics often act in synergy, increasing the effectiveness of the antibiotic.
Antiparasitic action
Several phytogenics have been confirmed as antiparasitic agents. Certain essential oils kill ciliated protozoa and flagellates by disrupting their cellular structure. In the case of nematodes, trematodes and leeches, phytochemicals damage their cuticle and their gastrointestinal tract. The mechanism of action of Myxozoans and Monogeneans is unknown.
Antioxidant and anti-inflammatory activity
In healthy animals, protection against the harmful effects of free radicals is achieved through maintaining a delicate equilibrium between the production of free radicals, that are needed for certain physiological processes, and their destruction through the antioxidant defense system. This equilibrium is called “the redox balance”.
Whenever the redox balance is disrupted, either due to an overproduction of free radicals or because of a malfunction of the antioxidant defense system, free radicals trigger a harmful chain reaction, called oxidative stress, that damages all kinds of biological molecules and starts inflammatory pathways.
A growing body of evidence in vitro and in vivo shows that some phytogenics are powerful antioxidants and can prevent and ameliorate the dysfunctions caused by oxidative stress.
On the other hand, inflammation is a complex biological reaction to a harmful event, such as the presence of oxidative stress, pathogens, toxins, or damaged cells. Although inflammation is a beneficial process aimed at the repair of the damaged tissue, when it persists, it may lead to disease and productivity losses. Intestinal inflammation is associated with poor nutrient absorption, the disruption of the gut barrier function and the diversion of nutrients and energy that would have been destined for growth toward the inflammatory reaction.
Some compounds derived from plants have anti-inflammatory effects, either thanks to their antioxidant activity or by blocking metabolic processes related to inflammation.
Stimulation of the immune system
Immunostimulants are compounds that stimulate the non-specific defense mechanisms in shrimp. When there is immune stimulation, the number of hemocytes circulating in the hemolymph increases.
Research has demonstrated that some plant-derived compounds increase the count of hemocytes by more than 60% in healthy animals and by more than 30% during diseases. Cellular immune responses and the secretion of immune-related enzymes, such as phagocytic activity, superoxide anion production, phenoloxidase, acid phosphatase, alkaline phosphatase and lysozyme activity are also increased.
Use of phytogenics in shrimp farming
Most of the studies on the application of phytogenics have been conducted with poultry and pigs. However, since fish and shrimp farming industries are constantly under threat due to the outbreak of infectious diseases, there is increasing interest in natural products, not only to replace antibiotics and promote productivity but also to prevent diseases. The detailed mechanisms of action of phytogenics on fish and shrimp have been little studied compared to other species.
The following are the main applications of phytogenics in shrimp farming, according to our field experience.
Productivity improvement
Application of plant-derived compounds results in better productive parameters, such as: • Yield of the crop increased by 15-35%. • 16-44% more survivability in healthy animals, and 35-78% more survivability during certain diseases. • 5-18% better final weight. • 3-10% better feed conversion ratio.
Successful start at the hatchery
Phytogenics in water-soluble forms, added to water tanks at the hatchery, help reduce the load of pathogenic bacteria, promote growth and enhance the survival rate.
Improvement of sensory quality and shelf life
The administration of certain phytogenics improves the sensory quality of shrimp meat by increasing the percentage of taste-related amino acids. On the other hand, antioxidant phytochemicals delay lipid oxidation and prolong shrimp’s shelf life.
Balance of gut flora
In recent years, it has been discovered that the digestive flora is involved in processes as diverse as feed digestion, immune response and inflammatory reactions. Phytogenics are able to modulate the composition of bacterial communities, positively impacting the functioning of the digestive system and the overall health of the animal.
Prevention of diseases
Outbreaks of infectious diseases are continuously endangering shrimp farming around the world. Much of the losses are induced by infections of bacterial origin and among the different types of pathogenic bacteria, the genus Vibrio stands out. Vibrio sp.
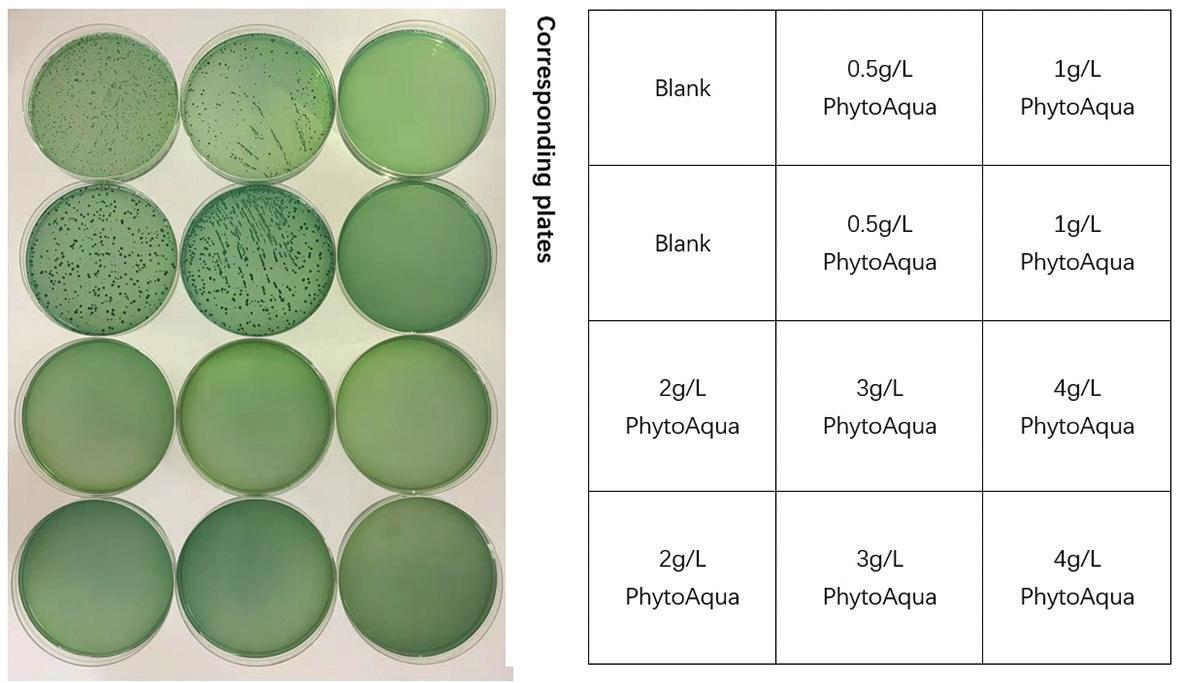
Figure 1. Culture in Vibrio parahaemolyticus agar, at 35°C for 24 hours. Inoculum of 5*104 CFU/plate. According to results, 0.5 g/L PhytoAqua slightly inhibited the growth of Vibrio parahaemolyticus, and 1 g/L PhytoAqua or higher more completely inhibited its growth.
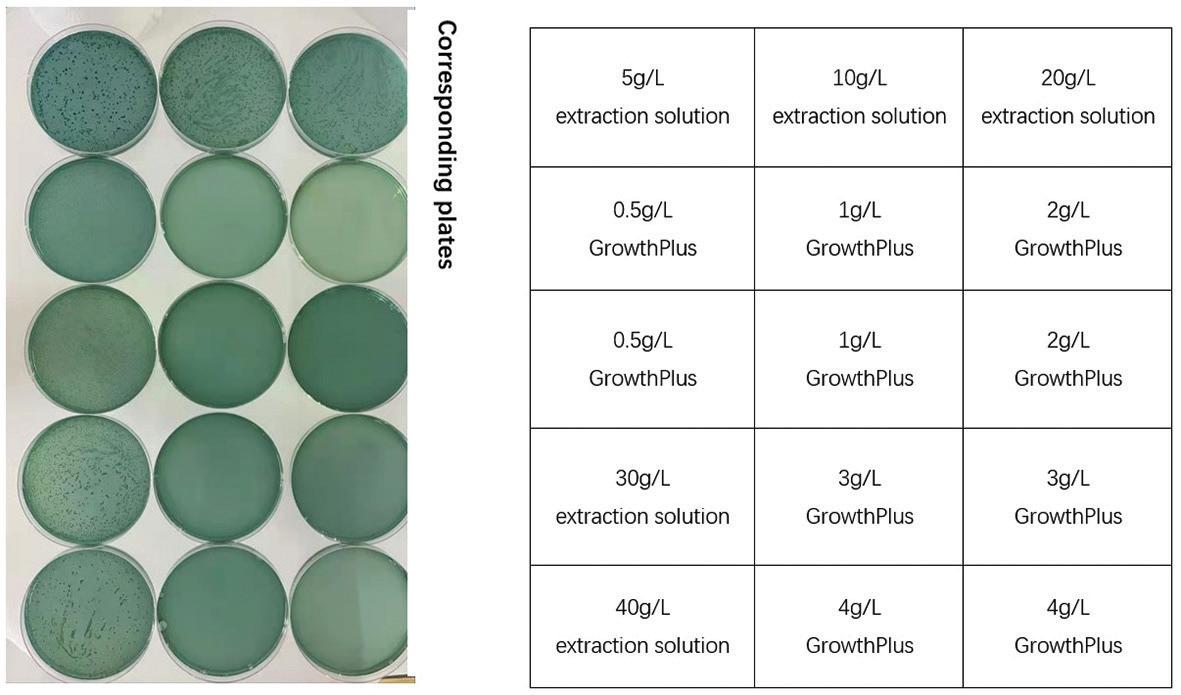
Figure 2. Culture in Vibrio parahaemolyticus agar, at 35°C for 24 hours. Inoculum of 3.7*105 CFU/plate. According to results, 0.5 g/L GrowthPlus significantly inhibited the growth of Vibrio parahaemolyticus, and 1 g/L GrowthPlus or higher more completely inhibited its growth.
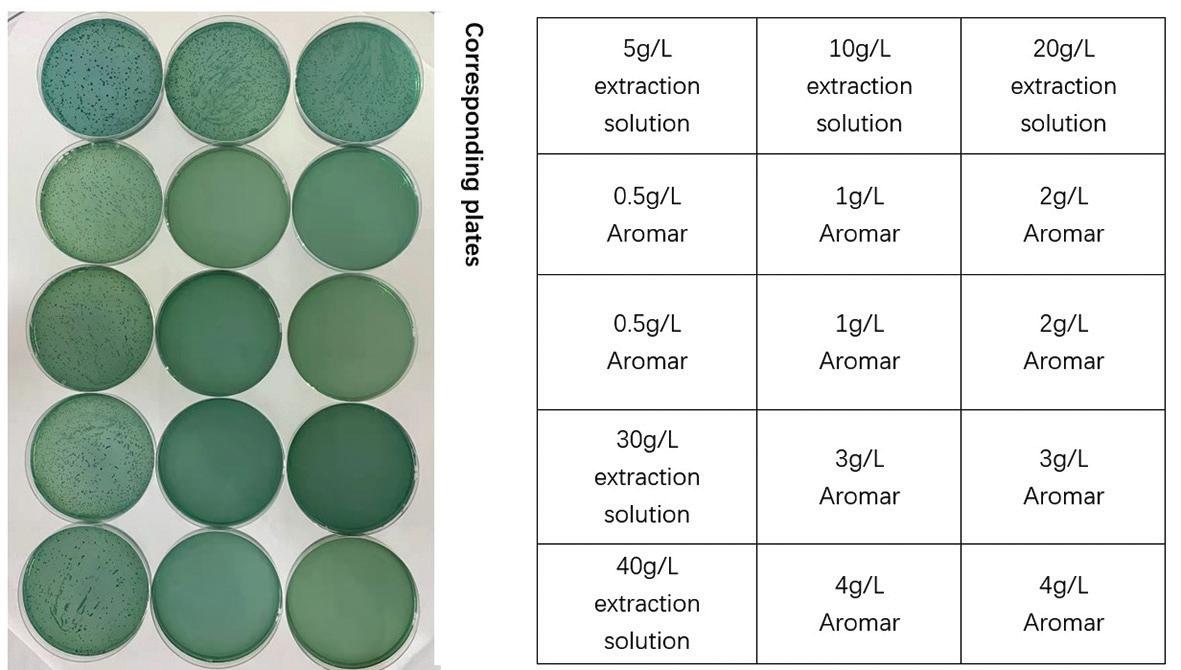
Figure 3. Culture in Vibrio parahaemolyticus agar, at 35°C for 24 hours. Inoculum of 3.7*105 CFU/plate. According to results, 0.5 g/L AroMar slightly inhibited the growth of Vibrio parahaemolyticus, and 1 g/L AroMar or higher more completely inhibited its growth.
are Gram-negative bacteria, which are found in seawater environments all around the world, and cause lesions, immune suppression, discoloration and death. Overuse of antibiotics to control vibriosis has led to drug resistance. Vibrio parahaemolyticus is the causative agent of hepatopancreas necrosis disease (AHPND). AHPND can cause up to 100% mortality within 20-30 days and is an emerging disease that has severely damaged the global shrimp industry. Figures 1-3 show a trial testing three products in vitro to prove their antibacterial effect against Vibrio parahaemolyticus, which lays the basis for their application in aquaculture. All three products contain phytogenics and permeabilizing agents to increase their effectiveness against Gram-negatives. Field trials that have not yet been published show that these products are effective in reducing the total number of Vibrio bacteria found in the hepatopancreas and intestine of white shrimp.
Conclusion
Plant-based additives have been used in aquaculture for relatively few years, and are still unknown to many farmers and nutritionists, but they are proving to have great potential, either to prevent disease, such as to replace antibiotics and promote growth. It is necessary to continue working, both in the investigation of their mechanisms of action and in the demonstration of their effects in the field, to achieve more scientific and targeted application.
More information: Ruby Yu
Research and Development PlusVet Animal Health E: yuhaixia@plusvet.cn
Teresa M. Agulles Teixidó
Director of Operations PlusVet Animal Health E: operations@plusvet.eu
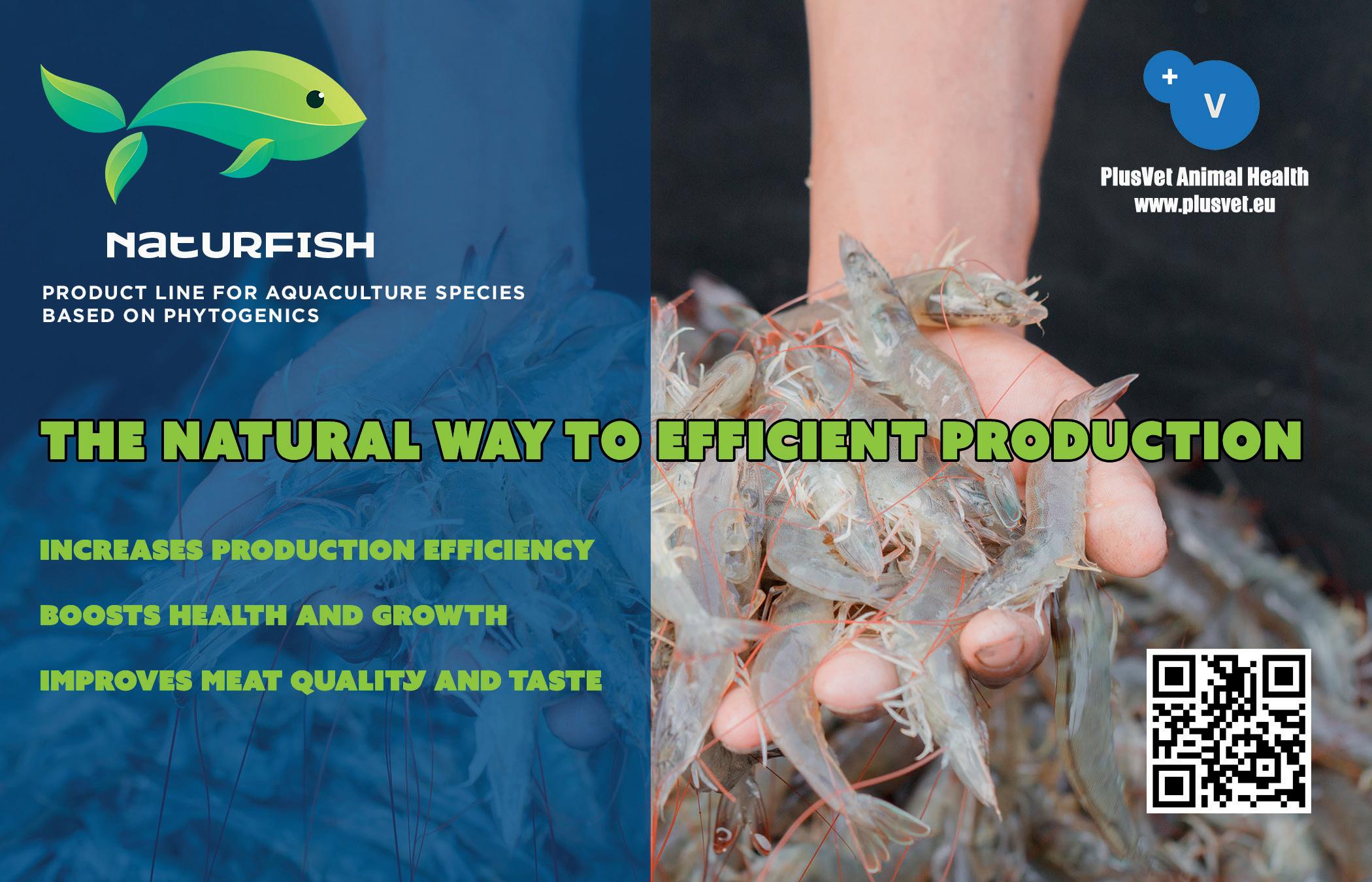