20 minute read
THE FUTURE OF INFECTIOUS DISEASES
from World + 3 Journal
by WorldPlus3
Next Article
Kitty Attwood asks how the nature of infectious diseases will change with 3ºC of global warming?'.
Our current global situation is impossible to ignore. The human population appears more vulnerable than ever before; not only to the impending effects of climate change but also in light of the current COVID-19 pandemic. These two seemingly unconnected realities are in fact more closely associated than they first appear. This poses both challenges and opportunities by creating a link between reducing the effects of climate change and reducing the effects of infectious diseases for human populations. The challenge we are facing is that the predicted effects of three degrees of global warming, including changes to precipitation, atmospheric and oceanic circulation patterns, will alter the global incidence prevalence of infectious diseases. This presents us with a global public health crisis as the distribution and seasonality of existing infectious diseases change in response to these factors around the world. In addition to the direct effect of climate change on infectious diseases, the societal processes of over-consumption and exploitation that threaten to drive this level of global warming will also influence global disease risk. As agriculture, forestry and other factors account for around a quarter of global emissions (IPCC “Climate Change 2014: Synthesis Report” 45), the processes of agricultural intensification and deforestation that encourage disease emergence are inextricably linked to climate change. It is therefore essential to engage in debates concerning changing global disease risks and the public health measures that can be put in place to mitigate against both epidemics and pandemics like COVID-19.
The evidence for climate-associated disease risk is clear: the International Panel on Climate Change (IPCC) published their Fifth Assessment Report in IPCC Climate Change 2014: Synthesis Report. Contribution of Working Groups I, II and III to the Fifth Assessment Report of the Intergovernmental Panel on Climate Change [Core Writing Team, R.K. Pachauri and L.A. Meyer (eds.)]. IPCC [2014]: p. 151 2014, which confirmed that the predicted temperature and rainfall changes associated with climate change will alter the spread of infectious diseases (IPCC “Climate Change 2014: Synthesis Report” 69).
“In addition to the direct effect of climate change on infectious diseases, the societal processes of over-consumption and exploitation that threaten to drive this level of global warming will also influence global disease risk.”
Climate change can affect infectious diseases in different ways, depending on the type of transmission. Vectorborne diseases (those that arise due to contact with a vector organisation, such as a mosquito), for example, are expected to change in geographical distribution and seasonality as changing climatic conditions alter the domains of vector species. Changes to precipitation patterns as well as snow and ice cover are also expected to alter water quality, potentially leading to changes to water-borne diseases. Both of these factors present particular challenges for vulnerable populations (ibid.). The IPCC’s more recent 1.5ºC report (IPCC “Global Warming of 1.5°C” 39) confirms that there is strong evidence that these changes are already happening: in Canada, there have been increased cases of Lyme disease, which is transmitted by tick bites (Smith et al. 727) and northern Europe has seen an increase in Vibrioemergence — a bacterial infection linked to ocean temperatures (Smith et al. 725). The report concluded with “very high confidence” that every additional degree of warming would increase the magnitude of these changes (IPCC “Global Warming of 1.5°C" 39). Although this warming could lead to the reduction of disease incidence in some areas, the potential for outbreaks of infectious diseases such as malaria, West Nile virus or dengue fever in areas that do not have the appropriate public health infrastructure is severe.
The Intergovernmental Panel on Climate Change (IPCC) is the United Nations body for assessing the science related to climate change. It was created to promote climate mitigation and adaption options.
Vector-borne diseases are particularly dependent on climatic conditions because the incidence of the disease (the rate of new case outbreaks in a population) is affected by the climate-sensitive factors of habitat suitability, abundance, distribution, activity and reproduction rates of the vector (Semenza and Menne 364). Transmission of malaria, for example, is dependent on the vector (the Anopheles mosquito) as well as the plasmodium parasite, which is the organism responsible for the infection in both mosquitoes and human hosts. A study that assessed malaria transmission suitability across different IPCC climate change possibilities found geographic shifts across all future climate scenarios, both in areas where cases of malaria are endemic (constant) and only seasonal (Ryan et al. 1). Taking into account future population growth, the study found that warming in line with the IPCC’s worst-case climate scenario (an average of 4.22ºC warming by 2080) would see 75.9 million additional people at risk of malaria in Eastern and Southern Africa by 2080 (ibid.). Even in a future with only 2.75ºC of warming by 2080, there would be emerging areas of endemic suitability for the Anopheles mosquito — many of these being in areas that currently have a low malaria risk status (Ryan et al. 9). This risks overwhelming countries that have populations with low immunity and poor investment in malaria prevention strategies such as treatment, education and bite-prevention resources. The reality is that the shifting suitability for malaria transmission will disproportionately affect economically vulnerable countries, because of socioeconomic factors such as building regulations, land-use and health care facilities that influence the risk of an epidemic (Semenza and Menne 368).
"Taking into account future population growth, the study found that warming in line with the IPCC’s worstcase climate scenario (an average of 4.22ºC warming by 2080) would see 75.9 million additional people at risk of malaria in Eastern and Southern Africa by 2080."
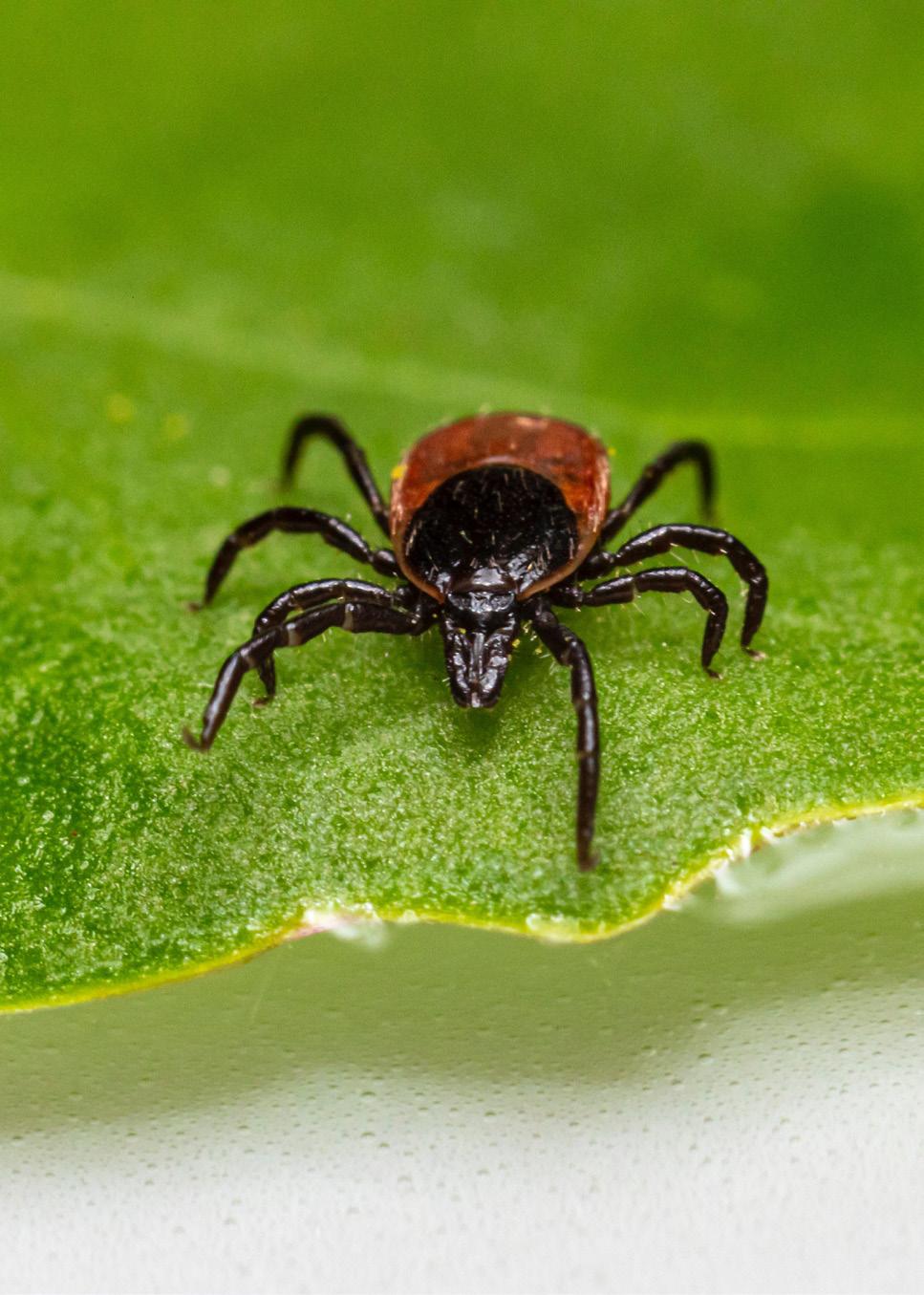
A tick
In Europe and North America, changes to the nature of vector-borne diseases are already being witnessed; since the 1980s, the prevalence of tick-borne diseases has increased due to the trend of longer summers and shorter winters (Semenza and Menne 368). Since increased temperature accelerates the development cycle, egg production and population density of ticks, climate models that predict longer, drier summers will correspond to a northward shift in tick-borne diseases such as Lyme disease (ibid.). However, vector-borne diseases are not the only type of infection that is being affected by climate change; the incidence and distribution of food-borne diseases are also changing. In the Baltic sea, rising sea surface temperatures are leading to the aforementioned increased vibrio emergence — a bacteria that causes gastroenteritis through contaminated seafood (Ebi et al. 5). Water-borne diseases will be similarly effected because outbreaks of diseases such as Cholera are also associated with water temperature (Semenza and Menne 370). Therefore, the current seasonal shifts of such diseases to higher latitudes in warmer months may extend in duration and extent in the future (ibid.).
The changes that will occur to existing infectious diseases are unfortunately only half of the problem; climate change and the associated process of land-use change will also alter disease emergence. Emerging infectious diseases (EIDs) are infections that newly appear in a population (Morse 7). Disease emergence is linked to ecological and demographic factors that promote human contact with previously unknown microbes, often via wildlife — a key example of this being the assumed origin of COVID-19: a wet market in Wuhan that sold wild animals and their meat (Li et al. 1202). The wildlife trade increases human contact with new pathogens via hunters, traders and consumers, whether for meat in countries such as China (where the wildlife trade is a $20 billion industry that employs around 15 million people), or for exotic pet industries in countries like the US (Dobson et al. 379). The risks of these industries are exacerbated by the lack of regulations and enforcement in many countries, causing animals to be kept in poor conditions that facilitate the spread of disease, and resulting in lack of health screening during import (ibid.). These interactions that are central to wildlife industries can have significant effects; human interaction with wildlife accounts for three-quarters of zoonotic emerging diseases (Loh et al. 432). Zoonotic diseases themselves (those passed to humans from animals) account for around two-thirds of all emerging infectious diseases (ibid.).
Another key factor that increases human contact with new pathogenic microbes is deforestation and the expansion of intensive agriculture — a major contributor to climate change. Since pathogen species (species that cause disease) follow the latitudinal trend of species richness, whereby there is greater diversity of species towards the equator, pathogen species richness is high where large areas of tropical rainforest are being deforested (Guégan et al. 5). Forest fragmentation increases the potential for pathogen ‘spillover’ into human systems due to the ‘edge effect’ where humans and their livestock come into contact with wildlife at the forest’s edge (Dobson et al. 379). This occurs as population growth and economic development drive settlements, agricultural activity and road networks further into forested land (ibid.). The frequency of outbreaks of infectious diseases along forest edges is affected by the length of the forest perimeter (ibid.). If the level of forest conversion is around 50%, there is maximum opportunity for an outbreak to occur due to increased forest perimeter length (ibid.). The recent emerging diseases of Ebola, Nipah, SARS and COVID-19 emerged in human populations through these processes; specifically being transferred to humans as bats feed nearer to human populations due to habitat disruption (ibid.). This demonstrates how disease emergence increases as humans increase stresses upon natural ecosystems.
The emergence of infectious diseases is thus related to social factors such as human demographics and behaviour, economic development, land-use, and international trade and commerce (Loh et al. 433). Many of these factors are intrinsically linked to climate change; deforestation alone is estimated to cause around 11% of global greenhouse gas emissions (IPCC “Global Warming of 1.5°C” 28). However, the link between EIDs and climate change is not limited to this correlative relationship; there is also a causal relationship between climatological variation and the ecological diversification of pathogen species (Hoberg and Brooks 2).
“The emergence of infectious diseases is thus related to social factors such as human demographics and behaviour, economic development, land-use, and international trade and commerce ”
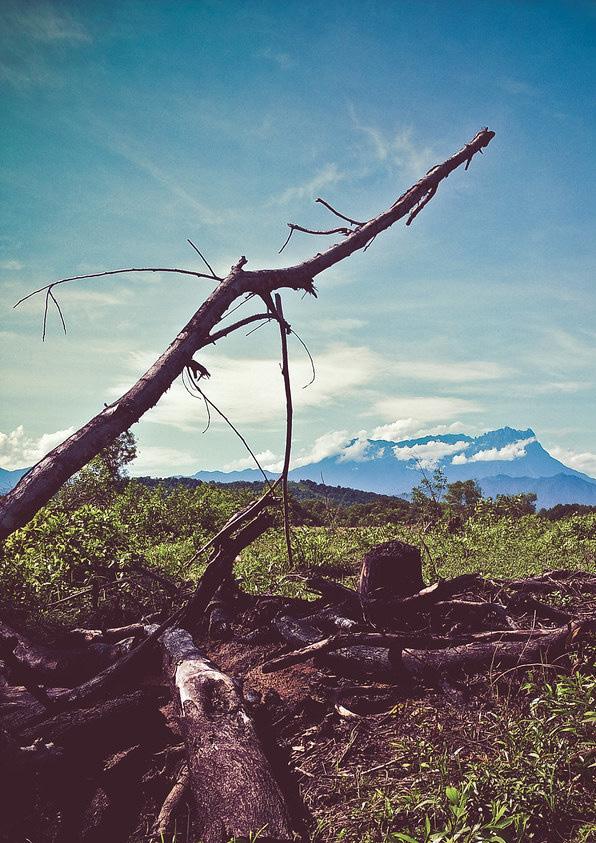
New developments in the ecological understanding of pathogen and host relationships show how significantly climate change can open new opportunities for disease emergence. Previously, pathogens were thought to be limited in both their evolution and spread to new hosts due to their coevolutionary relationships with host species. A coevolutionary relationship involves the simultaneous evolution of two species, restricting both the evolution of the pathogen and its ability to spread due to the specificity of this development process, thus limiting the emergence of infectious diseases (Brooks et al. 1). Pathogens were thus thought to be highly specialised species that have unique requirements and can only thrive under specific conditions (Brooks et al. 2). The new understanding is that pathogen species are not as limited to their current host as previously thought; instead there exists a much wider range that the pathogen could occupy if given the opportunity (Hoberg and Brooks 4). This theory, called the Stockholm Paradigm, means that pathogens do not require an unusual mutation to extend to a new host. Instead, a pathogen simply requires an opportunity to do so.
This new information is alarming because in an era of ecological transformation, the opportunities for pathogens to colonise new hosts are increasing. For example, evidence suggests that deforestation of closed forests in Central and West Africa created the opportunity for Ebola to move from its ‘natural host’ (fruit bats) to its new host (humans) along forest perimeters (Olivero et al. 1). Both the land-use change and direct climatic change expected to accompany global warming this century will only increase the potential opportunities for pathogens to occupy new hosts by altering global species distributions and ecosystem structures. This has the potential to be extremely destructive to biodiversity, human food systems and human health. The Stockholm Paradigm summarises how disease incidence is linked to our current global situation where the world is experiencing an unprecedented acceleration in population growth, landuse change, global connectivity and climate change. These changes are creating the perfect conditions for pathogens to diversify, rendering human populations extremely vulnerable to new infectious diseases.
In response to this global health challenge, it is essential to both mitigate these risks by addressing their causes, and adapt to them by reducing the impact of pathogen diversification. Monitoring, detection, education and policy development are all essential functions to prevent infectious disease spread and manage an outbreak. Many of these processes are aided by technological developments; for example, monitoring and planning can be achieved using digital datasets, geospatial modelling and satellite data (Ebi et al. 7). The demand for integrated technologies to assess trends and identify risk areas for infectious disease outbreaks could justify the pooling of large-scale environmental and epidemiological datasets (Semenza and Menne 372). These datasets could then be used to identify vulnerable populations and inform effective responses using statistical analysis. The growth in technology enabling this kind of analysis shows that we are more prepared than ever before to both prevent and respond to disease risks. Although extensive monitoring systems would require significant investment, the cost of disease outbreaks is usually much higher than the cost of preventing them. This fact seems undeniable when the UK alone has experienced a decline in GDP three times greater than that of the 2008 global financial crisis during the onset of the coronavirus outbreak in April (ONS). In light of the COVID-19 pandemic and its economic consequences, the long-term economic benefit of investing in infectious disease monitoring and prevention seems clear.
“Although extensive monitoring systems would require significant investment, the cost of disease outbreaks is usually much higher than the cost of preventing them.”
Co-evolution occurs when two or more species evolve alongside each other and so exert a selection pressure on the other. These reciprocal relationships may be predator/ prey (as for example a feedback loop of faster prey leading to faster predators leading to faster prey), but they can also be mutually beneficial and highly specialized. These mutualistic arrangements include the relationship between dairying ants and the aphids they ‘farm’, and most of the bacteria in your gut!
Investing in infectious disease prevention will also bring further benefits by reducing deforestation, wildlife exploitation and minimising climate change impacts (Dobson et al. 380). For example, the estimated annual cost of programmes to reduce deforestation and create disease surveillance systems in high-risk areas is between $17-26 billion, whereas the global cost of the COVID-19 pandemic was estimated to lie between $8-16 trillion in July. However, the economic case for deforestation reduction is only part of the picture; such initiatives must consider the needs of the populations within the countries they are implemented. This is essential to avoid repeating the negative consequences that have arisen as a result of previous attempts to reduce deforestation. The UN’s attempt to reduce deforestation via the ‘REDD+’ project, for example, is thought to have increased violence, dispossessed native peoples from their land and primarily affected local farmers rather than large corporations (Friends of the Earth 21). In order to prevent this result, initiatives must consider the root causes of rainforest conversion and engage in a less top-down approach.
Investment must also be directed towards further research, which can reveal more effective ways to monitor and predict infectious disease spread. For example, a 2015 study assessed a database of emerging infectious diseases, classing each by the transmission pathway of the EID (whether airborne, vector-borne or so on) and the suspected driver of the EID (such as landuse or human demographics and behaviour) (Loh et al. 435). The study found that the transmission pathway of the disease was dependent on the suspected EID driver (ibid.). This is significant because the type of transmission pathway affects the response that is needed to reduce the spread of the disease; for example, as COVID-19 is transmitted via contact and aerosols, important safety measures include regular hand-washing and using masks. In contrast, responses to vector-borne diseases are focused on vector control, such as through environmental management and the use of mosquito nets in the case of mosquitoborne diseases.
Understanding the link between EID driver and transmission pathway can also aid prediction, based on the potential disease drivers that are prevalent in an area. Furthermore, the same disease can follow different transmission pathways in different areas if the EID driver varies. For example, in Malaysia, Nipah virus is primarily spread by direct human contact with pigs, as the disease driver is agricultural intensification causing fruit bats to feed on fruit plantations near pig farms (Loh et al. 435). In contrast, in Bangladesh, Nipah virus is principally spread via food-borne transmission, specifically through the ingestion of fresh date palm sap that is contaminated by bat faeces (ibid.). This means that in Malaysia, effective Nipah virus control measures can focus on PPE and hand-washing, whereas food hygiene measures may be more effective in Bangladesh. Understanding the differences between EID drivers can thus enable a proactive rather than a reactive approach which is essential for effective intervention.
Investment and research are not the only methods for addressing the increasing incidence of infectious diseases. A comprehensive approach to infectious disease management requires extensive attitude shifts and changes to consumption patterns. Reducing meat consumption, eradicating animal cruelty and exploitation of natural habitats as well as placing restrictions on the wildlife trade are all critical. Key steps are already being taken— China has banned wild animal consumption since the outbreak of COVID-19—but greater funding and regulation is needed to enforce regulations (Dobson et al. 379). As with initiatives to reduce deforestation, efforts to reduce wild meat consumption must consider indigenous communities with diets that rely on wildlife protein (Dobson et al. 380).
Since antibiotic usage is pervasive in livestock production, reducing overall meat consumption also has the additional benefit of reducing antibiotic resistance, which is vital for maintaining essential treatments for bacterial infectious diseases (Cohen). Attitude shifts and investment thus have the potential to provide opportunities for the effective management of disease, whilst also creating further benefits, such as improving environmental conditions and promoting the ethical treatment of animals. Changes to the distribution of existing diseases may even create opportunities for disease eradication in some areas. For example, temperature increases across Africa may cause some areas (including coastal Western Africa and the Horn of Africa) to shift from continual to only seasonal suitability for malaria transmission (Ryan et al. 5). If interventions are concentrated in these areas, there is potential to eliminate the disease in certain countries.
"The same disease can follow different transmission pathways in different areas if the EID driver varies."
The nature of climate change and its effect on infectious diseases is intricate and will present correspondingly complex challenges in a world that is heading towards three degrees of global warming. However, if an informed, proactive approach is taken, potential opportunities exist within these changes. The unfolding COVID-19 crisis should be a wake-up call; our current treatment of the biosphere is changing the planet in ways we cannot predict. To address the public health crisis of shifting infectious disease prevalence, it is essential to minimise the effect of climate change and, if possible, avoid a scenario where three degrees of warming is a reality. At the same time, vital research and investment must be carried out to aid the prevention, monitoring and control of new and existing diseases. Measures to mitigate against climate change and disease go hand in hand; we must do both to ensure a safe future for both our planet and our health.
Complete Bibliography:
Brooks, Daniel R.; Hoberg, Eric P.; Boeger, Walter A. The Stockholm paradigm: climate change and emerging disease. Chicago. [2020].
Cohen, Nick. Surely the link between abusing animals and the world’s health is now clear. The Guardian [2020].
Dobson, Andrew P, Stuart L Pimm, Lee Hannah, Les Kaufman, Jorge A Ahumada, Amy W Ando, Aaron Bernstein, Jonah Busch, Peter Daszak, Jens Engelmann, Margaret F Kinnaird, Binbin V Li, Ted Loch-Temzelides, Thomas Lovejoy, Katarzyna Nowak, Patrick R Roehrdanz, and Mariana M Vale. “Ecology and Economics for Pandemic Prevention.” Science (New York, N.Y.) 369.6502 [2020]: pp. 379-381. Web.
Ebi, Kristie L., Nicholas H. Ogden, Jan C. Semenza, and Alistair Woodward. “Detecting and Attributing Health Burdens to Climate Change.” Environmental Health Perspectives 125.8 [2017]: 085004. Web.
Friends of the Earth. REDD: The realities in black and white. Friends of the Earth International [2010].
Guégan, Jean-François; Ayouba, Ahidjio; Cappelle, Julien; de Thoisy, Benoît. “Forests and emerging infectious diseases: unleashing the beast within.” Environmental Research Letters 15.8 [2020].
Hoberg, Eric P. P., and Brooks, Daniel R. R. “Evolution in Action: Climate Change, Biodiversity Dynamics and Emerging Infectious Disease.” Philosophical Transactions of the Royal Society of London. Series B, Biological Sciences 370.1665 [2015]. Web.
IPCC Climate Change 2014: Synthesis Report. Contribution of Working Groups I, II and III to the Fifth Assessment Report of the Intergovernmental Panel on Climate Change [Core Writing Team, R.K. Pachauri and L.A. Meyer (eds.)]. IPCC [2014]: p. 151
IPCC Global Warming of 1.5°C. An IPCC Special Report on the impacts of global warming of 1.5°C above pre-industrial levels and related global greenhouse gas emission pathways, in the context... 33
of strengthening the global response to the threat of climate change, sustainable development, and efforts to eradicate poverty [Masson-Delmotte, V., P. Zhai, H.-O. Pörtner, D. Roberts, J. Skea, P.R. Shukla, A. Pirani, W. Moufouma-Okia, C. Péan, R. Pidcock, S. Connors, J.B.R. Matthews, Y. Chen, X. Zhou, M.I. Gomis, E. Lonnoy, T. Maycock, M. Tignor, and T. Waterfield (eds.)]. In Press. [2018].
Li, Qun; Guan, Xuhua; Wu, Peng; Wang, Xiaoye; Zhou, Lei; Tong, Yeqing; Ren, Ruiqi; Leung, Kathy S.M; Lau, Eric H.Y; Wong, Jessica Y; Xing, Xuesen; Xiang, Nijuan; Wu, Yang; Li, Chao; Chen, Qi; Li, Dan; Liu, Tian; Zhao, Jing; Liu, Man; Tu, Wenxiao. “Early Transmission Dynamics in Wuhan, China, of Novel Coronavirus–Infected Pneumonia.” The New England Journal of Medicine. 382.13 [2020] pp. 1199–1207.
Loh, Elizabeth H; Zambrana-Torrelio, Carlos; Olival, Kevin J; Bogich, Tiffany L; Johnson, Christine K; Mazet, Jonna A. K; Karesh, William; Daszak, Peter “Targeting Transmission Pathways for Emerging Zoonotic Disease Surveillance and Control.” Vector-Borne and Zoonotic Diseases. 15.7 [2015] pp. 432–437.
Morse, S. S. “Factors in the Emergence of Infectious Diseases.” Emerging Infectious Diseases 1.1 [1995]: pp. 7-15. Web.
Olivero, Jesús; Fa, John; Real, Raimundo; Márquez, Ana; Farfán, Miguel; Vargas, J.; Gaveau, David; Salim, Mohammad; Park, Douglas; Suter, Jamison; King, Shona; Leendertz, Siv; Sheil, Douglas; Nasi, Robert “Recent Loss of Closed Forests Is Associated with Ebola Virus Disease Outbreaks.” Sci Rep 7.1 [2017]: p. 14291. Web.
ONS, 2020: Coronavirus and the impact on output in the UK economy: April 2020. Office for National Statistics. [2020] https://www.ons.gov. uk/economy/grossdomesticproductgdp/articles/ coronavirusandtheimpactonoutputintheukeconomy/ april2020. Accessed: 27/09/20.
Ryan, Sadie; Lippi, Catherine; Zermoglio, Fernanda. “Shifting Transmission Risk for Malaria in Africa with Climate Change: A Framework for Planning and Intervention.” Malaria Journal 19.1 [2020]: 170. Web.
Semenza, Jan and Menne, Bettina. “Climate change and infectious diseases in Europe.” The Lancet Infectious Diseases, 9.6 [2009] pp. 365–375.
Smith, K.R., A. Woodward, D. Campbell-Lendrum, D.D. Chadee, Y. Honda, Q. Liu, J.M. Olwoch, B. Revich, and R. Sauerborn. “Human health: impacts, adaptation, and co-benefits.” In: Climate Change 2014: Impacts, Adaptation, and Vulnerability. Part A: Global and Sectoral Aspects. Contribution of Working Group II to the Fifth Assessment Report of the Intergovernmental Panel on Climate Change. Field, C.B., V.R. Barros, D.J. Dokken, K.J. Mach, M.D. Mastrandrea, T.E. Bilir, M. Chatterjee, K.L. Ebi, Y.O. Estrada, R.C. Genova, B. Girma, E.S. Kissel, A.N. Levy, S. MacCracken, P.R. Mastrandrea, and L.L. White (eds.). Cambridge University Press, Cambridge [2014] pp. 709-754.
Co-evolution occurs when two or more species evolve alongside each other and so exert a selection pressure on the other. These reciprocal relationships may be predator/ prey (as for example a feedback loop of faster prey leading to faster predators leading to faster prey), but they can also be mutually beneficial and highly specialized. These mutualistic arrangements include the relationship between dairying ants and the aphids they ‘farm’, and most of the bacteria in your gut!