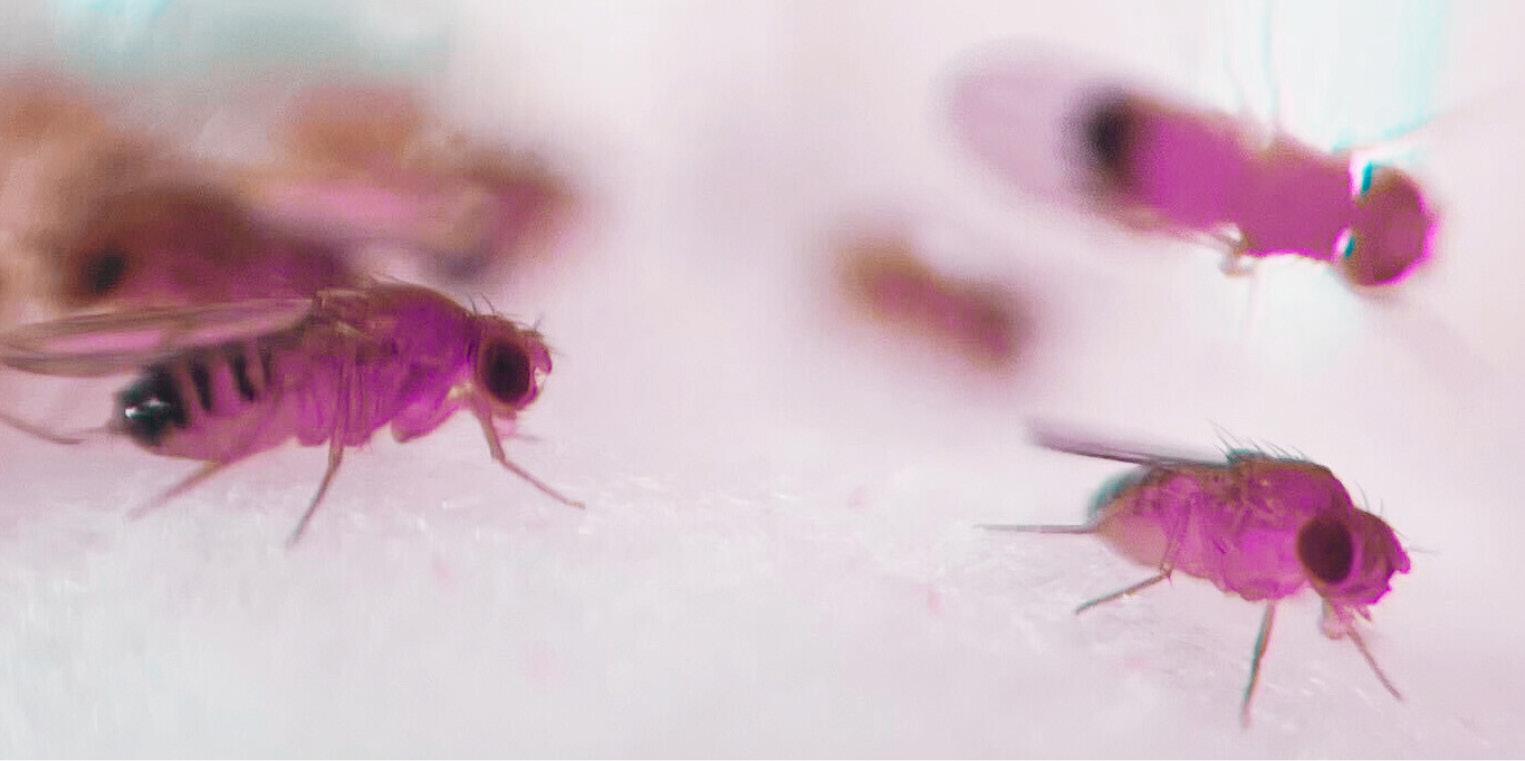
5 minute read
Backbone Optional: Invertebrate Models Bring New Insights to Modern Physiology
By Koustubh Vaze Research Fellow, Molecular & Integrative Physiology
The estimated number of plant and animal species on the earth stands over 8 million, but most of our understanding about how organisms’ function at the molecular and cellular level have come from a tiny minority of them - what are known in science as “model organisms”. The choice of a model organism is a trade-off among factors such as convenience, suitability of the organism for the research question, translational potential and many others. Animal species have been of special significance due to the potential of their findings in modeling human physiology and diseases. A broad range of animals from single celled protozoa to primates are in use as model organisms, but mice and rats account for >95% of all animals used in research s. Mouse (Mus musculus) is the preeminent genetic model to understand human physiology and disease due to its genetic and physiological similarity with humans than most other model organisms and the wide availability of genetic tools to manipulate their genes. Although the mouse has great translational potential, it is not always the best choice. The invertebrate genetic model animals like roundworm Caenorhabditis elegans, fruit fly Drosophila melanogaster and other emerging genetic models offer several advantages over the mouse model in many ways, making them powerful model systems under several circumstances.
Advertisement
Why study worms and flies?
Highly efficient methods for forward and reverse genetics, extensive genetic and genomic resources, ease of genome editing and availability of multiple alternative tools that enable control over spatiotem- poral manipulation of gene function in worms and fruit flies make them very attractive systems for the analysis of gene function. Their small size, short lifespan, ease of rearing and breeding in large numbers in a tiny space plus all the features of the genetic model allows scientists to find genes and study their function at a fraction of the cost and time than in any vertebrate model animal. Despite being evolutionarily distant, discoveries in worms and flies have repeatedly revealed the conserved molecular pathways demonstrating their remarkable genetic and physiological similarity to mammals including humans. While their nervous systems are relatively small, they are sophisticated with rich behavioral repertoires. Genetically-encoded tools can be used to manipulate and monitor neural function, enabling analysis of whole-brain neural activity and functional characterization of entire neural circuits underlying complex behaviors in free living animals. Together, these features make worms and flies excellent model systems for numerous biological processes and diseases in humans, and an efficient starting material in translational research.
Worm and fly labs at MIP
Many research groups at Molecular & Integrative Physiology (MIP) are successfully utilizing the power of worms and fruit flies to probe the molecular mechanisms underlying aging and sensory systems, and eventually testing the translatability of those findings in mice and humans.
Lee lab: Animals cope with environmental stresses by activating appropriate response mechanisms which not only develop stress resistance but also improve longevity. The Lee lab uses fly models to study stress response mechanisms involving Sestrins, a family of stress inducible proteins and autophagy.
Pletcher lab: The process of aging is sensitive to nutrient availability and many other environmental cues. The Pletcher lab is focusing on how fruit flies perceive nutrient availability and modulate aging, using a combination of genetic, biochemical and behavioral techniques. The conserved neurotransmitter serotonin is an important modulator of lifespan in specific nutritional contexts. Earlier study from Pletcher lab showed that visual detection of dead conspecifics shortens lifespan in fruit fly, and its dependence on serotonin signaling. A recent, exiting piece of work by Dr. Allyson Munneke, a fresh graduate and Dr. Tuhin Chakraborty, a research specialist from the Pletcher lab shows that serotonin receptor 5-HT2A mediated signaling is responsible for this lifespan shortening by promoting dietary protein intake, through the activation of 5-HT2A neurons in the brain. Together, these results provide a deeper mechanistic insight into how the perception of nutrient availability alters physiology and behavior to modulate lifespan.
Leiser lab: Intestinal expression of a xenobiotic detoxification enzyme Flavin-containing monoaoxygenase-2 (FMO-2) is necessary and sufficient for dietary restriction (DR) induced lifespan extension in worms. The Leiser lab is extensively investigating neurosensory pathways that induce the expression of FMO-2 and also its physiological role in extending lifespan. Two recent studies from the Leiser lab have uncovered the neuronal signaling pathways that contribute to FMO-2 induction during DR, as well as pathways downstream of FMO-2 that led to lifespan extension. Mere exposure to food smells during dietary restriction is sufficient to suppress the intestinal FMO-2 expression and reverse the lifespan extension. Miller et al. 2022 have shown that serotonin and dopamine receptor blockers can prevent food smell induced lifespan shortening under DR, and also identified chemosensory and enteric neurons possibly involved in the signaling. These results strongly suggest that DR induces intestinal FMO-2 expression by suppressing serotonergic and dopaminergic signaling, uncovering a mechanism underlying FMO-2 induction during DR. A further study by Choi et al. 2022 investigated the downstream pathways required for FMO-2 mediated lifespan extension. This study demonstrated that FMO-2 oxygenates tryptophan and rewires One Carbon Metabolism, resulting in a decrease in methylation flux which leads to lifespan extension.
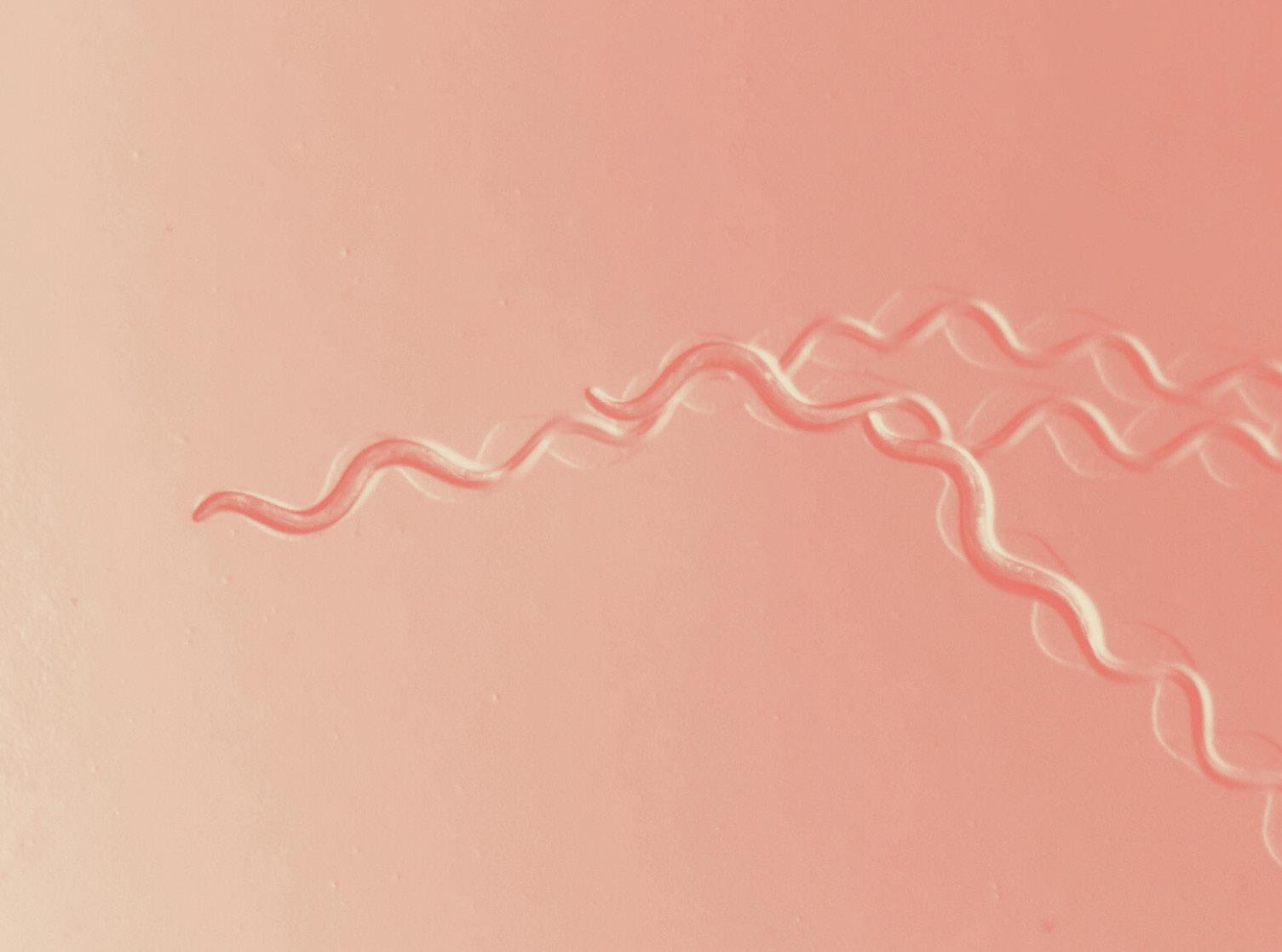
Truttmann Lab: The Truttmann lab is interested in how protein homeostasis is maintained and how proteotoxic stress leads to diseases of neurodegeneration and aging. The lab is particularly interested in protein chaperones that ensure proper folding of proteins or facilitate degradation when proteins are misfolded. Recent work by student Mirella Hernandez-Lima has shown that an important posttranslational modification called ampylation, regulates the production of a secreted factor called TGF beta, and effects growth and function of neurons in worms through altered TGF beta signalling.
Xu lab: The ability to sense and respond to environmental stimuli is critical for survival. The Xu lab uses a worm model to investigate the molecular mechanisms involved in detection, transduction and processing of diverse sensory modalities and their consequences for behaviors and aging. A genetic screen for regulators of worm photoreceptor LITE-1 in a 2022 study by Dr. Xinxing Zhang in the Xu lab identified genes coding for protein complex – the BBSome, best known for its role in maintaining ciliary function and named after its association with a ciliopathic disorder Bardet-Biedl syndrome (BBS). This study was the first ever demonstration of cilia-independent function for the BBSome suggesting that the symptoms of BBS may not entirely stem from the ciliary dysfunction; providing a new insight into the etiology of ciliopathies in general.
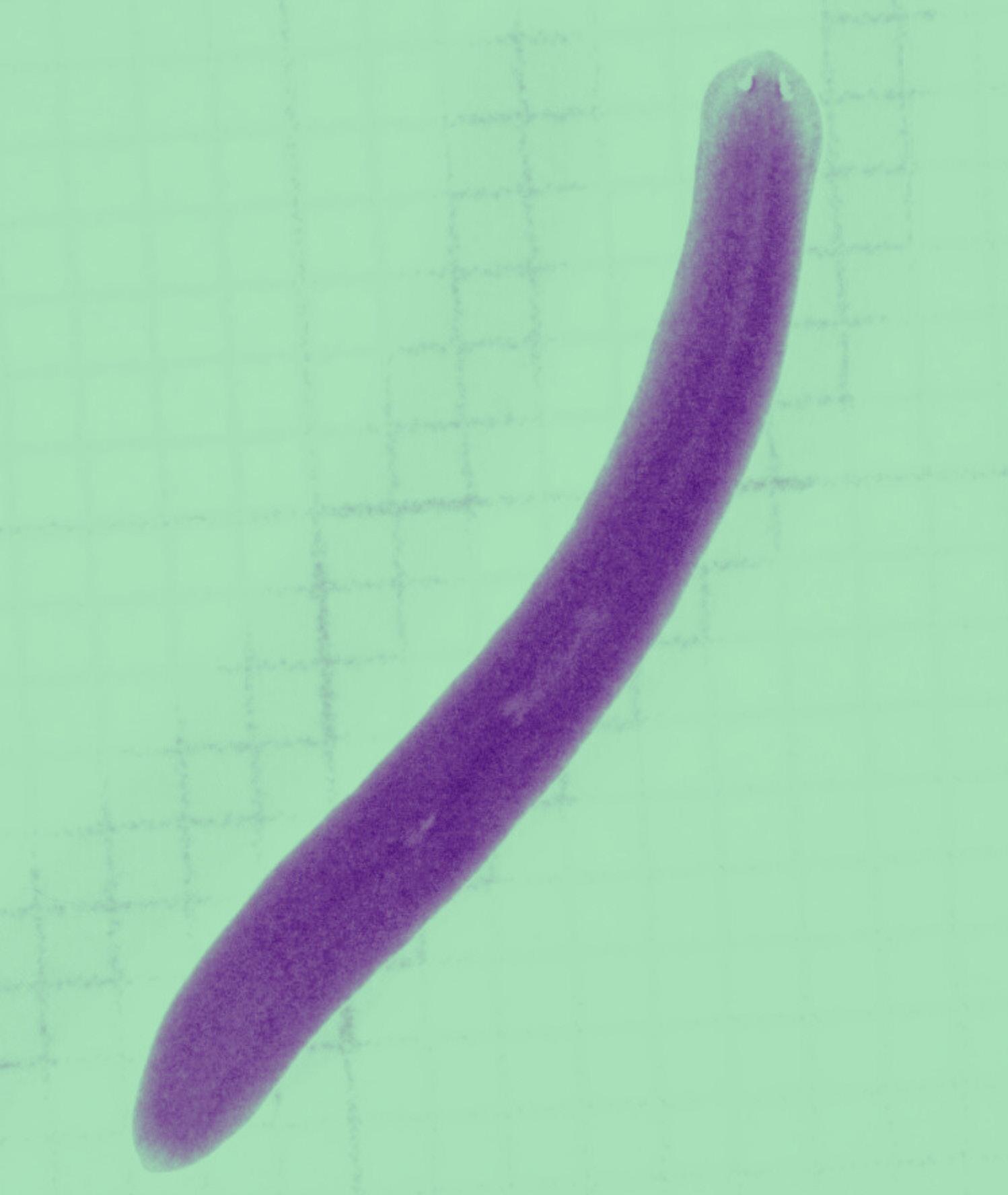
Expanding the model organism repertoire in MIP
Well established genetic model animals too have limitations. Often, established models are not the best suited to effectively address questions concerning many biological processes simply due the lack or inadequate expression of desired trait(s). Biologists are therefore turning many other animal species better suited for their research questions. Rapidly advancing sequencing and genome editing technologies have lead to the full genomes of these organisms being catalogued, their gene expression can now be studied using RNA sequencing, and their genes can be manipulated to study important physiological mechanisms.
Guo lab: The freshwater flatworm- planarian Schmidtea mediterranea is well known for its exceptional ability to regenerate and extreme longevity. Dr. Longhua Gu is one of the two new assistant professors hired in MIP in the last year. Dr. Guo is using planarian as a model to investigate mechanisms underlying extreme longevity and regenerative capacity, and how the two processes interact. Until recently, investigation of molecular mechanisms underlying interesting life history traits in the planarians were largely propelled by the RNA interference approach. The Guo lab is actively involved in building sophisticated genetic and genomic resources, and in exploring the molecular, cellular and developmental basis of its longevity and extreme regenerative capacity. Besides the planarians, Dr. Guo is also developing the Leopard gecko Eublepharis macularius, a favorite reptile pet as a vertebrate model of aging and tissue regeneration.
We are excited to see the growth of model organism research in MIP over the last decade. The growing number of model organisms used in physiological research are allowing for fundamental discoveries in how the nervous system works, how organs are maintained throughout an organism’s lifespan and key pathways that regulate overall health and longevity. We look forward to the continued creative research in MIP that combines new genetic tools, physiology, imaging, and behavior in these interesting invertebrate organisms that have surprisingly conserved mechanisms and similar physiology that underlie many aspects of human health.