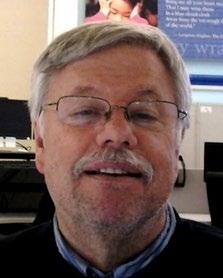
10 minute read
Student Agency and Equity
by NYSASCD
New York State P-12 Science Learning Standards: Student Agency and Equity in Contemporary Science Education With All Students
Gregory Borman, Theresa Ocol, Okhee Lee
Gregory Borman is the Director of Science for the New York City Department of Education (NYC DOE). He helped develop the New York State Strategic Plan for Science and is a member of the New York State Science Content Advisory Panel. His NYC DOE team is supporting the implementation of the New York State P-12 Science Learning Standards through professional learning, curriculum evaluation, and collaboration with higher education and informal science education institutions.
The previous generation of content standards was developed and implemented prior to the era of the No Child Left Behind Act of 2001. In the early 2010s, the current generation of content standards was released and, subsequently, adopted or adapted by states across the nation. Based on the current standards, the New York State P-12 Science Standards (NYS P-12 SLS) were adopted in December 2016. Implementation began in July 2017 but was delayed for 2 years due to the COVID-19 pandemic. The NYS P-12 SLS continue to be rolled out over the grade levels and bands (see the Revised April 2021 Science Implementation Timeline Map). State science assessments at Grades 5 and 8 and high school Regents Exams will be administrated gradually starting from the 2023-2024 school year.
In this article, we describe how contemporary approaches in science education, as represented in the NYS P-12 SLS, “flip” traditional approaches regarding two central questions: “What counts as science and science learning?” and “Who are science learners?” In response to these two questions, we describe how contemporary approaches center on student agency and student equity.
Contemporary Approaches in Science Education
The previous generation of science standards was based on the National Science Education Standards (National Research
Theresa Ocol, Ed.D. is the Senior Director of Content Areas with the Division of Multilingual Learners at the New York City Department of Education (NYC DOE). She focuses on designing, planning, and delivering professional learning opportunities and resources for science and English as a New Language educators from grades K-12. Theresa has worked in the NYC DOE for the last 17 years. Theresa embraces the notion that all ELLs/MLs bring assets to their science learning. Council, 1996). The New York State Learning Standards for Mathematics, Science and Technology were released in 1996, the same year that the National Science Education Standards were published. Since the mid-1990s, the national and state science standards hadn’t been updated to reflect new advances in science and engineering disciplines and new research on children’s abilities in learning science.
A Framework for K-12 Science Education (National Research Council, 2012) presented contemporary views on what counts as science and how children learn science, which provided the foundation for the development of the Next Generation Science Standards (NGSS Lead States, 2013b). Since the publication of the Framework and the release of the NGSS in the early 2010s, 20 states and the District of Columbia adopted the NGSS, and 27 states developed their own standards based on the Framework. The NYS P-12 SLS were based largely on the Framework and the NGSS. Contemporary approaches in science standards, including the NYS P-12 SLS, “flip” traditional approaches by centering on student agency and student equity, as shown in the following figure:
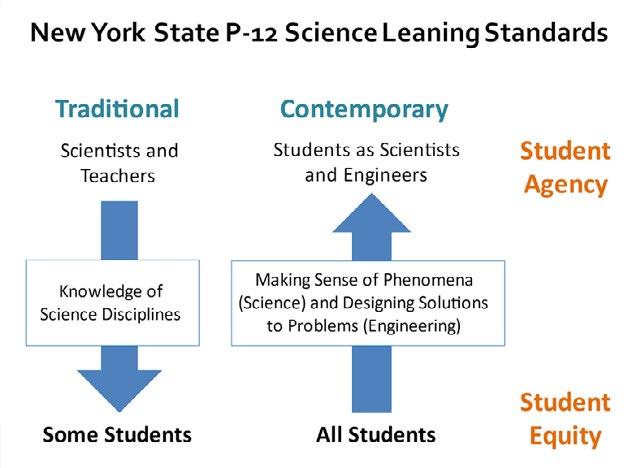
Figure I - Approaches in Science Education
Student Agency: What Counts as Science and Science Learning?
Traditionally, scientists and science educators defined the knowledge to be taught in school science. Science learning involved the accumulation of discrete elements of science knowledge (i.e., what knowledge is; knowledge-as-given). This canonical science knowledge was often presented through science textbooks, meaning that literacy skills (reading and writing) were a precursor or prerequisite to learning science. Canonical science knowledge was confirmed by school science lab investigations.
An instructional unit was organized around a science topic in a science discipline. Then, the body of science knowledge on this topic was broken down into a list of science concepts. A unit addressed a science topic, and lessons addressed related science concepts on the topic. A lesson or a lesson plan was designed for one class period of 45-60 minutes. A unit made sense from the perspective of a science discipline in that it addressed related science concepts, but not from the perspective of students who did not see the purpose in learning those science concepts. For example, a fifth-grade science unit on properties of matter and changes in matter addressed a list of science concepts, and lessons were developed around the concepts. In a lesson, to learn the concept of chemical change, students conducted the classic experiment of mixing baking soda with vinegar. They explained that, through a chemical reaction, a new substance is created in the form of a gas that inflates a balloon. Most students wondered how these science concepts would have any relevance to their lives or future careers.
In “flipping” traditional approaches, contemporary science education places student agency at the center. Students engage
Okhee Lee, Ed.D. is a professor in the Steinhardt School of Culture, Education, and Human Development at New York University. Her research involves integrating science, language, and computational thinking, with a focus on multilingual learners. Her latest work focuses on justice-centered STEM education to address pressing societal challenges using the case of the COVID-19 pandemic.
in science and engineering to make sense of phenomena and problems as scientists and engineers do in their professional work (i.e., what knowledge does; knowledge-in-use). To make sense of phenomena and problems, students engage in three-dimensional learning by blending science and engineering practices (e.g., ask questions, develop models, argue from evidence, construct explanations); crosscutting concepts (e.g., patterns, systems, structure and function, stability and change); and disciplinary core ideas in physical science, life science, Earth and space science, and engineering. Over time, students develop their science understanding coherently.
An instructional unit is organized around a phenomenon or problem. With teacher guidance, students decide on a driving question of the unit to guide their sensemaking of the phenomenon or problem. Lessons are developed around specific questions about aspects of the phenomenon or problem. A lesson is designed to answer a question about an aspect of the phenomenon or problem or to provide opportunities to investigate the phenomenon or problem in different contexts. A lesson typically involves a couple of class periods. For example, a fifth-grade science unit is framed around the phenomenon of large amounts of garbage that students produce every day in school, at home, and in the community (Lee et al., 2019). To make sense of the phenomenon, students decide on the driving question of the unit, “What happens to our garbage?” To answer specific questions about aspects of garbage, students engage in three-dimensional learning (specifically, disciplinary core ideas about properties of matter and changes in matter). In a lesson, students answer a question about the smell of garbage by conducting the classic experiment of mixing baking soda with vinegar. They explain that, through a chemical reaction, garbage materials mix and create a new substance in the form of a gas. This investigation helps students explain the smell of garbage as a new substance in the form of a gas. Throughout the unit, students answer the driving question about garbage and explain the phenomenon using disciplinary core ideas.
In both traditional and contemporary approaches, students may engage with the same lesson-level phenomena (e.g., mixing baking soda with vinegar) and science concepts (e.g., a new substance is created through a
chemical reaction). However, the purposes are different. Quinn (2021), chair of the Framework, describes the difference as follows:
Sometimes the questions that students ask, and the intended science concepts, also require intermediate investigations around more limited phenomena to elucidate aspects of the framing [anchoring] phenomenon. These activities look a bit more like traditional school science, but, if introduced appropriately, they differ from it in that these activities now have a context: students know they are doing this work to answer a question they have about the larger framing phenomenon (p. 868).
Moreover, in contemporary approaches, an instructional unit and lessons can be fluid and porous across science and engineering disciplines. Phenomena and problems do not draw distinct boundaries, as they are not inherently discipline specific. Instead, a (physical science); decomposition of some garbage materials (life science); a landfill harming soil, water, air, and organisms (Earth science); and the reduction of pollution from plastic bottles (engineering).
Student Equity: Who Are Science Learners?
Traditionally, students were viewed as receivers of science knowledge defined by scientists and science educators. School science was grounded in established bodies of knowledge in science disciplines. Traditional approaches resulted in only a small portion of students—“a select few”—coming to understand and appreciate how science knowledge is connected to form science disciplines—an understanding that is essential to move on to science careers. This small portion of students was, and continues to be, mostly white male students from higher socioeconomic backgrounds (McGee, 2016). However, science did not make sense to many students who did not come to see it as relevant to their lives or future careers.
In “flipping” traditional approaches, contemporary science education places student equity at the center. To promote sense-making with all students, phenomena
phenomenon or problem can be explained using core ideas across disciplines. For example, garbage can be explained in terms of structure and properties of garbage materials
and problems should be compelling to all students, especially those who may not see science and engineering as relevant to their lives or future careers (Lee, 2020). From the equity perspective, all students bring their cultural and linguistic resources to make sense of phenomena and problems. From a science perspective, students use science ideas as they investigate a driving question to explain a phenomenon. From an engineering perspective, students learn science in the context of solving an authentic problem.
For example, as students explain the driving question of the garbage unit (i.e., “What happens to our garbage?”), all students bring questions about aspects of the garbage based on their experiences in school, at home, and in the community. Students design investigations to answer questions, such as the following: How do we sort garbage into categories? Why do food materials disappear (i.e., decompose) but non-food materials do not disappear? What causes food materials to decompose? As students observe the large amounts of garbage they make, they become aware of the need to reduce the garbage produced. In addition, they design solutions to reduce pollution from plastic bottles that do not decompose and harm the environment. Eventually, students realize that landfills tend to affect minoritized communities and address systemic injustices in society.
Contemporary approaches in science education aim for “all standards, all students” (NGSS Lead States, 2013a). The ability to explain phenomena in science and design solutions to problems in engineering is essential for all students to navigate their lives and future careers. This ability is also important for students to be contributing members of their communities, as they use science to make informed, equitable decisions about societal challenges in their lives and communities. Students from diverse backgrounds have multiple points of entry to learning science, multiple ways of knowing, and multiple ways of communicating science ideas. Contemporary approaches respect the diversity of students’ experiences, recognize the merit of students’ science ideas, and promote the inclusion of all students in the science classroom.
REFERENCES
Lee, O. (2020). Making everyday phenomena phenomenal: Using phenomena to promote equity in science instruction:
Science and Children, 58(1), 56-61.
https://www.nsta.org/science-andchildren/science-and-childrenseptemberoctober-2020/makingeveryday-phenomena
Lee, O., Llosa, L., Grapin, S. E., Haas, A., & Goggins, M. (2019). Science and language integration with English learners: A conceptual framework guiding instructional materials development.
Science Education, 103(2), 317-337. https://doi.org/10.1002/sce.21498
McGee, E. O. (2016). Devalued Black and Latino racial identities: A byproduct of STEM college culture?
American Educational Research
Journal, 53(6), 1626-1662. https://doi. org/10.3102%2F0002831216676572
National Research Council. (1996).
National science education standards.
National Academies Press. https://nap. nationalacademies.org/download/4962
National Research Council. (2012). A framework for K-12 science education:
Practices, crosscutting concepts, and core ideas. The National Academies Press.
https://nap.nationalacademies.org/ download/13165
NGSS Lead States. (2013a). Appendix D–“All standards, all students”: Making the Next
Generation Science Standards accessible to all students. https://www.nextgenscience.
org/sites/default/files/Appendix%20
D%20Diversity%20and%20Equity%20 6-14-13.pdf
NGSS Lead States. (2013b). Next Generation
Science Standards: For states, by states. The
National Academies Press. https://nap.
nationalacademies.org/download/18290
Quinn, H. (2021). Commentary: The role of curriculum resources in promoting effective and equitable science learning.
Journal of Science Teacher Education, 32(7), 847-851. https://doi.org/10.1080/1