
13 minute read
Local engineers are part of multidisciplinary teams studying microplastic pollution (cover)
Local engineers are part of multidisciplinary teams studying microplastic pollution
by Steven Day, Christy Tyler, Samantha Romanick, James Roussie, James McGrath
Over the last decade there has been a lot of attention given to plastic waste that enters the environment. The waste generated from these durable, lightweight plastics behaves differently than materials of the past. It doesn’t degrade quickly and many polymers have a density that is less than or near enough to water that plastic can move through our water in ways that we’re only recently coming to understand. Plastic bags and six-pack rings affecting marine mammals captured attention decades ago and images of giant garbage patches in the oceans were circulated widely several years ago. More recently, our ideas about the many different ways that plastics can end up in our environment and affect the health of the ecosystem and humans has evolved from the concept that large quantities of waste ends up floating in the ocean.
The first change is our increased awareness of microplastics, some of which are manufactured at a small scale, and others of which are formed by the breakup of macro materials due to weathering, wear, and mechanical forces. These microparticles, classified as anything smaller than 5 mm, may not even be visible to the eye. Secondly, there is recent attention on freshwater systems, including rivers and lakes. Plastics behave differently in freshwater systems, in part because the density of the water itself is lower than ocean water, making it more likely that plastics will sink, rather than float. Third, there is a recent awareness that microplastics are small enough to travel suspended in water, or even air, and are ingested or inhaled by humans.

Local engineers and scientists are working to better understand how microplastics move through freshwater systems and affect ecosystems and human health. The participants in many separate projects collaborate through a microplastics workgroup, which includes close to thirty participants from seven different organizations. This workgroup comes together once a month to share recent findings in the literature as well as their own research on the topics of microplastics in our environment, human exposure to microplastics, and MP effects on wildlife and human health. Organizations represented in this group include Rochester Institute of Technology, University of Rochester, UR Medical Center, SiMPore Inc., Parverio Inc., Ithaca College, and Ithaca Area Wastewater Treatment Facility/Cornell University. Participants include high school students, undergraduate and graduate students, postdoctoral associates, professors, managers, and directors. The workgroup includes investigators conducting studies on: 1) the transport and ecological impact of MPs on the Greater Rochester Area’s many waterways; 2) the health impacts of MPs including the potentially toxic chemicals they can leach or adsorb; 3) the development of affordable and rapid methods to extract and identify microplastics in liquids and room air, and 4) the amount of microplastics people in Rochester are ingesting through drinking water or inhaling through room air. A few of these projects are described here.
What are the effects of these plastics on freshwater ecosystems, such as Lake Ontario?
The Laurentian Great Lakes span nearly 95,000 square miles and are the largest connected freshwater system in the world. They supply water for consumption, power, and recreation to the 30 million people that live in the Great Lakes basin. Matt Hoffman and others at RIT have estimated that only a small fraction (4 tons) of the approximately 2,500 tons of plastic that enter Lake Erie annually are floating on the surface and have shown similar data for Lake Ontario. An interdisciplinary team at RIT, led by Christy Tyler (an ecologist), and Hoffman (a mathematician), with Steven Day (mechanical engineer), Nathan Eddingsaas (a chemist), and Andre’ Hudson (a molecular biologist) is trying to understand the fate and impact of the “missing plastic”.
In addition to the mechanical and solar breakup of larger plastics into microplastics, there is a relationship to the biological environment. An ongoing project funded by the National Oceanic and Atmospheric Administration’s New York Sea Grant College Program is investigating the toxicity of the plastics on aquatic organisms as well as the biological effects on the particles.
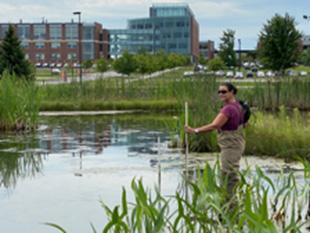
Microorganisms, including algae and bacteria, colonize plastic particles to create a biofilm. This added coating can change the effective density of the particle so that many plastics that initially float ultimately sink to the bottom and are retained in the ecosystem. The team placed samples of 6 different microplastics at both the surface and sediment of 5 local bodies of water and are collecting and analyzing them at 1, 4, and 12 months' time to see effects of aging in different environments. The team is also measuring how aquatic animals inhabiting the floor of these ecosystems such as aquatic worms and zebra mussels, help to bury some plastics within the sediment, making it more likely that this burial becomes their eventual fate.
The RIT team includes ecologists, modelers, chemists, and engineering faculty in the Kate Gleason College of Engineering at RIT. Dr. Steven Day, in the Biomedical Engineering department, became involved because of his background in fluid mechanics. Fluid mechanics is a field that’s traditionally been closely associated with engineering (every Mechanical, Chemical, Biomedical engineer took at least one course!), even though it has applications to all fields of science, including biology and environmental science. Day has experience with experimental methods in fluid mechanics, such as Particle Image Velocimetry and Particle Tracking Velocimetry, both of which deliberately add small plastic particles in order to visualize the motion of otherwise transparent fluids. Small (10-50 um) polystyrene particles are commonly used in these methods because their density and size make them so nearly buoyant that they travel with the flow, which means that they also easily move with the motion of water through our environment. These optical methods are ideally suited to measure the movement of plastic microparticles through water.
The team has found so far that aging and biofouling does lead to changes in the settling rate of plastics, with some materials changing from floating to sinking, and that these effects vary from location to location. They’ve also found that many plastics are toxic to aquatic worms, even those species capable of persisting in highly polluted ecosystems. However, the plastics appear to become slightly less toxic after aging in some environments, perhaps because additives leach into the water, but in other environments, the plastics increase in toxicity with aging, likely because other pollutants in the water associate with the plastic polymer. At the same time, these organisms were found to actively bury plastic fibers in the sediments, leading to long term retention in the sediment.
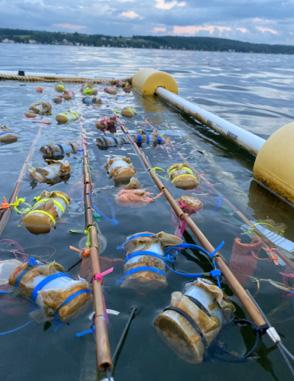
What are the effects on Human Health?
Recently, Dr. Samantha Romanick, founder of the local microplastics workgroup described above, was awarded a postdoctoral training fellowship in Pulmonary Medicine from the UofR Medical Center. Dr. Romanick’s research is examining the potential of inhalable plastic microfibers to trigger an inflammatory response in the lung. While a large number of studies have found microplastics in marine animals where they are known to do harm, these studies make it clear that humans are not not immune to the impacts of microplastic pollution. The potential impacts of microplastics on human health are only beginning to be understood, but plastic particles have been identified in a number of human tissues including the placenta, the colon, and the lung. Microplastics from the environment are ingested through contaminated food sources and inhaled through both indoor and outdoor air. It is estimated that humans are ingesting five grams of plastic a week, which is equivalent to the size of a plastic credit card, from contaminated sources such as drinking water, salt, seafood, beer, honey, tea, fast food, and rice. However, studies suggest that microplastics ingestion is minimal compared to inhalation of MFs, mainly from indoor environments. Carpeted homes, furniture, textiles, and clothing contribute to the amount of MFs found indoors. Considering humans spend 70-90% of their time indoors, studies suggest that vacuuming often, investing in natural fiber textiles and hard floors can minimize microfibers within the household.
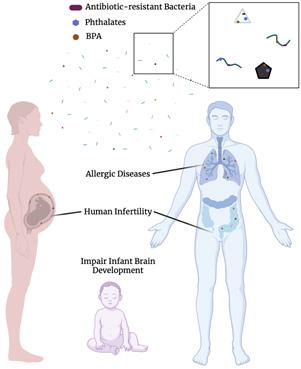
Even if the current impacts of microplastics on human health are small, humans add a record amount of durable plastics to the environment each year and so there is a serious concern for the future levels of microplastic consumption. And the concern is not just with the plastics themselves, but for the chemical pollutants with toxicological activity they can carry. For example, plastics have been shown to leach toxic chemicals such as BPA, dioxins, and phthalates, all of which have been shown to be harmful to human health. Studies suggest that human exposure to these contaminants may be linked to the rise in allergic diseases and phthalates can impair infant brain development. These contaminants are also considered endocrine disrupting chemicals, which affect human fertility. Microplastics may also serve as vectors of antibiotic-resistant bacteria and other harmful pathogens. All of the plastic polymers aged in the environment by the RIT team had evidence of antibiotic resistant bacteria in the biofilm. Altogether, these data suggest significant potential risks of microplastics for human health and warrant immediate investigation and mitigation.
Developing devices to aid in the collection and analysis of microplastics.
As the increasingly widespread prevalence of microplastics has been recognized, methods for detecting their presence, as well as their enumeration and compositional analysis, have undergone rapid development. At present, there are no widely accepted “gold standard” methods and professional bodies such as ISO and ASTM are both working on standardization of related protocols. Historically, initial efforts involved use of traditional optical microscopy techniques for observing microplastics that were manually picked out of samples of interest. This kind of manual retrieval was possible only for plastic particles that were several millimeters in length. For instance, plastic fibers have been plucked using tweezers from sediment or sand samples and then transferred to microscope slides. Subsequent efforts to study smaller microplastic particles have employed filtration with stainless steel sieves with cut-offs sizes at 20 and 200 µm commonly in use. In some cases, any captured microplastics could be directly visualized on these stainless-steel sieves, but smaller microplastics below 20 µm remained practically challenging to detect because the vast majority of commercially available filters with micron-scale cutoffs are also composed of synthetic polymers (e.g., polycarbonate or polytetrafluoroethylene). Researchers have thus been forced to employ more elaborate methods to discriminate sample-derived microplastics from the plastic background of lower cut-off filters.
Methods employed for enumerating and analyzing small microplastics less than 20 µm include chemical treatment of samples prior to filtration to remove endogenous organic substances (e.g. incubation in concentrated potassium hydroxide and/or hydrogen peroxide), followed by serial filtration through filters with decreasing cut-offs. Any organic materials that survive the harsh treatments are likely to be synthetic polymers. Two methods are in use presently for analyzing any plastics captured on this series of filters. First, washing the filters is used to release captured plastics, which are then transferred to appropriately reflective or background-silent substrates for spectroscopic analysis by Raman or infrared microscopy. Second, plastics can be stained in solution (prior to filtration) with a fluorescent dye that is selectively absorbed by synthetic polymers that survive the harsh chemical treatment. The most commonly used dye is Nile Red and presumably stains only synthetic polymers and not any inorganic materials present after chemical treatment and filtration. Owing to its bright fluorescence, Nile Red staining (coupled with the above workflow) greatly aids in discriminating non-plastic from plastic particles (see figure on page 13).
None of the above-described methods offers a onestep workflow in which the same filter can be used for both capture and analysis of microplastics. Several groups in Rochester are addressing this problem. SiMPore Inc. has designed MicroSlit Silicon Nitride Filters, with rectangular prism-shaped (rather than cylindrical) pores and has adapted their use for microplastic capture-and-analysis (see figure). These MicroSlit Filters offer precise cut-off (by the narrow dimension of their slit features) and overall high permeability since the slit pores can remain open as particles are captured and occupy other regions of the slits. SiMPore presently fabricates these filters at the Rochester Institute of Technology’s Semiconductor and Microsystems Fabrication Laboratory. Advantages offered by MicroSlit Filters are their high flow rates and plastic-free background that is compatible with currently in-use analyses such as optical microscopy (with Nile Red staining) and Raman and infrared spectroscopy. University of Rochester Prof. James McGrath (Dept. of Biomedical Engineering) used SiMPore’s MicroSlit Filters to survey the City of Rochester drinking water system from its production facility at Hemlock Lake through eight additional locations onto the University campus. McGrath and colleagues recently published their findings, observing increasing levels of particles (including microplastics) entrained in samples collected beyond the water production source at Hemlock Lake and were able to classify particles into fibers and nonfibers using machine learning algorithms. A nascent startup company, Parverio Inc., is commercializing microplastic testing services to consumers and water authorities based on the simplified capture-and-analyze methods enabled by SiMPore’s MicroSlit Filters.
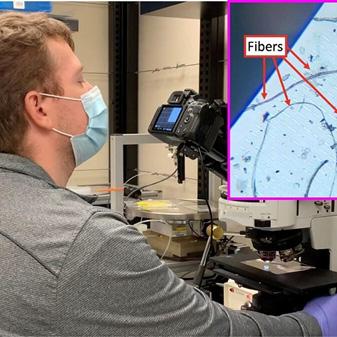
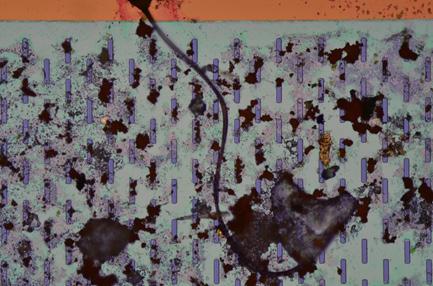
This is an exciting and dynamic field, spanning polymer chemistry, human behavior, environmental science, fluid mechanics, advanced manufacturing to both understand and address a serious emerging issue. There is often a role for engineers to participate and contribute both to the basic science and to eventual technologies. In our Rochester community, engineers are working alongside scientists and medical professionals to better understand all the ways that microplastics move through our environment and body, to build technologies to aid in the study of, and eventually to help propose solutions to mitigate negative effects of microplastics. The microplastic group demonstrates a great cooperation between local universities and industry. If you are in the Rochester community and want to contribute, please reach out.
Steven Day (Steven.Day@RIT.edu) is an Associate Professor in the Kate Gleason College of Engineering at RIT. He studies fluid mechanics of medical devices and biological systems and is only recently applying these methods to look at the motion of particles through our environment.
Steven Day (Steven.Day@RIT.edu) is an Associate Professor in the Kate Gleason College of Engineering at RIT. He studies fluid mechanics of medical devices and biological systems and is only recently applying these methods to look at the motion of particles through our environment.
Christy Tyler (actsbi@rit.edu) is an Associate Professor in the Thomas H. Gosnell School of Life Sciences at RIT. Dr. Tyler’s research focuses on ecology and biogeochemistry of aquatic ecosystems to understand the impact of emerging contaminants, invasive species, and urbanization and develop solutions for restoration.
Samantha Romanick (sromanic@ur.rochester.edu) is a postdoctoral fellow in the Biomedical Engineering Department at the University of Rochester, founder of the microplastics workgroup and studying the potential health effects microplastics pose to the human lung.
James Roussie (jroussie@simpore.com) is the Chief Scientific Officer and a co-founder of SiMPore Inc. The company develops and markets silicon nanomembranes for a variety of life science and material science applications. He recently served as the Principal Investigator on a NIH Phase I SBIR for application of silicon nanomembranes for the capture and analysis of environmental microplastics.
James McGrath (jmcgrath@bme.rochester.edu) is a Professor of Biomedical Engineering at the University of Rochester and a cofounder of SiMPore Inc. and Parverio Inc. His laboratory develops and applies ultrathin membrane technologies to solve problems in the biomedical and environmental sciences.