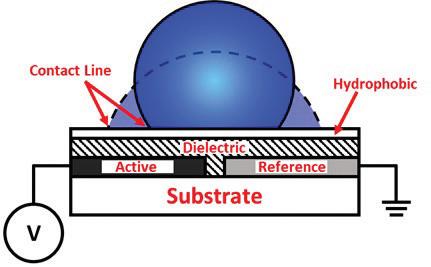
6 minute read
Droplet ejection for 3D digital microfluidics (Feature
Droplet ejection for 3D digital microfluidics
by Collin T. Burkhart, PhD Candidate, RIT
People around the world must frequently cope with a variety of health issues. Pathogens such as viruses, bacteria, and parasites can all cause diseases if they infect the body. For instance, the disease malaria is a result of a bite from a mosquito carrying Plasmodium falciparum parasites. Malaria can quickly become life-threatening and requires immediate treatment. Some pathogens, such as SARS-CoV-2, are also contagious and can spread from an infected person to an uninfected person through direct or even indirect contact. People can also be afflicted by a number of disorders, such as sickle cell disease, cystic fibrosis, and hemophilia. These types of conditions can all be traced to one or more genetic mutations that arise over time or are inherited at birth. Depending upon the particular genetic mutation, people can pass along a disorder to their children without necessarily having it themselves.
Diagnosis is a critical first step in the treatment of these conditions. Rapid and accurate diagnosis can improve outcomes by quickly identifying infected individuals so treatment can begin and further spread can be mitigated. Unfortunately, testing can be costly depending on the equipment, tools, and personnel required. As the cost of diagnostic testing increases, accessibility to high-quality health screening decreases. As a result, researchers have been developing new “lab-on-a-chip” devices for rapid, low-cost diagnostic testing. These devices seek to miniaturize and automate tests performed in traditional laboratories on individual palm-sized chips. The reduced form factor reduces reagent costs while providing faster results since chemicals have shorter diffusion lengths and higher surface area-to-volume ratios. These devices are also suitable for point-of-care testing, particularly in remote regions without access to traditional laboratories.
Digital microfluidic (DMF) devices are a class of lab-on-a-chip device where individual droplets are manipulated using electrical signals to perform diagnostic tests. These devices manipulate droplets via the electrowetting phenomenon. A typical device is built on a glass or silicon wafer substrate and consists of a grid of electrodes coated with different polymer layers (Fig. 1). Droplets rest over the coated electrodes which receive actuation signals to deform the droplets. Depending on the configuration, the circuit can be completed by a thinly coated top electrode, an inserted wire, or by one or more separate coplanar electrodes. The dielectric layer over the actuated electrodes delays the onset of electrolysis in the droplets, and hydrophobic coatings allow for more significant deformation during actuation. As voltage is Figure 1. Side-view diagram of an open coplanar electrowetting setup. applied, a radially outward “electrowetting force” spreads the droplet due to charge buildup along the three-phase contact line. This reduces the apparent contact angle of the droplet. Higher root-mean-square voltages cause more significant reductions up to a system-dependent saturation point.
By positioning droplets over multiple electrodes, electrowetting can be applied to select regions of the droplet. This can be used to change the shape on one side, while leaving the rest of the droplet relatively unaffected. The difference in curvature across the droplet gives rise to a pressure gradient that pulls droplets toward active electrodes. Electrowetting can be used in this way to position droplets over an entire array of electrodes. Electrowetting on dielectric devices can merge, split, or mix droplets. Sequences of these simple operations have been used to perform complex biomedical protocols, including: synthesizing chemicals, separating target particles, and running immunoassays. A generic electrode grid can be readily automated for a wide variety of applications.
Electrowetting on dielectric can also be used to eject droplets off of the device surface to pass them between two opposing electrode grids. The electrowetting response in millimeter-size, aqueous droplets is typically underdamped. As such, droplets reach a more spread position in the first milliseconds of actuation before settling to their steady-state position. The time for a droplet to reach its widest position is referred to as its spreading time. When a direct current (DC) actuation signal is applied for this duration, the droplet is allowed to maximize its surface
14
| The ROCHESTER ENGINEER SEPTEMBER 2020 feature article
energy. When the DC signal is released, the contact angle reverts toward its unactuated position causing the droplet to return toward its initial position. A capillary wave travels from the contact line to the apex of the droplet, and the excess surface energy can be sufficient to induce detachment. Combined with horizontal movement, this allows droplets to be routed in 3D space. This enables more sophisticated routing patterns that can minimize reagent cross-contamination and increase overall operation density in a given device footprint.
Electrowetting-based detachment is currently being studied in greater detail in the DMFL to understand how different factors impact droplet ejection in air (Figure 2). Droplets are actuated at increasing voltage until detachment is observed. Threshold voltages between cases are then compared to observe how a variable such as droplet size impacts the energy required for ejection. As detachment occurs in a matter of milliseconds, a high-speed camera records all trials. Frames are reviewed to determine the lowest voltage at which separation between the droplet and the device is visible. This voltage threshold is used as a metric to compare performance between trials. Results from testing are used to develop improved models for detachment that Figure 2. Experimental facility used to eject incorporate droplet size and electrode design. droplets via electrowetting. In testing thus far, salt solution droplets between 3 and 15 µL have been successfully detached from DMF devices (Figure 3). This has been done on single electrode devices with a ground wire and on coplanar multi-electrode devices. While previous coplanar detachment in air has required more complex actuation to induce perturbations in the droplet prior to detaching, droplets ejected here are detached using single pulses. This is attributed to the electrode geometry of the devices used (Figure 2 inset). The radial symmetry allows droplets to spread outward in all directions so minimal energy is wasted on azimuthal oscillations as the droplet is released. Gap space in the design is also kept to a minimum so a droplet on the device rests primarily over regions charged by the electrodes. Experimental results have shown that the detachment threshold increases with droplet size, contrary to previously reported findings that accounted only for surface energy in the detachment process. New models for ejection have been developed based on these results that now also account for changes in gravitational potential energy since the droplet’s center of mass rises throughout detachment.
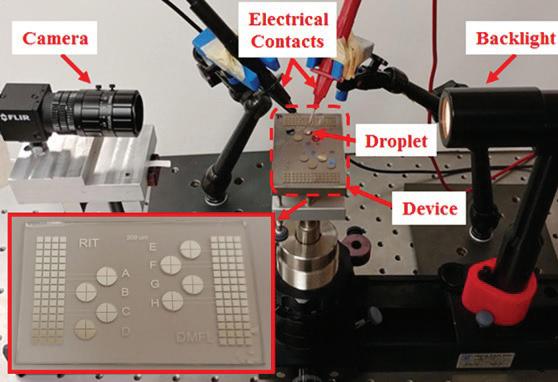
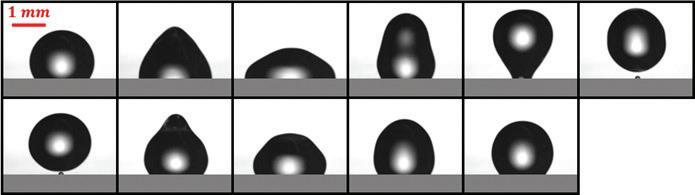
Understanding the droplet detachment process is critical in the development of new 3D DMF devices that can minimize crosscontamination Figure 3. Captured frames during the ejection of a 3 µL droplet of sodium chloride solution. in biological Frames are approximately 3.3 ms apart from left to right. applications. By manipulating the droplet and electrowetting device, actuation requirements can be minimized and detachment made more predictable. The next focus in the DMFL is studying the role of electrode geometry in greater detail. The findings of this investigation will be incorporated with previous results to develop more complete models of droplet ejection.
Mr. Collin Burkhart is an Engineering PhD candidate from the Discrete Microfluidics Laboratory at the Rochester Institute of Technology
feature article SEPTEMBER 2020 The ROCHESTER ENGINEER | 15